NUCLEIC ACIDS NUCLEOTIDES DNA RNA THE GENETIC CODE
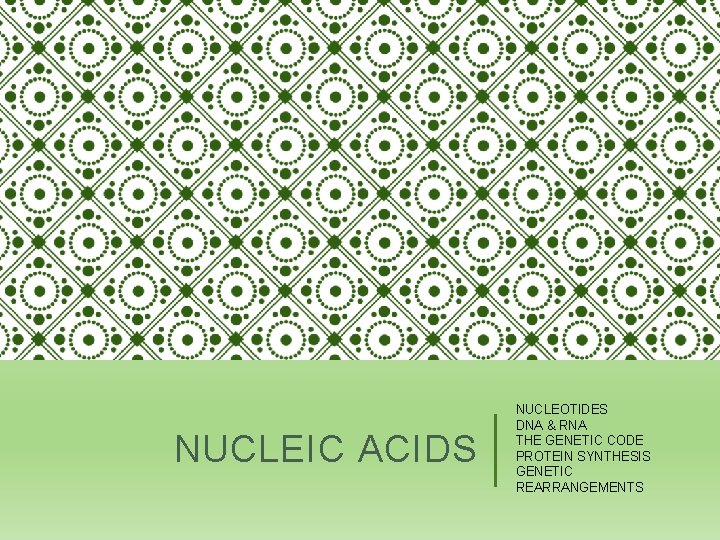
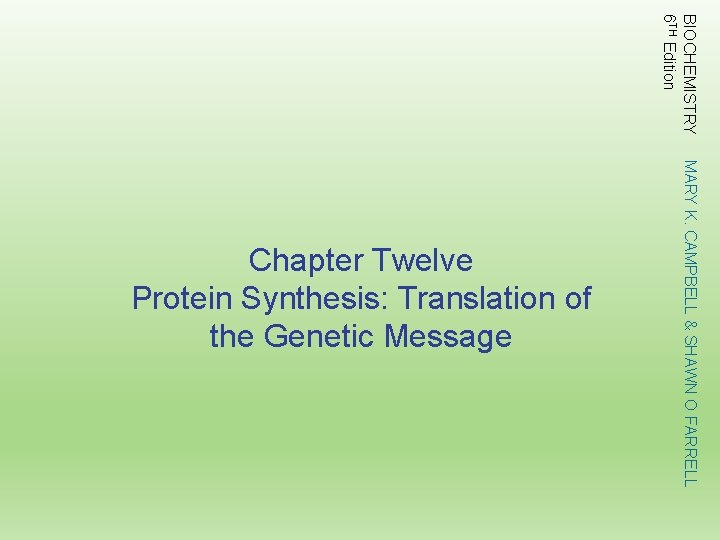
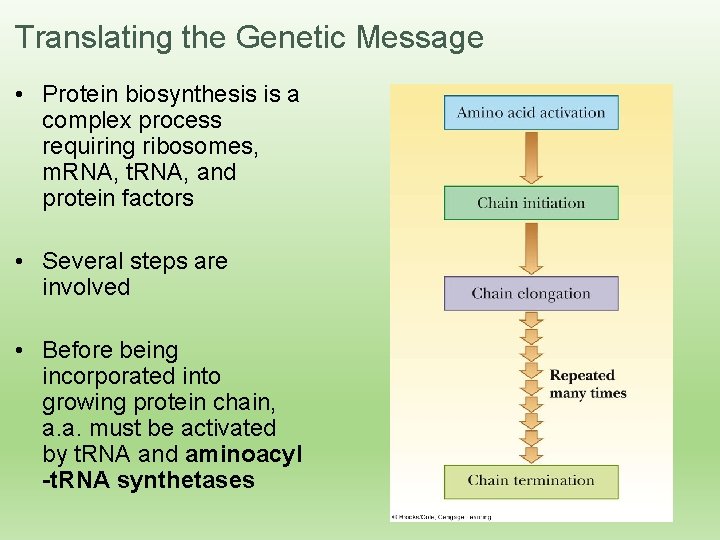
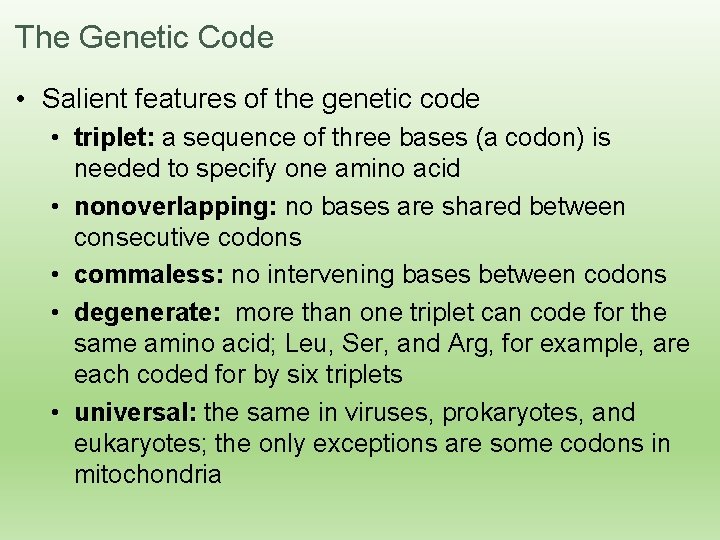
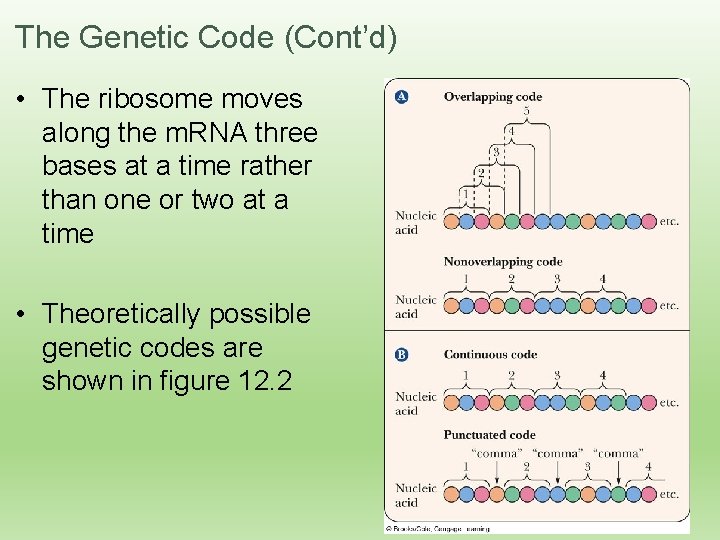
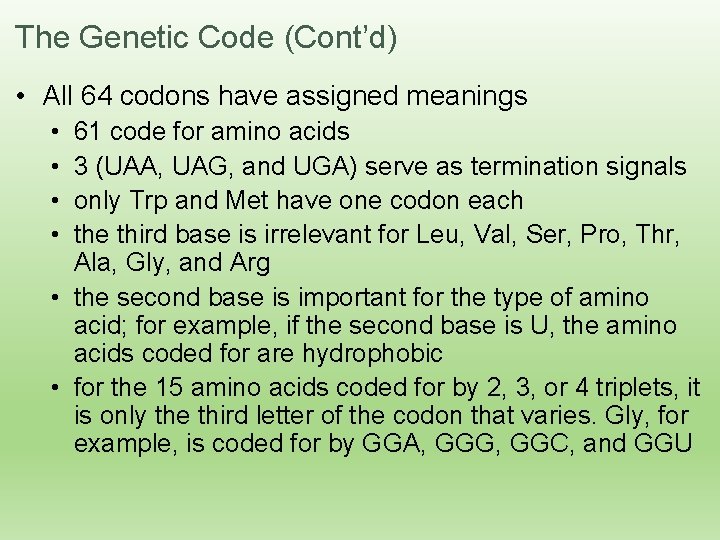
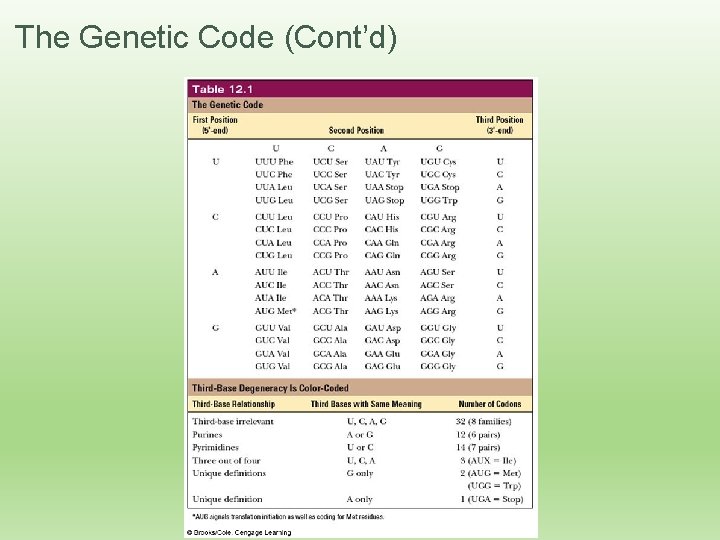
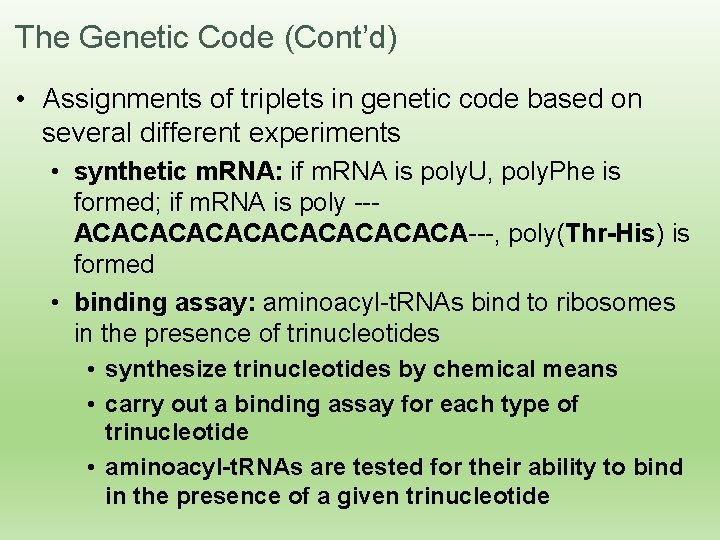
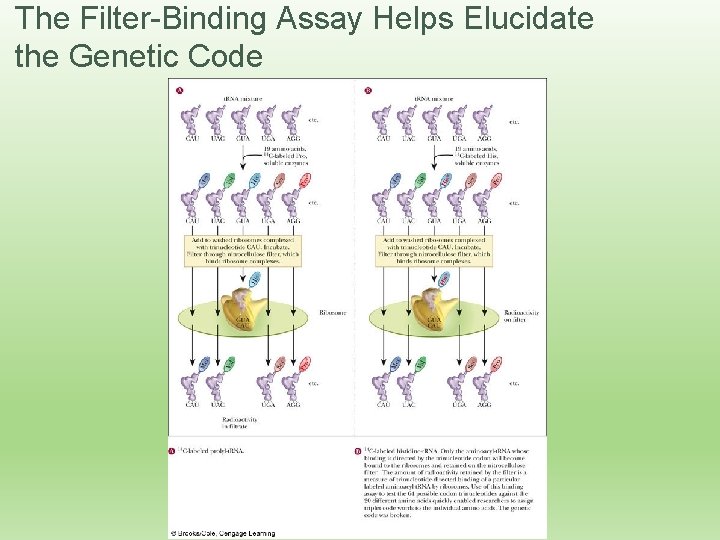
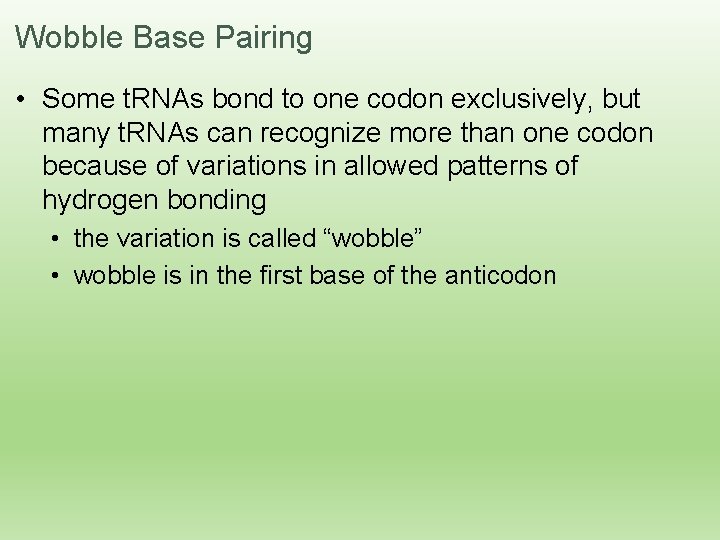
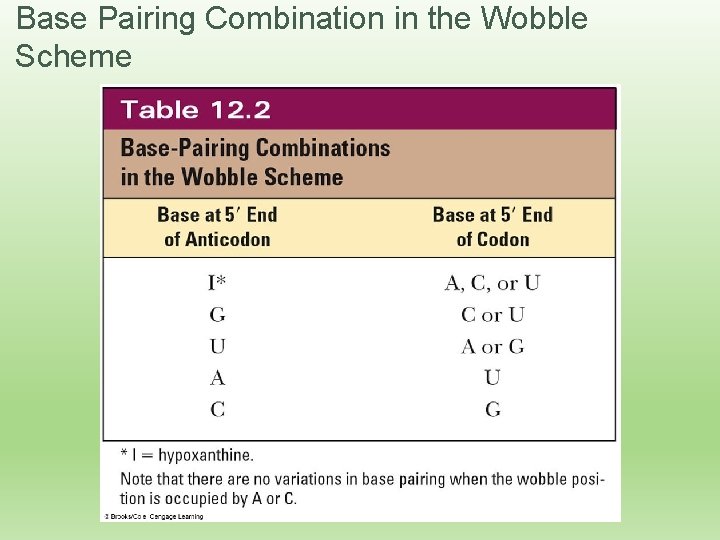
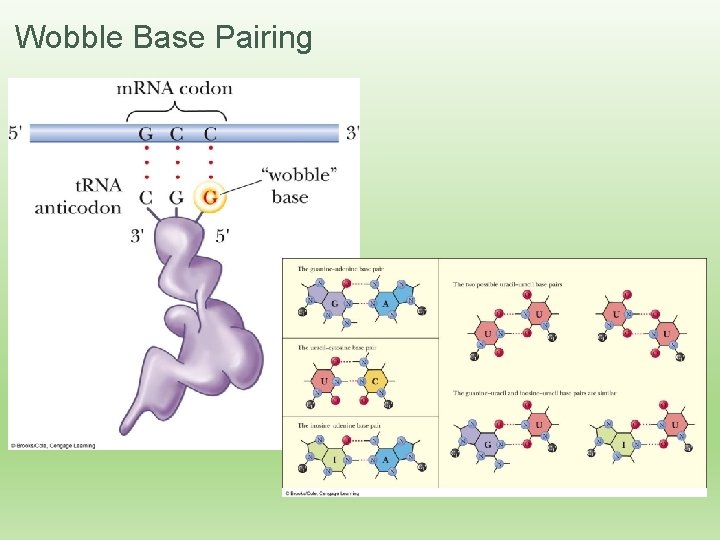
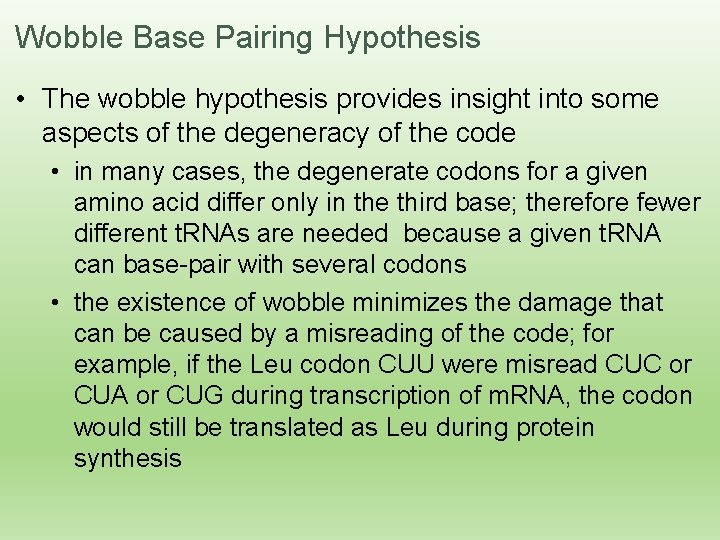
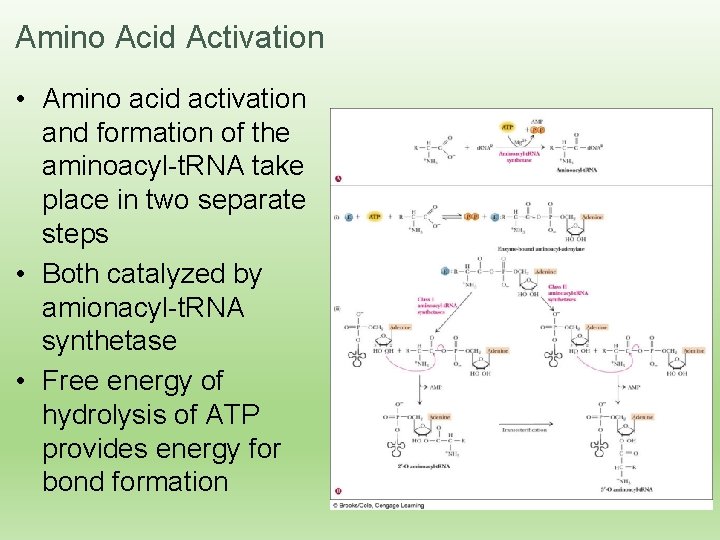
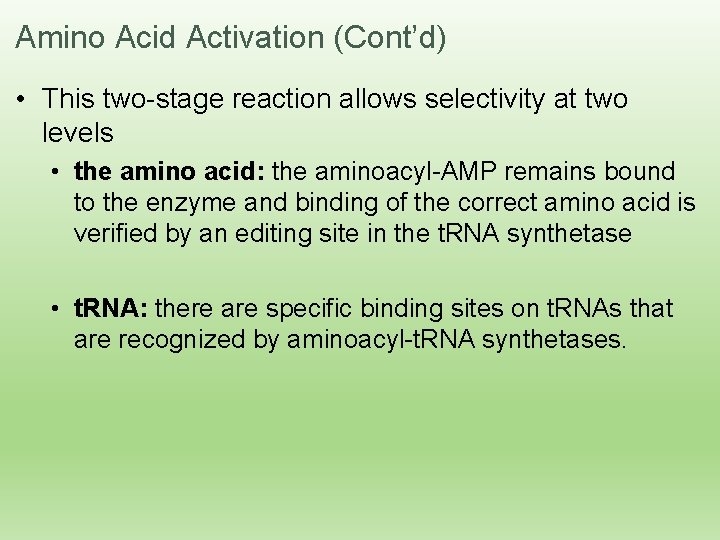
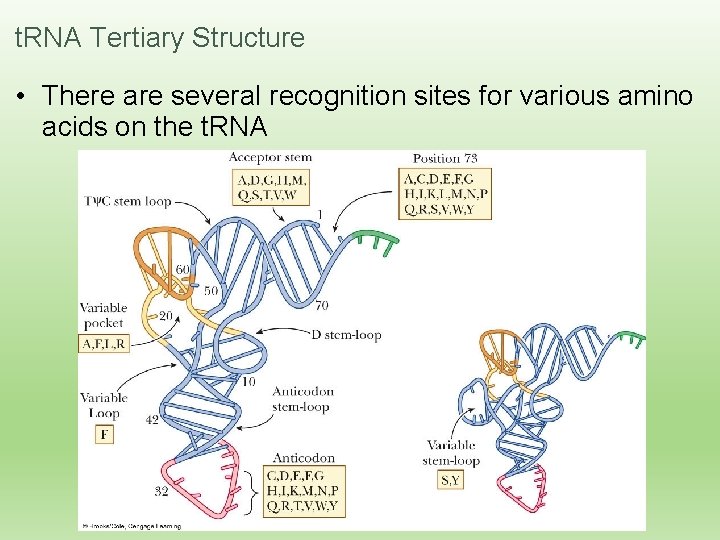
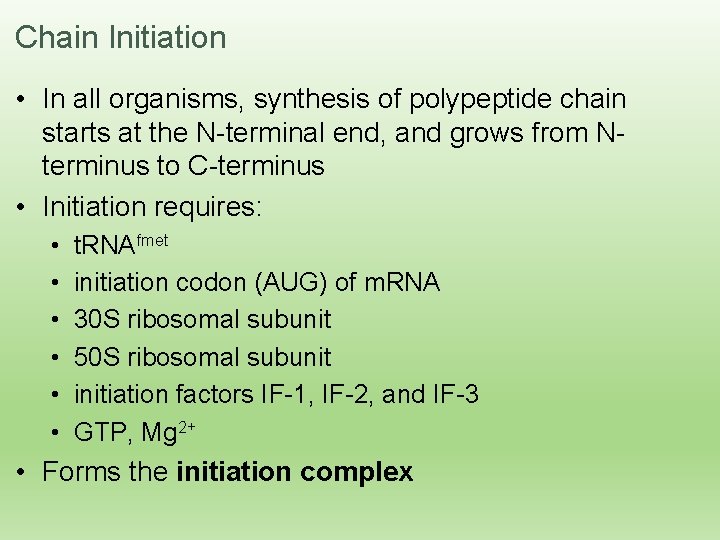
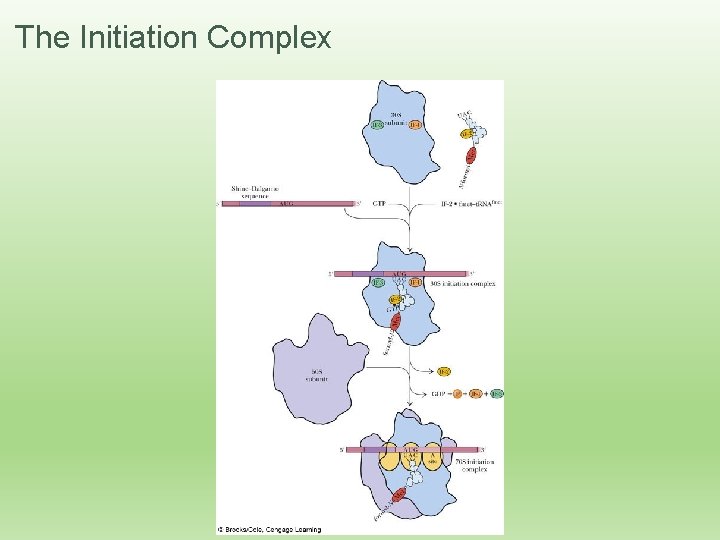
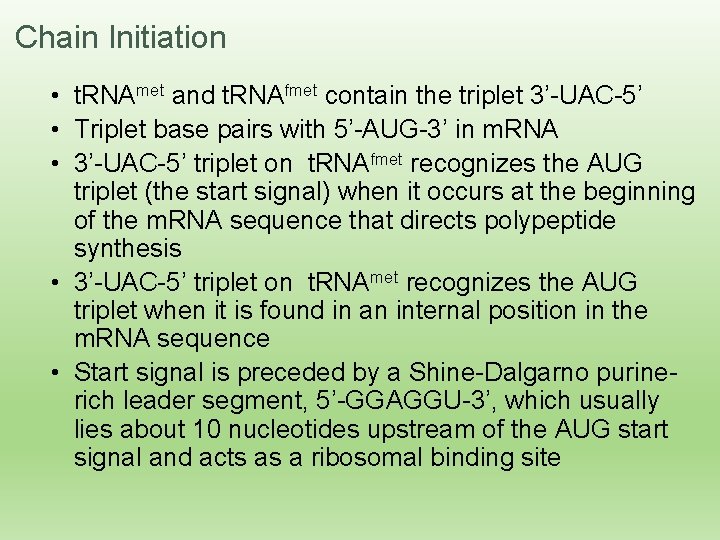
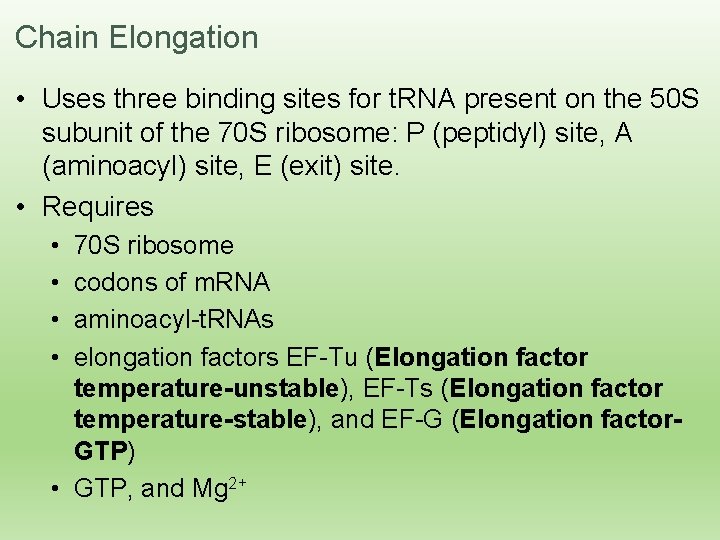
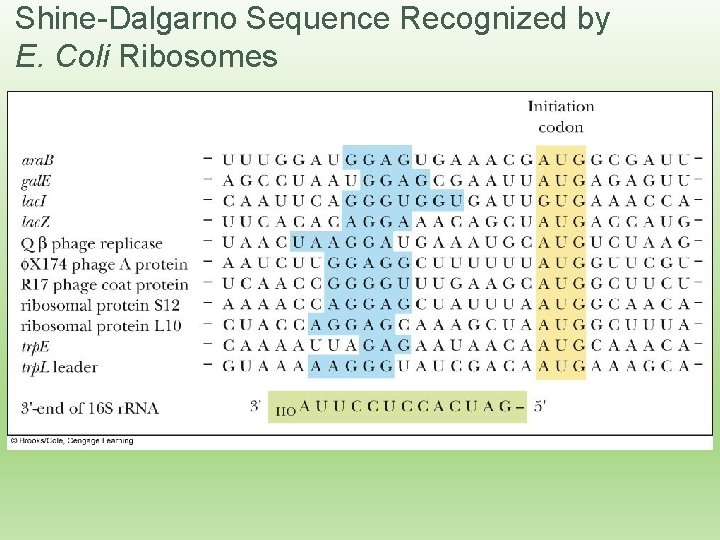
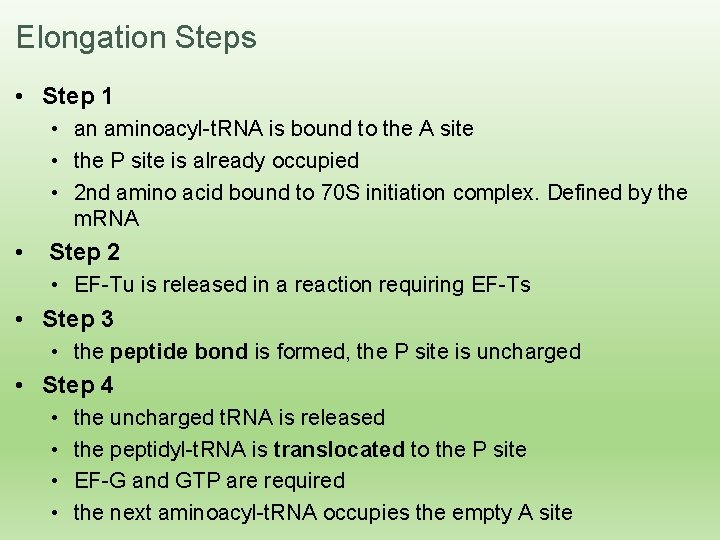
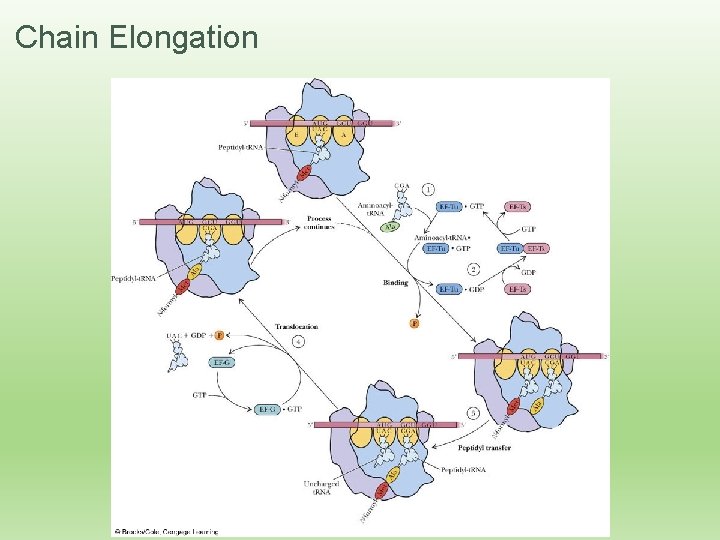
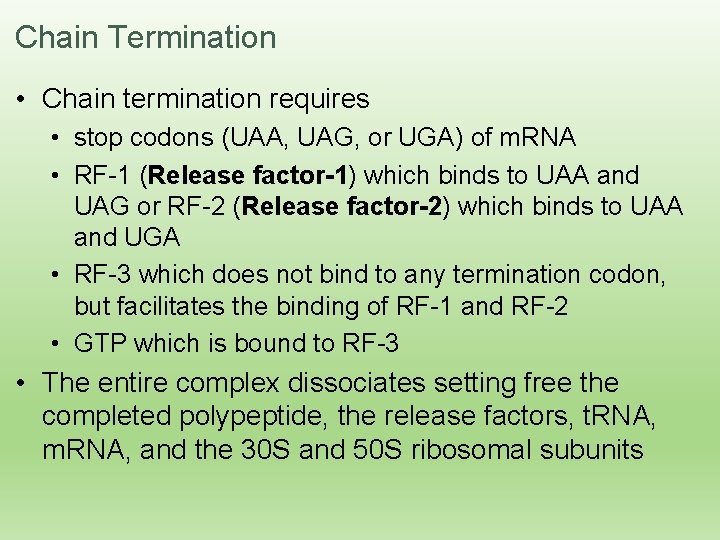
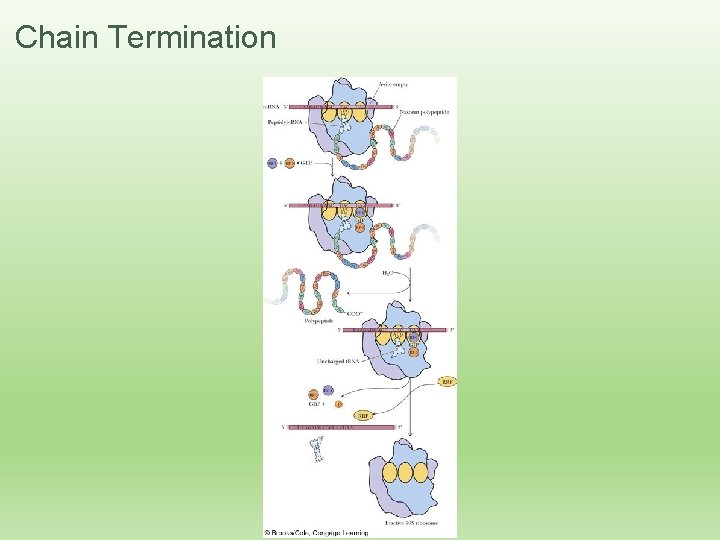
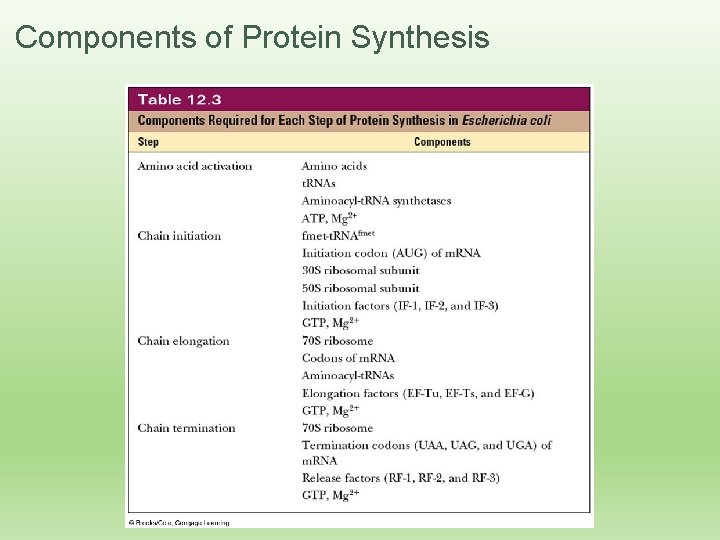
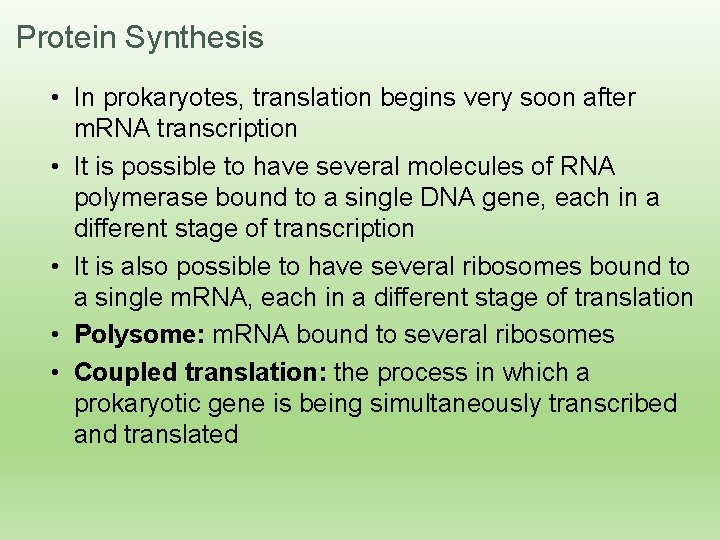
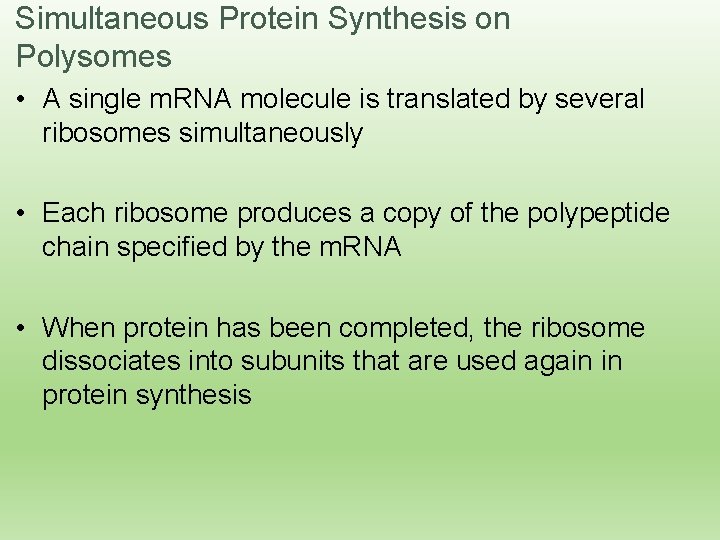
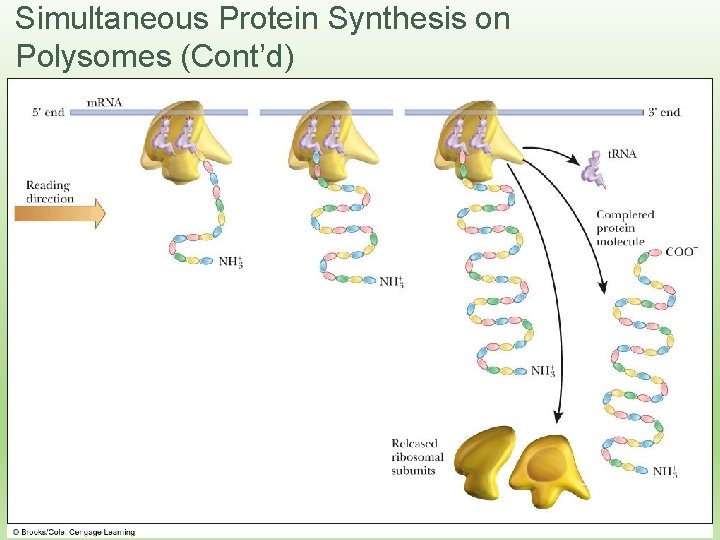
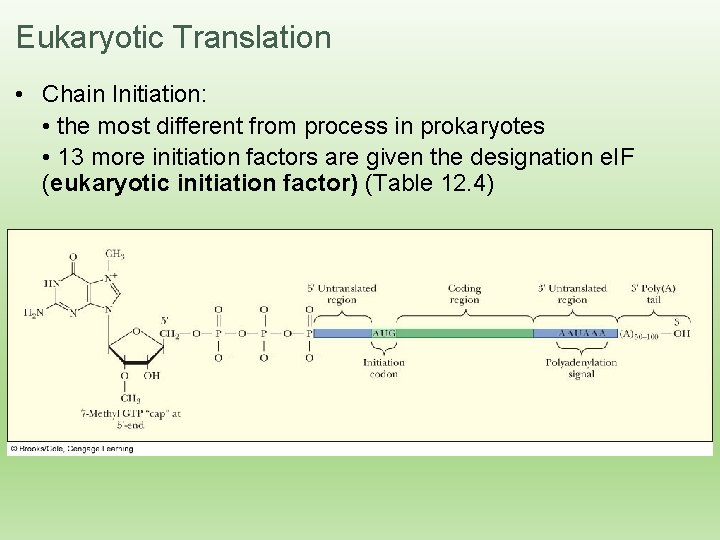
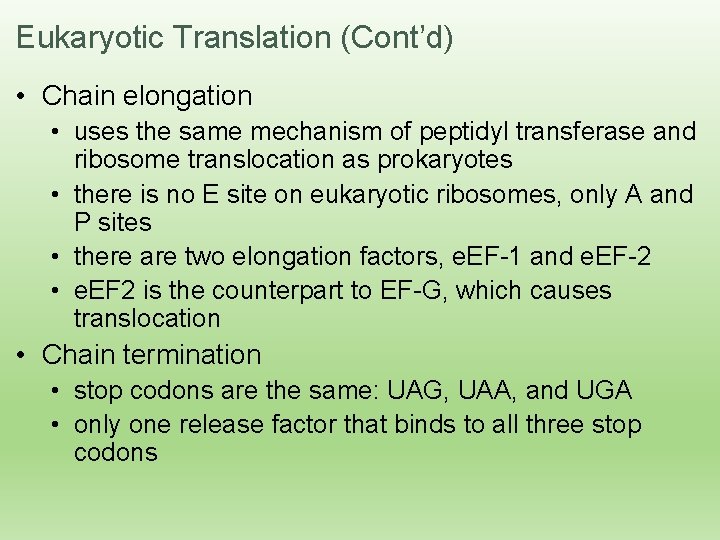
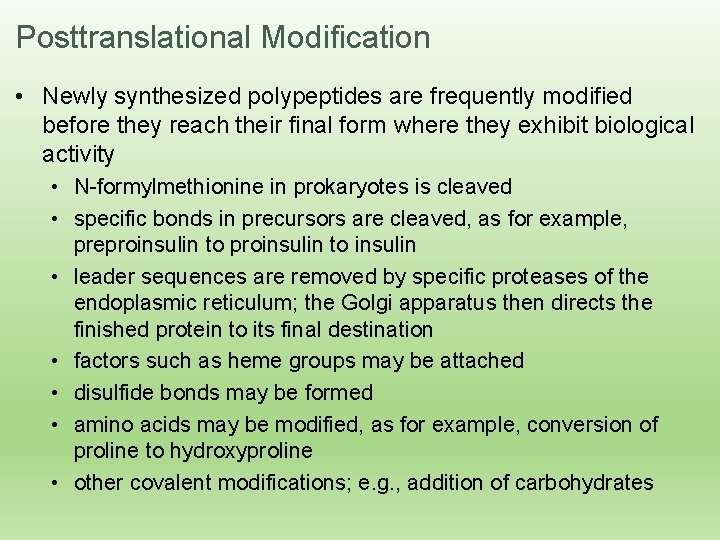
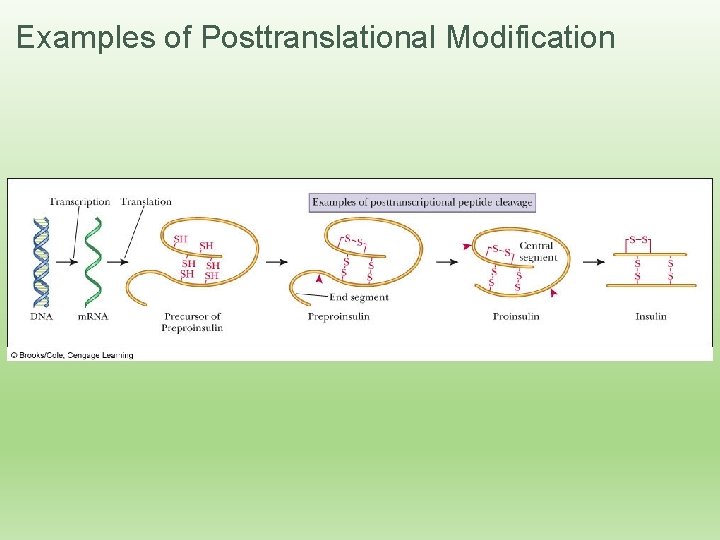
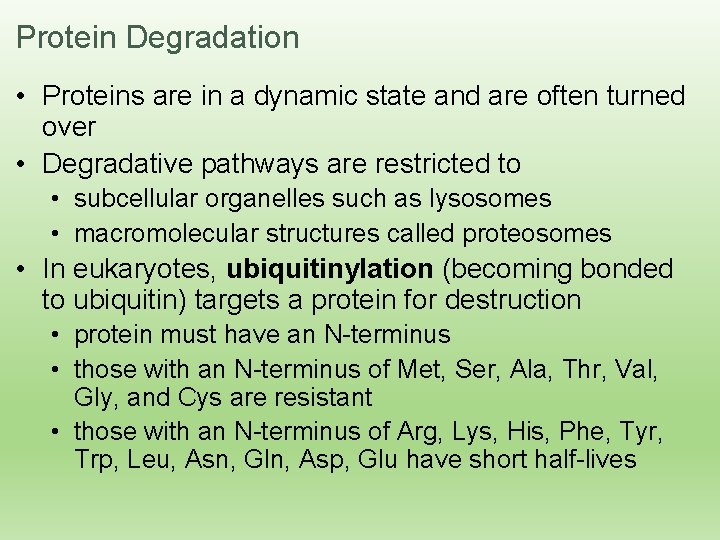
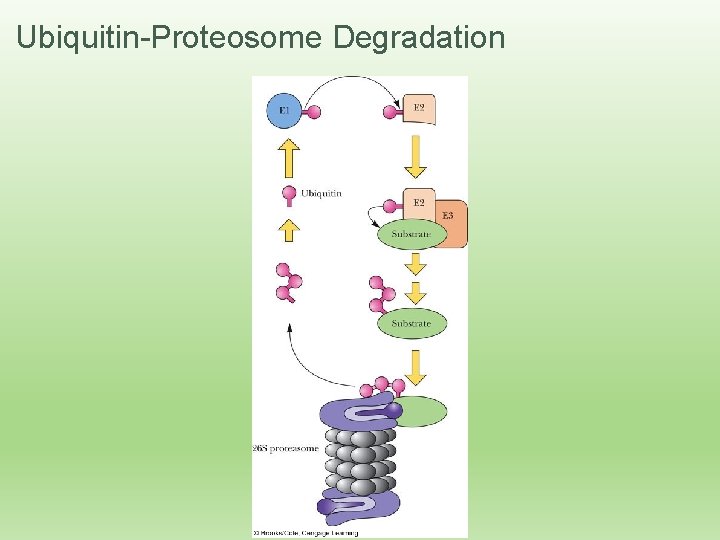
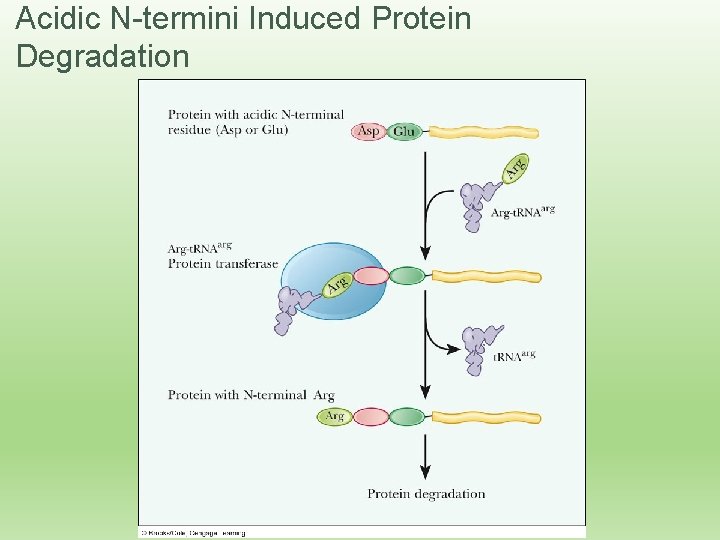
- Slides: 36
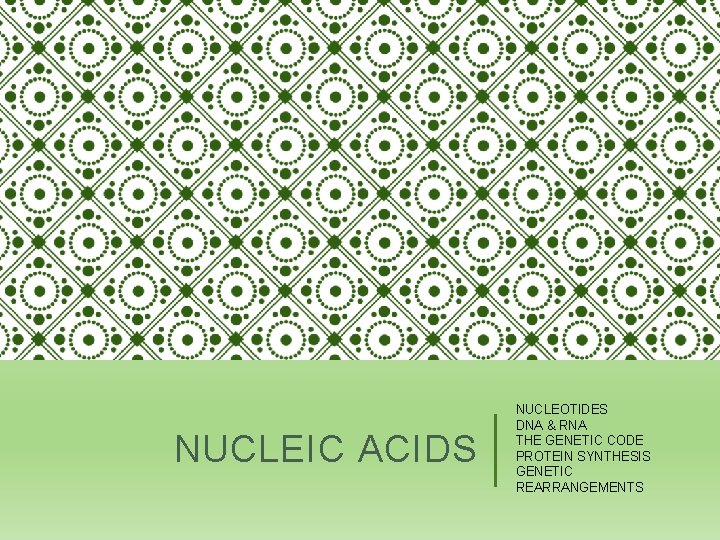
NUCLEIC ACIDS NUCLEOTIDES DNA & RNA THE GENETIC CODE PROTEIN SYNTHESIS GENETIC REARRANGEMENTS
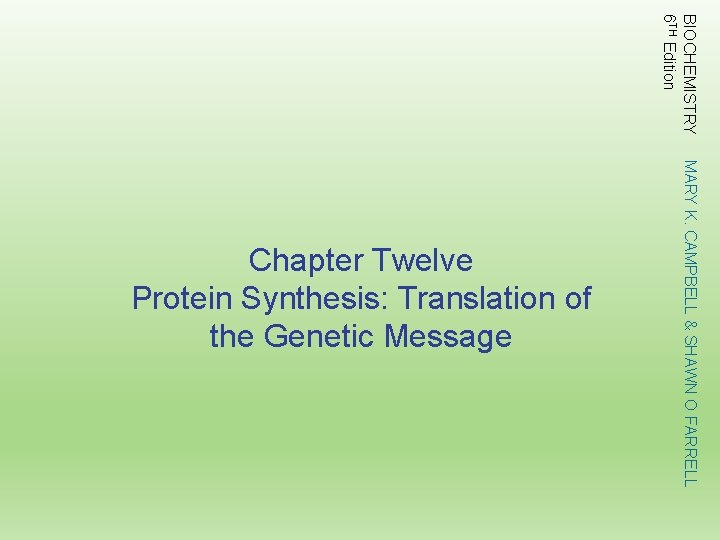
BIOCHEMISTRY 6 TH Edition MARY K. CAMPBELL & SHAWN O FARRELL Chapter Twelve Protein Synthesis: Translation of the Genetic Message
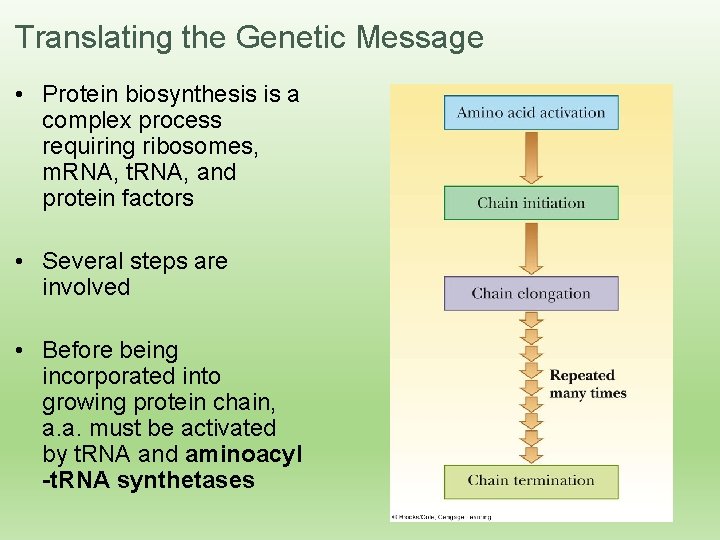
Translating the Genetic Message • Protein biosynthesis is a complex process requiring ribosomes, m. RNA, t. RNA, and protein factors • Several steps are involved • Before being incorporated into growing protein chain, a. a. must be activated by t. RNA and aminoacyl -t. RNA synthetases
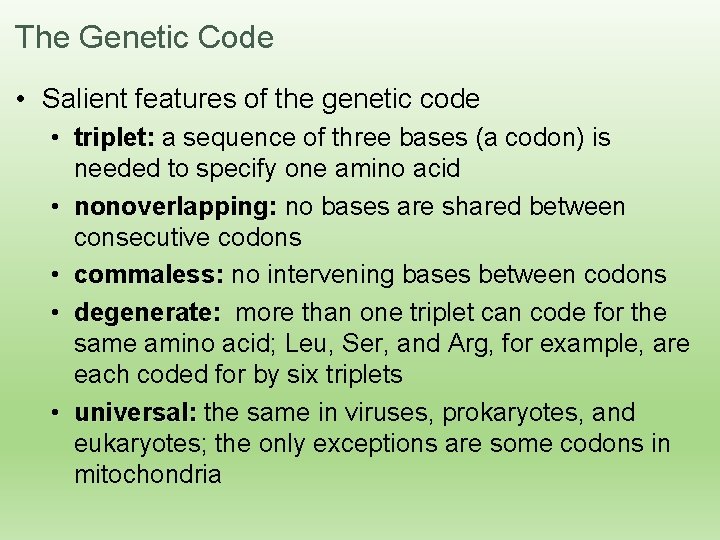
The Genetic Code • Salient features of the genetic code • triplet: a sequence of three bases (a codon) is needed to specify one amino acid • nonoverlapping: no bases are shared between consecutive codons • commaless: no intervening bases between codons • degenerate: more than one triplet can code for the same amino acid; Leu, Ser, and Arg, for example, are each coded for by six triplets • universal: the same in viruses, prokaryotes, and eukaryotes; the only exceptions are some codons in mitochondria
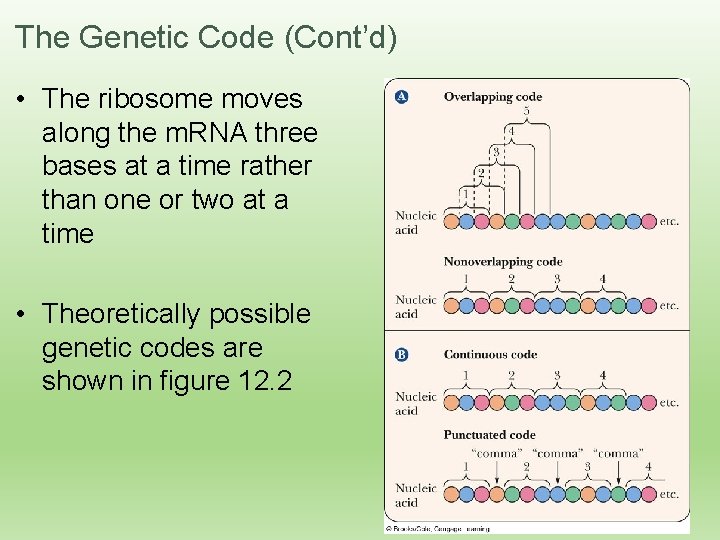
The Genetic Code (Cont’d) • The ribosome moves along the m. RNA three bases at a time rather than one or two at a time • Theoretically possible genetic codes are shown in figure 12. 2
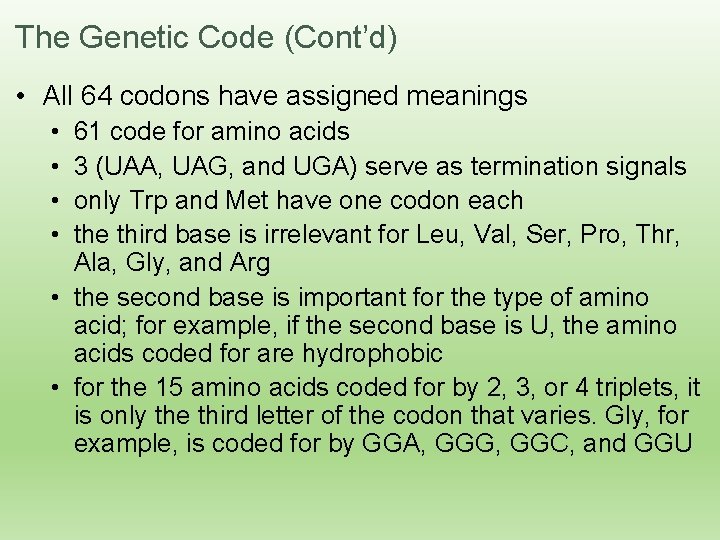
The Genetic Code (Cont’d) • All 64 codons have assigned meanings • • 61 code for amino acids 3 (UAA, UAG, and UGA) serve as termination signals only Trp and Met have one codon each the third base is irrelevant for Leu, Val, Ser, Pro, Thr, Ala, Gly, and Arg • the second base is important for the type of amino acid; for example, if the second base is U, the amino acids coded for are hydrophobic • for the 15 amino acids coded for by 2, 3, or 4 triplets, it is only the third letter of the codon that varies. Gly, for example, is coded for by GGA, GGG, GGC, and GGU
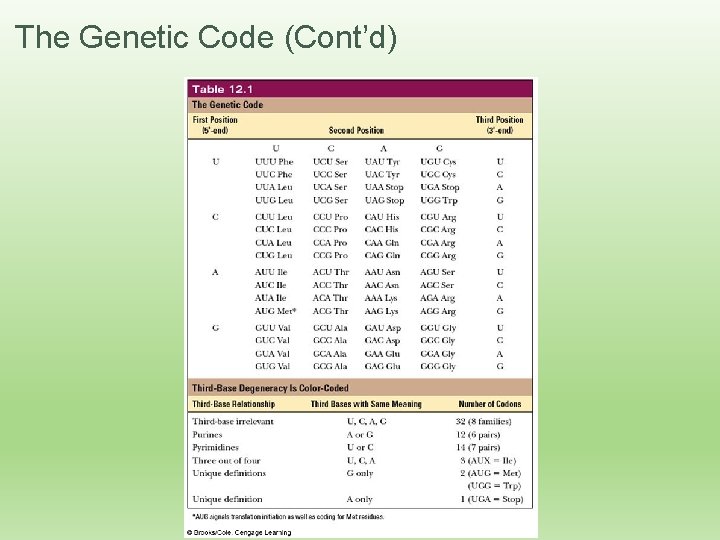
The Genetic Code (Cont’d)
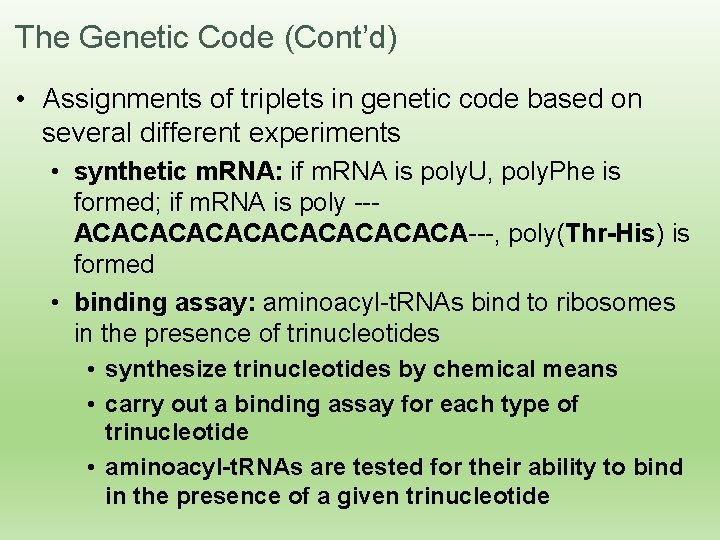
The Genetic Code (Cont’d) • Assignments of triplets in genetic code based on several different experiments • synthetic m. RNA: if m. RNA is poly. U, poly. Phe is formed; if m. RNA is poly --ACACACACACA---, poly(Thr-His) is formed • binding assay: aminoacyl-t. RNAs bind to ribosomes in the presence of trinucleotides • synthesize trinucleotides by chemical means • carry out a binding assay for each type of trinucleotide • aminoacyl-t. RNAs are tested for their ability to bind in the presence of a given trinucleotide
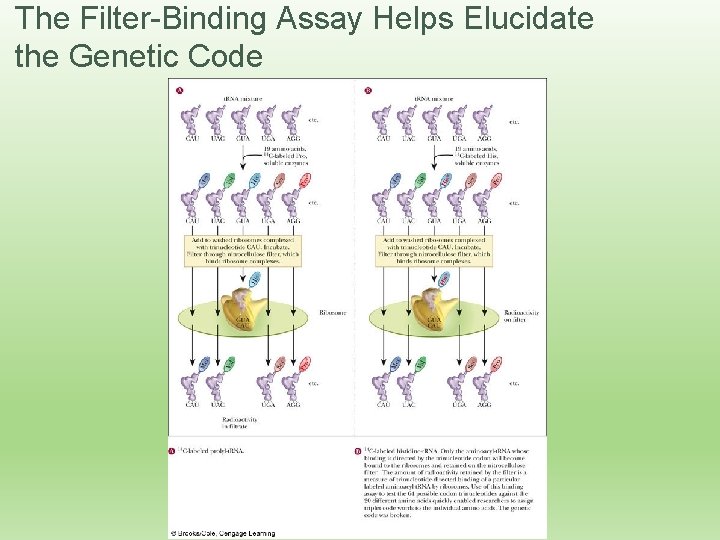
The Filter-Binding Assay Helps Elucidate the Genetic Code
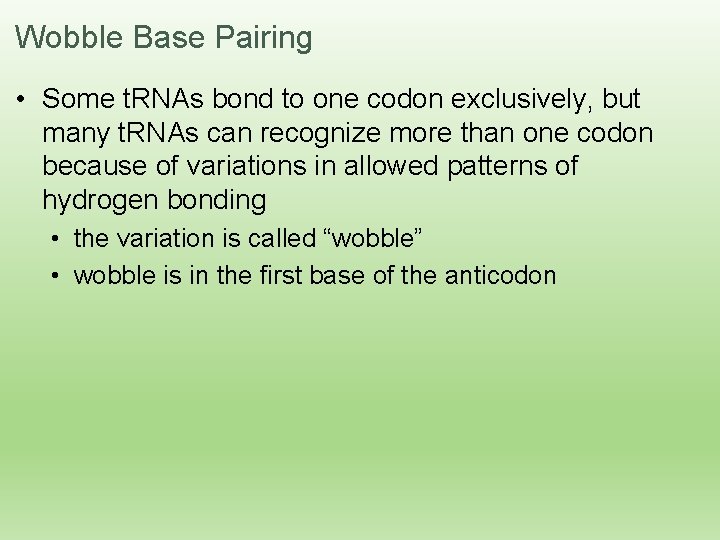
Wobble Base Pairing • Some t. RNAs bond to one codon exclusively, but many t. RNAs can recognize more than one codon because of variations in allowed patterns of hydrogen bonding • the variation is called “wobble” • wobble is in the first base of the anticodon
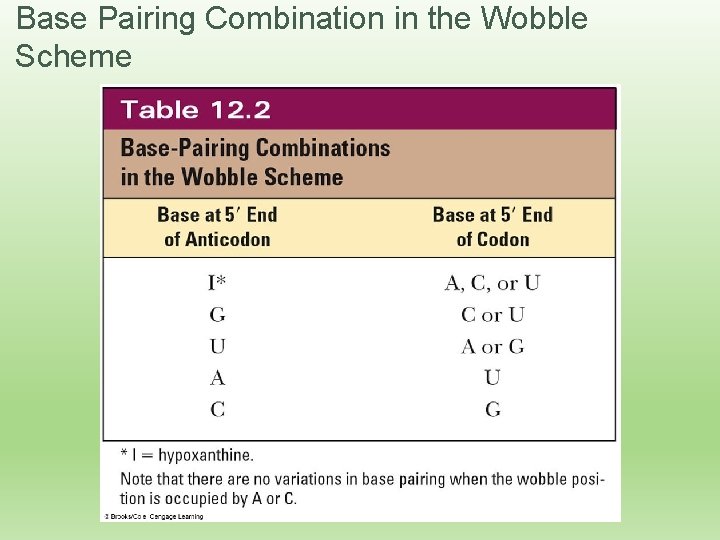
Base Pairing Combination in the Wobble Scheme
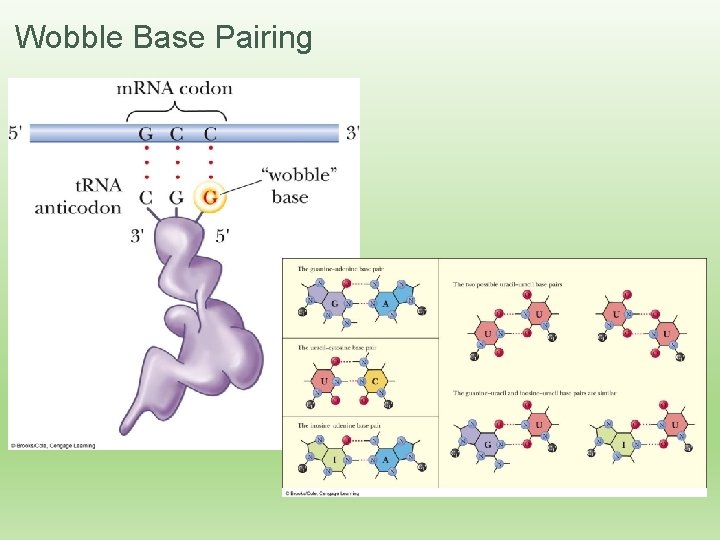
Wobble Base Pairing
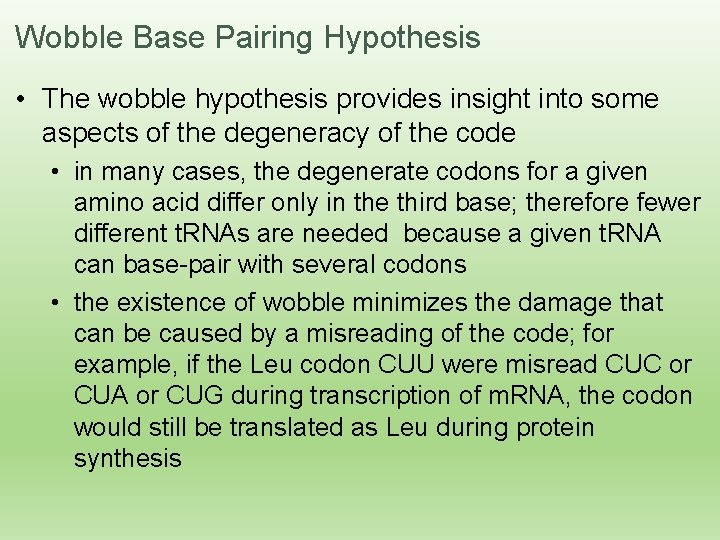
Wobble Base Pairing Hypothesis • The wobble hypothesis provides insight into some aspects of the degeneracy of the code • in many cases, the degenerate codons for a given amino acid differ only in the third base; therefore fewer different t. RNAs are needed because a given t. RNA can base-pair with several codons • the existence of wobble minimizes the damage that can be caused by a misreading of the code; for example, if the Leu codon CUU were misread CUC or CUA or CUG during transcription of m. RNA, the codon would still be translated as Leu during protein synthesis
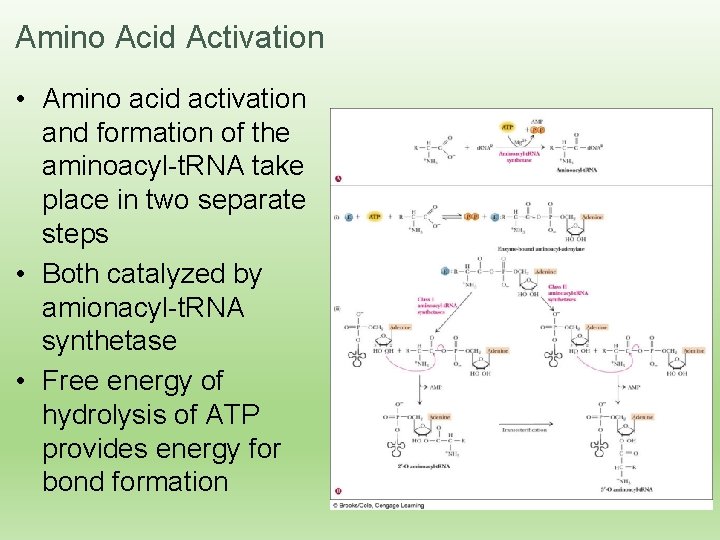
Amino Acid Activation • Amino acid activation and formation of the aminoacyl-t. RNA take place in two separate steps • Both catalyzed by amionacyl-t. RNA synthetase • Free energy of hydrolysis of ATP provides energy for bond formation
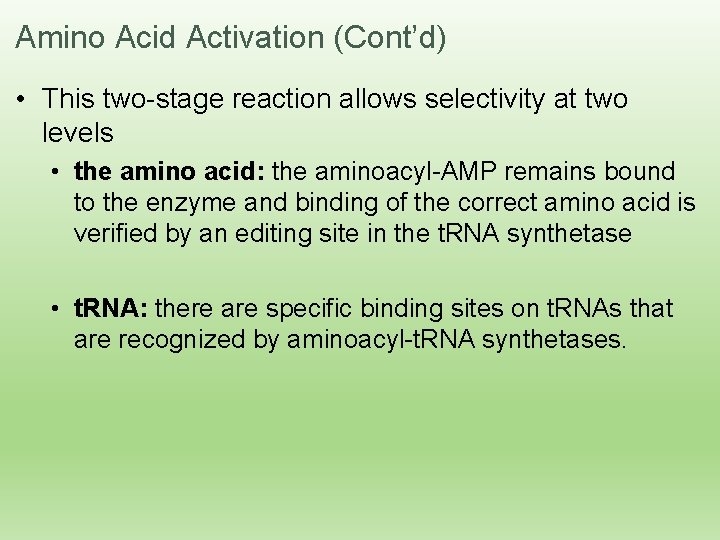
Amino Acid Activation (Cont’d) • This two-stage reaction allows selectivity at two levels • the amino acid: the aminoacyl-AMP remains bound to the enzyme and binding of the correct amino acid is verified by an editing site in the t. RNA synthetase • t. RNA: there are specific binding sites on t. RNAs that are recognized by aminoacyl-t. RNA synthetases.
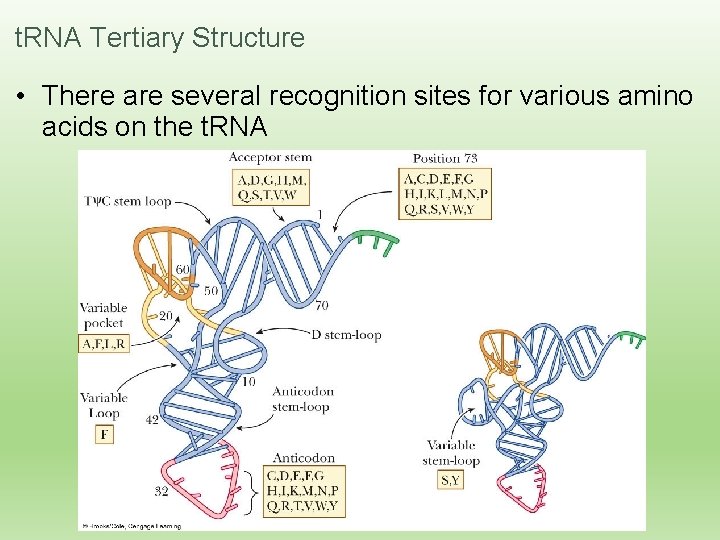
t. RNA Tertiary Structure • There are several recognition sites for various amino acids on the t. RNA
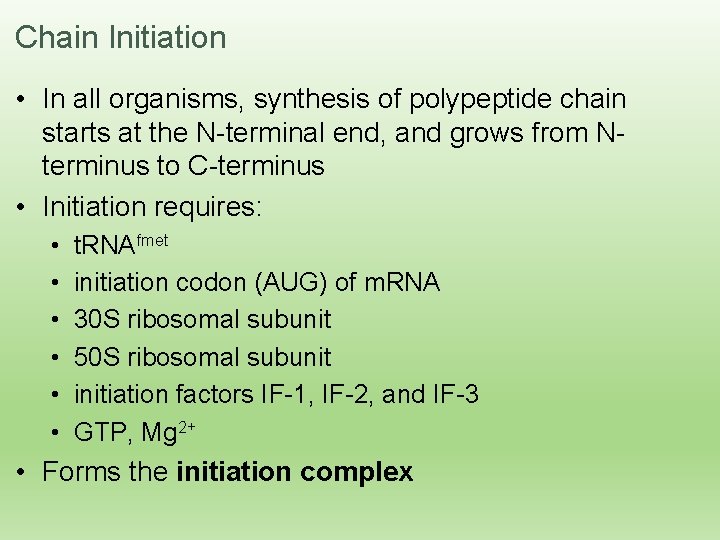
Chain Initiation • In all organisms, synthesis of polypeptide chain starts at the N-terminal end, and grows from Nterminus to C-terminus • Initiation requires: • • • t. RNAfmet initiation codon (AUG) of m. RNA 30 S ribosomal subunit 50 S ribosomal subunit initiation factors IF-1, IF-2, and IF-3 GTP, Mg 2+ • Forms the initiation complex
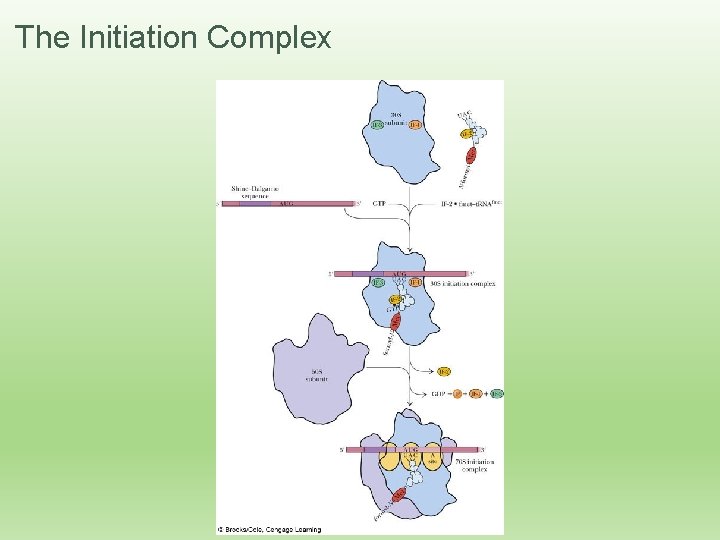
The Initiation Complex
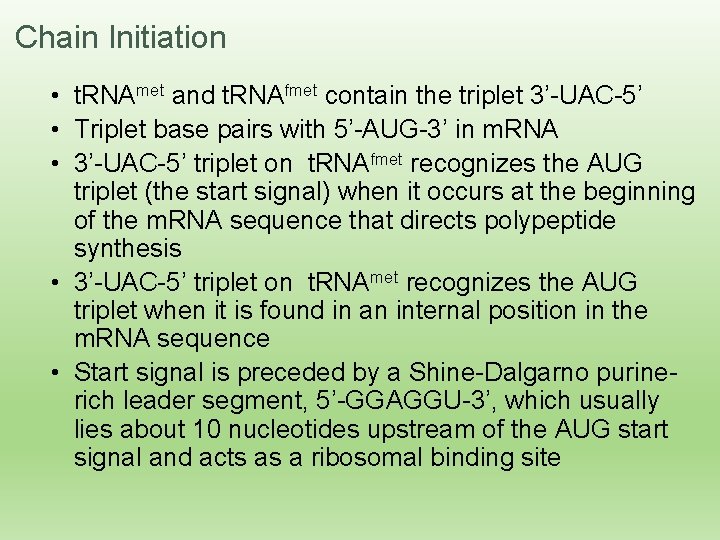
Chain Initiation • t. RNAmet and t. RNAfmet contain the triplet 3’-UAC-5’ • Triplet base pairs with 5’-AUG-3’ in m. RNA • 3’-UAC-5’ triplet on t. RNAfmet recognizes the AUG triplet (the start signal) when it occurs at the beginning of the m. RNA sequence that directs polypeptide synthesis • 3’-UAC-5’ triplet on t. RNAmet recognizes the AUG triplet when it is found in an internal position in the m. RNA sequence • Start signal is preceded by a Shine-Dalgarno purinerich leader segment, 5’-GGAGGU-3’, which usually lies about 10 nucleotides upstream of the AUG start signal and acts as a ribosomal binding site
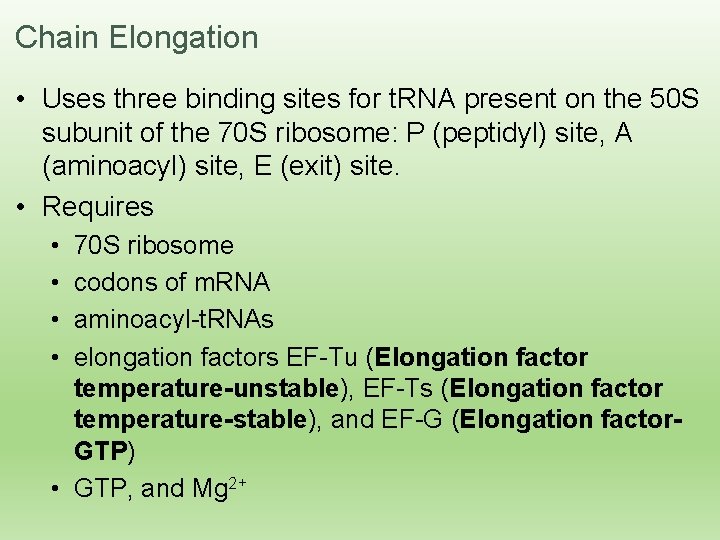
Chain Elongation • Uses three binding sites for t. RNA present on the 50 S subunit of the 70 S ribosome: P (peptidyl) site, A (aminoacyl) site, E (exit) site. • Requires • • 70 S ribosome codons of m. RNA aminoacyl-t. RNAs elongation factors EF-Tu (Elongation factor temperature-unstable), EF-Ts (Elongation factor temperature-stable), and EF-G (Elongation factor. GTP) • GTP, and Mg 2+
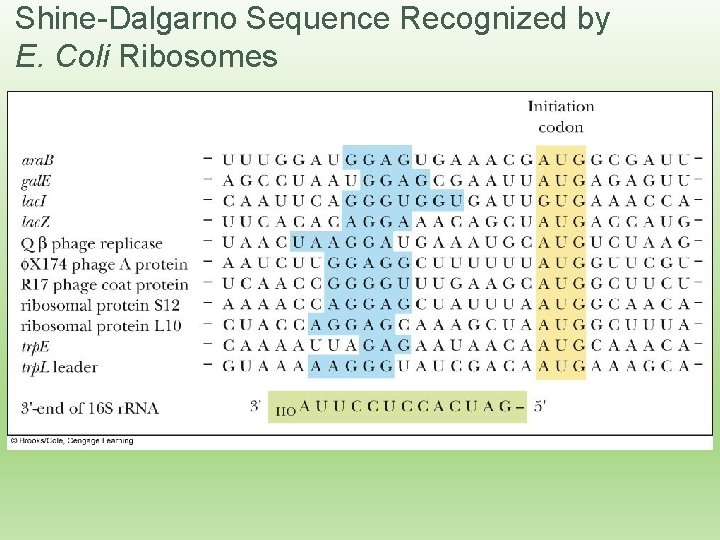
Shine-Dalgarno Sequence Recognized by E. Coli Ribosomes
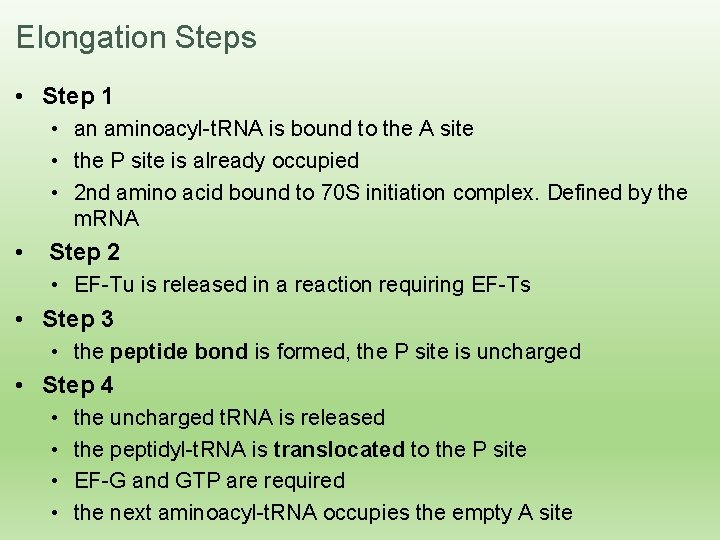
Elongation Steps • Step 1 • an aminoacyl-t. RNA is bound to the A site • the P site is already occupied • 2 nd amino acid bound to 70 S initiation complex. Defined by the m. RNA • Step 2 • EF-Tu is released in a reaction requiring EF-Ts • Step 3 • the peptide bond is formed, the P site is uncharged • Step 4 • • the uncharged t. RNA is released the peptidyl-t. RNA is translocated to the P site EF-G and GTP are required the next aminoacyl-t. RNA occupies the empty A site
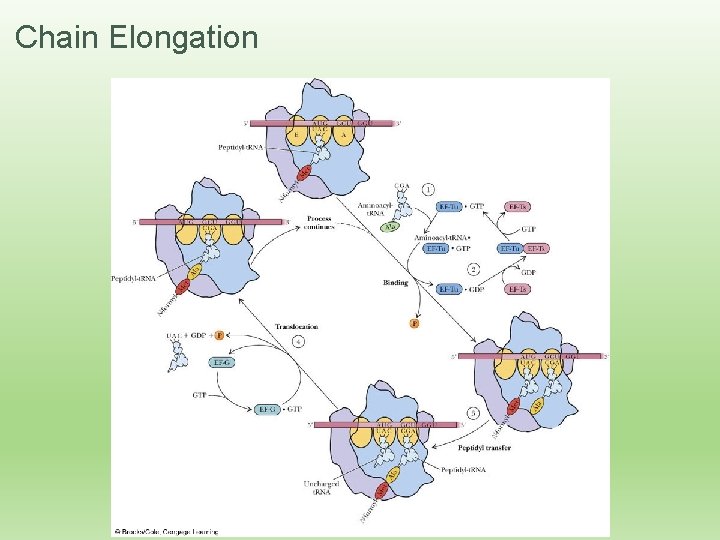
Chain Elongation
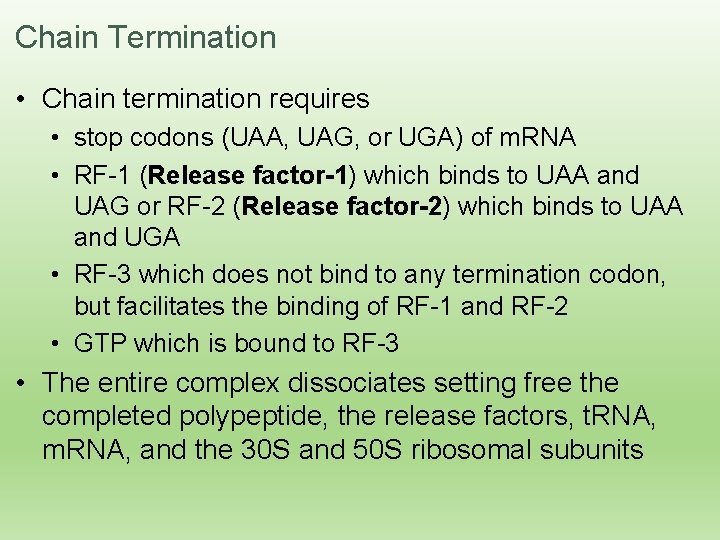
Chain Termination • Chain termination requires • stop codons (UAA, UAG, or UGA) of m. RNA • RF-1 (Release factor-1) which binds to UAA and UAG or RF-2 (Release factor-2) which binds to UAA and UGA • RF-3 which does not bind to any termination codon, but facilitates the binding of RF-1 and RF-2 • GTP which is bound to RF-3 • The entire complex dissociates setting free the completed polypeptide, the release factors, t. RNA, m. RNA, and the 30 S and 50 S ribosomal subunits
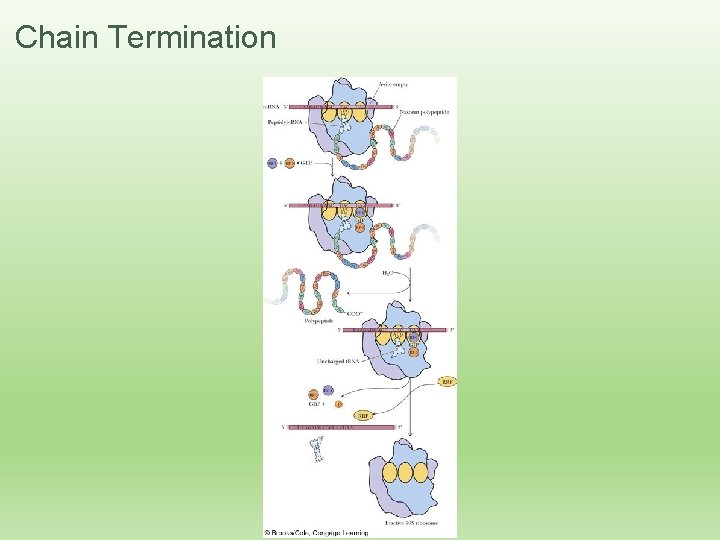
Chain Termination
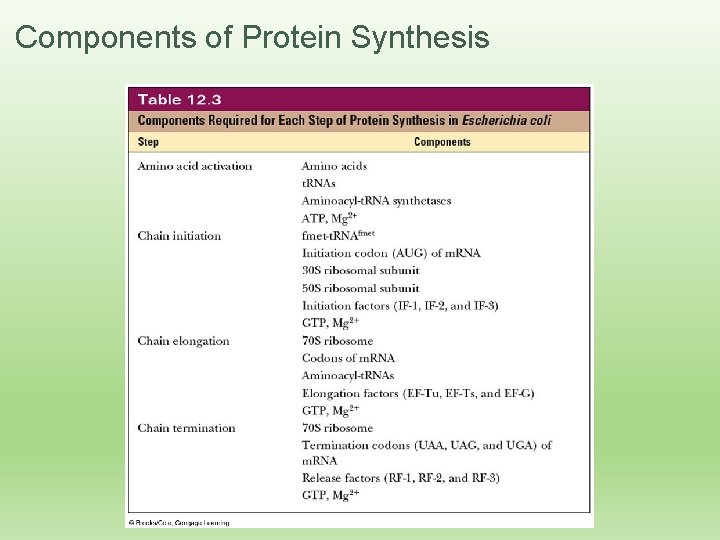
Components of Protein Synthesis
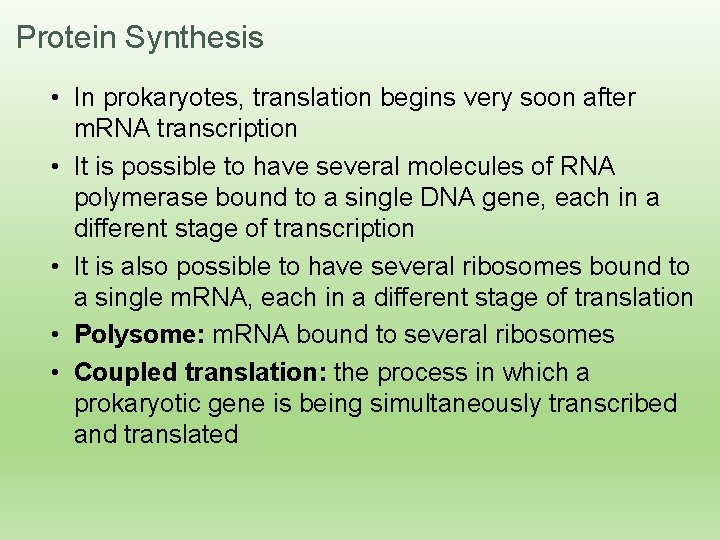
Protein Synthesis • In prokaryotes, translation begins very soon after m. RNA transcription • It is possible to have several molecules of RNA polymerase bound to a single DNA gene, each in a different stage of transcription • It is also possible to have several ribosomes bound to a single m. RNA, each in a different stage of translation • Polysome: m. RNA bound to several ribosomes • Coupled translation: the process in which a prokaryotic gene is being simultaneously transcribed and translated
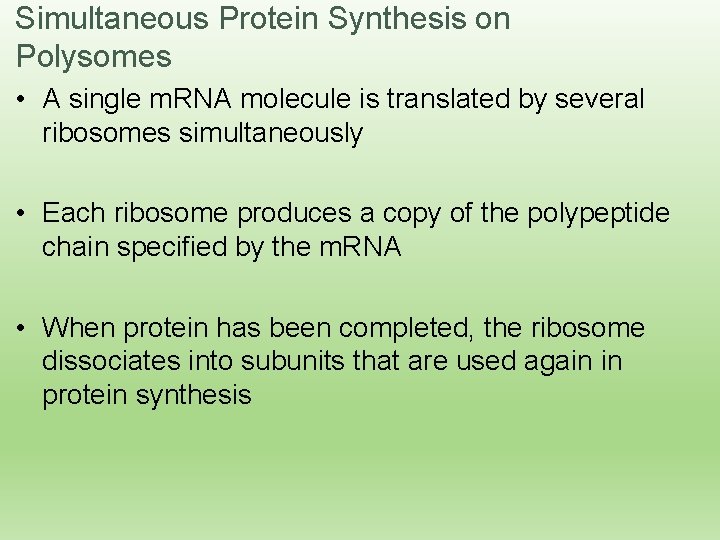
Simultaneous Protein Synthesis on Polysomes • A single m. RNA molecule is translated by several ribosomes simultaneously • Each ribosome produces a copy of the polypeptide chain specified by the m. RNA • When protein has been completed, the ribosome dissociates into subunits that are used again in protein synthesis
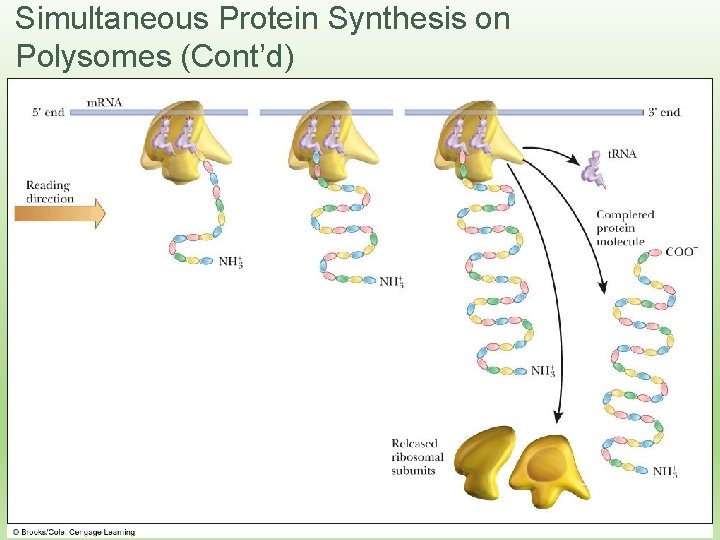
Simultaneous Protein Synthesis on Polysomes (Cont’d)
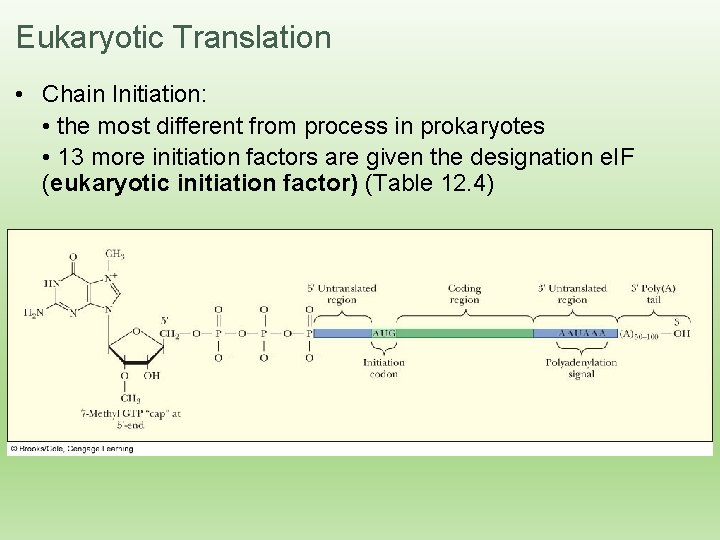
Eukaryotic Translation • Chain Initiation: • the most different from process in prokaryotes • 13 more initiation factors are given the designation e. IF (eukaryotic initiation factor) (Table 12. 4)
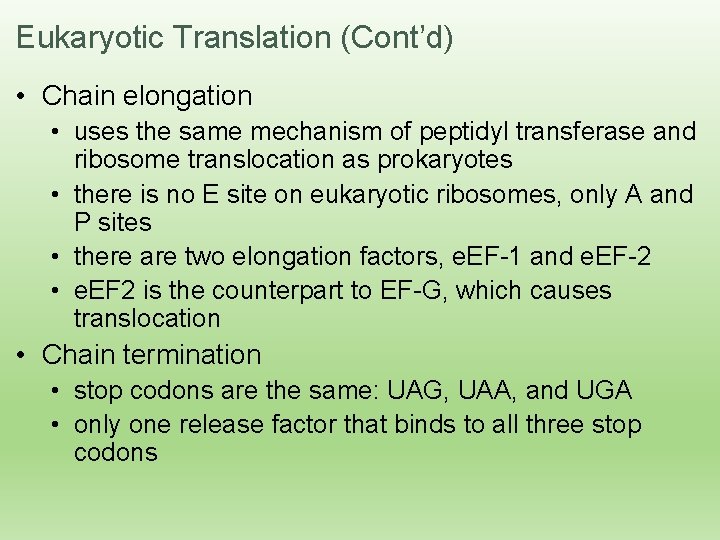
Eukaryotic Translation (Cont’d) • Chain elongation • uses the same mechanism of peptidyl transferase and ribosome translocation as prokaryotes • there is no E site on eukaryotic ribosomes, only A and P sites • there are two elongation factors, e. EF-1 and e. EF-2 • e. EF 2 is the counterpart to EF-G, which causes translocation • Chain termination • stop codons are the same: UAG, UAA, and UGA • only one release factor that binds to all three stop codons
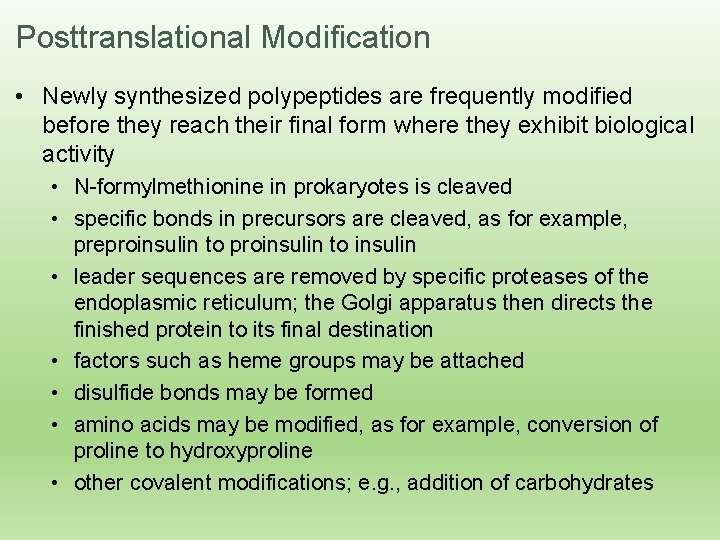
Posttranslational Modification • Newly synthesized polypeptides are frequently modified before they reach their final form where they exhibit biological activity • N-formylmethionine in prokaryotes is cleaved • specific bonds in precursors are cleaved, as for example, preproinsulin to insulin • leader sequences are removed by specific proteases of the endoplasmic reticulum; the Golgi apparatus then directs the finished protein to its final destination • factors such as heme groups may be attached • disulfide bonds may be formed • amino acids may be modified, as for example, conversion of proline to hydroxyproline • other covalent modifications; e. g. , addition of carbohydrates
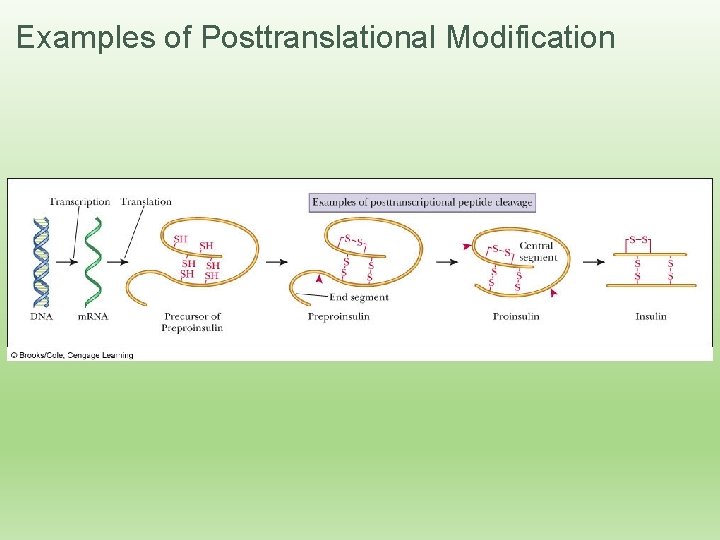
Examples of Posttranslational Modification
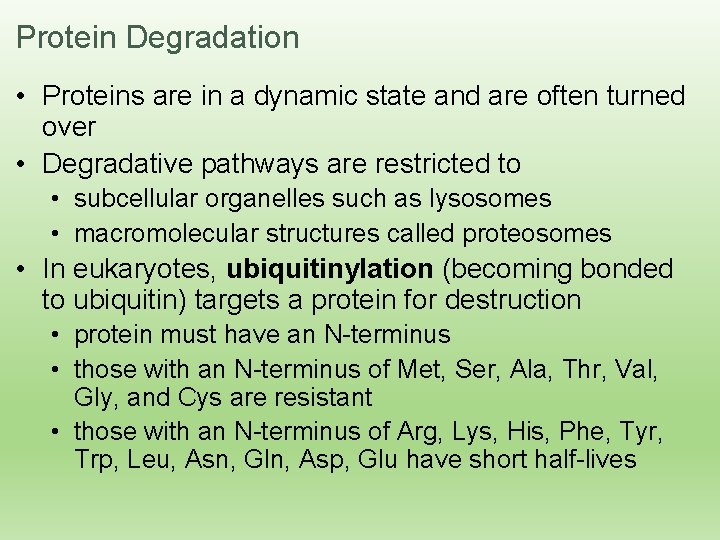
Protein Degradation • Proteins are in a dynamic state and are often turned over • Degradative pathways are restricted to • subcellular organelles such as lysosomes • macromolecular structures called proteosomes • In eukaryotes, ubiquitinylation (becoming bonded to ubiquitin) targets a protein for destruction • protein must have an N-terminus • those with an N-terminus of Met, Ser, Ala, Thr, Val, Gly, and Cys are resistant • those with an N-terminus of Arg, Lys, His, Phe, Tyr, Trp, Leu, Asn, Gln, Asp, Glu have short half-lives
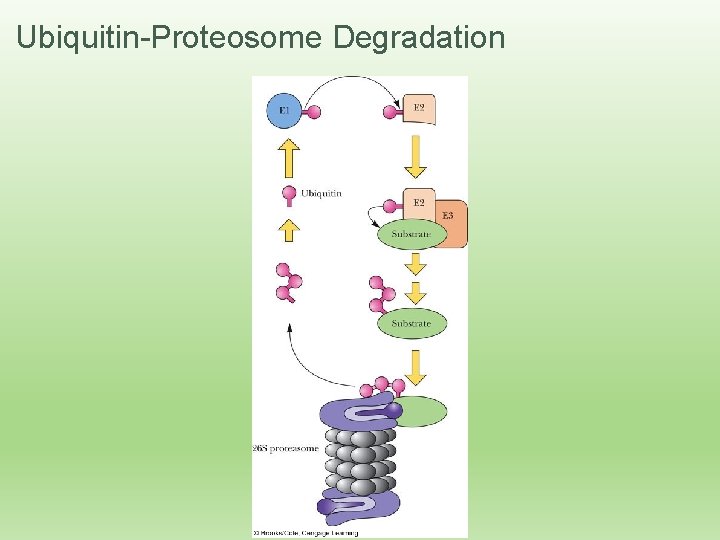
Ubiquitin-Proteosome Degradation
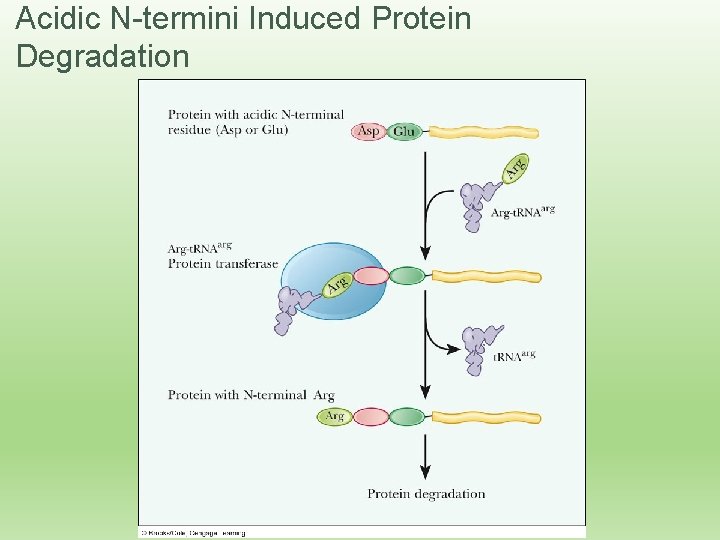
Acidic N-termini Induced Protein Degradation
Nucleotide sequence vs amino acid sequence
Dna and genes chapter 11
Four nucleotides of dna
Order of nucleotides in dna
When dna untwists, unzips, and nucleotides fill in.
Alloburinol
Hydoxyle
What is an anticodon
Nucleic acid building blocks
Nucleic acid structure
Function of nucleic acids
Nucleic acids are made up of
Dna rna
Importance of nucleic acid
Stores and transmits genetic (hereditary) information
Nucleic acid
Nucleic acid foods
Nucleic acids
Nature of nucleic acid
Dna molecule diagram labeled
Phosphodiester bonds
Nucleic acid dna structure
Nucleic acid dna structure
Trace the code genetic code table
Genetic drift definition
Genetic programming vs genetic algorithm
Genetic programming vs genetic algorithm
Gene flow vs genetic drift
What is the difference between genetic drift and gene flow
Unlike dna rna contains
Cytoplasm structure
Comparing dna and rna worksheet answers
Dna to protein steps
Dna to rna rules
Complete the following venn diagram
Dna and rna coloring worksheet
Rna base pairs vs dna