EP 06 Energy and Climate Change Dr JeanFrancois
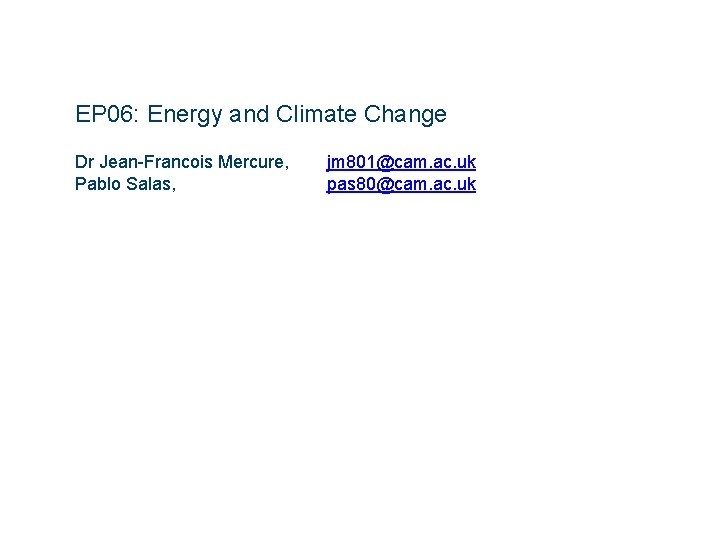
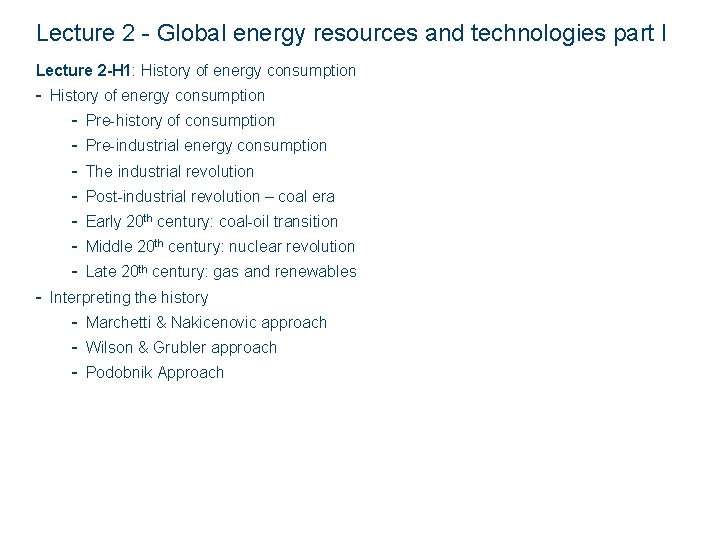
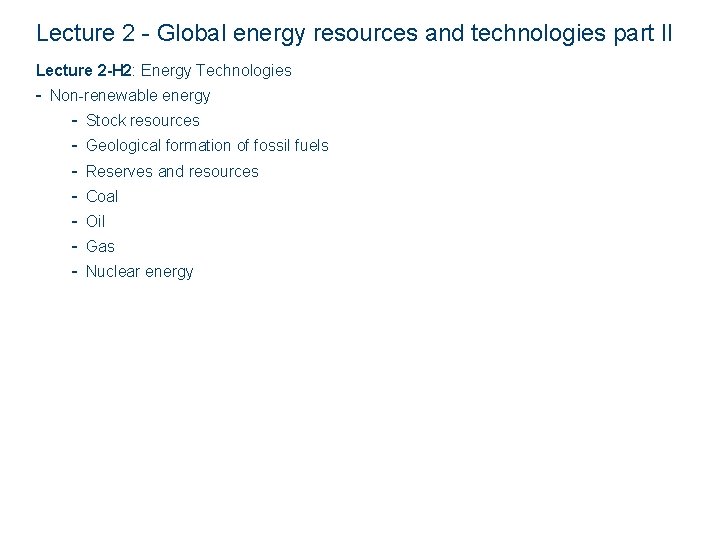
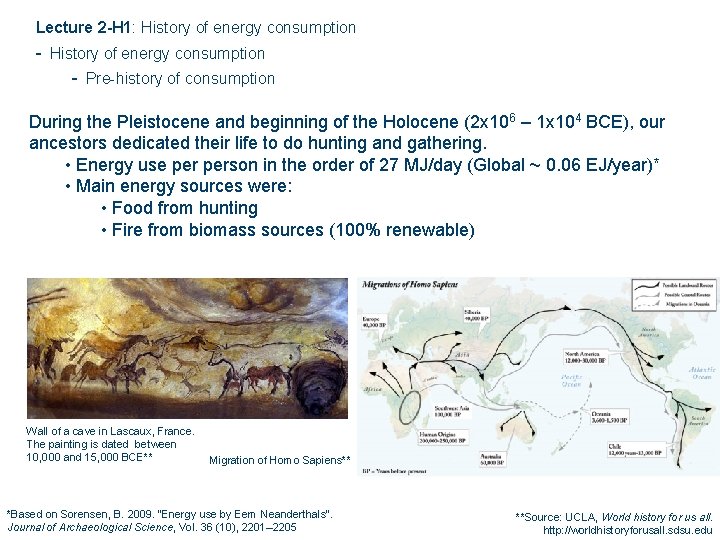
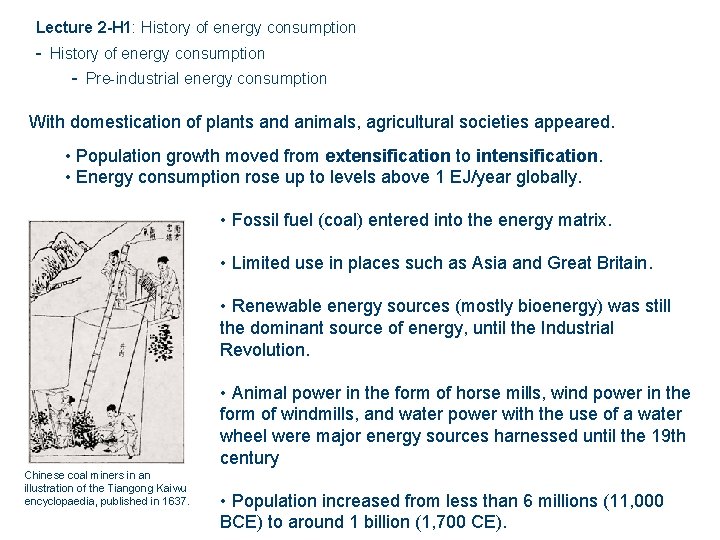
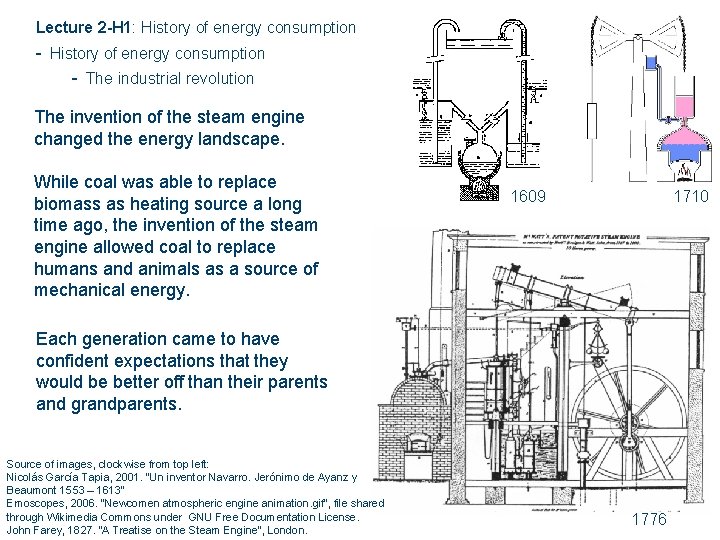
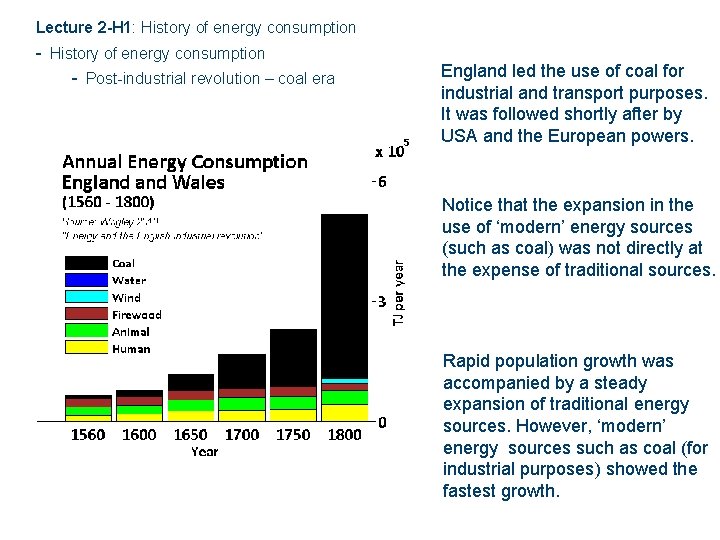
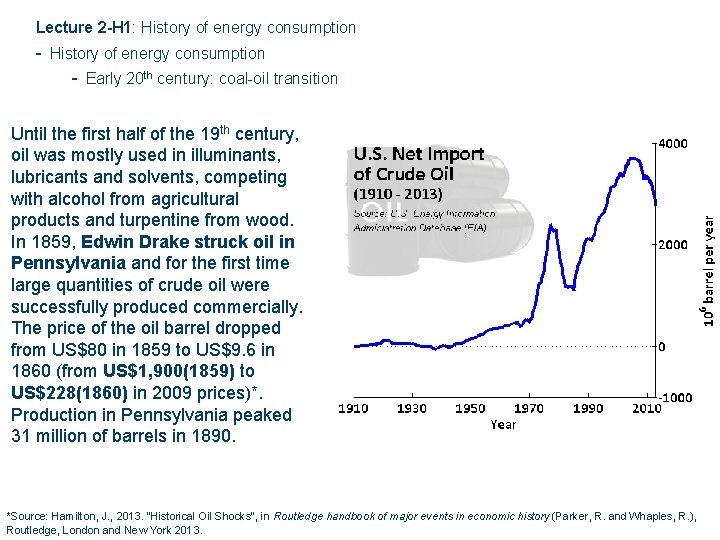
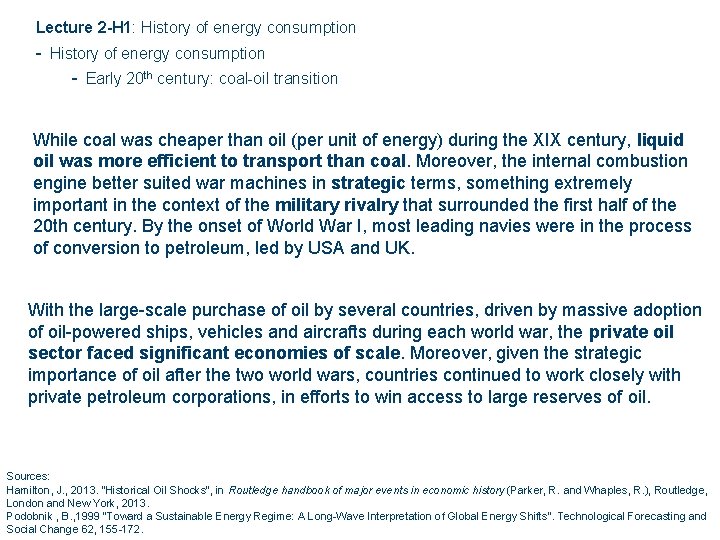
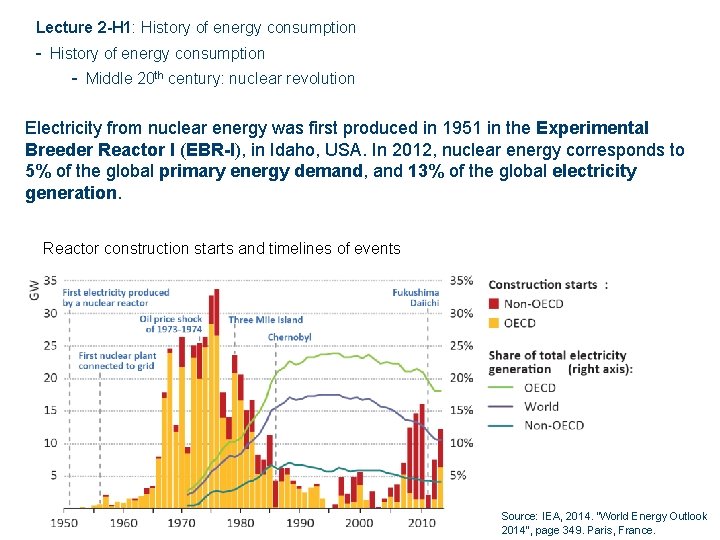
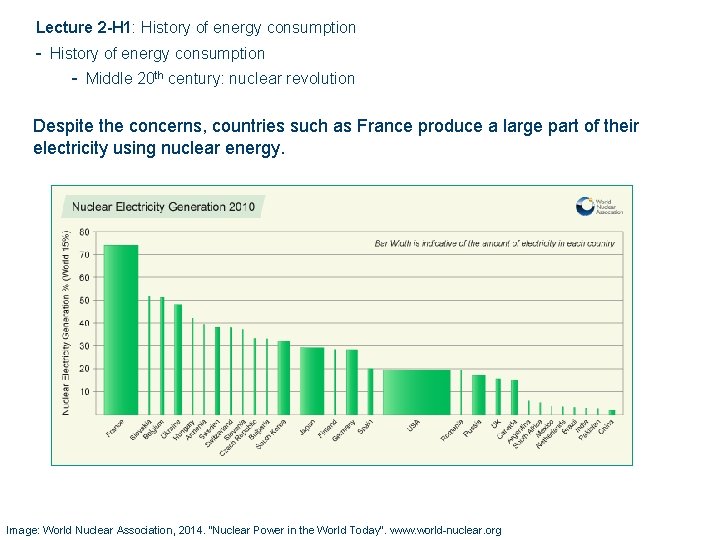
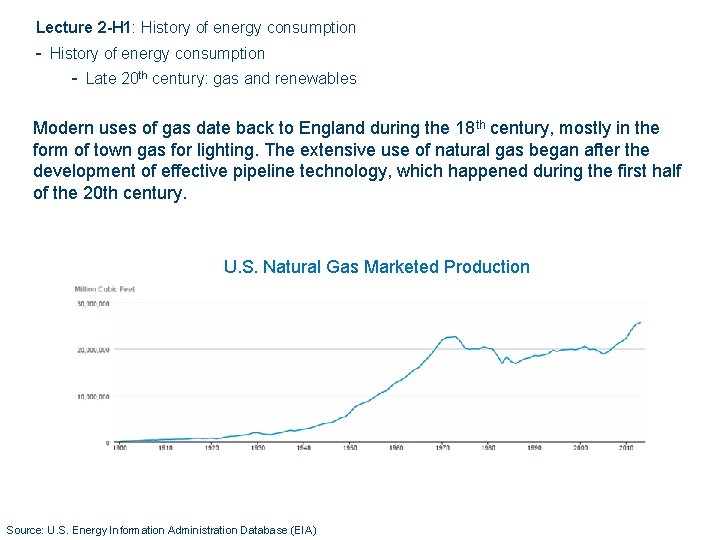
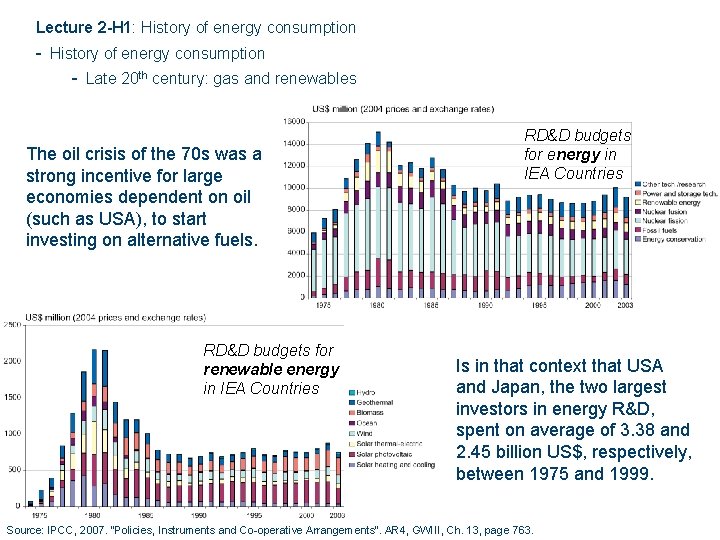
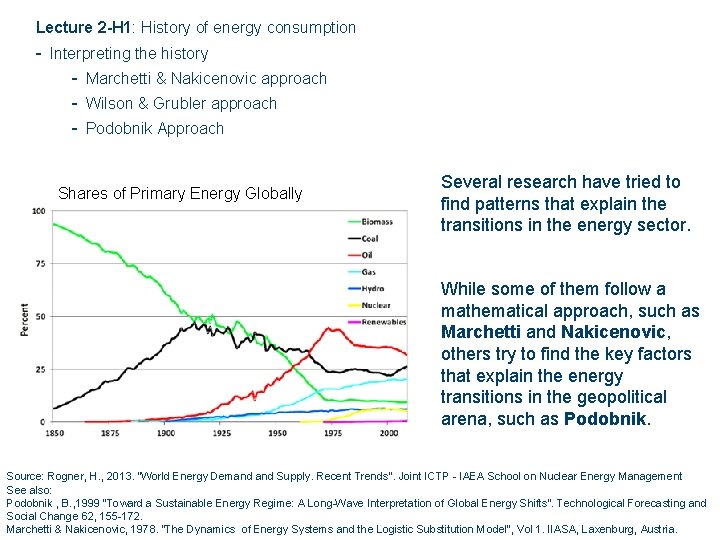
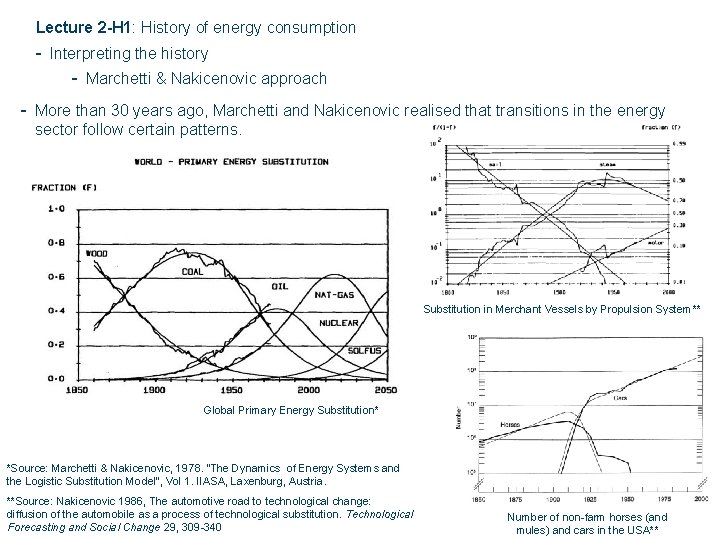
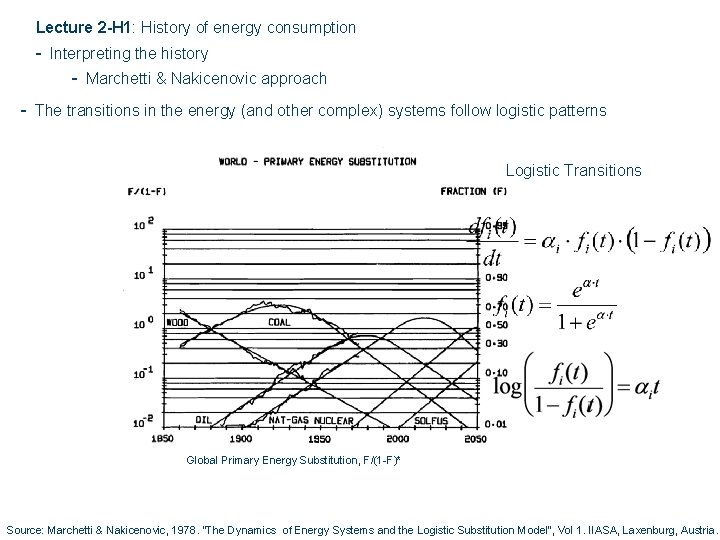
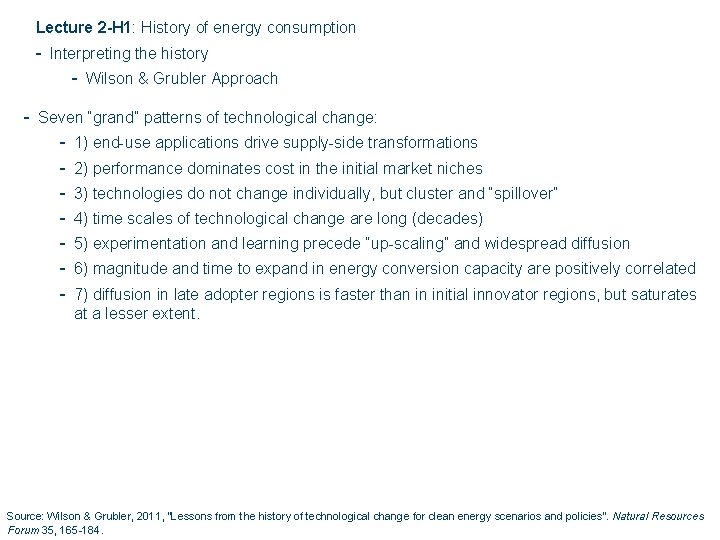
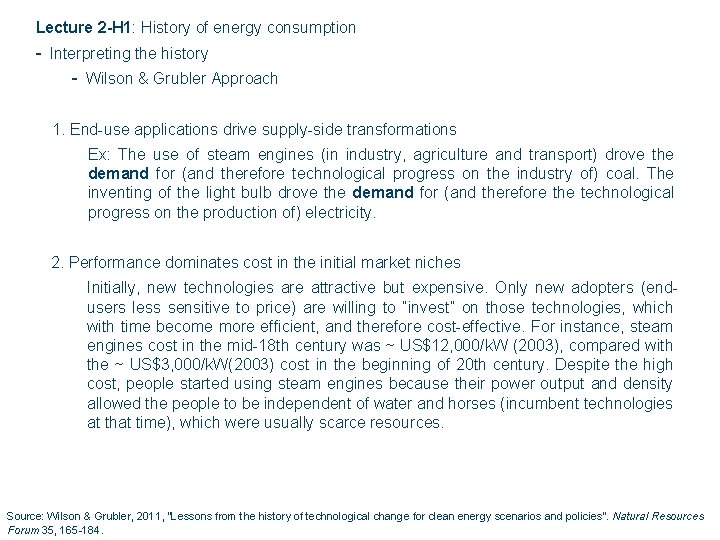
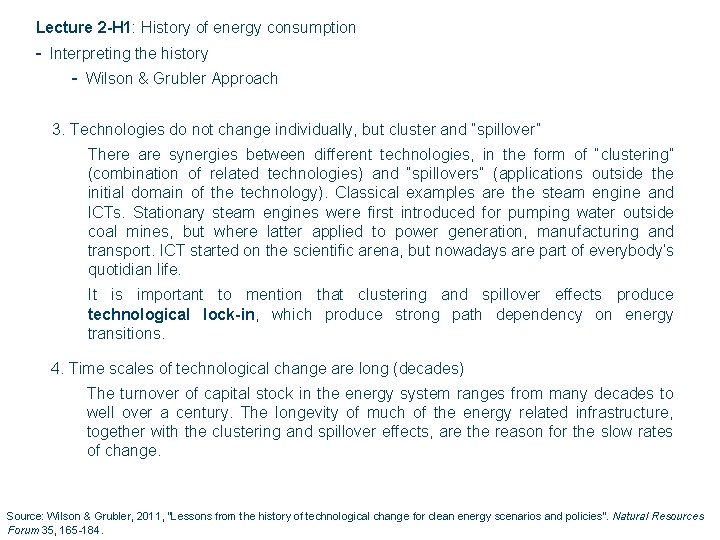
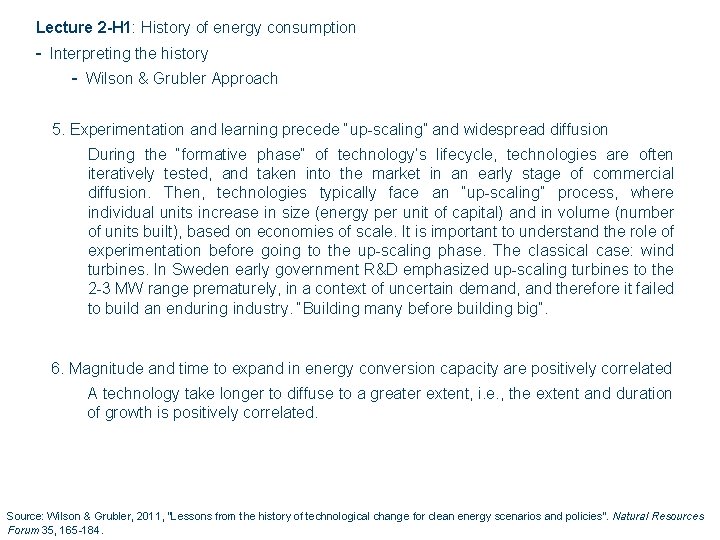
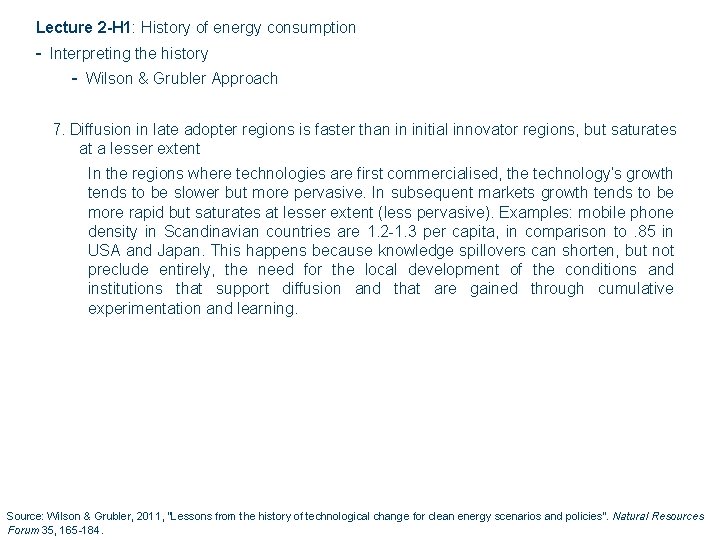
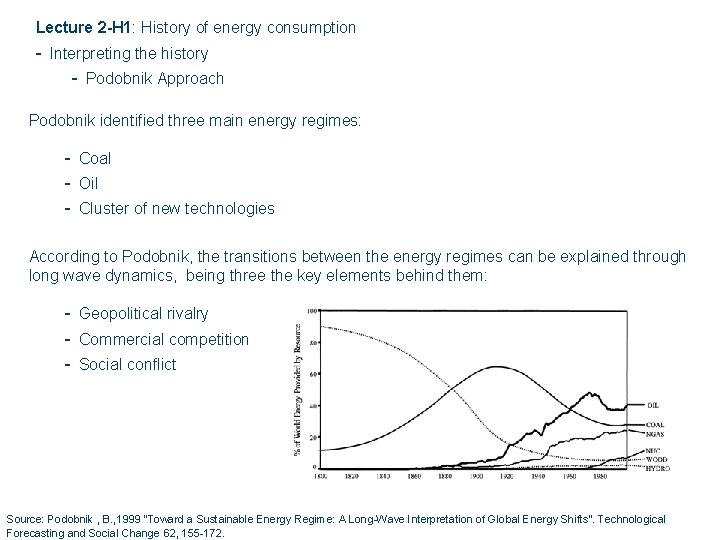
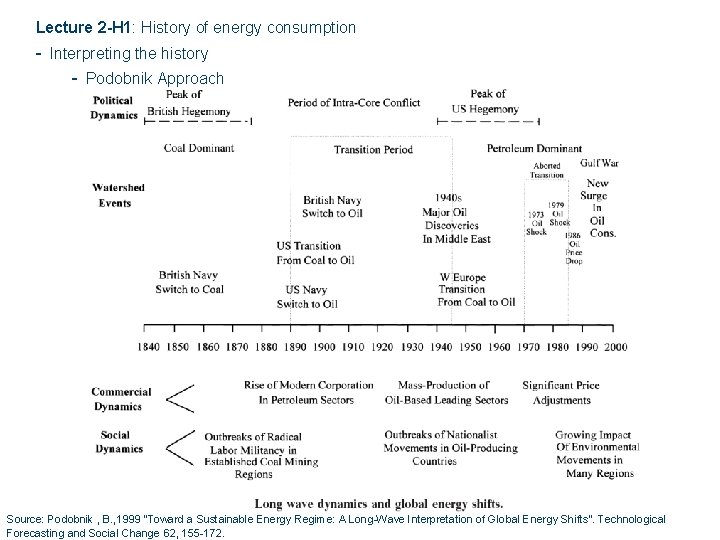
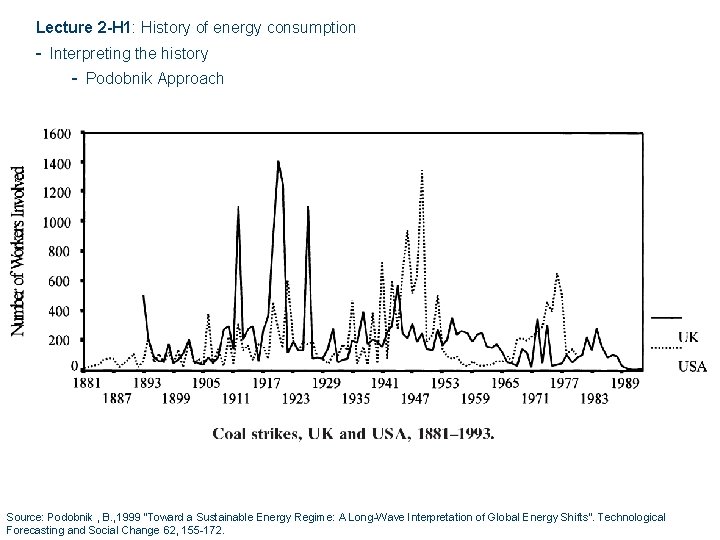
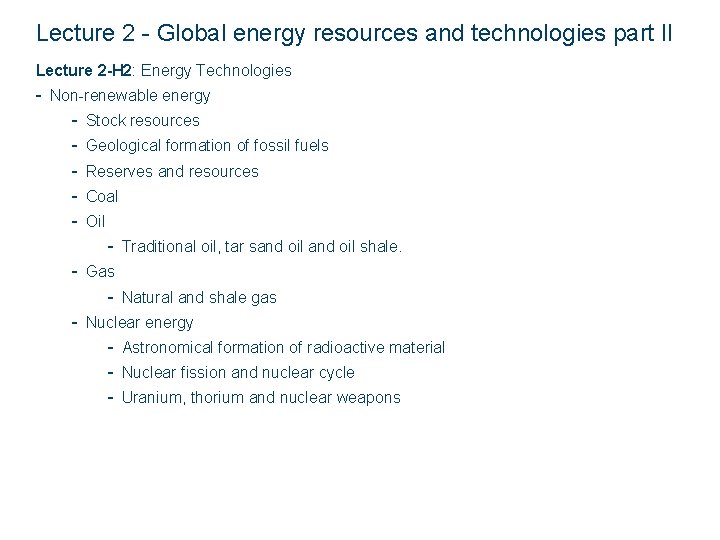
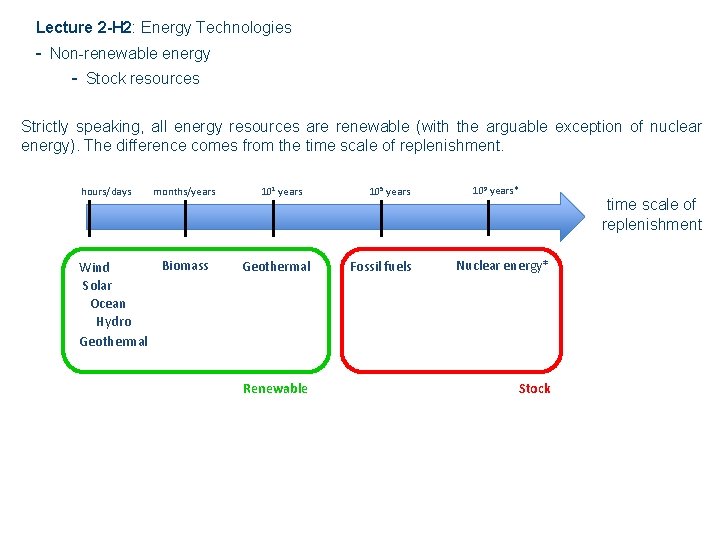
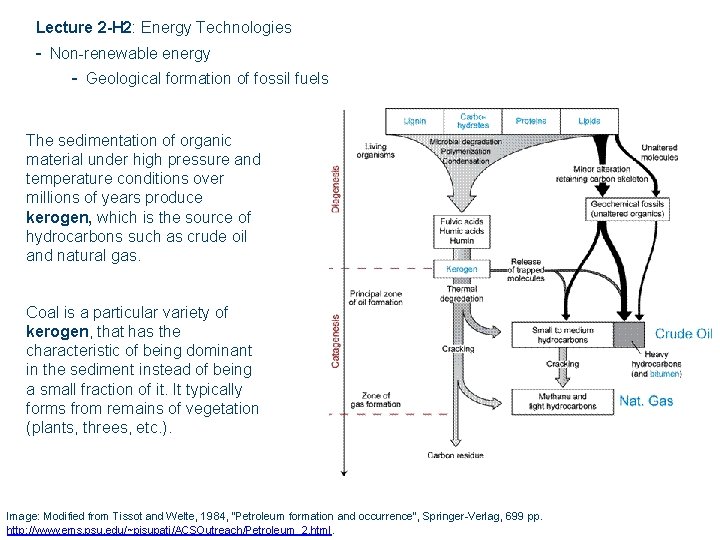
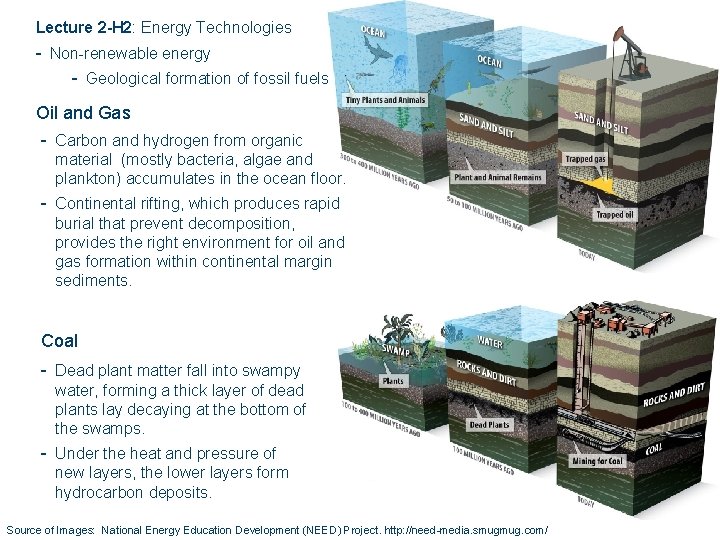
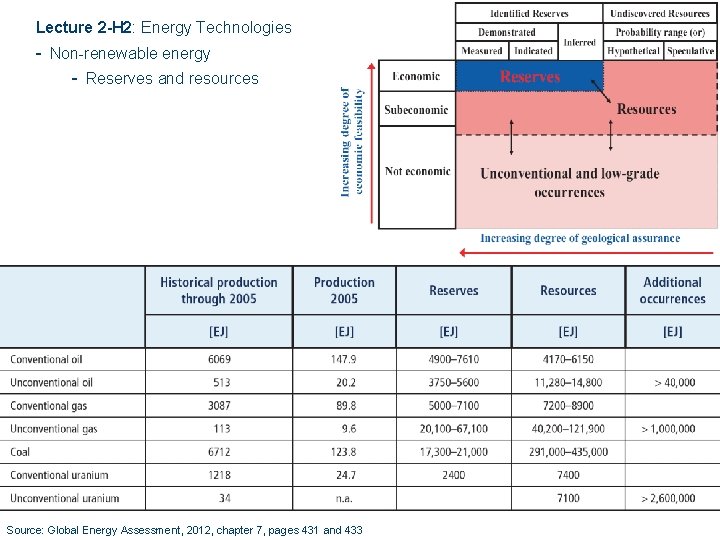
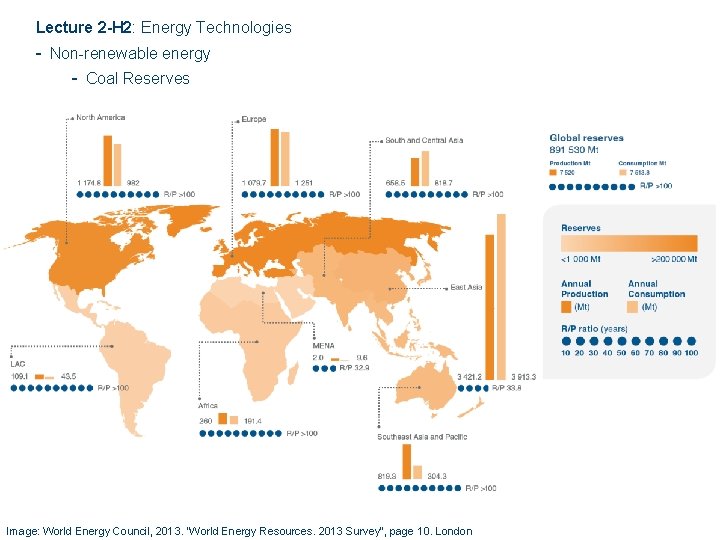
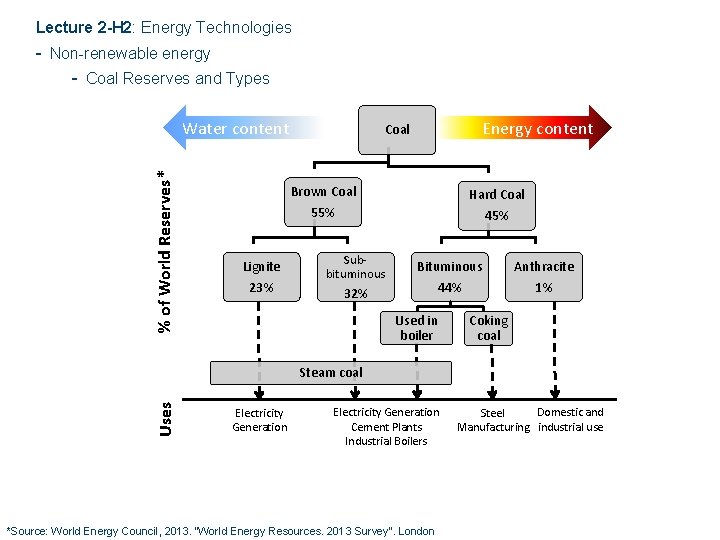
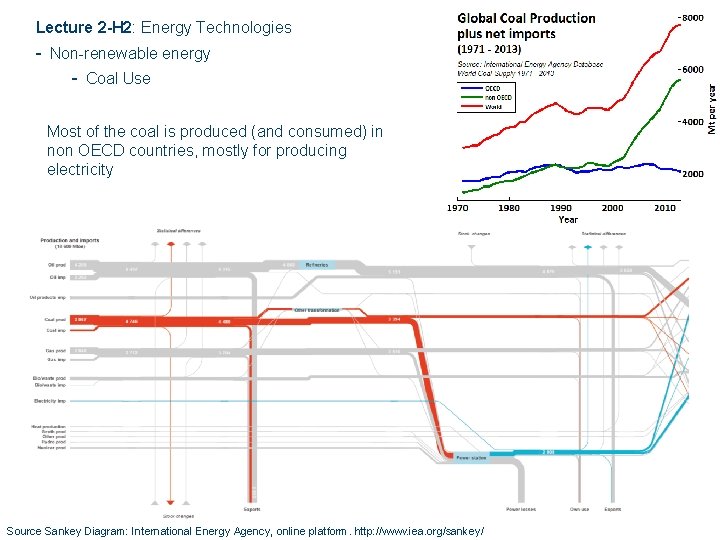
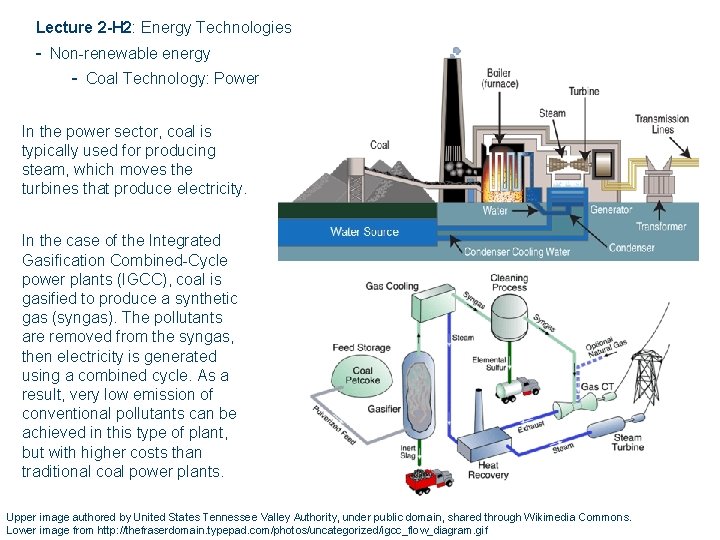
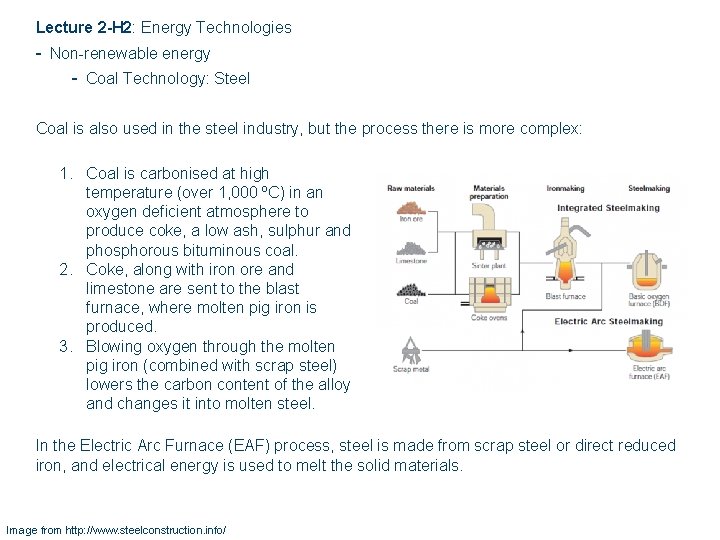
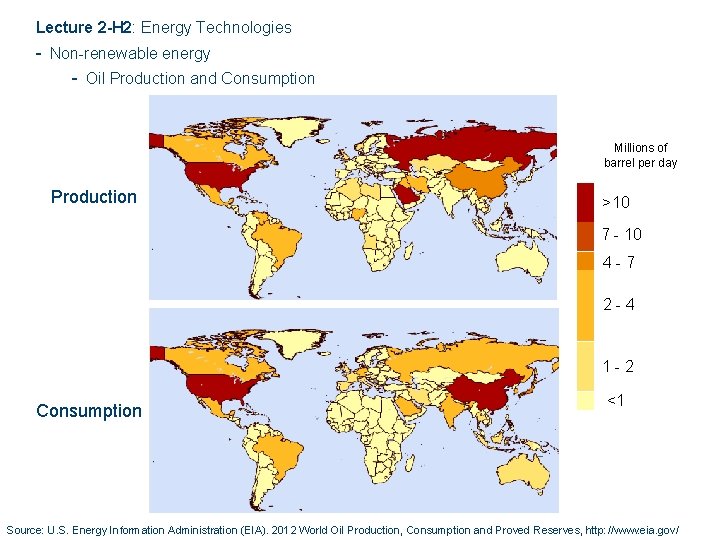
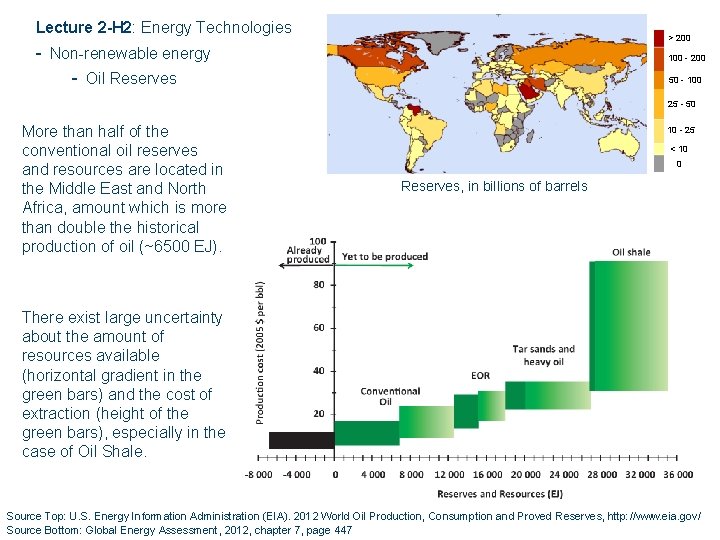
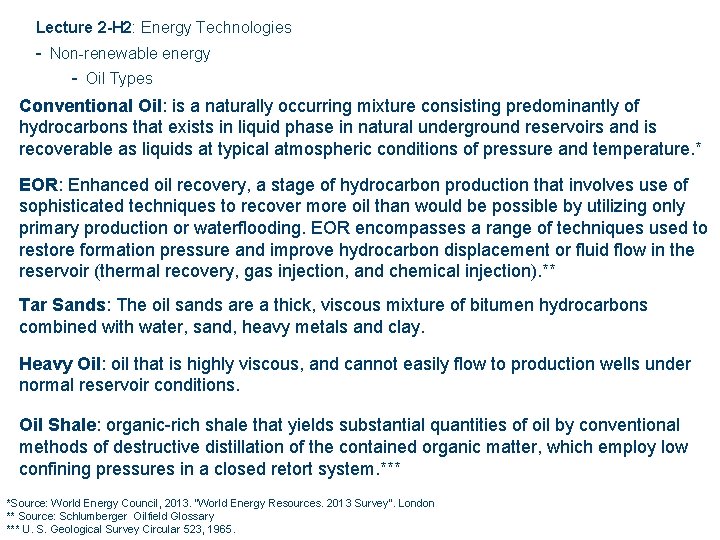
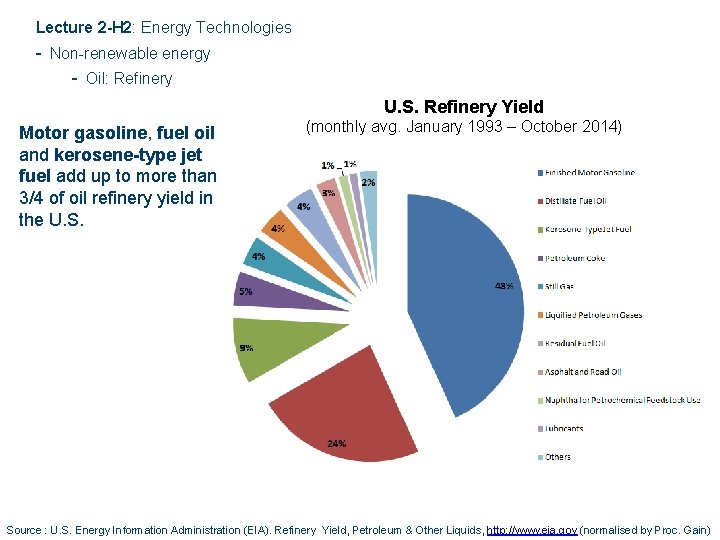
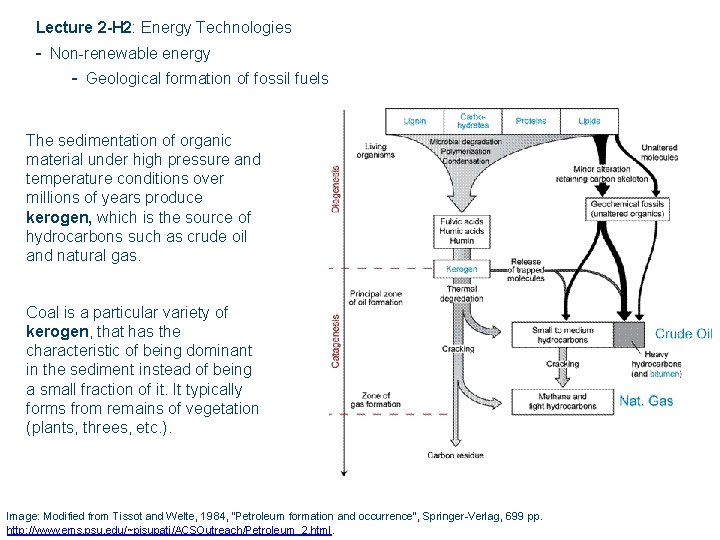
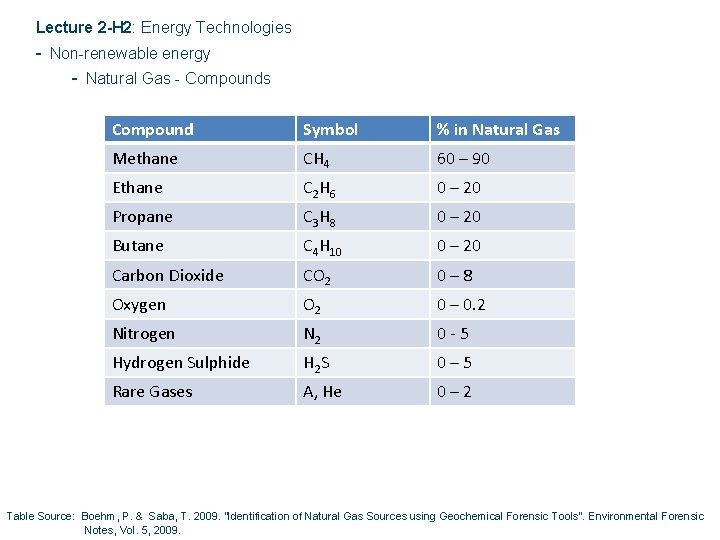
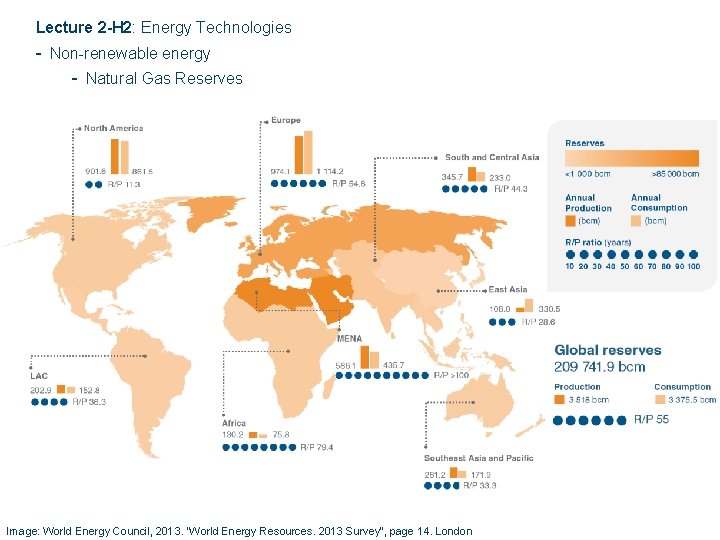
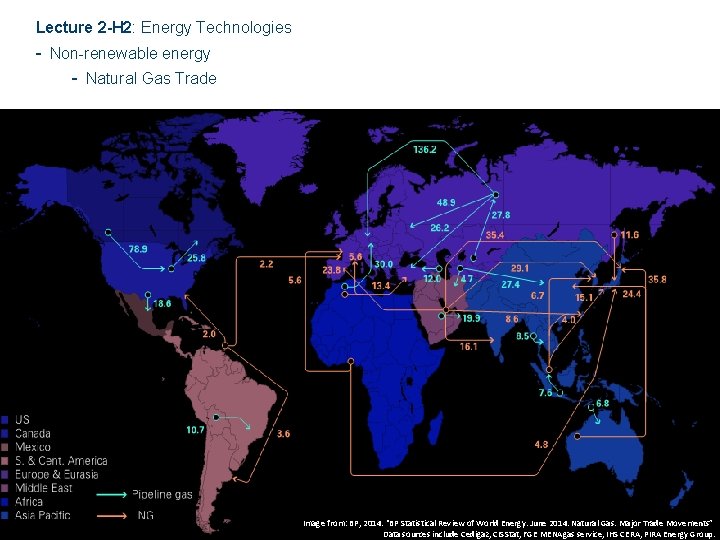
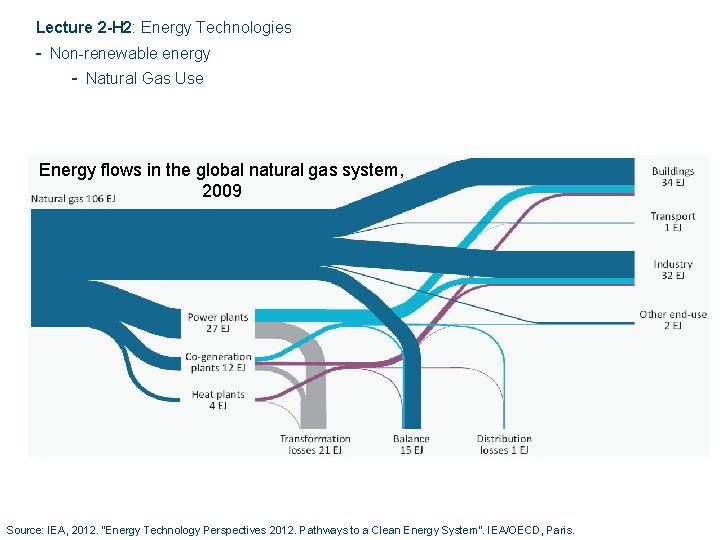
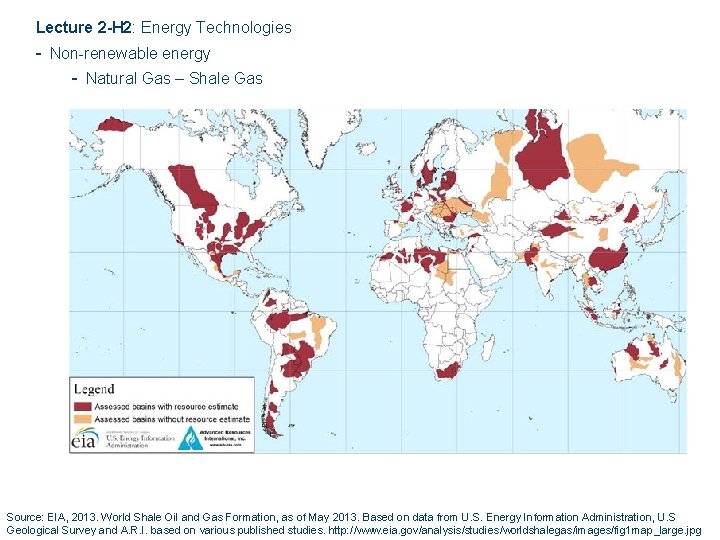
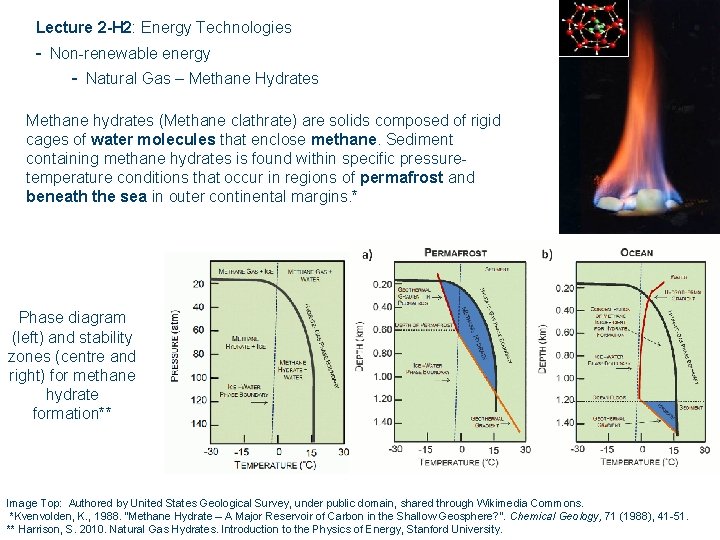
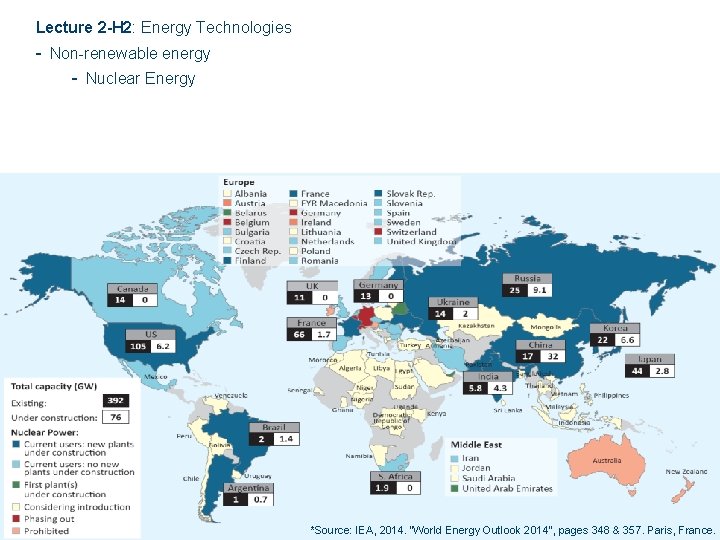
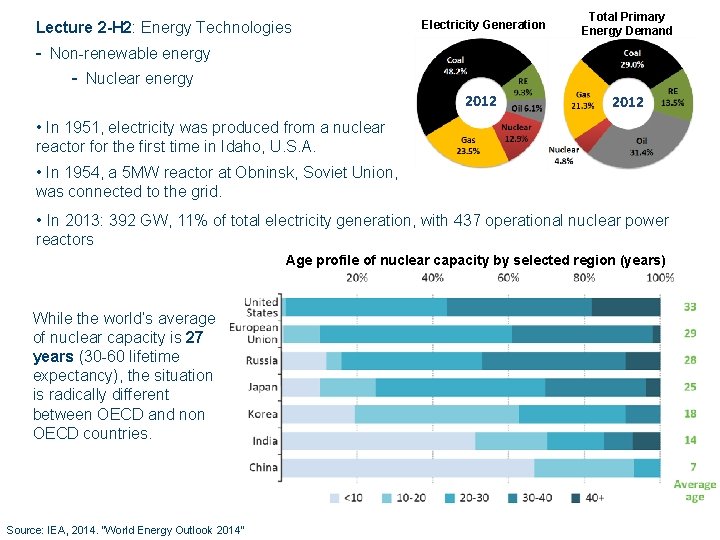
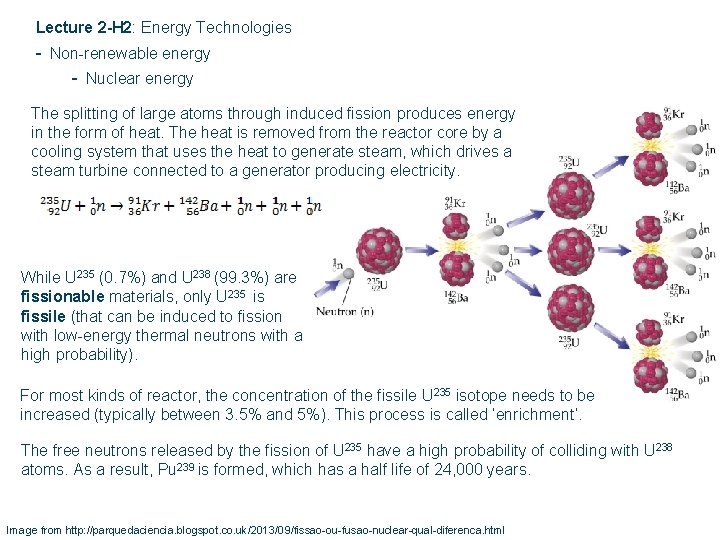
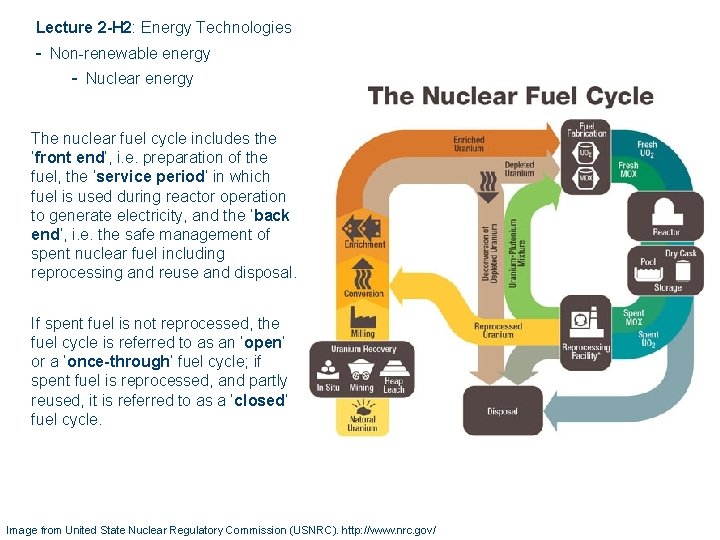
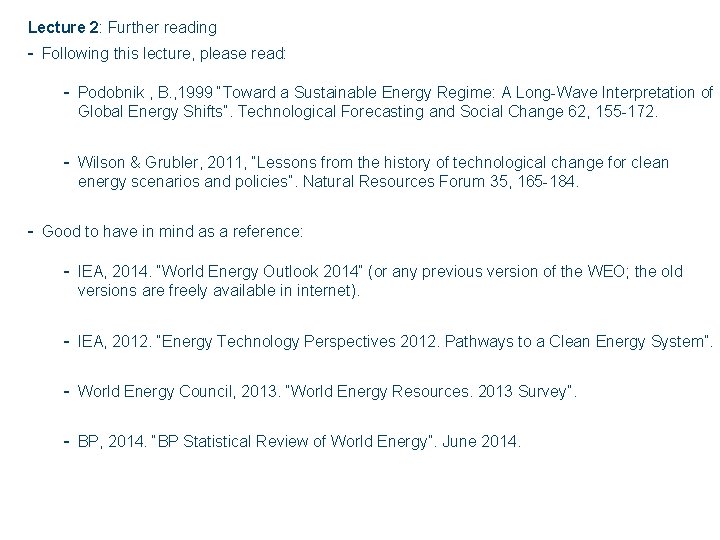
- Slides: 50
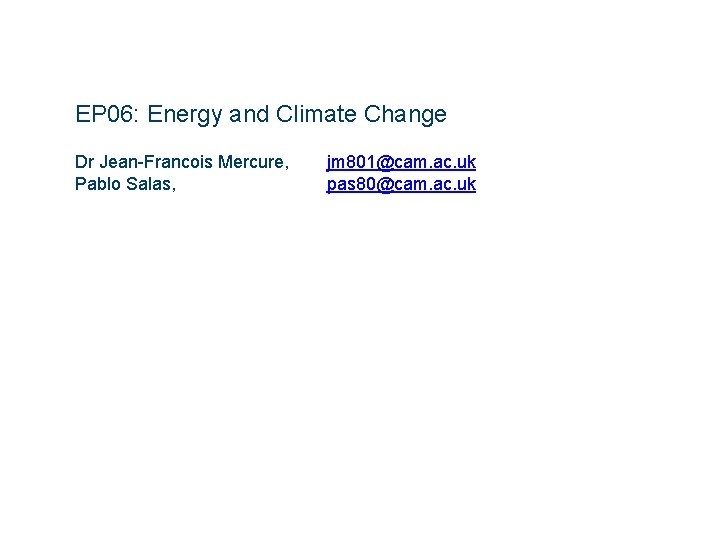
EP 06: Energy and Climate Change Dr Jean-Francois Mercure, Pablo Salas, jm 801@cam. ac. uk pas 80@cam. ac. uk
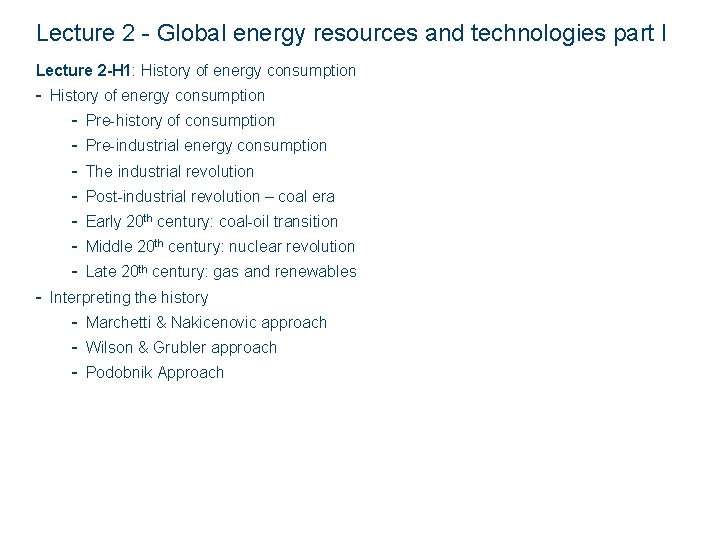
Lecture 2 - Global energy resources and technologies part I Lecture 2 -H 1: History of energy consumption - Pre-history of consumption - Pre-industrial energy consumption - The industrial revolution - Post-industrial revolution – coal era - Early 20 th century: coal-oil transition - Middle 20 th century: nuclear revolution - Late 20 th century: gas and renewables - Interpreting the history - Marchetti & Nakicenovic approach - Wilson & Grubler approach - Podobnik Approach
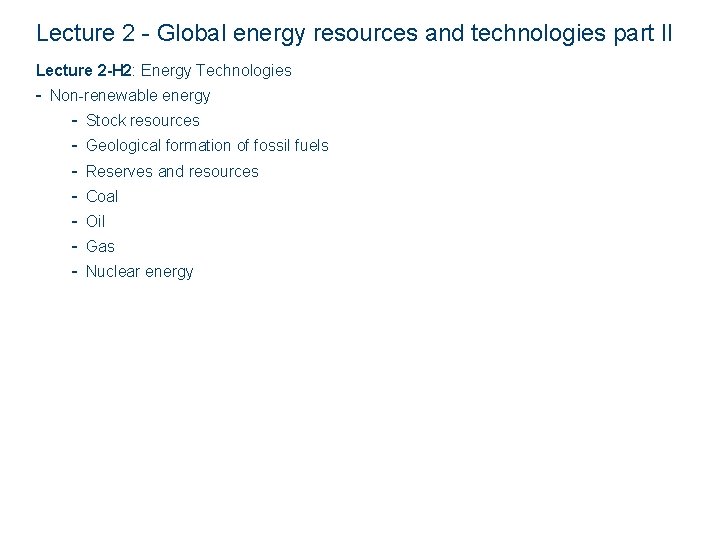
Lecture 2 - Global energy resources and technologies part II Lecture 2 -H 2: Energy Technologies - Non-renewable energy - Stock resources - Geological formation of fossil fuels - Reserves and resources - Coal - Oil - Gas - Nuclear energy
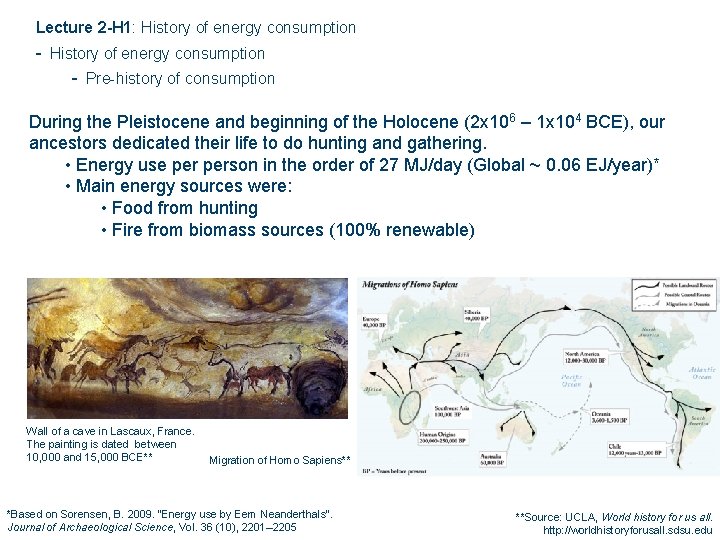
Lecture 2 -H 1: History of energy consumption - Pre-history of consumption During the Pleistocene and beginning of the Holocene (2 x 106 – 1 x 104 BCE), our ancestors dedicated their life to do hunting and gathering. • Energy use person in the order of 27 MJ/day (Global ~ 0. 06 EJ/year)* • Main energy sources were: • Food from hunting • Fire from biomass sources (100% renewable) Wall of a cave in Lascaux, France. The painting is dated between 10, 000 and 15, 000 BCE** Migration of Homo Sapiens** *Based on Sorensen, B. 2009. ”Energy use by Eem Neanderthals”. Journal of Archaeological Science, Vol. 36 (10), 2201– 2205 **Source: UCLA, World history for us all. http: //worldhistoryforusall. sdsu. edu
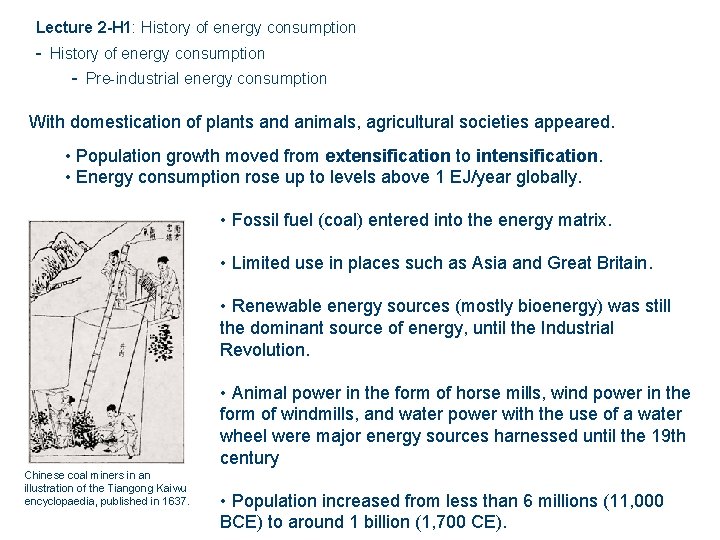
Lecture 2 -H 1: History of energy consumption - Pre-industrial energy consumption With domestication of plants and animals, agricultural societies appeared. • Population growth moved from extensification to intensification. • Energy consumption rose up to levels above 1 EJ/year globally. • Fossil fuel (coal) entered into the energy matrix. • Limited use in places such as Asia and Great Britain. • Renewable energy sources (mostly bioenergy) was still the dominant source of energy, until the Industrial Revolution. • Animal power in the form of horse mills, wind power in the form of windmills, and water power with the use of a water wheel were major energy sources harnessed until the 19 th century Chinese coal miners in an illustration of the Tiangong Kaiwu encyclopaedia, published in 1637. • Population increased from less than 6 millions (11, 000 BCE) to around 1 billion (1, 700 CE).
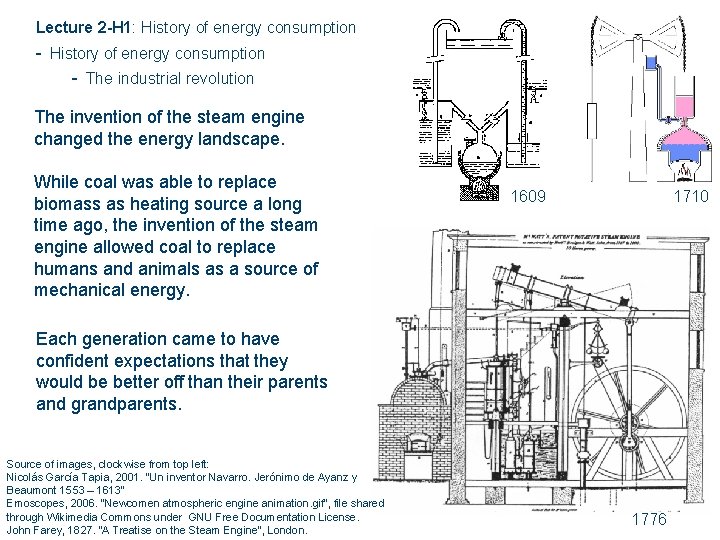
Lecture 2 -H 1: History of energy consumption - The industrial revolution The invention of the steam engine changed the energy landscape. While coal was able to replace biomass as heating source a long time ago, the invention of the steam engine allowed coal to replace humans and animals as a source of mechanical energy. 1609 1710 Each generation came to have confident expectations that they would be better off than their parents and grandparents. Source of images, clockwise from top left: Nicolás García Tapia, 2001. “Un inventor Navarro. Jerónimo de Ayanz y Beaumont 1553 – 1613” Emoscopes, 2006. “Newcomen atmospheric engine animation. gif”, file shared through Wikimedia Commons under GNU Free Documentation License. John Farey, 1827. “A Treatise on the Steam Engine”, London. 1776
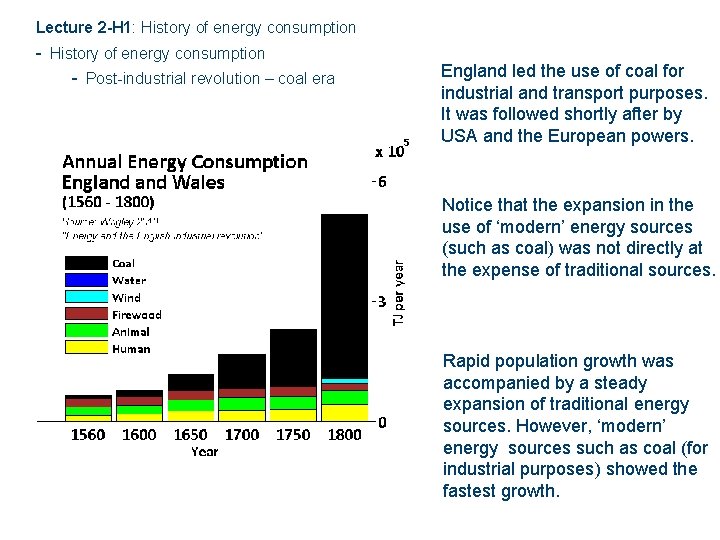
Lecture 2 -H 1: History of energy consumption - Post-industrial revolution – coal era England led the use of coal for industrial and transport purposes. It was followed shortly after by USA and the European powers. Notice that the expansion in the use of ‘modern’ energy sources (such as coal) was not directly at the expense of traditional sources. Rapid population growth was accompanied by a steady expansion of traditional energy sources. However, ‘modern’ energy sources such as coal (for industrial purposes) showed the fastest growth.
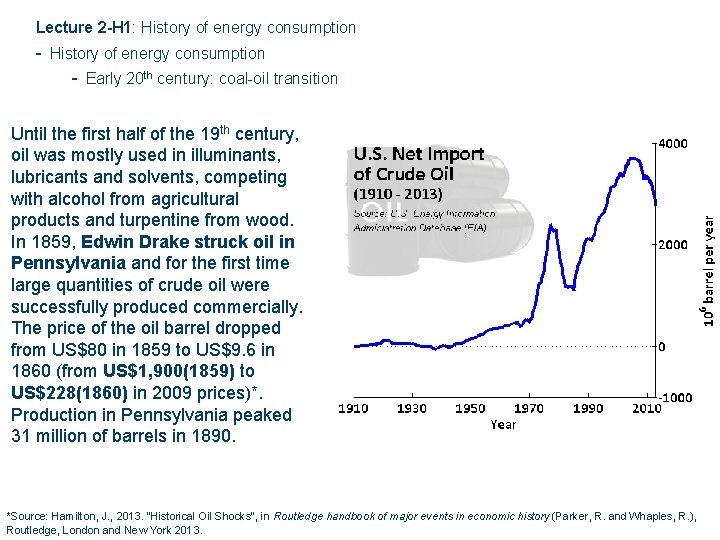
Lecture 2 -H 1: History of energy consumption - Early 20 th century: coal-oil transition Until the first half of the 19 th century, oil was mostly used in illuminants, lubricants and solvents, competing with alcohol from agricultural products and turpentine from wood. In 1859, Edwin Drake struck oil in Pennsylvania and for the first time large quantities of crude oil were successfully produced commercially. The price of the oil barrel dropped from US$80 in 1859 to US$9. 6 in 1860 (from US$1, 900(1859) to US$228(1860) in 2009 prices)*. Production in Pennsylvania peaked 31 million of barrels in 1890. *Source: Hamilton, J. , 2013. “Historical Oil Shocks”, in Routledge handbook of major events in economic history (Parker, R. and Whaples, R. ), Routledge, London and New York 2013.
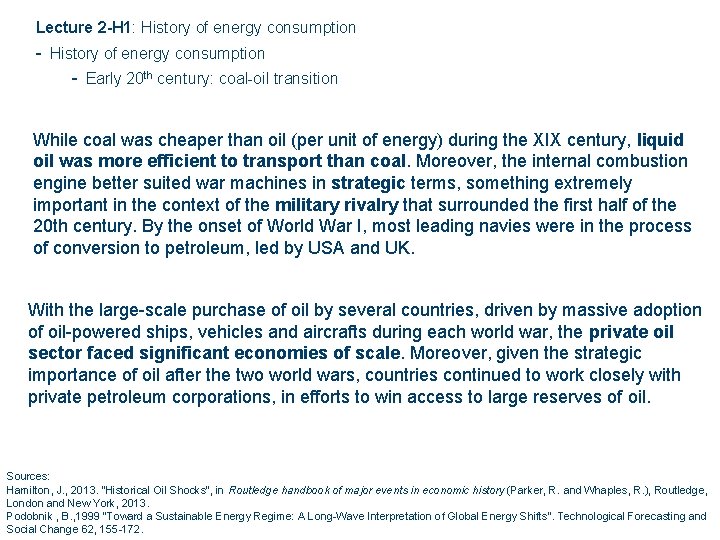
Lecture 2 -H 1: History of energy consumption - Early 20 th century: coal-oil transition While coal was cheaper than oil (per unit of energy) during the XIX century, liquid oil was more efficient to transport than coal. Moreover, the internal combustion engine better suited war machines in strategic terms, something extremely important in the context of the military rivalry that surrounded the first half of the 20 th century. By the onset of World War I, most leading navies were in the process of conversion to petroleum, led by USA and UK. With the large-scale purchase of oil by several countries, driven by massive adoption of oil-powered ships, vehicles and aircrafts during each world war, the private oil sector faced significant economies of scale. Moreover, given the strategic importance of oil after the two world wars, countries continued to work closely with private petroleum corporations, in efforts to win access to large reserves of oil. Sources: Hamilton, J. , 2013. “Historical Oil Shocks”, in Routledge handbook of major events in economic history (Parker, R. and Whaples, R. ), Routledge, London and New York, 2013. Podobnik , B. , 1999 “Toward a Sustainable Energy Regime: A Long-Wave Interpretation of Global Energy Shifts”. Technological Forecasting and Social Change 62, 155 -172.
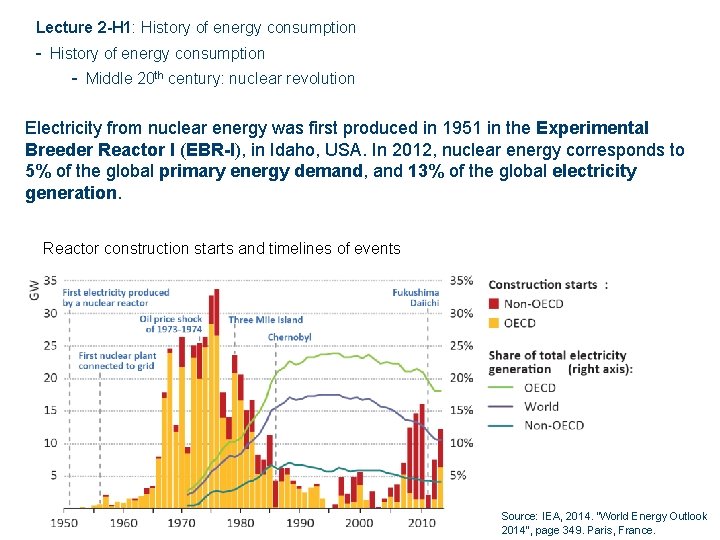
Lecture 2 -H 1: History of energy consumption - Middle 20 th century: nuclear revolution Electricity from nuclear energy was first produced in 1951 in the Experimental Breeder Reactor I (EBR-I), in Idaho, USA. In 2012, nuclear energy corresponds to 5% of the global primary energy demand, and 13% of the global electricity generation. Reactor construction starts and timelines of events Source: IEA, 2014. “World Energy Outlook 2014”, page 349. Paris, France.
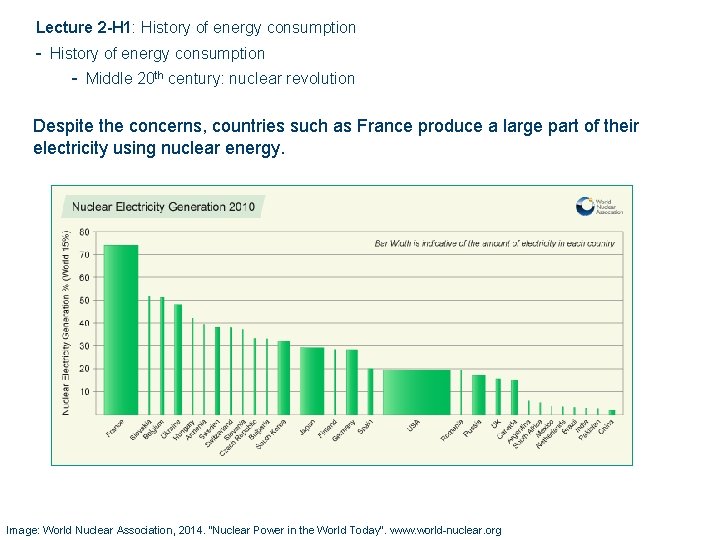
Lecture 2 -H 1: History of energy consumption - Middle 20 th century: nuclear revolution Despite the concerns, countries such as France produce a large part of their electricity using nuclear energy. Image: World Nuclear Association, 2014. “Nuclear Power in the World Today”. www. world-nuclear. org
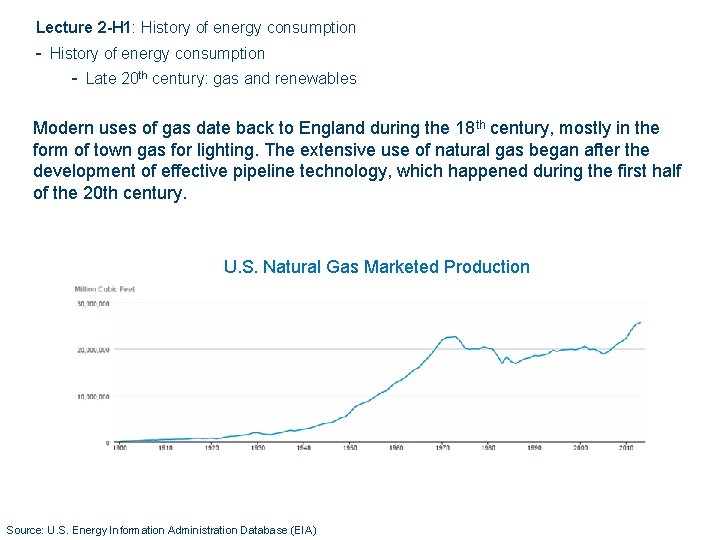
Lecture 2 -H 1: History of energy consumption - Late 20 th century: gas and renewables Modern uses of gas date back to England during the 18 th century, mostly in the form of town gas for lighting. The extensive use of natural gas began after the development of effective pipeline technology, which happened during the first half of the 20 th century. U. S. Natural Gas Marketed Production Source: U. S. Energy Information Administration Database (EIA)
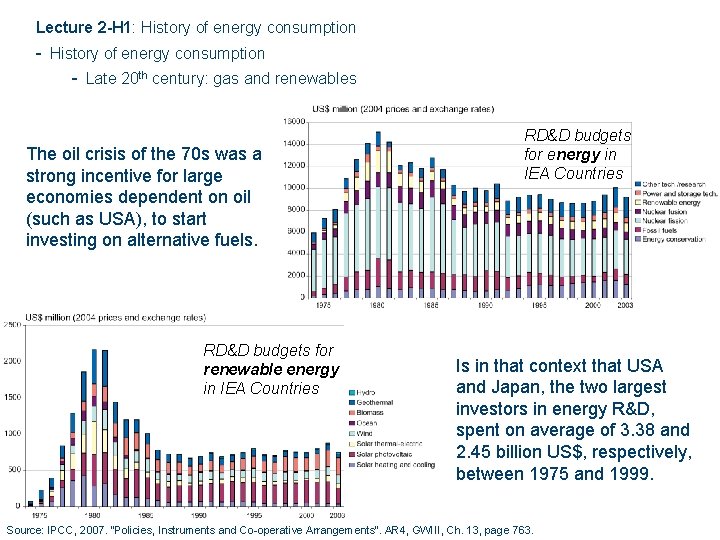
Lecture 2 -H 1: History of energy consumption - Late 20 th century: gas and renewables The oil crisis of the 70 s was a strong incentive for large economies dependent on oil (such as USA), to start investing on alternative fuels. RD&D budgets for renewable energy in IEA Countries RD&D budgets for energy in IEA Countries Is in that context that USA and Japan, the two largest investors in energy R&D, spent on average of 3. 38 and 2. 45 billion US$, respectively, between 1975 and 1999. Source: IPCC, 2007. “Policies, Instruments and Co-operative Arrangements”. AR 4, GWIII, Ch. 13, page 763.
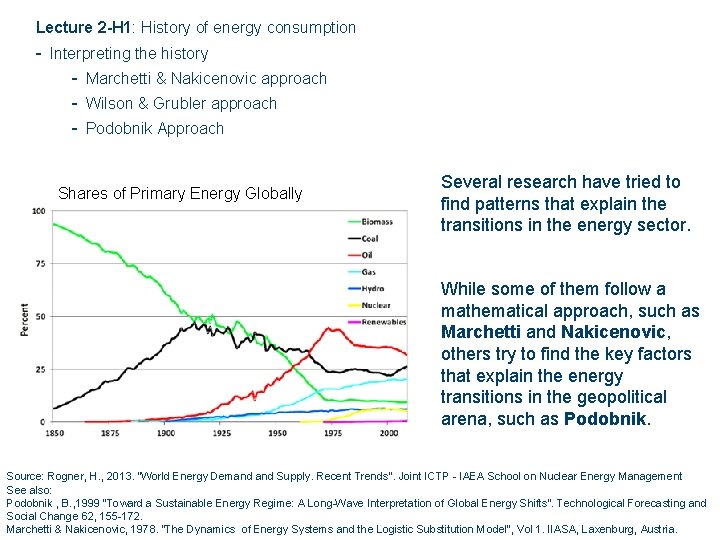
Lecture 2 -H 1: History of energy consumption - Interpreting the history - Marchetti & Nakicenovic approach - Wilson & Grubler approach - Podobnik Approach Shares of Primary Energy Globally Several research have tried to find patterns that explain the transitions in the energy sector. While some of them follow a mathematical approach, such as Marchetti and Nakicenovic, others try to find the key factors that explain the energy transitions in the geopolitical arena, such as Podobnik. Source: Rogner, H. , 2013. “World Energy Demand Supply. Recent Trends”. Joint ICTP - IAEA School on Nuclear Energy Management See also: Podobnik , B. , 1999 “Toward a Sustainable Energy Regime: A Long-Wave Interpretation of Global Energy Shifts”. Technological Forecasting and Social Change 62, 155 -172. Marchetti & Nakicenovic, 1978. “The Dynamics of Energy Systems and the Logistic Substitution Model”, Vol 1. IIASA, Laxenburg, Austria.
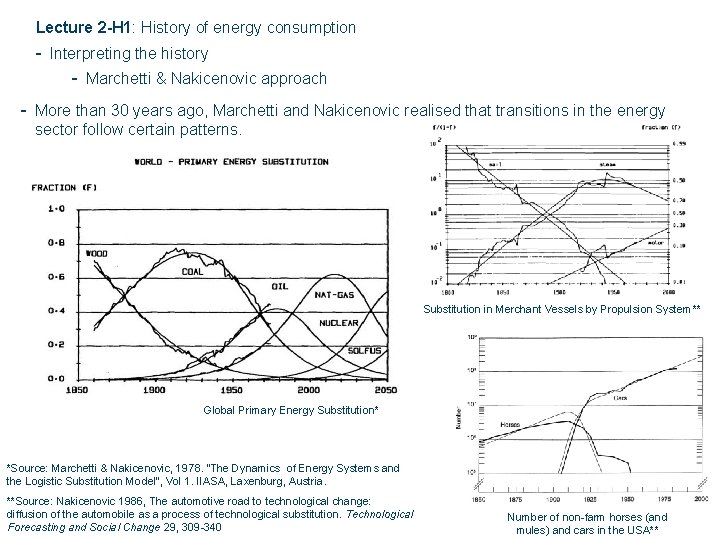
Lecture 2 -H 1: History of energy consumption - Interpreting the history - Marchetti & Nakicenovic approach - More than 30 years ago, Marchetti and Nakicenovic realised that transitions in the energy sector follow certain patterns. Substitution in Merchant Vessels by Propulsion System** Global Primary Energy Substitution* *Source: Marchetti & Nakicenovic, 1978. “The Dynamics of Energy Systems and the Logistic Substitution Model”, Vol 1. IIASA, Laxenburg, Austria. **Source: Nakicenovic 1986, The automotive road to technological change: diffusion of the automobile as a process of technological substitution. Technological Forecasting and Social Change 29, 309 -340 Number of non-farm horses (and mules) and cars in the USA**
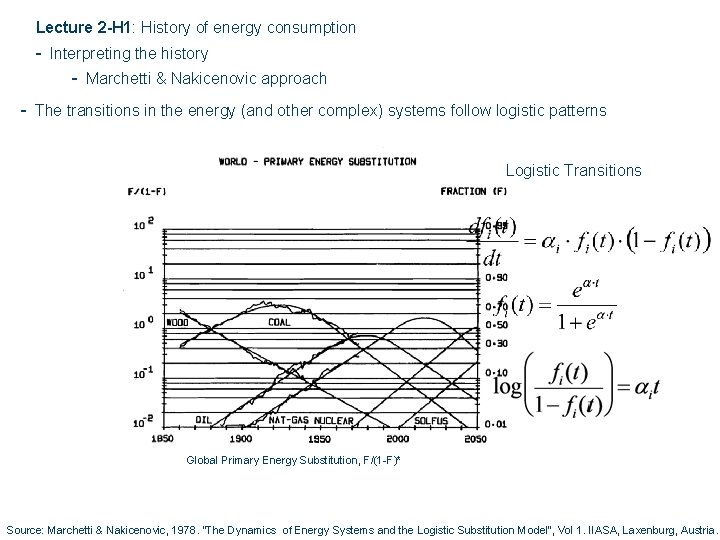
Lecture 2 -H 1: History of energy consumption - Interpreting the history - Marchetti & Nakicenovic approach - The transitions in the energy (and other complex) systems follow logistic patterns Logistic Transitions Global Primary Energy Substitution, F/(1 -F)* Source: Marchetti & Nakicenovic, 1978. “The Dynamics of Energy Systems and the Logistic Substitution Model”, Vol 1. IIASA, Laxenburg, Austria.
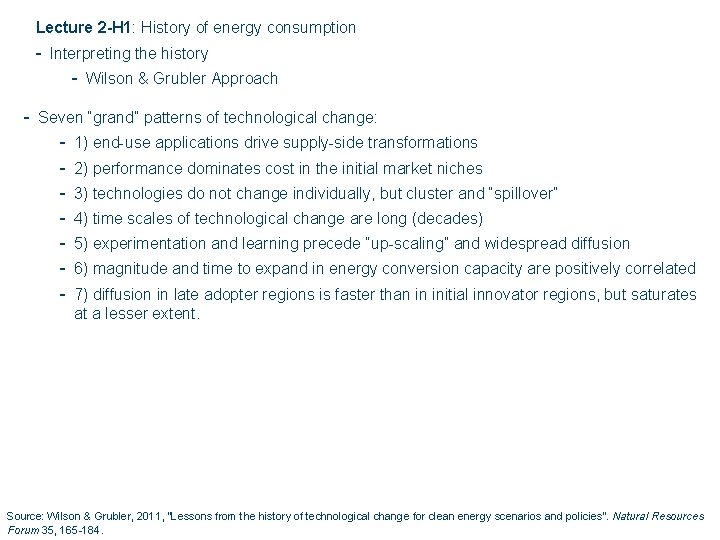
Lecture 2 -H 1: History of energy consumption - Interpreting the history - Wilson & Grubler Approach - Seven “grand” patterns of technological change: - 1) end-use applications drive supply-side transformations - 2) performance dominates cost in the initial market niches - 3) technologies do not change individually, but cluster and “spillover” - 4) time scales of technological change are long (decades) - 5) experimentation and learning precede “up-scaling” and widespread diffusion - 6) magnitude and time to expand in energy conversion capacity are positively correlated - 7) diffusion in late adopter regions is faster than in initial innovator regions, but saturates at a lesser extent. Source: Wilson & Grubler, 2011, “Lessons from the history of technological change for clean energy scenarios and policies”. Natural Resources Forum 35, 165 -184.
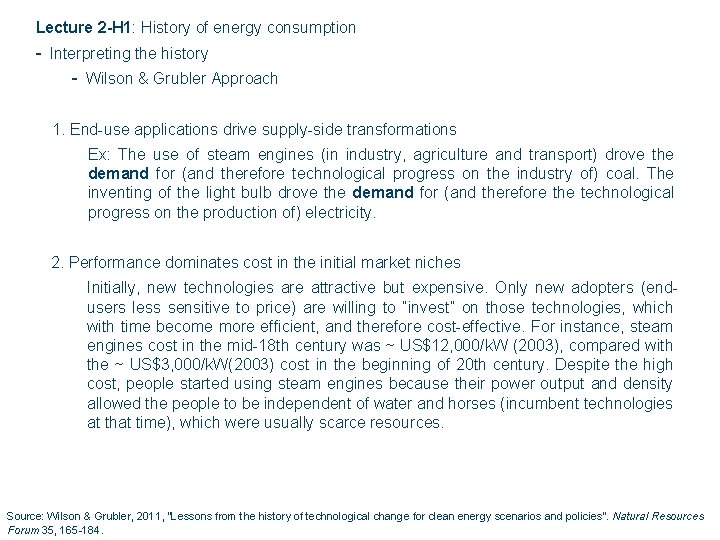
Lecture 2 -H 1: History of energy consumption - Interpreting the history - Wilson & Grubler Approach 1. End-use applications drive supply-side transformations Ex: The use of steam engines (in industry, agriculture and transport) drove the demand for (and therefore technological progress on the industry of) coal. The inventing of the light bulb drove the demand for (and therefore the technological progress on the production of) electricity. 2. Performance dominates cost in the initial market niches Initially, new technologies are attractive but expensive. Only new adopters (endusers less sensitive to price) are willing to “invest” on those technologies, which with time become more efficient, and therefore cost-effective. For instance, steam engines cost in the mid-18 th century was ~ US$12, 000/k. W (2003), compared with the ~ US$3, 000/k. W(2003) cost in the beginning of 20 th century. Despite the high cost, people started using steam engines because their power output and density allowed the people to be independent of water and horses (incumbent technologies at that time), which were usually scarce resources. Source: Wilson & Grubler, 2011, “Lessons from the history of technological change for clean energy scenarios and policies”. Natural Resources Forum 35, 165 -184.
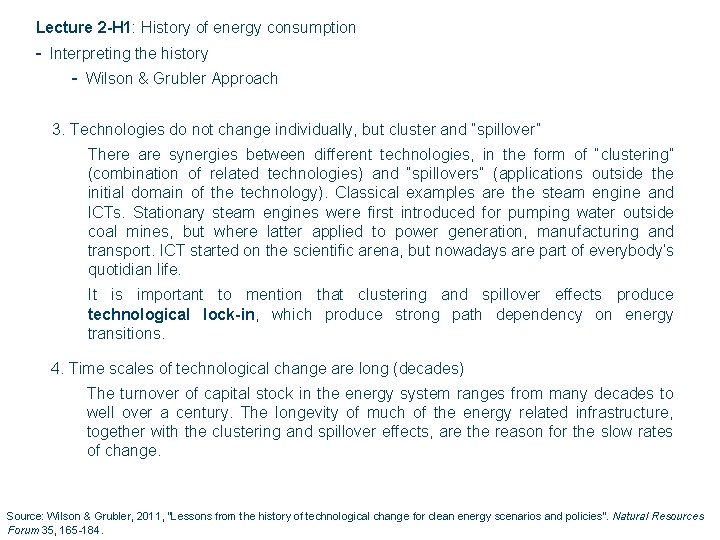
Lecture 2 -H 1: History of energy consumption - Interpreting the history - Wilson & Grubler Approach 3. Technologies do not change individually, but cluster and “spillover” There are synergies between different technologies, in the form of “clustering” (combination of related technologies) and “spillovers” (applications outside the initial domain of the technology). Classical examples are the steam engine and ICTs. Stationary steam engines were first introduced for pumping water outside coal mines, but where latter applied to power generation, manufacturing and transport. ICT started on the scientific arena, but nowadays are part of everybody’s quotidian life. It is important to mention that clustering and spillover effects produce technological lock-in, which produce strong path dependency on energy transitions. 4. Time scales of technological change are long (decades) The turnover of capital stock in the energy system ranges from many decades to well over a century. The longevity of much of the energy related infrastructure, together with the clustering and spillover effects, are the reason for the slow rates of change. Source: Wilson & Grubler, 2011, “Lessons from the history of technological change for clean energy scenarios and policies”. Natural Resources Forum 35, 165 -184.
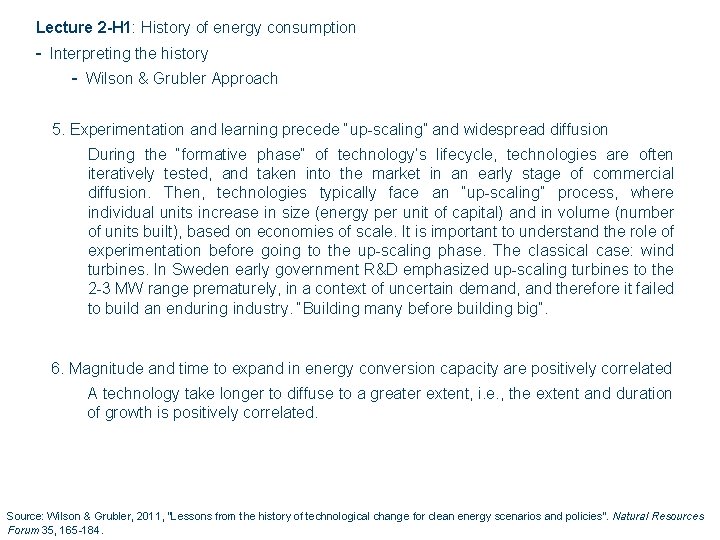
Lecture 2 -H 1: History of energy consumption - Interpreting the history - Wilson & Grubler Approach 5. Experimentation and learning precede “up-scaling” and widespread diffusion During the “formative phase” of technology’s lifecycle, technologies are often iteratively tested, and taken into the market in an early stage of commercial diffusion. Then, technologies typically face an “up-scaling” process, where individual units increase in size (energy per unit of capital) and in volume (number of units built), based on economies of scale. It is important to understand the role of experimentation before going to the up-scaling phase. The classical case: wind turbines. In Sweden early government R&D emphasized up-scaling turbines to the 2 -3 MW range prematurely, in a context of uncertain demand, and therefore it failed to build an enduring industry. “Building many before building big”. 6. Magnitude and time to expand in energy conversion capacity are positively correlated A technology take longer to diffuse to a greater extent, i. e. , the extent and duration of growth is positively correlated. Source: Wilson & Grubler, 2011, “Lessons from the history of technological change for clean energy scenarios and policies”. Natural Resources Forum 35, 165 -184.
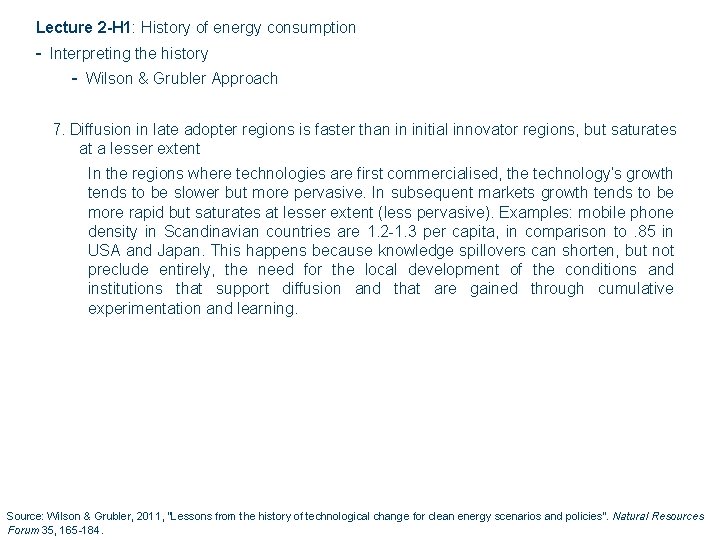
Lecture 2 -H 1: History of energy consumption - Interpreting the history - Wilson & Grubler Approach 7. Diffusion in late adopter regions is faster than in initial innovator regions, but saturates at a lesser extent In the regions where technologies are first commercialised, the technology’s growth tends to be slower but more pervasive. In subsequent markets growth tends to be more rapid but saturates at lesser extent (less pervasive). Examples: mobile phone density in Scandinavian countries are 1. 2 -1. 3 per capita, in comparison to. 85 in USA and Japan. This happens because knowledge spillovers can shorten, but not preclude entirely, the need for the local development of the conditions and institutions that support diffusion and that are gained through cumulative experimentation and learning. Source: Wilson & Grubler, 2011, “Lessons from the history of technological change for clean energy scenarios and policies”. Natural Resources Forum 35, 165 -184.
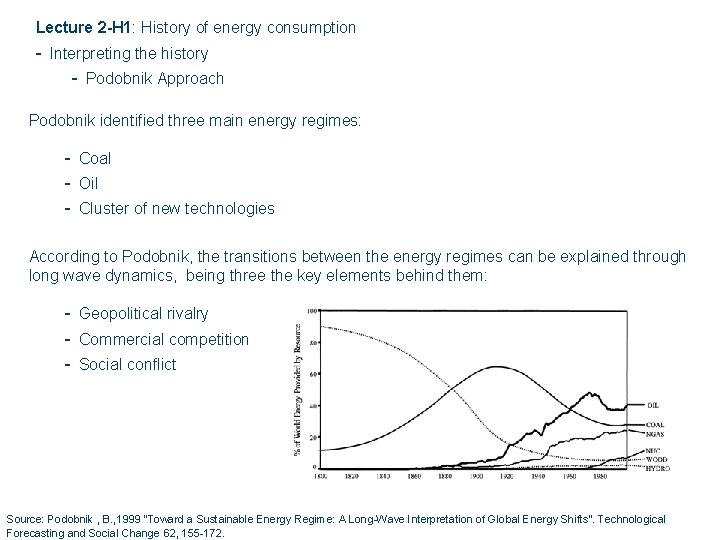
Lecture 2 -H 1: History of energy consumption - Interpreting the history - Podobnik Approach Podobnik identified three main energy regimes: - Coal - Oil - Cluster of new technologies According to Podobnik, the transitions between the energy regimes can be explained through long wave dynamics, being three the key elements behind them: - Geopolitical rivalry - Commercial competition - Social conflict Source: Podobnik , B. , 1999 “Toward a Sustainable Energy Regime: A Long-Wave Interpretation of Global Energy Shifts”. Technological Forecasting and Social Change 62, 155 -172.
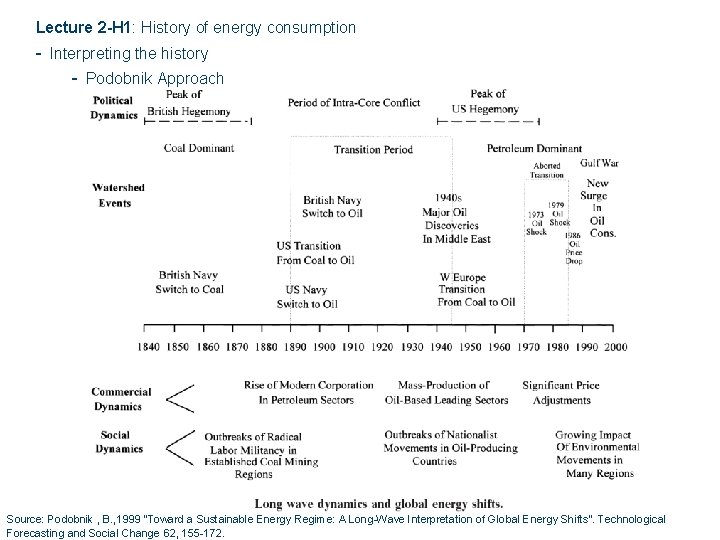
Lecture 2 -H 1: History of energy consumption - Interpreting the history - Podobnik Approach Source: Podobnik , B. , 1999 “Toward a Sustainable Energy Regime: A Long-Wave Interpretation of Global Energy Shifts”. Technological Forecasting and Social Change 62, 155 -172.
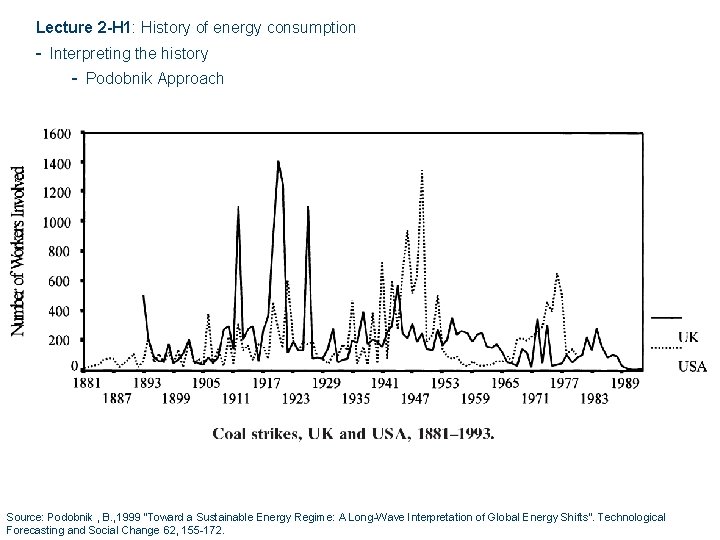
Lecture 2 -H 1: History of energy consumption - Interpreting the history - Podobnik Approach Source: Podobnik , B. , 1999 “Toward a Sustainable Energy Regime: A Long-Wave Interpretation of Global Energy Shifts”. Technological Forecasting and Social Change 62, 155 -172.
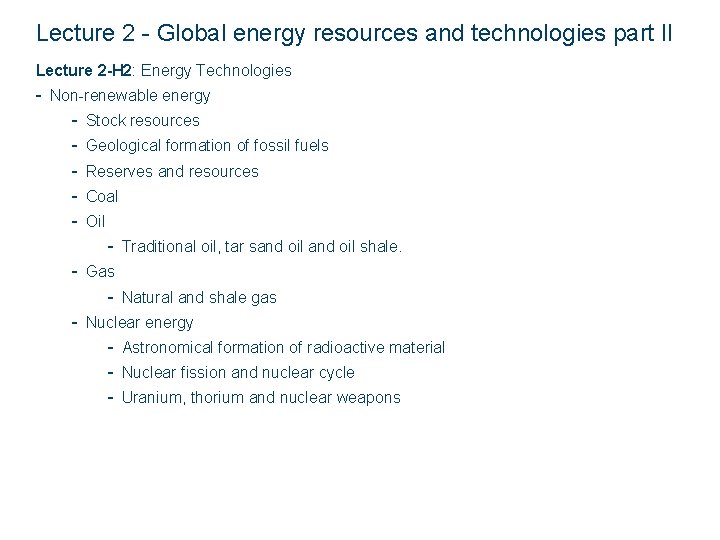
Lecture 2 - Global energy resources and technologies part II Lecture 2 -H 2: Energy Technologies - Non-renewable energy - Stock resources - Geological formation of fossil fuels - Reserves and resources - Coal - Oil - Traditional oil, tar sand oil shale. - Gas - Natural and shale gas - Nuclear energy - Astronomical formation of radioactive material - Nuclear fission and nuclear cycle - Uranium, thorium and nuclear weapons
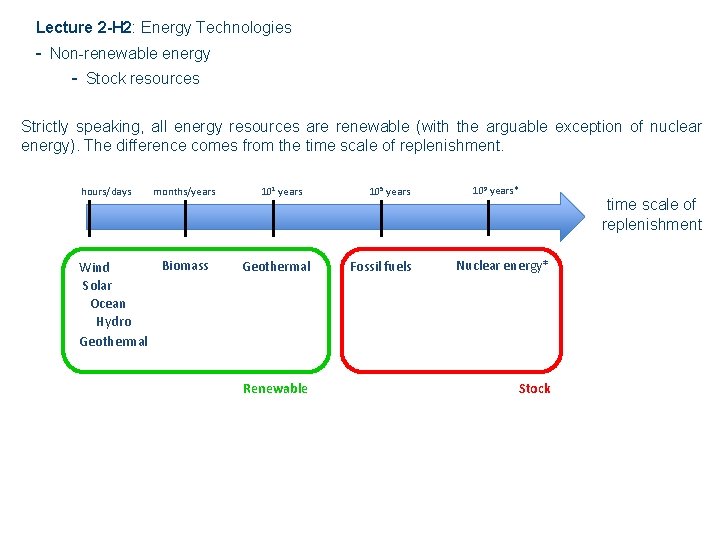
Lecture 2 -H 2: Energy Technologies - Non-renewable energy - Stock resources Strictly speaking, all energy resources are renewable (with the arguable exception of nuclear energy). The difference comes from the time scale of replenishment. hours/days Wind Solar Ocean Hydro Geothermal months/years Biomass 101 years Geothermal Renewable 105 years Fossil fuels 109 years* Nuclear energy* Stock time scale of replenishment
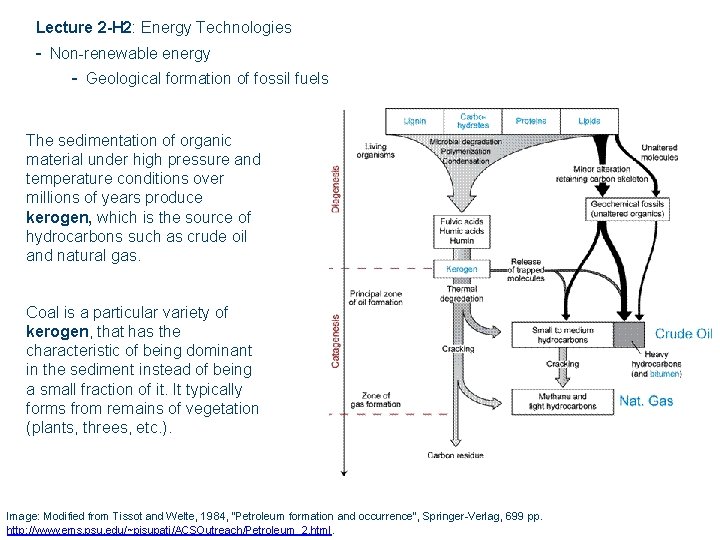
Lecture 2 -H 2: Energy Technologies - Non-renewable energy - Geological formation of fossil fuels The sedimentation of organic material under high pressure and temperature conditions over millions of years produce kerogen, which is the source of hydrocarbons such as crude oil and natural gas. Coal is a particular variety of kerogen, that has the characteristic of being dominant in the sediment instead of being a small fraction of it. It typically forms from remains of vegetation (plants, threes, etc. ). Image: Modified from Tissot and Welte, 1984, “Petroleum formation and occurrence”, Springer-Verlag, 699 pp. http: //www. ems. psu. edu/~pisupati/ACSOutreach/Petroleum_2. html.
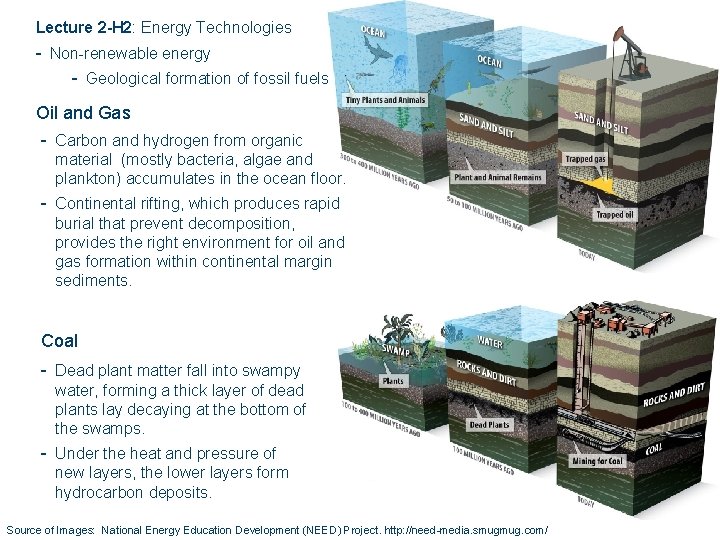
Lecture 2 -H 2: Energy Technologies - Non-renewable energy - Geological formation of fossil fuels Oil and Gas - Carbon and hydrogen from organic material (mostly bacteria, algae and plankton) accumulates in the ocean floor. - Continental rifting, which produces rapid burial that prevent decomposition, provides the right environment for oil and gas formation within continental margin sediments. Coal - Dead plant matter fall into swampy water, forming a thick layer of dead plants lay decaying at the bottom of the swamps. - Under the heat and pressure of new layers, the lower layers form hydrocarbon deposits. Source of Images: National Energy Education Development (NEED) Project. http: //need-media. smugmug. com/
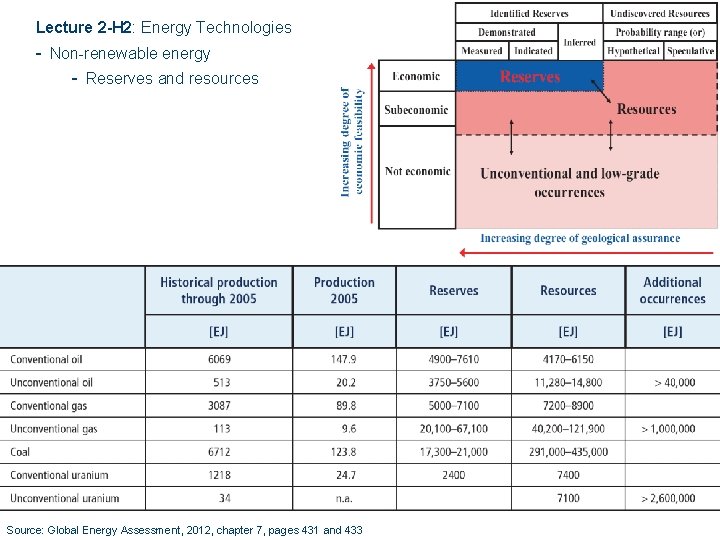
Lecture 2 -H 2: Energy Technologies - Non-renewable energy - Reserves and resources Source: Global Energy Assessment, 2012, chapter 7, pages 431 and 433
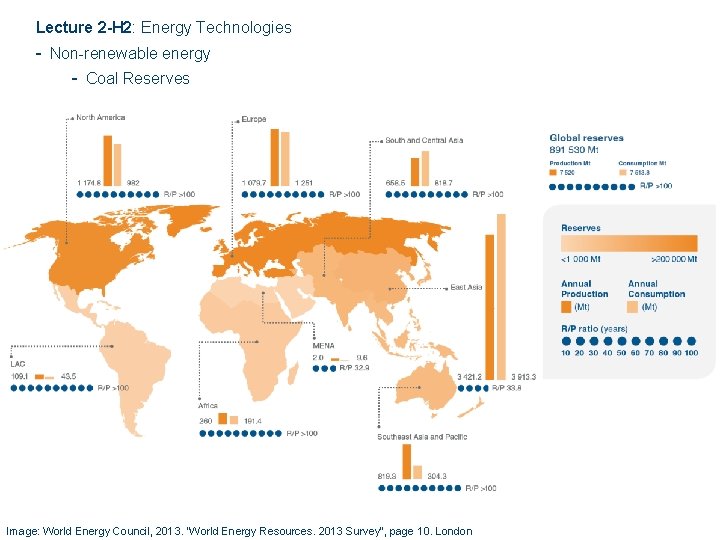
Lecture 2 -H 2: Energy Technologies - Non-renewable energy - Coal Reserves Image: World Energy Council, 2013. “World Energy Resources. 2013 Survey”, page 10. London
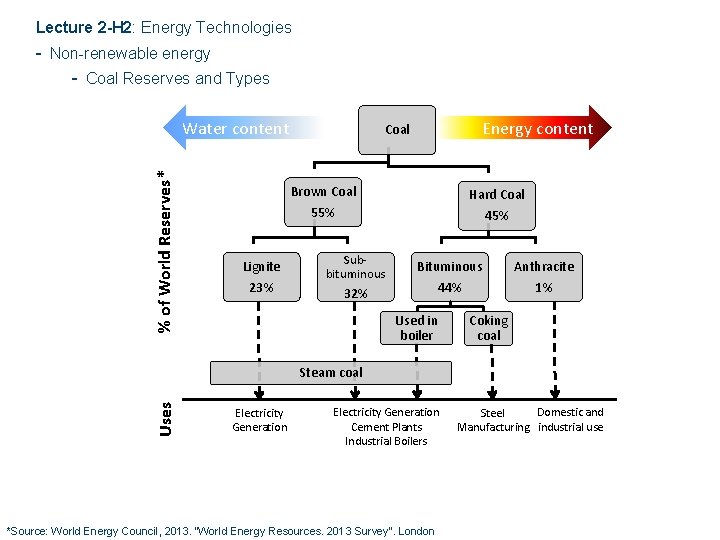
Lecture 2 -H 2: Energy Technologies - Non-renewable energy - Coal Reserves and Types % of World Reserves* Water content Lignite 23% Energy content Coal Brown Coal Hard Coal 55% 45% Subbituminous Bituminous Anthracite 44% 1% 32% Used in boiler Coking coal Uses Steam coal Electricity Generation Cement Plants Industrial Boilers *Source: World Energy Council, 2013. “World Energy Resources. 2013 Survey”. London Domestic and Steel Manufacturing industrial use
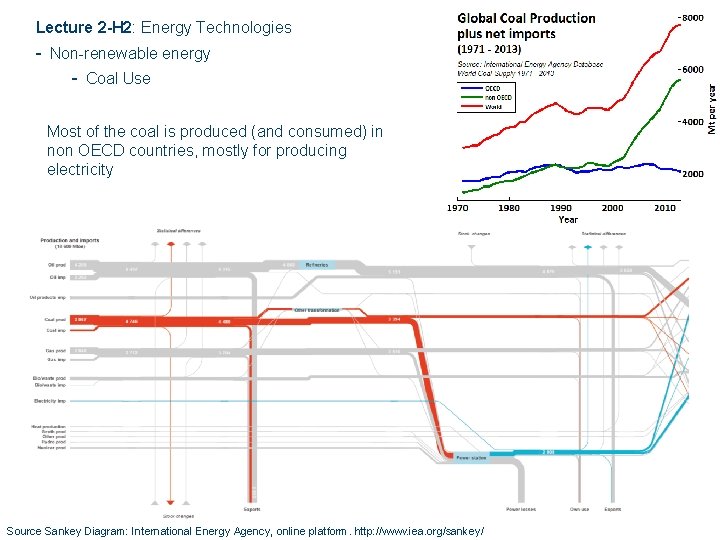
Lecture 2 -H 2: Energy Technologies - Non-renewable energy - Coal Use Most of the coal is produced (and consumed) in non OECD countries, mostly for producing electricity Source Sankey Diagram: International Energy Agency, online platform. http: //www. iea. org/sankey/
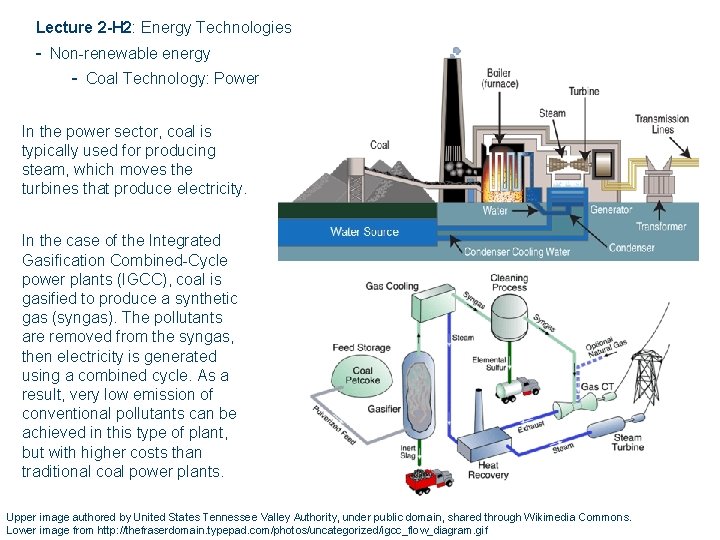
Lecture 2 -H 2: Energy Technologies - Non-renewable energy - Coal Technology: Power In the power sector, coal is typically used for producing steam, which moves the turbines that produce electricity. In the case of the Integrated Gasification Combined-Cycle power plants (IGCC), coal is gasified to produce a synthetic gas (syngas). The pollutants are removed from the syngas, then electricity is generated using a combined cycle. As a result, very low emission of conventional pollutants can be achieved in this type of plant, but with higher costs than traditional coal power plants. Upper image authored by United States Tennessee Valley Authority, under public domain, shared through Wikimedia Commons. Lower image from http: //thefraserdomain. typepad. com/photos/uncategorized/igcc_flow_diagram. gif
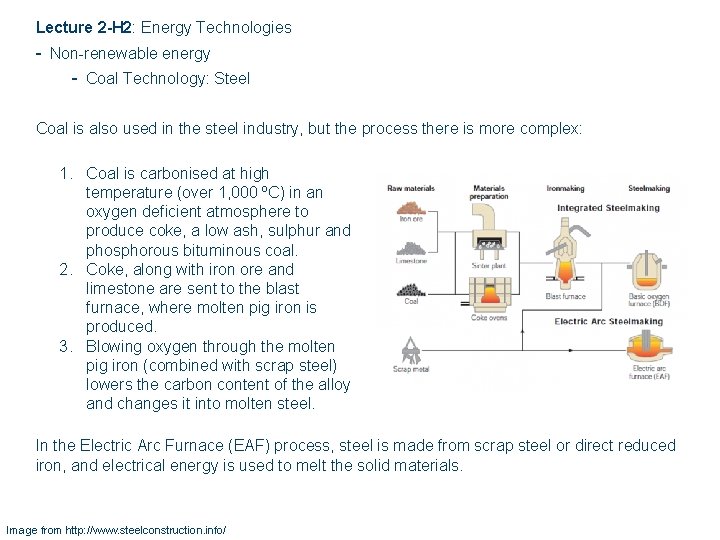
Lecture 2 -H 2: Energy Technologies - Non-renewable energy - Coal Technology: Steel Coal is also used in the steel industry, but the process there is more complex: 1. Coal is carbonised at high temperature (over 1, 000 ºC) in an oxygen deficient atmosphere to produce coke, a low ash, sulphur and phosphorous bituminous coal. 2. Coke, along with iron ore and limestone are sent to the blast furnace, where molten pig iron is produced. 3. Blowing oxygen through the molten pig iron (combined with scrap steel) lowers the carbon content of the alloy and changes it into molten steel. In the Electric Arc Furnace (EAF) process, steel is made from scrap steel or direct reduced iron, and electrical energy is used to melt the solid materials. Image from http: //www. steelconstruction. info/
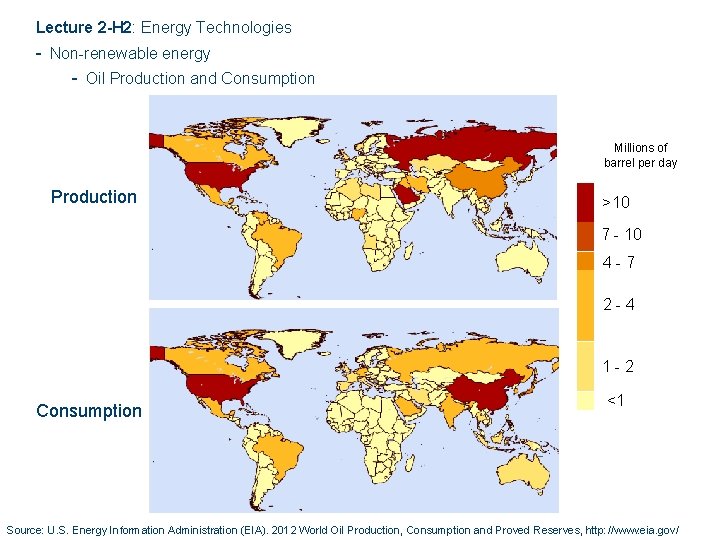
Lecture 2 -H 2: Energy Technologies - Non-renewable energy - Oil Production and Consumption Millions of barrel per day Production >10 7 - 10 4 -7 2 -4 1 -2 Consumption <1 Source: U. S. Energy Information Administration (EIA). 2012 World Oil Production, Consumption and Proved Reserves, http: //www. eia. gov/
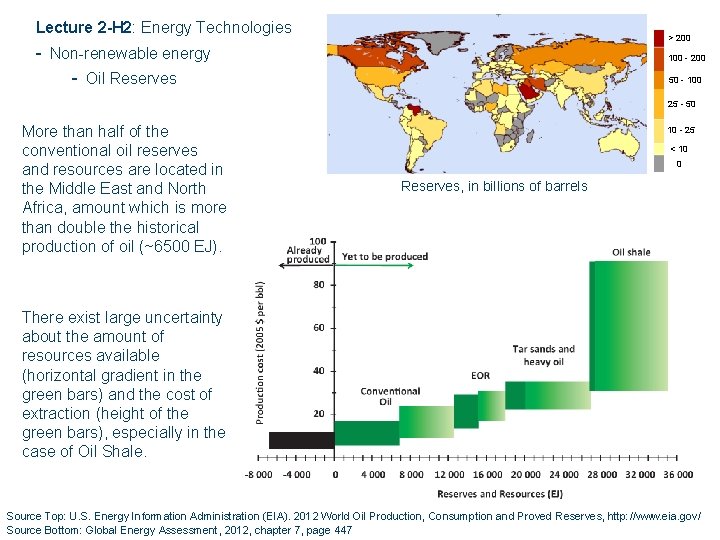
Lecture 2 -H 2: Energy Technologies > 200 - Non-renewable energy - Oil Reserves 100 - 200 50 - 100 25 - 50 More than half of the conventional oil reserves and resources are located in the Middle East and North Africa, amount which is more than double the historical production of oil (~6500 EJ). 10 - 25 < 10 0 Reserves, in billions of barrels There exist large uncertainty about the amount of resources available (horizontal gradient in the green bars) and the cost of extraction (height of the green bars), especially in the case of Oil Shale. Source Top: U. S. Energy Information Administration (EIA). 2012 World Oil Production, Consumption and Proved Reserves, http: //www. eia. gov/ Source Bottom: Global Energy Assessment, 2012, chapter 7, page 447
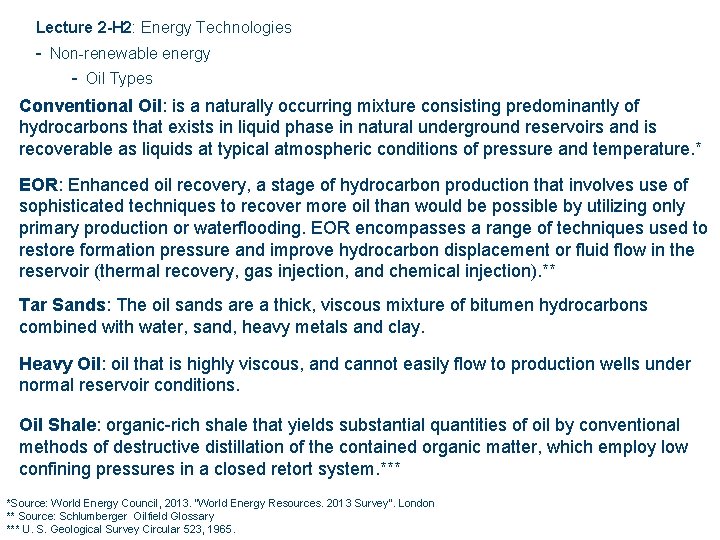
Lecture 2 -H 2: Energy Technologies - Non-renewable energy - Oil Types Conventional Oil: is a naturally occurring mixture consisting predominantly of hydrocarbons that exists in liquid phase in natural underground reservoirs and is recoverable as liquids at typical atmospheric conditions of pressure and temperature. * EOR: Enhanced oil recovery, a stage of hydrocarbon production that involves use of sophisticated techniques to recover more oil than would be possible by utilizing only primary production or waterflooding. EOR encompasses a range of techniques used to restore formation pressure and improve hydrocarbon displacement or fluid flow in the reservoir (thermal recovery, gas injection, and chemical injection). ** Tar Sands: The oil sands are a thick, viscous mixture of bitumen hydrocarbons combined with water, sand, heavy metals and clay. Heavy Oil: oil that is highly viscous, and cannot easily flow to production wells under normal reservoir conditions. Oil Shale: organic-rich shale that yields substantial quantities of oil by conventional methods of destructive distillation of the contained organic matter, which employ low confining pressures in a closed retort system. *** *Source: World Energy Council, 2013. “World Energy Resources. 2013 Survey”. London ** Source: Schlumberger Oilfield Glossary *** U. S. Geological Survey Circular 523, 1965.
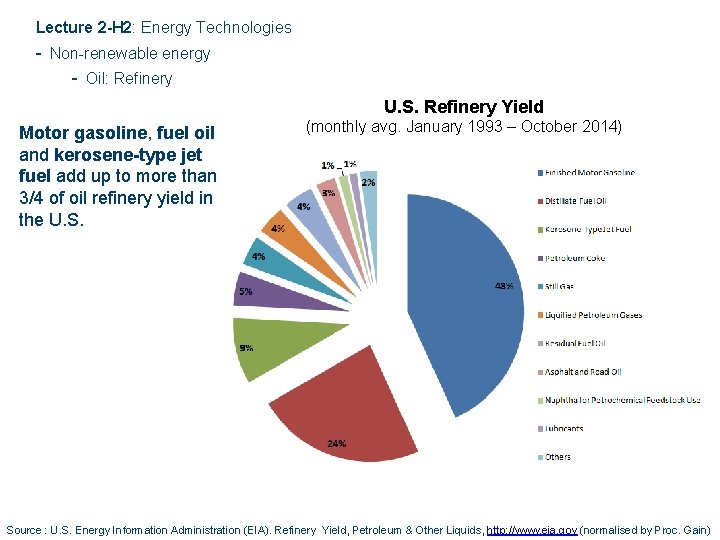
Lecture 2 -H 2: Energy Technologies - Non-renewable energy - Oil: Refinery U. S. Refinery Yield Motor gasoline, fuel oil and kerosene-type jet fuel add up to more than 3/4 of oil refinery yield in the U. S. (monthly avg. January 1993 – October 2014) Source : U. S. Energy Information Administration (EIA). Refinery Yield, Petroleum & Other Liquids, http: //www. eia. gov (normalised by Proc. Gain)
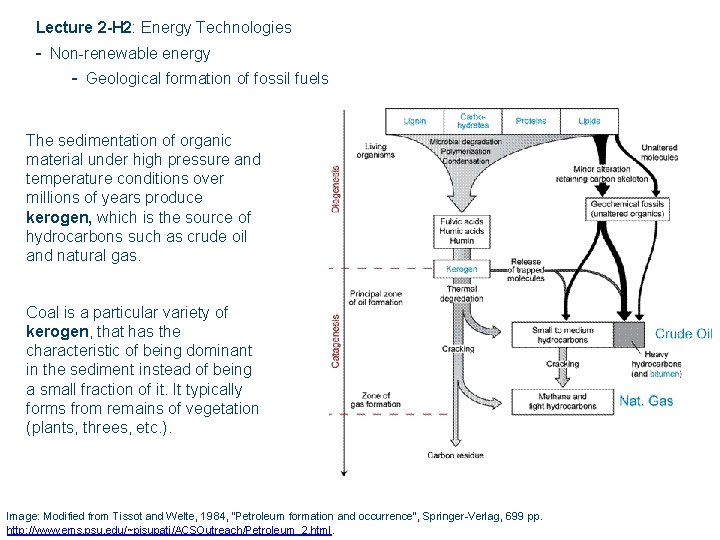
Lecture 2 -H 2: Energy Technologies - Non-renewable energy - Geological formation of fossil fuels The sedimentation of organic material under high pressure and temperature conditions over millions of years produce kerogen, which is the source of hydrocarbons such as crude oil and natural gas. Coal is a particular variety of kerogen, that has the characteristic of being dominant in the sediment instead of being a small fraction of it. It typically forms from remains of vegetation (plants, threes, etc. ). Image: Modified from Tissot and Welte, 1984, “Petroleum formation and occurrence”, Springer-Verlag, 699 pp. http: //www. ems. psu. edu/~pisupati/ACSOutreach/Petroleum_2. html.
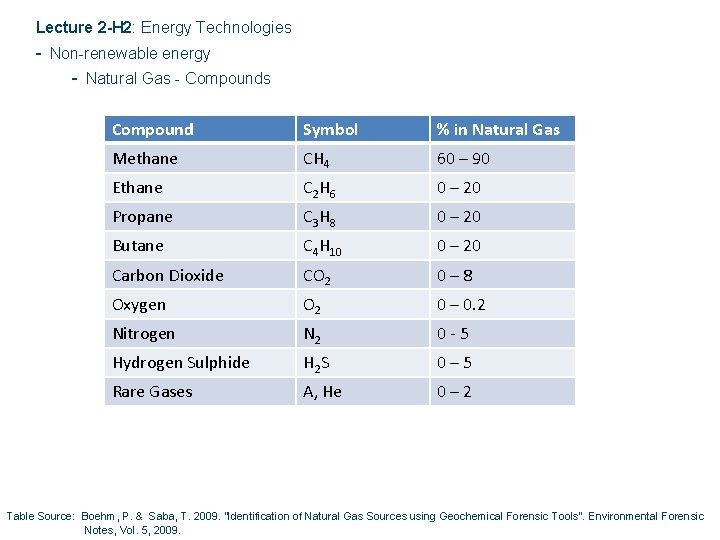
Lecture 2 -H 2: Energy Technologies - Non-renewable energy - Natural Gas - Compounds Compound Symbol % in Natural Gas Methane CH 4 60 – 90 Ethane C 2 H 6 0 – 20 Propane C 3 H 8 0 – 20 Butane C 4 H 10 0 – 20 Carbon Dioxide CO 2 0– 8 Oxygen O 2 0 – 0. 2 Nitrogen N 2 0 -5 Hydrogen Sulphide H 2 S 0– 5 Rare Gases A, He 0– 2 Table Source: Boehm, P. & Saba, T. 2009. “Identification of Natural Gas Sources using Geochemical Forensic Tools”. Environmental Forensic Notes, Vol. 5, 2009.
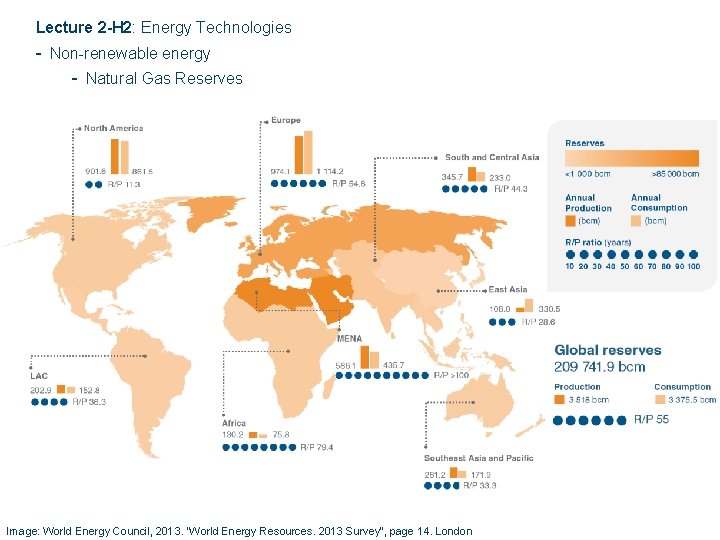
Lecture 2 -H 2: Energy Technologies - Non-renewable energy - Natural Gas Reserves Image: World Energy Council, 2013. “World Energy Resources. 2013 Survey”, page 14. London
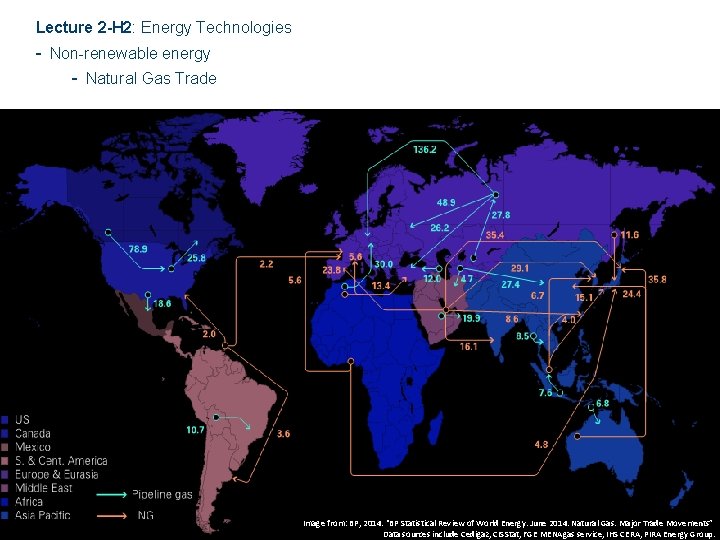
Lecture 2 -H 2: Energy Technologies - Non-renewable energy - Natural Gas Trade Image from: BP, 2014. “BP Statistical Review of World Energy. June 2014. Natural Gas. Major Trade Movements” Data sources include Cedigaz, CISStat, FGE MENAgas service, IHS CERA, PIRA Energy Group.
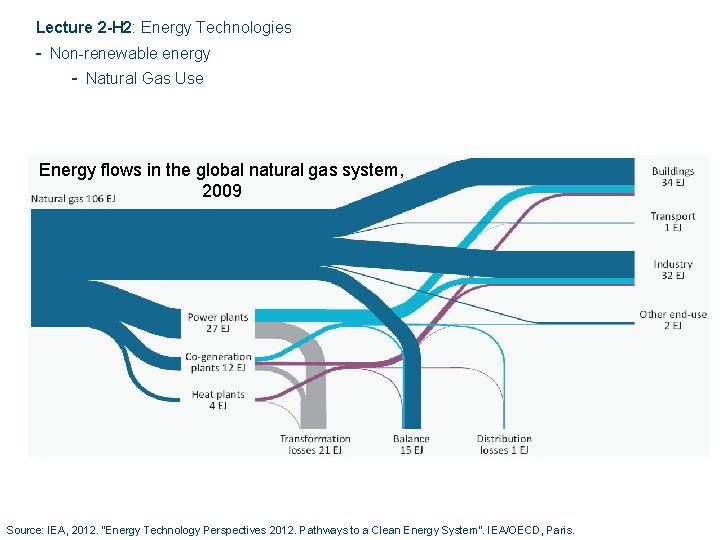
Lecture 2 -H 2: Energy Technologies - Non-renewable energy - Natural Gas Use Energy flows in the global natural gas system, 2009 Source: IEA, 2012. “Energy Technology Perspectives 2012. Pathways to a Clean Energy System”. IEA/OECD, Paris.
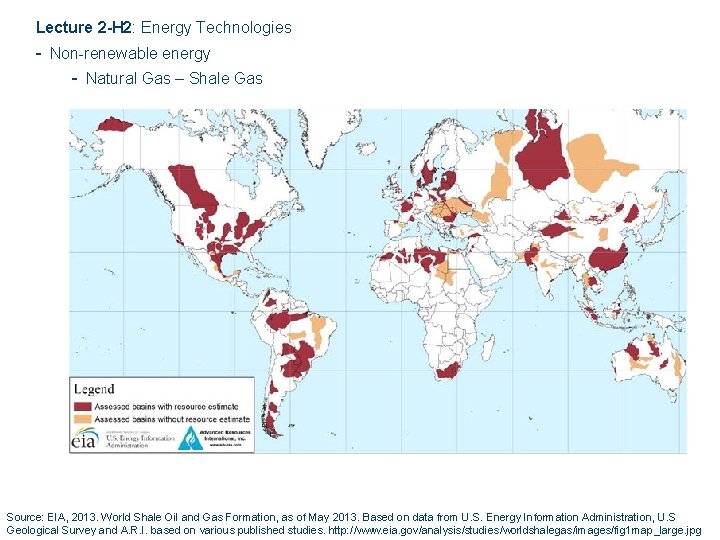
Lecture 2 -H 2: Energy Technologies - Non-renewable energy - Natural Gas – Shale Gas Source: EIA, 2013. World Shale Oil and Gas Formation, as of May 2013. Based on data from U. S. Energy Information Administration, U. S Geological Survey and A. R. I. based on various published studies. http: //www. eia. gov/analysis/studies/worldshalegas/images/fig 1 map_large. jpg
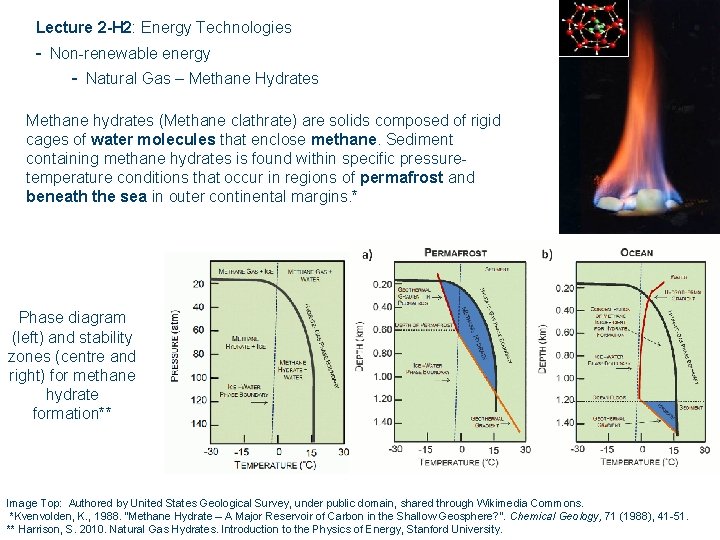
Lecture 2 -H 2: Energy Technologies - Non-renewable energy - Natural Gas – Methane Hydrates Methane hydrates (Methane clathrate) are solids composed of rigid cages of water molecules that enclose methane. Sediment containing methane hydrates is found within specific pressuretemperature conditions that occur in regions of permafrost and beneath the sea in outer continental margins. * Phase diagram (left) and stability zones (centre and right) for methane hydrate formation** Image Top: Authored by United States Geological Survey, under public domain, shared through Wikimedia Commons. *Kvenvolden, K. , 1988. “Methane Hydrate – A Major Reservoir of Carbon in the Shallow Geosphere? ”. Chemical Geology, 71 (1988), 41 -51. ** Harrison, S. 2010. Natural Gas Hydrates. Introduction to the Physics of Energy, Stanford University.
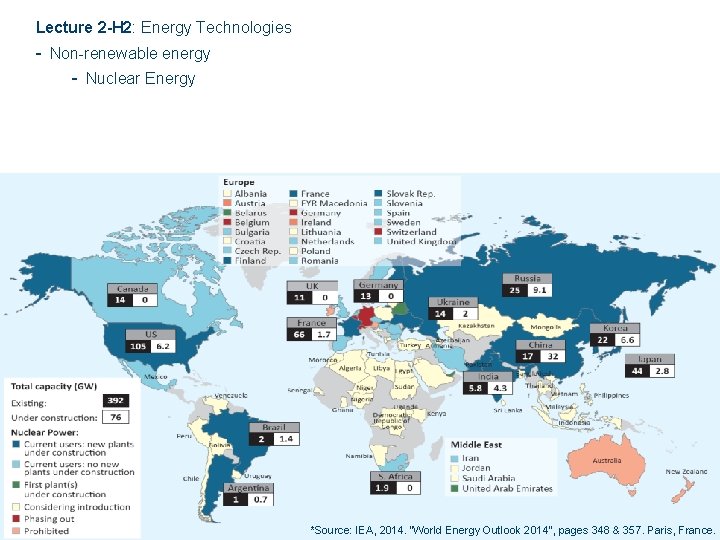
Lecture 2 -H 2: Energy Technologies - Non-renewable energy - Nuclear Energy *Source: IEA, 2014. “World Energy Outlook 2014”, pages 348 & 357. Paris, France.
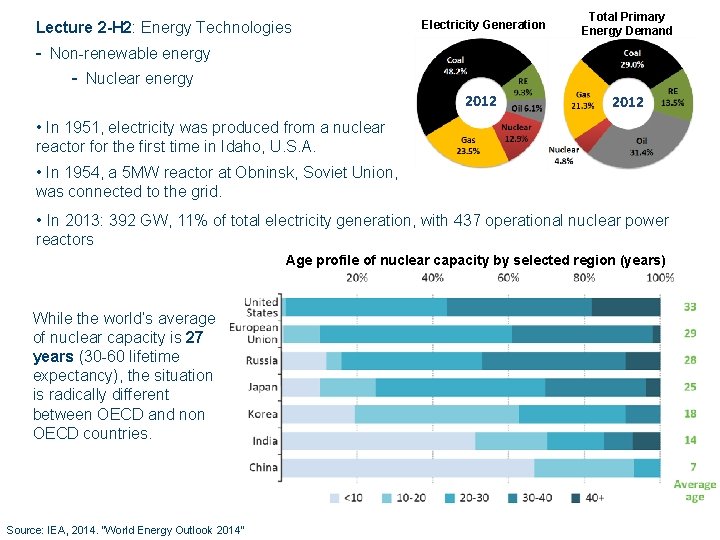
Lecture 2 -H 2: Energy Technologies Electricity Generation Total Primary Energy Demand 2012 - Non-renewable energy - Nuclear energy • In 1951, electricity was produced from a nuclear reactor for the first time in Idaho, U. S. A. • In 1954, a 5 MW reactor at Obninsk, Soviet Union, was connected to the grid. • In 2013: 392 GW, 11% of total electricity generation, with 437 operational nuclear power reactors Age profile of nuclear capacity by selected region (years) While the world’s average of nuclear capacity is 27 years (30 -60 lifetime expectancy), the situation is radically different between OECD and non OECD countries. Source: IEA, 2014. “World Energy Outlook 2014”
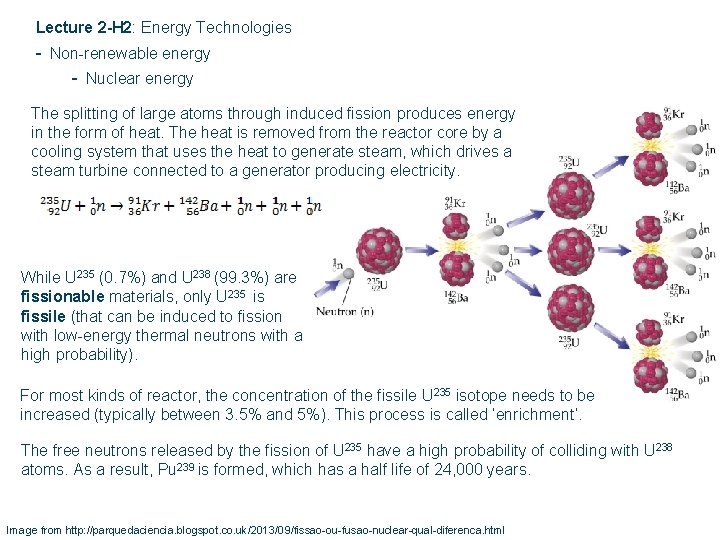
Lecture 2 -H 2: Energy Technologies - Non-renewable energy - Nuclear energy The splitting of large atoms through induced fission produces energy in the form of heat. The heat is removed from the reactor core by a cooling system that uses the heat to generate steam, which drives a steam turbine connected to a generator producing electricity. While U 235 (0. 7%) and U 238 (99. 3%) are fissionable materials, only U 235 is fissile (that can be induced to fission with low-energy thermal neutrons with a high probability). For most kinds of reactor, the concentration of the fissile U 235 isotope needs to be increased (typically between 3. 5% and 5%). This process is called ‘enrichment’. The free neutrons released by the fission of U 235 have a high probability of colliding with U 238 atoms. As a result, Pu 239 is formed, which has a half life of 24, 000 years. Image from http: //parquedaciencia. blogspot. co. uk/2013/09/fissao-ou-fusao-nuclear-qual-diferenca. html
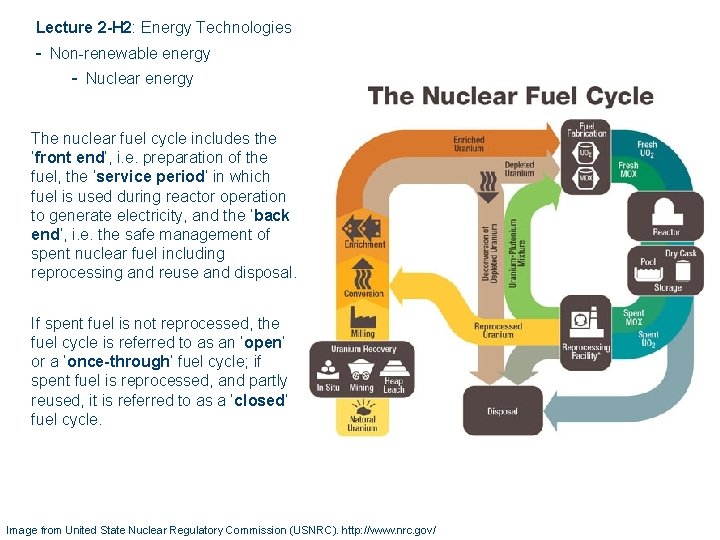
Lecture 2 -H 2: Energy Technologies - Non-renewable energy - Nuclear energy The nuclear fuel cycle includes the ‘front end’, i. e. preparation of the fuel, the ‘service period’ in which fuel is used during reactor operation to generate electricity, and the ‘back end’, i. e. the safe management of spent nuclear fuel including reprocessing and reuse and disposal. If spent fuel is not reprocessed, the fuel cycle is referred to as an ‘open’ or a ‘once-through’ fuel cycle; if spent fuel is reprocessed, and partly reused, it is referred to as a ‘closed’ fuel cycle. Image from United State Nuclear Regulatory Commission (USNRC). http: //www. nrc. gov/
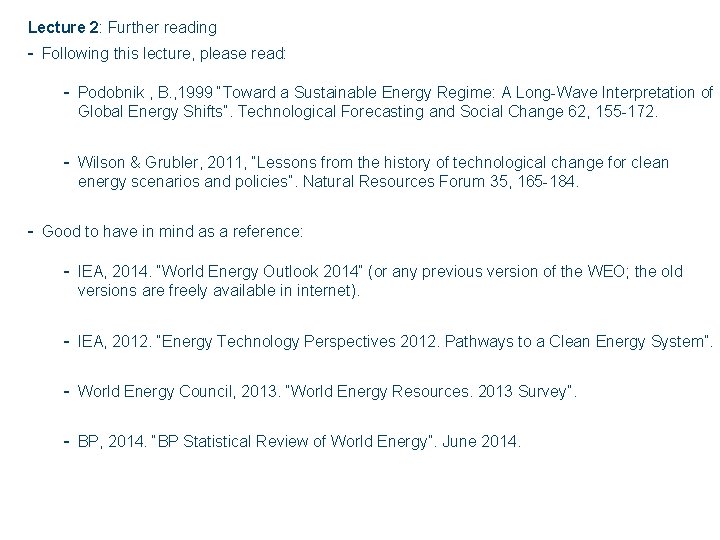
Lecture 2: Further reading - Following this lecture, please read: - Podobnik , B. , 1999 “Toward a Sustainable Energy Regime: A Long-Wave Interpretation of Global Energy Shifts”. Technological Forecasting and Social Change 62, 155 -172. - Wilson & Grubler, 2011, “Lessons from the history of technological change for clean energy scenarios and policies”. Natural Resources Forum 35, 165 -184. - Good to have in mind as a reference: - IEA, 2014. “World Energy Outlook 2014” (or any previous version of the WEO; the old versions are freely available in internet). - IEA, 2012. “Energy Technology Perspectives 2012. Pathways to a Clean Energy System”. - World Energy Council, 2013. “World Energy Resources. 2013 Survey”. - BP, 2014. “BP Statistical Review of World Energy”. June 2014.
Climate change 2014 mitigation of climate change
"energy efficiency" "climate change"
Strategy and future
"energy efficiency" "climate change"
"energy management" "climate change"
Climate change meaning and definition
Chapter 13 atmosphere and climate change section 1
Chapter 13 atmosphere and climate change
Energy energy transfer and general energy analysis
Energy energy transfer and general energy analysis
Persuasive essay about global warming
Karnataka state action plan on climate change
Brainpop ocean currents
Unit 9 climate change
Conclusion of climate change
Conclusion of climate change
Conclusion of climate change
Mathematics of climate change
Environmentalism definition ap world history
Climate change mitigation
414 climate change
Conclusion of climate change
Factors of climate change
Human causes of climate change
Conceptual framework
Uk climate change
Climate change interview
Factors effecting climate change
Conclusion of climate change
Conclusion of climate change
Climate change causing droughts
Climate change hypothesis
How global warming works
Financing education in a climate of change
Climate change pathos
Dan miller climate change
Un climate change
Rhetorical questions about climate change
Awgcc
Climate change
How does climate change affect us
Climate change national security threat
How climate change
Climate change sydney
Climate change activities
Prodipto ghosh
Mark southgate
Henrik svensmark
Climate change activities
Northwestern european countries
Impacts of climate change