Analytical Transmissions Electron Microscopy TEM Part I The
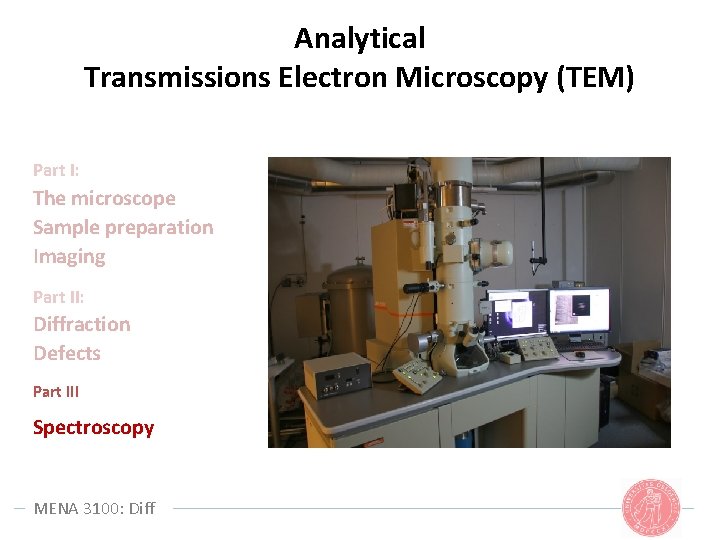
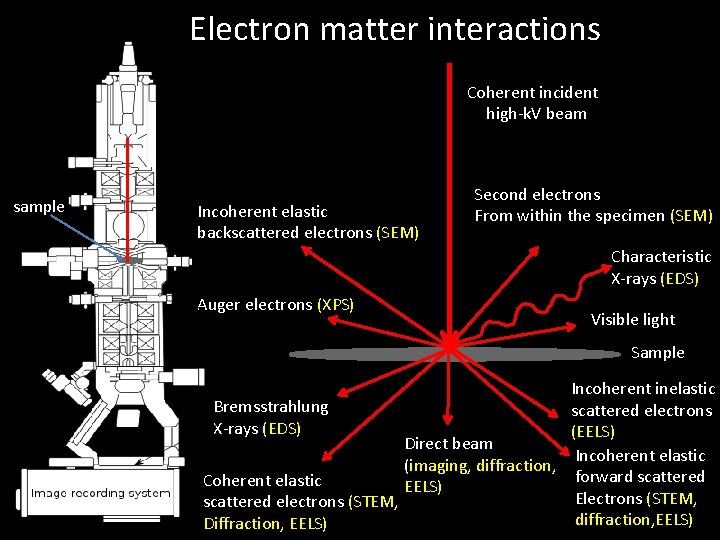
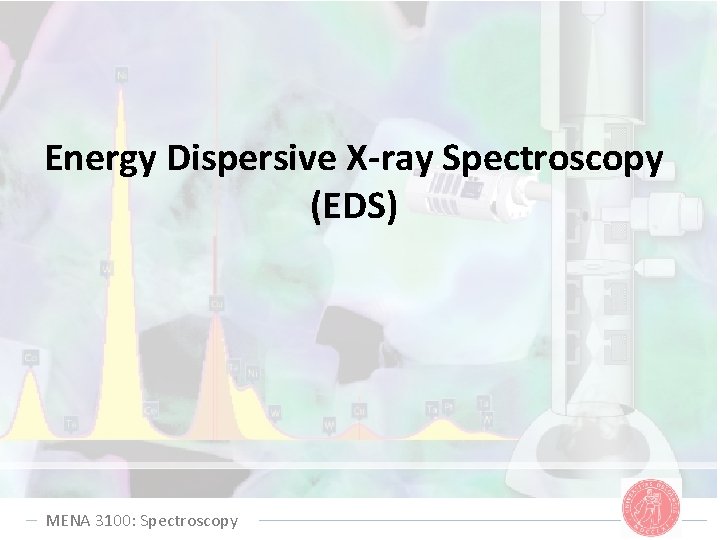
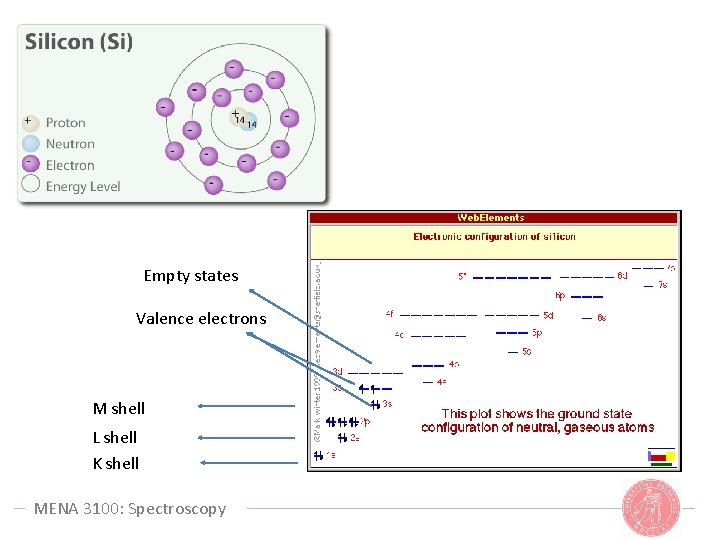
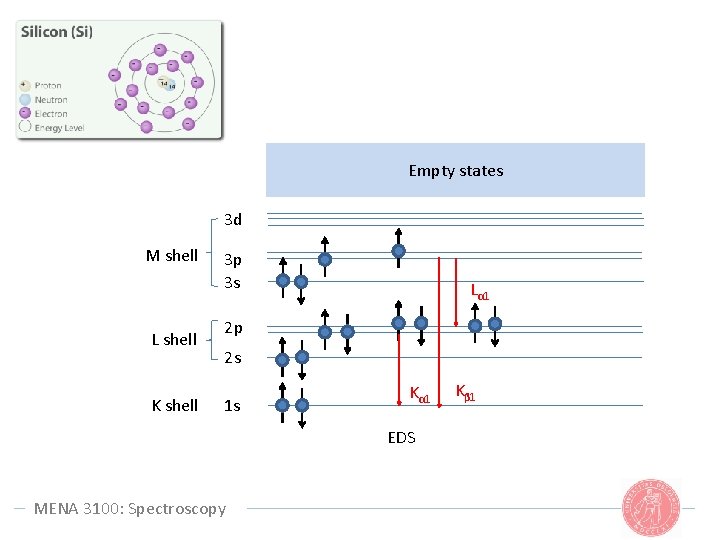
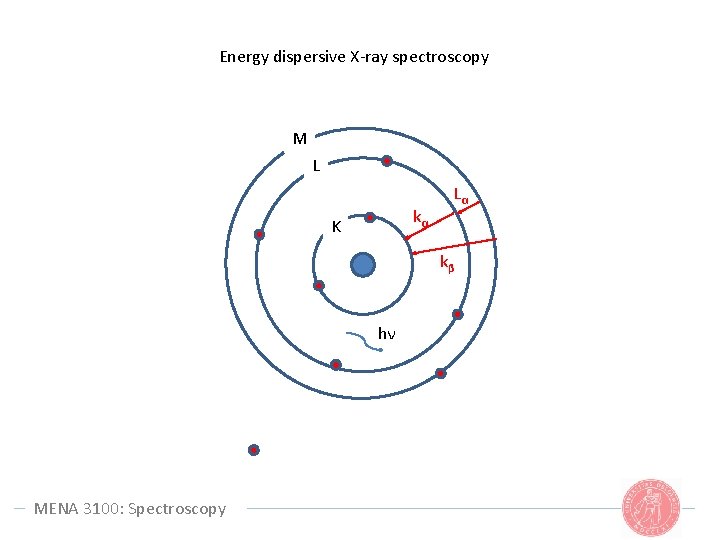
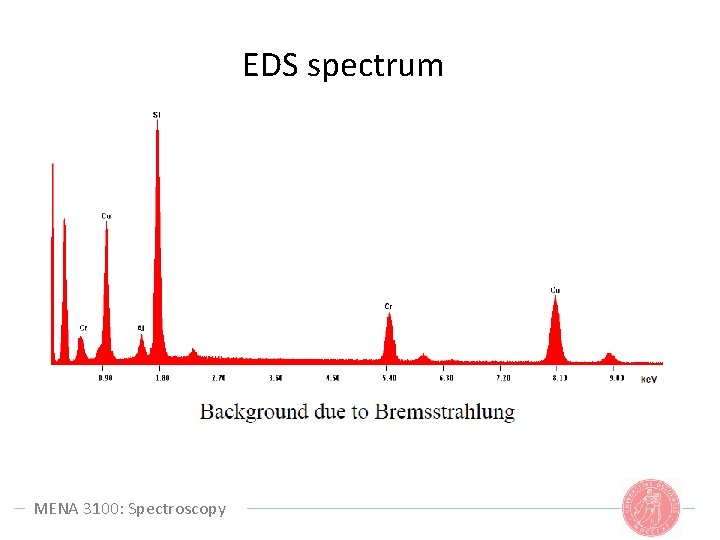
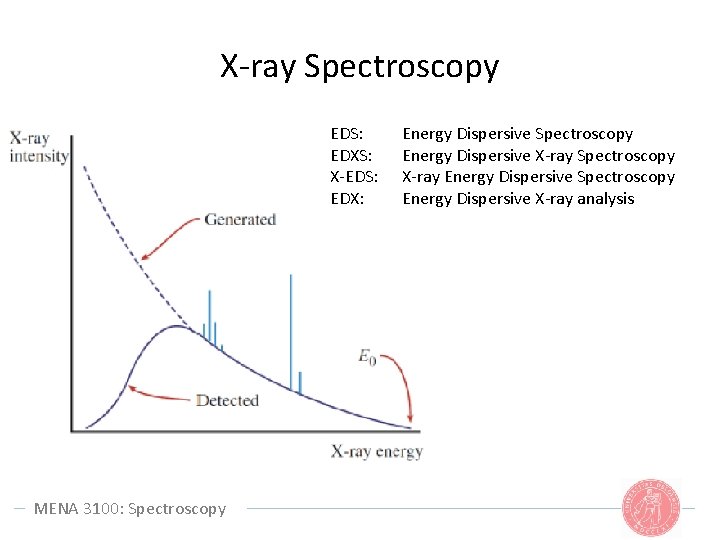
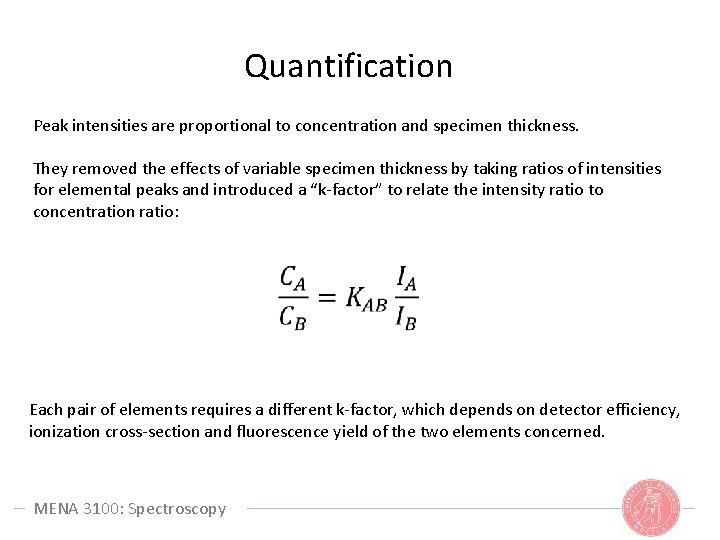
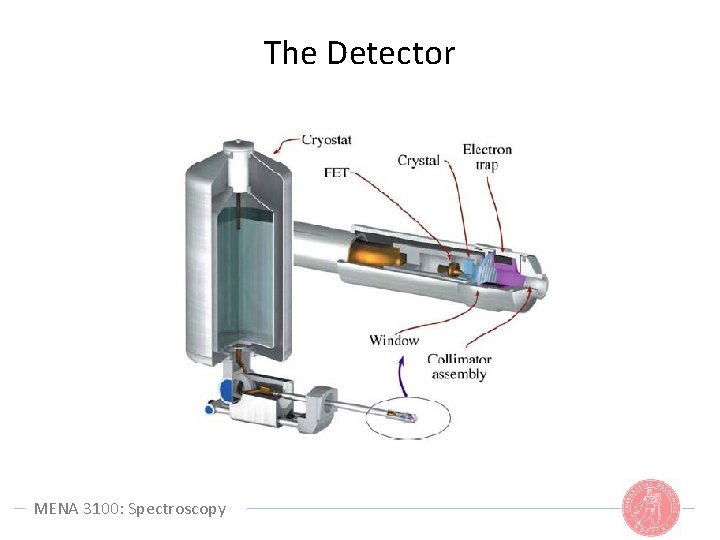
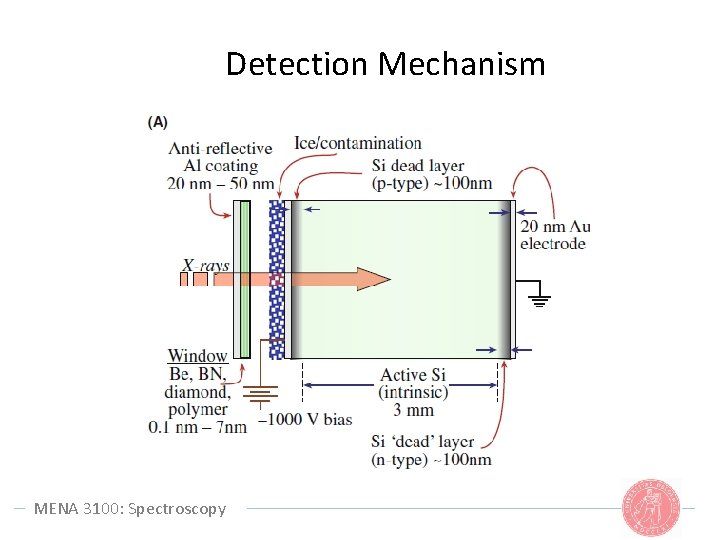
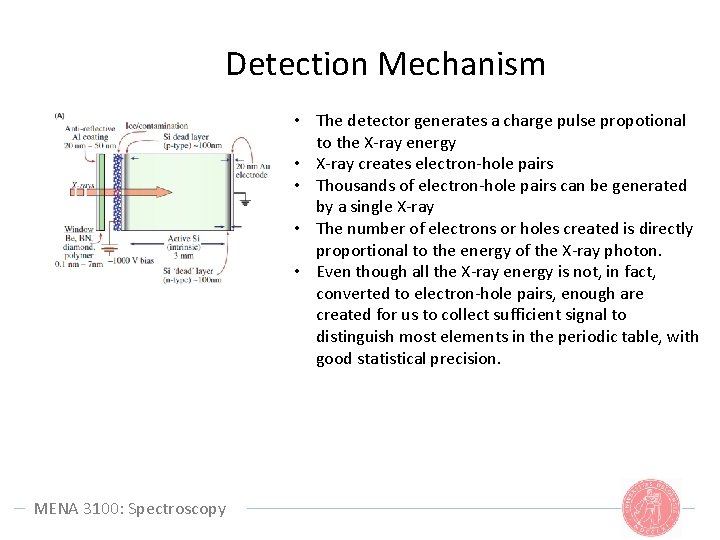
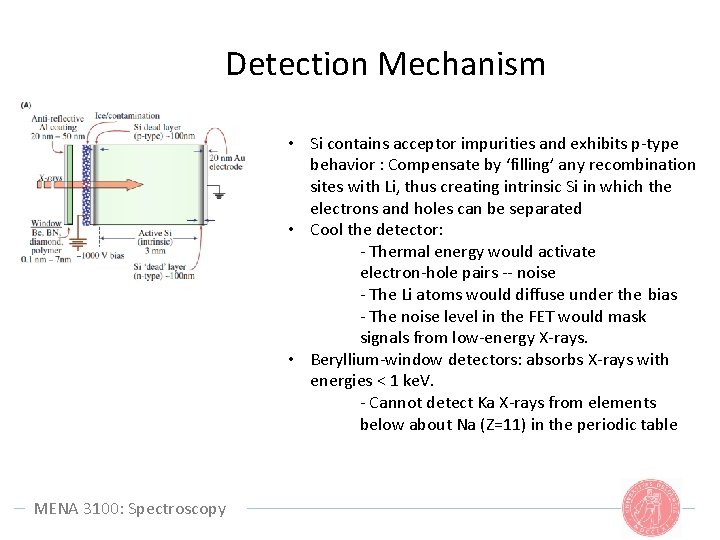
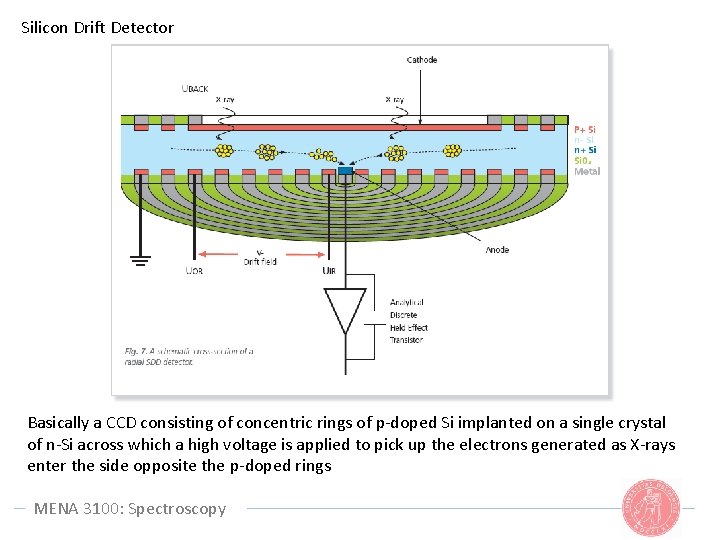
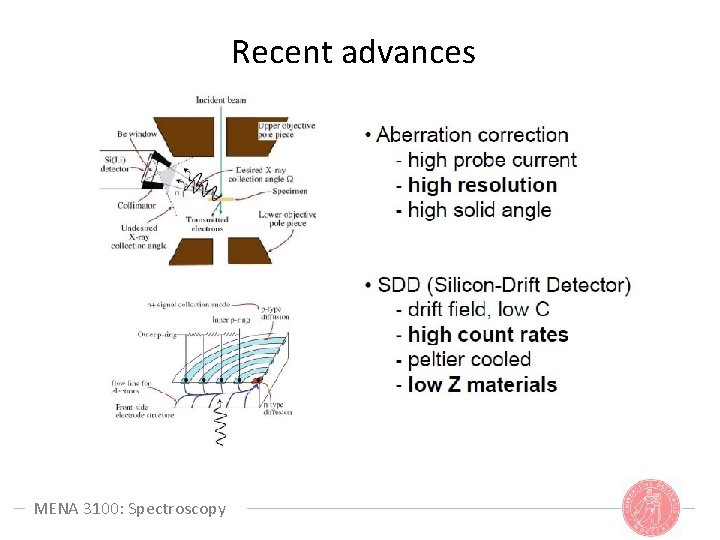
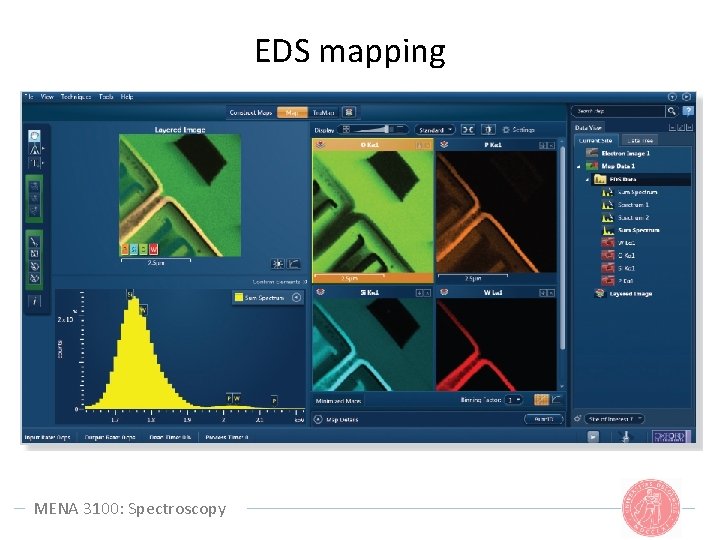
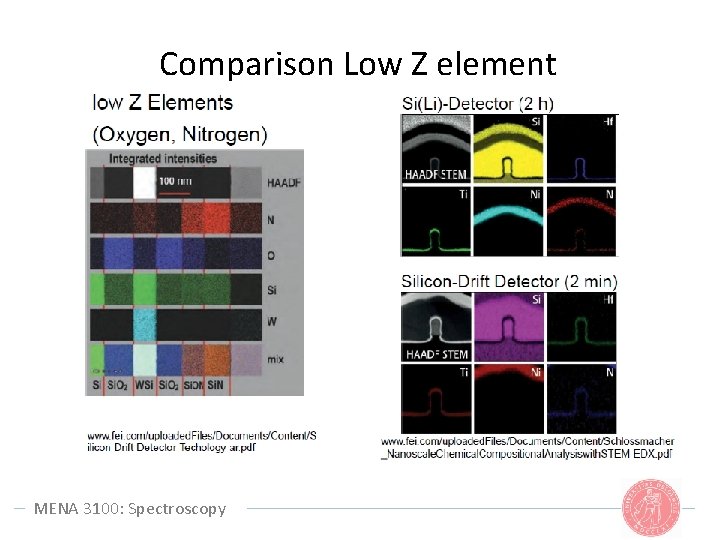
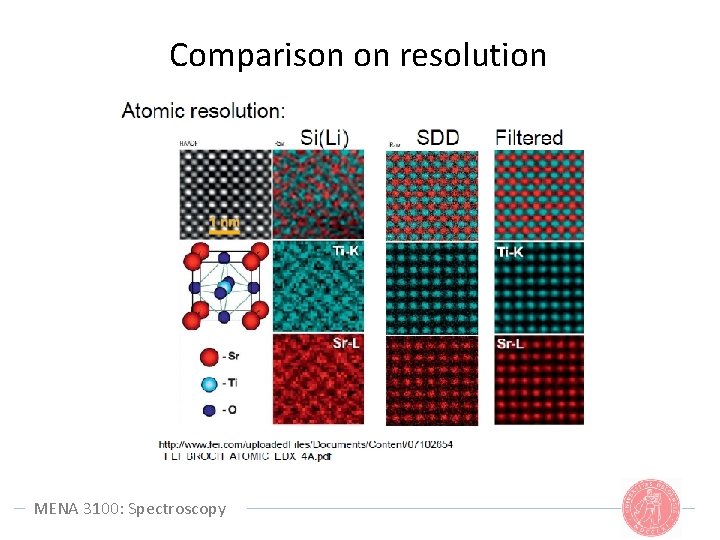
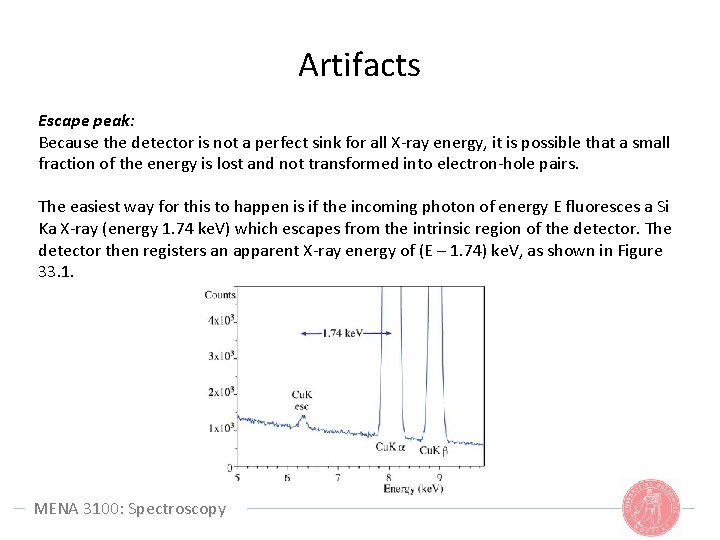
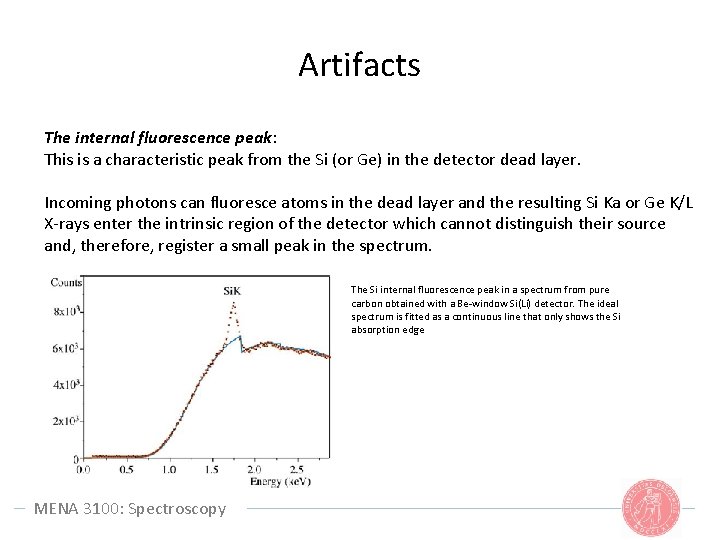
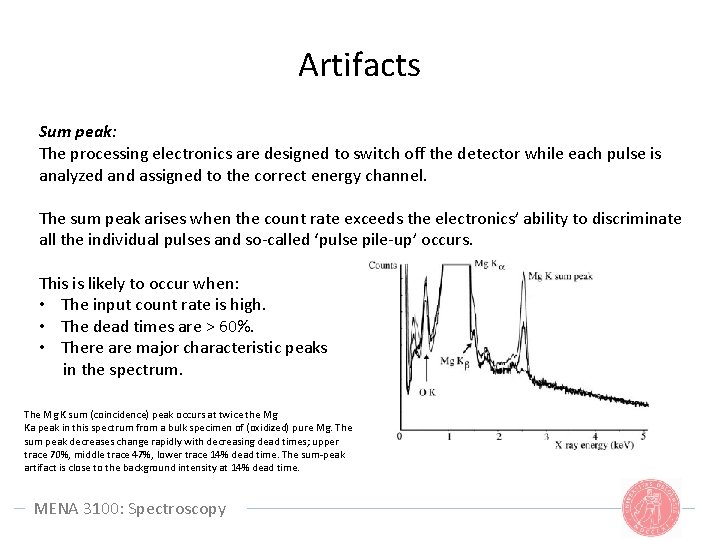
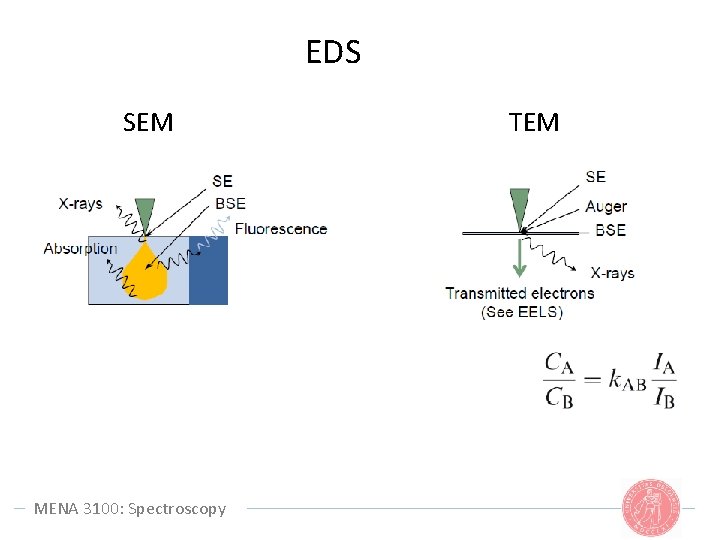
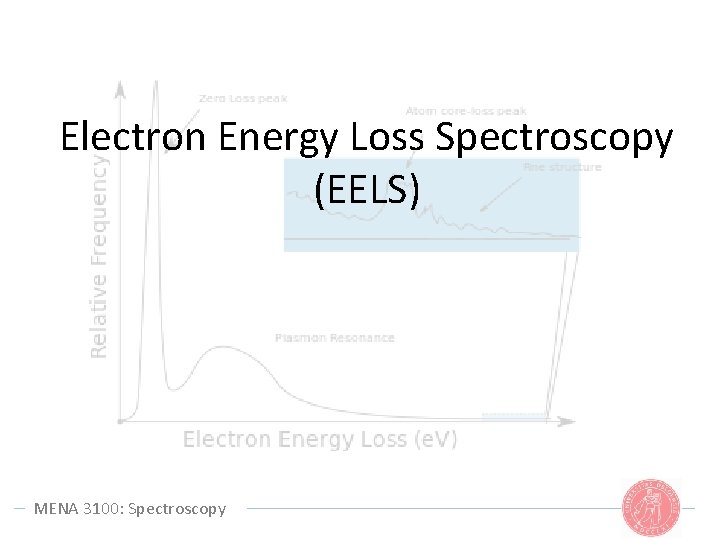
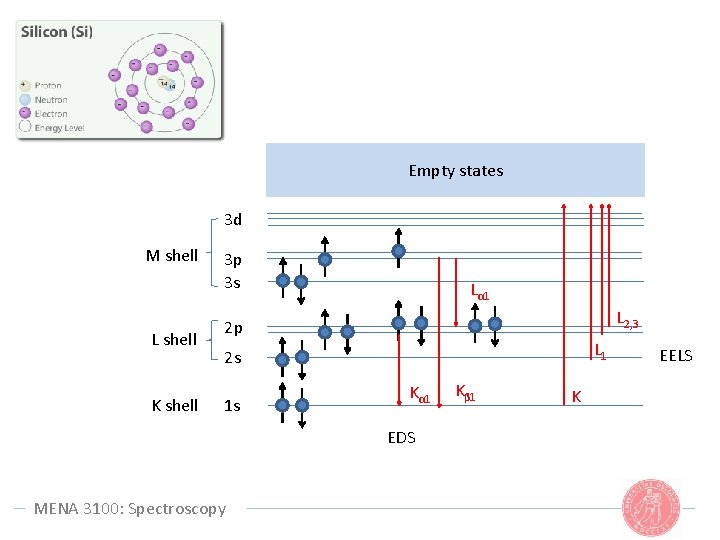
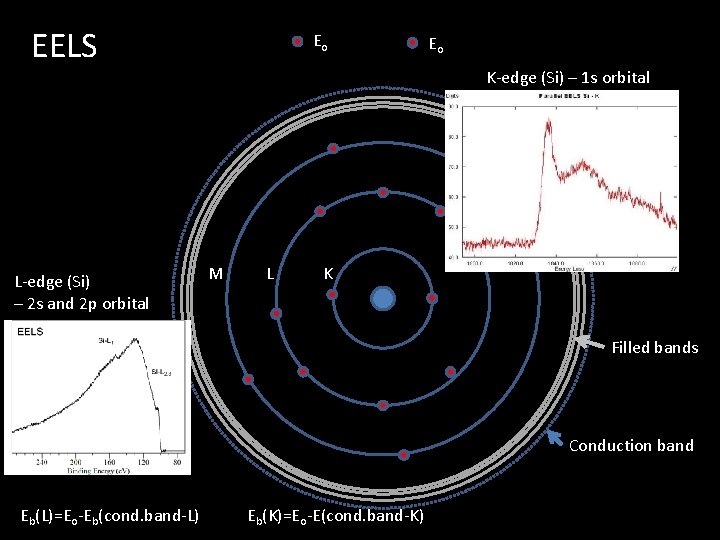
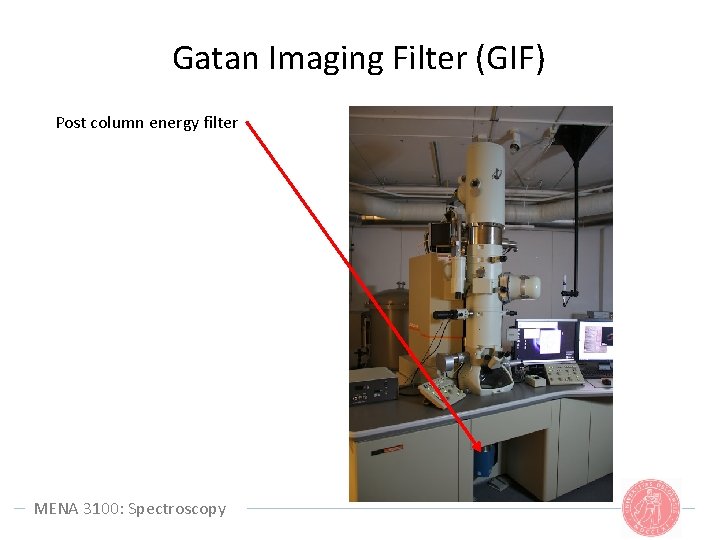
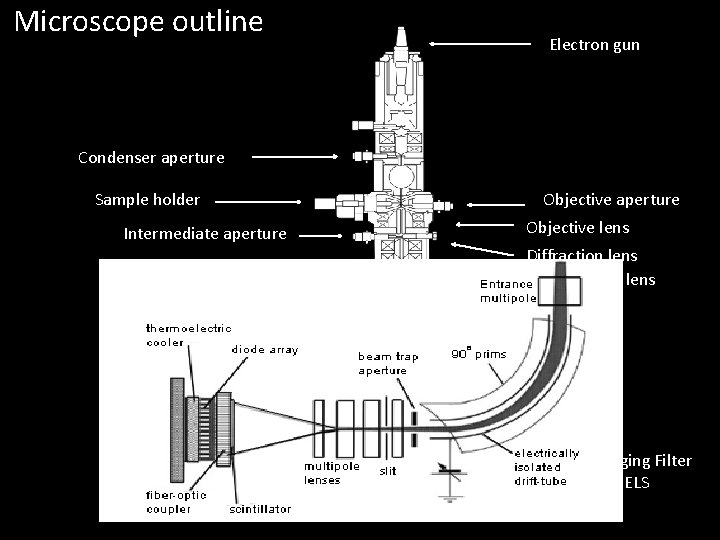
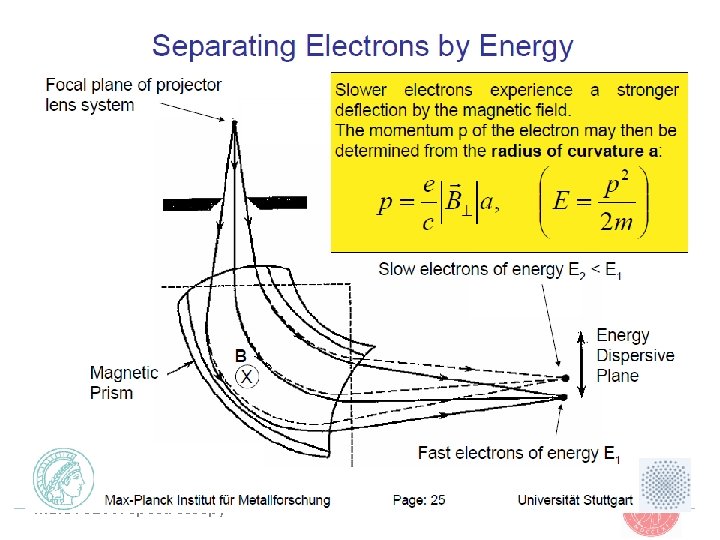
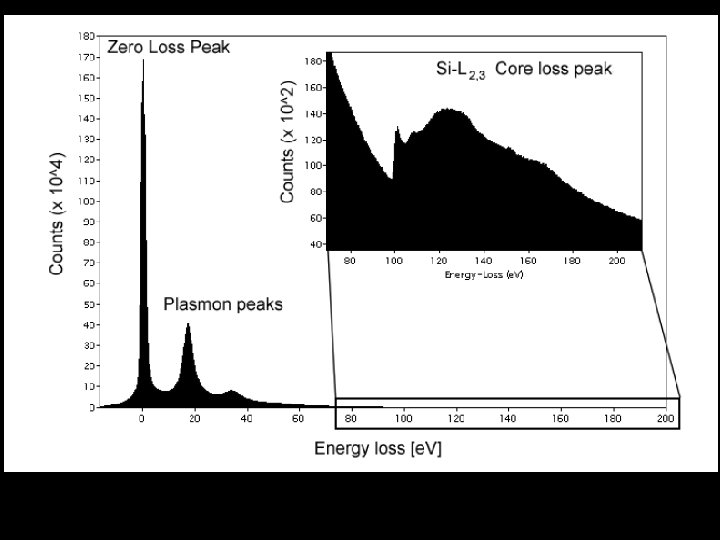
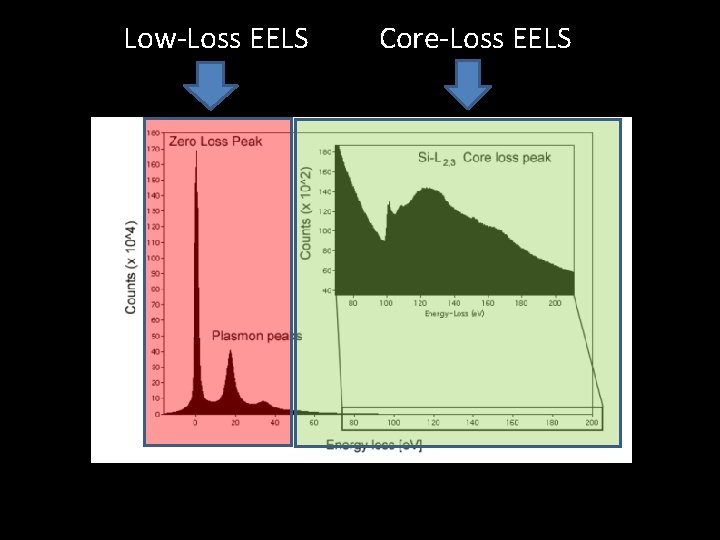
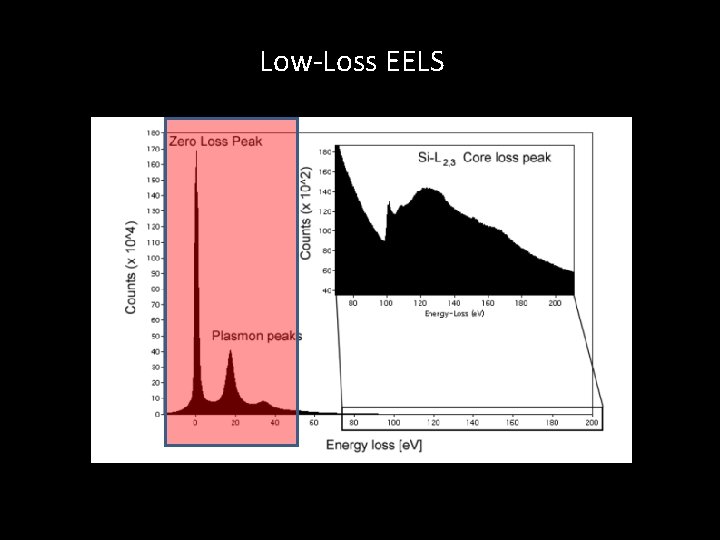
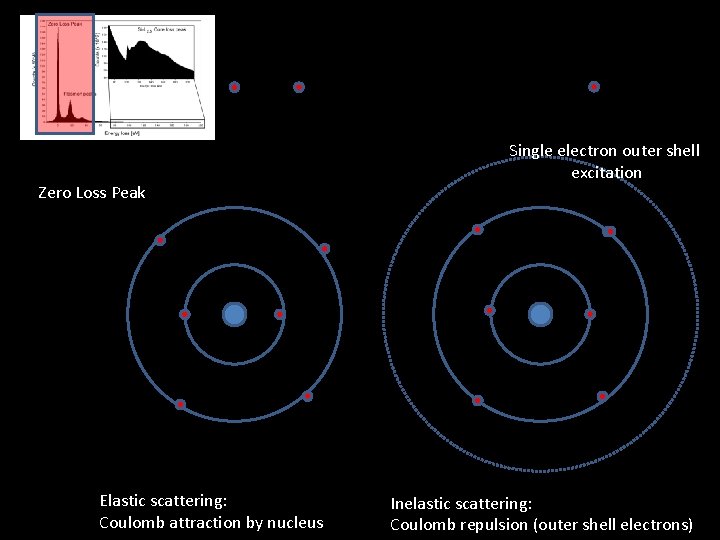
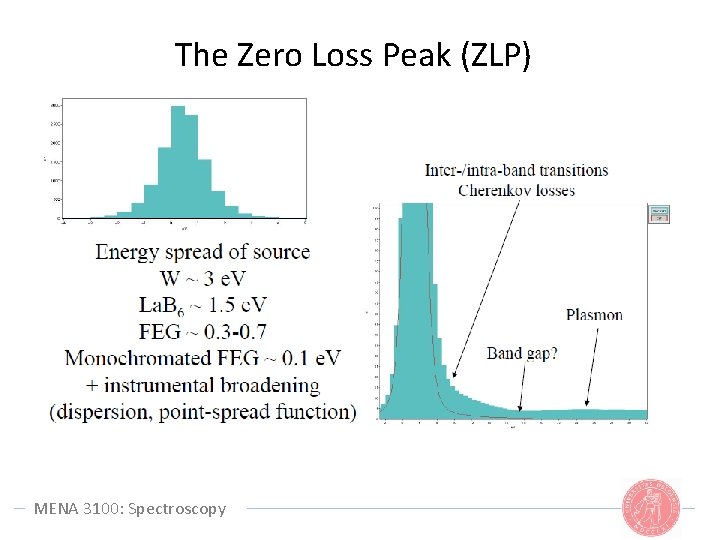
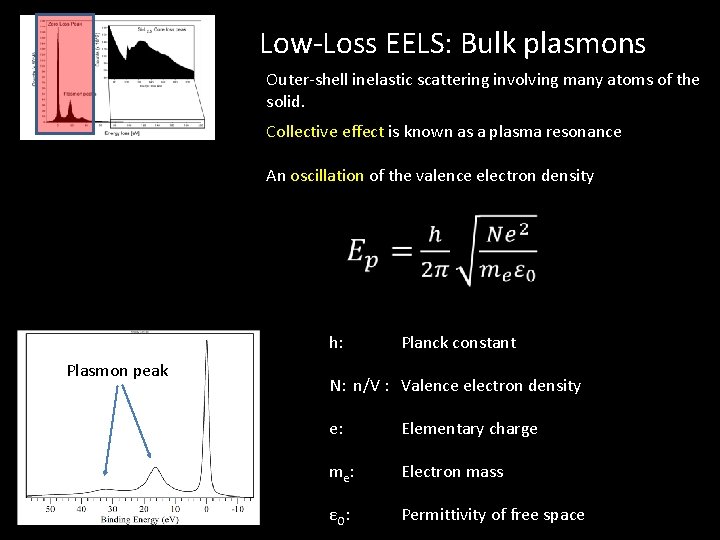
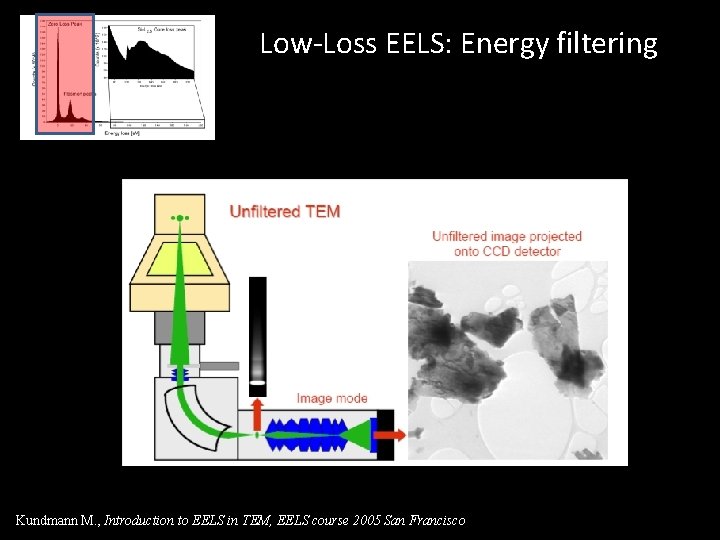
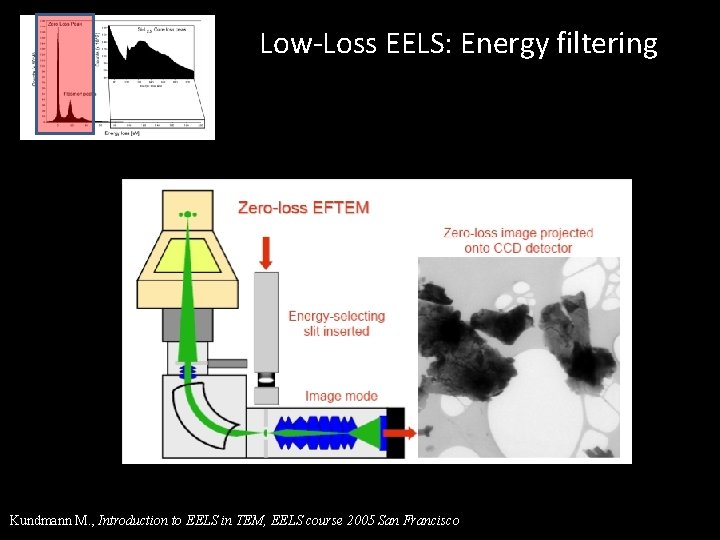
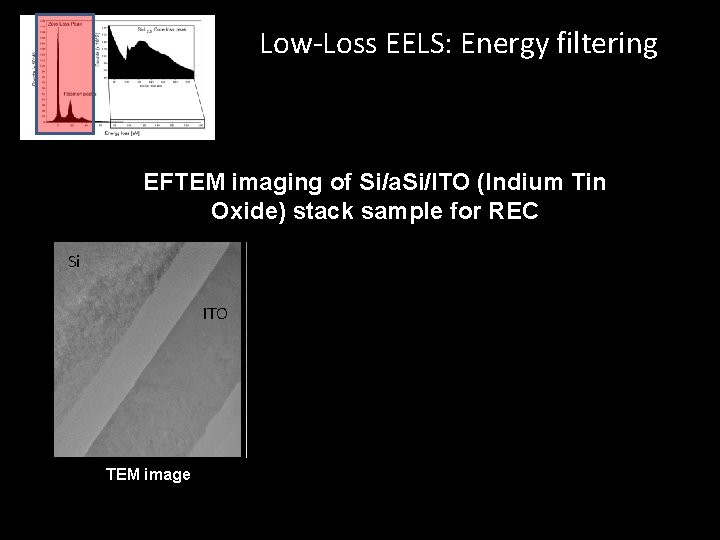
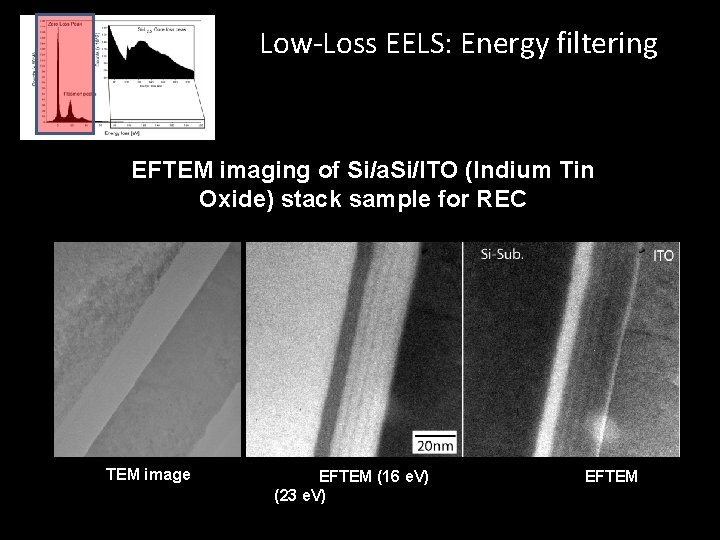
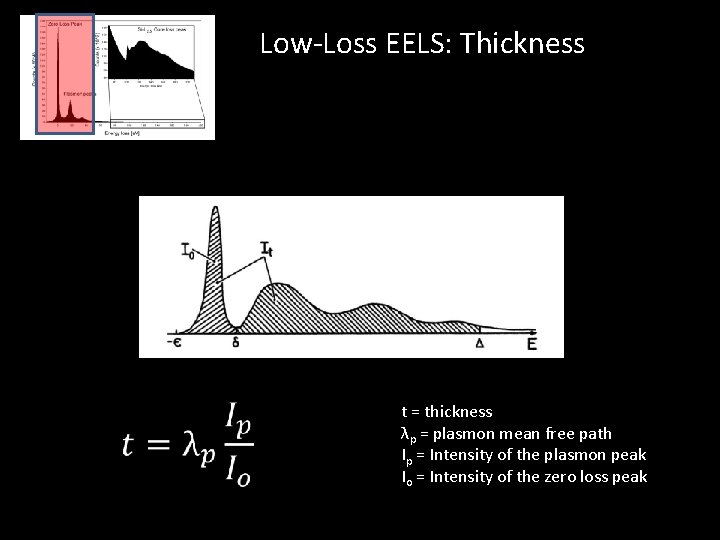
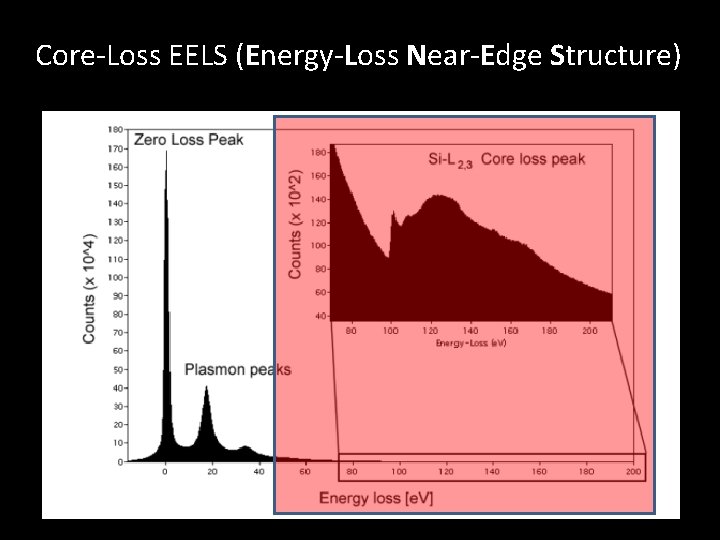
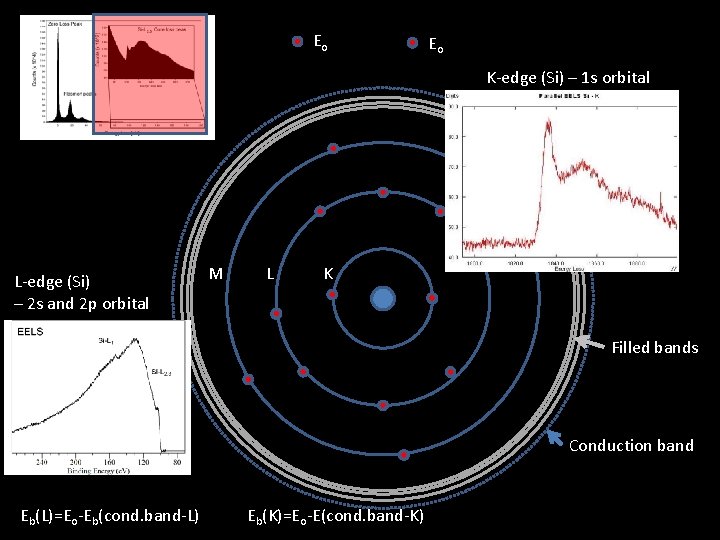
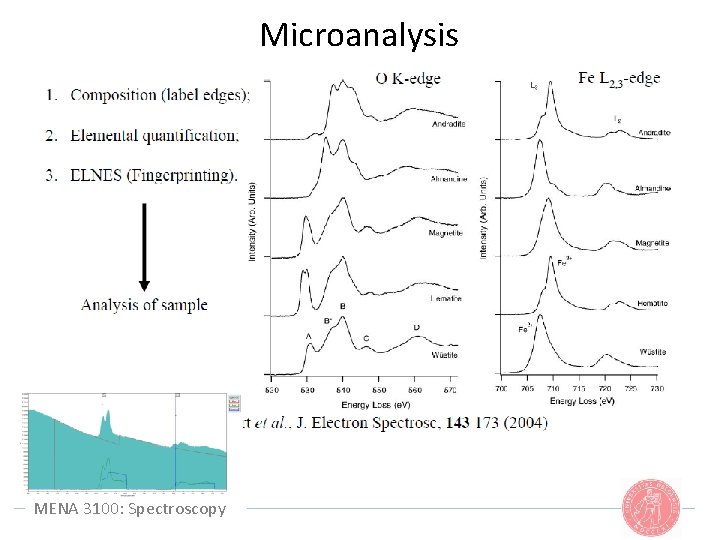
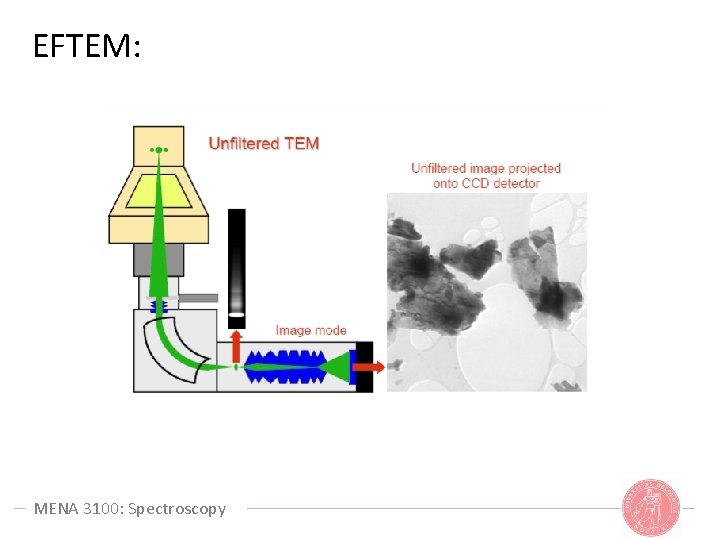
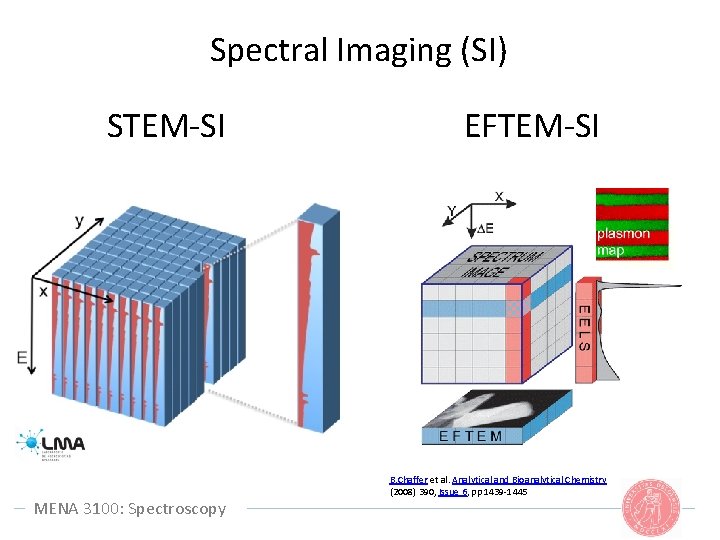
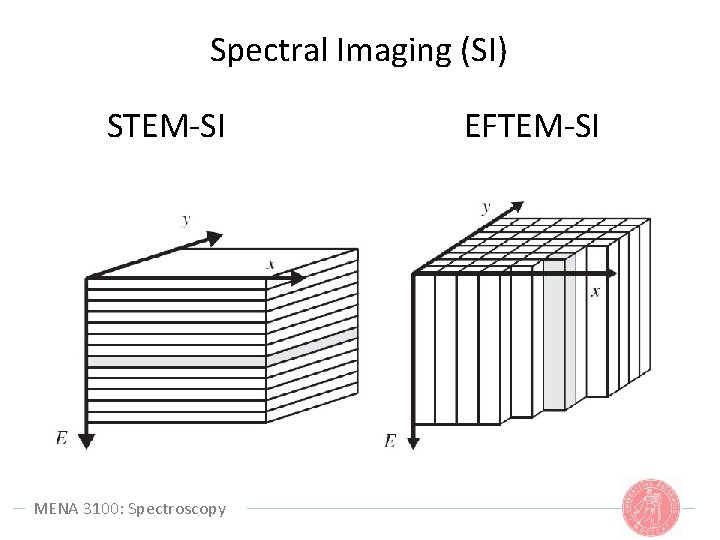
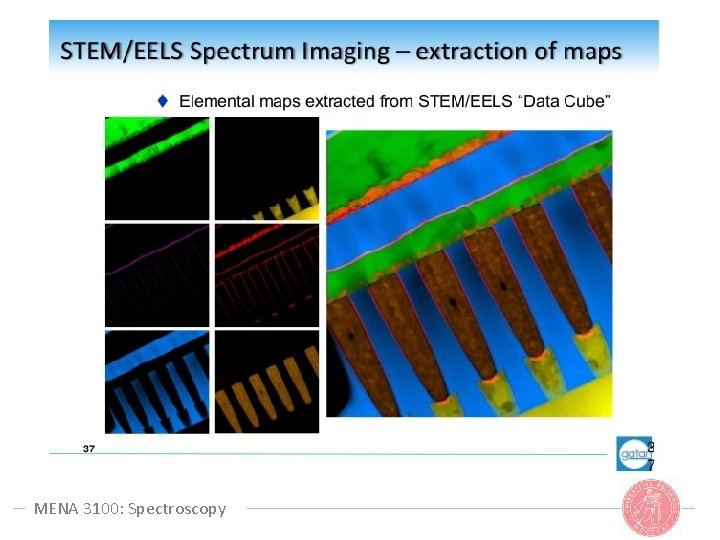
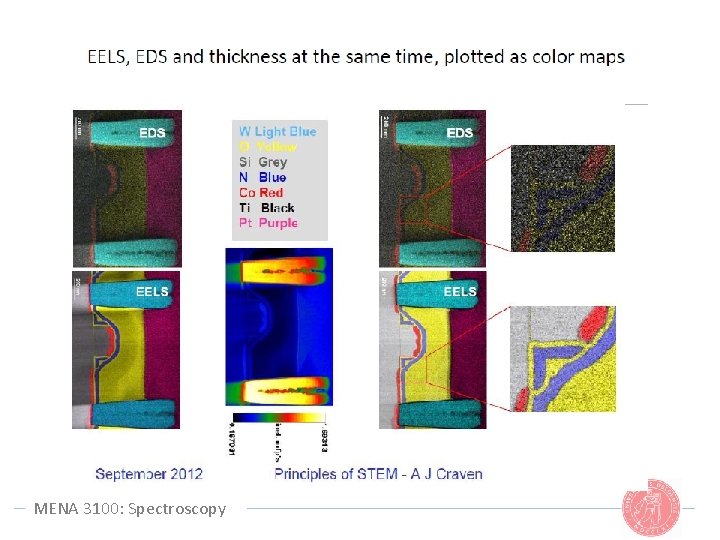
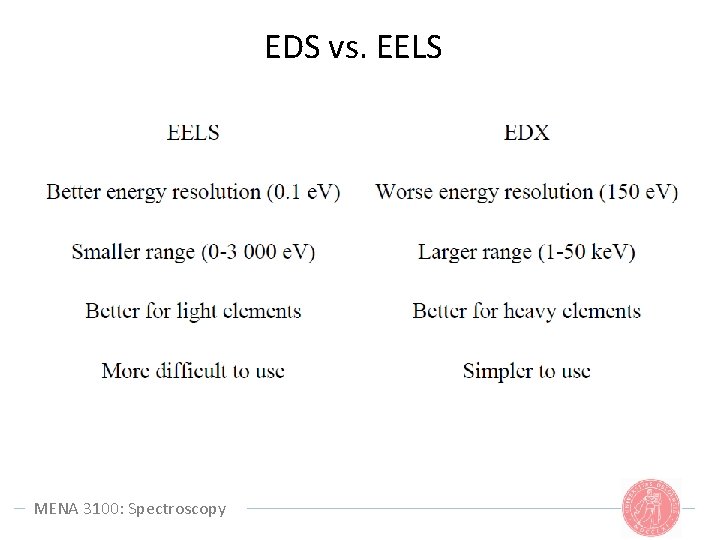
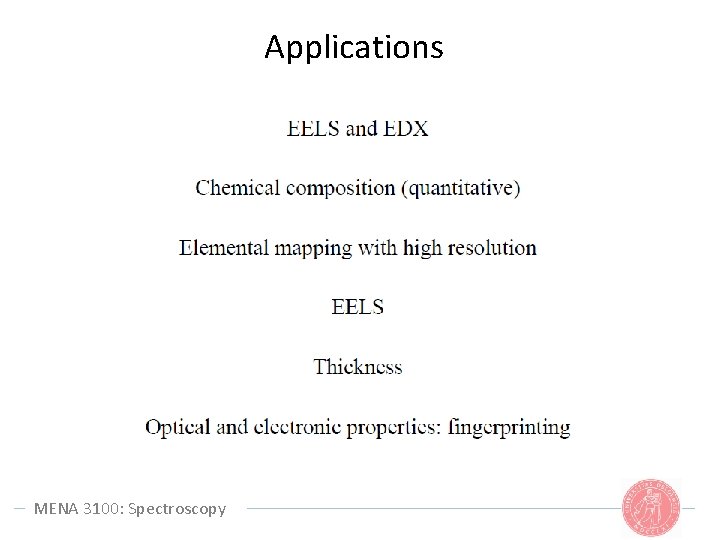
- Slides: 49
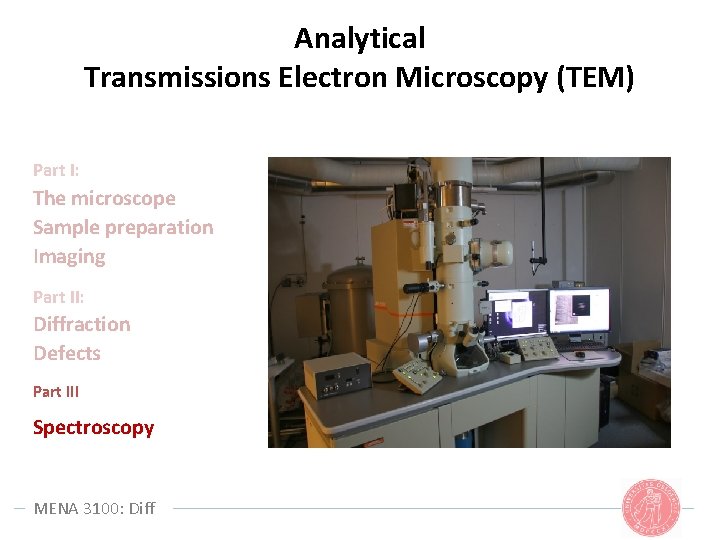
Analytical Transmissions Electron Microscopy (TEM) Part I: The microscope Sample preparation Imaging Part II: Diffraction Defects Part III Spectroscopy MENA 3100: Diff
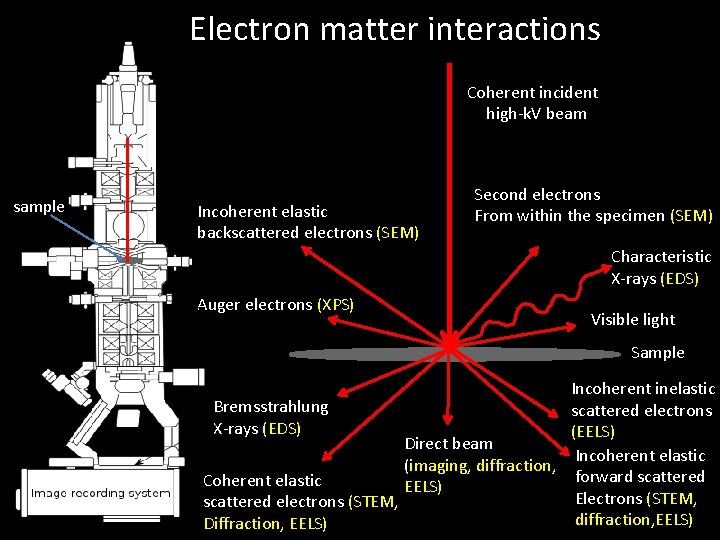
Electron matter interactions Coherent incident high-k. V beam sample Incoherent elastic backscattered electrons (SEM) Second electrons From within the specimen (SEM) Characteristic X-rays (EDS) Auger electrons (XPS) Visible light Sample Incoherent inelastic Bremsstrahlung scattered electrons X-rays (EDS) (EELS) Direct beam Incoherent elastic (imaging, diffraction, forward scattered Coherent elastic EELS) Electrons (STEM, scattered electrons (STEM, diffraction, EELS) Diffraction, EELS)
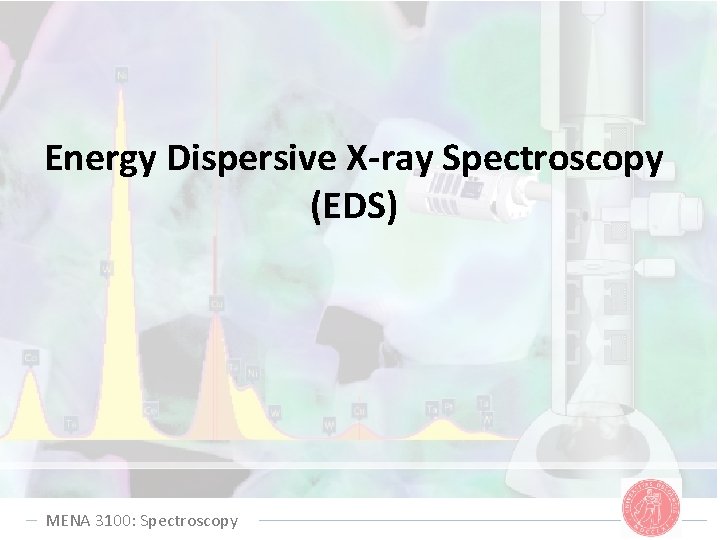
Energy Dispersive X-ray Spectroscopy (EDS) MENA 3100: Spectroscopy
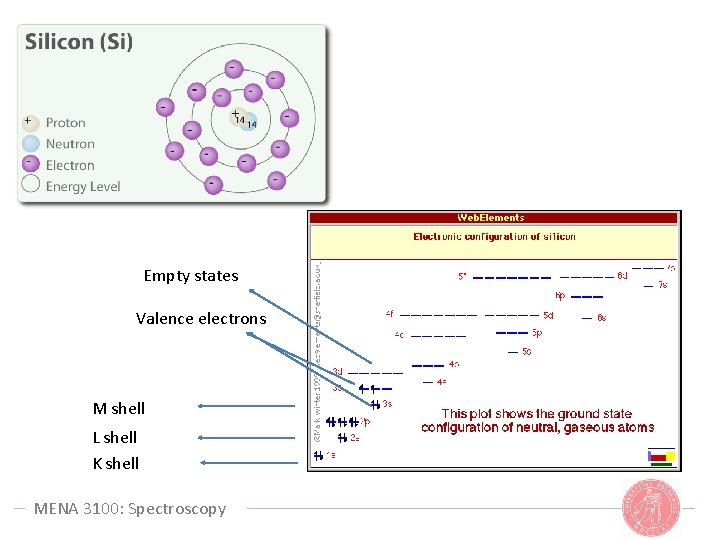
Empty states Valence electrons M shell L shell K shell MENA 3100: Spectroscopy
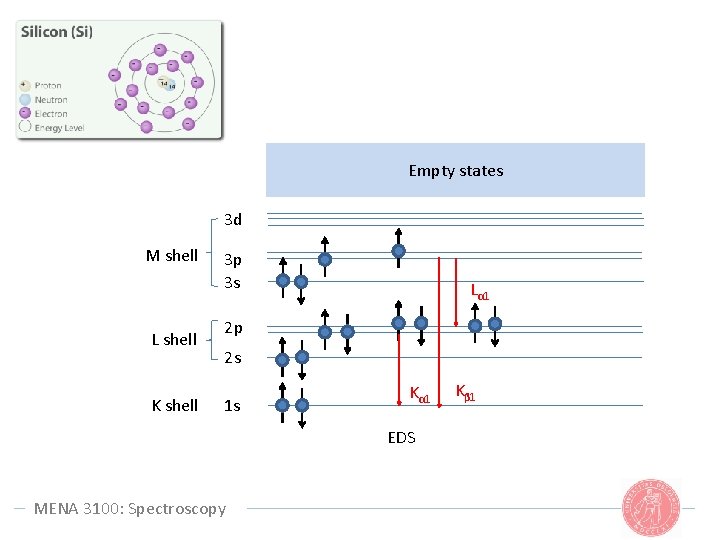
Empty states 3 d M shell L shell K shell 3 p 3 s Lα 1 2 p 2 s 1 s Kα 1 EDS MENA 3100: Spectroscopy Kβ 1
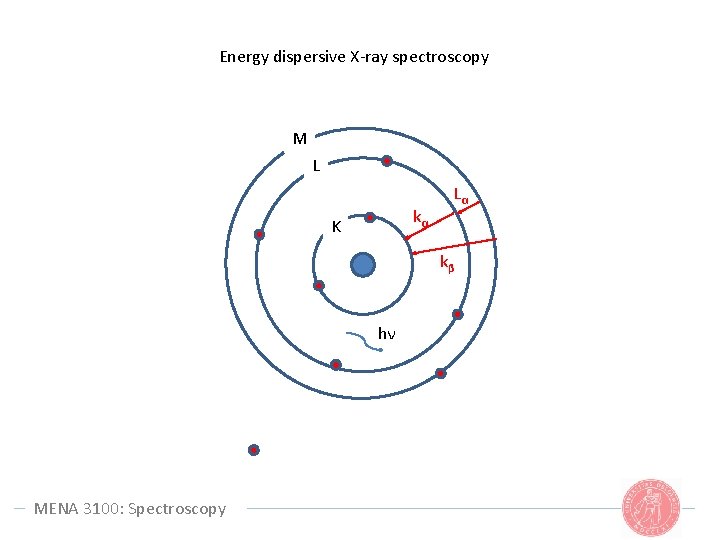
Energy dispersive X-ray spectroscopy M L kα K Lα kβ hν MENA 3100: Spectroscopy
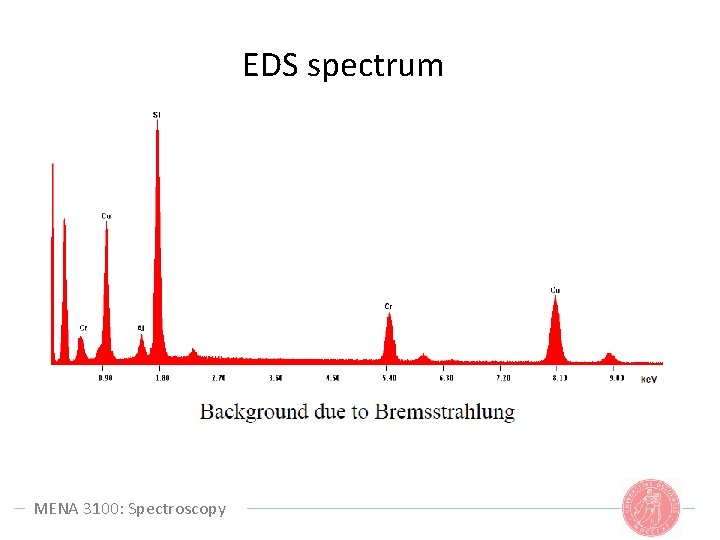
EDS spectrum MENA 3100: Spectroscopy
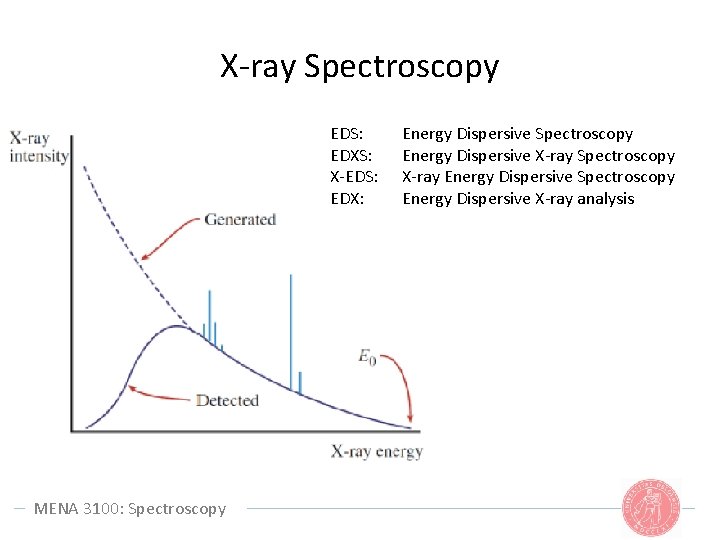
X-ray Spectroscopy EDS: EDXS: X-EDS: EDX: MENA 3100: Spectroscopy Energy Dispersive X-ray Spectroscopy X-ray Energy Dispersive Spectroscopy Energy Dispersive X-ray analysis
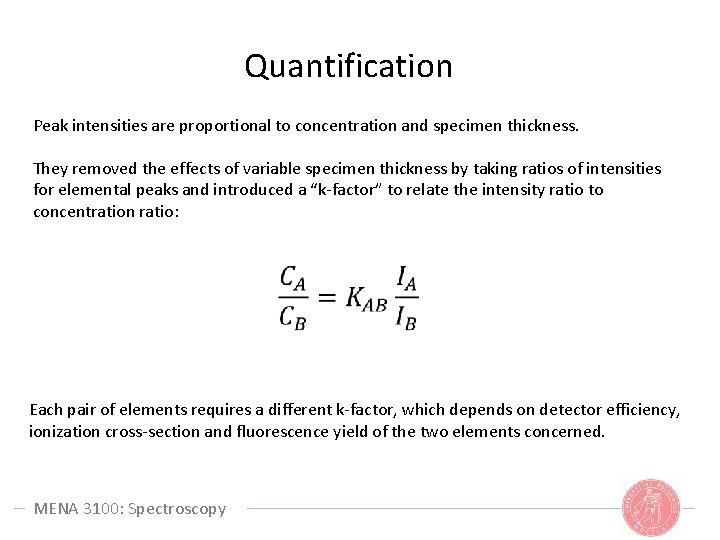
Quantification Peak intensities are proportional to concentration and specimen thickness. They removed the effects of variable specimen thickness by taking ratios of intensities for elemental peaks and introduced a “k-factor” to relate the intensity ratio to concentration ratio: Each pair of elements requires a different k-factor, which depends on detector efficiency, ionization cross-section and fluorescence yield of the two elements concerned. MENA 3100: Spectroscopy
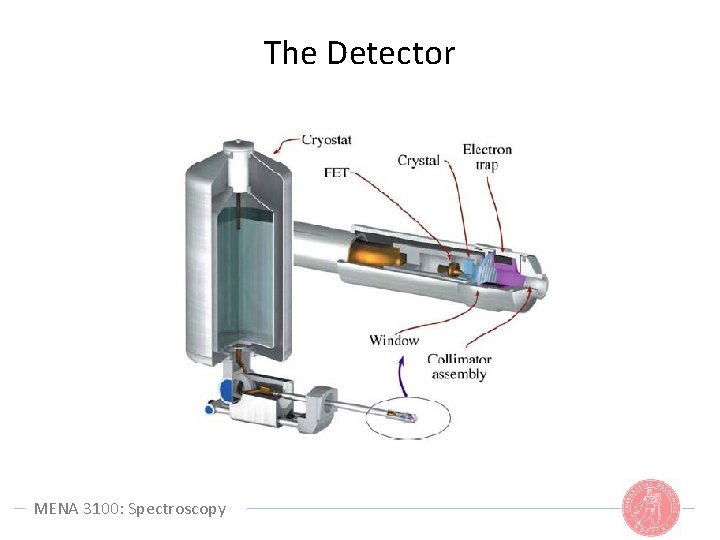
The Detector MENA 3100: Spectroscopy
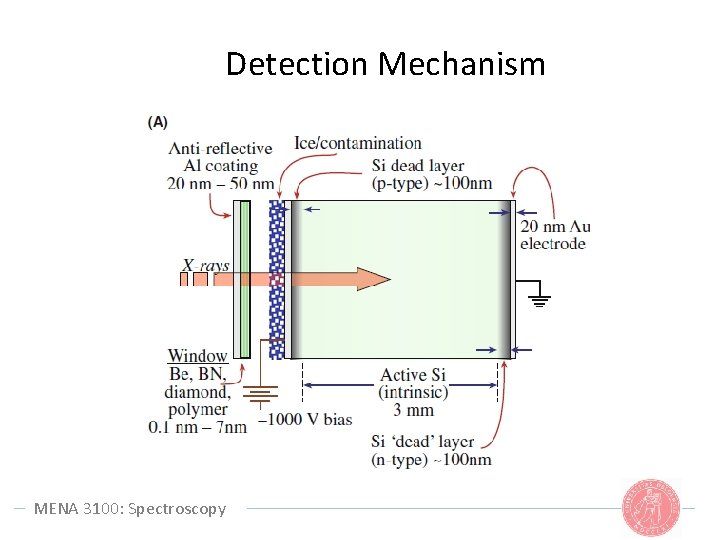
Detection Mechanism MENA 3100: Spectroscopy
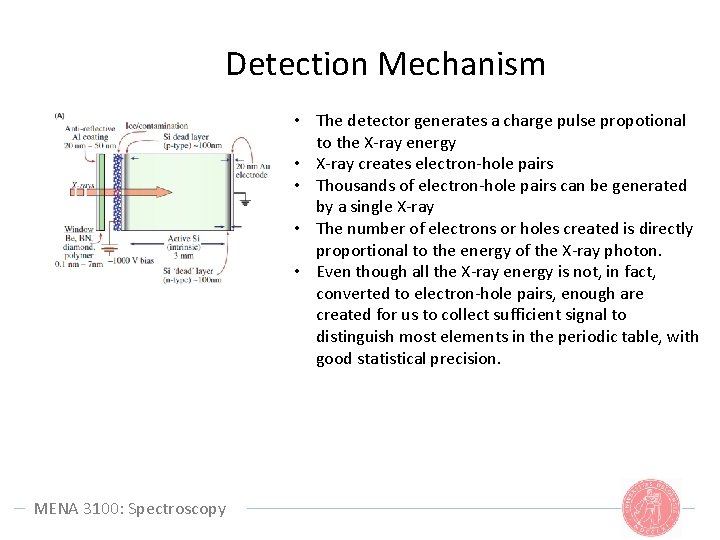
Detection Mechanism • The detector generates a charge pulse propotional to the X-ray energy • X-ray creates electron-hole pairs • Thousands of electron-hole pairs can be generated by a single X-ray • The number of electrons or holes created is directly proportional to the energy of the X-ray photon. • Even though all the X-ray energy is not, in fact, converted to electron-hole pairs, enough are created for us to collect sufficient signal to distinguish most elements in the periodic table, with good statistical precision. MENA 3100: Spectroscopy
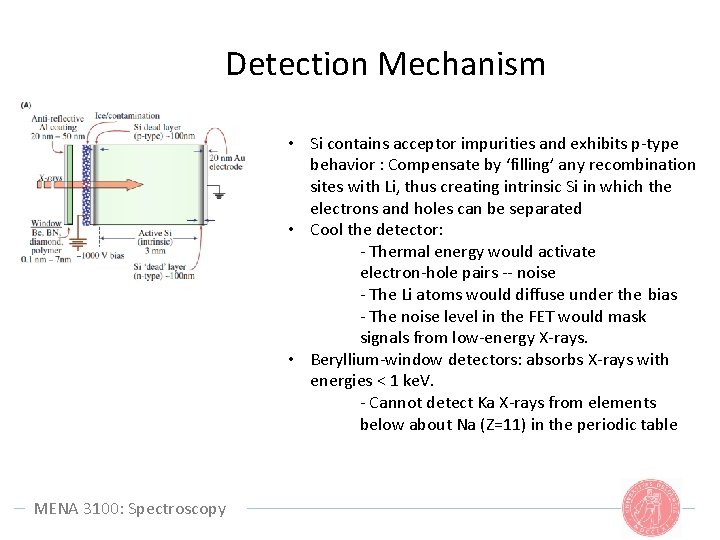
Detection Mechanism • Si contains acceptor impurities and exhibits p-type behavior : Compensate by ‘filling’ any recombination sites with Li, thus creating intrinsic Si in which the electrons and holes can be separated • Cool the detector: - Thermal energy would activate electron-hole pairs -- noise - The Li atoms would diffuse under the bias - The noise level in the FET would mask signals from low-energy X-rays. • Beryllium-window detectors: absorbs X-rays with energies < 1 ke. V. - Cannot detect Ka X-rays from elements below about Na (Z=11) in the periodic table MENA 3100: Spectroscopy
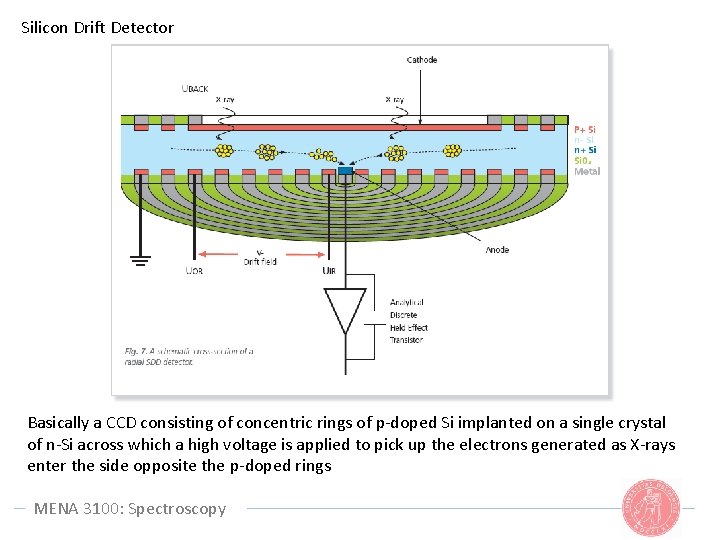
Silicon Drift Detector Basically a CCD consisting of concentric rings of p-doped Si implanted on a single crystal of n-Si across which a high voltage is applied to pick up the electrons generated as X-rays enter the side opposite the p-doped rings MENA 3100: Spectroscopy
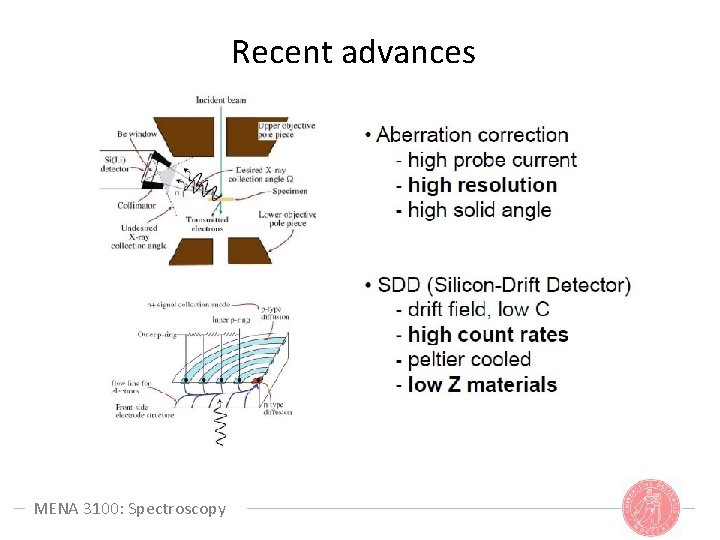
Recent advances MENA 3100: Spectroscopy
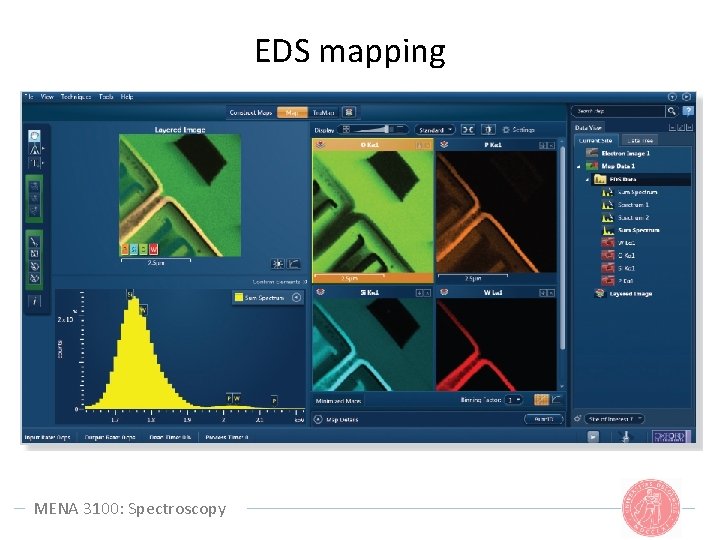
EDS mapping MENA 3100: Spectroscopy
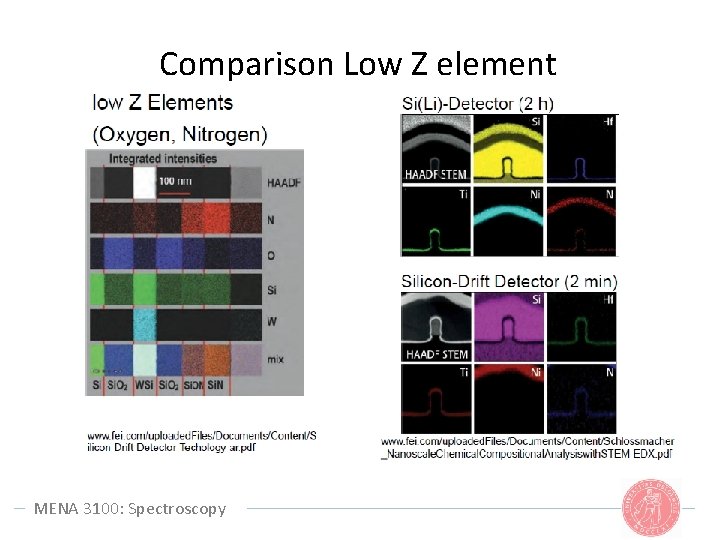
Comparison Low Z element MENA 3100: Spectroscopy
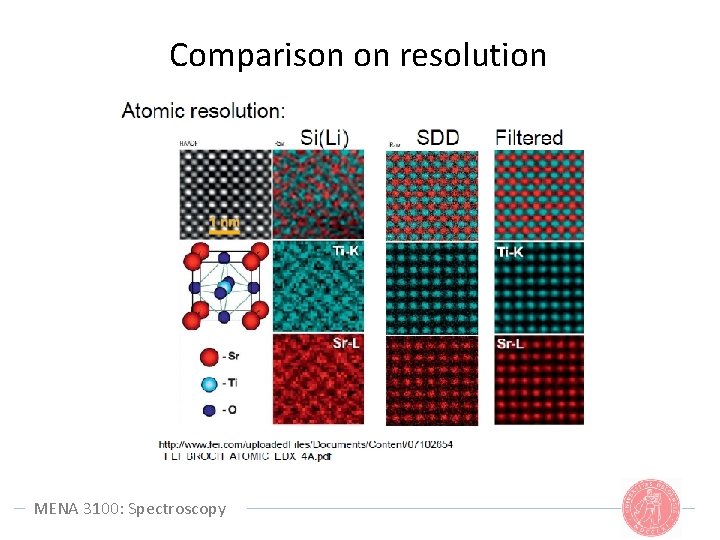
Comparison on resolution MENA 3100: Spectroscopy
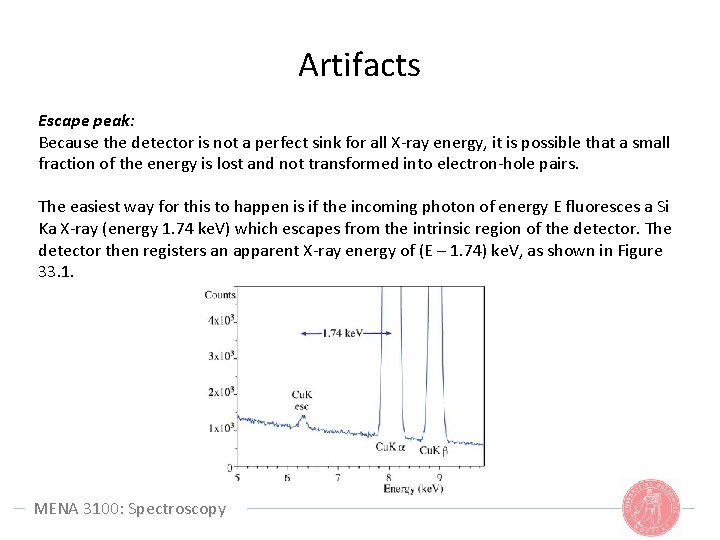
Artifacts Escape peak: Because the detector is not a perfect sink for all X-ray energy, it is possible that a small fraction of the energy is lost and not transformed into electron-hole pairs. The easiest way for this to happen is if the incoming photon of energy E fluoresces a Si Ka X-ray (energy 1. 74 ke. V) which escapes from the intrinsic region of the detector. The detector then registers an apparent X-ray energy of (E – 1. 74) ke. V, as shown in Figure 33. 1. MENA 3100: Spectroscopy
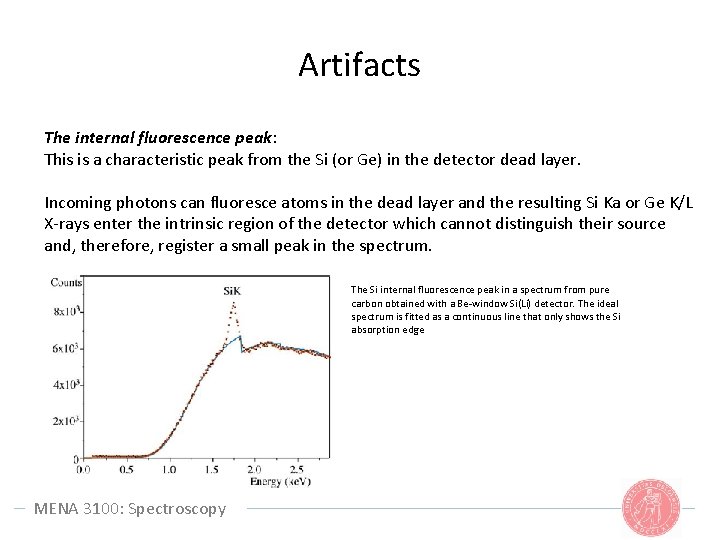
Artifacts The internal fluorescence peak: This is a characteristic peak from the Si (or Ge) in the detector dead layer. Incoming photons can fluoresce atoms in the dead layer and the resulting Si Ka or Ge K/L X-rays enter the intrinsic region of the detector which cannot distinguish their source and, therefore, register a small peak in the spectrum. The Si internal fluorescence peak in a spectrum from pure carbon obtained with a Be-window Si(Li) detector. The ideal spectrum is fitted as a continuous line that only shows the Si absorption edge MENA 3100: Spectroscopy
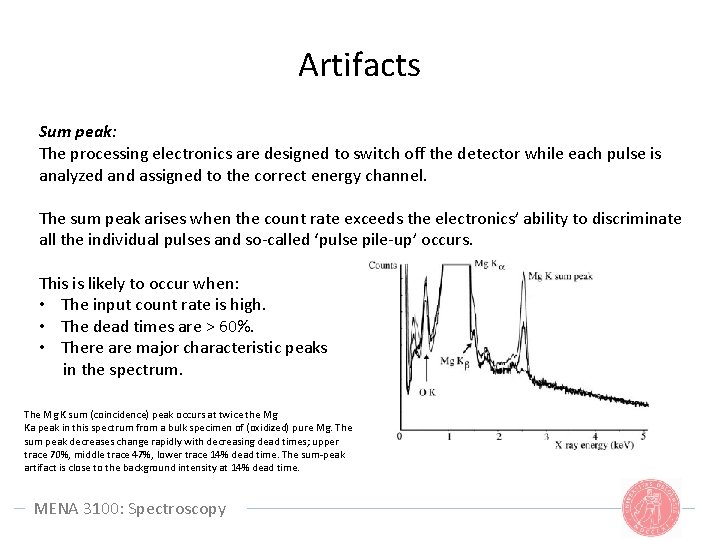
Artifacts Sum peak: The processing electronics are designed to switch off the detector while each pulse is analyzed and assigned to the correct energy channel. The sum peak arises when the count rate exceeds the electronics’ ability to discriminate all the individual pulses and so-called ‘pulse pile-up’ occurs. This is likely to occur when: • The input count rate is high. • The dead times are > 60%. • There are major characteristic peaks in the spectrum. The Mg K sum (coincidence) peak occurs at twice the Mg Ka peak in this spectrum from a bulk specimen of (oxidized) pure Mg. The sum peak decreases change rapidly with decreasing dead times; upper trace 70%, middle trace 47%, lower trace 14% dead time. The sum-peak artifact is close to the background intensity at 14% dead time. MENA 3100: Spectroscopy
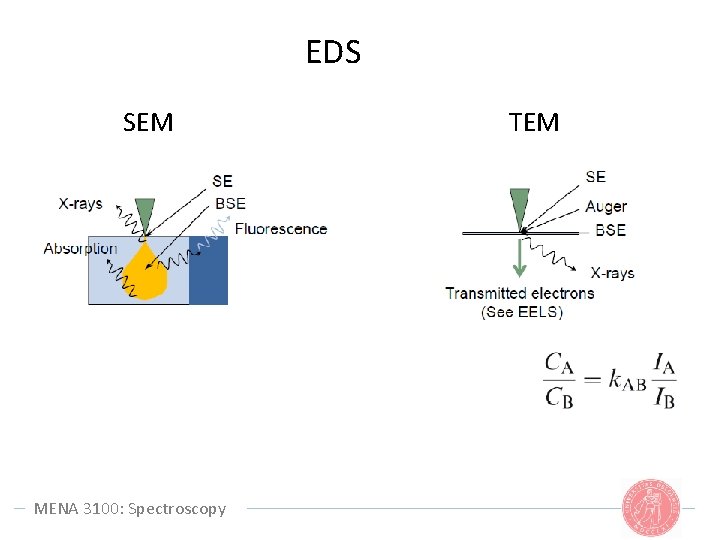
EDS SEM MENA 3100: Spectroscopy TEM
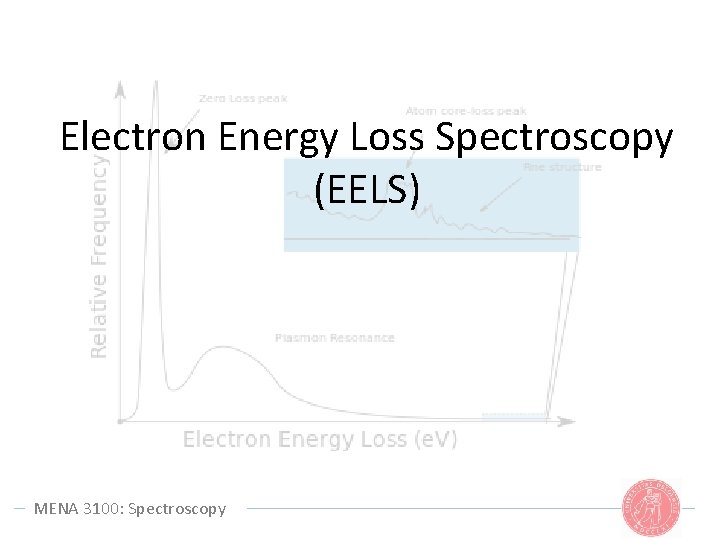
Electron Energy Loss Spectroscopy (EELS) MENA 3100: Spectroscopy
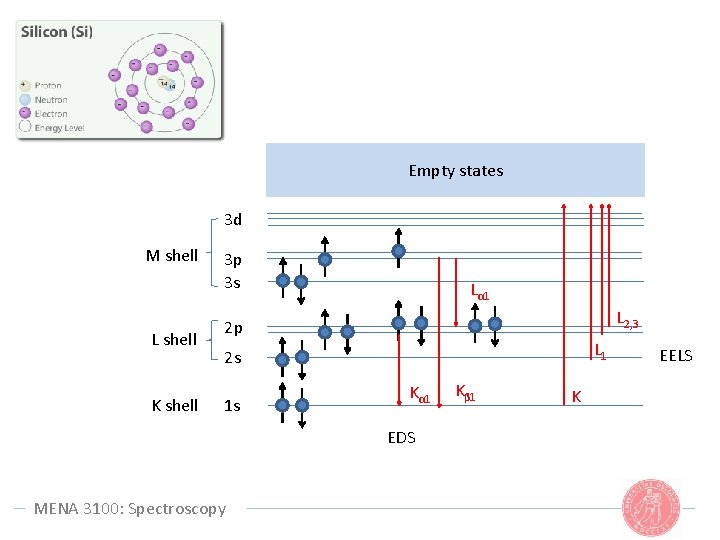
Empty states 3 d M shell L shell K shell 3 p 3 s Lα 1 L 2, 3 2 p L 1 2 s 1 s Kα 1 EDS MENA 3100: Spectroscopy Kβ 1 K EELS
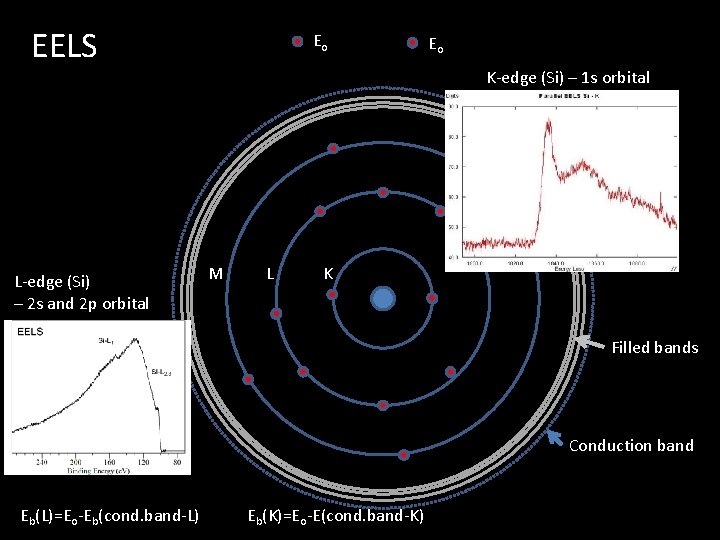
EELS L-edge (Si) – 2 s and 2 p orbital Eo Eo K-edge (Si) – 1 s orbital M L K Filled bands Conduction band Eb(L)=Eo-Eb(cond. band-L) Eb(K)=Eo-E(cond. band-K)
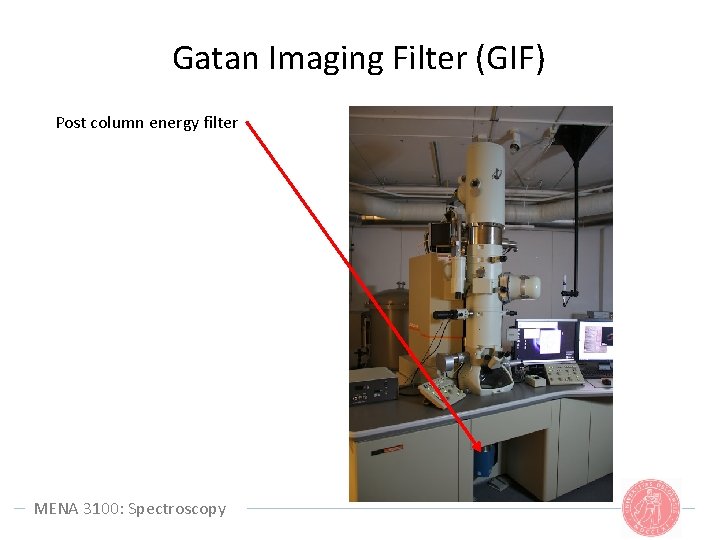
Gatan Imaging Filter (GIF) Post column energy filter MENA 3100: Spectroscopy
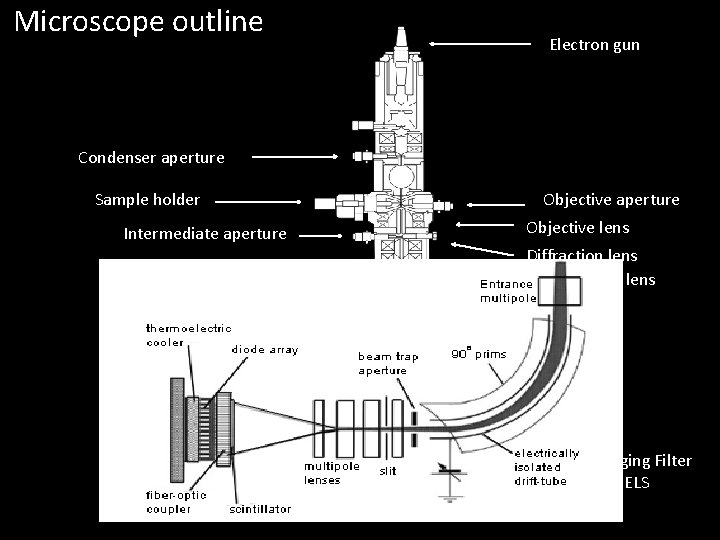
Microscope outline Electron gun Condenser aperture Sample holder Intermediate aperture Projector lenses Objective aperture Objective lens Diffraction lens Intermediate lens Fluorescent screen Gatan Imaging Filter For EELS
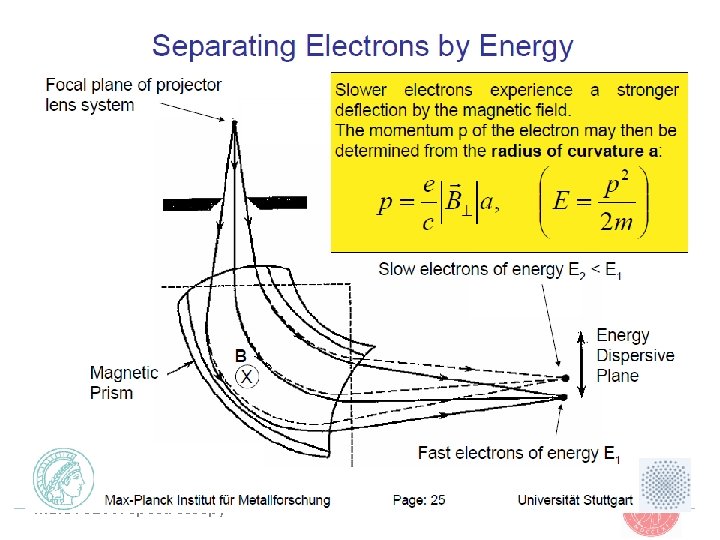
MENA 3100: Spectroscopy
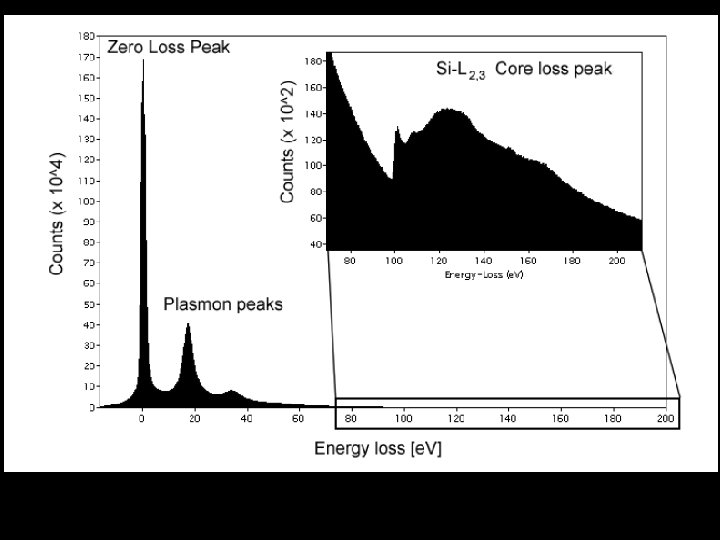
Projector crossover Viewing screen Slit Detector Multipole lenses 90 o magnetic prism Beam trap aperture
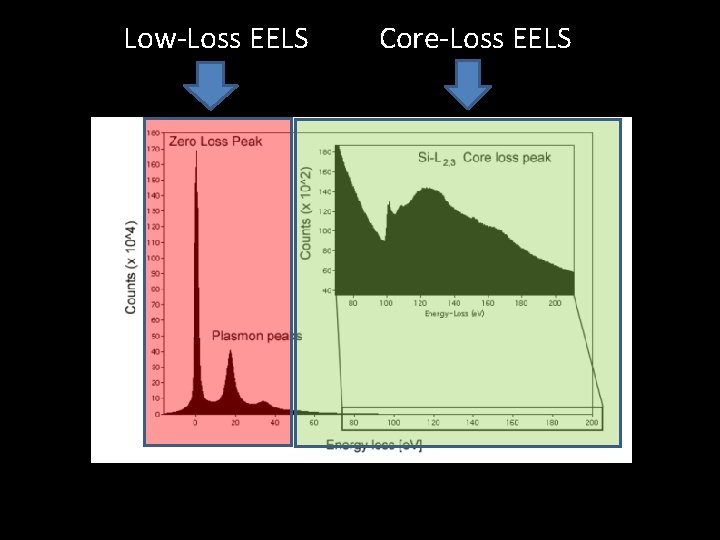
Low-Loss EELS Core-Loss EELS
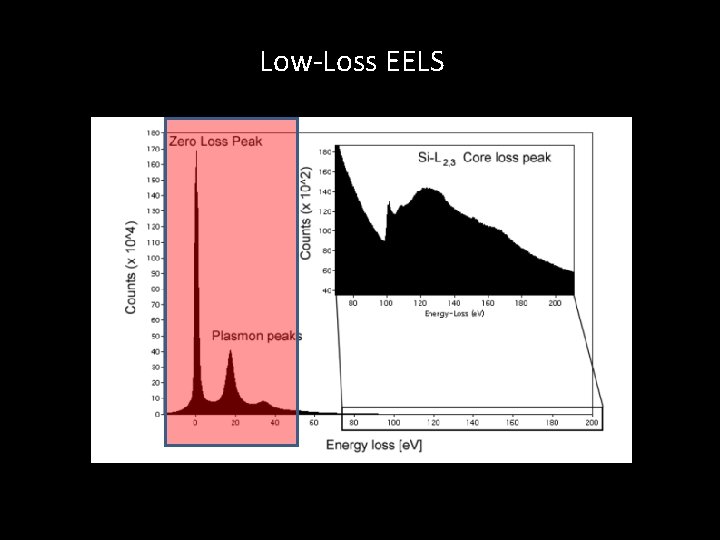
Low-Loss EELS
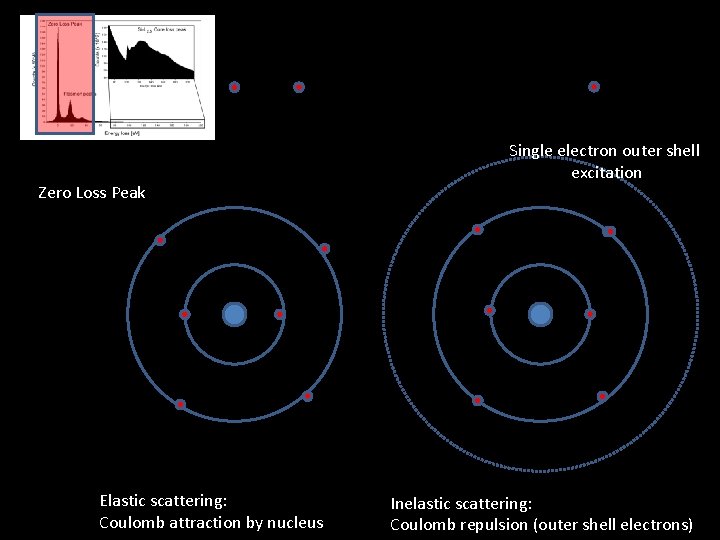
Zero Loss Peak Elastic scattering: Coulomb attraction by nucleus Single electron outer shell excitation Inelastic scattering: Coulomb repulsion (outer shell electrons)
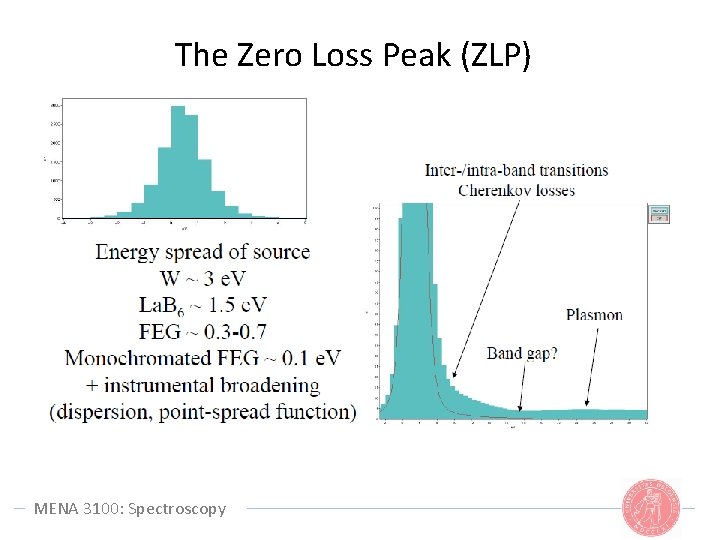
The Zero Loss Peak (ZLP) MENA 3100: Spectroscopy
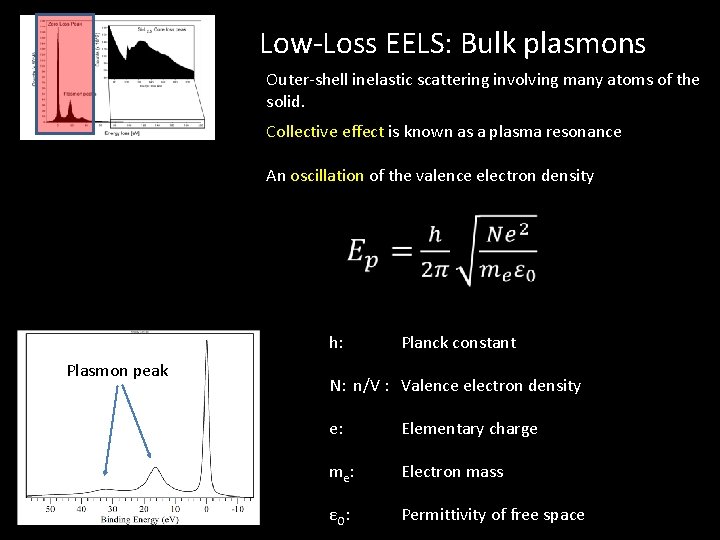
Low-Loss EELS: Bulk plasmons Outer-shell inelastic scattering involving many atoms of the solid. Collective effect is known as a plasma resonance An oscillation of the valence electron density h: Plasmon peak Planck constant N: n/V : Valence electron density e: Elementary charge me: Electron mass εO: Permittivity of free space
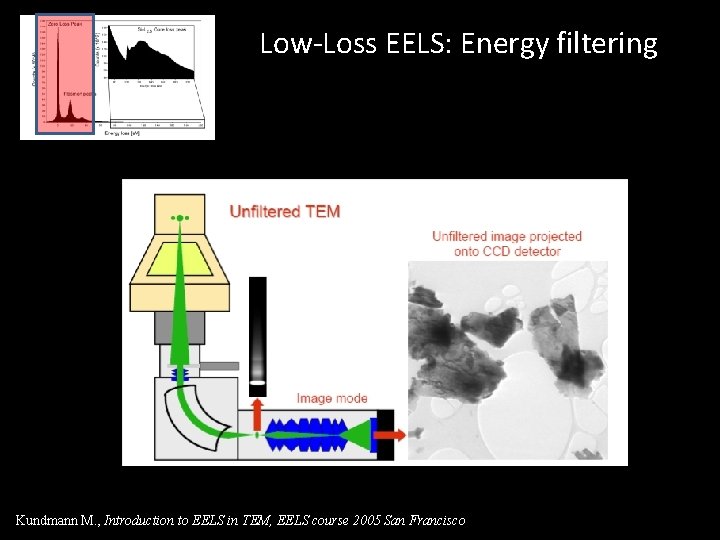
Low-Loss EELS: Energy filtering Kundmann M. , Introduction to EELS in TEM, EELS course 2005 San Francisco
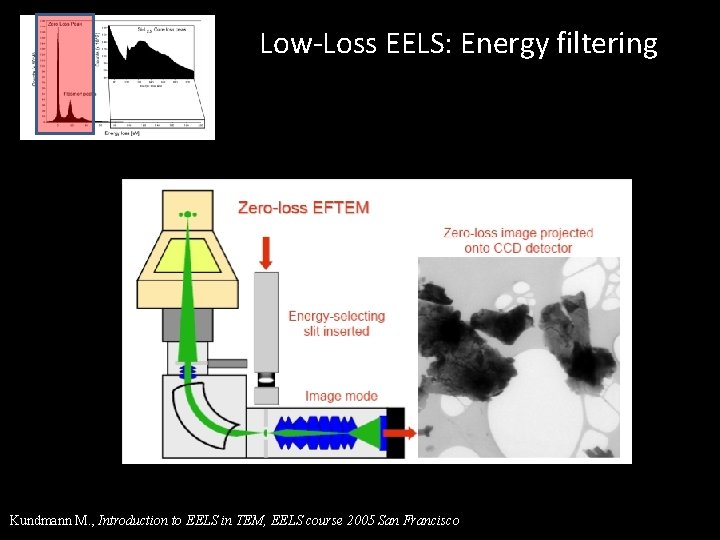
Low-Loss EELS: Energy filtering Kundmann M. , Introduction to EELS in TEM, EELS course 2005 San Francisco
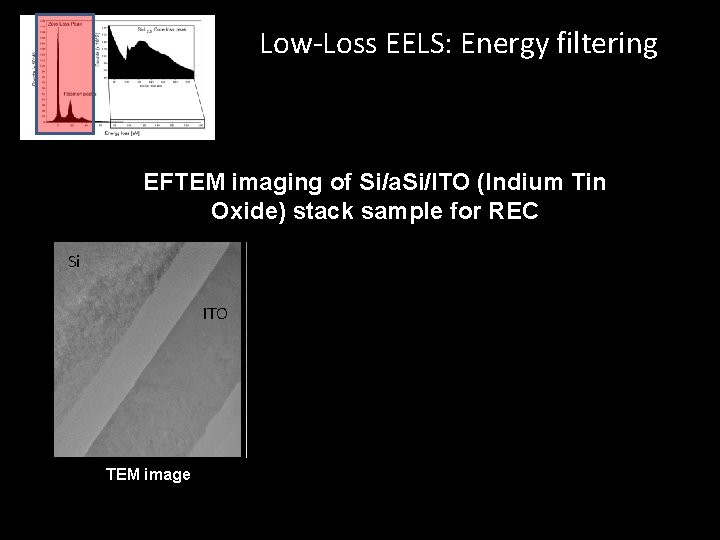
Low-Loss EELS: Energy filtering EFTEM imaging of Si/a. Si/ITO (Indium Tin Oxide) stack sample for REC Si ITO TEM image
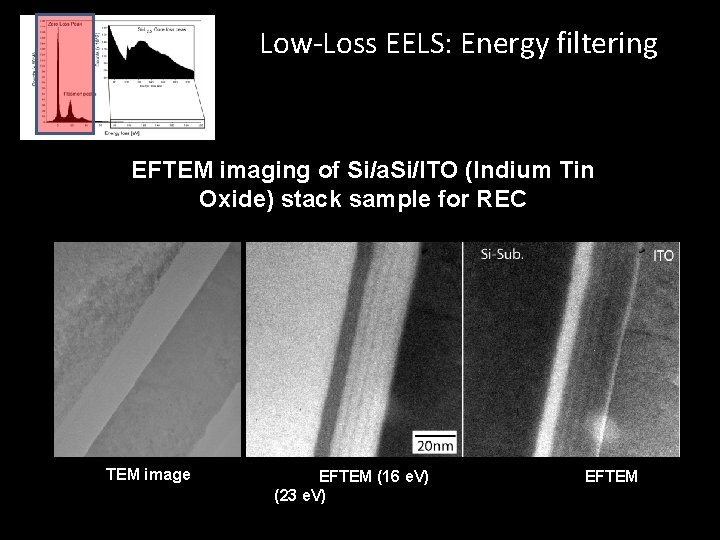
Low-Loss EELS: Energy filtering EFTEM imaging of Si/a. Si/ITO (Indium Tin Oxide) stack sample for REC TEM image EFTEM (16 e. V) (23 e. V) EFTEM
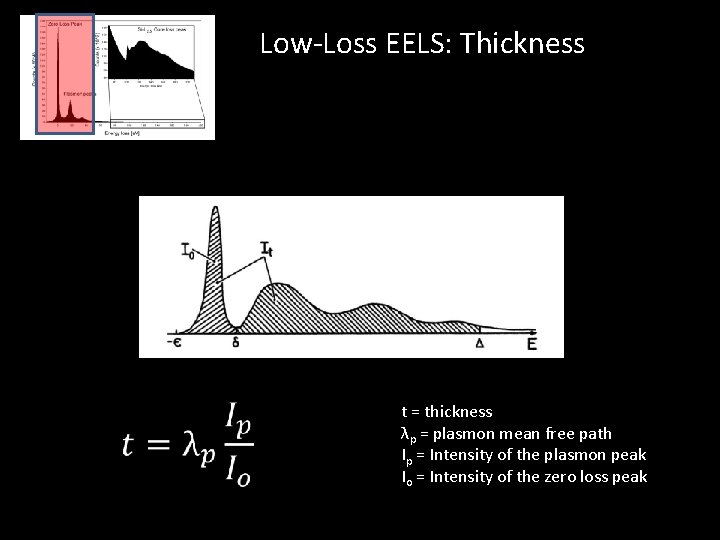
Low-Loss EELS: Thickness t = thickness λp = plasmon mean free path Ip = Intensity of the plasmon peak Io = Intensity of the zero loss peak
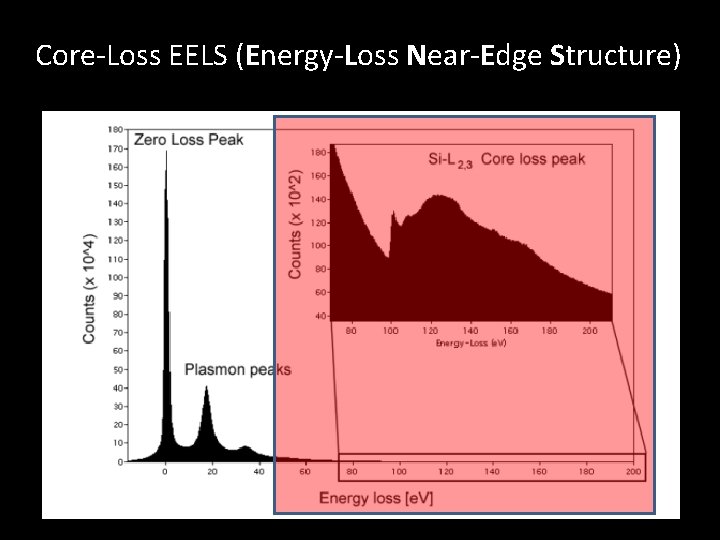
Core-Loss EELS (Energy-Loss Near-Edge Structure)
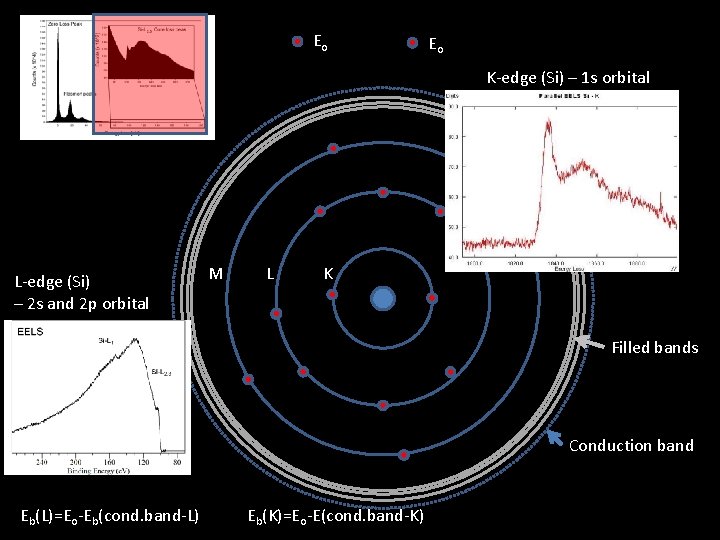
Eo Eo K-edge (Si) – 1 s orbital L-edge (Si) – 2 s and 2 p orbital M L K Filled bands Conduction band Eb(L)=Eo-Eb(cond. band-L) Eb(K)=Eo-E(cond. band-K)
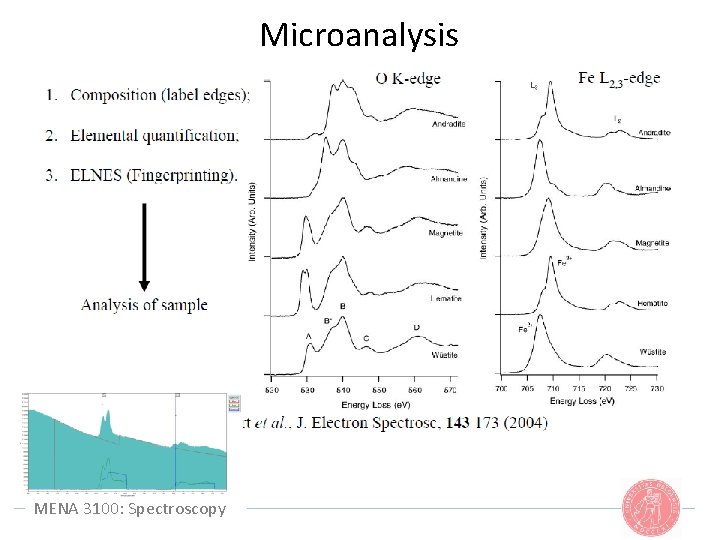
Microanalysis MENA 3100: Spectroscopy
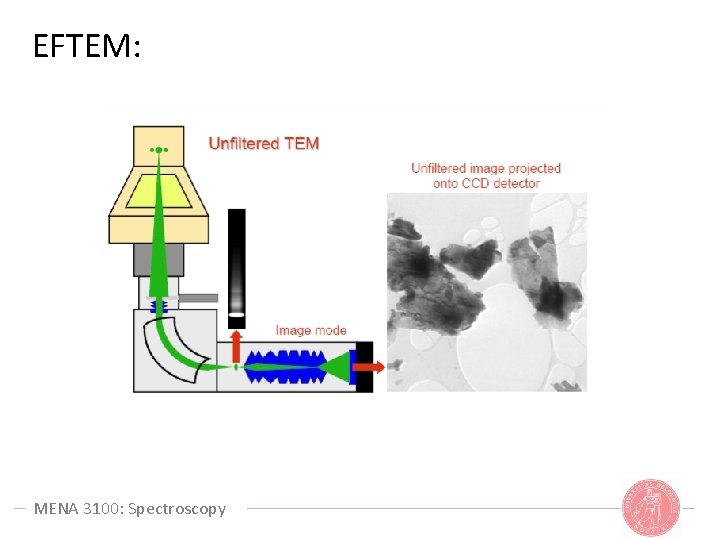
EFTEM: MENA 3100: Spectroscopy
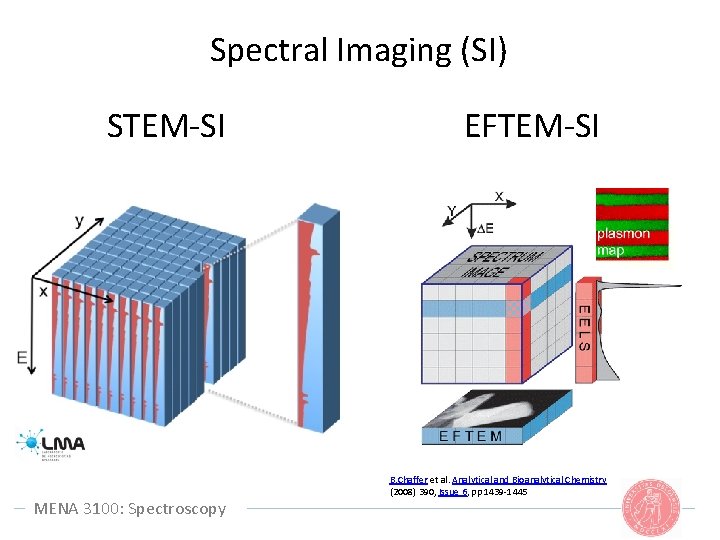
Spectral Imaging (SI) STEM-SI MENA 3100: Spectroscopy EFTEM-SI B. Chaffer et al. Analytical and Bioanalytical Chemistry (2008) 390, Issue 6, pp 1439 -1445
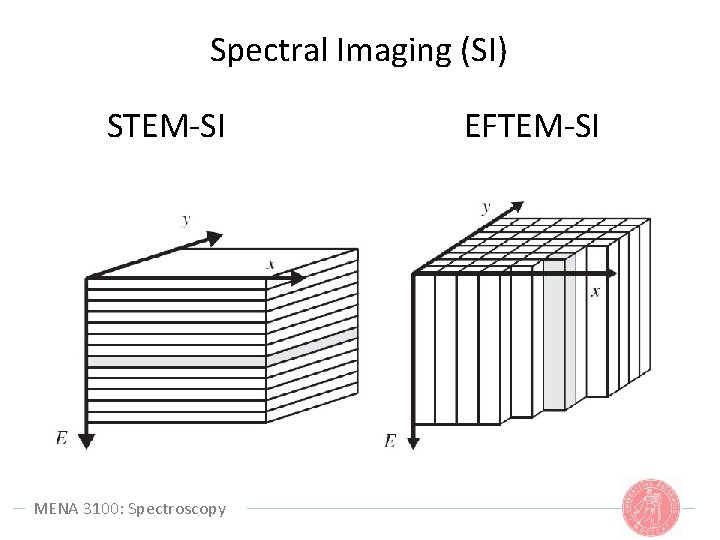
Spectral Imaging (SI) STEM-SI MENA 3100: Spectroscopy EFTEM-SI
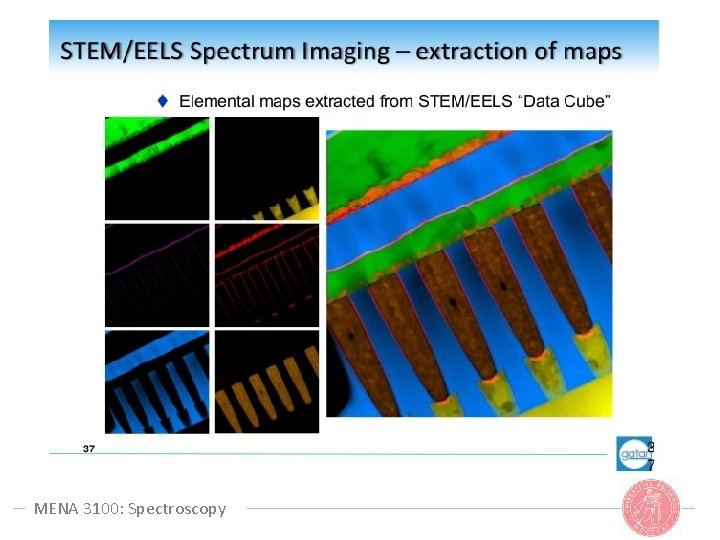
MENA 3100: Spectroscopy
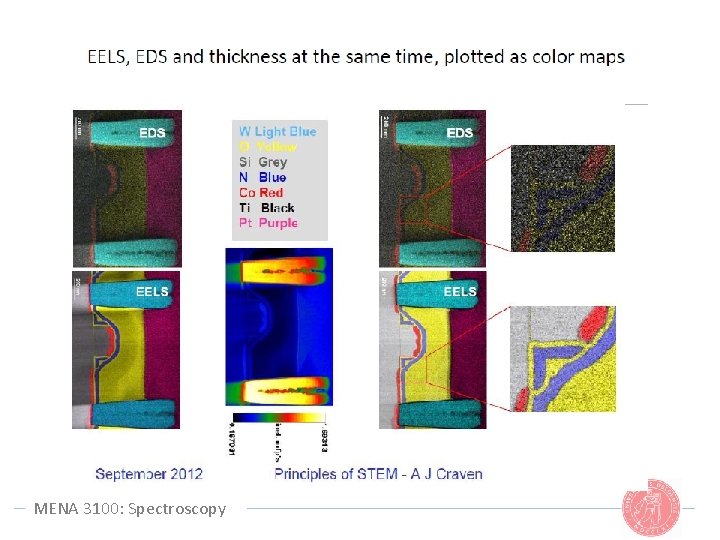
MENA 3100: Spectroscopy
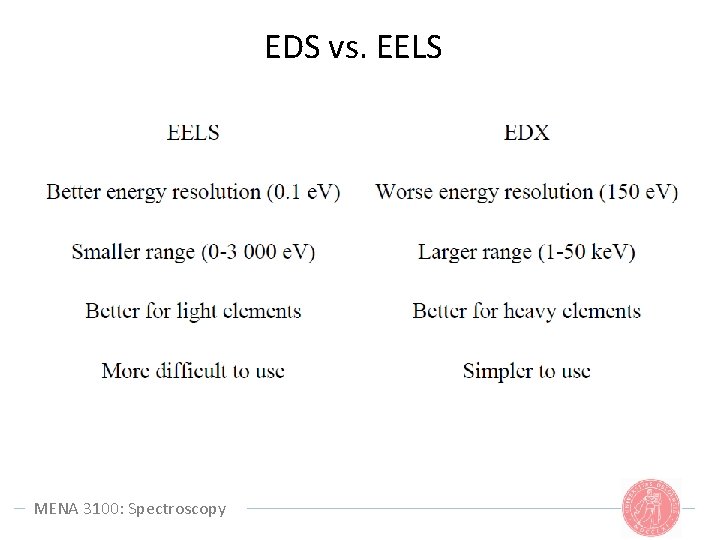
EDS vs. EELS MENA 3100: Spectroscopy
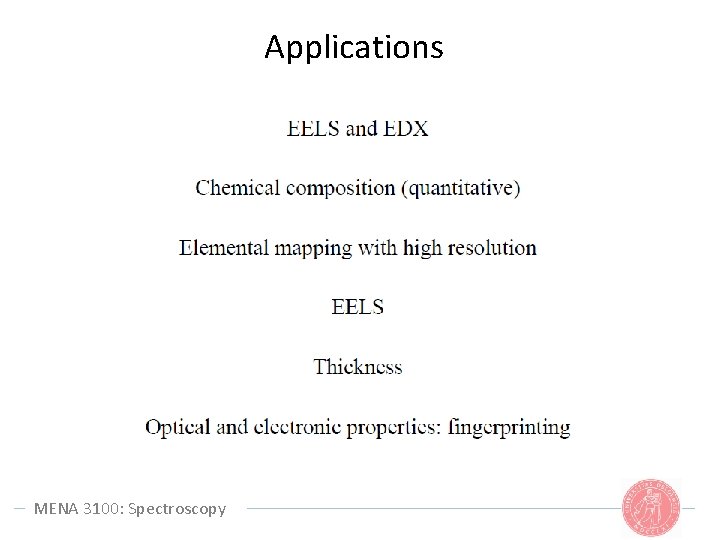
Applications MENA 3100: Spectroscopy
Electron microscopy data bank
Bacteriorhodopsin
Scanning electron microscopy
Quem tem ouvidos ouça quem tem olhos veja
Aquele que encontra um amigo encontra um tesouro versiculo
Tem gente que tem cheiro
Tem gente que tem cheiro de passarinho quando canta
Electron guns tem
Hiv transmissions
Mtved exemple
Les transmissions écrites et orales
Nicole antczak
Fluorescence microscopy
Spermatozoma
Dark ground microscopy
1674 microscopy
Microscopy tutor
Cast in stool microscopy
Differential interference microscopy
Types of microscopy
Light sources for fluorescence microscopy
Basic concepts of microscopy
Confocal fluorescence microscopy
Provider-performed microscopy test examples
Differential interference microscopy
Principle of phase contrast microscopy
Microscopy
Advantages of scanning probe microscopy
Scanning thermal microscopy
Photo emission microscopy failure analysis
What is forensic microscopy
Microscopy tutor
"dna microscopy"
Laser confocal microscopy
Phase contrast microscope advantages
Uses of light microscopes
Dic phase contrast
Oa module
Marc levoy
Microscopy
Dic microscopy
Types of microscopy
Multiphoton microscopy principle
Congestion vs hyperemia
Data recovery techniques
Biological evaluation of crude drugs
Urine microscopy pictures with names
Light sheet microscopy
Microscopy and measurement section 1-4 review
Stool dr test