UNIT 2 PRINCIPLES OF HEAT FLOW IN FLUIDS
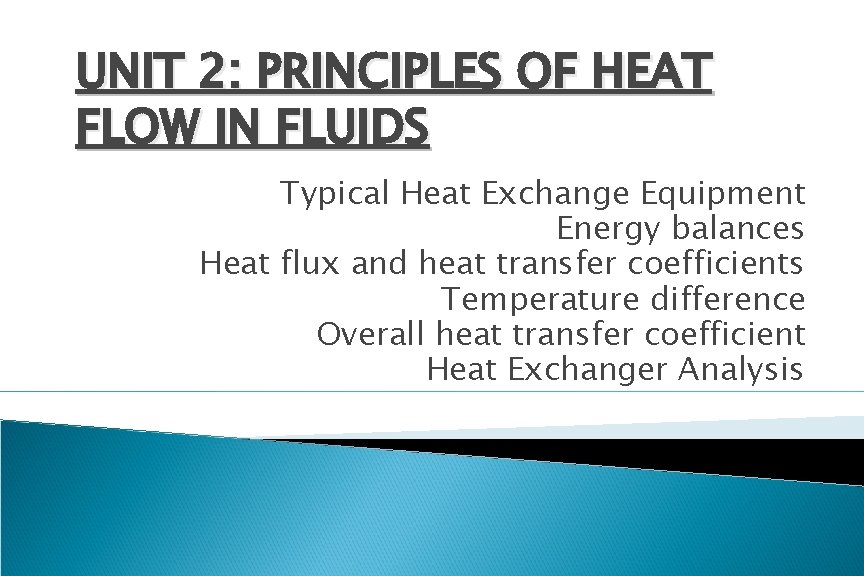
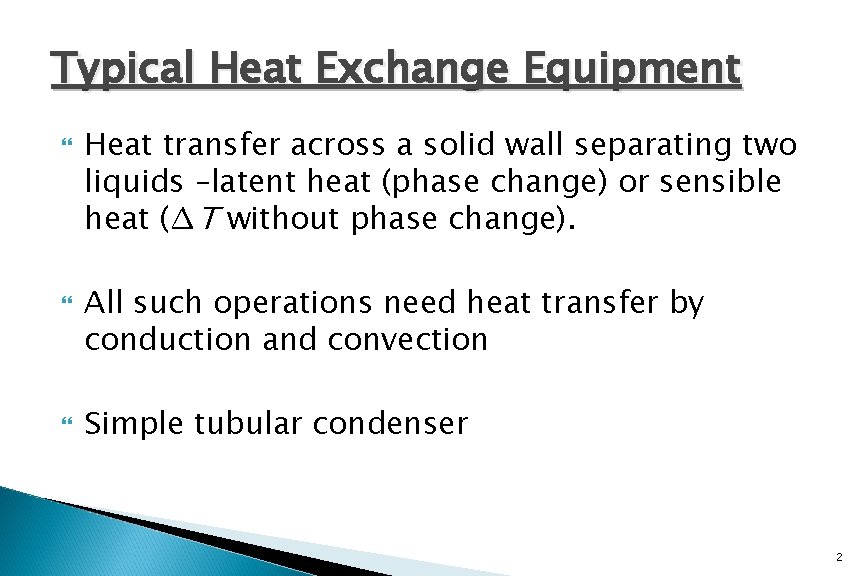
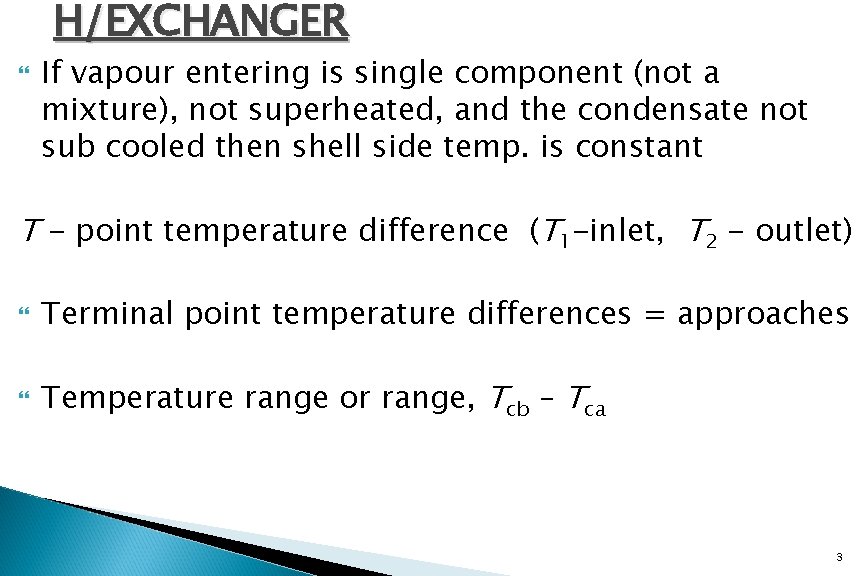
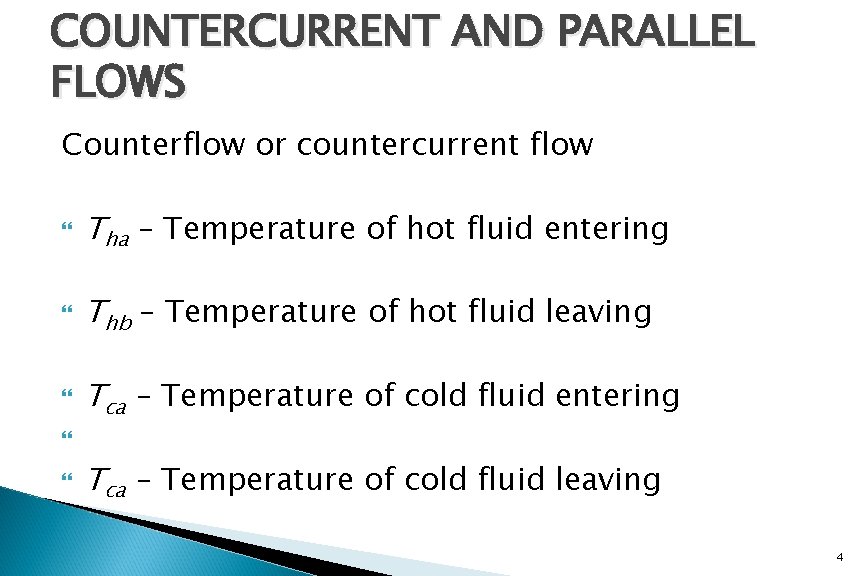
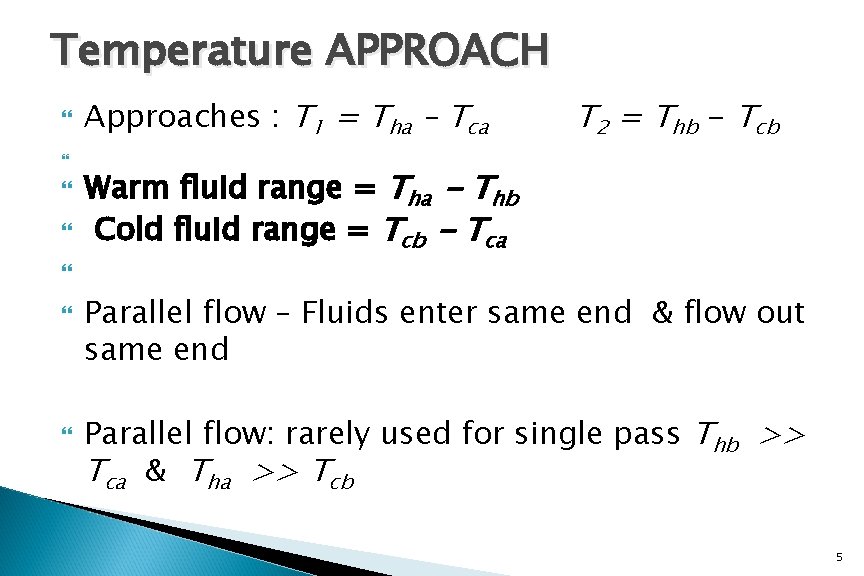
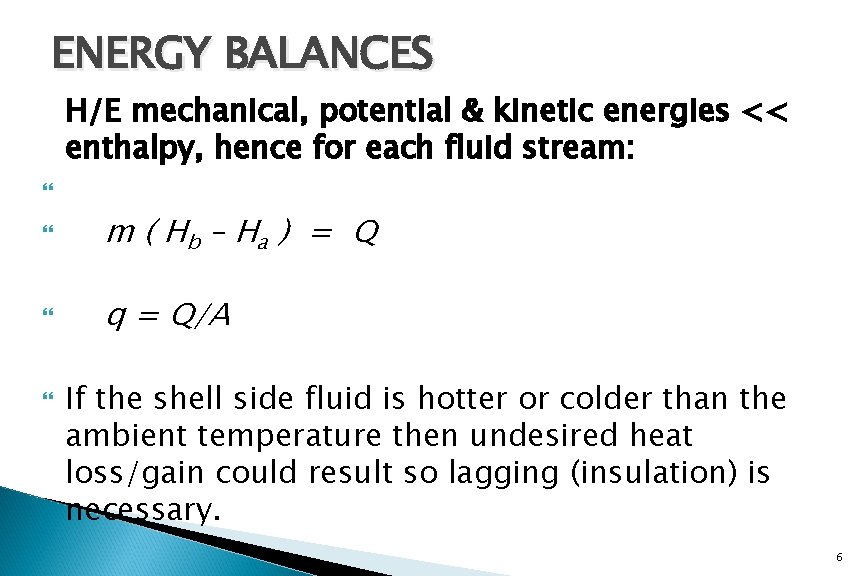
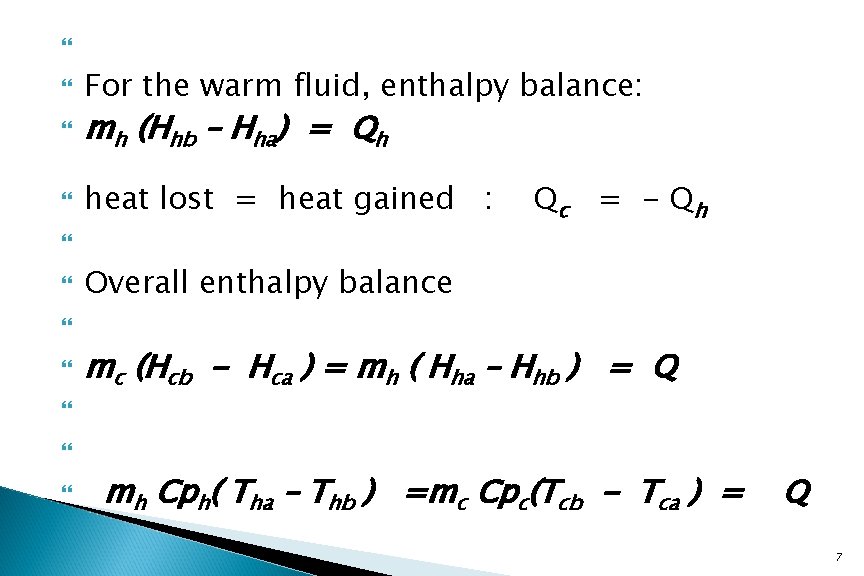
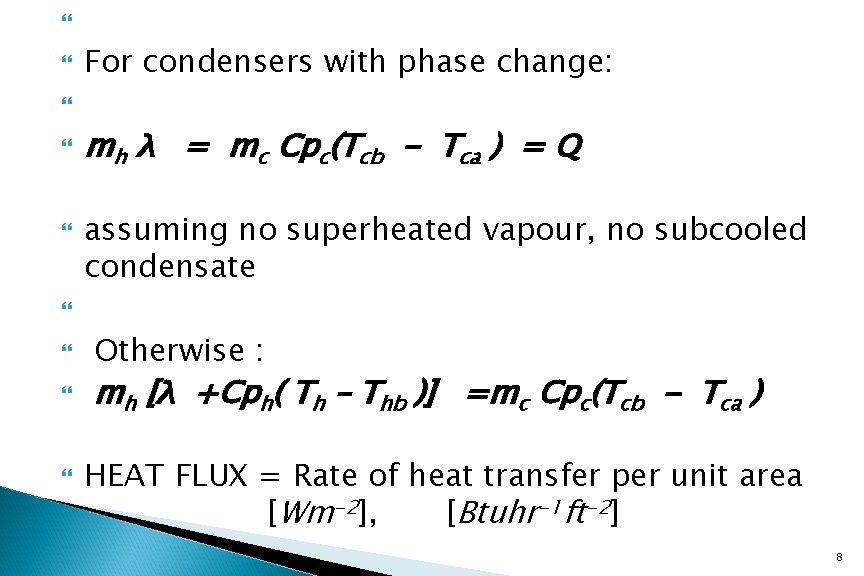
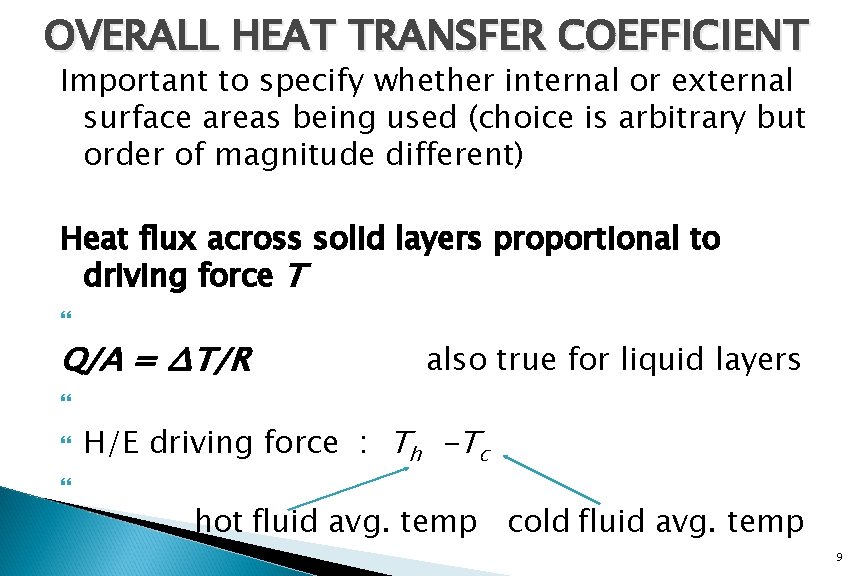
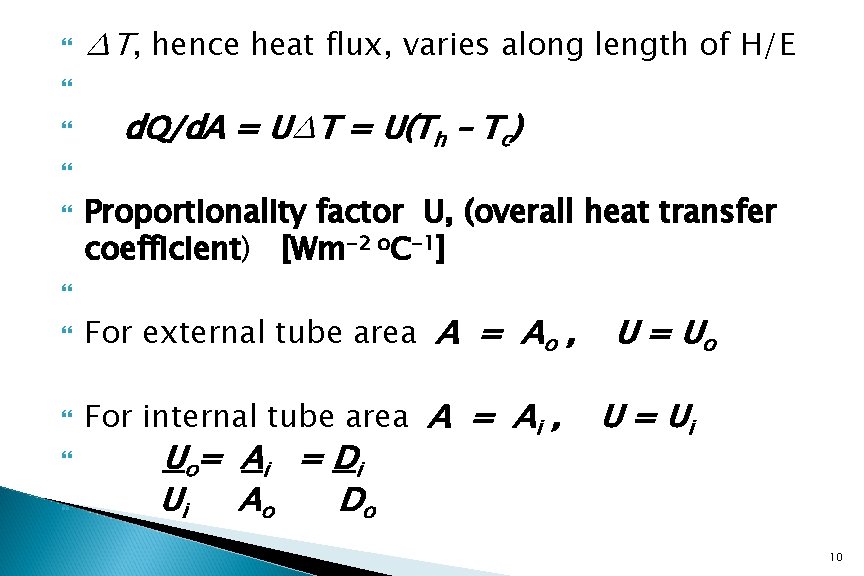
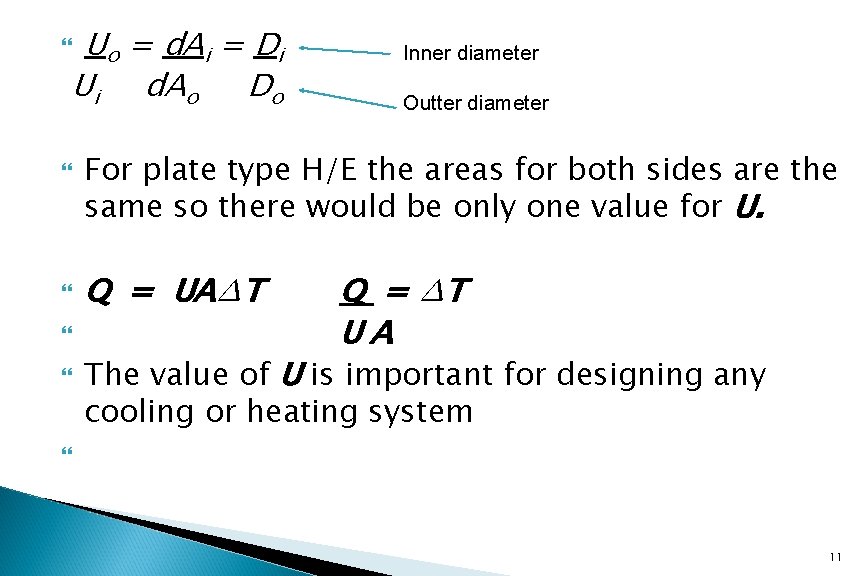
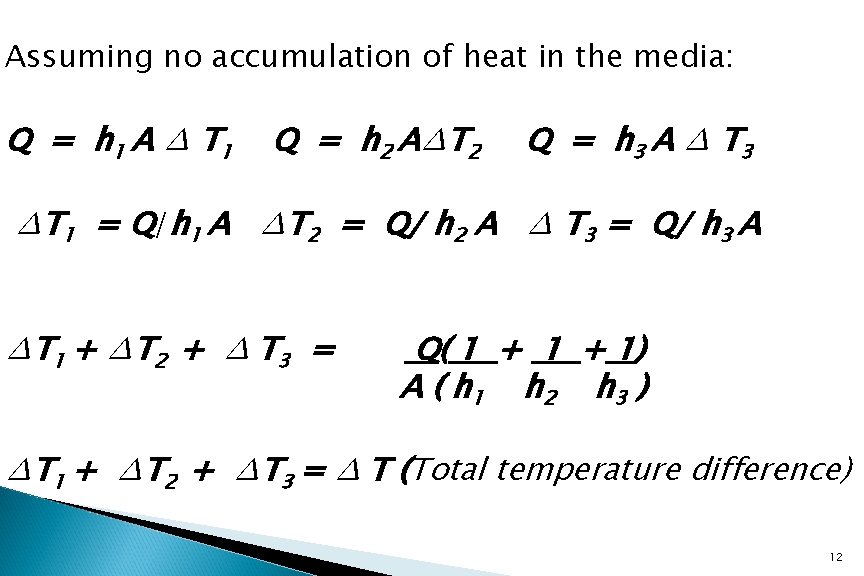
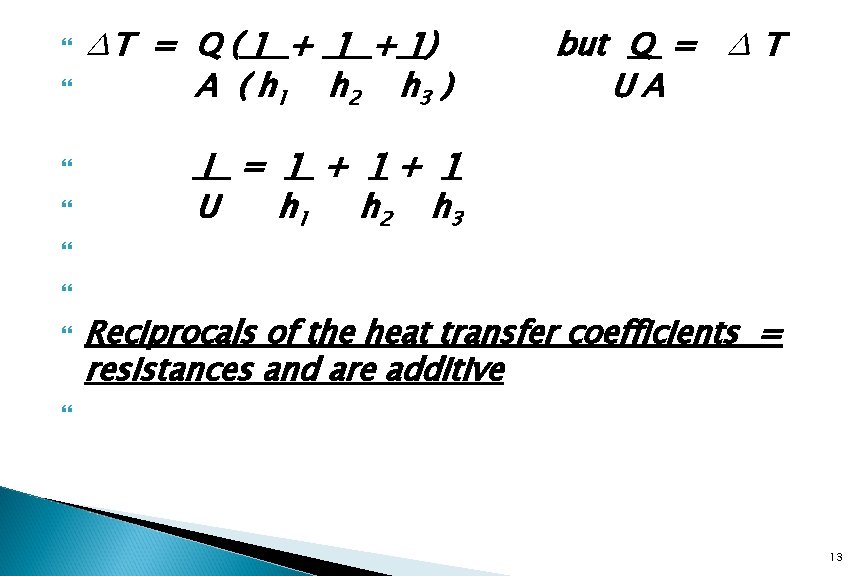
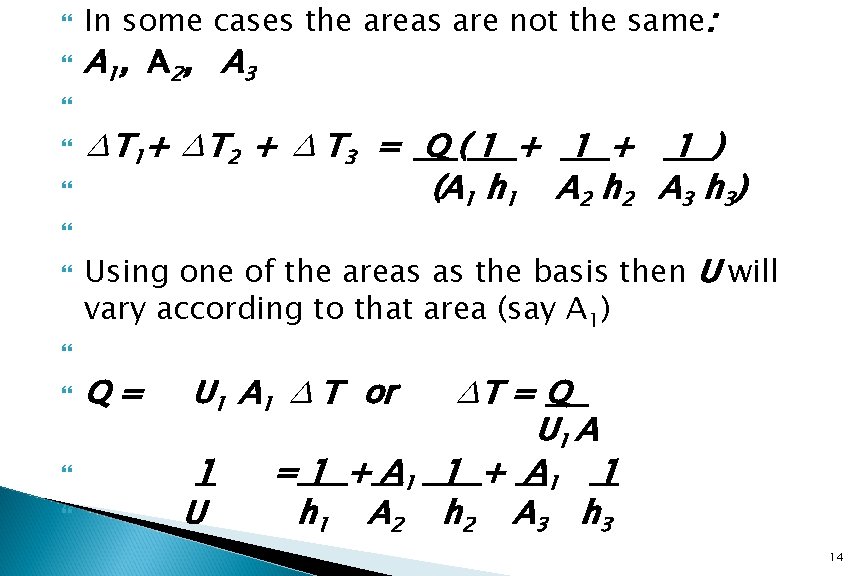
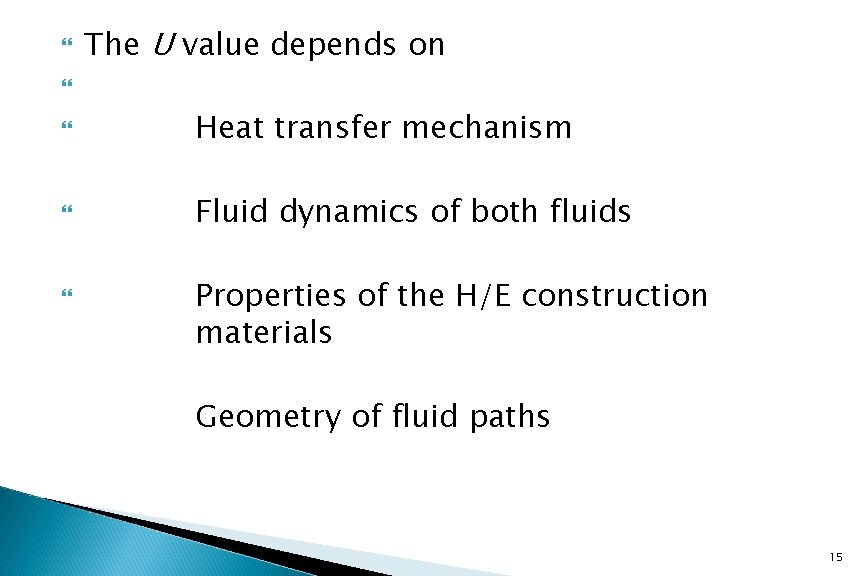
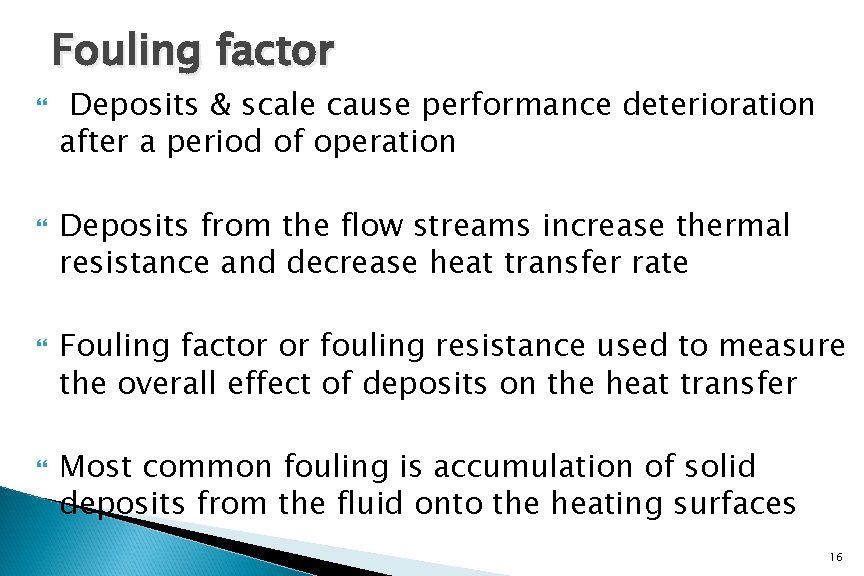
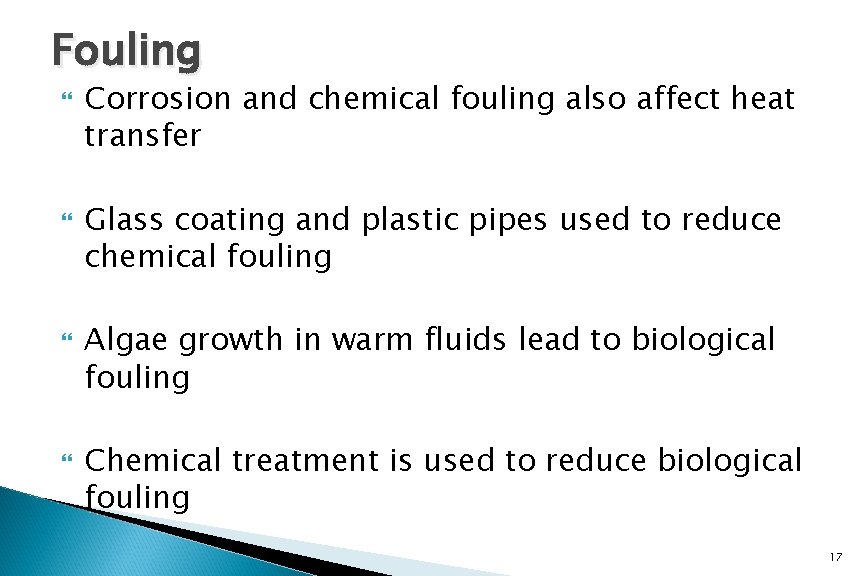
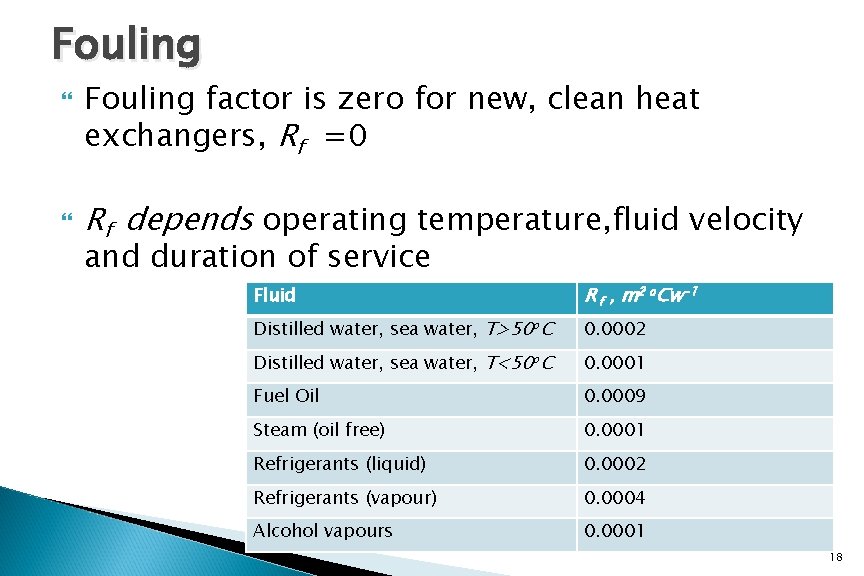
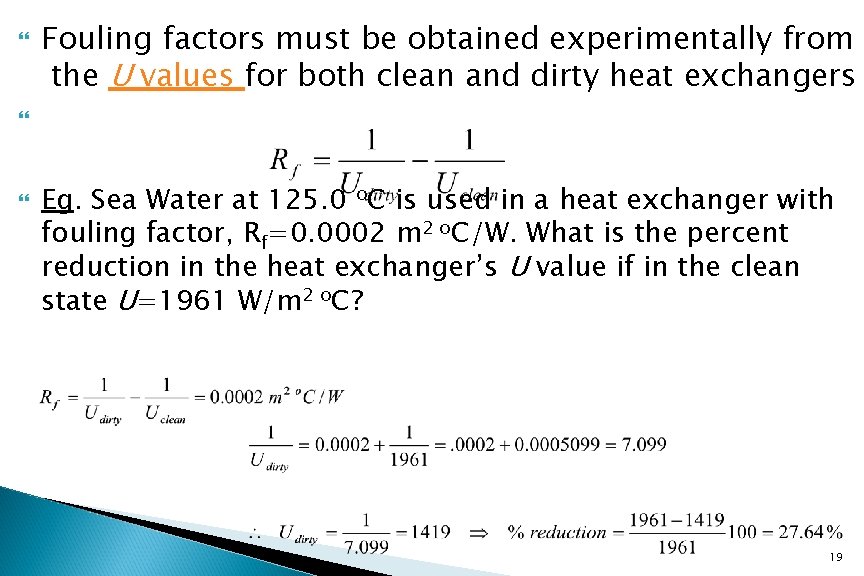
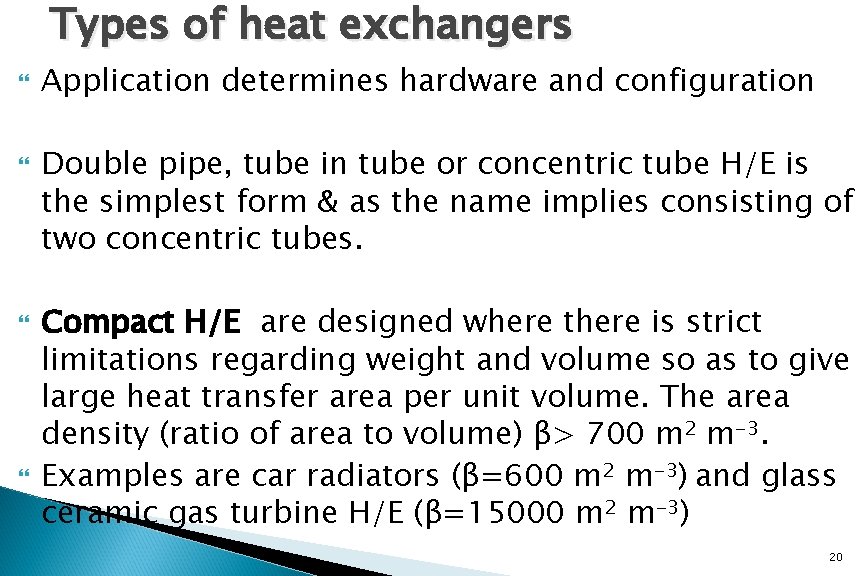
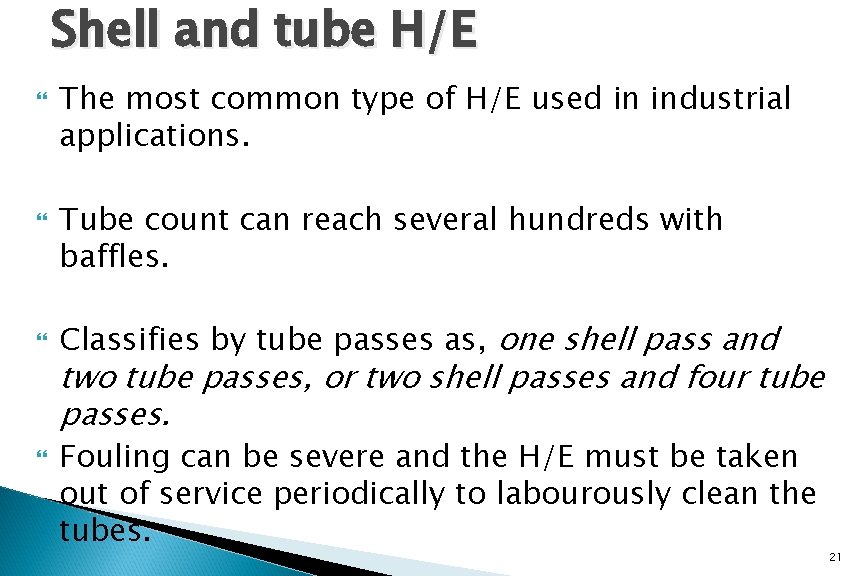
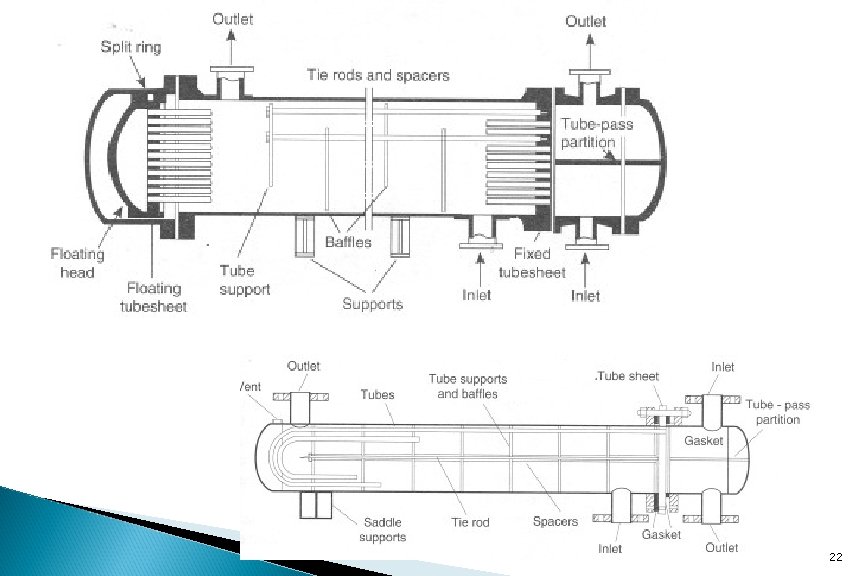
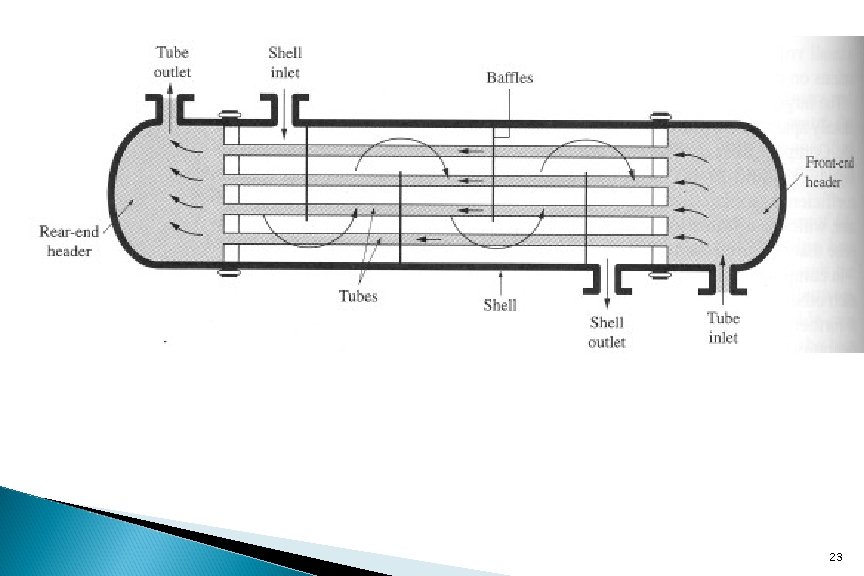
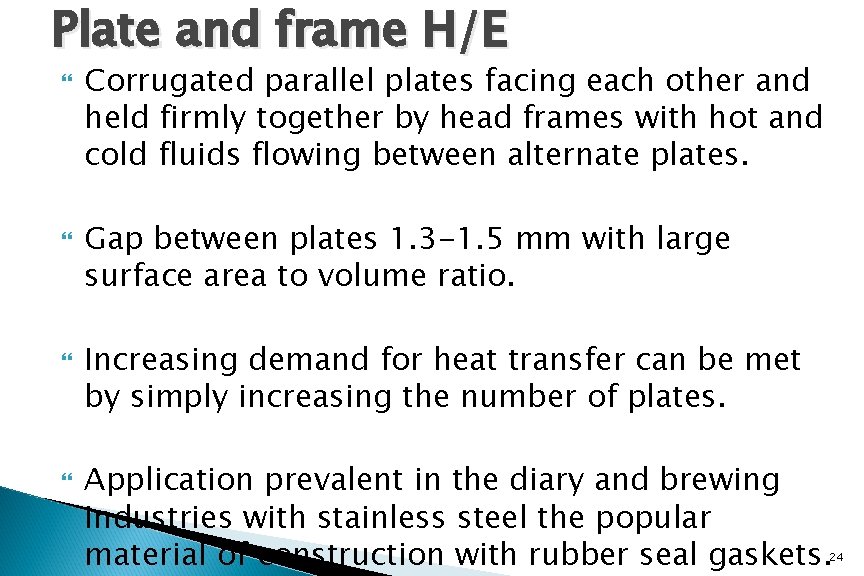
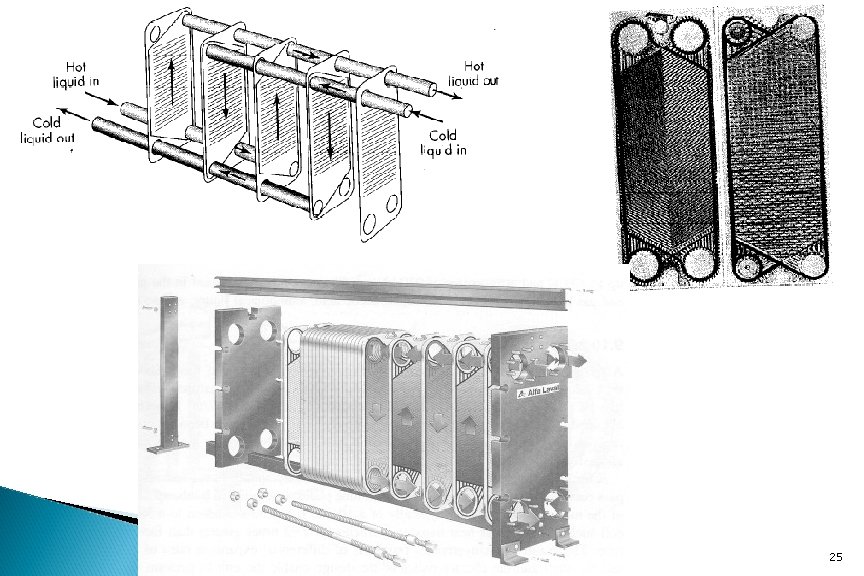
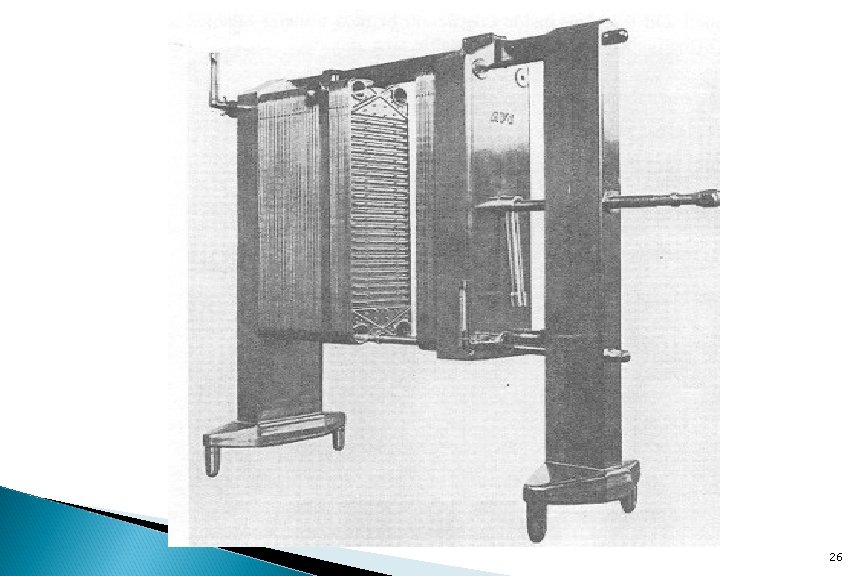
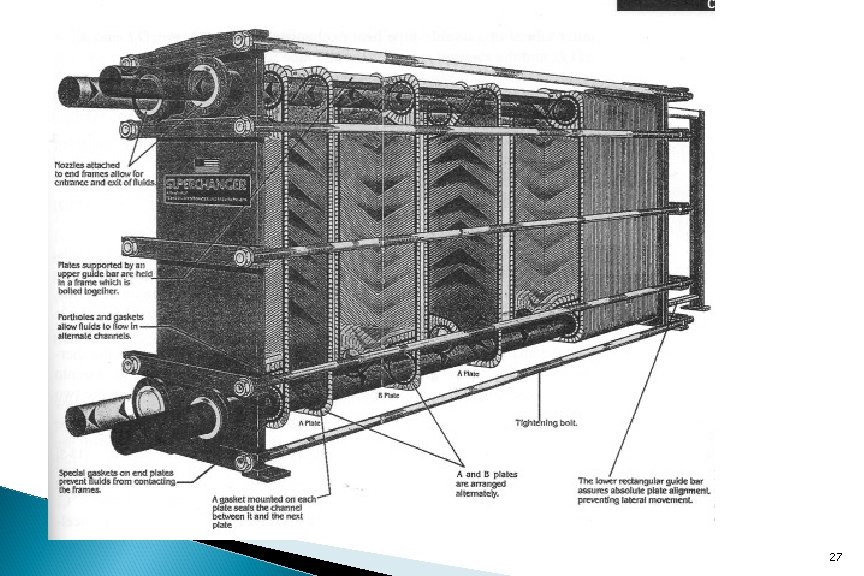
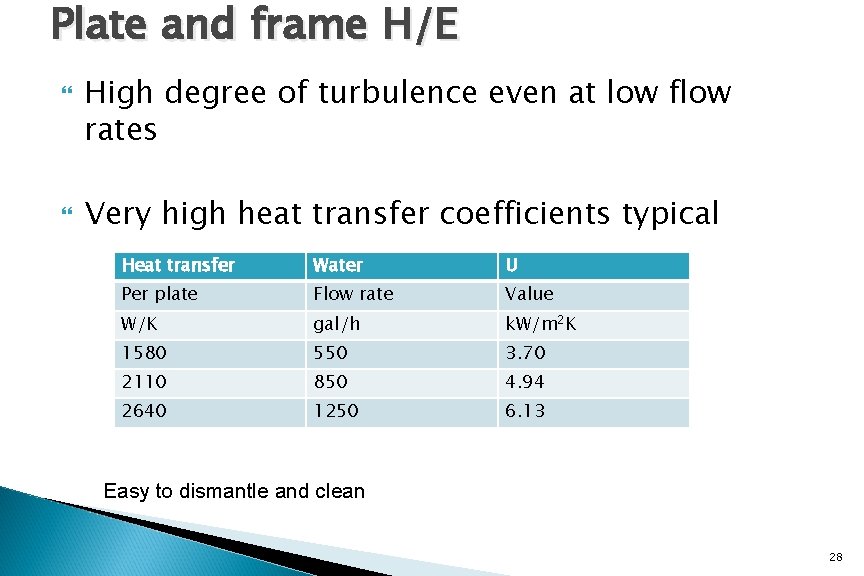
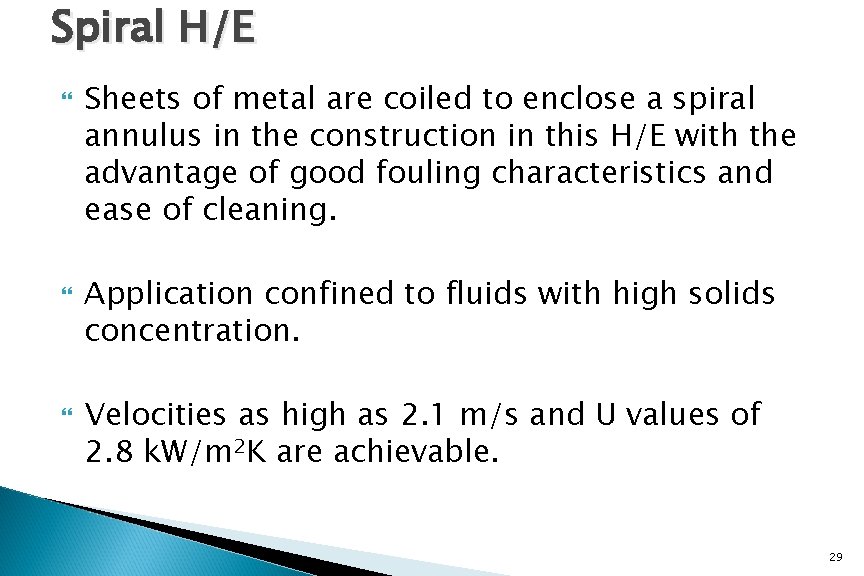
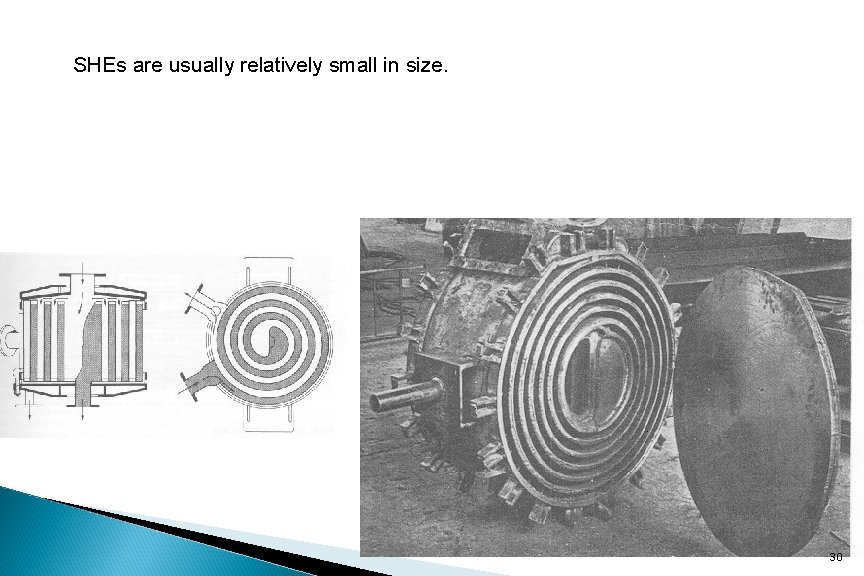
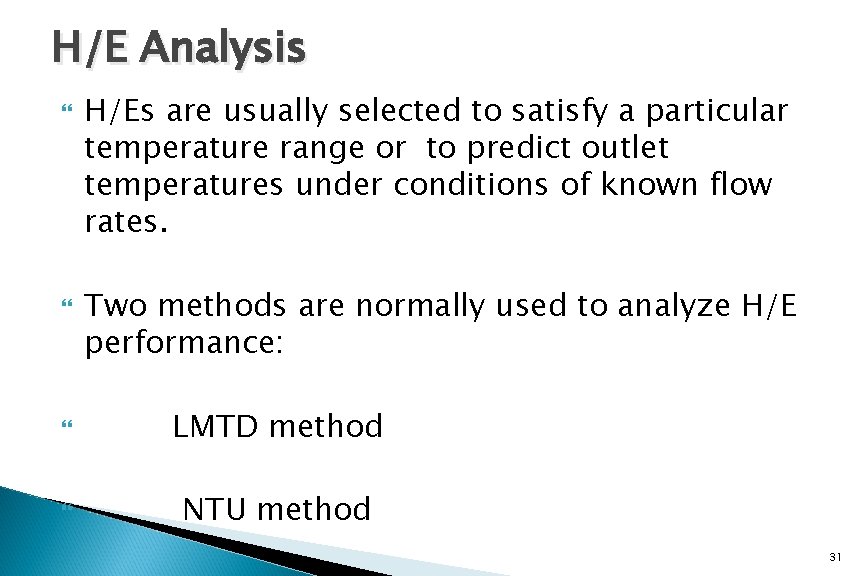
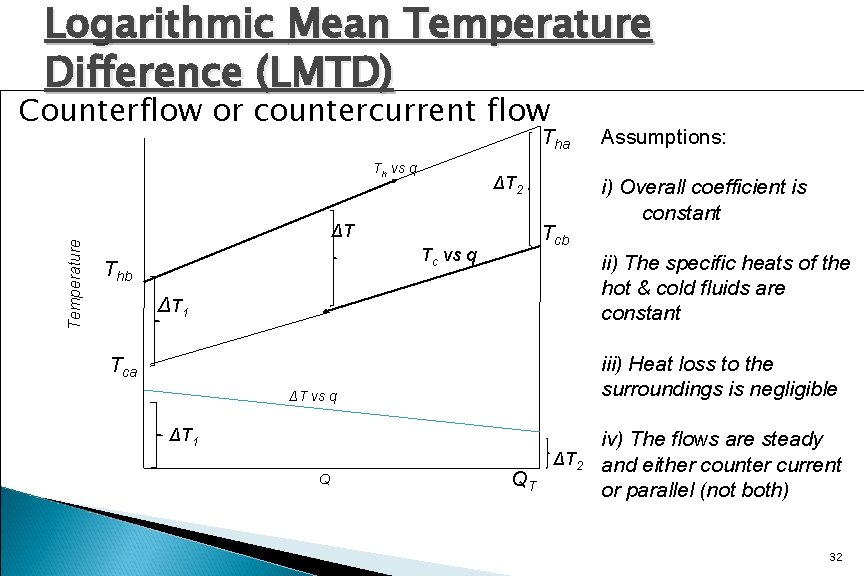
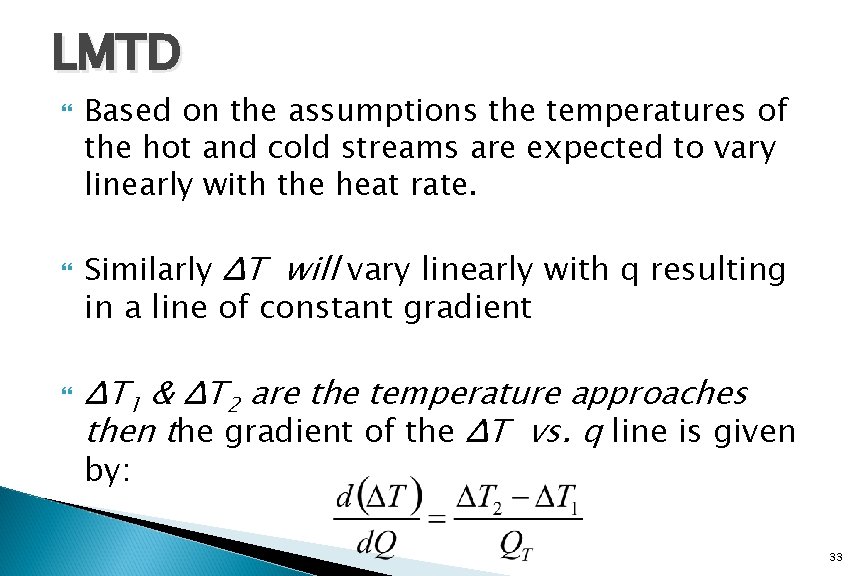
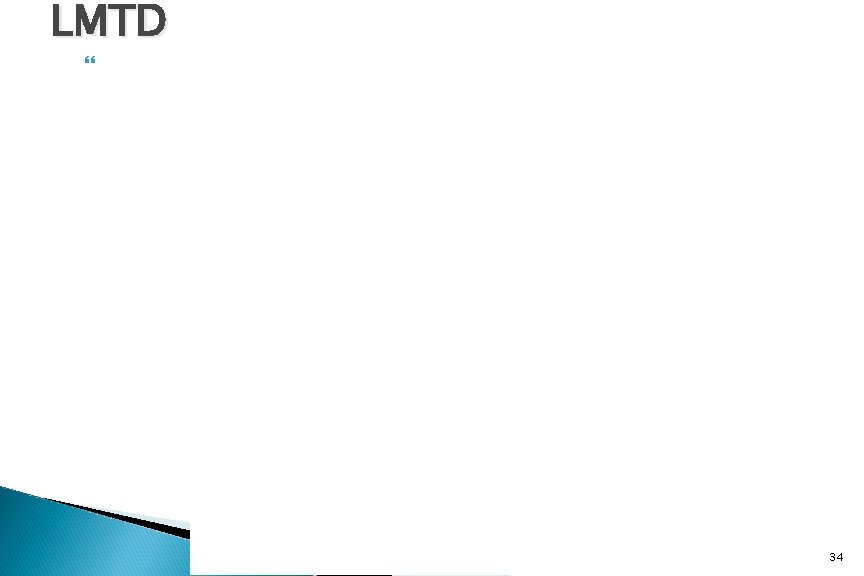
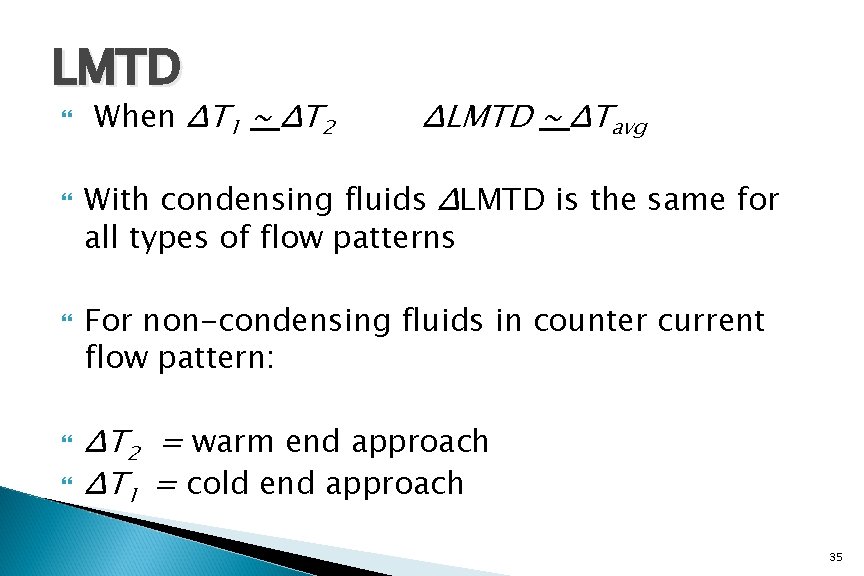
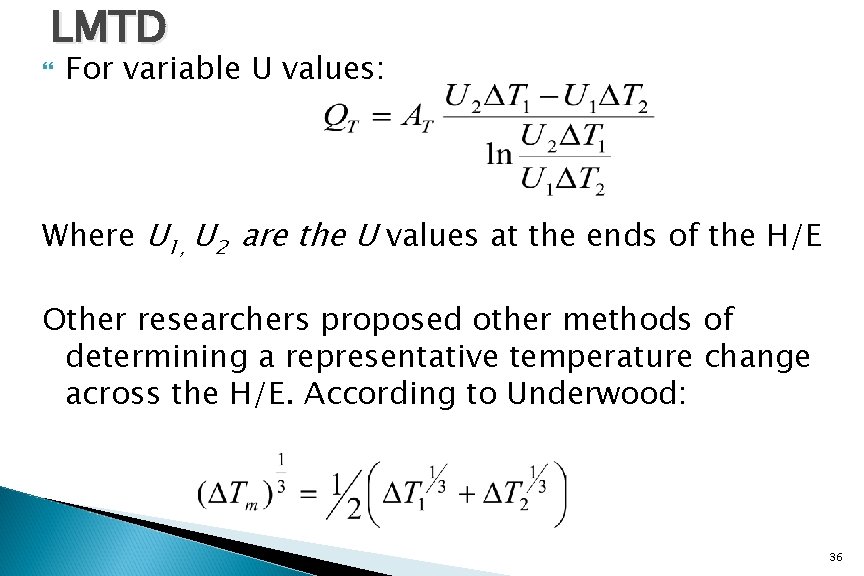
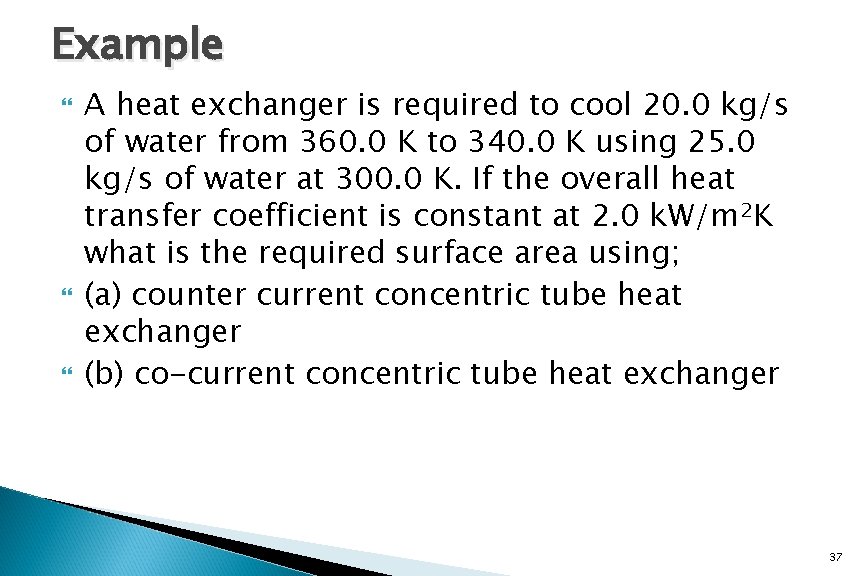
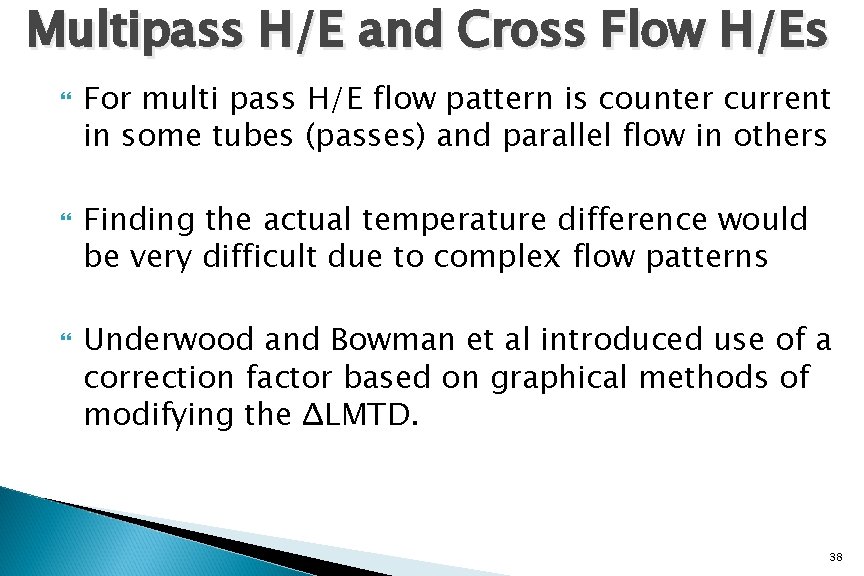
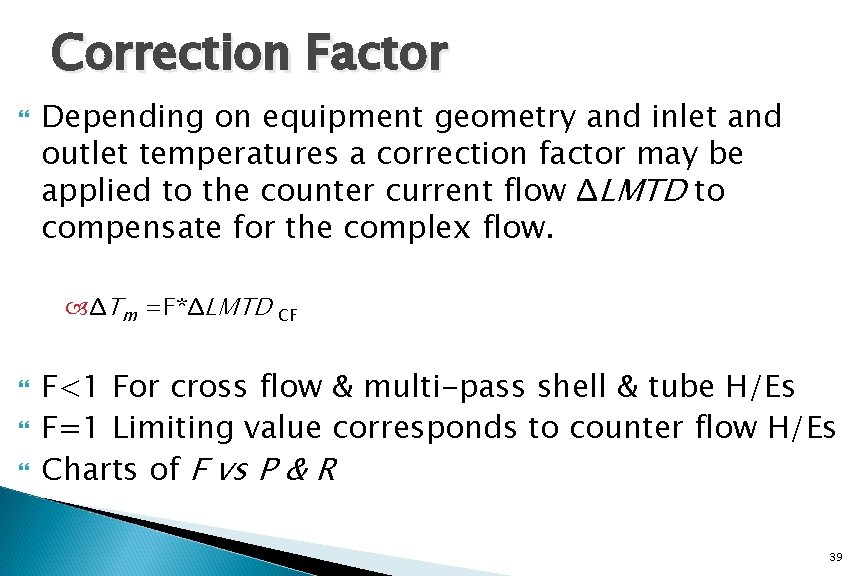
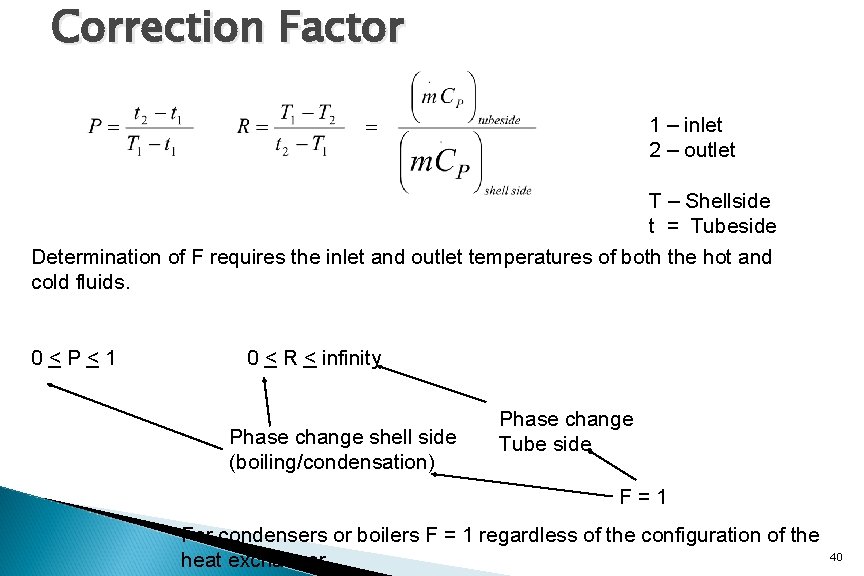
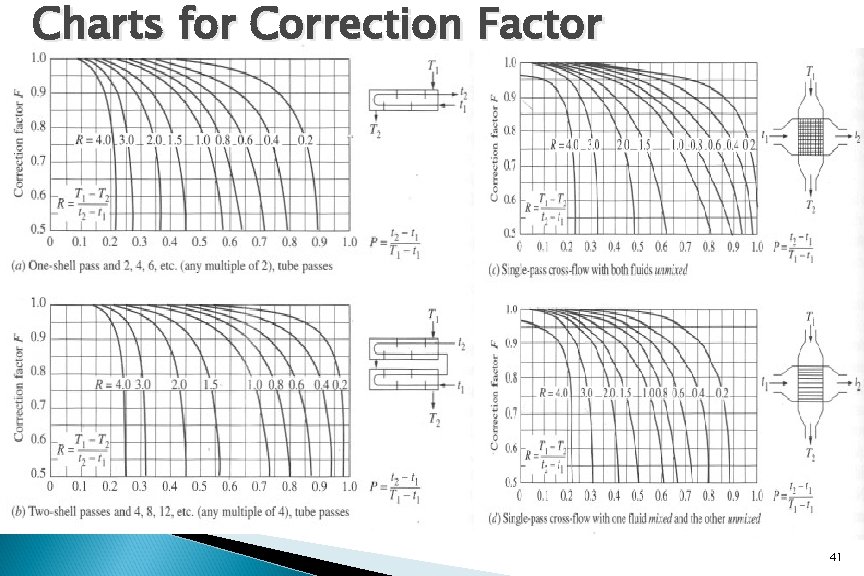
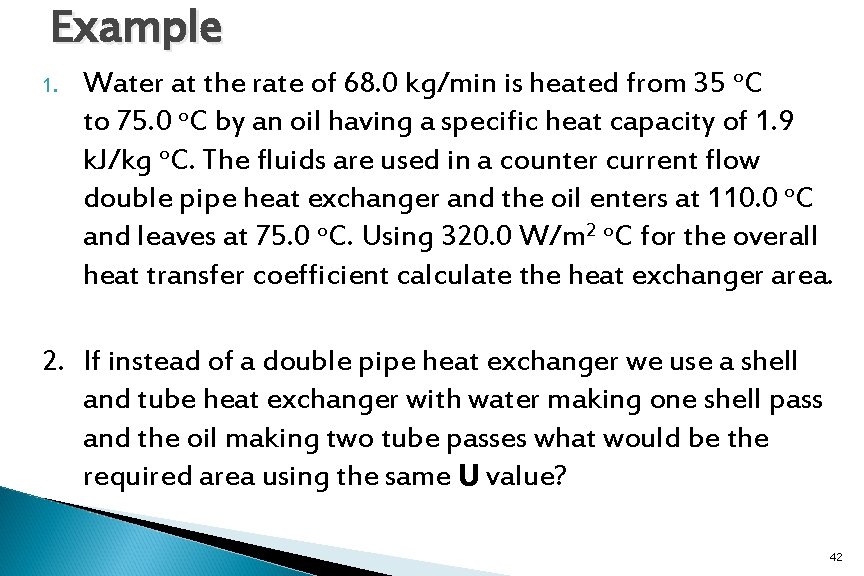
- Slides: 42
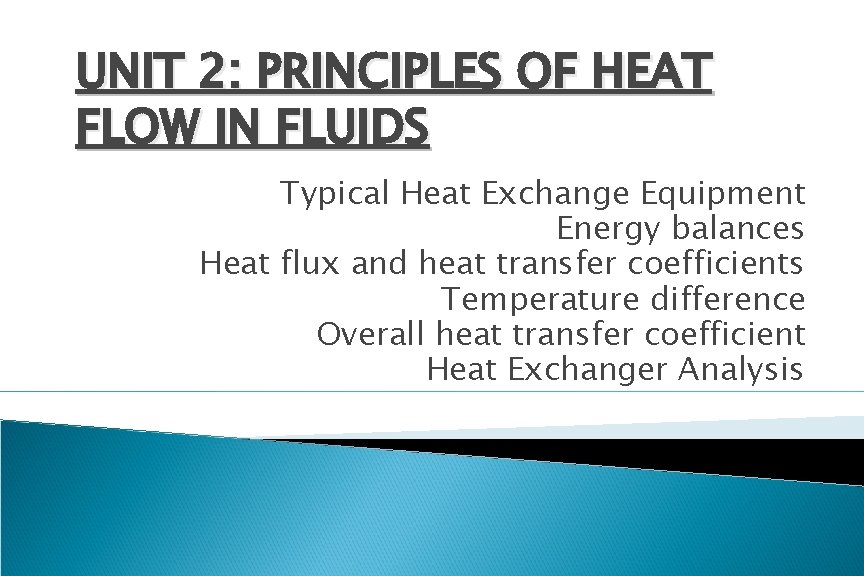
UNIT 2: PRINCIPLES OF HEAT FLOW IN FLUIDS Typical Heat Exchange Equipment Energy balances Heat flux and heat transfer coefficients Temperature difference Overall heat transfer coefficient Heat Exchanger Analysis
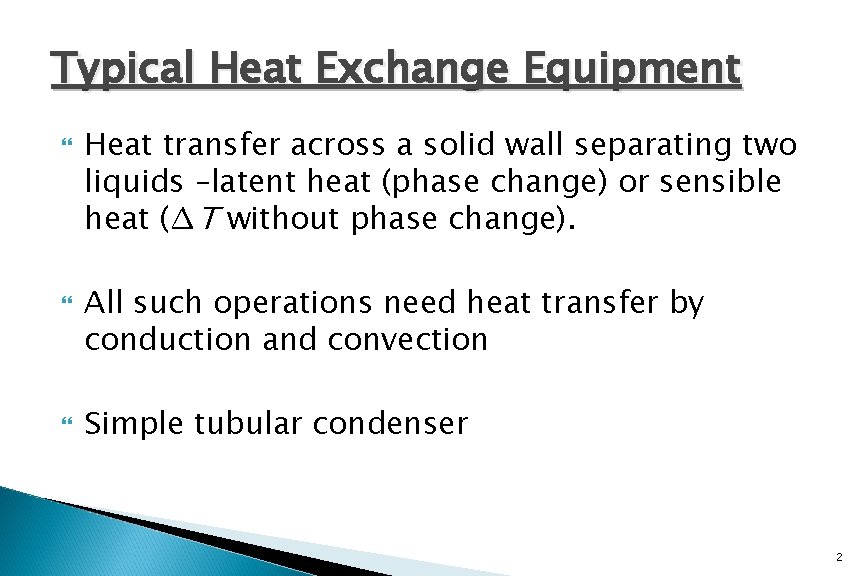
Typical Heat Exchange Equipment Heat transfer across a solid wall separating two liquids –latent heat (phase change) or sensible heat (∆T without phase change). All such operations need heat transfer by conduction and convection Simple tubular condenser 2
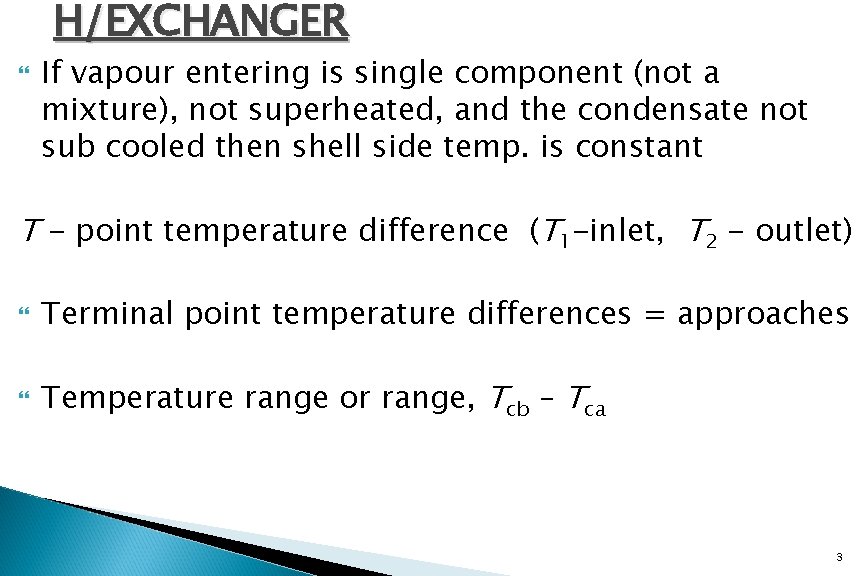
H/EXCHANGER If vapour entering is single component (not a mixture), not superheated, and the condensate not sub cooled then shell side temp. is constant T - point temperature difference (T 1 -inlet, T 2 - outlet) Terminal point temperature differences = approaches Temperature range or range, Tcb – Tca 3
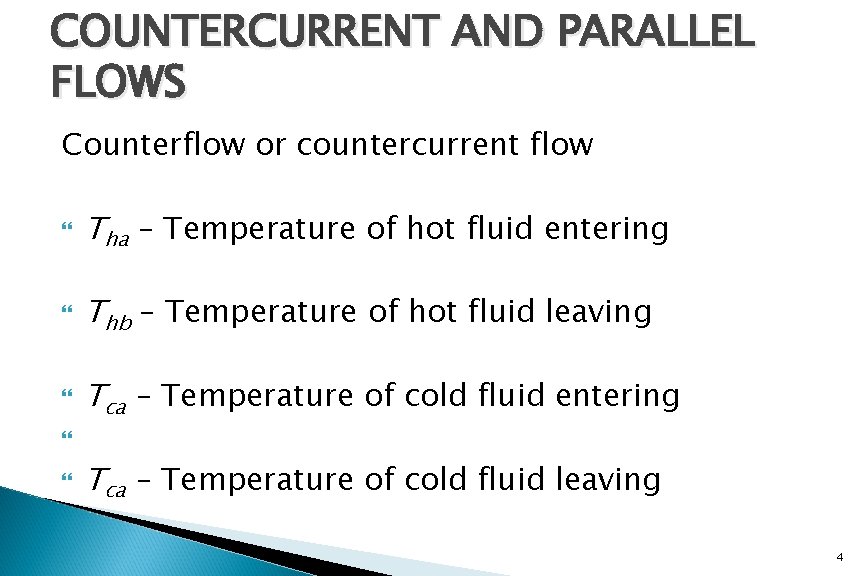
COUNTERCURRENT AND PARALLEL FLOWS Counterflow or countercurrent flow Tha – Temperature of hot fluid entering Thb – Temperature of hot fluid leaving Tca – Temperature of cold fluid entering Tca – Temperature of cold fluid leaving 4
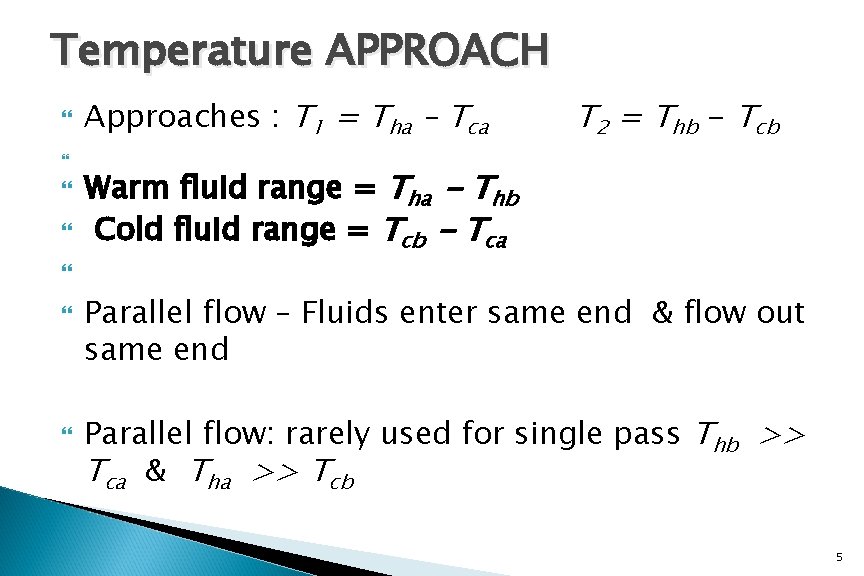
Temperature APPROACH Approaches : T 1 = Tha – Tca T 2 = Thb - Tcb Warm fluid range = Tha - Thb Cold fluid range = Tcb - Tca Parallel flow – Fluids enter same end & flow out same end Parallel flow: rarely used for single pass Thb >> Tca & Tha >> Tcb 5
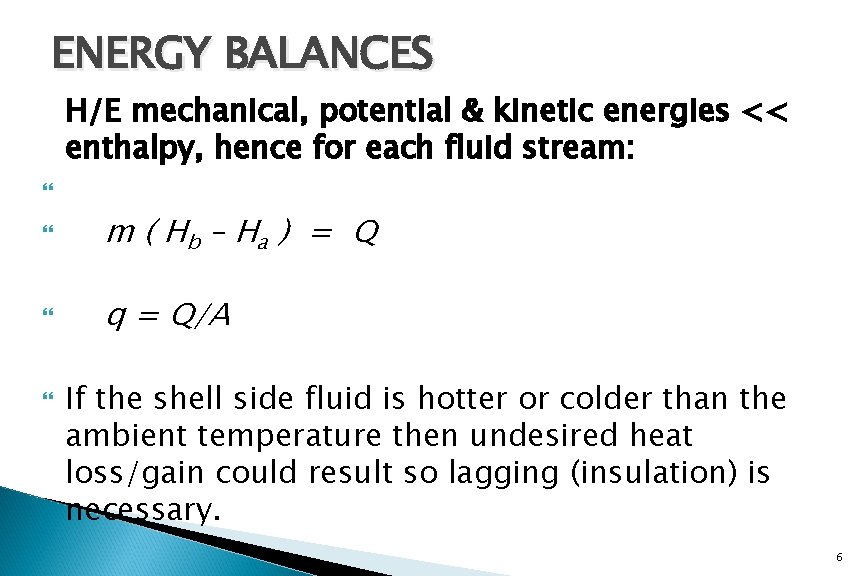
ENERGY BALANCES H/E mechanical, potential & kinetic energies << enthalpy, hence for each fluid stream: m ( H b – Ha ) = Q q = Q/A If the shell side fluid is hotter or colder than the ambient temperature then undesired heat loss/gain could result so lagging (insulation) is necessary. 6
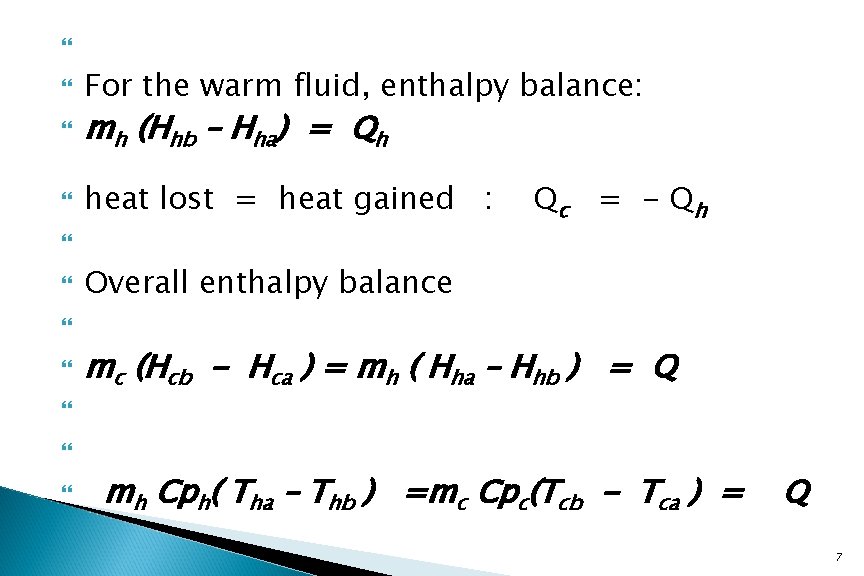
For the warm fluid, enthalpy balance: mh (Hhb – Hha) = Qh heat lost = heat gained : Overall enthalpy balance Q c = - Qh mc (Hcb - Hca ) = mh ( Hha – Hhb ) = Q mh Cph( Tha – Thb ) =mc Cpc(Tcb - Tca ) = Q 7
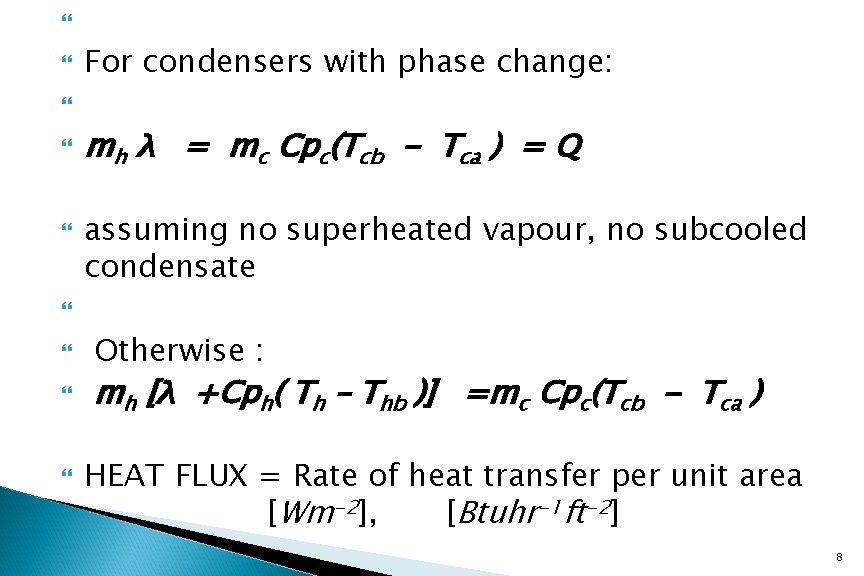
For condensers with phase change: mh λ = mc Cpc(Tcb - Tca ) = Q assuming no superheated vapour, no subcooled condensate Otherwise : mh [λ +Cph( Th – Thb )] =mc Cpc(Tcb - Tca ) HEAT FLUX = Rate of heat transfer per unit area [Wm-2], [Btuhr-1 ft-2] 8
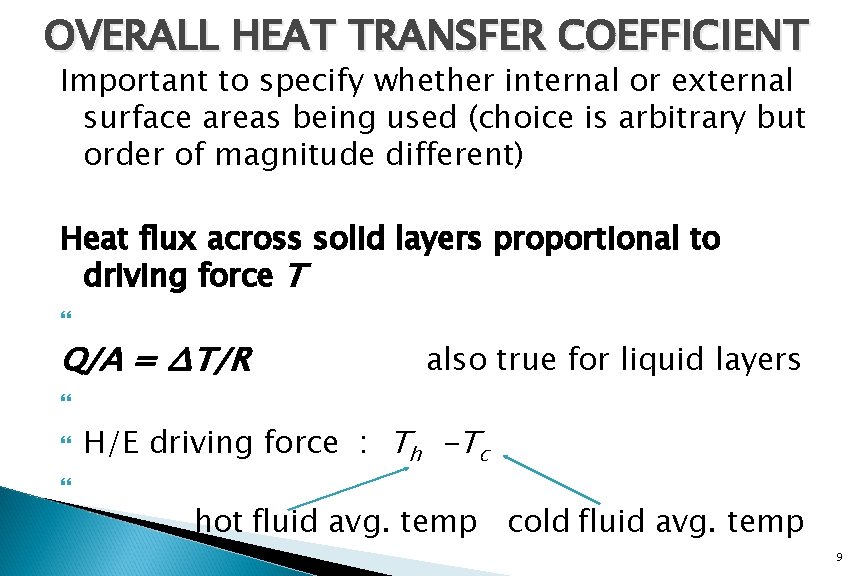
OVERALL HEAT TRANSFER COEFFICIENT Important to specify whether internal or external surface areas being used (choice is arbitrary but order of magnitude different) Heat flux across solid layers proportional to driving force T Q/A = ∆T/R also true for liquid layers H/E driving force : Th -Tc hot fluid avg. temp cold fluid avg. temp 9
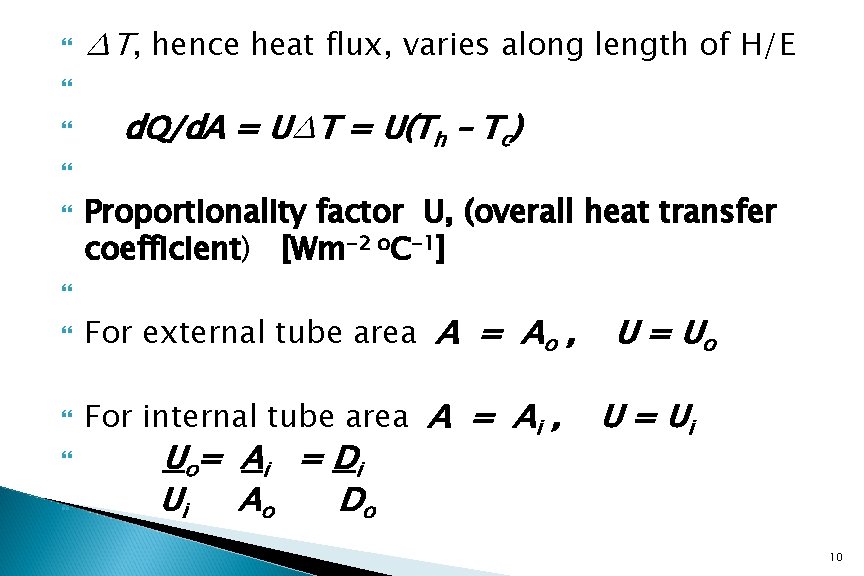
∆T, hence heat flux, varies along length of H/E d. Q/d. A = U∆T = U(Th – Tc) Proportionality factor U, (overall heat transfer coefficient) [Wm-2 o. C-1] For external tube area A = Ao , U = Uo For internal tube area A = Ai , U o = A i = Di Ui Ao Do U = Ui 10
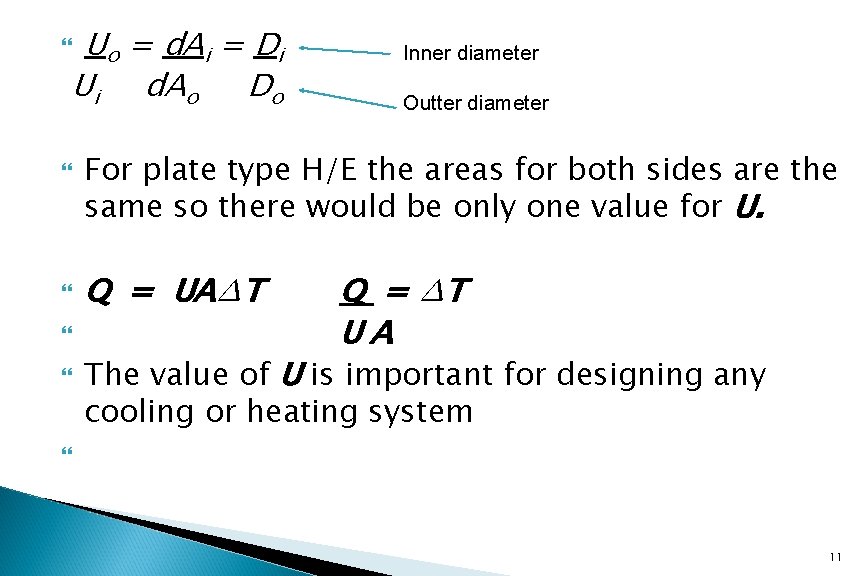
Uo = d. Ai = Di Ui d. Ao Do Inner diameter Outter diameter For plate type H/E the areas for both sides are the same so there would be only one value for U. Q = UA∆T Q = ∆T UA The value of U is important for designing any cooling or heating system 11
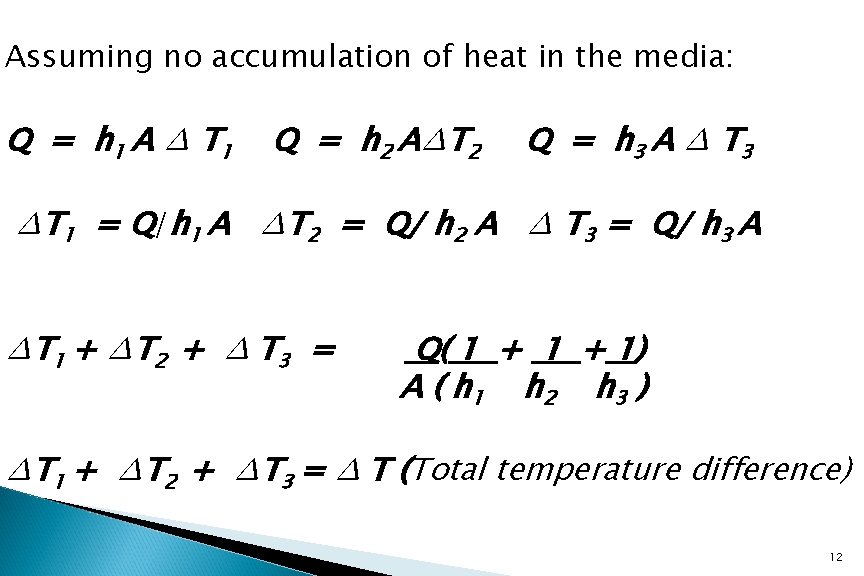
Assuming no accumulation of heat in the media: Q = h 1 A ∆ T 1 Q = h 2 A∆T 2 Q = h 3 A ∆ T 3 ∆T 1 = Q/h 1 A ∆T 2 = Q/ h 2 A ∆ T 3 = Q/ h 3 A ∆T 1 + ∆T 2 + ∆ T 3 = Q( 1 + 1) A ( h 1 h 2 h 3 ) ∆T 1 + ∆T 2 + ∆T 3 = ∆ T (Total temperature difference) 12
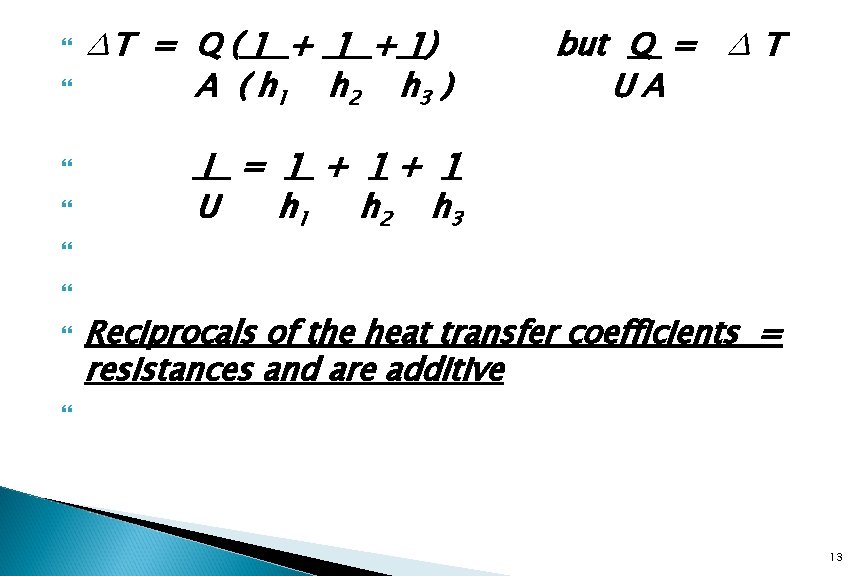
∆T = Q ( 1 + 1) A ( h 1 h 2 h 3 ) but Q = ∆ T UA I = 1 + 1+ 1 U h 1 h 2 h 3 Reciprocals of the heat transfer coefficients = resistances and are additive 13
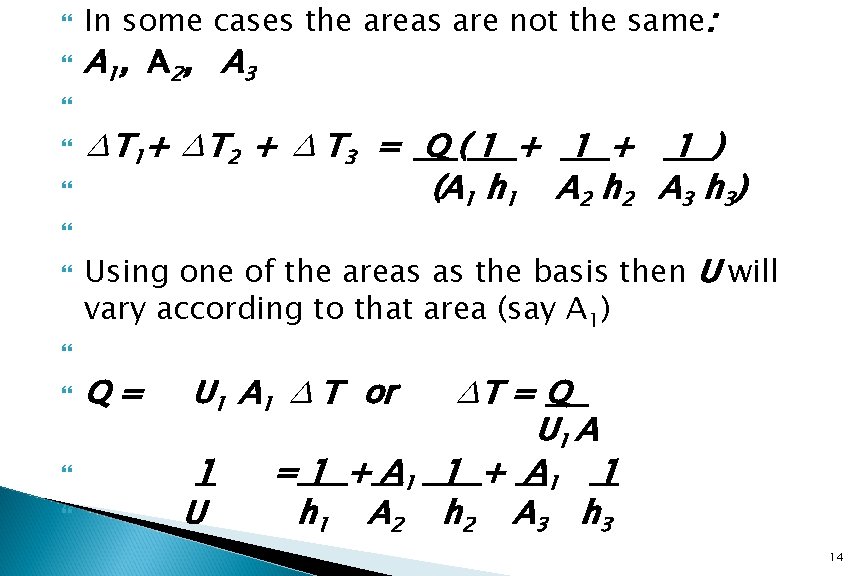
In some cases the areas are not the same: A 1, A 2, A 3 ∆T 1+ ∆T 2 + ∆ T 3 = Q ( 1 + 1 ) (A 1 h 1 A 2 h 2 A 3 h 3) Using one of the areas as the basis then U will vary according to that area (say A 1) Q= U 1 A 1 ∆ T or 1 U = 1 + A 1 h 1 A 2 ∆T = Q U 1 A 1 + A 1 1 h 2 A 3 h 3 14
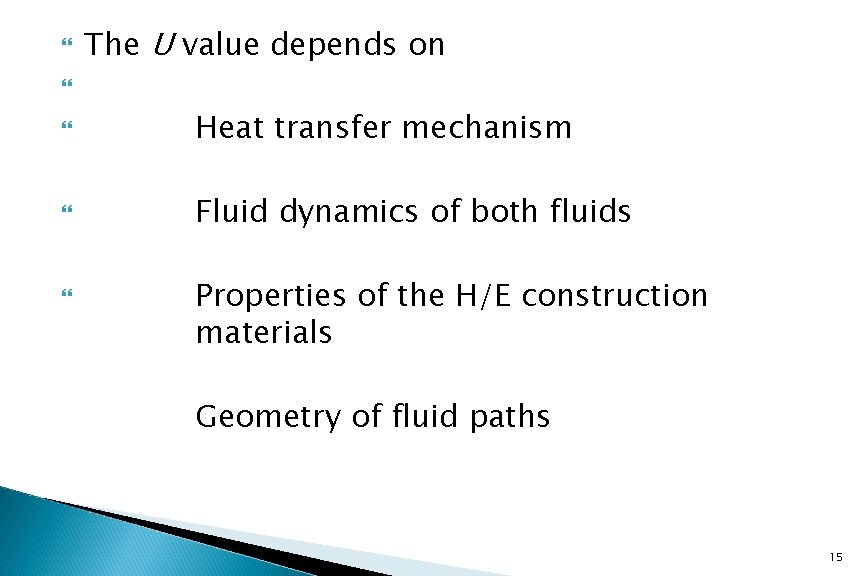
The U value depends on Heat transfer mechanism Fluid dynamics of both fluids Properties of the H/E construction materials Geometry of fluid paths 15
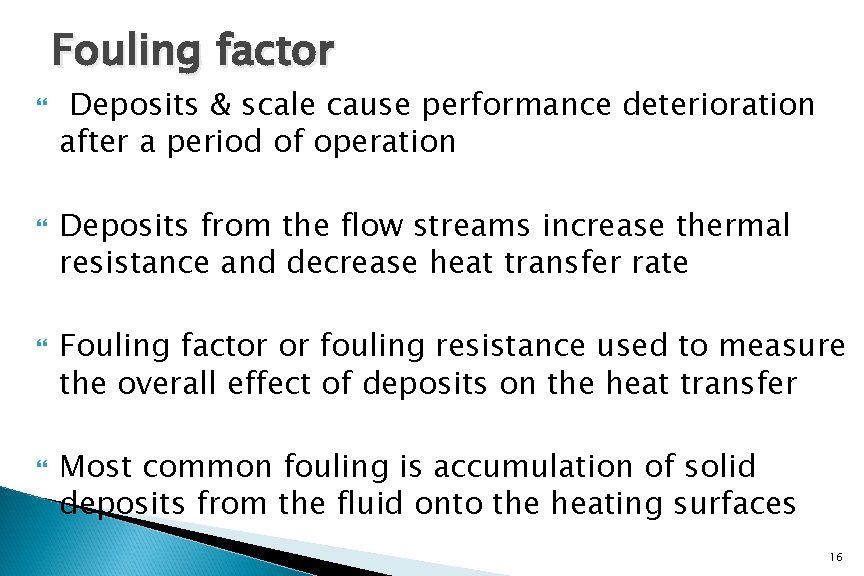
Fouling factor Deposits & scale cause performance deterioration after a period of operation Deposits from the flow streams increase thermal resistance and decrease heat transfer rate Fouling factor or fouling resistance used to measure the overall effect of deposits on the heat transfer Most common fouling is accumulation of solid deposits from the fluid onto the heating surfaces 16
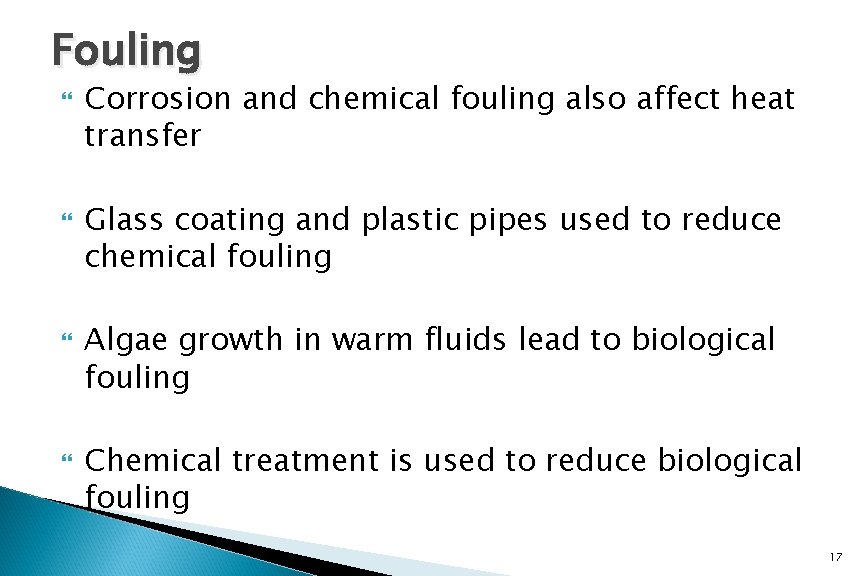
Fouling Corrosion and chemical fouling also affect heat transfer Glass coating and plastic pipes used to reduce chemical fouling Algae growth in warm fluids lead to biological fouling Chemical treatment is used to reduce biological fouling 17
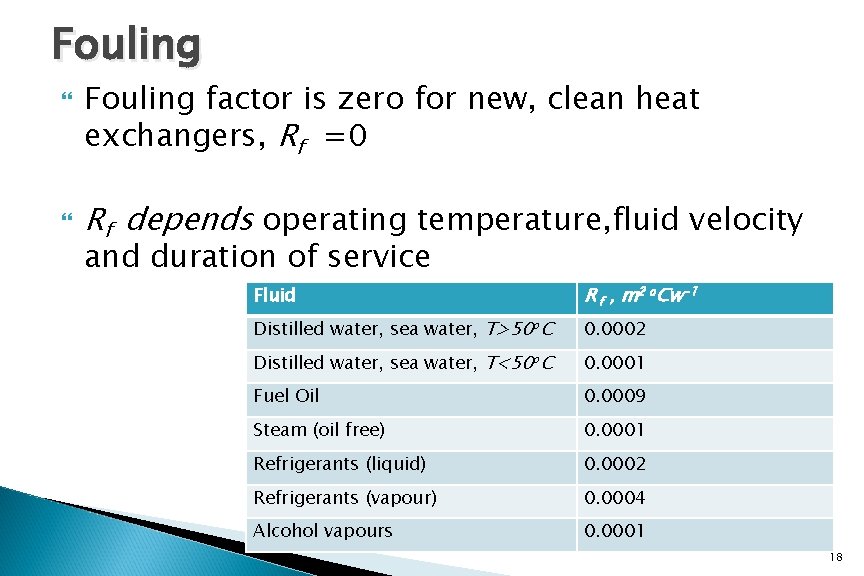
Fouling factor is zero for new, clean heat exchangers, Rf =0 Rf depends operating temperature, fluid velocity and duration of service Fluid R f , m 2 o. Cw-1 Distilled water, sea water, T>50 o. C 0. 0002 Distilled water, sea water, T<50 o. C 0. 0001 Fuel Oil 0. 0009 Steam (oil free) 0. 0001 Refrigerants (liquid) 0. 0002 Refrigerants (vapour) 0. 0004 Alcohol vapours 0. 0001 18
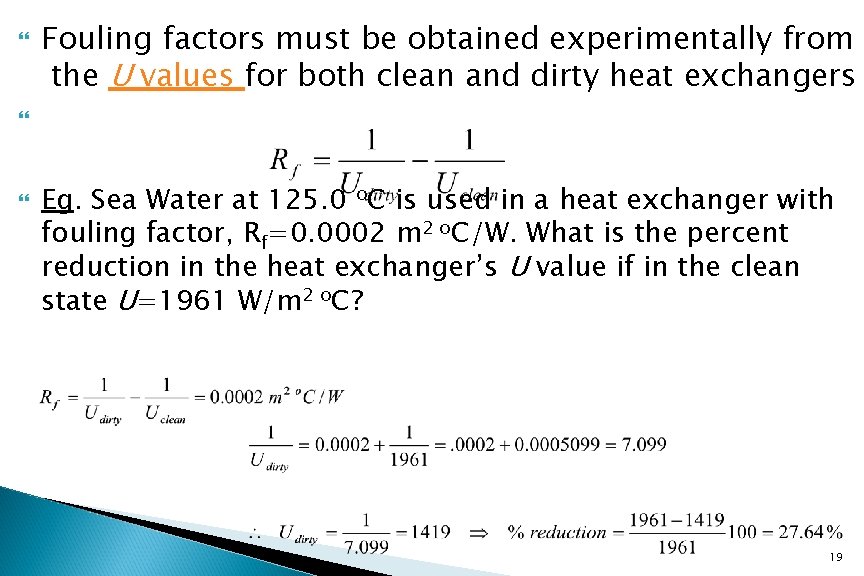
Fouling factors must be obtained experimentally from the U values for both clean and dirty heat exchangers Eg. Sea Water at 125. 0 o. C is used in a heat exchanger with fouling factor, Rf=0. 0002 m 2 o. C/W. What is the percent reduction in the heat exchanger’s U value if in the clean state U=1961 W/m 2 o. C? 19
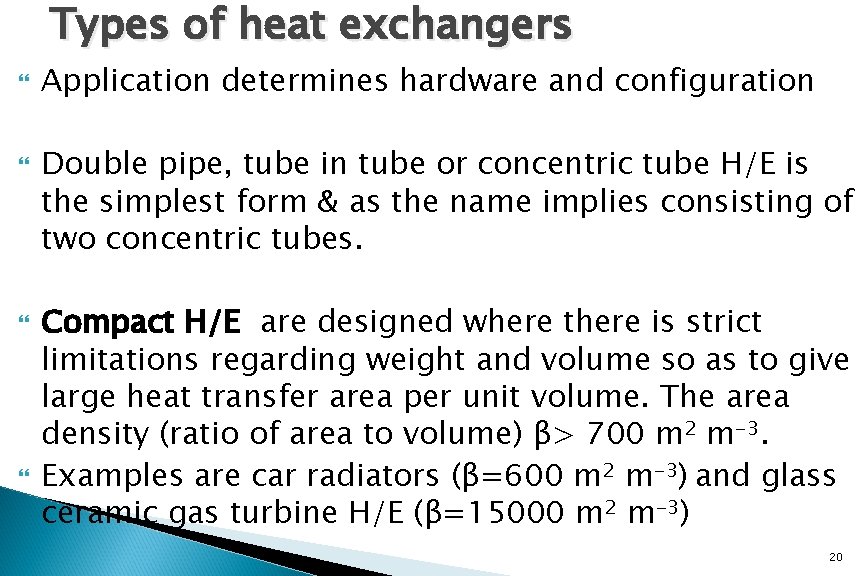
Types of heat exchangers Application determines hardware and configuration Double pipe, tube in tube or concentric tube H/E is the simplest form & as the name implies consisting of two concentric tubes. Compact H/E are designed where there is strict limitations regarding weight and volume so as to give large heat transfer area per unit volume. The area density (ratio of area to volume) β> 700 m 2 m-3. Examples are car radiators (β=600 m 2 m-3) and glass ceramic gas turbine H/E (β=15000 m 2 m-3) 20
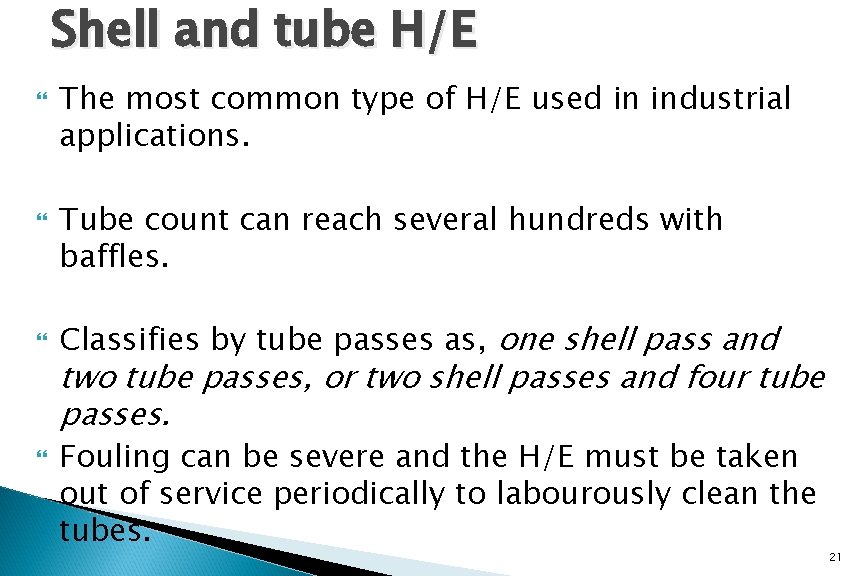
Shell and tube H/E The most common type of H/E used in industrial applications. Tube count can reach several hundreds with baffles. Classifies by tube passes as, one shell pass and two tube passes, or two shell passes and four tube passes. Fouling can be severe and the H/E must be taken out of service periodically to labourously clean the tubes. 21
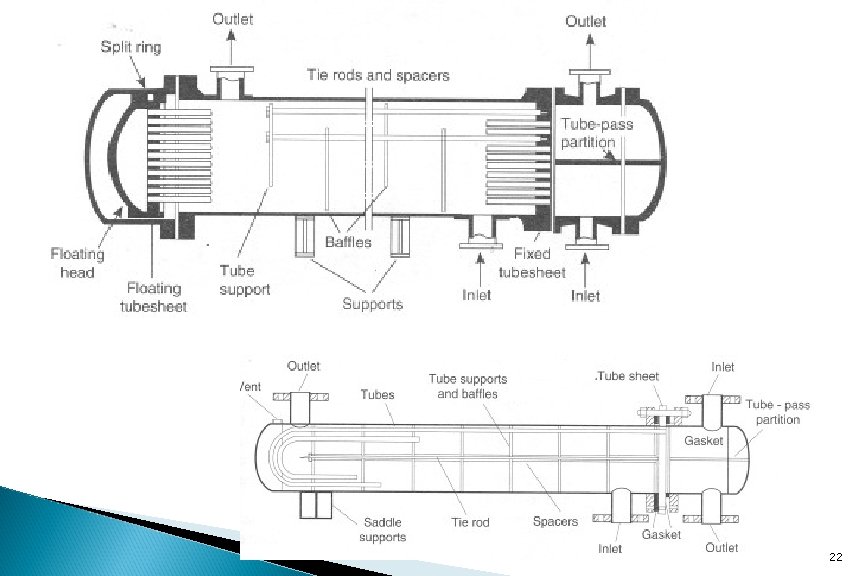
22
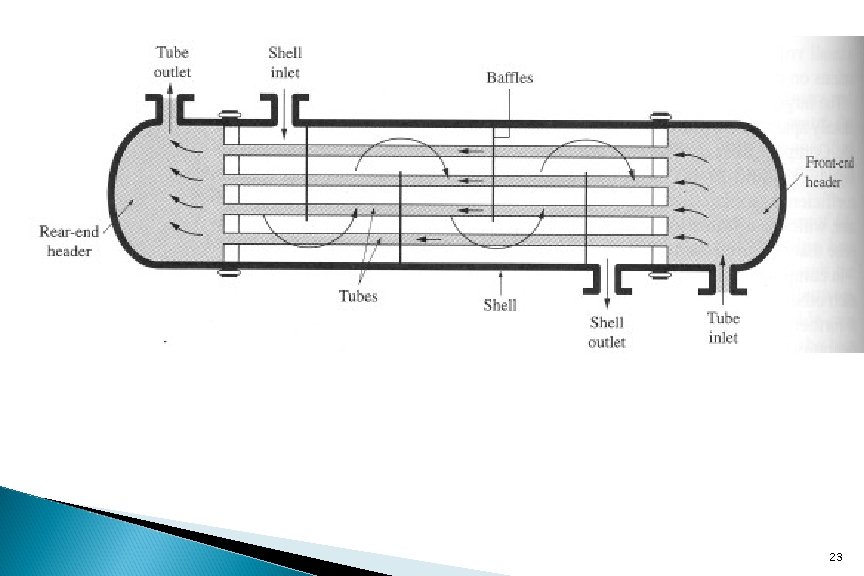
23
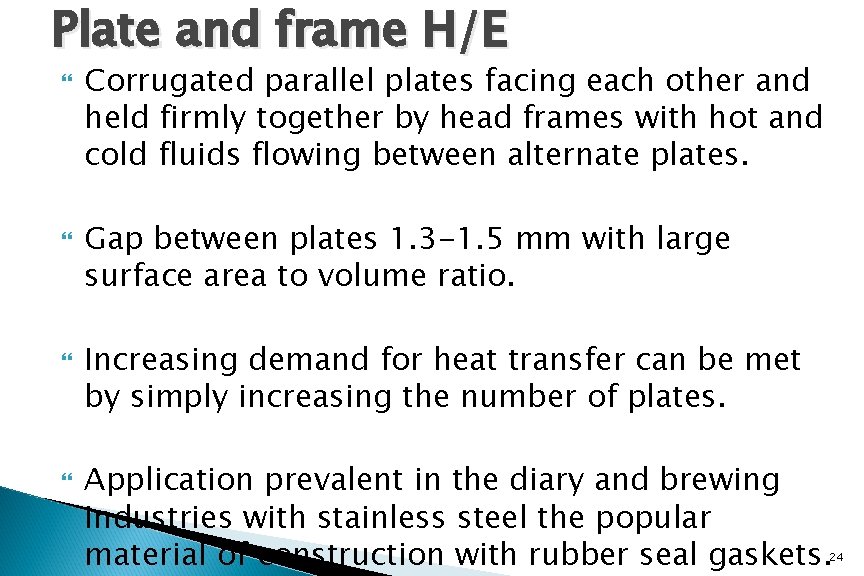
Plate and frame H/E Corrugated parallel plates facing each other and held firmly together by head frames with hot and cold fluids flowing between alternate plates. Gap between plates 1. 3 -1. 5 mm with large surface area to volume ratio. Increasing demand for heat transfer can be met by simply increasing the number of plates. Application prevalent in the diary and brewing industries with stainless steel the popular material of construction with rubber seal gaskets. 24
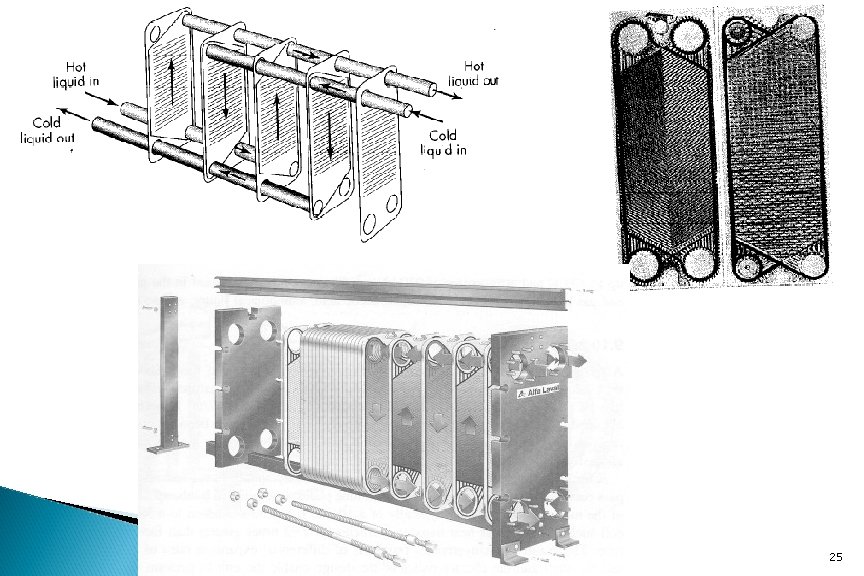
25
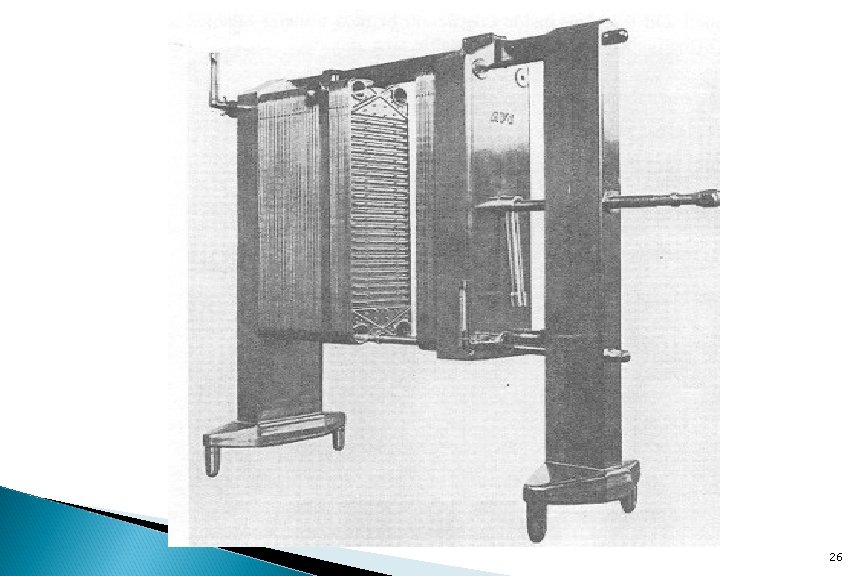
26
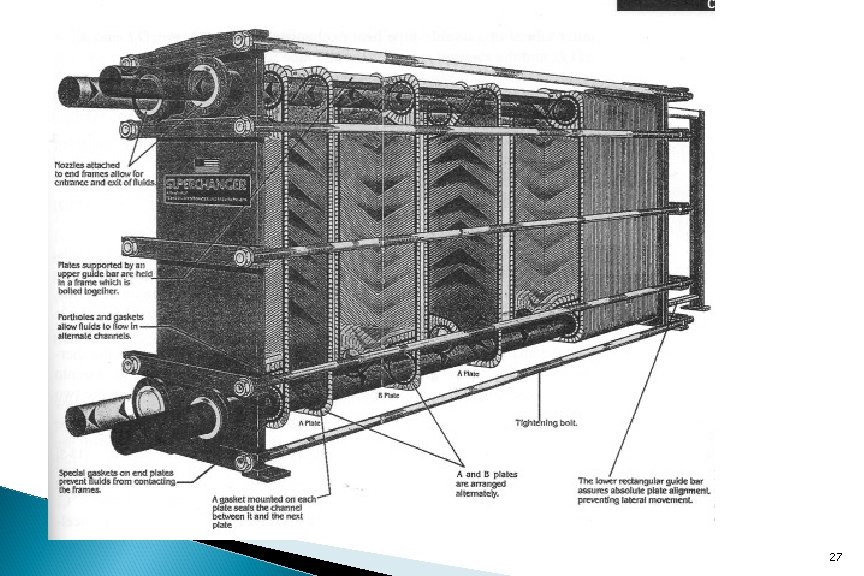
27
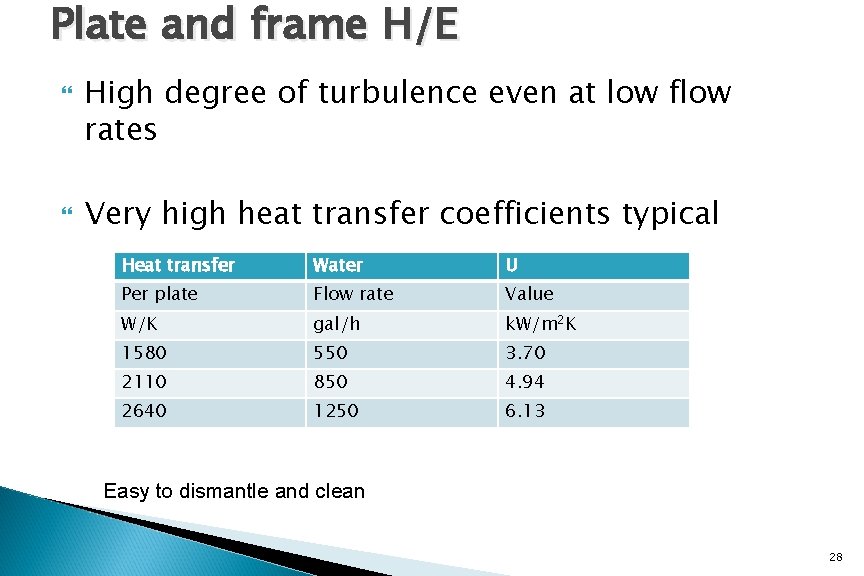
Plate and frame H/E High degree of turbulence even at low flow rates Very high heat transfer coefficients typical Heat transfer Water U Per plate Flow rate Value W/K gal/h k. W/m 2 K 1580 550 3. 70 2110 850 4. 94 2640 1250 6. 13 Easy to dismantle and clean 28
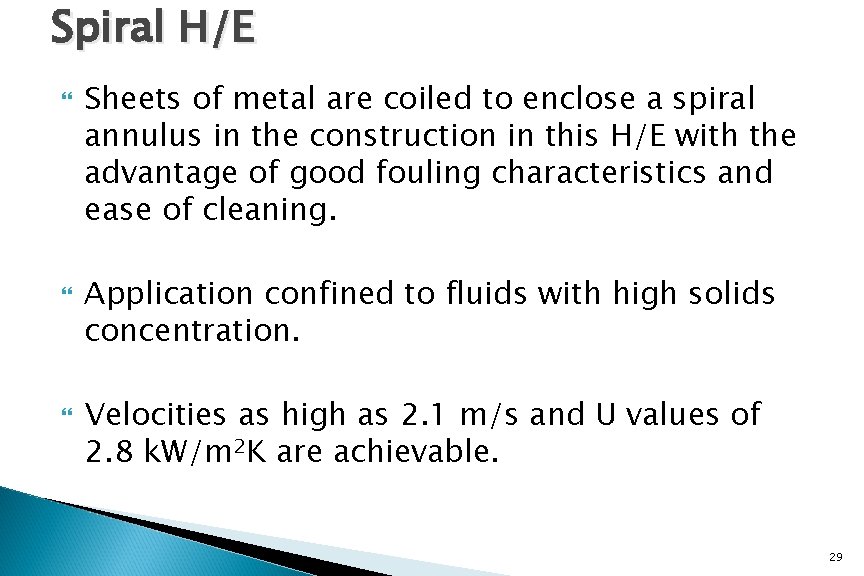
Spiral H/E Sheets of metal are coiled to enclose a spiral annulus in the construction in this H/E with the advantage of good fouling characteristics and ease of cleaning. Application confined to fluids with high solids concentration. Velocities as high as 2. 1 m/s and U values of 2. 8 k. W/m 2 K are achievable. 29
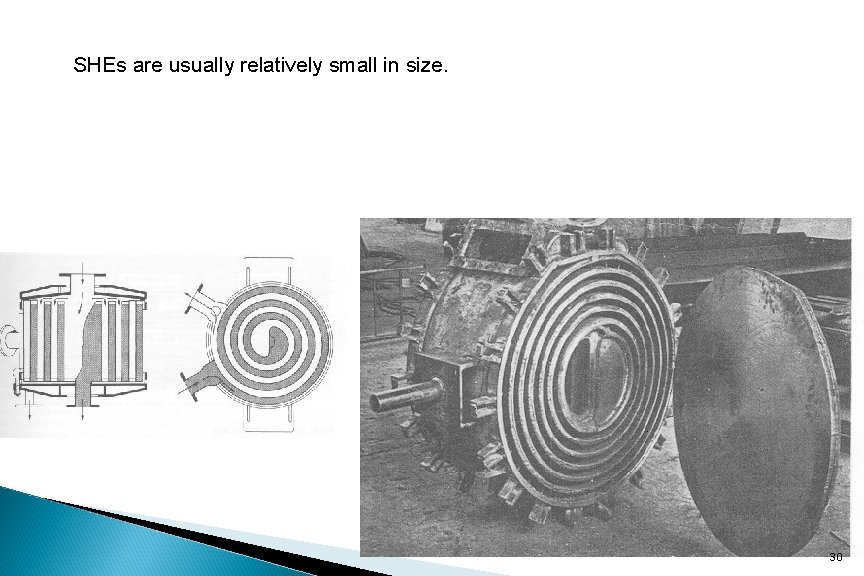
SHEs are usually relatively small in size. 30
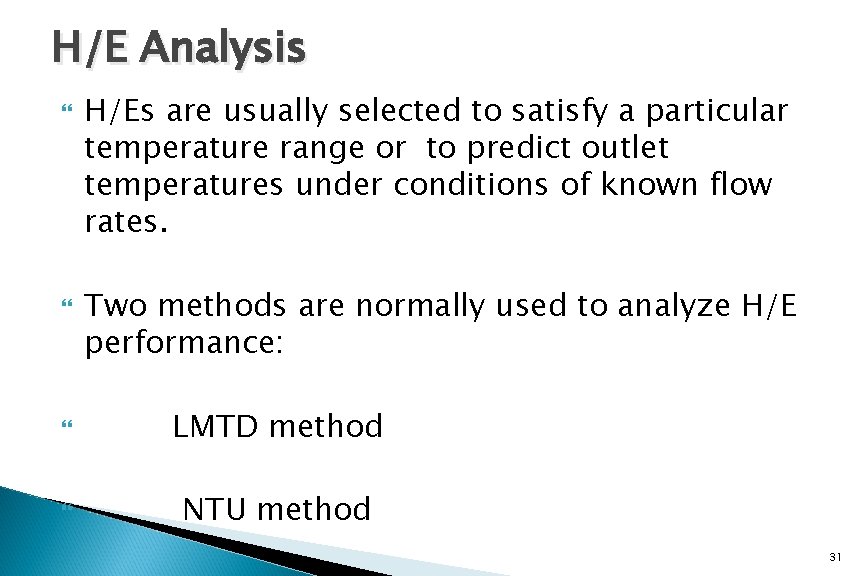
H/E Analysis H/Es are usually selected to satisfy a particular temperature range or to predict outlet temperatures under conditions of known flow rates. Two methods are normally used to analyze H/E performance: LMTD method NTU method 31
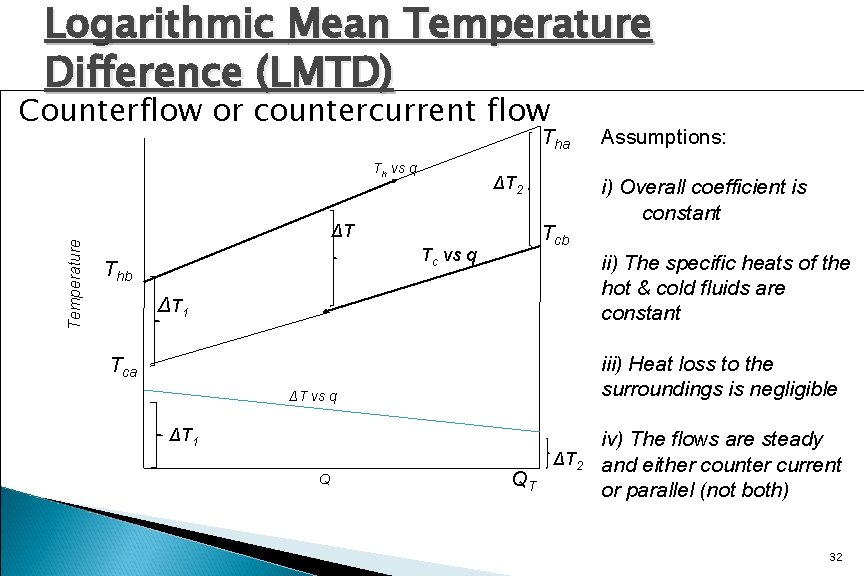
Logarithmic Mean Temperature Difference (LMTD) Counterflow or countercurrent flow Tha Temperature Th vs q ΔT 2 ΔT Tcb Tc vs q Thb i) Overall coefficient is constant ii) The specific heats of the hot & cold fluids are constant ΔT 1 iii) Heat loss to the surroundings is negligible Tca ΔT vs q ΔT 1 Q Assumptions: QT ΔT 2 iv) The flows are steady and either counter current or parallel (not both) 32
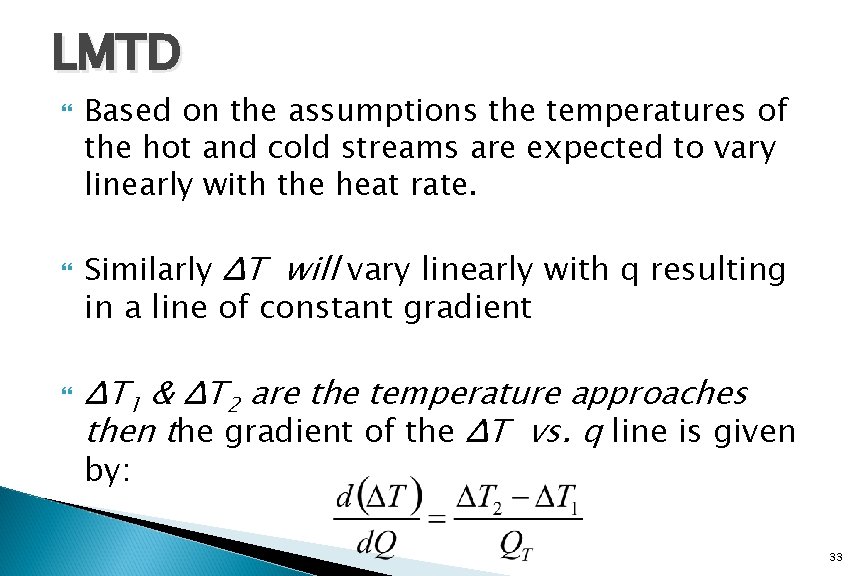
LMTD Based on the assumptions the temperatures of the hot and cold streams are expected to vary linearly with the heat rate. Similarly ΔT will vary linearly with q resulting in a line of constant gradient ΔT 1 & ΔT 2 are the temperature approaches then the gradient of the ΔT vs. q line is given by: 33
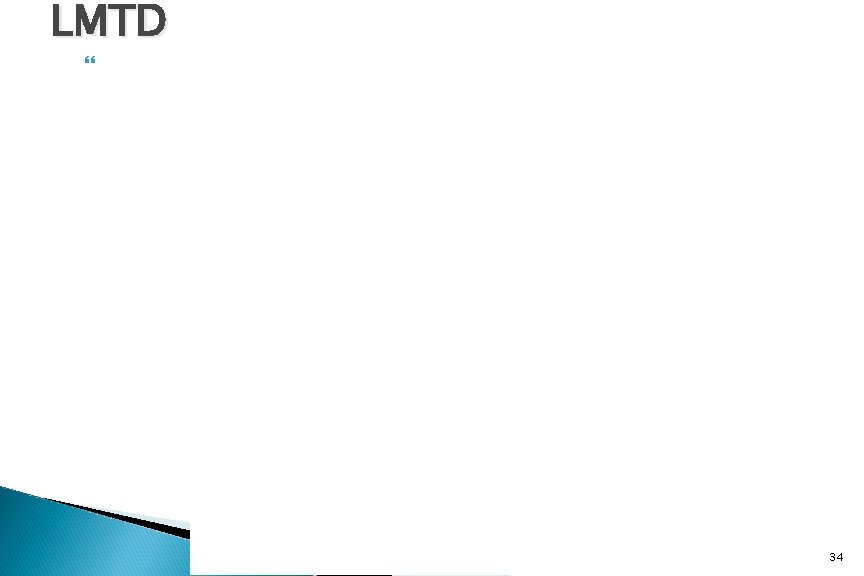
LMTD 34
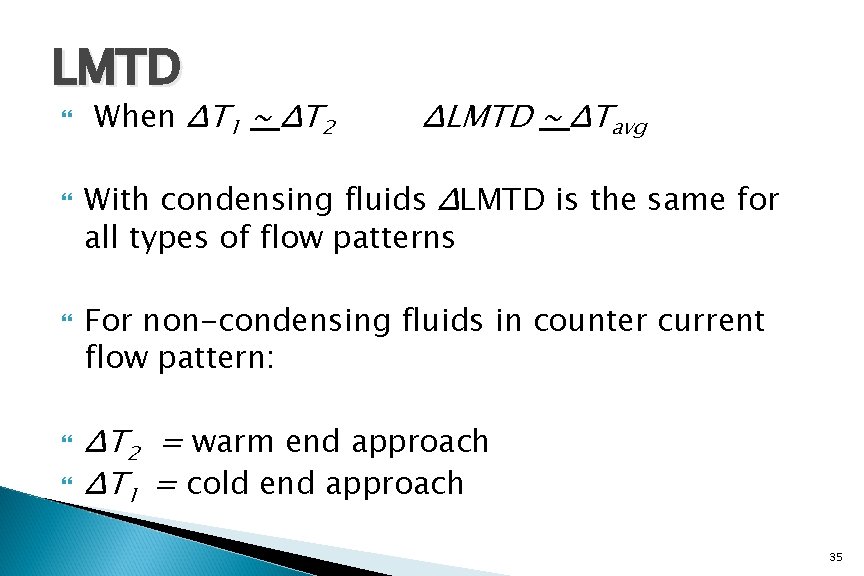
LMTD When ΔT 1 ~ ΔT 2 ΔLMTD ~ ΔTavg With condensing fluids ΔLMTD is the same for all types of flow patterns For non-condensing fluids in counter current flow pattern: ΔT 2 = warm end approach ΔT 1 = cold end approach 35
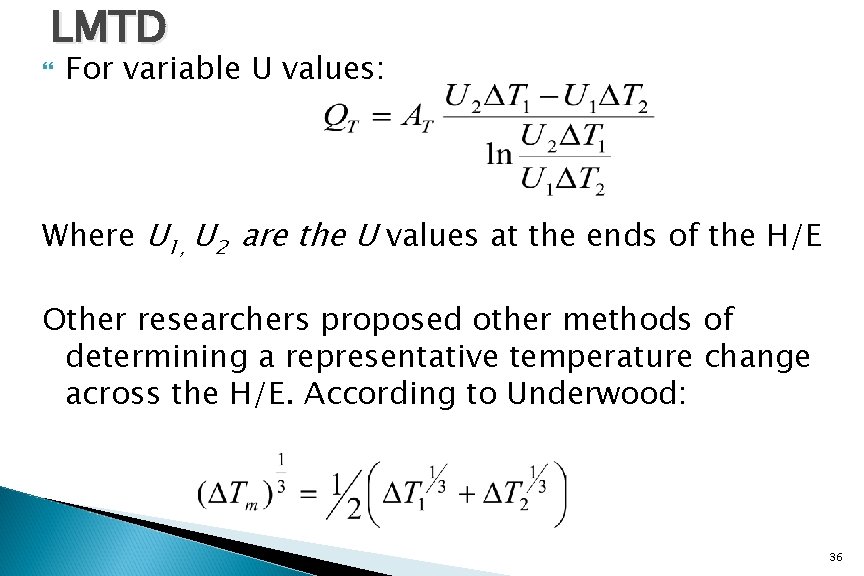
LMTD For variable U values: Where U 1, U 2 are the U values at the ends of the H/E Other researchers proposed other methods of determining a representative temperature change across the H/E. According to Underwood: 36
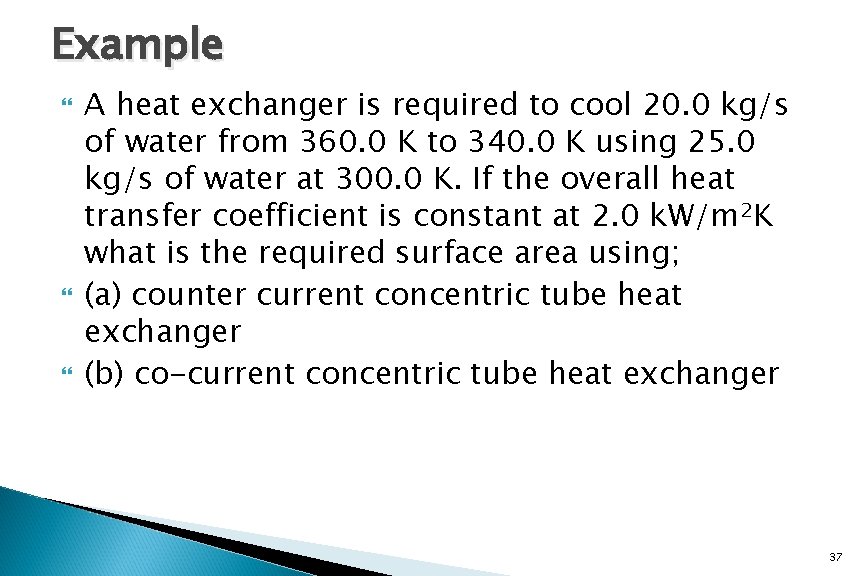
Example A heat exchanger is required to cool 20. 0 kg/s of water from 360. 0 K to 340. 0 K using 25. 0 kg/s of water at 300. 0 K. If the overall heat transfer coefficient is constant at 2. 0 k. W/m 2 K what is the required surface area using; (a) counter current concentric tube heat exchanger (b) co-current concentric tube heat exchanger 37
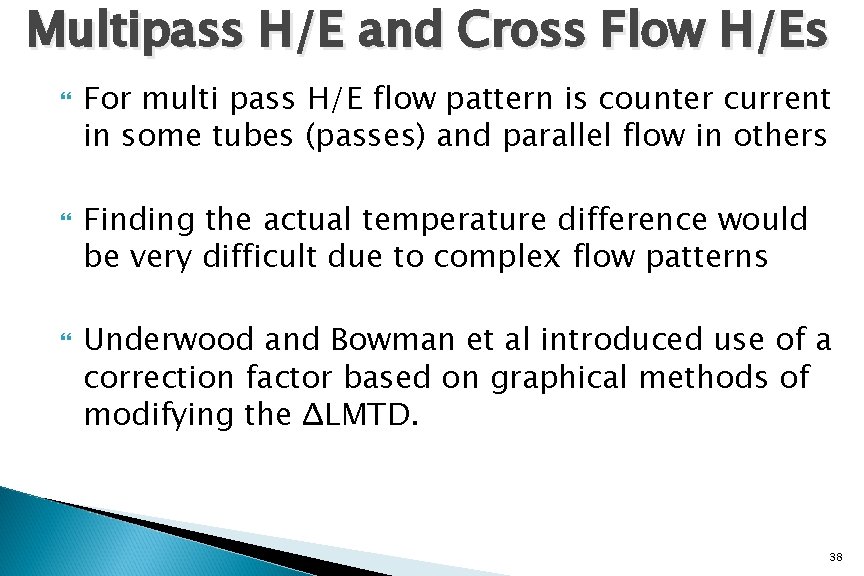
Multipass H/E and Cross Flow H/Es For multi pass H/E flow pattern is counter current in some tubes (passes) and parallel flow in others Finding the actual temperature difference would be very difficult due to complex flow patterns Underwood and Bowman et al introduced use of a correction factor based on graphical methods of modifying the ΔLMTD. 38
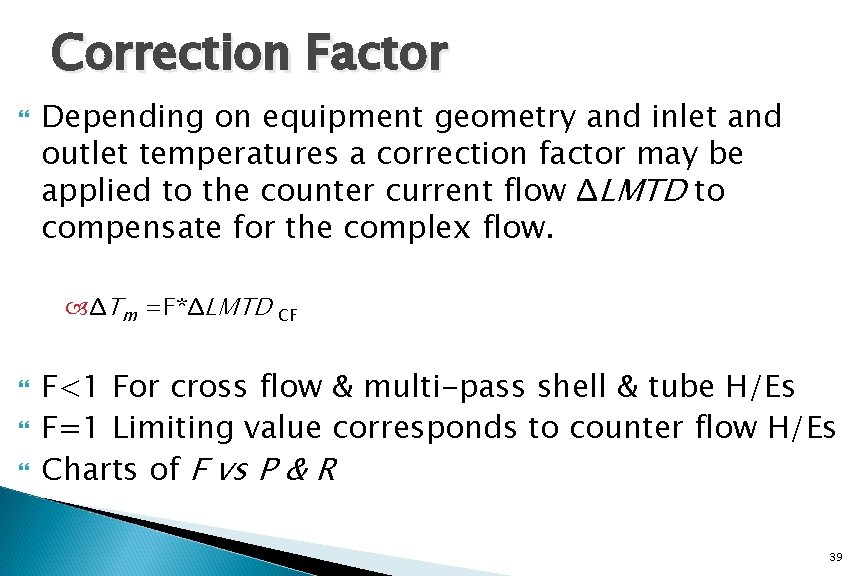
Correction Factor Depending on equipment geometry and inlet and outlet temperatures a correction factor may be applied to the counter current flow ΔLMTD to compensate for the complex flow. ΔTm =F*ΔLMTD CF F<1 For cross flow & multi-pass shell & tube H/Es F=1 Limiting value corresponds to counter flow H/Es Charts of F vs P & R 39
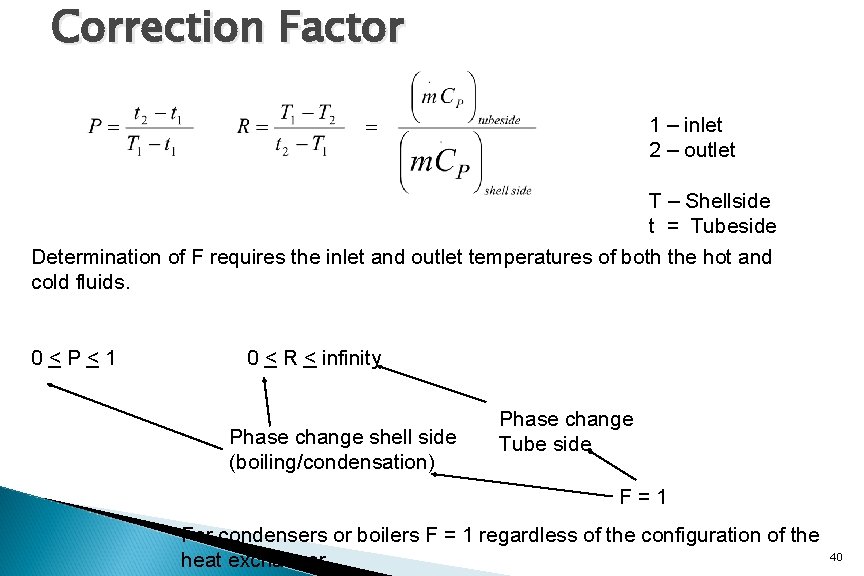
Correction Factor 1 – inlet 2 – outlet T – Shellside t = Tubeside Determination of F requires the inlet and outlet temperatures of both the hot and cold fluids. 0<P<1 0 < R < infinity Phase change shell side (boiling/condensation) Phase change Tube side F=1 For condensers or boilers F = 1 regardless of the configuration of the heat exchanger 40
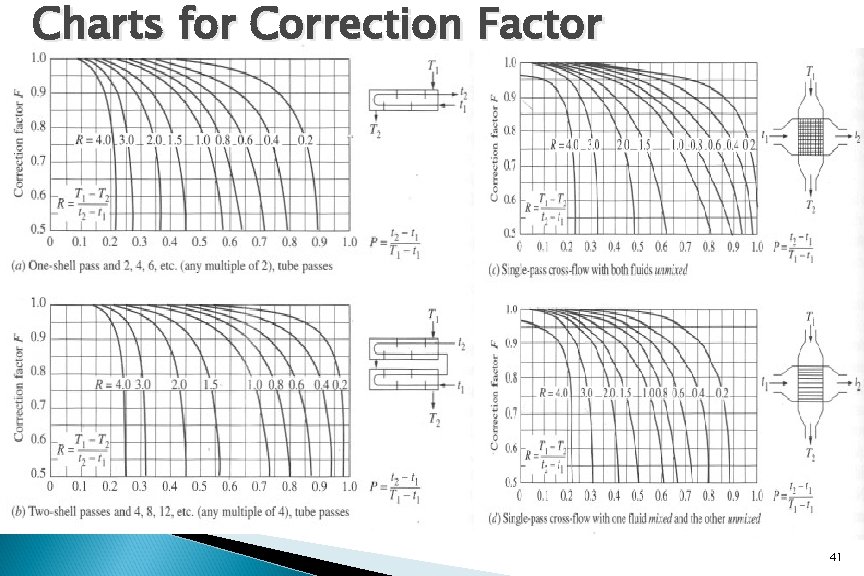
Charts for Correction Factor 41
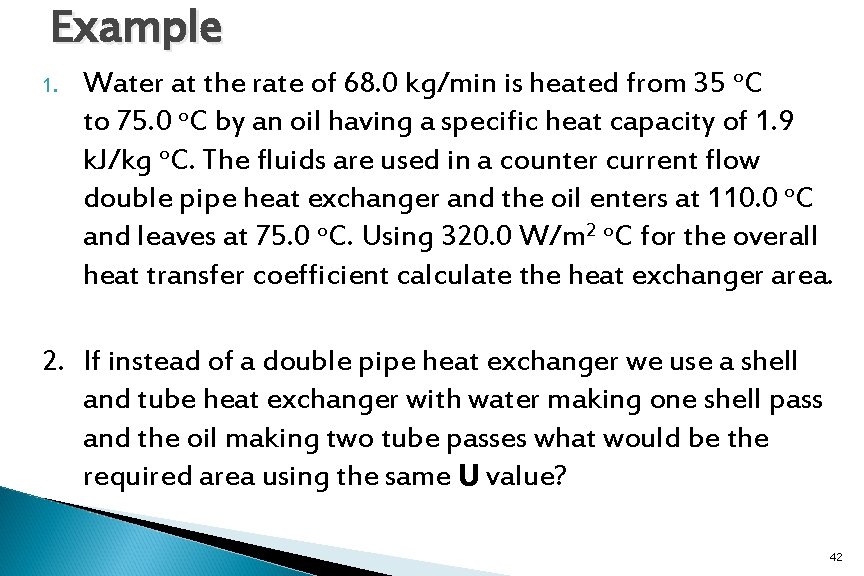
Example 1. Water at the rate of 68. 0 kg/min is heated from 35 o. C to 75. 0 o. C by an oil having a specific heat capacity of 1. 9 k. J/kg o. C. The fluids are used in a counter current flow double pipe heat exchanger and the oil enters at 110. 0 o. C and leaves at 75. 0 o. C. Using 320. 0 W/m 2 o. C for the overall heat transfer coefficient calculate the heat exchanger area. 2. If instead of a double pipe heat exchanger we use a shell and tube heat exchanger with water making one shell pass and the oil making two tube passes what would be the required area using the same U value? 42
Unit 10, unit 10 review tests, unit 10 general test
4:2:1 rule
Types of bases in suppositories
Buoyancyability
Fluids under pressure
Compressible and incompressible fluids
Fluids physics problems and solutions
Pediatric maintenance fluids 4-2-1 rule
Pediatric fluids 4 2 1
Fluid order chart
Australian standards for texture modified foods and fluids
Normal saline uses
Fluid at rest example
Colloid fluids
Crystalloid fluids examples
Colloid osmotic pressure
4/2/1 rule
4-2-1 maintenance fluids
Euler's formula fluid mechanics
Compressible and incompressible fluids
Hypotonic fluids
Apparent weight formula
Extracellular fluid and interstitial fluid
Compressible and incompressible fluids
Holliday segar
Regulation of body fluids
Anaesthesit
Enhancing thermal conductivity of fluids with nanoparticles
Pitot tube bernoulli equation
Volumetric properties of pure fluids
What is the upward force that fluids exert on all matter
The way you see your body is
Physics of fluids
Fluid mechanics
Fluids at rest
Discovering science 8
Viscosity fluid mechanics
Momentum fluid
Static fluids
Static fluids
Body fluid volume
Dilution principle of measuring body fluids
Iv fluid composition