Transcription Central Dogma Genes Sequence of DNA that
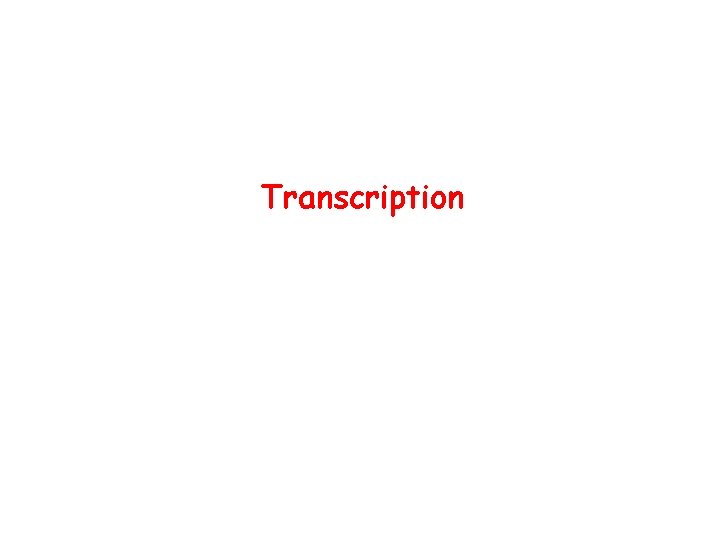
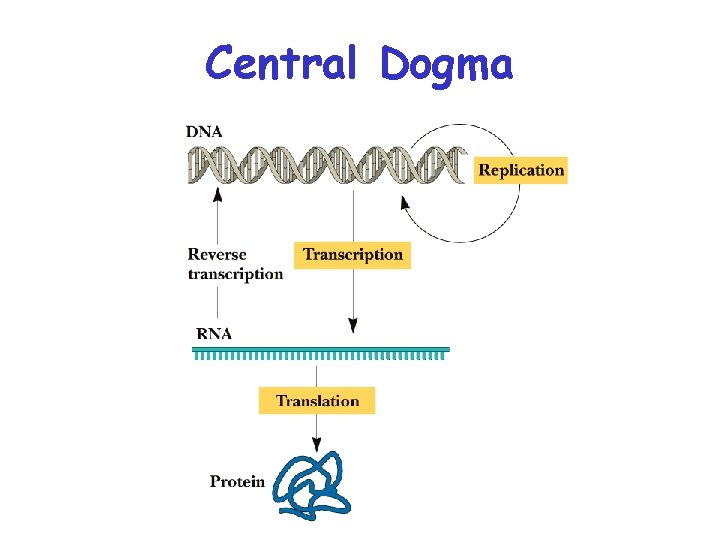
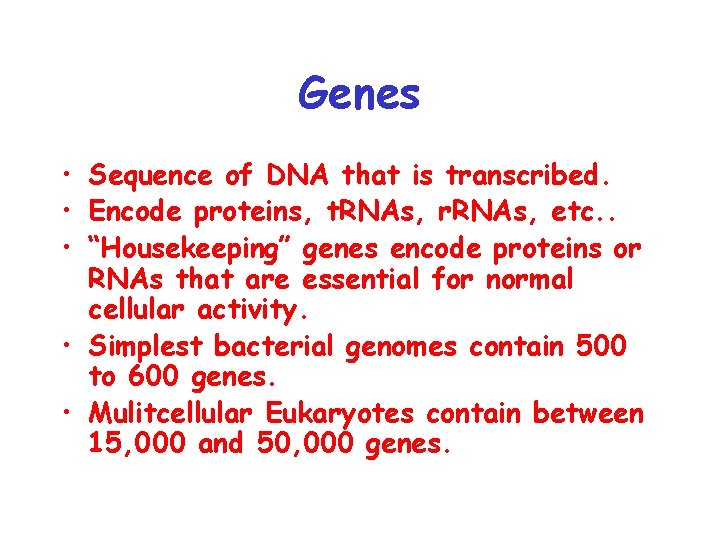
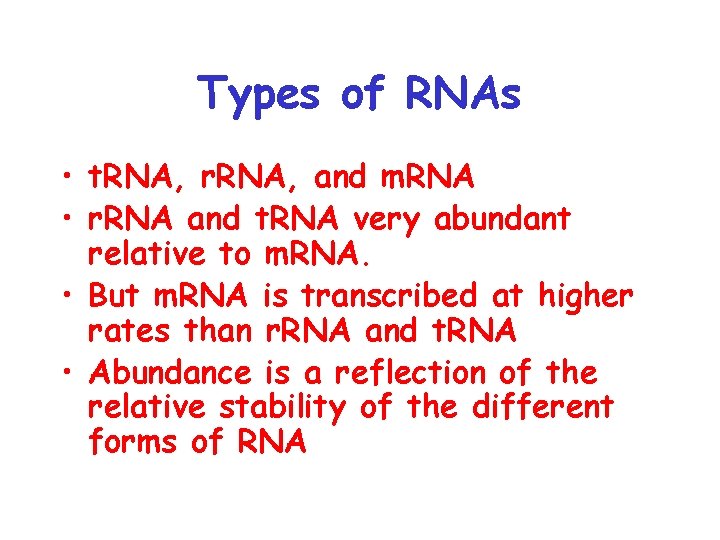
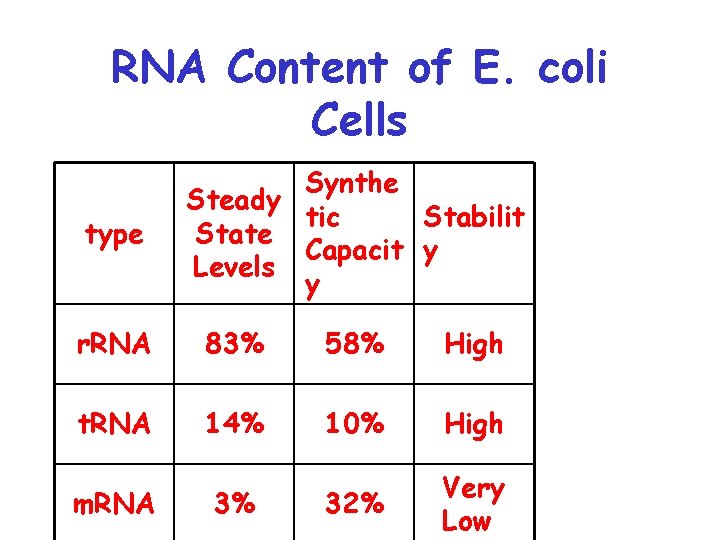
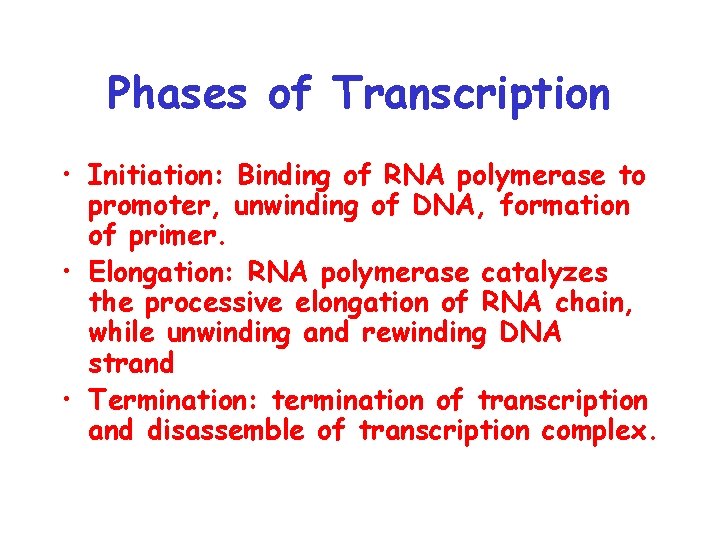
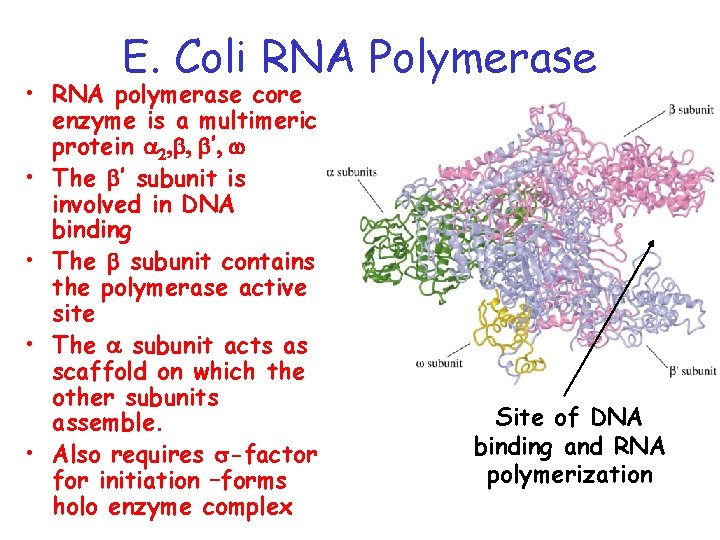
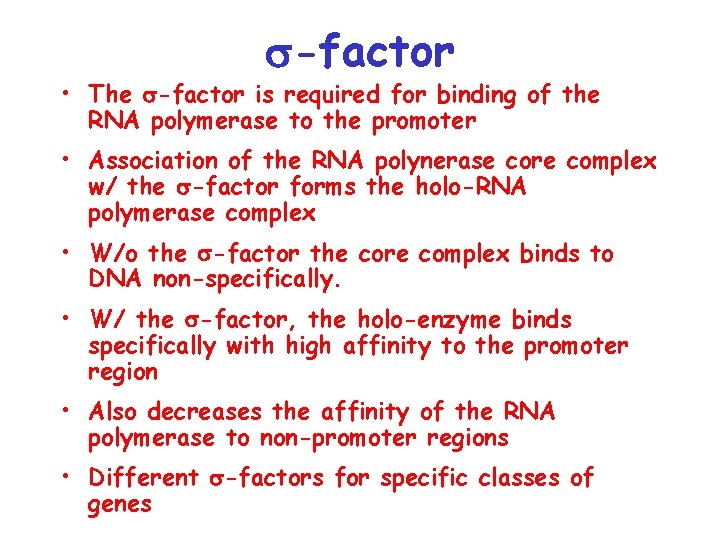
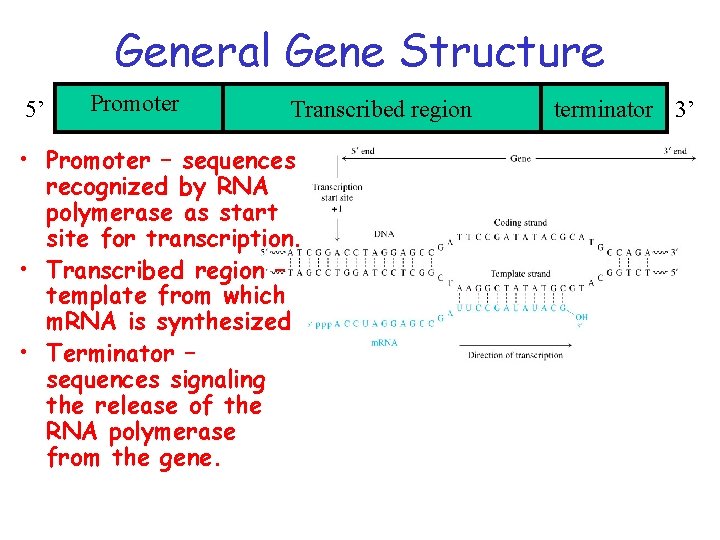
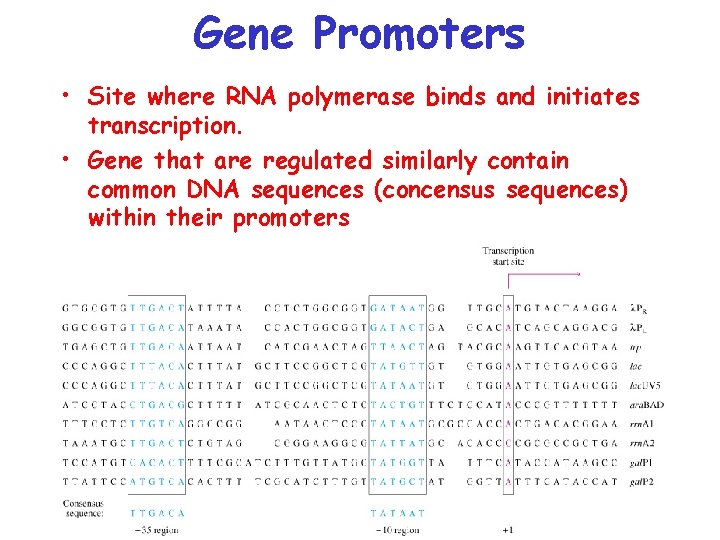
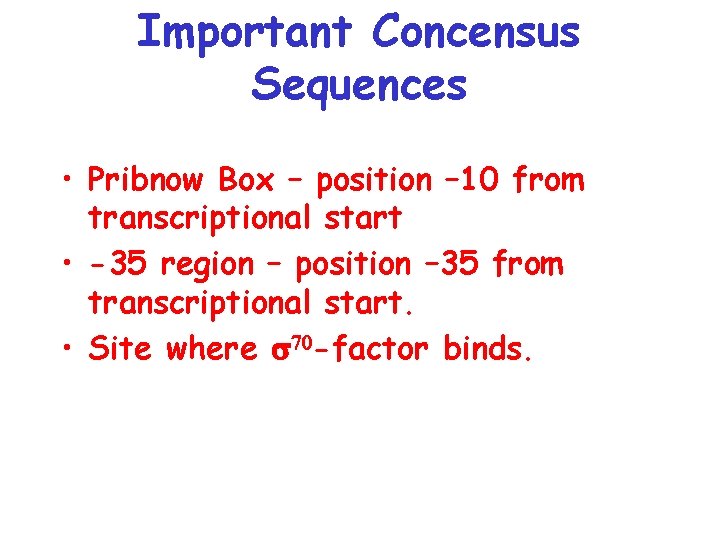
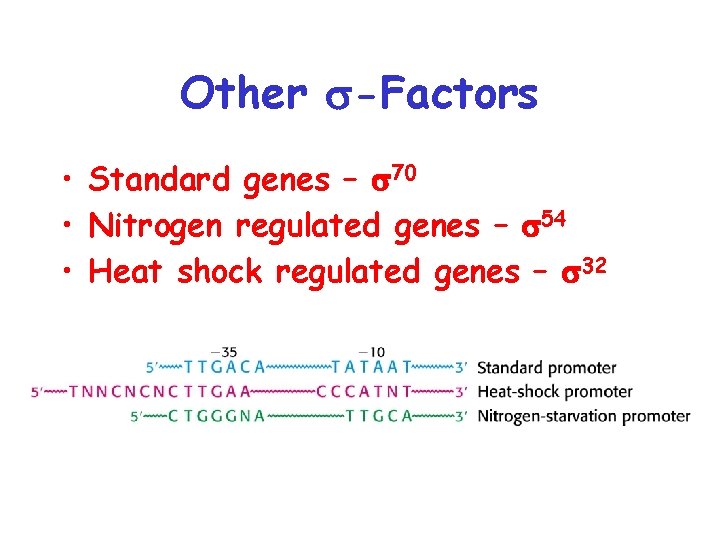
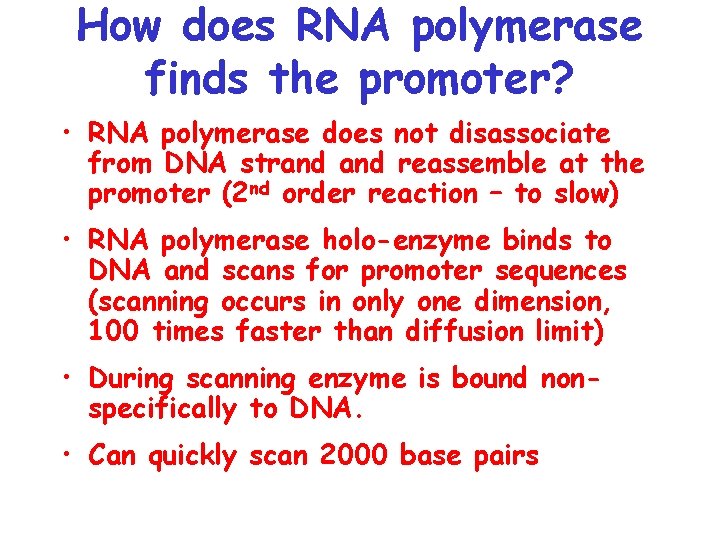
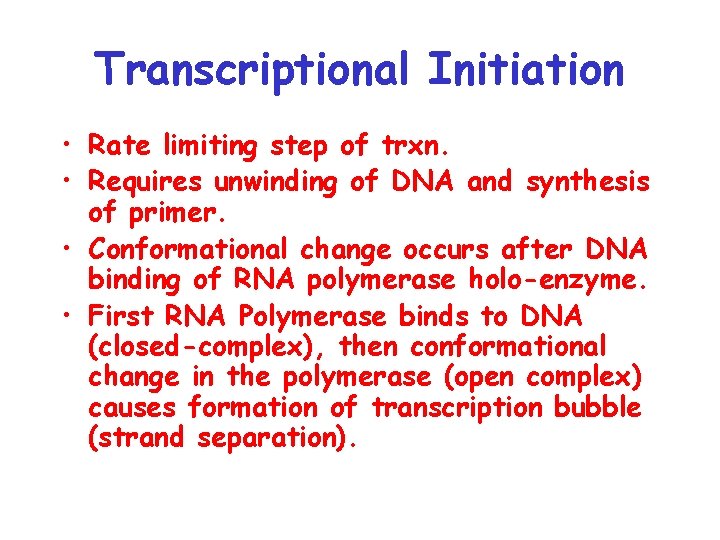
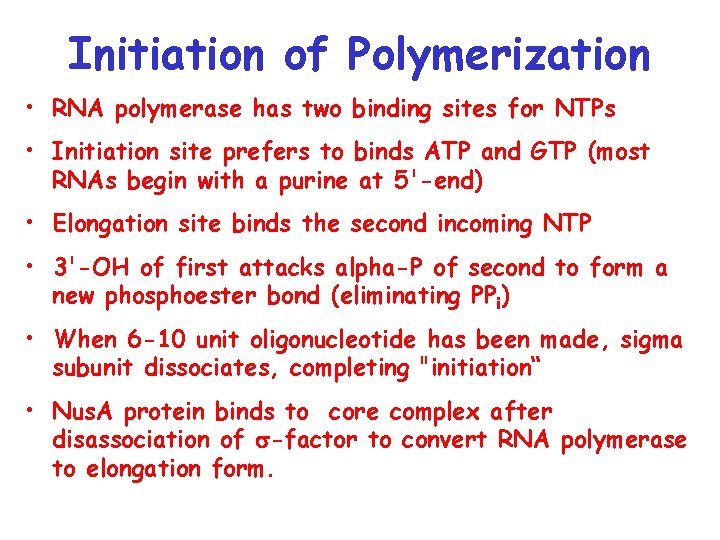
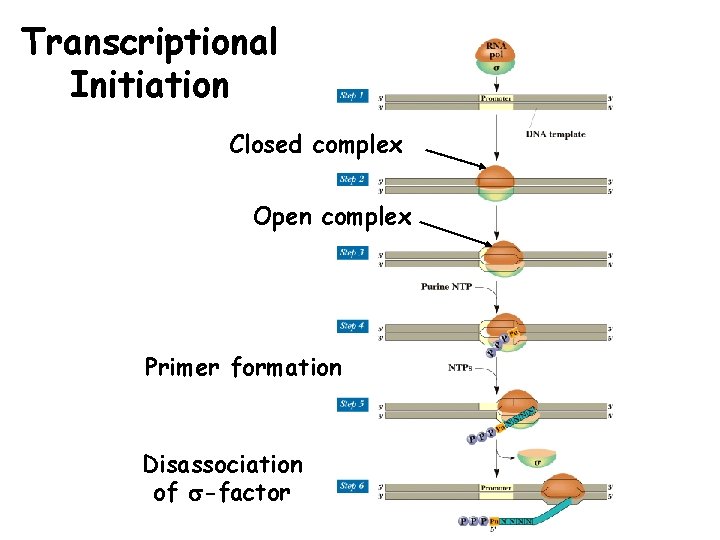
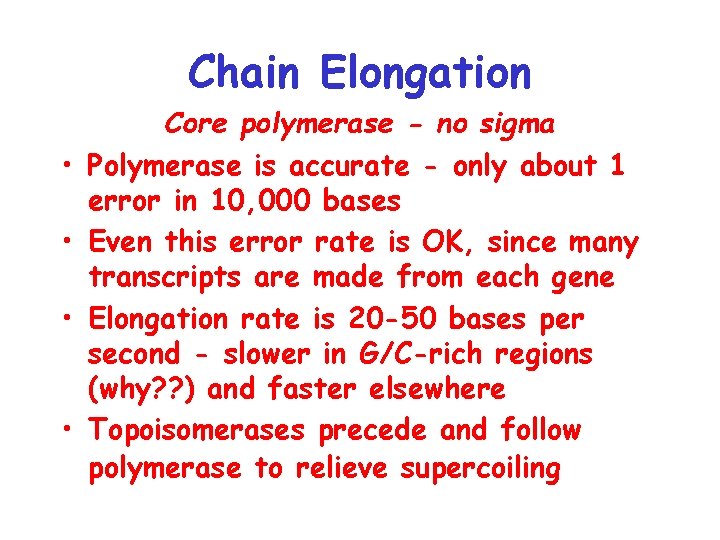
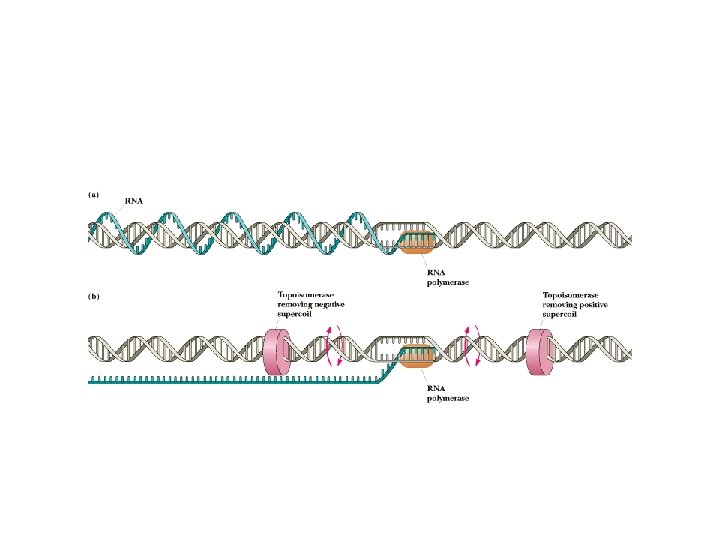
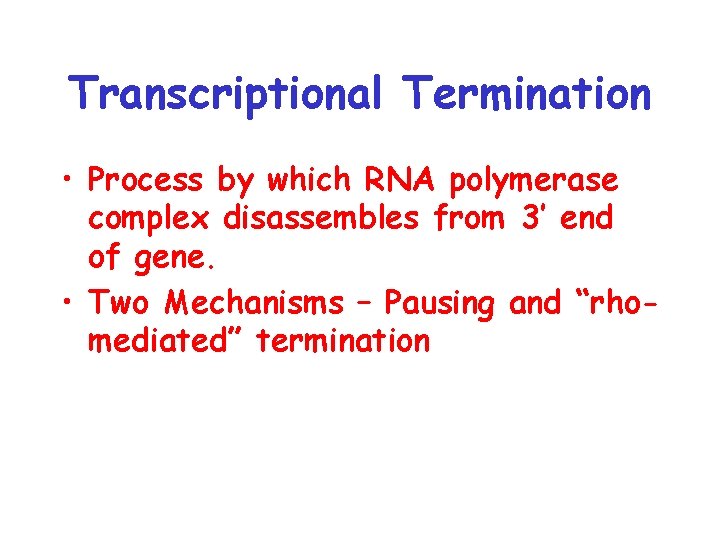
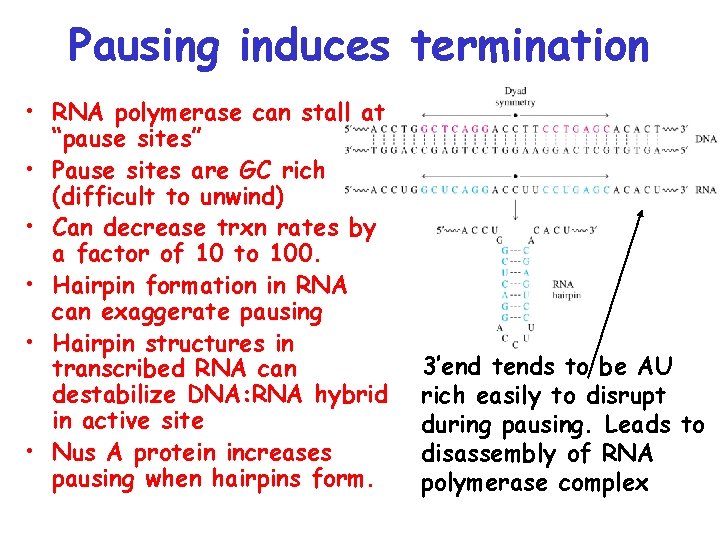
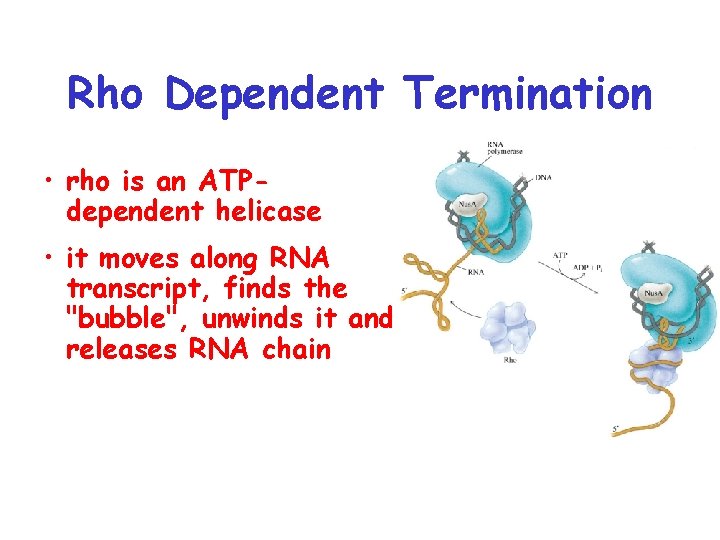
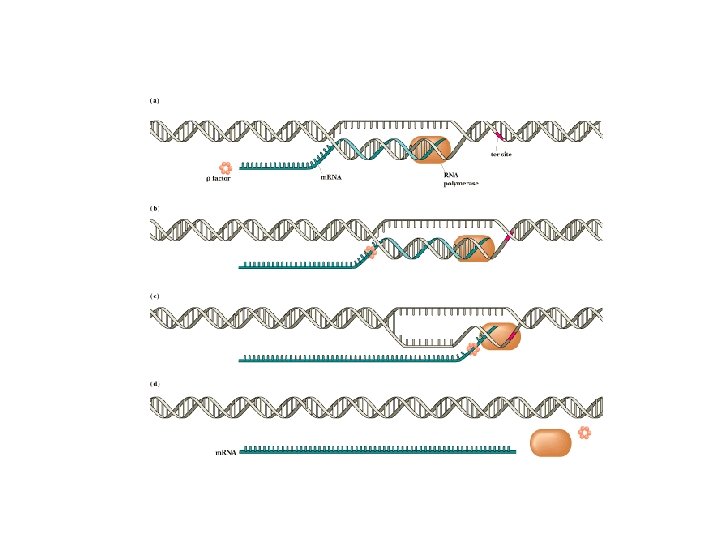
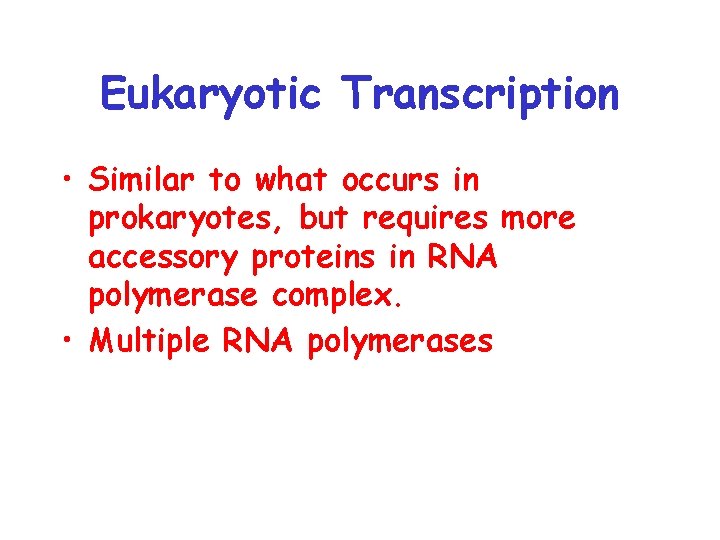
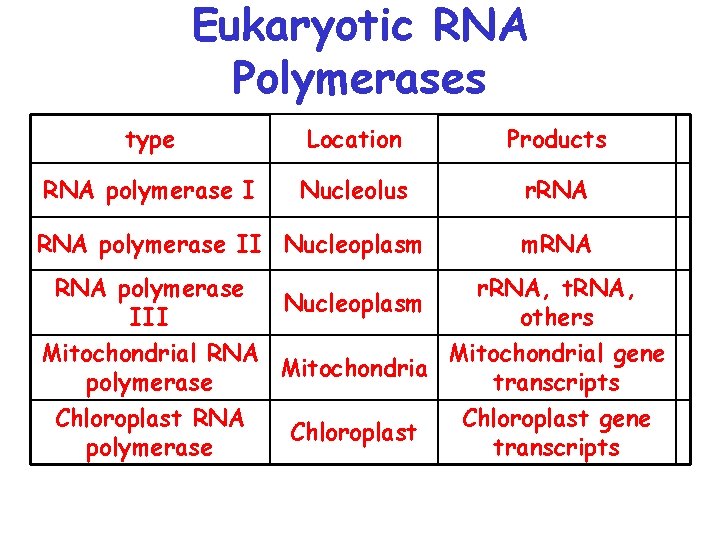
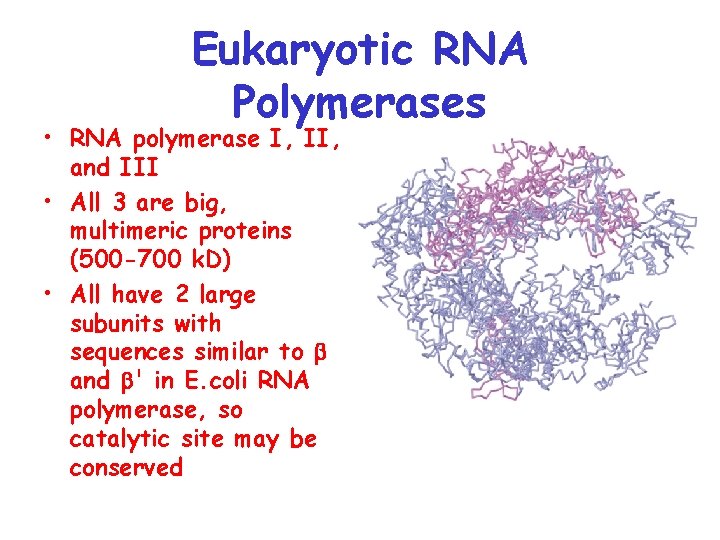
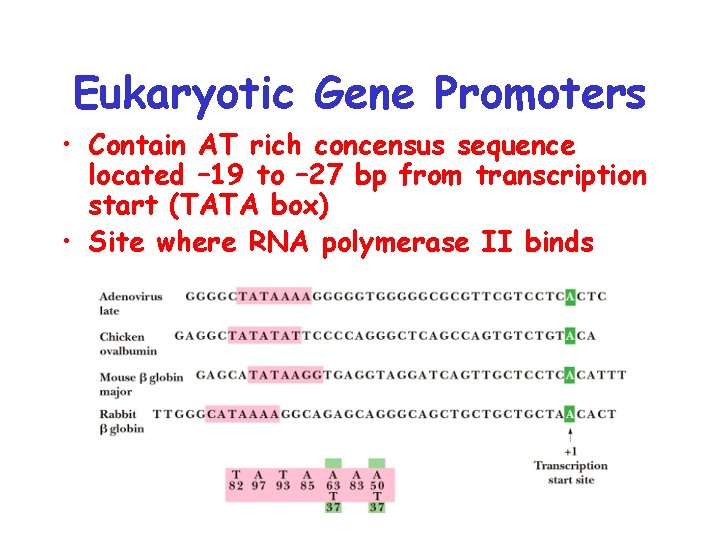
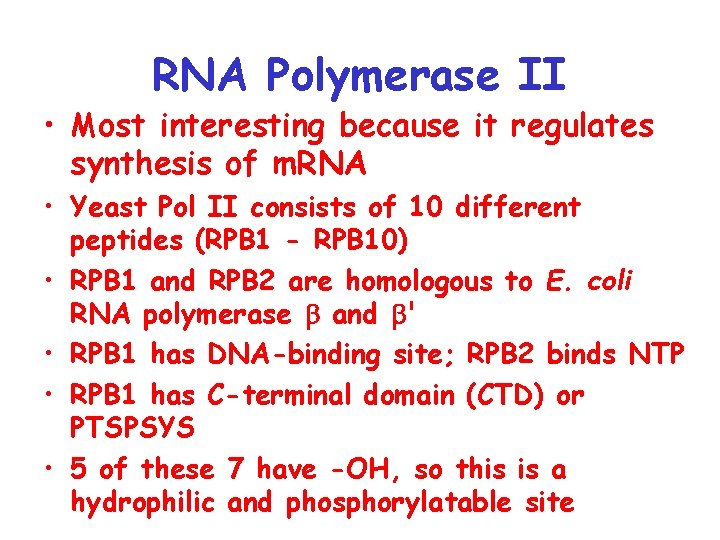
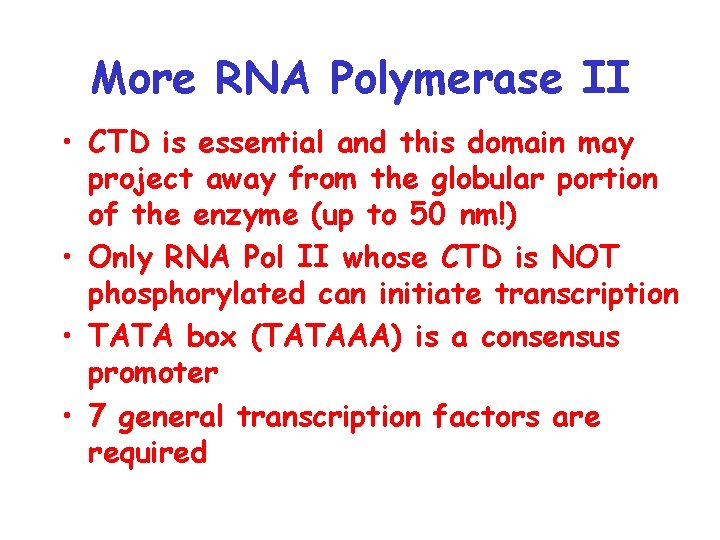
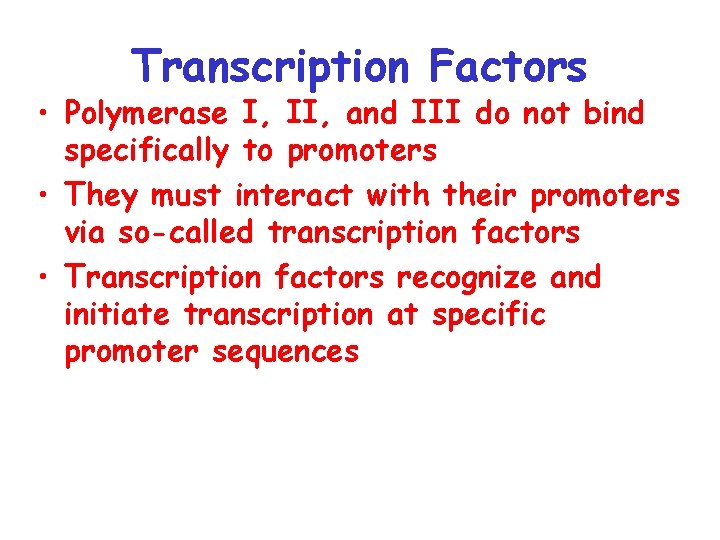
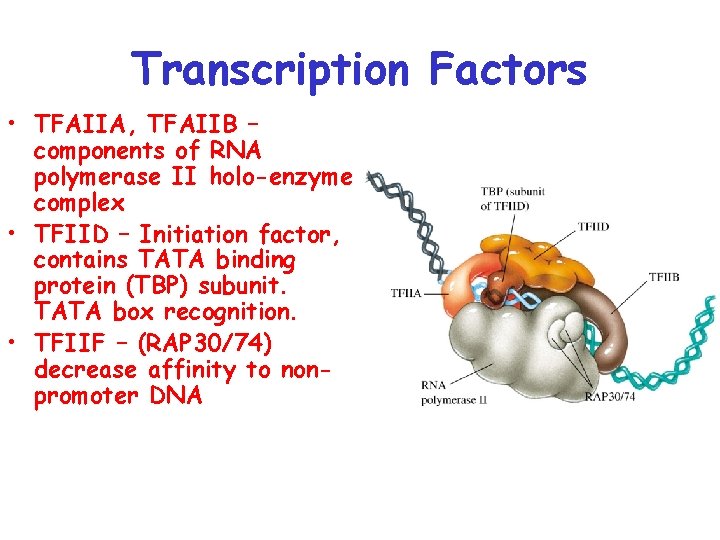
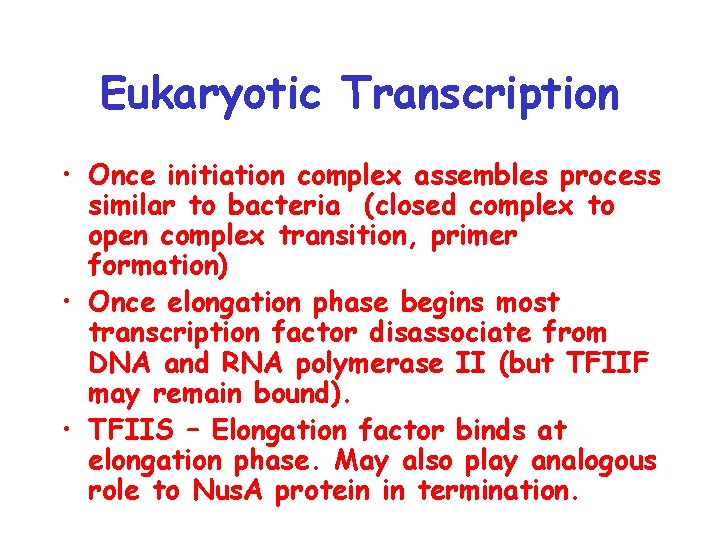
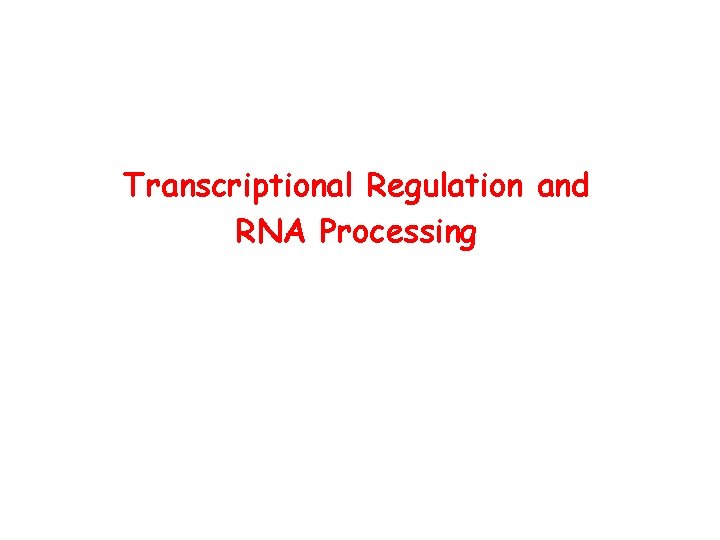
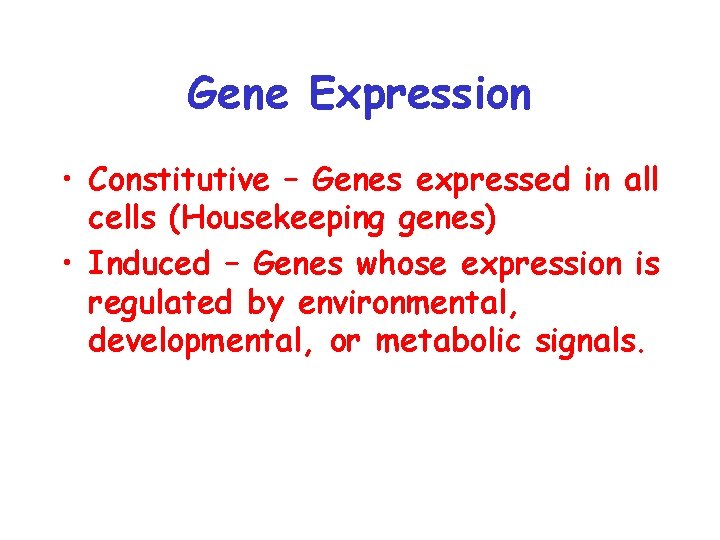
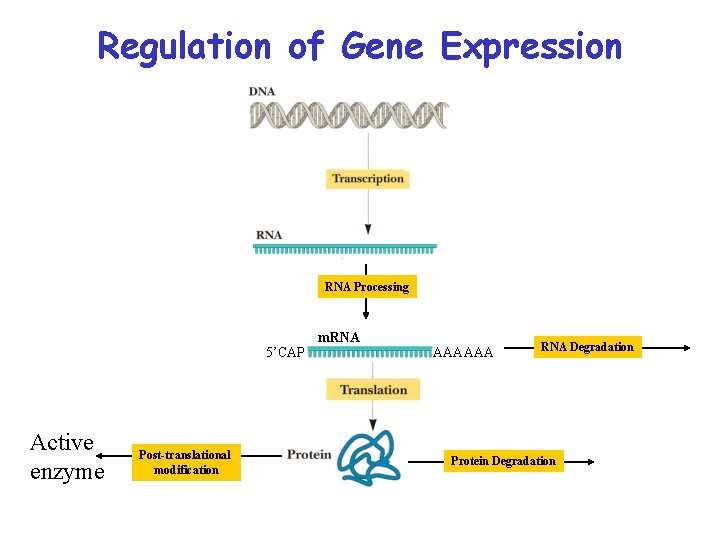
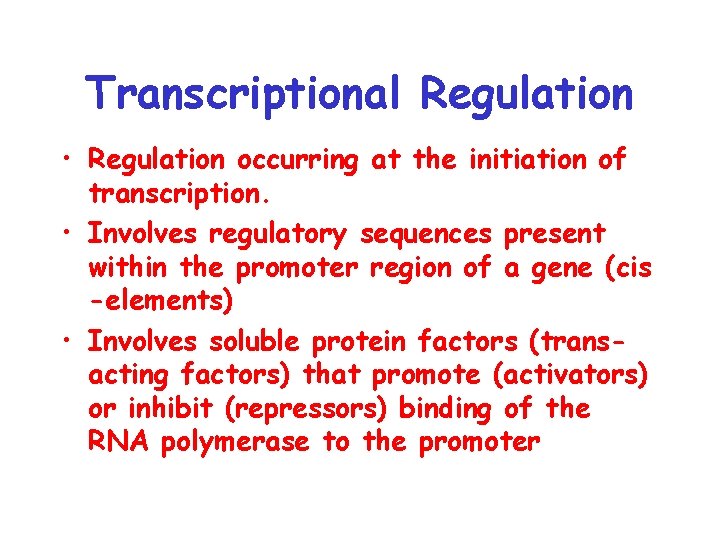
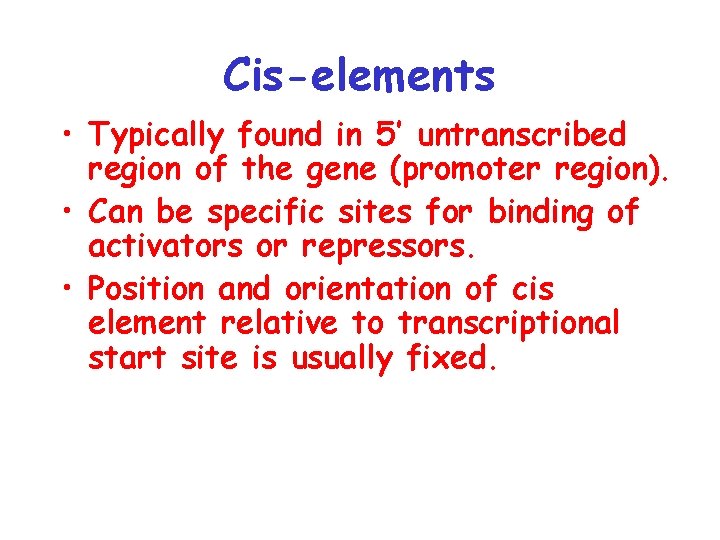
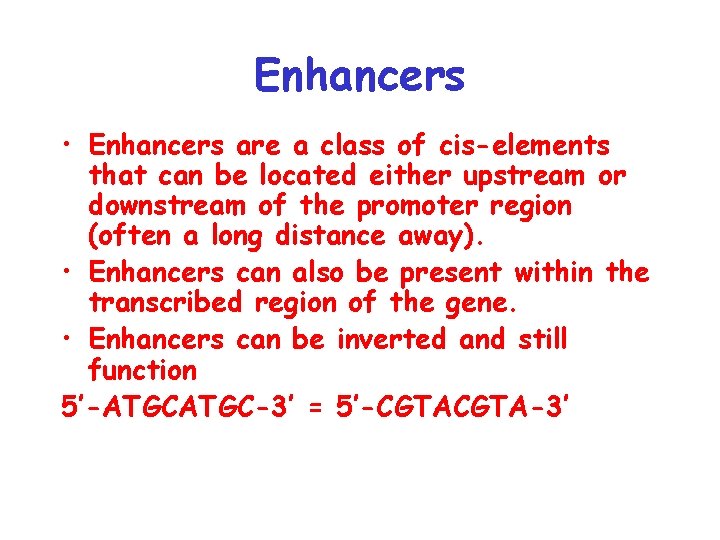
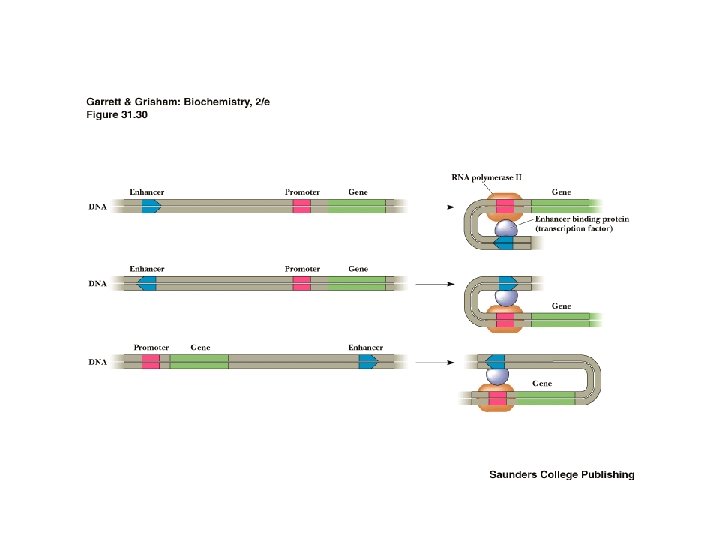
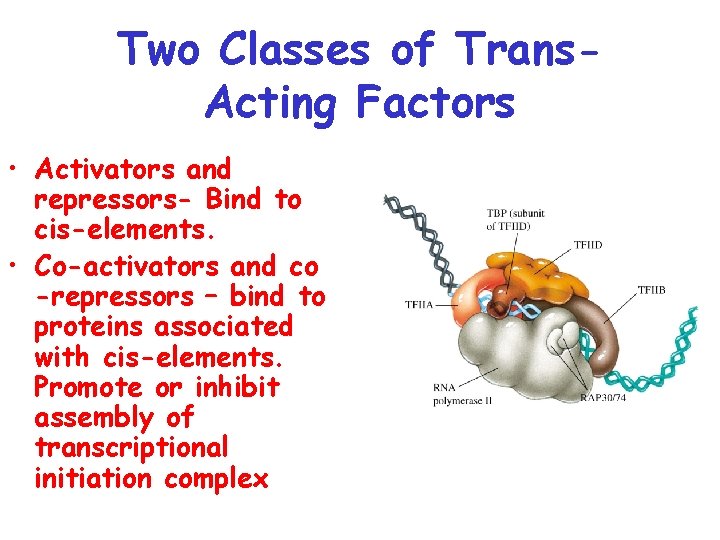
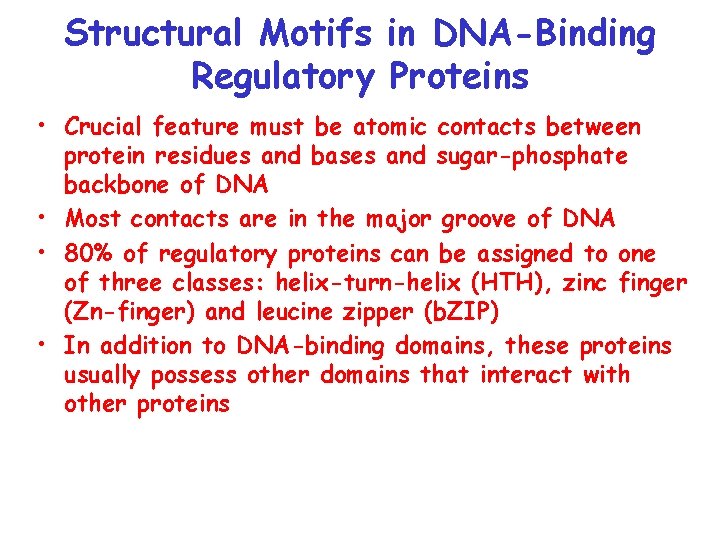
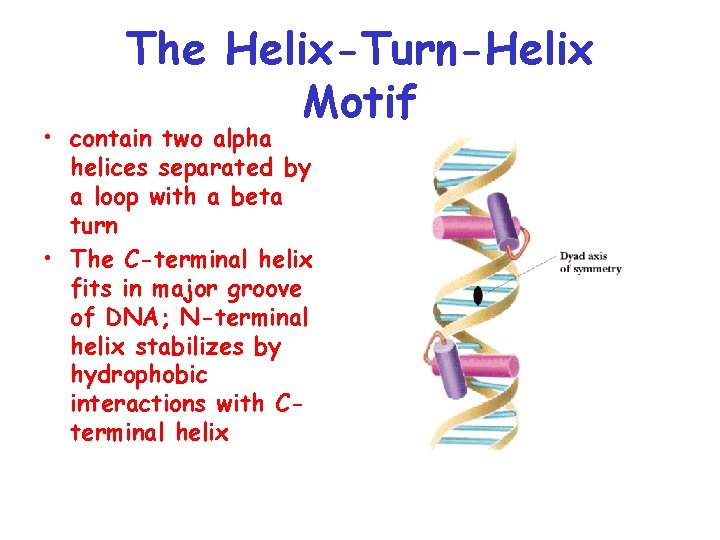
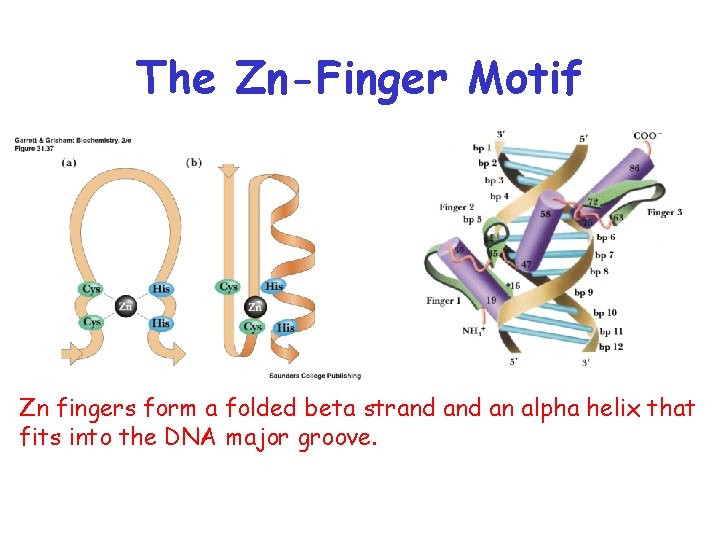
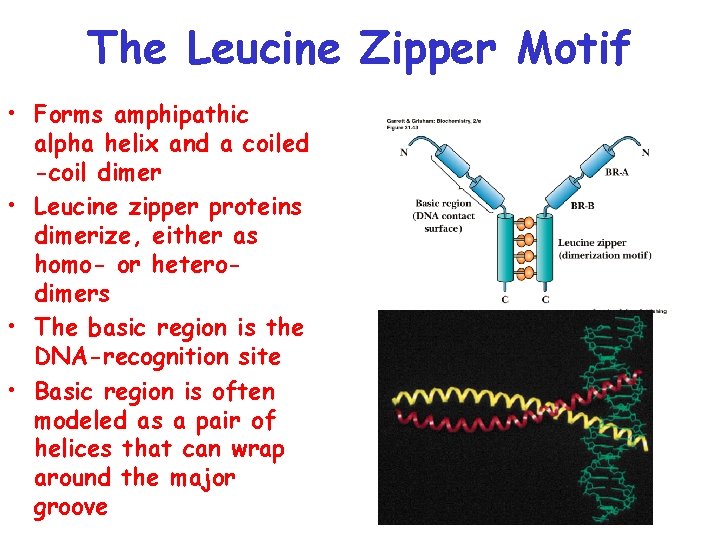
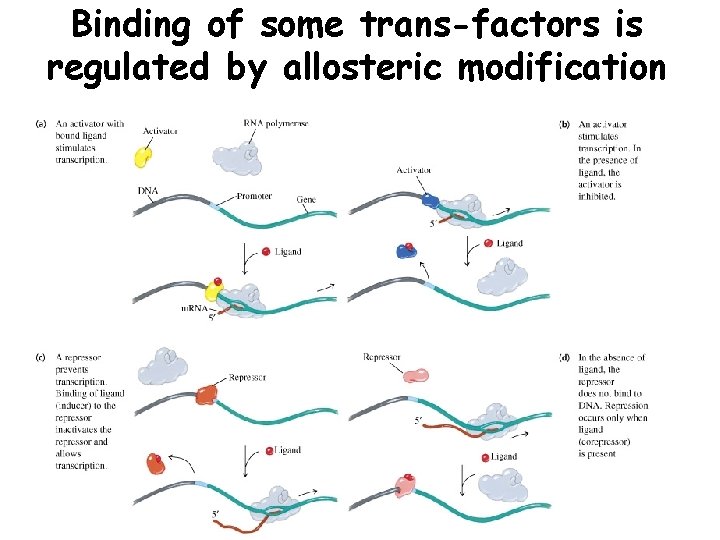
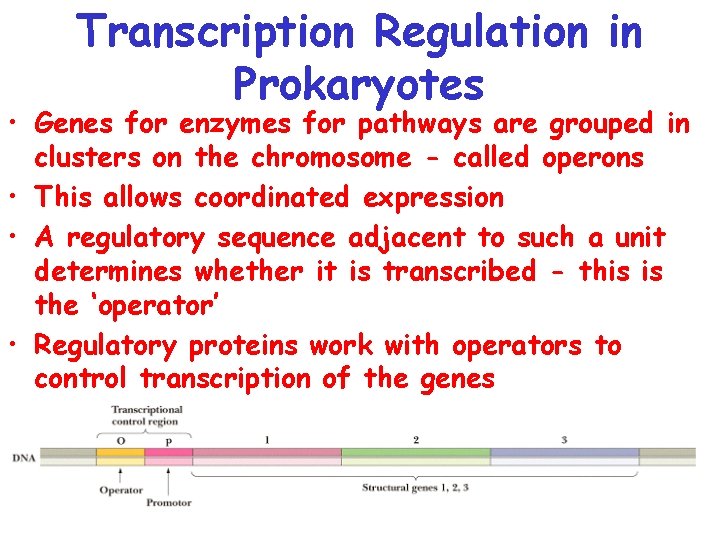
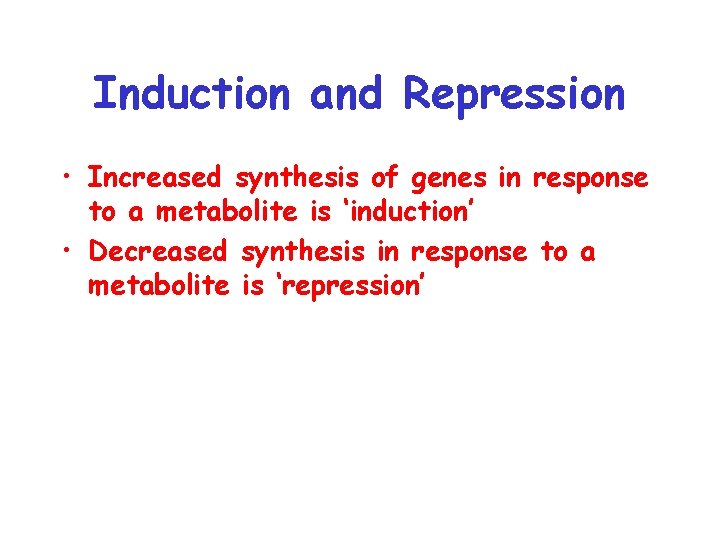
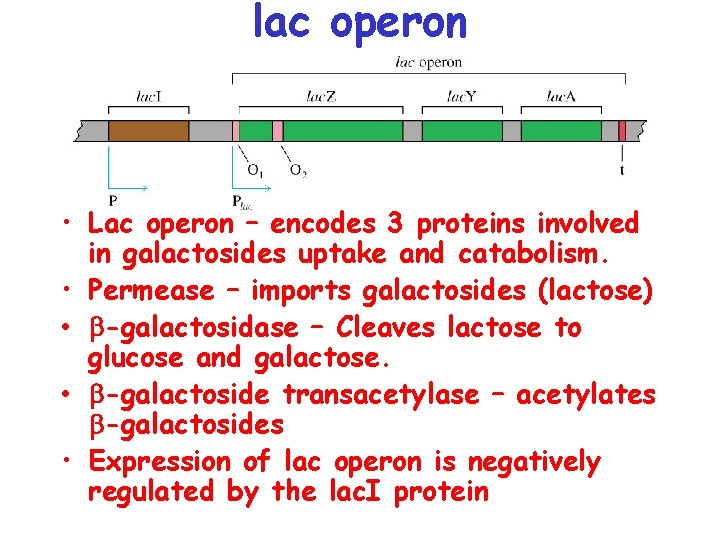
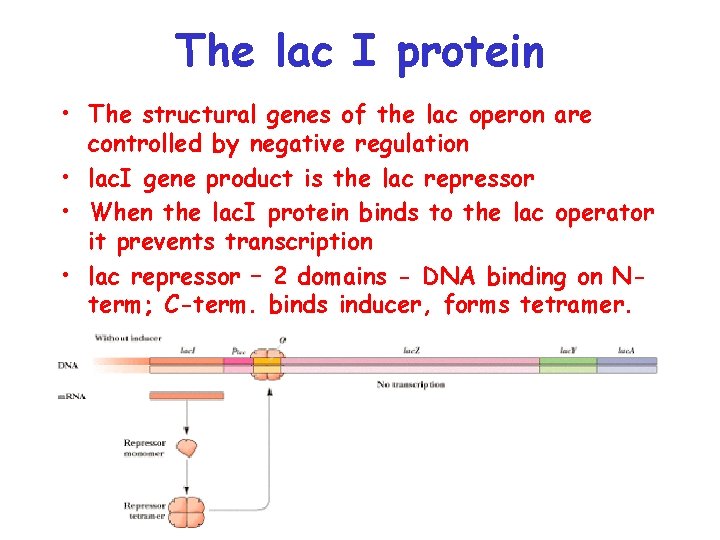
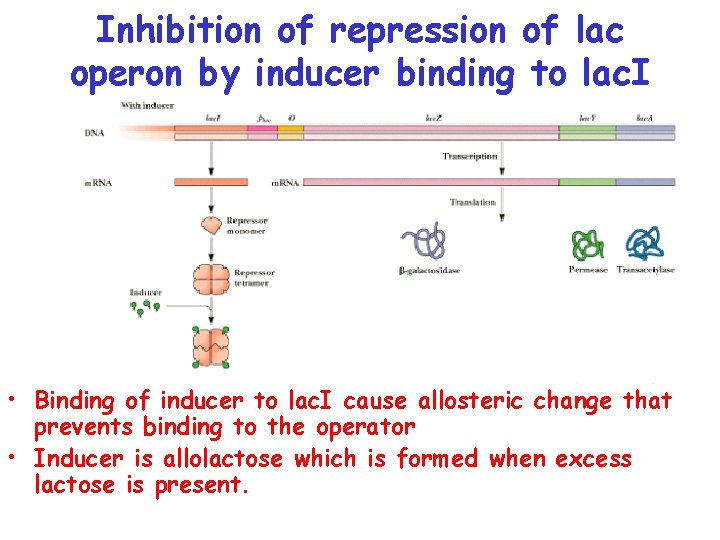
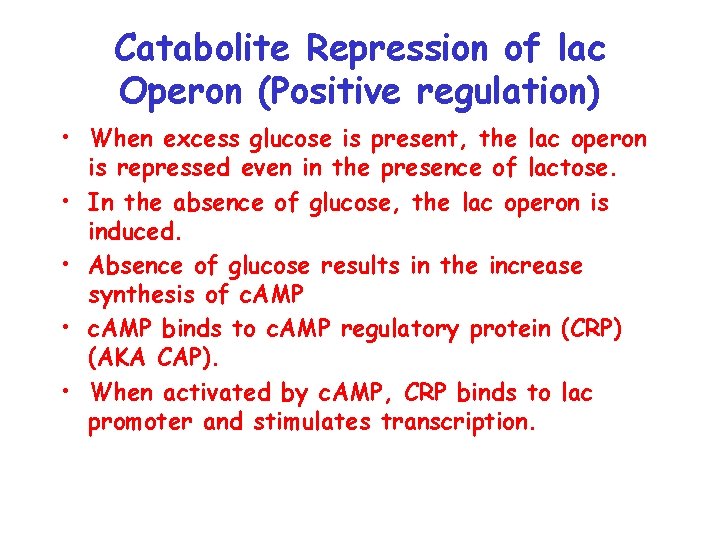
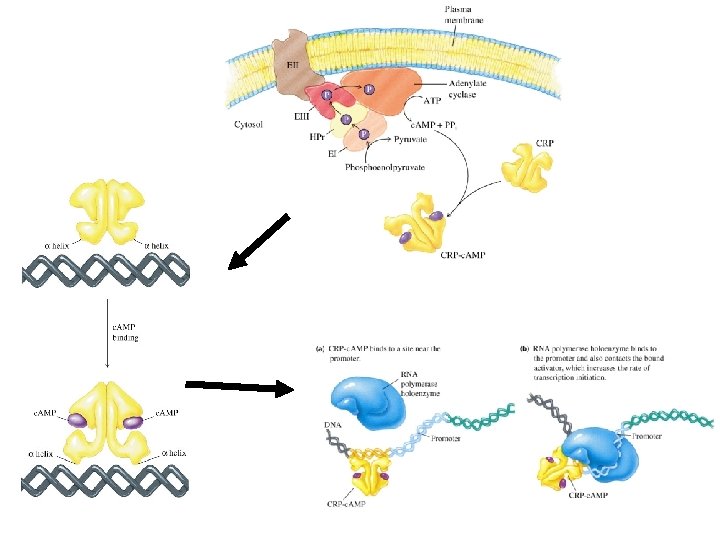
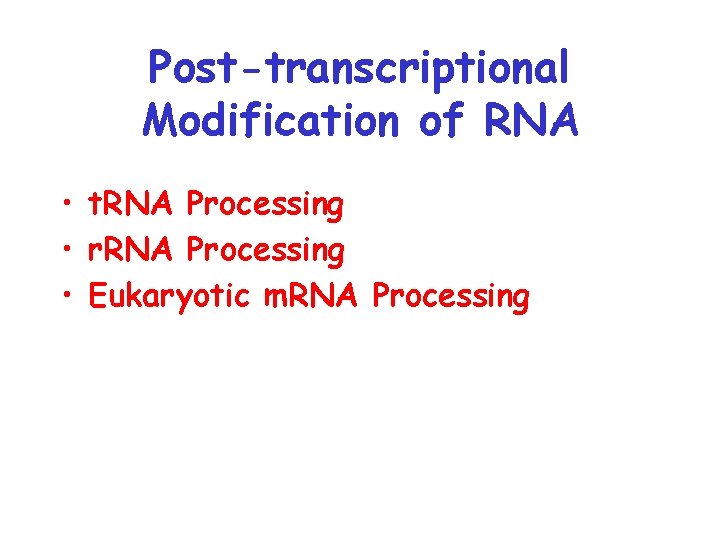
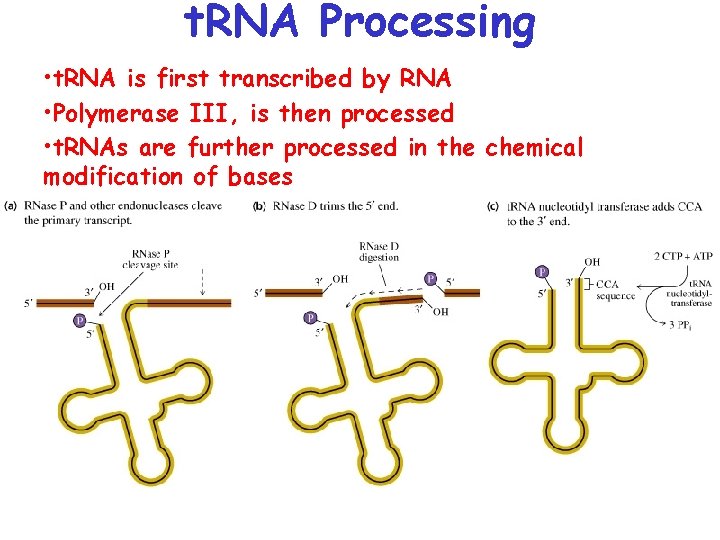
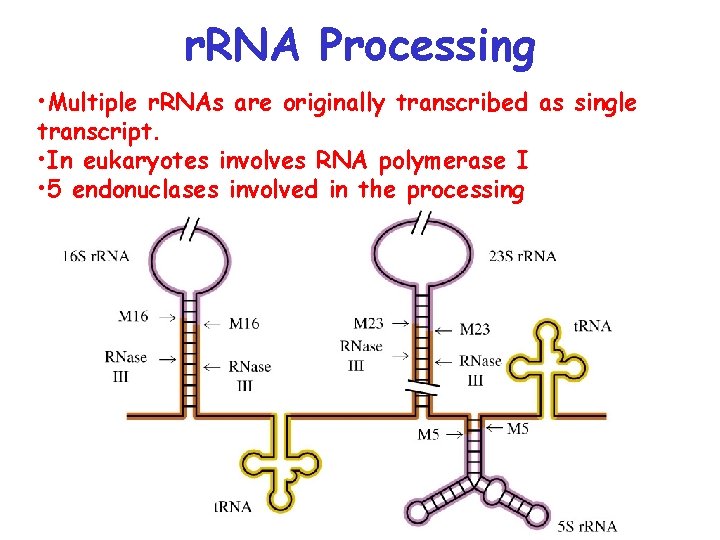
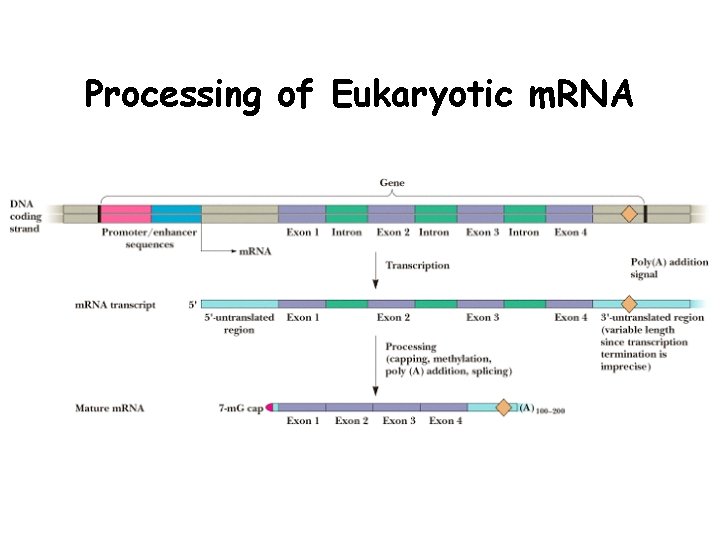
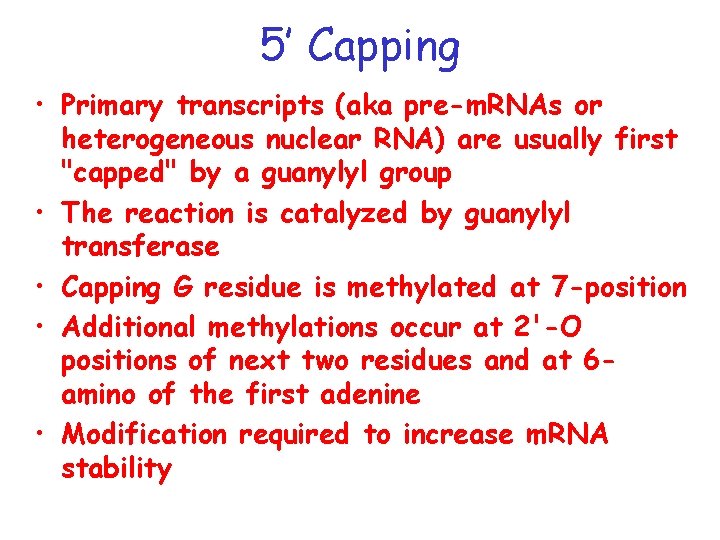
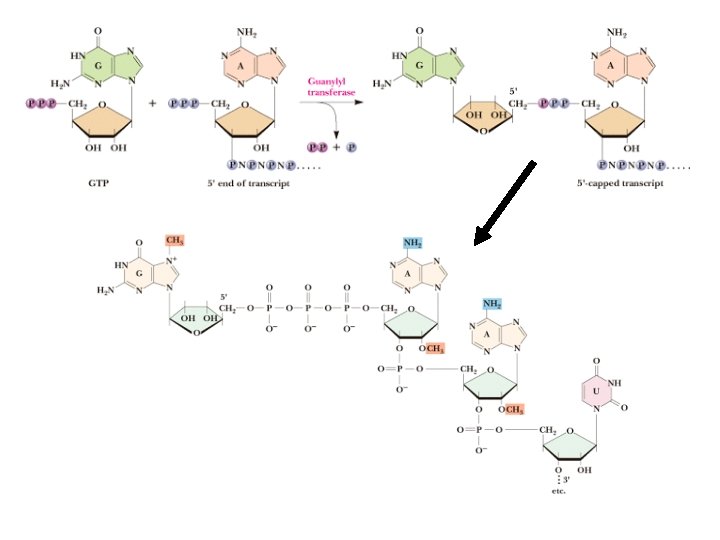
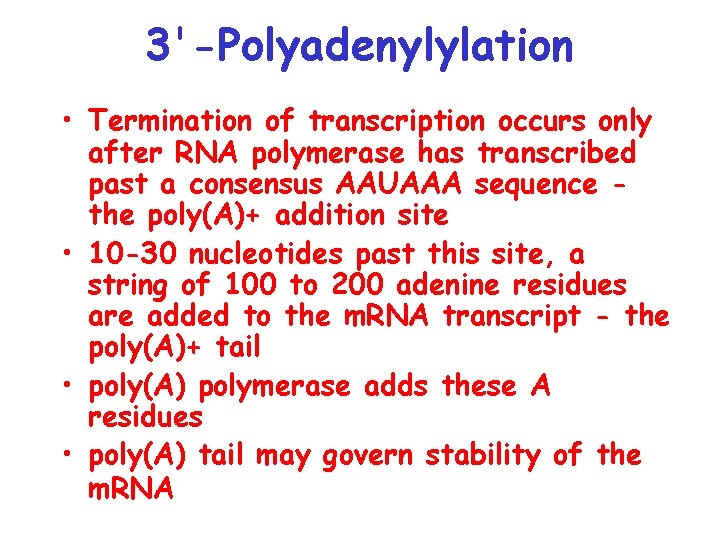
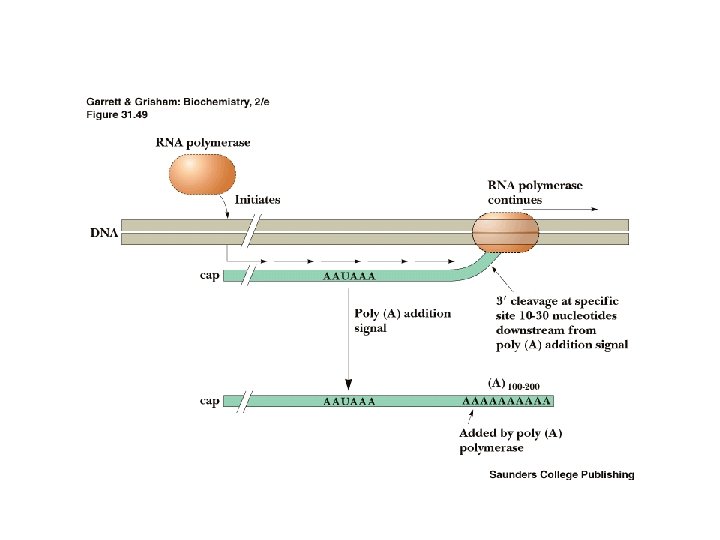
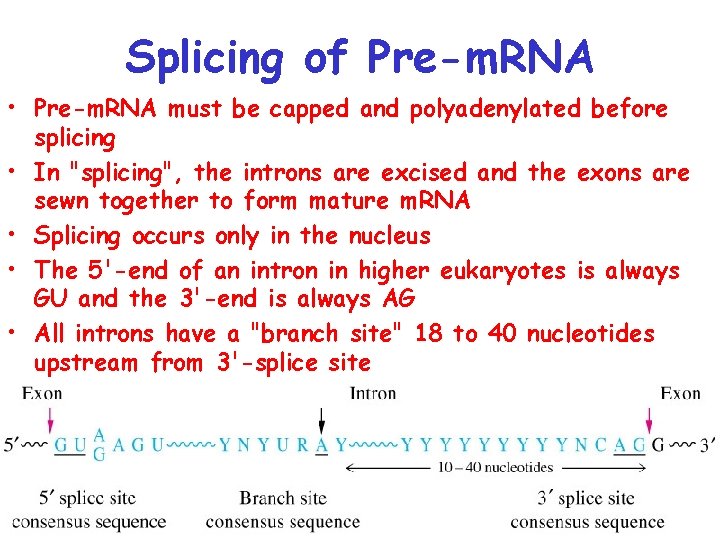
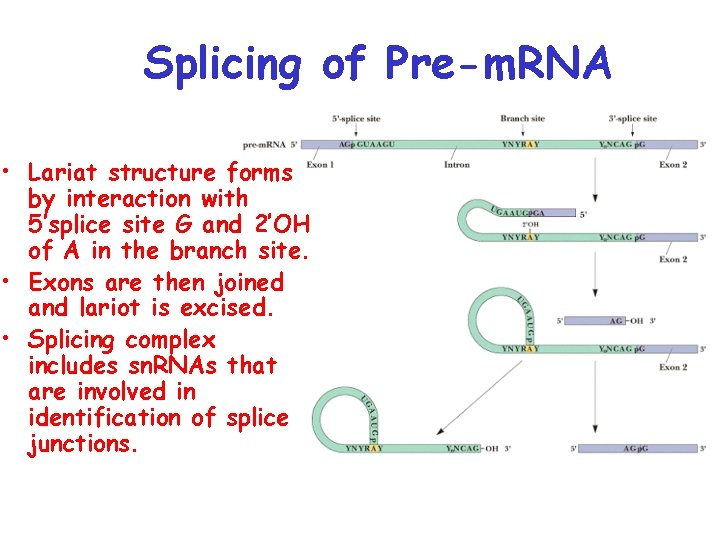
- Slides: 61
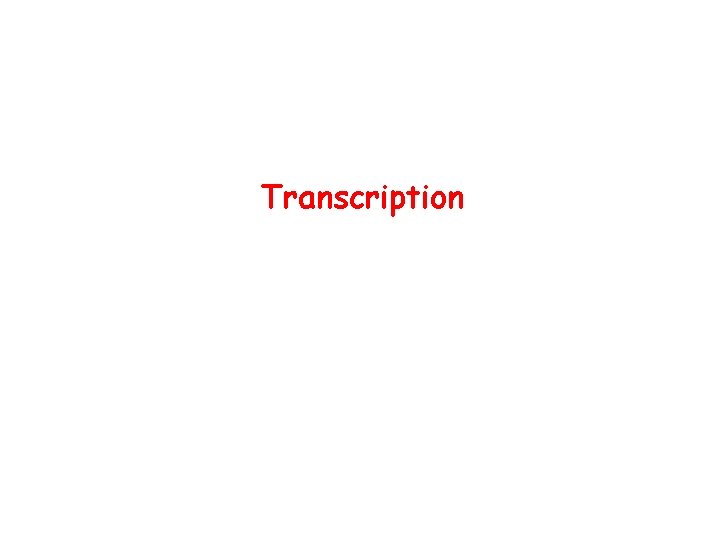
Transcription
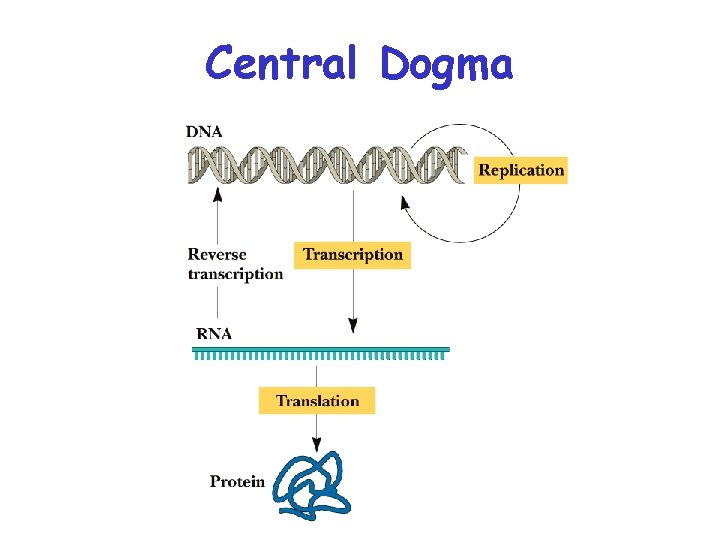
Central Dogma
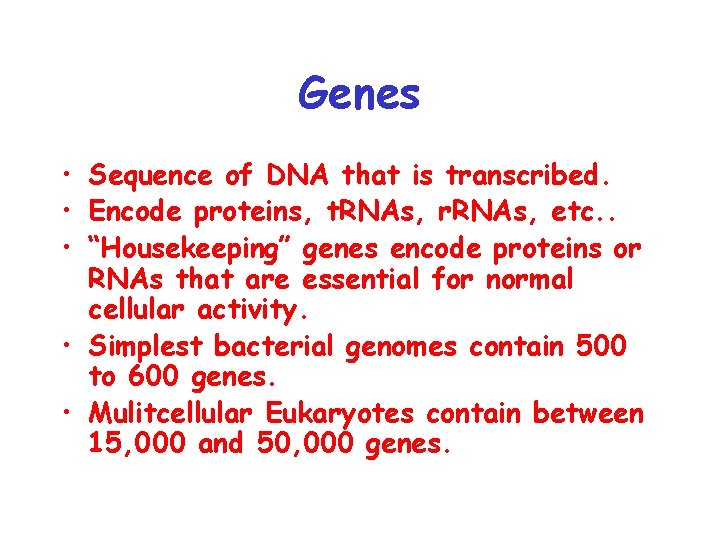
Genes • Sequence of DNA that is transcribed. • Encode proteins, t. RNAs, r. RNAs, etc. . • “Housekeeping” genes encode proteins or RNAs that are essential for normal cellular activity. • Simplest bacterial genomes contain 500 to 600 genes. • Mulitcellular Eukaryotes contain between 15, 000 and 50, 000 genes.
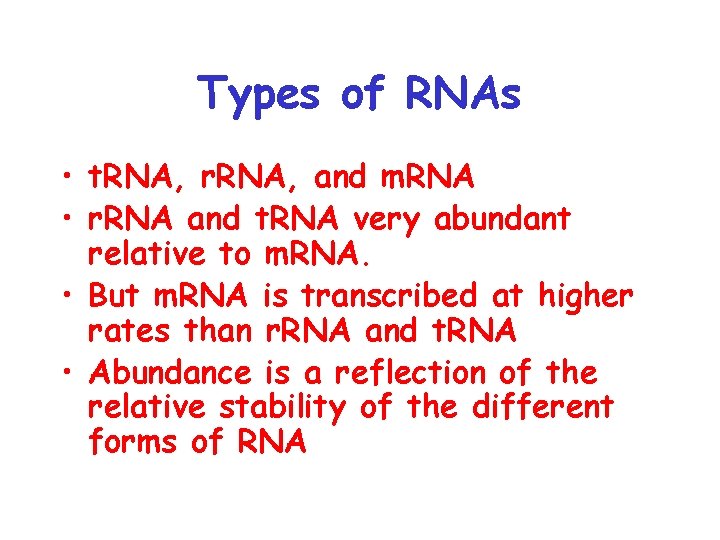
Types of RNAs • t. RNA, r. RNA, and m. RNA • r. RNA and t. RNA very abundant relative to m. RNA. • But m. RNA is transcribed at higher rates than r. RNA and t. RNA • Abundance is a reflection of the relative stability of the different forms of RNA
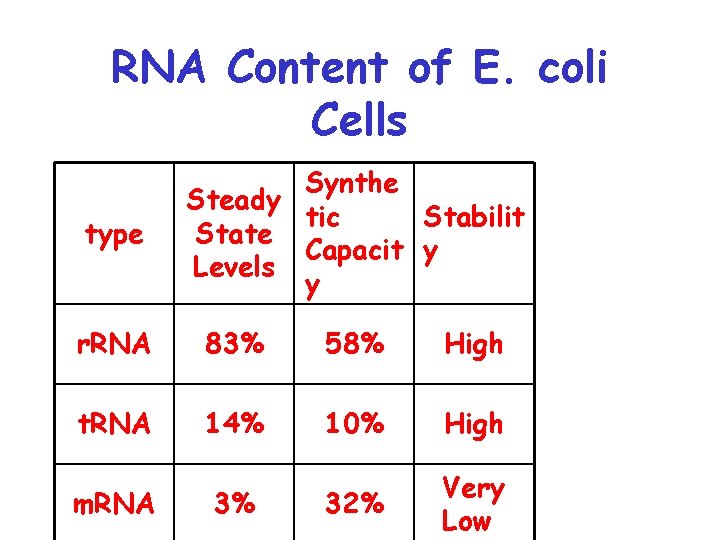
RNA Content of E. coli Cells type Synthe Steady tic Stabilit State Capacit y Levels y r. RNA 83% 58% High t. RNA 14% 10% High 32% Very Low m. RNA 3%
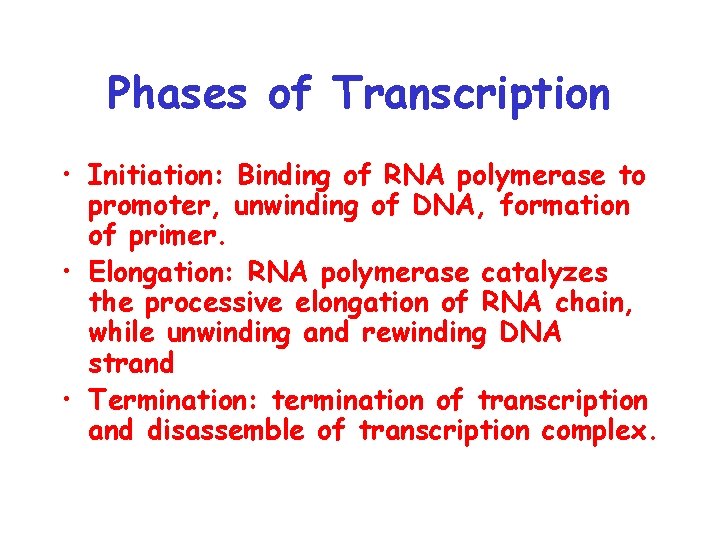
Phases of Transcription • Initiation: Binding of RNA polymerase to promoter, unwinding of DNA, formation of primer. • Elongation: RNA polymerase catalyzes the processive elongation of RNA chain, while unwinding and rewinding DNA strand • Termination: termination of transcription and disassemble of transcription complex.
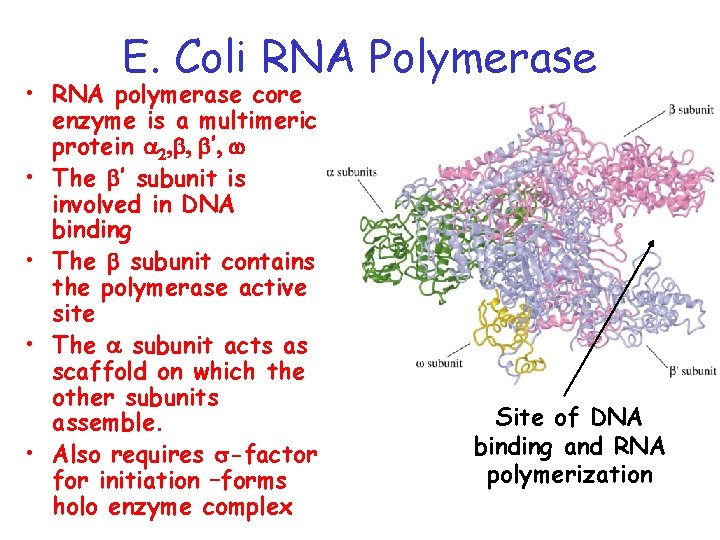
E. Coli RNA Polymerase • RNA polymerase core enzyme is a multimeric protein a 2, , ’, w • The ’ subunit is involved in DNA binding • The subunit contains the polymerase active site • The a subunit acts as scaffold on which the other subunits assemble. • Also requires s-factor for initiation –forms holo enzyme complex Site of DNA binding and RNA polymerization
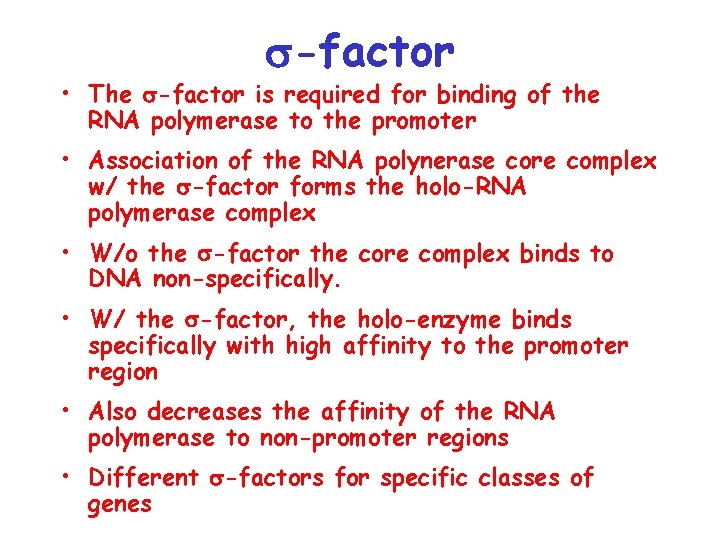
s-factor • The s-factor is required for binding of the RNA polymerase to the promoter • Association of the RNA polynerase core complex w/ the s-factor forms the holo-RNA polymerase complex • W/o the s-factor the core complex binds to DNA non-specifically. • W/ the s-factor, the holo-enzyme binds specifically with high affinity to the promoter region • Also decreases the affinity of the RNA polymerase to non-promoter regions • Different s-factors for specific classes of genes
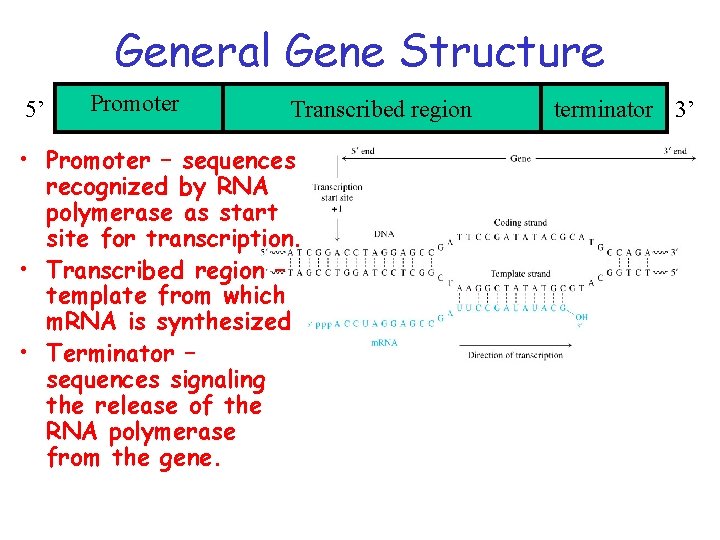
General Gene Structure 5’ Promoter Transcribed region • Promoter – sequences recognized by RNA polymerase as start site for transcription. • Transcribed region – template from which m. RNA is synthesized • Terminator – sequences signaling the release of the RNA polymerase from the gene. terminator 3’
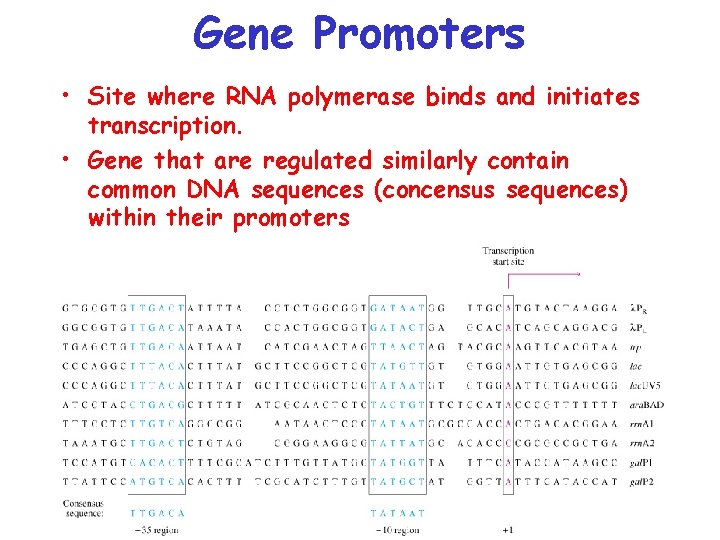
Gene Promoters • Site where RNA polymerase binds and initiates transcription. • Gene that are regulated similarly contain common DNA sequences (concensus sequences) within their promoters
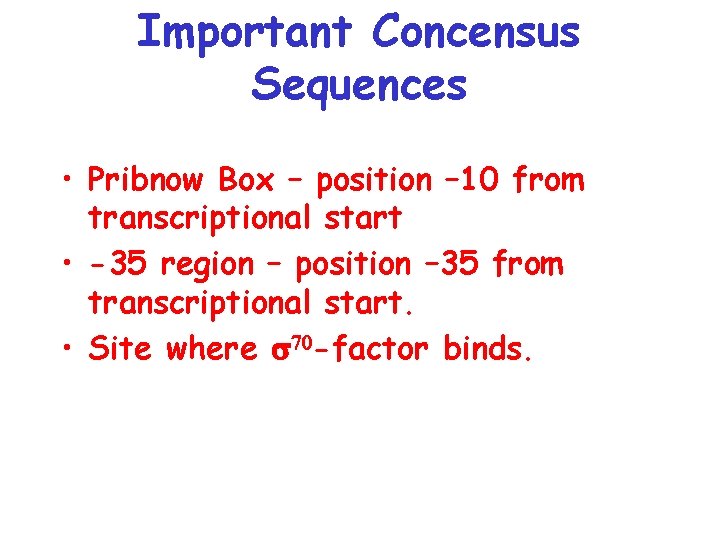
Important Concensus Sequences • Pribnow Box – position – 10 from transcriptional start • -35 region – position – 35 from transcriptional start. • Site where s 70 -factor binds.
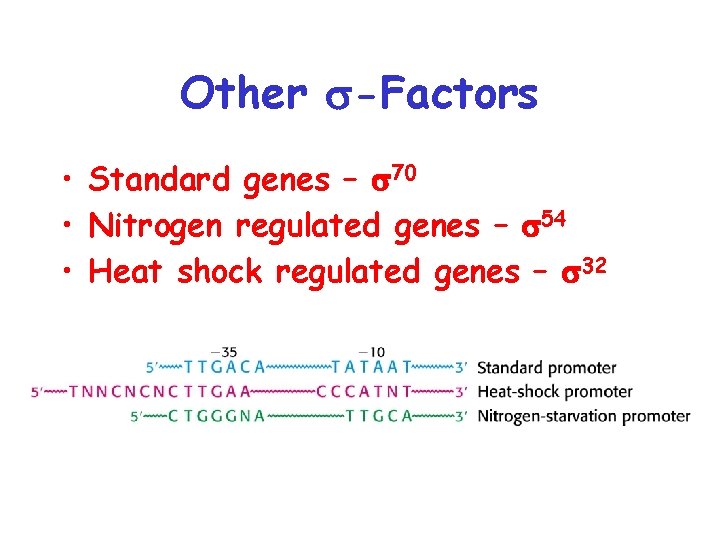
Other s-Factors • Standard genes – s 70 • Nitrogen regulated genes – s 54 • Heat shock regulated genes – s 32
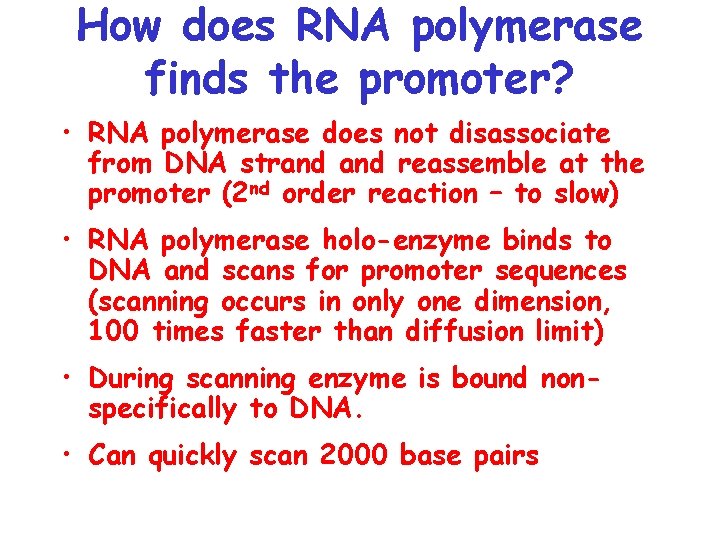
How does RNA polymerase finds the promoter? • RNA polymerase does not disassociate from DNA strand reassemble at the promoter (2 nd order reaction – to slow) • RNA polymerase holo-enzyme binds to DNA and scans for promoter sequences (scanning occurs in only one dimension, 100 times faster than diffusion limit) • During scanning enzyme is bound nonspecifically to DNA. • Can quickly scan 2000 base pairs
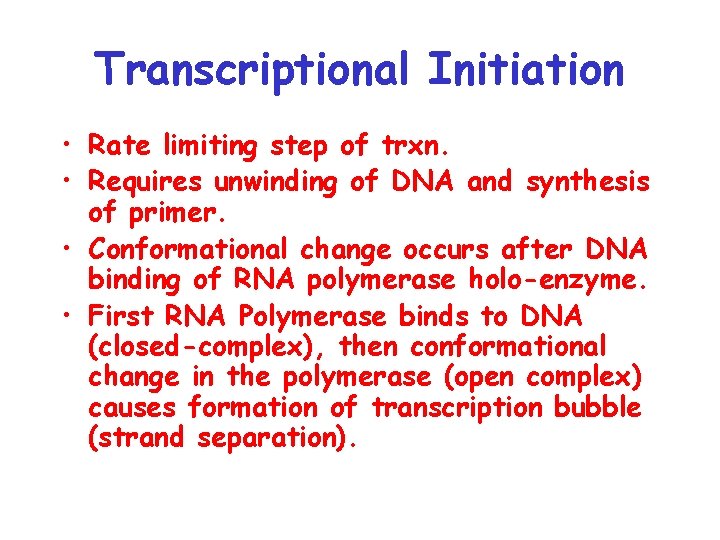
Transcriptional Initiation • Rate limiting step of trxn. • Requires unwinding of DNA and synthesis of primer. • Conformational change occurs after DNA binding of RNA polymerase holo-enzyme. • First RNA Polymerase binds to DNA (closed-complex), then conformational change in the polymerase (open complex) causes formation of transcription bubble (strand separation).
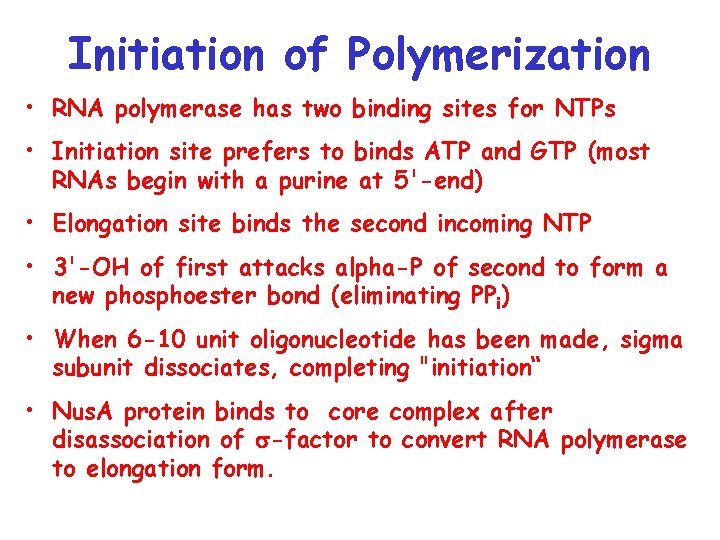
Initiation of Polymerization • RNA polymerase has two binding sites for NTPs • Initiation site prefers to binds ATP and GTP (most RNAs begin with a purine at 5'-end) • Elongation site binds the second incoming NTP • 3'-OH of first attacks alpha-P of second to form a new phosphoester bond (eliminating PPi) • When 6 -10 unit oligonucleotide has been made, sigma subunit dissociates, completing "initiation“ • Nus. A protein binds to core complex after disassociation of s-factor to convert RNA polymerase to elongation form.
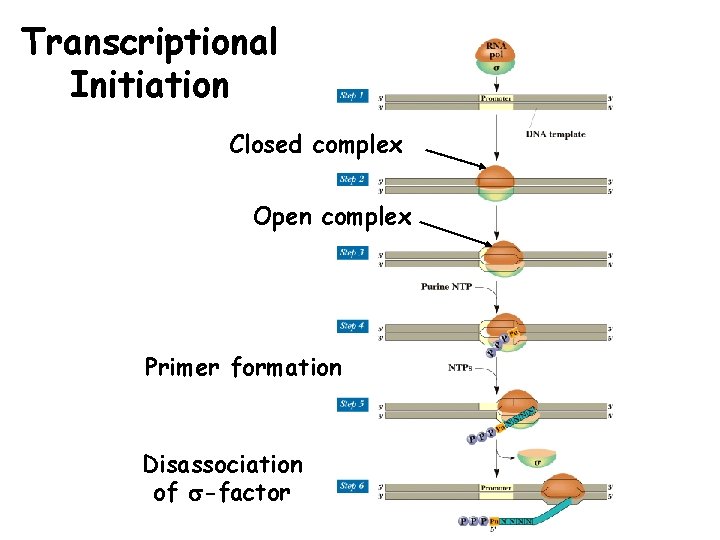
Transcriptional Initiation Closed complex Open complex Primer formation Disassociation of s-factor
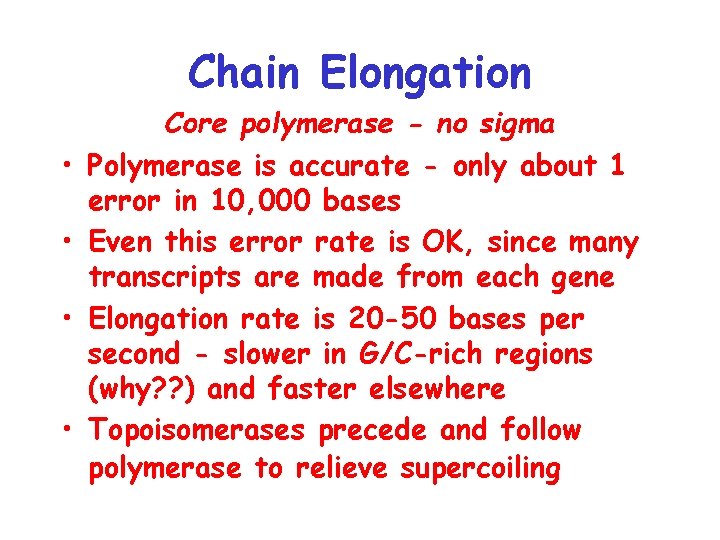
Chain Elongation • • Core polymerase - no sigma Polymerase is accurate - only about 1 error in 10, 000 bases Even this error rate is OK, since many transcripts are made from each gene Elongation rate is 20 -50 bases per second - slower in G/C-rich regions (why? ? ) and faster elsewhere Topoisomerases precede and follow polymerase to relieve supercoiling
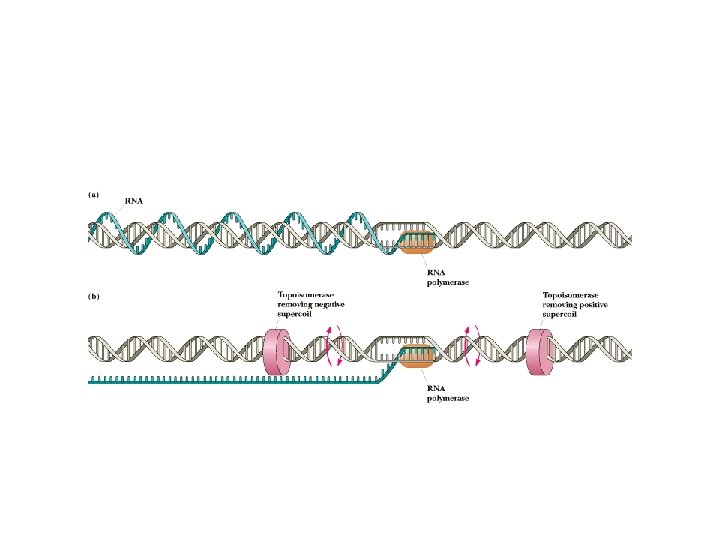
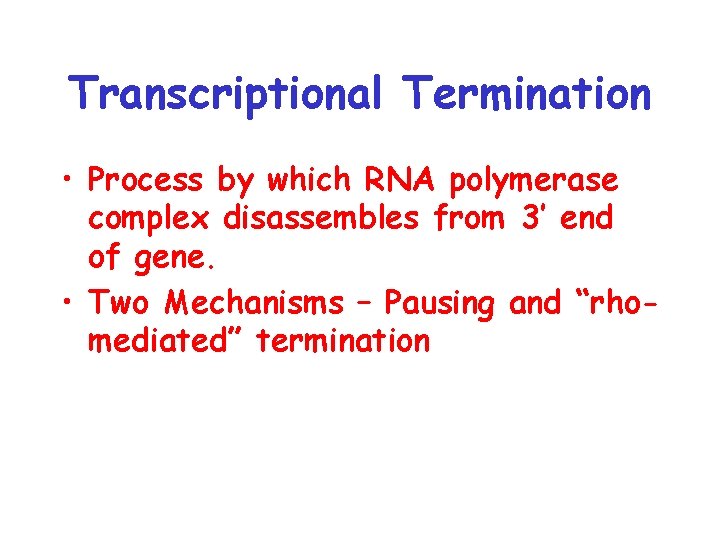
Transcriptional Termination • Process by which RNA polymerase complex disassembles from 3’ end of gene. • Two Mechanisms – Pausing and “rhomediated” termination
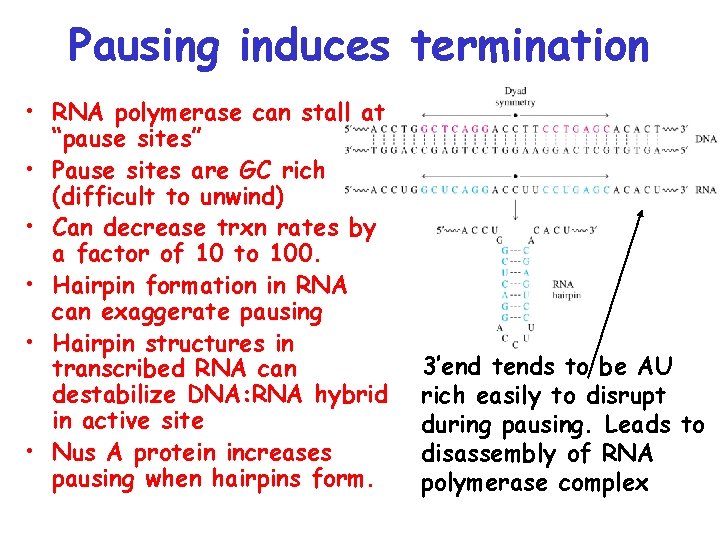
Pausing induces termination • RNA polymerase can stall at “pause sites” • Pause sites are GC rich (difficult to unwind) • Can decrease trxn rates by a factor of 10 to 100. • Hairpin formation in RNA can exaggerate pausing • Hairpin structures in transcribed RNA can destabilize DNA: RNA hybrid in active site • Nus A protein increases pausing when hairpins form. 3’end tends to be AU rich easily to disrupt during pausing. Leads to disassembly of RNA polymerase complex
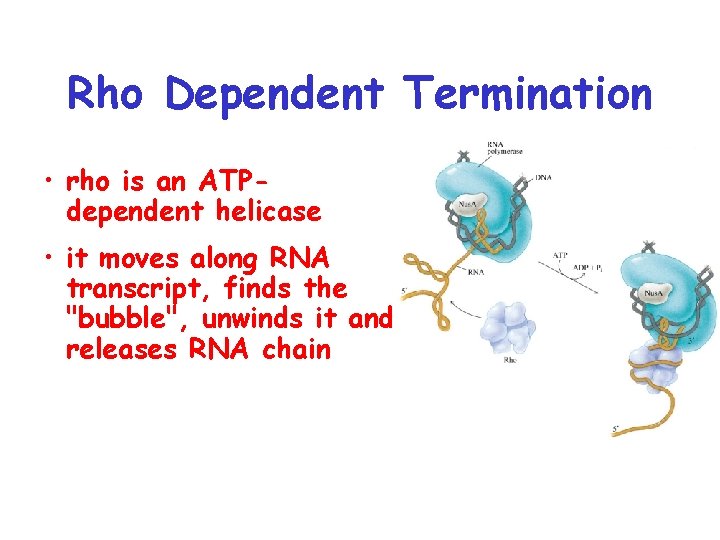
Rho Dependent Termination • rho is an ATPdependent helicase • it moves along RNA transcript, finds the "bubble", unwinds it and releases RNA chain
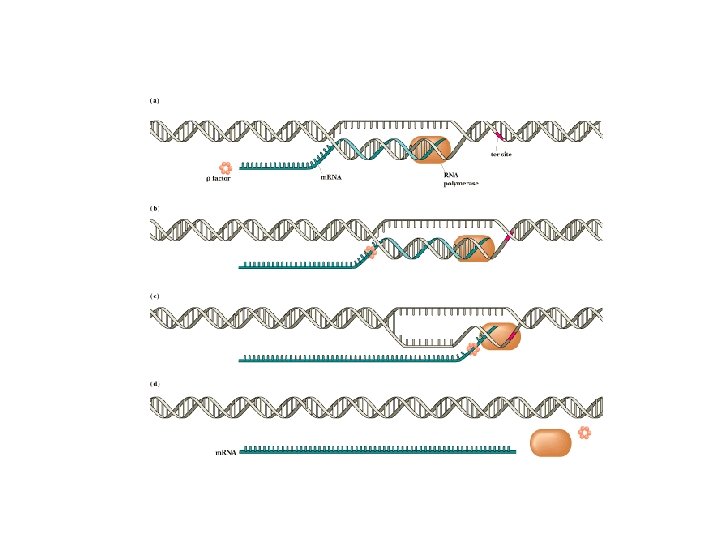
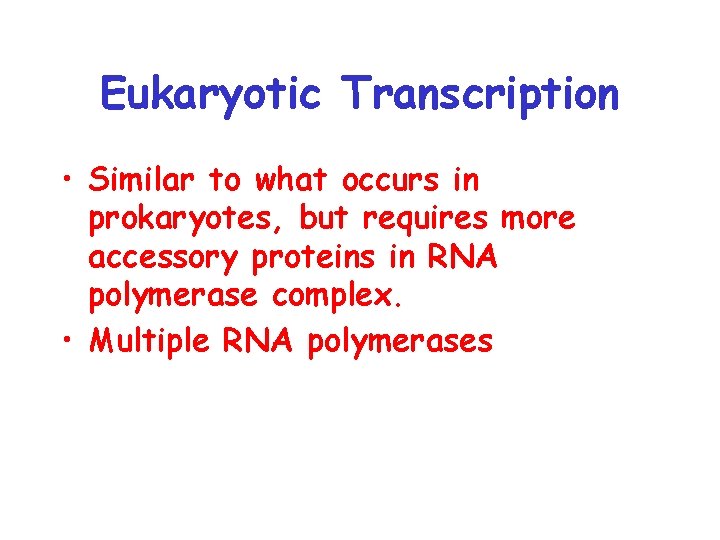
Eukaryotic Transcription • Similar to what occurs in prokaryotes, but requires more accessory proteins in RNA polymerase complex. • Multiple RNA polymerases
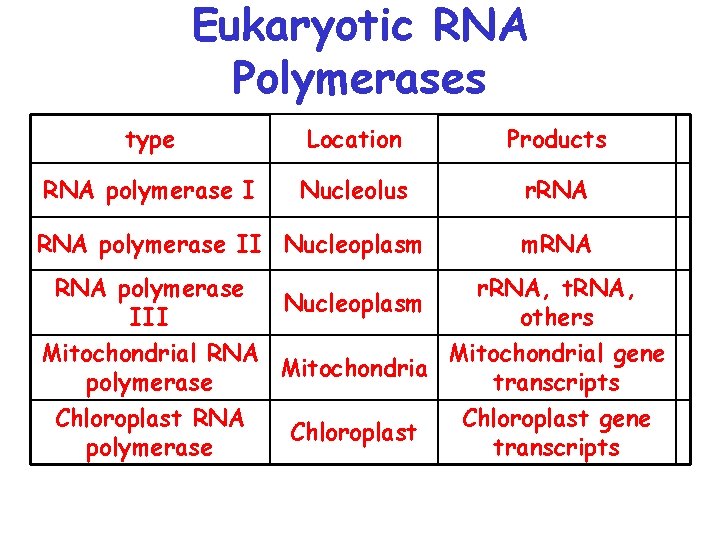
Eukaryotic RNA Polymerases type Location Products RNA polymerase I Nucleolus r. RNA polymerase II Nucleoplasm RNA polymerase III Nucleoplasm m. RNA r. RNA, t. RNA, others Mitochondrial RNA Mitochondrial gene Mitochondria polymerase transcripts Chloroplast RNA Chloroplast gene Chloroplast polymerase transcripts
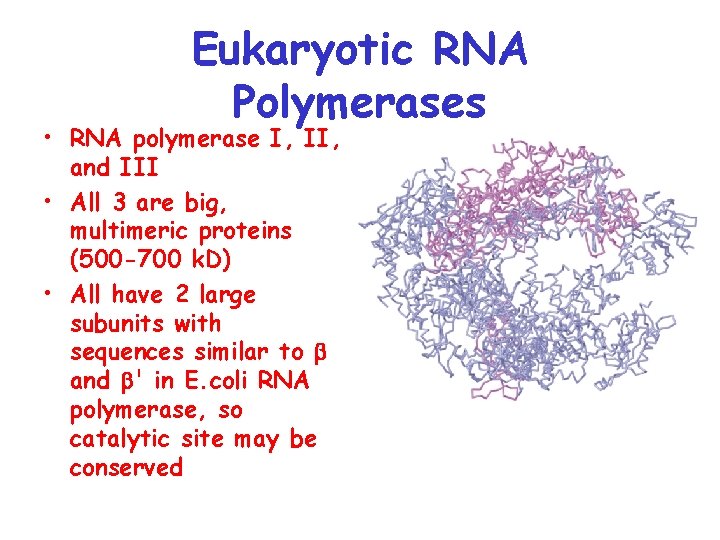
Eukaryotic RNA Polymerases • RNA polymerase I, II, and III • All 3 are big, multimeric proteins (500 -700 k. D) • All have 2 large subunits with sequences similar to and ' in E. coli RNA polymerase, so catalytic site may be conserved
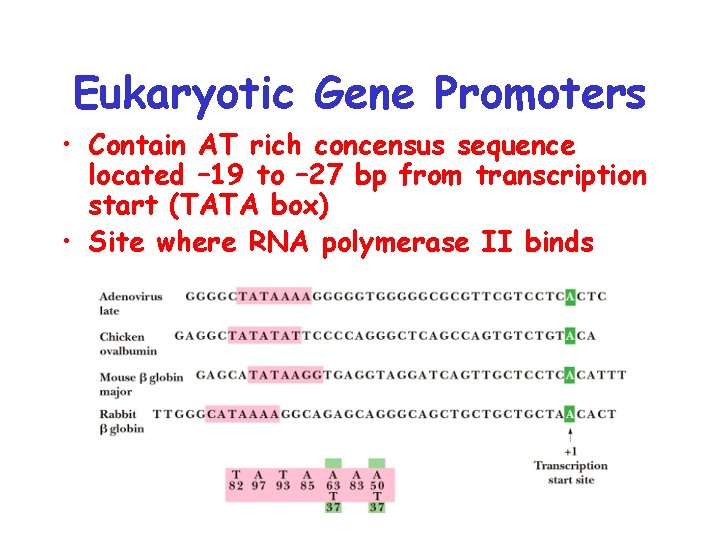
Eukaryotic Gene Promoters • Contain AT rich concensus sequence located – 19 to – 27 bp from transcription start (TATA box) • Site where RNA polymerase II binds
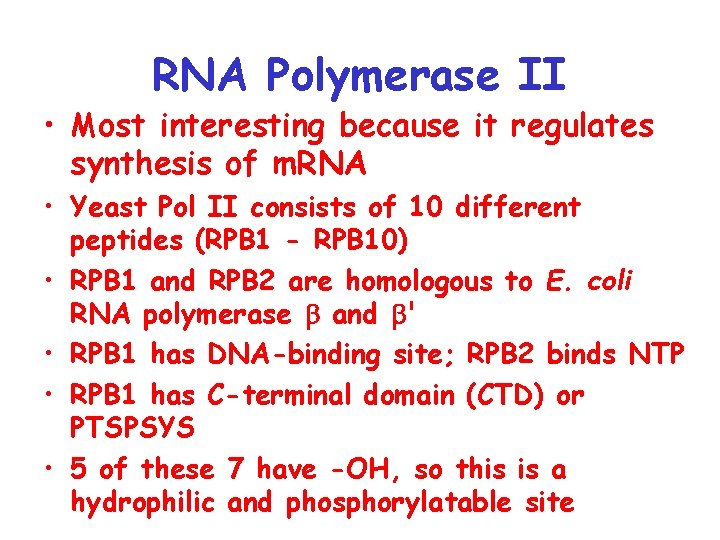
RNA Polymerase II • Most interesting because it regulates synthesis of m. RNA • Yeast Pol II consists of 10 different peptides (RPB 1 - RPB 10) • RPB 1 and RPB 2 are homologous to E. coli RNA polymerase and ' • RPB 1 has DNA-binding site; RPB 2 binds NTP • RPB 1 has C-terminal domain (CTD) or PTSPSYS • 5 of these 7 have -OH, so this is a hydrophilic and phosphorylatable site
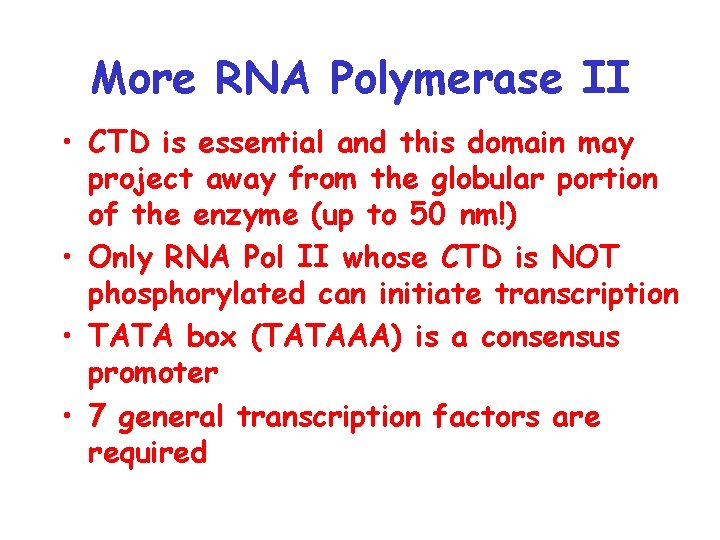
More RNA Polymerase II • CTD is essential and this domain may project away from the globular portion of the enzyme (up to 50 nm!) • Only RNA Pol II whose CTD is NOT phosphorylated can initiate transcription • TATA box (TATAAA) is a consensus promoter • 7 general transcription factors are required
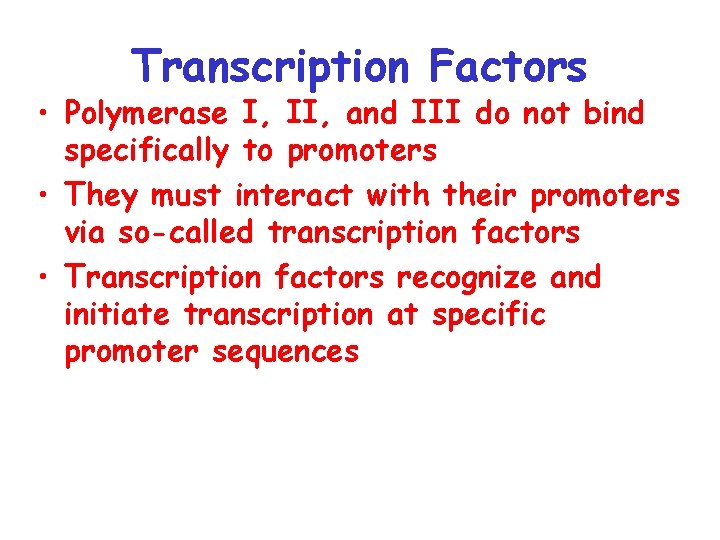
Transcription Factors • Polymerase I, II, and III do not bind specifically to promoters • They must interact with their promoters via so-called transcription factors • Transcription factors recognize and initiate transcription at specific promoter sequences
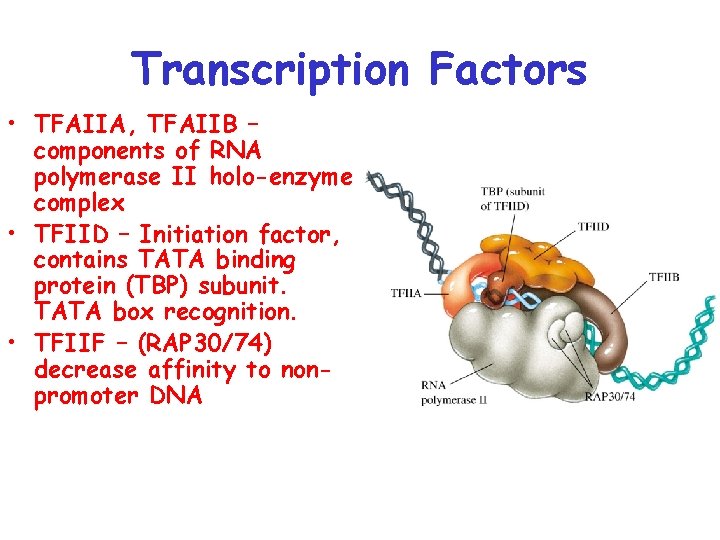
Transcription Factors • TFAIIA, TFAIIB – components of RNA polymerase II holo-enzyme complex • TFIID – Initiation factor, contains TATA binding protein (TBP) subunit. TATA box recognition. • TFIIF – (RAP 30/74) decrease affinity to nonpromoter DNA
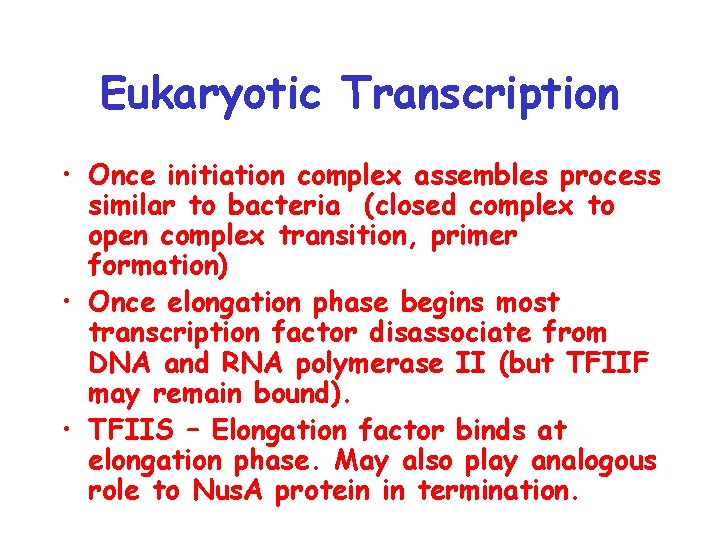
Eukaryotic Transcription • Once initiation complex assembles process similar to bacteria (closed complex to open complex transition, primer formation) • Once elongation phase begins most transcription factor disassociate from DNA and RNA polymerase II (but TFIIF may remain bound). • TFIIS – Elongation factor binds at elongation phase. May also play analogous role to Nus. A protein in termination.
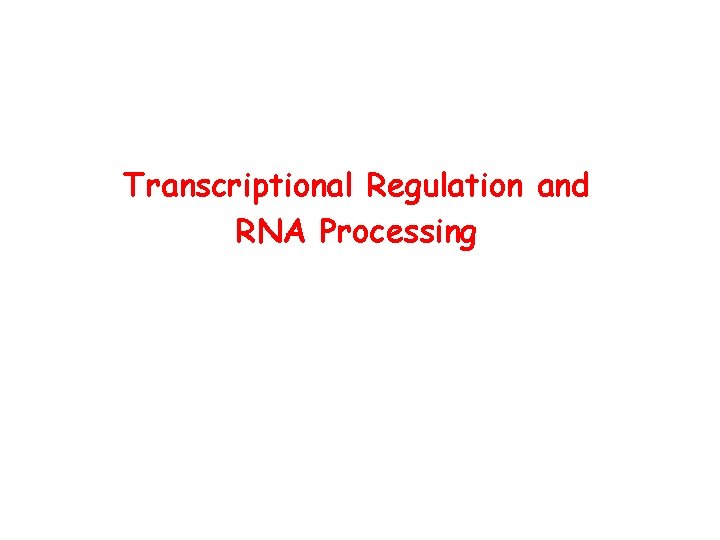
Transcriptional Regulation and RNA Processing
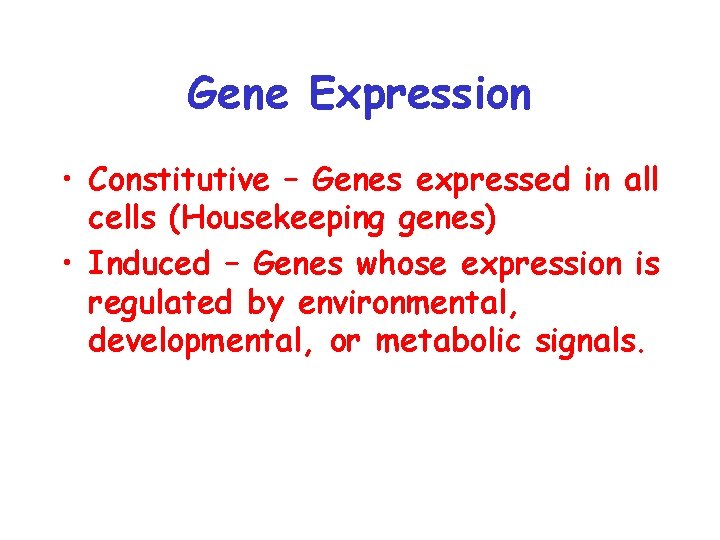
Gene Expression • Constitutive – Genes expressed in all cells (Housekeeping genes) • Induced – Genes whose expression is regulated by environmental, developmental, or metabolic signals.
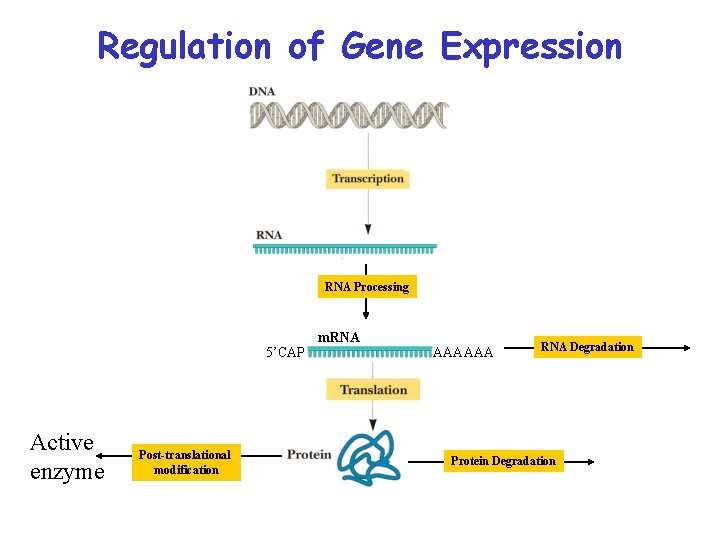
Regulation of Gene Expression RNA Processing 5’CAP Active enzyme Post-translational modification m. RNA AAAAAA RNA Degradation Protein Degradation
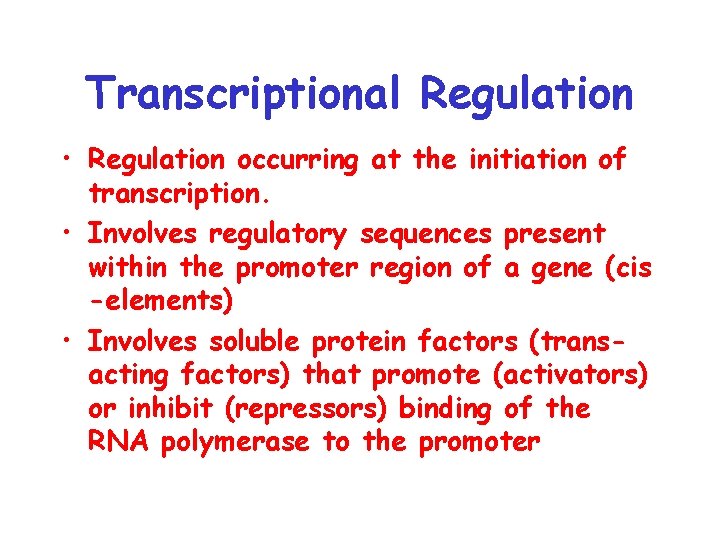
Transcriptional Regulation • Regulation occurring at the initiation of transcription. • Involves regulatory sequences present within the promoter region of a gene (cis -elements) • Involves soluble protein factors (transacting factors) that promote (activators) or inhibit (repressors) binding of the RNA polymerase to the promoter
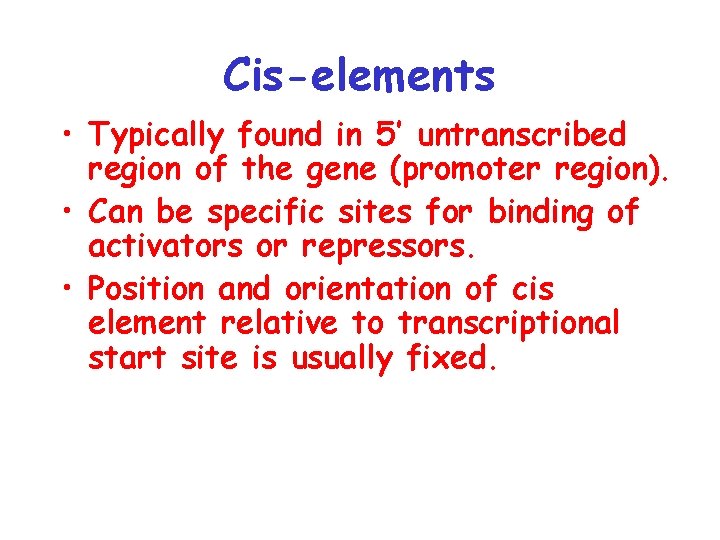
Cis-elements • Typically found in 5’ untranscribed region of the gene (promoter region). • Can be specific sites for binding of activators or repressors. • Position and orientation of cis element relative to transcriptional start site is usually fixed.
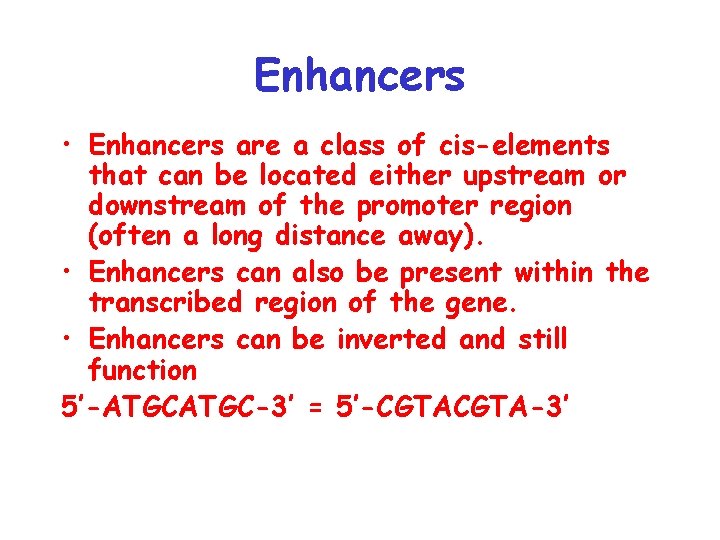
Enhancers • Enhancers are a class of cis-elements that can be located either upstream or downstream of the promoter region (often a long distance away). • Enhancers can also be present within the transcribed region of the gene. • Enhancers can be inverted and still function 5’-ATGC-3’ = 5’-CGTA-3’
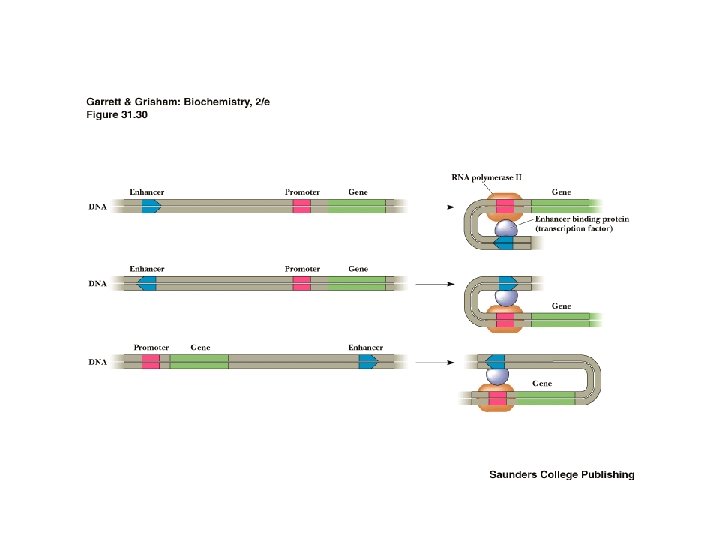
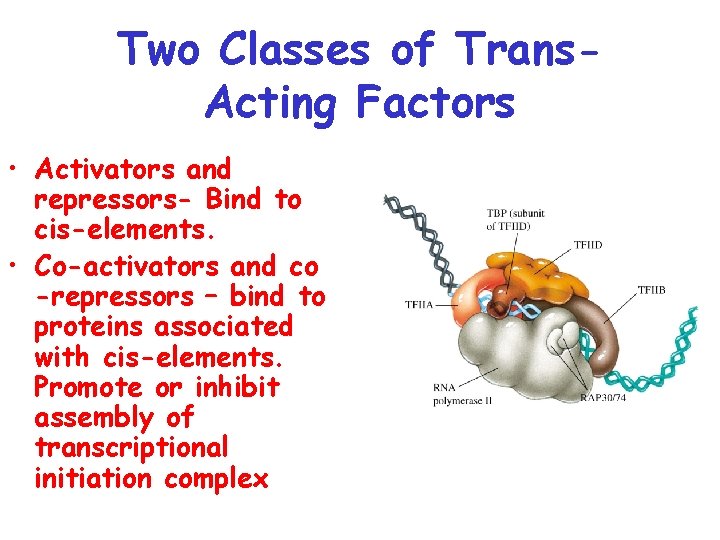
Two Classes of Trans. Acting Factors • Activators and repressors- Bind to cis-elements. • Co-activators and co -repressors – bind to proteins associated with cis-elements. Promote or inhibit assembly of transcriptional initiation complex
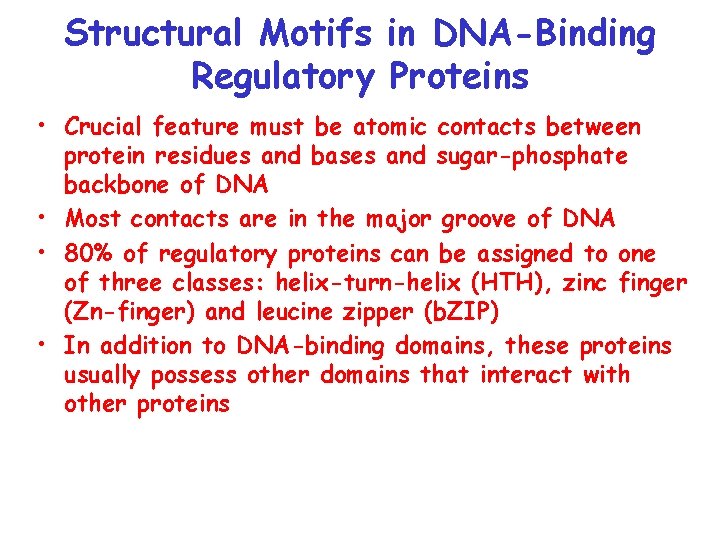
Structural Motifs in DNA-Binding Regulatory Proteins • Crucial feature must be atomic contacts between protein residues and bases and sugar-phosphate backbone of DNA • Most contacts are in the major groove of DNA • 80% of regulatory proteins can be assigned to one of three classes: helix-turn-helix (HTH), zinc finger (Zn-finger) and leucine zipper (b. ZIP) • In addition to DNA-binding domains, these proteins usually possess other domains that interact with other proteins
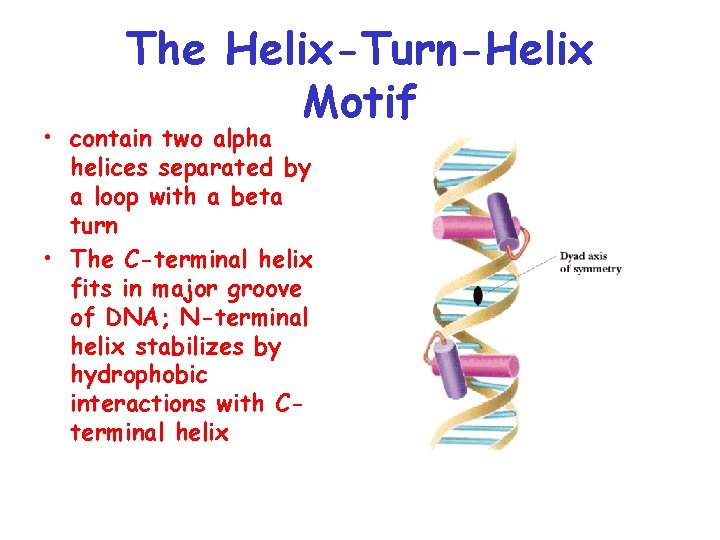
The Helix-Turn-Helix Motif • contain two alpha helices separated by a loop with a beta turn • The C-terminal helix fits in major groove of DNA; N-terminal helix stabilizes by hydrophobic interactions with Cterminal helix
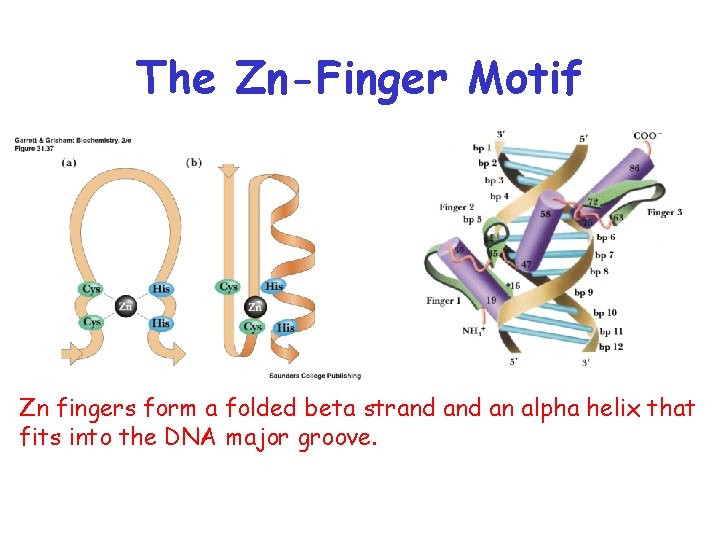
The Zn-Finger Motif Zn fingers form a folded beta strand an alpha helix that fits into the DNA major groove.
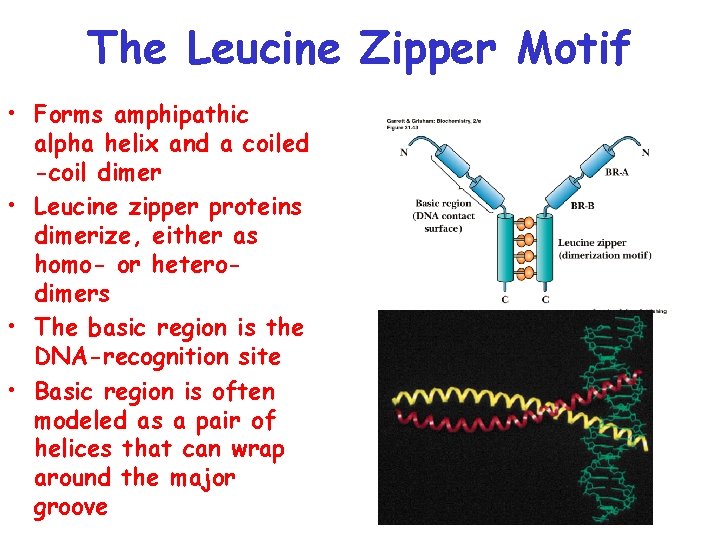
The Leucine Zipper Motif • Forms amphipathic alpha helix and a coiled -coil dimer • Leucine zipper proteins dimerize, either as homo- or heterodimers • The basic region is the DNA-recognition site • Basic region is often modeled as a pair of helices that can wrap around the major groove
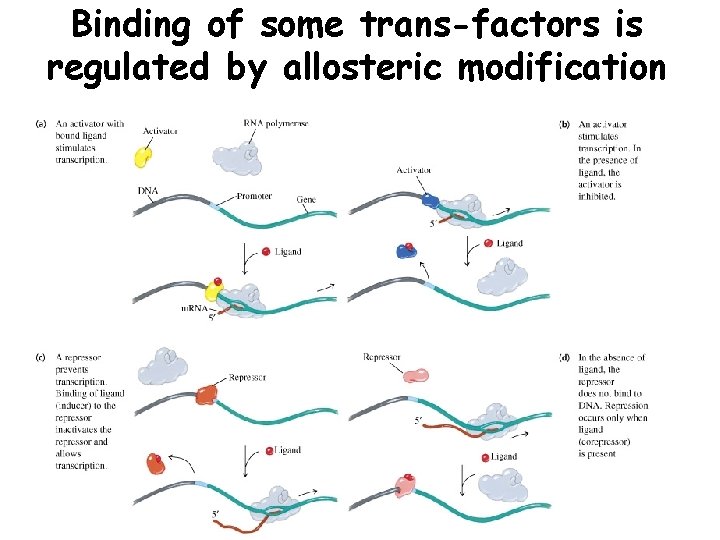
Binding of some trans-factors is regulated by allosteric modification
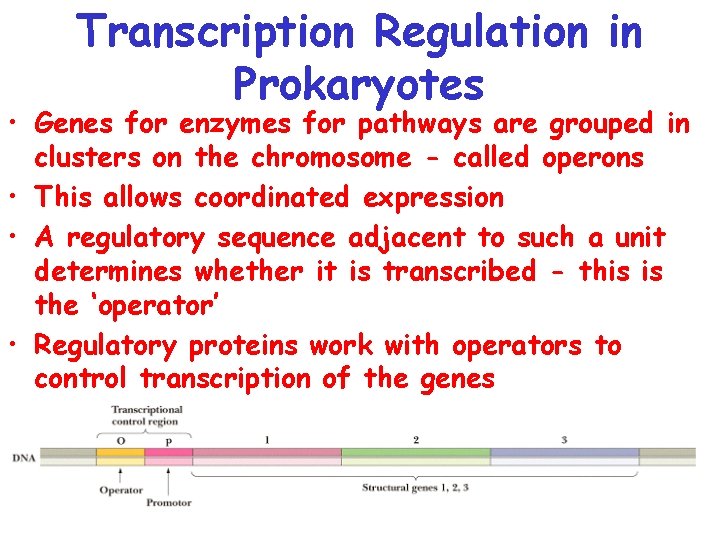
Transcription Regulation in Prokaryotes • Genes for enzymes for pathways are grouped in clusters on the chromosome - called operons • This allows coordinated expression • A regulatory sequence adjacent to such a unit determines whether it is transcribed - this is the ‘operator’ • Regulatory proteins work with operators to control transcription of the genes
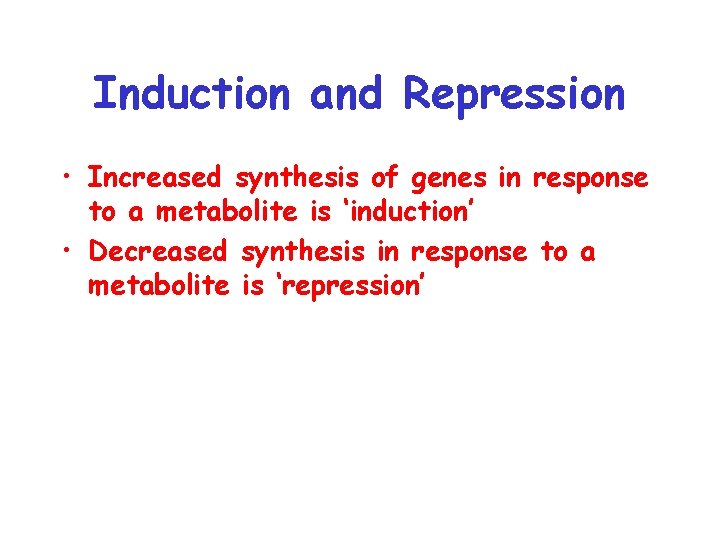
Induction and Repression • Increased synthesis of genes in response to a metabolite is ‘induction’ • Decreased synthesis in response to a metabolite is ‘repression’
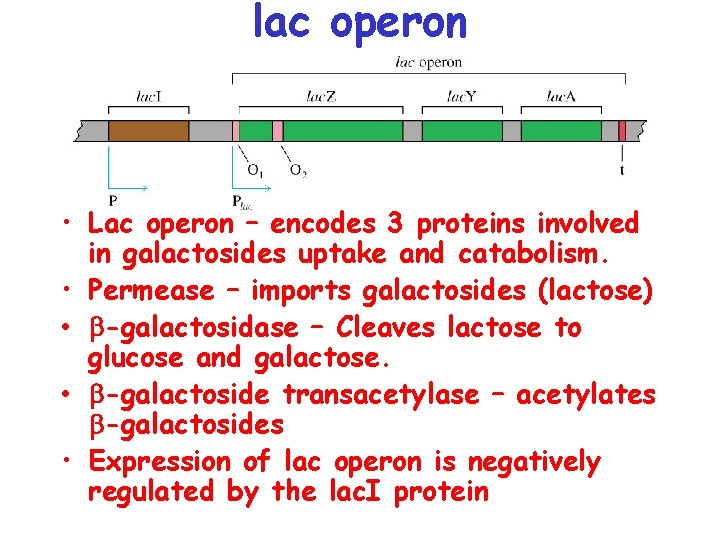
lac operon • Lac operon – encodes 3 proteins involved in galactosides uptake and catabolism. • Permease – imports galactosides (lactose) • -galactosidase – Cleaves lactose to glucose and galactose. • -galactoside transacetylase – acetylates -galactosides • Expression of lac operon is negatively regulated by the lac. I protein
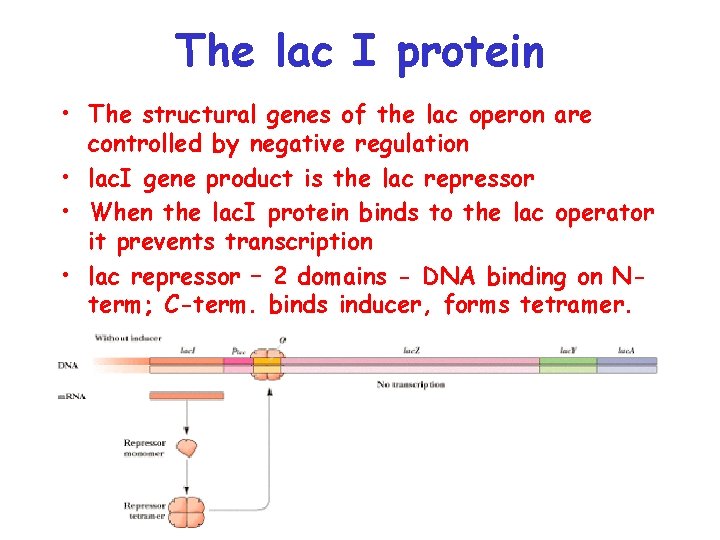
The lac I protein • The structural genes of the lac operon are controlled by negative regulation • lac. I gene product is the lac repressor • When the lac. I protein binds to the lac operator it prevents transcription • lac repressor – 2 domains - DNA binding on Nterm; C-term. binds inducer, forms tetramer.
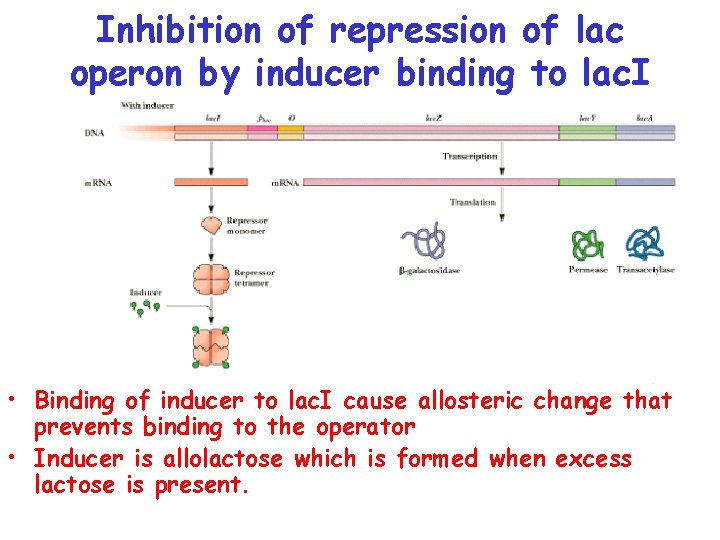
Inhibition of repression of lac operon by inducer binding to lac. I • Binding of inducer to lac. I cause allosteric change that prevents binding to the operator • Inducer is allolactose which is formed when excess lactose is present.
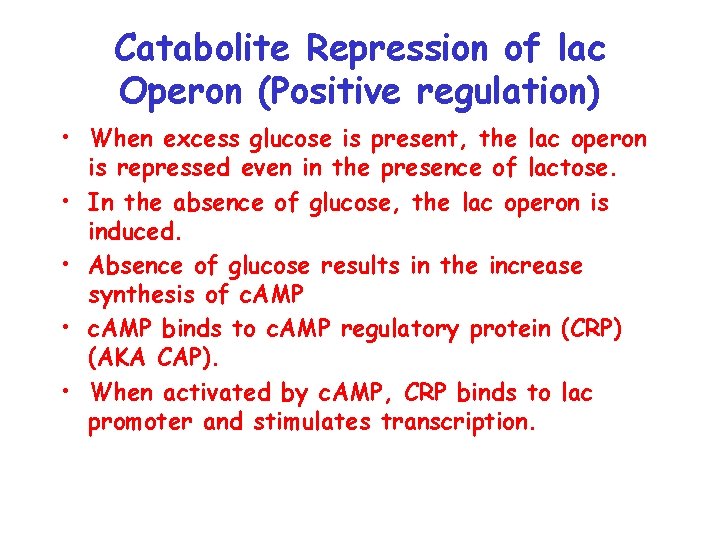
Catabolite Repression of lac Operon (Positive regulation) • When excess glucose is present, the lac operon is repressed even in the presence of lactose. • In the absence of glucose, the lac operon is induced. • Absence of glucose results in the increase synthesis of c. AMP • c. AMP binds to c. AMP regulatory protein (CRP) (AKA CAP). • When activated by c. AMP, CRP binds to lac promoter and stimulates transcription.
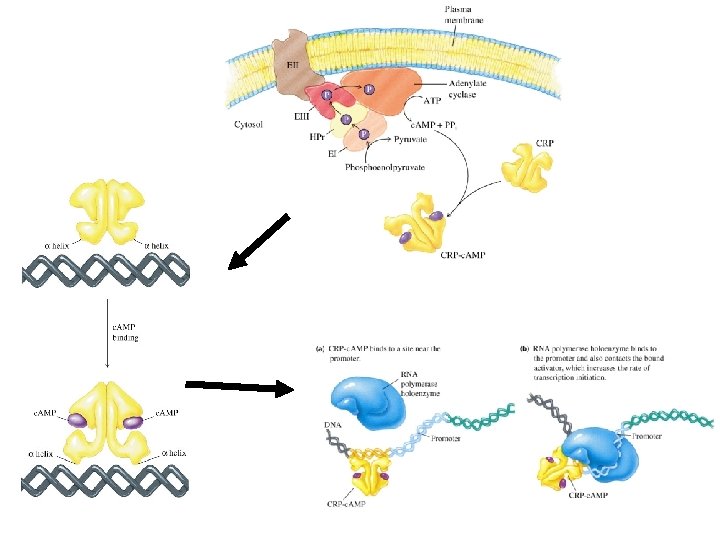
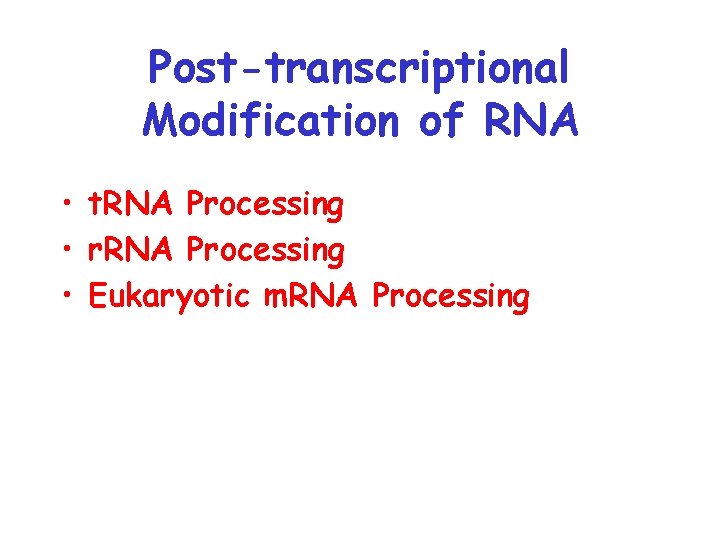
Post-transcriptional Modification of RNA • t. RNA Processing • r. RNA Processing • Eukaryotic m. RNA Processing
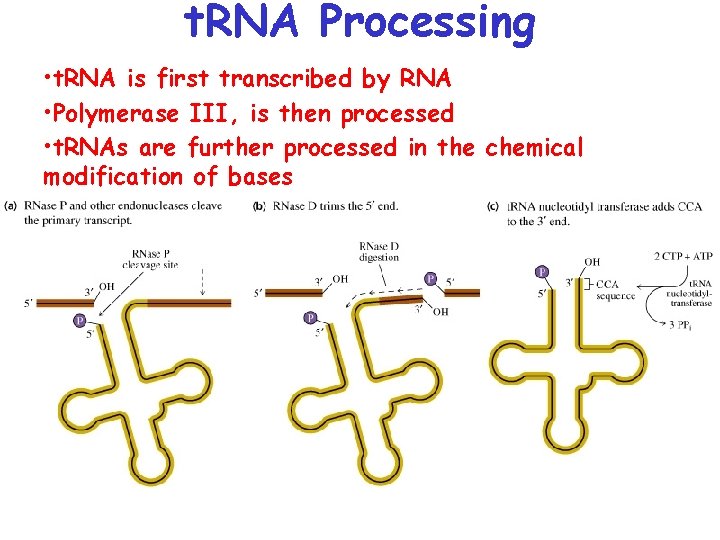
t. RNA Processing • t. RNA is first transcribed by RNA • Polymerase III, is then processed • t. RNAs are further processed in the chemical modification of bases
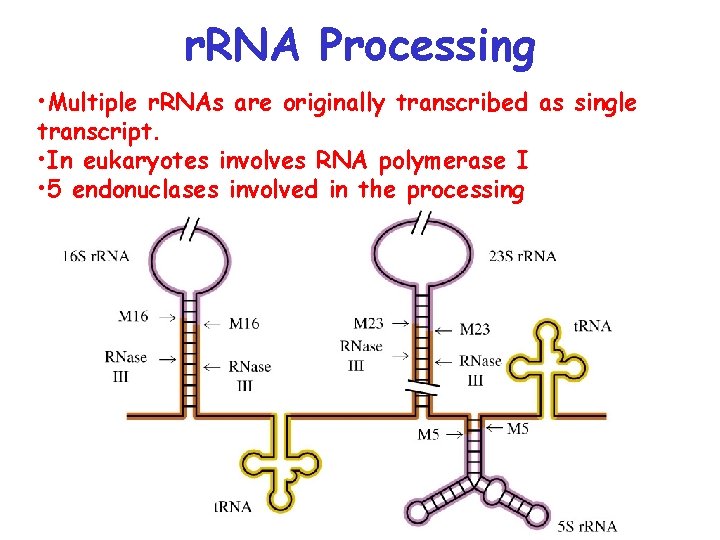
r. RNA Processing • Multiple r. RNAs are originally transcribed as single transcript. • In eukaryotes involves RNA polymerase I • 5 endonuclases involved in the processing
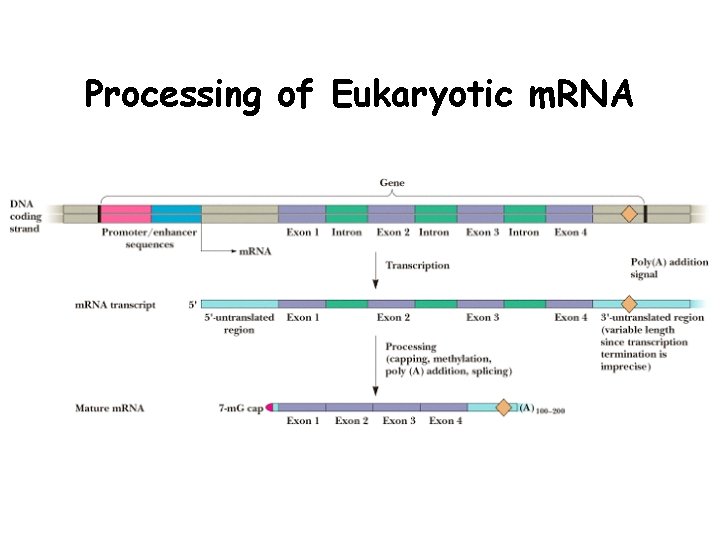
Processing of Eukaryotic m. RNA
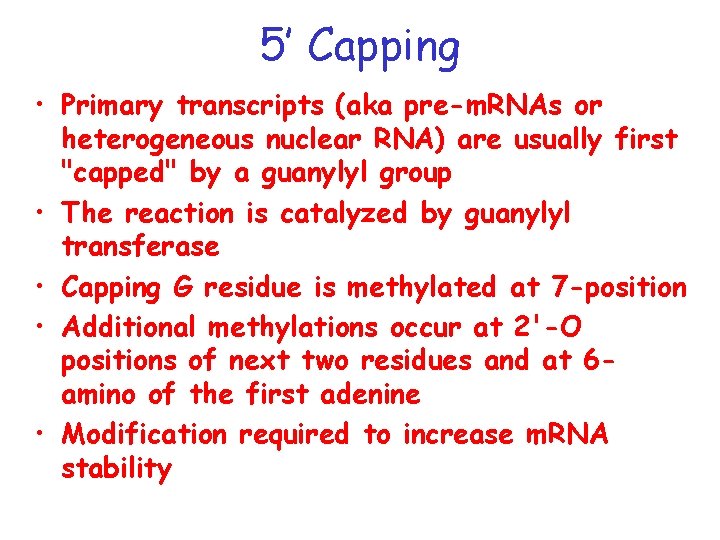
5’ Capping • Primary transcripts (aka pre-m. RNAs or heterogeneous nuclear RNA) are usually first "capped" by a guanylyl group • The reaction is catalyzed by guanylyl transferase • Capping G residue is methylated at 7 -position • Additional methylations occur at 2'-O positions of next two residues and at 6 amino of the first adenine • Modification required to increase m. RNA stability
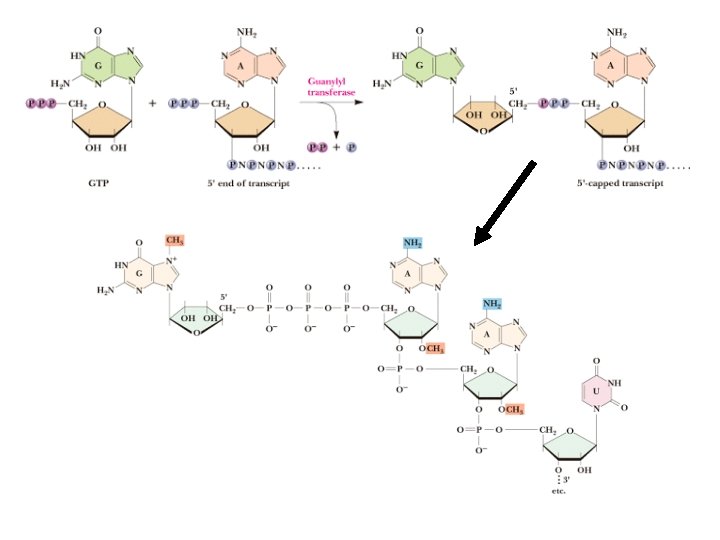
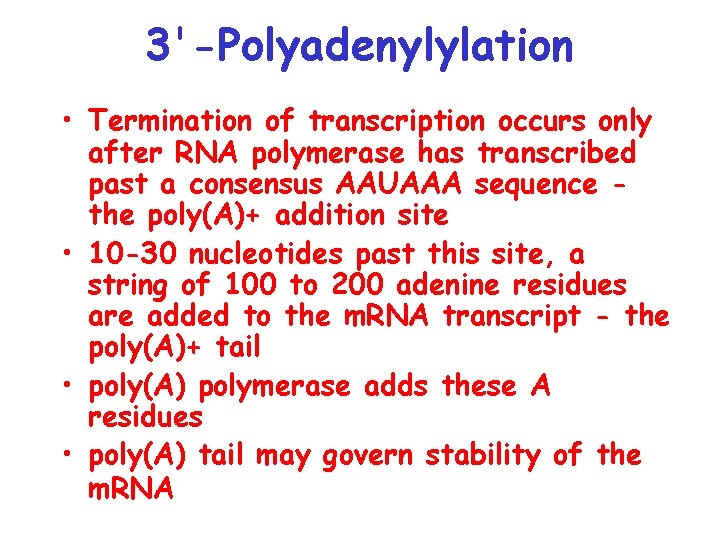
3'-Polyadenylylation • Termination of transcription occurs only after RNA polymerase has transcribed past a consensus AAUAAA sequence the poly(A)+ addition site • 10 -30 nucleotides past this site, a string of 100 to 200 adenine residues are added to the m. RNA transcript - the poly(A)+ tail • poly(A) polymerase adds these A residues • poly(A) tail may govern stability of the m. RNA
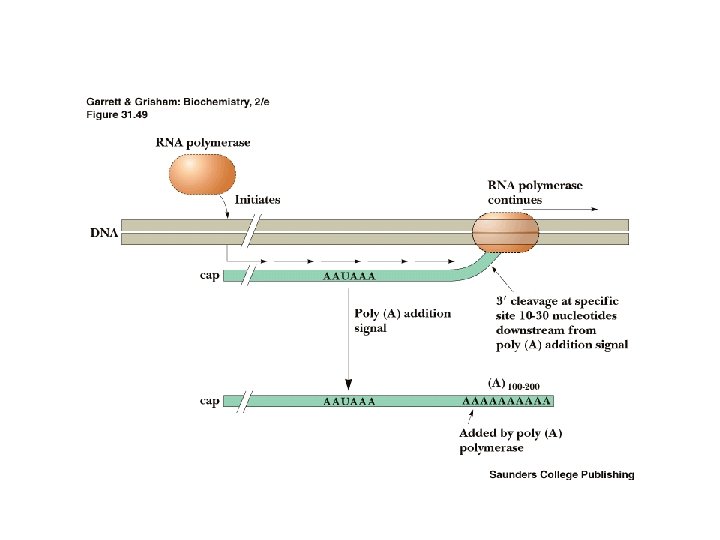
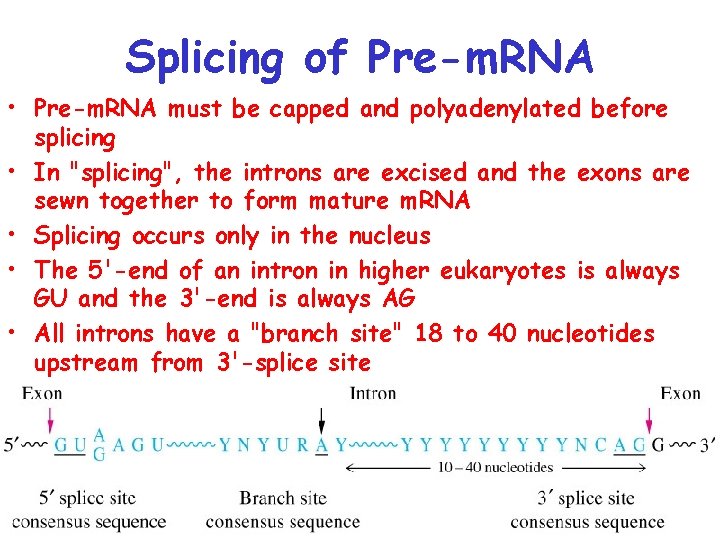
Splicing of Pre-m. RNA • Pre-m. RNA must be capped and polyadenylated before splicing • In "splicing", the introns are excised and the exons are sewn together to form mature m. RNA • Splicing occurs only in the nucleus • The 5'-end of an intron in higher eukaryotes is always GU and the 3'-end is always AG • All introns have a "branch site" 18 to 40 nucleotides upstream from 3'-splice site
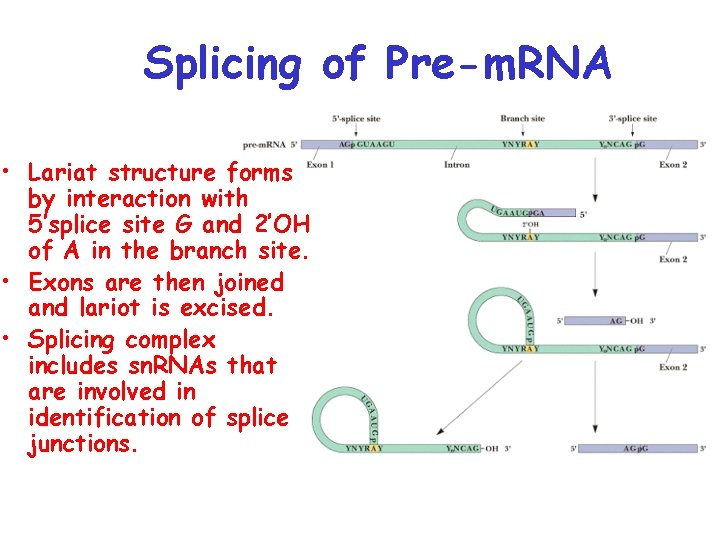
Splicing of Pre-m. RNA • Lariat structure forms by interaction with 5’splice site G and 2’OH of A in the branch site. • Exons are then joined and lariot is excised. • Splicing complex includes sn. RNAs that are involved in identification of splice junctions.
Dna to protein steps
Dna rna protein central dogma
Central dogma
Dogma
Linked genes and unlinked genes
Homeotic genes
Polygenic inheritance
Protein synthesis
Whats the central dogma of biology
Central dogma
Central dogma
Central dogma cartoon
Central dogma
Dogma central
Dogma central
Central dogma
Drug metabolism liver
Dogma central de la biología molecular
Control de la transcripción
Replication
Central dogma of biology
Biologia molecular
Central dogma diagram
Dogma central da biologia
Dna coloring transcription and translation
Rna
Dna transcription and translation
Dna to rna transcription
Dna replication transcription and translation
Dna transcription
Dna and transcription tutorial
Rho independent termination
Dna to rna transcription
Translation
Dna transcription
Dna transcription
Genes chromosomes and dna
Chapter 11 dna and genes
Dna, genes and chromosomes relationship
Dna and genes chapter 11
Genes chromosomes dna
What is the relationship between dna chromosomes and genes
What is a gene
Dna genes
Dna chromosomes genes diagram
Dna rna protein synthesis homework #2 dna replication
How to determine the sequence of genes along a chromosome
Phân độ lown
Block nhĩ thất độ 2 mobitz 2
Thơ thất ngôn tứ tuyệt đường luật
Thơ thất ngôn tứ tuyệt đường luật
Chiến lược kinh doanh quốc tế của walmart
Tìm vết của đường thẳng
Hãy nói thật ít để làm được nhiều
Tôn thất thuyết là ai
Gây tê cơ vuông thắt lưng
Sau thất bại ở hồ điển triệt
Replication fork
Bioflix activity dna replication lagging strand synthesis
Coding dna and non coding dna
Replication process
Haced todo lo que el os diga