Renal Replacement Therapy Childrens Healthcare of Atlanta Renal
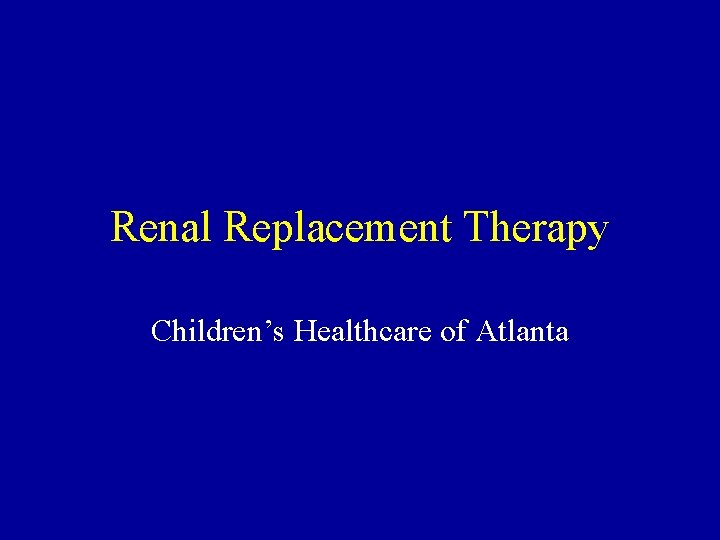
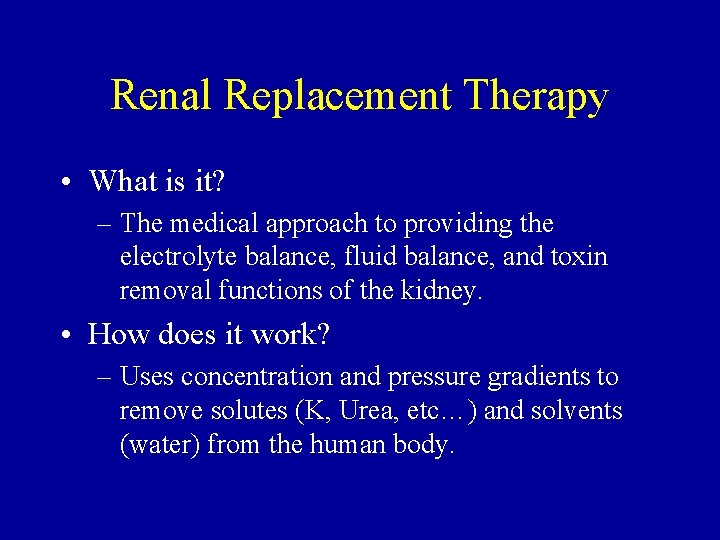
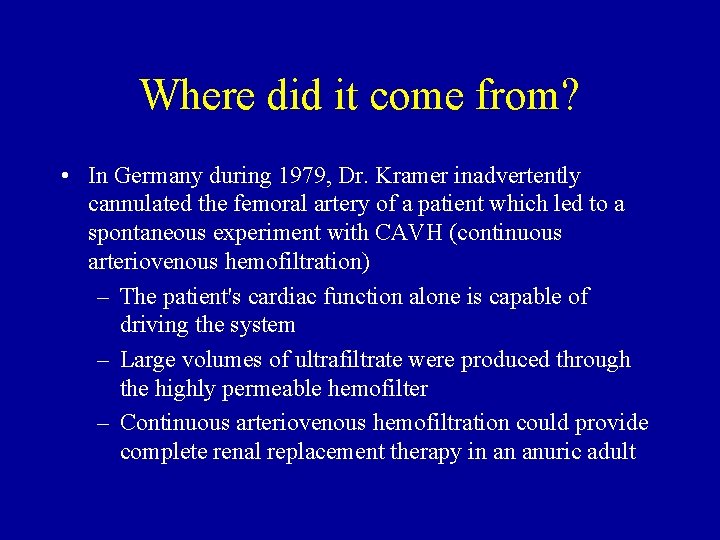
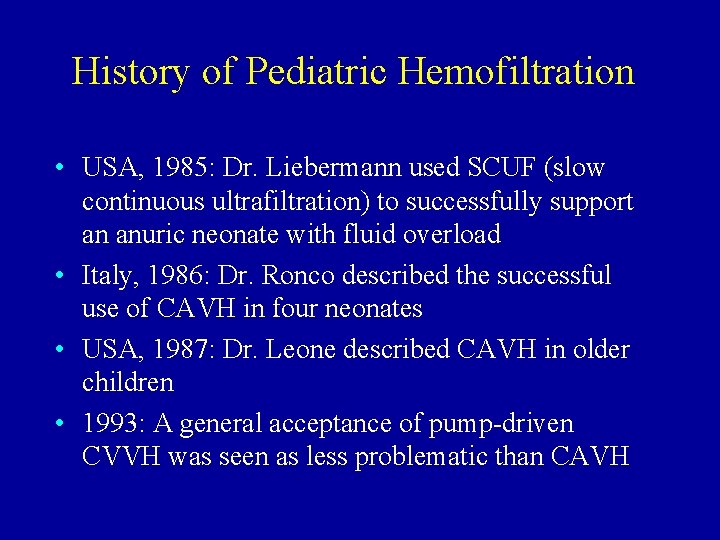
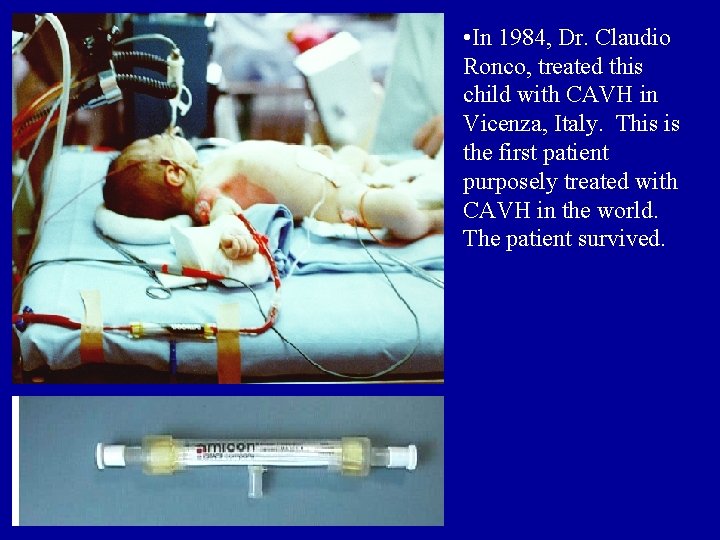
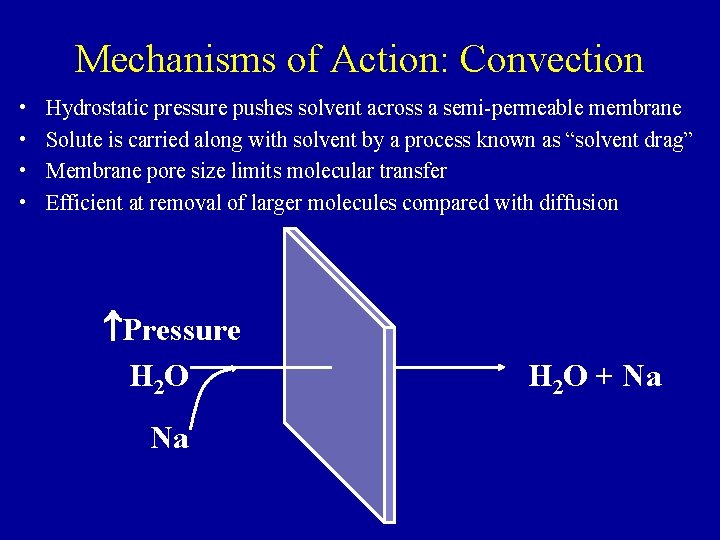
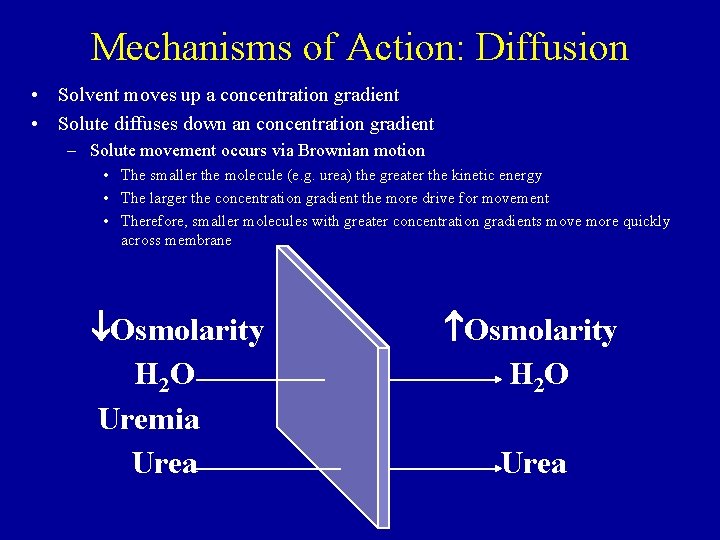
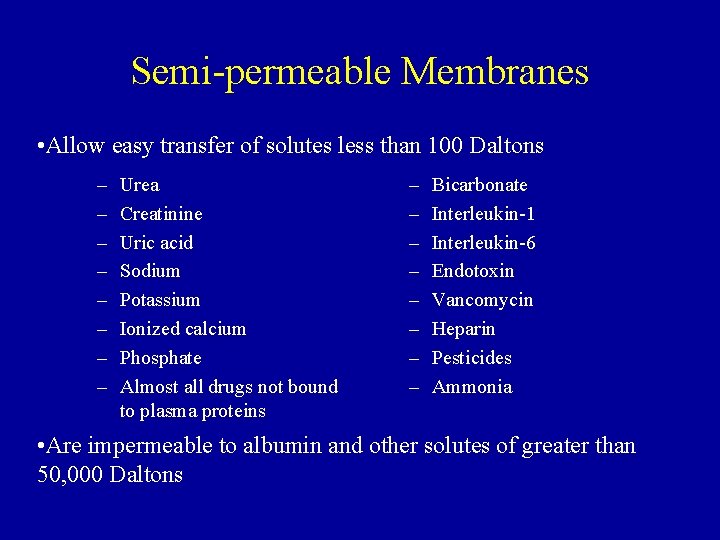
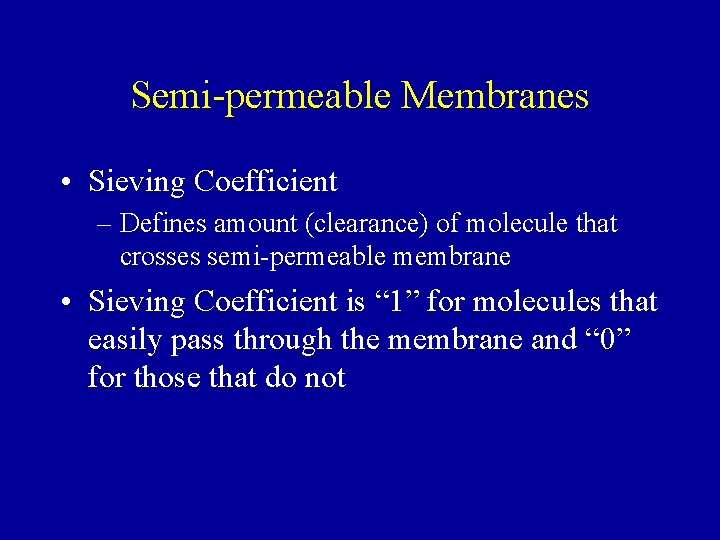
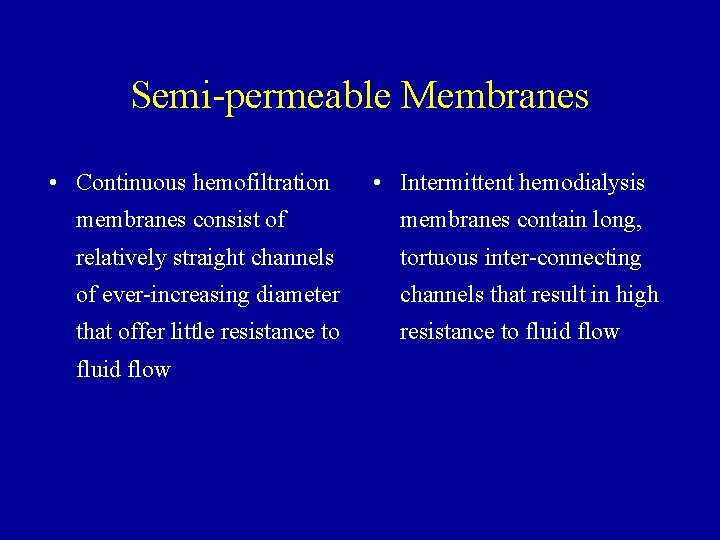
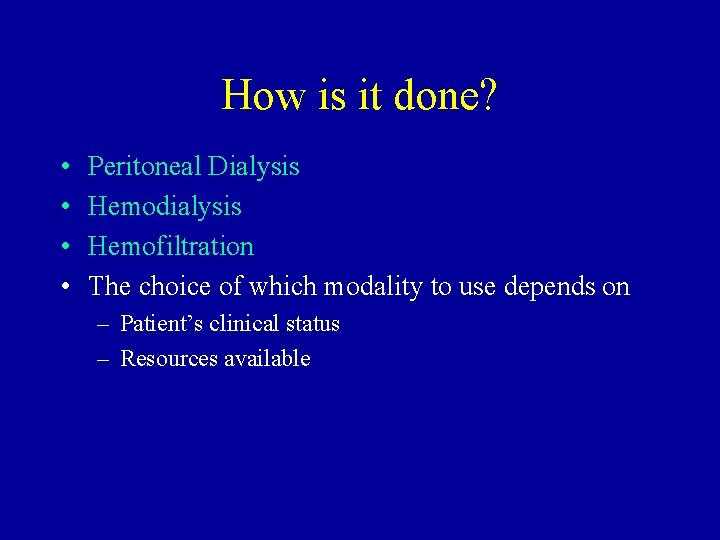
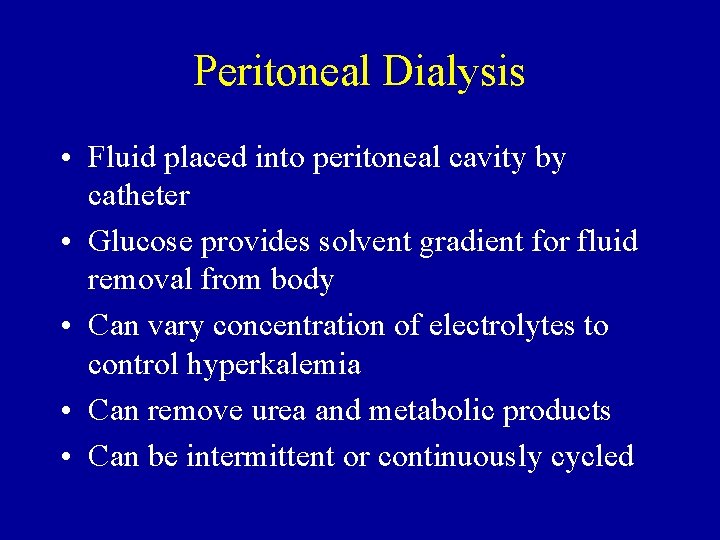
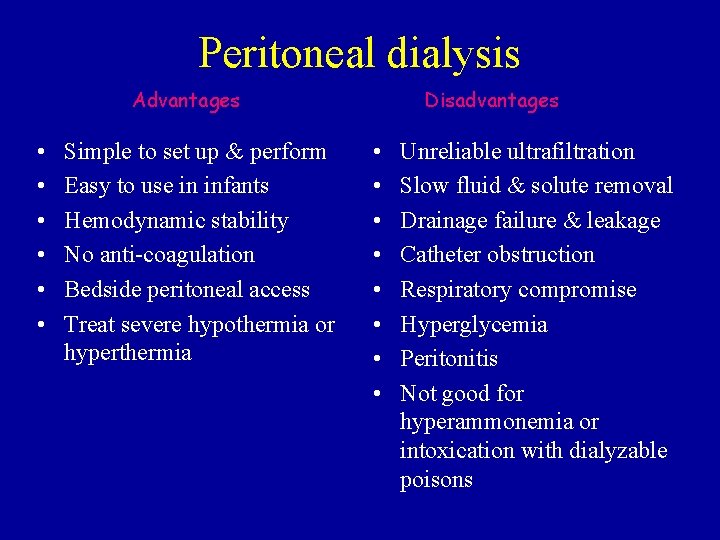
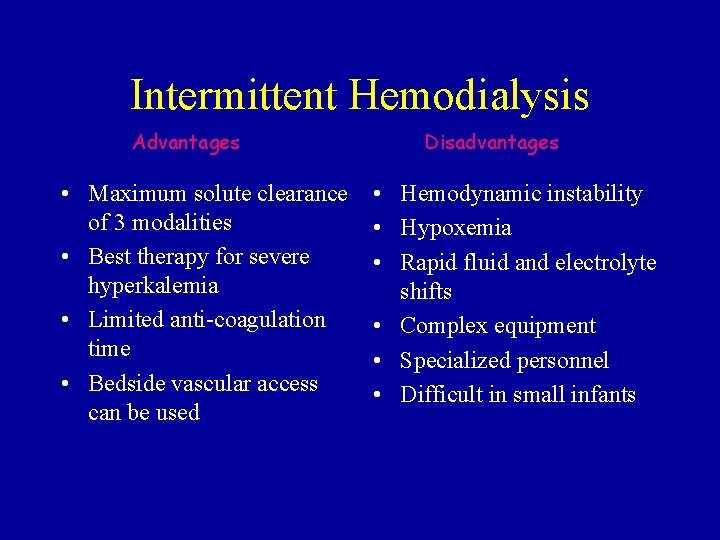
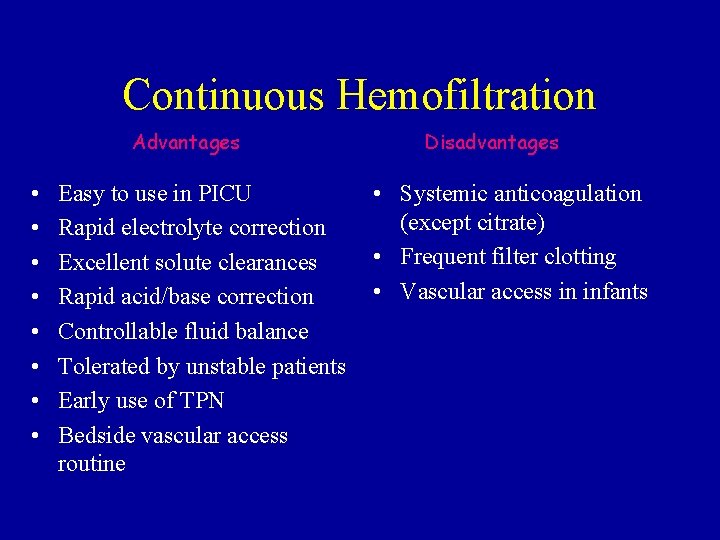
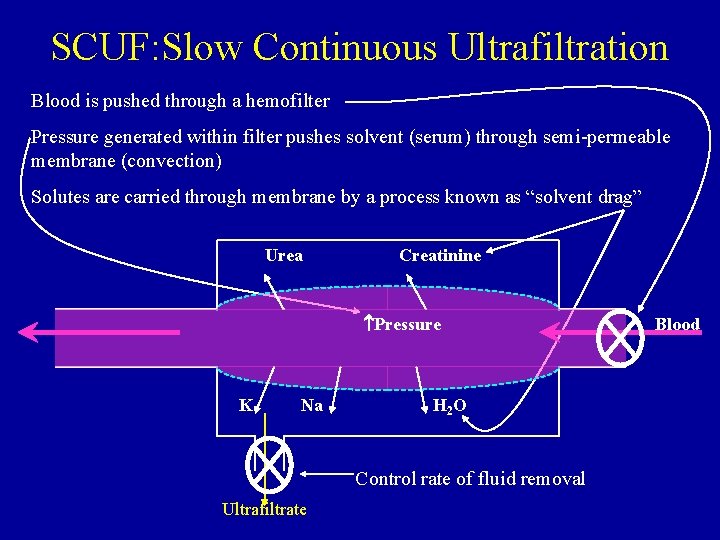
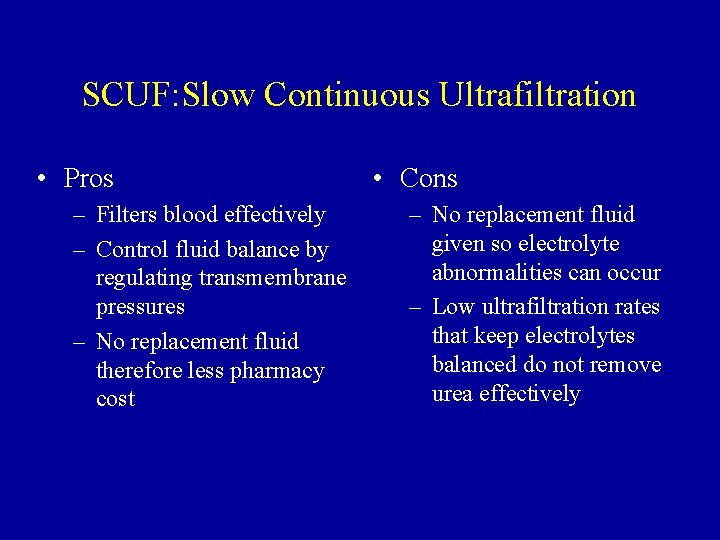
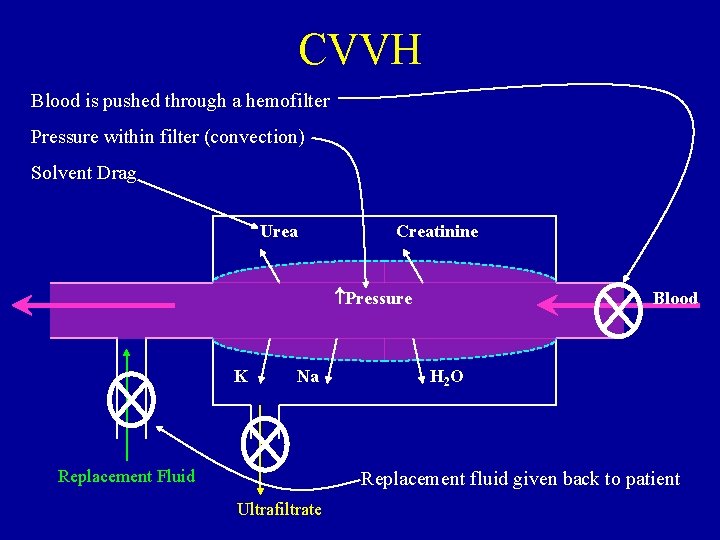
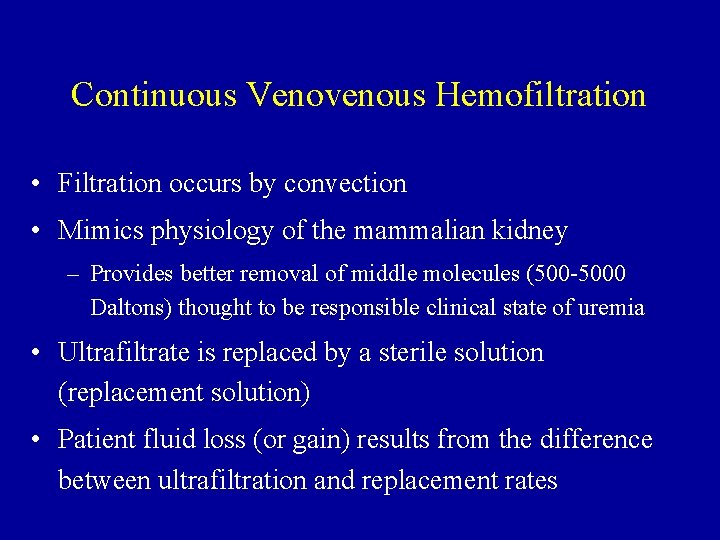
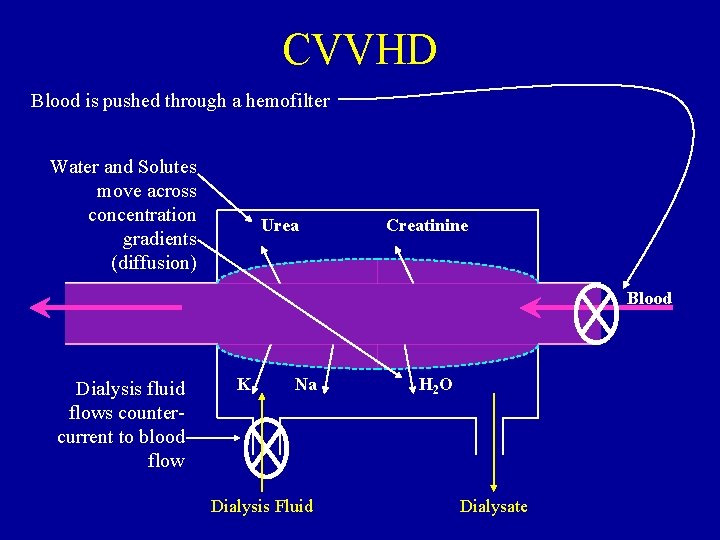
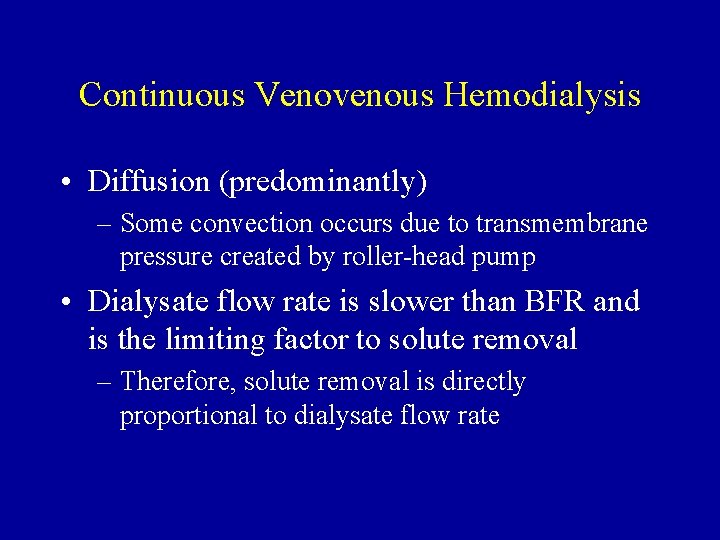
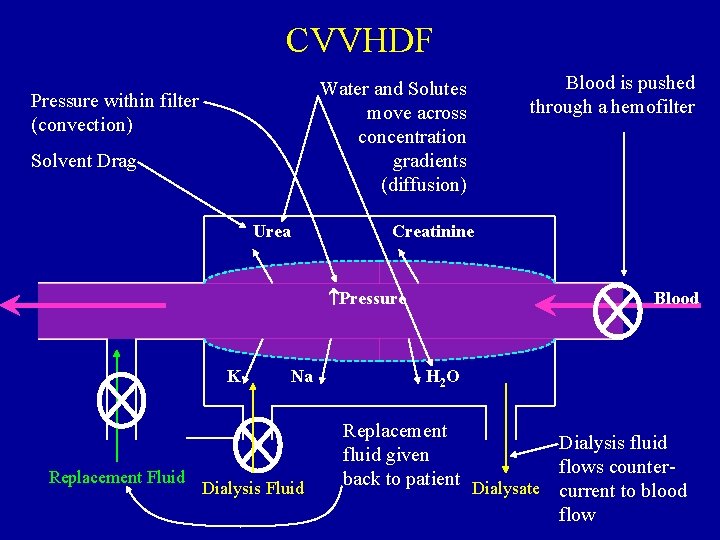
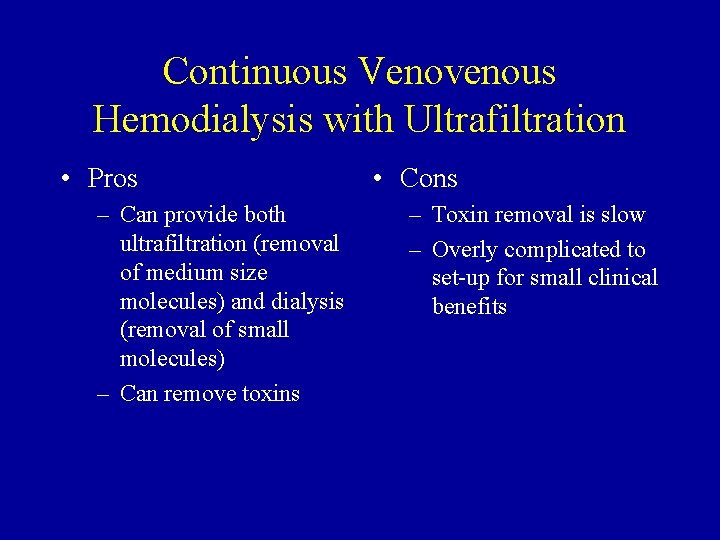
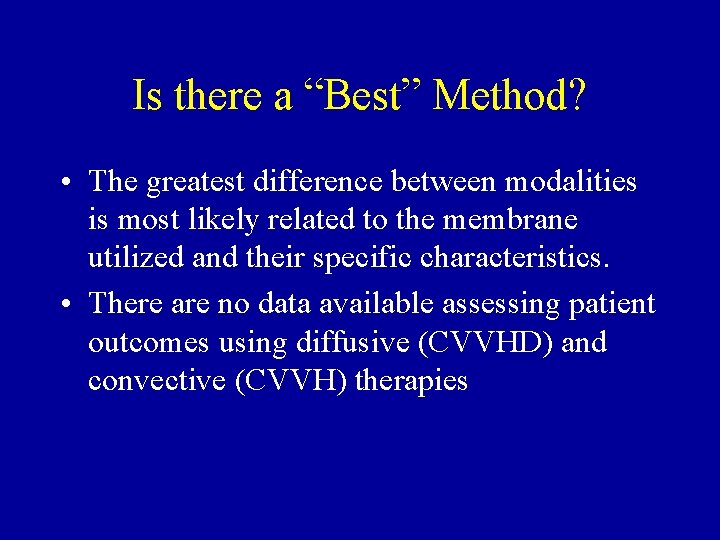
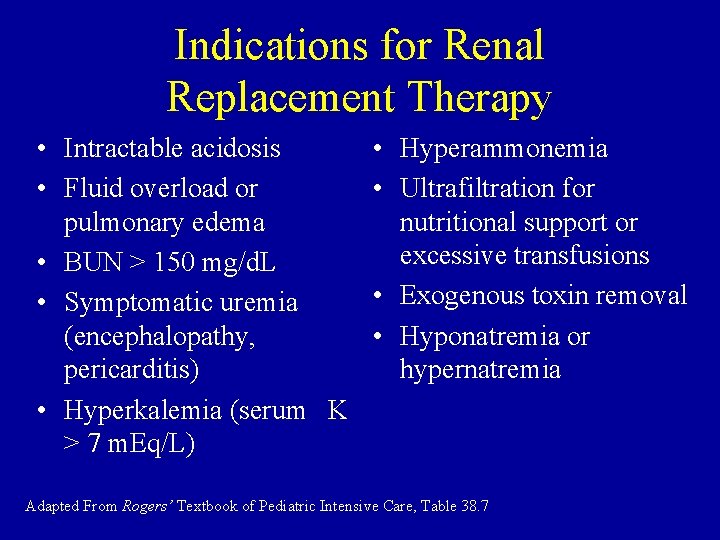
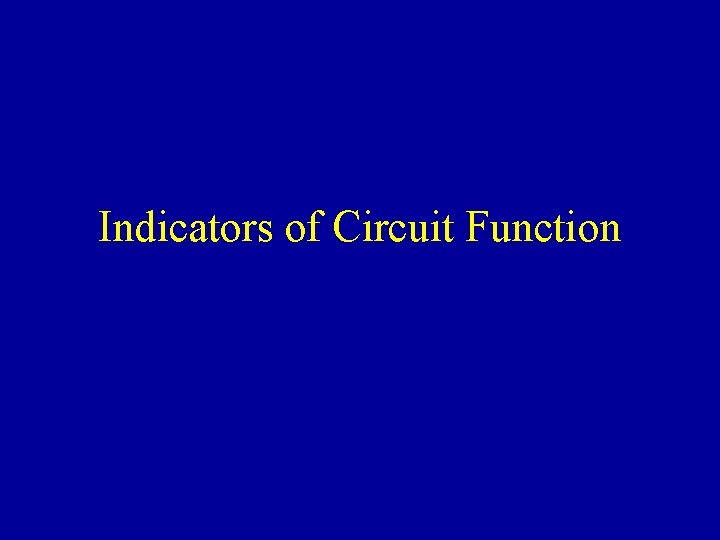
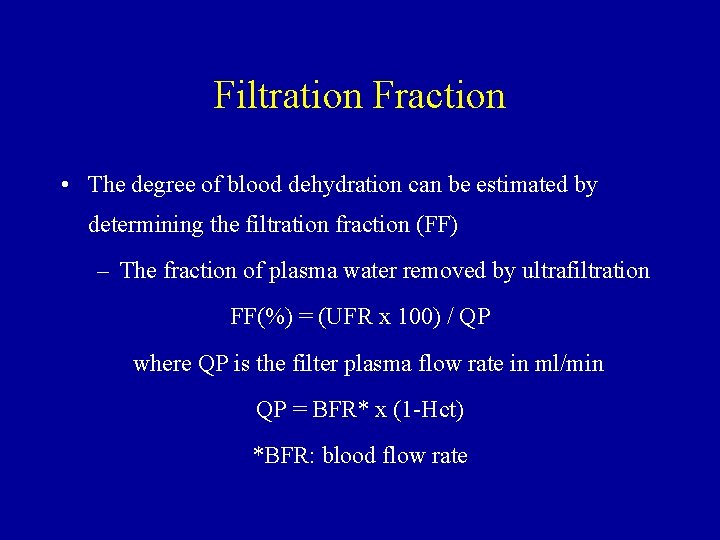
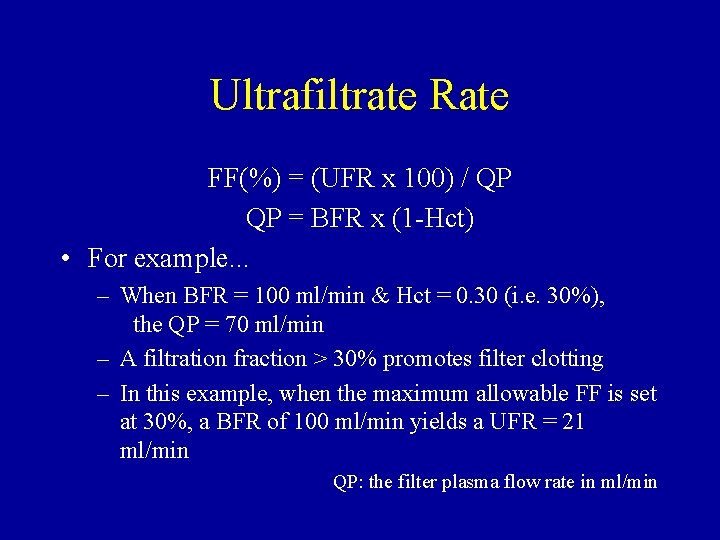
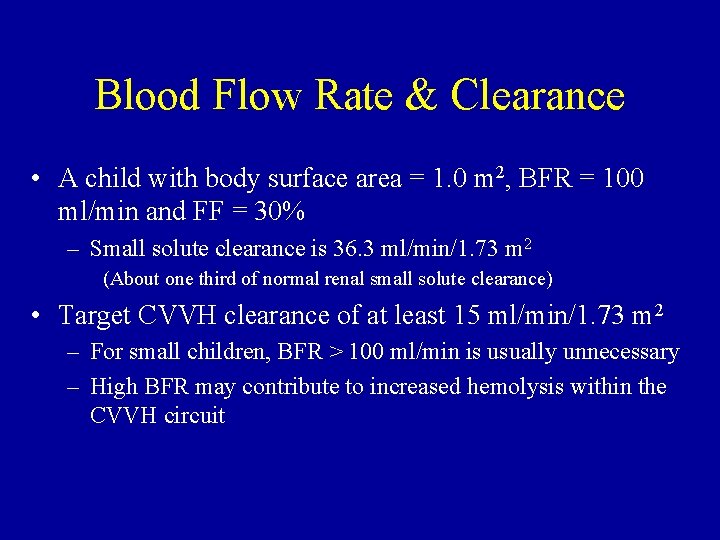
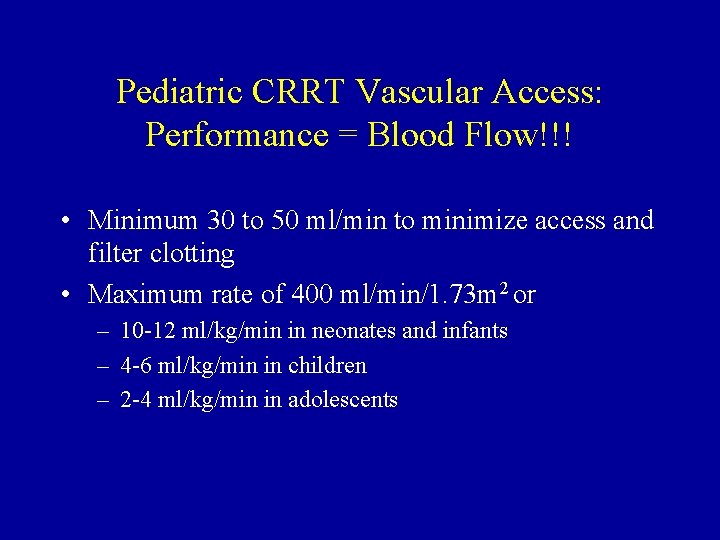
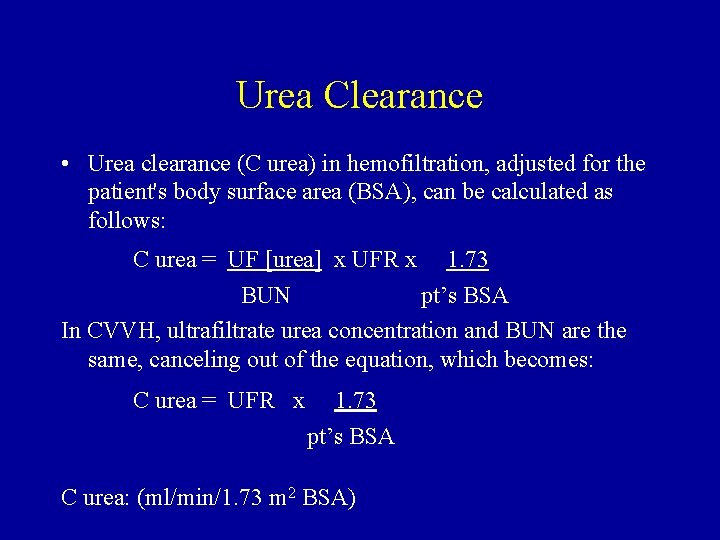
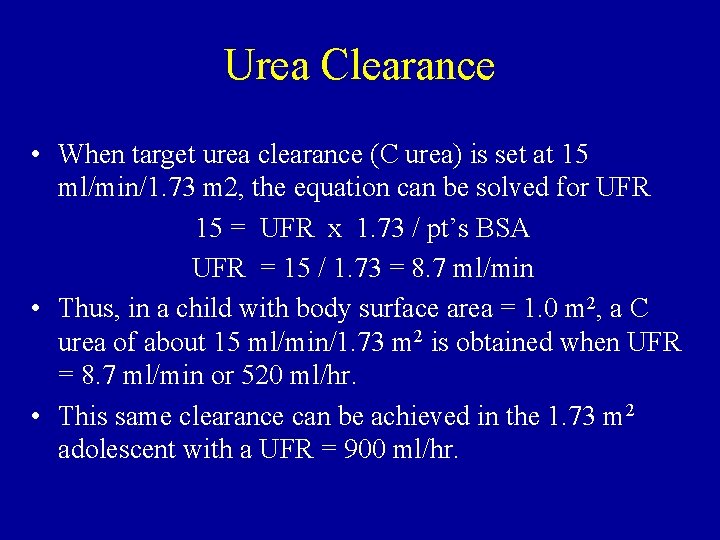
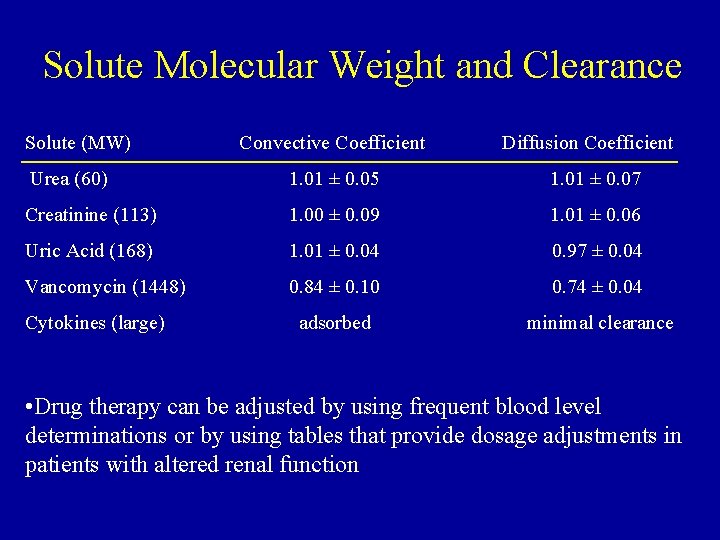
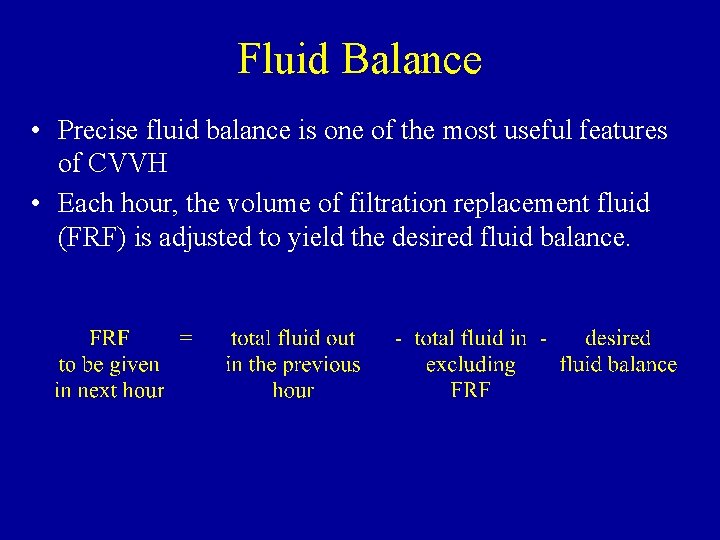
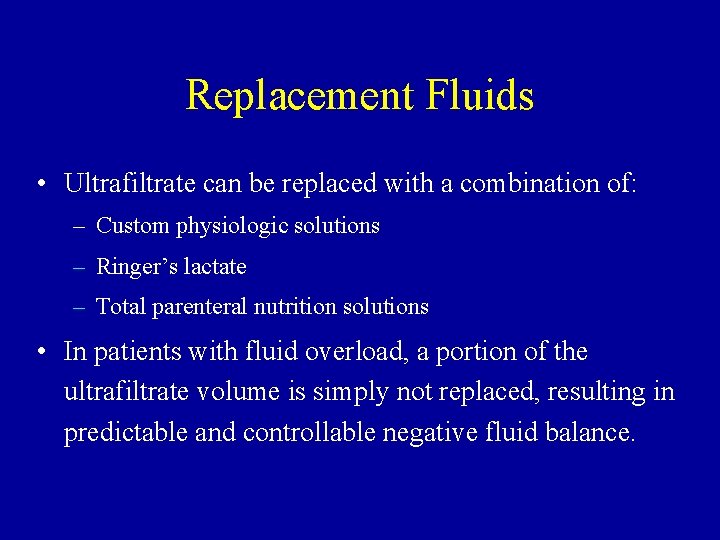
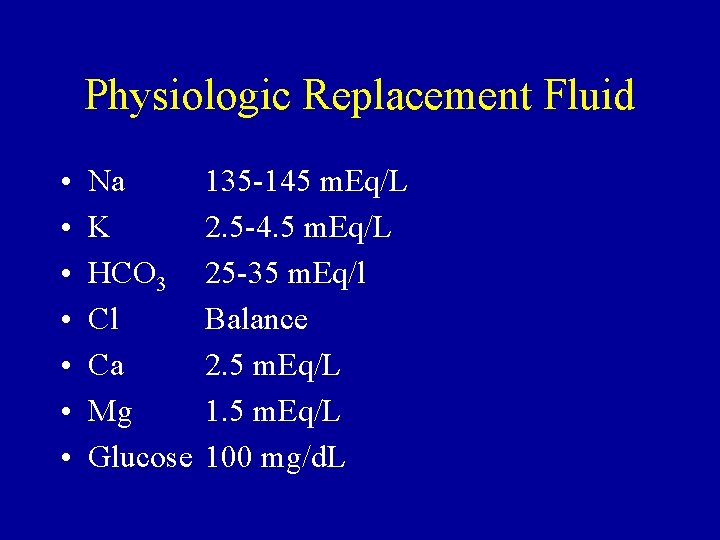
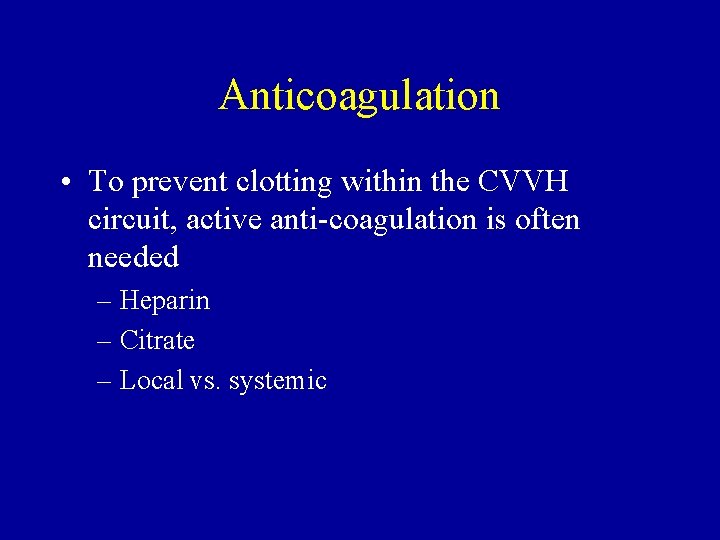
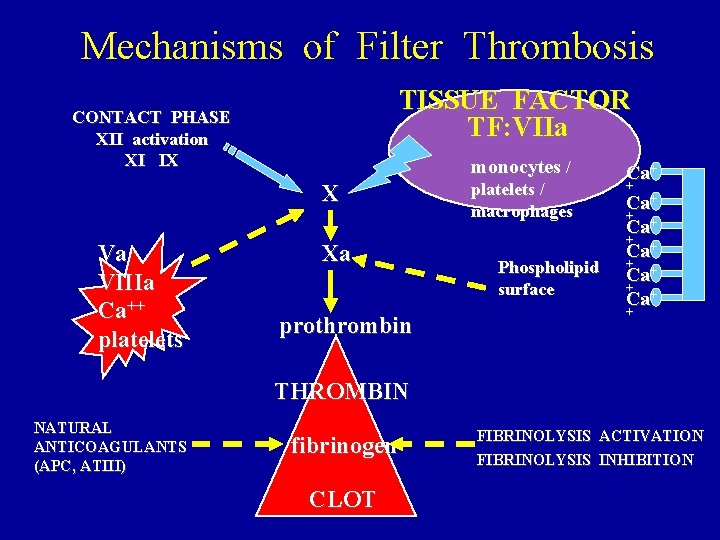
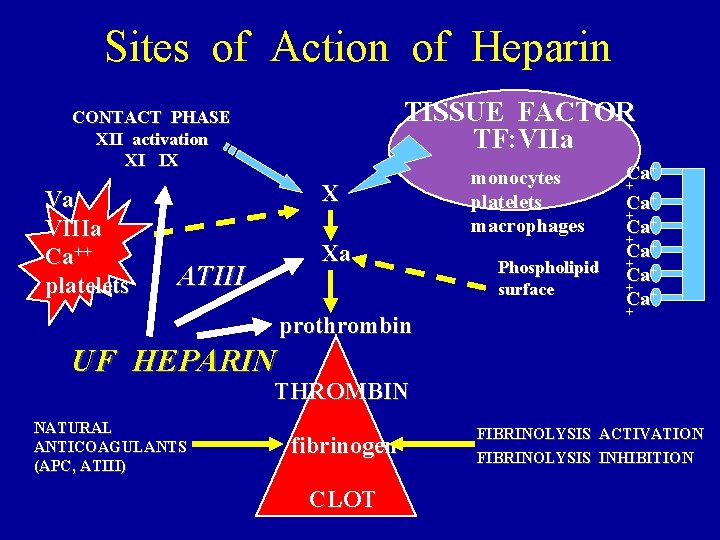
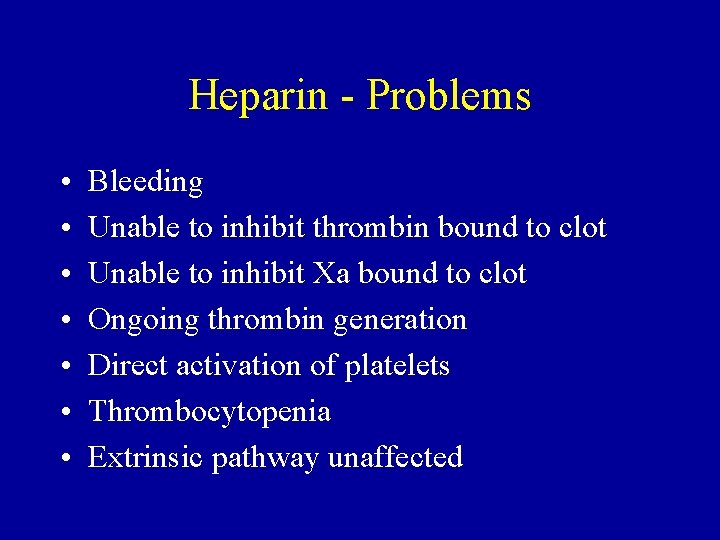
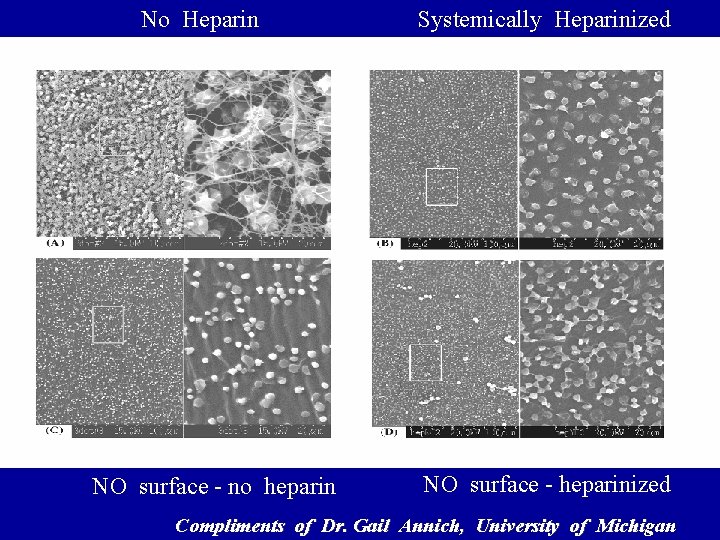
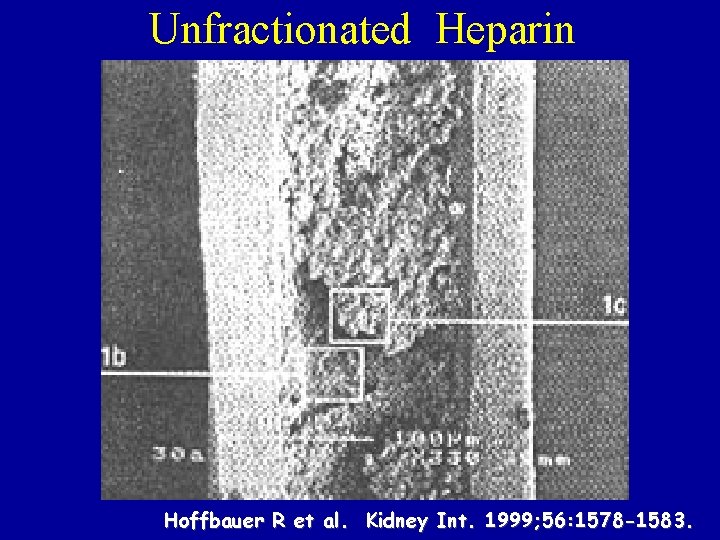
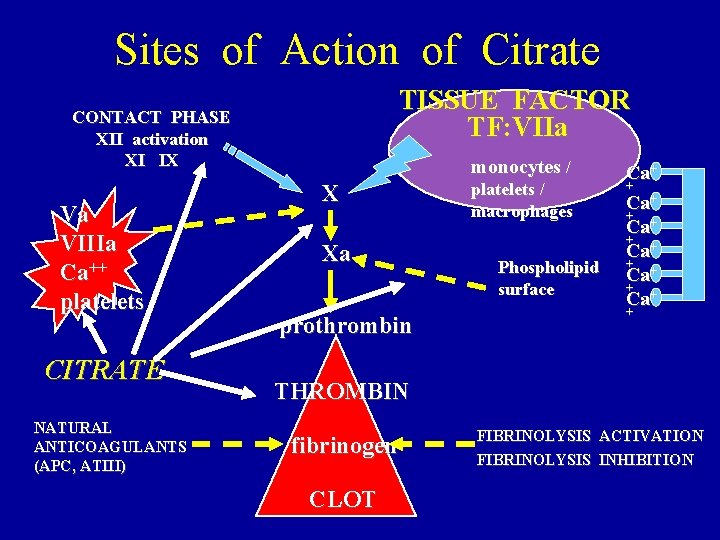
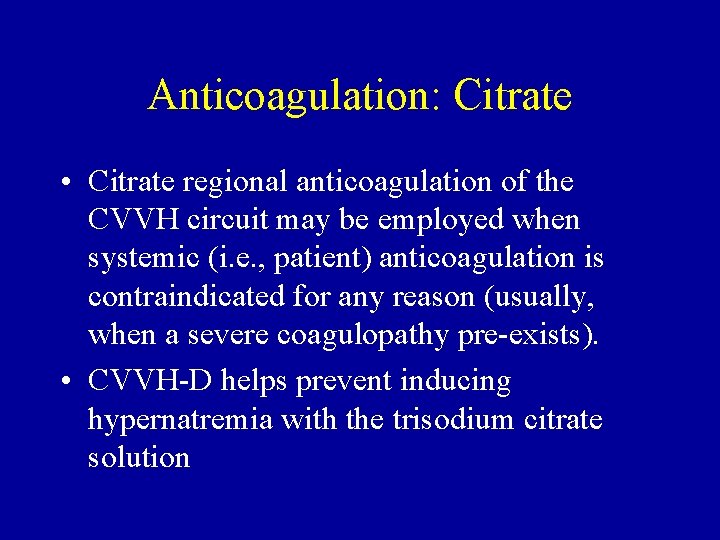
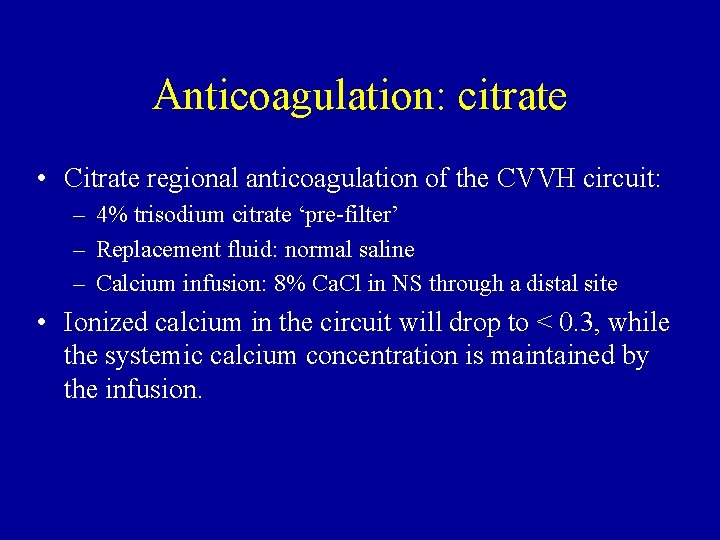
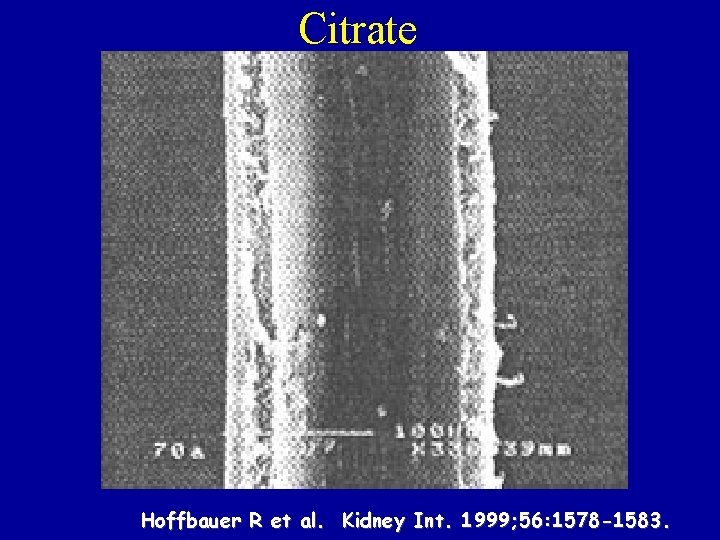
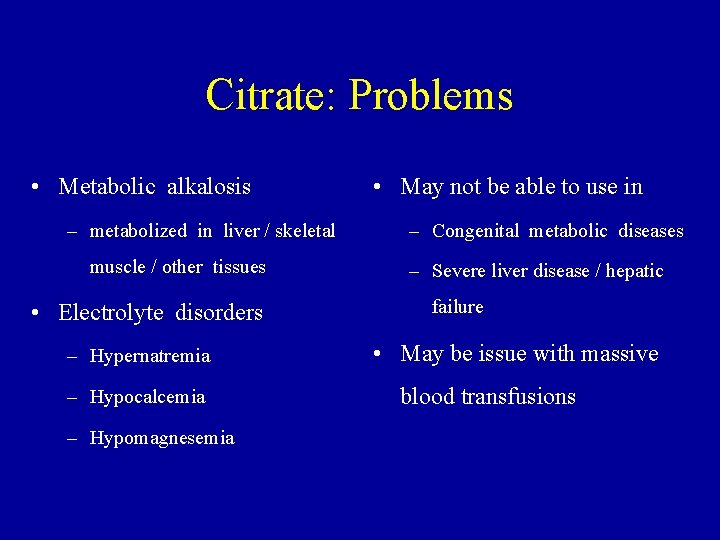
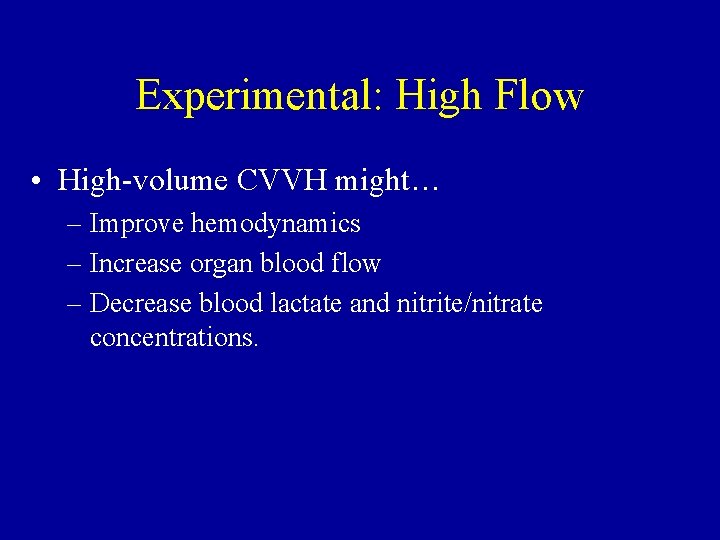
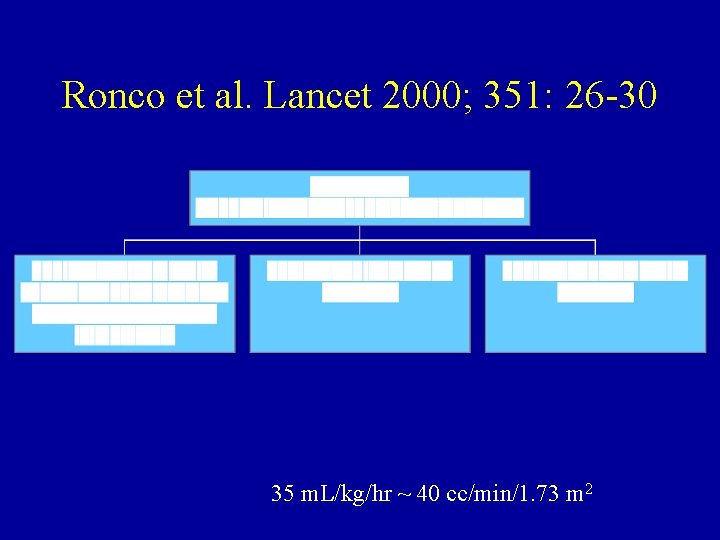
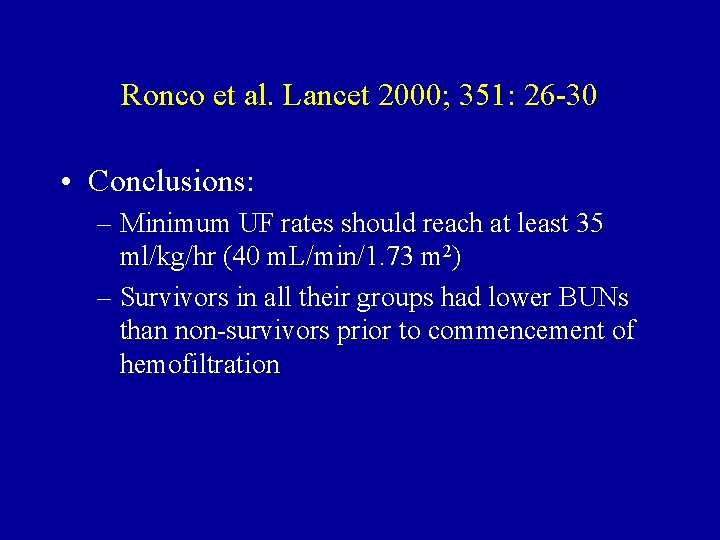
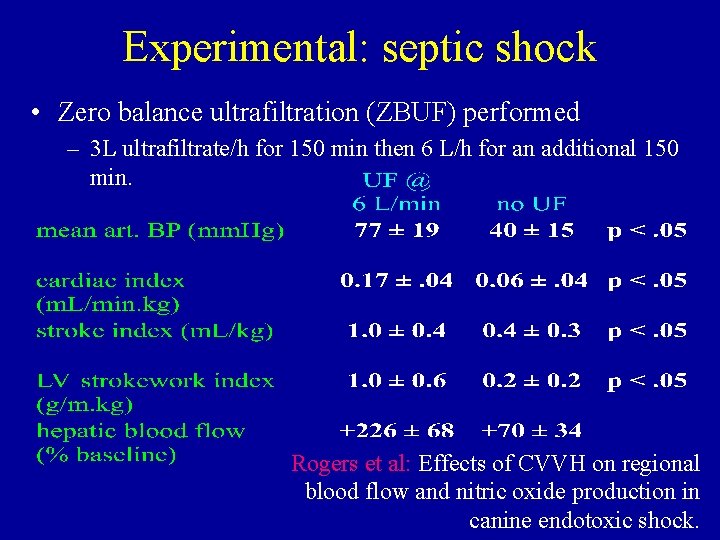
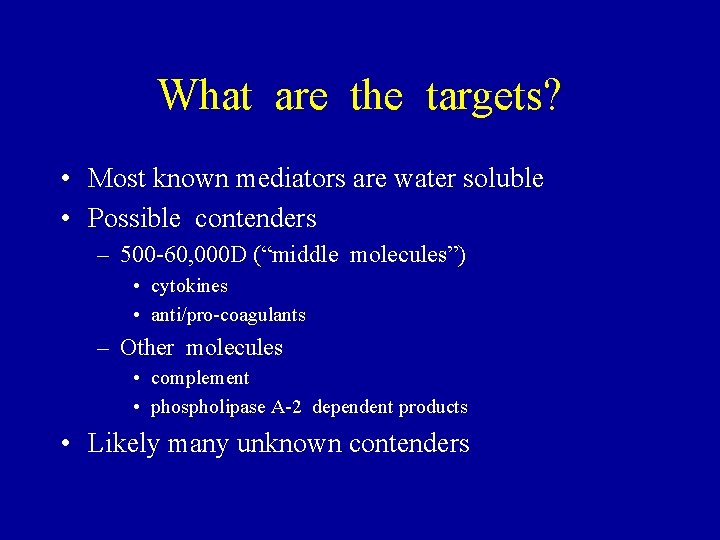
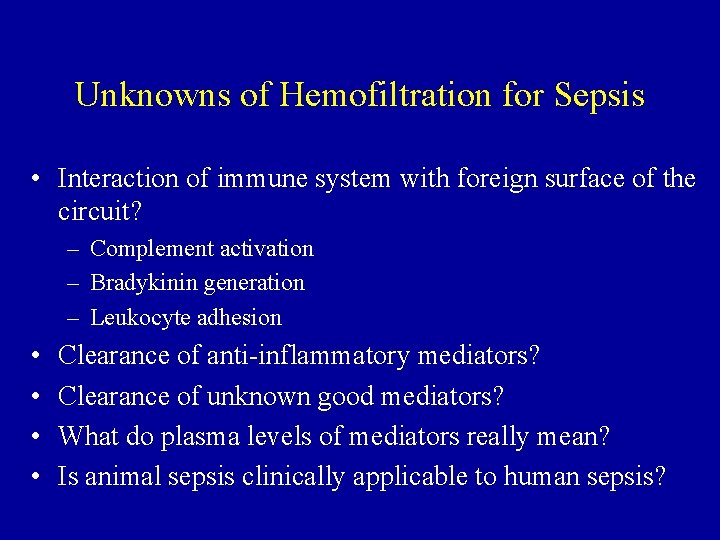
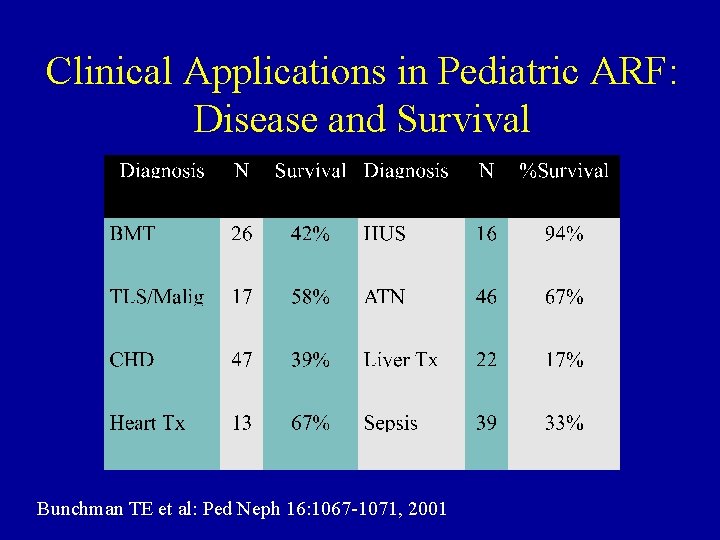
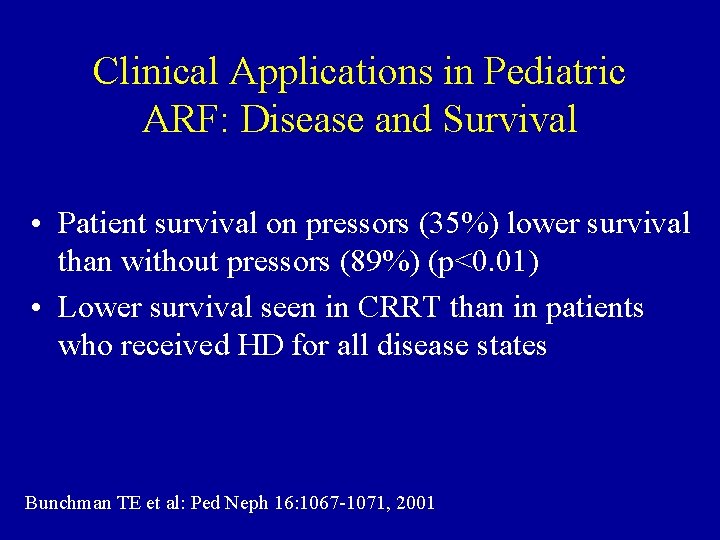
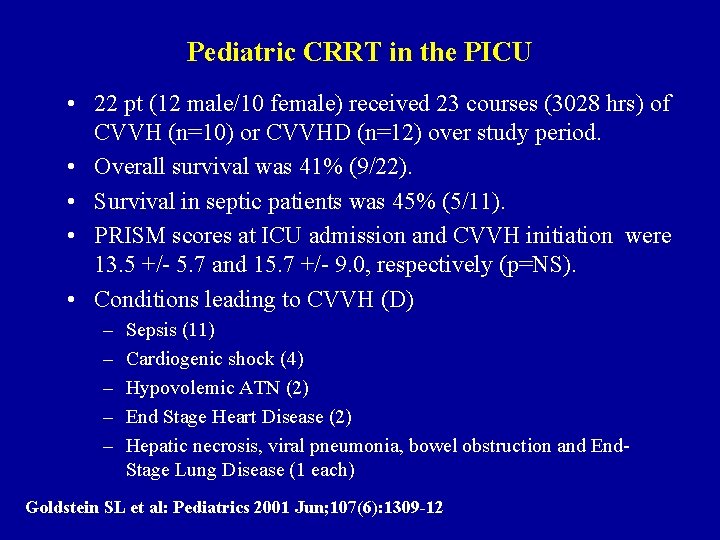
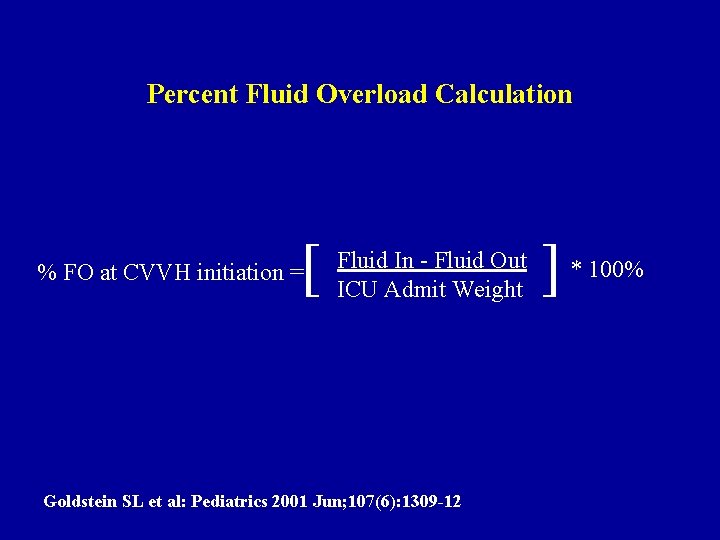
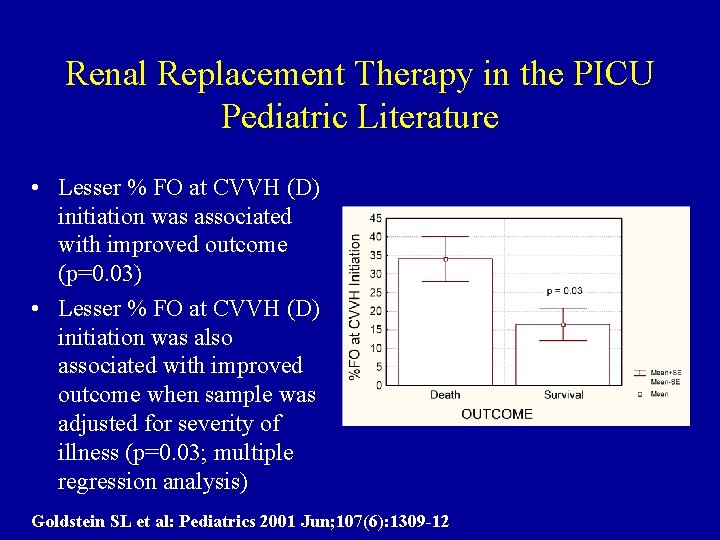
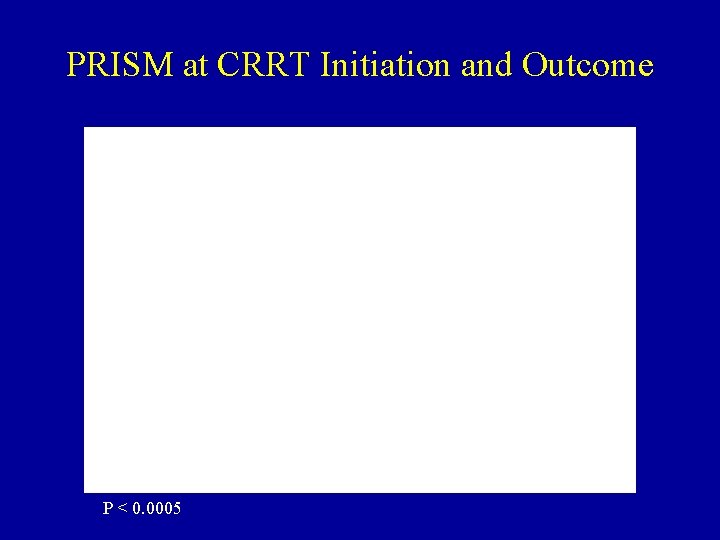
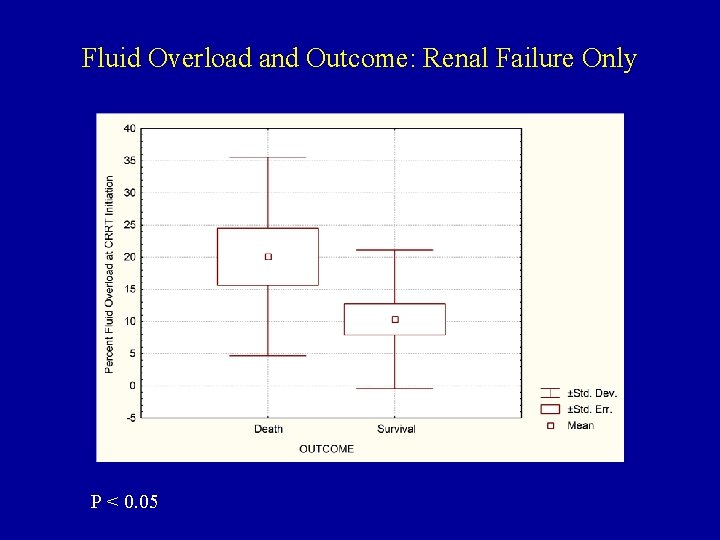
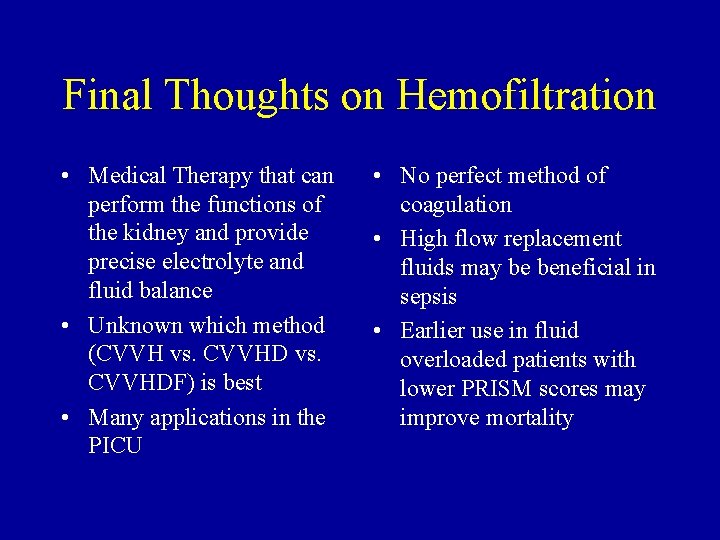
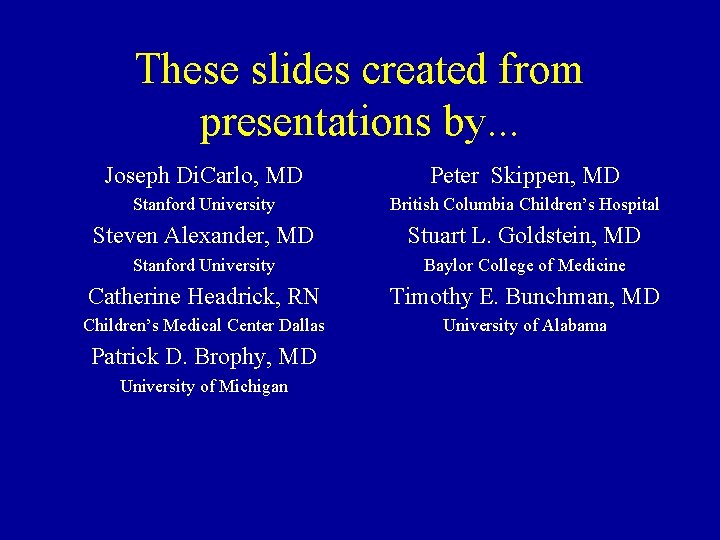
- Slides: 62
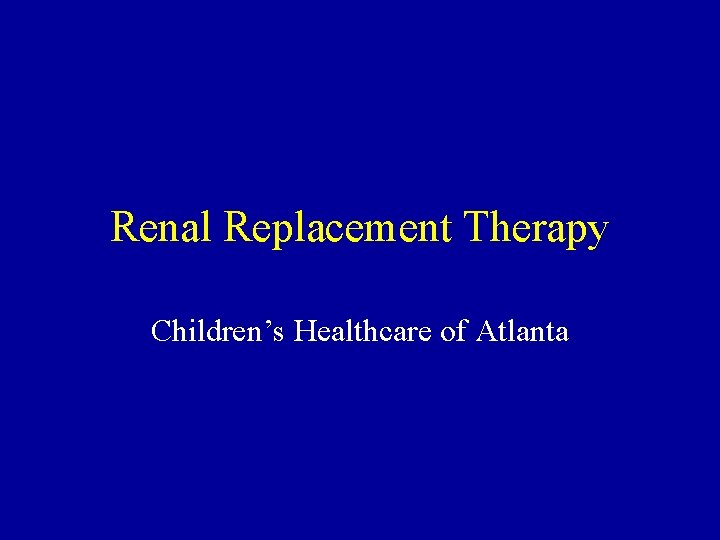
Renal Replacement Therapy Children’s Healthcare of Atlanta
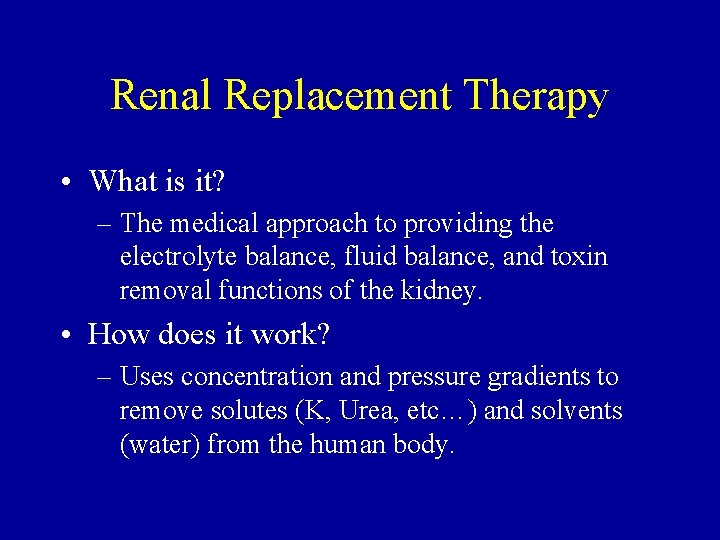
Renal Replacement Therapy • What is it? – The medical approach to providing the electrolyte balance, fluid balance, and toxin removal functions of the kidney. • How does it work? – Uses concentration and pressure gradients to remove solutes (K, Urea, etc…) and solvents (water) from the human body.
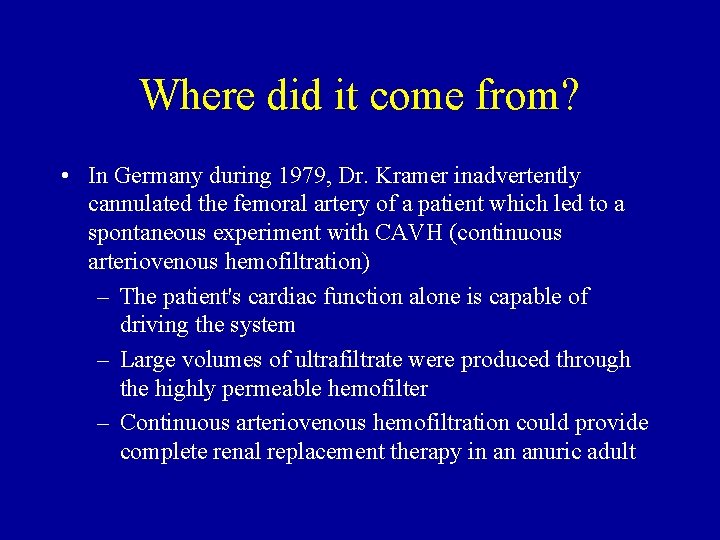
Where did it come from? • In Germany during 1979, Dr. Kramer inadvertently cannulated the femoral artery of a patient which led to a spontaneous experiment with CAVH (continuous arteriovenous hemofiltration) – The patient's cardiac function alone is capable of driving the system – Large volumes of ultrafiltrate were produced through the highly permeable hemofilter – Continuous arteriovenous hemofiltration could provide complete renal replacement therapy in an anuric adult
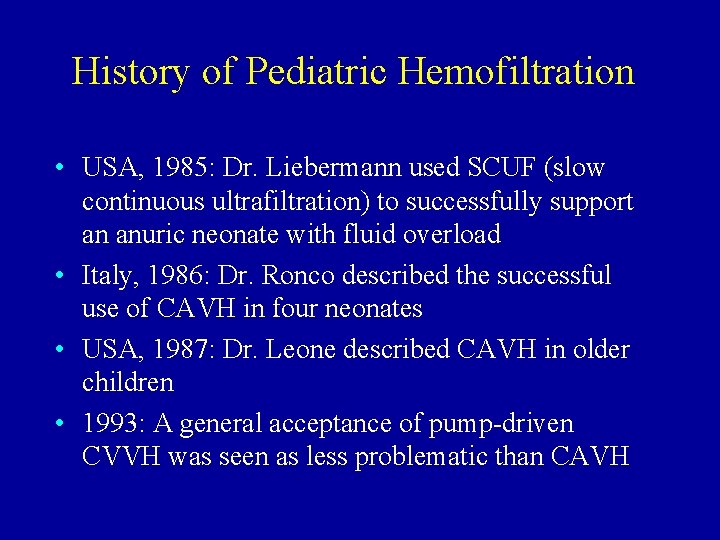
History of Pediatric Hemofiltration • USA, 1985: Dr. Liebermann used SCUF (slow continuous ultrafiltration) to successfully support an anuric neonate with fluid overload • Italy, 1986: Dr. Ronco described the successful use of CAVH in four neonates • USA, 1987: Dr. Leone described CAVH in older children • 1993: A general acceptance of pump-driven CVVH was seen as less problematic than CAVH
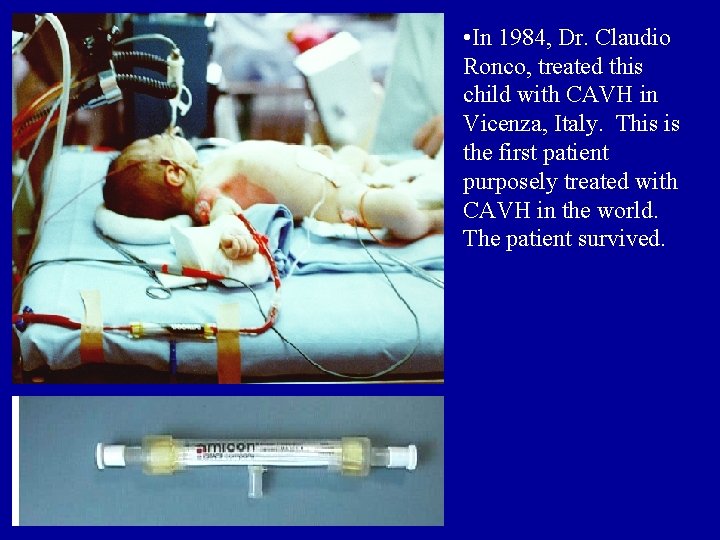
• In 1984, Dr. Claudio Ronco, treated this child with CAVH in Vicenza, Italy. This is the first patient purposely treated with CAVH in the world. The patient survived.
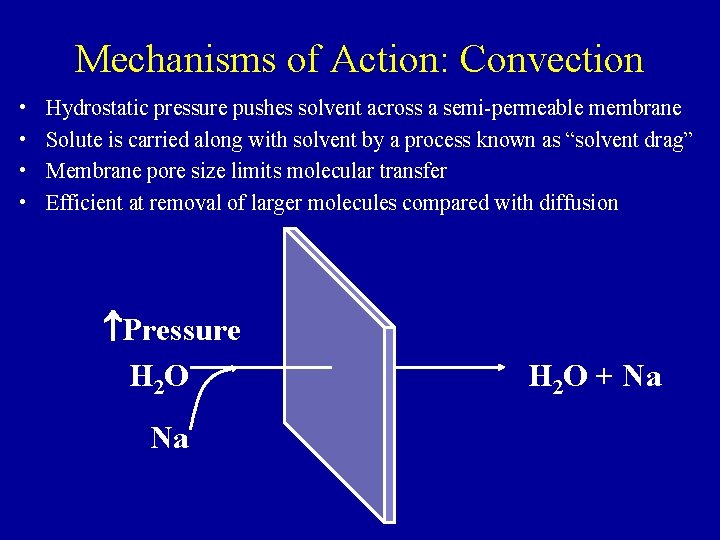
Mechanisms of Action: Convection • • Hydrostatic pressure pushes solvent across a semi-permeable membrane Solute is carried along with solvent by a process known as “solvent drag” Membrane pore size limits molecular transfer Efficient at removal of larger molecules compared with diffusion Pressure H 2 O Na H 2 O + Na
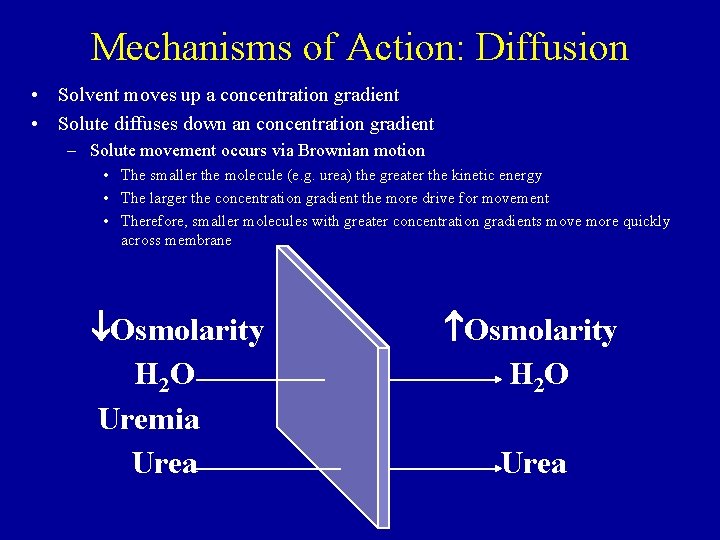
Mechanisms of Action: Diffusion • Solvent moves up a concentration gradient • Solute diffuses down an concentration gradient – Solute movement occurs via Brownian motion • The smaller the molecule (e. g. urea) the greater the kinetic energy • The larger the concentration gradient the more drive for movement • Therefore, smaller molecules with greater concentration gradients move more quickly across membrane Osmolarity H 2 O Uremia Urea Osmolarity H 2 O Urea
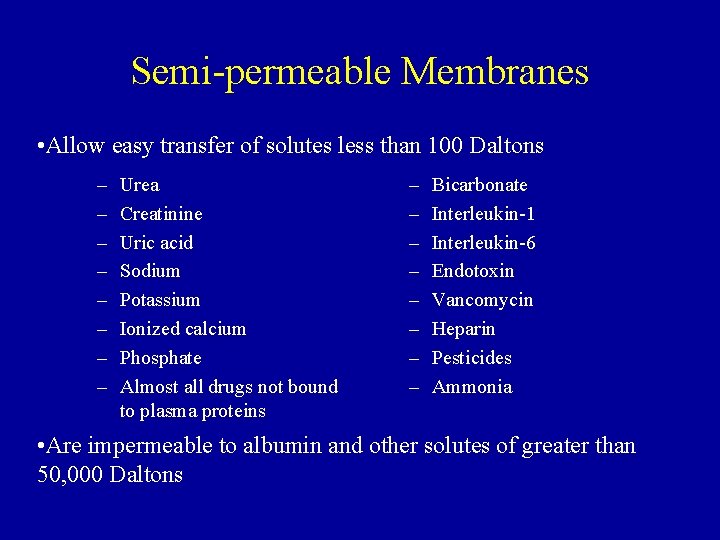
Semi-permeable Membranes • Allow easy transfer of solutes less than 100 Daltons – – – – Urea Creatinine Uric acid Sodium Potassium Ionized calcium Phosphate Almost all drugs not bound to plasma proteins – – – – Bicarbonate Interleukin-1 Interleukin-6 Endotoxin Vancomycin Heparin Pesticides Ammonia • Are impermeable to albumin and other solutes of greater than 50, 000 Daltons
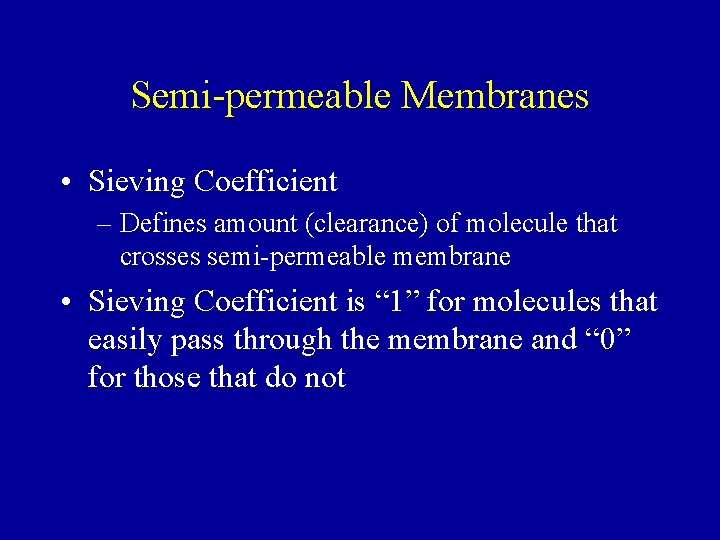
Semi-permeable Membranes • Sieving Coefficient – Defines amount (clearance) of molecule that crosses semi-permeable membrane • Sieving Coefficient is “ 1” for molecules that easily pass through the membrane and “ 0” for those that do not
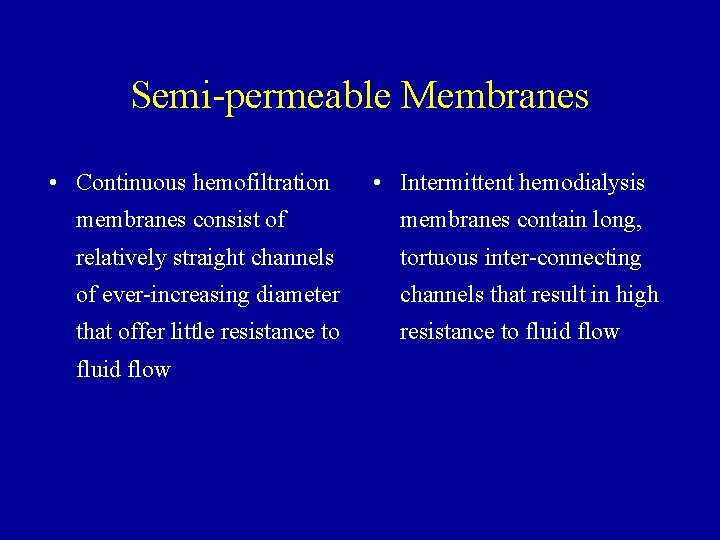
Semi-permeable Membranes • Continuous hemofiltration • Intermittent hemodialysis membranes consist of membranes contain long, relatively straight channels tortuous inter-connecting of ever-increasing diameter channels that result in high that offer little resistance to fluid flow
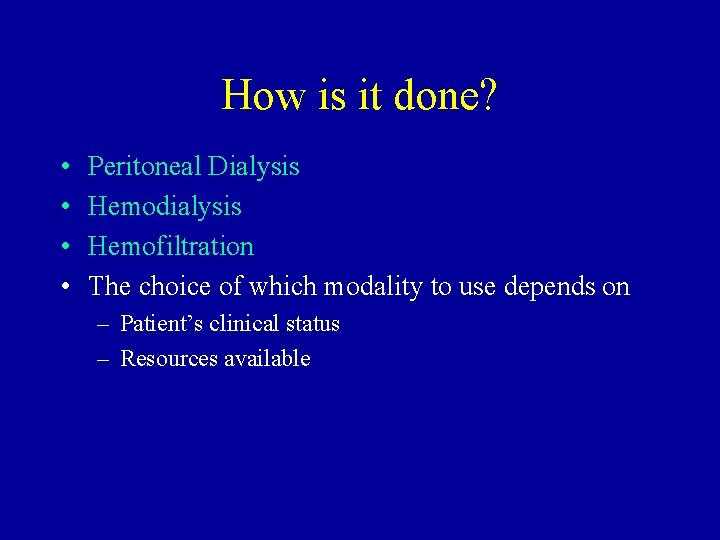
How is it done? • • Peritoneal Dialysis Hemodialysis Hemofiltration The choice of which modality to use depends on – Patient’s clinical status – Resources available
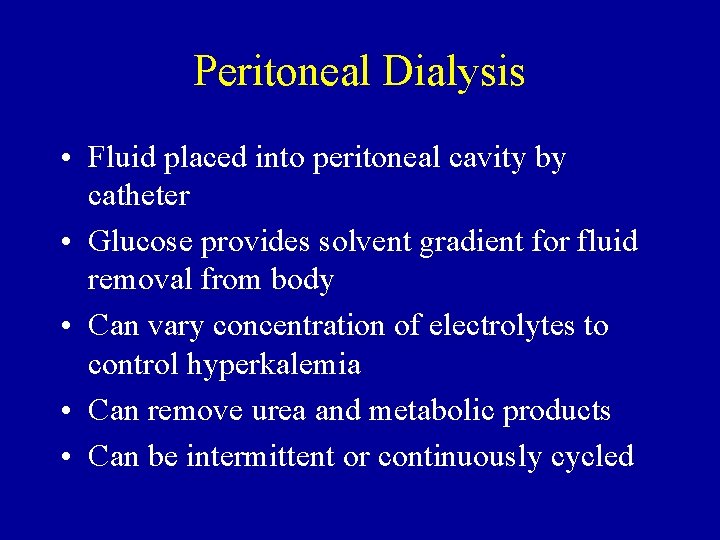
Peritoneal Dialysis • Fluid placed into peritoneal cavity by catheter • Glucose provides solvent gradient for fluid removal from body • Can vary concentration of electrolytes to control hyperkalemia • Can remove urea and metabolic products • Can be intermittent or continuously cycled
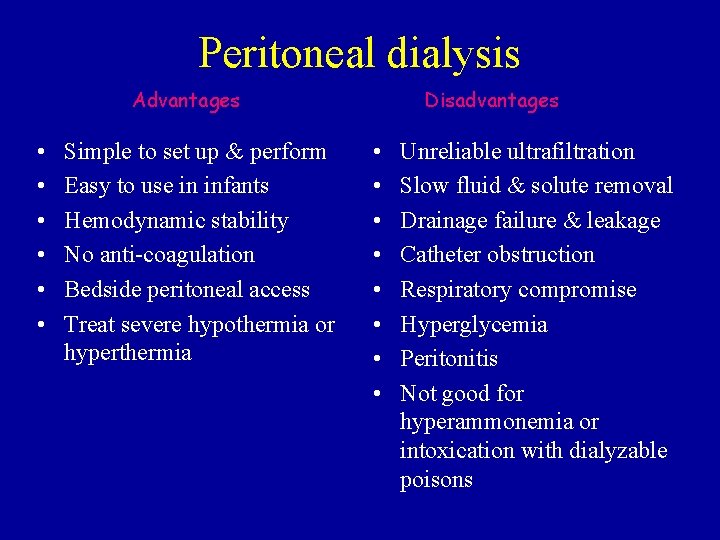
Peritoneal dialysis Advantages • • • Simple to set up & perform Easy to use in infants Hemodynamic stability No anti-coagulation Bedside peritoneal access Treat severe hypothermia or hyperthermia Disadvantages • • Unreliable ultrafiltration Slow fluid & solute removal Drainage failure & leakage Catheter obstruction Respiratory compromise Hyperglycemia Peritonitis Not good for hyperammonemia or intoxication with dialyzable poisons
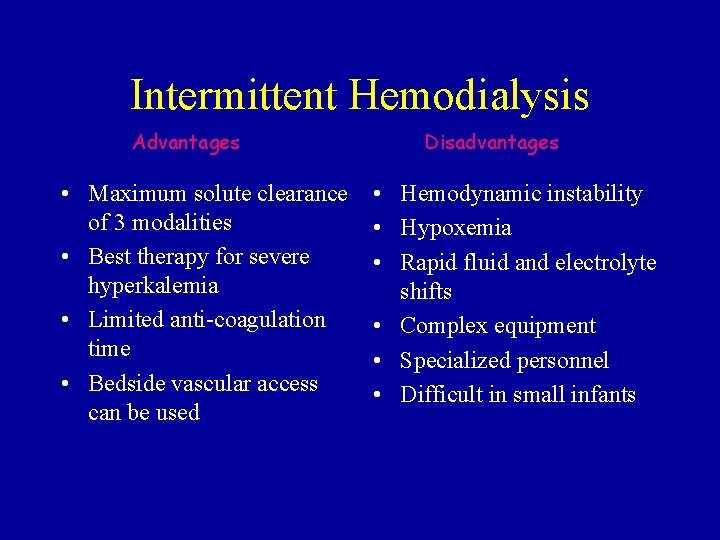
Intermittent Hemodialysis Advantages • Maximum solute clearance of 3 modalities • Best therapy for severe hyperkalemia • Limited anti-coagulation time • Bedside vascular access can be used Disadvantages • Hemodynamic instability • Hypoxemia • Rapid fluid and electrolyte shifts • Complex equipment • Specialized personnel • Difficult in small infants
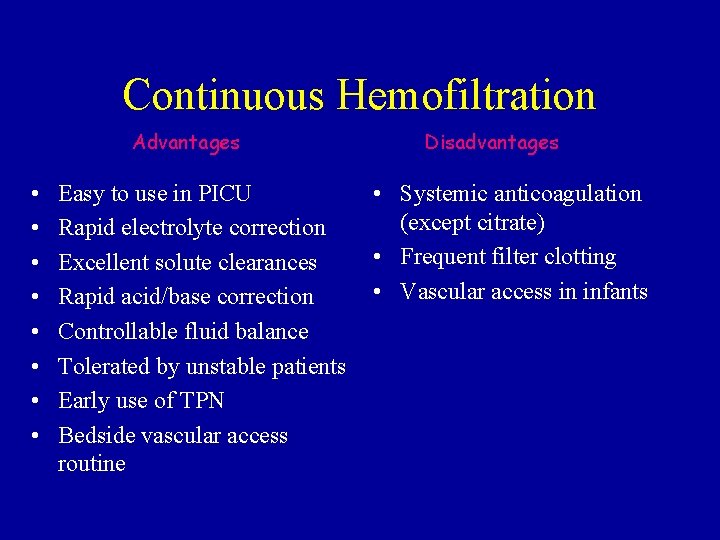
Continuous Hemofiltration Advantages • • Easy to use in PICU Rapid electrolyte correction Excellent solute clearances Rapid acid/base correction Controllable fluid balance Tolerated by unstable patients Early use of TPN Bedside vascular access routine Disadvantages • Systemic anticoagulation (except citrate) • Frequent filter clotting • Vascular access in infants
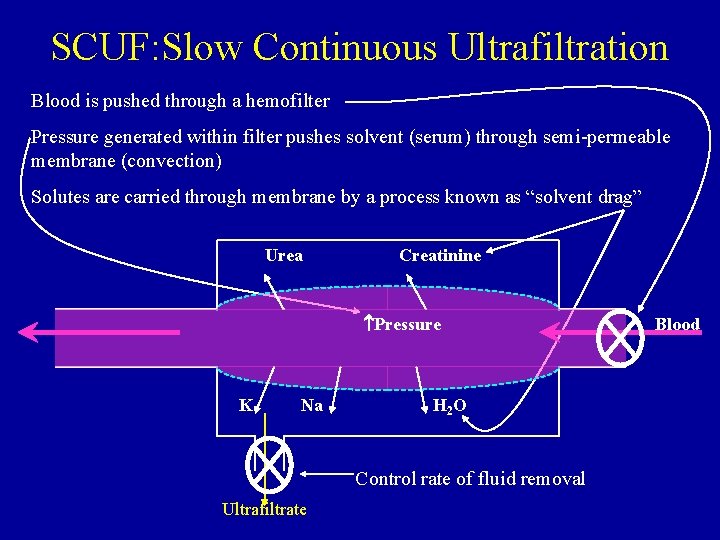
SCUF: Slow Continuous Ultrafiltration Blood is pushed through a hemofilter Pressure generated within filter pushes solvent (serum) through semi-permeable membrane (convection) Solutes are carried through membrane by a process known as “solvent drag” Urea Creatinine Pressure K Na H 2 O Control rate of fluid removal Ultrafiltrate Blood
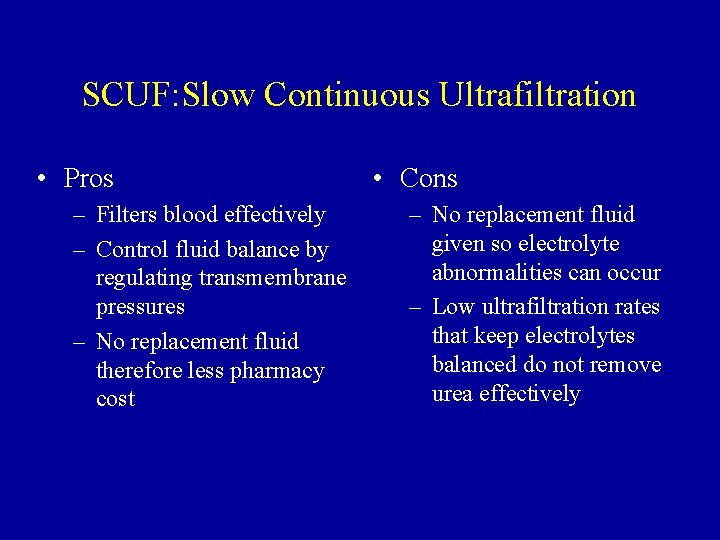
SCUF: Slow Continuous Ultrafiltration • Pros – Filters blood effectively – Control fluid balance by regulating transmembrane pressures – No replacement fluid therefore less pharmacy cost • Cons – No replacement fluid given so electrolyte abnormalities can occur – Low ultrafiltration rates that keep electrolytes balanced do not remove urea effectively
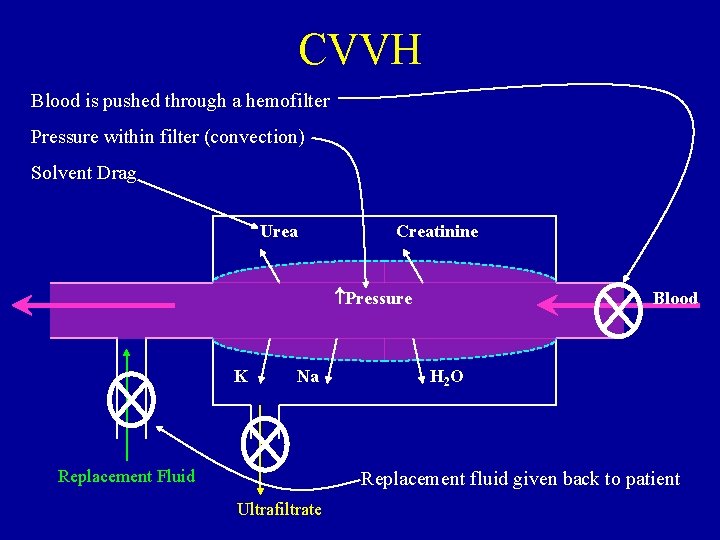
CVVH Blood is pushed through a hemofilter Pressure within filter (convection) Solvent Drag Urea Creatinine Pressure K Na Replacement Fluid Blood H 2 O Replacement fluid given back to patient Ultrafiltrate
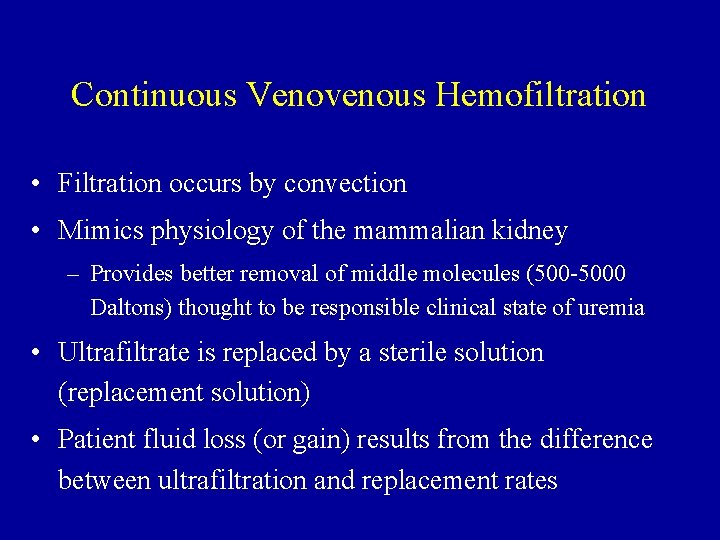
Continuous Venovenous Hemofiltration • Filtration occurs by convection • Mimics physiology of the mammalian kidney – Provides better removal of middle molecules (500 -5000 Daltons) thought to be responsible clinical state of uremia • Ultrafiltrate is replaced by a sterile solution (replacement solution) • Patient fluid loss (or gain) results from the difference between ultrafiltration and replacement rates
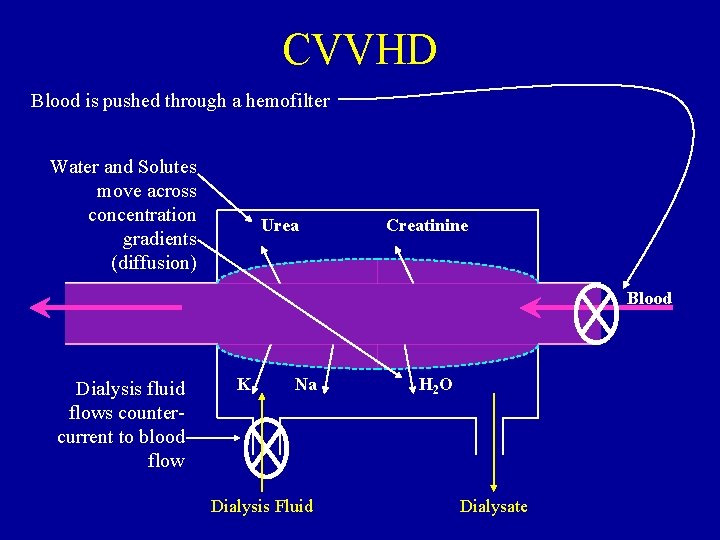
CVVHD Blood is pushed through a hemofilter Water and Solutes move across concentration gradients (diffusion) Urea Creatinine Blood Dialysis fluid flows countercurrent to blood flow K Na Dialysis Fluid H 2 O Dialysate
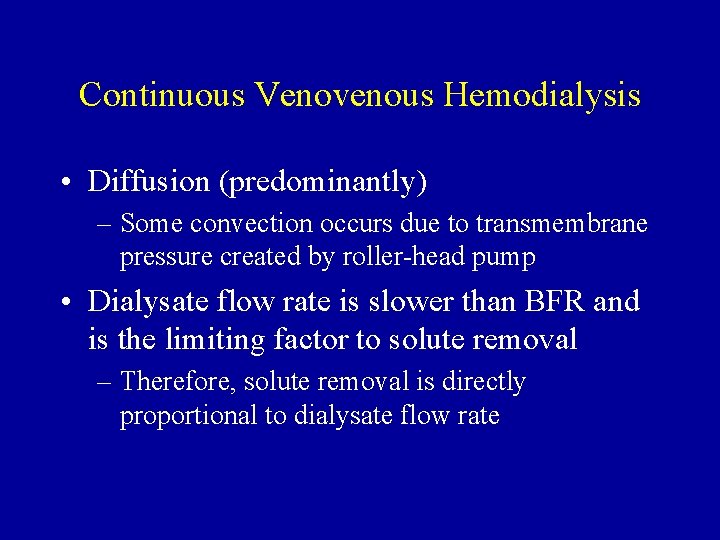
Continuous Venovenous Hemodialysis • Diffusion (predominantly) – Some convection occurs due to transmembrane pressure created by roller-head pump • Dialysate flow rate is slower than BFR and is the limiting factor to solute removal – Therefore, solute removal is directly proportional to dialysate flow rate
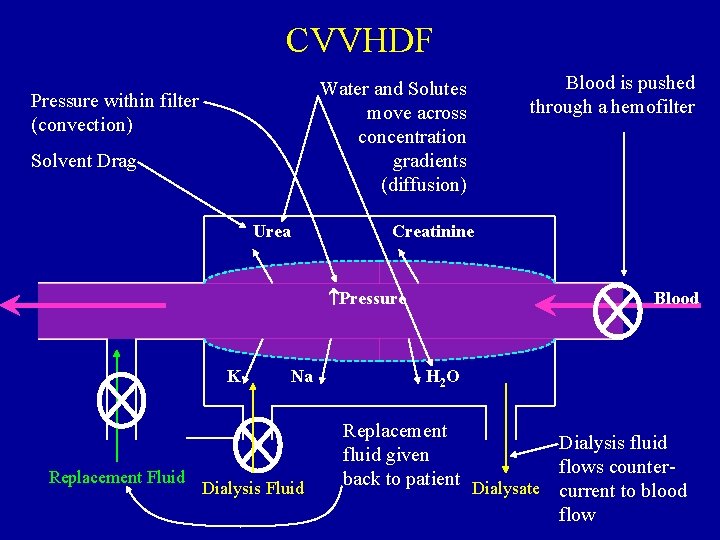
CVVHDF Water and Solutes move across concentration gradients (diffusion) Pressure within filter (convection) Solvent Drag Urea Creatinine Pressure K Replacement Fluid Na Dialysis Fluid Blood is pushed through a hemofilter Blood H 2 O Replacement Dialysis fluid given flows counterback to patient Dialysate current to blood flow
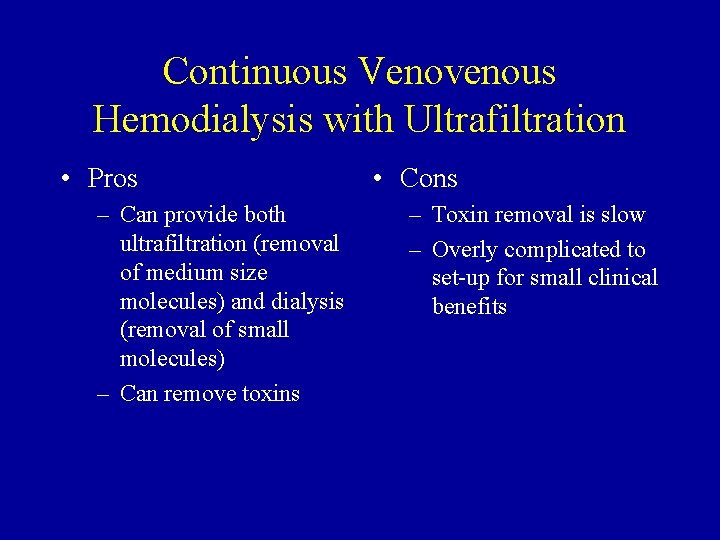
Continuous Venovenous Hemodialysis with Ultrafiltration • Pros – Can provide both ultrafiltration (removal of medium size molecules) and dialysis (removal of small molecules) – Can remove toxins • Cons – Toxin removal is slow – Overly complicated to set-up for small clinical benefits
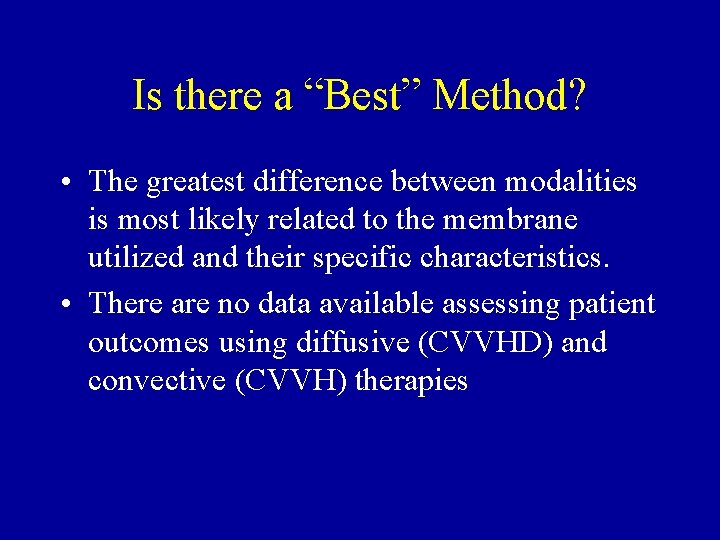
Is there a “Best” Method? • The greatest difference between modalities is most likely related to the membrane utilized and their specific characteristics. • There are no data available assessing patient outcomes using diffusive (CVVHD) and convective (CVVH) therapies
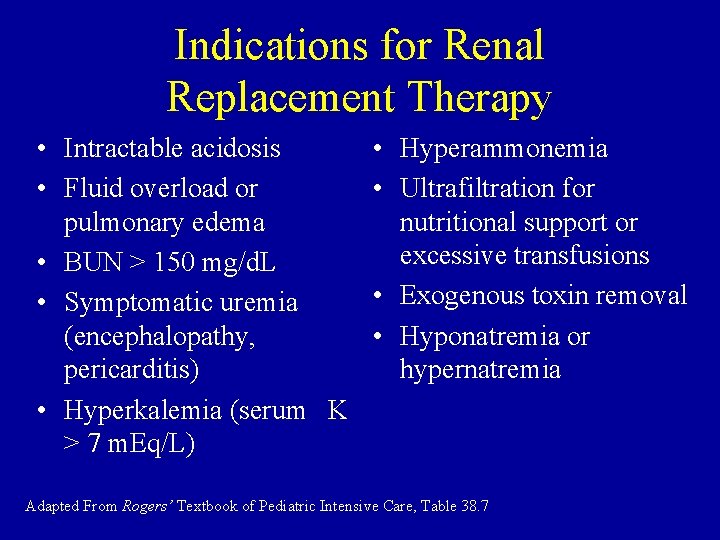
Indications for Renal Replacement Therapy • Intractable acidosis • Fluid overload or pulmonary edema • BUN > 150 mg/d. L • Symptomatic uremia (encephalopathy, pericarditis) • Hyperkalemia (serum K > 7 m. Eq/L) • Hyperammonemia • Ultrafiltration for nutritional support or excessive transfusions • Exogenous toxin removal • Hyponatremia or hypernatremia Adapted From Rogers’ Textbook of Pediatric Intensive Care, Table 38. 7
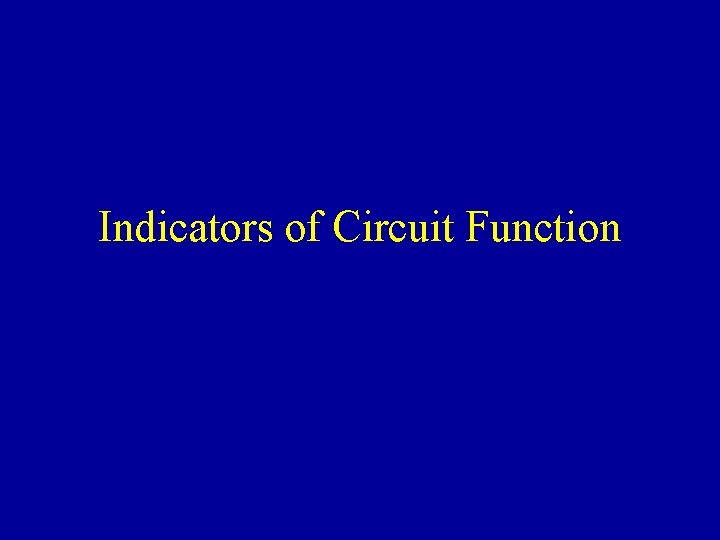
Indicators of Circuit Function
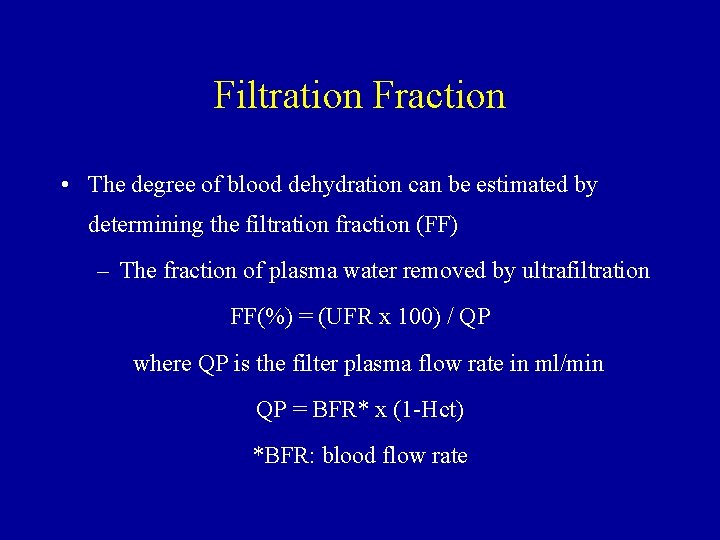
Filtration Fraction • The degree of blood dehydration can be estimated by determining the filtration fraction (FF) – The fraction of plasma water removed by ultrafiltration FF(%) = (UFR x 100) / QP where QP is the filter plasma flow rate in ml/min QP = BFR* x (1 -Hct) *BFR: blood flow rate
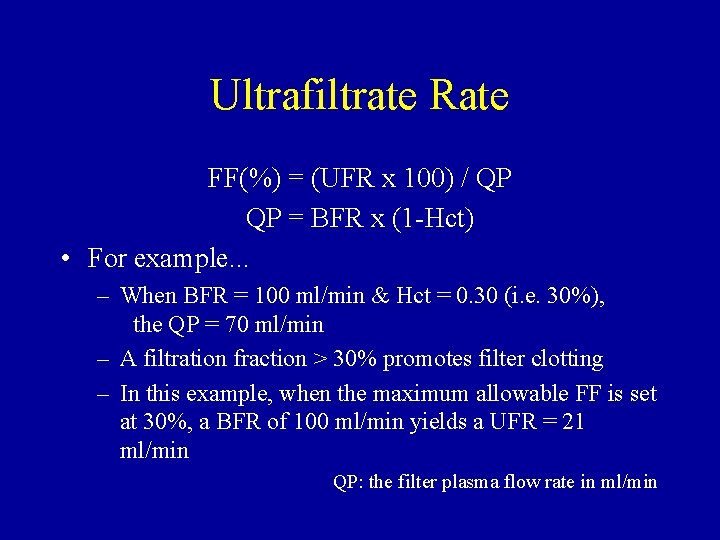
Ultrafiltrate Rate FF(%) = (UFR x 100) / QP QP = BFR x (1 -Hct) • For example. . . – When BFR = 100 ml/min & Hct = 0. 30 (i. e. 30%), the QP = 70 ml/min – A filtration fraction > 30% promotes filter clotting – In this example, when the maximum allowable FF is set at 30%, a BFR of 100 ml/min yields a UFR = 21 ml/min QP: the filter plasma flow rate in ml/min
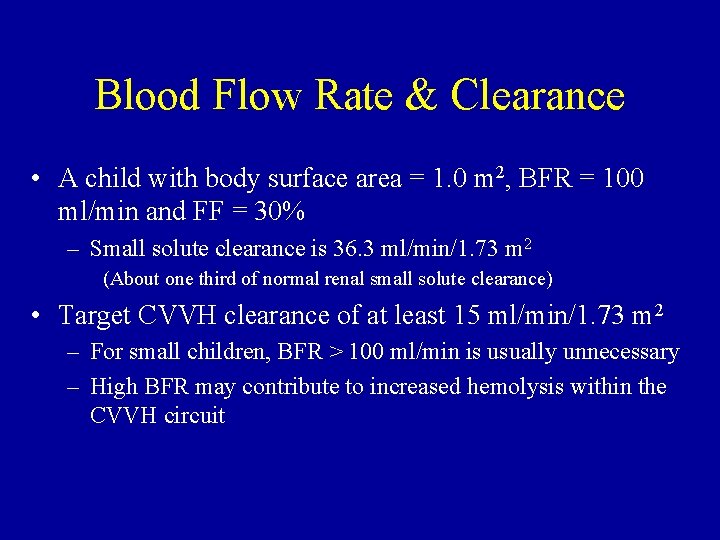
Blood Flow Rate & Clearance • A child with body surface area = 1. 0 m 2, BFR = 100 ml/min and FF = 30% – Small solute clearance is 36. 3 ml/min/1. 73 m 2 (About one third of normal renal small solute clearance) • Target CVVH clearance of at least 15 ml/min/1. 73 m 2 – For small children, BFR > 100 ml/min is usually unnecessary – High BFR may contribute to increased hemolysis within the CVVH circuit
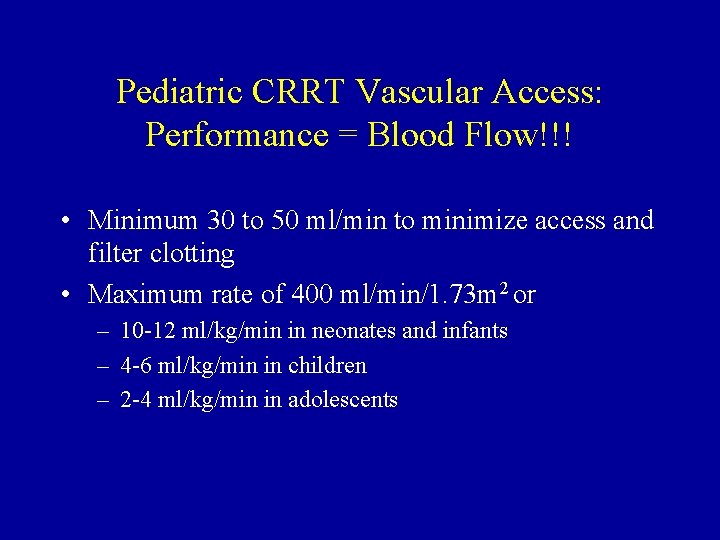
Pediatric CRRT Vascular Access: Performance = Blood Flow!!! • Minimum 30 to 50 ml/min to minimize access and filter clotting • Maximum rate of 400 ml/min/1. 73 m 2 or – 10 -12 ml/kg/min in neonates and infants – 4 -6 ml/kg/min in children – 2 -4 ml/kg/min in adolescents
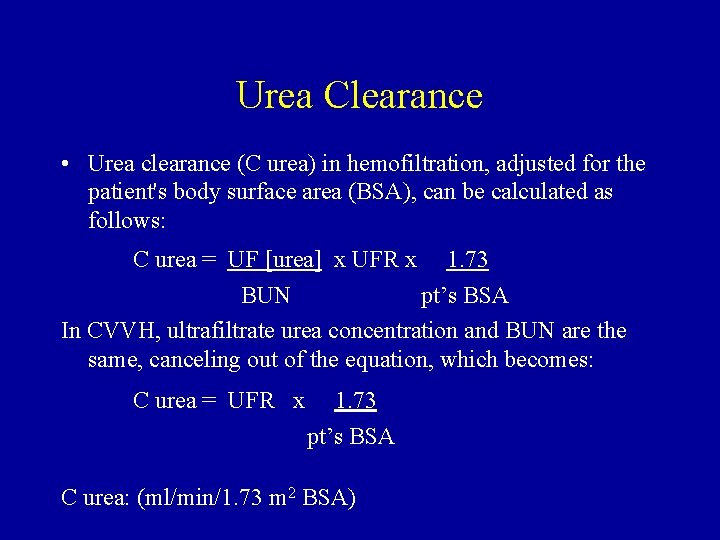
Urea Clearance • Urea clearance (C urea) in hemofiltration, adjusted for the patient's body surface area (BSA), can be calculated as follows: C urea = UF [urea] x UFR x 1. 73 BUN pt’s BSA In CVVH, ultrafiltrate urea concentration and BUN are the same, canceling out of the equation, which becomes: C urea = UFR x 1. 73 pt’s BSA C urea: (ml/min/1. 73 m 2 BSA)
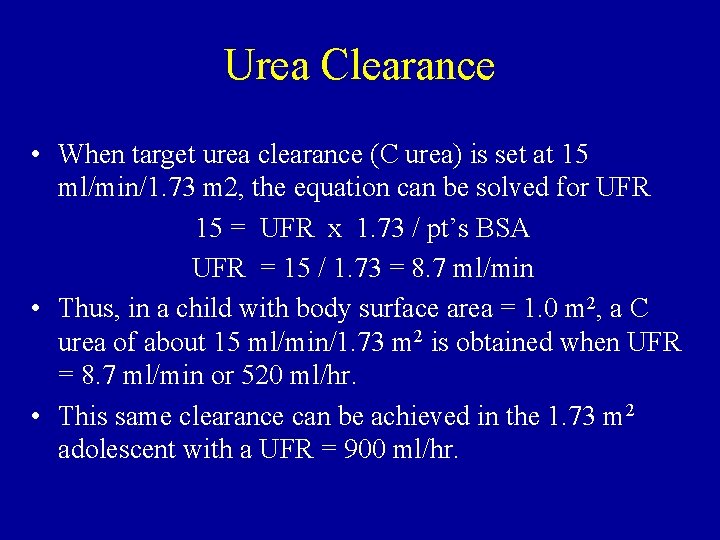
Urea Clearance • When target urea clearance (C urea) is set at 15 ml/min/1. 73 m 2, the equation can be solved for UFR 15 = UFR x 1. 73 / pt’s BSA UFR = 15 / 1. 73 = 8. 7 ml/min • Thus, in a child with body surface area = 1. 0 m 2, a C urea of about 15 ml/min/1. 73 m 2 is obtained when UFR = 8. 7 ml/min or 520 ml/hr. • This same clearance can be achieved in the 1. 73 m 2 adolescent with a UFR = 900 ml/hr.
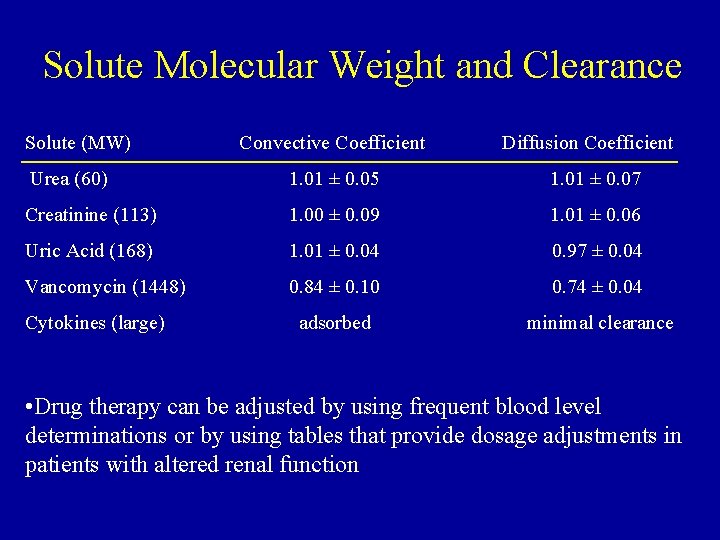
Solute Molecular Weight and Clearance Solute (MW) Convective Coefficient Diffusion Coefficient Urea (60) 1. 01 ± 0. 05 1. 01 ± 0. 07 Creatinine (113) 1. 00 ± 0. 09 1. 01 ± 0. 06 Uric Acid (168) 1. 01 ± 0. 04 0. 97 ± 0. 04 Vancomycin (1448) 0. 84 ± 0. 10 0. 74 ± 0. 04 adsorbed minimal clearance Cytokines (large) • Drug therapy can be adjusted by using frequent blood level determinations or by using tables that provide dosage adjustments in patients with altered renal function
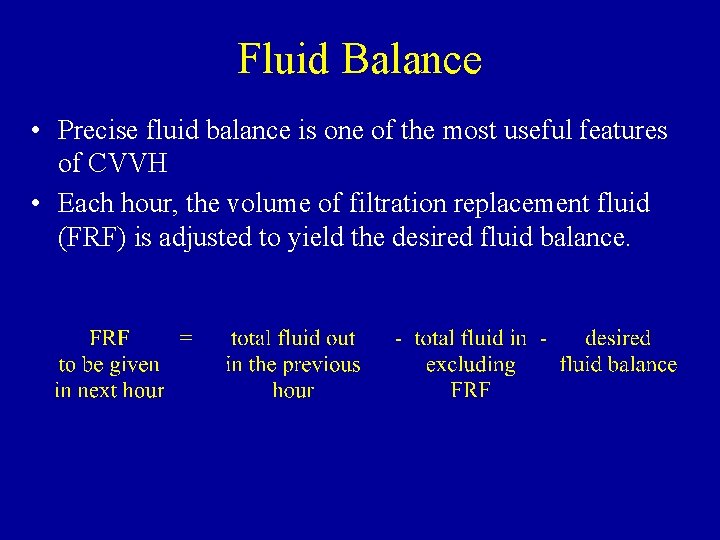
Fluid Balance • Precise fluid balance is one of the most useful features of CVVH • Each hour, the volume of filtration replacement fluid (FRF) is adjusted to yield the desired fluid balance.
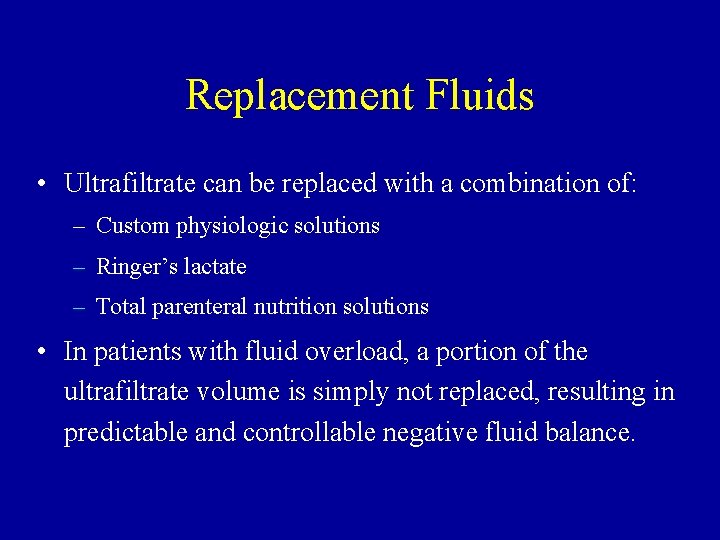
Replacement Fluids • Ultrafiltrate can be replaced with a combination of: – Custom physiologic solutions – Ringer’s lactate – Total parenteral nutrition solutions • In patients with fluid overload, a portion of the ultrafiltrate volume is simply not replaced, resulting in predictable and controllable negative fluid balance.
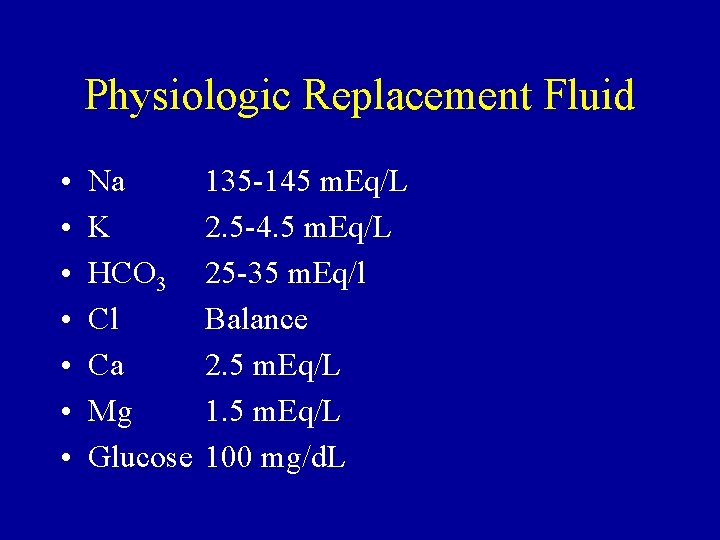
Physiologic Replacement Fluid • • Na K HCO 3 Cl Ca Mg Glucose 135 -145 m. Eq/L 2. 5 -4. 5 m. Eq/L 25 -35 m. Eq/l Balance 2. 5 m. Eq/L 100 mg/d. L
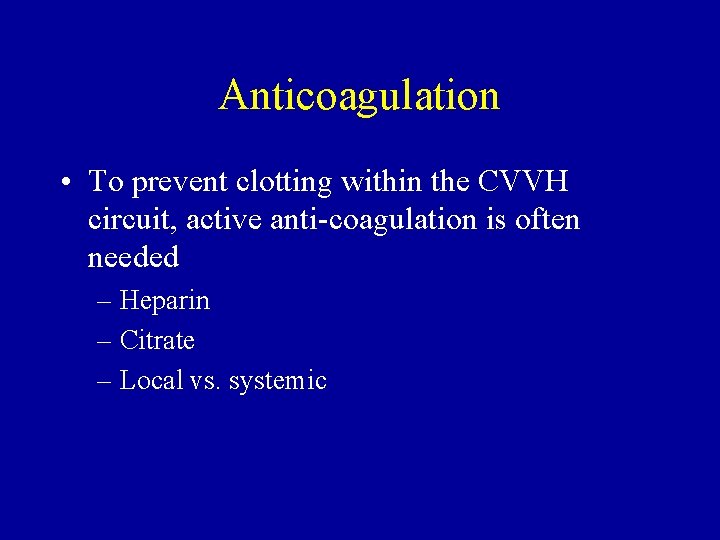
Anticoagulation • To prevent clotting within the CVVH circuit, active anti-coagulation is often needed – Heparin – Citrate – Local vs. systemic
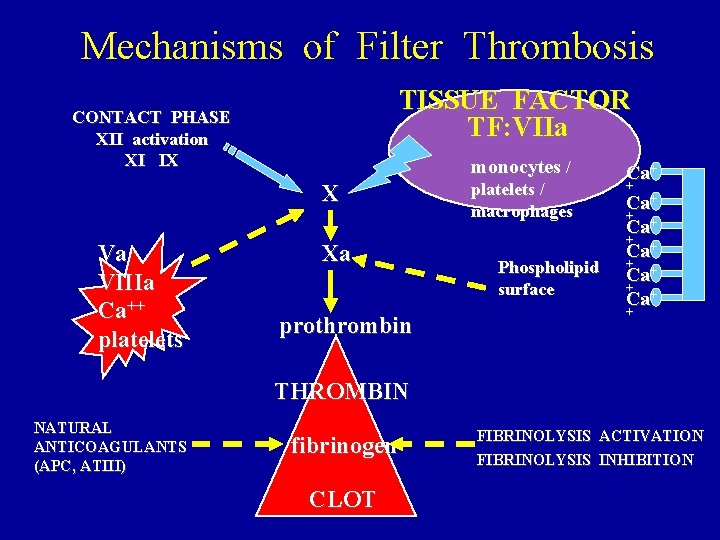
Mechanisms of Filter Thrombosis TISSUE FACTOR TF: VIIa CONTACT PHASE XII activation XI IX monocytes / X Va VIIIa Ca++ platelets Xa prothrombin platelets / macrophages Phospholipid surface Ca+ + + Ca + THROMBIN NATURAL ANTICOAGULANTS (APC, ATIII) fibrinogen CLOT FIBRINOLYSIS ACTIVATION FIBRINOLYSIS INHIBITION
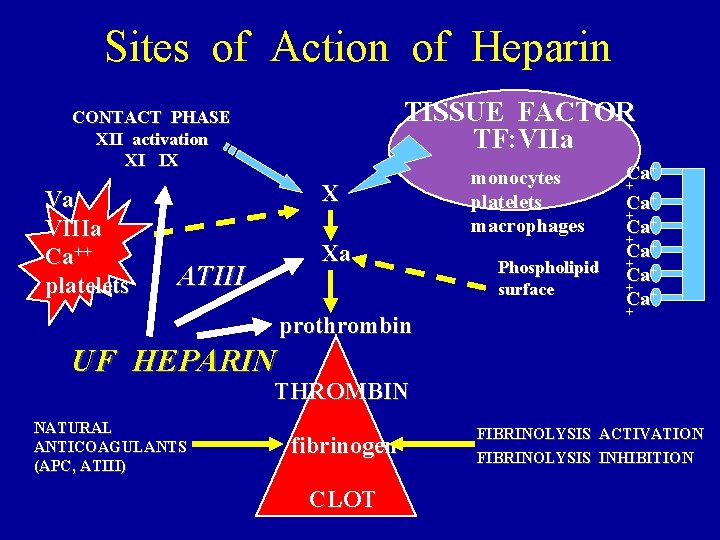
Sites of Action of Heparin TISSUE FACTOR TF: VIIa CONTACT PHASE XII activation XI IX Va VIIIa Ca++ platelets X Xa ATIII prothrombin monocytes platelets macrophages Phospholipid surface Ca+ + + Ca + UF HEPARIN THROMBIN NATURAL ANTICOAGULANTS (APC, ATIII) fibrinogen CLOT FIBRINOLYSIS ACTIVATION FIBRINOLYSIS INHIBITION
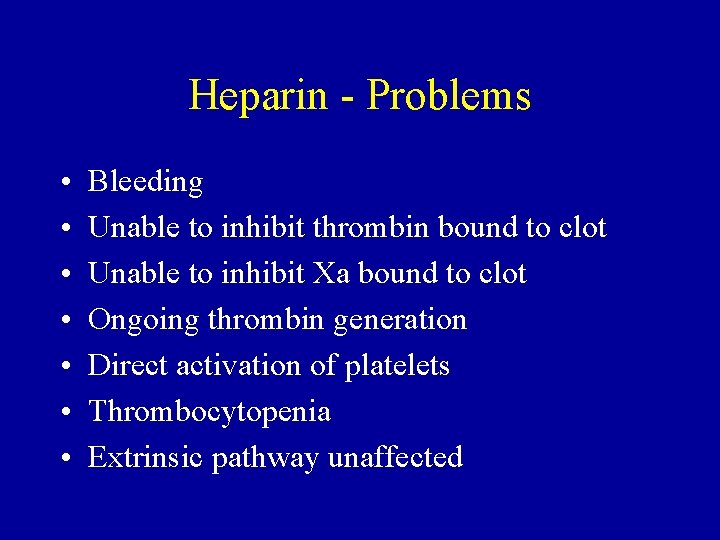
Heparin - Problems • • Bleeding Unable to inhibit thrombin bound to clot Unable to inhibit Xa bound to clot Ongoing thrombin generation Direct activation of platelets Thrombocytopenia Extrinsic pathway unaffected
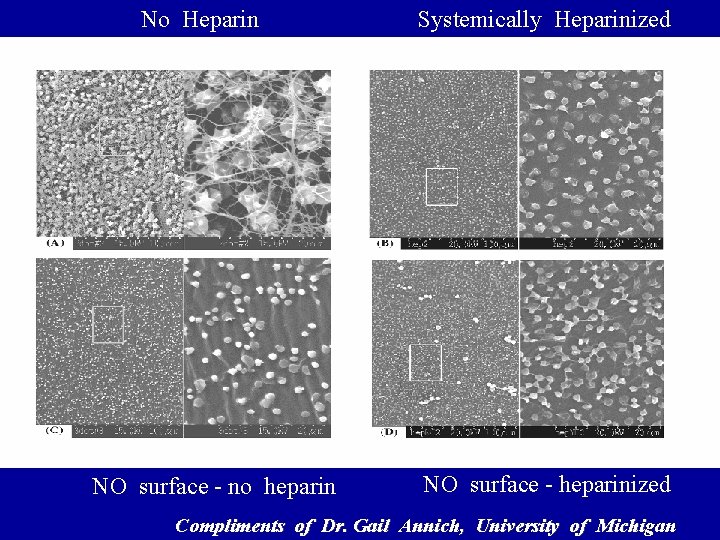
No Heparin NO surface - no heparin Systemically Heparinized NO surface - heparinized Compliments of Dr. Gail Annich, University of Michigan
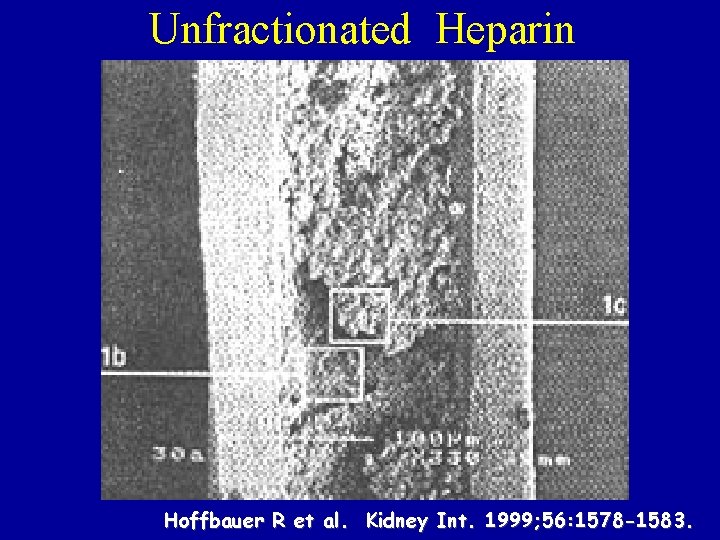
Unfractionated Heparin Hoffbauer R et al. Kidney Int. 1999; 56: 1578 -1583.
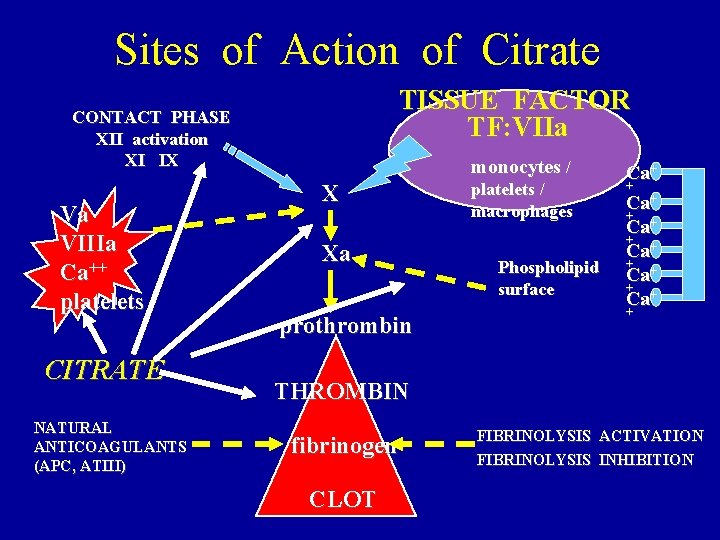
Sites of Action of Citrate TISSUE FACTOR TF: VIIa CONTACT PHASE XII activation XI IX Va VIIIa Ca++ platelets CITRATE NATURAL ANTICOAGULANTS (APC, ATIII) monocytes / X Xa prothrombin platelets / macrophages Phospholipid surface Ca+ + + Ca + THROMBIN fibrinogen CLOT FIBRINOLYSIS ACTIVATION FIBRINOLYSIS INHIBITION
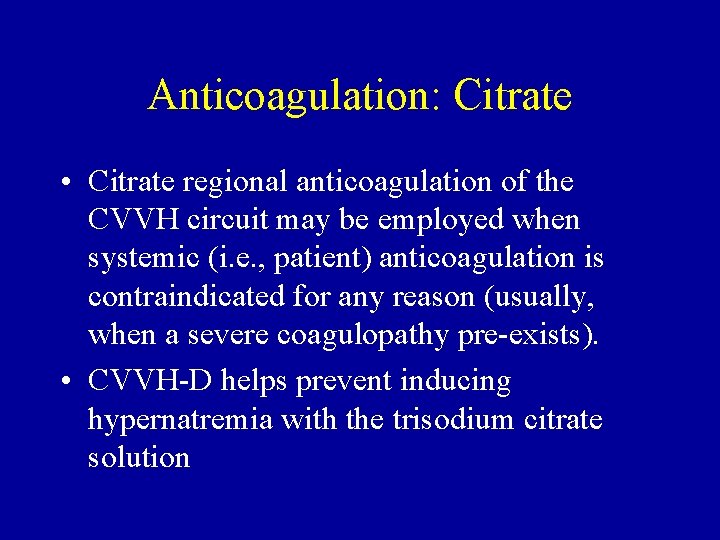
Anticoagulation: Citrate • Citrate regional anticoagulation of the CVVH circuit may be employed when systemic (i. e. , patient) anticoagulation is contraindicated for any reason (usually, when a severe coagulopathy pre-exists). • CVVH-D helps prevent inducing hypernatremia with the trisodium citrate solution
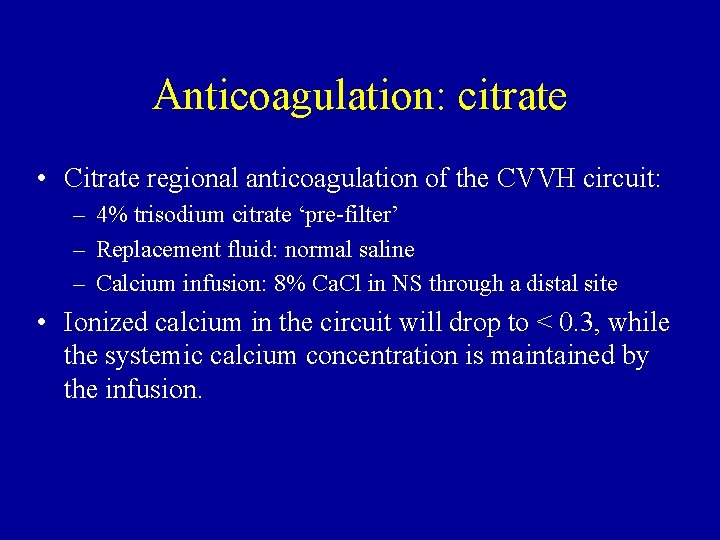
Anticoagulation: citrate • Citrate regional anticoagulation of the CVVH circuit: – 4% trisodium citrate ‘pre-filter’ – Replacement fluid: normal saline – Calcium infusion: 8% Ca. Cl in NS through a distal site • Ionized calcium in the circuit will drop to < 0. 3, while the systemic calcium concentration is maintained by the infusion.
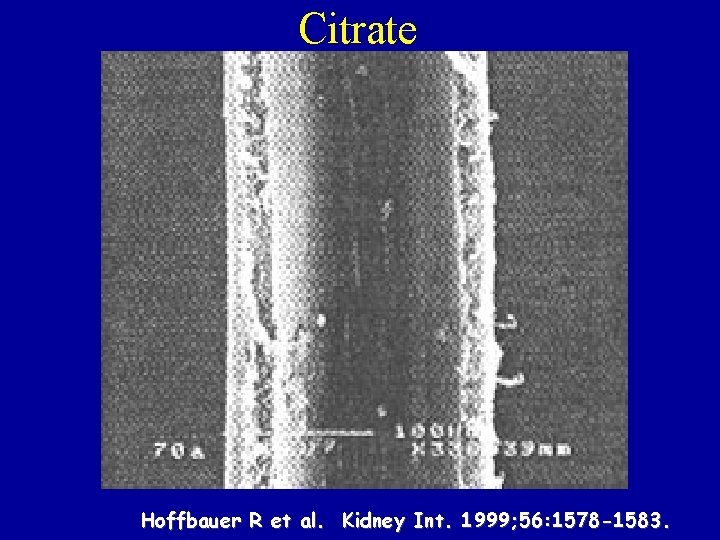
Citrate Hoffbauer R et al. Kidney Int. 1999; 56: 1578 -1583.
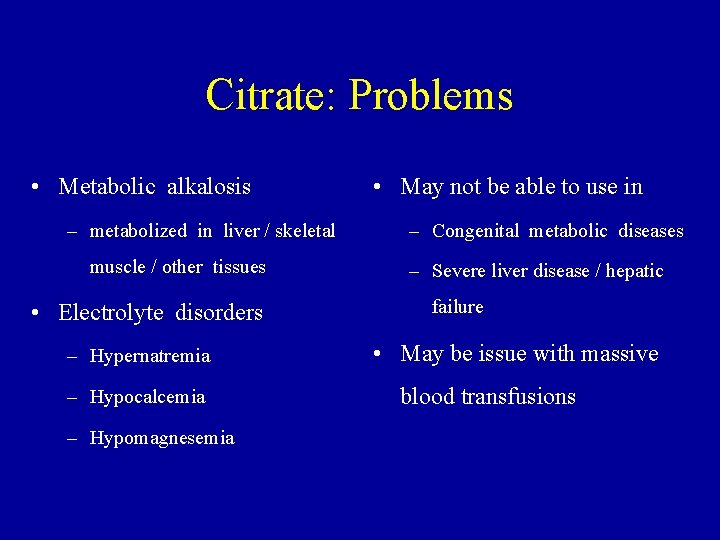
Citrate: Problems • Metabolic alkalosis – metabolized in liver / skeletal muscle / other tissues • Electrolyte disorders – Hypernatremia – Hypocalcemia – Hypomagnesemia • May not be able to use in – Congenital metabolic diseases – Severe liver disease / hepatic failure • May be issue with massive blood transfusions
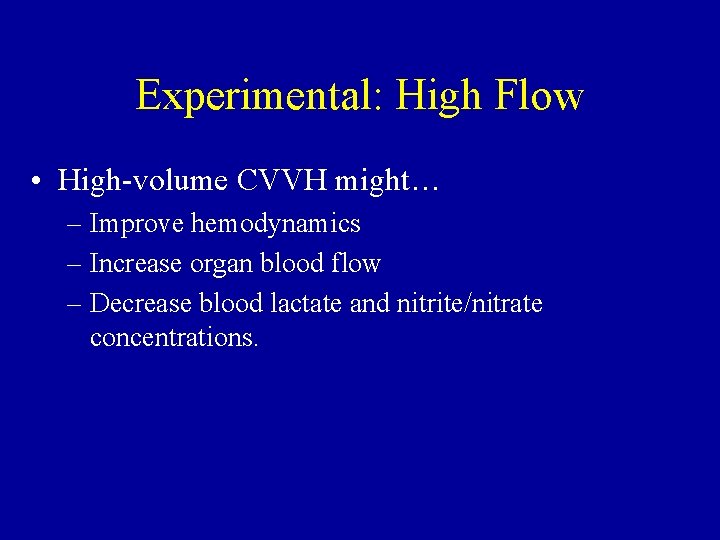
Experimental: High Flow • High-volume CVVH might… – Improve hemodynamics – Increase organ blood flow – Decrease blood lactate and nitrite/nitrate concentrations.
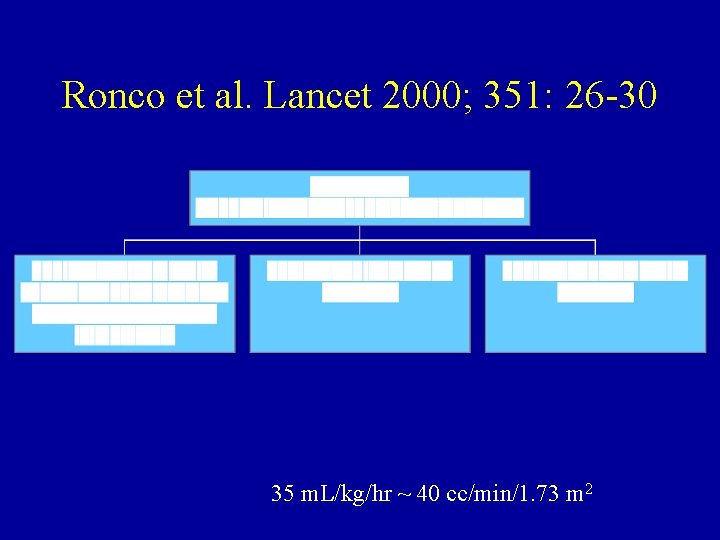
Ronco et al. Lancet 2000; 351: 26 -30 35 m. L/kg/hr ~ 40 cc/min/1. 73 m 2
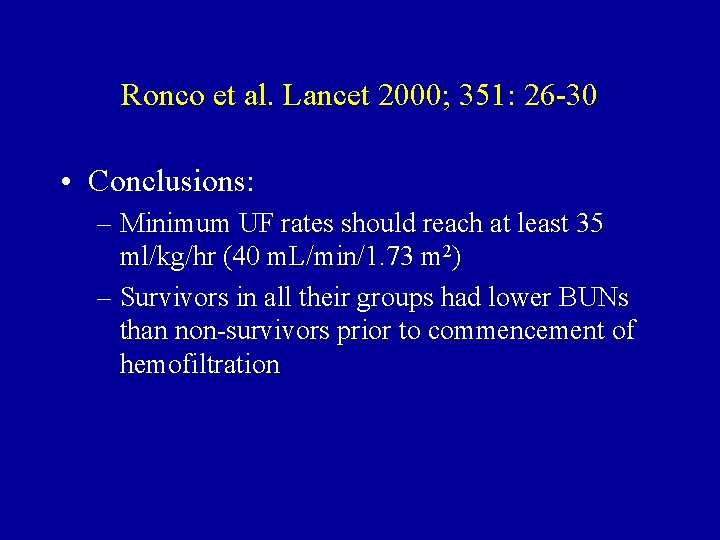
Ronco et al. Lancet 2000; 351: 26 -30 • Conclusions: – Minimum UF rates should reach at least 35 ml/kg/hr (40 m. L/min/1. 73 m 2) – Survivors in all their groups had lower BUNs than non-survivors prior to commencement of hemofiltration
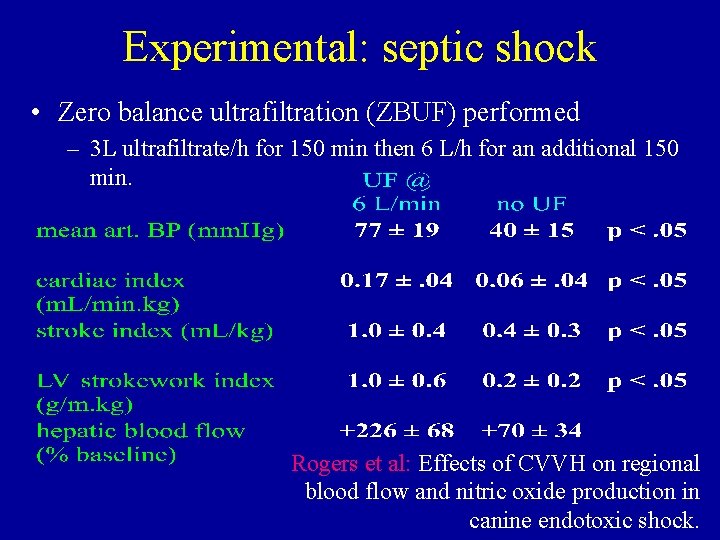
Experimental: septic shock • Zero balance ultrafiltration (ZBUF) performed – 3 L ultrafiltrate/h for 150 min then 6 L/h for an additional 150 min. Rogers et al: Effects of CVVH on regional blood flow and nitric oxide production in canine endotoxic shock.
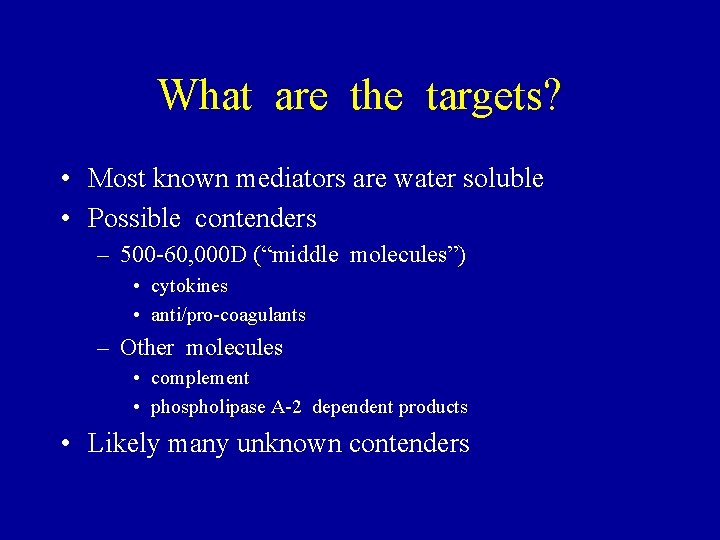
What are the targets? • Most known mediators are water soluble • Possible contenders – 500 -60, 000 D (“middle molecules”) • cytokines • anti/pro-coagulants – Other molecules • complement • phospholipase A-2 dependent products • Likely many unknown contenders
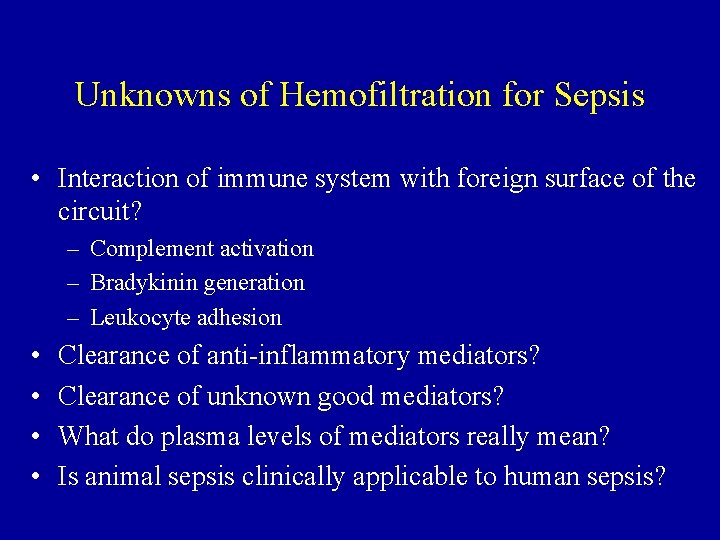
Unknowns of Hemofiltration for Sepsis • Interaction of immune system with foreign surface of the circuit? – Complement activation – Bradykinin generation – Leukocyte adhesion • • Clearance of anti-inflammatory mediators? Clearance of unknown good mediators? What do plasma levels of mediators really mean? Is animal sepsis clinically applicable to human sepsis?
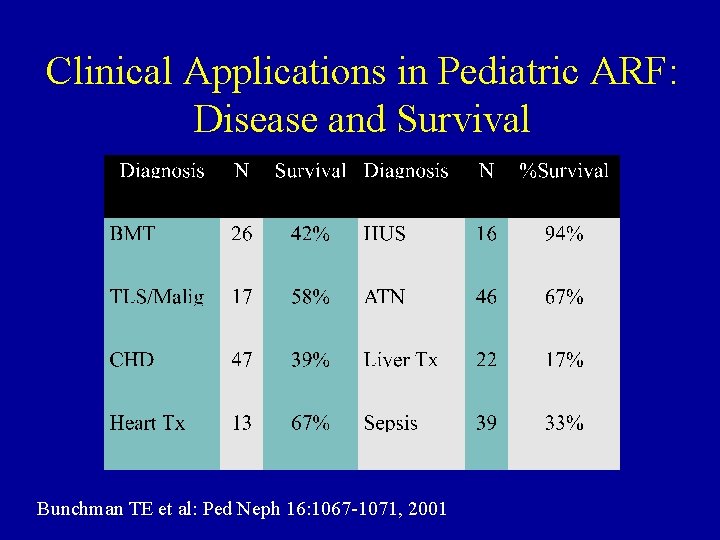
Clinical Applications in Pediatric ARF: Disease and Survival Bunchman TE et al: Ped Neph 16: 1067 -1071, 2001
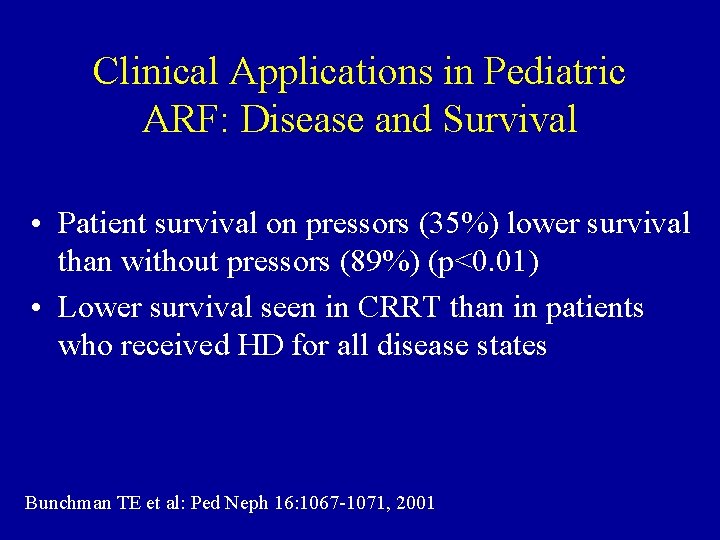
Clinical Applications in Pediatric ARF: Disease and Survival • Patient survival on pressors (35%) lower survival than without pressors (89%) (p<0. 01) • Lower survival seen in CRRT than in patients who received HD for all disease states Bunchman TE et al: Ped Neph 16: 1067 -1071, 2001
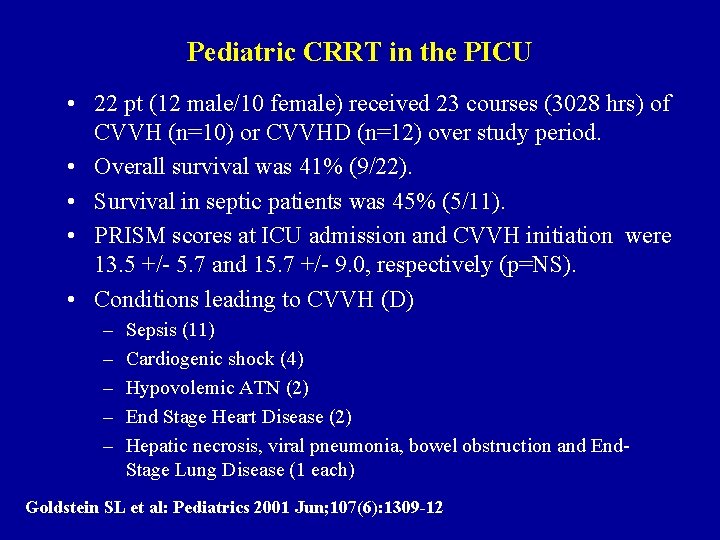
Pediatric CRRT in the PICU • 22 pt (12 male/10 female) received 23 courses (3028 hrs) of CVVH (n=10) or CVVHD (n=12) over study period. • Overall survival was 41% (9/22). • Survival in septic patients was 45% (5/11). • PRISM scores at ICU admission and CVVH initiation were 13. 5 +/- 5. 7 and 15. 7 +/- 9. 0, respectively (p=NS). • Conditions leading to CVVH (D) – – – Sepsis (11) Cardiogenic shock (4) Hypovolemic ATN (2) End Stage Heart Disease (2) Hepatic necrosis, viral pneumonia, bowel obstruction and End. Stage Lung Disease (1 each) Goldstein SL et al: Pediatrics 2001 Jun; 107(6): 1309 -12
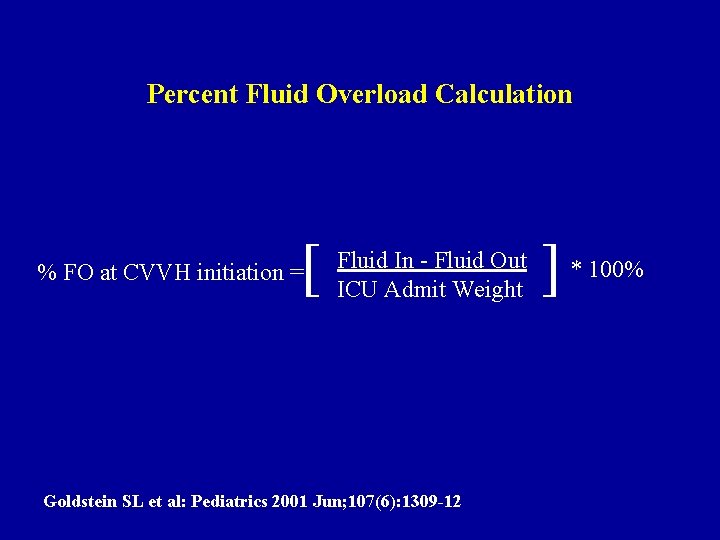
Percent Fluid Overload Calculation [ % FO at CVVH initiation = Fluid In - Fluid Out ICU Admit Weight Goldstein SL et al: Pediatrics 2001 Jun; 107(6): 1309 -12 ] * 100%
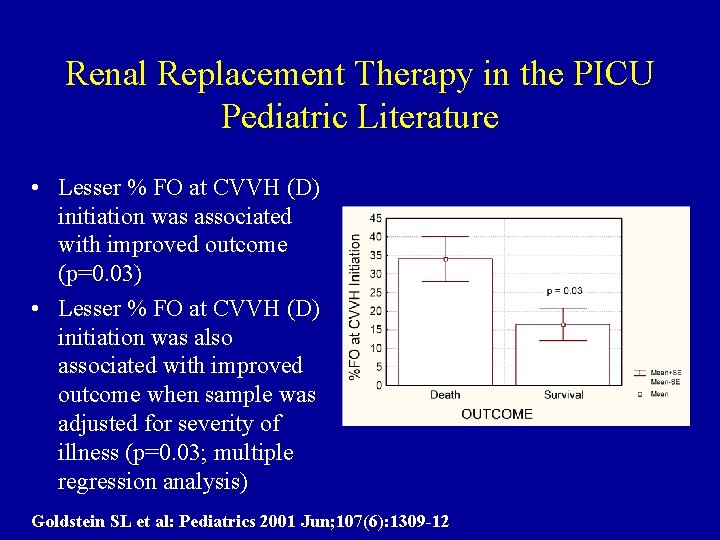
Renal Replacement Therapy in the PICU Pediatric Literature • Lesser % FO at CVVH (D) initiation was associated with improved outcome (p=0. 03) • Lesser % FO at CVVH (D) initiation was also associated with improved outcome when sample was adjusted for severity of illness (p=0. 03; multiple regression analysis) Goldstein SL et al: Pediatrics 2001 Jun; 107(6): 1309 -12
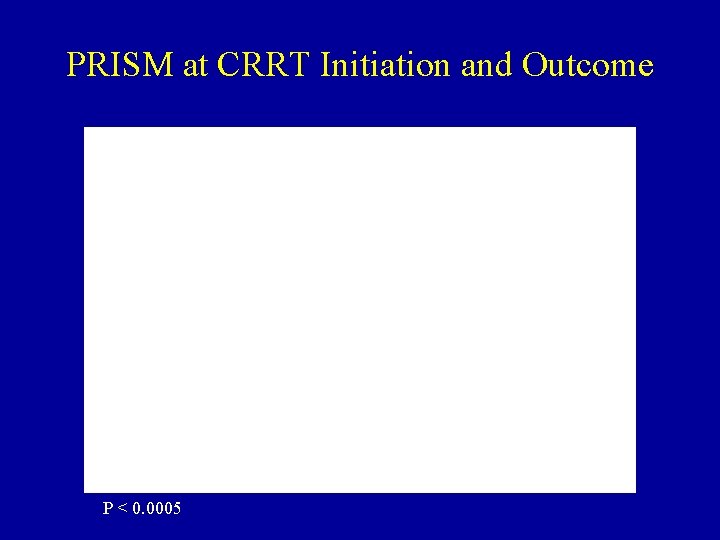
PRISM at CRRT Initiation and Outcome P < 0. 0005
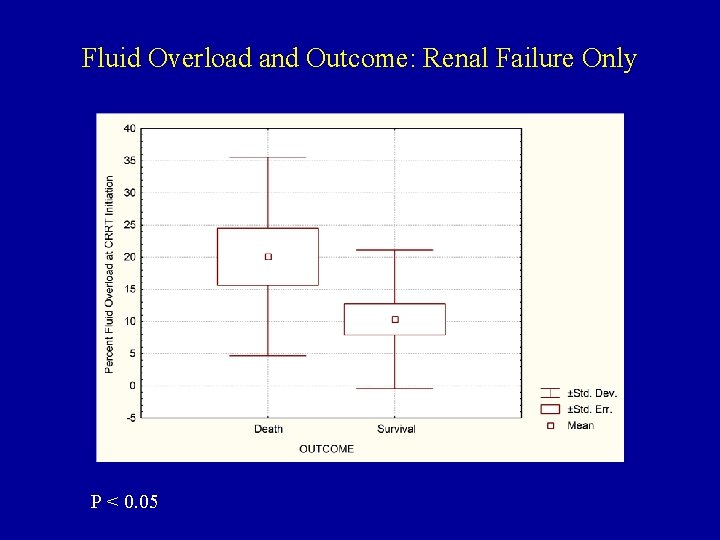
Fluid Overload and Outcome: Renal Failure Only P < 0. 05
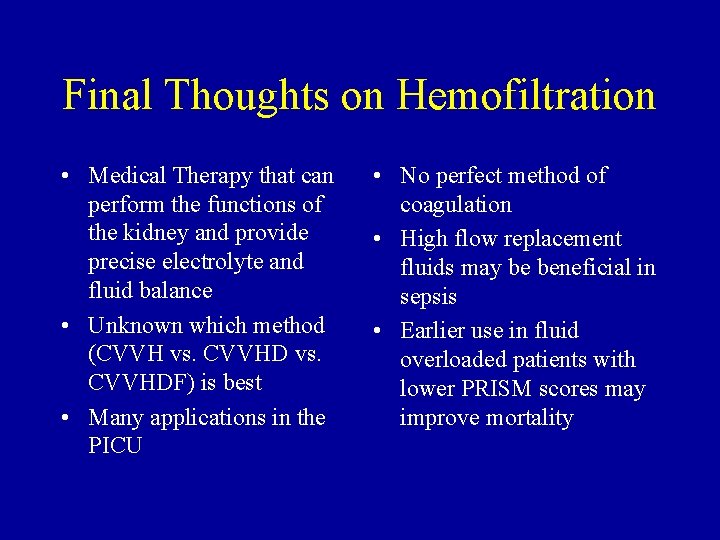
Final Thoughts on Hemofiltration • Medical Therapy that can perform the functions of the kidney and provide precise electrolyte and fluid balance • Unknown which method (CVVH vs. CVVHDF) is best • Many applications in the PICU • No perfect method of coagulation • High flow replacement fluids may be beneficial in sepsis • Earlier use in fluid overloaded patients with lower PRISM scores may improve mortality
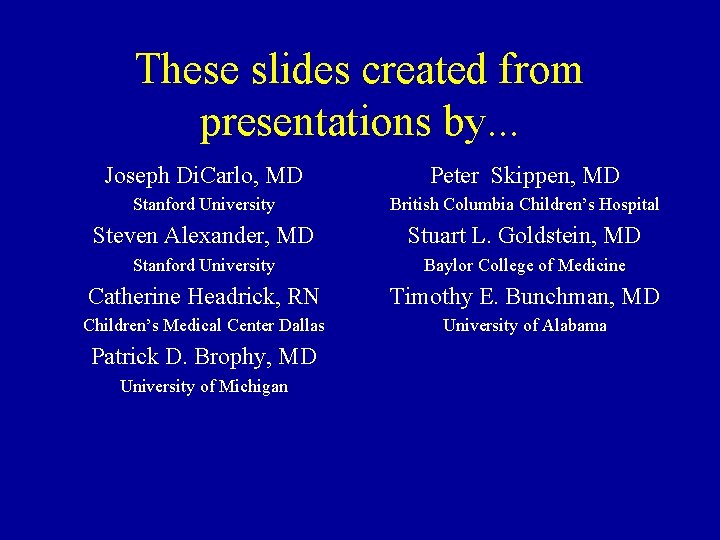
These slides created from presentations by. . . Joseph Di. Carlo, MD Peter Skippen, MD Stanford University British Columbia Children’s Hospital Steven Alexander, MD Stuart L. Goldstein, MD Stanford University Baylor College of Medicine Catherine Headrick, RN Timothy E. Bunchman, MD Children’s Medical Center Dallas University of Alabama Patrick D. Brophy, MD University of Michigan
Diagnostico etiologico
Res extra commercium
Aeiou renal replacement therapy
Combustion reaction formula
Renal corpuscle
Healthcare and the healthcare team chapter 2
Sports medicine definition
866-841-7659
Major physiological anions
Occupational therapy intervention plan for hip arthroplasty
Humanistic therapies aim to boost
Both psychoanalysis and humanistic therapy stress
Bioness integrated therapy system occupational therapy
Black childrens memorial
Childrens services walsall
World book day quiz
Low grade fever
Levine childrens
The childrens university of manchester
Moli texaschildrens
History of childrens literature
23 april international children's day turkey
Longman picture dictionary
Childrens services
Childrens christian fund
Colorado children's book award
Kirklees safeguarding childrens board
Stuart spencer recruitment
Federal reserve bank of atlanta jobs
Kronik pankreatit komplikasyonları
Atlanta rm clayton water reclamation plant
Atlanta watershed management
Envirotainer atlanta
Atlanta technical college dual enrollment
A duck flying due east passes over atlanta
Atlanta race riot of 1906
South river watershed alliance
Polyamory atlanta
Project walk atlanta
Trinity presbyterian church atlanta
What does the name marcus mean
Data quality atlanta
Clasificacion pancreatitis
Dr salomon aguilar medina
Koncept home
Aon hewitt careers
Haps score pancreatitis
Intrenchment creek
10 cent beer night streaker
"msa"
Modified atlanta classification
Prepaid legal opportunity
Pediatric urologist atlanta ga
Thomasova dlaha
Hilton garden inn atlanta aquarium
Motorcycle roundup in atlanta
Atlanta traffic report
Atlanta code enforcement
Balthazar kriterleri
Project hope atlanta
Rollator rental ga
Atlanta mission sweepstakes
Eeoc atlanta district office