Nanoscience and our Energy Future Present Primary Power
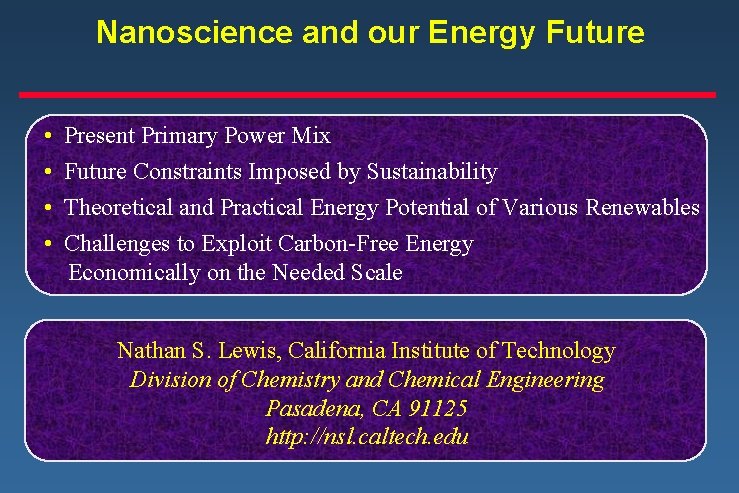
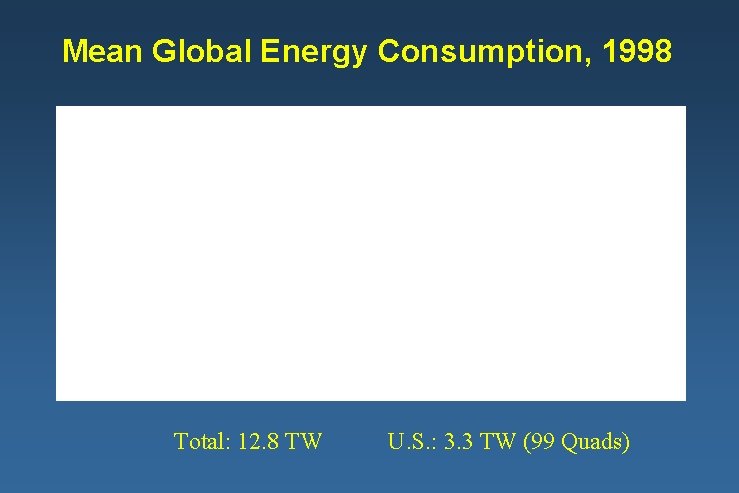
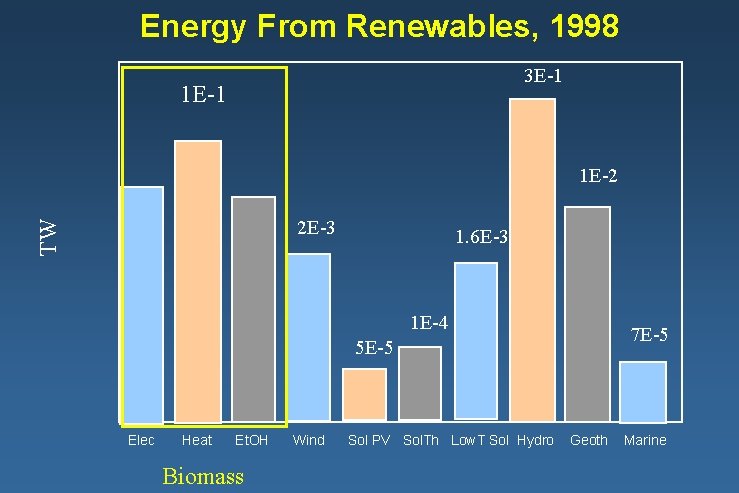
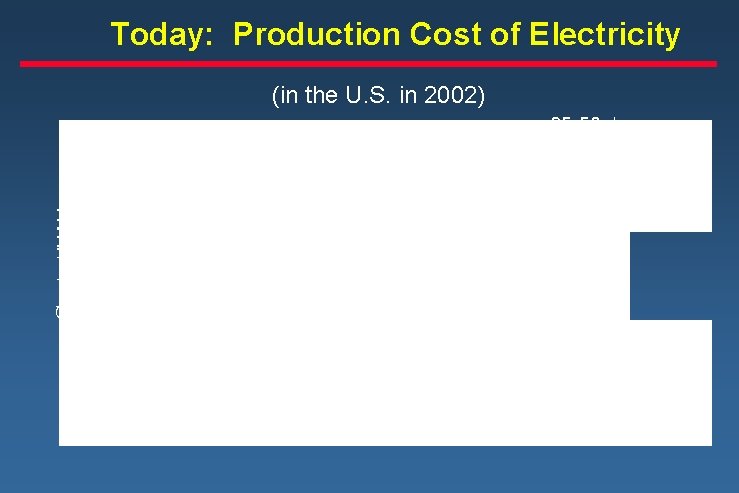
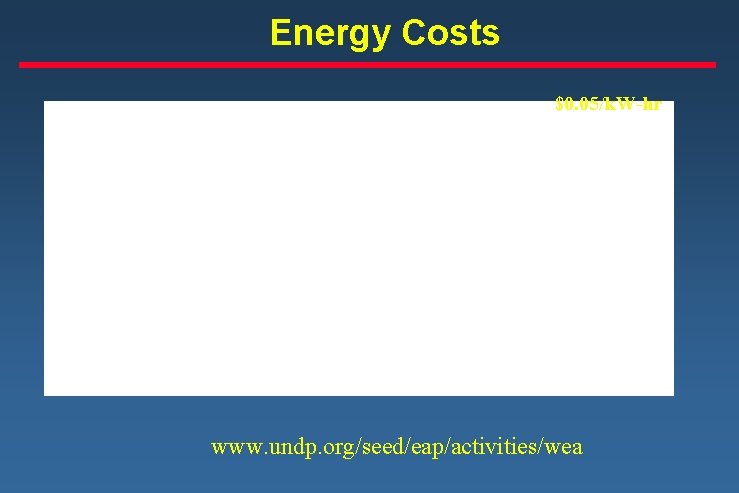
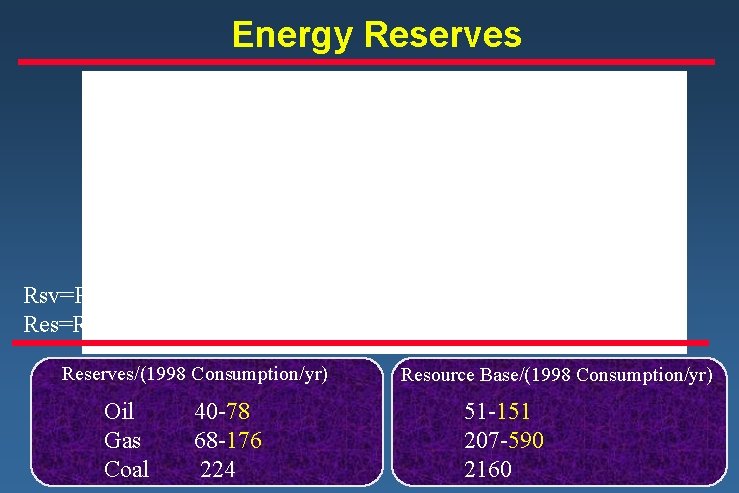
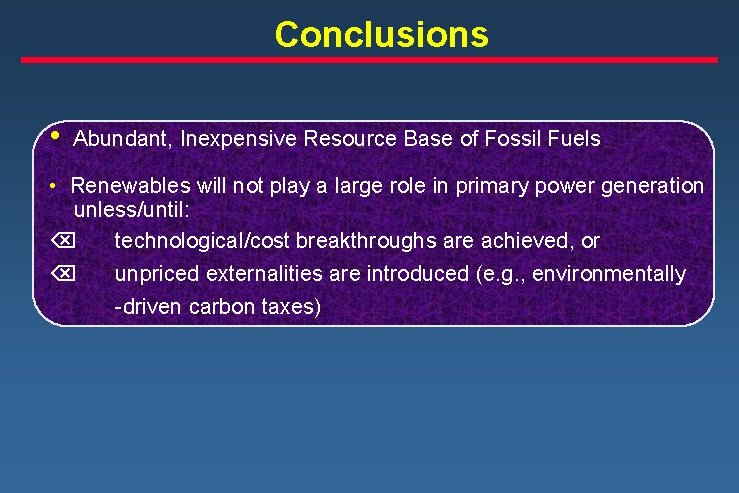
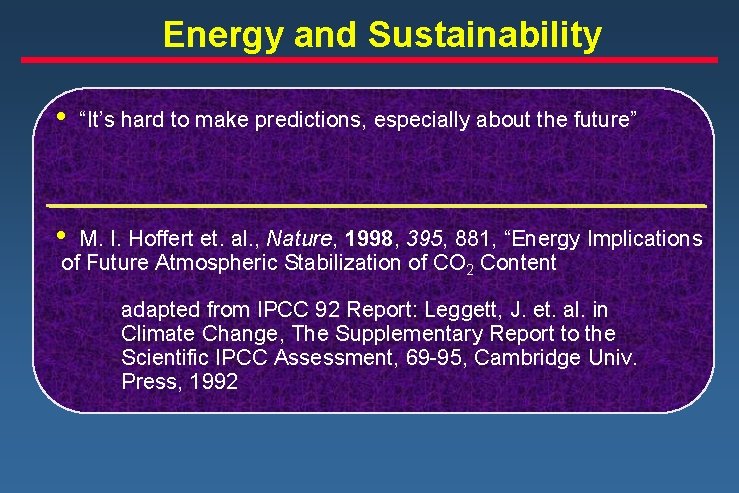
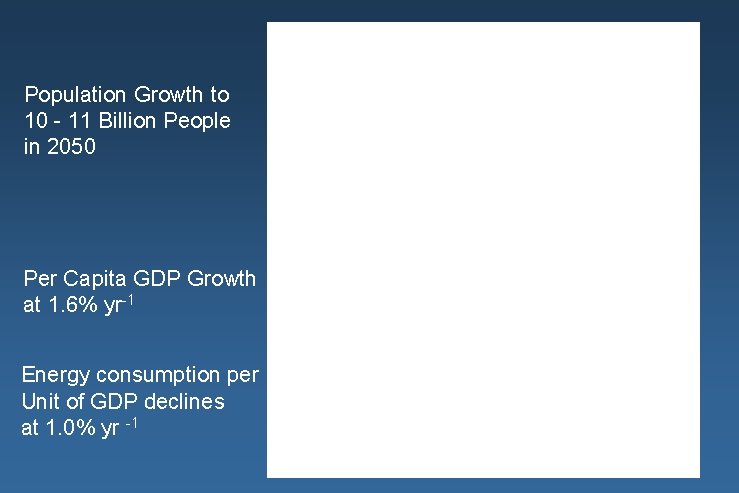
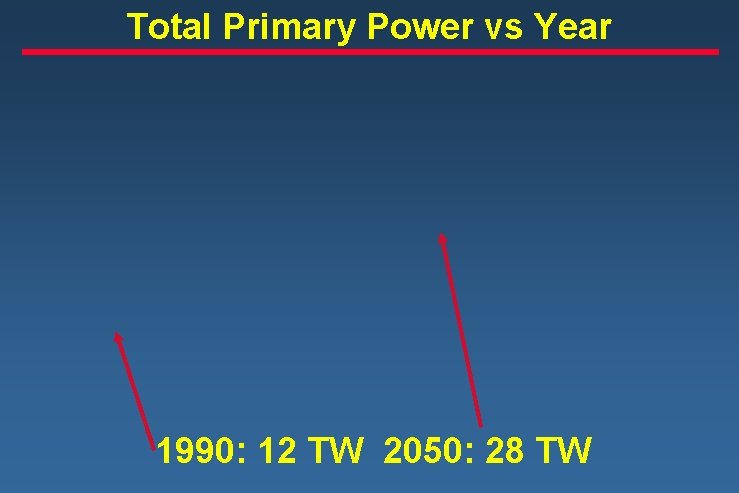
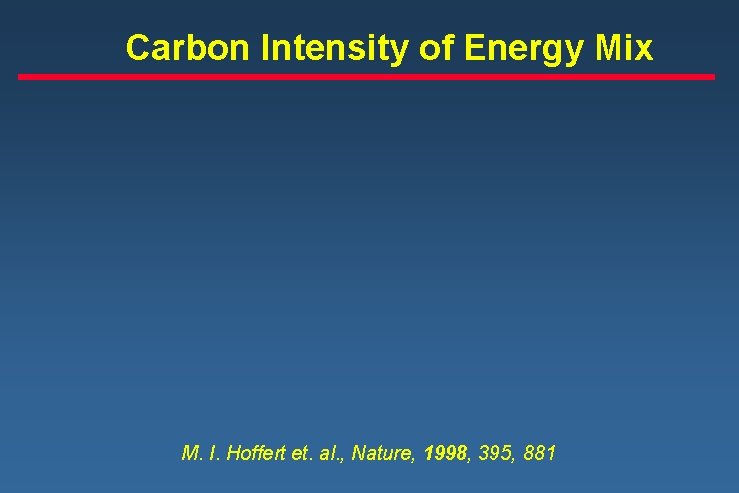
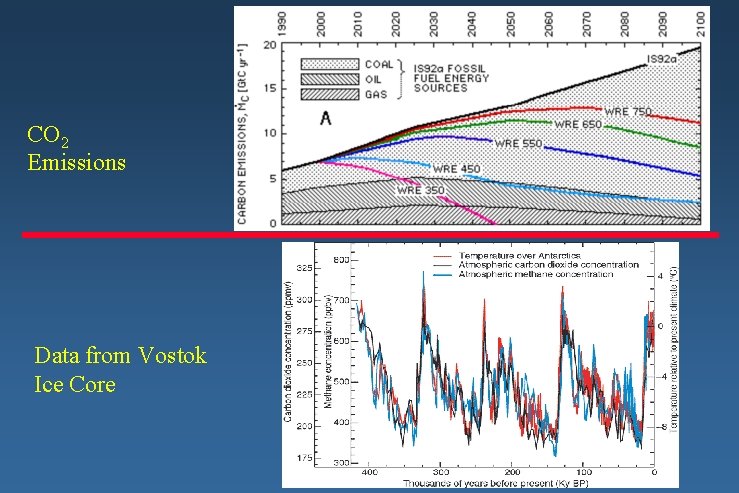
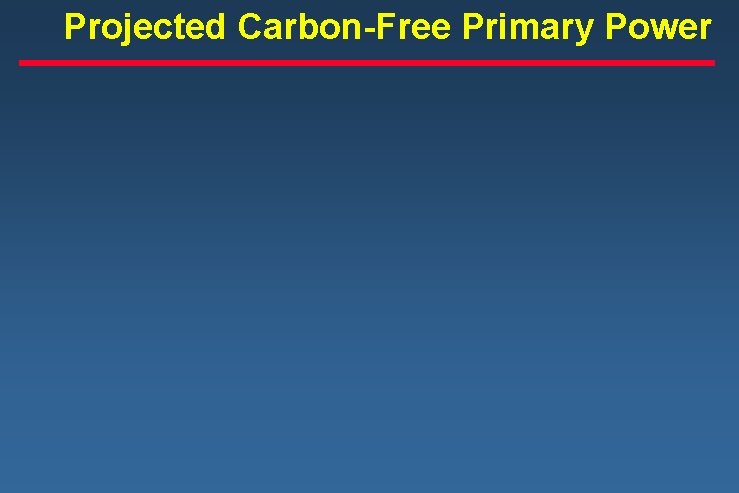
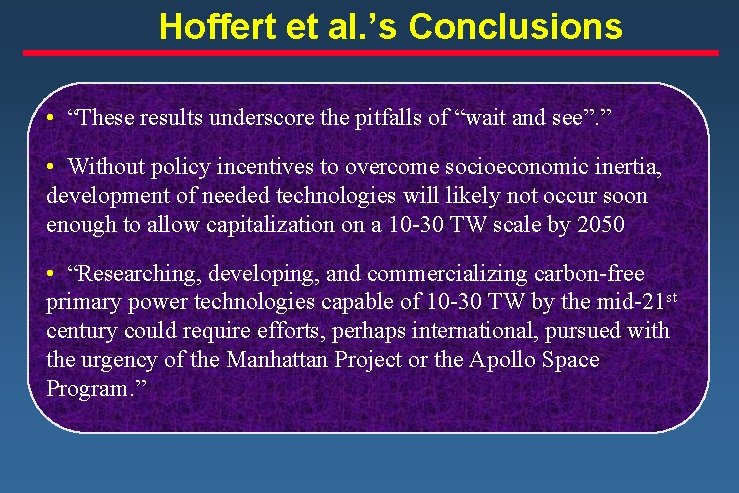
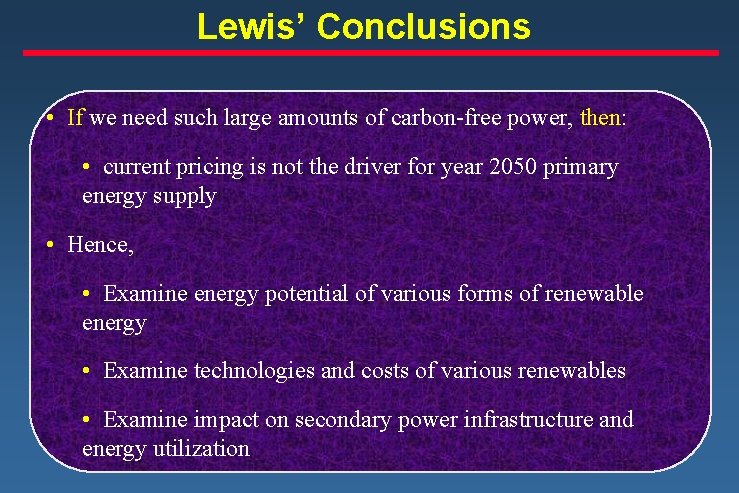
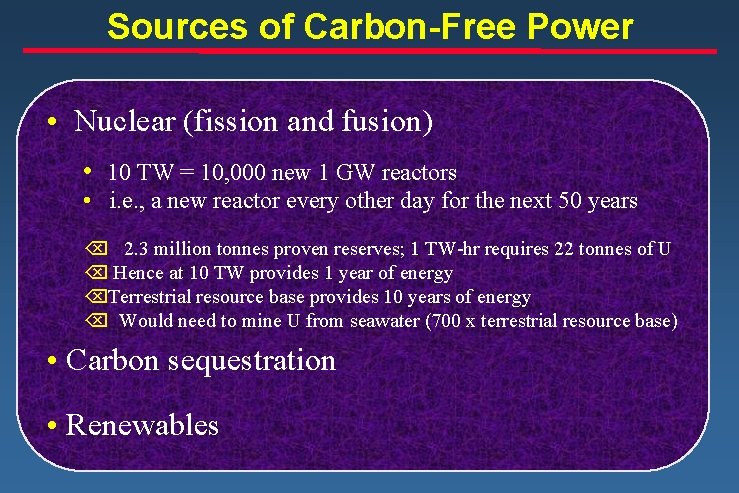
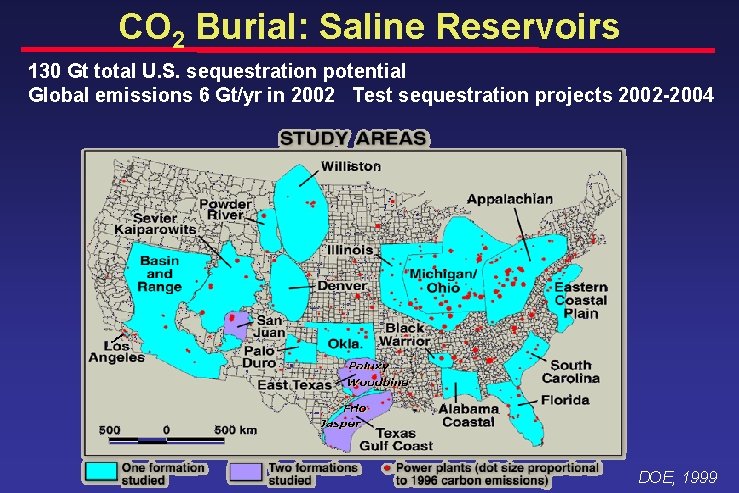
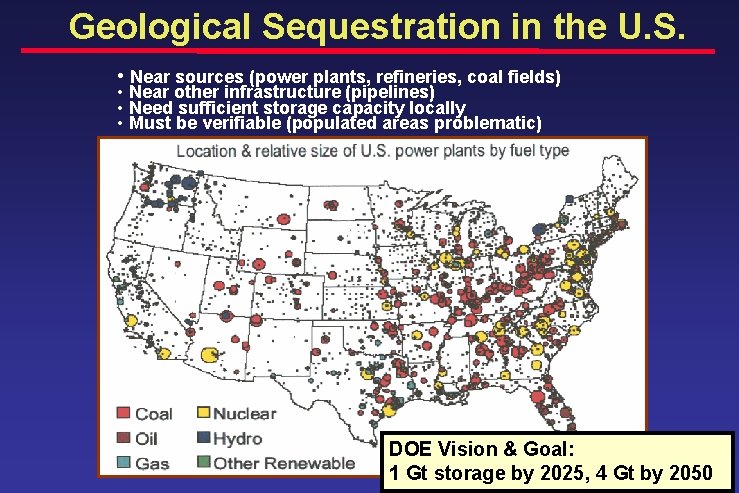
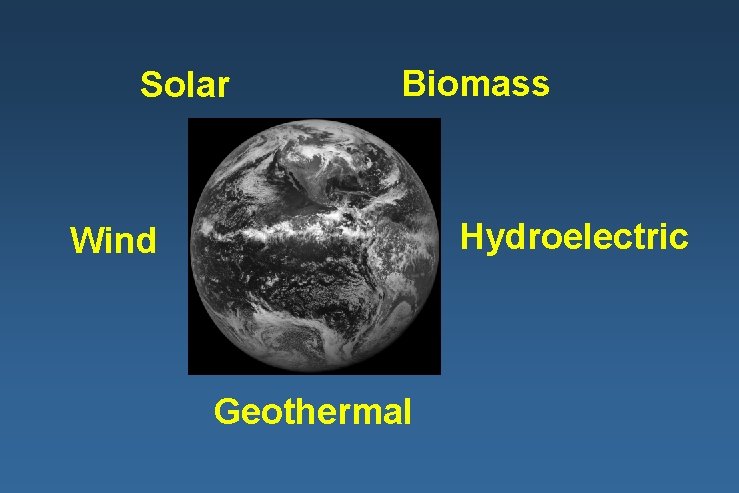
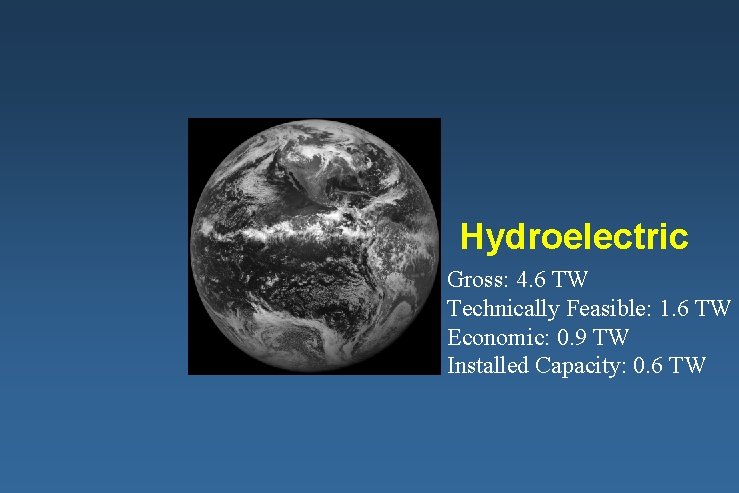
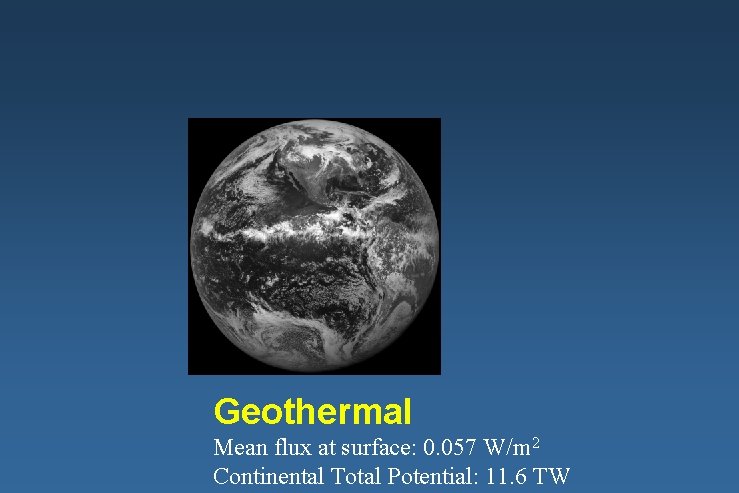
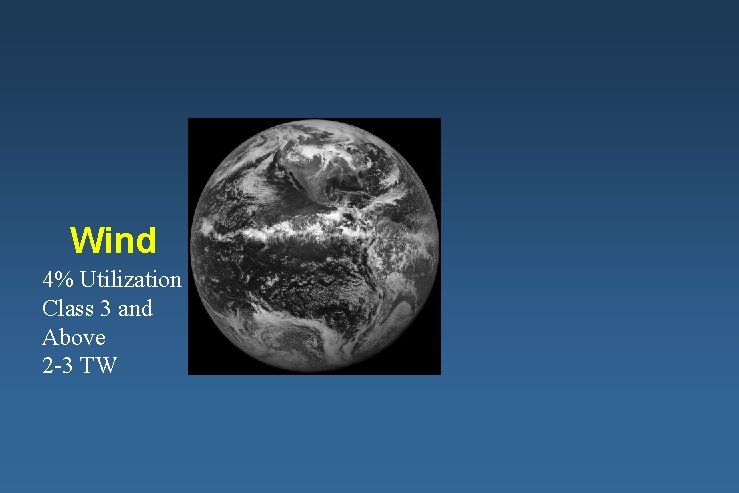
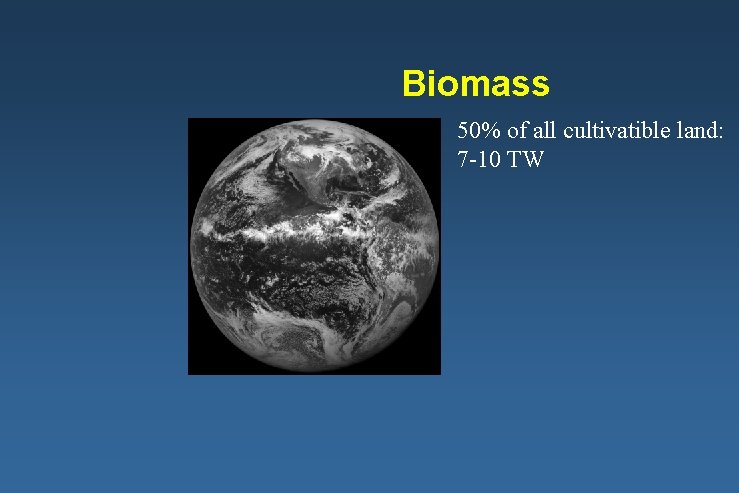
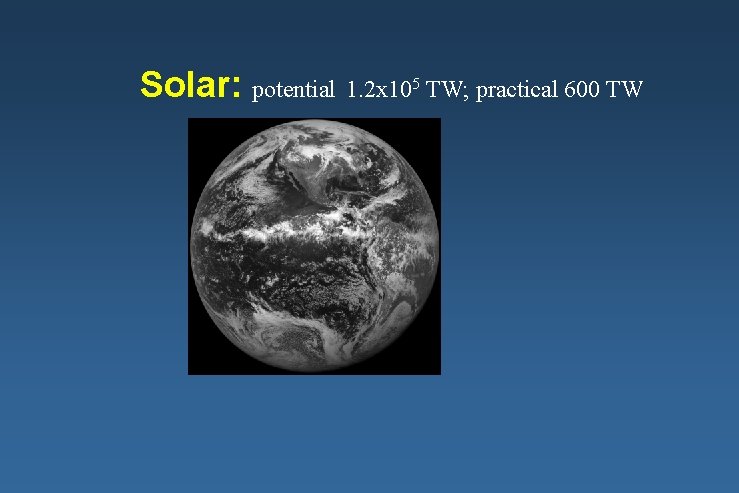
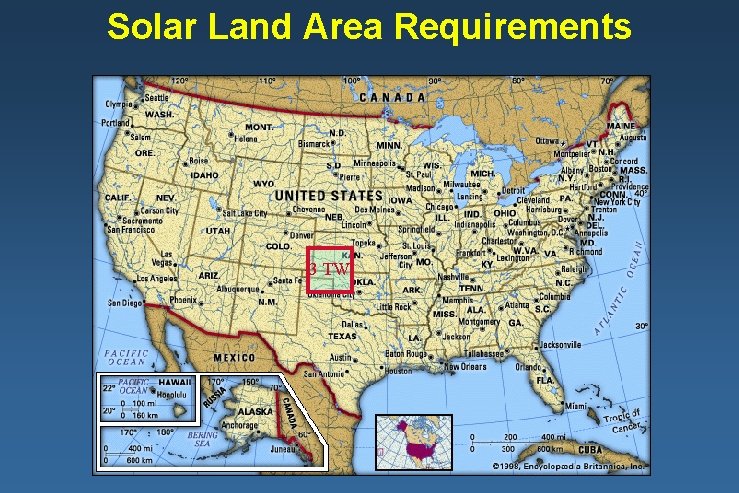
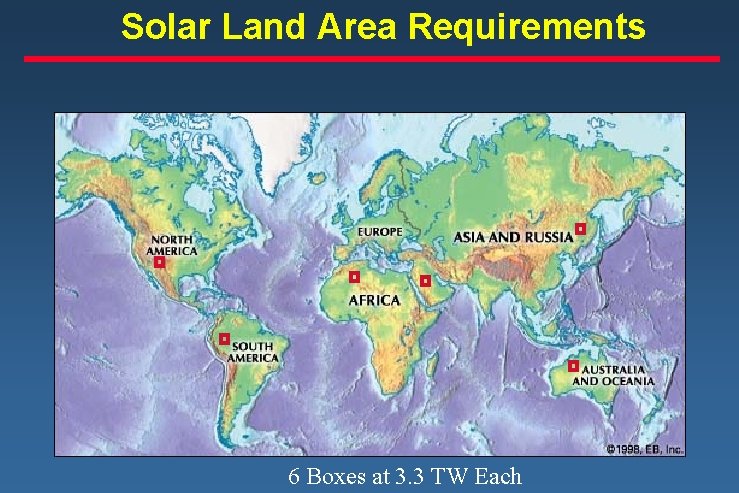
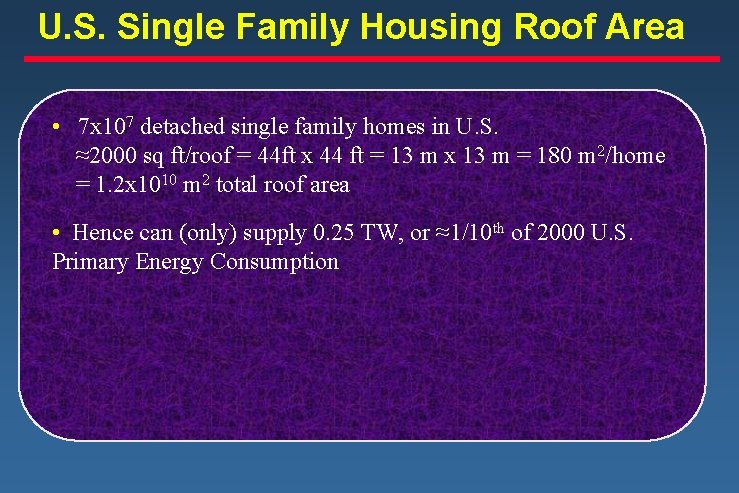
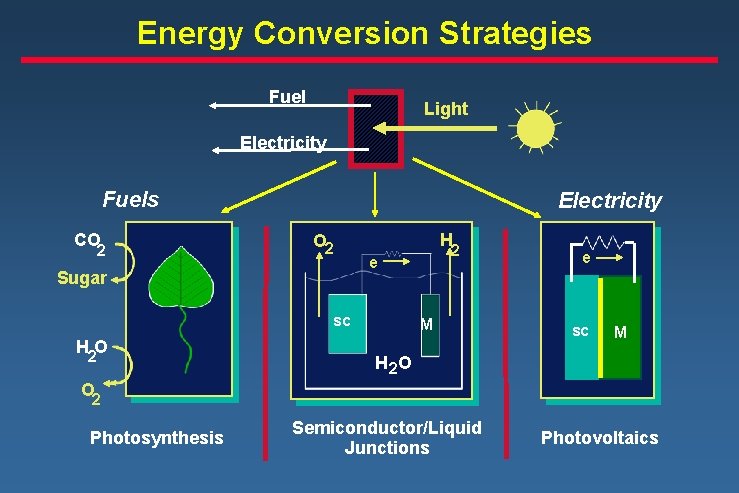
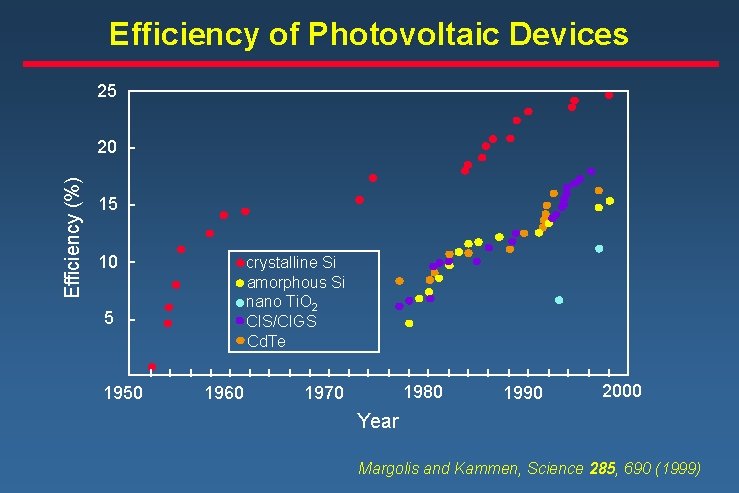
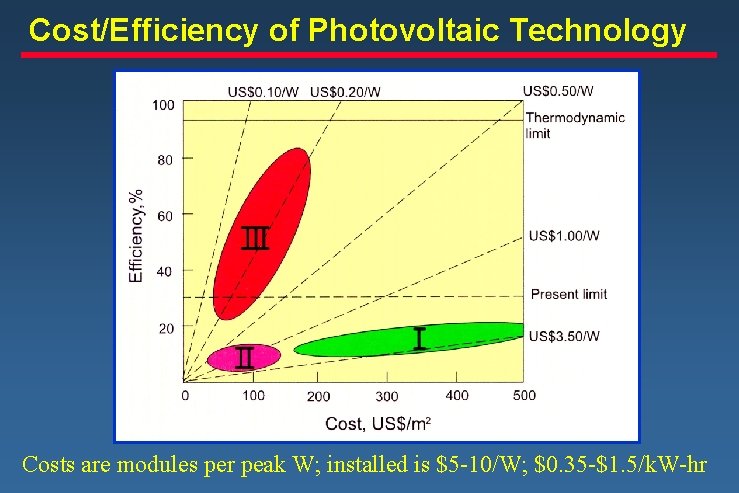
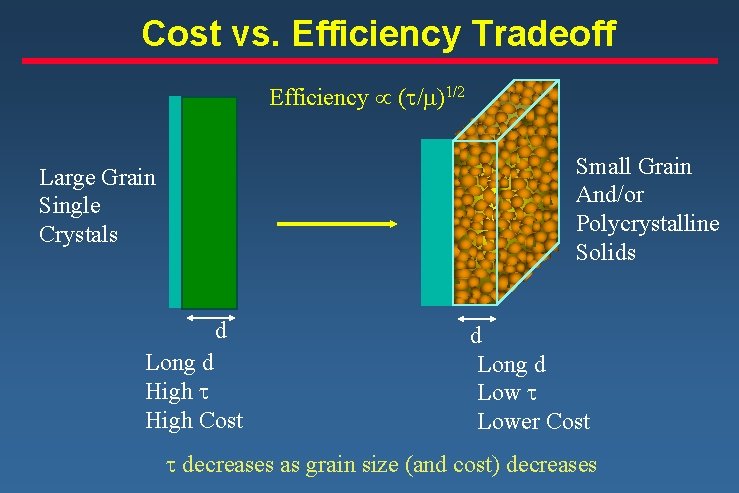
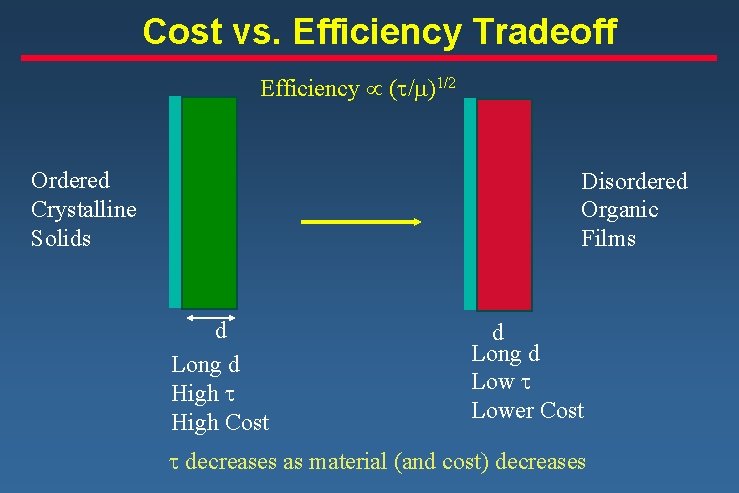
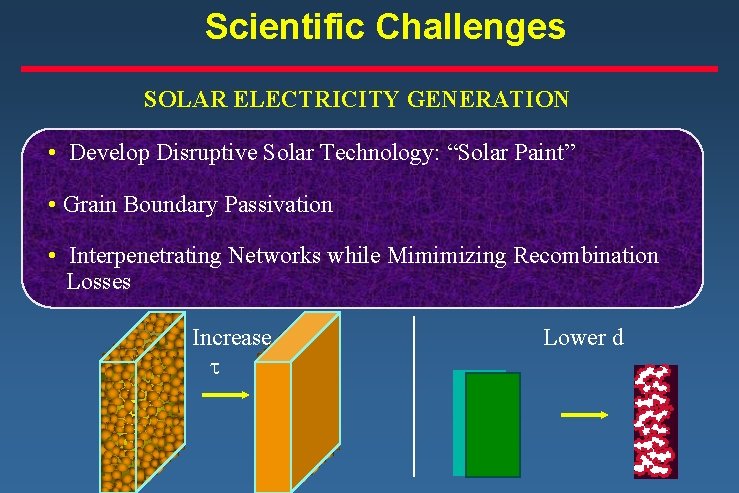
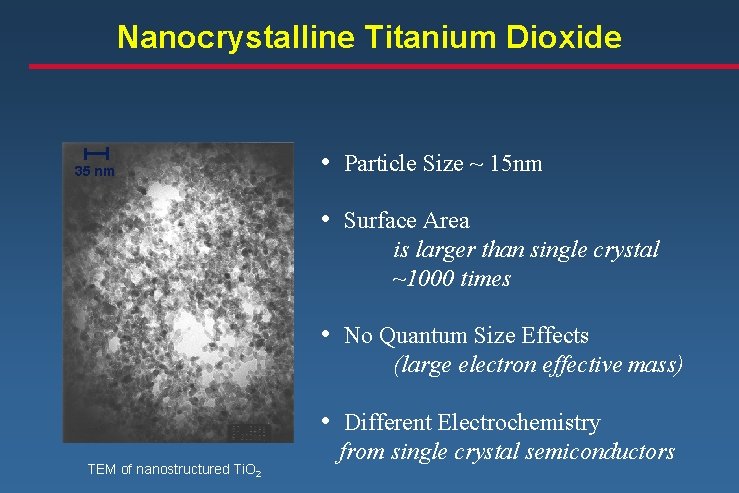
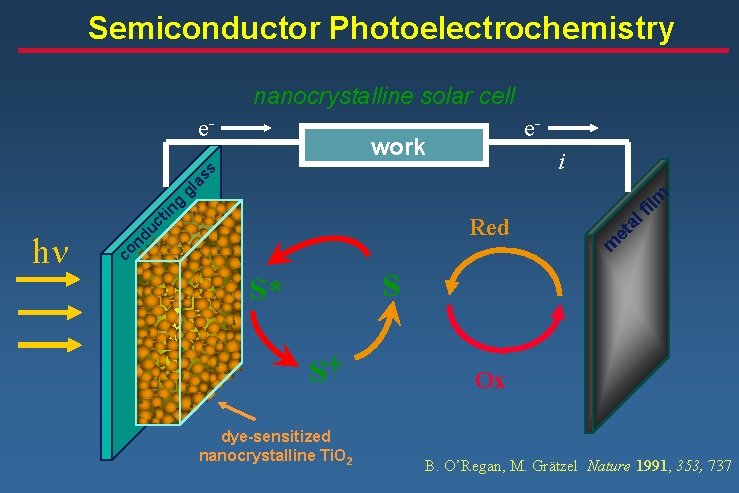
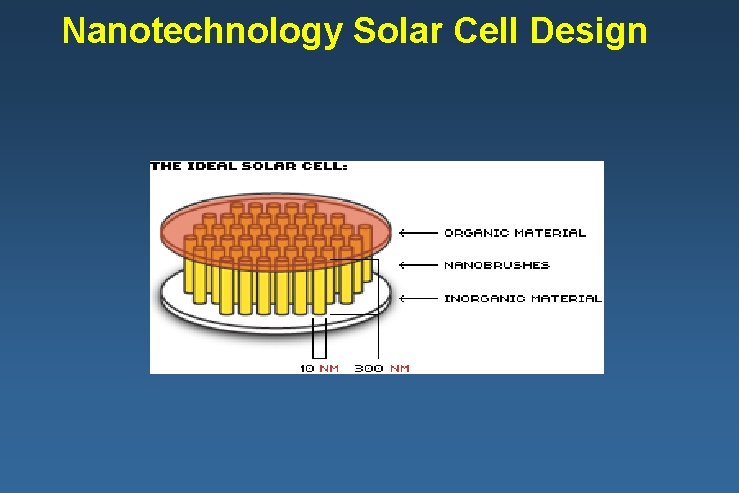
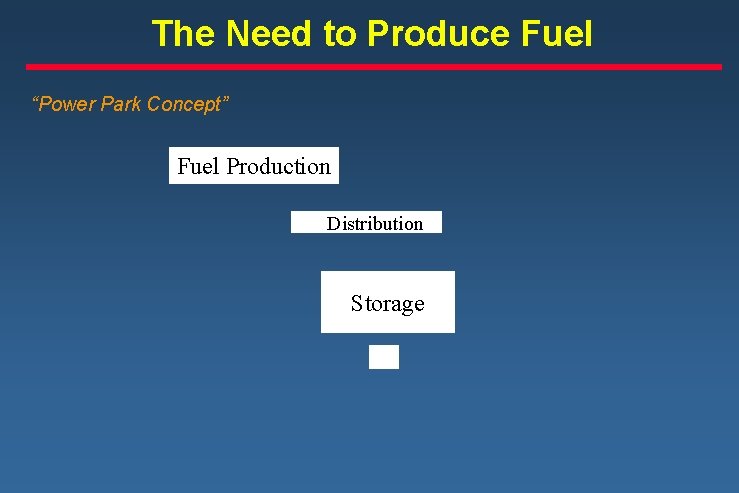
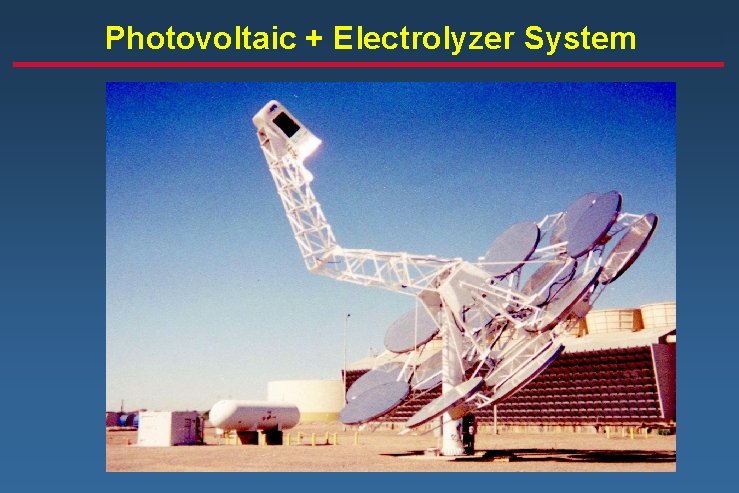
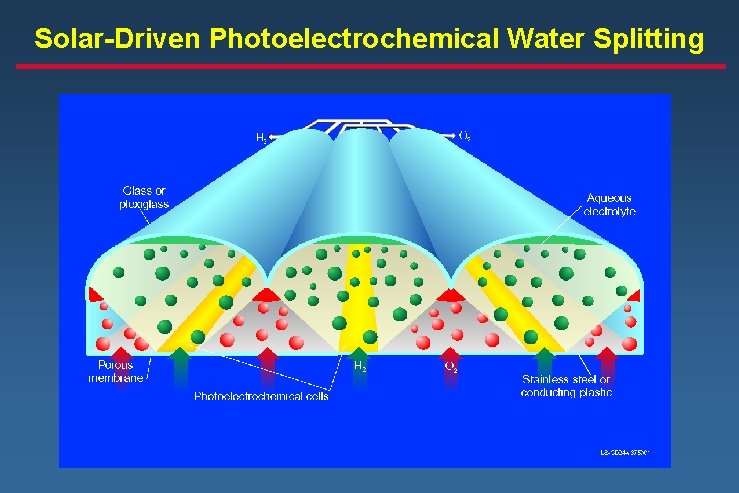
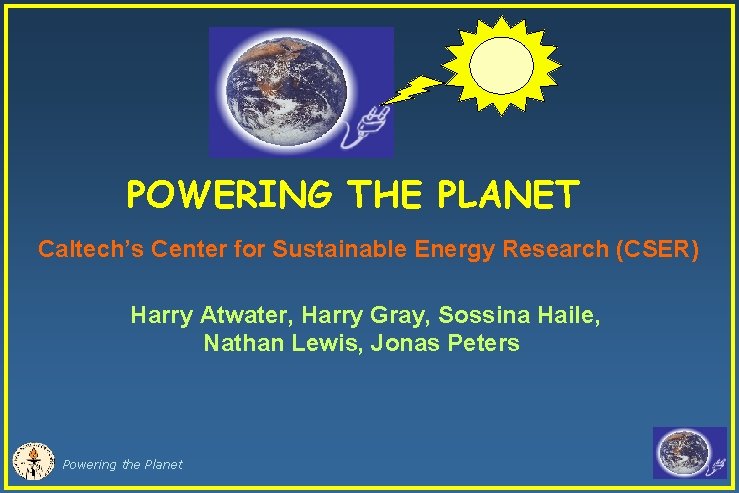
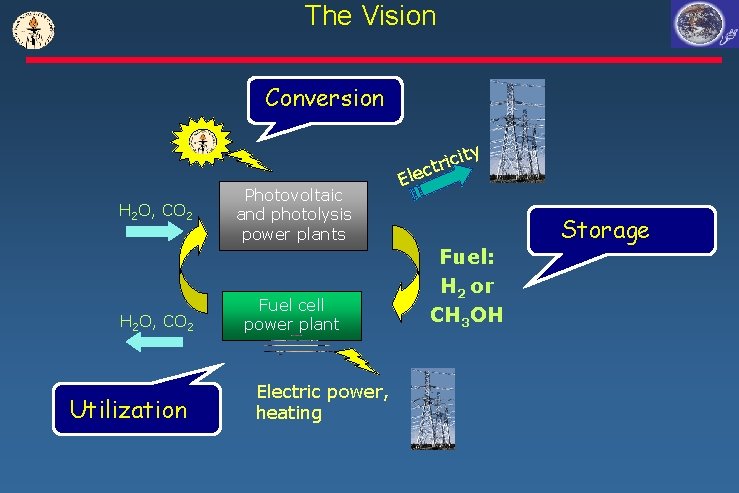
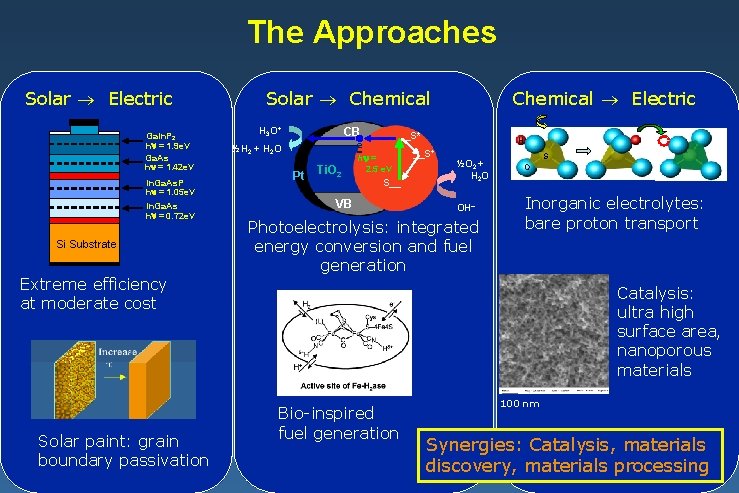
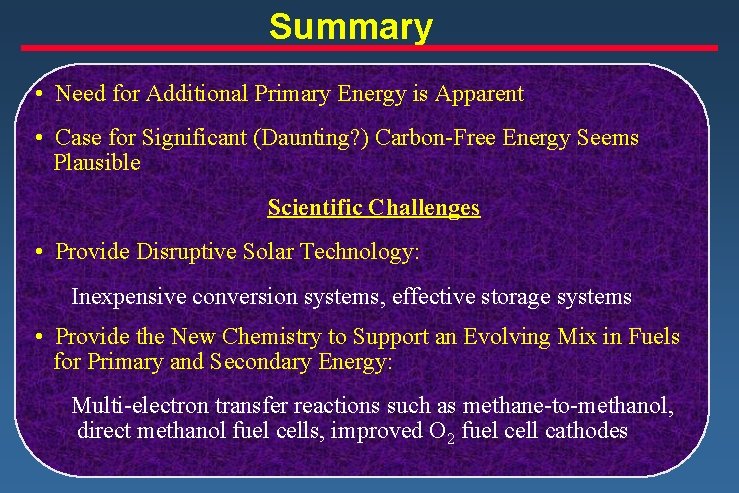
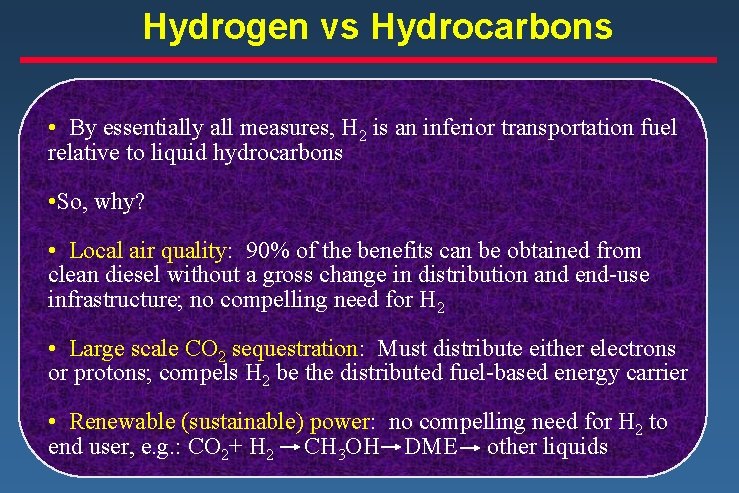
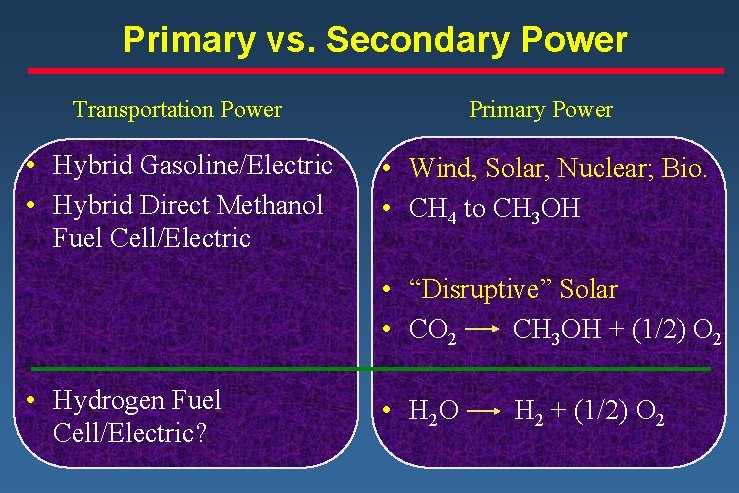
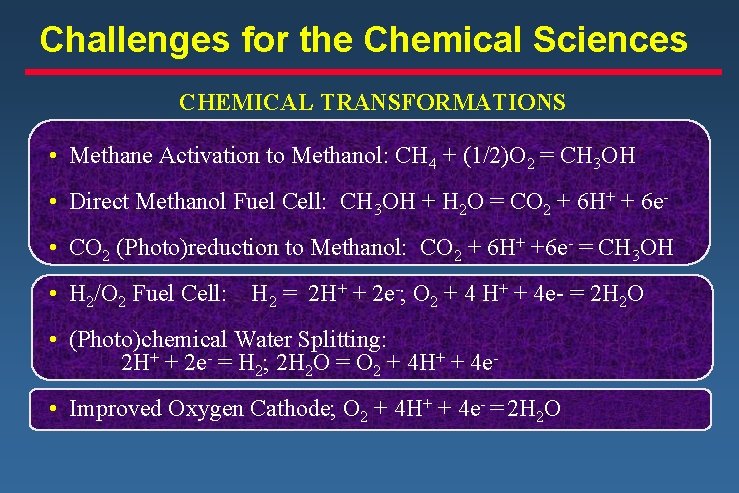
- Slides: 46
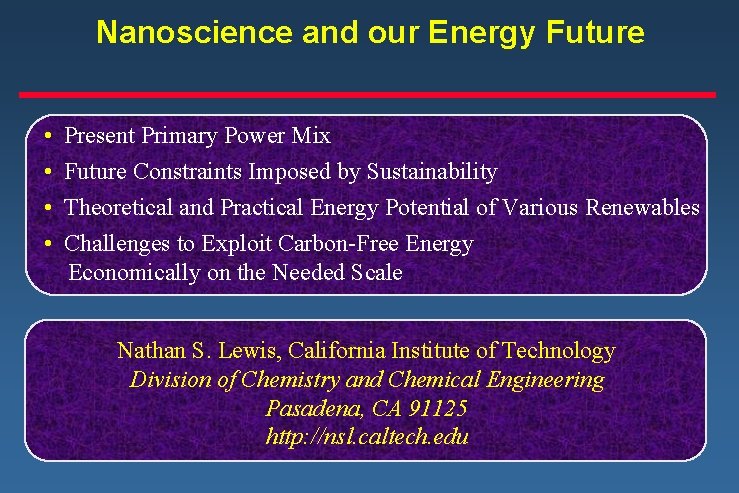
Nanoscience and our Energy Future • • Present Primary Power Mix Future Constraints Imposed by Sustainability Theoretical and Practical Energy Potential of Various Renewables Challenges to Exploit Carbon-Free Energy Economically on the Needed Scale Nathan S. Lewis, California Institute of Technology Division of Chemistry and Chemical Engineering Pasadena, CA 91125 http: //nsl. caltech. edu
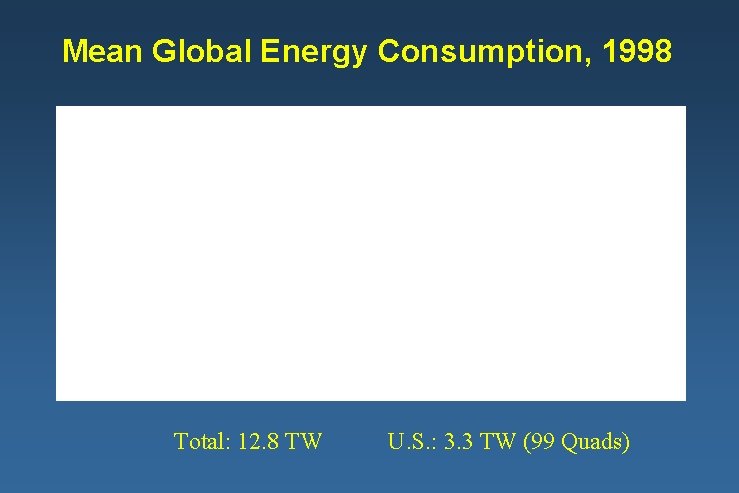
Mean Global Energy Consumption, 1998 Gas Total: 12. 8 TW Hydro Renew U. S. : 3. 3 TW (99 Quads)
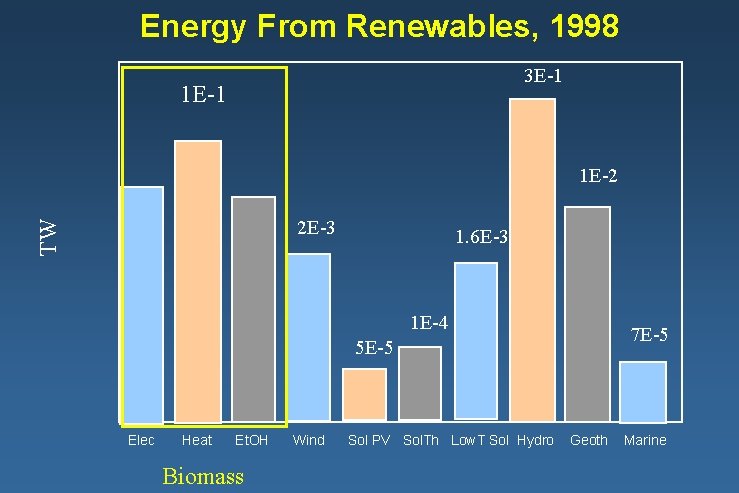
Energy From Renewables, 1998 3 E-1 1 E-2 TW 2 E-3 1. 6 E-3 1 E-4 7 E-5 5 E-5 Elec Heat Et. OH Biomass Wind Sol PV Sol. Th Low. T Sol Hydro Geoth Marine
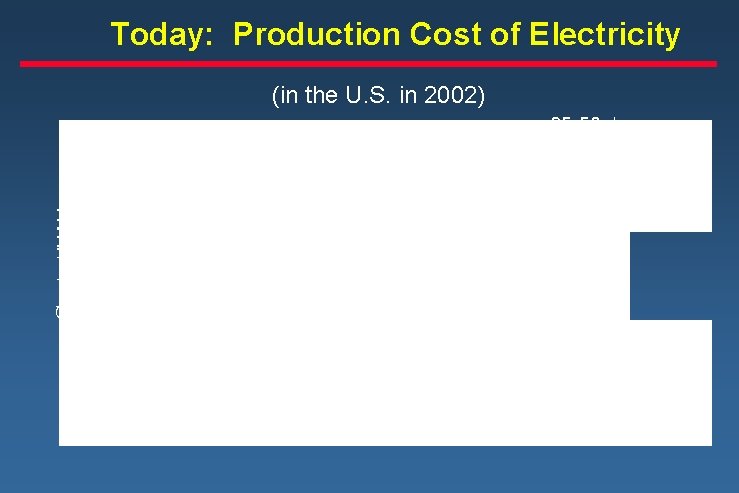
Today: Production Cost of Electricity (in the U. S. in 2002) Cost, ¢/k. W-hr 25 -50 ¢ 1 -4 ¢ 2. 3 -5. 0 ¢ 6 -8 ¢ 5 -7 ¢ 6 -7 ¢
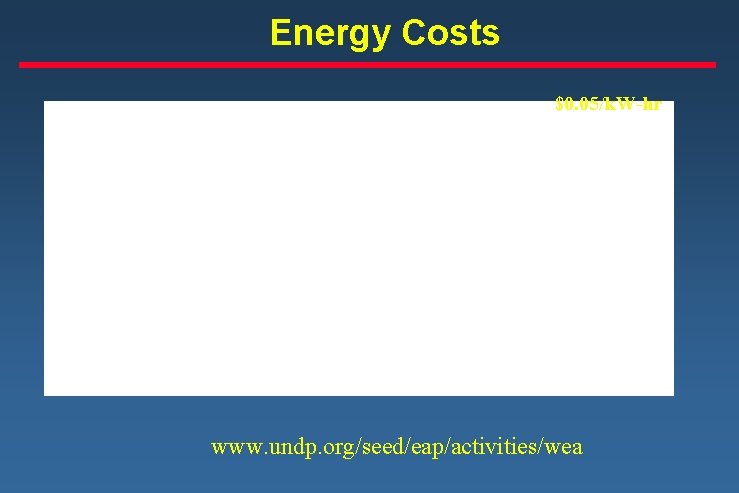
Energy Costs Europe Brazil $0. 05/k. W-hr www. undp. org/seed/eap/activities/wea
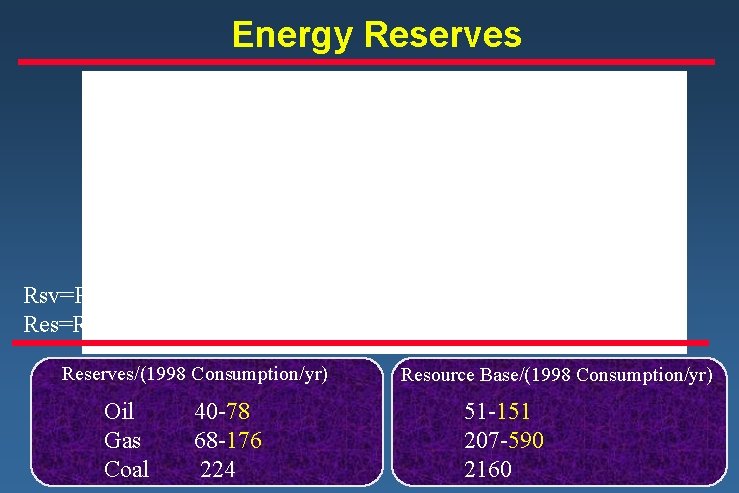
Energy Reserves Rsv=Reserves Res=Resources Reserves/(1998 Consumption/yr) Oil Gas Coal 40 -78 68 -176 224 Resource Base/(1998 Consumption/yr) 51 -151 207 -590 2160
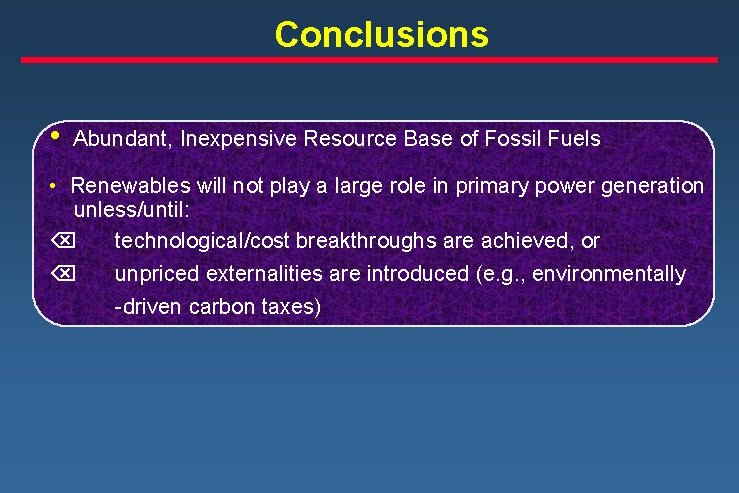
Conclusions • Abundant, Inexpensive Resource Base of Fossil Fuels • Renewables will not play a large role in primary power generation unless/until: Õ technological/cost breakthroughs are achieved, or Õ unpriced externalities are introduced (e. g. , environmentally -driven carbon taxes)
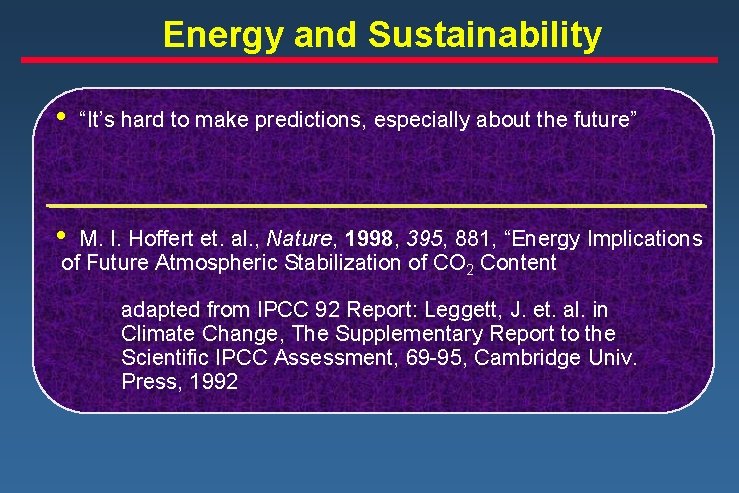
Energy and Sustainability • “It’s hard to make predictions, especially about the future” • M. I. Hoffert et. al. , Nature, 1998, 395, 881, “Energy Implications of Future Atmospheric Stabilization of CO 2 Content adapted from IPCC 92 Report: Leggett, J. et. al. in Climate Change, The Supplementary Report to the Scientific IPCC Assessment, 69 -95, Cambridge Univ. Press, 1992
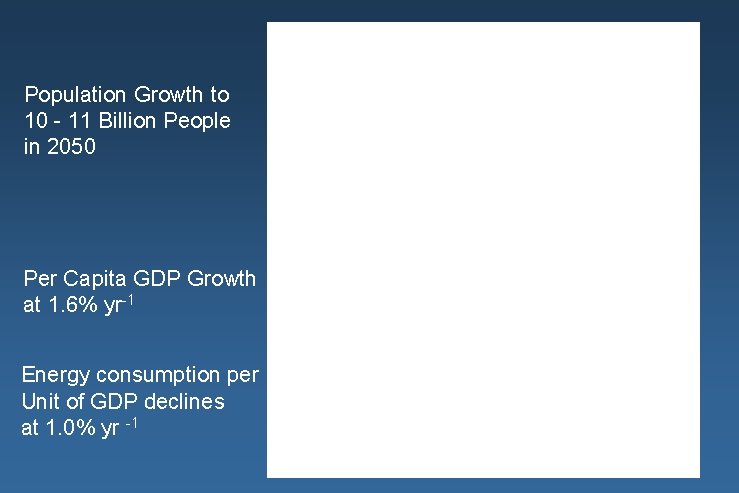
Population Growth to 10 - 11 Billion People in 2050 Per Capita GDP Growth at 1. 6% yr-1 Energy consumption per Unit of GDP declines at 1. 0% yr -1
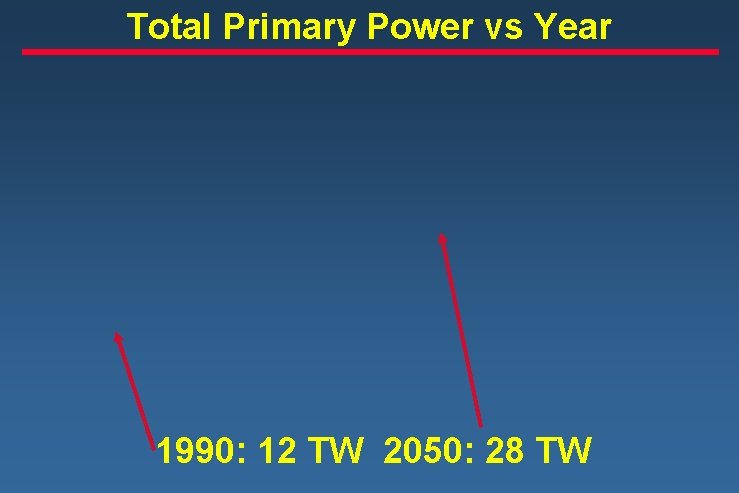
Total Primary Power vs Year 1990: 12 TW 2050: 28 TW
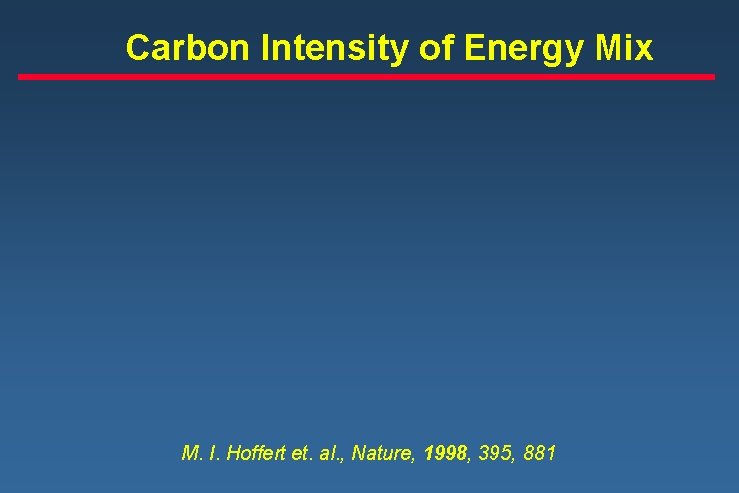
Carbon Intensity of Energy Mix M. I. Hoffert et. al. , Nature, 1998, 395, 881
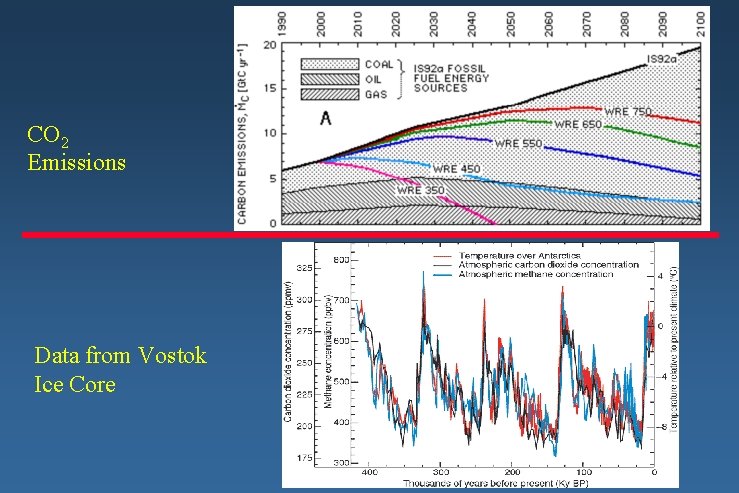
CO 2 Emissions Data from Vostok Ice Core
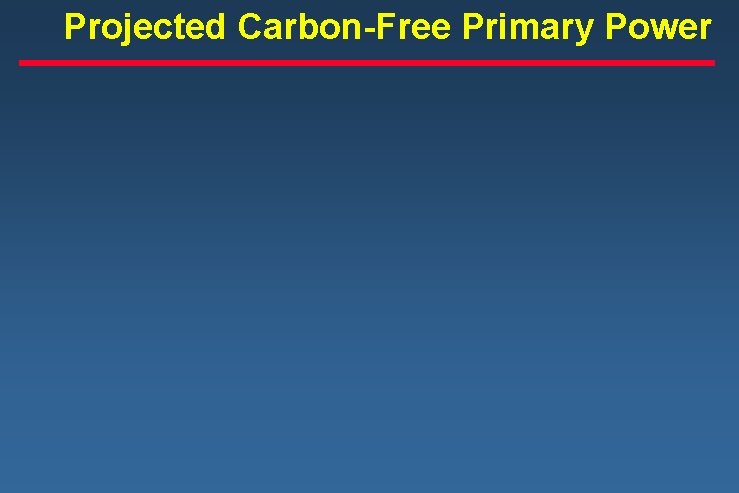
Projected Carbon-Free Primary Power
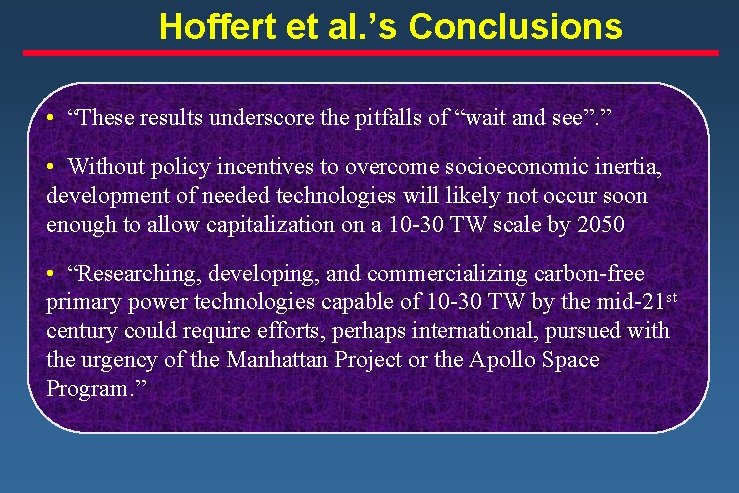
Hoffert et al. ’s Conclusions • “These results underscore the pitfalls of “wait and see”. ” • Without policy incentives to overcome socioeconomic inertia, development of needed technologies will likely not occur soon enough to allow capitalization on a 10 -30 TW scale by 2050 • “Researching, developing, and commercializing carbon-free primary power technologies capable of 10 -30 TW by the mid-21 st century could require efforts, perhaps international, pursued with the urgency of the Manhattan Project or the Apollo Space Program. ”
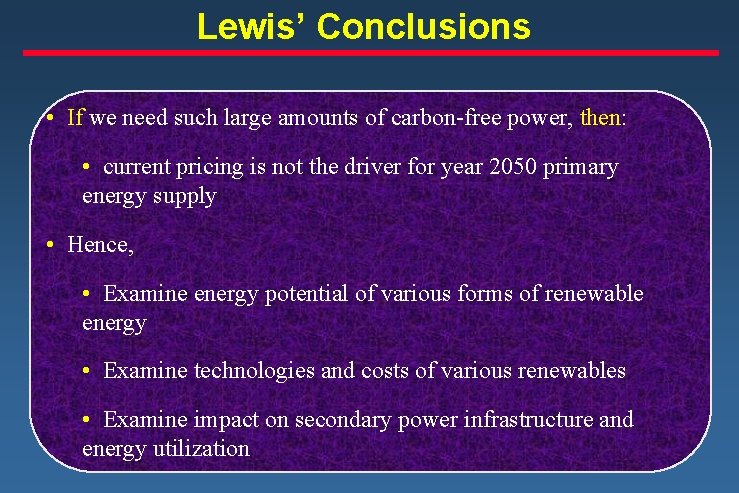
Lewis’ Conclusions • If we need such large amounts of carbon-free power, then: • current pricing is not the driver for year 2050 primary energy supply • Hence, • Examine energy potential of various forms of renewable energy • Examine technologies and costs of various renewables • Examine impact on secondary power infrastructure and energy utilization
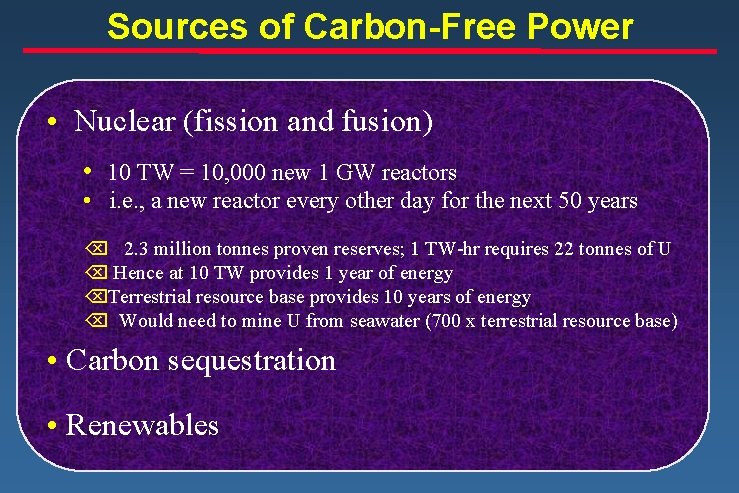
Sources of Carbon-Free Power • Nuclear (fission and fusion) • 10 TW = 10, 000 new 1 GW reactors • i. e. , a new reactor every other day for the next 50 years Õ 2. 3 million tonnes proven reserves; 1 TW-hr requires 22 tonnes of U Õ Hence at 10 TW provides 1 year of energy ÕTerrestrial resource base provides 10 years of energy Õ Would need to mine U from seawater (700 x terrestrial resource base) • Carbon sequestration • Renewables
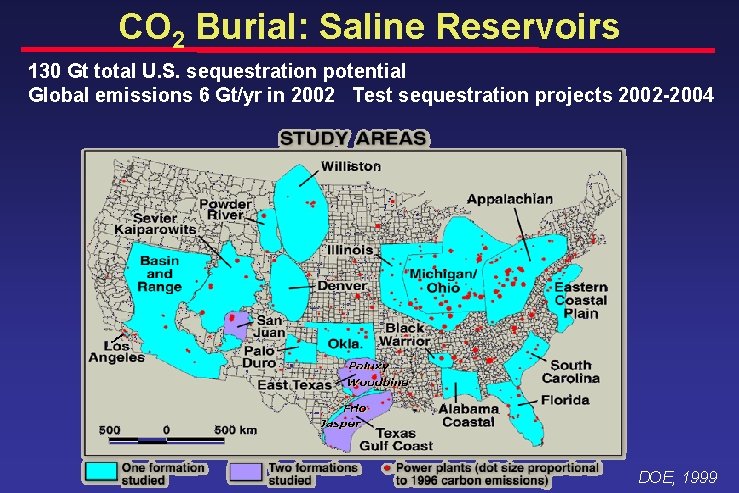
CO 2 Burial: Saline Reservoirs 130 Gt total U. S. sequestration potential Global emissions 6 Gt/yr in 2002 Test sequestration projects 2002 -2004 DOE, 1999
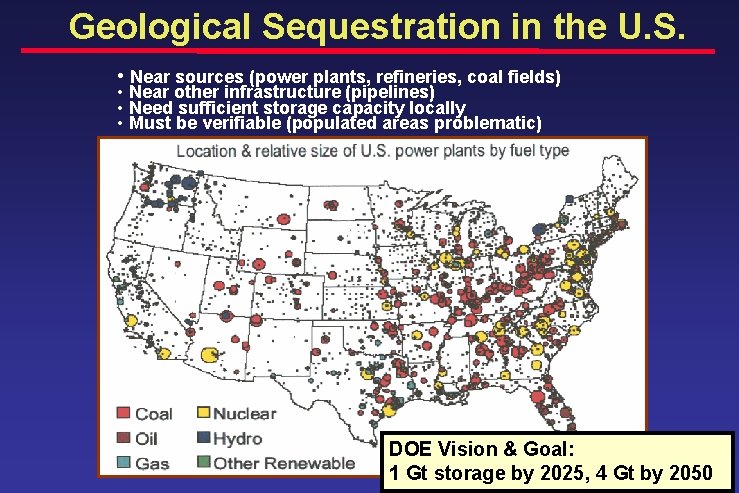
Geological Sequestration in the U. S. • Near sources (power plants, refineries, coal fields) • Near other infrastructure (pipelines) • Need sufficient storage capacity locally • Must be verifiable (populated areas problematic) DOE Vision & Goal: 1 Gt storage by 2025, 4 Gt by 2050
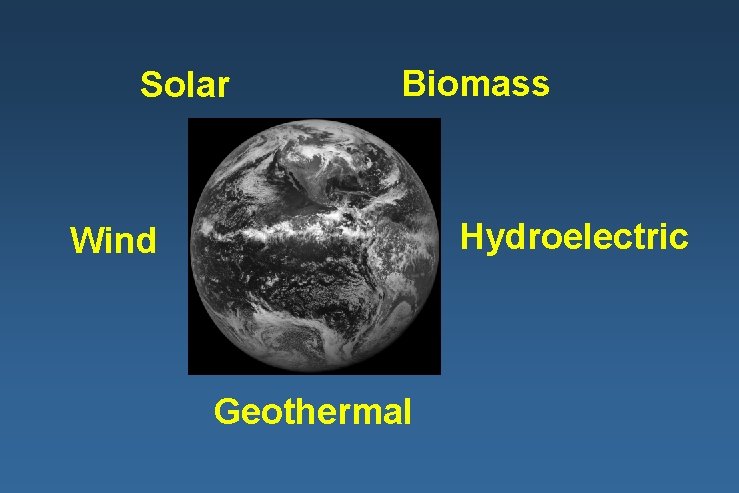
Solar Biomass Hydroelectric Wind Geothermal
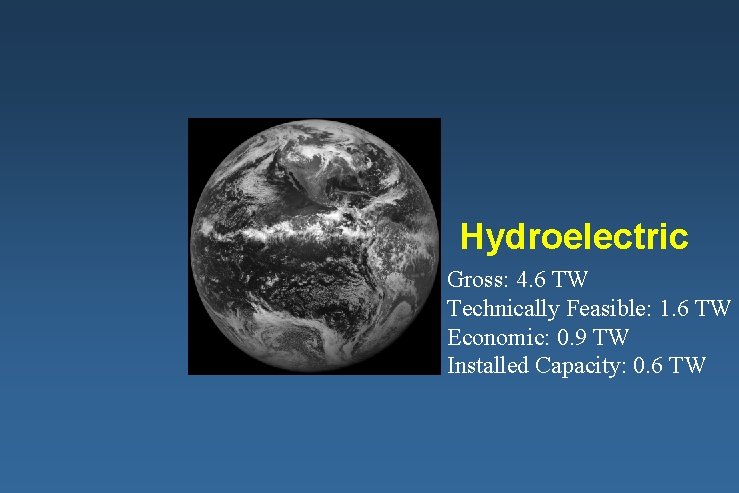
Hydroelectric Gross: 4. 6 TW Technically Feasible: 1. 6 TW Economic: 0. 9 TW Installed Capacity: 0. 6 TW
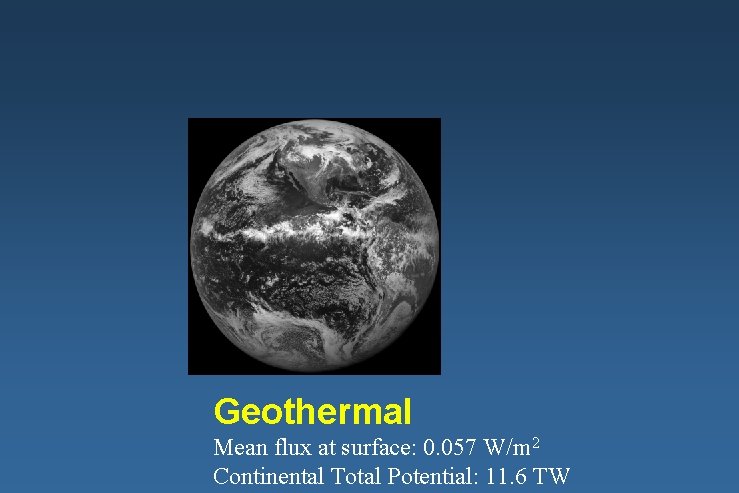
Geothermal Mean flux at surface: 0. 057 W/m 2 Continental Total Potential: 11. 6 TW
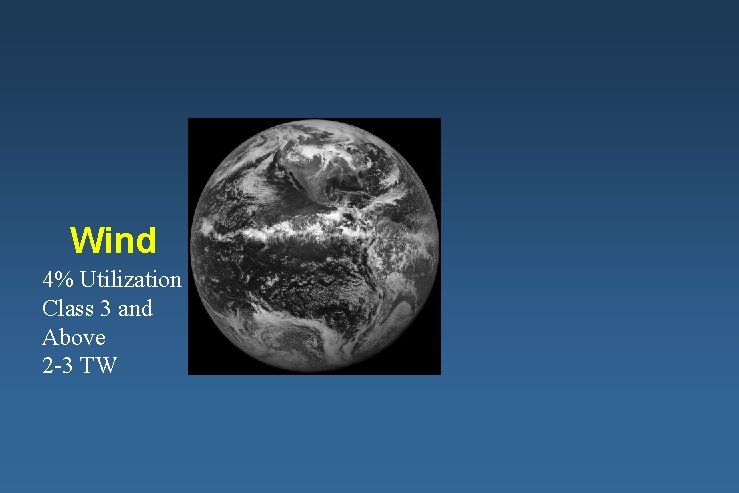
Wind 4% Utilization Class 3 and Above 2 -3 TW
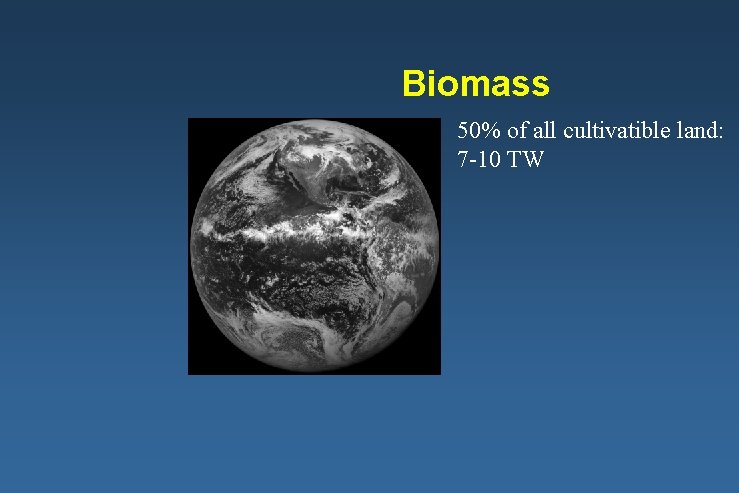
Biomass 50% of all cultivatible land: 7 -10 TW
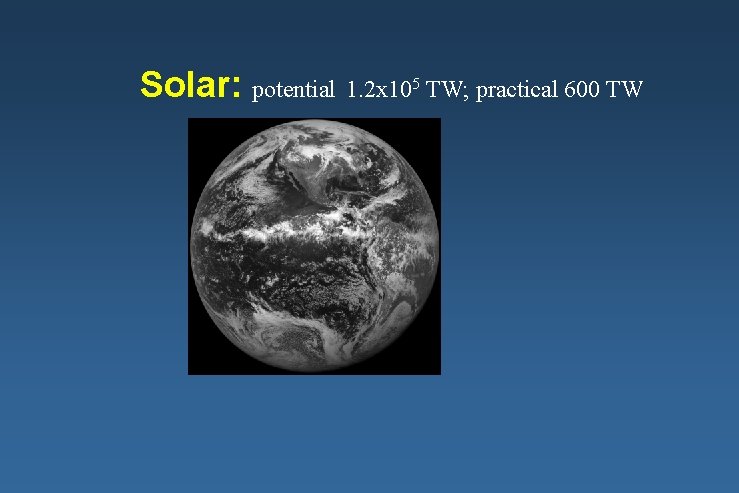
Solar: potential 1. 2 x 105 TW; practical 600 TW
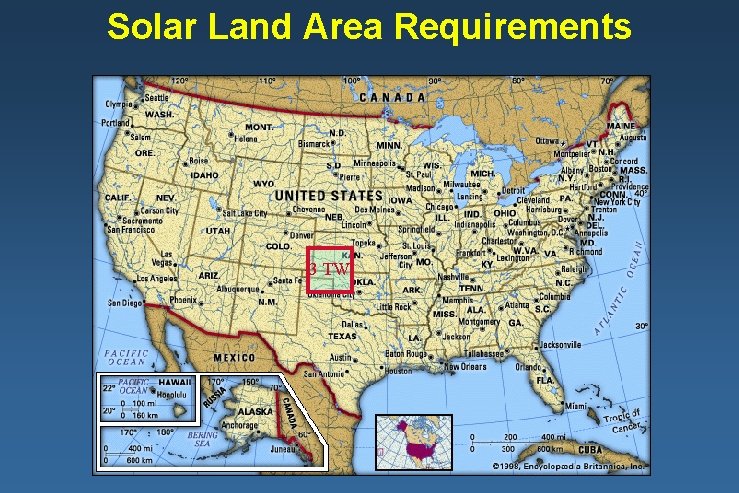
Solar Land Area Requirements 3 TW
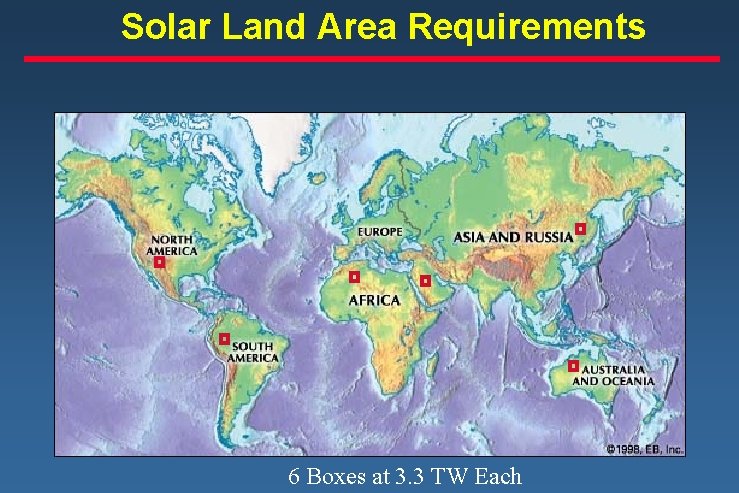
Solar Land Area Requirements 6 Boxes at 3. 3 TW Each
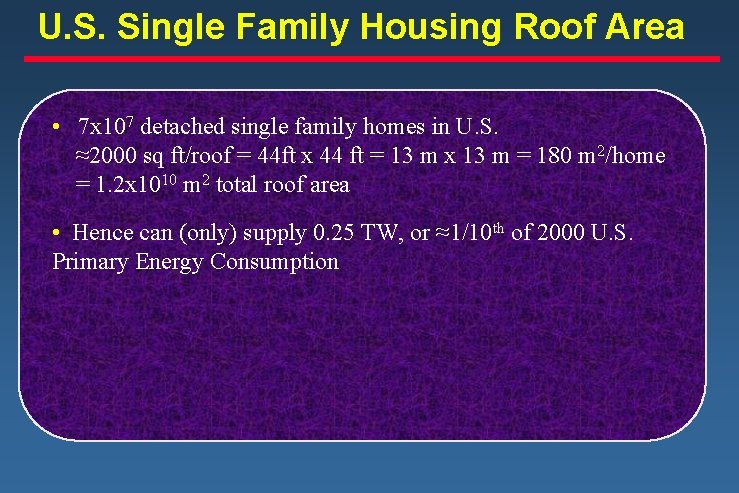
U. S. Single Family Housing Roof Area • 7 x 107 detached single family homes in U. S. ≈2000 sq ft/roof = 44 ft x 44 ft = 13 m x 13 m = 180 m 2/home = 1. 2 x 1010 m 2 total roof area • Hence can (only) supply 0. 25 TW, or ≈1/10 th of 2000 U. S. Primary Energy Consumption
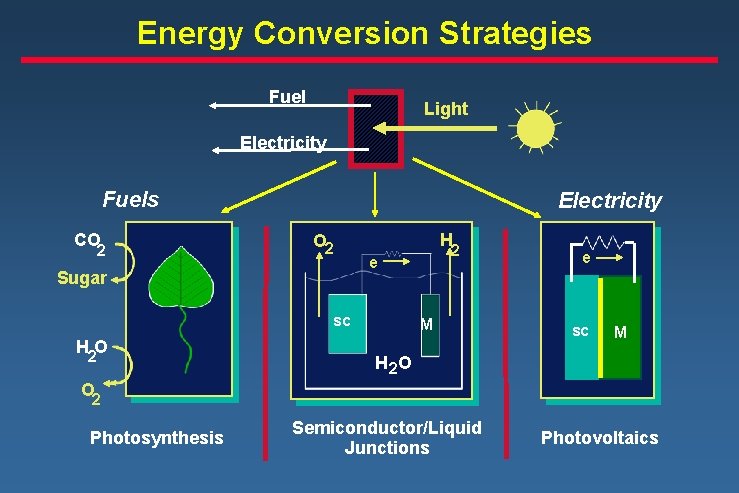
Energy Conversion Strategies Fuel Light Electricity Fuels CO 2 Electricity O 2 Sugar H sc H 2 O 2 e M e sc M H 2 O O 2 Photosynthesis Semiconductor/Liquid Junctions Photovoltaics
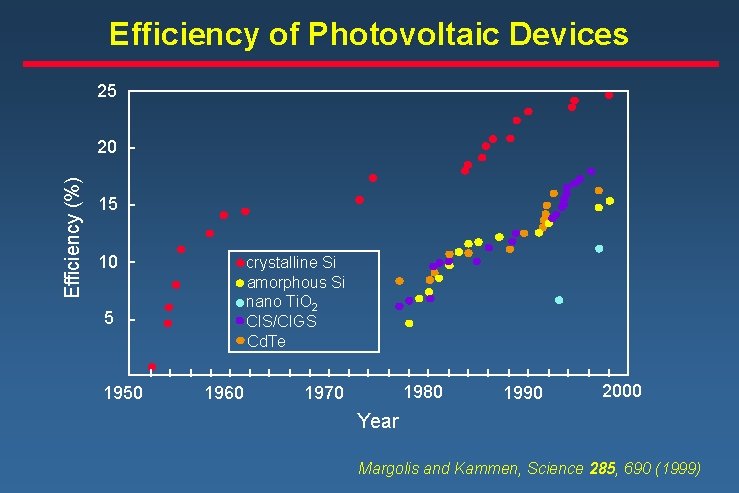
Efficiency of Photovoltaic Devices 25 Efficiency (%) 20 15 10 crystalline Si amorphous Si nano Ti. O 2 CIS/CIGS Cd. Te 5 1950 1960 1980 1970 1990 2000 Year Margolis and Kammen, Science 285, 690 (1999)
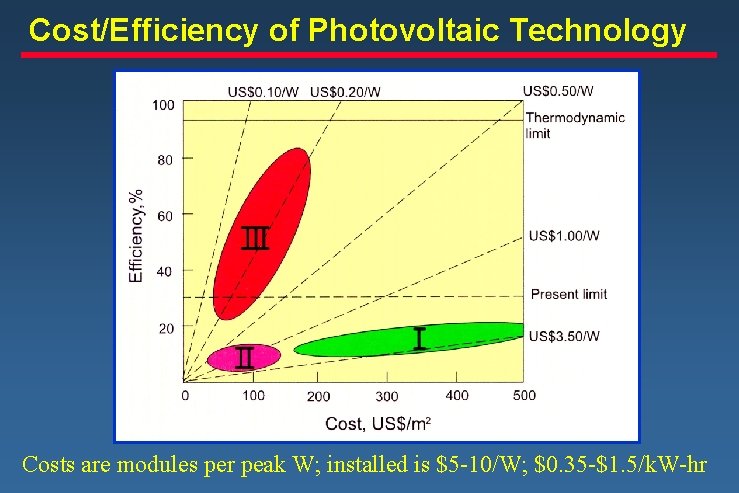
Cost/Efficiency of Photovoltaic Technology Costs are modules per peak W; installed is $5 -10/W; $0. 35 -$1. 5/k. W-hr
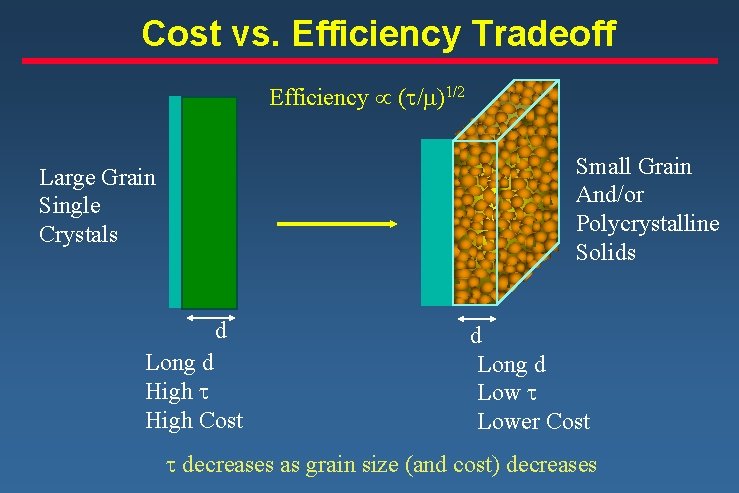
Cost vs. Efficiency Tradeoff Efficiency µ (t/m)1/2 Small Grain And/or Polycrystalline Solids Large Grain Single Crystals d Long d High t High Cost d Long d Low t Lower Cost t decreases as grain size (and cost) decreases
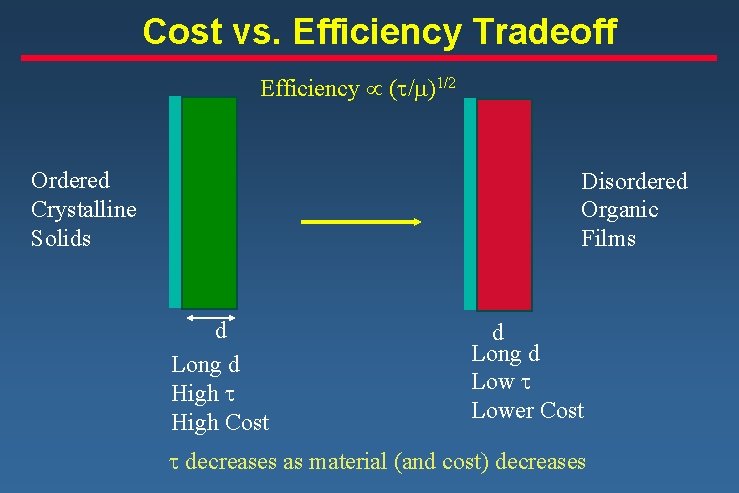
Cost vs. Efficiency Tradeoff Efficiency µ (t/m)1/2 Ordered Crystalline Solids Disordered Organic Films d Long d High t High Cost d Long d Low t Lower Cost t decreases as material (and cost) decreases
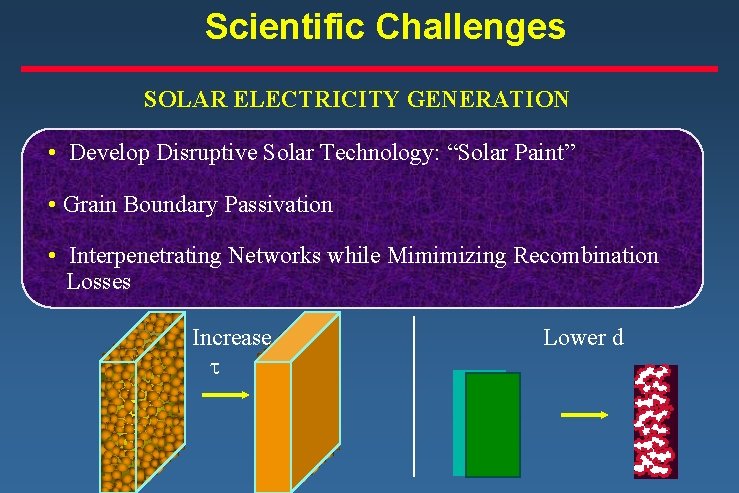
Scientific Challenges SOLAR ELECTRICITY GENERATION • Develop Disruptive Solar Technology: “Solar Paint” • Grain Boundary Passivation • Interpenetrating Networks while Mimimizing Recombination Losses Increase t Lower d
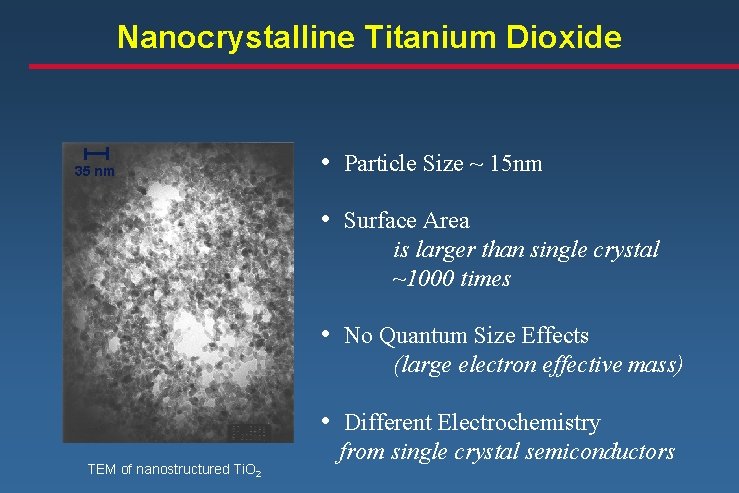
Nanocrystalline Titanium Dioxide 35 nm • Particle Size ~ 15 nm • Surface Area is larger than single crystal ~1000 times • No Quantum Size Effects (large electron effective mass) • Different Electrochemistry TEM of nanostructured Ti. O 2 from single crystal semiconductors
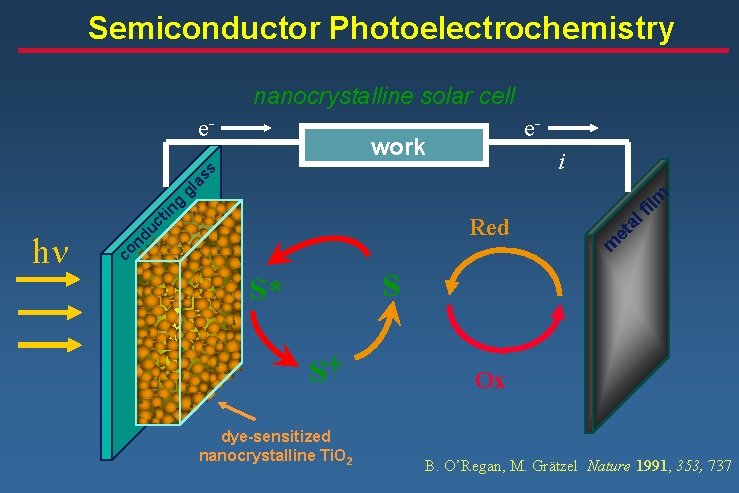
Semiconductor Photoelectrochemistry nanocrystalline solar cell e- e- work fil al m nd uc t co h Red et in g m gl a ss i S S* S+ dye-sensitized nanocrystalline Ti. O 2 Ox B. O’Regan, M. Grätzel Nature 1991, 353, 737
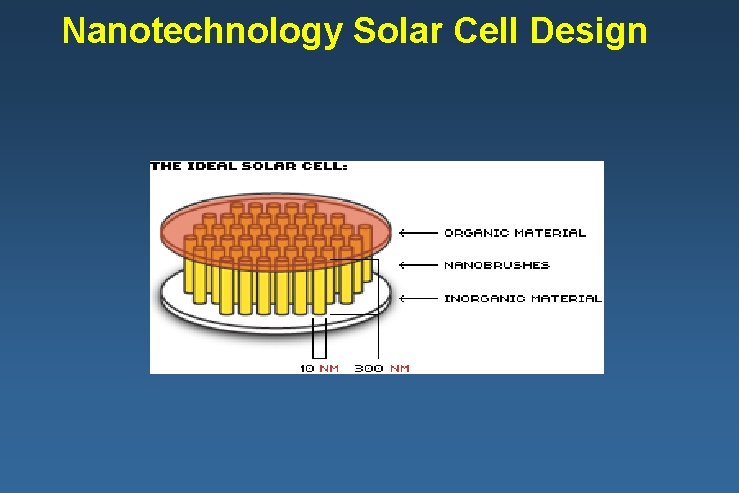
Nanotechnology Solar Cell Design
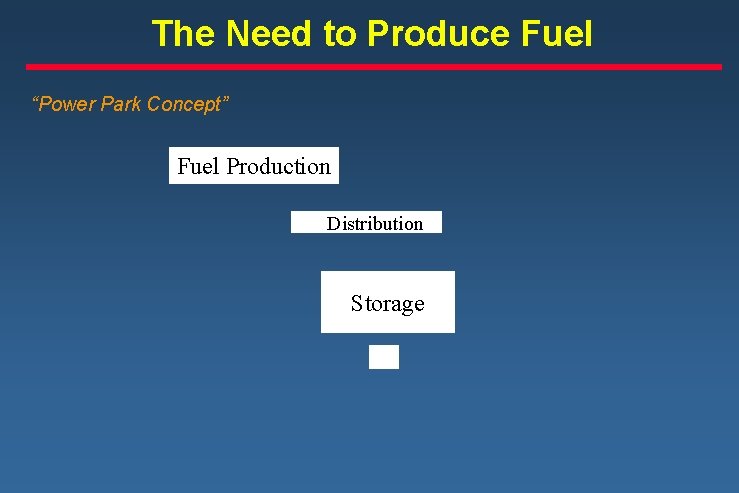
The Need to Produce Fuel “Power Park Concept” Fuel Production Distribution Storage
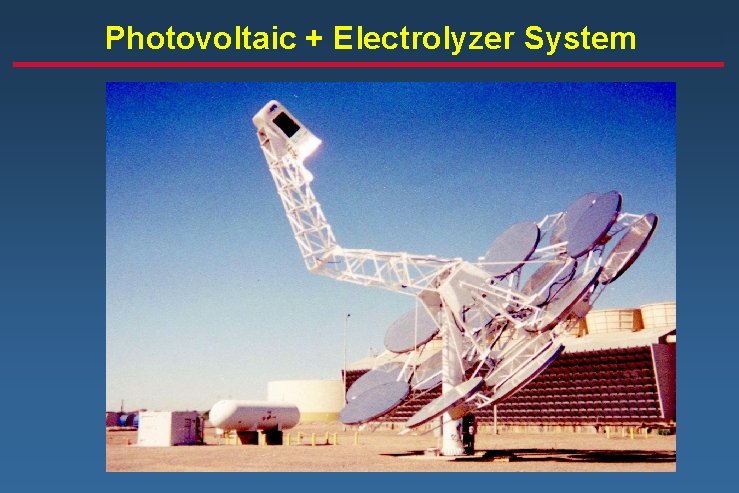
Photovoltaic + Electrolyzer System
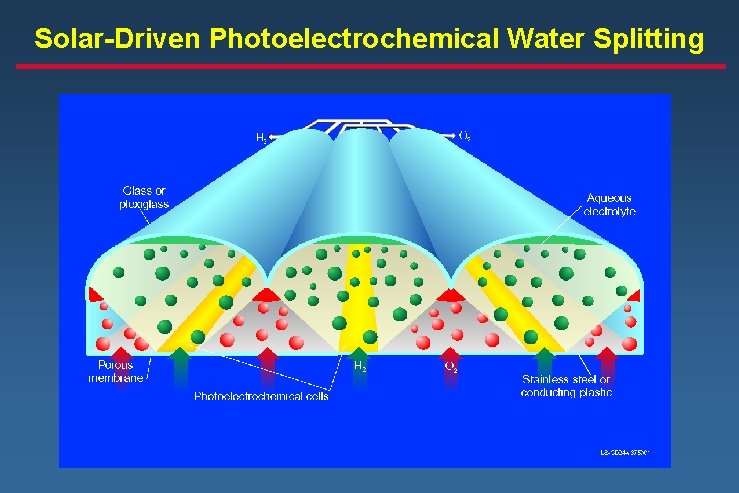
Solar-Driven Photoelectrochemical Water Splitting
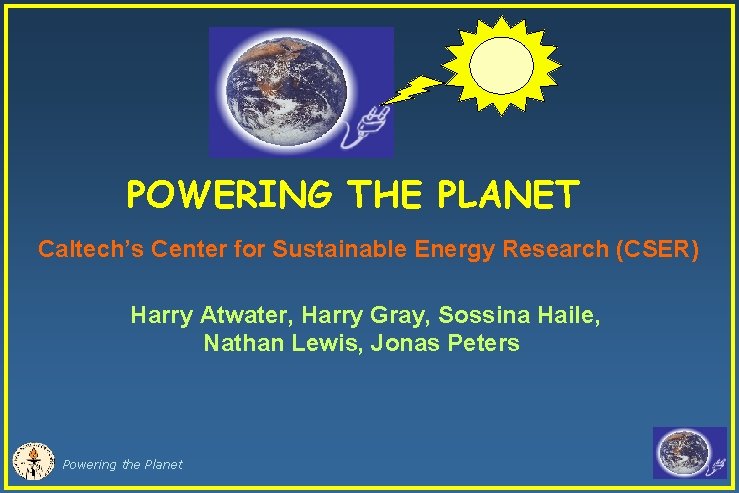
POWERING THE PLANET Caltech’s Center for Sustainable Energy Research (CSER) Harry Atwater, Harry Gray, Sossina Haile, Nathan Lewis, Jonas Peters Powering the Planet
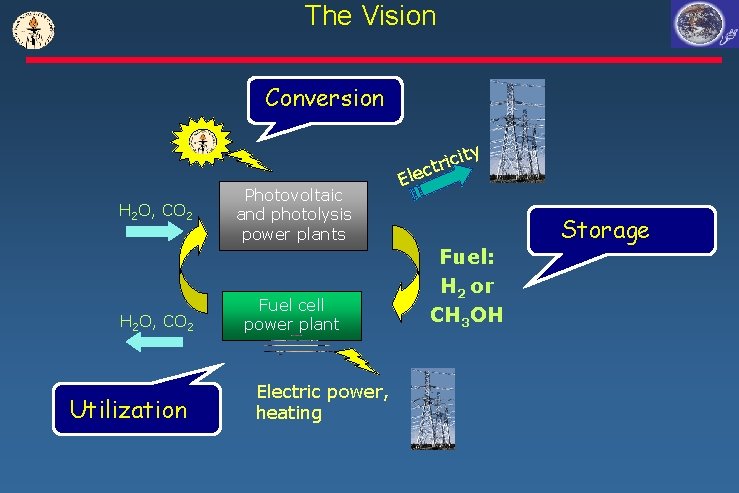
The Vision Conversion H 2 O, CO 2 Utilization Photovoltaic and photolysis power plants Fuel cell power plant Electric power, heating Ele ty ci i r t c Fuel: H 2 or CH 3 OH Storage
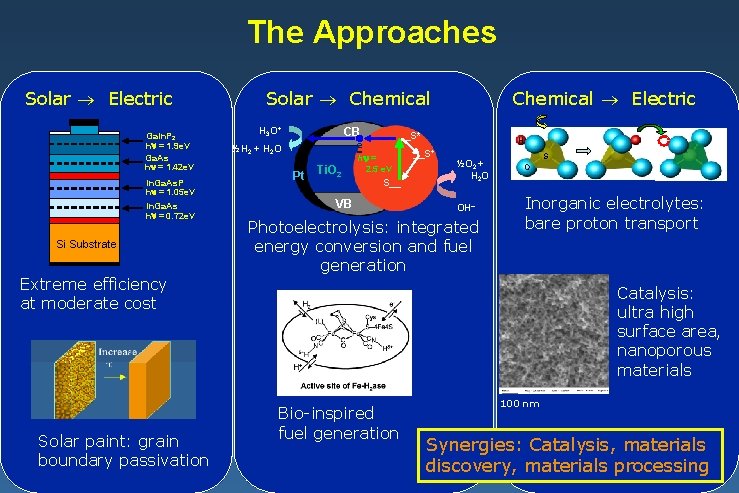
The Approaches Solar Electric Ga. In. P 2 h = 1. 9 e. V Ga. As h = 1. 42 e. V In. Ga. As. P h = 1. 05 e. V In. Ga. As h = 0. 72 e. V Si Substrate Extreme efficiency at moderate cost Solar paint: grain boundary passivation Chemical Electric Solar Chemical H 3 O+ CB ½H 2 + H 2 O e __S* Pt Ti. O 2 h = 2. 5 e. V S__ VB H __S+ ½O 2 + H 2 O OH Photoelectrolysis: integrated energy conversion and fuel generation S O Inorganic electrolytes: bare proton transport Catalysis: ultra high surface area, nanoporous materials Bio-inspired fuel generation 100 nm Synergies: Catalysis, materials discovery, materials processing
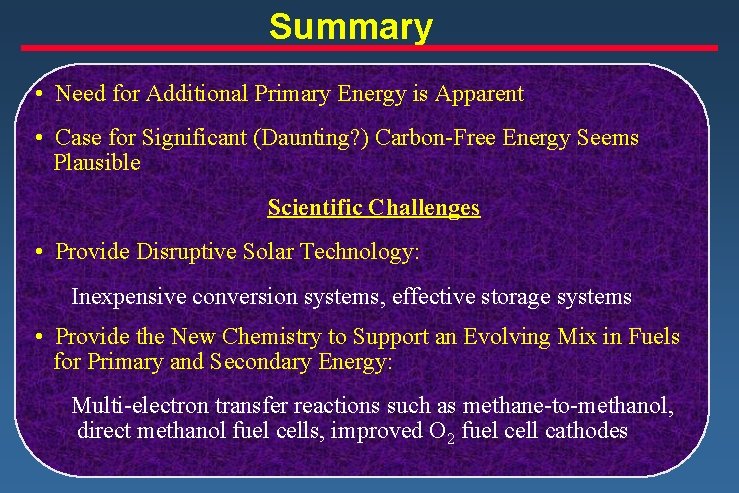
Summary • Need for Additional Primary Energy is Apparent • Case for Significant (Daunting? ) Carbon-Free Energy Seems Plausible Scientific Challenges • Provide Disruptive Solar Technology: Inexpensive conversion systems, effective storage systems • Provide the New Chemistry to Support an Evolving Mix in Fuels for Primary and Secondary Energy: Multi-electron transfer reactions such as methane-to-methanol, direct methanol fuel cells, improved O 2 fuel cell cathodes
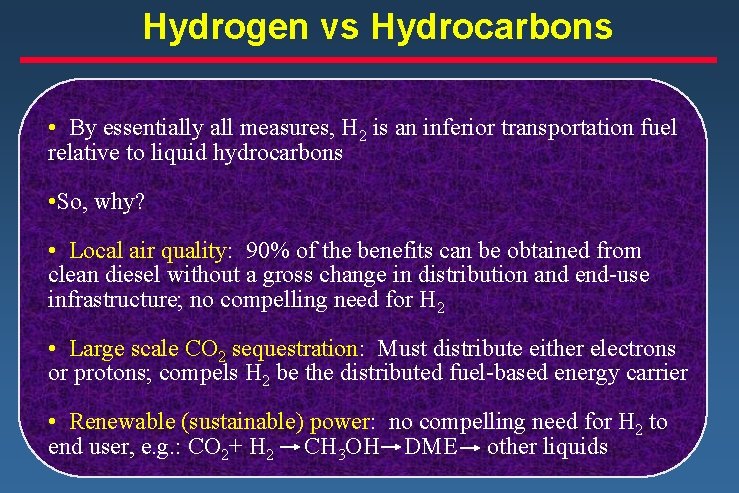
Hydrogen vs Hydrocarbons • By essentially all measures, H 2 is an inferior transportation fuel relative to liquid hydrocarbons • So, why? • Local air quality: 90% of the benefits can be obtained from clean diesel without a gross change in distribution and end-use infrastructure; no compelling need for H 2 • Large scale CO 2 sequestration: Must distribute either electrons or protons; compels H 2 be the distributed fuel-based energy carrier • Renewable (sustainable) power: no compelling need for H 2 to end user, e. g. : CO 2+ H 2 CH 3 OH DME other liquids
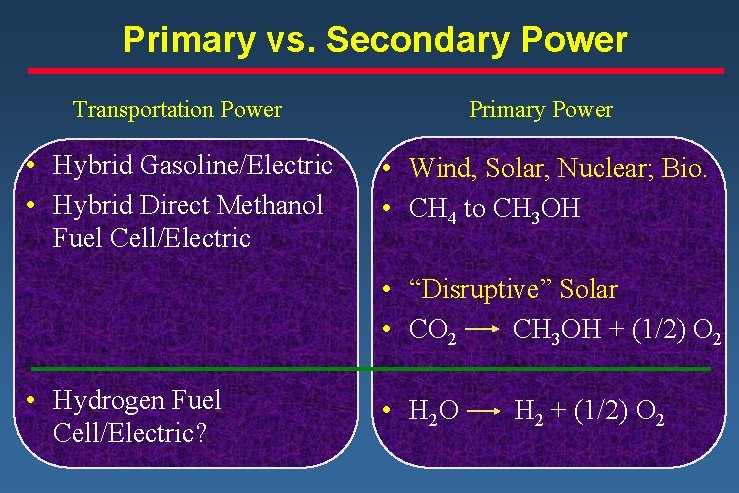
Primary vs. Secondary Power Transportation Power Primary Power • Hybrid Gasoline/Electric • Hybrid Direct Methanol Fuel Cell/Electric • Wind, Solar, Nuclear; Bio. • CH 4 to CH 3 OH • “Disruptive” Solar • CO 2 CH 3 OH + (1/2) O 2 • Hydrogen Fuel Cell/Electric? • H 2 O H 2 + (1/2) O 2
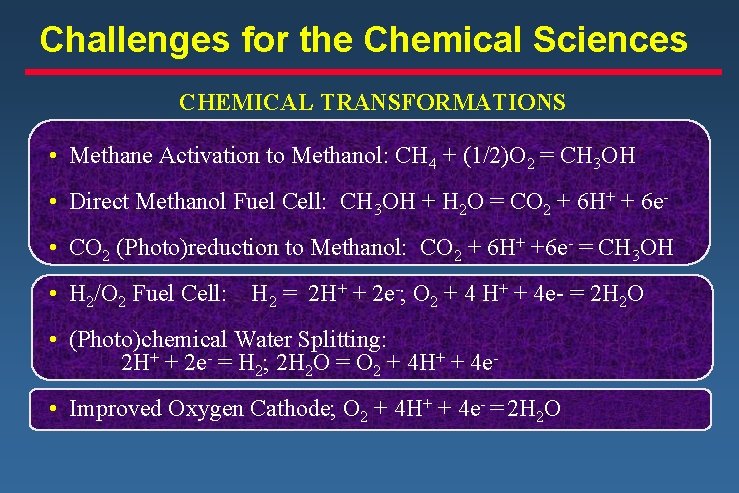
Challenges for the Chemical Sciences CHEMICAL TRANSFORMATIONS • Methane Activation to Methanol: CH 4 + (1/2)O 2 = CH 3 OH • Direct Methanol Fuel Cell: CH 3 OH + H 2 O = CO 2 + 6 H+ + 6 e • CO 2 (Photo)reduction to Methanol: CO 2 + 6 H+ +6 e- = CH 3 OH • H 2/O 2 Fuel Cell: H 2 = 2 H+ + 2 e-; O 2 + 4 H+ + 4 e- = 2 H 2 O • (Photo)chemical Water Splitting: 2 H+ + 2 e- = H 2; 2 H 2 O = O 2 + 4 H+ + 4 e • Improved Oxygen Cathode; O 2 + 4 H+ + 4 e- = 2 H 2 O
Difference between nanoscience and nanotechnology
Sciwo
Disadvantage of nanotechnology
Our census our future
Our census our future
Our future is in our hands quotes
Nanoscience
Nanoscience
Advantages of nanoparticles gcse
Nanoscience
Nanoscience
Future continuous vs future perfect exercises
Future perfect continuous and simple
Primary energy and secondary energy
Primary energy and secondary energy
Future continuous tense
Power trianlge
Energy energy transfer and general energy analysis
Energy energy transfer and general energy analysis
Present simple, past simple, future simple
Past simple future
Present simple present continuous past simple future simple
Past continuous passive
Passive of present perfect
Simple past simple present simple future
Passive eat
Awareness of ourselves and our environment is:
Our awareness of ourselves and our environment is called
Our awareness of ourselves and our environment
Awareness of ourselves and our environment is
Present simple present continuous and present perfect
Future perfect future continuous exercises
Tense
Future plans and finished future actions
Future continuous vs future perfect
Future continuous and future perfect objasnjenje
Thinking affects our language which then affects our
Ok 313
Our life is what our thoughts make it
We bow our hearts we bend our knees
Our life is what our thoughts make it
Money madness written by
God our father christ our brother
Wish in the present
Powering our future
Our future hope
Missionary prayer poem