Miller Indices 1 Equivalent Planes h k l
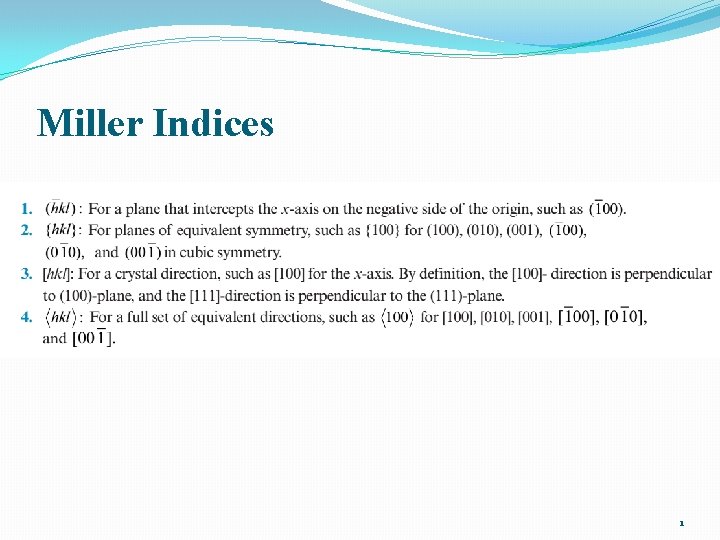
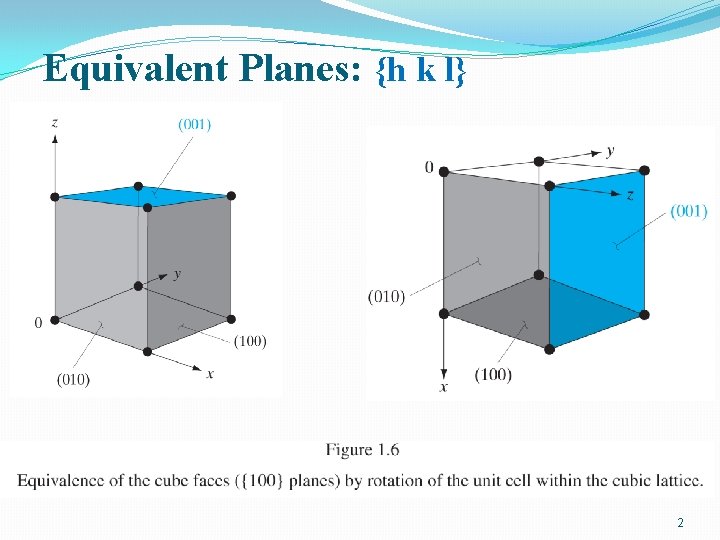
![Crystal Directions: [h k l] 3 Crystal Directions: [h k l] 3](https://slidetodoc.com/presentation_image_h2/94cc0c572c292a84b8b7866ce8b9efb2/image-3.jpg)
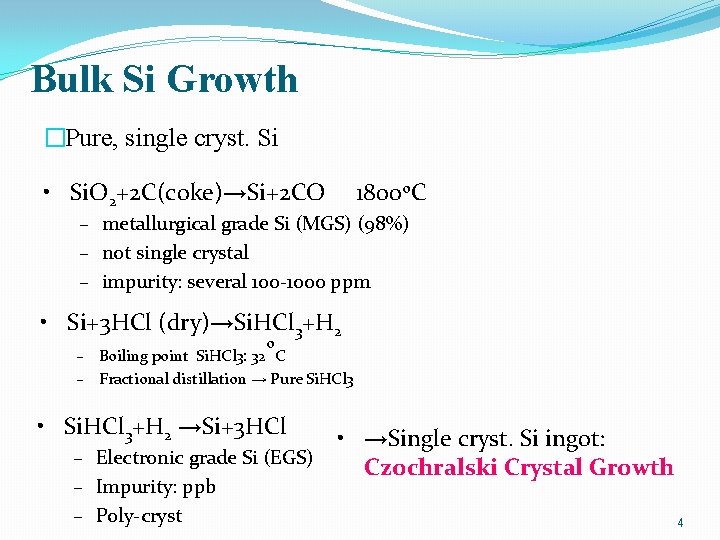
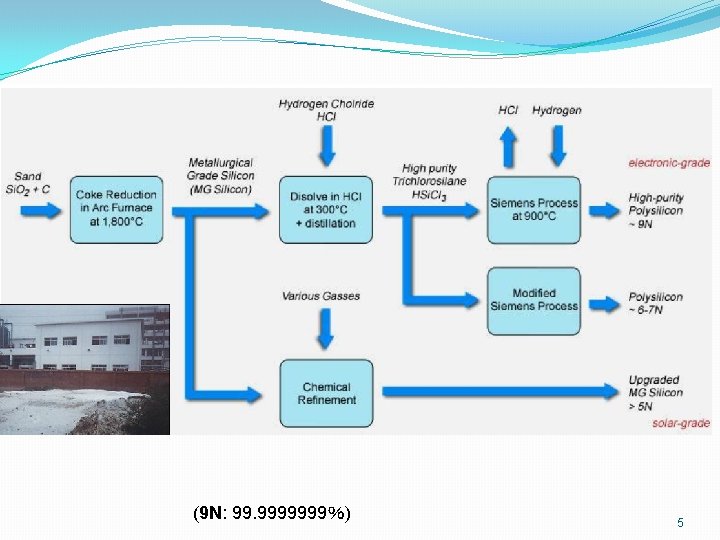
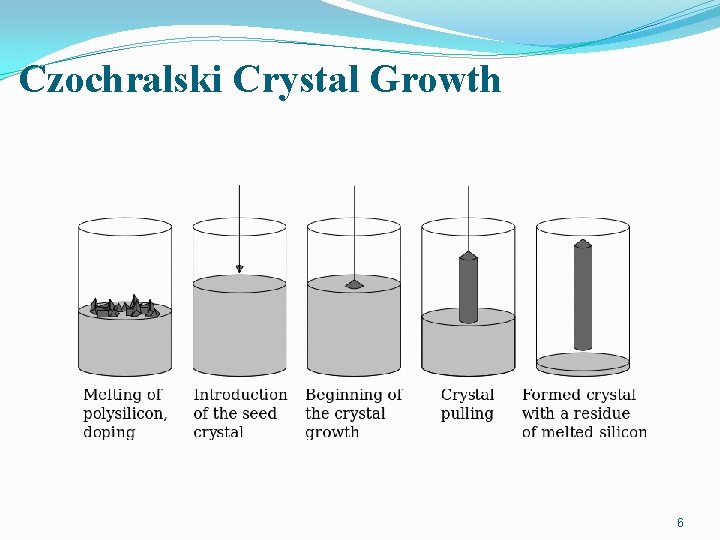
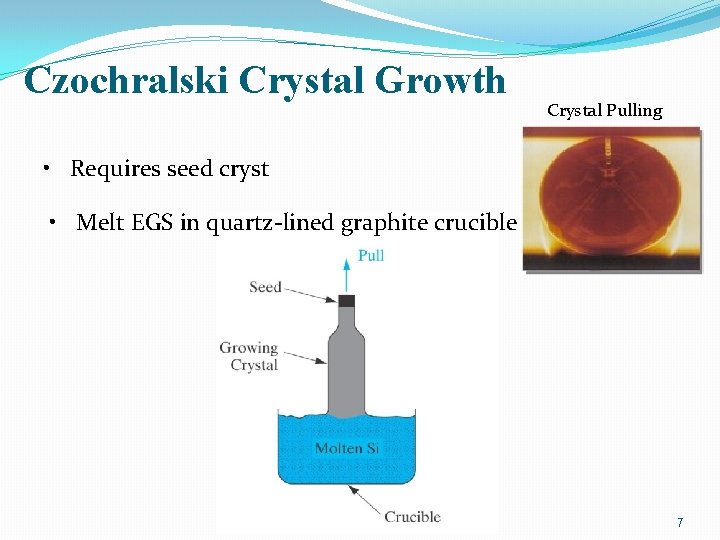
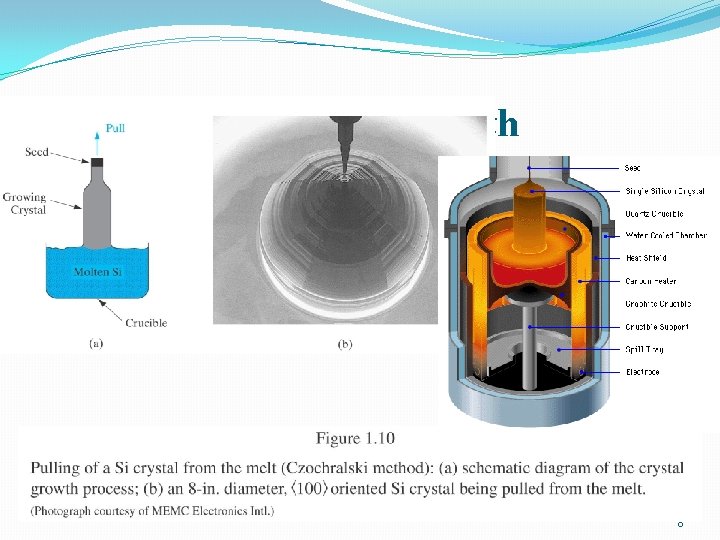
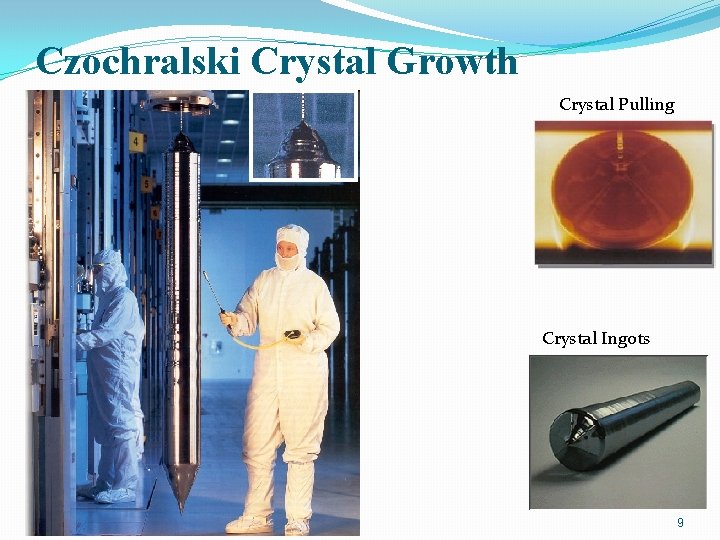
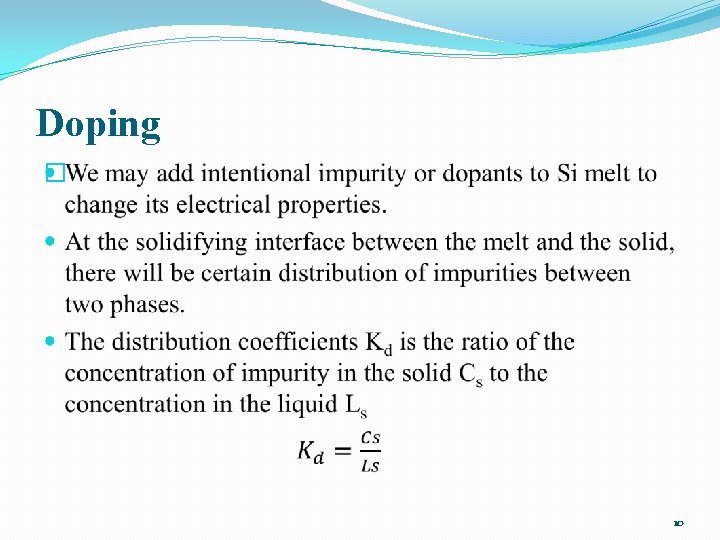
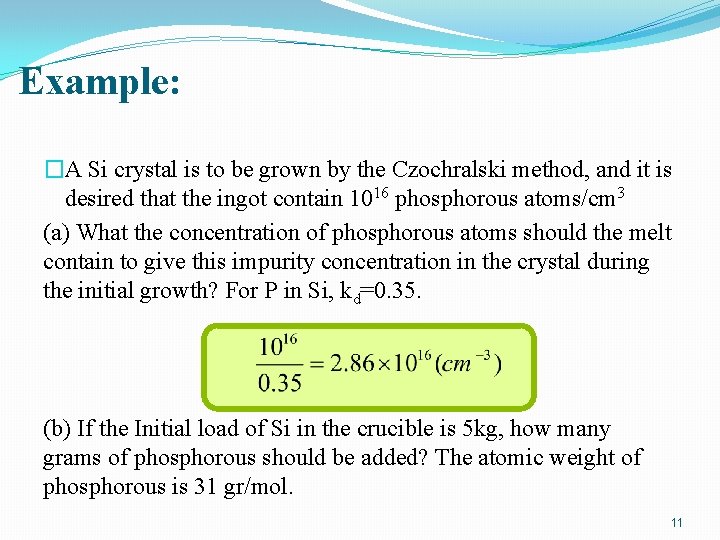
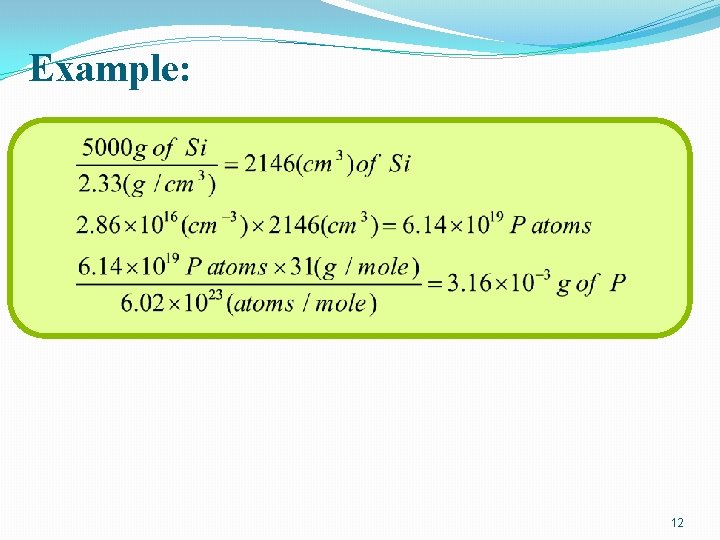
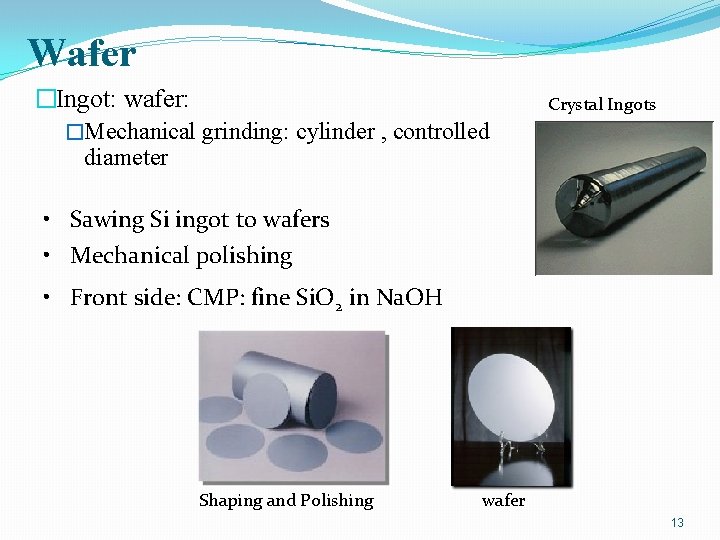
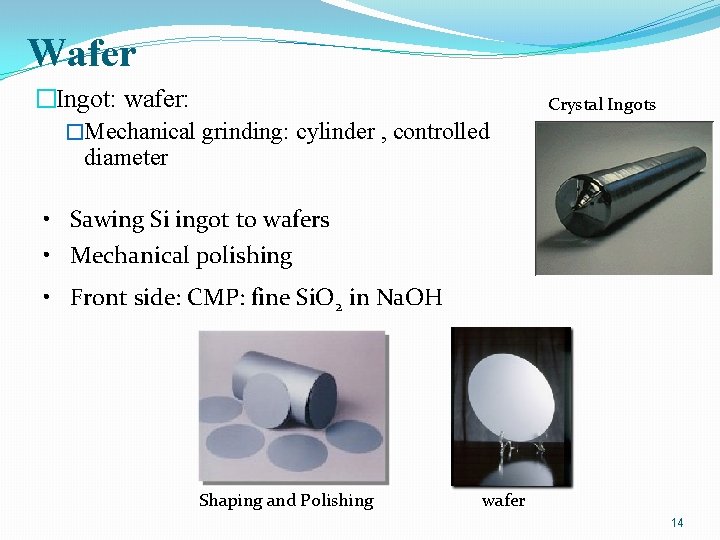
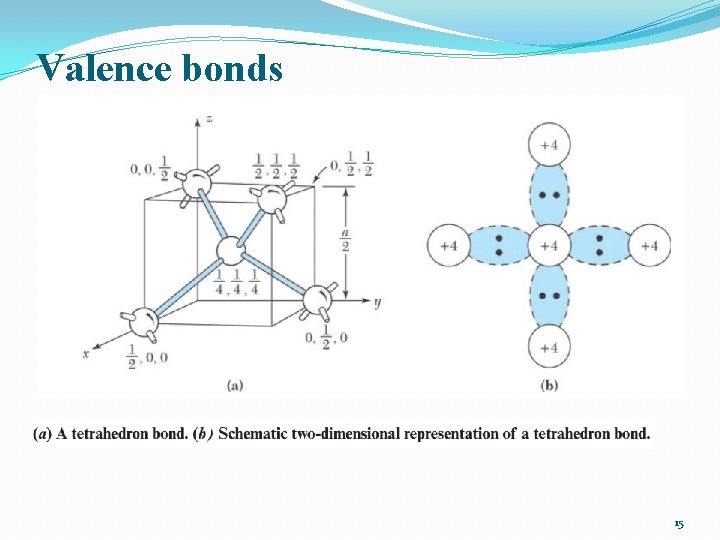
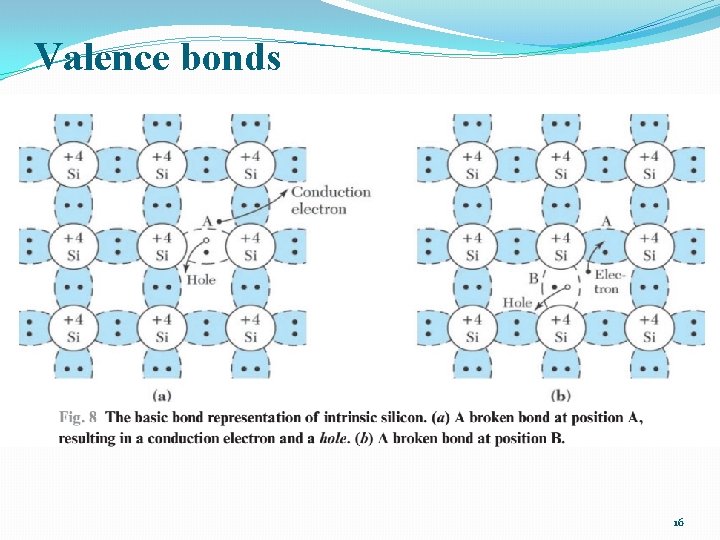
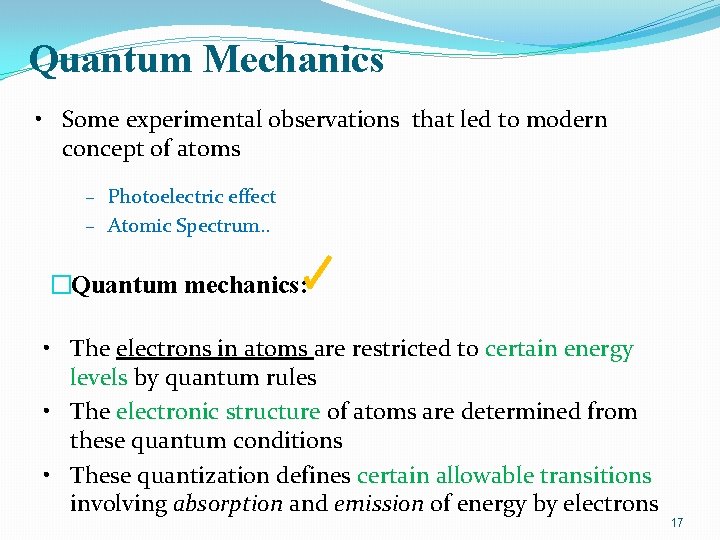
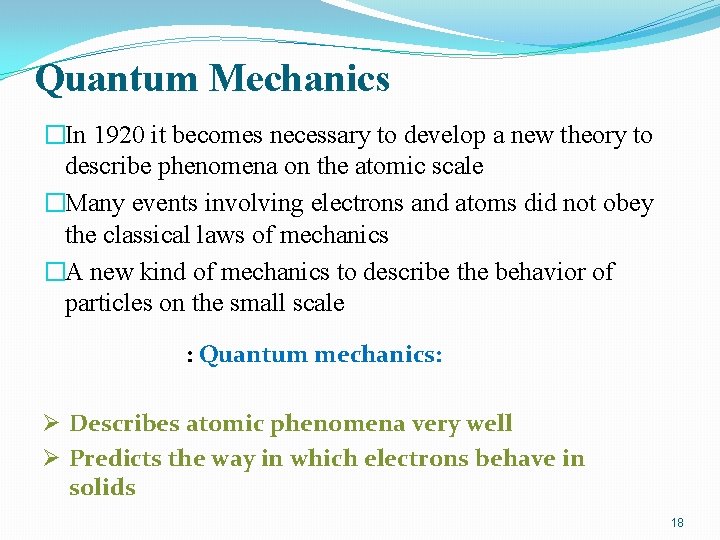
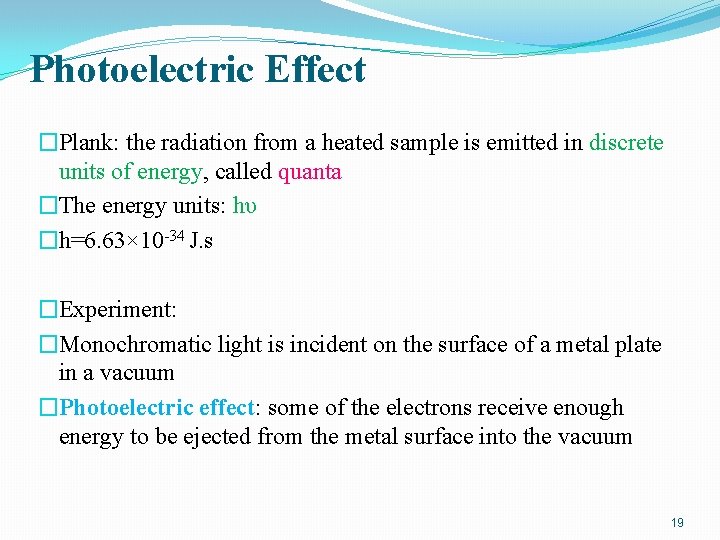
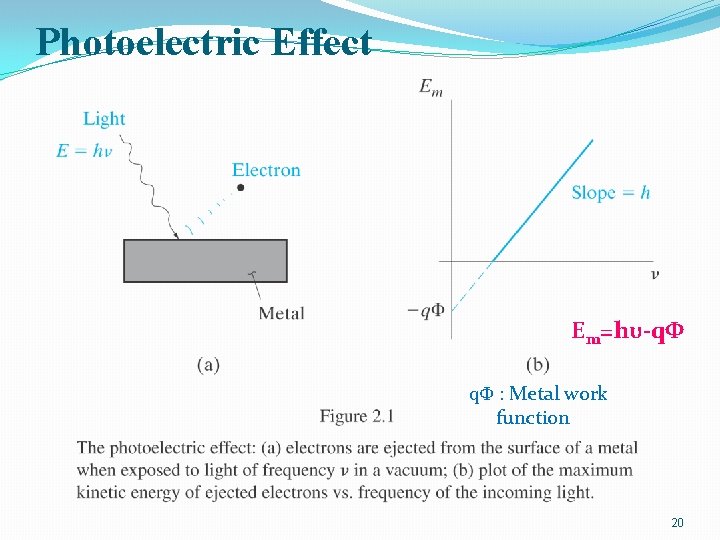
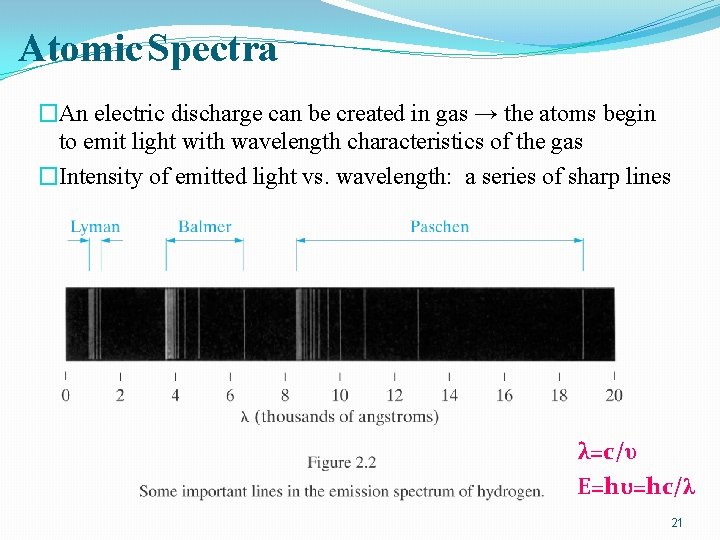
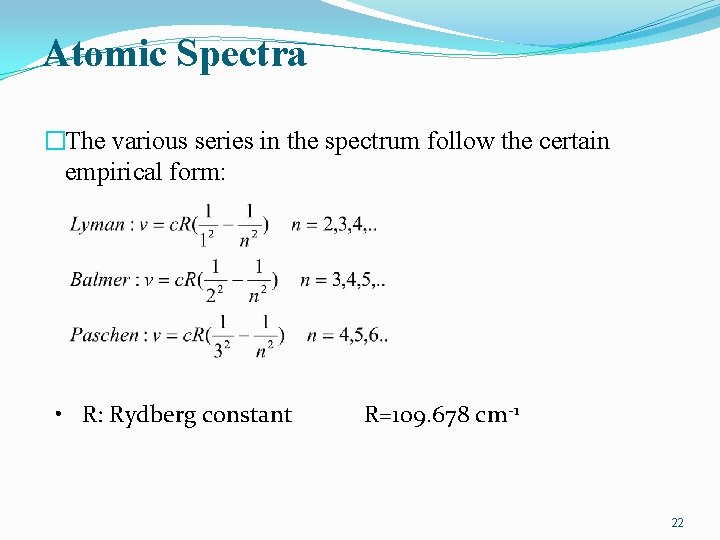
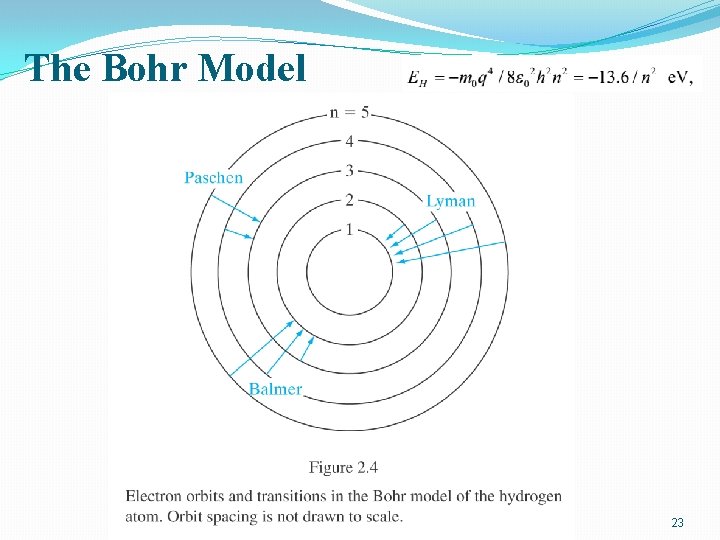
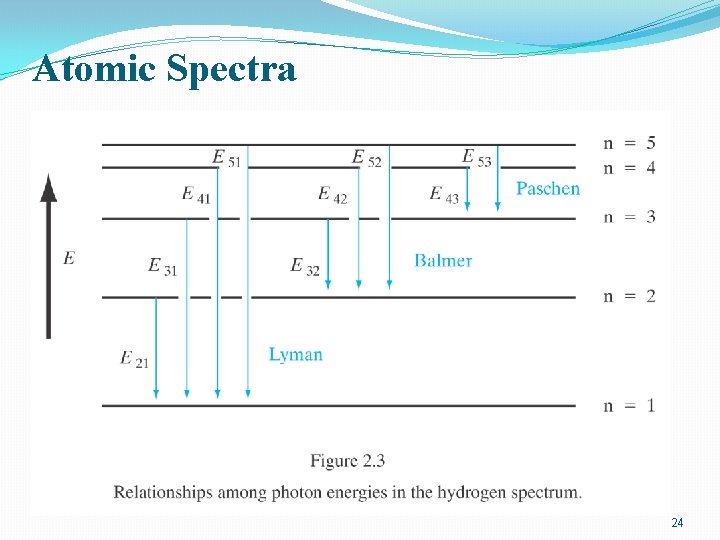
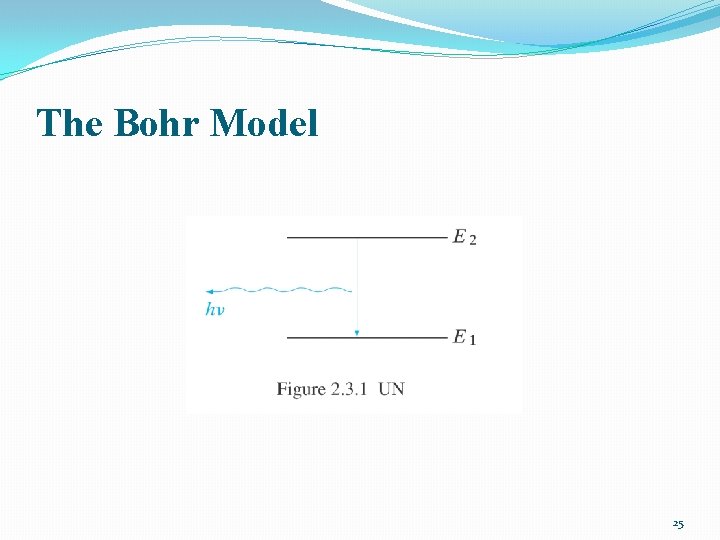
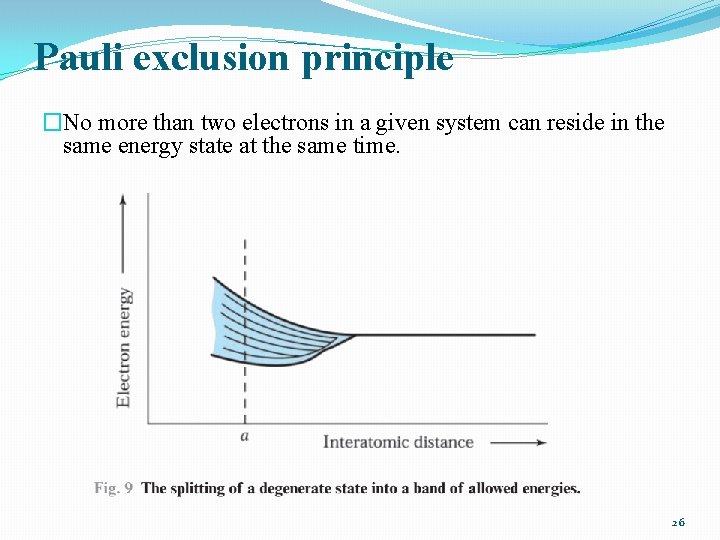
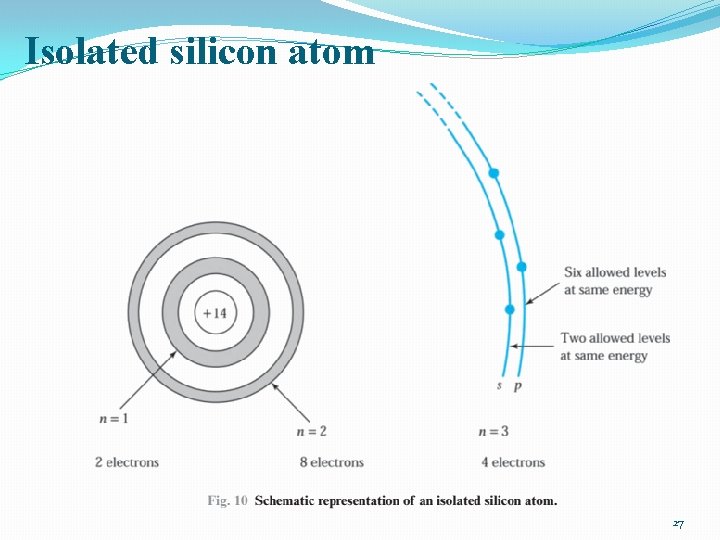
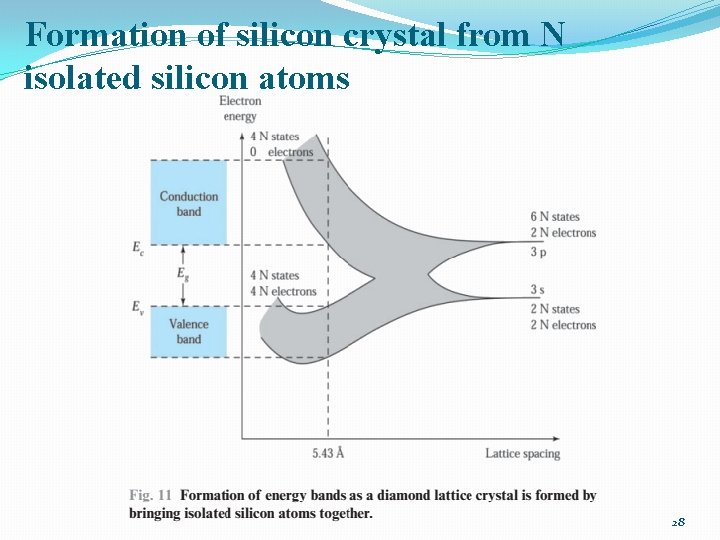
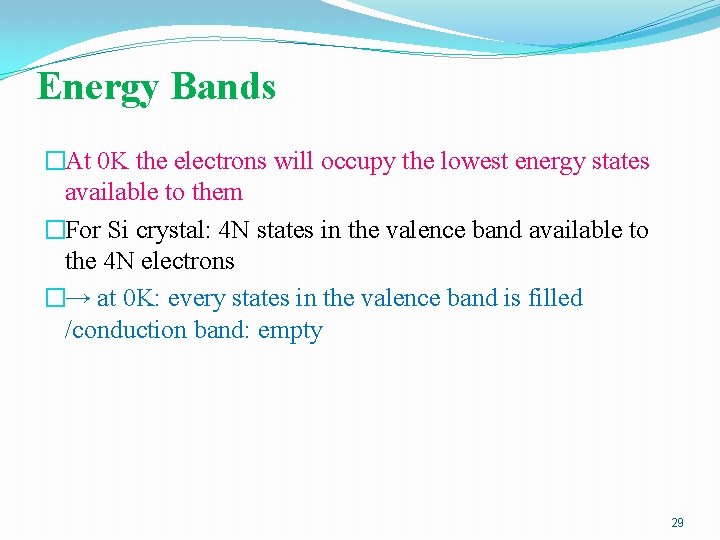
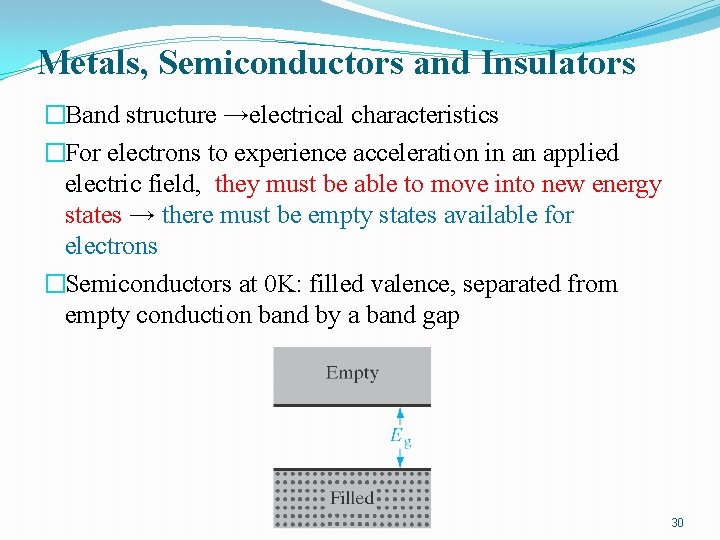
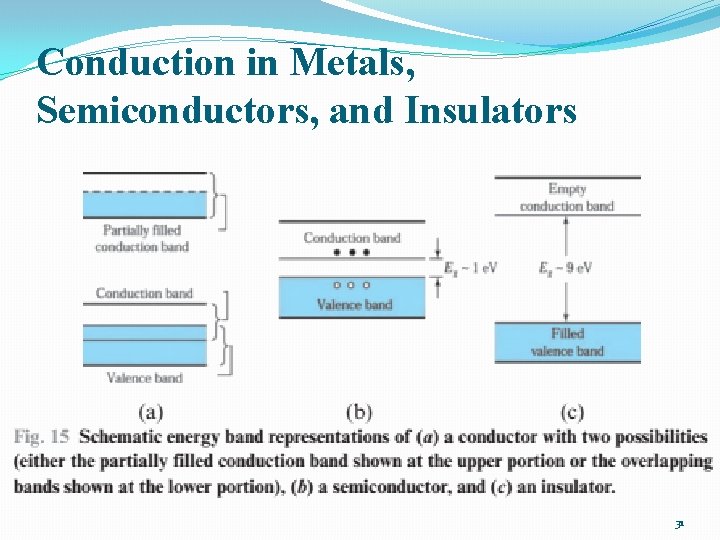
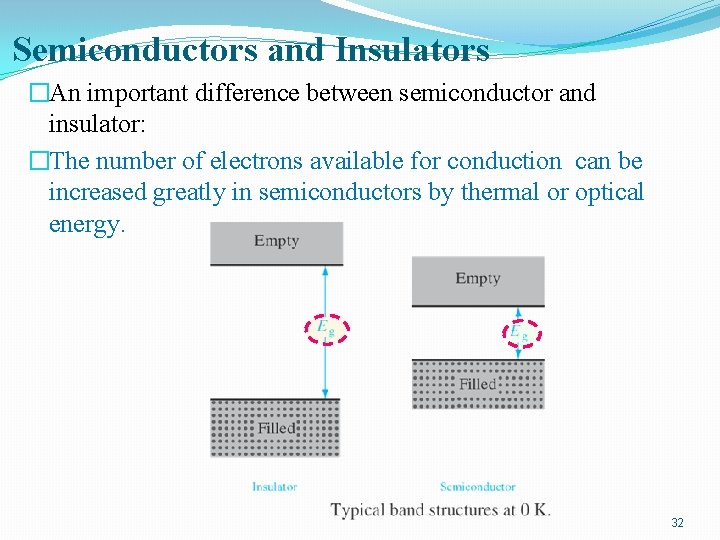
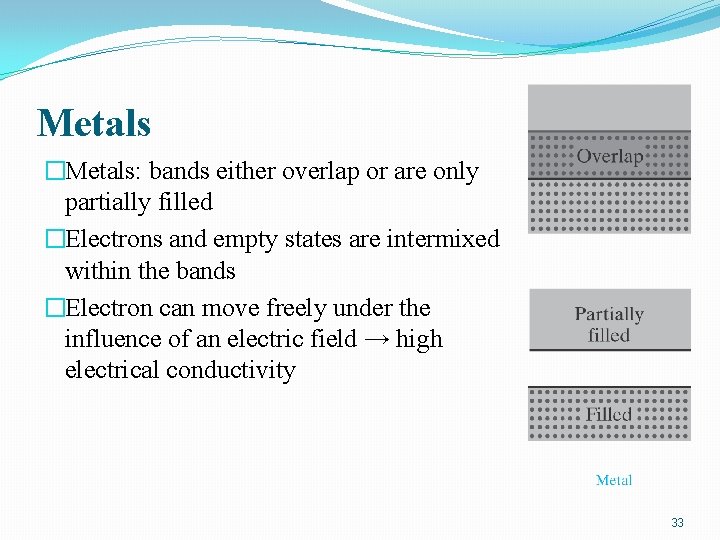
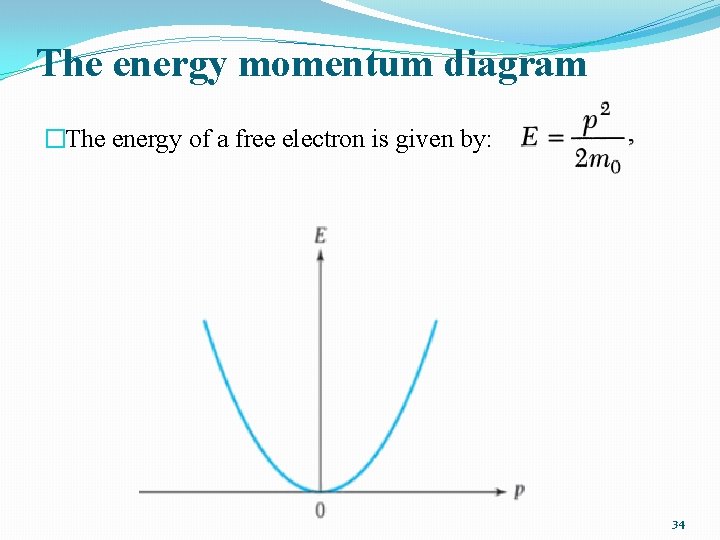
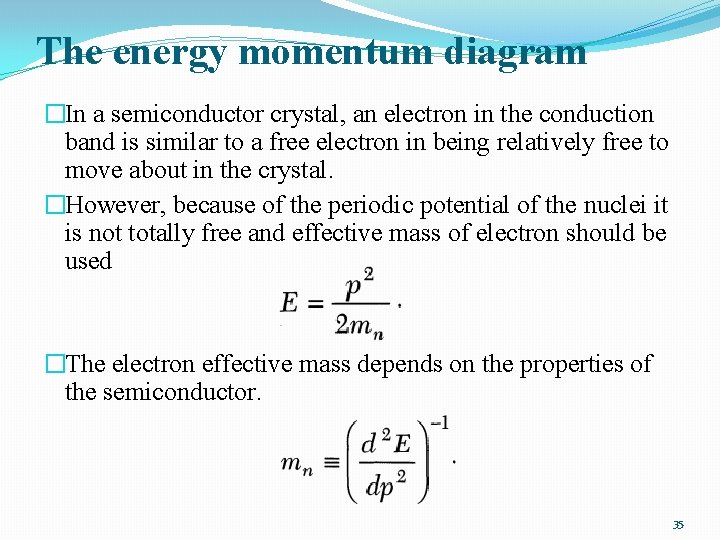
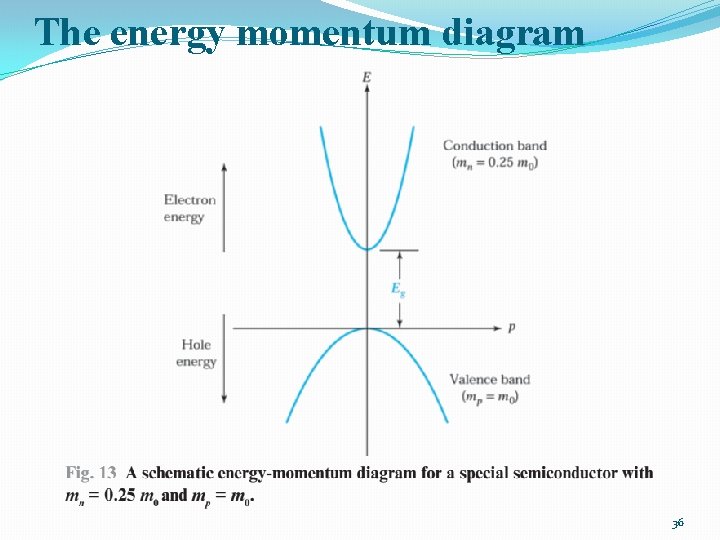
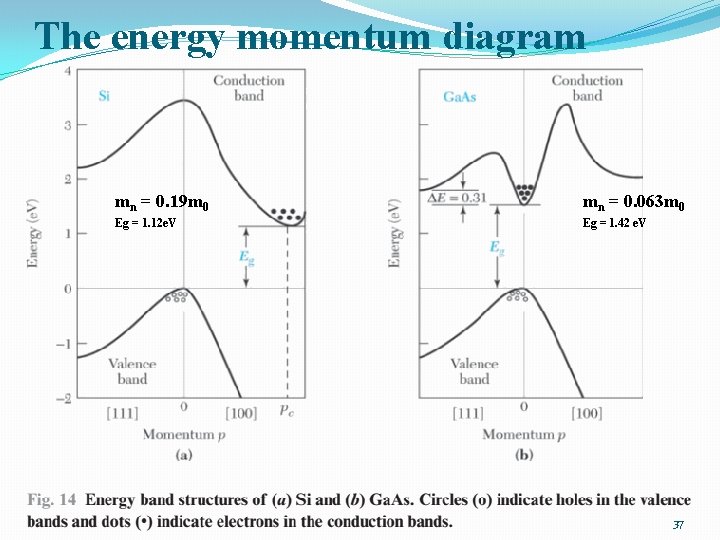
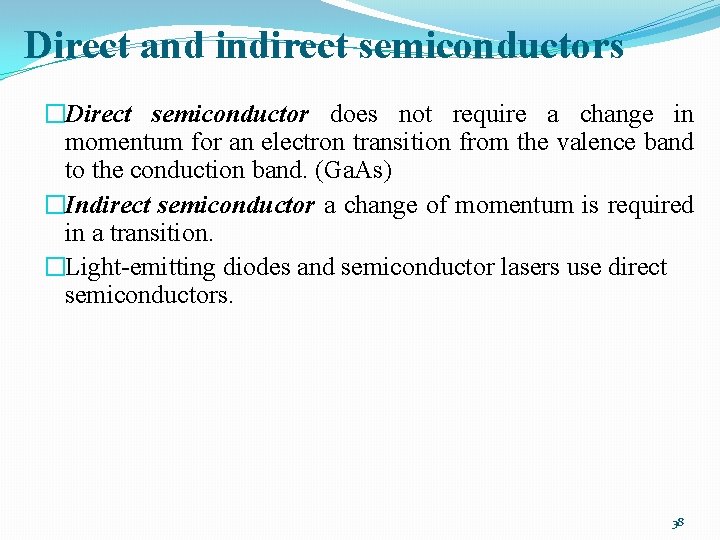
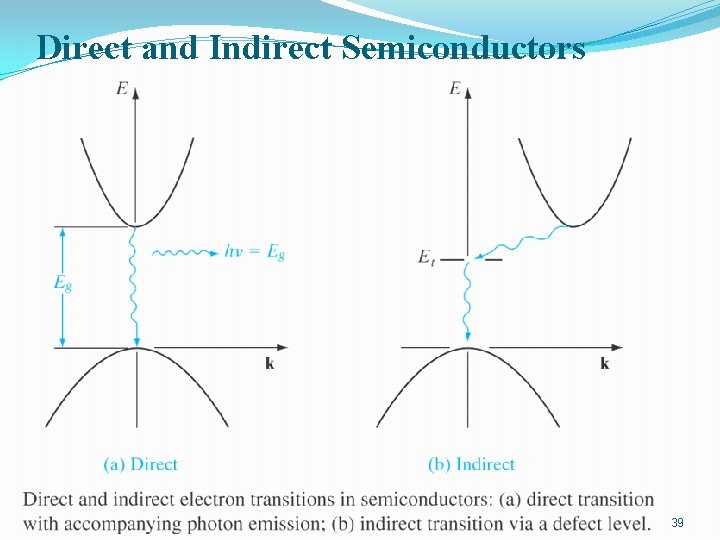
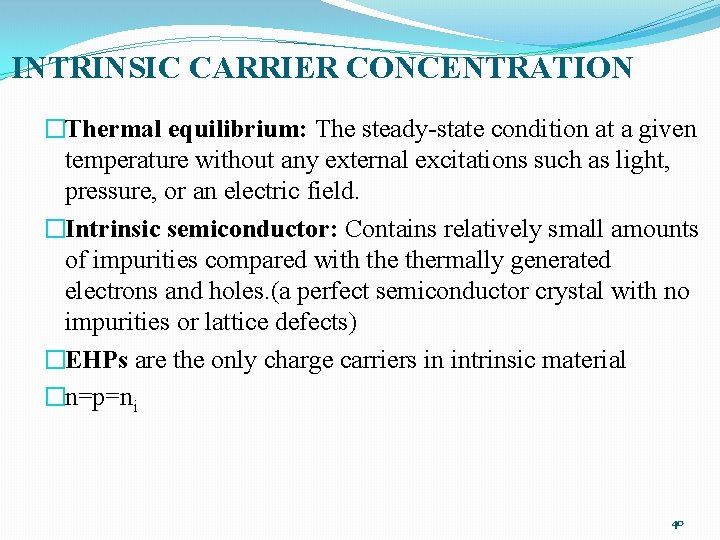
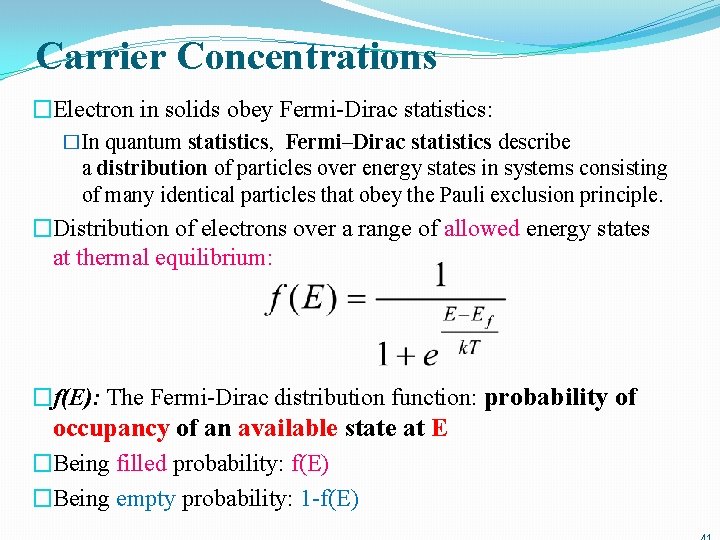
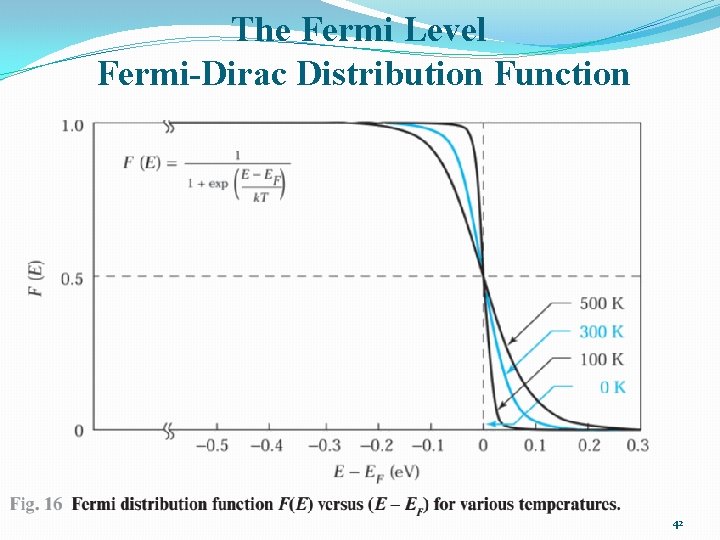
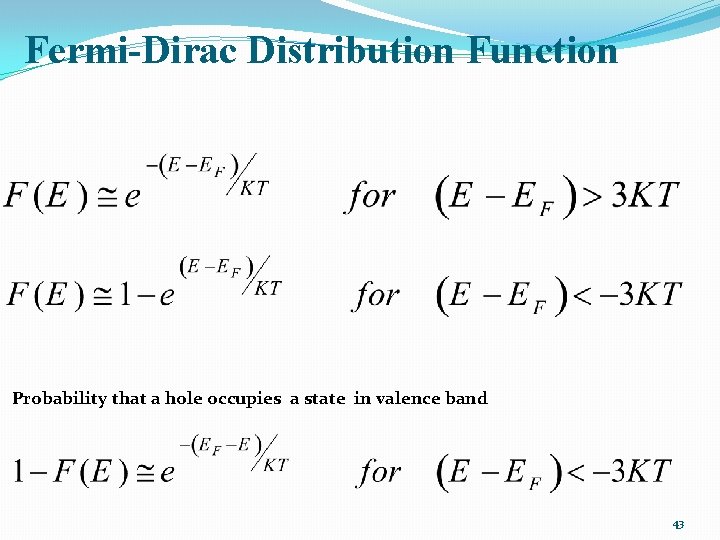
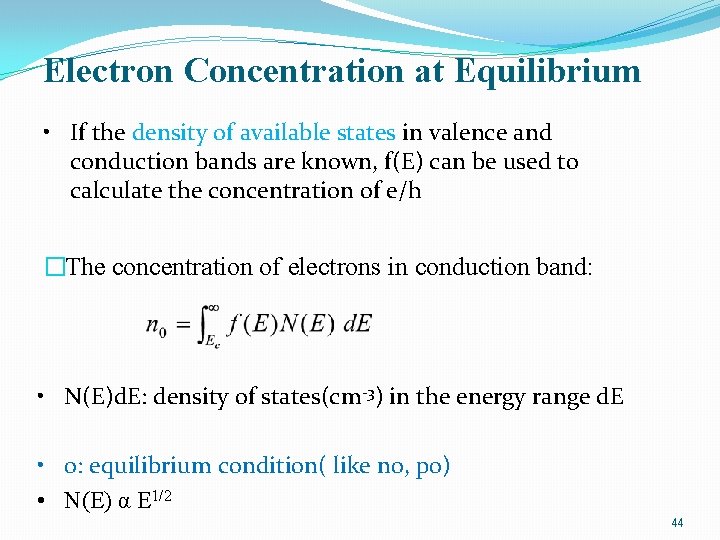
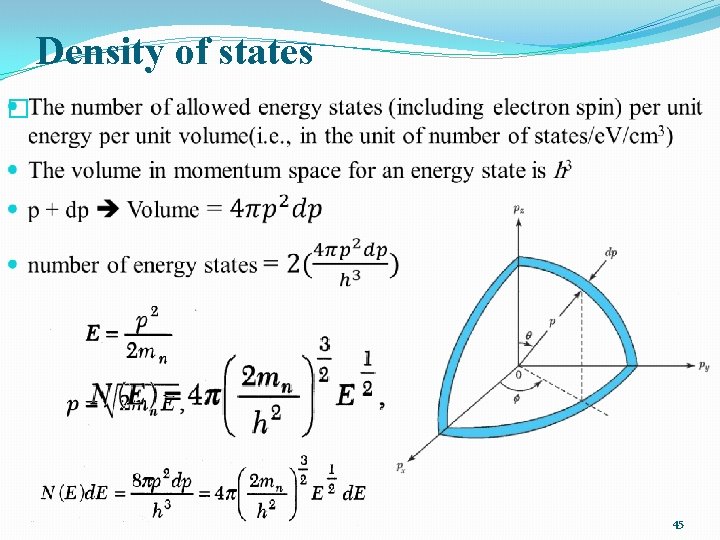
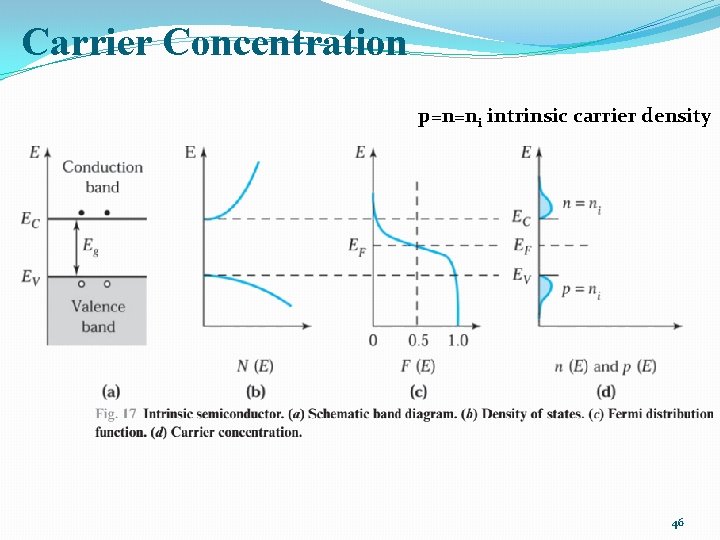
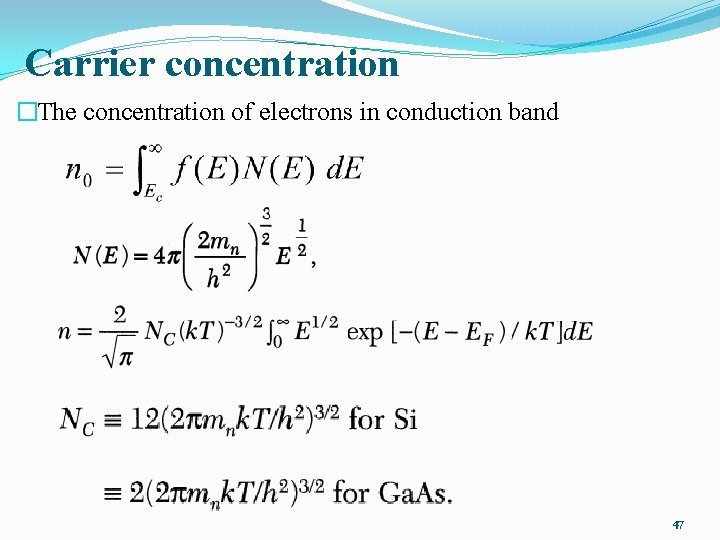
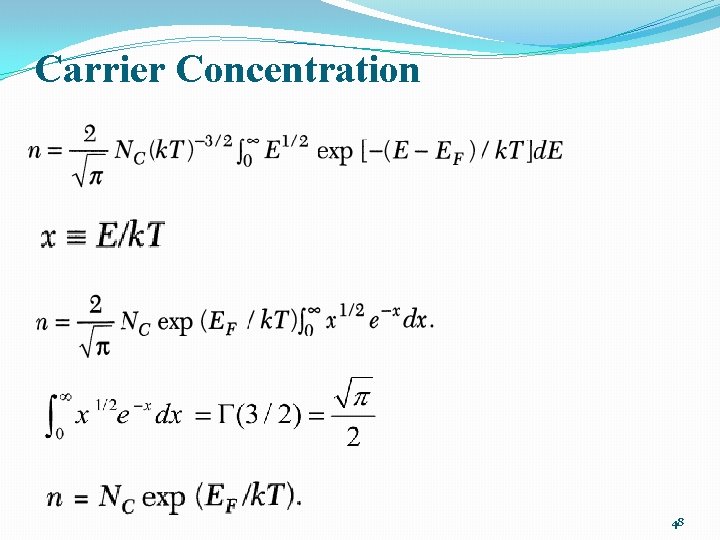
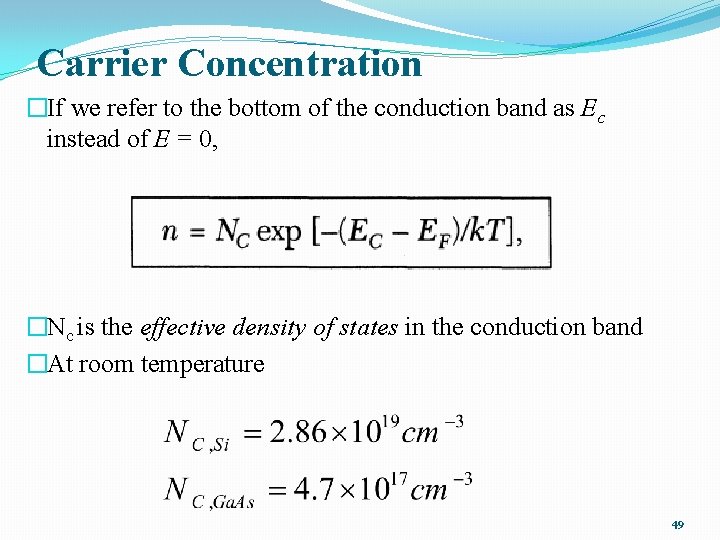
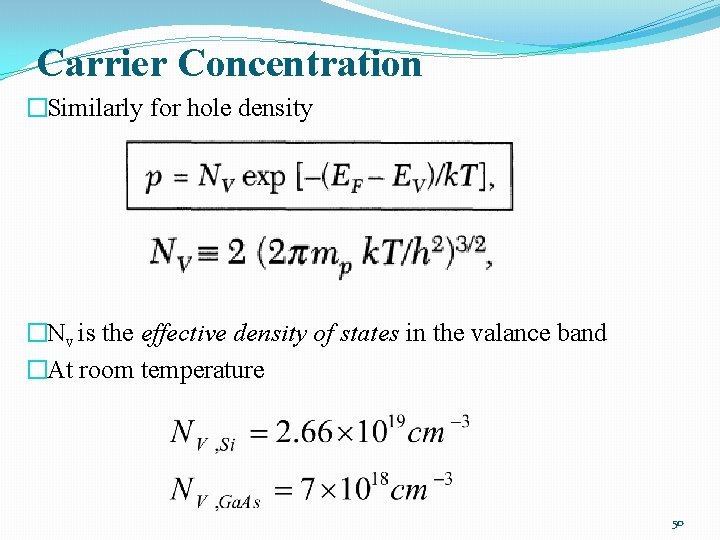
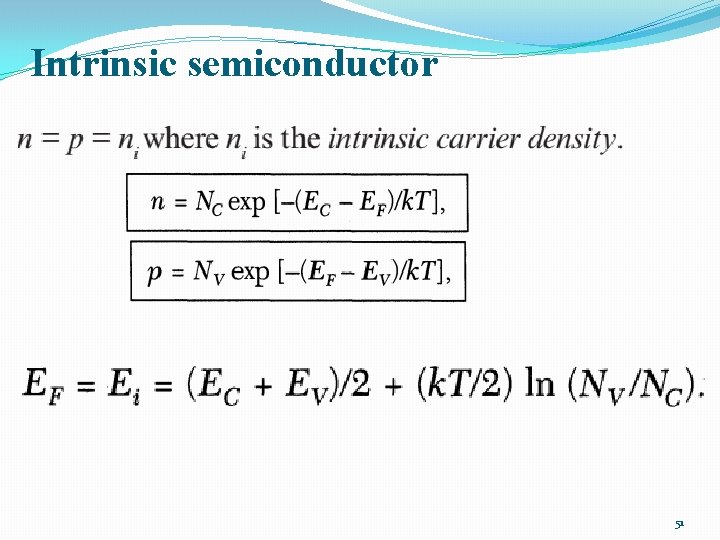
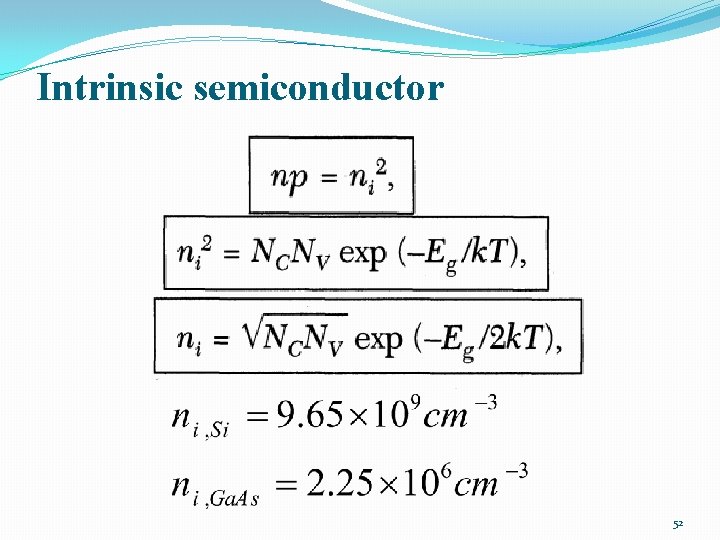
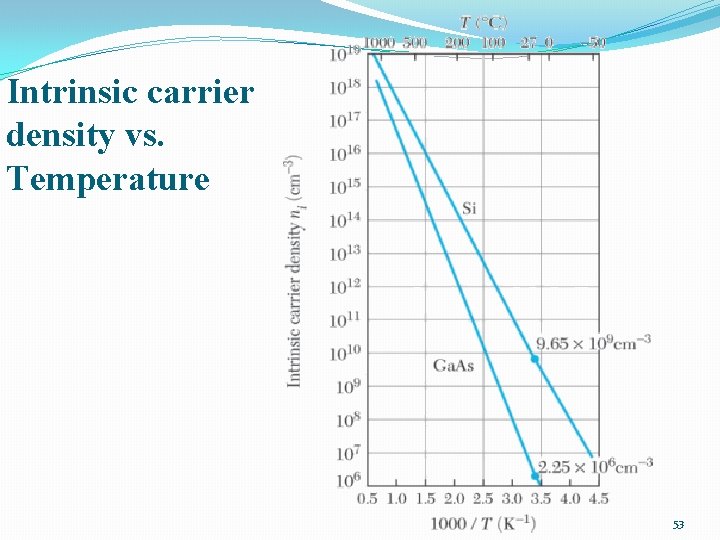
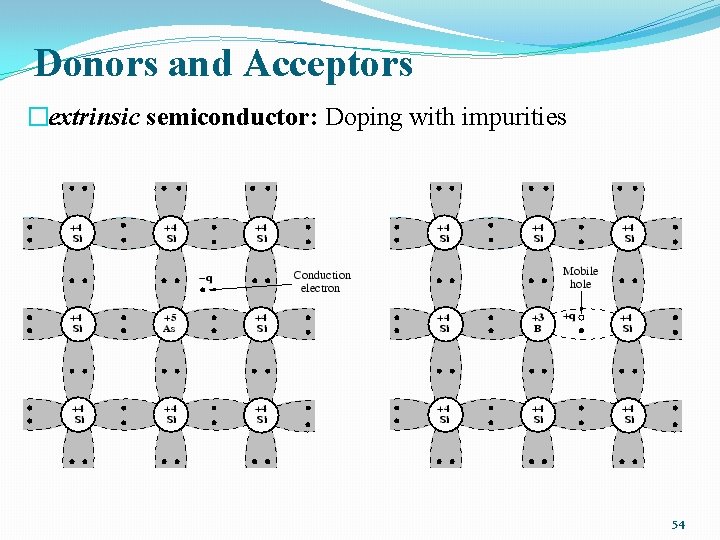
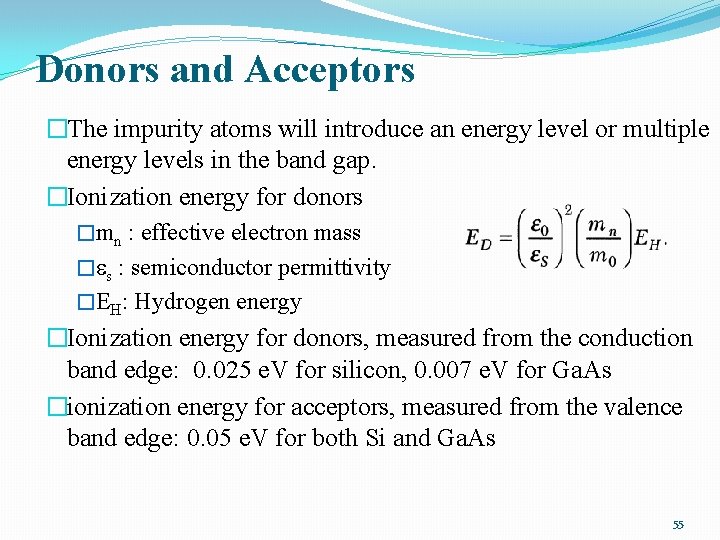
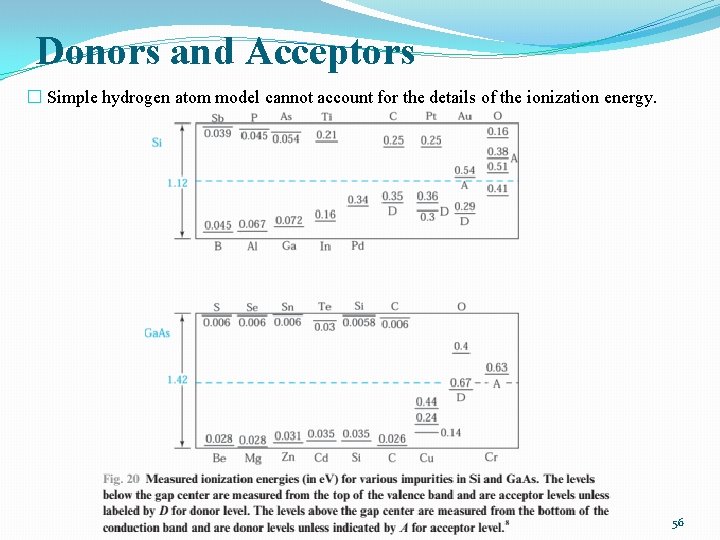
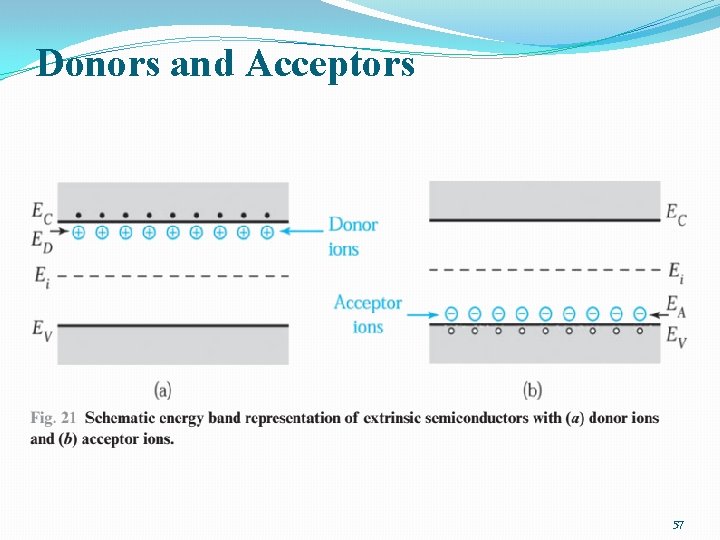
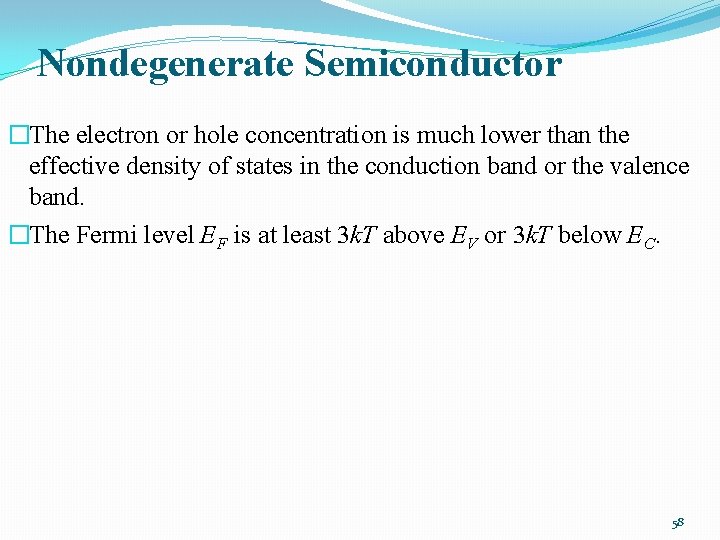
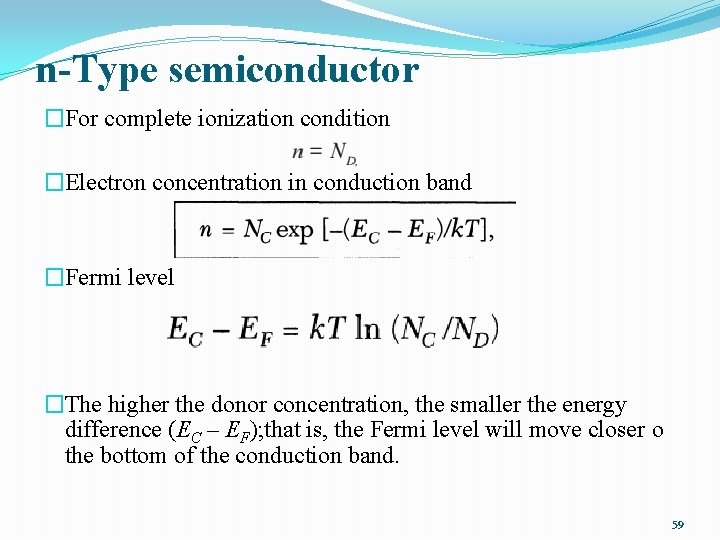
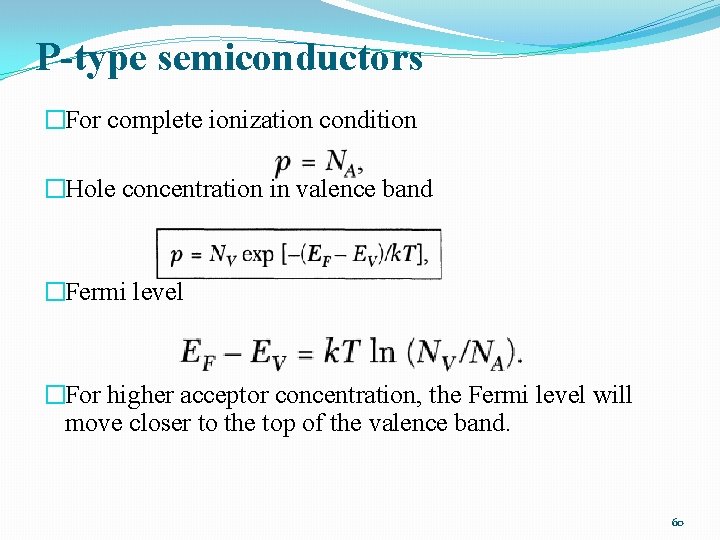
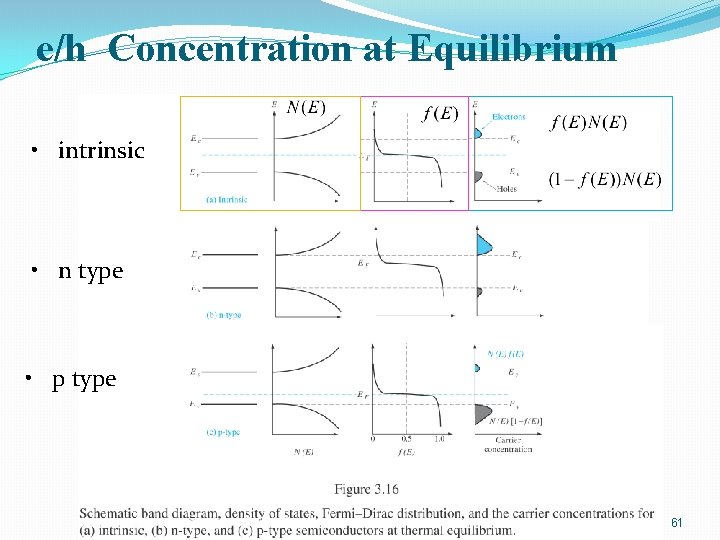
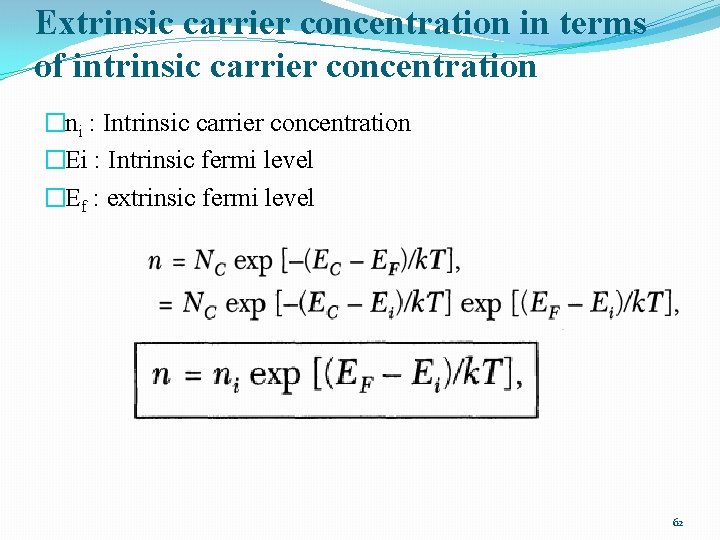
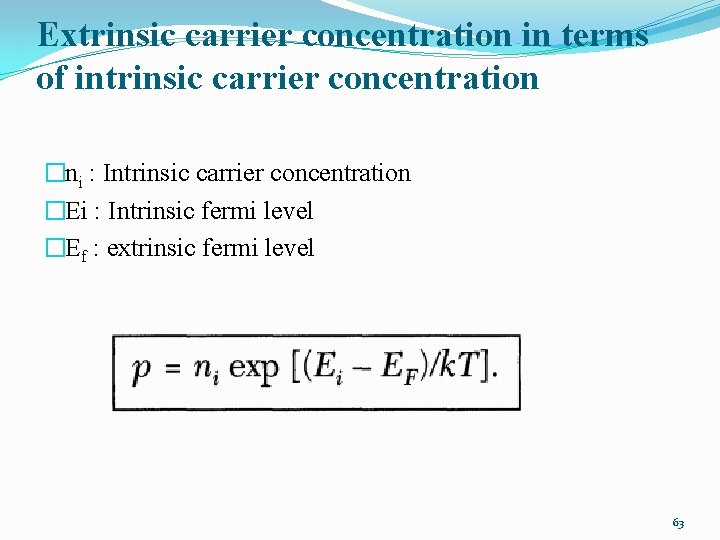
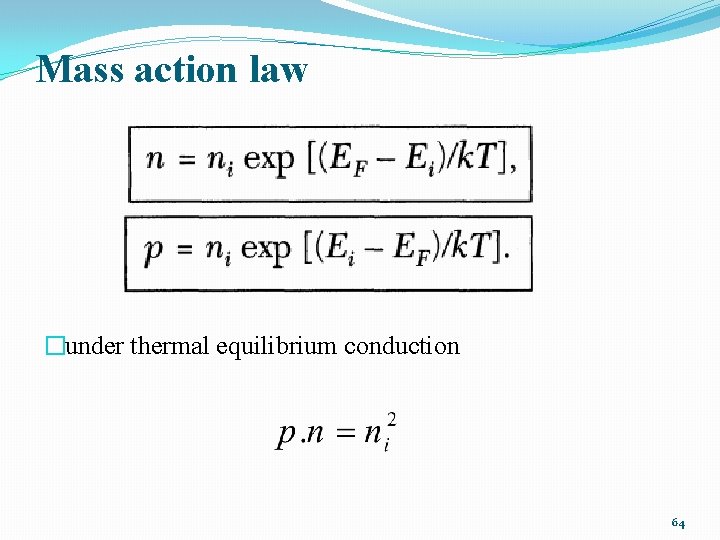
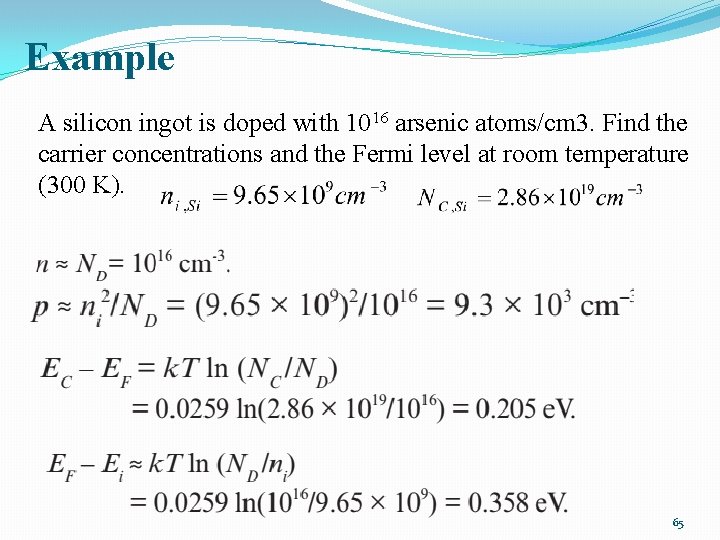
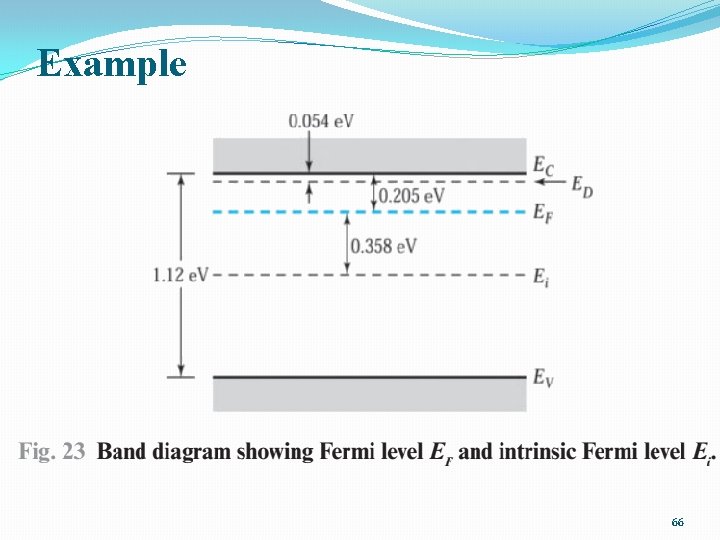
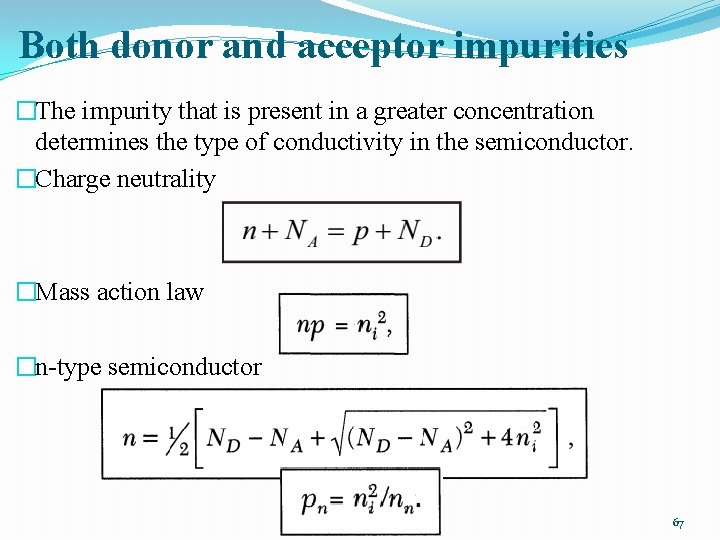
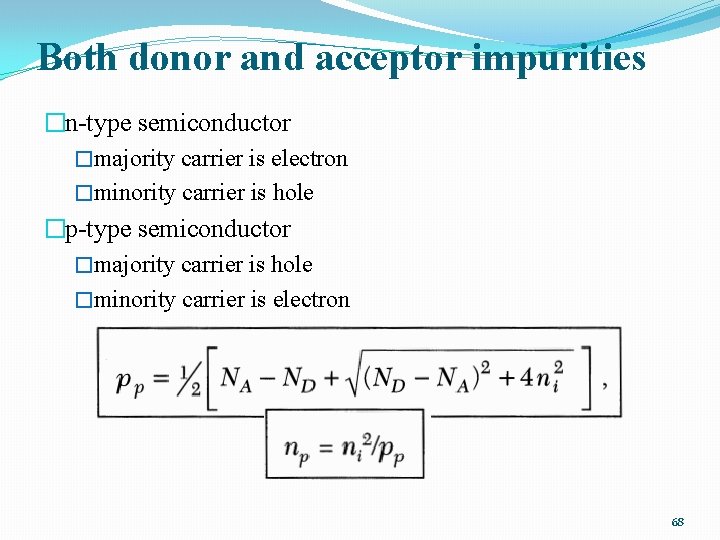
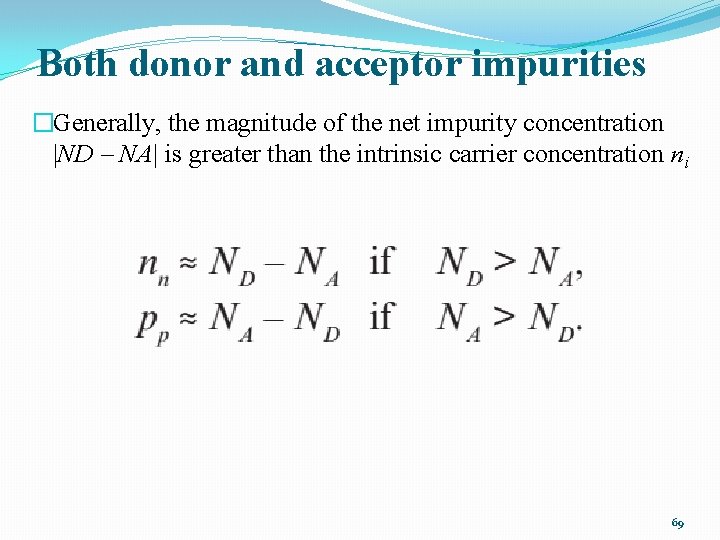
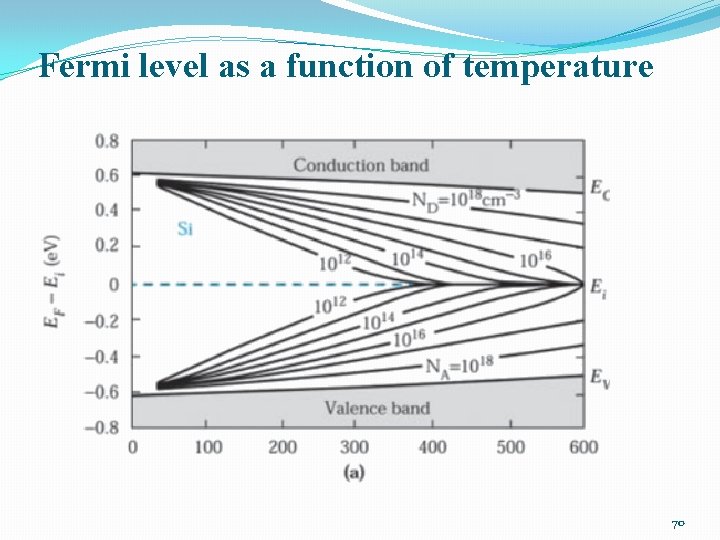
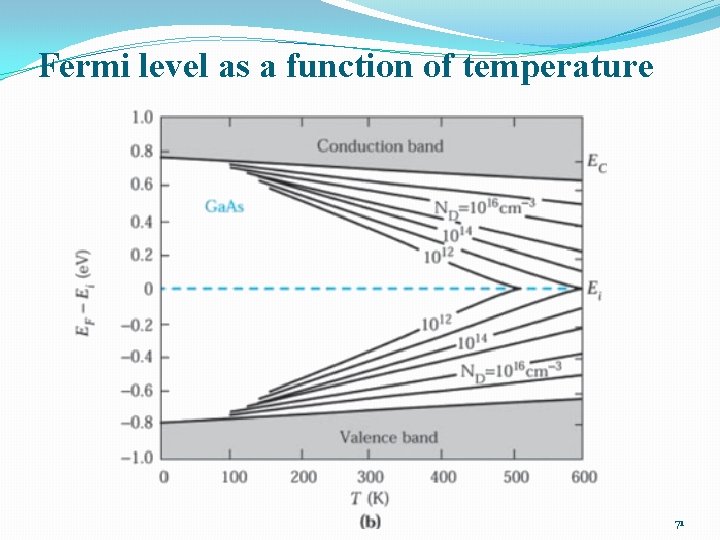
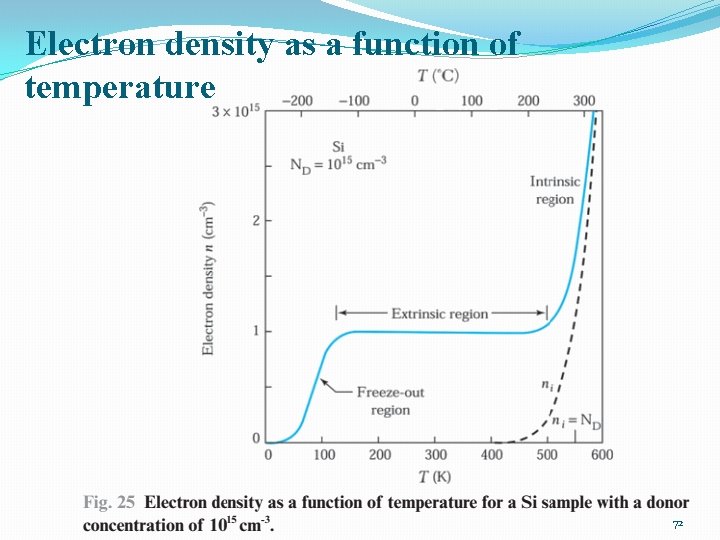
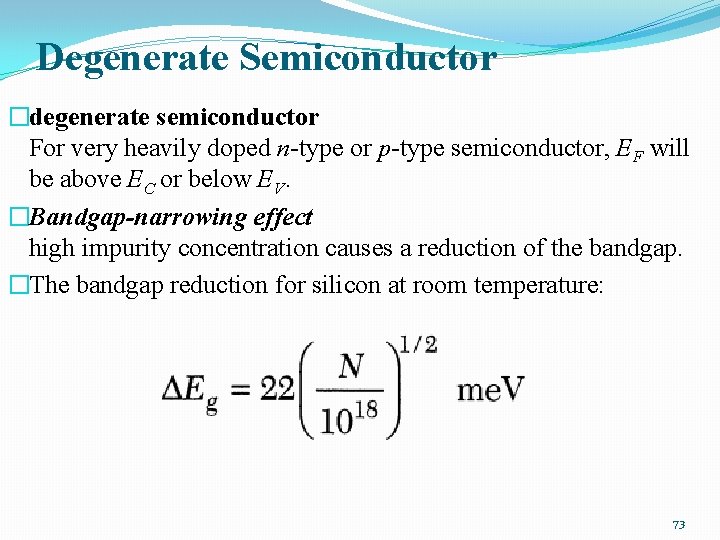
- Slides: 73
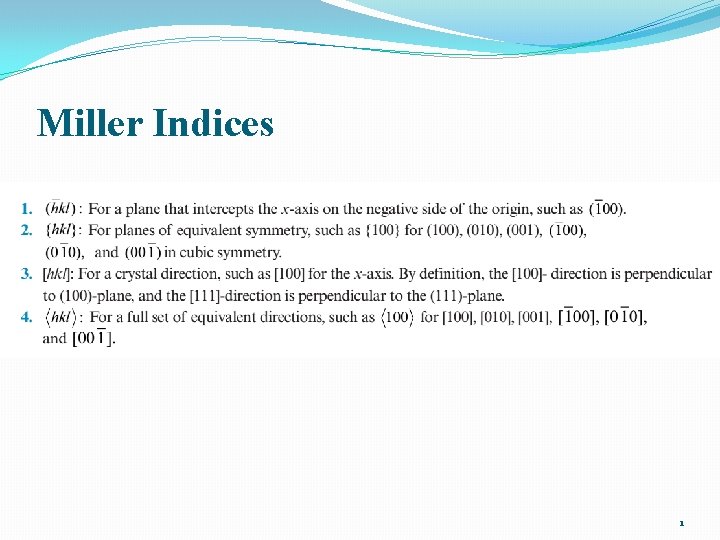
Miller Indices 1
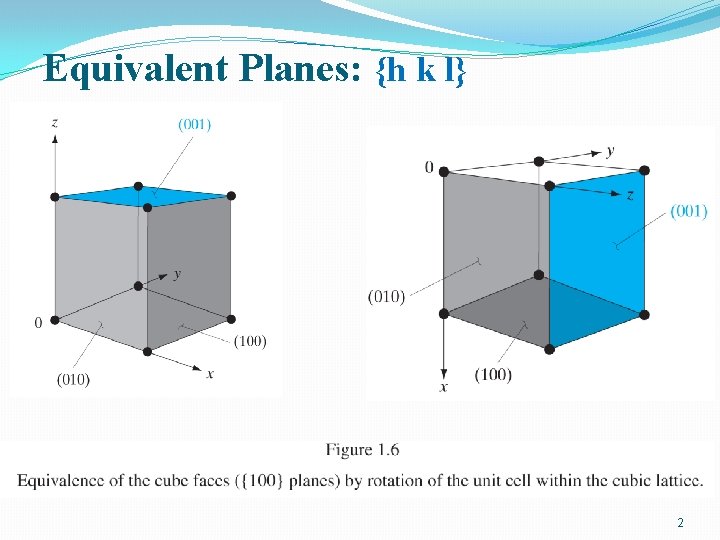
Equivalent Planes: {h k l} 2
![Crystal Directions h k l 3 Crystal Directions: [h k l] 3](https://slidetodoc.com/presentation_image_h2/94cc0c572c292a84b8b7866ce8b9efb2/image-3.jpg)
Crystal Directions: [h k l] 3
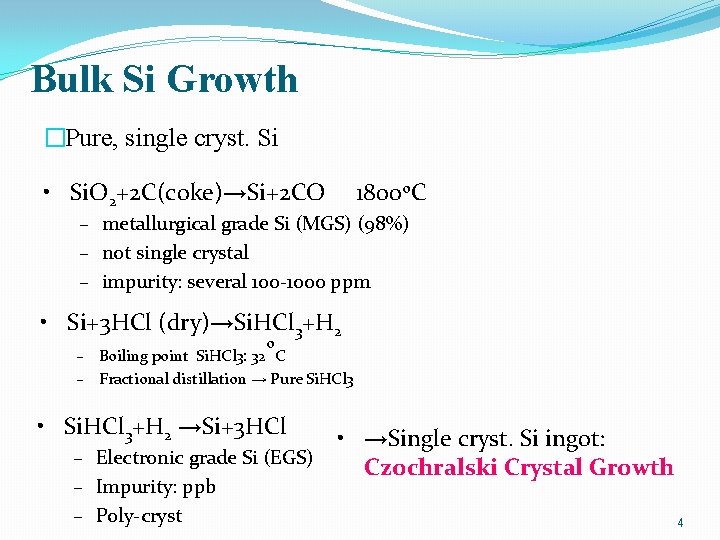
Bulk Si Growth �Pure, single cryst. Si • Si. O 2+2 C(coke)→Si+2 CO 1800 o. C – metallurgical grade Si (MGS) (98%) – not single crystal – impurity: several 100 -1000 ppm • Si+3 HCl (dry)→Si. HCl 3+H 2 o – Boiling point Si. HCl 3: 32 C – Fractional distillation → Pure Si. HCl 3 • Si. HCl 3+H 2 →Si+3 HCl • →Single cryst. Si ingot: – Electronic grade Si (EGS) Czochralski Crystal Growth – Impurity: ppb – Poly-cryst 4
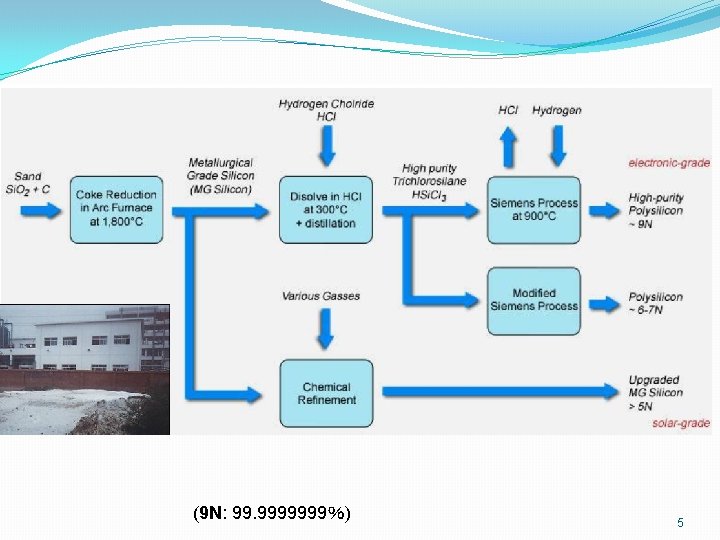
From Sand to Silicon (9 N: 99. 9999999%) 5
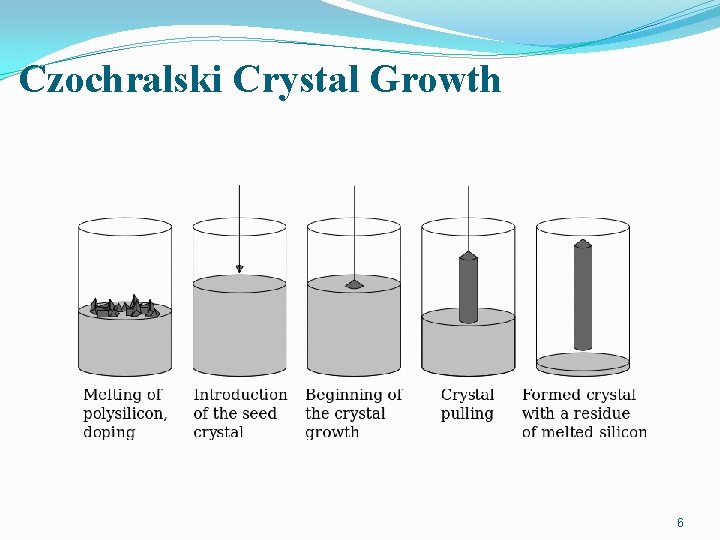
Czochralski Crystal Growth 6
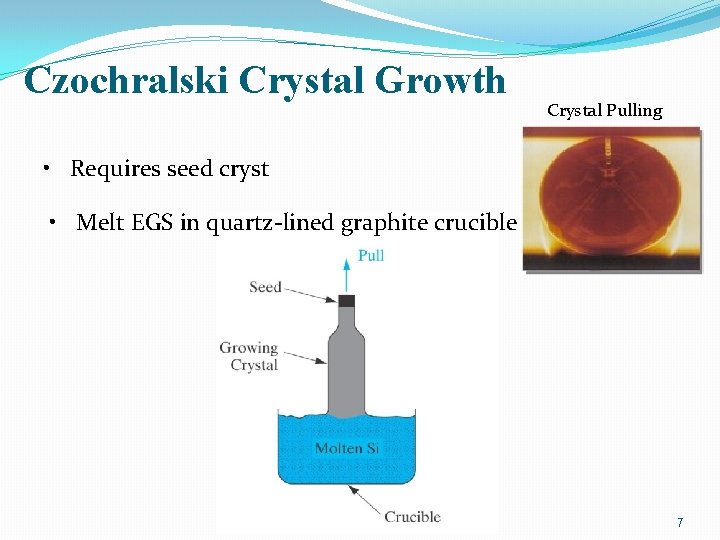
Czochralski Crystal Growth Crystal Pulling • Requires seed cryst • Melt EGS in quartz-lined graphite crucible 7
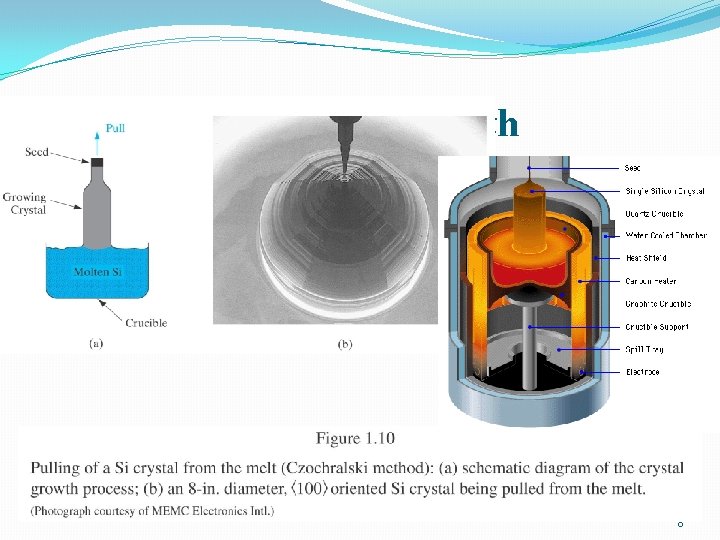
Czochralski Crystal Growth 8
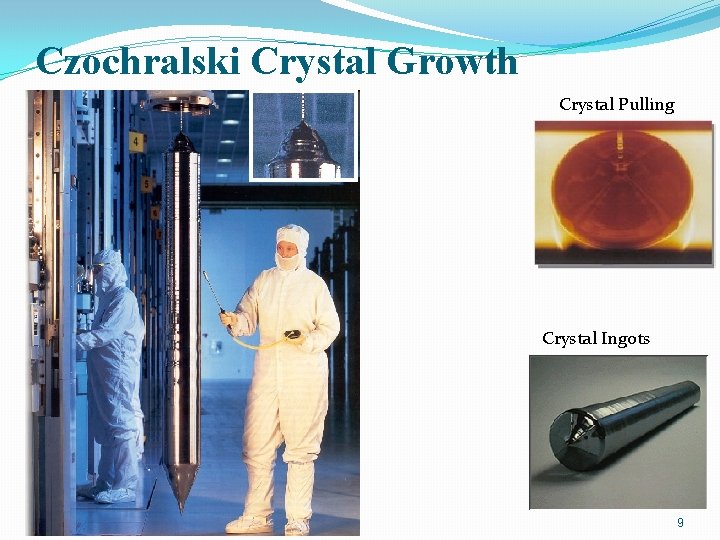
Czochralski Crystal Growth Crystal Pulling Crystal Ingots 9
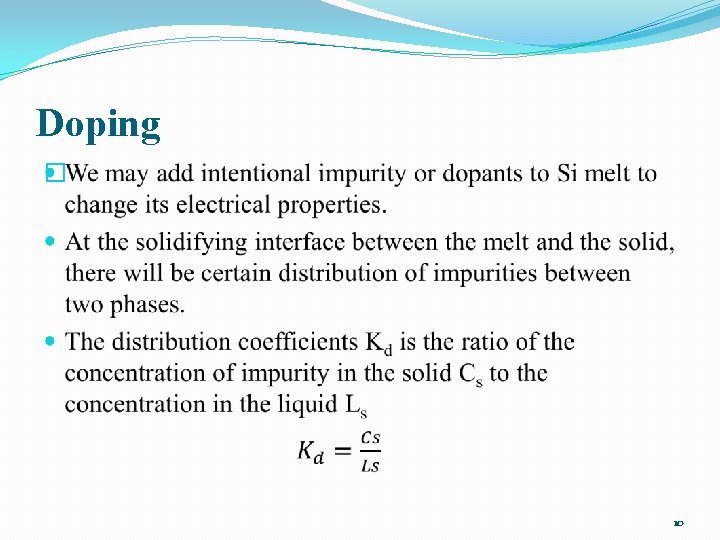
Doping � 10
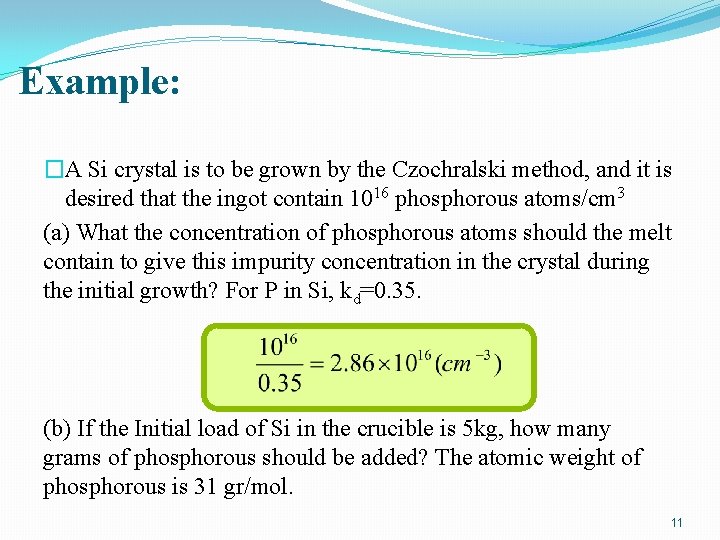
Example: �A Si crystal is to be grown by the Czochralski method, and it is desired that the ingot contain 1016 phosphorous atoms/cm 3 (a) What the concentration of phosphorous atoms should the melt contain to give this impurity concentration in the crystal during the initial growth? For P in Si, kd=0. 35. (b) If the Initial load of Si in the crucible is 5 kg, how many grams of phosphorous should be added? The atomic weight of phosphorous is 31 gr/mol. 11
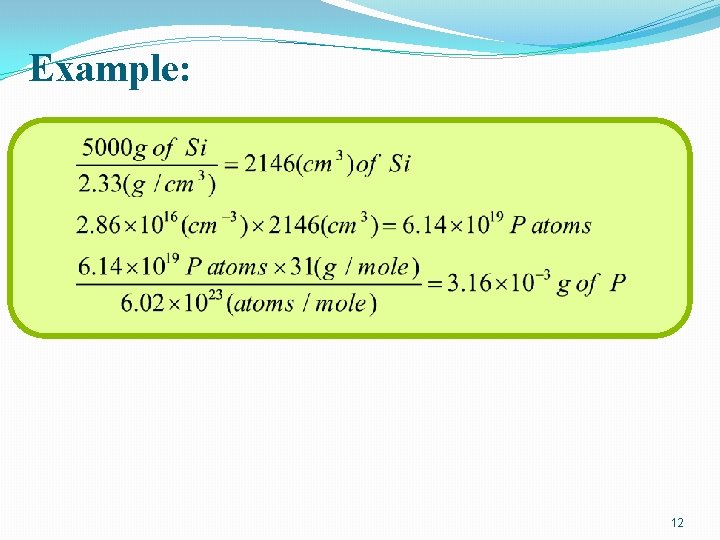
Example: 12
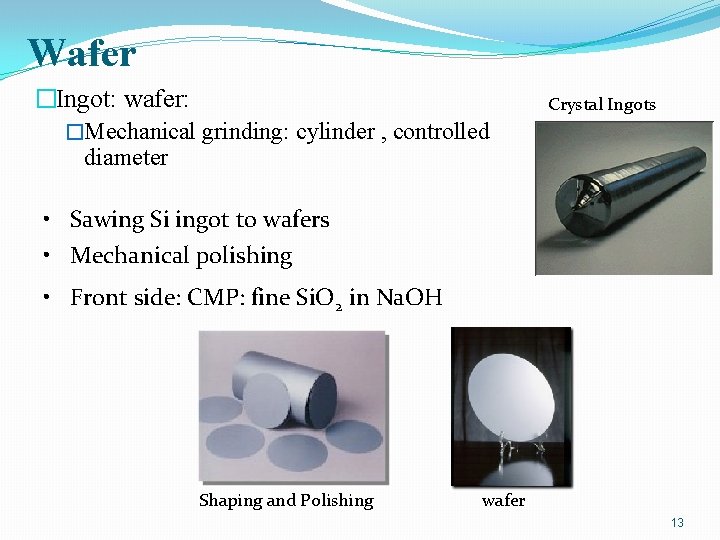
Wafer �Ingot: wafer: �Mechanical grinding: cylinder , controlled diameter Crystal Ingots • Sawing Si ingot to wafers • Mechanical polishing • Front side: CMP: fine Si. O 2 in Na. OH Shaping and Polishing wafer 13
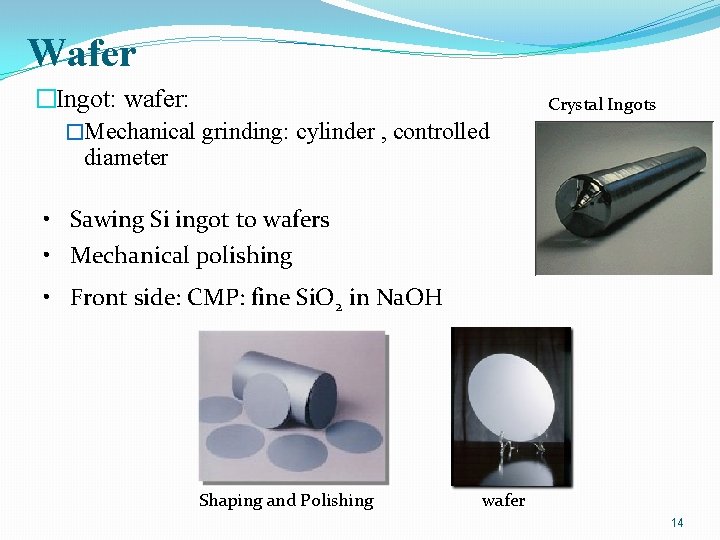
Wafer �Ingot: wafer: �Mechanical grinding: cylinder , controlled diameter Crystal Ingots • Sawing Si ingot to wafers • Mechanical polishing • Front side: CMP: fine Si. O 2 in Na. OH Shaping and Polishing wafer 14
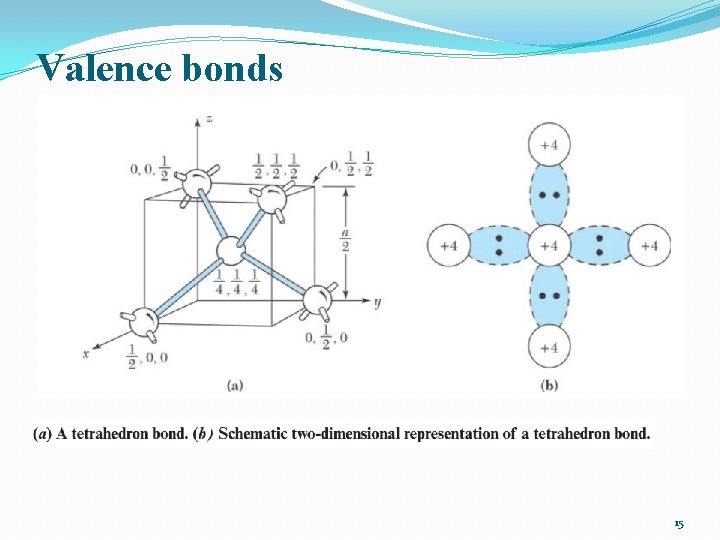
Valence bonds 15
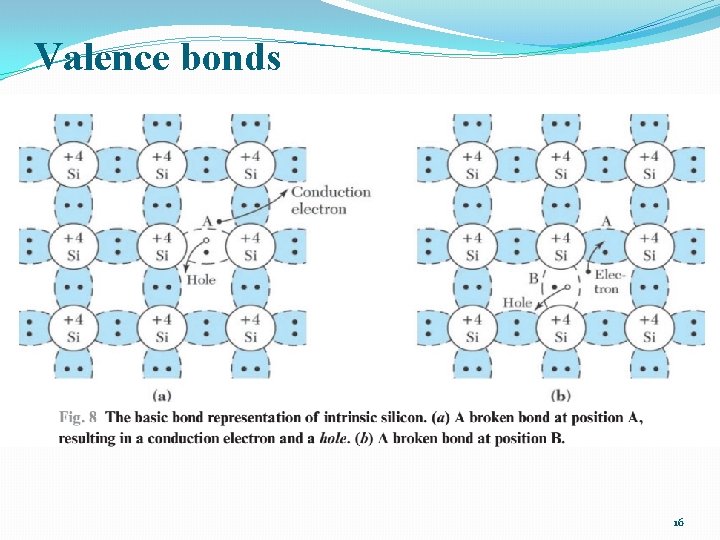
Valence bonds 16
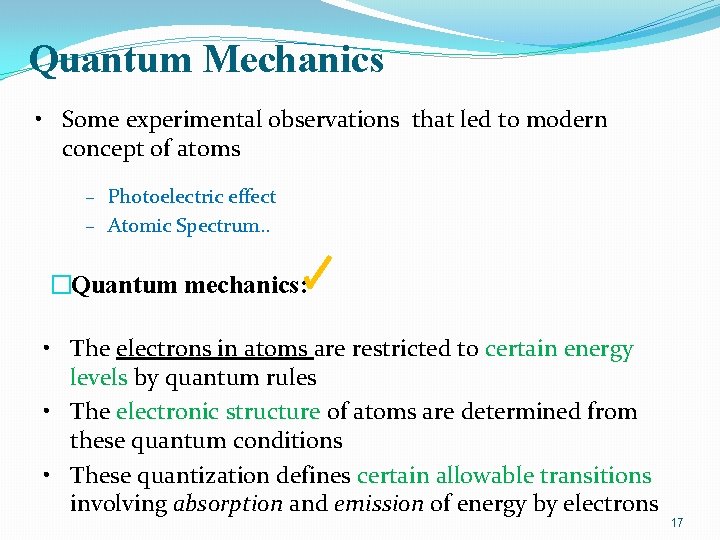
Quantum Mechanics • Some experimental observations that led to modern concept of atoms – Photoelectric effect – Atomic Spectrum. . �Quantum mechanics: • The electrons in atoms are restricted to certain energy levels by quantum rules • The electronic structure of atoms are determined from these quantum conditions • These quantization defines certain allowable transitions involving absorption and emission of energy by electrons 17
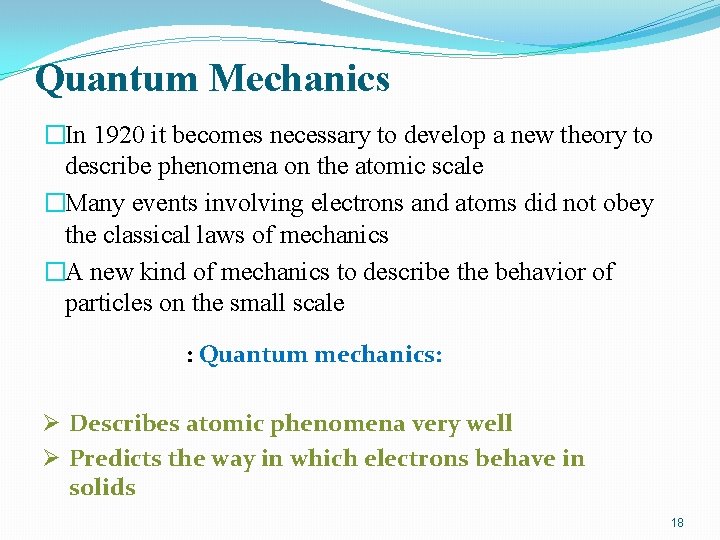
Quantum Mechanics �In 1920 it becomes necessary to develop a new theory to describe phenomena on the atomic scale �Many events involving electrons and atoms did not obey the classical laws of mechanics �A new kind of mechanics to describe the behavior of particles on the small scale : Quantum mechanics: Ø Describes atomic phenomena very well Ø Predicts the way in which electrons behave in solids 18
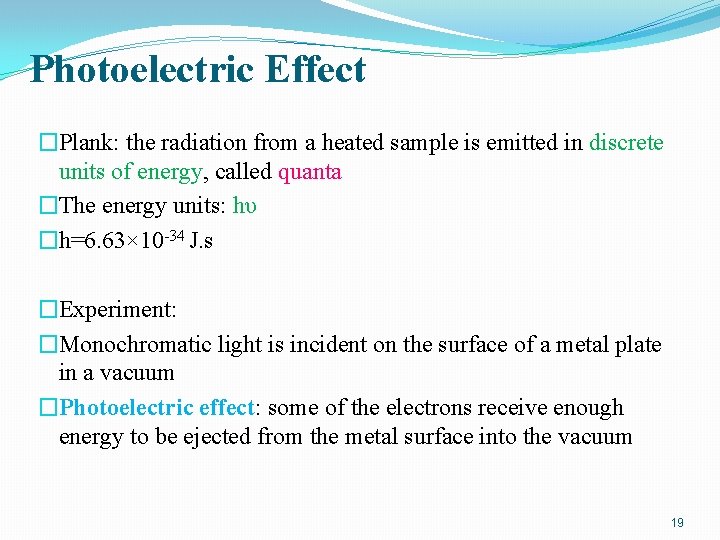
Photoelectric Effect �Plank: the radiation from a heated sample is emitted in discrete units of energy, called quanta �The energy units: hυ �h=6. 63× 10 -34 J. s �Experiment: �Monochromatic light is incident on the surface of a metal plate in a vacuum �Photoelectric effect: some of the electrons receive enough energy to be ejected from the metal surface into the vacuum 19
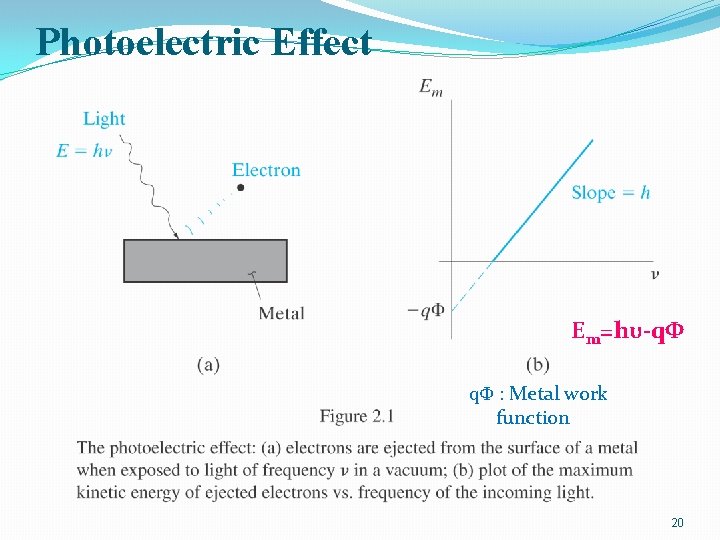
Photoelectric Effect Em=hυ-qΦ qΦ : Metal work function 20
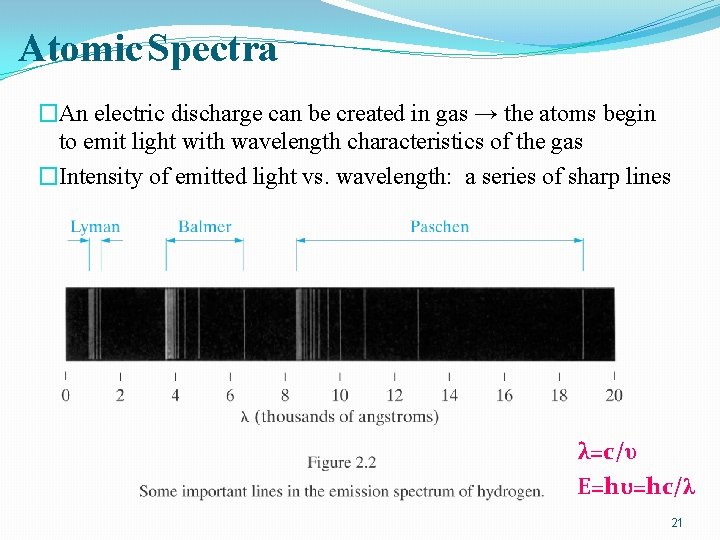
Atomic Spectra �An electric discharge can be created in gas → the atoms begin to emit light with wavelength characteristics of the gas �Intensity of emitted light vs. wavelength: a series of sharp lines λ=c/υ E=hυ=hc/λ 21
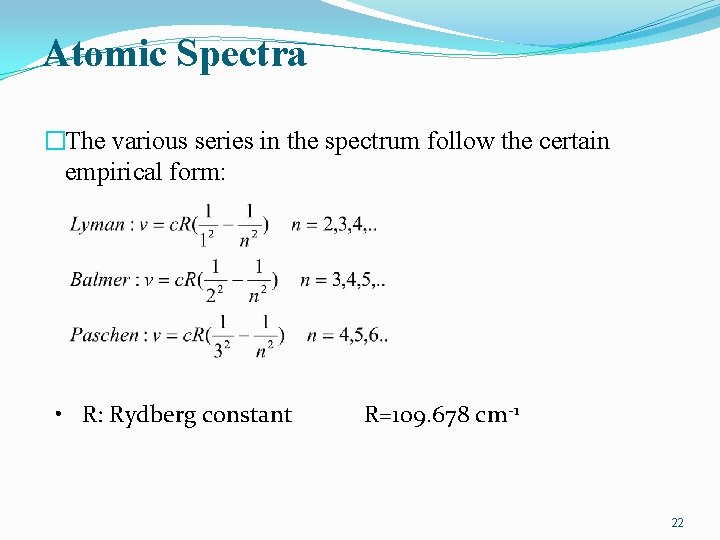
Atomic Spectra �The various series in the spectrum follow the certain empirical form: • R: Rydberg constant R=109. 678 cm-1 22
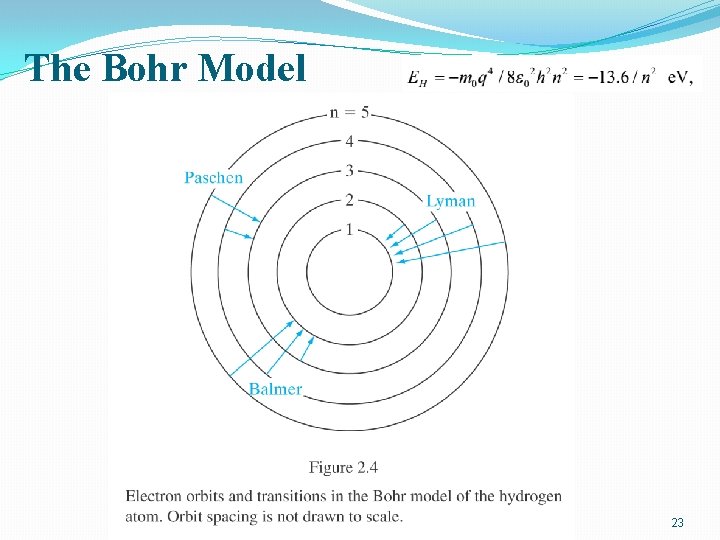
The Bohr Model 23
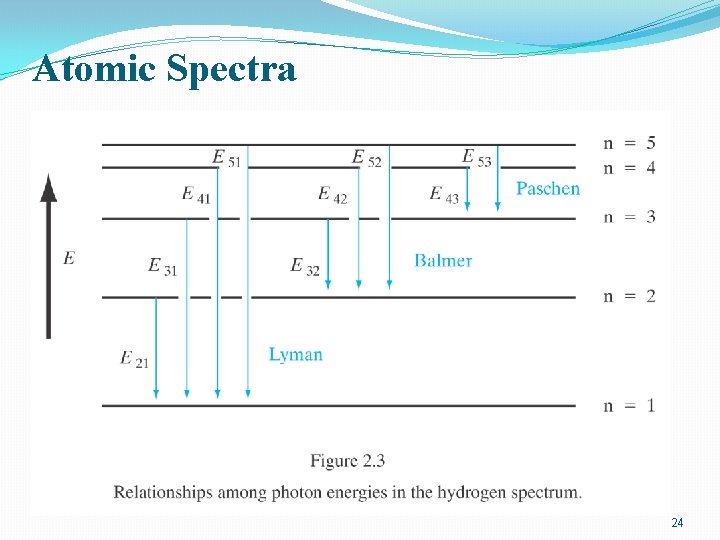
Atomic Spectra 24
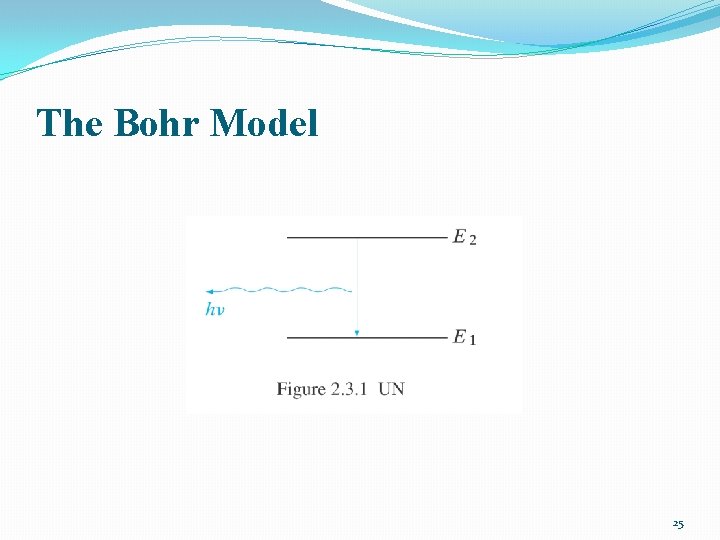
The Bohr Model 25
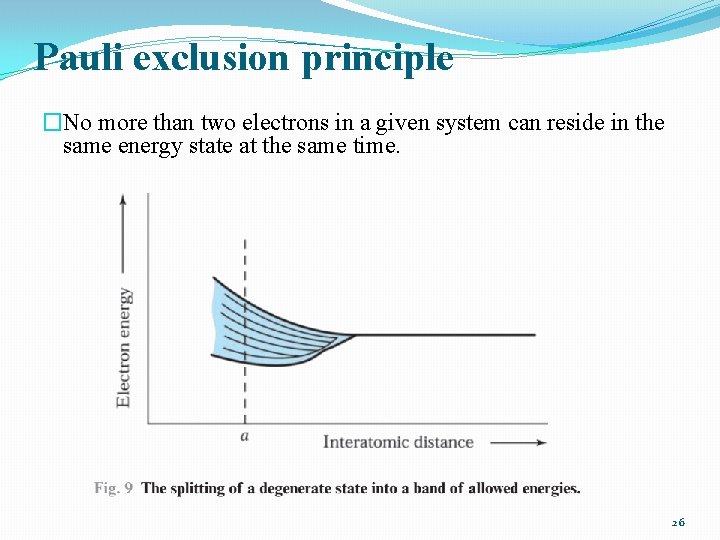
Pauli exclusion principle �No more than two electrons in a given system can reside in the same energy state at the same time. 26
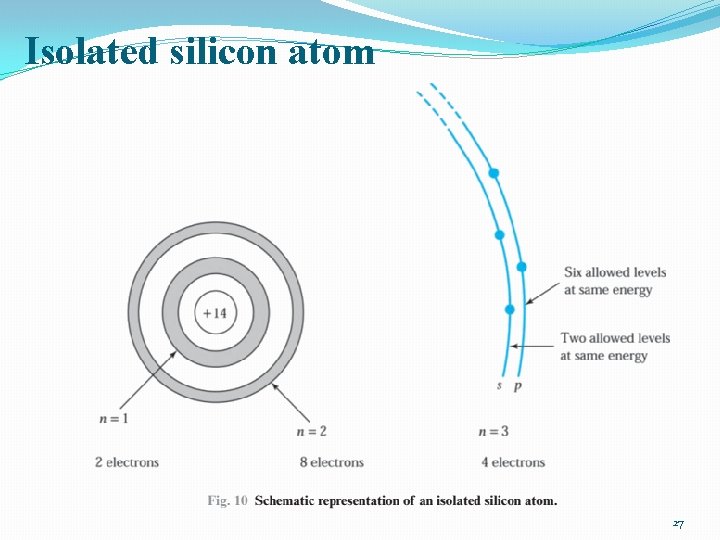
Isolated silicon atom 27
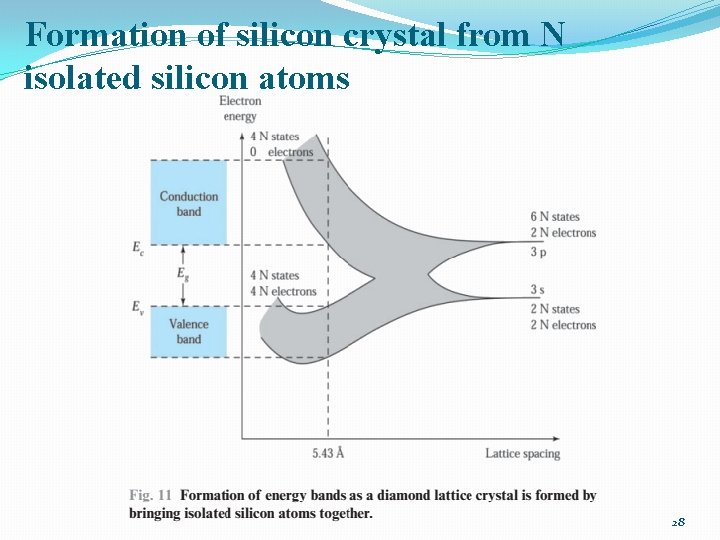
Formation of silicon crystal from N isolated silicon atoms 28
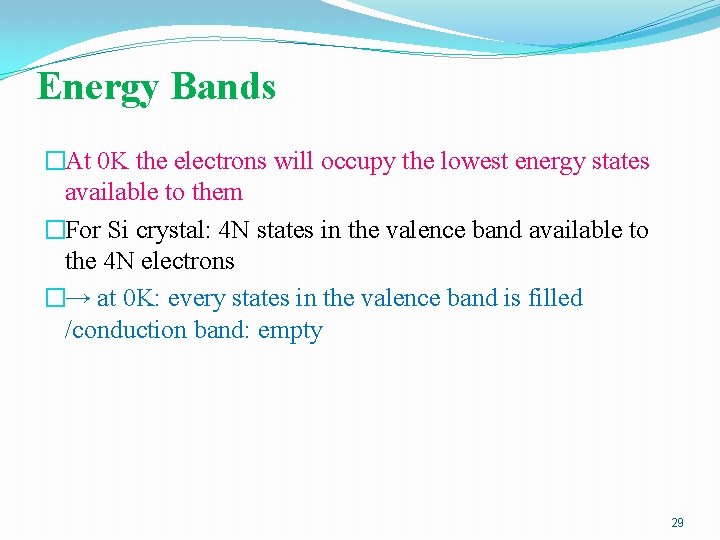
Energy Bands �At 0 K the electrons will occupy the lowest energy states available to them �For Si crystal: 4 N states in the valence band available to the 4 N electrons �→ at 0 K: every states in the valence band is filled /conduction band: empty 29
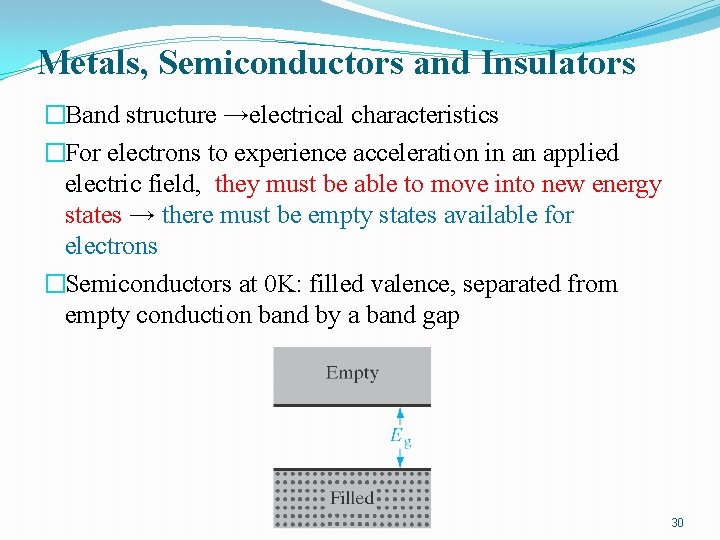
Metals, Semiconductors and Insulators �Band structure →electrical characteristics �For electrons to experience acceleration in an applied electric field, they must be able to move into new energy states → there must be empty states available for electrons �Semiconductors at 0 K: filled valence, separated from empty conduction band by a band gap 30
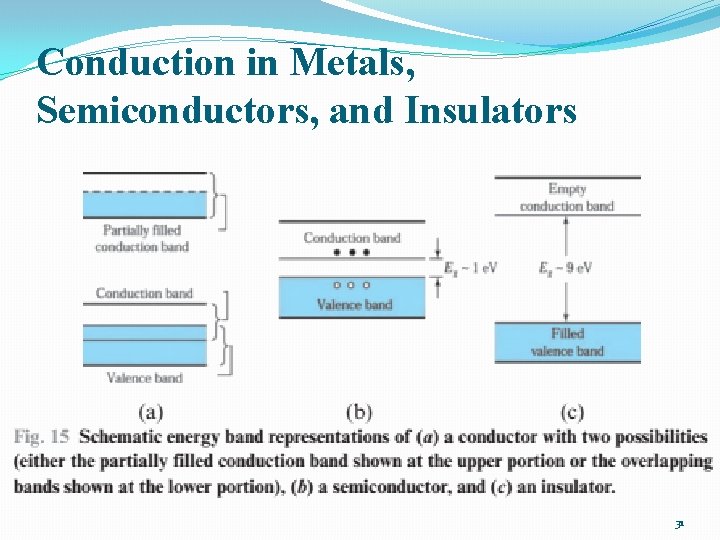
Conduction in Metals, Semiconductors, and Insulators 31
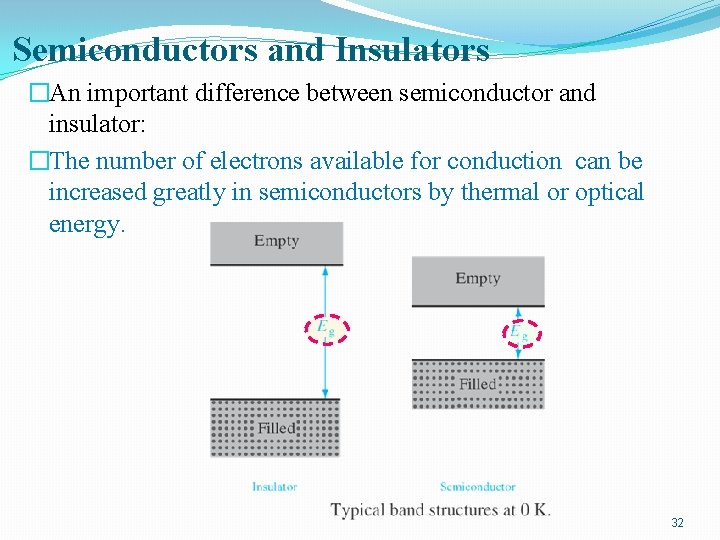
Semiconductors and Insulators �An important difference between semiconductor and insulator: �The number of electrons available for conduction can be increased greatly in semiconductors by thermal or optical energy. 32
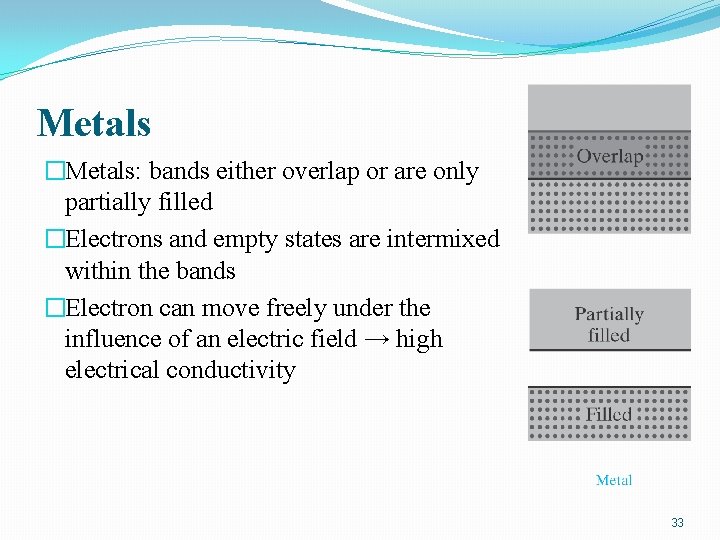
Metals �Metals: bands either overlap or are only partially filled �Electrons and empty states are intermixed within the bands �Electron can move freely under the influence of an electric field → high electrical conductivity 33
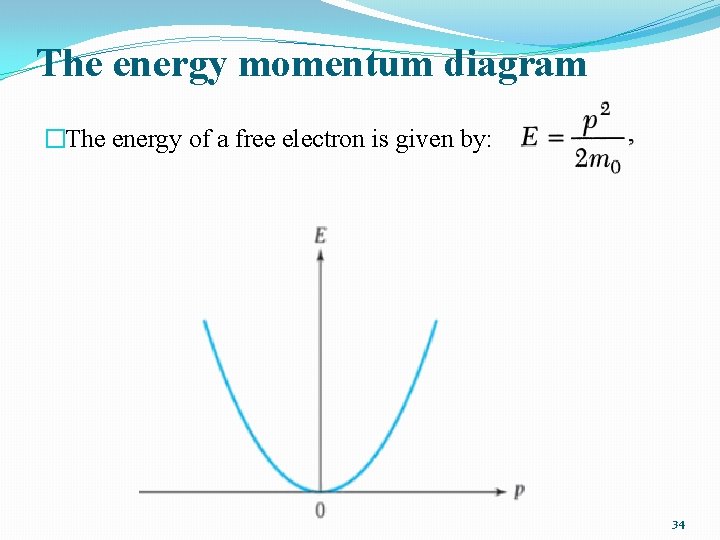
The energy momentum diagram �The energy of a free electron is given by: 34
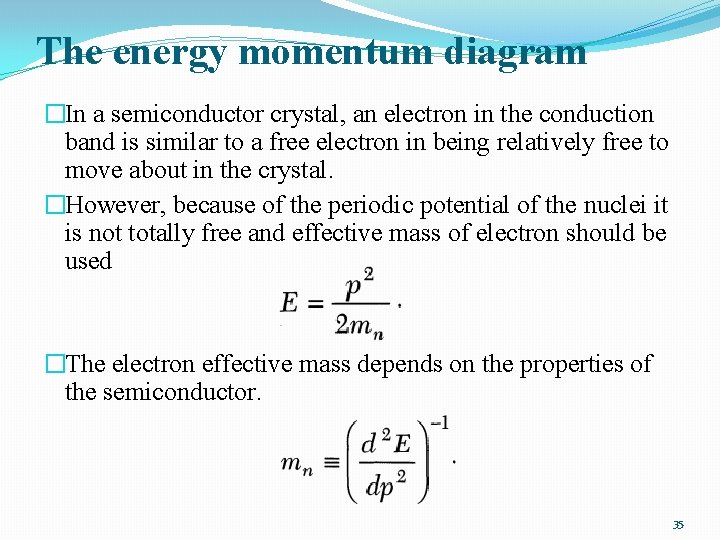
The energy momentum diagram �In a semiconductor crystal, an electron in the conduction band is similar to a free electron in being relatively free to move about in the crystal. �However, because of the periodic potential of the nuclei it is not totally free and effective mass of electron should be used �The electron effective mass depends on the properties of the semiconductor. 35
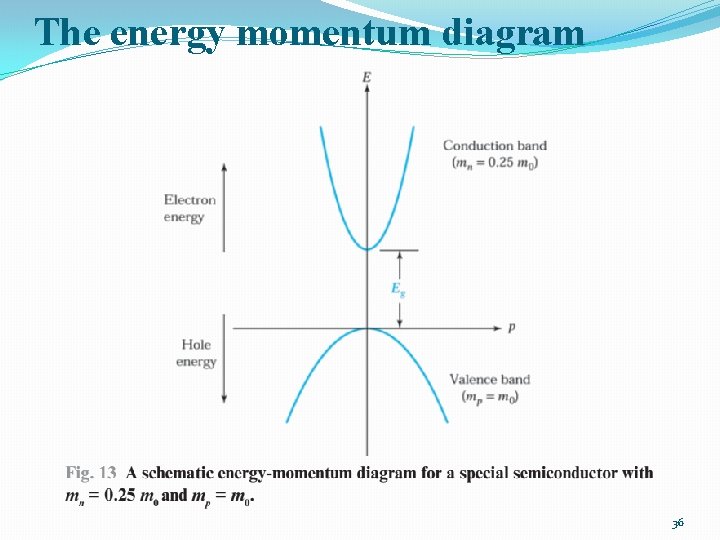
The energy momentum diagram 36
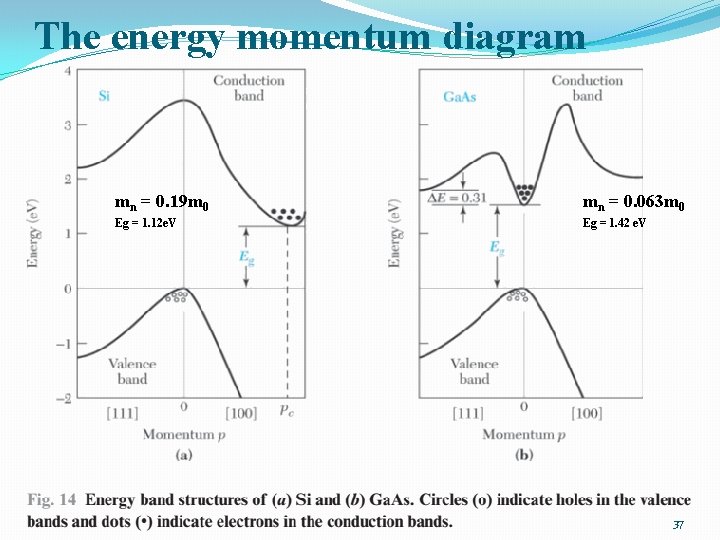
The energy momentum diagram mn = 0. 19 m 0 mn = 0. 063 m 0 Eg = 1. 12 e. V Eg = 1. 42 e. V 37
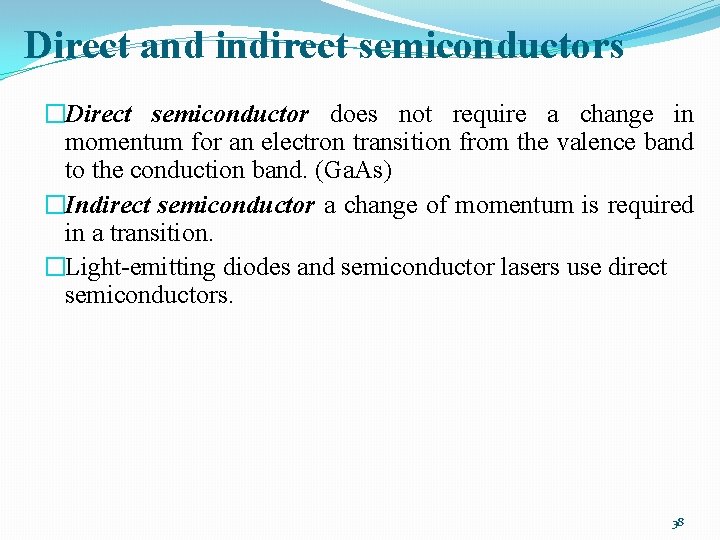
Direct and indirect semiconductors �Direct semiconductor does not require a change in momentum for an electron transition from the valence band to the conduction band. (Ga. As) �Indirect semiconductor a change of momentum is required in a transition. �Light-emitting diodes and semiconductor lasers use direct semiconductors. 38
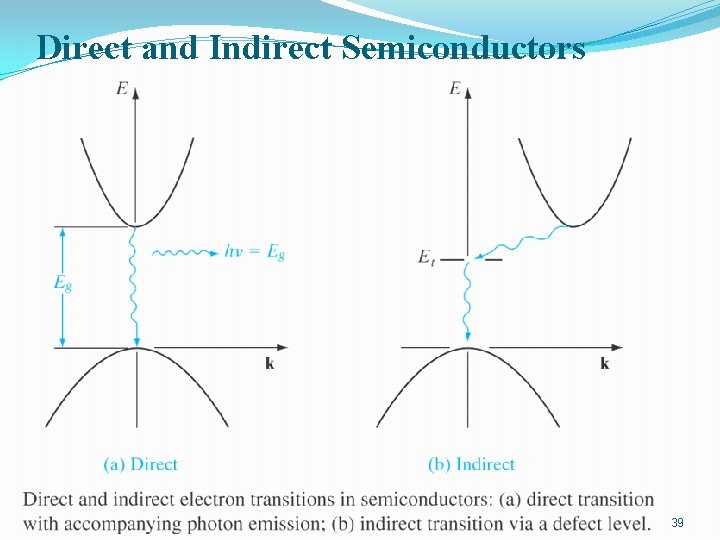
Direct and Indirect Semiconductors 39
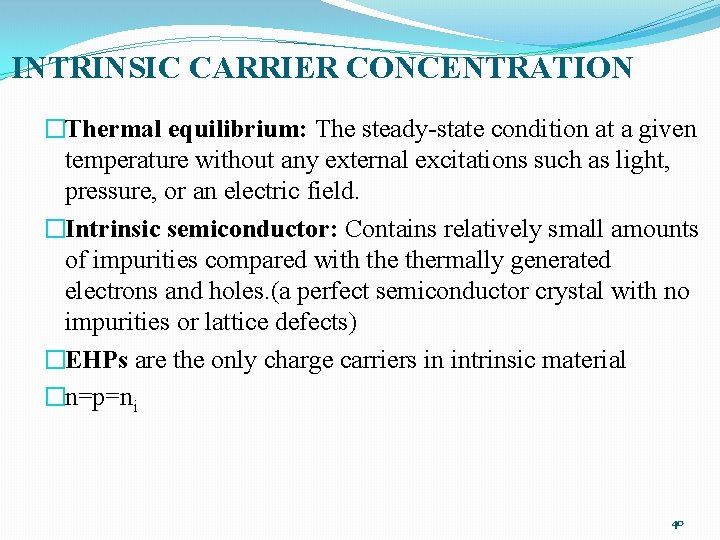
INTRINSIC CARRIER CONCENTRATION �Thermal equilibrium: The steady-state condition at a given temperature without any external excitations such as light, pressure, or an electric field. �Intrinsic semiconductor: Contains relatively small amounts of impurities compared with thermally generated electrons and holes. (a perfect semiconductor crystal with no impurities or lattice defects) �EHPs are the only charge carriers in intrinsic material �n=p=ni 40
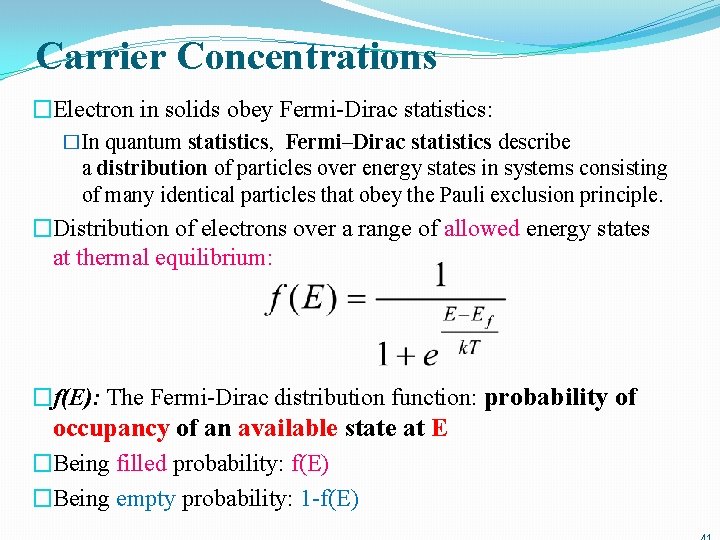
Carrier Concentrations �Electron in solids obey Fermi-Dirac statistics: �In quantum statistics, Fermi–Dirac statistics describe a distribution of particles over energy states in systems consisting of many identical particles that obey the Pauli exclusion principle. �Distribution of electrons over a range of allowed energy states at thermal equilibrium: �f(E): The Fermi-Dirac distribution function: probability of occupancy of an available state at E �Being filled probability: f(E) �Being empty probability: 1 -f(E)
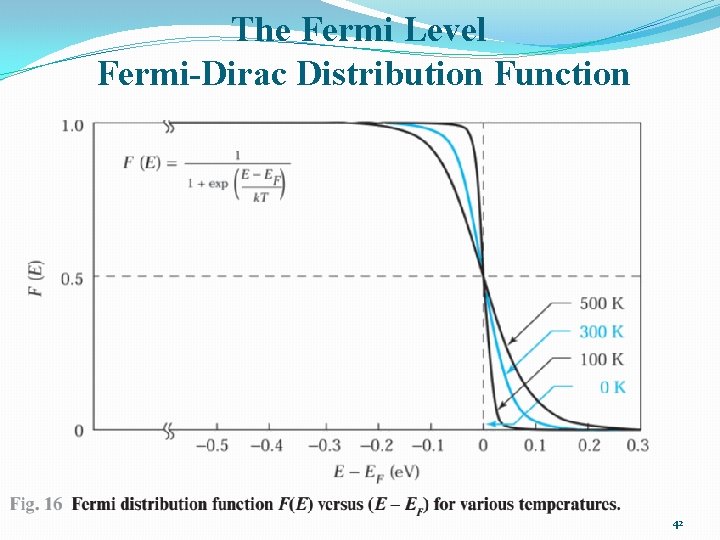
The Fermi Level Fermi-Dirac Distribution Function 42
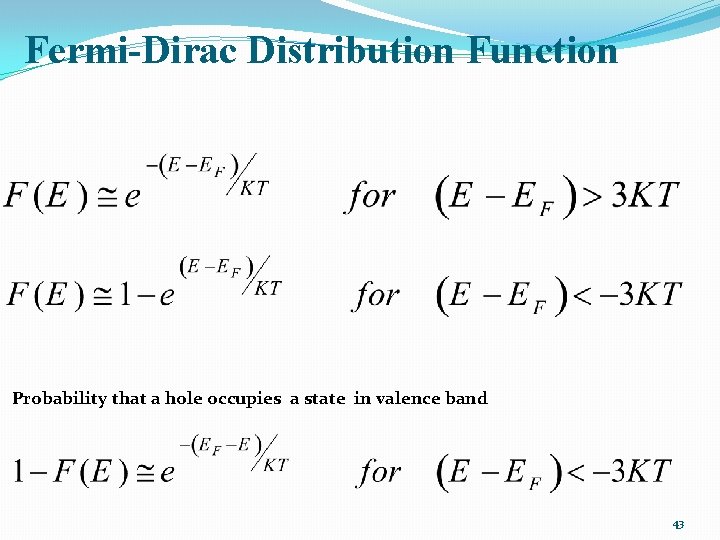
Fermi-Dirac Distribution Function Probability that a hole occupies a state in valence band 43
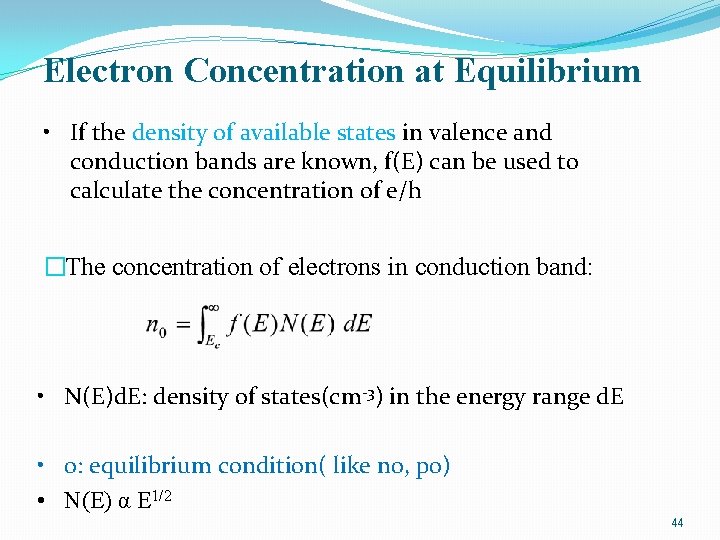
Electron Concentration at Equilibrium • If the density of available states in valence and conduction bands are known, f(E) can be used to calculate the concentration of e/h �The concentration of electrons in conduction band: • N(E)d. E: density of states(cm-3) in the energy range d. E • 0: equilibrium condition( like n 0, p 0) • N(E) α E 1/2 44
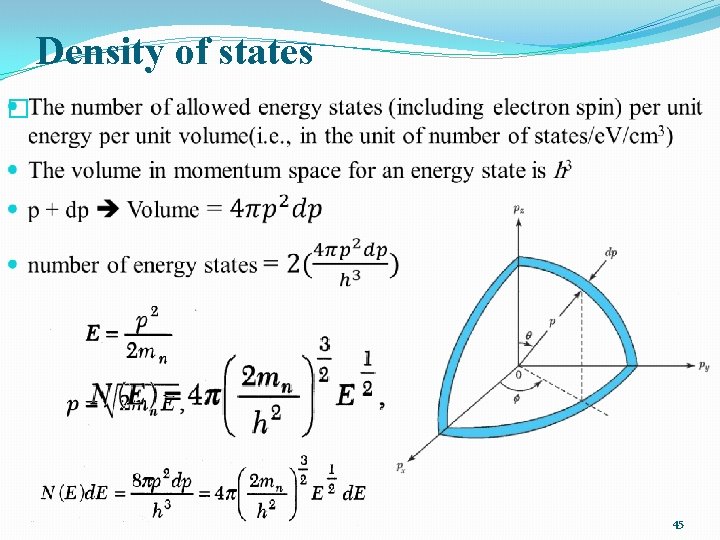
Density of states � 45
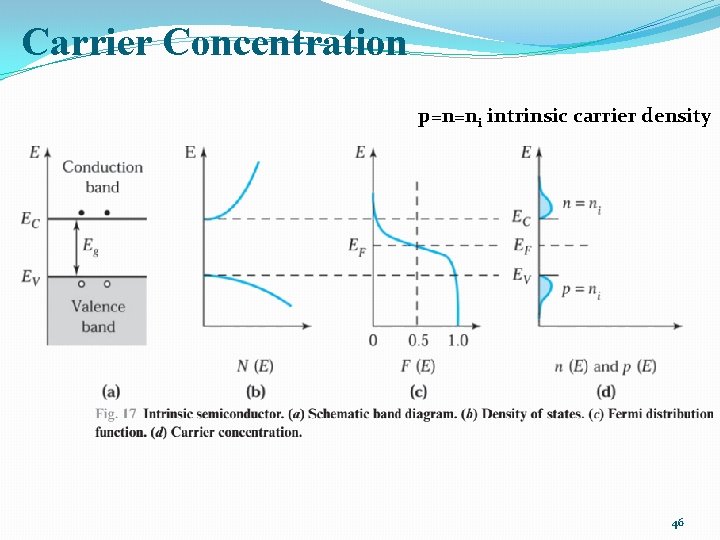
Carrier Concentration p=n=ni intrinsic carrier density 46
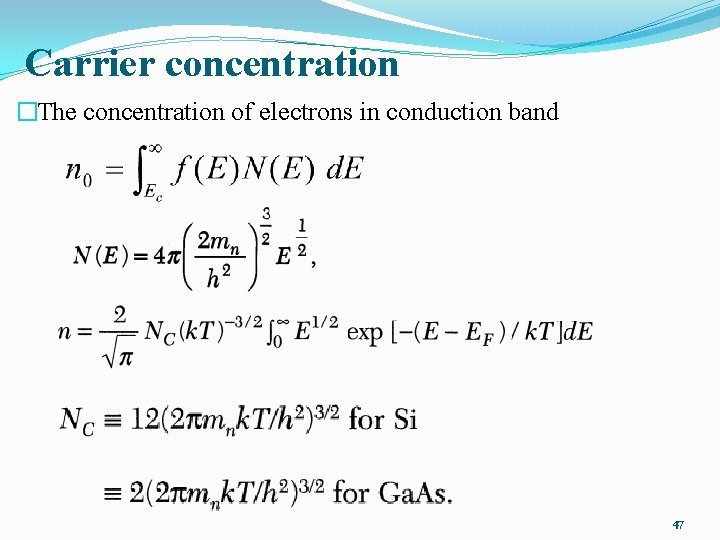
Carrier concentration �The concentration of electrons in conduction band 47
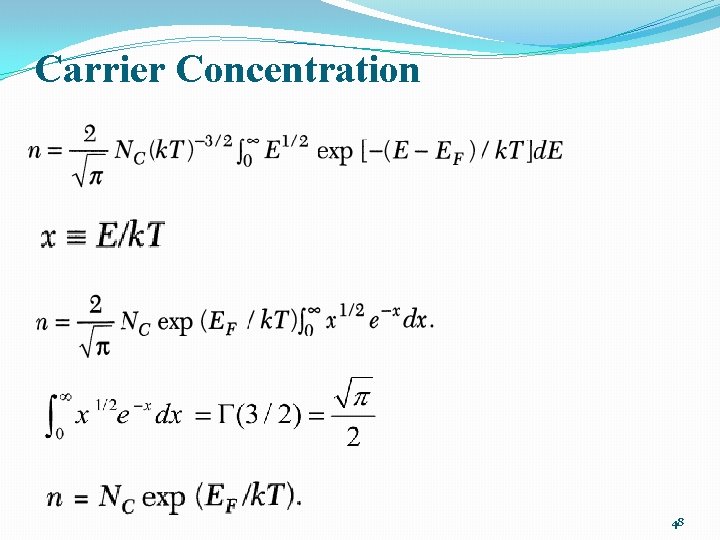
Carrier Concentration 48
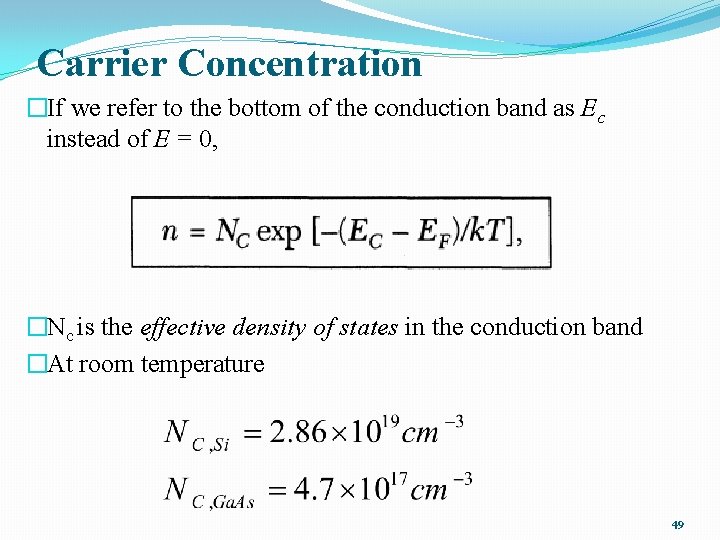
Carrier Concentration �If we refer to the bottom of the conduction band as Ec instead of E = 0, �Nc is the effective density of states in the conduction band �At room temperature 49
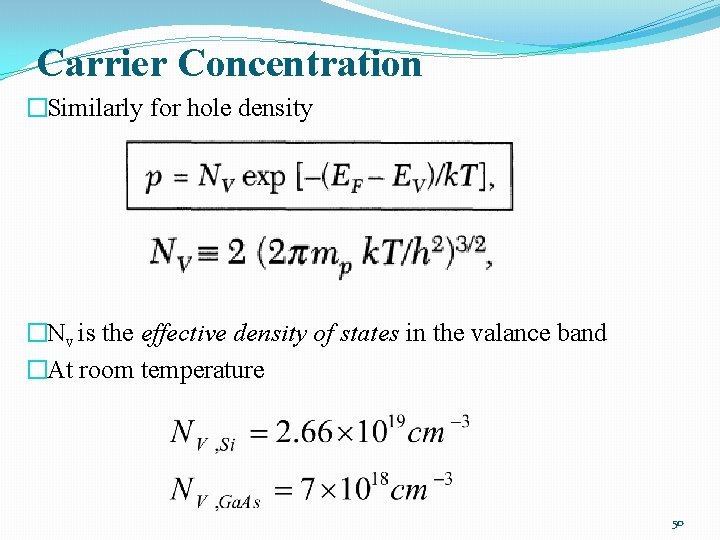
Carrier Concentration �Similarly for hole density �Nv is the effective density of states in the valance band �At room temperature 50
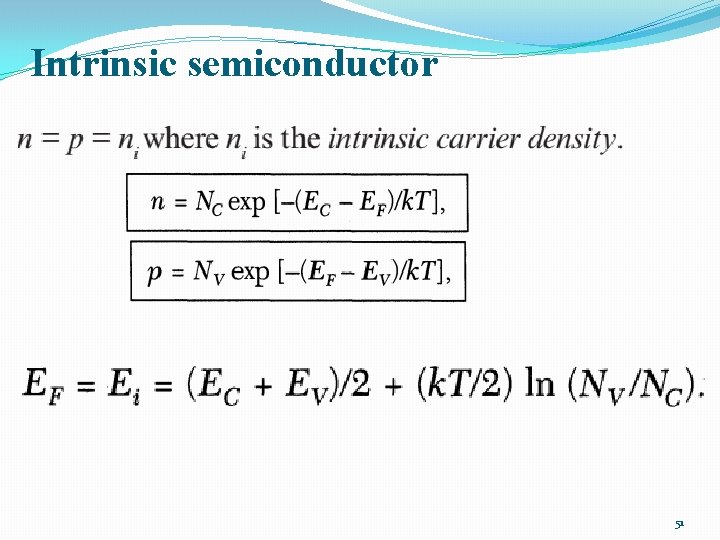
Intrinsic semiconductor 51
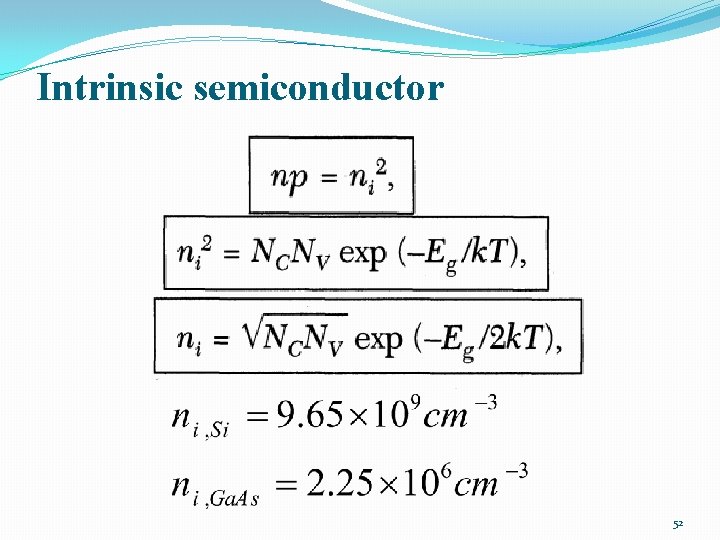
Intrinsic semiconductor 52
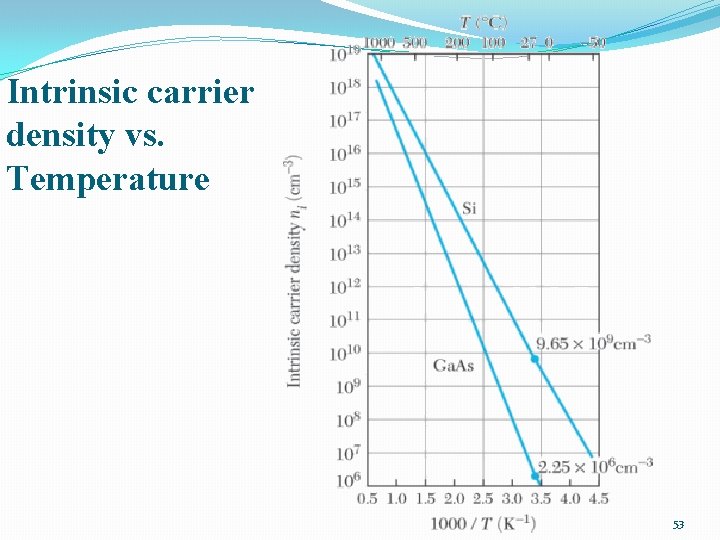
Intrinsic carrier density vs. Temperature 53
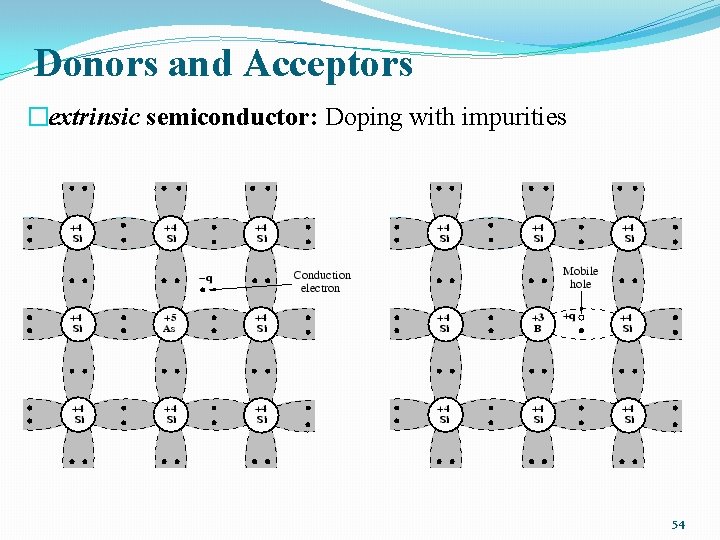
Donors and Acceptors �extrinsic semiconductor: Doping with impurities 54
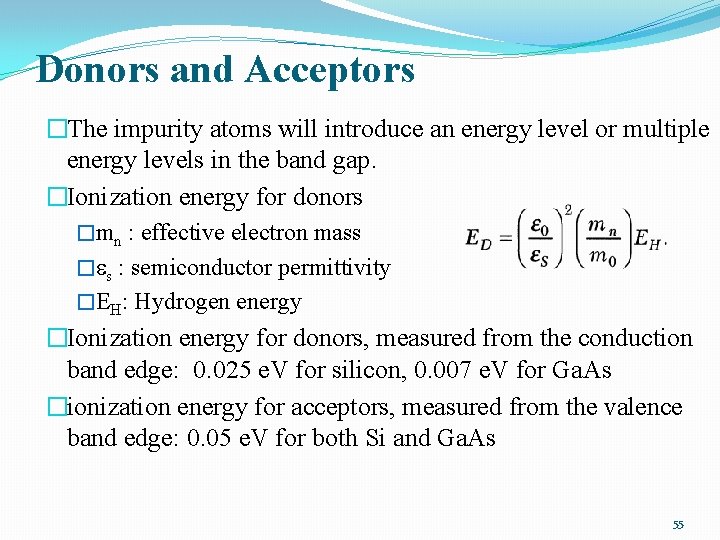
Donors and Acceptors �The impurity atoms will introduce an energy level or multiple energy levels in the band gap. �Ionization energy for donors �mn : effective electron mass � s : semiconductor permittivity �EH: Hydrogen energy �Ionization energy for donors, measured from the conduction band edge: 0. 025 e. V for silicon, 0. 007 e. V for Ga. As �ionization energy for acceptors, measured from the valence band edge: 0. 05 e. V for both Si and Ga. As 55
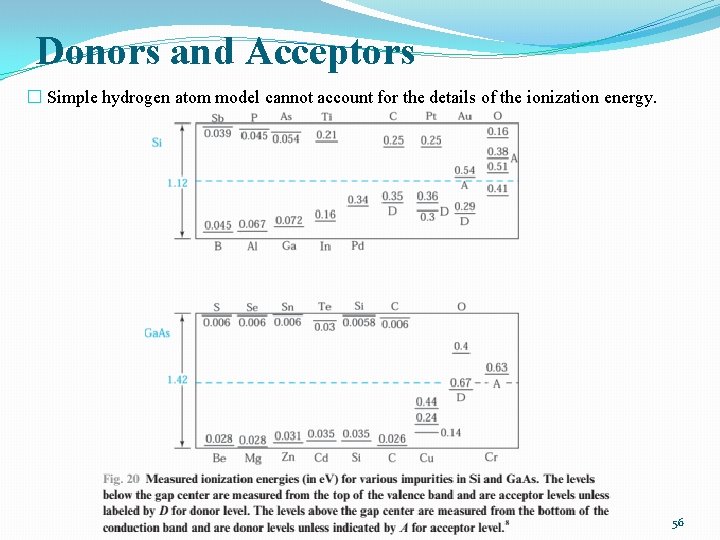
Donors and Acceptors � Simple hydrogen atom model cannot account for the details of the ionization energy. 56
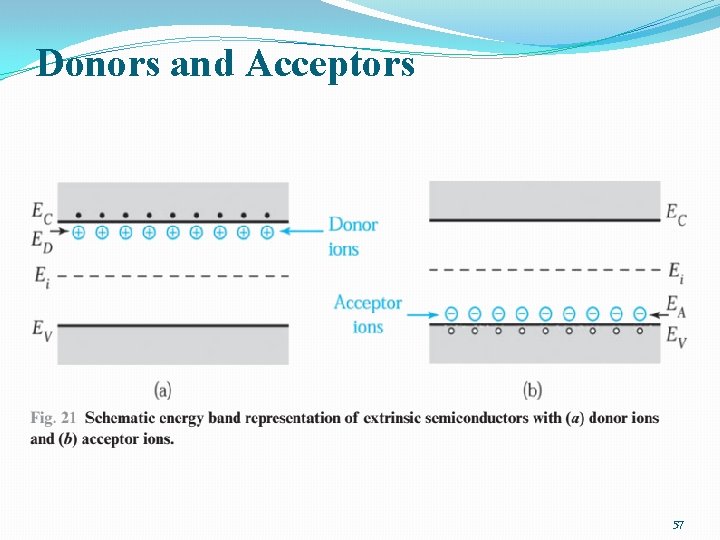
Donors and Acceptors 57
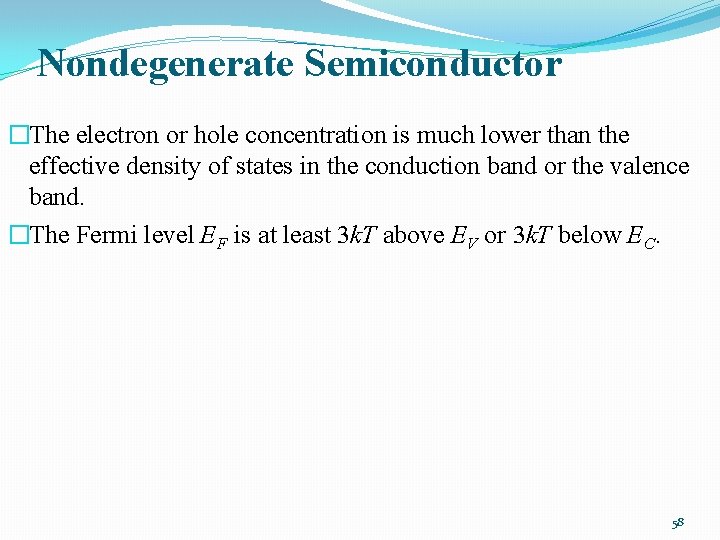
Nondegenerate Semiconductor �The electron or hole concentration is much lower than the effective density of states in the conduction band or the valence band. �The Fermi level EF is at least 3 k. T above EV or 3 k. T below EC. 58
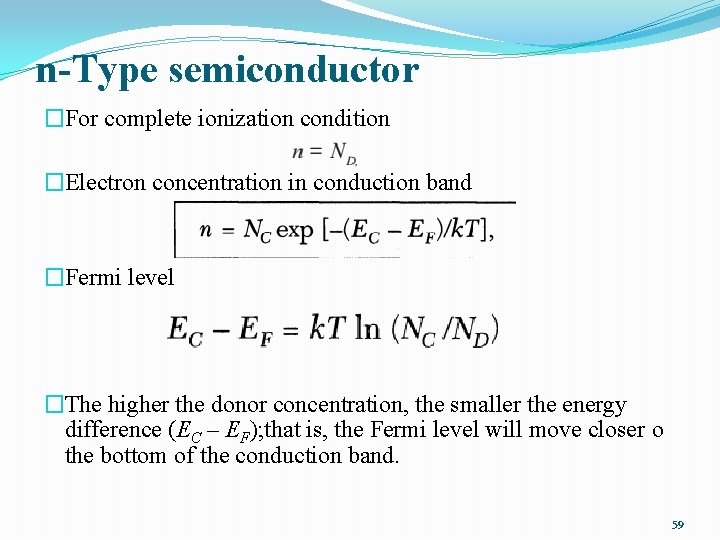
n-Type semiconductor �For complete ionization condition �Electron concentration in conduction band �Fermi level �The higher the donor concentration, the smaller the energy difference (EC – EF); that is, the Fermi level will move closer o the bottom of the conduction band. 59
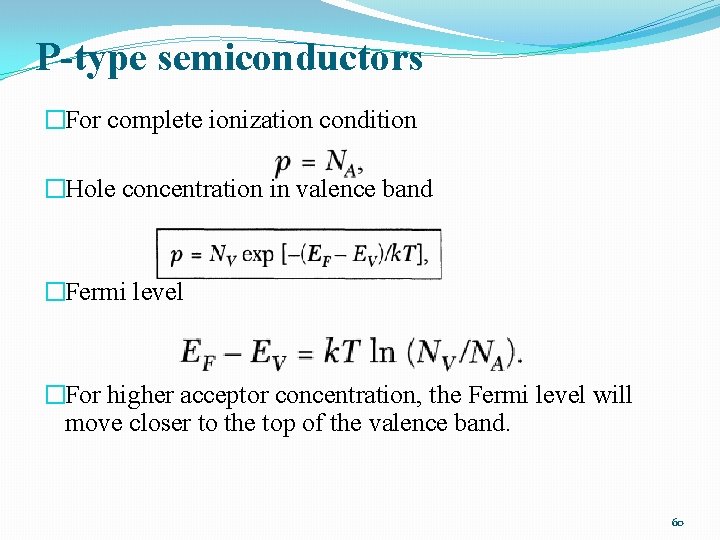
P-type semiconductors �For complete ionization condition �Hole concentration in valence band �Fermi level �For higher acceptor concentration, the Fermi level will move closer to the top of the valence band. 60
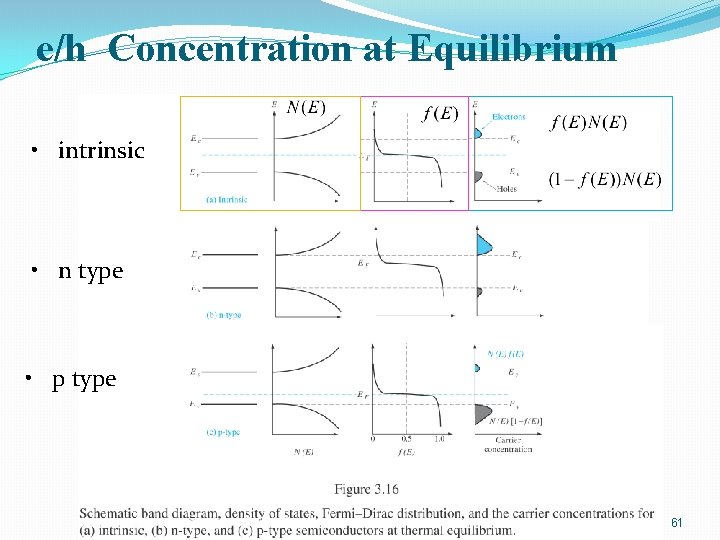
e/h Concentration at Equilibrium • intrinsic • n type • p type 61
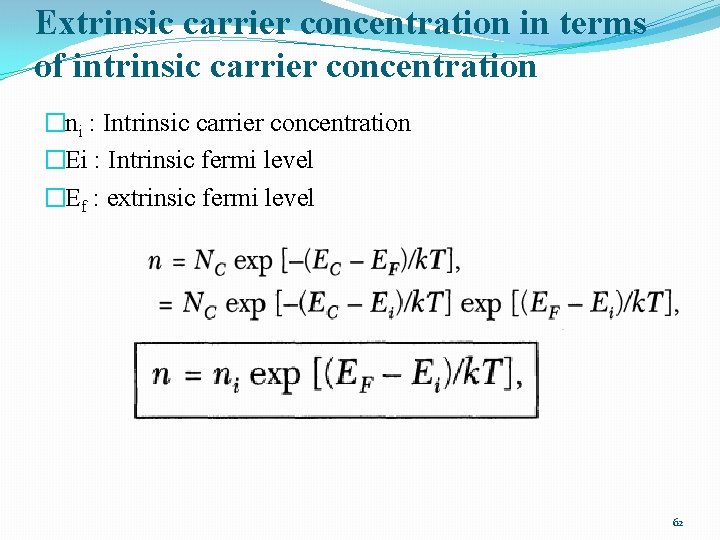
Extrinsic carrier concentration in terms of intrinsic carrier concentration �ni : Intrinsic carrier concentration �Ei : Intrinsic fermi level �Ef : extrinsic fermi level 62
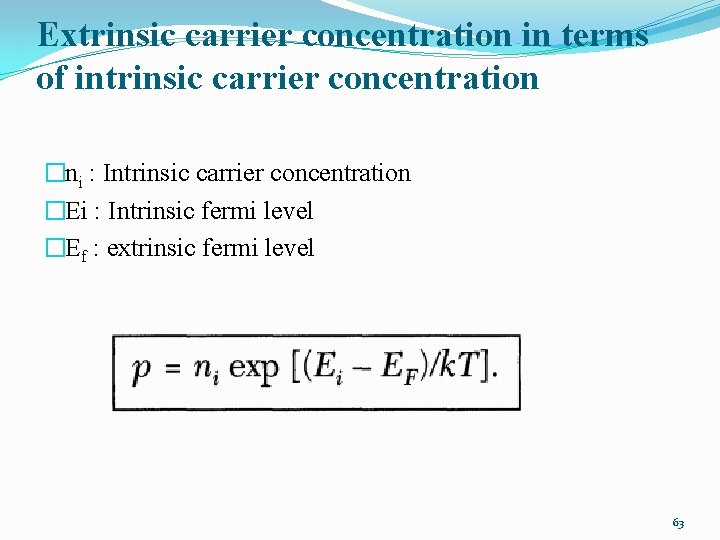
Extrinsic carrier concentration in terms of intrinsic carrier concentration �ni : Intrinsic carrier concentration �Ei : Intrinsic fermi level �Ef : extrinsic fermi level 63
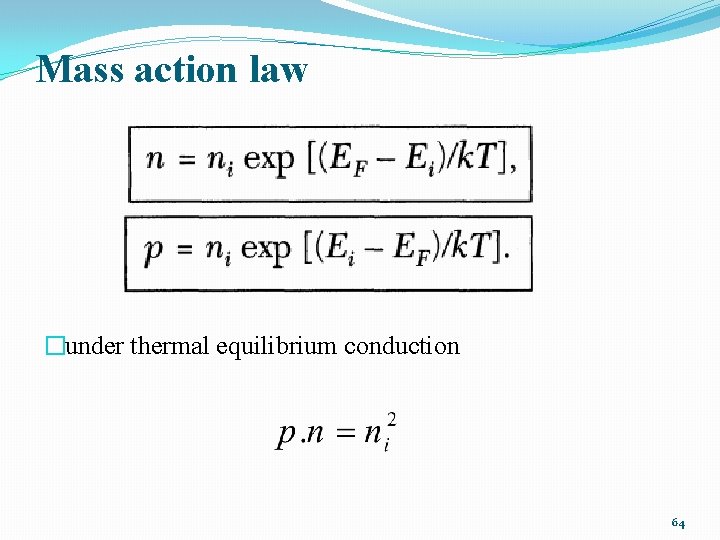
Mass action law �under thermal equilibrium conduction 64
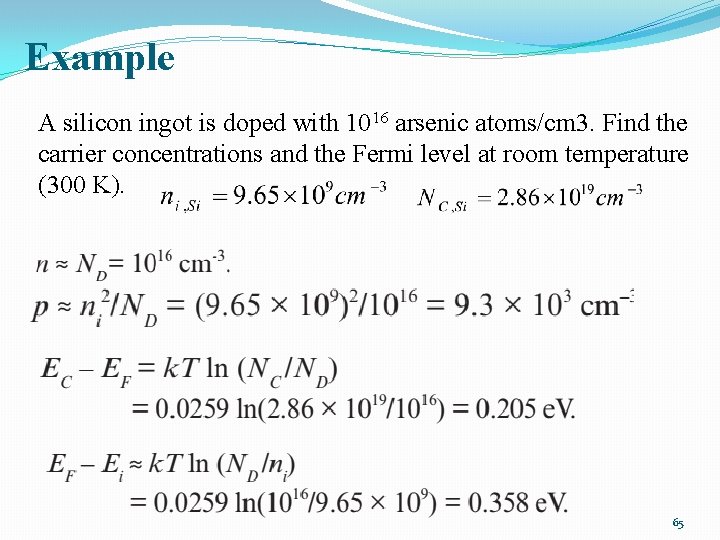
Example A silicon ingot is doped with 1016 arsenic atoms/cm 3. Find the carrier concentrations and the Fermi level at room temperature (300 K). 65
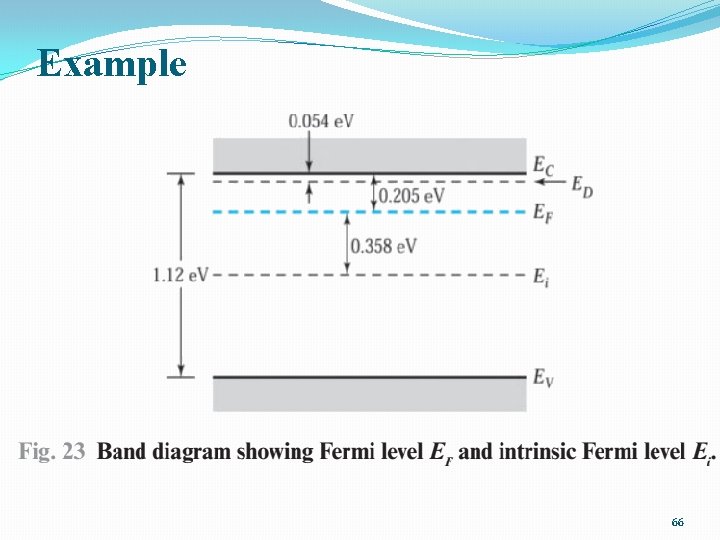
Example 66
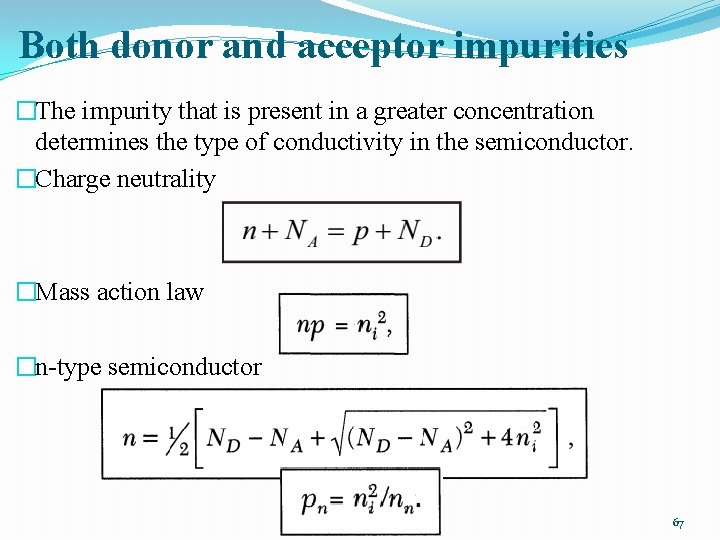
Both donor and acceptor impurities �The impurity that is present in a greater concentration determines the type of conductivity in the semiconductor. �Charge neutrality �Mass action law �n-type semiconductor 67
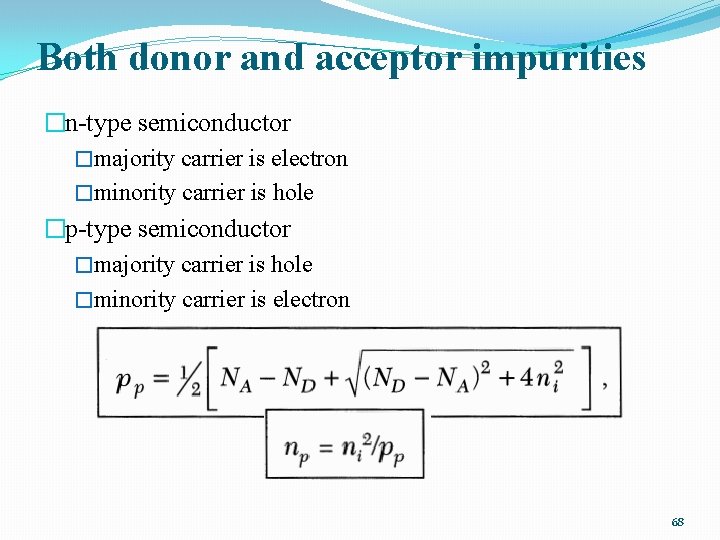
Both donor and acceptor impurities �n-type semiconductor �majority carrier is electron �minority carrier is hole �p-type semiconductor �majority carrier is hole �minority carrier is electron 68
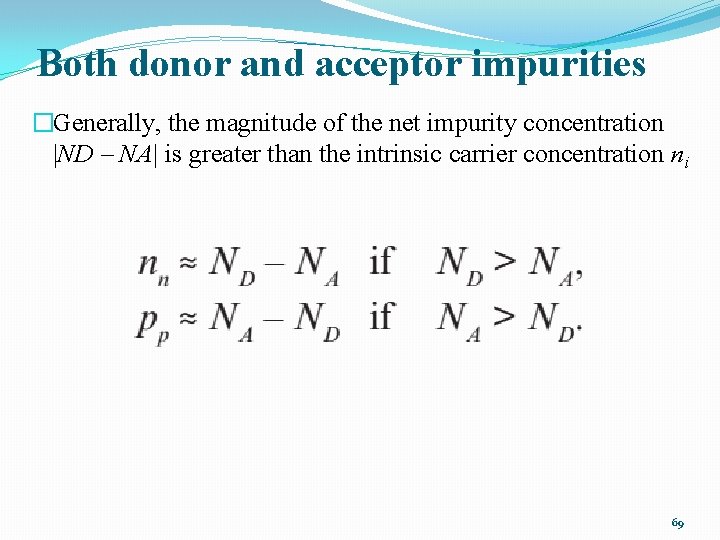
Both donor and acceptor impurities �Generally, the magnitude of the net impurity concentration |ND – NA| is greater than the intrinsic carrier concentration ni 69
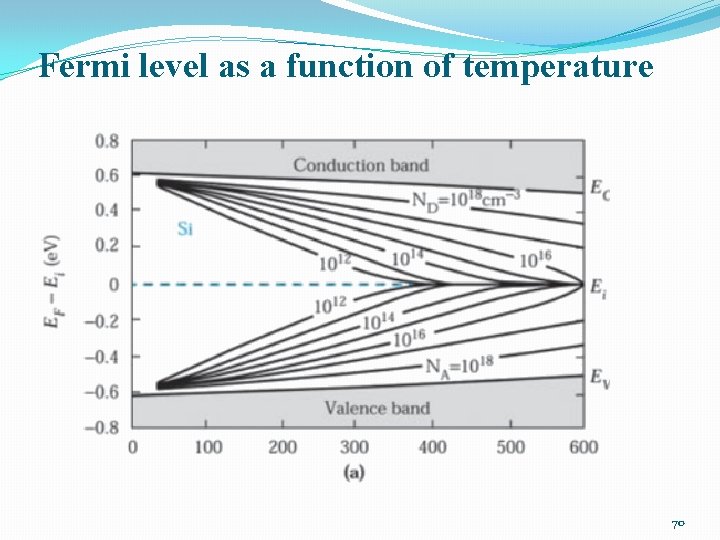
Fermi level as a function of temperature 70
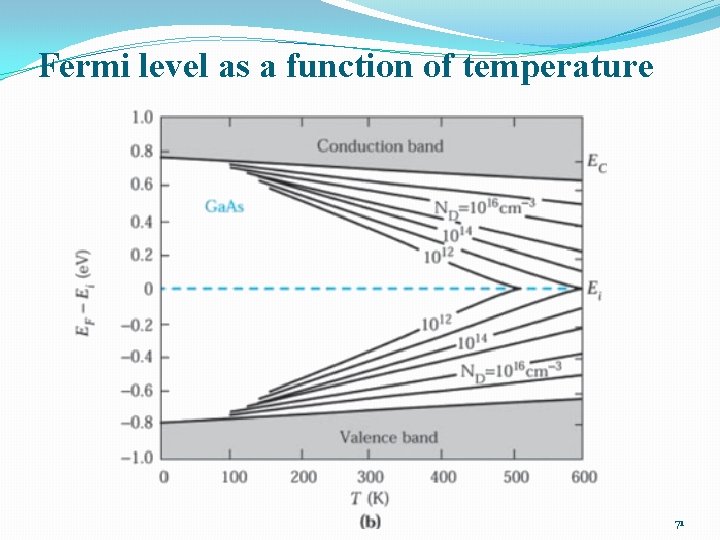
Fermi level as a function of temperature 71
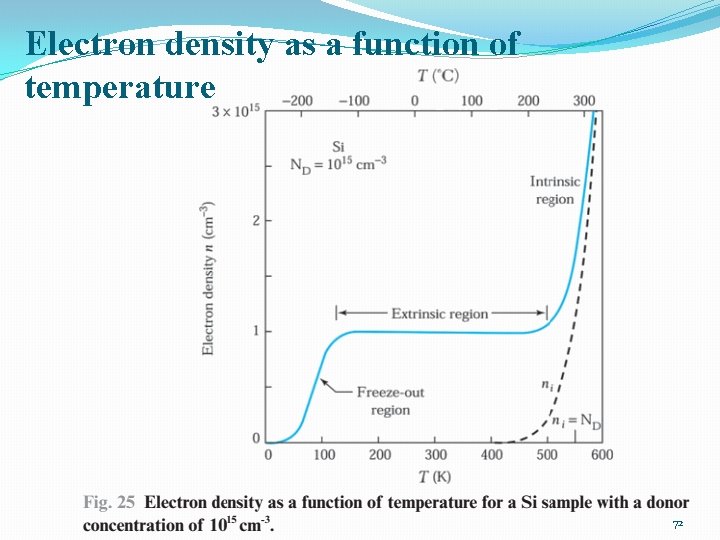
Electron density as a function of temperature 72
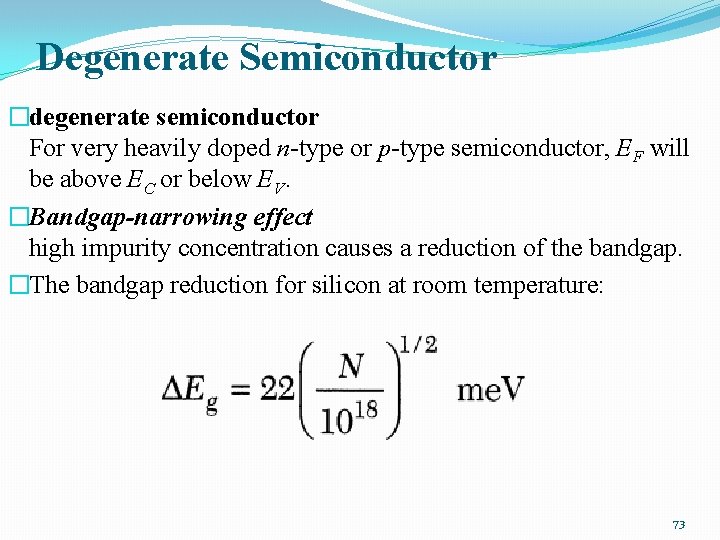
Degenerate Semiconductor �degenerate semiconductor For very heavily doped n-type or p-type semiconductor, EF will be above EC or below EV. �Bandgap-narrowing effect high impurity concentration causes a reduction of the bandgap. �The bandgap reduction for silicon at room temperature: 73
110 miller indices
Miller indices 111
Miller indices 210
Primitive cell
Crystallographic directions hexagonal crystals
Miller indices
Miller indices of a plane are represented as
Miller indices visualizer
Crystallographic direction
Diamond structure apf
Miller indices visualizer
Frederica miller
Miller indices examples
What directions
Miller indices
Dbu writing center
Medical plaza miller 131 miller street
Indices rules nat 5
Peso especifico
Properties of indices
Indices law
Indices with brackets
Negative reciprocal
Simplify 6xaxa
Rate of infiltration formula
Significado de cuento
Bei biological exposure index
Sem modification indices
Array indices must be positive integers or logical values
Solving logarithmic equations
Indices gcse questions
Indices worksheet
Plasticity index chart of soil
Vsg en capilar
Indices gcse questions
Les indice de subjectivité
Fractional indices
Expanding multiple brackets
Ligne faitiere des cretes
Sic notarial
Antecedent moisture condition
Jackfruit maturity indices
Poches para tubérositaires
Geotecnia
Indices multiplication rule
Formula leucocitaria normal
Type de texte
Fit indices
Indices answers
Stoxx blue chip
Préservation des traces et indices
Insertar indice word 2007
Futuros sobre indices
Indices ferricos
Options on stock indices and currencies
The refractive indices of crown glass prism for c d f
Log and indices rules
Indices law
Expression equivalent
Manœuvre arrache botte
Fractions equivalent to 1/4
Summation of moment
Use source transformation to find v0 in the circuit below
Fractions equivalent to 3/8
Lexical equivalent
Erf requirements for teacher 3 2020
Logical equivalence examples
Dc analysis of transistor circuits
Central lymphoid organs
Find the equivalent resistance
Equivalent fractions investigation
Dynamic equivalence and formal equivalence
Coal fired boiler efficiency calculation
Proper subset