Maximum Entropy Maximum Entropy Production and their Application
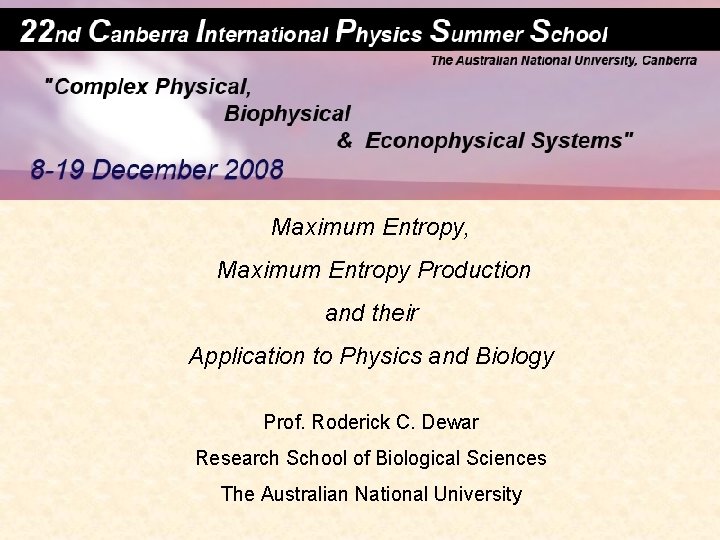
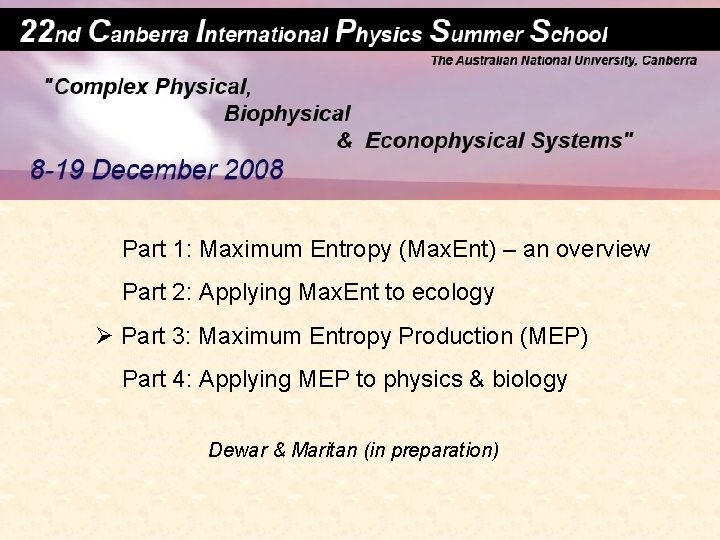
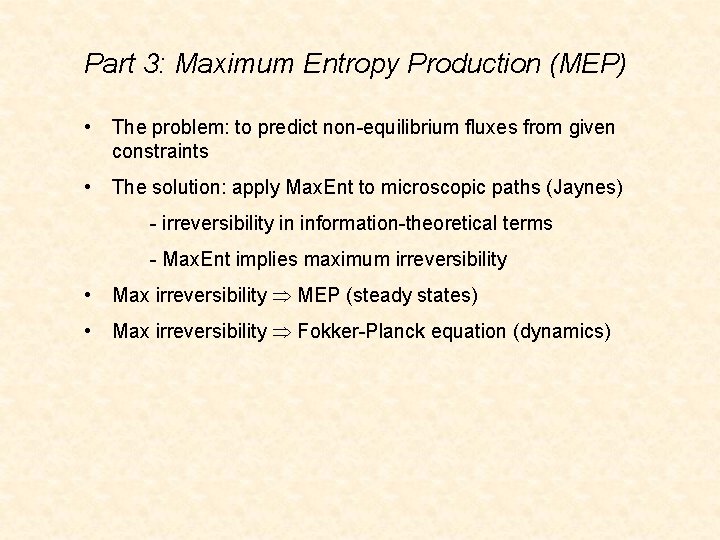
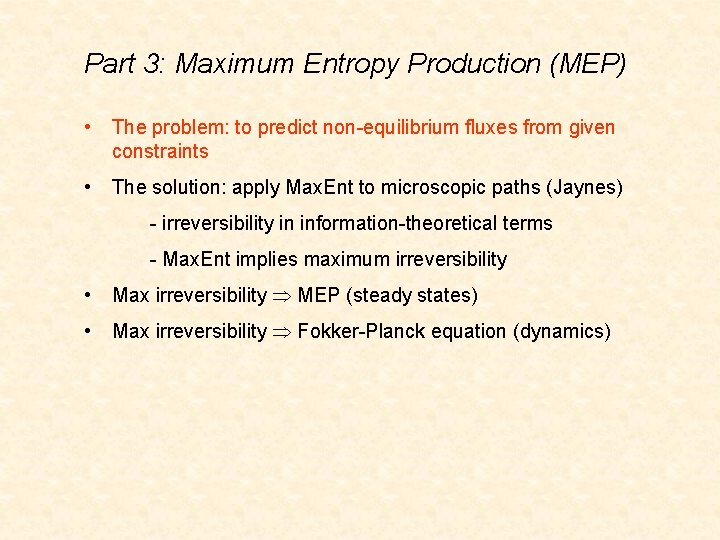
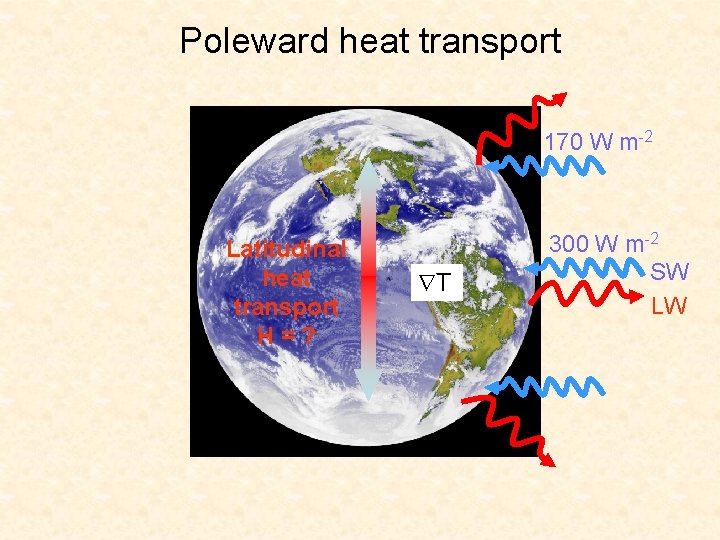
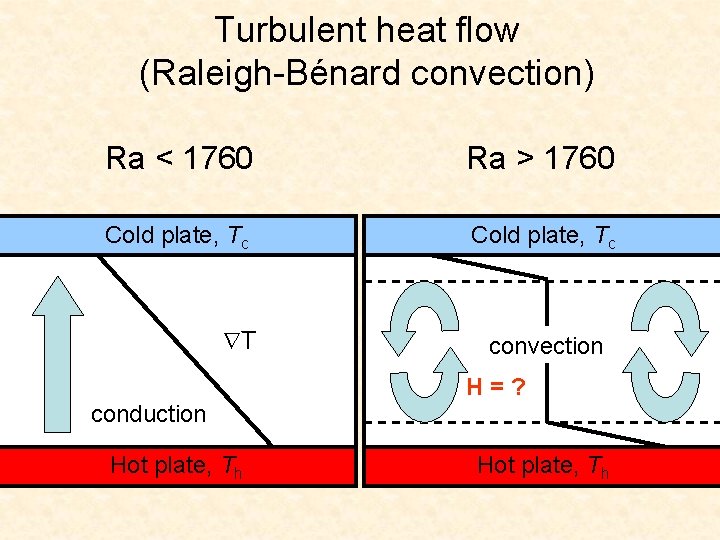
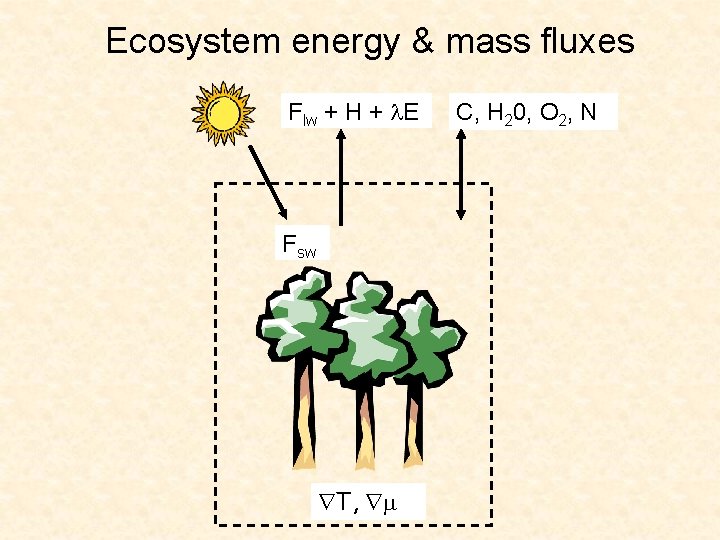
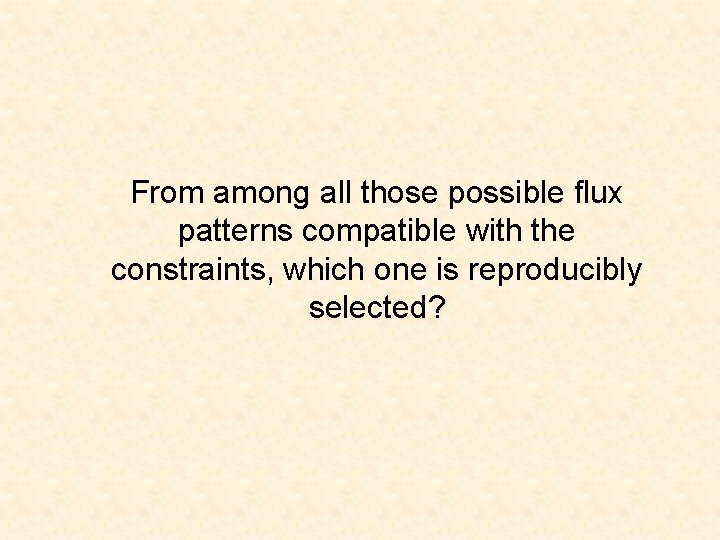
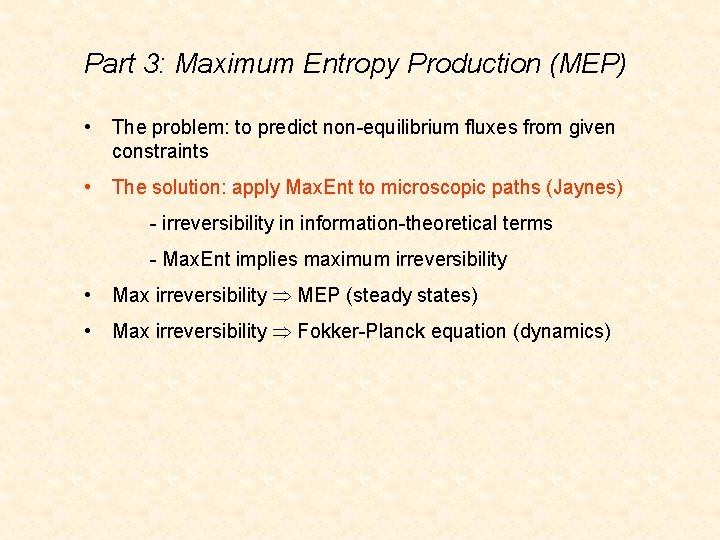
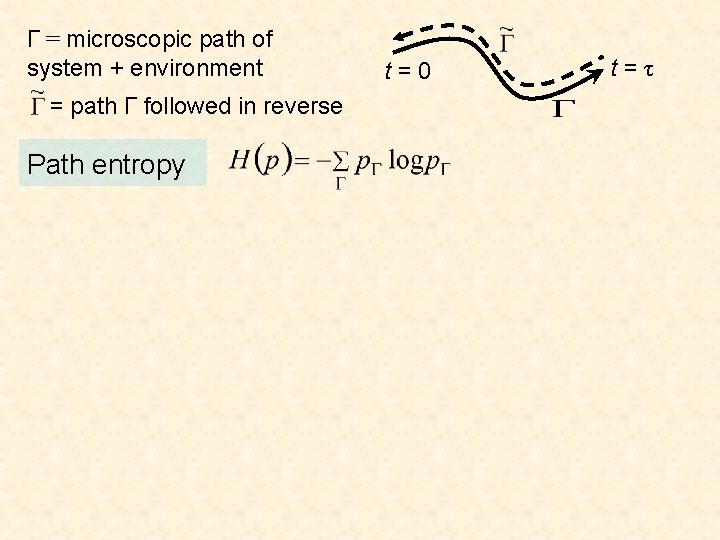
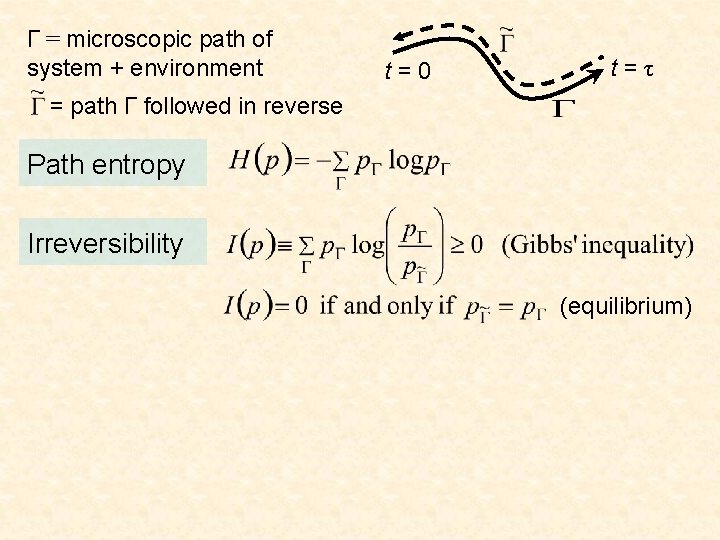
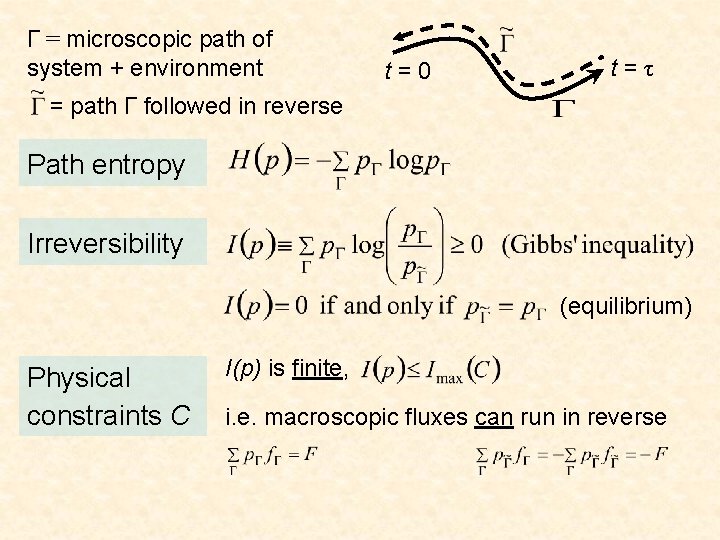
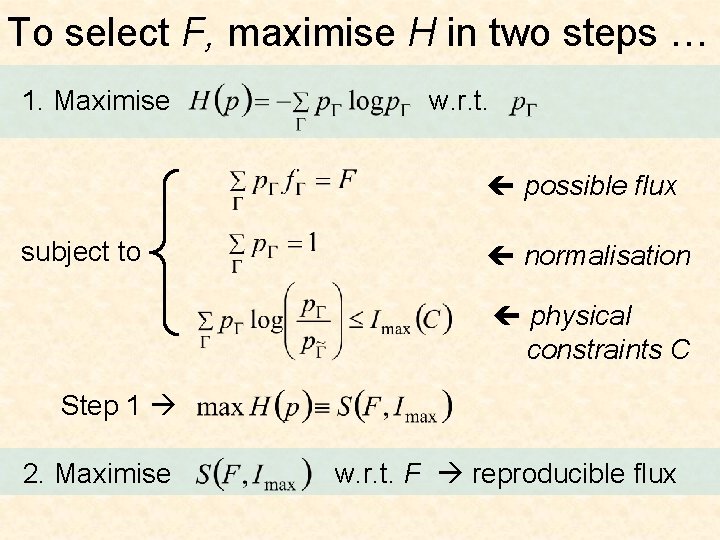
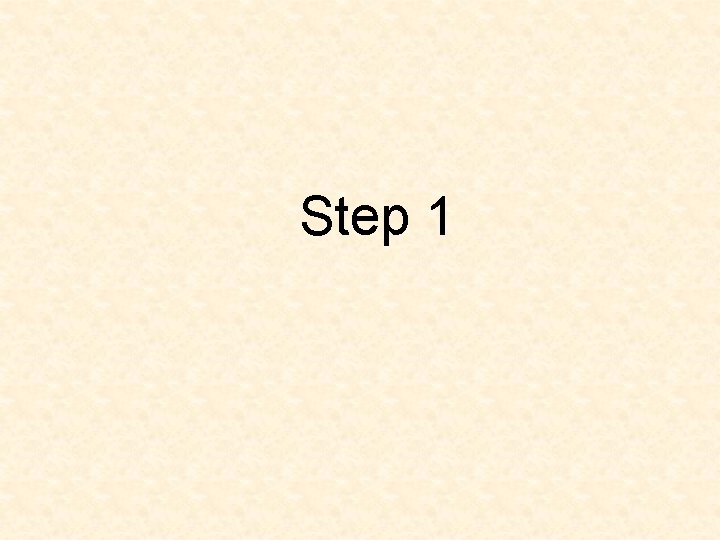
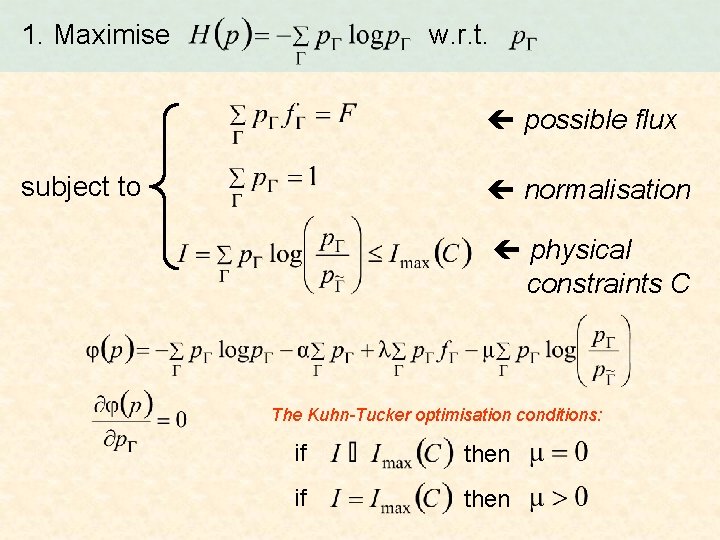
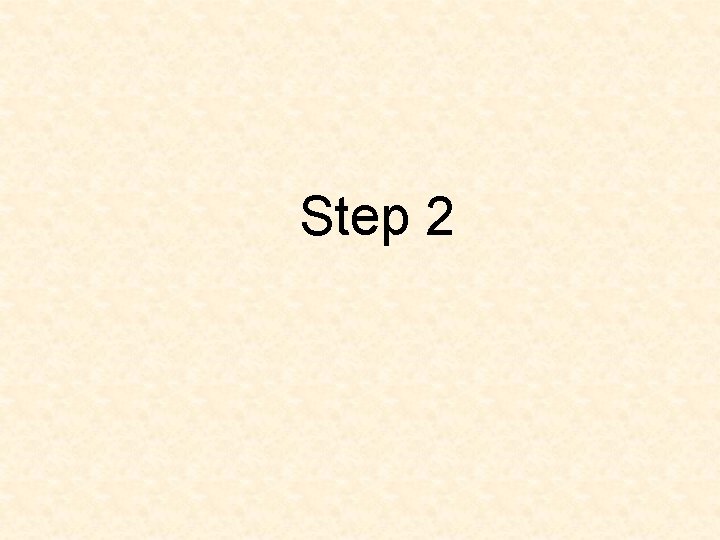
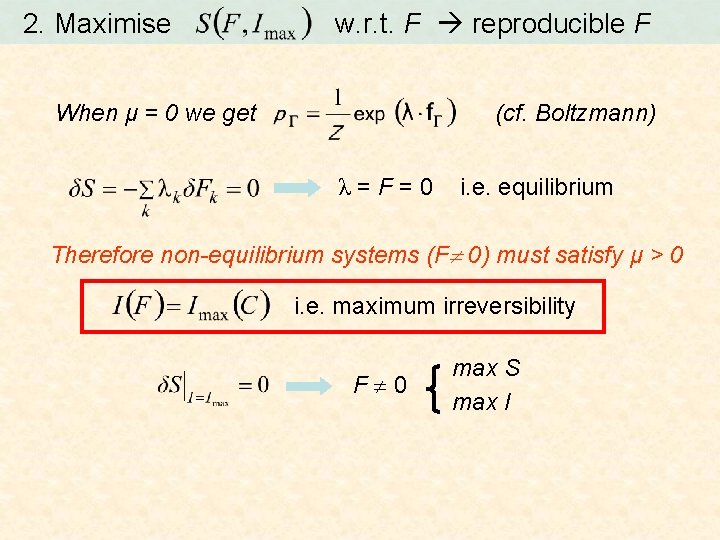
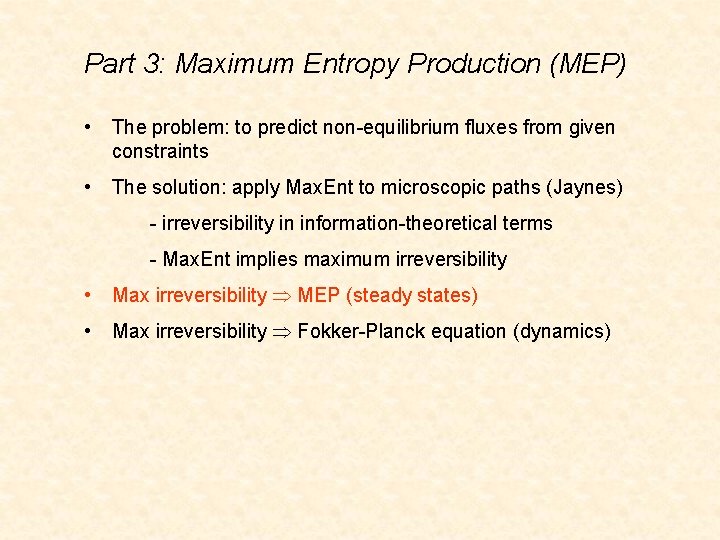
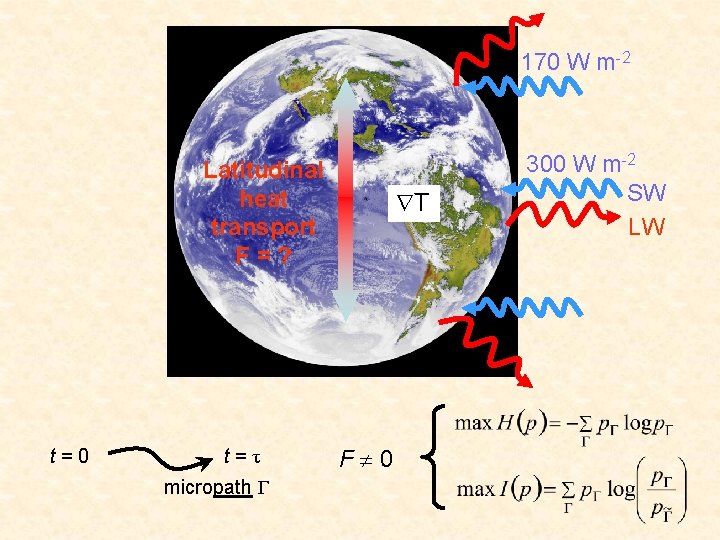
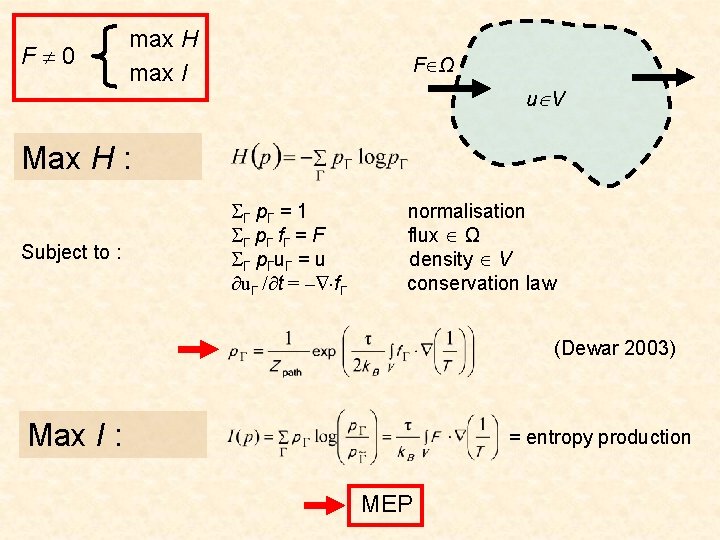
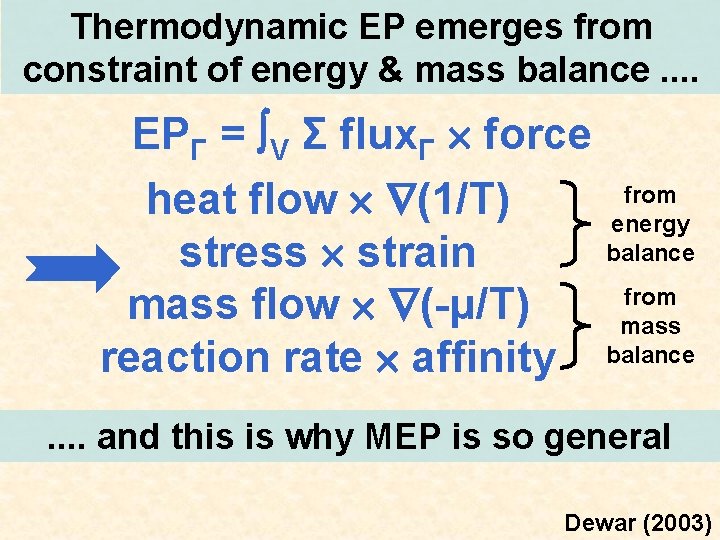
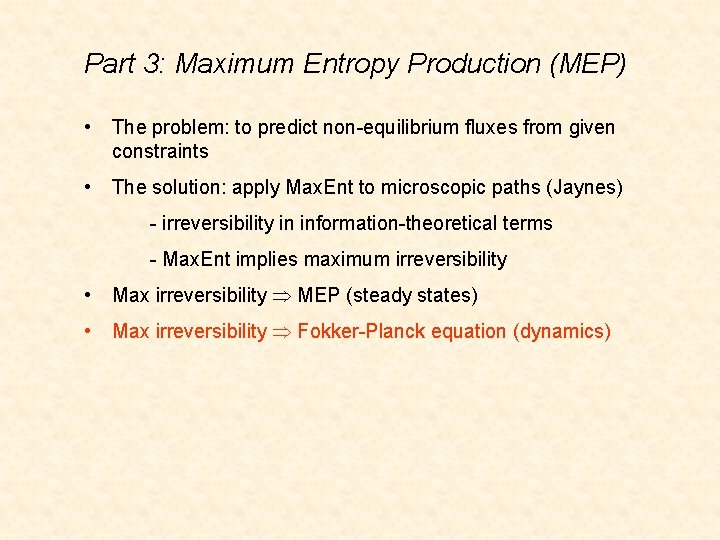
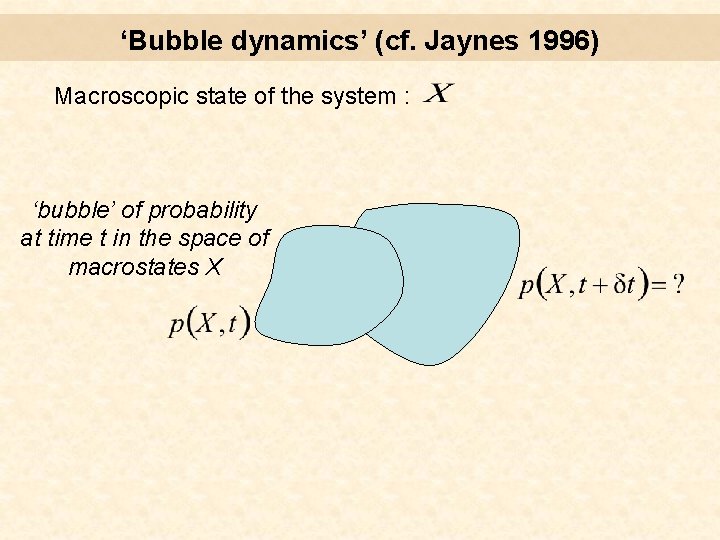
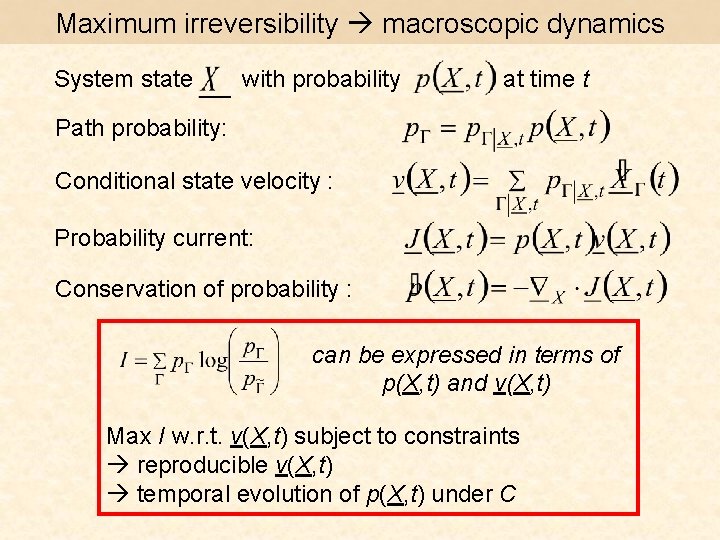
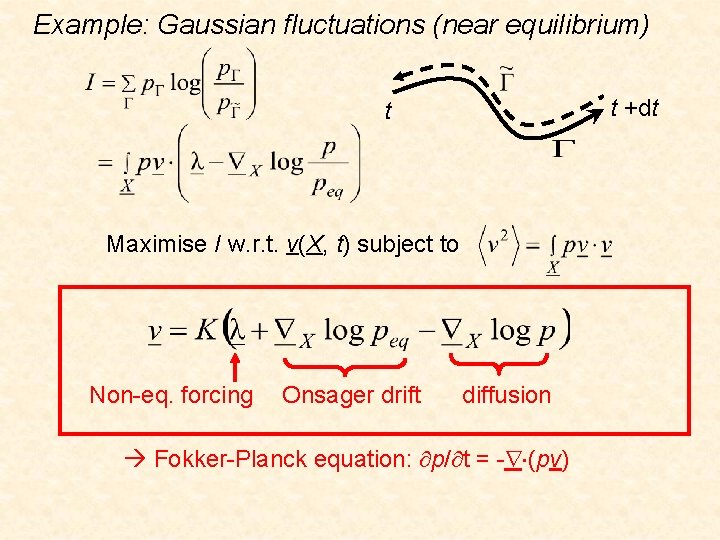
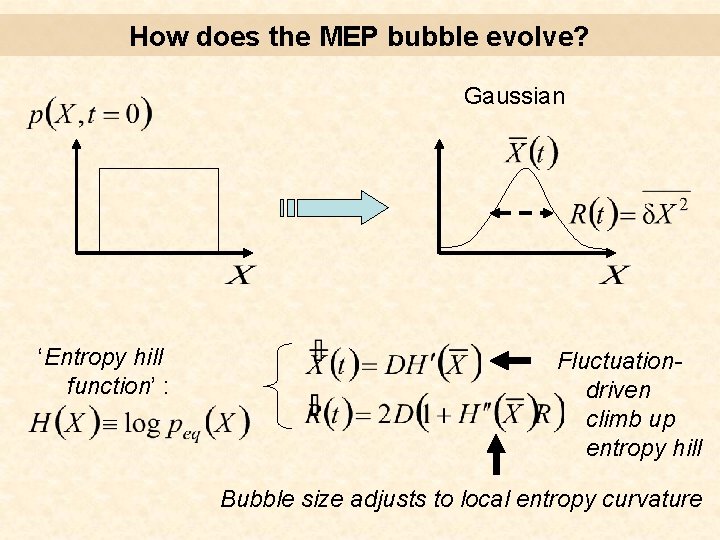
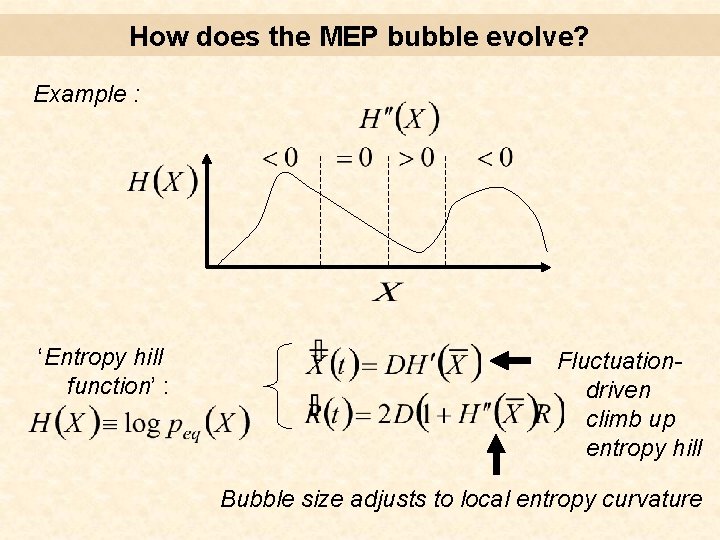
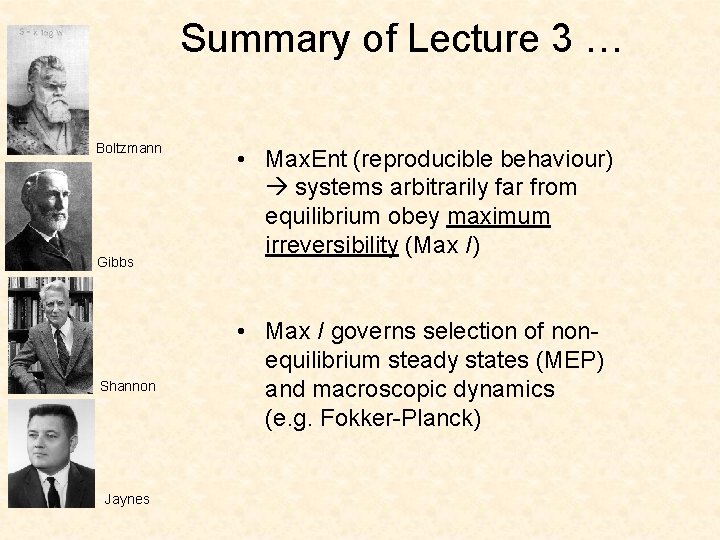
- Slides: 28
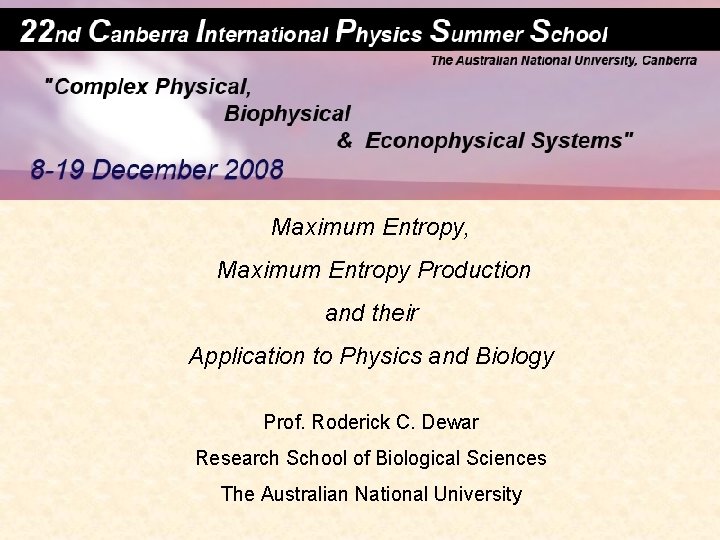
Maximum Entropy, Maximum Entropy Production and their Application to Physics and Biology Prof. Roderick C. Dewar Research School of Biological Sciences The Australian National University
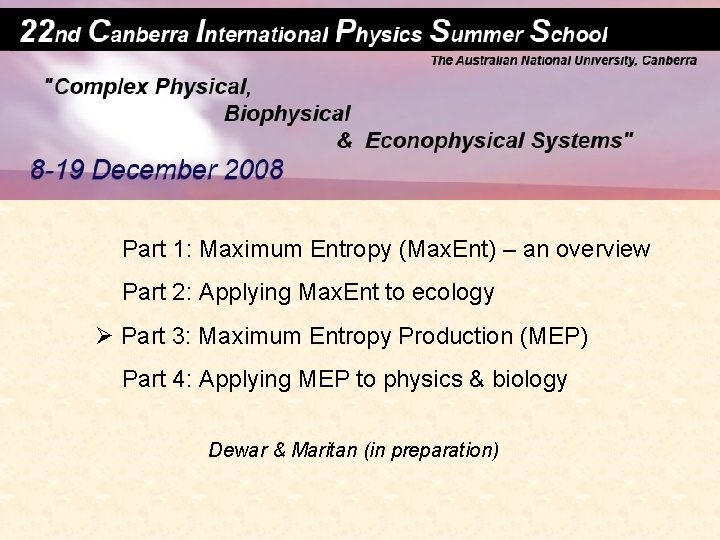
Part 1: Maximum Entropy (Max. Ent) – an overview Part 2: Applying Max. Ent to ecology Ø Part 3: Maximum Entropy Production (MEP) Part 4: Applying MEP to physics & biology Dewar & Maritan (in preparation)
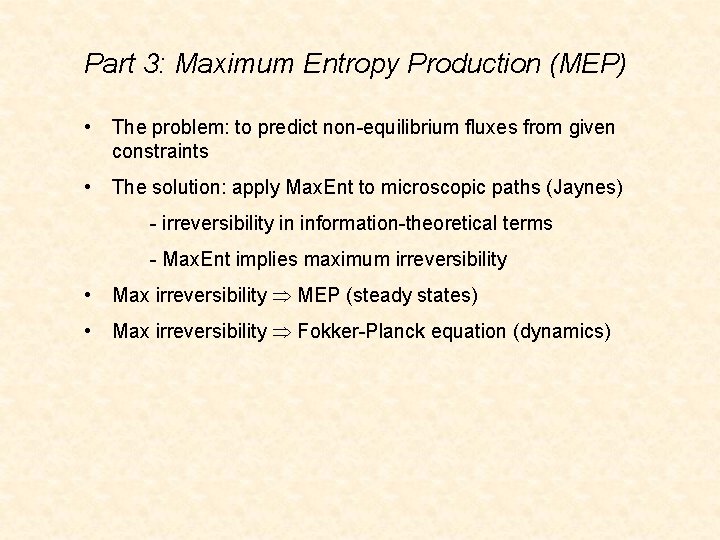
Part 3: Maximum Entropy Production (MEP) • The problem: to predict non-equilibrium fluxes from given constraints • The solution: apply Max. Ent to microscopic paths (Jaynes) - irreversibility in information-theoretical terms - Max. Ent implies maximum irreversibility • Max irreversibility MEP (steady states) • Max irreversibility Fokker-Planck equation (dynamics)
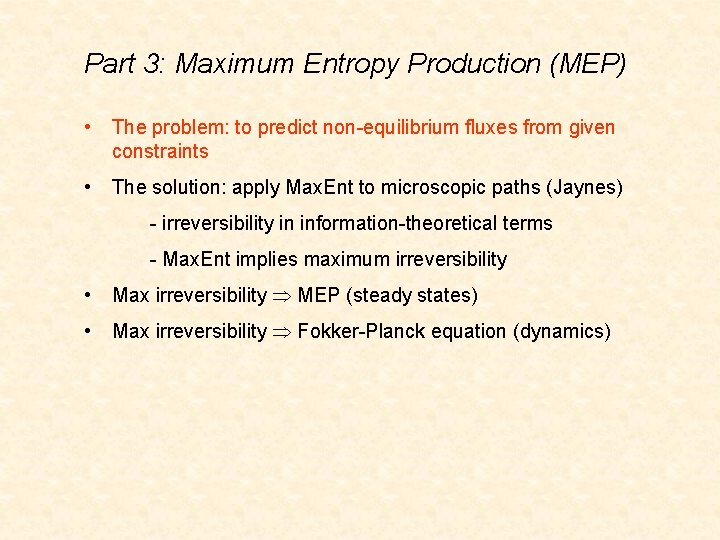
Part 3: Maximum Entropy Production (MEP) • The problem: to predict non-equilibrium fluxes from given constraints • The solution: apply Max. Ent to microscopic paths (Jaynes) - irreversibility in information-theoretical terms - Max. Ent implies maximum irreversibility • Max irreversibility MEP (steady states) • Max irreversibility Fokker-Planck equation (dynamics)
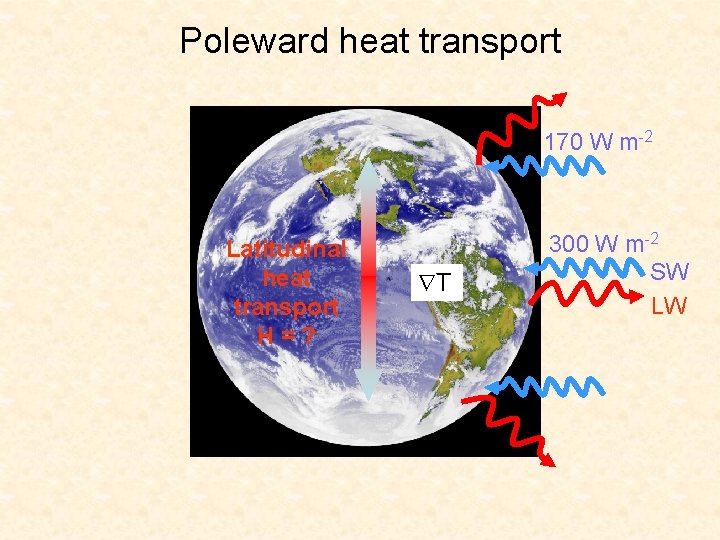
Poleward heat transport 170 W m-2 Latitudinal heat transport H=? T 300 W m-2 SW LW
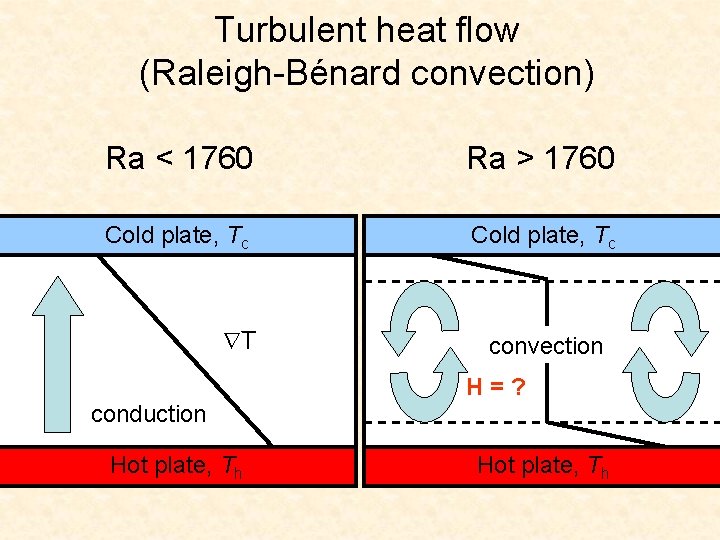
Turbulent heat flow (Raleigh-Bénard convection) Ra < 1760 Ra > 1760 Cold plate, Tc T convection H=? conduction Hot plate, Th
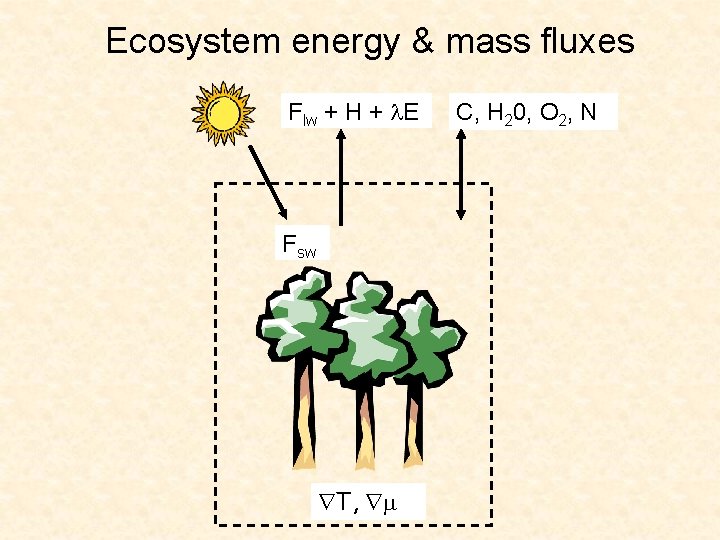
Ecosystem energy & mass fluxes Flw + H + E Fsw T, C, H 20, O 2, N
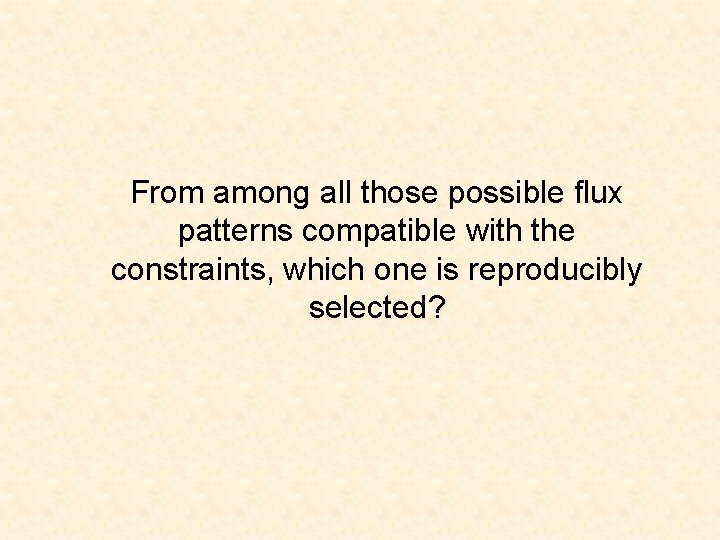
From among all those possible flux patterns compatible with the constraints, which one is reproducibly selected?
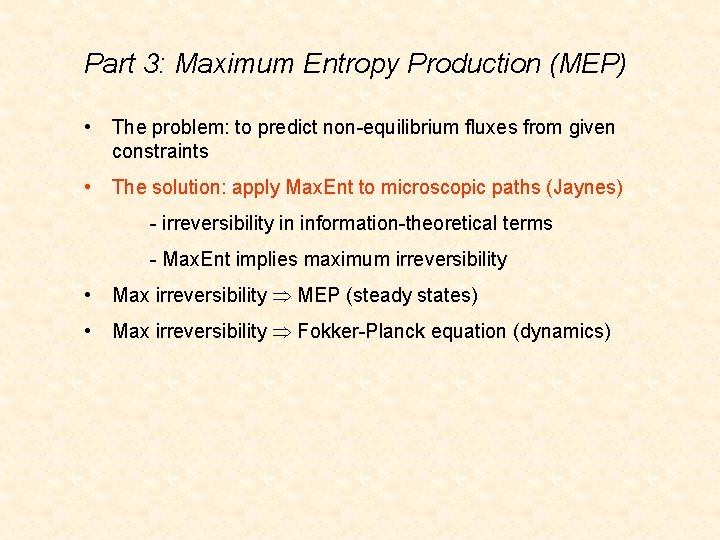
Part 3: Maximum Entropy Production (MEP) • The problem: to predict non-equilibrium fluxes from given constraints • The solution: apply Max. Ent to microscopic paths (Jaynes) - irreversibility in information-theoretical terms - Max. Ent implies maximum irreversibility • Max irreversibility MEP (steady states) • Max irreversibility Fokker-Planck equation (dynamics)
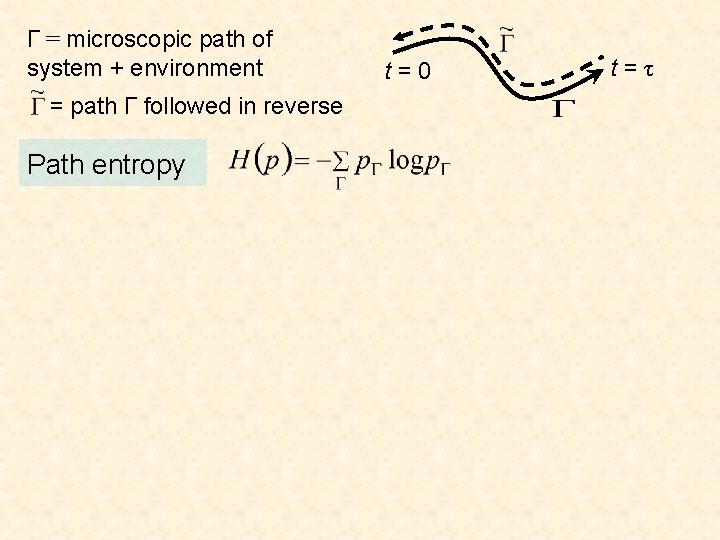
Γ = microscopic path of system + environment = path Γ followed in reverse Path entropy t=0 t=τ
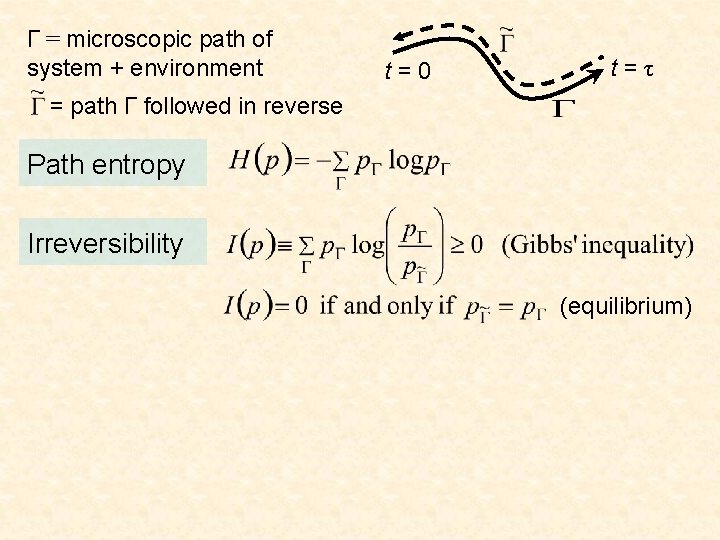
Γ = microscopic path of system + environment t=0 t=τ = path Γ followed in reverse Path entropy Irreversibility (equilibrium)
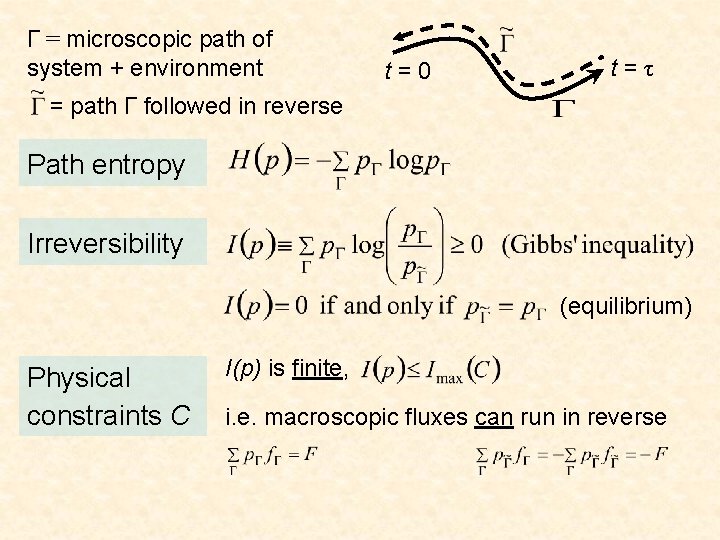
Γ = microscopic path of system + environment t=0 t=τ = path Γ followed in reverse Path entropy Irreversibility (equilibrium) Physical constraints C I(p) is finite, i. e. macroscopic fluxes can run in reverse
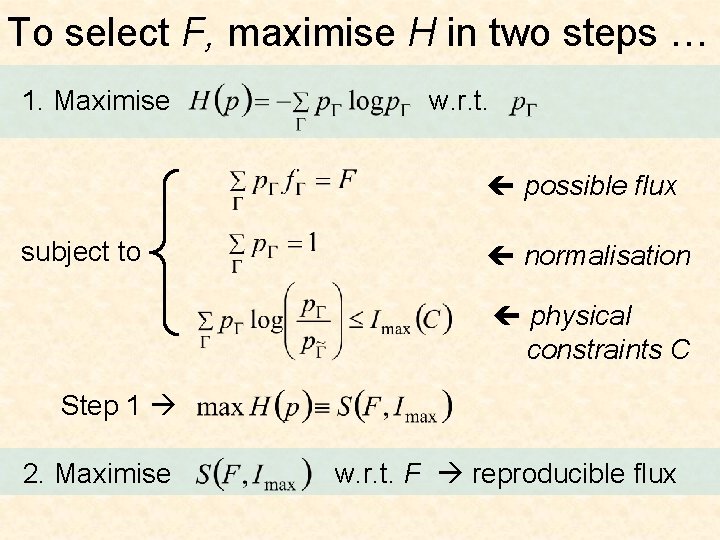
To select F, maximise H in two steps … 1. Maximise w. r. t. possible flux subject to normalisation physical constraints C Step 1 2. Maximise w. r. t. F reproducible flux
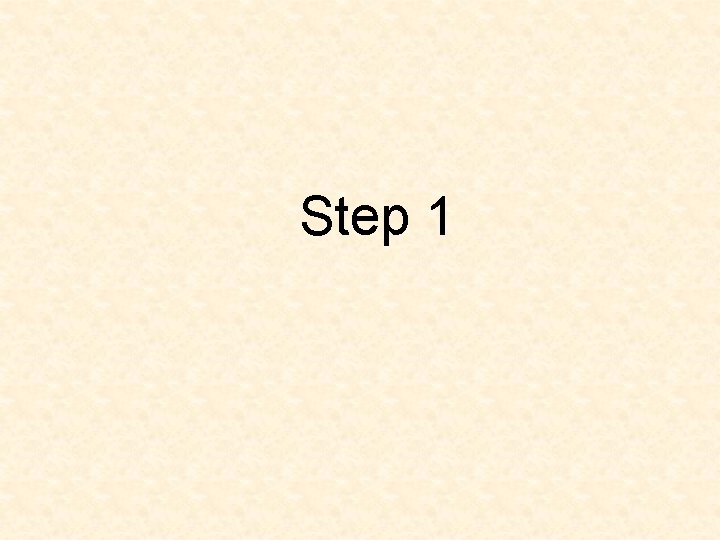
Step 1
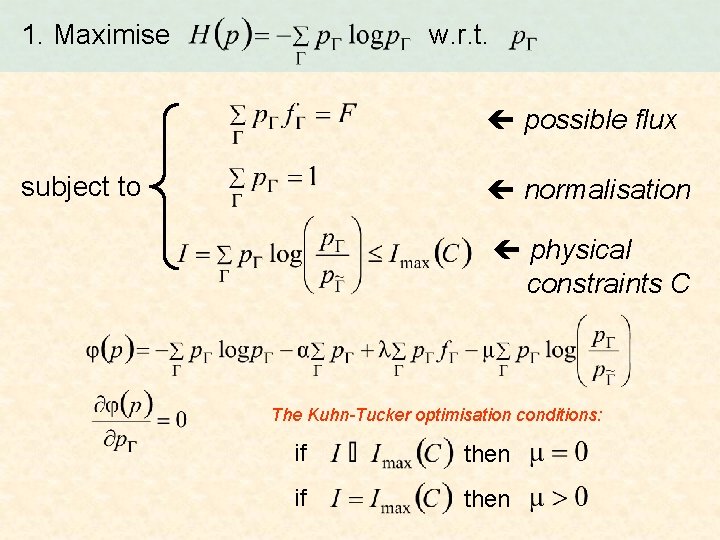
1. Maximise w. r. t. possible flux subject to normalisation physical constraints C The Kuhn-Tucker optimisation conditions: if then
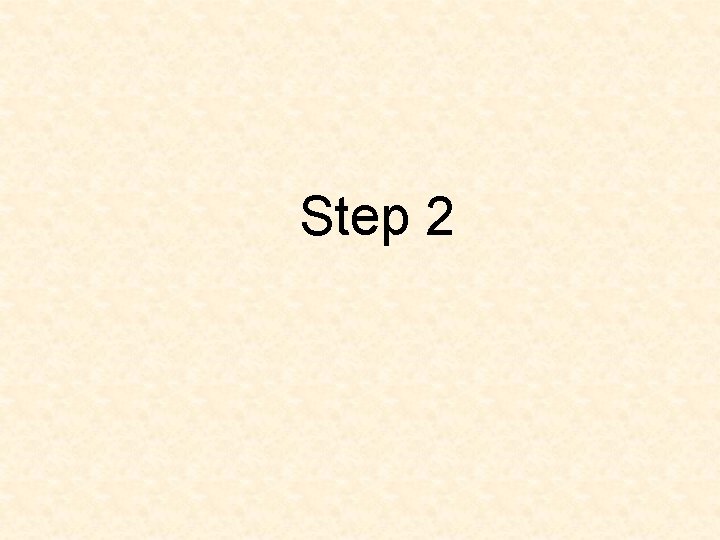
Step 2
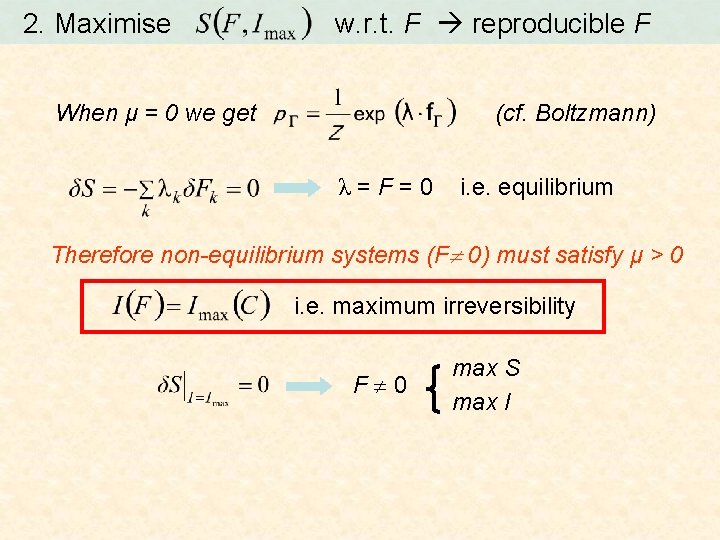
2. Maximise w. r. t. F reproducible F When μ = 0 we get (cf. Boltzmann) λ=F=0 i. e. equilibrium Therefore non-equilibrium systems (F 0) must satisfy μ > 0 i. e. maximum irreversibility F 0 max S max I
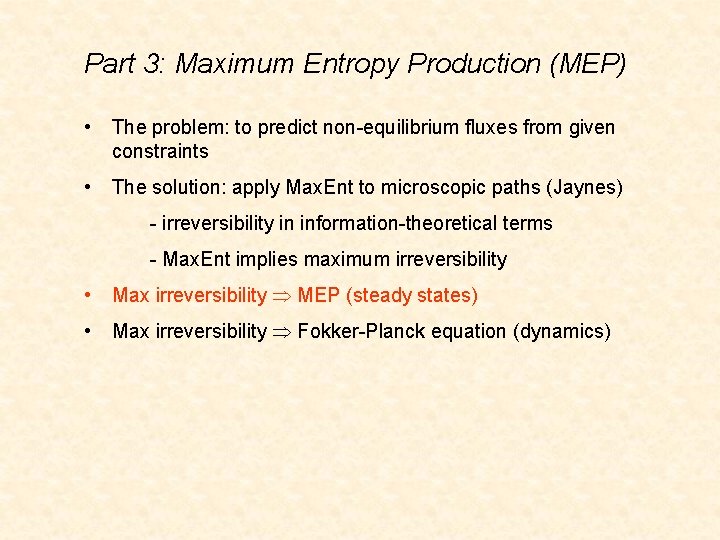
Part 3: Maximum Entropy Production (MEP) • The problem: to predict non-equilibrium fluxes from given constraints • The solution: apply Max. Ent to microscopic paths (Jaynes) - irreversibility in information-theoretical terms - Max. Ent implies maximum irreversibility • Max irreversibility MEP (steady states) • Max irreversibility Fokker-Planck equation (dynamics)
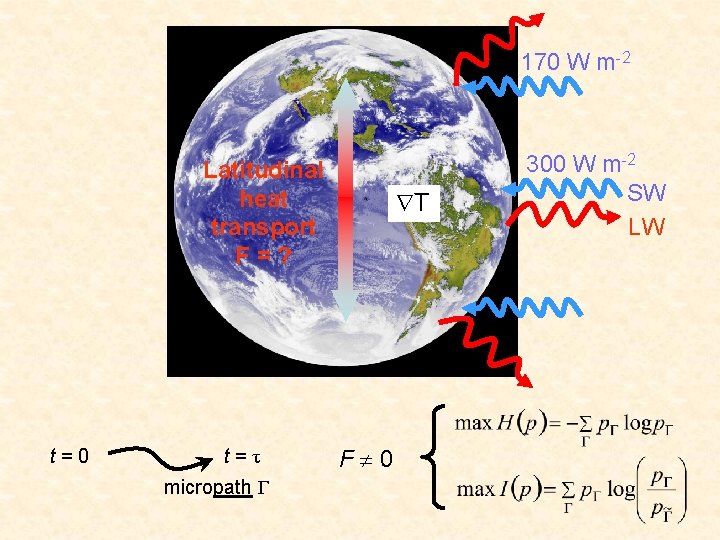
170 W m-2 Latitudinal heat transport F=? t=0 t=τ micropath Γ T F 0 300 W m-2 SW LW
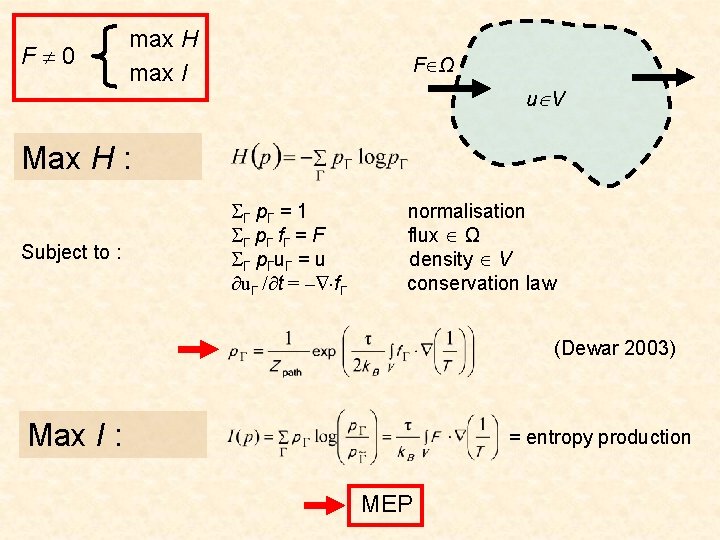
F 0 max H max I F Ω u V Max H : Subject to : Γ pΓ = 1 Γ pΓ f Γ = F Γ pΓuΓ = u uΓ / t = – fΓ normalisation flux Ω density V conservation law (Dewar 2003) Max I : = entropy production MEP
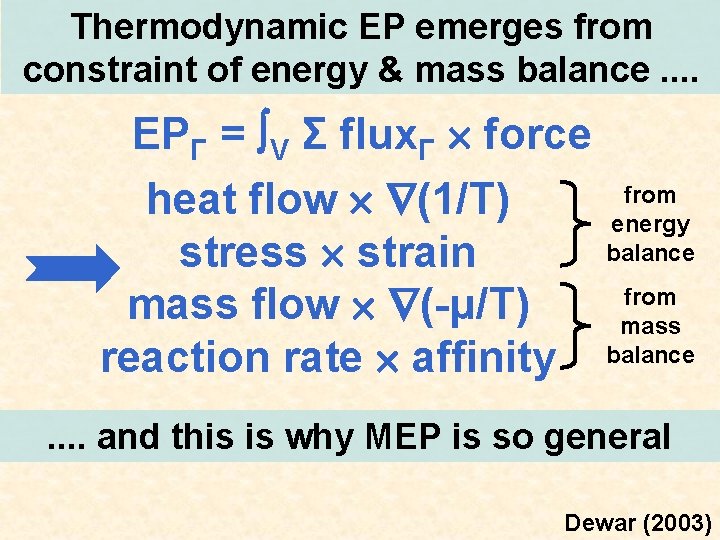
Thermodynamic EP emerges from constraint of energy & mass balance. . EPΓ = V Σ fluxΓ force heat flow (1/T) stress strain mass flow (-μ/T) reaction rate affinity from energy balance from mass balance . . and this is why MEP is so general Dewar (2003)
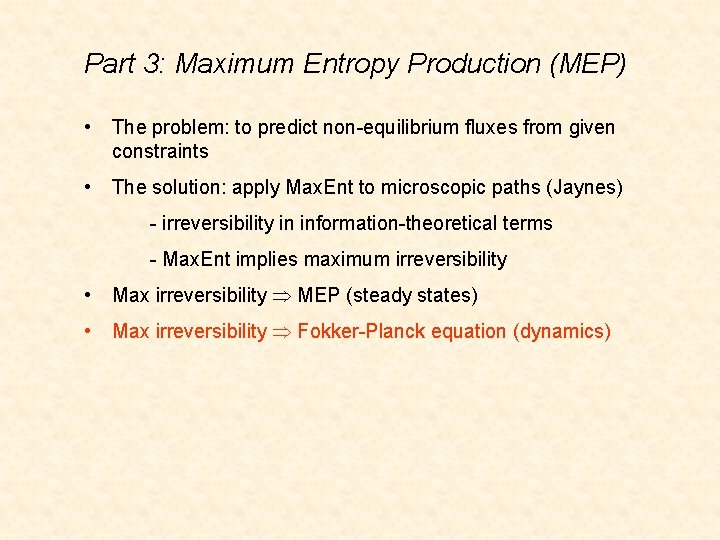
Part 3: Maximum Entropy Production (MEP) • The problem: to predict non-equilibrium fluxes from given constraints • The solution: apply Max. Ent to microscopic paths (Jaynes) - irreversibility in information-theoretical terms - Max. Ent implies maximum irreversibility • Max irreversibility MEP (steady states) • Max irreversibility Fokker-Planck equation (dynamics)
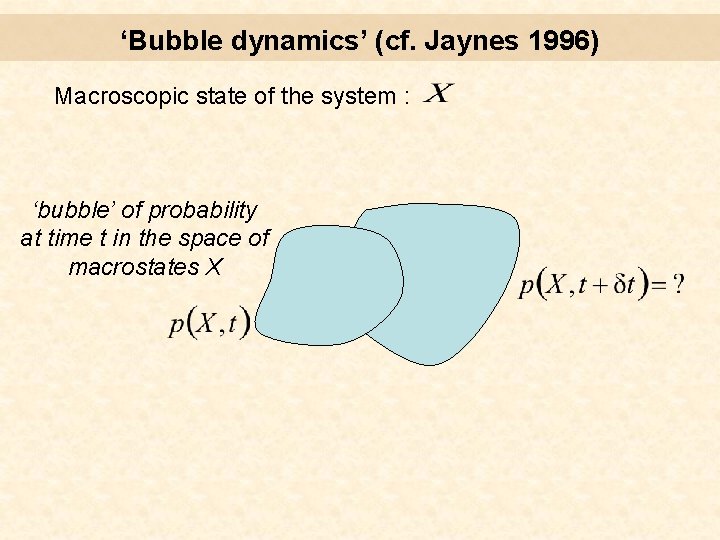
‘Bubble dynamics’ (cf. Jaynes 1996) Macroscopic state of the system : ‘bubble’ of probability at time t in the space of macrostates X
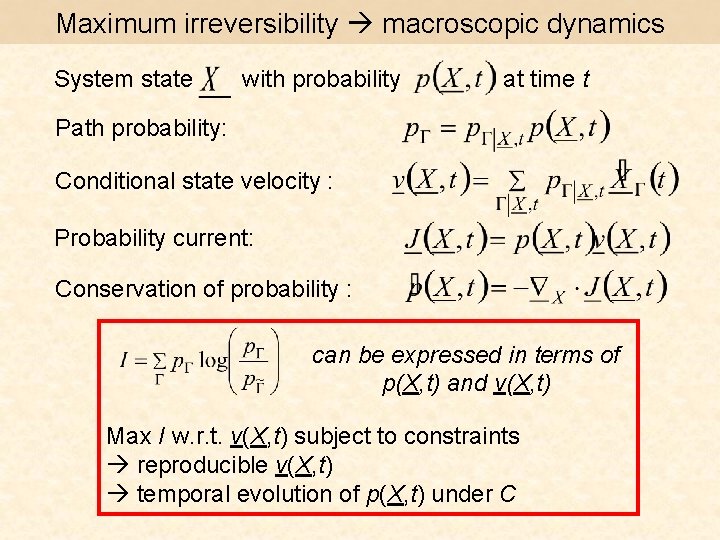
Maximum irreversibility macroscopic dynamics System state with probability at time t Path probability: Conditional state velocity : Probability current: Conservation of probability : can be expressed in terms of p(X, t) and v(X, t) Max I w. r. t. v(X, t) subject to constraints reproducible v(X, t) temporal evolution of p(X, t) under C
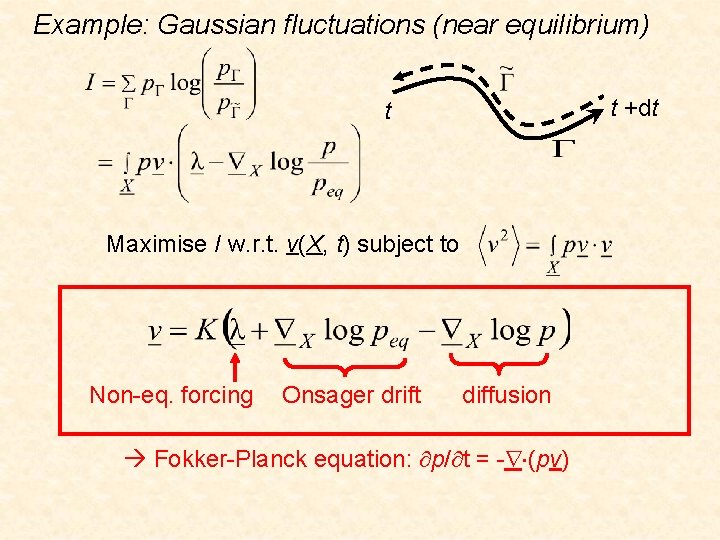
Example: Gaussian fluctuations (near equilibrium) t +dt t Maximise I w. r. t. v(X, t) subject to Non-eq. forcing Onsager drift diffusion Fokker-Planck equation: p/ t = - (pv)
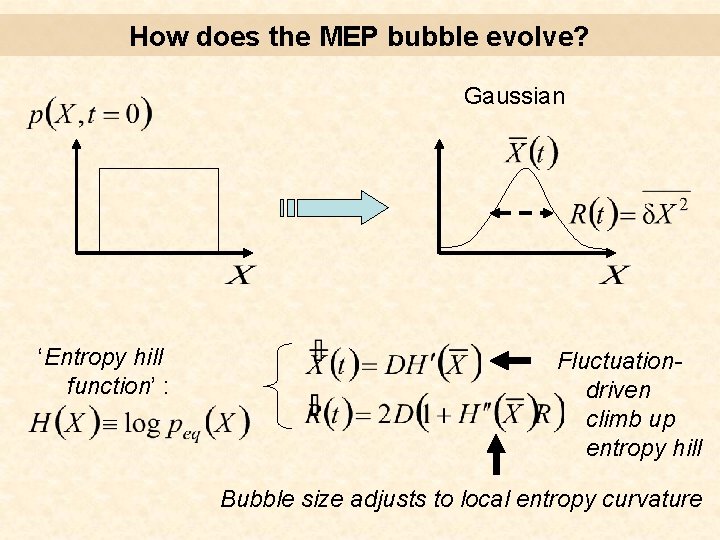
How does the MEP bubble evolve? Gaussian ‘Entropy hill function’ : Fluctuationdriven climb up entropy hill Bubble size adjusts to local entropy curvature
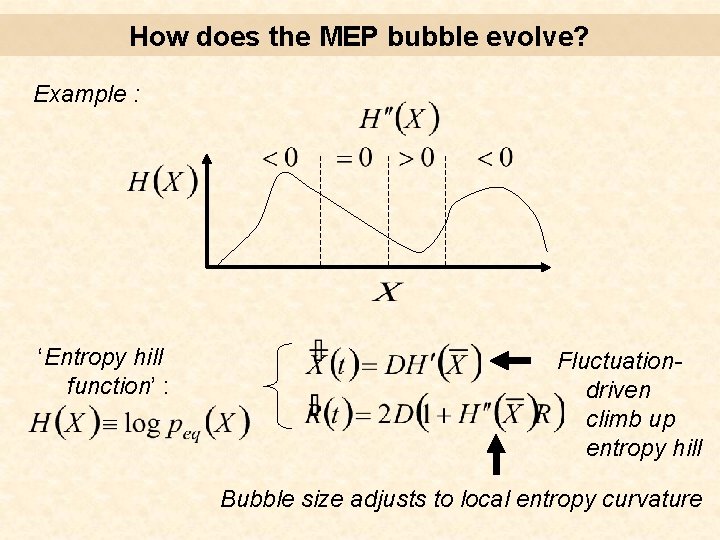
How does the MEP bubble evolve? Example : ‘Entropy hill function’ : Fluctuationdriven climb up entropy hill Bubble size adjusts to local entropy curvature
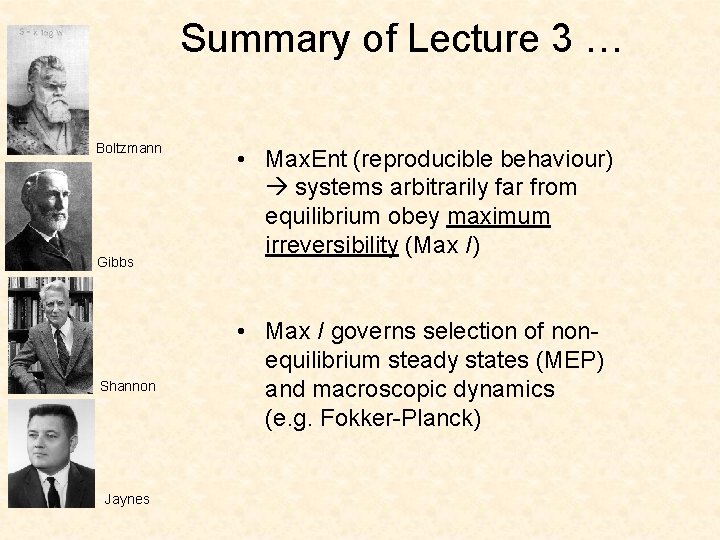
Summary of Lecture 3 … Boltzmann Gibbs Shannon Jaynes • Max. Ent (reproducible behaviour) systems arbitrarily far from equilibrium obey maximum irreversibility (Max I) • Max I governs selection of nonequilibrium steady states (MEP) and macroscopic dynamics (e. g. Fokker-Planck)
Pre-production, production, post-production
Minimum enthalpy maximum entropy
Absolute min and max
Maximum likelihood vs maximum parsimony
Maximum likelihood vs maximum parsimony
Maximum power transfer calculator
Application framework in android architecture
Romeo prologue
Absolute entropy
Entropy and heat transfer
What is enthalpy and entropy
Negative delta g
Relationship between entropy and free energy
Ap chemistry spontaneity entropy and free energy
Gibbs free energy non standard conditions
Entropy order parameters and complexity
Maximum mode of 8086
Entropy equation temperature
Increase in entropy principle
Nilai entropi propana
Reversible and irreversible process
Formula for entropy change
Entropy is scalar or vector
δhsys
Thermodynamics
Entropy change formula
Entropy in bits
Huffman coding entropy
Entropy balance for closed system