High Level Triggering Fred Wickens High Level Triggering
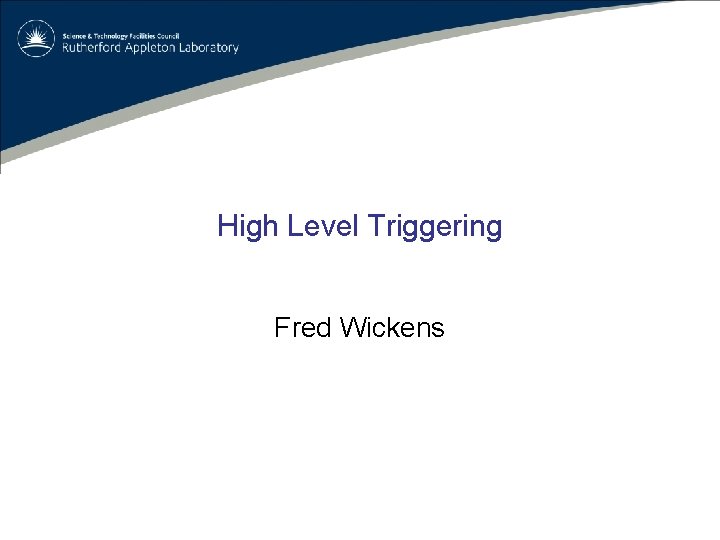
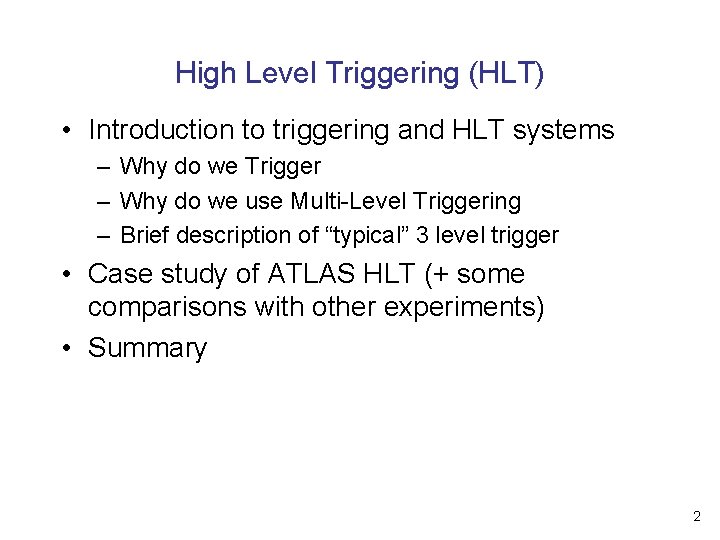
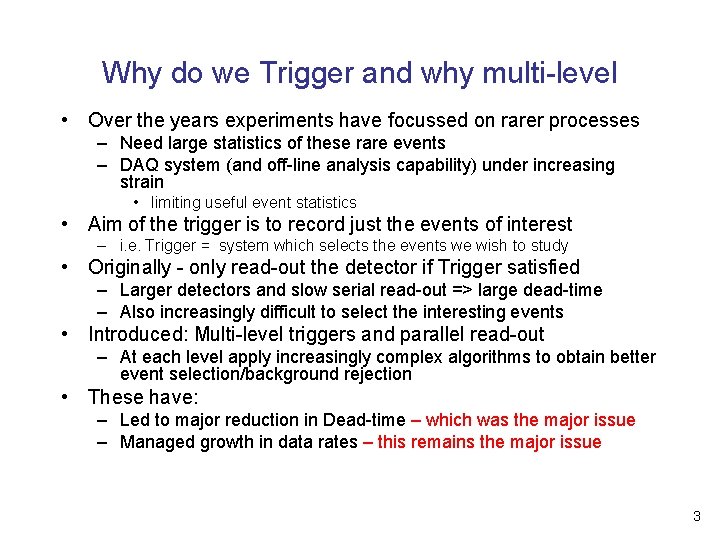
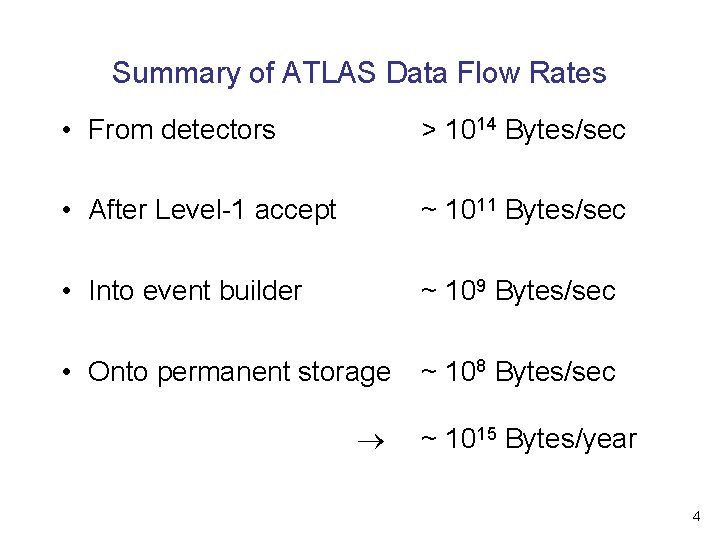
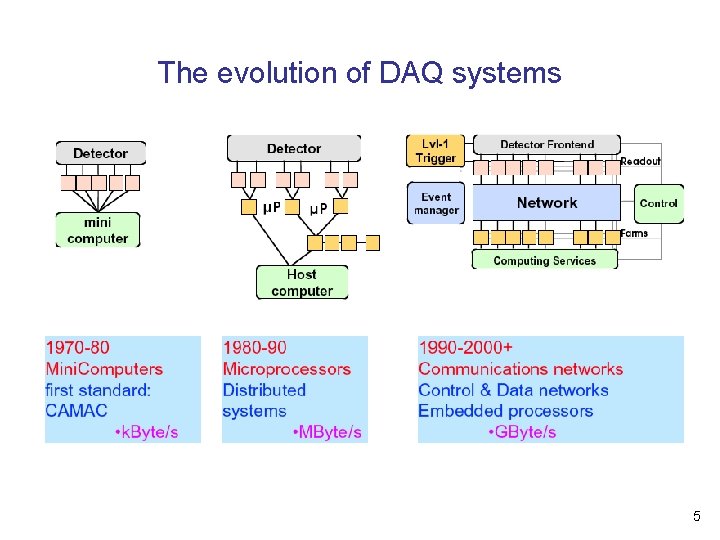
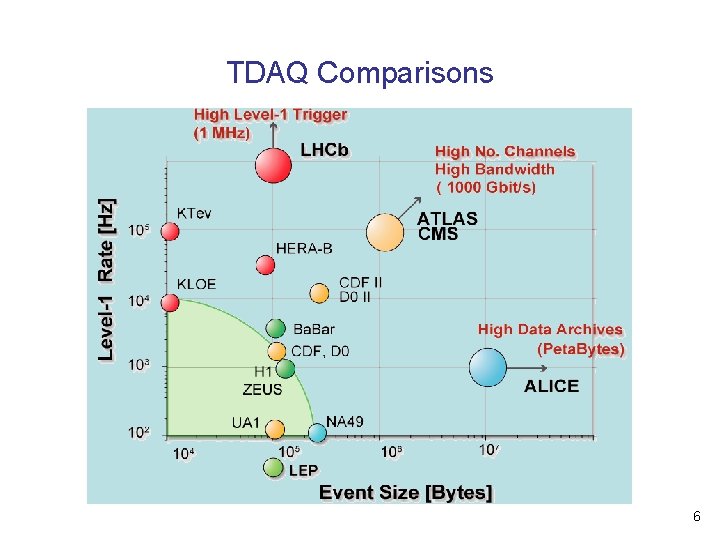
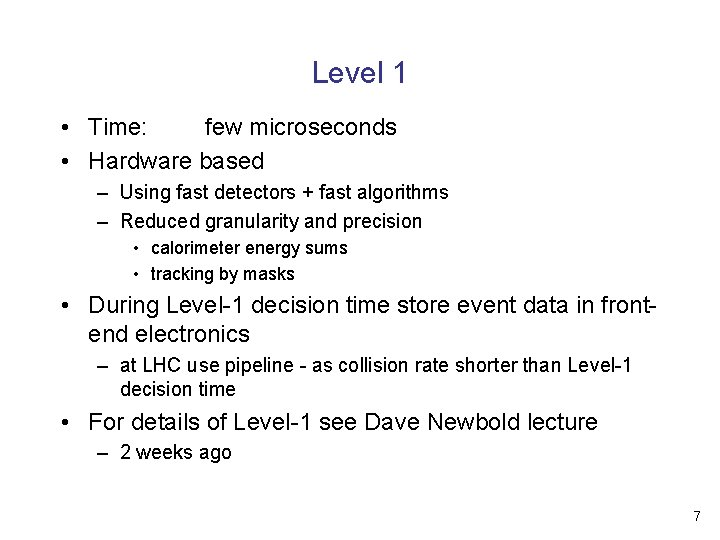
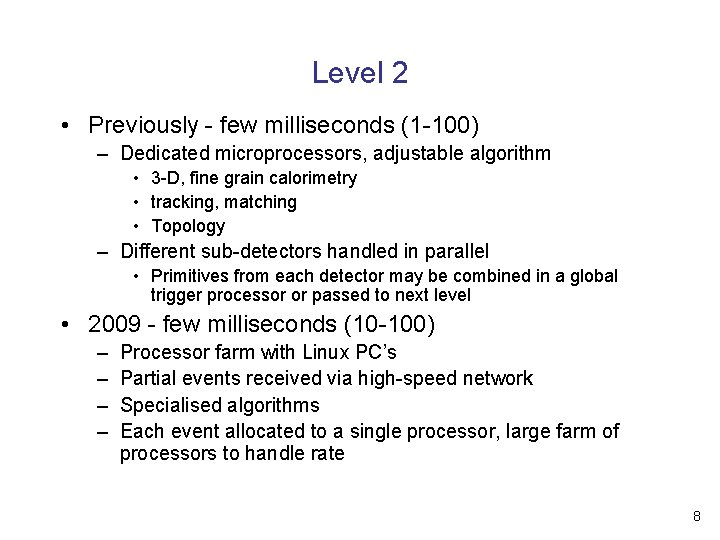
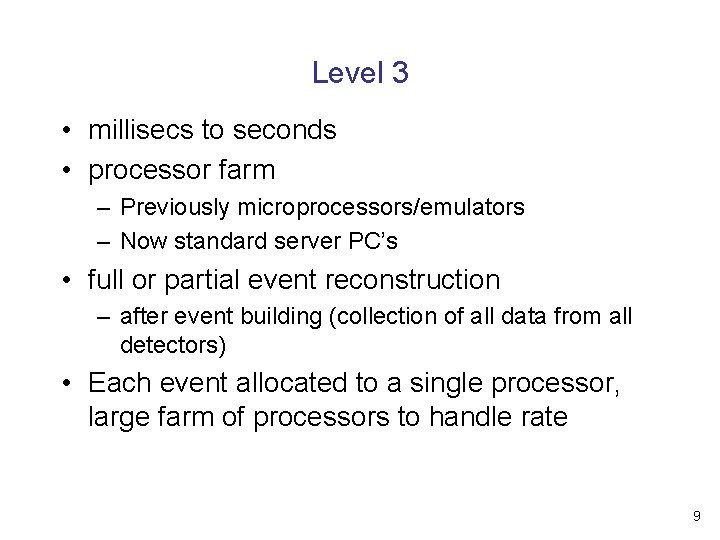
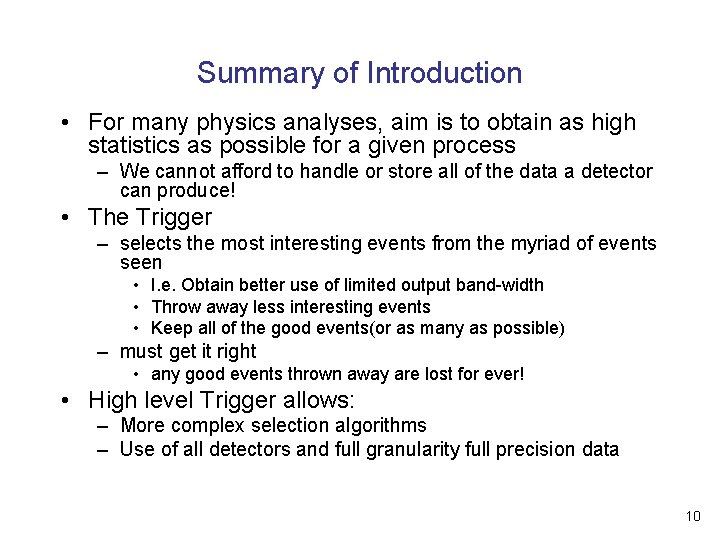
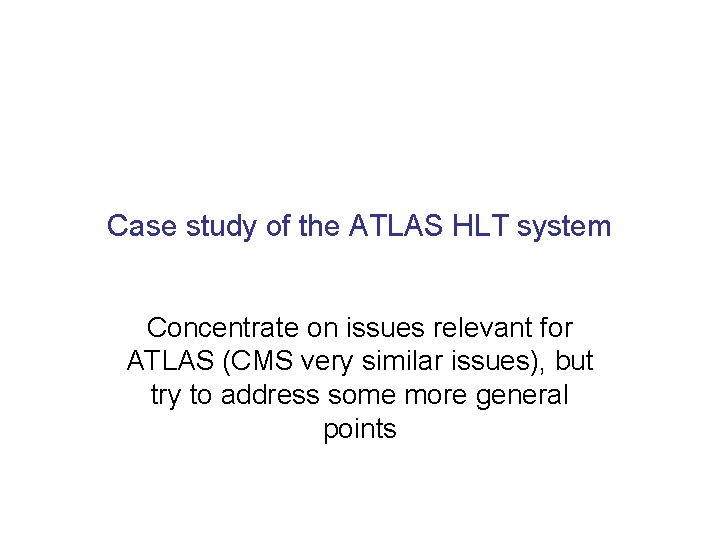
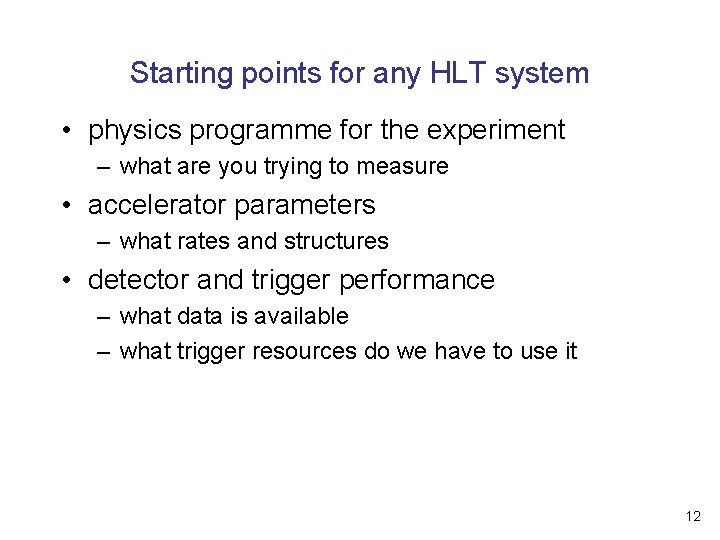
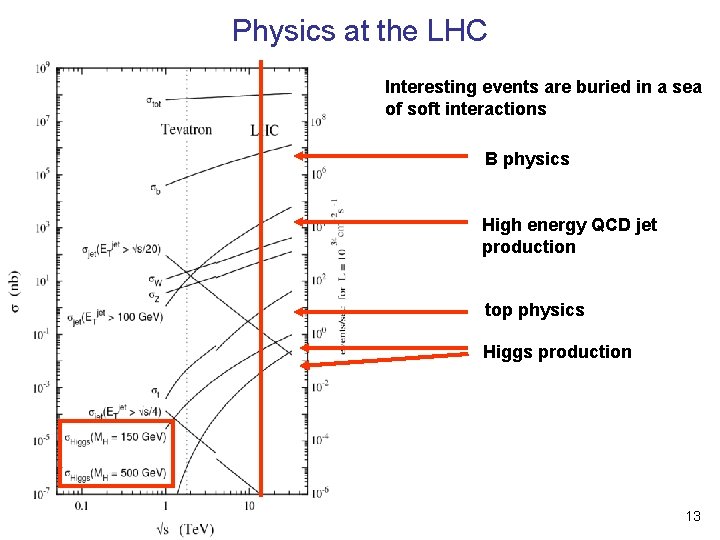
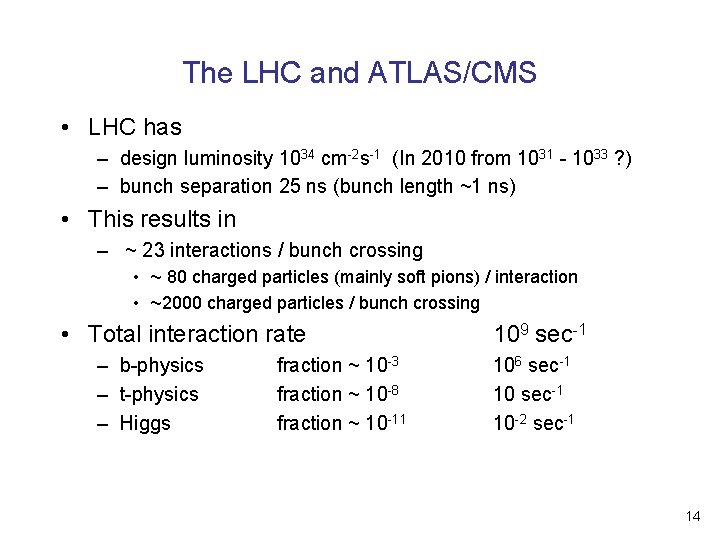
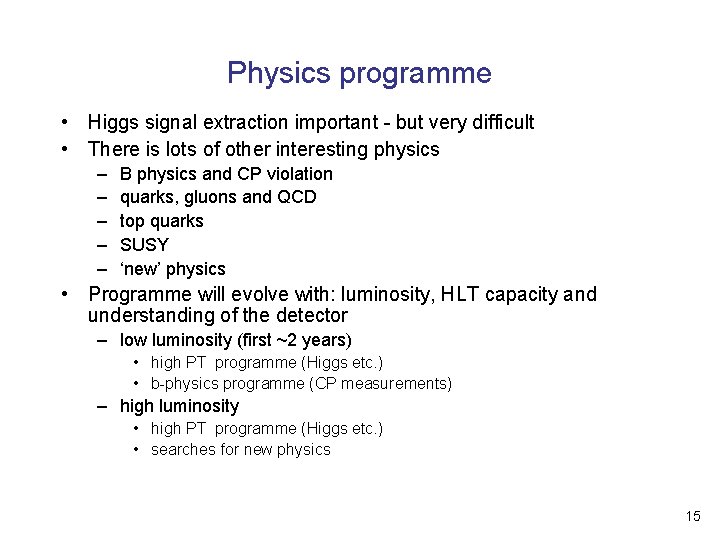
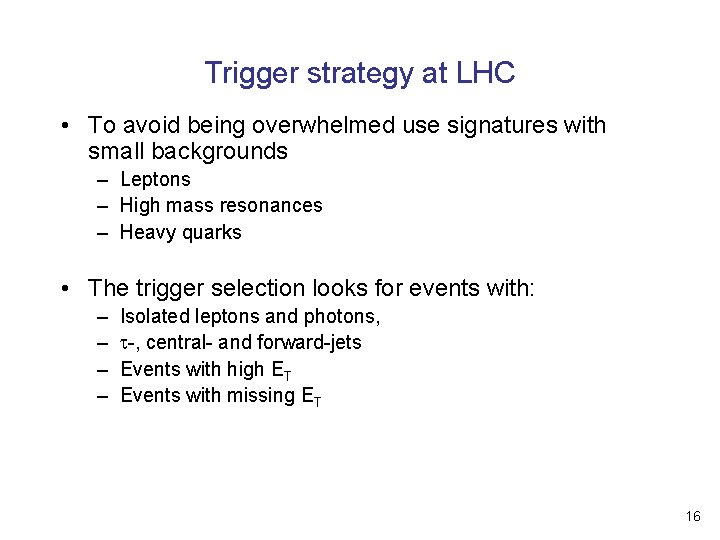
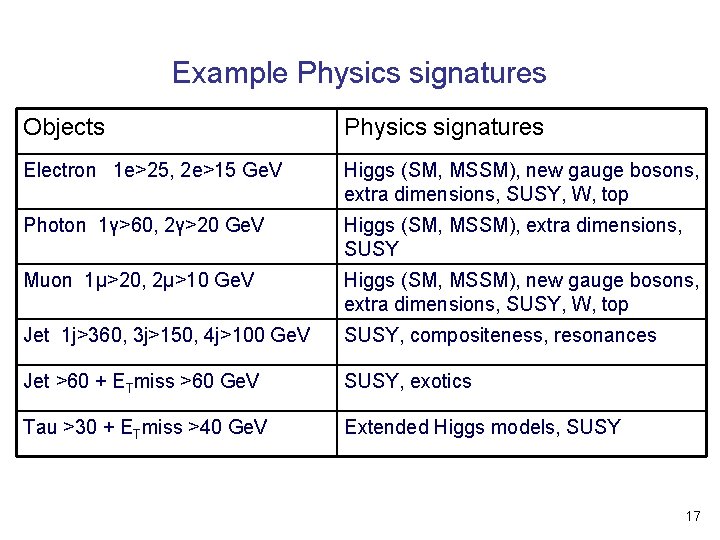
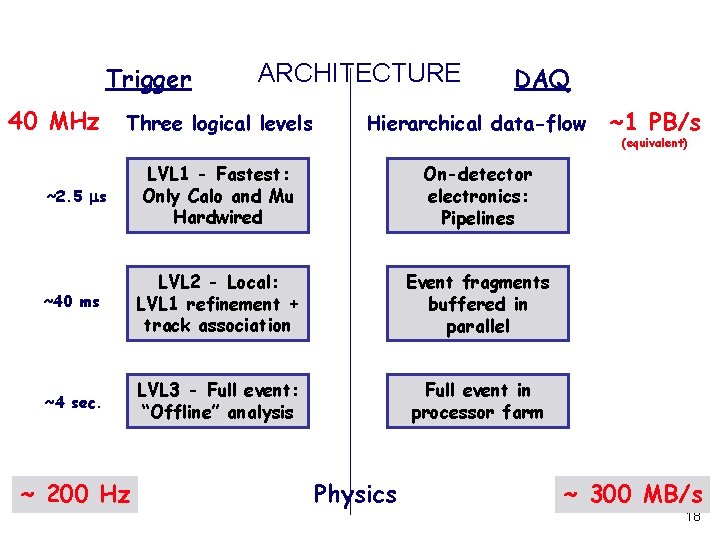
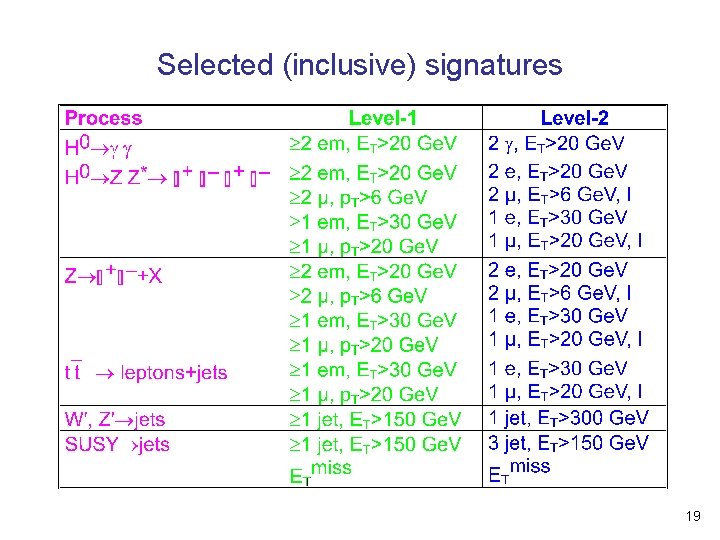
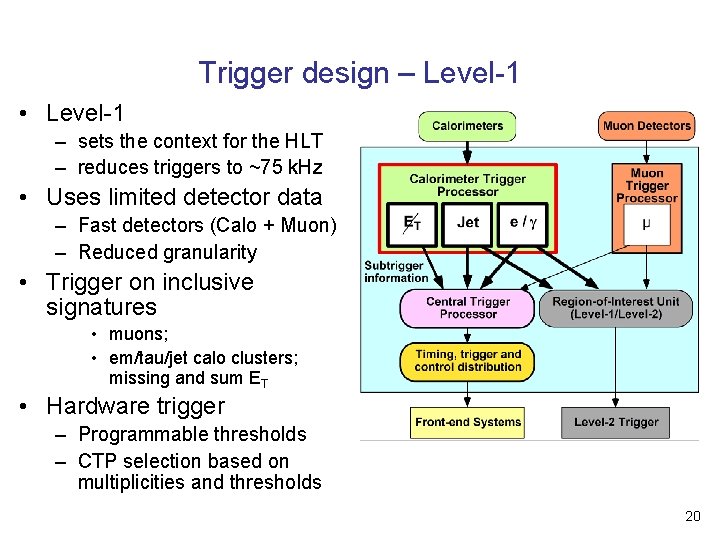
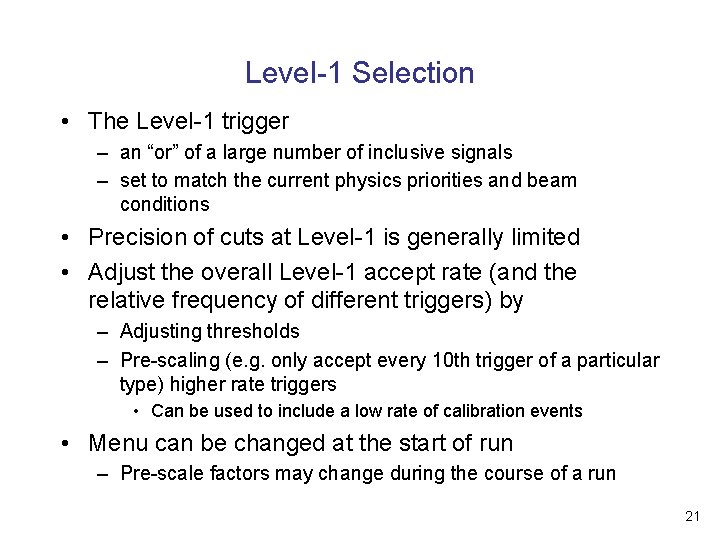
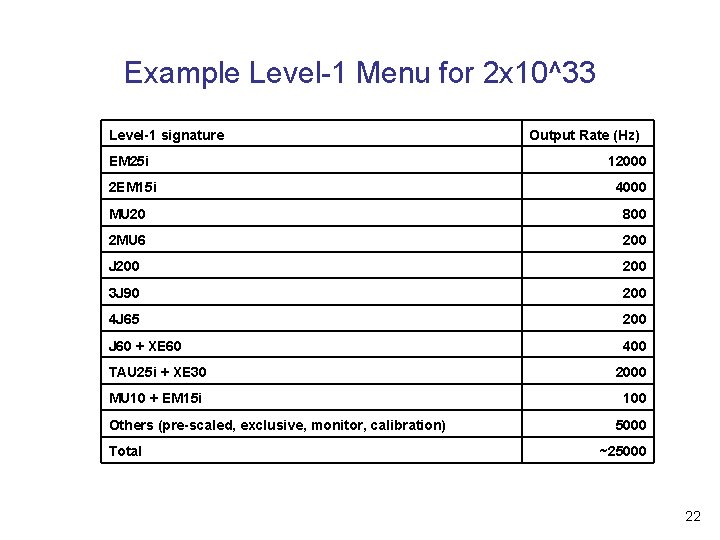
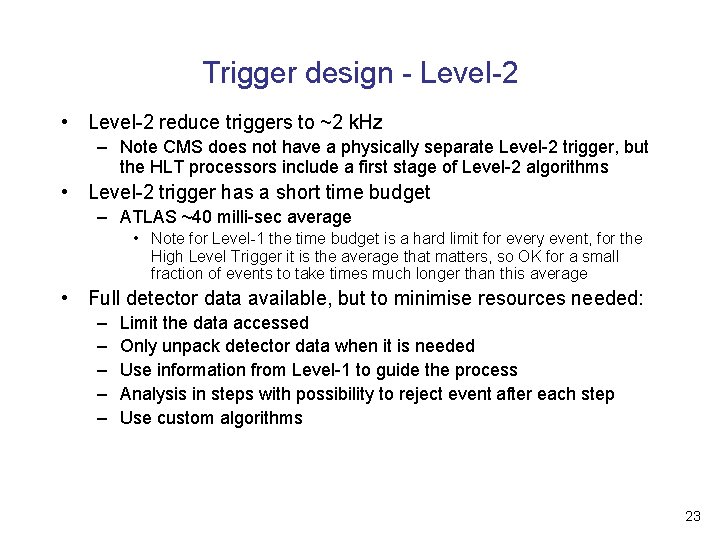
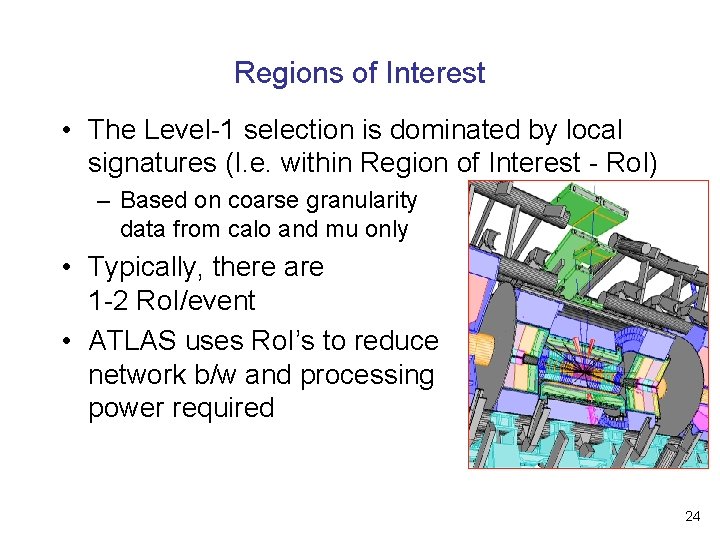
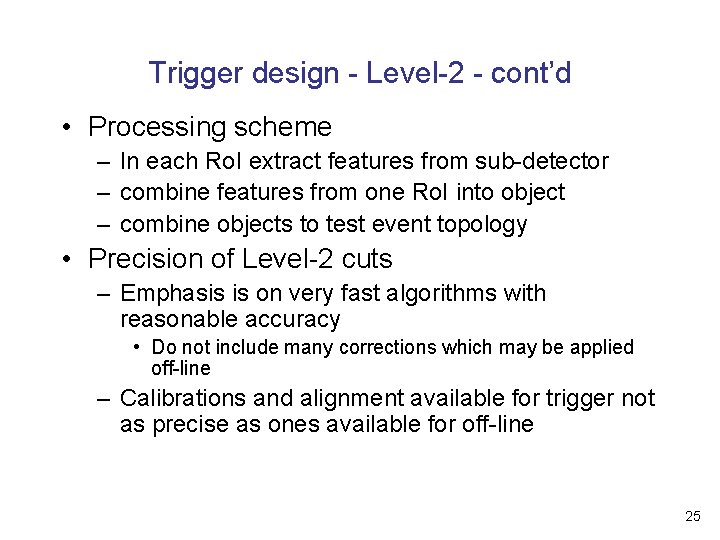
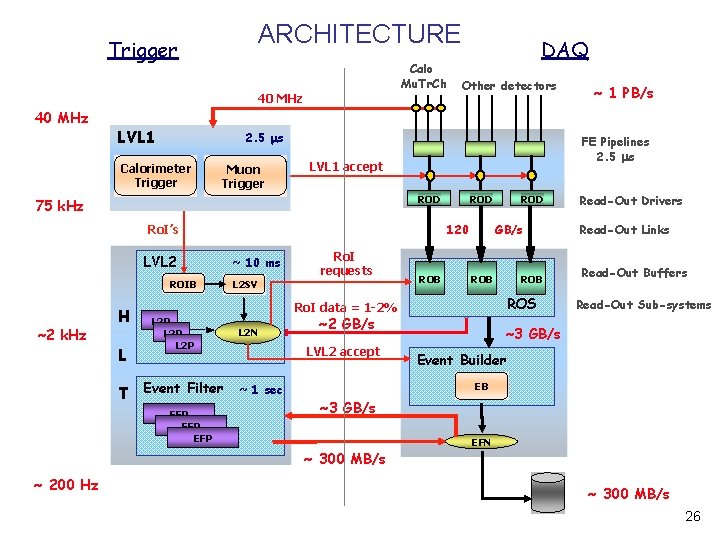
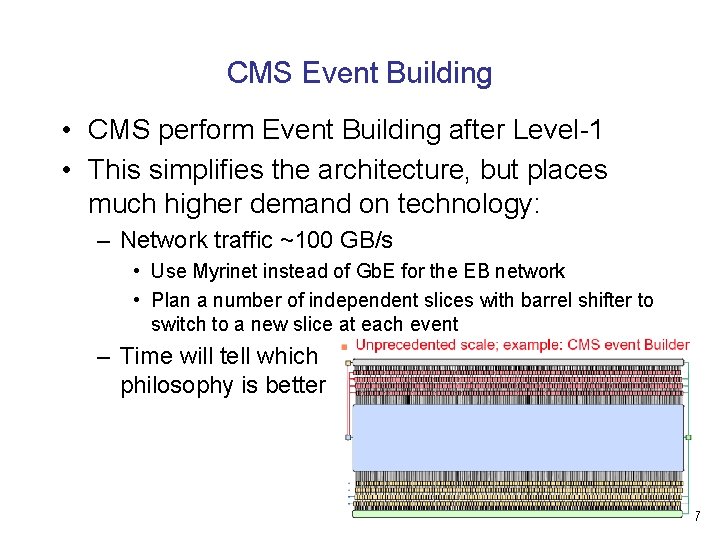
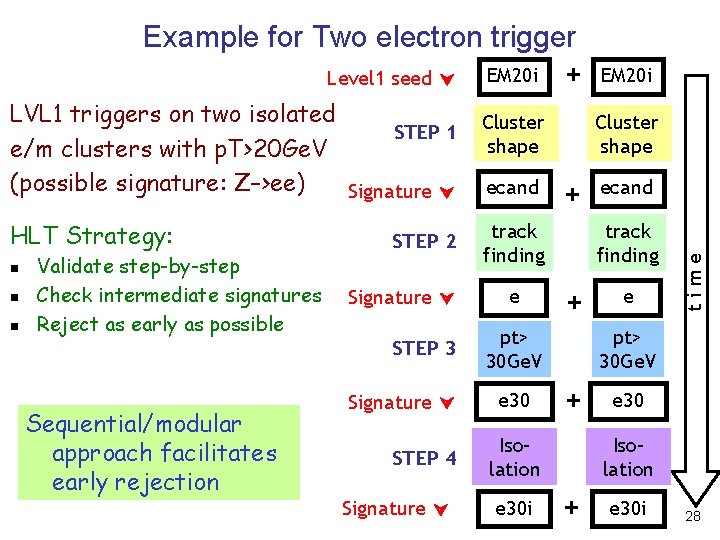
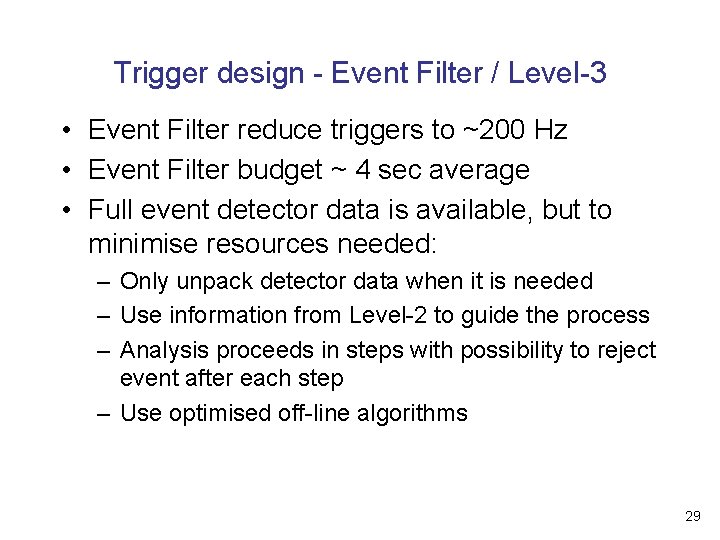
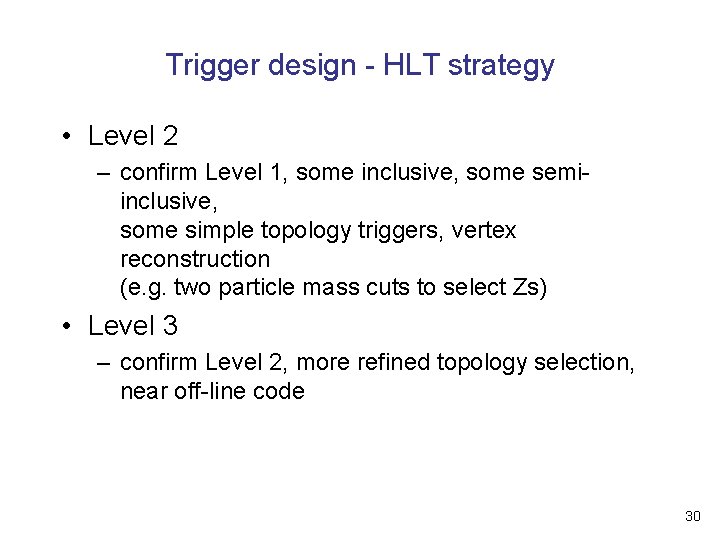
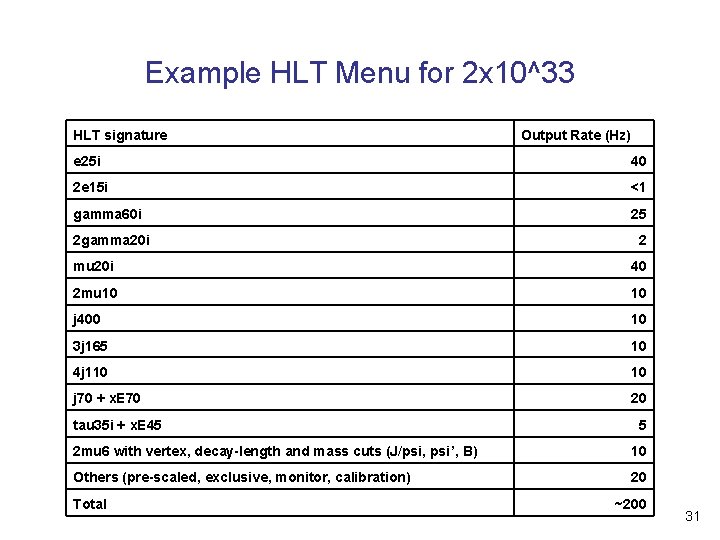
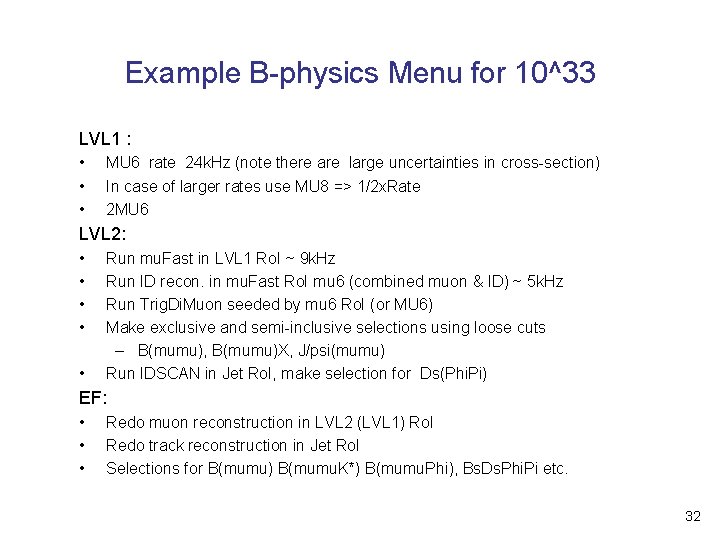
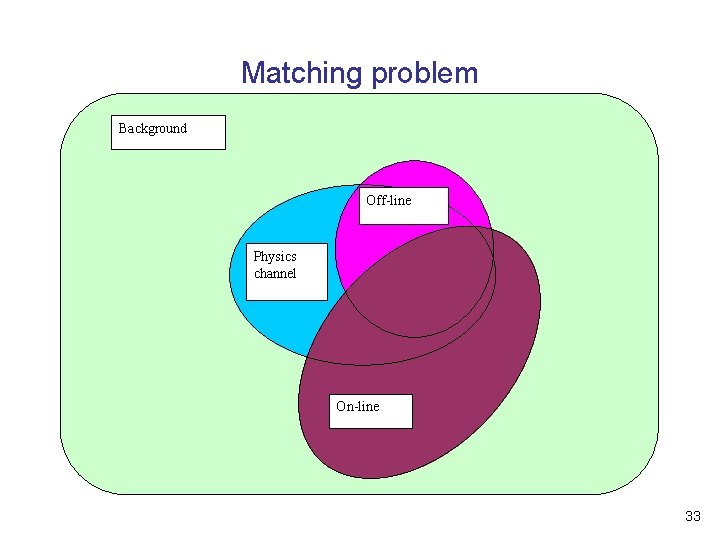
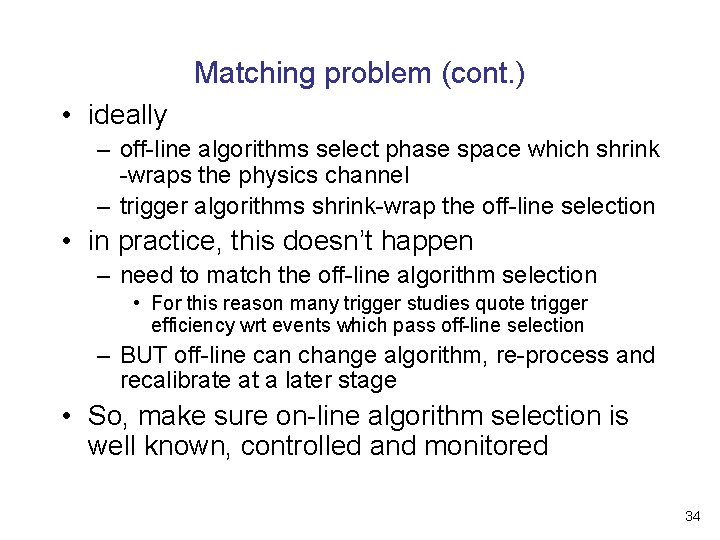
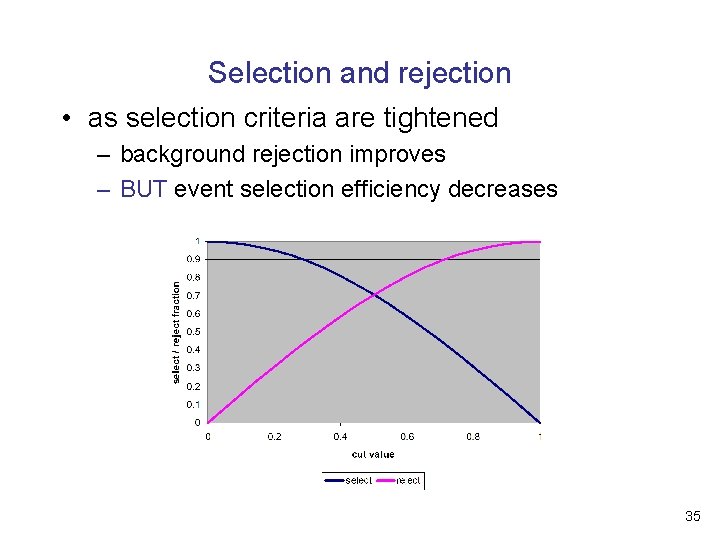
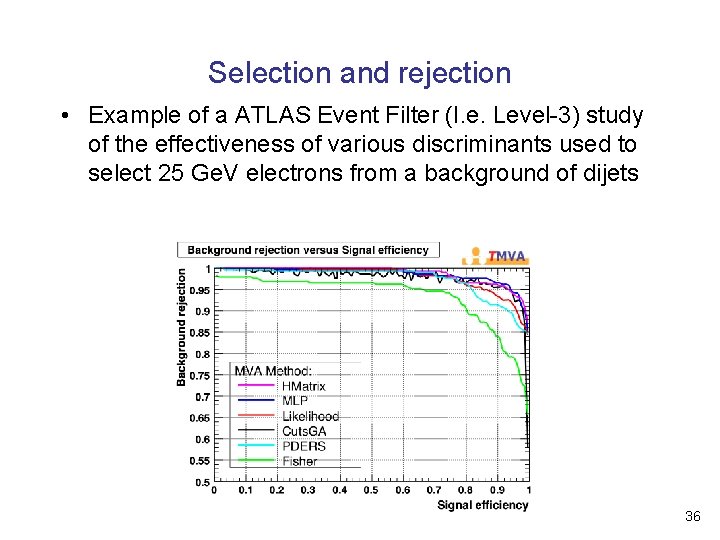
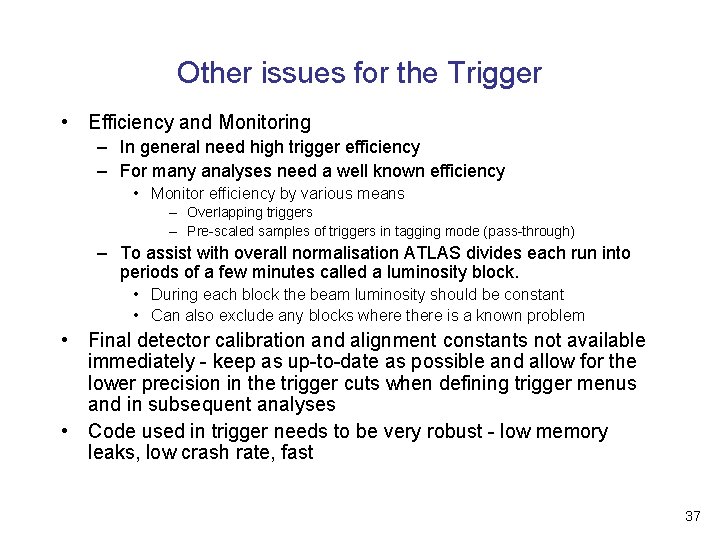
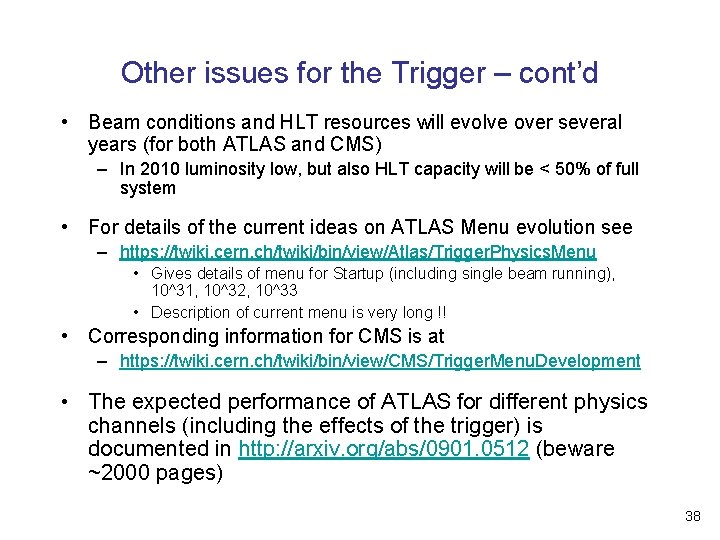
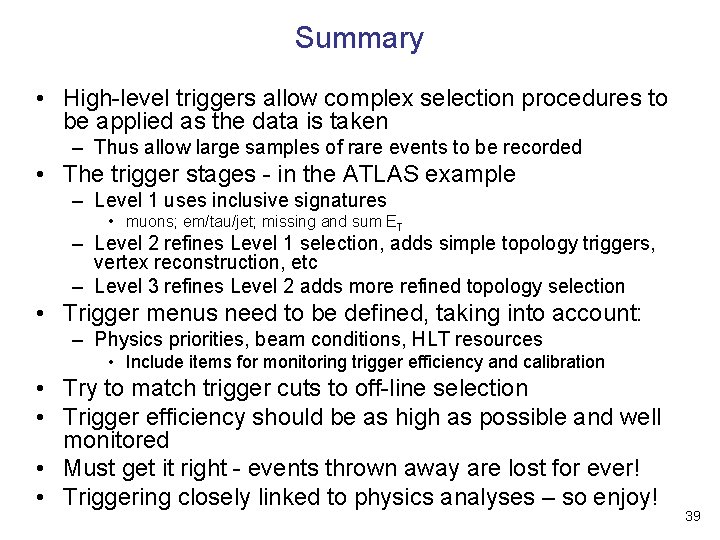
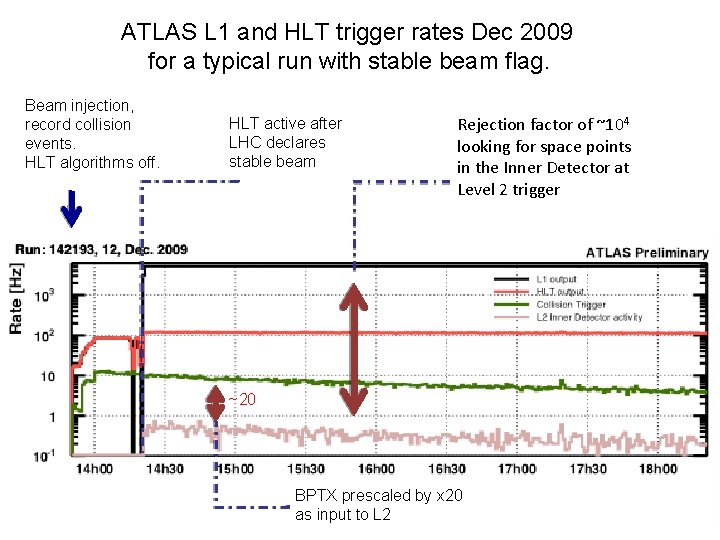
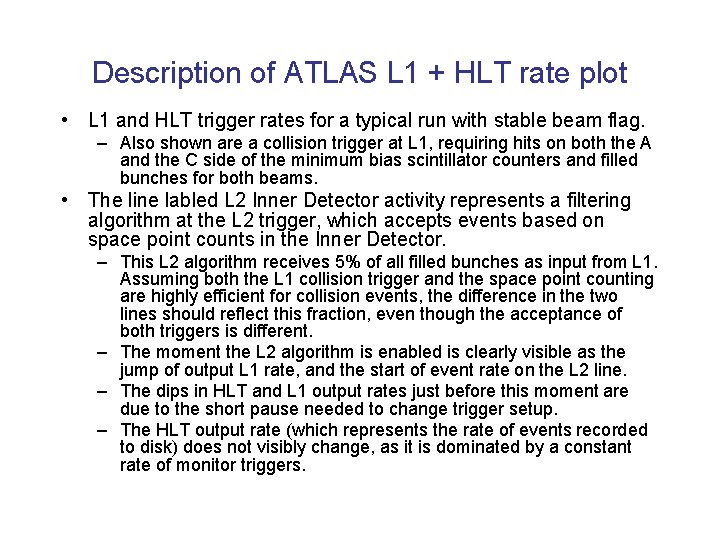
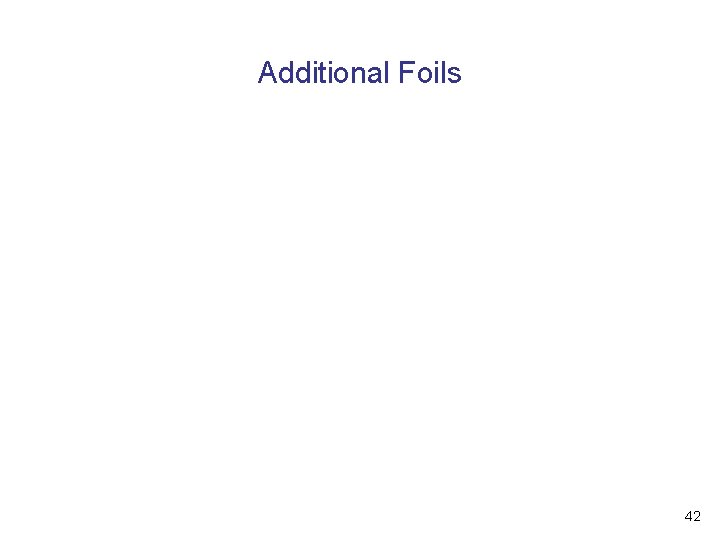
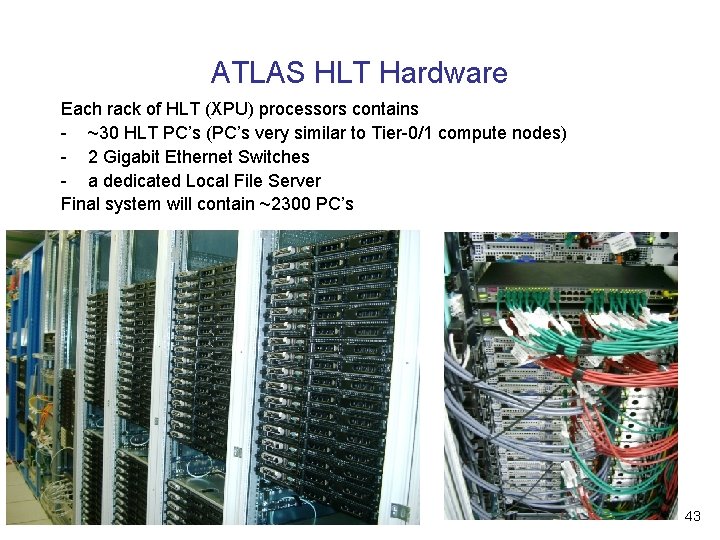
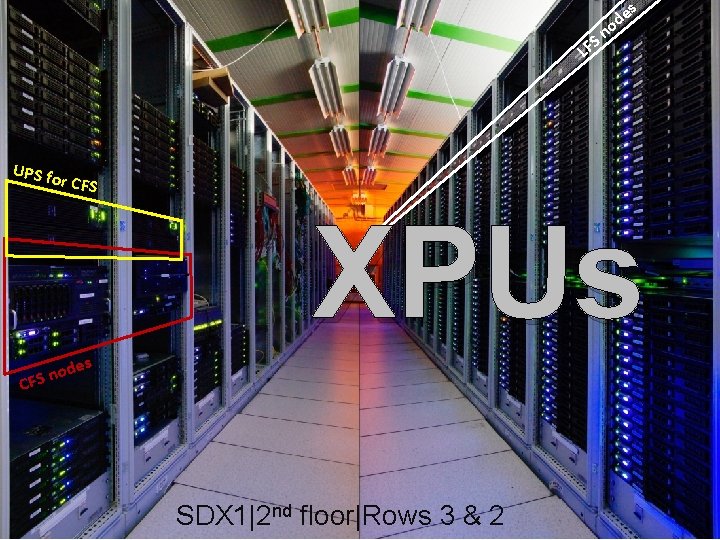
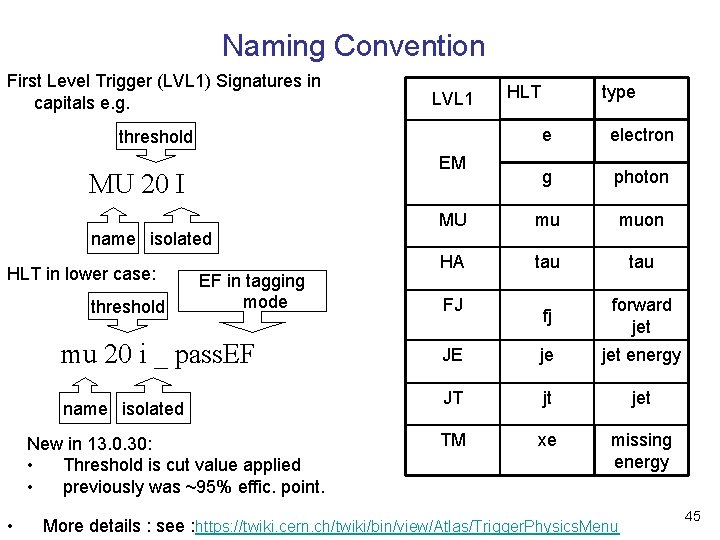
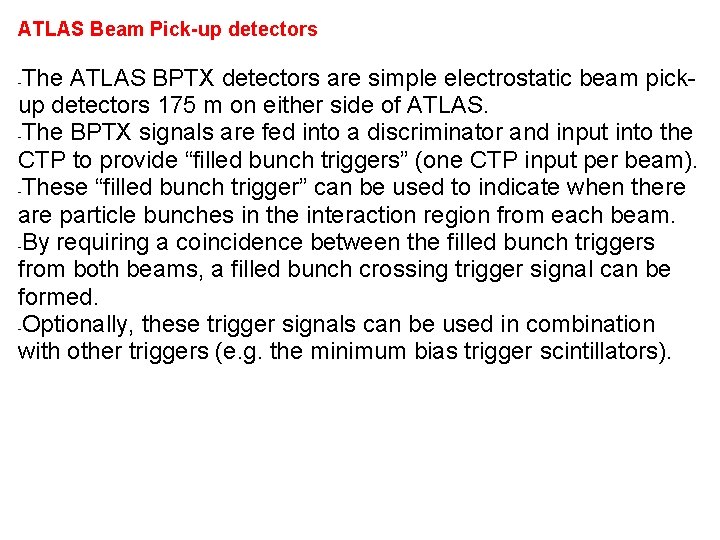
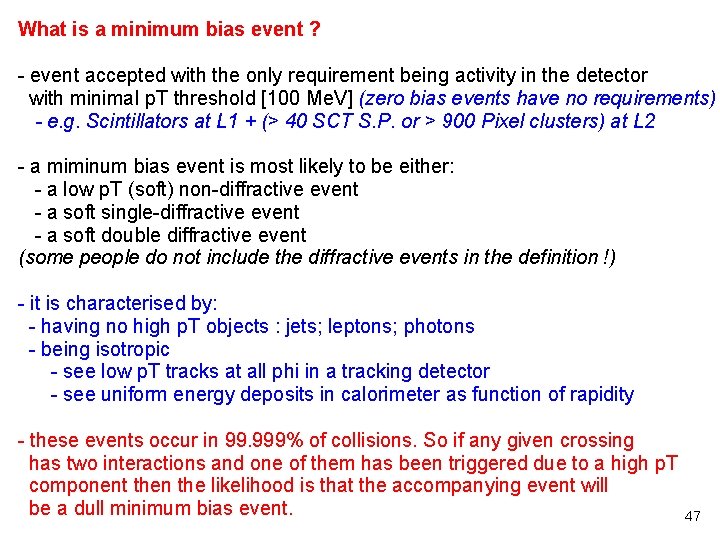
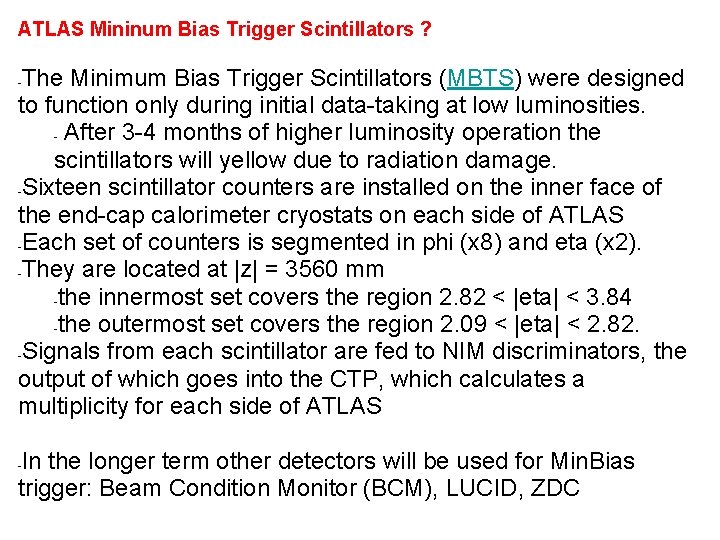
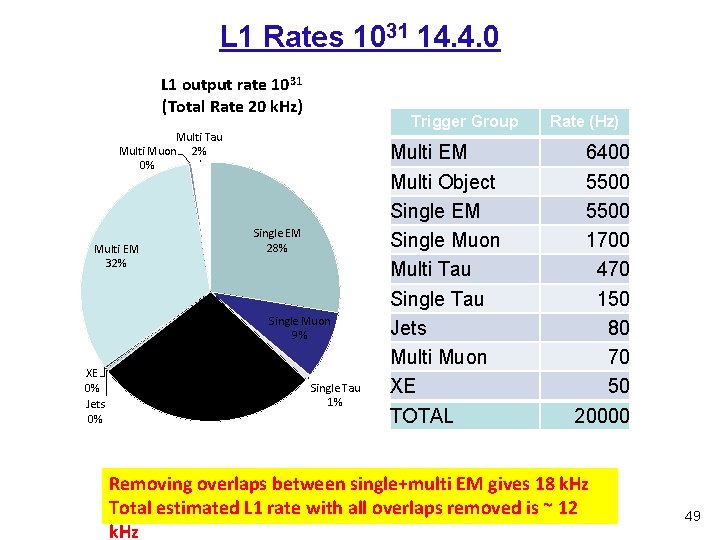
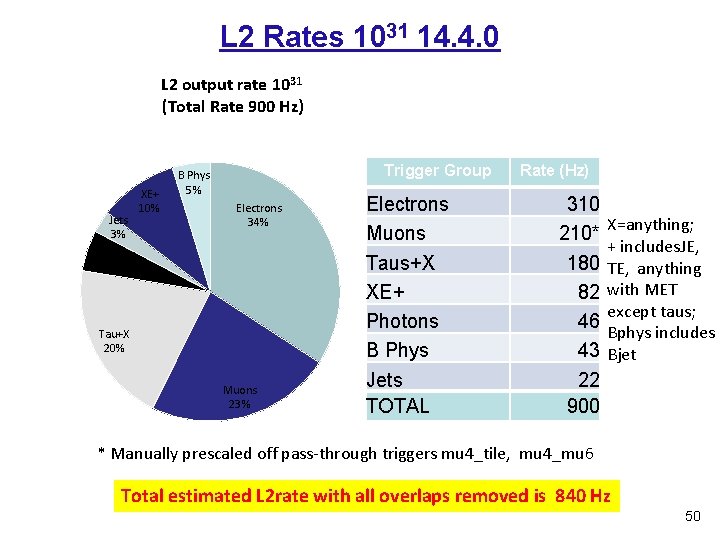
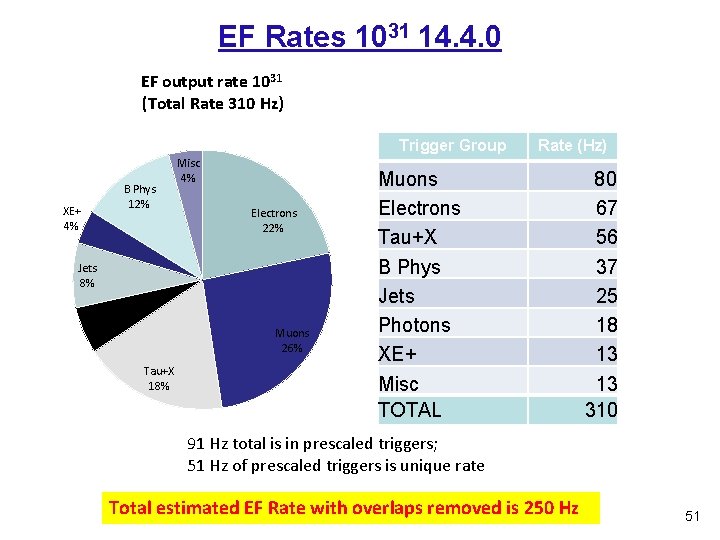
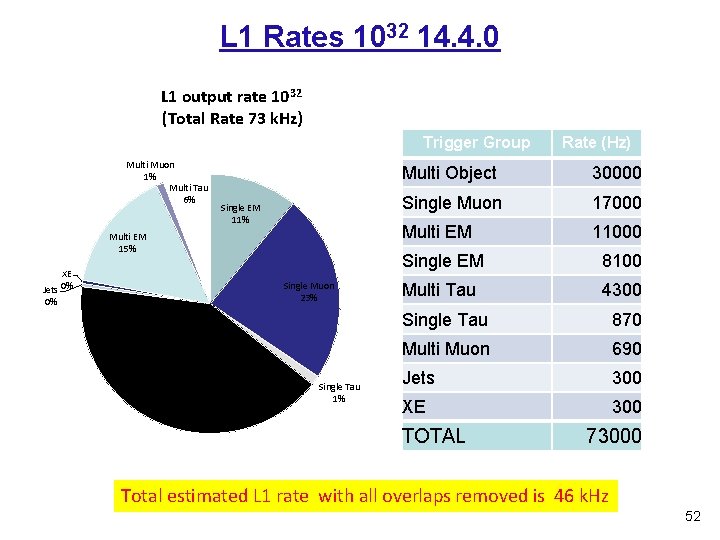
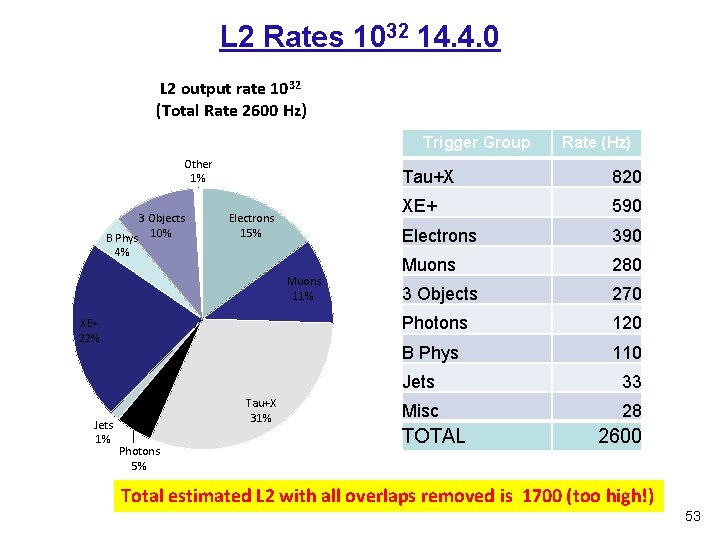
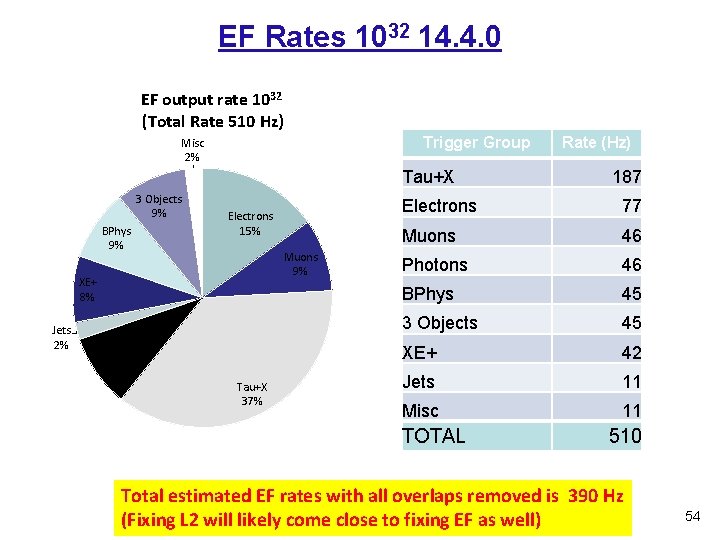
- Slides: 54
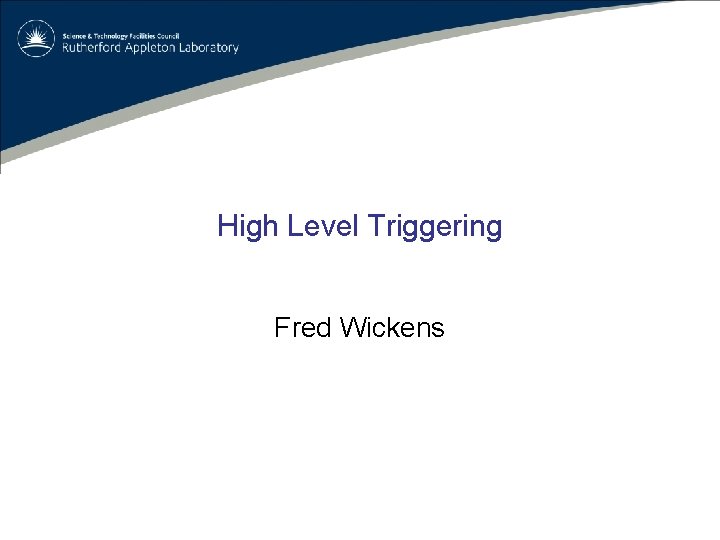
High Level Triggering Fred Wickens
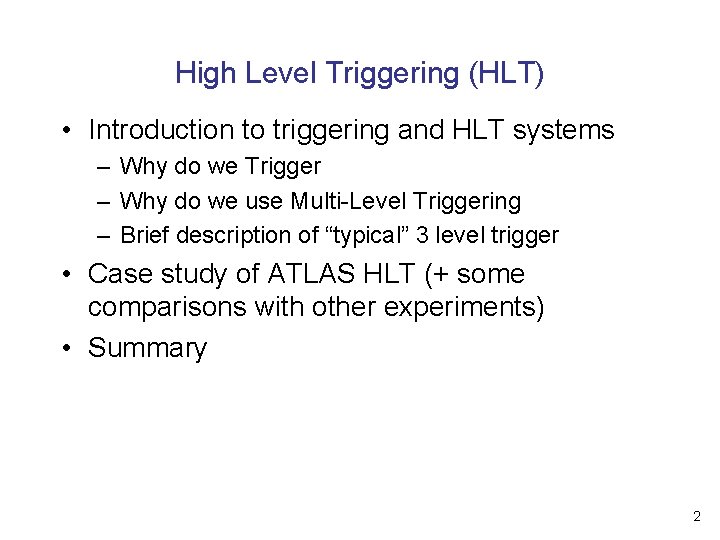
High Level Triggering (HLT) • Introduction to triggering and HLT systems – Why do we Trigger – Why do we use Multi-Level Triggering – Brief description of “typical” 3 level trigger • Case study of ATLAS HLT (+ some comparisons with other experiments) • Summary 2
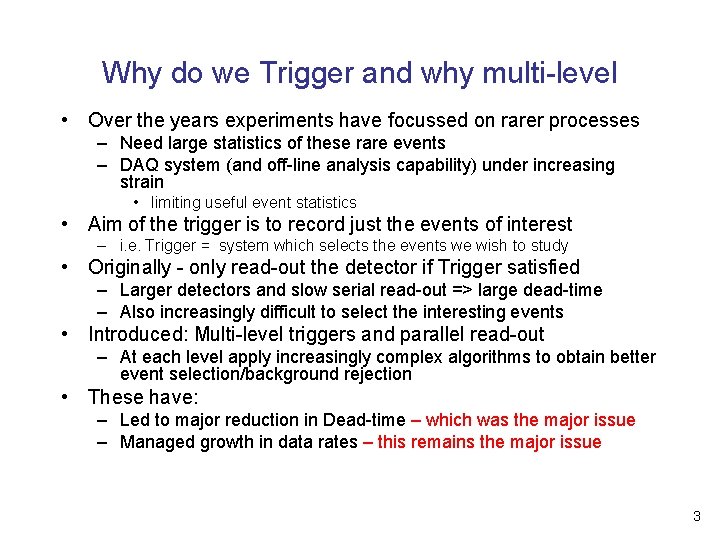
Why do we Trigger and why multi-level • Over the years experiments have focussed on rarer processes – Need large statistics of these rare events – DAQ system (and off-line analysis capability) under increasing strain • limiting useful event statistics • Aim of the trigger is to record just the events of interest – i. e. Trigger = system which selects the events we wish to study • Originally - only read-out the detector if Trigger satisfied – Larger detectors and slow serial read-out => large dead-time – Also increasingly difficult to select the interesting events • Introduced: Multi-level triggers and parallel read-out – At each level apply increasingly complex algorithms to obtain better event selection/background rejection • These have: – Led to major reduction in Dead-time – which was the major issue – Managed growth in data rates – this remains the major issue 3
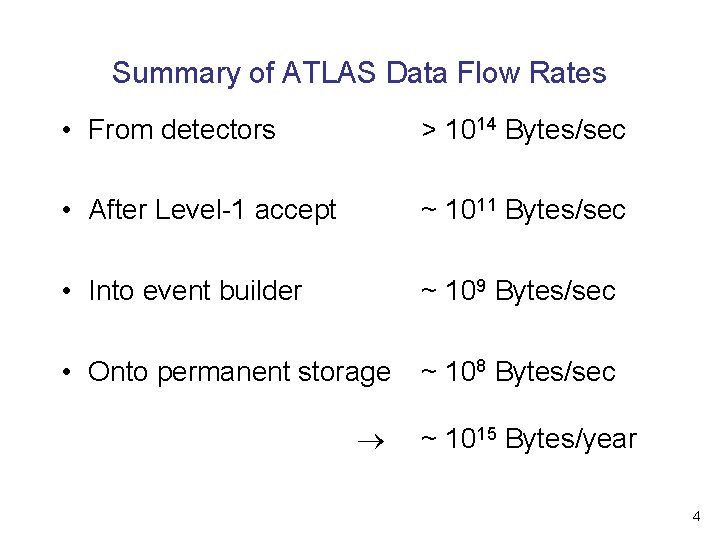
Summary of ATLAS Data Flow Rates • From detectors > 1014 Bytes/sec • After Level-1 accept ~ 1011 Bytes/sec • Into event builder ~ 109 Bytes/sec • Onto permanent storage ~ 108 Bytes/sec ~ 1015 Bytes/year 4
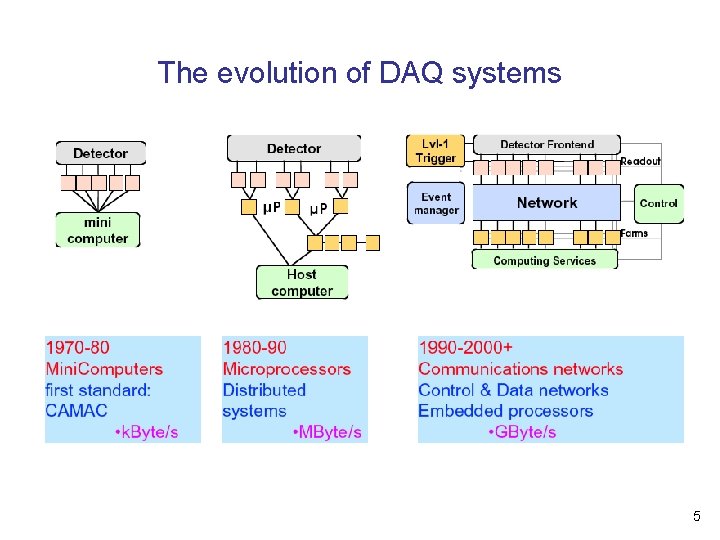
The evolution of DAQ systems 5
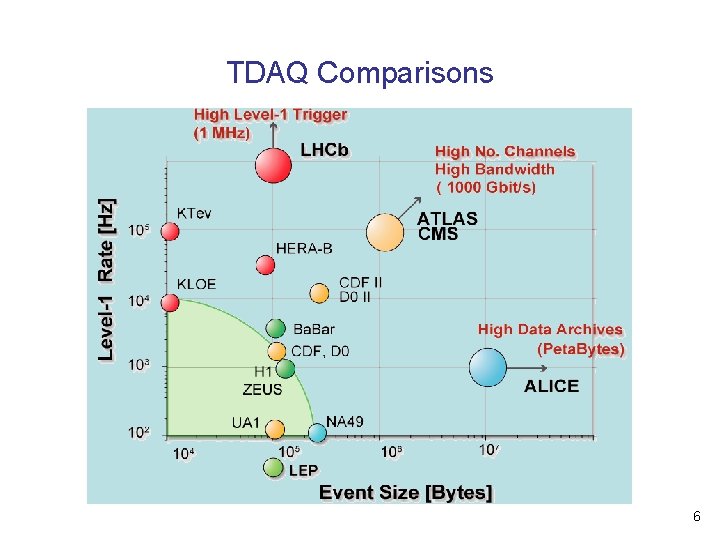
TDAQ Comparisons 6
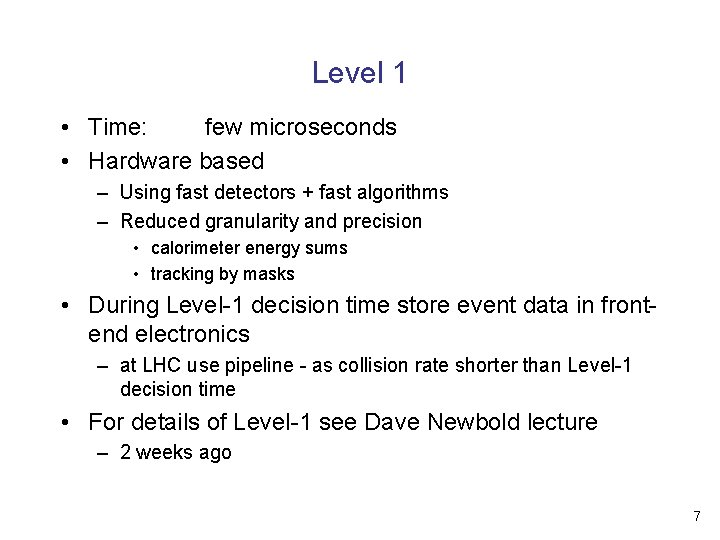
Level 1 • Time: few microseconds • Hardware based – Using fast detectors + fast algorithms – Reduced granularity and precision • calorimeter energy sums • tracking by masks • During Level-1 decision time store event data in frontend electronics – at LHC use pipeline - as collision rate shorter than Level-1 decision time • For details of Level-1 see Dave Newbold lecture – 2 weeks ago 7
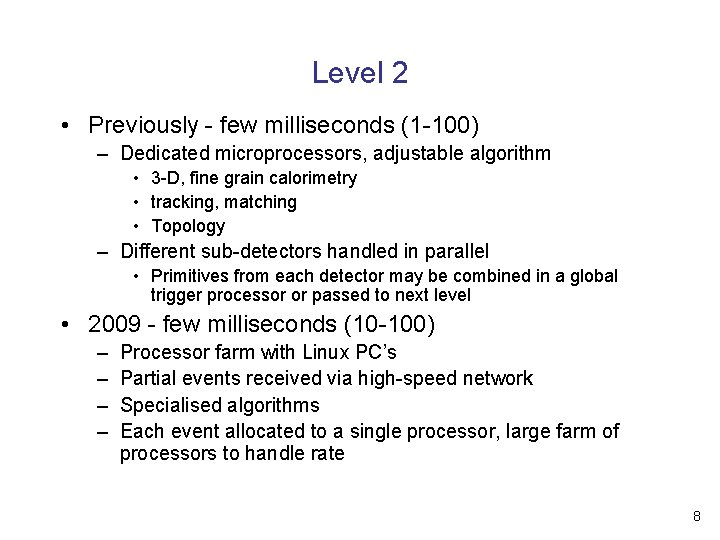
Level 2 • Previously - few milliseconds (1 -100) – Dedicated microprocessors, adjustable algorithm • 3 -D, fine grain calorimetry • tracking, matching • Topology – Different sub-detectors handled in parallel • Primitives from each detector may be combined in a global trigger processor or passed to next level • 2009 - few milliseconds (10 -100) – – Processor farm with Linux PC’s Partial events received via high-speed network Specialised algorithms Each event allocated to a single processor, large farm of processors to handle rate 8
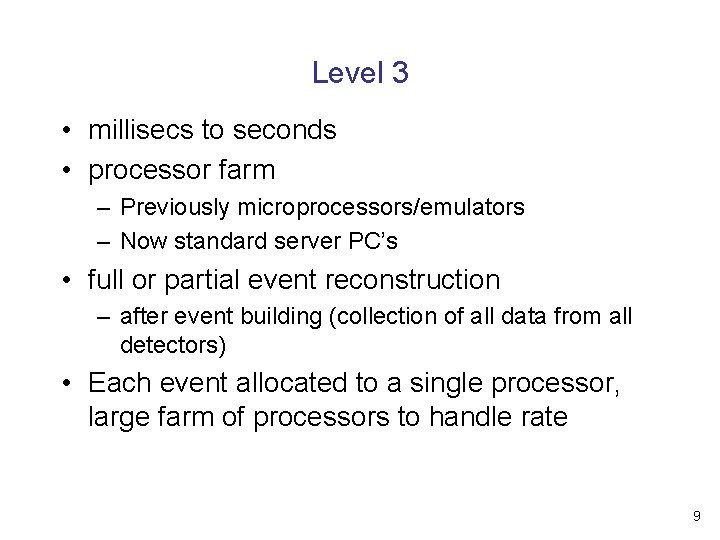
Level 3 • millisecs to seconds • processor farm – Previously microprocessors/emulators – Now standard server PC’s • full or partial event reconstruction – after event building (collection of all data from all detectors) • Each event allocated to a single processor, large farm of processors to handle rate 9
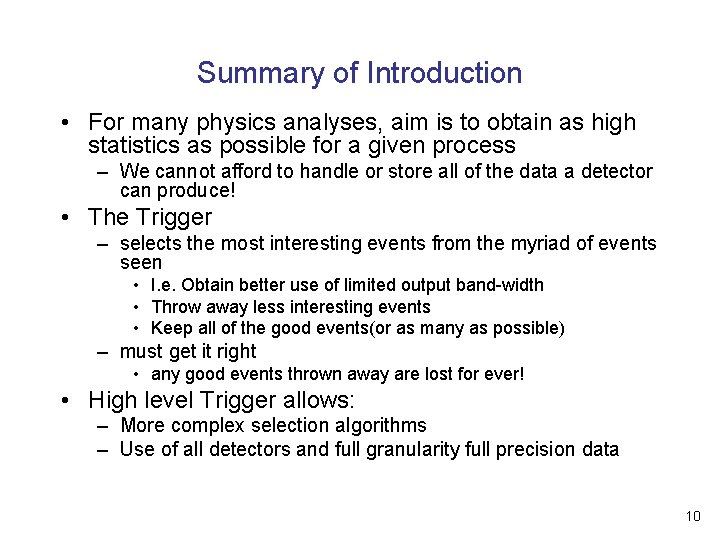
Summary of Introduction • For many physics analyses, aim is to obtain as high statistics as possible for a given process – We cannot afford to handle or store all of the data a detector can produce! • The Trigger – selects the most interesting events from the myriad of events seen • I. e. Obtain better use of limited output band-width • Throw away less interesting events • Keep all of the good events(or as many as possible) – must get it right • any good events thrown away are lost for ever! • High level Trigger allows: – More complex selection algorithms – Use of all detectors and full granularity full precision data 10
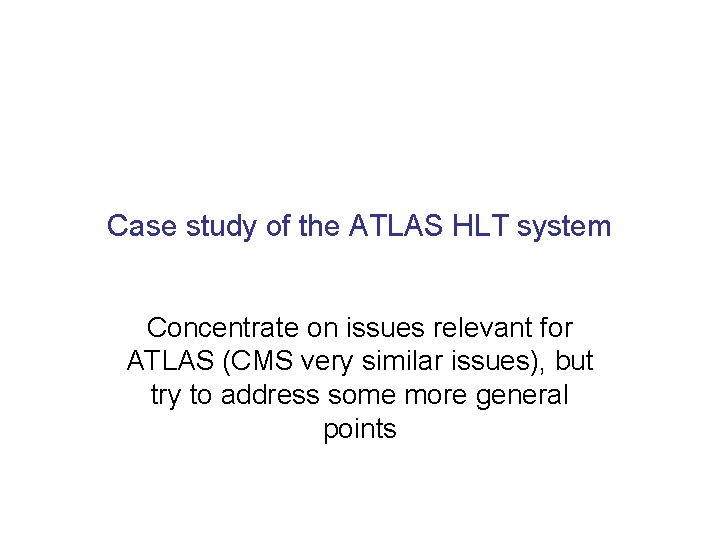
Case study of the ATLAS HLT system Concentrate on issues relevant for ATLAS (CMS very similar issues), but try to address some more general points
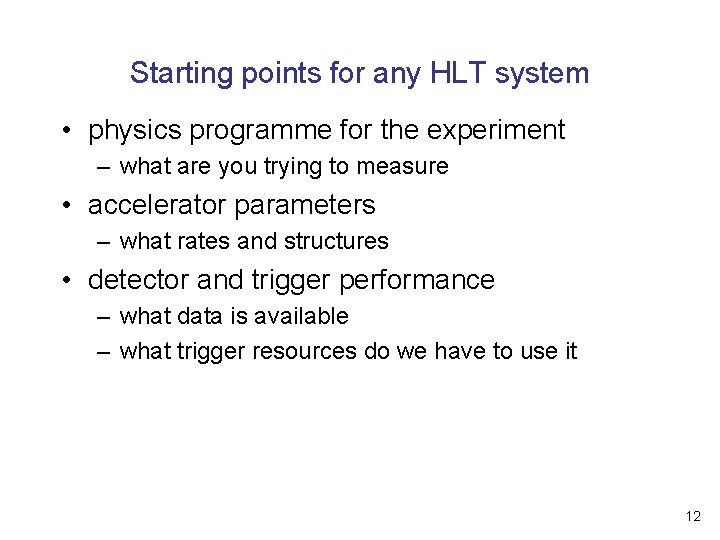
Starting points for any HLT system • physics programme for the experiment – what are you trying to measure • accelerator parameters – what rates and structures • detector and trigger performance – what data is available – what trigger resources do we have to use it 12
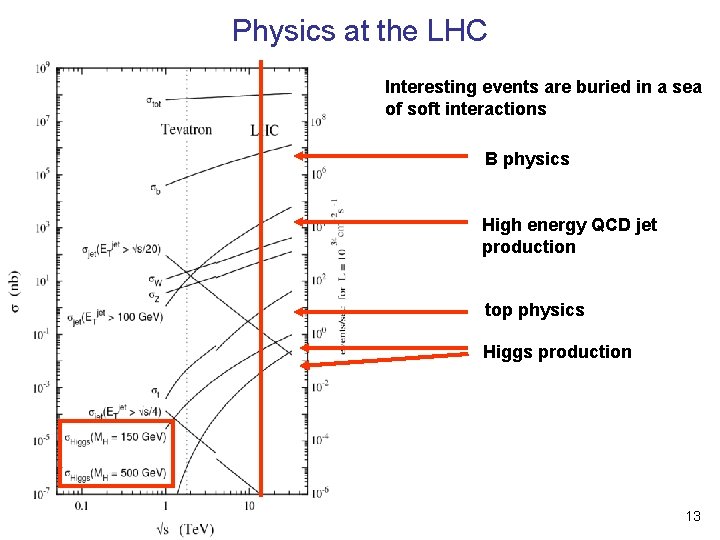
Physics at the LHC Interesting events are buried in a sea of soft interactions B physics High energy QCD jet production top physics Higgs production 13
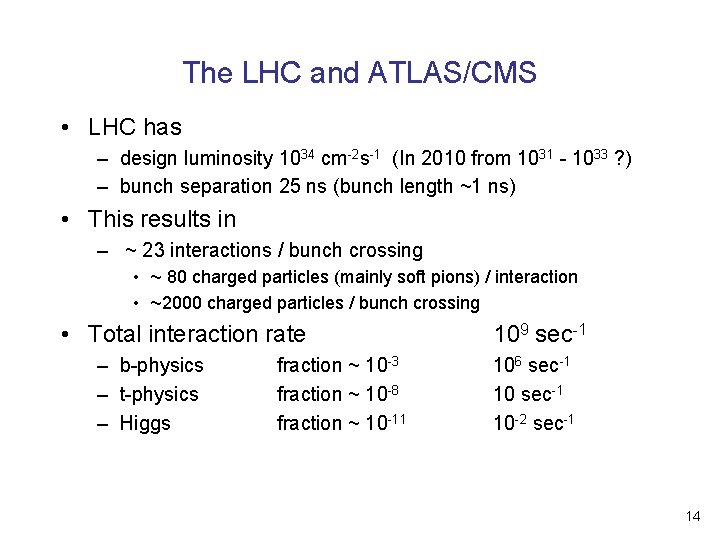
The LHC and ATLAS/CMS • LHC has – design luminosity 1034 cm-2 s-1 (In 2010 from 1031 - 1033 ? ) – bunch separation 25 ns (bunch length ~1 ns) • This results in – ~ 23 interactions / bunch crossing • ~ 80 charged particles (mainly soft pions) / interaction • ~2000 charged particles / bunch crossing • Total interaction rate – b-physics – t-physics – Higgs fraction ~ 10 -3 fraction ~ 10 -8 fraction ~ 10 -11 109 sec-1 106 sec-1 10 -2 sec-1 14
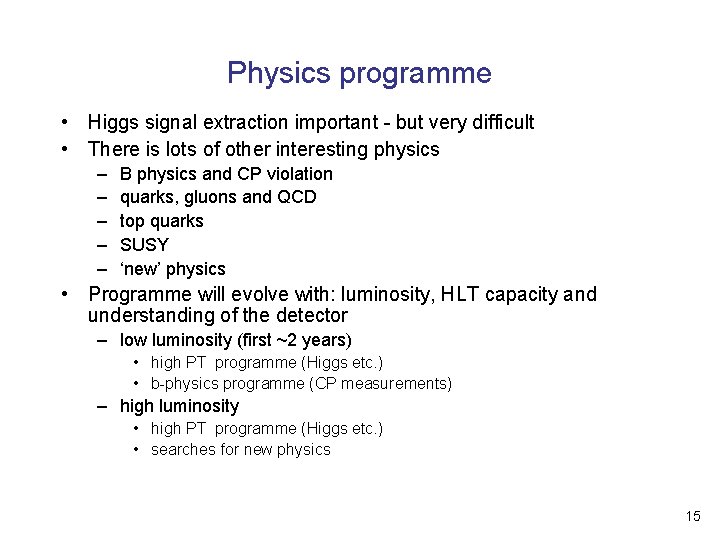
Physics programme • Higgs signal extraction important - but very difficult • There is lots of other interesting physics – – – B physics and CP violation quarks, gluons and QCD top quarks SUSY ‘new’ physics • Programme will evolve with: luminosity, HLT capacity and understanding of the detector – low luminosity (first ~2 years) • high PT programme (Higgs etc. ) • b-physics programme (CP measurements) – high luminosity • high PT programme (Higgs etc. ) • searches for new physics 15
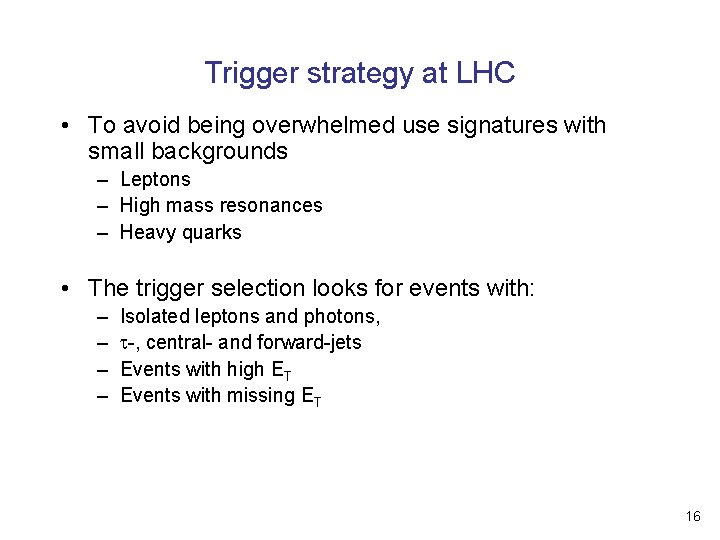
Trigger strategy at LHC • To avoid being overwhelmed use signatures with small backgrounds – Leptons – High mass resonances – Heavy quarks • The trigger selection looks for events with: – – Isolated leptons and photons, -, central- and forward-jets Events with high ET Events with missing ET 16
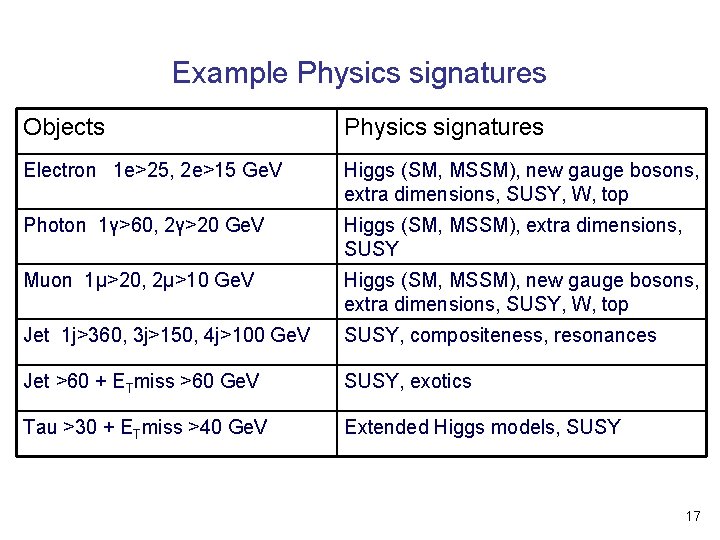
Example Physics signatures Objects Physics signatures Electron 1 e>25, 2 e>15 Ge. V Higgs (SM, MSSM), new gauge bosons, extra dimensions, SUSY, W, top Photon 1γ>60, 2γ>20 Ge. V Higgs (SM, MSSM), extra dimensions, SUSY Muon 1μ>20, 2μ>10 Ge. V Higgs (SM, MSSM), new gauge bosons, extra dimensions, SUSY, W, top Jet 1 j>360, 3 j>150, 4 j>100 Ge. V SUSY, compositeness, resonances Jet >60 + ETmiss >60 Ge. V SUSY, exotics Tau >30 + ETmiss >40 Ge. V Extended Higgs models, SUSY 17
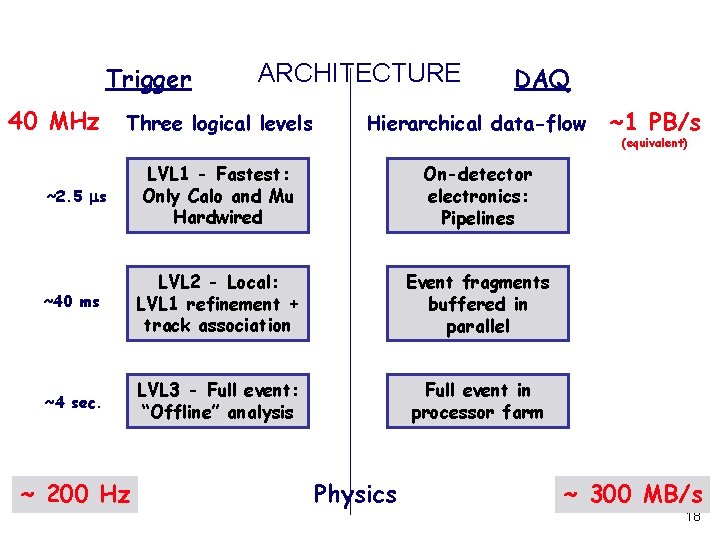
Trigger 40 MHz ARCHITECTURE DAQ Three logical levels Hierarchical data-flow LVL 1 - Fastest: Only Calo and Mu Hardwired On-detector electronics: Pipelines ~40 ms LVL 2 - Local: LVL 1 refinement + track association Event fragments buffered in parallel ~4 sec. LVL 3 - Full event: “Offline” analysis Full event in processor farm ~2. 5 ms ~ 200 Hz Physics ~1 PB/s (equivalent) ~ 300 MB/s 18
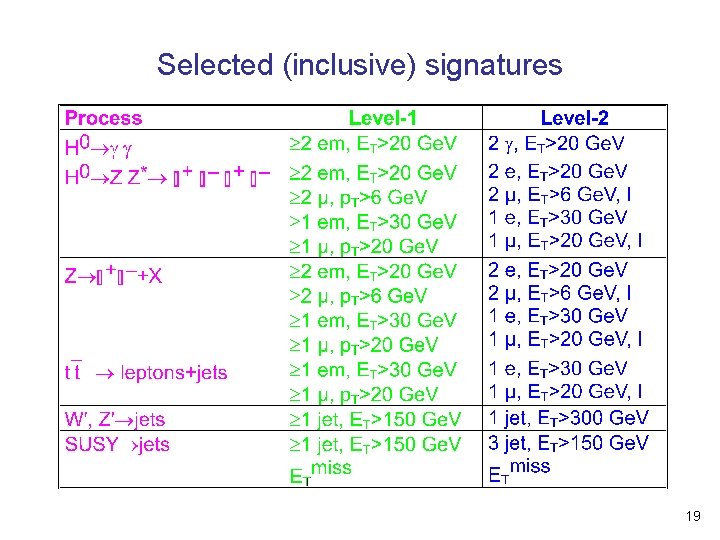
Selected (inclusive) signatures 19
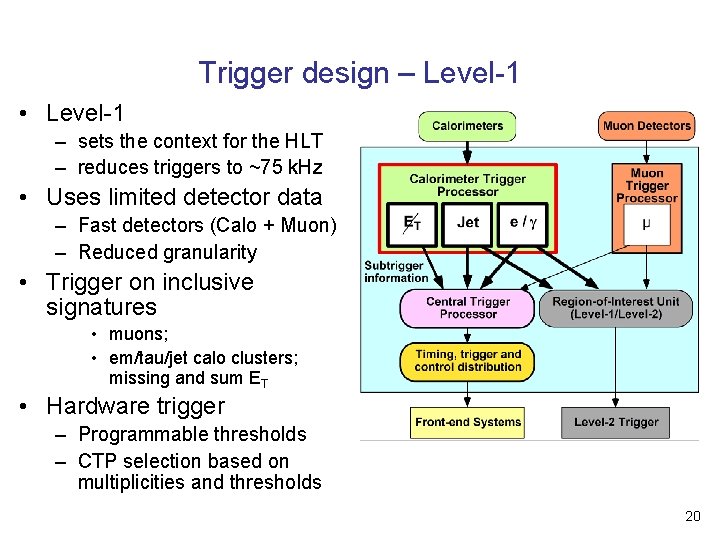
Trigger design – Level-1 • Level-1 – sets the context for the HLT – reduces triggers to ~75 k. Hz • Uses limited detector data – Fast detectors (Calo + Muon) – Reduced granularity • Trigger on inclusive signatures • muons; • em/tau/jet calo clusters; missing and sum ET • Hardware trigger – Programmable thresholds – CTP selection based on multiplicities and thresholds 20
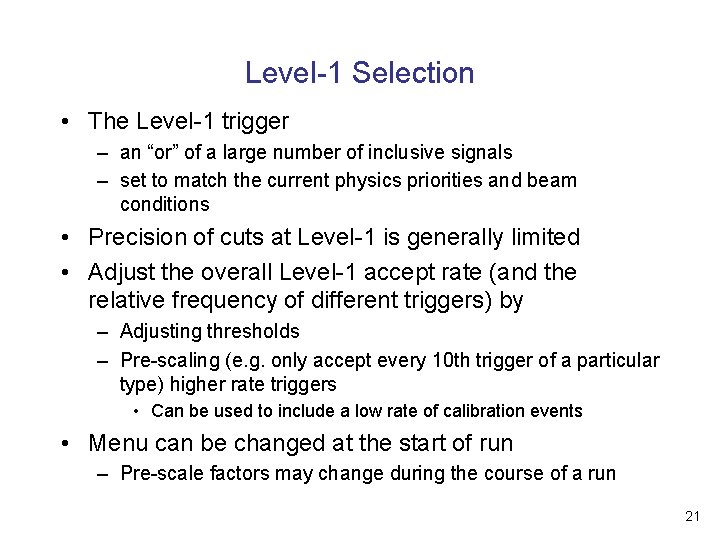
Level-1 Selection • The Level-1 trigger – an “or” of a large number of inclusive signals – set to match the current physics priorities and beam conditions • Precision of cuts at Level-1 is generally limited • Adjust the overall Level-1 accept rate (and the relative frequency of different triggers) by – Adjusting thresholds – Pre-scaling (e. g. only accept every 10 th trigger of a particular type) higher rate triggers • Can be used to include a low rate of calibration events • Menu can be changed at the start of run – Pre-scale factors may change during the course of a run 21
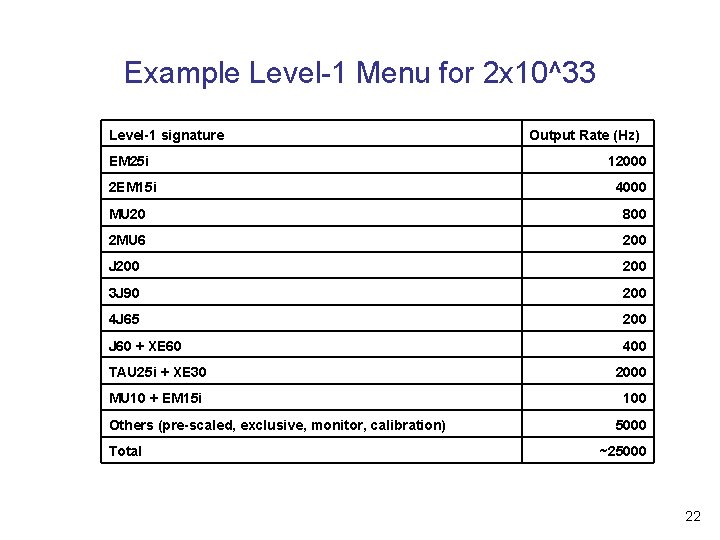
Example Level-1 Menu for 2 x 10^33 Level-1 signature Output Rate (Hz) EM 25 i 12000 2 EM 15 i 4000 MU 20 800 2 MU 6 200 J 200 3 J 90 200 4 J 65 200 J 60 + XE 60 400 TAU 25 i + XE 30 2000 MU 10 + EM 15 i 100 Others (pre-scaled, exclusive, monitor, calibration) Total 5000 ~25000 22
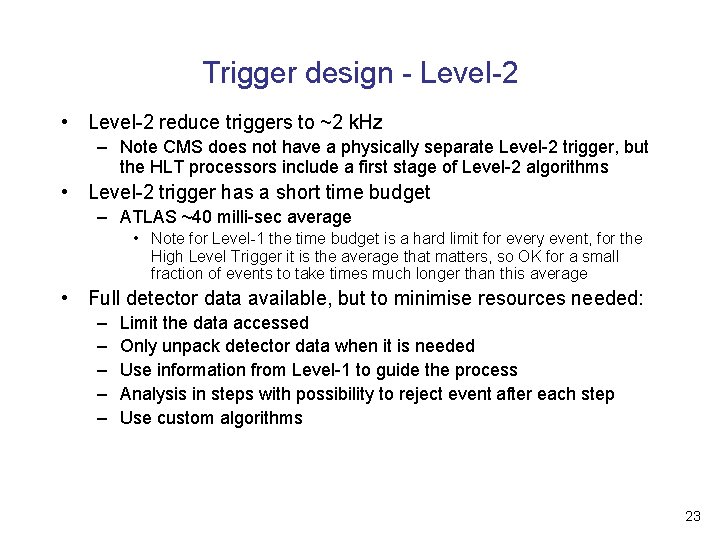
Trigger design - Level-2 • Level-2 reduce triggers to ~2 k. Hz – Note CMS does not have a physically separate Level-2 trigger, but the HLT processors include a first stage of Level-2 algorithms • Level-2 trigger has a short time budget – ATLAS ~40 milli-sec average • Note for Level-1 the time budget is a hard limit for every event, for the High Level Trigger it is the average that matters, so OK for a small fraction of events to take times much longer than this average • Full detector data available, but to minimise resources needed: – – – Limit the data accessed Only unpack detector data when it is needed Use information from Level-1 to guide the process Analysis in steps with possibility to reject event after each step Use custom algorithms 23
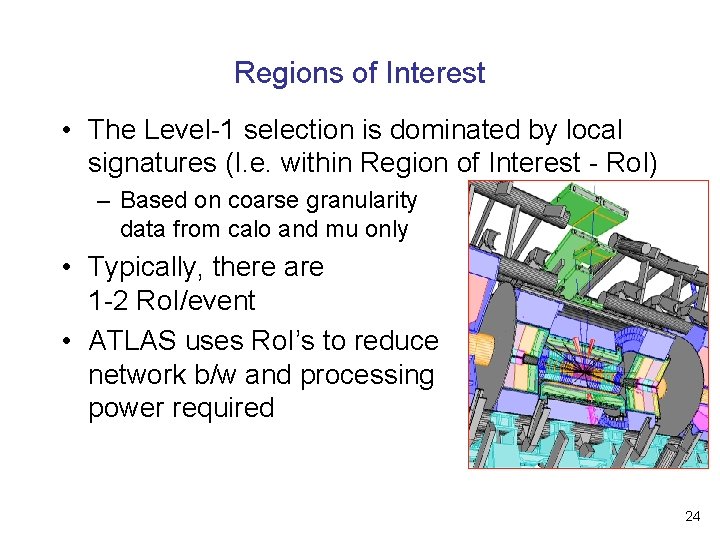
Regions of Interest • The Level-1 selection is dominated by local signatures (I. e. within Region of Interest - Ro. I) – Based on coarse granularity data from calo and mu only • Typically, there are 1 -2 Ro. I/event • ATLAS uses Ro. I’s to reduce network b/w and processing power required 24
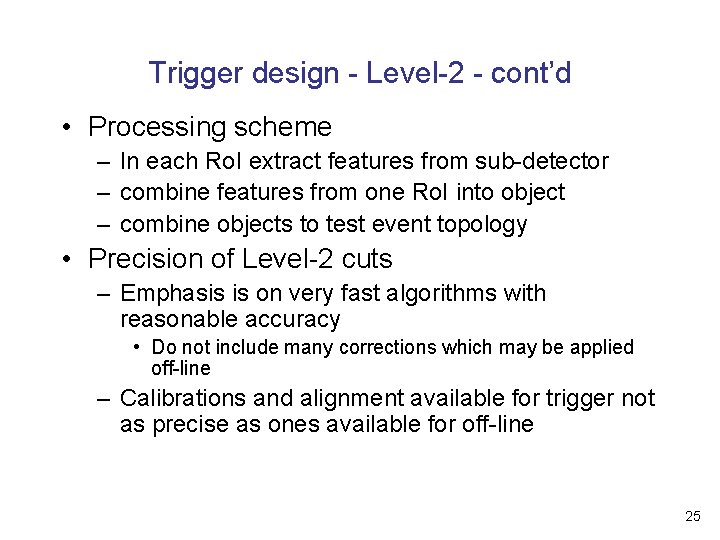
Trigger design - Level-2 - cont’d • Processing scheme – In each Ro. I extract features from sub-detector – combine features from one Ro. I into object – combine objects to test event topology • Precision of Level-2 cuts – Emphasis is on very fast algorithms with reasonable accuracy • Do not include many corrections which may be applied off-line – Calibrations and alignment available for trigger not as precise as ones available for off-line 25
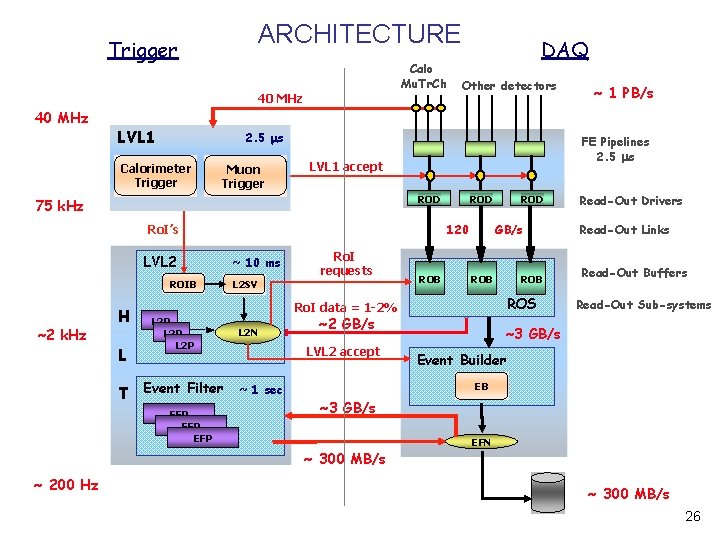
ARCHITECTURE Trigger Calo Mu. Tr. Ch 40 MHz LVL 1 Muon Trigger ROD ROIB L ROD 120 Ro. I’s LVL 2 ~ 10 ms Ro. I requests L 2 SV ROB ROD GB/s ROB ROS Ro. I data = 1 -2% L 2 P T Event Filter EFP EFP L 2 N ~2 GB/s LVL 2 accept ~ 1 sec ~ 1 PB/s FE Pipelines 2. 5 ms LVL 1 accept 75 k. Hz ~2 k. Hz Other detectors 2. 5 ms Calorimeter Trigger H DAQ Read-Out Drivers Read-Out Links Read-Out Buffers Read-Out Sub-systems ~3 GB/s Event Builder EB ~3 GB/s EFN ~ 300 MB/s ~ 200 Hz ~ 300 MB/s 26
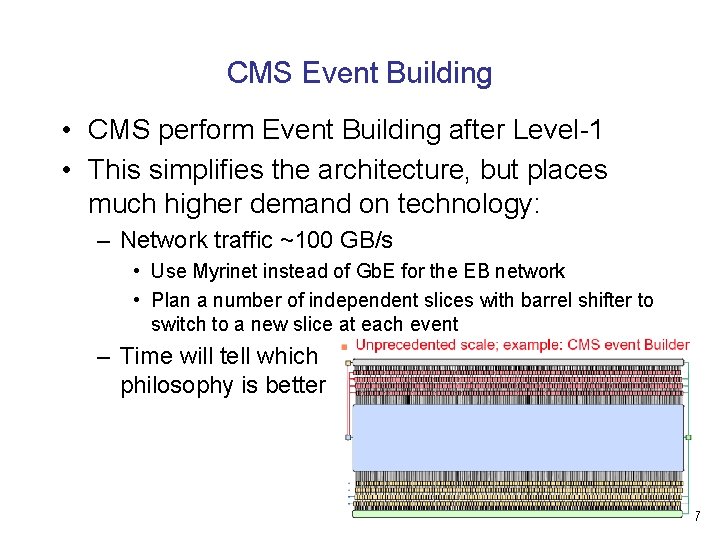
CMS Event Building • CMS perform Event Building after Level-1 • This simplifies the architecture, but places much higher demand on technology: – Network traffic ~100 GB/s • Use Myrinet instead of Gb. E for the EB network • Plan a number of independent slices with barrel shifter to switch to a new slice at each event – Time will tell which philosophy is better 27
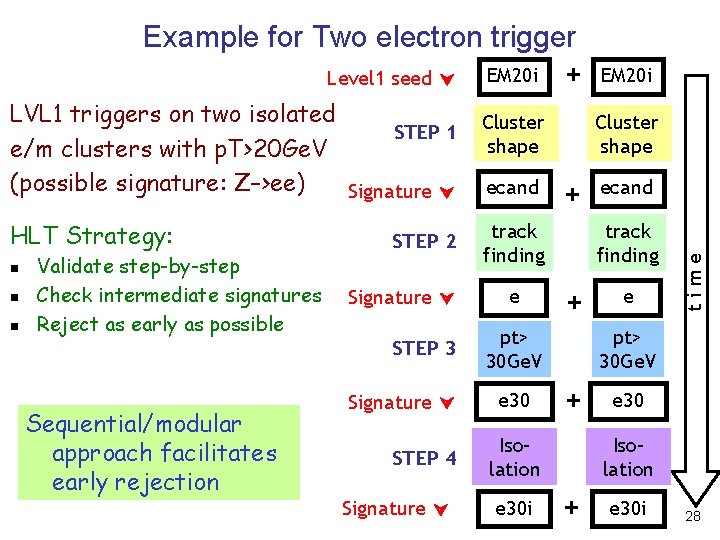
Example for Two electron trigger Level 1 seed EM 20 i + EM 20 i HLT Strategy: n n n Validate step-by-step Check intermediate signatures Reject as early as possible Sequential/modular approach facilitates early rejection STEP 2 Signature STEP 3 Signature STEP 4 Signature track finding e track finding + pt> 30 Ge. V e 30 pt> 30 Ge. V + Iso– lation e 30 i e time LVL 1 triggers on two isolated Cluster STEP 1 shape e/m clusters with p. T>20 Ge. V (possible signature: Z–>ee) ecand + ecand Signature e 30 Iso– lation + e 30 i 28
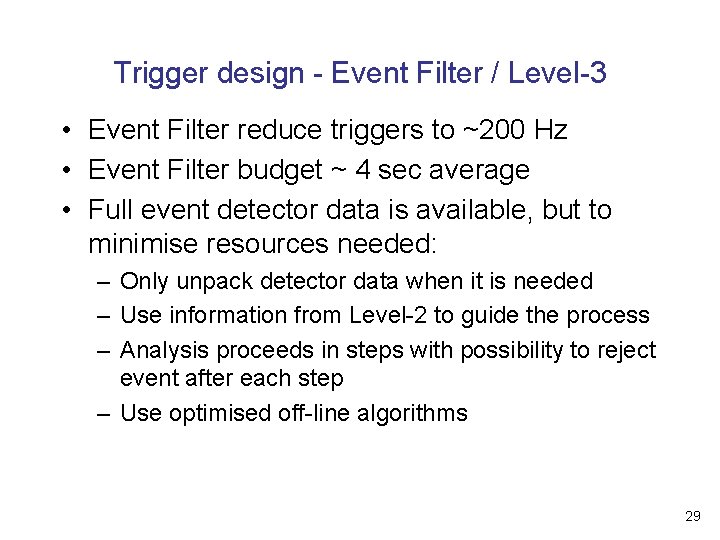
Trigger design - Event Filter / Level-3 • Event Filter reduce triggers to ~200 Hz • Event Filter budget ~ 4 sec average • Full event detector data is available, but to minimise resources needed: – Only unpack detector data when it is needed – Use information from Level-2 to guide the process – Analysis proceeds in steps with possibility to reject event after each step – Use optimised off-line algorithms 29
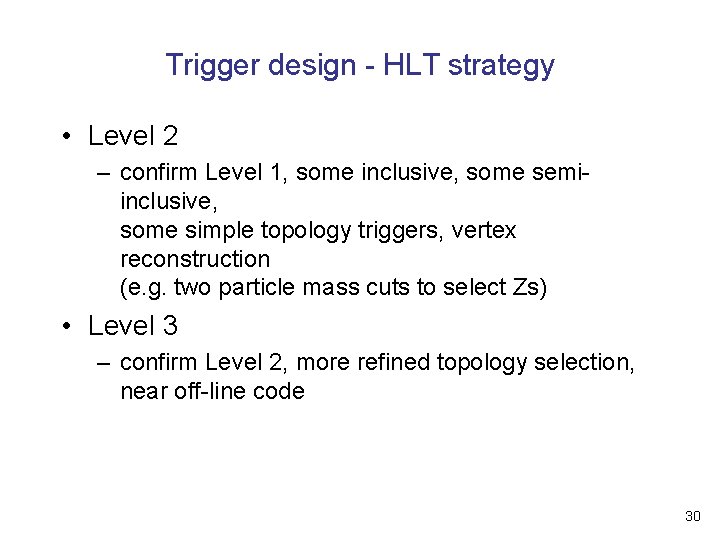
Trigger design - HLT strategy • Level 2 – confirm Level 1, some inclusive, some semiinclusive, some simple topology triggers, vertex reconstruction (e. g. two particle mass cuts to select Zs) • Level 3 – confirm Level 2, more refined topology selection, near off-line code 30
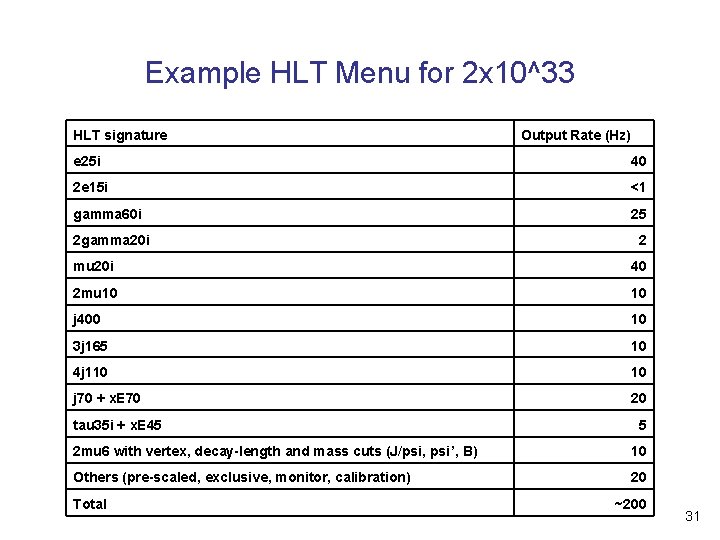
Example HLT Menu for 2 x 10^33 HLT signature Output Rate (Hz) e 25 i 40 2 e 15 i <1 gamma 60 i 25 2 gamma 20 i 2 mu 20 i 40 2 mu 10 10 j 400 10 3 j 165 10 4 j 110 10 j 70 + x. E 70 20 tau 35 i + x. E 45 5 2 mu 6 with vertex, decay-length and mass cuts (J/psi, psi’, B) 10 Others (pre-scaled, exclusive, monitor, calibration) 20 Total ~200 31
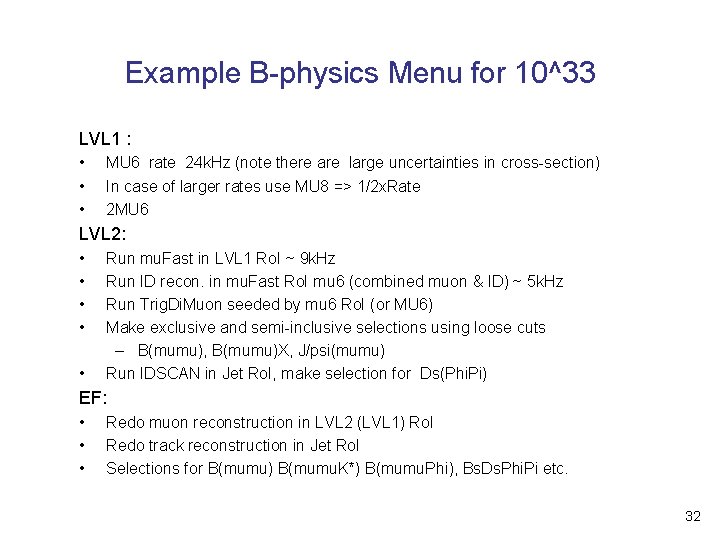
Example B-physics Menu for 10^33 LVL 1 : • • • MU 6 rate 24 k. Hz (note there are large uncertainties in cross-section) In case of larger rates use MU 8 => 1/2 x. Rate 2 MU 6 LVL 2: • • • Run mu. Fast in LVL 1 Ro. I ~ 9 k. Hz Run ID recon. in mu. Fast Ro. I mu 6 (combined muon & ID) ~ 5 k. Hz Run Trig. Di. Muon seeded by mu 6 Ro. I (or MU 6) Make exclusive and semi-inclusive selections using loose cuts – B(mumu), B(mumu)X, J/psi(mumu) Run IDSCAN in Jet Ro. I, make selection for Ds(Phi. Pi) EF: • • • Redo muon reconstruction in LVL 2 (LVL 1) Ro. I Redo track reconstruction in Jet Ro. I Selections for B(mumu) B(mumu. K*) B(mumu. Phi), Bs. Ds. Phi. Pi etc. 32
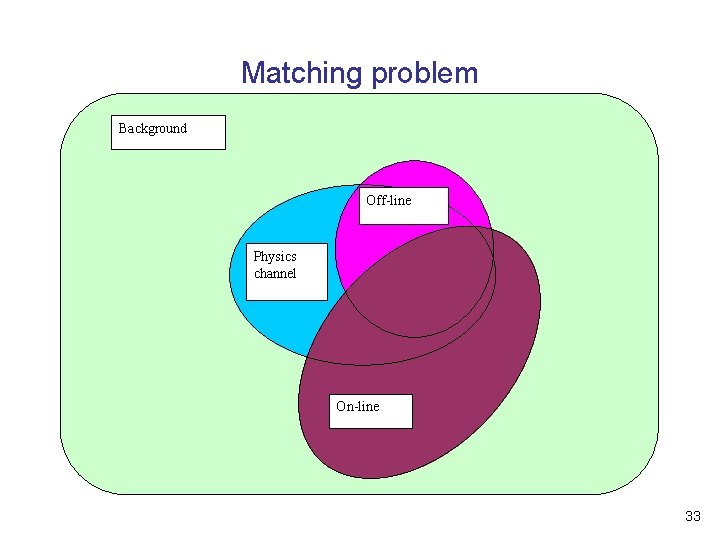
Matching problem Background Off-line Physics channel On-line 33
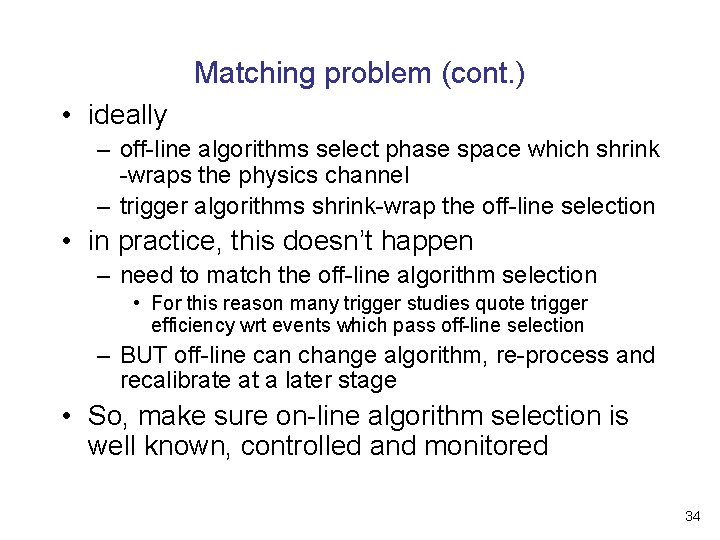
Matching problem (cont. ) • ideally – off-line algorithms select phase space which shrink -wraps the physics channel – trigger algorithms shrink-wrap the off-line selection • in practice, this doesn’t happen – need to match the off-line algorithm selection • For this reason many trigger studies quote trigger efficiency wrt events which pass off-line selection – BUT off-line can change algorithm, re-process and recalibrate at a later stage • So, make sure on-line algorithm selection is well known, controlled and monitored 34
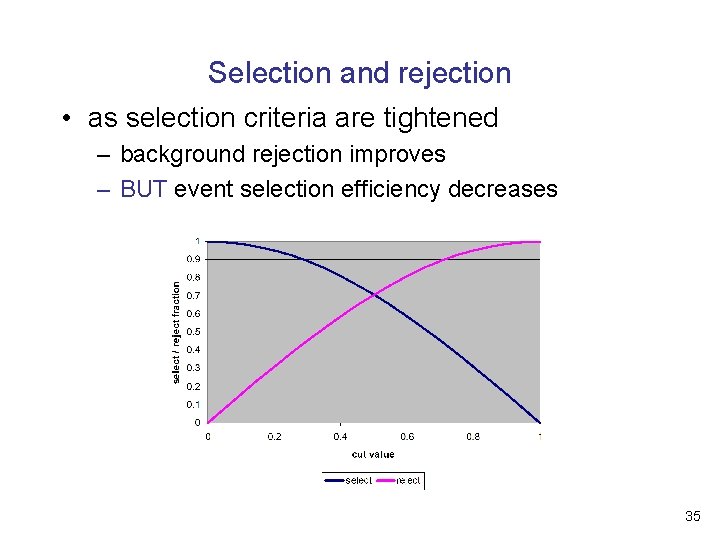
Selection and rejection • as selection criteria are tightened – background rejection improves – BUT event selection efficiency decreases 35
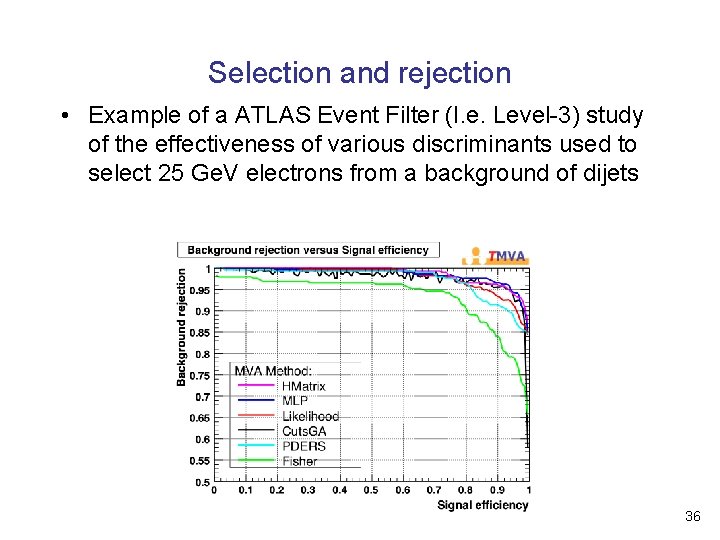
Selection and rejection • Example of a ATLAS Event Filter (I. e. Level-3) study of the effectiveness of various discriminants used to select 25 Ge. V electrons from a background of dijets 36
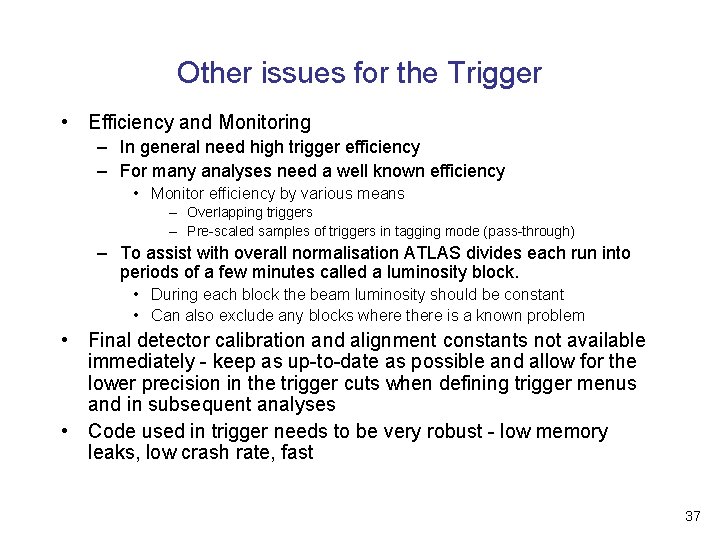
Other issues for the Trigger • Efficiency and Monitoring – In general need high trigger efficiency – For many analyses need a well known efficiency • Monitor efficiency by various means – Overlapping triggers – Pre-scaled samples of triggers in tagging mode (pass-through) – To assist with overall normalisation ATLAS divides each run into periods of a few minutes called a luminosity block. • During each block the beam luminosity should be constant • Can also exclude any blocks where there is a known problem • Final detector calibration and alignment constants not available immediately - keep as up-to-date as possible and allow for the lower precision in the trigger cuts when defining trigger menus and in subsequent analyses • Code used in trigger needs to be very robust - low memory leaks, low crash rate, fast 37
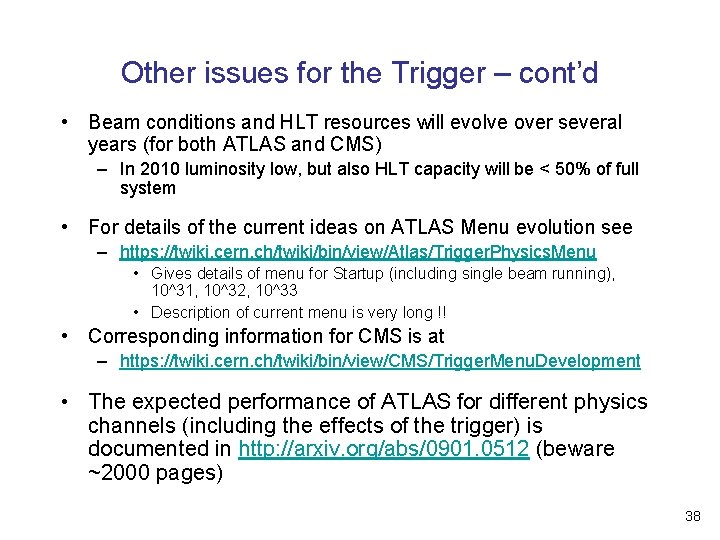
Other issues for the Trigger – cont’d • Beam conditions and HLT resources will evolve over several years (for both ATLAS and CMS) – In 2010 luminosity low, but also HLT capacity will be < 50% of full system • For details of the current ideas on ATLAS Menu evolution see – https: //twiki. cern. ch/twiki/bin/view/Atlas/Trigger. Physics. Menu • Gives details of menu for Startup (including single beam running), 10^31, 10^32, 10^33 • Description of current menu is very long !! • Corresponding information for CMS is at – https: //twiki. cern. ch/twiki/bin/view/CMS/Trigger. Menu. Development • The expected performance of ATLAS for different physics channels (including the effects of the trigger) is documented in http: //arxiv. org/abs/0901. 0512 (beware ~2000 pages) 38
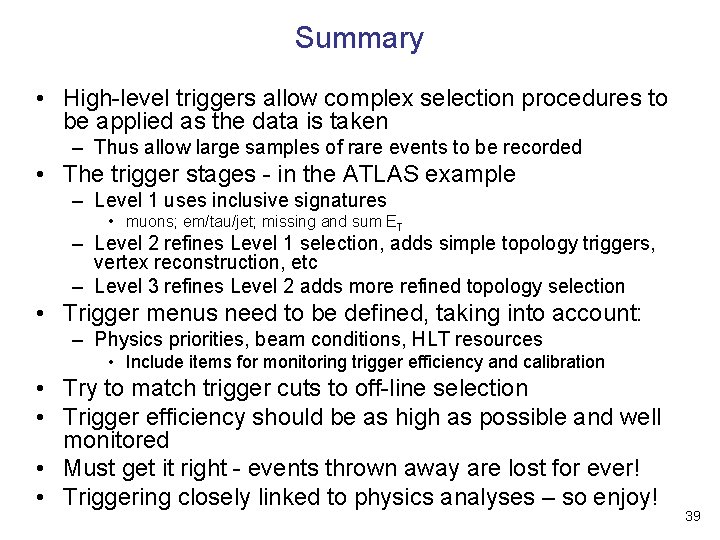
Summary • High-level triggers allow complex selection procedures to be applied as the data is taken – Thus allow large samples of rare events to be recorded • The trigger stages - in the ATLAS example – Level 1 uses inclusive signatures • muons; em/tau/jet; missing and sum ET – Level 2 refines Level 1 selection, adds simple topology triggers, vertex reconstruction, etc – Level 3 refines Level 2 adds more refined topology selection • Trigger menus need to be defined, taking into account: – Physics priorities, beam conditions, HLT resources • Include items for monitoring trigger efficiency and calibration • Try to match trigger cuts to off-line selection • Trigger efficiency should be as high as possible and well monitored • Must get it right - events thrown away are lost for ever! • Triggering closely linked to physics analyses – so enjoy! 39
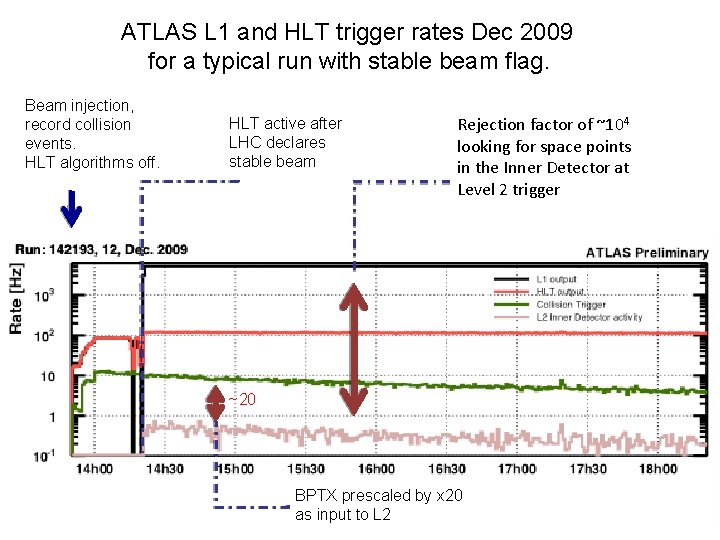
ATLAS L 1 and HLT trigger rates Dec 2009 for a typical run with stable beam flag. Beam injection, record collision events. HLT algorithms off. HLT active after LHC declares stable beam Rejection factor of ~104 looking for space points in the Inner Detector at Level 2 trigger ~20 BPTX prescaled by x 20 as input to L 2
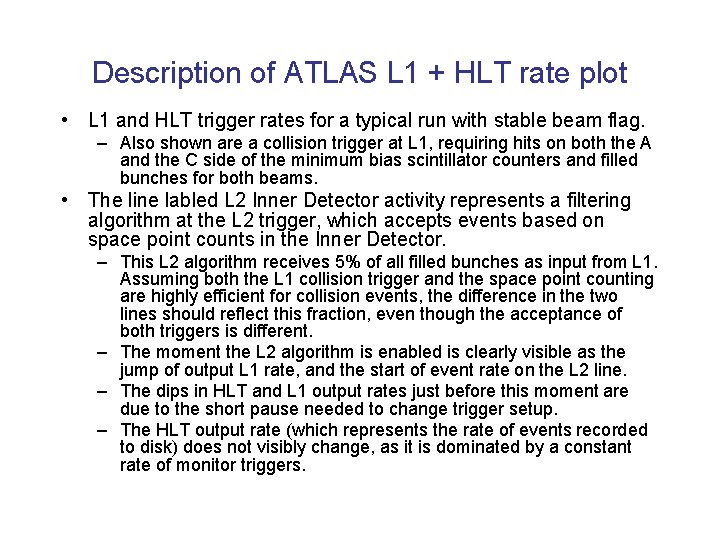
Description of ATLAS L 1 + HLT rate plot • L 1 and HLT trigger rates for a typical run with stable beam flag. – Also shown are a collision trigger at L 1, requiring hits on both the A and the C side of the minimum bias scintillator counters and filled bunches for both beams. • The line labled L 2 Inner Detector activity represents a filtering algorithm at the L 2 trigger, which accepts events based on space point counts in the Inner Detector. – This L 2 algorithm receives 5% of all filled bunches as input from L 1. Assuming both the L 1 collision trigger and the space point counting are highly efficient for collision events, the difference in the two lines should reflect this fraction, even though the acceptance of both triggers is different. – The moment the L 2 algorithm is enabled is clearly visible as the jump of output L 1 rate, and the start of event rate on the L 2 line. – The dips in HLT and L 1 output rates just before this moment are due to the short pause needed to change trigger setup. – The HLT output rate (which represents the rate of events recorded to disk) does not visibly change, as it is dominated by a constant rate of monitor triggers.
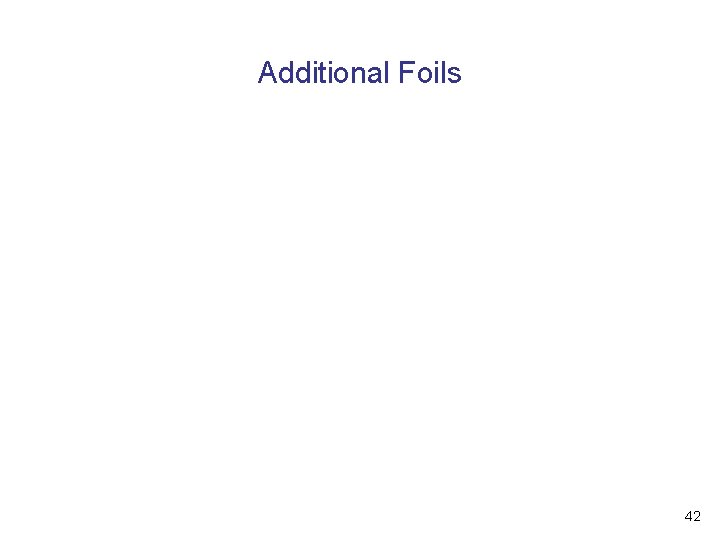
Additional Foils 42
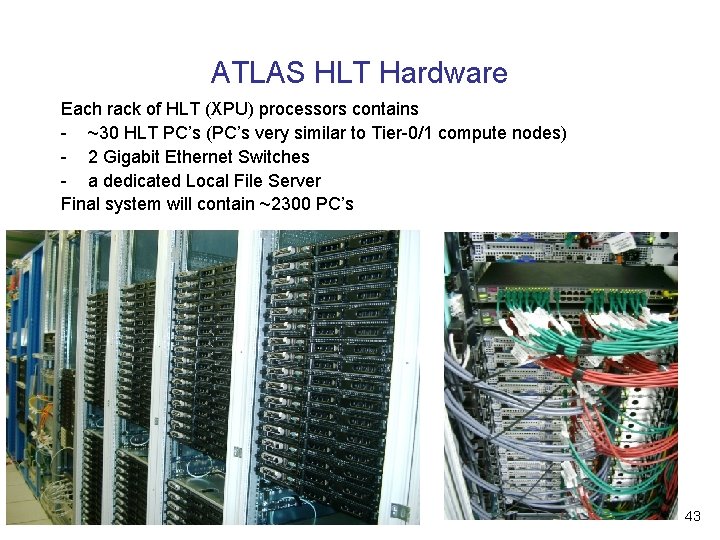
ATLAS HLT Hardware Each rack of HLT (XPU) processors contains - ~30 HLT PC’s (PC’s very similar to Tier-0/1 compute nodes) - 2 Gigabit Ethernet Switches - a dedicated Local File Server Final system will contain ~2300 PC’s 43
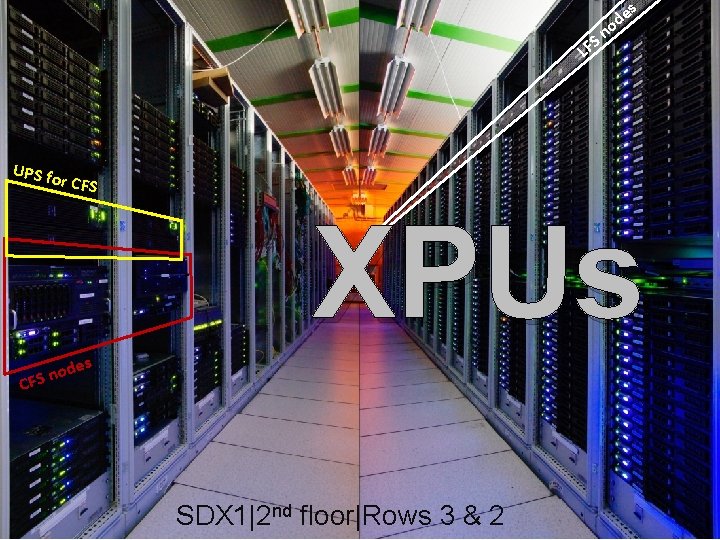
s e d o Sn LF UPS fo r CFS XPUs des o n FS C SDX 1|2 nd floor|Rows 3 & 2 44
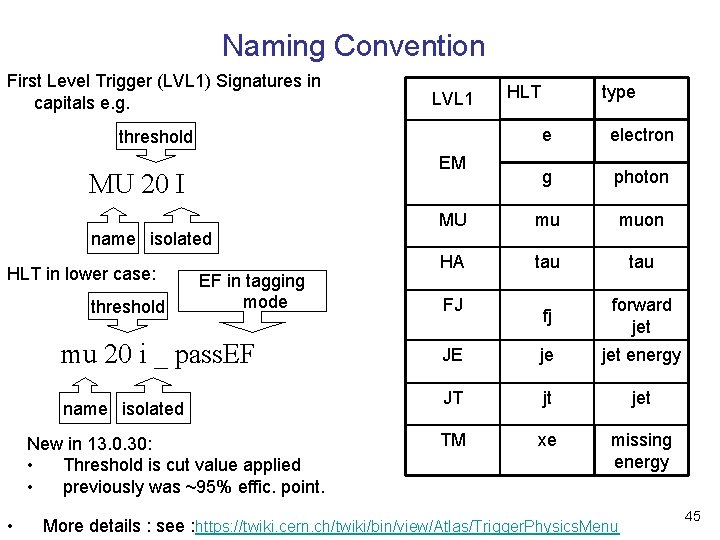
Naming Convention First Level Trigger (LVL 1) Signatures in capitals e. g. LVL 1 electron g photon MU mu muon HA tau fj forward jet JE je jet energy JT jt jet TM xe missing energy EM name isolated HLT in lower case: threshold EF in tagging mode mu 20 i _ pass. EF name isolated New in 13. 0. 30: • Threshold is cut value applied • previously was ~95% effic. point. • type e threshold MU 20 I HLT FJ More details : see : https: //twiki. cern. ch/twiki/bin/view/Atlas/Trigger. Physics. Menu 45
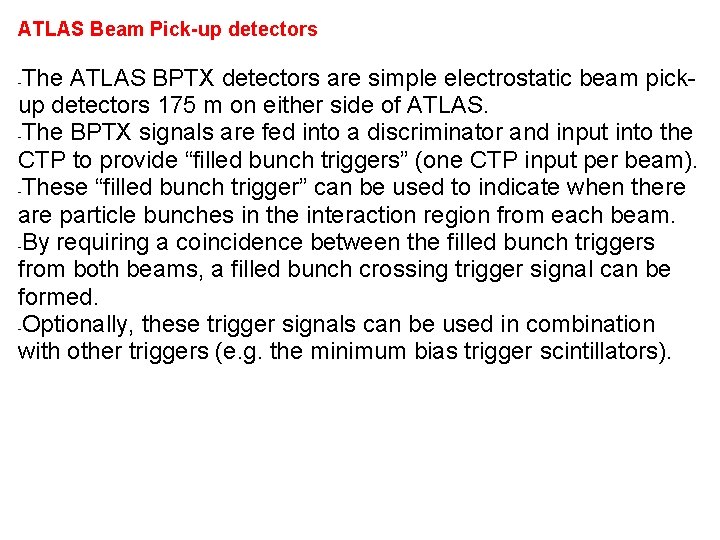
ATLAS Beam Pick-up detectors The ATLAS BPTX detectors are simple electrostatic beam pickup detectors 175 m on either side of ATLAS. -The BPTX signals are fed into a discriminator and input into the CTP to provide “filled bunch triggers” (one CTP input per beam). -These “filled bunch trigger” can be used to indicate when there are particle bunches in the interaction region from each beam. -By requiring a coincidence between the filled bunch triggers from both beams, a filled bunch crossing trigger signal can be formed. -Optionally, these trigger signals can be used in combination with other triggers (e. g. the minimum bias trigger scintillators). -
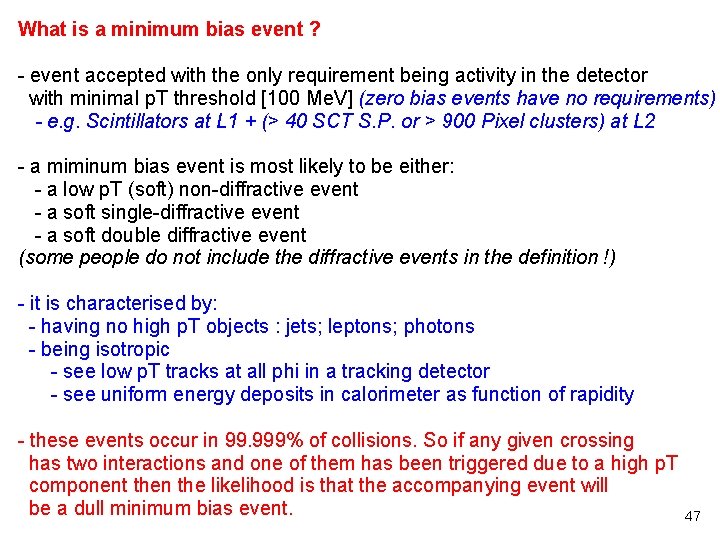
What is a minimum bias event ? - event accepted with the only requirement being activity in the detector with minimal p. T threshold [100 Me. V] (zero bias events have no requirements) - e. g. Scintillators at L 1 + (> 40 SCT S. P. or > 900 Pixel clusters) at L 2 - a miminum bias event is most likely to be either: - a low p. T (soft) non-diffractive event - a soft single-diffractive event - a soft double diffractive event (some people do not include the diffractive events in the definition !) - it is characterised by: - having no high p. T objects : jets; leptons; photons - being isotropic - see low p. T tracks at all phi in a tracking detector - see uniform energy deposits in calorimeter as function of rapidity - these events occur in 99. 999% of collisions. So if any given crossing has two interactions and one of them has been triggered due to a high p. T component then the likelihood is that the accompanying event will be a dull minimum bias event. 47
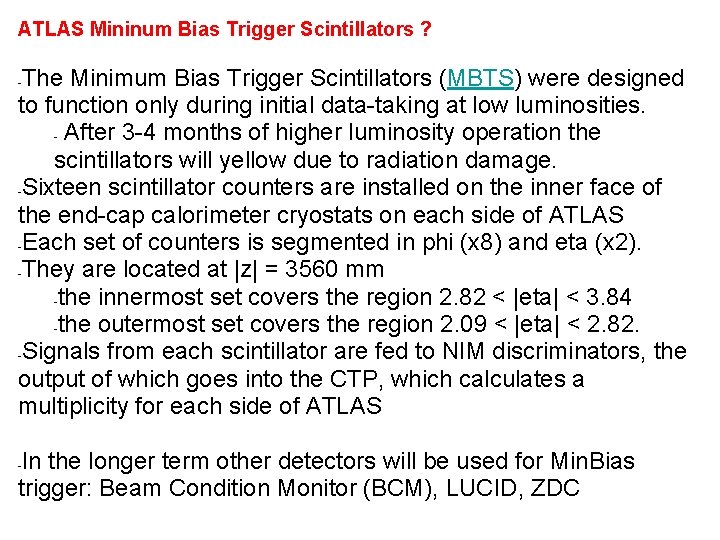
ATLAS Mininum Bias Trigger Scintillators ? The Minimum Bias Trigger Scintillators (MBTS) were designed to function only during initial data-taking at low luminosities. - After 3 -4 months of higher luminosity operation the scintillators will yellow due to radiation damage. -Sixteen scintillator counters are installed on the inner face of the end-cap calorimeter cryostats on each side of ATLAS -Each set of counters is segmented in phi (x 8) and eta (x 2). -They are located at |z| = 3560 mm -the innermost set covers the region 2. 82 < |eta| < 3. 84 -the outermost set covers the region 2. 09 < |eta| < 2. 82. -Signals from each scintillator are fed to NIM discriminators, the output of which goes into the CTP, which calculates a multiplicity for each side of ATLAS - In the longer term other detectors will be used for Min. Bias trigger: Beam Condition Monitor (BCM), LUCID, ZDC -
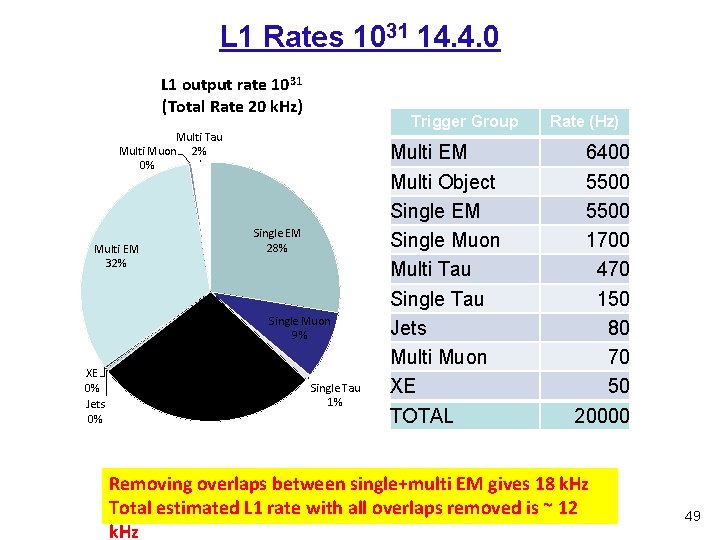
L 1 Rates 1031 14. 4. 0 L 1 output rate 1031 (Total Rate 20 k. Hz) Trigger Group Multi Tau Multi Muon 2% 0% Single EM 28% Multi EM 32% Single Muon 9% XE 0% Jets 0% Multi Object 28% Single Tau 1% Multi EM Multi Object Single EM Single Muon Multi Tau Single Tau Jets Multi Muon XE TOTAL Rate (Hz) 6400 5500 1700 470 150 80 70 50 20000 Removing overlaps between single+multi EM gives 18 k. Hz Total estimated L 1 rate with all overlaps removed is ~ 12 k. Hz 49
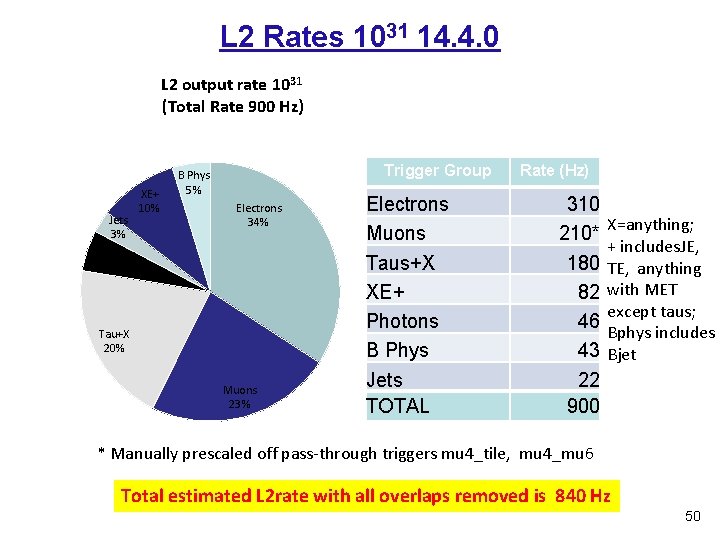
L 2 Rates 1031 14. 4. 0 L 2 output rate 1031 (Total Rate 900 Hz) Jets 3% XE+ 10% Trigger Group B Phys 5% Electrons 34% Photons 5% Tau+X 20% Muons 23% Electrons Muons Taus+X XE+ Photons B Phys Jets TOTAL Rate (Hz) 310 210* 180 82 46 43 22 900 X=anything; + includes. JE, TE, anything with MET except taus; Bphys includes Bjet * Manually prescaled off pass-through triggers mu 4_tile, mu 4_mu 6 Total estimated L 2 rate with all overlaps removed is 840 Hz 50
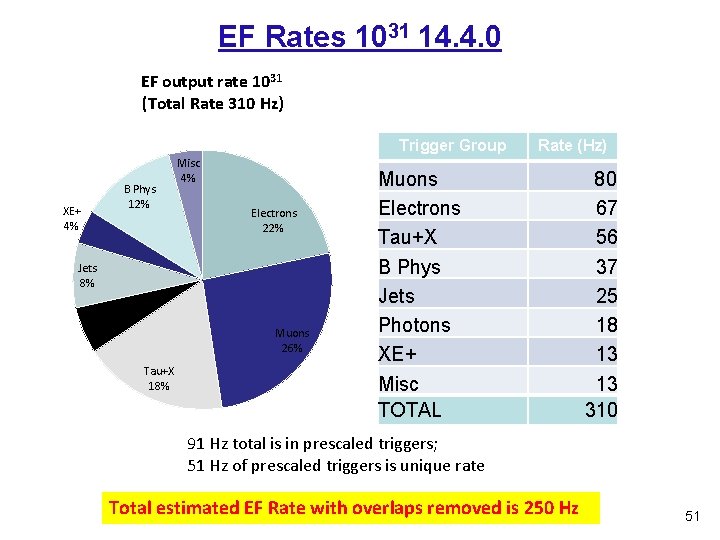
EF Rates 1031 14. 4. 0 EF output rate 1031 (Total Rate 310 Hz) Trigger Group B Phys 12% XE+ 4% Misc 4% Electrons 22% Jets 8% Photons 6% Muons 26% Tau+X 18% Rate (Hz) Muons Electrons Tau+X B Phys Jets Photons XE+ Misc TOTAL 80 67 56 37 25 18 13 13 310 91 Hz total is in prescaled triggers; 51 Hz of prescaled triggers is unique rate Total estimated EF Rate with overlaps removed is 250 Hz 51
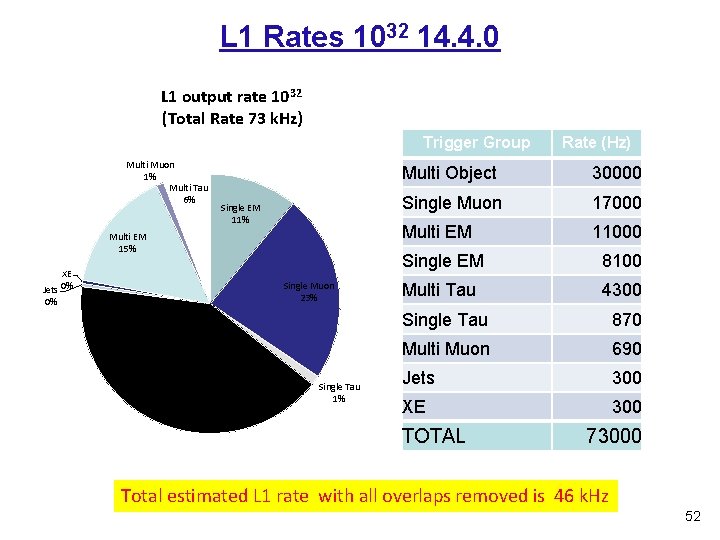
L 1 Rates 1032 14. 4. 0 L 1 output rate 1032 (Total Rate 73 k. Hz) Trigger Group Multi Muon 1% Multi Tau 6% Single EM 11% Multi EM 15% XE 0% Jets Single Muon 23% 0% Multi Object 41% Single Tau 1% Rate (Hz) Multi Object 30000 Single Muon 17000 Multi EM 11000 Single EM 8100 Multi Tau 4300 Single Tau 870 Multi Muon 690 Jets 300 XE 300 TOTAL 73000 Total estimated L 1 rate with all overlaps removed is 46 k. Hz 52
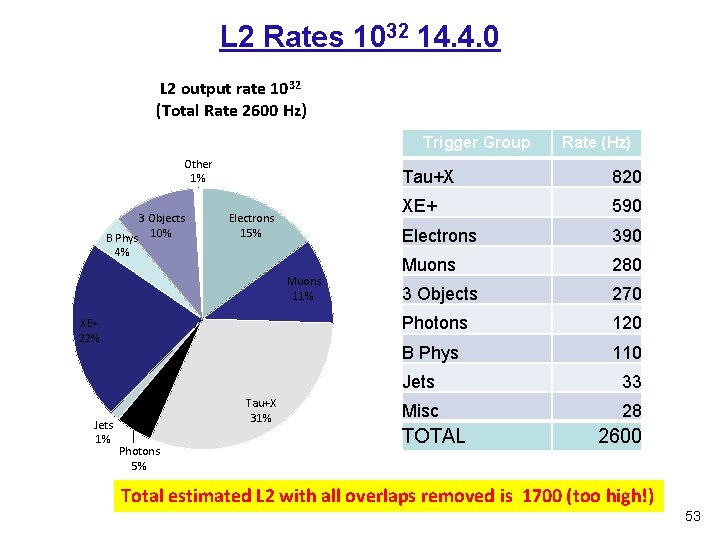
L 2 Rates 1032 14. 4. 0 L 2 output rate 1032 (Total Rate 2600 Hz) Trigger Group Other 1% 3 Objects B Phys 10% Electrons 15% 4% Muons 11% XE+ 22% Jets 1% Tau+X 31% Photons 5% Rate (Hz) Tau+X 820 XE+ 590 Electrons 390 Muons 280 3 Objects 270 Photons 120 B Phys 110 Jets 33 Misc 28 TOTAL 2600 Total estimated L 2 with all overlaps removed is 1700 (too high!) 53
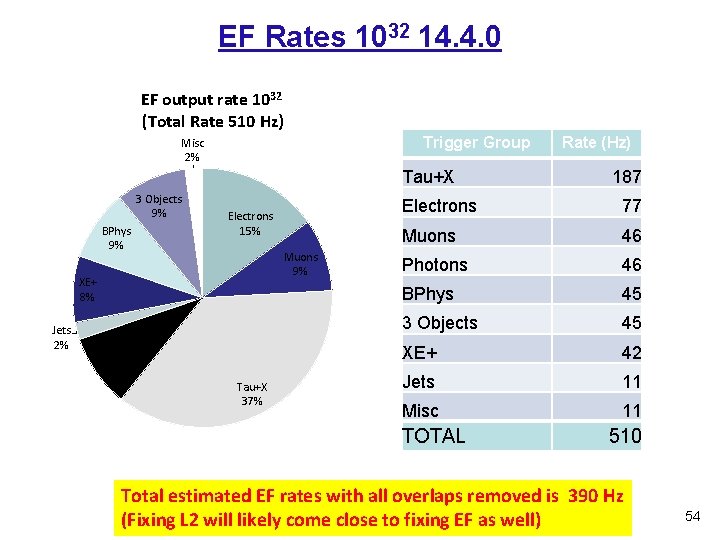
EF Rates 1032 14. 4. 0 EF output rate 1032 (Total Rate 510 Hz) Trigger Group Misc 2% Tau+X 3 Objects 9% BPhys 9% Electrons 15% Muons 9% XE+ 8% Jets 2% Photons 9% Tau+X 37% Rate (Hz) 187 Electrons 77 Muons 46 Photons 46 BPhys 45 3 Objects 45 XE+ 42 Jets 11 Misc 11 TOTAL 510 Total estimated EF rates with all overlaps removed is 390 Hz (Fixing L 2 will likely come close to fixing EF as well) 54
Edge triggering and level triggering
T flip flop truth table
Wickens et al fruit meat and professions
Memory definition
Chase and simon 1973
Wickens
Multiple resource theory
Reverse triggering
Intrinsic standoff ratio of ujt formula
What is a flip flop
Beneficial ownership triggering events
Combining random variables calculator
Rcp hlsl
Fred langford
Fred van horzen
King philip coughed on fred and he got sick
Taxonomy is a branch of science which
Optical bloch equations two-level system
Liderazgo consciente kofman
Fred jones positive classroom discipline
Mary golda ross
Fred ndembi
Intermittent schedule of reinforcement
Fred aminzadeh
Fred vultee
Horizontal answer
Fred h garc
Vpl 03-14
Fred pollack
The fred factor
Fred kizito
Fred w066
Fred kucharski
Fred van dorp
Halloween poem kenn nesbitt
Fred zwarts
Fred principle
Fred zwicky
Fred galusha
Short paragraph with pronouns
Fredslåten barn i alla länder
Who is the modifier of nebular hypothesis in 1796?
Sanborn fred factor download
Red rhythms read write inc
Debate
Fred gottheil
Fred noble
Fred doherty
Fred kniffen housing styles
A child sits upright in a wagon which is moving
Cultural landscape example ap human geography
Fred sigernes
Fred sigernes
Fred sigernes
Fred raab