Enzyme Kinetics Chapter 8 Kinetics Study of rxn
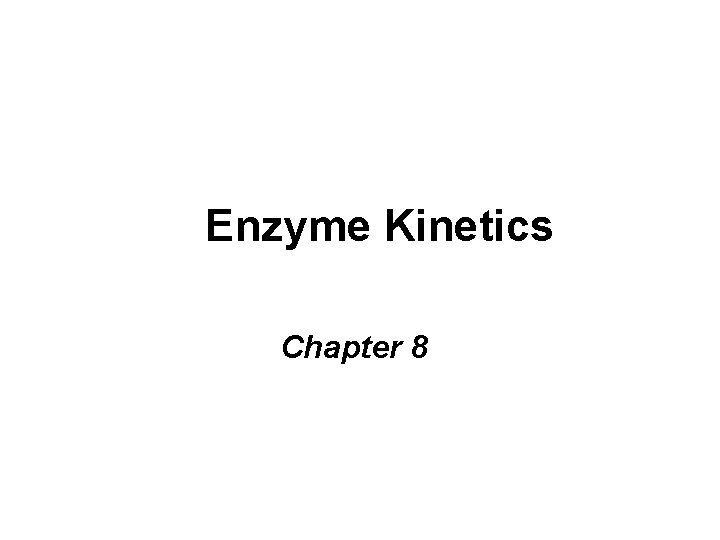
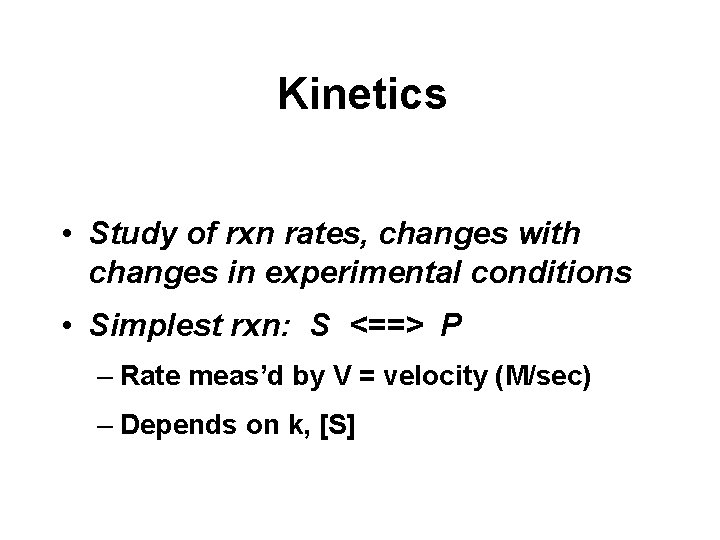
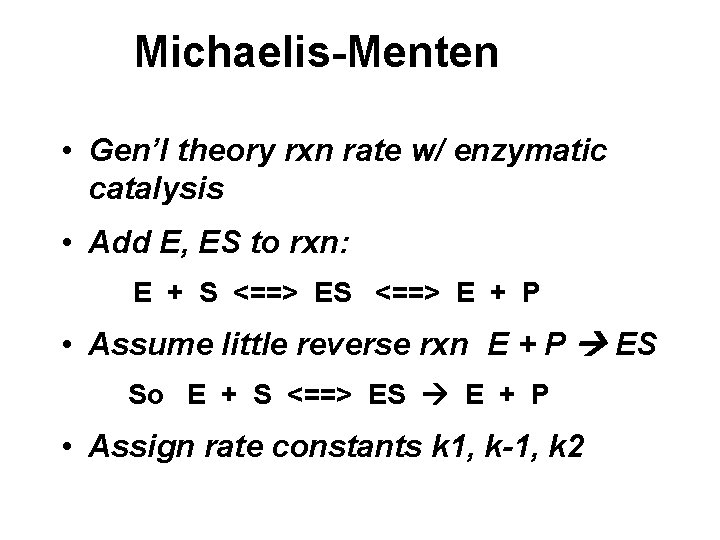
![• Assume Vo condition: [S] >>> [E] – Since S used up during • Assume Vo condition: [S] >>> [E] – Since S used up during](https://slidetodoc.com/presentation_image_h2/6e5ad7c6f2de2c489a78f799edfa6cdc/image-4.jpg)
![Exper’l findings: – As incr [S], V incr’s linearly up to some max V Exper’l findings: – As incr [S], V incr’s linearly up to some max V](https://slidetodoc.com/presentation_image_h2/6e5ad7c6f2de2c489a78f799edfa6cdc/image-5.jpg)
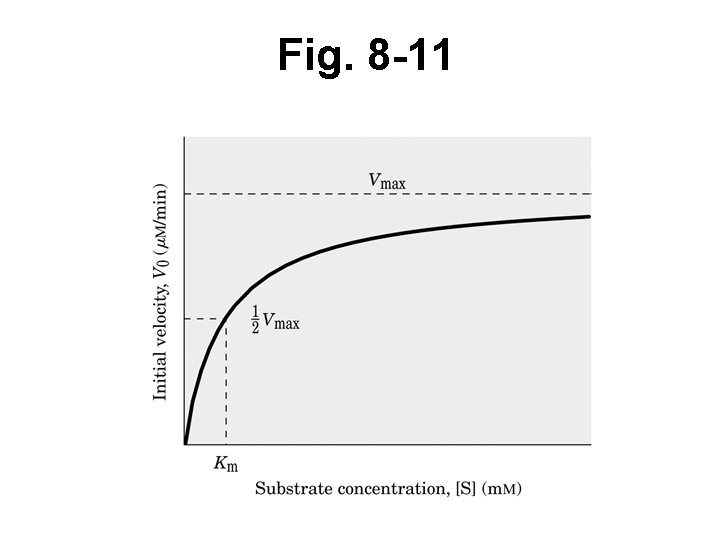
![M-M relates [E], [S], [P] exper’ly provable variables • New constant: KM = (k M-M relates [E], [S], [P] exper’ly provable variables • New constant: KM = (k](https://slidetodoc.com/presentation_image_h2/6e5ad7c6f2de2c489a78f799edfa6cdc/image-7.jpg)
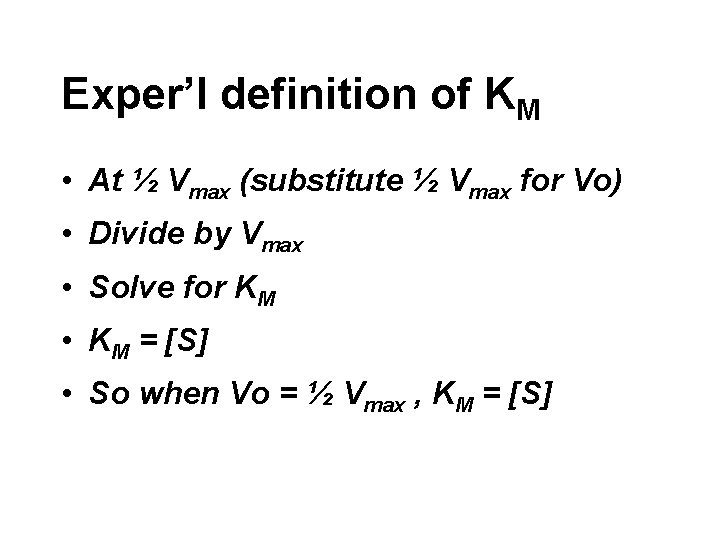
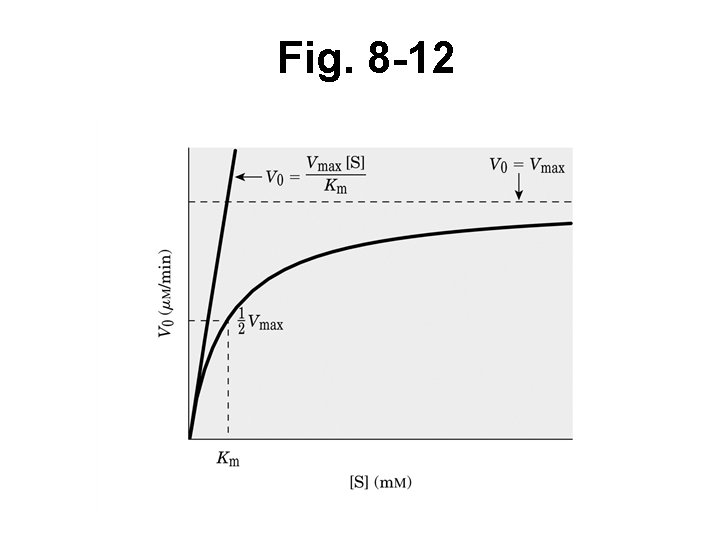
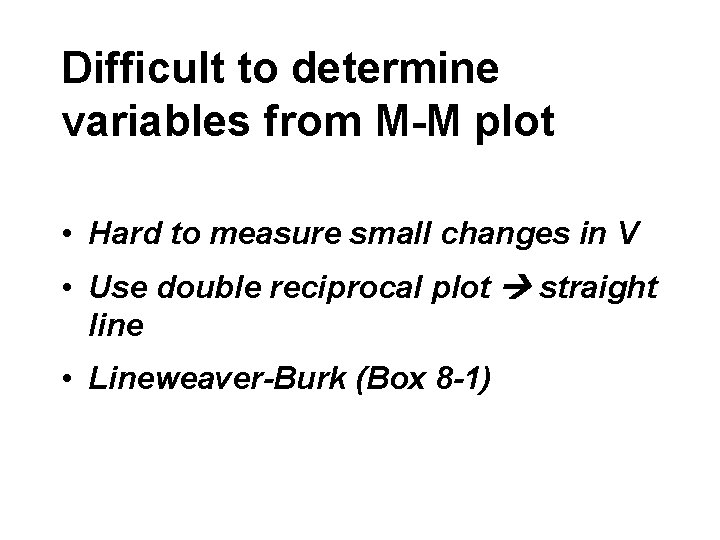
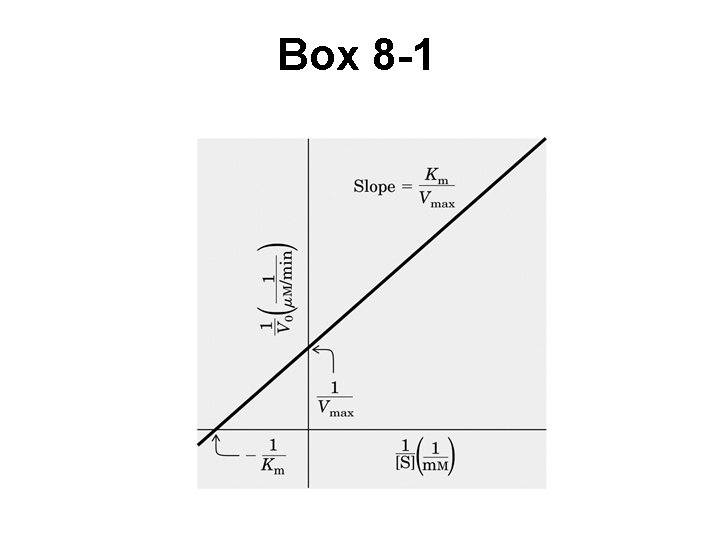
![KM (Table 8 -6) • [S] at which ½ enz active sites filled • KM (Table 8 -6) • [S] at which ½ enz active sites filled •](https://slidetodoc.com/presentation_image_h2/6e5ad7c6f2de2c489a78f799edfa6cdc/image-12.jpg)
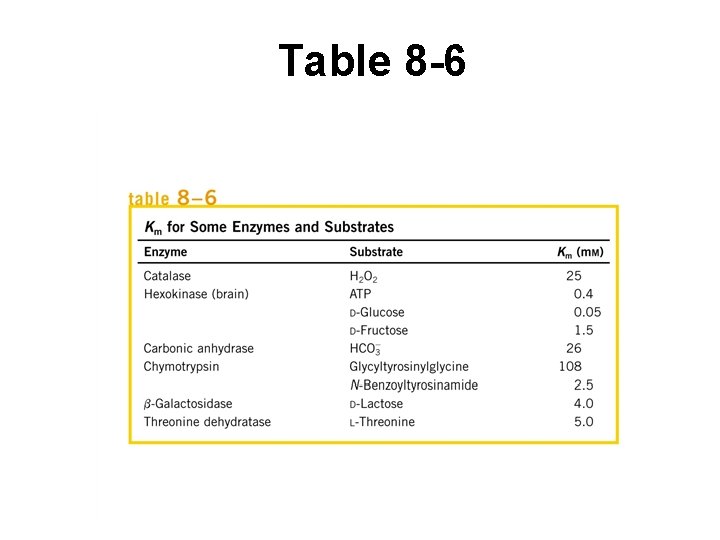
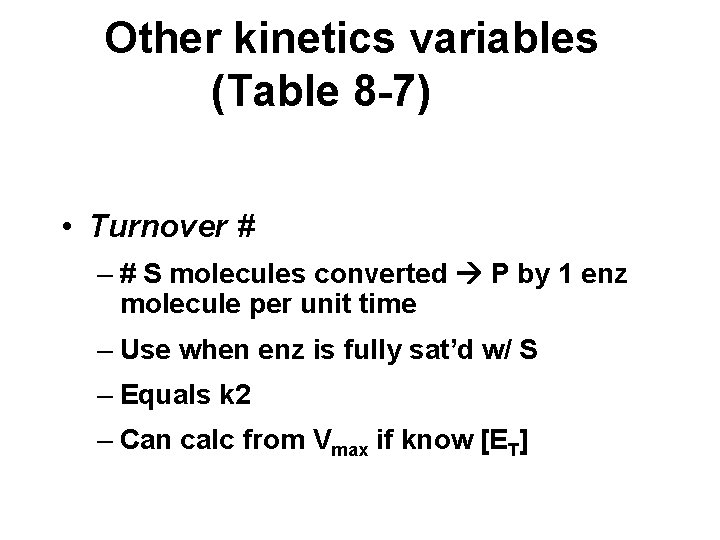
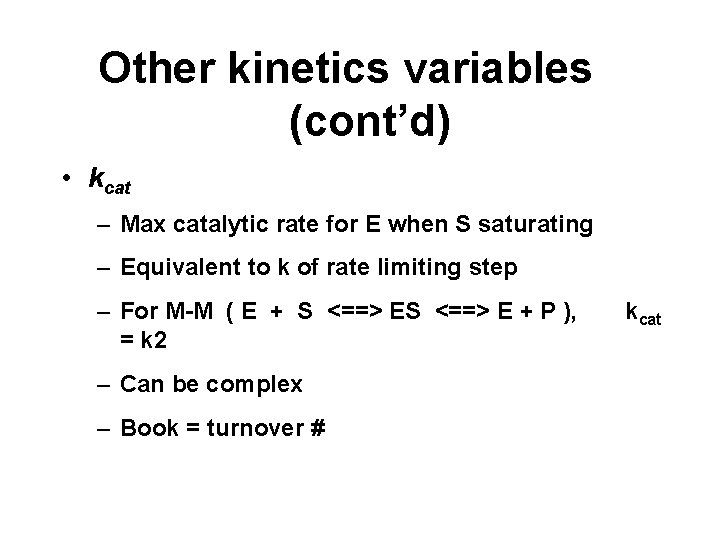
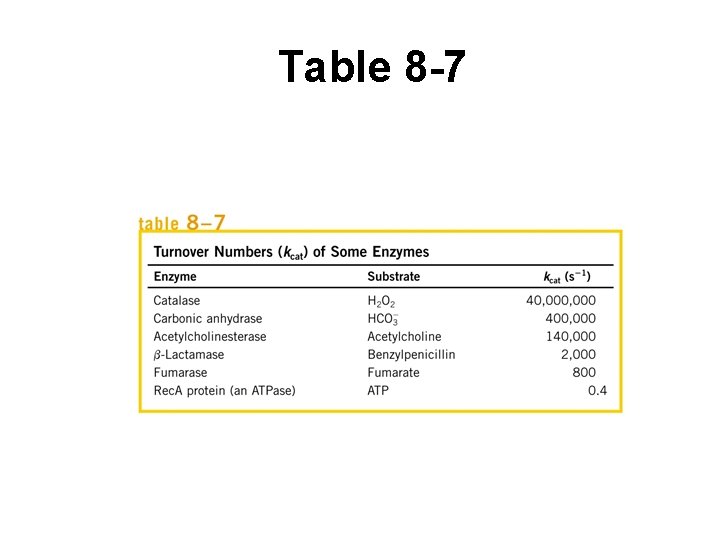
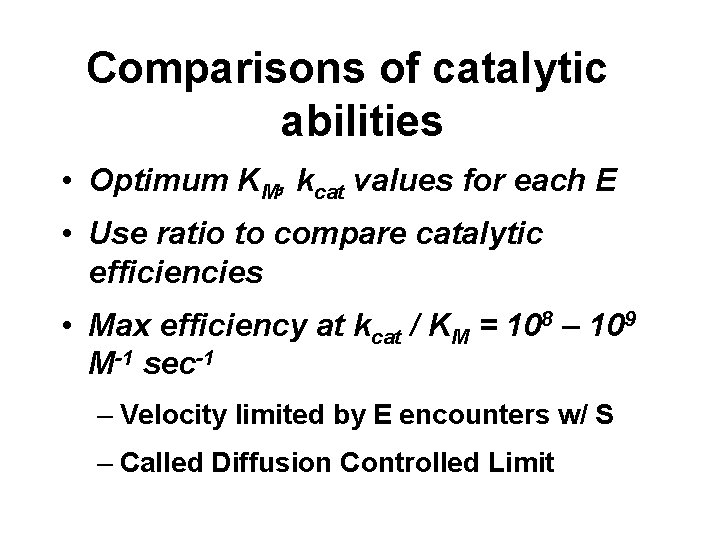
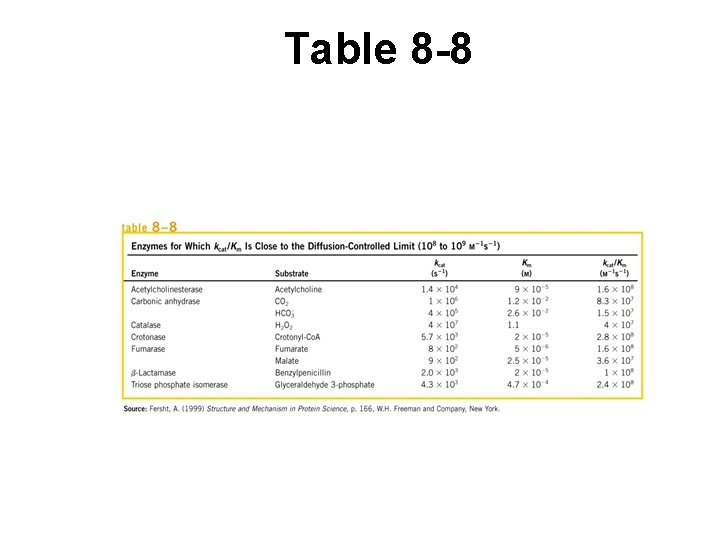
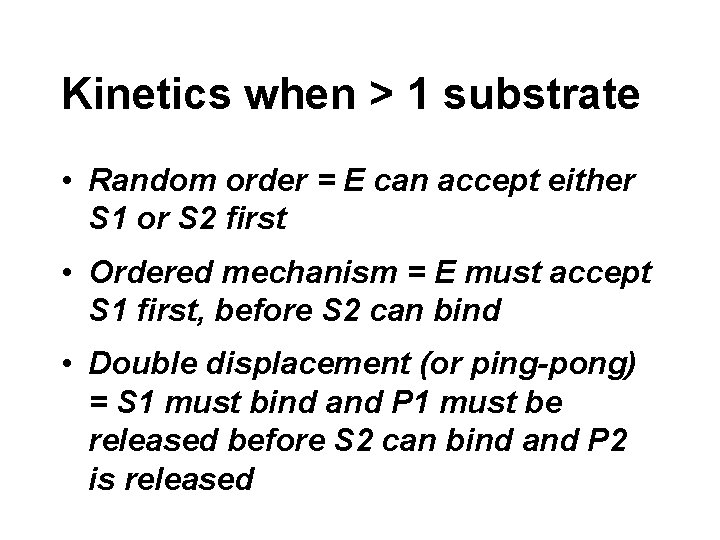
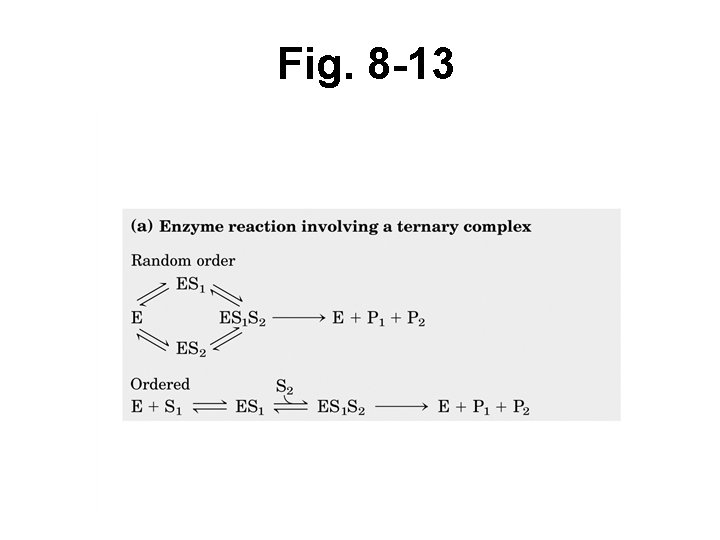
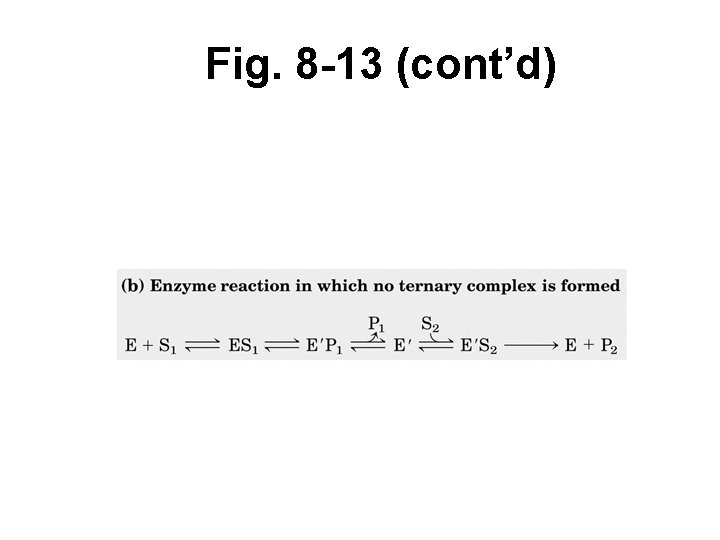
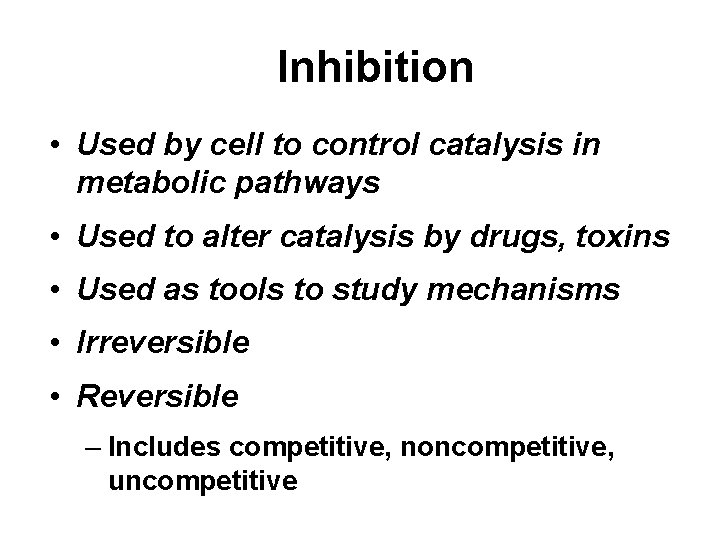
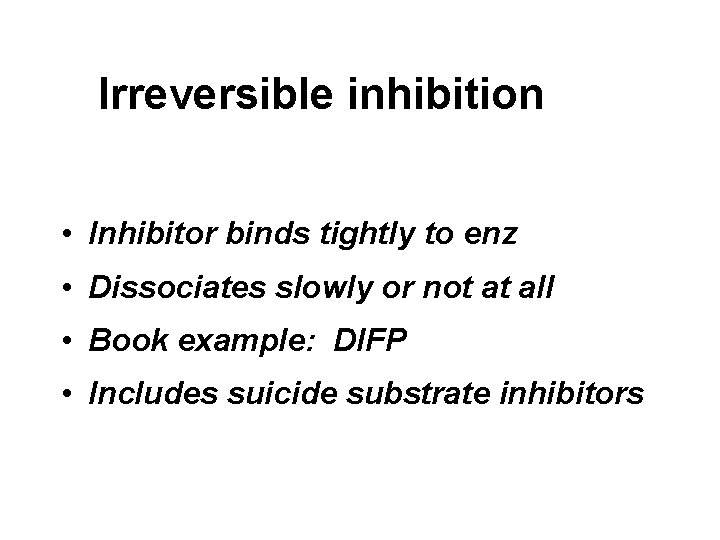
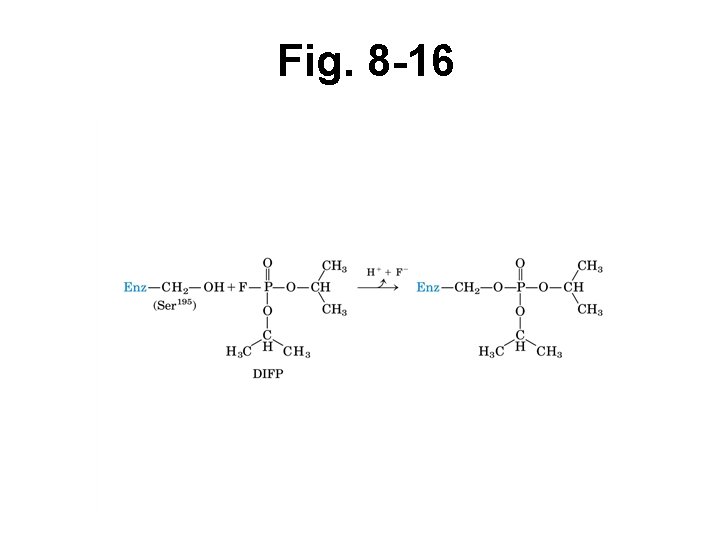
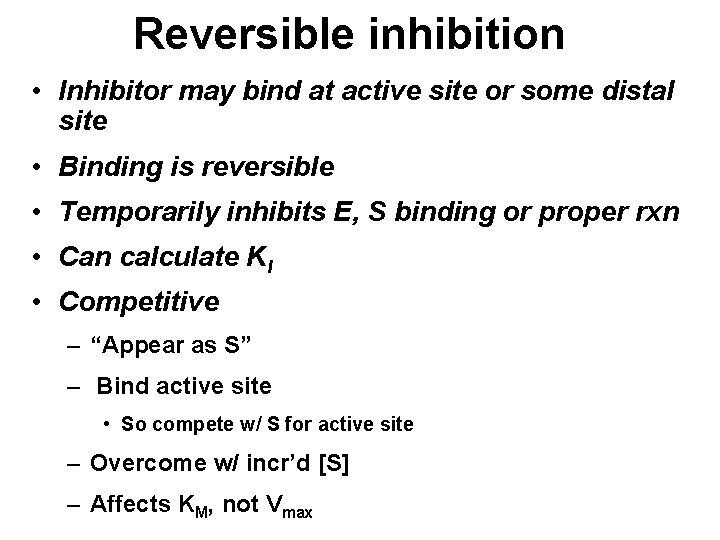
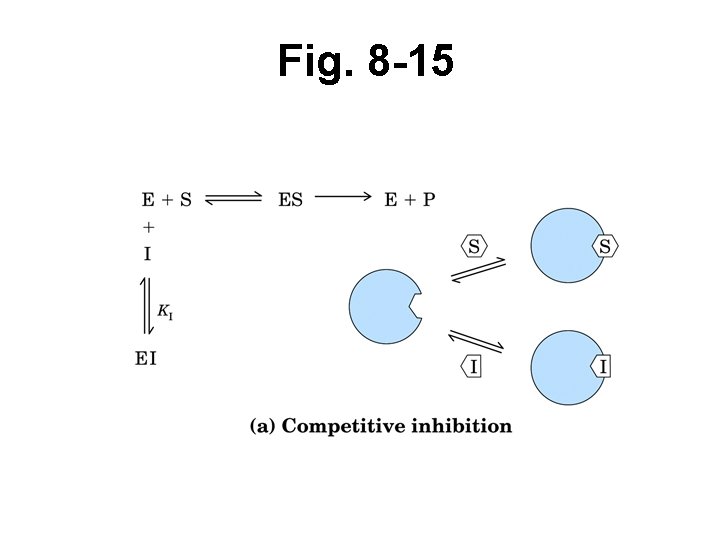
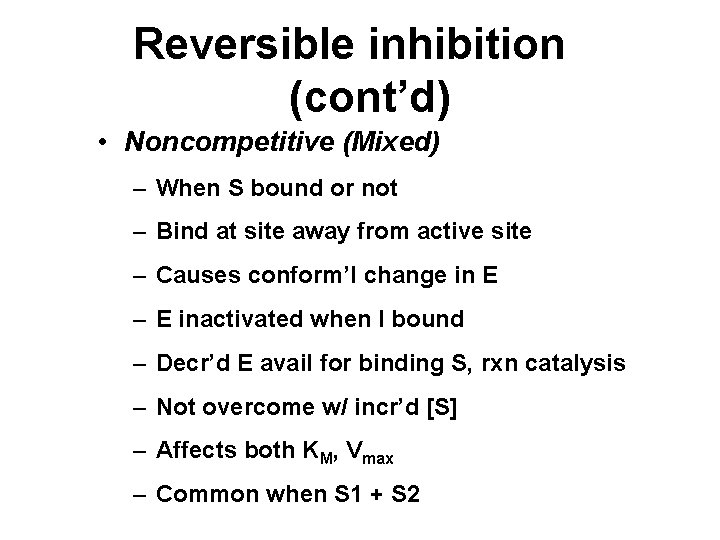
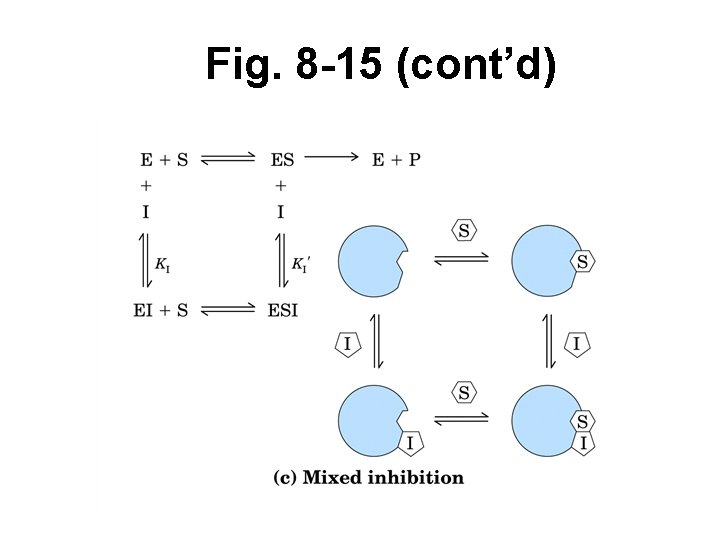
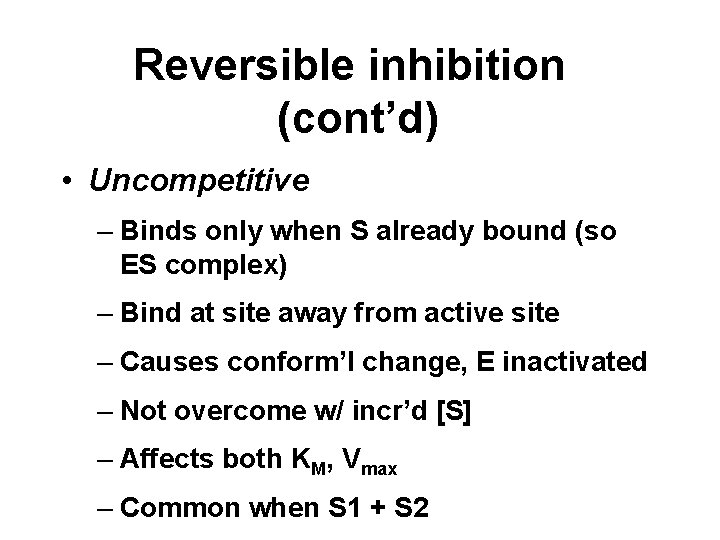
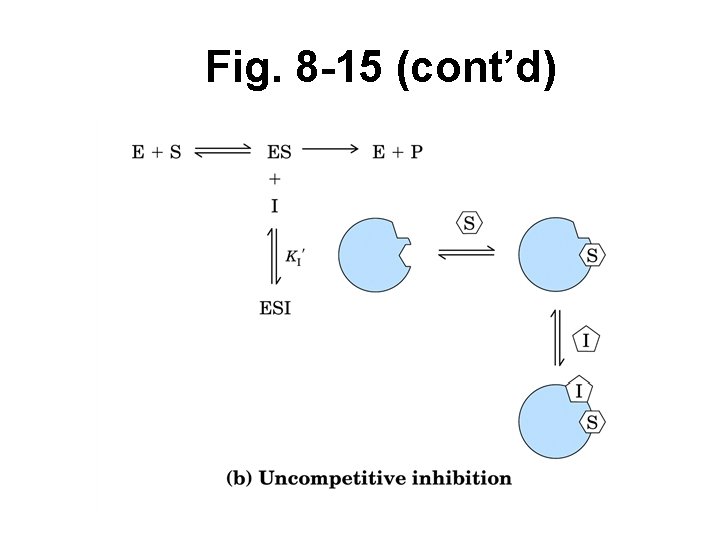
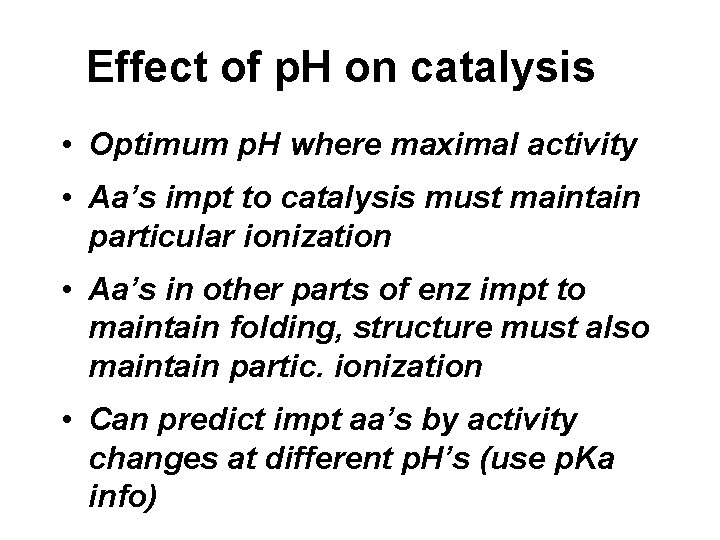
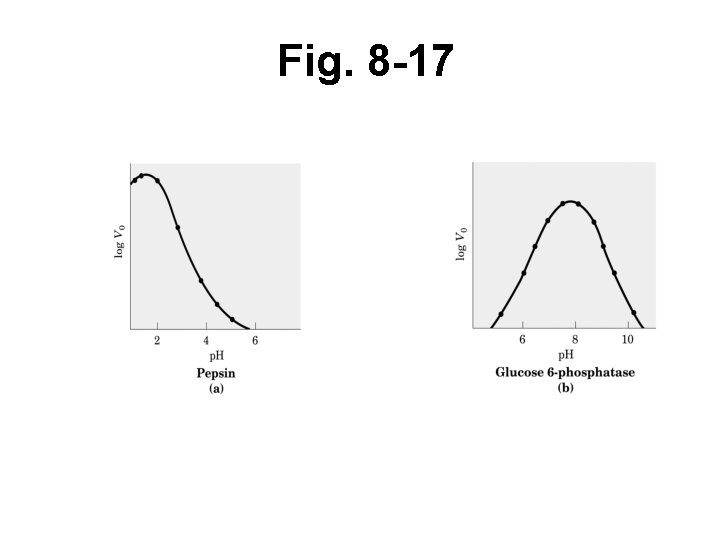
- Slides: 32
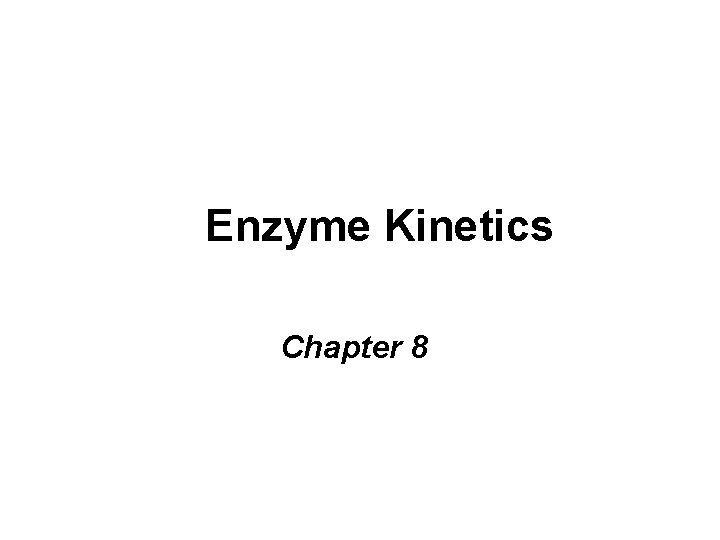
Enzyme Kinetics Chapter 8
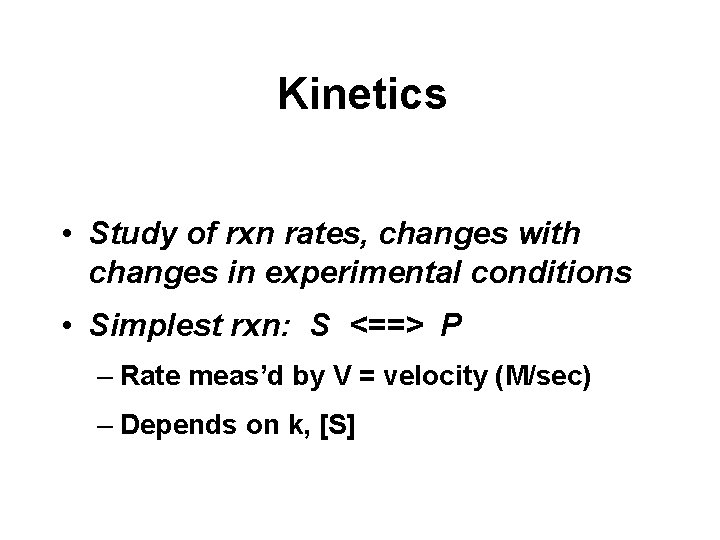
Kinetics • Study of rxn rates, changes with changes in experimental conditions • Simplest rxn: S <==> P – Rate meas’d by V = velocity (M/sec) – Depends on k, [S]
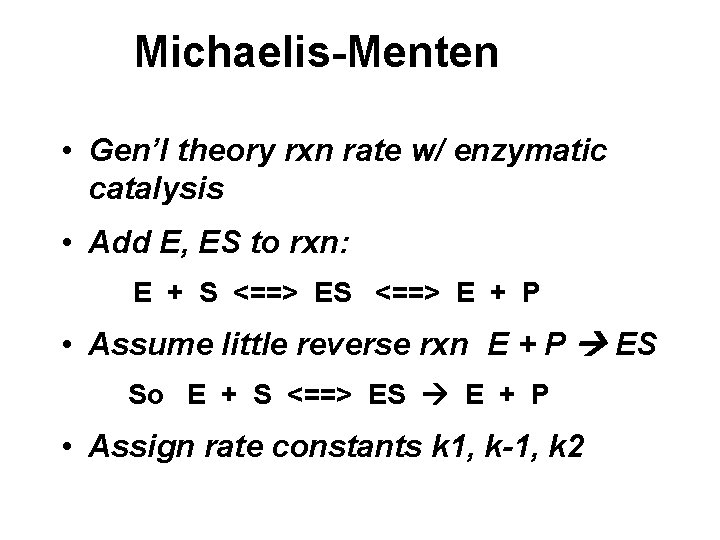
Michaelis-Menten • Gen’l theory rxn rate w/ enzymatic catalysis • Add E, ES to rxn: E + S <==> E + P • Assume little reverse rxn E + P ES So E + S <==> ES E + P • Assign rate constants k 1, k-1, k 2
![Assume Vo condition S E Since S used up during • Assume Vo condition: [S] >>> [E] – Since S used up during](https://slidetodoc.com/presentation_image_h2/6e5ad7c6f2de2c489a78f799edfa6cdc/image-4.jpg)
• Assume Vo condition: [S] >>> [E] – Since S used up during rxn, can’t be limiting • Assume: – All E goes to ES • Assume fixed amt enzyme – If all E ES, will see max rate of P formed – At steady state, rate form’n ES = rate breakdown ES
![Experl findings As incr S V incrs linearly up to some max V Exper’l findings: – As incr [S], V incr’s linearly up to some max V](https://slidetodoc.com/presentation_image_h2/6e5ad7c6f2de2c489a78f799edfa6cdc/image-5.jpg)
Exper’l findings: – As incr [S], V incr’s linearly up to some max V – At max V, little V incr regardless of [S] added
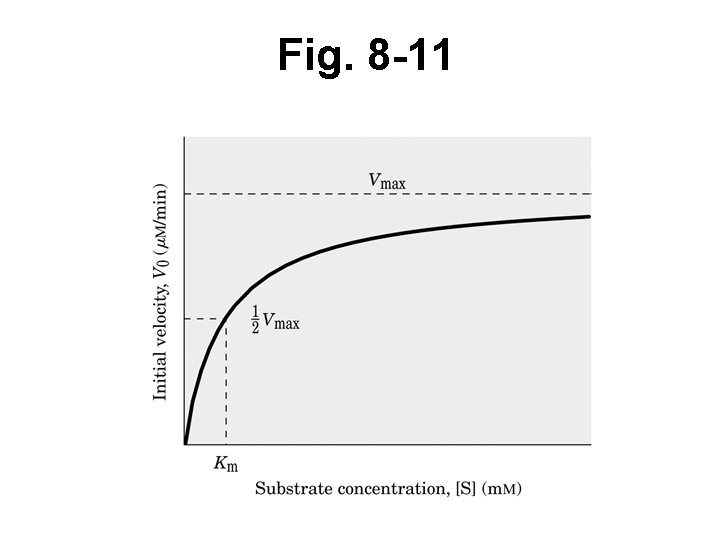
Fig. 8 -11
![MM relates E S P experly provable variables New constant KM k M-M relates [E], [S], [P] exper’ly provable variables • New constant: KM = (k](https://slidetodoc.com/presentation_image_h2/6e5ad7c6f2de2c489a78f799edfa6cdc/image-7.jpg)
M-M relates [E], [S], [P] exper’ly provable variables • New constant: KM = (k 2 + k-1) / k 1 • M-M eq’n: • Vo = (Vmax [S]) / (KM + [S]) • Quantitative relationship between – Initial velocity – Max rate of rxn – Initial [S]
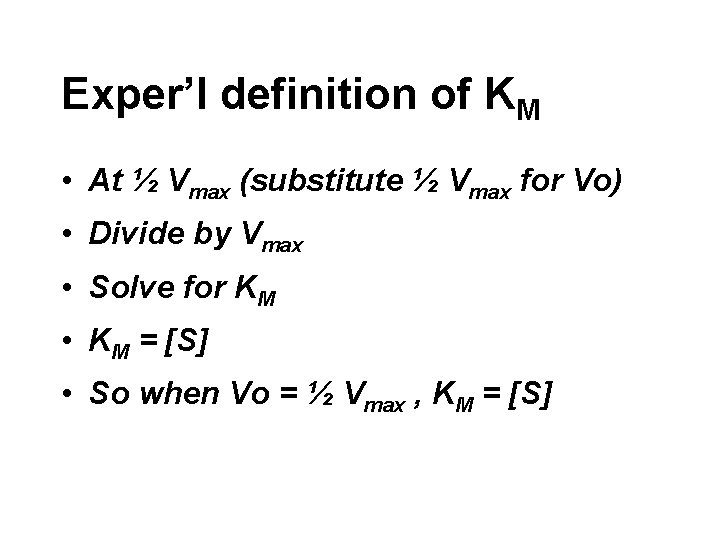
Exper’l definition of KM • At ½ Vmax (substitute ½ Vmax for Vo) • Divide by Vmax • Solve for KM • KM = [S] • So when Vo = ½ Vmax , KM = [S]
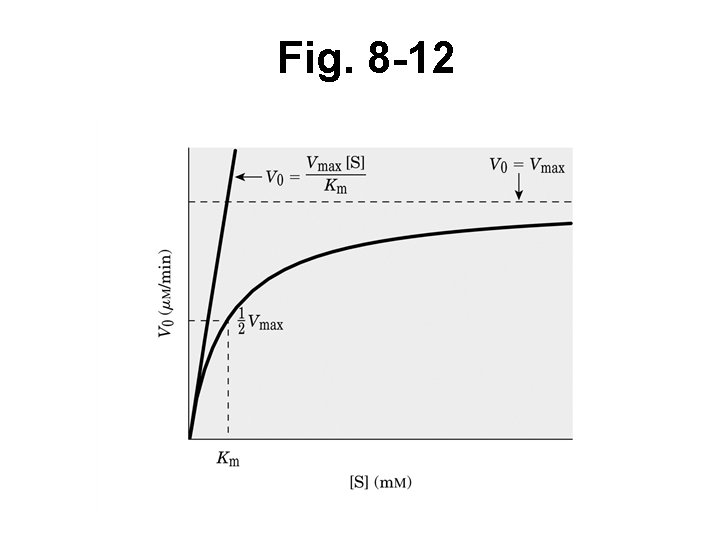
Fig. 8 -12
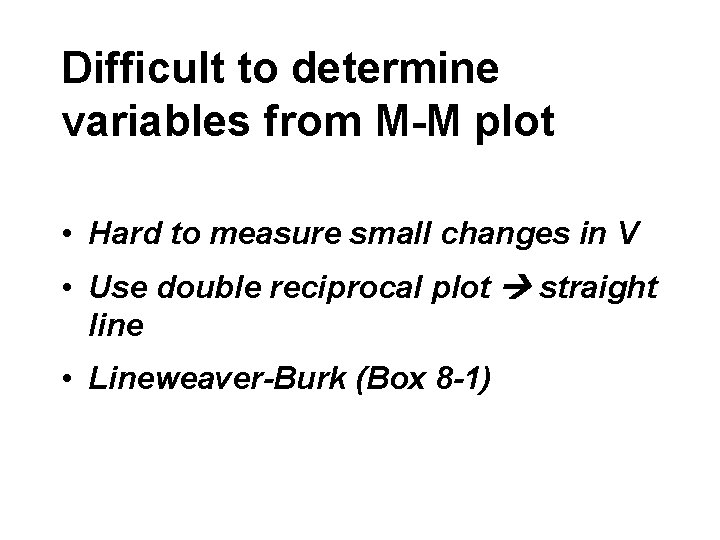
Difficult to determine variables from M-M plot • Hard to measure small changes in V • Use double reciprocal plot straight line • Lineweaver-Burk (Box 8 -1)
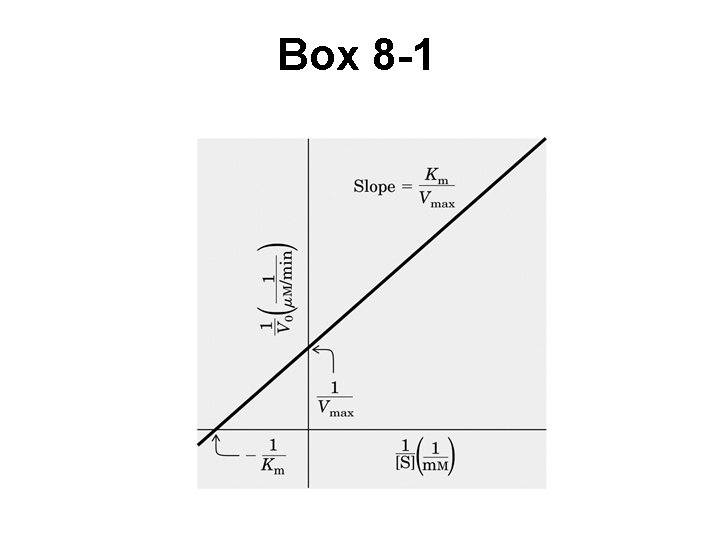
Box 8 -1
![KM Table 8 6 S at which ½ enz active sites filled KM (Table 8 -6) • [S] at which ½ enz active sites filled •](https://slidetodoc.com/presentation_image_h2/6e5ad7c6f2de2c489a78f799edfa6cdc/image-12.jpg)
KM (Table 8 -6) • [S] at which ½ enz active sites filled • Related to rate constants • In living cells, value close to [S] for that E – Commonly enz active sites NOT saturated w/ S • May describe affinity of E for S ONLY if k-1 >>> k 2 – Right half of rxn equation negligible – KM = k-1 / k 1 – Describes rate form’n, breakdown of ES – Here, KM value indicates strength of binding E-S – In real life, system is more complex
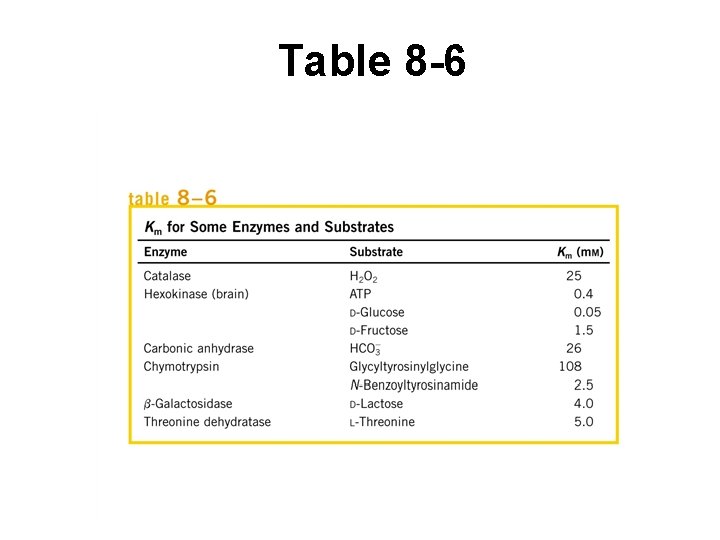
Table 8 -6
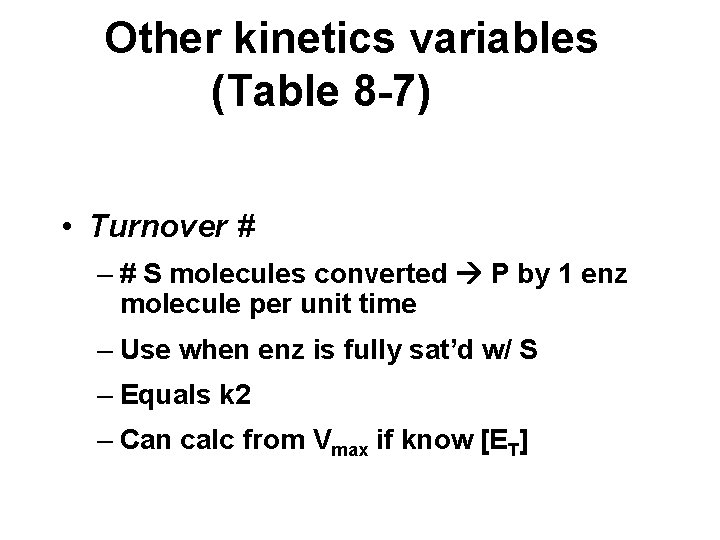
Other kinetics variables (Table 8 -7) • Turnover # – # S molecules converted P by 1 enz molecule per unit time – Use when enz is fully sat’d w/ S – Equals k 2 – Can calc from Vmax if know [ET]
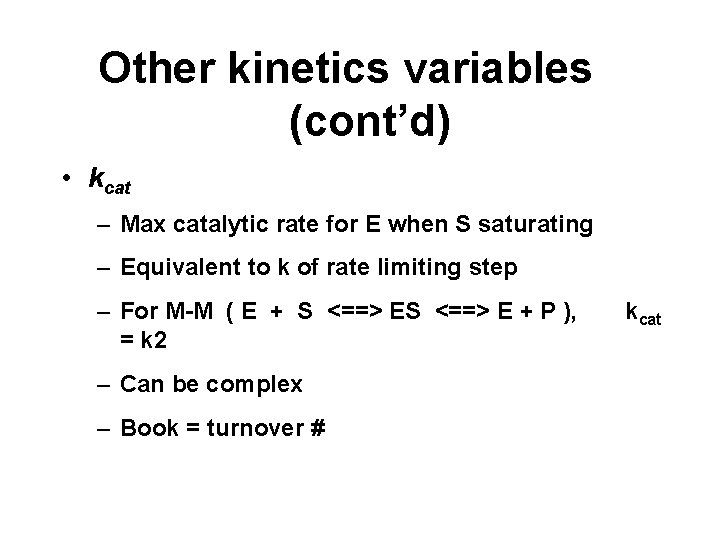
Other kinetics variables (cont’d) • kcat – Max catalytic rate for E when S saturating – Equivalent to k of rate limiting step – For M-M ( E + S <==> E + P ), = k 2 – Can be complex – Book = turnover # kcat
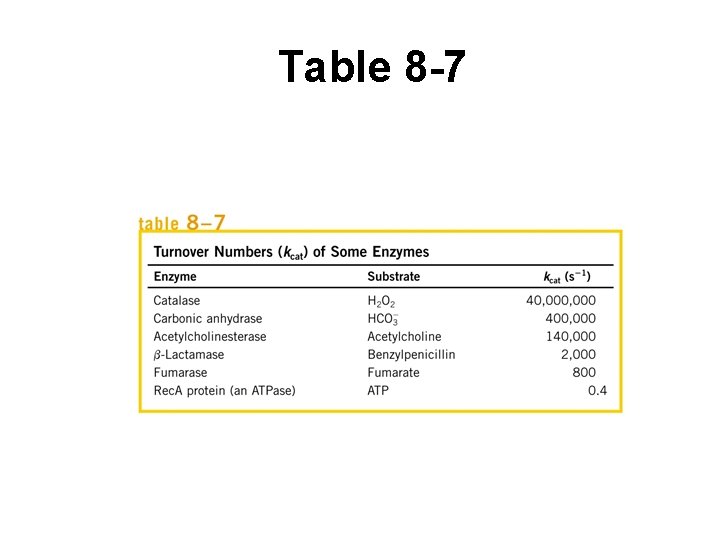
Table 8 -7
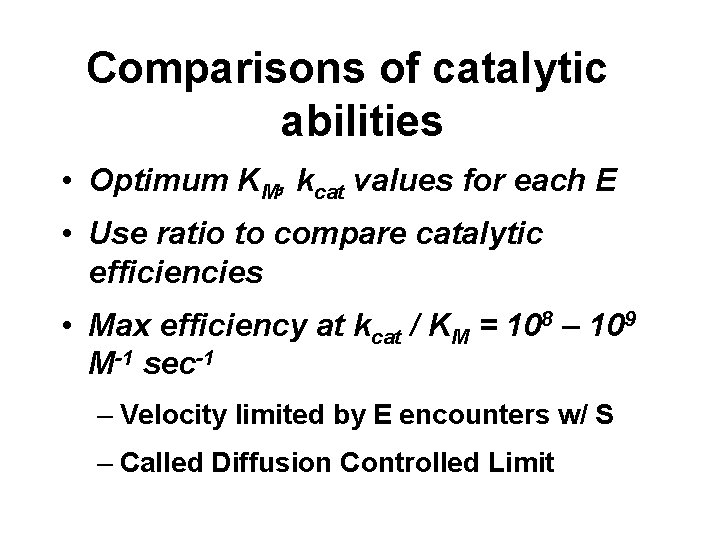
Comparisons of catalytic abilities • Optimum KM, kcat values for each E • Use ratio to compare catalytic efficiencies • Max efficiency at kcat / KM = 108 – 109 M-1 sec-1 – Velocity limited by E encounters w/ S – Called Diffusion Controlled Limit
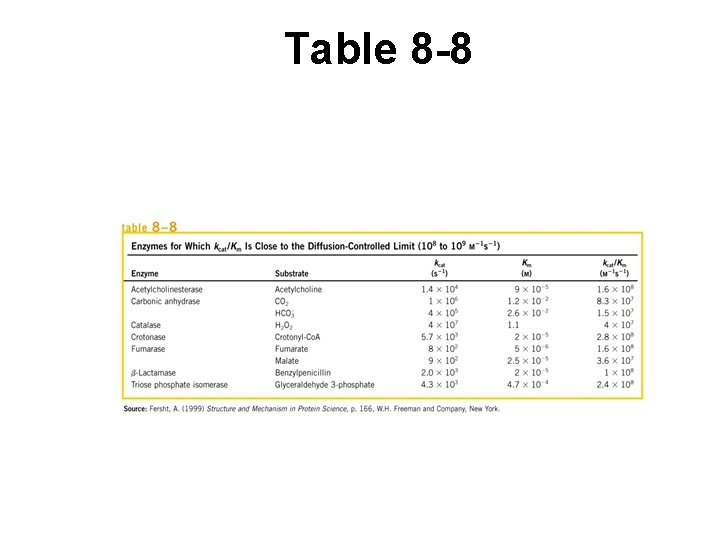
Table 8 -8
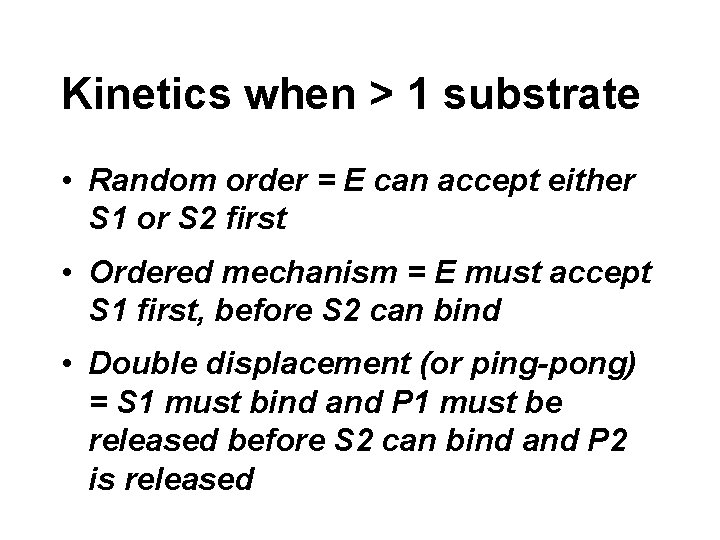
Kinetics when > 1 substrate • Random order = E can accept either S 1 or S 2 first • Ordered mechanism = E must accept S 1 first, before S 2 can bind • Double displacement (or ping-pong) = S 1 must bind and P 1 must be released before S 2 can bind and P 2 is released
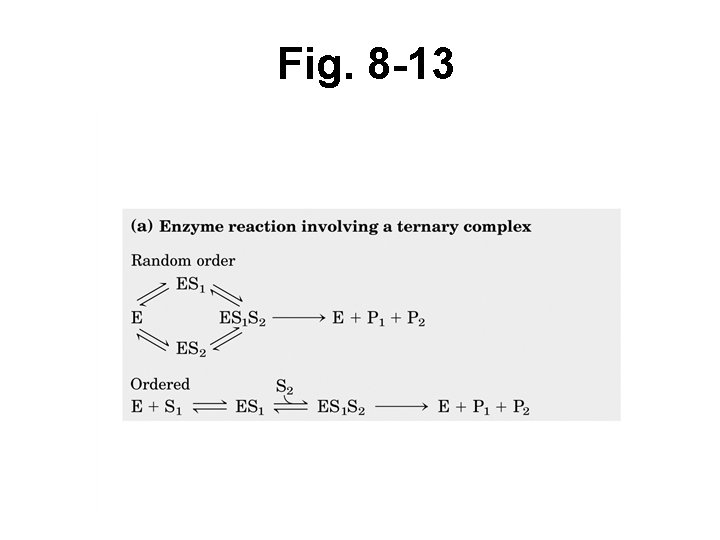
Fig. 8 -13
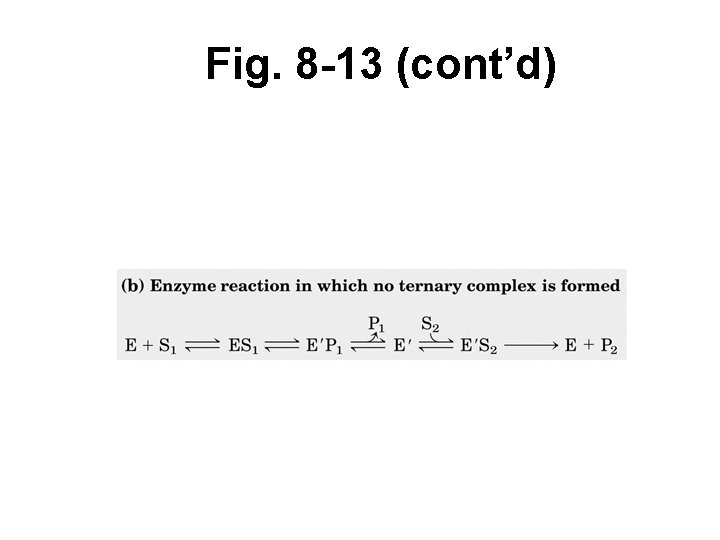
Fig. 8 -13 (cont’d)
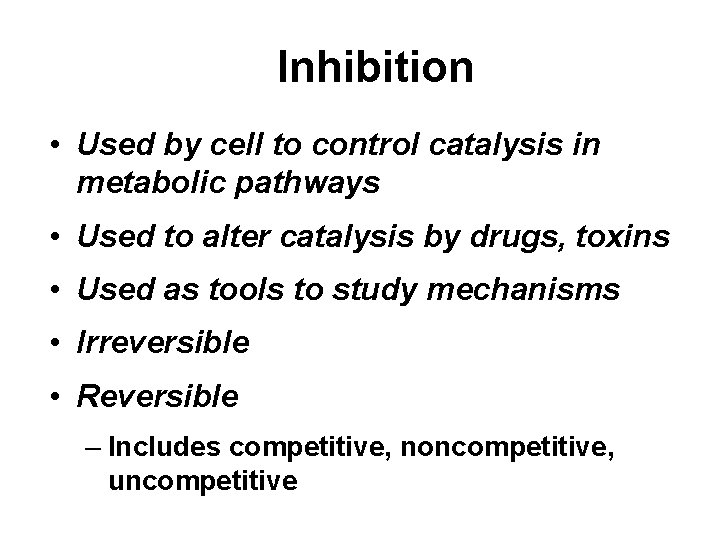
Inhibition • Used by cell to control catalysis in metabolic pathways • Used to alter catalysis by drugs, toxins • Used as tools to study mechanisms • Irreversible • Reversible – Includes competitive, noncompetitive, uncompetitive
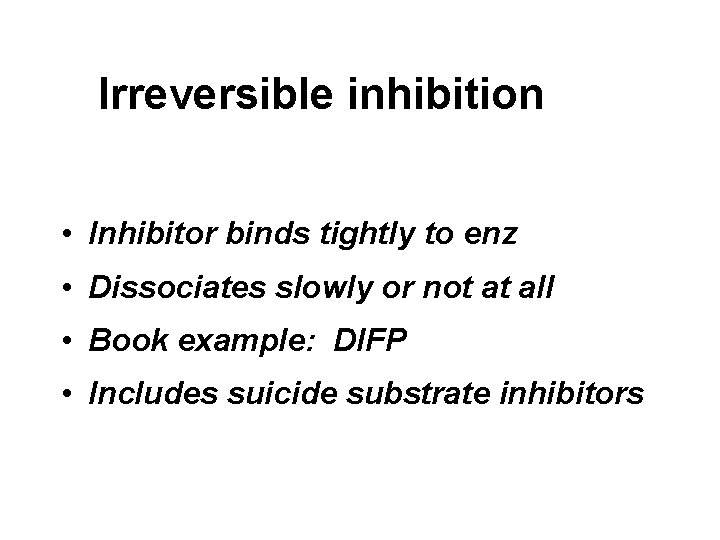
Irreversible inhibition • Inhibitor binds tightly to enz • Dissociates slowly or not at all • Book example: DIFP • Includes suicide substrate inhibitors
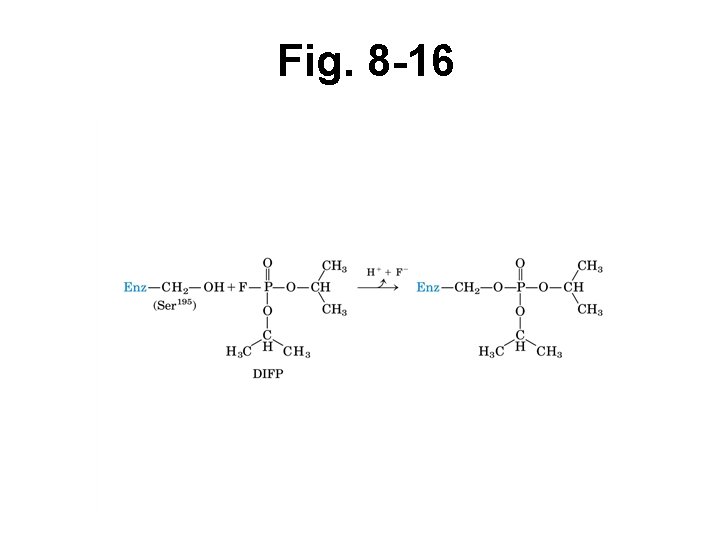
Fig. 8 -16
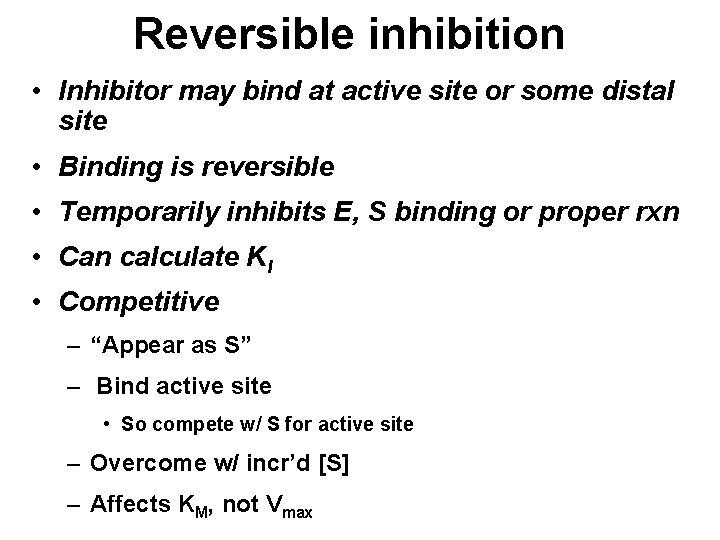
Reversible inhibition • Inhibitor may bind at active site or some distal site • Binding is reversible • Temporarily inhibits E, S binding or proper rxn • Can calculate KI • Competitive – “Appear as S” – Bind active site • So compete w/ S for active site – Overcome w/ incr’d [S] – Affects KM, not Vmax
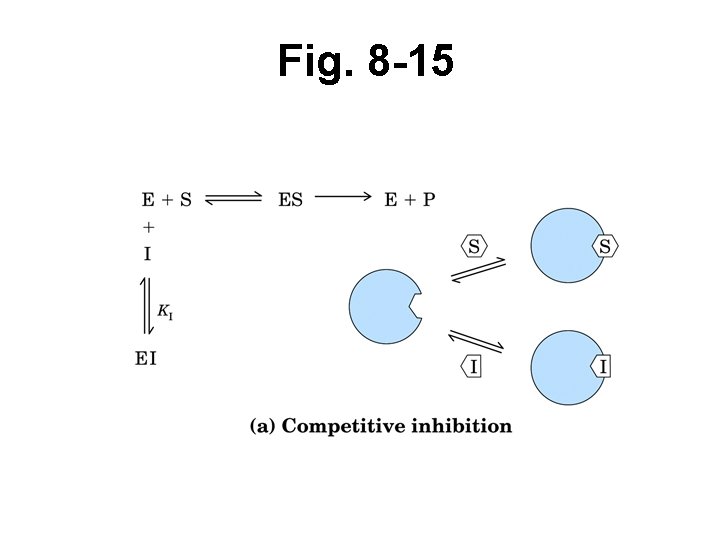
Fig. 8 -15
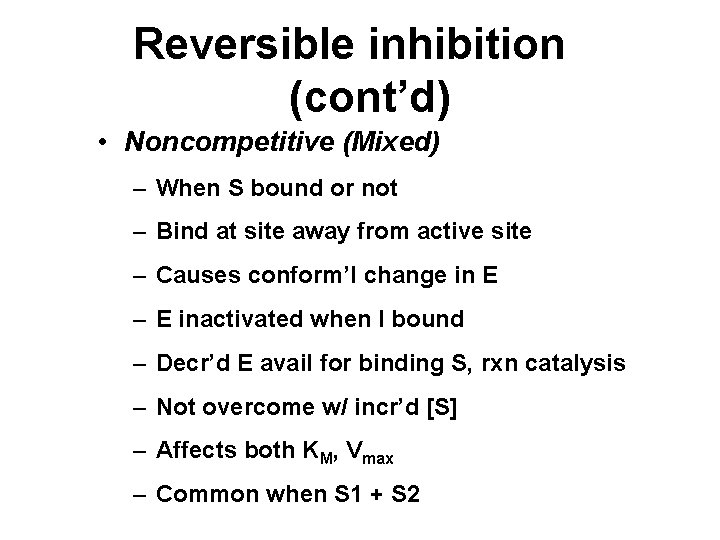
Reversible inhibition (cont’d) • Noncompetitive (Mixed) – When S bound or not – Bind at site away from active site – Causes conform’l change in E – E inactivated when I bound – Decr’d E avail for binding S, rxn catalysis – Not overcome w/ incr’d [S] – Affects both KM, Vmax – Common when S 1 + S 2
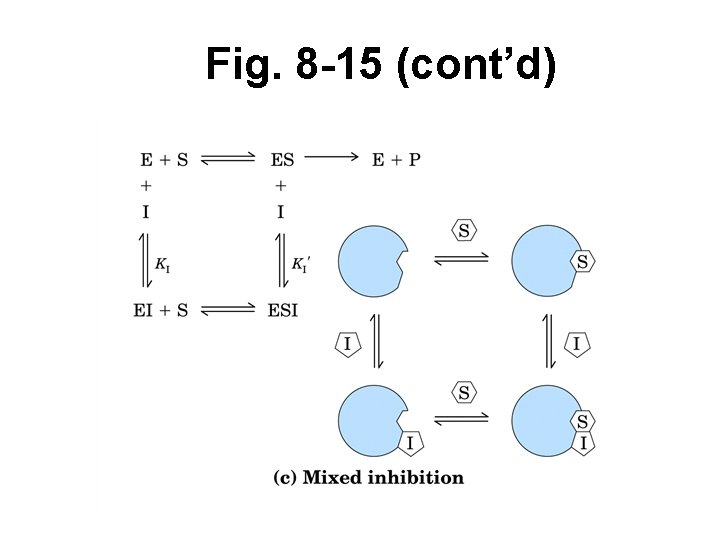
Fig. 8 -15 (cont’d)
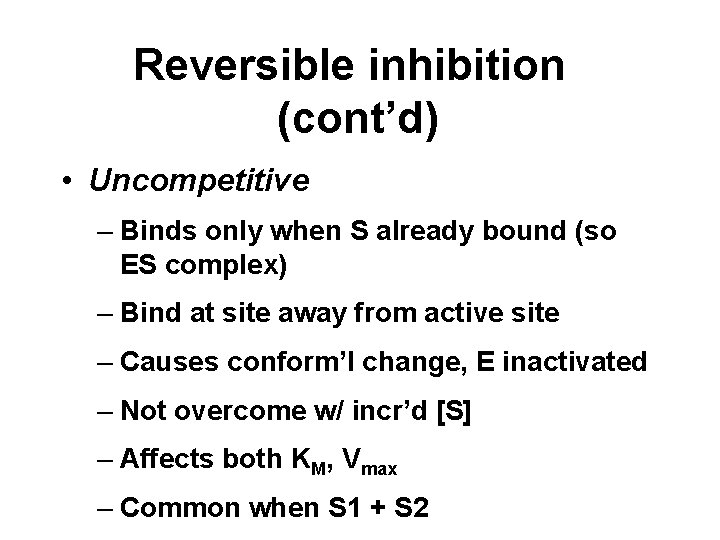
Reversible inhibition (cont’d) • Uncompetitive – Binds only when S already bound (so ES complex) – Bind at site away from active site – Causes conform’l change, E inactivated – Not overcome w/ incr’d [S] – Affects both KM, Vmax – Common when S 1 + S 2
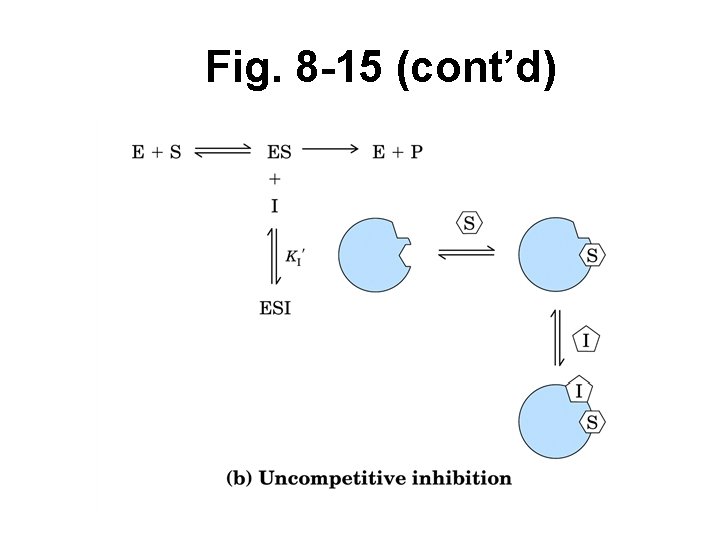
Fig. 8 -15 (cont’d)
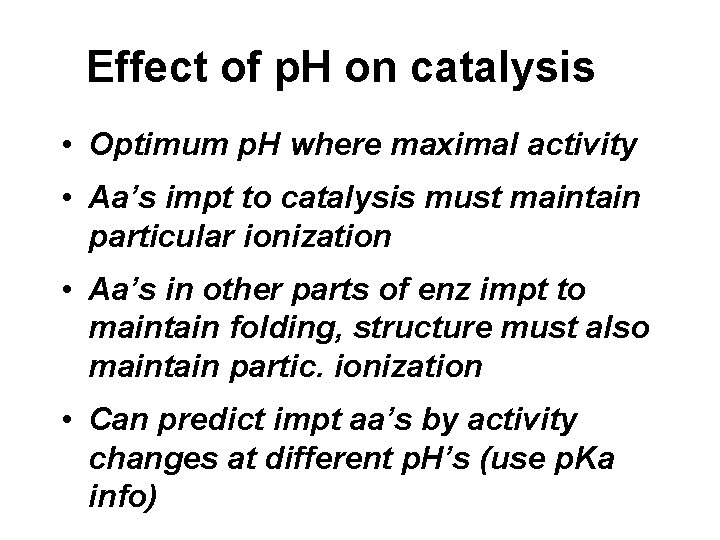
Effect of p. H on catalysis • Optimum p. H where maximal activity • Aa’s impt to catalysis must maintain particular ionization • Aa’s in other parts of enz impt to maintain folding, structure must also maintain partic. ionization • Can predict impt aa’s by activity changes at different p. H’s (use p. Ka info)
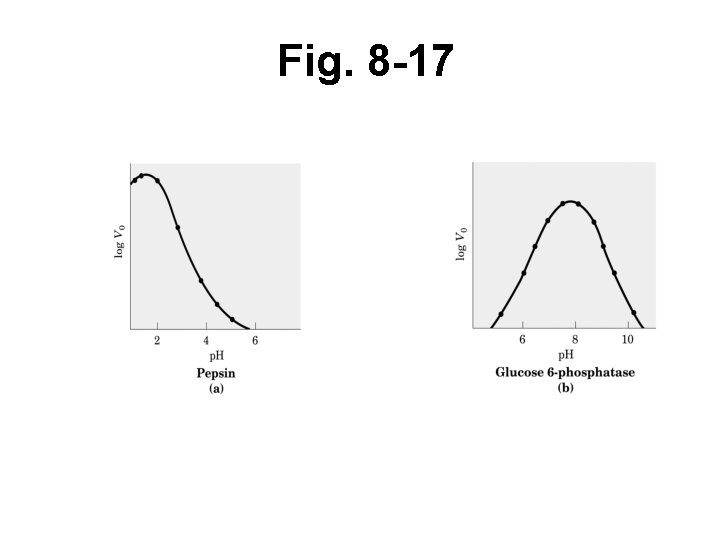
Fig. 8 -17
Xn+1=rxn(1-xn) explicacion
Enzyme catalyze
Steady state biochemistry
Enzyme kinetics
Molecularity
Kinetics and equilibrium
First order kinetics
Plane kinetics of rigid bodies
Difference between 1st order and zero order kinetics
First order drug elimination
Collision theory of kinetics
Chemistry grade 11 unit 4 chemical kinetics
Kinetics flotation chemicals
Chemical kinetics half life
Chemical kinetics definition
Planar kinetics of a rigid body force and acceleration
Kinetics of rigid bodies engineering mechanics
Kinetics of a particle force and acceleration
Ap chemistry kinetics
Kinetics of crystal violet fading
Octet kinetics
Kinetics of particles newton's second law
Chemical kinetics experiment
Kinetics of a particle: impulse and momentum
Dynafit software
Hemotology
Kinematics of rigid bodies problems and solutions
Data kinetics ltd
Vmax equation
Planar kinetics of a rigid body work and energy
Kinetics reaction
Applications of chemical kinetics
Growth kinetics