Chapter 9 Cellular Respiration Harvesting Chemical Energy Power
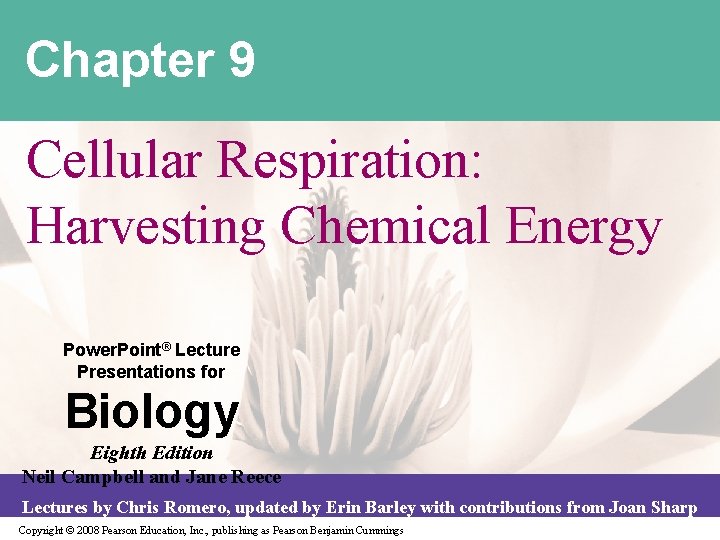
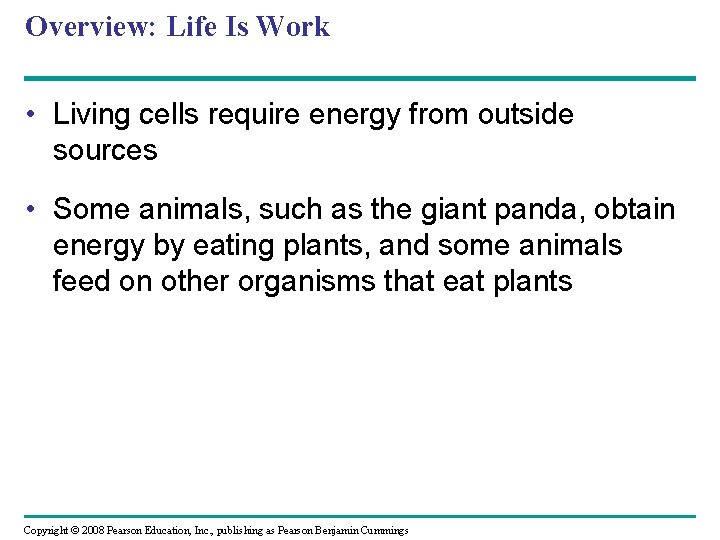
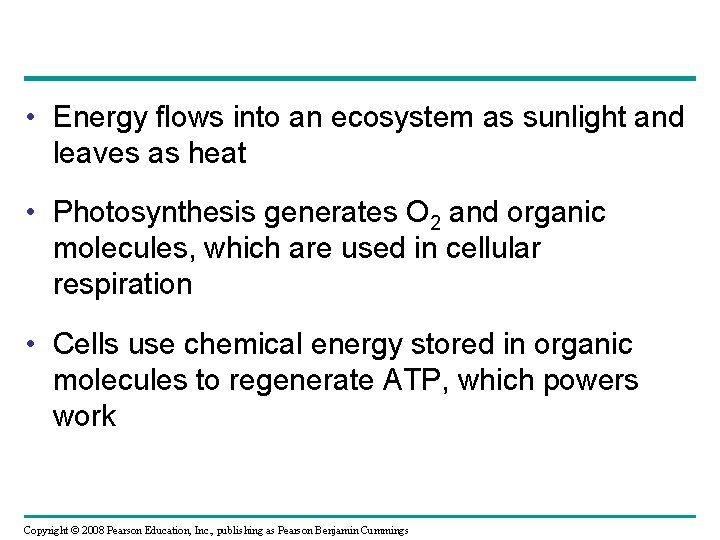
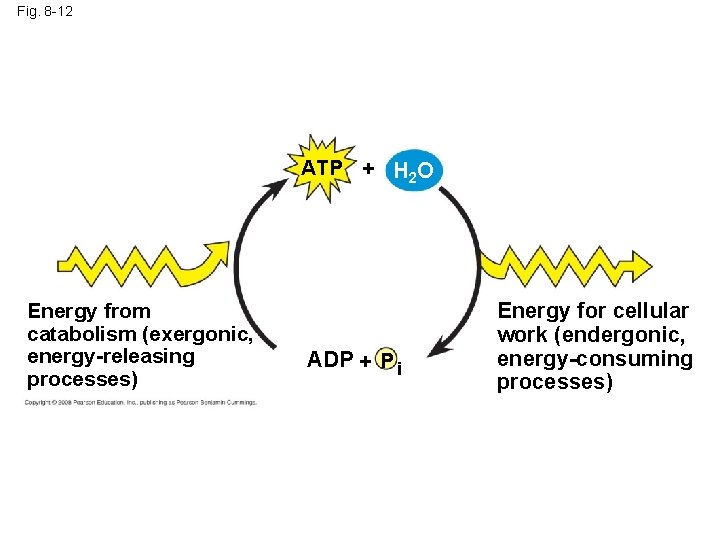
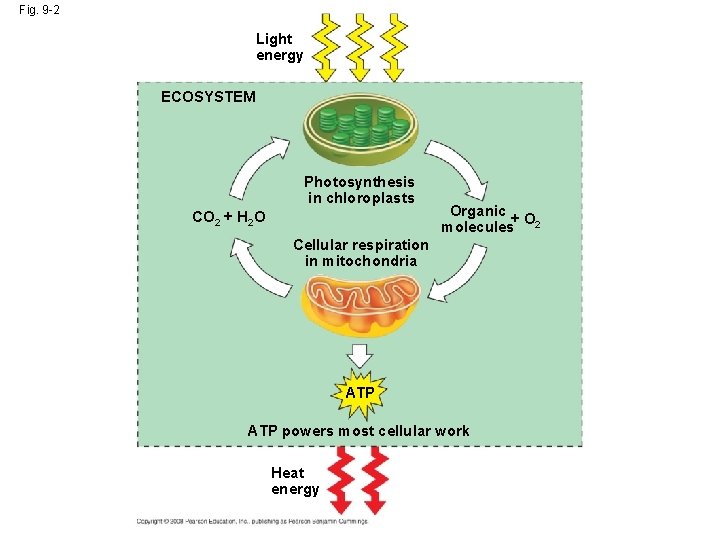
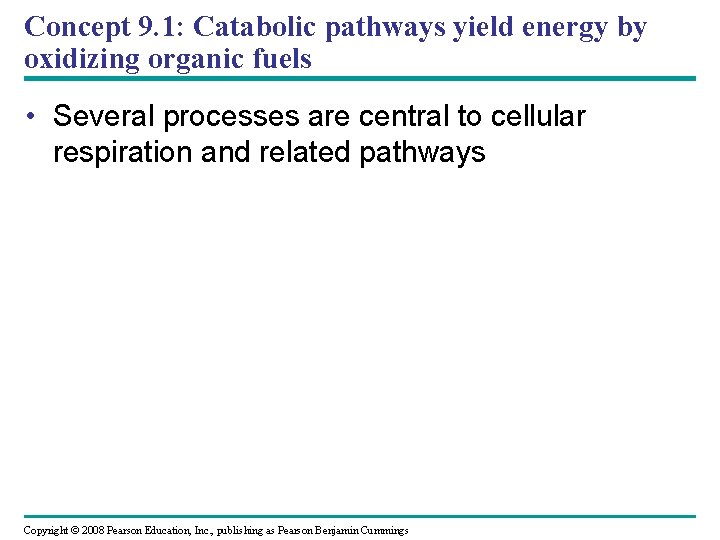
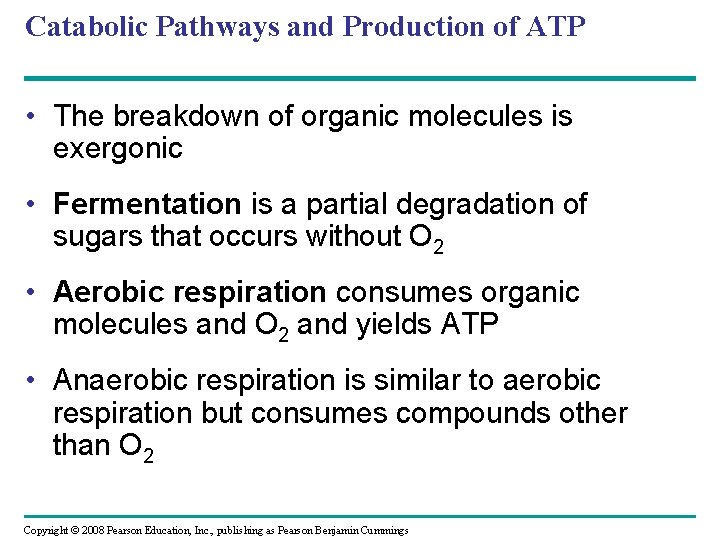
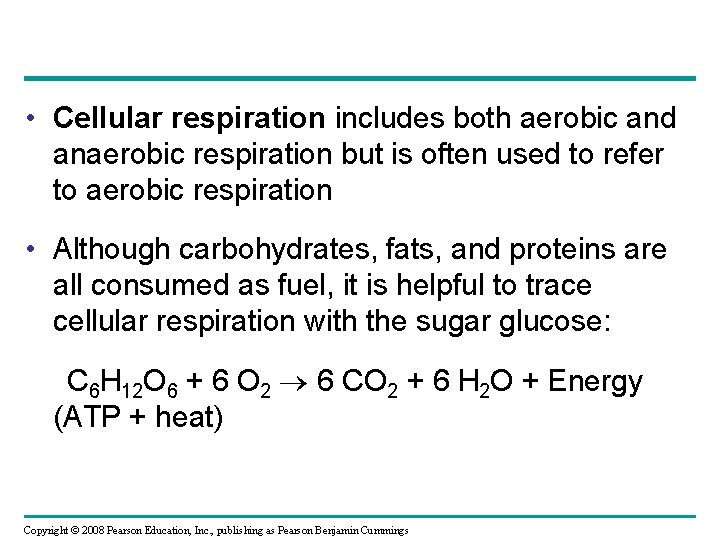
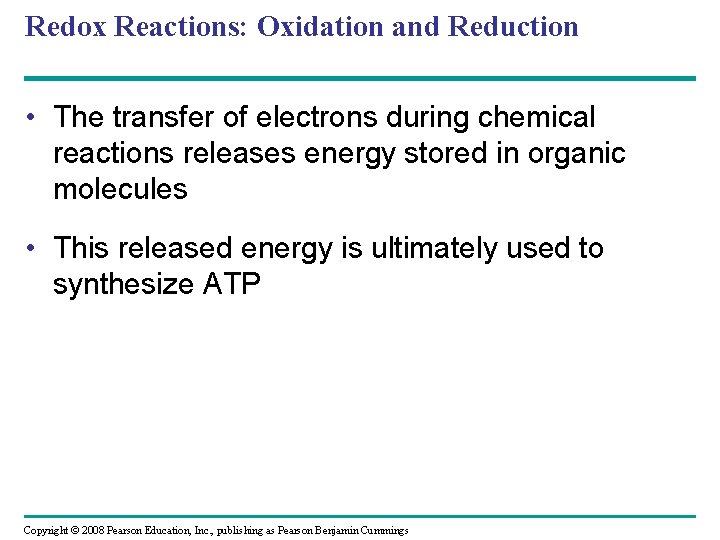
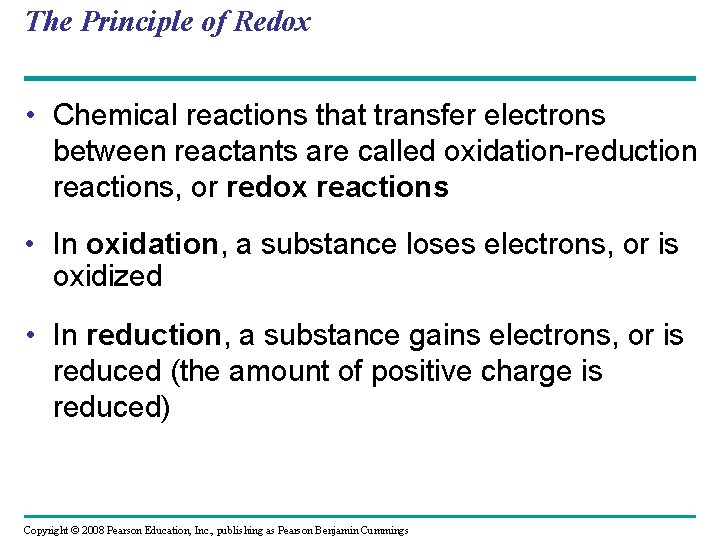
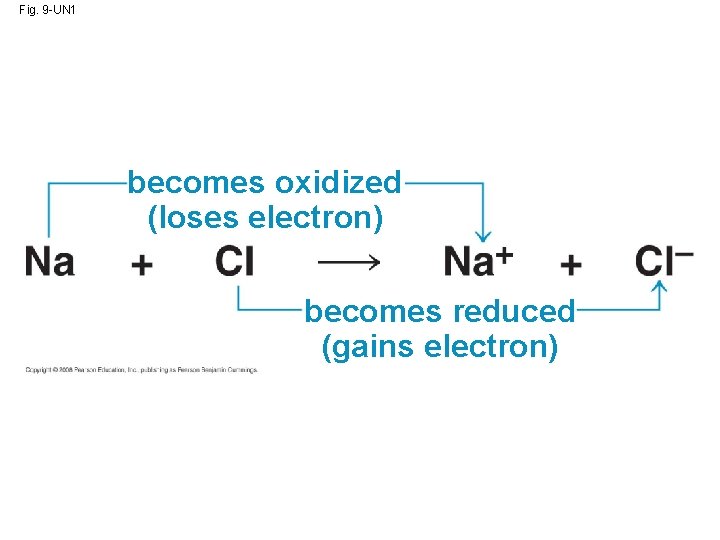
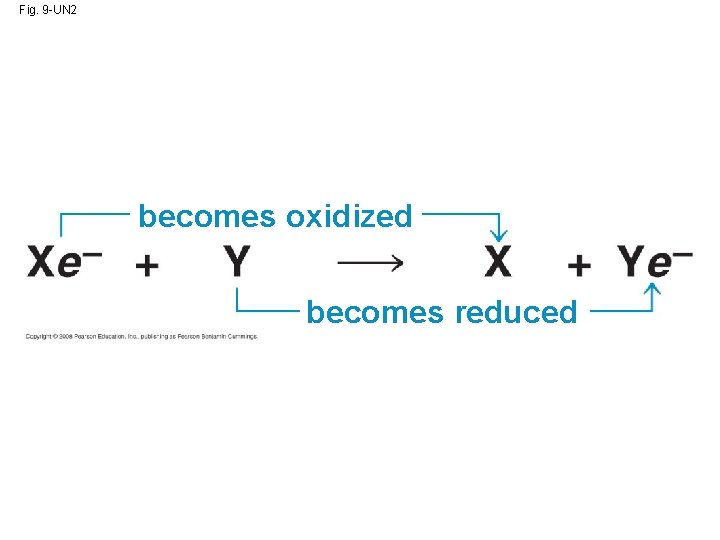
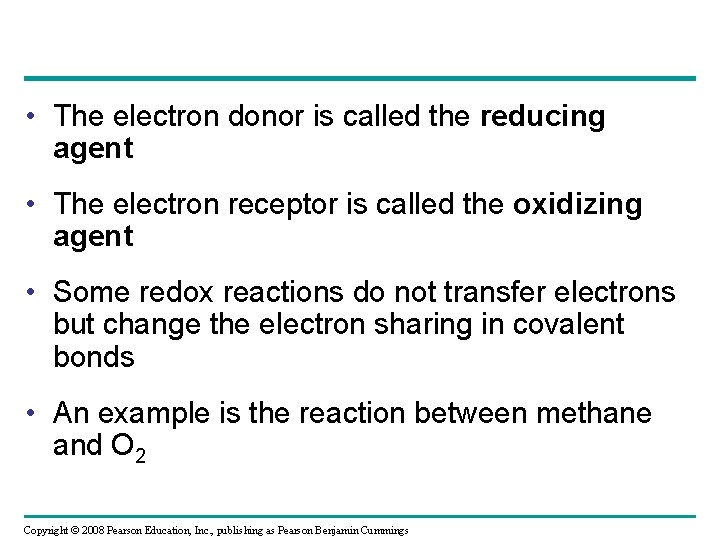
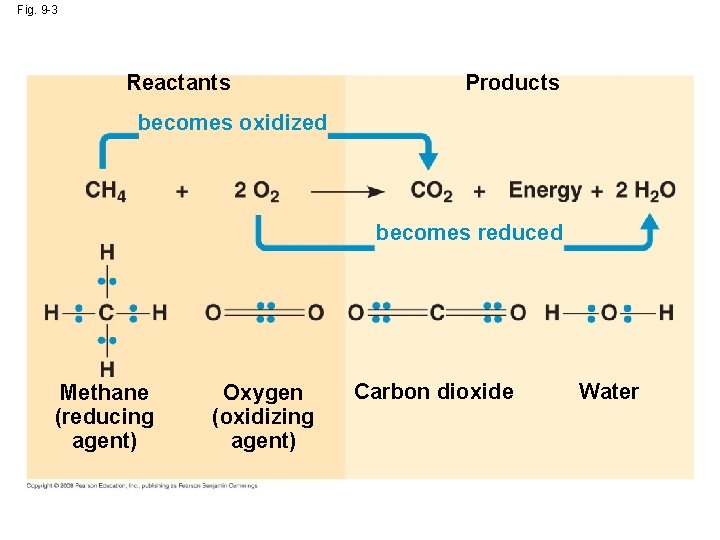
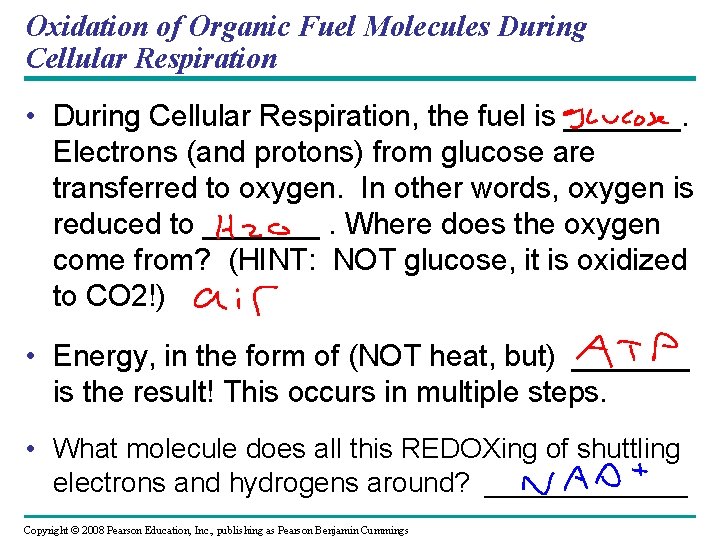
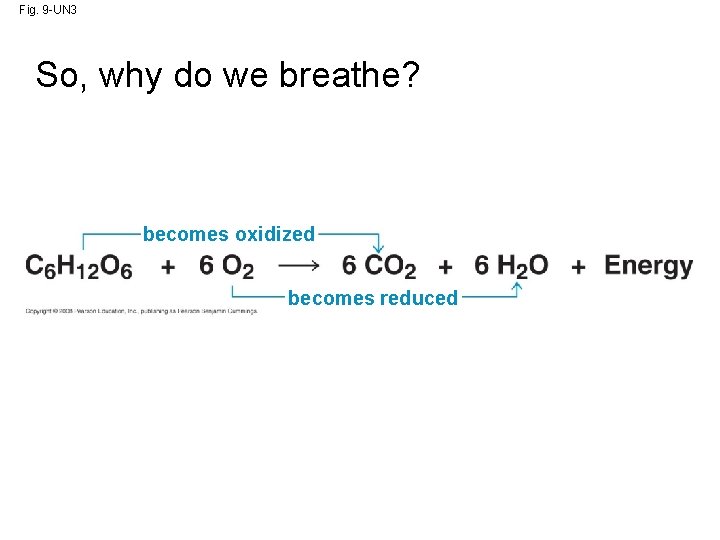
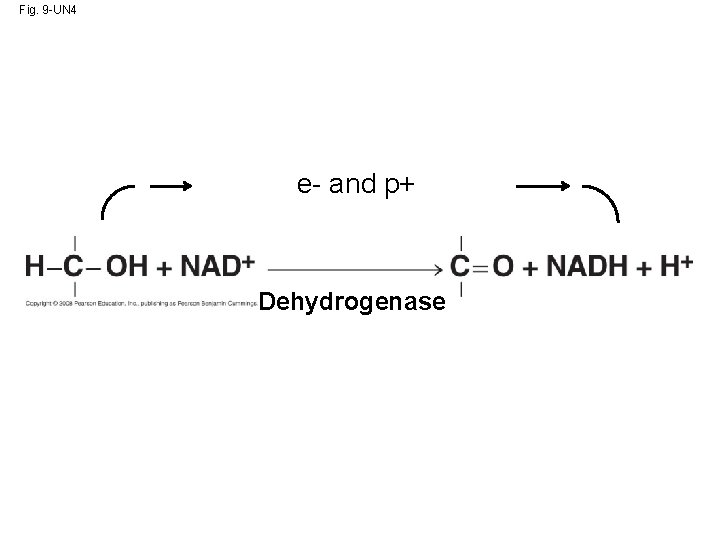
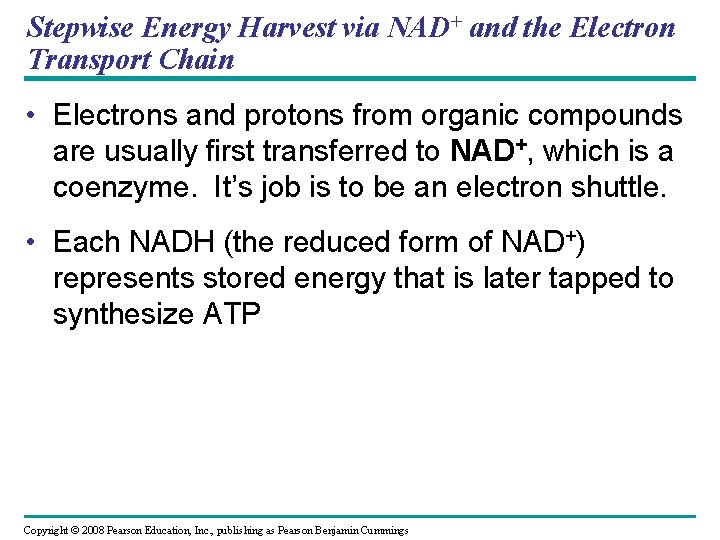
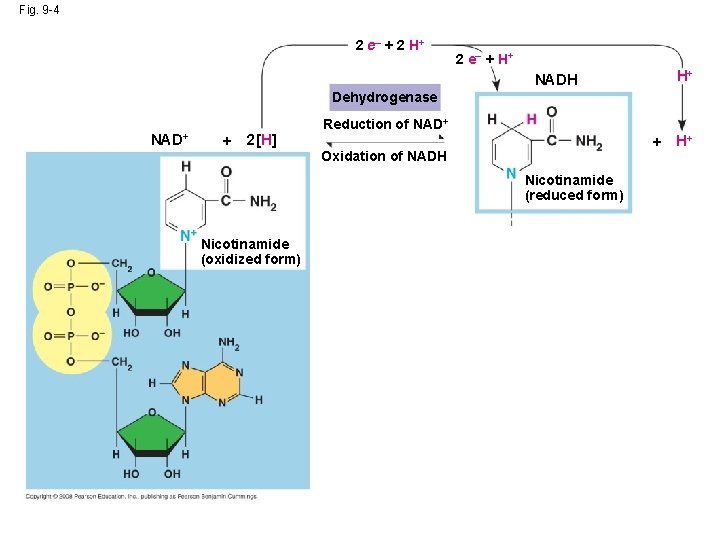
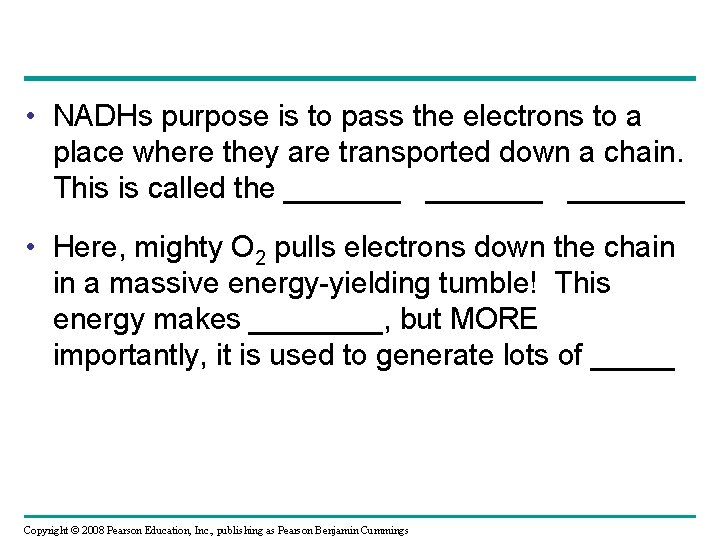
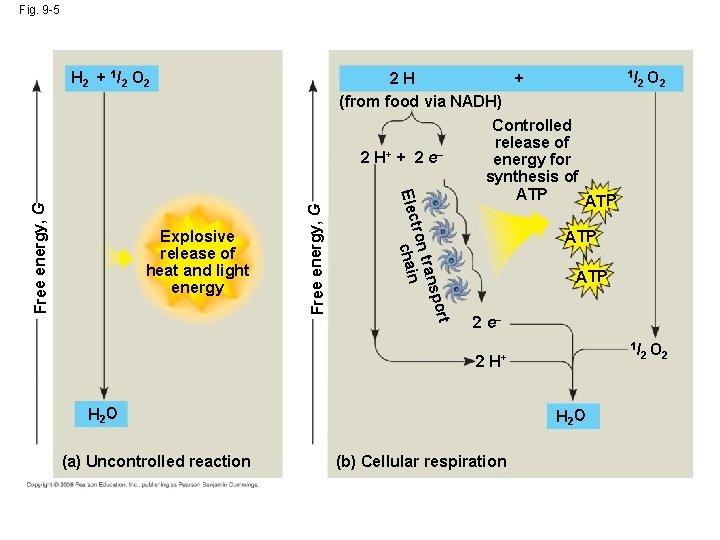
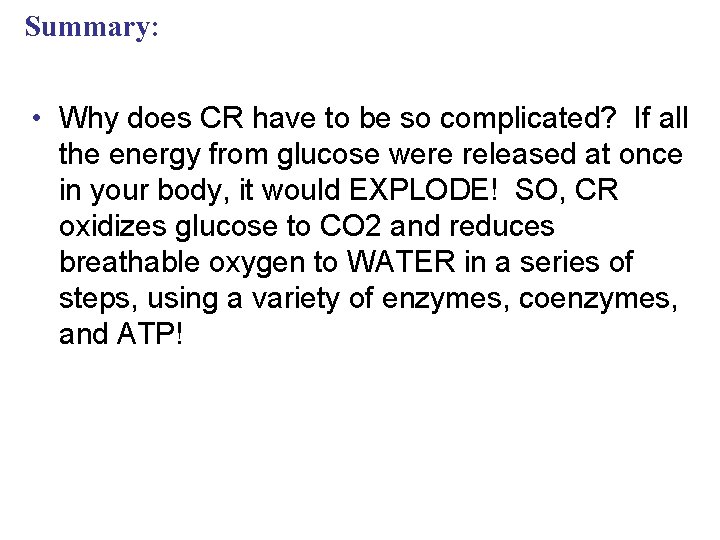
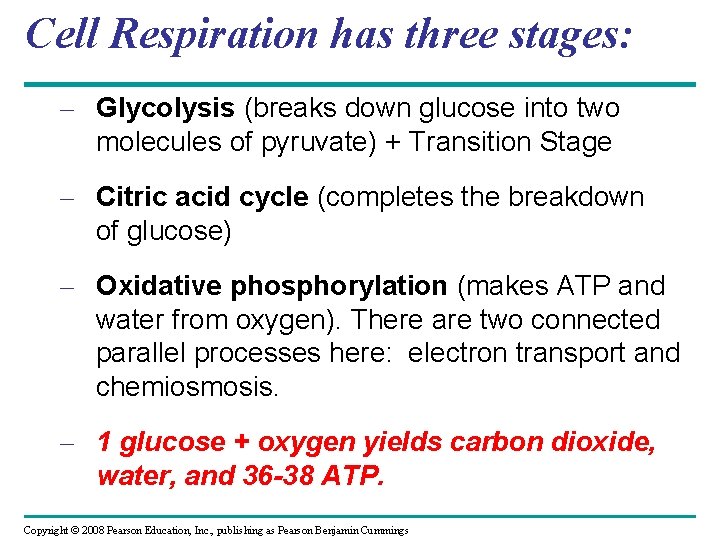
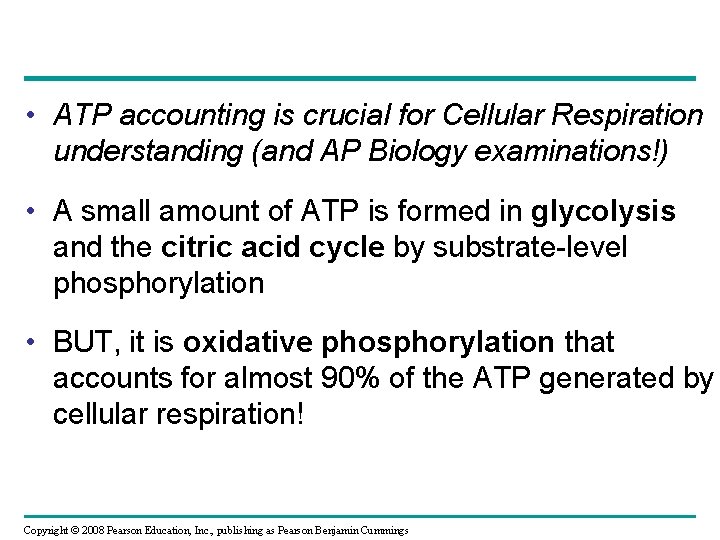
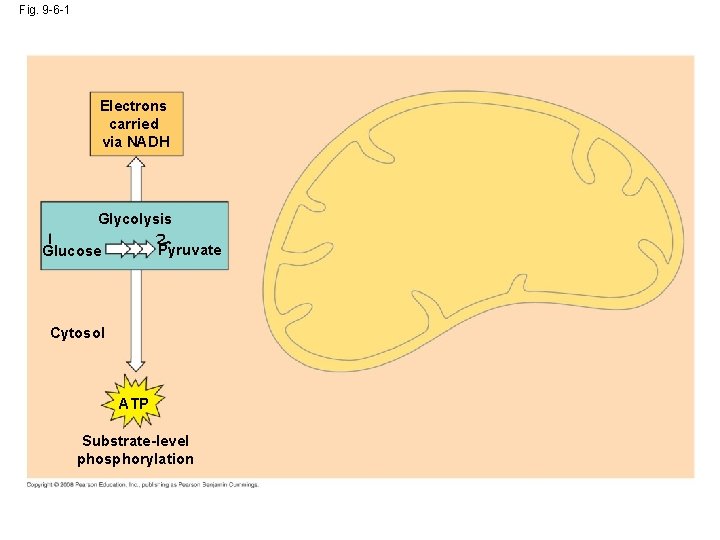
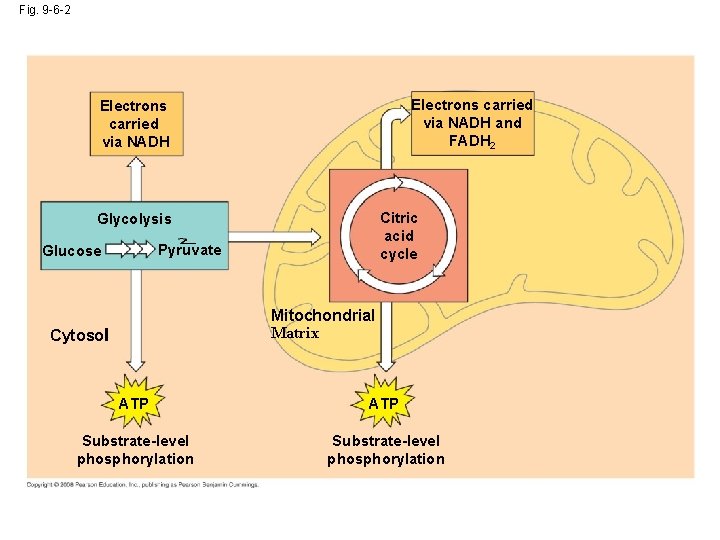
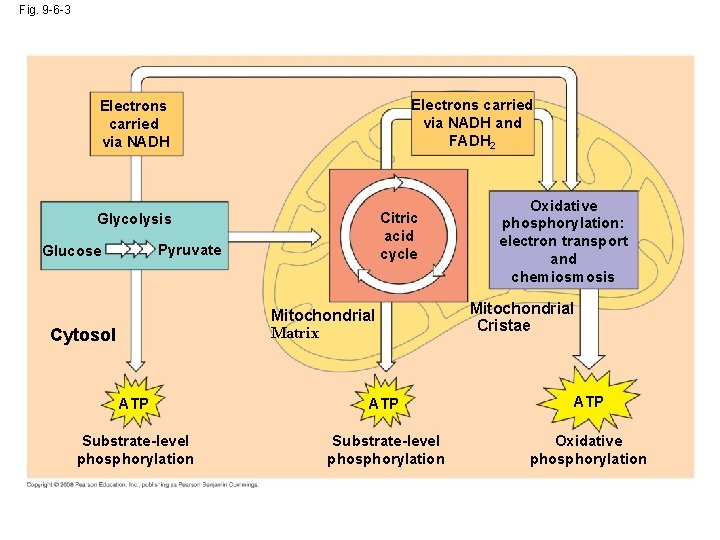
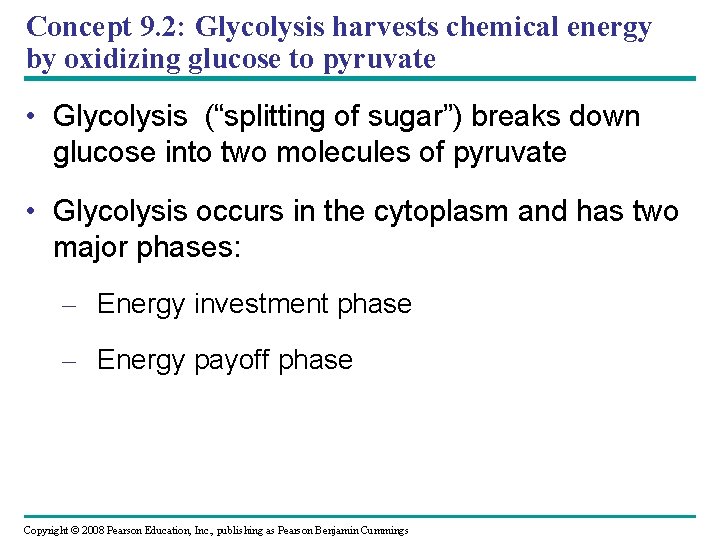
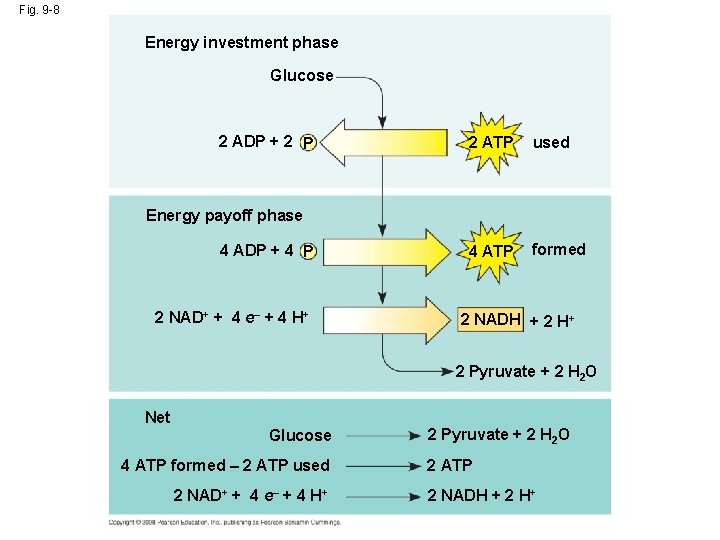
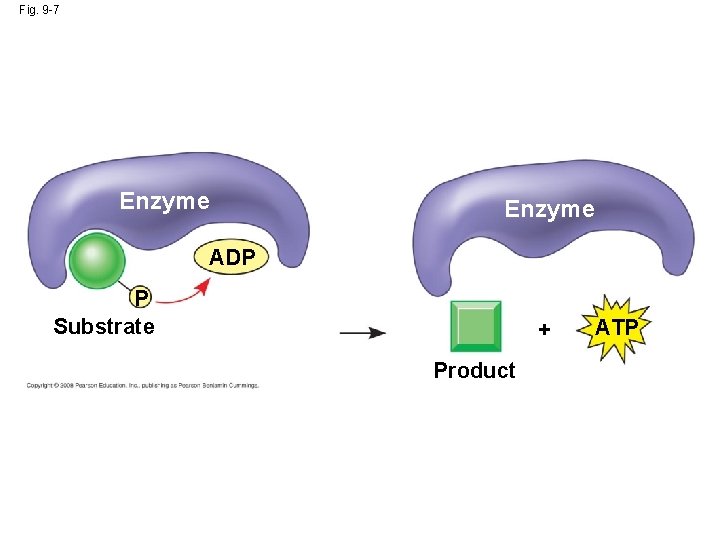
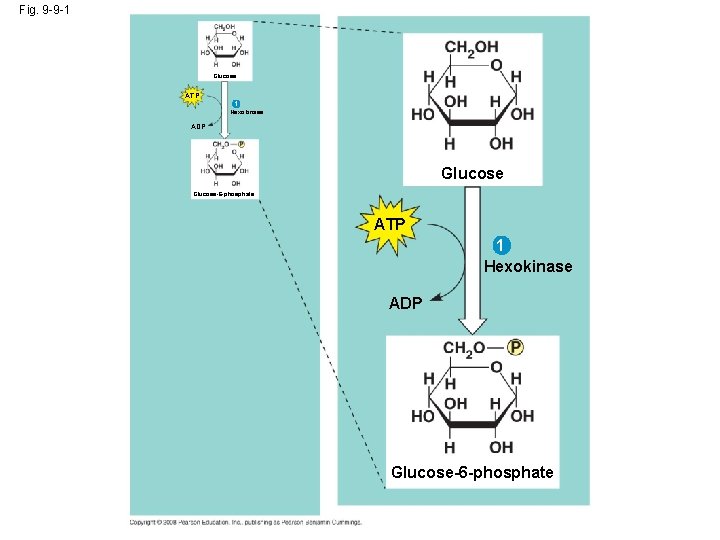
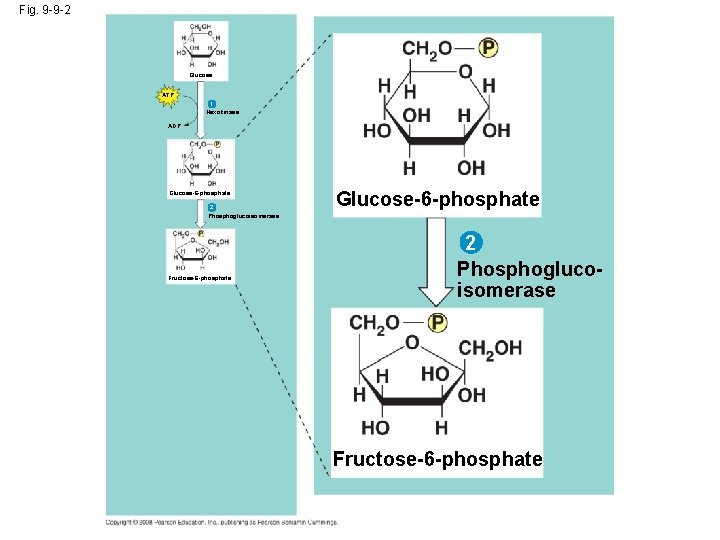
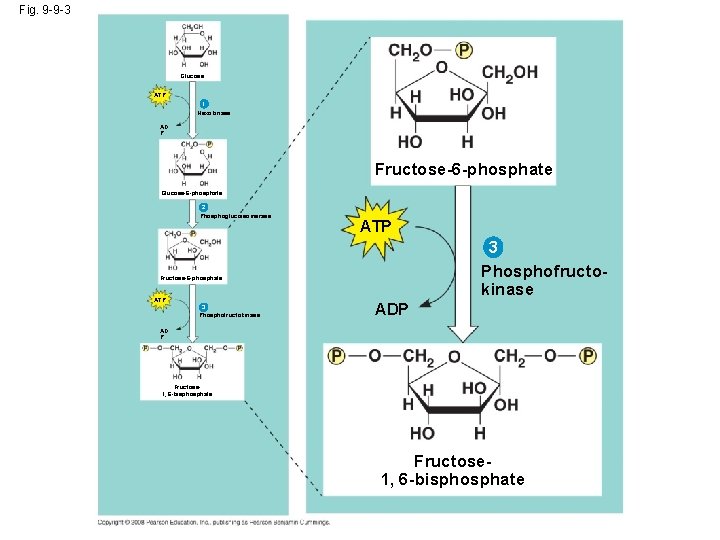
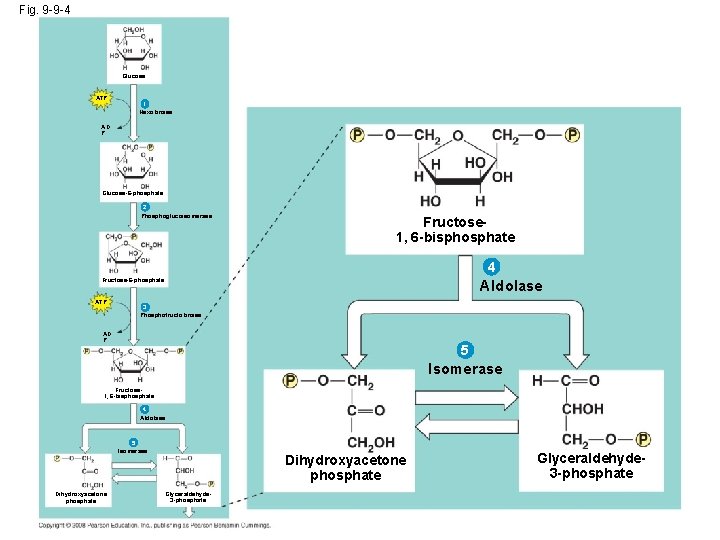
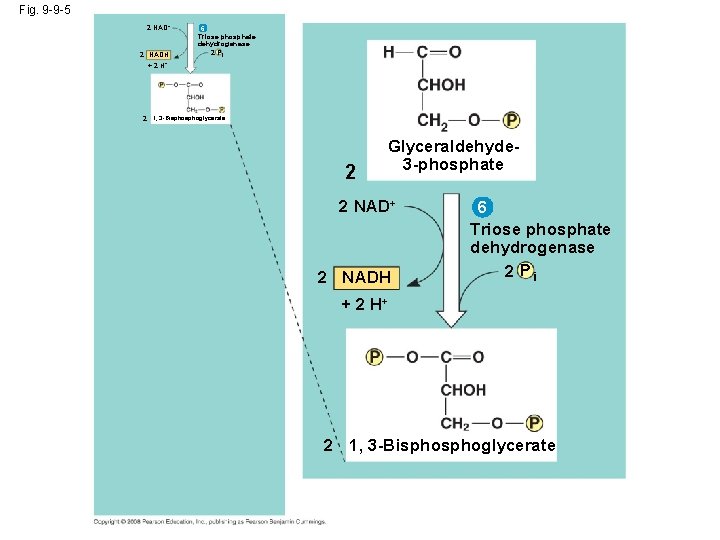
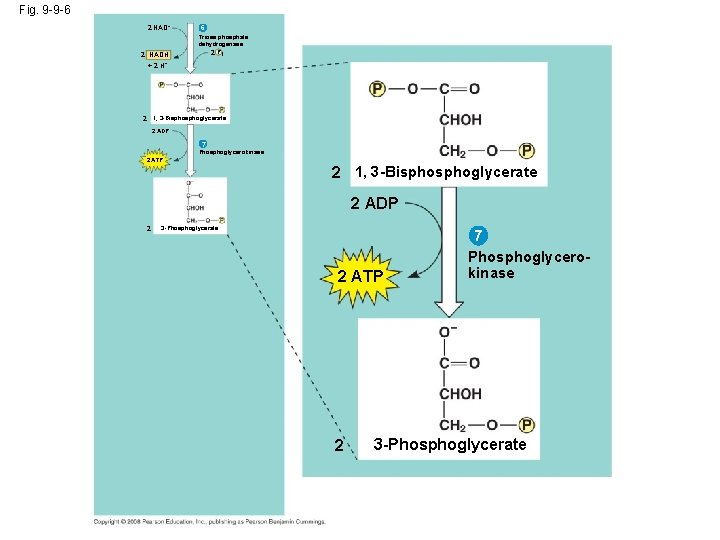
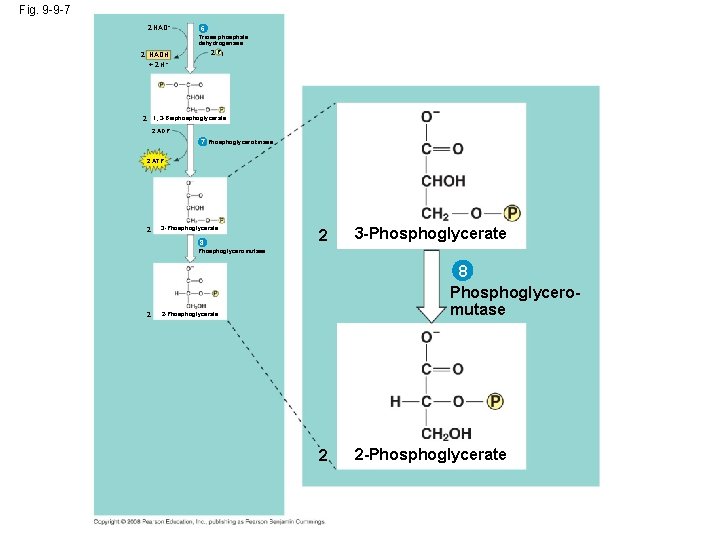
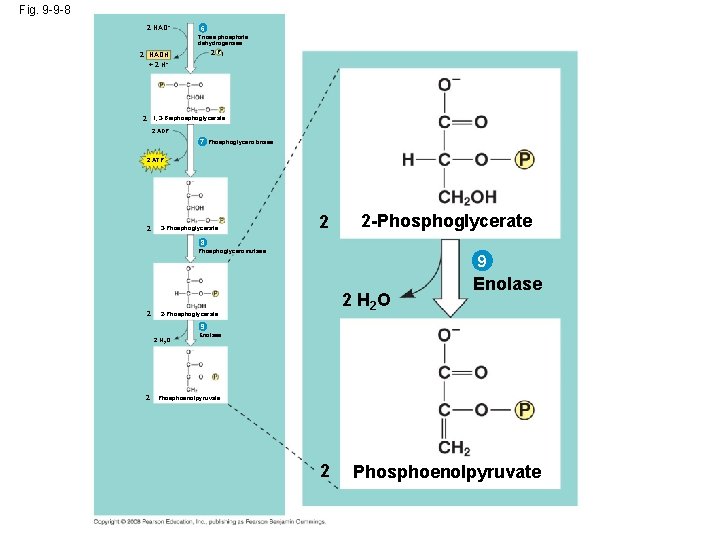
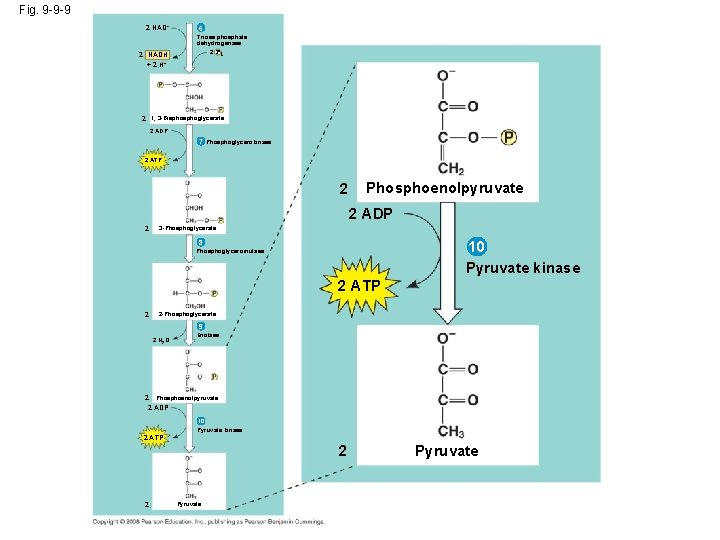
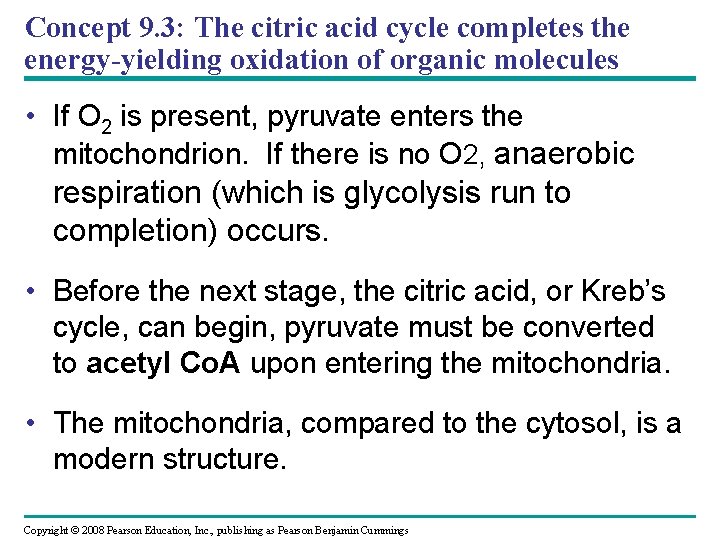
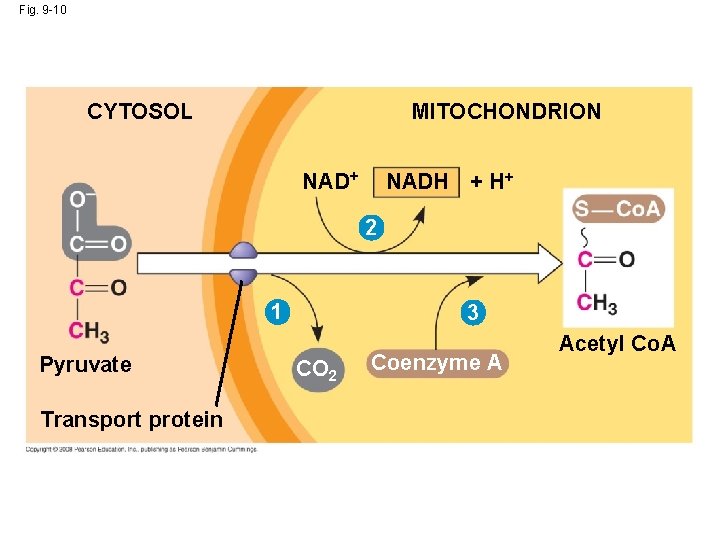
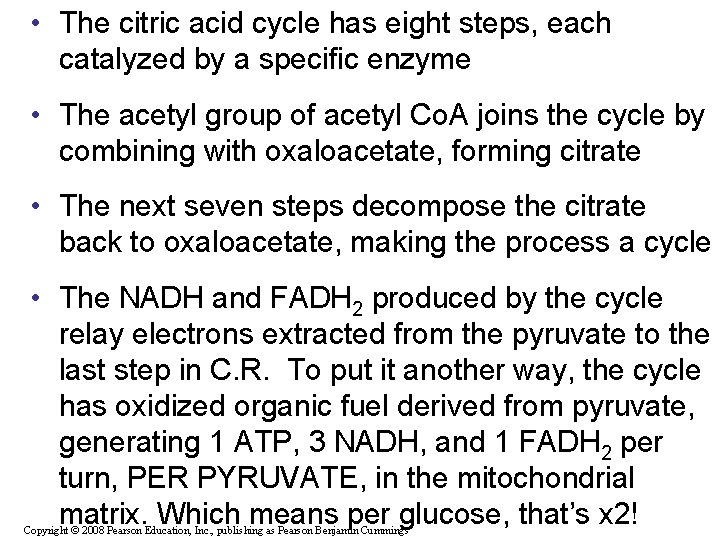
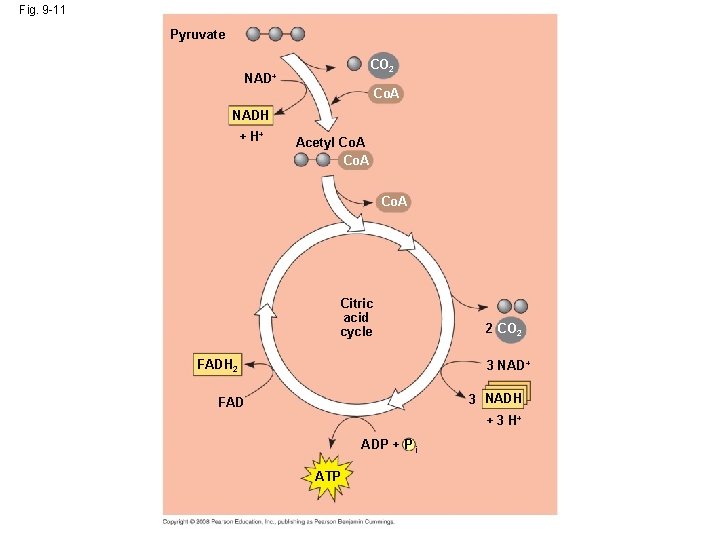
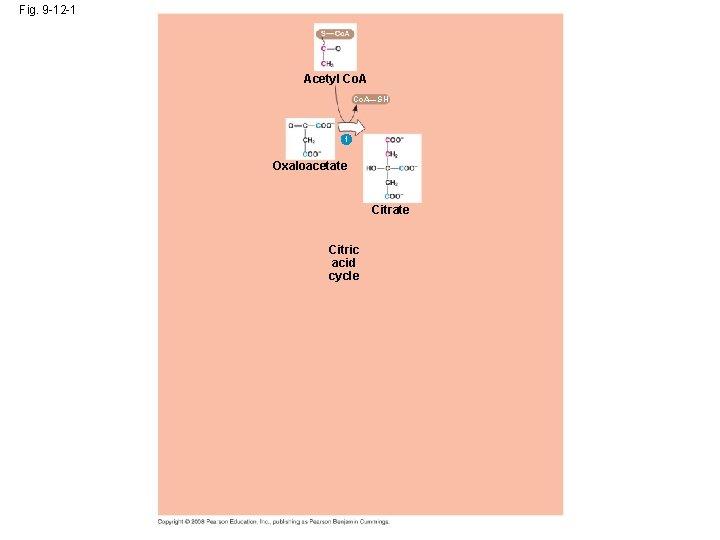
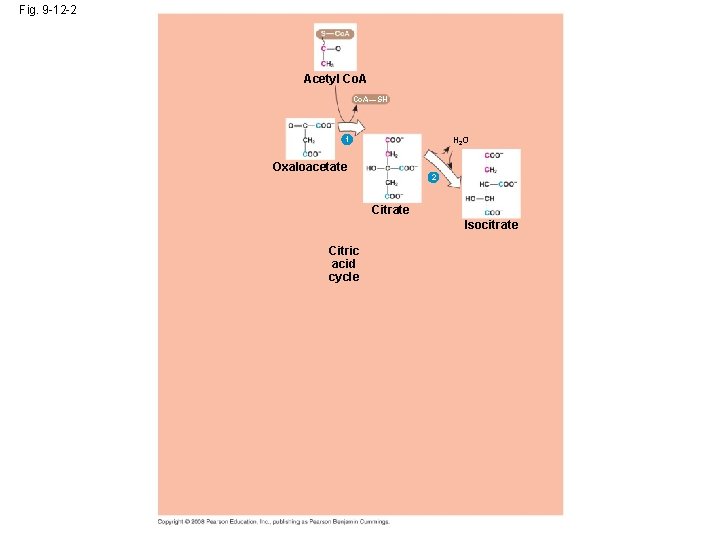
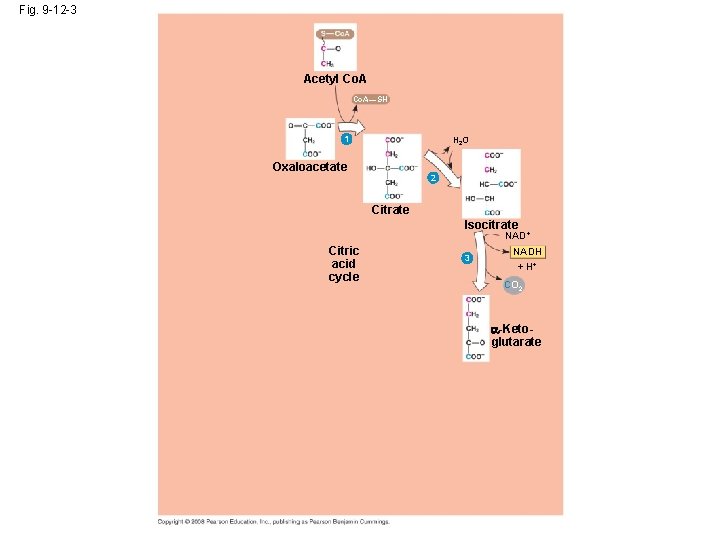
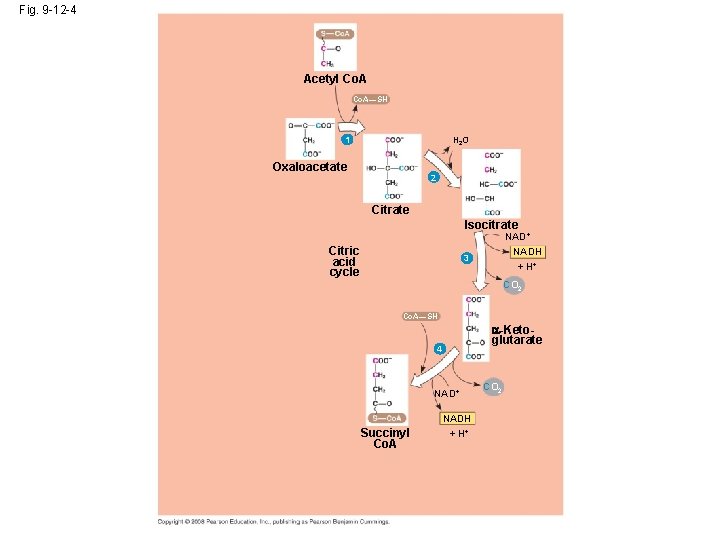
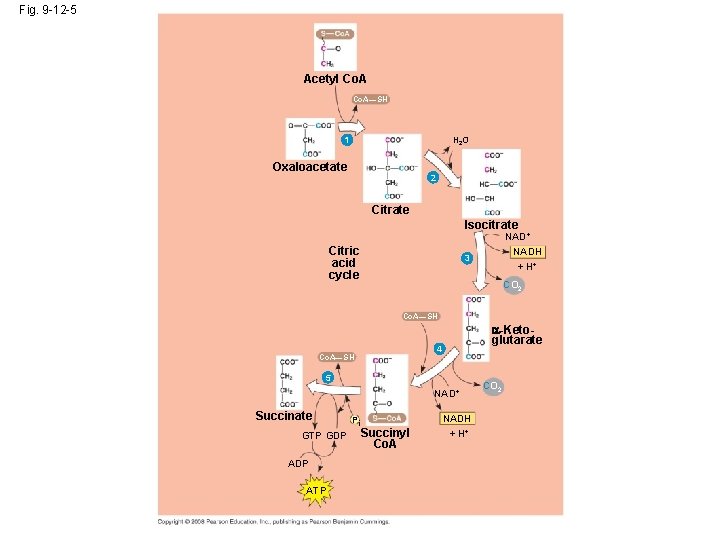
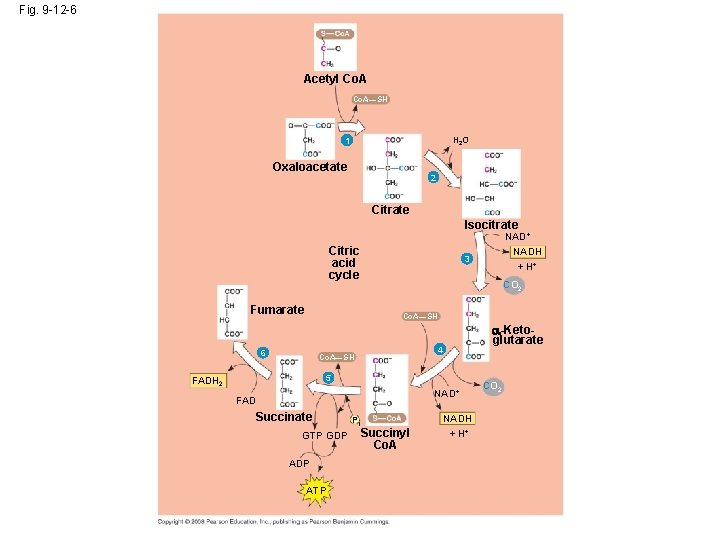
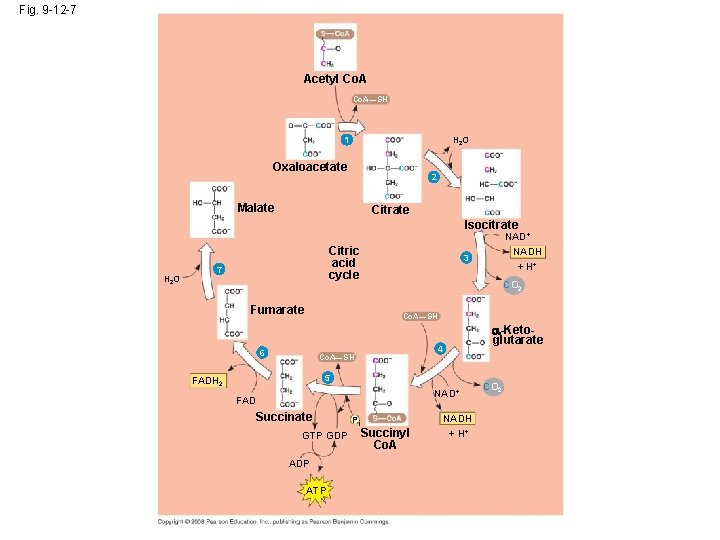
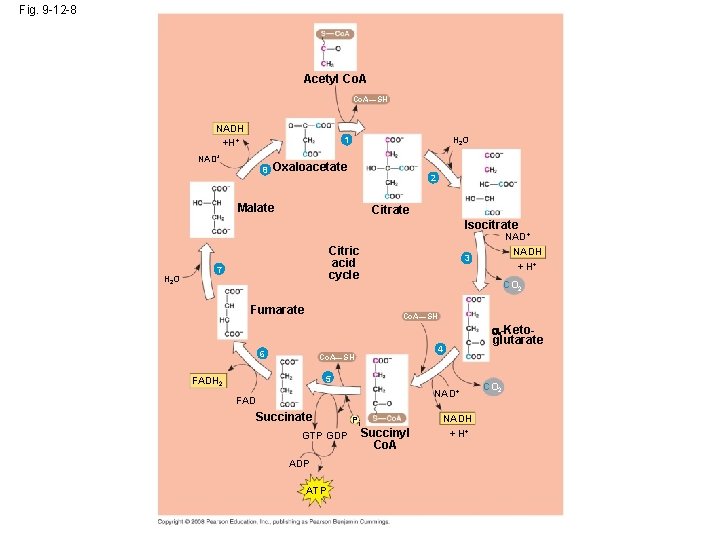
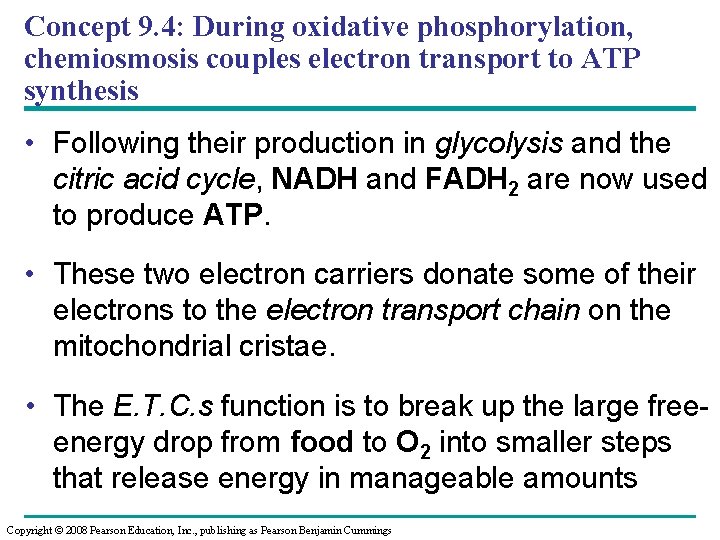
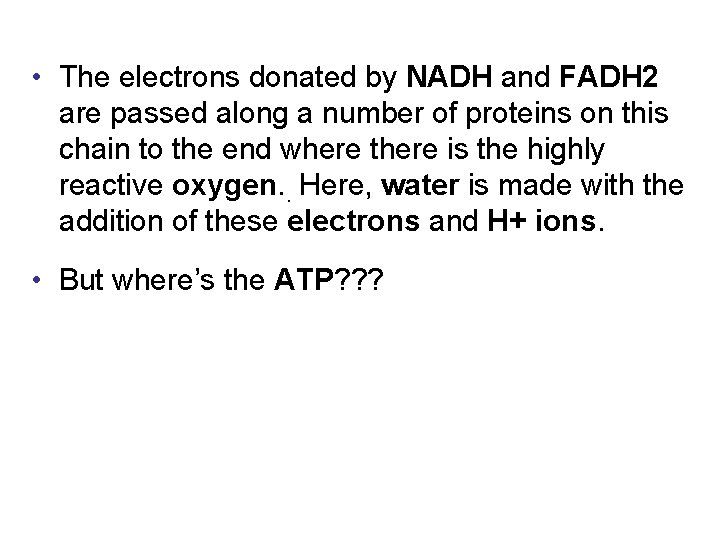
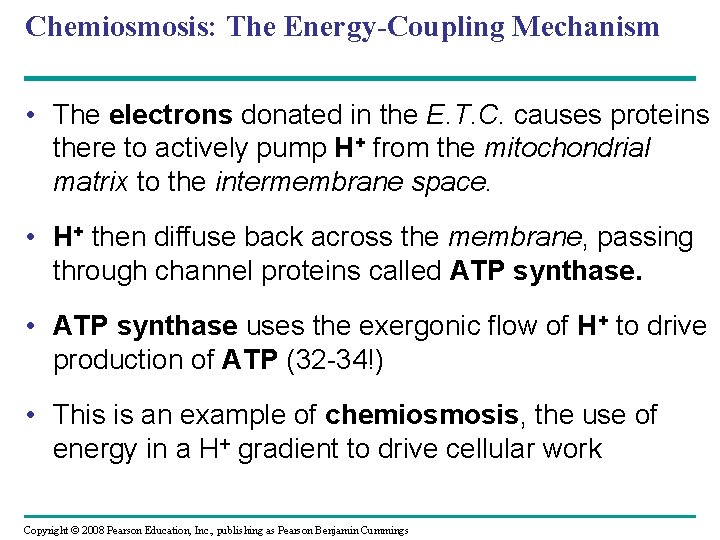
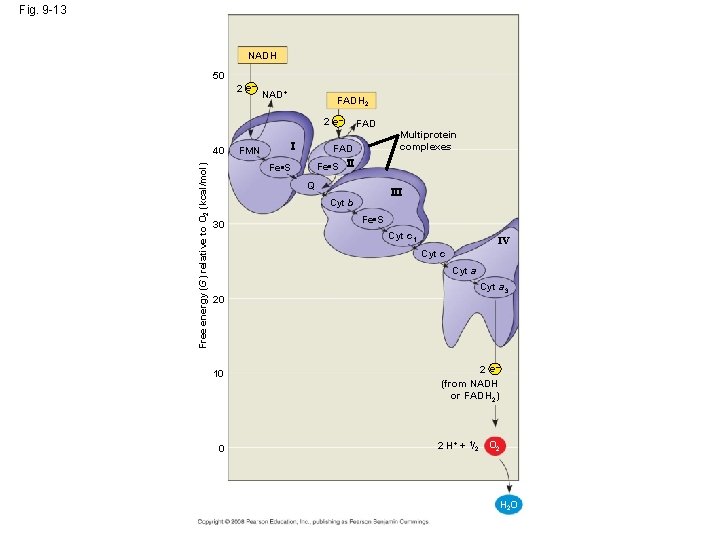
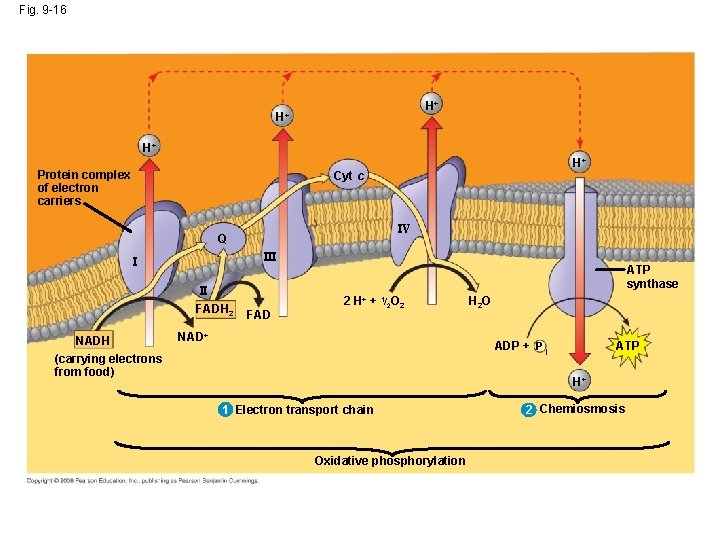
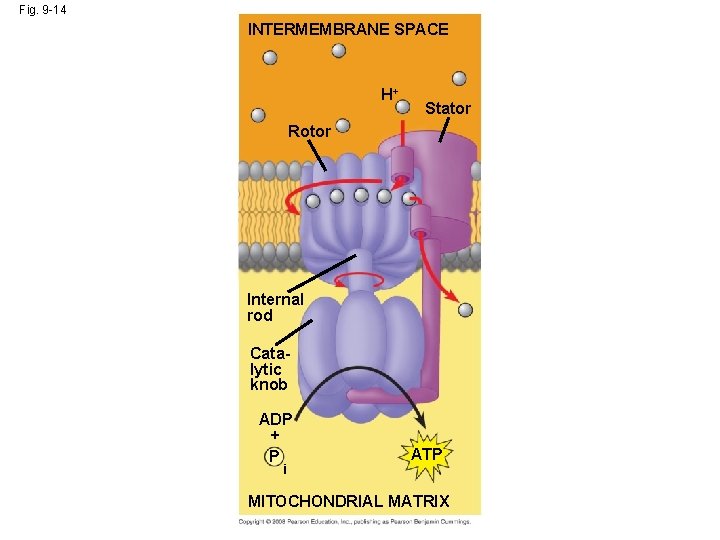
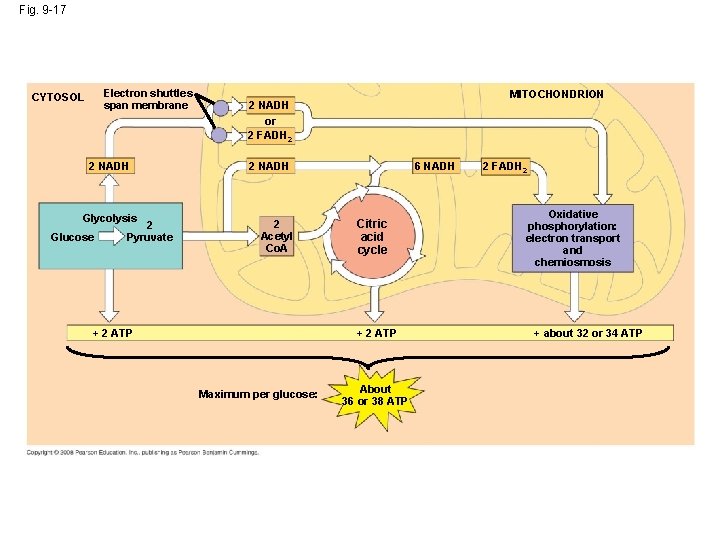
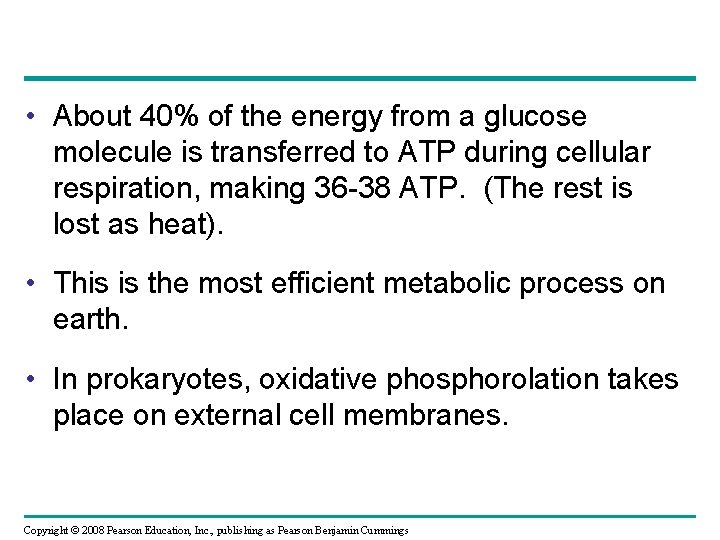
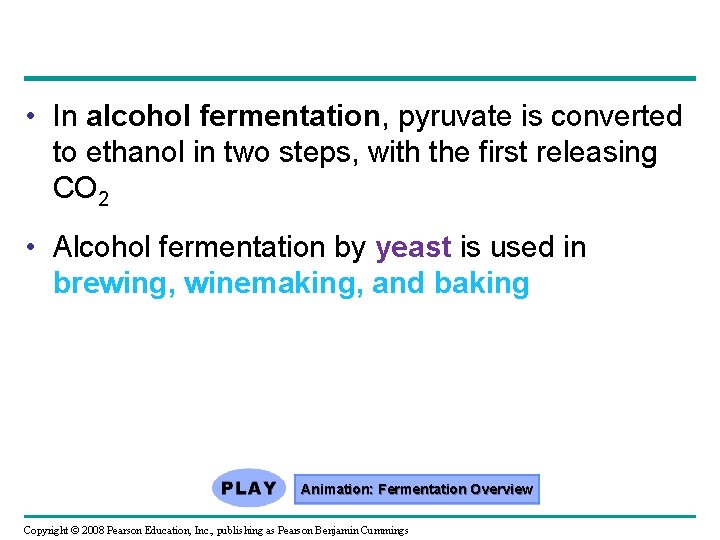
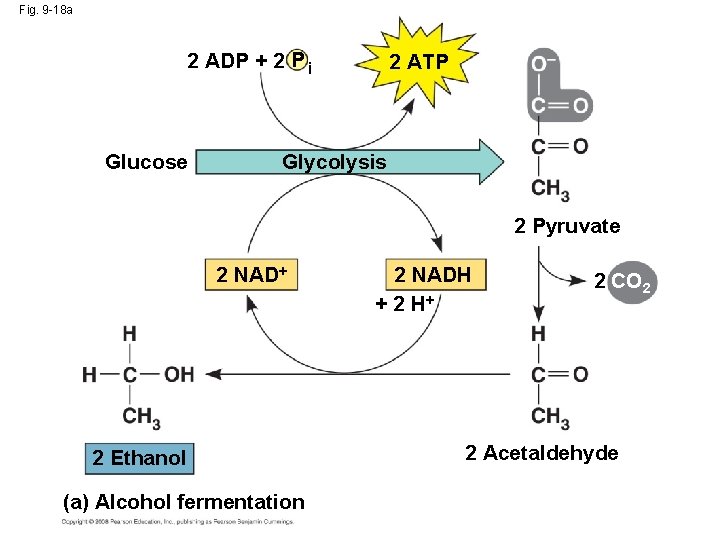
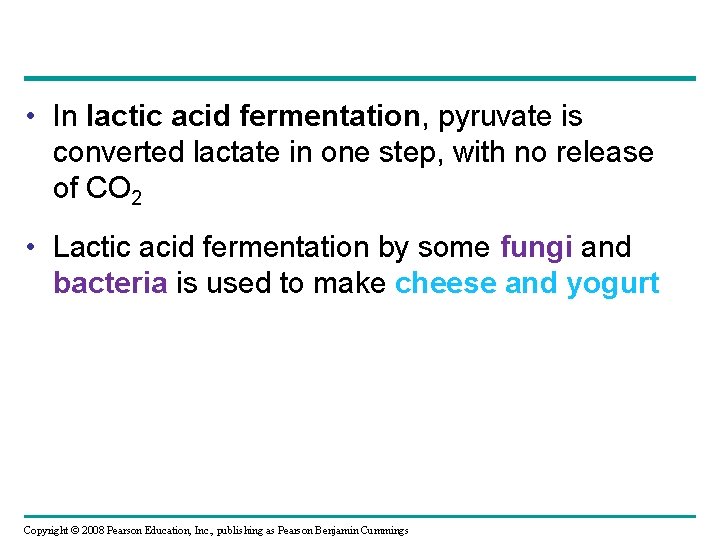
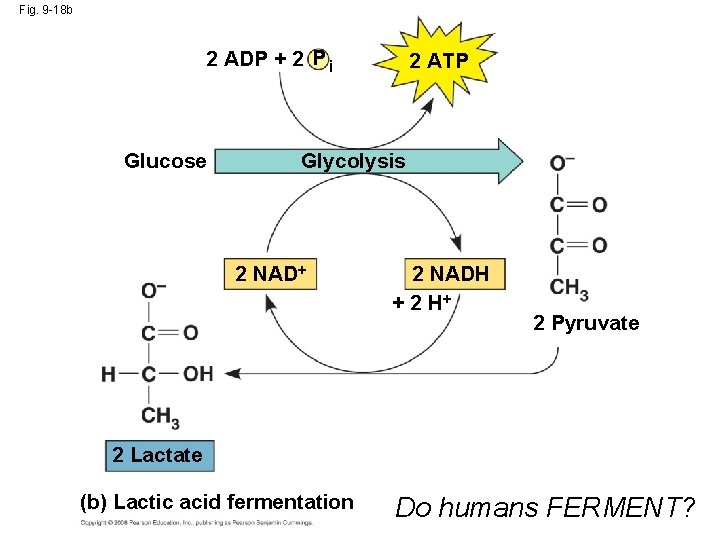
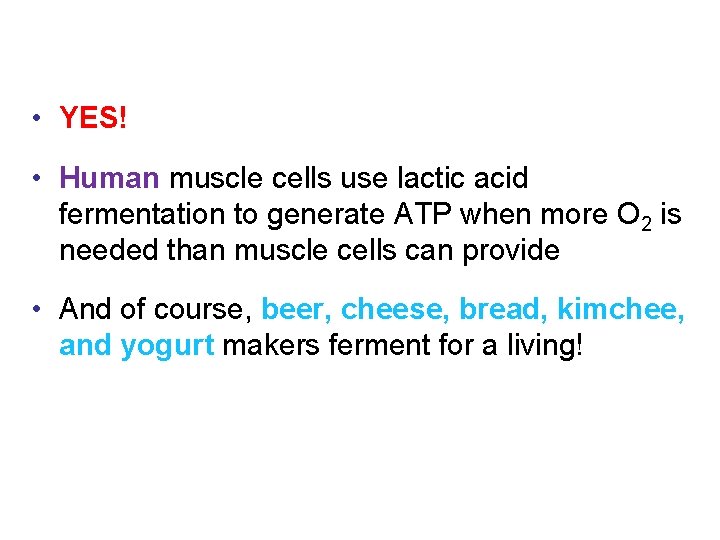
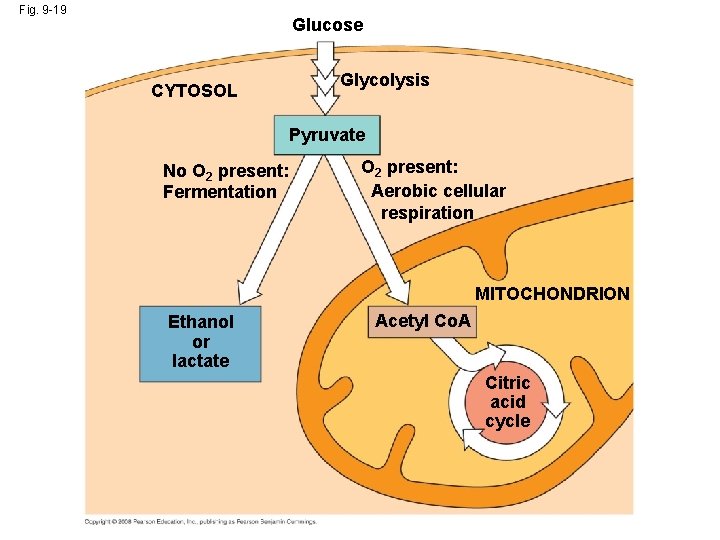
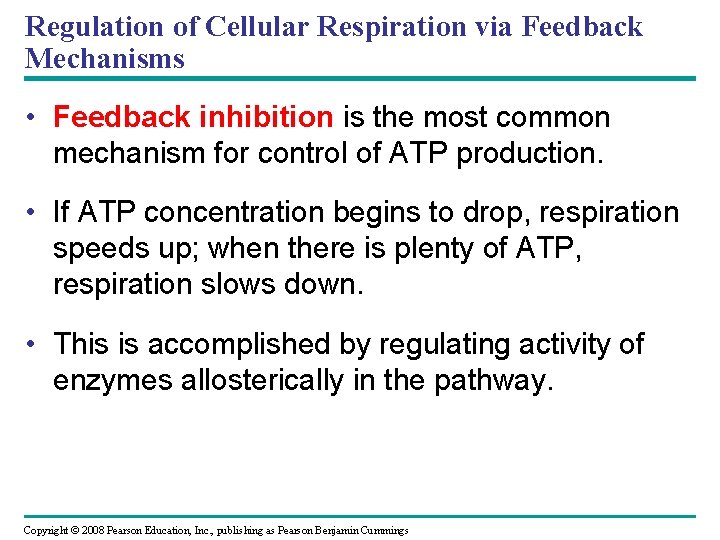
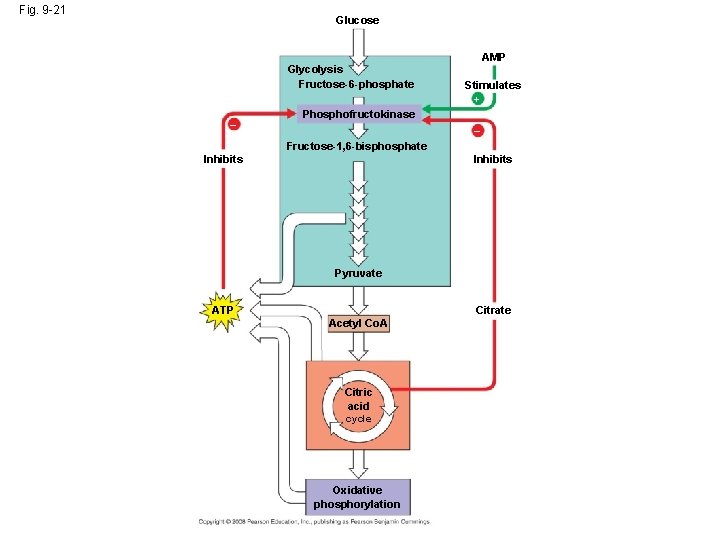
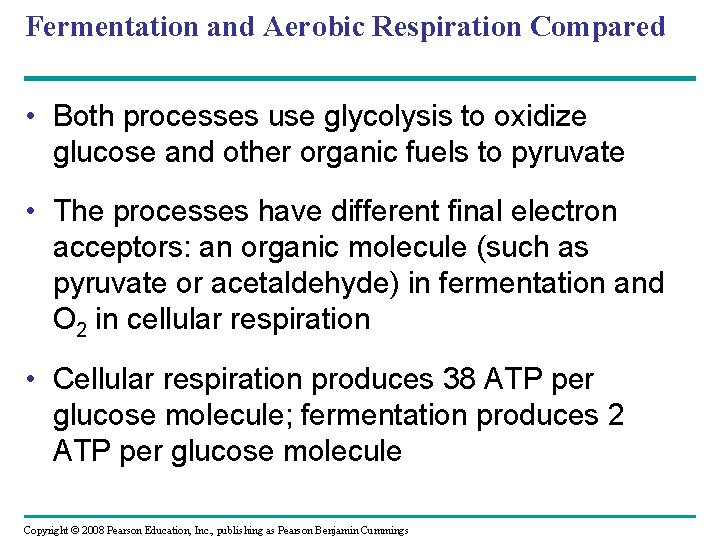
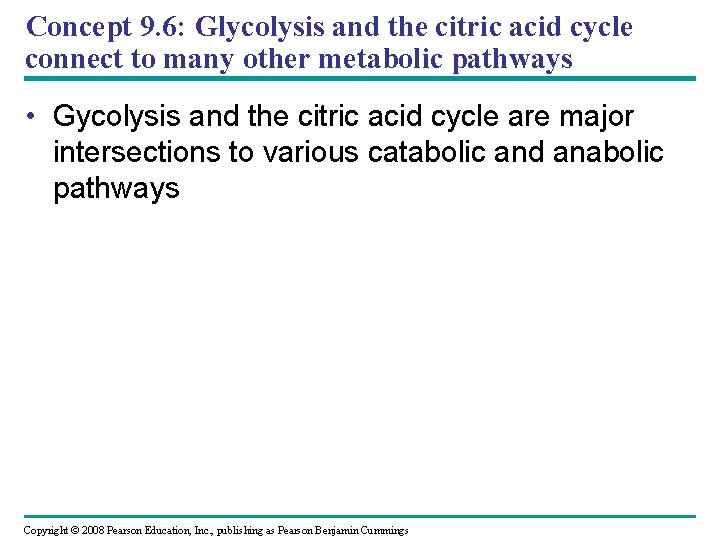
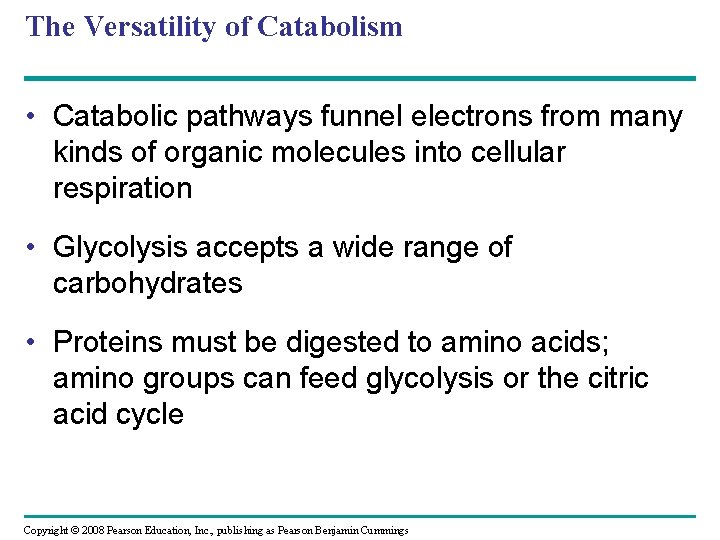
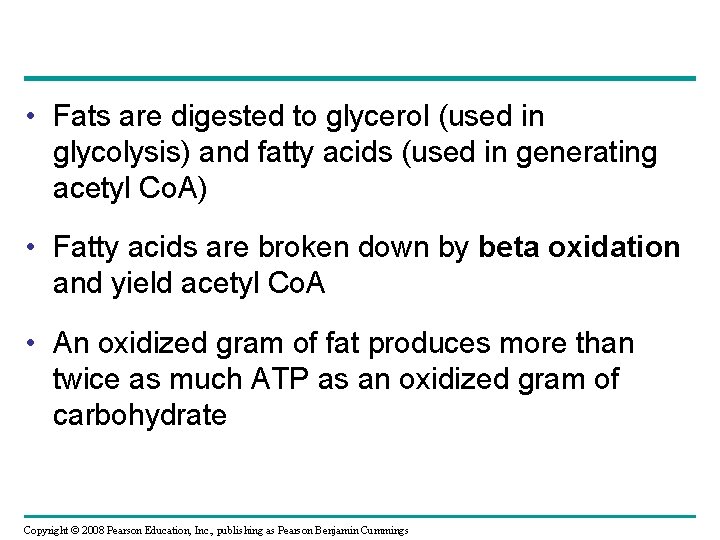
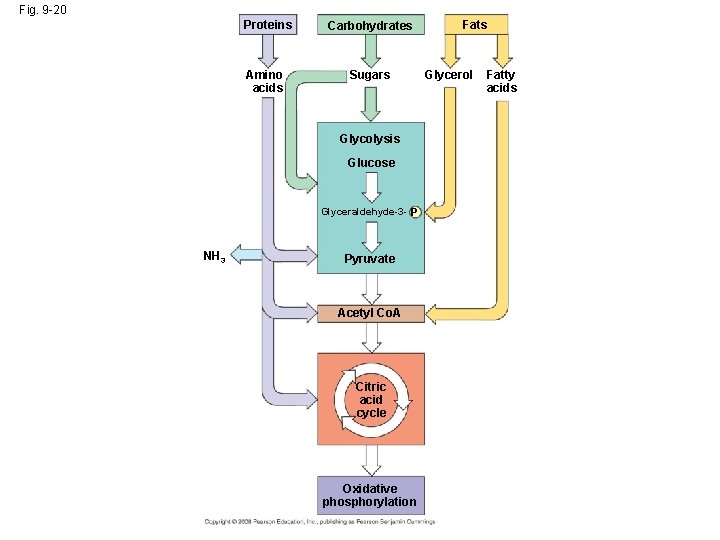
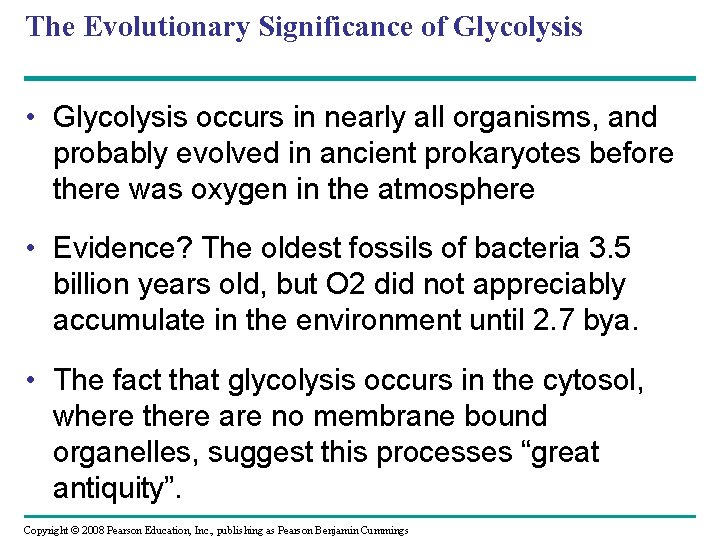
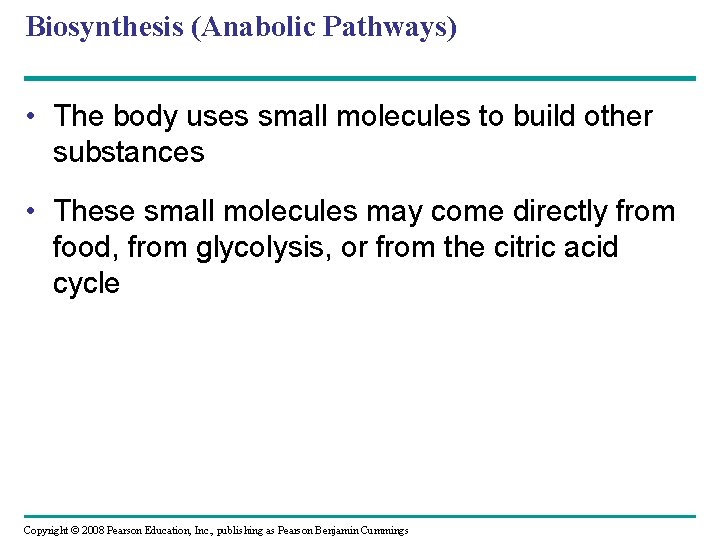
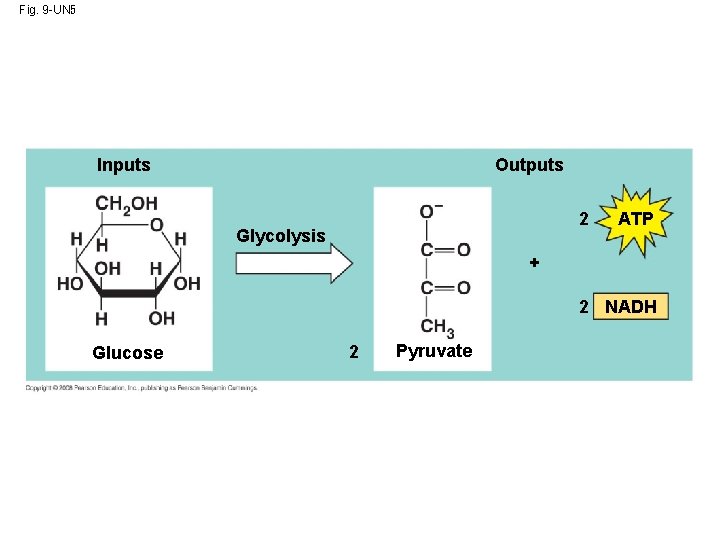
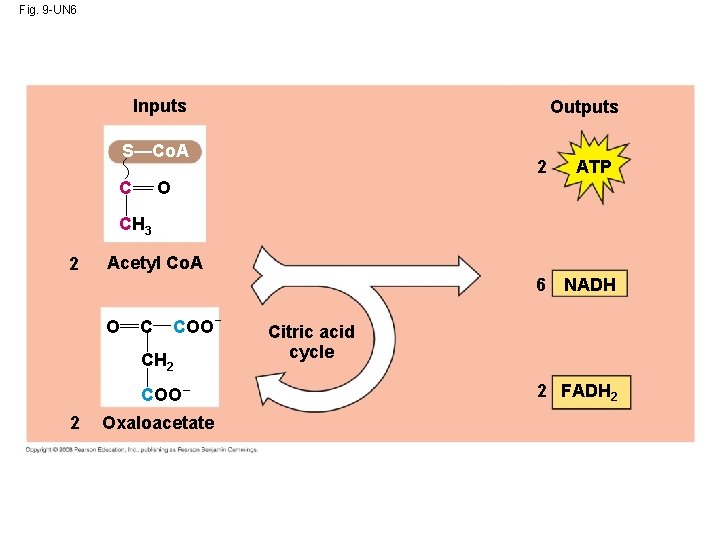
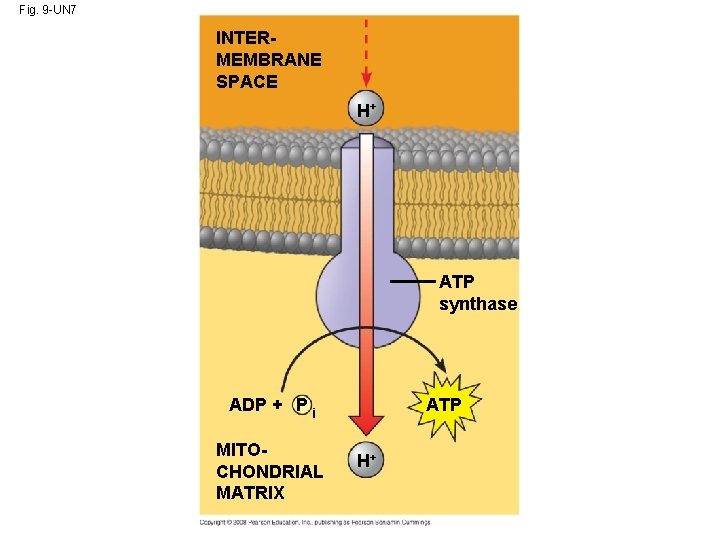
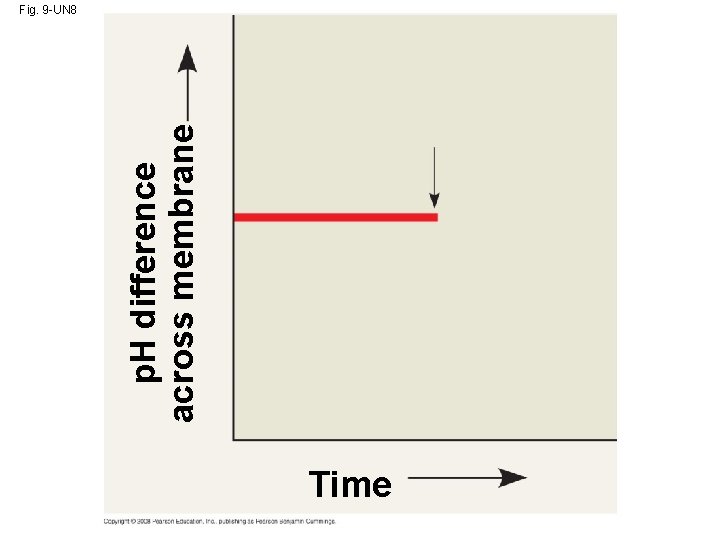
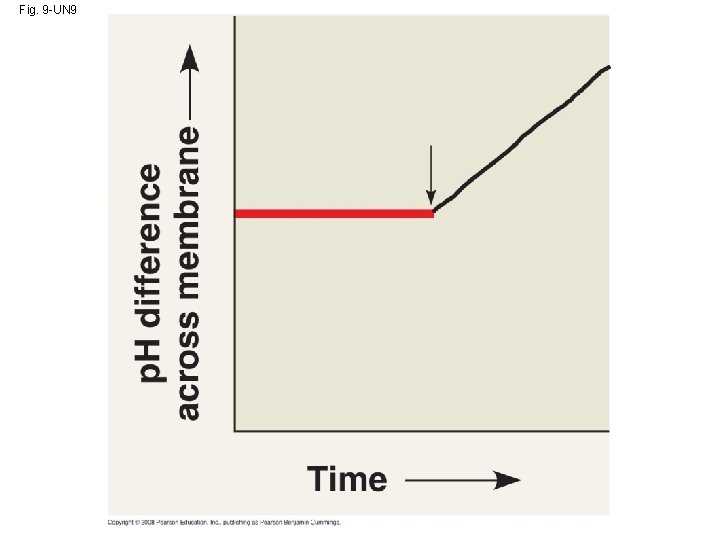
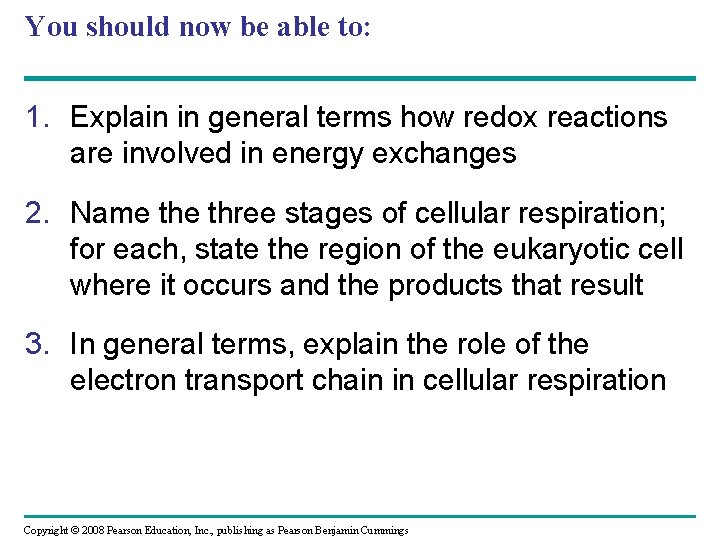
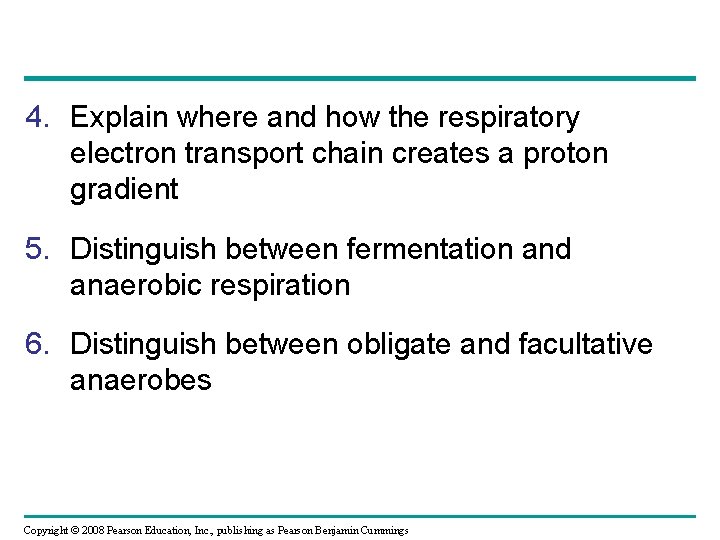
- Slides: 81
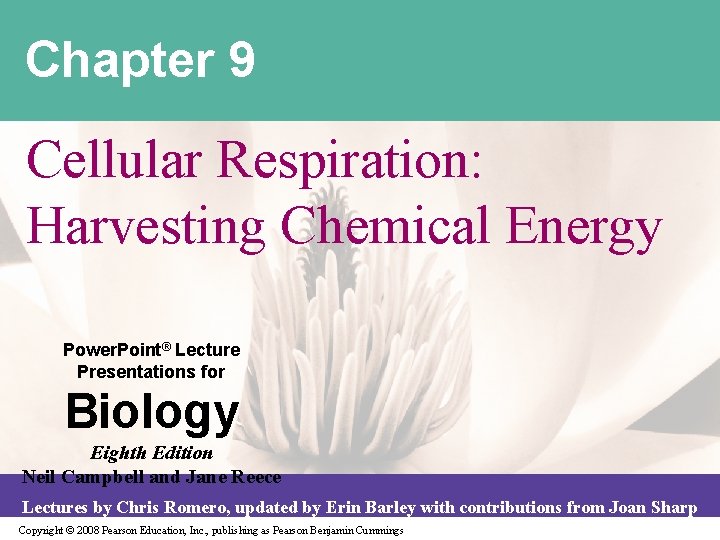
Chapter 9 Cellular Respiration: Harvesting Chemical Energy Power. Point® Lecture Presentations for Biology Eighth Edition Neil Campbell and Jane Reece Lectures by Chris Romero, updated by Erin Barley with contributions from Joan Sharp Copyright © 2008 Pearson Education, Inc. , publishing as Pearson Benjamin Cummings
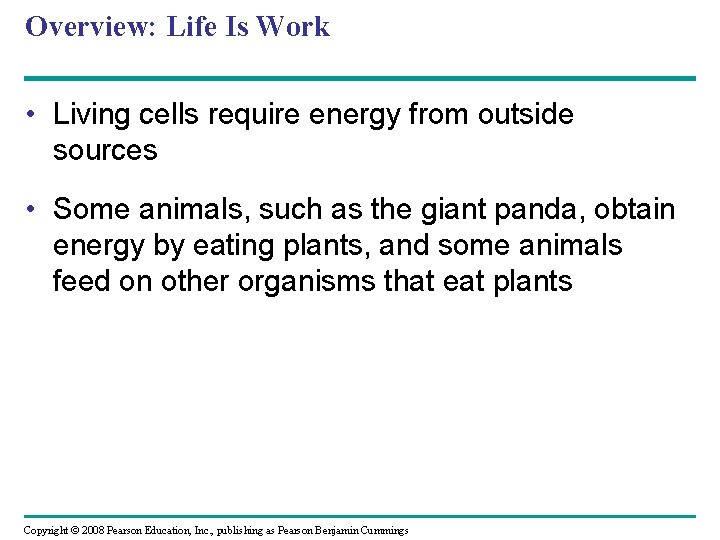
Overview: Life Is Work • Living cells require energy from outside sources • Some animals, such as the giant panda, obtain energy by eating plants, and some animals feed on other organisms that eat plants Copyright © 2008 Pearson Education, Inc. , publishing as Pearson Benjamin Cummings
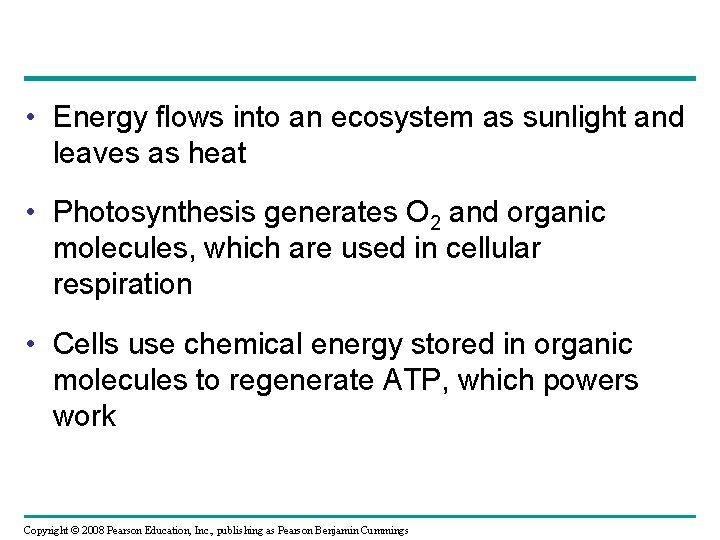
• Energy flows into an ecosystem as sunlight and leaves as heat • Photosynthesis generates O 2 and organic molecules, which are used in cellular respiration • Cells use chemical energy stored in organic molecules to regenerate ATP, which powers work Copyright © 2008 Pearson Education, Inc. , publishing as Pearson Benjamin Cummings
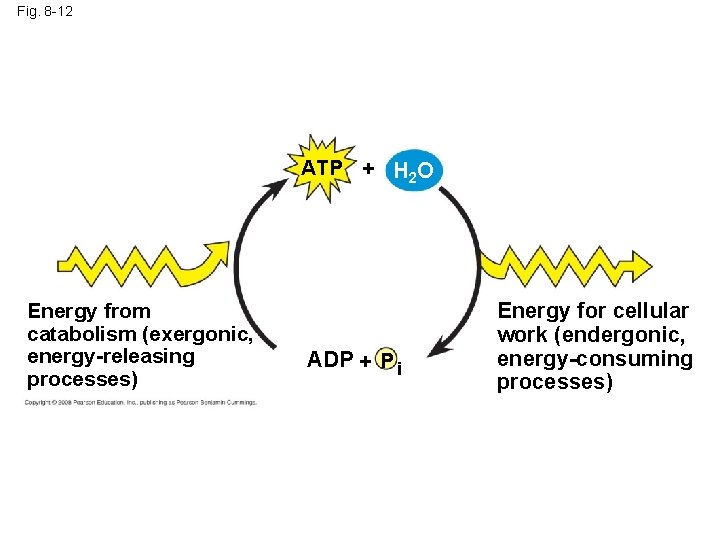
Fig. 8 -12 ATP + H 2 O Energy from catabolism (exergonic, energy-releasing processes) ADP + P i Energy for cellular work (endergonic, energy-consuming processes)
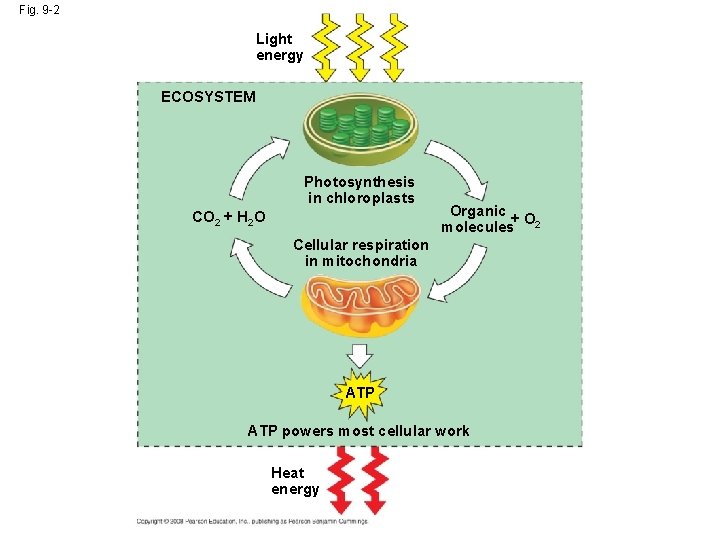
Fig. 9 -2 Light energy ECOSYSTEM Photosynthesis in chloroplasts CO 2 + H 2 O Organic +O molecules 2 Cellular respiration in mitochondria ATP powers most cellular work Heat energy
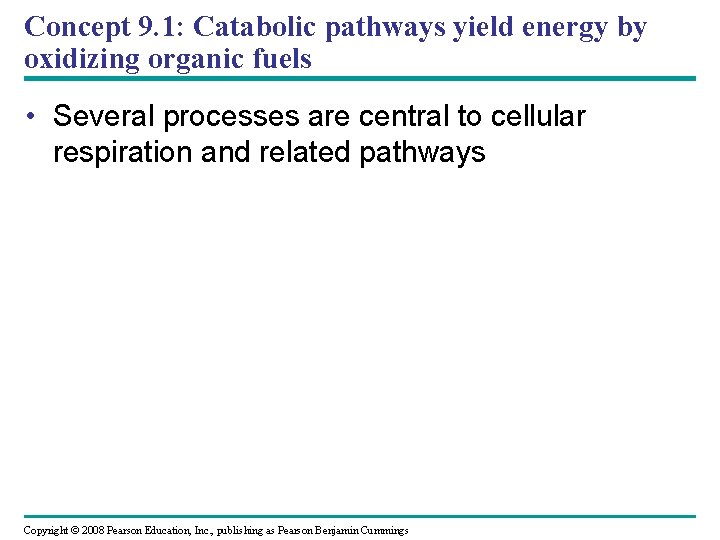
Concept 9. 1: Catabolic pathways yield energy by oxidizing organic fuels • Several processes are central to cellular respiration and related pathways Copyright © 2008 Pearson Education, Inc. , publishing as Pearson Benjamin Cummings
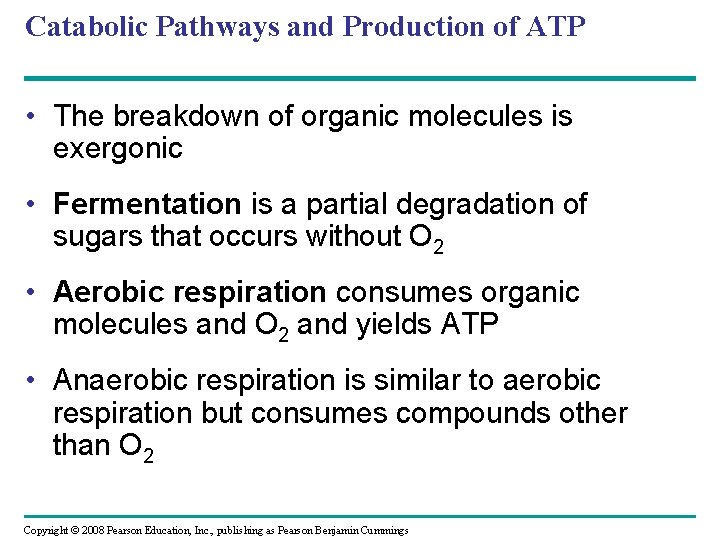
Catabolic Pathways and Production of ATP • The breakdown of organic molecules is exergonic • Fermentation is a partial degradation of sugars that occurs without O 2 • Aerobic respiration consumes organic molecules and O 2 and yields ATP • Anaerobic respiration is similar to aerobic respiration but consumes compounds other than O 2 Copyright © 2008 Pearson Education, Inc. , publishing as Pearson Benjamin Cummings
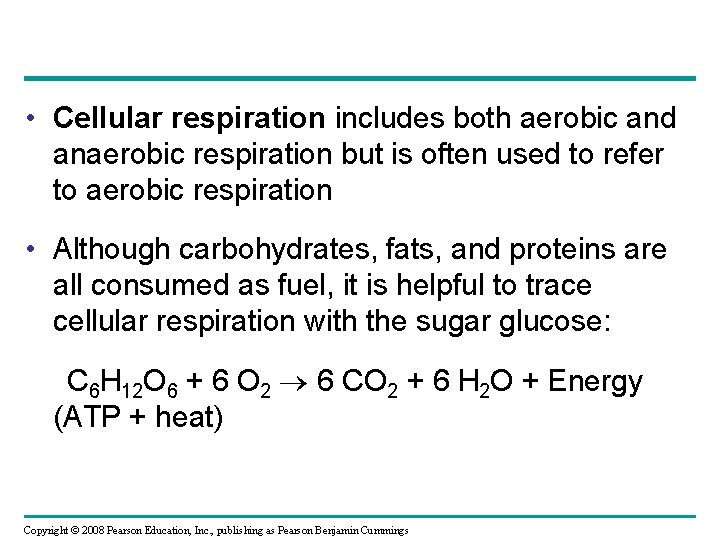
• Cellular respiration includes both aerobic and anaerobic respiration but is often used to refer to aerobic respiration • Although carbohydrates, fats, and proteins are all consumed as fuel, it is helpful to trace cellular respiration with the sugar glucose: C 6 H 12 O 6 + 6 O 2 6 CO 2 + 6 H 2 O + Energy (ATP + heat) Copyright © 2008 Pearson Education, Inc. , publishing as Pearson Benjamin Cummings
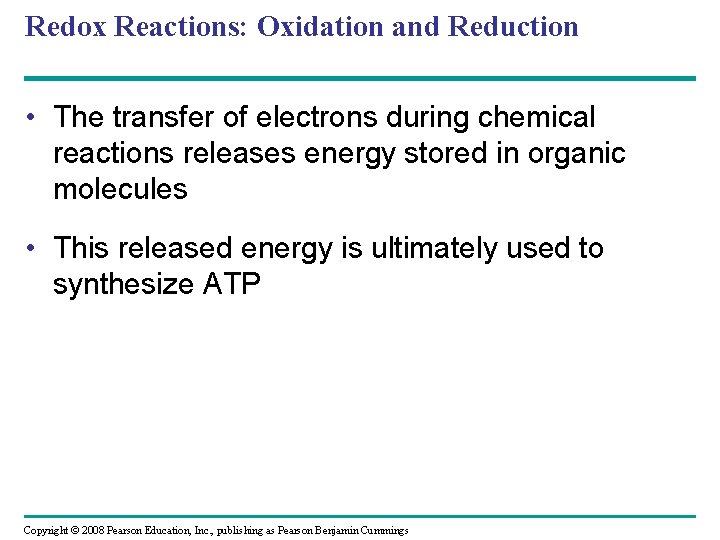
Redox Reactions: Oxidation and Reduction • The transfer of electrons during chemical reactions releases energy stored in organic molecules • This released energy is ultimately used to synthesize ATP Copyright © 2008 Pearson Education, Inc. , publishing as Pearson Benjamin Cummings
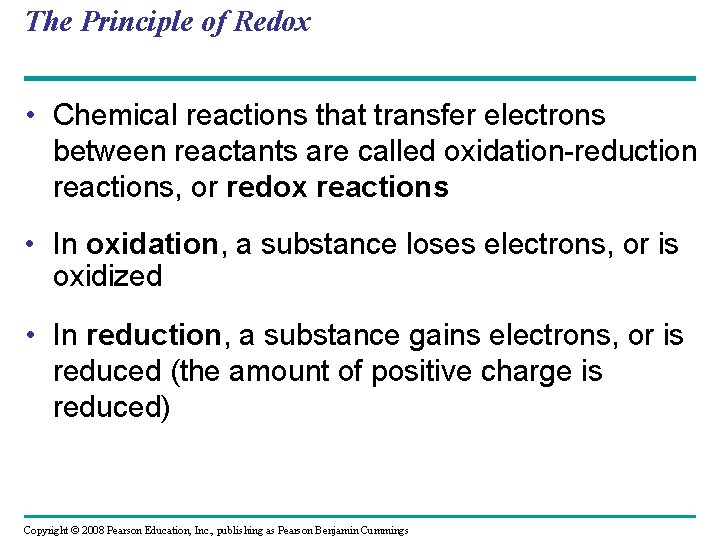
The Principle of Redox • Chemical reactions that transfer electrons between reactants are called oxidation-reduction reactions, or redox reactions • In oxidation, a substance loses electrons, or is oxidized • In reduction, a substance gains electrons, or is reduced (the amount of positive charge is reduced) Copyright © 2008 Pearson Education, Inc. , publishing as Pearson Benjamin Cummings
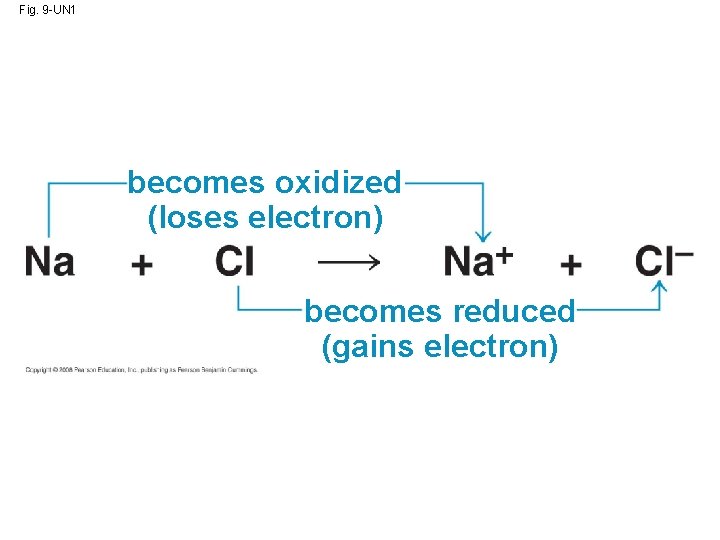
Fig. 9 -UN 1 becomes oxidized (loses electron) becomes reduced (gains electron)
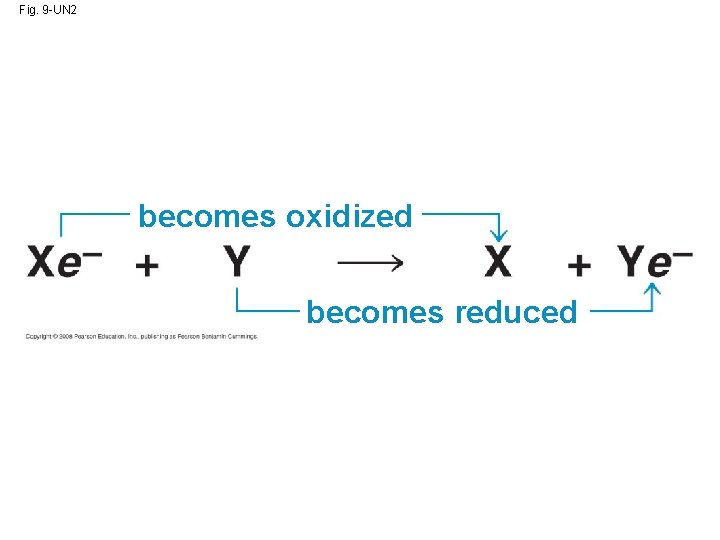
Fig. 9 -UN 2 becomes oxidized becomes reduced
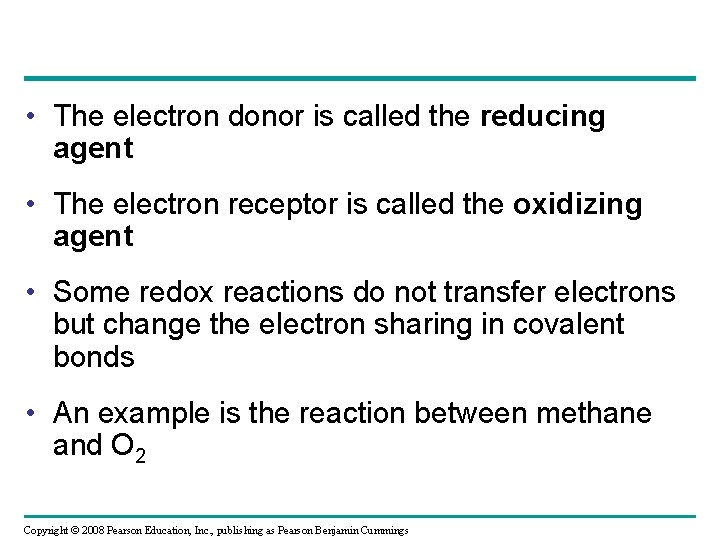
• The electron donor is called the reducing agent • The electron receptor is called the oxidizing agent • Some redox reactions do not transfer electrons but change the electron sharing in covalent bonds • An example is the reaction between methane and O 2 Copyright © 2008 Pearson Education, Inc. , publishing as Pearson Benjamin Cummings
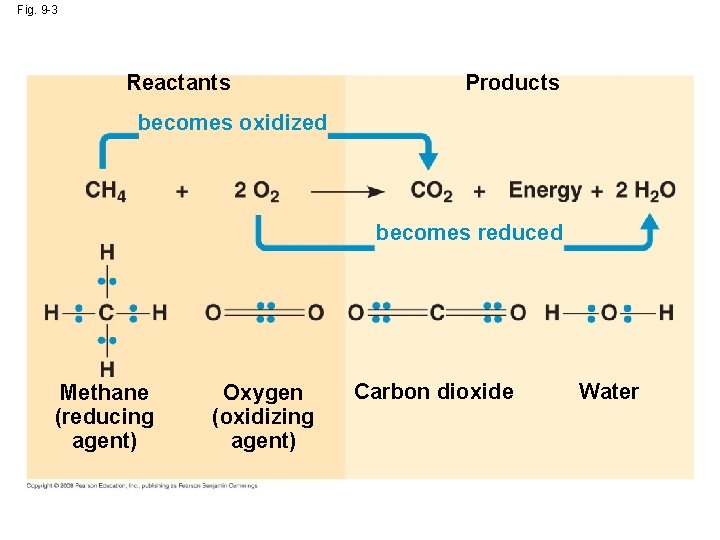
Fig. 9 -3 Reactants Products becomes oxidized becomes reduced Methane (reducing agent) Oxygen (oxidizing agent) Carbon dioxide Water
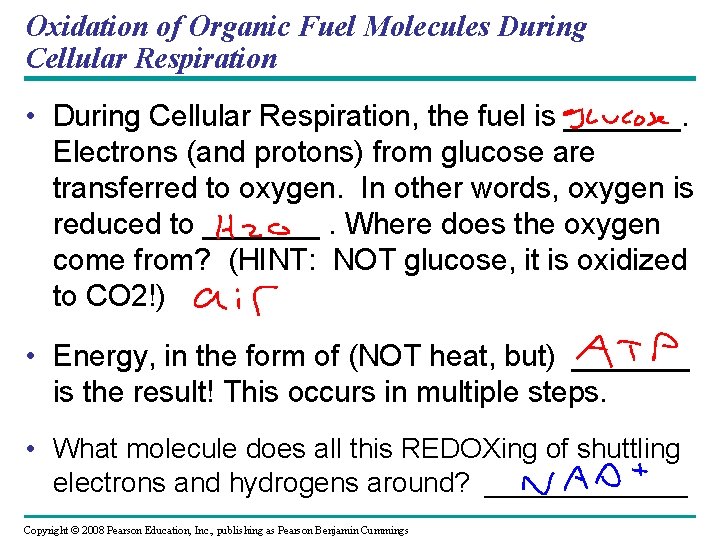
Oxidation of Organic Fuel Molecules During Cellular Respiration • During Cellular Respiration, the fuel is _______. Electrons (and protons) from glucose are transferred to oxygen. In other words, oxygen is reduced to _______. Where does the oxygen come from? (HINT: NOT glucose, it is oxidized to CO 2!) • Energy, in the form of (NOT heat, but) _______ is the result! This occurs in multiple steps. • What molecule does all this REDOXing of shuttling electrons and hydrogens around? _______ Copyright © 2008 Pearson Education, Inc. , publishing as Pearson Benjamin Cummings
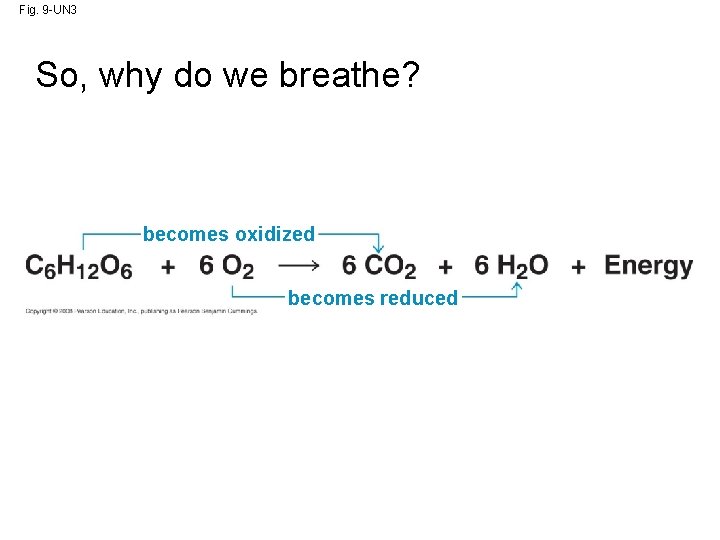
Fig. 9 -UN 3 So, why do we breathe? becomes oxidized becomes reduced
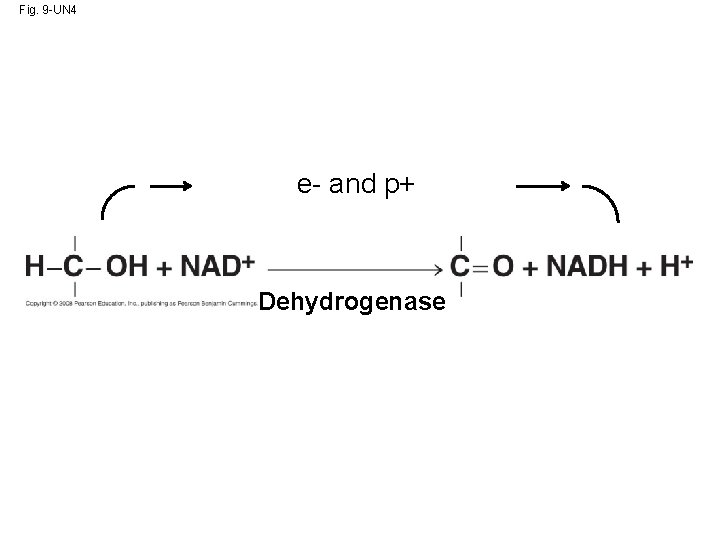
Fig. 9 -UN 4 e- and p+ Dehydrogenase
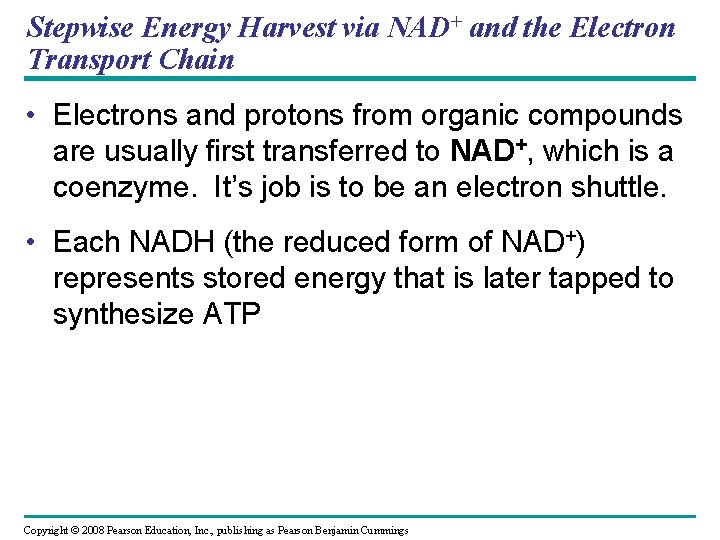
Stepwise Energy Harvest via NAD+ and the Electron Transport Chain • Electrons and protons from organic compounds are usually first transferred to NAD+, which is a coenzyme. It’s job is to be an electron shuttle. • Each NADH (the reduced form of NAD+) represents stored energy that is later tapped to synthesize ATP Copyright © 2008 Pearson Education, Inc. , publishing as Pearson Benjamin Cummings
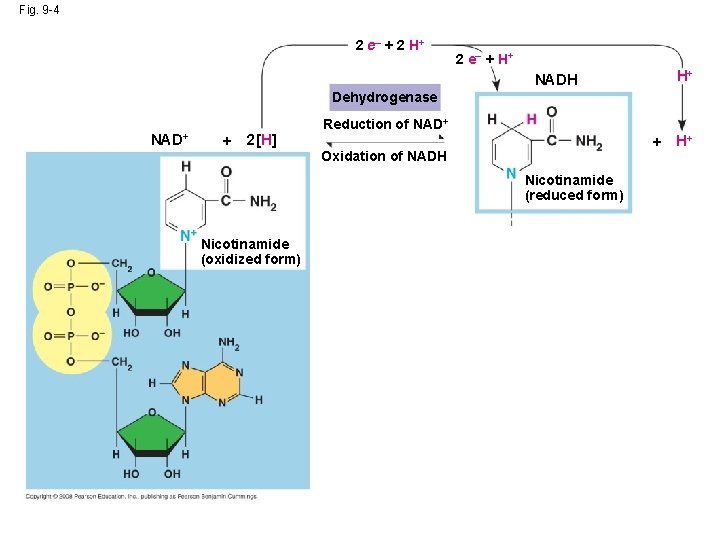
Fig. 9 -4 2 e– + 2 H+ 2 e– + H+ NADH H+ Dehydrogenase NAD+ + 2[H] Reduction of NAD+ + H+ Oxidation of NADH Nicotinamide (reduced form) Nicotinamide (oxidized form)
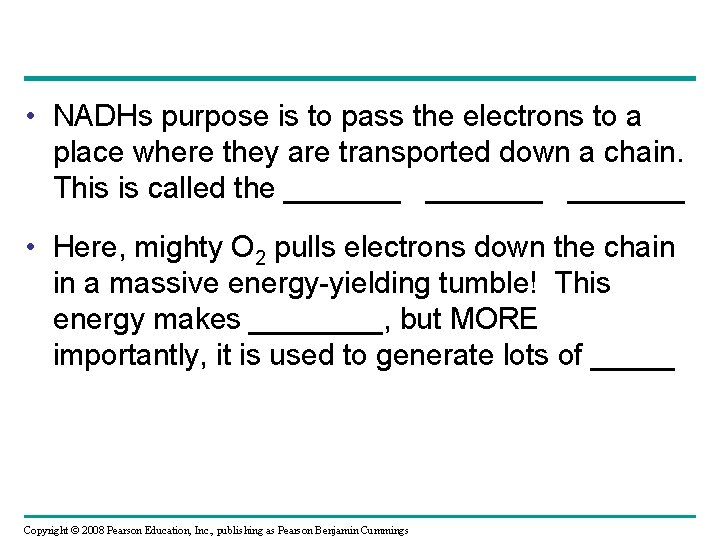
• NADHs purpose is to pass the electrons to a place where they are transported down a chain. This is called the _______ • Here, mighty O 2 pulls electrons down the chain in a massive energy-yielding tumble! This energy makes ____, but MORE importantly, it is used to generate lots of _____ Copyright © 2008 Pearson Education, Inc. , publishing as Pearson Benjamin Cummings
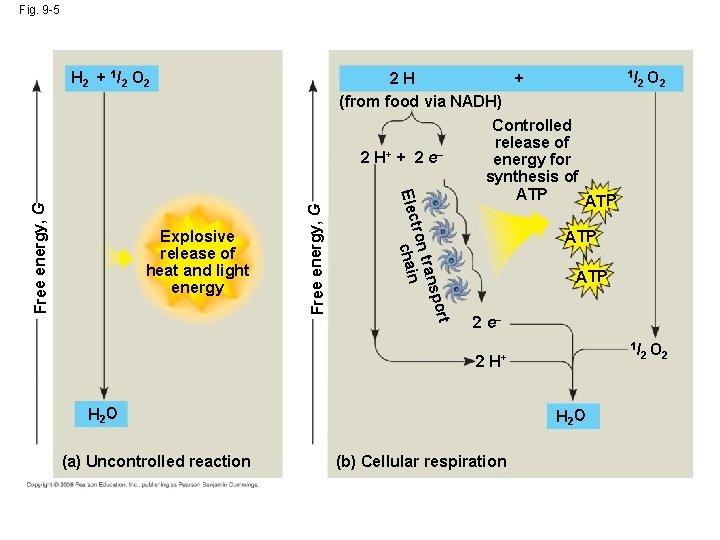
Fig. 9 -5 ort Free energy, G Explosive release of heat and light energy + 2 H (from food via NADH) Controlled release of + – 2 H + 2 e energy for synthesis of ATP sp tran tron Elec chain Free energy, G H 2 + 1 / 2 O 2 ATP 2 e– 2 1/ H+ H 2 O (a) Uncontrolled reaction 1/ H 2 O (b) Cellular respiration 2 O 2
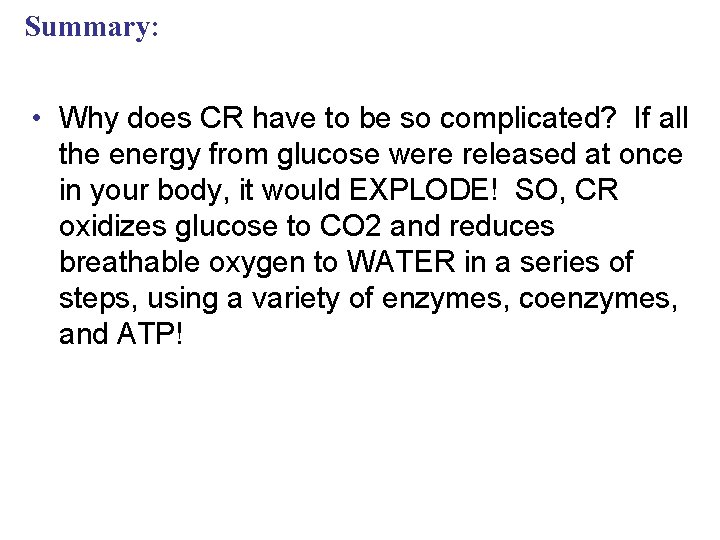
Summary: • Why does CR have to be so complicated? If all the energy from glucose were released at once in your body, it would EXPLODE! SO, CR oxidizes glucose to CO 2 and reduces breathable oxygen to WATER in a series of steps, using a variety of enzymes, coenzymes, and ATP!
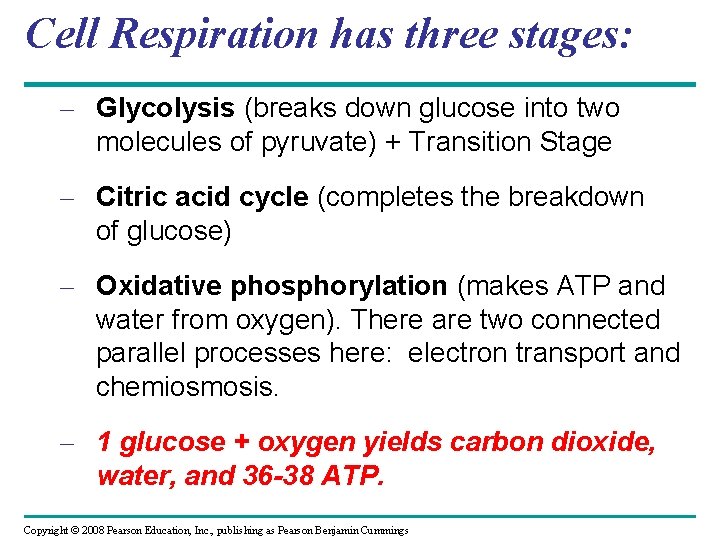
Cell Respiration has three stages: – Glycolysis (breaks down glucose into two molecules of pyruvate) + Transition Stage – Citric acid cycle (completes the breakdown of glucose) – Oxidative phosphorylation (makes ATP and water from oxygen). There are two connected parallel processes here: electron transport and chemiosmosis. – 1 glucose + oxygen yields carbon dioxide, water, and 36 -38 ATP. Copyright © 2008 Pearson Education, Inc. , publishing as Pearson Benjamin Cummings
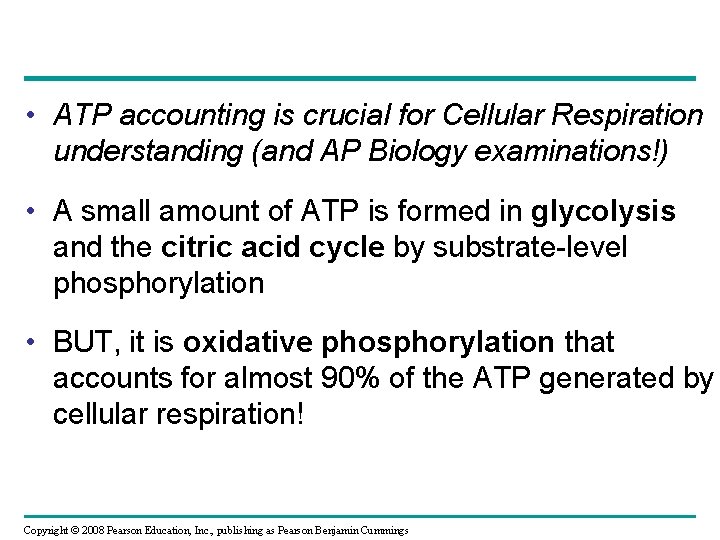
• ATP accounting is crucial for Cellular Respiration understanding (and AP Biology examinations!) • A small amount of ATP is formed in glycolysis and the citric acid cycle by substrate-level phosphorylation • BUT, it is oxidative phosphorylation that accounts for almost 90% of the ATP generated by cellular respiration! Copyright © 2008 Pearson Education, Inc. , publishing as Pearson Benjamin Cummings
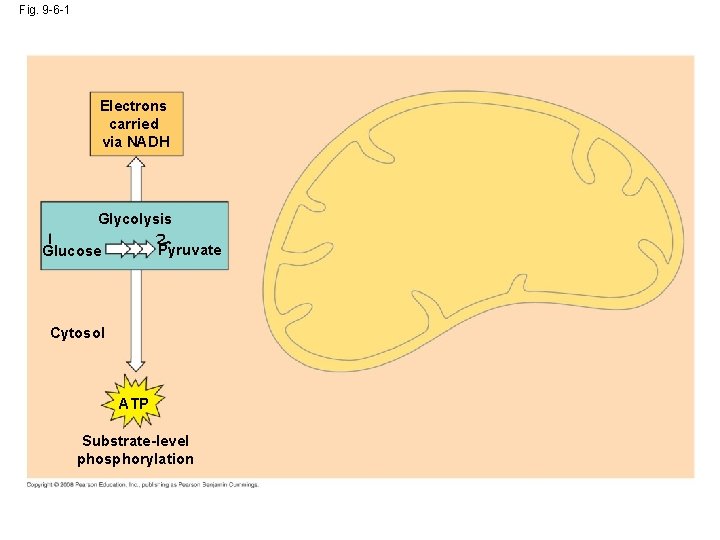
Fig. 9 -6 -1 Electrons carried via NADH Glycolysis Pyruvate Glucose Cytosol ATP Substrate-level phosphorylation
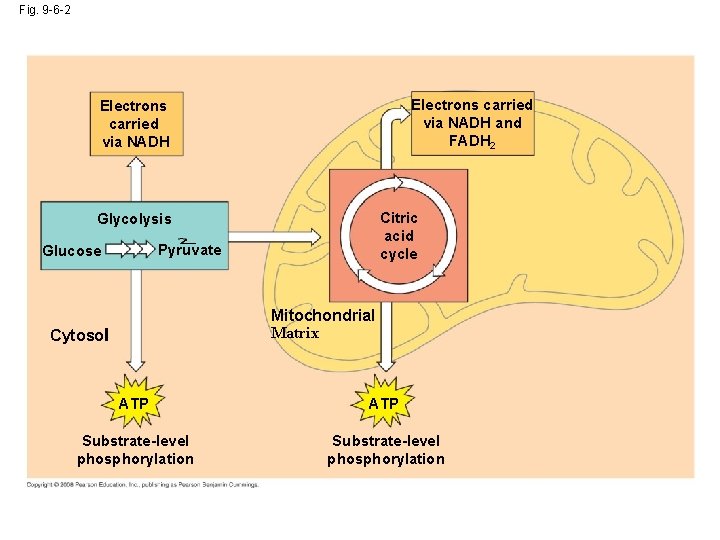
Fig. 9 -6 -2 Electrons carried via NADH and FADH 2 Electrons carried via NADH Citric acid cycle Glycolysis Pyruvate Glucose Mitochondrial Matrix Cytosol ATP Substrate-level phosphorylation
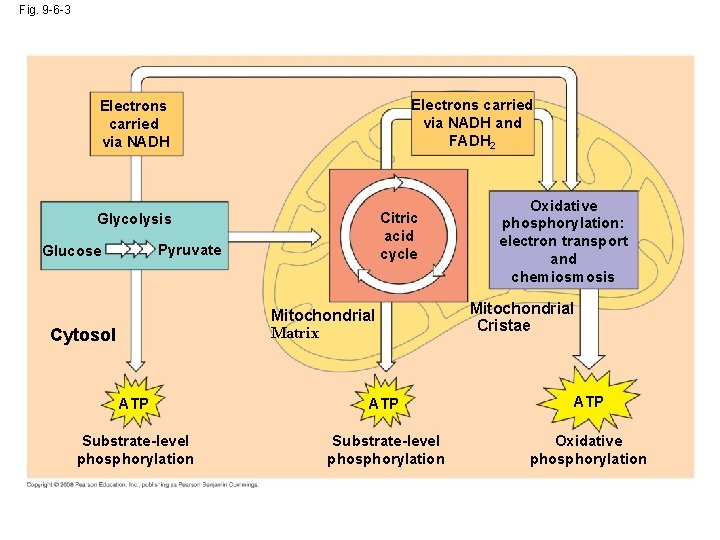
Fig. 9 -6 -3 Electrons carried via NADH and FADH 2 Electrons carried via NADH Citric acid cycle Glycolysis Pyruvate Glucose Mitochondrial Matrix Cytosol Oxidative phosphorylation: electron transport and chemiosmosis Mitochondrial Cristae ATP ATP Substrate-level phosphorylation Oxidative phosphorylation
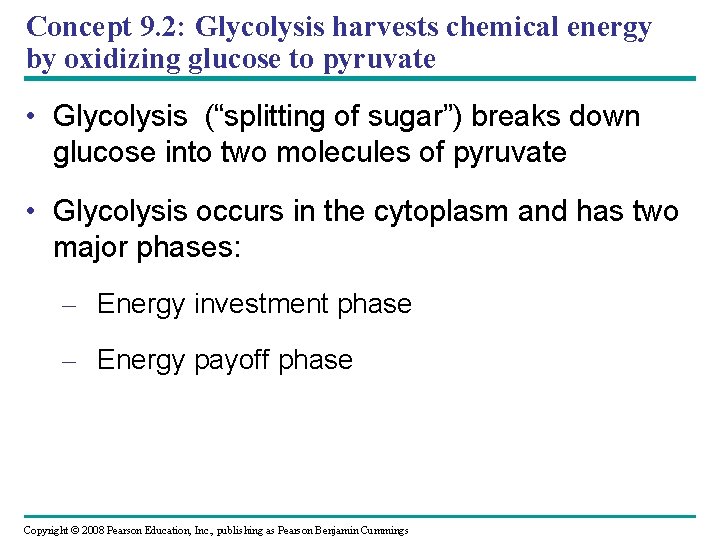
Concept 9. 2: Glycolysis harvests chemical energy by oxidizing glucose to pyruvate • Glycolysis (“splitting of sugar”) breaks down glucose into two molecules of pyruvate • Glycolysis occurs in the cytoplasm and has two major phases: – Energy investment phase – Energy payoff phase Copyright © 2008 Pearson Education, Inc. , publishing as Pearson Benjamin Cummings
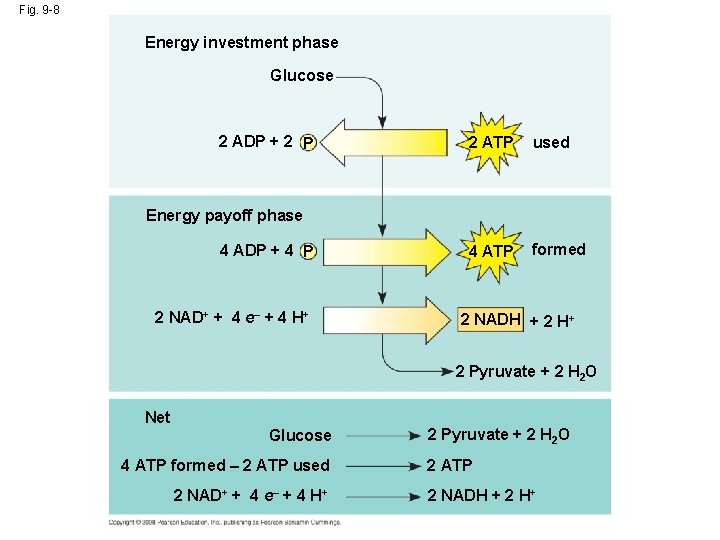
Fig. 9 -8 Energy investment phase Glucose 2 ADP + 2 P 2 ATP used 4 ATP formed Energy payoff phase 4 ADP + 4 P 2 NAD+ + 4 e– + 4 H+ 2 NADH + 2 H+ 2 Pyruvate + 2 H 2 O Net Glucose 4 ATP formed – 2 ATP used 2 NAD+ + 4 e– + 4 H+ 2 Pyruvate + 2 H 2 O 2 ATP 2 NADH + 2 H+
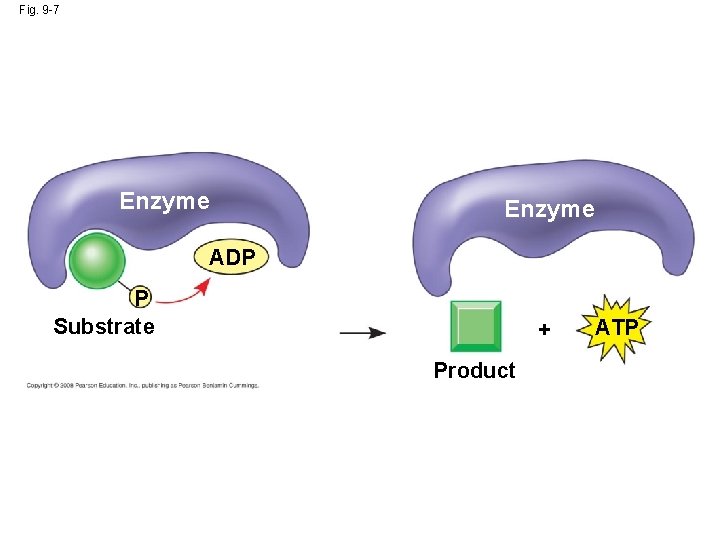
Fig. 9 -7 Enzyme ADP P Substrate + Product ATP
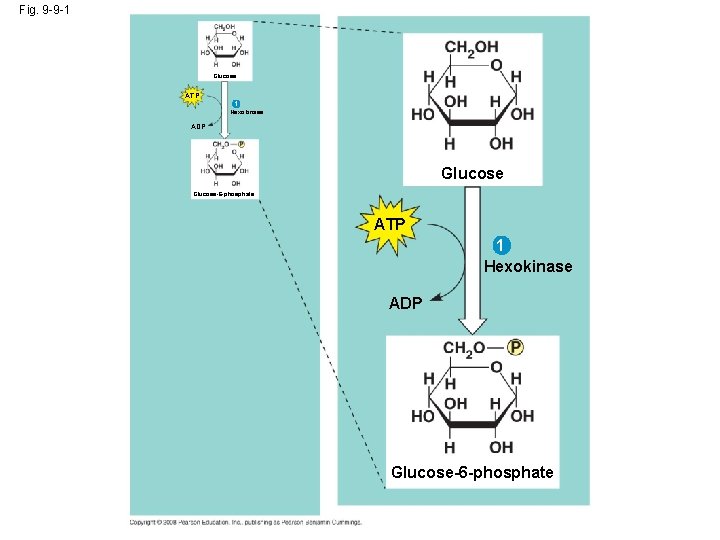
Fig. 9 -9 -1 Glucose ATP 1 Hexokinase ADP Glucose-6 -phosphate
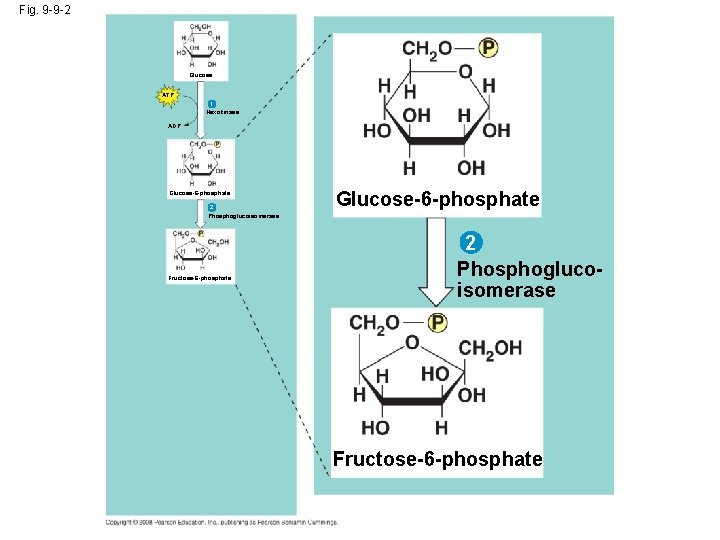
Fig. 9 -9 -2 Glucose ATP 1 Hexokinase ADP Glucose-6 -phosphate 2 Phosphoglucoisomerase Fructose-6 -phosphate
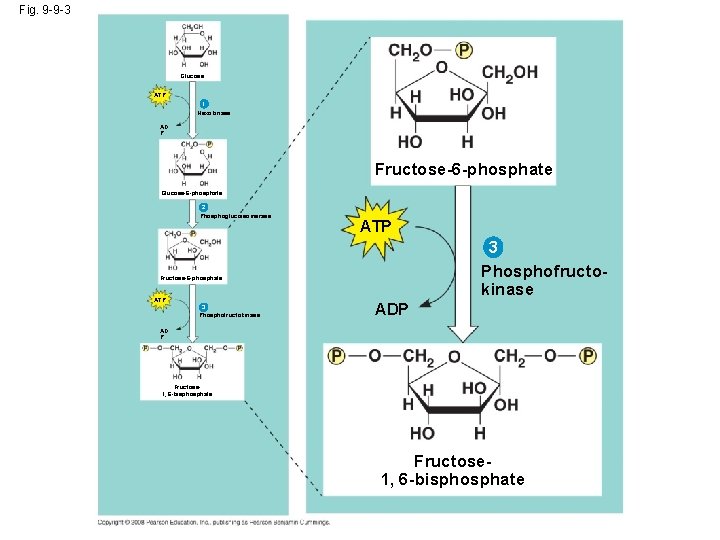
Fig. 9 -9 -3 Glucose ATP 1 Hexokinase AD P Fructose-6 -phosphate Glucose-6 -phosphate 2 Phosphoglucoisomerase ATP 3 Phosphofructokinase Fructose-6 -phosphate ATP 3 Phosphofructokinase ADP AD P Fructose 1, 6 -bisphosphate
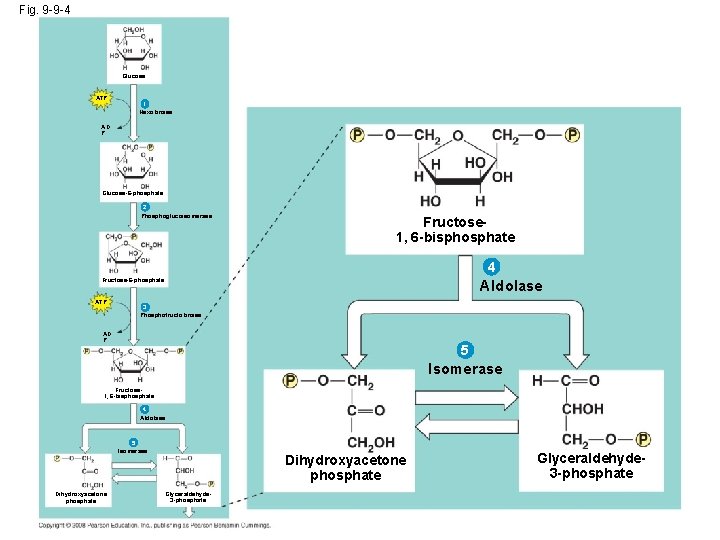
Fig. 9 -9 -4 Glucose ATP 1 Hexokinase AD P Glucose-6 -phosphate 2 Phosphoglucoisomerase Fructose 1, 6 -bisphosphate 4 Fructose-6 -phosphate ATP Aldolase 3 Phosphofructokinase AD P 5 Isomerase Fructose 1, 6 -bisphosphate 4 Aldolase 5 Isomerase Dihydroxyacetone phosphate Glyceraldehyde 3 -phosphate
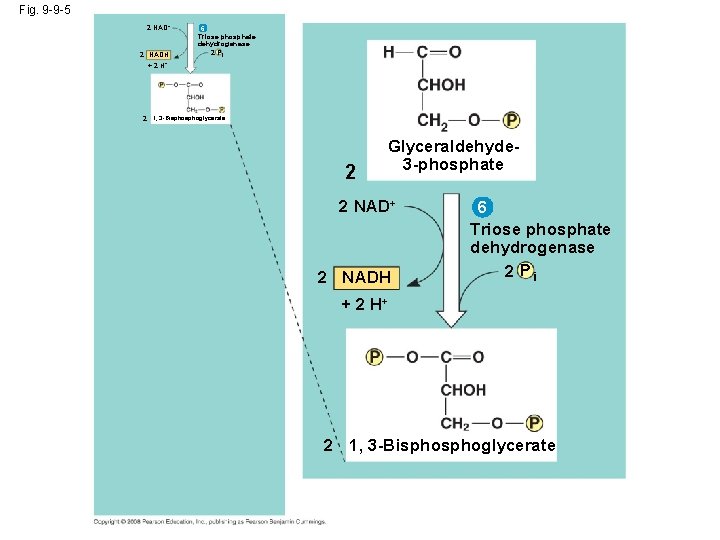
Fig. 9 -9 -5 2 NAD+ 2 NADH + 2 H+ 6 Triose phosphate dehydrogenase 2 Pi 2 1, 3 -Bisphoglycerate 2 Glyceraldehyde 3 -phosphate 2 NAD+ 2 NADH 6 Triose phosphate dehydrogenase 2 Pi + 2 H+ 2 1, 3 -Bisphoglycerate
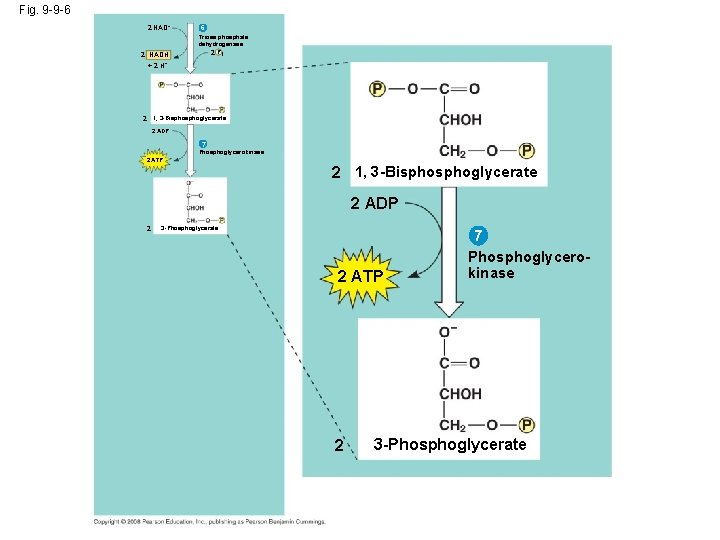
Fig. 9 -9 -6 2 NAD+ 2 NADH + 2 H+ 6 Triose phosphate dehydrogenase 2 Pi 2 1, 3 -Bisphoglycerate 2 ADP 7 Phosphoglycerokinase 2 ATP 2 1, 3 -Bisphoglycerate 2 ADP 2 3 -Phosphoglycerate 2 ATP 2 7 Phosphoglycerokinase 3 -Phosphoglycerate
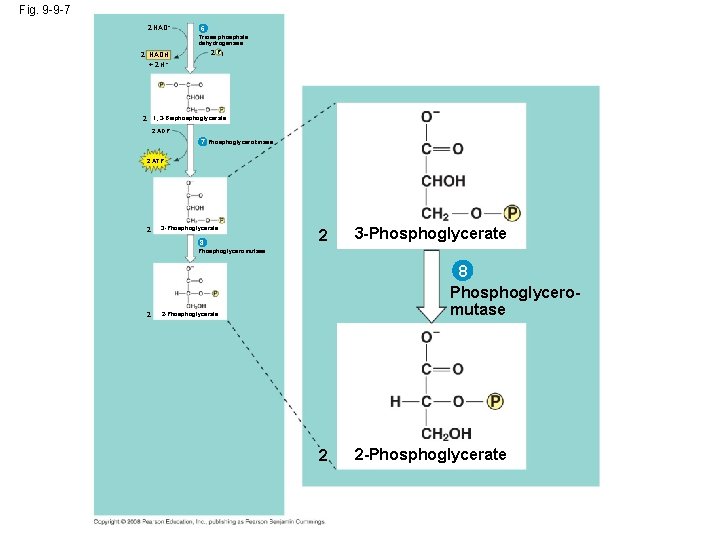
Fig. 9 -9 -7 2 NAD+ 2 NADH + 2 H+ 6 Triose phosphate dehydrogenase 2 Pi 2 1, 3 -Bisphoglycerate 2 ADP 7 Phosphoglycerokinase 2 ATP 2 3 -Phosphoglycerate 8 2 3 -Phosphoglycerate Phosphoglyceromutase 2 8 Phosphoglyceromutase 2 -Phosphoglycerate 2 2 -Phosphoglycerate
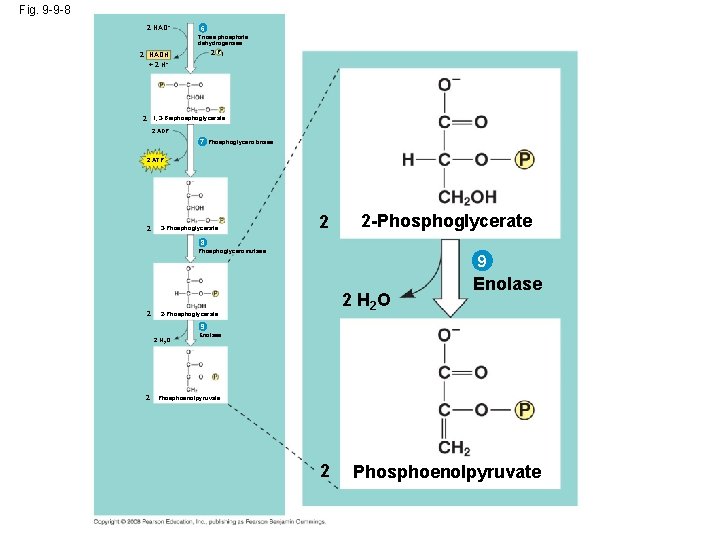
Fig. 9 -9 -8 2 NAD+ 2 NADH + 2 H+ 6 Triose phosphate dehydrogenase 2 Pi 2 1, 3 -Bisphoglycerate 2 ADP 7 Phosphoglycerokinase 2 ATP 2 3 -Phosphoglycerate 2 2 -Phosphoglycerate 8 Phosphoglyceromutase 2 9 2 H 2 O 2 -Phosphoglycerate Enolase 9 2 H 2 O 2 Enolase Phosphoenolpyruvate 2 Phosphoenolpyruvate
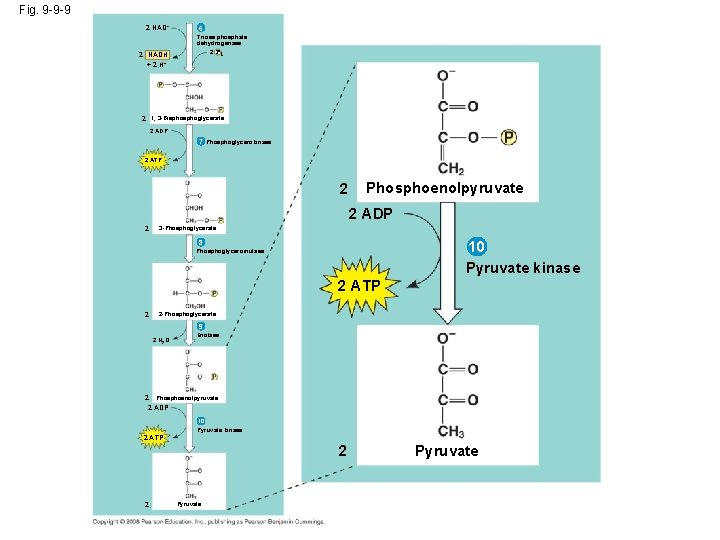
Fig. 9 -9 -9 2 NAD+ 2 NADH + 2 H+ 6 Triose phosphate dehydrogenase 2 Pi 2 1, 3 -Bisphoglycerate 2 ADP 7 Phosphoglycerokinase 2 ATP 2 Phosphoenolpyruvate 2 ADP 2 3 -Phosphoglycerate 8 10 Pyruvate kinase Phosphoglyceromutase 2 ATP 2 2 -Phosphoglycerate 9 2 H 2 O Enolase 2 Phosphoenolpyruvate 2 ADP 10 Pyruvate kinase 2 ATP 2 2 Pyruvate
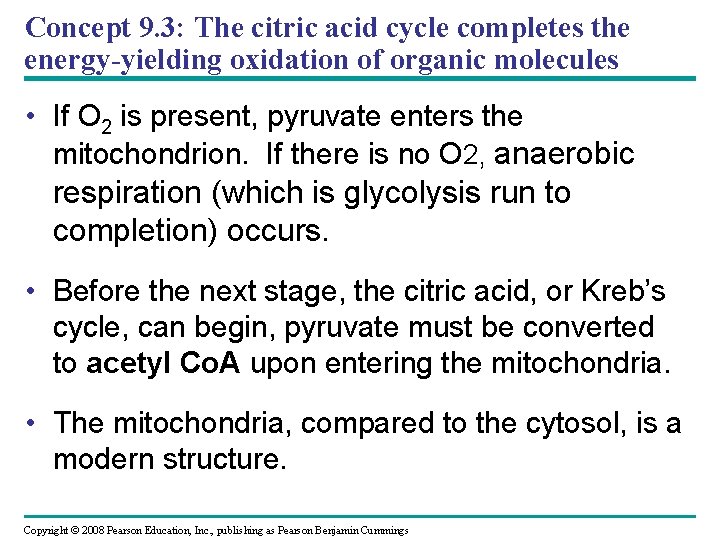
Concept 9. 3: The citric acid cycle completes the energy-yielding oxidation of organic molecules • If O 2 is present, pyruvate enters the mitochondrion. If there is no O 2, anaerobic respiration (which is glycolysis run to completion) occurs. • Before the next stage, the citric acid, or Kreb’s cycle, can begin, pyruvate must be converted to acetyl Co. A upon entering the mitochondria. • The mitochondria, compared to the cytosol, is a modern structure. Copyright © 2008 Pearson Education, Inc. , publishing as Pearson Benjamin Cummings
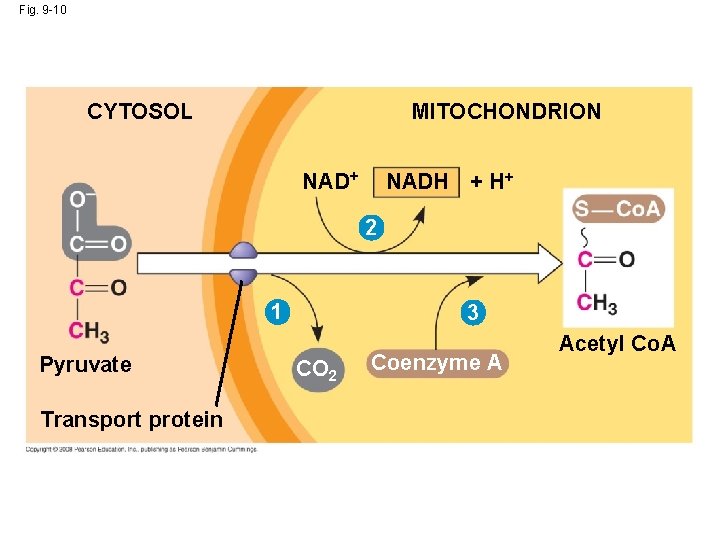
Fig. 9 -10 CYTOSOL MITOCHONDRION NAD+ NADH + H+ 2 1 Pyruvate Transport protein 3 CO 2 Coenzyme A Acetyl Co. A
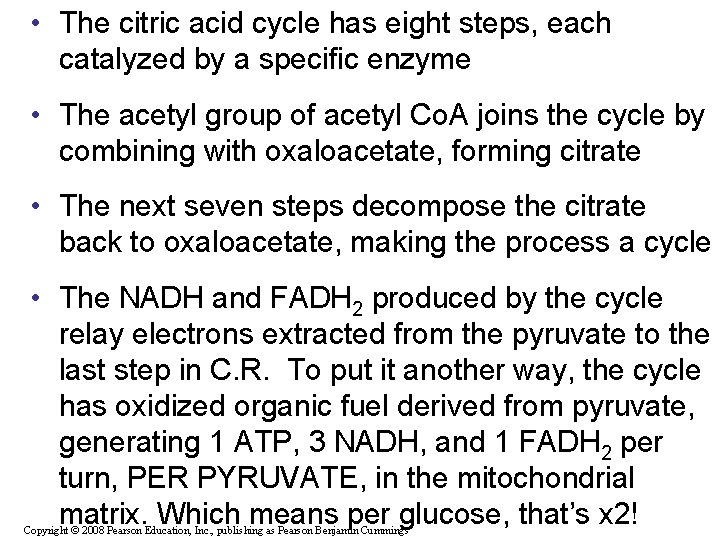
• The citric acid cycle has eight steps, each catalyzed by a specific enzyme • The acetyl group of acetyl Co. A joins the cycle by combining with oxaloacetate, forming citrate • The next seven steps decompose the citrate back to oxaloacetate, making the process a cycle • The NADH and FADH 2 produced by the cycle relay electrons extracted from the pyruvate to the last step in C. R. To put it another way, the cycle has oxidized organic fuel derived from pyruvate, generating 1 ATP, 3 NADH, and 1 FADH 2 per turn, PER PYRUVATE, in the mitochondrial matrix. Which means per glucose, that’s x 2! Copyright © 2008 Pearson Education, Inc. , publishing as Pearson Benjamin Cummings
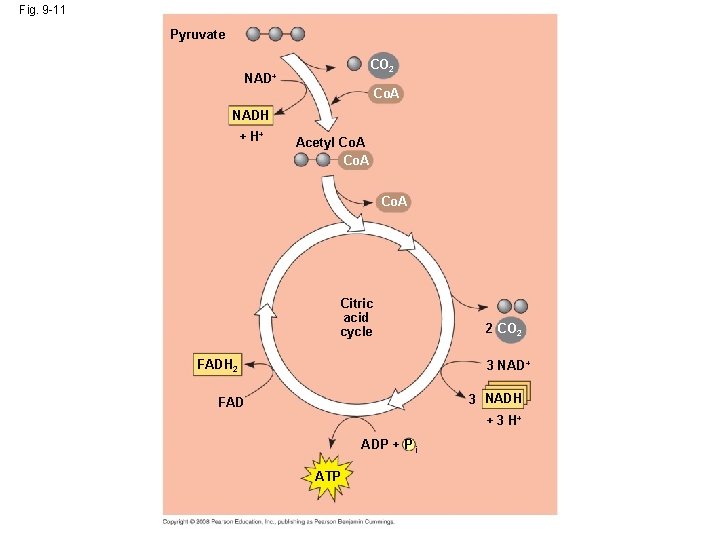
Fig. 9 -11 Pyruvate CO 2 NAD+ Co. A NADH + H+ Acetyl Co. A Citric acid cycle FADH 2 2 CO 2 3 NAD+ 3 NADH FAD + 3 H+ ADP + P i ATP
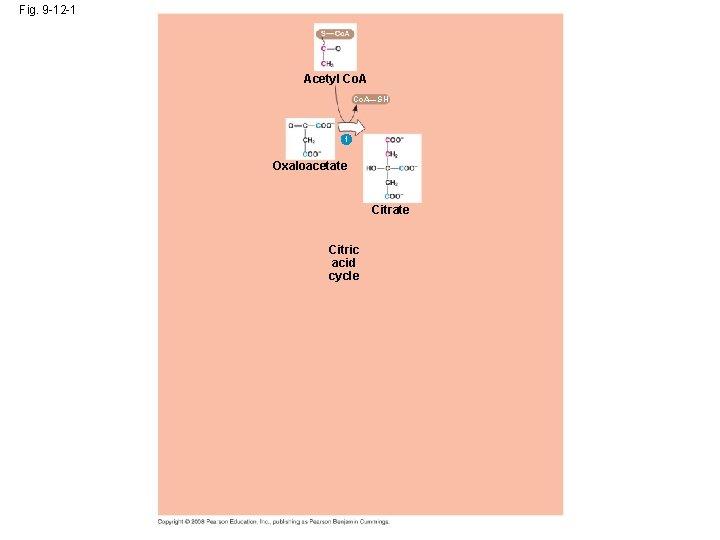
Fig. 9 -12 -1 Acetyl Co. A—SH 1 Oxaloacetate Citric acid cycle
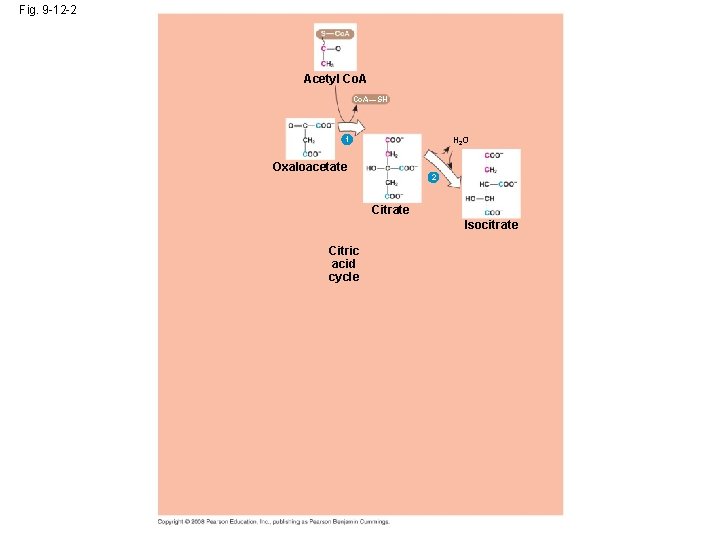
Fig. 9 -12 -2 Acetyl Co. A—SH H 2 O 1 Oxaloacetate 2 Citrate Isocitrate Citric acid cycle
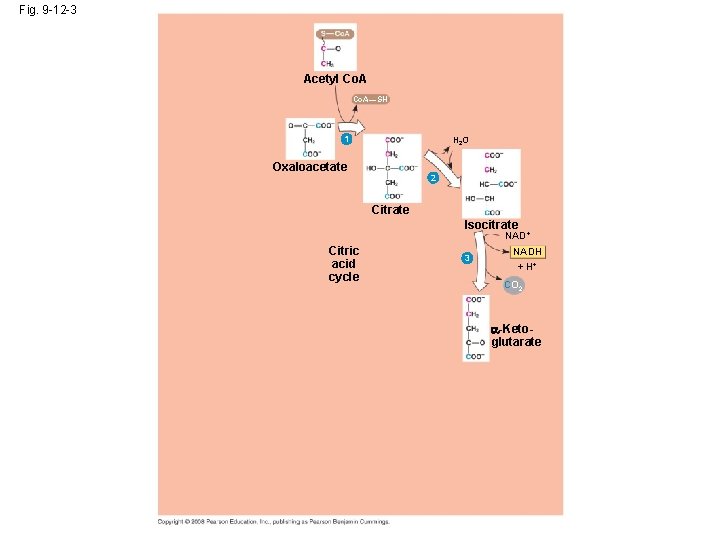
Fig. 9 -12 -3 Acetyl Co. A—SH 1 H 2 O Oxaloacetate 2 Citrate Isocitrate NAD+ Citric acid cycle 3 NADH + H+ CO 2 -Ketoglutarate
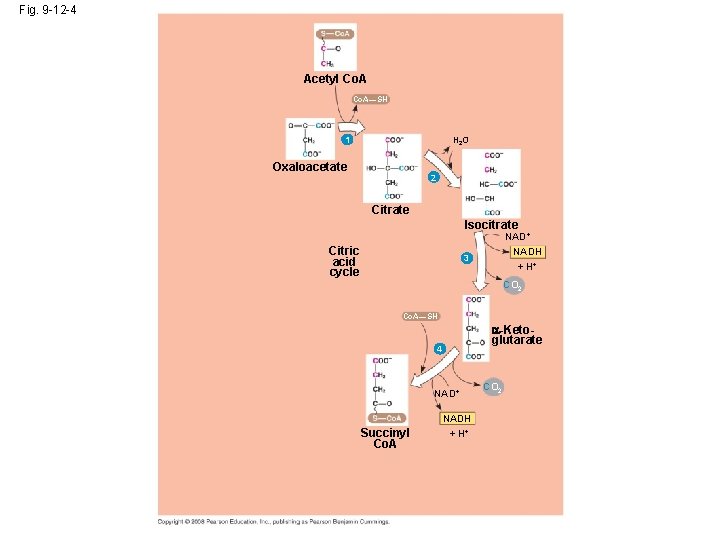
Fig. 9 -12 -4 Acetyl Co. A—SH 1 H 2 O Oxaloacetate 2 Citrate Isocitrate NAD+ Citric acid cycle NADH + H+ 3 CO 2 Co. A—SH -Ketoglutarate 4 NAD+ Succinyl Co. A NADH + H+ CO 2
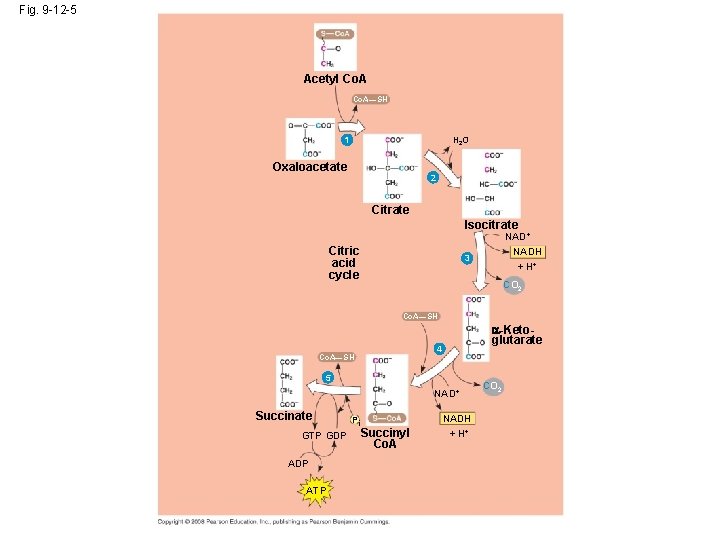
Fig. 9 -12 -5 Acetyl Co. A—SH 1 H 2 O Oxaloacetate 2 Citrate Isocitrate NAD+ Citric acid cycle NADH + H+ 3 CO 2 Co. A—SH -Ketoglutarate 4 Co. A—SH 5 NAD+ Succinate GTP GDP ATP Pi Succinyl Co. A NADH + H+ CO 2
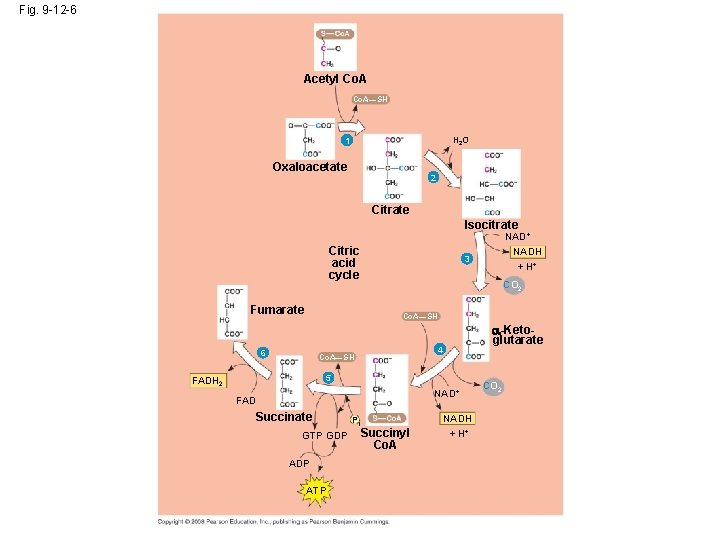
Fig. 9 -12 -6 Acetyl Co. A—SH H 2 O 1 Oxaloacetate 2 Citrate Isocitrate NAD+ Citric acid cycle Fumarate NADH + H+ 3 CO 2 Co. A—SH 6 -Ketoglutarate 4 Co. A—SH 5 FADH 2 NAD+ FAD Succinate GTP GDP ATP Pi Succinyl Co. A NADH + H+ CO 2
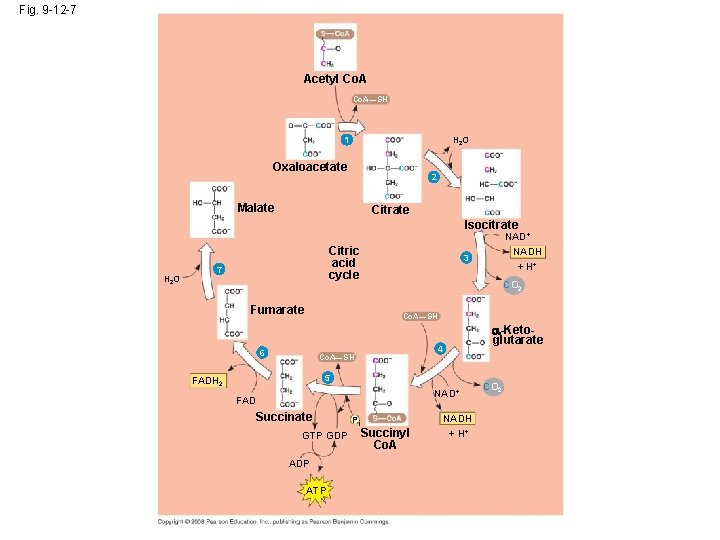
Fig. 9 -12 -7 Acetyl Co. A—SH H 2 O 1 Oxaloacetate 2 Malate Citrate Isocitrate NAD+ H 2 O Citric acid cycle 7 Fumarate NADH + H+ 3 CO 2 Co. A—SH 6 -Ketoglutarate 4 Co. A—SH 5 FADH 2 NAD+ FAD Succinate GTP GDP ATP Pi Succinyl Co. A NADH + H+ CO 2
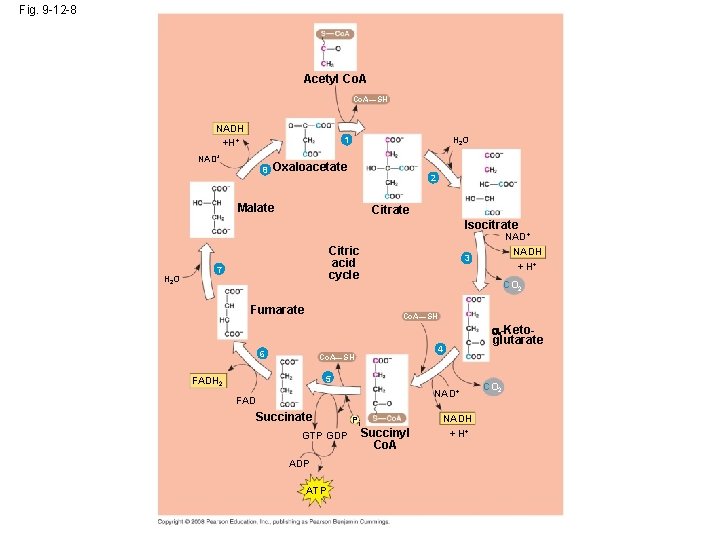
Fig. 9 -12 -8 Acetyl Co. A—SH NADH +H+ H 2 O 1 NAD+ 8 Oxaloacetate 2 Malate Citrate Isocitrate NAD+ H 2 O Citric acid cycle 7 Fumarate NADH + H+ 3 CO 2 Co. A—SH 6 -Ketoglutarate 4 Co. A—SH 5 FADH 2 NAD+ FAD Succinate GTP GDP ATP Pi Succinyl Co. A NADH + H+ CO 2
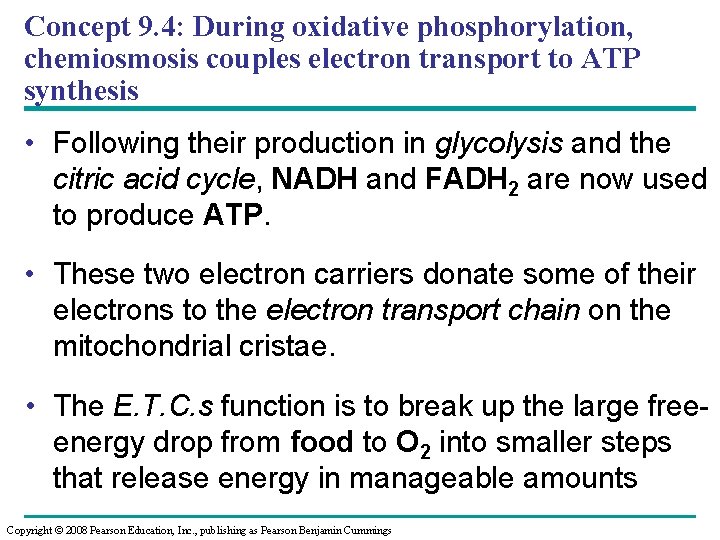
Concept 9. 4: During oxidative phosphorylation, chemiosmosis couples electron transport to ATP synthesis • Following their production in glycolysis and the citric acid cycle, NADH and FADH 2 are now used to produce ATP. • These two electron carriers donate some of their electrons to the electron transport chain on the mitochondrial cristae. • The E. T. C. s function is to break up the large freeenergy drop from food to O 2 into smaller steps that release energy in manageable amounts Copyright © 2008 Pearson Education, Inc. , publishing as Pearson Benjamin Cummings
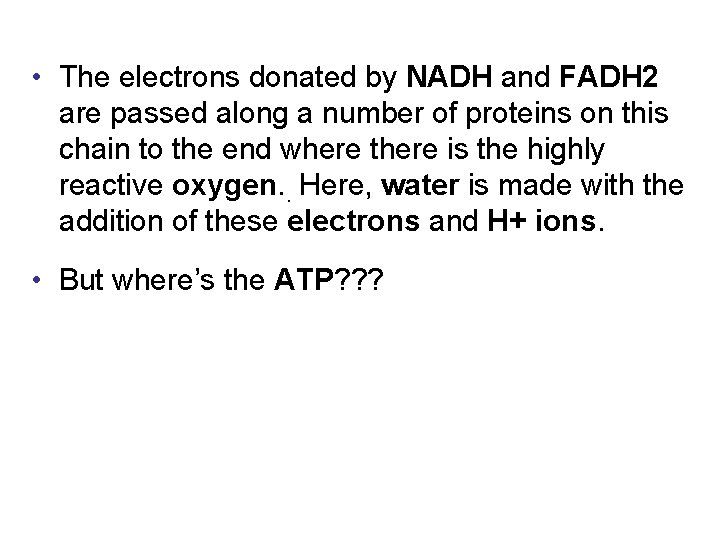
• The electrons donated by NADH and FADH 2 are passed along a number of proteins on this chain to the end where there is the highly reactive oxygen. . Here, water is made with the addition of these electrons and H+ ions. • But where’s the ATP? ? ?
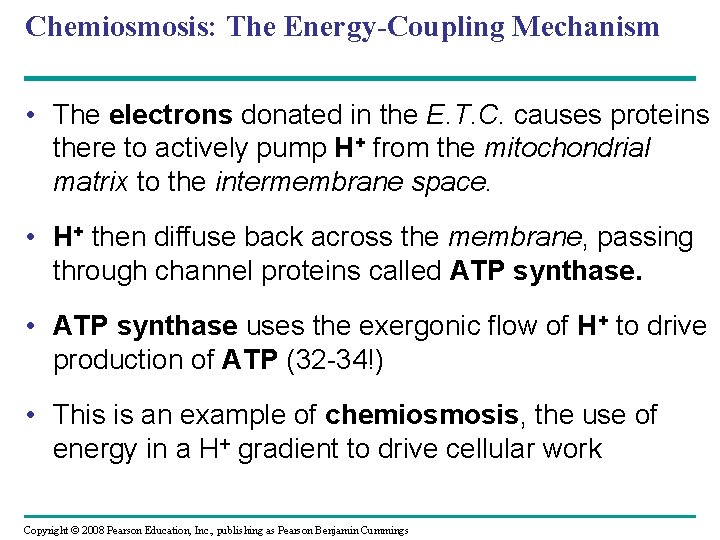
Chemiosmosis: The Energy-Coupling Mechanism • The electrons donated in the E. T. C. causes proteins there to actively pump H+ from the mitochondrial matrix to the intermembrane space. • H+ then diffuse back across the membrane, passing through channel proteins called ATP synthase. • ATP synthase uses the exergonic flow of H+ to drive production of ATP (32 -34!) • This is an example of chemiosmosis, the use of energy in a H+ gradient to drive cellular work Copyright © 2008 Pearson Education, Inc. , publishing as Pearson Benjamin Cummings
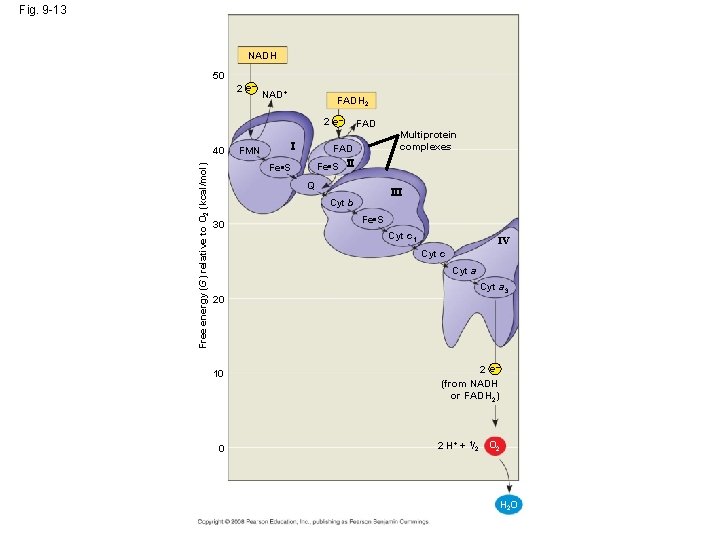
Fig. 9 -13 NADH 50 2 e– NAD+ FADH 2 2 e– Free energy (G) relative to O 2 (kcal/mol) 40 FMN FAD Multiprotein complexes FAD Fe • S Q Cyt b 30 Fe • S Cyt c 1 IV Cyt c Cyt a 20 10 0 Cyt a 3 2 e– (from NADH or FADH 2) 2 H+ + 1/2 O 2 H 2 O
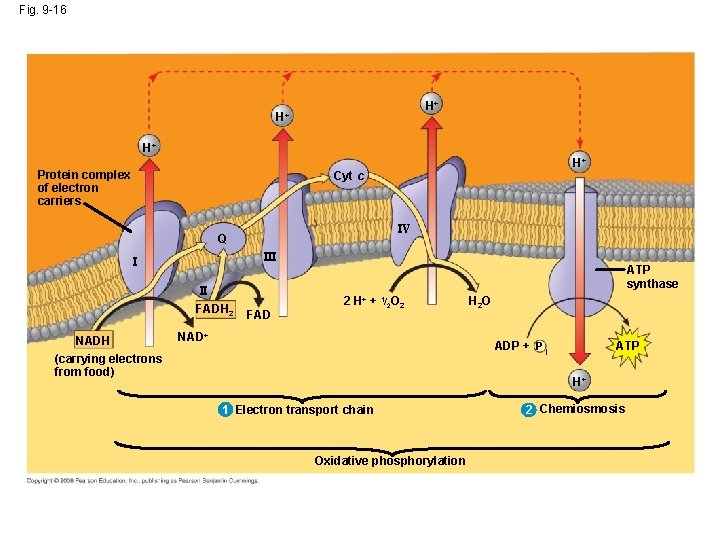
Fig. 9 -16 H+ H+ H+ Protein complex of electron carriers H+ Cyt c V Q FADH 2 NADH ATP synthase FAD 2 H+ + 1/2 O 2 NAD+ H 2 O ADP + P i (carrying electrons from food) ATP H+ 1 Electron transport chain Oxidative phosphorylation 2 Chemiosmosis
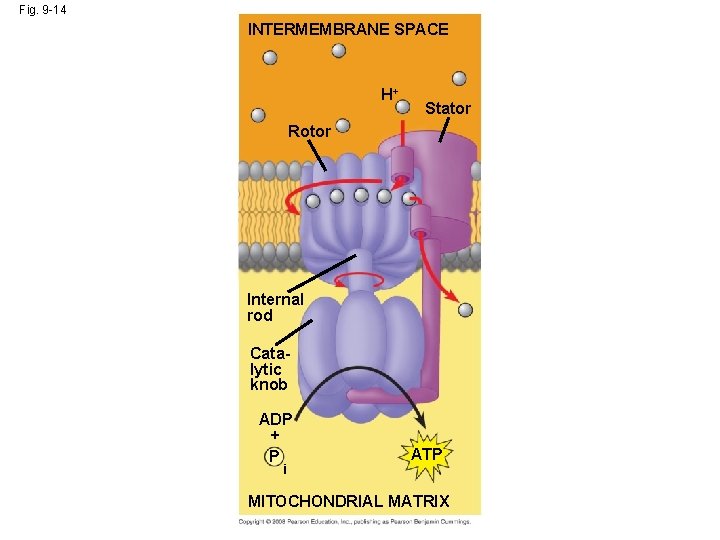
Fig. 9 -14 INTERMEMBRANE SPACE H+ Stator Rotor Internal rod Catalytic knob ADP + P i ATP MITOCHONDRIAL MATRIX
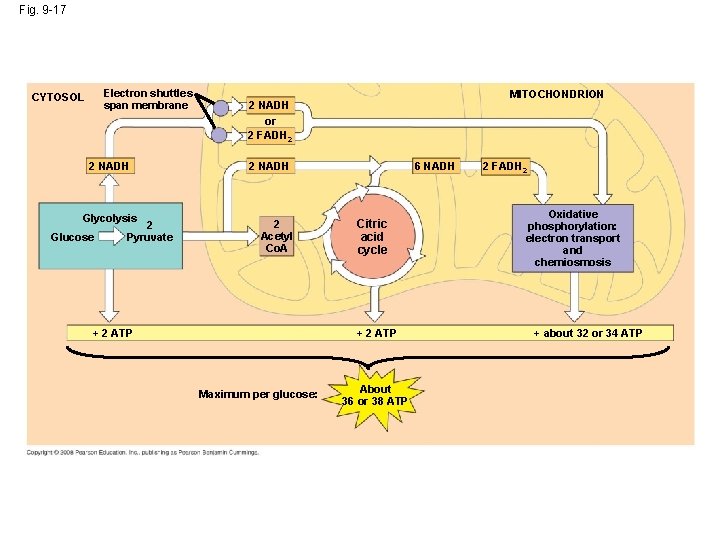
Fig. 9 -17 Electron shuttles span membrane CYTOSOL 2 NADH Glycolysis Glucose 2 Pyruvate MITOCHONDRION 2 NADH or 2 FADH 2 6 NADH 2 Acetyl Co. A + 2 ATP Citric acid cycle + 2 ATP Maximum per glucose: About 36 or 38 ATP 2 FADH 2 Oxidative phosphorylation: electron transport and chemiosmosis + about 32 or 34 ATP
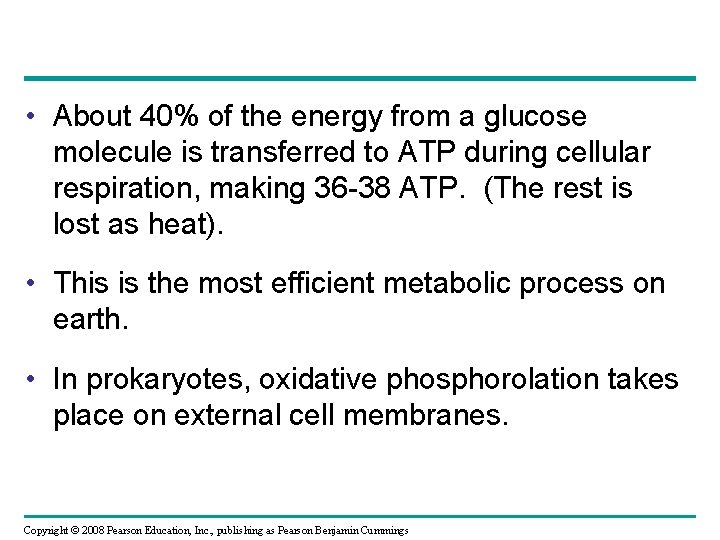
• About 40% of the energy from a glucose molecule is transferred to ATP during cellular respiration, making 36 -38 ATP. (The rest is lost as heat). • This is the most efficient metabolic process on earth. • In prokaryotes, oxidative phosphorolation takes place on external cell membranes. Copyright © 2008 Pearson Education, Inc. , publishing as Pearson Benjamin Cummings
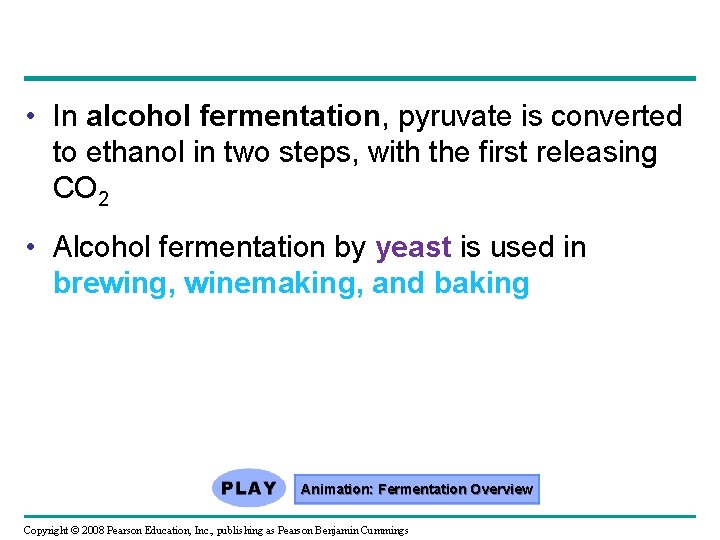
• In alcohol fermentation, pyruvate is converted to ethanol in two steps, with the first releasing CO 2 • Alcohol fermentation by yeast is used in brewing, winemaking, and baking Animation: Fermentation Overview Copyright © 2008 Pearson Education, Inc. , publishing as Pearson Benjamin Cummings
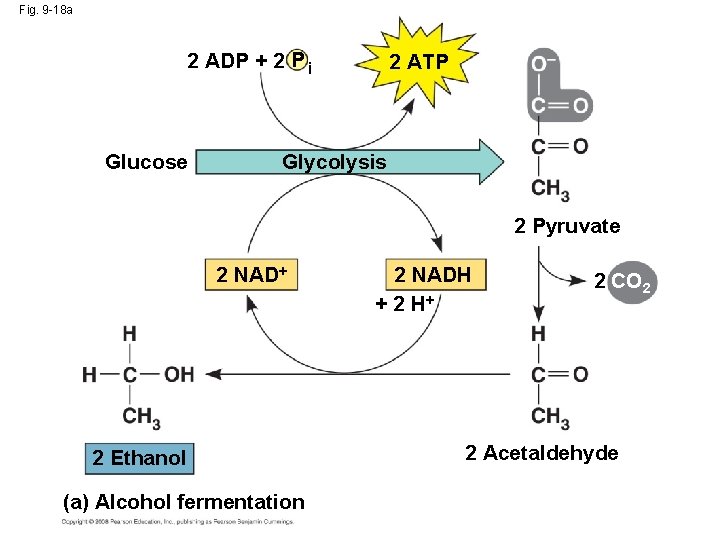
Fig. 9 -18 a 2 ADP + 2 P i Glucose 2 ATP Glycolysis 2 Pyruvate 2 NAD+ 2 Ethanol (a) Alcohol fermentation 2 NADH + 2 H+ 2 CO 2 2 Acetaldehyde
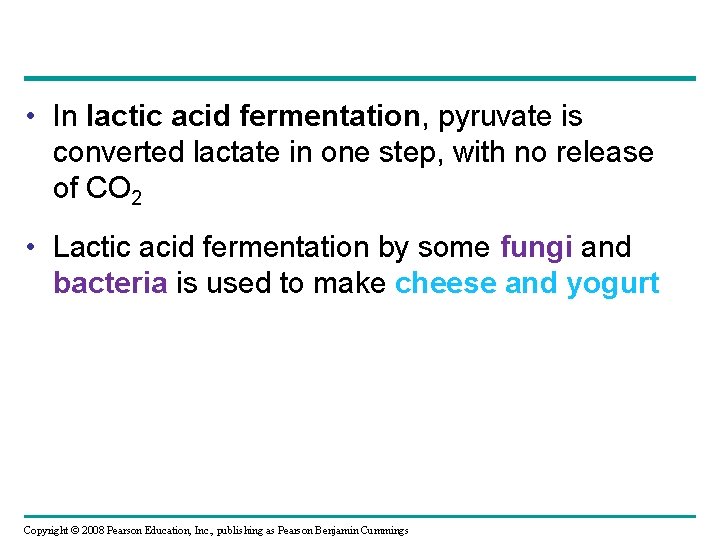
• In lactic acid fermentation, pyruvate is converted lactate in one step, with no release of CO 2 • Lactic acid fermentation by some fungi and bacteria is used to make cheese and yogurt Copyright © 2008 Pearson Education, Inc. , publishing as Pearson Benjamin Cummings
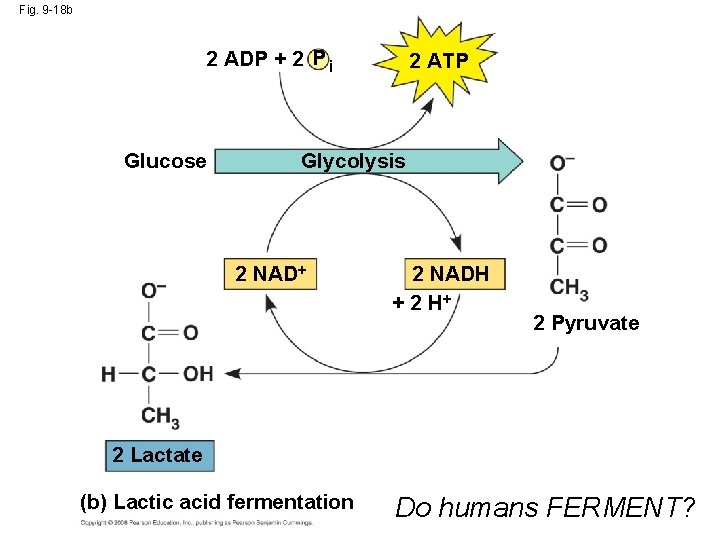
Fig. 9 -18 b 2 ADP + 2 P i Glucose 2 ATP Glycolysis 2 NAD+ 2 NADH + 2 H+ 2 Pyruvate 2 Lactate (b) Lactic acid fermentation Do humans FERMENT?
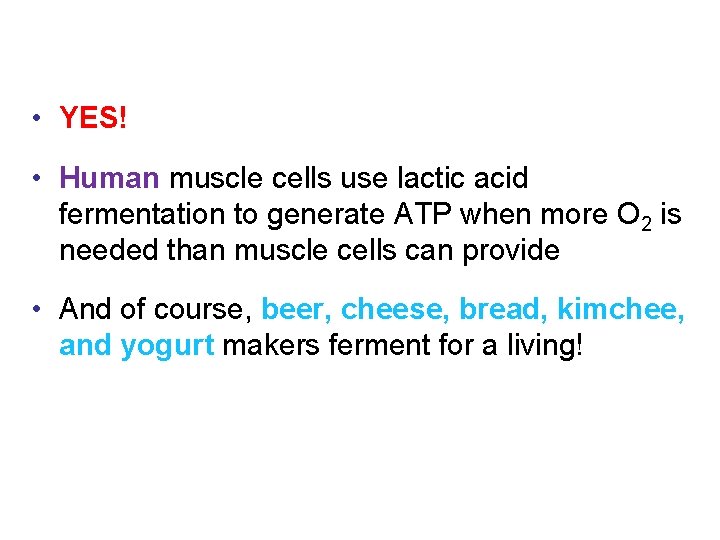
• YES! • Human muscle cells use lactic acid fermentation to generate ATP when more O 2 is needed than muscle cells can provide • And of course, beer, cheese, bread, kimchee, and yogurt makers ferment for a living!
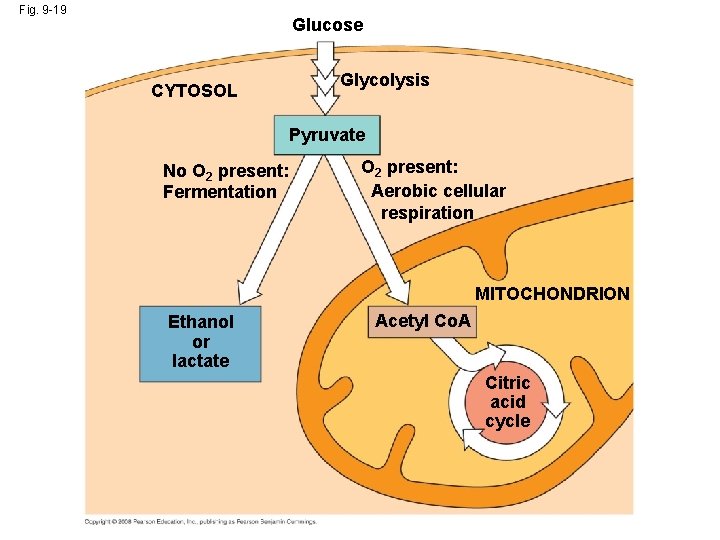
Fig. 9 -19 Glucose CYTOSOL Glycolysis Pyruvate No O 2 present: Fermentation O 2 present: Aerobic cellular respiration MITOCHONDRION Ethanol or lactate Acetyl Co. A Citric acid cycle
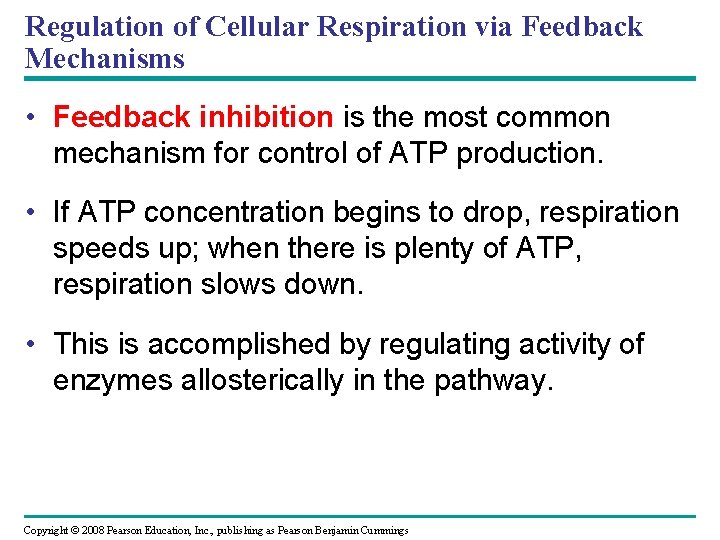
Regulation of Cellular Respiration via Feedback Mechanisms • Feedback inhibition is the most common mechanism for control of ATP production. • If ATP concentration begins to drop, respiration speeds up; when there is plenty of ATP, respiration slows down. • This is accomplished by regulating activity of enzymes allosterically in the pathway. Copyright © 2008 Pearson Education, Inc. , publishing as Pearson Benjamin Cummings
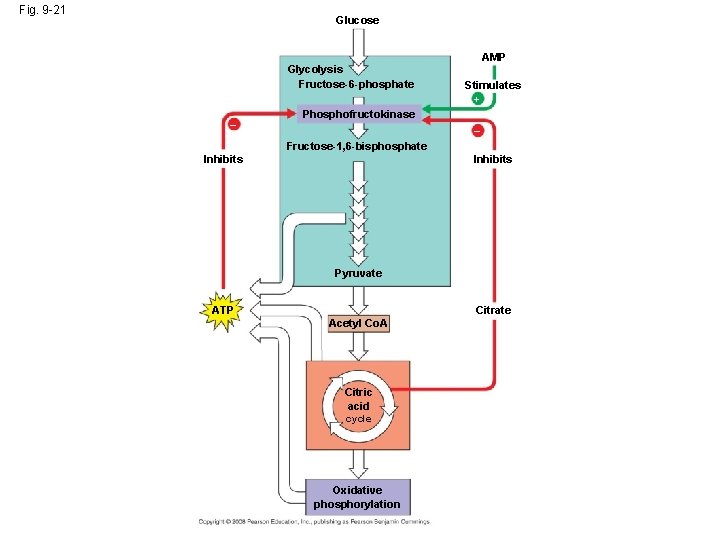
Fig. 9 -21 Glucose Glycolysis Fructose-6 -phosphate – AMP Stimulates + Phosphofructokinase – Fructose-1, 6 -bisphosphate Inhibits Pyruvate ATP Citrate Acetyl Co. A Citric acid cycle Oxidative phosphorylation
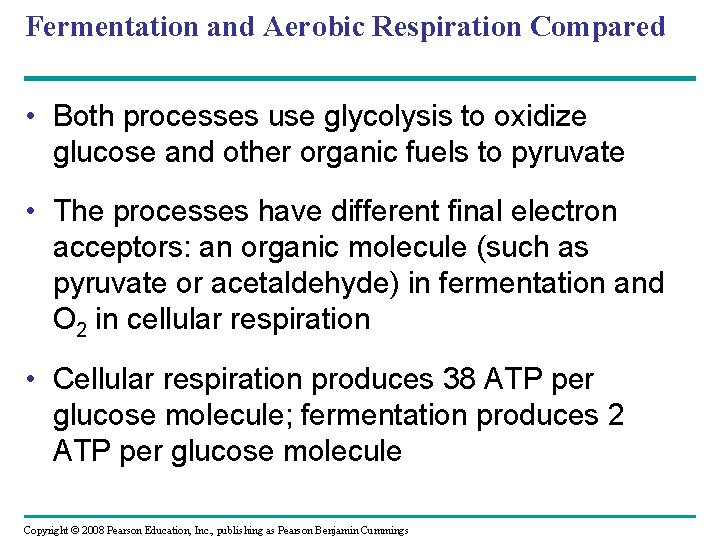
Fermentation and Aerobic Respiration Compared • Both processes use glycolysis to oxidize glucose and other organic fuels to pyruvate • The processes have different final electron acceptors: an organic molecule (such as pyruvate or acetaldehyde) in fermentation and O 2 in cellular respiration • Cellular respiration produces 38 ATP per glucose molecule; fermentation produces 2 ATP per glucose molecule Copyright © 2008 Pearson Education, Inc. , publishing as Pearson Benjamin Cummings
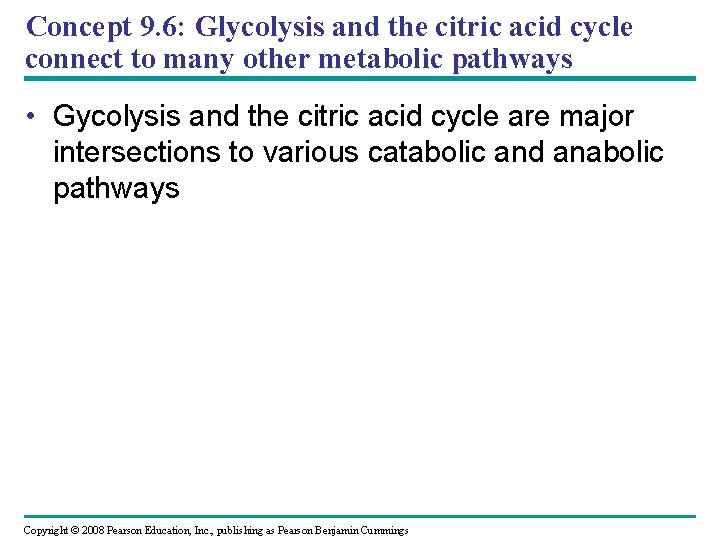
Concept 9. 6: Glycolysis and the citric acid cycle connect to many other metabolic pathways • Gycolysis and the citric acid cycle are major intersections to various catabolic and anabolic pathways Copyright © 2008 Pearson Education, Inc. , publishing as Pearson Benjamin Cummings
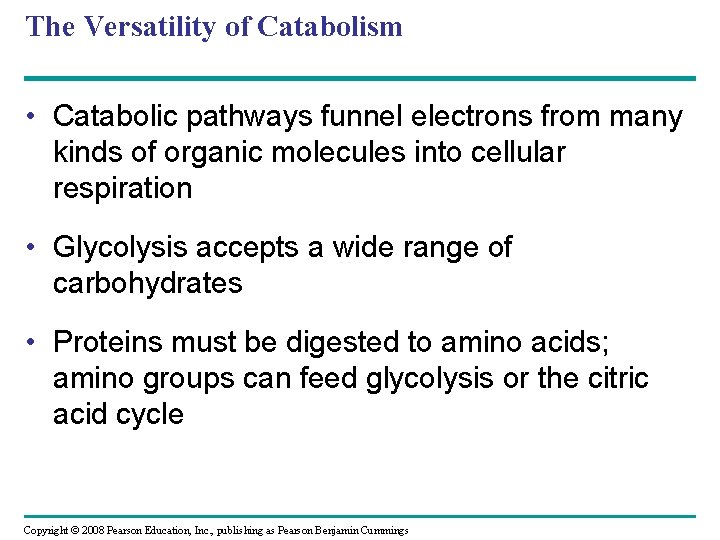
The Versatility of Catabolism • Catabolic pathways funnel electrons from many kinds of organic molecules into cellular respiration • Glycolysis accepts a wide range of carbohydrates • Proteins must be digested to amino acids; amino groups can feed glycolysis or the citric acid cycle Copyright © 2008 Pearson Education, Inc. , publishing as Pearson Benjamin Cummings
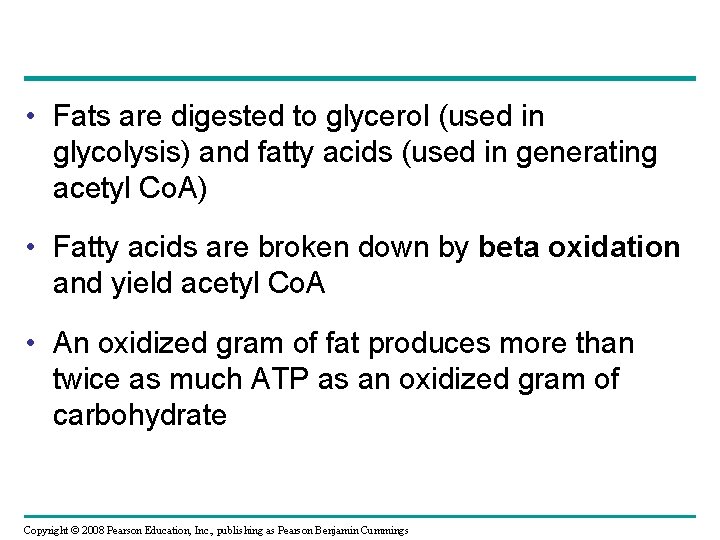
• Fats are digested to glycerol (used in glycolysis) and fatty acids (used in generating acetyl Co. A) • Fatty acids are broken down by beta oxidation and yield acetyl Co. A • An oxidized gram of fat produces more than twice as much ATP as an oxidized gram of carbohydrate Copyright © 2008 Pearson Education, Inc. , publishing as Pearson Benjamin Cummings
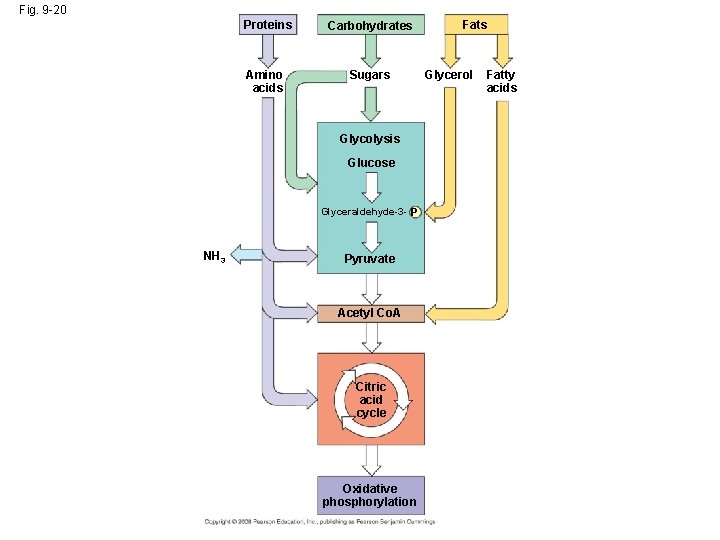
Fig. 9 -20 Proteins Amino acids Carbohydrates Sugars Glycolysis Glucose Glyceraldehyde-3 - P NH 3 Pyruvate Acetyl Co. A Citric acid cycle Oxidative phosphorylation Fats Glycerol Fatty acids
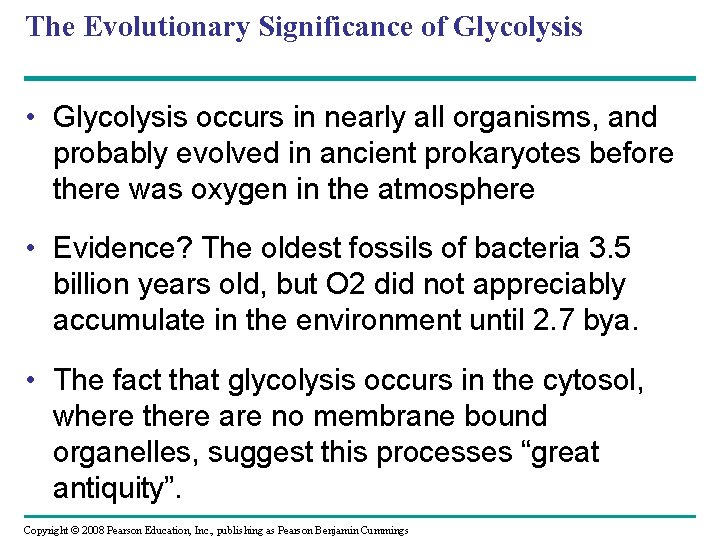
The Evolutionary Significance of Glycolysis • Glycolysis occurs in nearly all organisms, and probably evolved in ancient prokaryotes before there was oxygen in the atmosphere • Evidence? The oldest fossils of bacteria 3. 5 billion years old, but O 2 did not appreciably accumulate in the environment until 2. 7 bya. • The fact that glycolysis occurs in the cytosol, where there are no membrane bound organelles, suggest this processes “great antiquity”. Copyright © 2008 Pearson Education, Inc. , publishing as Pearson Benjamin Cummings
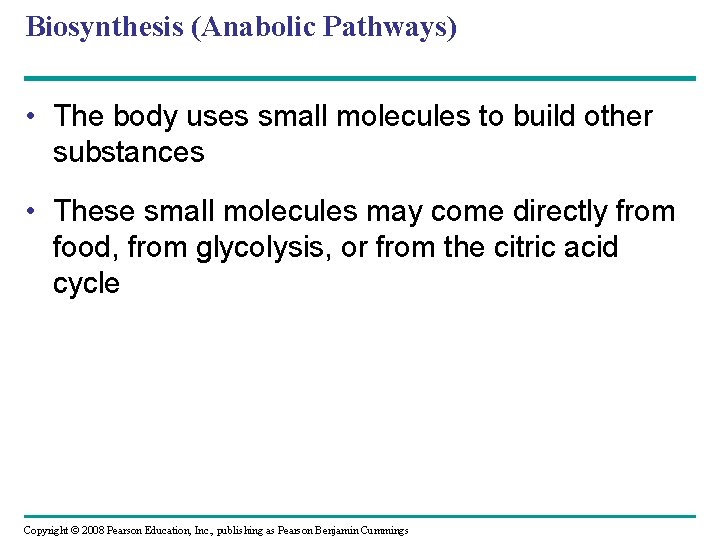
Biosynthesis (Anabolic Pathways) • The body uses small molecules to build other substances • These small molecules may come directly from food, from glycolysis, or from the citric acid cycle Copyright © 2008 Pearson Education, Inc. , publishing as Pearson Benjamin Cummings
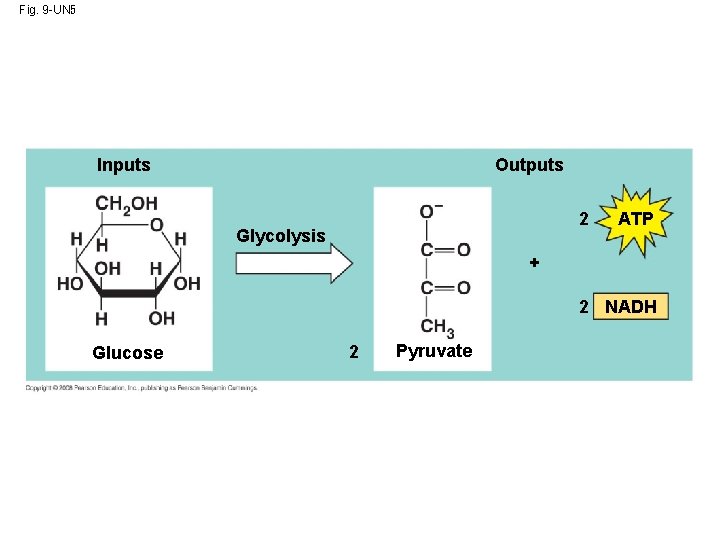
Fig. 9 -UN 5 Outputs Inputs 2 Glycolysis ATP + 2 NADH Glucose 2 Pyruvate
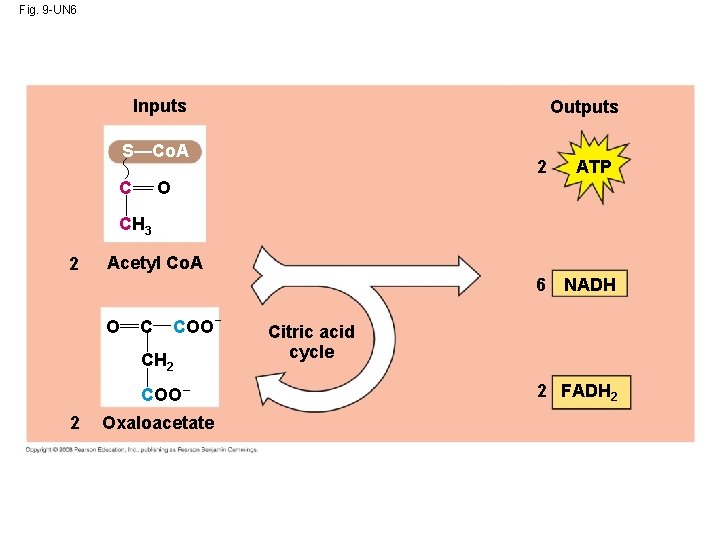
Fig. 9 -UN 6 Inputs Outputs S—Co. A C 2 ATP O CH 3 2 Acetyl Co. A 6 NADH O C COO CH 2 COO 2 Oxaloacetate Citric acid cycle 2 FADH 2
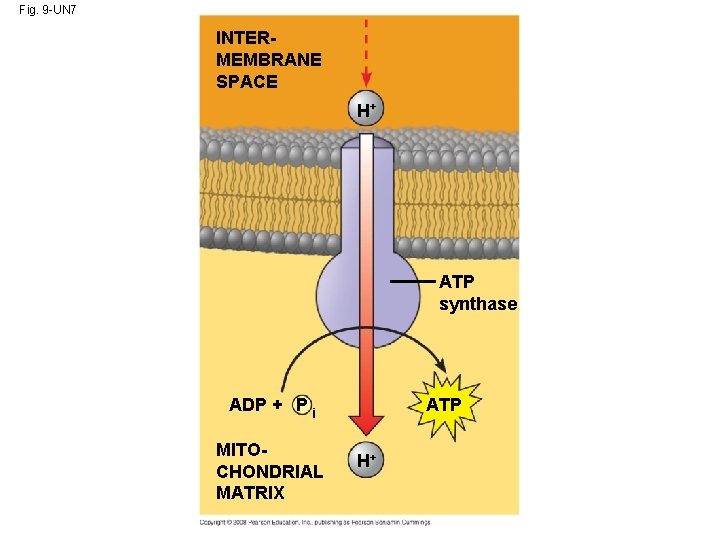
Fig. 9 -UN 7 INTERMEMBRANE SPACE H+ ATP synthase ADP + P i MITOCHONDRIAL MATRIX ATP H+
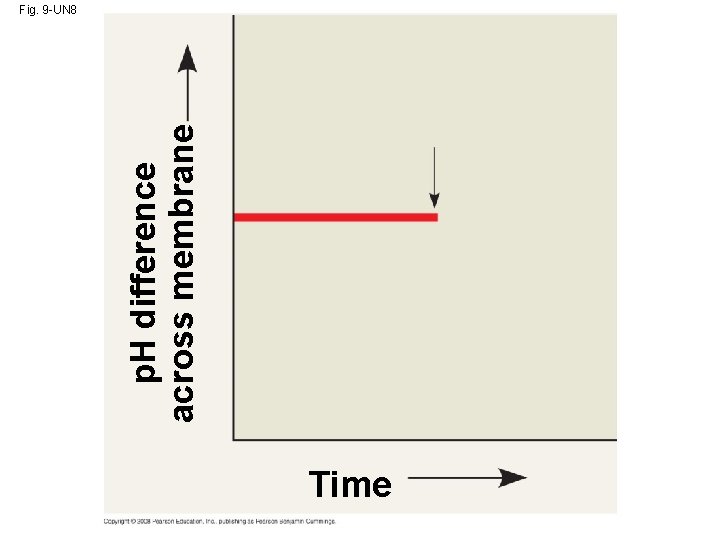
p. H difference across membrane Fig. 9 -UN 8 Time
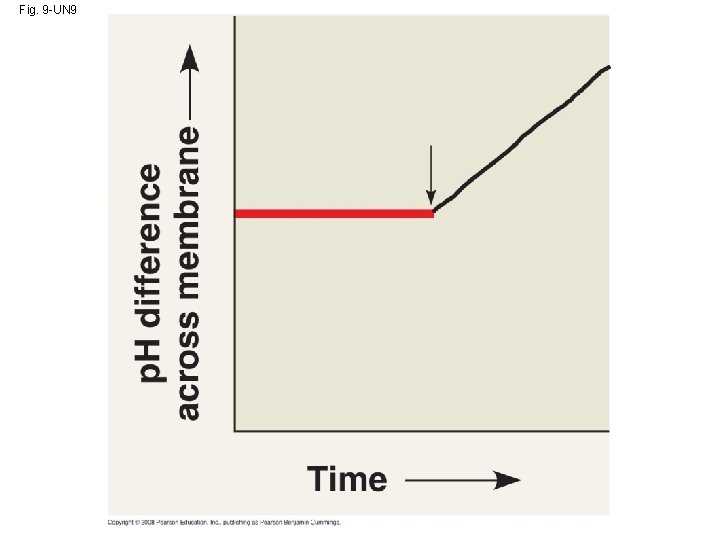
Fig. 9 -UN 9
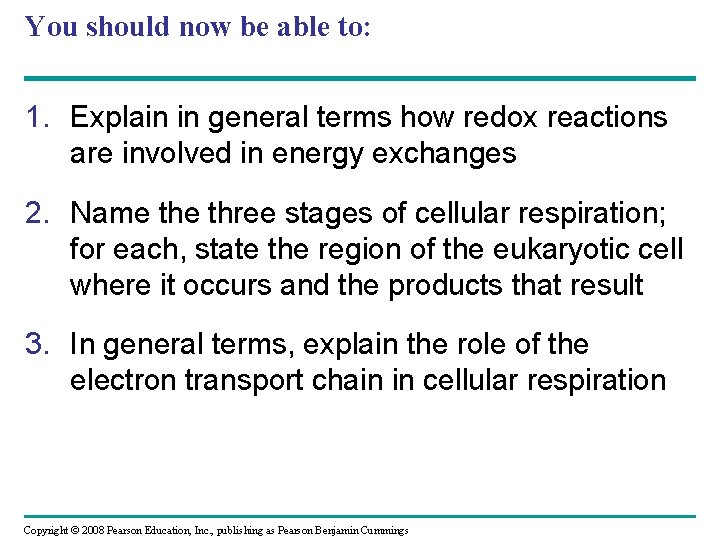
You should now be able to: 1. Explain in general terms how redox reactions are involved in energy exchanges 2. Name three stages of cellular respiration; for each, state the region of the eukaryotic cell where it occurs and the products that result 3. In general terms, explain the role of the electron transport chain in cellular respiration Copyright © 2008 Pearson Education, Inc. , publishing as Pearson Benjamin Cummings
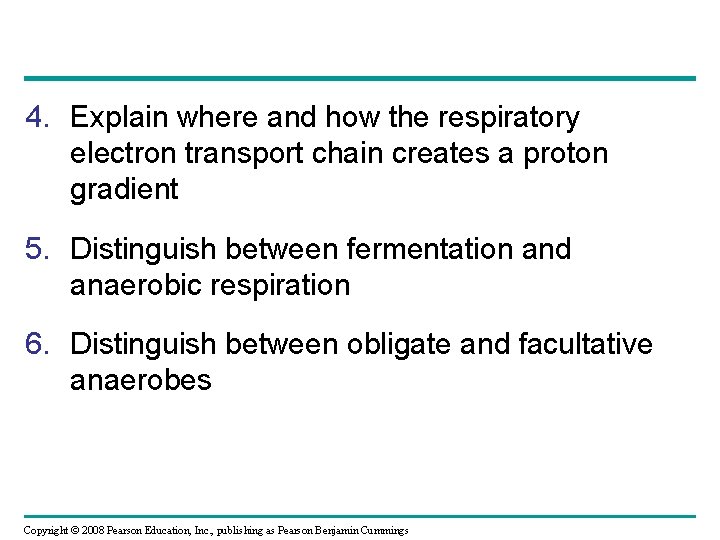
4. Explain where and how the respiratory electron transport chain creates a proton gradient 5. Distinguish between fermentation and anaerobic respiration 6. Distinguish between obligate and facultative anaerobes Copyright © 2008 Pearson Education, Inc. , publishing as Pearson Benjamin Cummings
The stages of cellular respiration
Chapter 9 cellular respiration harvesting chemical energy
Chapter 9: cellular respiration: harvesting chemical energy
Cellular respiration harvesting chemical energy
Chemical formula for cellular respiration
What is the equation for cellular respiration
What is the chemical formula of cellular respiration
Energy flow in cellular respiration
Energy flow in cellular respiration
Cellular respiration obtaining energy from food
Cellular respiration obtaining energy from food
Chapter 8 section 3: cellular respiration
Section 1 how organisms obtain energy
Redox reaction in cellular respiration
What is the correct equation for cellular respiration?
Cellular respiration steps
Types of respiration
Define fermentation
Why is cellular respiration important
Complementary processes
Amloplast
Photosynthesis formula
Starting materials for cellular respiration
What happens during glycolysis
Overview of cellular respiration
Aerobic respiration overview
Cellular respiration
Total atp produced in cellular respiration
Lab bench cellular respiration
What is the word equation for cellular respiration
Higher human biology cellular respiration
Cellular respiration reactants
Cellular respiration releases
Starting materials for cellular respiration
Redox reaction in cellular respiration
Where in the cell does cellular respiration occur
Role of cellular respiration
Cellular respiration releases
Cellular respiration redox
Crash course glycolysis
Aerobic cellular respiration equation
Cellular respiration process diagram
Overall reaction of cellular respiration
Cellular respiration occurs in
Electron transport chain cellular respiration
Where does cellular respiration take place
Function of cellular respiration
Cellular respiration endothermic or exothermic
Overall reaction of cellular respiration
Formula for photosynthesis and cellular respiration
Snails and elodea virtual lab
Pmat cell cycle
Difference between autotroph and heterotroph
Cellular respiration goal
Cellular respiration fact
Road map for cellular respiration
Cellular respiration table
Starting materials for cellular respiration
Photosynthesis and cellular respiration diagram
Cellular respiration foldable
Stages of cellular respiration diagram
Cellular respiration
Cellular respiration pearson
Labeled mitochondria cellular respiration
Cellular respiration
Cellular respiration
Cellular respiration organelle
Where does the oxygen that we breathe come from edpuzzle
Concept 5 cellular respiration
Cellular respiration modle
Section 3 cellular respiration
Photosynthesis and cellular respiration
Total atp produced in cellular respiration
Which organism is an autotroph
Wood badge ticket examples
Glucose cellular respiration
Section 3 cellular respiration worksheet answers
Steps 1
Cellular respiration song
What is produced in cellular respiration
What type of cell performs cellular respiration
Copyright