Chapter 8 Wind and Weather Wind Wind The
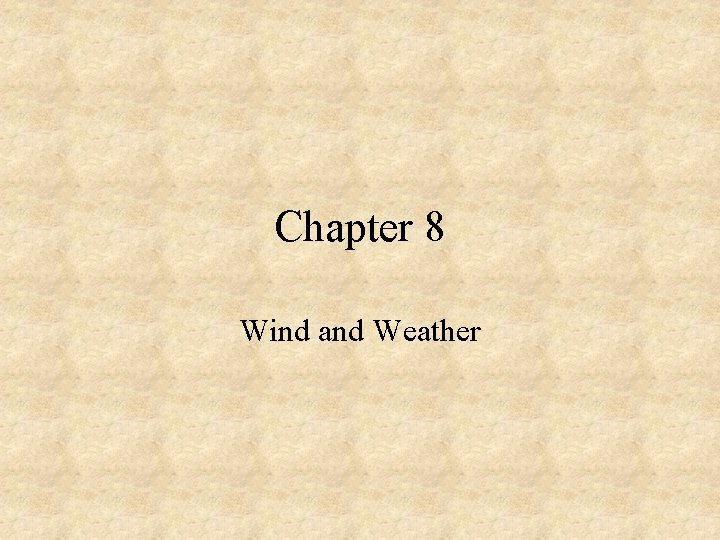
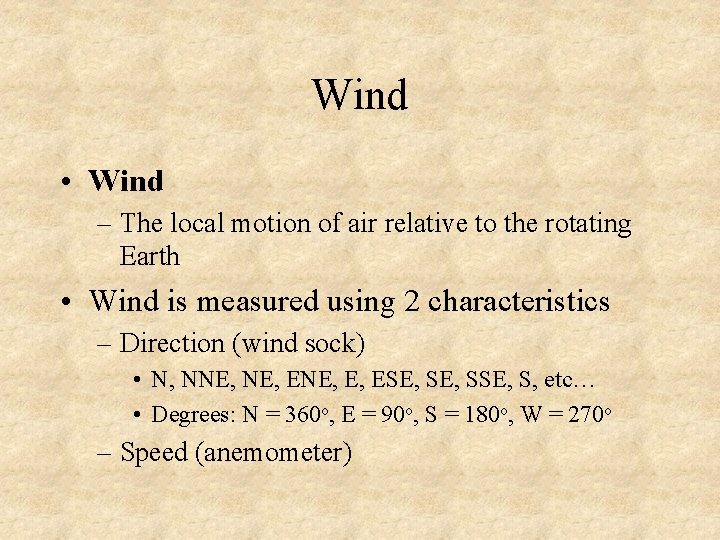
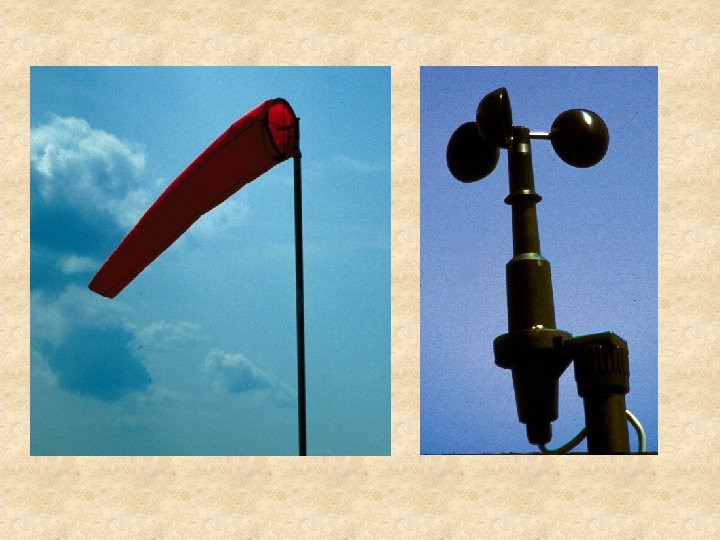
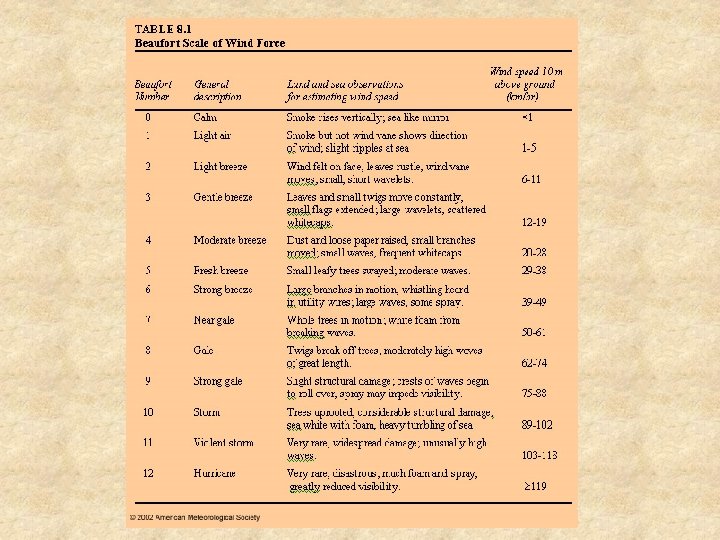
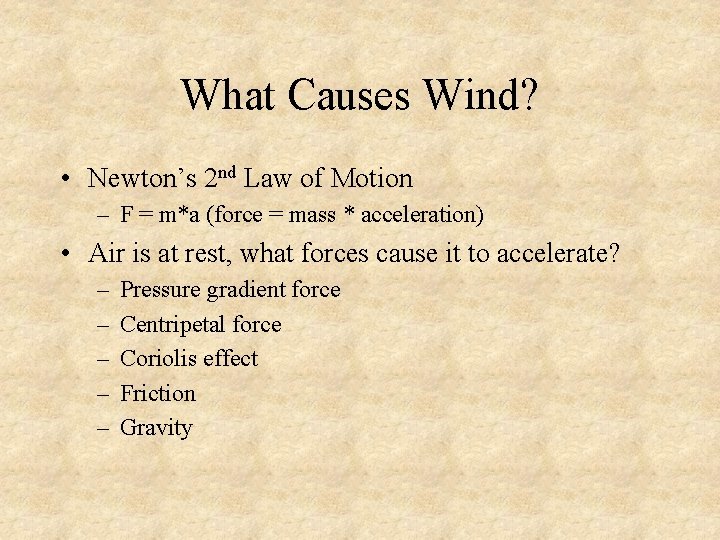
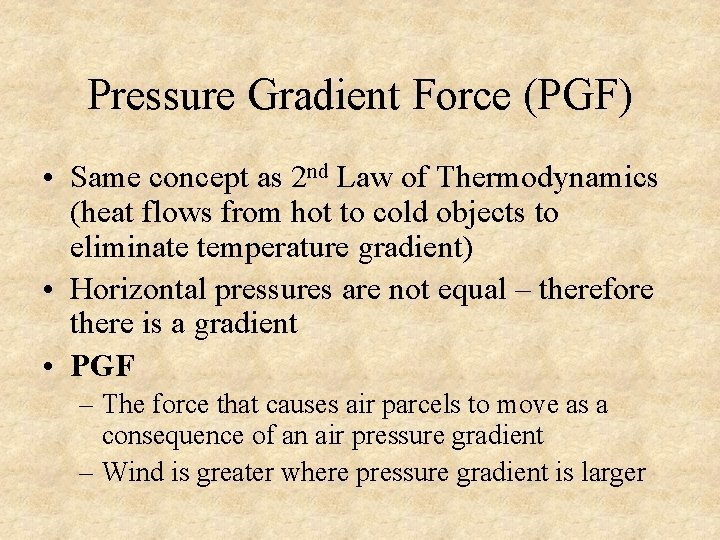
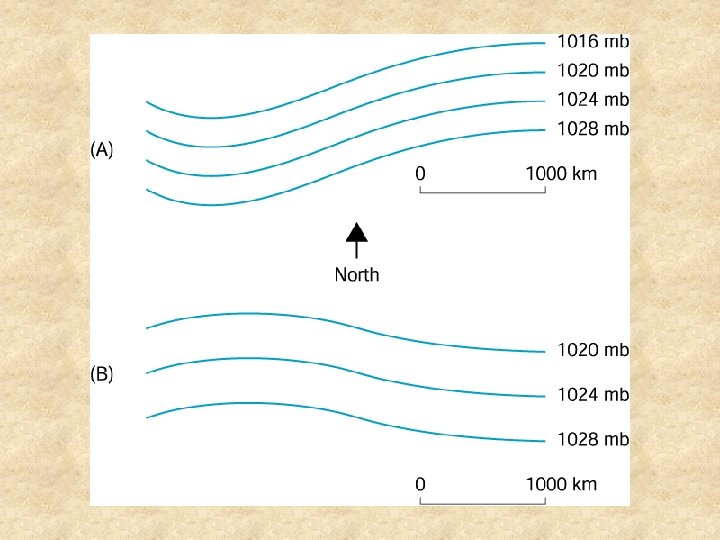
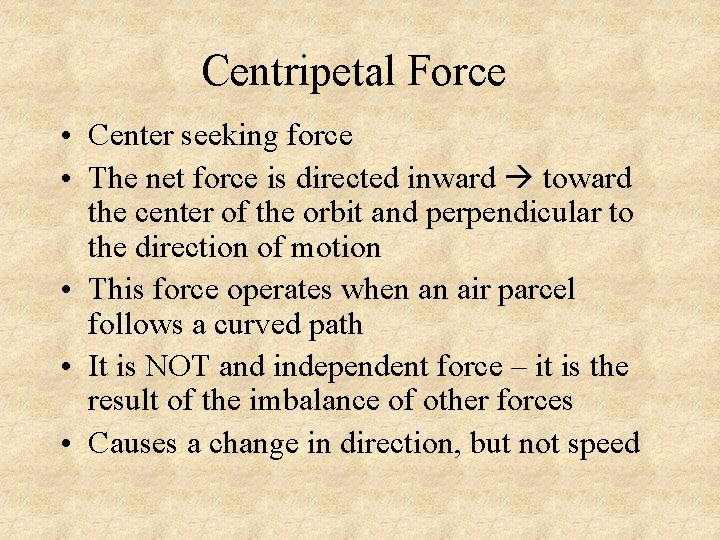
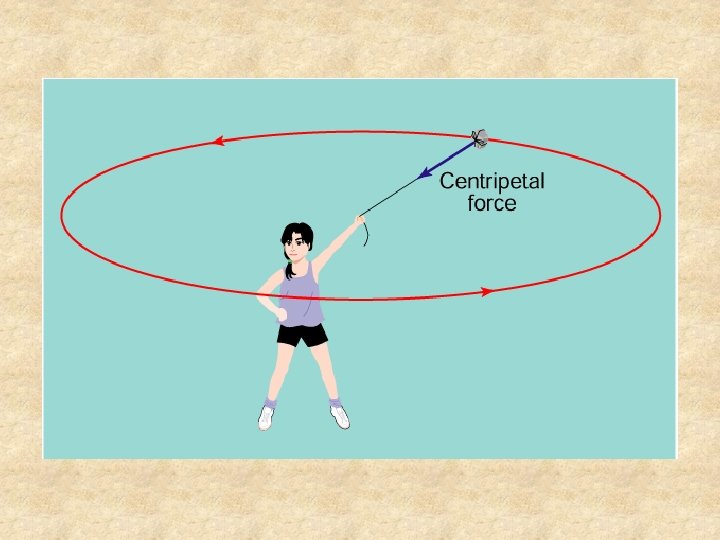
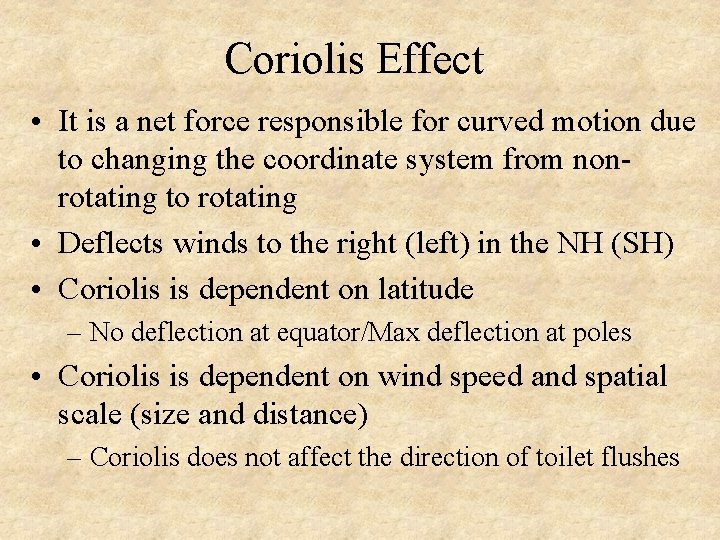
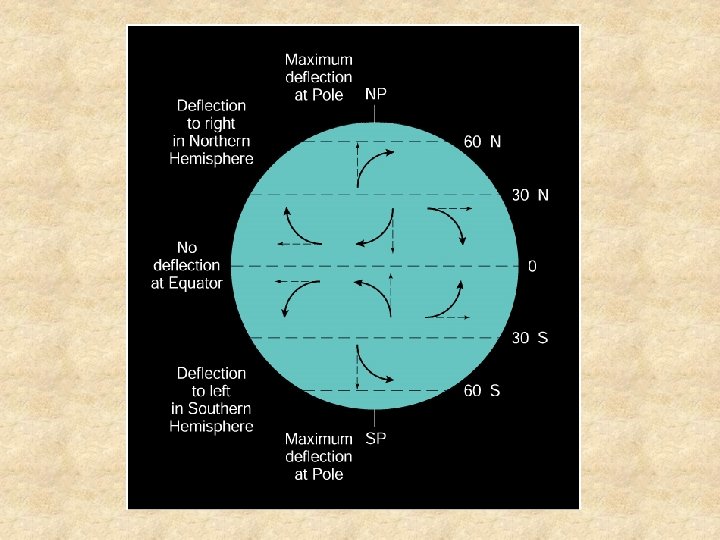
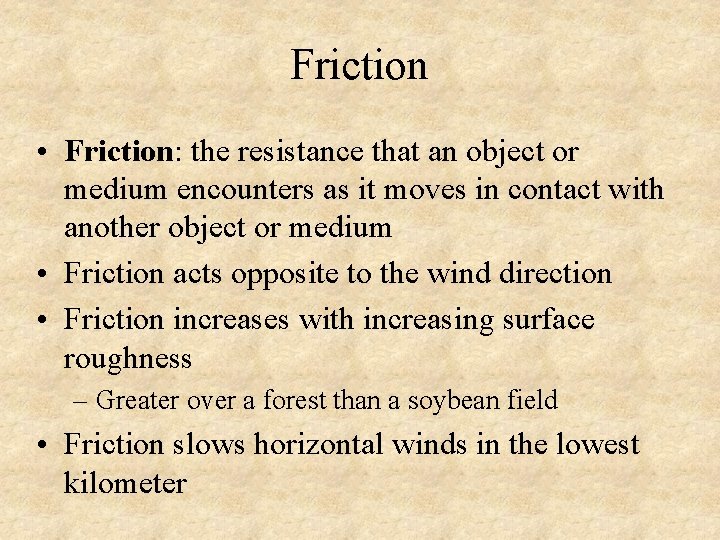
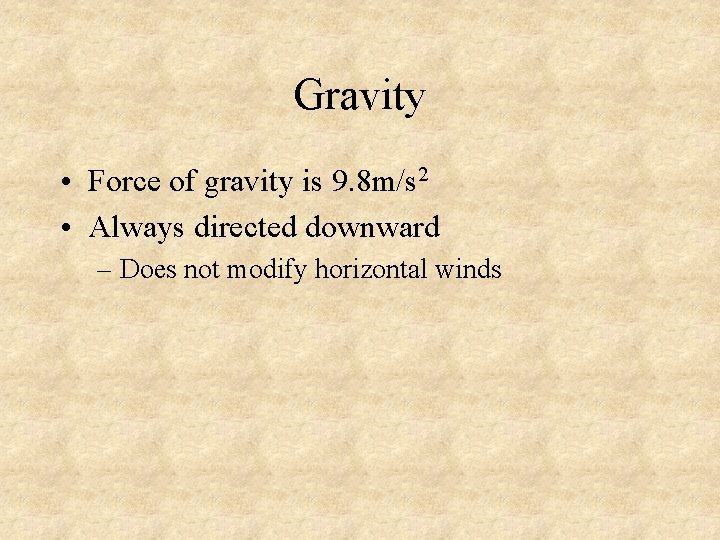
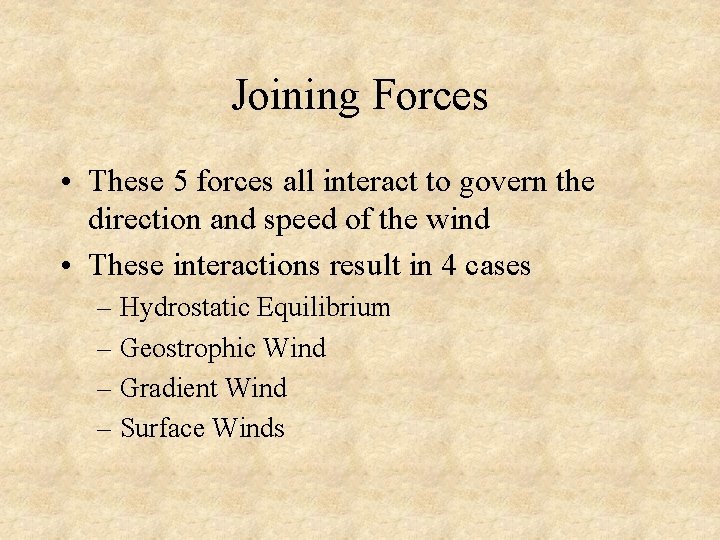
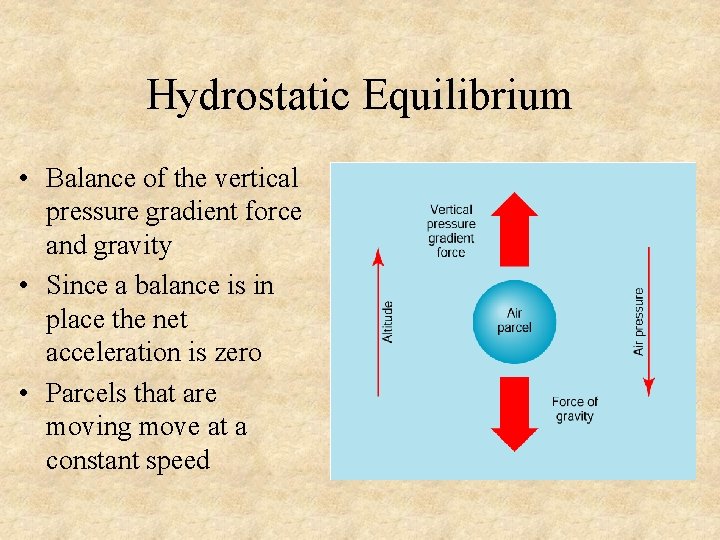
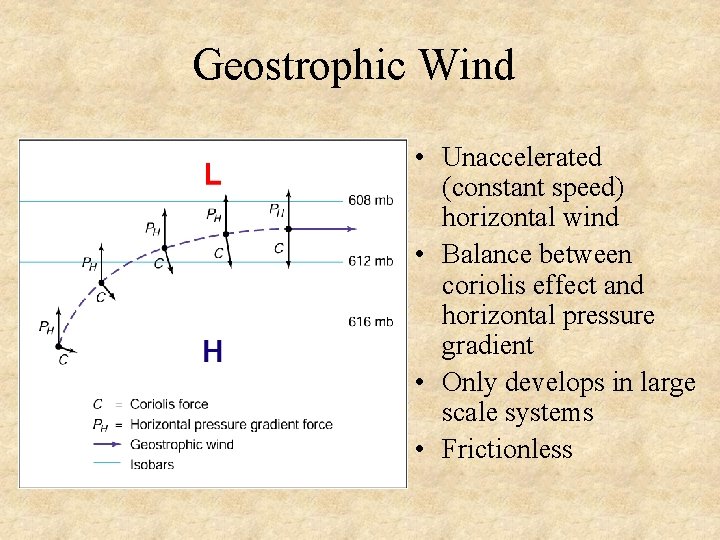
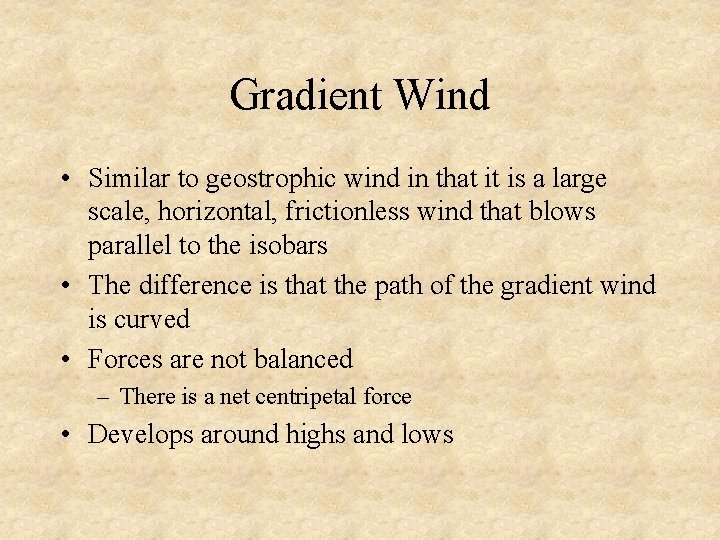
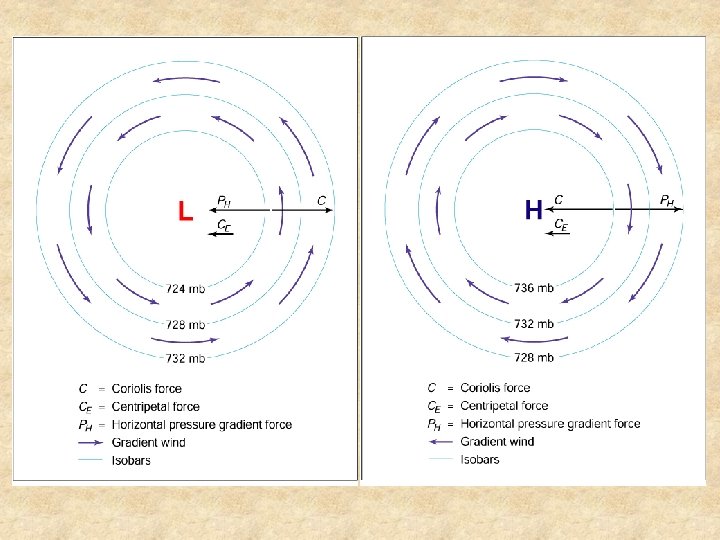
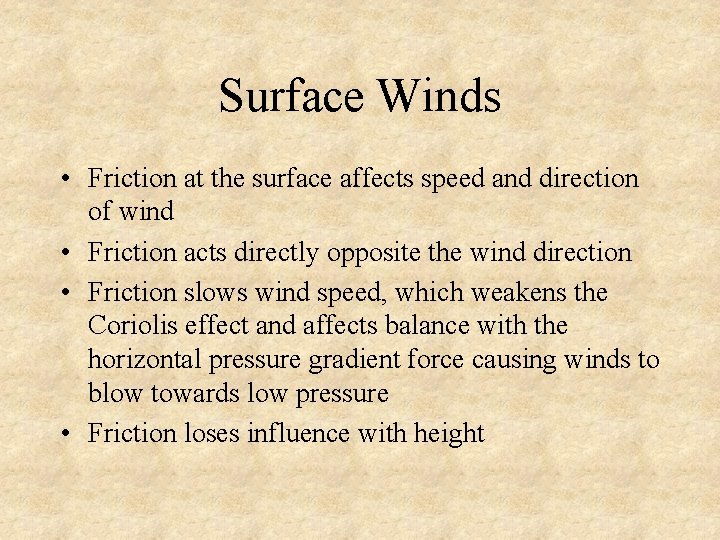
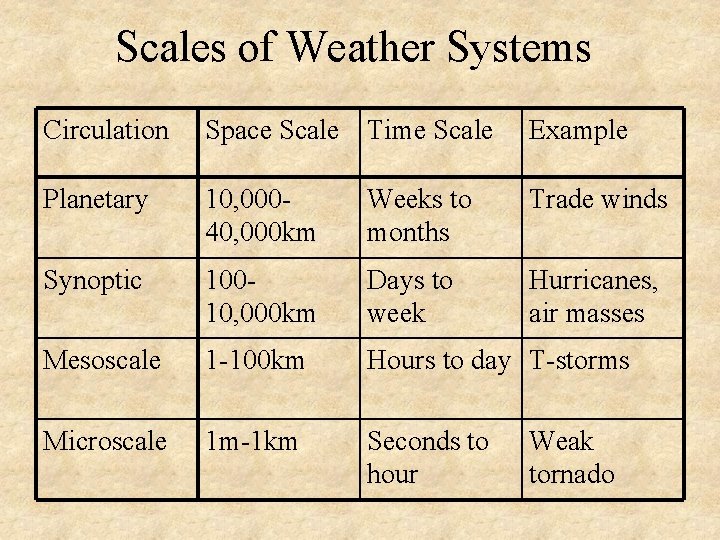
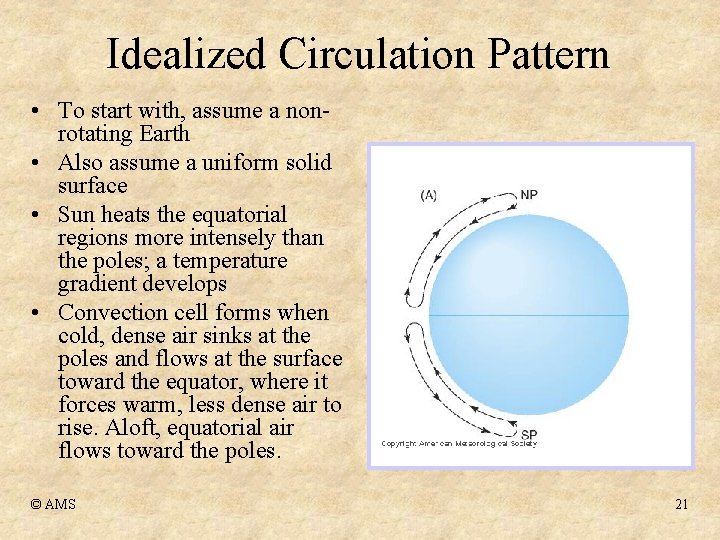
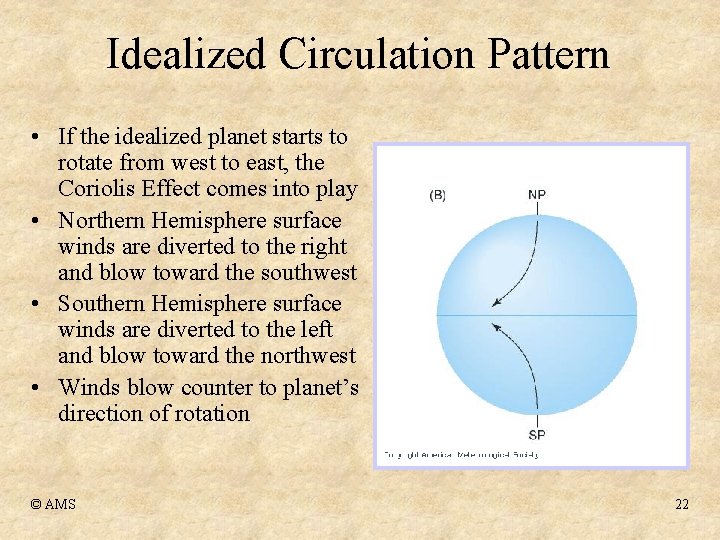
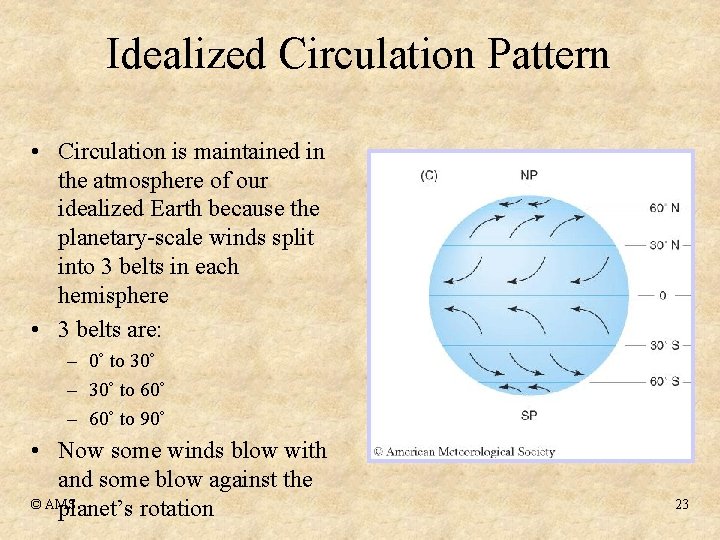
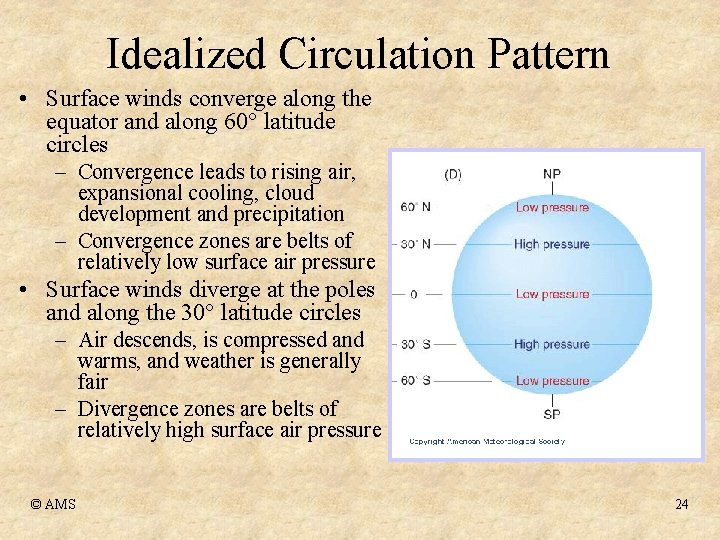
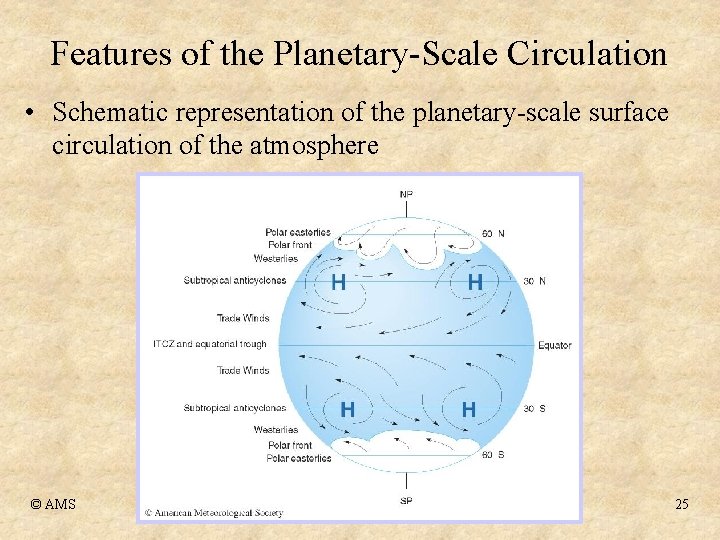
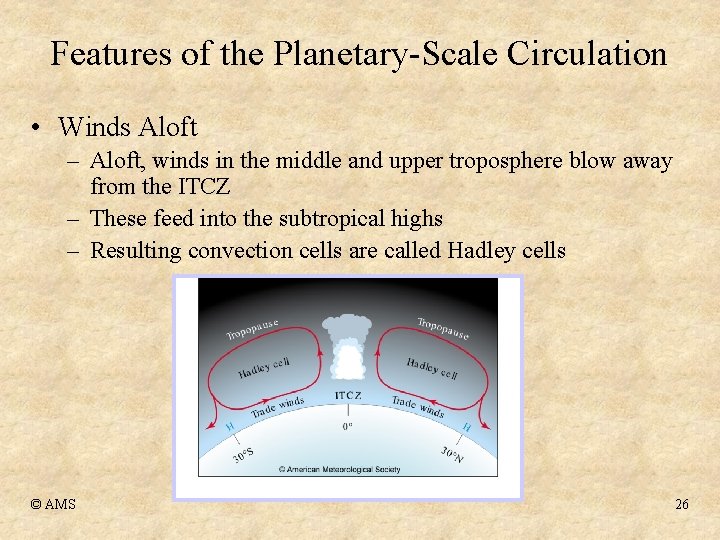
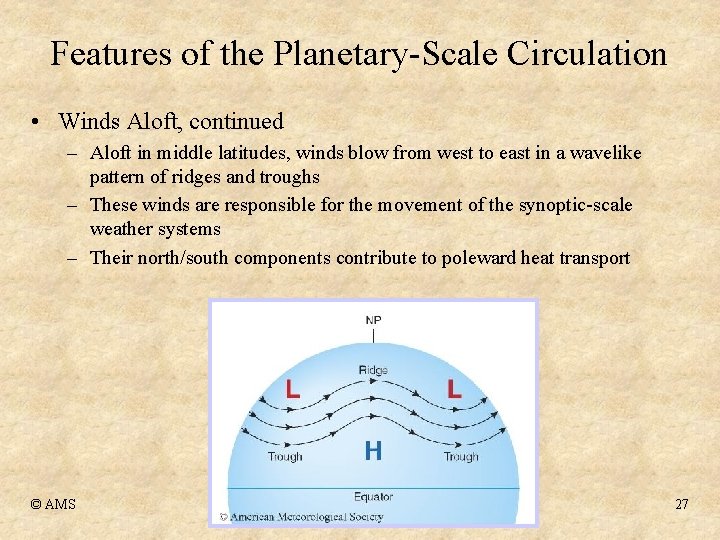
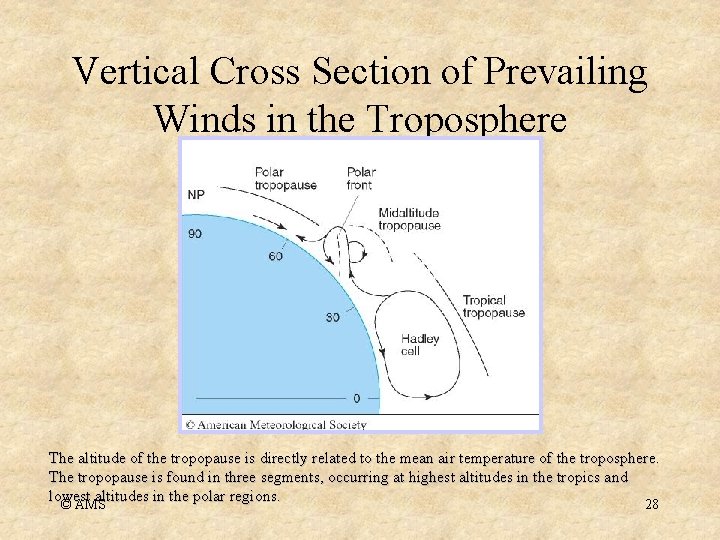
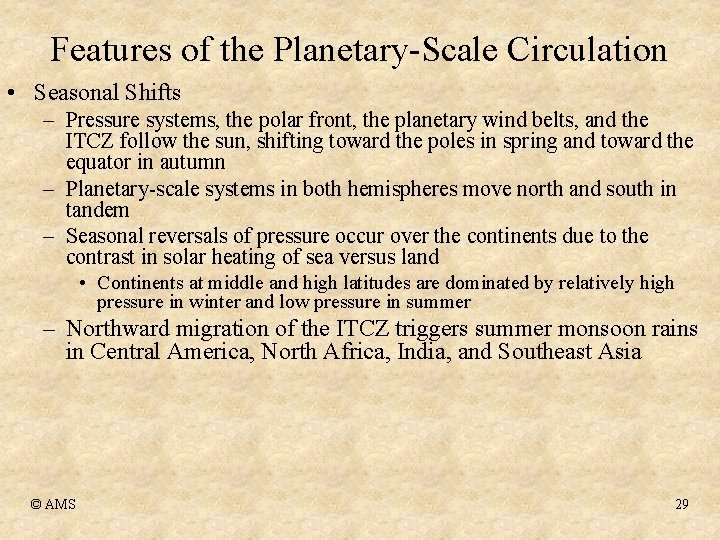
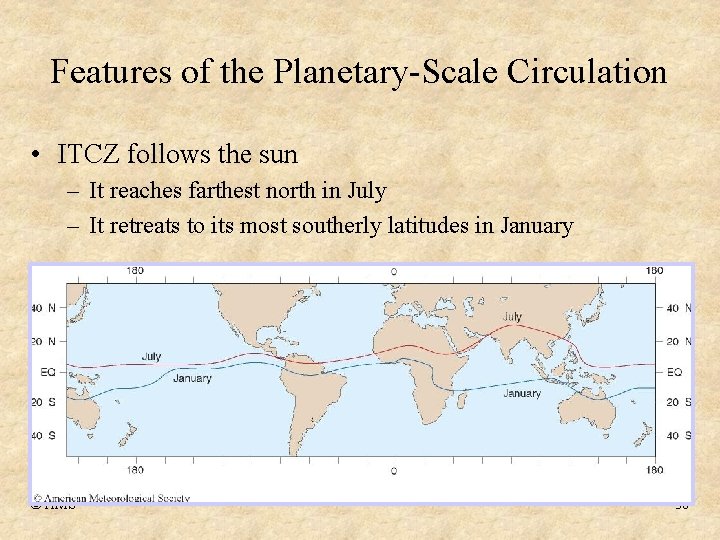
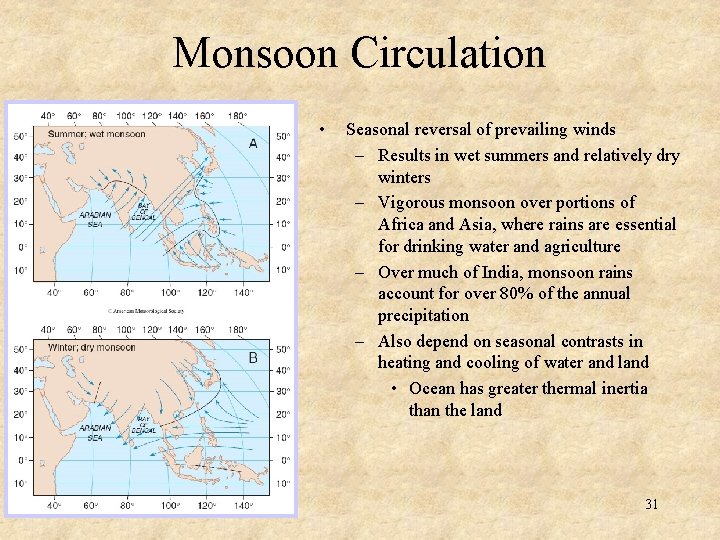
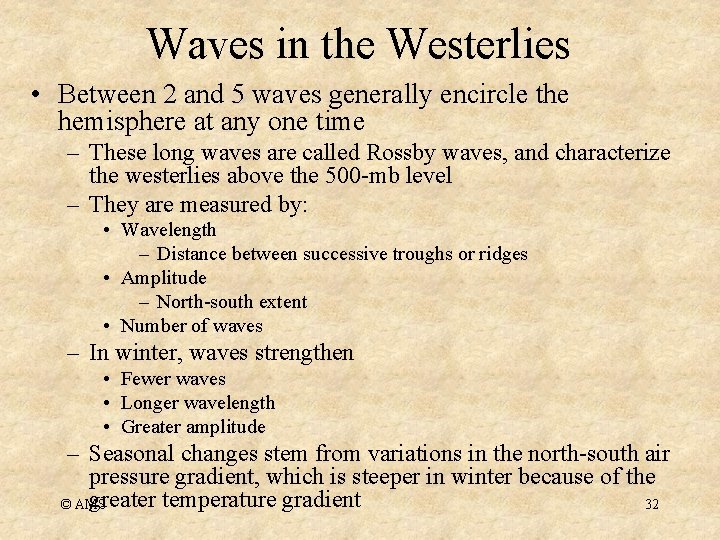
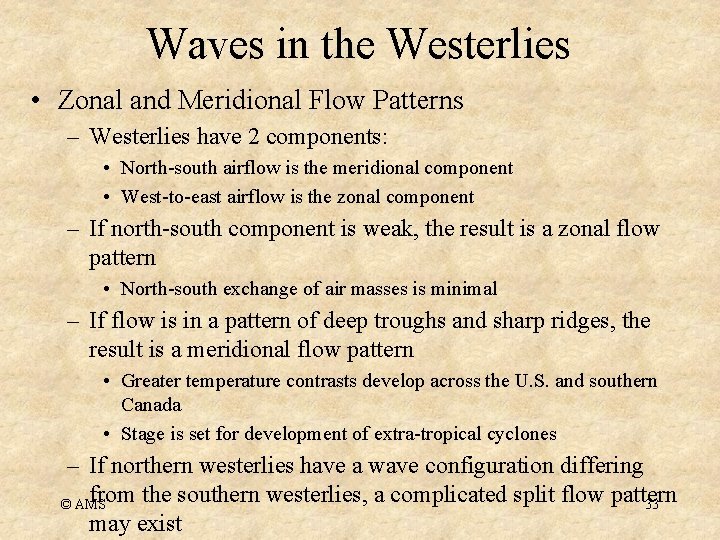
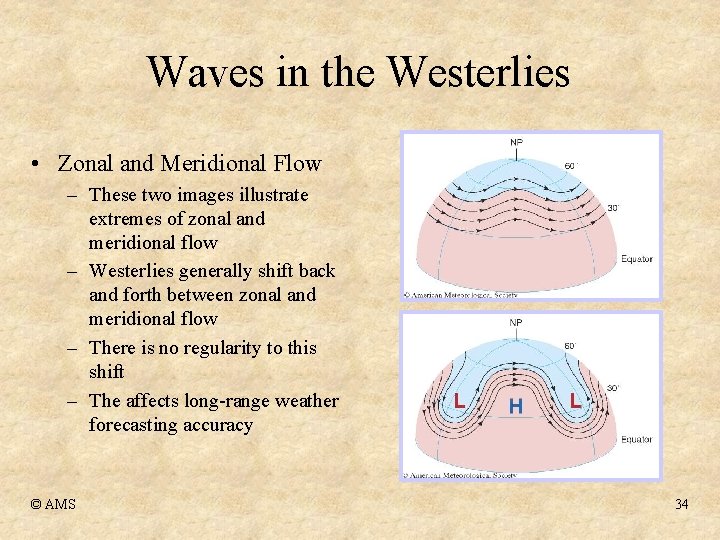
- Slides: 34
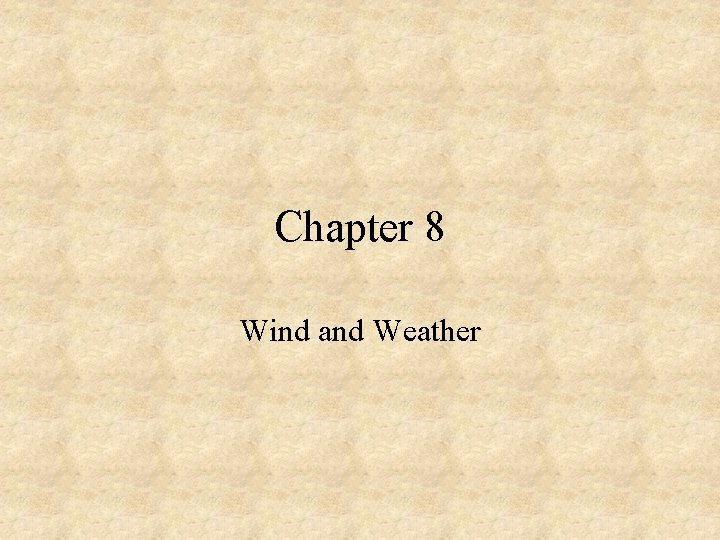
Chapter 8 Wind and Weather
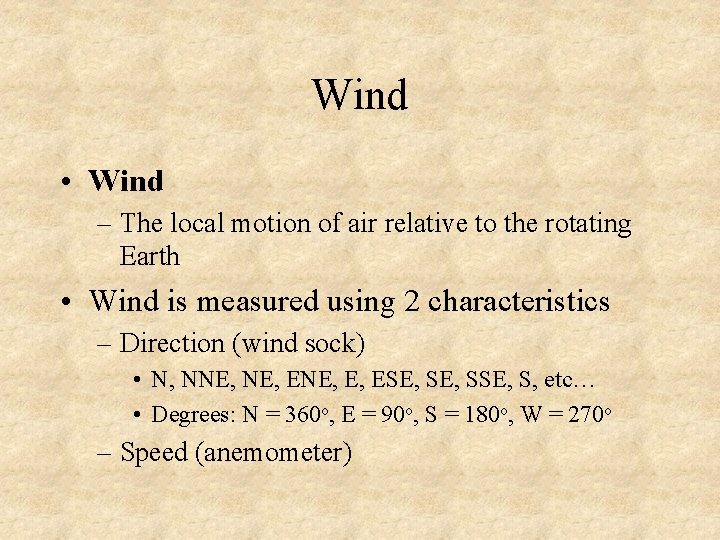
Wind • Wind – The local motion of air relative to the rotating Earth • Wind is measured using 2 characteristics – Direction (wind sock) • N, NNE, ENE, E, ESE, SSE, S, etc… • Degrees: N = 360 o, E = 90 o, S = 180 o, W = 270 o – Speed (anemometer)
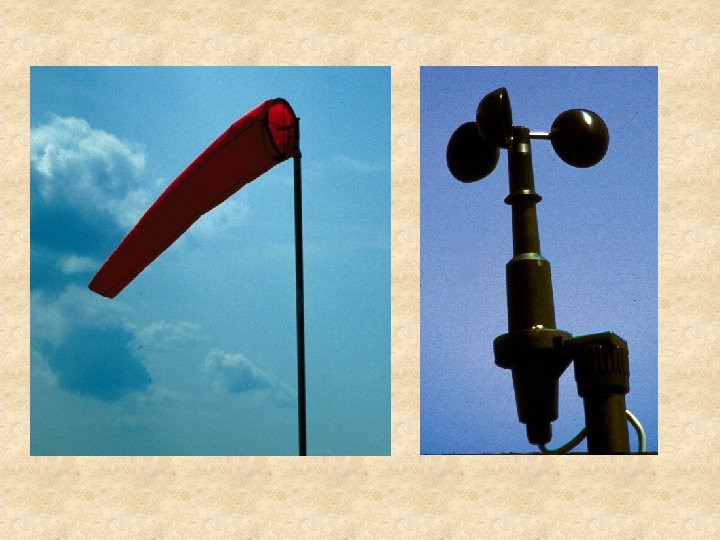
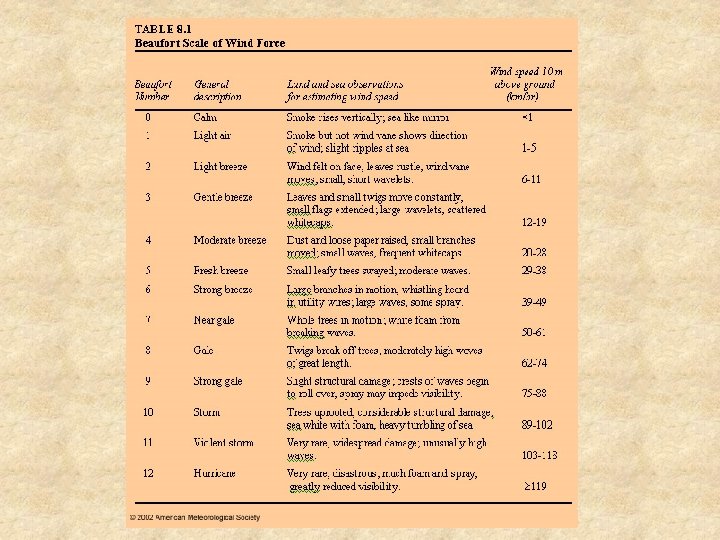
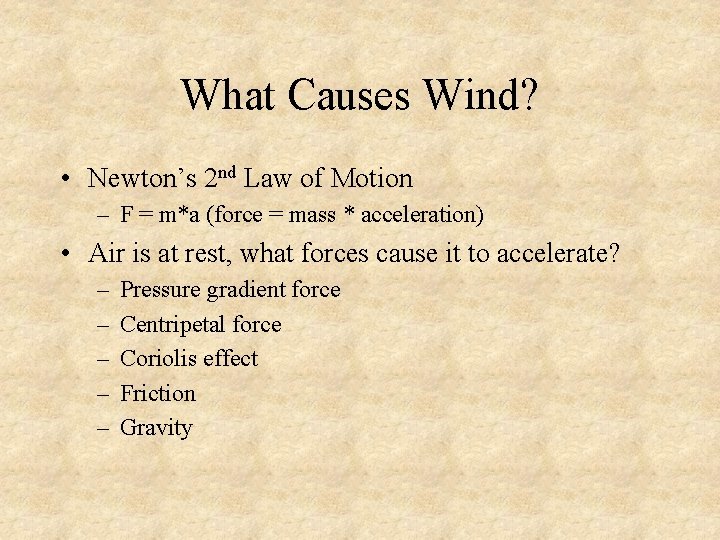
What Causes Wind? • Newton’s 2 nd Law of Motion – F = m*a (force = mass * acceleration) • Air is at rest, what forces cause it to accelerate? – – – Pressure gradient force Centripetal force Coriolis effect Friction Gravity
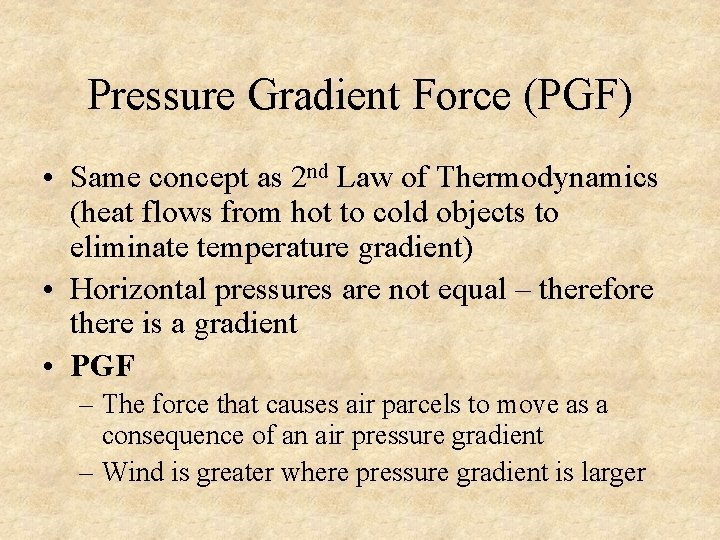
Pressure Gradient Force (PGF) • Same concept as 2 nd Law of Thermodynamics (heat flows from hot to cold objects to eliminate temperature gradient) • Horizontal pressures are not equal – therefore there is a gradient • PGF – The force that causes air parcels to move as a consequence of an air pressure gradient – Wind is greater where pressure gradient is larger
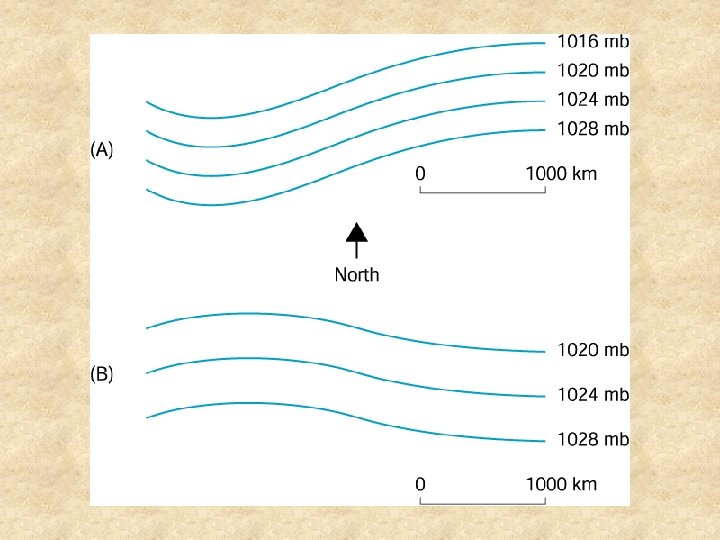
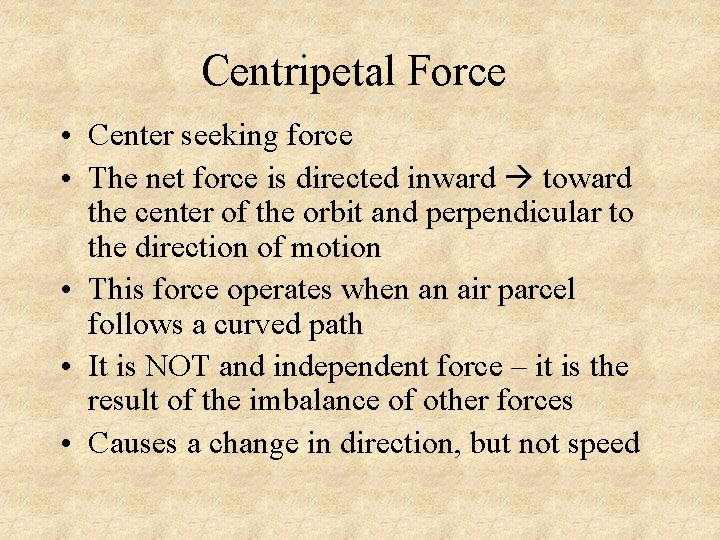
Centripetal Force • Center seeking force • The net force is directed inward toward the center of the orbit and perpendicular to the direction of motion • This force operates when an air parcel follows a curved path • It is NOT and independent force – it is the result of the imbalance of other forces • Causes a change in direction, but not speed
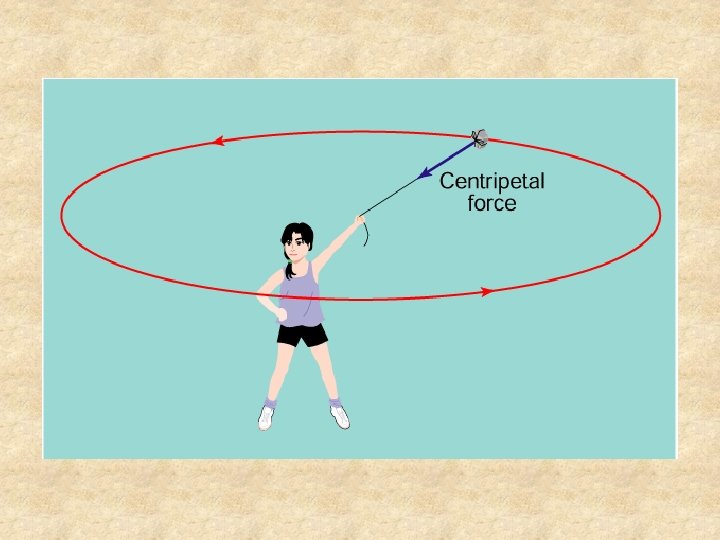
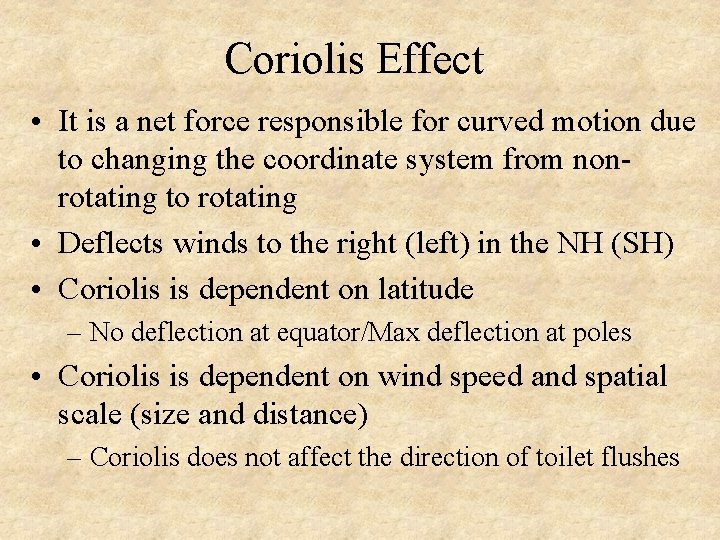
Coriolis Effect • It is a net force responsible for curved motion due to changing the coordinate system from nonrotating to rotating • Deflects winds to the right (left) in the NH (SH) • Coriolis is dependent on latitude – No deflection at equator/Max deflection at poles • Coriolis is dependent on wind speed and spatial scale (size and distance) – Coriolis does not affect the direction of toilet flushes
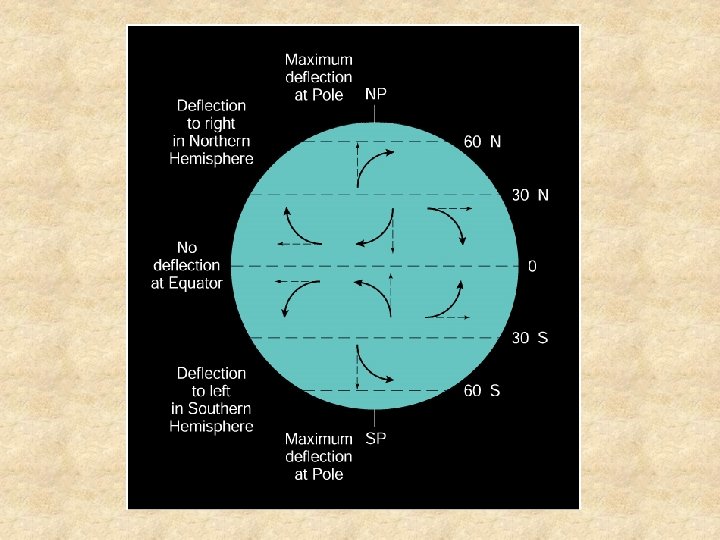
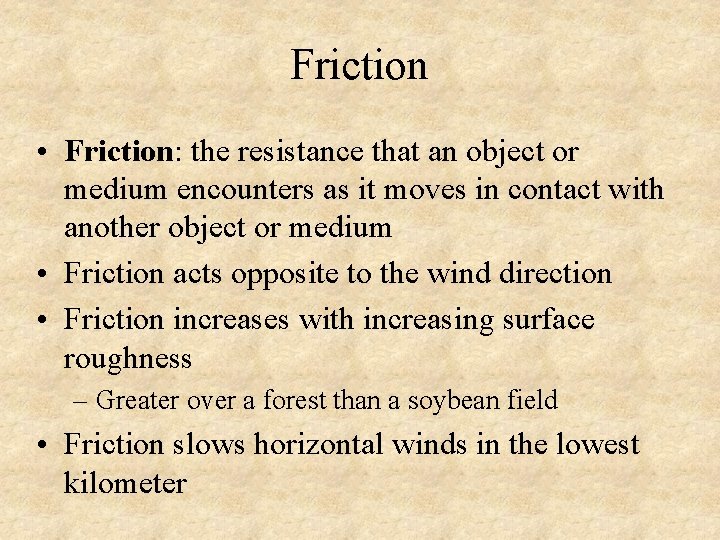
Friction • Friction: the resistance that an object or medium encounters as it moves in contact with another object or medium • Friction acts opposite to the wind direction • Friction increases with increasing surface roughness – Greater over a forest than a soybean field • Friction slows horizontal winds in the lowest kilometer
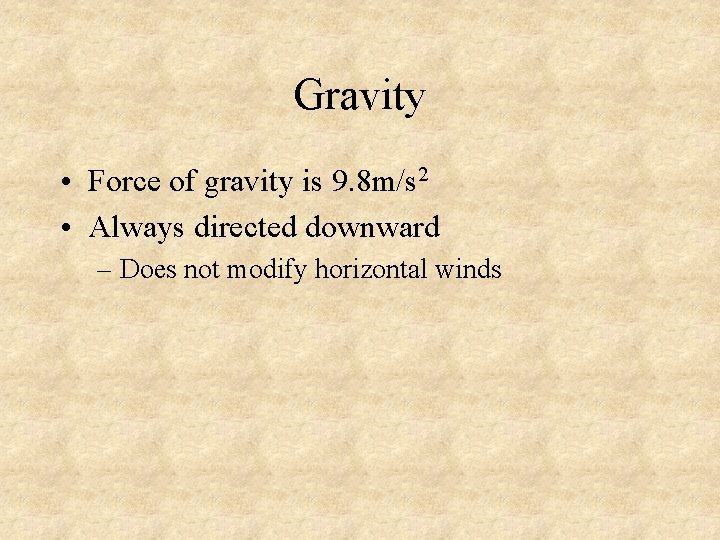
Gravity • Force of gravity is 9. 8 m/s 2 • Always directed downward – Does not modify horizontal winds
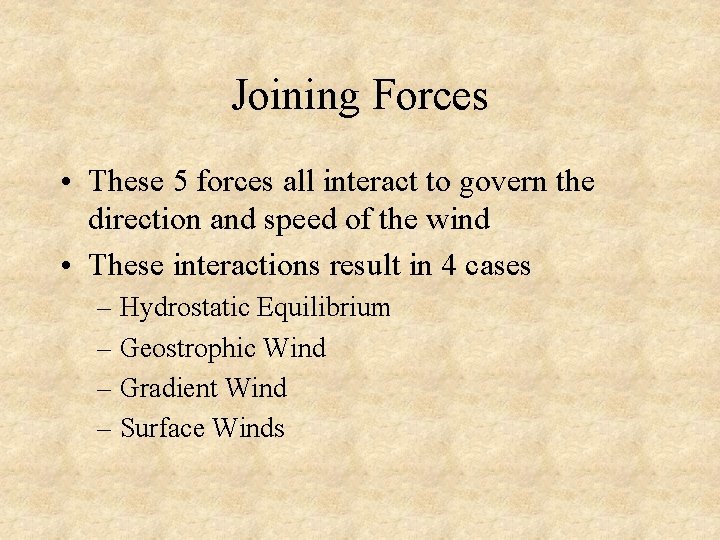
Joining Forces • These 5 forces all interact to govern the direction and speed of the wind • These interactions result in 4 cases – Hydrostatic Equilibrium – Geostrophic Wind – Gradient Wind – Surface Winds
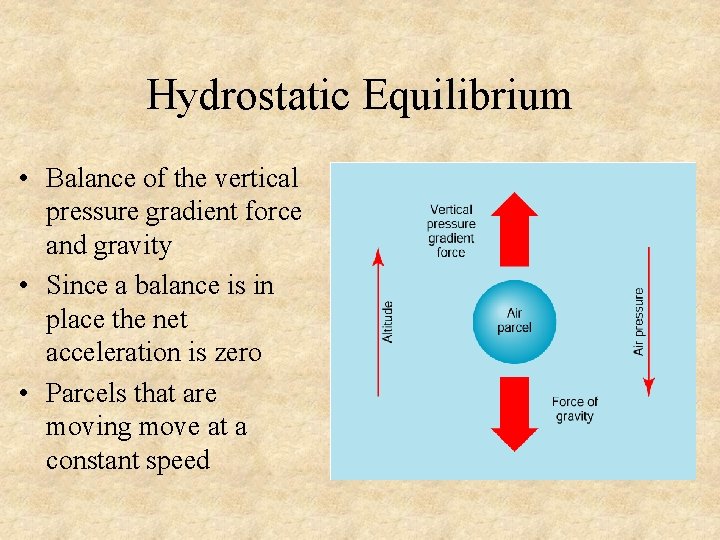
Hydrostatic Equilibrium • Balance of the vertical pressure gradient force and gravity • Since a balance is in place the net acceleration is zero • Parcels that are moving move at a constant speed
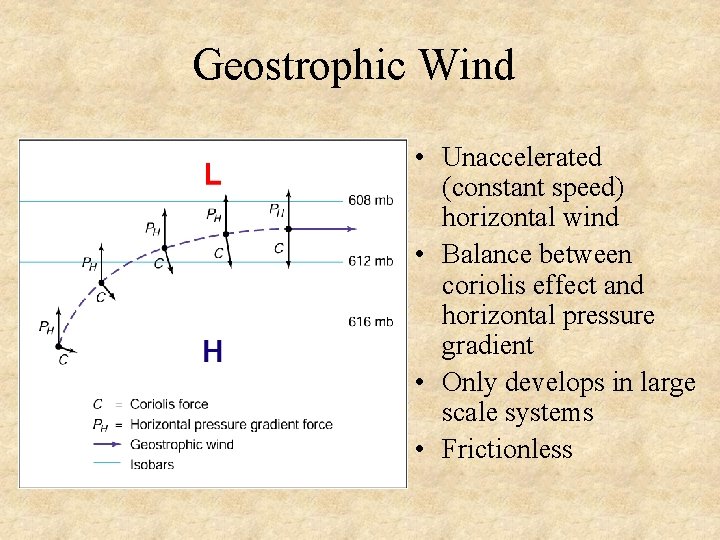
Geostrophic Wind • Unaccelerated (constant speed) horizontal wind • Balance between coriolis effect and horizontal pressure gradient • Only develops in large scale systems • Frictionless
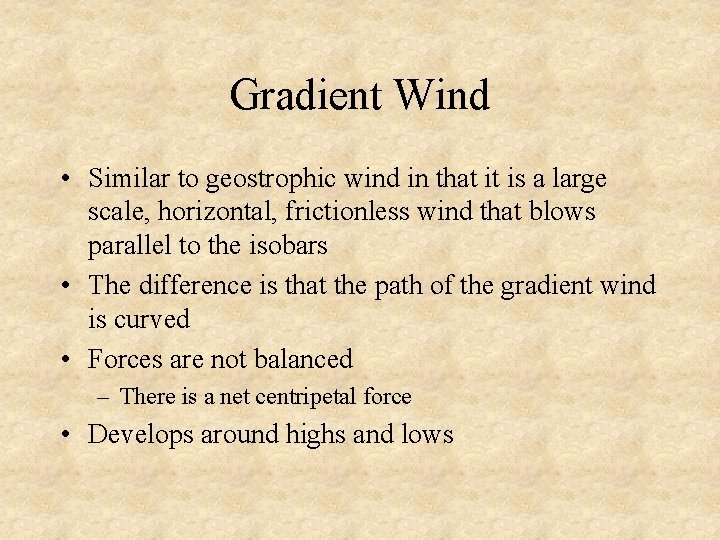
Gradient Wind • Similar to geostrophic wind in that it is a large scale, horizontal, frictionless wind that blows parallel to the isobars • The difference is that the path of the gradient wind is curved • Forces are not balanced – There is a net centripetal force • Develops around highs and lows
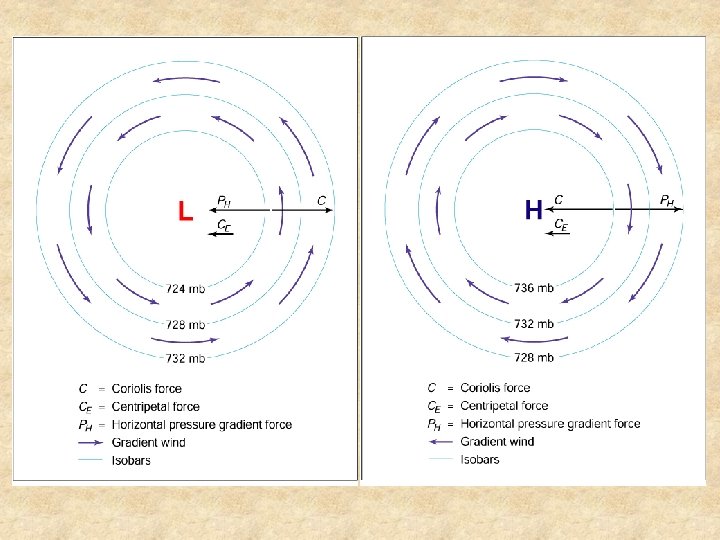
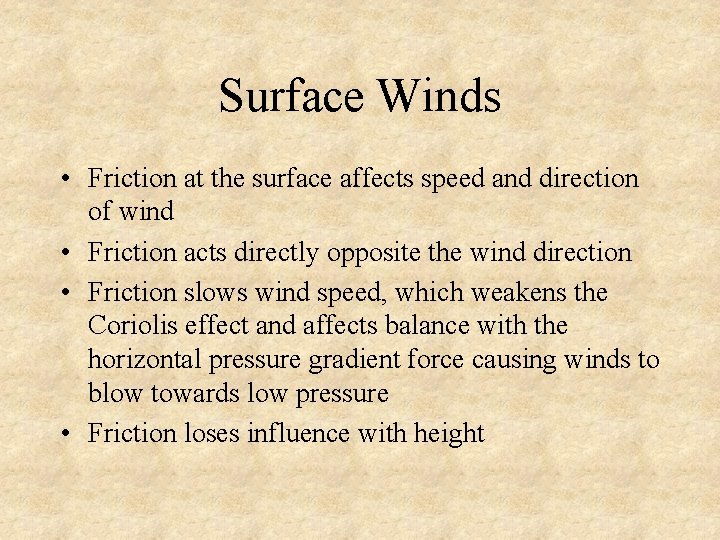
Surface Winds • Friction at the surface affects speed and direction of wind • Friction acts directly opposite the wind direction • Friction slows wind speed, which weakens the Coriolis effect and affects balance with the horizontal pressure gradient force causing winds to blow towards low pressure • Friction loses influence with height
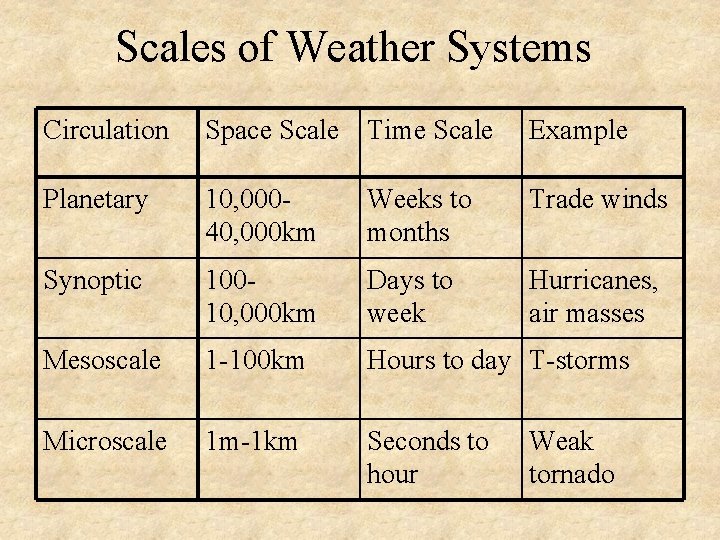
Scales of Weather Systems Circulation Space Scale Time Scale Example Planetary 10, 00040, 000 km Weeks to months Trade winds Synoptic 10010, 000 km Days to week Hurricanes, air masses Mesoscale 1 -100 km Hours to day T-storms Microscale 1 m-1 km Seconds to hour Weak tornado
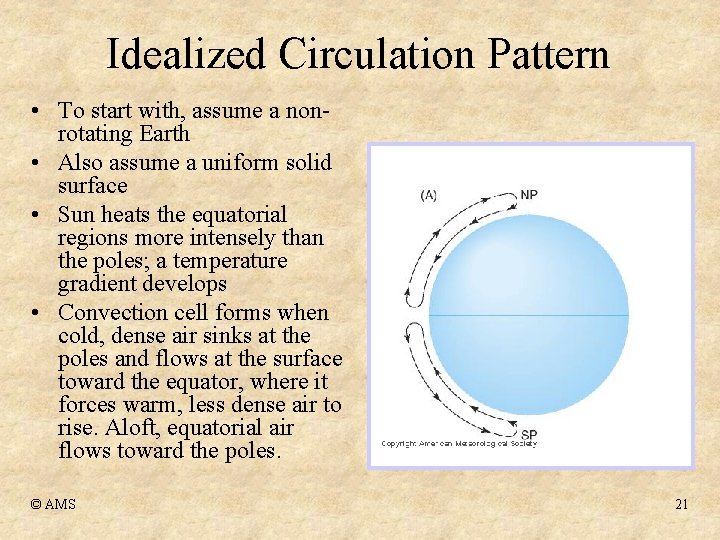
Idealized Circulation Pattern • To start with, assume a nonrotating Earth • Also assume a uniform solid surface • Sun heats the equatorial regions more intensely than the poles; a temperature gradient develops • Convection cell forms when cold, dense air sinks at the poles and flows at the surface toward the equator, where it forces warm, less dense air to rise. Aloft, equatorial air flows toward the poles. © AMS 21
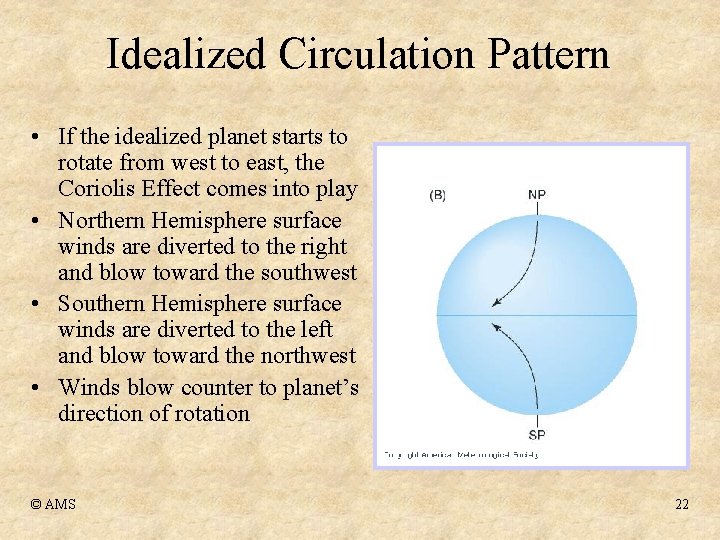
Idealized Circulation Pattern • If the idealized planet starts to rotate from west to east, the Coriolis Effect comes into play • Northern Hemisphere surface winds are diverted to the right and blow toward the southwest • Southern Hemisphere surface winds are diverted to the left and blow toward the northwest • Winds blow counter to planet’s direction of rotation © AMS 22
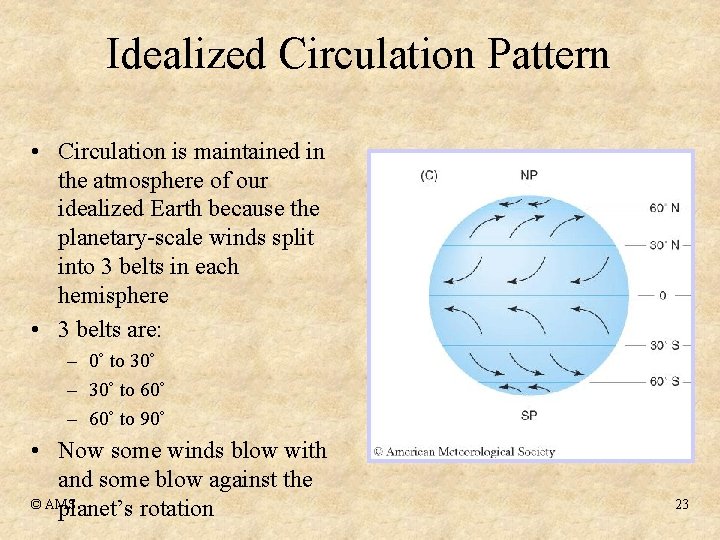
Idealized Circulation Pattern • Circulation is maintained in the atmosphere of our idealized Earth because the planetary-scale winds split into 3 belts in each hemisphere • 3 belts are: – 0° to 30° – 30° to 60° – 60° to 90° • Now some winds blow with and some blow against the © AMS planet’s rotation 23
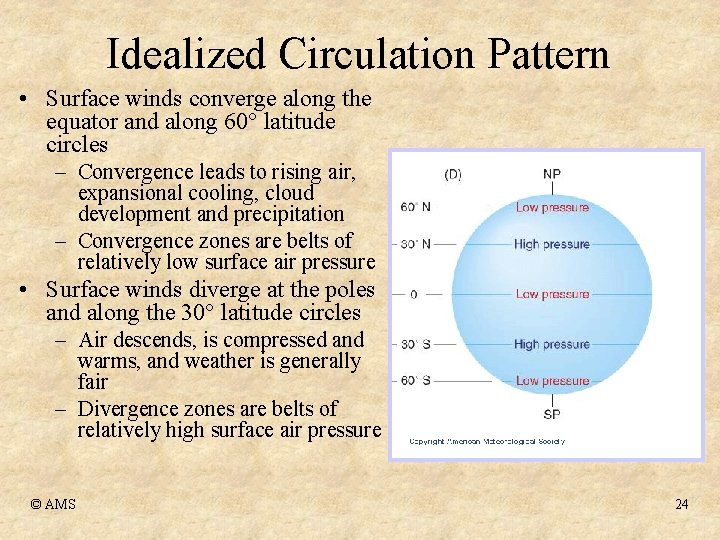
Idealized Circulation Pattern • Surface winds converge along the equator and along 60° latitude circles – Convergence leads to rising air, expansional cooling, cloud development and precipitation – Convergence zones are belts of relatively low surface air pressure • Surface winds diverge at the poles and along the 30° latitude circles – Air descends, is compressed and warms, and weather is generally fair – Divergence zones are belts of relatively high surface air pressure © AMS 24
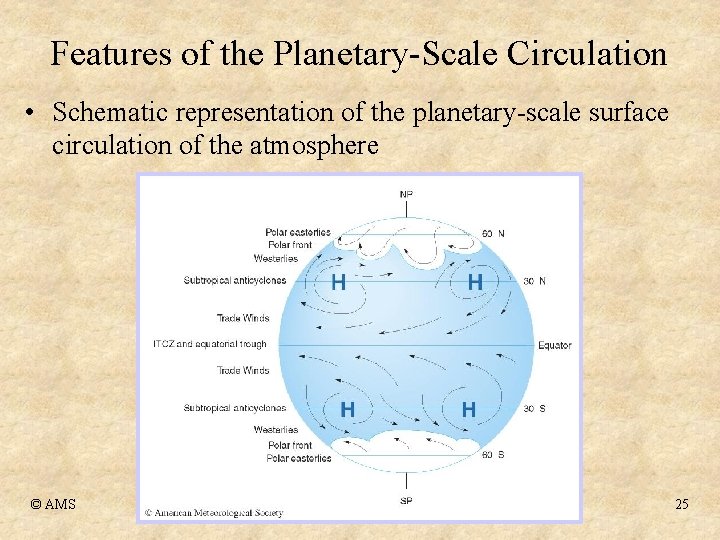
Features of the Planetary-Scale Circulation • Schematic representation of the planetary-scale surface circulation of the atmosphere © AMS 25
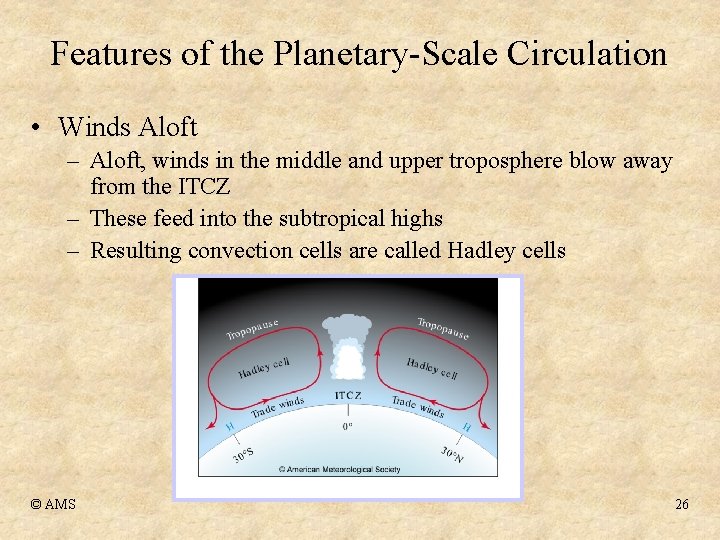
Features of the Planetary-Scale Circulation • Winds Aloft – Aloft, winds in the middle and upper troposphere blow away from the ITCZ – These feed into the subtropical highs – Resulting convection cells are called Hadley cells © AMS 26
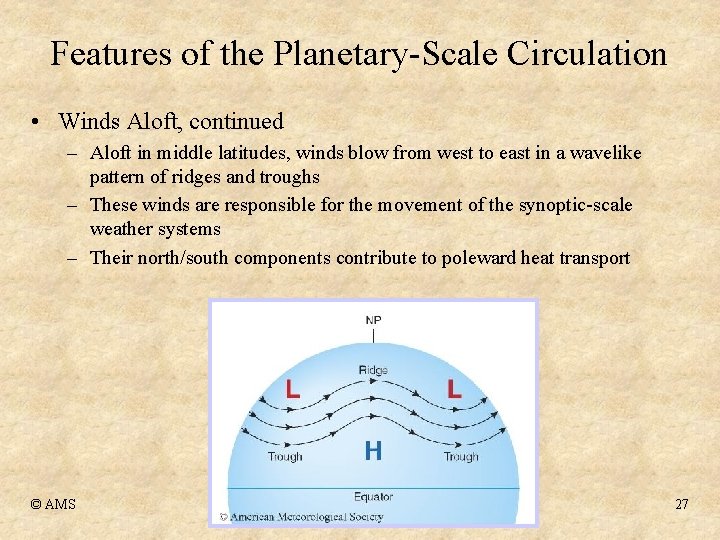
Features of the Planetary-Scale Circulation • Winds Aloft, continued – Aloft in middle latitudes, winds blow from west to east in a wavelike pattern of ridges and troughs – These winds are responsible for the movement of the synoptic-scale weather systems – Their north/south components contribute to poleward heat transport © AMS 27
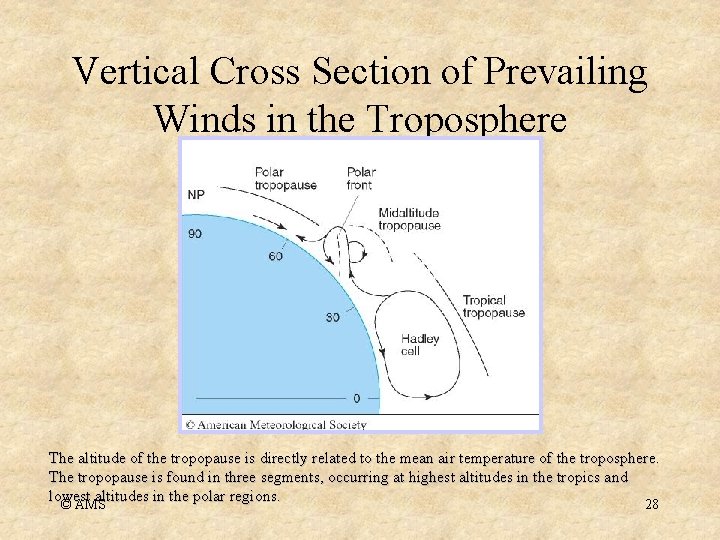
Vertical Cross Section of Prevailing Winds in the Troposphere The altitude of the tropopause is directly related to the mean air temperature of the troposphere. The tropopause is found in three segments, occurring at highest altitudes in the tropics and lowest altitudes in the polar regions. © AMS 28
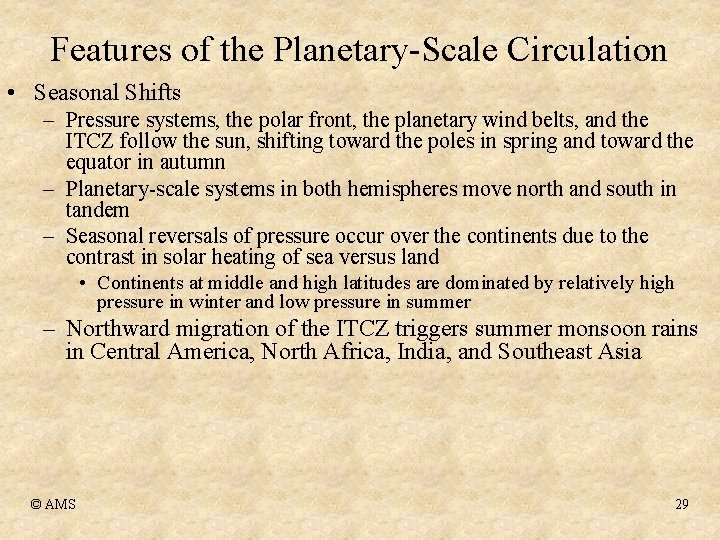
Features of the Planetary-Scale Circulation • Seasonal Shifts – Pressure systems, the polar front, the planetary wind belts, and the ITCZ follow the sun, shifting toward the poles in spring and toward the equator in autumn – Planetary-scale systems in both hemispheres move north and south in tandem – Seasonal reversals of pressure occur over the continents due to the contrast in solar heating of sea versus land • Continents at middle and high latitudes are dominated by relatively high pressure in winter and low pressure in summer – Northward migration of the ITCZ triggers summer monsoon rains in Central America, North Africa, India, and Southeast Asia © AMS 29
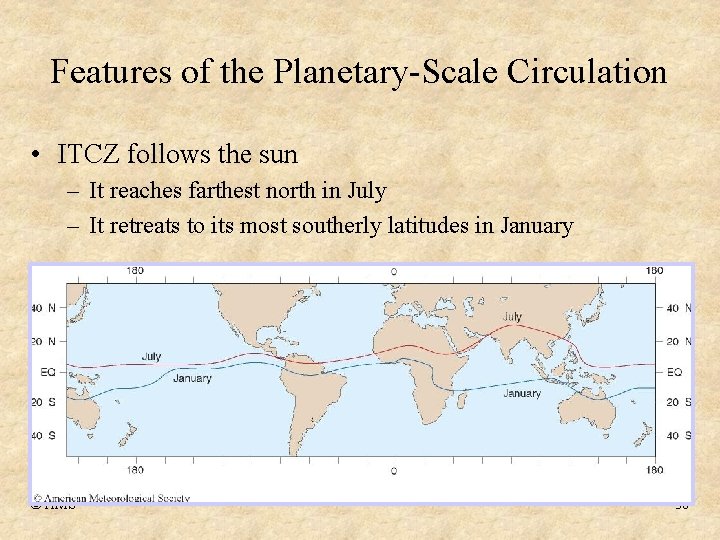
Features of the Planetary-Scale Circulation • ITCZ follows the sun – It reaches farthest north in July – It retreats to its most southerly latitudes in January © AMS 30
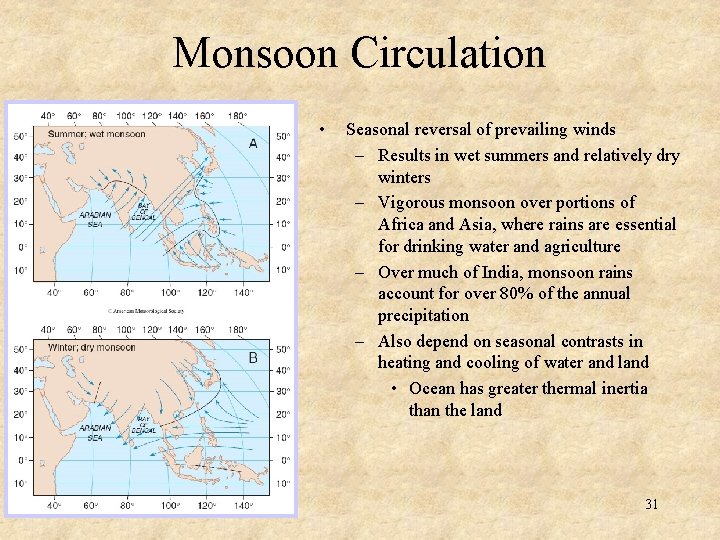
Monsoon Circulation • © AMS Seasonal reversal of prevailing winds – Results in wet summers and relatively dry winters – Vigorous monsoon over portions of Africa and Asia, where rains are essential for drinking water and agriculture – Over much of India, monsoon rains account for over 80% of the annual precipitation – Also depend on seasonal contrasts in heating and cooling of water and land • Ocean has greater thermal inertia than the land 31
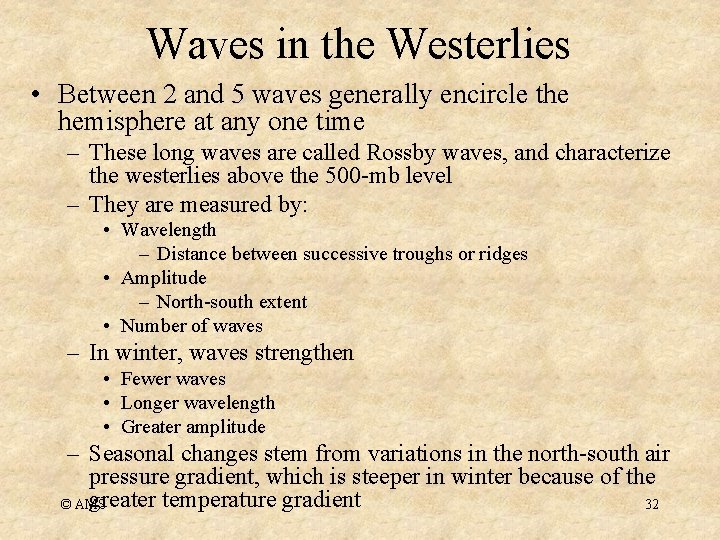
Waves in the Westerlies • Between 2 and 5 waves generally encircle the hemisphere at any one time – These long waves are called Rossby waves, and characterize the westerlies above the 500 -mb level – They are measured by: • Wavelength – Distance between successive troughs or ridges • Amplitude – North-south extent • Number of waves – In winter, waves strengthen • Fewer waves • Longer wavelength • Greater amplitude – Seasonal changes stem from variations in the north-south air pressure gradient, which is steeper in winter because of the greater temperature gradient © AMS 32
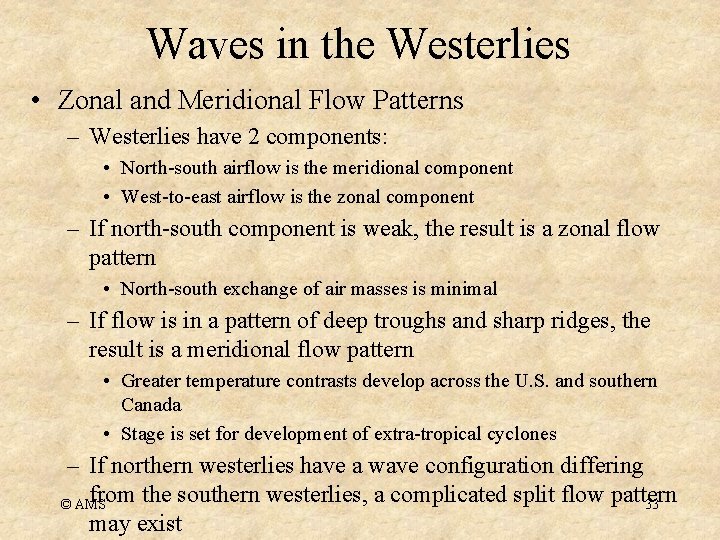
Waves in the Westerlies • Zonal and Meridional Flow Patterns – Westerlies have 2 components: • North-south airflow is the meridional component • West-to-east airflow is the zonal component – If north-south component is weak, the result is a zonal flow pattern • North-south exchange of air masses is minimal – If flow is in a pattern of deep troughs and sharp ridges, the result is a meridional flow pattern • Greater temperature contrasts develop across the U. S. and southern Canada • Stage is set for development of extra-tropical cyclones – If northern westerlies have a wave configuration differing from the southern westerlies, a complicated split flow pattern © AMS 33 may exist
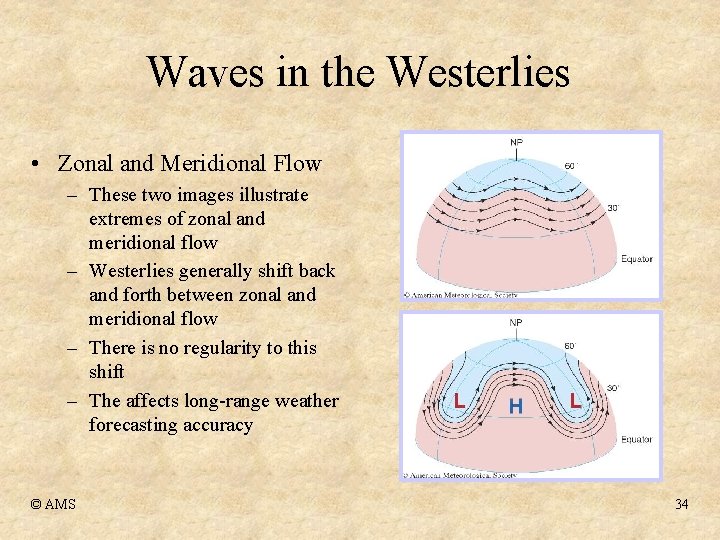
Waves in the Westerlies • Zonal and Meridional Flow – These two images illustrate extremes of zonal and meridional flow – Westerlies generally shift back and forth between zonal and meridional flow – There is no regularity to this shift – The affects long-range weather forecasting accuracy © AMS 34
How does wind affect weather and climate
How do prevailing winds affect climate
Weather symbols chart
Whether the weather is cold tongue twister
Poem on seasons
Its stormy
Whether the weather is fine
Heavy weather by weather report
Capital weather gang weather wall
Station model weather symbols
Amca 500-l
Kurshalter
Hát kết hợp bộ gõ cơ thể
Slidetodoc
Bổ thể
Tỉ lệ cơ thể trẻ em
Voi kéo gỗ như thế nào
Glasgow thang điểm
Hát lên người ơi alleluia
Các môn thể thao bắt đầu bằng tiếng bóng
Thế nào là hệ số cao nhất
Các châu lục và đại dương trên thế giới
Công của trọng lực
Trời xanh đây là của chúng ta thể thơ
Mật thư anh em như thể tay chân
Phép trừ bù
Phản ứng thế ankan
Các châu lục và đại dương trên thế giới
Thể thơ truyền thống
Quá trình desamine hóa có thể tạo ra
Một số thể thơ truyền thống
Cái miệng nó xinh thế chỉ nói điều hay thôi
Vẽ hình chiếu vuông góc của vật thể sau
Nguyên nhân của sự mỏi cơ sinh 8
đặc điểm cơ thể của người tối cổ