Ch 14 Translation Messenger RNA Transfer RNA Attachment
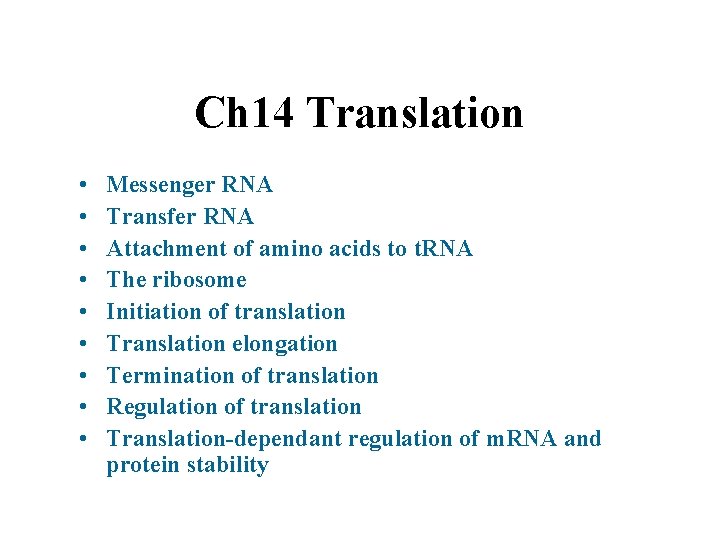
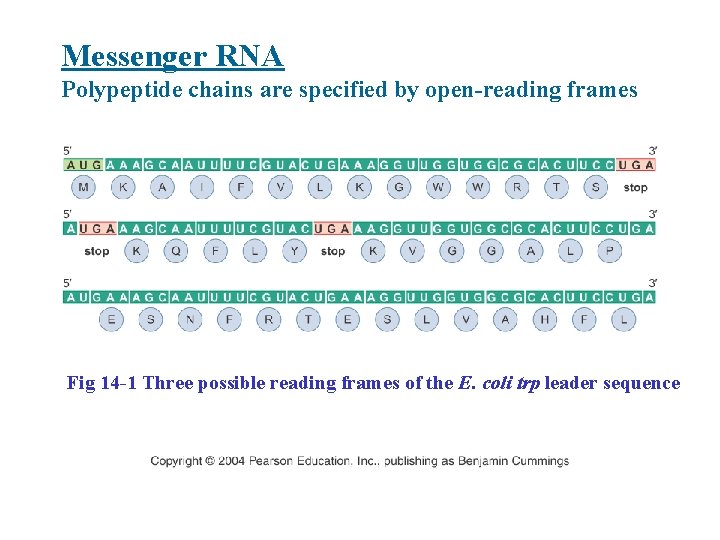
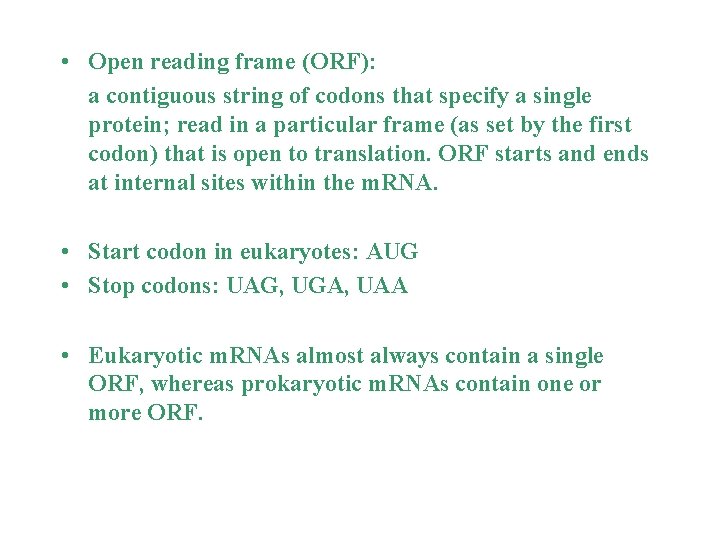
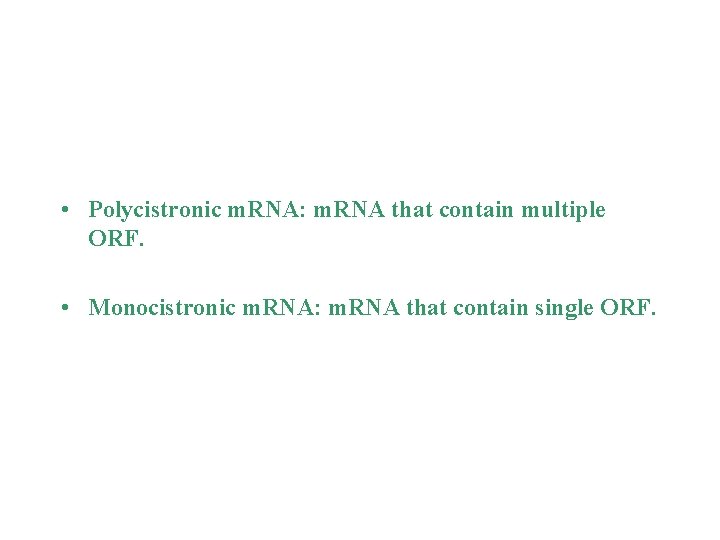
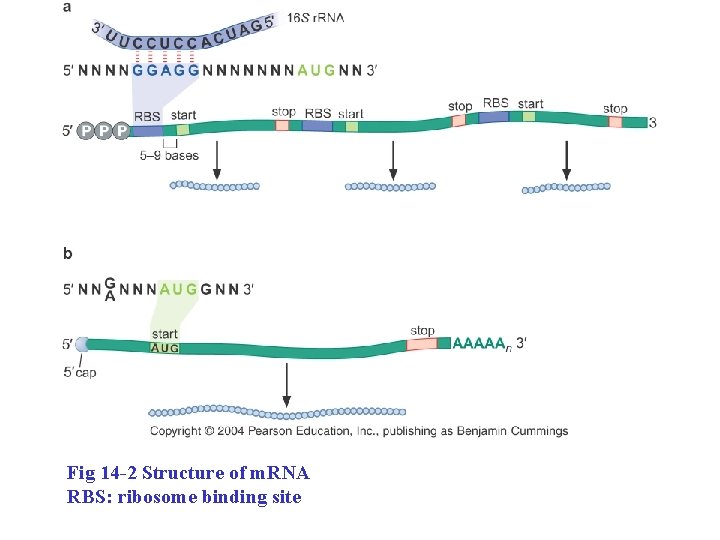
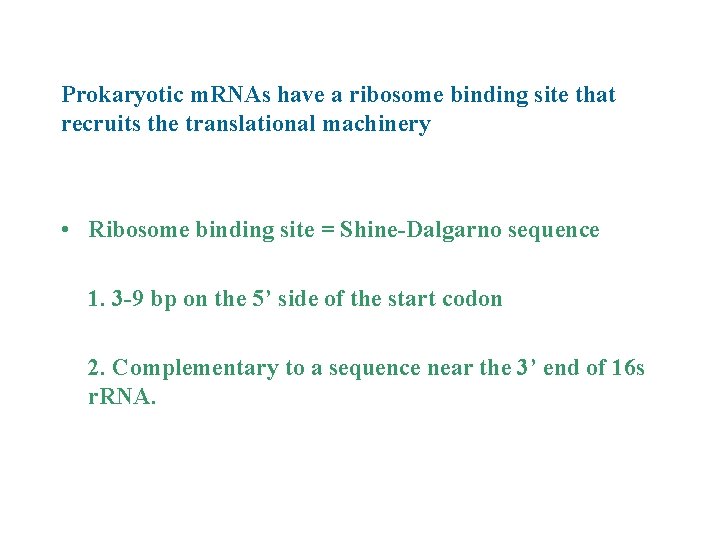
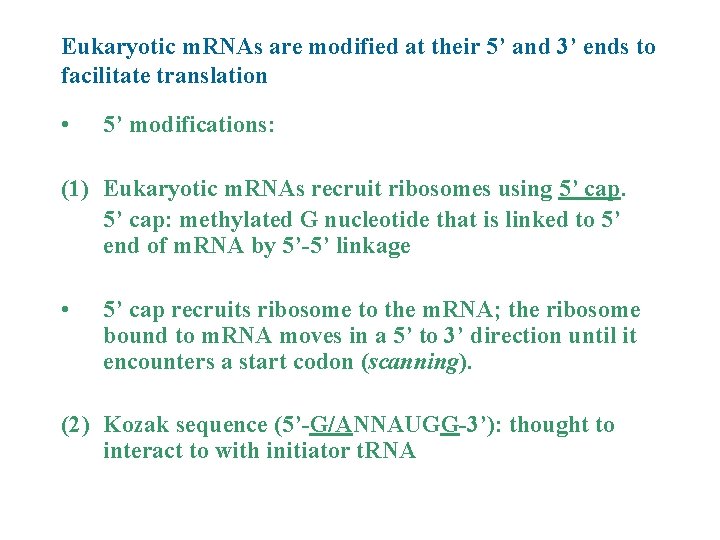
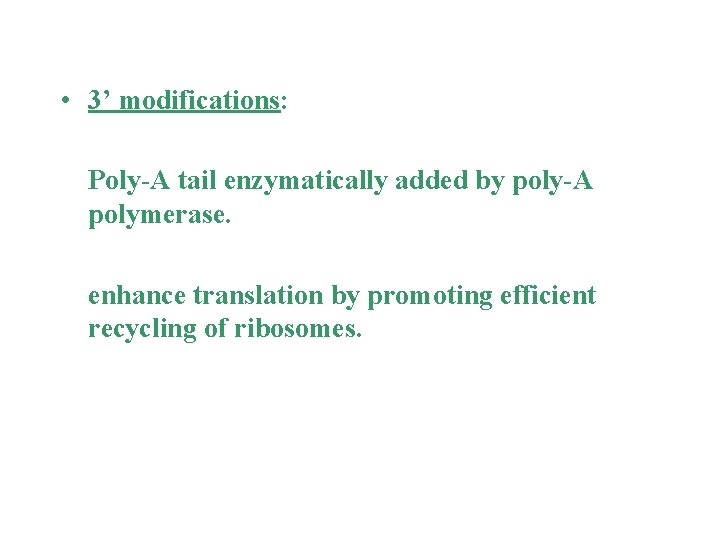
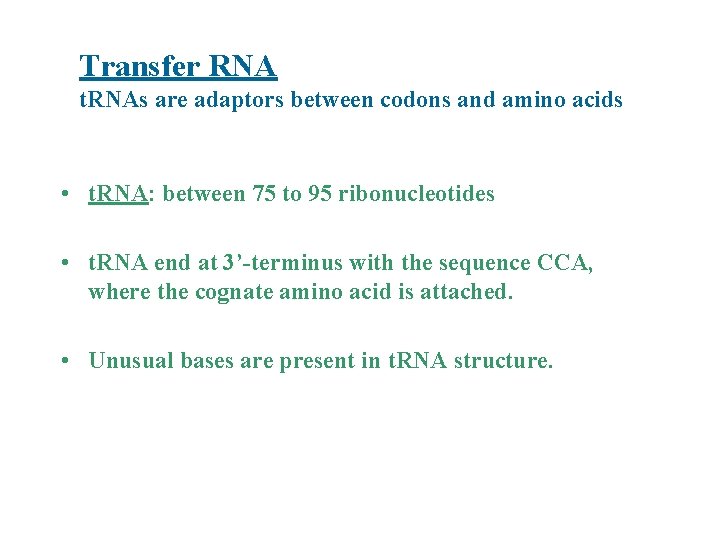
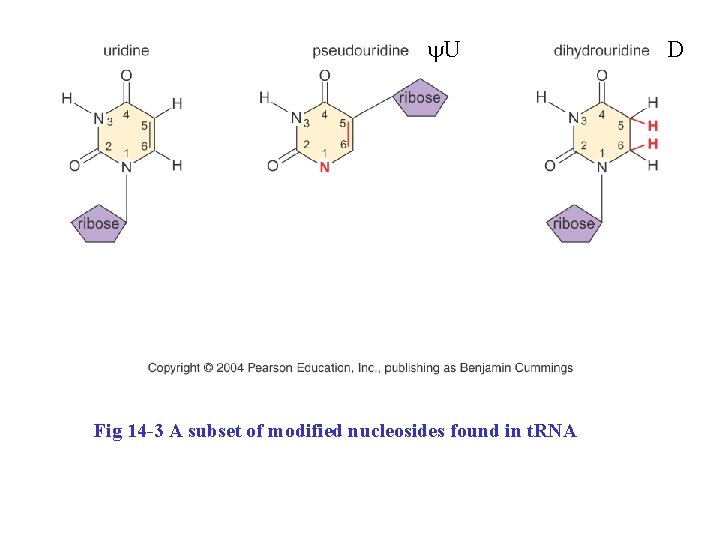
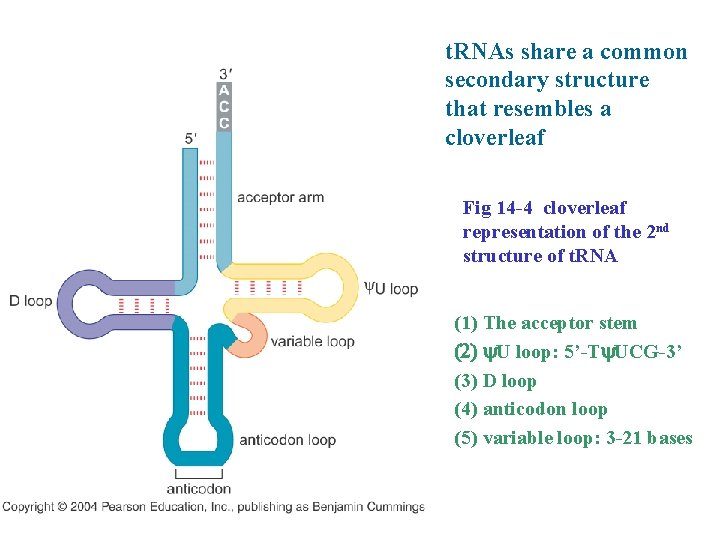
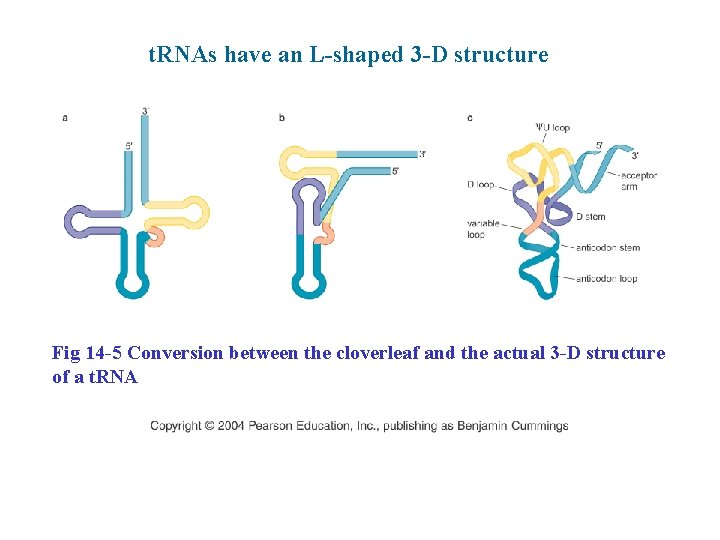
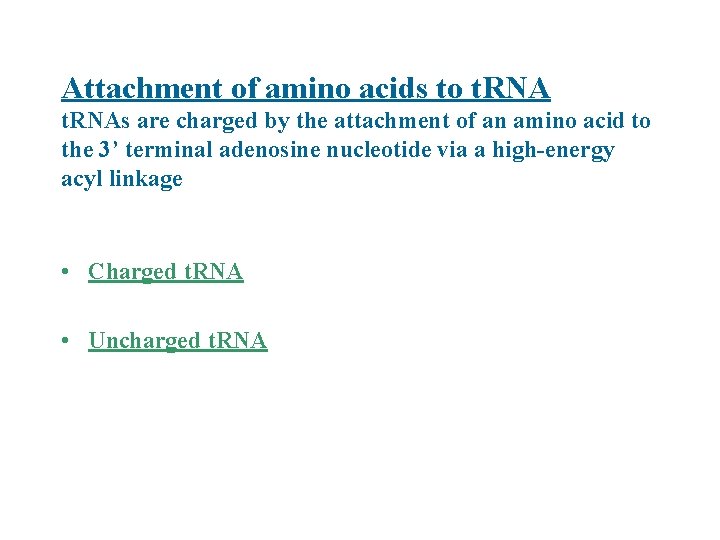
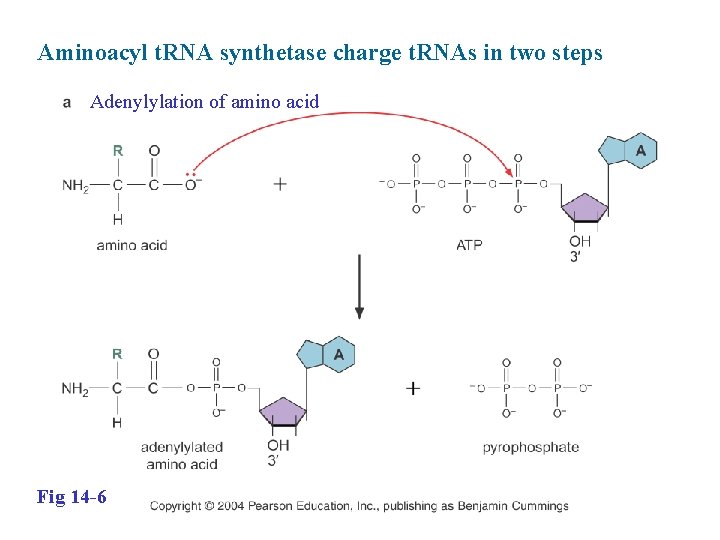
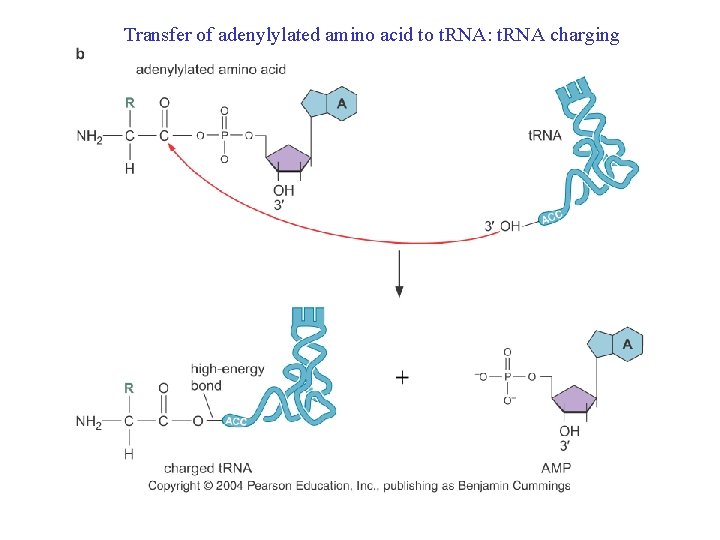
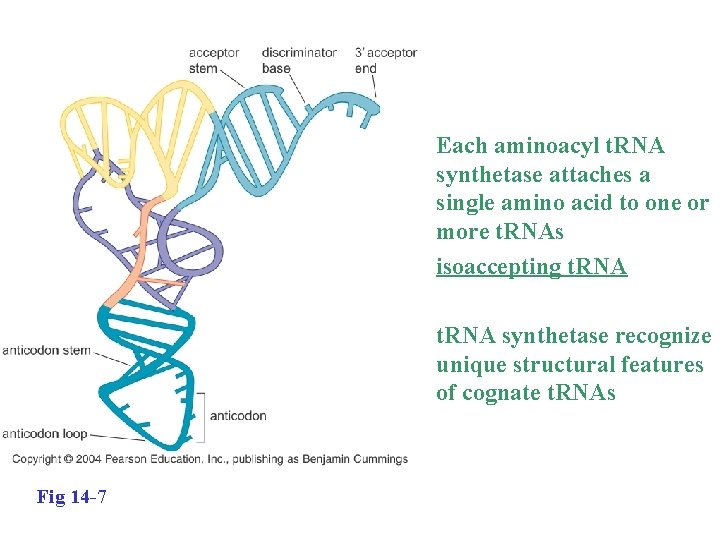
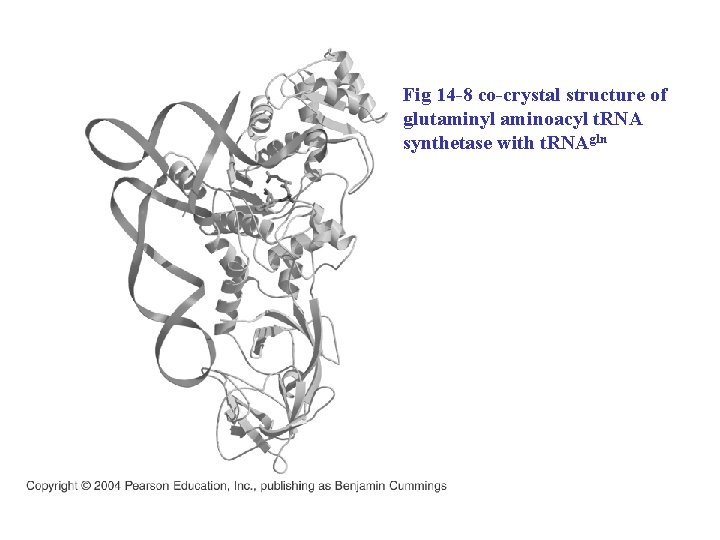
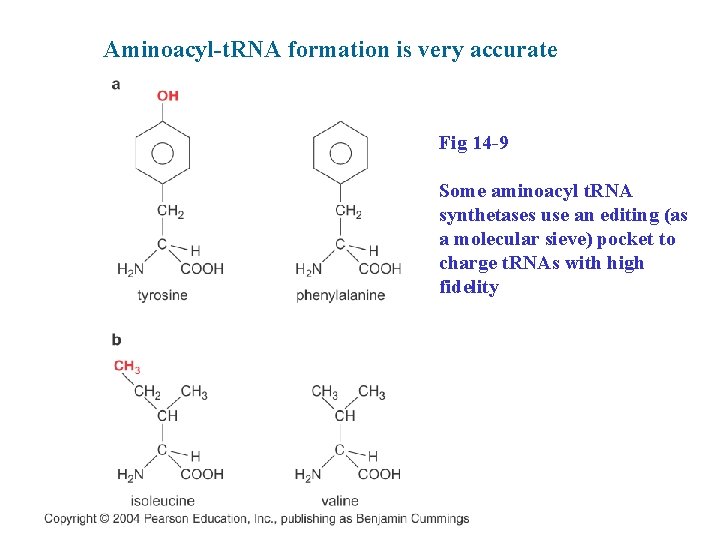
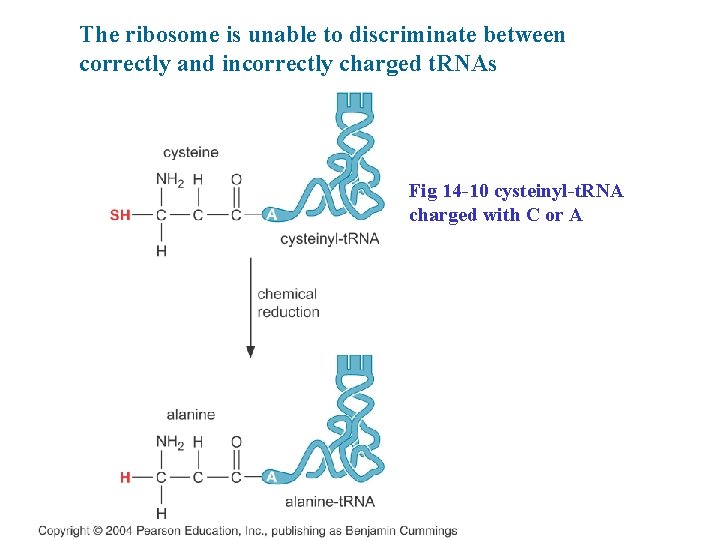
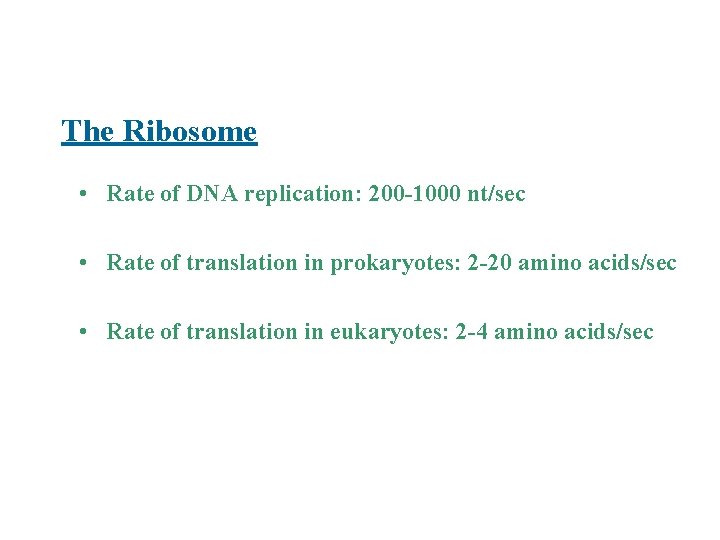
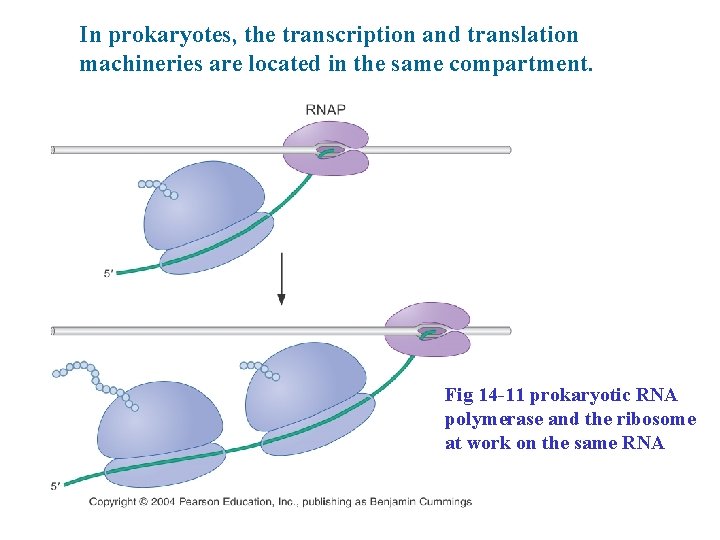
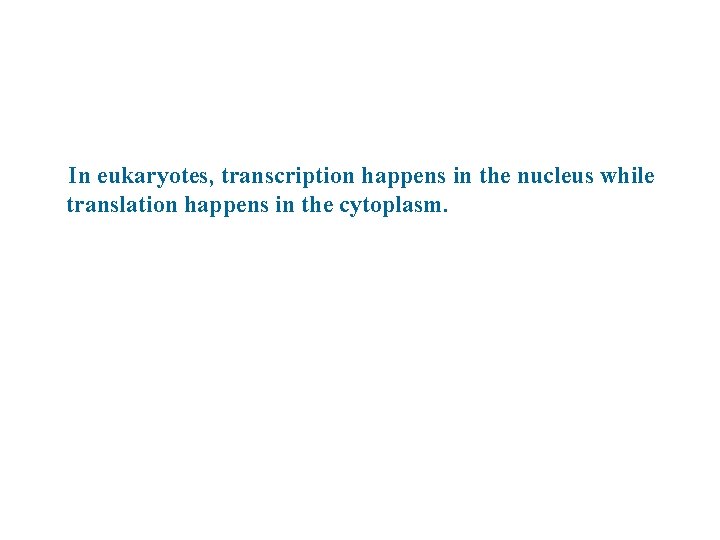
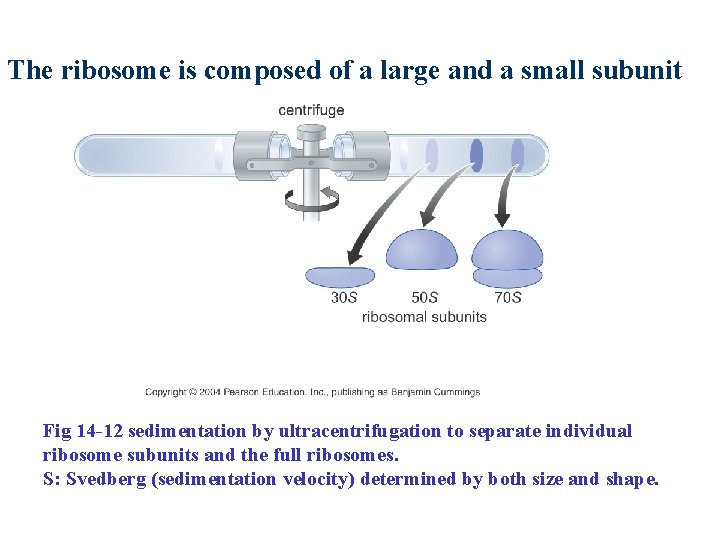
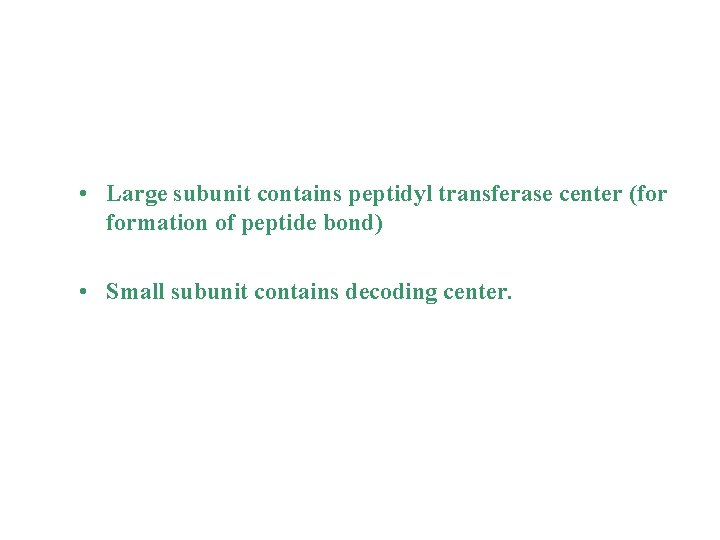
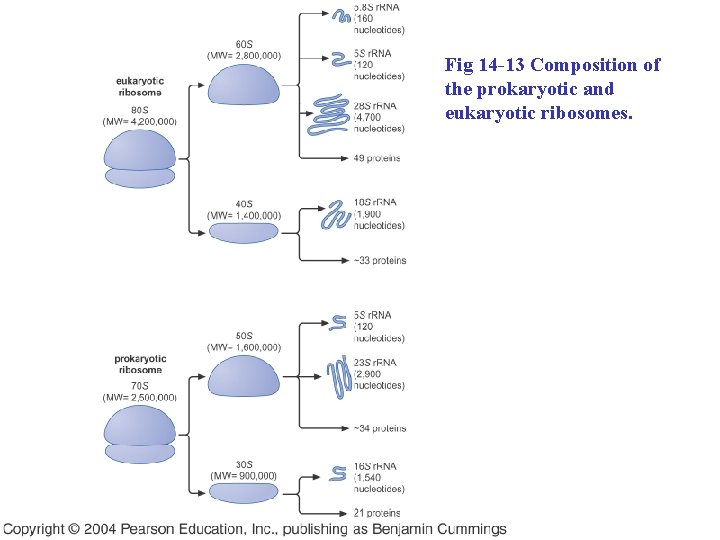
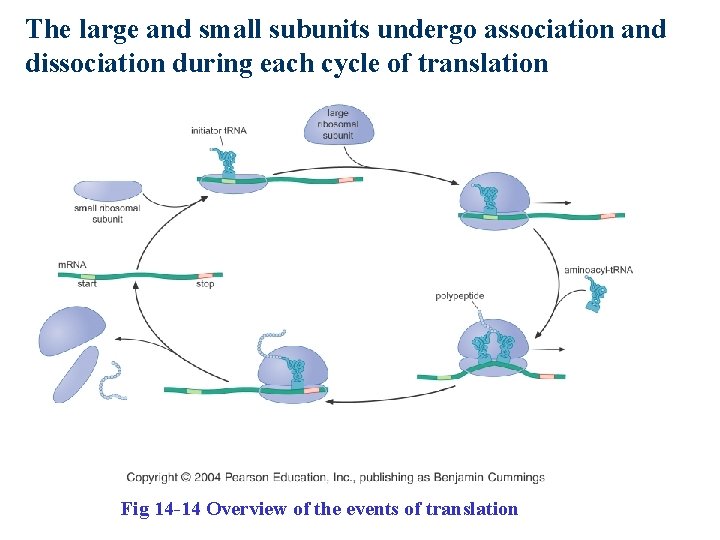
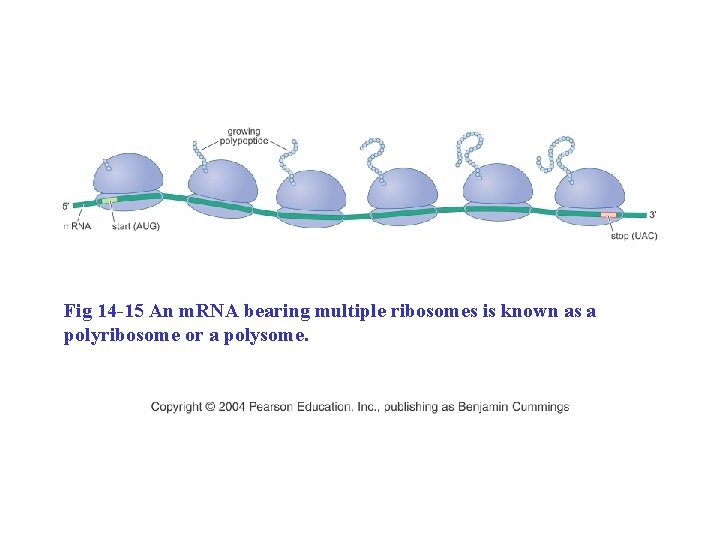
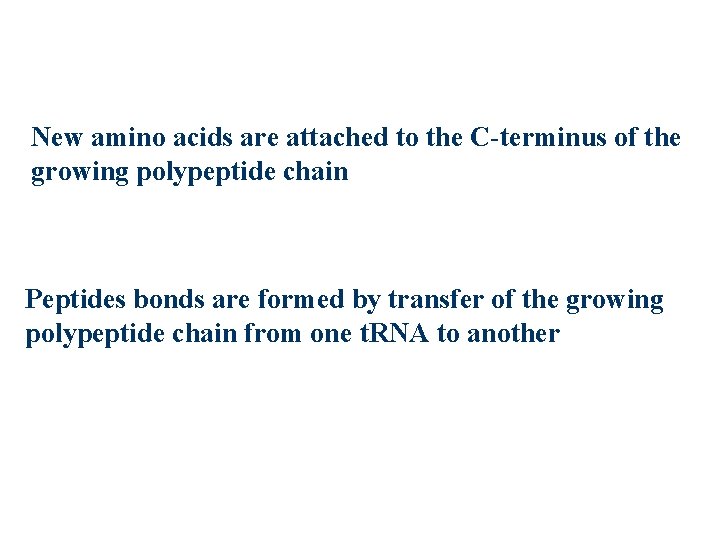
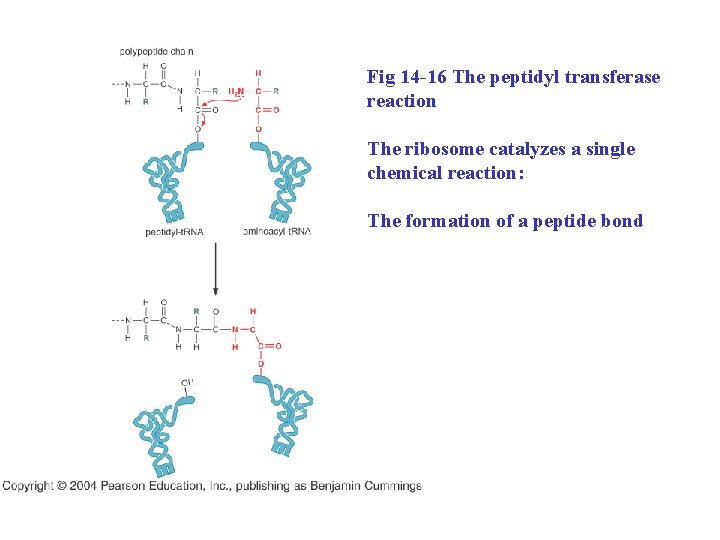
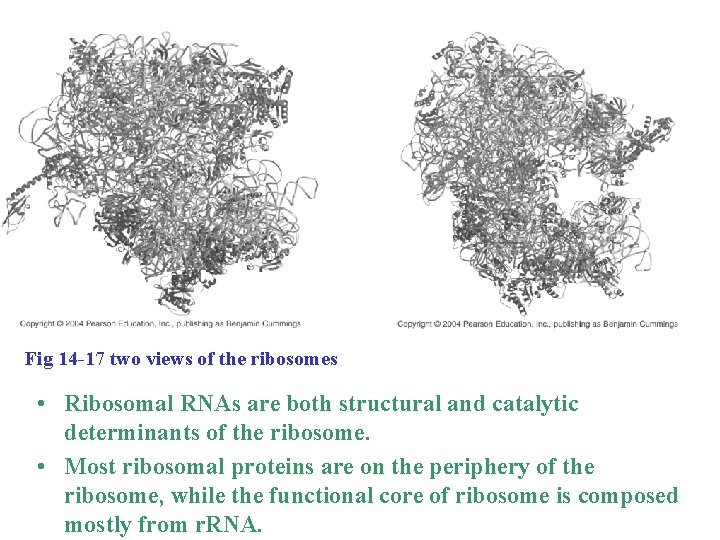
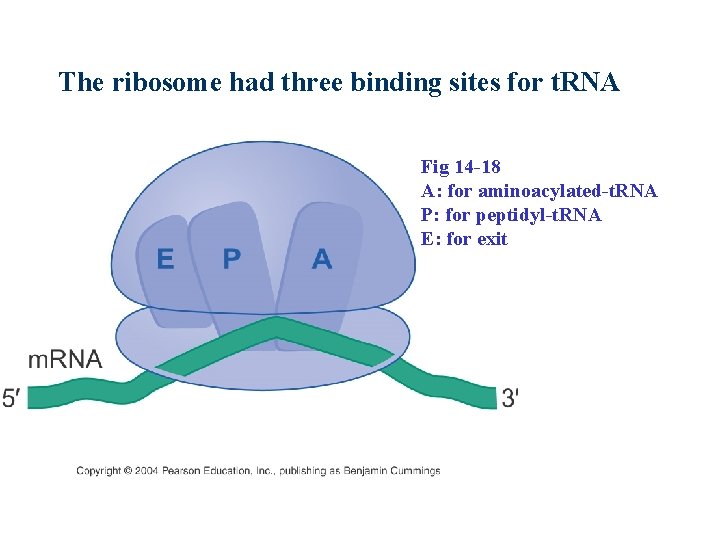
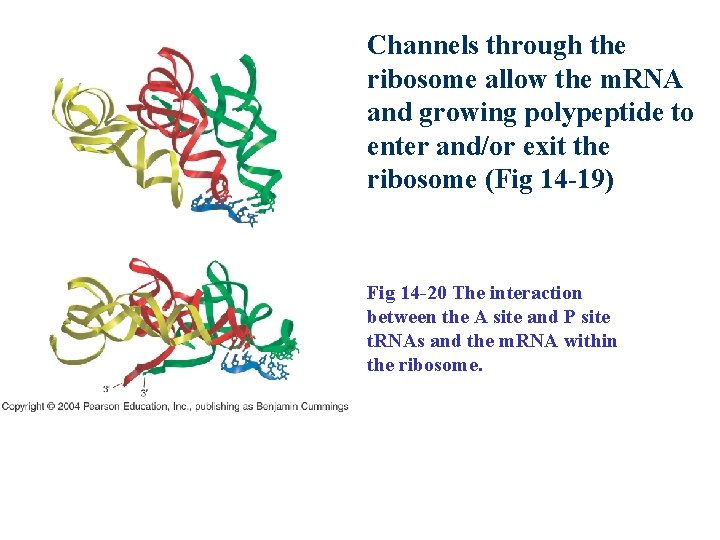
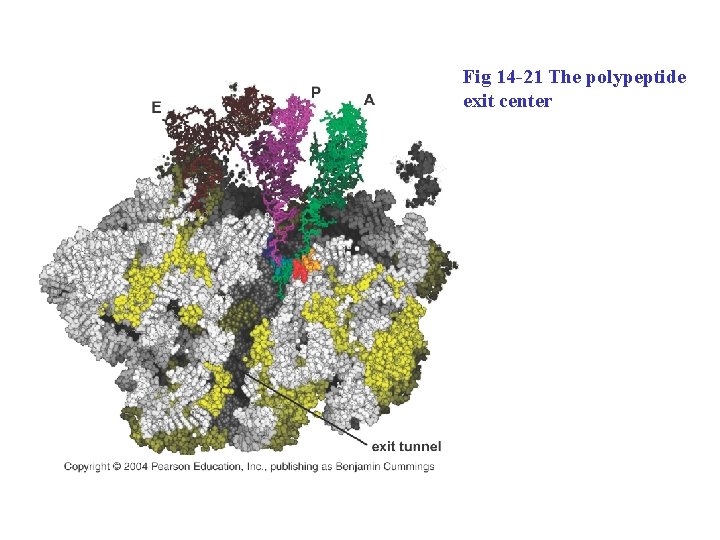
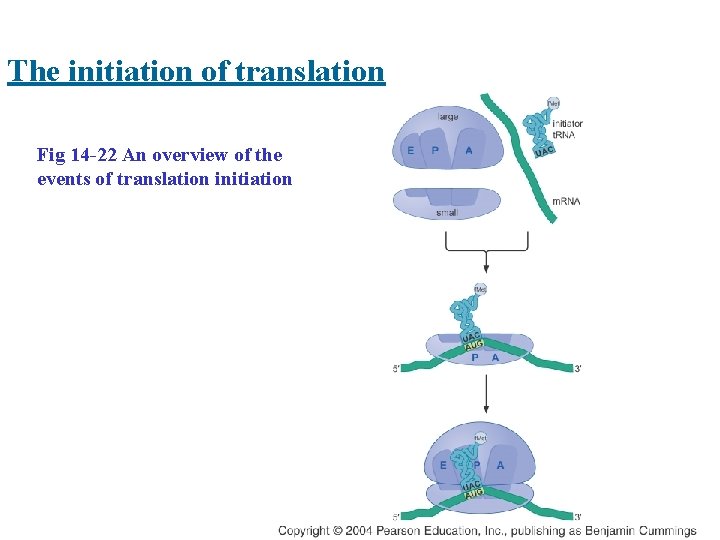
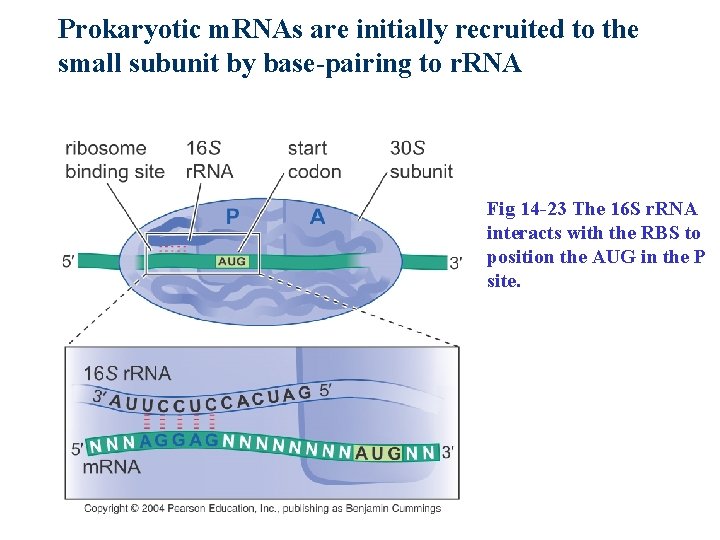
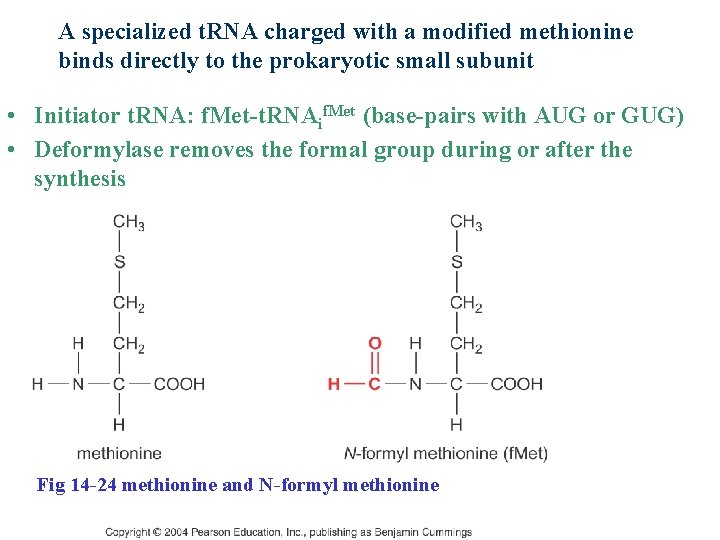
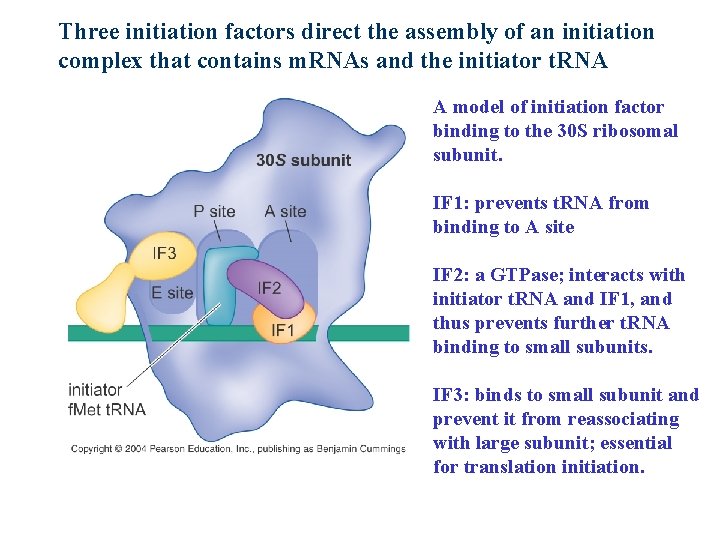
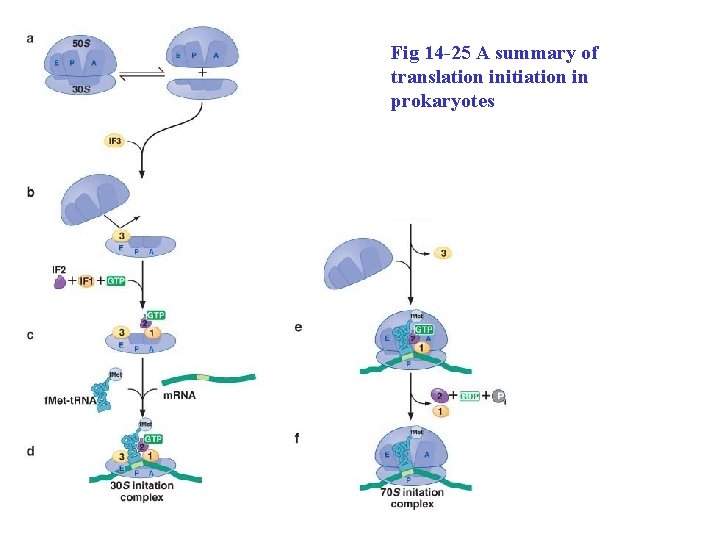
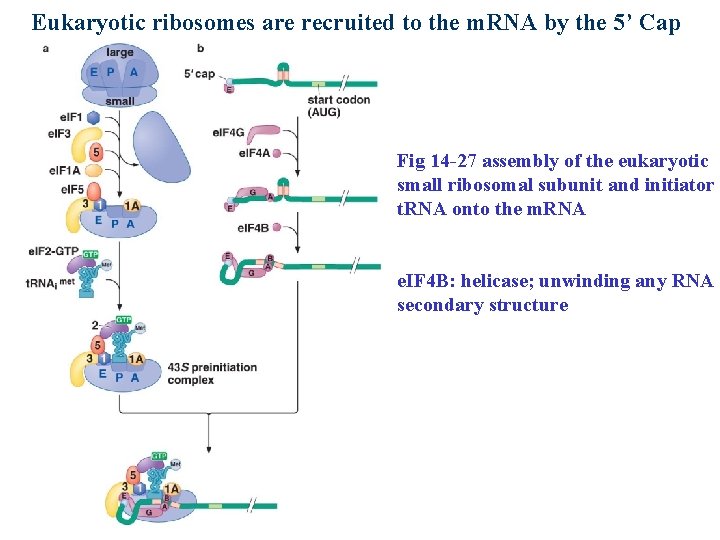
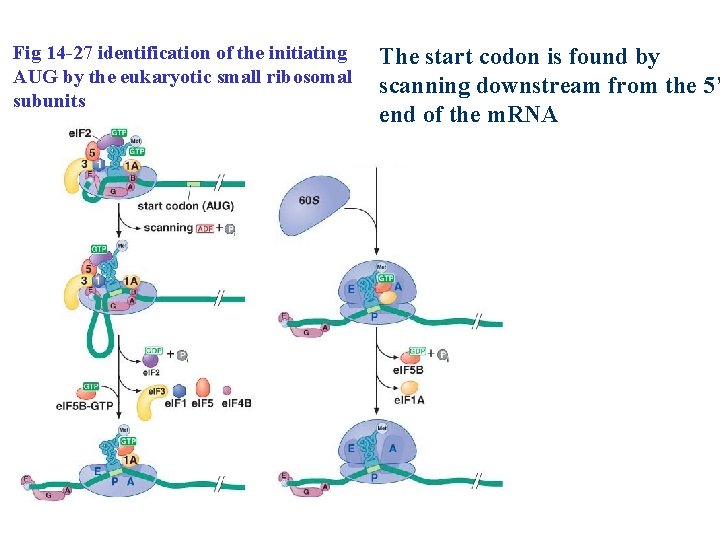
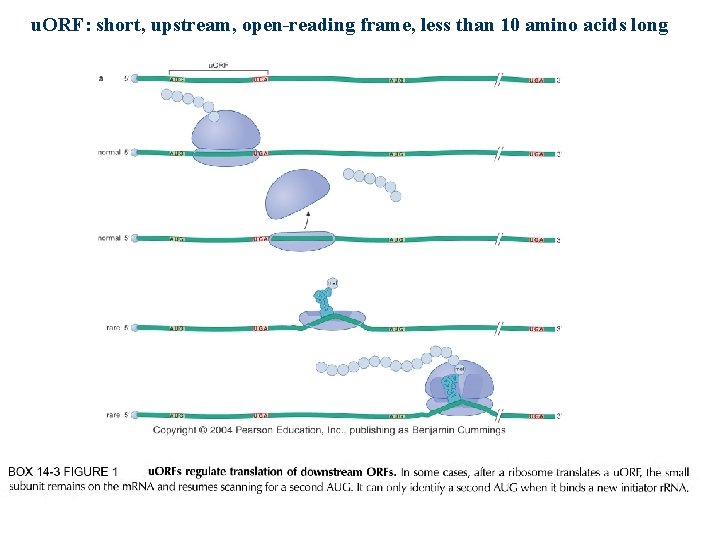
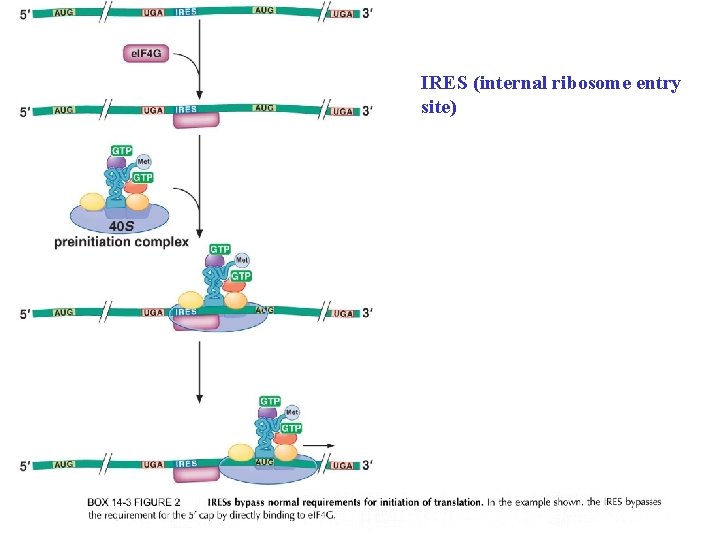
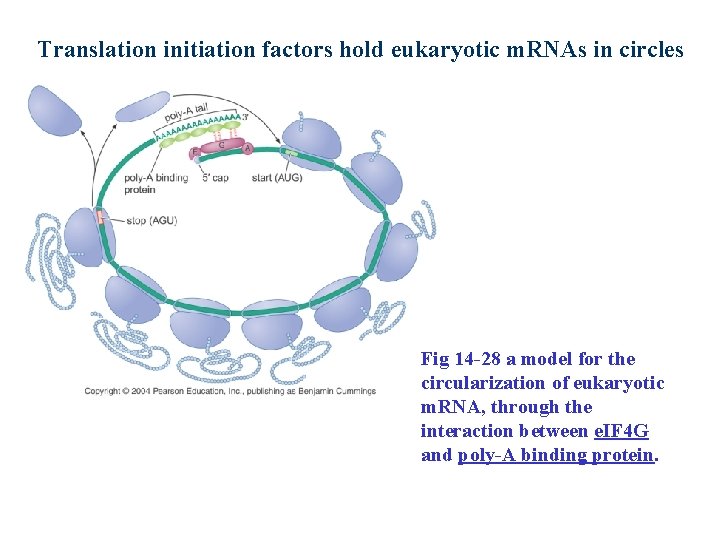
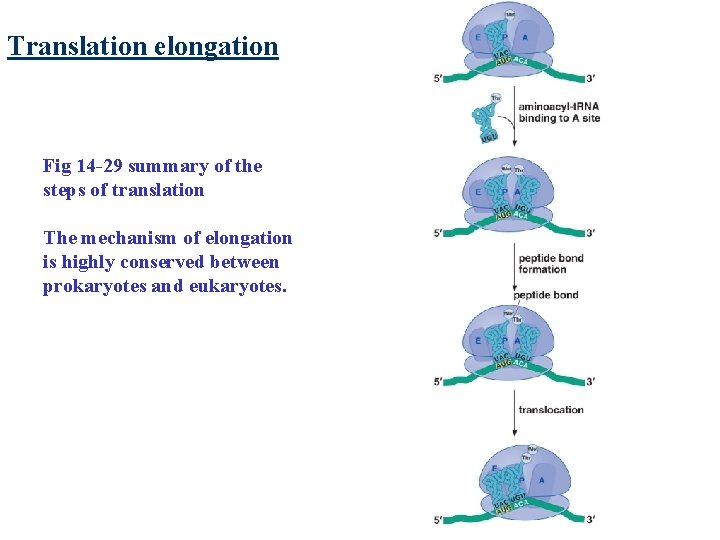
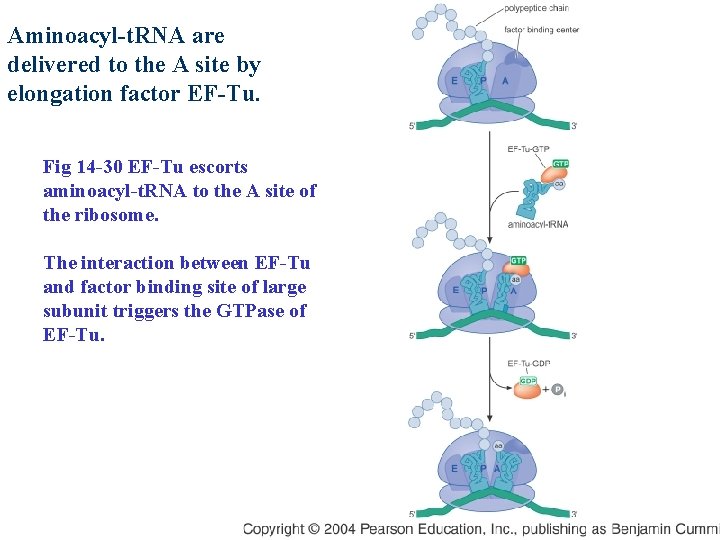
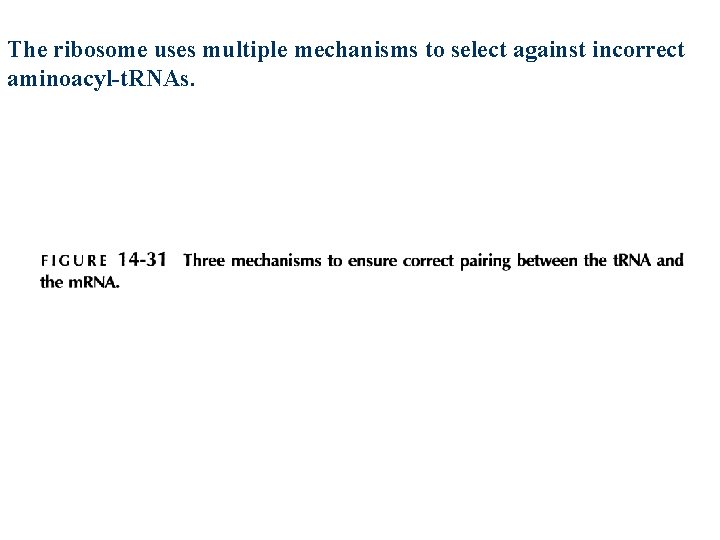
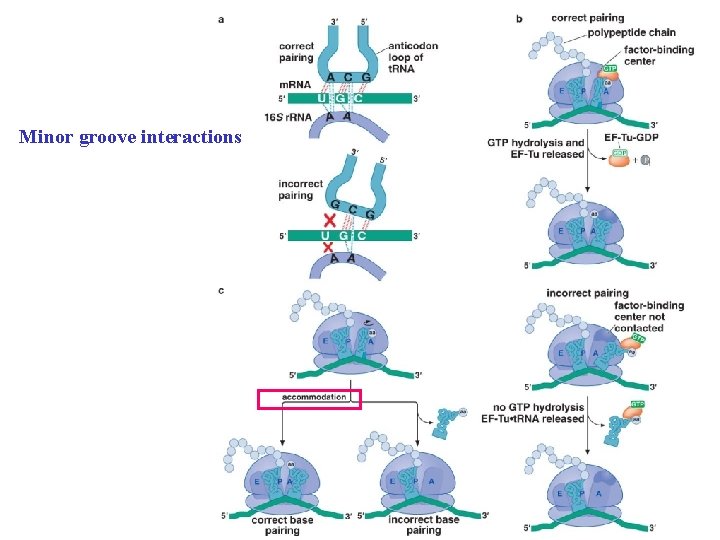
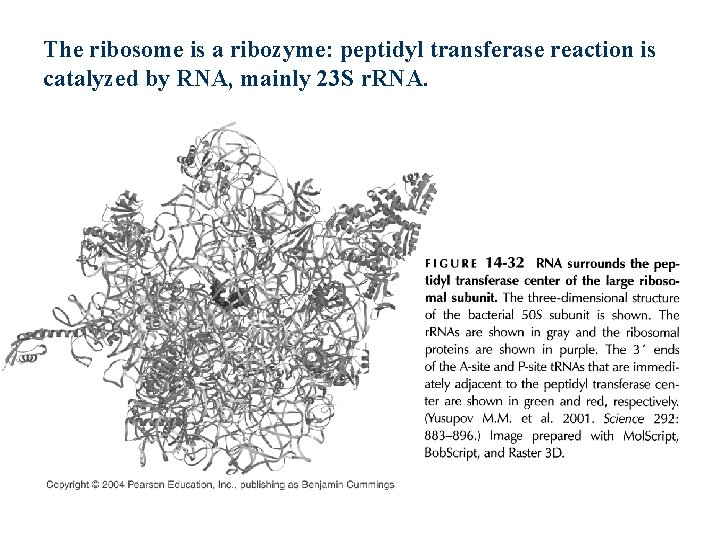
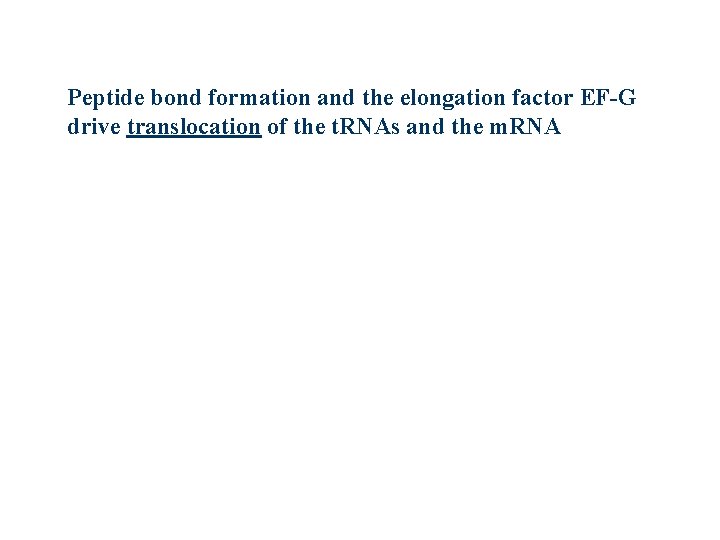
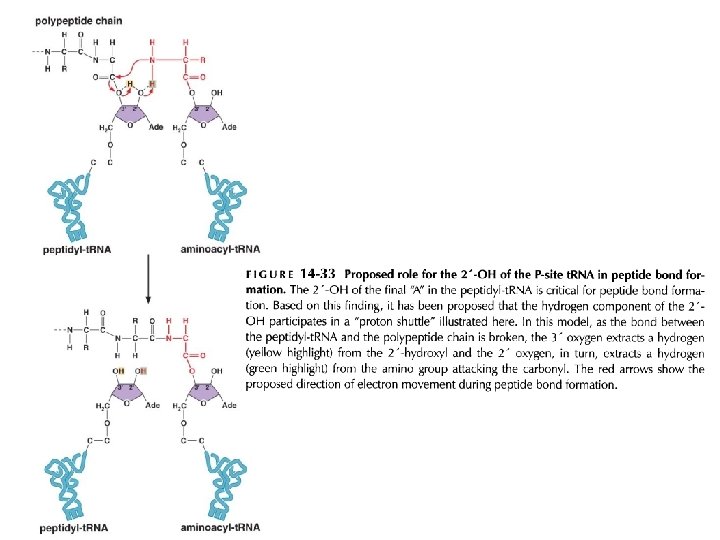
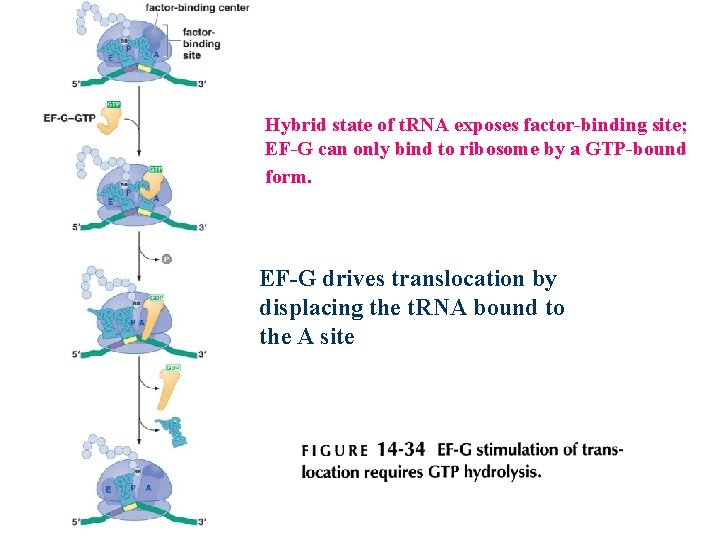
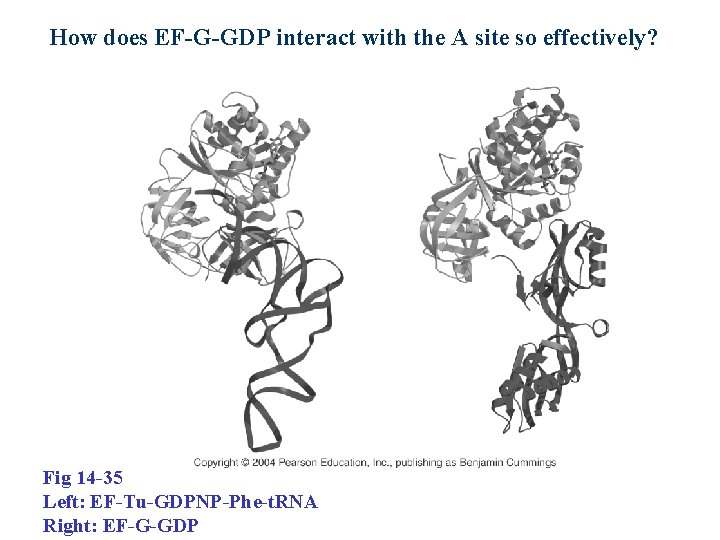
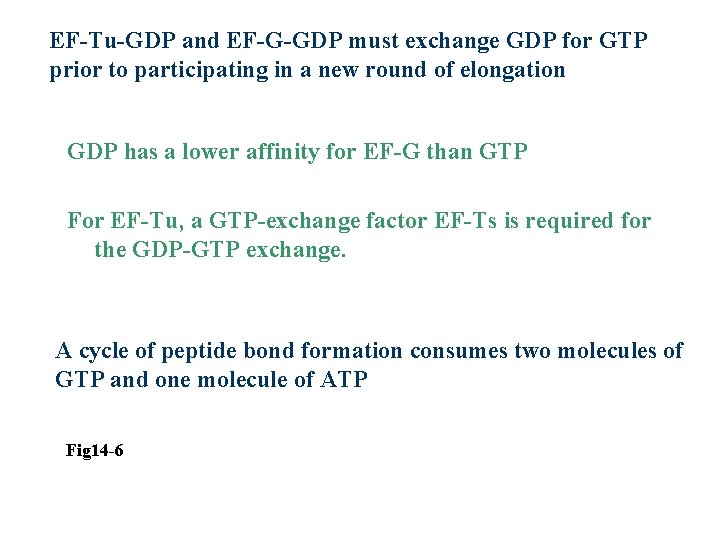
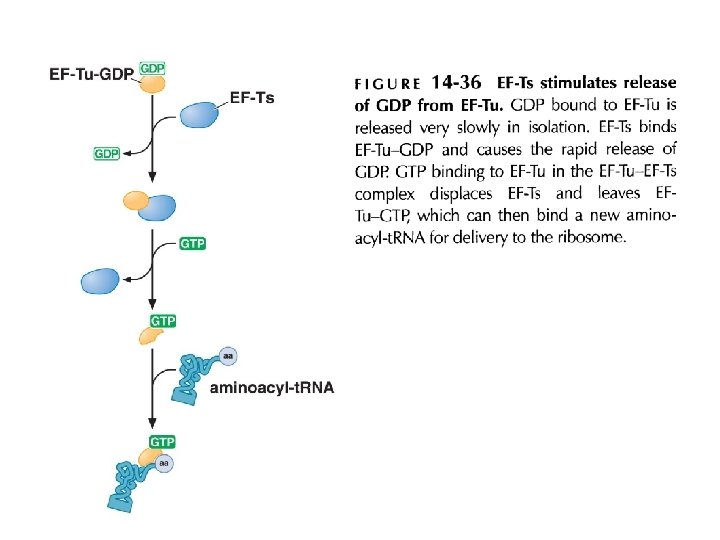
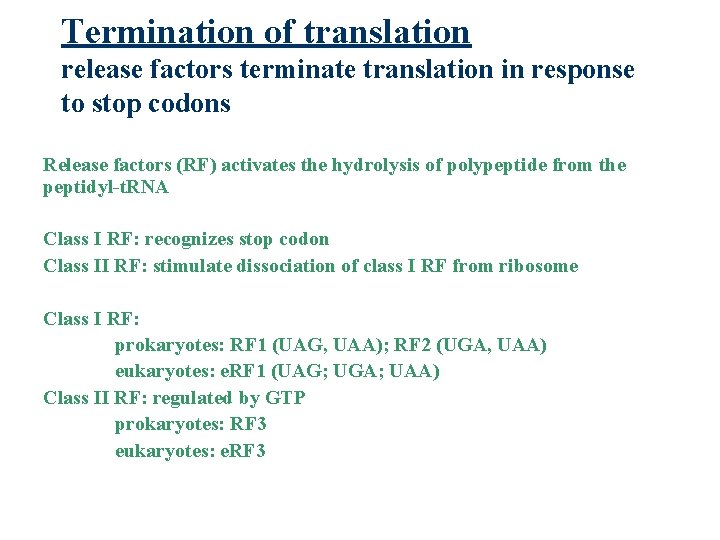
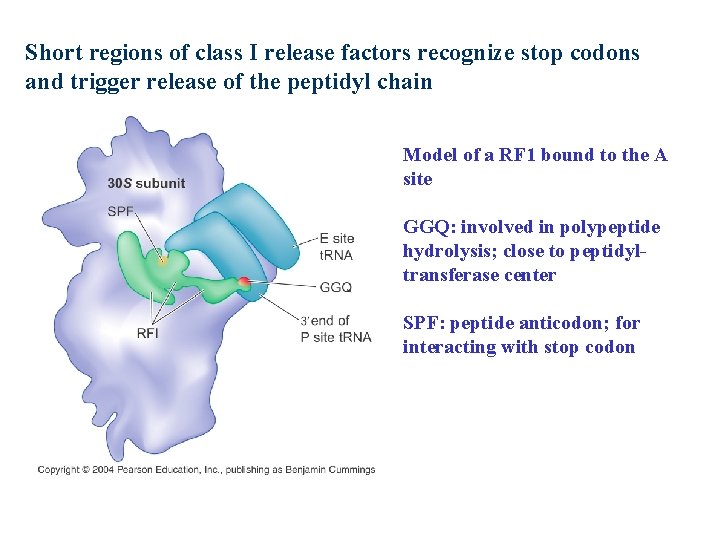
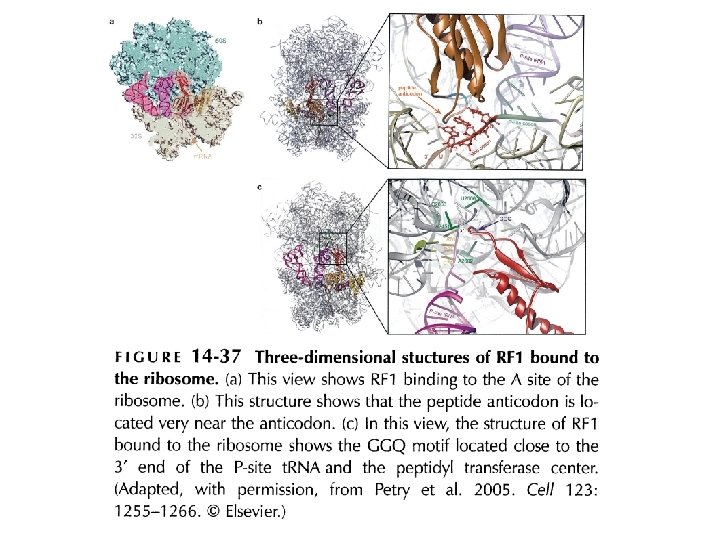
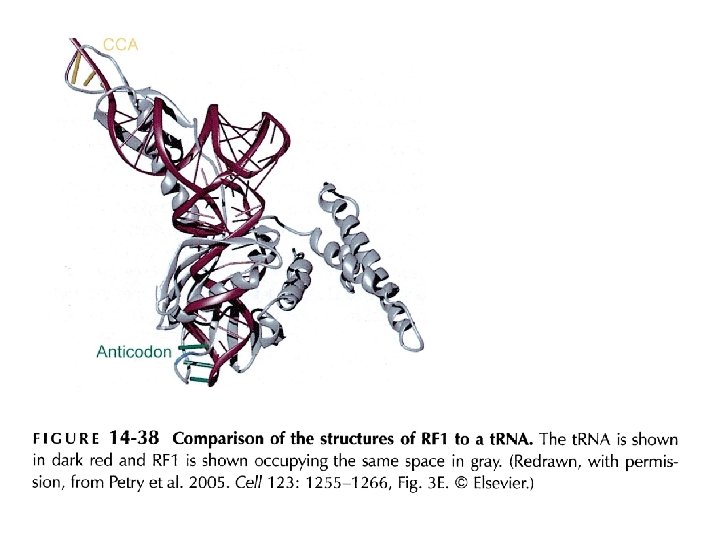
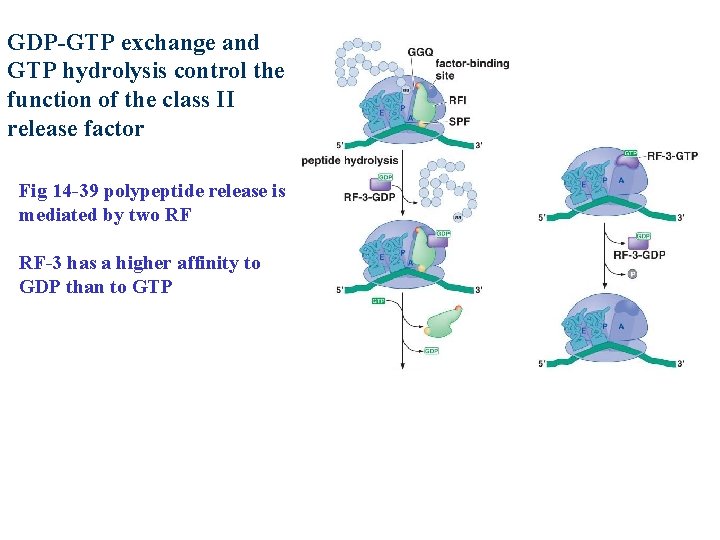
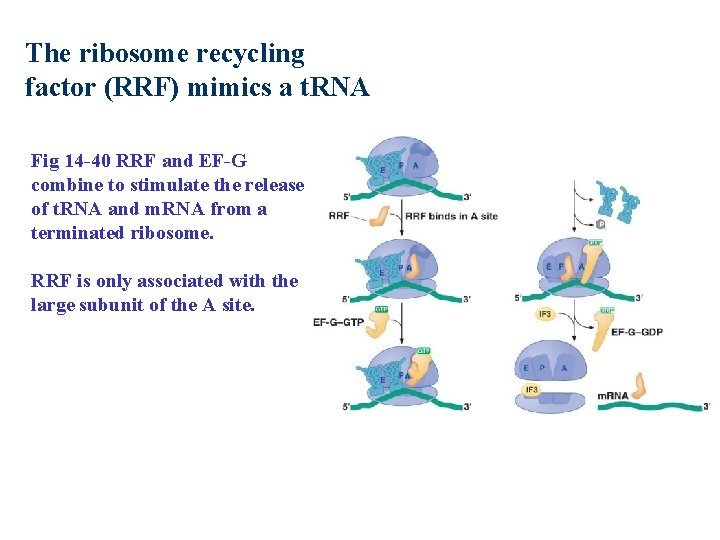
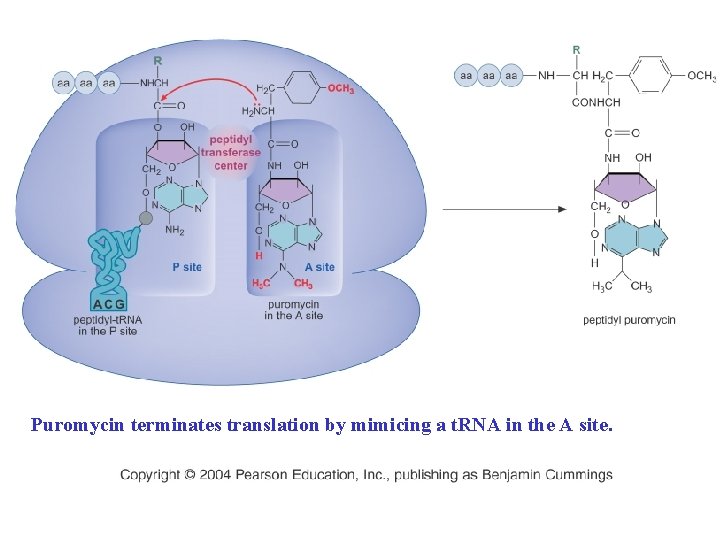
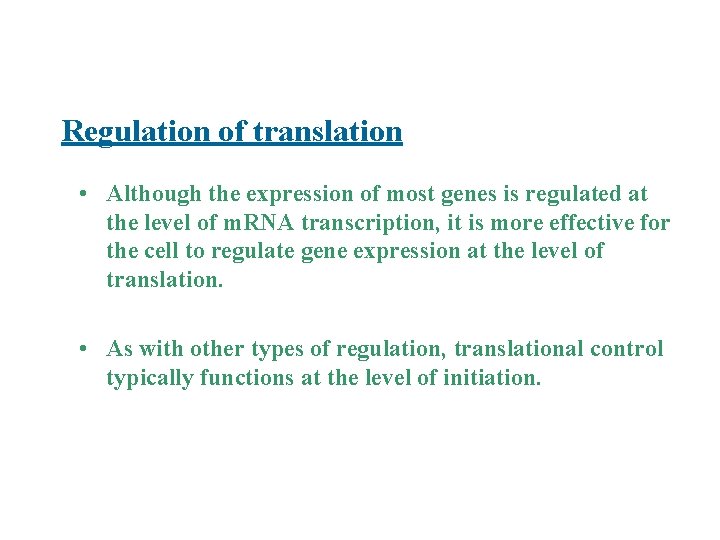
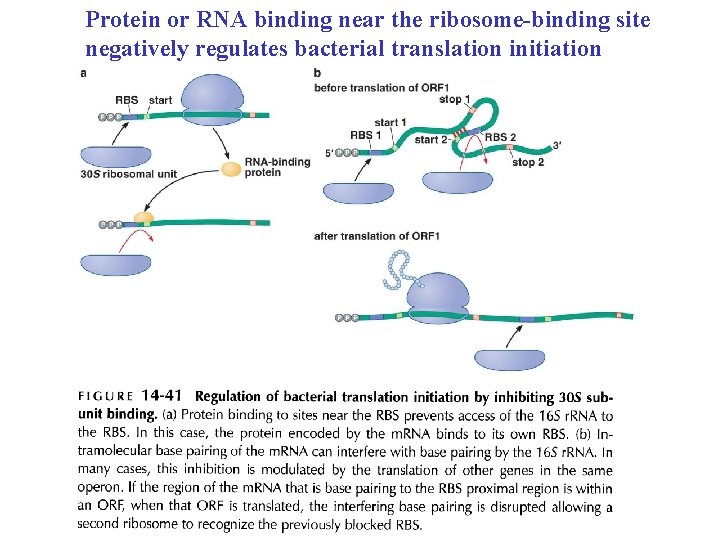
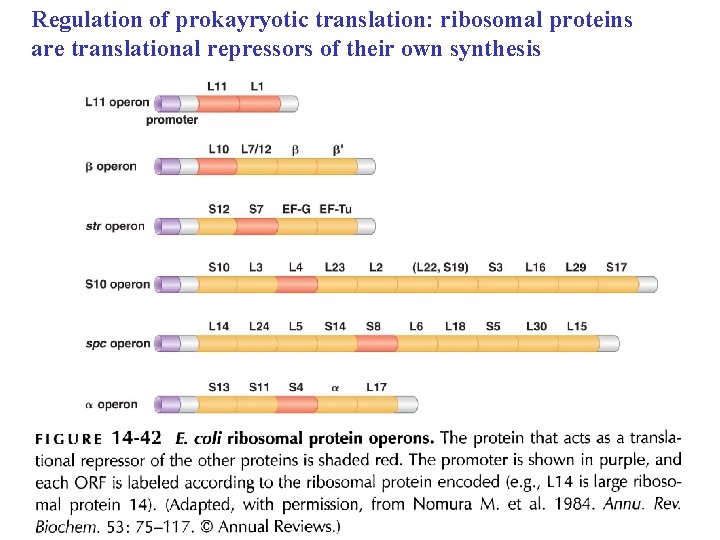
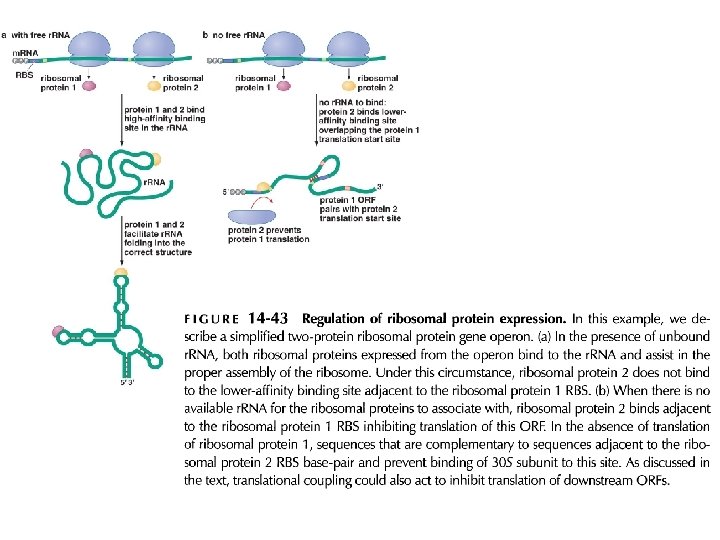
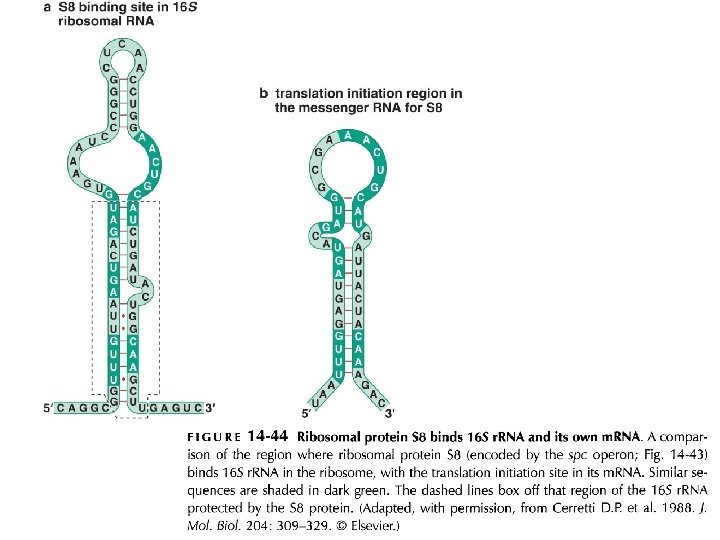
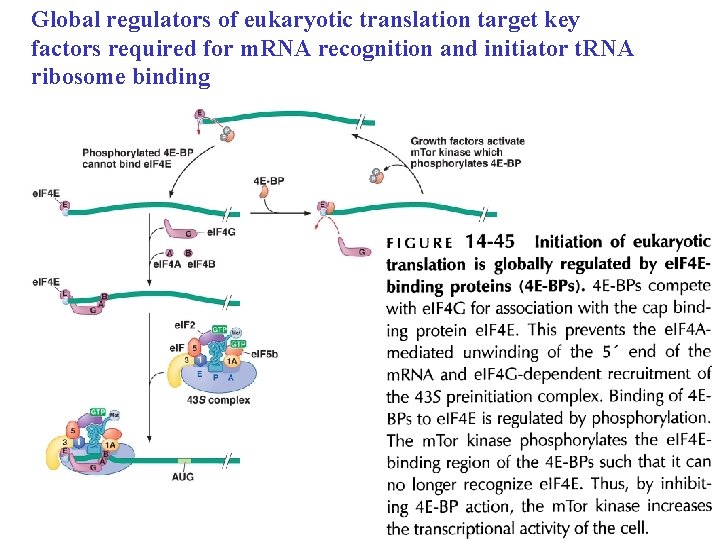
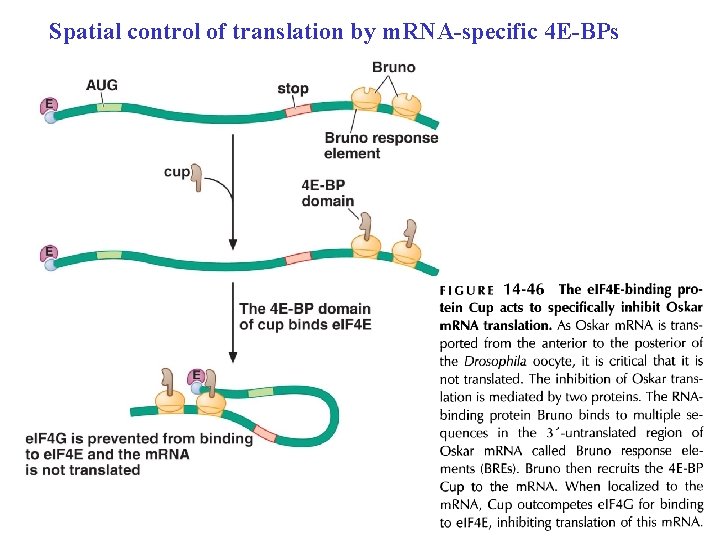
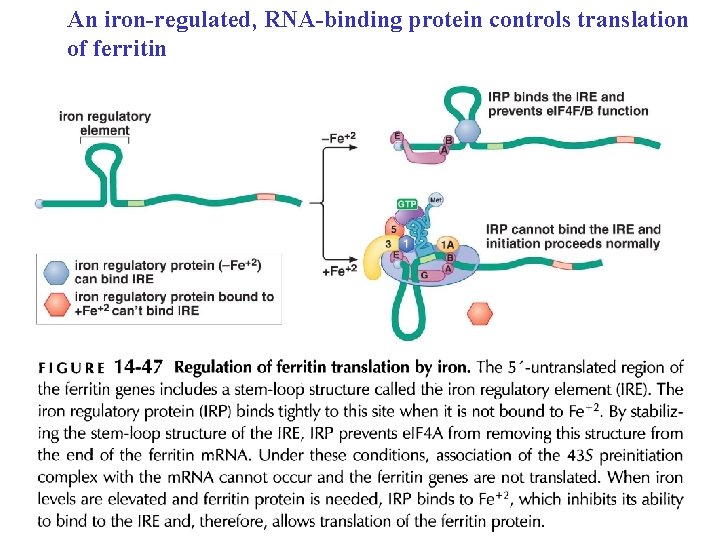
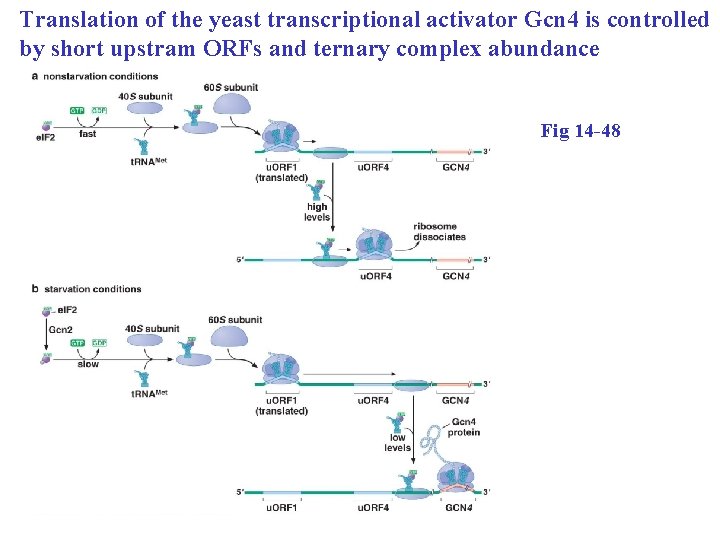
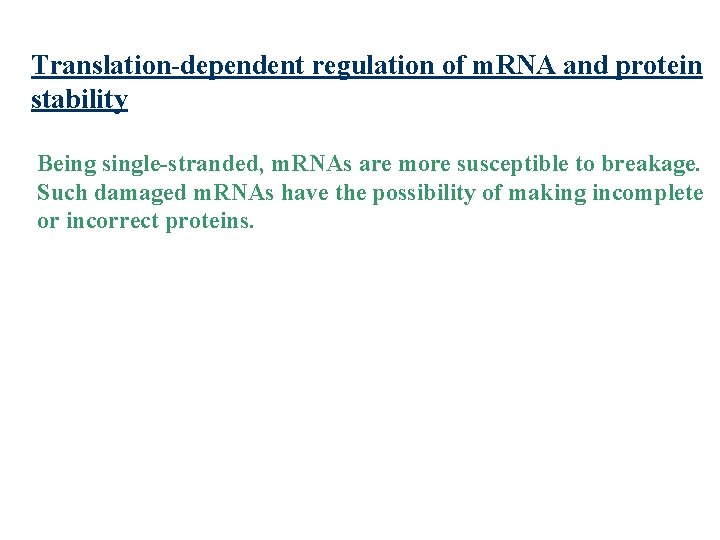
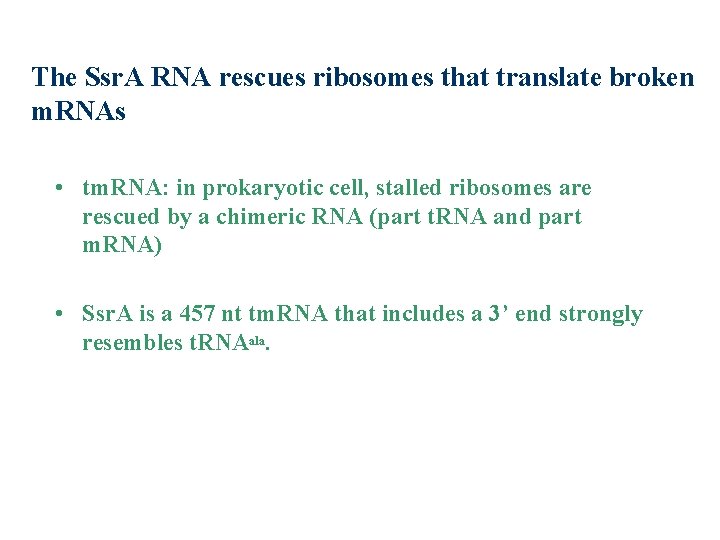
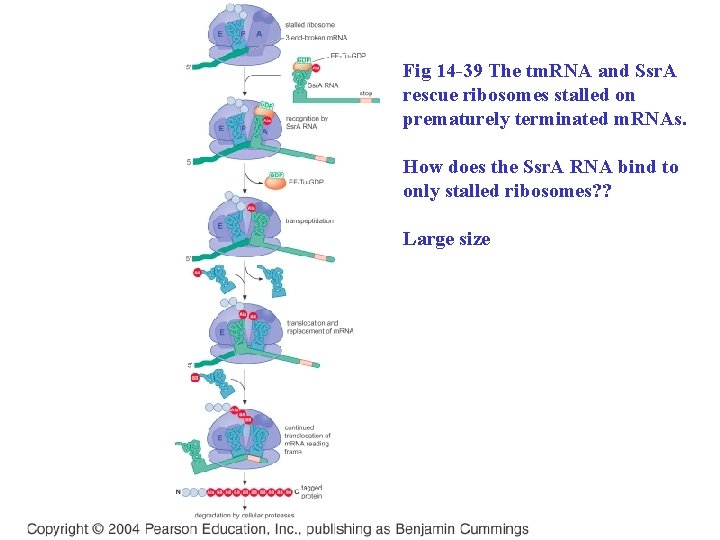
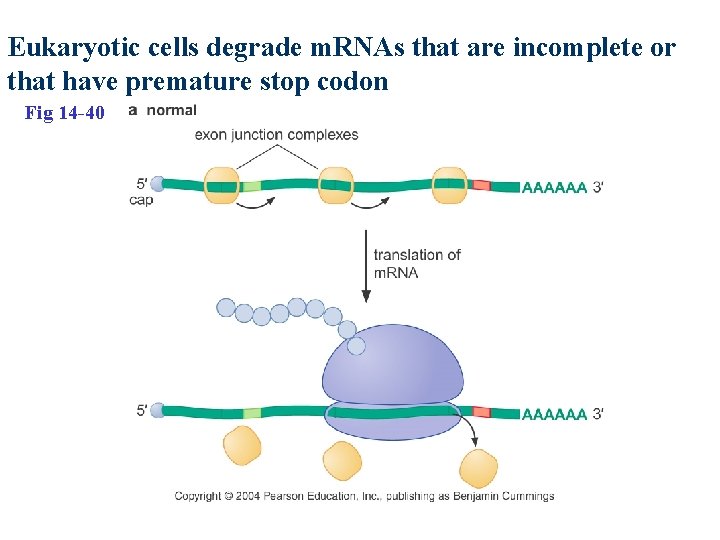
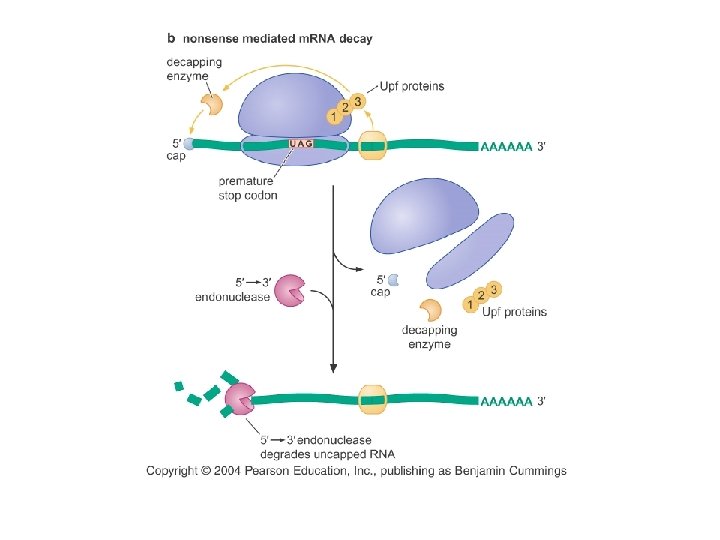
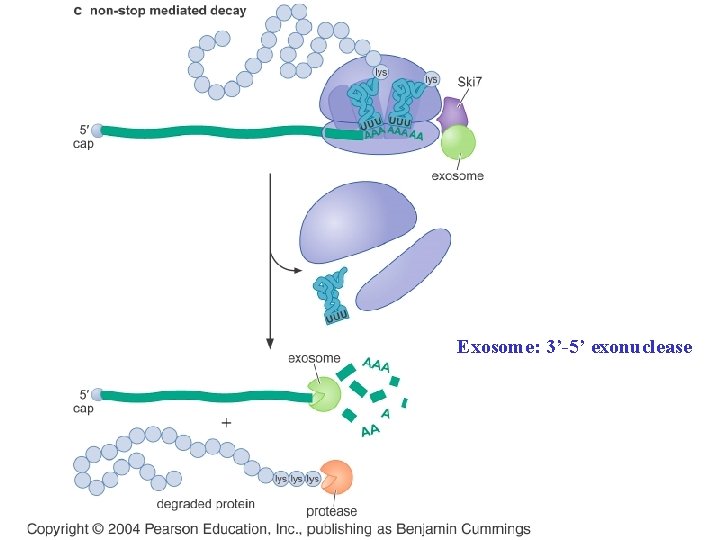
- Slides: 76
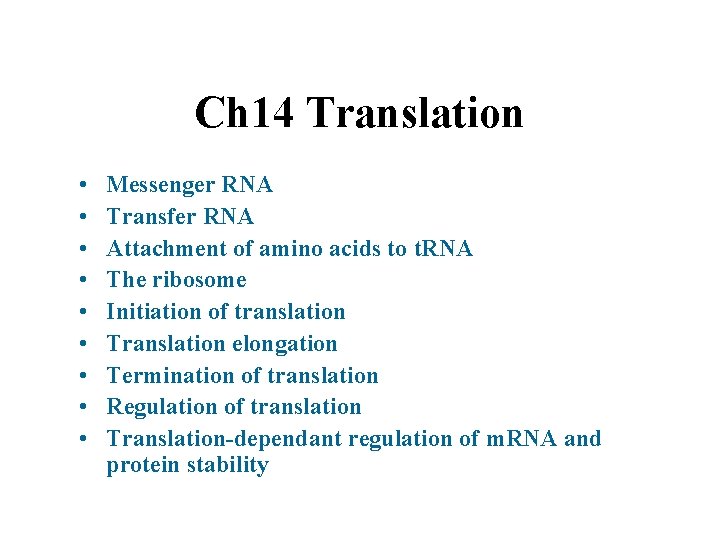
Ch 14 Translation • • • Messenger RNA Transfer RNA Attachment of amino acids to t. RNA The ribosome Initiation of translation Translation elongation Termination of translation Regulation of translation Translation-dependant regulation of m. RNA and protein stability
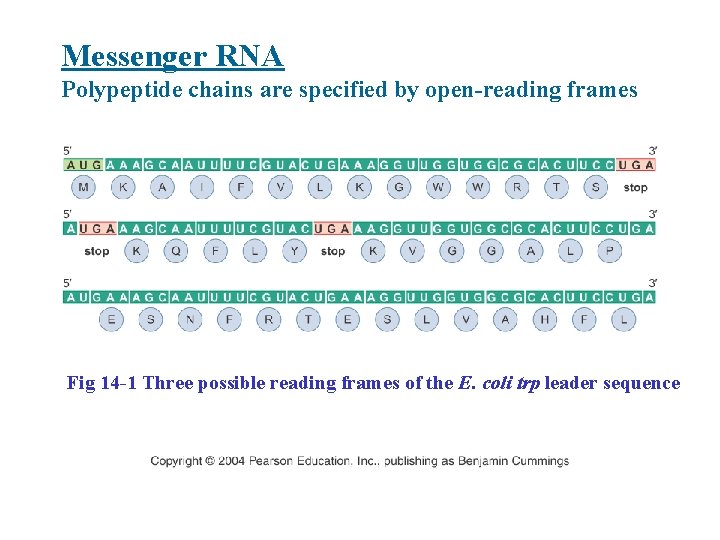
Messenger RNA Polypeptide chains are specified by open-reading frames Fig 14 -1 Three possible reading frames of the E. coli trp leader sequence
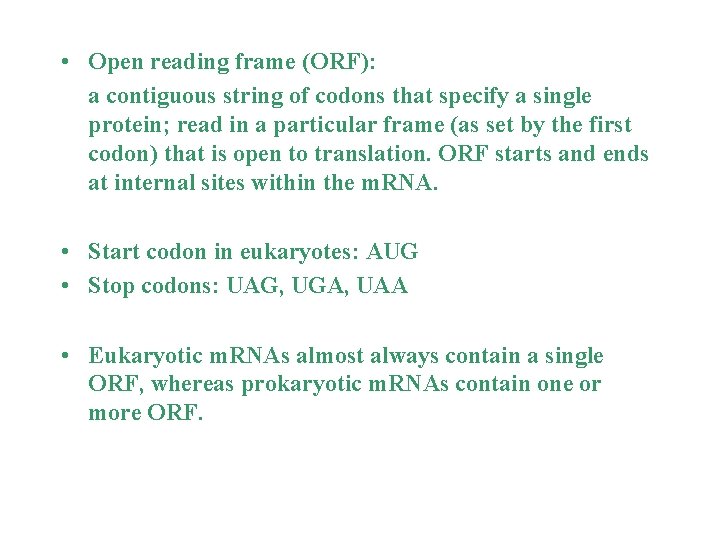
• Open reading frame (ORF): a contiguous string of codons that specify a single protein; read in a particular frame (as set by the first codon) that is open to translation. ORF starts and ends at internal sites within the m. RNA. • Start codon in eukaryotes: AUG • Stop codons: UAG, UGA, UAA • Eukaryotic m. RNAs almost always contain a single ORF, whereas prokaryotic m. RNAs contain one or more ORF.
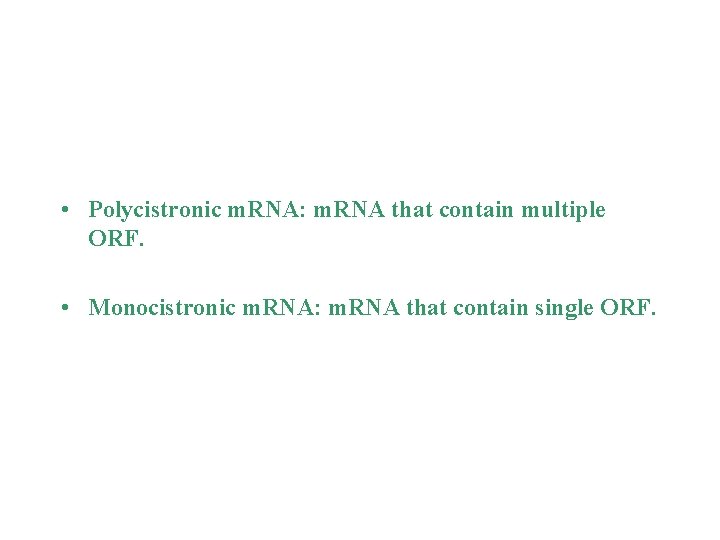
• Polycistronic m. RNA: m. RNA that contain multiple ORF. • Monocistronic m. RNA: m. RNA that contain single ORF.
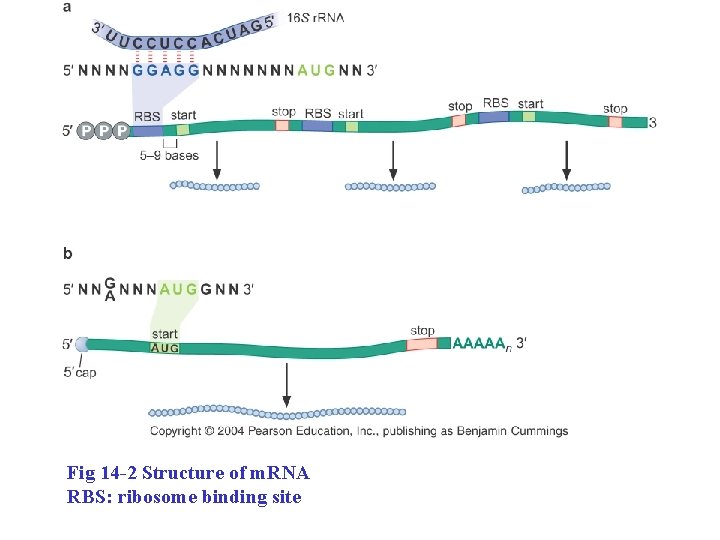
Fig 14 -2 Structure of m. RNA RBS: ribosome binding site
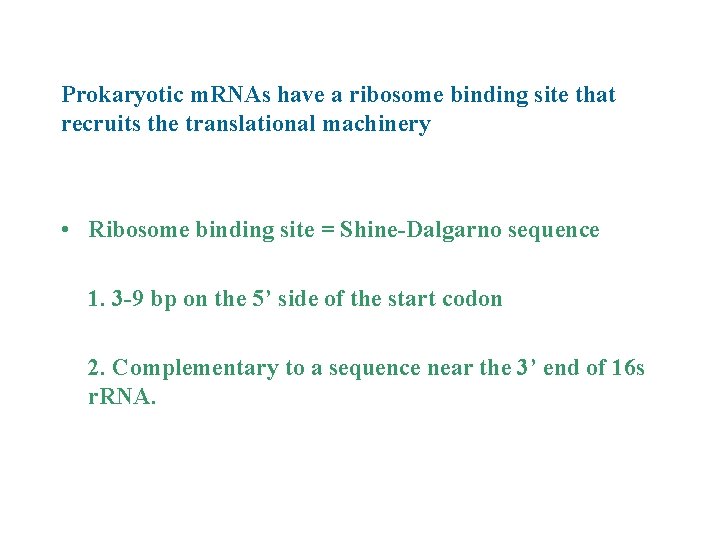
Prokaryotic m. RNAs have a ribosome binding site that recruits the translational machinery • Ribosome binding site = Shine-Dalgarno sequence 1. 3 -9 bp on the 5’ side of the start codon 2. Complementary to a sequence near the 3’ end of 16 s r. RNA.
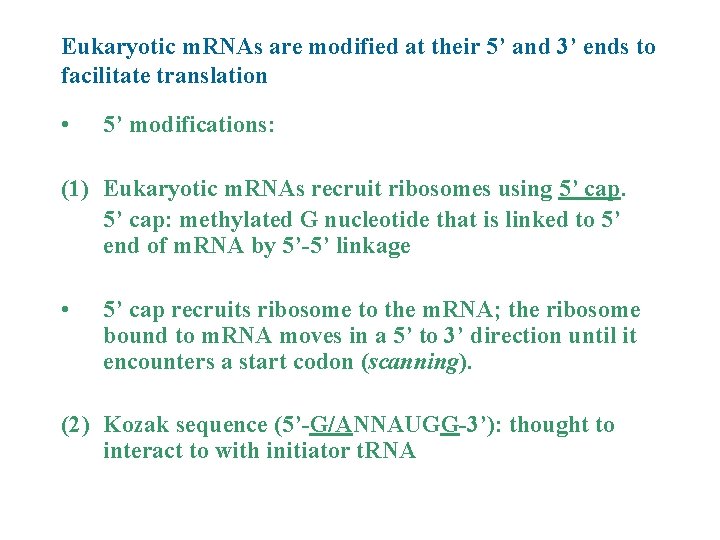
Eukaryotic m. RNAs are modified at their 5’ and 3’ ends to facilitate translation • 5’ modifications: (1) Eukaryotic m. RNAs recruit ribosomes using 5’ cap: methylated G nucleotide that is linked to 5’ end of m. RNA by 5’-5’ linkage • 5’ cap recruits ribosome to the m. RNA; the ribosome bound to m. RNA moves in a 5’ to 3’ direction until it encounters a start codon (scanning). (2) Kozak sequence (5’-G/ANNAUGG-3’): thought to interact to with initiator t. RNA
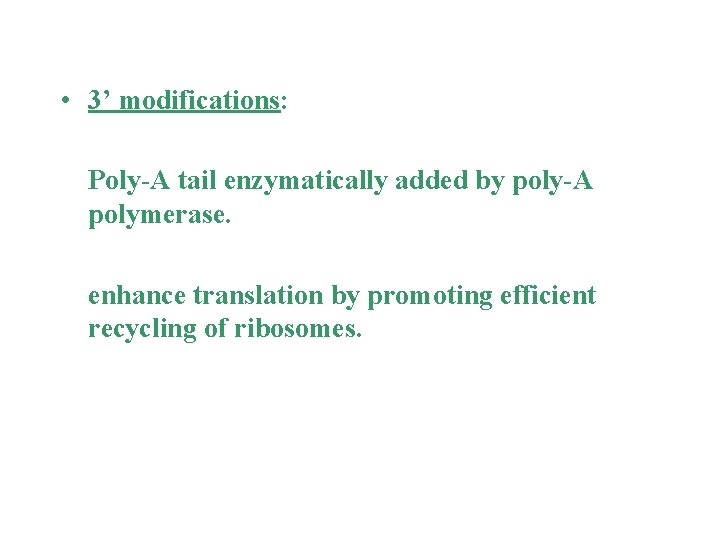
• 3’ modifications: Poly-A tail enzymatically added by poly-A polymerase. enhance translation by promoting efficient recycling of ribosomes.
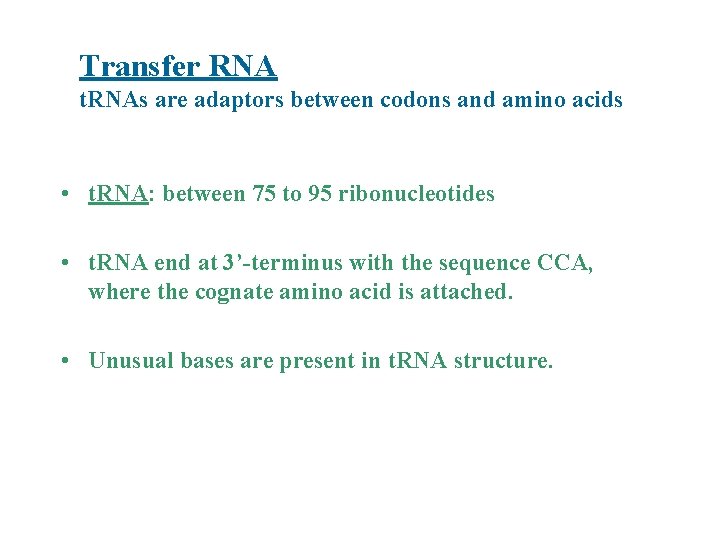
Transfer RNA t. RNAs are adaptors between codons and amino acids • t. RNA: between 75 to 95 ribonucleotides • t. RNA end at 3’-terminus with the sequence CCA, where the cognate amino acid is attached. • Unusual bases are present in t. RNA structure.
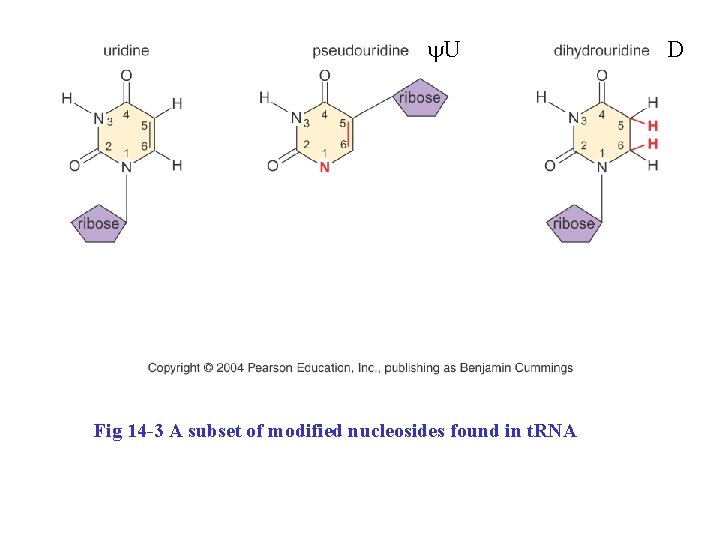
y. U Fig 14 -3 A subset of modified nucleosides found in t. RNA D
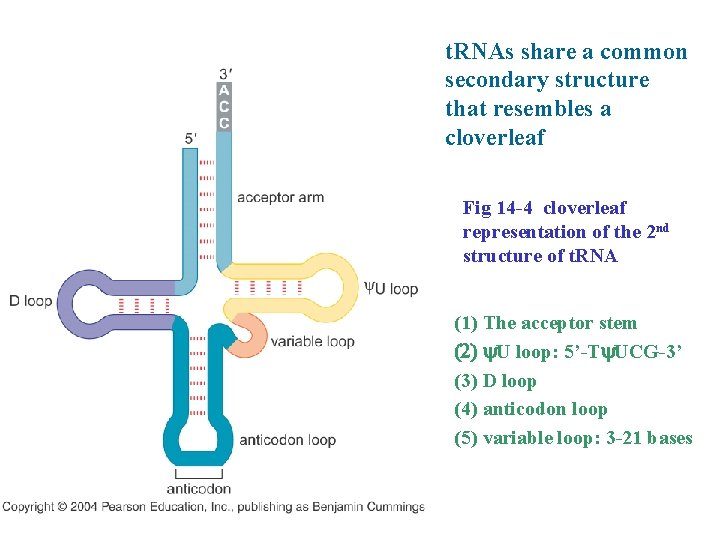
t. RNAs share a common secondary structure that resembles a cloverleaf Fig 14 -4 cloverleaf representation of the 2 nd structure of t. RNA (1) The acceptor stem (2) y. U loop: 5’-Ty. UCG-3’ (3) D loop (4) anticodon loop (5) variable loop: 3 -21 bases
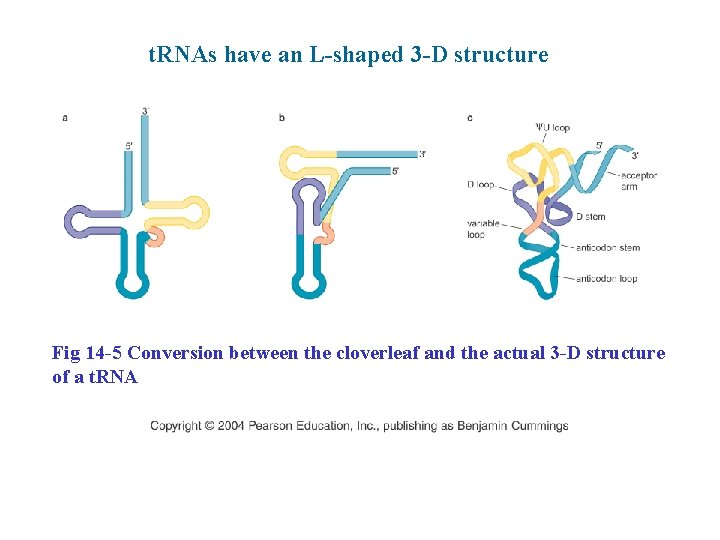
t. RNAs have an L-shaped 3 -D structure Fig 14 -5 Conversion between the cloverleaf and the actual 3 -D structure of a t. RNA
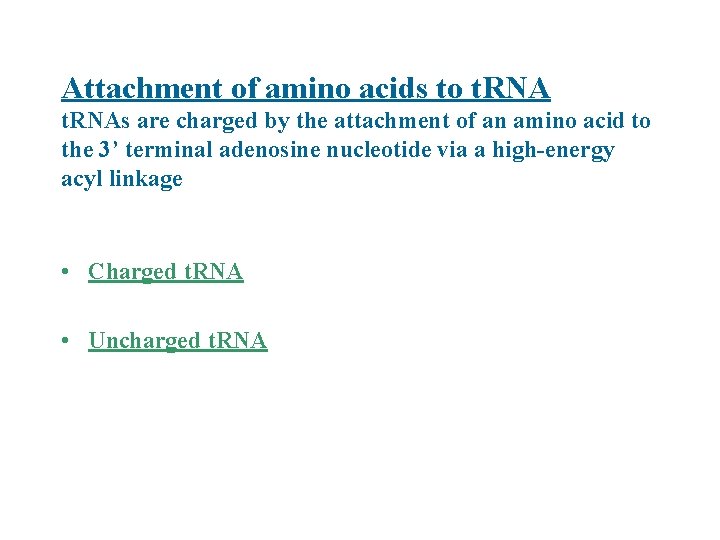
Attachment of amino acids to t. RNAs are charged by the attachment of an amino acid to the 3’ terminal adenosine nucleotide via a high-energy acyl linkage • Charged t. RNA • Uncharged t. RNA
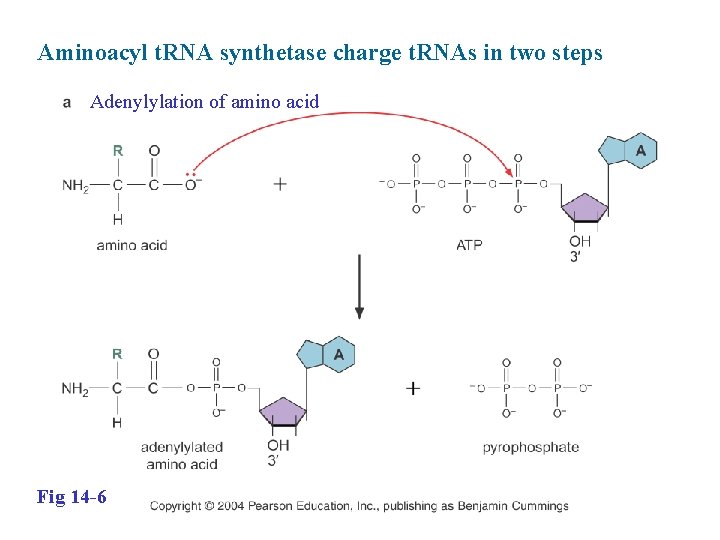
Aminoacyl t. RNA synthetase charge t. RNAs in two steps Adenylylation of amino acid Fig 14 -6
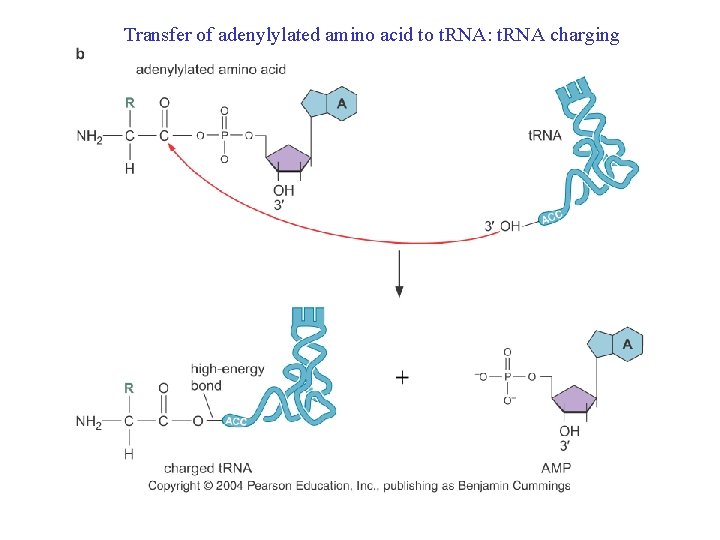
Transfer of adenylylated amino acid to t. RNA: t. RNA charging
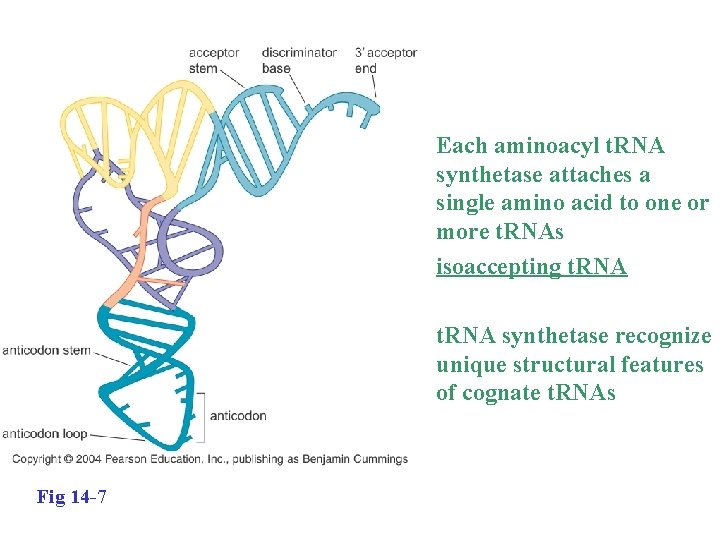
• Each aminoacyl t. RNA synthetase attaches a single amino acid to one or more t. RNAs isoaccepting t. RNA • t. RNA synthetase recognize unique structural features of cognate t. RNAs Fig 14 -7
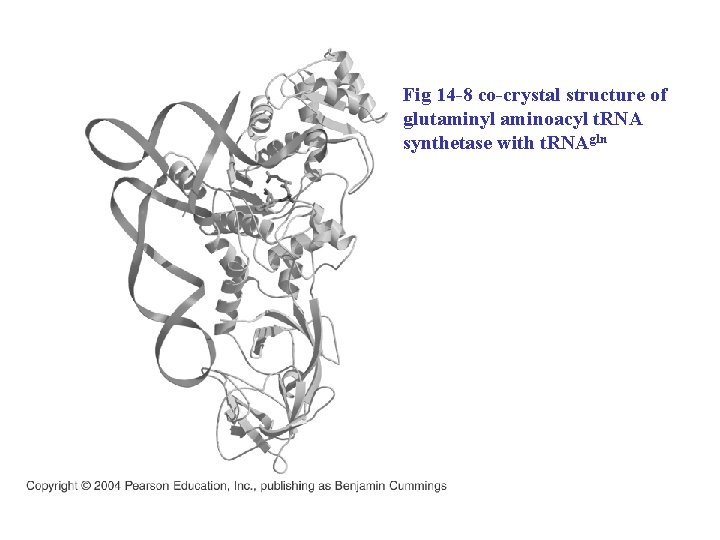
Fig 14 -8 co-crystal structure of glutaminyl aminoacyl t. RNA synthetase with t. RNAgln
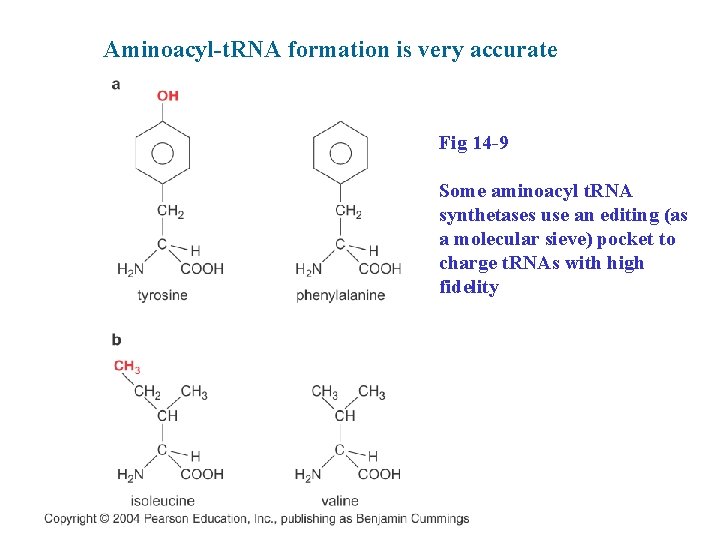
Aminoacyl-t. RNA formation is very accurate Fig 14 -9 Some aminoacyl t. RNA synthetases use an editing (as a molecular sieve) pocket to charge t. RNAs with high fidelity
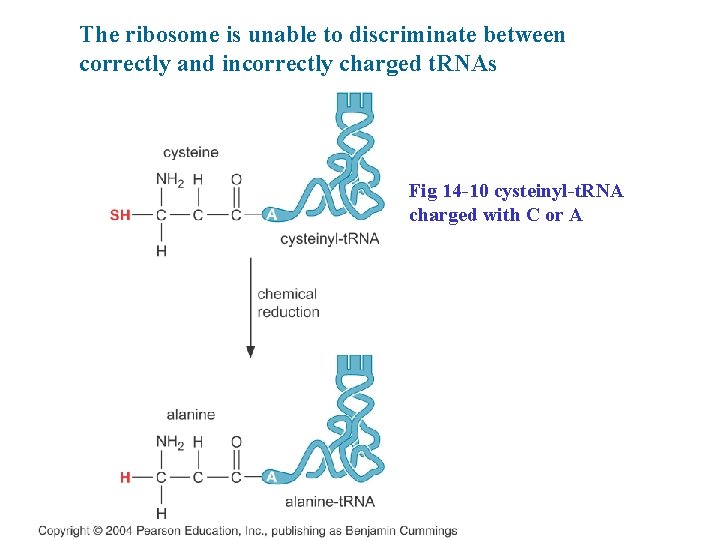
The ribosome is unable to discriminate between correctly and incorrectly charged t. RNAs Fig 14 -10 cysteinyl-t. RNA charged with C or A
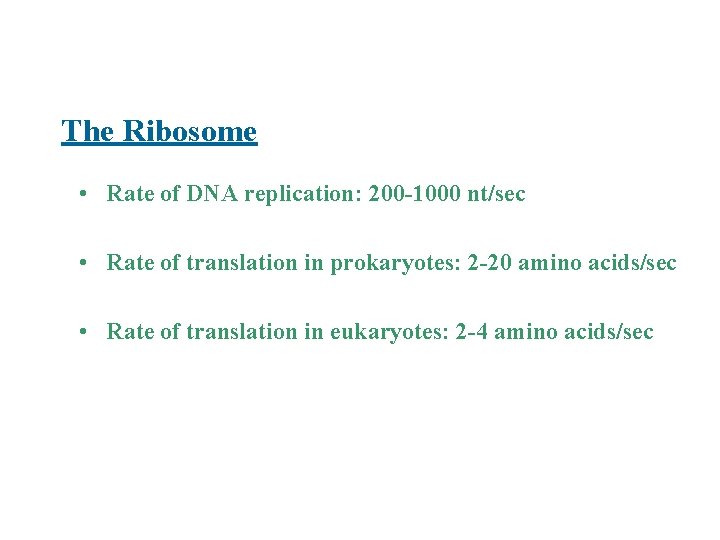
The Ribosome • Rate of DNA replication: 200 -1000 nt/sec • Rate of translation in prokaryotes: 2 -20 amino acids/sec • Rate of translation in eukaryotes: 2 -4 amino acids/sec
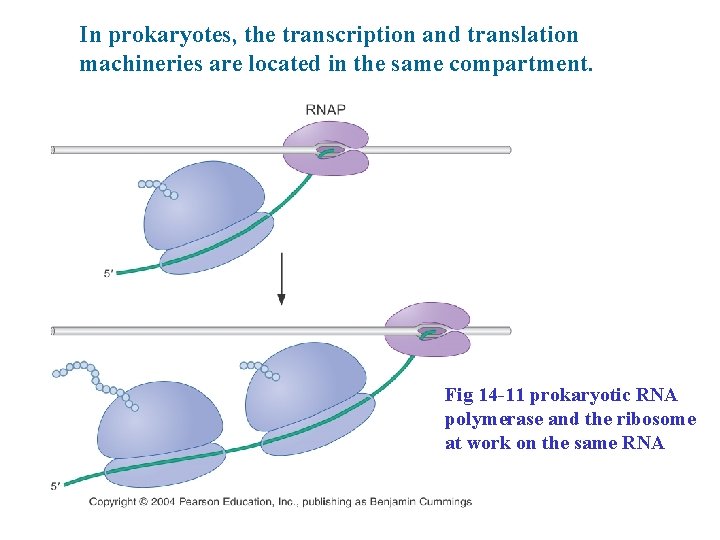
In prokaryotes, the transcription and translation machineries are located in the same compartment. Fig 14 -11 prokaryotic RNA polymerase and the ribosome at work on the same RNA
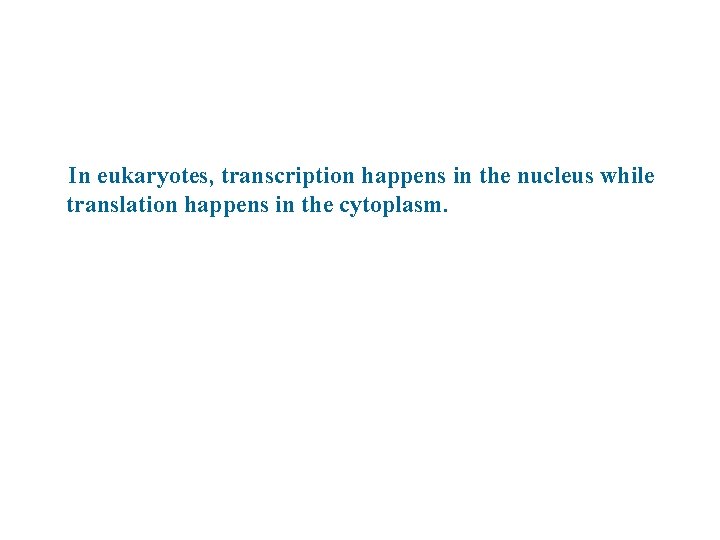
In eukaryotes, transcription happens in the nucleus while translation happens in the cytoplasm.
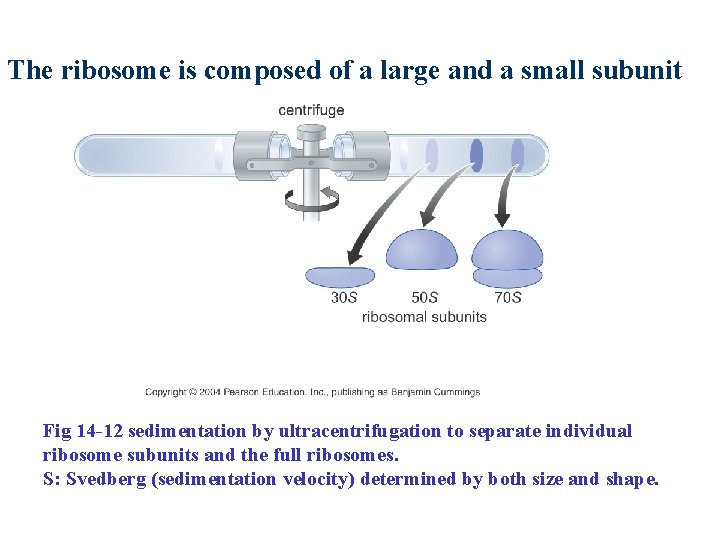
The ribosome is composed of a large and a small subunit Fig 14 -12 sedimentation by ultracentrifugation to separate individual ribosome subunits and the full ribosomes. S: Svedberg (sedimentation velocity) determined by both size and shape.
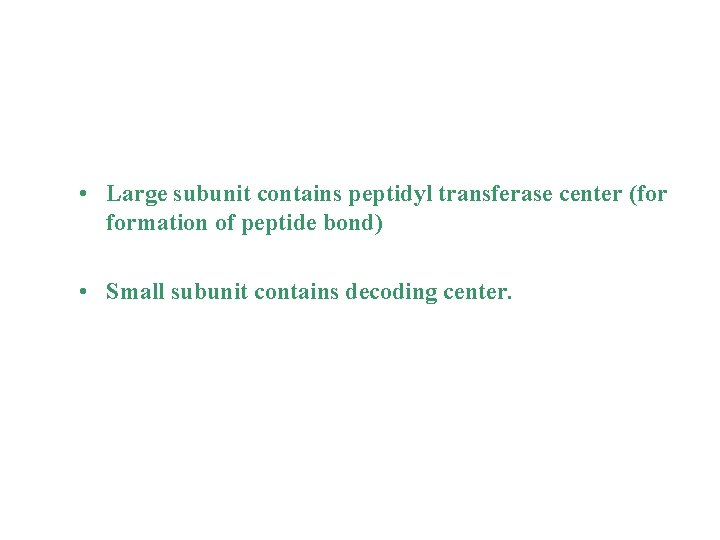
• Large subunit contains peptidyl transferase center (for formation of peptide bond) • Small subunit contains decoding center.
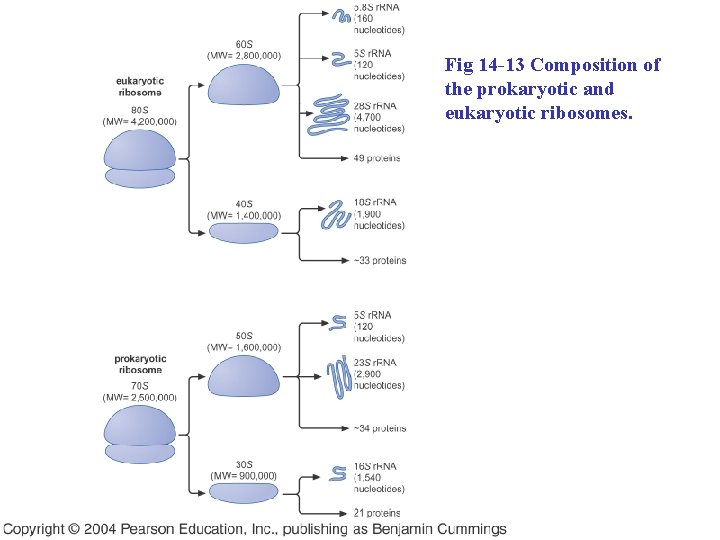
Fig 14 -13 Composition of the prokaryotic and eukaryotic ribosomes.
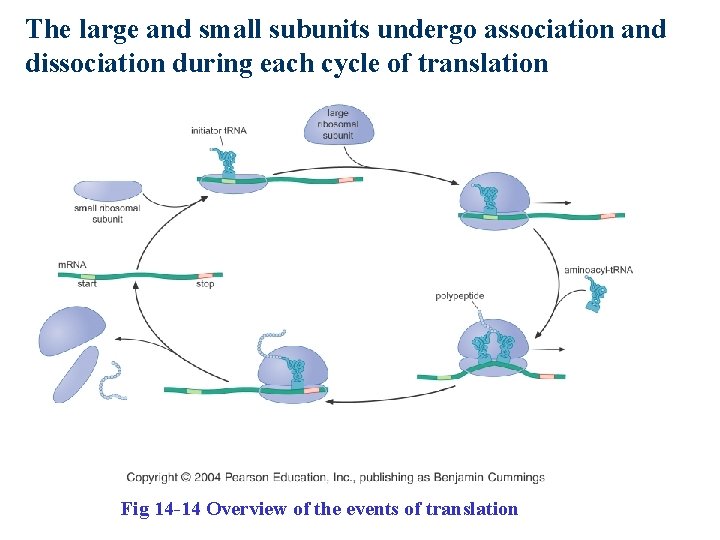
The large and small subunits undergo association and dissociation during each cycle of translation Fig 14 -14 Overview of the events of translation
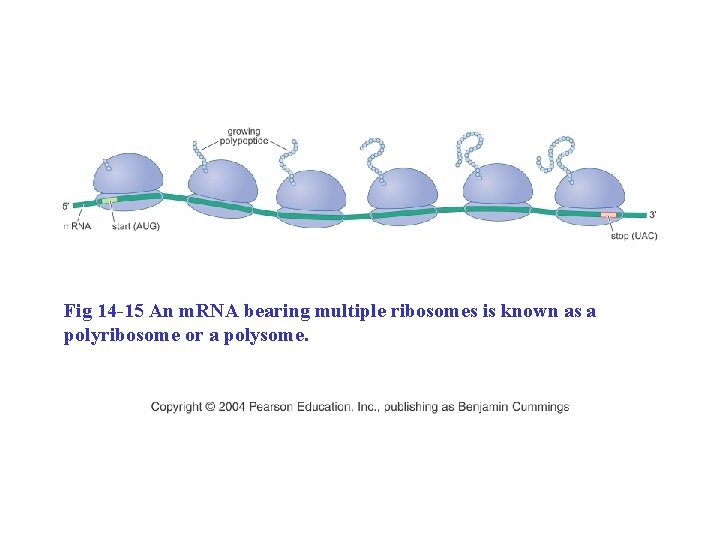
Fig 14 -15 An m. RNA bearing multiple ribosomes is known as a polyribosome or a polysome.
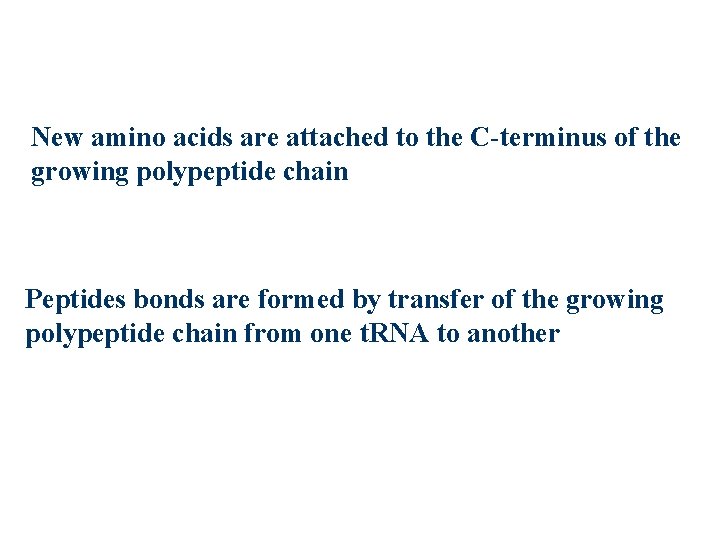
New amino acids are attached to the C-terminus of the growing polypeptide chain Peptides bonds are formed by transfer of the growing polypeptide chain from one t. RNA to another
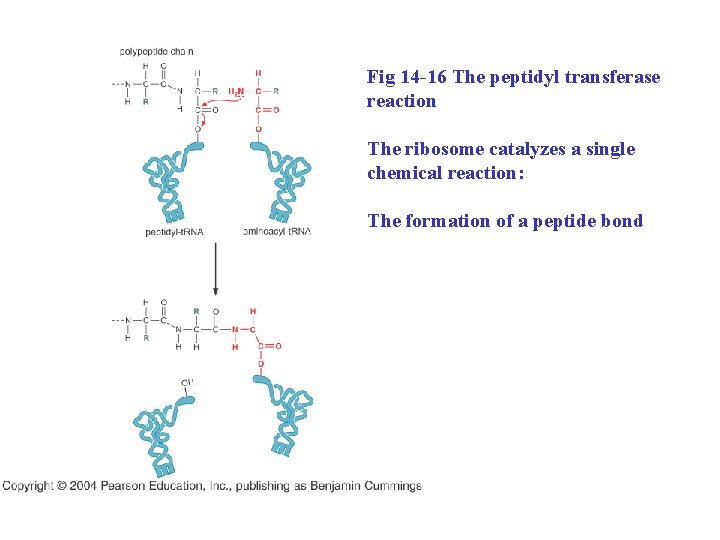
Fig 14 -16 The peptidyl transferase reaction The ribosome catalyzes a single chemical reaction: The formation of a peptide bond
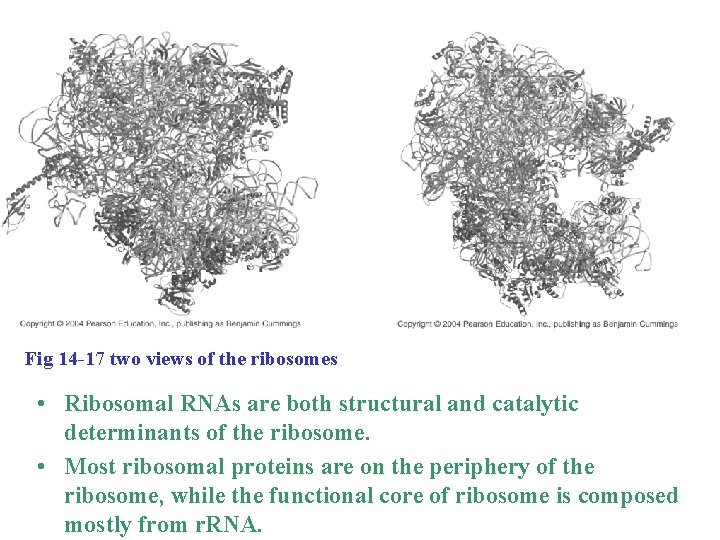
Fig 14 -17 two views of the ribosomes • Ribosomal RNAs are both structural and catalytic determinants of the ribosome. • Most ribosomal proteins are on the periphery of the ribosome, while the functional core of ribosome is composed mostly from r. RNA.
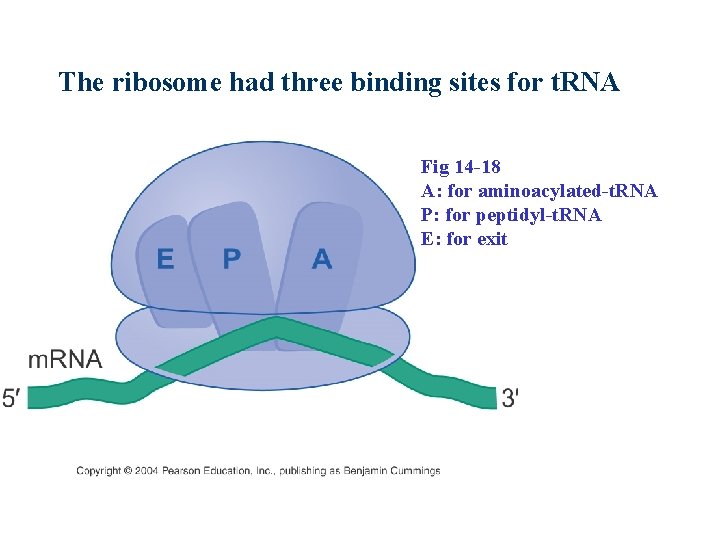
The ribosome had three binding sites for t. RNA Fig 14 -18 A: for aminoacylated-t. RNA P: for peptidyl-t. RNA E: for exit
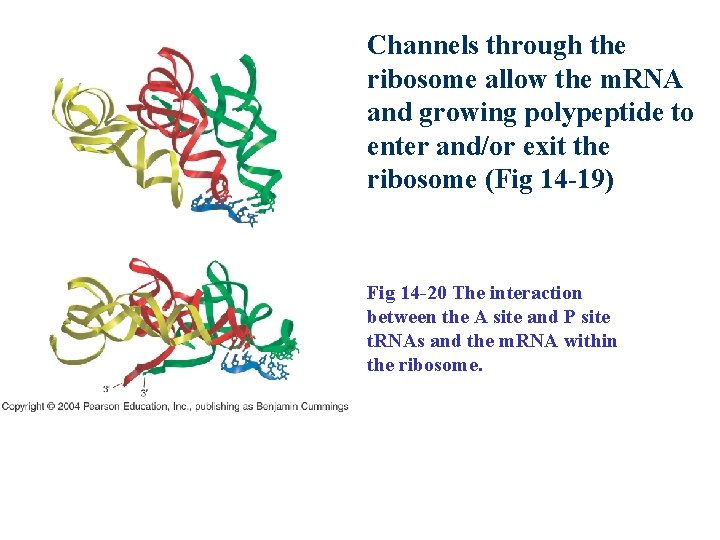
Channels through the ribosome allow the m. RNA and growing polypeptide to enter and/or exit the ribosome (Fig 14 -19) Fig 14 -20 The interaction between the A site and P site t. RNAs and the m. RNA within the ribosome.
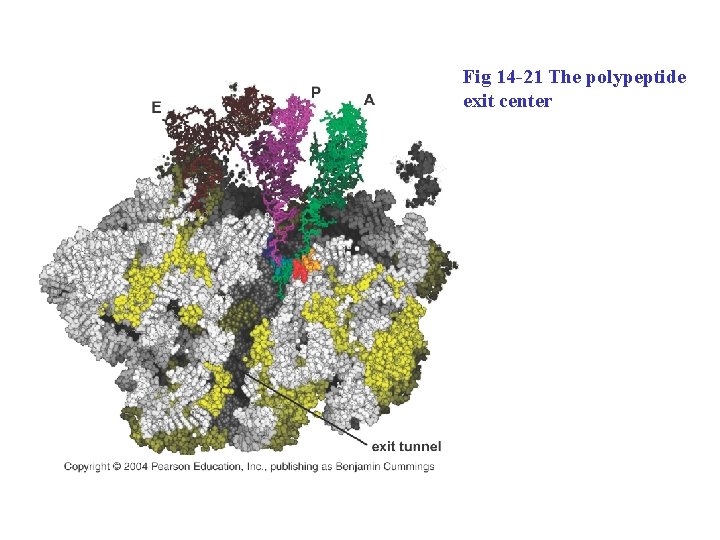
Fig 14 -21 The polypeptide exit center
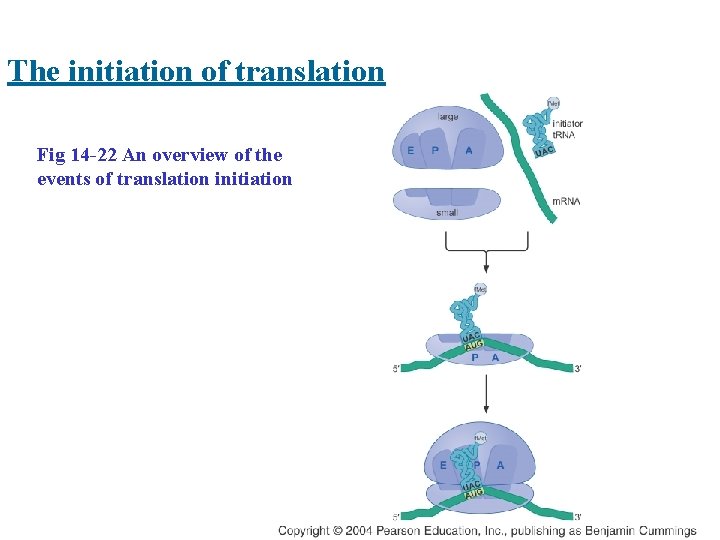
The initiation of translation Fig 14 -22 An overview of the events of translation initiation
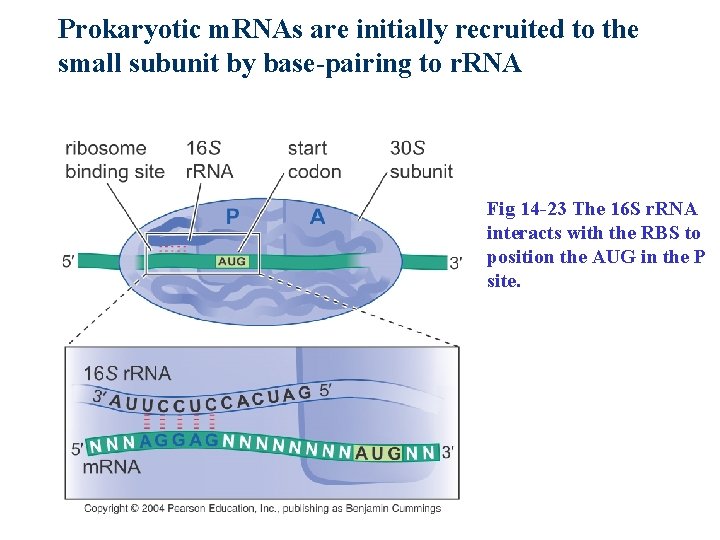
Prokaryotic m. RNAs are initially recruited to the small subunit by base-pairing to r. RNA Fig 14 -23 The 16 S r. RNA interacts with the RBS to position the AUG in the P site.
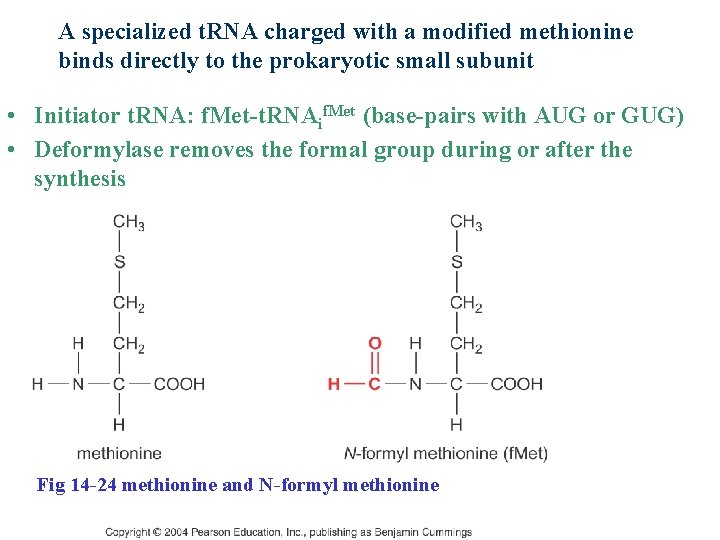
A specialized t. RNA charged with a modified methionine binds directly to the prokaryotic small subunit • Initiator t. RNA: f. Met-t. RNAif. Met (base-pairs with AUG or GUG) • Deformylase removes the formal group during or after the synthesis Fig 14 -24 methionine and N-formyl methionine
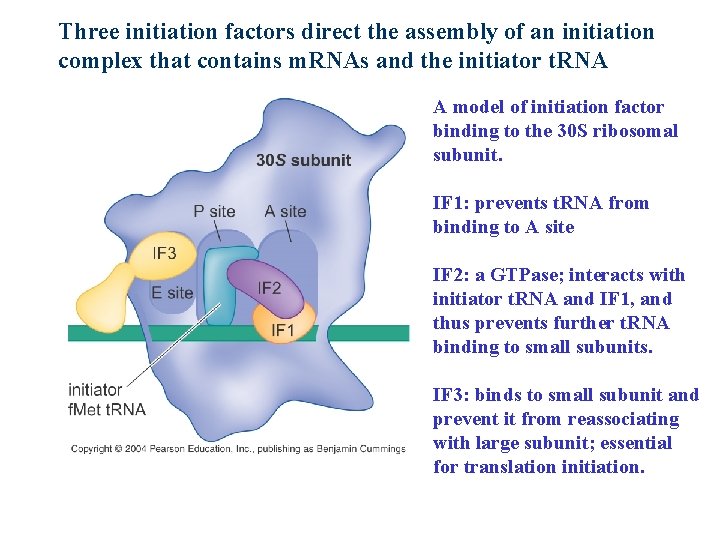
Three initiation factors direct the assembly of an initiation complex that contains m. RNAs and the initiator t. RNA A model of initiation factor binding to the 30 S ribosomal subunit. IF 1: prevents t. RNA from binding to A site IF 2: a GTPase; interacts with initiator t. RNA and IF 1, and thus prevents further t. RNA binding to small subunits. IF 3: binds to small subunit and prevent it from reassociating with large subunit; essential for translation initiation.
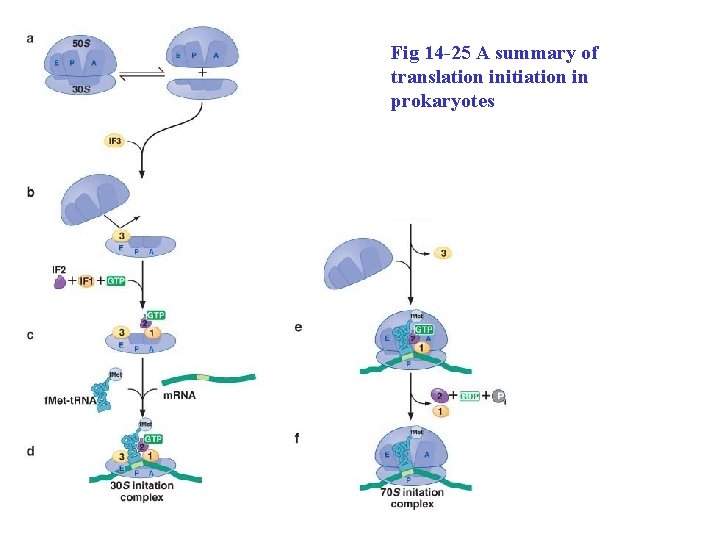
Fig 14 -25 A summary of translation initiation in prokaryotes
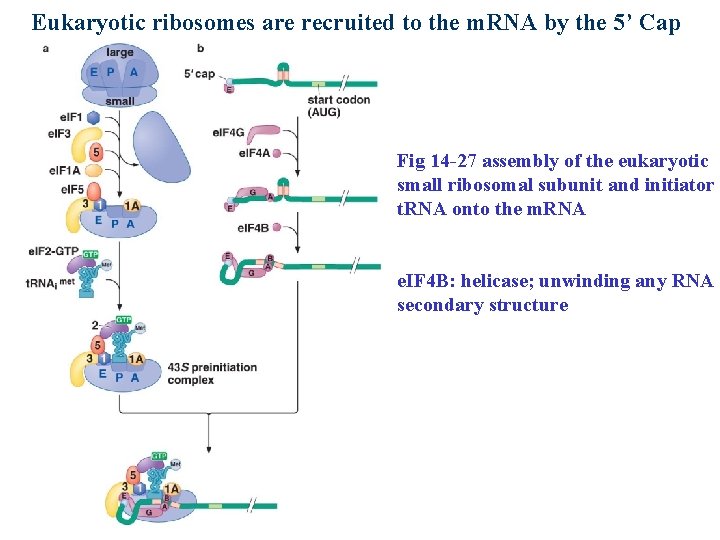
Eukaryotic ribosomes are recruited to the m. RNA by the 5’ Cap Fig 14 -27 assembly of the eukaryotic small ribosomal subunit and initiator t. RNA onto the m. RNA e. IF 4 B: helicase; unwinding any RNA secondary structure
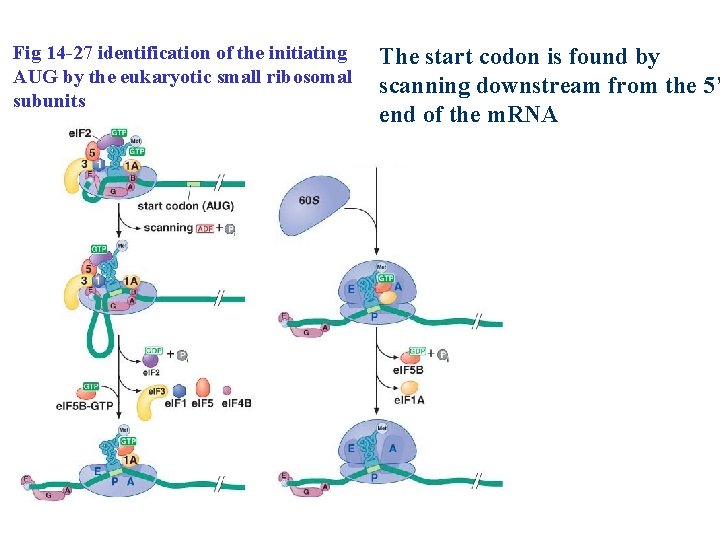
Fig 14 -27 identification of the initiating AUG by the eukaryotic small ribosomal subunits The start codon is found by scanning downstream from the 5’ end of the m. RNA
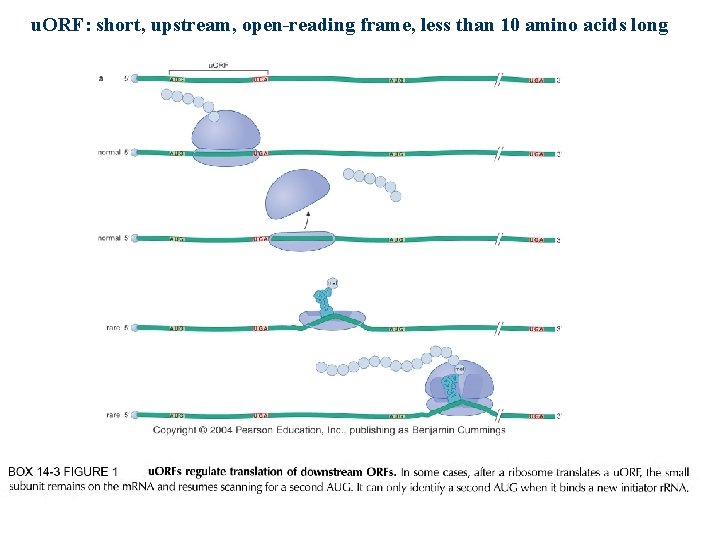
u. ORF: short, upstream, open-reading frame, less than 10 amino acids long
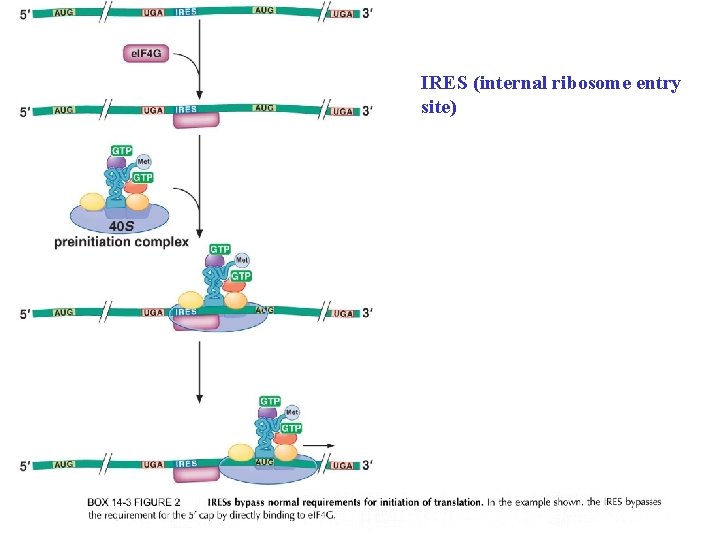
IRES (internal ribosome entry site)
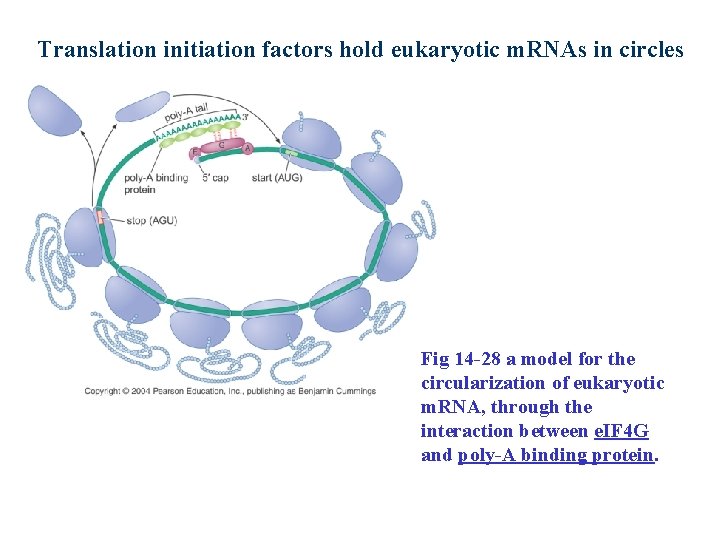
Translation initiation factors hold eukaryotic m. RNAs in circles Fig 14 -28 a model for the circularization of eukaryotic m. RNA, through the interaction between e. IF 4 G and poly-A binding protein.
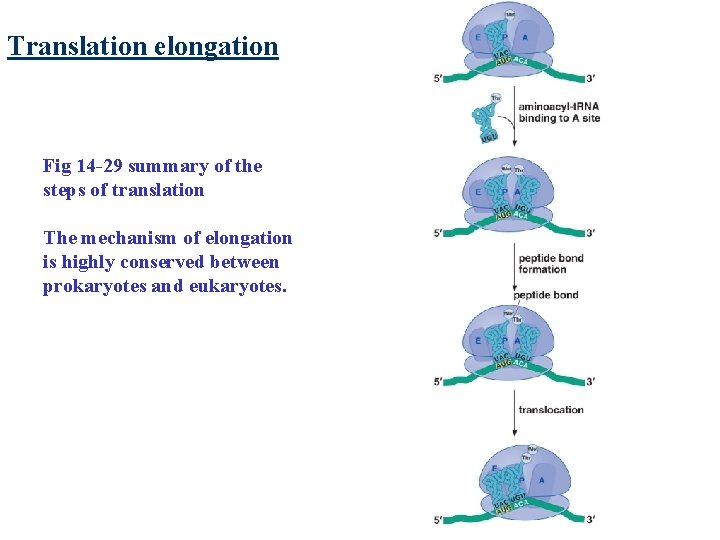
Translation elongation Fig 14 -29 summary of the steps of translation The mechanism of elongation is highly conserved between prokaryotes and eukaryotes.
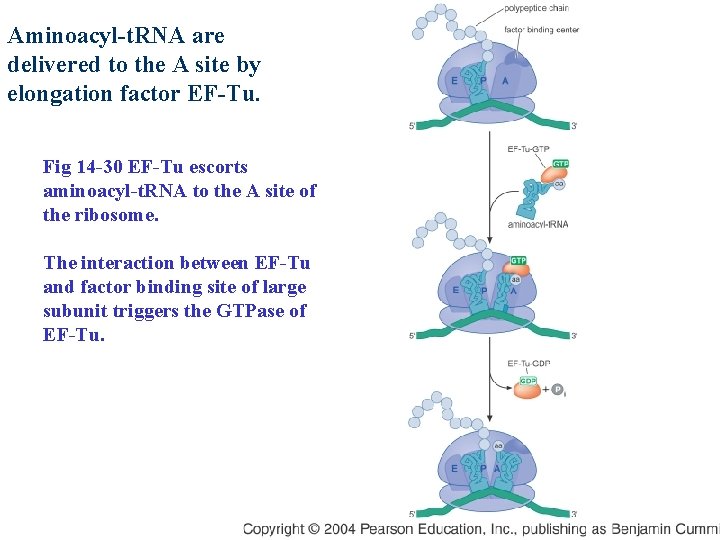
Aminoacyl-t. RNA are delivered to the A site by elongation factor EF-Tu. Fig 14 -30 EF-Tu escorts aminoacyl-t. RNA to the A site of the ribosome. The interaction between EF-Tu and factor binding site of large subunit triggers the GTPase of EF-Tu.
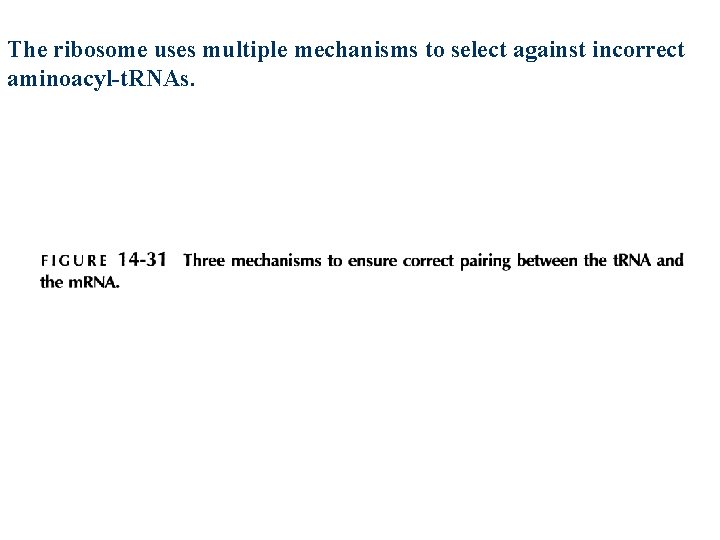
The ribosome uses multiple mechanisms to select against incorrect aminoacyl-t. RNAs.
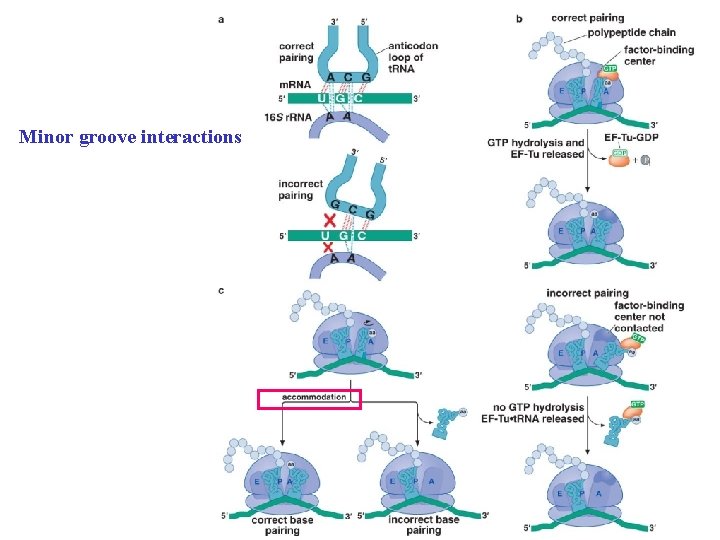
Minor groove interactions
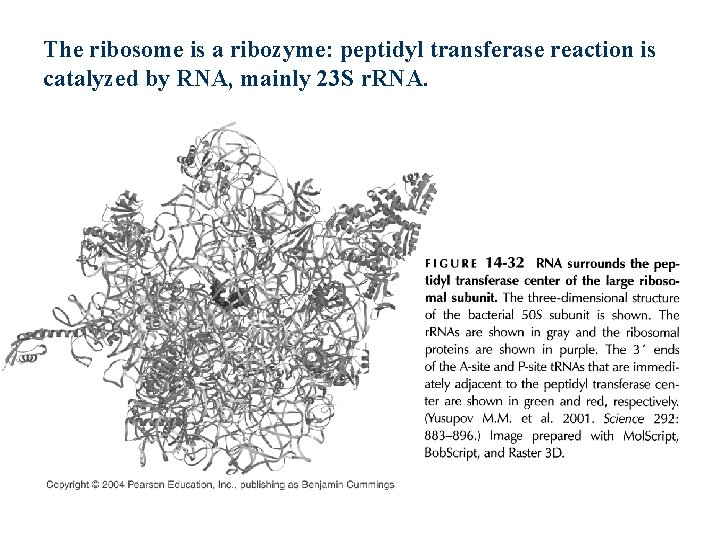
The ribosome is a ribozyme: peptidyl transferase reaction is catalyzed by RNA, mainly 23 S r. RNA.
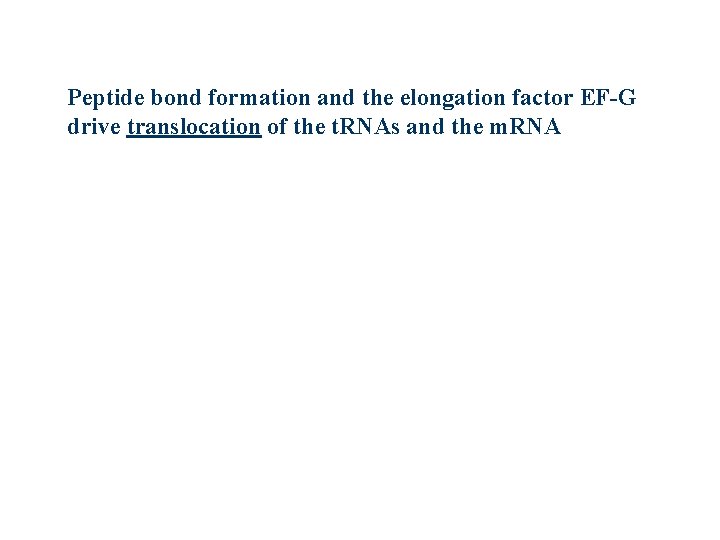
Peptide bond formation and the elongation factor EF-G drive translocation of the t. RNAs and the m. RNA
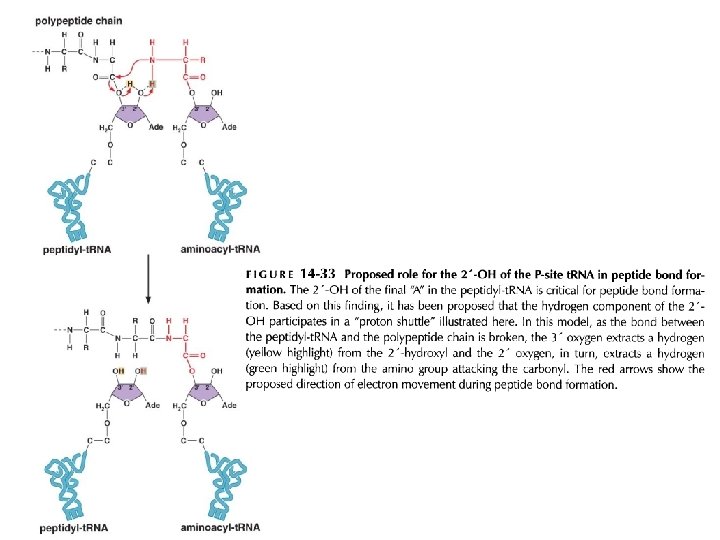
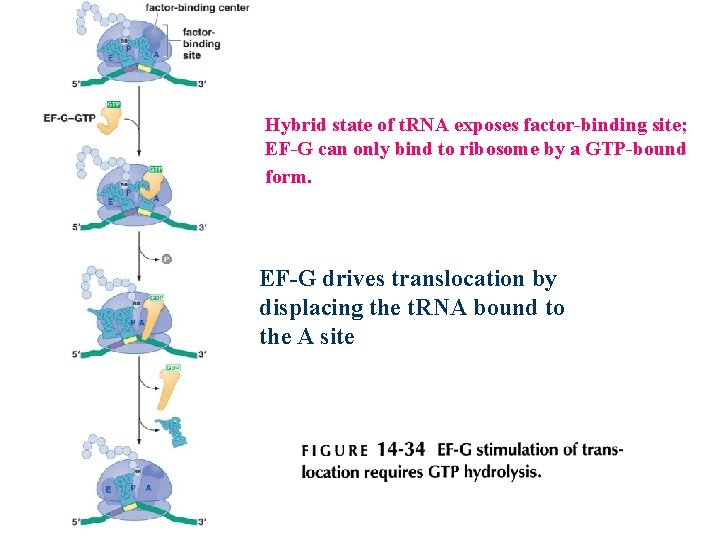
Hybrid state of t. RNA exposes factor-binding site; EF-G can only bind to ribosome by a GTP-bound form. EF-G drives translocation by displacing the t. RNA bound to the A site
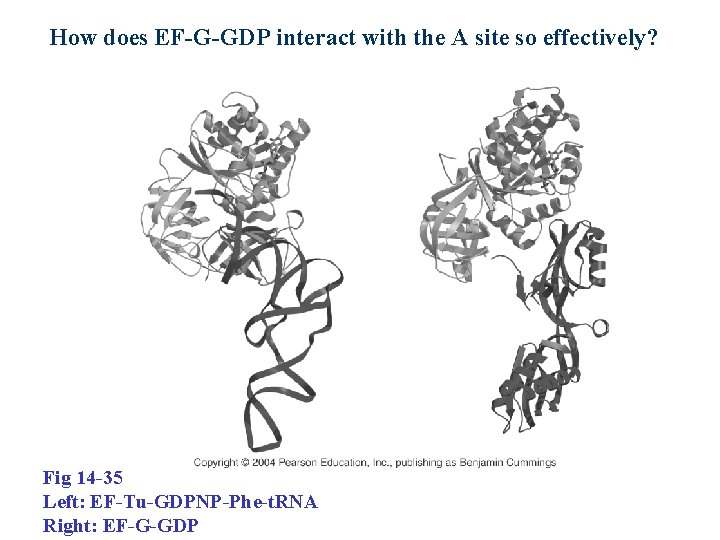
How does EF-G-GDP interact with the A site so effectively? Fig 14 -35 Left: EF-Tu-GDPNP-Phe-t. RNA Right: EF-G-GDP
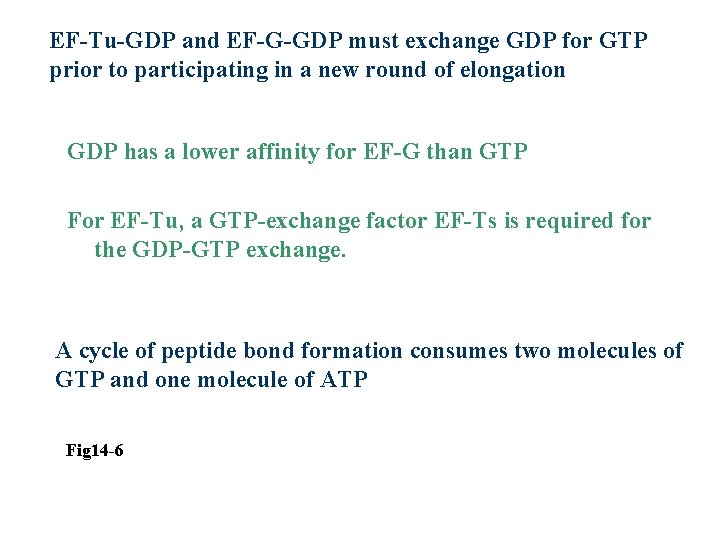
EF-Tu-GDP and EF-G-GDP must exchange GDP for GTP prior to participating in a new round of elongation GDP has a lower affinity for EF-G than GTP For EF-Tu, a GTP-exchange factor EF-Ts is required for the GDP-GTP exchange. A cycle of peptide bond formation consumes two molecules of GTP and one molecule of ATP Fig 14 -6
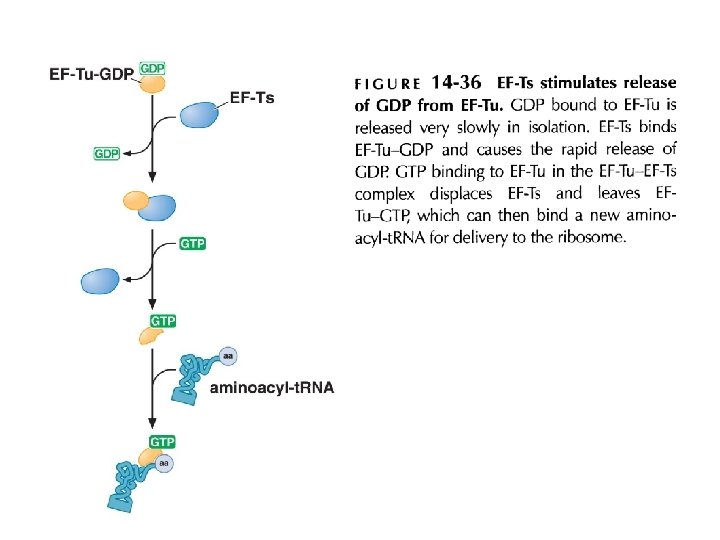
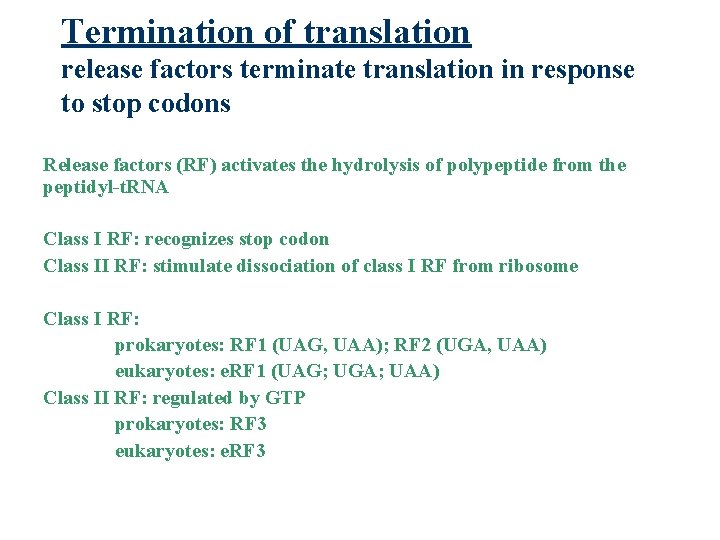
Termination of translation release factors terminate translation in response to stop codons Release factors (RF) activates the hydrolysis of polypeptide from the peptidyl-t. RNA Class I RF: recognizes stop codon Class II RF: stimulate dissociation of class I RF from ribosome Class I RF: prokaryotes: RF 1 (UAG, UAA); RF 2 (UGA, UAA) eukaryotes: e. RF 1 (UAG; UGA; UAA) Class II RF: regulated by GTP prokaryotes: RF 3 eukaryotes: e. RF 3
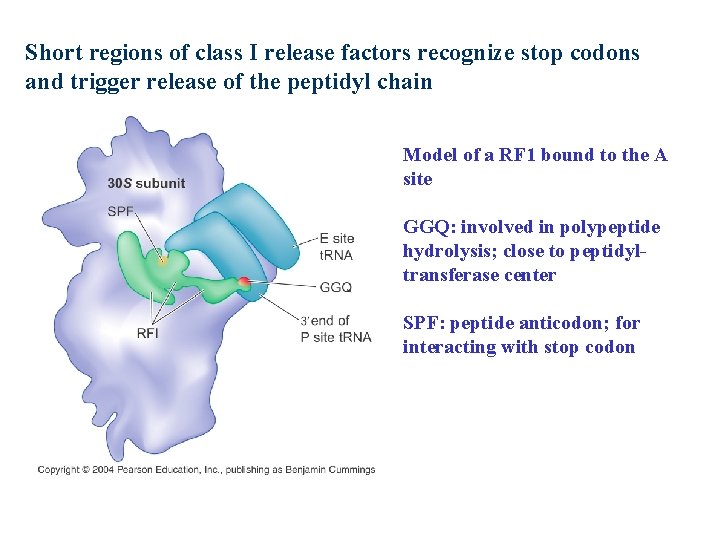
Short regions of class I release factors recognize stop codons and trigger release of the peptidyl chain Model of a RF 1 bound to the A site GGQ: involved in polypeptide hydrolysis; close to peptidyltransferase center SPF: peptide anticodon; for interacting with stop codon
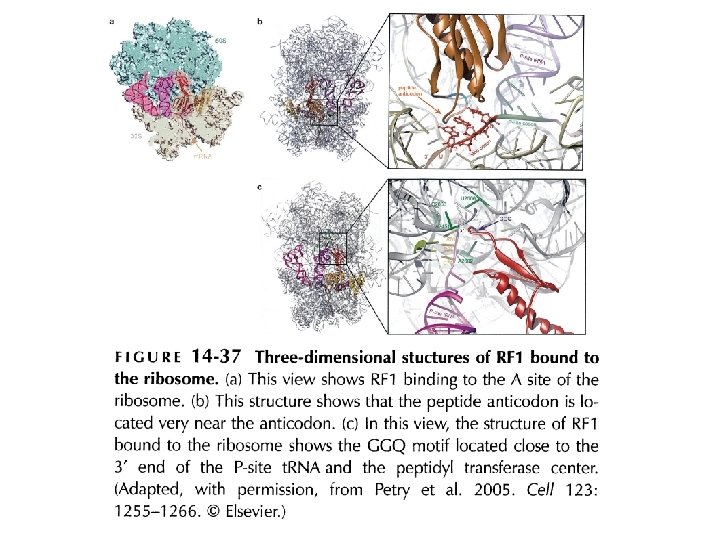
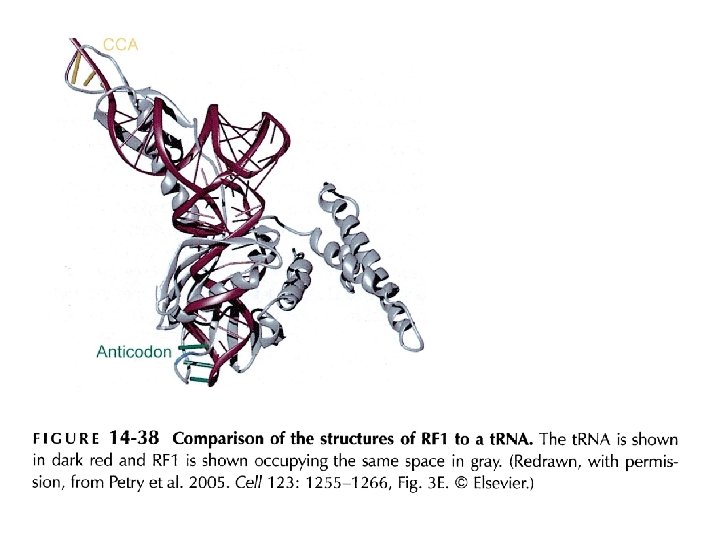
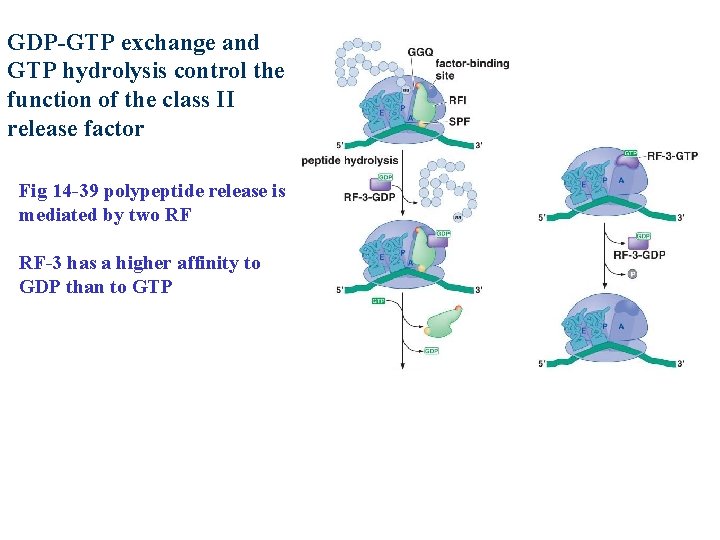
GDP-GTP exchange and GTP hydrolysis control the function of the class II release factor Fig 14 -39 polypeptide release is mediated by two RF RF-3 has a higher affinity to GDP than to GTP
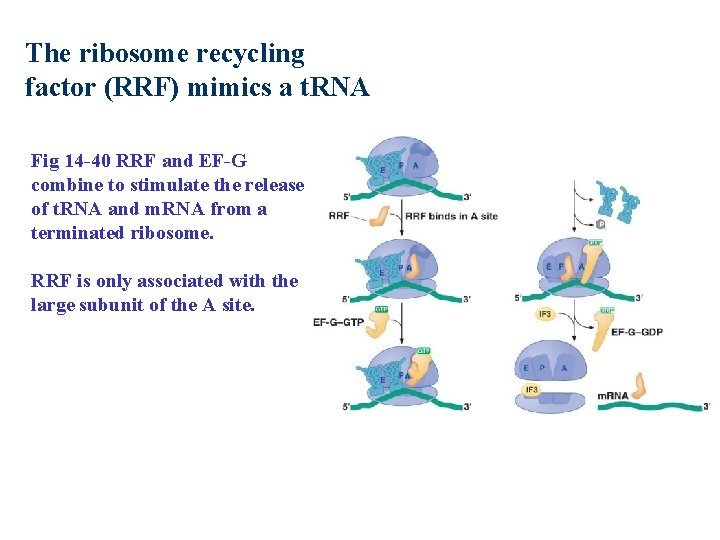
The ribosome recycling factor (RRF) mimics a t. RNA Fig 14 -40 RRF and EF-G combine to stimulate the release of t. RNA and m. RNA from a terminated ribosome. RRF is only associated with the large subunit of the A site.
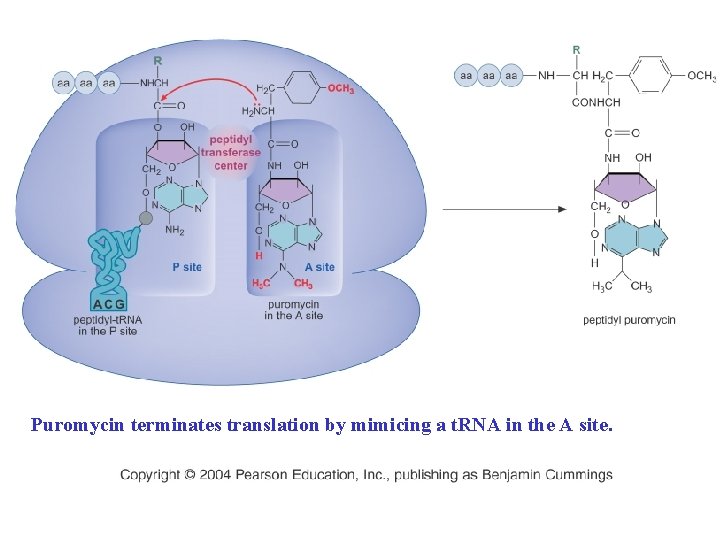
Puromycin terminates translation by mimicing a t. RNA in the A site.
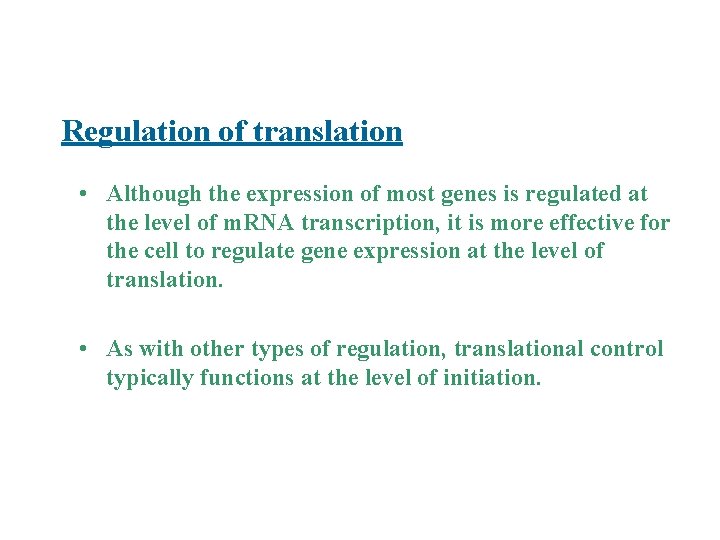
Regulation of translation • Although the expression of most genes is regulated at the level of m. RNA transcription, it is more effective for the cell to regulate gene expression at the level of translation. • As with other types of regulation, translational control typically functions at the level of initiation.
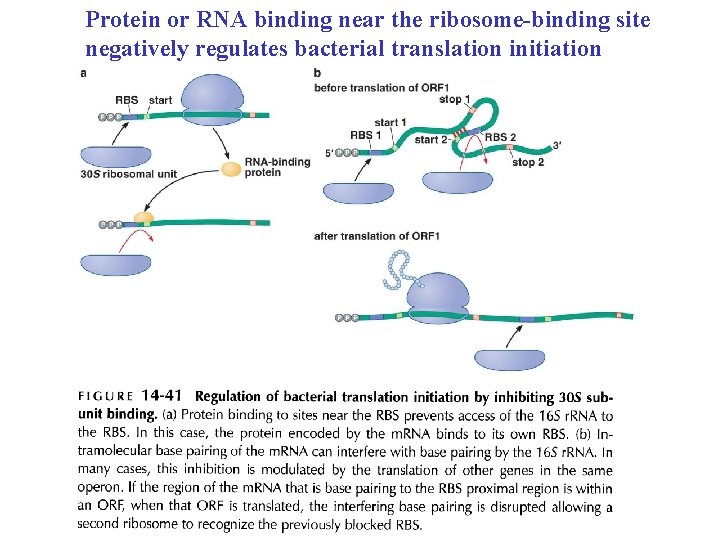
Protein or RNA binding near the ribosome-binding site negatively regulates bacterial translation initiation
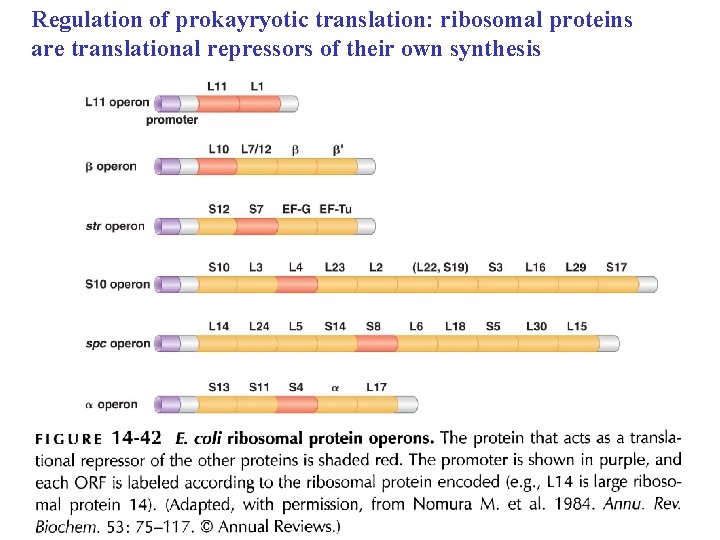
Regulation of prokayryotic translation: ribosomal proteins are translational repressors of their own synthesis
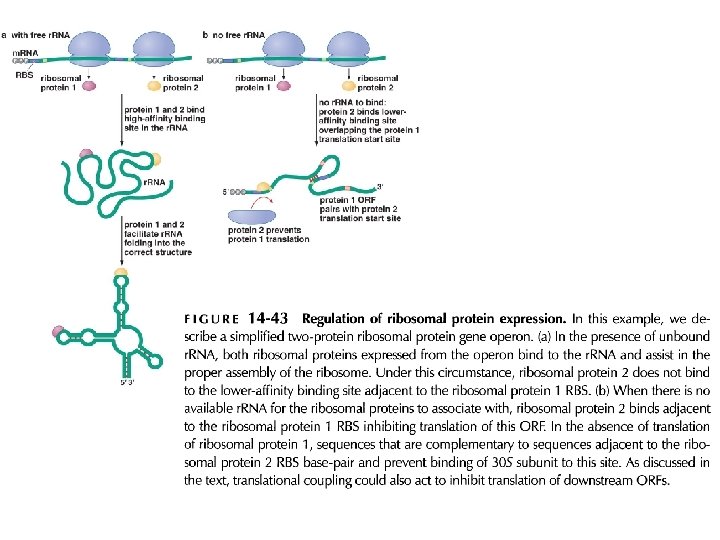
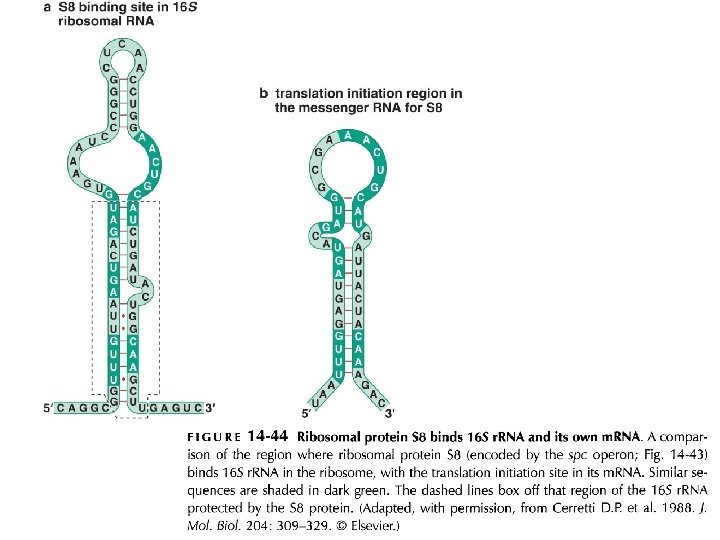
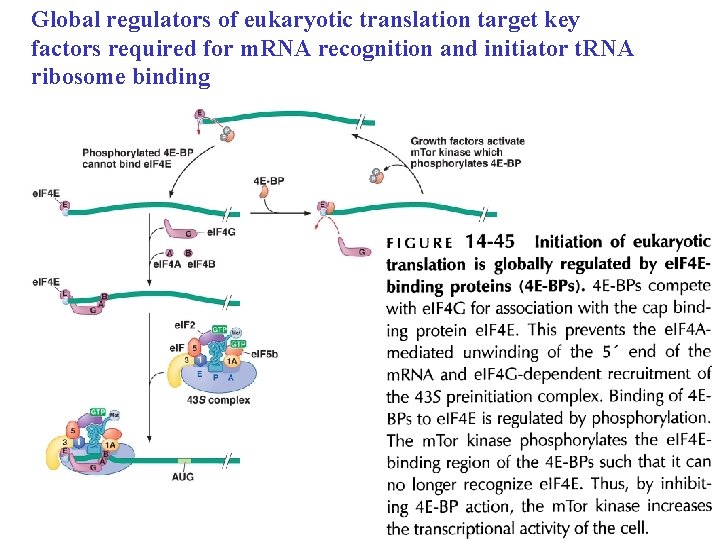
Global regulators of eukaryotic translation target key factors required for m. RNA recognition and initiator t. RNA ribosome binding
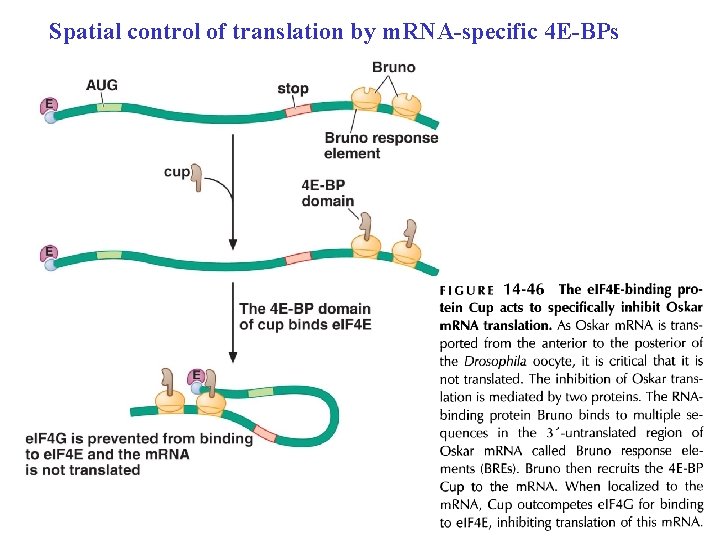
Spatial control of translation by m. RNA-specific 4 E-BPs
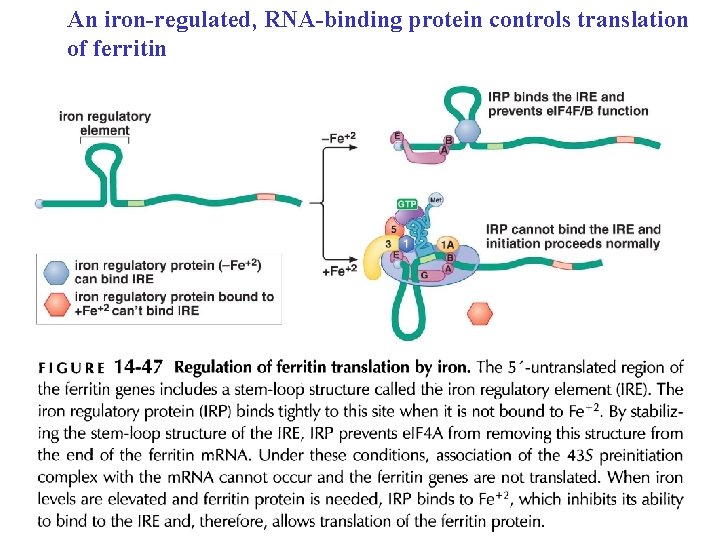
An iron-regulated, RNA-binding protein controls translation of ferritin
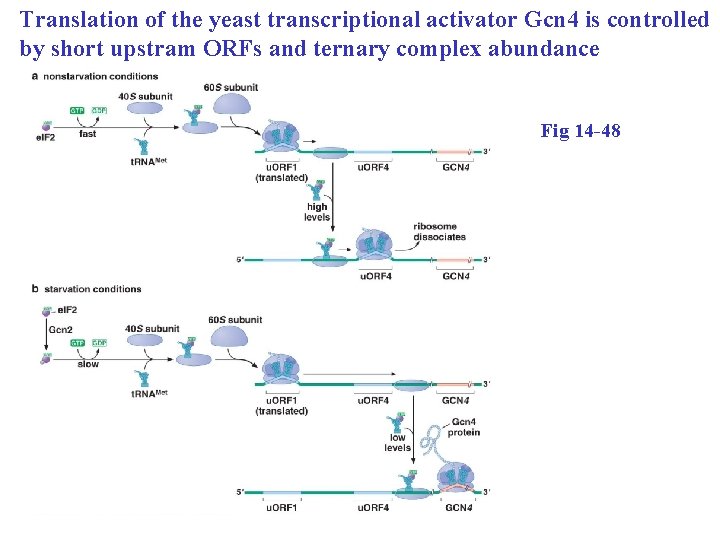
Translation of the yeast transcriptional activator Gcn 4 is controlled by short upstram ORFs and ternary complex abundance Fig 14 -48
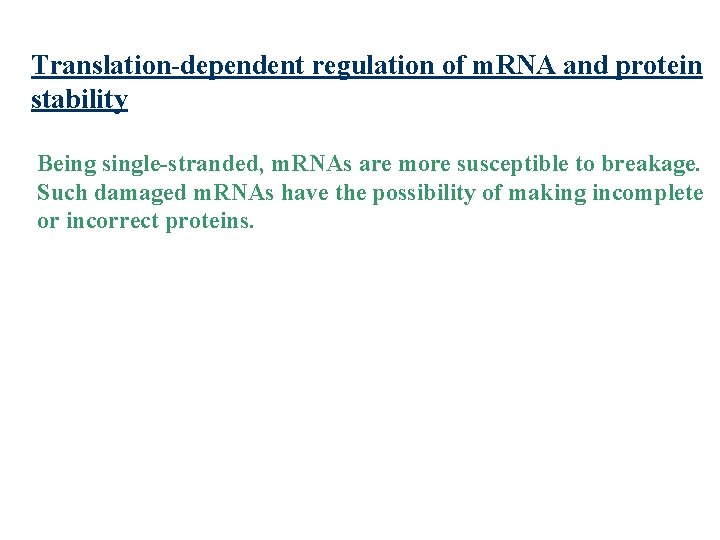
Translation-dependent regulation of m. RNA and protein stability Being single-stranded, m. RNAs are more susceptible to breakage. Such damaged m. RNAs have the possibility of making incomplete or incorrect proteins.
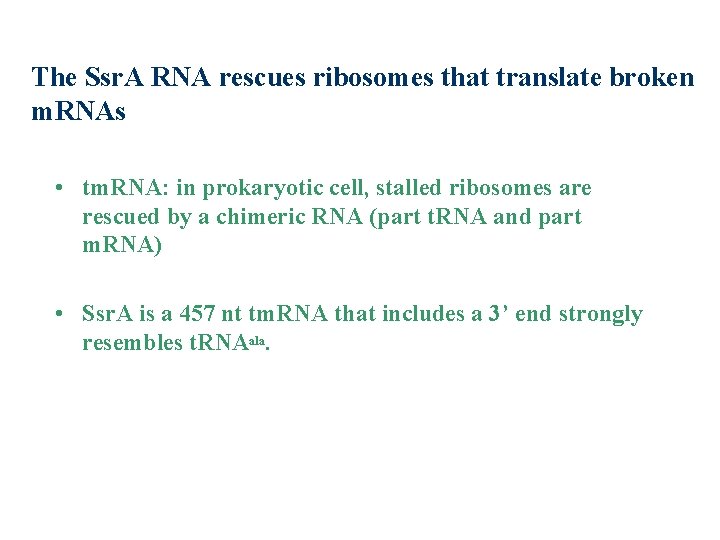
The Ssr. A RNA rescues ribosomes that translate broken m. RNAs • tm. RNA: in prokaryotic cell, stalled ribosomes are rescued by a chimeric RNA (part t. RNA and part m. RNA) • Ssr. A is a 457 nt tm. RNA that includes a 3’ end strongly resembles t. RNAala.
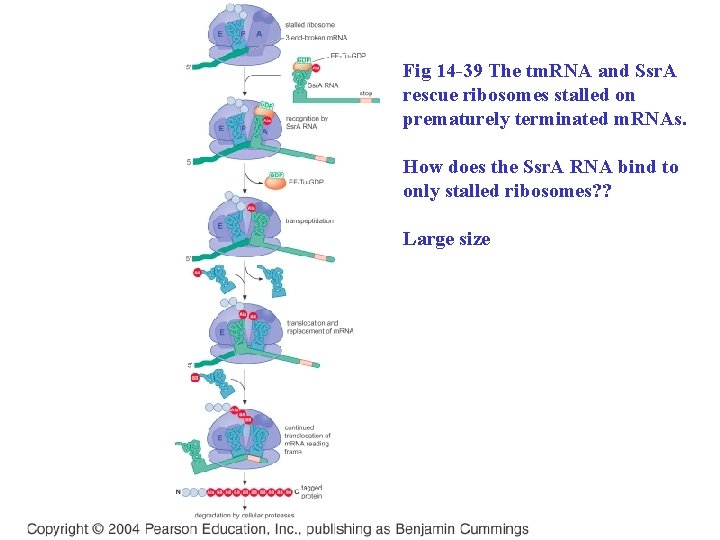
Fig 14 -39 The tm. RNA and Ssr. A rescue ribosomes stalled on prematurely terminated m. RNAs. How does the Ssr. A RNA bind to only stalled ribosomes? ? Large size
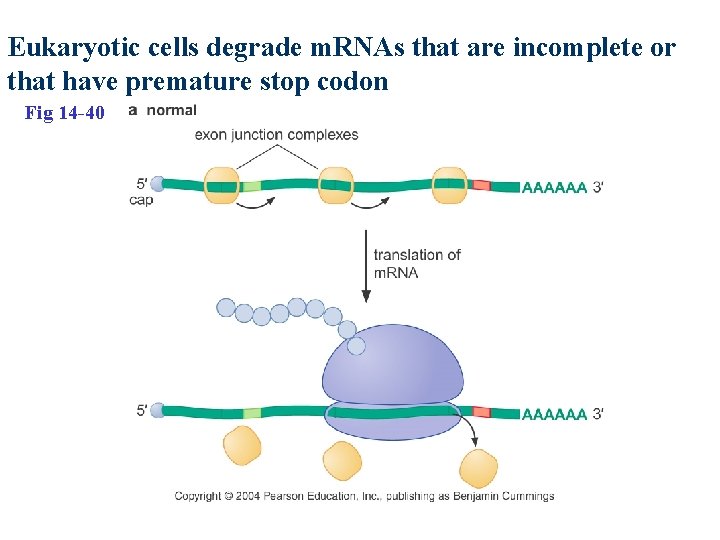
Eukaryotic cells degrade m. RNAs that are incomplete or that have premature stop codon Fig 14 -40
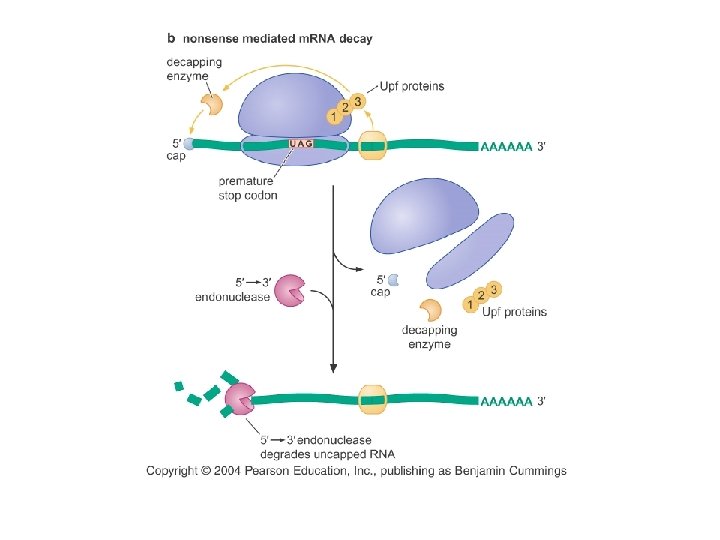
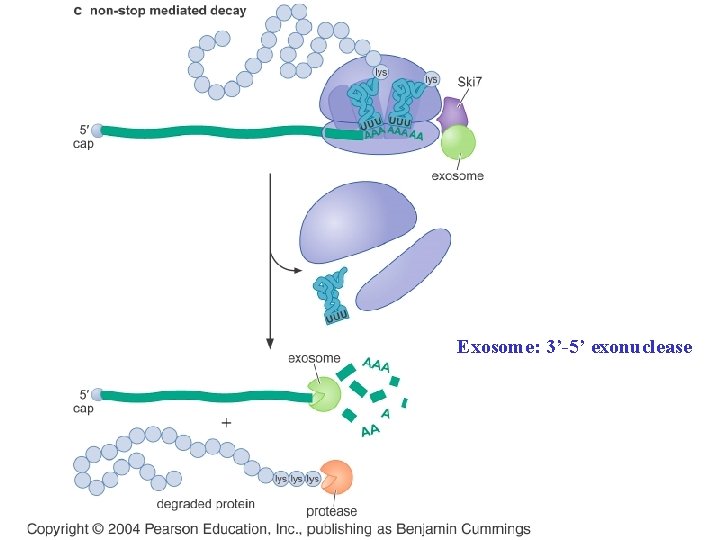
Exosome: 3’-5’ exonuclease
Messenger rna sequence
Codon chart
Messenger rna sequence
Protein synthesis
Polymeric gene interaction
Unlike dnarna contains
Protein synthesis
Rna transfer
Rna transfer
Compare and contrast dna and rna.
Dogma msc
Section 12 3 rna and protein synthesis
Northern blotting
Semantic theory
Transformation of functions
Cisco voice translation rule
Noun phrases
Is a disturbance that transfers energy from place to place
Pidgin facebook messenger
Calculator messenger
Gh
Kairos messenger
Nasza klasa blox
Second messenger hypothesis
Msn messenger chat rooms
What is jocasta
Squiggle messenger
What are the two main parts of a lipid?
Yahoo messenger
Web msn messenger live
Secondary messenger
Indeed in the messenger of allah
The messenger characterisation ed
I miss you allah
Messenger
Talk to nineveh messenger in the city
Msn 7 5
Analytical paragraph
Secure communication platform
Histamine
Messenger
I am the messenger spades summary
Greek messenger
Messenger web
Messenger
Messenger of god planet
Last messenger of god
2 cor 12:7-10
The messenger trainer
Rsview 32
Calculator messenger
Dynamic messenger
Instant messenger msn
Healthy emotional attachment
Objectives of attachment
Insert attachment
Attachment reflex
Rejection attachment
Stress breaker in rpd images
Outline and evaluate the learning theory of attachment
Function of the external oblique
Self concept attachment
Primitive defense mechanisms
Crittenden dmm
Secure attachment
Ainsworth et al 1978
Lateral position
Dismissive avoidant
Anxious attachment style
Internal occlusal rest
Disk attachment adalah
Employee attachment
What is ansa cervicalis
Schiller's potassium iodide test for attached gingiva
Outline and evaluate bowlby's monotropic theory 8 marks
Stress breaker dental bridge
Attachment styles in relationships