Aquatic Ecology Freshwater Part 2 Prof Dr N
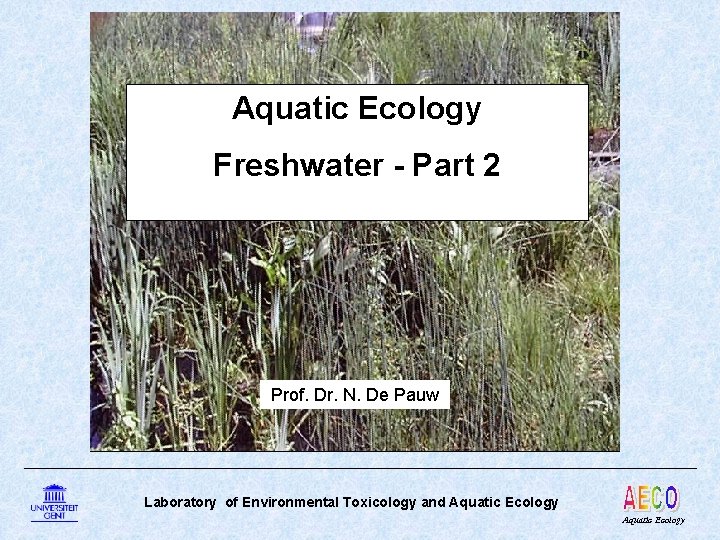
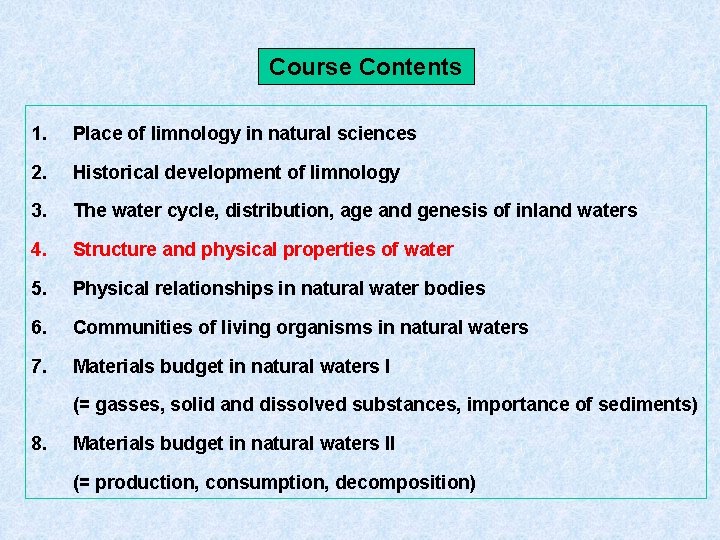
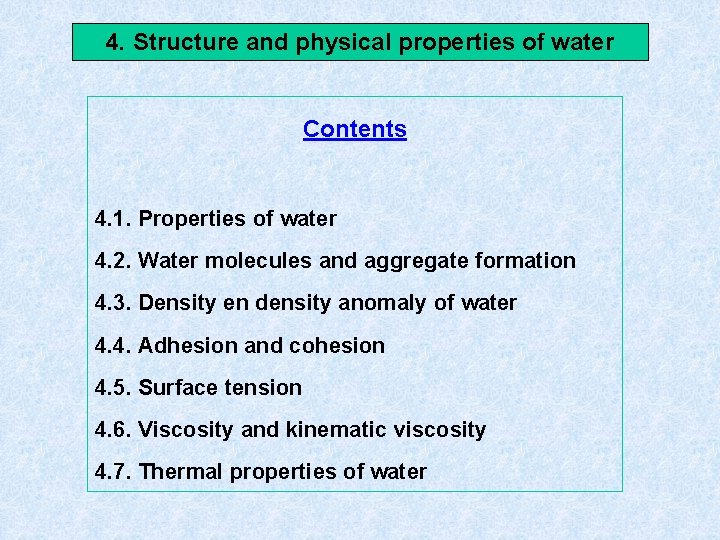
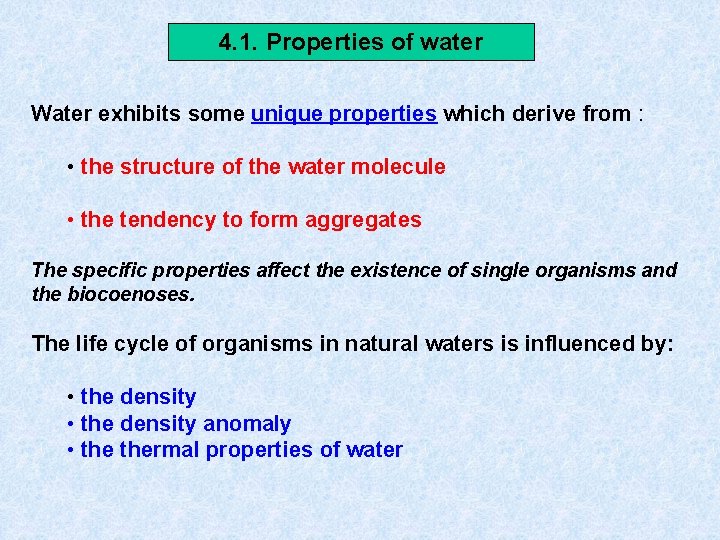
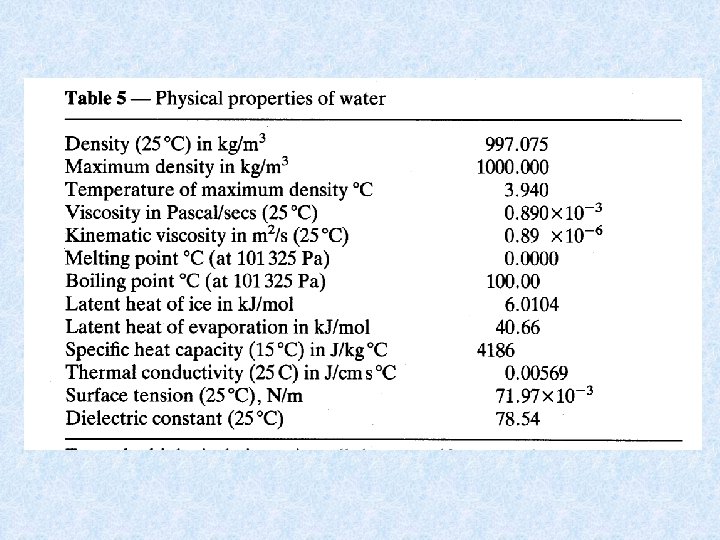
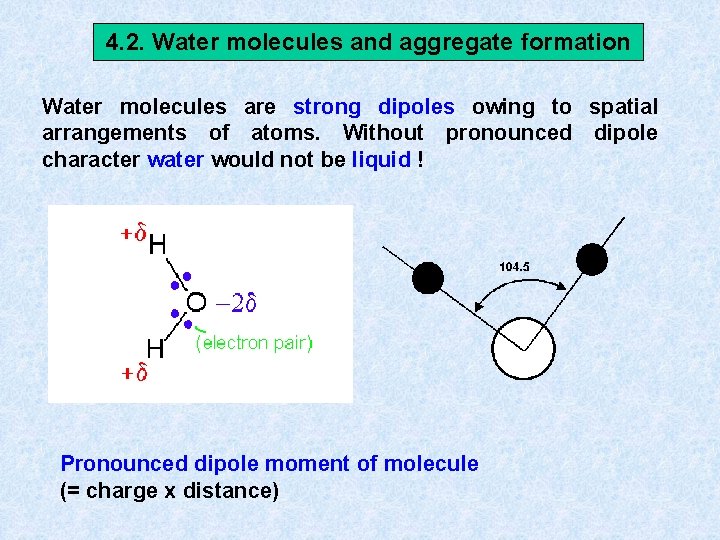
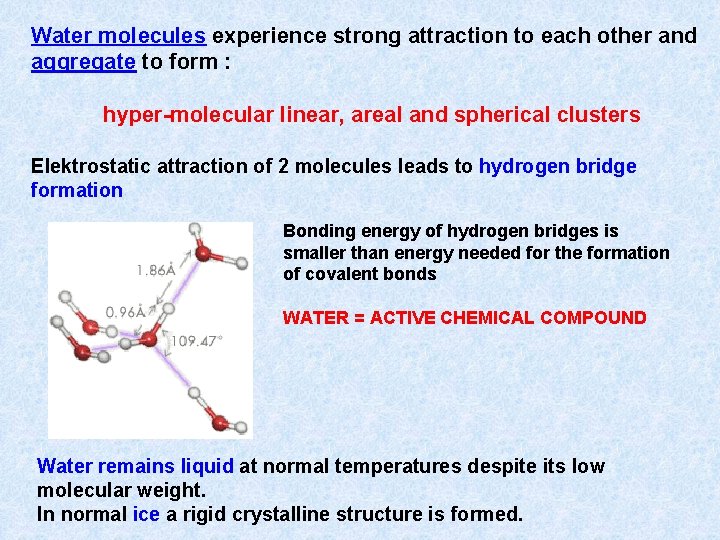
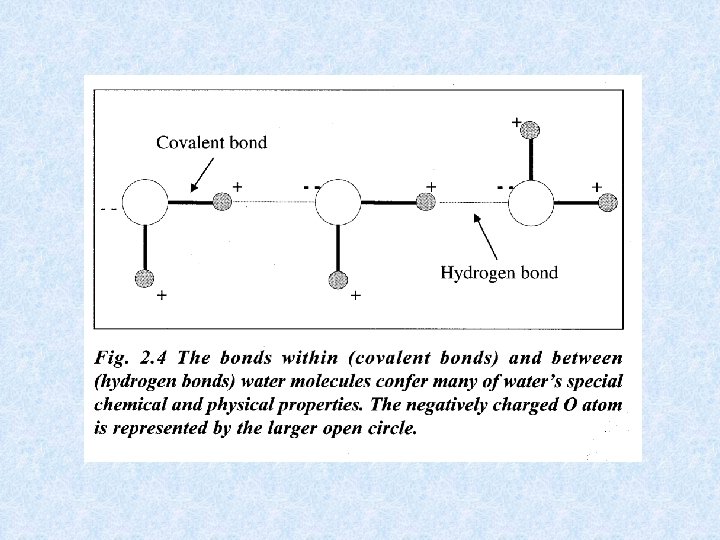
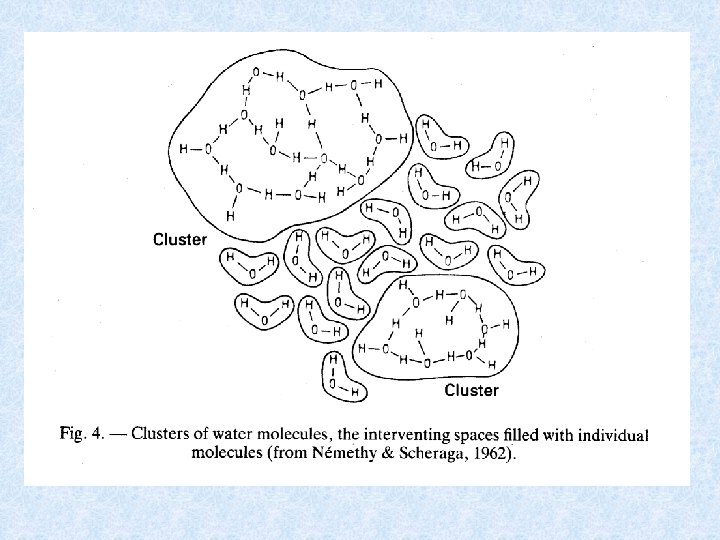
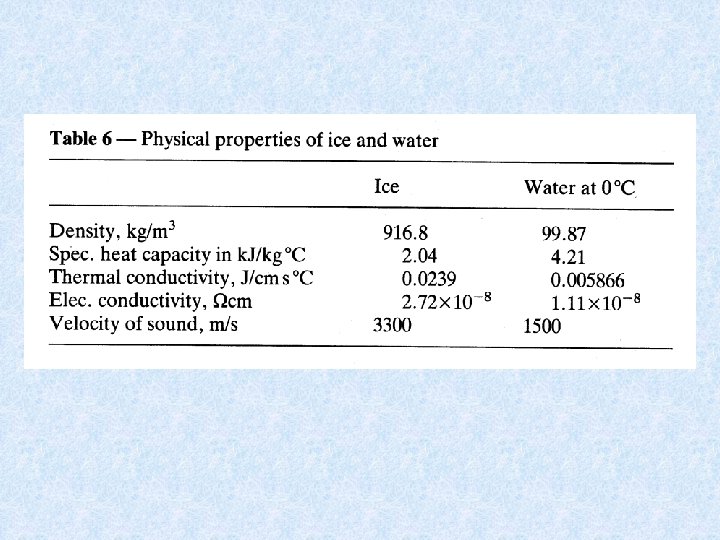
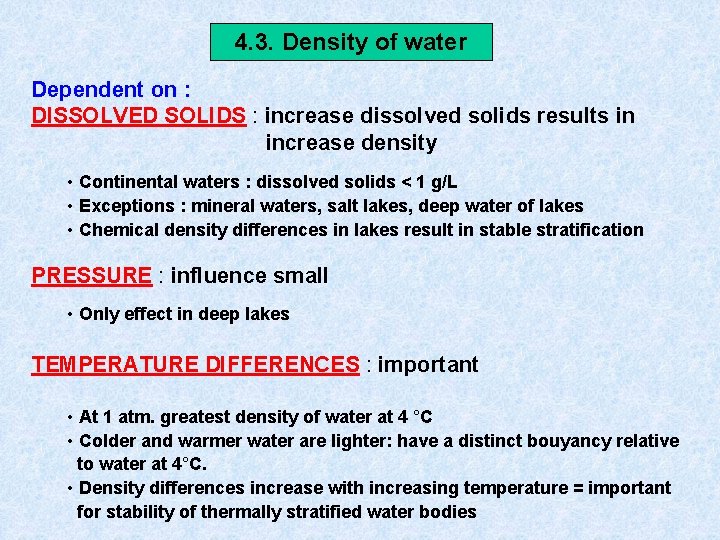
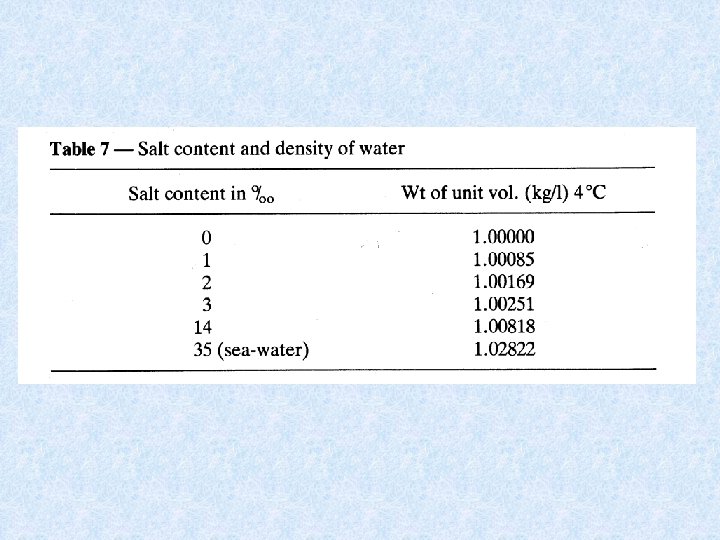
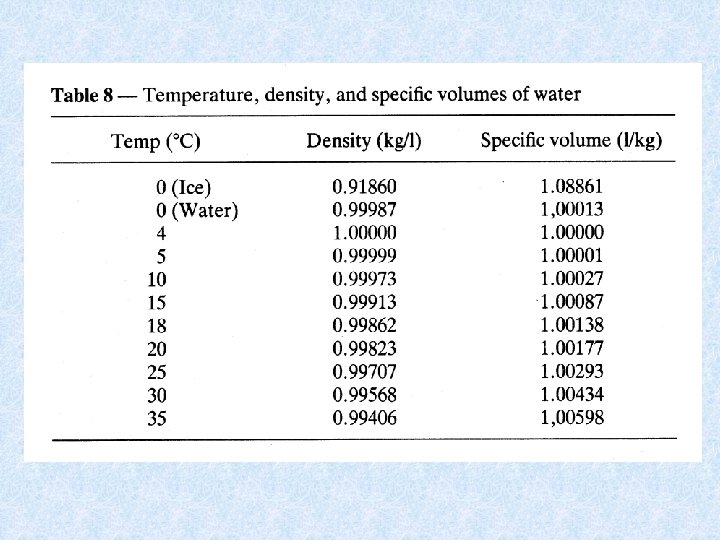
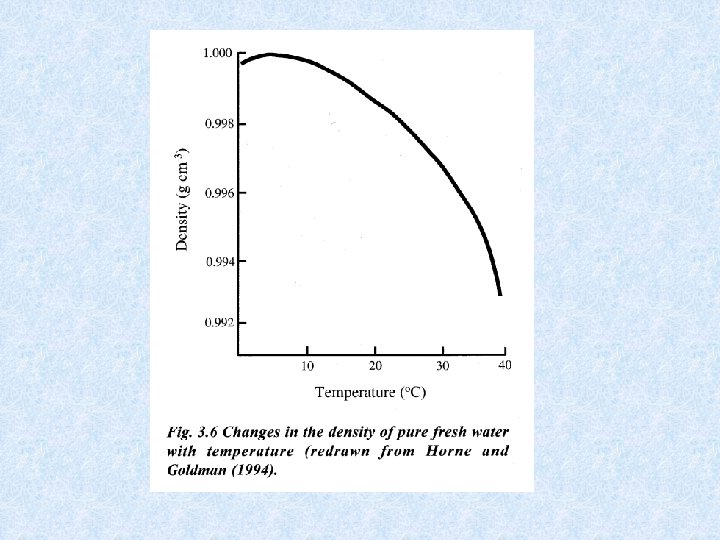
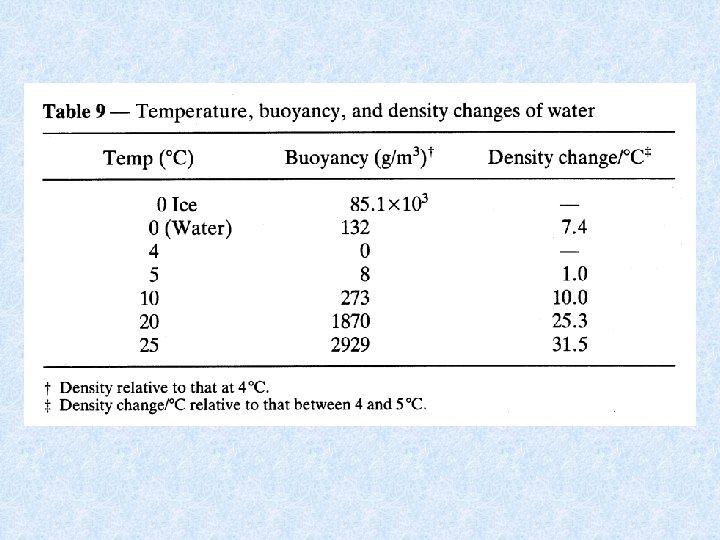
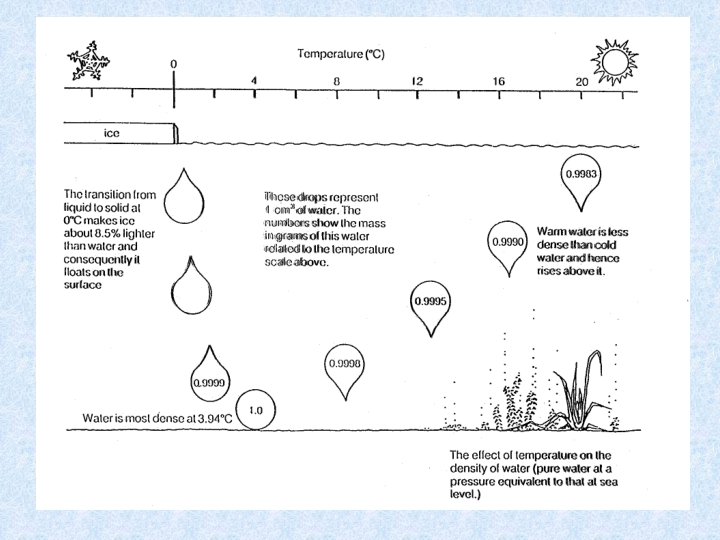
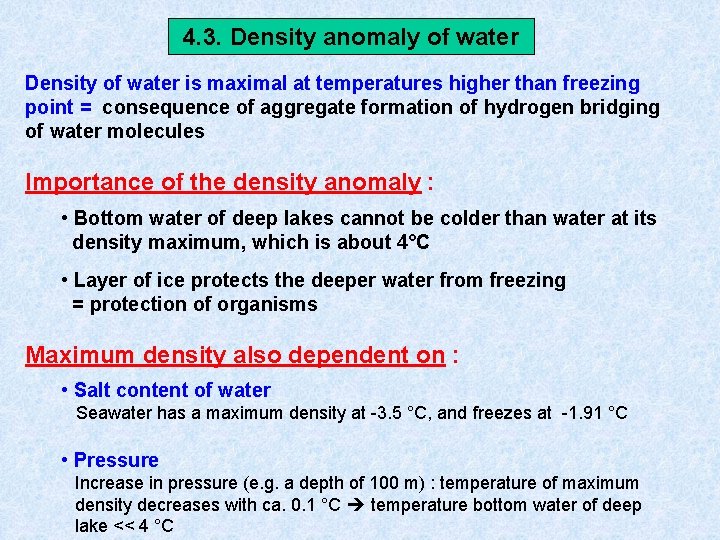
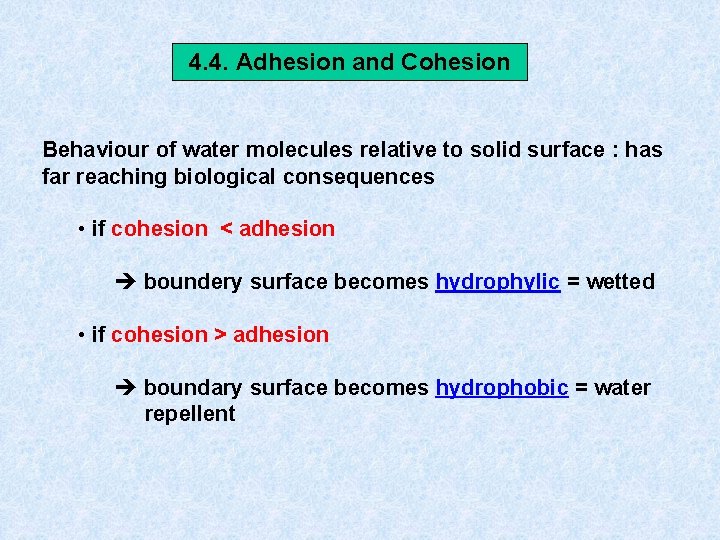
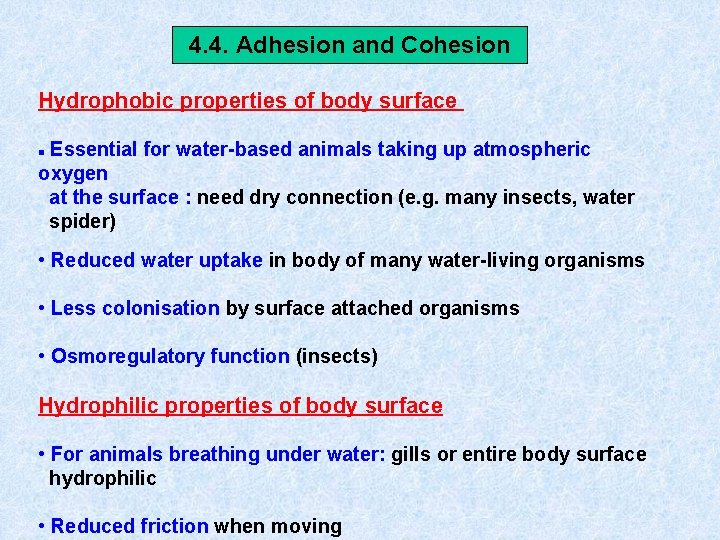
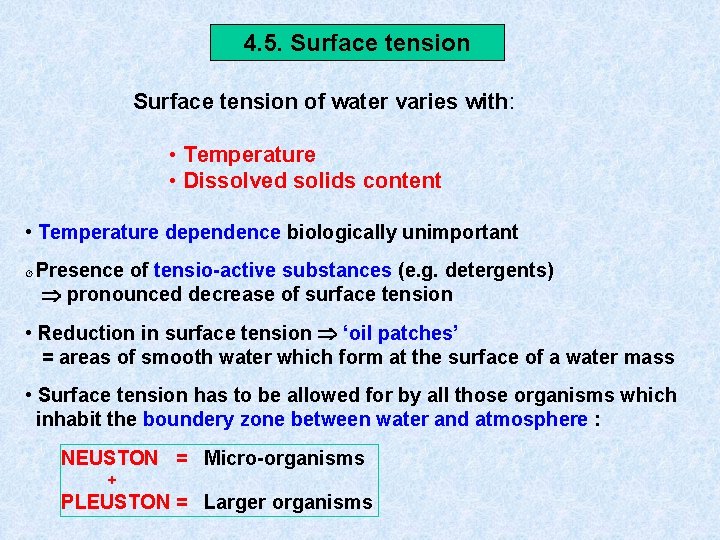
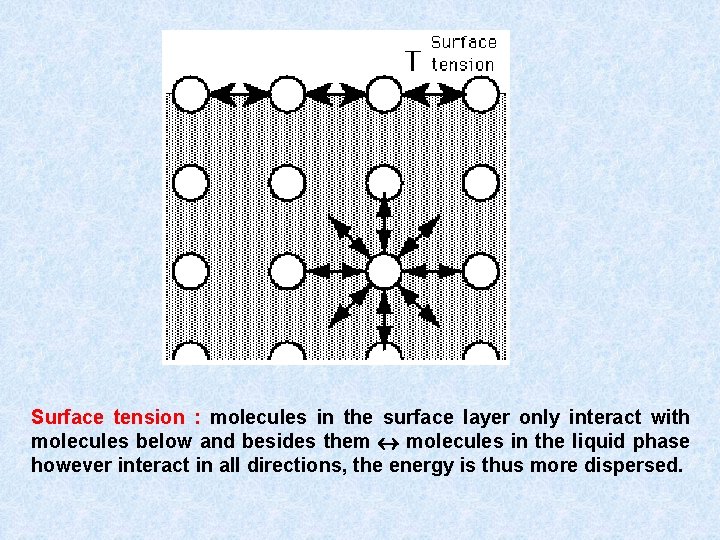
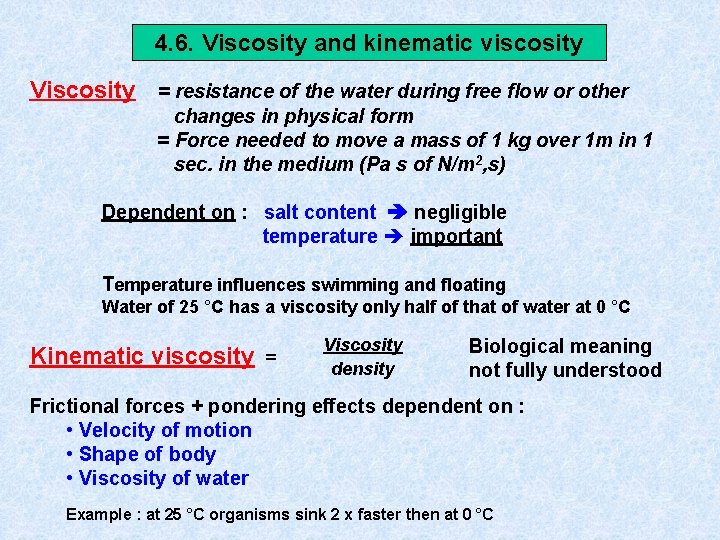
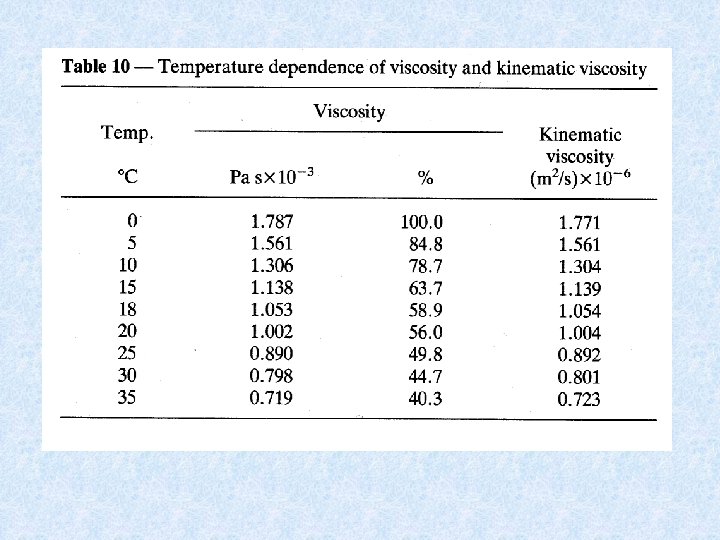
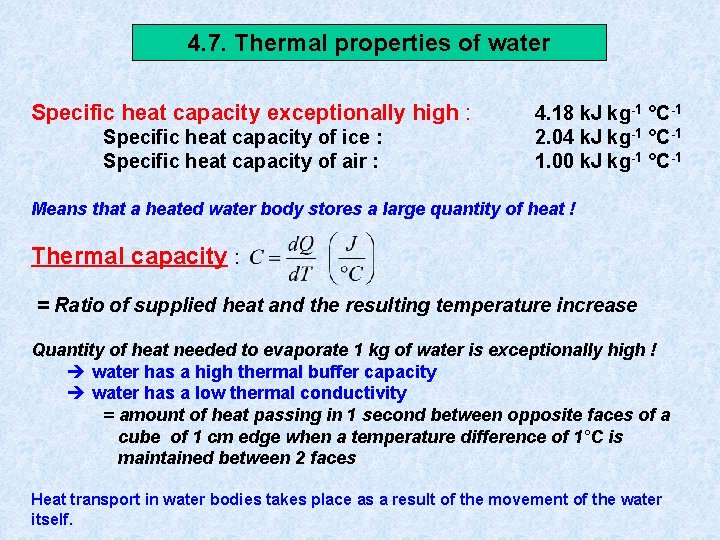
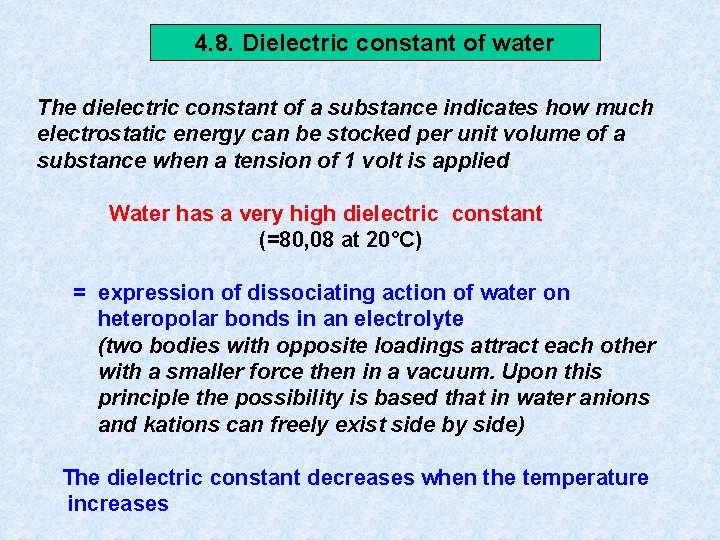
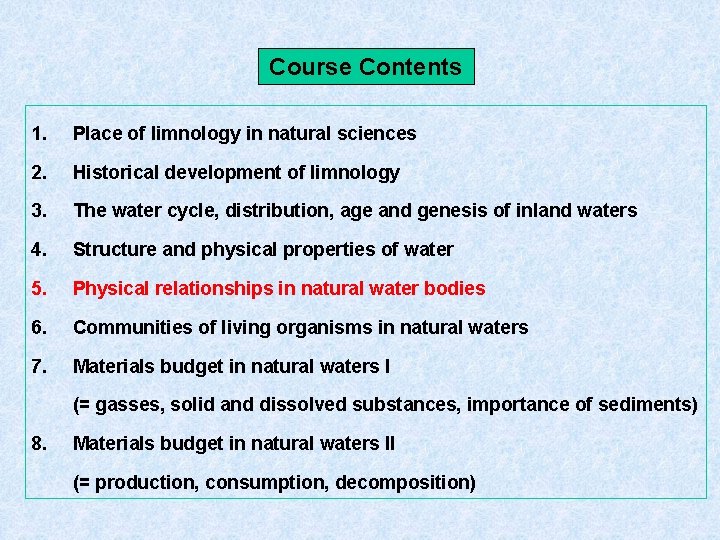
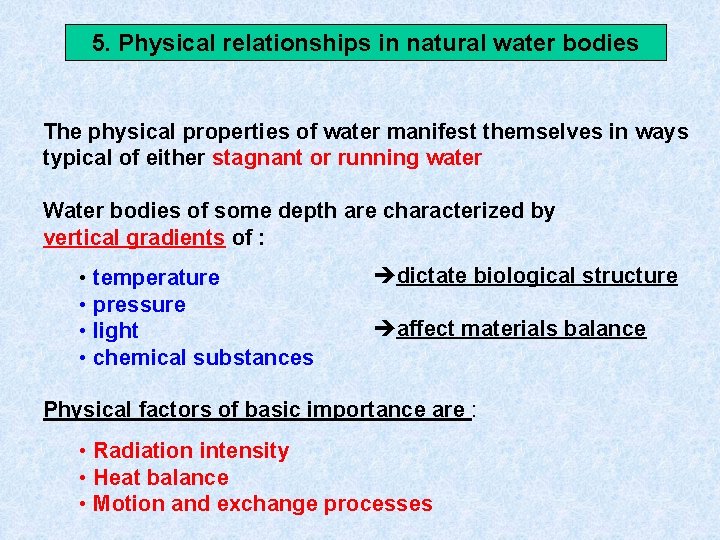
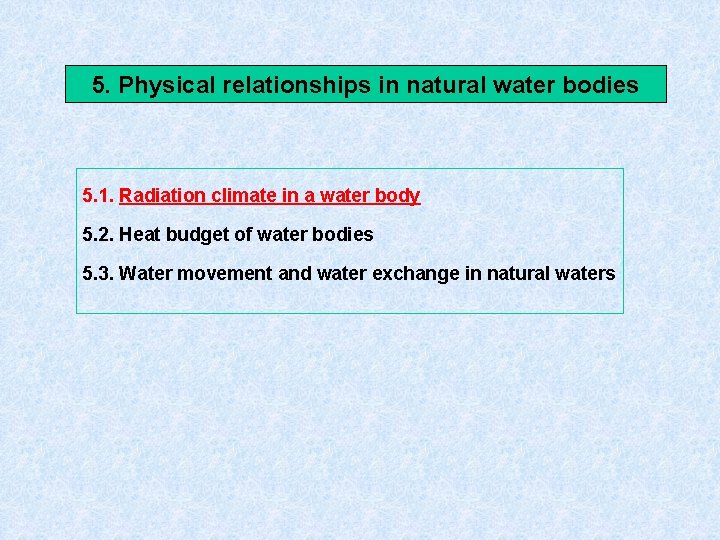
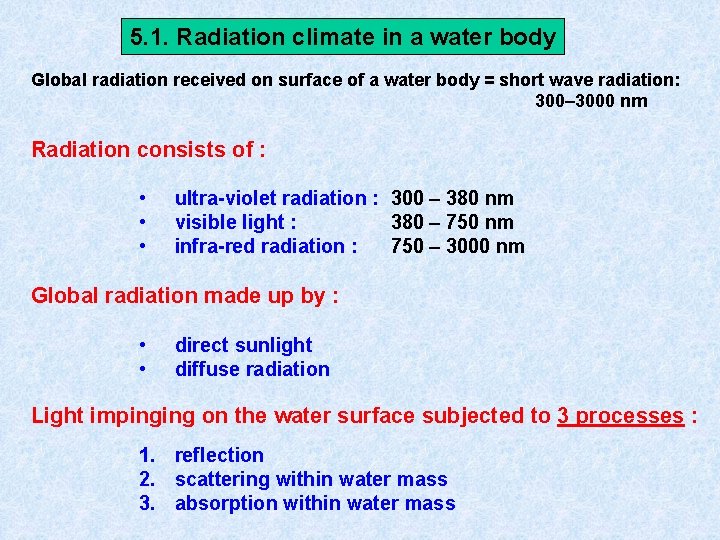
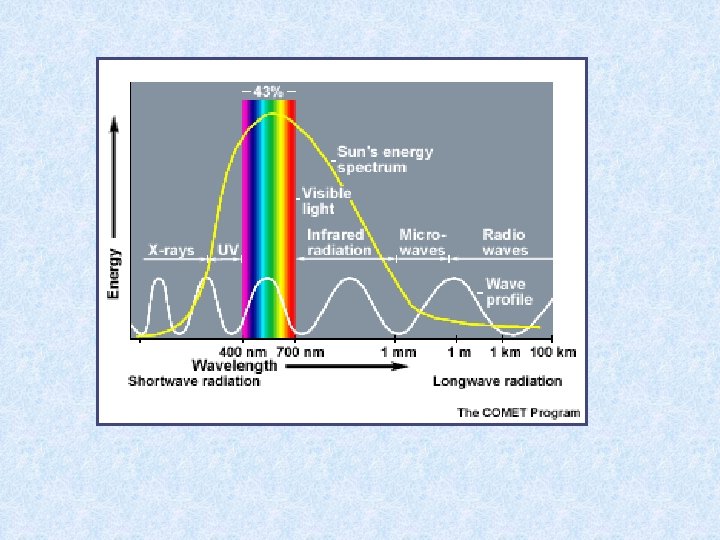
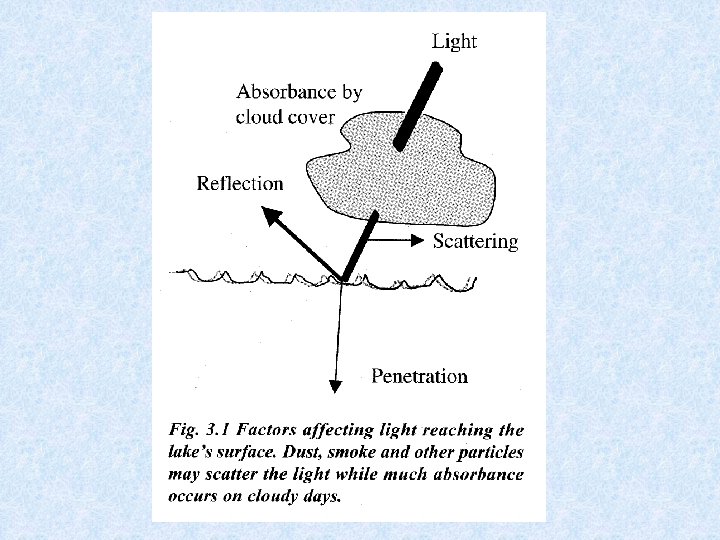
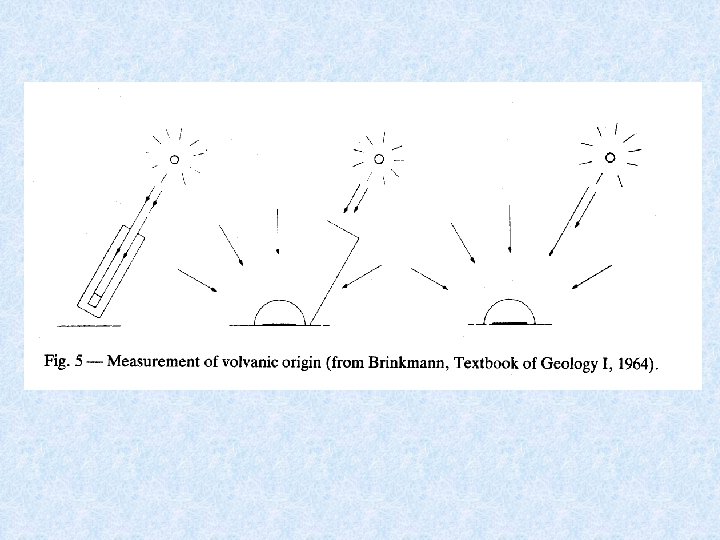
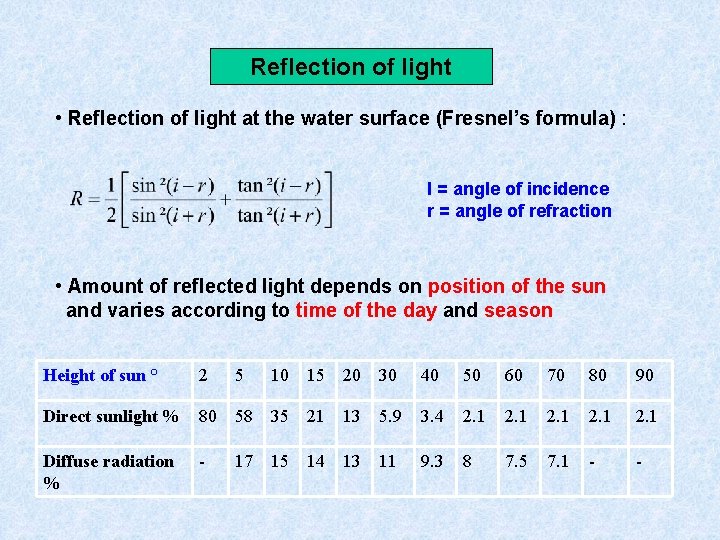
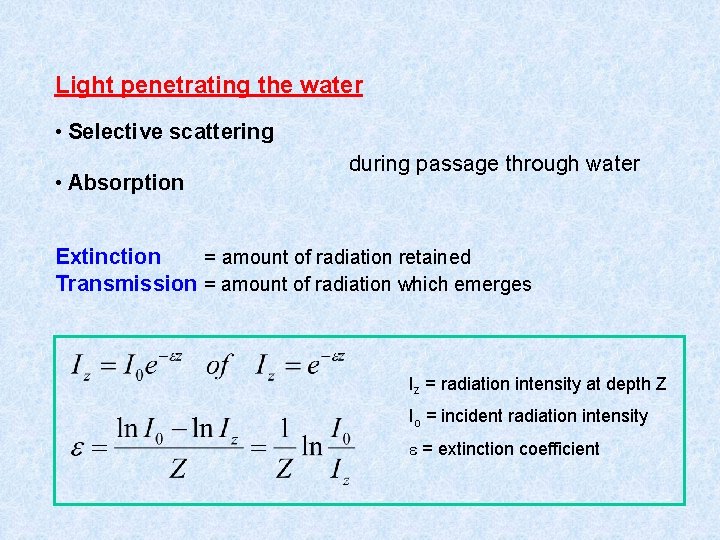
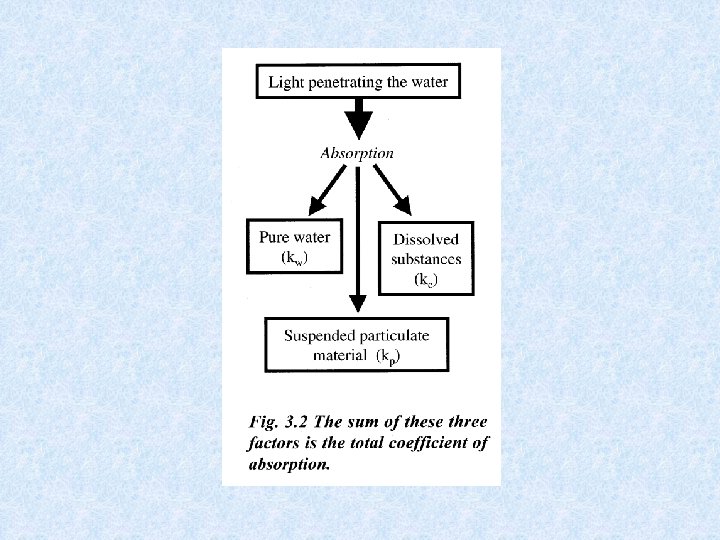
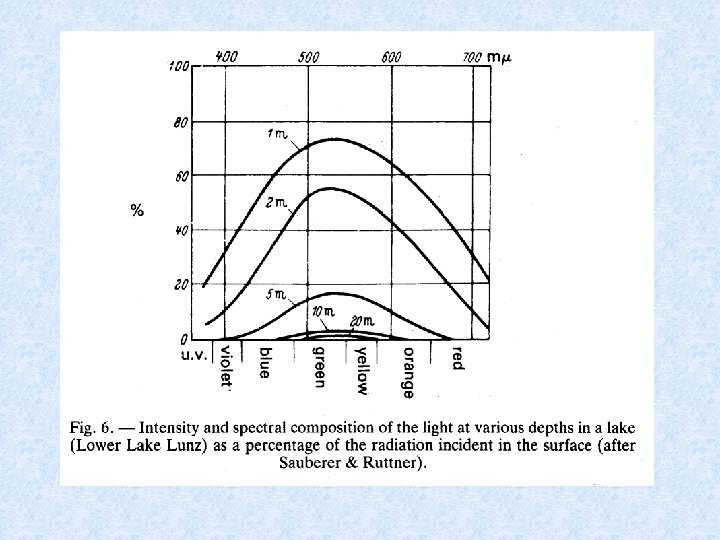
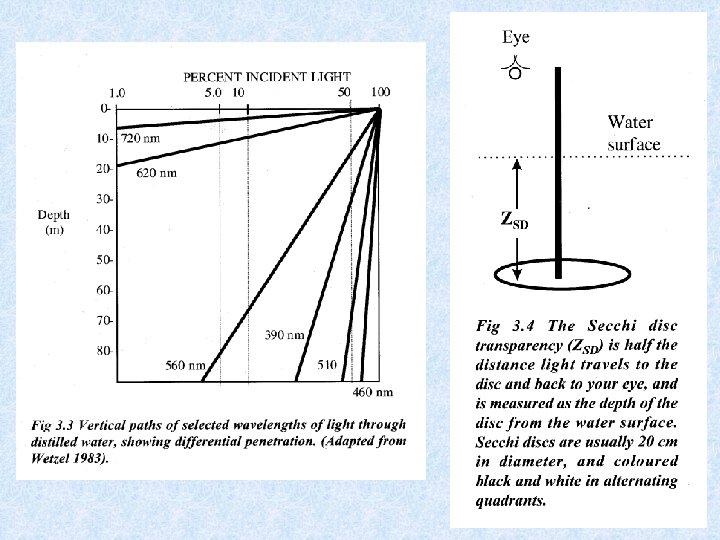
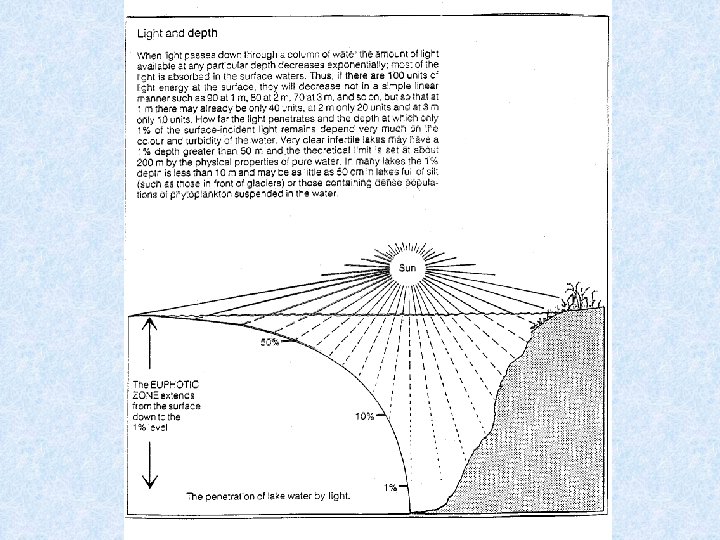
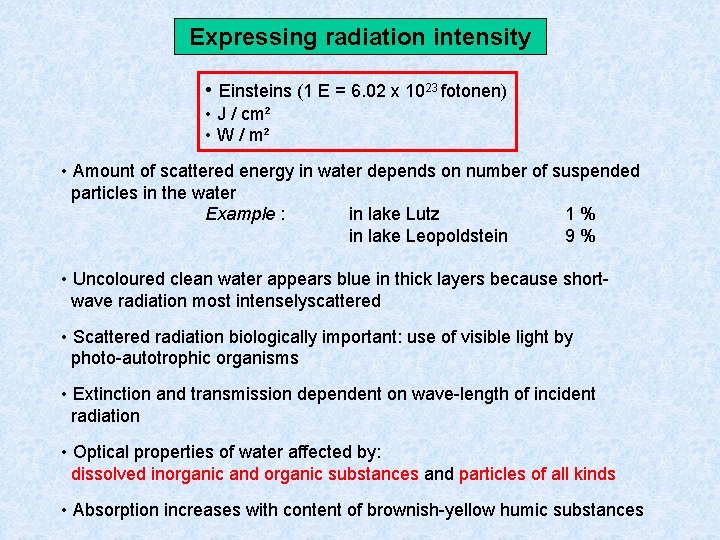
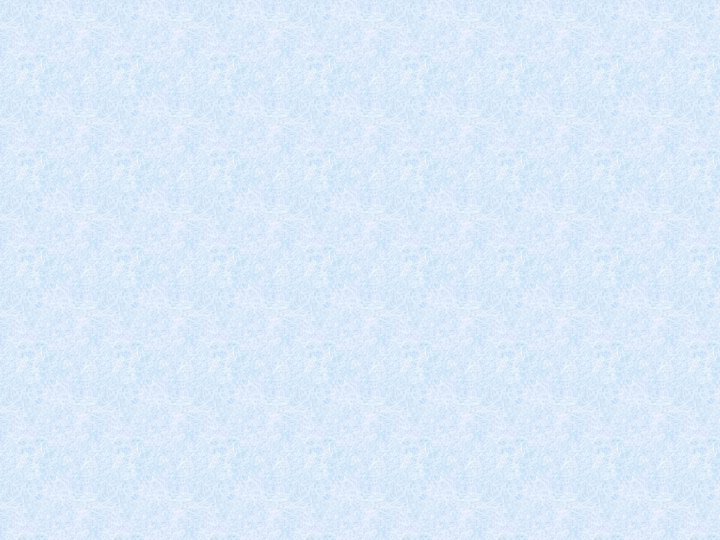
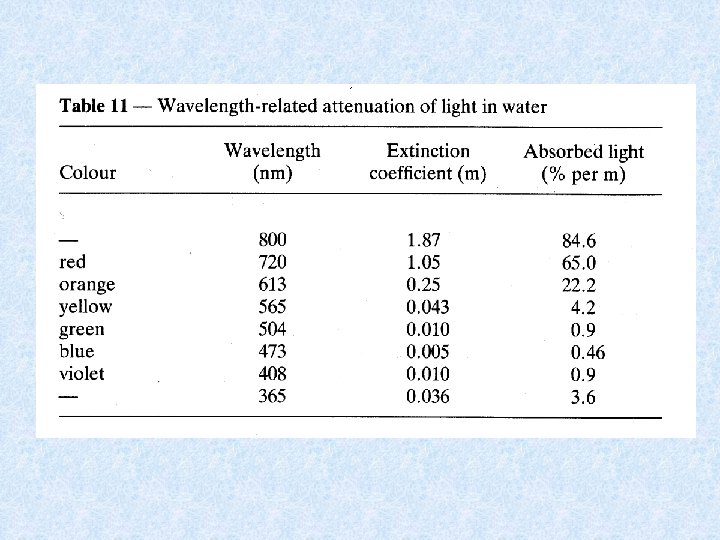
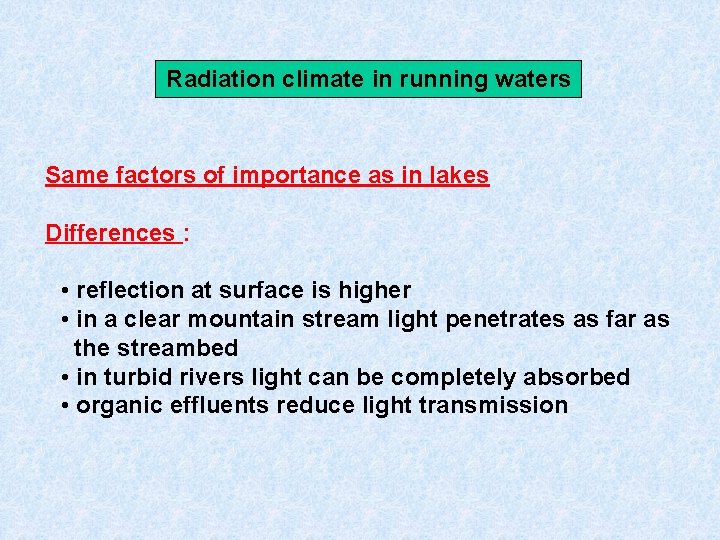
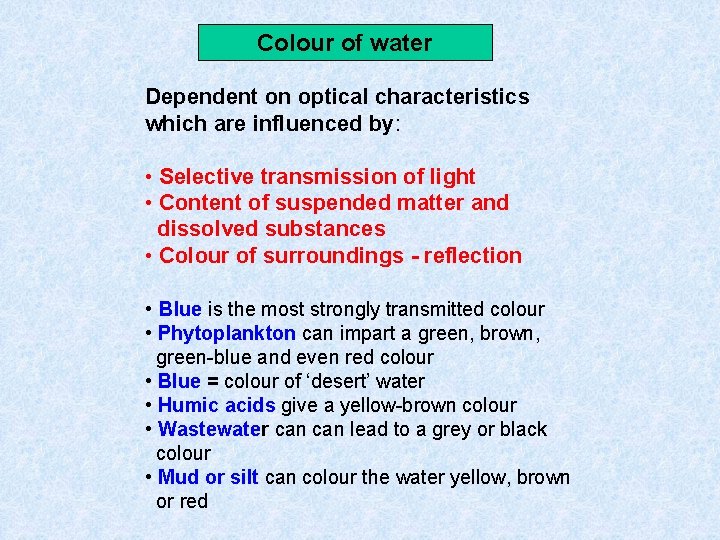
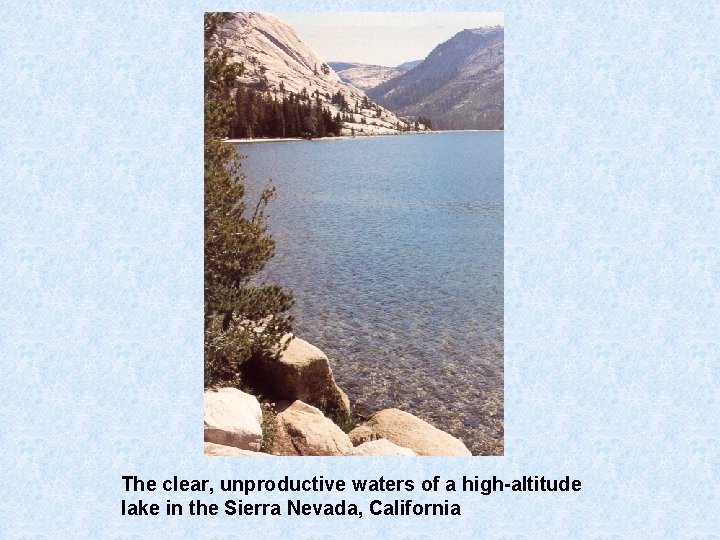
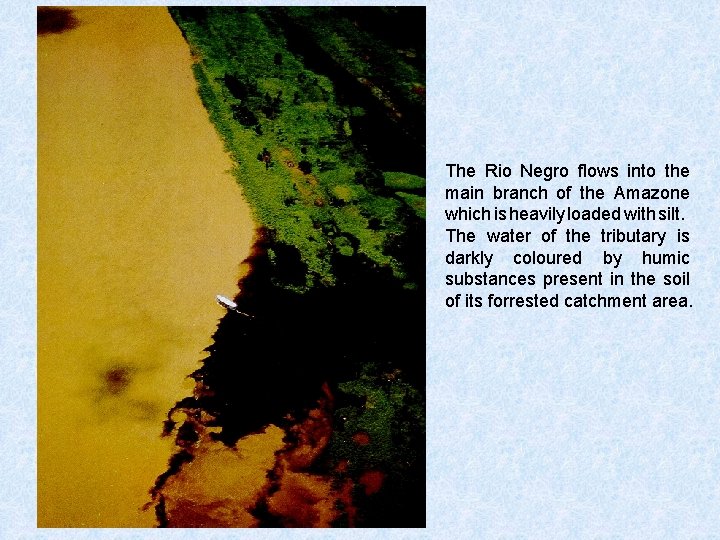
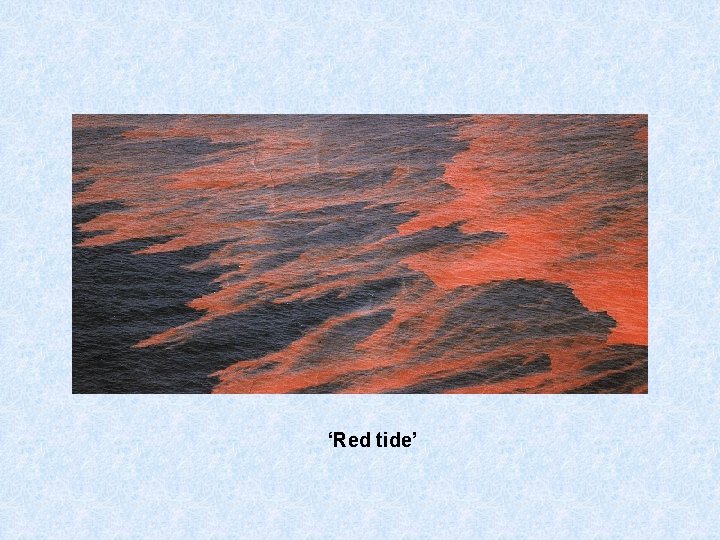
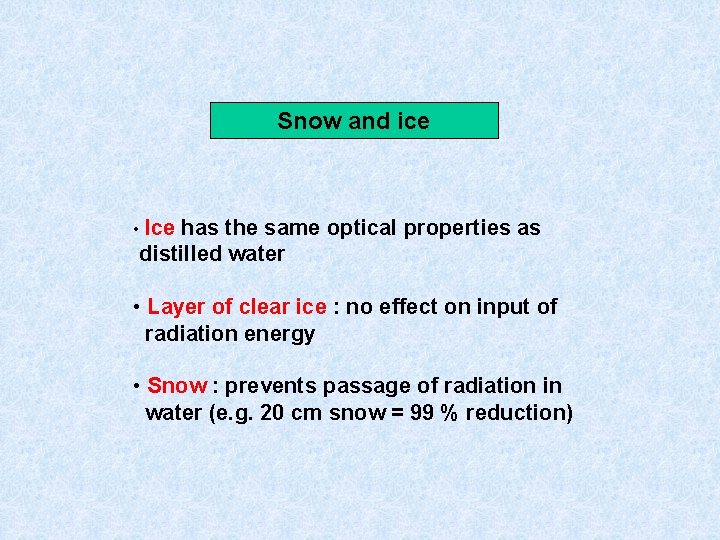
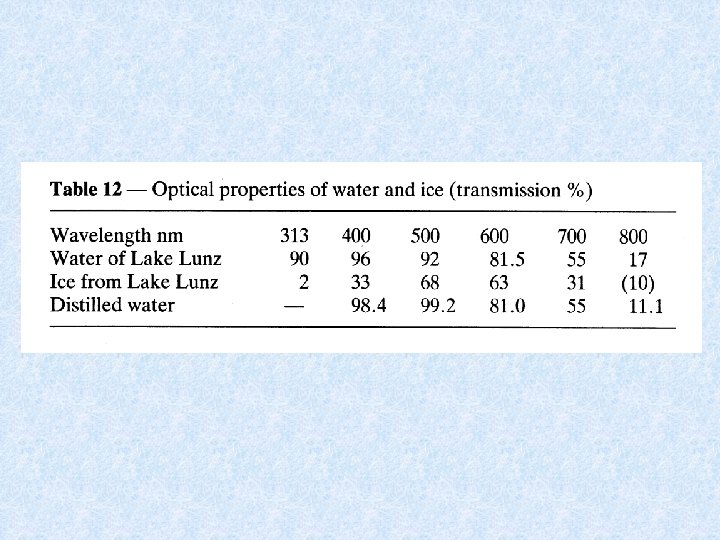
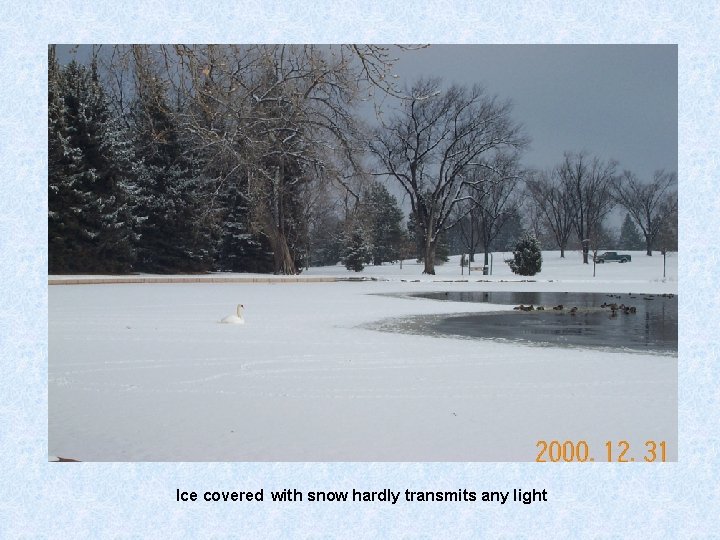
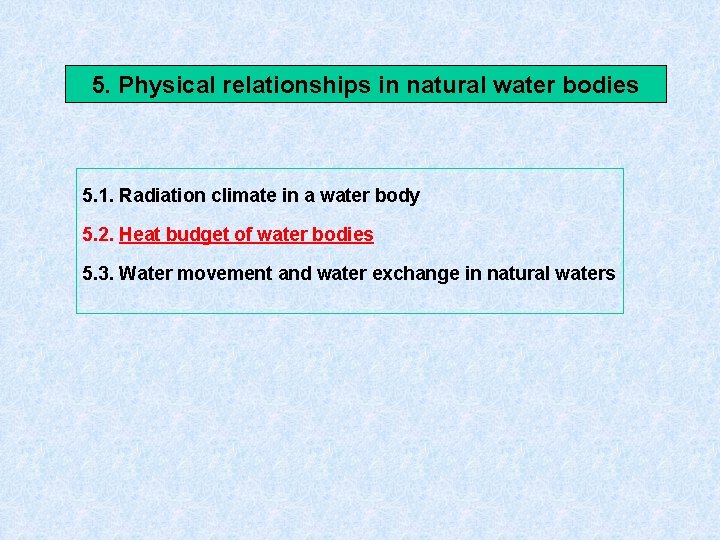
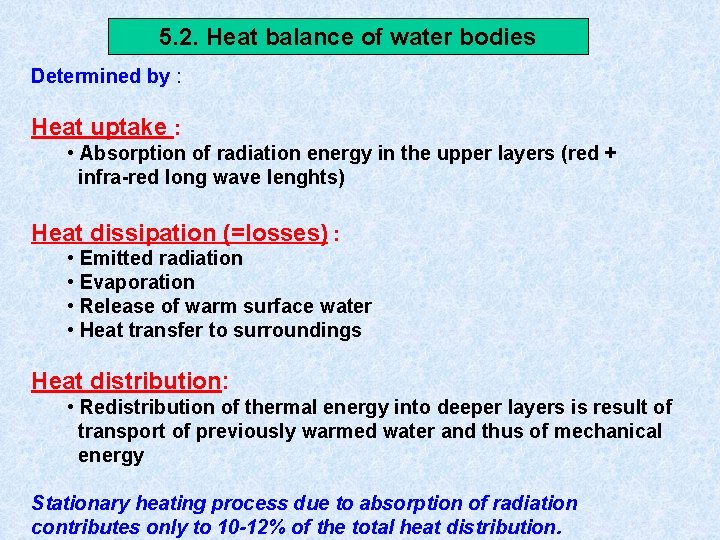
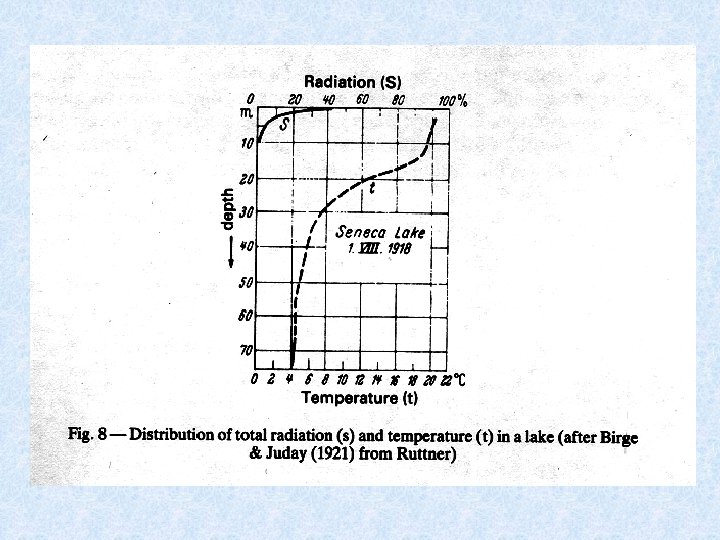
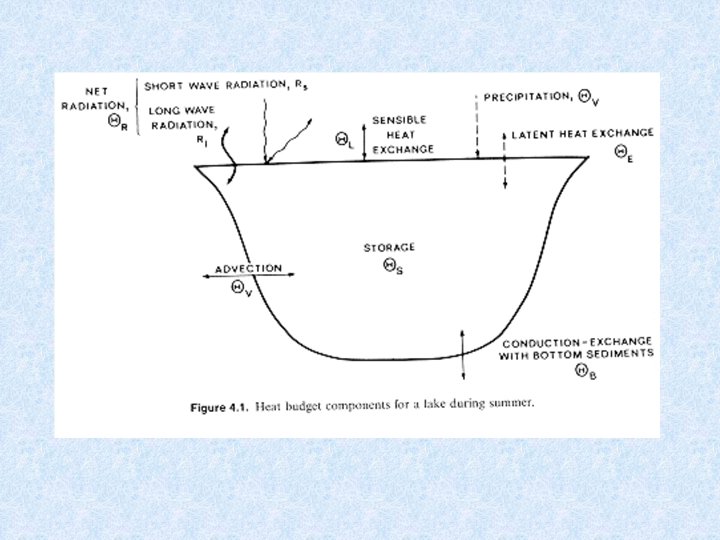
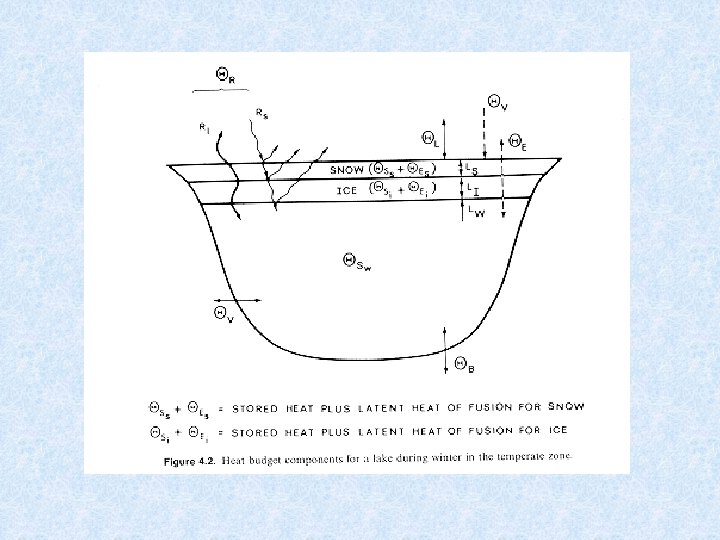
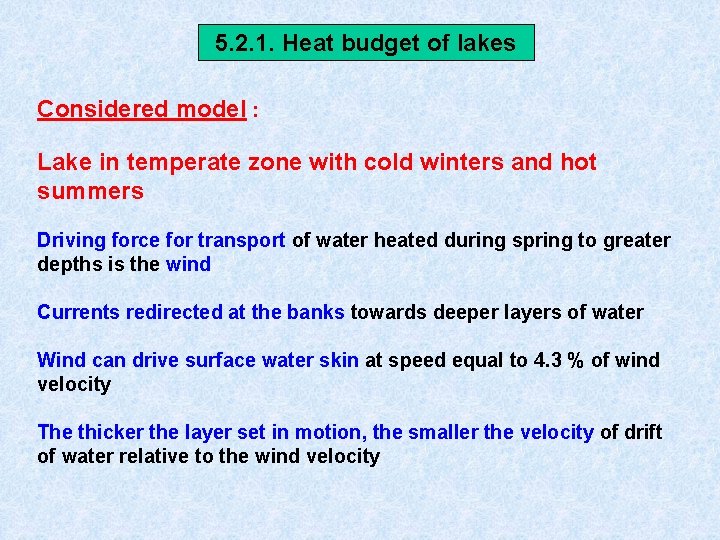
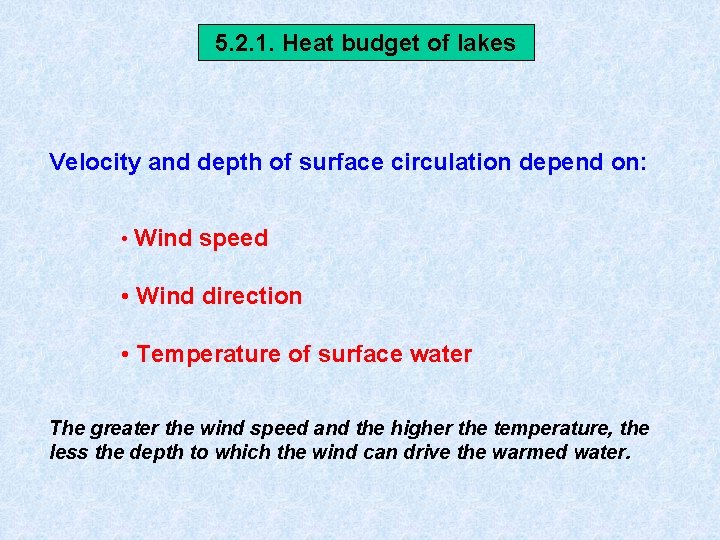
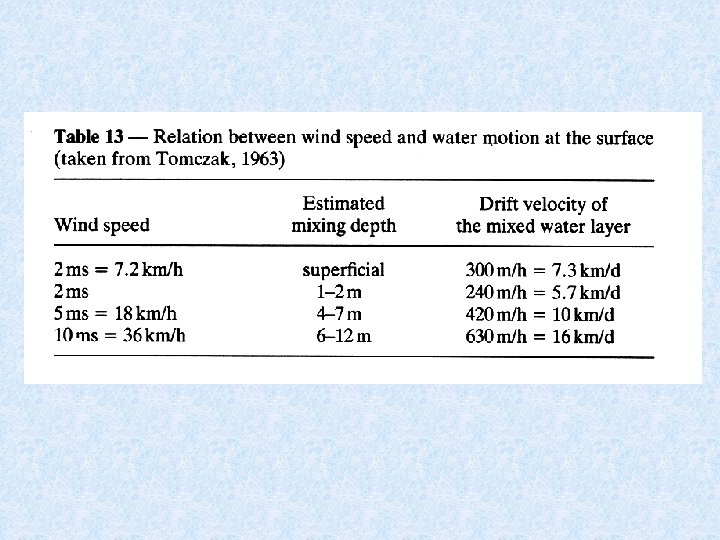
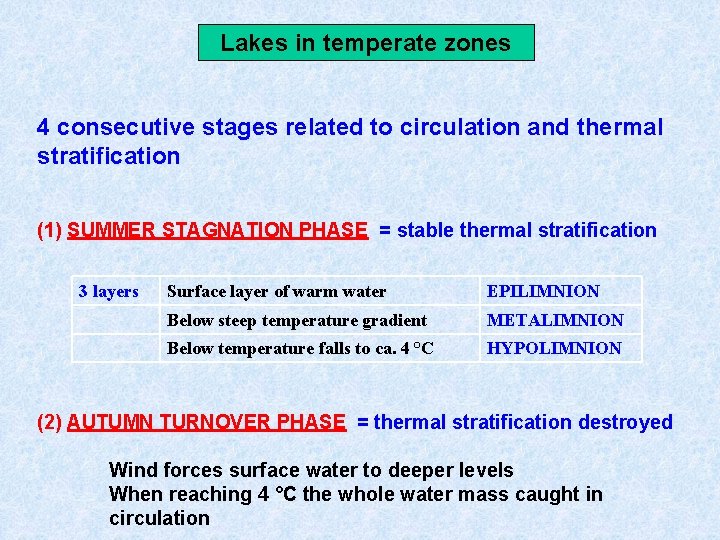
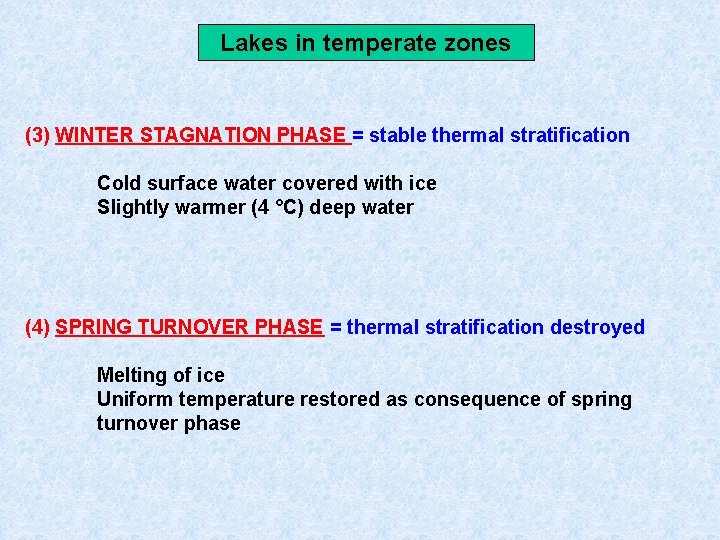
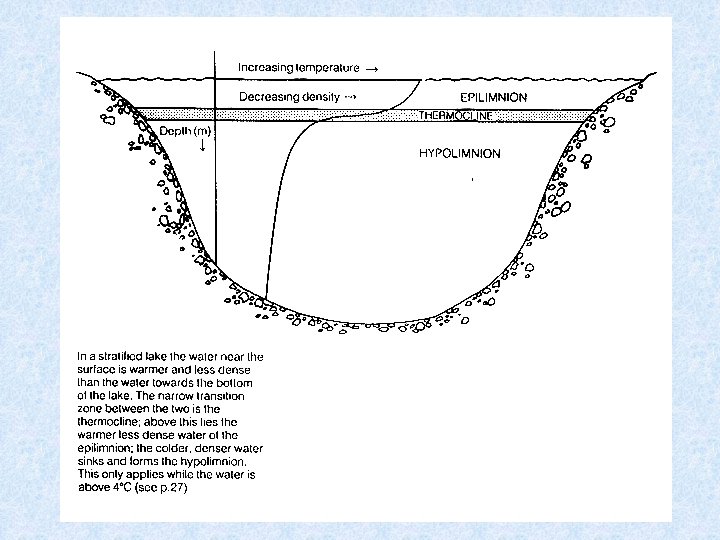
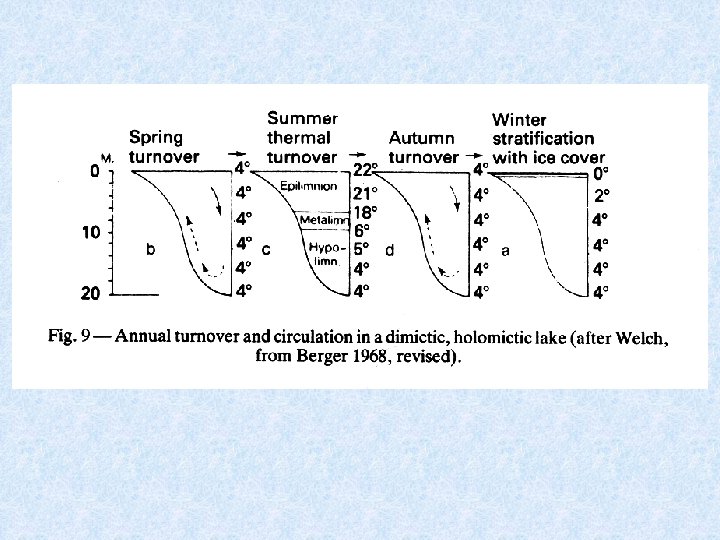
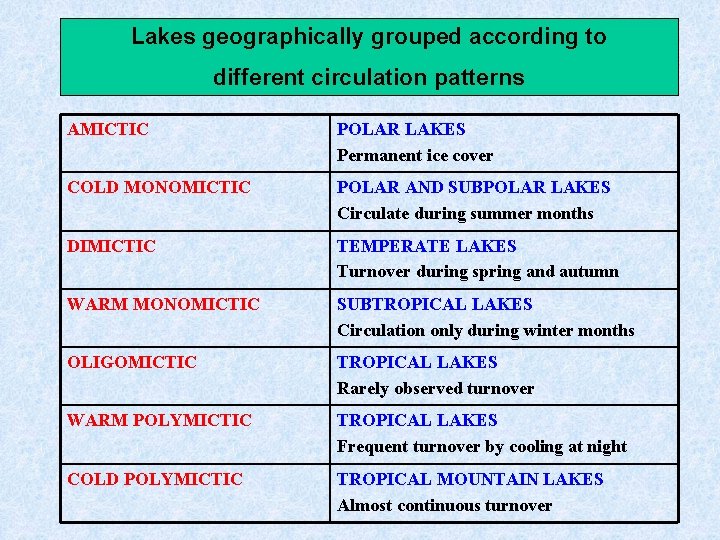
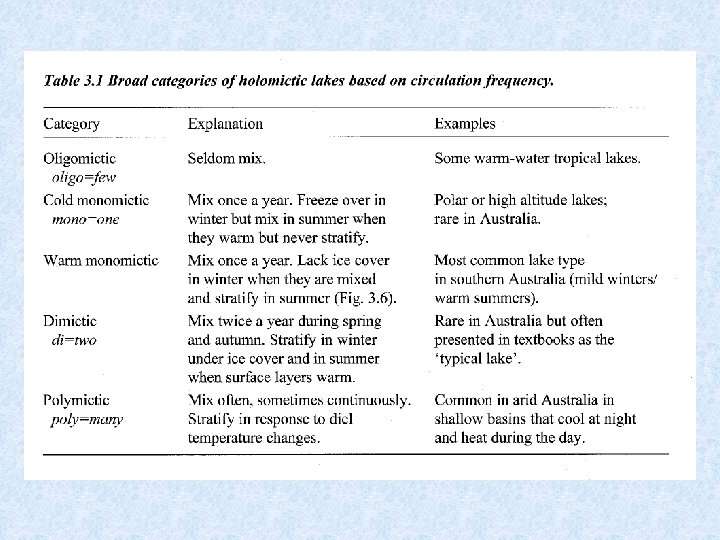
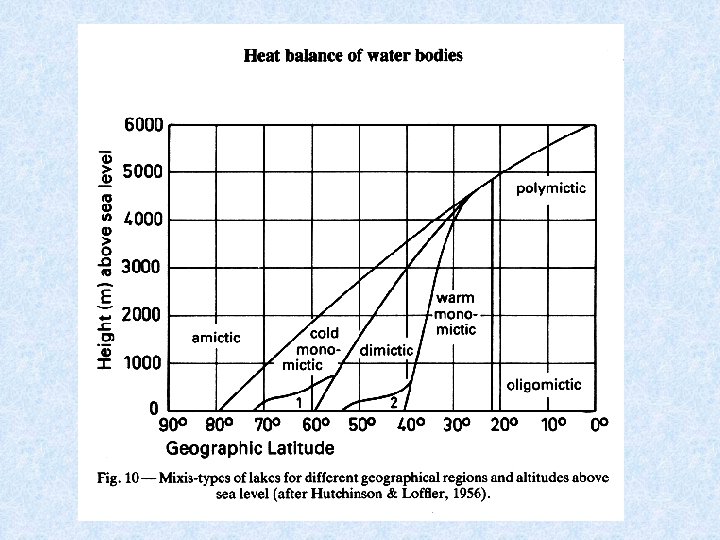
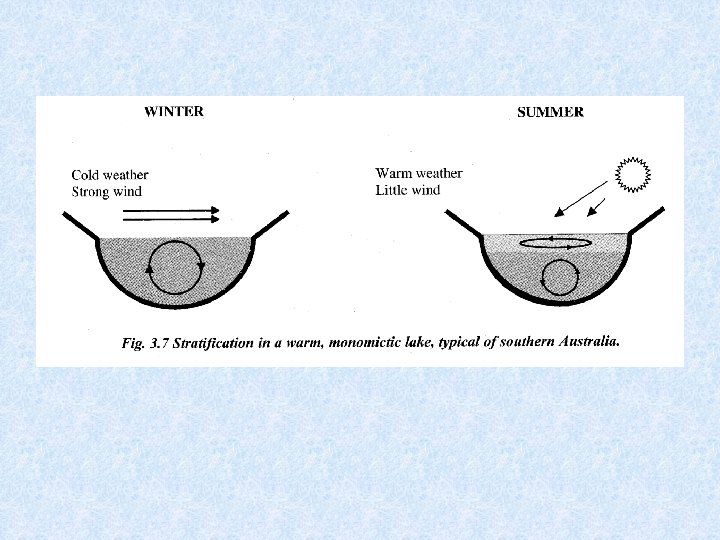
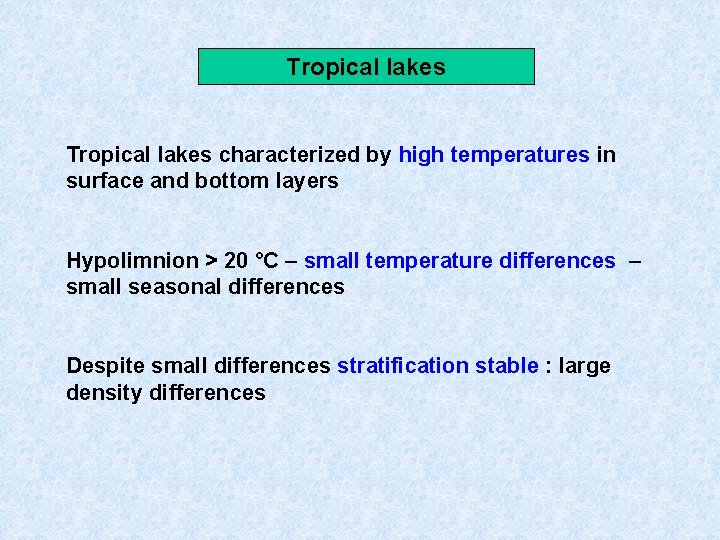
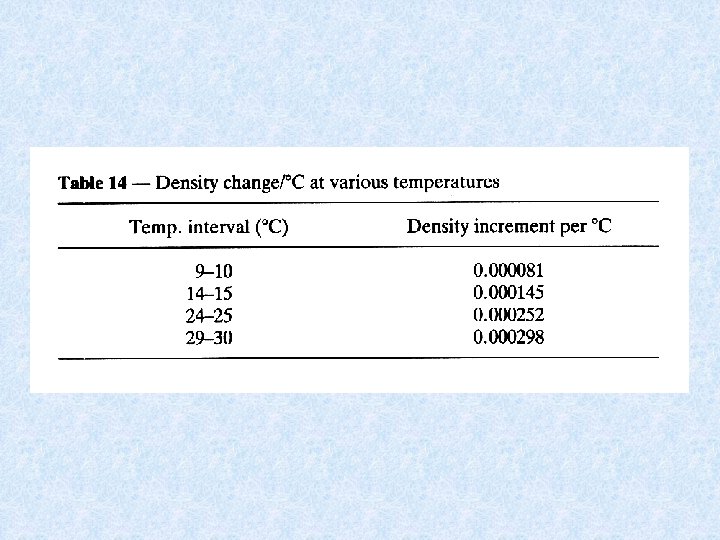
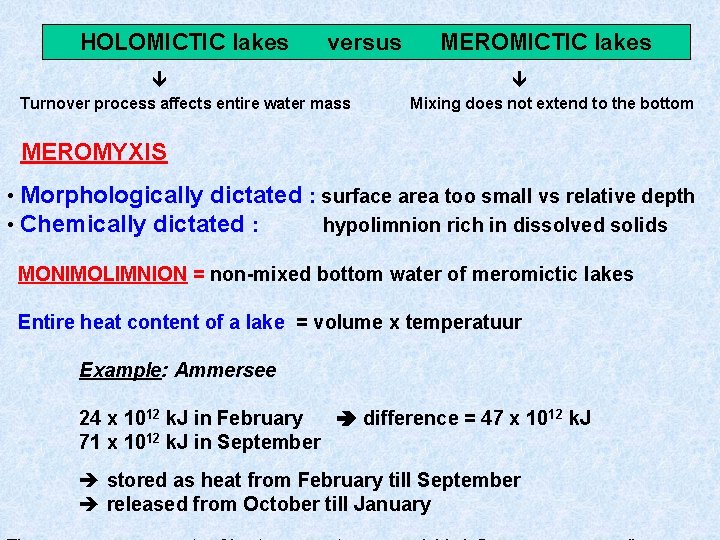
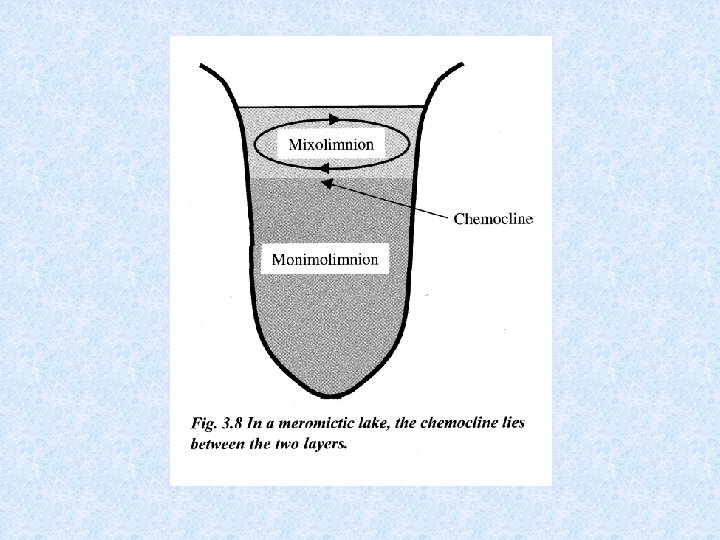
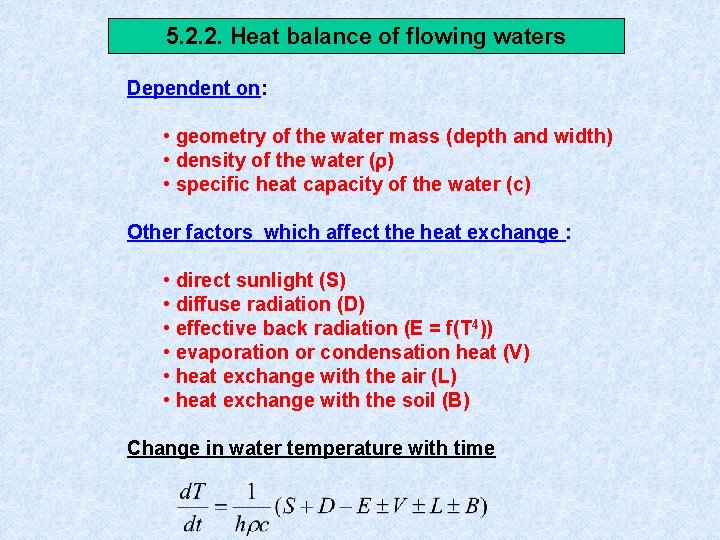
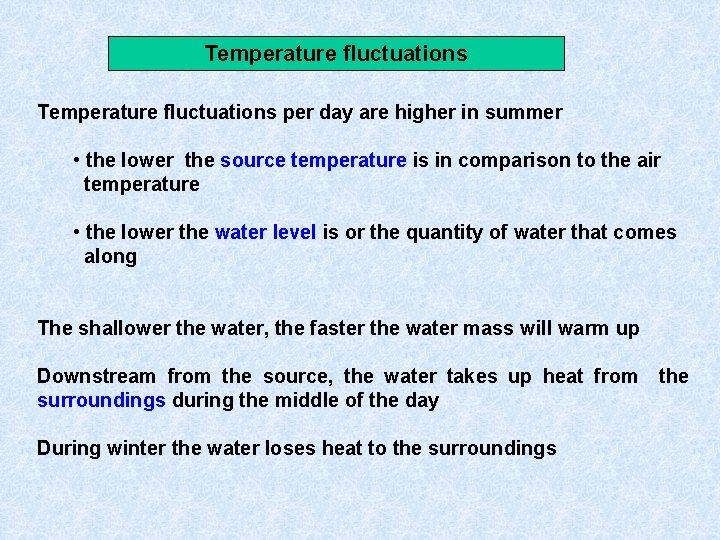
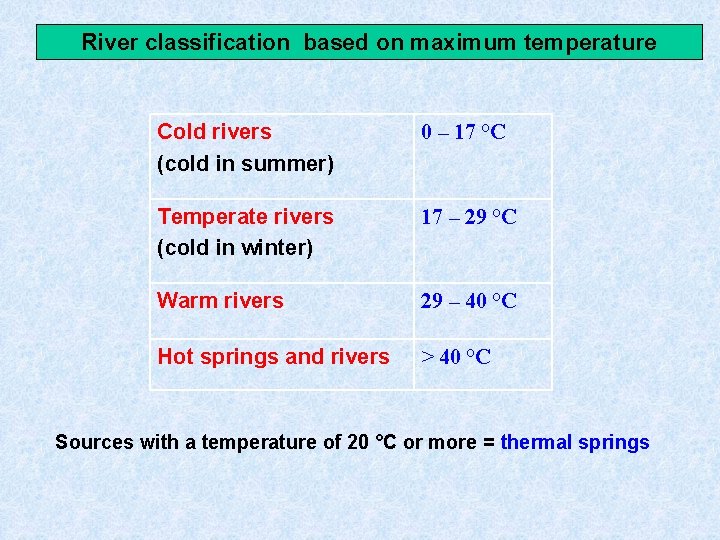
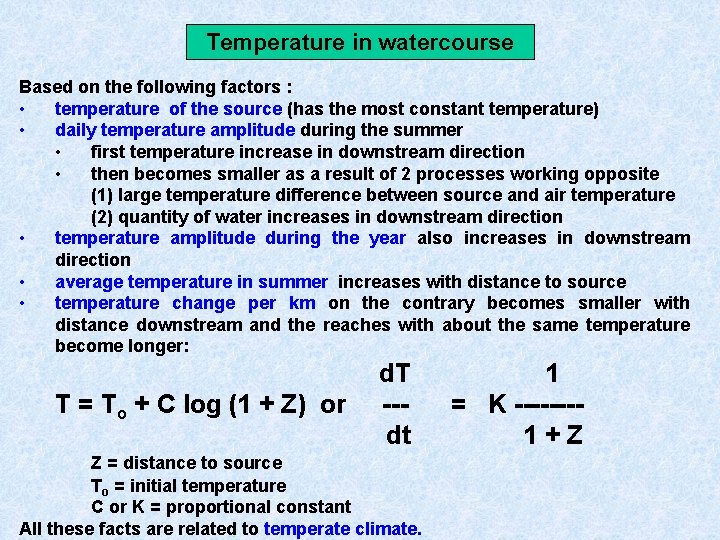
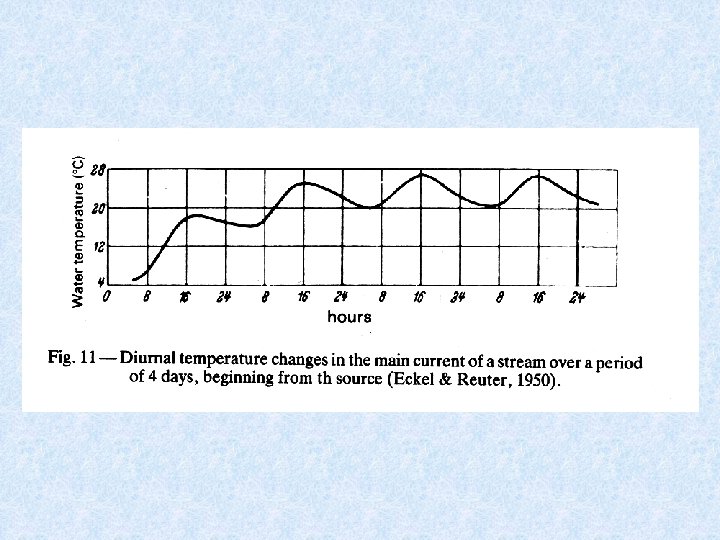
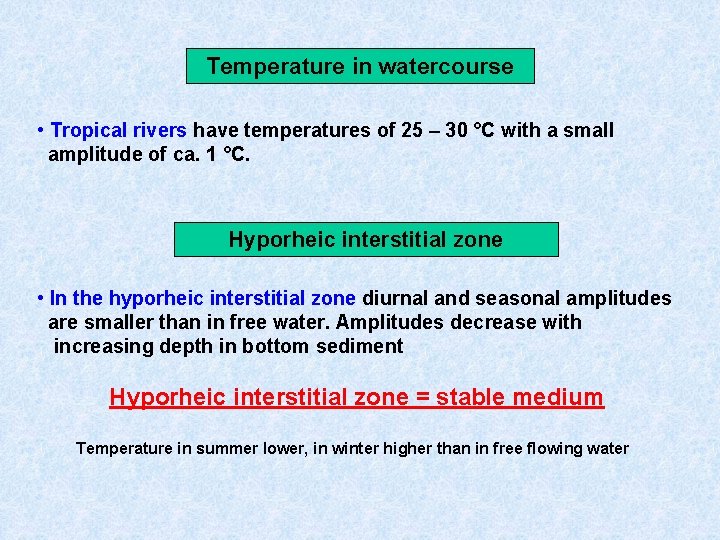
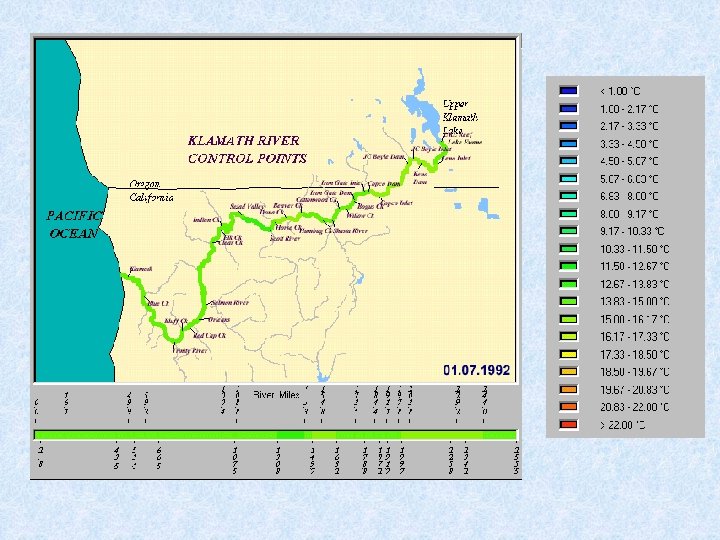
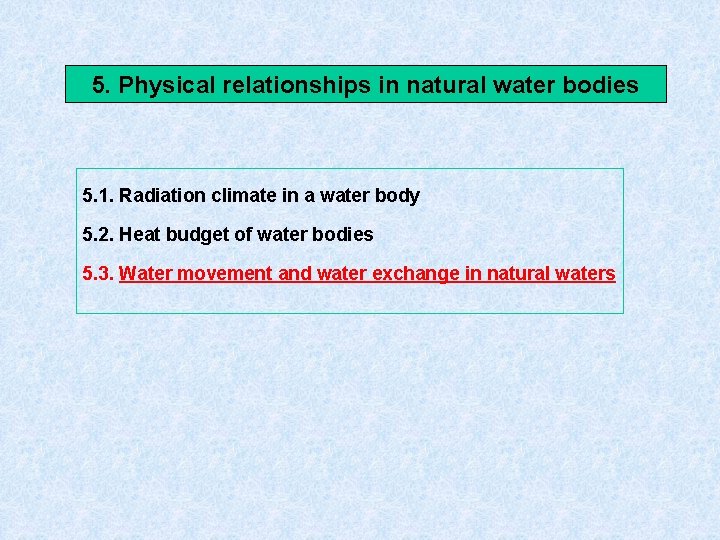
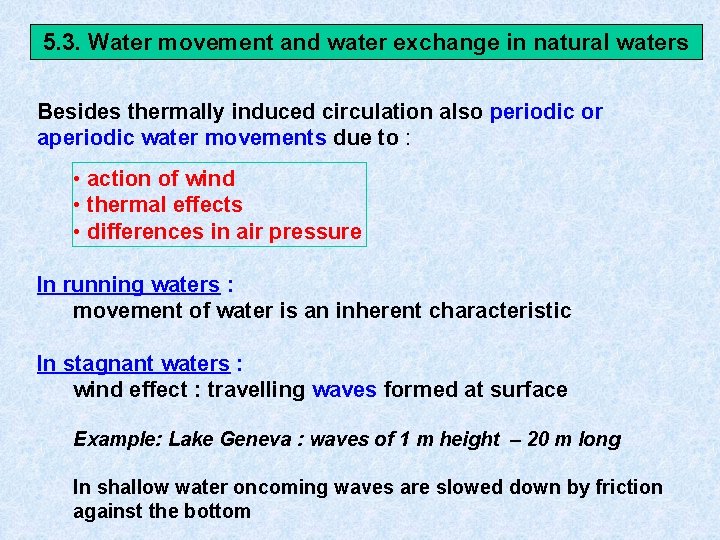
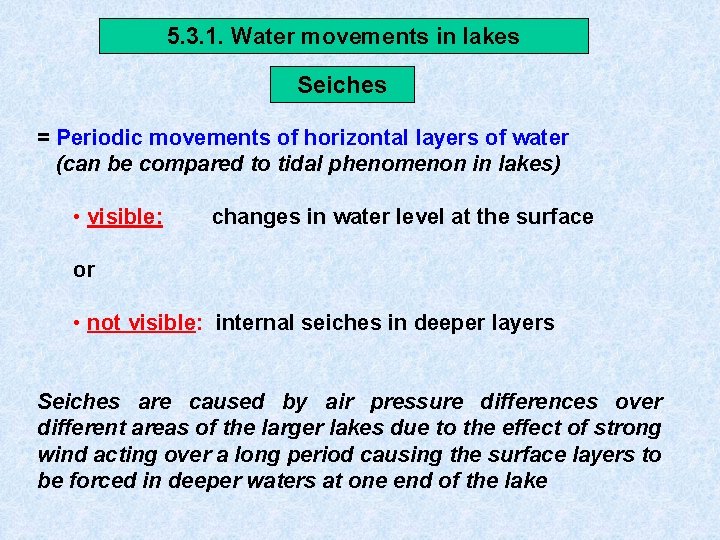
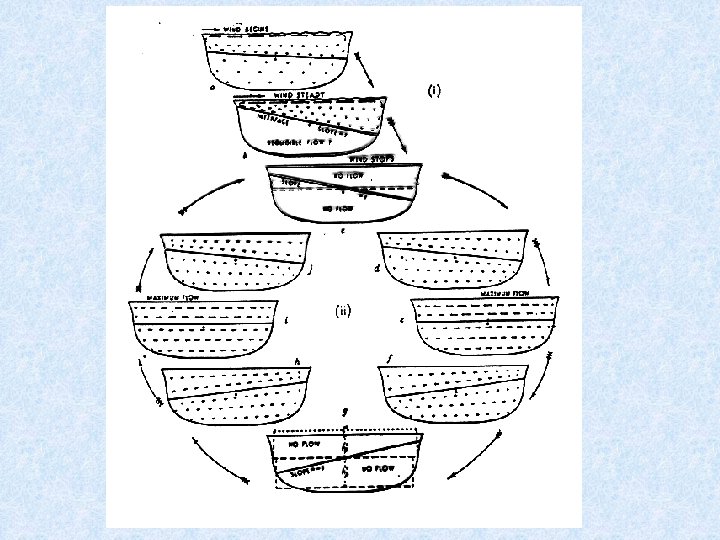
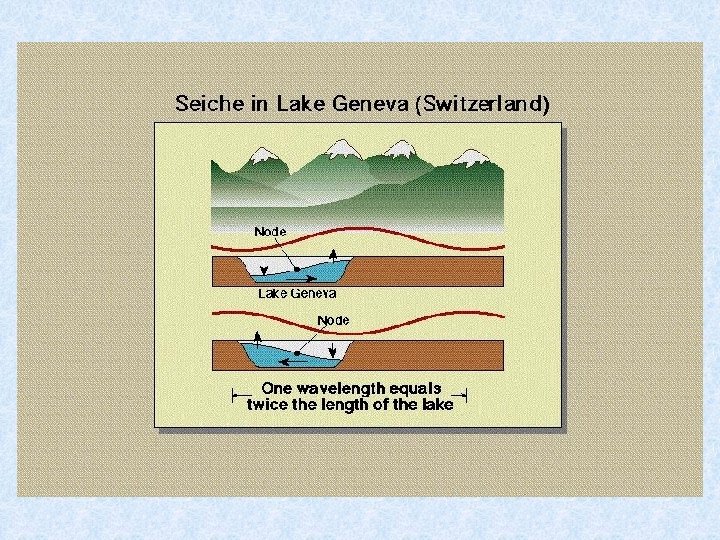
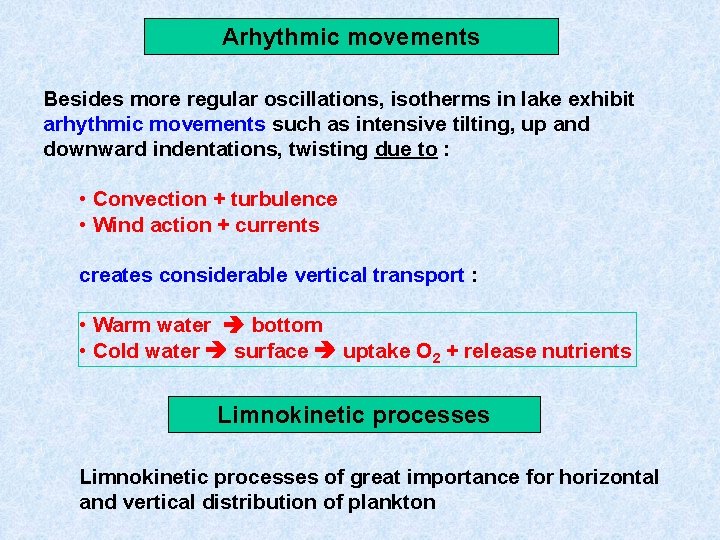
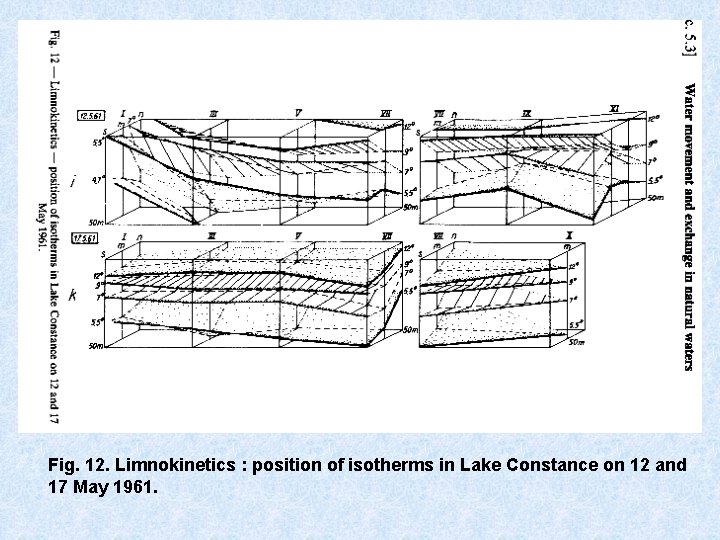
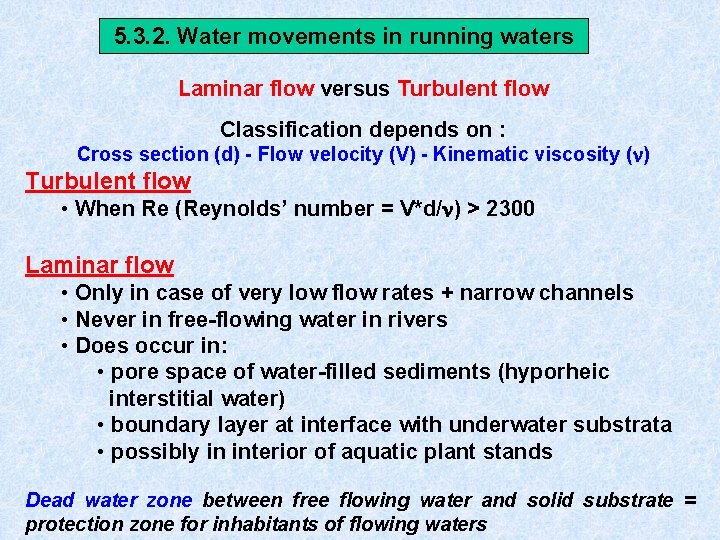
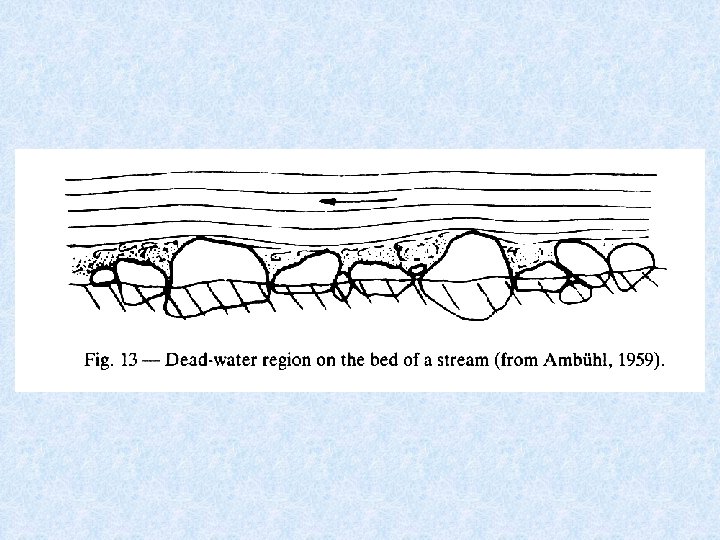
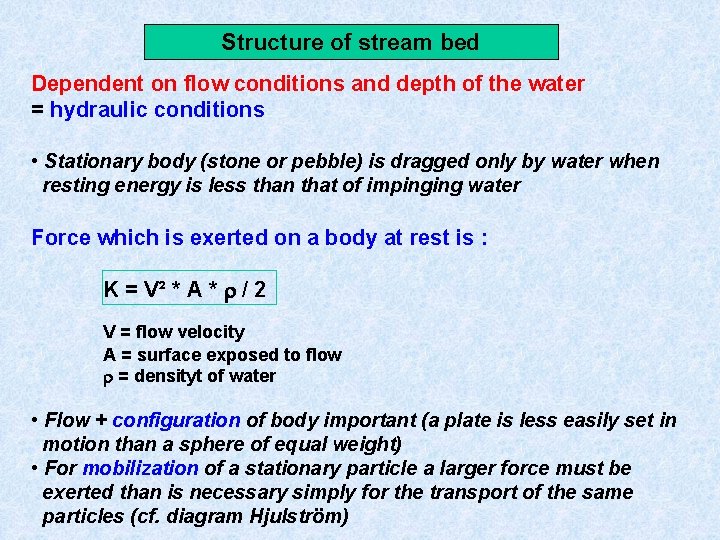
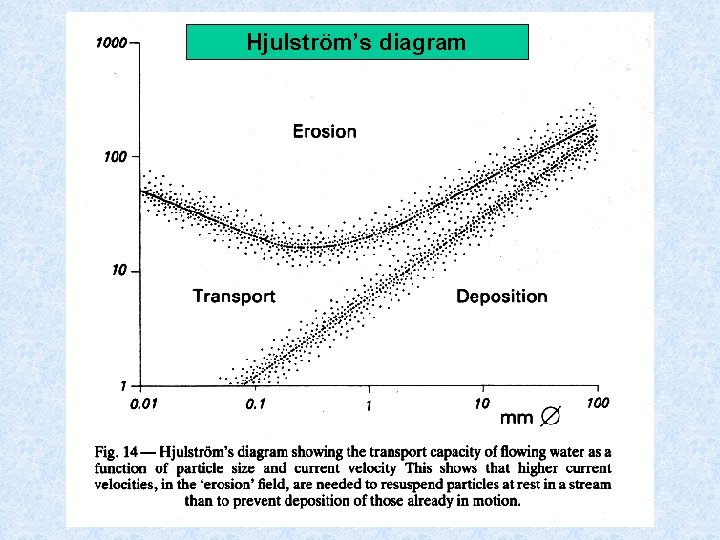
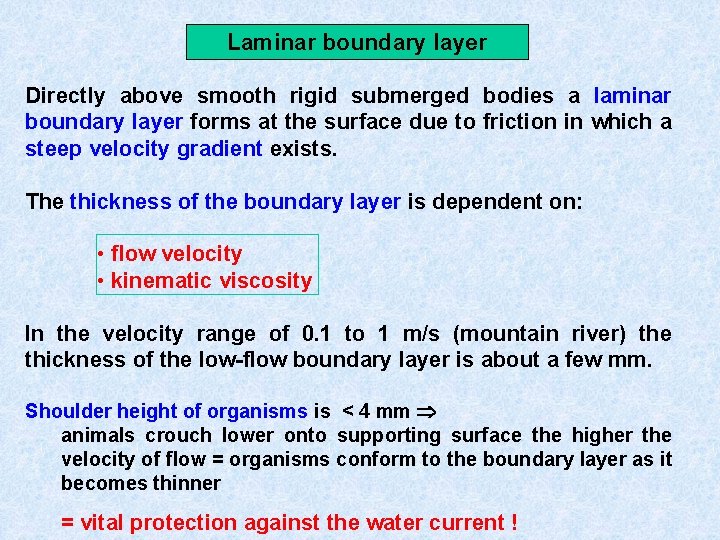
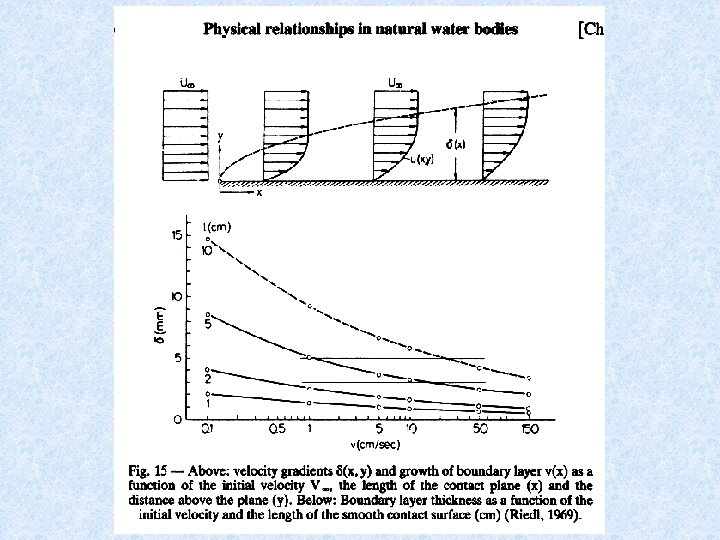
- Slides: 89
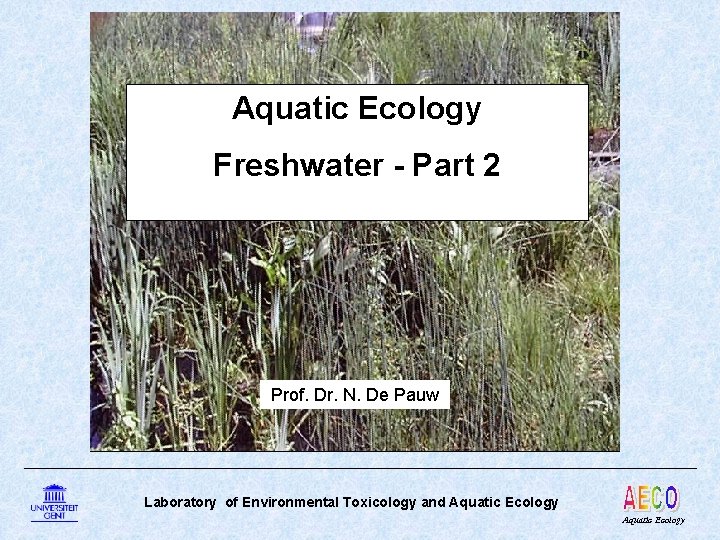
Aquatic Ecology Freshwater - Part 2 Prof. Dr. N. De Pauw Laboratory of Environmental Toxicology and Aquatic Ecology
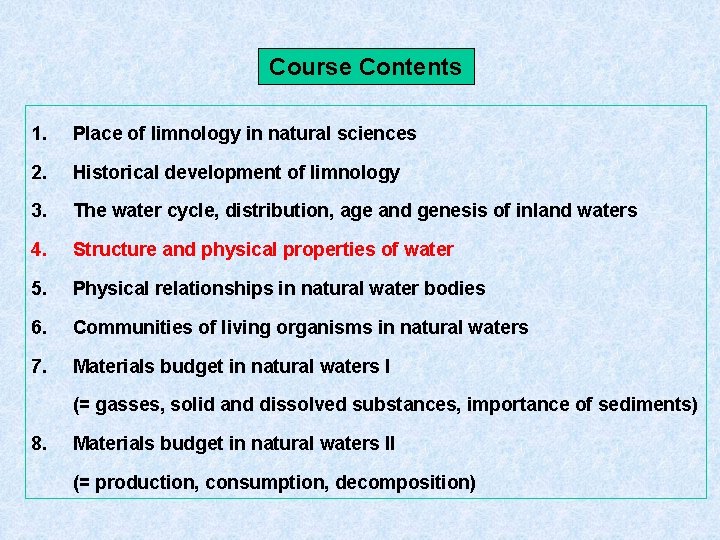
Course Contents 1. Place of limnology in natural sciences 2. Historical development of limnology 3. The water cycle, distribution, age and genesis of inland waters 4. Structure and physical properties of water 5. Physical relationships in natural water bodies 6. Communities of living organisms in natural waters 7. Materials budget in natural waters I (= gasses, solid and dissolved substances, importance of sediments) 8. Materials budget in natural waters II (= production, consumption, decomposition)
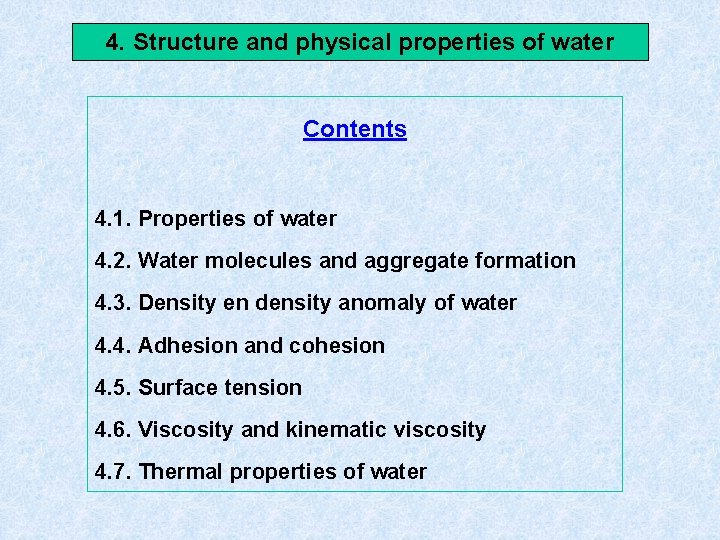
4. Structure and physical properties of water Contents 4. 1. Properties of water 4. 2. Water molecules and aggregate formation 4. 3. Density en density anomaly of water 4. 4. Adhesion and cohesion 4. 5. Surface tension 4. 6. Viscosity and kinematic viscosity 4. 7. Thermal properties of water
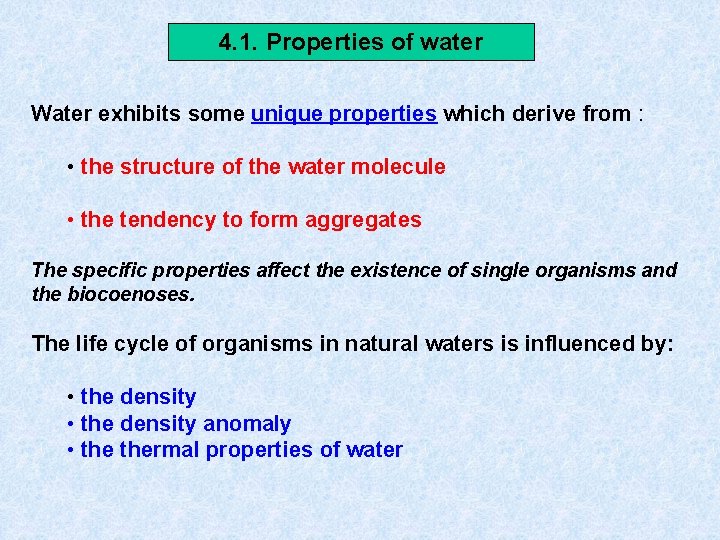
4. 1. Properties of water Water exhibits some unique properties which derive from : • the structure of the water molecule • the tendency to form aggregates The specific properties affect the existence of single organisms and the biocoenoses. The life cycle of organisms in natural waters is influenced by: • the density anomaly • thermal properties of water
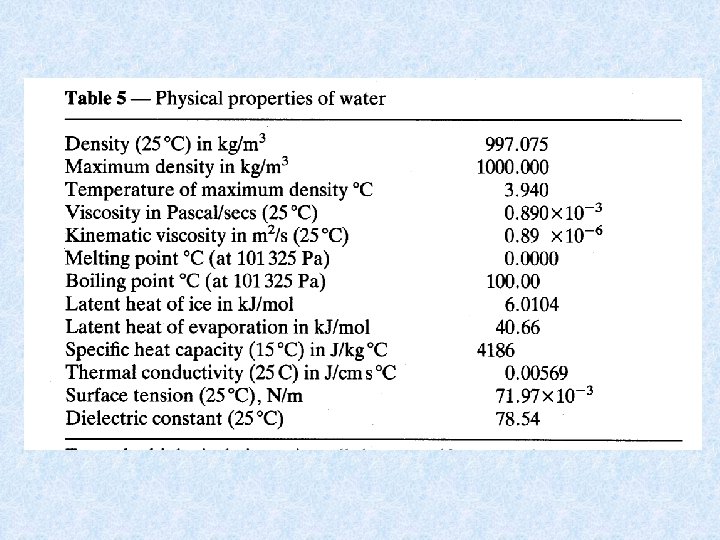
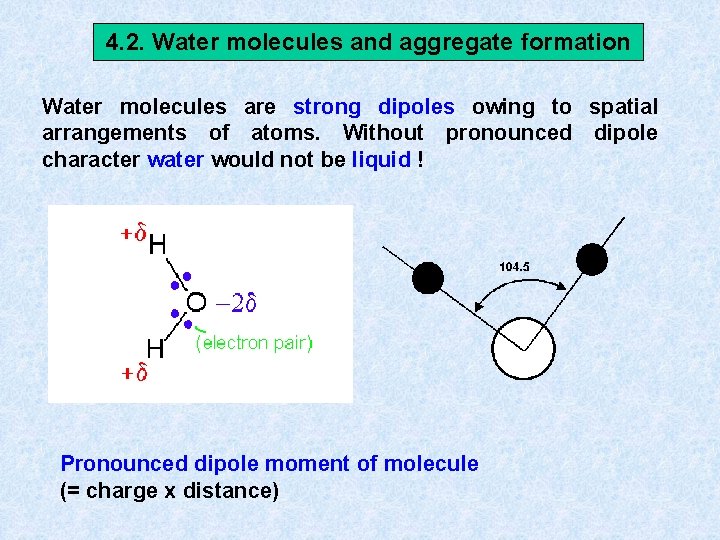
4. 2. Water molecules and aggregate formation Water molecules are strong dipoles owing to spatial arrangements of atoms. Without pronounced dipole character water would not be liquid ! Pronounced dipole moment of molecule (= charge x distance)
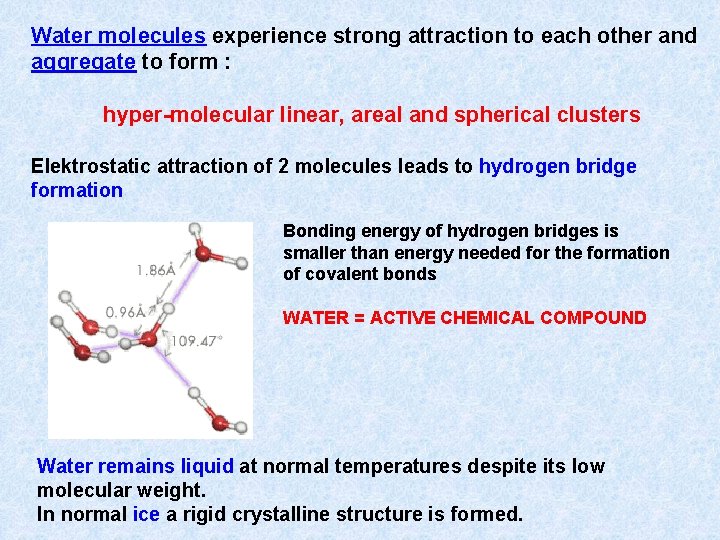
Water molecules experience strong attraction to each other and aggregate to form : hyper-molecular linear, areal and spherical clusters Elektrostatic attraction of 2 molecules leads to hydrogen bridge formation Bonding energy of hydrogen bridges is smaller than energy needed for the formation of covalent bonds WATER = ACTIVE CHEMICAL COMPOUND Water remains liquid at normal temperatures despite its low molecular weight. In normal ice a rigid crystalline structure is formed.
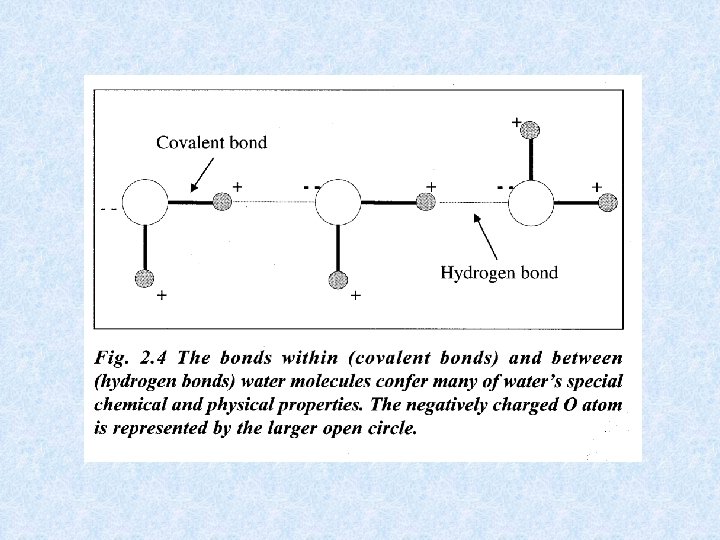
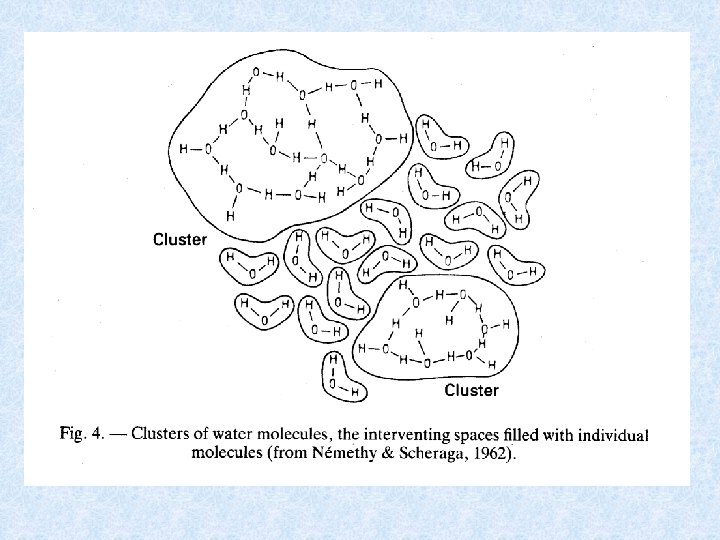
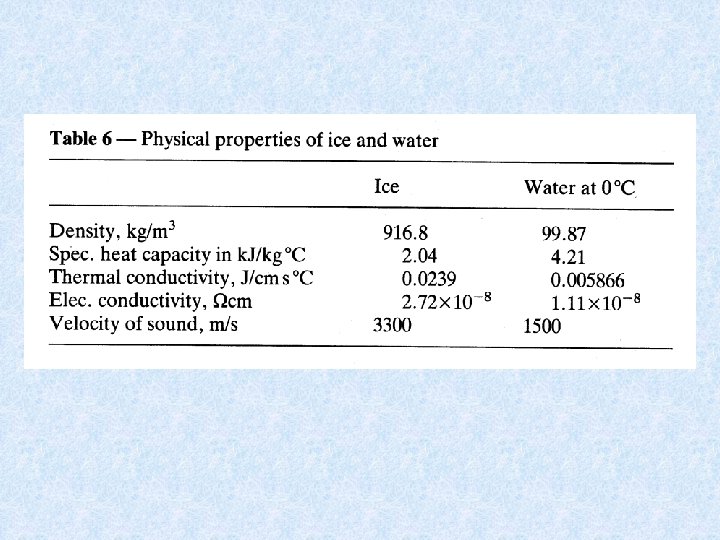
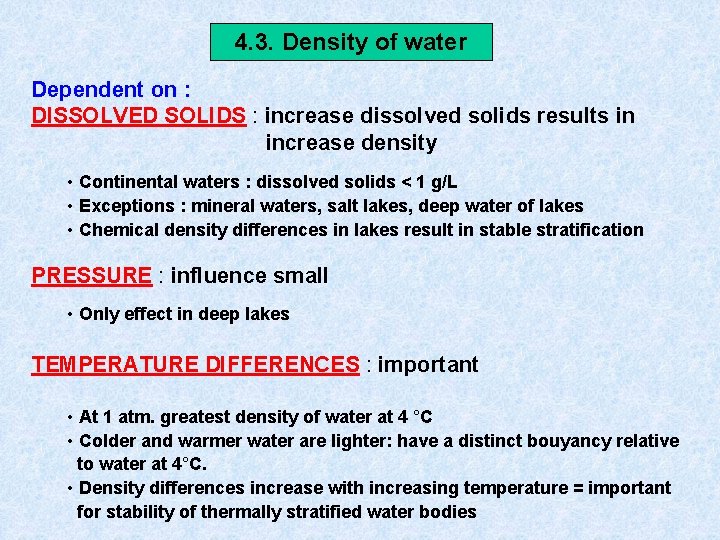
4. 3. Density of water Dependent on : DISSOLVED SOLIDS : increase dissolved solids results in increase density • Continental waters : dissolved solids < 1 g/L • Exceptions : mineral waters, salt lakes, deep water of lakes • Chemical density differences in lakes result in stable stratification PRESSURE : influence small • Only effect in deep lakes TEMPERATURE DIFFERENCES : important • At 1 atm. greatest density of water at 4 °C • Colder and warmer water are lighter: have a distinct bouyancy relative to water at 4°C. • Density differences increase with increasing temperature = important for stability of thermally stratified water bodies
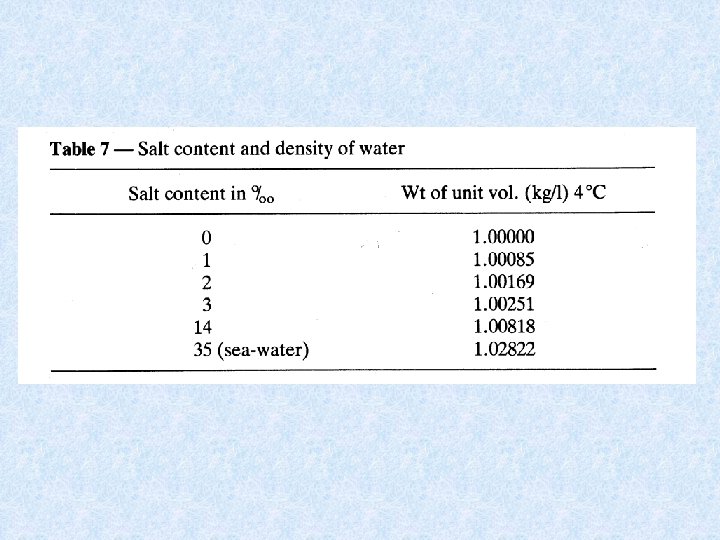
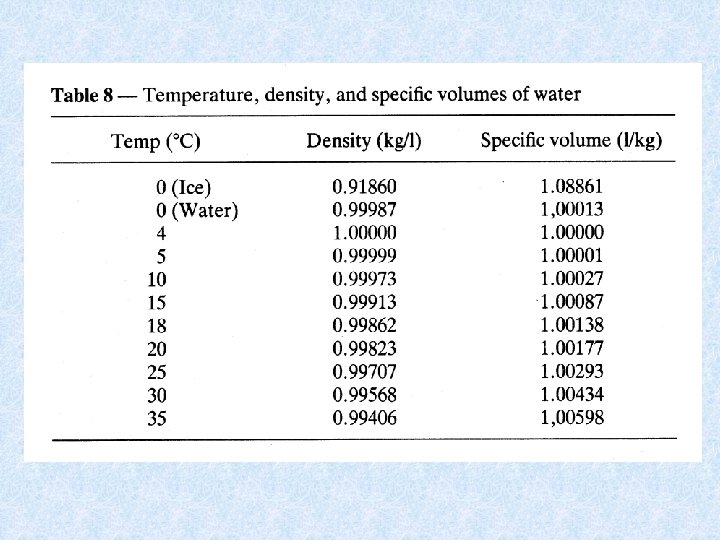
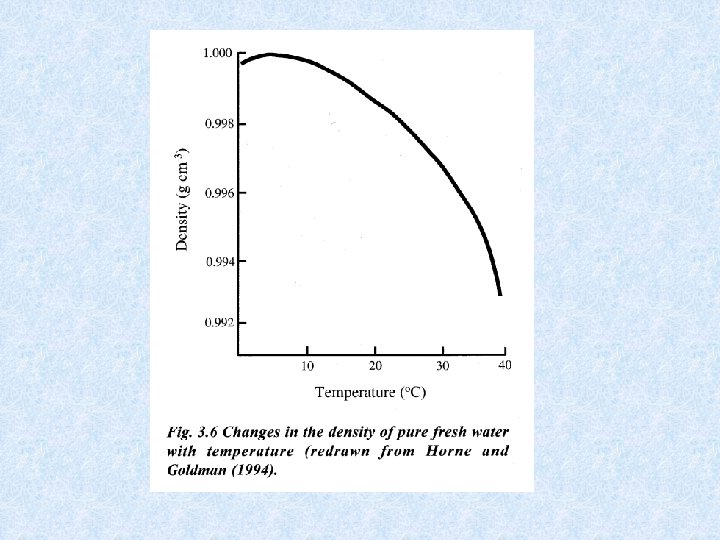
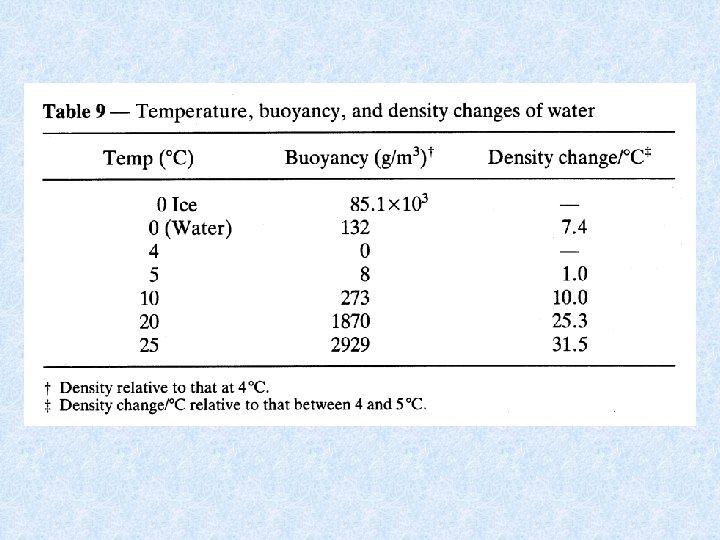
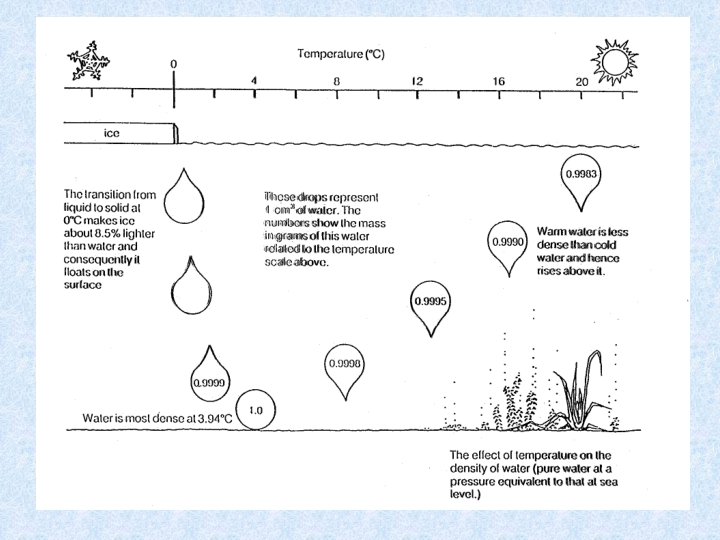
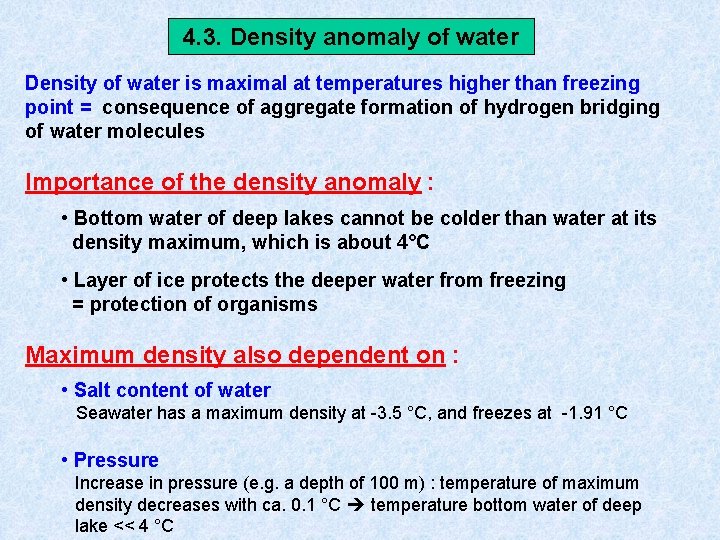
4. 3. Density anomaly of water Density of water is maximal at temperatures higher than freezing point = consequence of aggregate formation of hydrogen bridging of water molecules Importance of the density anomaly : • Bottom water of deep lakes cannot be colder than water at its density maximum, which is about 4°C • Layer of ice protects the deeper water from freezing = protection of organisms Maximum density also dependent on : • Salt content of water Seawater has a maximum density at -3. 5 °C, and freezes at -1. 91 °C • Pressure Increase in pressure (e. g. a depth of 100 m) : temperature of maximum density decreases with ca. 0. 1 °C temperature bottom water of deep lake << 4 °C
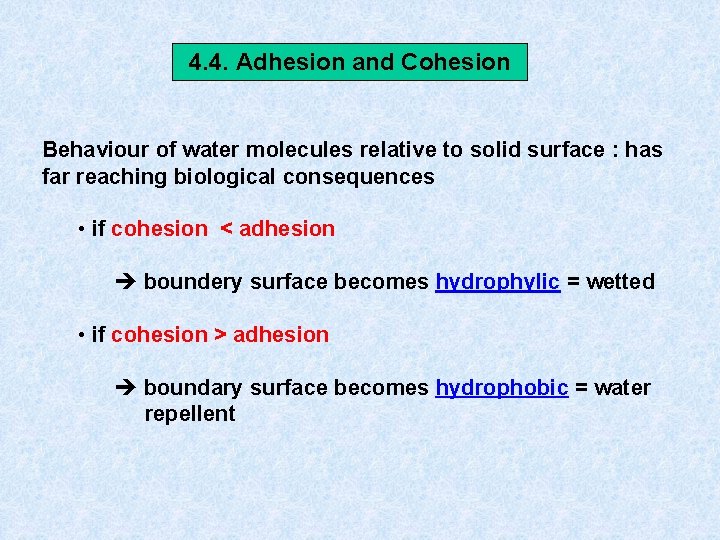
4. 4. Adhesion and Cohesion Behaviour of water molecules relative to solid surface : has far reaching biological consequences • if cohesion < adhesion boundery surface becomes hydrophylic = wetted • if cohesion > adhesion boundary surface becomes hydrophobic = water repellent
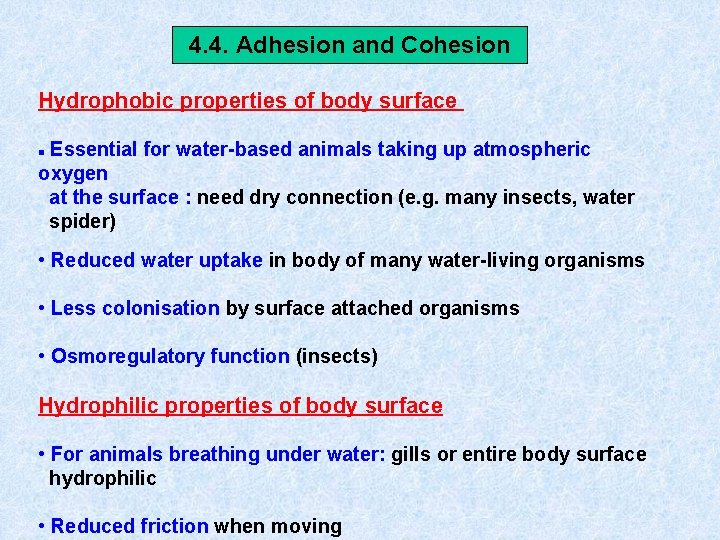
4. 4. Adhesion and Cohesion Hydrophobic properties of body surface Essential for water-based animals taking up atmospheric oxygen at the surface : need dry connection (e. g. many insects, water spider) n • Reduced water uptake in body of many water-living organisms • Less colonisation by surface attached organisms • Osmoregulatory function (insects) Hydrophilic properties of body surface • For animals breathing under water: gills or entire body surface hydrophilic • Reduced friction when moving
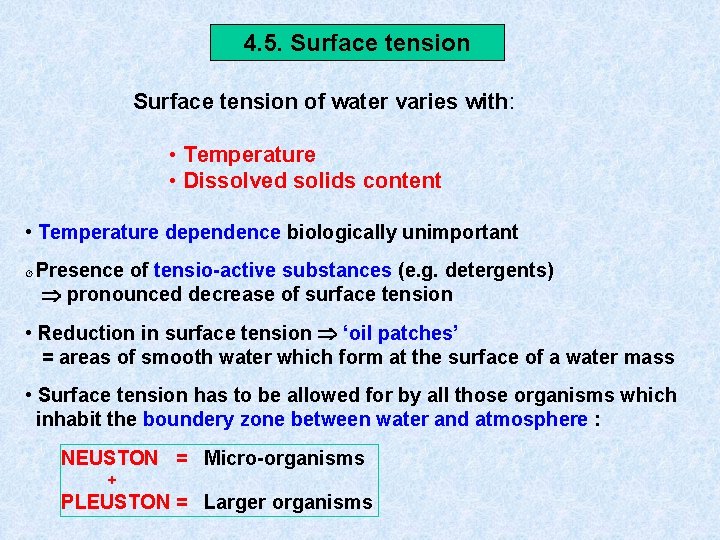
4. 5. Surface tension of water varies with: • Temperature • Dissolved solids content • Temperature dependence biologically unimportant ¾ Presence of tensio-active substances (e. g. detergents) pronounced decrease of surface tension • Reduction in surface tension ‘oil patches’ = areas of smooth water which form at the surface of a water mass • Surface tension has to be allowed for by all those organisms which inhabit the boundery zone between water and atmosphere : NEUSTON = Micro-organisms + PLEUSTON = Larger organisms
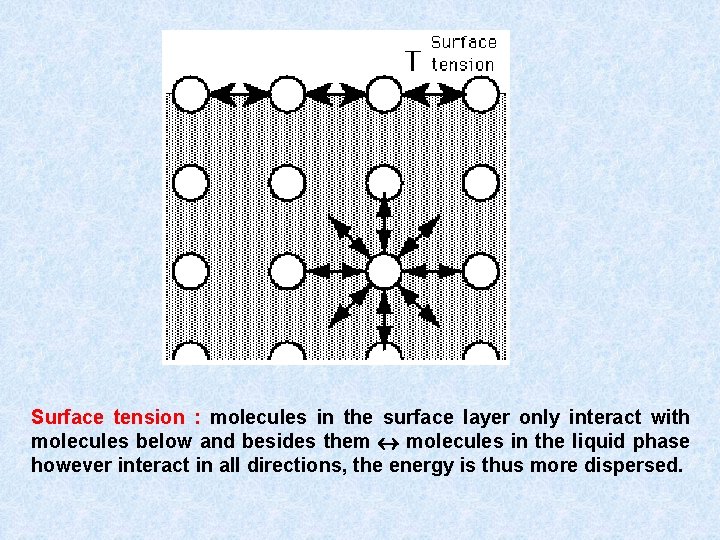
Surface tension : molecules in the surface layer only interact with molecules below and besides them molecules in the liquid phase however interact in all directions, the energy is thus more dispersed.
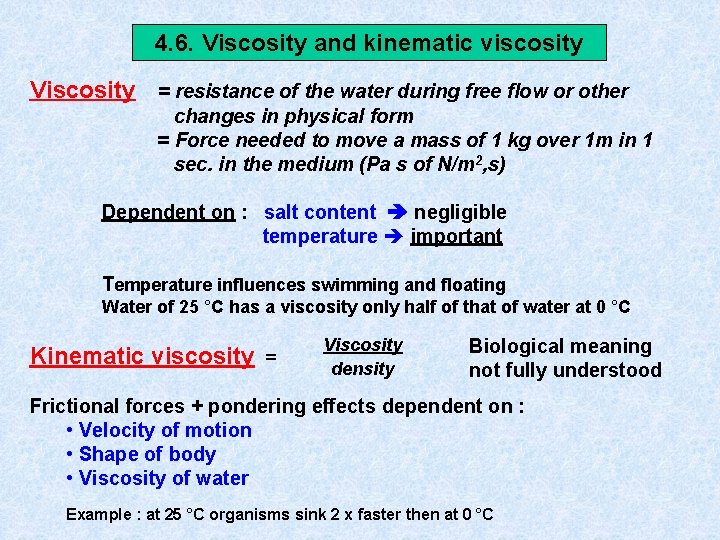
4. 6. Viscosity and kinematic viscosity Viscosity = resistance of the water during free flow or other changes in physical form = Force needed to move a mass of 1 kg over 1 m in 1 sec. in the medium (Pa s of N/m 2, s) Dependent on : salt content negligible temperature important Temperature influences swimming and floating Water of 25 °C has a viscosity only half of that of water at 0 °C Kinematic viscosity = Viscosity density Biological meaning not fully understood Frictional forces + pondering effects dependent on : • Velocity of motion • Shape of body • Viscosity of water Example : at 25 °C organisms sink 2 x faster then at 0 °C
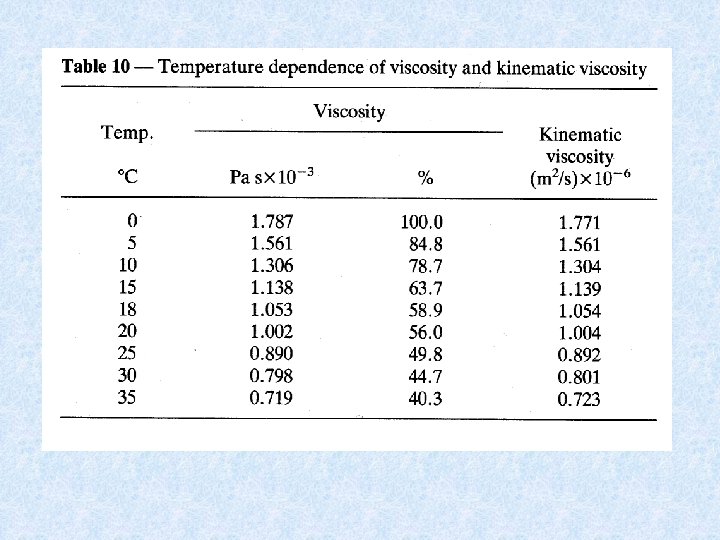
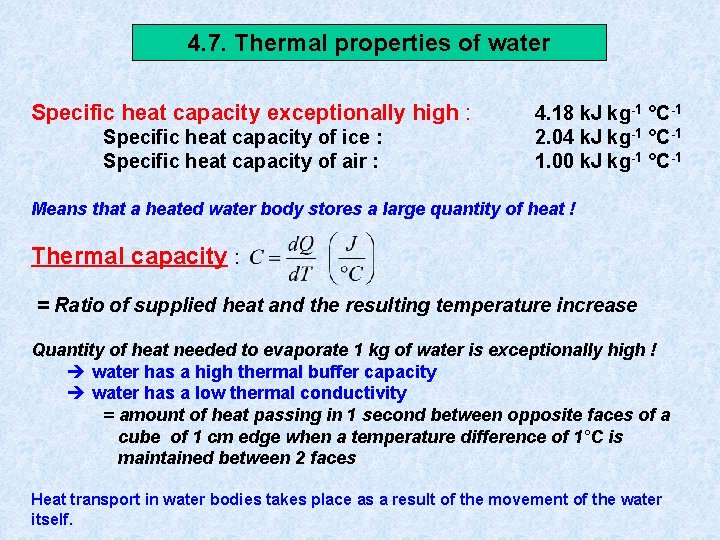
4. 7. Thermal properties of water Specific heat capacity exceptionally high : Specific heat capacity of ice : Specific heat capacity of air : 4. 18 k. J kg-1 °C-1 2. 04 k. J kg-1 °C-1 1. 00 k. J kg-1 °C-1 Means that a heated water body stores a large quantity of heat ! Thermal capacity : = Ratio of supplied heat and the resulting temperature increase Quantity of heat needed to evaporate 1 kg of water is exceptionally high ! water has a high thermal buffer capacity water has a low thermal conductivity = amount of heat passing in 1 second between opposite faces of a cube of 1 cm edge when a temperature difference of 1°C is maintained between 2 faces Heat transport in water bodies takes place as a result of the movement of the water itself.
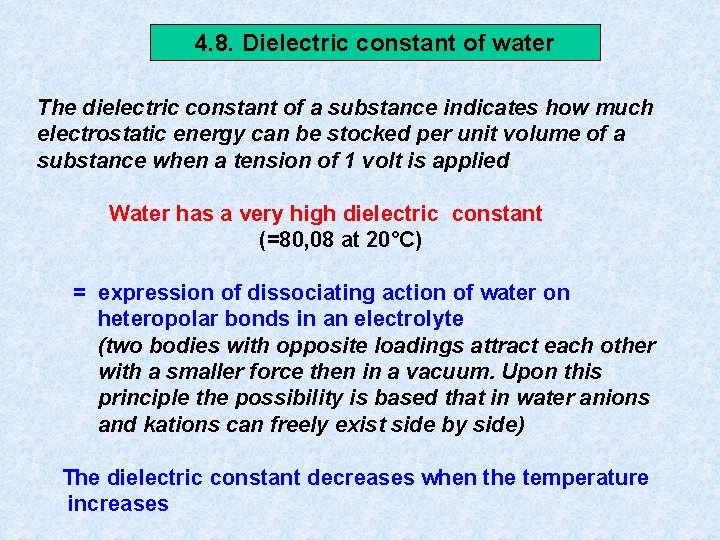
4. 8. Dielectric constant of water The dielectric constant of a substance indicates how much electrostatic energy can be stocked per unit volume of a substance when a tension of 1 volt is applied Water has a very high dielectric constant (=80, 08 at 20°C) = expression of dissociating action of water on heteropolar bonds in an electrolyte (two bodies with opposite loadings attract each other with a smaller force then in a vacuum. Upon this principle the possibility is based that in water anions and kations can freely exist side by side) The dielectric constant decreases when the temperature increases
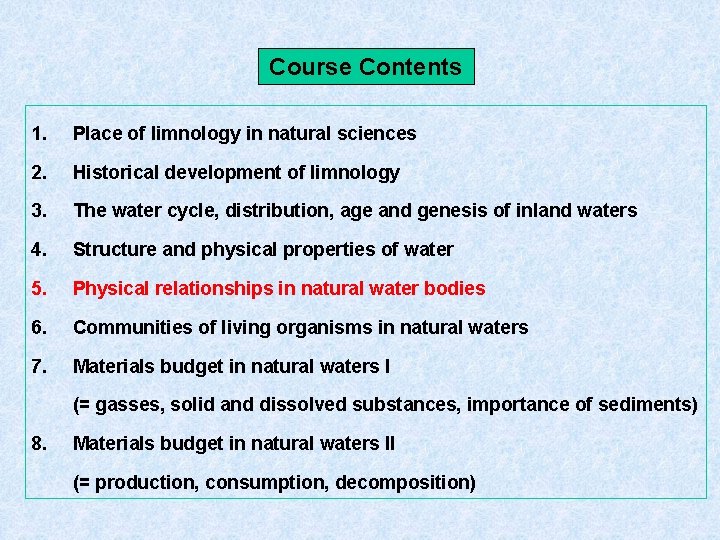
Course Contents 1. Place of limnology in natural sciences 2. Historical development of limnology 3. The water cycle, distribution, age and genesis of inland waters 4. Structure and physical properties of water 5. Physical relationships in natural water bodies 6. Communities of living organisms in natural waters 7. Materials budget in natural waters I (= gasses, solid and dissolved substances, importance of sediments) 8. Materials budget in natural waters II (= production, consumption, decomposition)
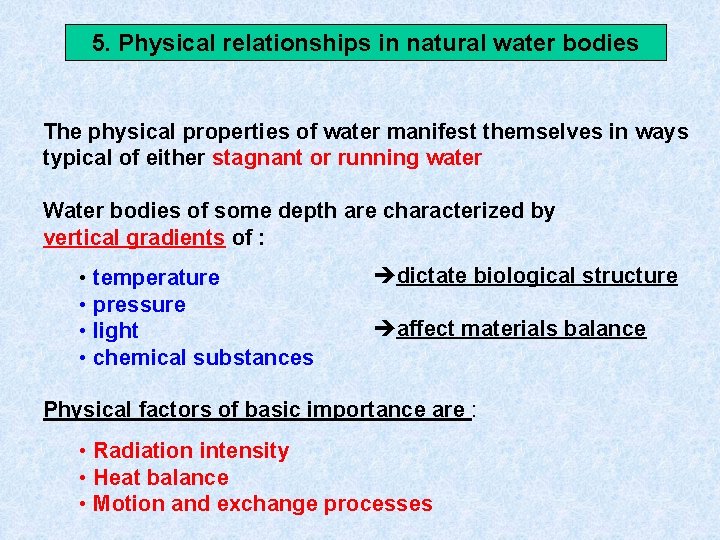
5. Physical relationships in natural water bodies The physical properties of water manifest themselves in ways typical of either stagnant or running water Water bodies of some depth are characterized by vertical gradients of : • temperature • pressure • light • chemical substances dictate biological structure affect materials balance Physical factors of basic importance are : • Radiation intensity • Heat balance • Motion and exchange processes
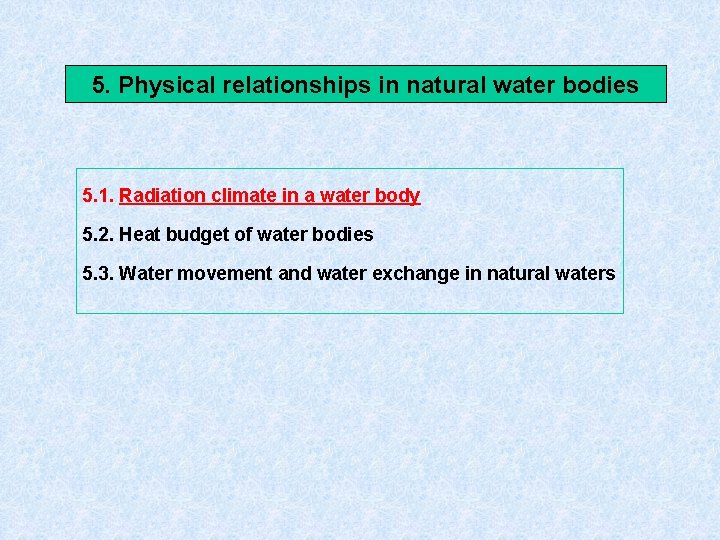
5. Physical relationships in natural water bodies 5. 1. Radiation climate in a water body 5. 2. Heat budget of water bodies 5. 3. Water movement and water exchange in natural waters
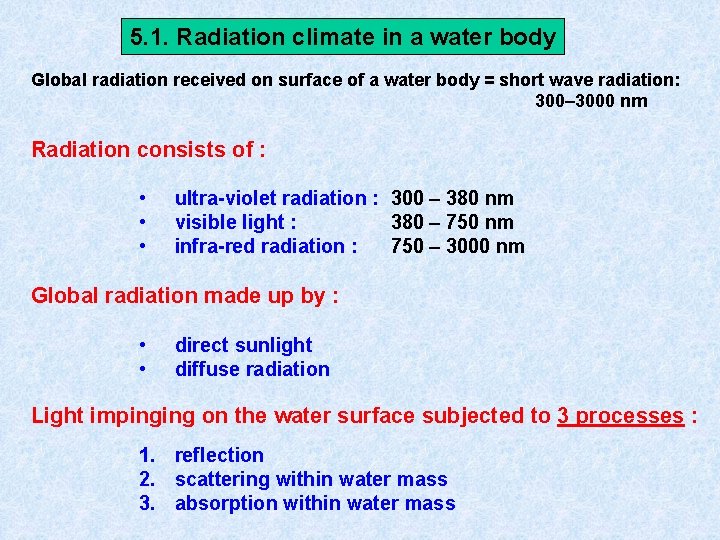
5. 1. Radiation climate in a water body Global radiation received on surface of a water body = short wave radiation: 300– 3000 nm Radiation consists of : • • • ultra-violet radiation : 300 – 380 nm visible light : 380 – 750 nm infra-red radiation : 750 – 3000 nm Global radiation made up by : • • direct sunlight diffuse radiation Light impinging on the water surface subjected to 3 processes : 1. reflection 2. scattering within water mass 3. absorption within water mass
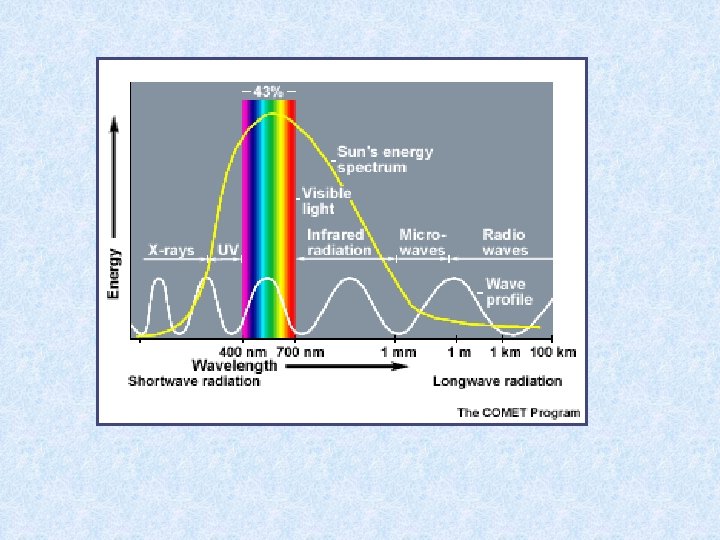
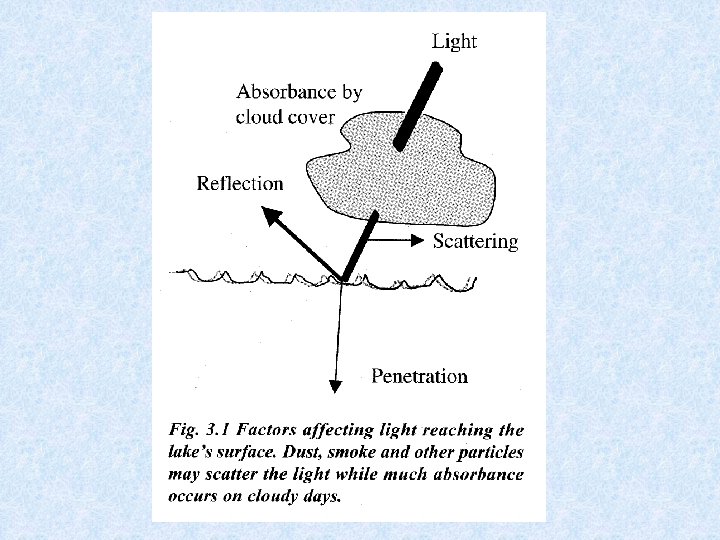
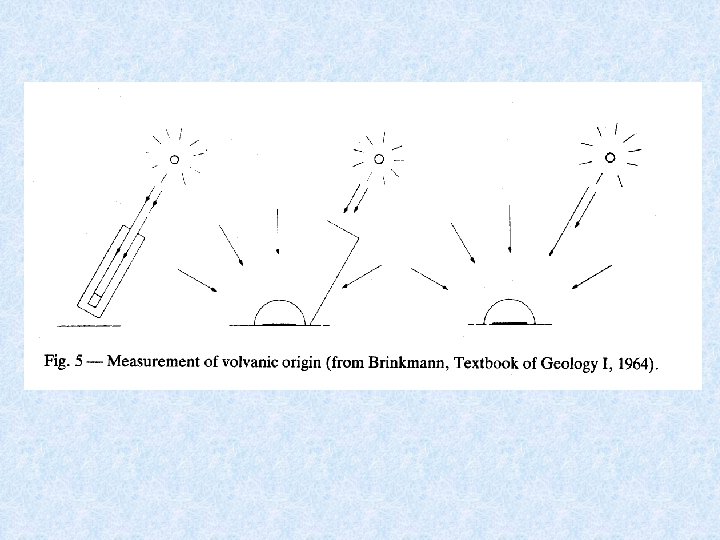
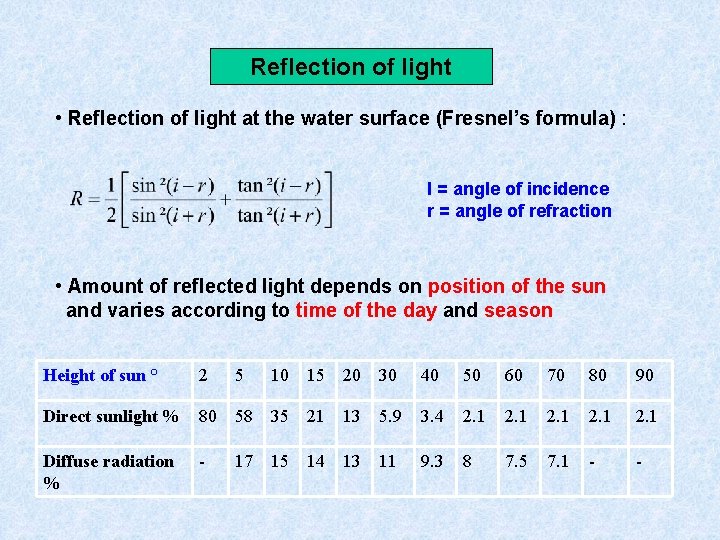
Reflection of light • Reflection of light at the water surface (Fresnel’s formula) : I = angle of incidence r = angle of refraction • Amount of reflected light depends on position of the sun and varies according to time of the day and season Height of sun ° 2 5 10 15 20 30 40 50 60 70 80 90 Direct sunlight % 80 58 35 21 13 5. 9 3. 4 2. 1 2. 1 Diffuse radiation % - 17 15 14 13 11 9. 3 8 7. 5 7. 1 - -
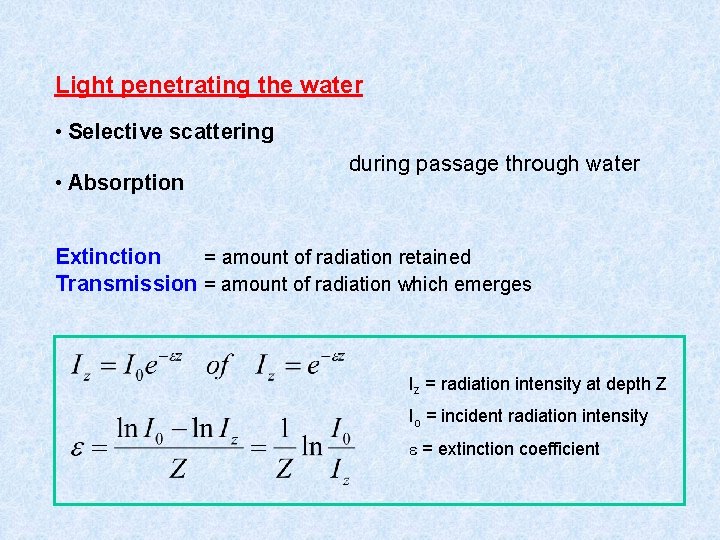
Light penetrating the water • Selective scattering • Absorption during passage through water Extinction = amount of radiation retained Transmission = amount of radiation which emerges Iz = radiation intensity at depth Z Io = incident radiation intensity = extinction coefficient
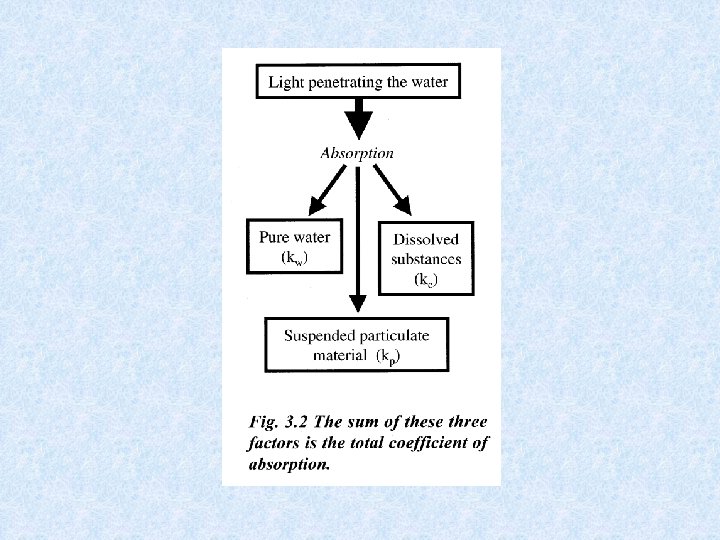
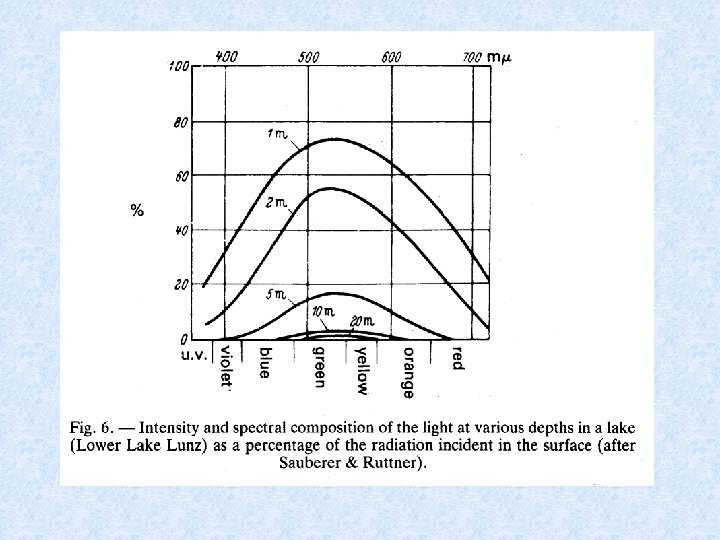
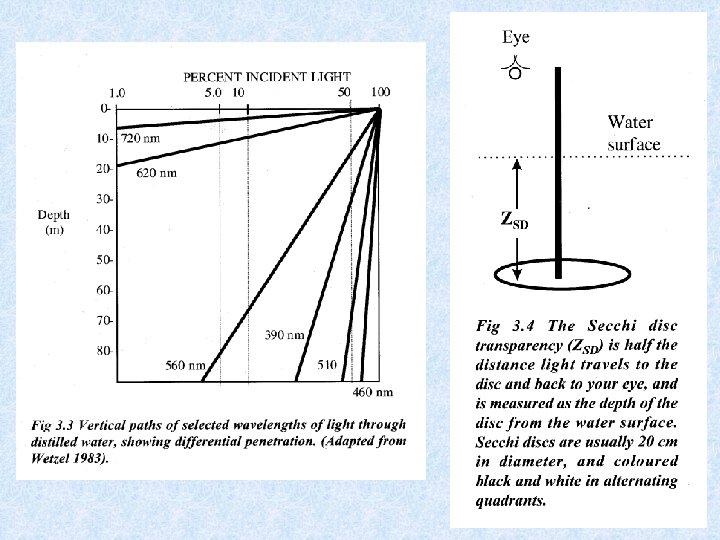
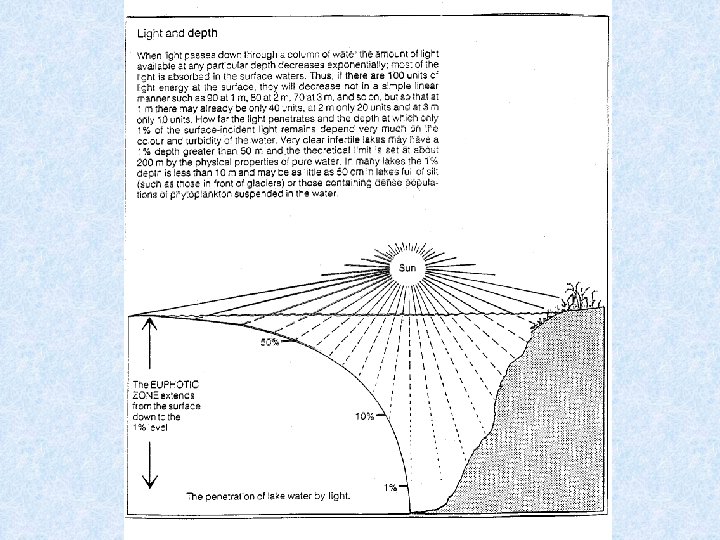
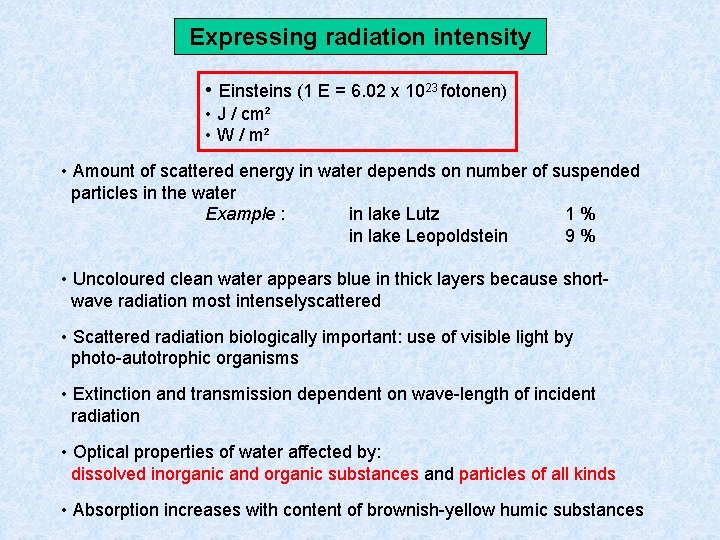
Expressing radiation intensity • Einsteins (1 E = 6. 02 x 1023 fotonen) • J / cm² • W / m² • Amount of scattered energy in water depends on number of suspended particles in the water Example : in lake Lutz 1% in lake Leopoldstein 9% • Uncoloured clean water appears blue in thick layers because shortwave radiation most intenselyscattered • Scattered radiation biologically important: use of visible light by photo-autotrophic organisms • Extinction and transmission dependent on wave-length of incident radiation • Optical properties of water affected by: dissolved inorganic and organic substances and particles of all kinds • Absorption increases with content of brownish-yellow humic substances
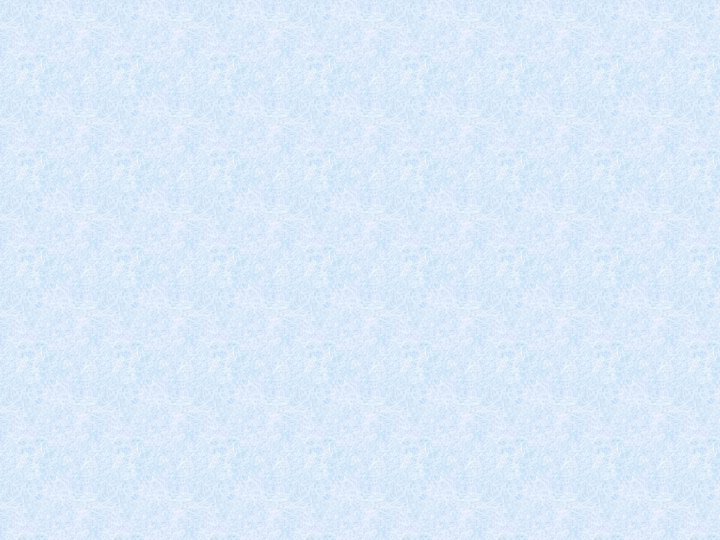
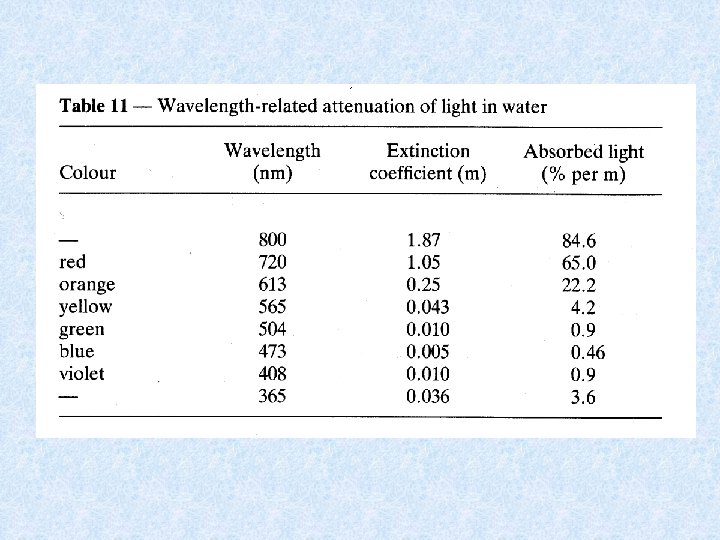
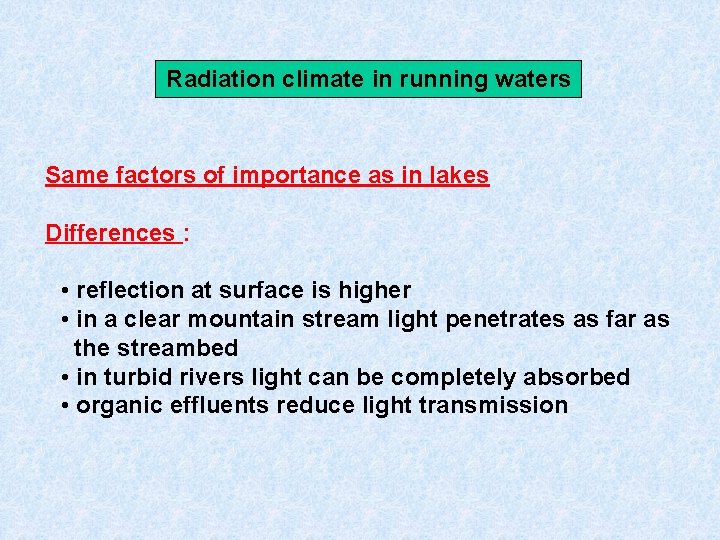
Radiation climate in running waters Same factors of importance as in lakes Differences : • reflection at surface is higher • in a clear mountain stream light penetrates as far as the streambed • in turbid rivers light can be completely absorbed • organic effluents reduce light transmission
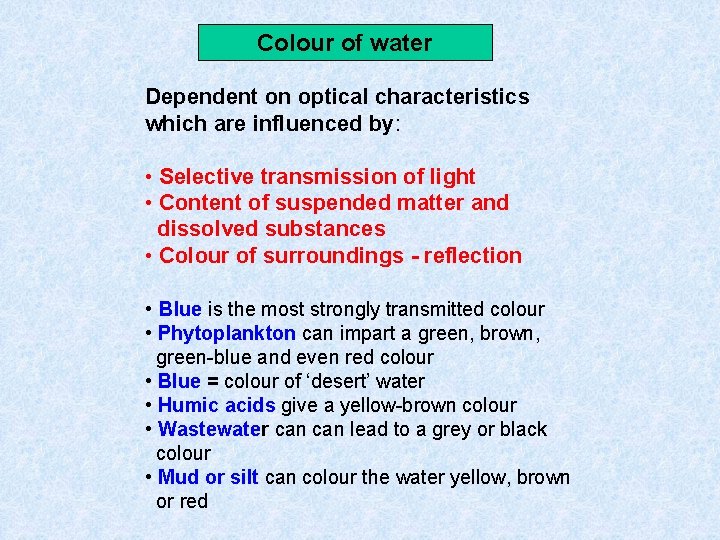
Colour of water Dependent on optical characteristics which are influenced by: • Selective transmission of light • Content of suspended matter and dissolved substances • Colour of surroundings - reflection • Blue is the most strongly transmitted colour • Phytoplankton can impart a green, brown, green-blue and even red colour • Blue = colour of ‘desert’ water • Humic acids give a yellow-brown colour • Wastewater can lead to a grey or black colour • Mud or silt can colour the water yellow, brown or red
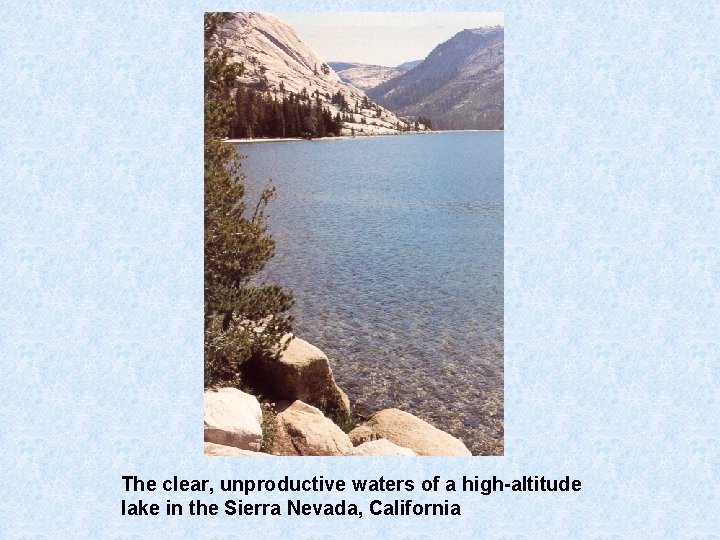
The clear, unproductive waters of a high-altitude lake in the Sierra Nevada, California
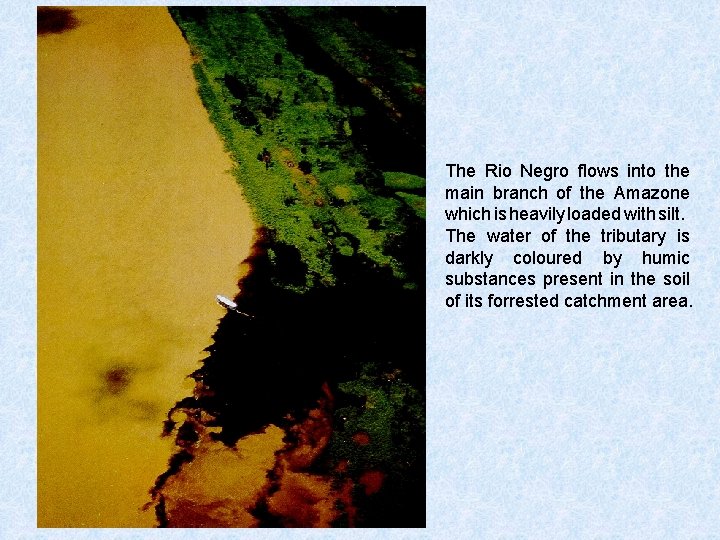
The Rio Negro flows into the main branch of the Amazone which is heavily loaded with silt. The water of the tributary is darkly coloured by humic substances present in the soil of its forrested catchment area.
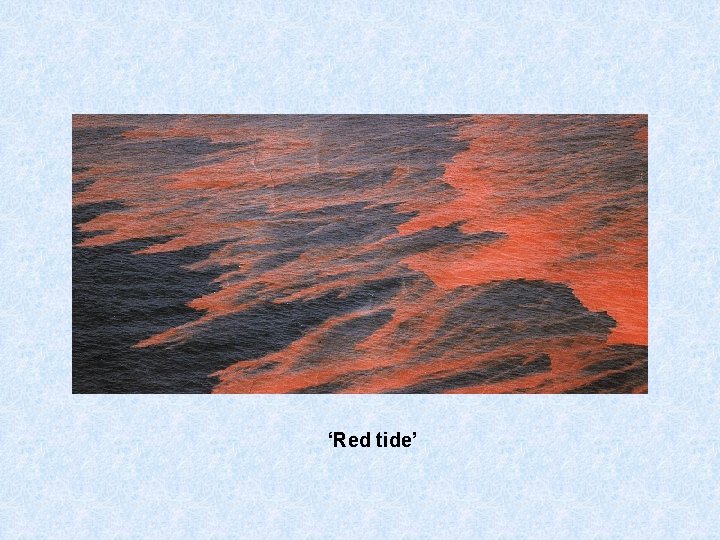
‘Red tide’
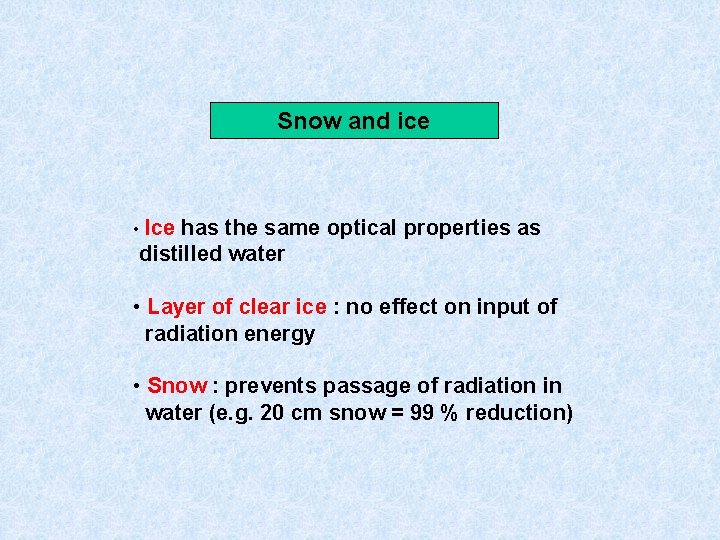
Snow and ice • Ice has the same optical properties as distilled water • Layer of clear ice : no effect on input of radiation energy • Snow : prevents passage of radiation in water (e. g. 20 cm snow = 99 % reduction)
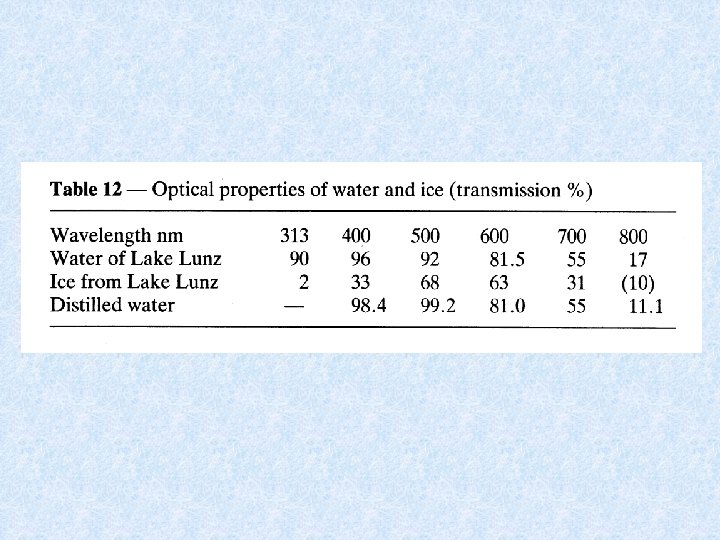
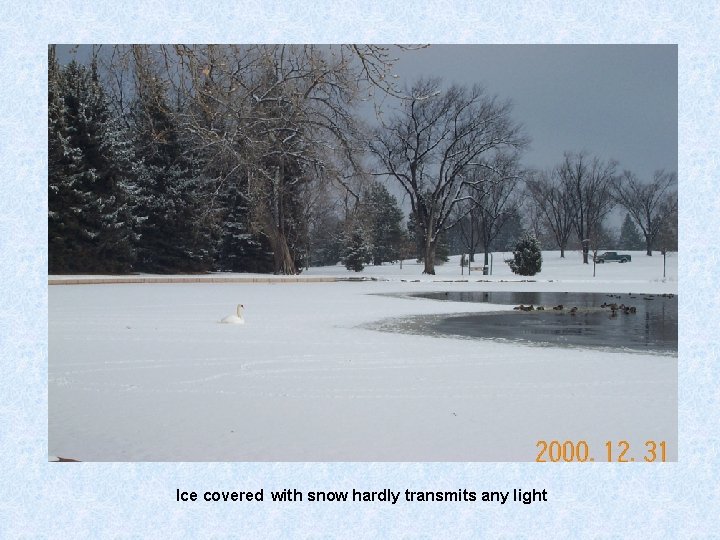
Ice covered with snow hardly transmits any light
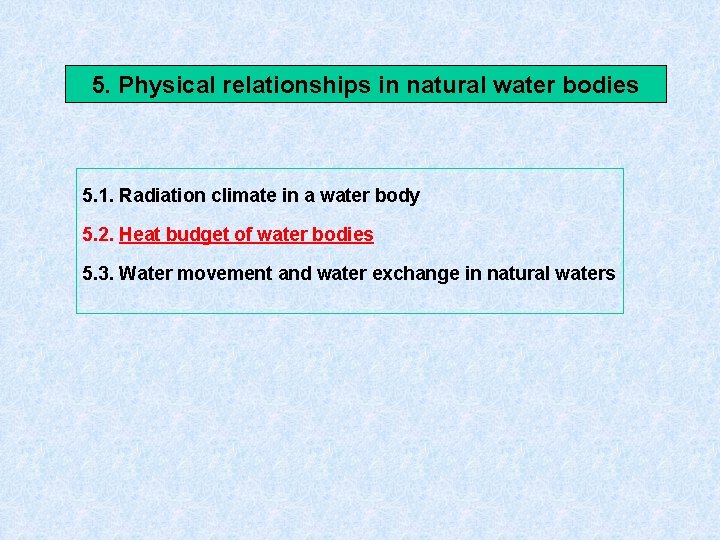
5. Physical relationships in natural water bodies 5. 1. Radiation climate in a water body 5. 2. Heat budget of water bodies 5. 3. Water movement and water exchange in natural waters
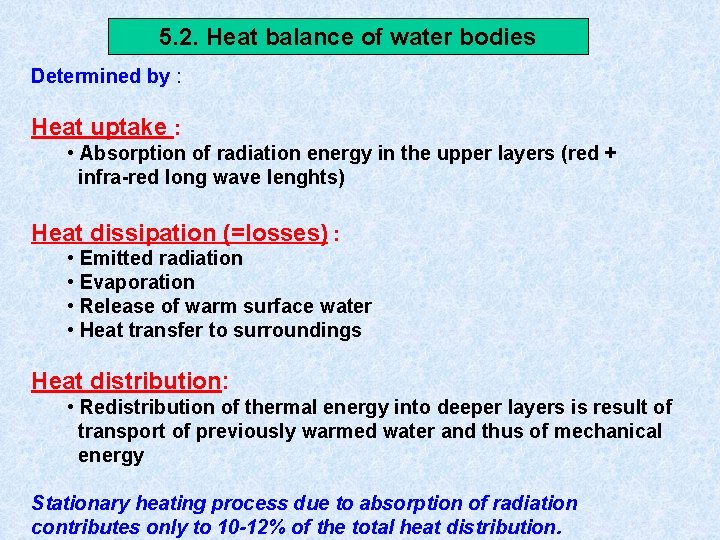
5. 2. Heat balance of water bodies Determined by : Heat uptake : • Absorption of radiation energy in the upper layers (red + infra-red long wave lenghts) Heat dissipation (=losses) : • Emitted radiation • Evaporation • Release of warm surface water • Heat transfer to surroundings Heat distribution: • Redistribution of thermal energy into deeper layers is result of transport of previously warmed water and thus of mechanical energy Stationary heating process due to absorption of radiation contributes only to 10 -12% of the total heat distribution.
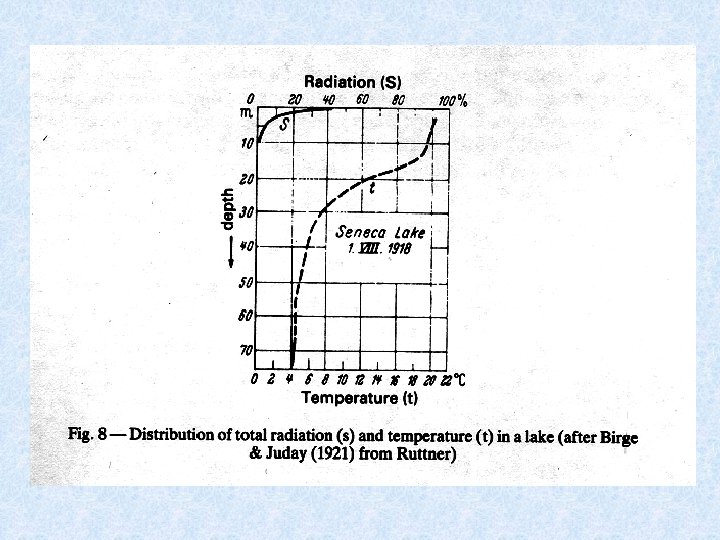
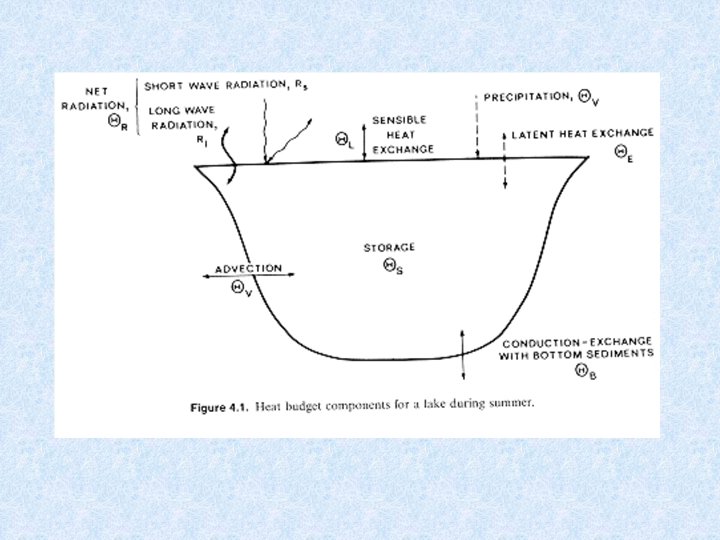
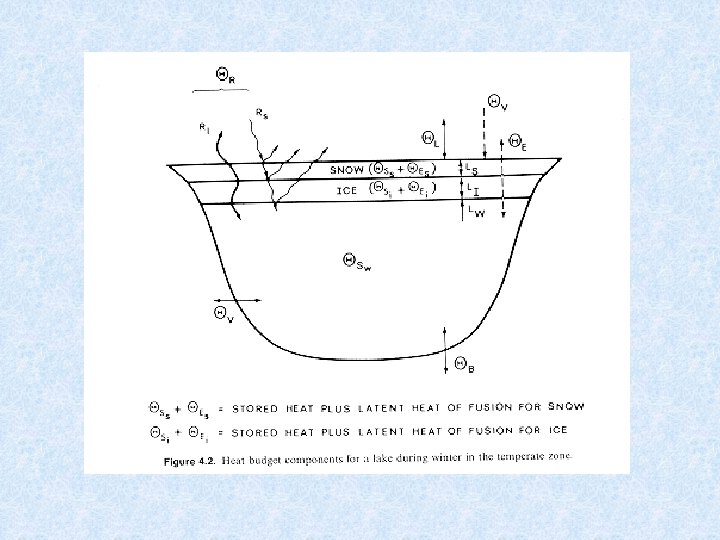
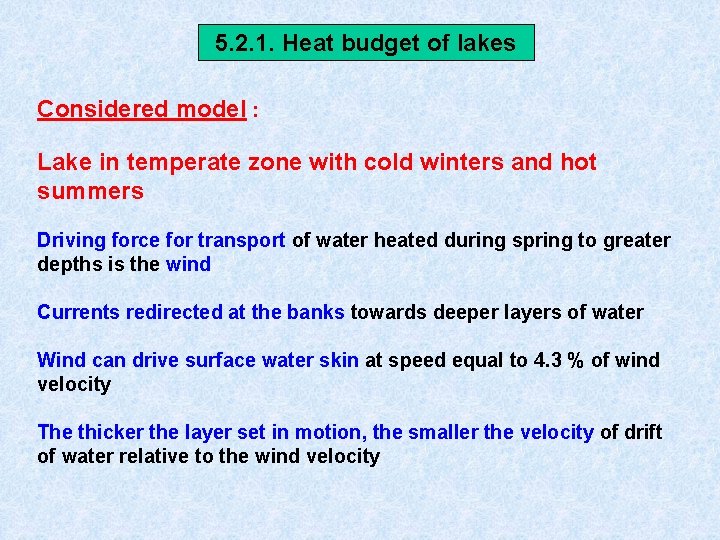
5. 2. 1. Heat budget of lakes Considered model : Lake in temperate zone with cold winters and hot summers Driving force for transport of water heated during spring to greater depths is the wind Currents redirected at the banks towards deeper layers of water Wind can drive surface water skin at speed equal to 4. 3 % of wind velocity The thicker the layer set in motion, the smaller the velocity of drift of water relative to the wind velocity
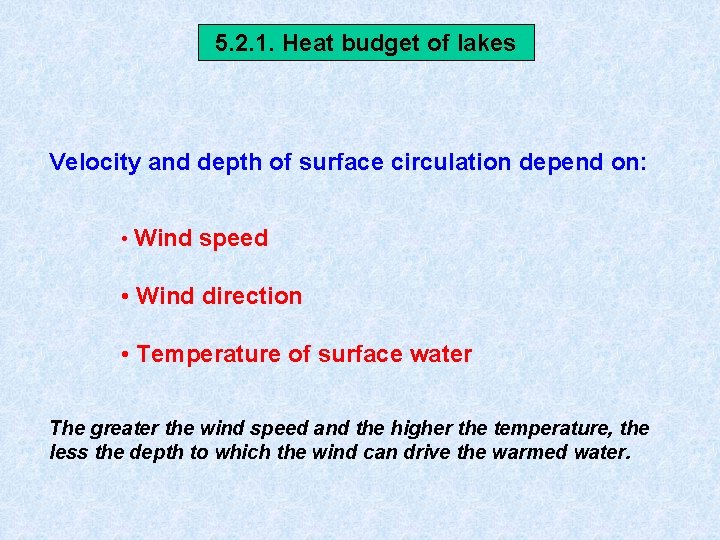
5. 2. 1. Heat budget of lakes Velocity and depth of surface circulation depend on: • Wind speed • Wind direction • Temperature of surface water The greater the wind speed and the higher the temperature, the less the depth to which the wind can drive the warmed water.
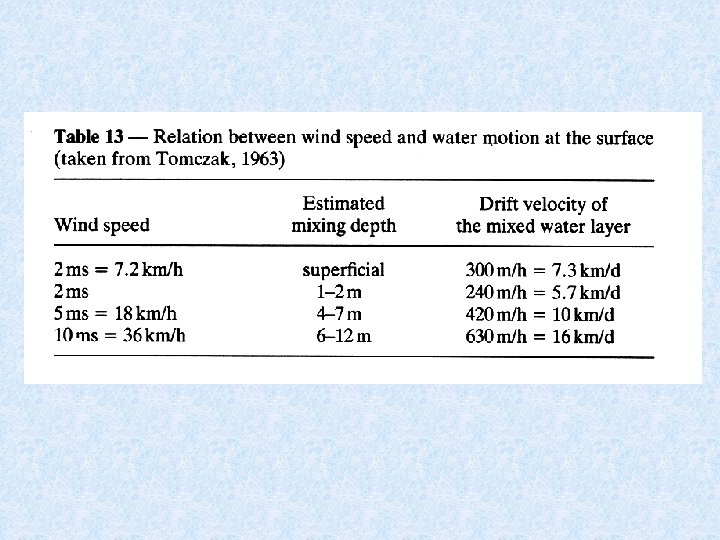
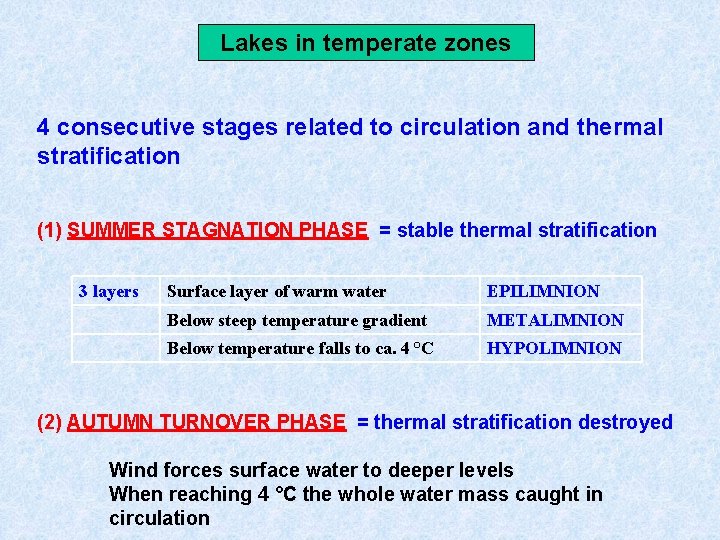
Lakes in temperate zones 4 consecutive stages related to circulation and thermal stratification (1) SUMMER STAGNATION PHASE = stable thermal stratification 3 layers Surface layer of warm water EPILIMNION Below steep temperature gradient METALIMNION Below temperature falls to ca. 4 °C HYPOLIMNION (2) AUTUMN TURNOVER PHASE = thermal stratification destroyed Wind forces surface water to deeper levels When reaching 4 °C the whole water mass caught in circulation
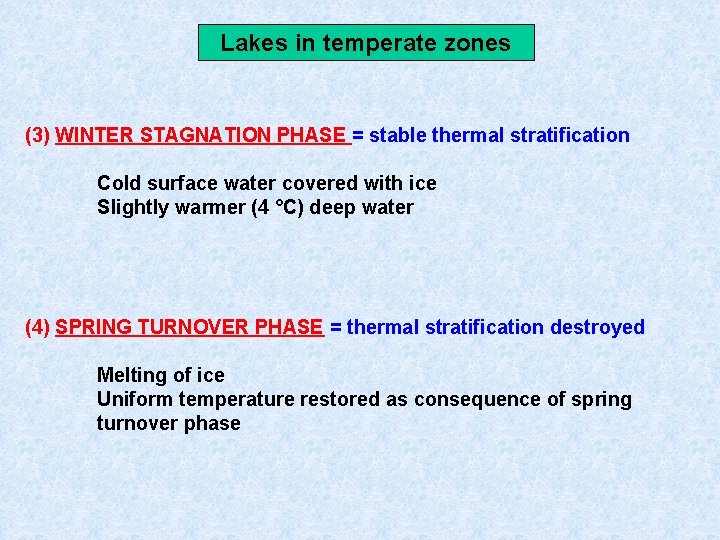
Lakes in temperate zones (3) WINTER STAGNATION PHASE = stable thermal stratification Cold surface water covered with ice Slightly warmer (4 °C) deep water (4) SPRING TURNOVER PHASE = thermal stratification destroyed Melting of ice Uniform temperature restored as consequence of spring turnover phase
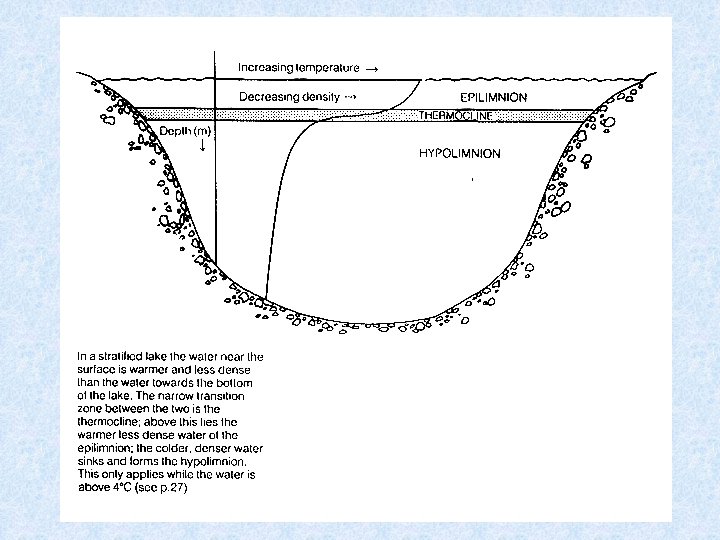
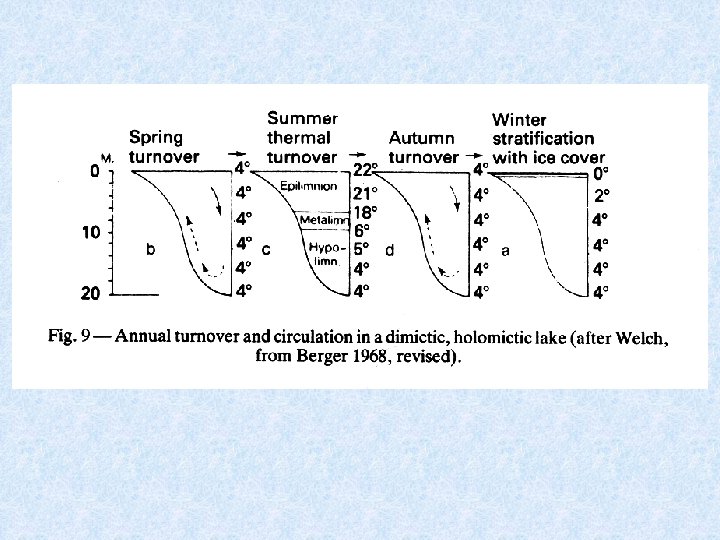
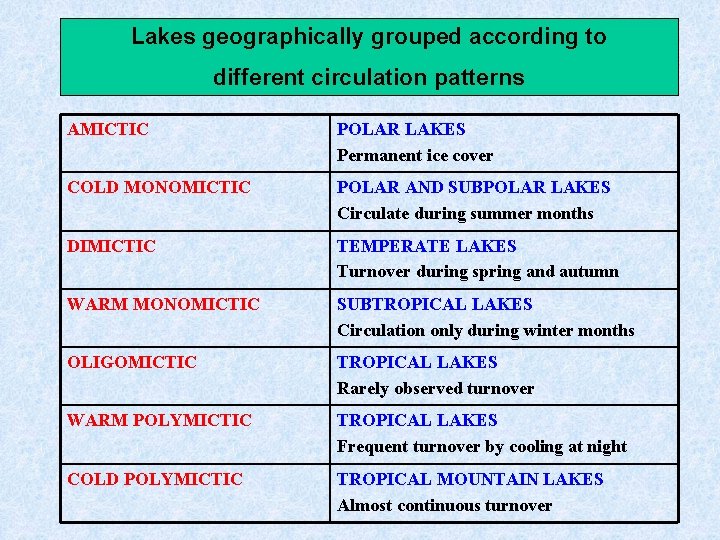
Lakes geographically grouped according to different circulation patterns AMICTIC POLAR LAKES Permanent ice cover COLD MONOMICTIC POLAR AND SUBPOLAR LAKES Circulate during summer months DIMICTIC TEMPERATE LAKES Turnover during spring and autumn WARM MONOMICTIC SUBTROPICAL LAKES Circulation only during winter months OLIGOMICTIC TROPICAL LAKES Rarely observed turnover WARM POLYMICTIC TROPICAL LAKES Frequent turnover by cooling at night COLD POLYMICTIC TROPICAL MOUNTAIN LAKES Almost continuous turnover
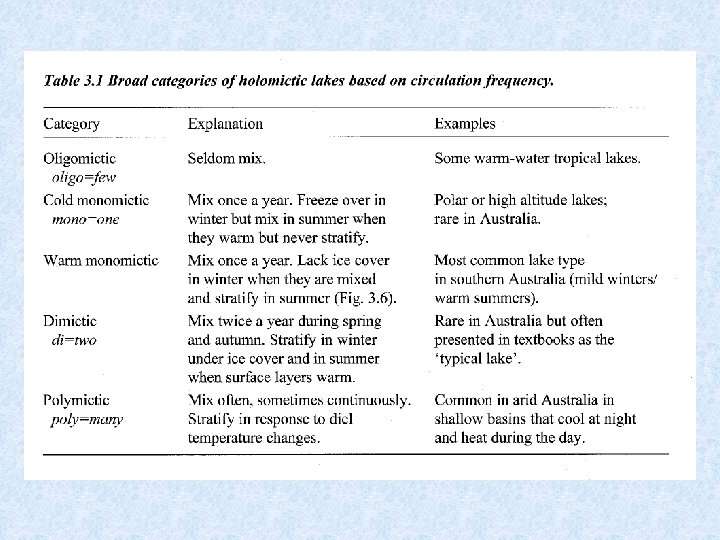
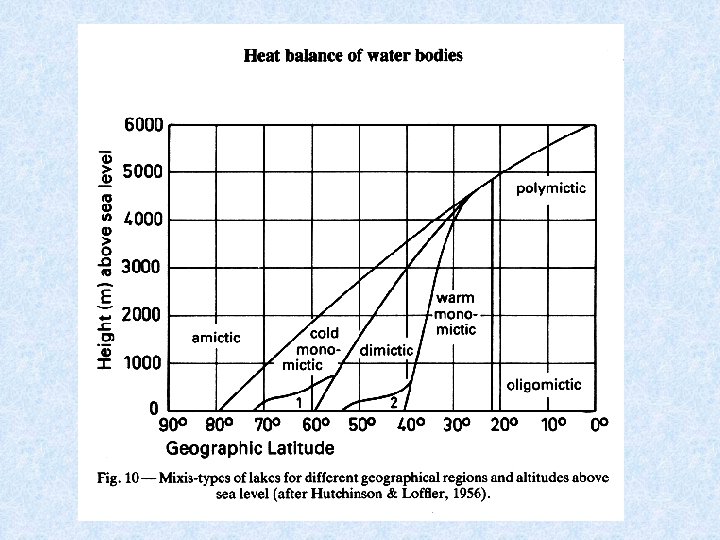
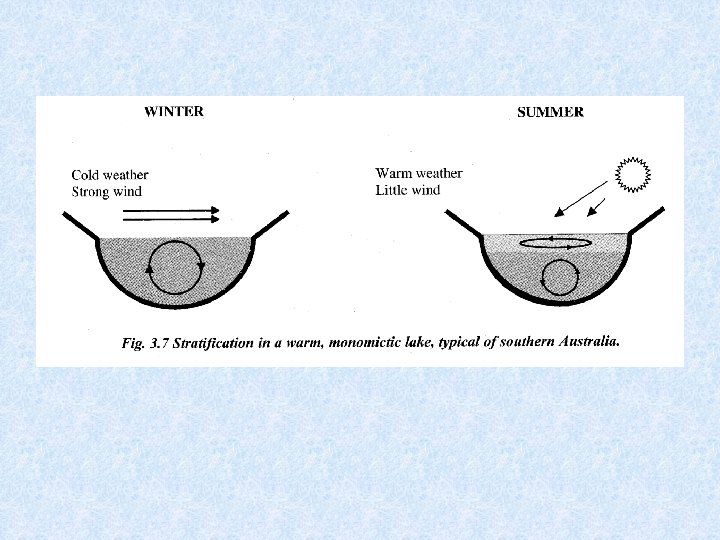
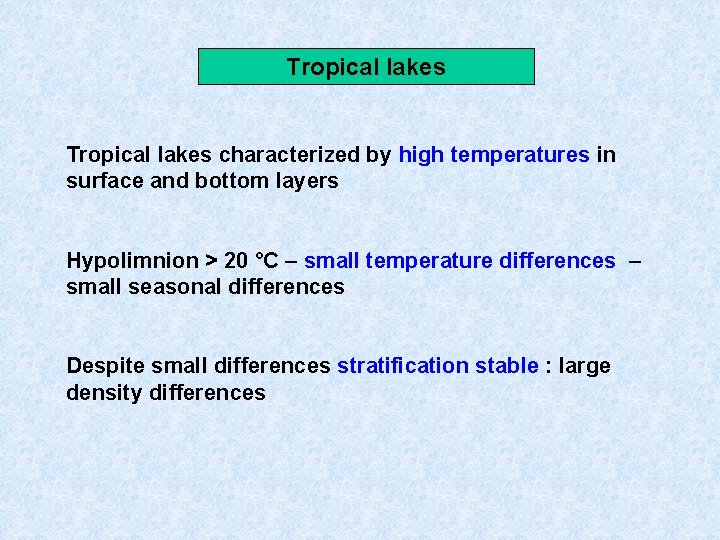
Tropical lakes characterized by high temperatures in surface and bottom layers Hypolimnion > 20 °C – small temperature differences – small seasonal differences Despite small differences stratification stable : large density differences
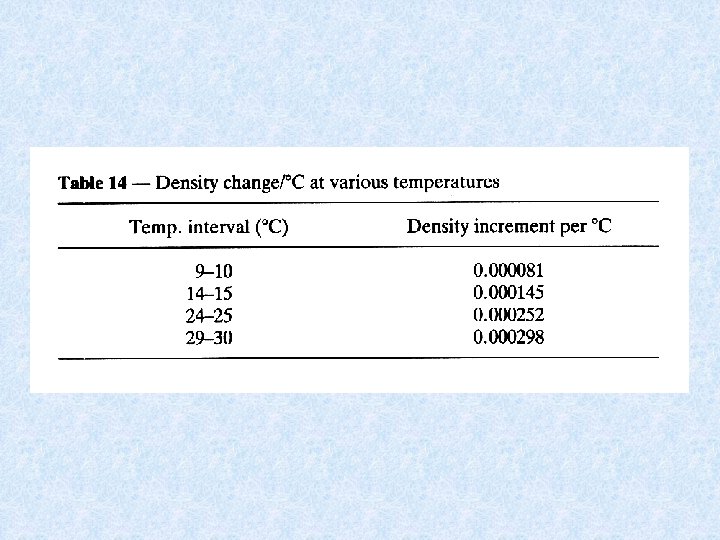
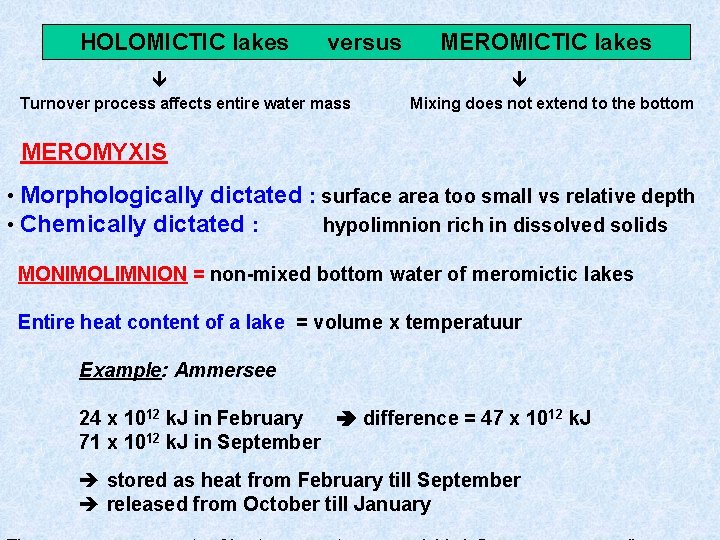
HOLOMICTIC lakes versus Turnover process affects entire water mass MEROMICTIC lakes Mixing does not extend to the bottom MEROMYXIS • Morphologically dictated : surface area too small vs relative depth • Chemically dictated : hypolimnion rich in dissolved solids MONIMOLIMNION = non-mixed bottom water of meromictic lakes Entire heat content of a lake = volume x temperatuur Example: Ammersee 24 x 1012 k. J in February difference = 47 x 1012 k. J 71 x 1012 k. J in September stored as heat from February till September released from October till January
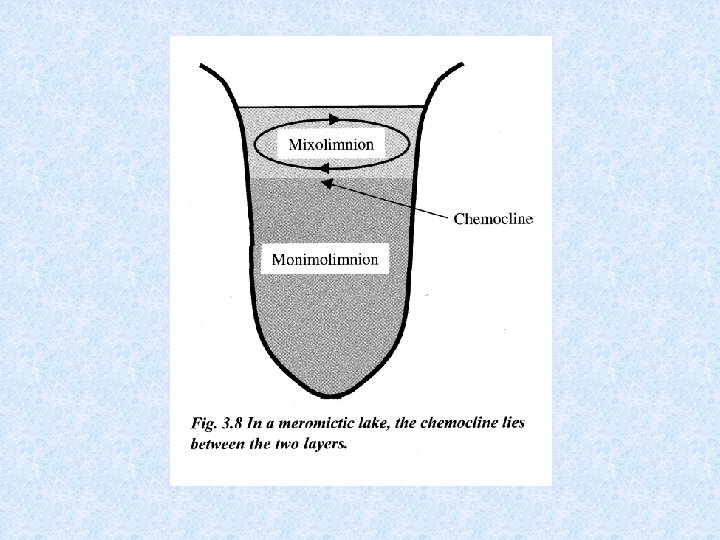
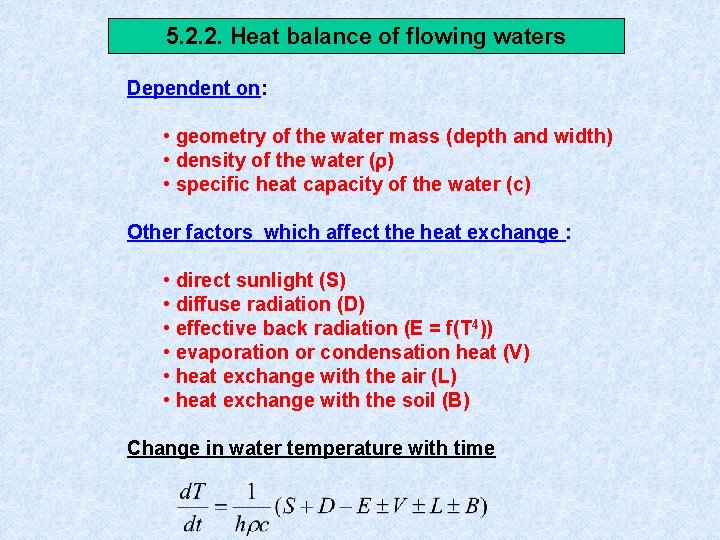
5. 2. 2. Heat balance of flowing waters Dependent on: • geometry of the water mass (depth and width) • density of the water ( ) • specific heat capacity of the water (c) Other factors which affect the heat exchange : • direct sunlight (S) • diffuse radiation (D) • effective back radiation (E = f(T 4)) • evaporation or condensation heat (V) • heat exchange with the air (L) • heat exchange with the soil (B) Change in water temperature with time
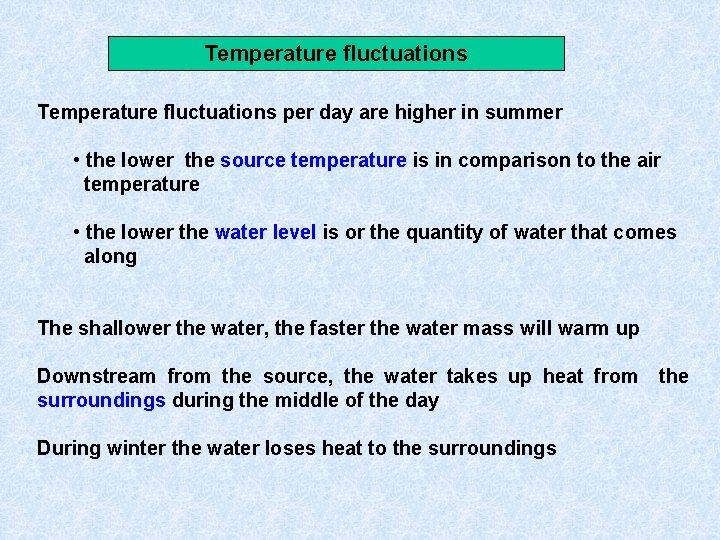
Temperature fluctuations per day are higher in summer • the lower the source temperature is in comparison to the air temperature • the lower the water level is or the quantity of water that comes along The shallower the water, the faster the water mass will warm up Downstream from the source, the water takes up heat from surroundings during the middle of the day During winter the water loses heat to the surroundings the
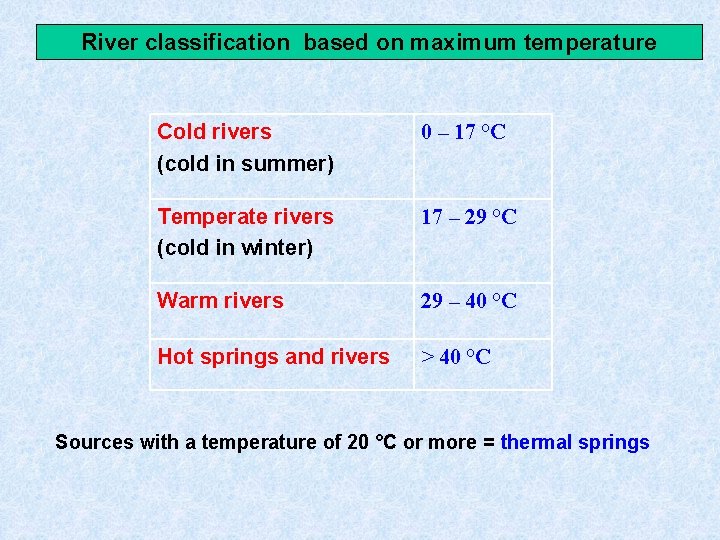
River classification based on maximum temperature Cold rivers (cold in summer) 0 – 17 °C Temperate rivers (cold in winter) 17 – 29 °C Warm rivers 29 – 40 °C Hot springs and rivers > 40 °C Sources with a temperature of 20 °C or more = thermal springs
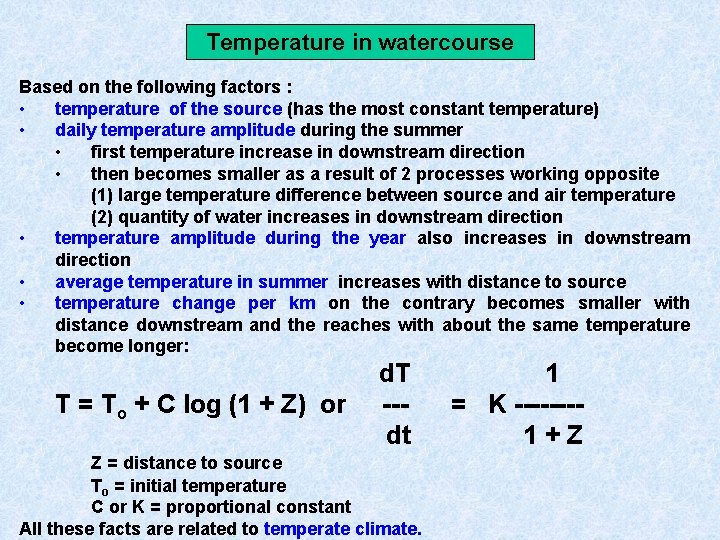
Temperature in watercourse Based on the following factors : • temperature of the source (has the most constant temperature) • daily temperature amplitude during the summer • first temperature increase in downstream direction • then becomes smaller as a result of 2 processes working opposite (1) large temperature difference between source and air temperature (2) quantity of water increases in downstream direction • temperature amplitude during the year also increases in downstream direction • average temperature in summer increases with distance to source • temperature change per km on the contrary becomes smaller with distance downstream and the reaches with about the same temperature become longer: T = To + C log (1 + Z) or d. T --dt Z = distance to source To = initial temperature C or K = proportional constant All these facts are related to temperate climate. 1 = K -------1+Z
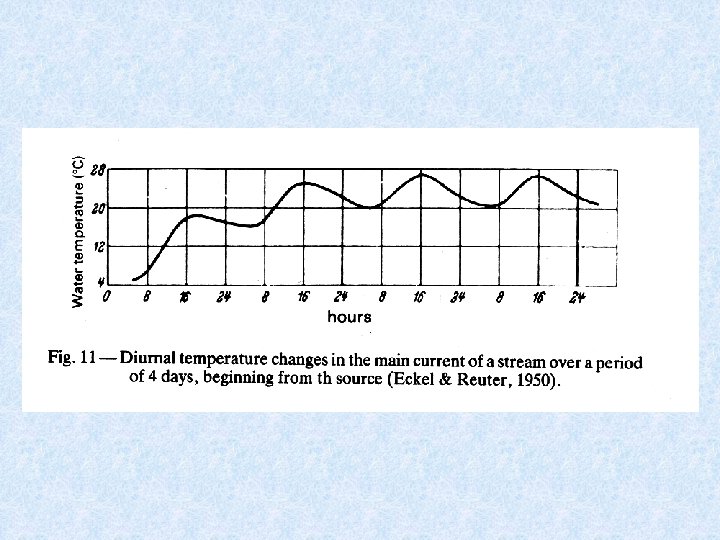
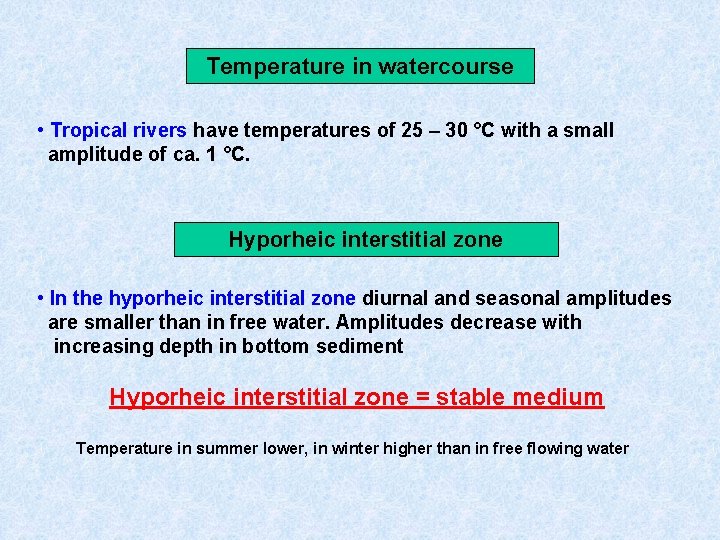
Temperature in watercourse • Tropical rivers have temperatures of 25 – 30 °C with a small amplitude of ca. 1 °C. Hyporheic interstitial zone • In the hyporheic interstitial zone diurnal and seasonal amplitudes are smaller than in free water. Amplitudes decrease with increasing depth in bottom sediment Hyporheic interstitial zone = stable medium Temperature in summer lower, in winter higher than in free flowing water
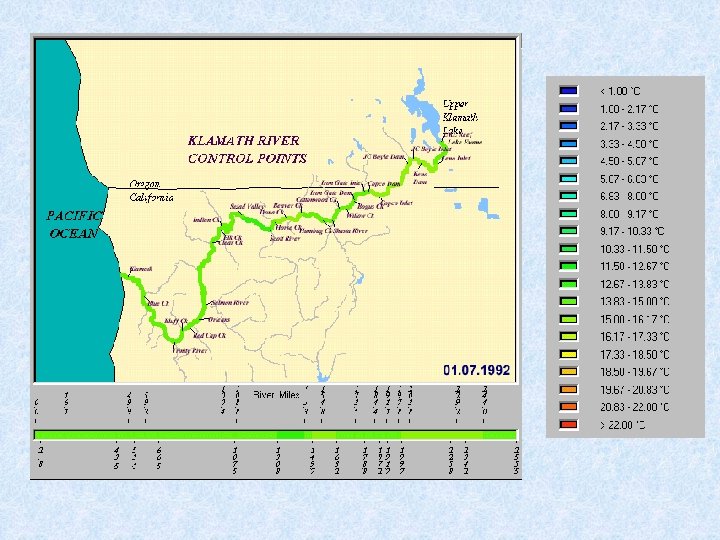
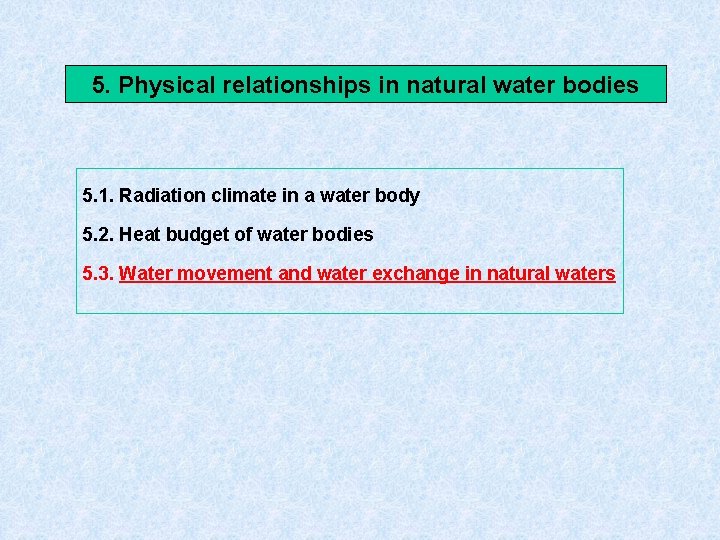
5. Physical relationships in natural water bodies 5. 1. Radiation climate in a water body 5. 2. Heat budget of water bodies 5. 3. Water movement and water exchange in natural waters
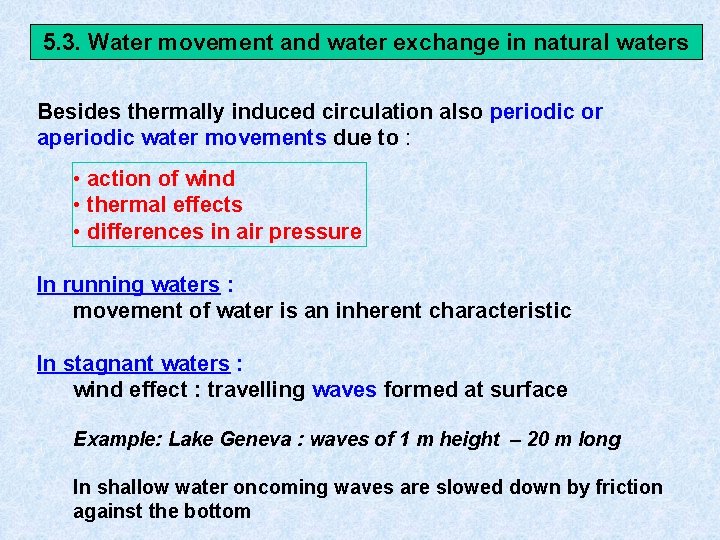
5. 3. Water movement and water exchange in natural waters Besides thermally induced circulation also periodic or aperiodic water movements due to : • action of wind • thermal effects • differences in air pressure In running waters : movement of water is an inherent characteristic In stagnant waters : wind effect : travelling waves formed at surface Example: Lake Geneva : waves of 1 m height – 20 m long In shallow water oncoming waves are slowed down by friction against the bottom
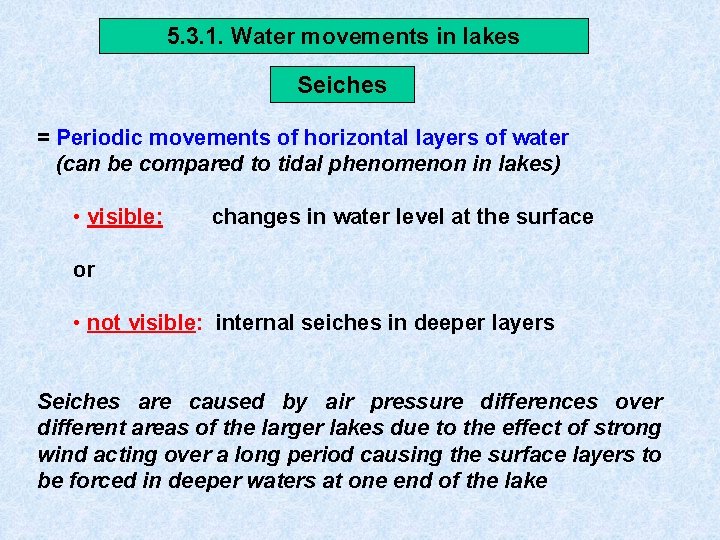
5. 3. 1. Water movements in lakes Seiches = Periodic movements of horizontal layers of water (can be compared to tidal phenomenon in lakes) • visible: changes in water level at the surface or • not visible: internal seiches in deeper layers Seiches are caused by air pressure differences over different areas of the larger lakes due to the effect of strong wind acting over a long period causing the surface layers to be forced in deeper waters at one end of the lake
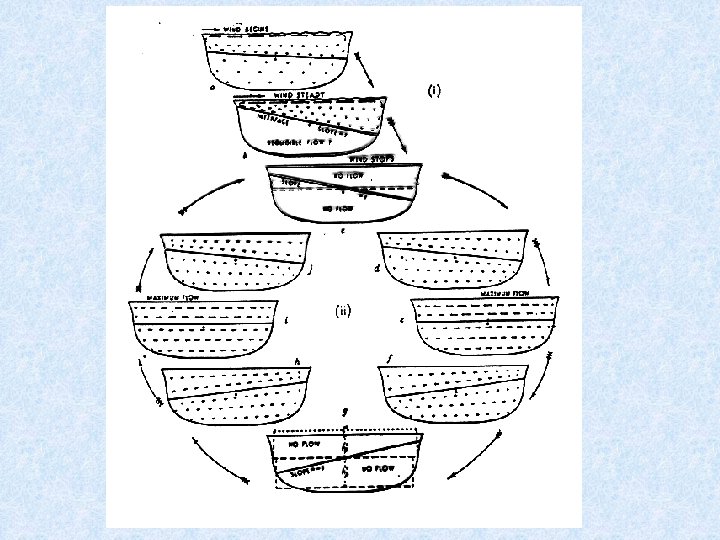
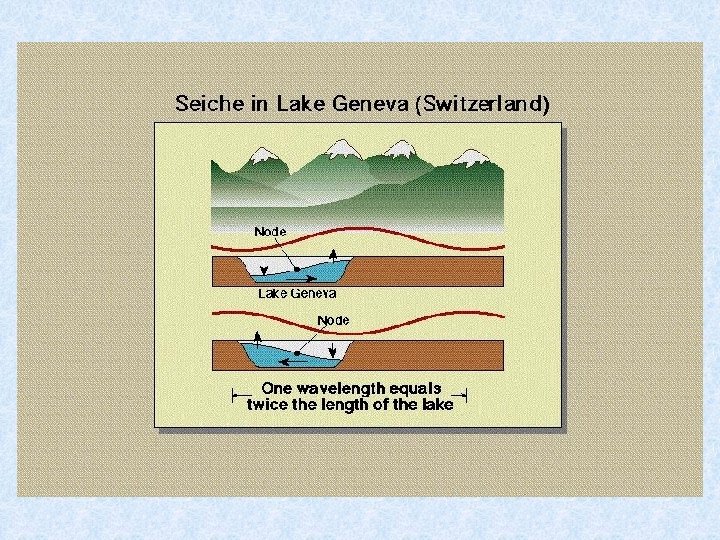
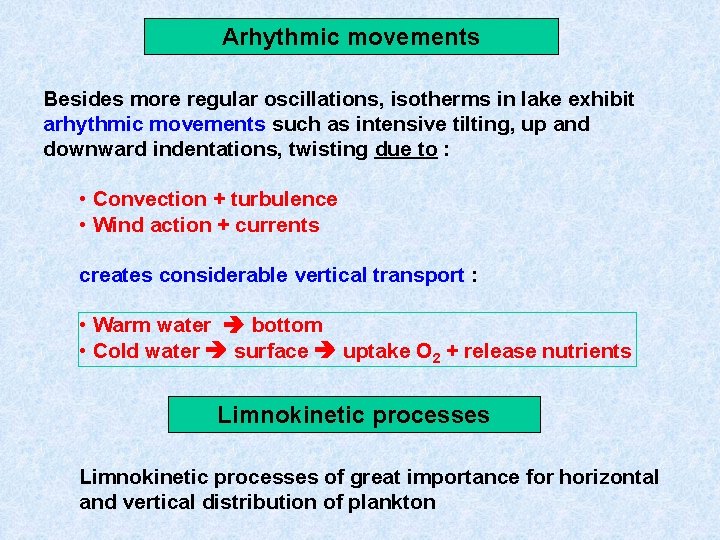
Arhythmic movements Besides more regular oscillations, isotherms in lake exhibit arhythmic movements such as intensive tilting, up and downward indentations, twisting due to : • Convection + turbulence • Wind action + currents creates considerable vertical transport : • Warm water bottom • Cold water surface uptake O 2 + release nutrients Limnokinetic processes of great importance for horizontal and vertical distribution of plankton
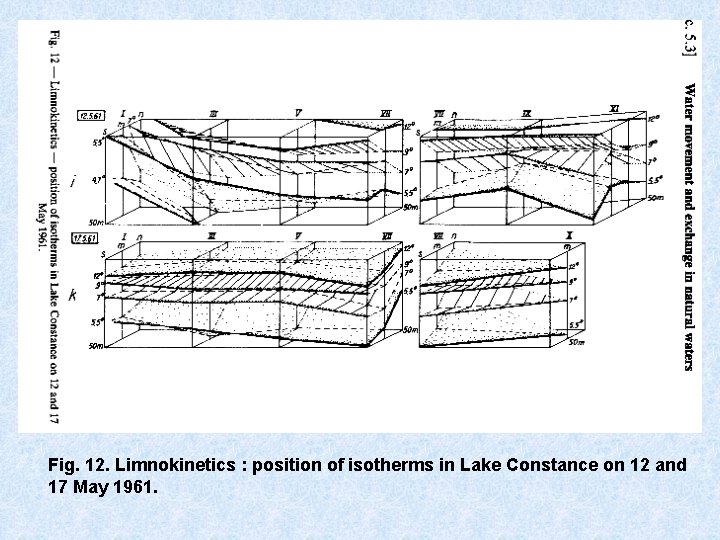
Fig. 12. Limnokinetics : position of isotherms in Lake Constance on 12 and 17 May 1961.
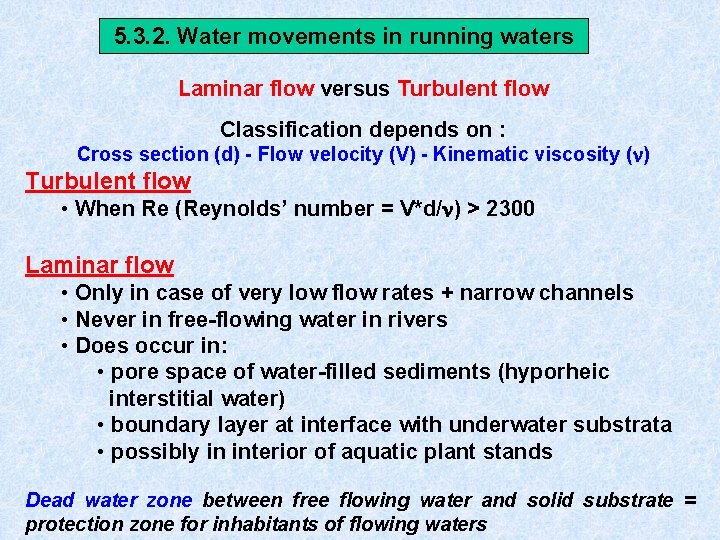
5. 3. 2. Water movements in running waters Laminar flow versus Turbulent flow Classification depends on : Cross section (d) - Flow velocity (V) - Kinematic viscosity ( ) Turbulent flow • When Re (Reynolds’ number = V*d/ ) > 2300 Laminar flow • Only in case of very low flow rates + narrow channels • Never in free-flowing water in rivers • Does occur in: • pore space of water-filled sediments (hyporheic interstitial water) • boundary layer at interface with underwater substrata • possibly in interior of aquatic plant stands Dead water zone between free flowing water and solid substrate = protection zone for inhabitants of flowing waters
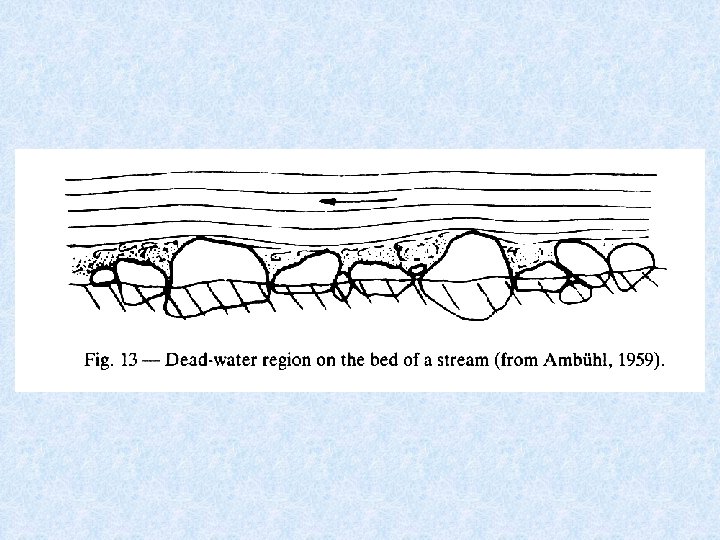
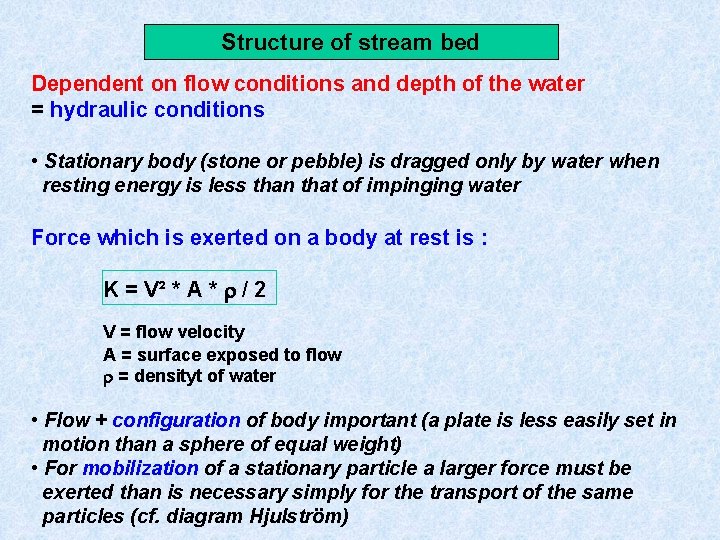
Structure of stream bed Dependent on flow conditions and depth of the water = hydraulic conditions • Stationary body (stone or pebble) is dragged only by water when resting energy is less than that of impinging water Force which is exerted on a body at rest is : K = V² * A * / 2 V = flow velocity A = surface exposed to flow = densityt of water • Flow + configuration of body important (a plate is less easily set in motion than a sphere of equal weight) • For mobilization of a stationary particle a larger force must be exerted than is necessary simply for the transport of the same particles (cf. diagram Hjulström)
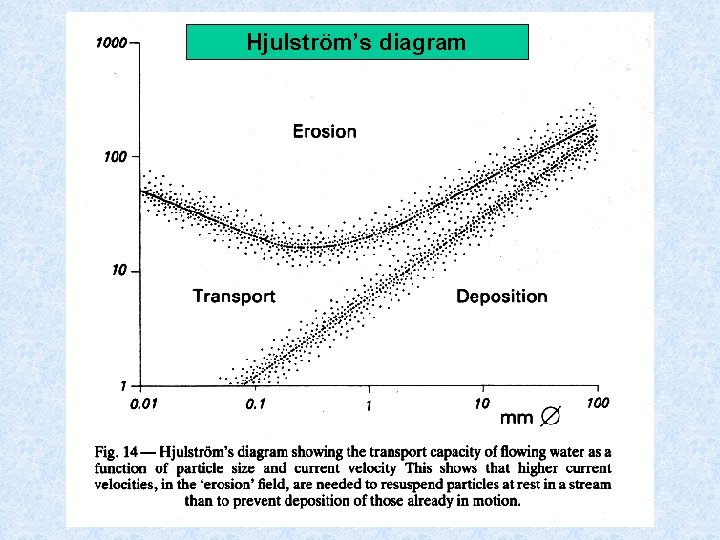
Hjulström’s diagram
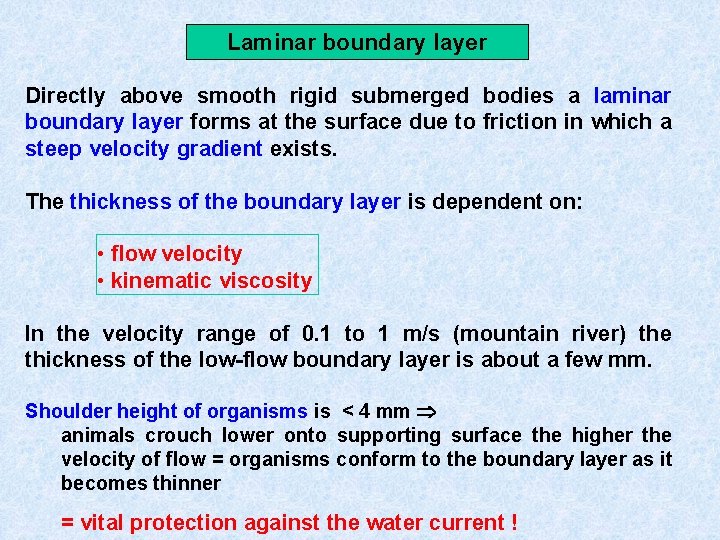
Laminar boundary layer Directly above smooth rigid submerged bodies a laminar boundary layer forms at the surface due to friction in which a steep velocity gradient exists. The thickness of the boundary layer is dependent on: • flow velocity • kinematic viscosity In the velocity range of 0. 1 to 1 m/s (mountain river) the thickness of the low-flow boundary layer is about a few mm. Shoulder height of organisms is < 4 mm animals crouch lower onto supporting surface the higher the velocity of flow = organisms conform to the boundary layer as it becomes thinner = vital protection against the water current !
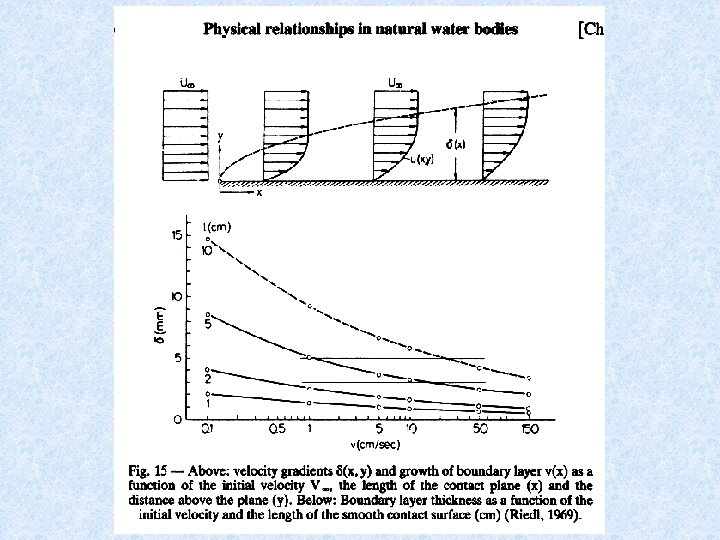
Aquatic ecology definition
Community level ecology
Ecology part 1
Freshwater zones diagram
Biome foldable
Freshwater echinoderms
Freshwater biomes climate
River with tributaries
Wetland ecosystem definition
Freshwater animals and adaptations
Laticotids
Chapter 14 water resources answer key
Climate of freshwater wetlands
Abiotic factors in freshwater
Latitude in climate
Freshwater
Upstate freshwater institute
Endangered animals of north america
Food web analysis activity
Freshwater basin
Texas freshwater fish identification
Saltwater fish in freshwater explode
Texas freshwater fish species
Map of freshwater biome
Mardu graw
Freshwater zones diagram
Describe freshwater
Dwa adalah
Pie chart of freshwater and saltwater
Description of freshwater
Human impact on freshwater ecosystems
Freshwater animal adaptations
Freshwater fish of belize
Freshwater fish marketing
Many freshwater invertebrates eliminate ammonia by
Freshwater biomes facts
Zones of lake ecosystem
Nbsc meaning
Non living things in temperate forest
Freshwater tertiary consumers
Minitab adalah
Technical object description example
Addition symbol
Parts of a bar counter
Part to part ratio definition
The part of a shadow surrounding the darkest part
Part part whole
Aquatic food chain
Planktivore
Profundal zone
Terrestrial vs aquatic food production systems
Aquatic biomes examples
Describe aquatic ecosystem
Why biomes are important
Interest grabber
Connecting the concepts: aquatic biomes
Wolffia
What kingdom is photosynthetic aquatic and unicellular
Aquatic areas
Eco bottle project
4-4 aquatic ecosystems
Aquatic
Aquatic organism passage
Bioma air laut
Aquatic
Aquatic science definition
Hydr words
Code.org minecraft aquatic
History of aquatic science
Chapter 7 aquatic ecosystems
Chapter 3 lesson 3 biomes and aquatic ecosystems
National aquatic resources research and development agency
What are the two main types of aquatic ecosystems
Are protists terrestrial or aquatic
Aquatic food chain
Otters are semi aquatic
Biomes and aquatic ecosystems
Morphological adaptations in aquatic plants
Section 4-4 aquatic ecosystems answer key
Section 4-4 aquatic ecosystems
Arlington masters swimming
Oie aquatic animal health code
Grazing food chain
Lesson outline lesson 2 aquatic ecosystems answer key
Transpiration in aquatic plants
Estero bay aquatic preserve
Ap environmental science aquatic biomes
The chaparral biome is best characterized by _______.
Aquatic biotechnology
Ecosystem webquest