VIII DNA Function Replication VIII DNA Function Replication
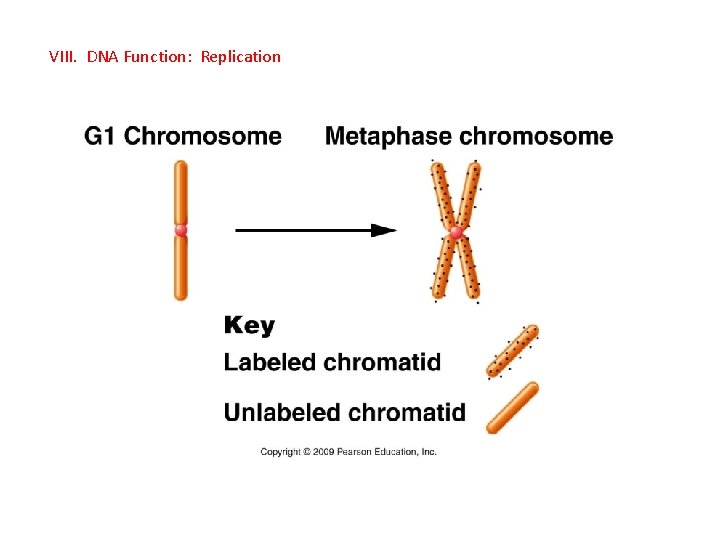
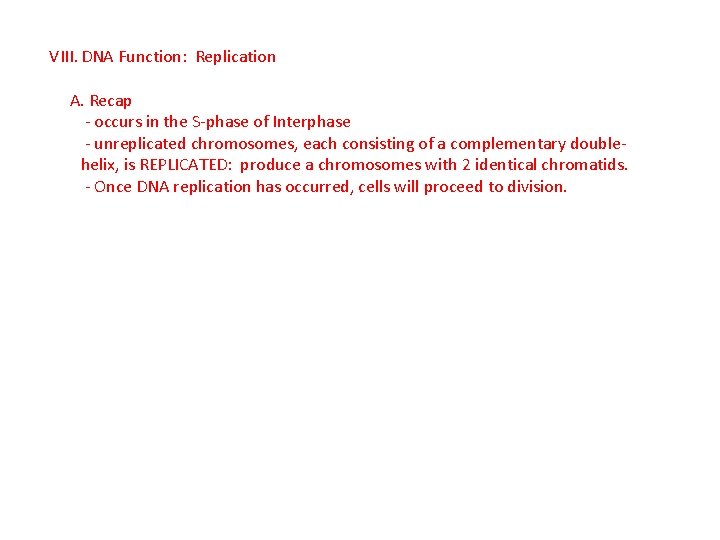
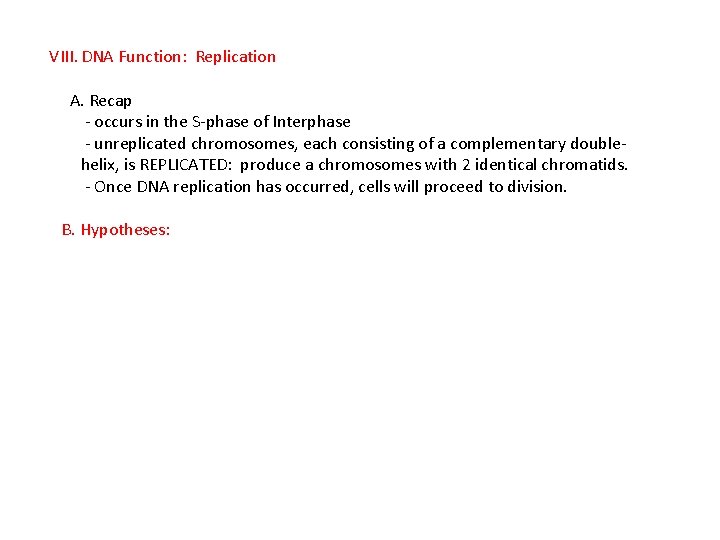
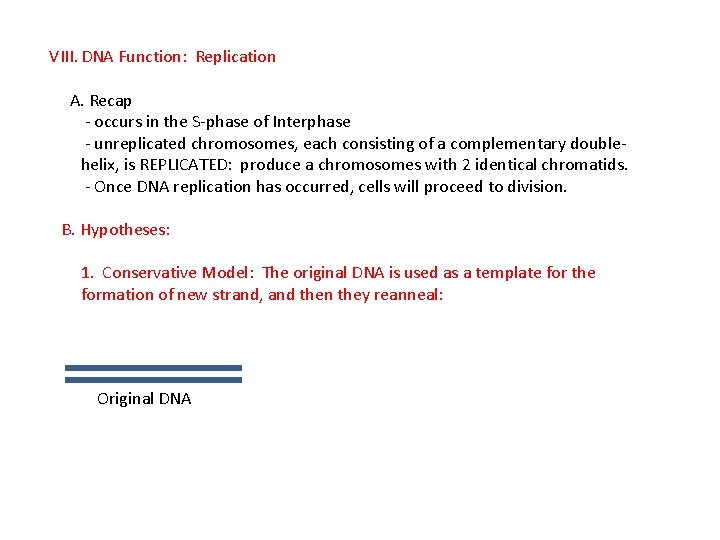
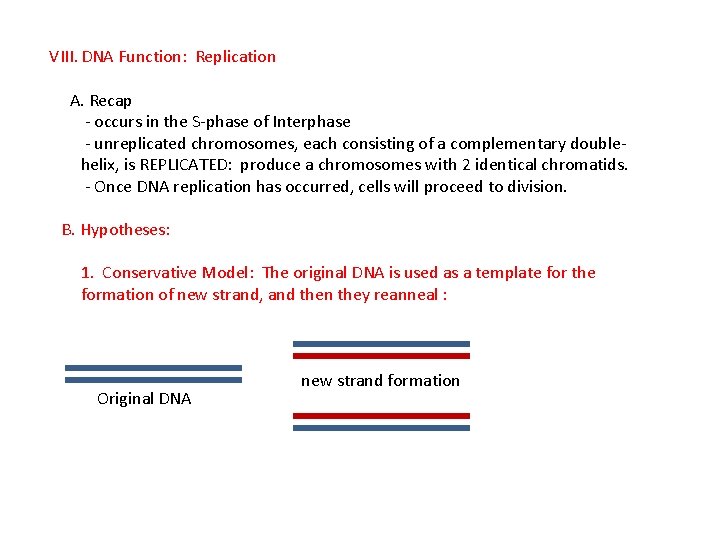
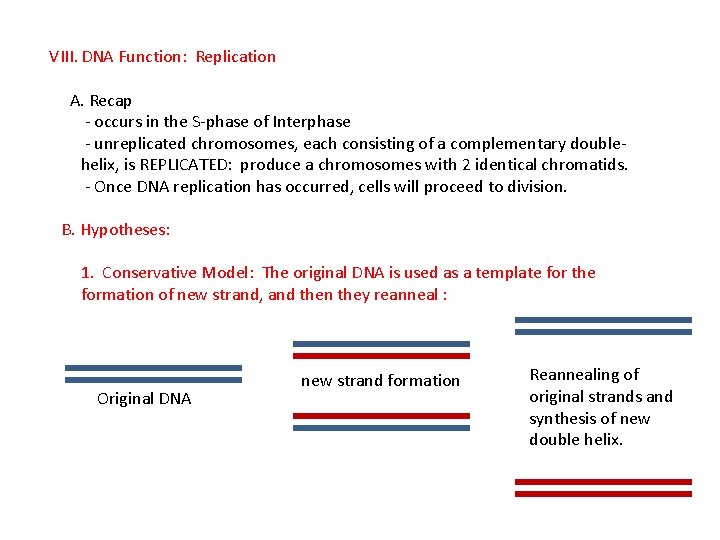
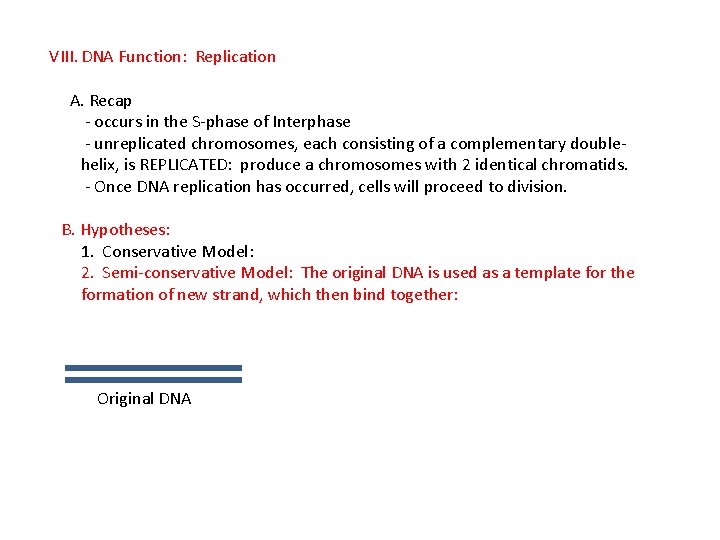
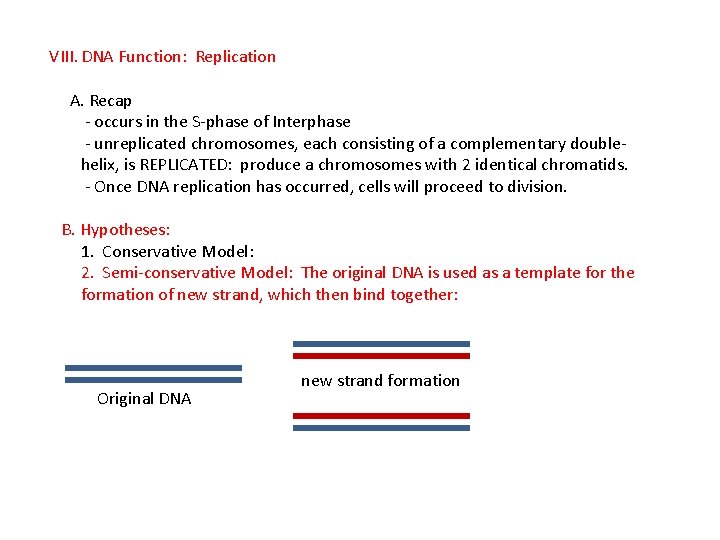
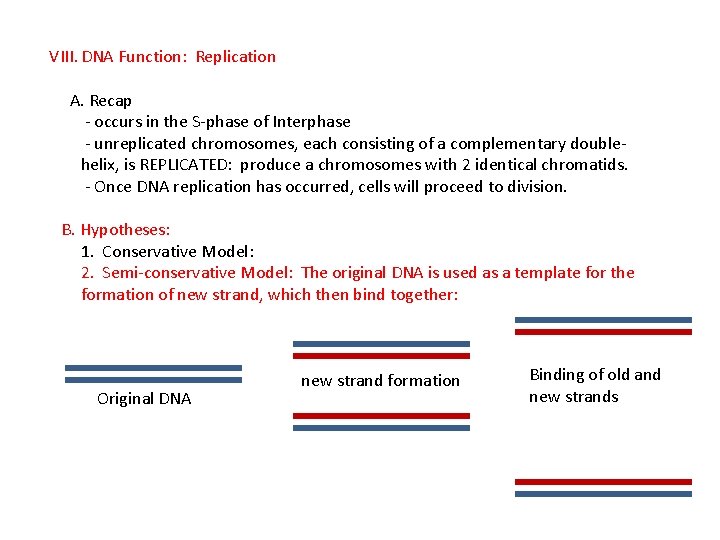
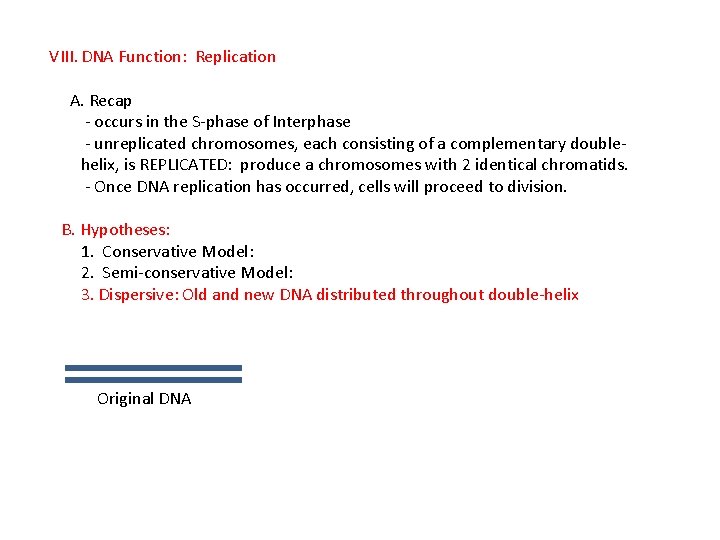
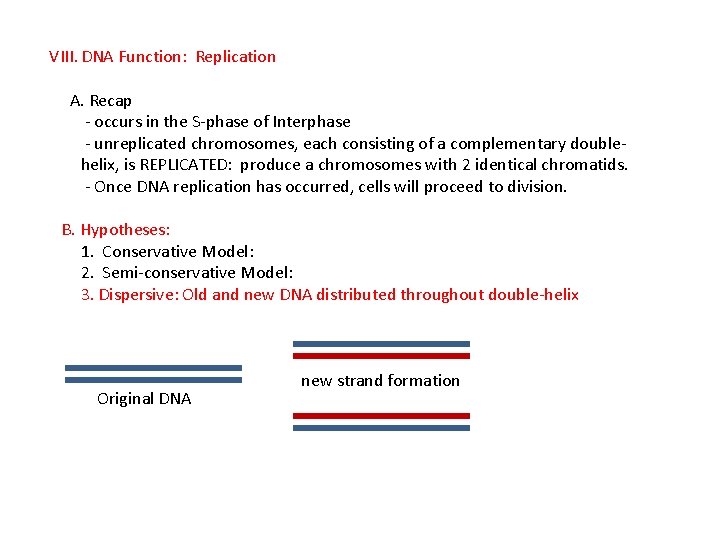
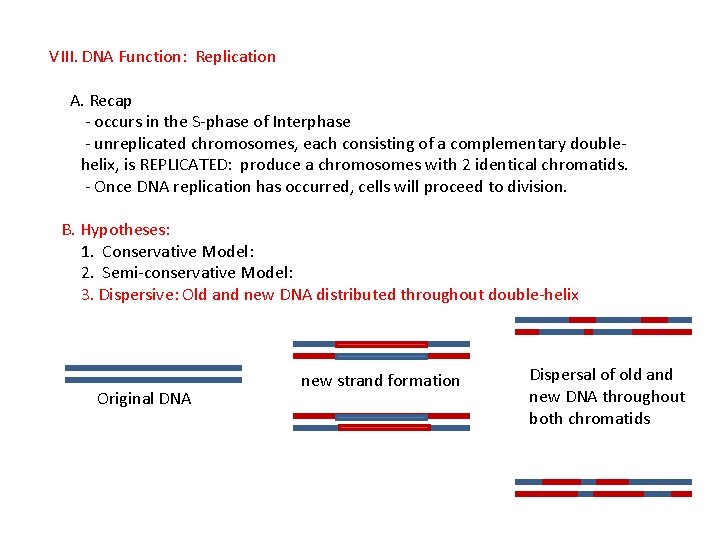
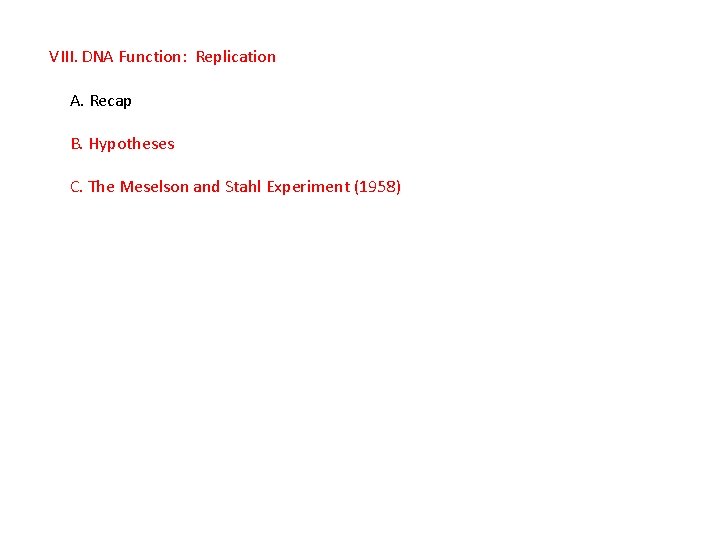
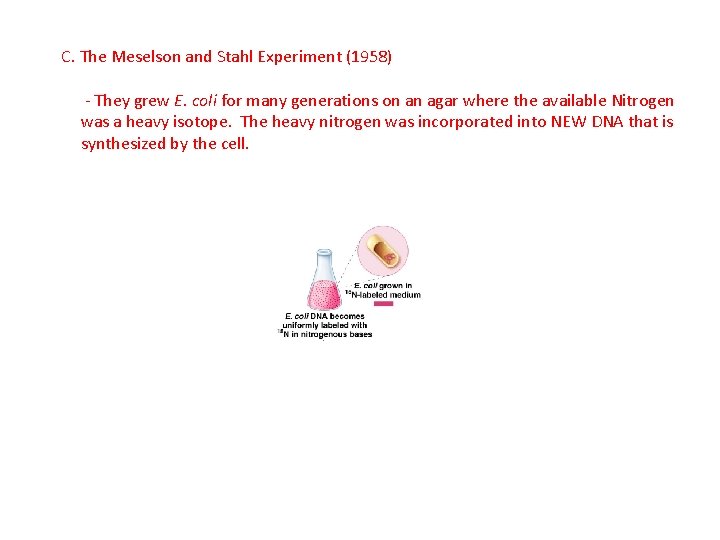
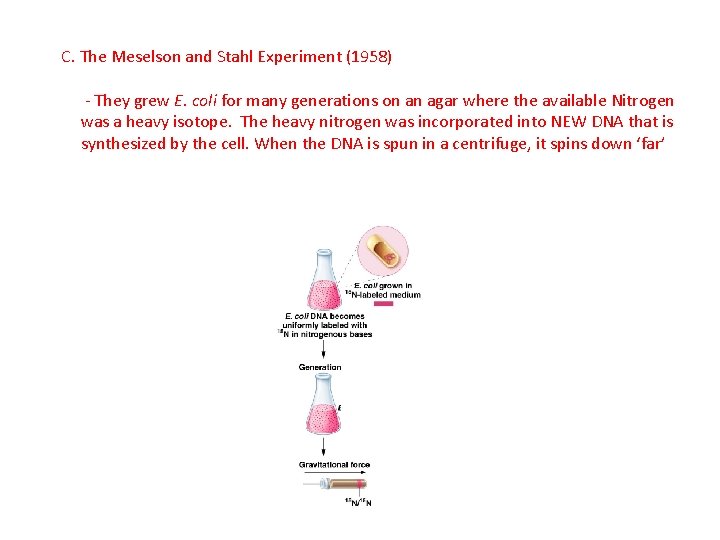
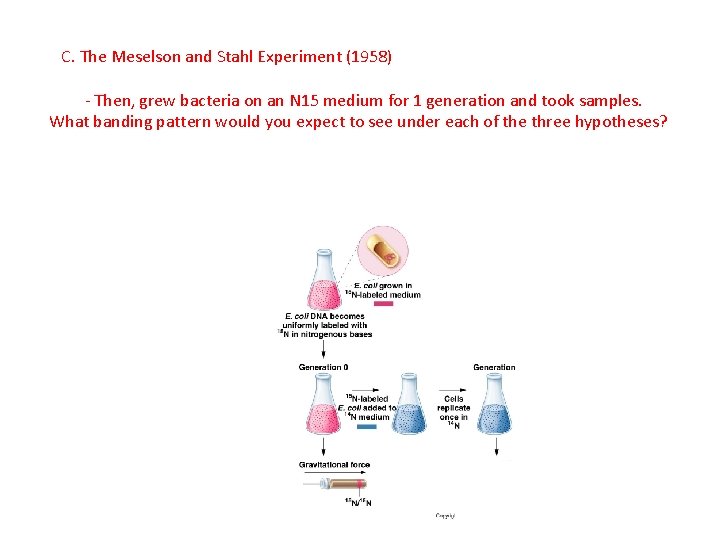
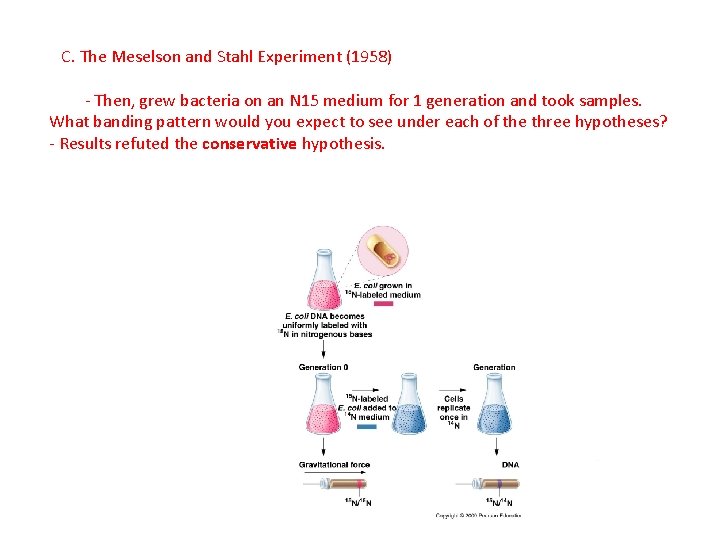
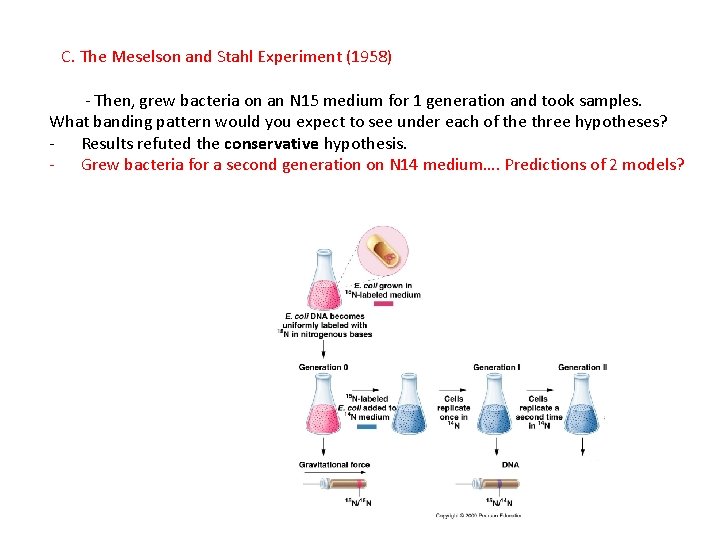
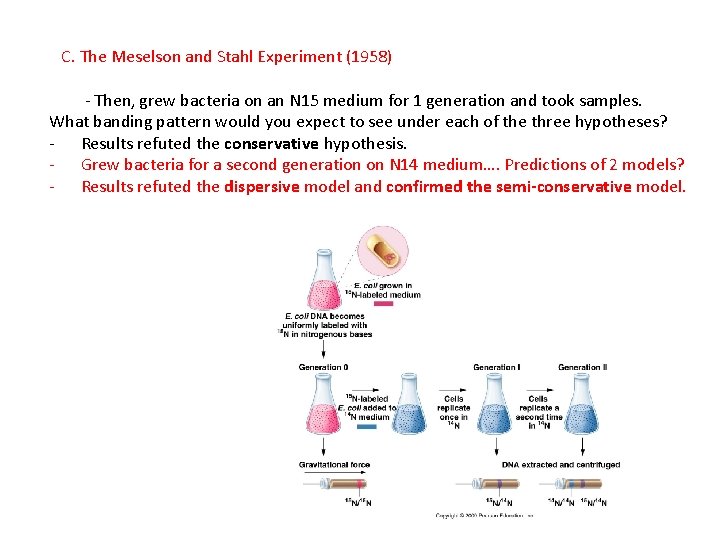
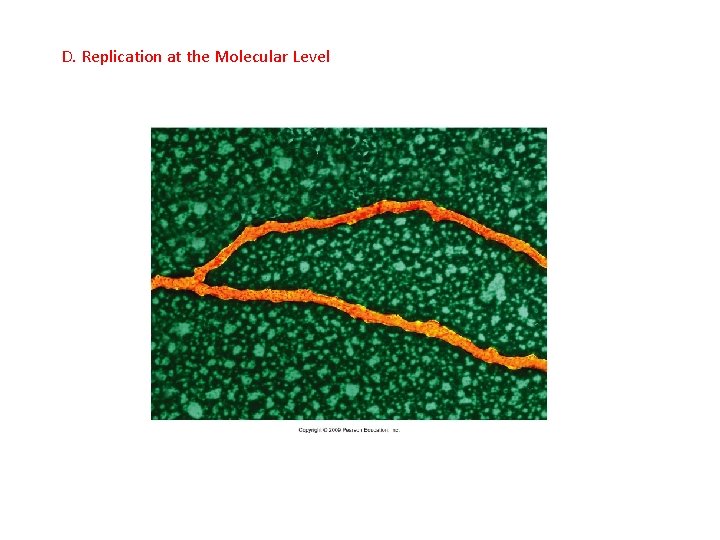
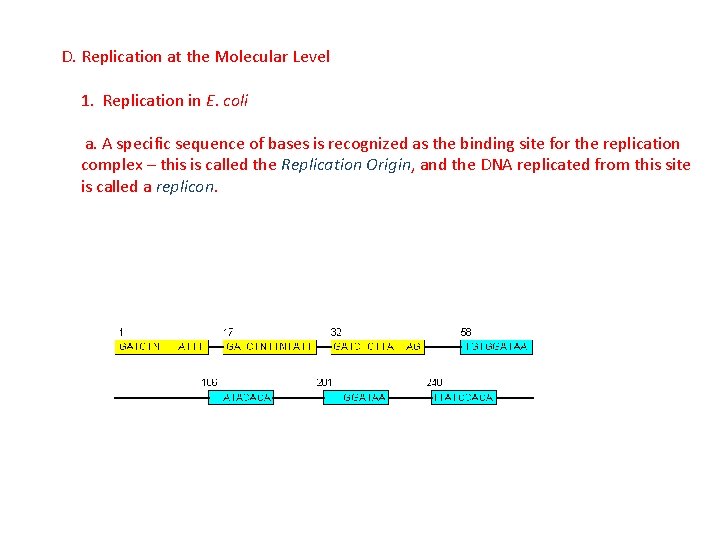
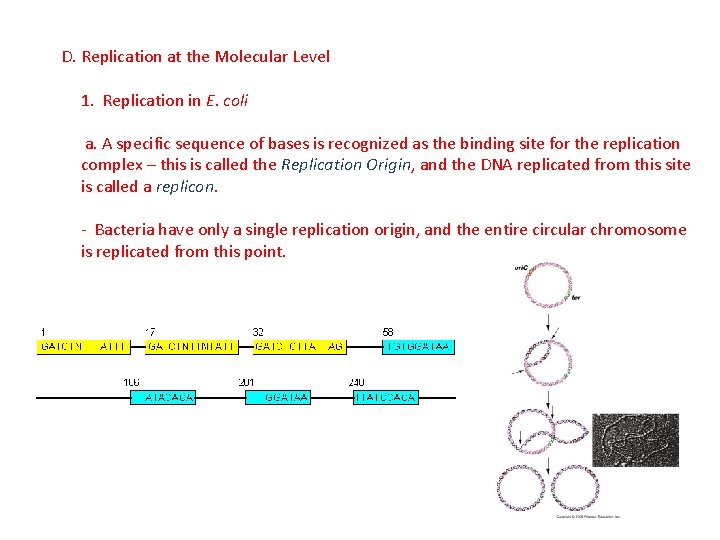
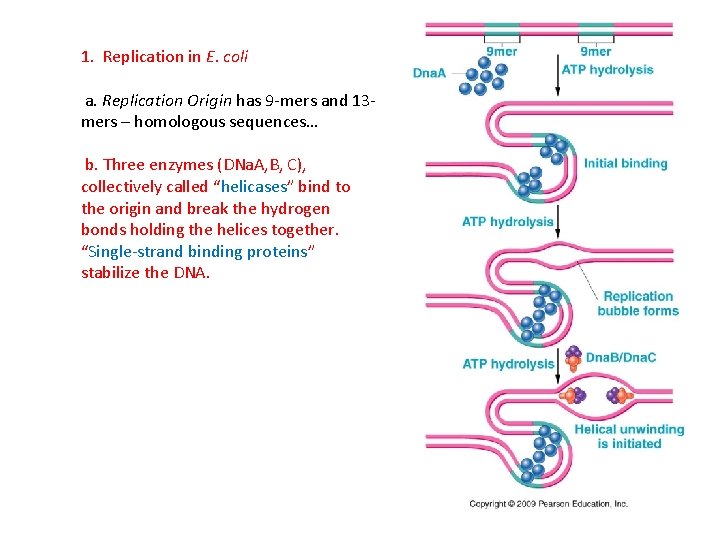
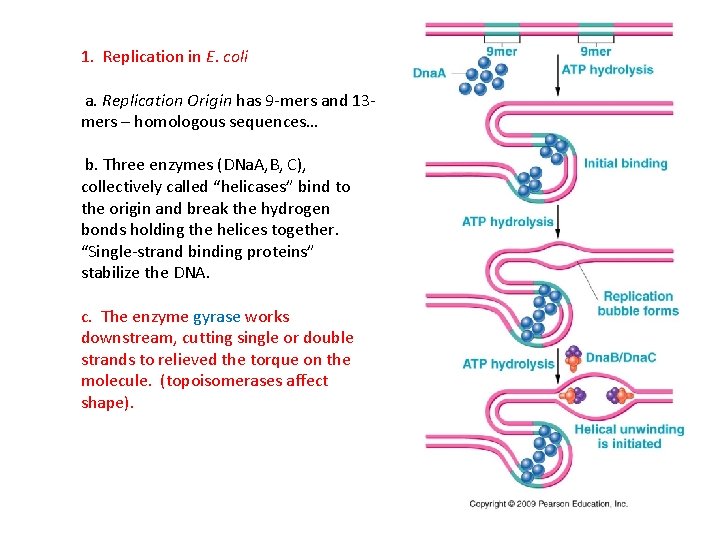
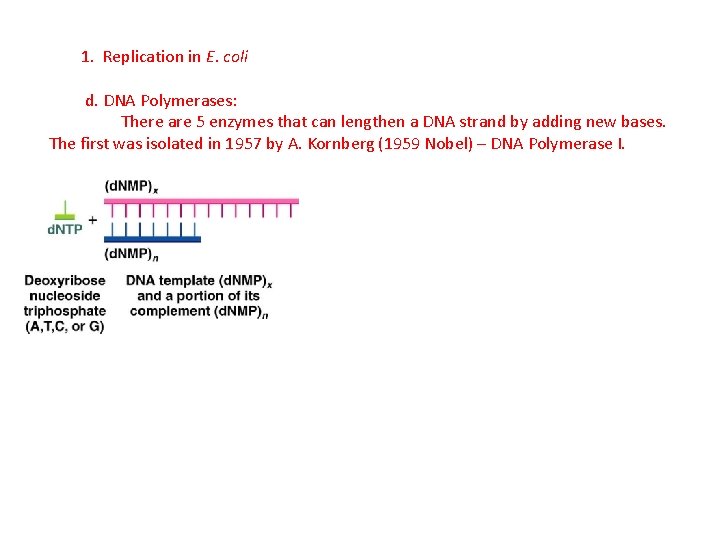
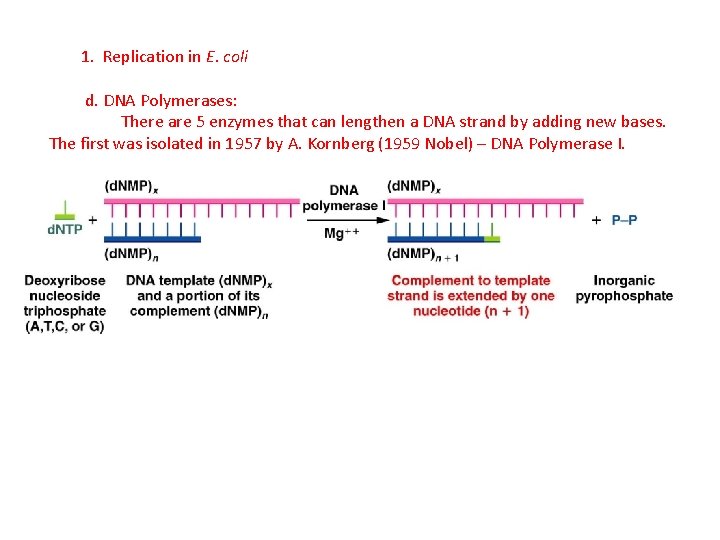
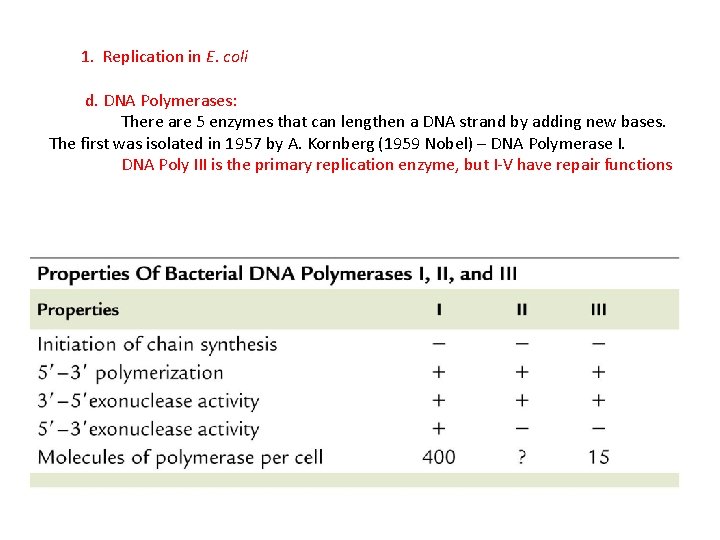
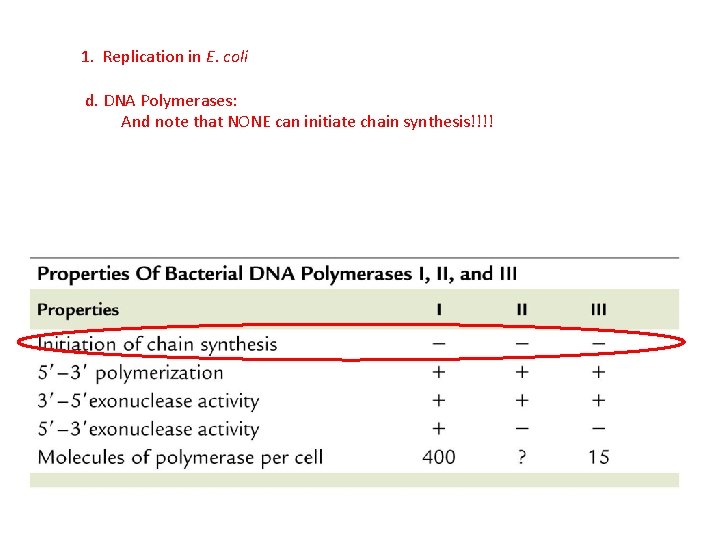
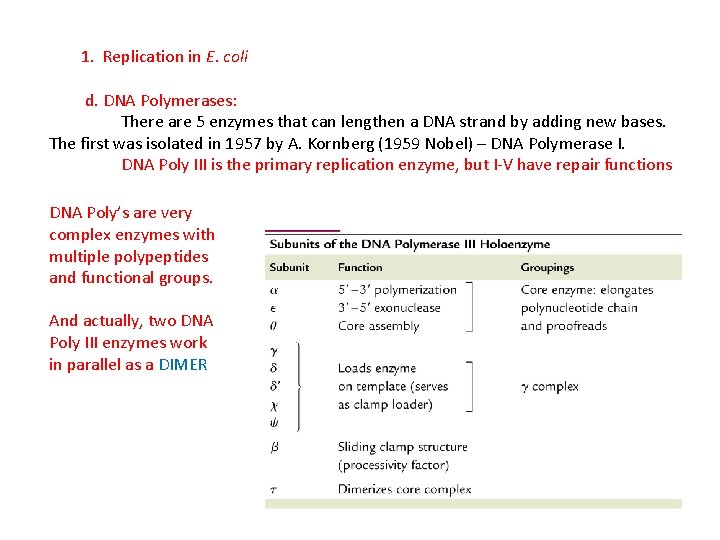
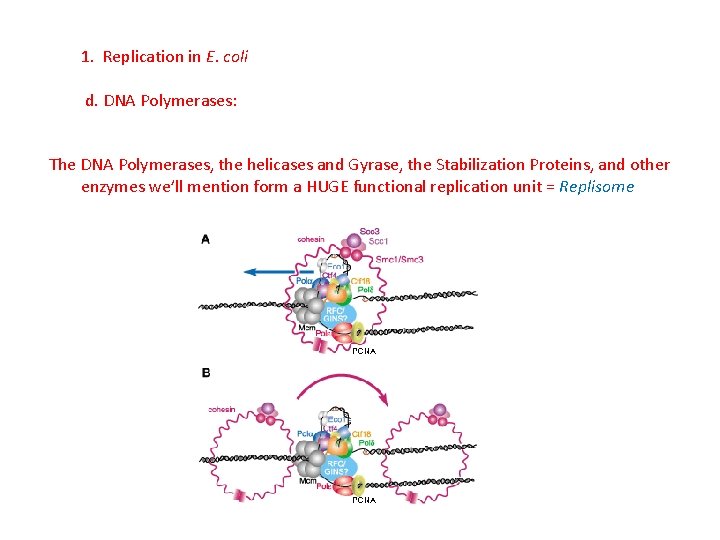
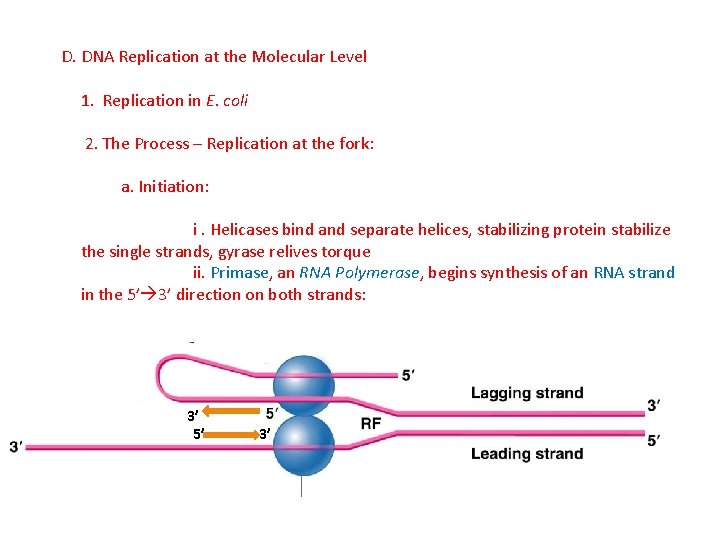
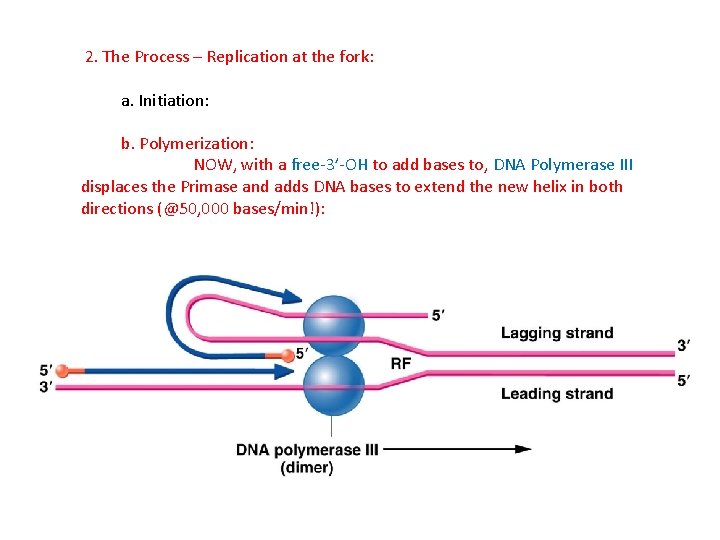
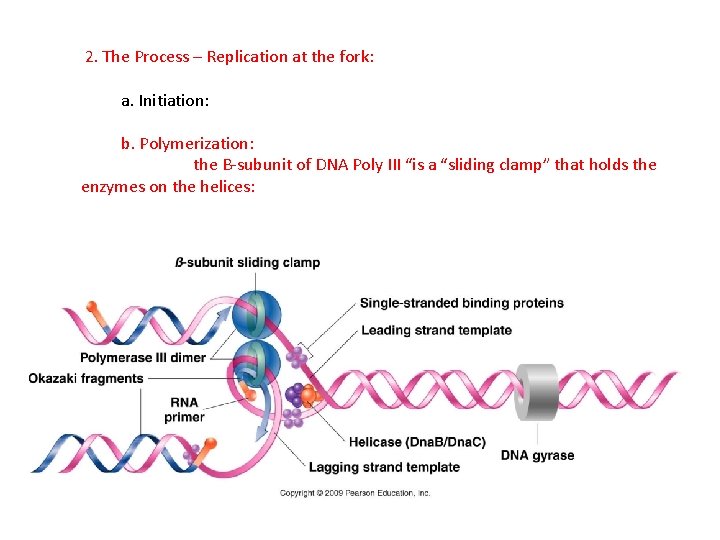
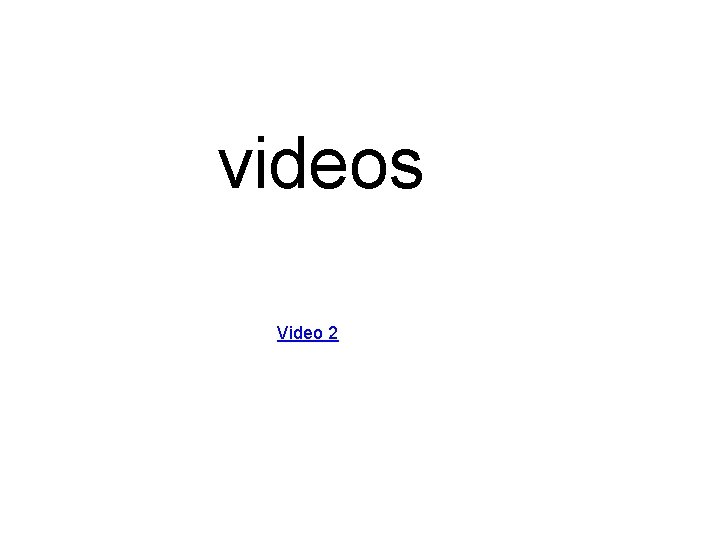
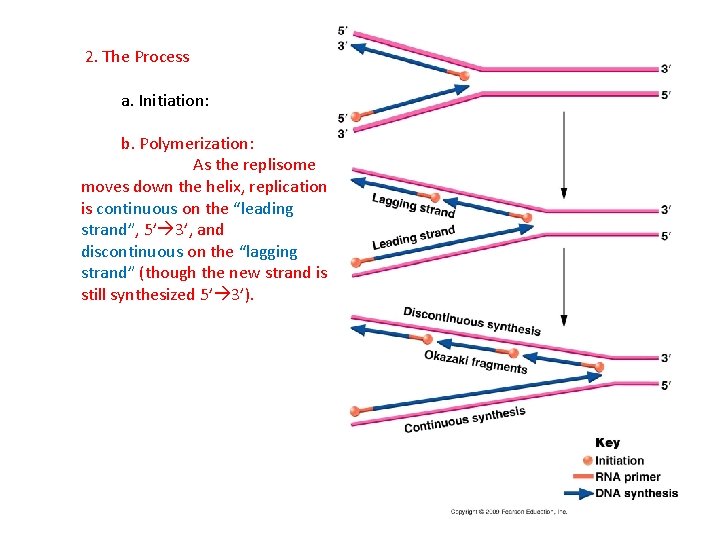
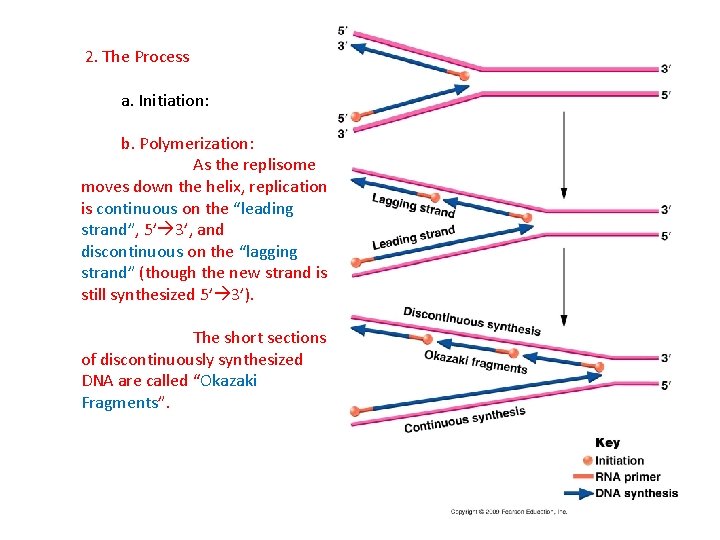
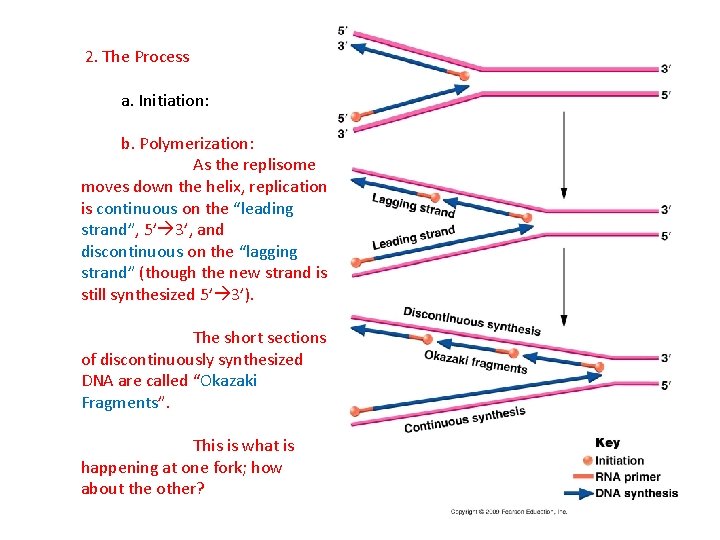
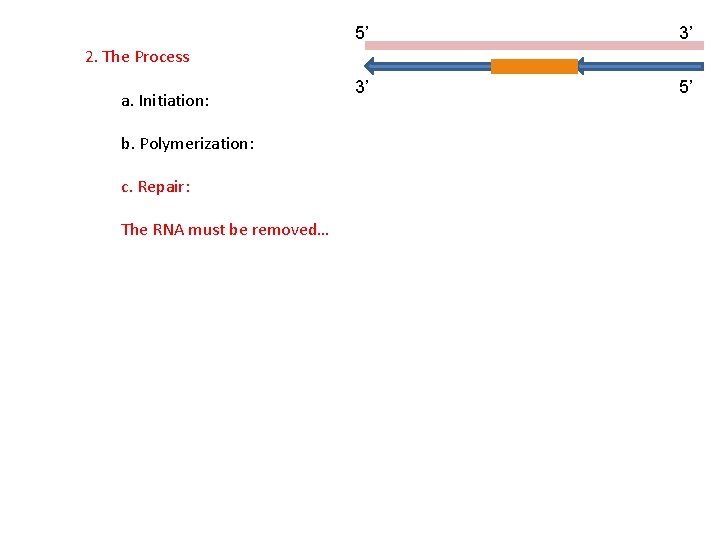
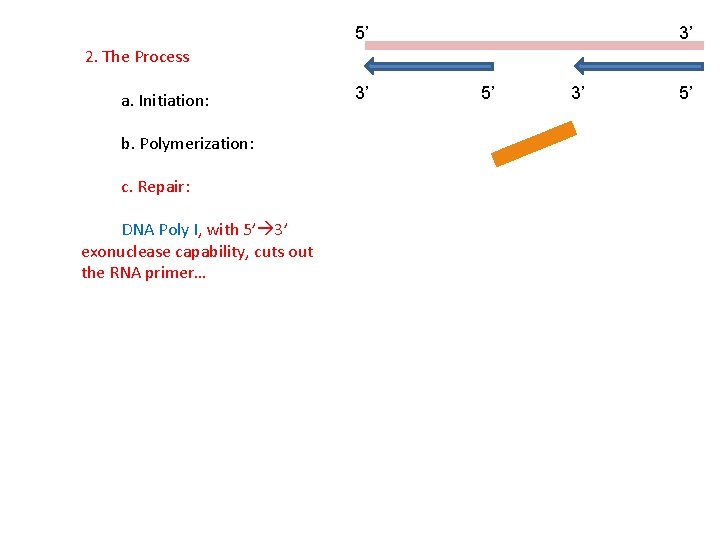
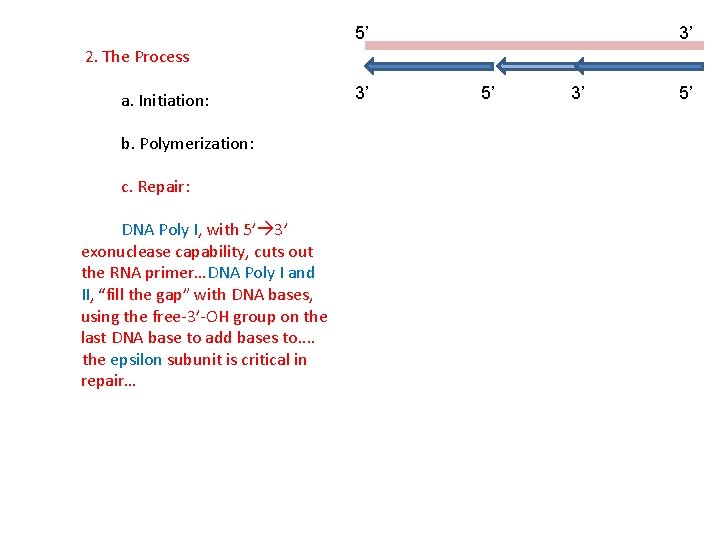
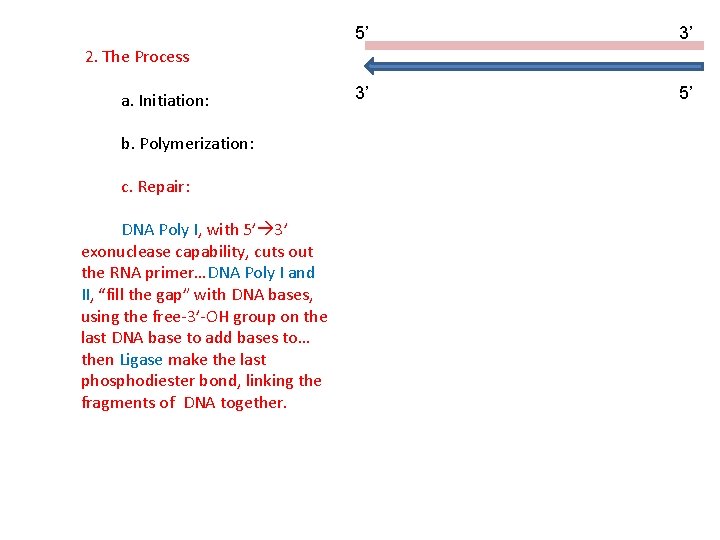
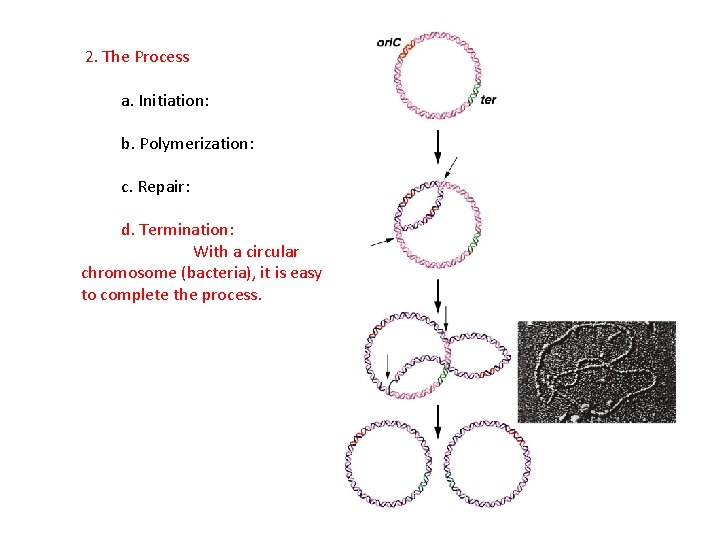
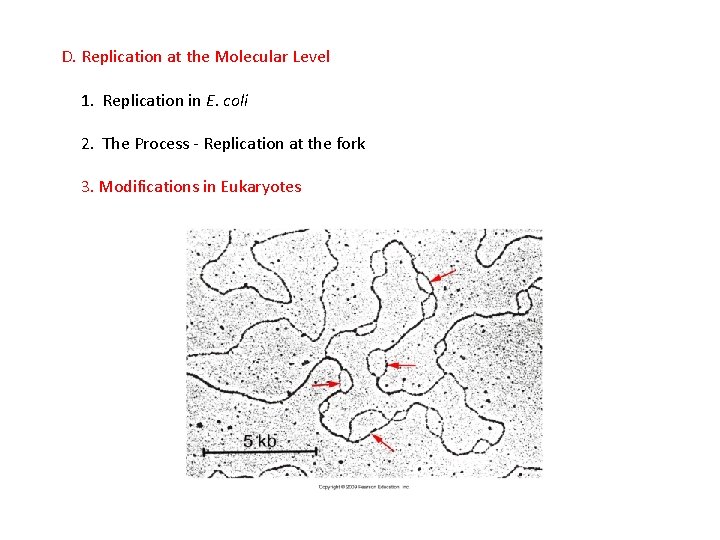
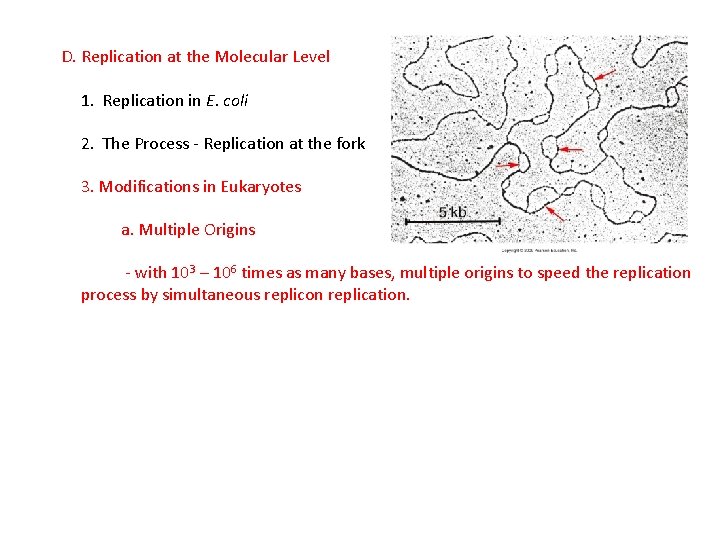
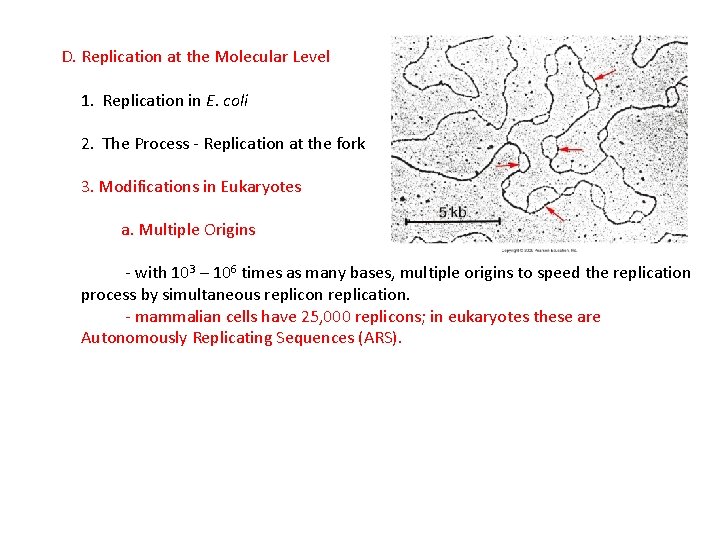
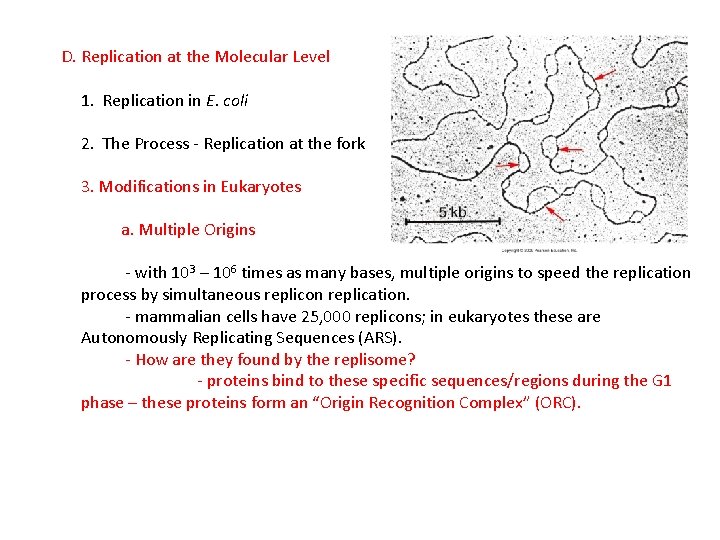
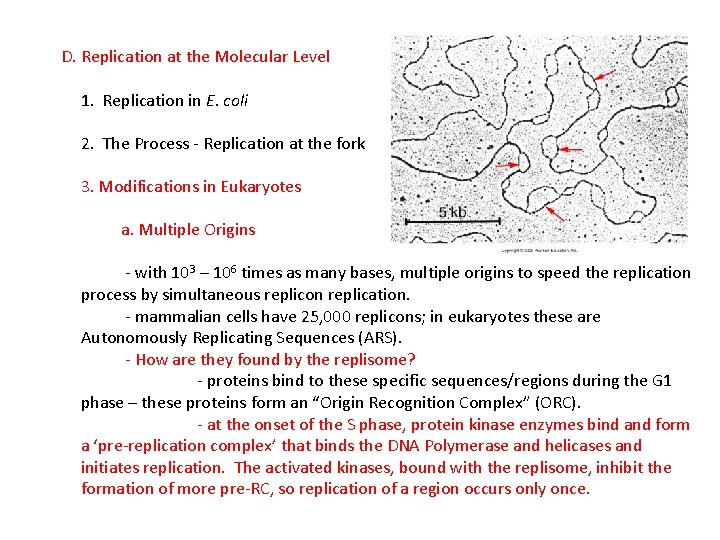
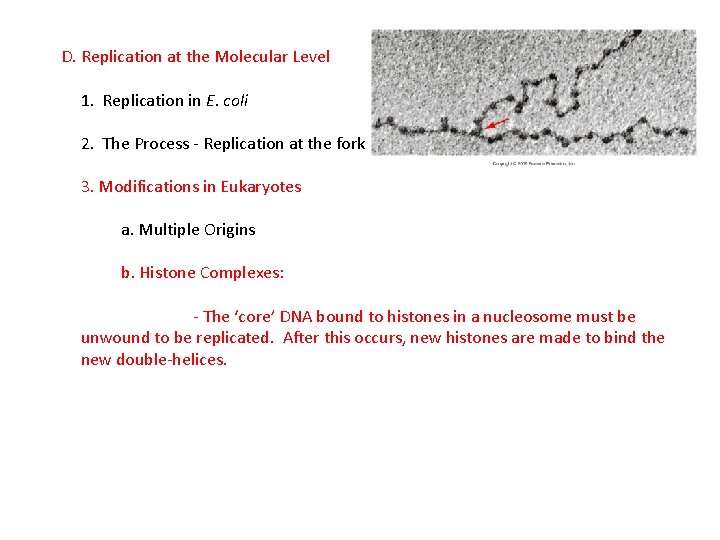
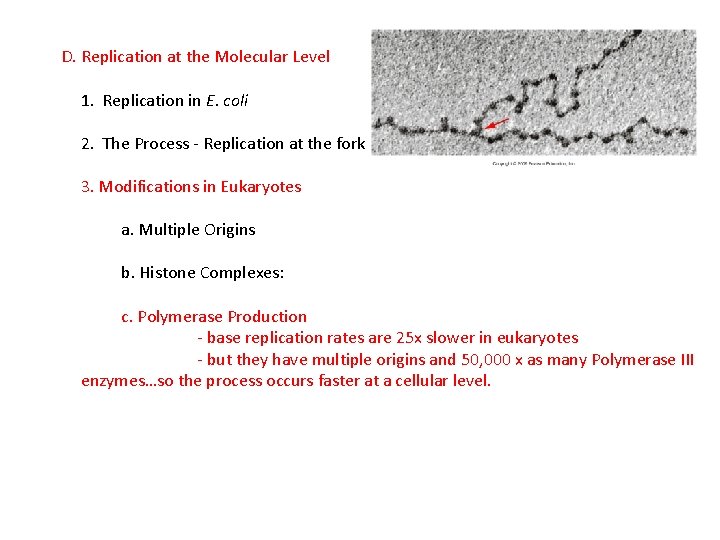
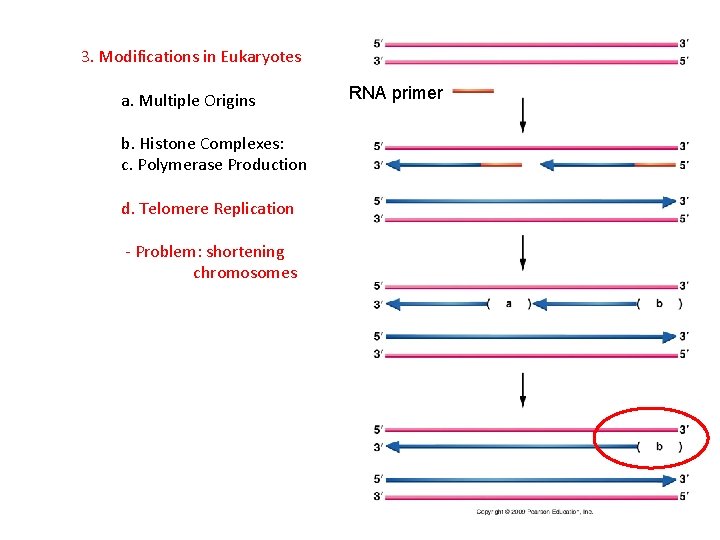
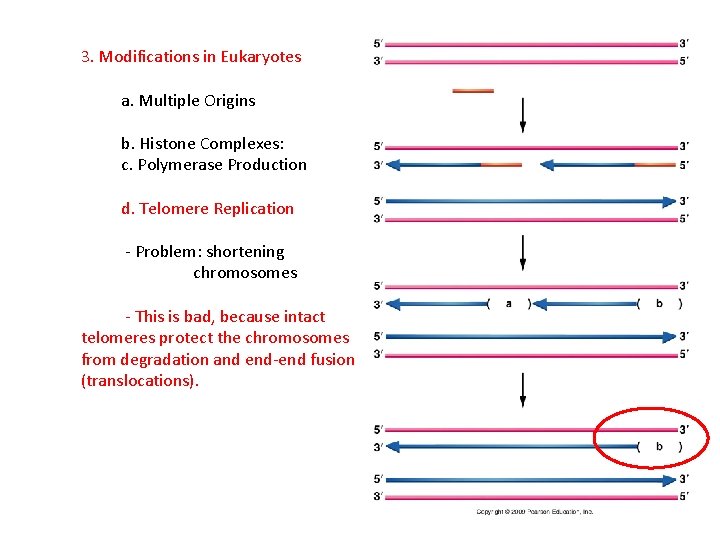
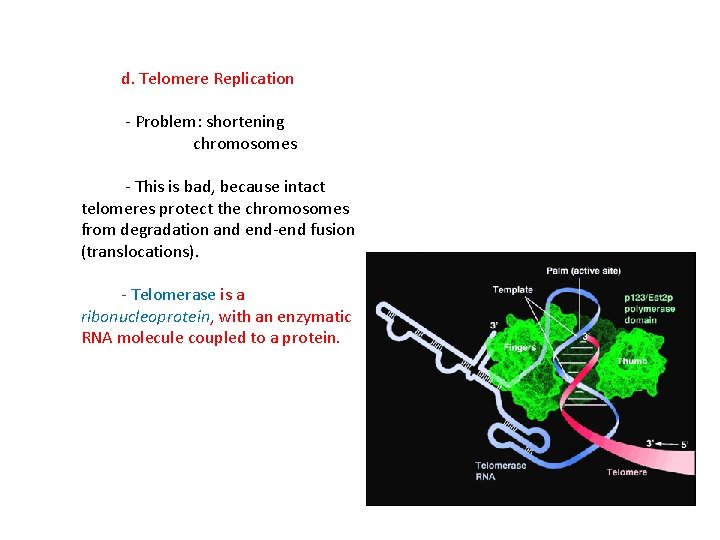
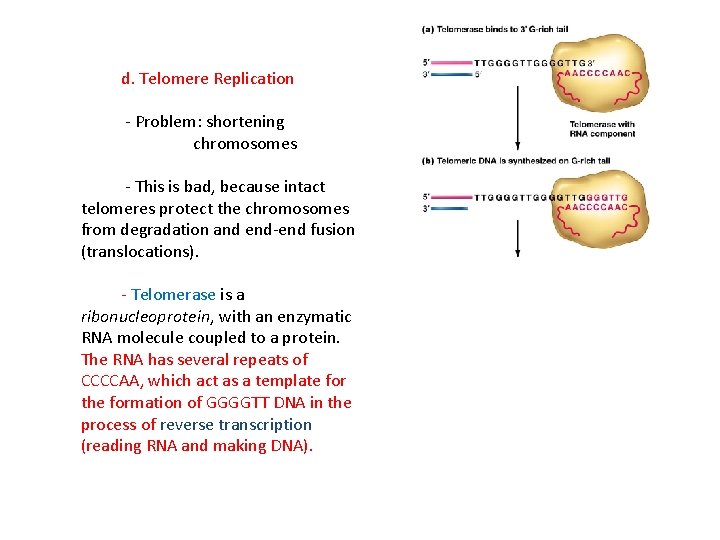
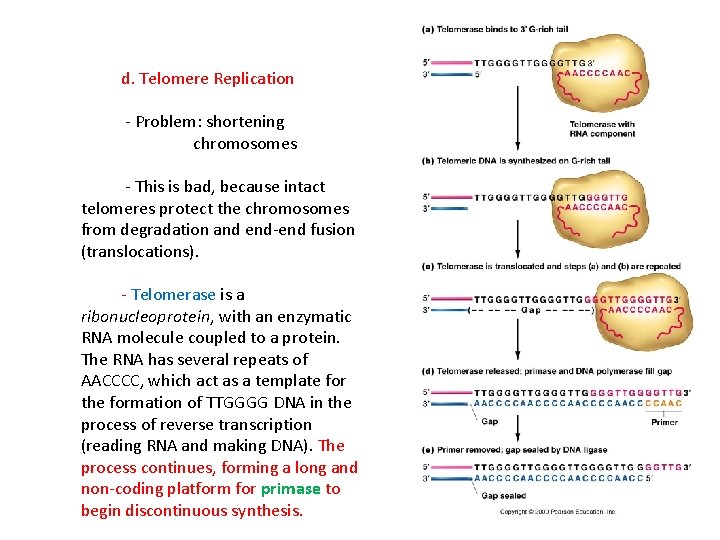
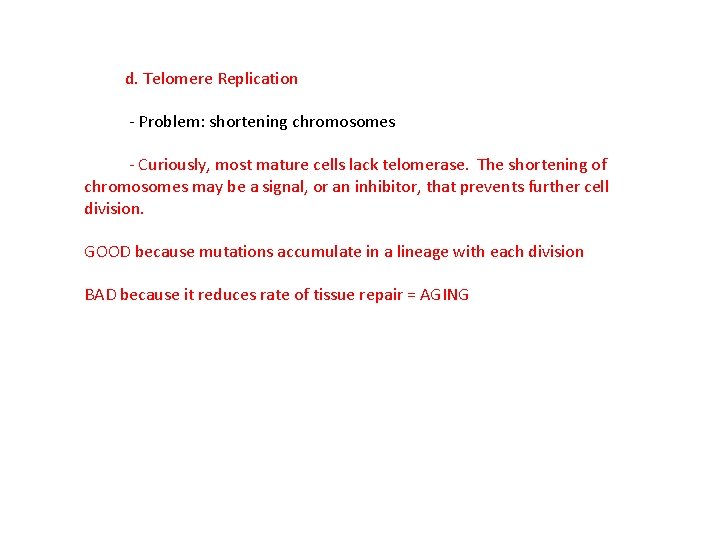
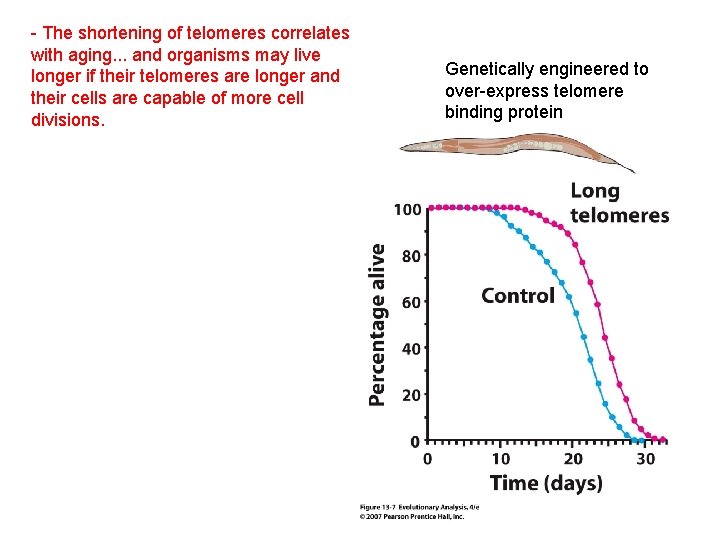
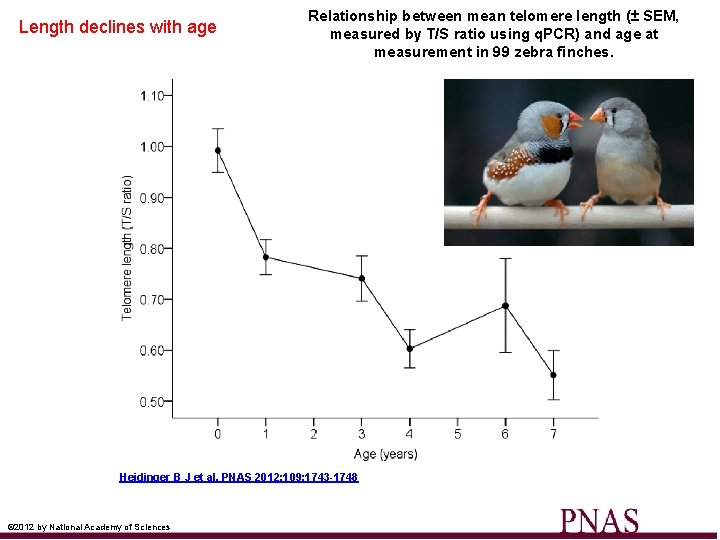
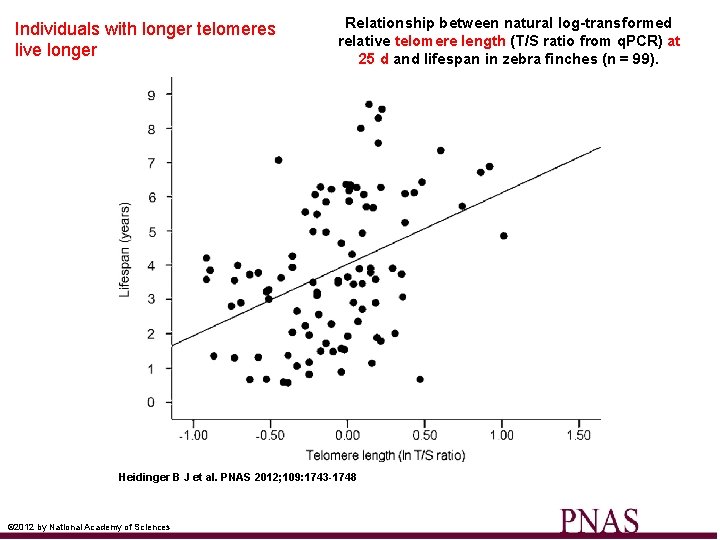
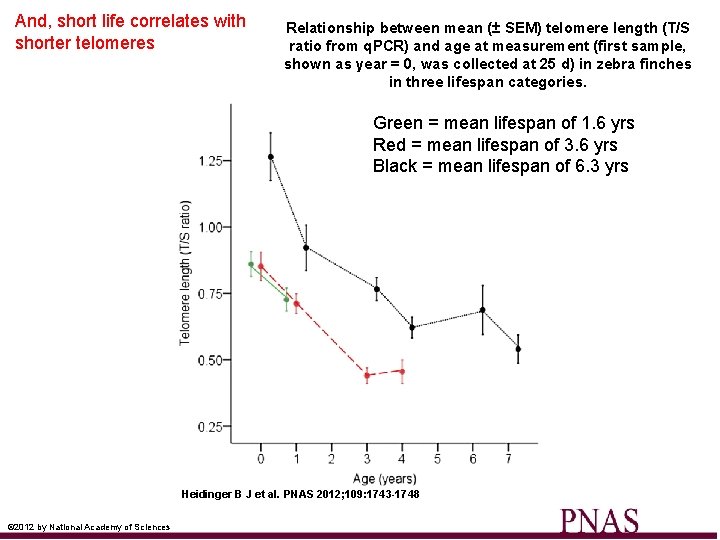
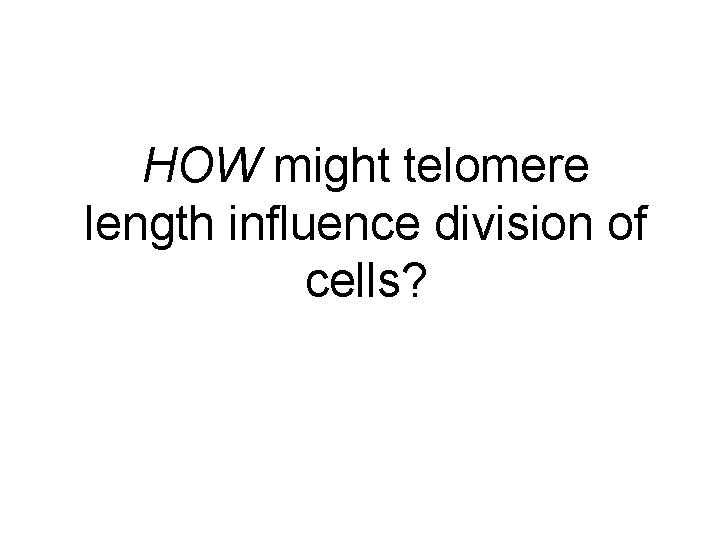
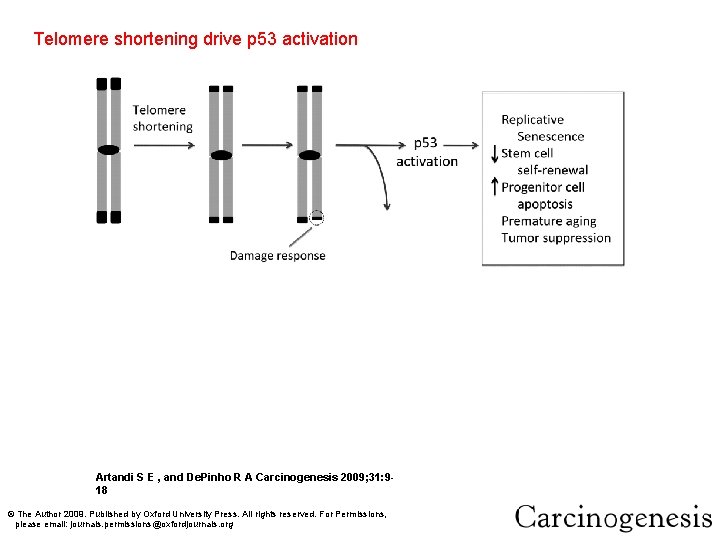
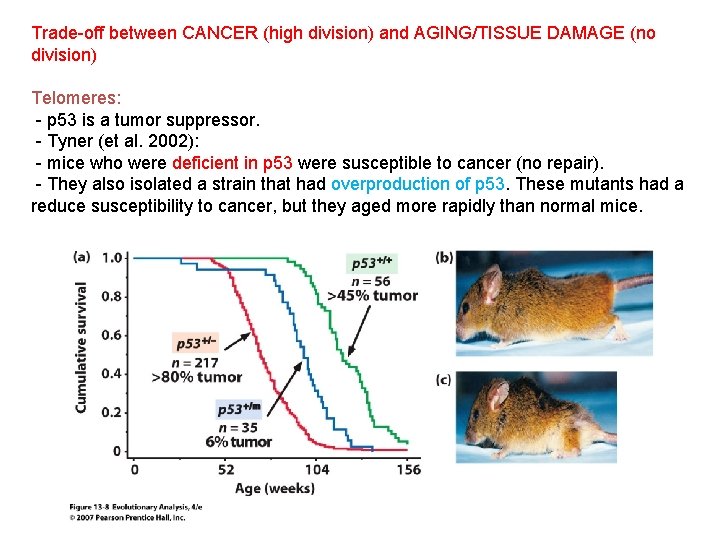
- Slides: 62
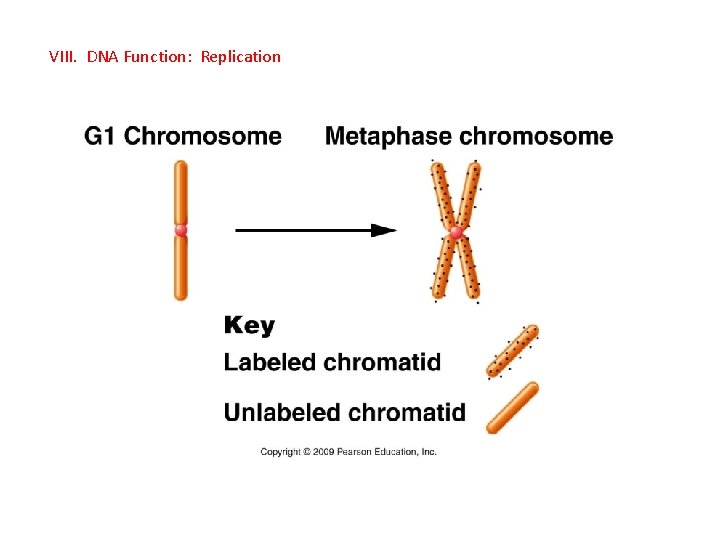
VIII. DNA Function: Replication
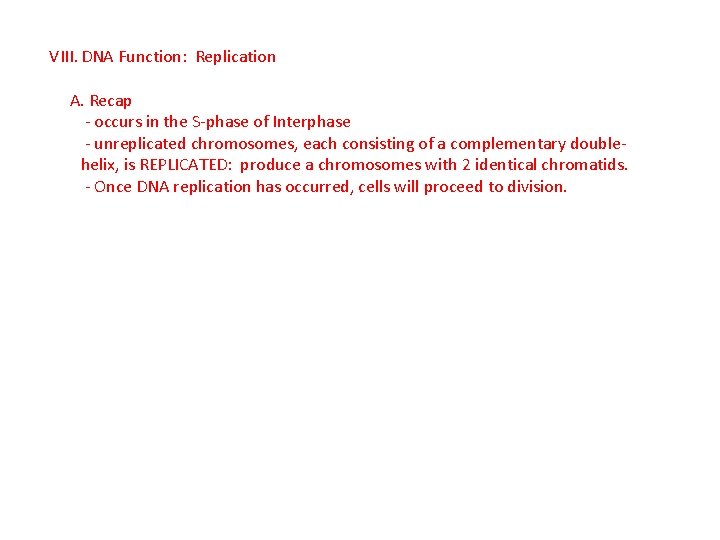
VIII. DNA Function: Replication A. Recap - occurs in the S-phase of Interphase - unreplicated chromosomes, each consisting of a complementary doublehelix, is REPLICATED: produce a chromosomes with 2 identical chromatids. - Once DNA replication has occurred, cells will proceed to division.
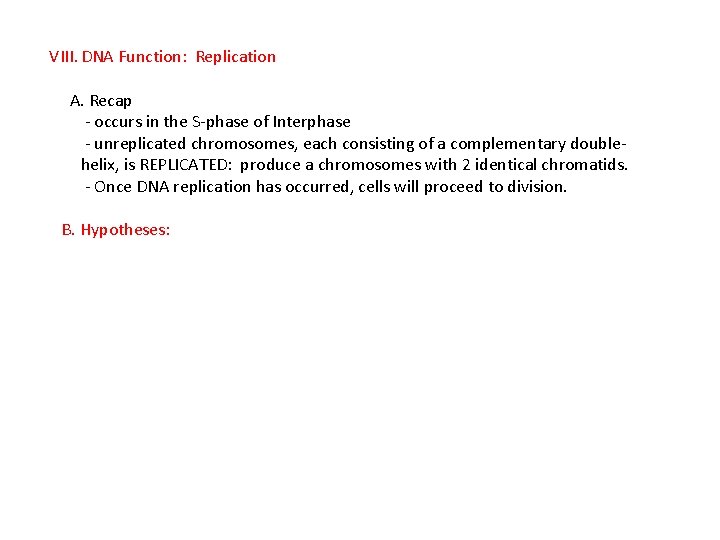
VIII. DNA Function: Replication A. Recap - occurs in the S-phase of Interphase - unreplicated chromosomes, each consisting of a complementary doublehelix, is REPLICATED: produce a chromosomes with 2 identical chromatids. - Once DNA replication has occurred, cells will proceed to division. B. Hypotheses:
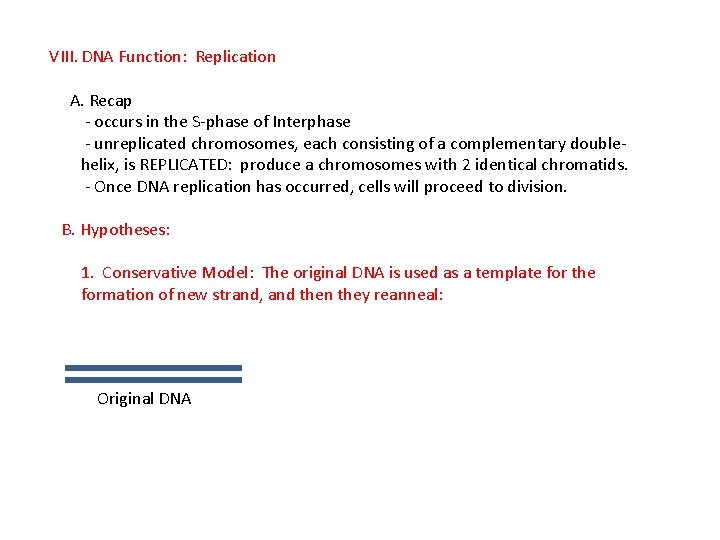
VIII. DNA Function: Replication A. Recap - occurs in the S-phase of Interphase - unreplicated chromosomes, each consisting of a complementary doublehelix, is REPLICATED: produce a chromosomes with 2 identical chromatids. - Once DNA replication has occurred, cells will proceed to division. B. Hypotheses: 1. Conservative Model: The original DNA is used as a template for the formation of new strand, and then they reanneal: Original DNA new strand formation Reannealing of original strands and synthesis of new double helix.
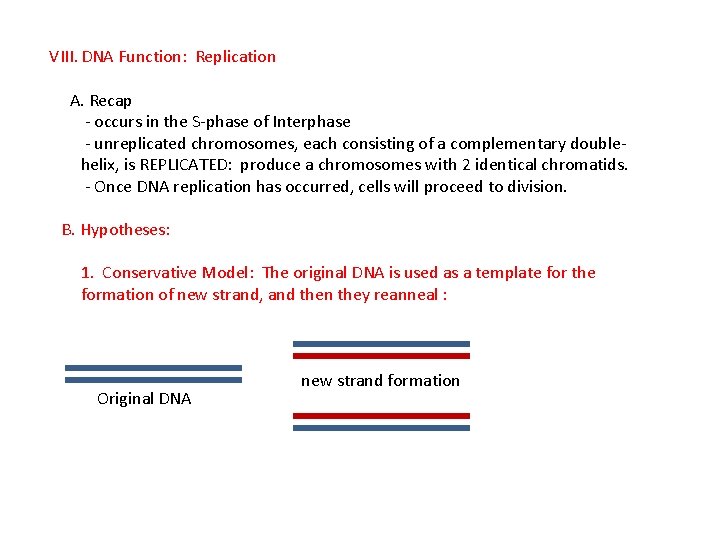
VIII. DNA Function: Replication A. Recap - occurs in the S-phase of Interphase - unreplicated chromosomes, each consisting of a complementary doublehelix, is REPLICATED: produce a chromosomes with 2 identical chromatids. - Once DNA replication has occurred, cells will proceed to division. B. Hypotheses: 1. Conservative Model: The original DNA is used as a template for the formation of new strand, and then they reanneal : Original DNA new strand formation Reannealing of original strands and synthesis of new double helix.
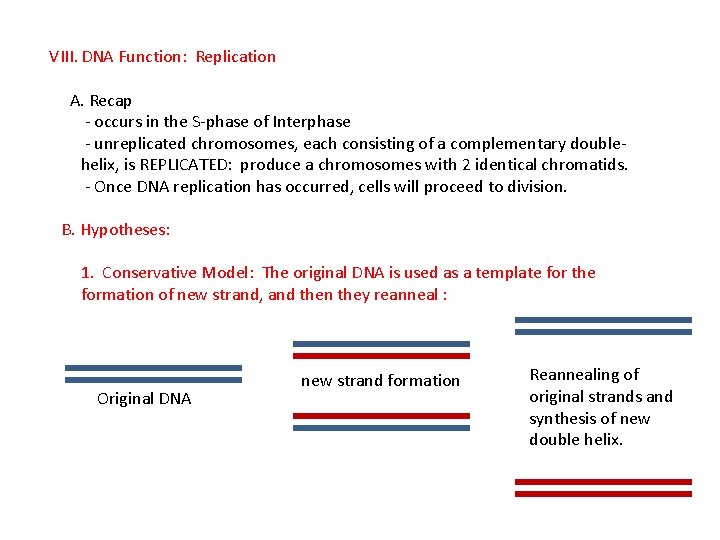
VIII. DNA Function: Replication A. Recap - occurs in the S-phase of Interphase - unreplicated chromosomes, each consisting of a complementary doublehelix, is REPLICATED: produce a chromosomes with 2 identical chromatids. - Once DNA replication has occurred, cells will proceed to division. B. Hypotheses: 1. Conservative Model: The original DNA is used as a template for the formation of new strand, and then they reanneal : Original DNA new strand formation Reannealing of original strands and synthesis of new double helix.
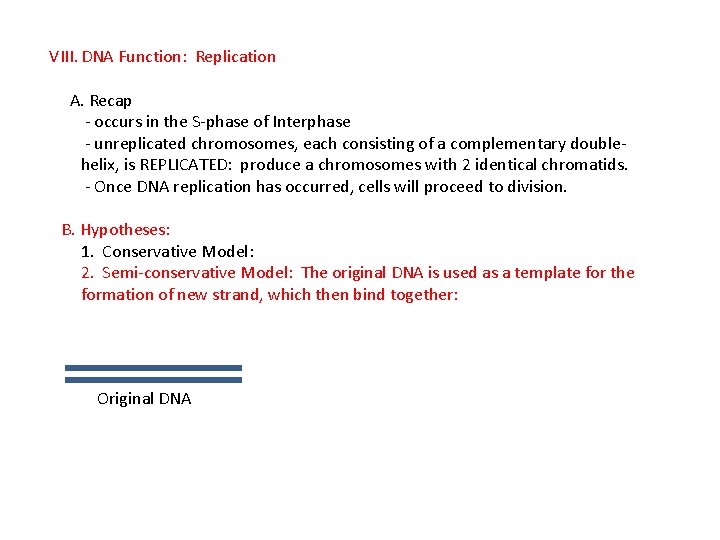
VIII. DNA Function: Replication A. Recap - occurs in the S-phase of Interphase - unreplicated chromosomes, each consisting of a complementary doublehelix, is REPLICATED: produce a chromosomes with 2 identical chromatids. - Once DNA replication has occurred, cells will proceed to division. B. Hypotheses: 1. Conservative Model: 2. Semi-conservative Model: The original DNA is used as a template for the formation of new strand, which then bind together: Original DNA new strand formation Binding of old and new strands
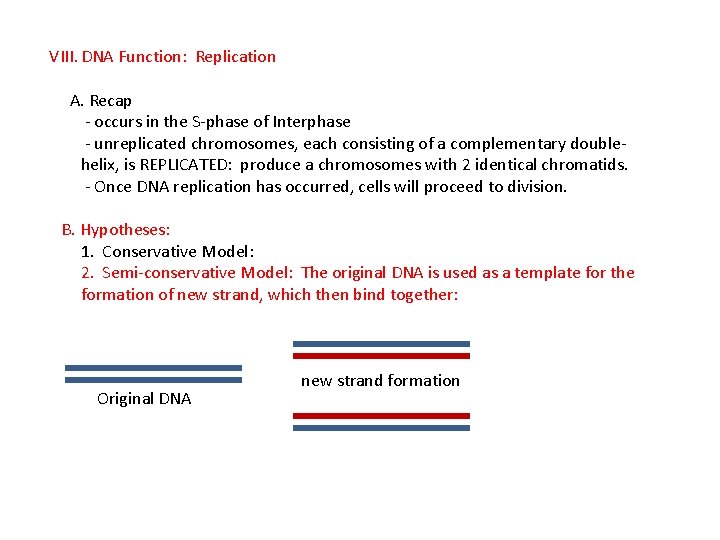
VIII. DNA Function: Replication A. Recap - occurs in the S-phase of Interphase - unreplicated chromosomes, each consisting of a complementary doublehelix, is REPLICATED: produce a chromosomes with 2 identical chromatids. - Once DNA replication has occurred, cells will proceed to division. B. Hypotheses: 1. Conservative Model: 2. Semi-conservative Model: The original DNA is used as a template for the formation of new strand, which then bind together: Original DNA new strand formation Binding of old and new strands
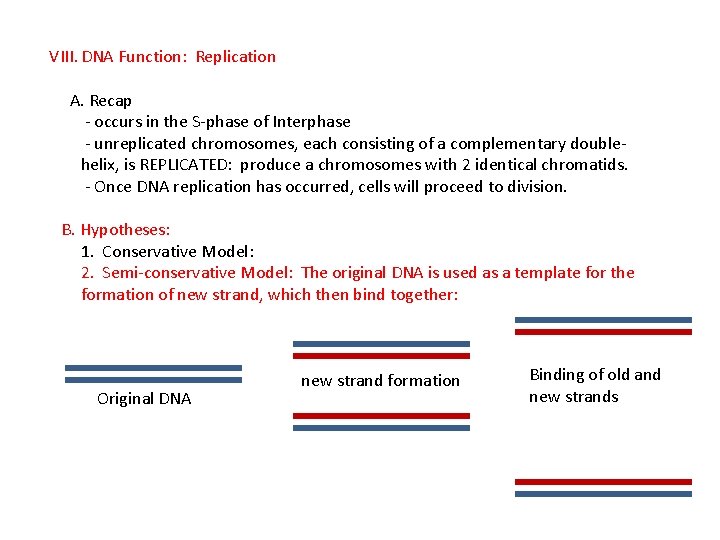
VIII. DNA Function: Replication A. Recap - occurs in the S-phase of Interphase - unreplicated chromosomes, each consisting of a complementary doublehelix, is REPLICATED: produce a chromosomes with 2 identical chromatids. - Once DNA replication has occurred, cells will proceed to division. B. Hypotheses: 1. Conservative Model: 2. Semi-conservative Model: The original DNA is used as a template for the formation of new strand, which then bind together: Original DNA new strand formation Binding of old and new strands
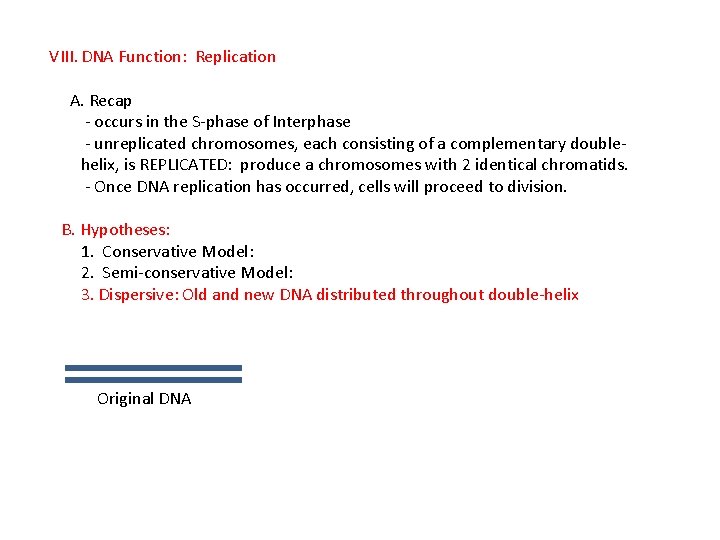
VIII. DNA Function: Replication A. Recap - occurs in the S-phase of Interphase - unreplicated chromosomes, each consisting of a complementary doublehelix, is REPLICATED: produce a chromosomes with 2 identical chromatids. - Once DNA replication has occurred, cells will proceed to division. B. Hypotheses: 1. Conservative Model: 2. Semi-conservative Model: 3. Dispersive: Old and new DNA distributed throughout double-helix Original DNA new strand formation Dispersal of old and new DNA throughout both chromatids
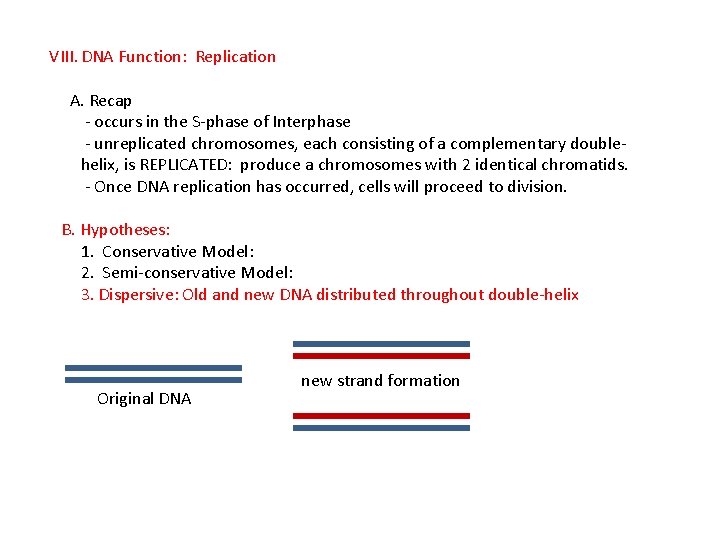
VIII. DNA Function: Replication A. Recap - occurs in the S-phase of Interphase - unreplicated chromosomes, each consisting of a complementary doublehelix, is REPLICATED: produce a chromosomes with 2 identical chromatids. - Once DNA replication has occurred, cells will proceed to division. B. Hypotheses: 1. Conservative Model: 2. Semi-conservative Model: 3. Dispersive: Old and new DNA distributed throughout double-helix Original DNA new strand formation Dispersal of old and new DNA throughout both chromatids
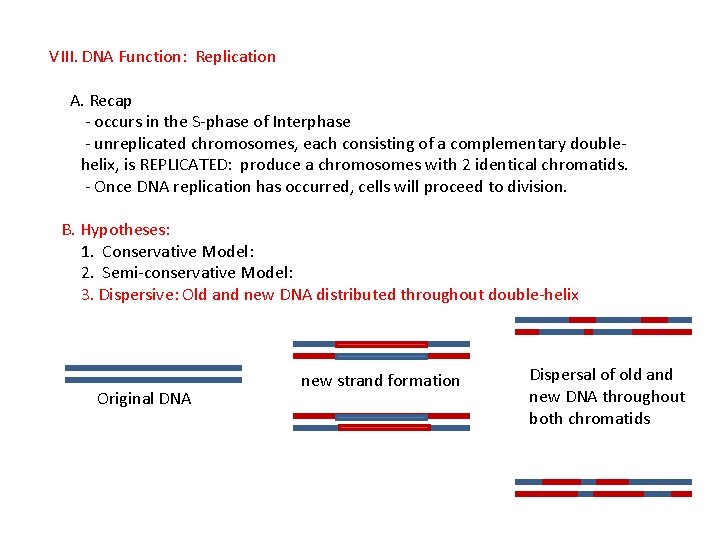
VIII. DNA Function: Replication A. Recap - occurs in the S-phase of Interphase - unreplicated chromosomes, each consisting of a complementary doublehelix, is REPLICATED: produce a chromosomes with 2 identical chromatids. - Once DNA replication has occurred, cells will proceed to division. B. Hypotheses: 1. Conservative Model: 2. Semi-conservative Model: 3. Dispersive: Old and new DNA distributed throughout double-helix Original DNA new strand formation Dispersal of old and new DNA throughout both chromatids
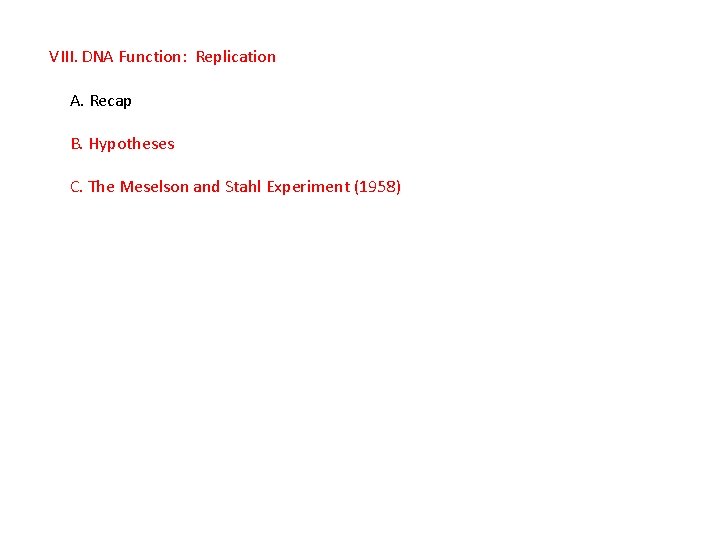
VIII. DNA Function: Replication A. Recap B. Hypotheses C. The Meselson and Stahl Experiment (1958)
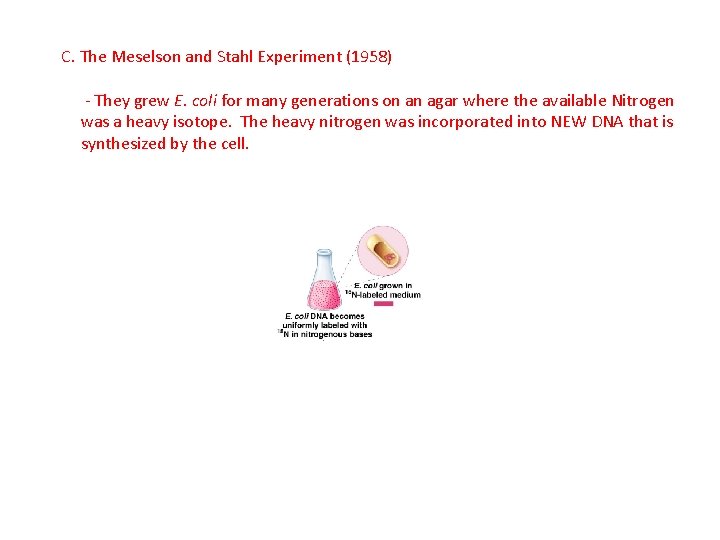
C. The Meselson and Stahl Experiment (1958) - They grew E. coli for many generations on an agar where the available Nitrogen was a heavy isotope. The heavy nitrogen was incorporated into NEW DNA that is synthesized by the cell.
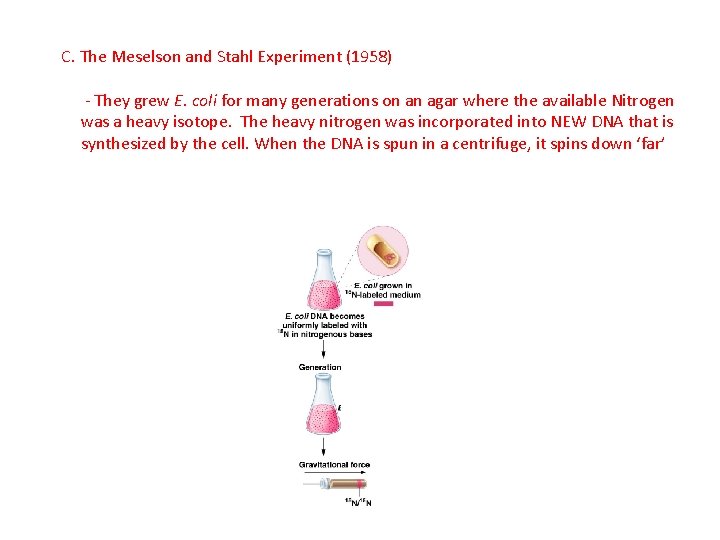
C. The Meselson and Stahl Experiment (1958) - They grew E. coli for many generations on an agar where the available Nitrogen was a heavy isotope. The heavy nitrogen was incorporated into NEW DNA that is synthesized by the cell. When the DNA is spun in a centrifuge, it spins down ‘far’
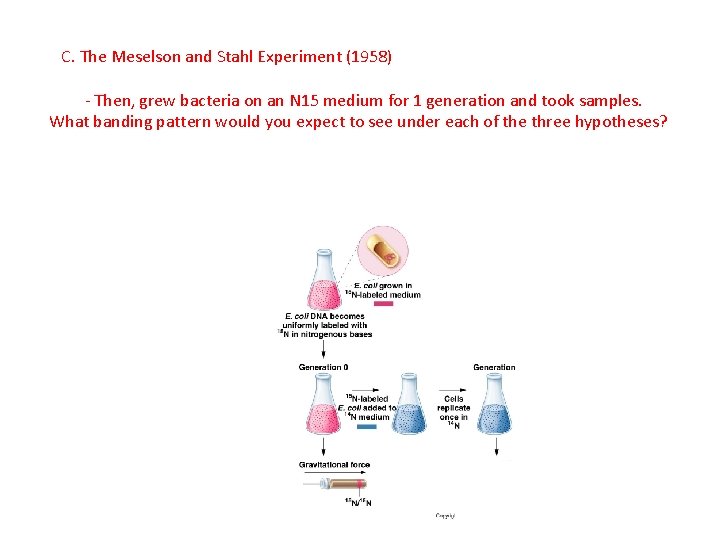
C. The Meselson and Stahl Experiment (1958) - Then, grew bacteria on an N 15 medium for 1 generation and took samples. What banding pattern would you expect to see under each of the three hypotheses?
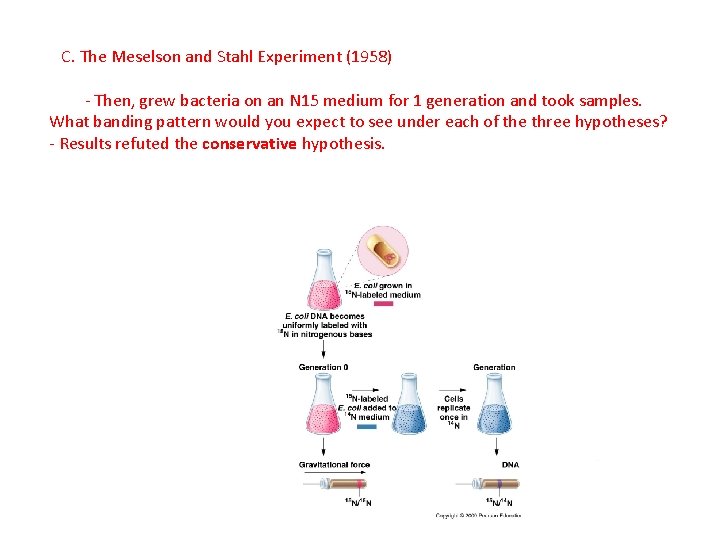
C. The Meselson and Stahl Experiment (1958) - Then, grew bacteria on an N 15 medium for 1 generation and took samples. What banding pattern would you expect to see under each of the three hypotheses? - Results refuted the conservative hypothesis.
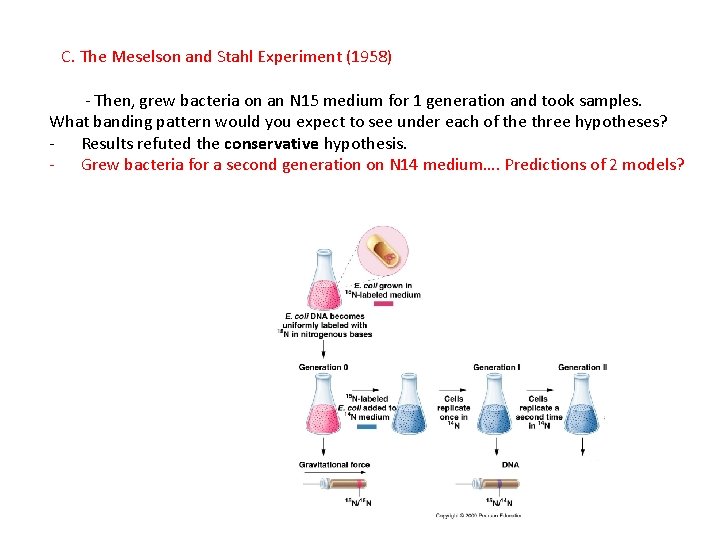
C. The Meselson and Stahl Experiment (1958) - Then, grew bacteria on an N 15 medium for 1 generation and took samples. What banding pattern would you expect to see under each of the three hypotheses? - Results refuted the conservative hypothesis. - Grew bacteria for a second generation on N 14 medium…. Predictions of 2 models?
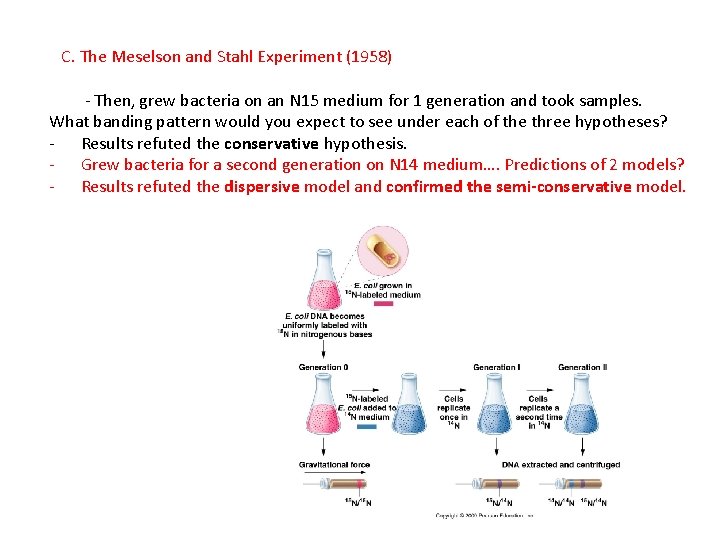
C. The Meselson and Stahl Experiment (1958) - Then, grew bacteria on an N 15 medium for 1 generation and took samples. What banding pattern would you expect to see under each of the three hypotheses? - Results refuted the conservative hypothesis. - Grew bacteria for a second generation on N 14 medium…. Predictions of 2 models? - Results refuted the dispersive model and confirmed the semi-conservative model.
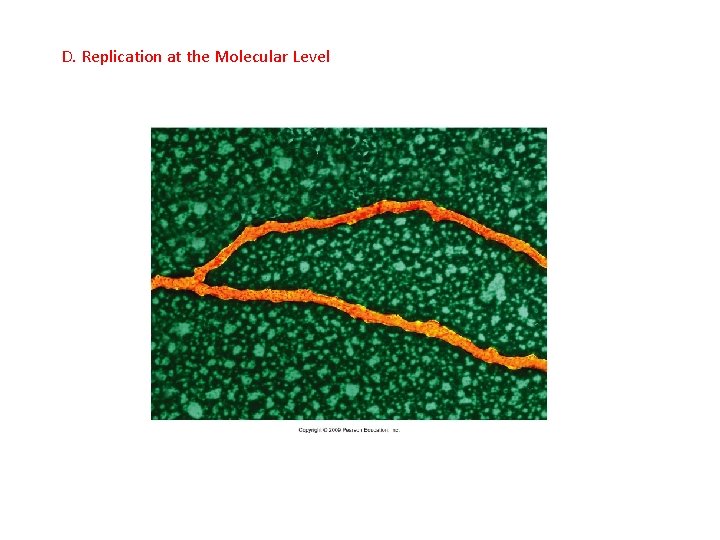
D. Replication at the Molecular Level
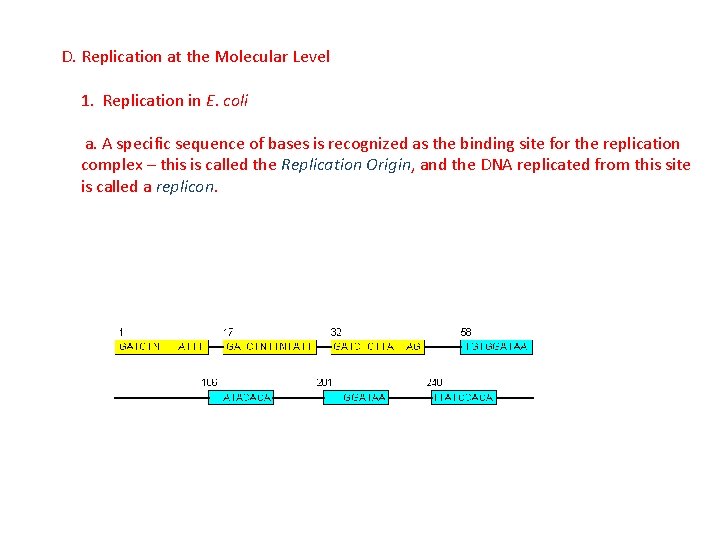
D. Replication at the Molecular Level 1. Replication in E. coli a. A specific sequence of bases is recognized as the binding site for the replication complex – this is called the Replication Origin, and the DNA replicated from this site is called a replicon.
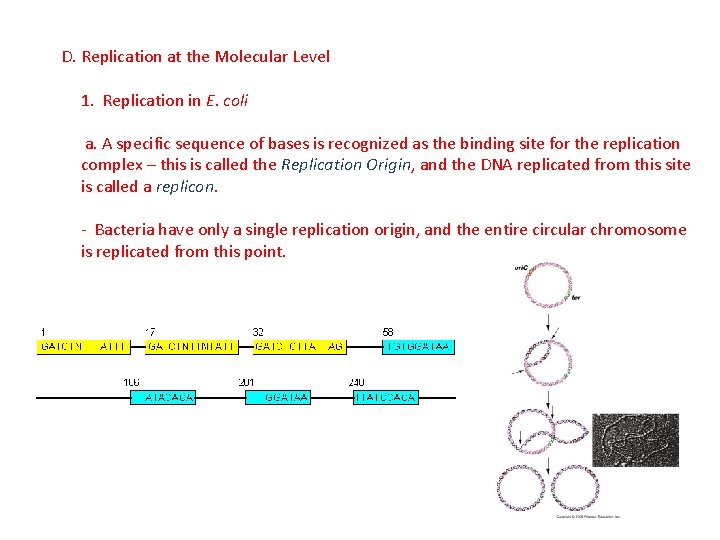
D. Replication at the Molecular Level 1. Replication in E. coli a. A specific sequence of bases is recognized as the binding site for the replication complex – this is called the Replication Origin, and the DNA replicated from this site is called a replicon. - Bacteria have only a single replication origin, and the entire circular chromosome is replicated from this point.
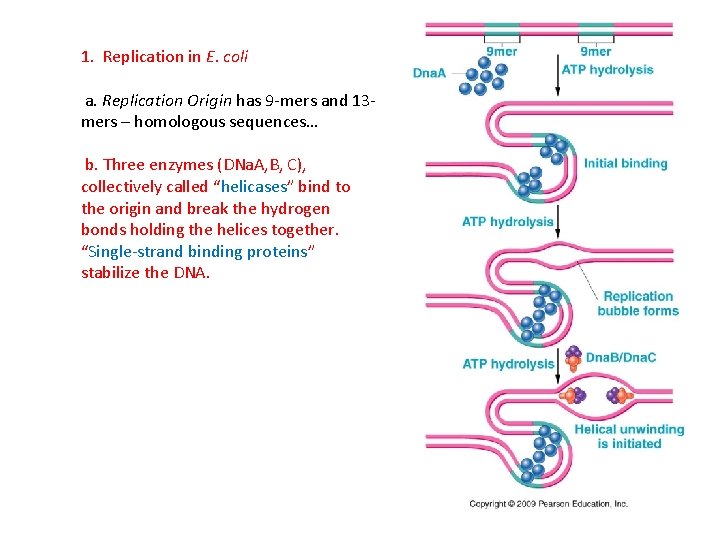
1. Replication in E. coli a. Replication Origin has 9 -mers and 13 mers – homologous sequences… b. Three enzymes (DNa. A, B, C), collectively called “helicases” bind to the origin and break the hydrogen bonds holding the helices together. “Single-strand binding proteins” stabilize the DNA.
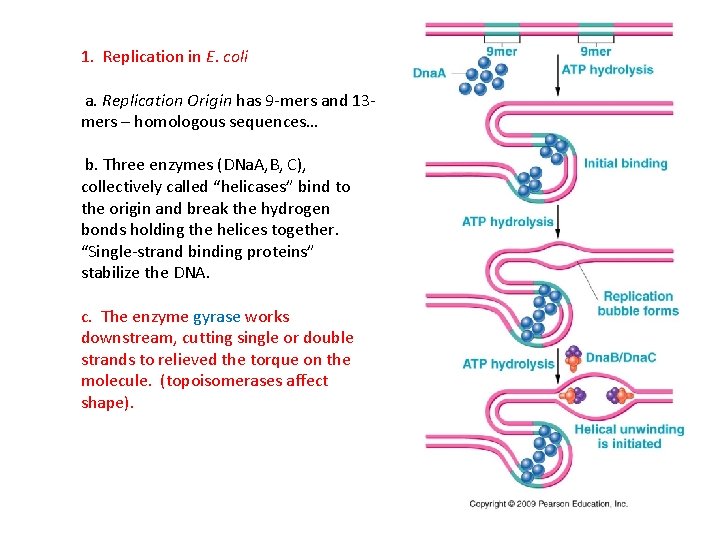
1. Replication in E. coli a. Replication Origin has 9 -mers and 13 mers – homologous sequences… b. Three enzymes (DNa. A, B, C), collectively called “helicases” bind to the origin and break the hydrogen bonds holding the helices together. “Single-strand binding proteins” stabilize the DNA. c. The enzyme gyrase works downstream, cutting single or double strands to relieved the torque on the molecule. (topoisomerases affect shape).
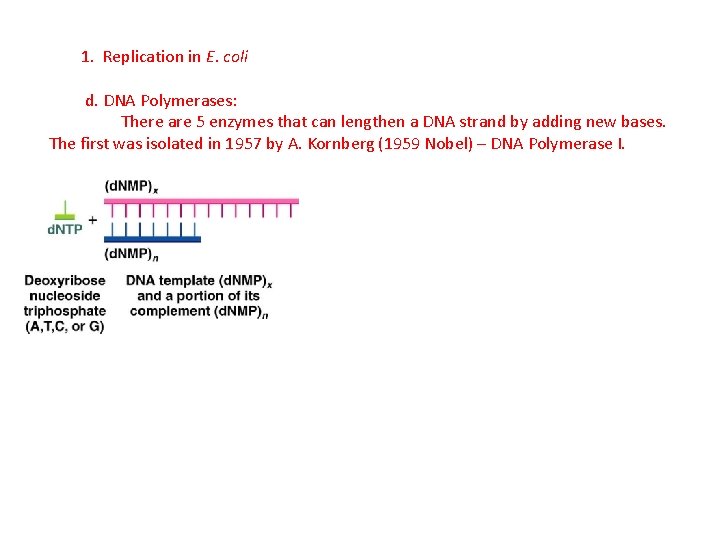
1. Replication in E. coli d. DNA Polymerases: There are 5 enzymes that can lengthen a DNA strand by adding new bases. The first was isolated in 1957 by A. Kornberg (1959 Nobel) – DNA Polymerase I.
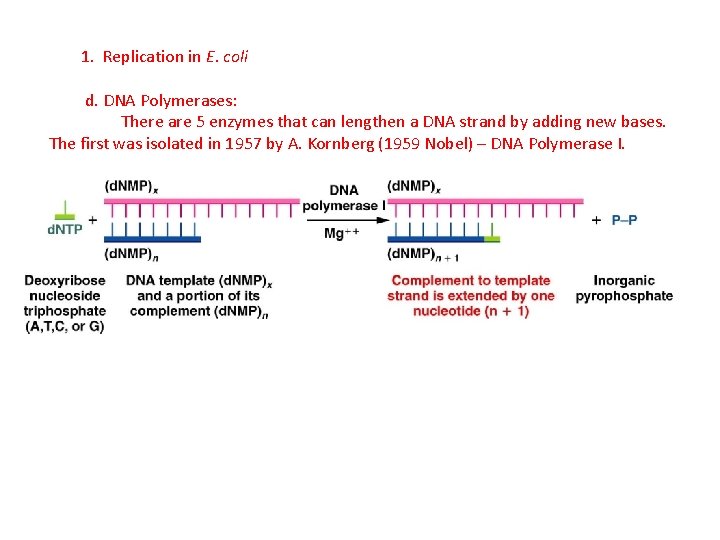
1. Replication in E. coli d. DNA Polymerases: There are 5 enzymes that can lengthen a DNA strand by adding new bases. The first was isolated in 1957 by A. Kornberg (1959 Nobel) – DNA Polymerase I.
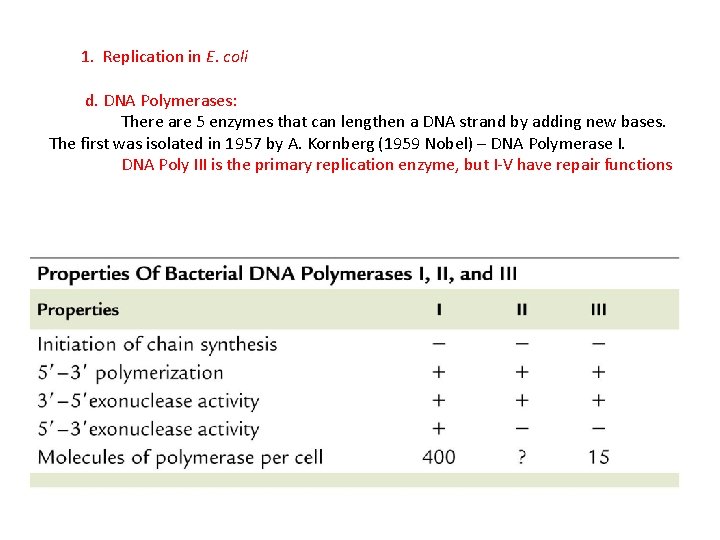
1. Replication in E. coli d. DNA Polymerases: There are 5 enzymes that can lengthen a DNA strand by adding new bases. The first was isolated in 1957 by A. Kornberg (1959 Nobel) – DNA Polymerase I. DNA Poly III is the primary replication enzyme, but I-V have repair functions
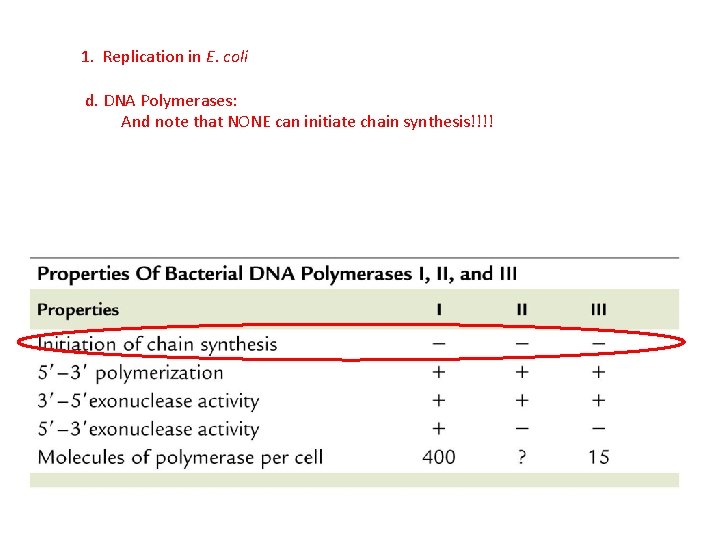
1. Replication in E. coli d. DNA Polymerases: And note that NONE can initiate chain synthesis!!!!
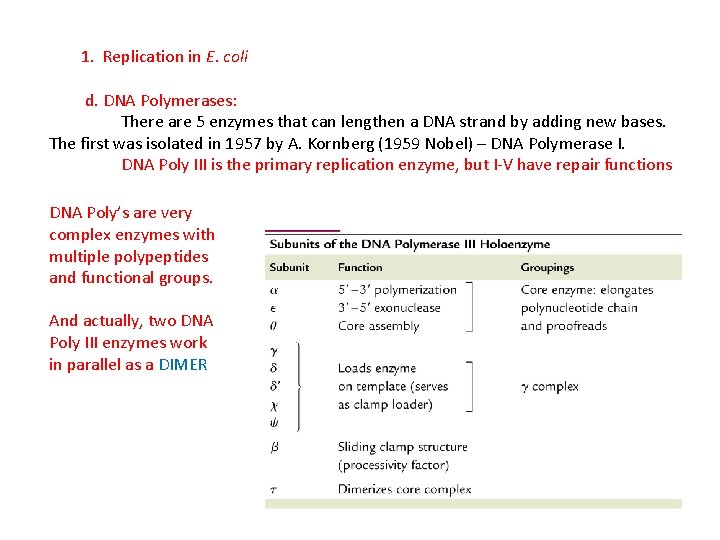
1. Replication in E. coli d. DNA Polymerases: There are 5 enzymes that can lengthen a DNA strand by adding new bases. The first was isolated in 1957 by A. Kornberg (1959 Nobel) – DNA Polymerase I. DNA Poly III is the primary replication enzyme, but I-V have repair functions DNA Poly’s are very complex enzymes with multiple polypeptides and functional groups. And actually, two DNA Poly III enzymes work in parallel as a DIMER
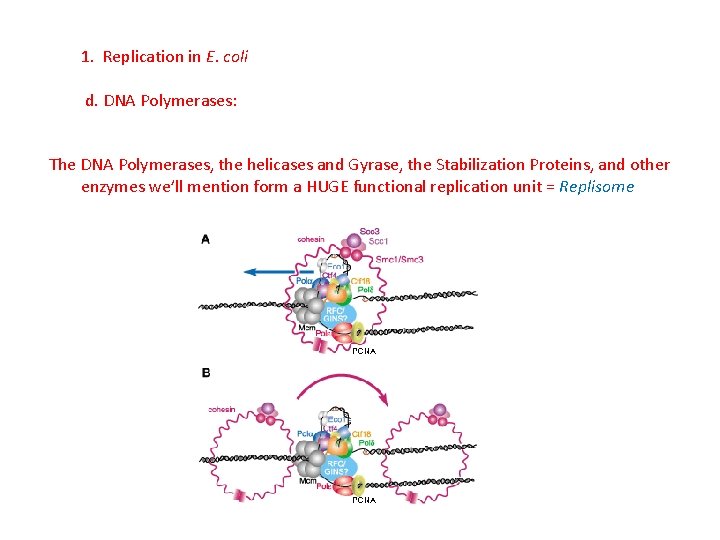
1. Replication in E. coli d. DNA Polymerases: The DNA Polymerases, the helicases and Gyrase, the Stabilization Proteins, and other enzymes we’ll mention form a HUGE functional replication unit = Replisome
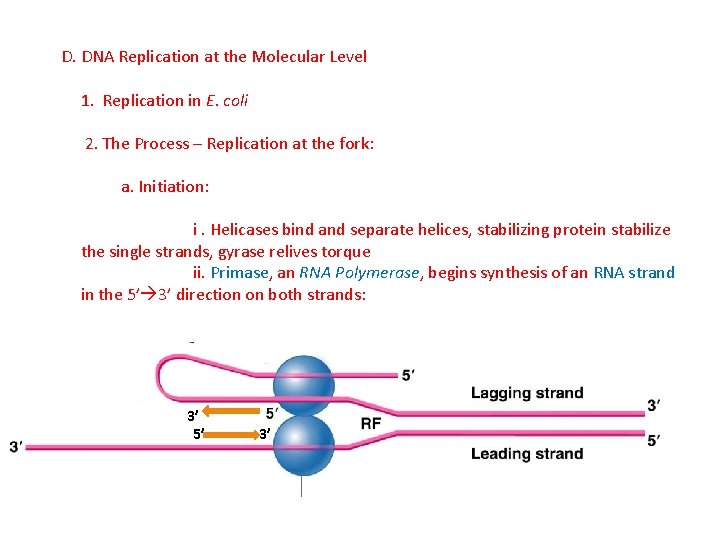
D. DNA Replication at the Molecular Level 1. Replication in E. coli 2. The Process – Replication at the fork: a. Initiation: i. Helicases bind and separate helices, stabilizing protein stabilize the single strands, gyrase relives torque ii. Primase, an RNA Polymerase, begins synthesis of an RNA strand in the 5’ 3’ direction on both strands: 3’ 5’ 3’
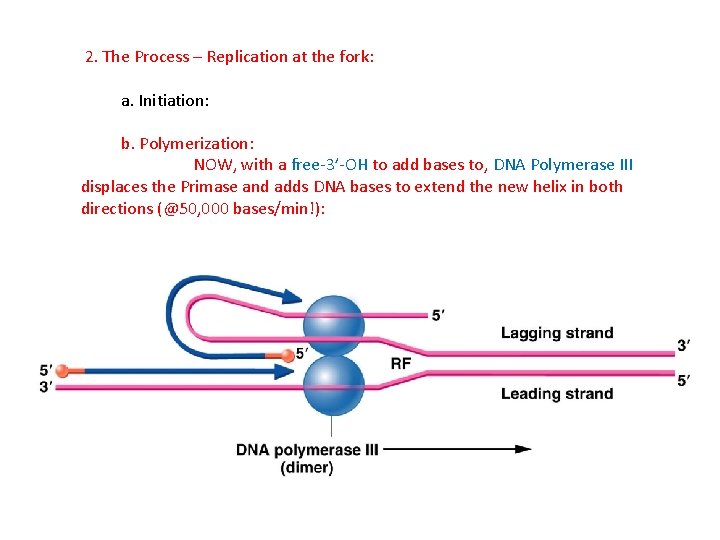
2. The Process – Replication at the fork: a. Initiation: b. Polymerization: NOW, with a free-3’-OH to add bases to, DNA Polymerase III displaces the Primase and adds DNA bases to extend the new helix in both directions (@50, 000 bases/min!):
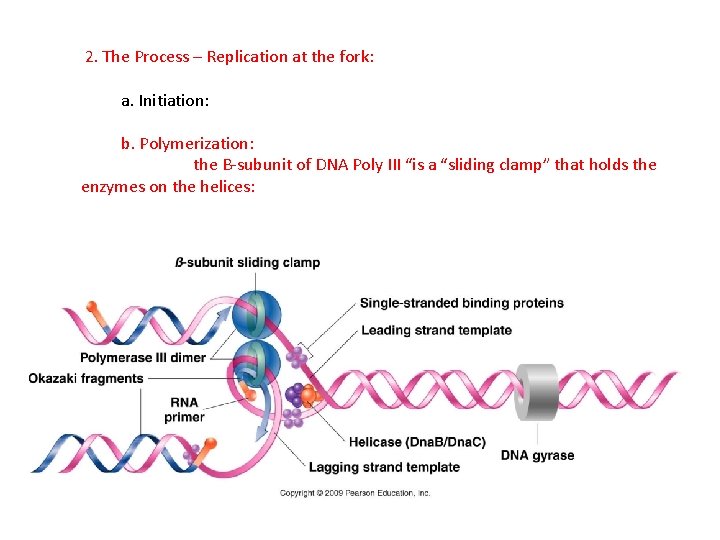
2. The Process – Replication at the fork: a. Initiation: b. Polymerization: the B-subunit of DNA Poly III “is a “sliding clamp” that holds the enzymes on the helices:
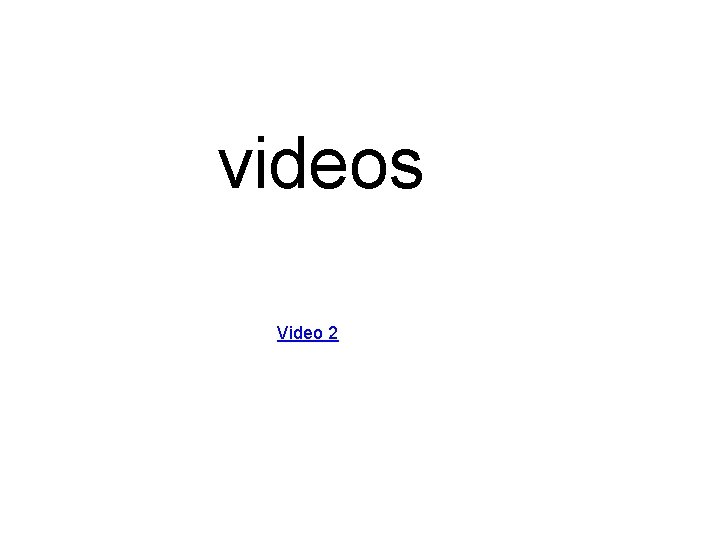
videos Video 2
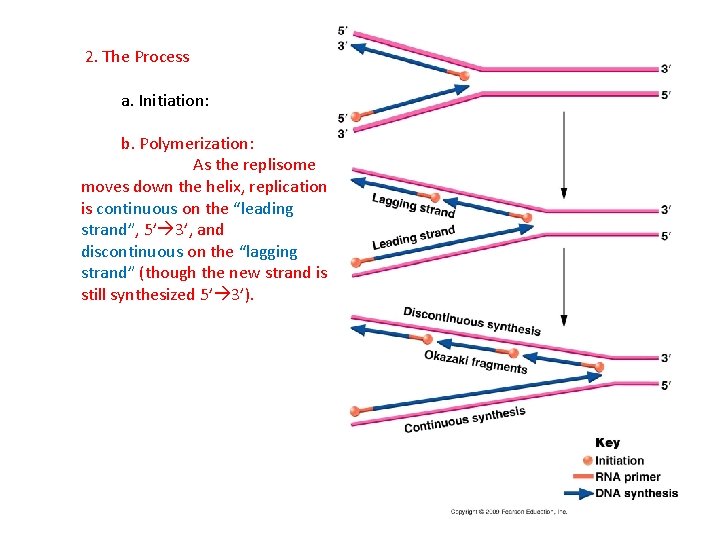
2. The Process a. Initiation: b. Polymerization: As the replisome moves down the helix, replication is continuous on the “leading strand”, 5’ 3’, and discontinuous on the “lagging strand” (though the new strand is still synthesized 5’ 3’).
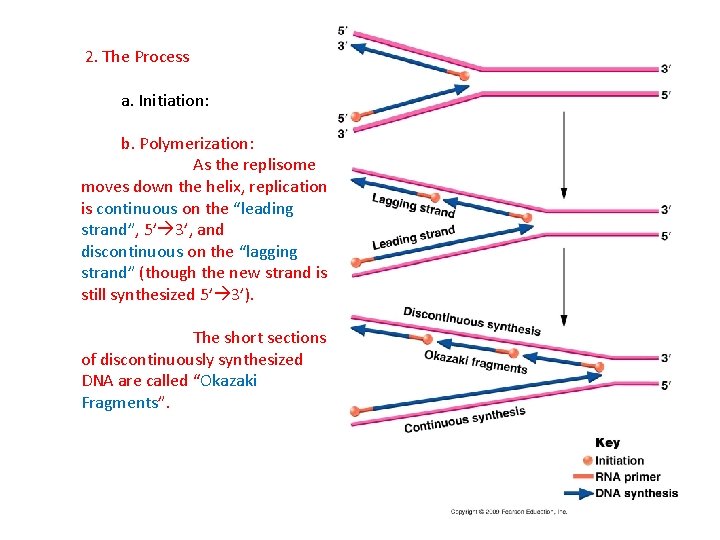
2. The Process a. Initiation: b. Polymerization: As the replisome moves down the helix, replication is continuous on the “leading strand”, 5’ 3’, and discontinuous on the “lagging strand” (though the new strand is still synthesized 5’ 3’). The short sections of discontinuously synthesized DNA are called “Okazaki Fragments”.
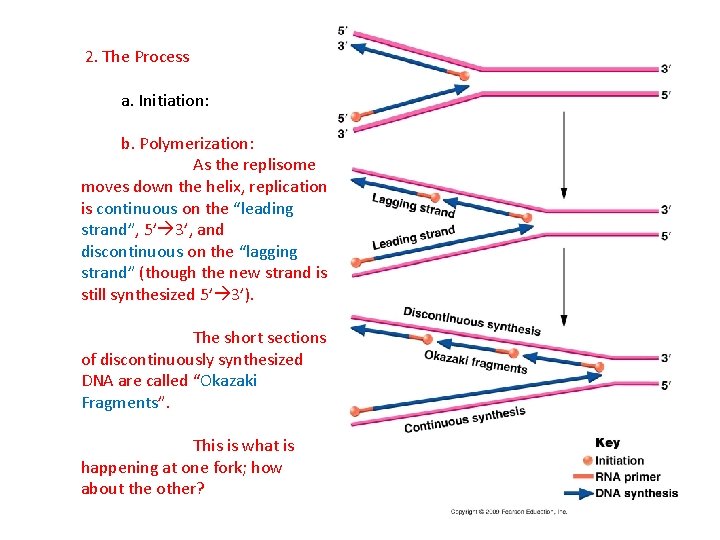
2. The Process a. Initiation: b. Polymerization: As the replisome moves down the helix, replication is continuous on the “leading strand”, 5’ 3’, and discontinuous on the “lagging strand” (though the new strand is still synthesized 5’ 3’). The short sections of discontinuously synthesized DNA are called “Okazaki Fragments”. This is what is happening at one fork; how about the other?
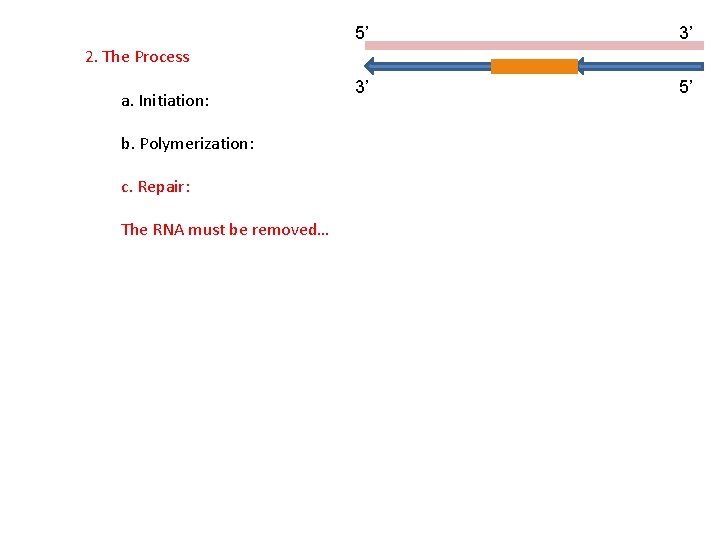
5’ 3’ 3’ 5’ 2. The Process a. Initiation: b. Polymerization: c. Repair: The RNA must be removed…
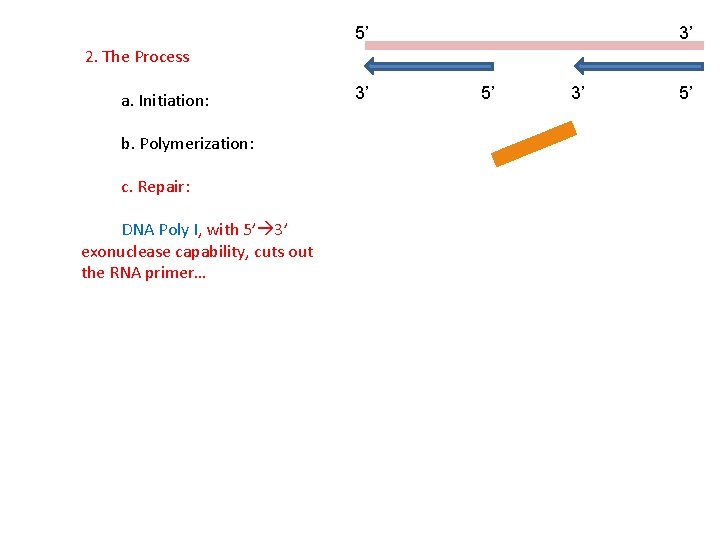
5’ 3’ 2. The Process a. Initiation: b. Polymerization: c. Repair: DNA Poly I, with 5’ 3’ exonuclease capability, cuts out the RNA primer… 3’ 5’
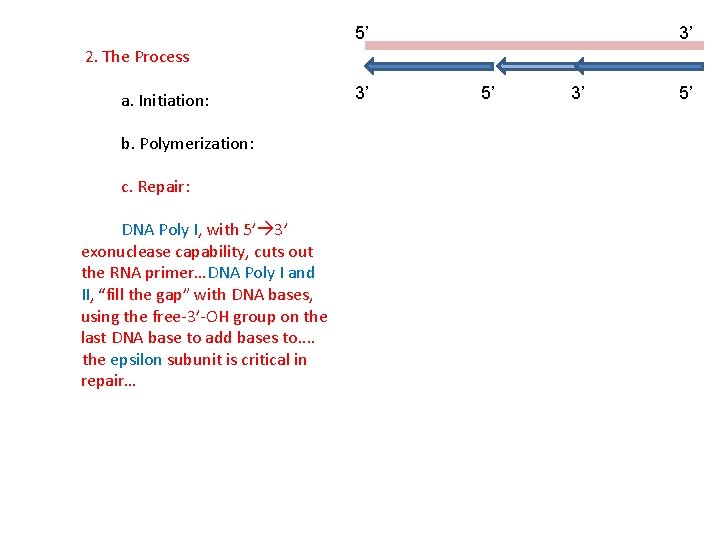
5’ 3’ 2. The Process a. Initiation: b. Polymerization: c. Repair: DNA Poly I, with 5’ 3’ exonuclease capability, cuts out the RNA primer…DNA Poly I and II, “fill the gap” with DNA bases, using the free-3’-OH group on the last DNA base to add bases to. . the epsilon subunit is critical in repair… 3’ 5’
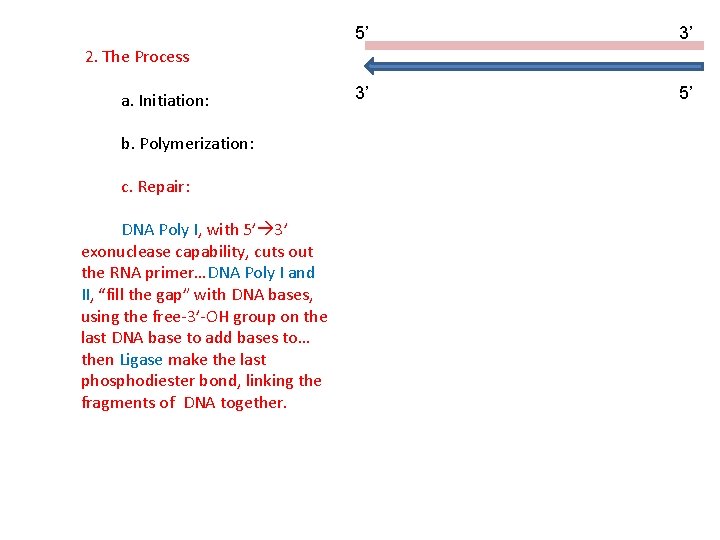
5’ 3’ 3’ 5’ 2. The Process a. Initiation: b. Polymerization: c. Repair: DNA Poly I, with 5’ 3’ exonuclease capability, cuts out the RNA primer…DNA Poly I and II, “fill the gap” with DNA bases, using the free-3’-OH group on the last DNA base to add bases to… then Ligase make the last phosphodiester bond, linking the fragments of DNA together.
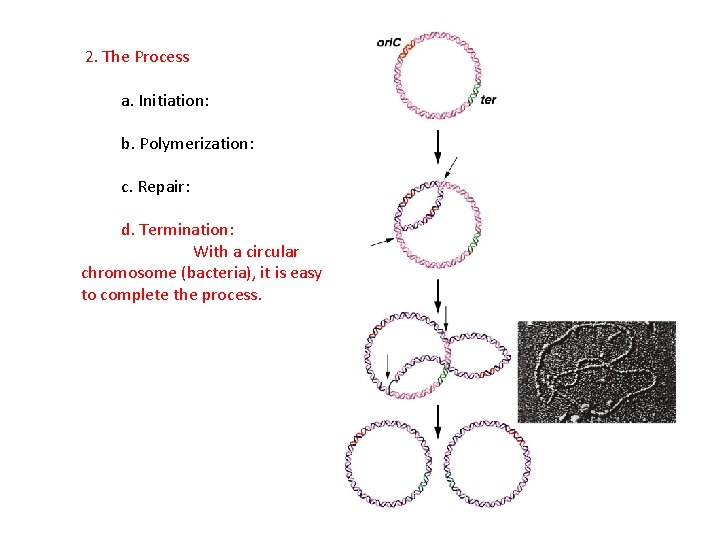
2. The Process a. Initiation: b. Polymerization: c. Repair: d. Termination: With a circular chromosome (bacteria), it is easy to complete the process.
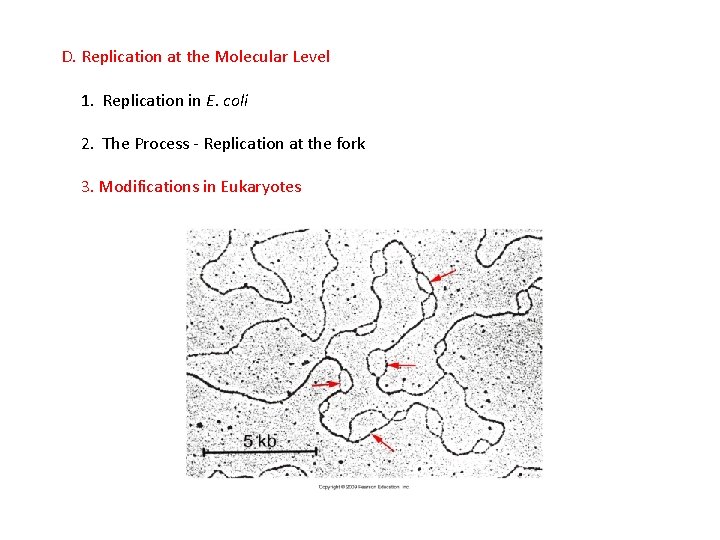
D. Replication at the Molecular Level 1. Replication in E. coli 2. The Process - Replication at the fork 3. Modifications in Eukaryotes
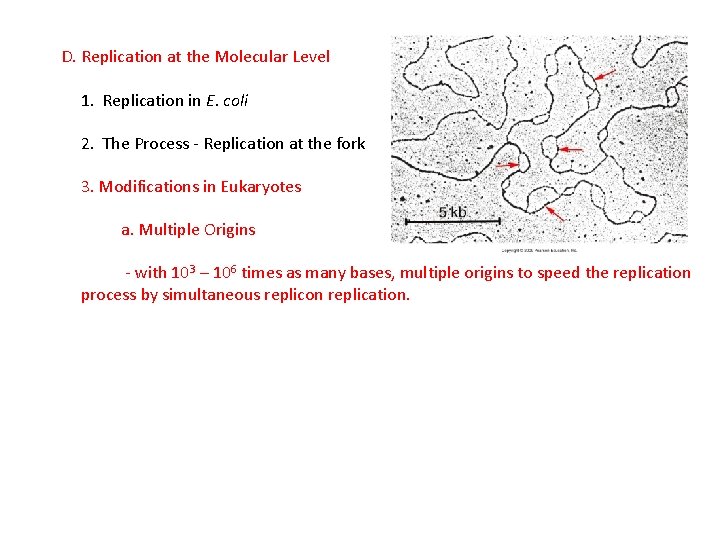
D. Replication at the Molecular Level 1. Replication in E. coli 2. The Process - Replication at the fork 3. Modifications in Eukaryotes a. Multiple Origins - with 103 – 106 times as many bases, multiple origins to speed the replication process by simultaneous replicon replication.
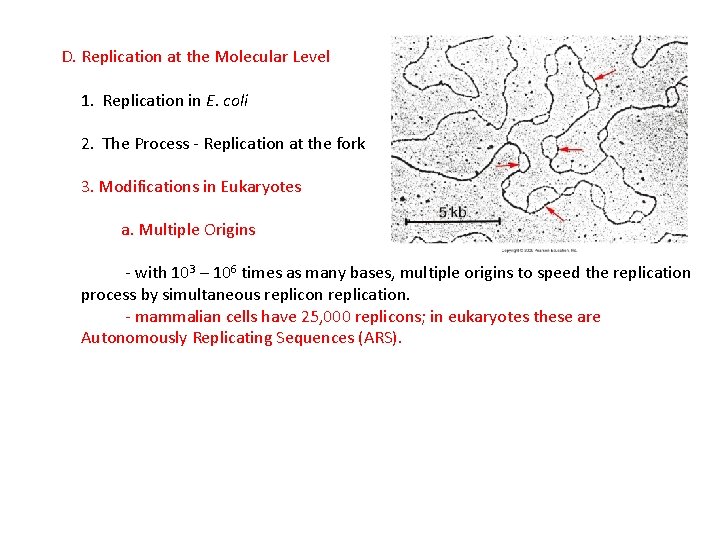
D. Replication at the Molecular Level 1. Replication in E. coli 2. The Process - Replication at the fork 3. Modifications in Eukaryotes a. Multiple Origins - with 103 – 106 times as many bases, multiple origins to speed the replication process by simultaneous replicon replication. - mammalian cells have 25, 000 replicons; in eukaryotes these are Autonomously Replicating Sequences (ARS).
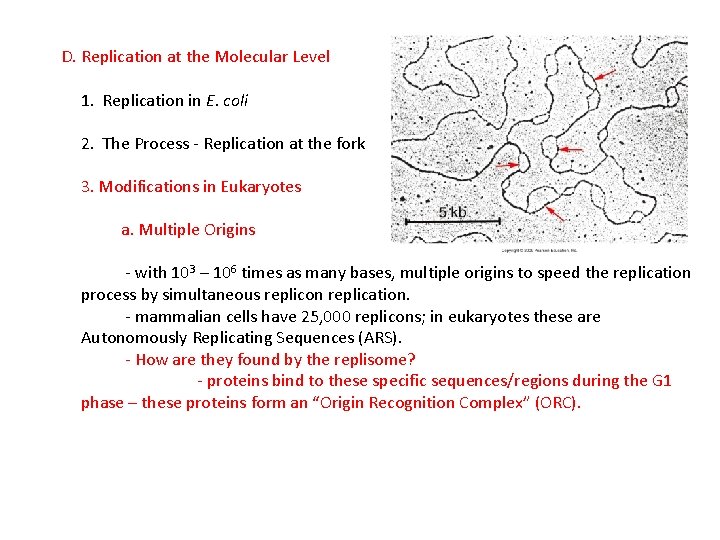
D. Replication at the Molecular Level 1. Replication in E. coli 2. The Process - Replication at the fork 3. Modifications in Eukaryotes a. Multiple Origins - with 103 – 106 times as many bases, multiple origins to speed the replication process by simultaneous replicon replication. - mammalian cells have 25, 000 replicons; in eukaryotes these are Autonomously Replicating Sequences (ARS). - How are they found by the replisome? - proteins bind to these specific sequences/regions during the G 1 phase – these proteins form an “Origin Recognition Complex” (ORC).
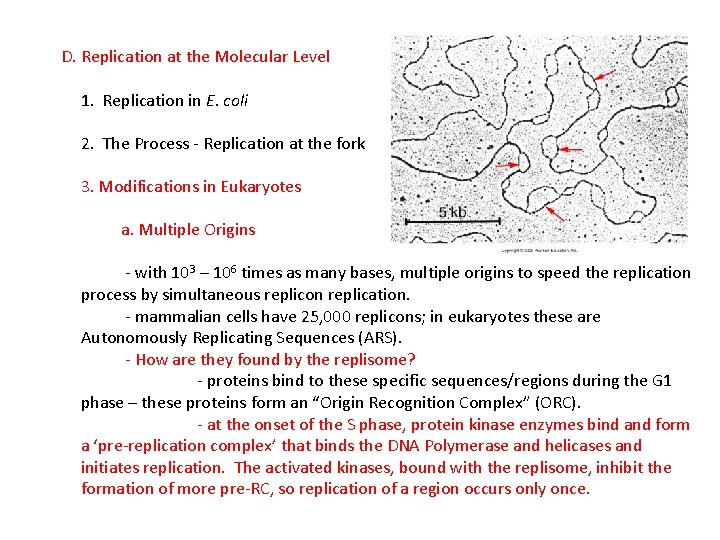
D. Replication at the Molecular Level 1. Replication in E. coli 2. The Process - Replication at the fork 3. Modifications in Eukaryotes a. Multiple Origins - with 103 – 106 times as many bases, multiple origins to speed the replication process by simultaneous replicon replication. - mammalian cells have 25, 000 replicons; in eukaryotes these are Autonomously Replicating Sequences (ARS). - How are they found by the replisome? - proteins bind to these specific sequences/regions during the G 1 phase – these proteins form an “Origin Recognition Complex” (ORC). - at the onset of the S phase, protein kinase enzymes bind and form a ‘pre-replication complex’ that binds the DNA Polymerase and helicases and initiates replication. The activated kinases, bound with the replisome, inhibit the formation of more pre-RC, so replication of a region occurs only once.
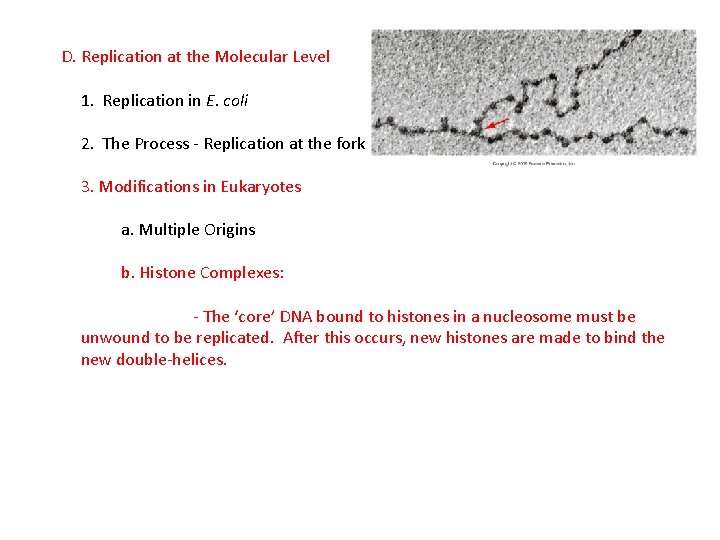
D. Replication at the Molecular Level 1. Replication in E. coli 2. The Process - Replication at the fork 3. Modifications in Eukaryotes a. Multiple Origins b. Histone Complexes: - The ‘core’ DNA bound to histones in a nucleosome must be unwound to be replicated. After this occurs, new histones are made to bind the new double-helices.
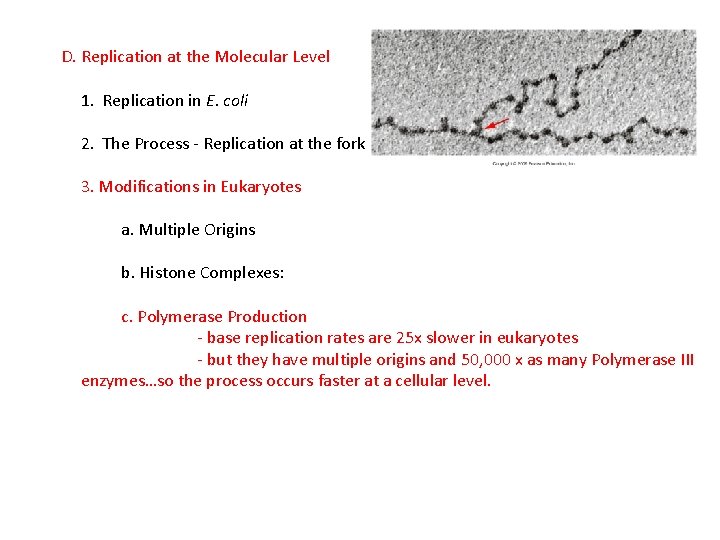
D. Replication at the Molecular Level 1. Replication in E. coli 2. The Process - Replication at the fork 3. Modifications in Eukaryotes a. Multiple Origins b. Histone Complexes: c. Polymerase Production - base replication rates are 25 x slower in eukaryotes - but they have multiple origins and 50, 000 x as many Polymerase III enzymes…so the process occurs faster at a cellular level.
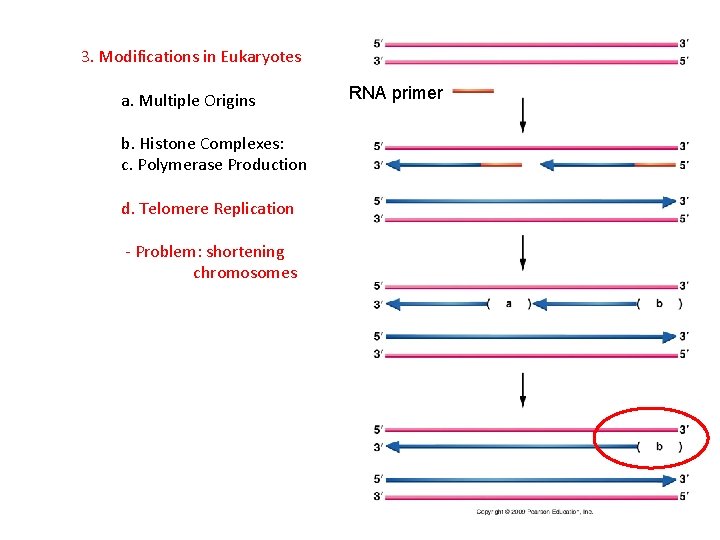
3. Modifications in Eukaryotes a. Multiple Origins b. Histone Complexes: c. Polymerase Production d. Telomere Replication - Problem: shortening chromosomes RNA primer
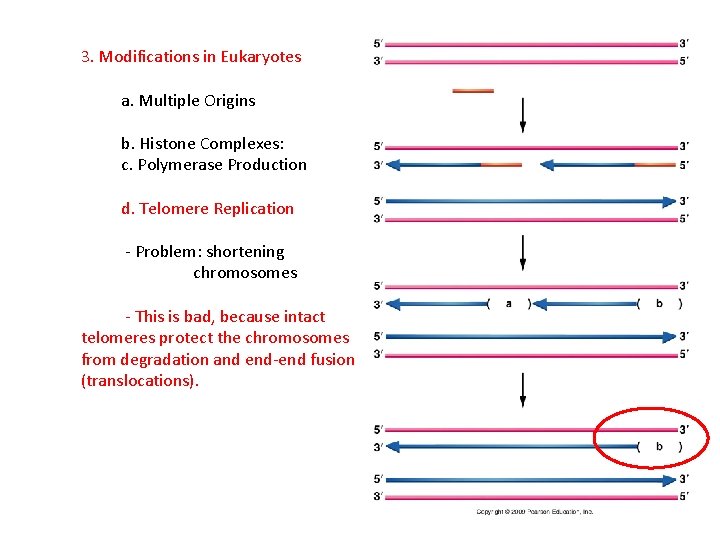
3. Modifications in Eukaryotes a. Multiple Origins b. Histone Complexes: c. Polymerase Production d. Telomere Replication - Problem: shortening chromosomes - This is bad, because intact telomeres protect the chromosomes from degradation and end-end fusion (translocations).
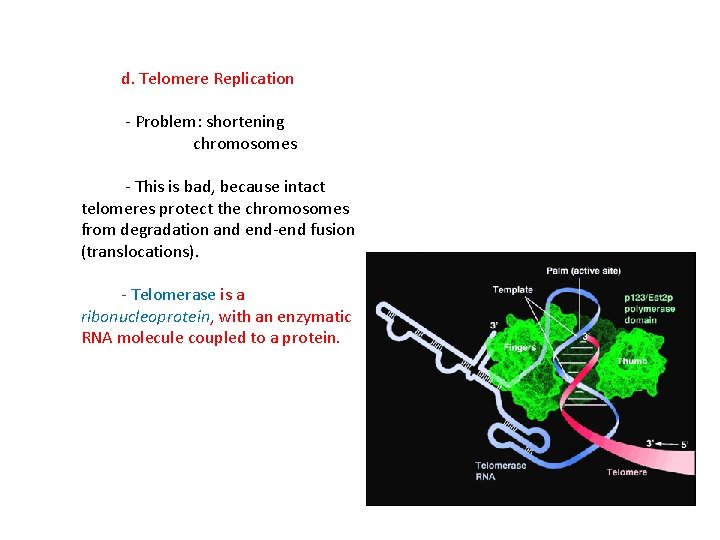
d. Telomere Replication - Problem: shortening chromosomes - This is bad, because intact telomeres protect the chromosomes from degradation and end-end fusion (translocations). - Telomerase is a ribonucleoprotein, with an enzymatic RNA molecule coupled to a protein.
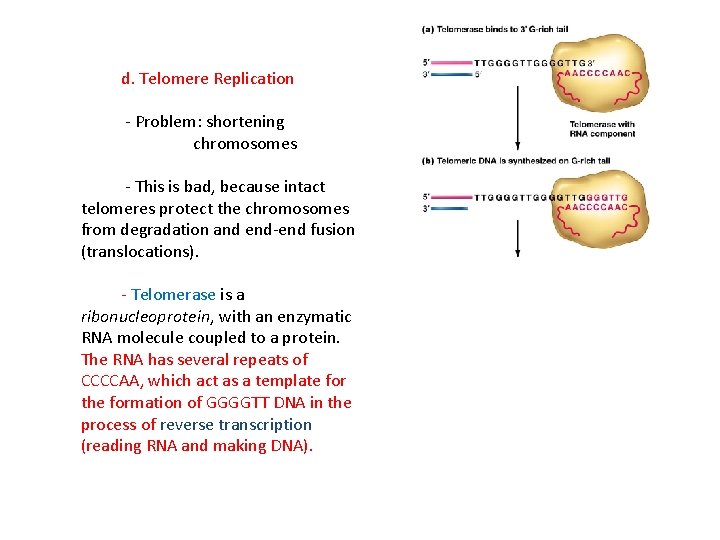
d. Telomere Replication - Problem: shortening chromosomes - This is bad, because intact telomeres protect the chromosomes from degradation and end-end fusion (translocations). - Telomerase is a ribonucleoprotein, with an enzymatic RNA molecule coupled to a protein. The RNA has several repeats of CCCCAA, which act as a template for the formation of GGGGTT DNA in the process of reverse transcription (reading RNA and making DNA).
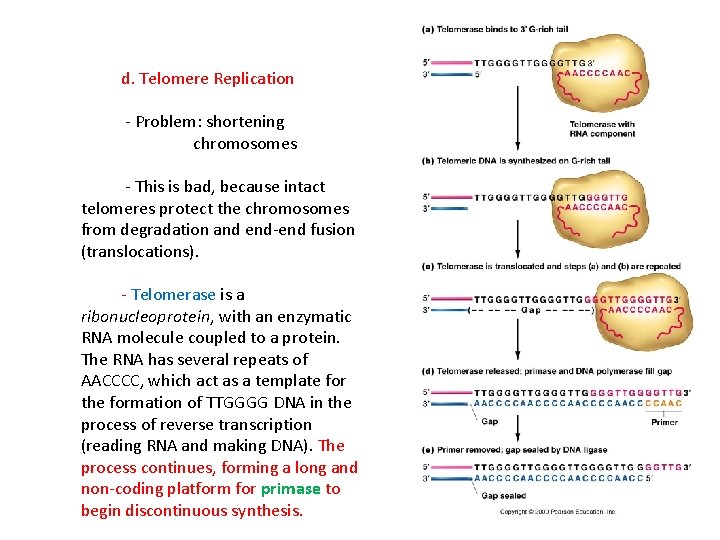
d. Telomere Replication - Problem: shortening chromosomes - This is bad, because intact telomeres protect the chromosomes from degradation and end-end fusion (translocations). - Telomerase is a ribonucleoprotein, with an enzymatic RNA molecule coupled to a protein. The RNA has several repeats of AACCCC, which act as a template for the formation of TTGGGG DNA in the process of reverse transcription (reading RNA and making DNA). The process continues, forming a long and non-coding platform for primase to begin discontinuous synthesis.
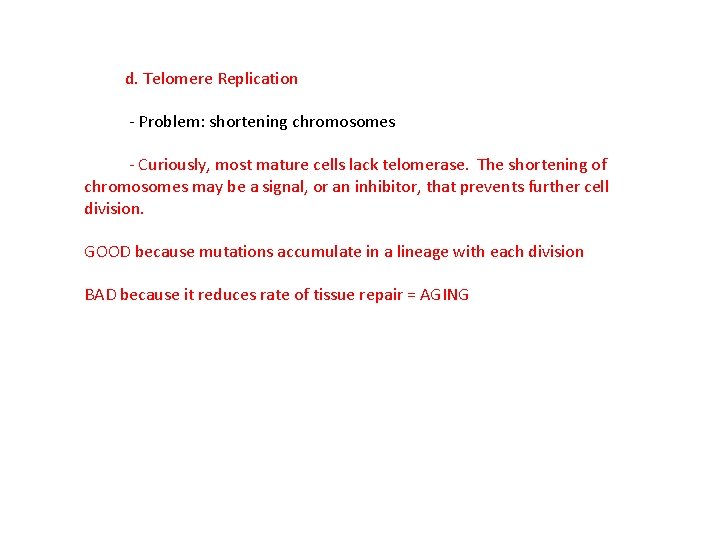
d. Telomere Replication - Problem: shortening chromosomes - Curiously, most mature cells lack telomerase. The shortening of chromosomes may be a signal, or an inhibitor, that prevents further cell division. GOOD because mutations accumulate in a lineage with each division BAD because it reduces rate of tissue repair = AGING
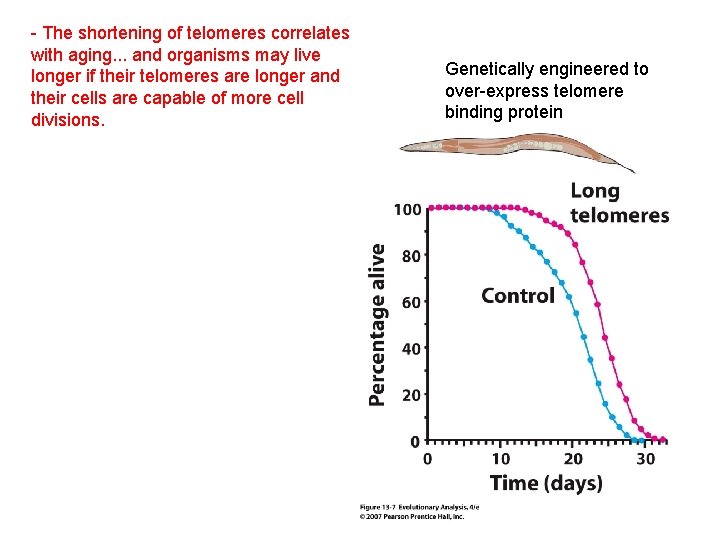
- The shortening of telomeres correlates with aging. . . and organisms may live longer if their telomeres are longer and their cells are capable of more cell divisions. Genetically engineered to over-express telomere binding protein
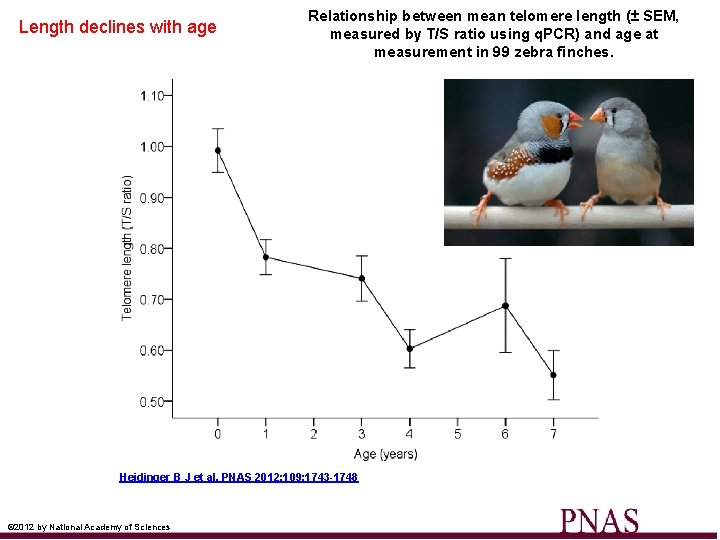
Length declines with age Relationship between mean telomere length (± SEM, measured by T/S ratio using q. PCR) and age at measurement in 99 zebra finches. Heidinger B J et al. PNAS 2012; 109: 1743 -1748 © 2012 by National Academy of Sciences
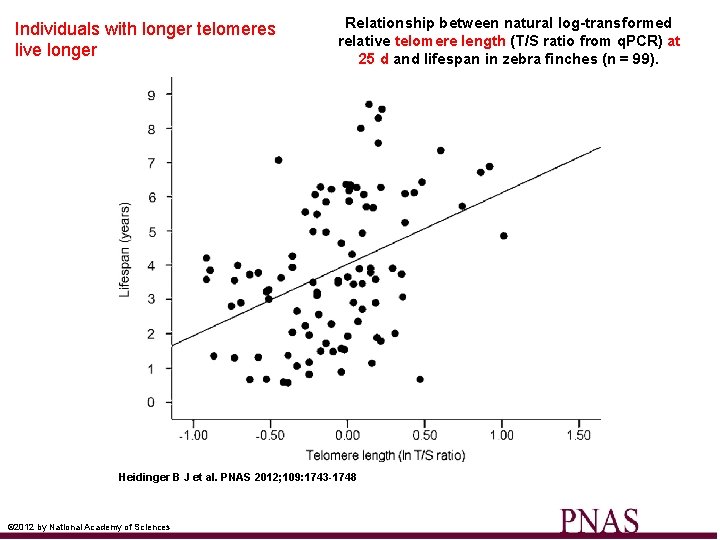
Individuals with longer telomeres live longer Relationship between natural log-transformed relative telomere length (T/S ratio from q. PCR) at 25 d and lifespan in zebra finches (n = 99). Heidinger B J et al. PNAS 2012; 109: 1743 -1748 © 2012 by National Academy of Sciences
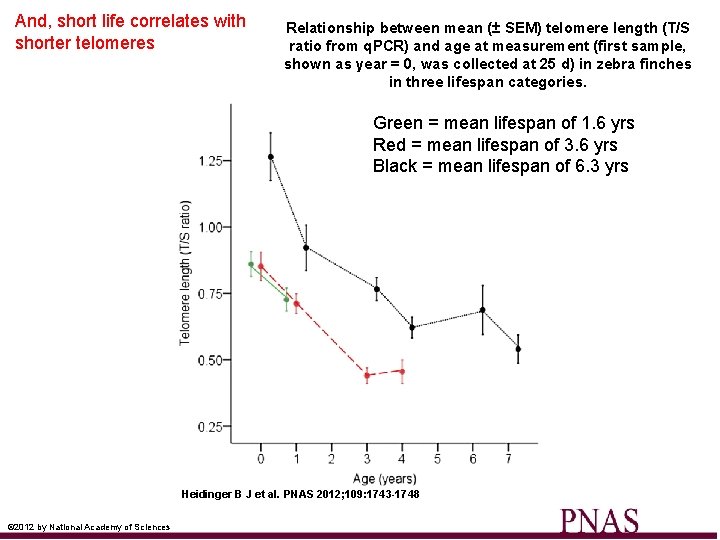
And, short life correlates with shorter telomeres Relationship between mean (± SEM) telomere length (T/S ratio from q. PCR) and age at measurement (first sample, shown as year = 0, was collected at 25 d) in zebra finches in three lifespan categories. Green = mean lifespan of 1. 6 yrs Red = mean lifespan of 3. 6 yrs Black = mean lifespan of 6. 3 yrs Heidinger B J et al. PNAS 2012; 109: 1743 -1748 © 2012 by National Academy of Sciences
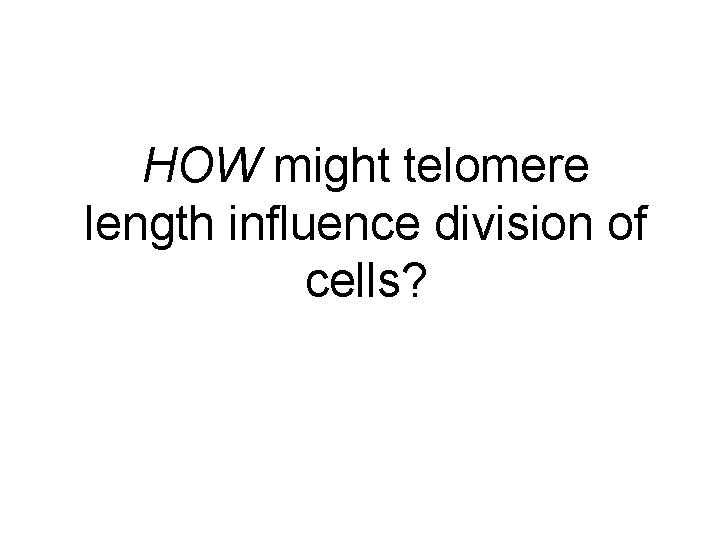
HOW might telomere length influence division of cells?
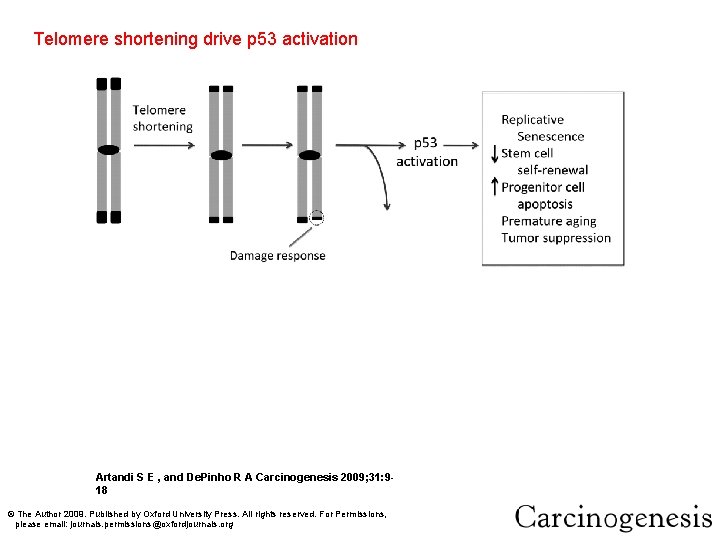
Telomere shortening activates p 53 activation and drives formation of epithelial cancers through gene Telomere shortening drive p 53 amplification and deletion. Artandi S E , and De. Pinho R A Carcinogenesis 2009; 31: 918 © The Author 2009. Published by Oxford University Press. All rights reserved. For Permissions, please email: journals. permissions@oxfordjournals. org
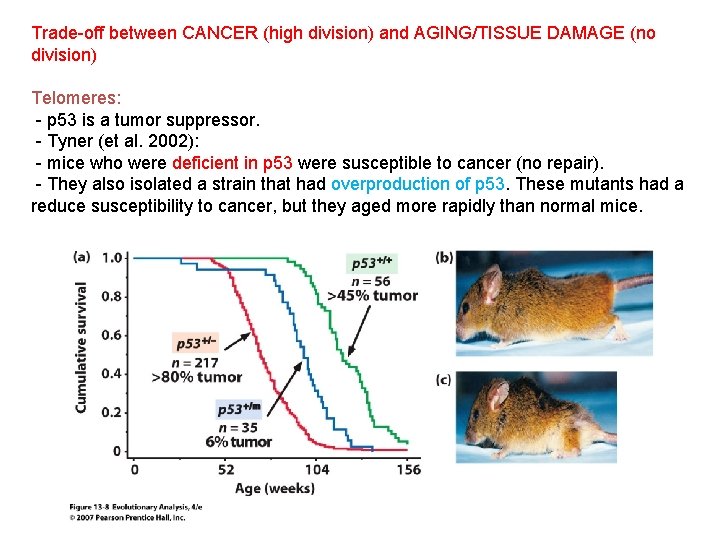
Trade-off between CANCER (high division) and AGING/TISSUE DAMAGE (no division) Telomeres: - p 53 is a tumor suppressor. - Tyner (et al. 2002): - mice who were deficient in p 53 were susceptible to cancer (no repair). - They also isolated a strain that had overproduction of p 53. These mutants had a reduce susceptibility to cancer, but they aged more rapidly than normal mice.
Bioflix activity dna replication lagging strand synthesis
Function of dna polymerase 3
Dna rna protein synthesis homework #2 dna replication
Haploid vs diploid
Amino acid decoder
Dna replication is semi-conservative
Okazaki fragments
Polyribosomes
Dna structure and replication packet answer key
Major enzymes in dna replication
Antiparallel in dna replication
Dna replication jeopardy
Dna replication fork
Dna replication vs pcr
What is this name
Kim foglia dna replication
Bioflix dna replication
Restaurant analogy
Dna replication fork
Dna replication transcription and translation
Replication of dna higher biology
Why is dna replication considered semiconservative
Dna replication pearson
Direction of helicase
Dna replication in bacteria occurs
Dna replication
Helicaee
Dna structure and replication
Dna replication comic strip
Dna replication facts
Accuracy of dna replication
Bioflix dna replication
Dna replication
Dna synthesis at replication fork
Dna replication phschool
Chapter 13 the molecular basis of inheritance
Importance of dna replication
What are the enzymes involved in dna replication
If replicated what will be the complementary strand
Dna replication fork
Dna replication
Dna replication
Dna primase
Replication fork
Orc dna replication
Significance of dna replication
Dna replication fork diagram
Dna replication
The replication bubble and antiparallel elongation
Summary of dna replication
Dna replication animation mcgraw hill
Friedrich miescher discovery
The principle enzyme involved in dna replication is called
Dna replication bubble animation
Dna replication is considered semiconservative because
Bioflix dna replication
Coding dna and non coding dna
Aqua destillata pro injectione
Kegunaan xenon
King henry viii family tree
Nervios vagos
Why did henry viii behead his wives
King louis xvi family tree