Timing Performance of MicroChannel Plates and other detectors
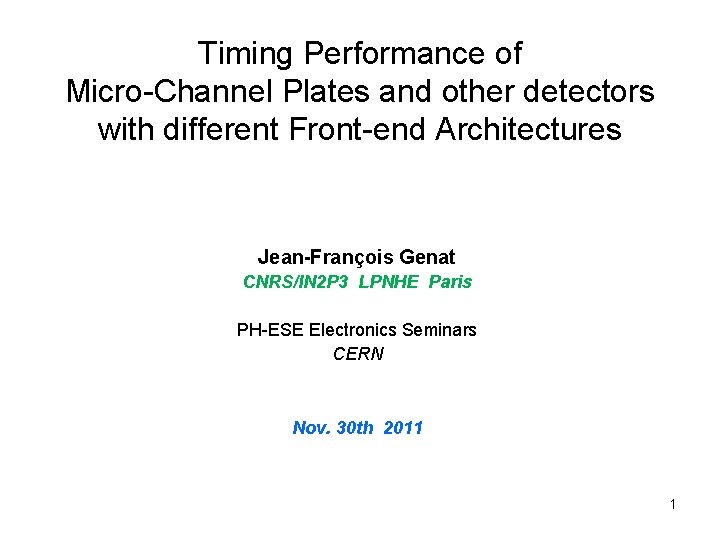
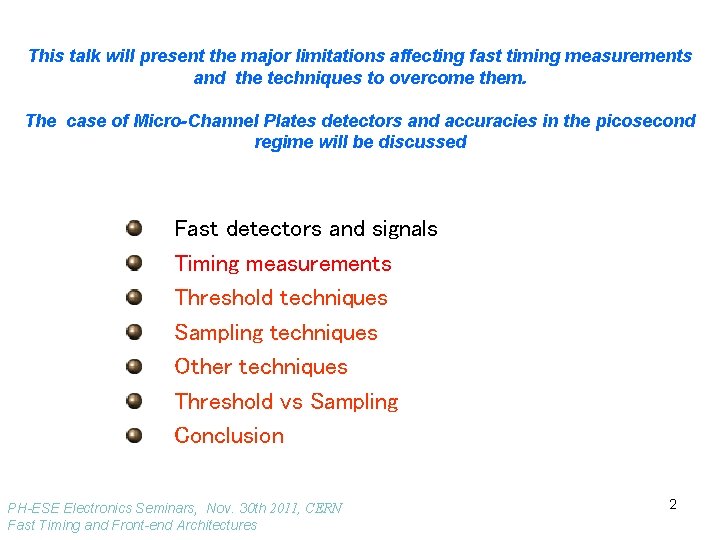
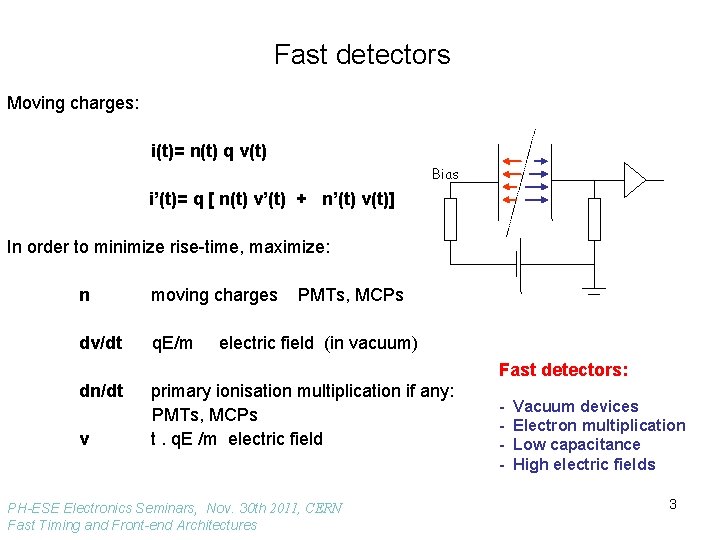
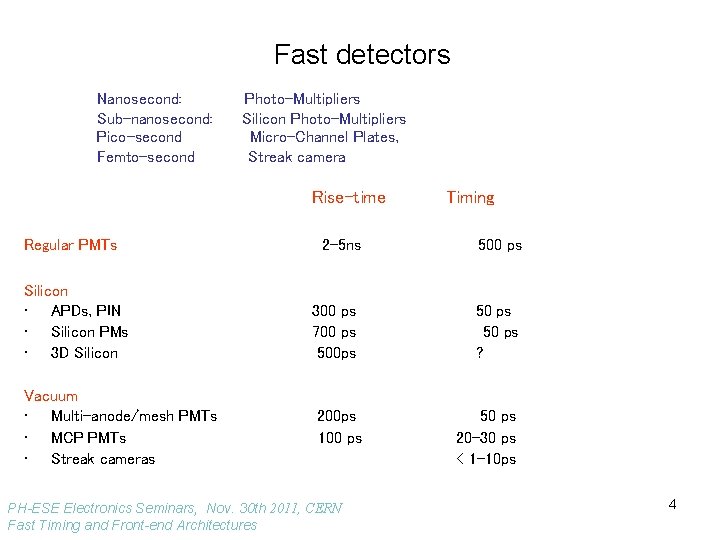
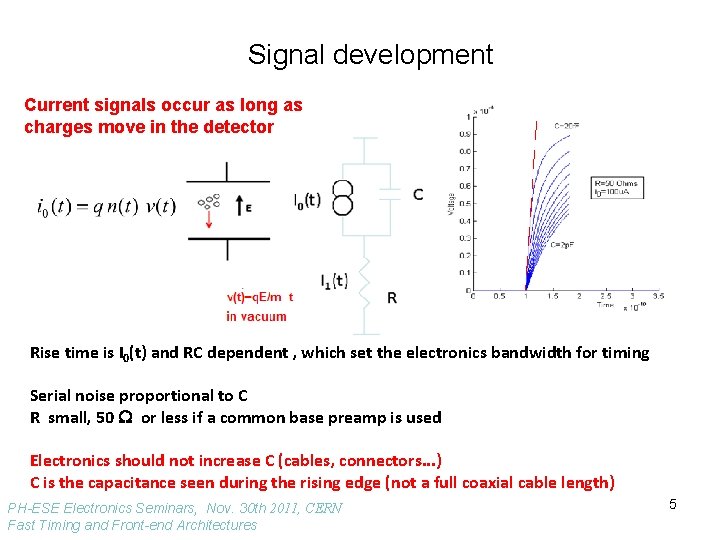
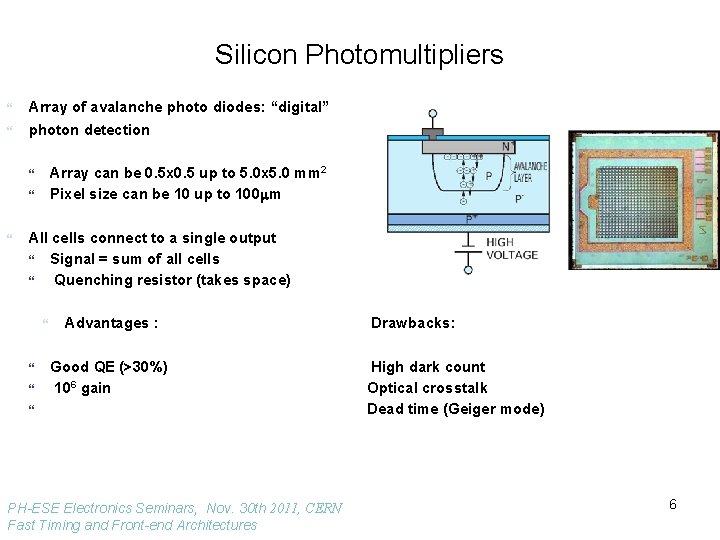
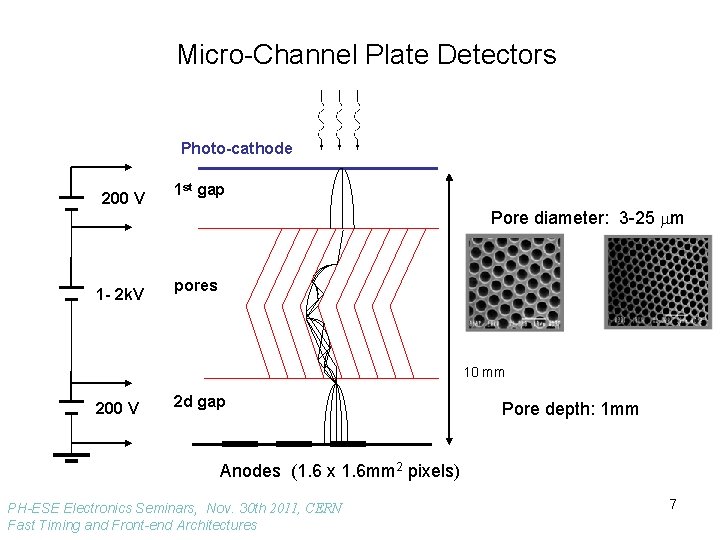
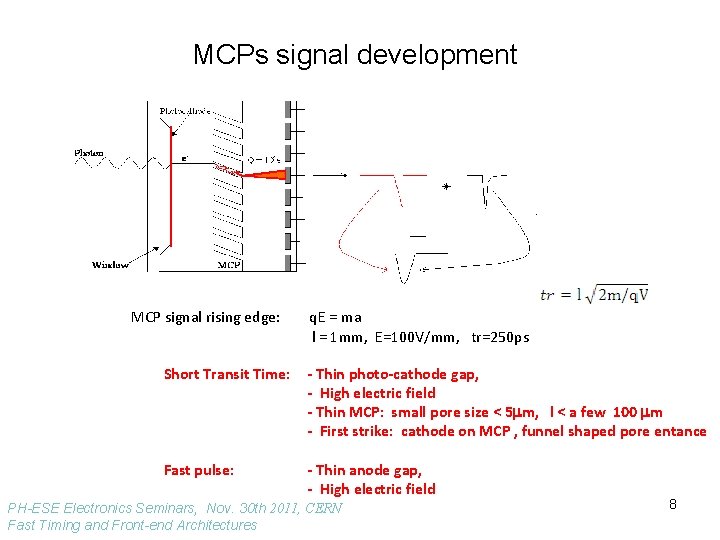
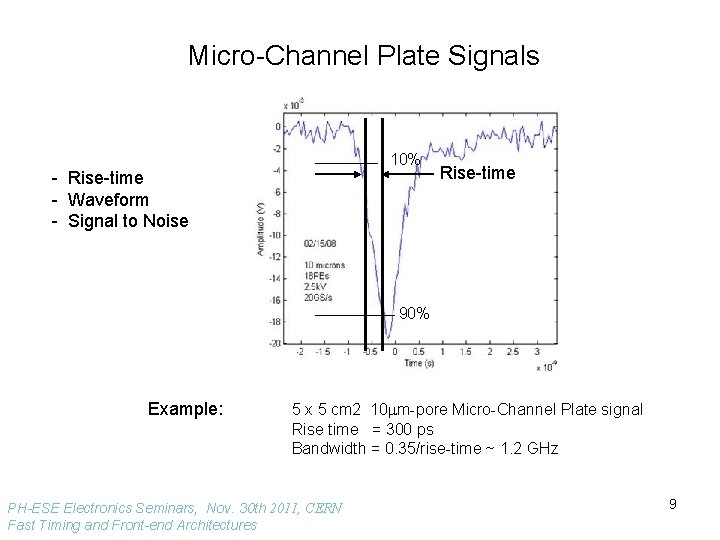
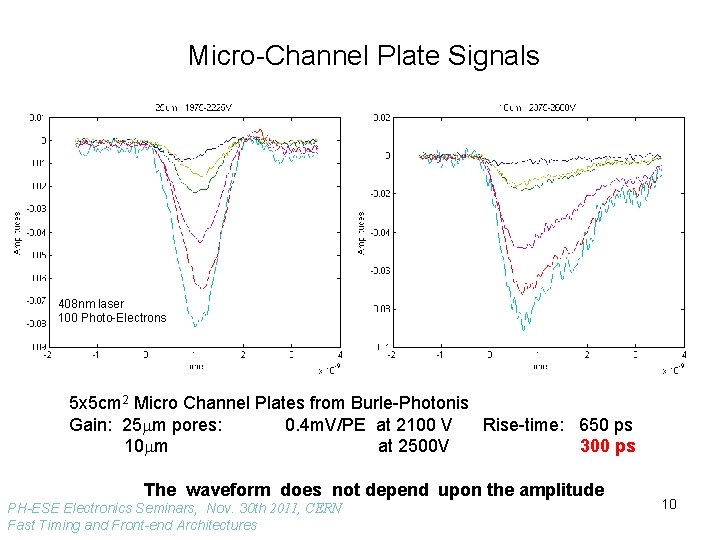
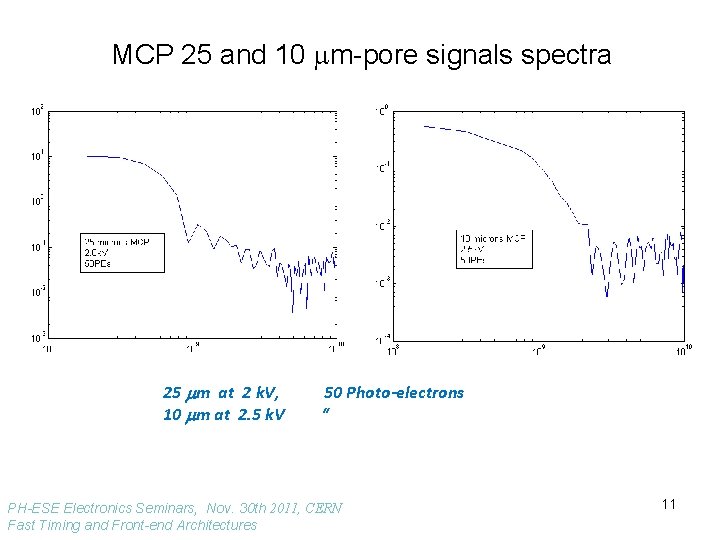
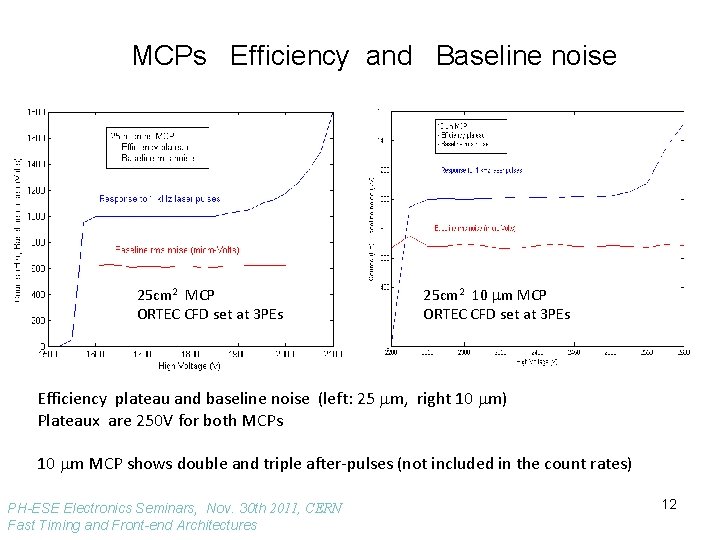
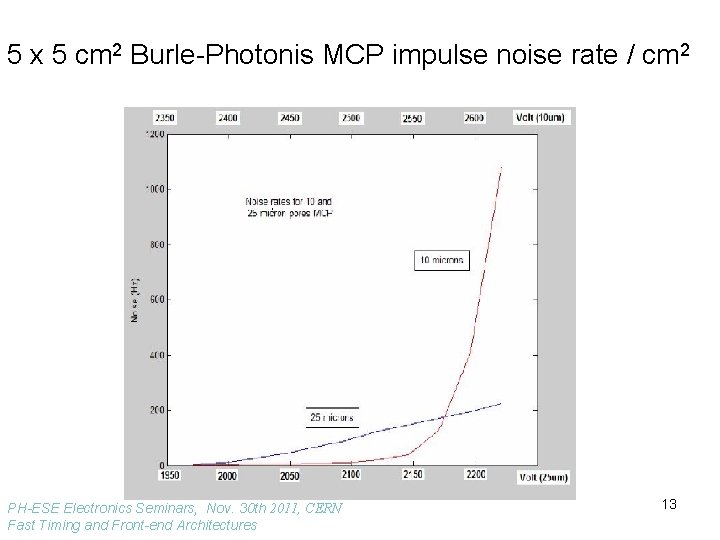
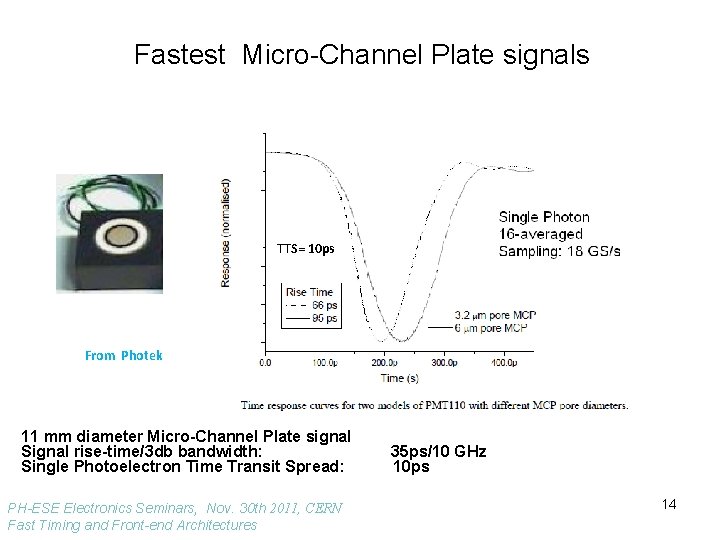
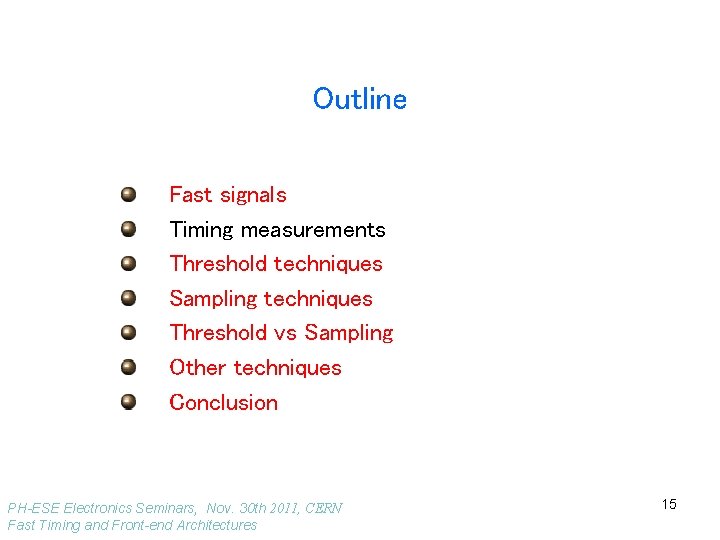
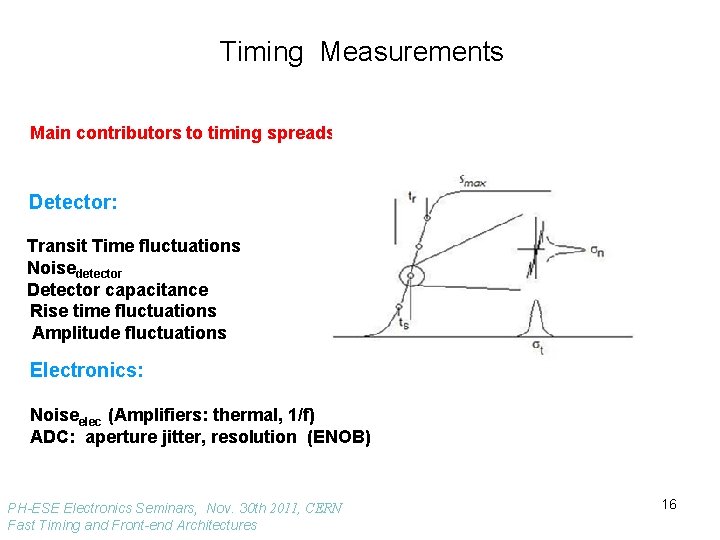
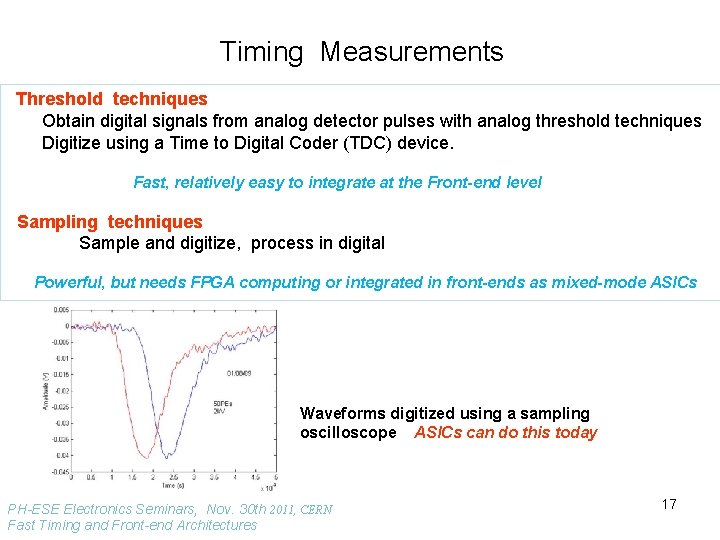
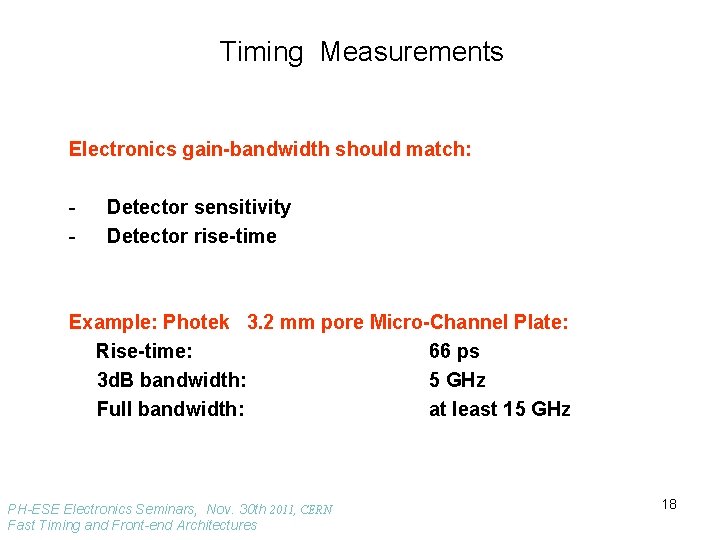
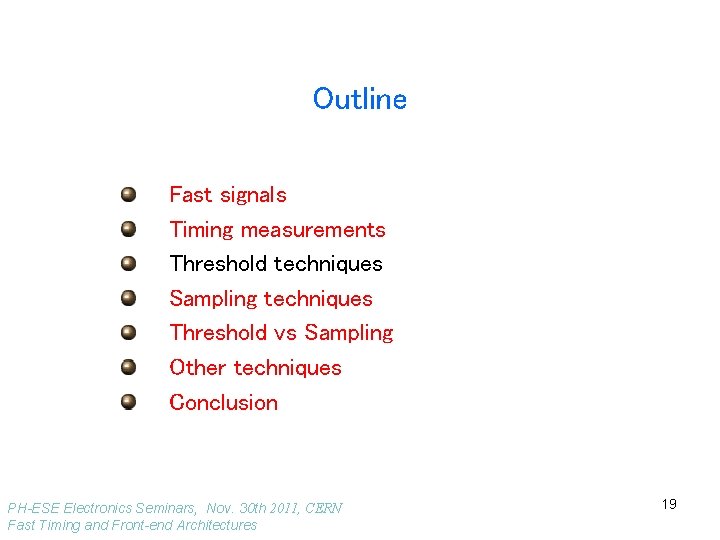
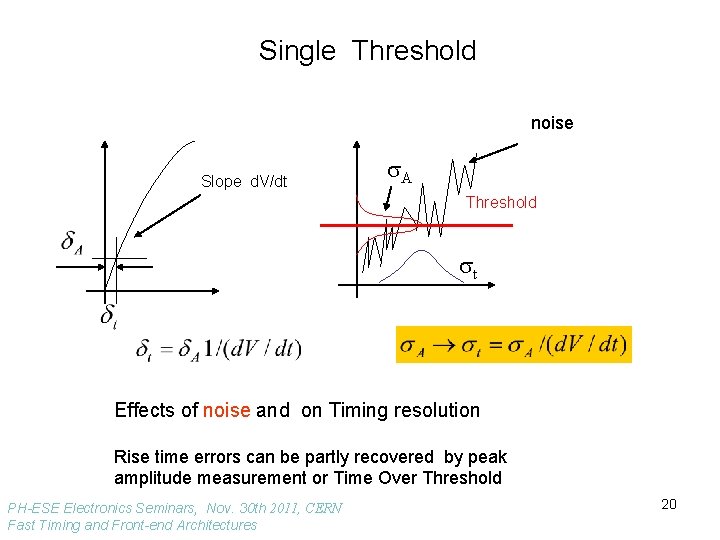
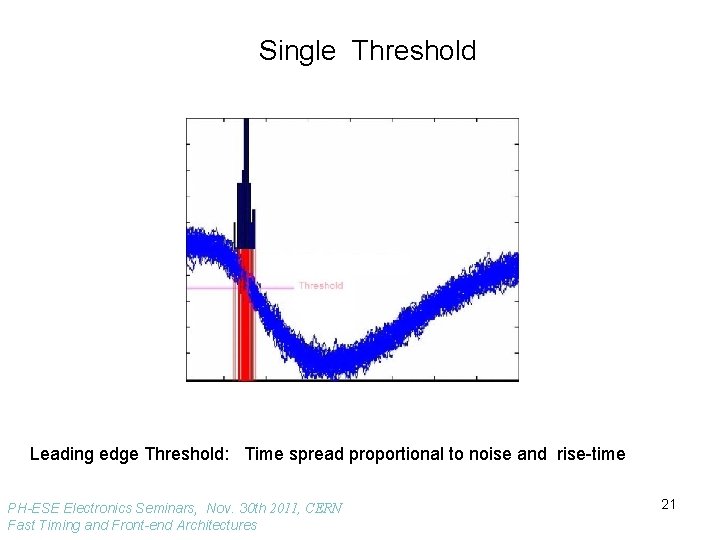
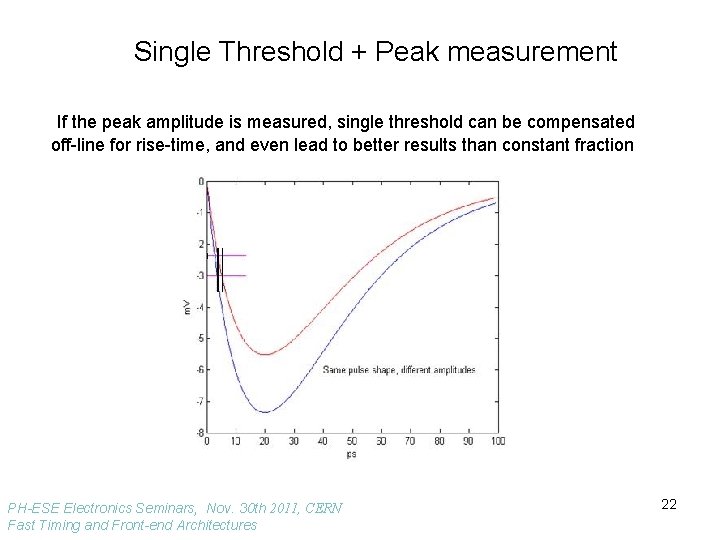
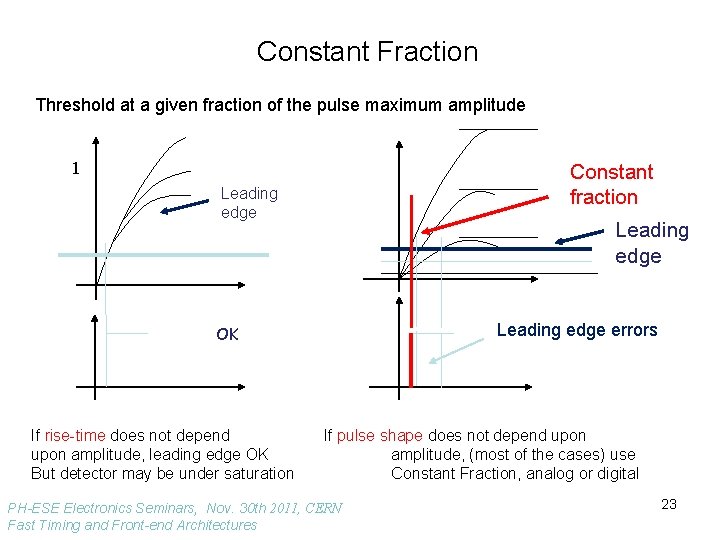
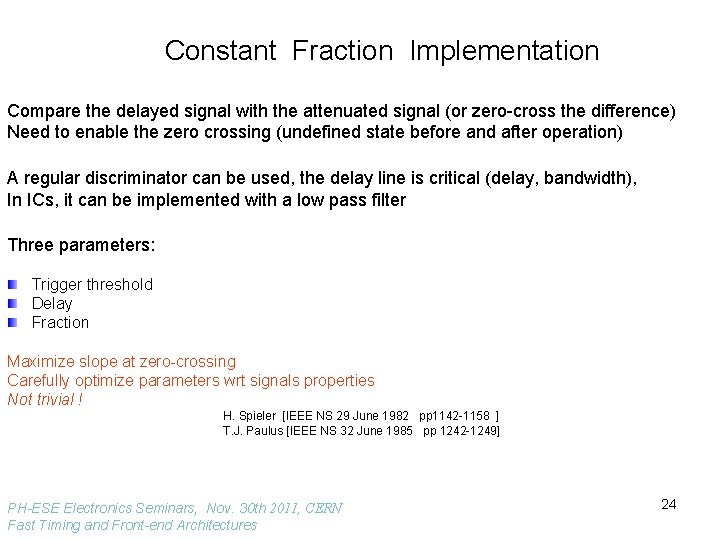
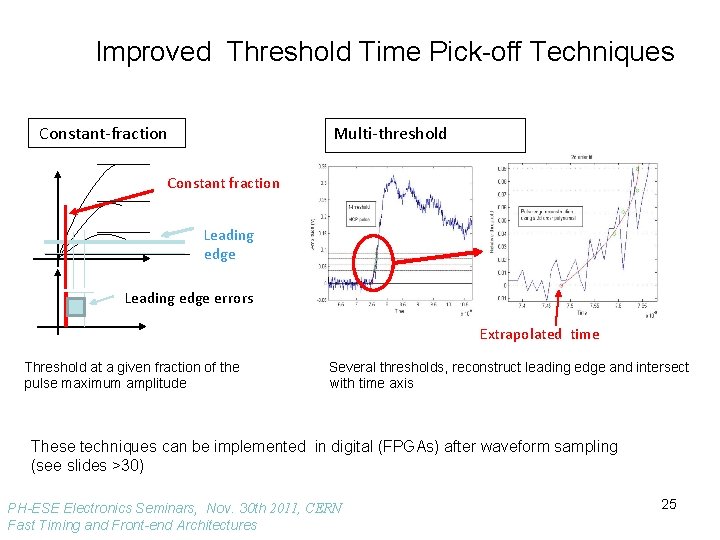
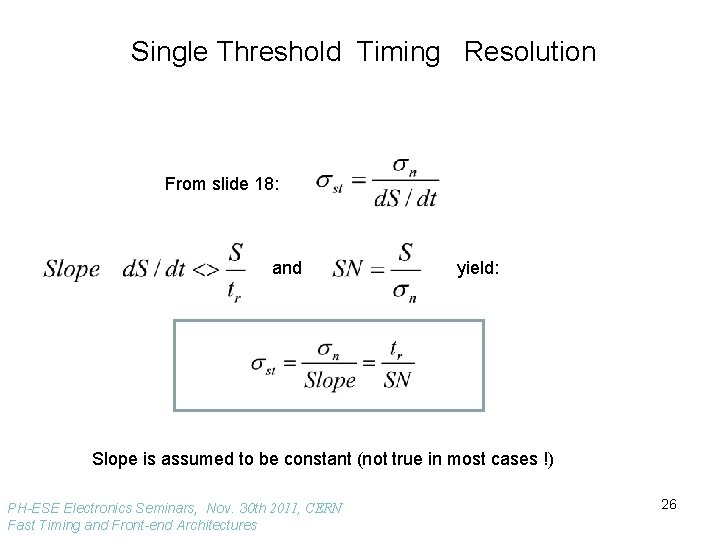
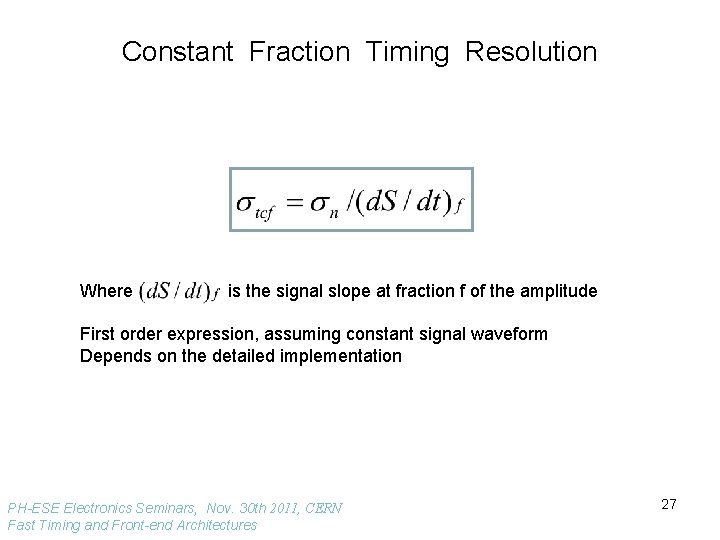
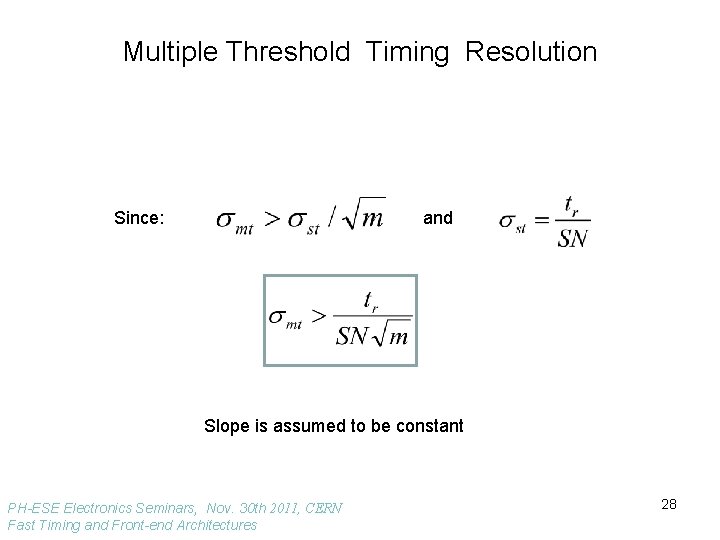
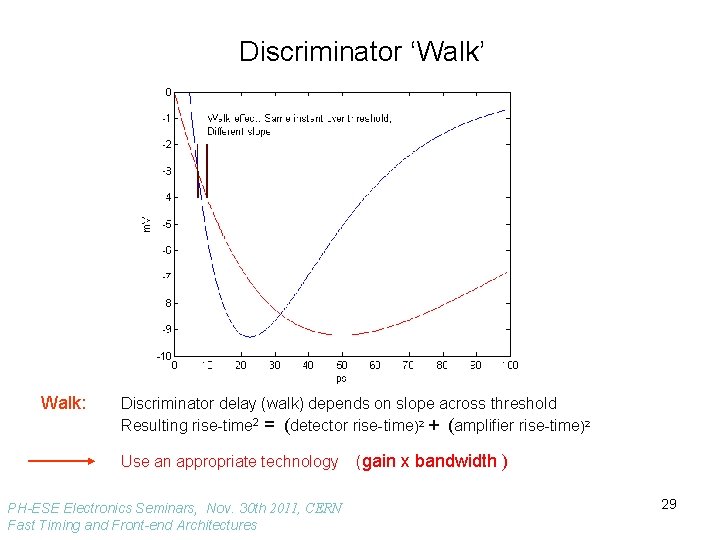
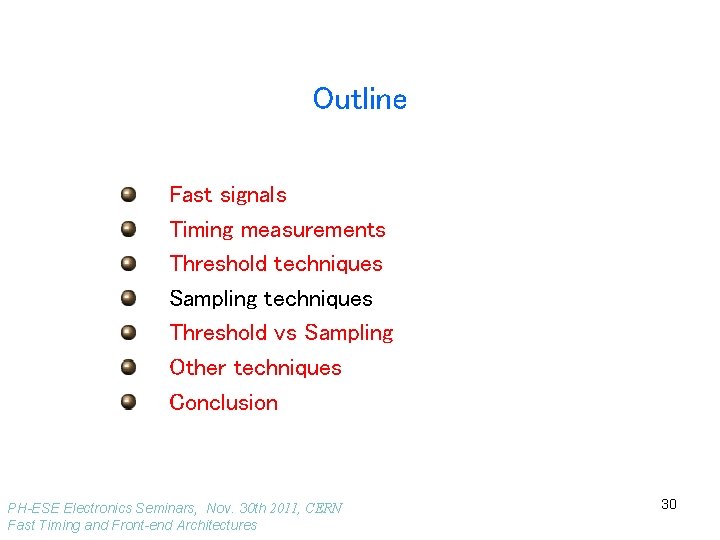
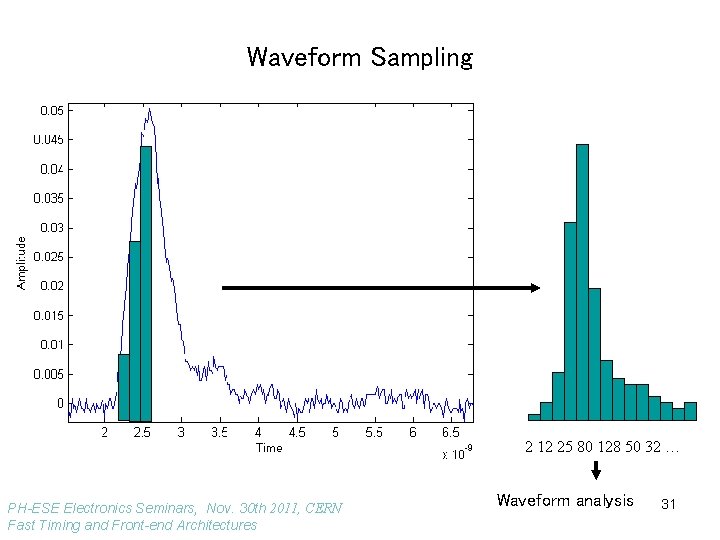
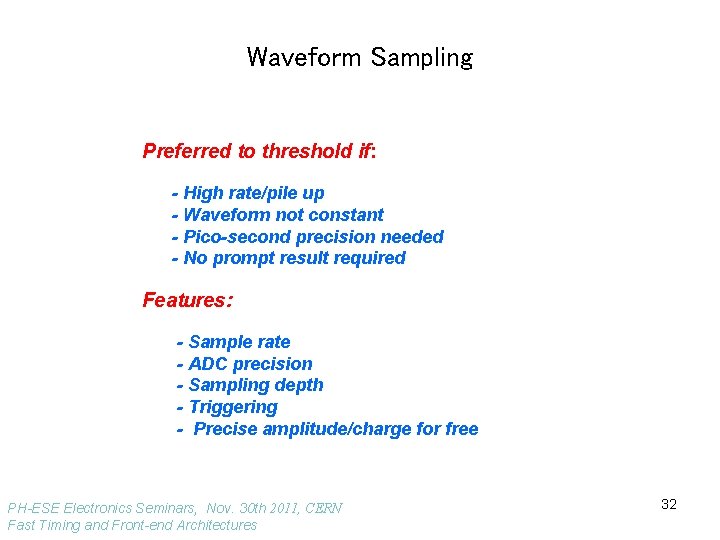
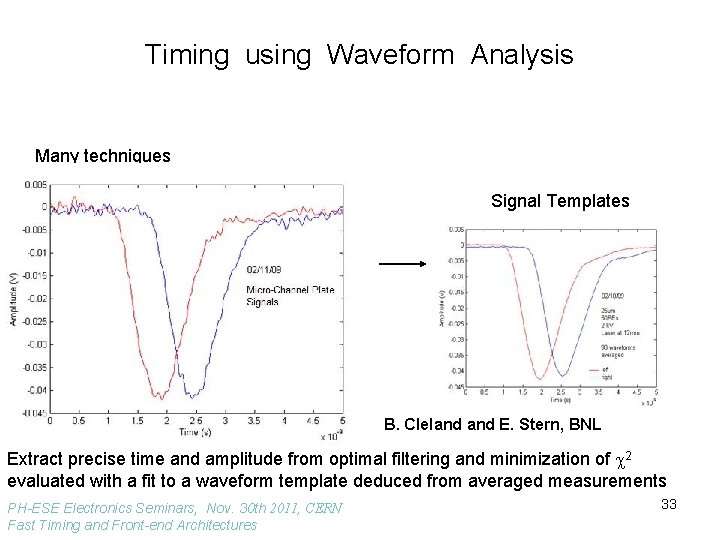
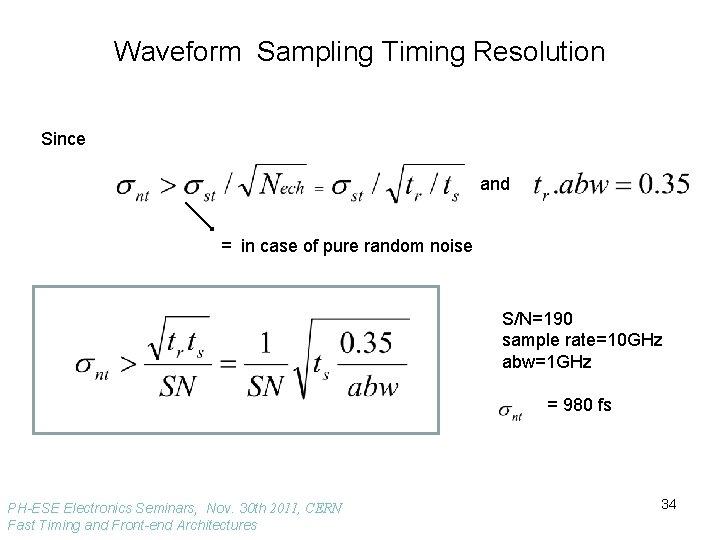
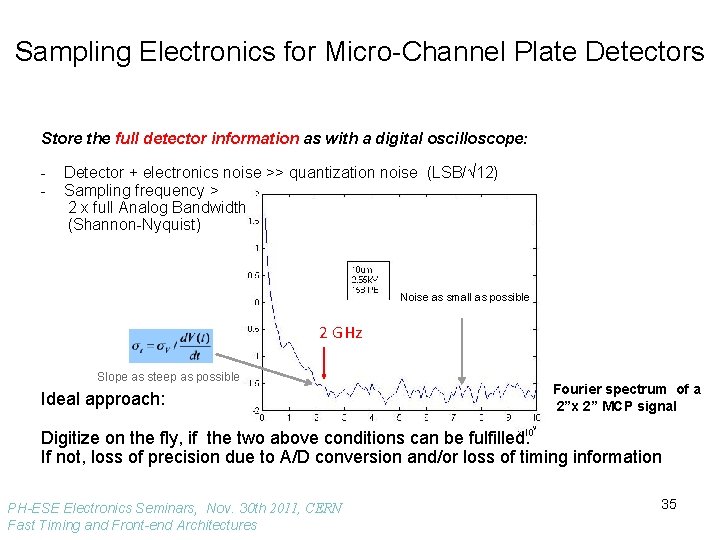
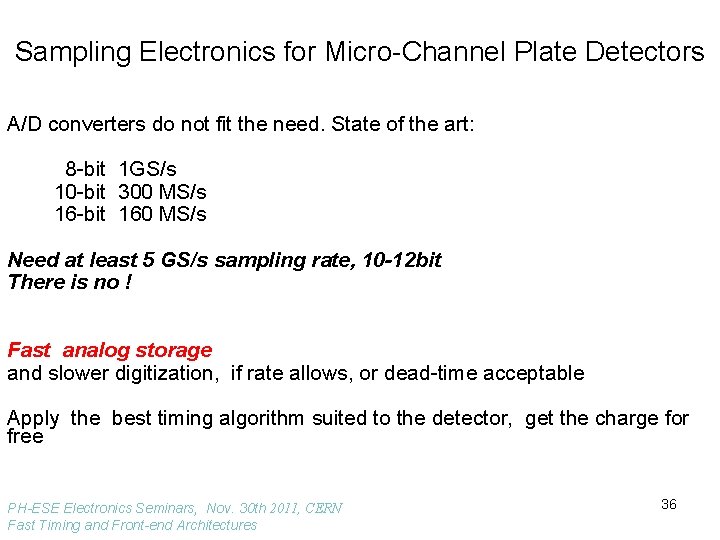
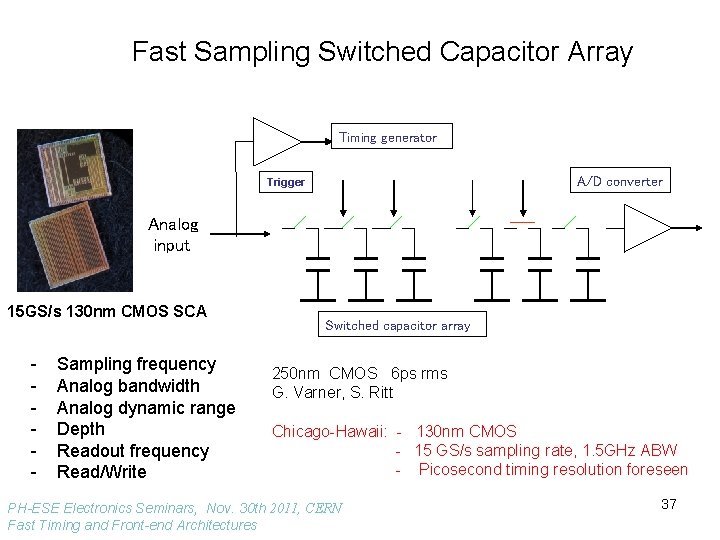
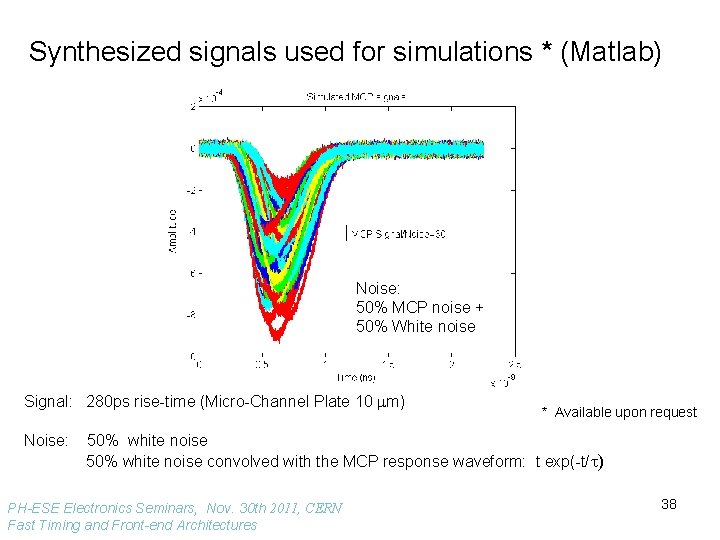
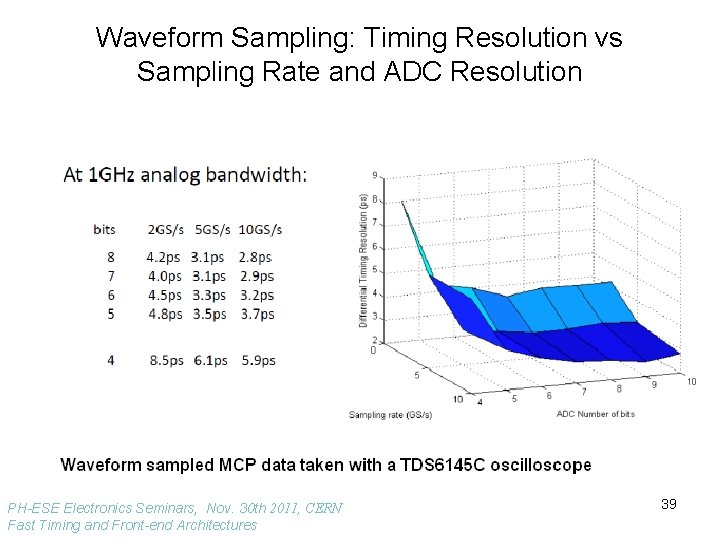
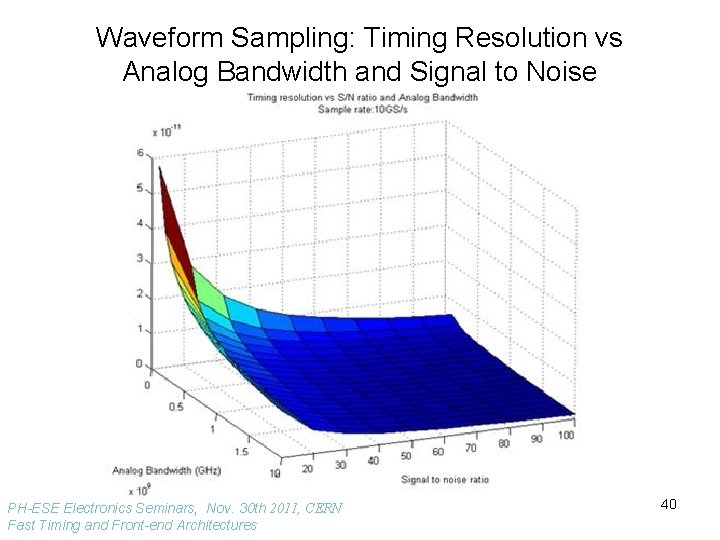
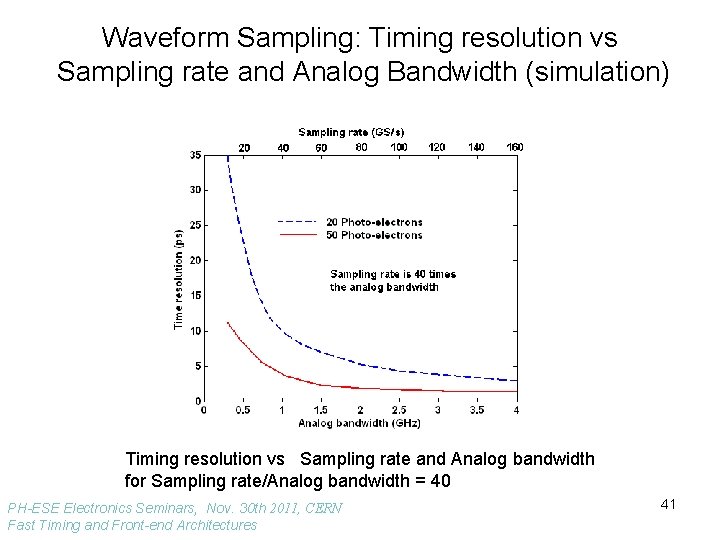
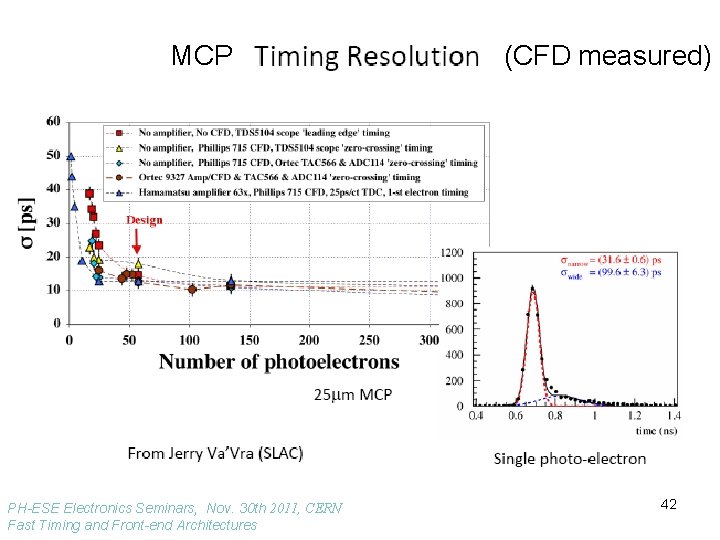
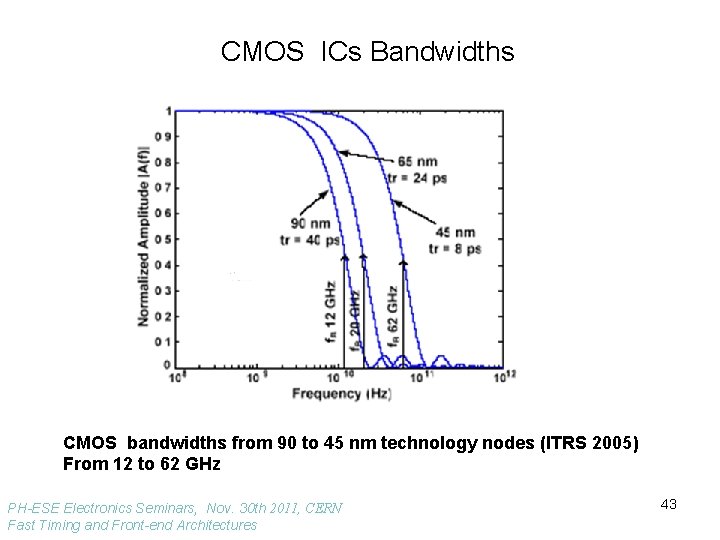
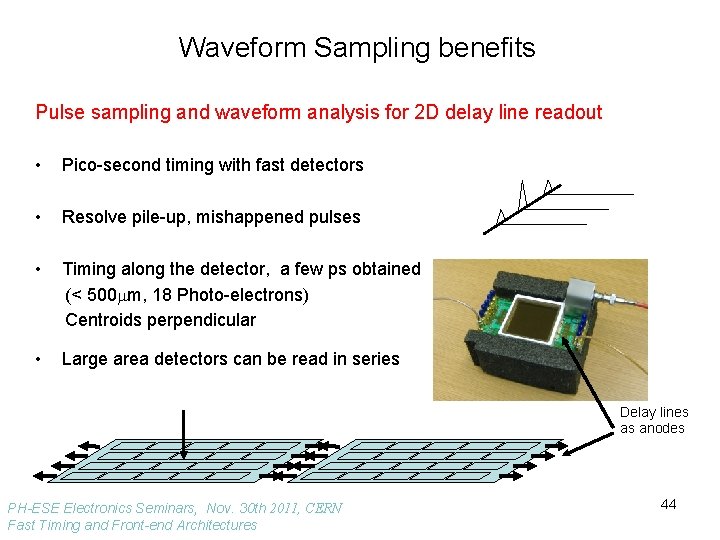
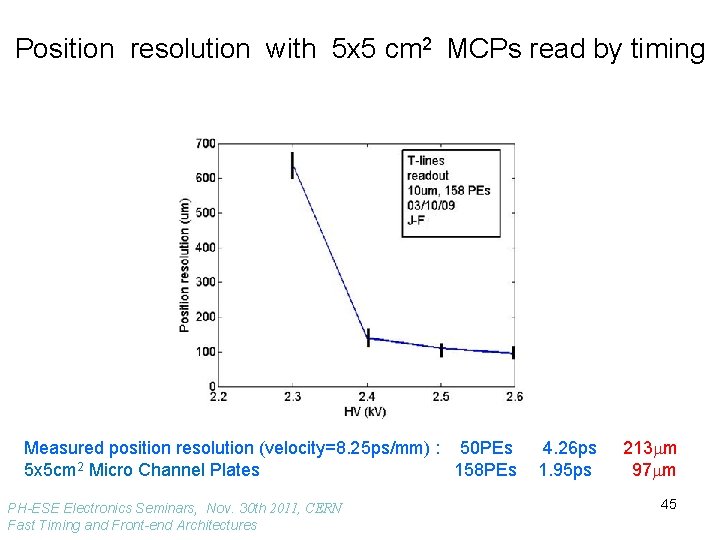
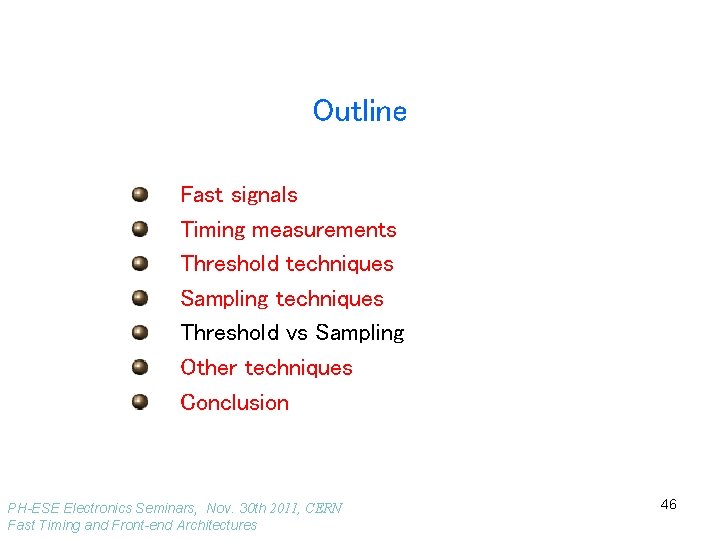
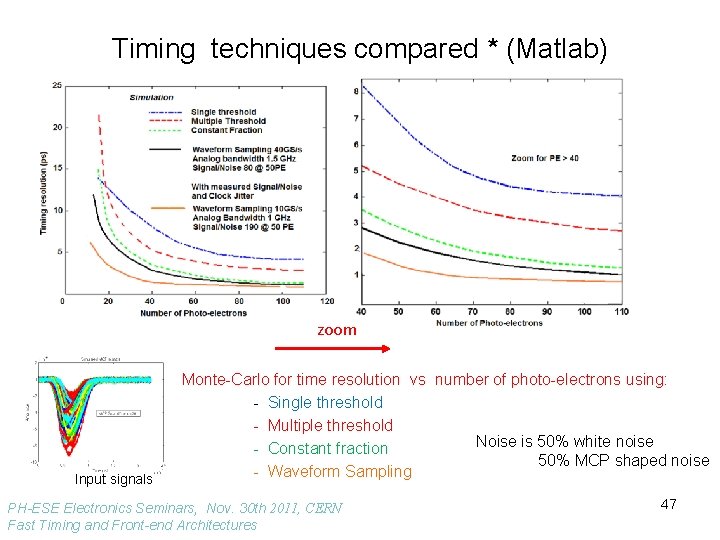
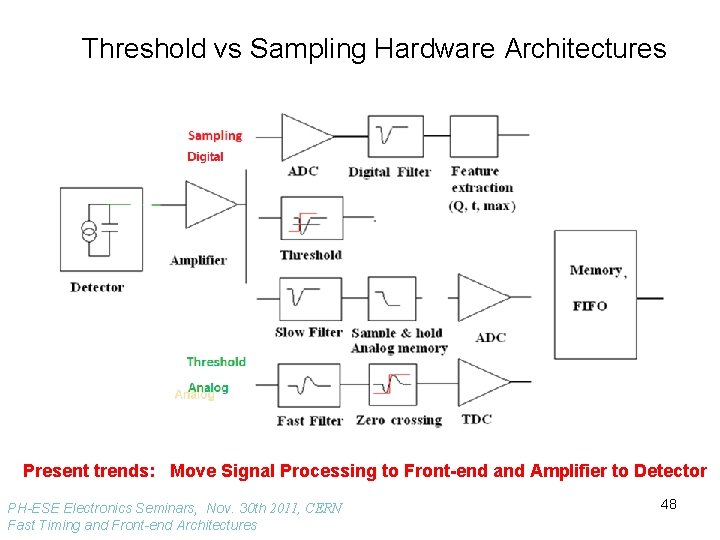
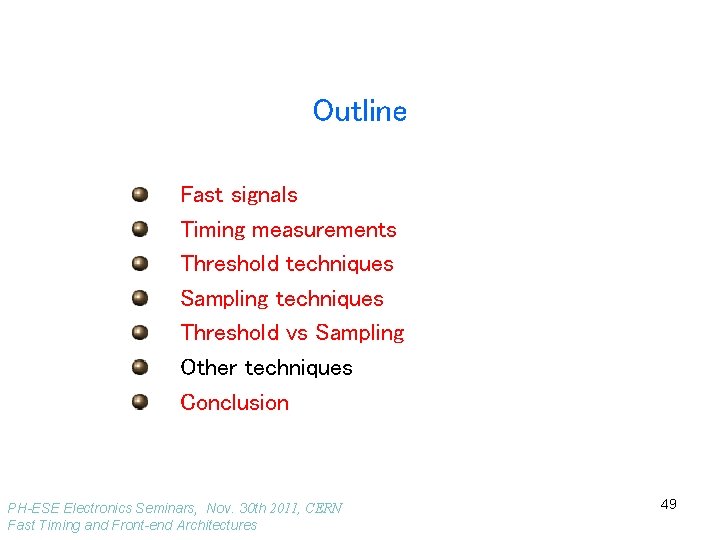
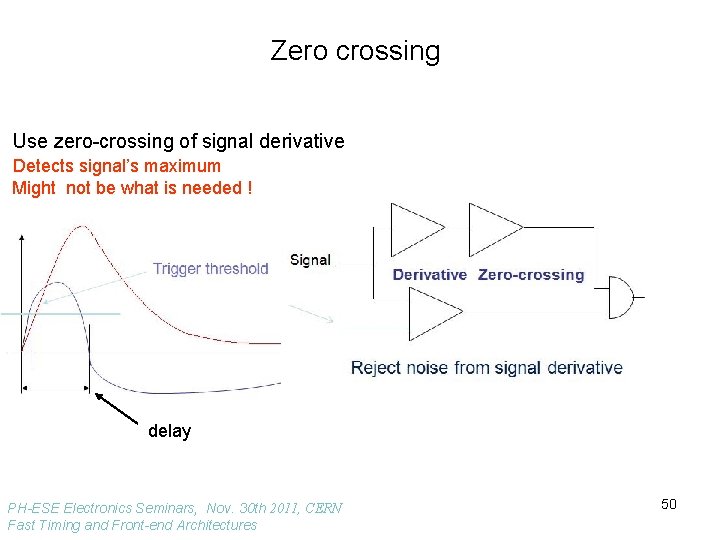
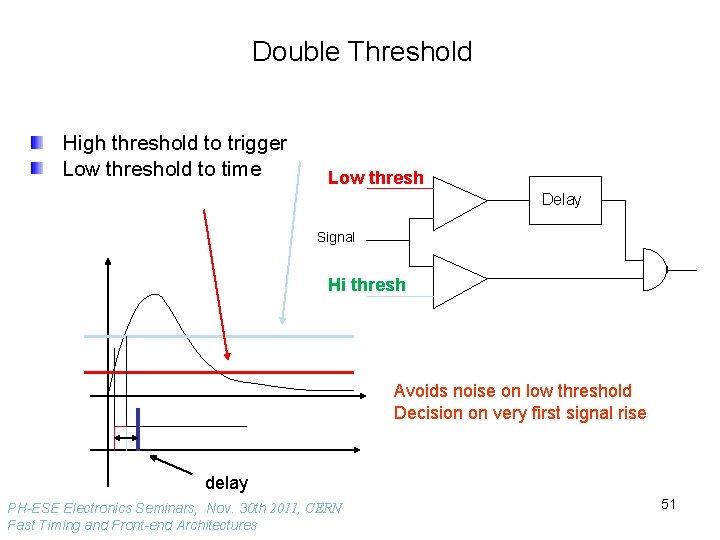
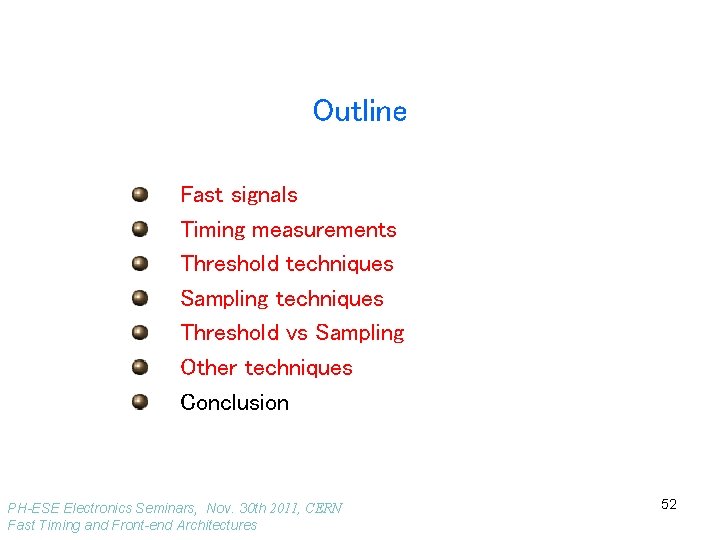
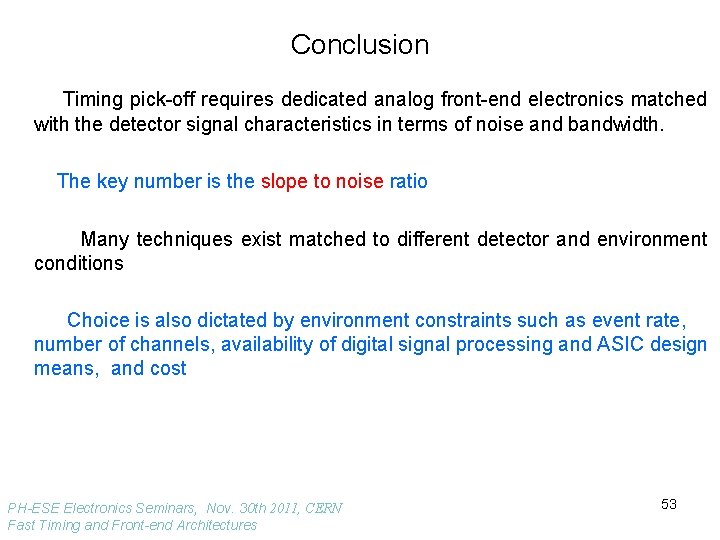
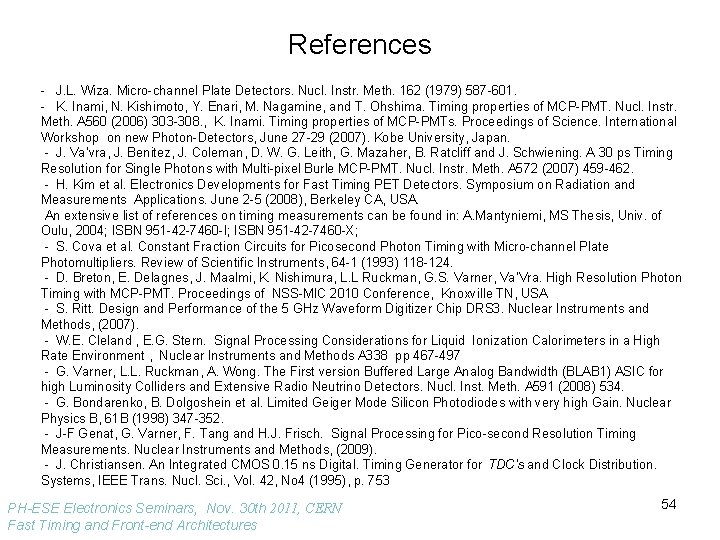
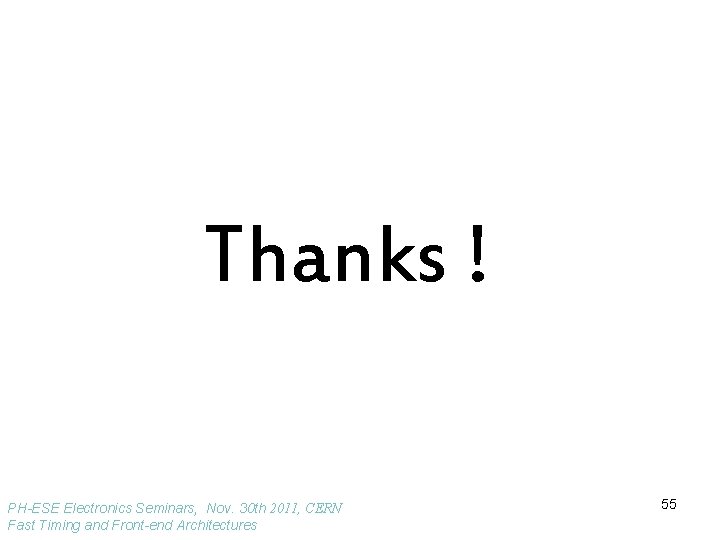
- Slides: 55
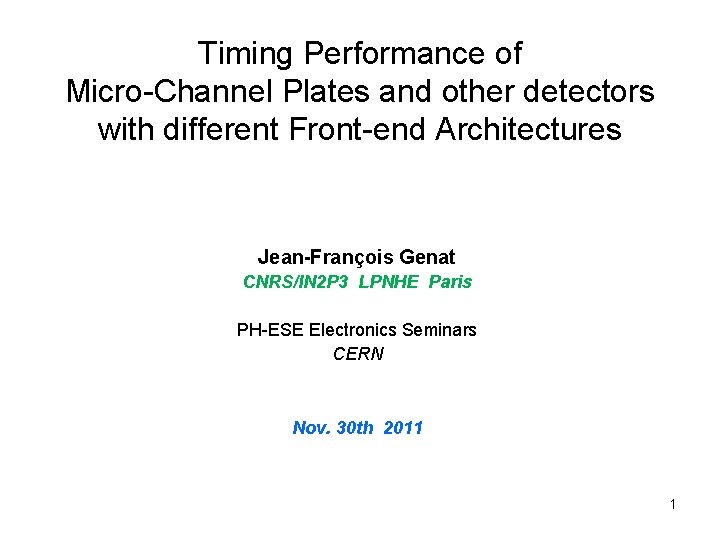
Timing Performance of Micro-Channel Plates and other detectors with different Front-end Architectures Jean-François Genat CNRS/IN 2 P 3 LPNHE Paris PH-ESE Electronics Seminars CERN Nov. 30 th 2011 1
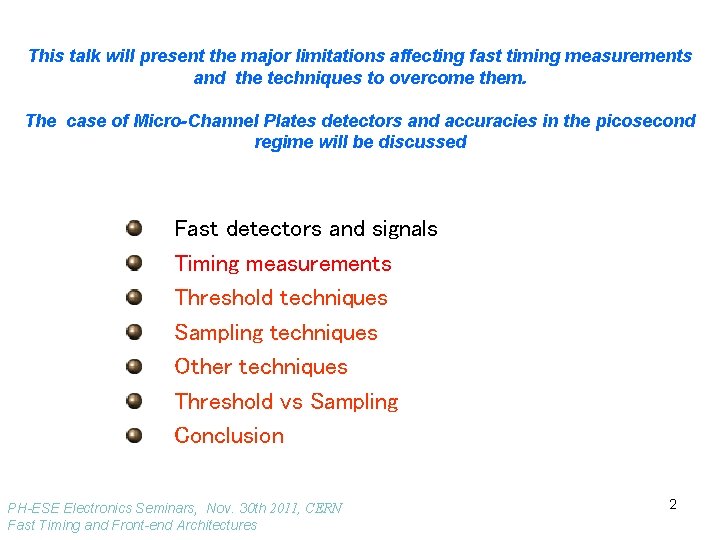
This talk will present the major limitations affecting fast timing measurements and the techniques to overcome them. The case of Micro-Channel Plates detectors and accuracies in the picosecond regime will be discussed Fast detectors and signals Timing measurements Threshold techniques Sampling techniques Other techniques Threshold vs Sampling Conclusion PH-ESE Electronics Seminars, Nov. 30 th 2011, CERN Fast Timing and Front-end Architectures 2
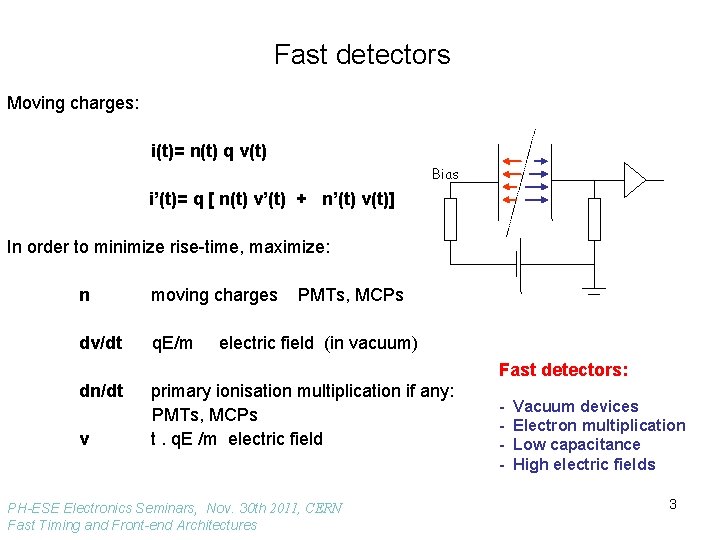
Fast detectors Moving charges: i(t)= n(t) q v(t) Bias i’(t)= q [ n(t) v’(t) + n’(t) v(t)] In order to minimize rise-time, maximize: n moving charges dv/dt q. E/m PMTs, MCPs electric field (in vacuum) Fast detectors: dn/dt v primary ionisation multiplication if any: PMTs, MCPs t. q. E /m electric field PH-ESE Electronics Seminars, Nov. 30 th 2011, CERN Fast Timing and Front-end Architectures - Vacuum devices Electron multiplication Low capacitance High electric fields 3
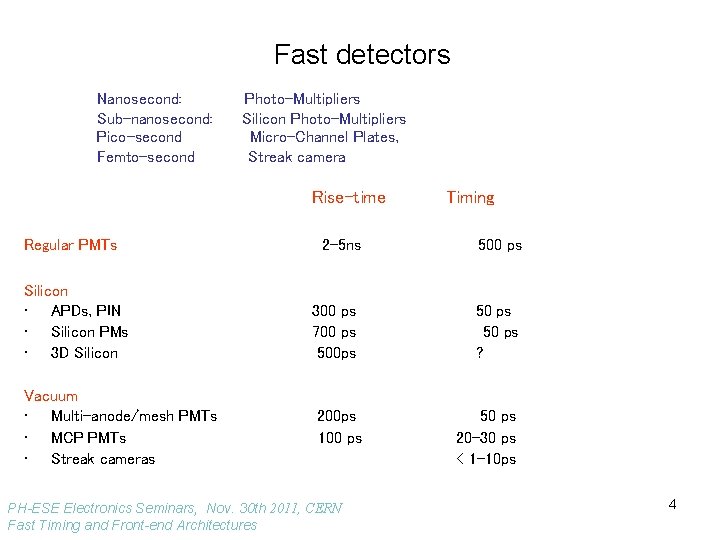
Fast detectors Nanosecond: Sub-nanosecond: Pico-second Femto-second Photo-Multipliers Silicon Photo-Multipliers Micro-Channel Plates, Streak camera Rise-time Regular PMTs Silicon • APDs, PIN • Silicon PMs • 3 D Silicon Vacuum • Multi-anode/mesh PMTs • MCP PMTs • Streak cameras 2 -5 ns 300 ps 700 ps 500 ps 200 ps 100 ps PH-ESE Electronics Seminars, Nov. 30 th 2011, CERN Fast Timing and Front-end Architectures Timing 500 ps 50 ps ? 50 ps 20 -30 ps < 1 -10 ps 4
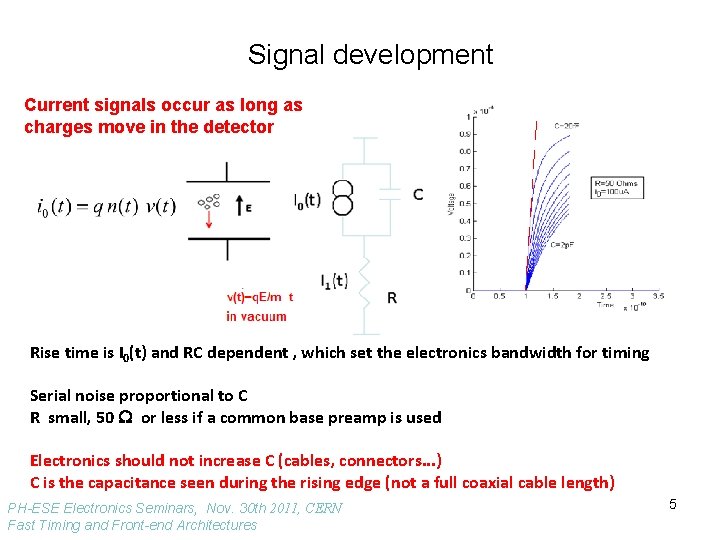
Signal development Current signals occur as long as charges move in the detector Rise time is I 0(t) and RC dependent , which set the electronics bandwidth for timing Serial noise proportional to C R small, 50 W or less if a common base preamp is used Electronics should not increase C (cables, connectors. . . ) C is the capacitance seen during the rising edge (not a full coaxial cable length) PH-ESE Electronics Seminars, Nov. 30 th 2011, CERN Fast Timing and Front-end Architectures 5
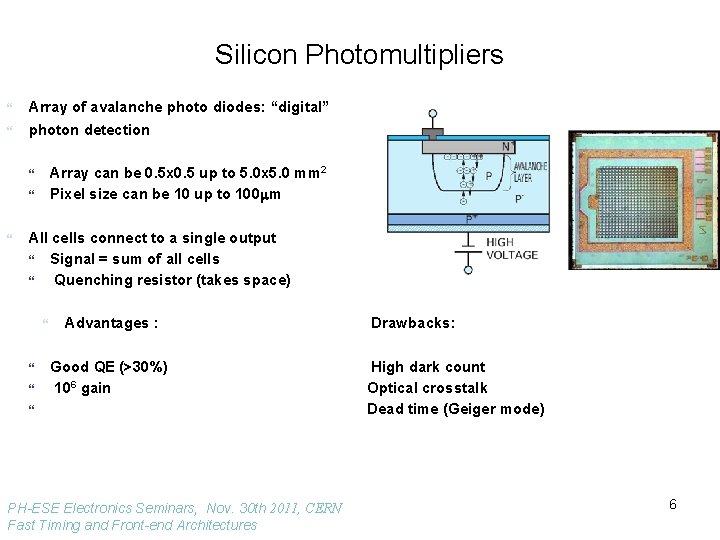
Silicon Photomultipliers Array of avalanche photo diodes: “digital” photon detection Array can be 0. 5 x 0. 5 up to 5. 0 x 5. 0 mm 2 Pixel size can be 10 up to 100 mm All cells connect to a single output Signal = sum of all cells Quenching resistor (takes space) Advantages : Good QE (>30%) 106 gain PH-ESE Electronics Seminars, Nov. 30 th 2011, CERN Fast Timing and Front-end Architectures Drawbacks: High dark count Optical crosstalk Dead time (Geiger mode) 6
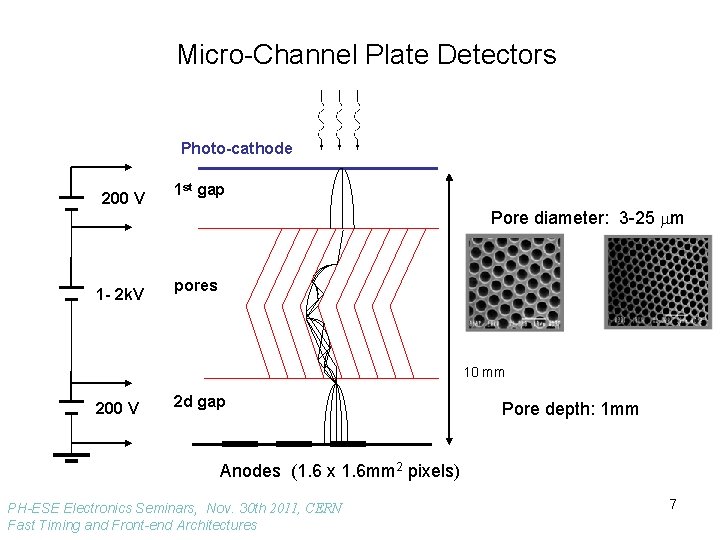
Micro-Channel Plate Detectors Photo-cathode 200 V 1 - 2 k. V 1 st gap Pore diameter: 3 -25 m pores 10 mm 200 V 2 d gap Pore depth: 1 mm Anodes (1. 6 x 1. 6 mm 2 pixels) PH-ESE Electronics Seminars, Nov. 30 th 2011, CERN Fast Timing and Front-end Architectures 7
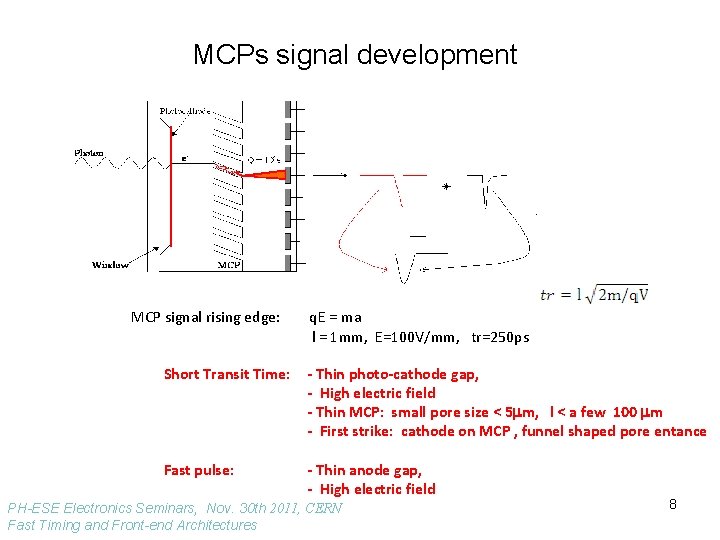
MCPs signal development MCP signal rising edge: q. E = ma l = 1 mm, E=100 V/mm, tr=250 ps Short Transit Time: - Thin photo-cathode gap, - High electric field - Thin MCP: small pore size < 5 mm, l < a few 100 mm - First strike: cathode on MCP , funnel shaped pore entance Fast pulse: - Thin anode gap, - High electric field PH-ESE Electronics Seminars, Nov. 30 th 2011, CERN Fast Timing and Front-end Architectures 8
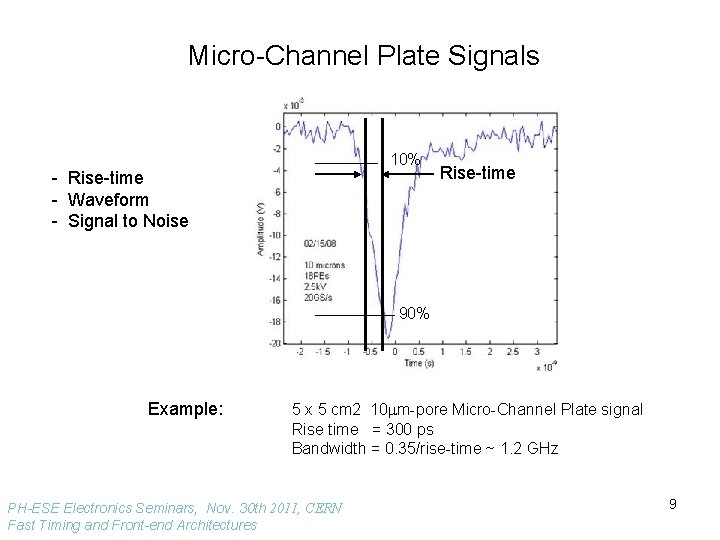
Micro-Channel Plate Signals 10% - Rise-time - Waveform - Signal to Noise Rise-time 90% Example: 5 x 5 cm 2 10 m-pore Micro-Channel Plate signal Rise time = 300 ps Bandwidth = 0. 35/rise-time ~ 1. 2 GHz PH-ESE Electronics Seminars, Nov. 30 th 2011, CERN Fast Timing and Front-end Architectures 9
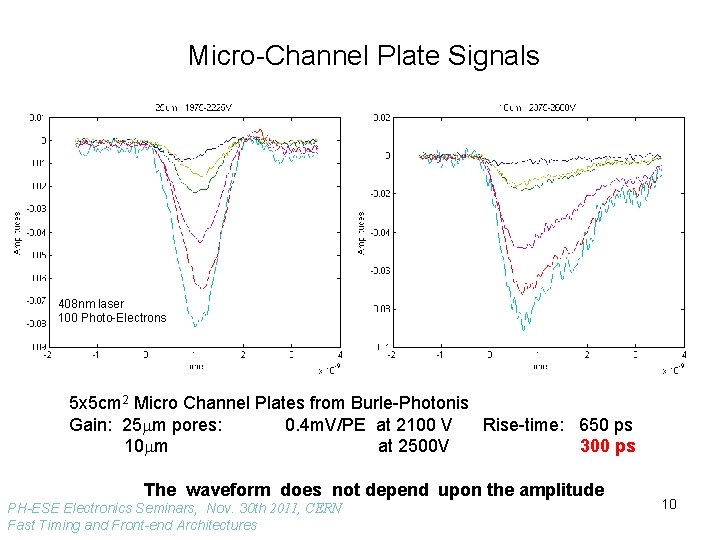
Micro-Channel Plate Signals 408 nm laser 100 Photo-Electrons 5 x 5 cm 2 Micro Channel Plates from Burle-Photonis Gain: 25 m pores: 0. 4 m. V/PE at 2100 V Rise-time: 650 ps 10 m at 2500 V 300 ps The waveform does not depend upon the amplitude PH-ESE Electronics Seminars, Nov. 30 th 2011, CERN Fast Timing and Front-end Architectures 10
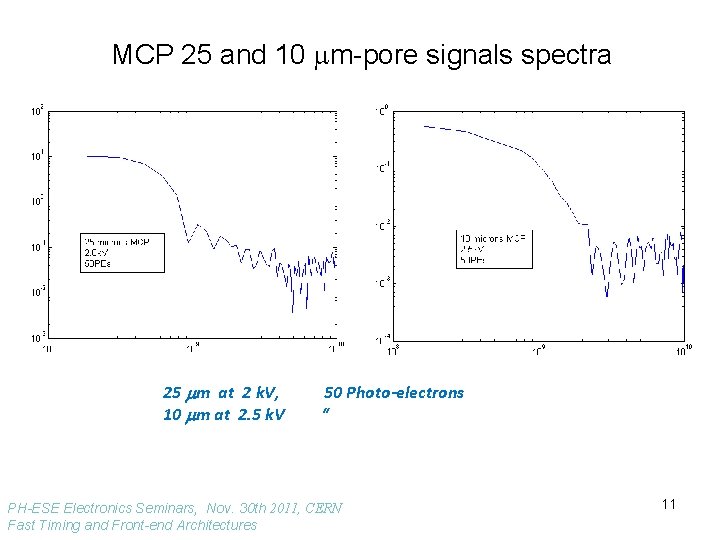
MCP 25 and 10 m-pore signals spectra 25 mm at 2 k. V, 10 mm at 2. 5 k. V 50 Photo-electrons “ PH-ESE Electronics Seminars, Nov. 30 th 2011, CERN Fast Timing and Front-end Architectures 11
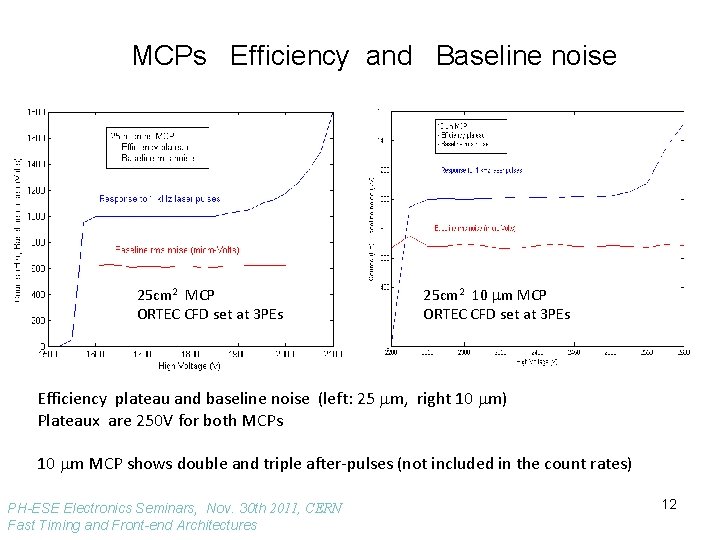
MCPs Efficiency and Baseline noise 25 cm 2 MCP ORTEC CFD set at 3 PEs 25 cm 2 10 m MCP ORTEC CFD set at 3 PEs Efficiency plateau and baseline noise (left: 25 m, right 10 m) Plateaux are 250 V for both MCPs 10 m MCP shows double and triple after-pulses (not included in the count rates) PH-ESE Electronics Seminars, Nov. 30 th 2011, CERN Fast Timing and Front-end Architectures 12
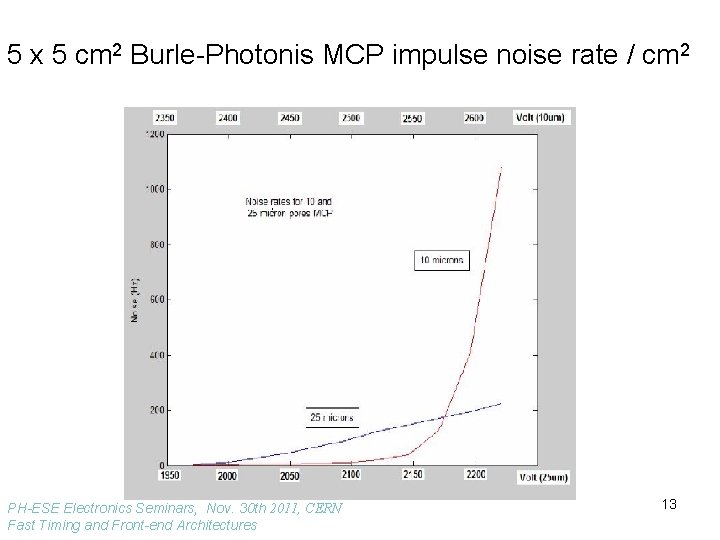
MCP 5 x 5 cm 2 Burle-Photonis MCP impulse noise rate / cm 2 PH-ESE Electronics Seminars, Nov. 30 th 2011, CERN Fast Timing and Front-end Architectures 13
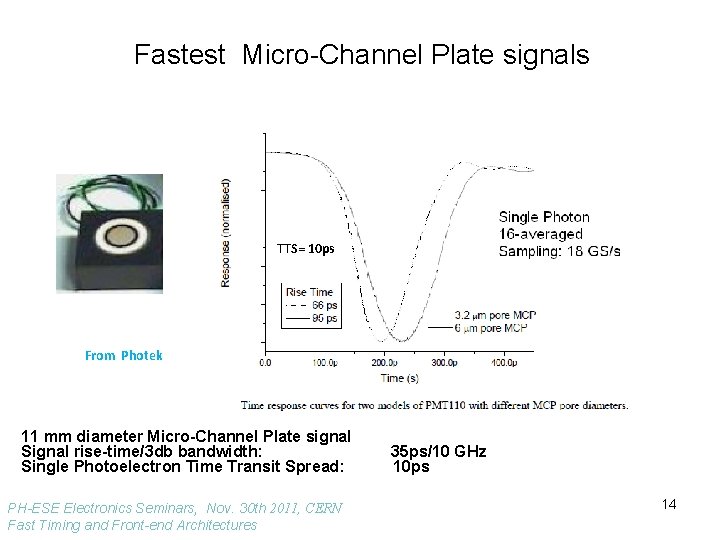
Fastest Micro-Channel Plate signals TTS= 10 ps From Photek 11 mm diameter Micro-Channel Plate signal Signal rise-time/3 db bandwidth: Single Photoelectron Time Transit Spread: PH-ESE Electronics Seminars, Nov. 30 th 2011, CERN Fast Timing and Front-end Architectures 35 ps/10 GHz 10 ps 14
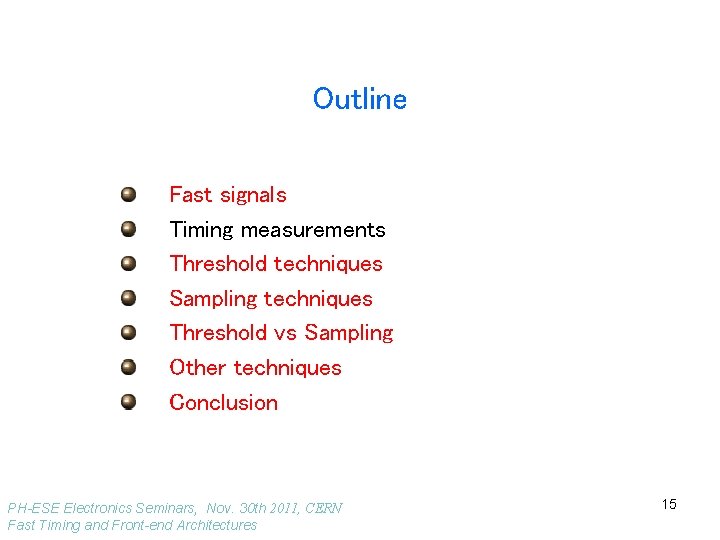
Outline Fast signals Timing measurements Threshold techniques Sampling techniques Threshold vs Sampling Other techniques Conclusion PH-ESE Electronics Seminars, Nov. 30 th 2011, CERN Fast Timing and Front-end Architectures 15
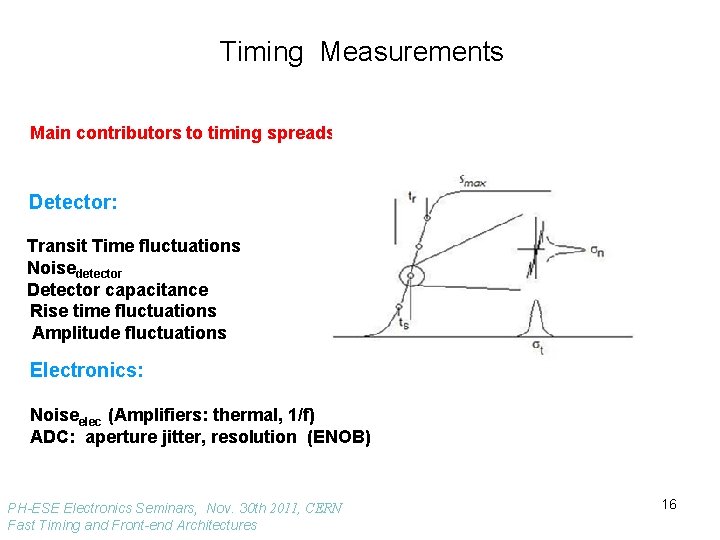
Timing Measurements Main contributors to timing spreads: Detector: Transit Time fluctuations Noisedetector Detector capacitance Rise time fluctuations Amplitude fluctuations Electronics: Noiseelec (Amplifiers: thermal, 1/f) ADC: aperture jitter, resolution (ENOB) PH-ESE Electronics Seminars, Nov. 30 th 2011, CERN Fast Timing and Front-end Architectures 16
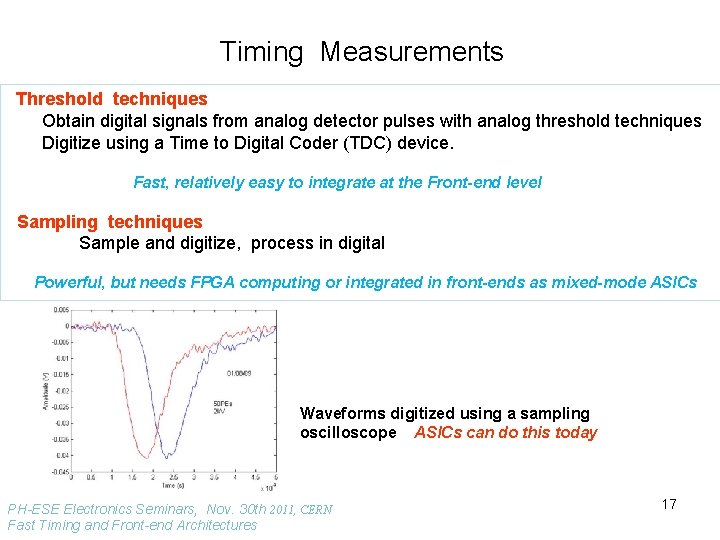
Timing Measurements Threshold techniques Obtain digital signals from analog detector pulses with analog threshold techniques Digitize using a Time to Digital Coder (TDC) device. Fast, relatively easy to integrate at the Front-end level Sampling techniques Sample and digitize, process in digital Powerful, but needs FPGA computing or integrated in front-ends as mixed-mode ASICs Waveforms digitized using a sampling oscilloscope ASICs can do this today PH-ESE Electronics Seminars, Nov. 30 th 2011, CERN Fast Timing and Front-end Architectures 17
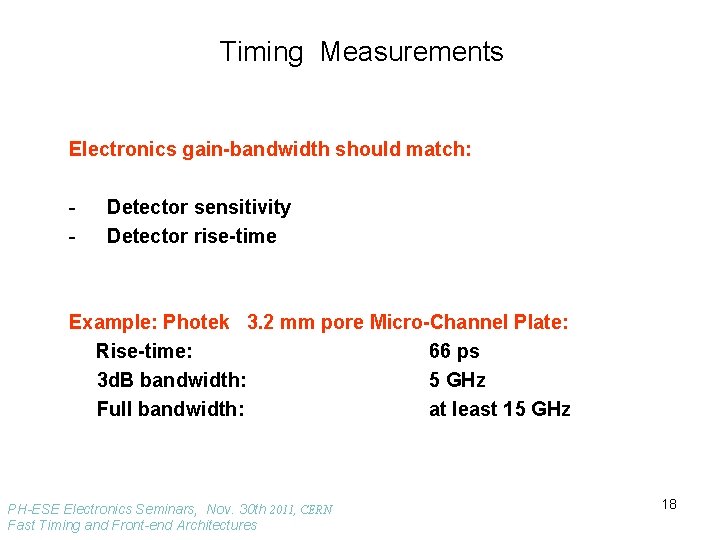
Timing Measurements Electronics gain-bandwidth should match: - Detector sensitivity Detector rise-time Example: Photek 3. 2 mm pore Micro-Channel Plate: Rise-time: 66 ps 3 d. B bandwidth: 5 GHz Full bandwidth: at least 15 GHz PH-ESE Electronics Seminars, Nov. 30 th 2011, CERN Fast Timing and Front-end Architectures 18
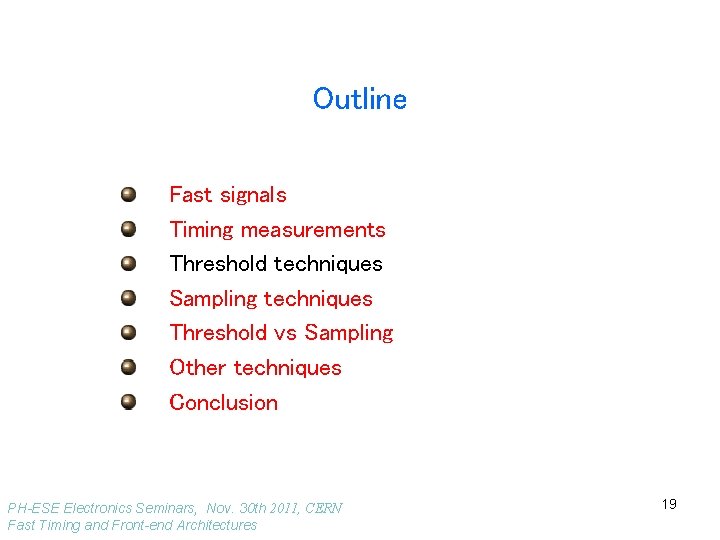
Outline Fast signals Timing measurements Threshold techniques Sampling techniques Threshold vs Sampling Other techniques Conclusion PH-ESE Electronics Seminars, Nov. 30 th 2011, CERN Fast Timing and Front-end Architectures 19
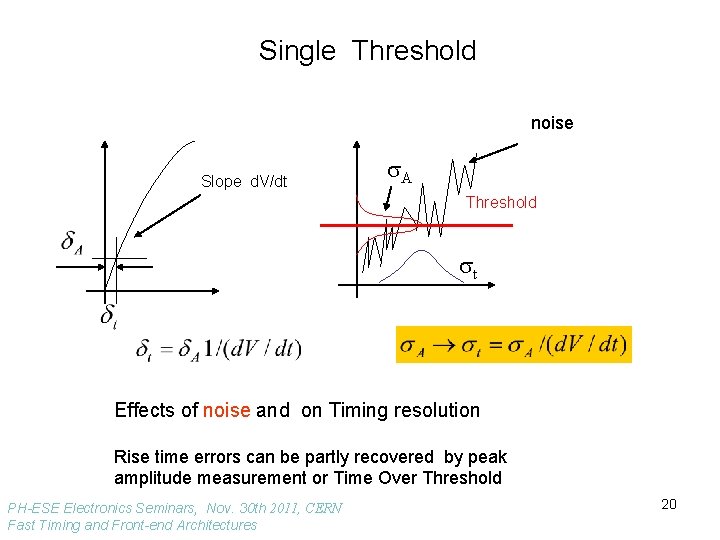
Single Threshold noise Slope d. V/dt s. A Threshold st Effects of noise and on Timing resolution Rise time errors can be partly recovered by peak amplitude measurement or Time Over Threshold PH-ESE Electronics Seminars, Nov. 30 th 2011, CERN Fast Timing and Front-end Architectures 20
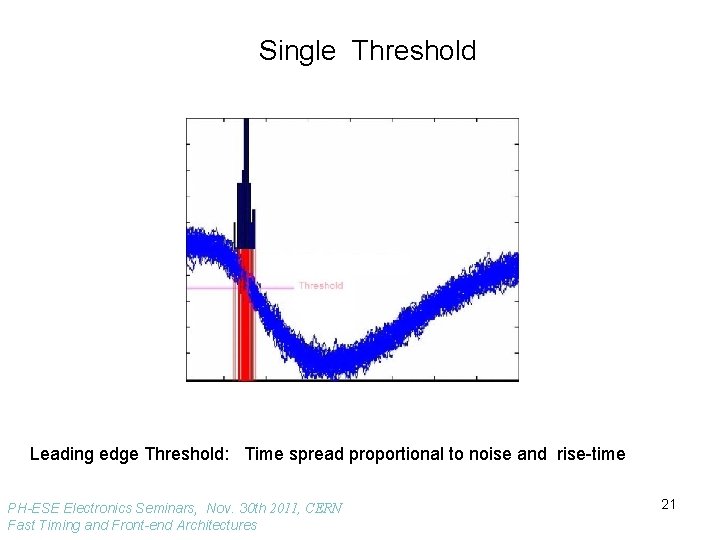
Single Threshold Leading edge Threshold: Time spread proportional to noise and rise-time PH-ESE Electronics Seminars, Nov. 30 th 2011, CERN Fast Timing and Front-end Architectures 21
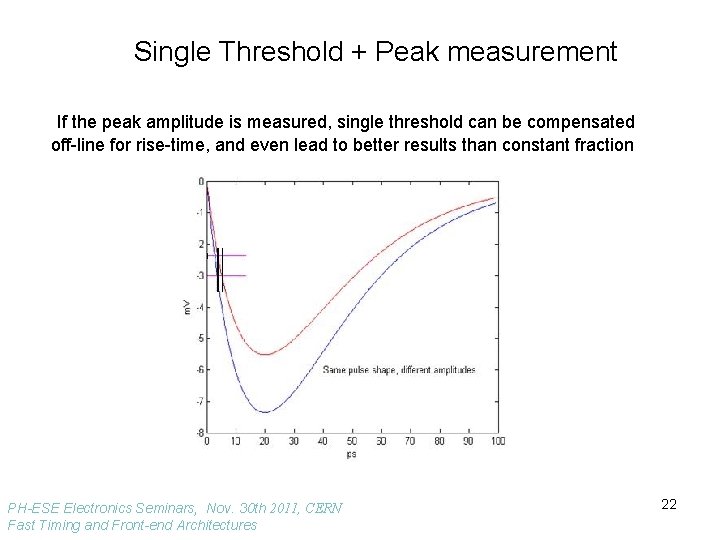
Single Threshold + Peak measurement If the peak amplitude is measured, single threshold can be compensated off-line for rise-time, and even lead to better results than constant fraction PH-ESE Electronics Seminars, Nov. 30 th 2011, CERN Fast Timing and Front-end Architectures 22
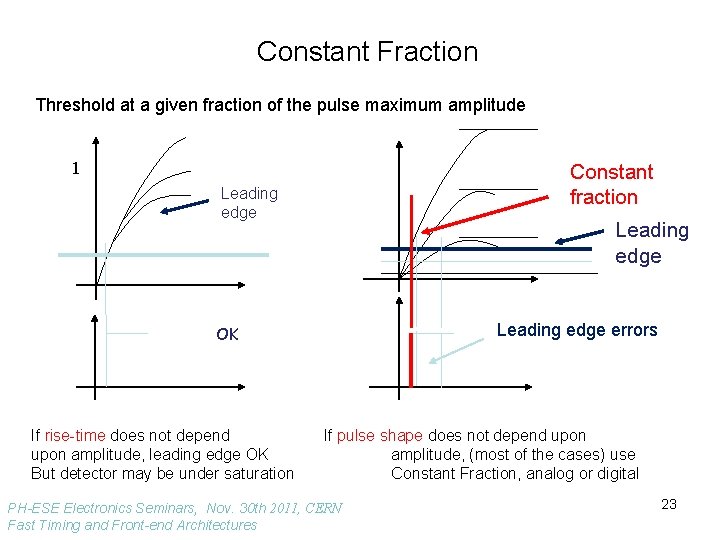
Constant Fraction Threshold at a given fraction of the pulse maximum amplitude 1 Constant fraction Leading edge errors OK If rise-time does not depend upon amplitude, leading edge OK But detector may be under saturation If pulse shape does not depend upon amplitude, (most of the cases) use Constant Fraction, analog or digital PH-ESE Electronics Seminars, Nov. 30 th 2011, CERN Fast Timing and Front-end Architectures 23
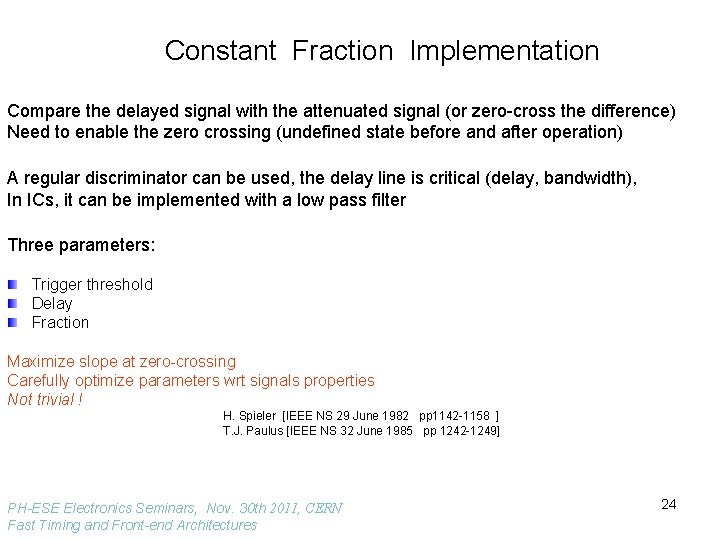
Constant Fraction Implementation Compare the delayed signal with the attenuated signal (or zero-cross the difference) Need to enable the zero crossing (undefined state before and after operation) A regular discriminator can be used, the delay line is critical (delay, bandwidth), In ICs, it can be implemented with a low pass filter Three parameters: Trigger threshold Delay Fraction Maximize slope at zero-crossing Carefully optimize parameters wrt signals properties Not trivial ! H. Spieler [IEEE NS 29 June 1982 pp 1142 -1158 ] T. J. Paulus [IEEE NS 32 June 1985 pp 1242 -1249] PH-ESE Electronics Seminars, Nov. 30 th 2011, CERN Fast Timing and Front-end Architectures 24
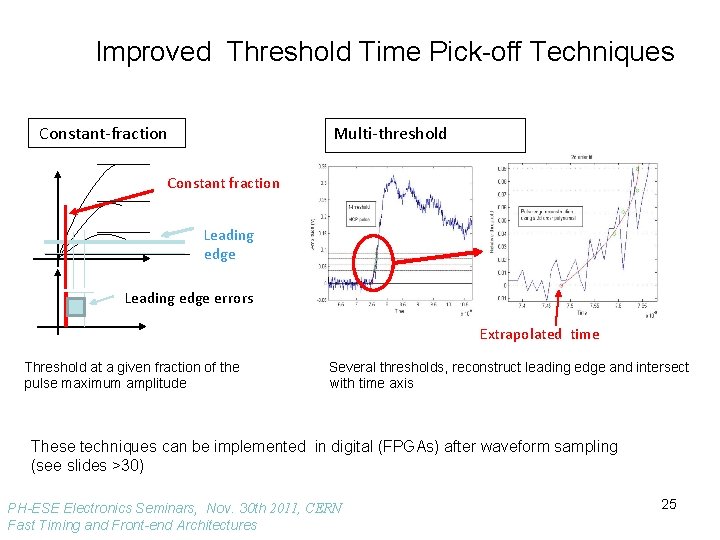
Improved Threshold Time Pick-off Techniques Constant-fraction Multi-threshold Constant fraction Leading edge errors Extrapolated time Threshold at a given fraction of the pulse maximum amplitude Several thresholds, reconstruct leading edge and intersect with time axis These techniques can be implemented in digital (FPGAs) after waveform sampling (see slides >30) PH-ESE Electronics Seminars, Nov. 30 th 2011, CERN Fast Timing and Front-end Architectures 25
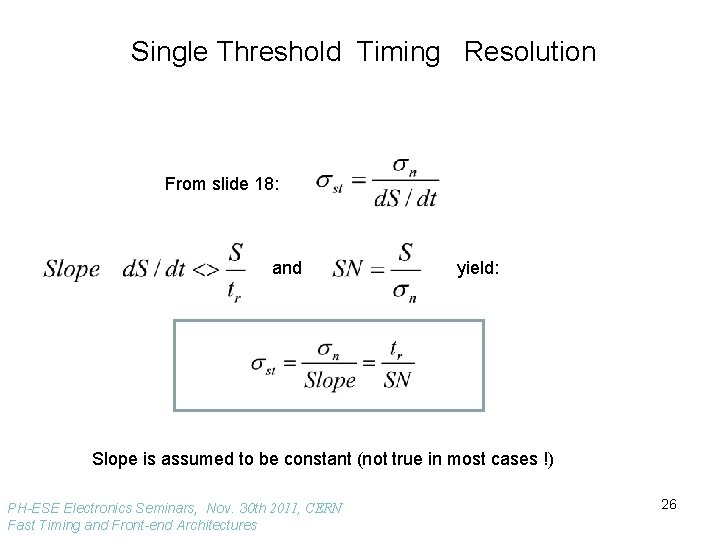
Single Threshold Timing Resolution From slide 18: and yield: Slope is assumed to be constant (not true in most cases !) PH-ESE Electronics Seminars, Nov. 30 th 2011, CERN Fast Timing and Front-end Architectures 26
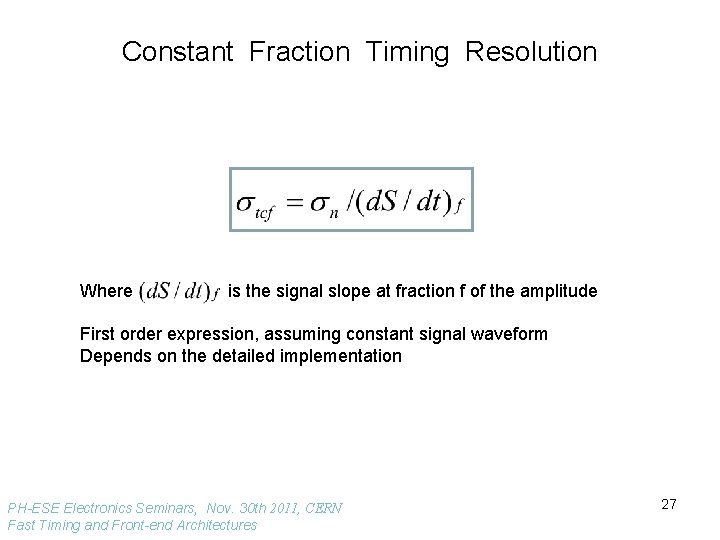
Constant Fraction Timing Resolution Where is the signal slope at fraction f of the amplitude First order expression, assuming constant signal waveform Depends on the detailed implementation PH-ESE Electronics Seminars, Nov. 30 th 2011, CERN Fast Timing and Front-end Architectures 27
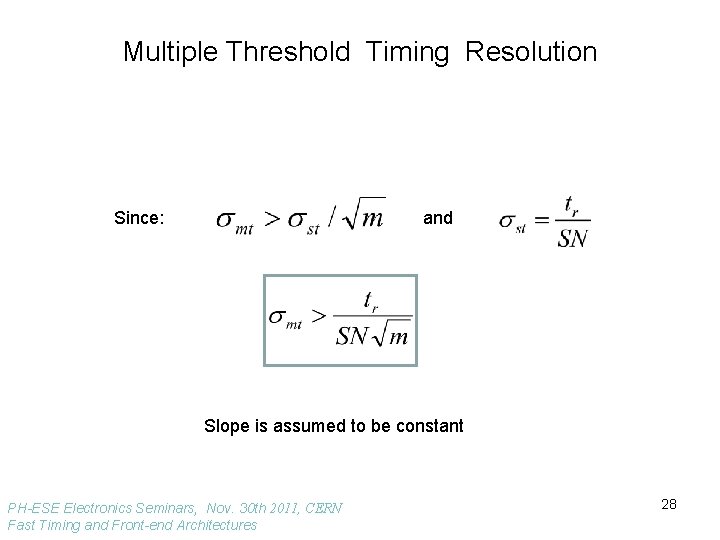
Multiple Threshold Timing Resolution Since: and Slope is assumed to be constant PH-ESE Electronics Seminars, Nov. 30 th 2011, CERN Fast Timing and Front-end Architectures 28
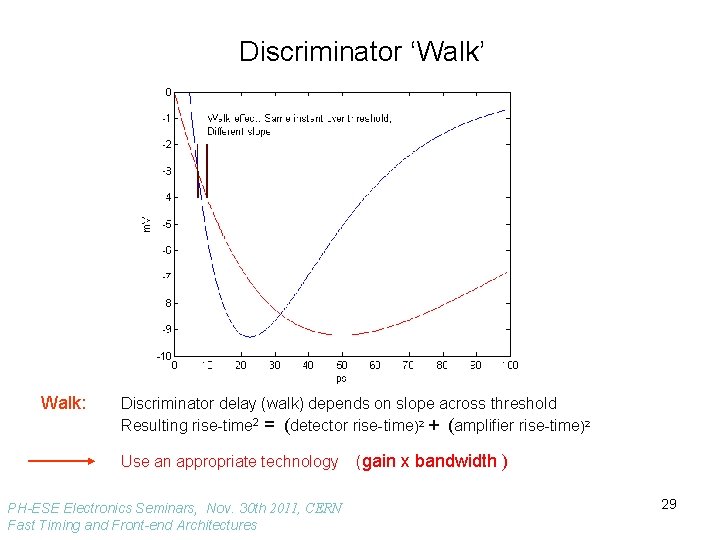
Discriminator ‘Walk’ Walk: Discriminator delay (walk) depends on slope across threshold Resulting rise-time 2 = (detector rise-time)2 + (amplifier rise-time)2 Use an appropriate technology PH-ESE Electronics Seminars, Nov. 30 th 2011, CERN Fast Timing and Front-end Architectures (gain x bandwidth ) 29
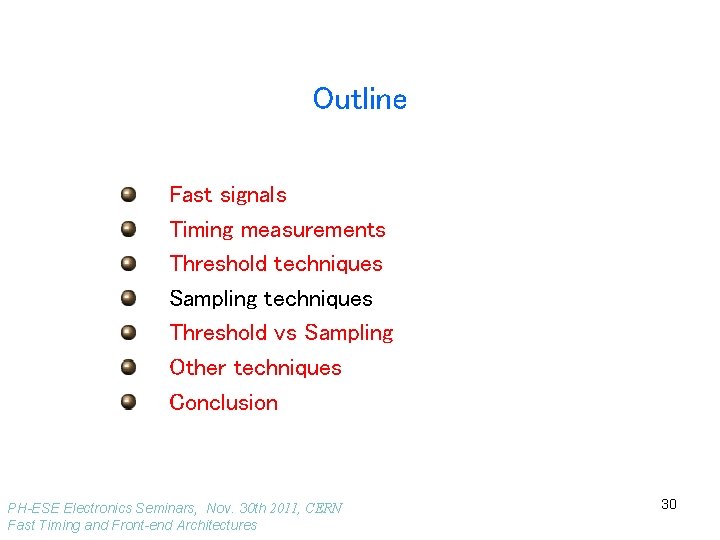
Outline Fast signals Timing measurements Threshold techniques Sampling techniques Threshold vs Sampling Other techniques Conclusion PH-ESE Electronics Seminars, Nov. 30 th 2011, CERN Fast Timing and Front-end Architectures 30
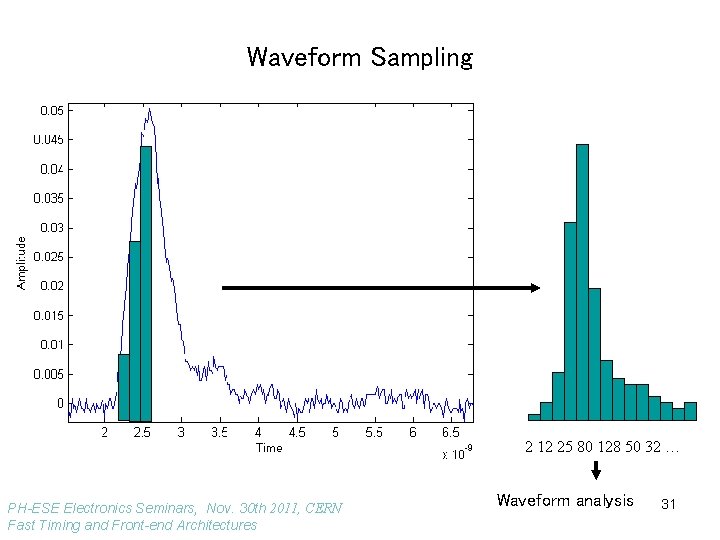
Waveform Sampling 2 12 25 80 128 50 32 … PH-ESE Electronics Seminars, Nov. 30 th 2011, CERN Fast Timing and Front-end Architectures Waveform analysis 31
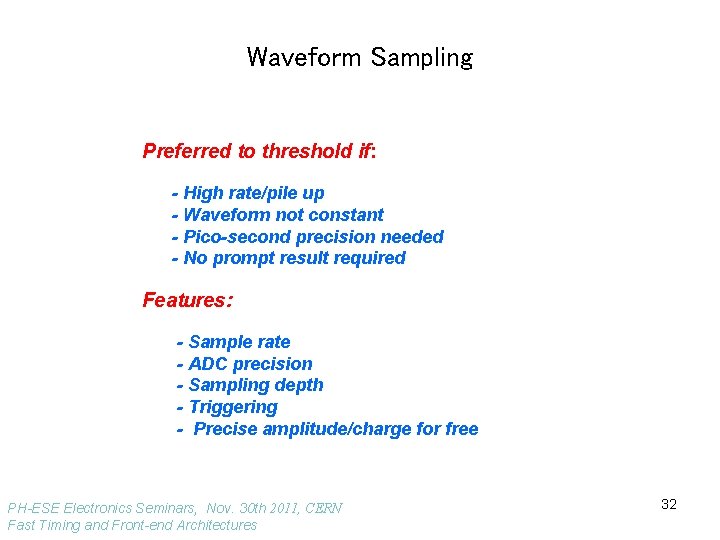
Waveform Sampling Preferred to threshold if: - High rate/pile up - Waveform not constant - Pico-second precision needed - No prompt result required Features: - Sample rate - ADC precision - Sampling depth - Triggering - Precise amplitude/charge for free PH-ESE Electronics Seminars, Nov. 30 th 2011, CERN Fast Timing and Front-end Architectures 32
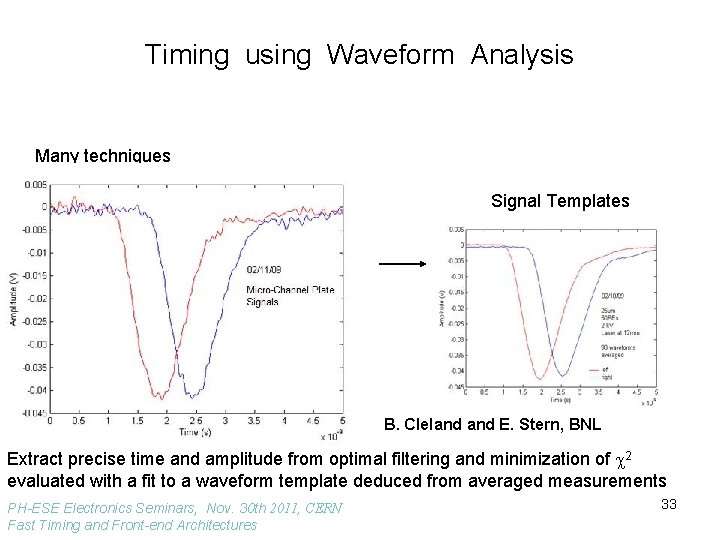
Timing using Waveform Analysis Many techniques Signal Templates Real MCP Laser data B. Cleland E. Stern, BNL Extract precise time and amplitude from optimal filtering and minimization of c 2 evaluated with a fit to a waveform template deduced from averaged measurements PH-ESE Electronics Seminars, Nov. 30 th 2011, CERN Fast Timing and Front-end Architectures 33
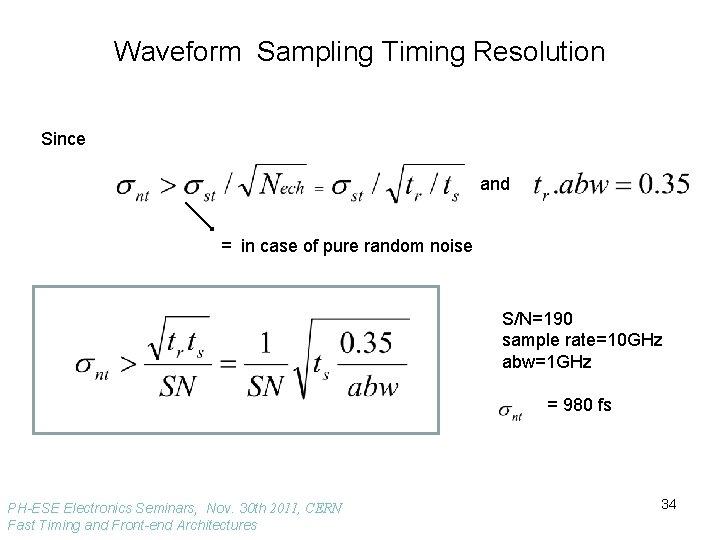
Waveform Sampling Timing Resolution Since and = in case of pure random noise S/N=190 sample rate=10 GHz abw=1 GHz = 980 fs PH-ESE Electronics Seminars, Nov. 30 th 2011, CERN Fast Timing and Front-end Architectures 34
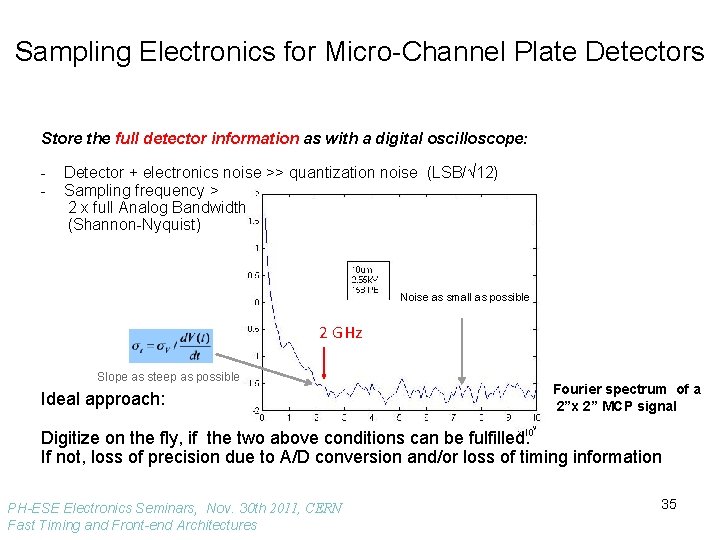
Sampling Electronics for Micro-Channel Plate Detectors Store the full detector information as with a digital oscilloscope: - Detector + electronics noise >> quantization noise (LSB/√ 12) Sampling frequency > 2 x full Analog Bandwidth (Shannon-Nyquist) Noise as small as possible 2 GHz Slope as steep as possible Ideal approach: Fourier spectrum of a 2”x 2” MCP signal Digitize on the fly, if the two above conditions can be fulfilled. If not, loss of precision due to A/D conversion and/or loss of timing information PH-ESE Electronics Seminars, Nov. 30 th 2011, CERN Fast Timing and Front-end Architectures 35
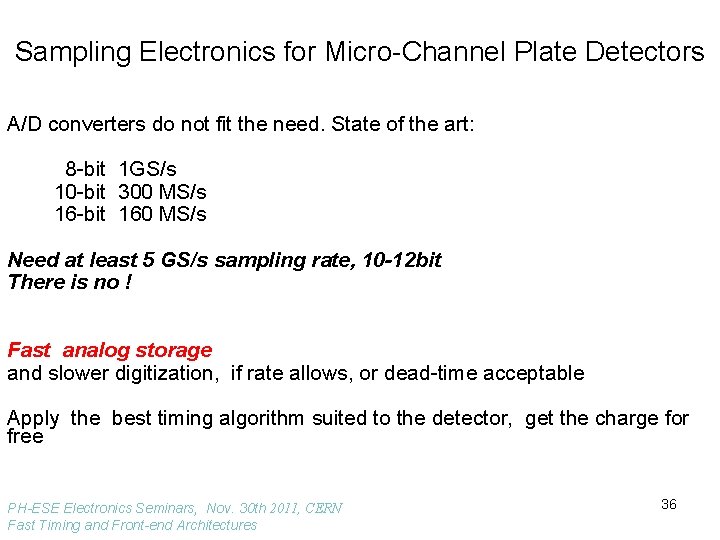
Sampling Electronics for Micro-Channel Plate Detectors A/D converters do not fit the need. State of the art: 8 -bit 1 GS/s 10 -bit 300 MS/s 16 -bit 160 MS/s Need at least 5 GS/s sampling rate, 10 -12 bit There is no ! Fast analog storage and slower digitization, if rate allows, or dead-time acceptable Apply the best timing algorithm suited to the detector, get the charge for free PH-ESE Electronics Seminars, Nov. 30 th 2011, CERN Fast Timing and Front-end Architectures 36
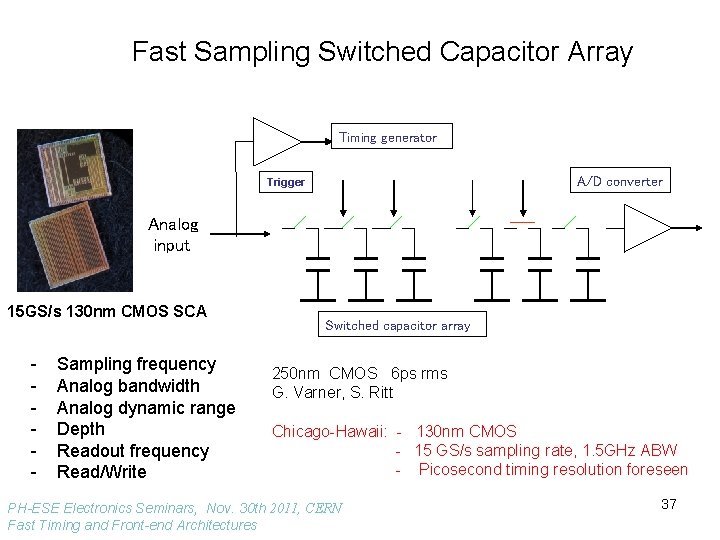
Fast Sampling Switched Capacitor Array Timing generator A/D converter Trigger Analog input 15 GS/s 130 nm CMOS SCA - Sampling frequency Analog bandwidth Analog dynamic range Depth Readout frequency Read/Write Switched capacitor array 250 nm CMOS 6 ps rms G. Varner, S. Ritt Chicago-Hawaii: - 130 nm CMOS - 15 GS/s sampling rate, 1. 5 GHz ABW - Picosecond timing resolution foreseen PH-ESE Electronics Seminars, Nov. 30 th 2011, CERN Fast Timing and Front-end Architectures 37
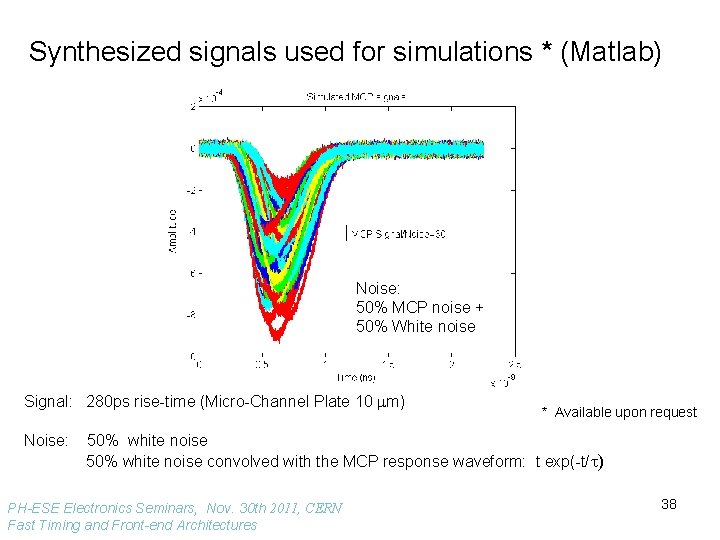
Synthesized signals used for simulations * (Matlab) Noise: 50% MCP noise + 50% White noise Signal: 280 ps rise-time (Micro-Channel Plate 10 m) Noise: * Available upon request 50% white noise convolved with the MCP response waveform: t exp(-t/t) PH-ESE Electronics Seminars, Nov. 30 th 2011, CERN Fast Timing and Front-end Architectures 38
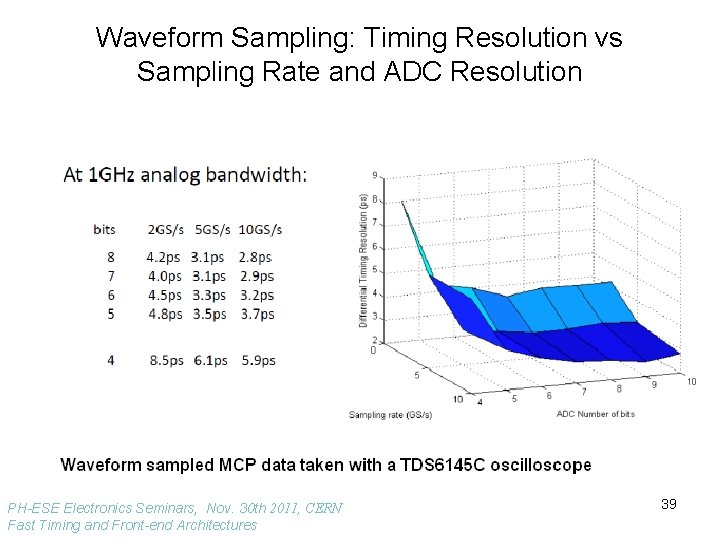
Waveform Sampling: Timing Resolution vs Sampling Rate and ADC Resolution PH-ESE Electronics Seminars, Nov. 30 th 2011, CERN Fast Timing and Front-end Architectures 39
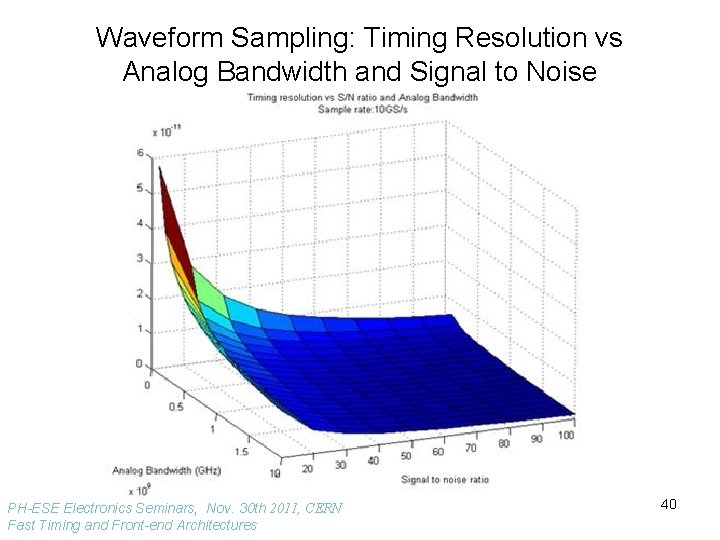
Waveform Sampling: Timing Resolution vs Analog Bandwidth and Signal to Noise PH-ESE Electronics Seminars, Nov. 30 th 2011, CERN Fast Timing and Front-end Architectures 40
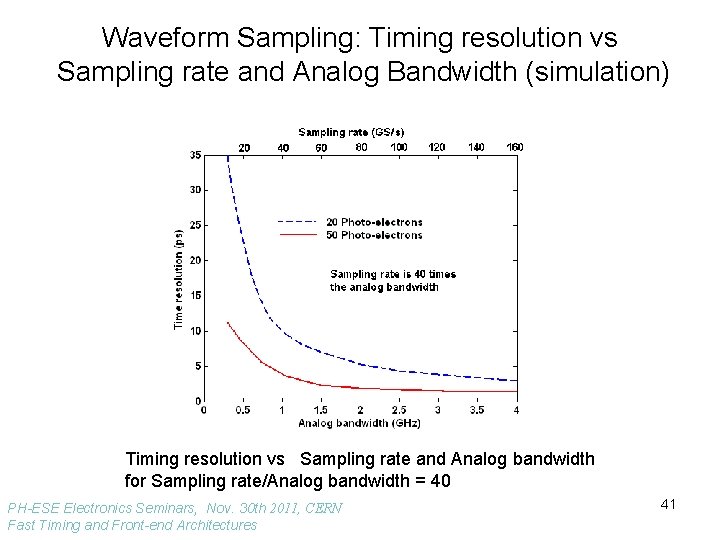
Waveform Sampling: Timing resolution vs Sampling rate and Analog Bandwidth (simulation) Timing resolution vs Sampling rate and Analog bandwidth for Sampling rate/Analog bandwidth = 40 PH-ESE Electronics Seminars, Nov. 30 th 2011, CERN Fast Timing and Front-end Architectures 41
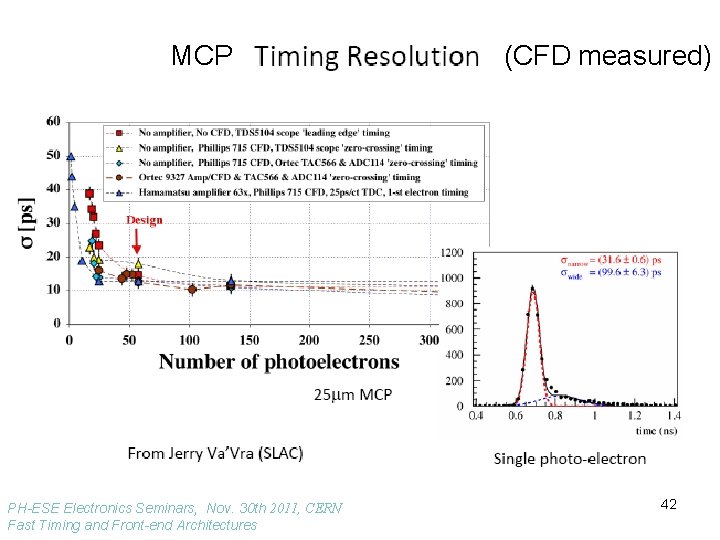
MCP Walk: (CFD measured) Discriminator delay (walk) depends on slope across threshold Resulting rise-time 2 = (detector rise-time)2 + (amplifier rise-time)2 Use an appropriate technology PH-ESE Electronics Seminars, Nov. 30 th 2011, CERN Fast Timing and Front-end Architectures (gain x bandwidth ) 42
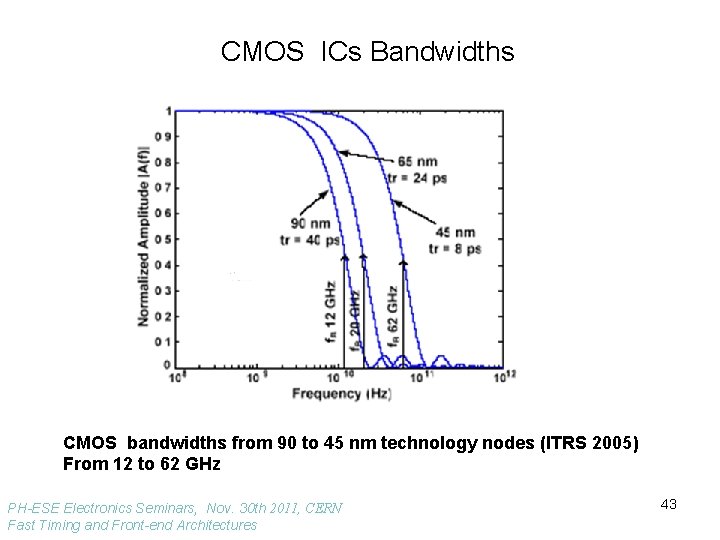
CMOS ICs Bandwidths CMOS bandwidths from 90 to 45 nm technology nodes (ITRS 2005) From 12 to 62 GHz PH-ESE Electronics Seminars, Nov. 30 th 2011, CERN Fast Timing and Front-end Architectures 43
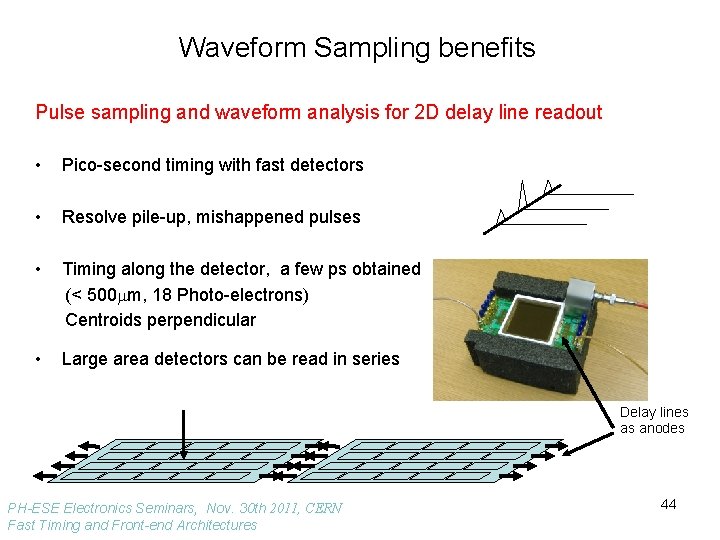
Waveform Sampling benefits Pulse sampling and waveform analysis for 2 D delay line readout • Pico-second timing with fast detectors • Resolve pile-up, mishappened pulses • Timing along the detector, a few ps obtained (< 500 m, 18 Photo-electrons) Centroids perpendicular • Large area detectors can be read in series Delay lines as anodes PH-ESE Electronics Seminars, Nov. 30 th 2011, CERN Fast Timing and Front-end Architectures 44
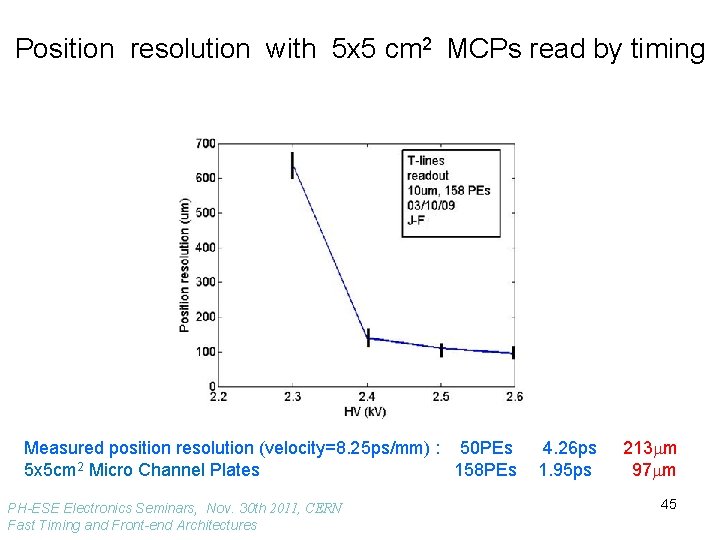
Position resolution with 5 x 5 cm 2 MCPs read by timing Measured position resolution (velocity=8. 25 ps/mm) : 50 PEs 5 x 5 cm 2 Micro Channel Plates 158 PEs PH-ESE Electronics Seminars, Nov. 30 th 2011, CERN Fast Timing and Front-end Architectures 4. 26 ps 1. 95 ps 213 m 97 m 45
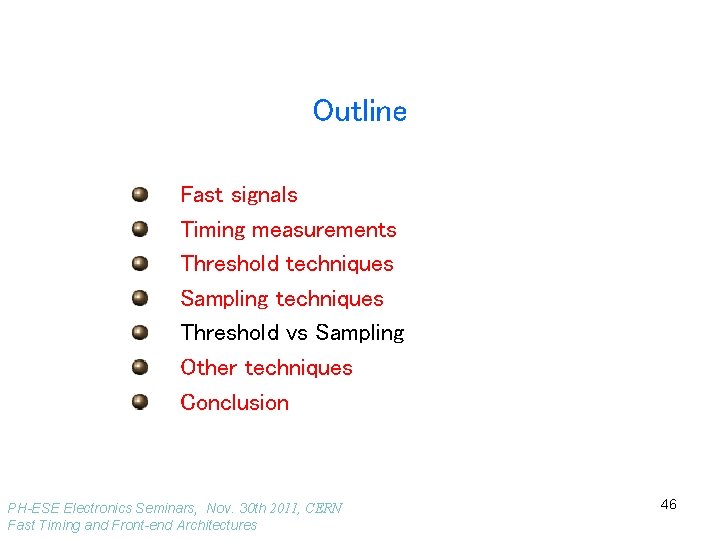
Outline Fast signals Timing measurements Threshold techniques Sampling techniques Threshold vs Sampling Other techniques Conclusion PH-ESE Electronics Seminars, Nov. 30 th 2011, CERN Fast Timing and Front-end Architectures 46
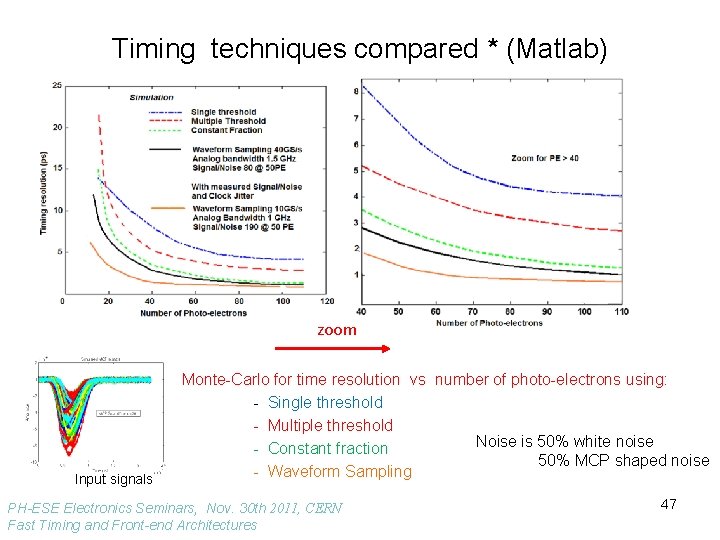
Timing techniques compared * (Matlab) zoom Input signals Monte-Carlo for time resolution vs number of photo-electrons using: - Single threshold - Multiple threshold Noise is 50% white noise - Constant fraction 50% MCP shaped noise - Waveform Sampling PH-ESE Electronics Seminars, Nov. 30 th 2011, CERN Fast Timing and Front-end Architectures 47
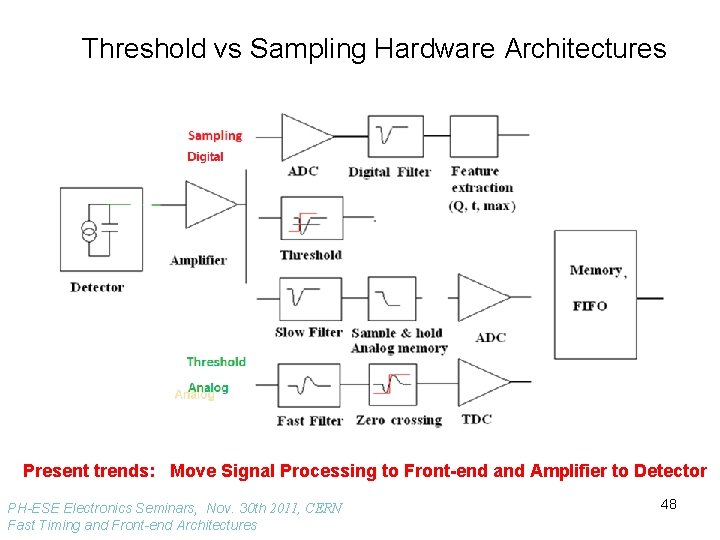
Threshold vs Sampling Hardware Architectures Present trends: Move Signal Processing to Front-end and Amplifier to Detector PH-ESE Electronics Seminars, Nov. 30 th 2011, CERN Fast Timing and Front-end Architectures 48
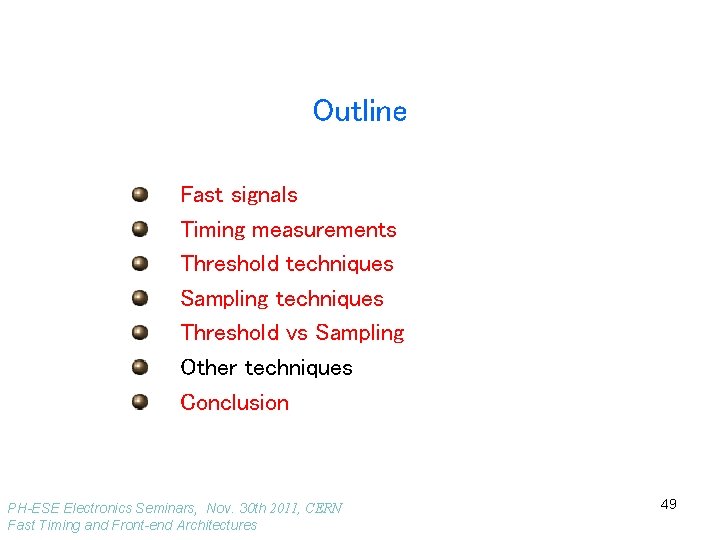
Outline Fast signals Timing measurements Threshold techniques Sampling techniques Threshold vs Sampling Other techniques Conclusion PH-ESE Electronics Seminars, Nov. 30 th 2011, CERN Fast Timing and Front-end Architectures 49
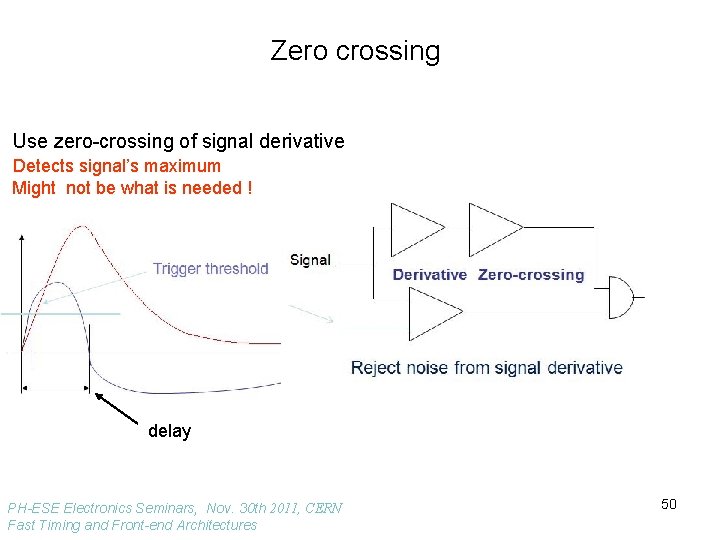
Zero crossing Use zero-crossing of signal derivative Detects signal’s maximum Might not be what is needed ! Signal Reject noise from signal derivative delay PH-ESE Electronics Seminars, Nov. 30 th 2011, CERN Fast Timing and Front-end Architectures 50
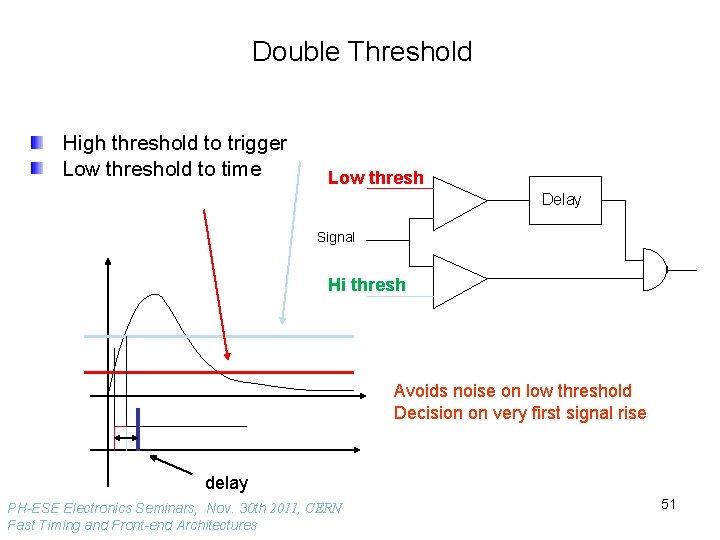
Double Threshold High threshold to trigger Low threshold to time Low thresh Delay Signal Hi thresh Avoids noise on low threshold Decision on very first signal rise delay PH-ESE Electronics Seminars, Nov. 30 th 2011, CERN Fast Timing and Front-end Architectures 51
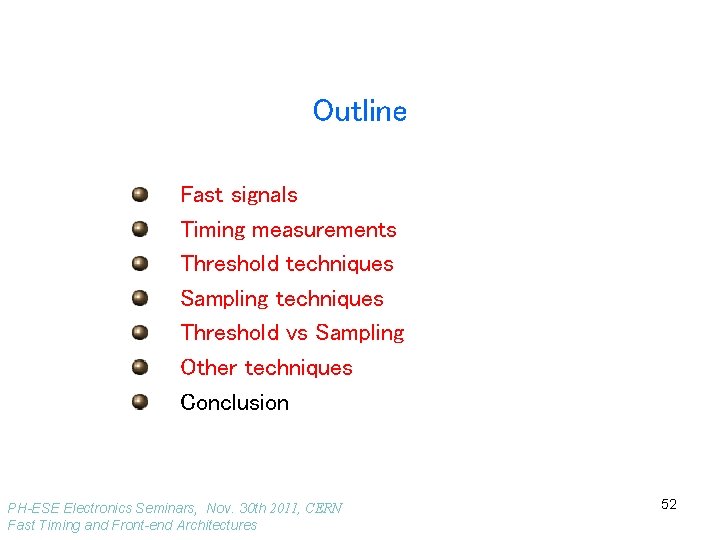
Outline Fast signals Timing measurements Threshold techniques Sampling techniques Threshold vs Sampling Other techniques Conclusion PH-ESE Electronics Seminars, Nov. 30 th 2011, CERN Fast Timing and Front-end Architectures 52
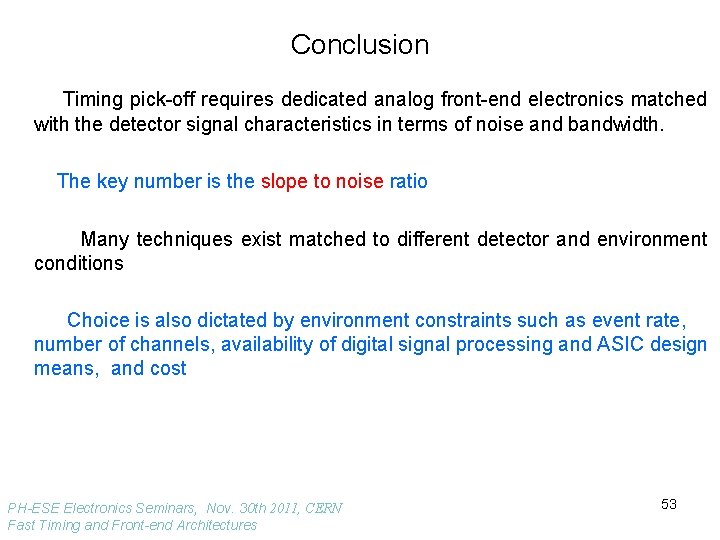
Conclusion Timing pick-off requires dedicated analog front-end electronics matched with the detector signal characteristics in terms of noise and bandwidth. The key number is the slope to noise ratio Many techniques exist matched to different detector and environment conditions Choice is also dictated by environment constraints such as event rate, number of channels, availability of digital signal processing and ASIC design means, and cost PH-ESE Electronics Seminars, Nov. 30 th 2011, CERN Fast Timing and Front-end Architectures 53
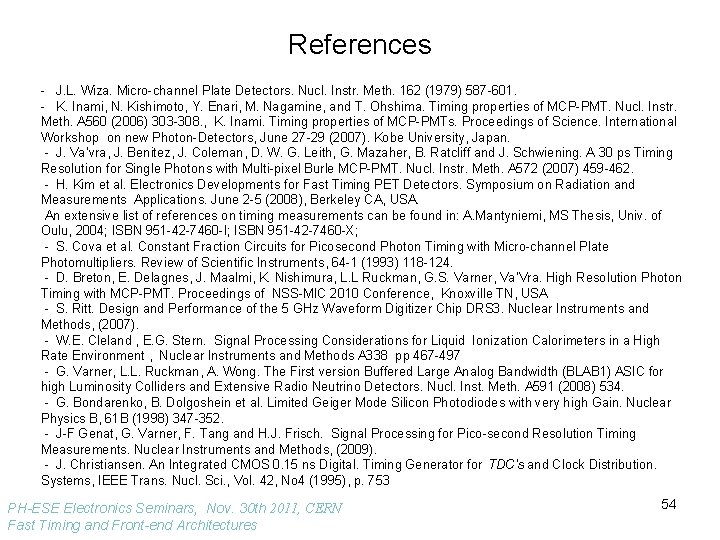
References - J. L. Wiza. Micro-channel Plate Detectors. Nucl. Instr. Meth. 162 (1979) 587 -601. - K. Inami, N. Kishimoto, Y. Enari, M. Nagamine, and T. Ohshima. Timing properties of MCP-PMT. Nucl. Instr. Meth. A 560 (2006) 303 -308. , K. Inami. Timing properties of MCP-PMTs. Proceedings of Science. International Workshop on new Photon-Detectors, June 27 -29 (2007). Kobe University, Japan. - J. Va’vra, J. Benitez, J. Coleman, D. W. G. Leith, G. Mazaher, B. Ratcliff and J. Schwiening. A 30 ps Timing Resolution for Single Photons with Multi-pixel Burle MCP-PMT. Nucl. Instr. Meth. A 572 (2007) 459 -462. - H. Kim et al. Electronics Developments for Fast Timing PET Detectors. Symposium on Radiation and Measurements Applications. June 2 -5 (2008), Berkeley CA, USA. An extensive list of references on timing measurements can be found in: A. Mantyniemi, MS Thesis, Univ. of Oulu, 2004; ISBN 951 -42 -7460 -I; ISBN 951 -42 -7460 -X; - S. Cova et al. Constant Fraction Circuits for Picosecond Photon Timing with Micro-channel Plate Photomultipliers. Review of Scientific Instruments, 64 -1 (1993) 118 -124. - D. Breton, E. Delagnes, J. Maalmi, K. Nishimura, L. L Ruckman, G. S. Varner, Va’Vra. High Resolution Photon Timing with MCP-PMT. Proceedings of NSS-MIC 2010 Conference, Knoxville TN, USA - S. Ritt. Design and Performance of the 5 GHz Waveform Digitizer Chip DRS 3. Nuclear Instruments and Methods, (2007). - W. E. Cleland , E. G. Stern. Signal Processing Considerations for Liquid Ionization Calorimeters in a High Rate Environment , Nuclear Instruments and Methods A 338 pp 467 -497 - G. Varner, L. L. Ruckman, A. Wong. The First version Buffered Large Analog Bandwidth (BLAB 1) ASIC for high Luminosity Colliders and Extensive Radio Neutrino Detectors. Nucl. Inst. Meth. A 591 (2008) 534. - G. Bondarenko, B. Dolgoshein et al. Limited Geiger Mode Silicon Photodiodes with very high Gain. Nuclear Physics B, 61 B (1998) 347 -352. - J-F Genat, G. Varner, F. Tang and H. J. Frisch. Signal Processing for Pico-second Resolution Timing Measurements. Nuclear Instruments and Methods, (2009). - J. Christiansen. An Integrated CMOS 0. 15 ns Digital. Timing Generator for TDC's and Clock Distribution. Systems, IEEE Trans. Nucl. Sci. , Vol. 42, No 4 (1995), p. 753 PH-ESE Electronics Seminars, Nov. 30 th 2011, CERN Fast Timing and Front-end Architectures 54
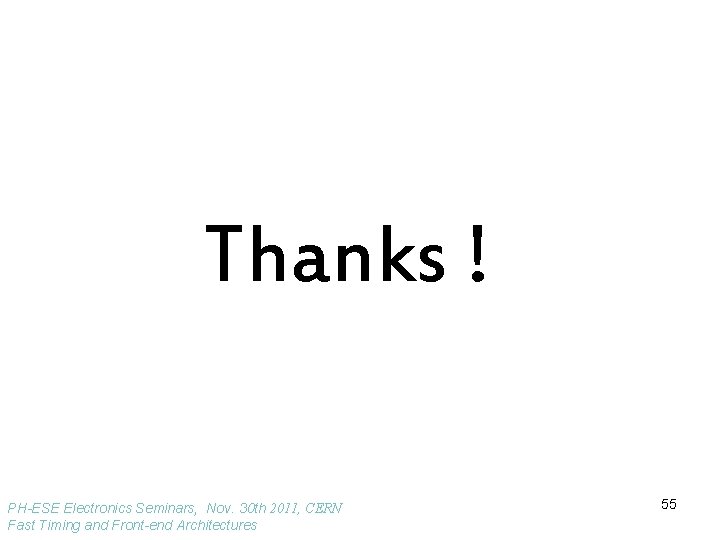
Thanks ! PH-ESE Electronics Seminars, Nov. 30 th 2011, CERN Fast Timing and Front-end Architectures 55
Microchannel devices
Delay lock loop
Choose my plat.gov
Plates that move towards each other
Plates that move towards each other
Where are feature detectors located
Feature detectors ap psychology
Rhmd: evasion-resilient hardware malware detectors
Feature detectors
Frontier detectors for frontier physics
Yodsawalai chodpathumwan
What is thermal detector
Streaming current
Vhv voltage detectors
Photo detectors
Nuclear detectors
Adsorption chromatography
Giant wave detectors murmurs universe
Wave could hear murmurs across universe
Types of positions
Carriers and catch plates
Bars rating scale
Performance management vs performance appraisal
2018 jcids manual
Ring of fire tectonic plates
Stramenophile
Ascomycetes diagram
Grand banks earthquake of 1929
Rules for serving food
Pronto plate
South dakota sellers permit
What happens when two oceanic plates collide
Different plate boundaries
What causes the plates to move
The place where two plates meet
Places where plates move apart
Real life divergent boundary
Constructive plate margin
Endoskeleton of spiny skinned animals are
V
What is this
Cd 7
Romanche trench
What is a modifier
Transform fault
Le master plates
Three major layers of earth
Euglenoids
Tectonic plates with names
Investigative question
Definition of chromatography
High performance liquid chromatography principle
Number of plates chromatography
Adjusted retention time
Chapter 13 smaw horizontal position
Tectonic plates bbc bitesize