The main isotope of Ti has mass 48
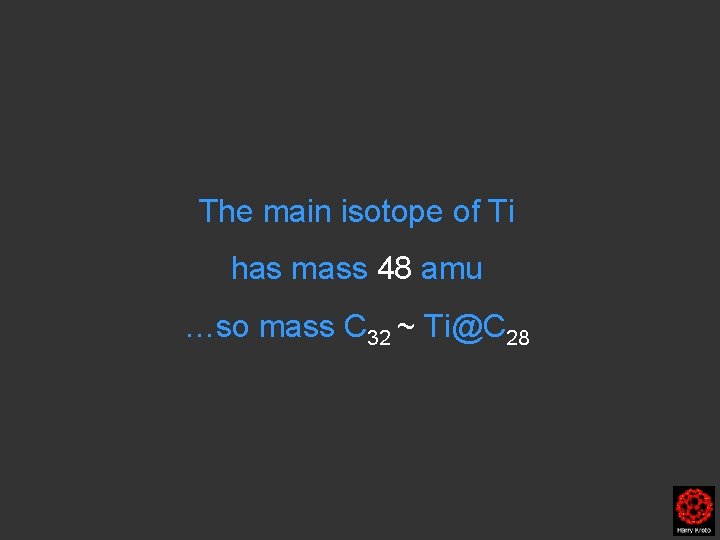
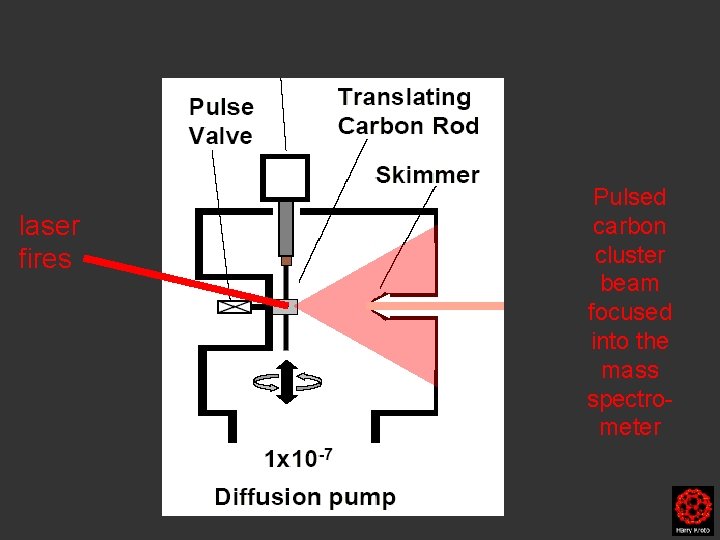
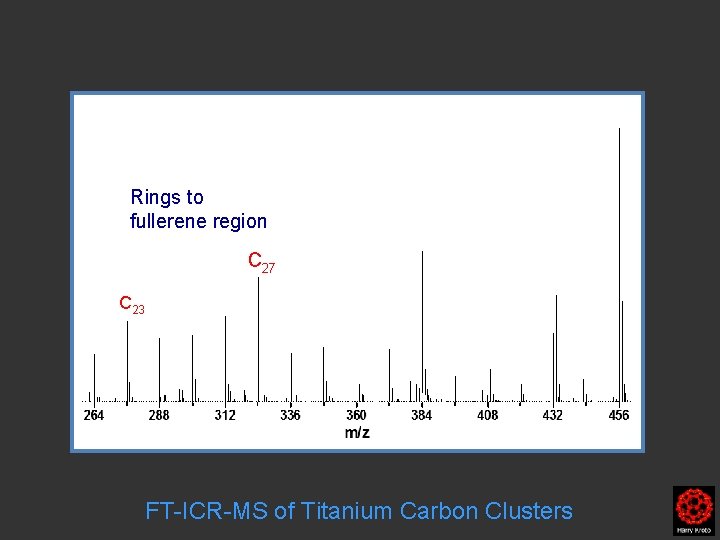
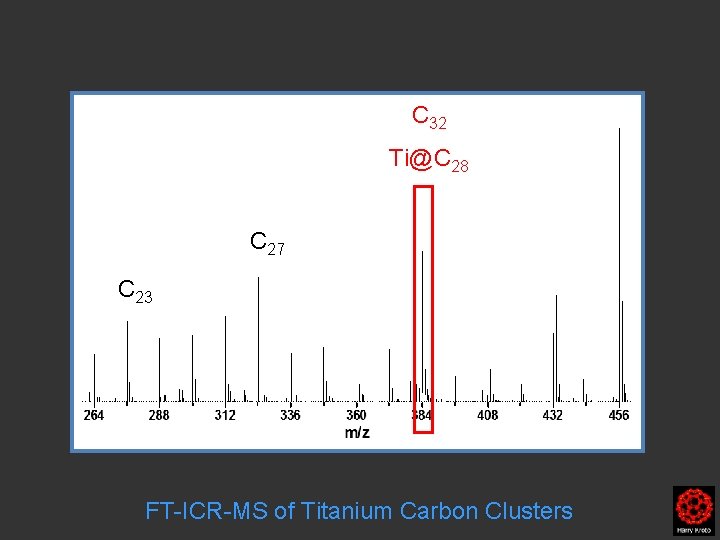
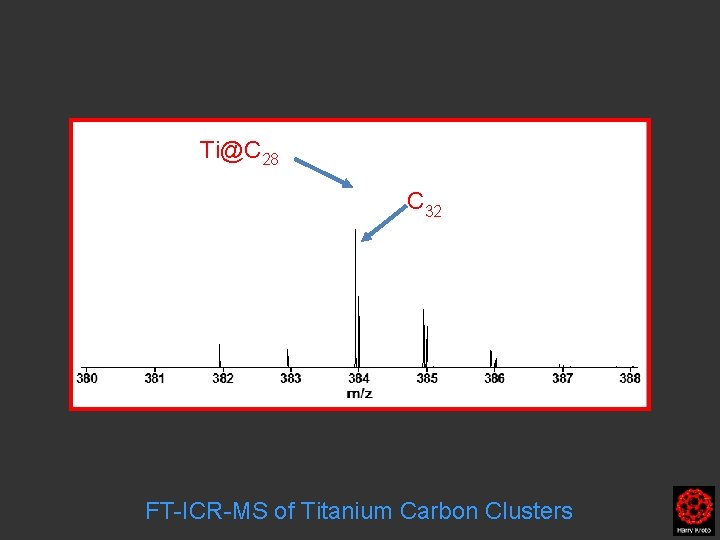
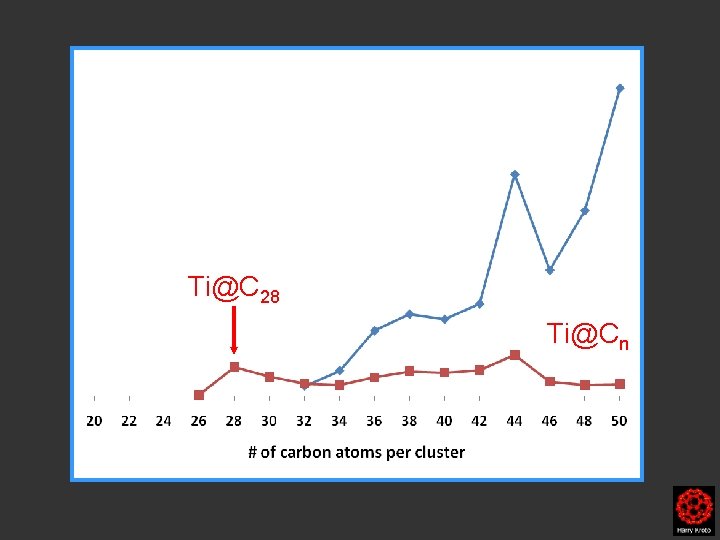
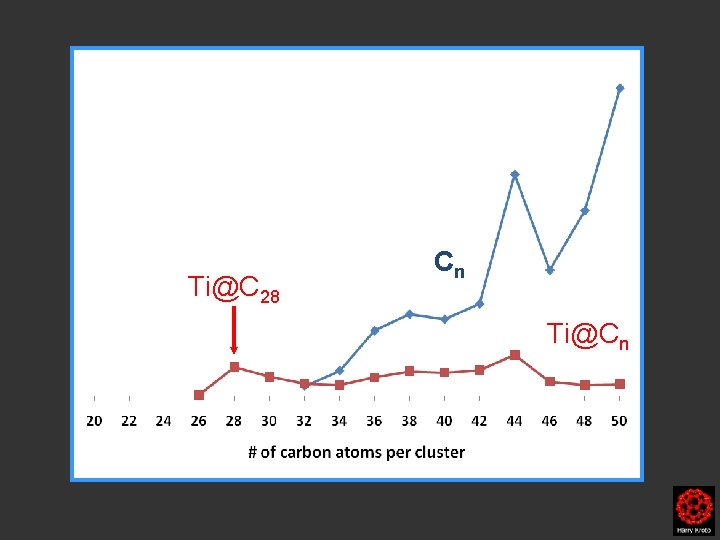
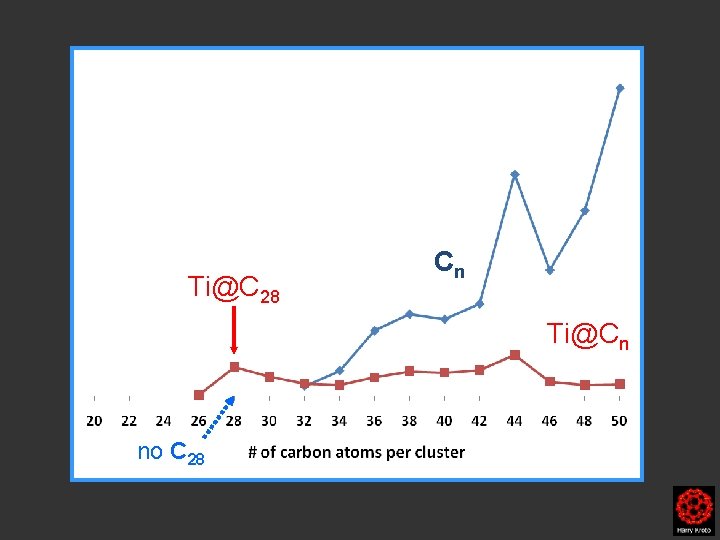
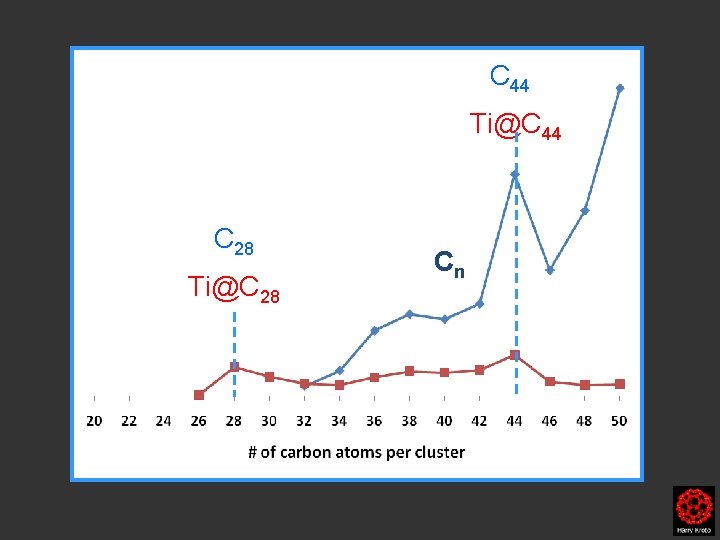
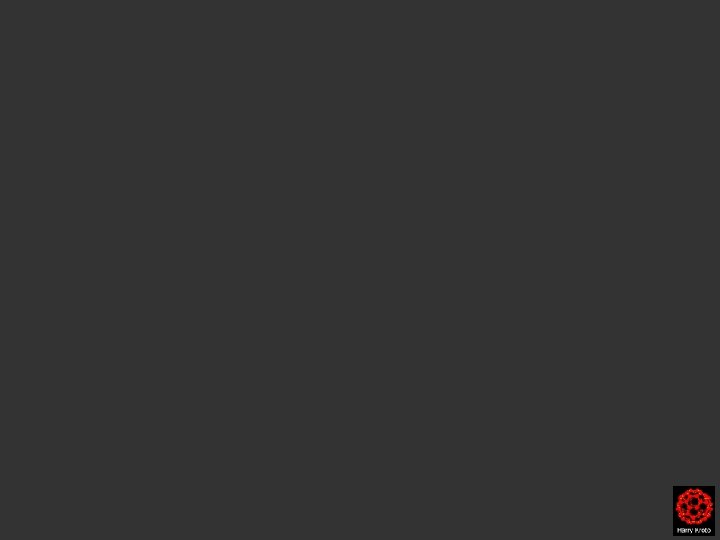
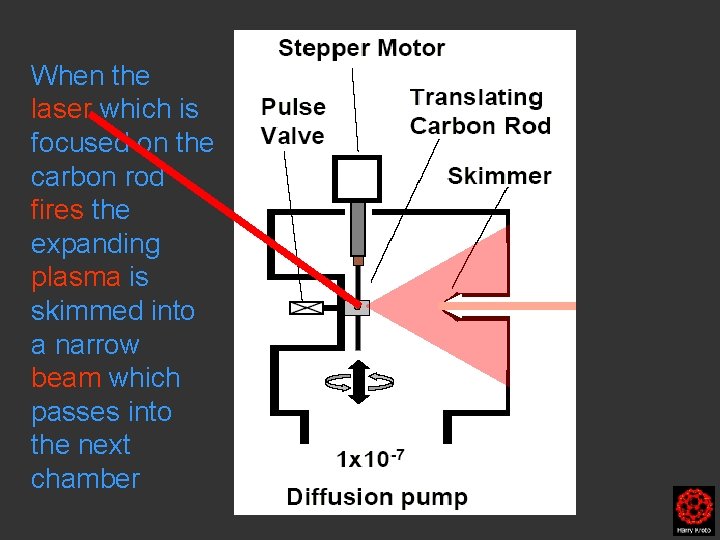
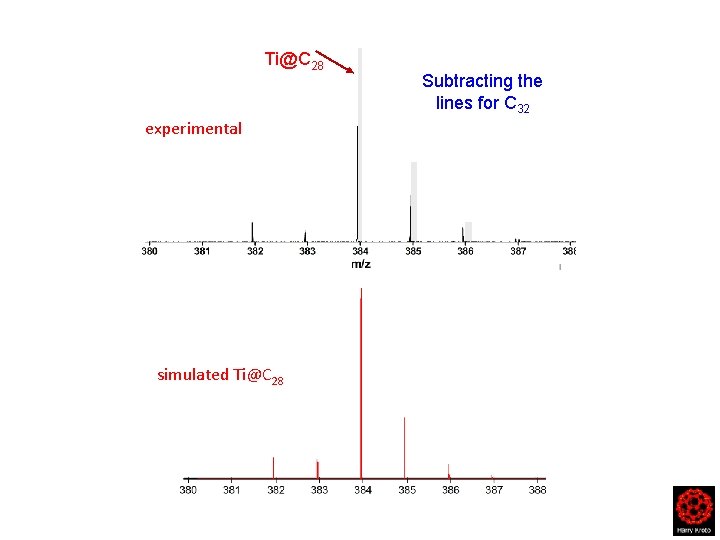
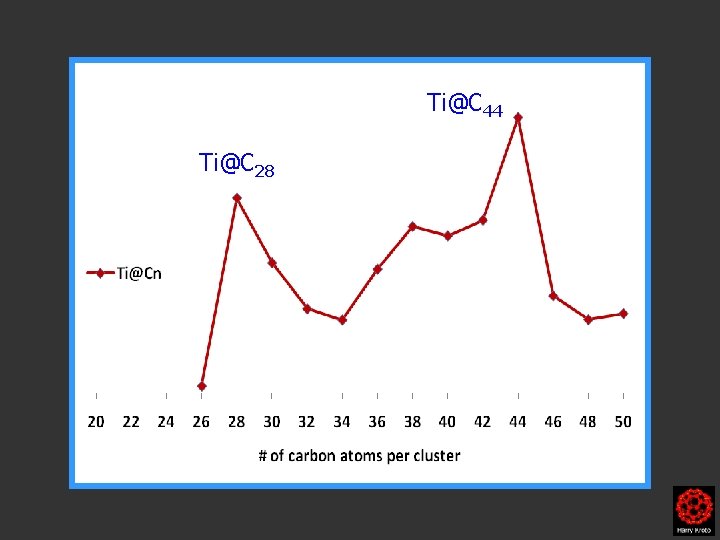
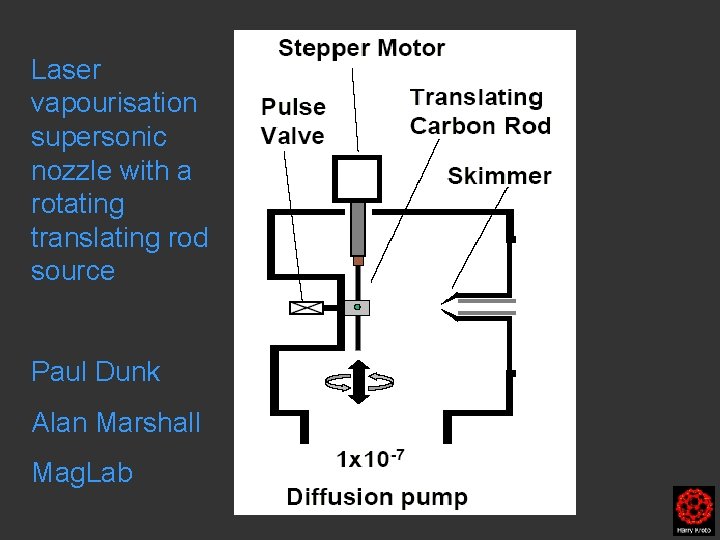
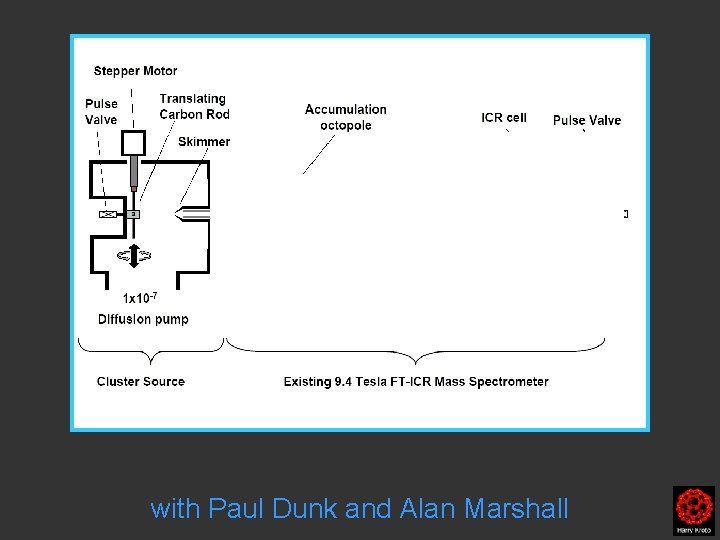
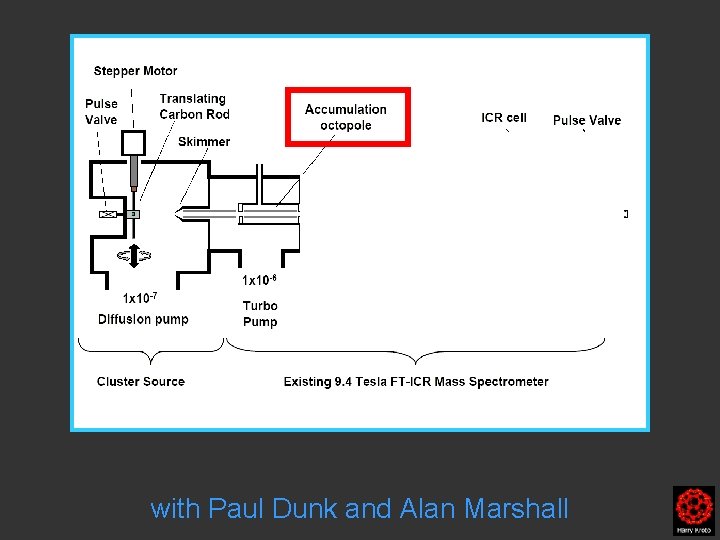
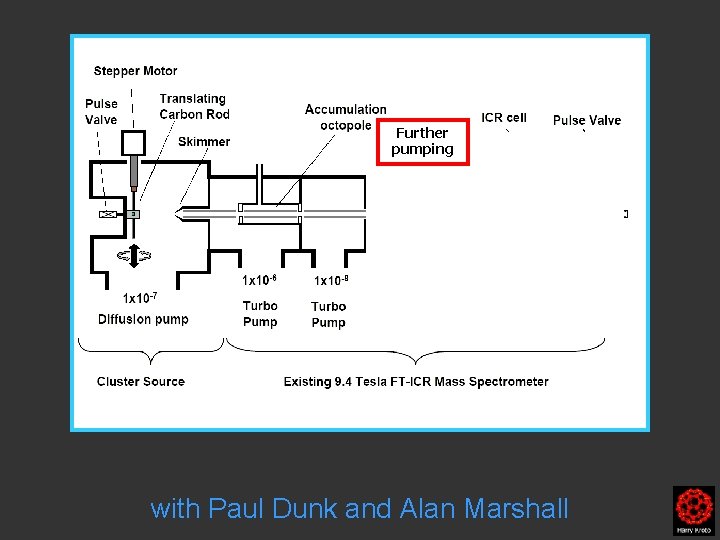
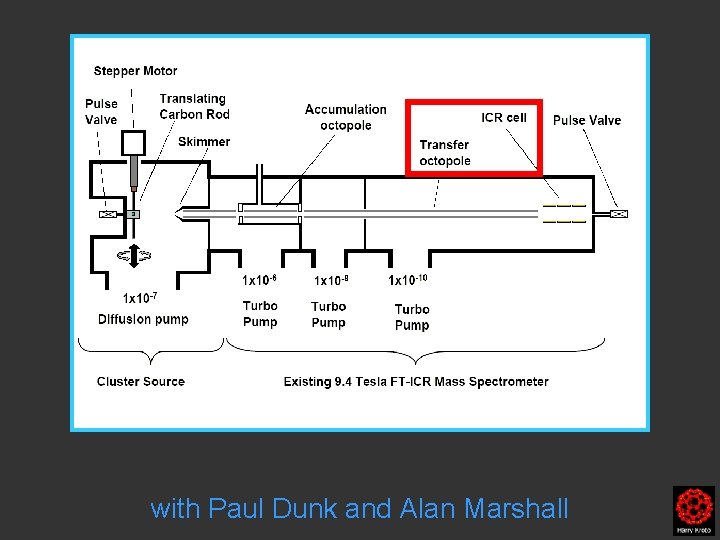
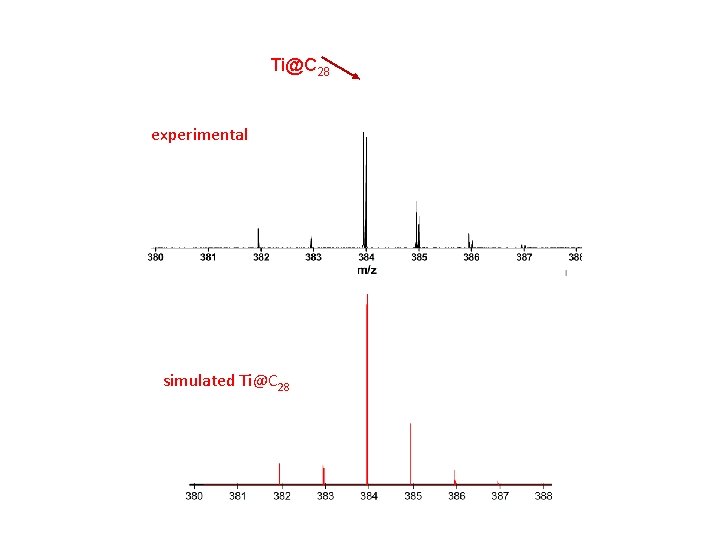
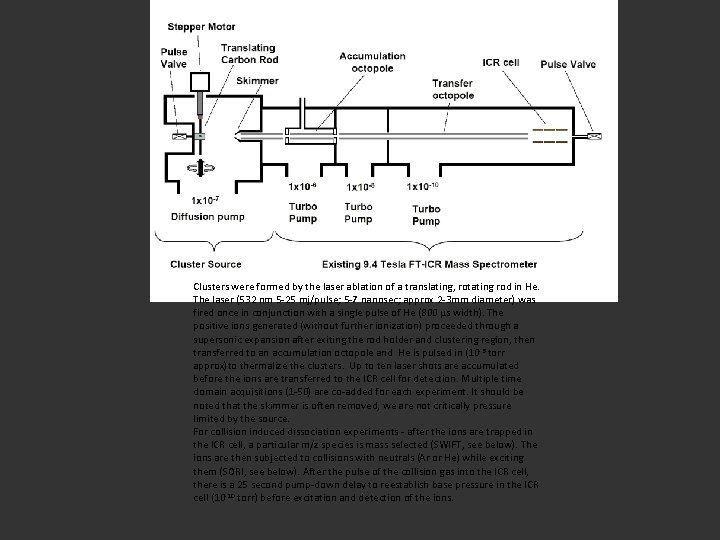
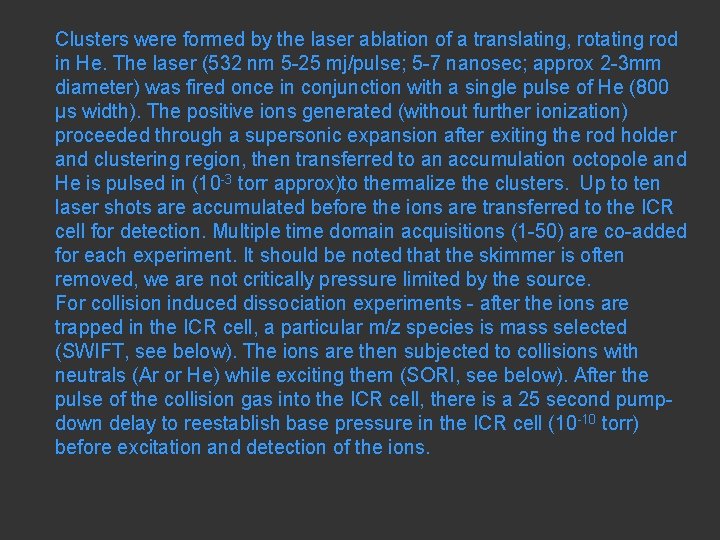
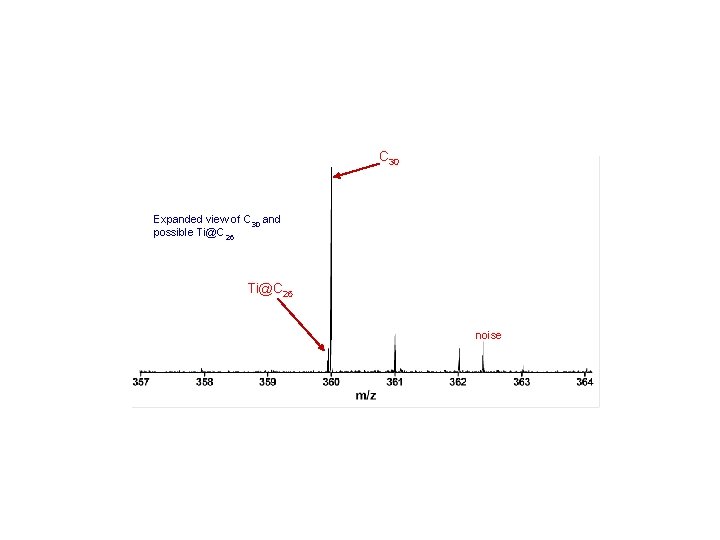
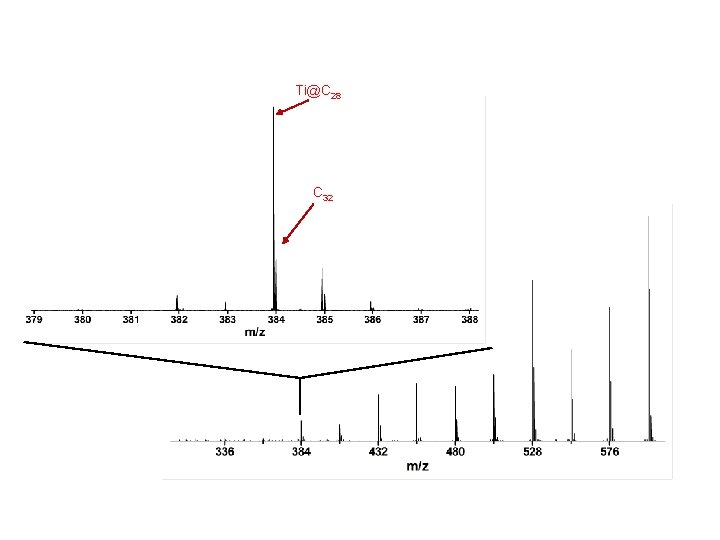
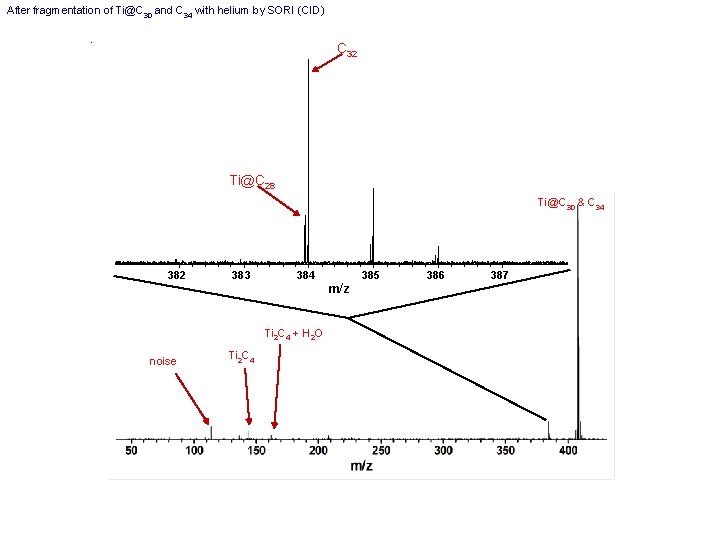
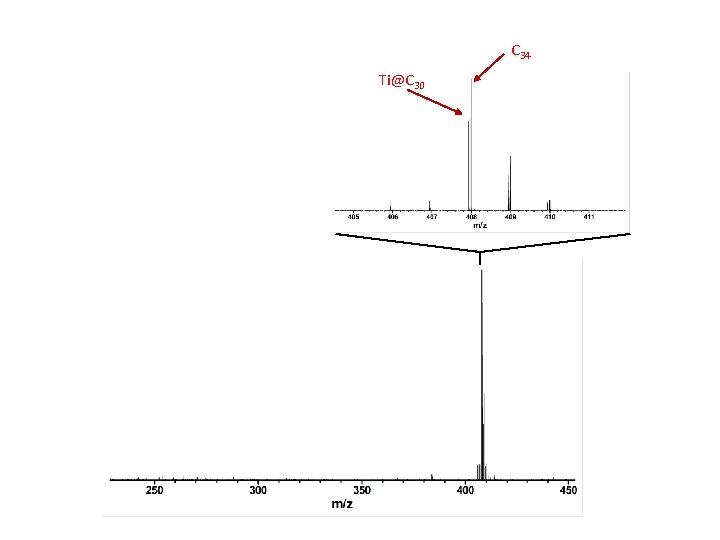
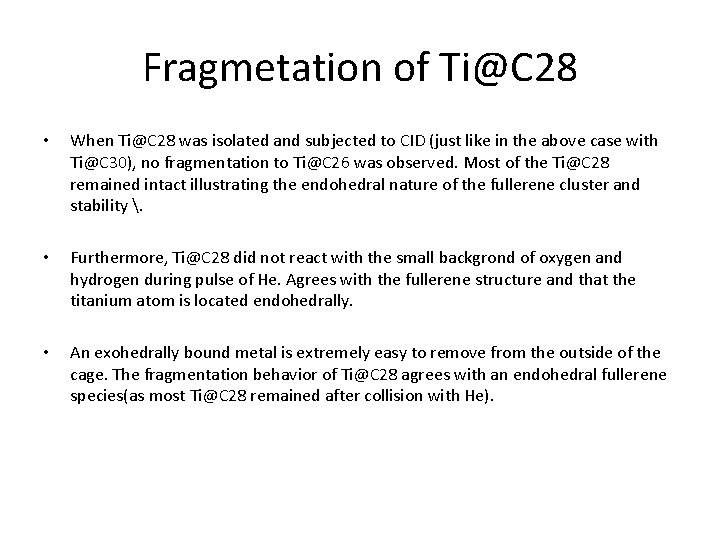
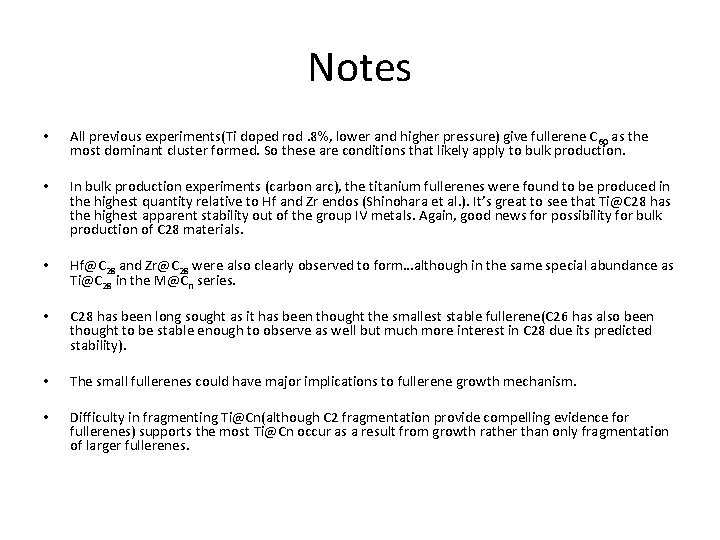
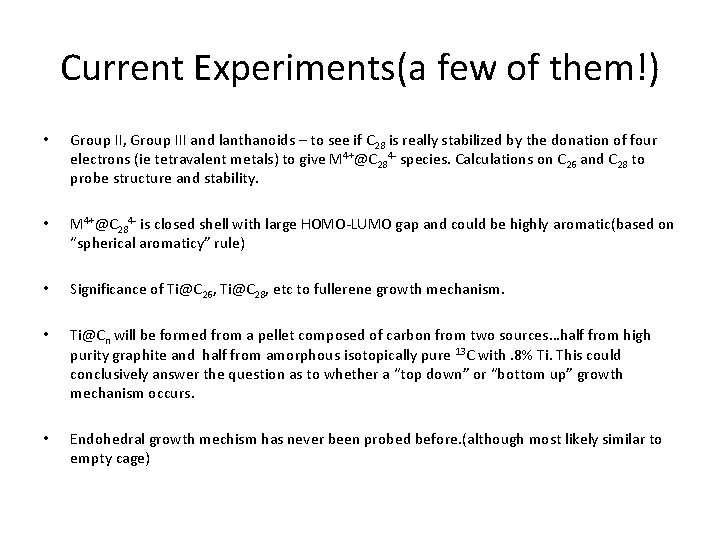
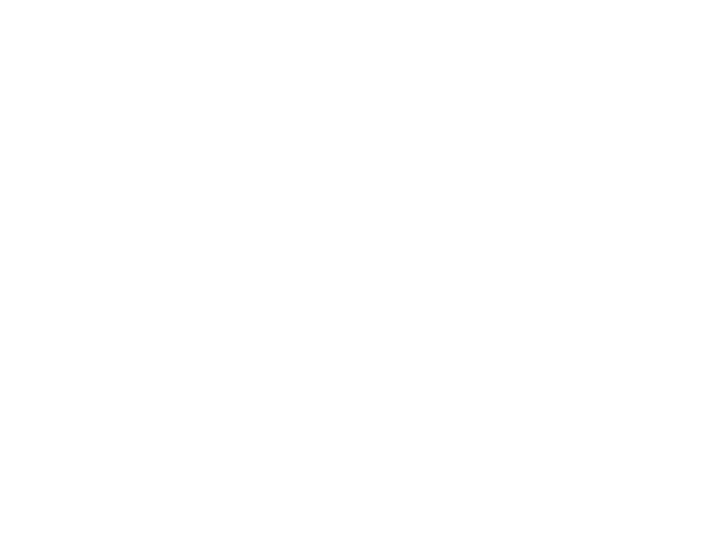
- Slides: 29
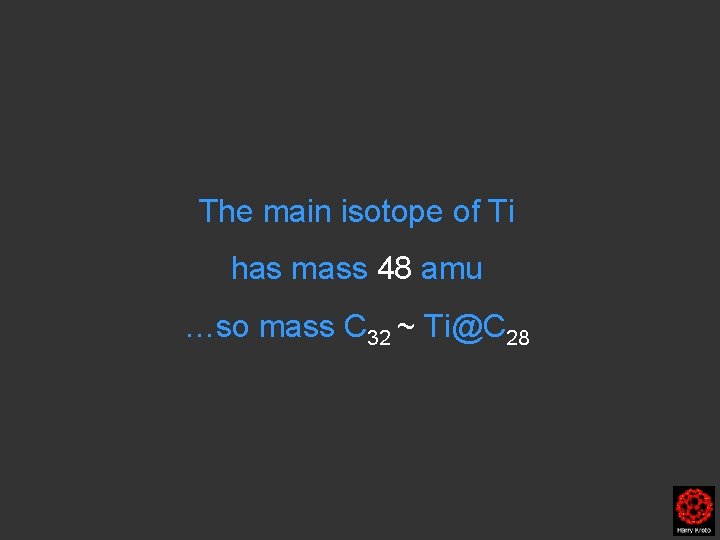
The main isotope of Ti has mass 48 amu …so mass C 32 ~ Ti@C 28
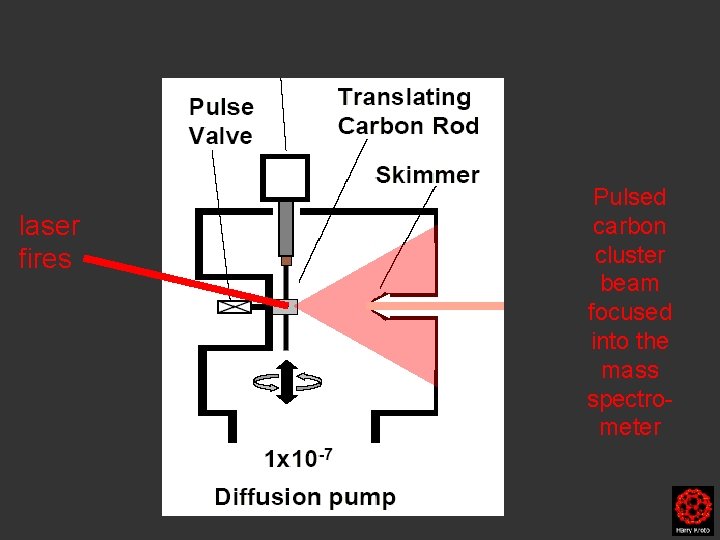
laser fires Pulsed carbon cluster beam focused into the mass spectrometer
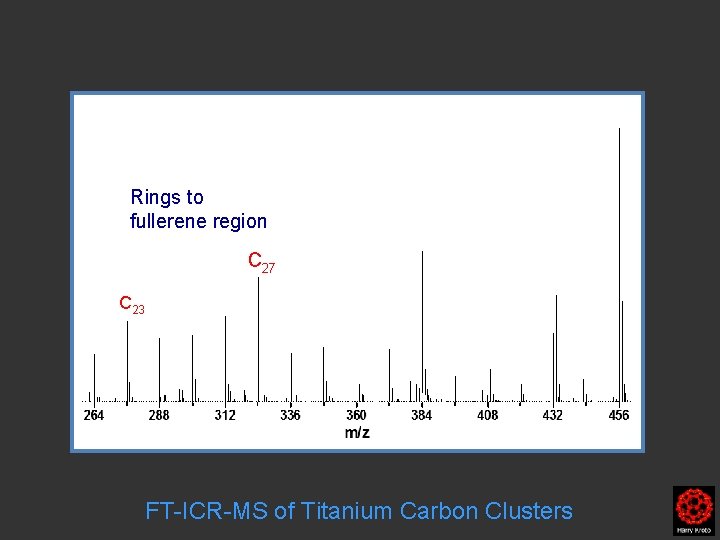
Rings to fullerene region C 27 C 23 FT-ICR-MS of Titanium Carbon Clusters
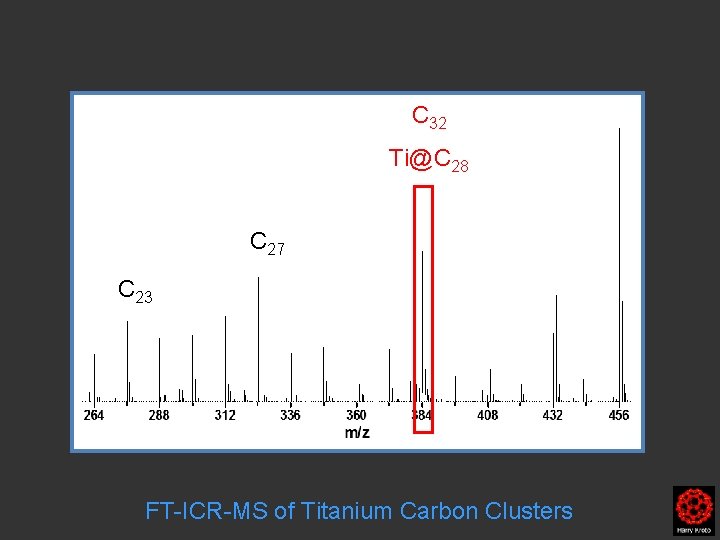
C 32 Ti@C 28 C 27 C 23 FT-ICR-MS of Titanium Carbon Clusters
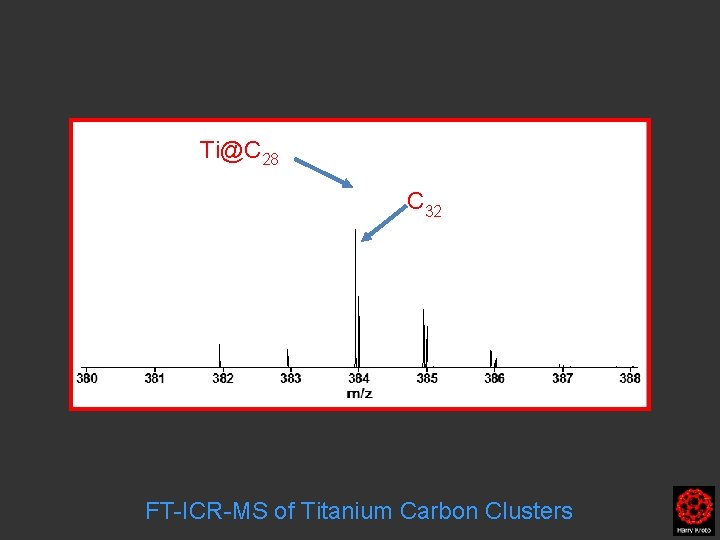
Ti@C 28 C 32 FT-ICR-MS of Titanium Carbon Clusters
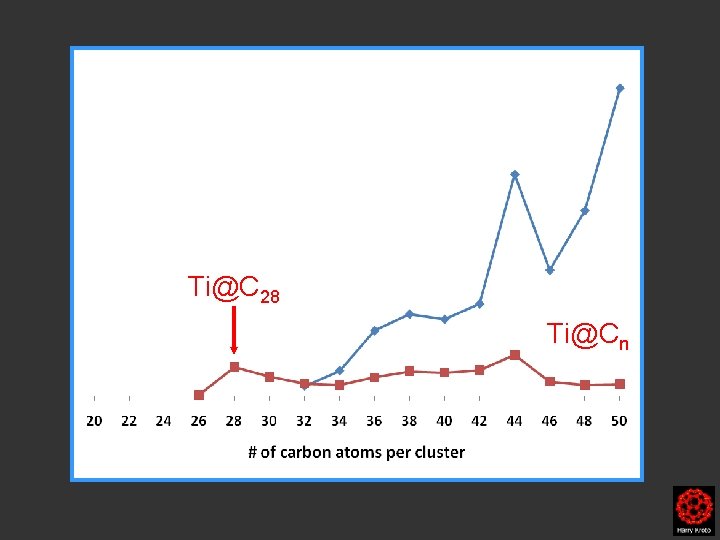
Ti@C 28 Ti@Cn
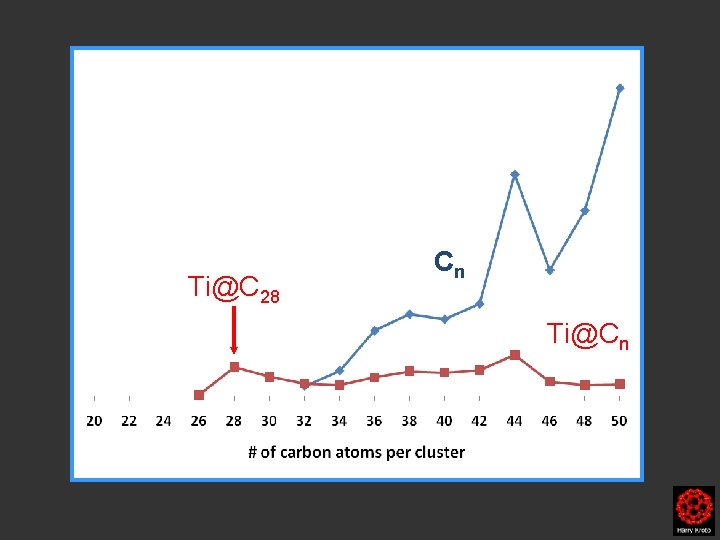
Ti@C 28 Cn Ti@Cn
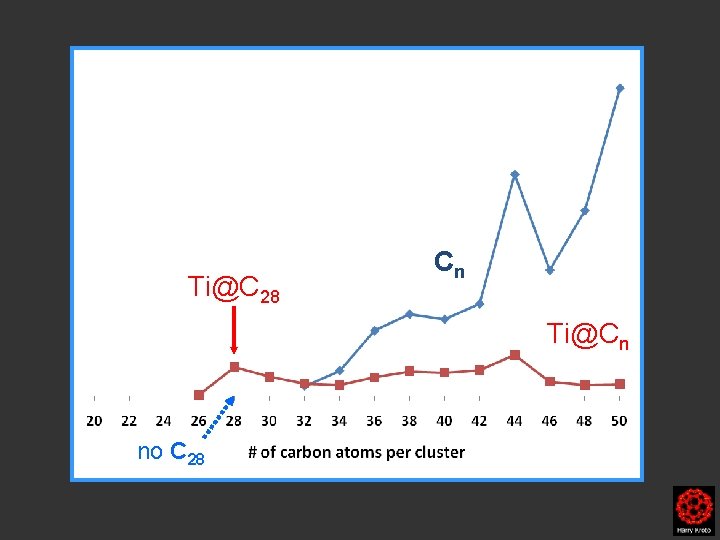
Ti@C 28 Cn Ti@Cn no C 28
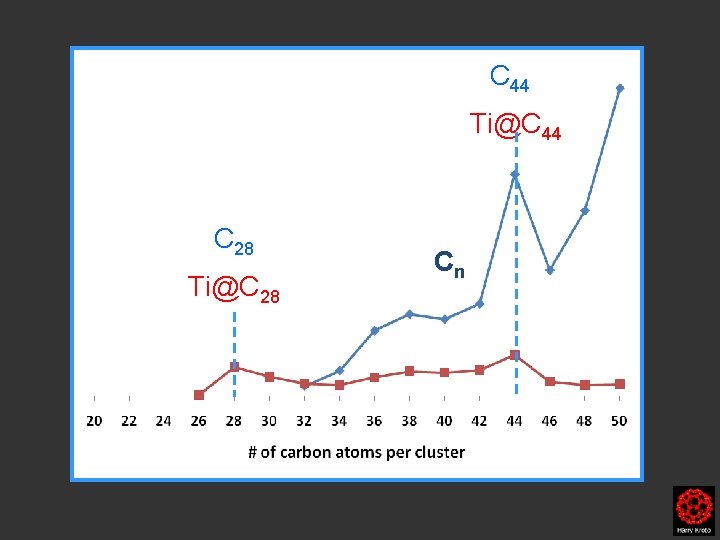
C 44 Ti@C 44 C 28 Ti@C 28 Cn
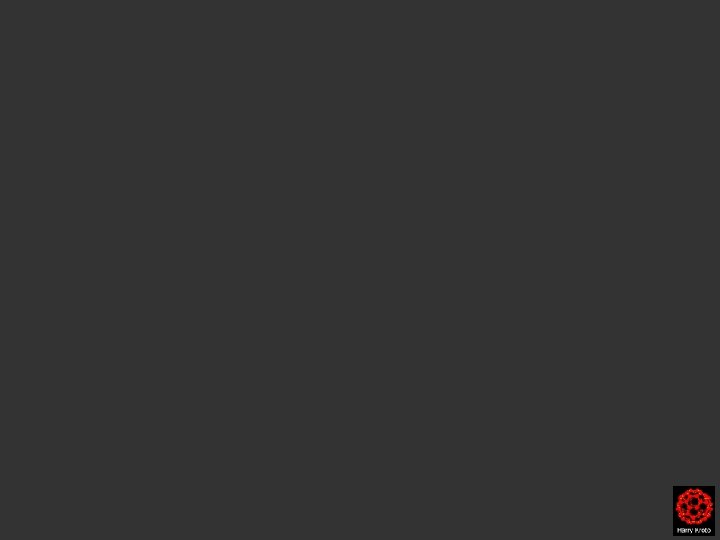
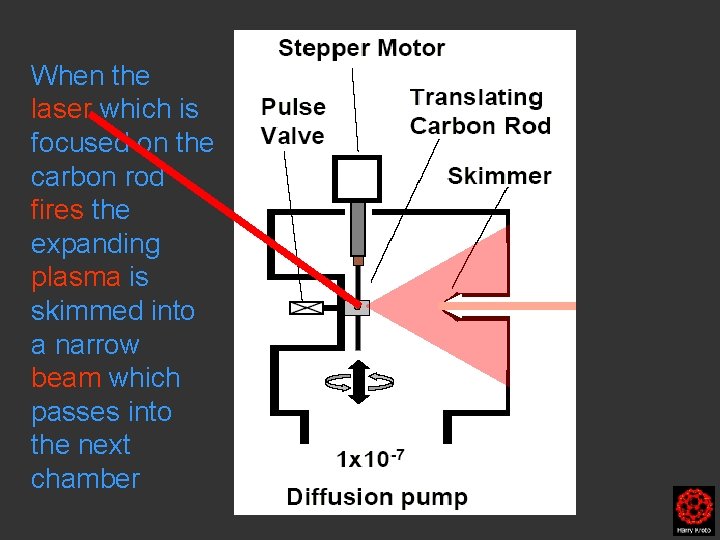
When the laser which is focused on the carbon rod fires the expanding plasma is skimmed into a narrow beam which passes into the next chamber
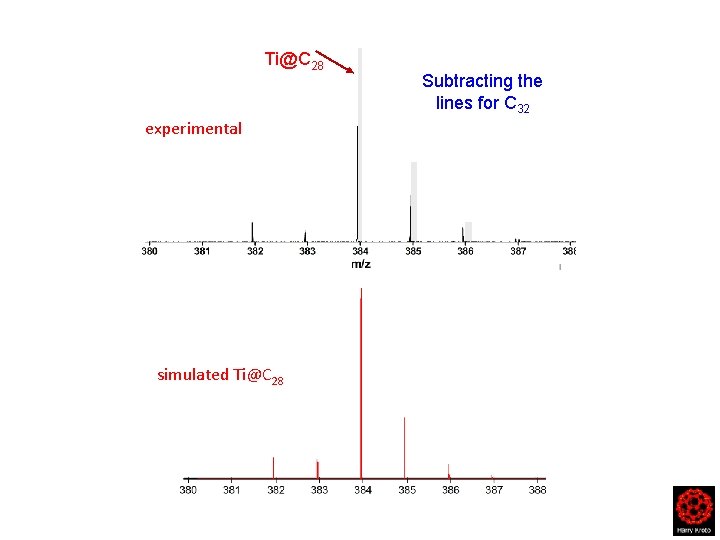
Ti@C 28 experimental simulated Ti@C 28 Subtracting the lines for C 32
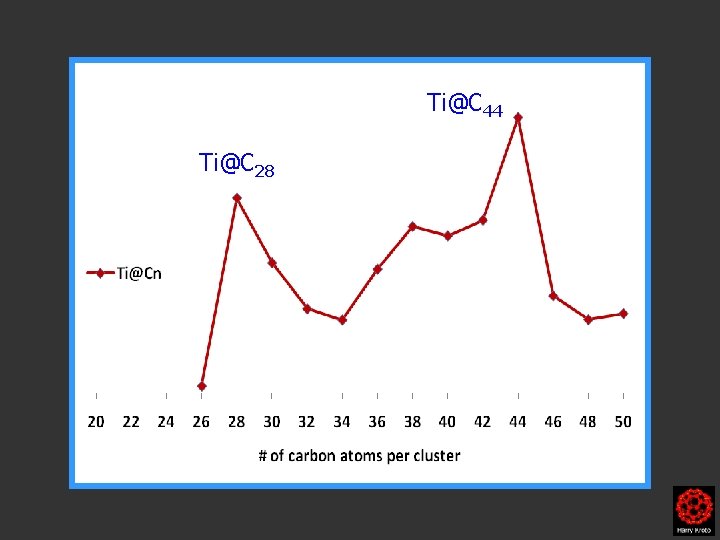
Ti@C 44 Ti@C 28
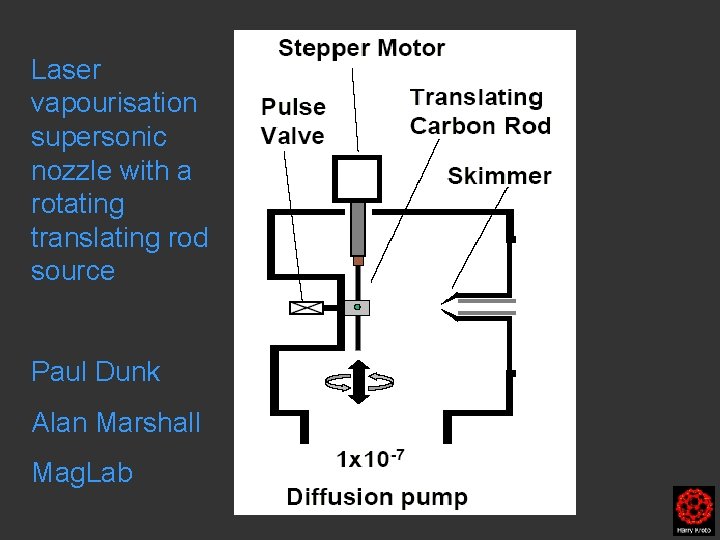
Laser vapourisation supersonic nozzle with a rotating translating rod source Paul Dunk Alan Marshall Mag. Lab
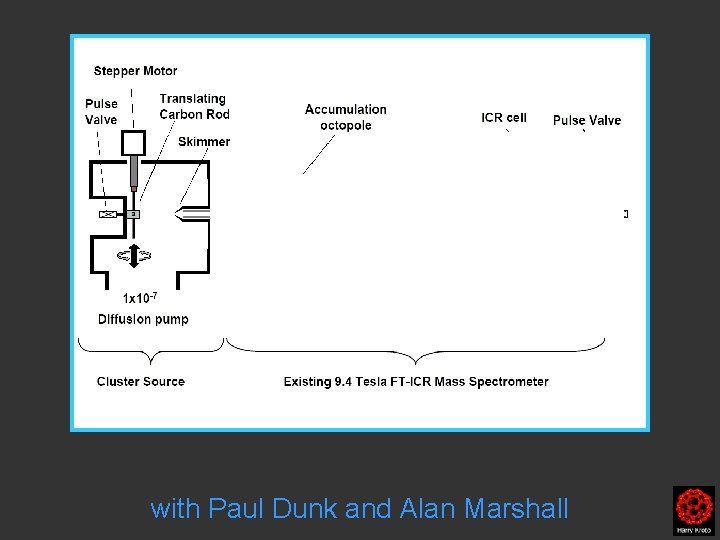
with Paul Dunk and Alan Marshall
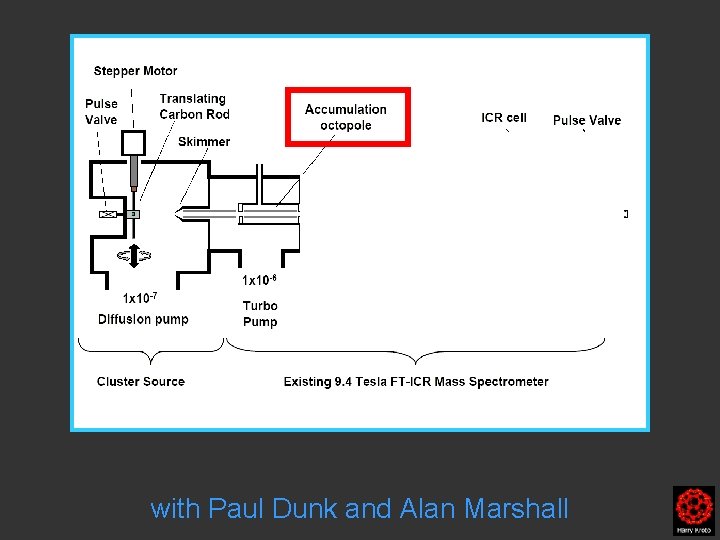
with Paul Dunk and Alan Marshall
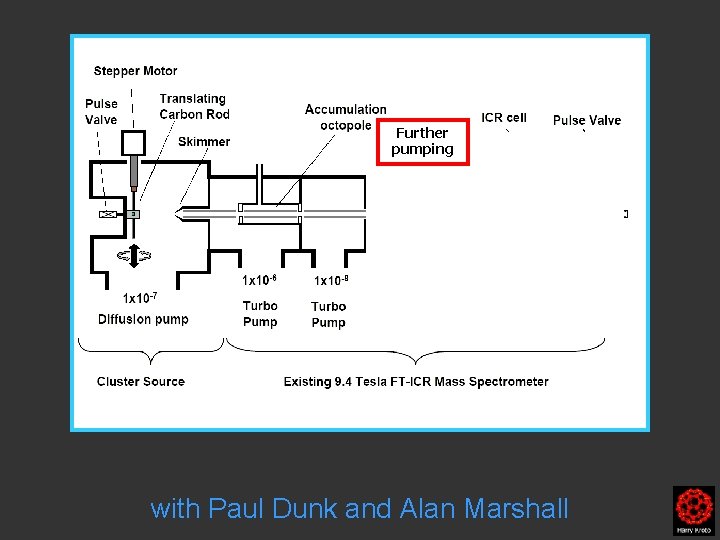
Further pumping with Paul Dunk and Alan Marshall
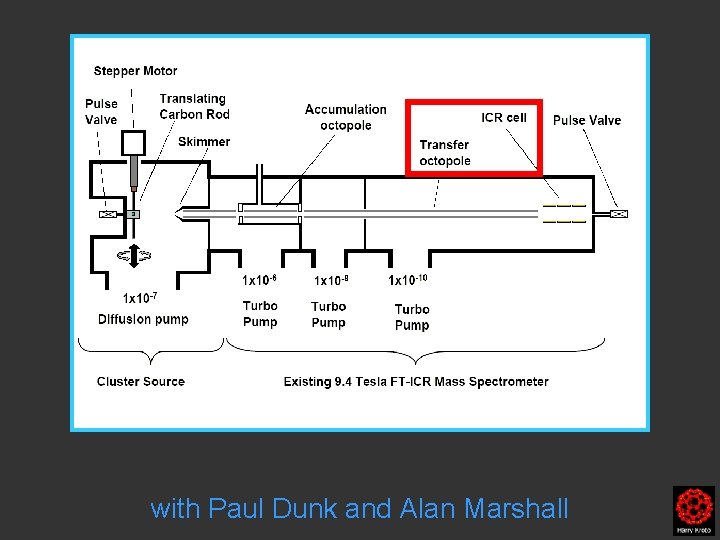
with Paul Dunk and Alan Marshall
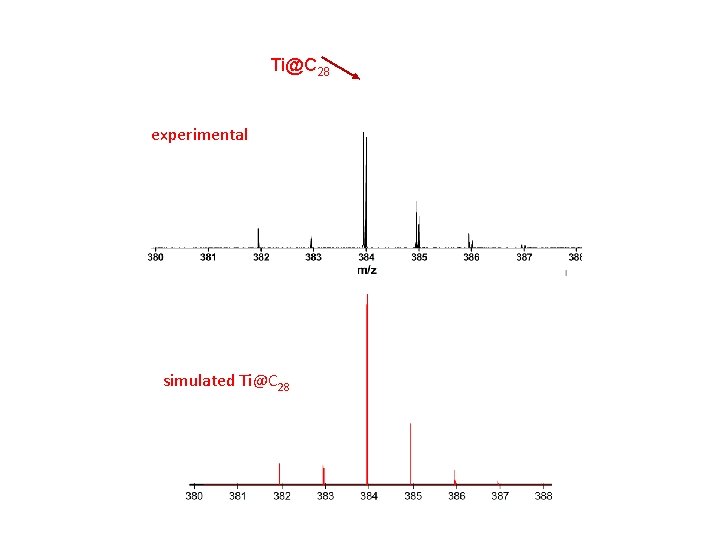
Ti@C 28 experimental simulated Ti@C 28
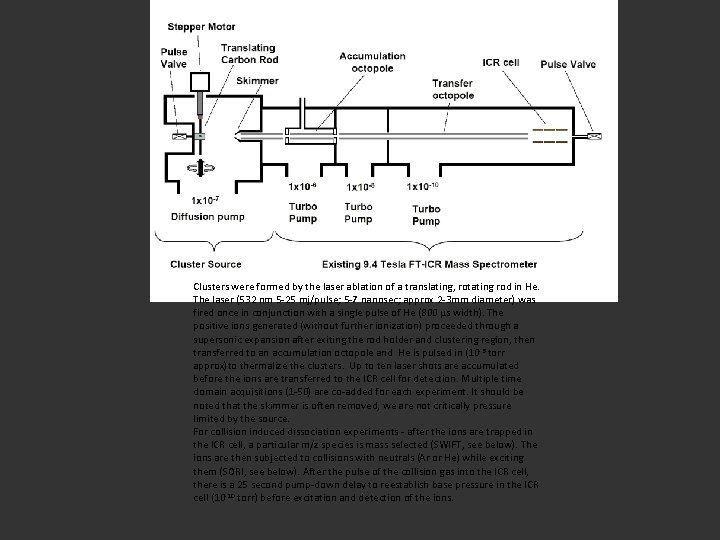
Clusters were formed by the laser ablation of a translating, rotating rod in He. The laser (532 nm 5 -25 mj/pulse; 5 -7 nanosec; approx 2 -3 mm diameter) was fired once in conjunction with a single pulse of He (800 μs width). The positive ions generated (without further ionization) proceeded through a supersonic expansion after exiting the rod holder and clustering region, then transferred to an accumulation octopole and He is pulsed in (10 -3 torr approx)to thermalize the clusters. Up to ten laser shots are accumulated before the ions are transferred to the ICR cell for detection. Multiple time domain acquisitions (1 -50) are co-added for each experiment. It should be noted that the skimmer is often removed, we are not critically pressure limited by the source. For collision induced dissociation experiments - after the ions are trapped in the ICR cell, a particular m/z species is mass selected (SWIFT, see below). The ions are then subjected to collisions with neutrals (Ar or He) while exciting them (SORI, see below). After the pulse of the collision gas into the ICR cell, there is a 25 second pump-down delay to reestablish base pressure in the ICR cell (10 -10 torr) before excitation and detection of the ions.
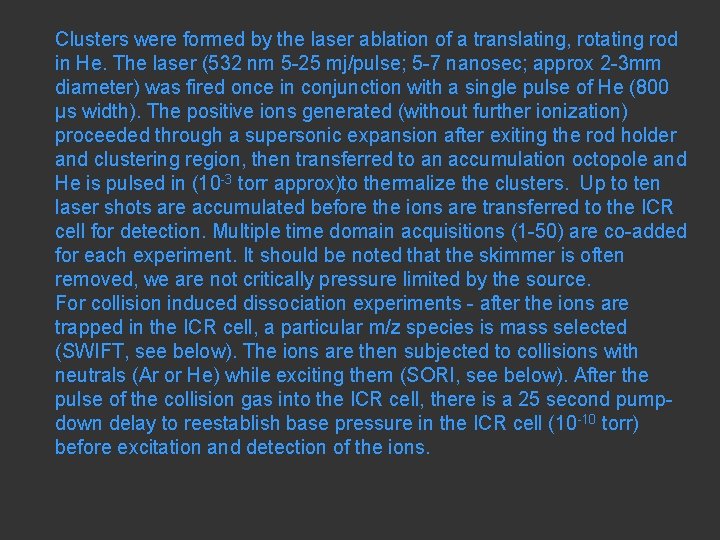
Clusters were formed by the laser ablation of a translating, rotating rod in He. The laser (532 nm 5 -25 mj/pulse; 5 -7 nanosec; approx 2 -3 mm diameter) was fired once in conjunction with a single pulse of He (800 μs width). The positive ions generated (without further ionization) proceeded through a supersonic expansion after exiting the rod holder and clustering region, then transferred to an accumulation octopole and He is pulsed in (10 -3 torr approx)to thermalize the clusters. Up to ten laser shots are accumulated before the ions are transferred to the ICR cell for detection. Multiple time domain acquisitions (1 -50) are co-added for each experiment. It should be noted that the skimmer is often removed, we are not critically pressure limited by the source. For collision induced dissociation experiments - after the ions are trapped in the ICR cell, a particular m/z species is mass selected (SWIFT, see below). The ions are then subjected to collisions with neutrals (Ar or He) while exciting them (SORI, see below). After the pulse of the collision gas into the ICR cell, there is a 25 second pumpdown delay to reestablish base pressure in the ICR cell (10 -10 torr) before excitation and detection of the ions.
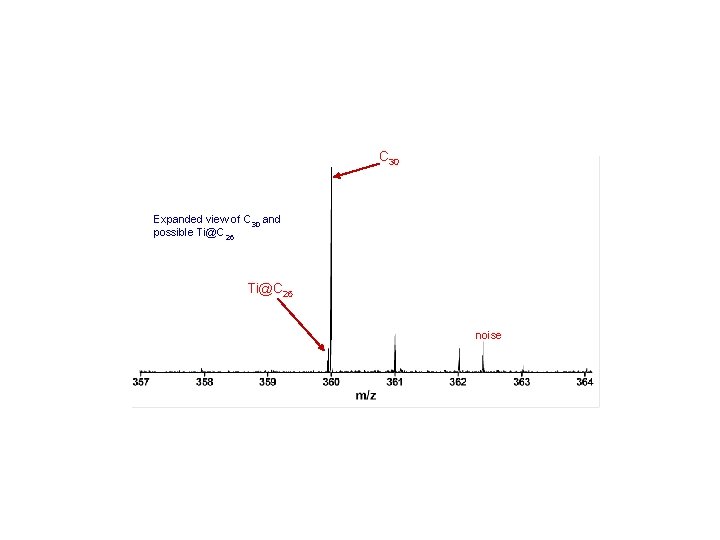
C 30 Expanded view of C 30 and possible Ti@C 26 noise
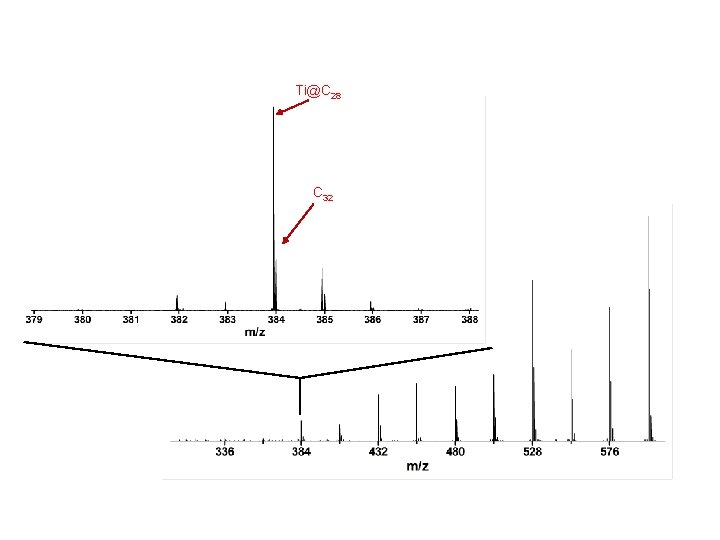
Ti@C 28 C 32
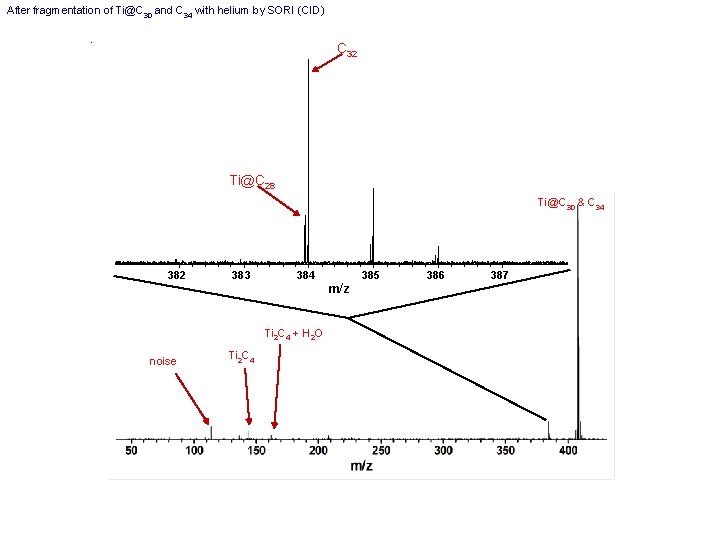
After fragmentation of Ti@C 30 and C 34 with helium by SORI (CID) C 32 Ti@C 28 Ti@C 30 & C 34 382 383 384 Ti 2 C 4 + H 2 O noise Ti 2 C 4 m/z 385 386 387
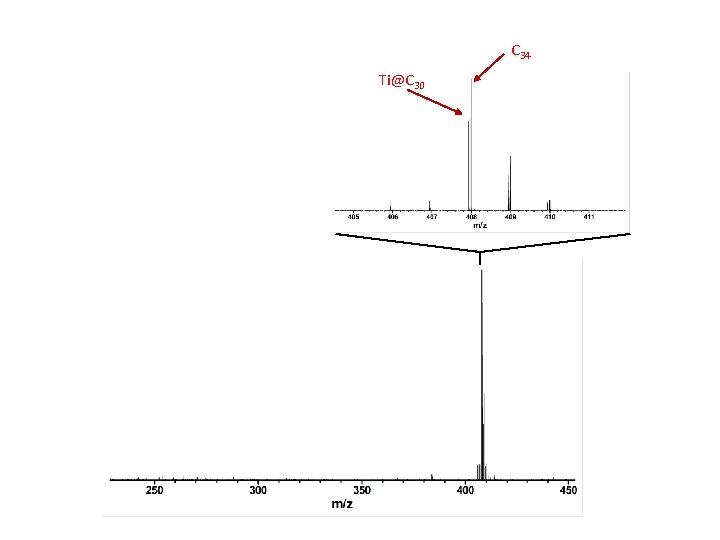
C 34 Ti@C 30
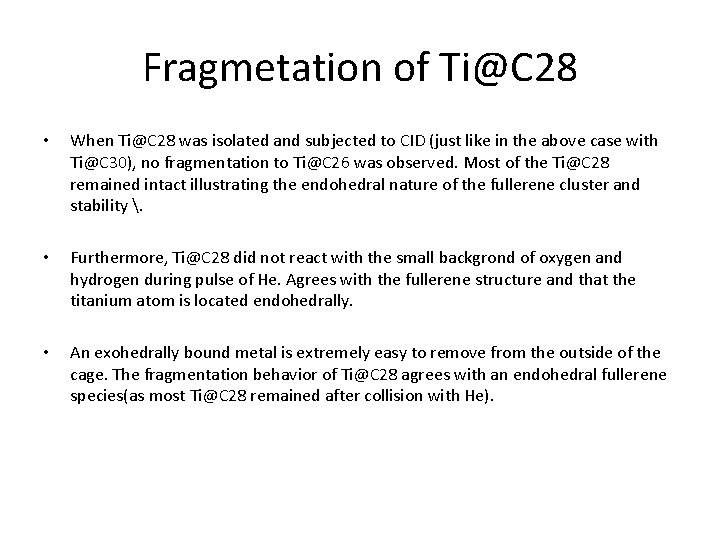
Fragmetation of Ti@C 28 • When Ti@C 28 was isolated and subjected to CID (just like in the above case with Ti@C 30), no fragmentation to Ti@C 26 was observed. Most of the Ti@C 28 remained intact illustrating the endohedral nature of the fullerene cluster and stability . • Furthermore, Ti@C 28 did not react with the small backgrond of oxygen and hydrogen during pulse of He. Agrees with the fullerene structure and that the titanium atom is located endohedrally. • An exohedrally bound metal is extremely easy to remove from the outside of the cage. The fragmentation behavior of Ti@C 28 agrees with an endohedral fullerene species(as most Ti@C 28 remained after collision with He).
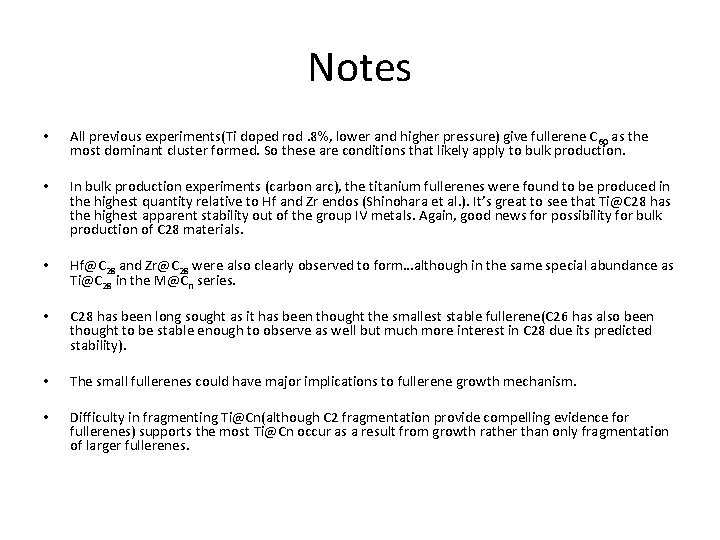
Notes • All previous experiments(Ti doped rod. 8%, lower and higher pressure) give fullerene C 60 as the most dominant cluster formed. So these are conditions that likely apply to bulk production. • In bulk production experiments (carbon arc), the titanium fullerenes were found to be produced in the highest quantity relative to Hf and Zr endos (Shinohara et al. ). It’s great to see that Ti@C 28 has the highest apparent stability out of the group IV metals. Again, good news for possibility for bulk production of C 28 materials. • Hf@C 28 and Zr@C 28 were also clearly observed to form…although in the same special abundance as Ti@C 28 in the M@Cn series. • C 28 has been long sought as it has been thought the smallest stable fullerene(C 26 has also been thought to be stable enough to observe as well but much more interest in C 28 due its predicted stability). • The small fullerenes could have major implications to fullerene growth mechanism. • Difficulty in fragmenting Ti@Cn(although C 2 fragmentation provide compelling evidence for fullerenes) supports the most Ti@Cn occur as a result from growth rather than only fragmentation of larger fullerenes.
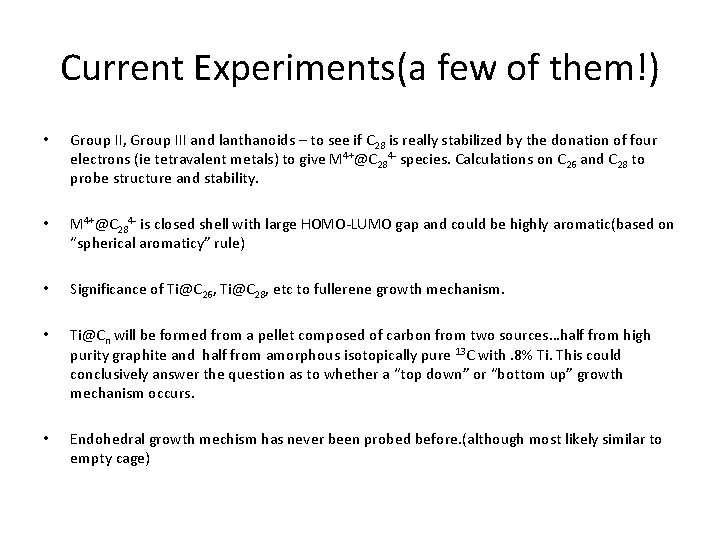
Current Experiments(a few of them!) • Group II, Group III and lanthanoids – to see if C 28 is really stabilized by the donation of four electrons (ie tetravalent metals) to give M 4+@C 284 - species. Calculations on C 26 and C 28 to probe structure and stability. • M 4+@C 284 - is closed shell with large HOMO-LUMO gap and could be highly aromatic(based on “spherical aromaticy” rule) • Significance of Ti@C 26, Ti@C 28, etc to fullerene growth mechanism. • Ti@Cn will be formed from a pellet composed of carbon from two sources…half from high purity graphite and half from amorphous isotopically pure 13 C with. 8% Ti. This could conclusively answer the question as to whether a “top down” or “bottom up” growth mechanism occurs. • Endohedral growth mechism has never been probed before. (although most likely similar to empty cage)
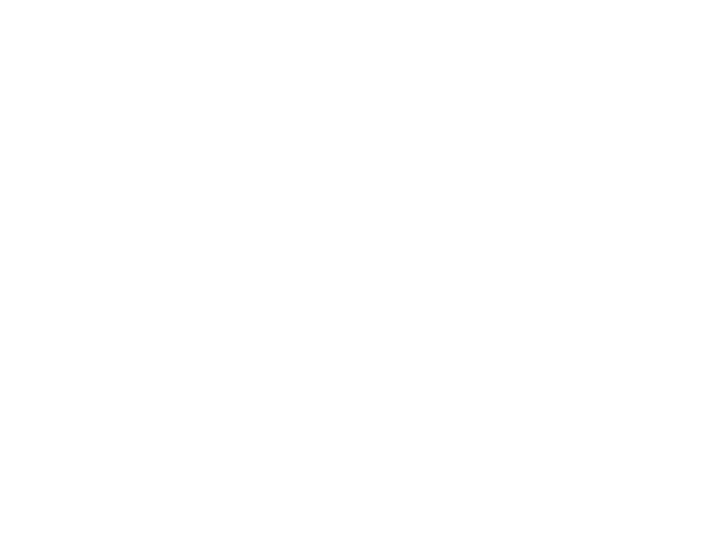
Frank has an eraser it has a mass of 4g
Ti-48 isotope
What is
Calculation of radioactive decay
Isotopic notation
Whats carbon dating
Isotope charge
Sn-117 isotope
Neon isotopic notation
How to calculate average atomic mass
Isotope notation for iron
How to calculate abundance of isotopes
Proton fun fact
Proton symbol
32s isotope
Rule of 12 in thyroid
Thyroid isotope scan
26mg isotope
136ba isotope
Xe-126 isotope
Ferrocene
Isotopic notation
Whats an isotope
Isotopes labeled
The isotope atoms differ in *
What is the name of this isotope?
Zr-96 isotope
What is the name of this isotope?
Isotope
Oxygen isotope ratio