Tectonic change 2 Theme 2 Investigating Tectonic and
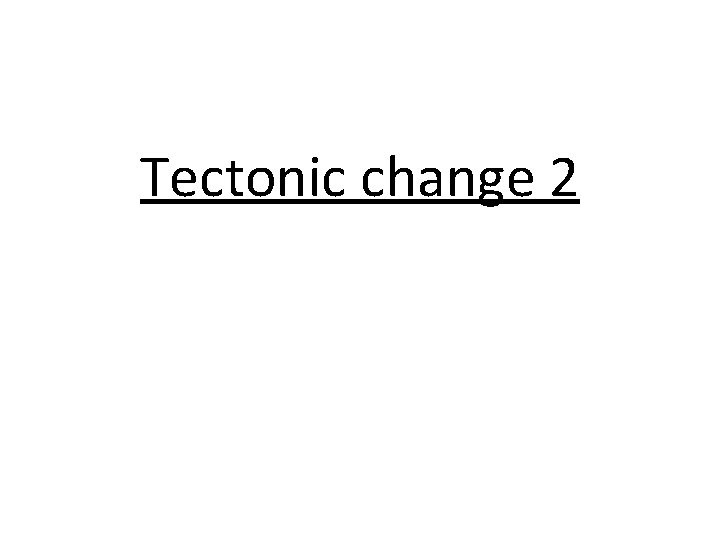
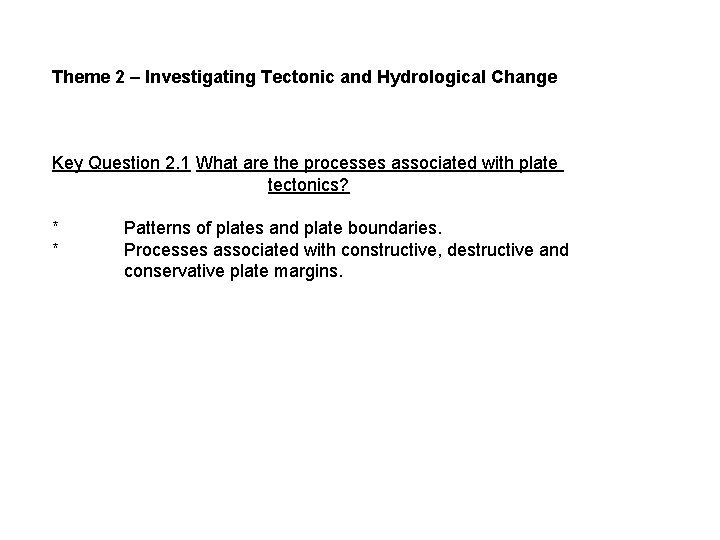
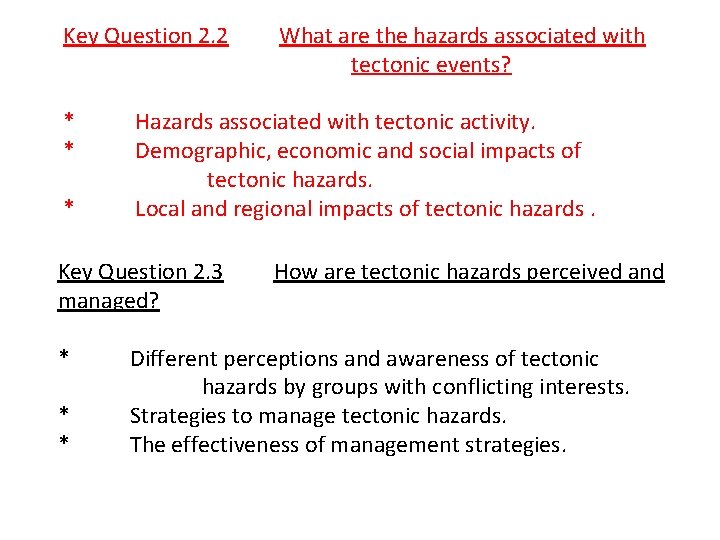
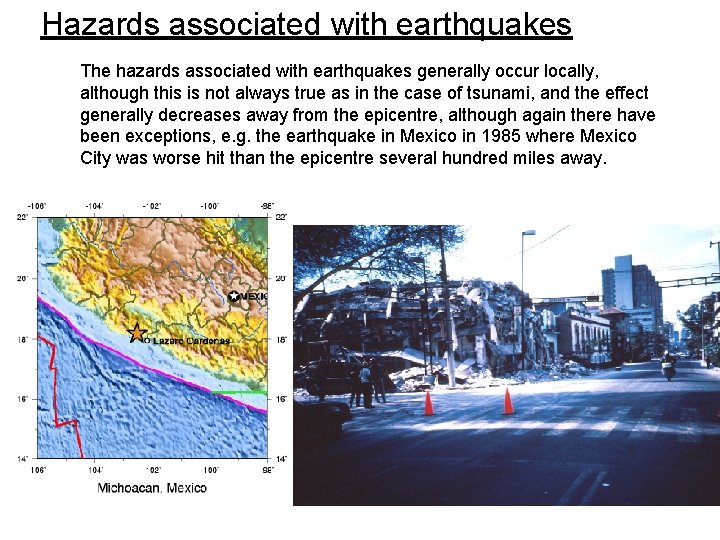
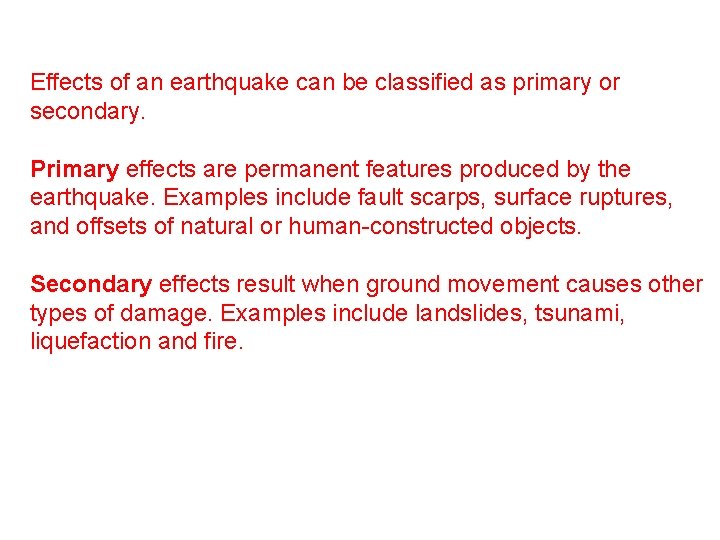
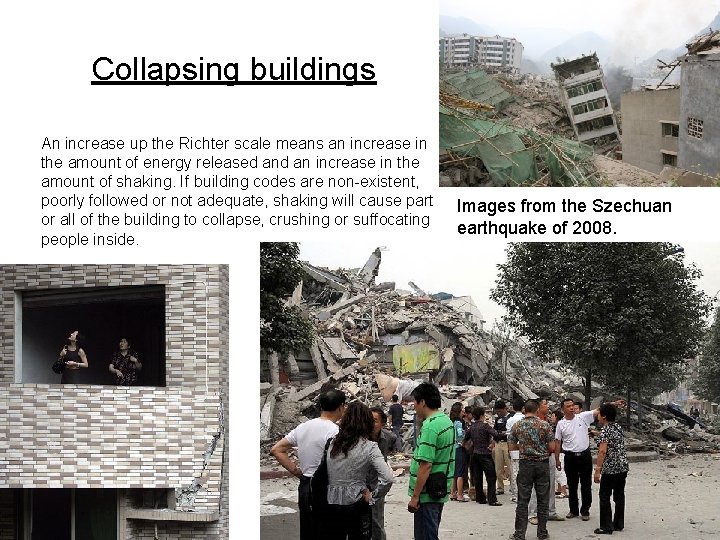
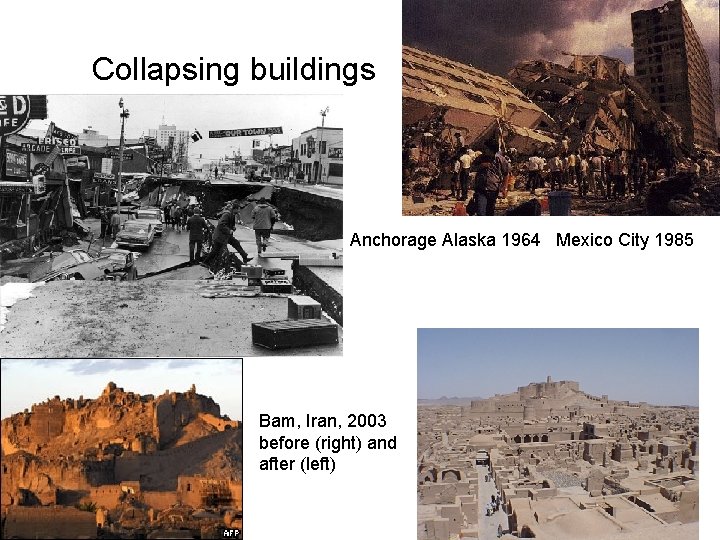
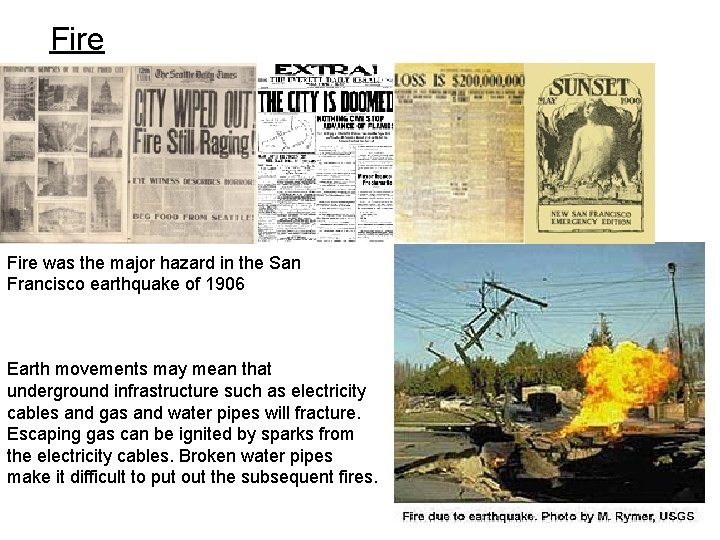
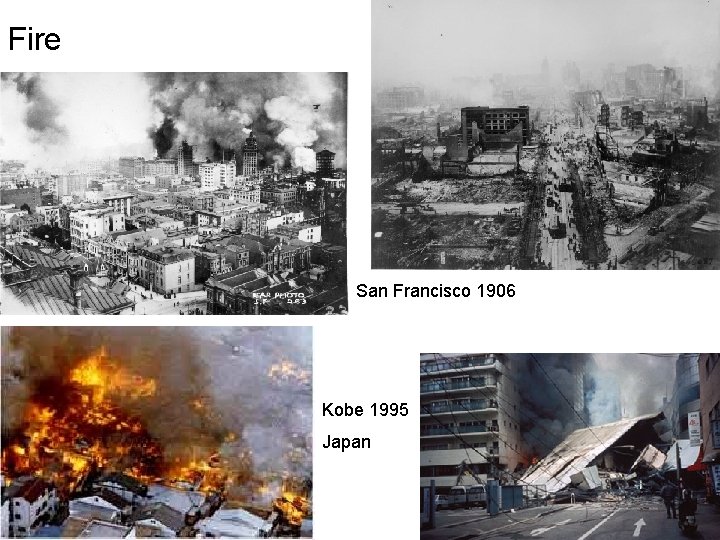
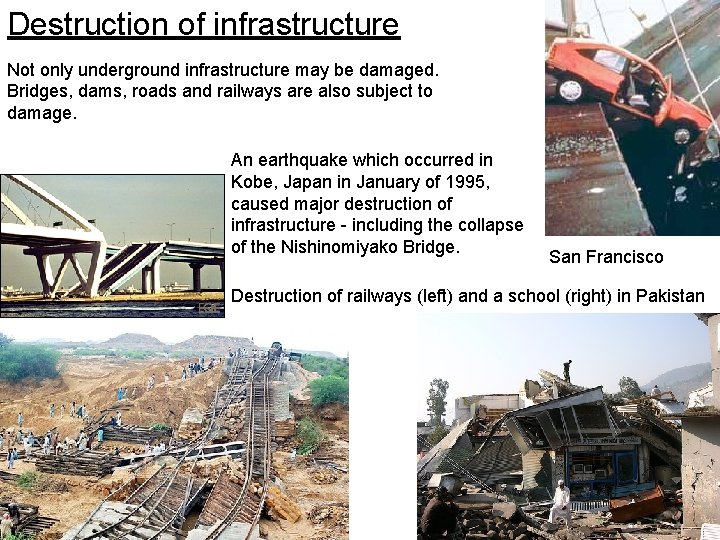
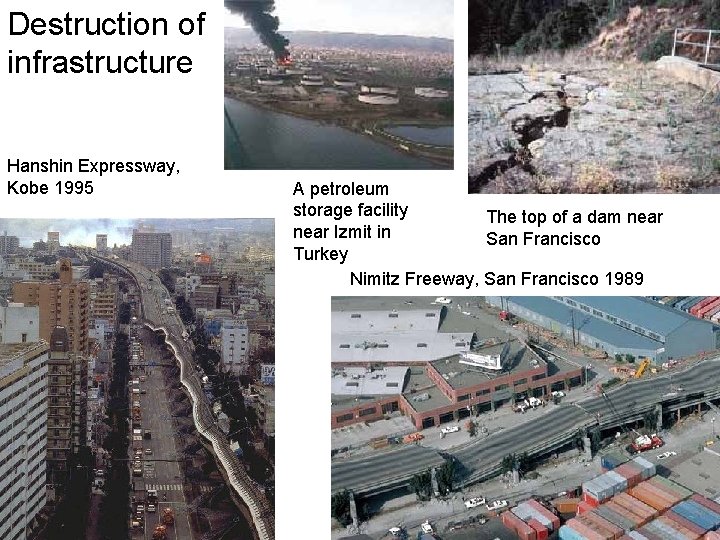
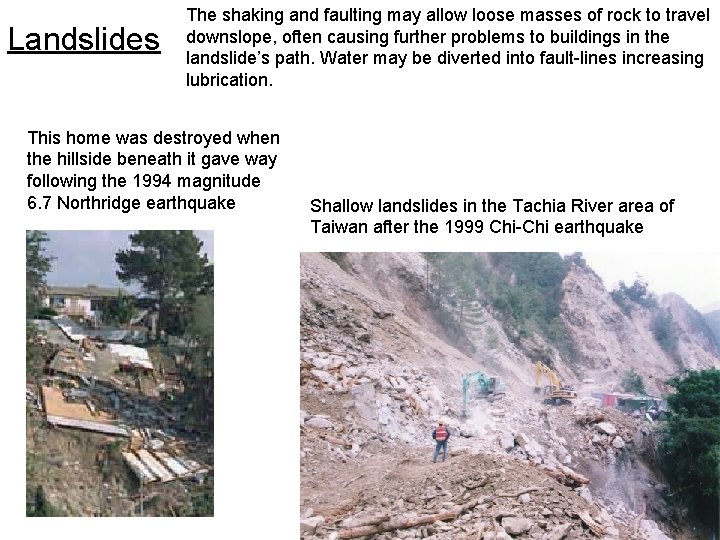
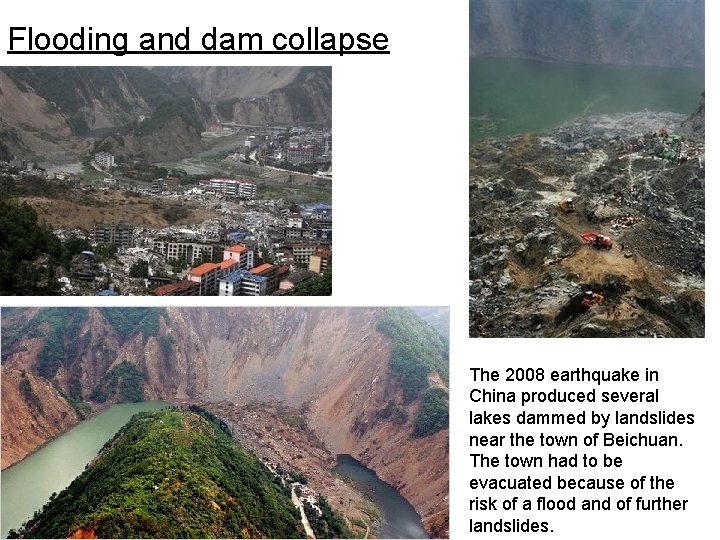
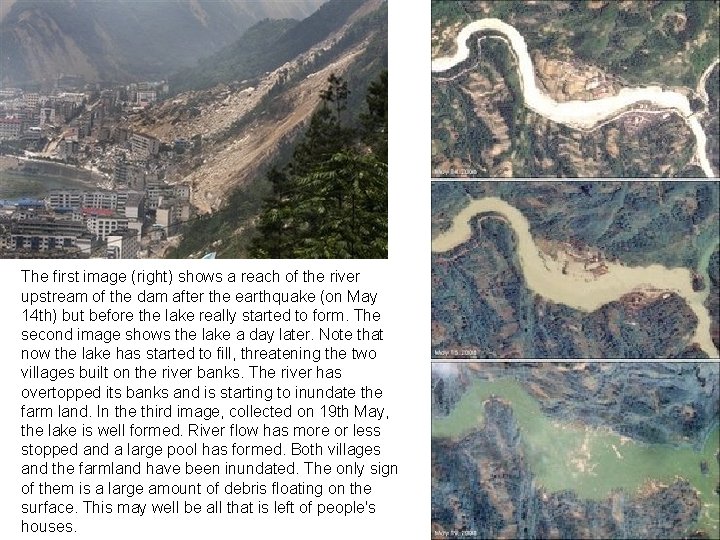
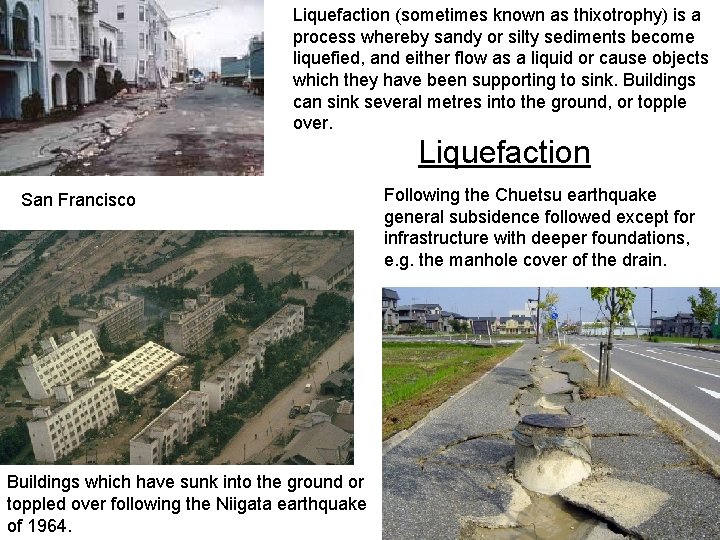
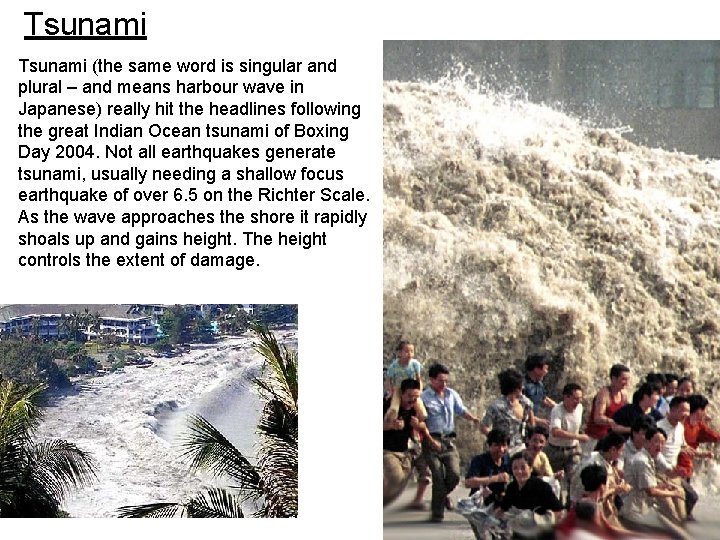
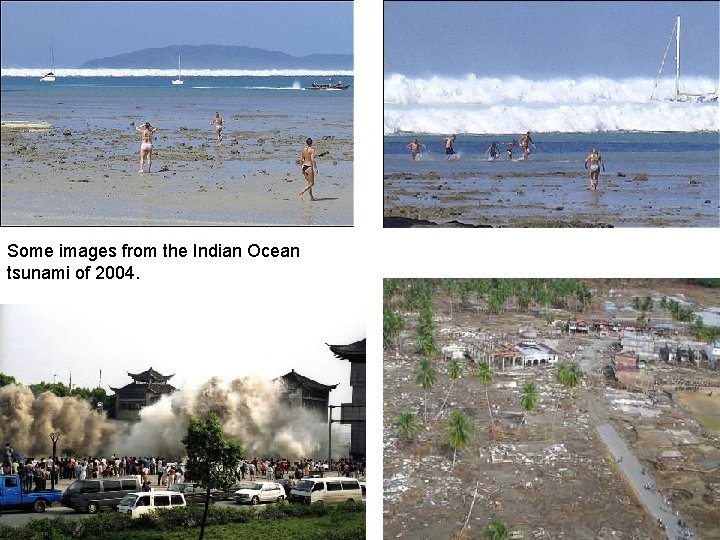
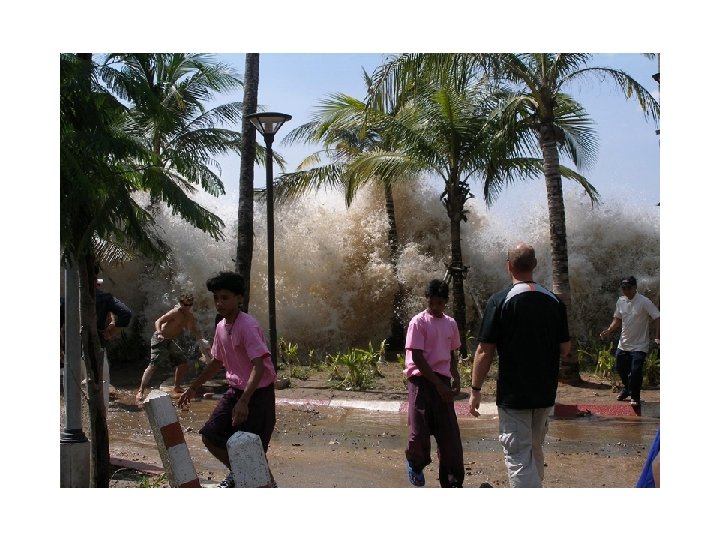
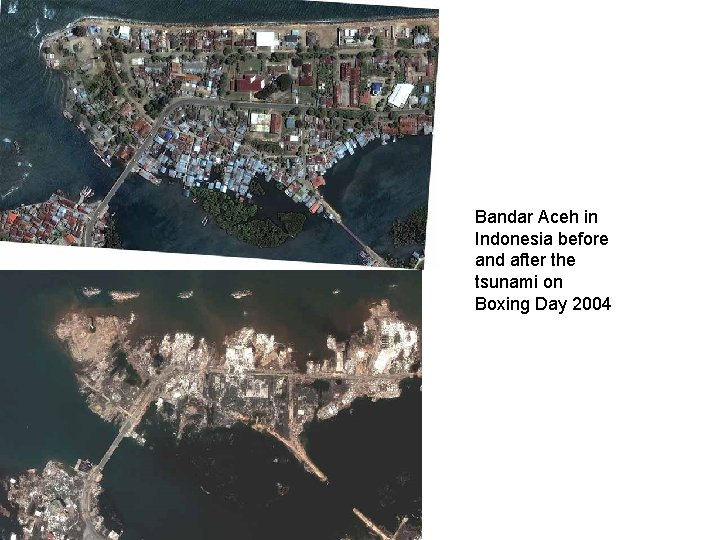
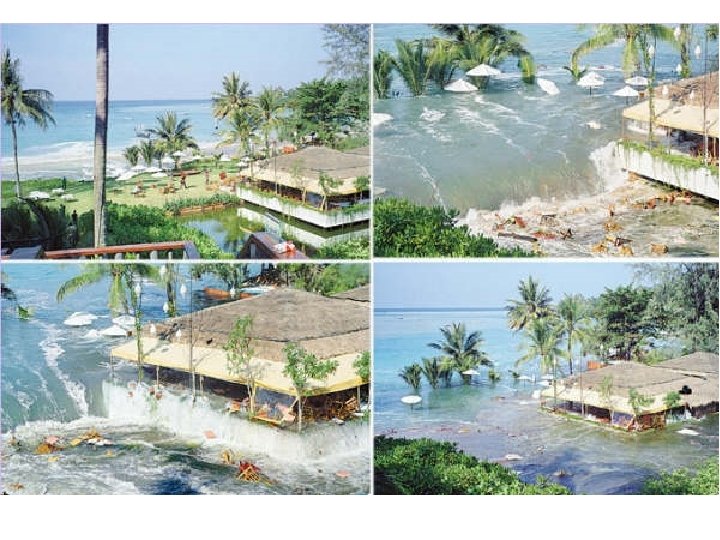
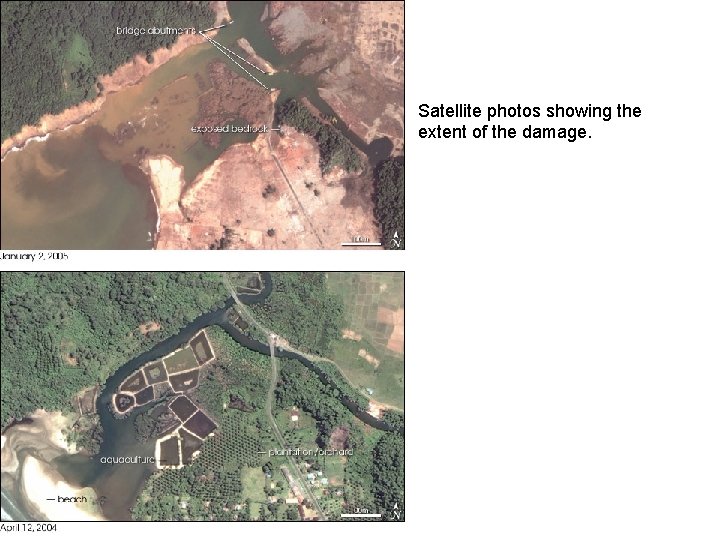
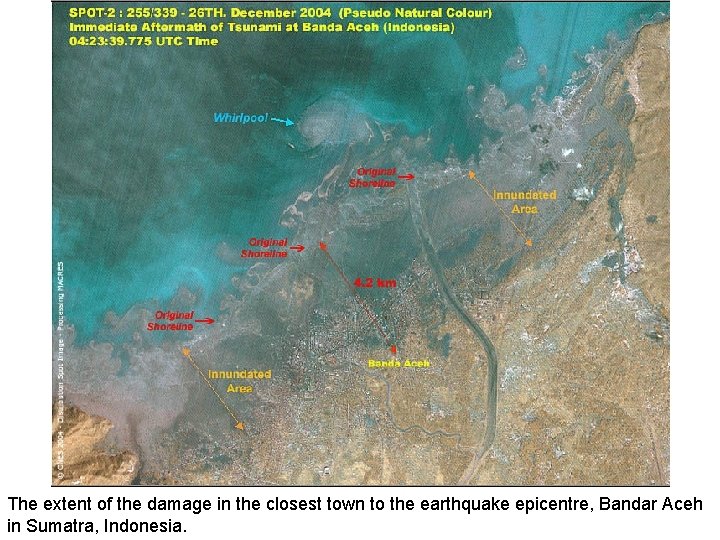
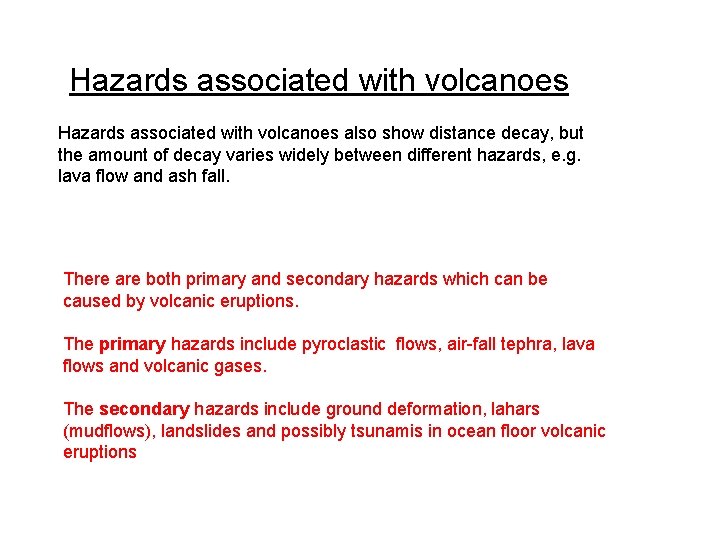
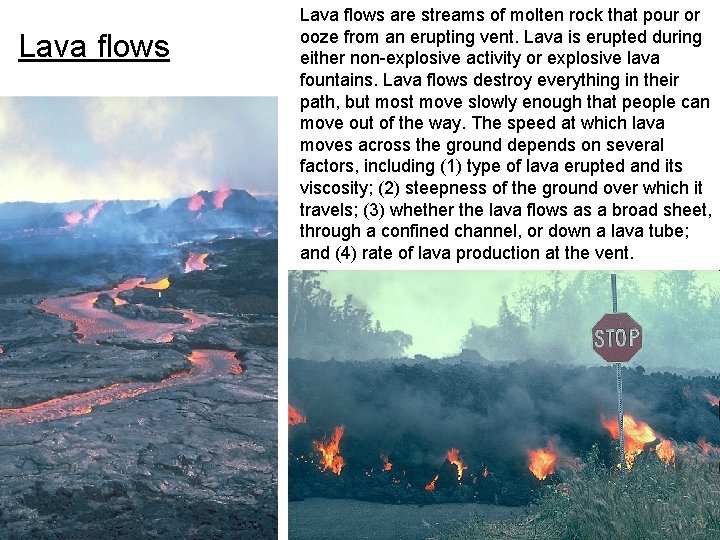
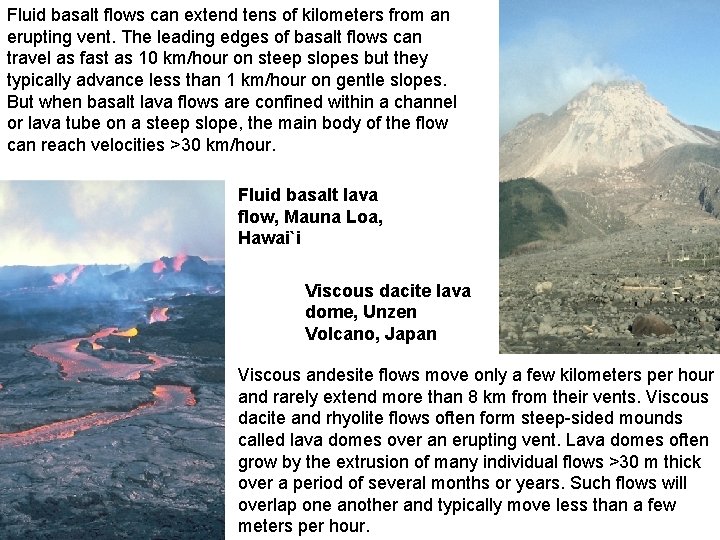
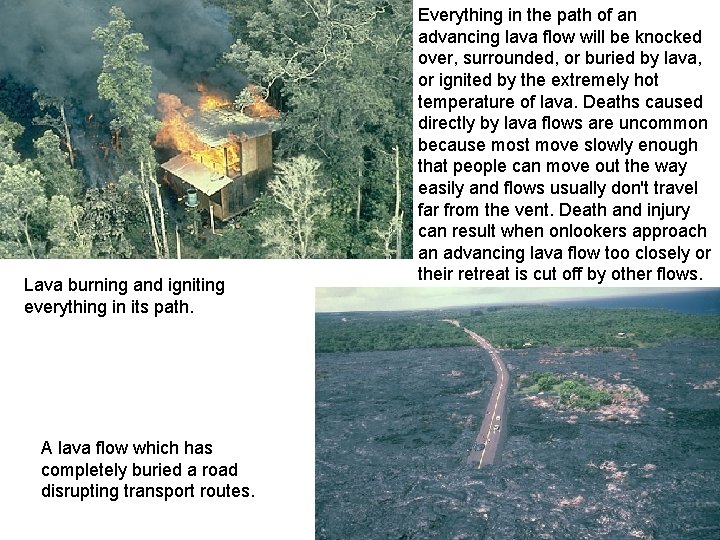
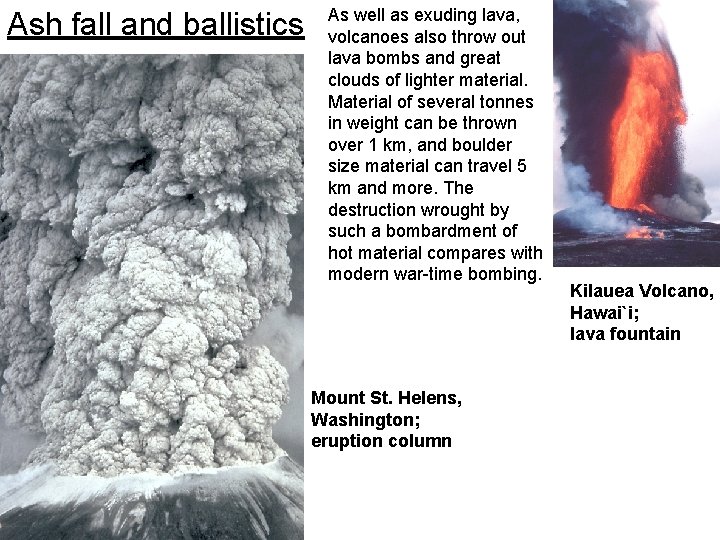
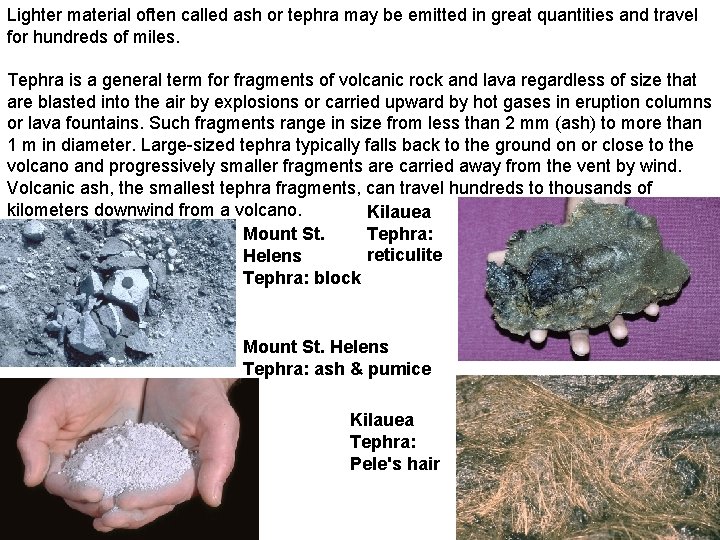
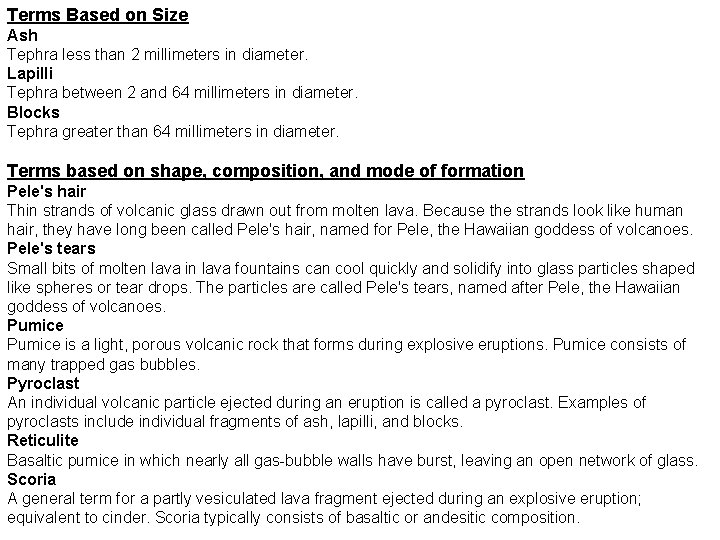
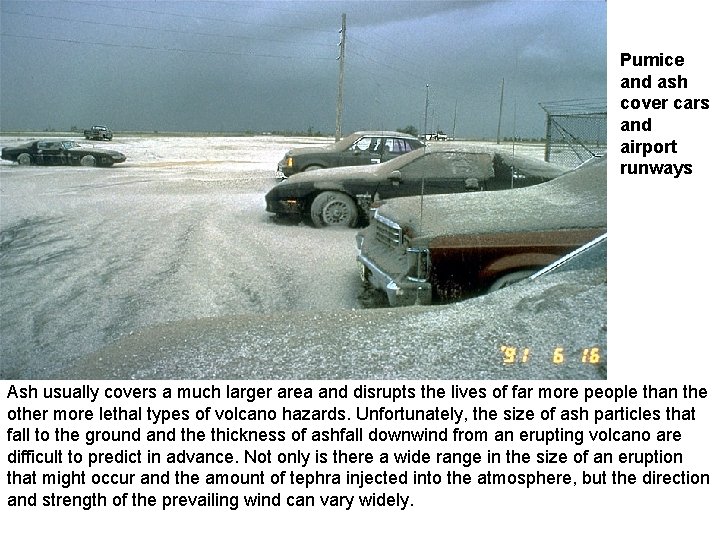
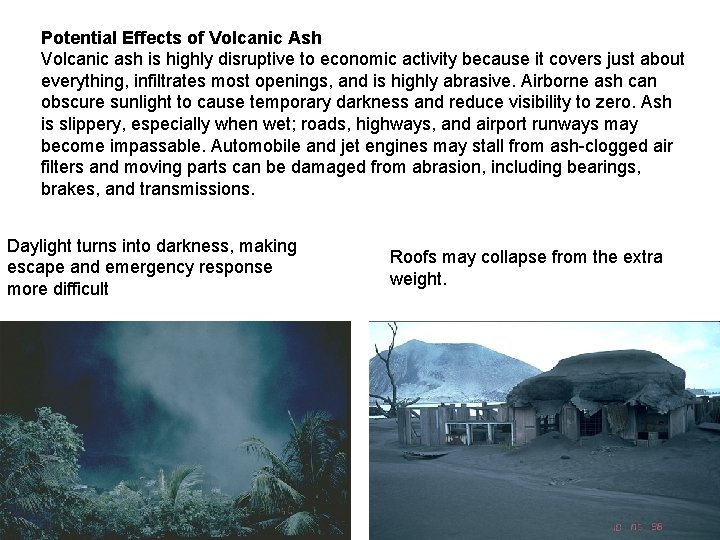
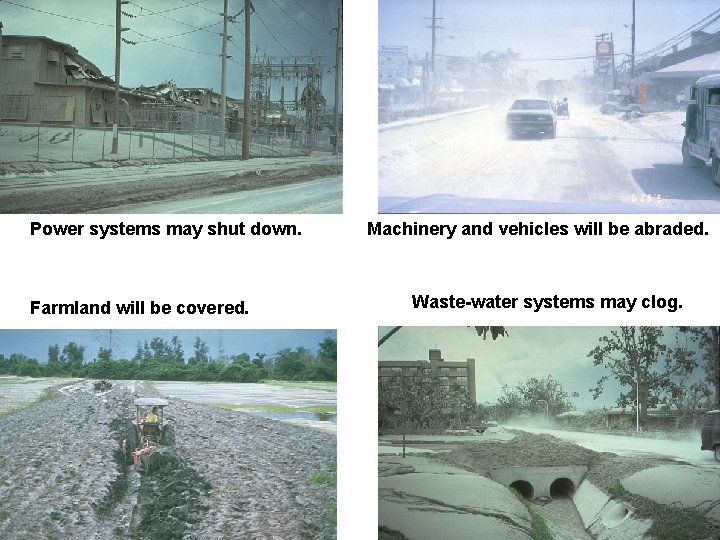
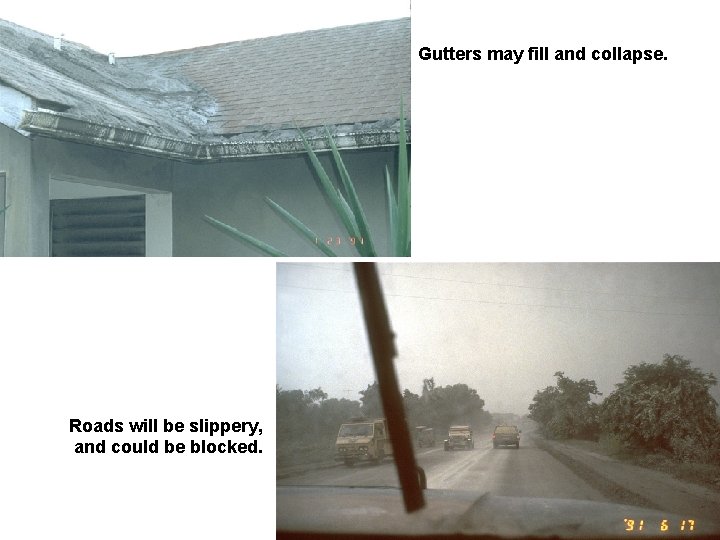
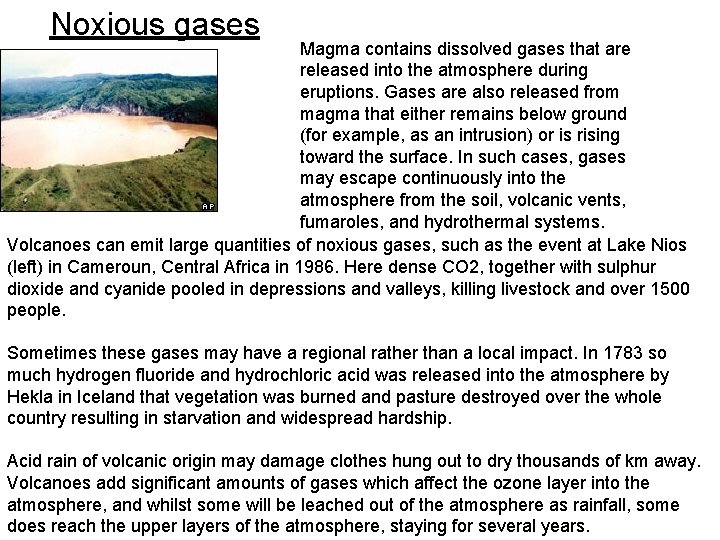
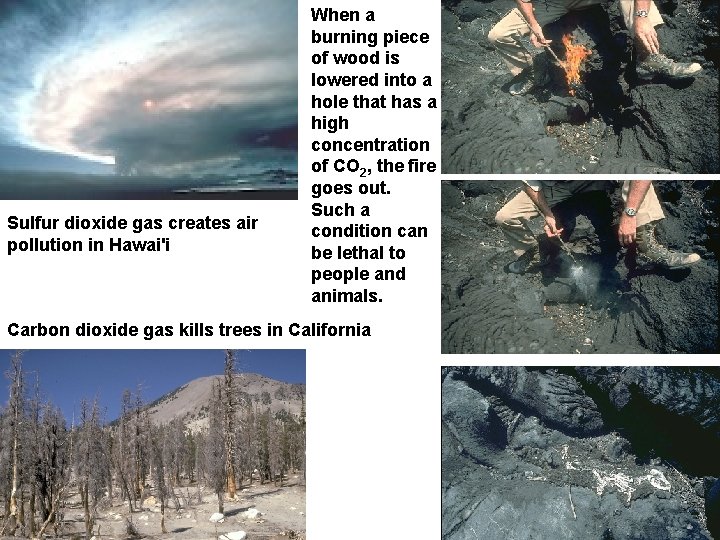
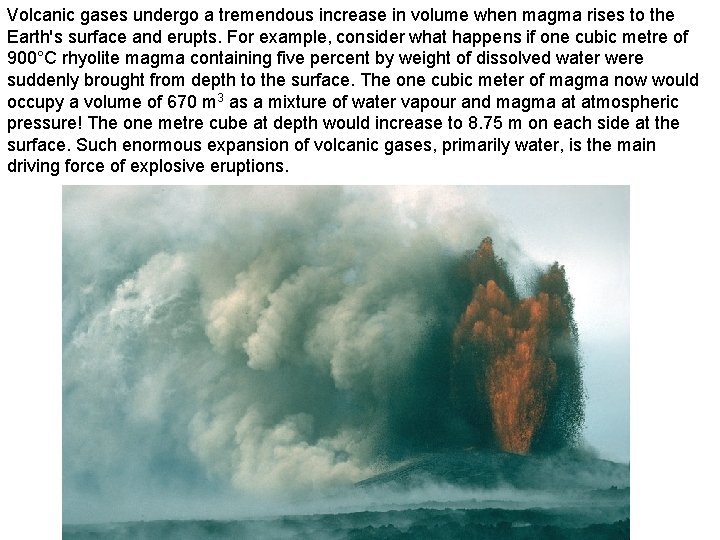
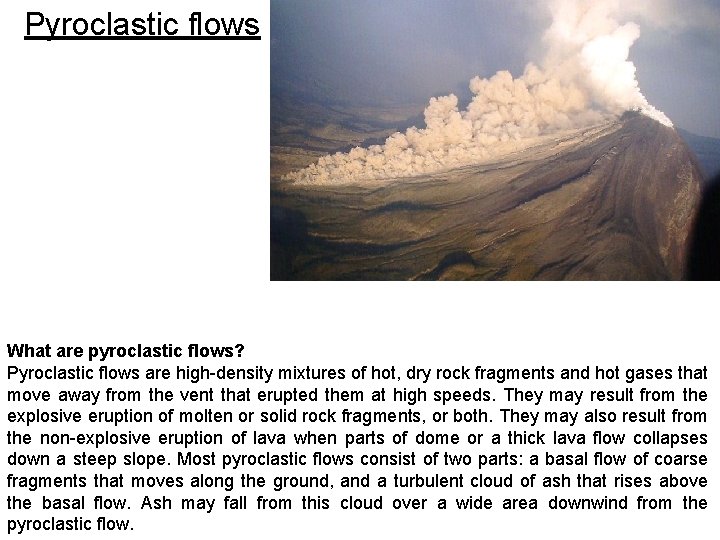
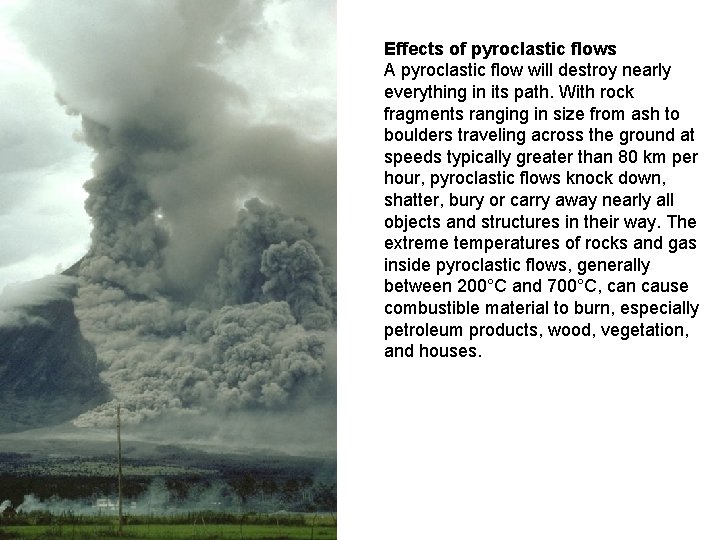
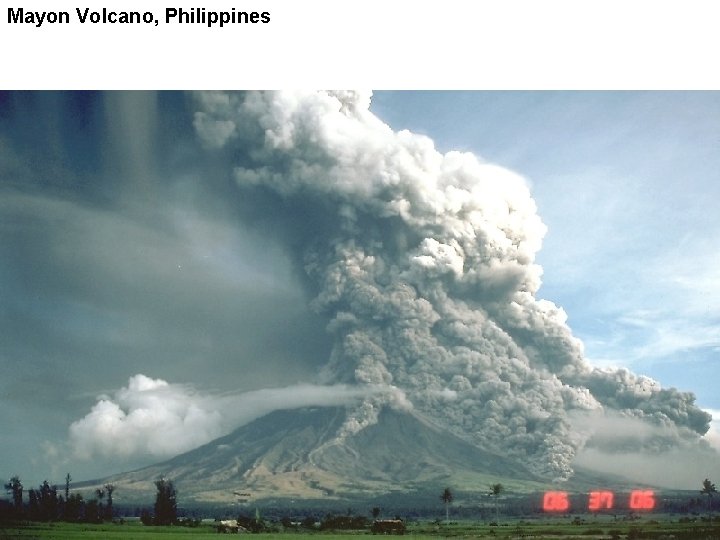
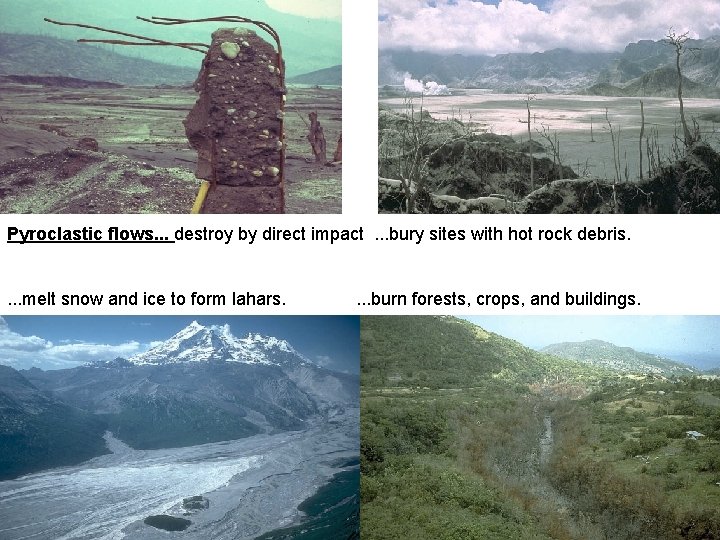
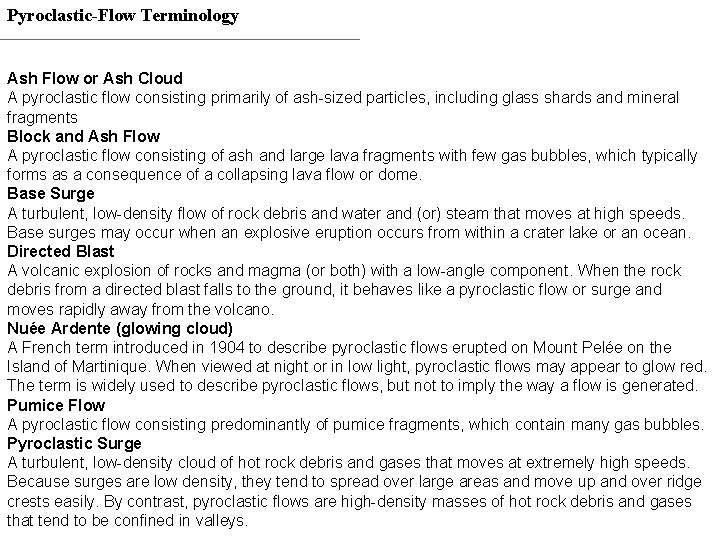
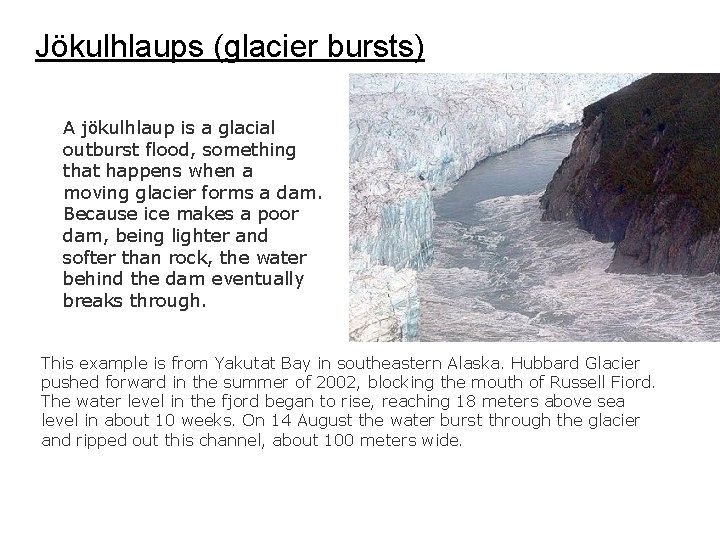
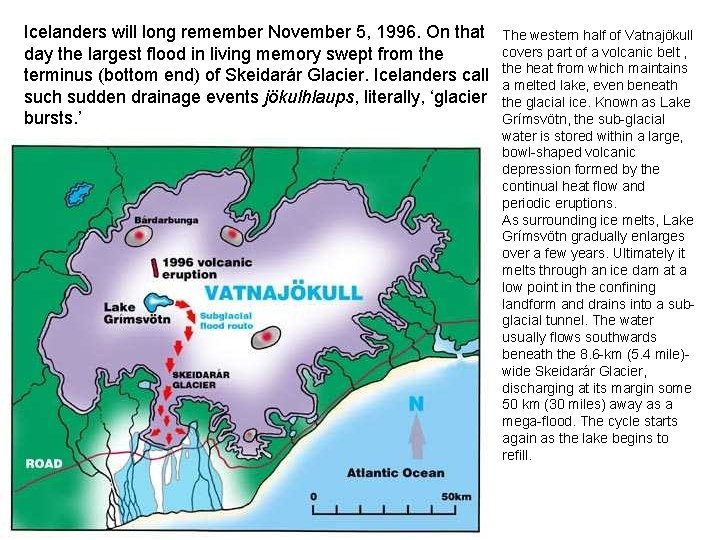
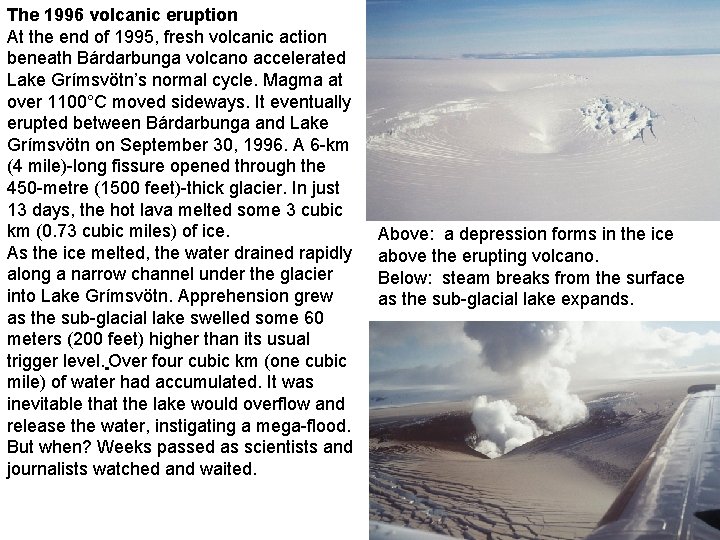
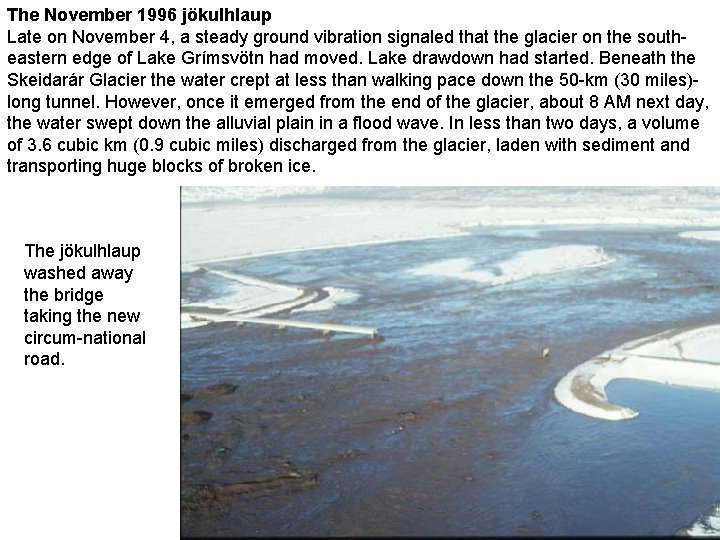
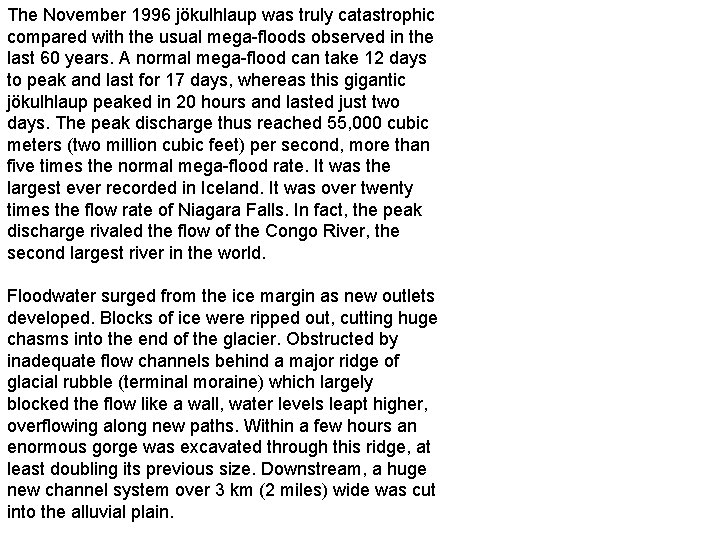
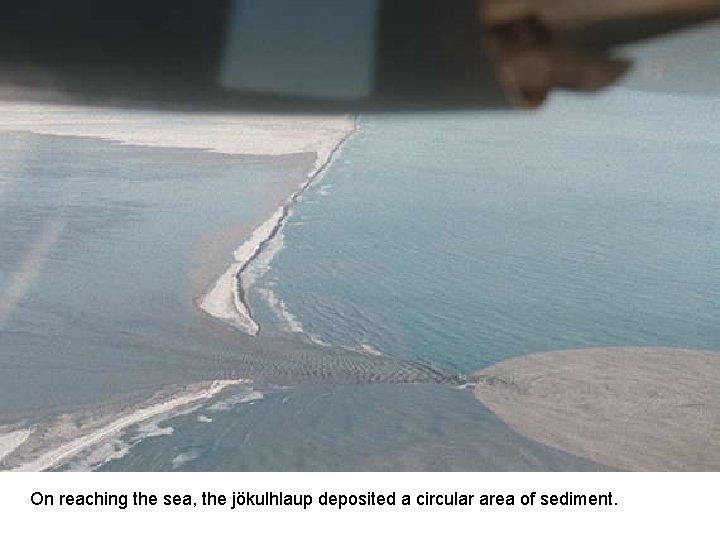
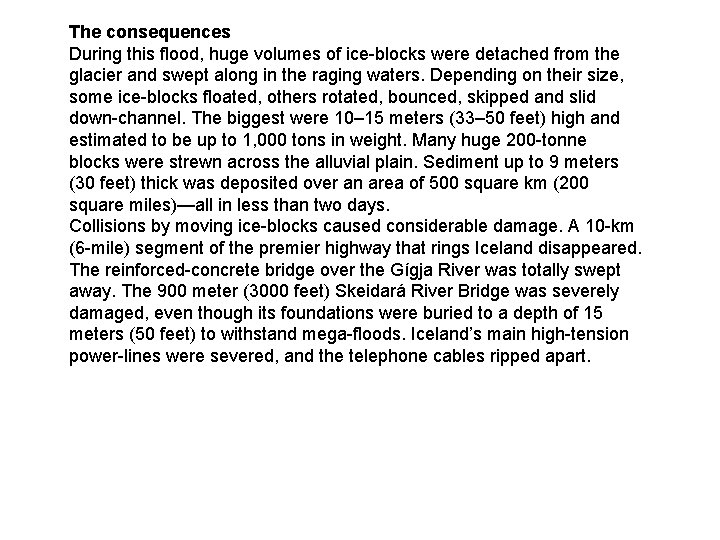
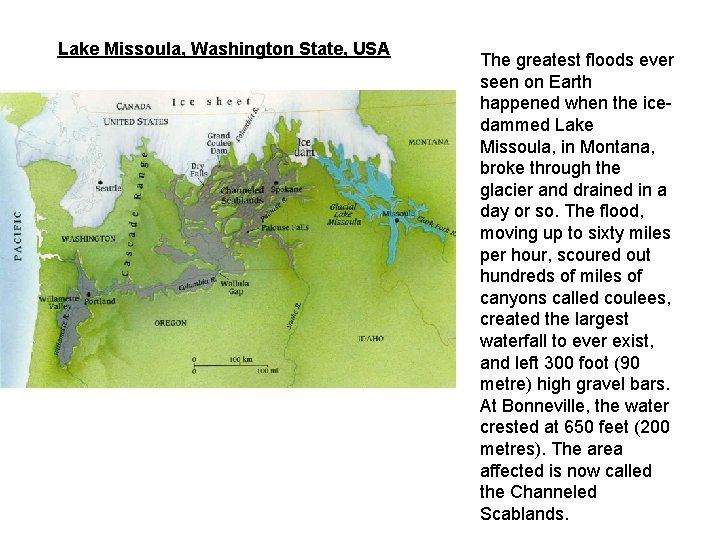
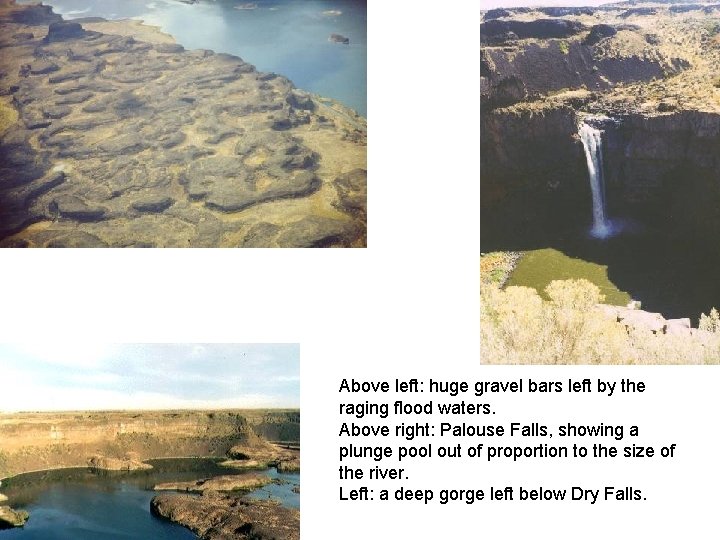
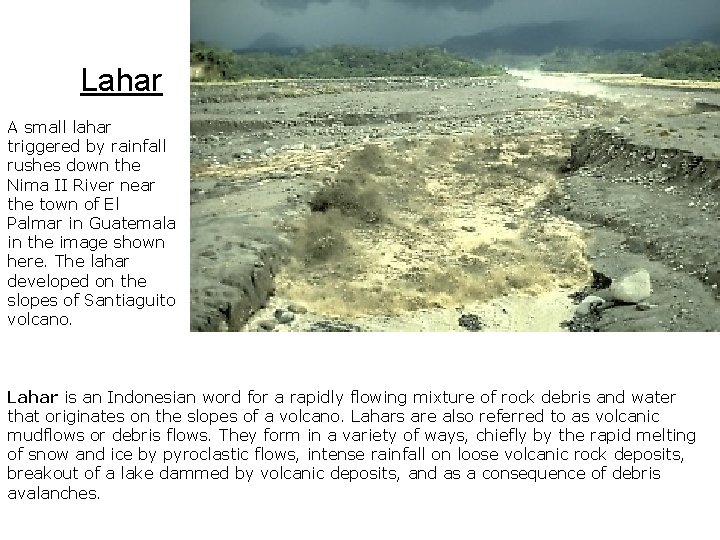
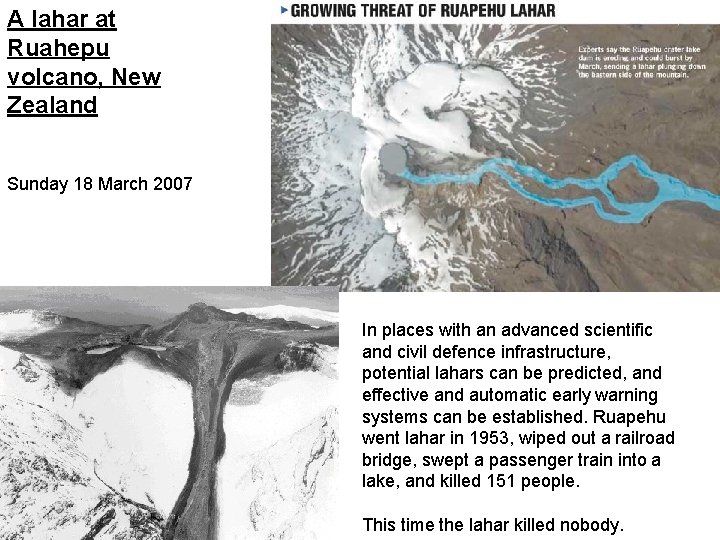
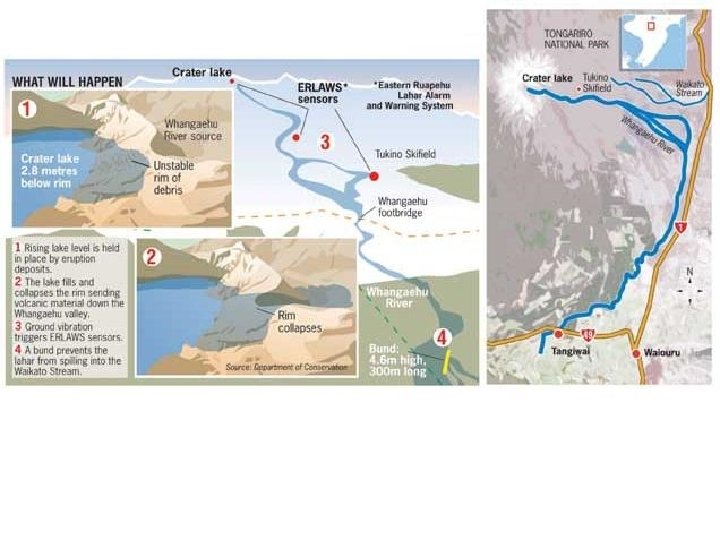
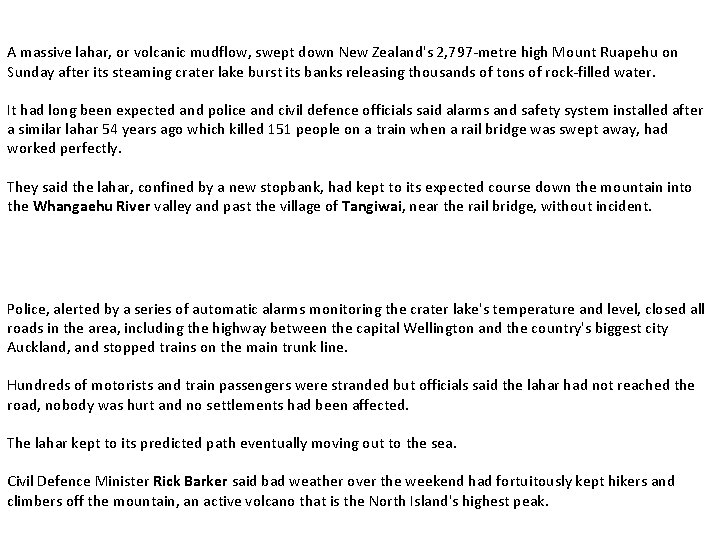
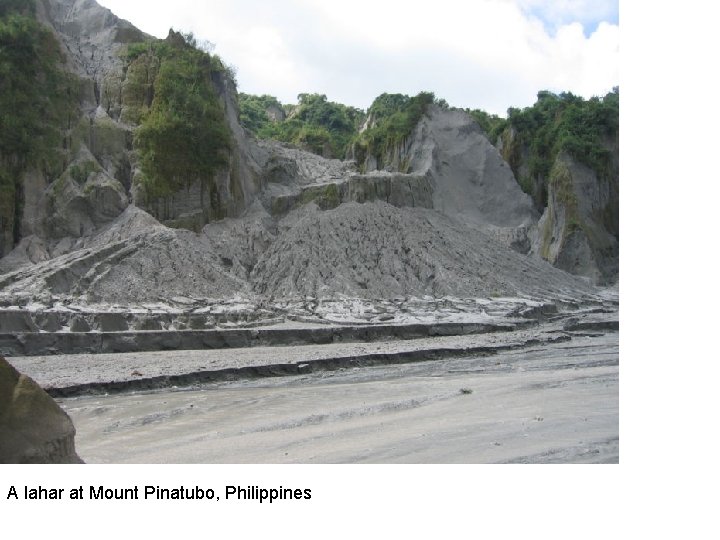
![Impacts of tectonic hazards [1] Demographic, [2] Economic, [3] Social 2008 Sichuan earthquake Date Impacts of tectonic hazards [1] Demographic, [2] Economic, [3] Social 2008 Sichuan earthquake Date](https://slidetodoc.com/presentation_image_h/d020a7159aa58ff4c55a30a84261b44e/image-56.jpg)
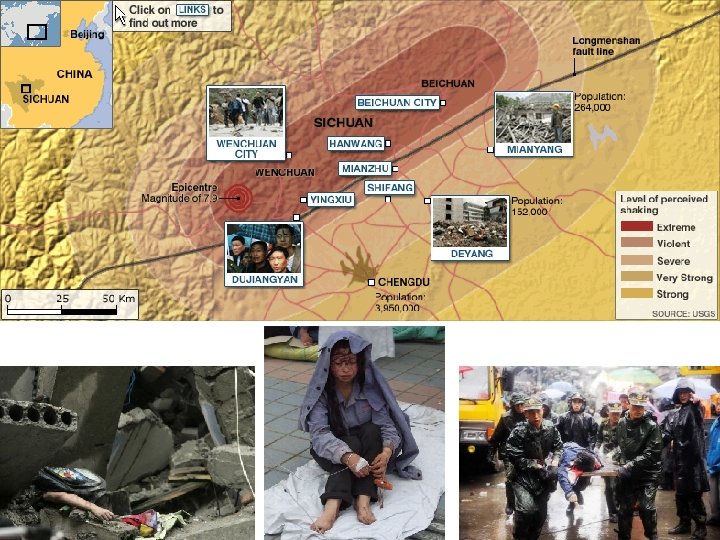
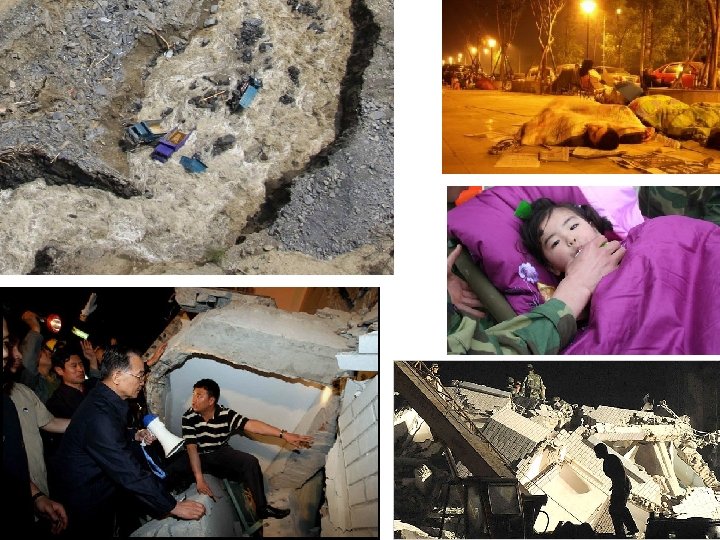
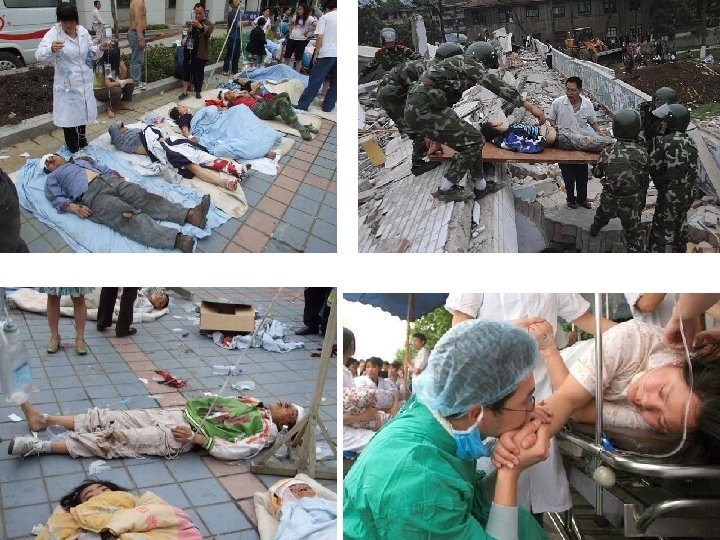
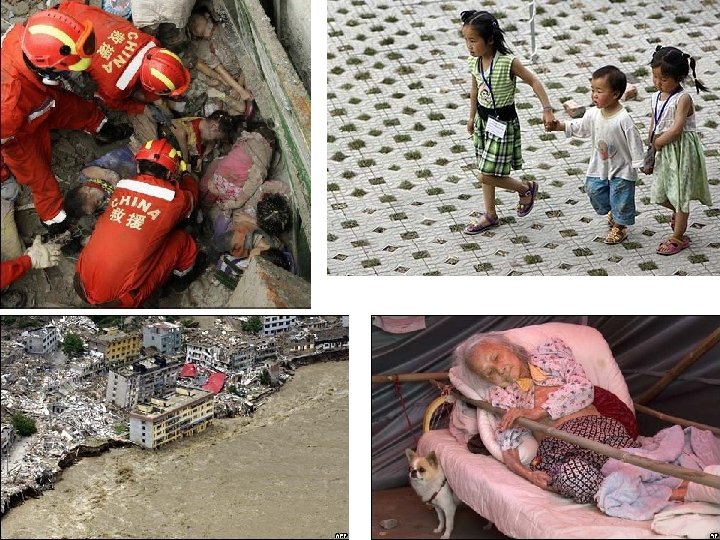
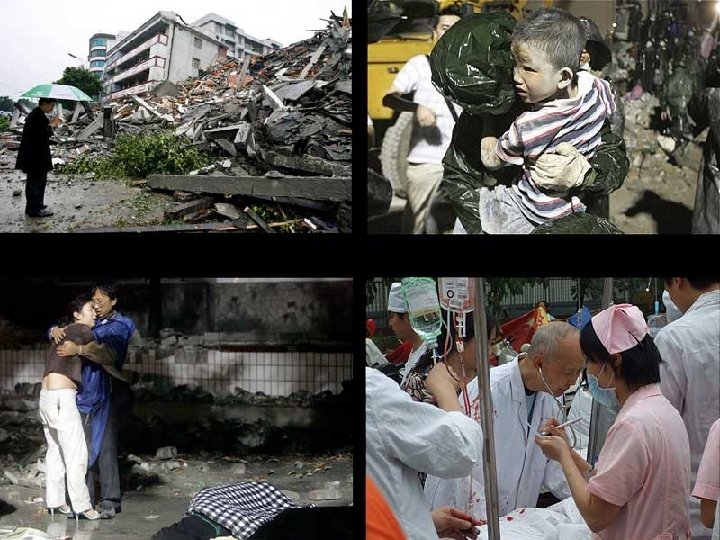
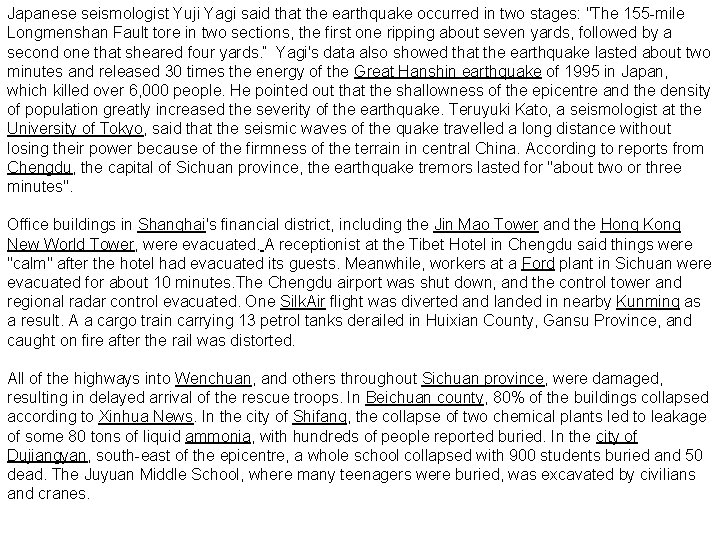
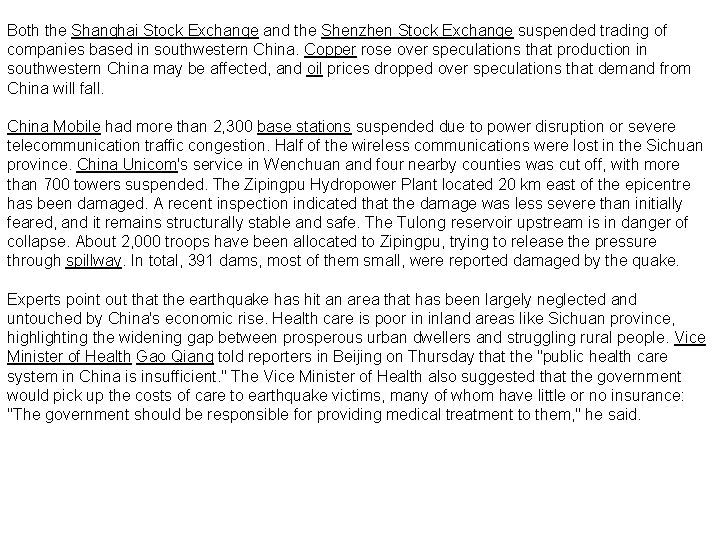
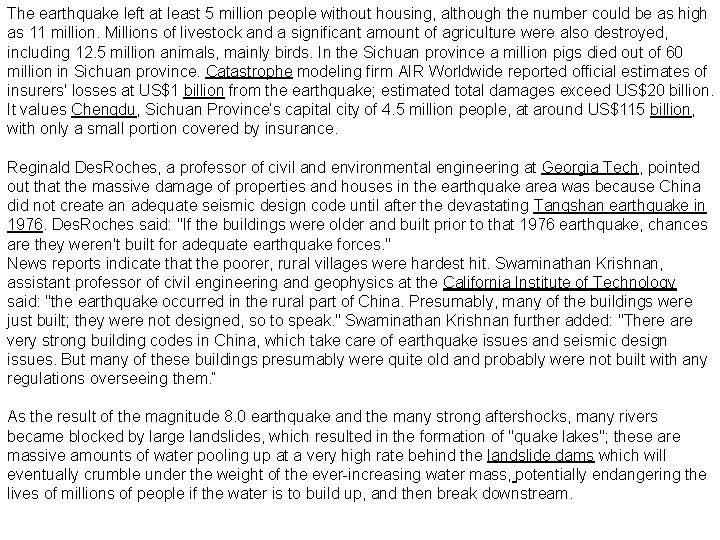
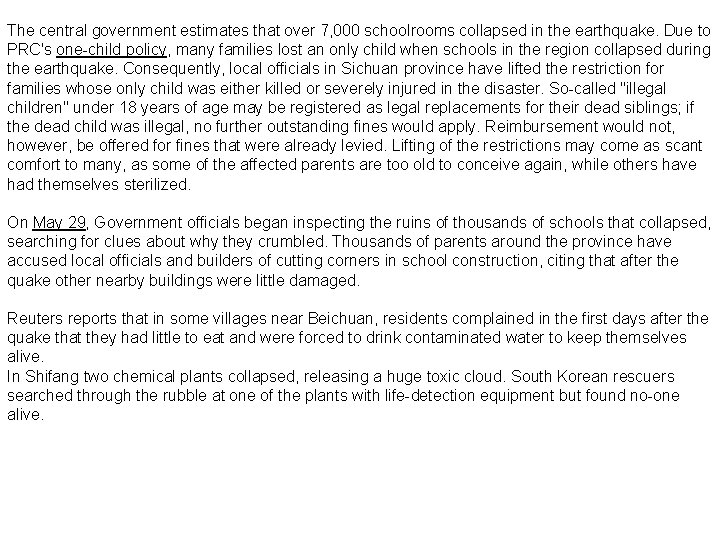
![Local and regional impacts of tectonic hazards Case study [1] Montserrat The impact of Local and regional impacts of tectonic hazards Case study [1] Montserrat The impact of](https://slidetodoc.com/presentation_image_h/d020a7159aa58ff4c55a30a84261b44e/image-66.jpg)
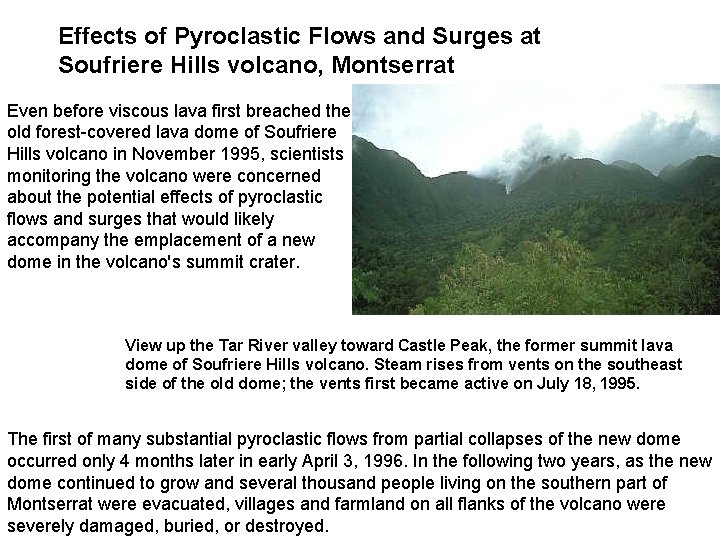
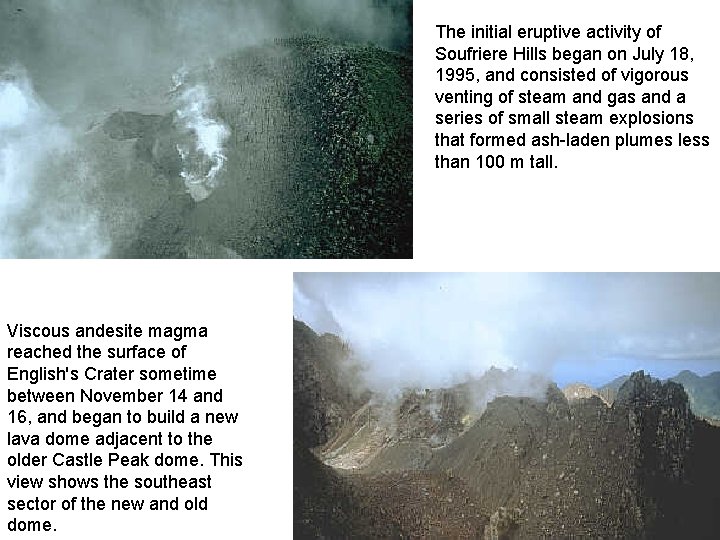
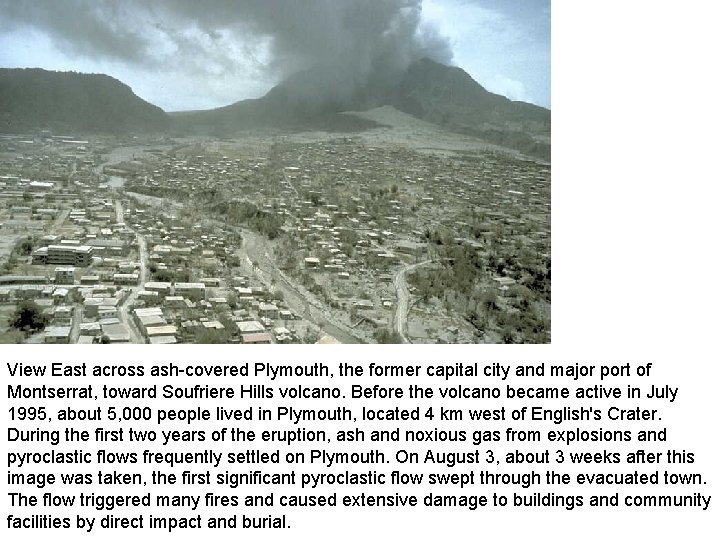
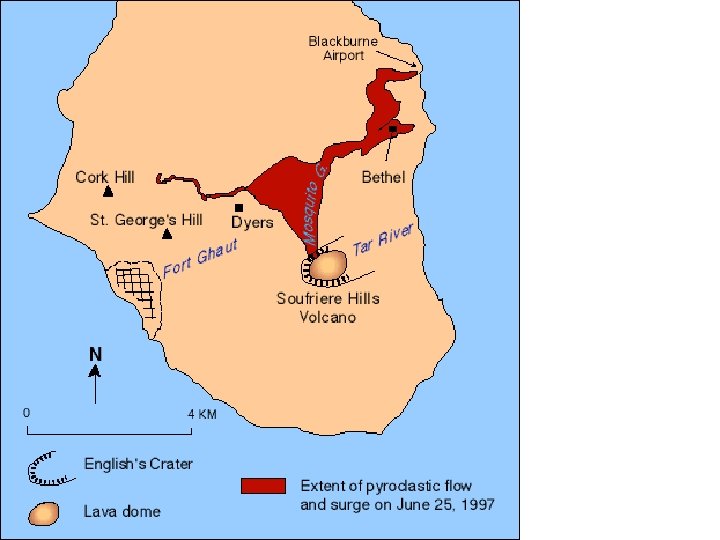
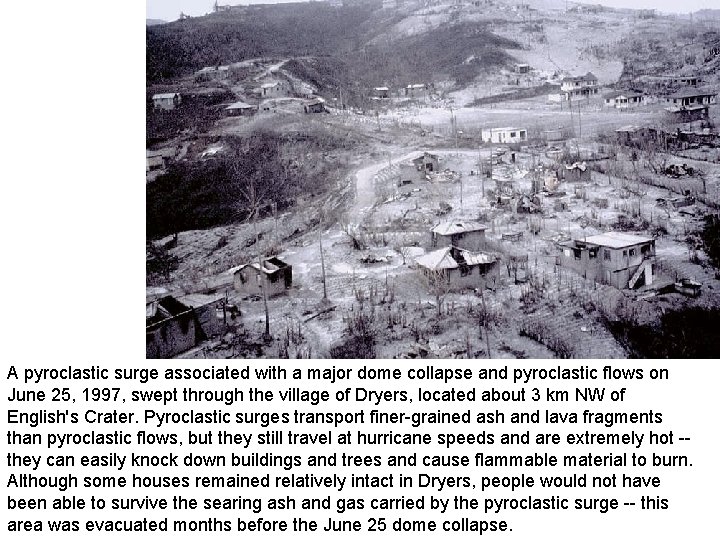
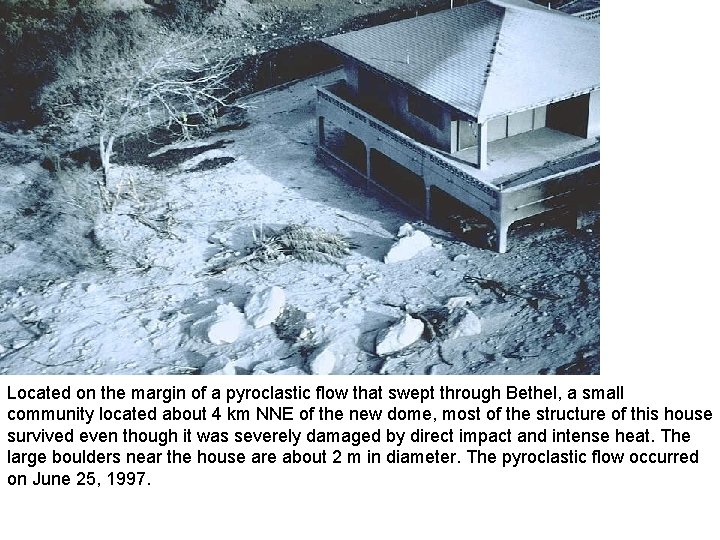
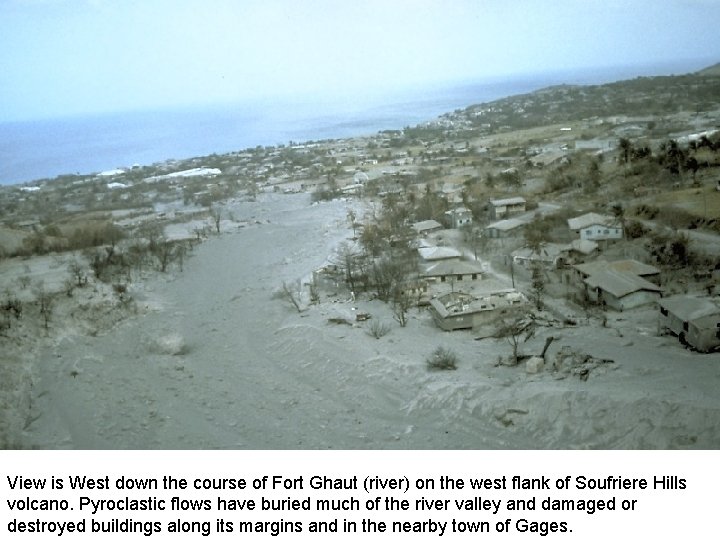
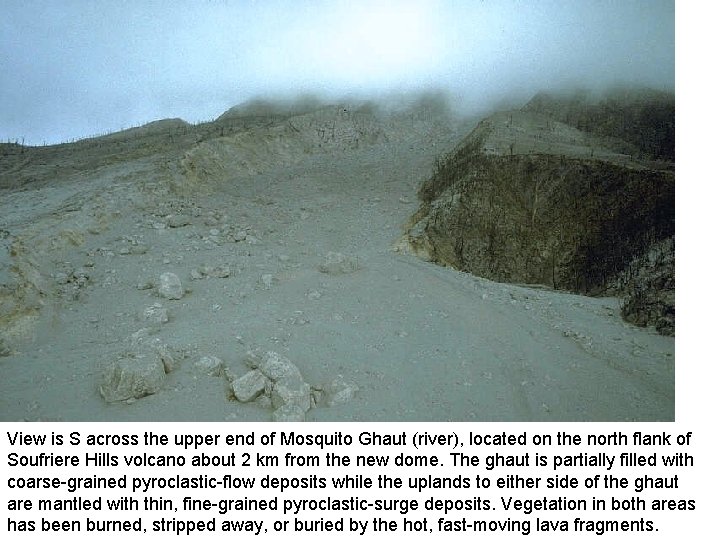
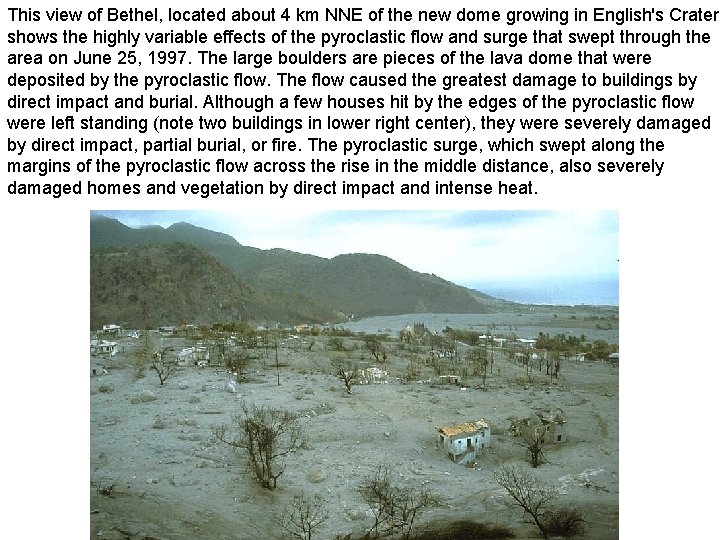
![Local and regional impacts of tectonic hazards Case study [2] Nevado del Ruiz The Local and regional impacts of tectonic hazards Case study [2] Nevado del Ruiz The](https://slidetodoc.com/presentation_image_h/d020a7159aa58ff4c55a30a84261b44e/image-76.jpg)
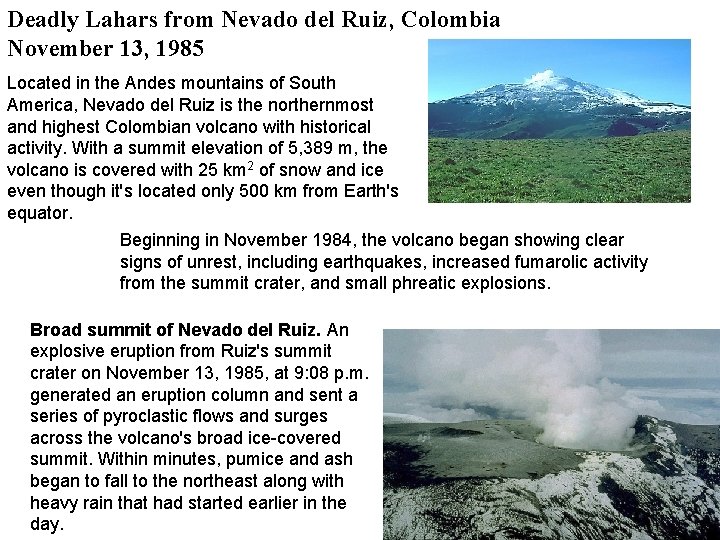
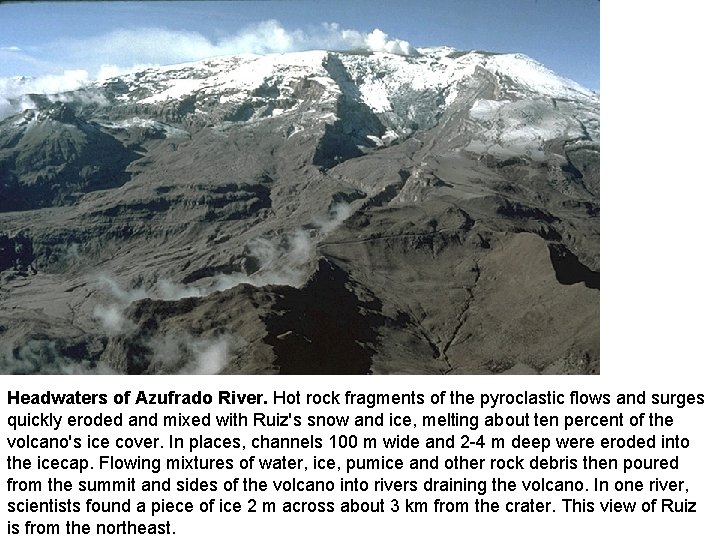
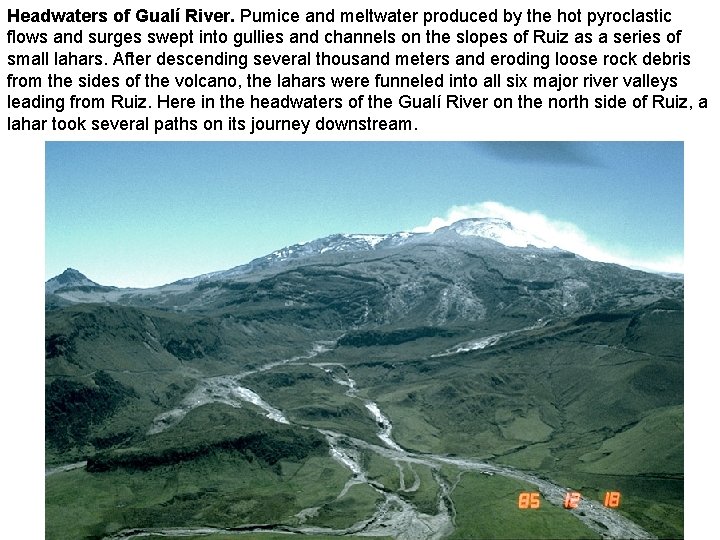
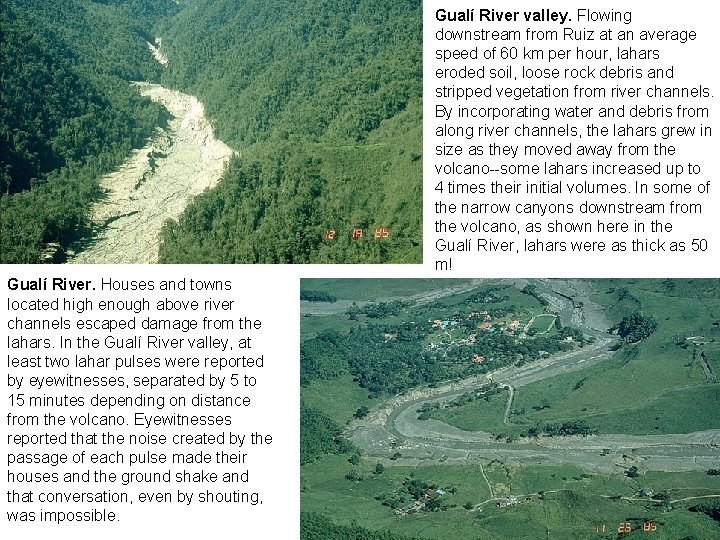
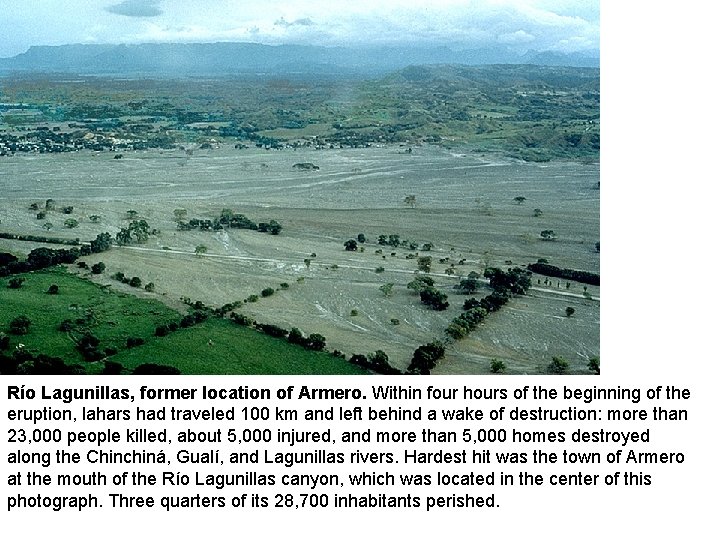
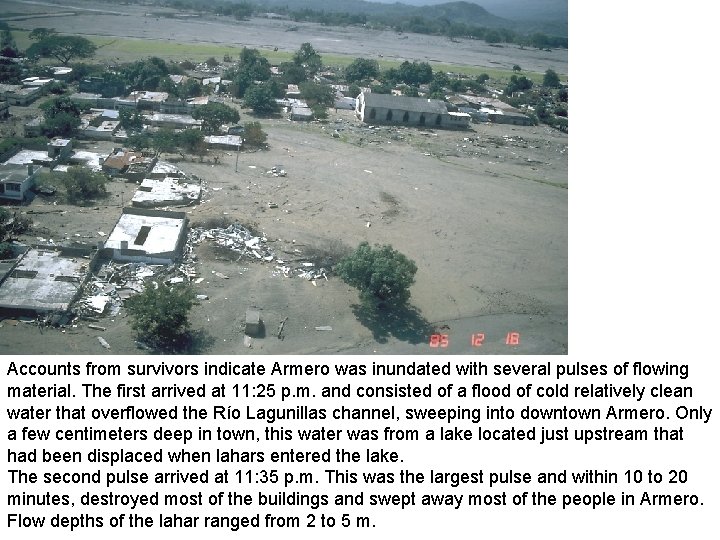
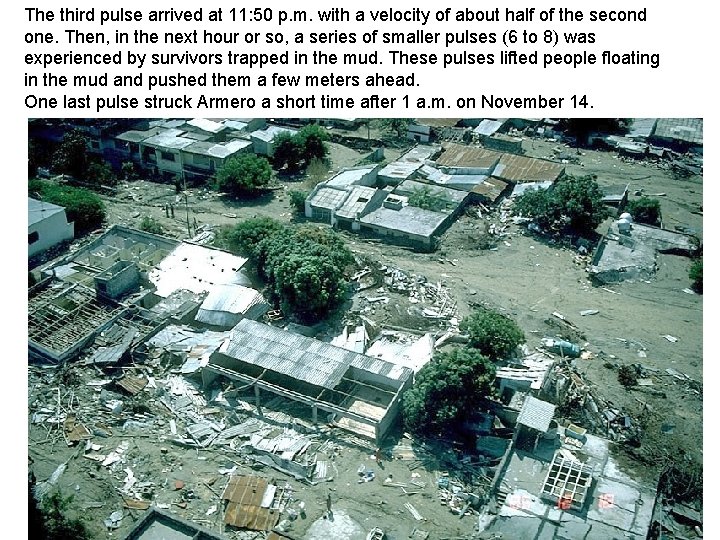
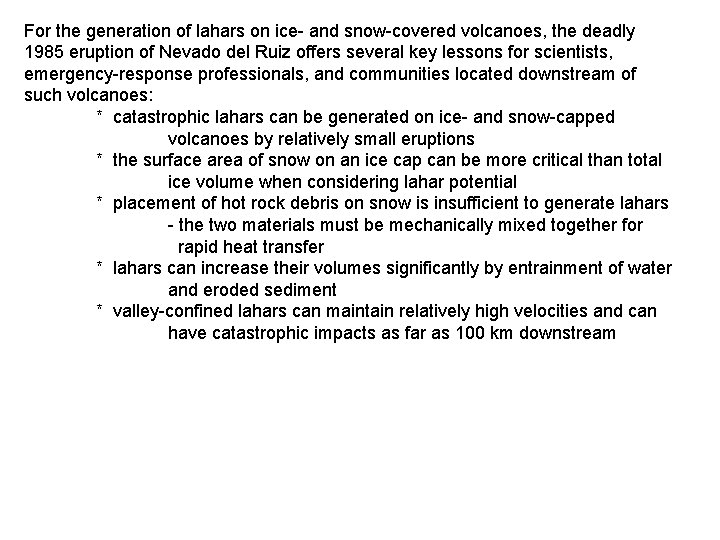
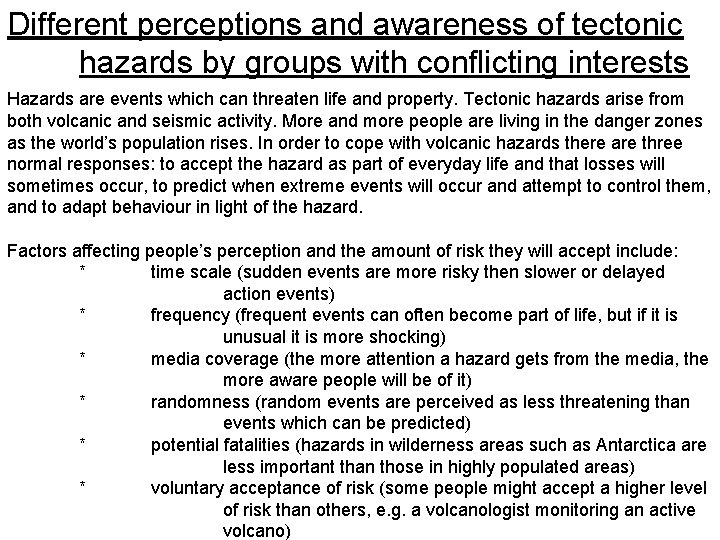
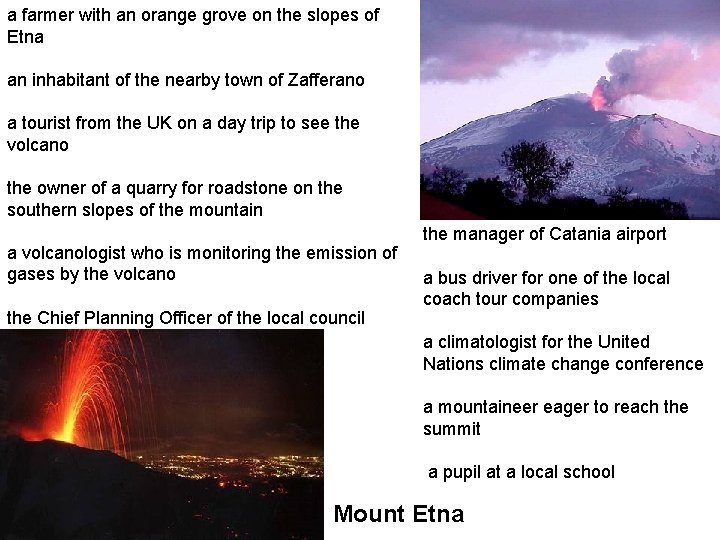
- Slides: 86
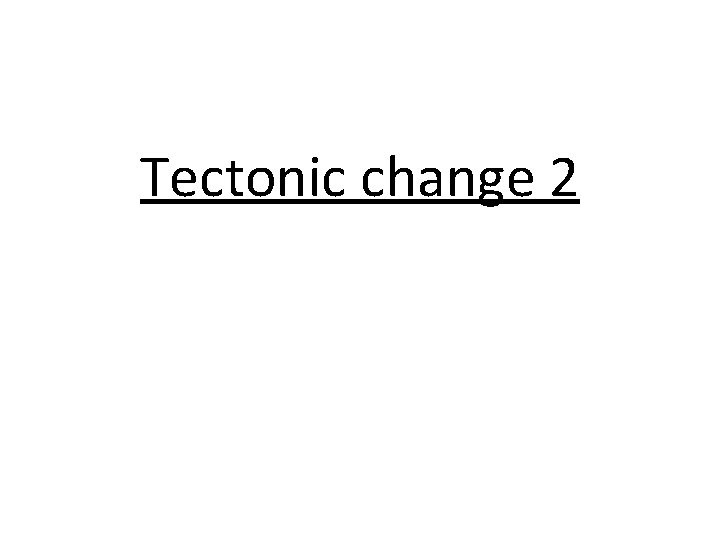
Tectonic change 2
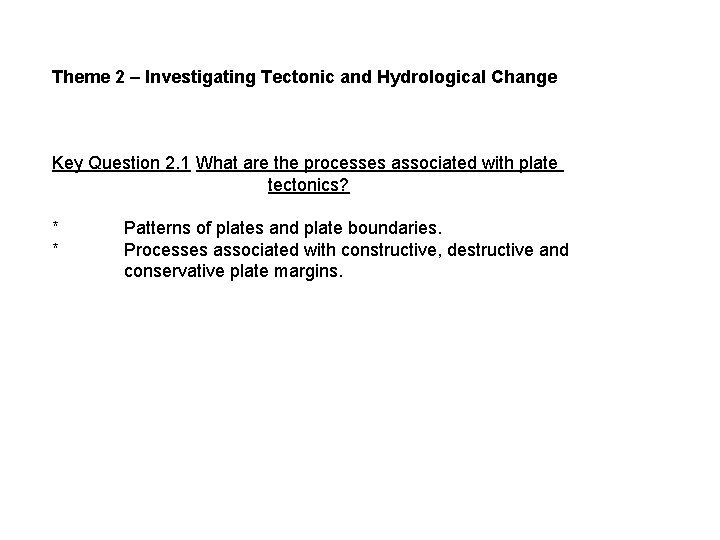
Theme 2 – Investigating Tectonic and Hydrological Change Key Question 2. 1 What are the processes associated with plate tectonics? * Patterns of plates and plate boundaries. * Processes associated with constructive, destructive and conservative plate margins.
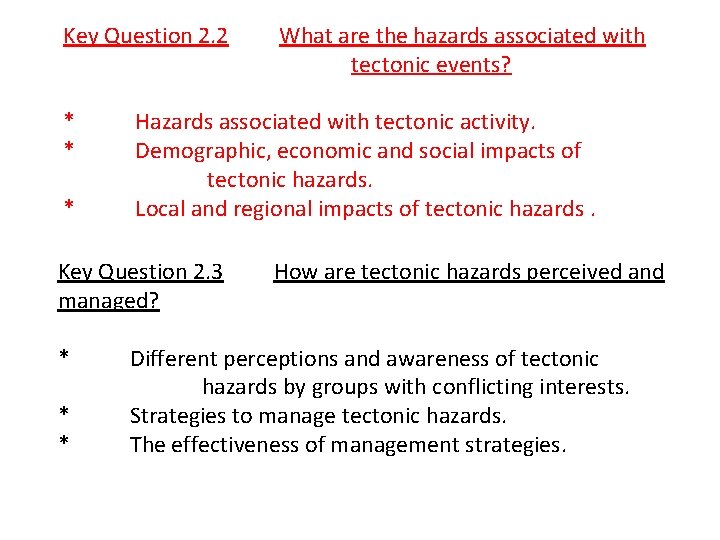
Key Question 2. 2 * * * Hazards associated with tectonic activity. Demographic, economic and social impacts of tectonic hazards. Local and regional impacts of tectonic hazards. Key Question 2. 3 managed? * * * What are the hazards associated with tectonic events? How are tectonic hazards perceived and Different perceptions and awareness of tectonic hazards by groups with conflicting interests. Strategies to manage tectonic hazards. The effectiveness of management strategies.
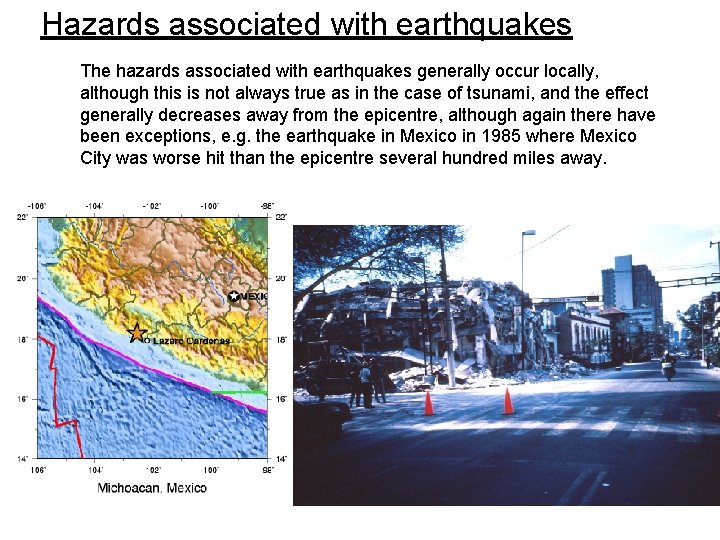
Hazards associated with earthquakes The hazards associated with earthquakes generally occur locally, although this is not always true as in the case of tsunami, and the effect generally decreases away from the epicentre, although again there have been exceptions, e. g. the earthquake in Mexico in 1985 where Mexico City was worse hit than the epicentre several hundred miles away.
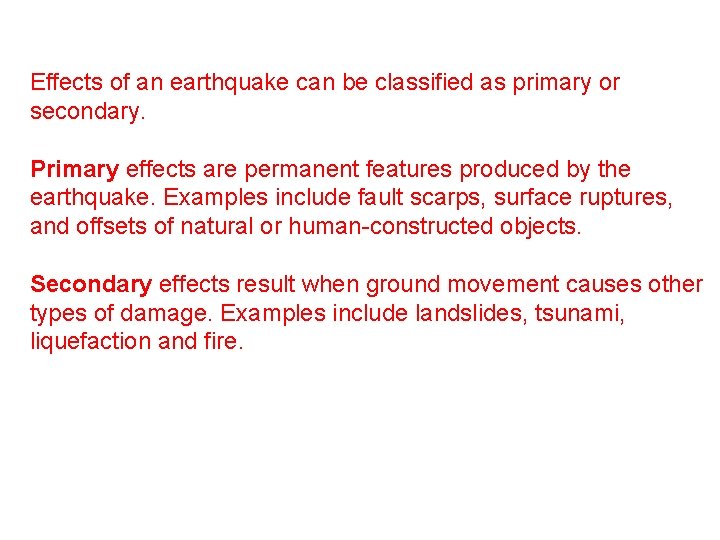
Effects of an earthquake can be classified as primary or secondary. Primary effects are permanent features produced by the earthquake. Examples include fault scarps, surface ruptures, and offsets of natural or human-constructed objects. Secondary effects result when ground movement causes other types of damage. Examples include landslides, tsunami, liquefaction and fire.
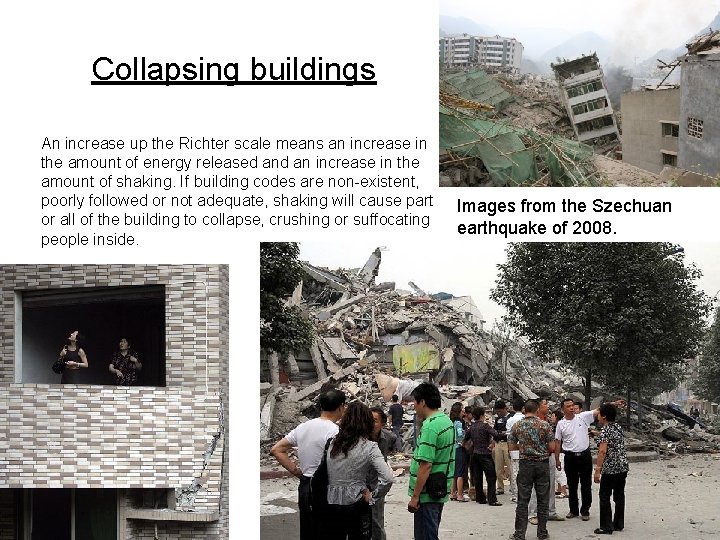
Collapsing buildings An increase up the Richter scale means an increase in the amount of energy released an increase in the amount of shaking. If building codes are non-existent, poorly followed or not adequate, shaking will cause part or all of the building to collapse, crushing or suffocating people inside. Images from the Szechuan earthquake of 2008.
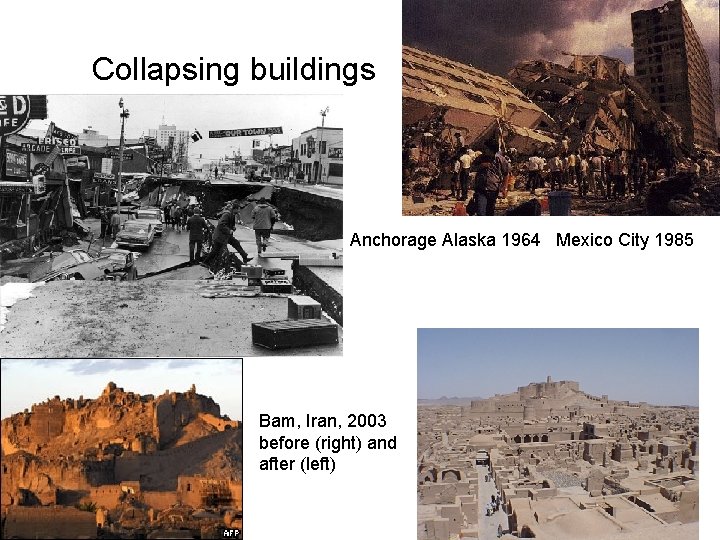
Collapsing buildings Anchorage Alaska 1964 Mexico City 1985 Bam, Iran, 2003 before (right) and after (left)
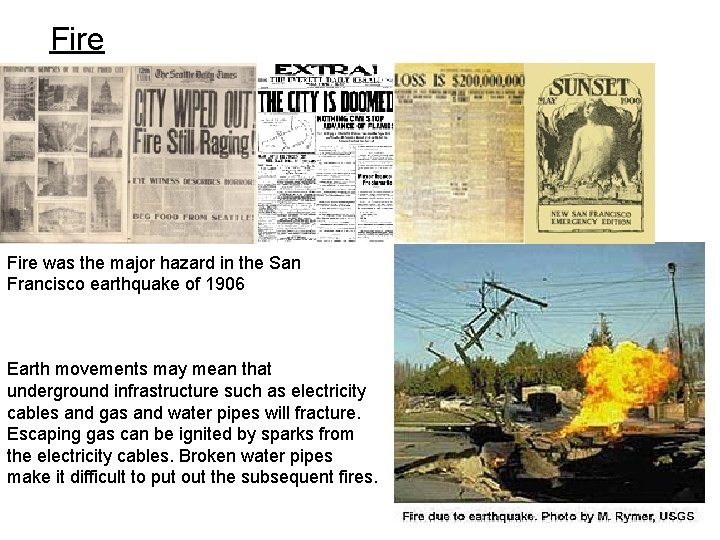
Fire was the major hazard in the San Francisco earthquake of 1906 Earth movements may mean that underground infrastructure such as electricity cables and gas and water pipes will fracture. Escaping gas can be ignited by sparks from the electricity cables. Broken water pipes make it difficult to put out the subsequent fires.
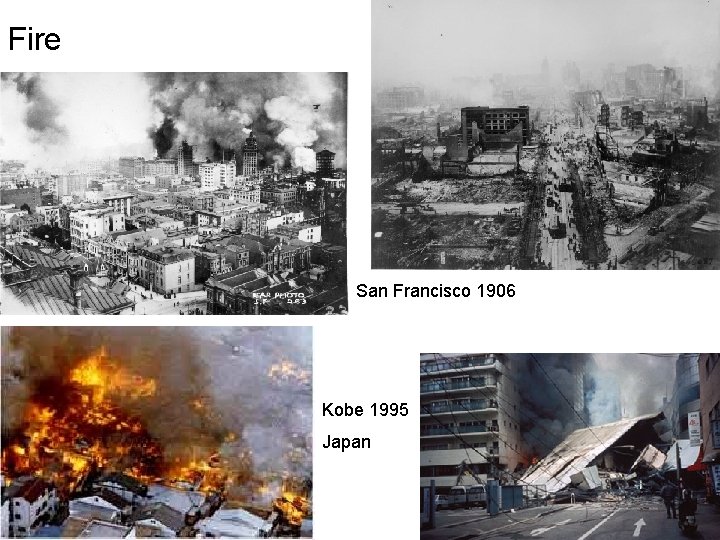
Fire San Francisco 1906 Kobe 1995 Japan
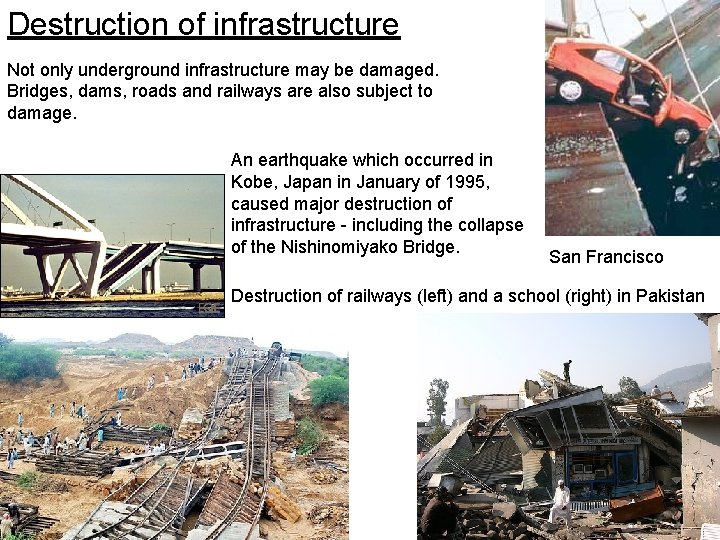
Destruction of infrastructure Not only underground infrastructure may be damaged. Bridges, dams, roads and railways are also subject to damage. An earthquake which occurred in Kobe, Japan in January of 1995, caused major destruction of infrastructure - including the collapse of the Nishinomiyako Bridge. San Francisco Destruction of railways (left) and a school (right) in Pakistan
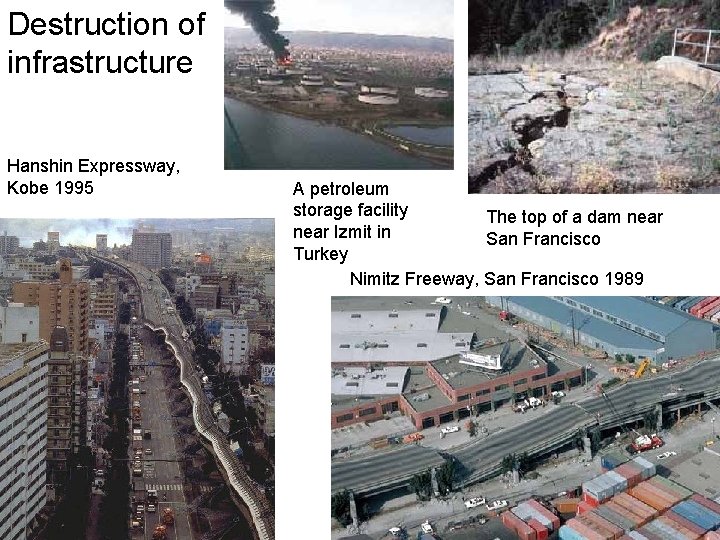
Destruction of infrastructure Hanshin Expressway, Kobe 1995 A petroleum storage facility The top of a dam near Izmit in San Francisco Turkey Nimitz Freeway, San Francisco 1989
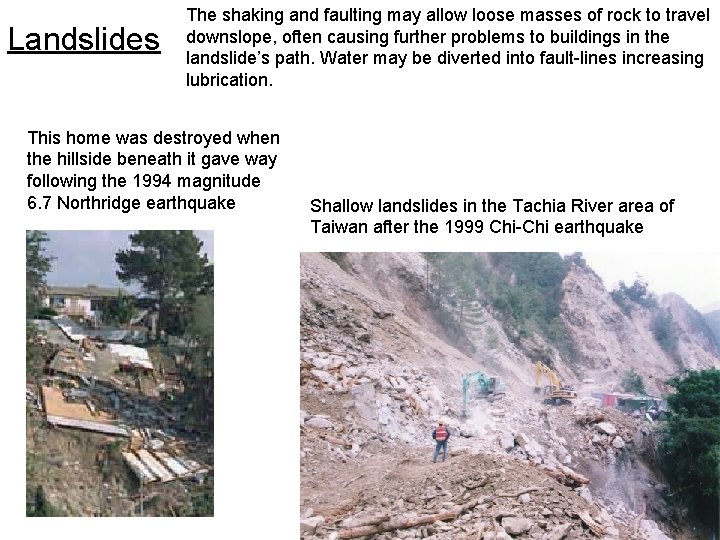
Landslides The shaking and faulting may allow loose masses of rock to travel downslope, often causing further problems to buildings in the landslide’s path. Water may be diverted into fault-lines increasing lubrication. This home was destroyed when the hillside beneath it gave way following the 1994 magnitude 6. 7 Northridge earthquake Shallow landslides in the Tachia River area of Taiwan after the 1999 Chi-Chi earthquake
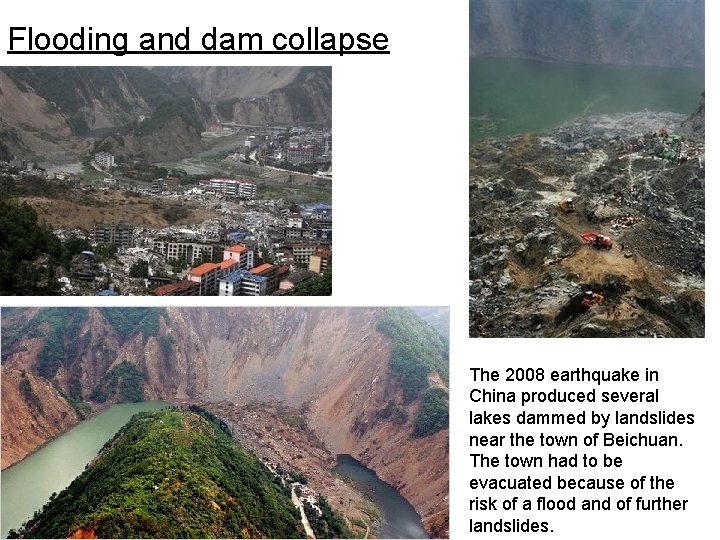
Flooding and dam collapse The 2008 earthquake in China produced several lakes dammed by landslides near the town of Beichuan. The town had to be evacuated because of the risk of a flood and of further landslides.
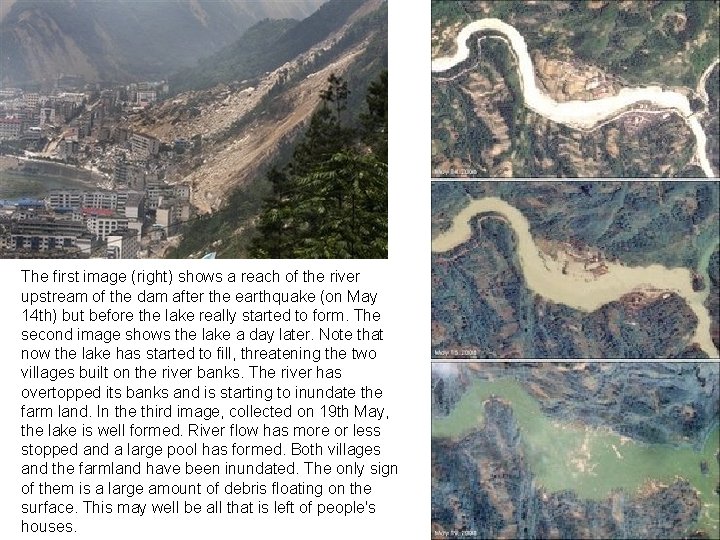
The first image (right) shows a reach of the river upstream of the dam after the earthquake (on May 14 th) but before the lake really started to form. The second image shows the lake a day later. Note that now the lake has started to fill, threatening the two villages built on the river banks. The river has overtopped its banks and is starting to inundate the farm land. In the third image, collected on 19 th May, the lake is well formed. River flow has more or less stopped and a large pool has formed. Both villages and the farmland have been inundated. The only sign of them is a large amount of debris floating on the surface. This may well be all that is left of people's houses.
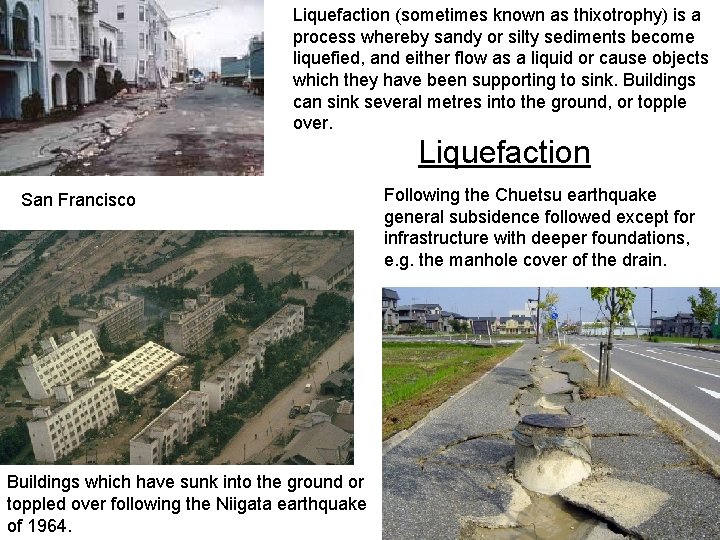
Liquefaction (sometimes known as thixotrophy) is a process whereby sandy or silty sediments become liquefied, and either flow as a liquid or cause objects which they have been supporting to sink. Buildings can sink several metres into the ground, or topple over. Liquefaction San Francisco Buildings which have sunk into the ground or toppled over following the Niigata earthquake of 1964. Following the Chuetsu earthquake general subsidence followed except for infrastructure with deeper foundations, e. g. the manhole cover of the drain.
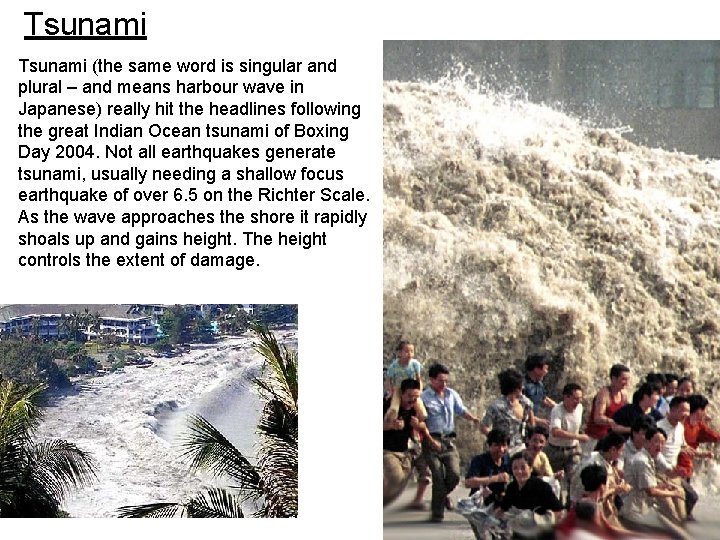
Tsunami (the same word is singular and plural – and means harbour wave in Japanese) really hit the headlines following the great Indian Ocean tsunami of Boxing Day 2004. Not all earthquakes generate tsunami, usually needing a shallow focus earthquake of over 6. 5 on the Richter Scale. As the wave approaches the shore it rapidly shoals up and gains height. The height controls the extent of damage.
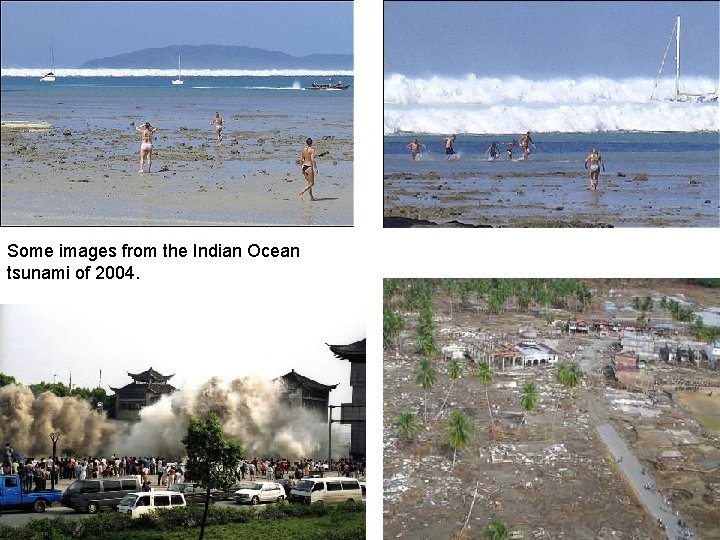
Some images from the Indian Ocean tsunami of 2004.
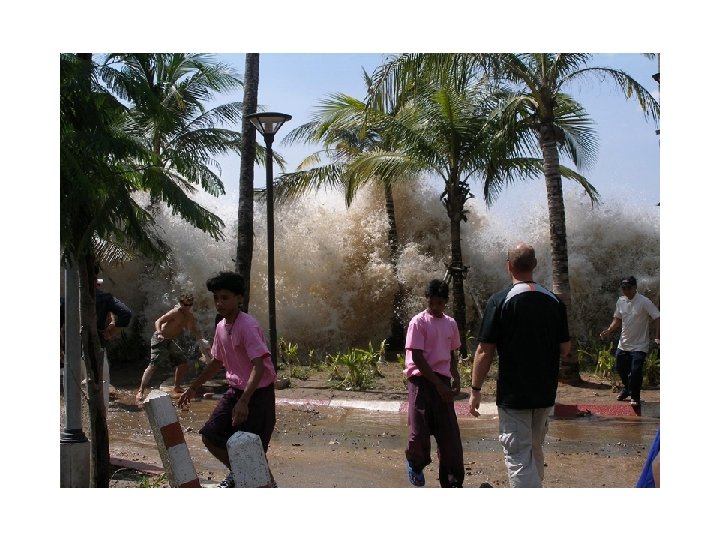
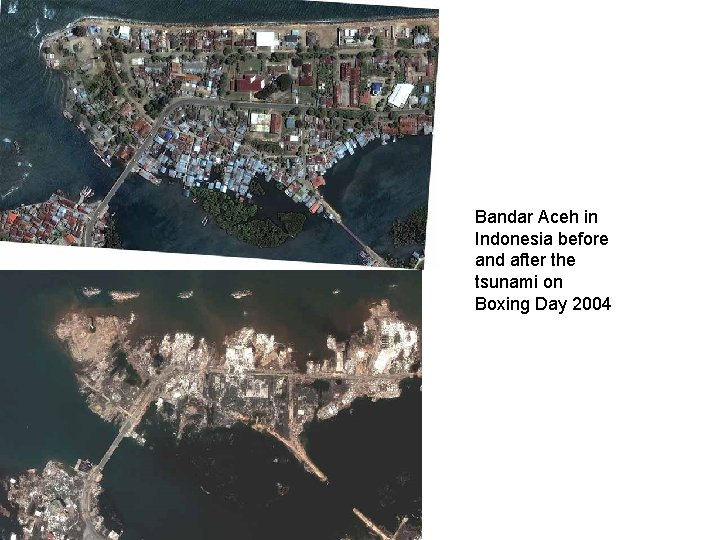
Bandar Aceh in Indonesia before and after the tsunami on Boxing Day 2004
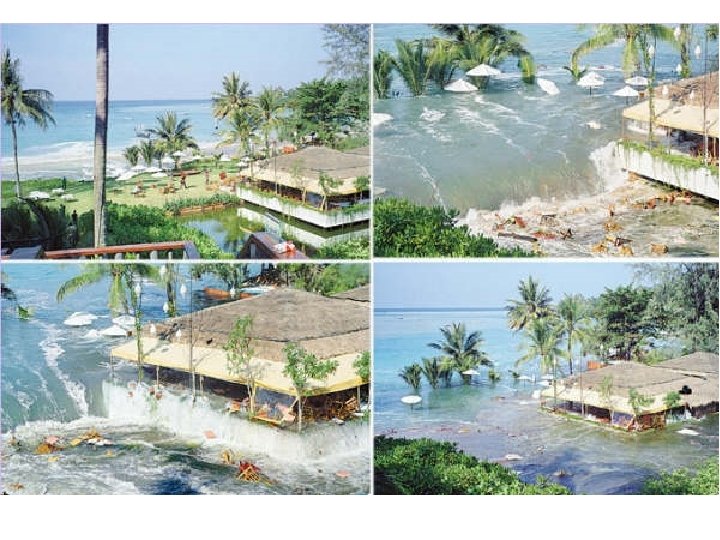
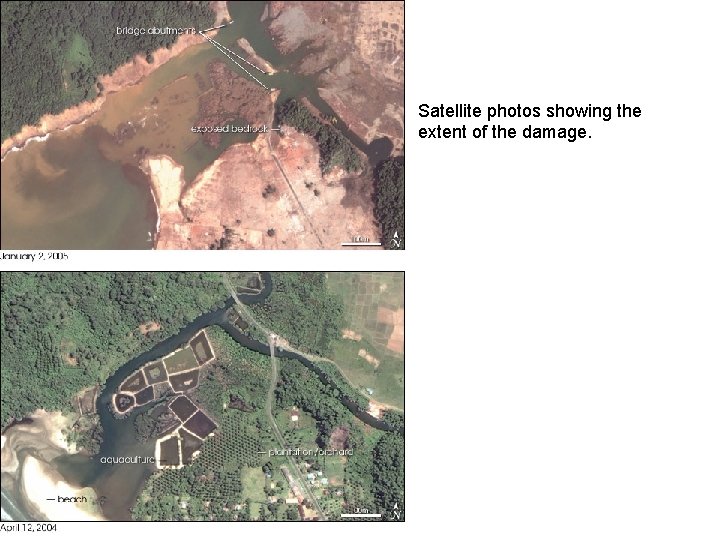
Satellite photos showing the extent of the damage.
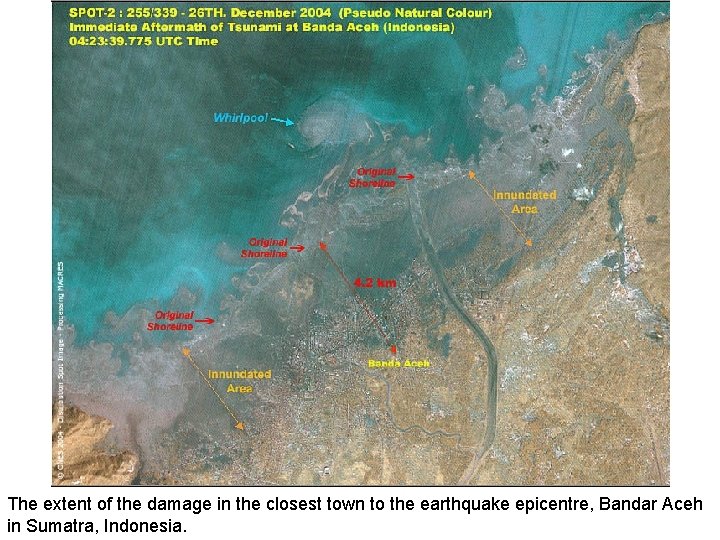
The extent of the damage in the closest town to the earthquake epicentre, Bandar Aceh in Sumatra, Indonesia.
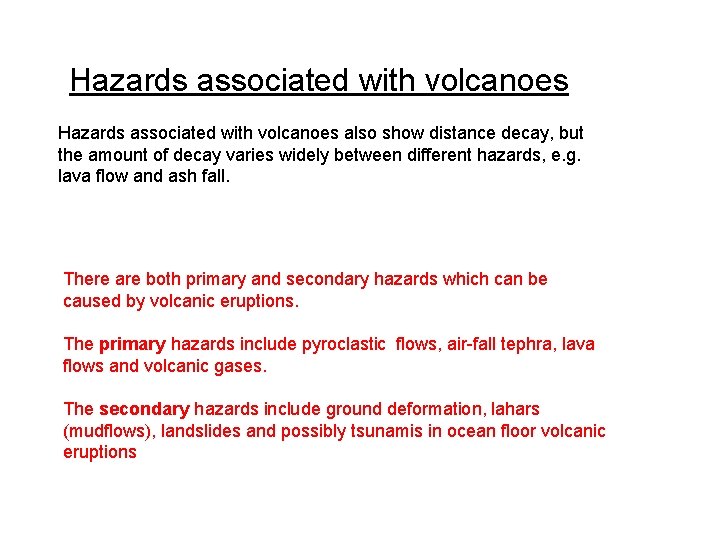
Hazards associated with volcanoes also show distance decay, but the amount of decay varies widely between different hazards, e. g. lava flow and ash fall. There are both primary and secondary hazards which can be caused by volcanic eruptions. The primary hazards include pyroclastic flows, air-fall tephra, lava flows and volcanic gases. The secondary hazards include ground deformation, lahars (mudflows), landslides and possibly tsunamis in ocean floor volcanic eruptions
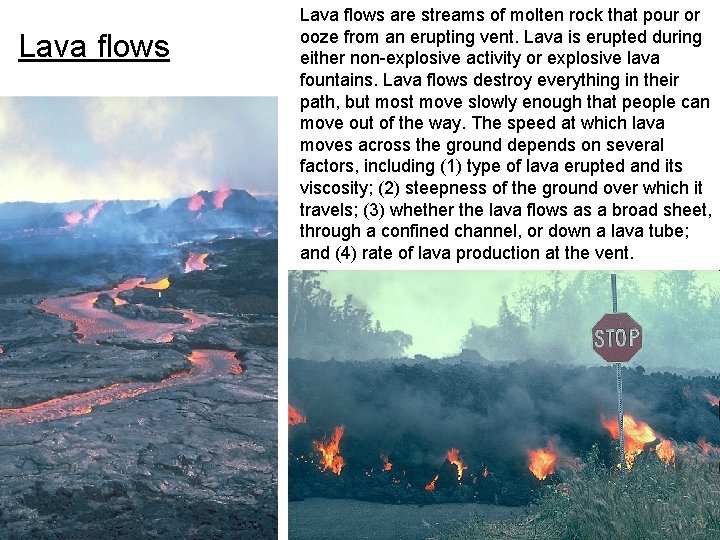
Lava flows are streams of molten rock that pour or ooze from an erupting vent. Lava is erupted during either non-explosive activity or explosive lava fountains. Lava flows destroy everything in their path, but most move slowly enough that people can move out of the way. The speed at which lava moves across the ground depends on several factors, including (1) type of lava erupted and its viscosity; (2) steepness of the ground over which it travels; (3) whether the lava flows as a broad sheet, through a confined channel, or down a lava tube; and (4) rate of lava production at the vent.
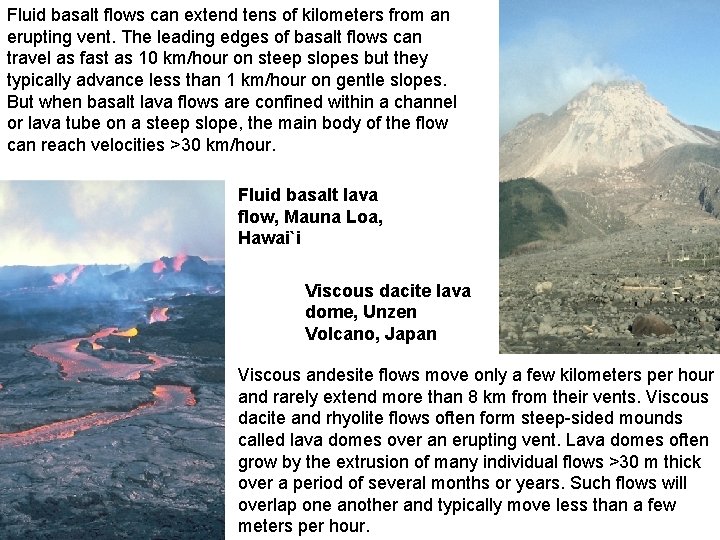
Fluid basalt flows can extend tens of kilometers from an erupting vent. The leading edges of basalt flows can travel as fast as 10 km/hour on steep slopes but they typically advance less than 1 km/hour on gentle slopes. But when basalt lava flows are confined within a channel or lava tube on a steep slope, the main body of the flow can reach velocities >30 km/hour. Fluid basalt lava flow, Mauna Loa, Hawai`i Viscous dacite lava dome, Unzen Volcano, Japan Viscous andesite flows move only a few kilometers per hour and rarely extend more than 8 km from their vents. Viscous dacite and rhyolite flows often form steep-sided mounds called lava domes over an erupting vent. Lava domes often grow by the extrusion of many individual flows >30 m thick over a period of several months or years. Such flows will overlap one another and typically move less than a few meters per hour.
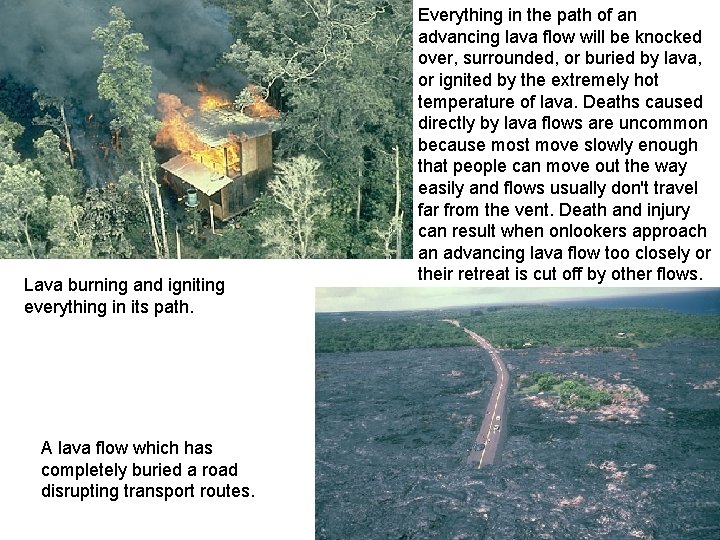
Lava burning and igniting everything in its path. A lava flow which has completely buried a road disrupting transport routes. Everything in the path of an advancing lava flow will be knocked over, surrounded, or buried by lava, or ignited by the extremely hot temperature of lava. Deaths caused directly by lava flows are uncommon because most move slowly enough that people can move out the way easily and flows usually don't travel far from the vent. Death and injury can result when onlookers approach an advancing lava flow too closely or their retreat is cut off by other flows.
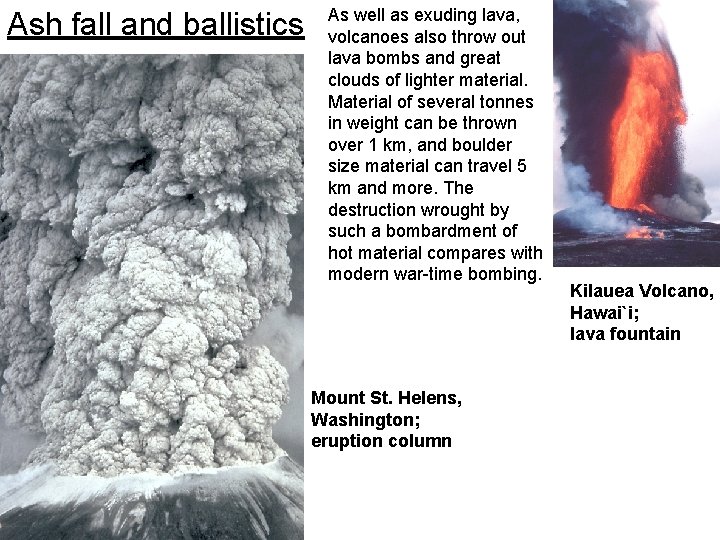
Ash fall and ballistics As well as exuding lava, volcanoes also throw out lava bombs and great clouds of lighter material. Material of several tonnes in weight can be thrown over 1 km, and boulder size material can travel 5 km and more. The destruction wrought by such a bombardment of hot material compares with modern war-time bombing. Mount St. Helens, Washington; eruption column Kilauea Volcano, Hawai`i; lava fountain
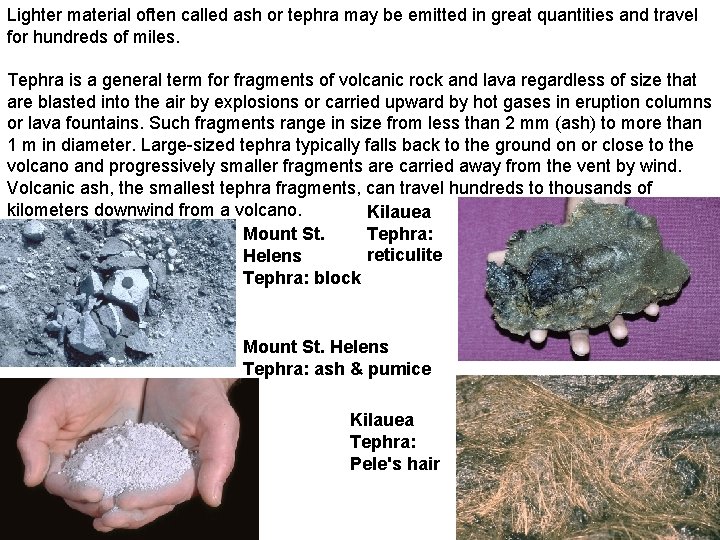
Lighter material often called ash or tephra may be emitted in great quantities and travel for hundreds of miles. Tephra is a general term for fragments of volcanic rock and lava regardless of size that are blasted into the air by explosions or carried upward by hot gases in eruption columns or lava fountains. Such fragments range in size from less than 2 mm (ash) to more than 1 m in diameter. Large-sized tephra typically falls back to the ground on or close to the volcano and progressively smaller fragments are carried away from the vent by wind. Volcanic ash, the smallest tephra fragments, can travel hundreds to thousands of kilometers downwind from a volcano. Kilauea Tephra: Mount St. reticulite Helens Tephra: block Mount St. Helens Tephra: ash & pumice Kilauea Tephra: Pele's hair
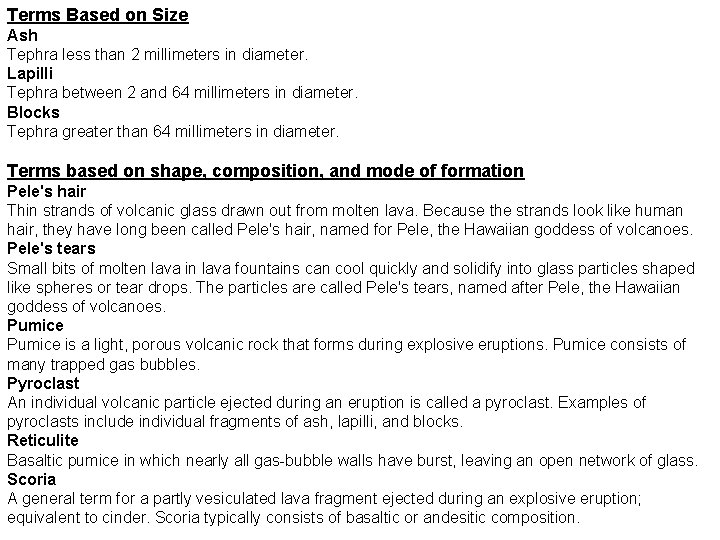
Terms Based on Size Ash Tephra less than 2 millimeters in diameter. Lapilli Tephra between 2 and 64 millimeters in diameter. Blocks Tephra greater than 64 millimeters in diameter. Terms based on shape, composition, and mode of formation Pele's hair Thin strands of volcanic glass drawn out from molten lava. Because the strands look like human hair, they have long been called Pele's hair, named for Pele, the Hawaiian goddess of volcanoes. Pele's tears Small bits of molten lava in lava fountains can cool quickly and solidify into glass particles shaped like spheres or tear drops. The particles are called Pele's tears, named after Pele, the Hawaiian goddess of volcanoes. Pumice is a light, porous volcanic rock that forms during explosive eruptions. Pumice consists of many trapped gas bubbles. Pyroclast An individual volcanic particle ejected during an eruption is called a pyroclast. Examples of pyroclasts include individual fragments of ash, lapilli, and blocks. Reticulite Basaltic pumice in which nearly all gas-bubble walls have burst, leaving an open network of glass. Scoria A general term for a partly vesiculated lava fragment ejected during an explosive eruption; equivalent to cinder. Scoria typically consists of basaltic or andesitic composition.
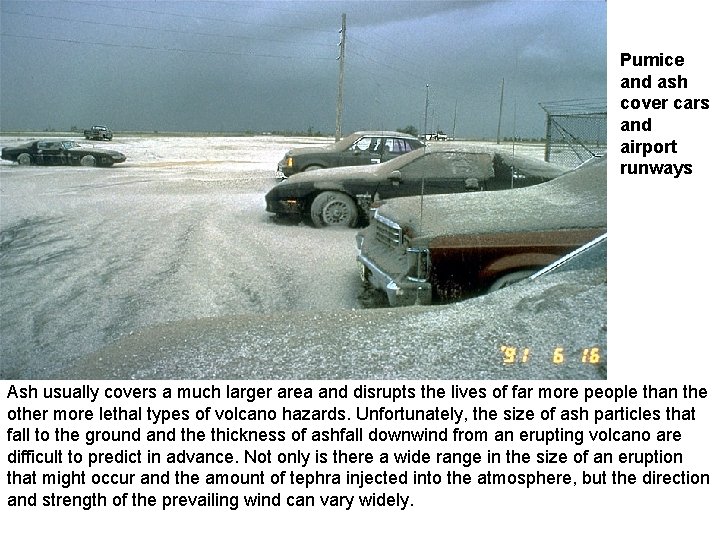
Pumice and ash cover cars and airport runways Ash usually covers a much larger area and disrupts the lives of far more people than the other more lethal types of volcano hazards. Unfortunately, the size of ash particles that fall to the ground and the thickness of ashfall downwind from an erupting volcano are difficult to predict in advance. Not only is there a wide range in the size of an eruption that might occur and the amount of tephra injected into the atmosphere, but the direction and strength of the prevailing wind can vary widely.
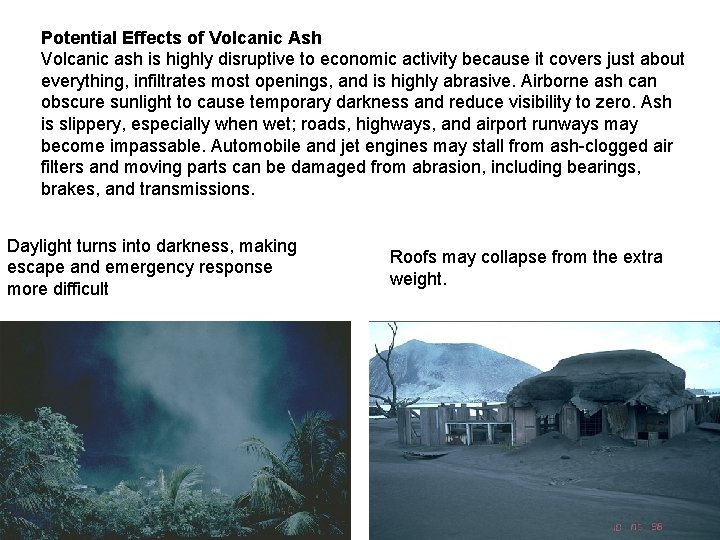
Potential Effects of Volcanic Ash Volcanic ash is highly disruptive to economic activity because it covers just about everything, infiltrates most openings, and is highly abrasive. Airborne ash can obscure sunlight to cause temporary darkness and reduce visibility to zero. Ash is slippery, especially when wet; roads, highways, and airport runways may become impassable. Automobile and jet engines may stall from ash-clogged air filters and moving parts can be damaged from abrasion, including bearings, brakes, and transmissions. Daylight turns into darkness, making escape and emergency response more difficult Roofs may collapse from the extra weight.
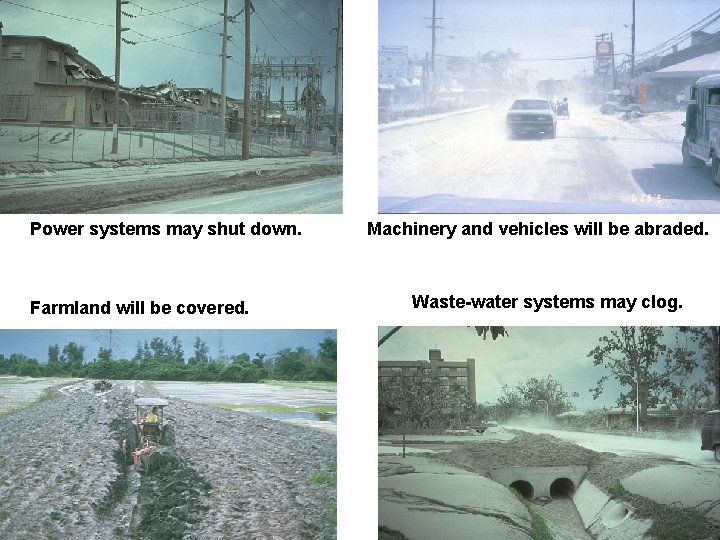
Power systems may shut down. Farmland will be covered. Machinery and vehicles will be abraded. Waste-water systems may clog.
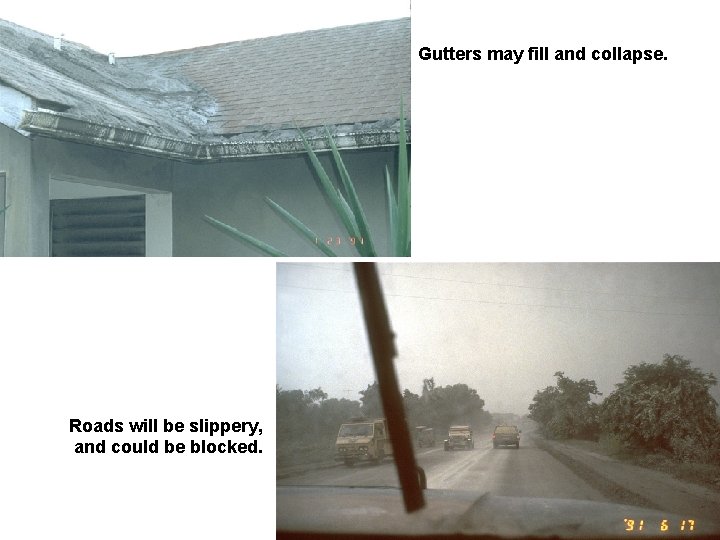
Gutters may fill and collapse. Roads will be slippery, and could be blocked.
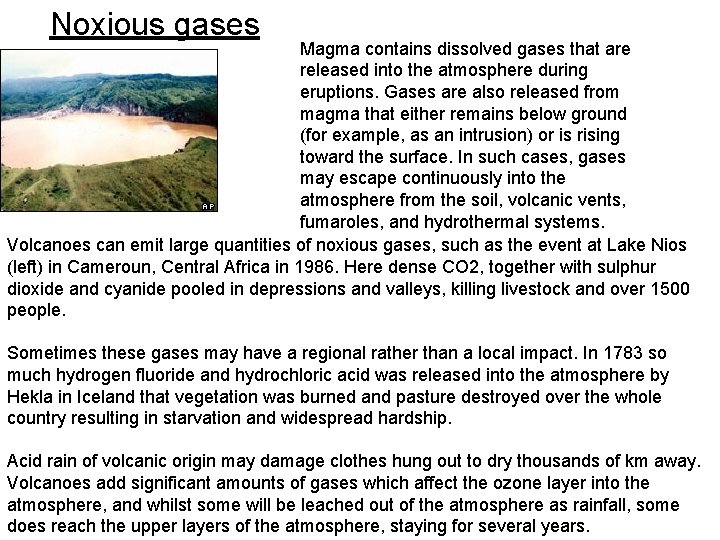
Noxious gases Magma contains dissolved gases that are released into the atmosphere during eruptions. Gases are also released from magma that either remains below ground (for example, as an intrusion) or is rising toward the surface. In such cases, gases may escape continuously into the atmosphere from the soil, volcanic vents, fumaroles, and hydrothermal systems. Volcanoes can emit large quantities of noxious gases, such as the event at Lake Nios (left) in Cameroun, Central Africa in 1986. Here dense CO 2, together with sulphur dioxide and cyanide pooled in depressions and valleys, killing livestock and over 1500 people. Sometimes these gases may have a regional rather than a local impact. In 1783 so much hydrogen fluoride and hydrochloric acid was released into the atmosphere by Hekla in Iceland that vegetation was burned and pasture destroyed over the whole country resulting in starvation and widespread hardship. Acid rain of volcanic origin may damage clothes hung out to dry thousands of km away. Volcanoes add significant amounts of gases which affect the ozone layer into the atmosphere, and whilst some will be leached out of the atmosphere as rainfall, some does reach the upper layers of the atmosphere, staying for several years.
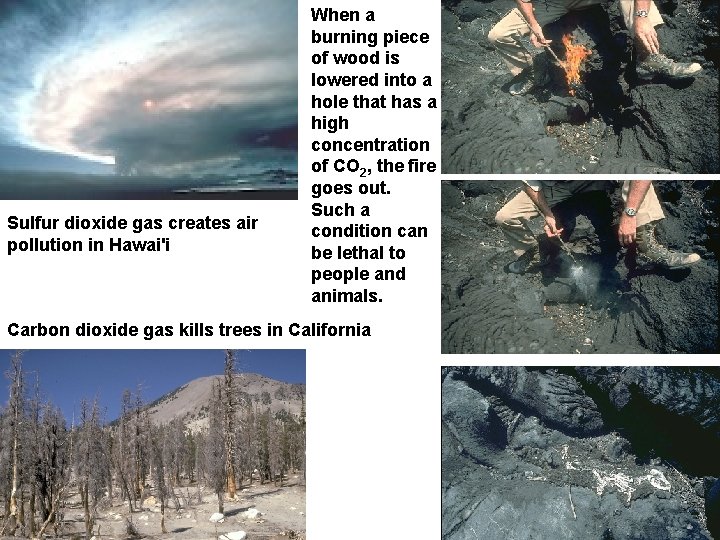
Sulfur dioxide gas creates air pollution in Hawai'i When a burning piece of wood is lowered into a hole that has a high concentration of CO 2, the fire goes out. Such a condition can be lethal to people and animals. Carbon dioxide gas kills trees in California
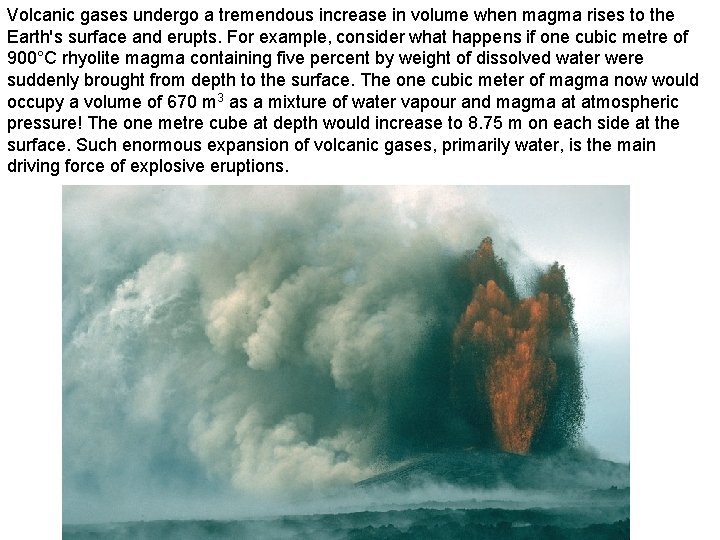
Volcanic gases undergo a tremendous increase in volume when magma rises to the Earth's surface and erupts. For example, consider what happens if one cubic metre of 900°C rhyolite magma containing five percent by weight of dissolved water were suddenly brought from depth to the surface. The one cubic meter of magma now would occupy a volume of 670 m 3 as a mixture of water vapour and magma at atmospheric pressure! The one metre cube at depth would increase to 8. 75 m on each side at the surface. Such enormous expansion of volcanic gases, primarily water, is the main driving force of explosive eruptions.
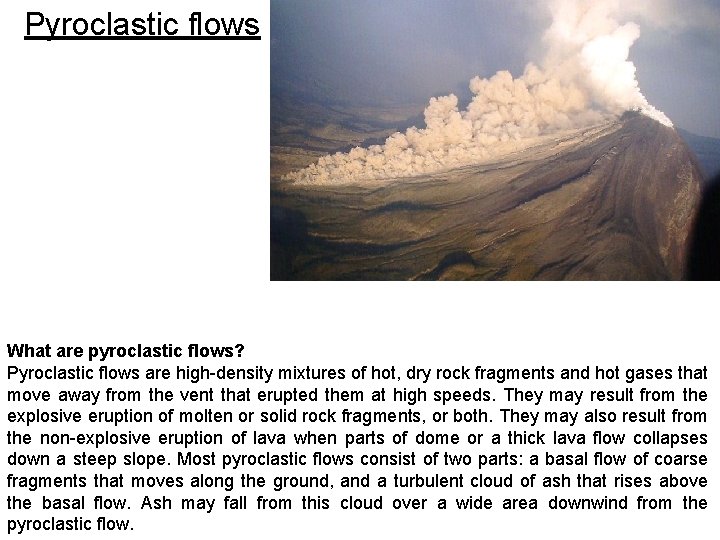
Pyroclastic flows What are pyroclastic flows? Pyroclastic flows are high-density mixtures of hot, dry rock fragments and hot gases that move away from the vent that erupted them at high speeds. They may result from the explosive eruption of molten or solid rock fragments, or both. They may also result from the non-explosive eruption of lava when parts of dome or a thick lava flow collapses down a steep slope. Most pyroclastic flows consist of two parts: a basal flow of coarse fragments that moves along the ground, and a turbulent cloud of ash that rises above the basal flow. Ash may fall from this cloud over a wide area downwind from the pyroclastic flow.
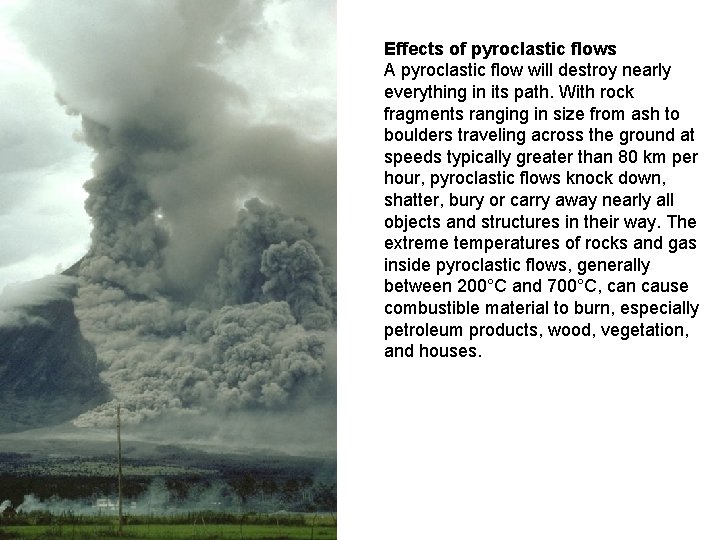
Effects of pyroclastic flows A pyroclastic flow will destroy nearly everything in its path. With rock fragments ranging in size from ash to boulders traveling across the ground at speeds typically greater than 80 km per hour, pyroclastic flows knock down, shatter, bury or carry away nearly all objects and structures in their way. The extreme temperatures of rocks and gas inside pyroclastic flows, generally between 200°C and 700°C, can cause combustible material to burn, especially petroleum products, wood, vegetation, and houses.
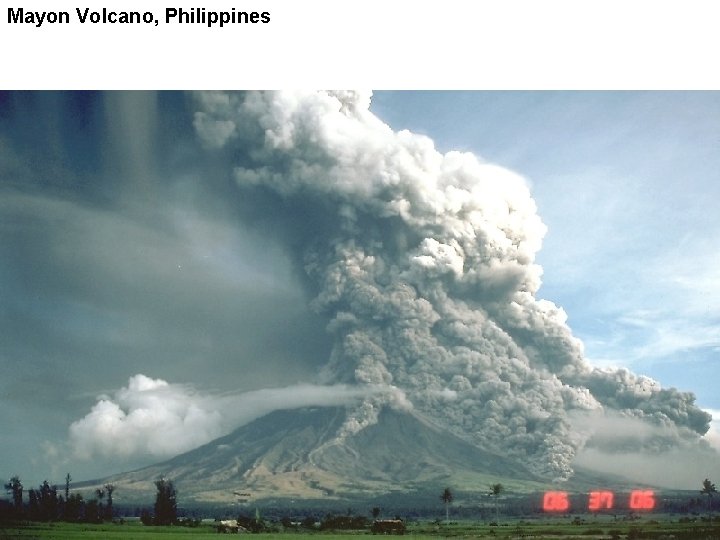
Mayon Volcano, Philippines
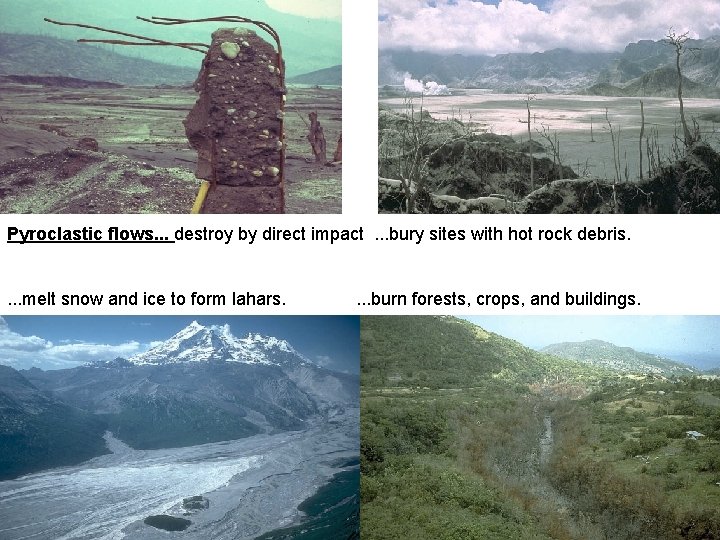
Pyroclastic flows. . . destroy by direct impact . . . bury sites with hot rock debris. . . . melt snow and ice to form lahars. . . . burn forests, crops, and buildings.
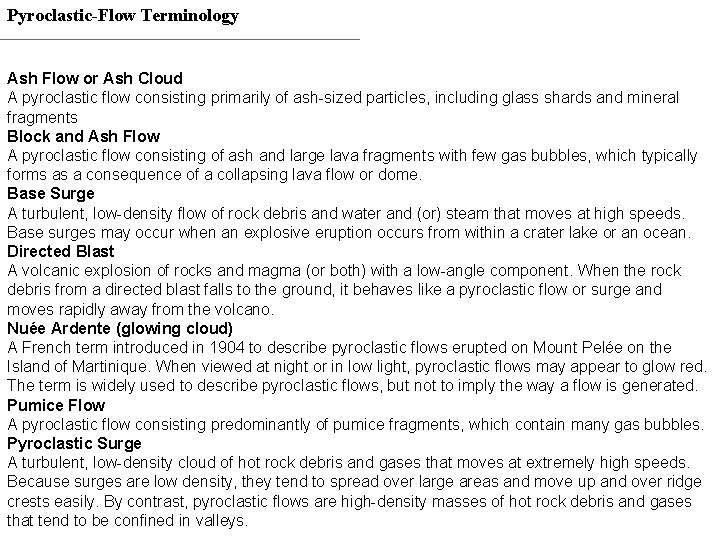
Pyroclastic-Flow Terminology Ash Flow or Ash Cloud A pyroclastic flow consisting primarily of ash-sized particles, including glass shards and mineral fragments Block and Ash Flow A pyroclastic flow consisting of ash and large lava fragments with few gas bubbles, which typically forms as a consequence of a collapsing lava flow or dome. Base Surge A turbulent, low-density flow of rock debris and water and (or) steam that moves at high speeds. Base surges may occur when an explosive eruption occurs from within a crater lake or an ocean. Directed Blast A volcanic explosion of rocks and magma (or both) with a low-angle component. When the rock debris from a directed blast falls to the ground, it behaves like a pyroclastic flow or surge and moves rapidly away from the volcano. Nuée Ardente (glowing cloud) A French term introduced in 1904 to describe pyroclastic flows erupted on Mount Pelée on the Island of Martinique. When viewed at night or in low light, pyroclastic flows may appear to glow red. The term is widely used to describe pyroclastic flows, but not to imply the way a flow is generated. Pumice Flow A pyroclastic flow consisting predominantly of pumice fragments, which contain many gas bubbles. Pyroclastic Surge A turbulent, low-density cloud of hot rock debris and gases that moves at extremely high speeds. Because surges are low density, they tend to spread over large areas and move up and over ridge crests easily. By contrast, pyroclastic flows are high-density masses of hot rock debris and gases that tend to be confined in valleys.
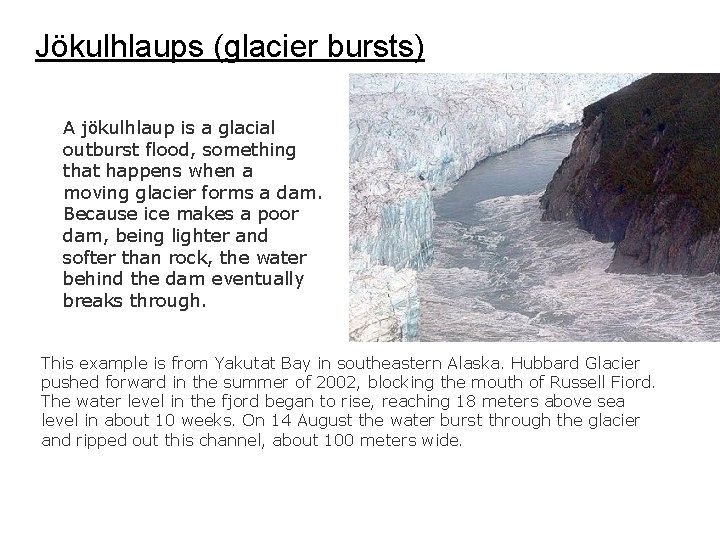
Jökulhlaups (glacier bursts) A jökulhlaup is a glacial outburst flood, something that happens when a moving glacier forms a dam. Because ice makes a poor dam, being lighter and softer than rock, the water behind the dam eventually breaks through. This example is from Yakutat Bay in southeastern Alaska. Hubbard Glacier pushed forward in the summer of 2002, blocking the mouth of Russell Fiord. The water level in the fjord began to rise, reaching 18 meters above sea level in about 10 weeks. On 14 August the water burst through the glacier and ripped out this channel, about 100 meters wide.
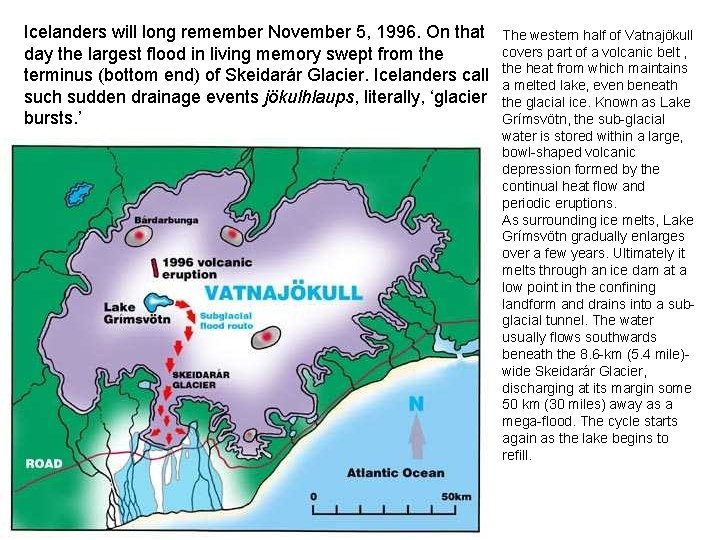
Icelanders will long remember November 5, 1996. On that day the largest flood in living memory swept from the terminus (bottom end) of Skeidarár Glacier. Icelanders call such sudden drainage events jökulhlaups, literally, ‘glacier bursts. ’ The western half of Vatnajökull covers part of a volcanic belt , the heat from which maintains a melted lake, even beneath the glacial ice. Known as Lake Grímsvötn, the sub-glacial water is stored within a large, bowl-shaped volcanic depression formed by the continual heat flow and periodic eruptions. As surrounding ice melts, Lake Grímsvötn gradually enlarges over a few years. Ultimately it melts through an ice dam at a low point in the confining landform and drains into a subglacial tunnel. The water usually flows southwards beneath the 8. 6 -km (5. 4 mile)wide Skeidarár Glacier, discharging at its margin some 50 km (30 miles) away as a mega-flood. The cycle starts again as the lake begins to refill.
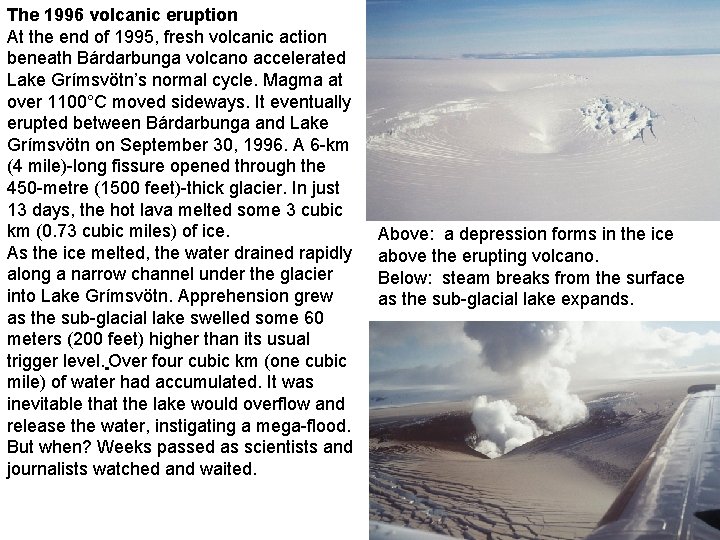
The 1996 volcanic eruption At the end of 1995, fresh volcanic action beneath Bárdarbunga volcano accelerated Lake Grímsvötn’s normal cycle. Magma at over 1100°C moved sideways. It eventually erupted between Bárdarbunga and Lake Grímsvötn on September 30, 1996. A 6 -km (4 mile)-long fissure opened through the 450 -metre (1500 feet)-thick glacier. In just 13 days, the hot lava melted some 3 cubic km (0. 73 cubic miles) of ice. As the ice melted, the water drained rapidly along a narrow channel under the glacier into Lake Grímsvötn. Apprehension grew as the sub-glacial lake swelled some 60 meters (200 feet) higher than its usual trigger level. Over four cubic km (one cubic mile) of water had accumulated. It was inevitable that the lake would overflow and release the water, instigating a mega-flood. But when? Weeks passed as scientists and journalists watched and waited. Above: a depression forms in the ice above the erupting volcano. Below: steam breaks from the surface as the sub-glacial lake expands.
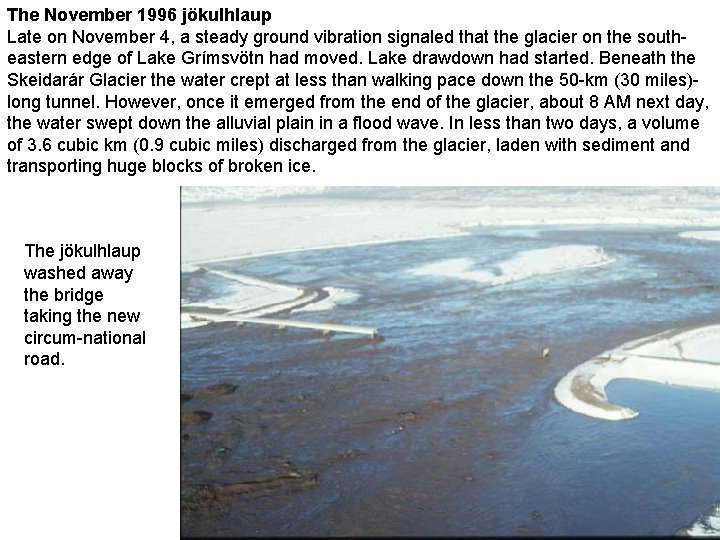
The November 1996 jökulhlaup Late on November 4, a steady ground vibration signaled that the glacier on the southeastern edge of Lake Grímsvötn had moved. Lake drawdown had started. Beneath the Skeidarár Glacier the water crept at less than walking pace down the 50 -km (30 miles)long tunnel. However, once it emerged from the end of the glacier, about 8 AM next day, the water swept down the alluvial plain in a flood wave. In less than two days, a volume of 3. 6 cubic km (0. 9 cubic miles) discharged from the glacier, laden with sediment and transporting huge blocks of broken ice. The jökulhlaup washed away the bridge taking the new circum-national road.
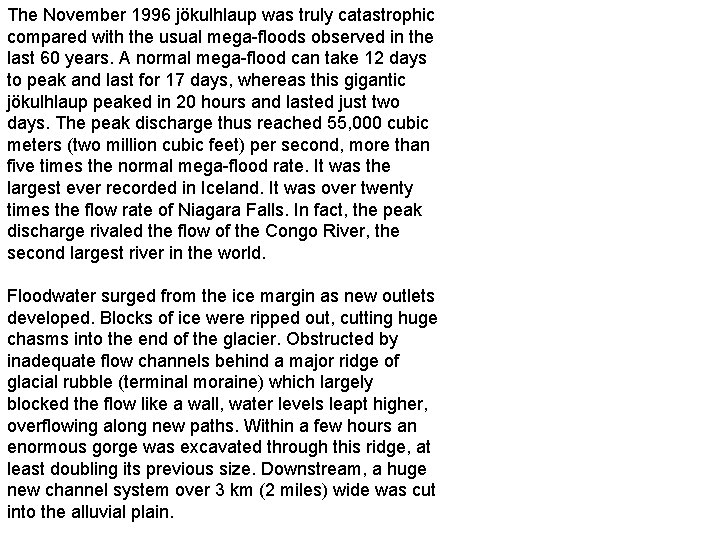
The November 1996 jökulhlaup was truly catastrophic compared with the usual mega-floods observed in the last 60 years. A normal mega-flood can take 12 days to peak and last for 17 days, whereas this gigantic jökulhlaup peaked in 20 hours and lasted just two days. The peak discharge thus reached 55, 000 cubic meters (two million cubic feet) per second, more than five times the normal mega-flood rate. It was the largest ever recorded in Iceland. It was over twenty times the flow rate of Niagara Falls. In fact, the peak discharge rivaled the flow of the Congo River, the second largest river in the world. Floodwater surged from the ice margin as new outlets developed. Blocks of ice were ripped out, cutting huge chasms into the end of the glacier. Obstructed by inadequate flow channels behind a major ridge of glacial rubble (terminal moraine) which largely blocked the flow like a wall, water levels leapt higher, overflowing along new paths. Within a few hours an enormous gorge was excavated through this ridge, at least doubling its previous size. Downstream, a huge new channel system over 3 km (2 miles) wide was cut into the alluvial plain.
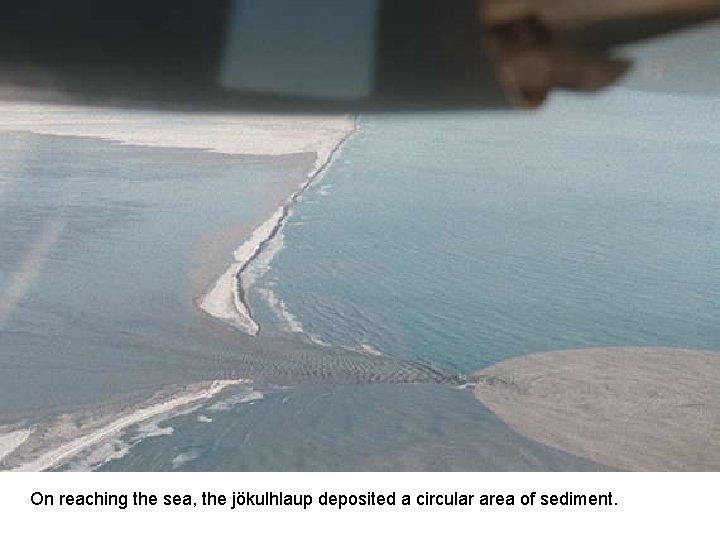
On reaching the sea, the jökulhlaup deposited a circular area of sediment.
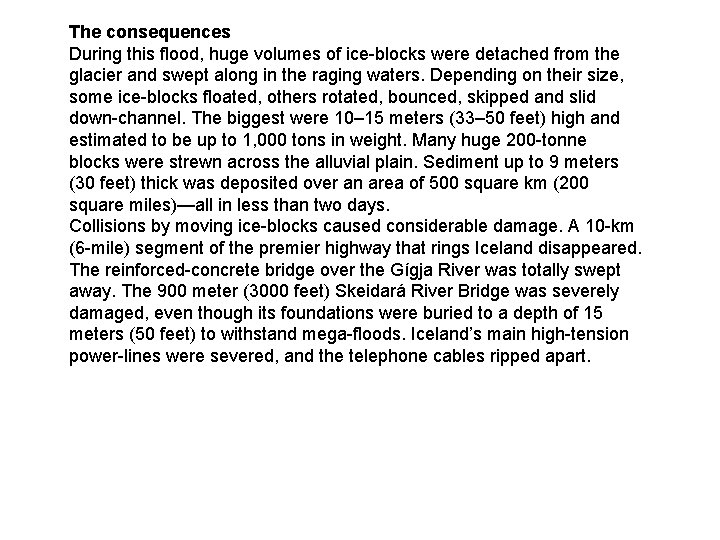
The consequences During this flood, huge volumes of ice-blocks were detached from the glacier and swept along in the raging waters. Depending on their size, some ice-blocks floated, others rotated, bounced, skipped and slid down-channel. The biggest were 10– 15 meters (33– 50 feet) high and estimated to be up to 1, 000 tons in weight. Many huge 200 -tonne blocks were strewn across the alluvial plain. Sediment up to 9 meters (30 feet) thick was deposited over an area of 500 square km (200 square miles)—all in less than two days. Collisions by moving ice-blocks caused considerable damage. A 10 -km (6 -mile) segment of the premier highway that rings Iceland disappeared. The reinforced-concrete bridge over the Gígja River was totally swept away. The 900 meter (3000 feet) Skeidará River Bridge was severely damaged, even though its foundations were buried to a depth of 15 meters (50 feet) to withstand mega-floods. Iceland’s main high-tension power-lines were severed, and the telephone cables ripped apart.
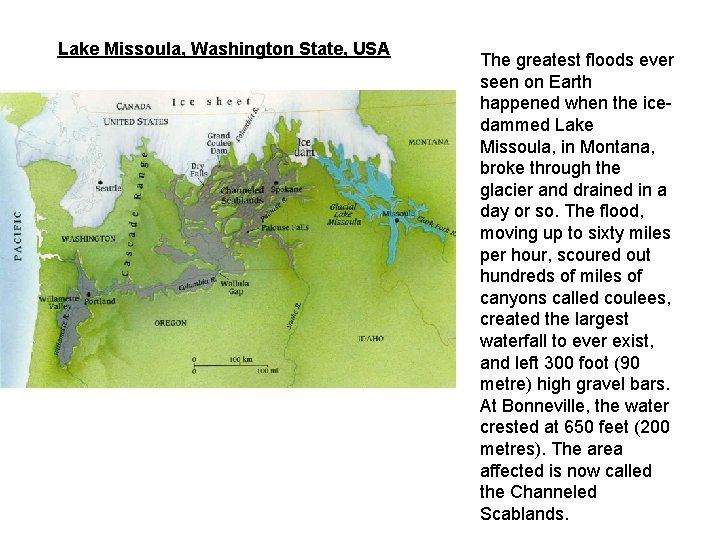
Lake Missoula, Washington State, USA The greatest floods ever seen on Earth happened when the icedammed Lake Missoula, in Montana, broke through the glacier and drained in a day or so. The flood, moving up to sixty miles per hour, scoured out hundreds of miles of canyons called coulees, created the largest waterfall to ever exist, and left 300 foot (90 metre) high gravel bars. At Bonneville, the water crested at 650 feet (200 metres). The area affected is now called the Channeled Scablands.
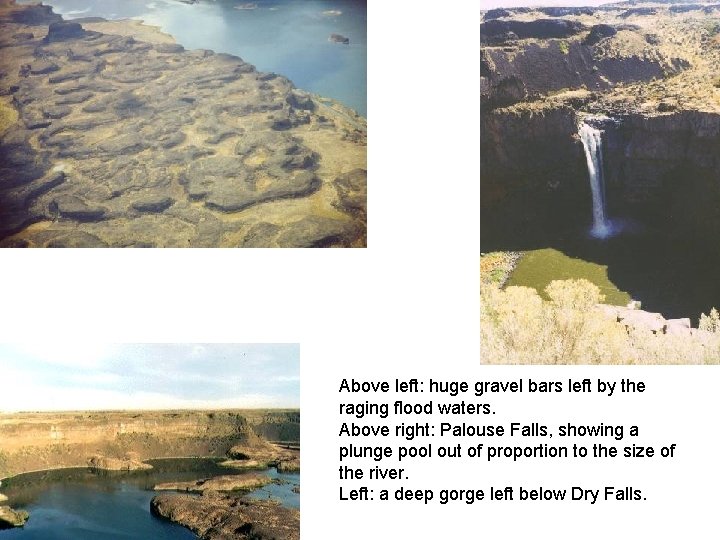
Above left: huge gravel bars left by the raging flood waters. Above right: Palouse Falls, showing a plunge pool out of proportion to the size of the river. Left: a deep gorge left below Dry Falls.
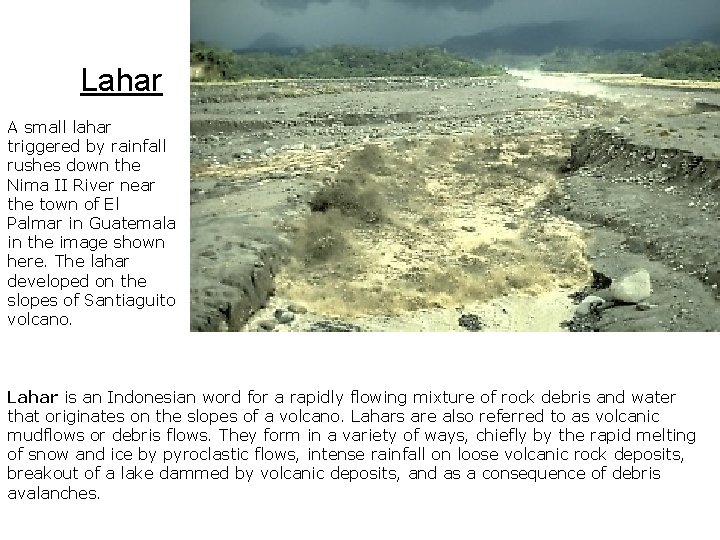
Lahar A small lahar triggered by rainfall rushes down the Nima II River near the town of El Palmar in Guatemala in the image shown here. The lahar developed on the slopes of Santiaguito volcano. Lahar is an Indonesian word for a rapidly flowing mixture of rock debris and water that originates on the slopes of a volcano. Lahars are also referred to as volcanic mudflows or debris flows. They form in a variety of ways, chiefly by the rapid melting of snow and ice by pyroclastic flows, intense rainfall on loose volcanic rock deposits, breakout of a lake dammed by volcanic deposits, and as a consequence of debris avalanches.
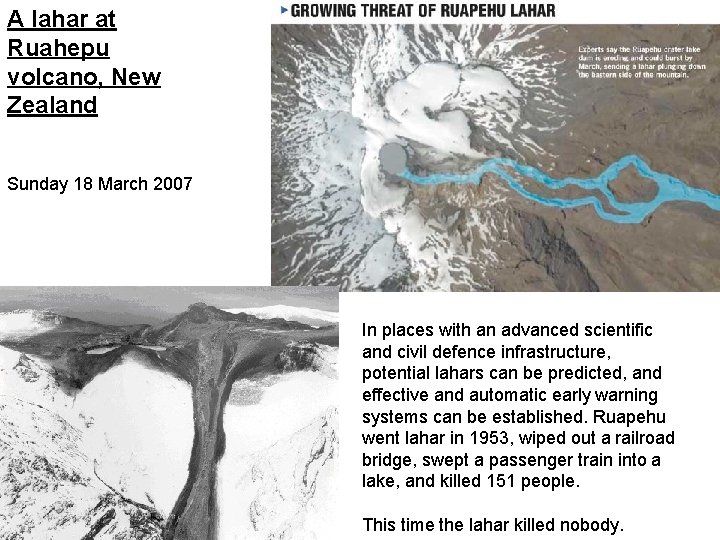
A lahar at Ruahepu volcano, New Zealand Sunday 18 March 2007 In places with an advanced scientific and civil defence infrastructure, potential lahars can be predicted, and effective and automatic early warning systems can be established. Ruapehu went lahar in 1953, wiped out a railroad bridge, swept a passenger train into a lake, and killed 151 people. This time the lahar killed nobody.
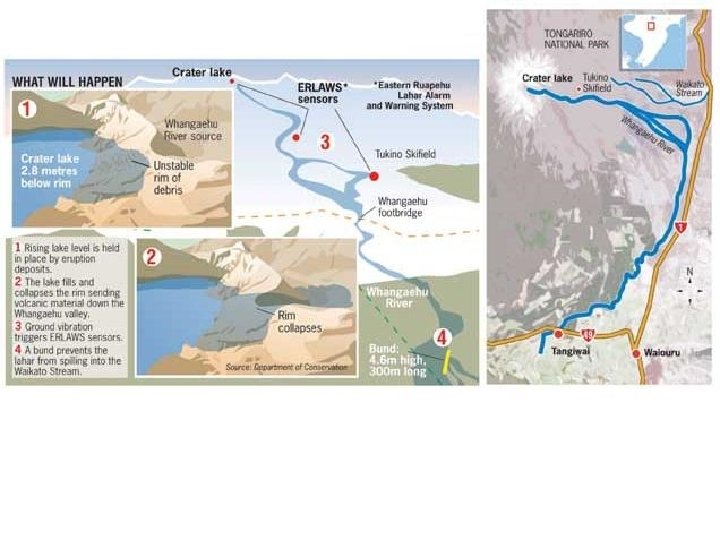
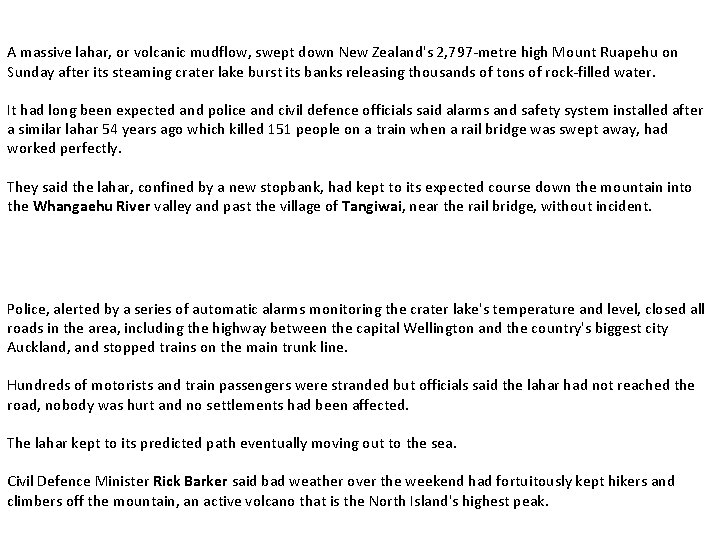
A massive lahar, or volcanic mudflow, swept down New Zealand's 2, 797 -metre high Mount Ruapehu on Sunday after its steaming crater lake burst its banks releasing thousands of tons of rock-filled water. It had long been expected and police and civil defence officials said alarms and safety system installed after a similar lahar 54 years ago which killed 151 people on a train when a rail bridge was swept away, had worked perfectly. They said the lahar, confined by a new stopbank, had kept to its expected course down the mountain into the Whangaehu River valley and past the village of Tangiwai, near the rail bridge, without incident. Police, alerted by a series of automatic alarms monitoring the crater lake's temperature and level, closed all roads in the area, including the highway between the capital Wellington and the country's biggest city Auckland, and stopped trains on the main trunk line. Hundreds of motorists and train passengers were stranded but officials said the lahar had not reached the road, nobody was hurt and no settlements had been affected. The lahar kept to its predicted path eventually moving out to the sea. Civil Defence Minister Rick Barker said bad weather over the weekend had fortuitously kept hikers and climbers off the mountain, an active volcano that is the North Island's highest peak.
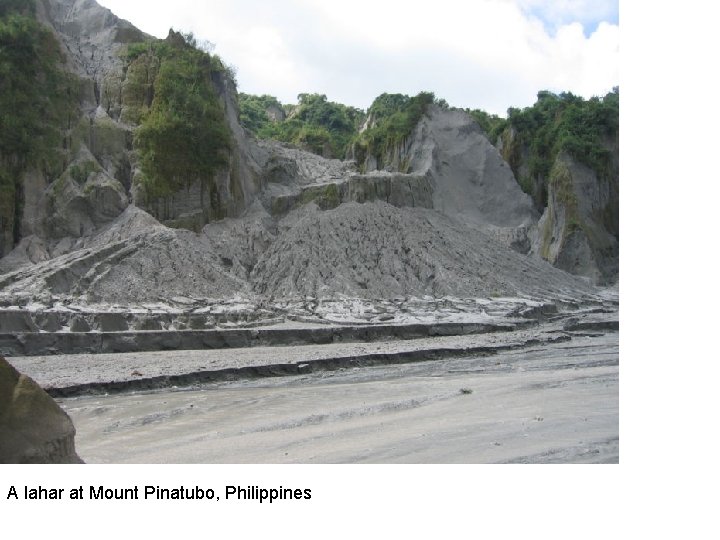
A lahar at Mount Pinatubo, Philippines
![Impacts of tectonic hazards 1 Demographic 2 Economic 3 Social 2008 Sichuan earthquake Date Impacts of tectonic hazards [1] Demographic, [2] Economic, [3] Social 2008 Sichuan earthquake Date](https://slidetodoc.com/presentation_image_h/d020a7159aa58ff4c55a30a84261b44e/image-56.jpg)
Impacts of tectonic hazards [1] Demographic, [2] Economic, [3] Social 2008 Sichuan earthquake Date May 12, 2008 Magnitude 8. 0 Richter Scale Depth: 19 kilometres (12 miles) Epicenter location: 31° 01′ 16″N 103° 22′ 01″E� / � 31. 021, 103. 367� (Yingxiu, Wenchuan, Ngawa in Sichuan Province) Countries/regions affected China Max. Intensity: X - Disastrous Aftershocks: 134 to 258 major, over 21, 568 total Casualties: 69, 197 dead (19 th deadliest earthquake of all time) 374, 176 injured 18, 238 missing (as of 16 July, 2008) The earthquake was felt as far away as Beijing (1, 500 kilometres (932 mi) away) and Shanghai (1, 700 kilometres (1, 056 mi) away), where office buildings swayed with the tremor. Approximately 15 million people lived in the affected area.
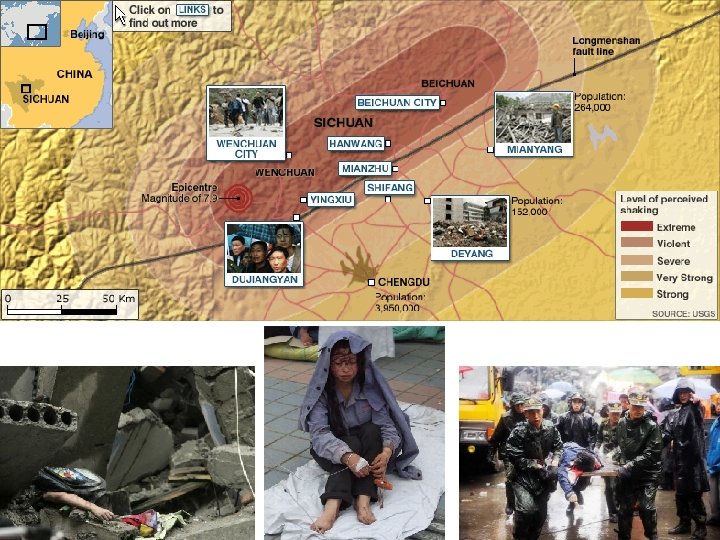
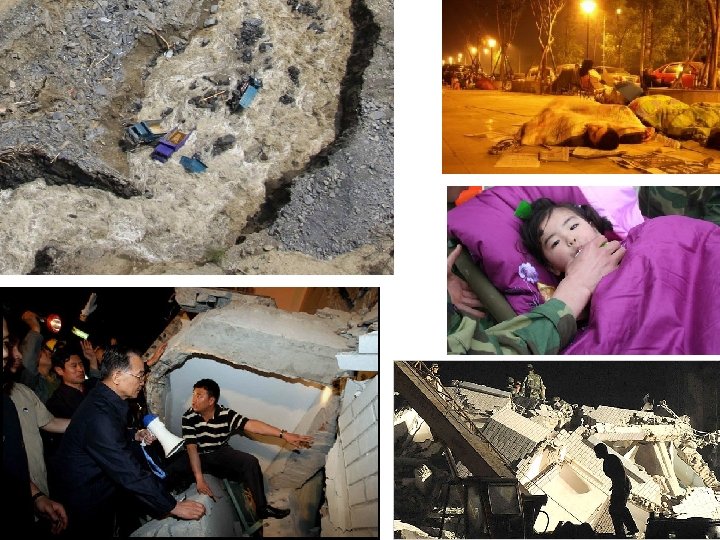
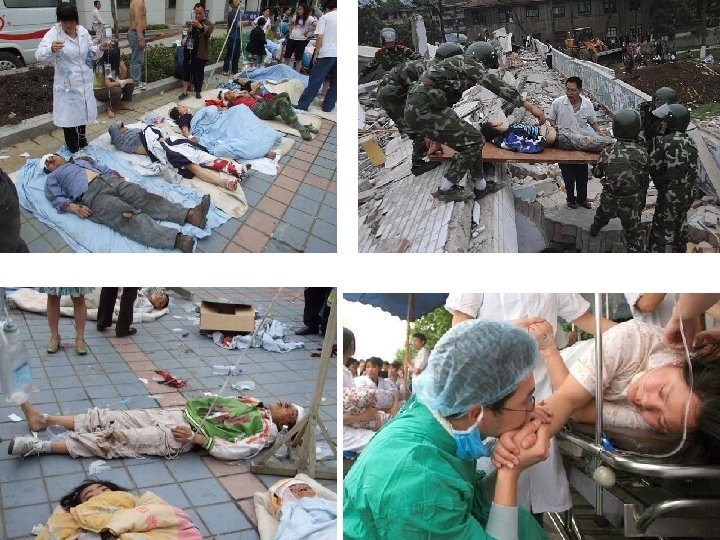
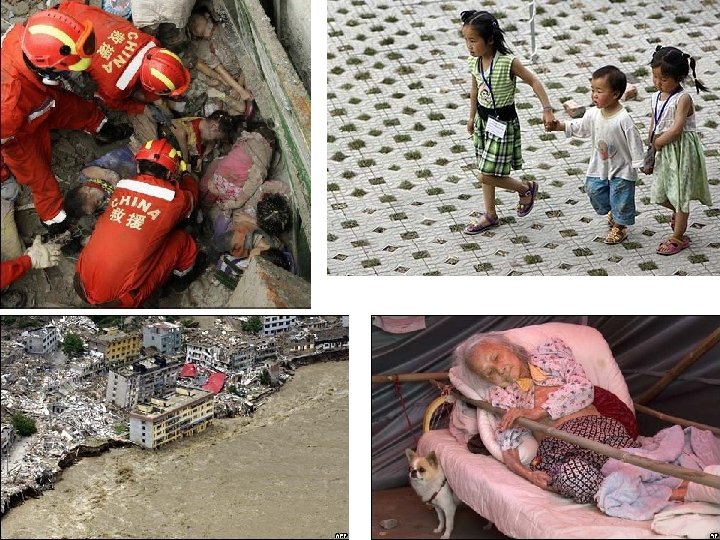
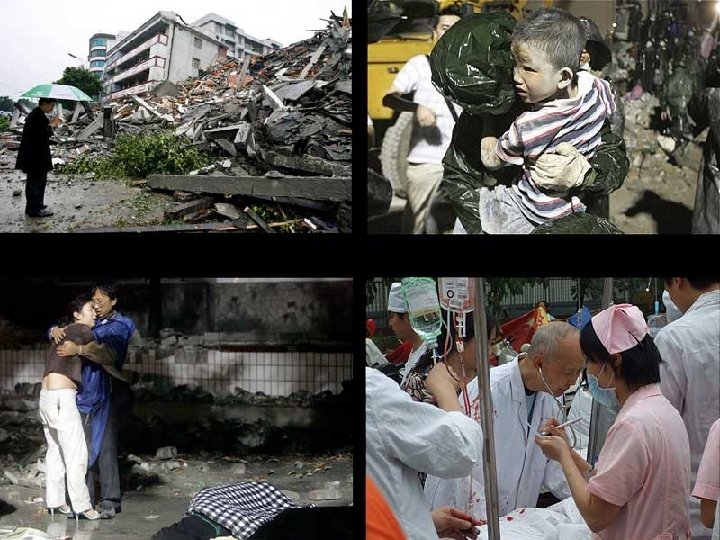
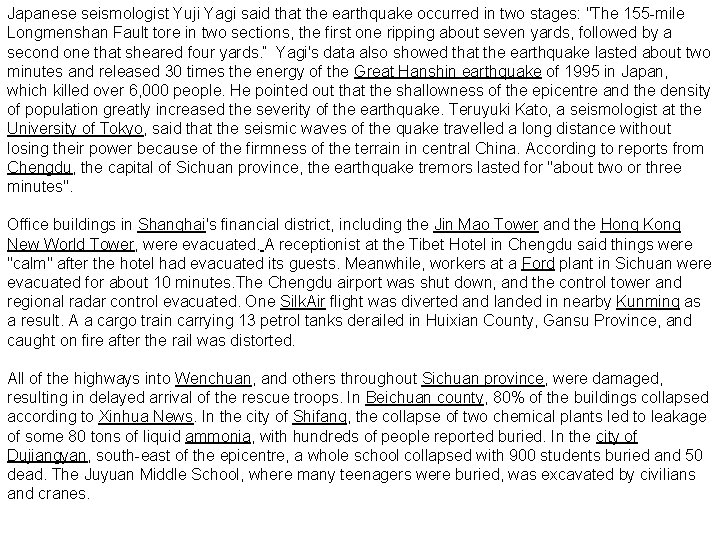
Japanese seismologist Yuji Yagi said that the earthquake occurred in two stages: "The 155 -mile Longmenshan Fault tore in two sections, the first one ripping about seven yards, followed by a second one that sheared four yards. “ Yagi's data also showed that the earthquake lasted about two minutes and released 30 times the energy of the Great Hanshin earthquake of 1995 in Japan, which killed over 6, 000 people. He pointed out that the shallowness of the epicentre and the density of population greatly increased the severity of the earthquake. Teruyuki Kato, a seismologist at the University of Tokyo, said that the seismic waves of the quake travelled a long distance without losing their power because of the firmness of the terrain in central China. According to reports from Chengdu, the capital of Sichuan province, the earthquake tremors lasted for "about two or three minutes". Office buildings in Shanghai's financial district, including the Jin Mao Tower and the Hong Kong New World Tower, were evacuated. A receptionist at the Tibet Hotel in Chengdu said things were "calm" after the hotel had evacuated its guests. Meanwhile, workers at a Ford plant in Sichuan were evacuated for about 10 minutes. The Chengdu airport was shut down, and the control tower and regional radar control evacuated. One Silk. Air flight was diverted and landed in nearby Kunming as a result. A a cargo train carrying 13 petrol tanks derailed in Huixian County, Gansu Province, and caught on fire after the rail was distorted. All of the highways into Wenchuan, and others throughout Sichuan province, were damaged, resulting in delayed arrival of the rescue troops. In Beichuan county, 80% of the buildings collapsed according to Xinhua News. In the city of Shifang, the collapse of two chemical plants led to leakage of some 80 tons of liquid ammonia, with hundreds of people reported buried. In the city of Dujiangyan, south-east of the epicentre, a whole school collapsed with 900 students buried and 50 dead. The Juyuan Middle School, where many teenagers were buried, was excavated by civilians and cranes.
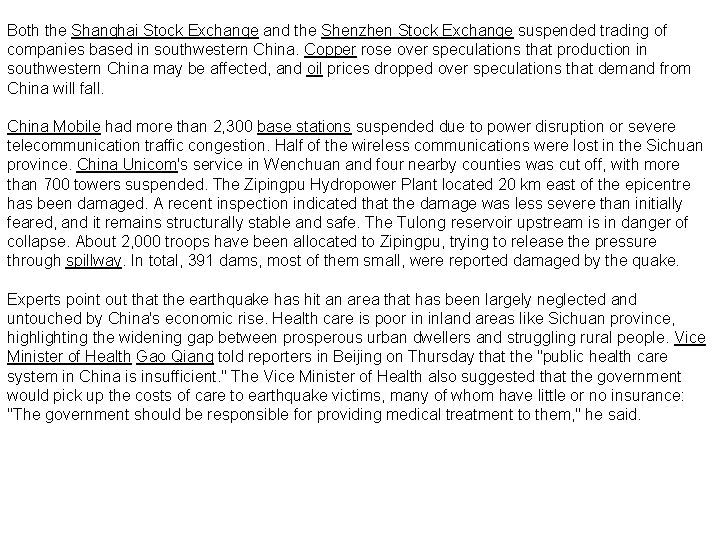
Both the Shanghai Stock Exchange and the Shenzhen Stock Exchange suspended trading of companies based in southwestern China. Copper rose over speculations that production in southwestern China may be affected, and oil prices dropped over speculations that demand from China will fall. China Mobile had more than 2, 300 base stations suspended due to power disruption or severe telecommunication traffic congestion. Half of the wireless communications were lost in the Sichuan province. China Unicom's service in Wenchuan and four nearby counties was cut off, with more than 700 towers suspended. The Zipingpu Hydropower Plant located 20 km east of the epicentre has been damaged. A recent inspection indicated that the damage was less severe than initially feared, and it remains structurally stable and safe. The Tulong reservoir upstream is in danger of collapse. About 2, 000 troops have been allocated to Zipingpu, trying to release the pressure through spillway. In total, 391 dams, most of them small, were reported damaged by the quake. Experts point out that the earthquake has hit an area that has been largely neglected and untouched by China's economic rise. Health care is poor in inland areas like Sichuan province, highlighting the widening gap between prosperous urban dwellers and struggling rural people. Vice Minister of Health Gao Qiang told reporters in Beijing on Thursday that the "public health care system in China is insufficient. " The Vice Minister of Health also suggested that the government would pick up the costs of care to earthquake victims, many of whom have little or no insurance: "The government should be responsible for providing medical treatment to them, " he said.
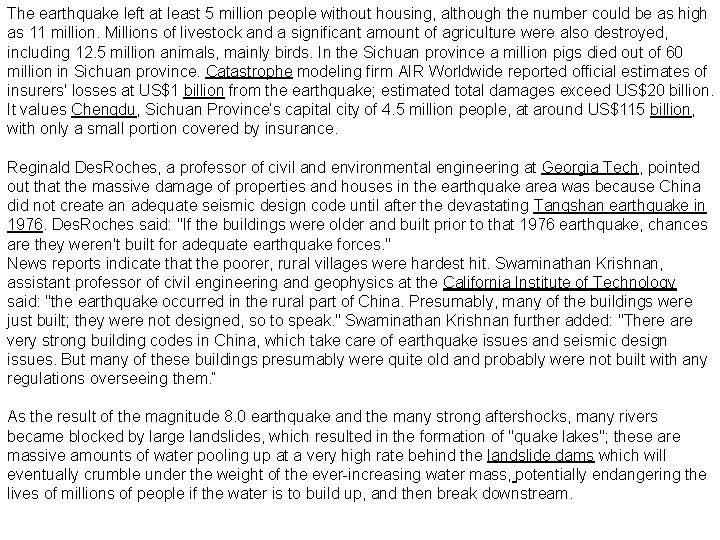
The earthquake left at least 5 million people without housing, although the number could be as high as 11 million. Millions of livestock and a significant amount of agriculture were also destroyed, including 12. 5 million animals, mainly birds. In the Sichuan province a million pigs died out of 60 million in Sichuan province. Catastrophe modeling firm AIR Worldwide reported official estimates of insurers' losses at US$1 billion from the earthquake; estimated total damages exceed US$20 billion. It values Chengdu, Sichuan Province’s capital city of 4. 5 million people, at around US$115 billion, with only a small portion covered by insurance. Reginald Des. Roches, a professor of civil and environmental engineering at Georgia Tech, pointed out that the massive damage of properties and houses in the earthquake area was because China did not create an adequate seismic design code until after the devastating Tangshan earthquake in 1976. Des. Roches said: "If the buildings were older and built prior to that 1976 earthquake, chances are they weren't built for adequate earthquake forces. " News reports indicate that the poorer, rural villages were hardest hit. Swaminathan Krishnan, assistant professor of civil engineering and geophysics at the California Institute of Technology said: "the earthquake occurred in the rural part of China. Presumably, many of the buildings were just built; they were not designed, so to speak. " Swaminathan Krishnan further added: "There are very strong building codes in China, which take care of earthquake issues and seismic design issues. But many of these buildings presumably were quite old and probably were not built with any regulations overseeing them. “ As the result of the magnitude 8. 0 earthquake and the many strong aftershocks, many rivers became blocked by large landslides, which resulted in the formation of "quake lakes"; these are massive amounts of water pooling up at a very high rate behind the landslide dams which will eventually crumble under the weight of the ever-increasing water mass, potentially endangering the lives of millions of people if the water is to build up, and then break downstream.
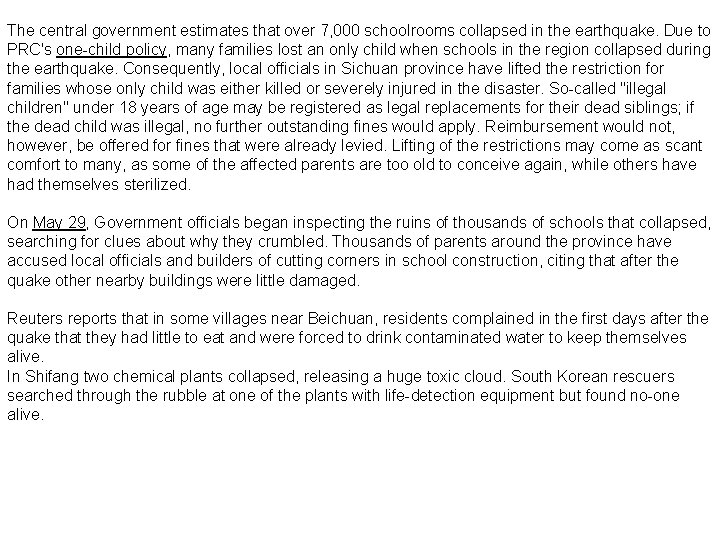
The central government estimates that over 7, 000 schoolrooms collapsed in the earthquake. Due to PRC's one-child policy, many families lost an only child when schools in the region collapsed during the earthquake. Consequently, local officials in Sichuan province have lifted the restriction for families whose only child was either killed or severely injured in the disaster. So-called "illegal children" under 18 years of age may be registered as legal replacements for their dead siblings; if the dead child was illegal, no further outstanding fines would apply. Reimbursement would not, however, be offered for fines that were already levied. Lifting of the restrictions may come as scant comfort to many, as some of the affected parents are too old to conceive again, while others have had themselves sterilized. On May 29, Government officials began inspecting the ruins of thousands of schools that collapsed, searching for clues about why they crumbled. Thousands of parents around the province have accused local officials and builders of cutting corners in school construction, citing that after the quake other nearby buildings were little damaged. Reuters reports that in some villages near Beichuan, residents complained in the first days after the quake that they had little to eat and were forced to drink contaminated water to keep themselves alive. In Shifang two chemical plants collapsed, releasing a huge toxic cloud. South Korean rescuers searched through the rubble at one of the plants with life-detection equipment but found no-one alive.
![Local and regional impacts of tectonic hazards Case study 1 Montserrat The impact of Local and regional impacts of tectonic hazards Case study [1] Montserrat The impact of](https://slidetodoc.com/presentation_image_h/d020a7159aa58ff4c55a30a84261b44e/image-66.jpg)
Local and regional impacts of tectonic hazards Case study [1] Montserrat The impact of pyroclastic surges and ashfall
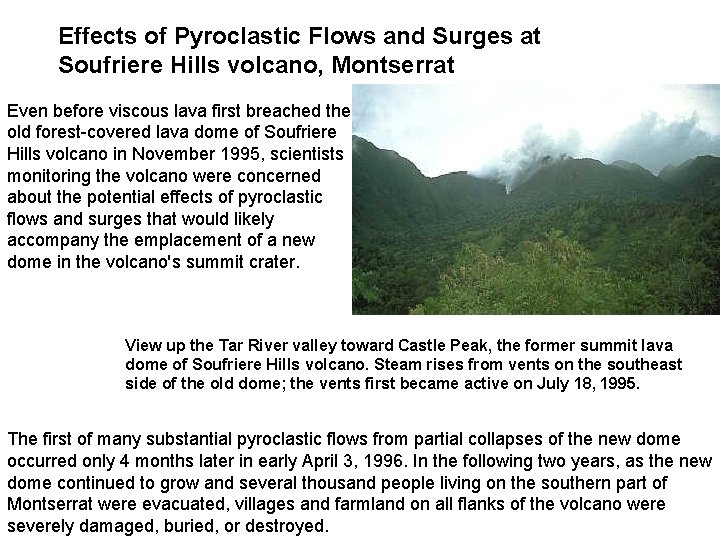
Effects of Pyroclastic Flows and Surges at Soufriere Hills volcano, Montserrat Even before viscous lava first breached the old forest-covered lava dome of Soufriere Hills volcano in November 1995, scientists monitoring the volcano were concerned about the potential effects of pyroclastic flows and surges that would likely accompany the emplacement of a new dome in the volcano's summit crater. View up the Tar River valley toward Castle Peak, the former summit lava dome of Soufriere Hills volcano. Steam rises from vents on the southeast side of the old dome; the vents first became active on July 18, 1995. The first of many substantial pyroclastic flows from partial collapses of the new dome occurred only 4 months later in early April 3, 1996. In the following two years, as the new dome continued to grow and several thousand people living on the southern part of Montserrat were evacuated, villages and farmland on all flanks of the volcano were severely damaged, buried, or destroyed.
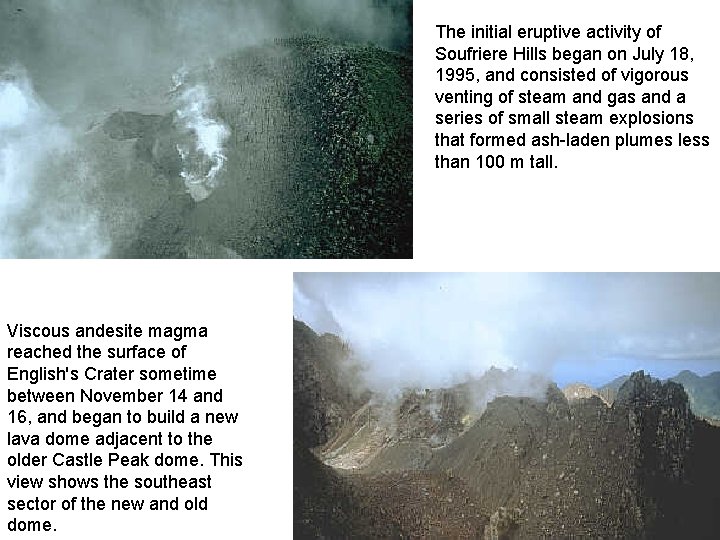
The initial eruptive activity of Soufriere Hills began on July 18, 1995, and consisted of vigorous venting of steam and gas and a series of small steam explosions that formed ash-laden plumes less than 100 m tall. Viscous andesite magma reached the surface of English's Crater sometime between November 14 and 16, and began to build a new lava dome adjacent to the older Castle Peak dome. This view shows the southeast sector of the new and old dome.
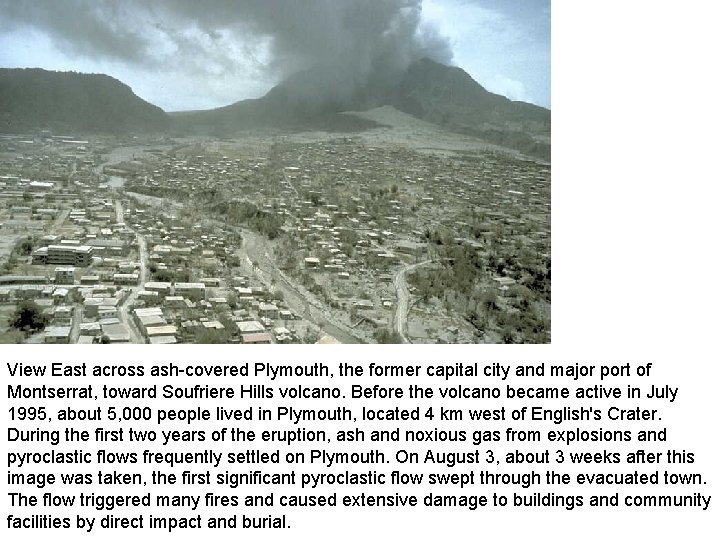
View East across ash-covered Plymouth, the former capital city and major port of Montserrat, toward Soufriere Hills volcano. Before the volcano became active in July 1995, about 5, 000 people lived in Plymouth, located 4 km west of English's Crater. During the first two years of the eruption, ash and noxious gas from explosions and pyroclastic flows frequently settled on Plymouth. On August 3, about 3 weeks after this image was taken, the first significant pyroclastic flow swept through the evacuated town. The flow triggered many fires and caused extensive damage to buildings and community facilities by direct impact and burial.
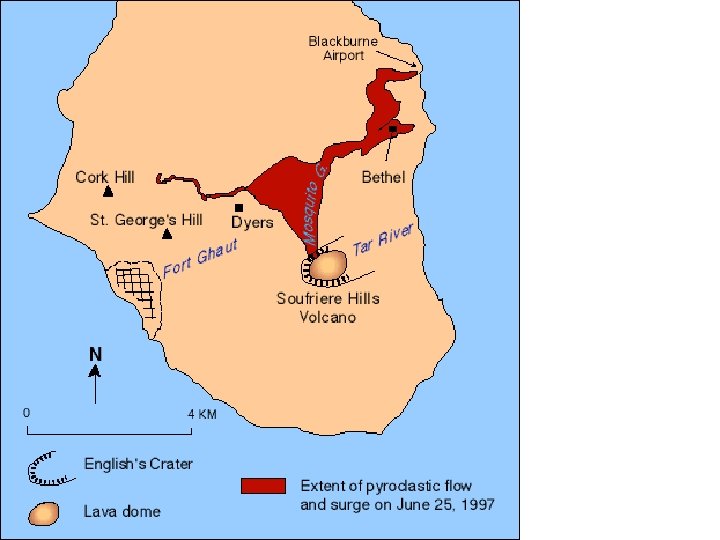
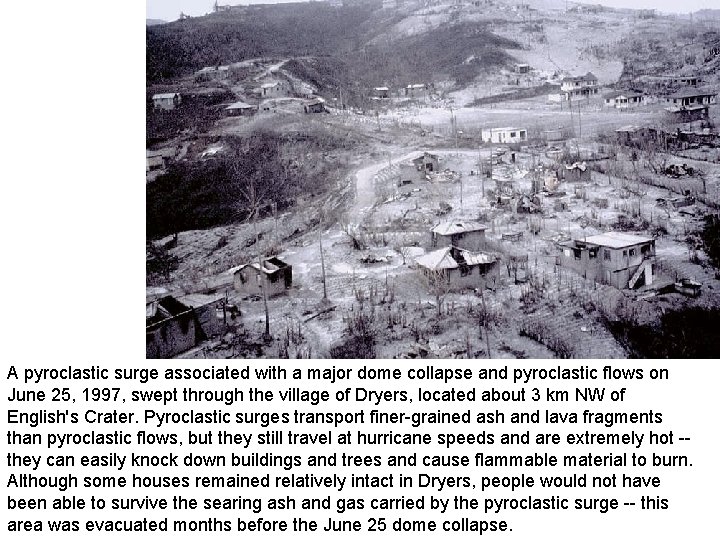
A pyroclastic surge associated with a major dome collapse and pyroclastic flows on June 25, 1997, swept through the village of Dryers, located about 3 km NW of English's Crater. Pyroclastic surges transport finer-grained ash and lava fragments than pyroclastic flows, but they still travel at hurricane speeds and are extremely hot -- they can easily knock down buildings and trees and cause flammable material to burn. Although some houses remained relatively intact in Dryers, people would not have been able to survive the searing ash and gas carried by the pyroclastic surge -- this area was evacuated months before the June 25 dome collapse.
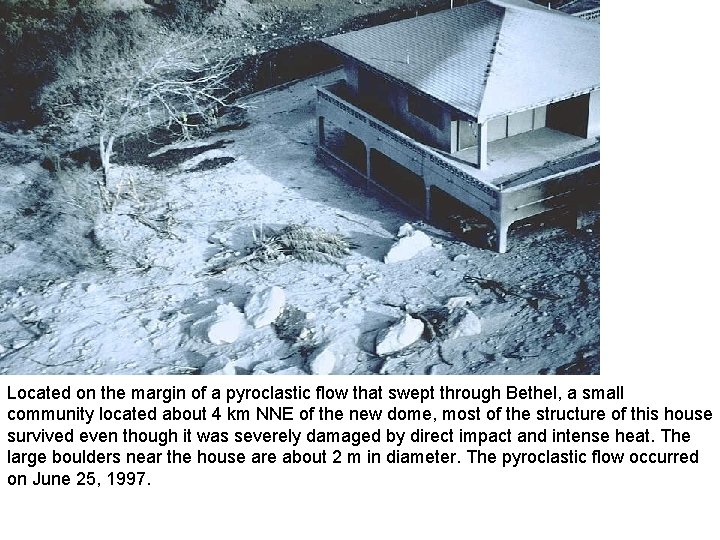
Located on the margin of a pyroclastic flow that swept through Bethel, a small community located about 4 km NNE of the new dome, most of the structure of this house survived even though it was severely damaged by direct impact and intense heat. The large boulders near the house are about 2 m in diameter. The pyroclastic flow occurred on June 25, 1997.
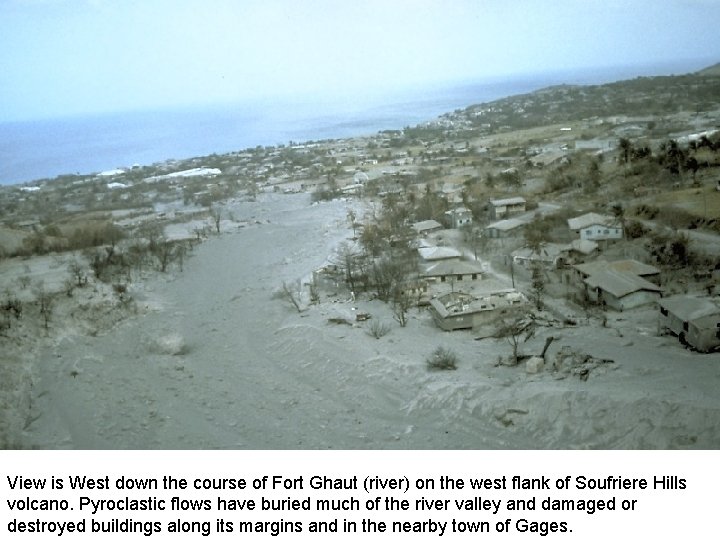
View is West down the course of Fort Ghaut (river) on the west flank of Soufriere Hills volcano. Pyroclastic flows have buried much of the river valley and damaged or destroyed buildings along its margins and in the nearby town of Gages.
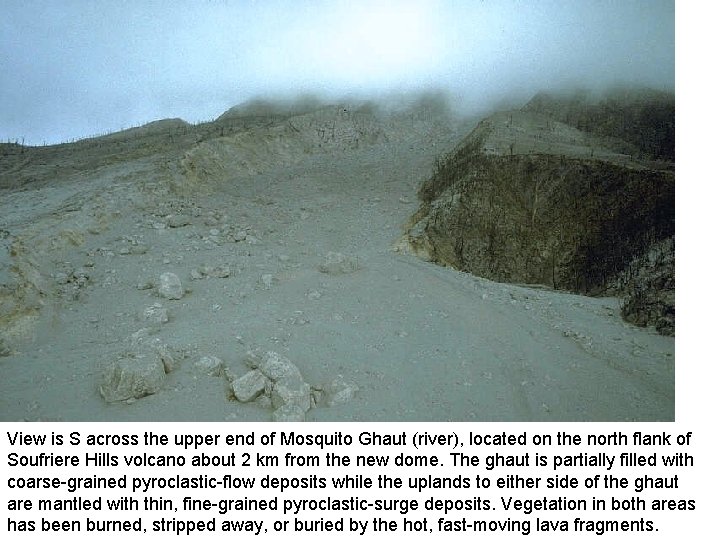
View is S across the upper end of Mosquito Ghaut (river), located on the north flank of Soufriere Hills volcano about 2 km from the new dome. The ghaut is partially filled with coarse-grained pyroclastic-flow deposits while the uplands to either side of the ghaut are mantled with thin, fine-grained pyroclastic-surge deposits. Vegetation in both areas has been burned, stripped away, or buried by the hot, fast-moving lava fragments.
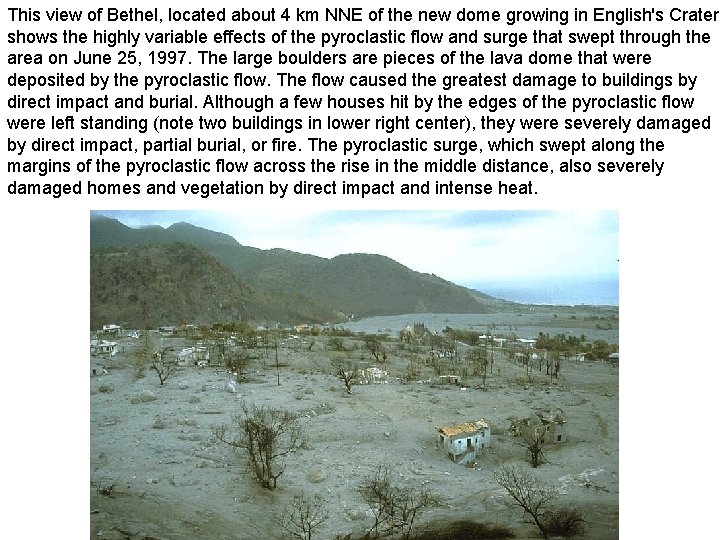
This view of Bethel, located about 4 km NNE of the new dome growing in English's Crater, shows the highly variable effects of the pyroclastic flow and surge that swept through the area on June 25, 1997. The large boulders are pieces of the lava dome that were deposited by the pyroclastic flow. The flow caused the greatest damage to buildings by direct impact and burial. Although a few houses hit by the edges of the pyroclastic flow were left standing (note two buildings in lower right center), they were severely damaged by direct impact, partial burial, or fire. The pyroclastic surge, which swept along the margins of the pyroclastic flow across the rise in the middle distance, also severely damaged homes and vegetation by direct impact and intense heat.
![Local and regional impacts of tectonic hazards Case study 2 Nevado del Ruiz The Local and regional impacts of tectonic hazards Case study [2] Nevado del Ruiz The](https://slidetodoc.com/presentation_image_h/d020a7159aa58ff4c55a30a84261b44e/image-76.jpg)
Local and regional impacts of tectonic hazards Case study [2] Nevado del Ruiz The impact of lahar in Colombia
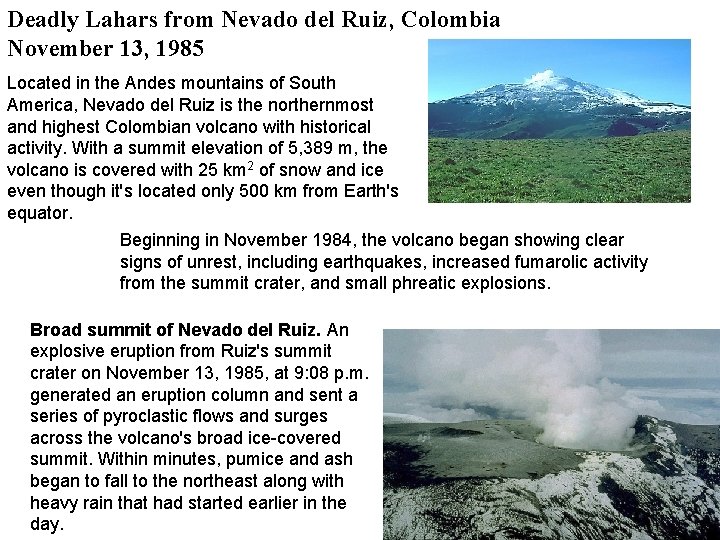
Deadly Lahars from Nevado del Ruiz, Colombia November 13, 1985 Located in the Andes mountains of South America, Nevado del Ruiz is the northernmost and highest Colombian volcano with historical activity. With a summit elevation of 5, 389 m, the volcano is covered with 25 km 2 of snow and ice even though it's located only 500 km from Earth's equator. Beginning in November 1984, the volcano began showing clear signs of unrest, including earthquakes, increased fumarolic activity from the summit crater, and small phreatic explosions. Broad summit of Nevado del Ruiz. An explosive eruption from Ruiz's summit crater on November 13, 1985, at 9: 08 p. m. generated an eruption column and sent a series of pyroclastic flows and surges across the volcano's broad ice-covered summit. Within minutes, pumice and ash began to fall to the northeast along with heavy rain that had started earlier in the day.
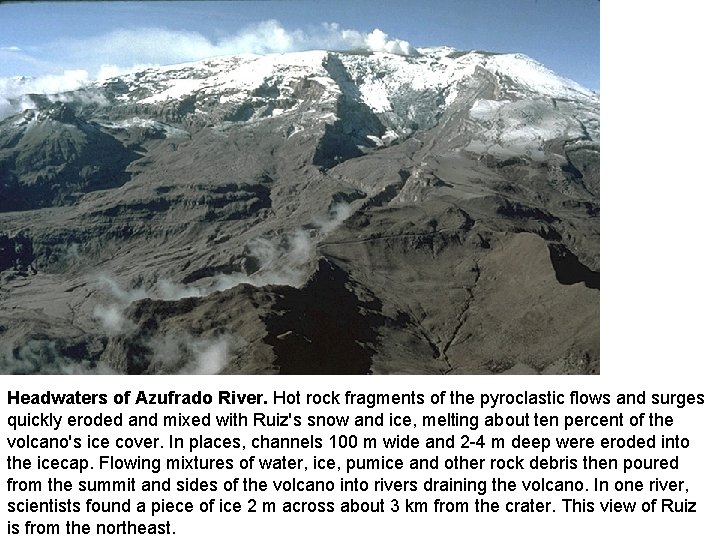
Headwaters of Azufrado River. Hot rock fragments of the pyroclastic flows and surges quickly eroded and mixed with Ruiz's snow and ice, melting about ten percent of the volcano's ice cover. In places, channels 100 m wide and 2 -4 m deep were eroded into the icecap. Flowing mixtures of water, ice, pumice and other rock debris then poured from the summit and sides of the volcano into rivers draining the volcano. In one river, scientists found a piece of ice 2 m across about 3 km from the crater. This view of Ruiz is from the northeast.
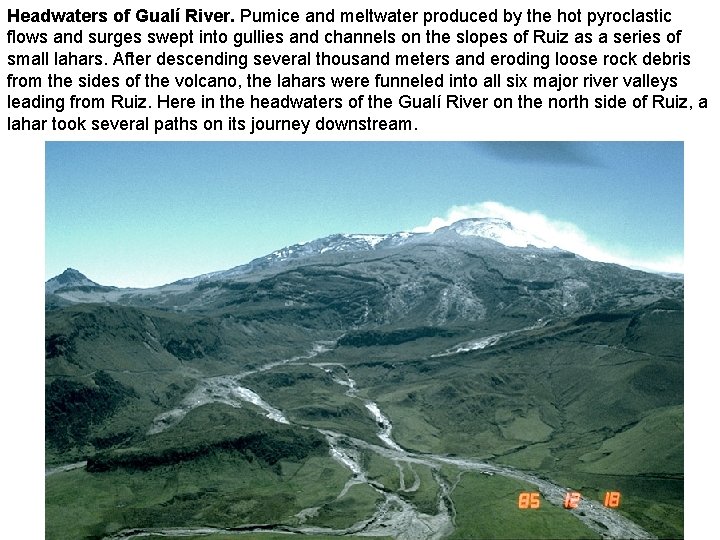
Headwaters of Gualí River. Pumice and meltwater produced by the hot pyroclastic flows and surges swept into gullies and channels on the slopes of Ruiz as a series of small lahars. After descending several thousand meters and eroding loose rock debris from the sides of the volcano, the lahars were funneled into all six major river valleys leading from Ruiz. Here in the headwaters of the Gualí River on the north side of Ruiz, a lahar took several paths on its journey downstream.
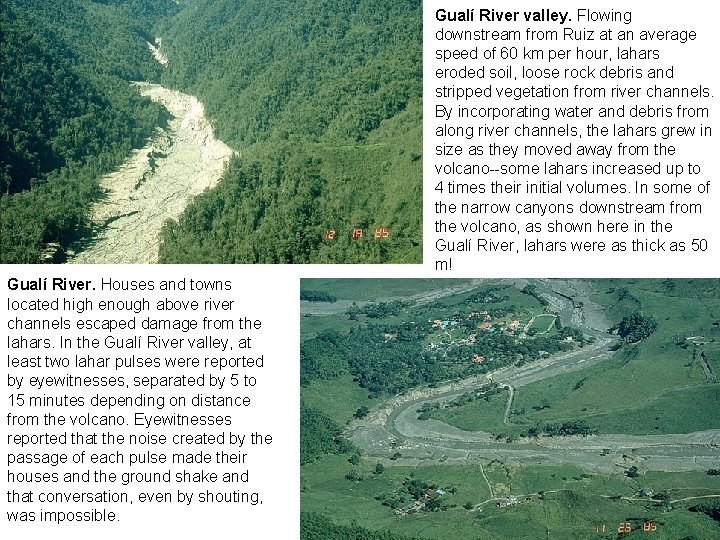
Gualí River valley. Flowing downstream from Ruiz at an average speed of 60 km per hour, lahars eroded soil, loose rock debris and stripped vegetation from river channels. By incorporating water and debris from along river channels, the lahars grew in size as they moved away from the volcano--some lahars increased up to 4 times their initial volumes. In some of the narrow canyons downstream from the volcano, as shown here in the Gualí River, lahars were as thick as 50 m! Gualí River. Houses and towns located high enough above river channels escaped damage from the lahars. In the Gualí River valley, at least two lahar pulses were reported by eyewitnesses, separated by 5 to 15 minutes depending on distance from the volcano. Eyewitnesses reported that the noise created by the passage of each pulse made their houses and the ground shake and that conversation, even by shouting, was impossible.
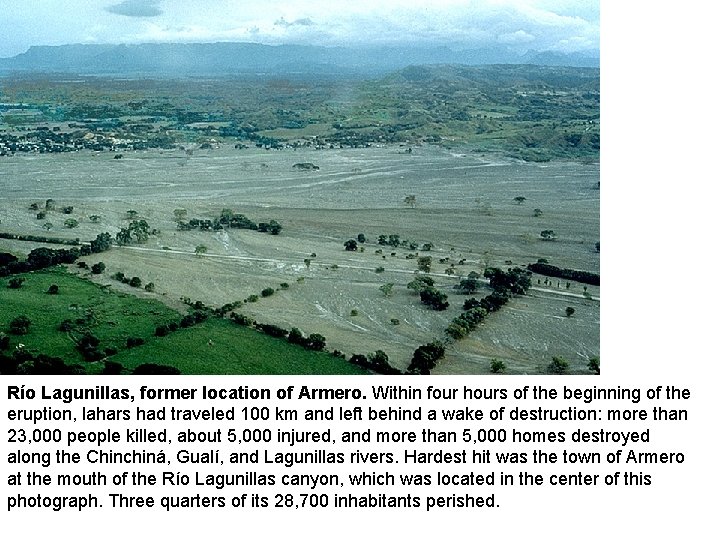
Río Lagunillas, former location of Armero. Within four hours of the beginning of the eruption, lahars had traveled 100 km and left behind a wake of destruction: more than 23, 000 people killed, about 5, 000 injured, and more than 5, 000 homes destroyed along the Chinchiná, Gualí, and Lagunillas rivers. Hardest hit was the town of Armero at the mouth of the Río Lagunillas canyon, which was located in the center of this photograph. Three quarters of its 28, 700 inhabitants perished.
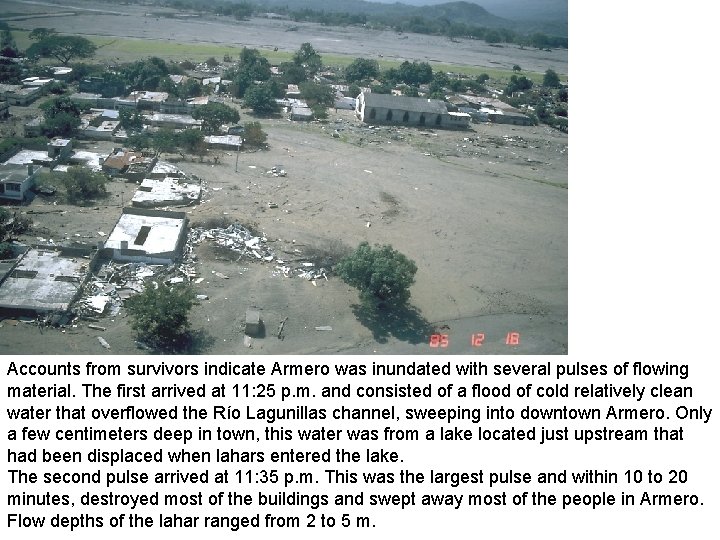
Accounts from survivors indicate Armero was inundated with several pulses of flowing material. The first arrived at 11: 25 p. m. and consisted of a flood of cold relatively clean water that overflowed the Río Lagunillas channel, sweeping into downtown Armero. Only a few centimeters deep in town, this water was from a lake located just upstream that had been displaced when lahars entered the lake. The second pulse arrived at 11: 35 p. m. This was the largest pulse and within 10 to 20 minutes, destroyed most of the buildings and swept away most of the people in Armero. Flow depths of the lahar ranged from 2 to 5 m.
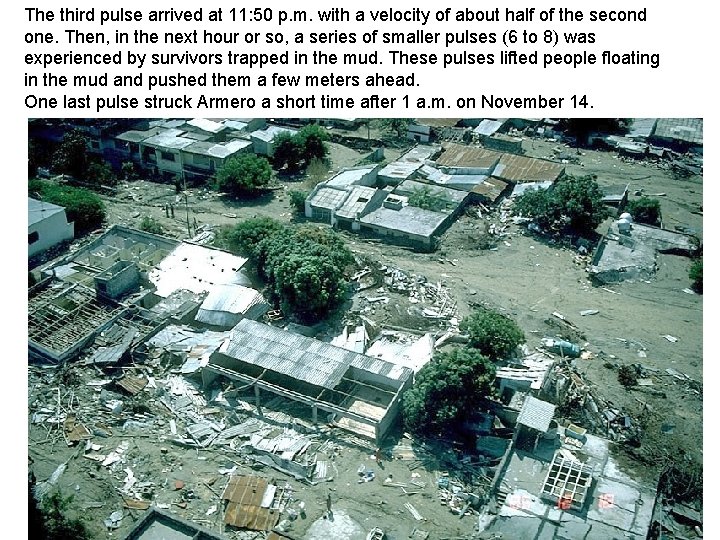
The third pulse arrived at 11: 50 p. m. with a velocity of about half of the second one. Then, in the next hour or so, a series of smaller pulses (6 to 8) was experienced by survivors trapped in the mud. These pulses lifted people floating in the mud and pushed them a few meters ahead. One last pulse struck Armero a short time after 1 a. m. on November 14.
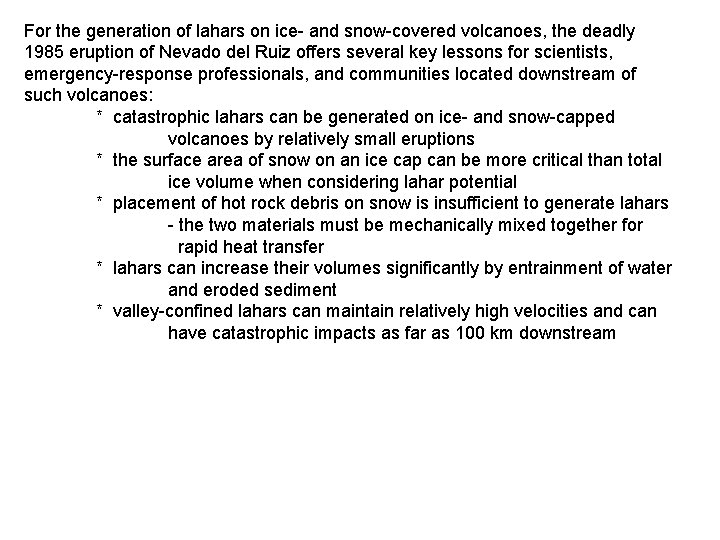
For the generation of lahars on ice- and snow-covered volcanoes, the deadly 1985 eruption of Nevado del Ruiz offers several key lessons for scientists, emergency-response professionals, and communities located downstream of such volcanoes: * catastrophic lahars can be generated on ice- and snow-capped volcanoes by relatively small eruptions * the surface area of snow on an ice cap can be more critical than total ice volume when considering lahar potential * placement of hot rock debris on snow is insufficient to generate lahars - the two materials must be mechanically mixed together for rapid heat transfer * lahars can increase their volumes significantly by entrainment of water and eroded sediment * valley-confined lahars can maintain relatively high velocities and can have catastrophic impacts as far as 100 km downstream
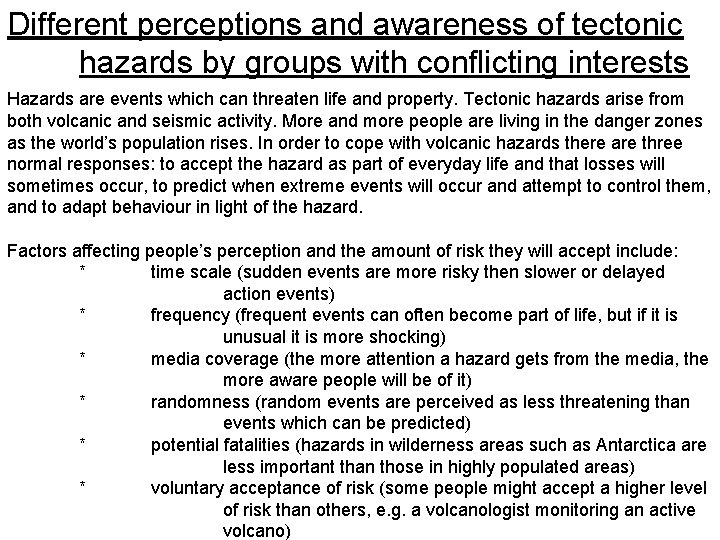
Different perceptions and awareness of tectonic hazards by groups with conflicting interests Hazards are events which can threaten life and property. Tectonic hazards arise from both volcanic and seismic activity. More and more people are living in the danger zones as the world’s population rises. In order to cope with volcanic hazards there are three normal responses: to accept the hazard as part of everyday life and that losses will sometimes occur, to predict when extreme events will occur and attempt to control them, and to adapt behaviour in light of the hazard. Factors affecting people’s perception and the amount of risk they will accept include: * time scale (sudden events are more risky then slower or delayed action events) * frequency (frequent events can often become part of life, but if it is unusual it is more shocking) * media coverage (the more attention a hazard gets from the media, the more aware people will be of it) * randomness (random events are perceived as less threatening than events which can be predicted) * potential fatalities (hazards in wilderness areas such as Antarctica are less important than those in highly populated areas) * voluntary acceptance of risk (some people might accept a higher level of risk than others, e. g. a volcanologist monitoring an active volcano)
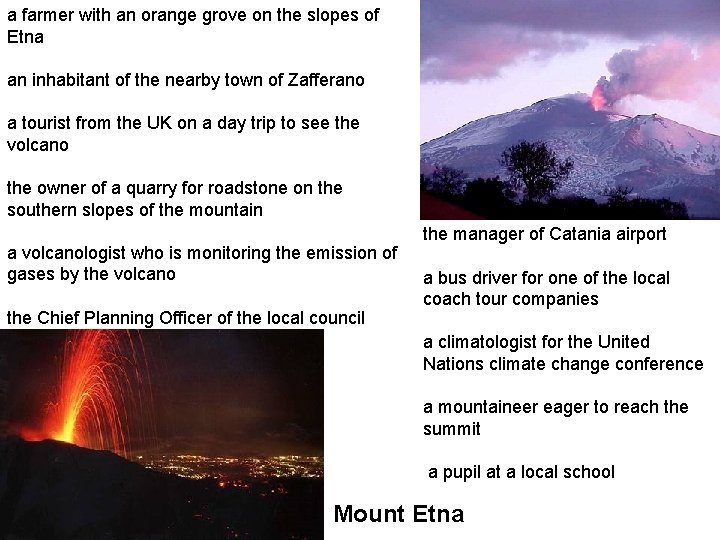
a farmer with an orange grove on the slopes of Etna an inhabitant of the nearby town of Zafferano a tourist from the UK on a day trip to see the volcano the owner of a quarry for roadstone on the southern slopes of the mountain a volcanologist who is monitoring the emission of gases by the volcano the Chief Planning Officer of the local council the manager of Catania airport a bus driver for one of the local coach tour companies a climatologist for the United Nations climate change conference a mountaineer eager to reach the summit a pupil at a local school Mount Etna
Investigating and making a case for drug diversion
Cell theory contributors
Investigating the properties of sound
Investigating science hsc
Investigating graphs of polynomial functions
Investigating graphs of functions for their properties
Investigating customer service
Investigating the world of work. lesson 1
Investigating graphs of polynomial functions
Unit 14 investigating customer service assignment 2
Investigating the graph of an inequality
Digital graphics to entertain
Investigating polynomials
Investigating system requirements
Science 14 module 1 answer key
What is a confluence in a river
6-7 investigating graphs of polynomial functions
Investigating quadratics
Investigating rivers
Thomas edison lesson
Methods of investigating behaviour
Investigating conductors lab report
What is this shape
Language meaning
Investigating system requirements
Specific heat capacity question
Suppose your class is investigating the weights of snickers
Difference between theme and genre
What is the difference between theme and universal theme?
Example of chemical change
Absolute change and relative change formula
Whats the difference between a chemical and physical change
Change in supply and change in quantity supplied
Which is an example of a physical change
Rocks change due to temperature and pressure change
Physical change vs chemical change venn diagram
First order change adalah
Primary and secondary effects of a tectonic hazard
Primary volcanic hazards
What is the theme for theme for english b
What is a universal theme?
Is cutting paper a physical change
Is an integer subtracted by an integer always an integer
Input and output market
Enagic comp plan
Reactive change is change that
Spare change physical versus chemical change
How does a physical change differ from a chemical change
Chemical change in baking
Is chopping firewood a physical or chemical change
Climate change 2014 mitigation of climate change
Ring of fire hotspots
Hazard profile examples
Plate tectonics vs continental drift
7 tectonic plates
Plunging anticline
Tectonic joints
Grand banks earthquake of 1929
Plunging anticline
How the continents fit together
Plate tectonic theory vs continental drift
What causes plates to move? *
Collision plate boundaries
Transform boundary oreo
Mt fuji plate boundary type
Transform fault
Tectonic plates with names
Investigative question
Joints geology
Tectonic setting of stratovolcanoes
Plate tectonics definition apes
Tectonic plates bbc bitesize
Tectonic plates
Tectonic plates interact at places called plate
Tectonic plates move
Collision plate margin diagram
Tectonic plates move
What tectonic plate is michigan on
East pacific rise
Tectonic plates quizizz
Plate tectonics with oreos
Gizmos building pangea
Pompeii the last day bbc
The plate tectonic system is driven by *
Tectonic
What causes tectonic plates to move? *
Geomorphology