Population Genetics Copyright The Mc GrawHill Companies Inc
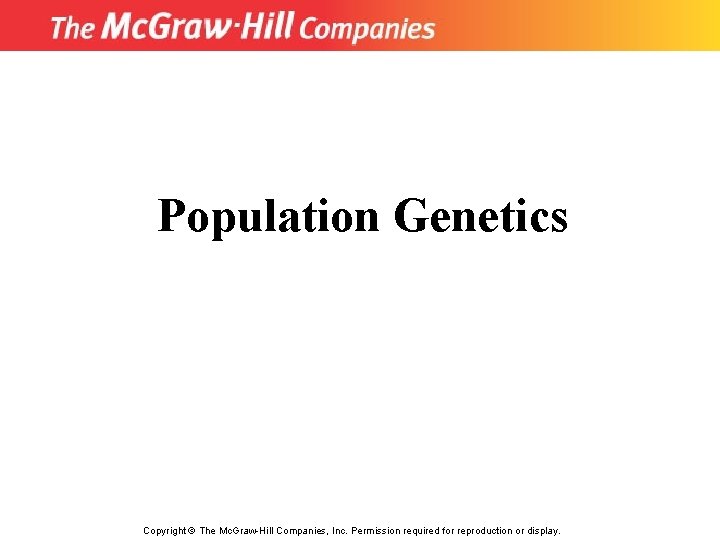
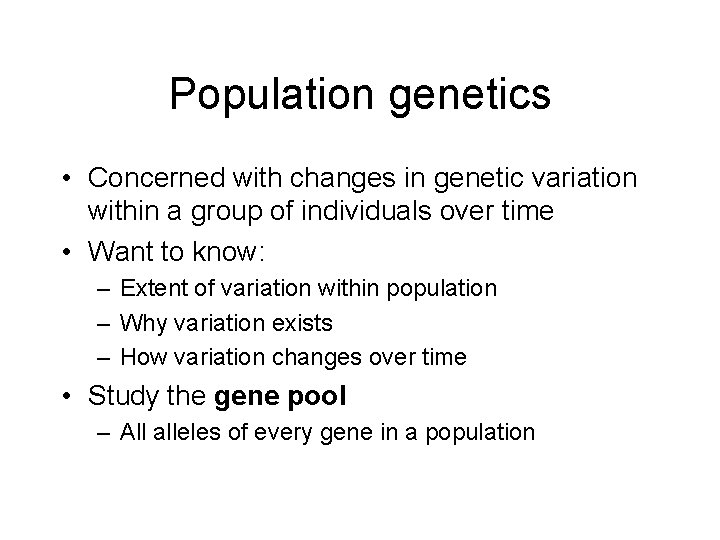
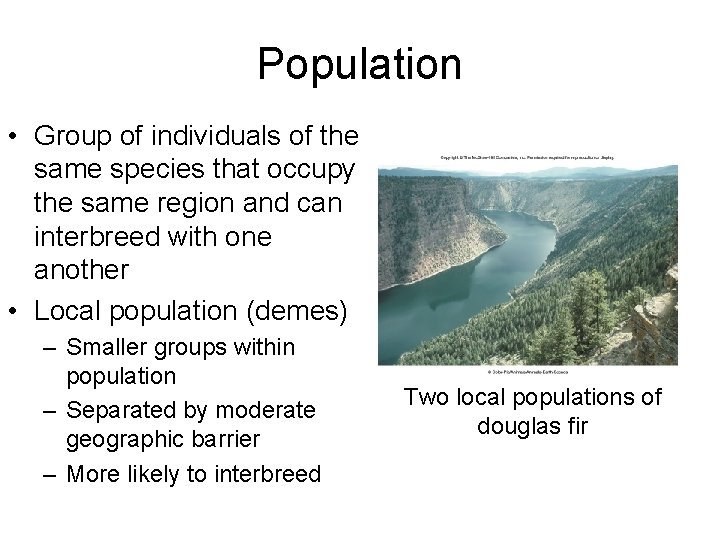
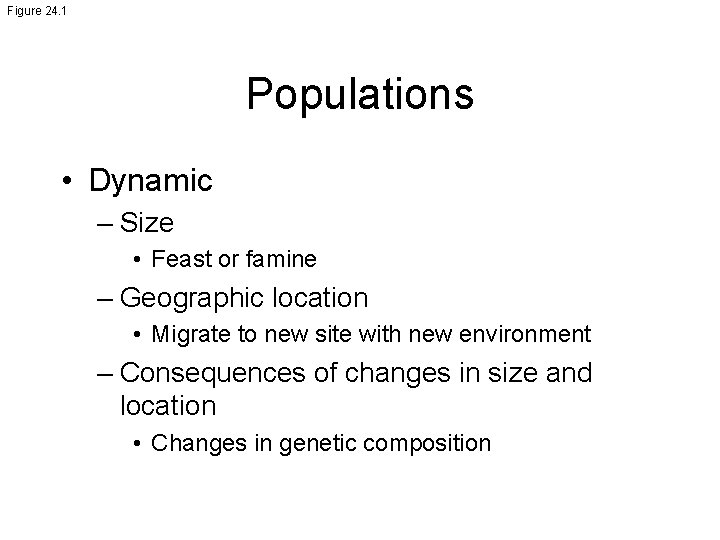
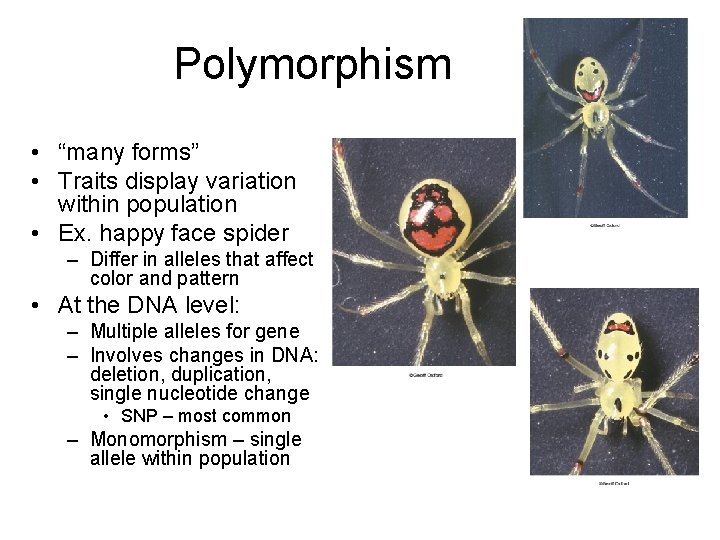
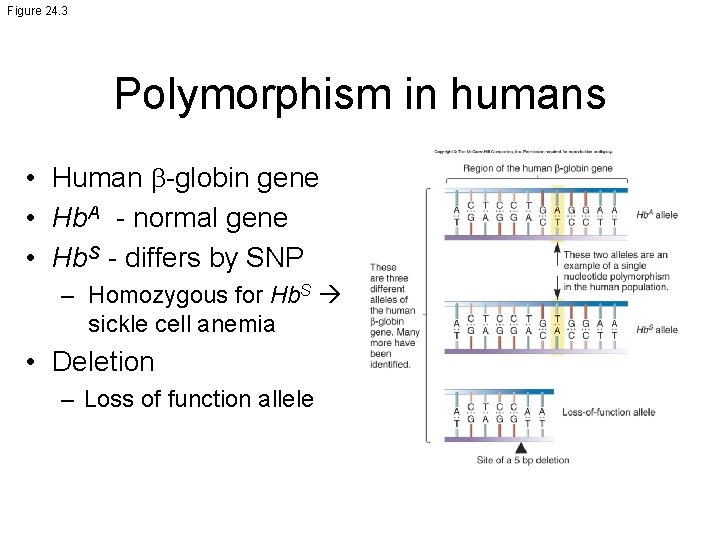
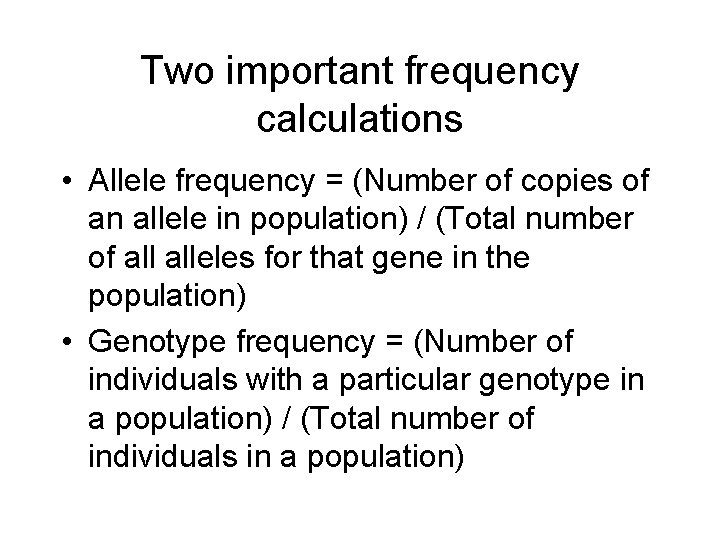
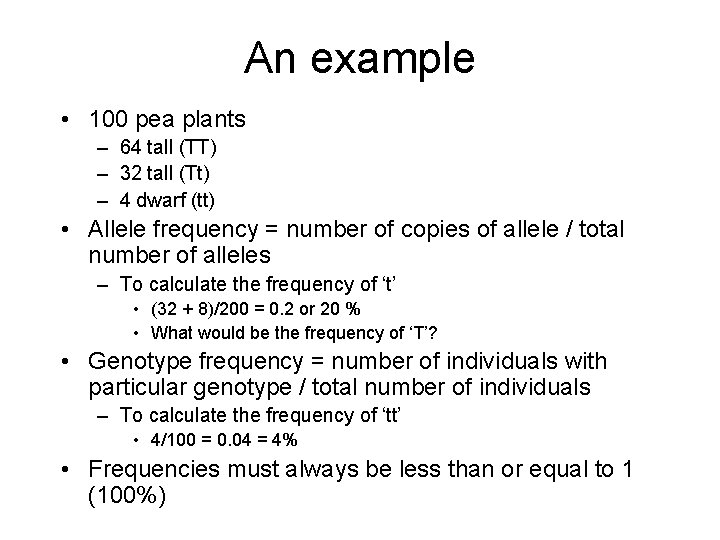
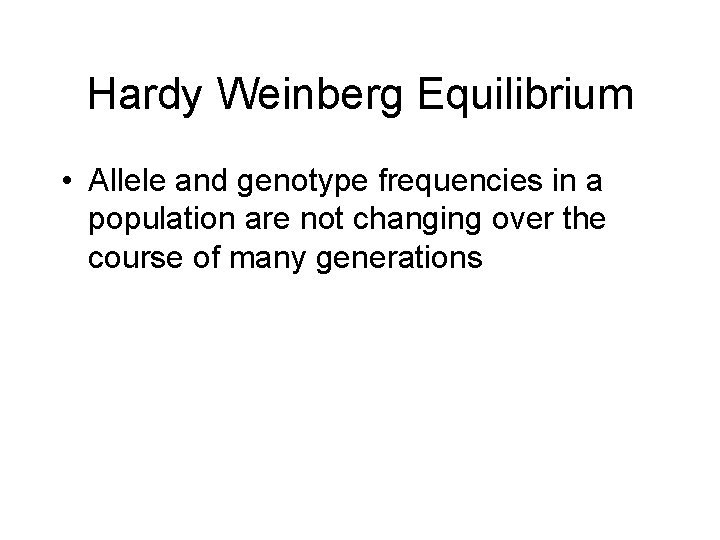
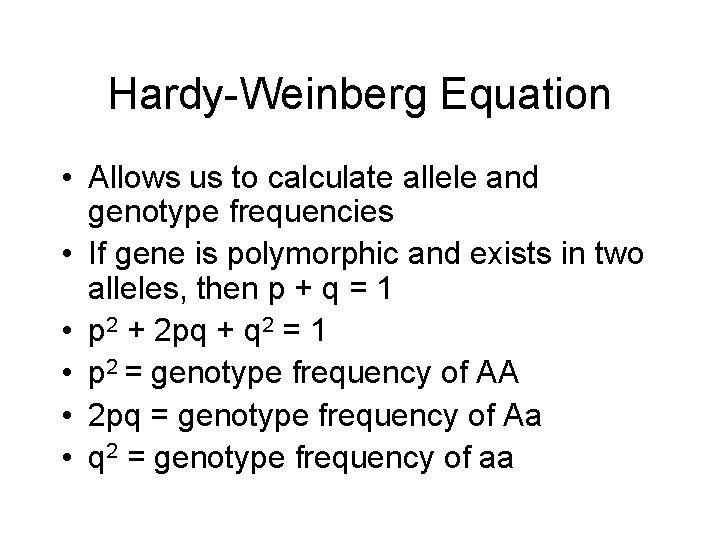
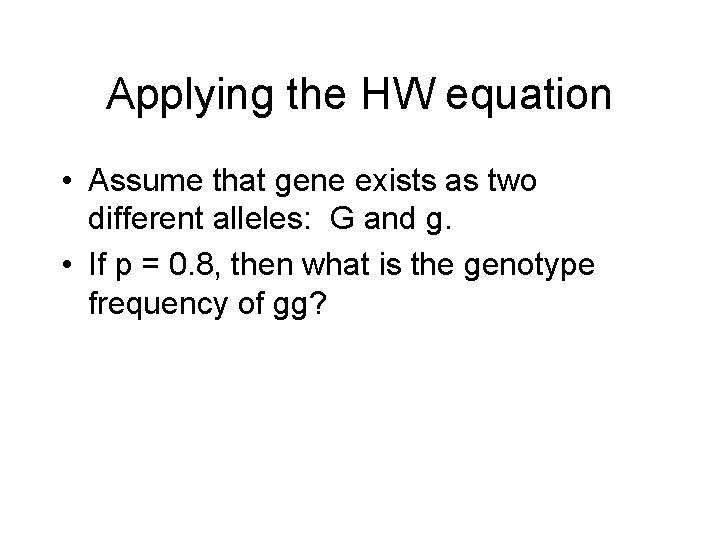
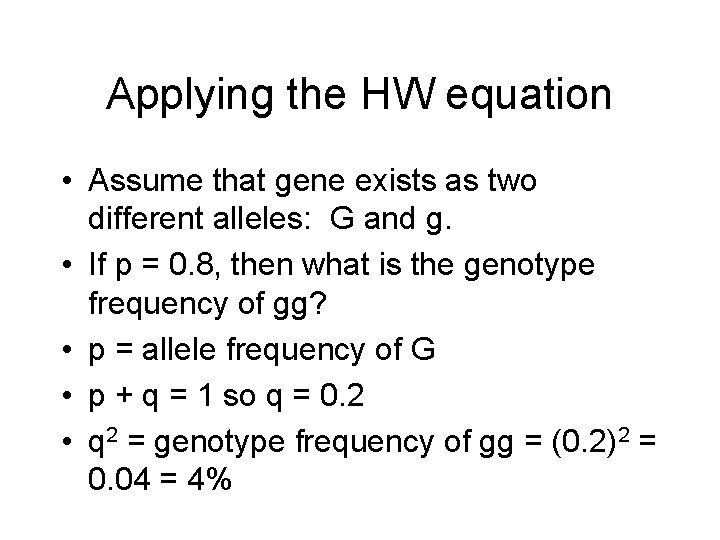
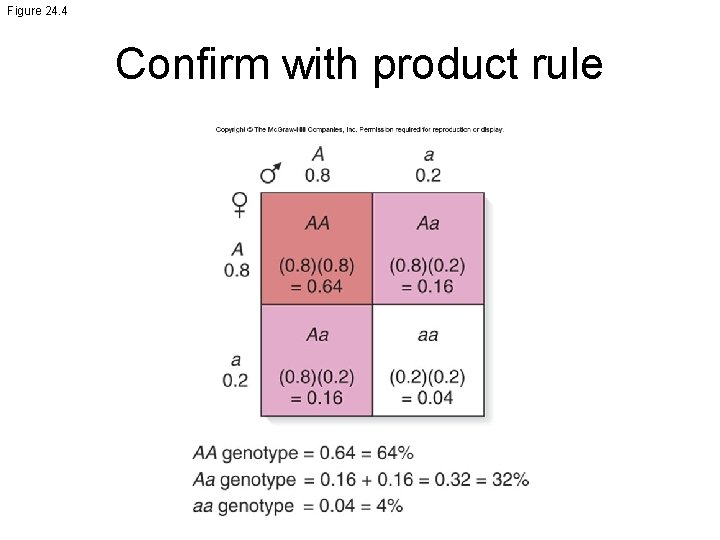
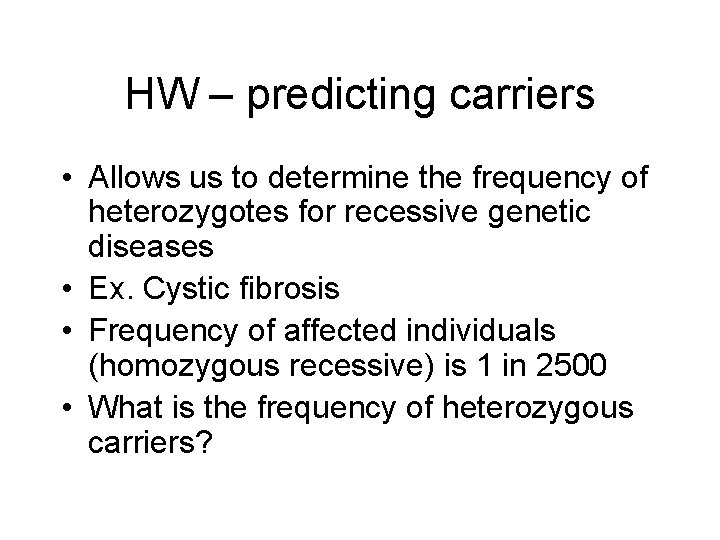
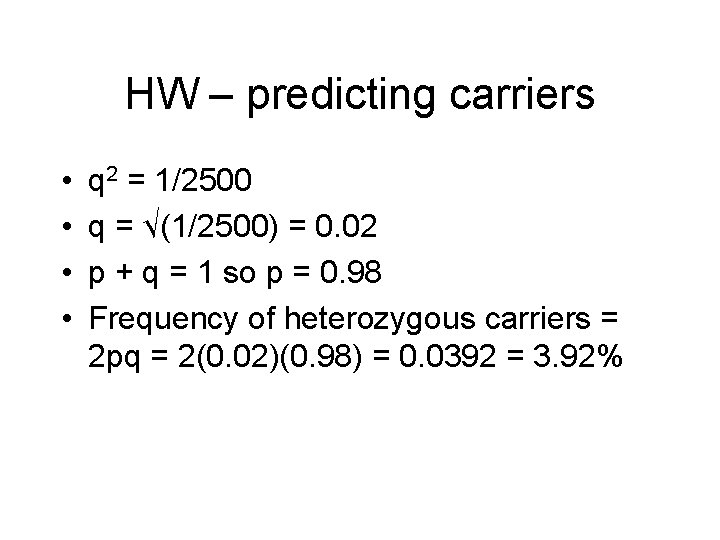
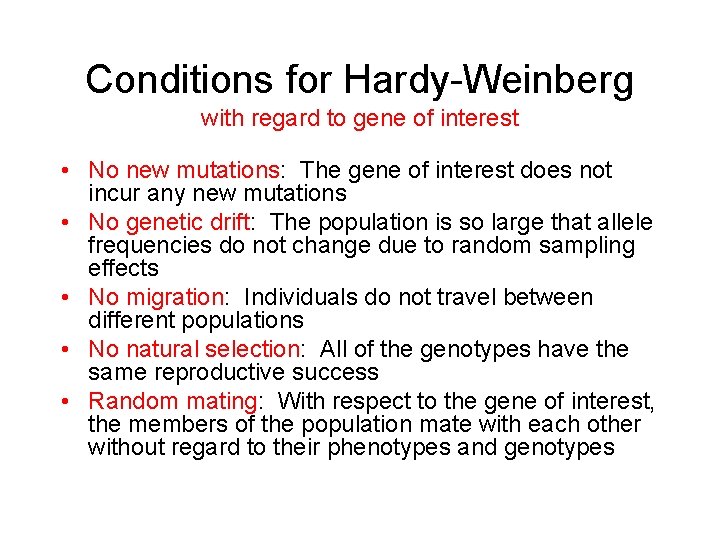
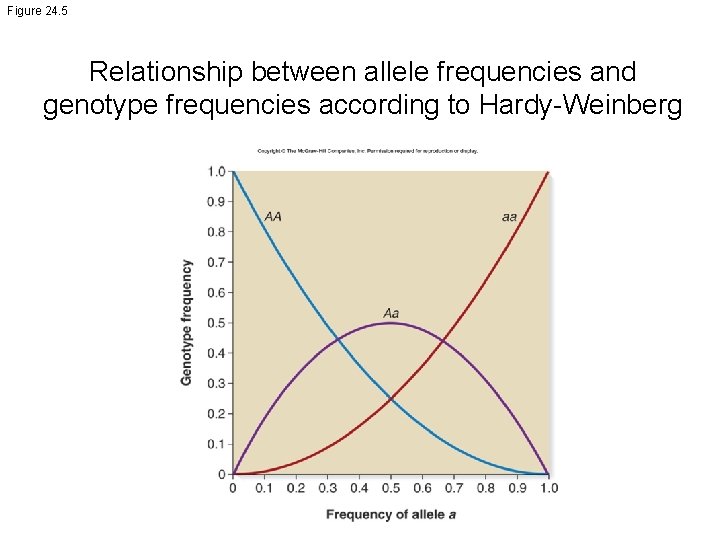
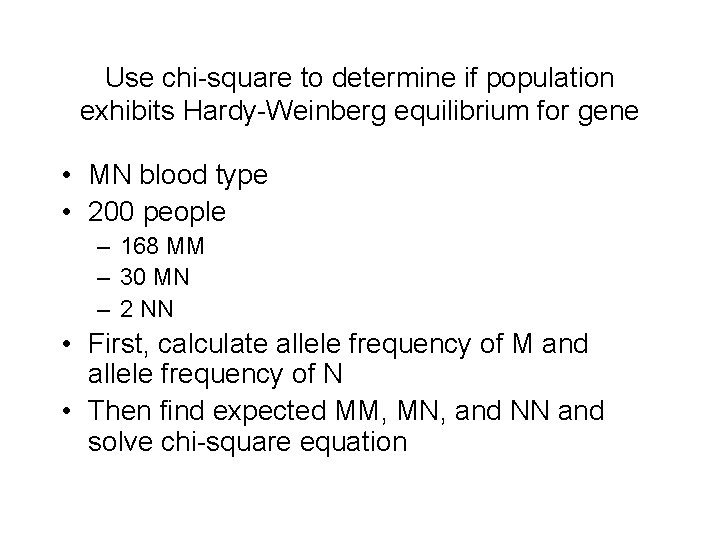
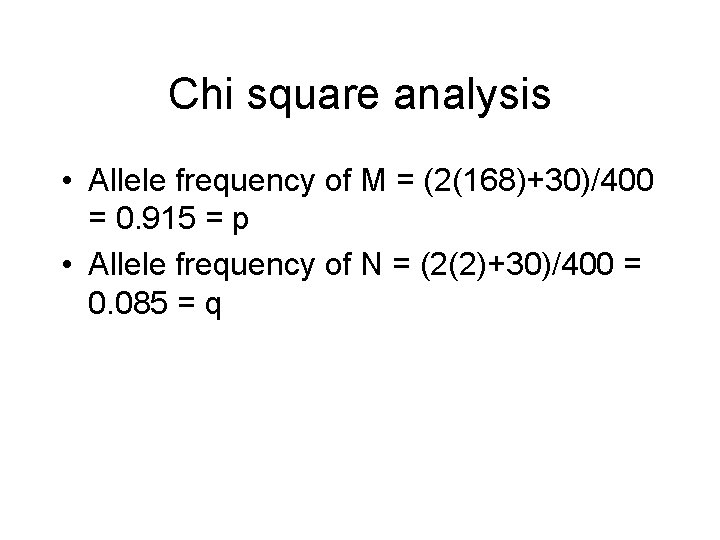
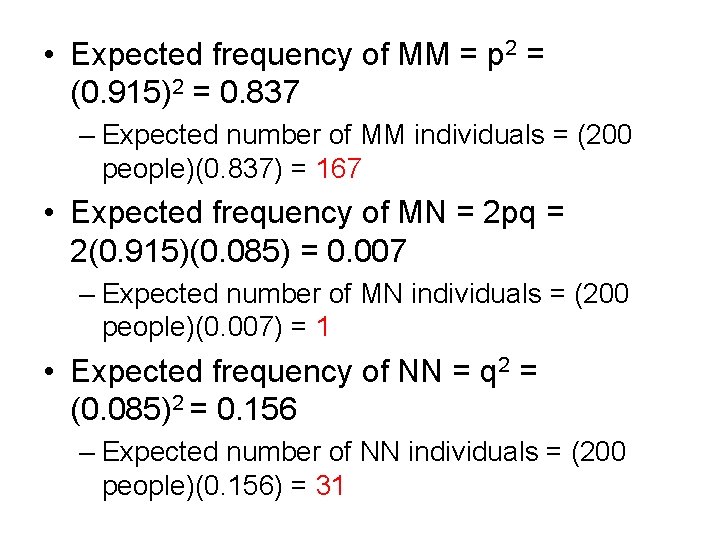
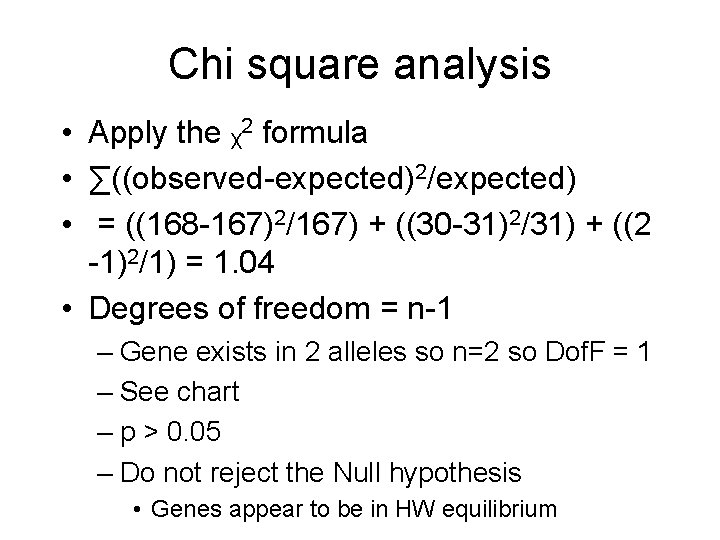
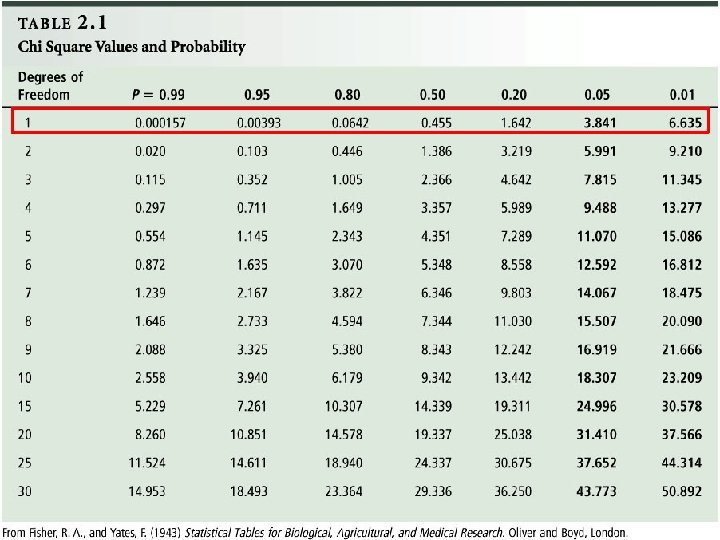
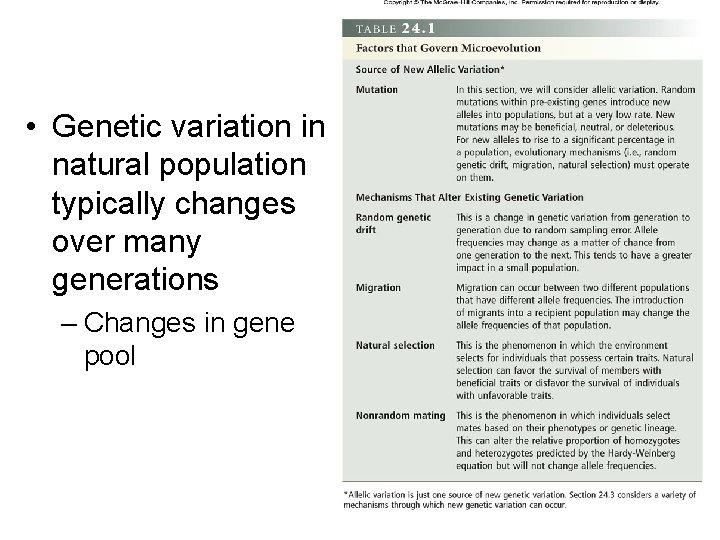
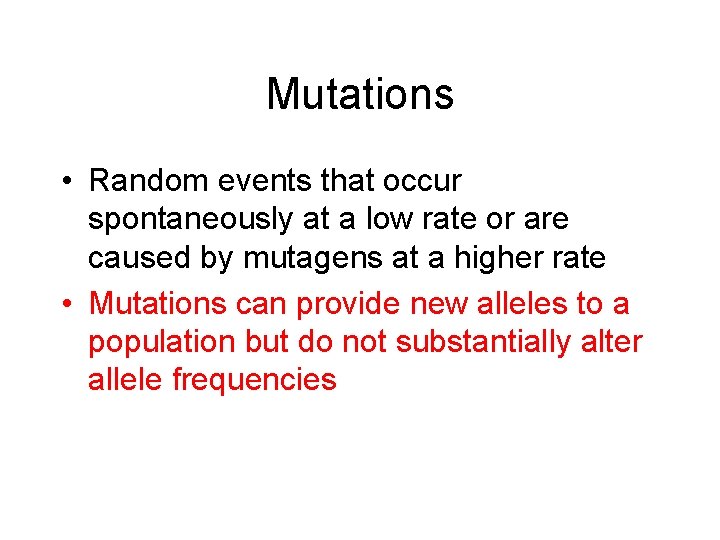
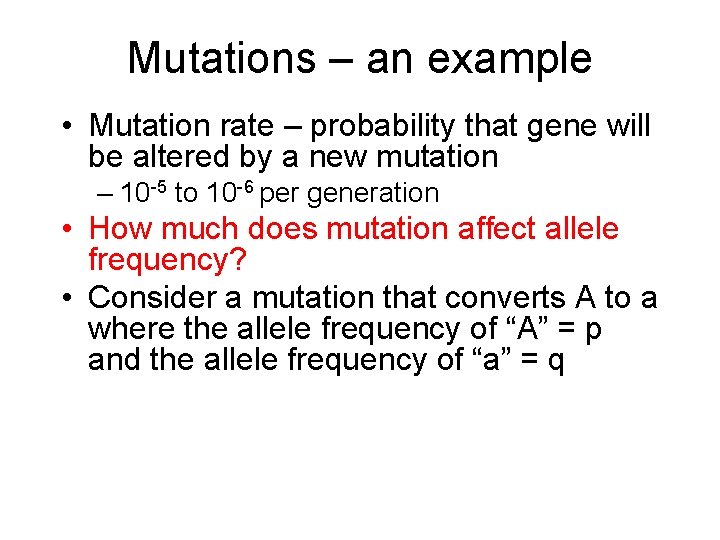
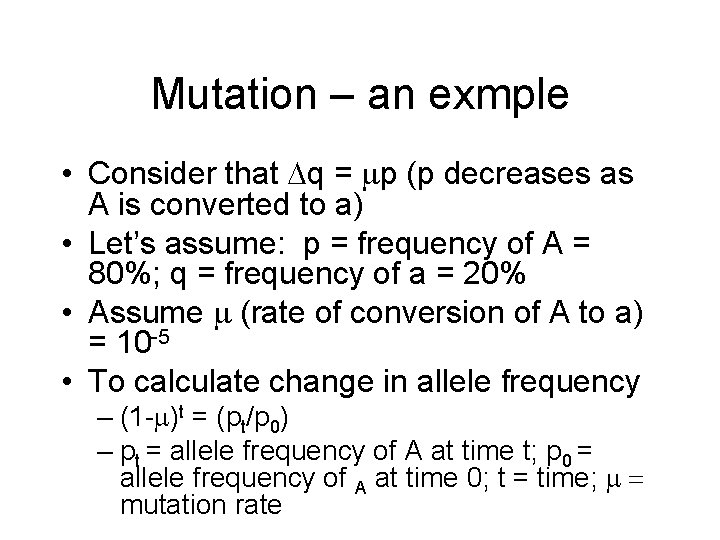
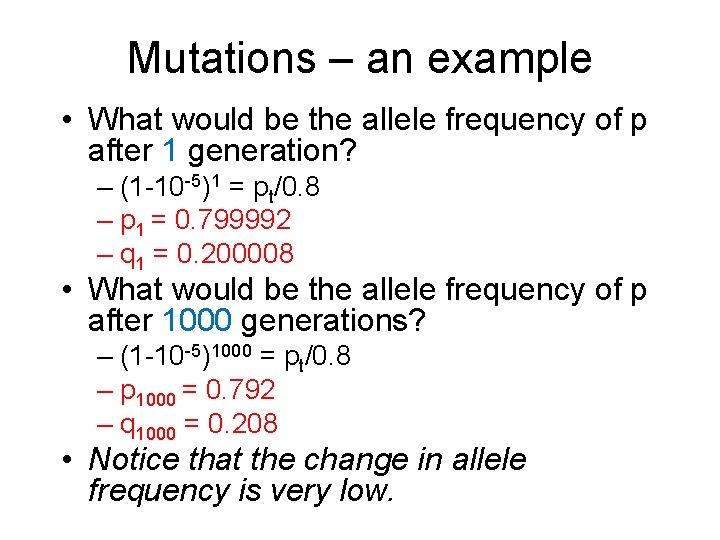
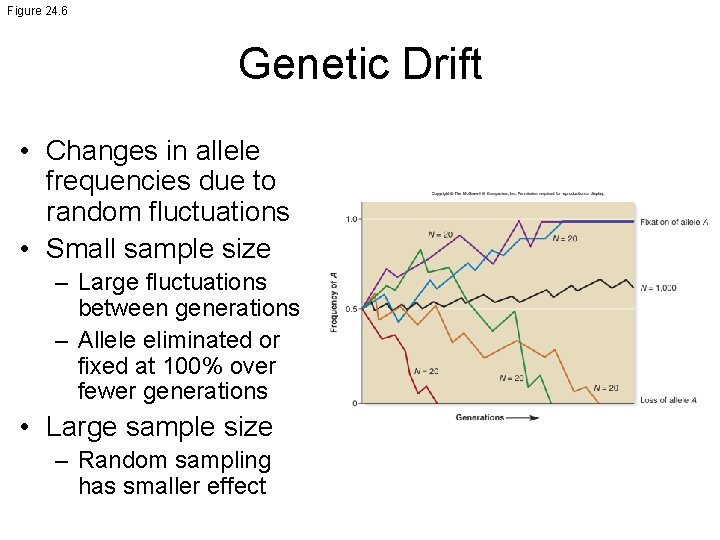
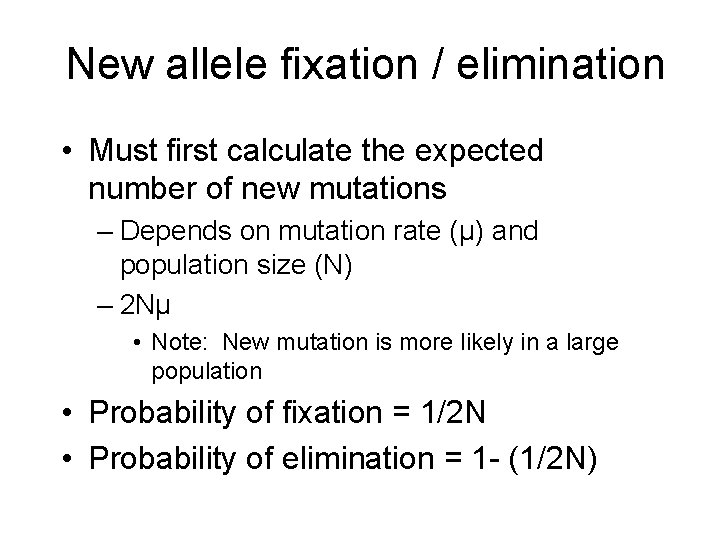
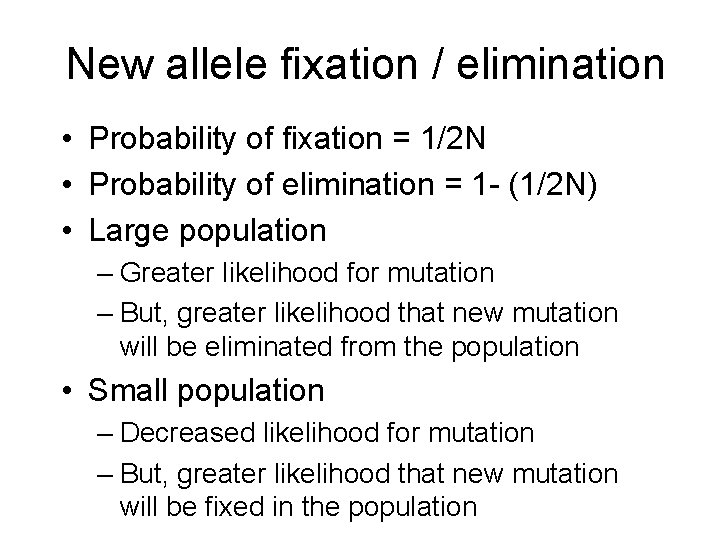
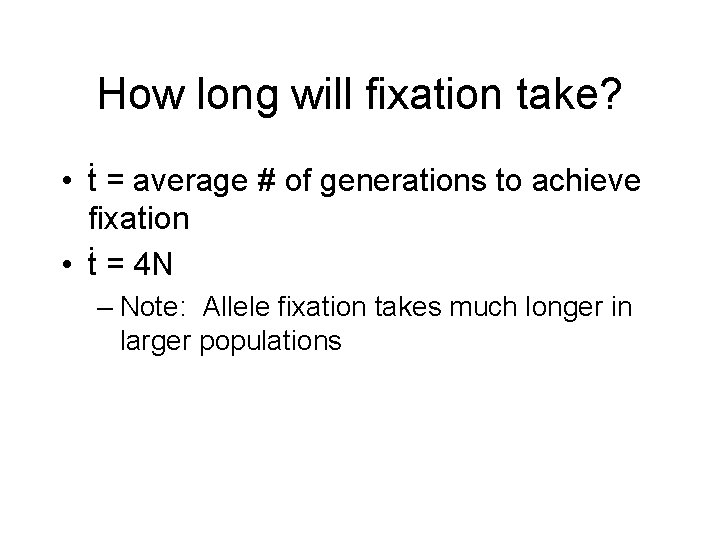
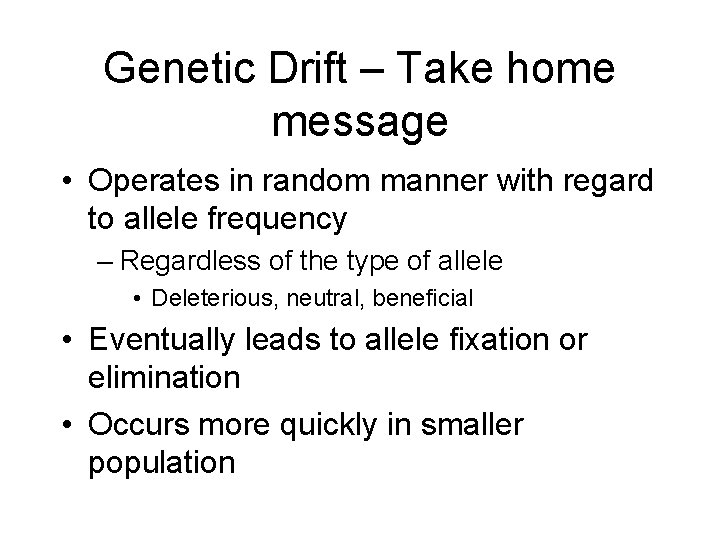
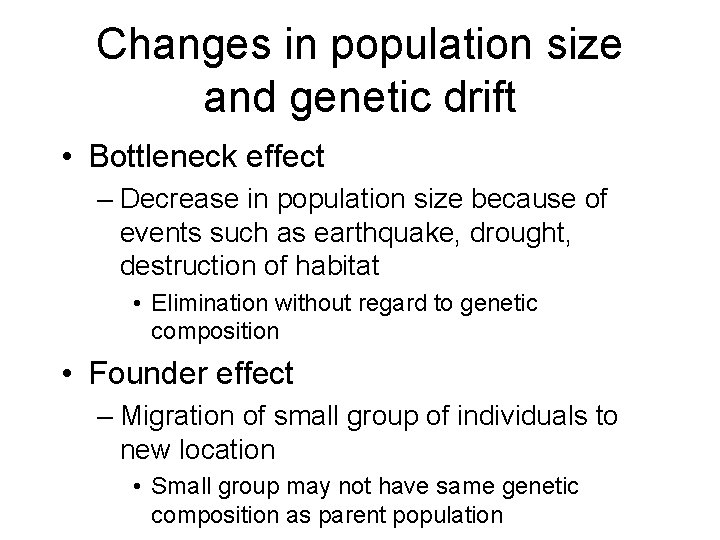
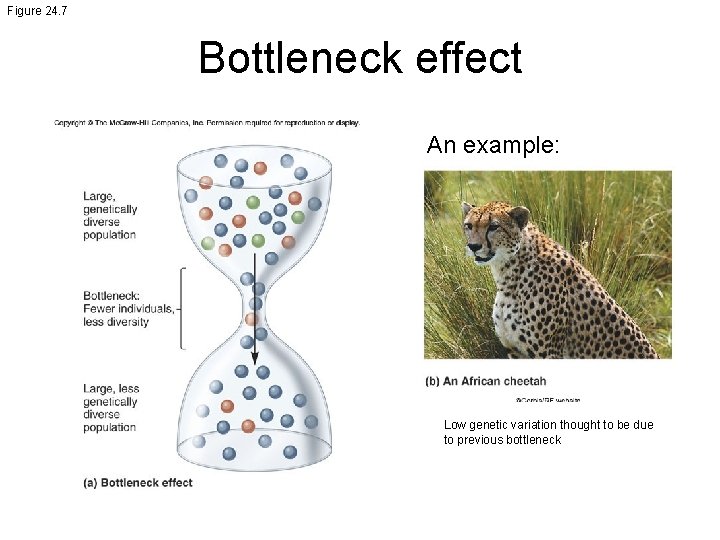
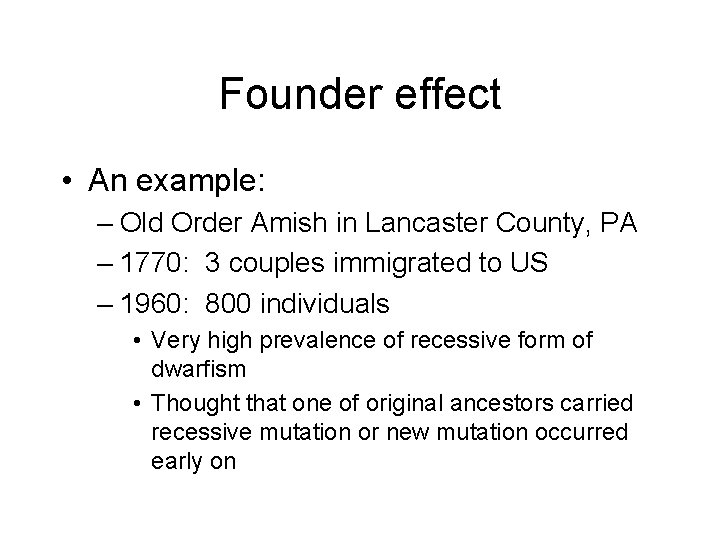
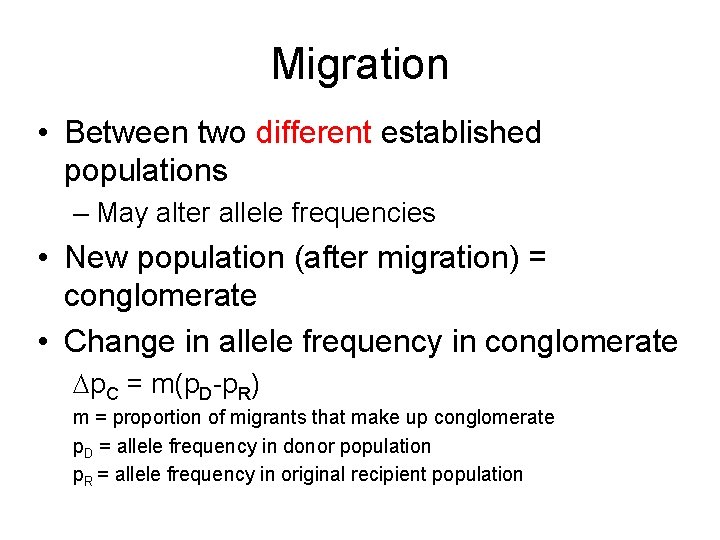
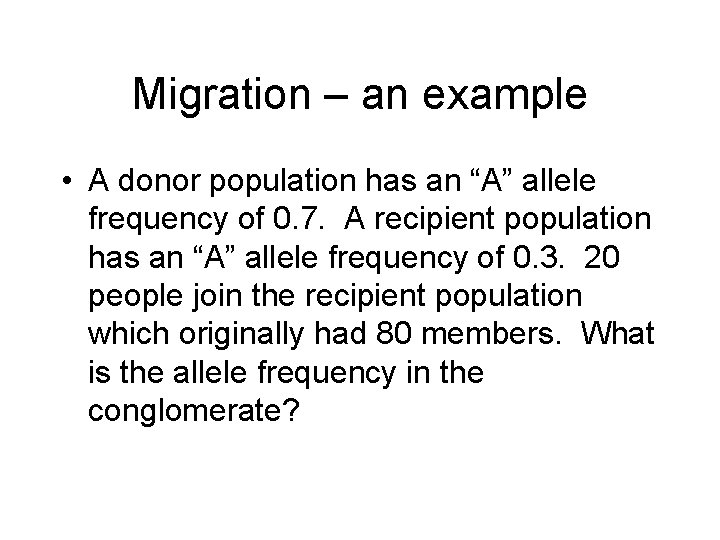
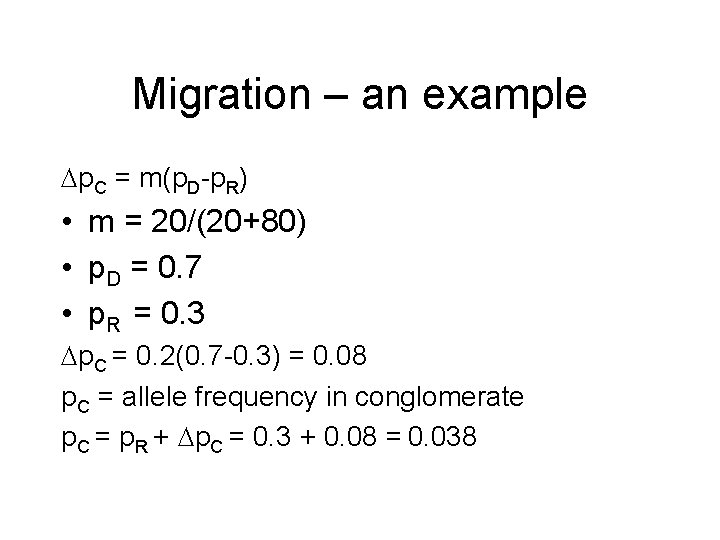
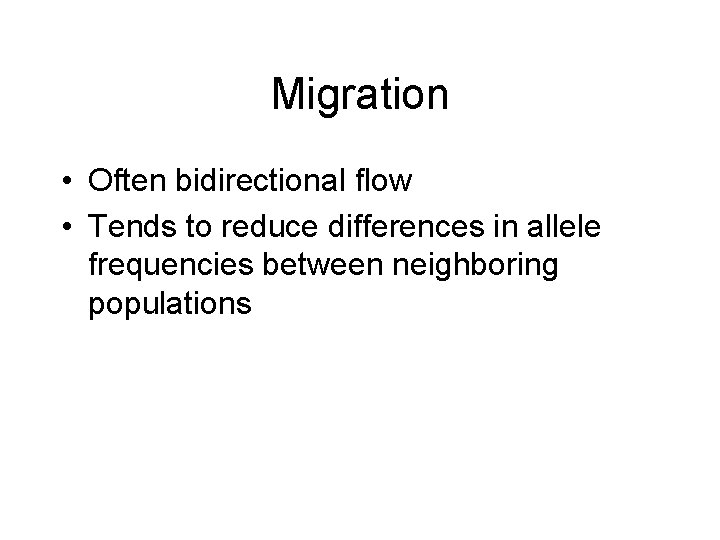
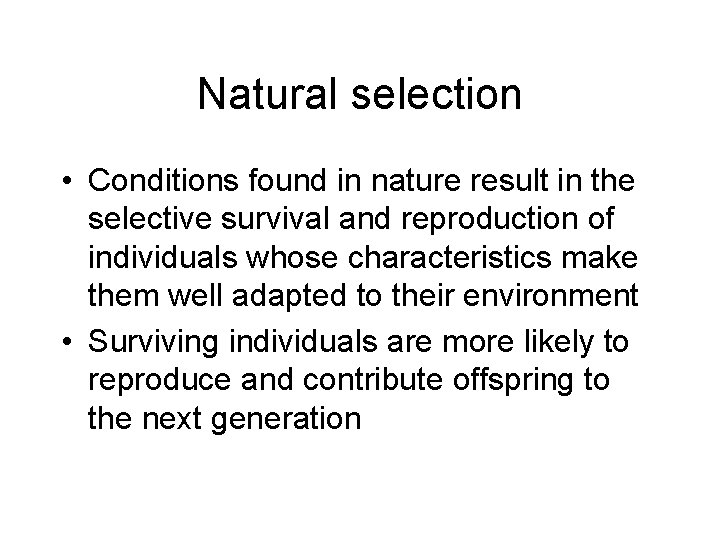
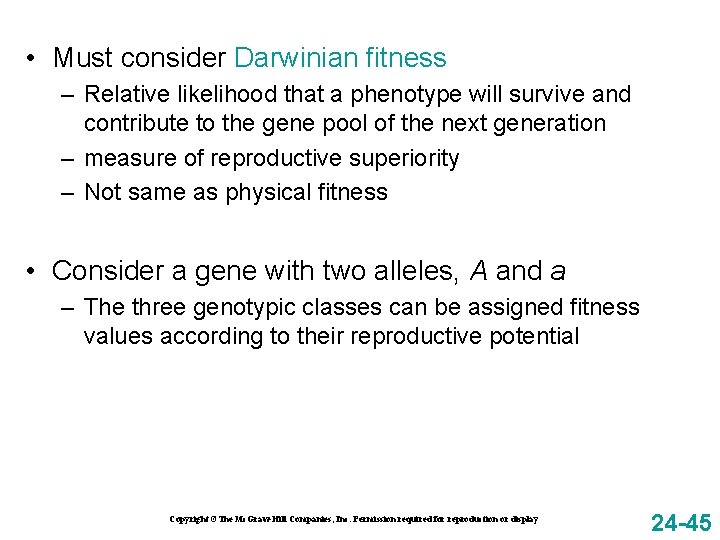
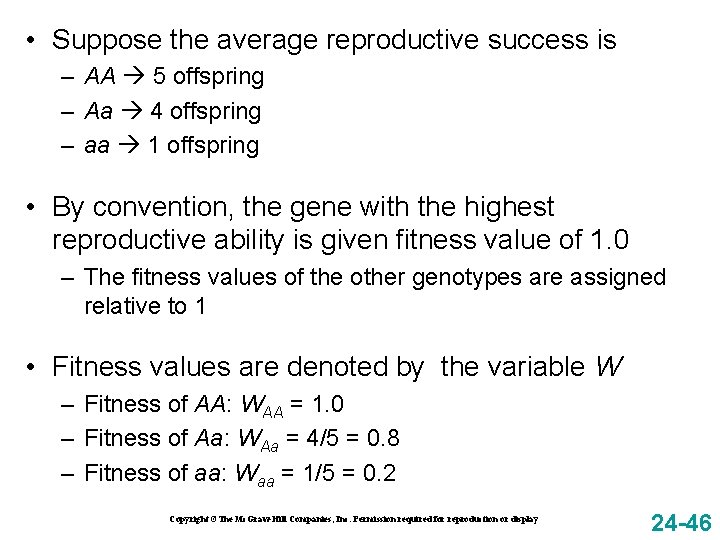
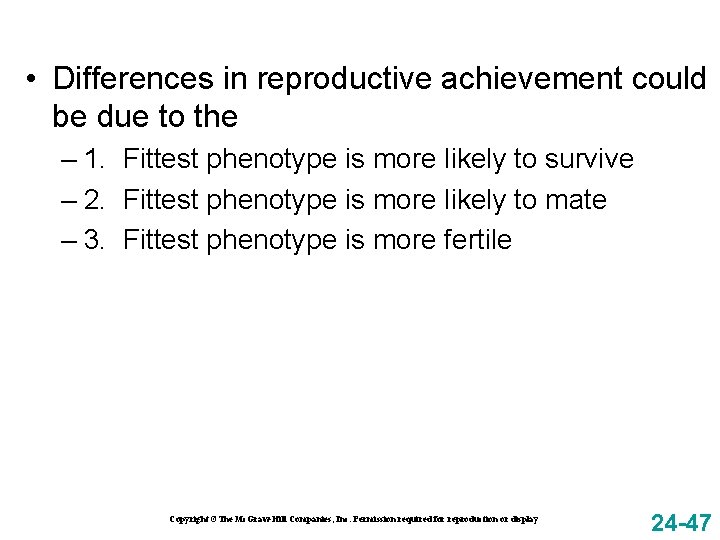
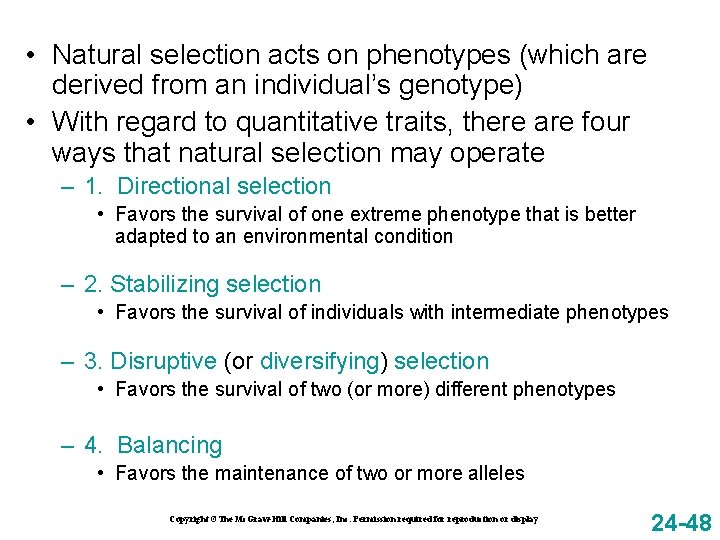
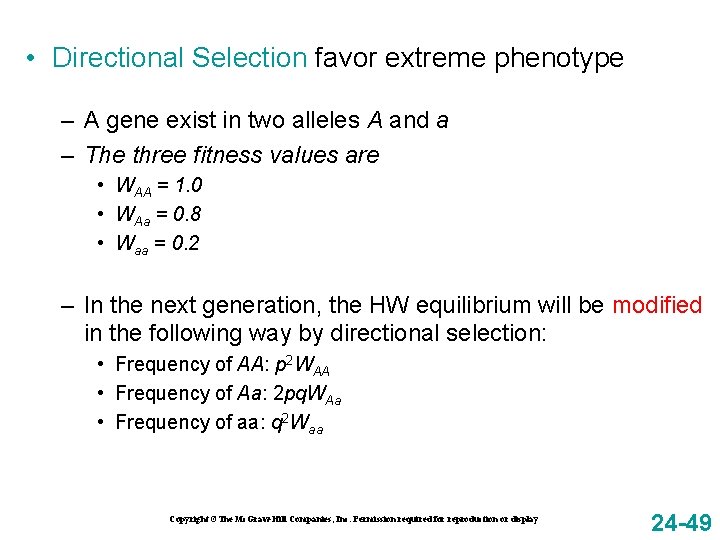
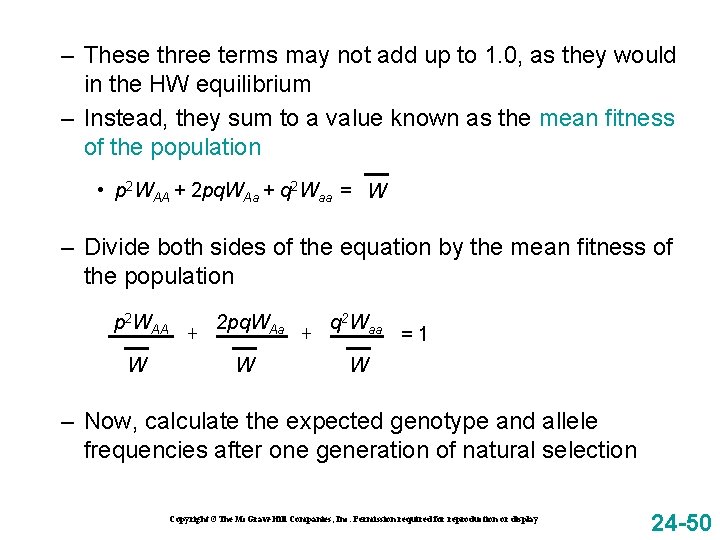
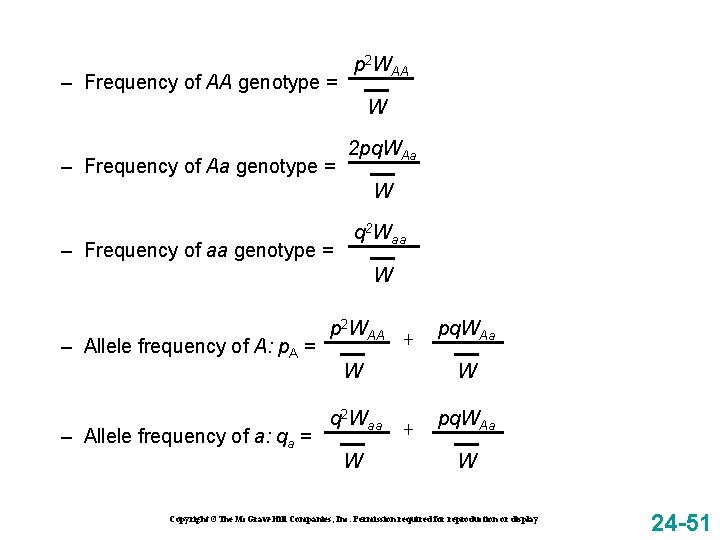
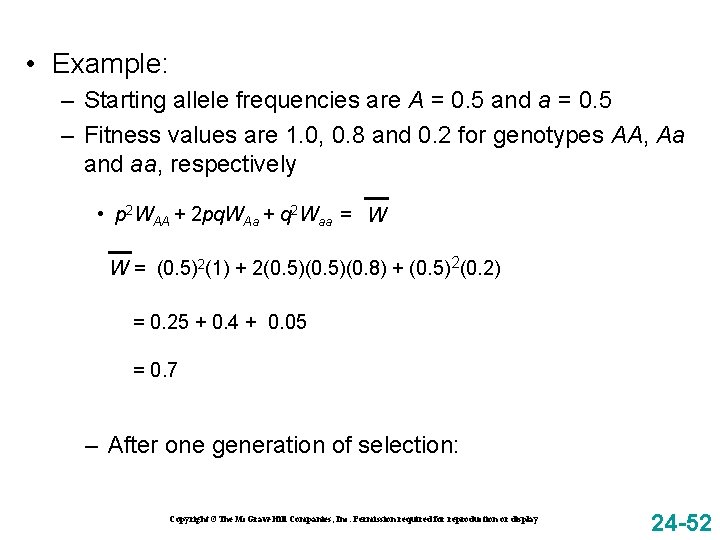
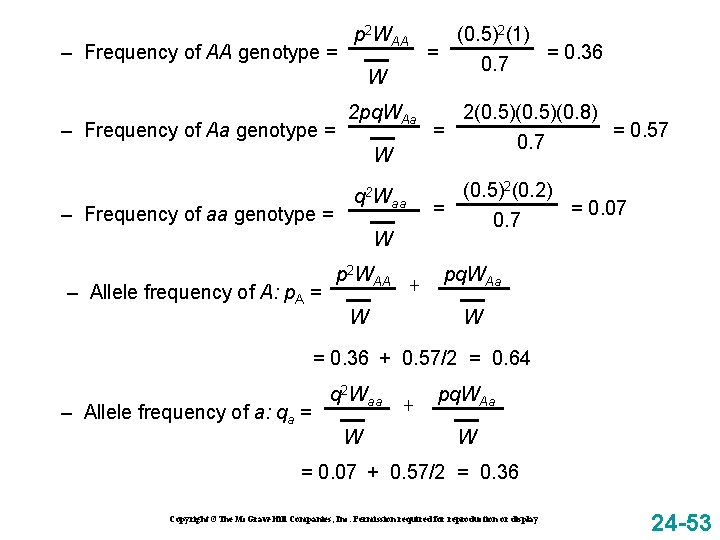
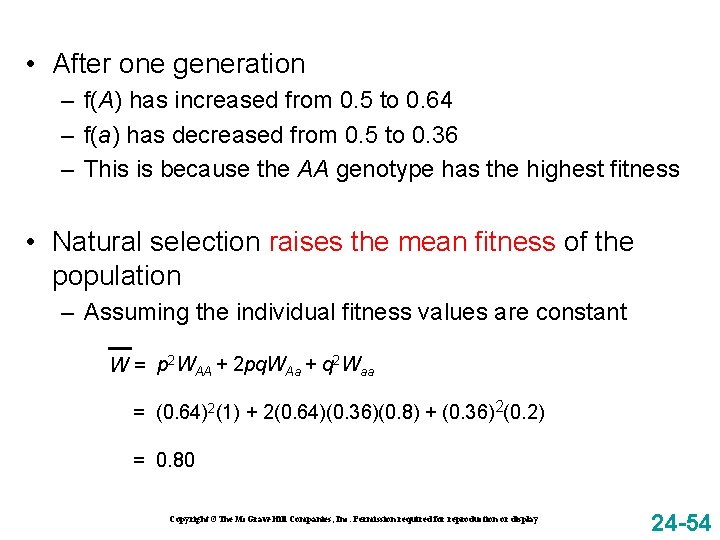
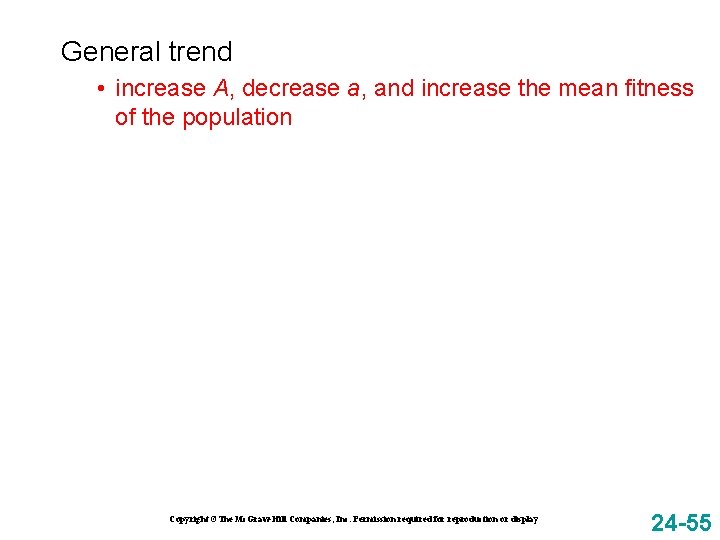
- Slides: 51
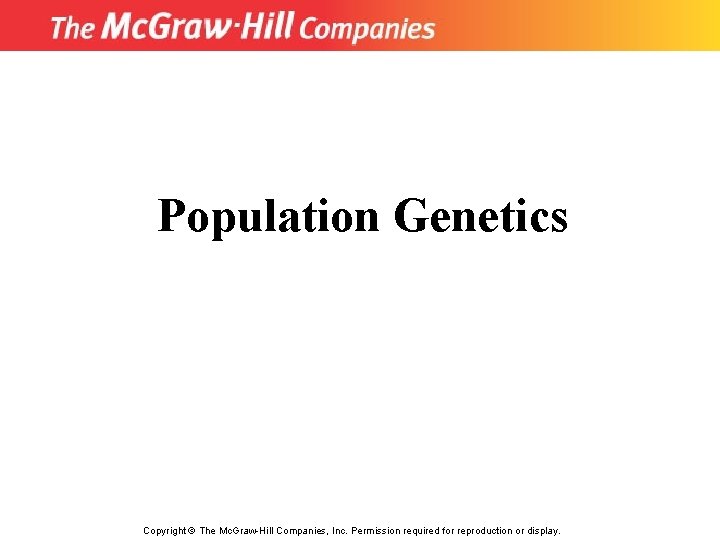
Population Genetics Copyright © The Mc. Graw-Hill Companies, Inc. Permission required for reproduction or display.
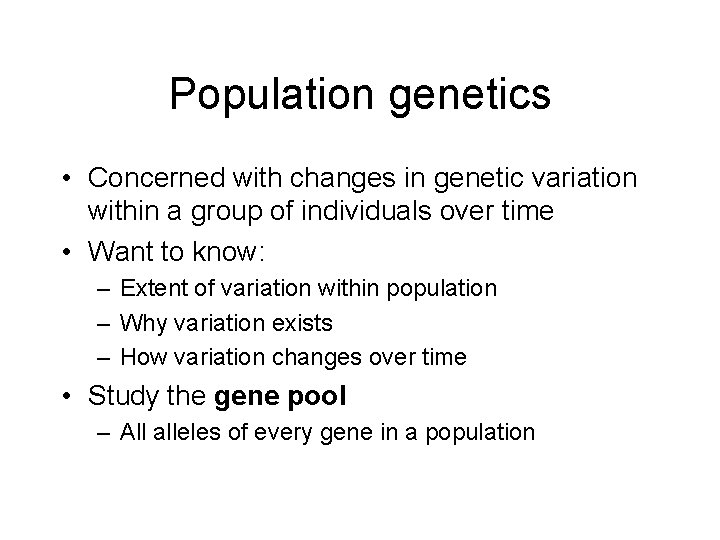
Population genetics • Concerned with changes in genetic variation within a group of individuals over time • Want to know: – Extent of variation within population – Why variation exists – How variation changes over time • Study the gene pool – All alleles of every gene in a population
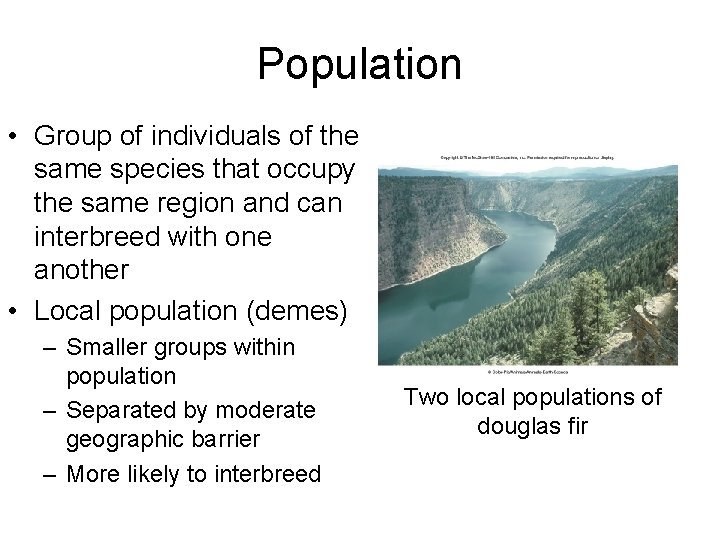
Population • Group of individuals of the same species that occupy the same region and can interbreed with one another • Local population (demes) – Smaller groups within population – Separated by moderate geographic barrier – More likely to interbreed Two local populations of douglas fir
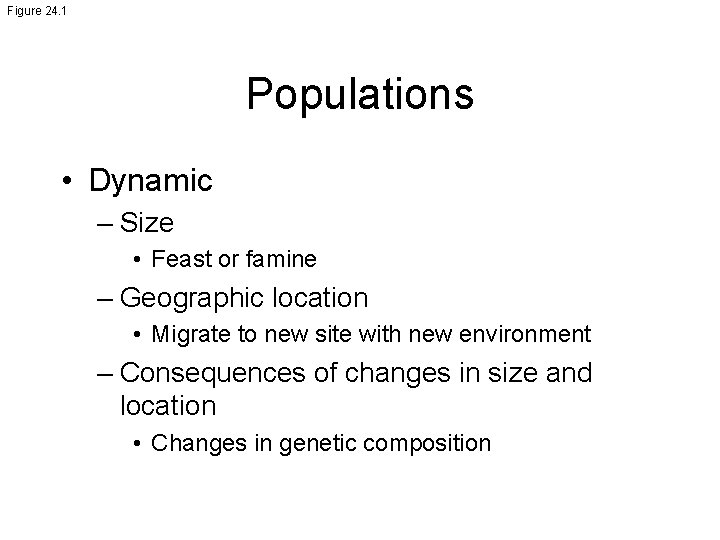
Figure 24. 1 Populations • Dynamic – Size • Feast or famine – Geographic location • Migrate to new site with new environment – Consequences of changes in size and location • Changes in genetic composition
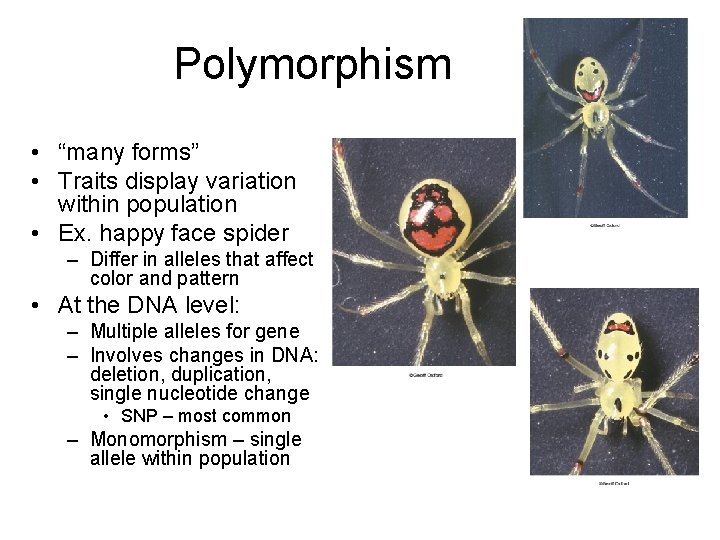
Polymorphism • “many forms” • Traits display variation within population • Ex. happy face spider – Differ in alleles that affect color and pattern • At the DNA level: – Multiple alleles for gene – Involves changes in DNA: deletion, duplication, single nucleotide change • SNP – most common – Monomorphism – single allele within population
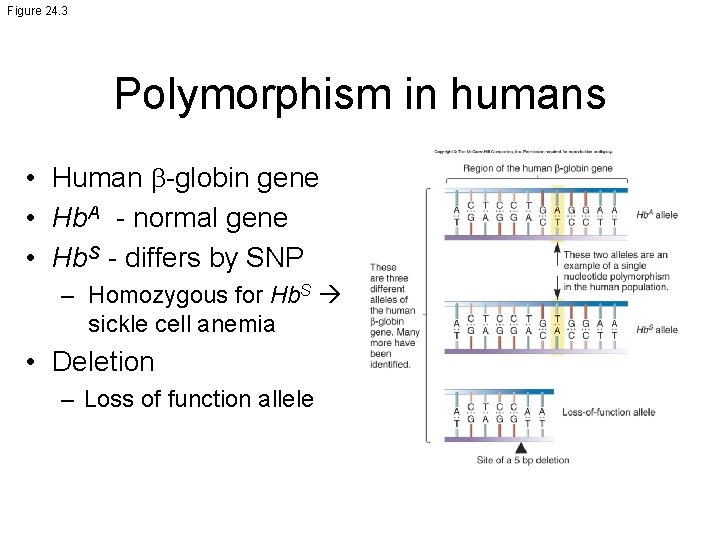
Figure 24. 3 Polymorphism in humans • Human b-globin gene • Hb. A - normal gene • Hb. S - differs by SNP – Homozygous for Hb. S sickle cell anemia • Deletion – Loss of function allele
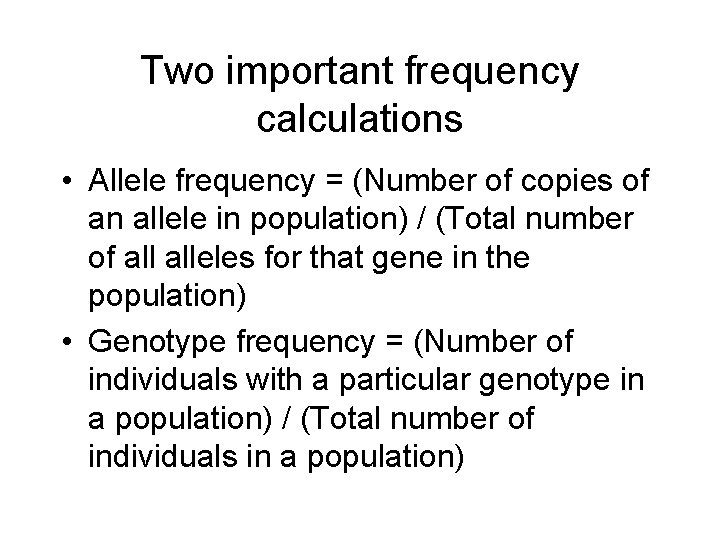
Two important frequency calculations • Allele frequency = (Number of copies of an allele in population) / (Total number of alleles for that gene in the population) • Genotype frequency = (Number of individuals with a particular genotype in a population) / (Total number of individuals in a population)
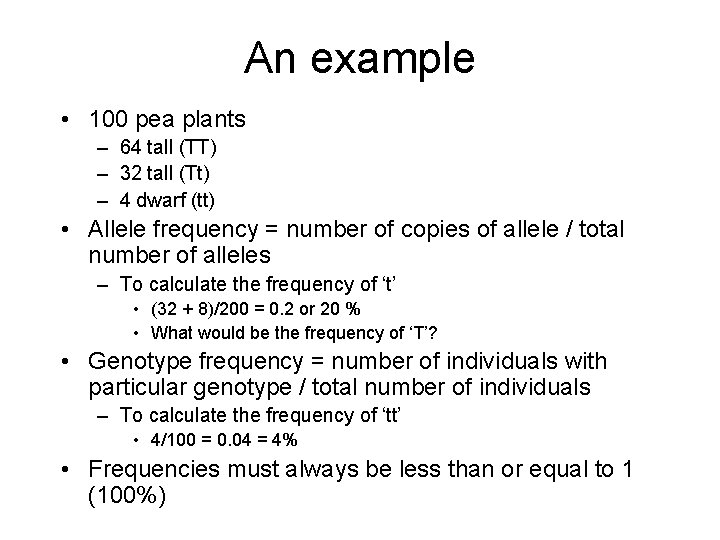
An example • 100 pea plants – 64 tall (TT) – 32 tall (Tt) – 4 dwarf (tt) • Allele frequency = number of copies of allele / total number of alleles – To calculate the frequency of ‘t’ • (32 + 8)/200 = 0. 2 or 20 % • What would be the frequency of ‘T’? • Genotype frequency = number of individuals with particular genotype / total number of individuals – To calculate the frequency of ‘tt’ • 4/100 = 0. 04 = 4% • Frequencies must always be less than or equal to 1 (100%)
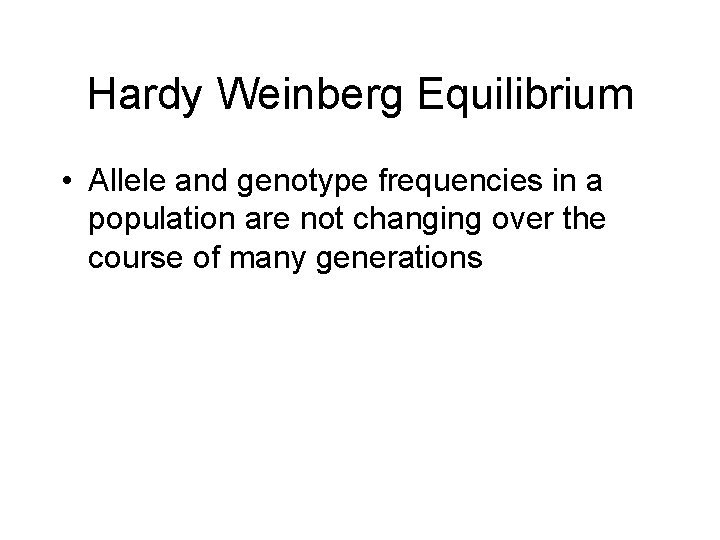
Hardy Weinberg Equilibrium • Allele and genotype frequencies in a population are not changing over the course of many generations
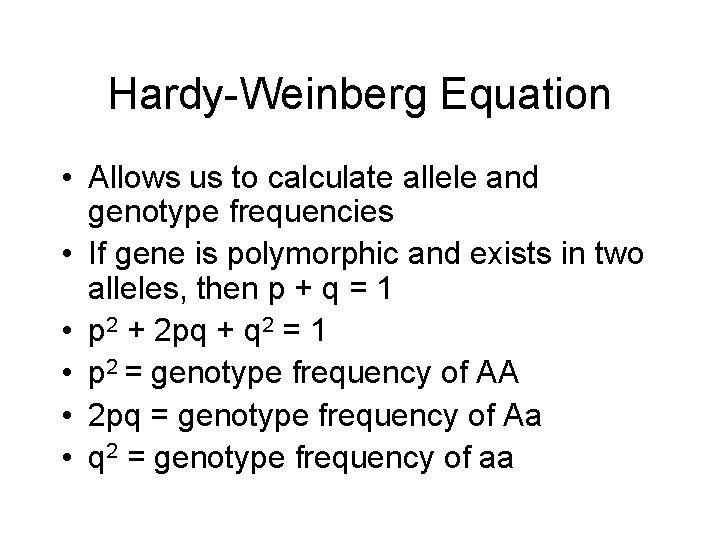
Hardy-Weinberg Equation • Allows us to calculate allele and genotype frequencies • If gene is polymorphic and exists in two alleles, then p + q = 1 • p 2 + 2 pq + q 2 = 1 • p 2 = genotype frequency of AA • 2 pq = genotype frequency of Aa • q 2 = genotype frequency of aa
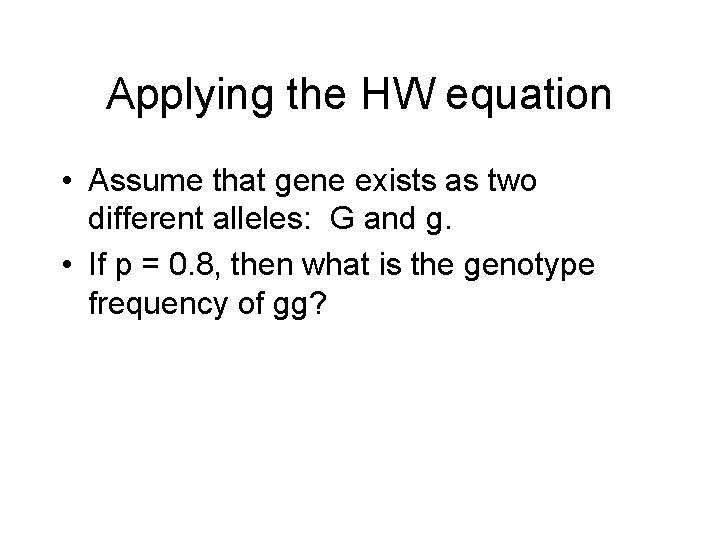
Applying the HW equation • Assume that gene exists as two different alleles: G and g. • If p = 0. 8, then what is the genotype frequency of gg?
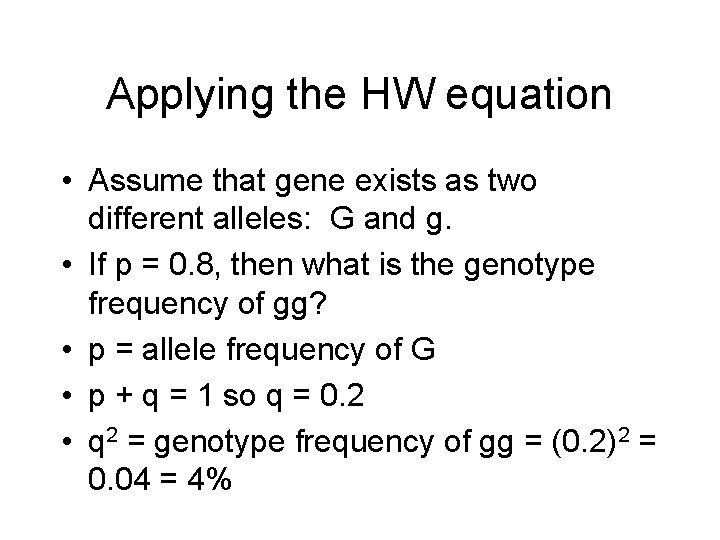
Applying the HW equation • Assume that gene exists as two different alleles: G and g. • If p = 0. 8, then what is the genotype frequency of gg? • p = allele frequency of G • p + q = 1 so q = 0. 2 • q 2 = genotype frequency of gg = (0. 2)2 = 0. 04 = 4%
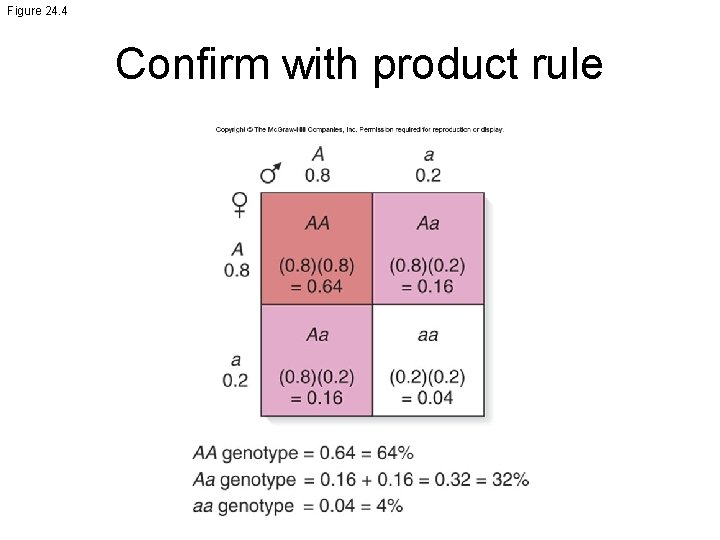
Figure 24. 4 Confirm with product rule
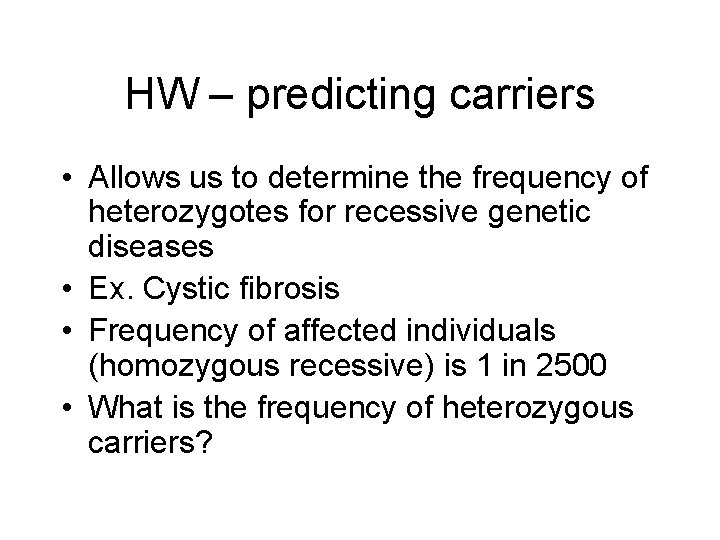
HW – predicting carriers • Allows us to determine the frequency of heterozygotes for recessive genetic diseases • Ex. Cystic fibrosis • Frequency of affected individuals (homozygous recessive) is 1 in 2500 • What is the frequency of heterozygous carriers?
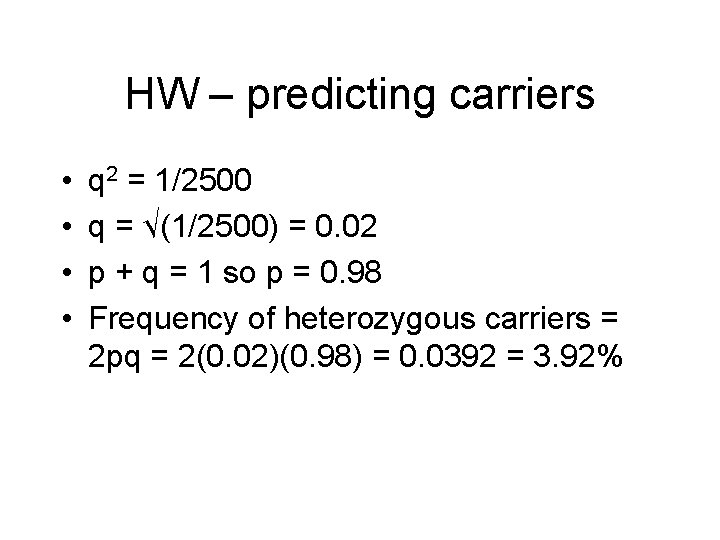
HW – predicting carriers • • q 2 = 1/2500 q = √(1/2500) = 0. 02 p + q = 1 so p = 0. 98 Frequency of heterozygous carriers = 2 pq = 2(0. 02)(0. 98) = 0. 0392 = 3. 92%
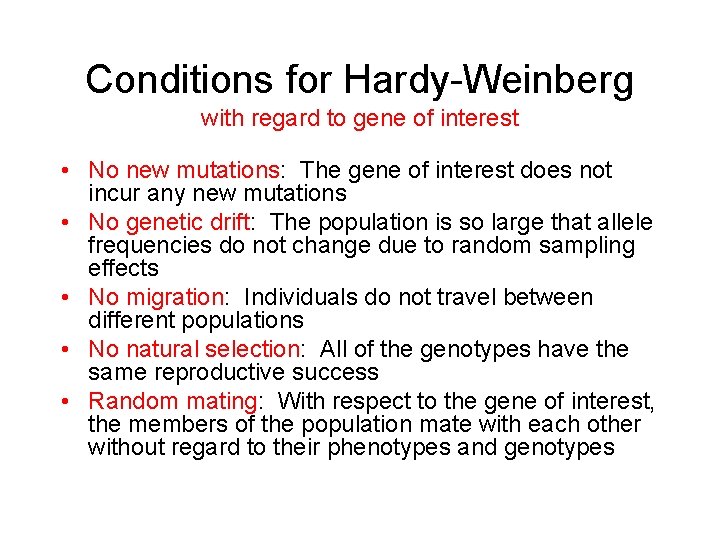
Conditions for Hardy-Weinberg with regard to gene of interest • No new mutations: The gene of interest does not incur any new mutations • No genetic drift: The population is so large that allele frequencies do not change due to random sampling effects • No migration: Individuals do not travel between different populations • No natural selection: All of the genotypes have the same reproductive success • Random mating: With respect to the gene of interest, the members of the population mate with each other without regard to their phenotypes and genotypes
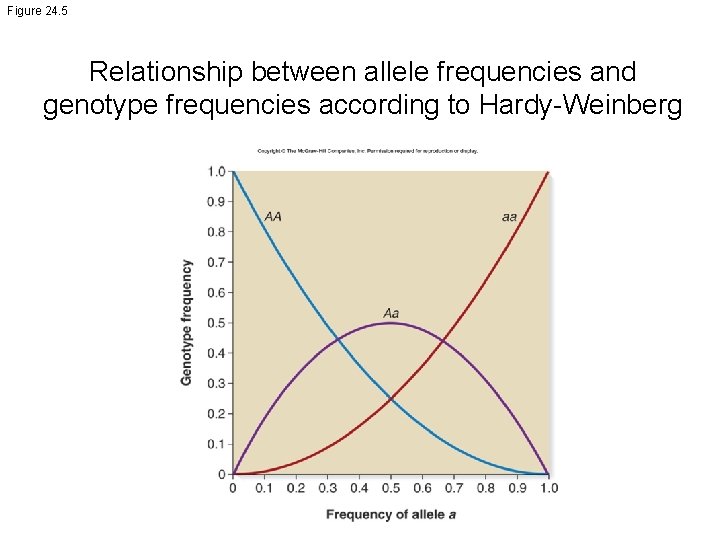
Figure 24. 5 Relationship between allele frequencies and genotype frequencies according to Hardy-Weinberg
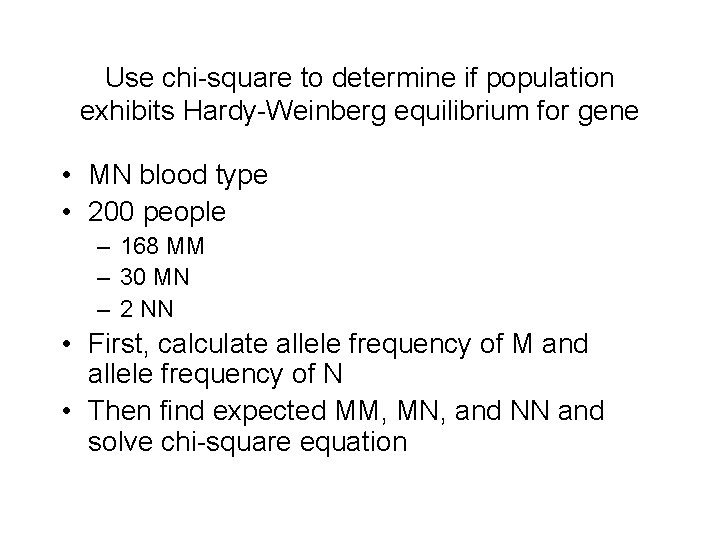
Use chi-square to determine if population exhibits Hardy-Weinberg equilibrium for gene • MN blood type • 200 people – 168 MM – 30 MN – 2 NN • First, calculate allele frequency of M and allele frequency of N • Then find expected MM, MN, and NN and solve chi-square equation
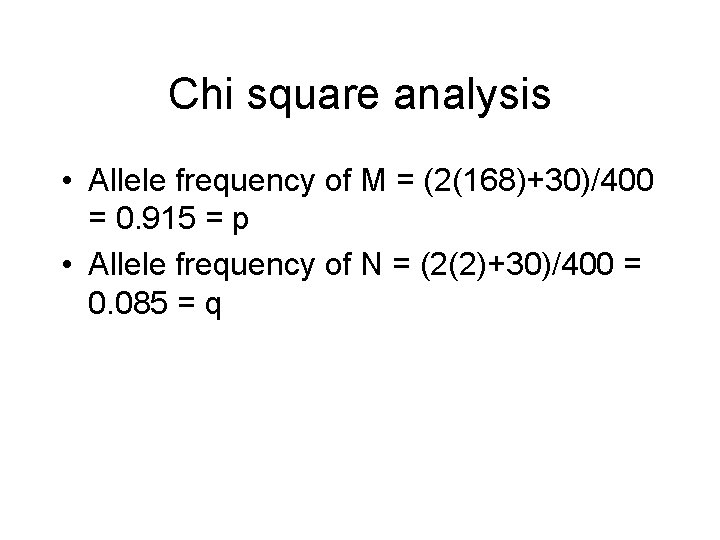
Chi square analysis • Allele frequency of M = (2(168)+30)/400 = 0. 915 = p • Allele frequency of N = (2(2)+30)/400 = 0. 085 = q
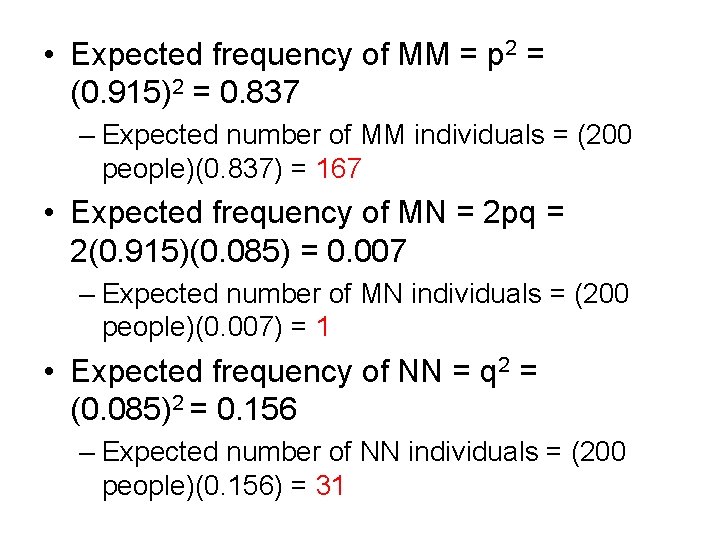
• Expected frequency of MM = p 2 = (0. 915)2 = 0. 837 – Expected number of MM individuals = (200 people)(0. 837) = 167 • Expected frequency of MN = 2 pq = 2(0. 915)(0. 085) = 0. 007 – Expected number of MN individuals = (200 people)(0. 007) = 1 • Expected frequency of NN = q 2 = (0. 085)2 = 0. 156 – Expected number of NN individuals = (200 people)(0. 156) = 31
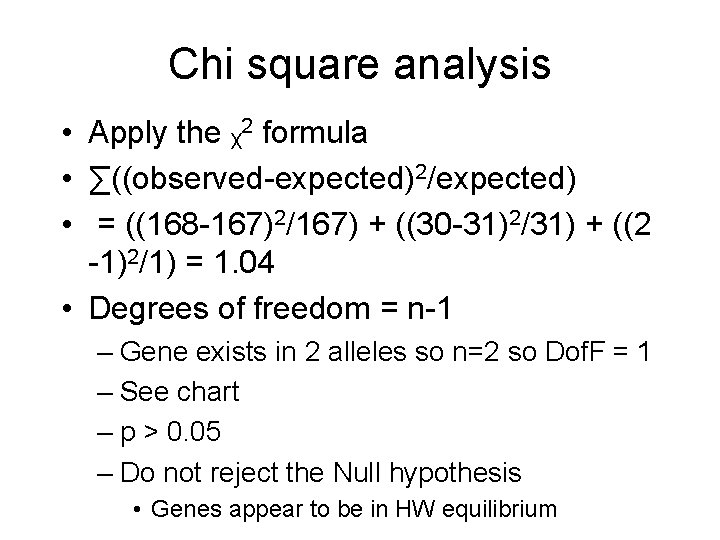
Chi square analysis • Apply the ᵪ 2 formula • ∑((observed-expected)2/expected) • = ((168 -167)2/167) + ((30 -31)2/31) + ((2 -1)2/1) = 1. 04 • Degrees of freedom = n-1 – Gene exists in 2 alleles so n=2 so Dof. F = 1 – See chart – p > 0. 05 – Do not reject the Null hypothesis • Genes appear to be in HW equilibrium
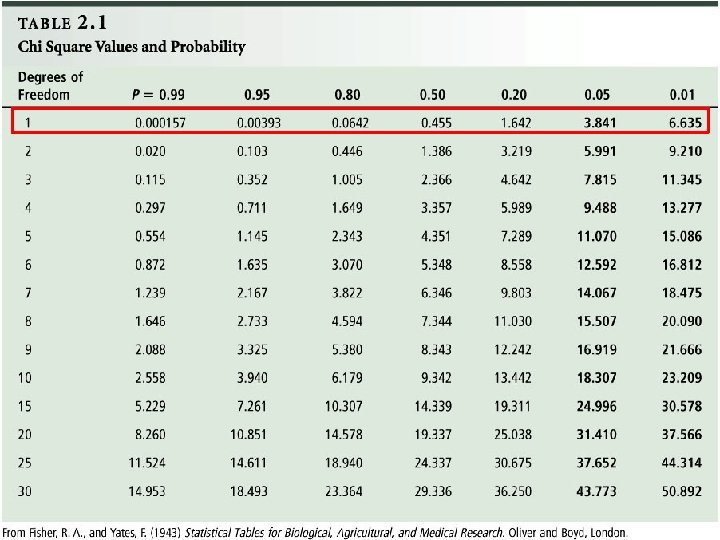
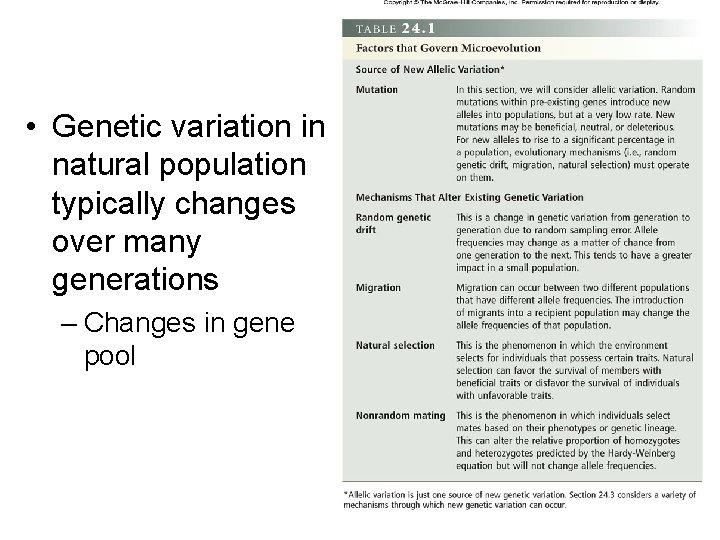
• Genetic variation in natural population typically changes over many generations – Changes in gene pool
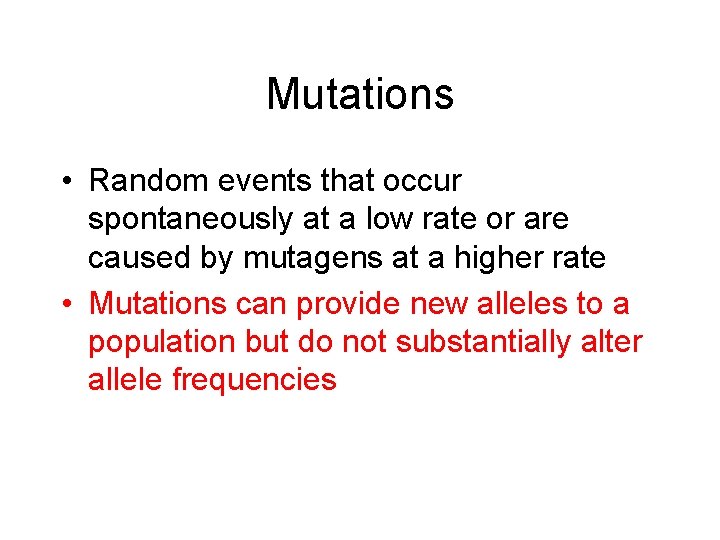
Mutations • Random events that occur spontaneously at a low rate or are caused by mutagens at a higher rate • Mutations can provide new alleles to a population but do not substantially alter allele frequencies
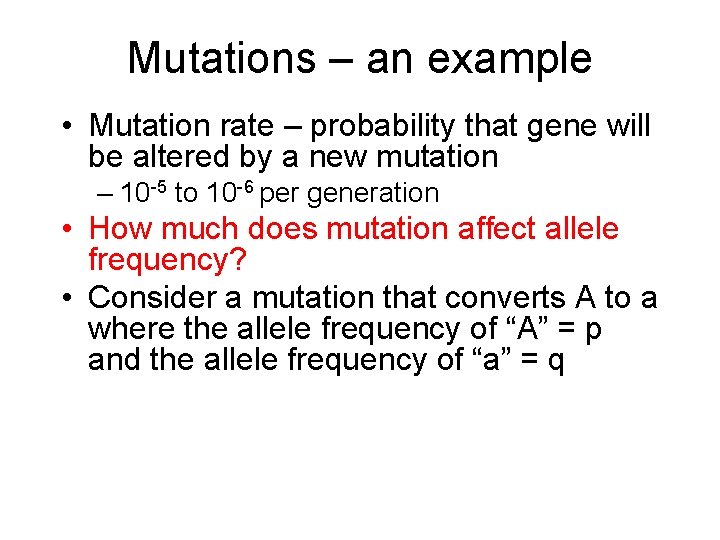
Mutations – an example • Mutation rate – probability that gene will be altered by a new mutation – 10 -5 to 10 -6 per generation • How much does mutation affect allele frequency? • Consider a mutation that converts A to a where the allele frequency of “A” = p and the allele frequency of “a” = q
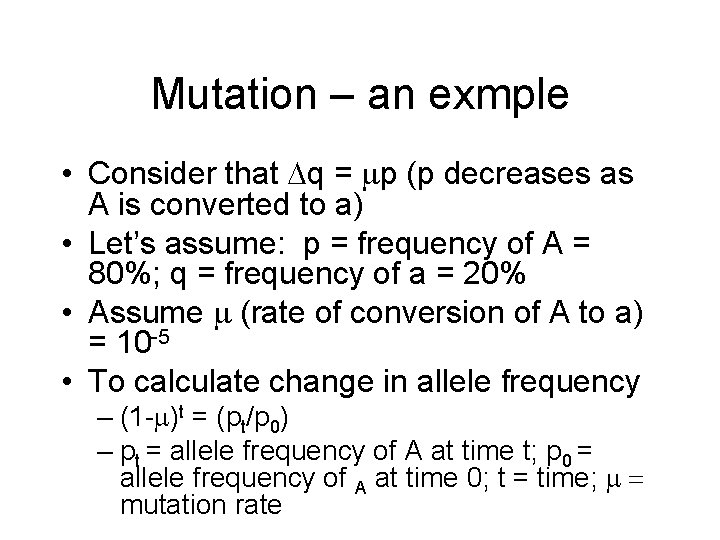
Mutation – an exmple • Consider that Dq = mp (p decreases as A is converted to a) • Let’s assume: p = frequency of A = 80%; q = frequency of a = 20% • Assume m (rate of conversion of A to a) = 10 -5 • To calculate change in allele frequency – (1 -m)t = (pt/p 0) – pt = allele frequency of A at time t; p 0 = allele frequency of A at time 0; t = time; m = mutation rate
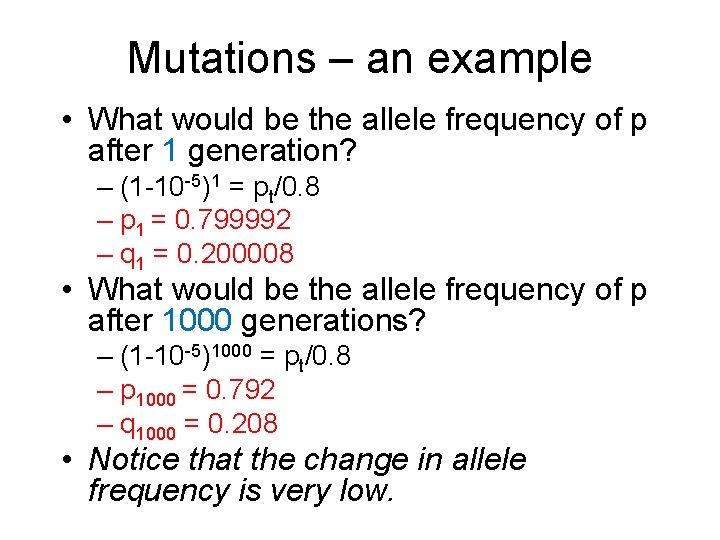
Mutations – an example • What would be the allele frequency of p after 1 generation? – (1 -10 -5)1 = pt/0. 8 – p 1 = 0. 799992 – q 1 = 0. 200008 • What would be the allele frequency of p after 1000 generations? – (1 -10 -5)1000 = pt/0. 8 – p 1000 = 0. 792 – q 1000 = 0. 208 • Notice that the change in allele frequency is very low.
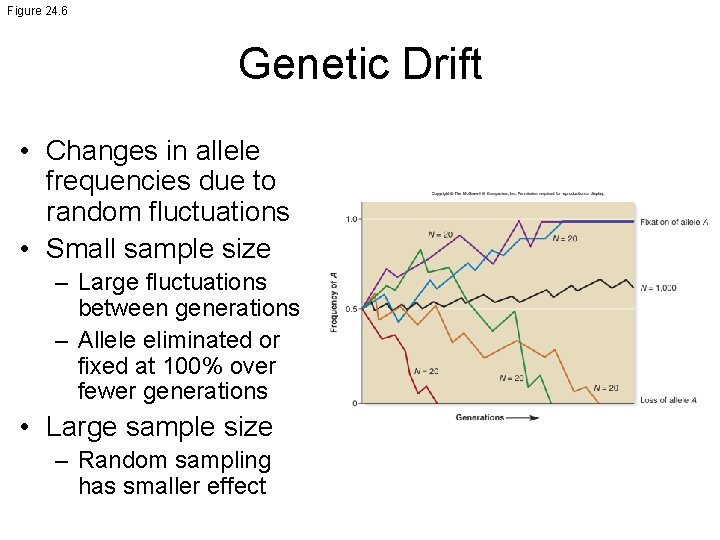
Figure 24. 6 Genetic Drift • Changes in allele frequencies due to random fluctuations • Small sample size – Large fluctuations between generations – Allele eliminated or fixed at 100% over fewer generations • Large sample size – Random sampling has smaller effect
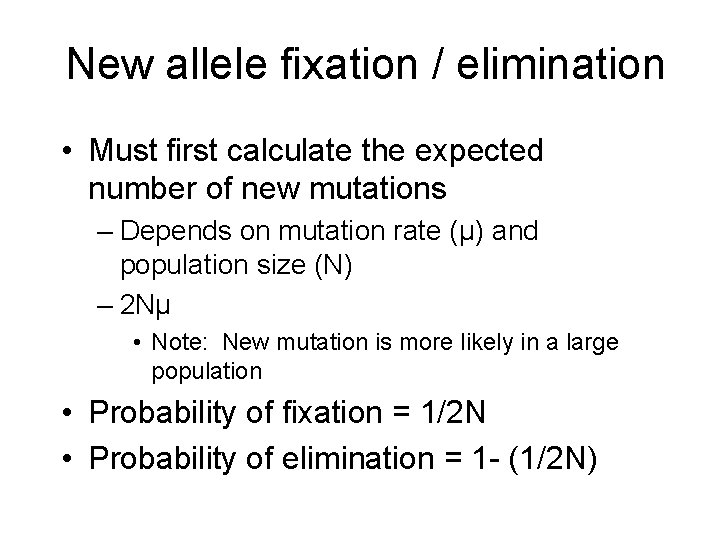
New allele fixation / elimination • Must first calculate the expected number of new mutations – Depends on mutation rate (μ) and population size (N) – 2 Nμ • Note: New mutation is more likely in a large population • Probability of fixation = 1/2 N • Probability of elimination = 1 - (1/2 N)
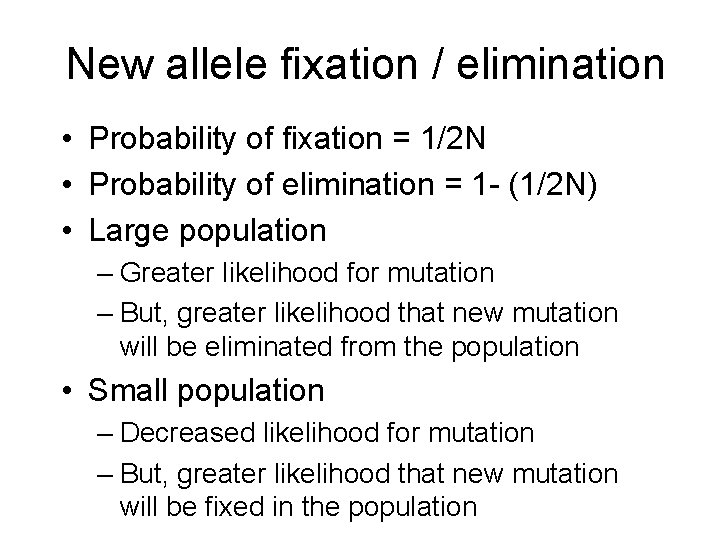
New allele fixation / elimination • Probability of fixation = 1/2 N • Probability of elimination = 1 - (1/2 N) • Large population – Greater likelihood for mutation – But, greater likelihood that new mutation will be eliminated from the population • Small population – Decreased likelihood for mutation – But, greater likelihood that new mutation will be fixed in the population
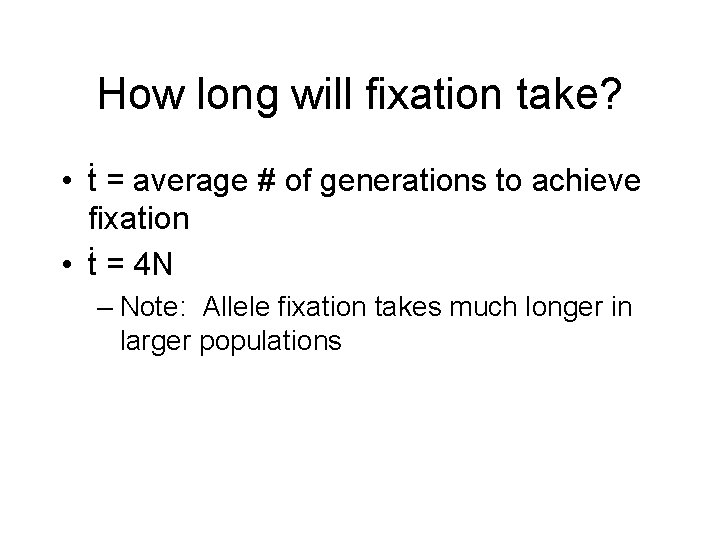
How long will fixation take? • ṫ = average # of generations to achieve fixation • ṫ = 4 N – Note: Allele fixation takes much longer in larger populations
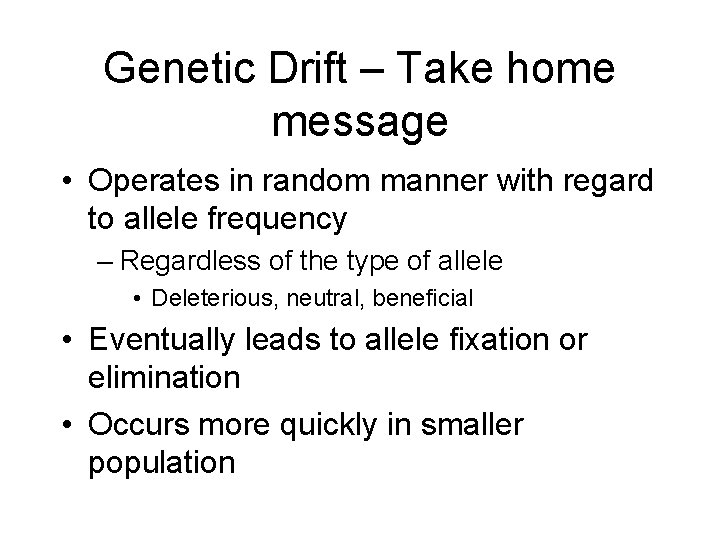
Genetic Drift – Take home message • Operates in random manner with regard to allele frequency – Regardless of the type of allele • Deleterious, neutral, beneficial • Eventually leads to allele fixation or elimination • Occurs more quickly in smaller population
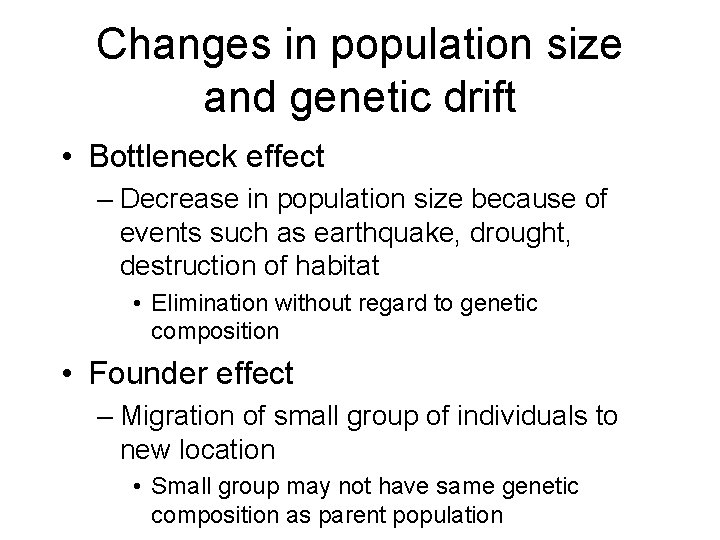
Changes in population size and genetic drift • Bottleneck effect – Decrease in population size because of events such as earthquake, drought, destruction of habitat • Elimination without regard to genetic composition • Founder effect – Migration of small group of individuals to new location • Small group may not have same genetic composition as parent population
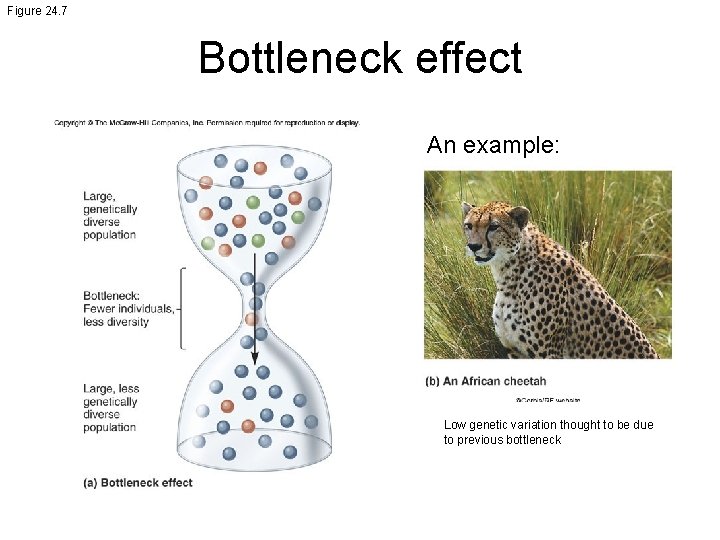
Figure 24. 7 Bottleneck effect An example: Low genetic variation thought to be due to previous bottleneck
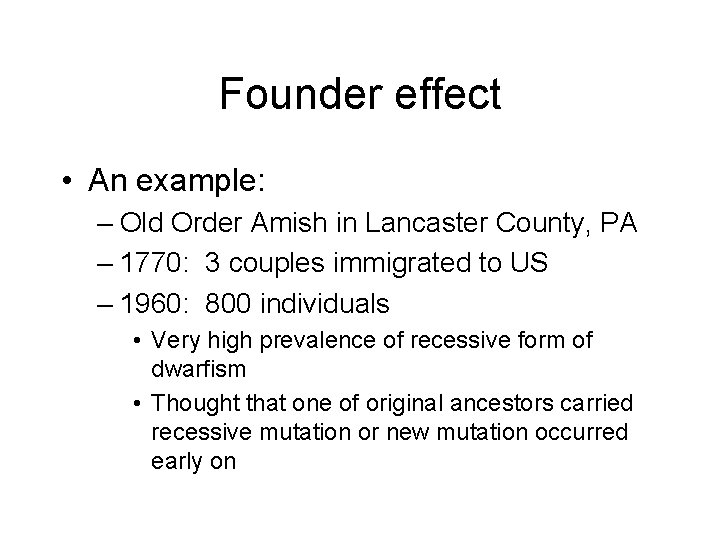
Founder effect • An example: – Old Order Amish in Lancaster County, PA – 1770: 3 couples immigrated to US – 1960: 800 individuals • Very high prevalence of recessive form of dwarfism • Thought that one of original ancestors carried recessive mutation or new mutation occurred early on
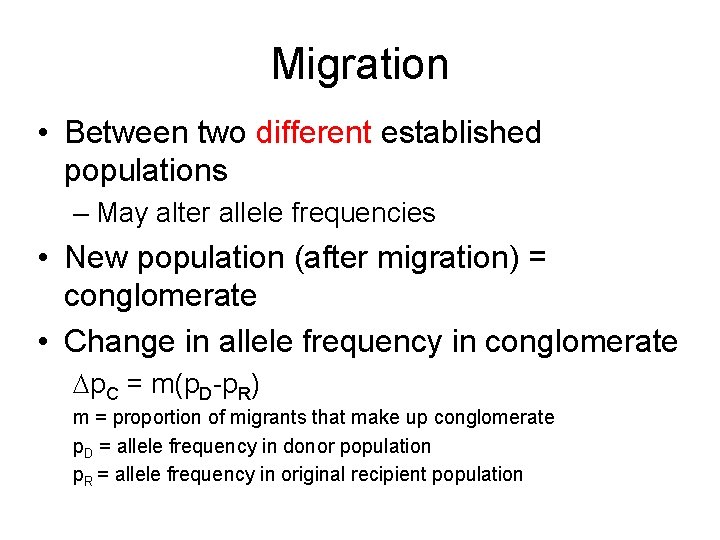
Migration • Between two different established populations – May alter allele frequencies • New population (after migration) = conglomerate • Change in allele frequency in conglomerate Dp. C = m(p. D-p. R) m = proportion of migrants that make up conglomerate p. D = allele frequency in donor population p. R = allele frequency in original recipient population
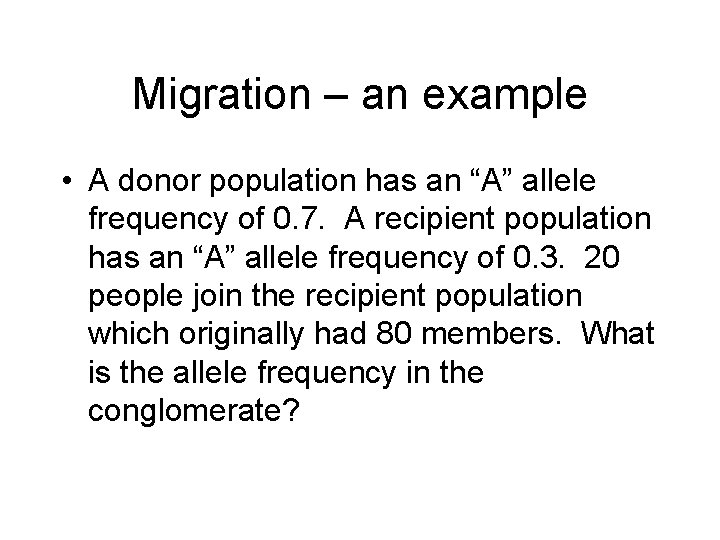
Migration – an example • A donor population has an “A” allele frequency of 0. 7. A recipient population has an “A” allele frequency of 0. 3. 20 people join the recipient population which originally had 80 members. What is the allele frequency in the conglomerate?
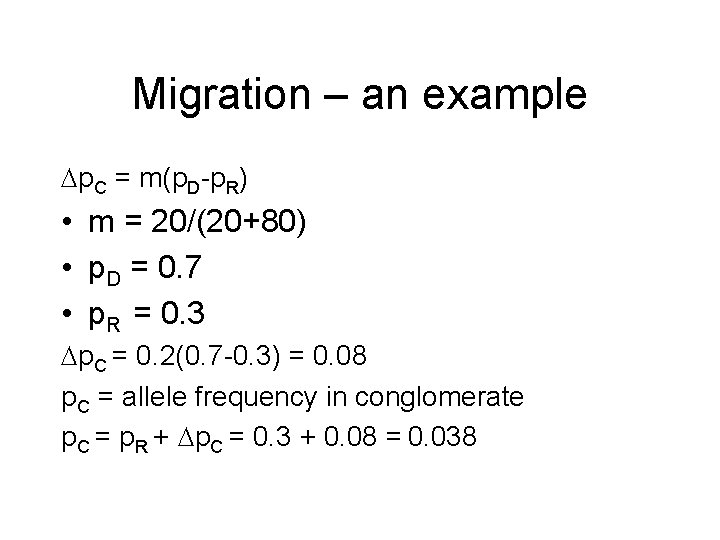
Migration – an example Dp. C = m(p. D-p. R) • m = 20/(20+80) • p. D = 0. 7 • p. R = 0. 3 Dp. C = 0. 2(0. 7 -0. 3) = 0. 08 p. C = allele frequency in conglomerate p. C = p. R + Dp. C = 0. 3 + 0. 08 = 0. 038
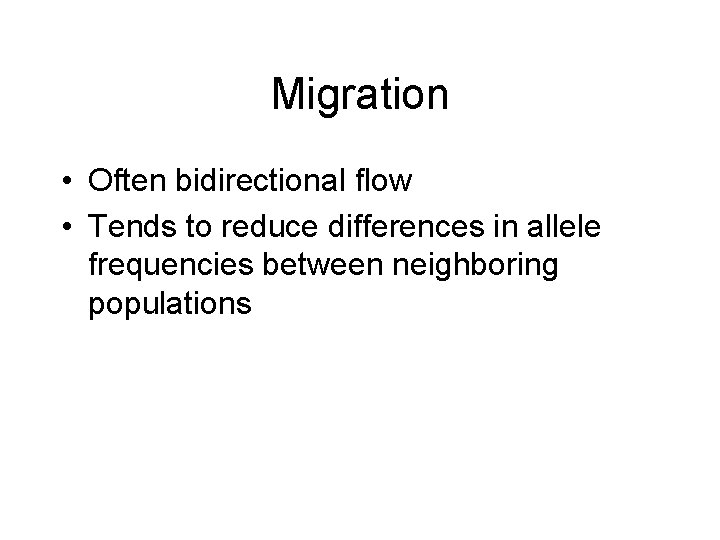
Migration • Often bidirectional flow • Tends to reduce differences in allele frequencies between neighboring populations
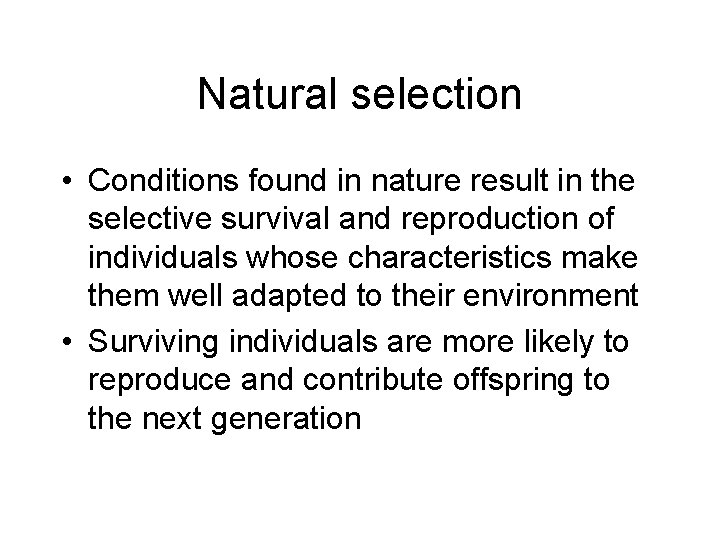
Natural selection • Conditions found in nature result in the selective survival and reproduction of individuals whose characteristics make them well adapted to their environment • Surviving individuals are more likely to reproduce and contribute offspring to the next generation
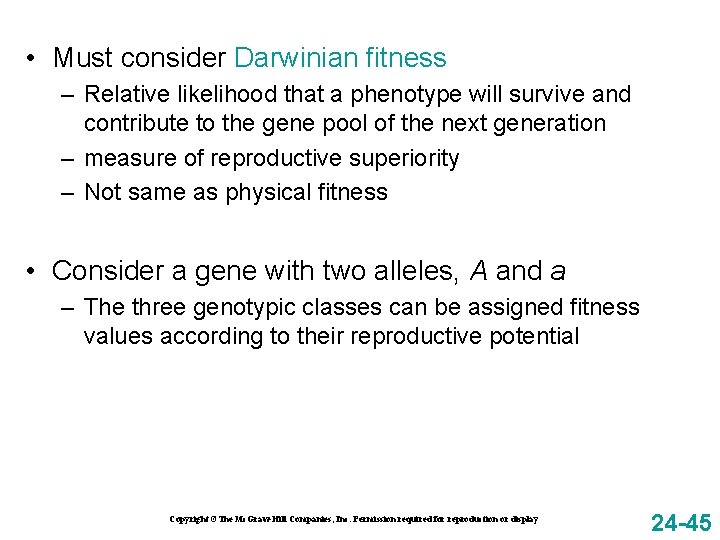
• Must consider Darwinian fitness – Relative likelihood that a phenotype will survive and contribute to the gene pool of the next generation – measure of reproductive superiority – Not same as physical fitness • Consider a gene with two alleles, A and a – The three genotypic classes can be assigned fitness values according to their reproductive potential Copyright ©The Mc. Graw-Hill Companies, Inc. Permission required for reproduction or display 24 -45
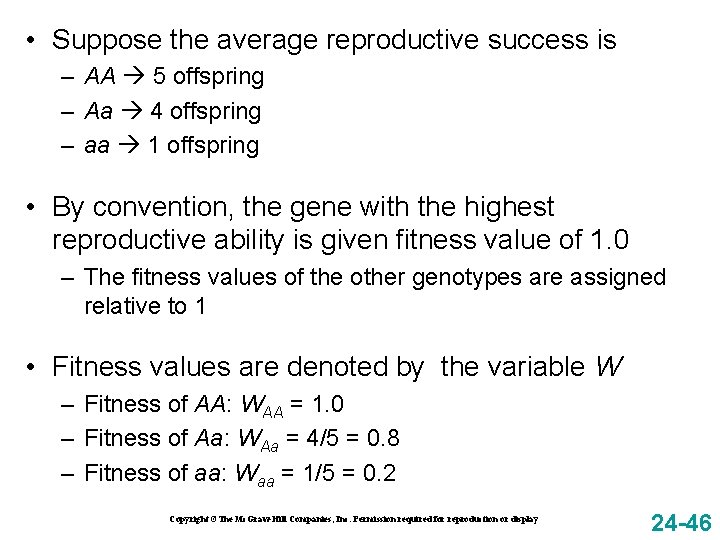
• Suppose the average reproductive success is – AA 5 offspring – Aa 4 offspring – aa 1 offspring • By convention, the gene with the highest reproductive ability is given fitness value of 1. 0 – The fitness values of the other genotypes are assigned relative to 1 • Fitness values are denoted by the variable W – Fitness of AA: WAA = 1. 0 – Fitness of Aa: WAa = 4/5 = 0. 8 – Fitness of aa: Waa = 1/5 = 0. 2 Copyright ©The Mc. Graw-Hill Companies, Inc. Permission required for reproduction or display 24 -46
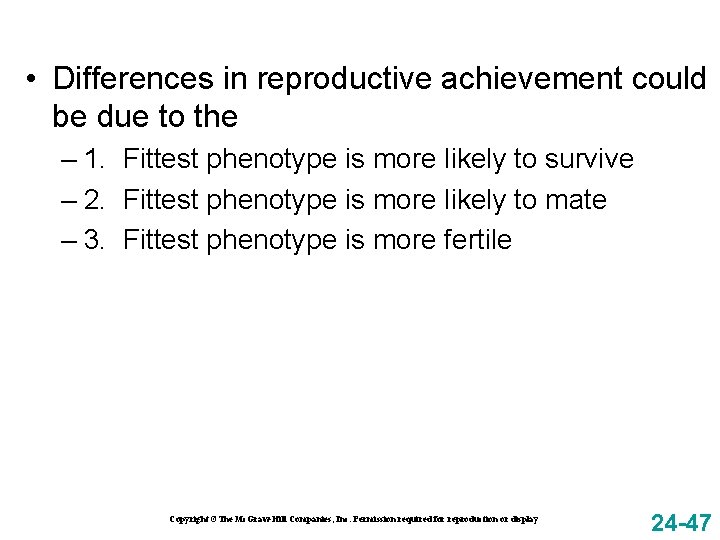
• Differences in reproductive achievement could be due to the – 1. Fittest phenotype is more likely to survive – 2. Fittest phenotype is more likely to mate – 3. Fittest phenotype is more fertile Copyright ©The Mc. Graw-Hill Companies, Inc. Permission required for reproduction or display 24 -47
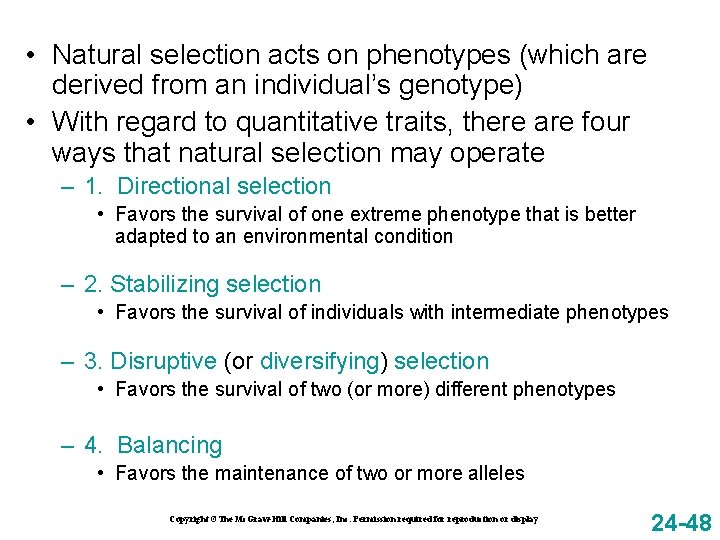
• Natural selection acts on phenotypes (which are derived from an individual’s genotype) • With regard to quantitative traits, there are four ways that natural selection may operate – 1. Directional selection • Favors the survival of one extreme phenotype that is better adapted to an environmental condition – 2. Stabilizing selection • Favors the survival of individuals with intermediate phenotypes – 3. Disruptive (or diversifying) selection • Favors the survival of two (or more) different phenotypes – 4. Balancing • Favors the maintenance of two or more alleles Copyright ©The Mc. Graw-Hill Companies, Inc. Permission required for reproduction or display 24 -48
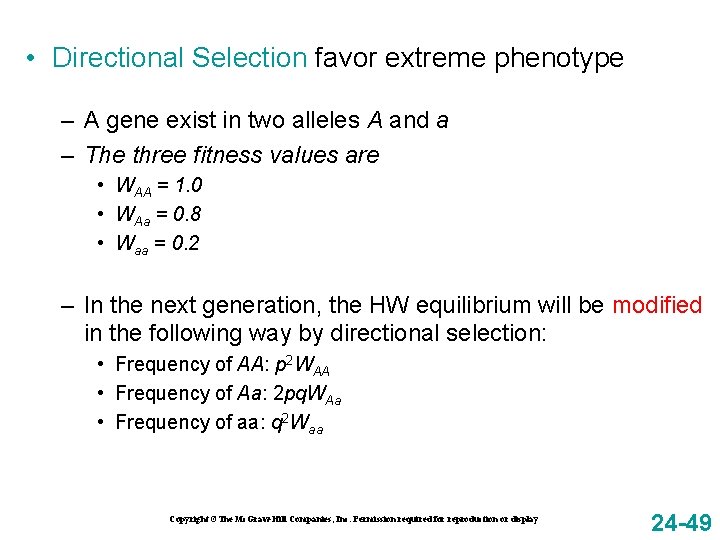
• Directional Selection favor extreme phenotype – A gene exist in two alleles A and a – The three fitness values are • WAA = 1. 0 • WAa = 0. 8 • Waa = 0. 2 – In the next generation, the HW equilibrium will be modified in the following way by directional selection: • Frequency of AA: p 2 WAA • Frequency of Aa: 2 pq. WAa • Frequency of aa: q 2 Waa Copyright ©The Mc. Graw-Hill Companies, Inc. Permission required for reproduction or display 24 -49
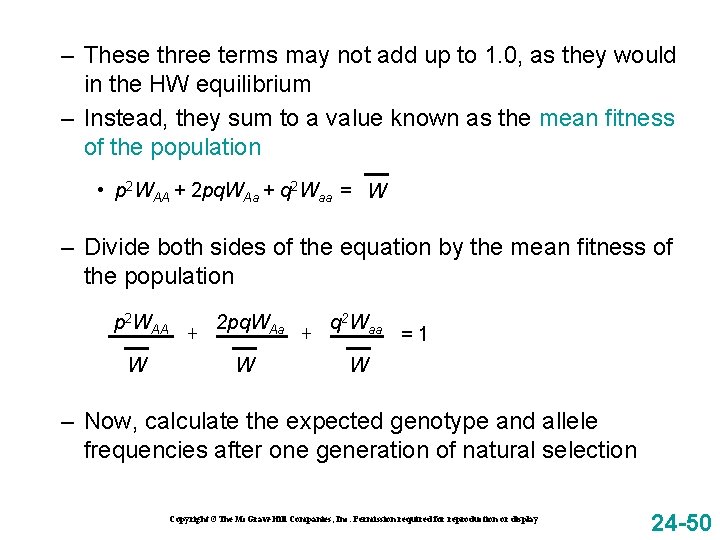
– These three terms may not add up to 1. 0, as they would in the HW equilibrium – Instead, they sum to a value known as the mean fitness of the population • p 2 WAA + 2 pq. WAa + q 2 Waa = W – Divide both sides of the equation by the mean fitness of the population p 2 WAA W + 2 pq. WAa W + q 2 Waa =1 W – Now, calculate the expected genotype and allele frequencies after one generation of natural selection Copyright ©The Mc. Graw-Hill Companies, Inc. Permission required for reproduction or display 24 -50
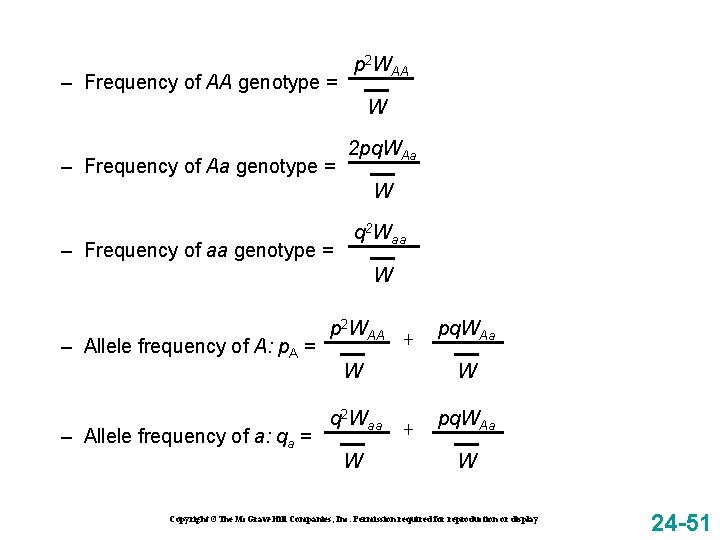
– Frequency of AA genotype = p 2 WAA W – Frequency of Aa genotype = 2 pq. WAa W – Frequency of aa genotype = q 2 Waa W – Allele frequency of A: p. A = – Allele frequency of a: qa = p 2 WAA + W q 2 Waa W pq. WAa W + pq. WAa W Copyright ©The Mc. Graw-Hill Companies, Inc. Permission required for reproduction or display 24 -51
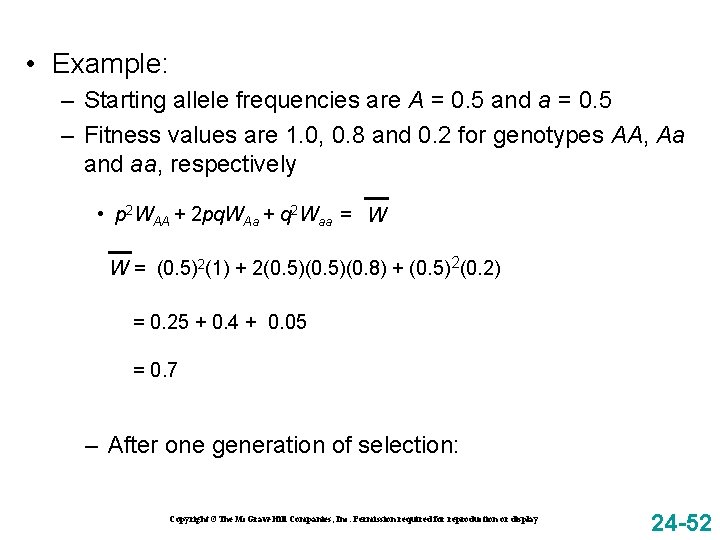
• Example: – Starting allele frequencies are A = 0. 5 and a = 0. 5 – Fitness values are 1. 0, 0. 8 and 0. 2 for genotypes AA, Aa and aa, respectively • p 2 WAA + 2 pq. WAa + q 2 Waa = W W = (0. 5)2(1) + 2(0. 5)(0. 8) + (0. 5)2(0. 2) = 0. 25 + 0. 4 + 0. 05 = 0. 7 – After one generation of selection: Copyright ©The Mc. Graw-Hill Companies, Inc. Permission required for reproduction or display 24 -52
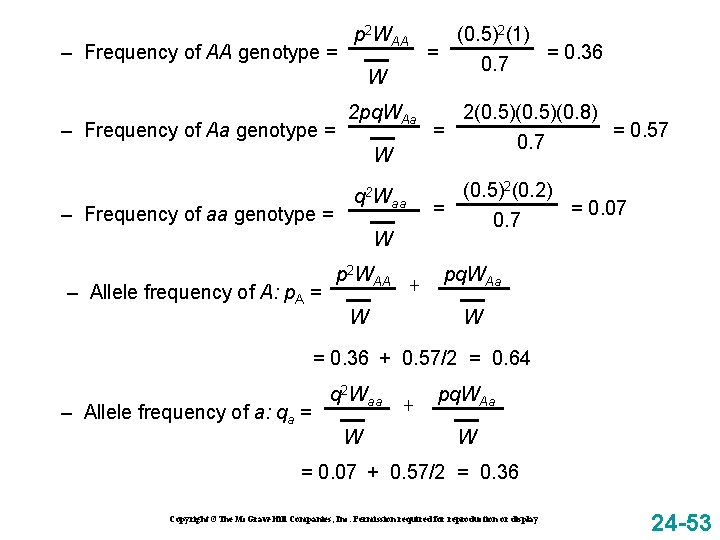
– Frequency of AA genotype = p 2 WAA (0. 5)2(1) = = 0. 36 0. 7 W – Frequency of Aa genotype = 2 pq. WAa = 2(0. 5)(0. 8) 0. 7 W – Frequency of aa genotype = (0. 5)2(0. 2) = = 0. 07 0. 7 q 2 Waa W – Allele frequency of A: p. A = p 2 WAA = 0. 57 + W pq. WAa W = 0. 36 + 0. 57/2 = 0. 64 – Allele frequency of a: qa = q 2 Waa W + pq. WAa W = 0. 07 + 0. 57/2 = 0. 36 Copyright ©The Mc. Graw-Hill Companies, Inc. Permission required for reproduction or display 24 -53
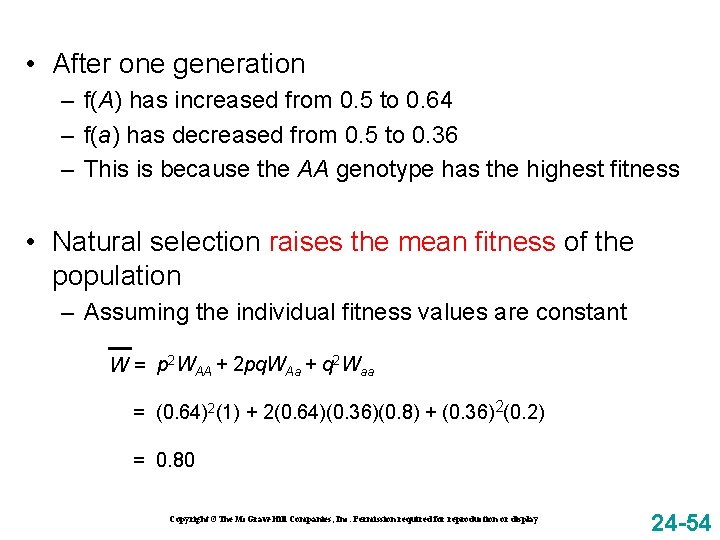
• After one generation – f(A) has increased from 0. 5 to 0. 64 – f(a) has decreased from 0. 5 to 0. 36 – This is because the AA genotype has the highest fitness • Natural selection raises the mean fitness of the population – Assuming the individual fitness values are constant W = p 2 WAA + 2 pq. WAa + q 2 Waa = (0. 64)2(1) + 2(0. 64)(0. 36)(0. 8) + (0. 36)2(0. 2) = 0. 80 Copyright ©The Mc. Graw-Hill Companies, Inc. Permission required for reproduction or display 24 -54
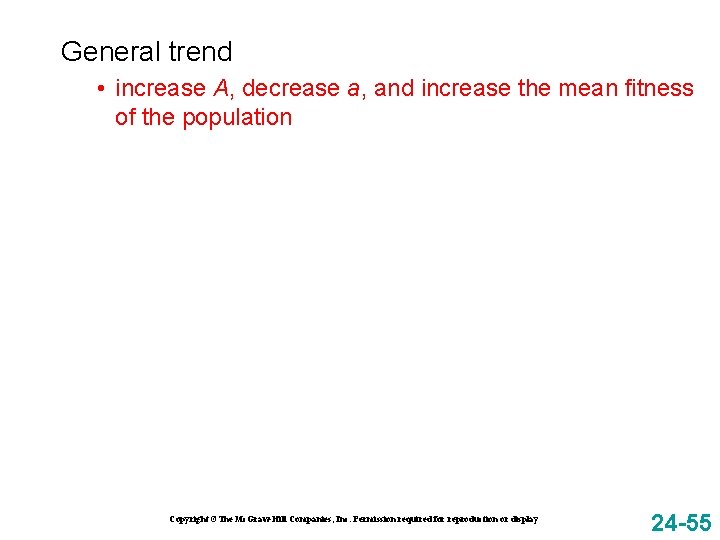
General trend • increase A, decrease a, and increase the mean fitness of the population Copyright ©The Mc. Graw-Hill Companies, Inc. Permission required for reproduction or display 24 -55
作業管理精簡版13版解答
Grawhill
Grawhill
Mc grawhill
Grawhill
Mc grawhill
Grawhill
Grawhill
Mc grawhill
Genetics
Population genetics
Similar
Population genetics definition
Population genetics
Population genetics and speciation worksheet answer key
Population genetics
Pearson education limited 2017
Copyright 2009 pearson education inc
2005 pearson prentice hall inc
Copyright 2010 pearson education inc
Copyright goodheart-willcox co. inc
Copyright pearson education inc
Copyright 2010 pearson education inc
Copyright 2010 pearson education inc
Copyright pearson education inc
2009 pearson education inc
Copyright 2009 pearson education inc
Copyright 2010 pearson education inc
2018 pearson education inc
Composition copyright example
Copyright by pearson education inc. answers
2010 pearson education inc
Copyright 2009 pearson education inc
Copyright 2010 pearson education inc
2014 pearson education inc
Intext:"copyright (c) tektronix, inc." "printer status"
Copyright 2010 pearson education inc
2015 pearson education inc
2008 pearson education inc
Copyright 2009 pearson education inc
Copyright 2009 pearson education inc
2010 pearson education inc
Copyright 2010 pearson education inc
Population ecology section 1 population dynamics
Chapter 4 section 1 population dynamics study guide
Study guide chapter 4 section 1 population dynamics
Section 1 population dynamics answer key
Thế nào là hệ số cao nhất
Thiếu nhi thế giới liên hoan
đặc điểm cơ thể của người tối cổ
Mật thư tọa độ 5x5