Physics 736 Experimental Methods in Nuclear Particle and
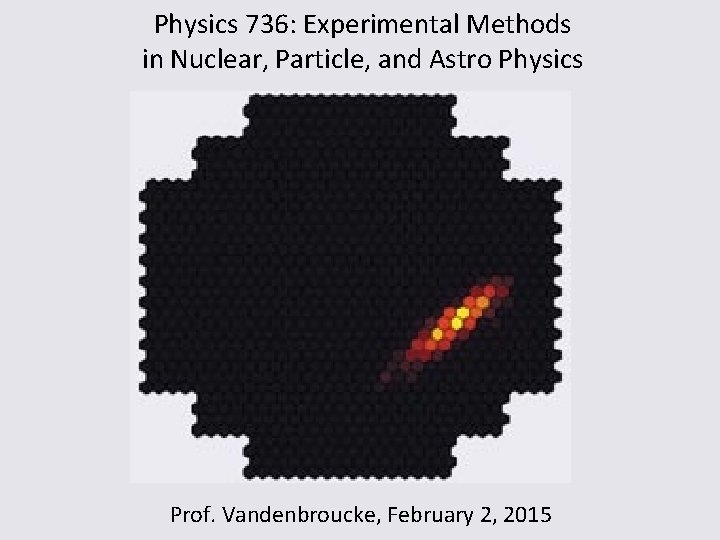
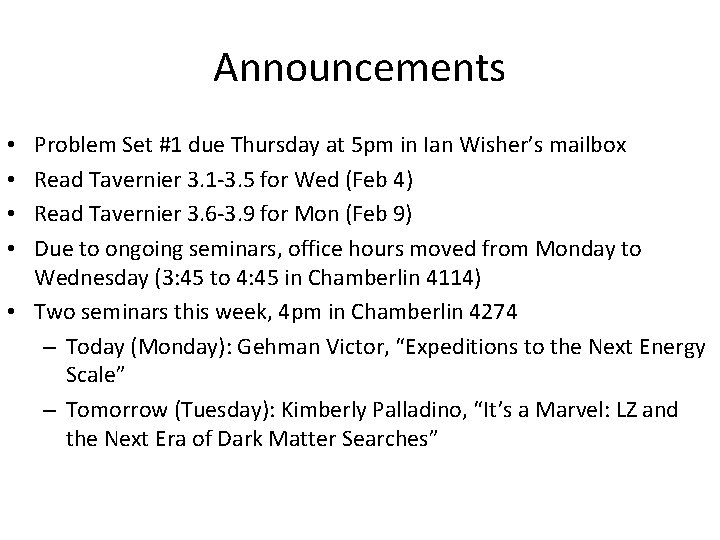
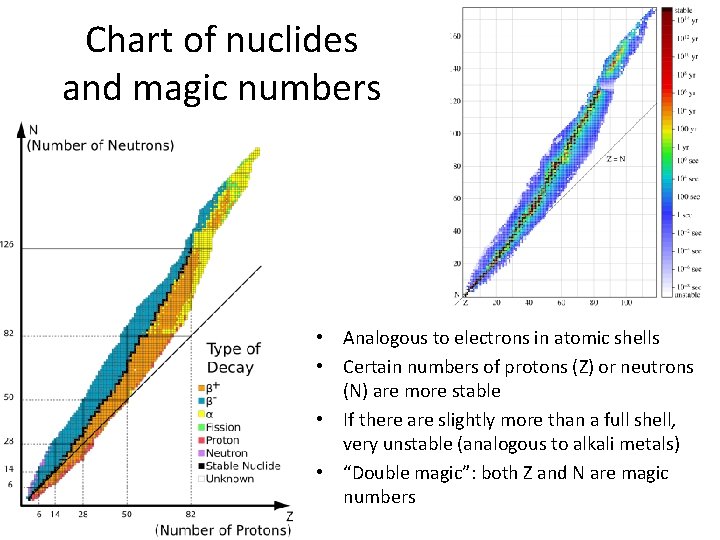
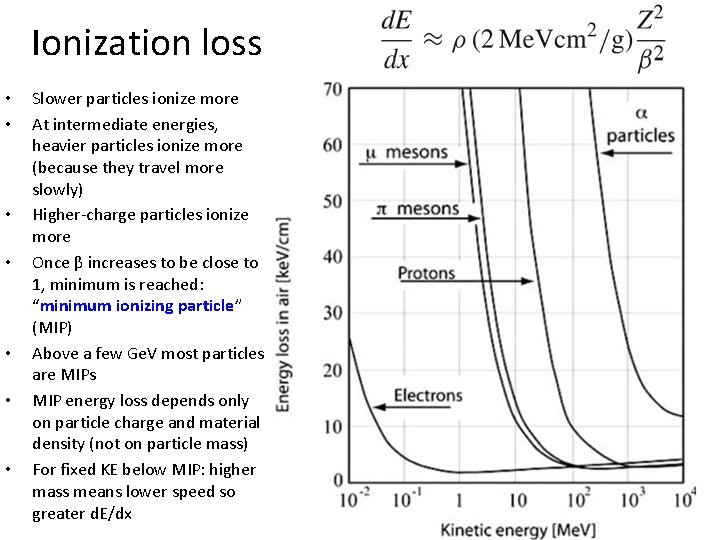
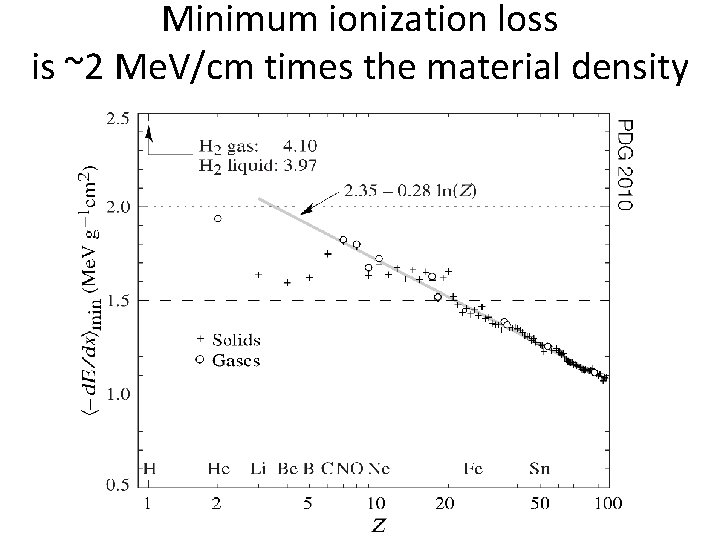
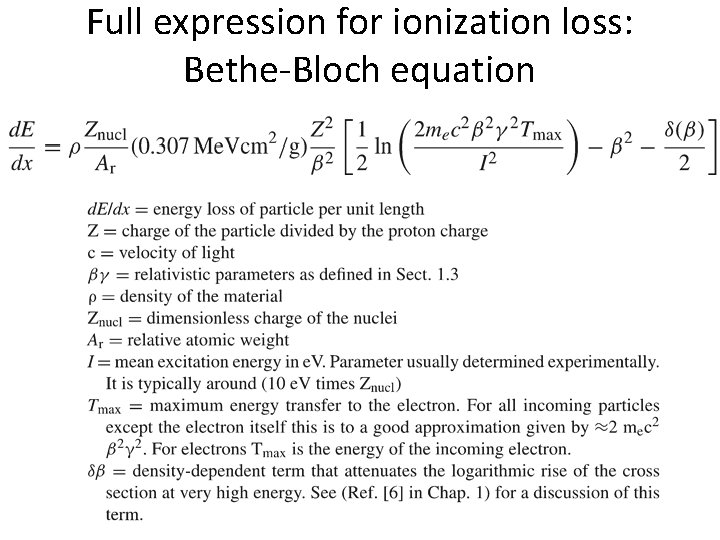
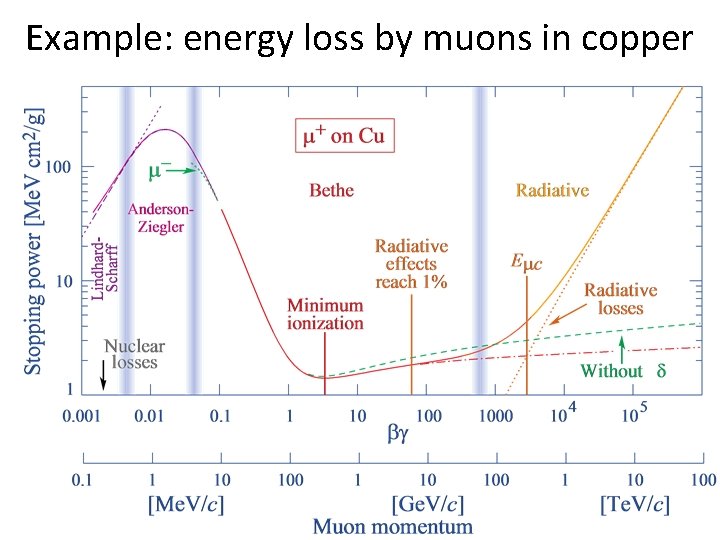
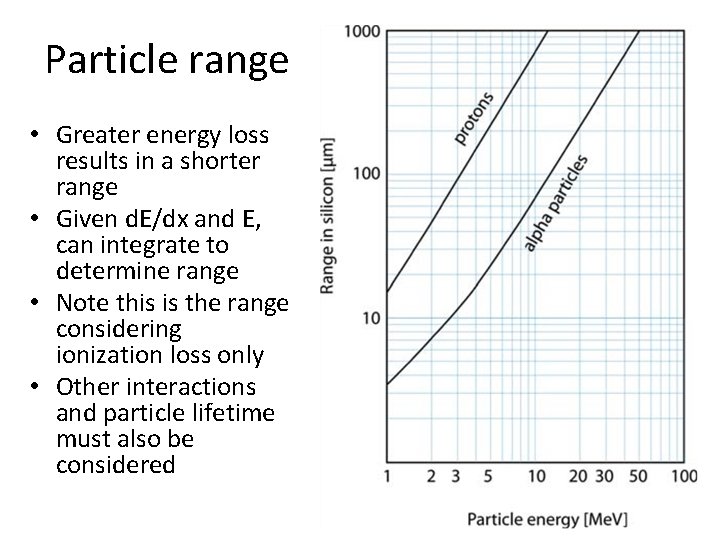
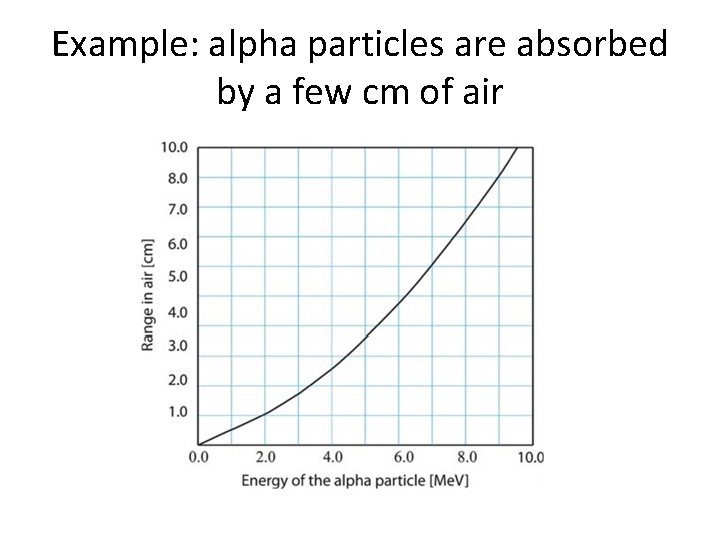
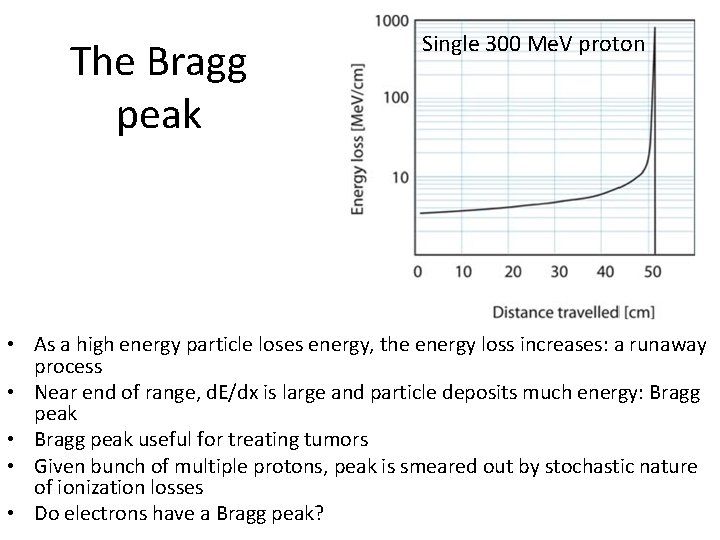
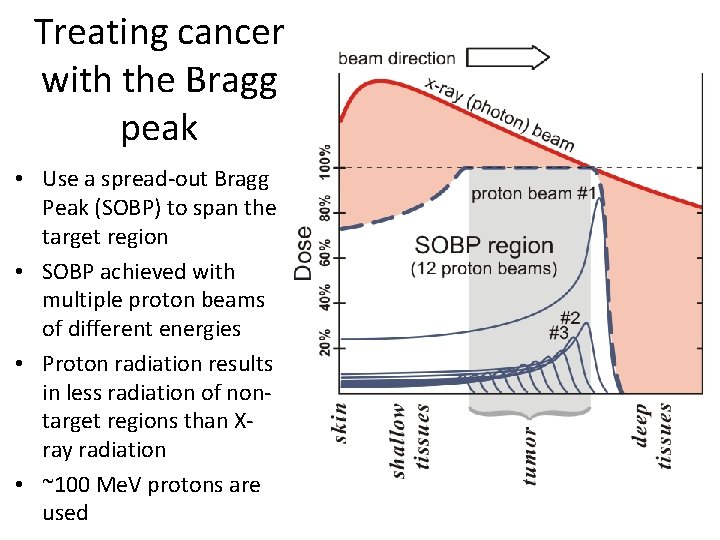
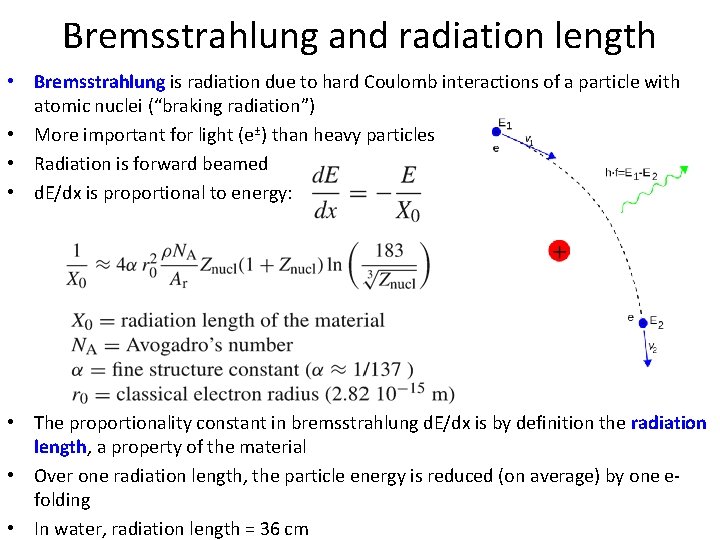
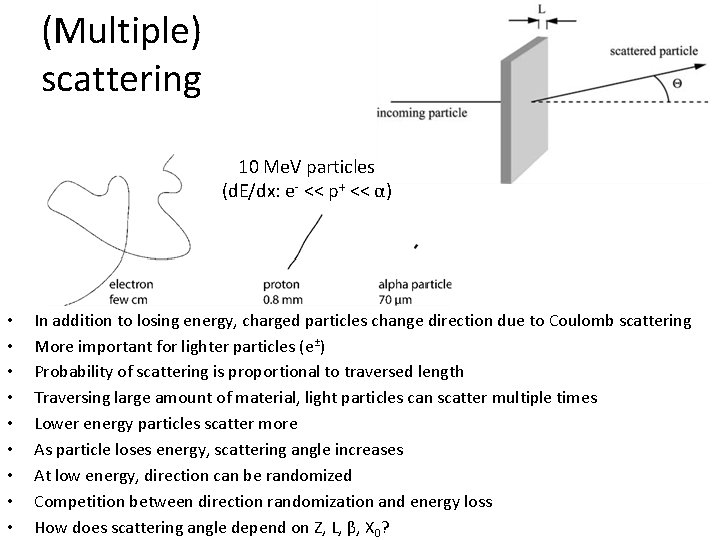
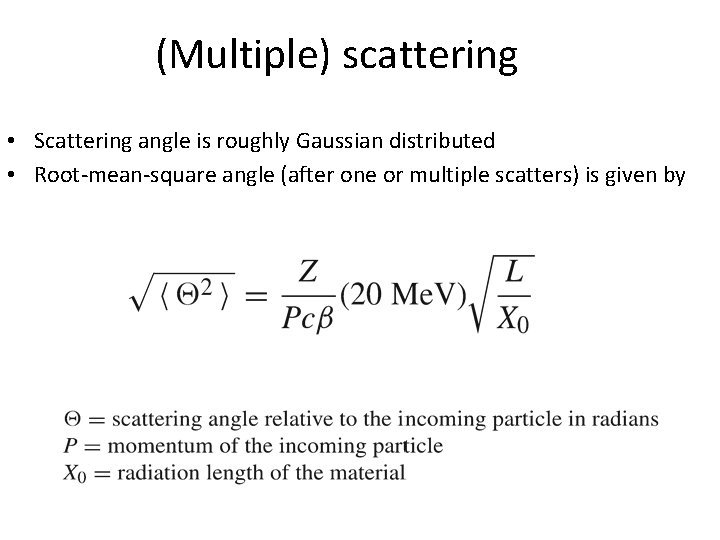
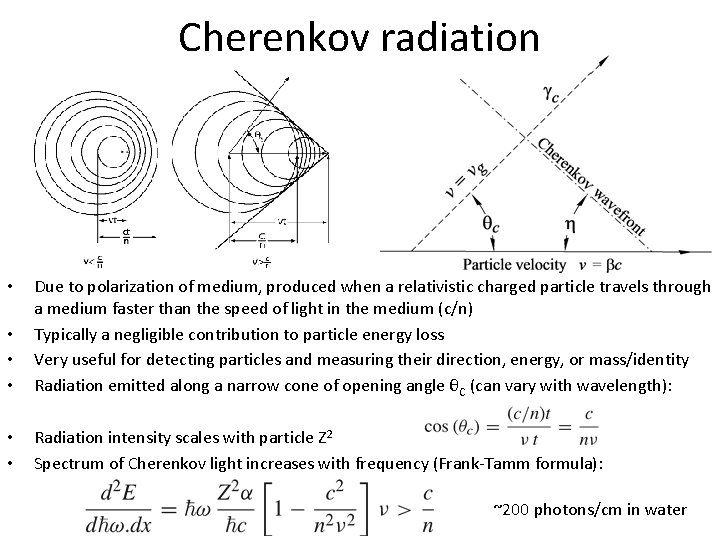
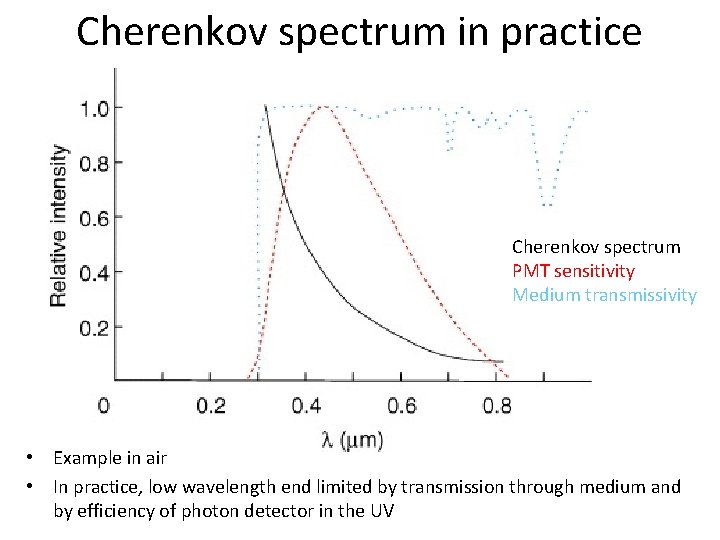
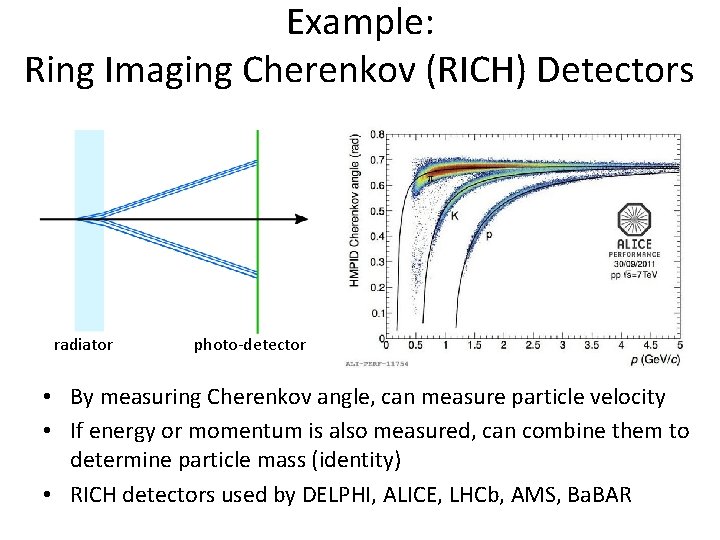
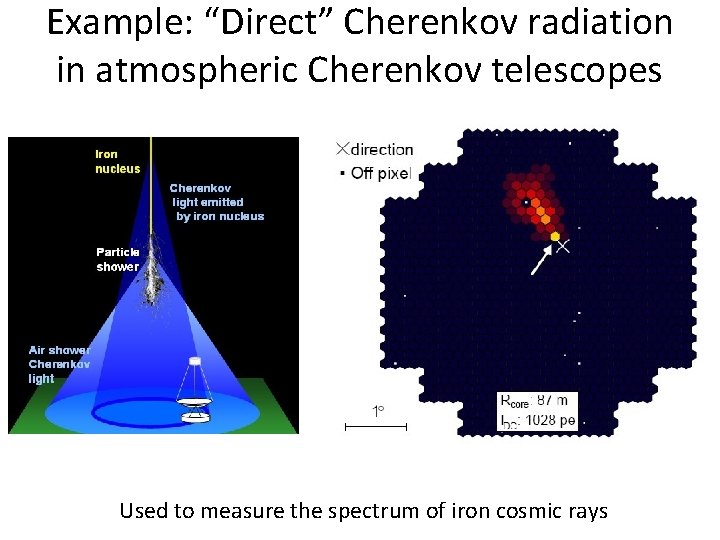
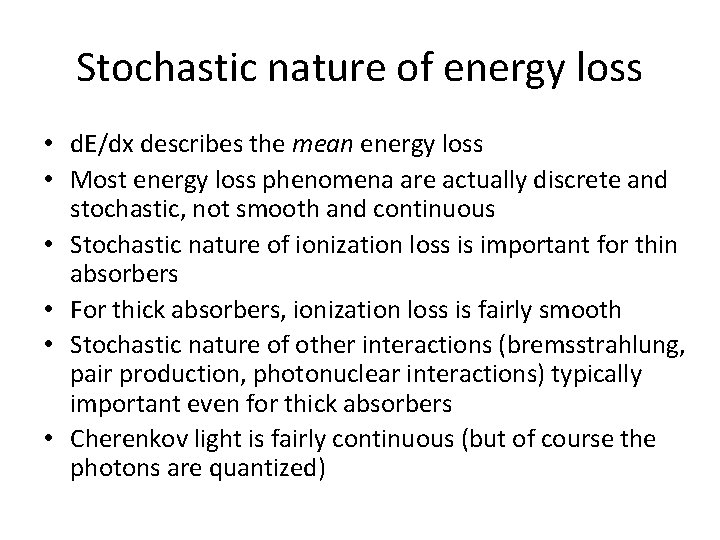
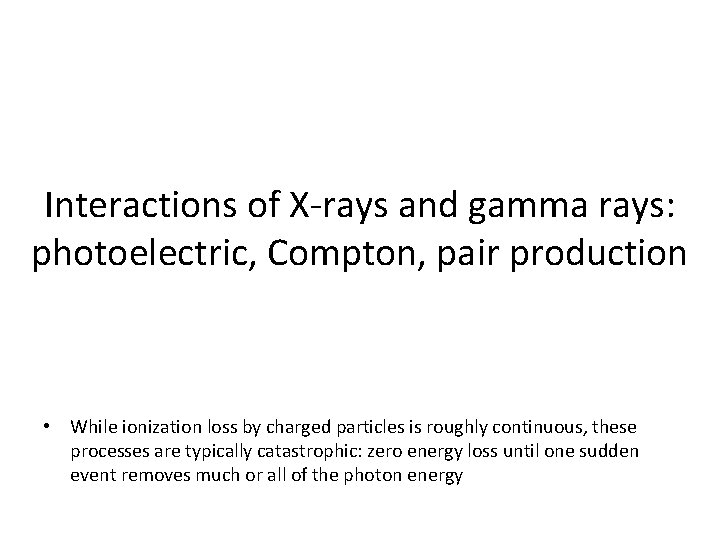
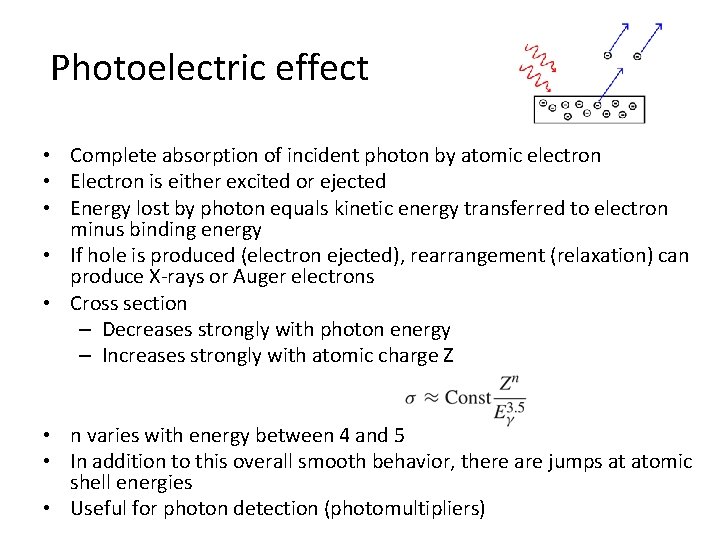
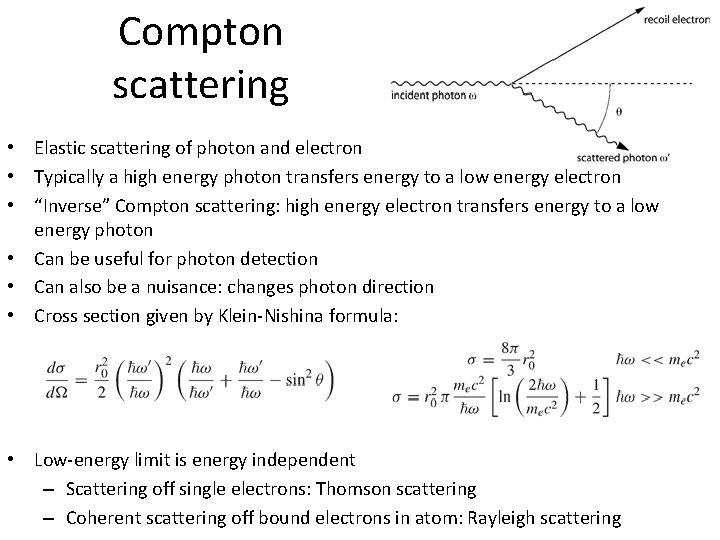
- Slides: 22
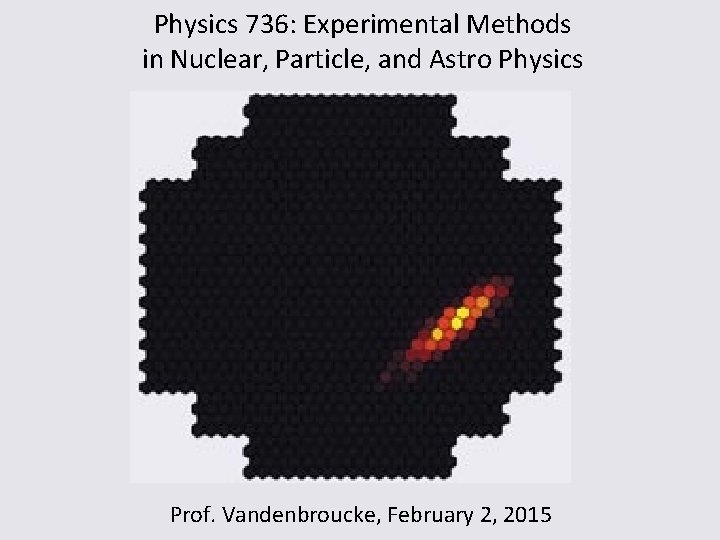
Physics 736: Experimental Methods in Nuclear, Particle, and Astro Physics Prof. Vandenbroucke, February 2, 2015
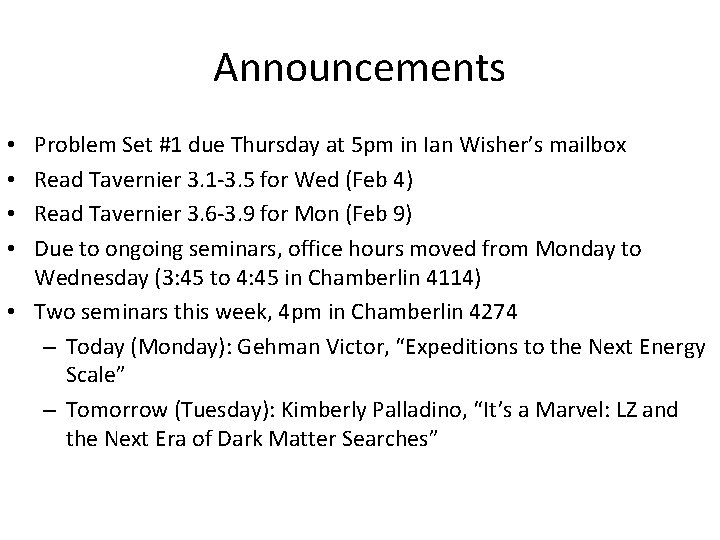
Announcements Problem Set #1 due Thursday at 5 pm in Ian Wisher’s mailbox Read Tavernier 3. 1 -3. 5 for Wed (Feb 4) Read Tavernier 3. 6 -3. 9 for Mon (Feb 9) Due to ongoing seminars, office hours moved from Monday to Wednesday (3: 45 to 4: 45 in Chamberlin 4114) • Two seminars this week, 4 pm in Chamberlin 4274 – Today (Monday): Gehman Victor, “Expeditions to the Next Energy Scale” – Tomorrow (Tuesday): Kimberly Palladino, “It’s a Marvel: LZ and the Next Era of Dark Matter Searches” • •
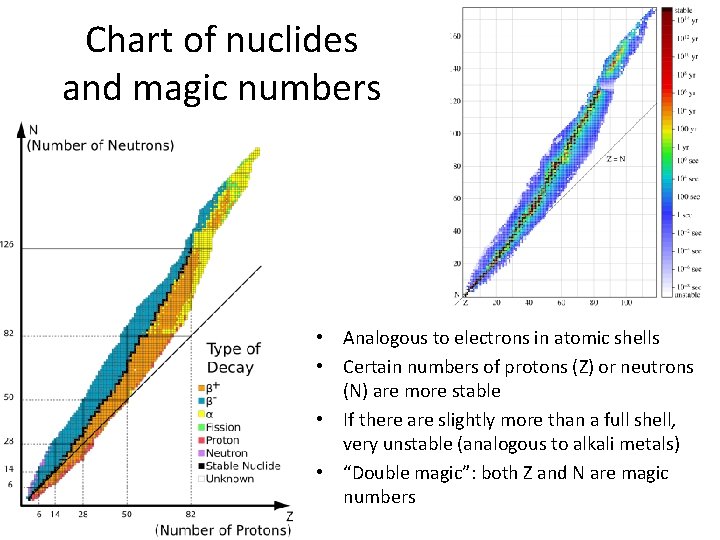
Chart of nuclides and magic numbers • Analogous to electrons in atomic shells • Certain numbers of protons (Z) or neutrons (N) are more stable • If there are slightly more than a full shell, very unstable (analogous to alkali metals) • “Double magic”: both Z and N are magic numbers
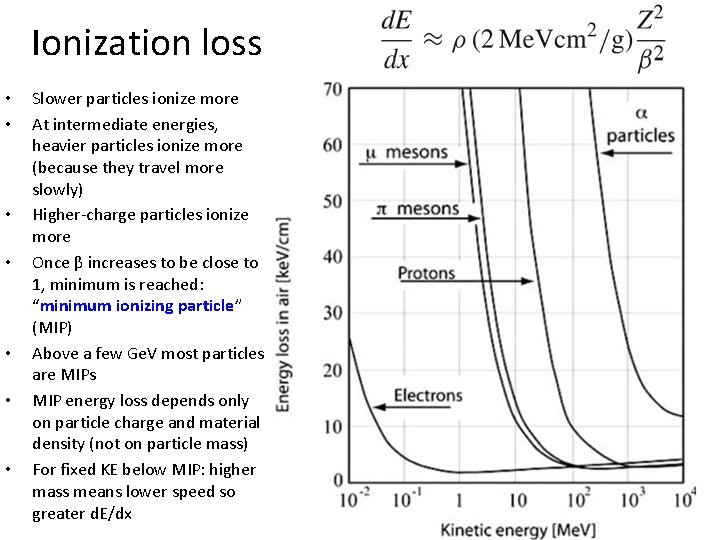
Ionization loss • • Slower particles ionize more At intermediate energies, heavier particles ionize more (because they travel more slowly) Higher-charge particles ionize more Once β increases to be close to 1, minimum is reached: “minimum ionizing particle” (MIP) Above a few Ge. V most particles are MIPs MIP energy loss depends only on particle charge and material density (not on particle mass) For fixed KE below MIP: higher mass means lower speed so greater d. E/dx
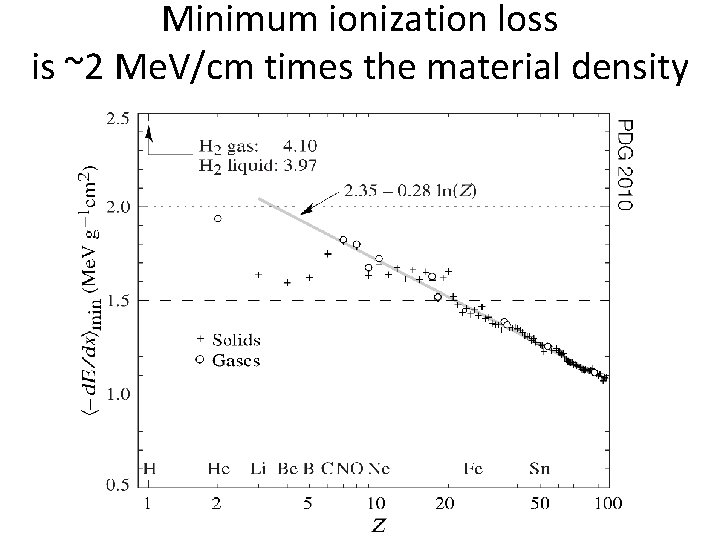
Minimum ionization loss is ~2 Me. V/cm times the material density
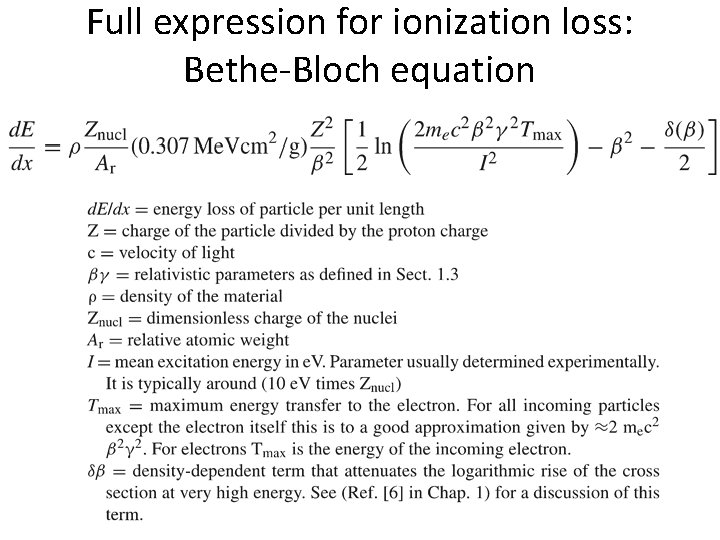
Full expression for ionization loss: Bethe-Bloch equation
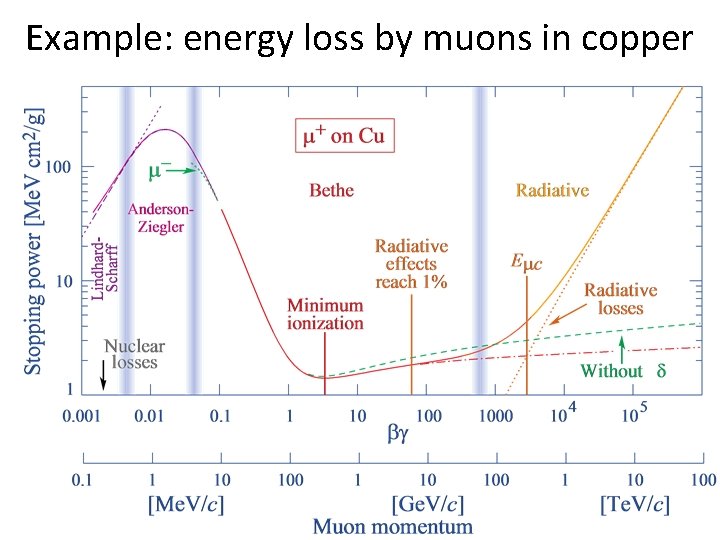
Example: energy loss by muons in copper
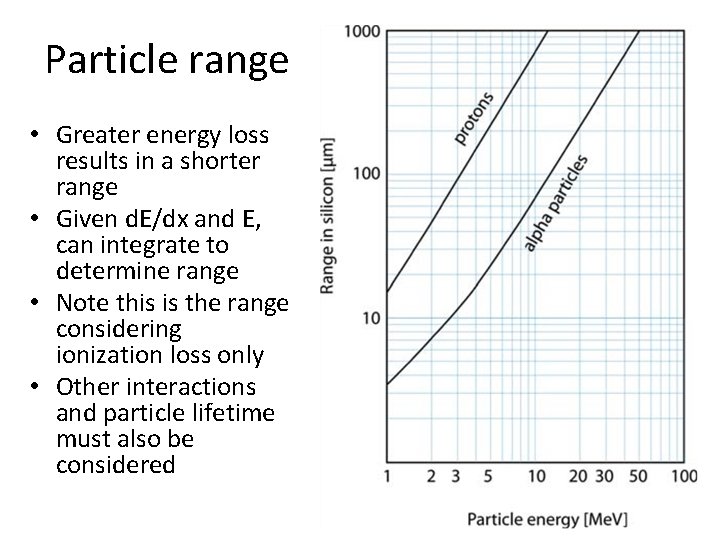
Particle range • Greater energy loss results in a shorter range • Given d. E/dx and E, can integrate to determine range • Note this is the range considering ionization loss only • Other interactions and particle lifetime must also be considered
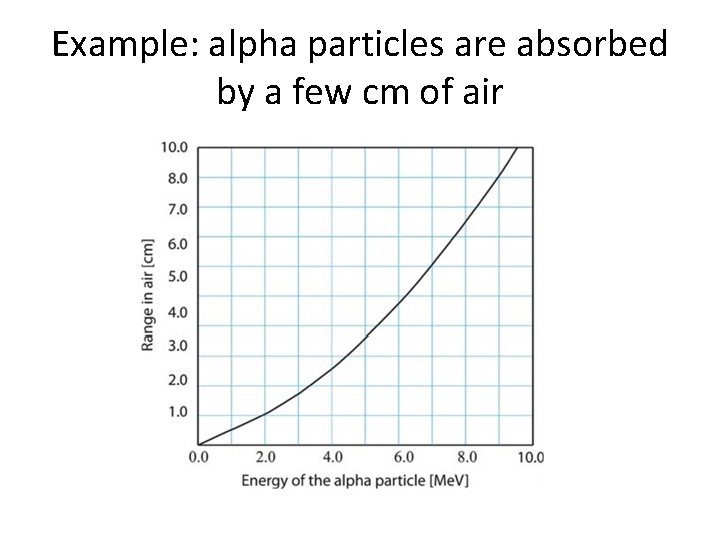
Example: alpha particles are absorbed by a few cm of air
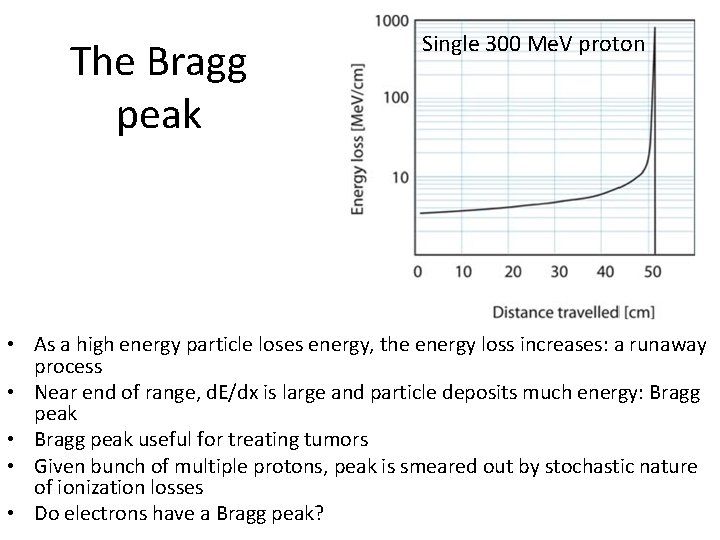
The Bragg peak Single 300 Me. V proton • As a high energy particle loses energy, the energy loss increases: a runaway process • Near end of range, d. E/dx is large and particle deposits much energy: Bragg peak • Bragg peak useful for treating tumors • Given bunch of multiple protons, peak is smeared out by stochastic nature of ionization losses • Do electrons have a Bragg peak?
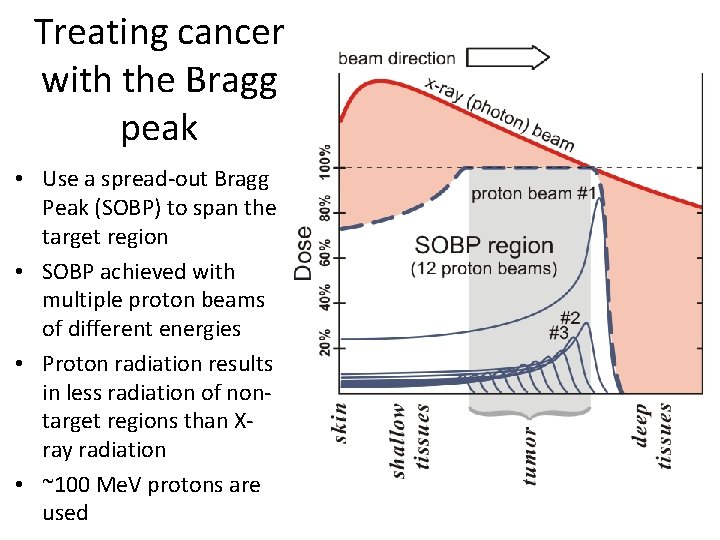
Treating cancer with the Bragg peak • Use a spread-out Bragg Peak (SOBP) to span the target region • SOBP achieved with multiple proton beams of different energies • Proton radiation results in less radiation of nontarget regions than Xray radiation • ~100 Me. V protons are used
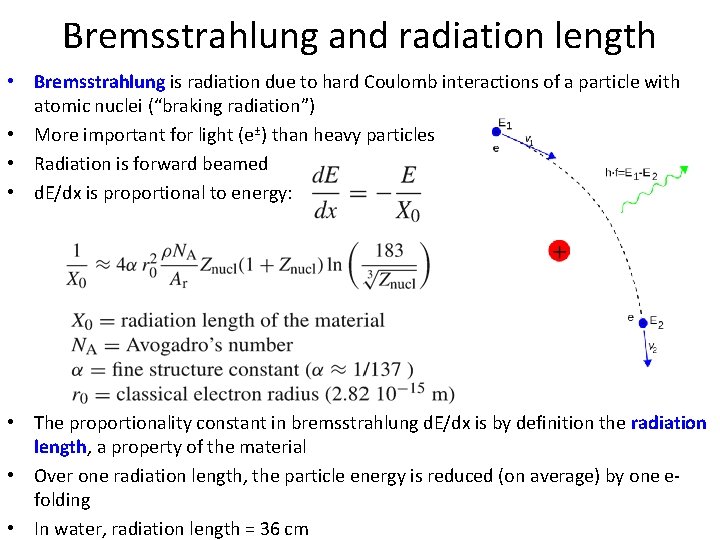
Bremsstrahlung and radiation length • Bremsstrahlung is radiation due to hard Coulomb interactions of a particle with atomic nuclei (“braking radiation”) • More important for light (e±) than heavy particles • Radiation is forward beamed • d. E/dx is proportional to energy: • The proportionality constant in bremsstrahlung d. E/dx is by definition the radiation length, a property of the material • Over one radiation length, the particle energy is reduced (on average) by one efolding • In water, radiation length = 36 cm
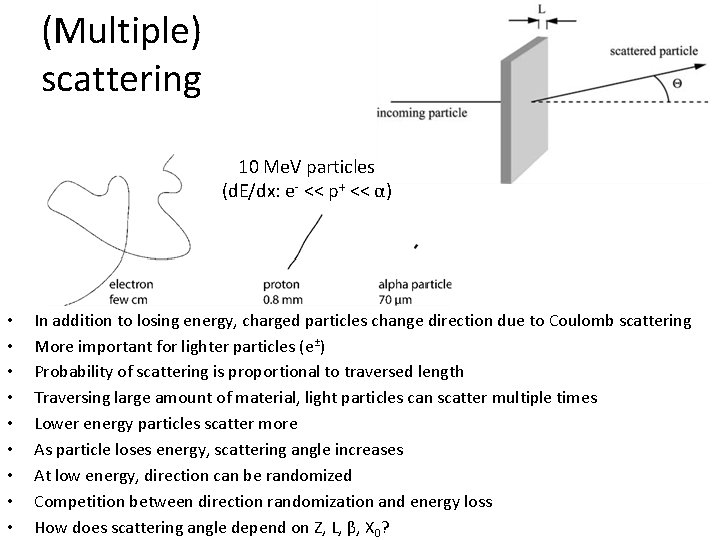
(Multiple) scattering 10 Me. V particles (d. E/dx: e- << p+ << α) • • • In addition to losing energy, charged particles change direction due to Coulomb scattering More important for lighter particles (e±) Probability of scattering is proportional to traversed length Traversing large amount of material, light particles can scatter multiple times Lower energy particles scatter more As particle loses energy, scattering angle increases At low energy, direction can be randomized Competition between direction randomization and energy loss How does scattering angle depend on Z, L, β, X 0?
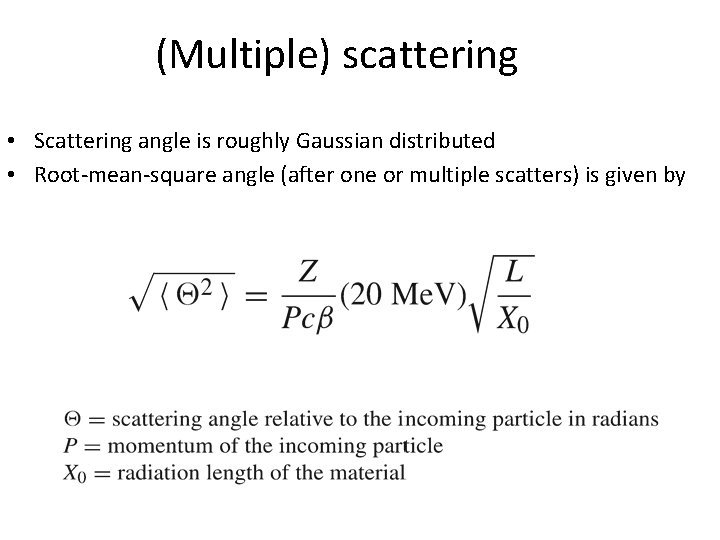
(Multiple) scattering • Scattering angle is roughly Gaussian distributed • Root-mean-square angle (after one or multiple scatters) is given by
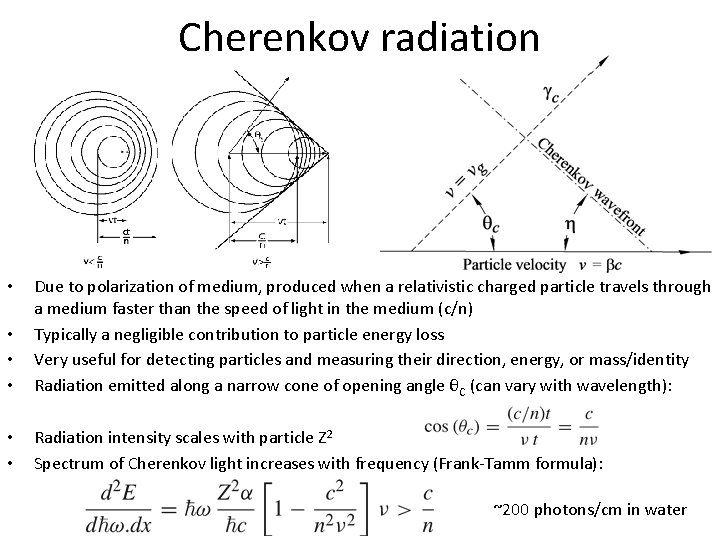
Cherenkov radiation • • • Due to polarization of medium, produced when a relativistic charged particle travels through a medium faster than the speed of light in the medium (c/n) Typically a negligible contribution to particle energy loss Very useful for detecting particles and measuring their direction, energy, or mass/identity Radiation emitted along a narrow cone of opening angle θC (can vary with wavelength): • • Radiation intensity scales with particle Z 2 Spectrum of Cherenkov light increases with frequency (Frank-Tamm formula): • ~200 photons/cm in water
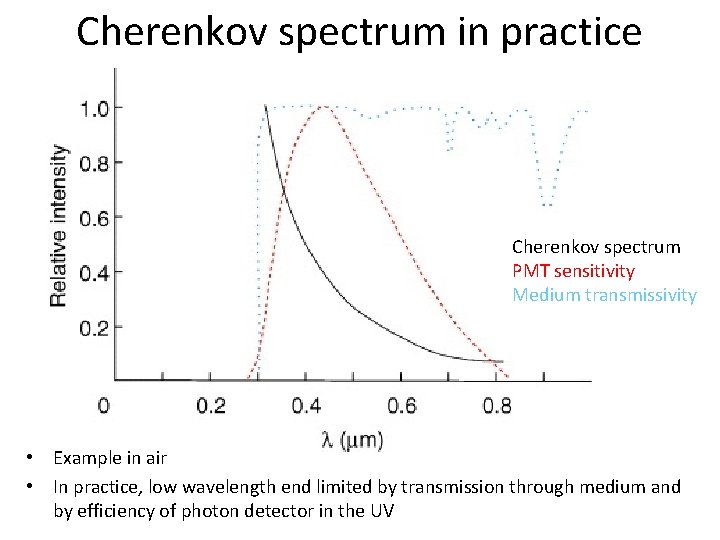
Cherenkov spectrum in practice Cherenkov spectrum PMT sensitivity Medium transmissivity • Example in air • In practice, low wavelength end limited by transmission through medium and by efficiency of photon detector in the UV
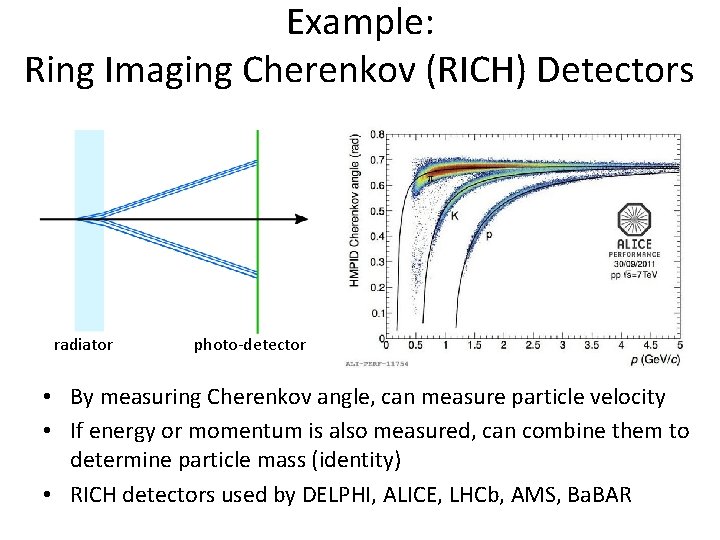
Example: Ring Imaging Cherenkov (RICH) Detectors radiator photo-detector • By measuring Cherenkov angle, can measure particle velocity • If energy or momentum is also measured, can combine them to determine particle mass (identity) • RICH detectors used by DELPHI, ALICE, LHCb, AMS, Ba. BAR
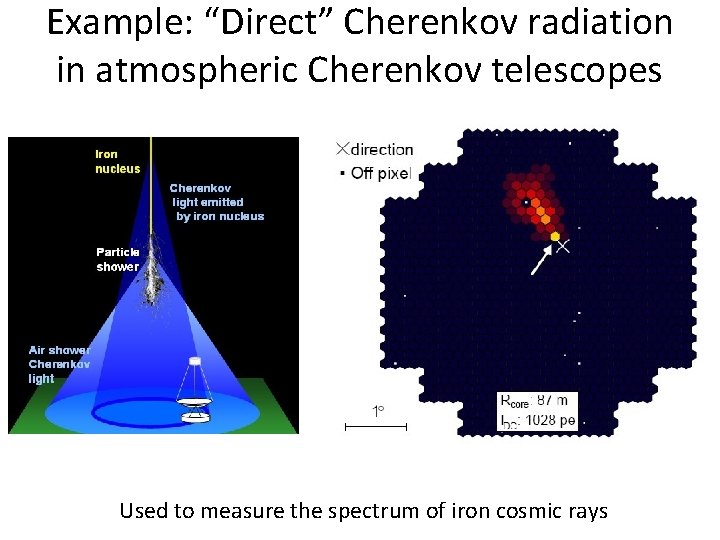
Example: “Direct” Cherenkov radiation in atmospheric Cherenkov telescopes Used to measure the spectrum of iron cosmic rays
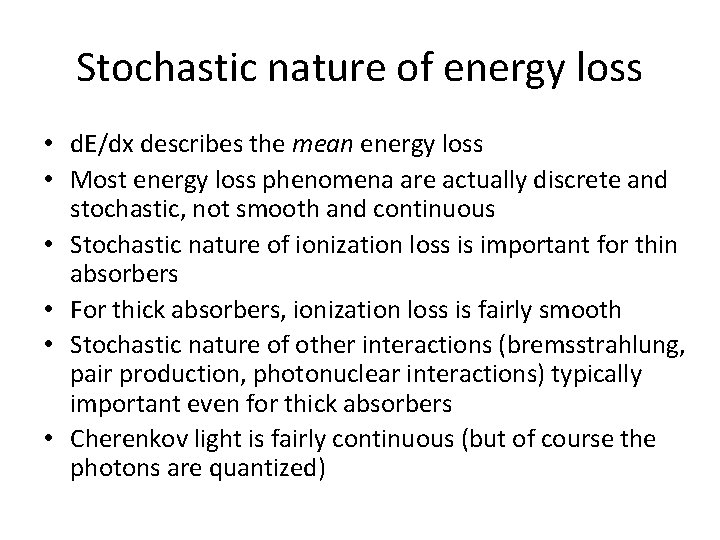
Stochastic nature of energy loss • d. E/dx describes the mean energy loss • Most energy loss phenomena are actually discrete and stochastic, not smooth and continuous • Stochastic nature of ionization loss is important for thin absorbers • For thick absorbers, ionization loss is fairly smooth • Stochastic nature of other interactions (bremsstrahlung, pair production, photonuclear interactions) typically important even for thick absorbers • Cherenkov light is fairly continuous (but of course the photons are quantized)
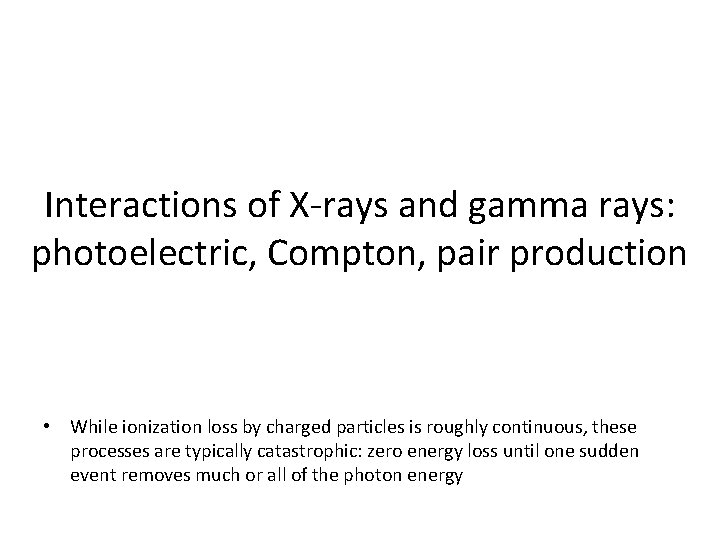
Interactions of X-rays and gamma rays: photoelectric, Compton, pair production • While ionization loss by charged particles is roughly continuous, these processes are typically catastrophic: zero energy loss until one sudden event removes much or all of the photon energy
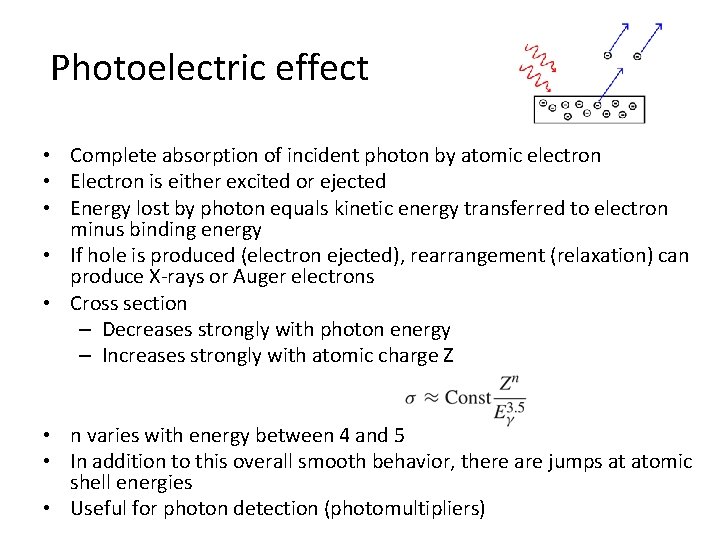
Photoelectric effect • Complete absorption of incident photon by atomic electron • Electron is either excited or ejected • Energy lost by photon equals kinetic energy transferred to electron minus binding energy • If hole is produced (electron ejected), rearrangement (relaxation) can produce X-rays or Auger electrons • Cross section – Decreases strongly with photon energy – Increases strongly with atomic charge Z • n varies with energy between 4 and 5 • In addition to this overall smooth behavior, there are jumps at atomic shell energies • Useful for photon detection (photomultipliers)
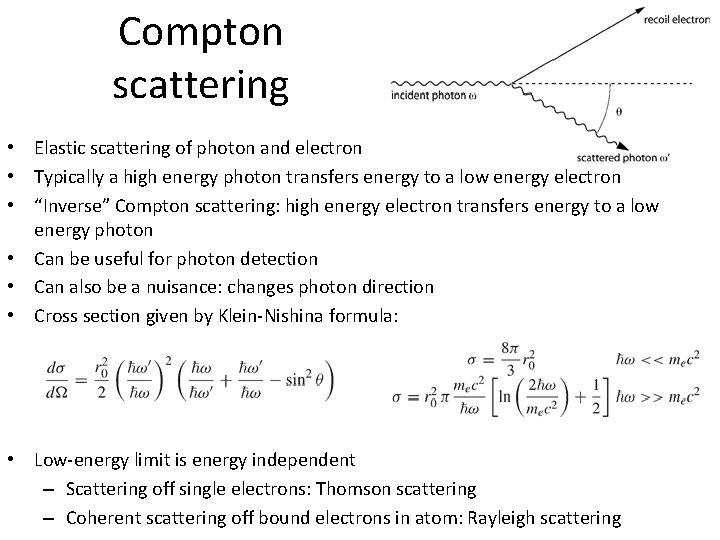
Compton scattering • Elastic scattering of photon and electron • Typically a high energy photon transfers energy to a low energy electron • “Inverse” Compton scattering: high energy electron transfers energy to a low energy photon • Can be useful for photon detection • Can also be a nuisance: changes photon direction • Cross section given by Klein-Nishina formula: • Low-energy limit is energy independent – Scattering off single electrons: Thomson scattering – Coherent scattering off bound electrons in atom: Rayleigh scattering
736 b payments
Experiment 736
736
736
Lesson 15 nuclear quest nuclear reactions
Fisión nuclear vs fision nuclear
Experimental vs non experimental
Research approaches and designs
Experimental vs non experimental research
Experimental vs nonexperimental research
Experimental vs non experimental
Quantum and nuclear physics
Experimental physics and industrial control system
"controls engineering"
Experimental physics and industrial control system
Pmt particle physics
Mark thomson particle physics
Particle physics
Particle physics practice quiz
Particle physics timeline
Monte carlo simulation particle physics
Cern particle physics
European laboratory for particle physics