Physics 736 Experimental Methods in Nuclear Particle and
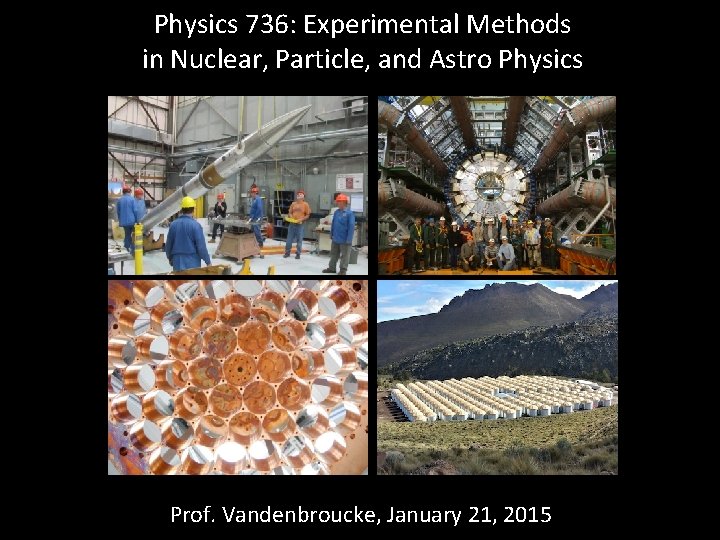
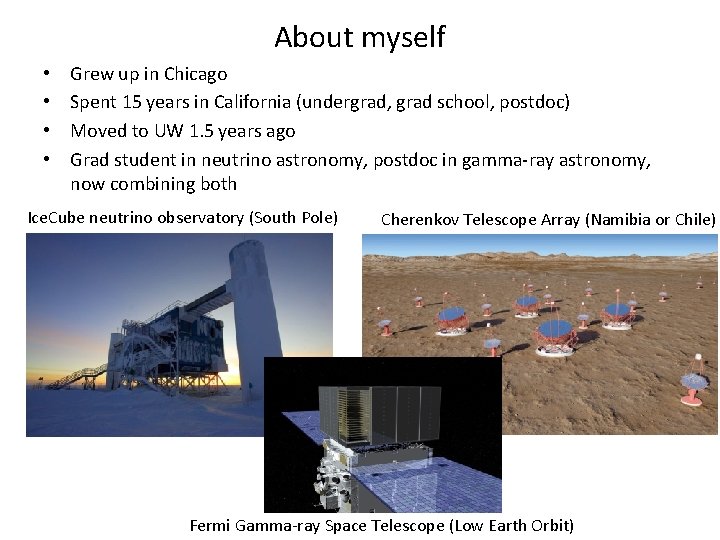
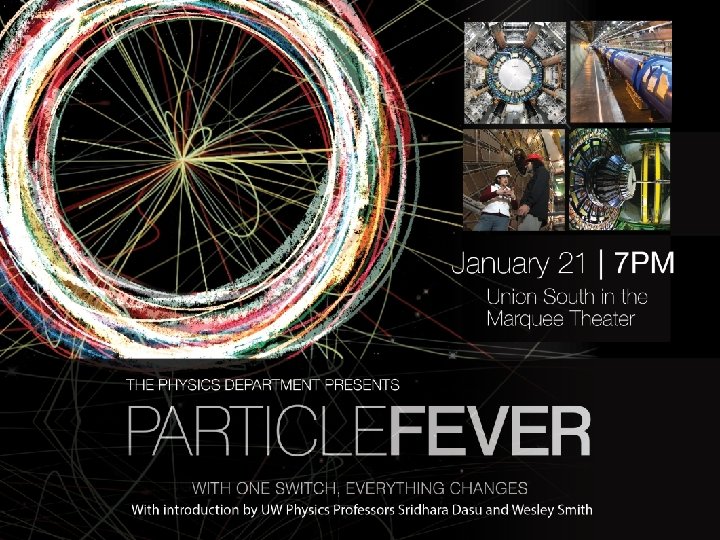
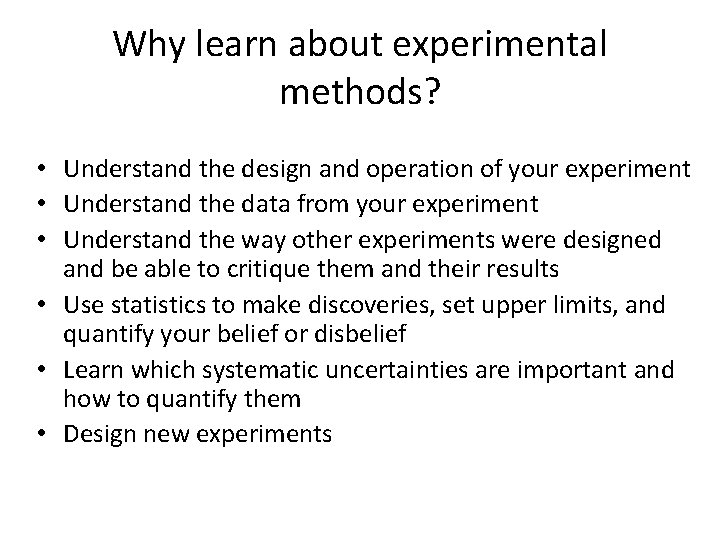
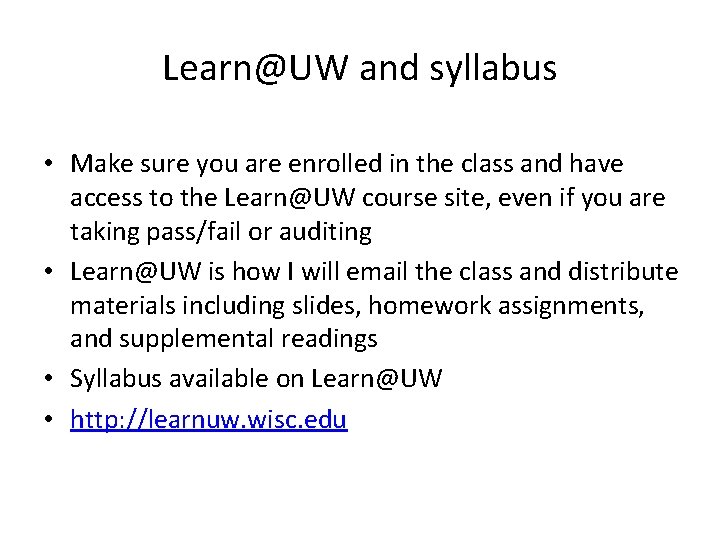
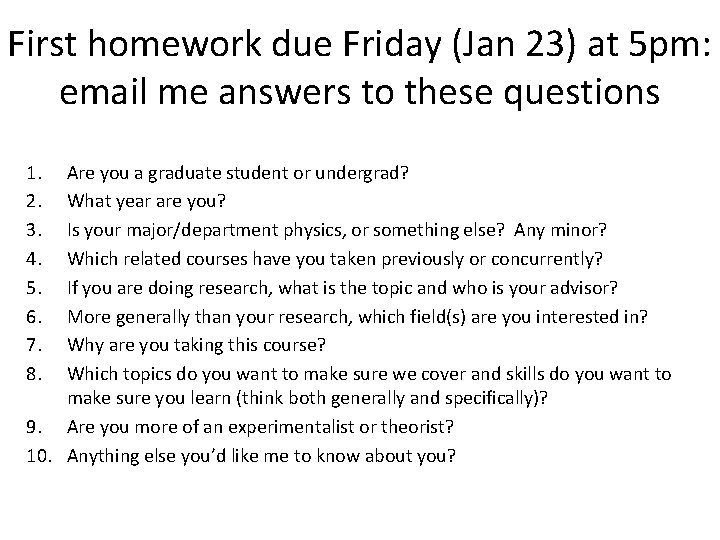
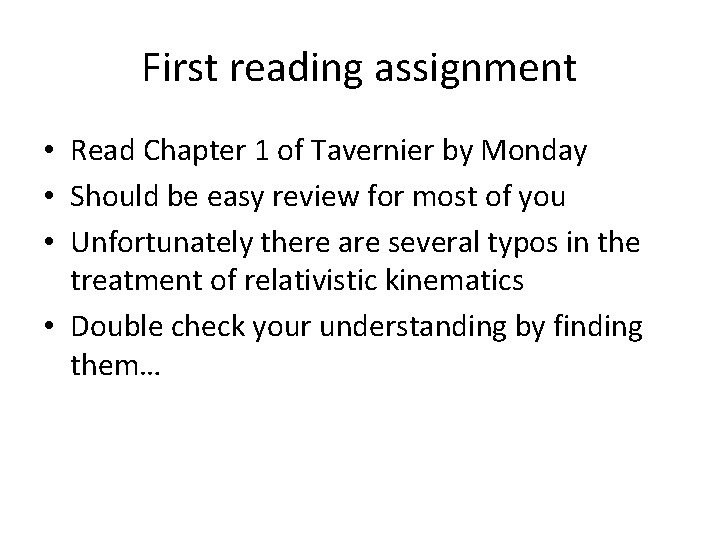
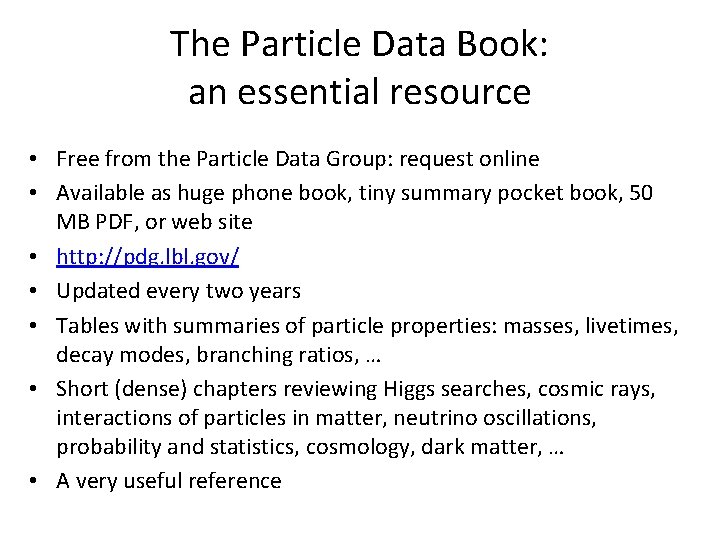
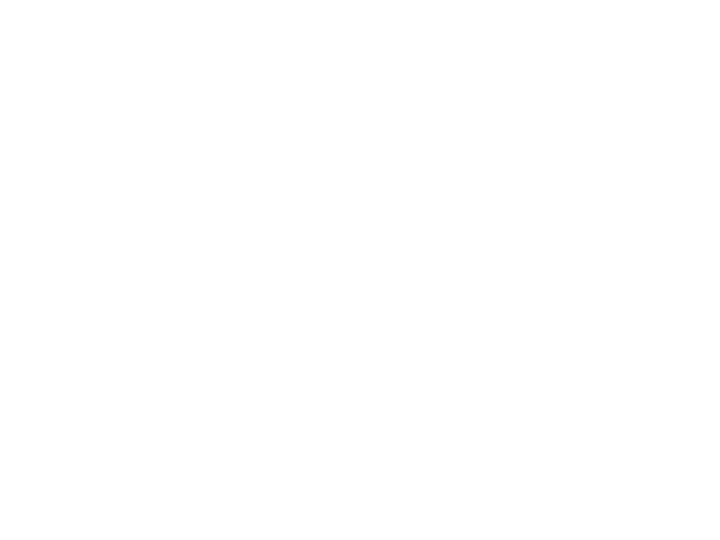
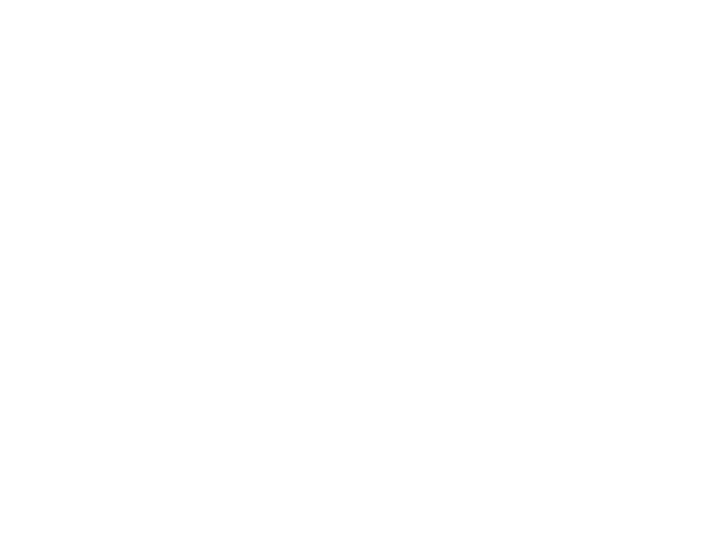
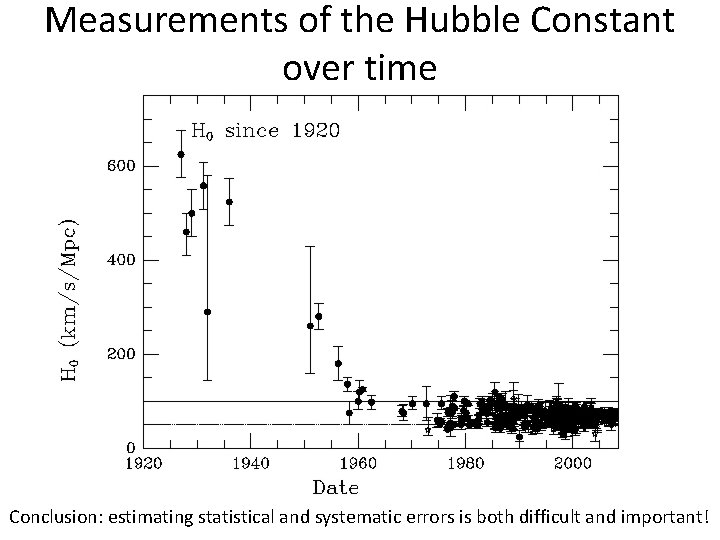
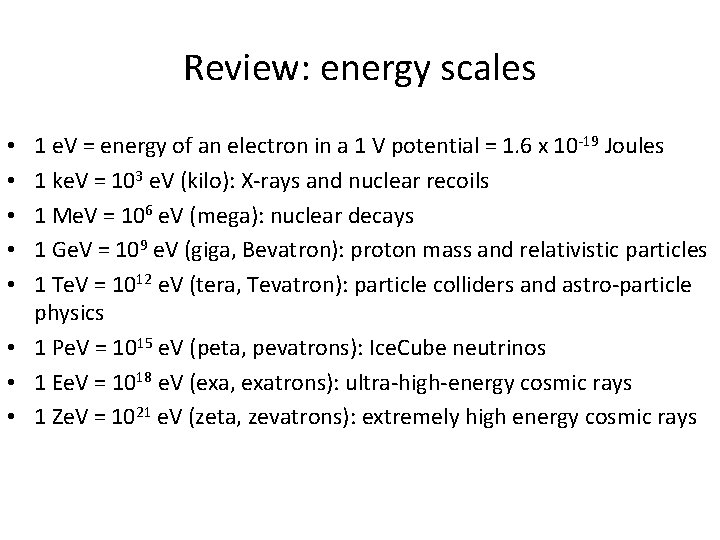
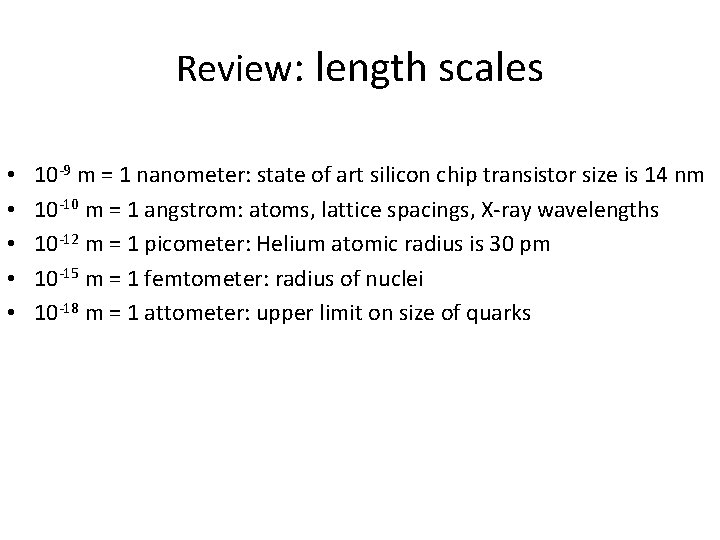
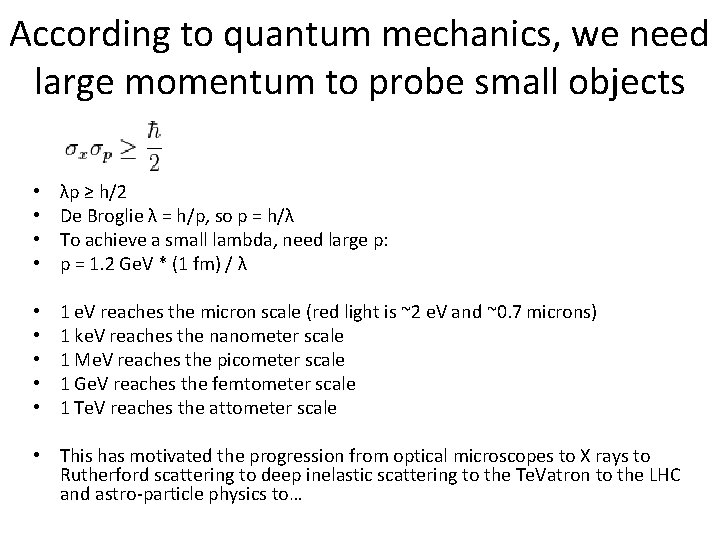
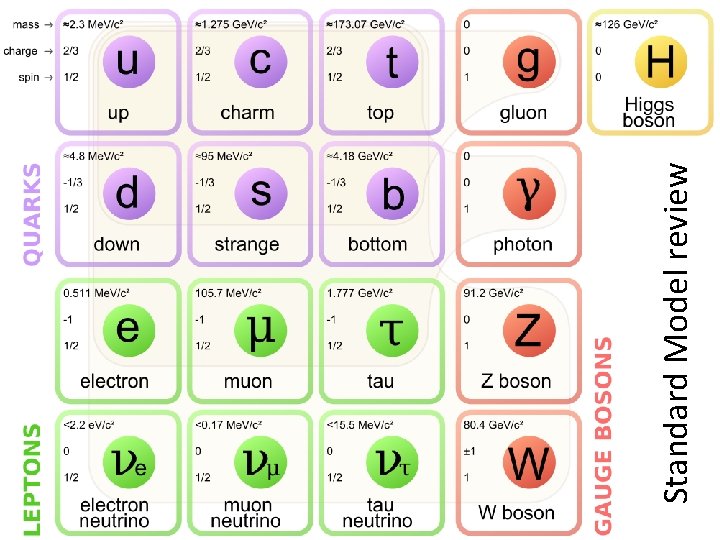
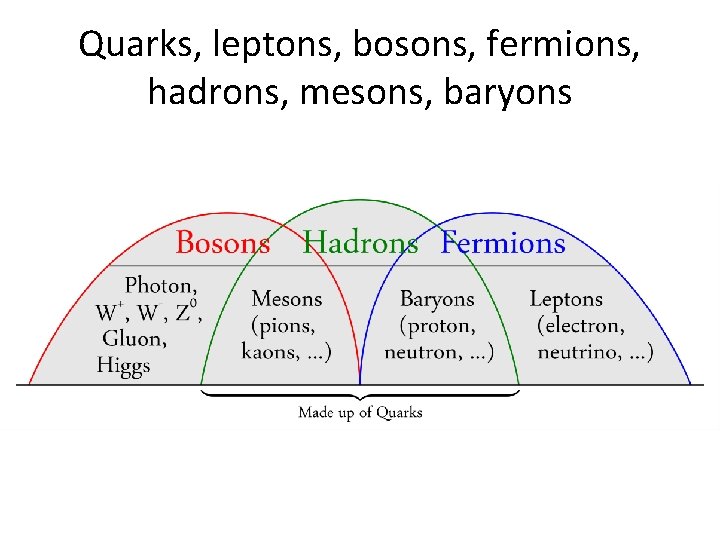
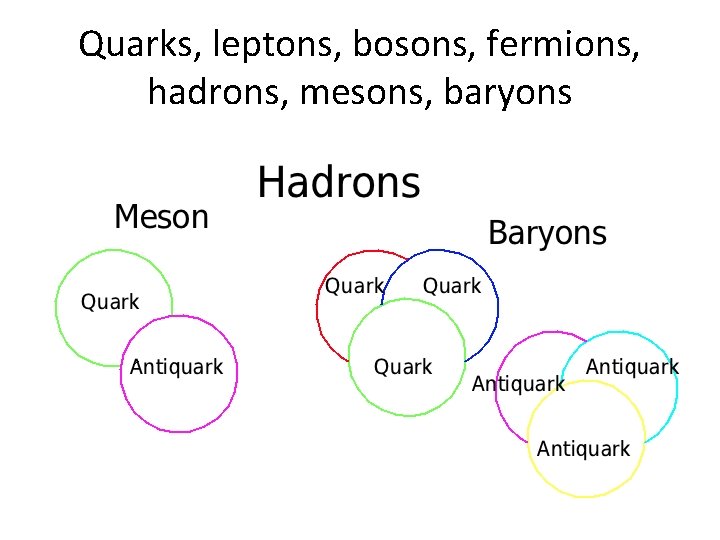
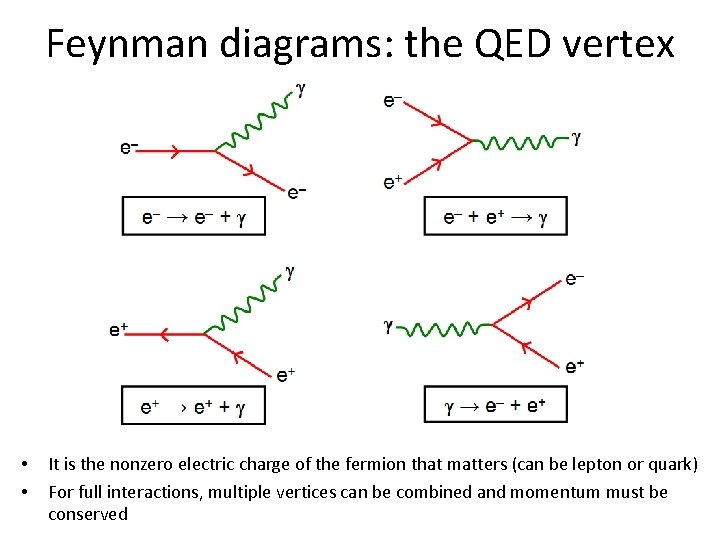
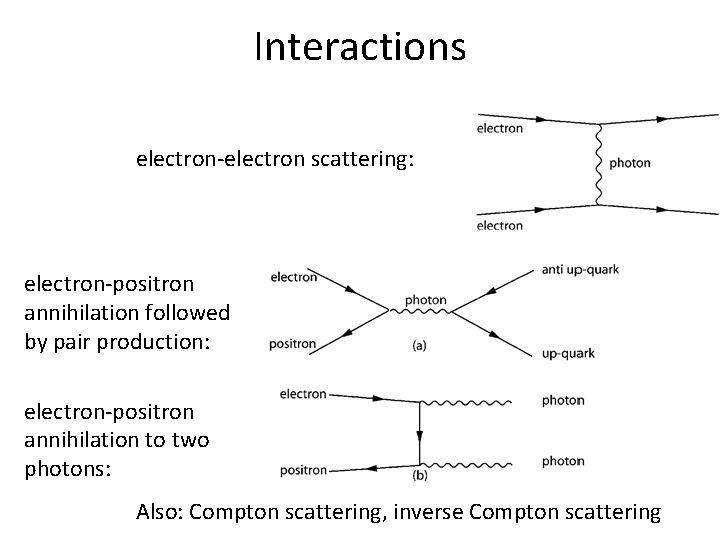
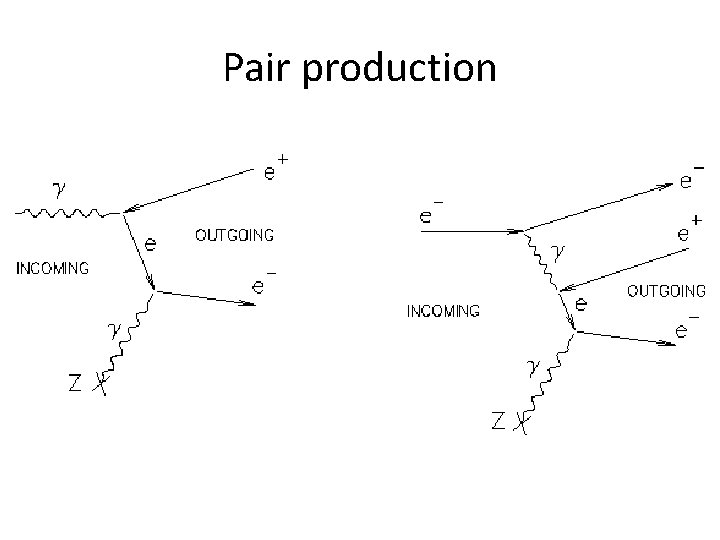
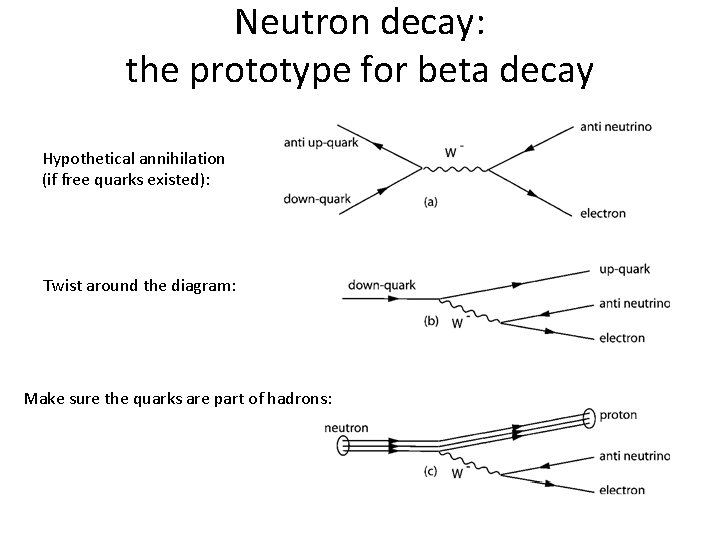
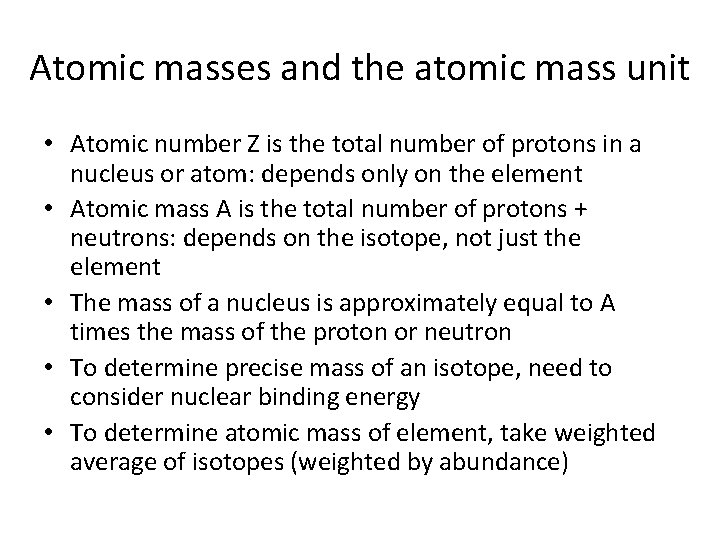
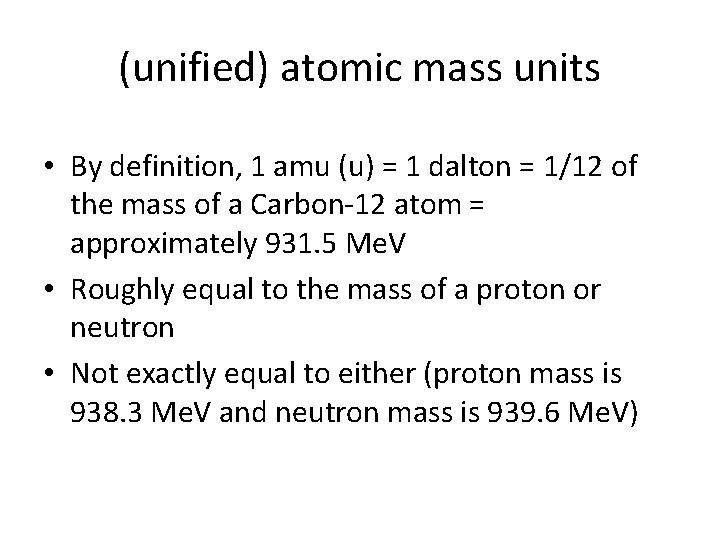
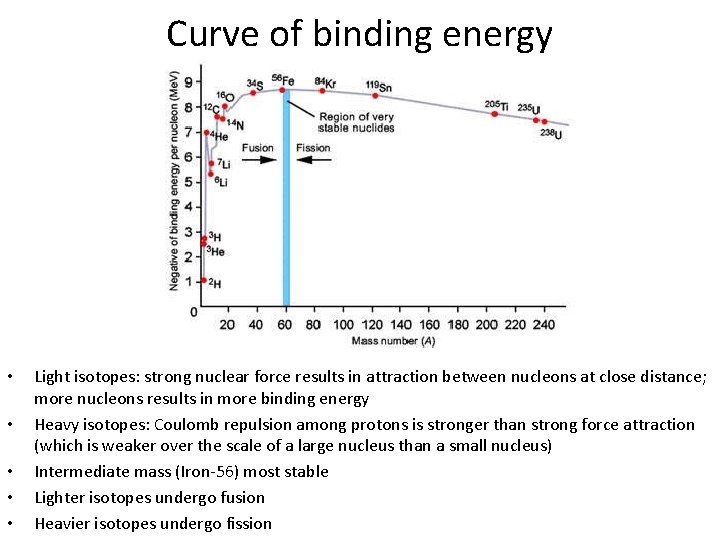
- Slides: 24
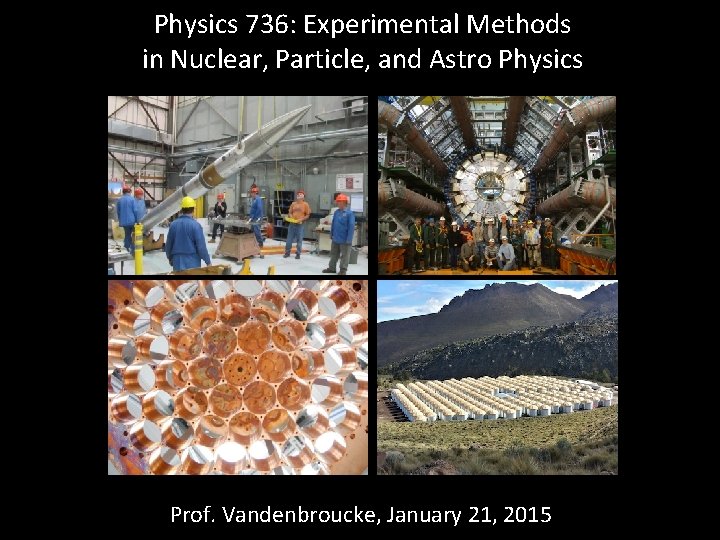
Physics 736: Experimental Methods in Nuclear, Particle, and Astro Physics Prof. Vandenbroucke, January 21, 2015
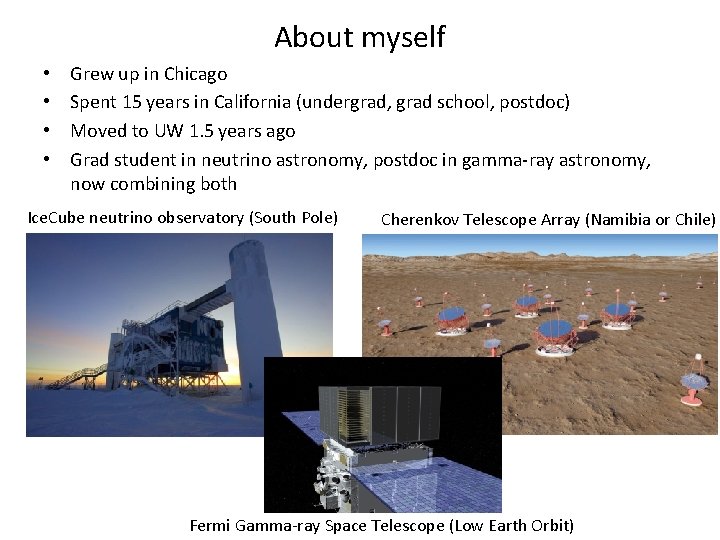
About myself • • Grew up in Chicago Spent 15 years in California (undergrad, grad school, postdoc) Moved to UW 1. 5 years ago Grad student in neutrino astronomy, postdoc in gamma-ray astronomy, now combining both Ice. Cube neutrino observatory (South Pole) Cherenkov Telescope Array (Namibia or Chile) Fermi Gamma-ray Space Telescope (Low Earth Orbit)
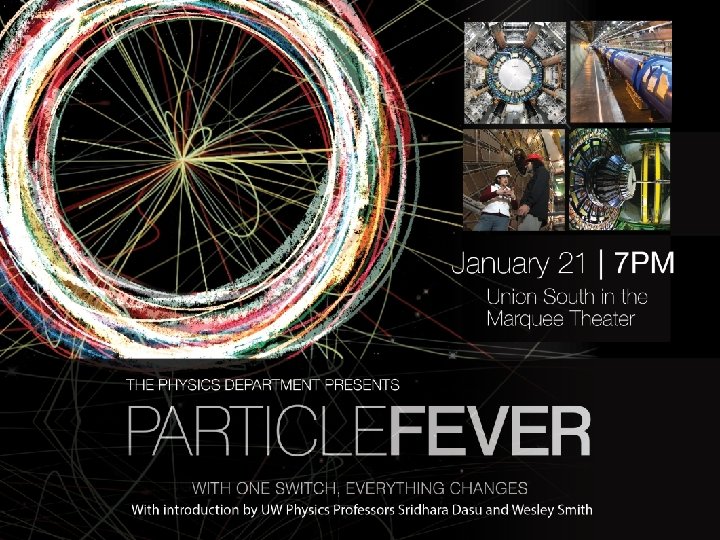
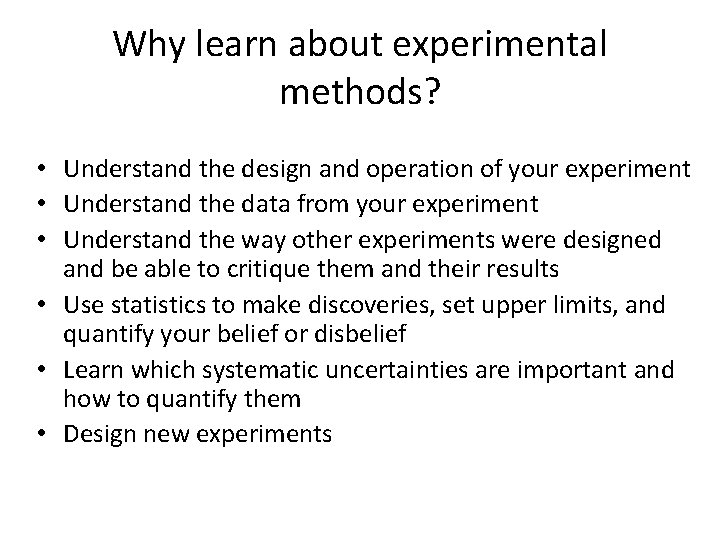
Why learn about experimental methods? • Understand the design and operation of your experiment • Understand the data from your experiment • Understand the way other experiments were designed and be able to critique them and their results • Use statistics to make discoveries, set upper limits, and quantify your belief or disbelief • Learn which systematic uncertainties are important and how to quantify them • Design new experiments
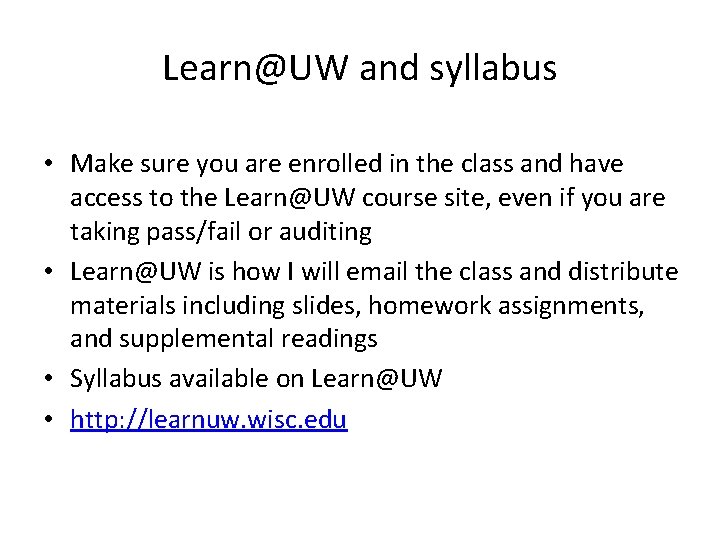
Learn@UW and syllabus • Make sure you are enrolled in the class and have access to the Learn@UW course site, even if you are taking pass/fail or auditing • Learn@UW is how I will email the class and distribute materials including slides, homework assignments, and supplemental readings • Syllabus available on Learn@UW • http: //learnuw. wisc. edu
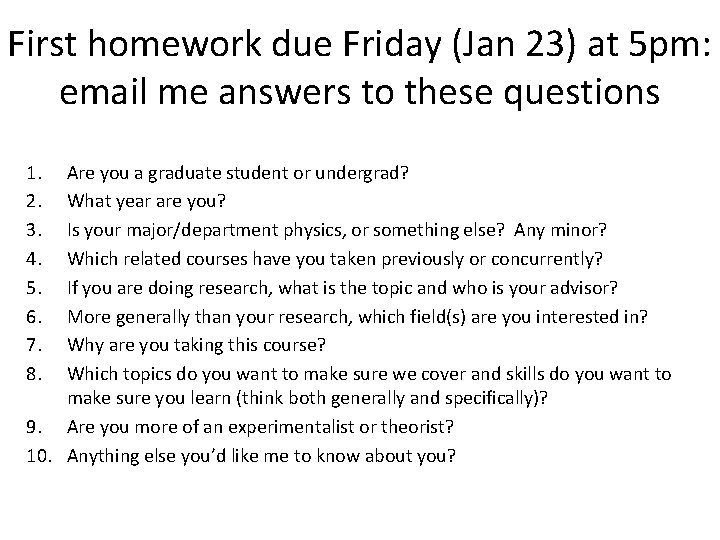
First homework due Friday (Jan 23) at 5 pm: email me answers to these questions 1. 2. 3. 4. 5. 6. 7. 8. Are you a graduate student or undergrad? What year are you? Is your major/department physics, or something else? Any minor? Which related courses have you taken previously or concurrently? If you are doing research, what is the topic and who is your advisor? More generally than your research, which field(s) are you interested in? Why are you taking this course? Which topics do you want to make sure we cover and skills do you want to make sure you learn (think both generally and specifically)? 9. Are you more of an experimentalist or theorist? 10. Anything else you’d like me to know about you?
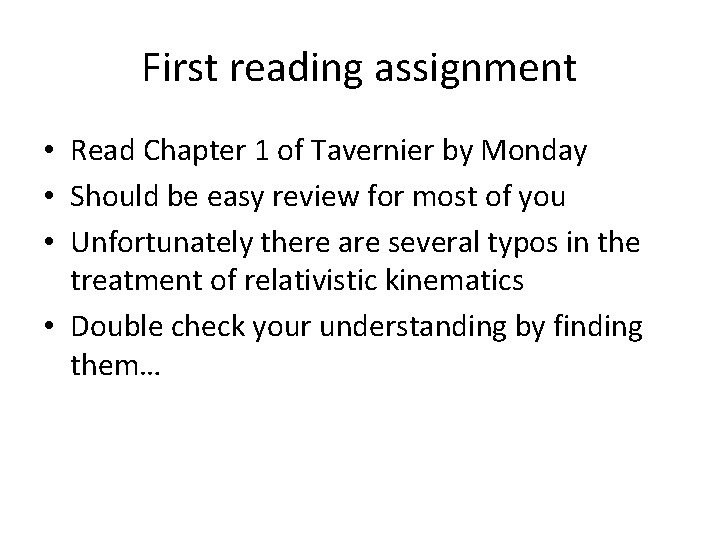
First reading assignment • Read Chapter 1 of Tavernier by Monday • Should be easy review for most of you • Unfortunately there are several typos in the treatment of relativistic kinematics • Double check your understanding by finding them…
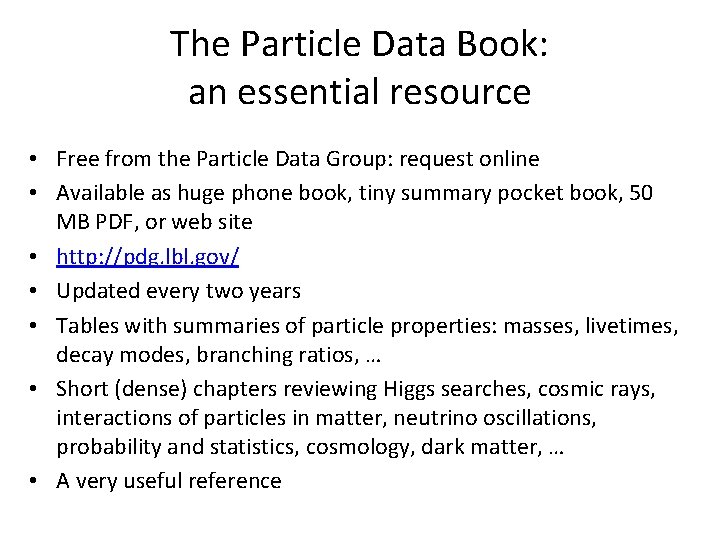
The Particle Data Book: an essential resource • Free from the Particle Data Group: request online • Available as huge phone book, tiny summary pocket book, 50 MB PDF, or web site • http: //pdg. lbl. gov/ • Updated every two years • Tables with summaries of particle properties: masses, livetimes, decay modes, branching ratios, … • Short (dense) chapters reviewing Higgs searches, cosmic rays, interactions of particles in matter, neutrino oscillations, probability and statistics, cosmology, dark matter, … • A very useful reference
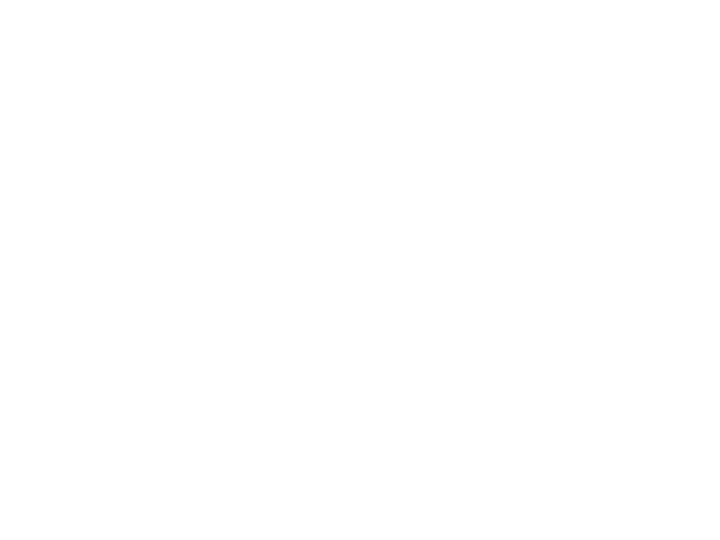
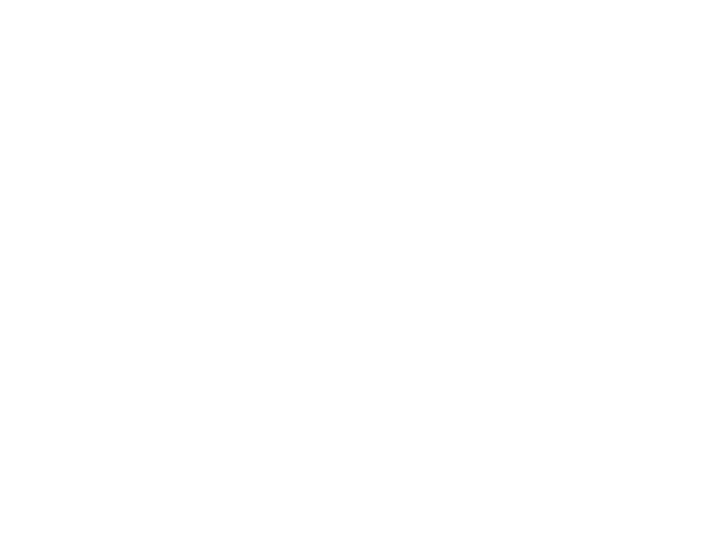
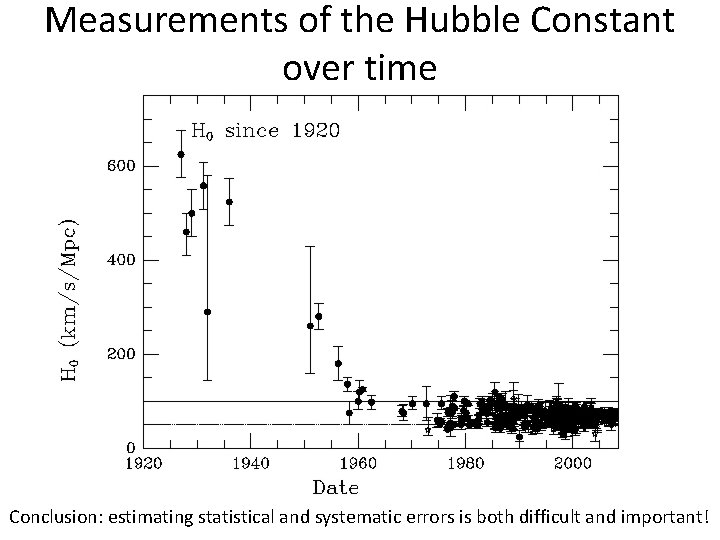
Measurements of the Hubble Constant over time Conclusion: estimating statistical and systematic errors is both difficult and important!
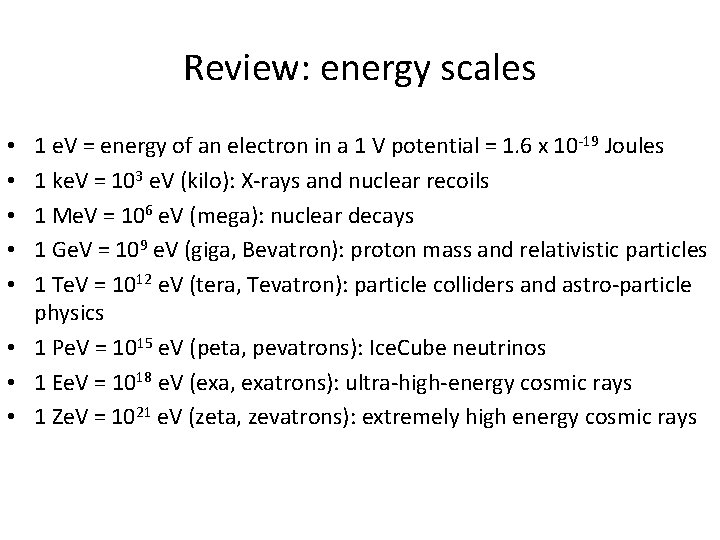
Review: energy scales 1 e. V = energy of an electron in a 1 V potential = 1. 6 x 10 -19 Joules 1 ke. V = 103 e. V (kilo): X-rays and nuclear recoils 1 Me. V = 106 e. V (mega): nuclear decays 1 Ge. V = 109 e. V (giga, Bevatron): proton mass and relativistic particles 1 Te. V = 1012 e. V (tera, Tevatron): particle colliders and astro-particle physics • 1 Pe. V = 1015 e. V (peta, pevatrons): Ice. Cube neutrinos • 1 Ee. V = 1018 e. V (exa, exatrons): ultra-high-energy cosmic rays • 1 Ze. V = 1021 e. V (zeta, zevatrons): extremely high energy cosmic rays • • •
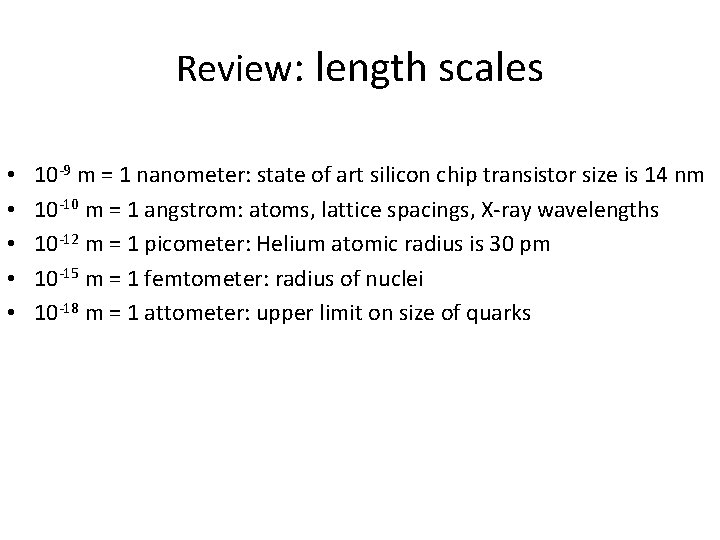
Review: length scales • • • 10 -9 m = 1 nanometer: state of art silicon chip transistor size is 14 nm 10 -10 m = 1 angstrom: atoms, lattice spacings, X-ray wavelengths 10 -12 m = 1 picometer: Helium atomic radius is 30 pm 10 -15 m = 1 femtometer: radius of nuclei 10 -18 m = 1 attometer: upper limit on size of quarks
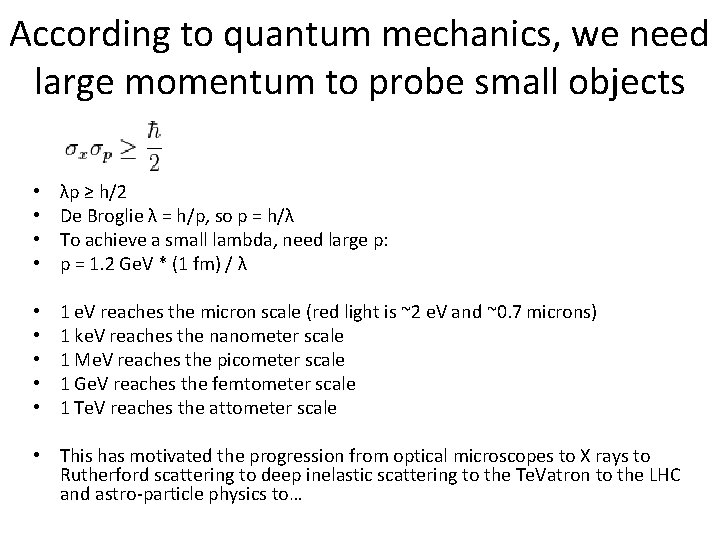
According to quantum mechanics, we need large momentum to probe small objects • • λp ≥ h/2 De Broglie λ = h/p, so p = h/λ To achieve a small lambda, need large p: p = 1. 2 Ge. V * (1 fm) / λ • • • 1 e. V reaches the micron scale (red light is ~2 e. V and ~0. 7 microns) 1 ke. V reaches the nanometer scale 1 Me. V reaches the picometer scale 1 Ge. V reaches the femtometer scale 1 Te. V reaches the attometer scale • This has motivated the progression from optical microscopes to X rays to Rutherford scattering to deep inelastic scattering to the Te. Vatron to the LHC and astro-particle physics to…
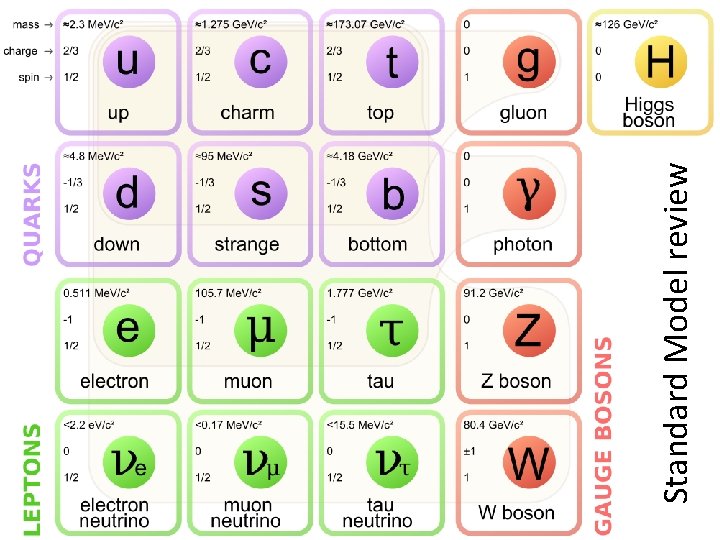
Standard Model review
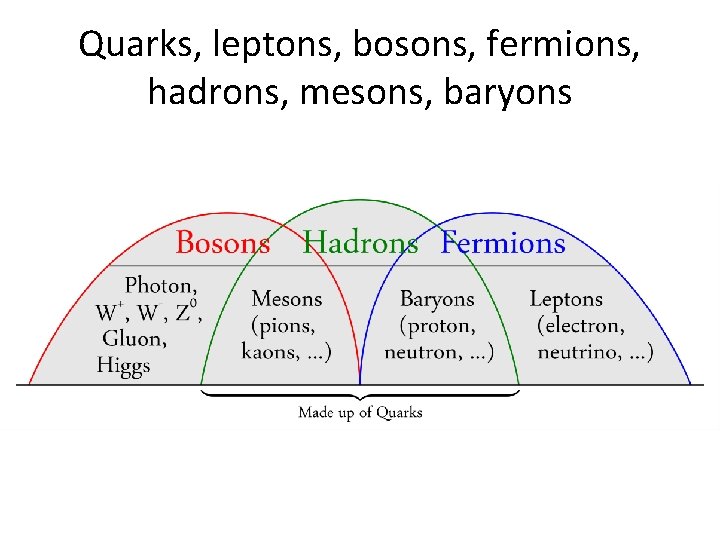
Quarks, leptons, bosons, fermions, hadrons, mesons, baryons
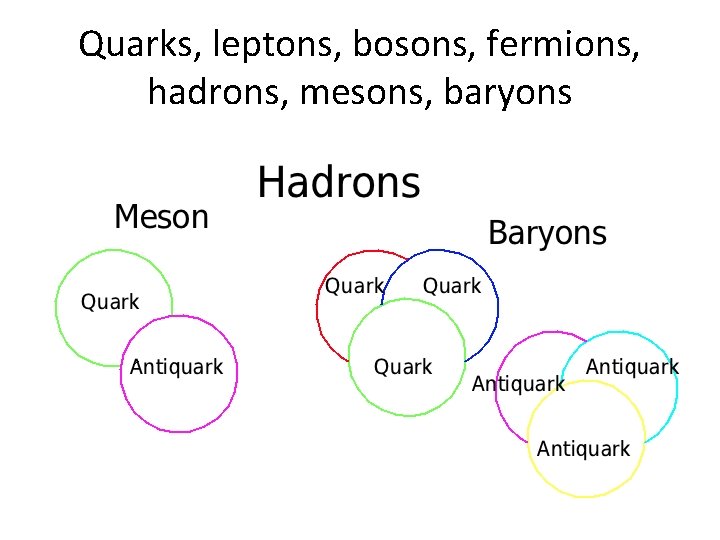
Quarks, leptons, bosons, fermions, hadrons, mesons, baryons
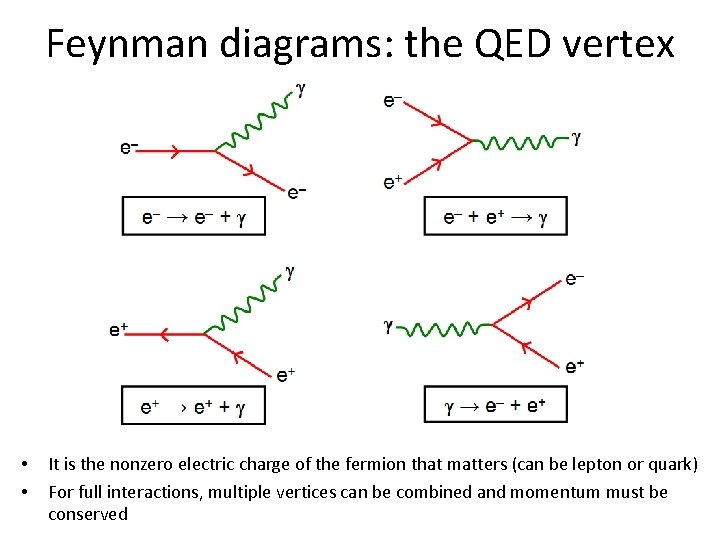
Feynman diagrams: the QED vertex • • It is the nonzero electric charge of the fermion that matters (can be lepton or quark) For full interactions, multiple vertices can be combined and momentum must be conserved
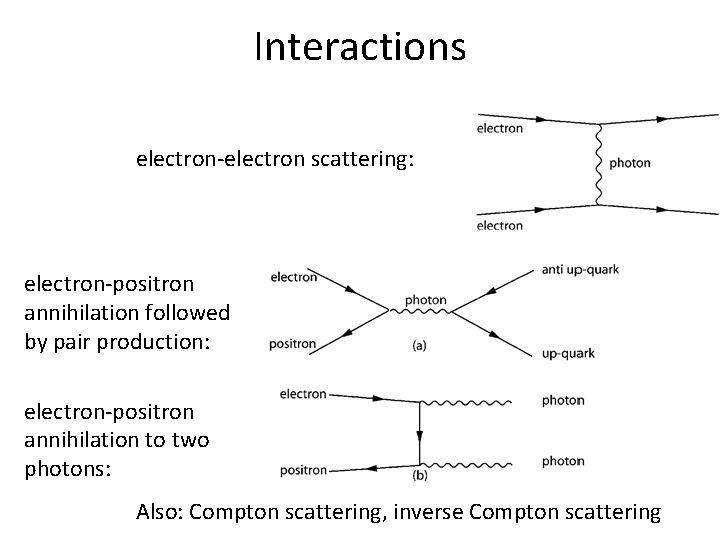
Interactions electron-electron scattering: electron-positron annihilation followed by pair production: electron-positron annihilation to two photons: Also: Compton scattering, inverse Compton scattering
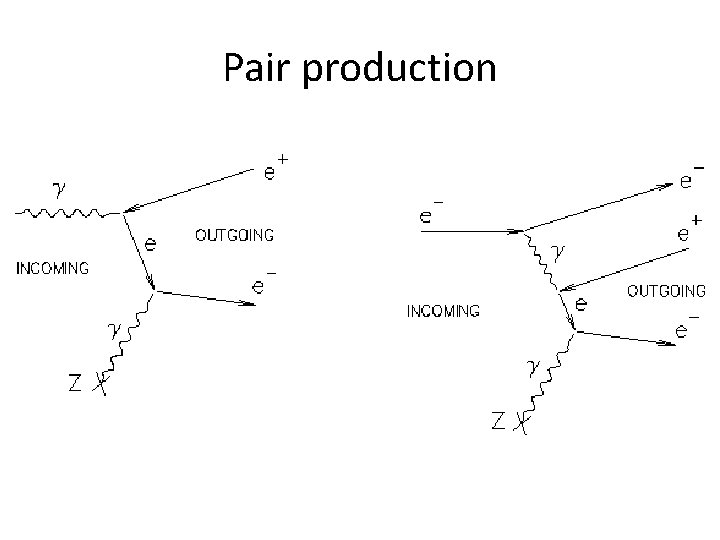
Pair production
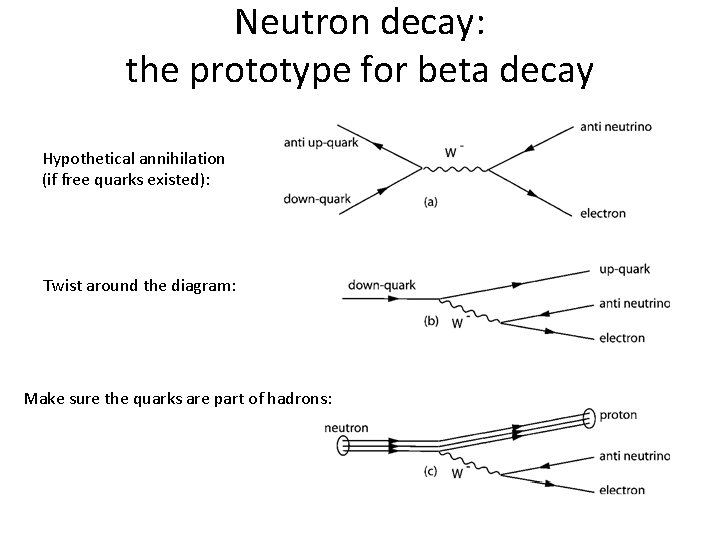
Neutron decay: the prototype for beta decay Hypothetical annihilation (if free quarks existed): Twist around the diagram: Make sure the quarks are part of hadrons:
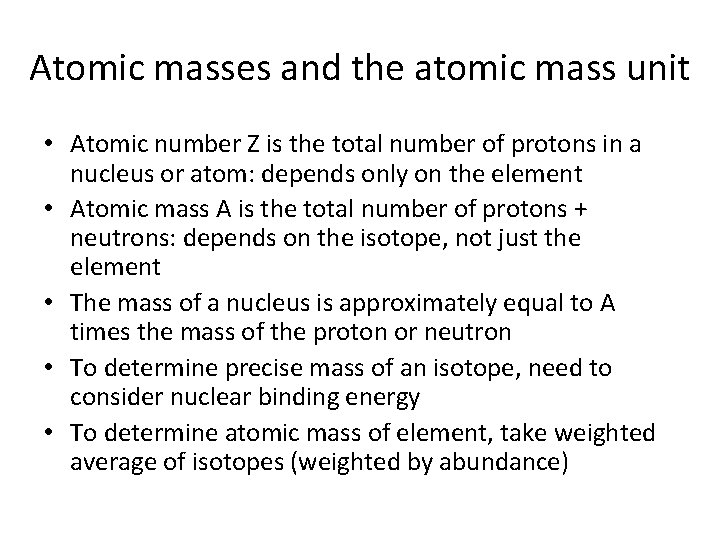
Atomic masses and the atomic mass unit • Atomic number Z is the total number of protons in a nucleus or atom: depends only on the element • Atomic mass A is the total number of protons + neutrons: depends on the isotope, not just the element • The mass of a nucleus is approximately equal to A times the mass of the proton or neutron • To determine precise mass of an isotope, need to consider nuclear binding energy • To determine atomic mass of element, take weighted average of isotopes (weighted by abundance)
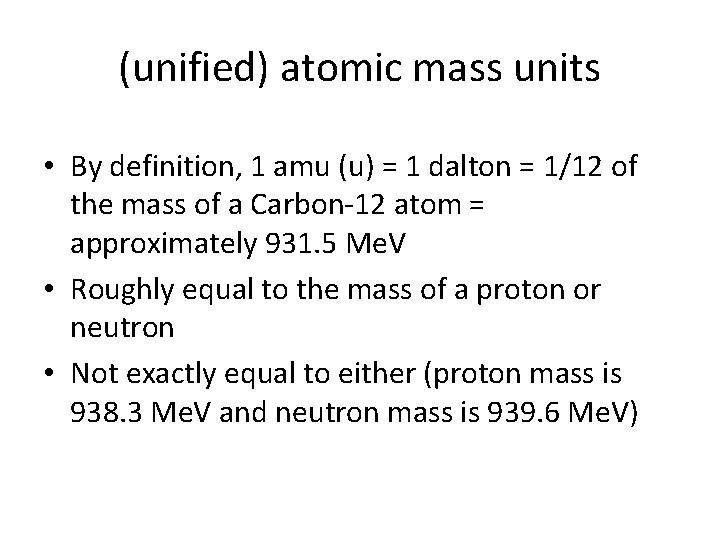
(unified) atomic mass units • By definition, 1 amu (u) = 1 dalton = 1/12 of the mass of a Carbon-12 atom = approximately 931. 5 Me. V • Roughly equal to the mass of a proton or neutron • Not exactly equal to either (proton mass is 938. 3 Me. V and neutron mass is 939. 6 Me. V)
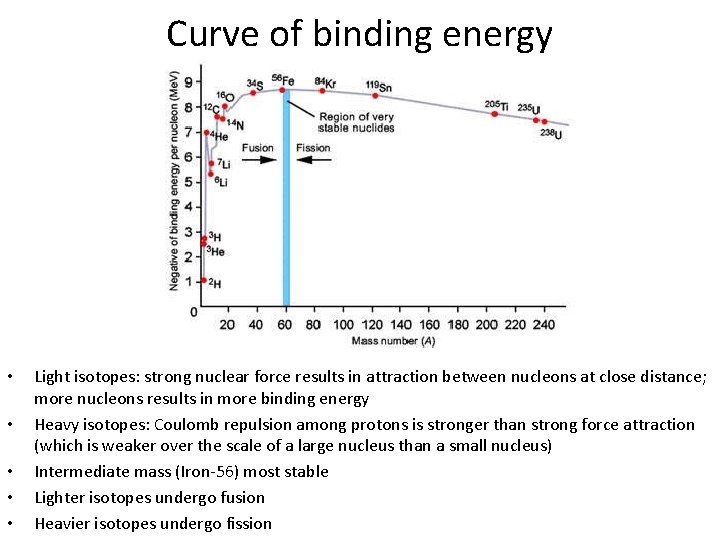
Curve of binding energy • • • Light isotopes: strong nuclear force results in attraction between nucleons at close distance; more nucleons results in more binding energy Heavy isotopes: Coulomb repulsion among protons is stronger than strong force attraction (which is weaker over the scale of a large nucleus than a small nucleus) Intermediate mass (Iron-56) most stable Lighter isotopes undergo fusion Heavier isotopes undergo fission
736 b payments
Experiment 736
736
Cherenkov spectrum
Lesson 15 nuclear quest nuclear reactions
Fisión nuclear vs fision nuclear
Experimental vs non experimental
Descriptive vs correlational vs experimental research
Disadvantages of experimental research
Non experimental design vs experimental
Experimental vs non experimental
Quantum and nuclear physics
Experimental physics and industrial control system
"controls engineering"
Experimental physics and industrial control system
Pmt particle physics
Mark thomson modern particle physics
Particle physics
Particle physics practice quiz
Particle physics timeline
Concezio bozzi
Cern particle physics
European laboratory for particle physics
Cern particle physics
Particle physics