Physics 736 Experimental Methods in Nuclear Particle and
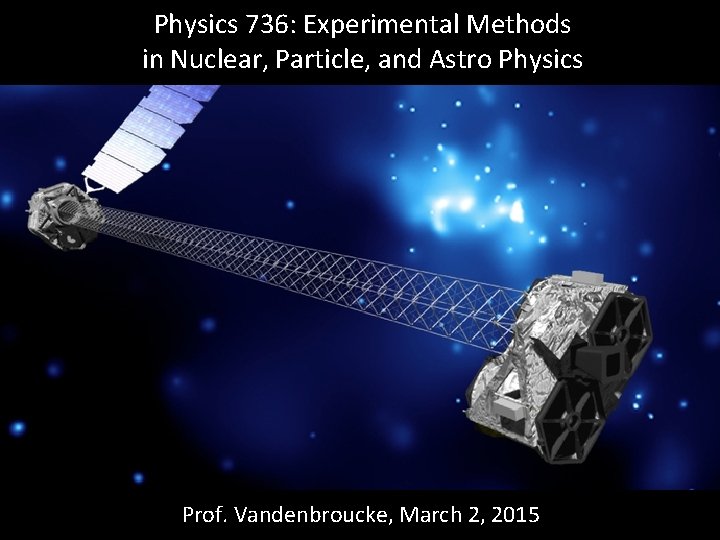
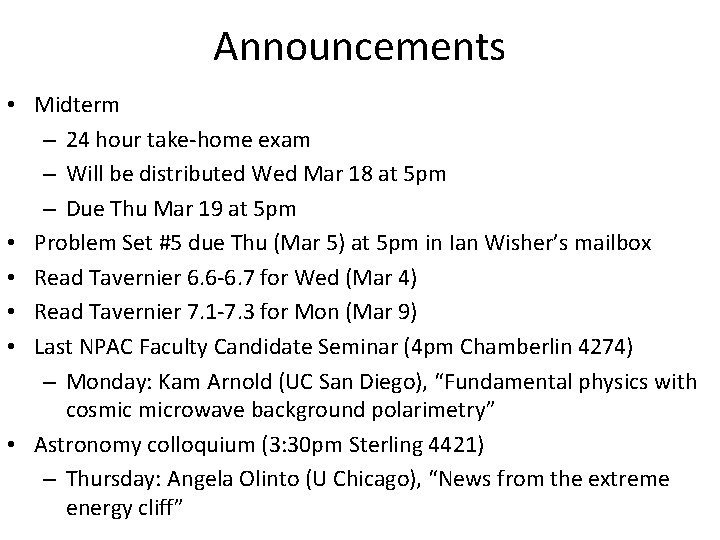
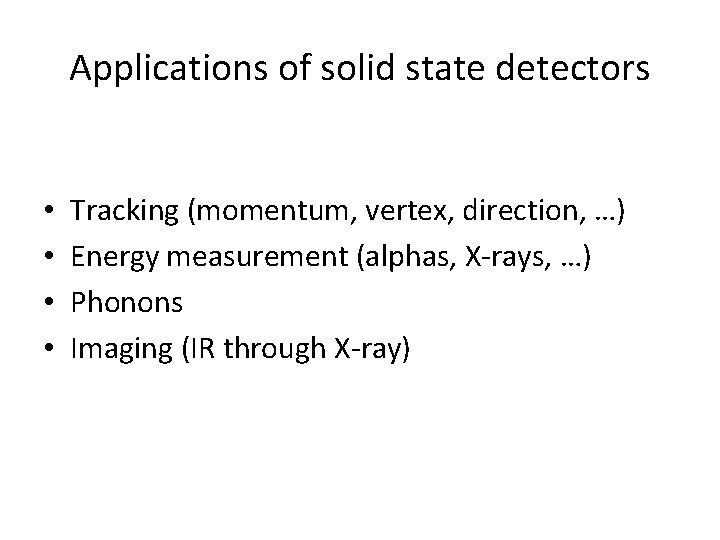
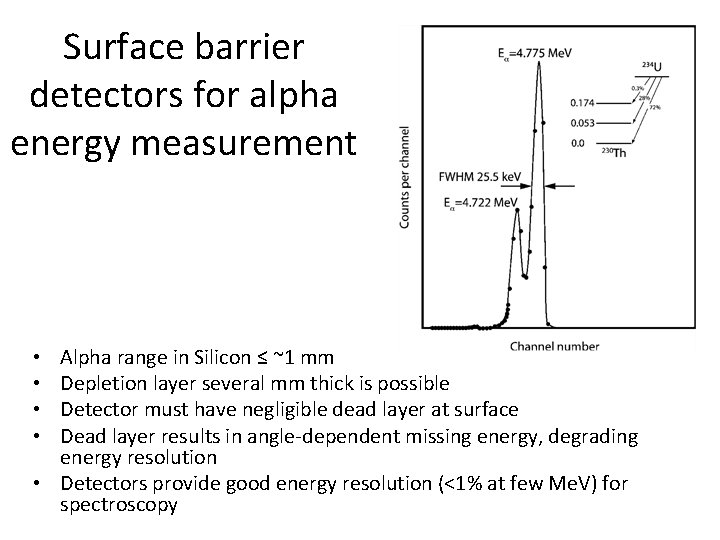
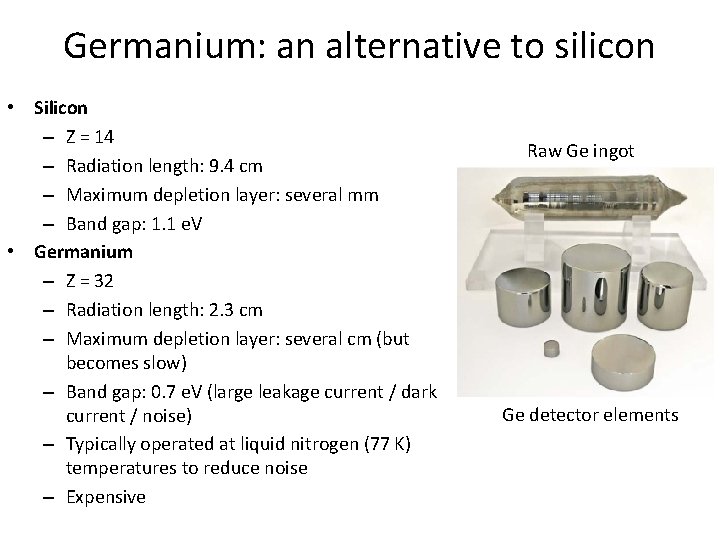
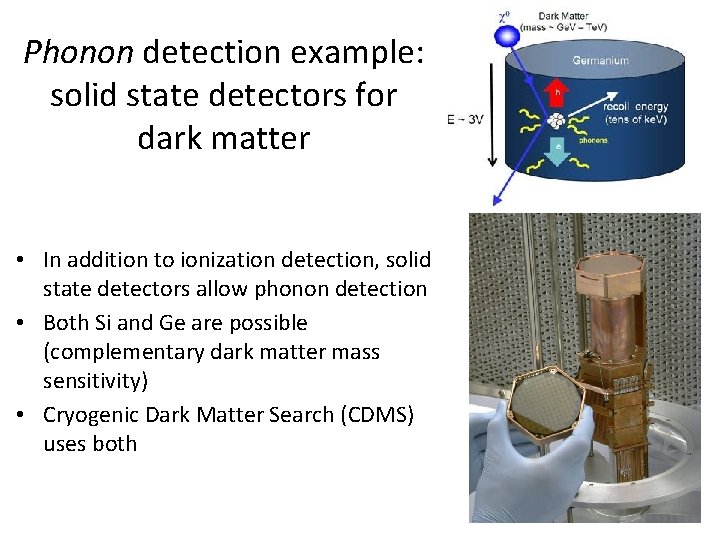
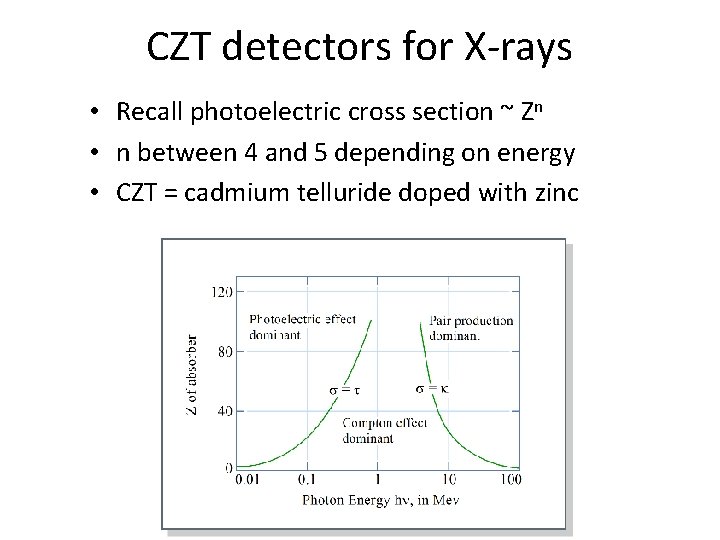
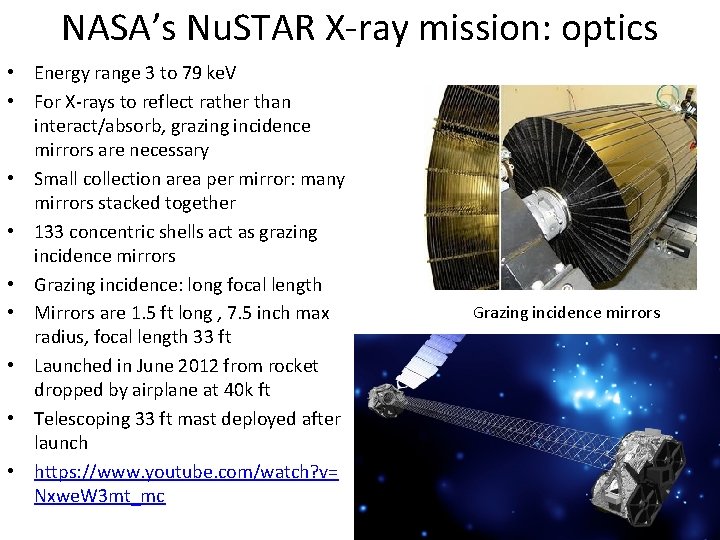
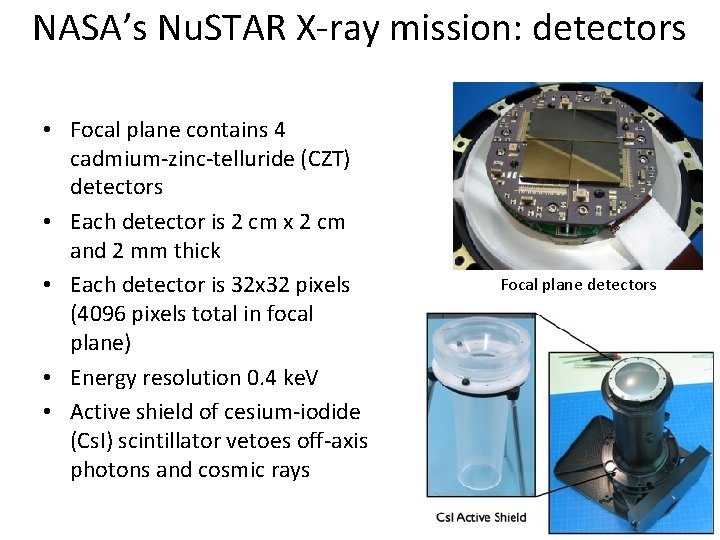
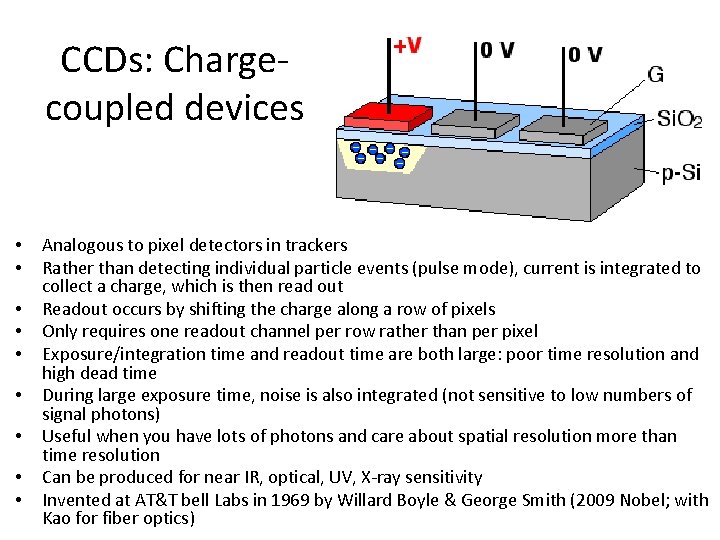
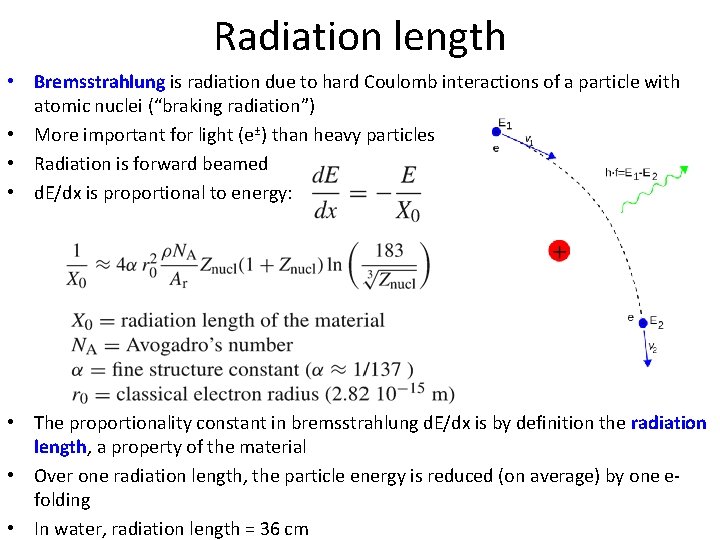
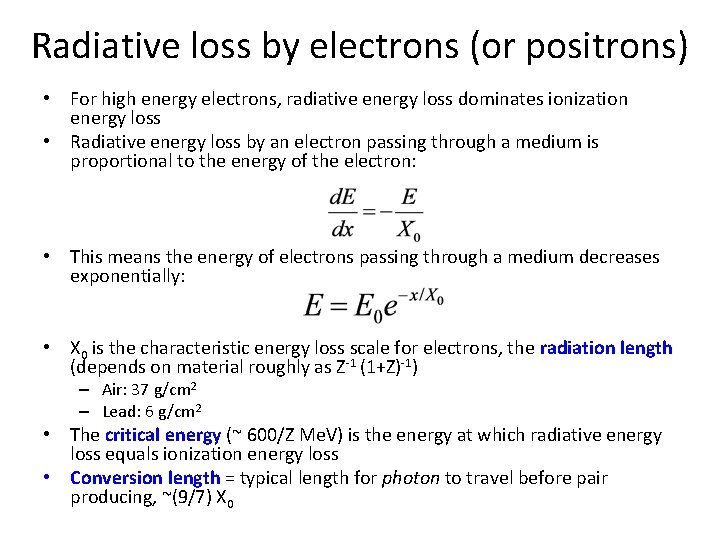
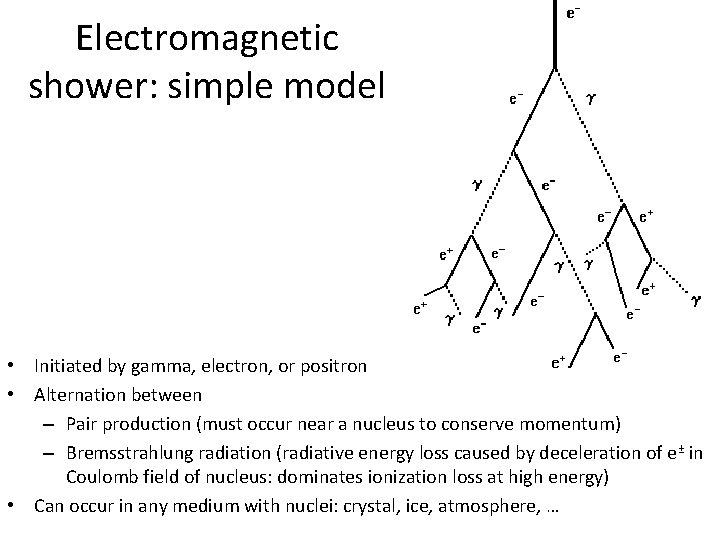
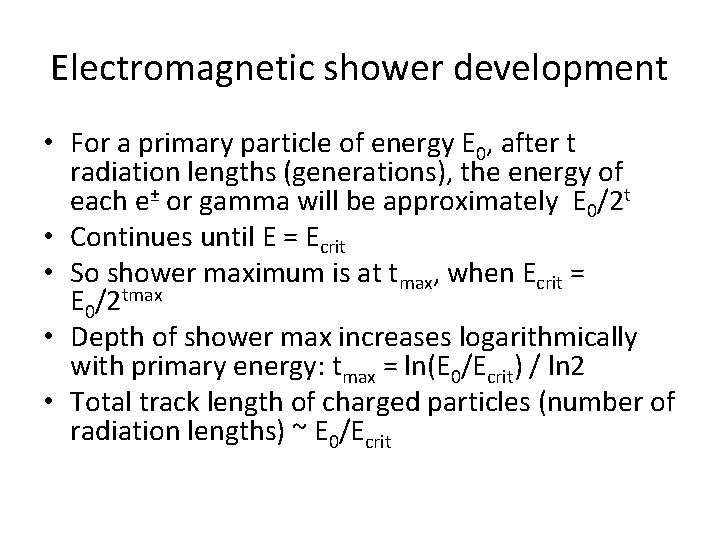
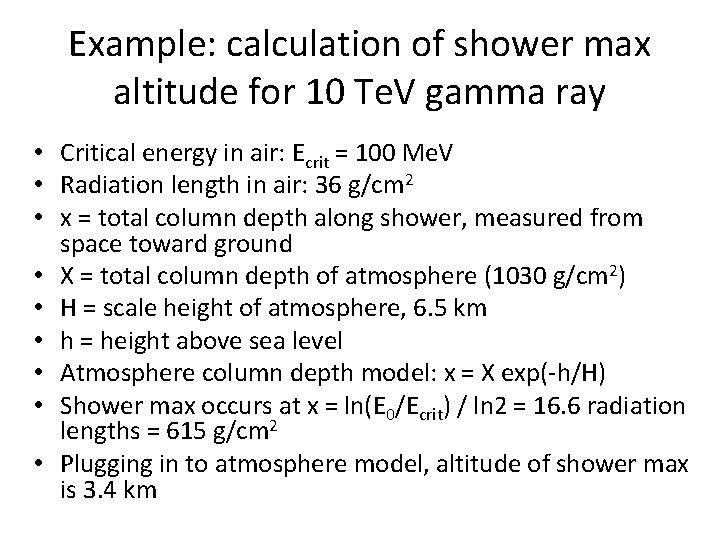
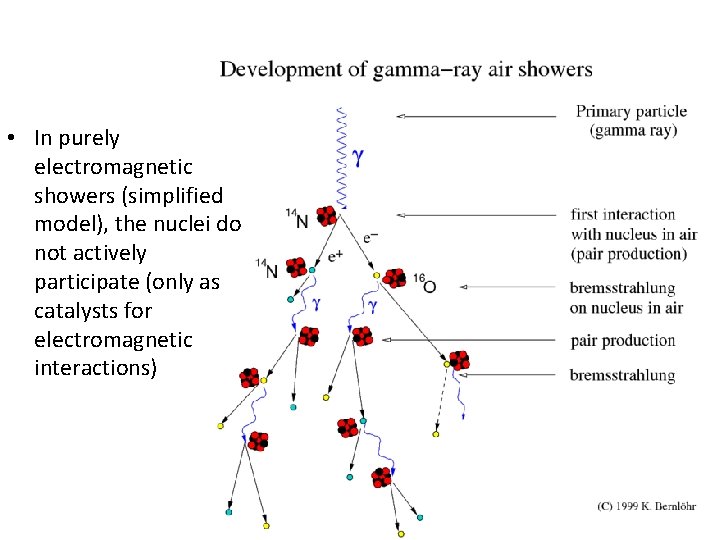
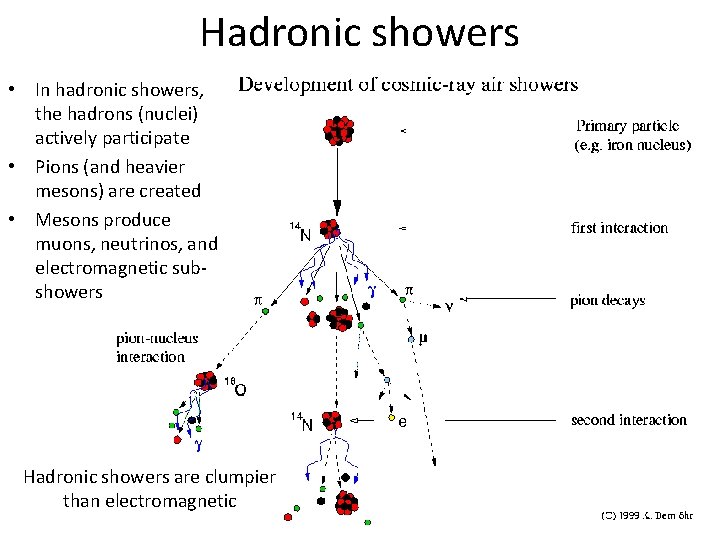
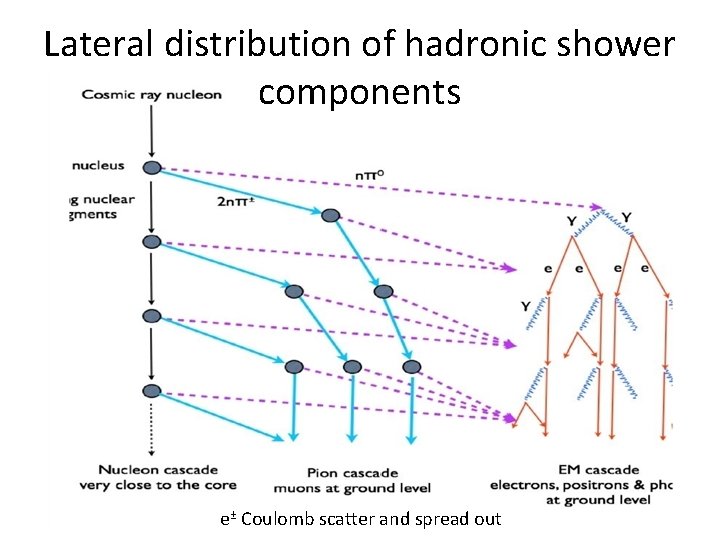
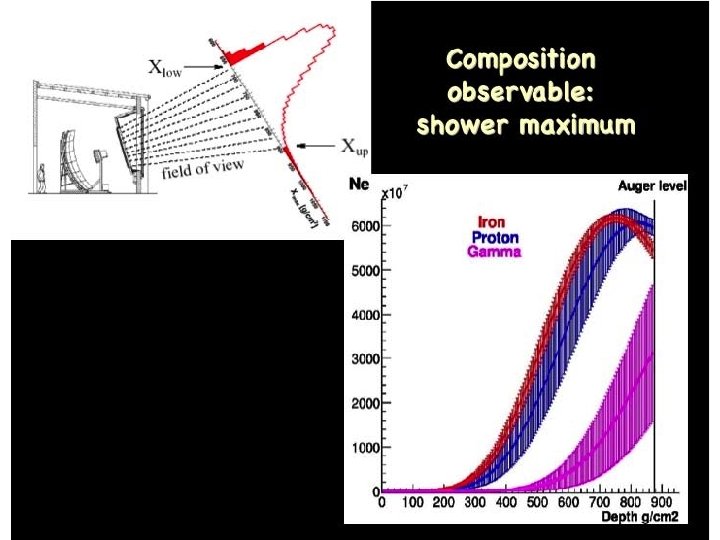
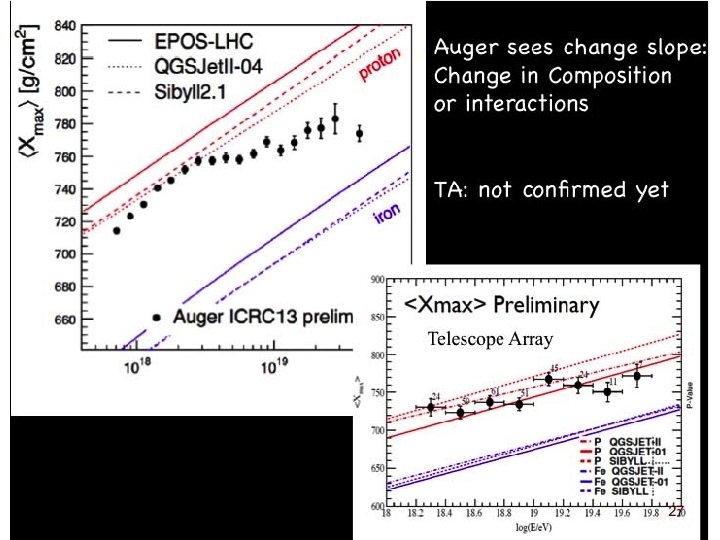
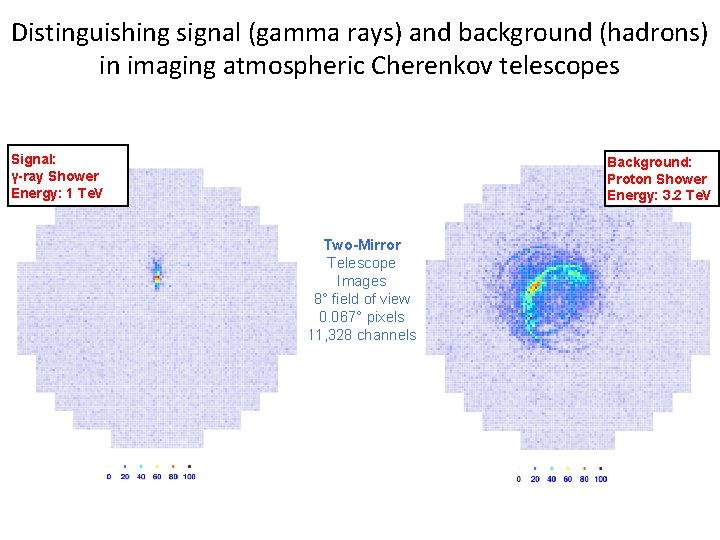
- Slides: 21
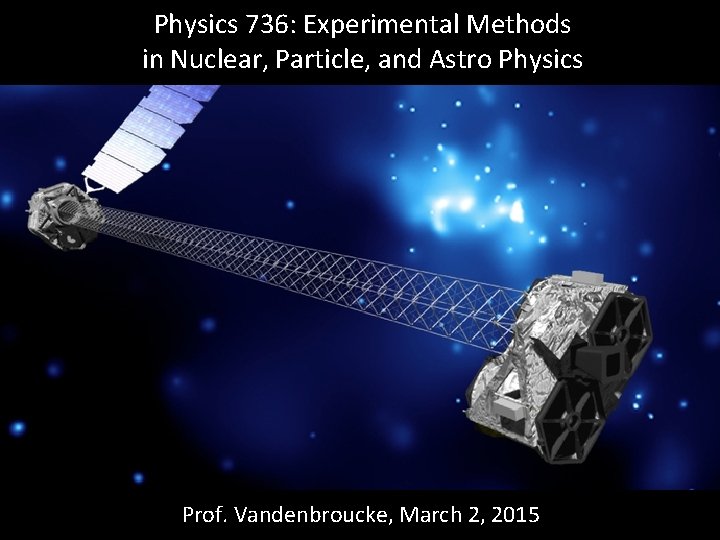
Physics 736: Experimental Methods in Nuclear, Particle, and Astro Physics Prof. Vandenbroucke, March 2, 2015
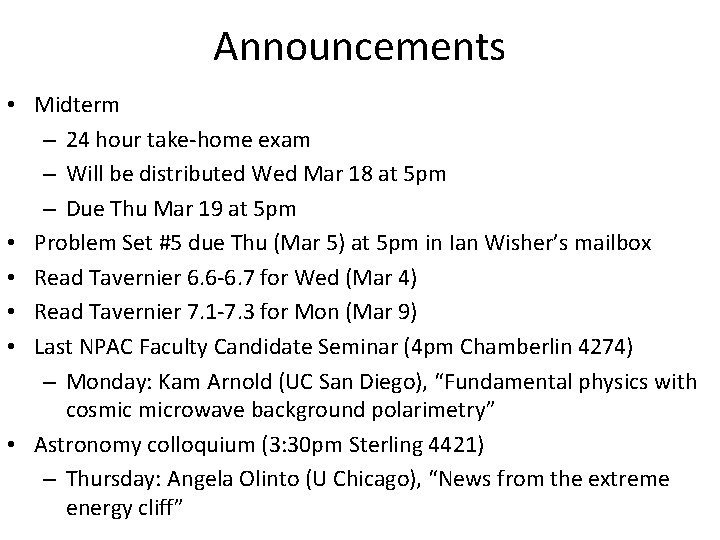
Announcements • Midterm – 24 hour take-home exam – Will be distributed Wed Mar 18 at 5 pm – Due Thu Mar 19 at 5 pm • Problem Set #5 due Thu (Mar 5) at 5 pm in Ian Wisher’s mailbox • Read Tavernier 6. 6 -6. 7 for Wed (Mar 4) • Read Tavernier 7. 1 -7. 3 for Mon (Mar 9) • Last NPAC Faculty Candidate Seminar (4 pm Chamberlin 4274) – Monday: Kam Arnold (UC San Diego), “Fundamental physics with cosmic microwave background polarimetry” • Astronomy colloquium (3: 30 pm Sterling 4421) – Thursday: Angela Olinto (U Chicago), “News from the extreme energy cliff”
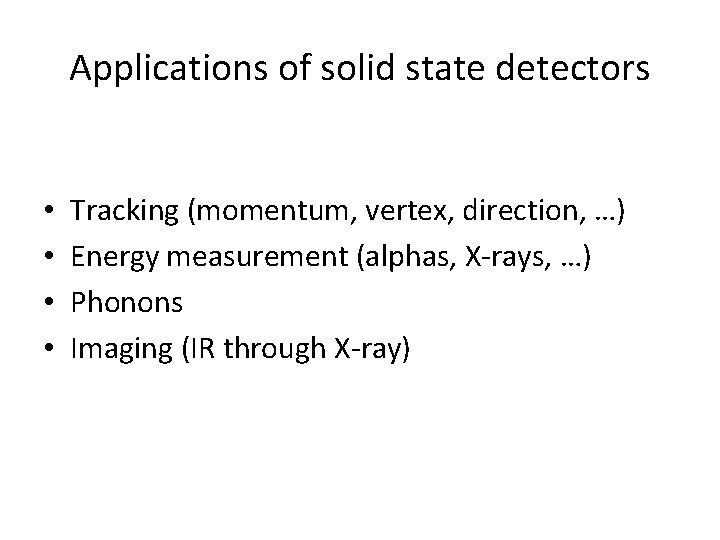
Applications of solid state detectors • • Tracking (momentum, vertex, direction, …) Energy measurement (alphas, X-rays, …) Phonons Imaging (IR through X-ray)
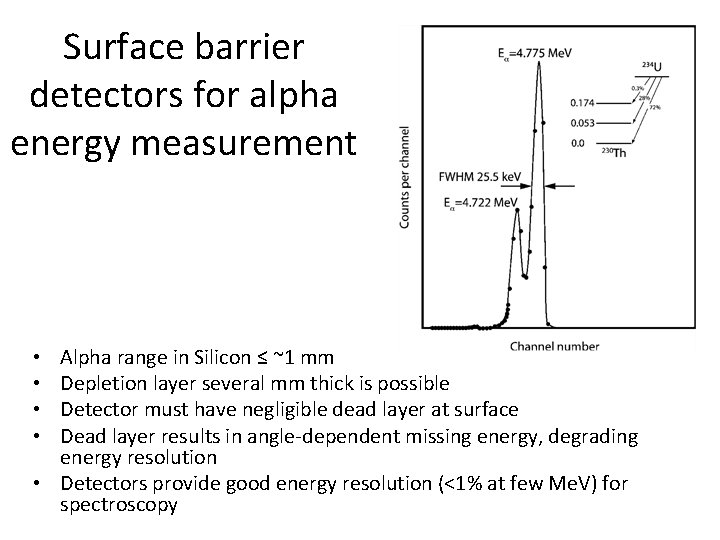
Surface barrier detectors for alpha energy measurement Alpha range in Silicon ≤ ~1 mm Depletion layer several mm thick is possible Detector must have negligible dead layer at surface Dead layer results in angle-dependent missing energy, degrading energy resolution • Detectors provide good energy resolution (<1% at few Me. V) for spectroscopy • •
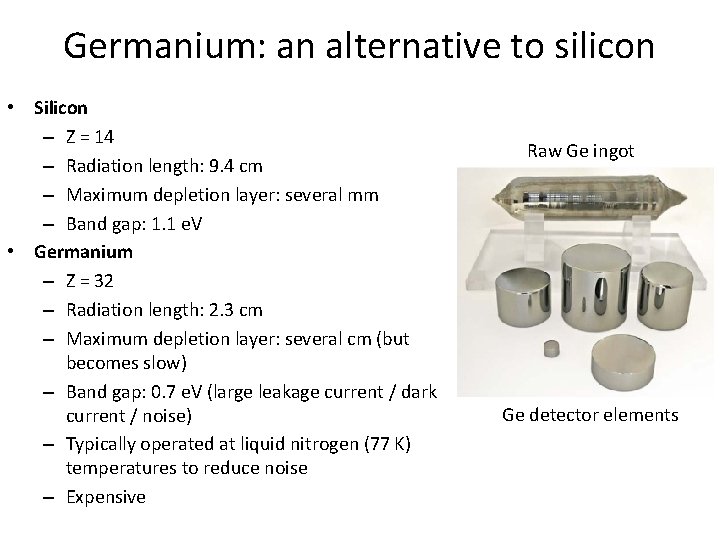
Germanium: an alternative to silicon • Silicon – Z = 14 – Radiation length: 9. 4 cm – Maximum depletion layer: several mm – Band gap: 1. 1 e. V • Germanium – Z = 32 – Radiation length: 2. 3 cm – Maximum depletion layer: several cm (but becomes slow) – Band gap: 0. 7 e. V (large leakage current / dark current / noise) – Typically operated at liquid nitrogen (77 K) temperatures to reduce noise – Expensive Raw Ge ingot Ge detector elements
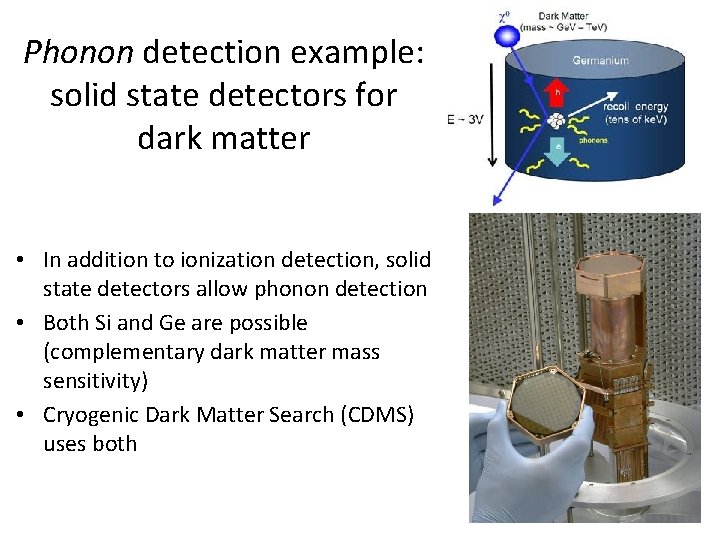
Phonon detection example: solid state detectors for dark matter • In addition to ionization detection, solid state detectors allow phonon detection • Both Si and Ge are possible (complementary dark matter mass sensitivity) • Cryogenic Dark Matter Search (CDMS) uses both
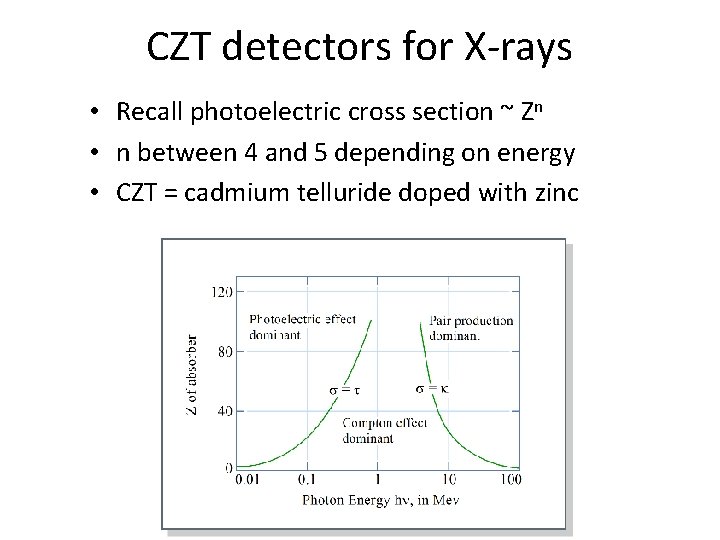
CZT detectors for X-rays • Recall photoelectric cross section ~ Zn • n between 4 and 5 depending on energy • CZT = cadmium telluride doped with zinc
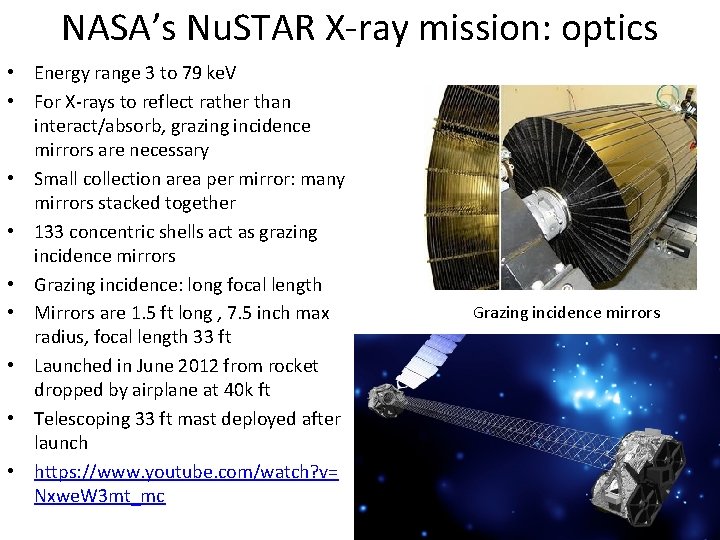
NASA’s Nu. STAR X-ray mission: optics • Energy range 3 to 79 ke. V • For X-rays to reflect rather than interact/absorb, grazing incidence mirrors are necessary • Small collection area per mirror: many mirrors stacked together • 133 concentric shells act as grazing incidence mirrors • Grazing incidence: long focal length • Mirrors are 1. 5 ft long , 7. 5 inch max radius, focal length 33 ft • Launched in June 2012 from rocket dropped by airplane at 40 k ft • Telescoping 33 ft mast deployed after launch • https: //www. youtube. com/watch? v= Nxwe. W 3 mt_mc Grazing incidence mirrors
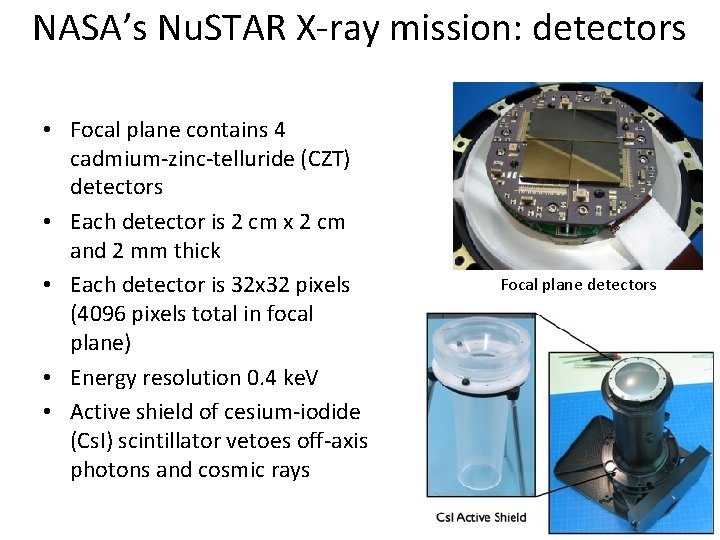
NASA’s Nu. STAR X-ray mission: detectors • Focal plane contains 4 cadmium-zinc-telluride (CZT) detectors • Each detector is 2 cm x 2 cm and 2 mm thick • Each detector is 32 x 32 pixels (4096 pixels total in focal plane) • Energy resolution 0. 4 ke. V • Active shield of cesium-iodide (Cs. I) scintillator vetoes off-axis photons and cosmic rays Focal plane detectors
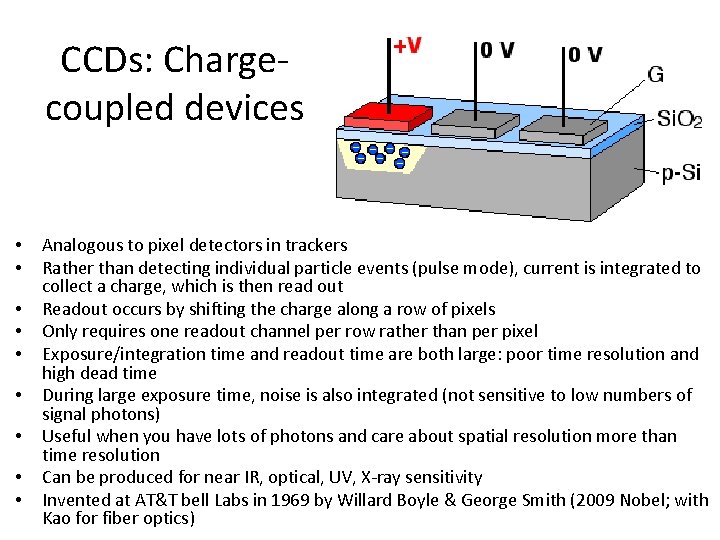
CCDs: Chargecoupled devices • • • Analogous to pixel detectors in trackers Rather than detecting individual particle events (pulse mode), current is integrated to collect a charge, which is then read out Readout occurs by shifting the charge along a row of pixels Only requires one readout channel per row rather than per pixel Exposure/integration time and readout time are both large: poor time resolution and high dead time During large exposure time, noise is also integrated (not sensitive to low numbers of signal photons) Useful when you have lots of photons and care about spatial resolution more than time resolution Can be produced for near IR, optical, UV, X-ray sensitivity Invented at AT&T bell Labs in 1969 by Willard Boyle & George Smith (2009 Nobel; with Kao for fiber optics)
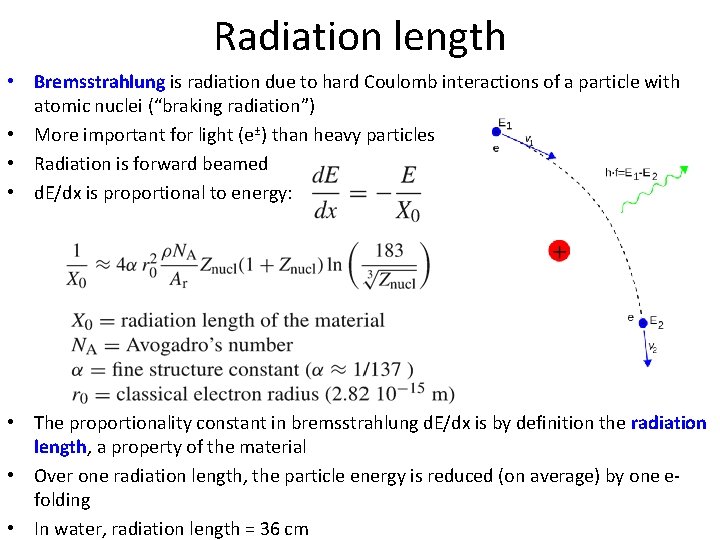
Radiation length • Bremsstrahlung is radiation due to hard Coulomb interactions of a particle with atomic nuclei (“braking radiation”) • More important for light (e±) than heavy particles • Radiation is forward beamed • d. E/dx is proportional to energy: • The proportionality constant in bremsstrahlung d. E/dx is by definition the radiation length, a property of the material • Over one radiation length, the particle energy is reduced (on average) by one efolding • In water, radiation length = 36 cm
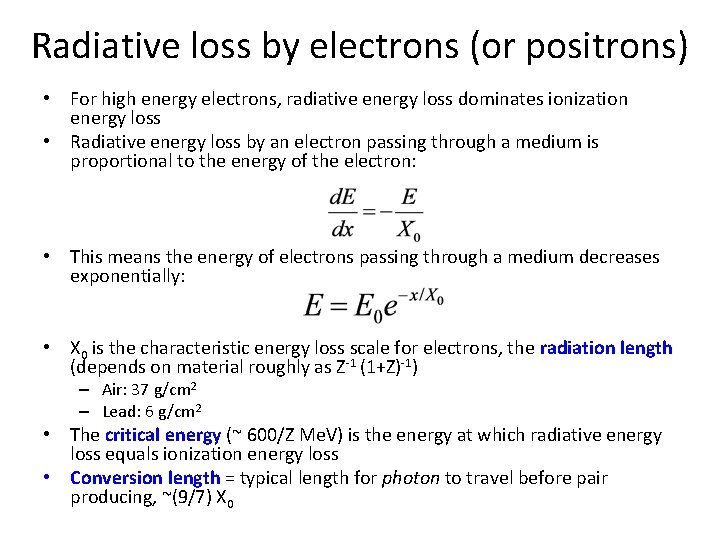
Radiative loss by electrons (or positrons) • For high energy electrons, radiative energy loss dominates ionization energy loss • Radiative energy loss by an electron passing through a medium is proportional to the energy of the electron: • This means the energy of electrons passing through a medium decreases exponentially: • X 0 is the characteristic energy loss scale for electrons, the radiation length (depends on material roughly as Z-1 (1+Z)-1) – Air: 37 g/cm 2 – Lead: 6 g/cm 2 • The critical energy (~ 600/Z Me. V) is the energy at which radiative energy loss equals ionization energy loss • Conversion length = typical length for photon to travel before pair producing, ~(9/7) X 0
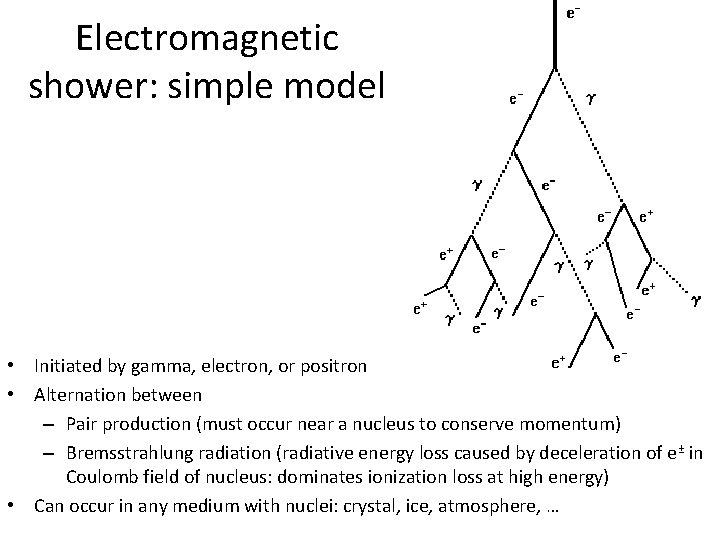
Electromagnetic shower: simple model • Initiated by gamma, electron, or positron • Alternation between – Pair production (must occur near a nucleus to conserve momentum) – Bremsstrahlung radiation (radiative energy loss caused by deceleration of e ± in Coulomb field of nucleus: dominates ionization loss at high energy) • Can occur in any medium with nuclei: crystal, ice, atmosphere, …
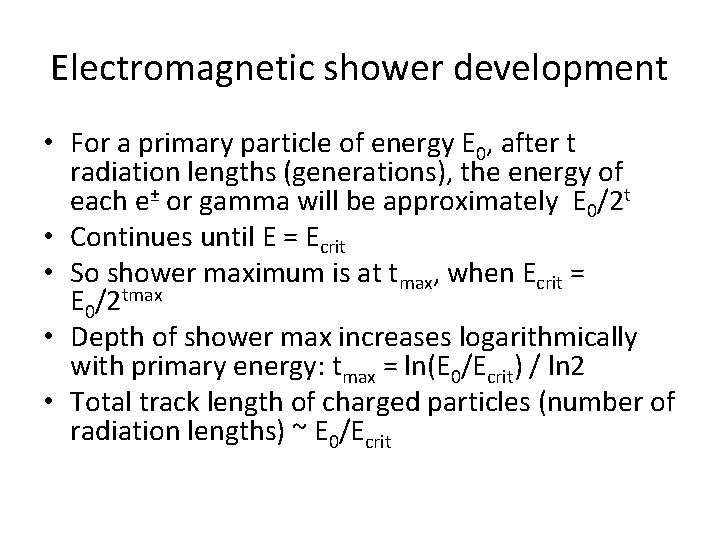
Electromagnetic shower development • For a primary particle of energy E 0, after t radiation lengths (generations), the energy of each e± or gamma will be approximately E 0/2 t • Continues until E = Ecrit • So shower maximum is at tmax, when Ecrit = E 0/2 tmax • Depth of shower max increases logarithmically with primary energy: tmax = ln(E 0/Ecrit) / ln 2 • Total track length of charged particles (number of radiation lengths) ~ E 0/Ecrit
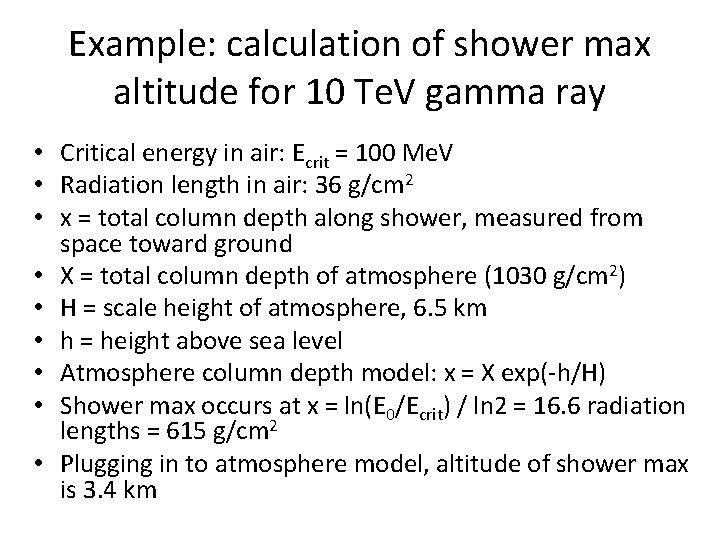
Example: calculation of shower max altitude for 10 Te. V gamma ray • Critical energy in air: Ecrit = 100 Me. V • Radiation length in air: 36 g/cm 2 • x = total column depth along shower, measured from space toward ground • X = total column depth of atmosphere (1030 g/cm 2) • H = scale height of atmosphere, 6. 5 km • h = height above sea level • Atmosphere column depth model: x = X exp(-h/H) • Shower max occurs at x = ln(E 0/Ecrit) / ln 2 = 16. 6 radiation lengths = 615 g/cm 2 • Plugging in to atmosphere model, altitude of shower max is 3. 4 km
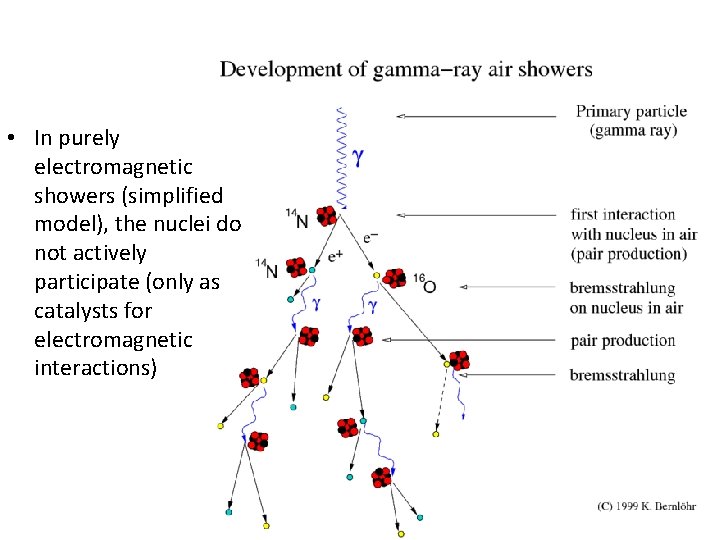
• In purely electromagnetic showers (simplified model), the nuclei do not actively participate (only as catalysts for electromagnetic interactions)
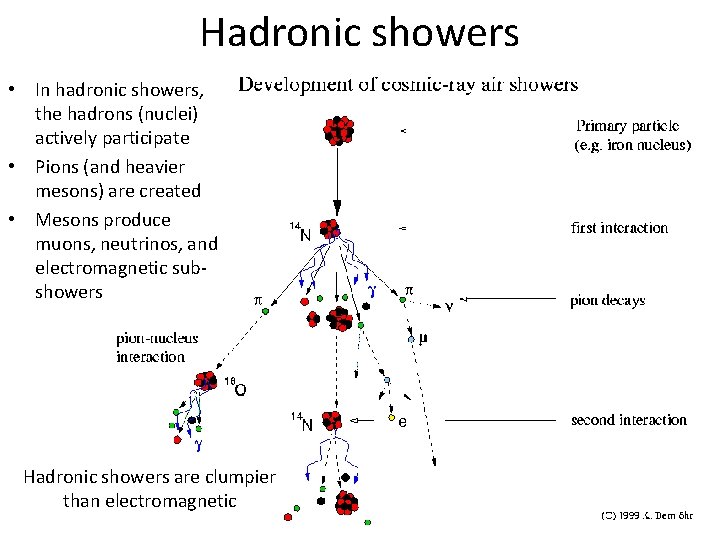
Hadronic showers • In hadronic showers, the hadrons (nuclei) actively participate • Pions (and heavier mesons) are created • Mesons produce muons, neutrinos, and electromagnetic subshowers Hadronic showers are clumpier than electromagnetic
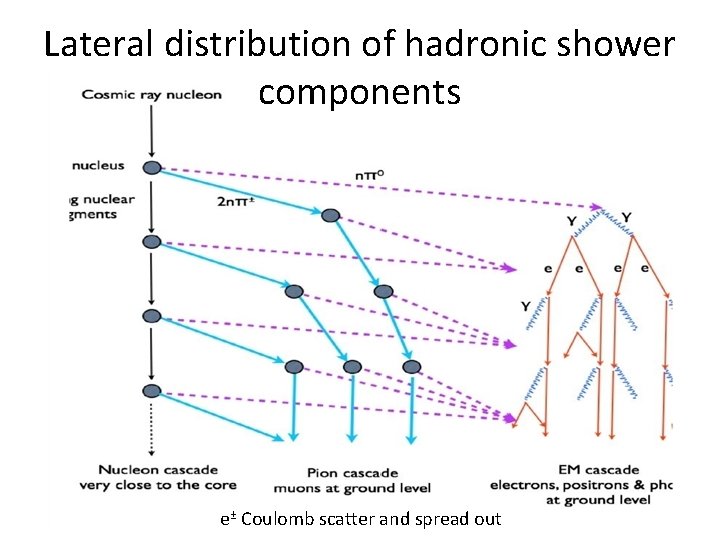
Lateral distribution of hadronic shower components e± Coulomb scatter and spread out
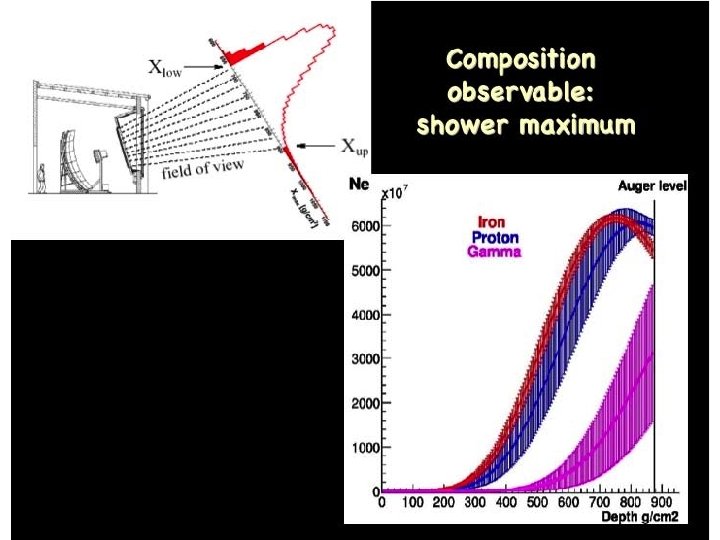
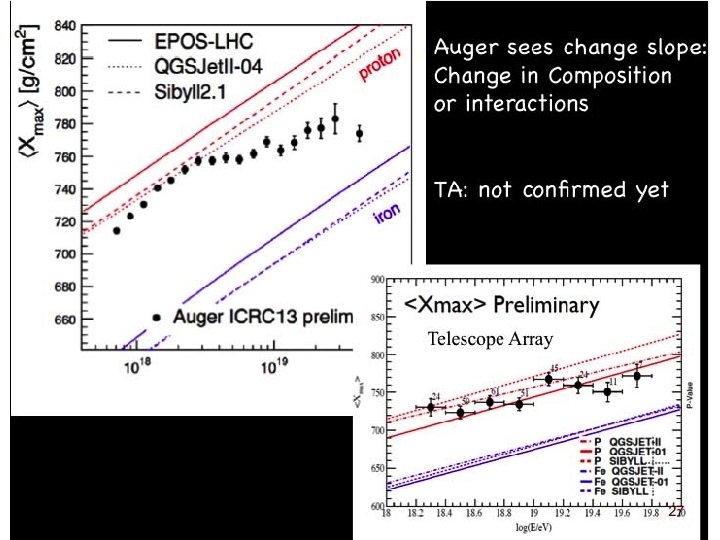
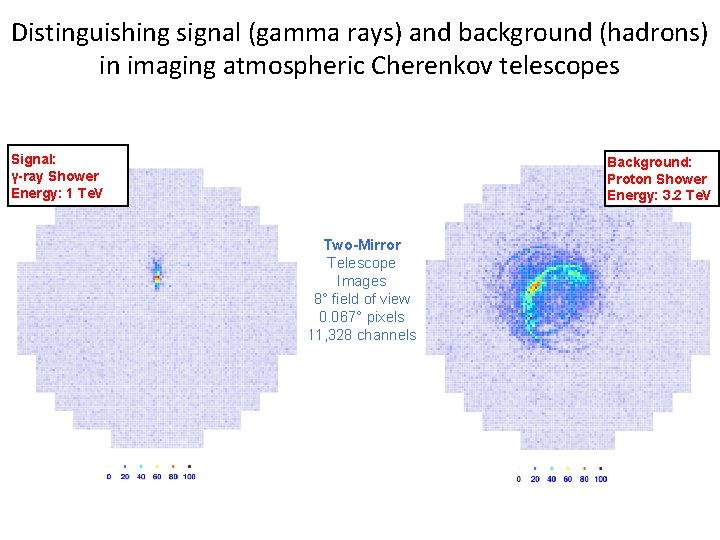
Distinguishing signal (gamma rays) and background (hadrons) in imaging atmospheric Cherenkov telescopes Signal: γ-ray Shower Energy: 1 Te. V Background: Proton Shower Energy: 3. 2 Te. V Two-Mirror Telescope Images 8° field of view 0. 067° pixels 11, 328 channels
736b payments
Experiment 736
736
Cherenkov spectrum
Lesson 15 nuclear quest nuclear reactions
Fisión nuclear vs fision nuclear
Experimental vs non experimental
Descriptive studies
Experimental vs non experimental research
Experimental vs non experimental
Nonexperimental study
Physics topic 12
Experimental physics and industrial control system
L
Experimental physics and industrial control system
Pmt particle physics
Mark thomson modern particle physics
Particle physics
Particle physics practice quiz
Particle physics timeline
Monte carlo simulation particle physics
Cern particle physics