Physics 736 Experimental Methods in Nuclear Particle and
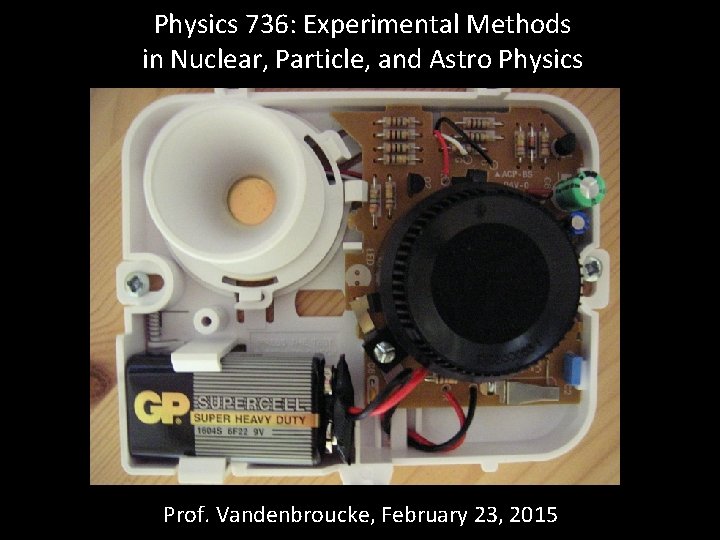
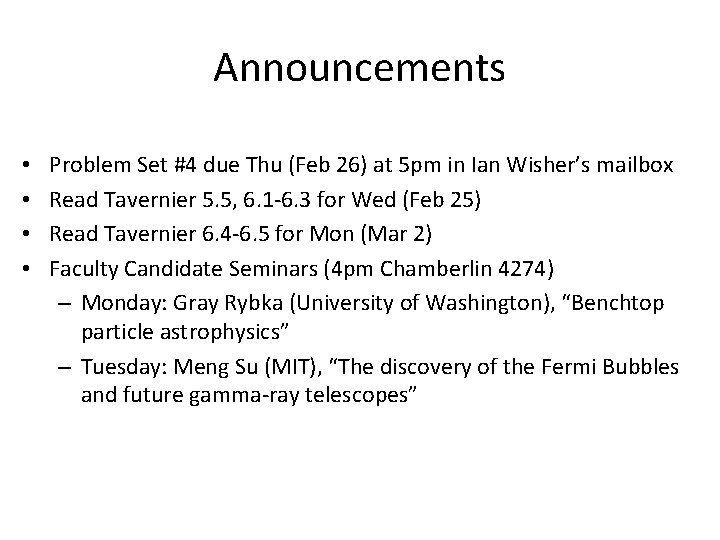
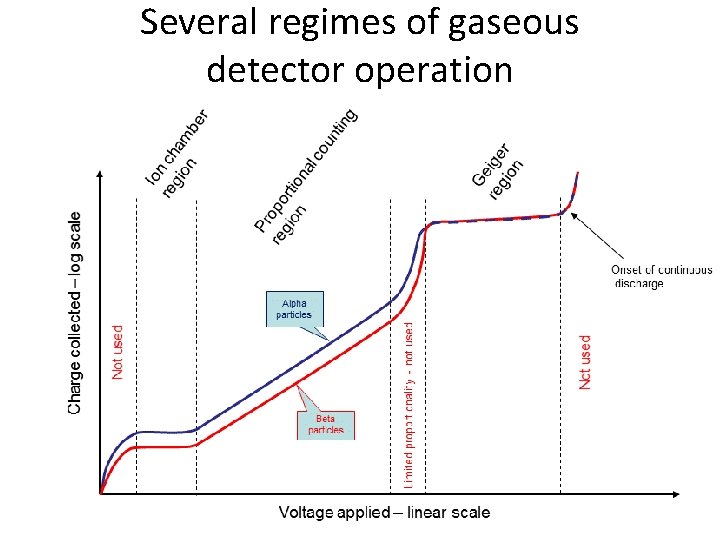
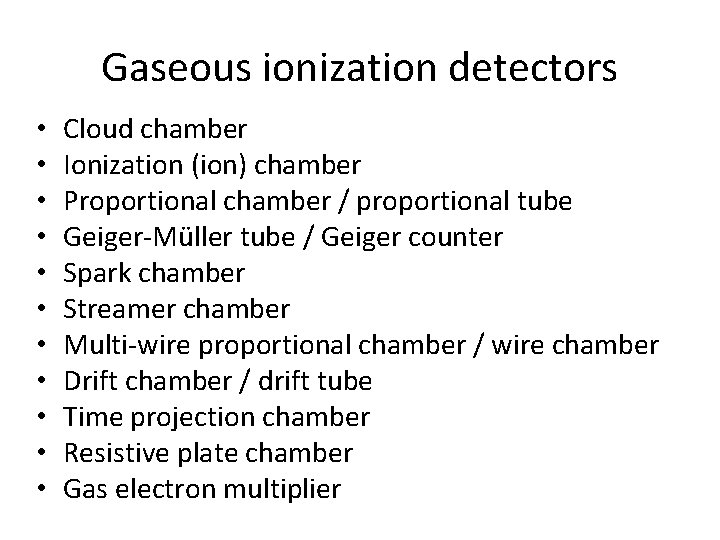
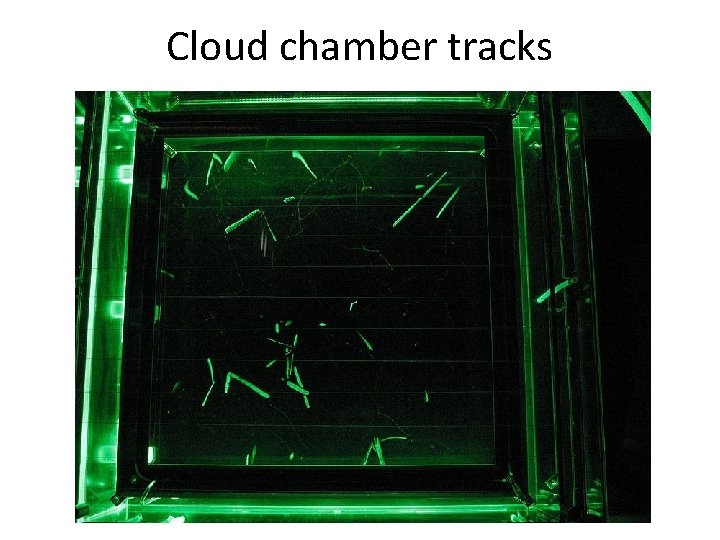
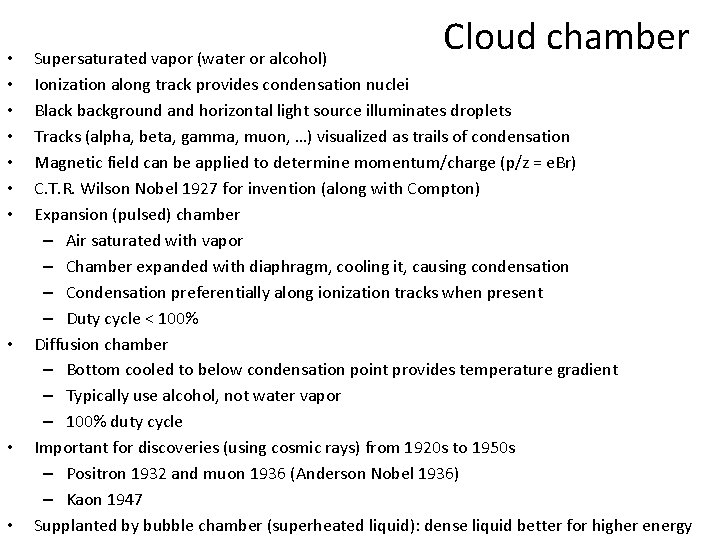
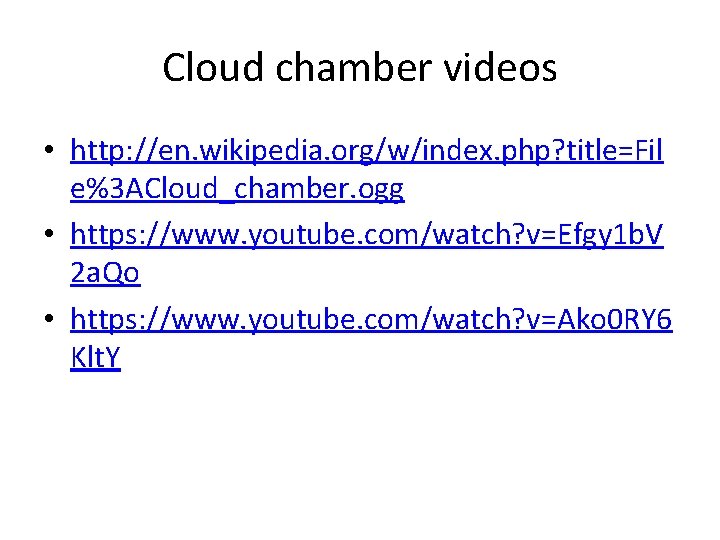
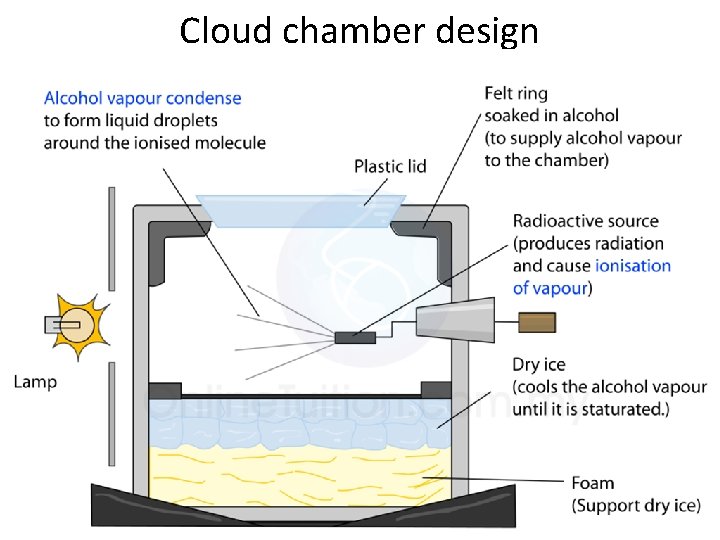
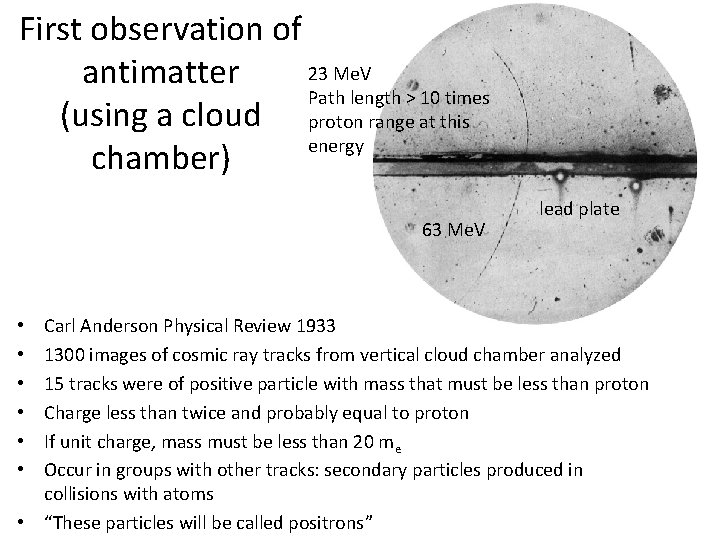
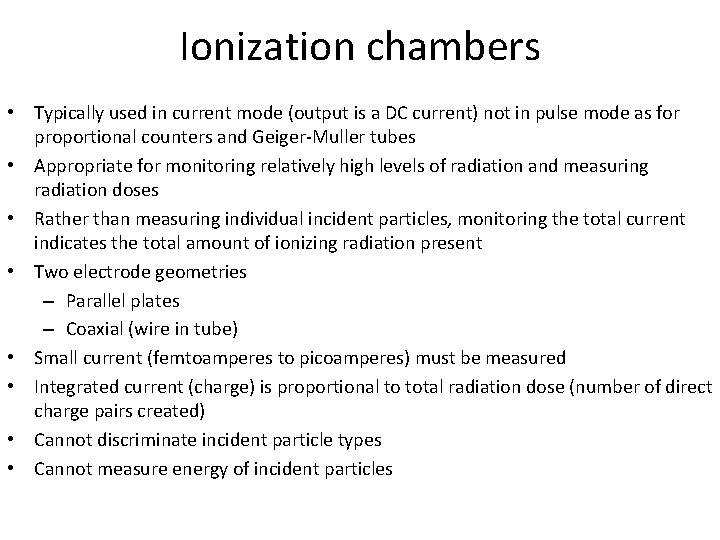
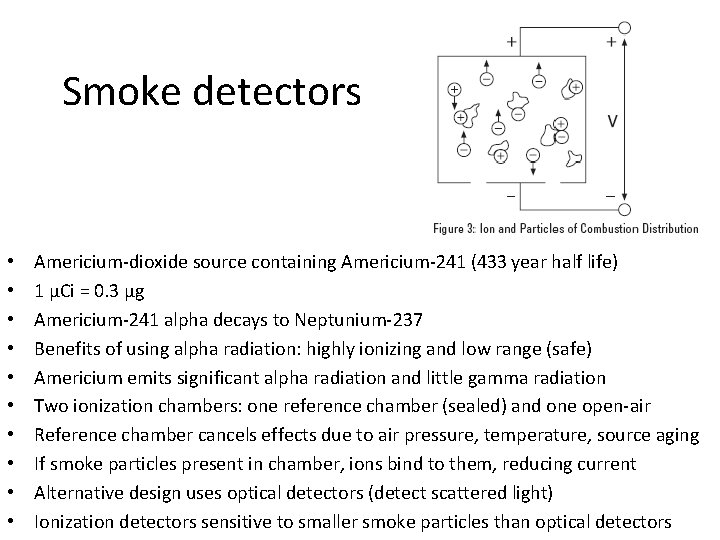
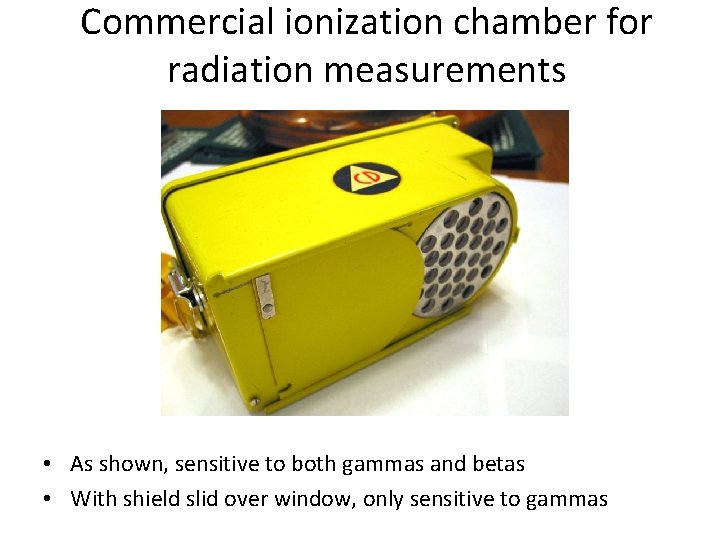
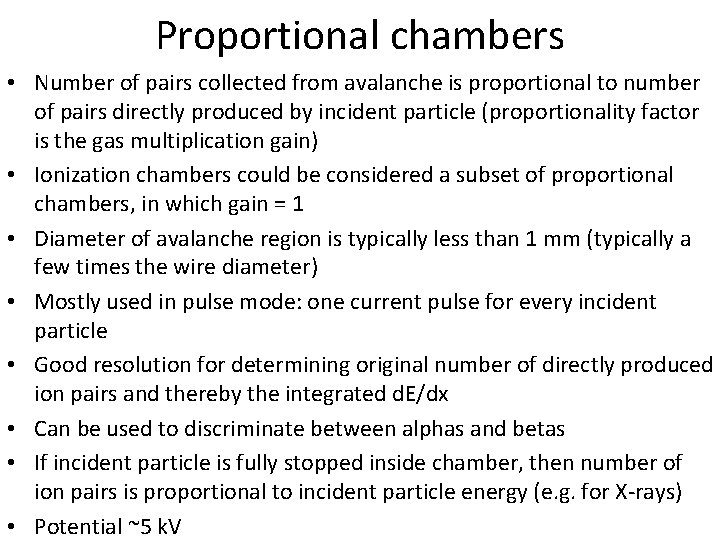
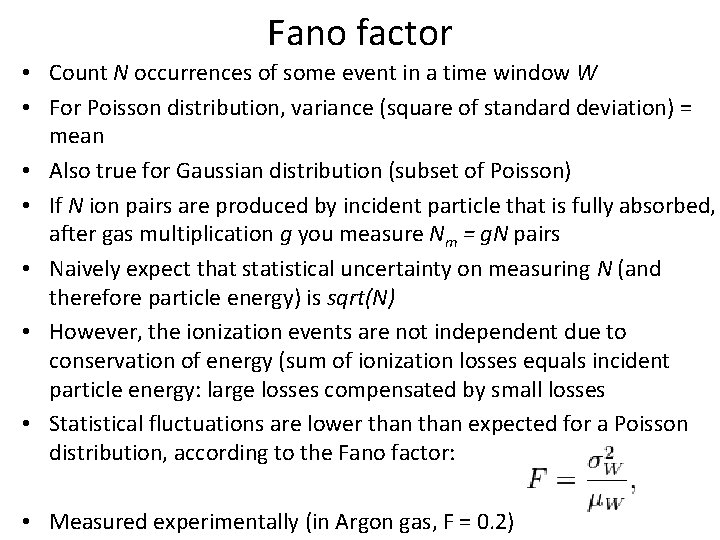
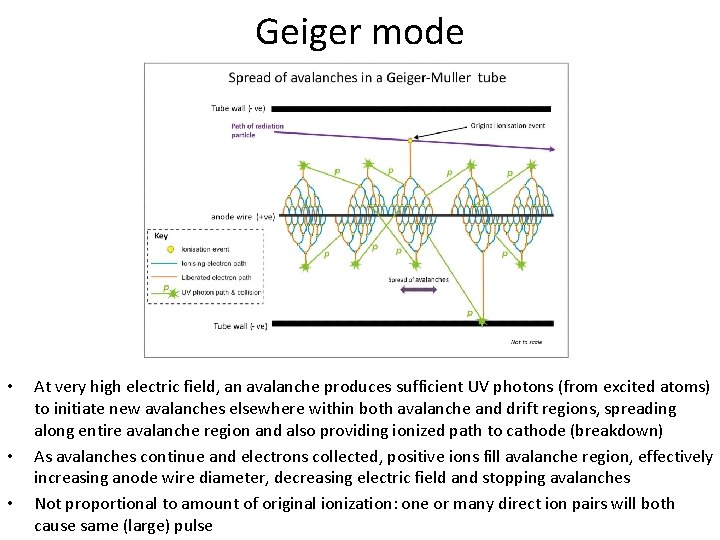
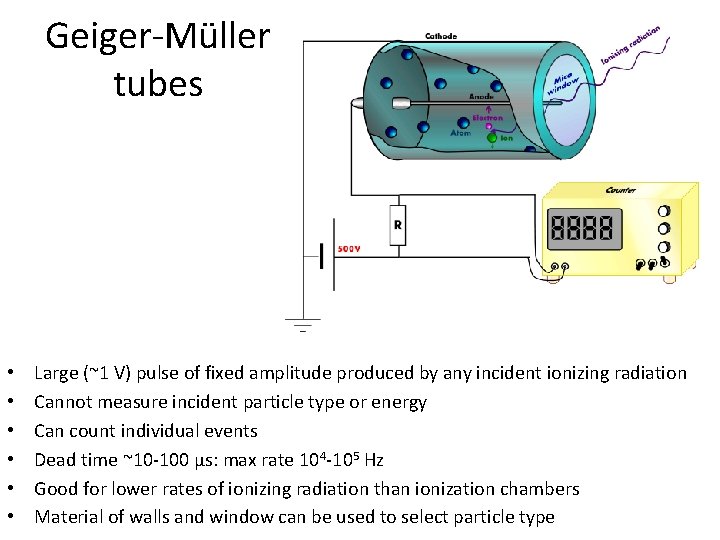
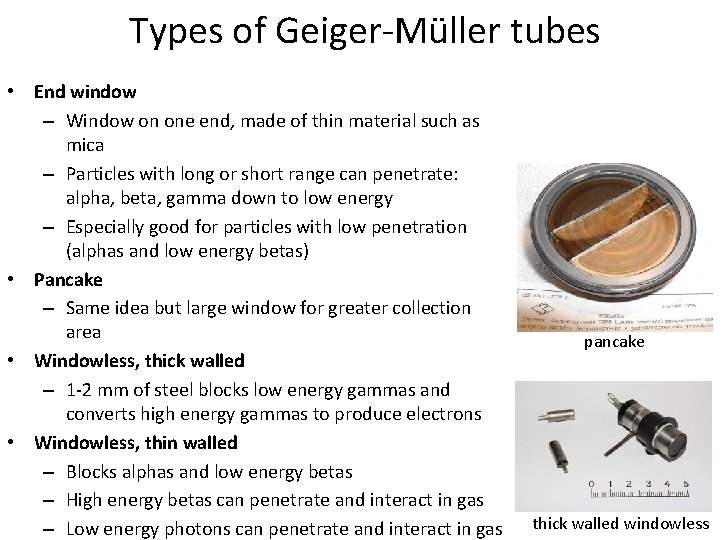
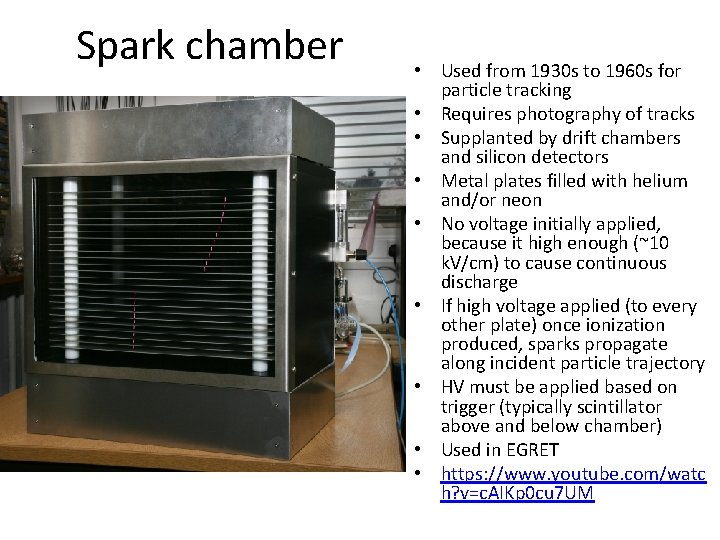
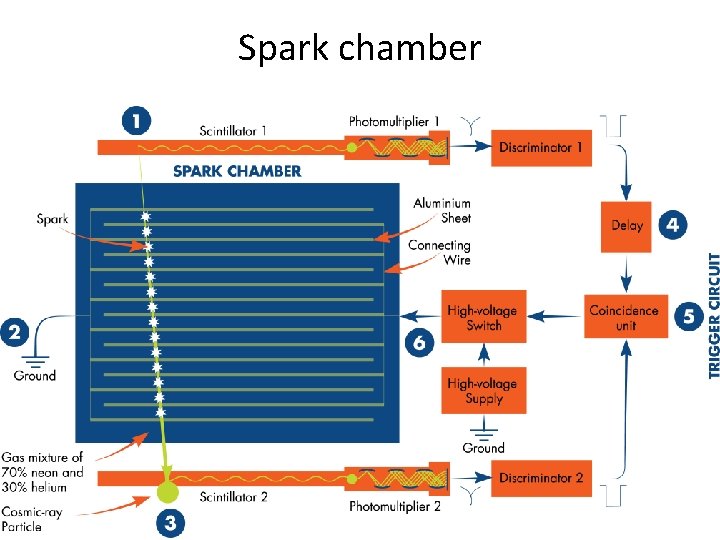
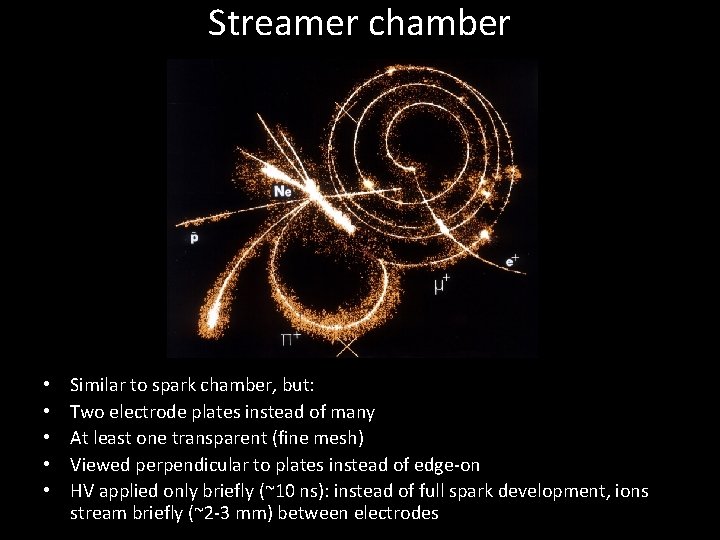
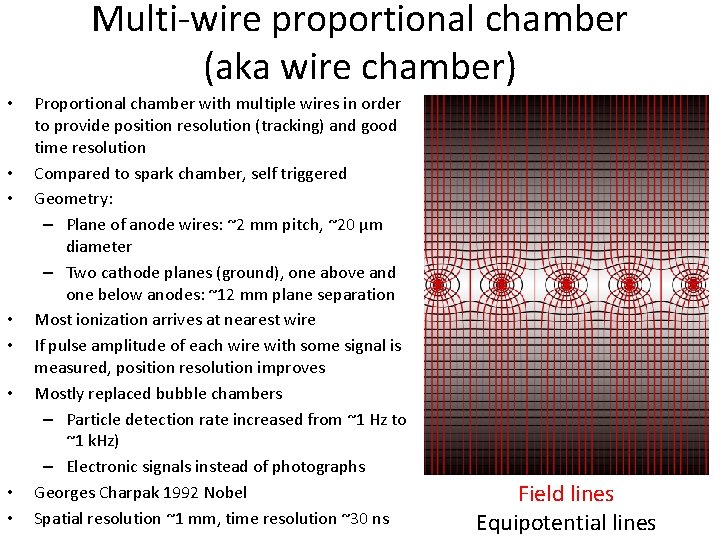
- Slides: 21
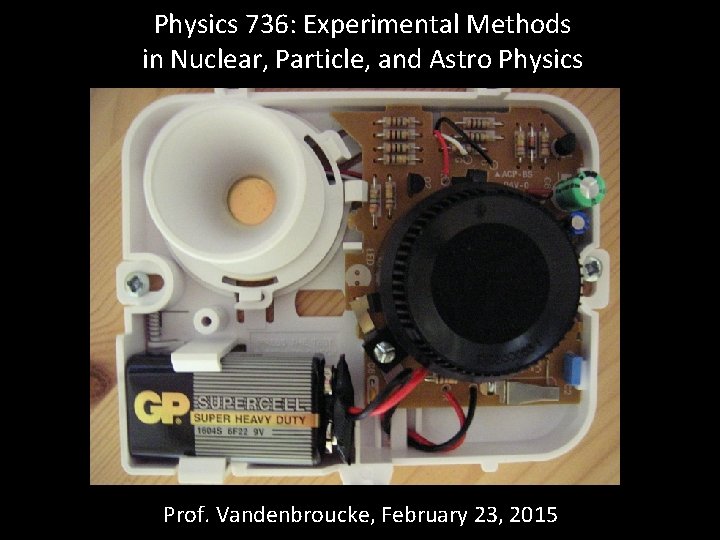
Physics 736: Experimental Methods in Nuclear, Particle, and Astro Physics Prof. Vandenbroucke, February 23, 2015
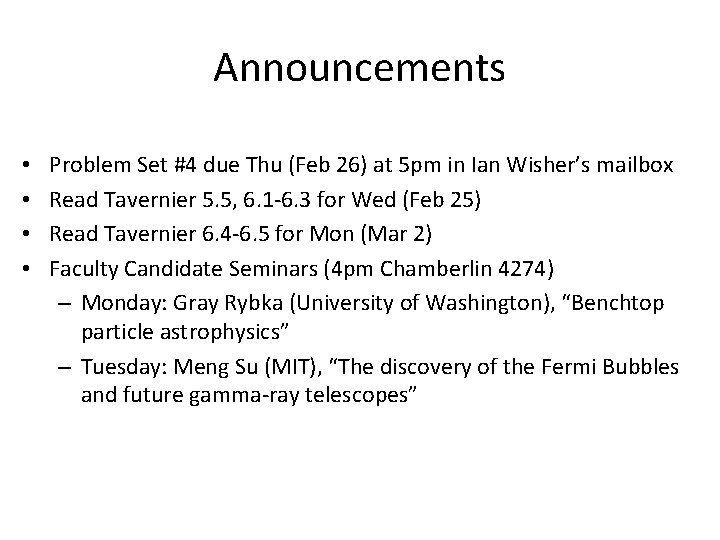
Announcements • • Problem Set #4 due Thu (Feb 26) at 5 pm in Ian Wisher’s mailbox Read Tavernier 5. 5, 6. 1 -6. 3 for Wed (Feb 25) Read Tavernier 6. 4 -6. 5 for Mon (Mar 2) Faculty Candidate Seminars (4 pm Chamberlin 4274) – Monday: Gray Rybka (University of Washington), “Benchtop particle astrophysics” – Tuesday: Meng Su (MIT), “The discovery of the Fermi Bubbles and future gamma-ray telescopes”
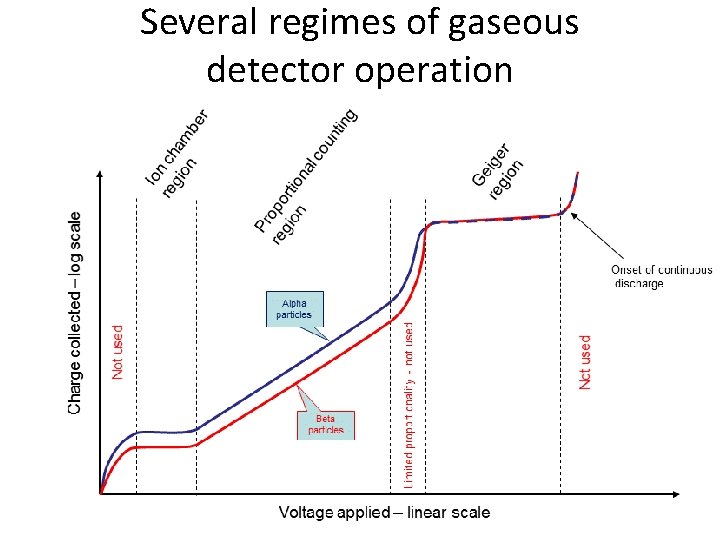
Several regimes of gaseous detector operation
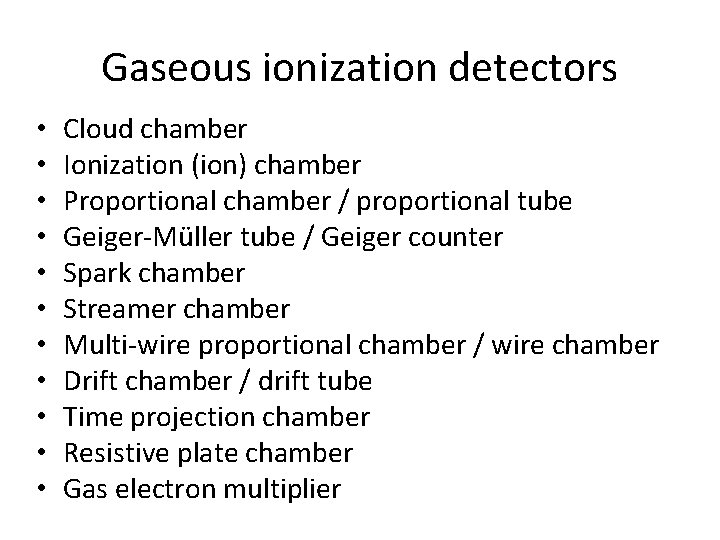
Gaseous ionization detectors • • • Cloud chamber Ionization (ion) chamber Proportional chamber / proportional tube Geiger-Müller tube / Geiger counter Spark chamber Streamer chamber Multi-wire proportional chamber / wire chamber Drift chamber / drift tube Time projection chamber Resistive plate chamber Gas electron multiplier
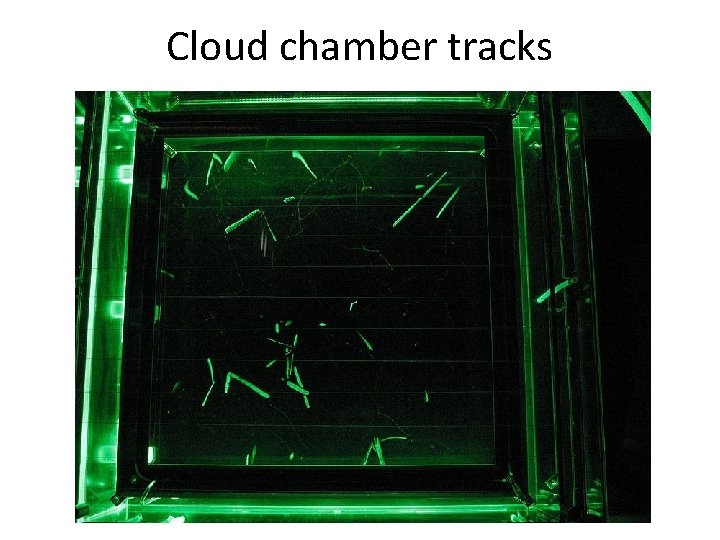
Cloud chamber tracks
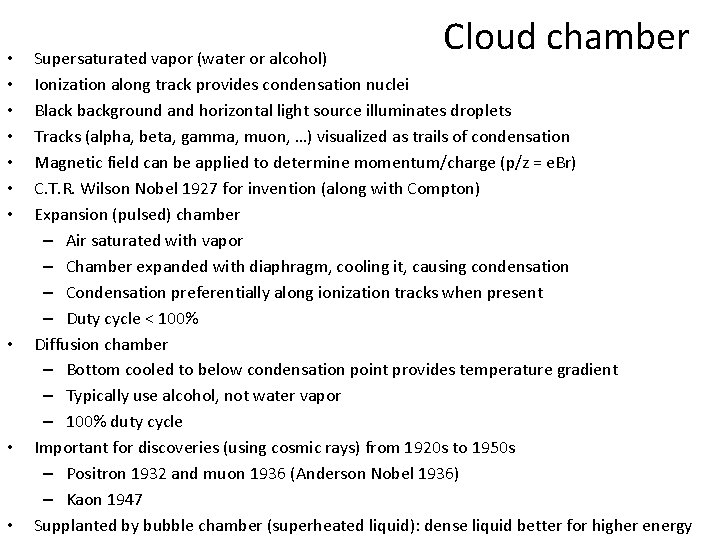
• • • Cloud chamber Supersaturated vapor (water or alcohol) Ionization along track provides condensation nuclei Black background and horizontal light source illuminates droplets Tracks (alpha, beta, gamma, muon, …) visualized as trails of condensation Magnetic field can be applied to determine momentum/charge (p/z = e. Br) C. T. R. Wilson Nobel 1927 for invention (along with Compton) Expansion (pulsed) chamber – Air saturated with vapor – Chamber expanded with diaphragm, cooling it, causing condensation – Condensation preferentially along ionization tracks when present – Duty cycle < 100% Diffusion chamber – Bottom cooled to below condensation point provides temperature gradient – Typically use alcohol, not water vapor – 100% duty cycle Important for discoveries (using cosmic rays) from 1920 s to 1950 s – Positron 1932 and muon 1936 (Anderson Nobel 1936) – Kaon 1947 Supplanted by bubble chamber (superheated liquid): dense liquid better for higher energy
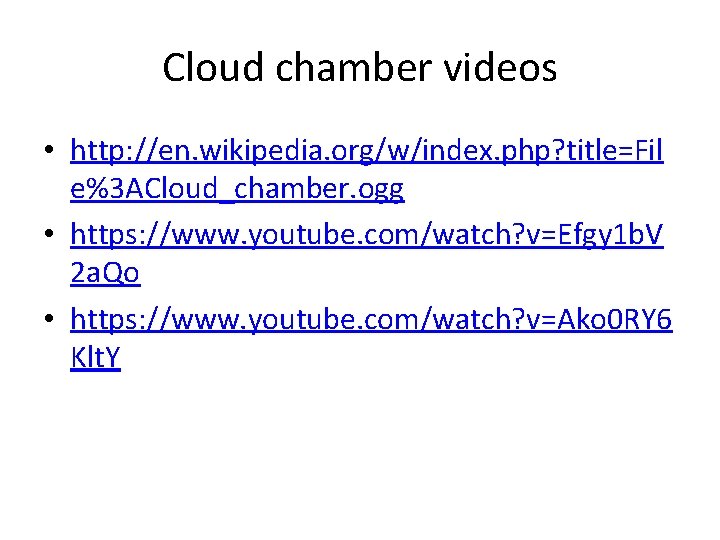
Cloud chamber videos • http: //en. wikipedia. org/w/index. php? title=Fil e%3 ACloud_chamber. ogg • https: //www. youtube. com/watch? v=Efgy 1 b. V 2 a. Qo • https: //www. youtube. com/watch? v=Ako 0 RY 6 Klt. Y
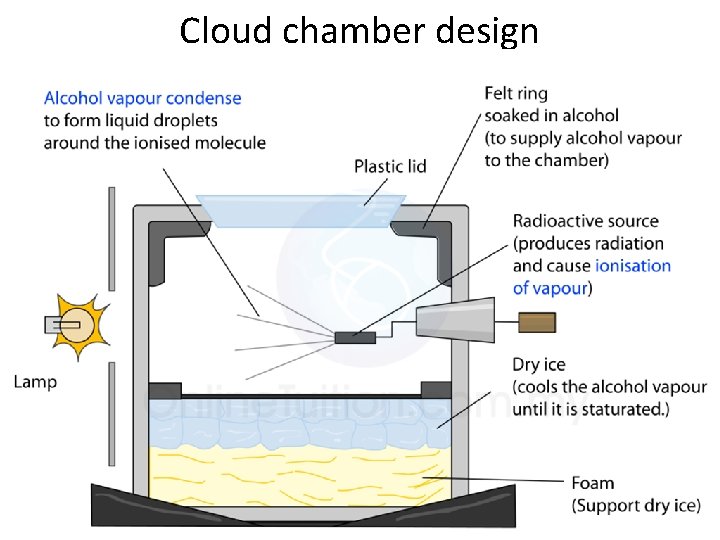
Cloud chamber design
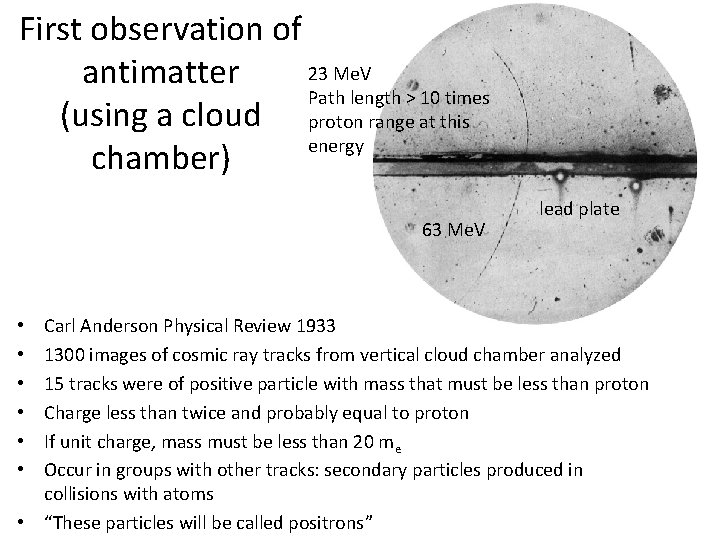
First observation of 23 Me. V antimatter Path length > 10 times (using a cloud proton range at this energy chamber) 63 Me. V lead plate Carl Anderson Physical Review 1933 1300 images of cosmic ray tracks from vertical cloud chamber analyzed 15 tracks were of positive particle with mass that must be less than proton Charge less than twice and probably equal to proton If unit charge, mass must be less than 20 me Occur in groups with other tracks: secondary particles produced in collisions with atoms • “These particles will be called positrons” • • •
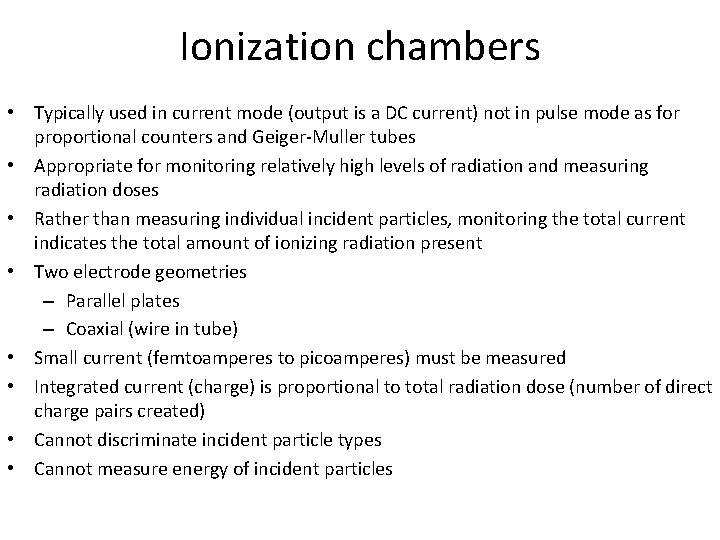
Ionization chambers • Typically used in current mode (output is a DC current) not in pulse mode as for proportional counters and Geiger-Muller tubes • Appropriate for monitoring relatively high levels of radiation and measuring radiation doses • Rather than measuring individual incident particles, monitoring the total current indicates the total amount of ionizing radiation present • Two electrode geometries – Parallel plates – Coaxial (wire in tube) • Small current (femtoamperes to picoamperes) must be measured • Integrated current (charge) is proportional to total radiation dose (number of direct charge pairs created) • Cannot discriminate incident particle types • Cannot measure energy of incident particles
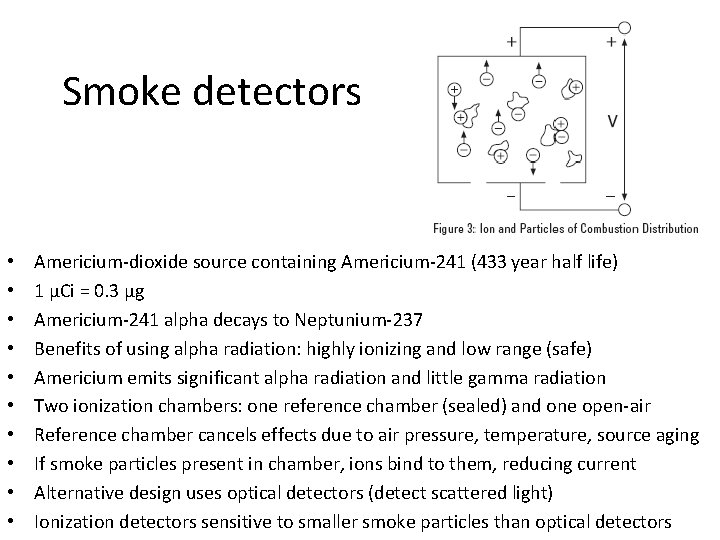
Smoke detectors • • • Americium-dioxide source containing Americium-241 (433 year half life) 1 µCi = 0. 3 µg Americium-241 alpha decays to Neptunium-237 Benefits of using alpha radiation: highly ionizing and low range (safe) Americium emits significant alpha radiation and little gamma radiation Two ionization chambers: one reference chamber (sealed) and one open-air Reference chamber cancels effects due to air pressure, temperature, source aging If smoke particles present in chamber, ions bind to them, reducing current Alternative design uses optical detectors (detect scattered light) Ionization detectors sensitive to smaller smoke particles than optical detectors
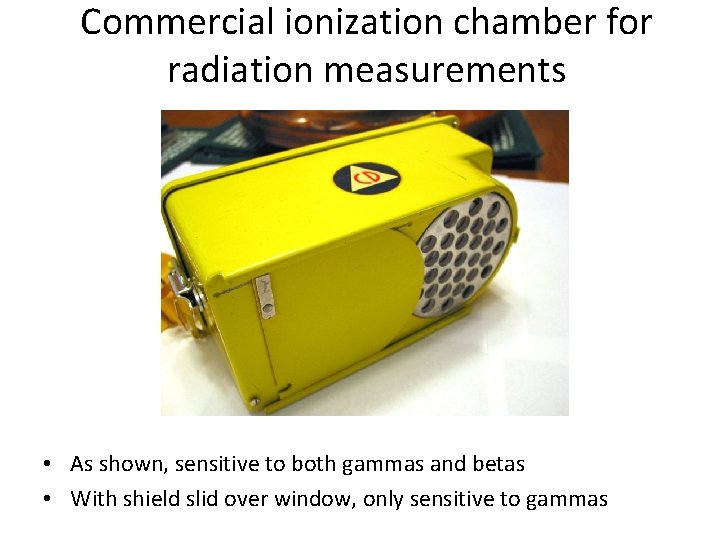
Commercial ionization chamber for radiation measurements • As shown, sensitive to both gammas and betas • With shield slid over window, only sensitive to gammas
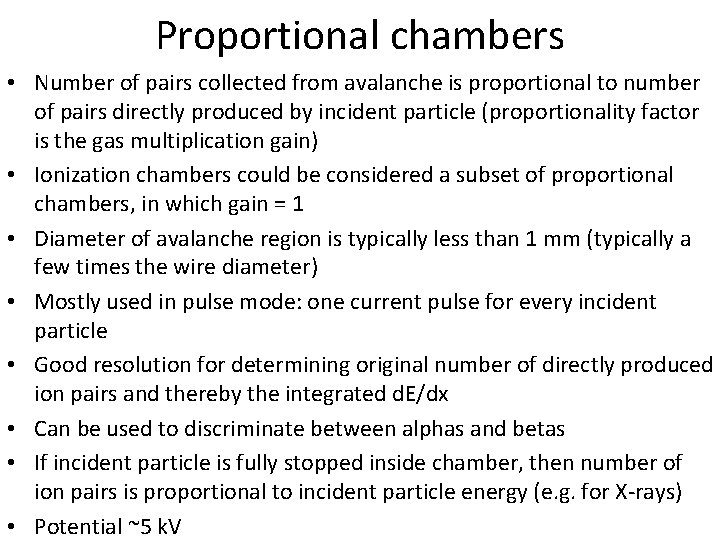
Proportional chambers • Number of pairs collected from avalanche is proportional to number of pairs directly produced by incident particle (proportionality factor is the gas multiplication gain) • Ionization chambers could be considered a subset of proportional chambers, in which gain = 1 • Diameter of avalanche region is typically less than 1 mm (typically a few times the wire diameter) • Mostly used in pulse mode: one current pulse for every incident particle • Good resolution for determining original number of directly produced ion pairs and thereby the integrated d. E/dx • Can be used to discriminate between alphas and betas • If incident particle is fully stopped inside chamber, then number of ion pairs is proportional to incident particle energy (e. g. for X-rays) • Potential ~5 k. V
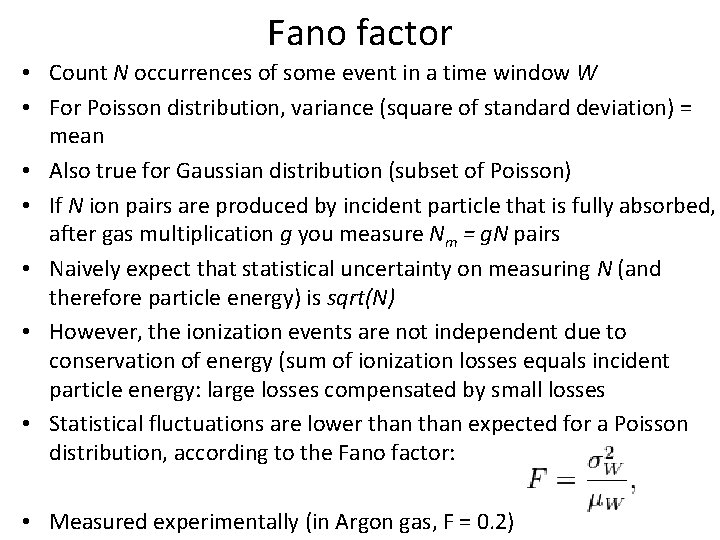
Fano factor • Count N occurrences of some event in a time window W • For Poisson distribution, variance (square of standard deviation) = mean • Also true for Gaussian distribution (subset of Poisson) • If N ion pairs are produced by incident particle that is fully absorbed, after gas multiplication g you measure Nm = g. N pairs • Naively expect that statistical uncertainty on measuring N (and therefore particle energy) is sqrt(N) • However, the ionization events are not independent due to conservation of energy (sum of ionization losses equals incident particle energy: large losses compensated by small losses • Statistical fluctuations are lower than expected for a Poisson distribution, according to the Fano factor: • Measured experimentally (in Argon gas, F = 0. 2)
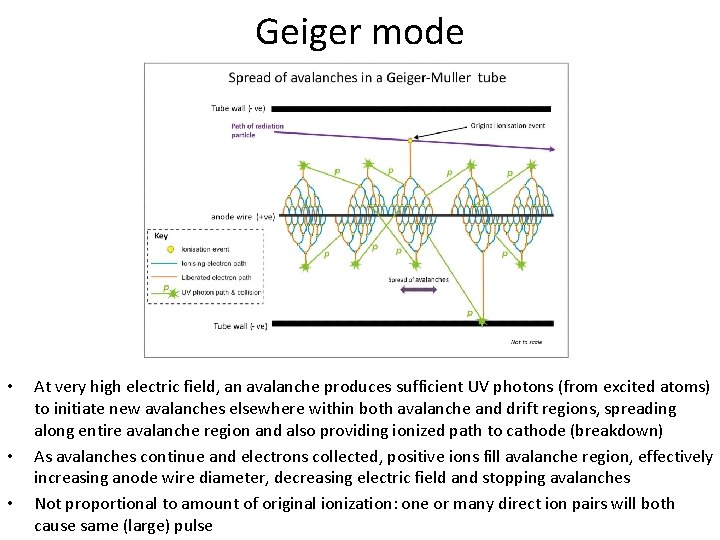
Geiger mode • • • At very high electric field, an avalanche produces sufficient UV photons (from excited atoms) to initiate new avalanches elsewhere within both avalanche and drift regions, spreading along entire avalanche region and also providing ionized path to cathode (breakdown) As avalanches continue and electrons collected, positive ions fill avalanche region, effectively increasing anode wire diameter, decreasing electric field and stopping avalanches Not proportional to amount of original ionization: one or many direct ion pairs will both cause same (large) pulse
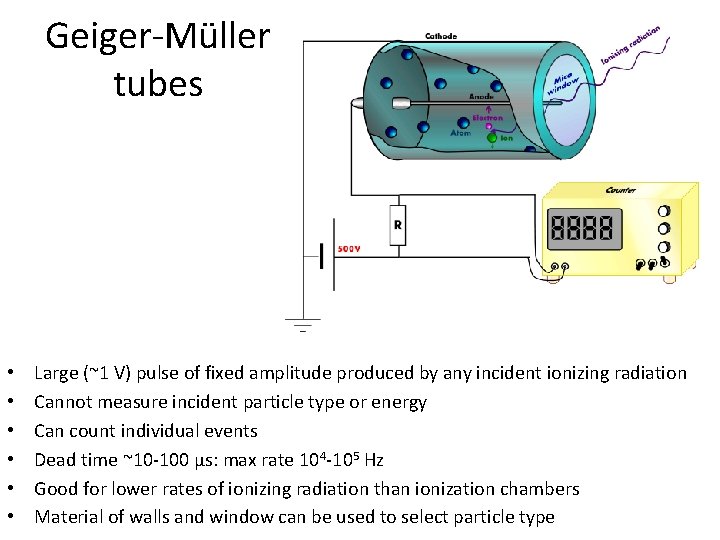
Geiger-Müller tubes • • • Large (~1 V) pulse of fixed amplitude produced by any incident ionizing radiation Cannot measure incident particle type or energy Can count individual events Dead time ~10 -100 µs: max rate 104 -105 Hz Good for lower rates of ionizing radiation than ionization chambers Material of walls and window can be used to select particle type
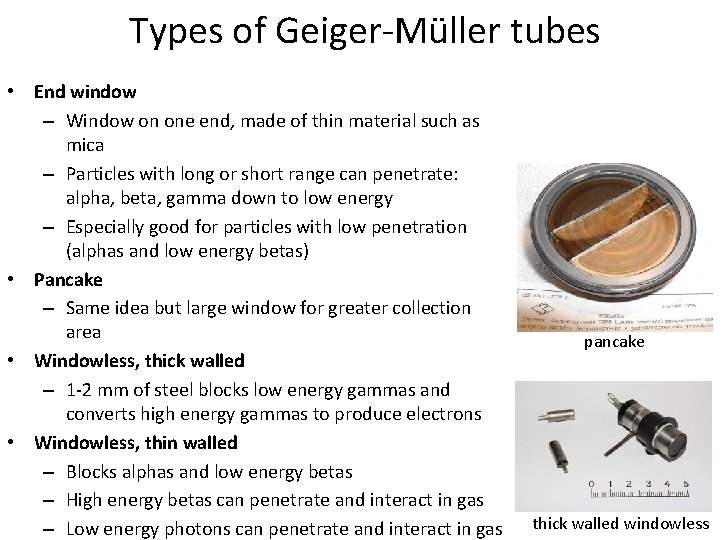
Types of Geiger-Müller tubes • End window – Window on one end, made of thin material such as mica – Particles with long or short range can penetrate: alpha, beta, gamma down to low energy – Especially good for particles with low penetration (alphas and low energy betas) • Pancake – Same idea but large window for greater collection area • Windowless, thick walled – 1 -2 mm of steel blocks low energy gammas and converts high energy gammas to produce electrons • Windowless, thin walled – Blocks alphas and low energy betas – High energy betas can penetrate and interact in gas – Low energy photons can penetrate and interact in gas pancake thick walled windowless
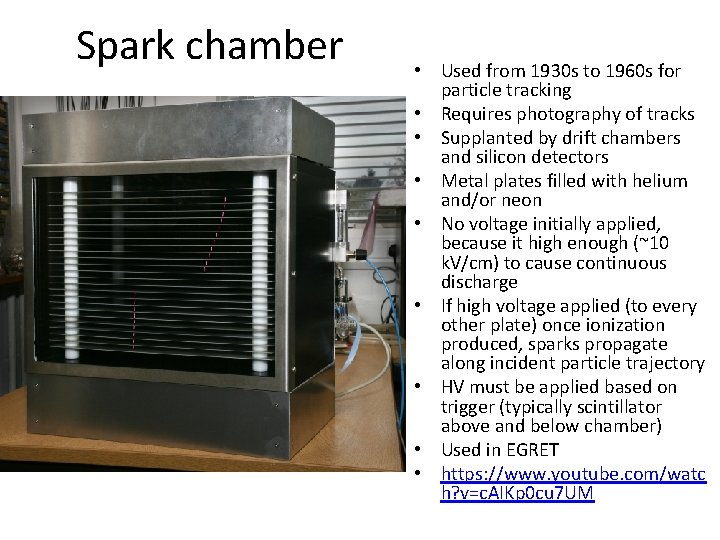
Spark chamber • Used from 1930 s to 1960 s for particle tracking • Requires photography of tracks • Supplanted by drift chambers and silicon detectors • Metal plates filled with helium and/or neon • No voltage initially applied, because it high enough (~10 k. V/cm) to cause continuous discharge • If high voltage applied (to every other plate) once ionization produced, sparks propagate along incident particle trajectory • HV must be applied based on trigger (typically scintillator above and below chamber) • Used in EGRET • https: //www. youtube. com/watc h? v=c. AIKp 0 cu 7 UM
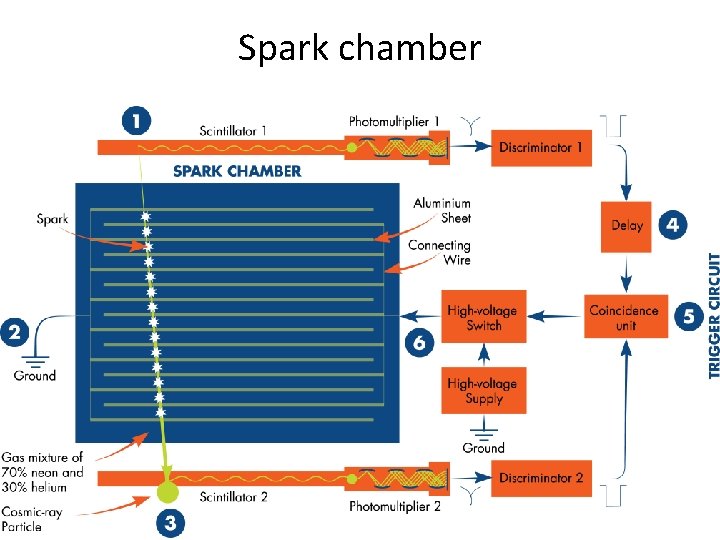
Spark chamber
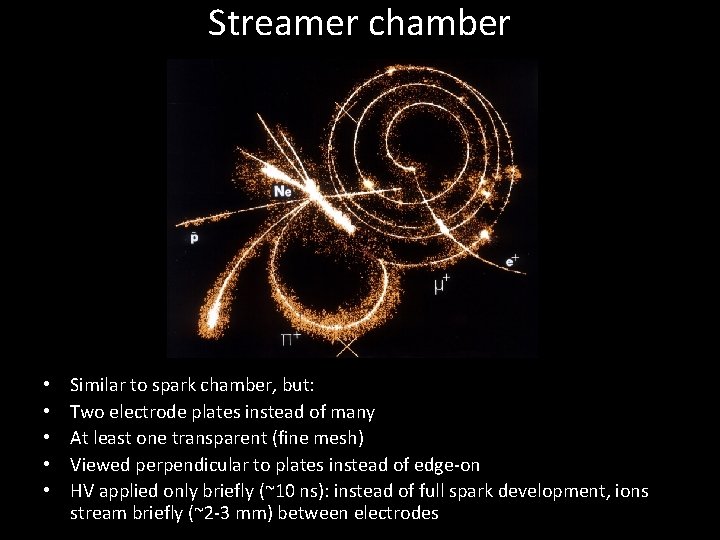
Streamer chamber • • • Similar to spark chamber, but: Two electrode plates instead of many At least one transparent (fine mesh) Viewed perpendicular to plates instead of edge-on HV applied only briefly (~10 ns): instead of full spark development, ions stream briefly (~2 -3 mm) between electrodes
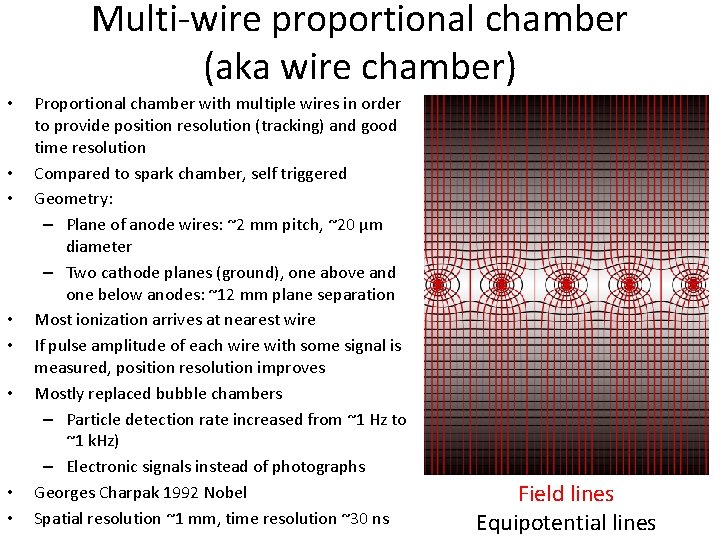
Multi-wire proportional chamber (aka wire chamber) • • Proportional chamber with multiple wires in order to provide position resolution (tracking) and good time resolution Compared to spark chamber, self triggered Geometry: – Plane of anode wires: ~2 mm pitch, ~20 µm diameter – Two cathode planes (ground), one above and one below anodes: ~12 mm plane separation Most ionization arrives at nearest wire If pulse amplitude of each wire with some signal is measured, position resolution improves Mostly replaced bubble chambers – Particle detection rate increased from ~1 Hz to ~1 k. Hz) – Electronic signals instead of photographs Georges Charpak 1992 Nobel Spatial resolution ~1 mm, time resolution ~30 ns Field lines Equipotential lines
Section 736(b) payments
Experiment 736
736
736
Lesson 15 nuclear quest nuclear reactions
Fisión nuclear vs fision nuclear
Experimental vs non experimental
Descriptive studies
Experimental vs non experimental research
Experimental vs non experimental
Experimental vs non experimental
Quantum and nuclear physics
Experimental physics and industrial control system
L
Experimental physics and industrial control system
Pmt particle physics
Mark thomson modern particle physics
Particle physics
Particle physics practice quiz
Particle physics timeline
Concezio bozzi
Cern particle physics