Physics 736 Experimental Methods in Nuclear Particle and
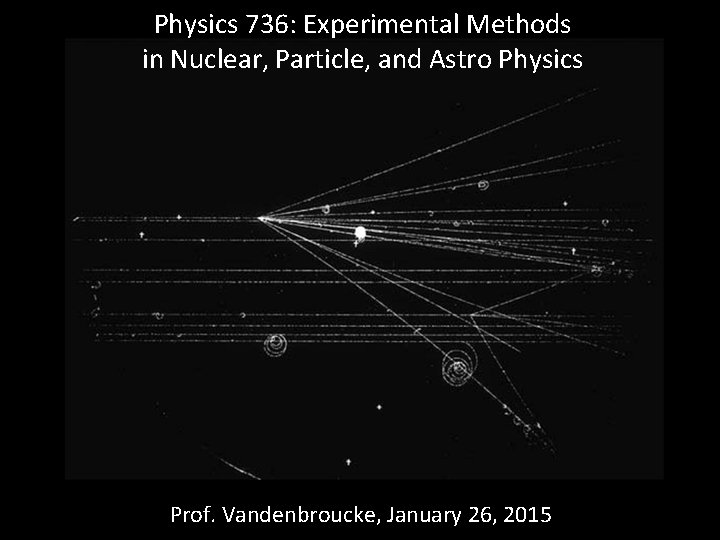
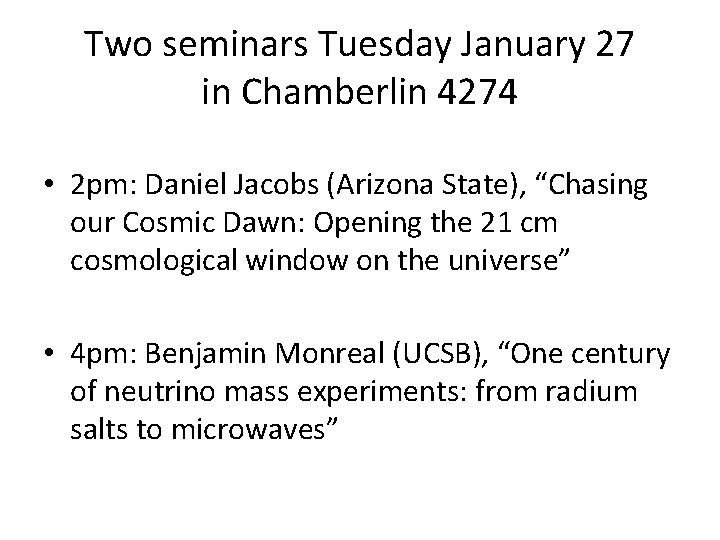
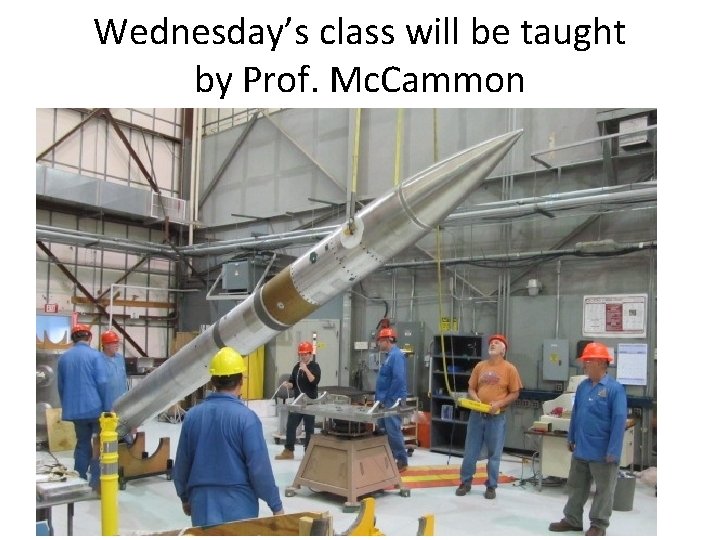
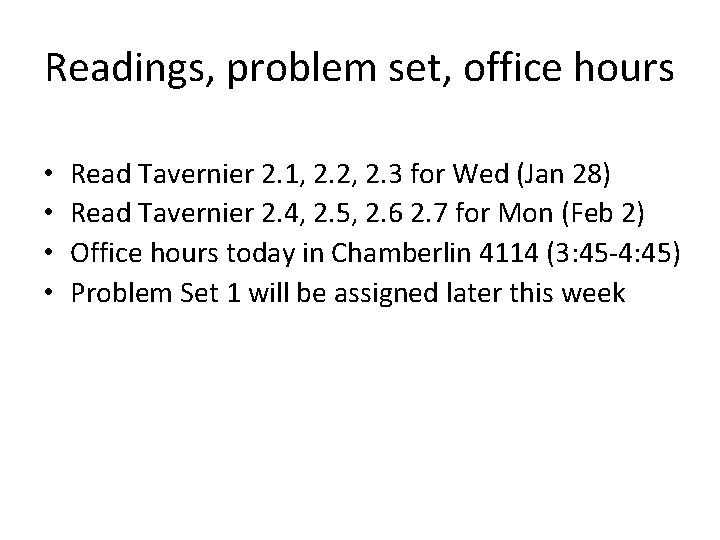
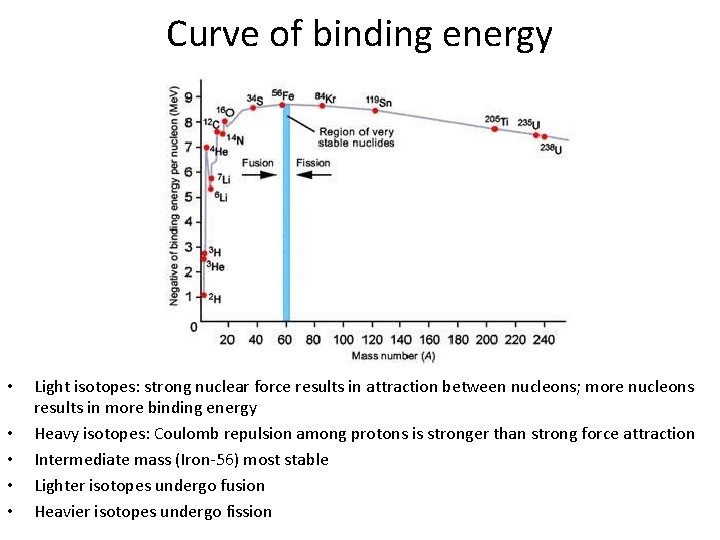
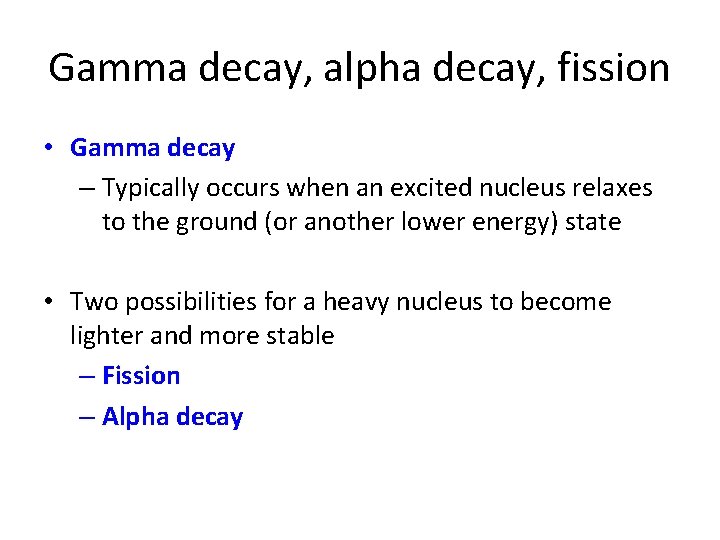
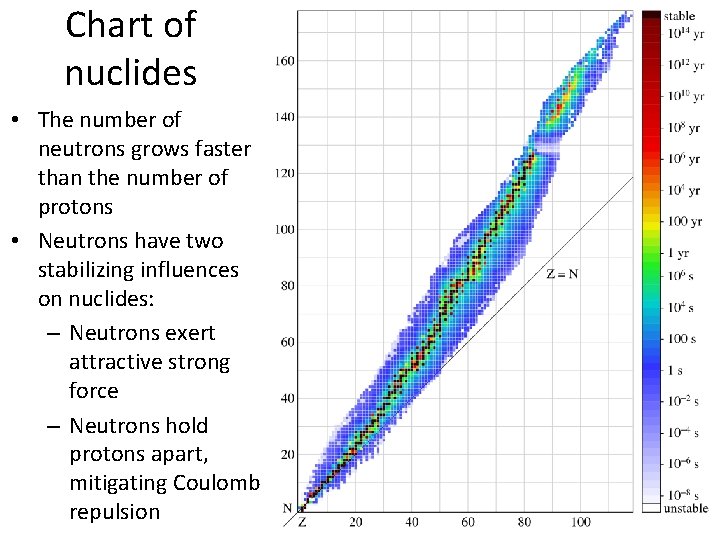
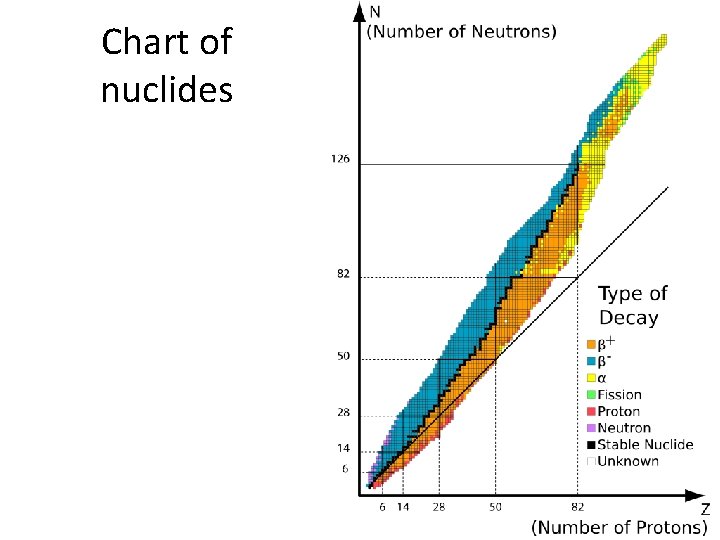
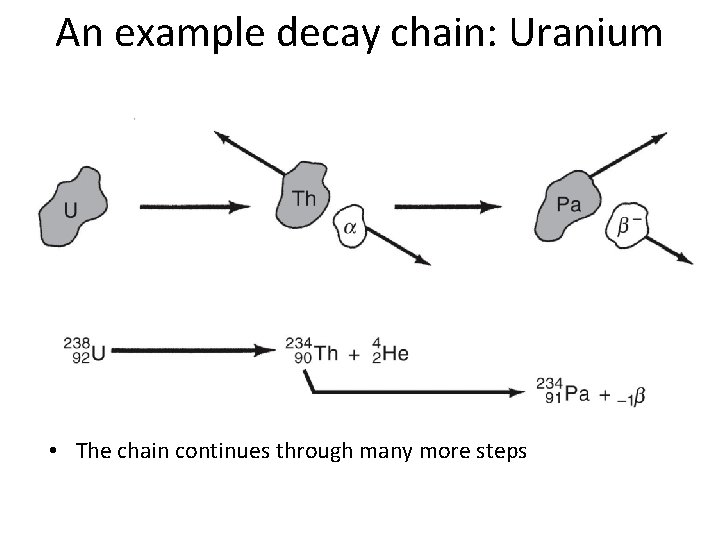
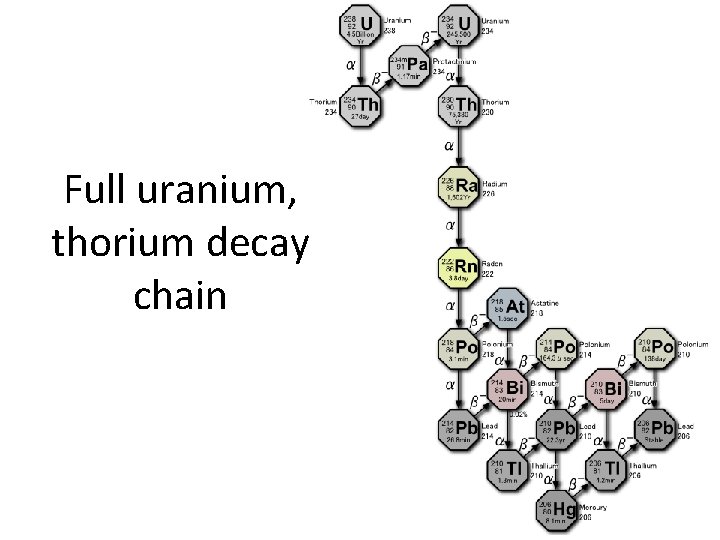
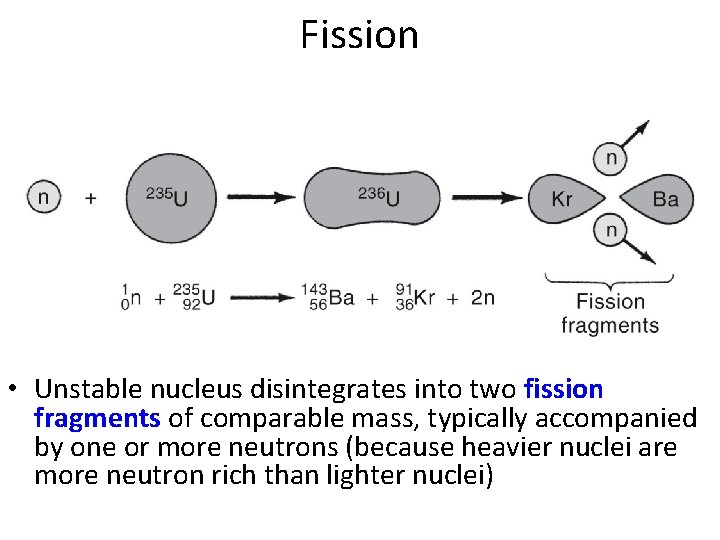
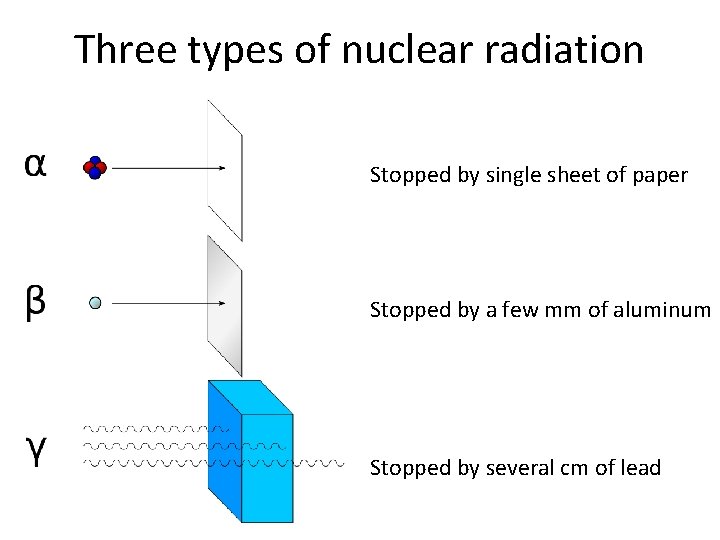
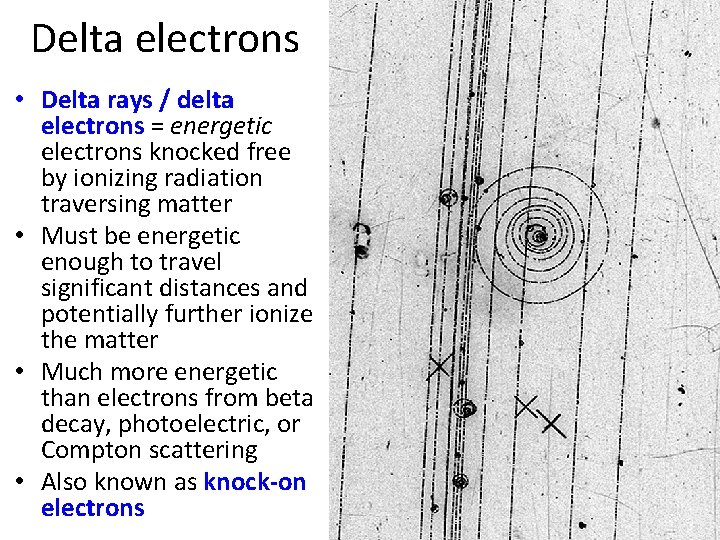
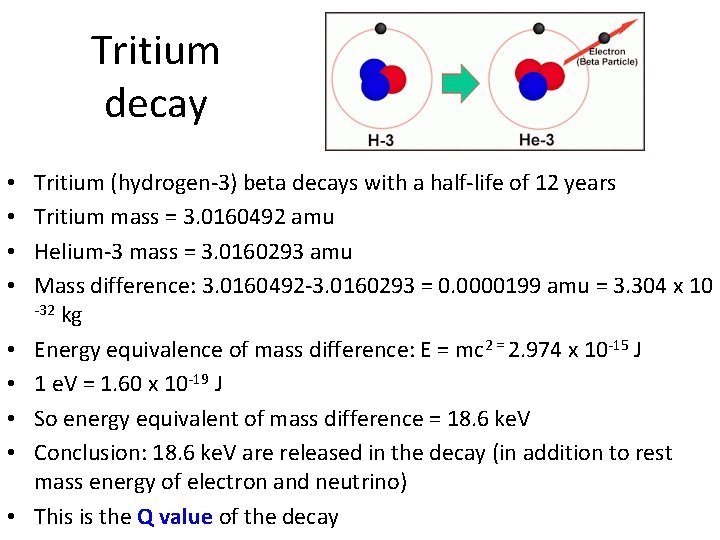
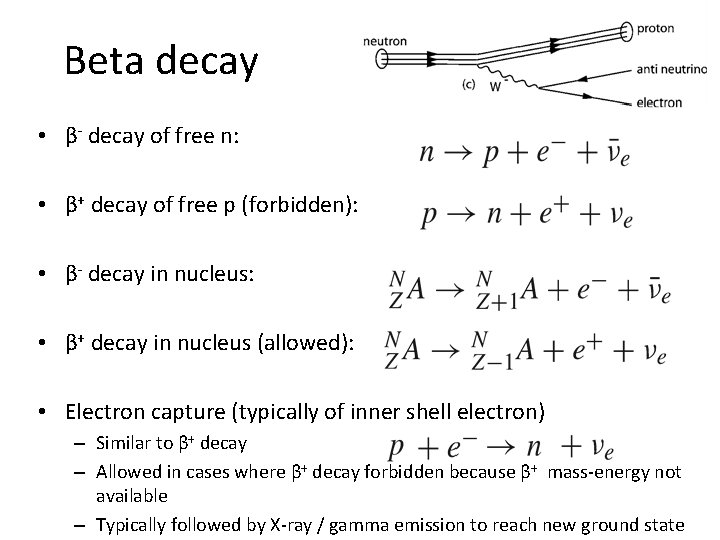
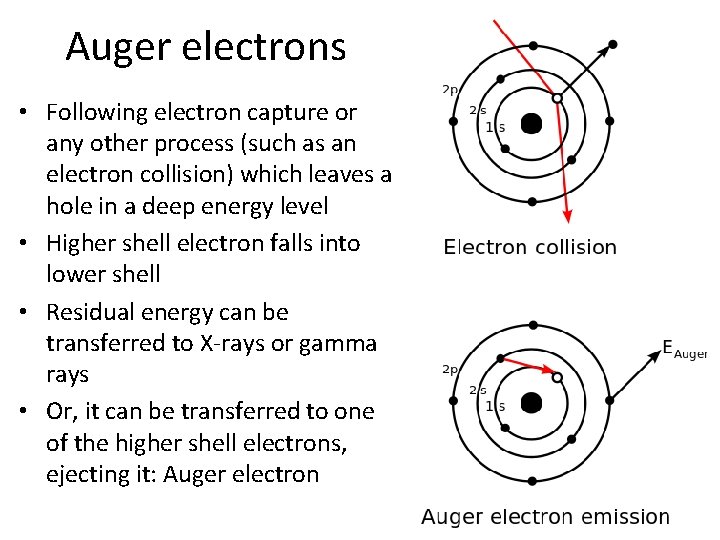
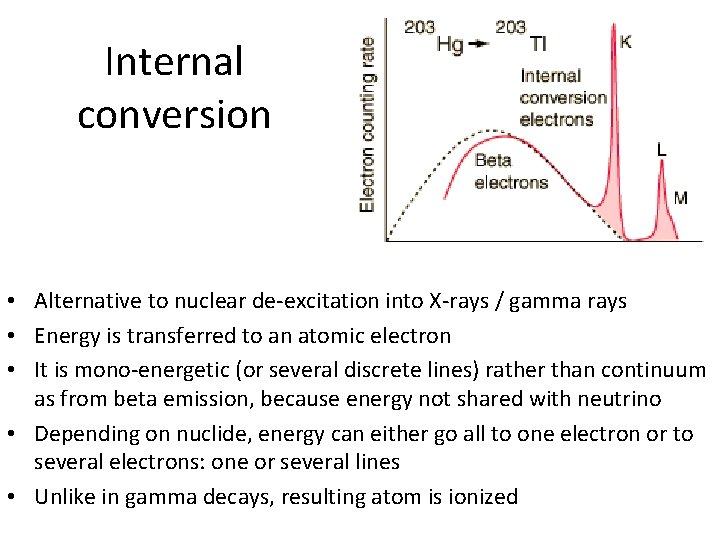
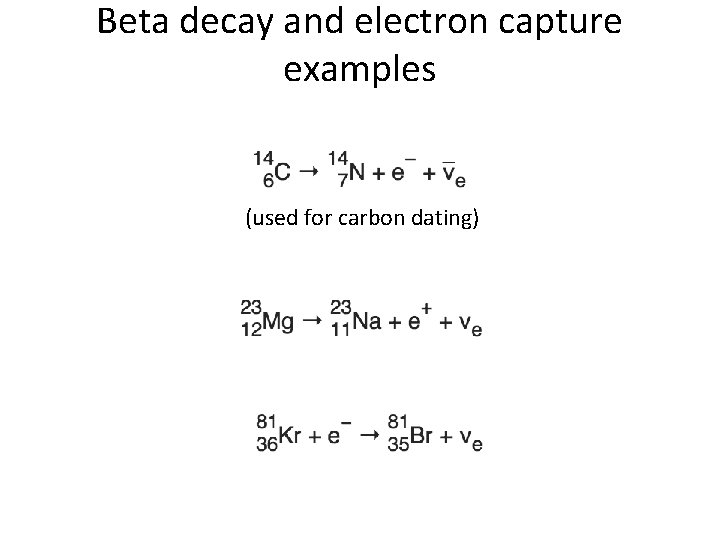
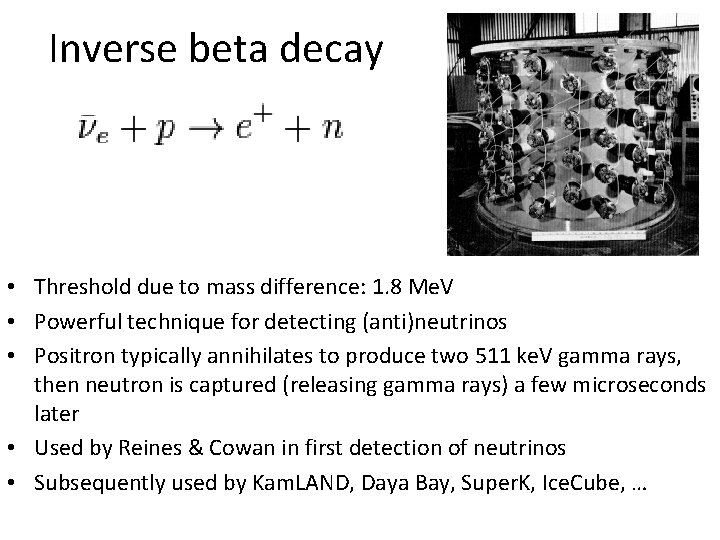
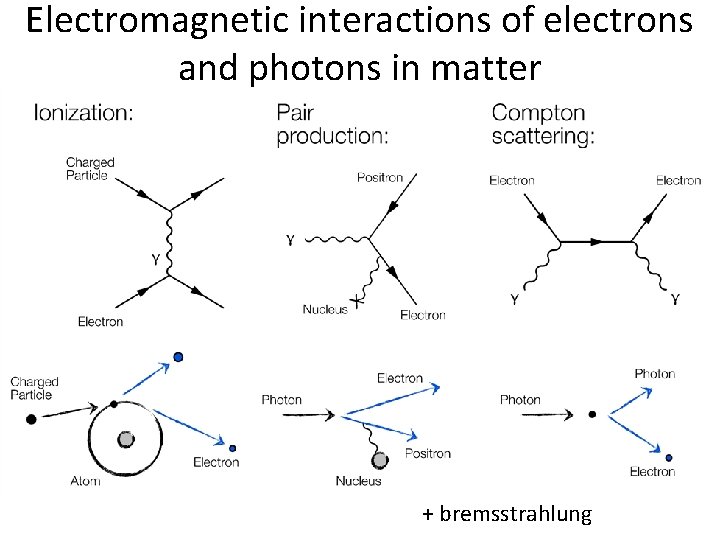
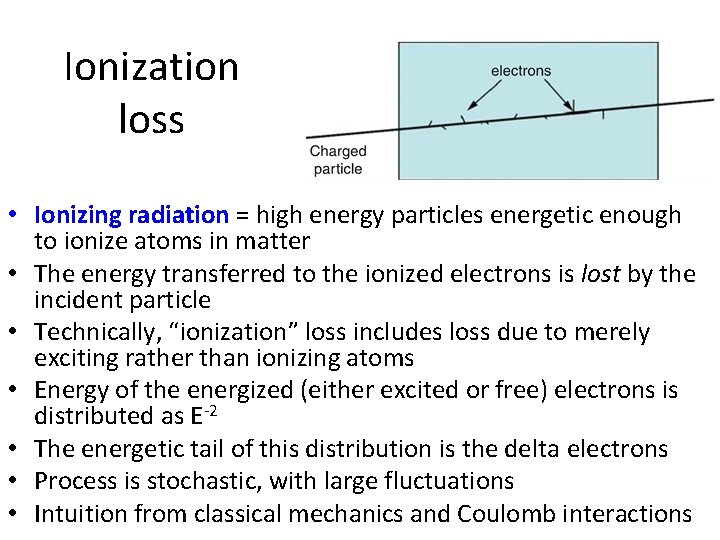
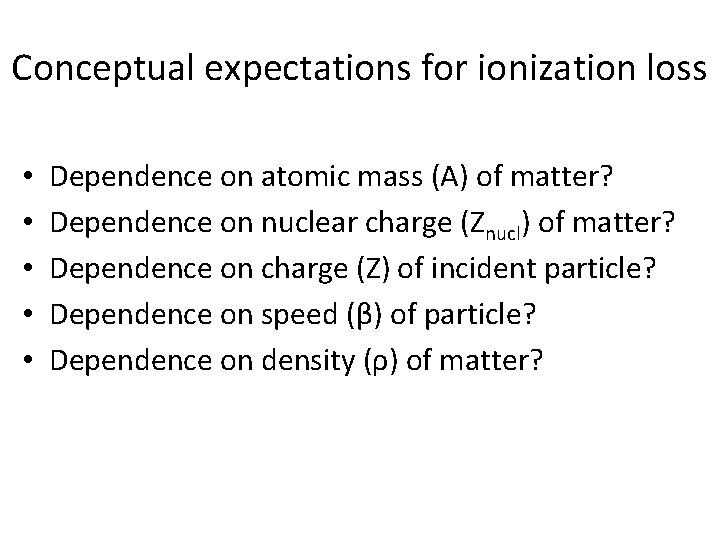
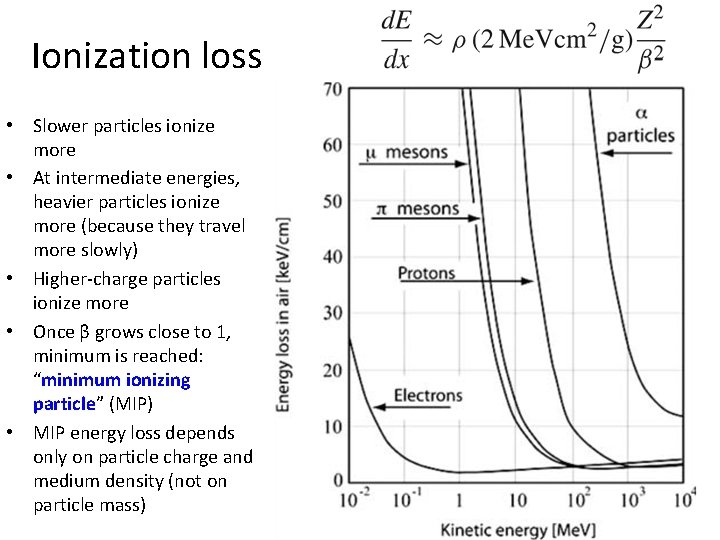
- Slides: 23
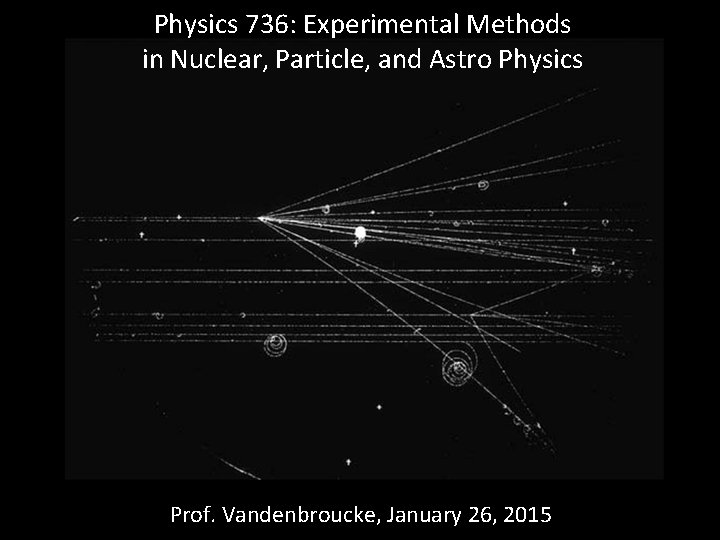
Physics 736: Experimental Methods in Nuclear, Particle, and Astro Physics Prof. Vandenbroucke, January 26, 2015
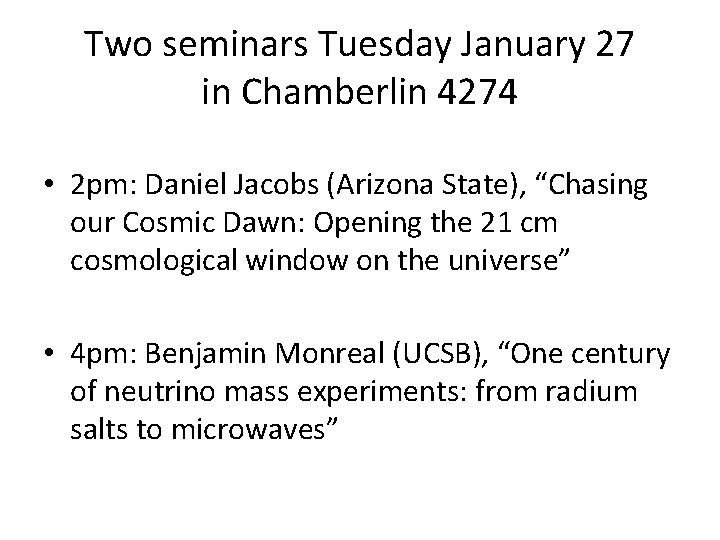
Two seminars Tuesday January 27 in Chamberlin 4274 • 2 pm: Daniel Jacobs (Arizona State), “Chasing our Cosmic Dawn: Opening the 21 cm cosmological window on the universe” • 4 pm: Benjamin Monreal (UCSB), “One century of neutrino mass experiments: from radium salts to microwaves”
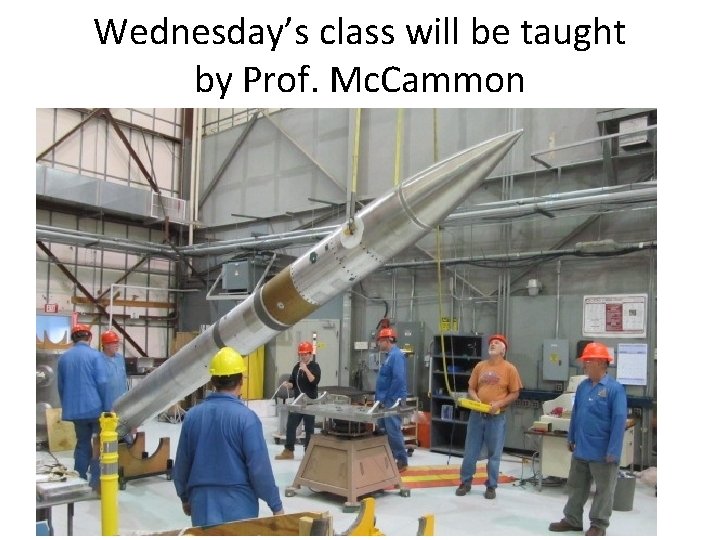
Wednesday’s class will be taught by Prof. Mc. Cammon
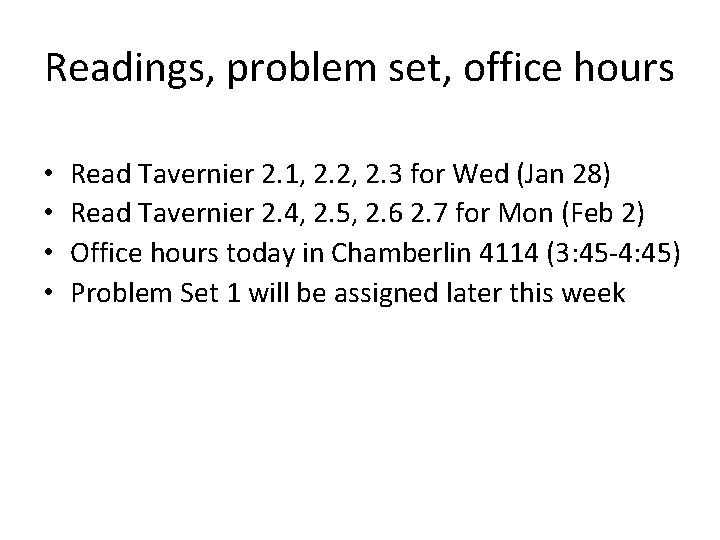
Readings, problem set, office hours • • Read Tavernier 2. 1, 2. 2, 2. 3 for Wed (Jan 28) Read Tavernier 2. 4, 2. 5, 2. 6 2. 7 for Mon (Feb 2) Office hours today in Chamberlin 4114 (3: 45 -4: 45) Problem Set 1 will be assigned later this week
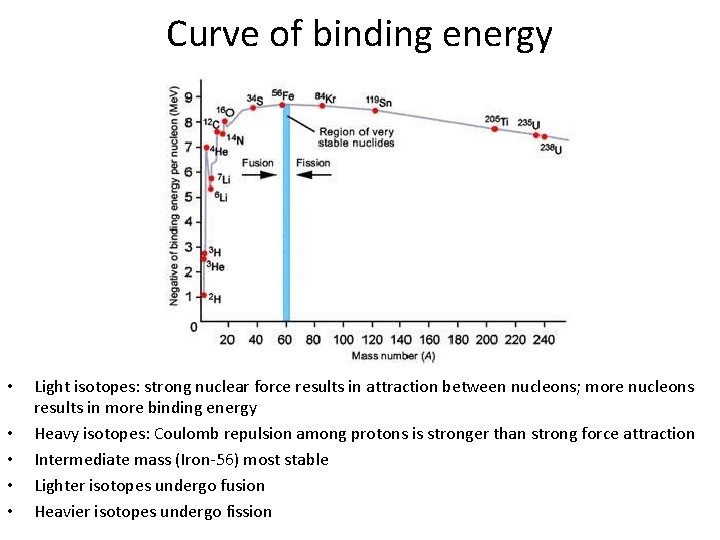
Curve of binding energy • • • Light isotopes: strong nuclear force results in attraction between nucleons; more nucleons results in more binding energy Heavy isotopes: Coulomb repulsion among protons is stronger than strong force attraction Intermediate mass (Iron-56) most stable Lighter isotopes undergo fusion Heavier isotopes undergo fission
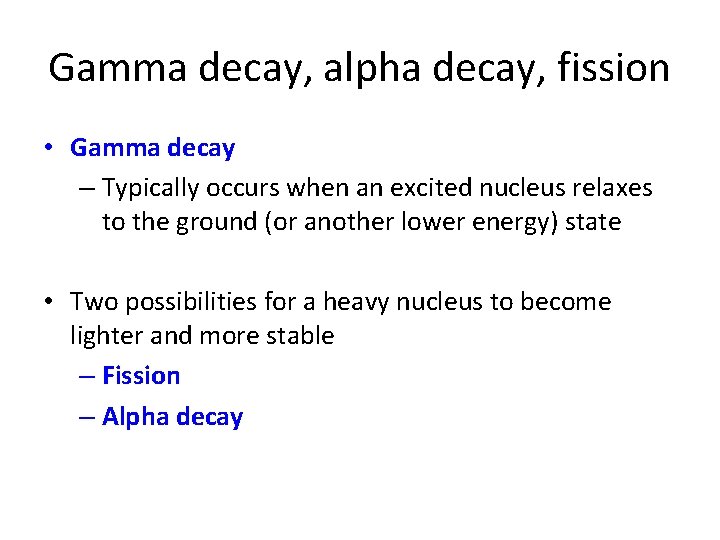
Gamma decay, alpha decay, fission • Gamma decay – Typically occurs when an excited nucleus relaxes to the ground (or another lower energy) state • Two possibilities for a heavy nucleus to become lighter and more stable – Fission – Alpha decay
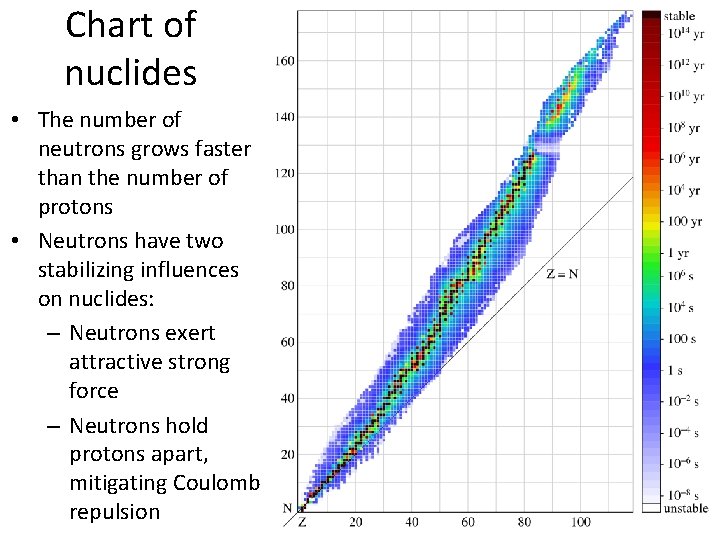
Chart of nuclides • The number of neutrons grows faster than the number of protons • Neutrons have two stabilizing influences on nuclides: – Neutrons exert attractive strong force – Neutrons hold protons apart, mitigating Coulomb repulsion
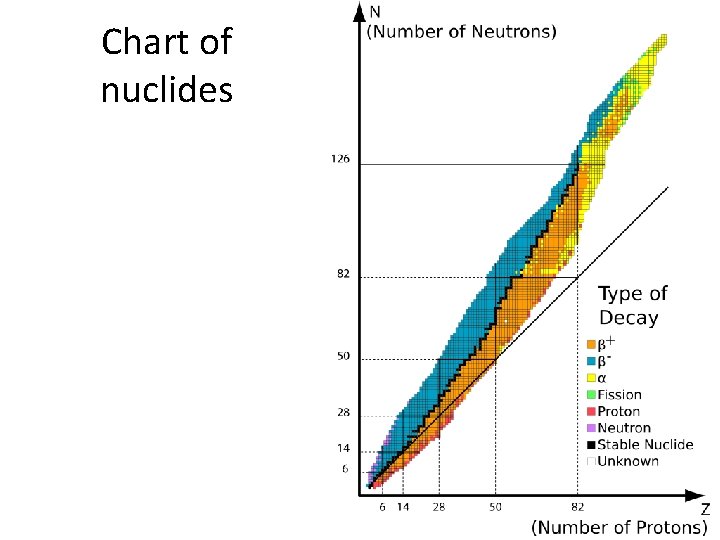
Chart of nuclides
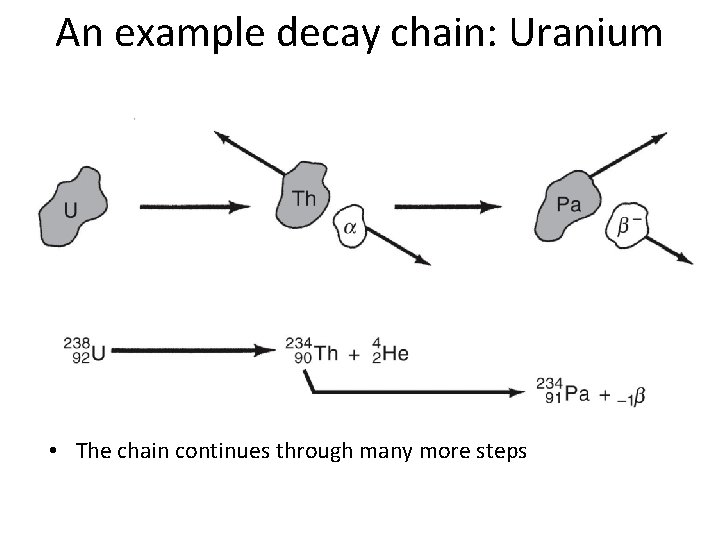
An example decay chain: Uranium • The chain continues through many more steps
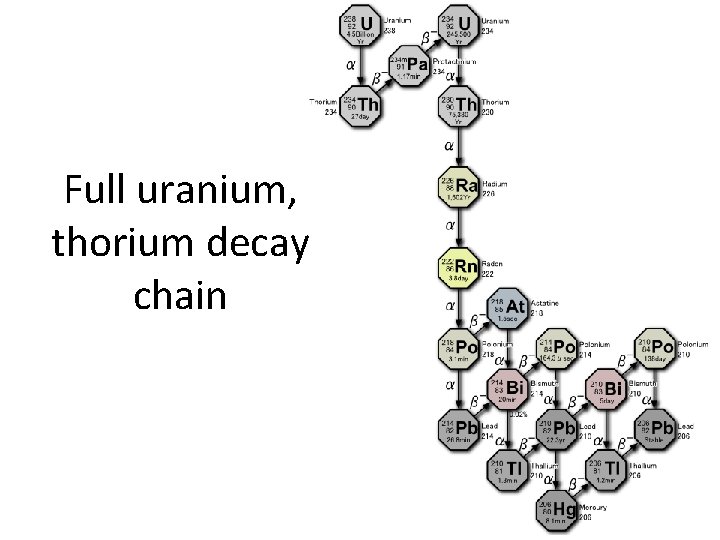
Full uranium, thorium decay chain
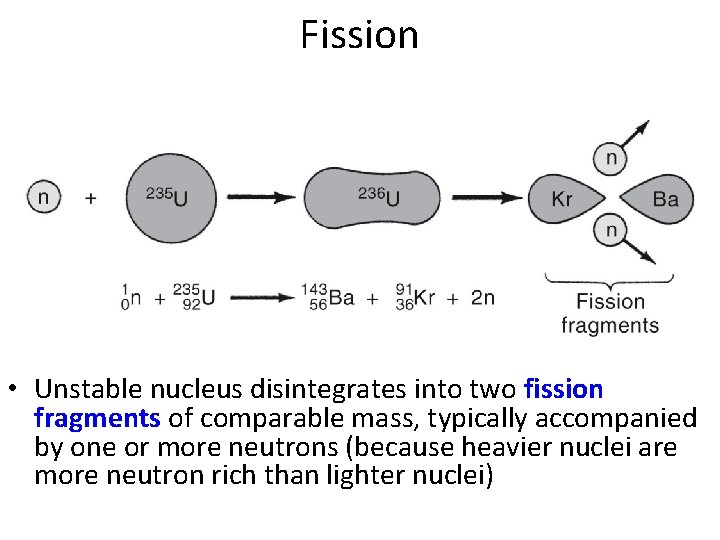
Fission • Unstable nucleus disintegrates into two fission fragments of comparable mass, typically accompanied by one or more neutrons (because heavier nuclei are more neutron rich than lighter nuclei)
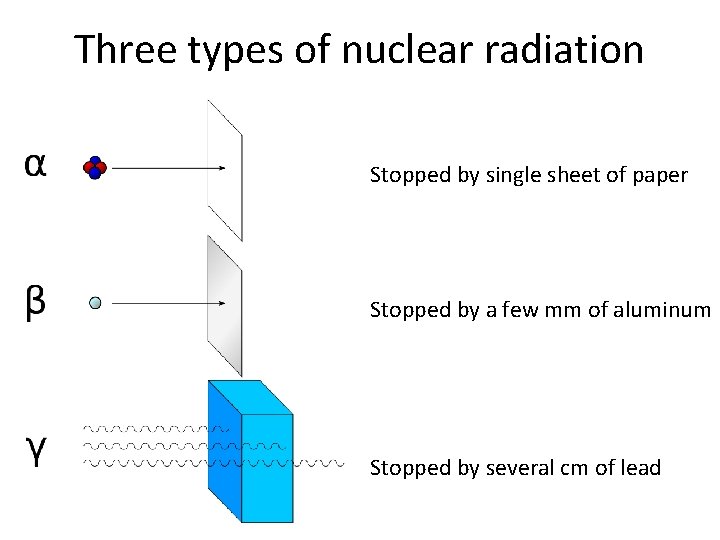
Three types of nuclear radiation Stopped by single sheet of paper Stopped by a few mm of aluminum Stopped by several cm of lead
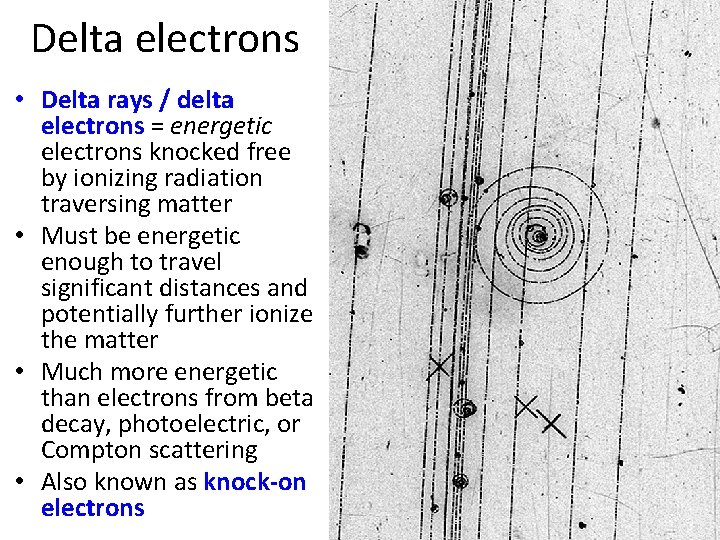
Delta electrons • Delta rays / delta electrons = energetic electrons knocked free by ionizing radiation traversing matter • Must be energetic enough to travel significant distances and potentially further ionize the matter • Much more energetic than electrons from beta decay, photoelectric, or Compton scattering • Also known as knock-on electrons
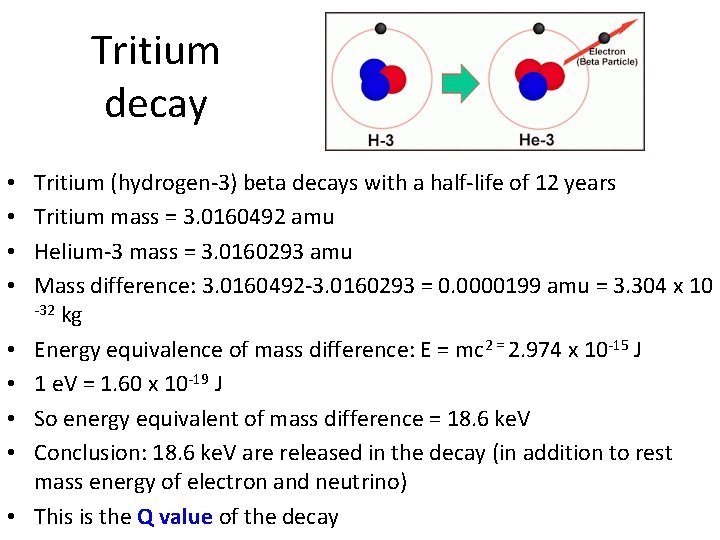
Tritium decay • • • Tritium (hydrogen-3) beta decays with a half-life of 12 years Tritium mass = 3. 0160492 amu Helium-3 mass = 3. 0160293 amu Mass difference: 3. 0160492 -3. 0160293 = 0. 0000199 amu = 3. 304 x 10 -32 kg Energy equivalence of mass difference: E = mc 2 = 2. 974 x 10 -15 J 1 e. V = 1. 60 x 10 -19 J So energy equivalent of mass difference = 18. 6 ke. V Conclusion: 18. 6 ke. V are released in the decay (in addition to rest mass energy of electron and neutrino) This is the Q value of the decay
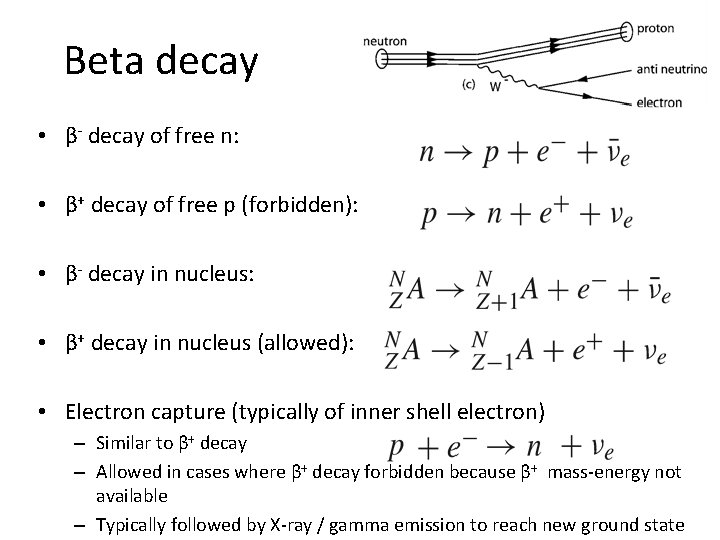
Beta decay • β- decay of free n: • β+ decay of free p (forbidden): • β- decay in nucleus: • β+ decay in nucleus (allowed): • Electron capture (typically of inner shell electron) – Similar to β+ decay – Allowed in cases where β+ decay forbidden because β+ mass-energy not available – Typically followed by X-ray / gamma emission to reach new ground state
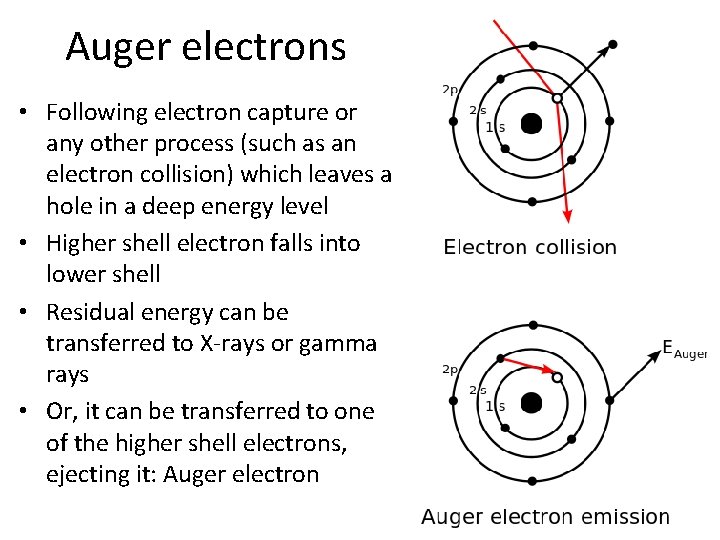
Auger electrons • Following electron capture or any other process (such as an electron collision) which leaves a hole in a deep energy level • Higher shell electron falls into lower shell • Residual energy can be transferred to X-rays or gamma rays • Or, it can be transferred to one of the higher shell electrons, ejecting it: Auger electron
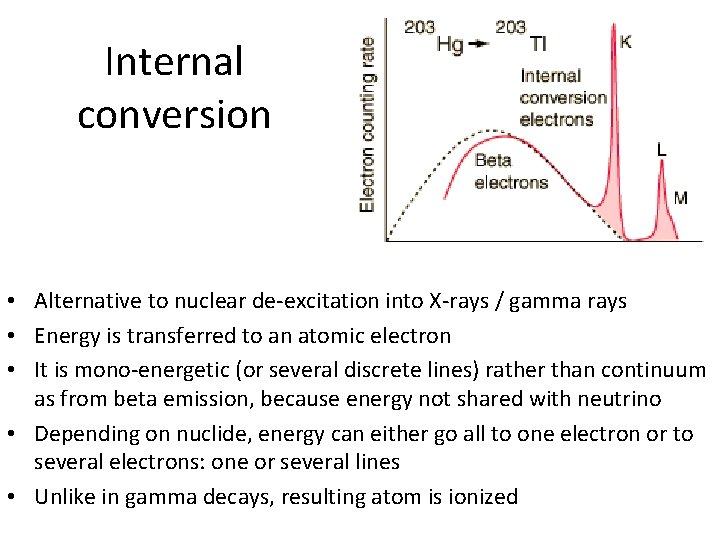
Internal conversion • Alternative to nuclear de-excitation into X-rays / gamma rays • Energy is transferred to an atomic electron • It is mono-energetic (or several discrete lines) rather than continuum as from beta emission, because energy not shared with neutrino • Depending on nuclide, energy can either go all to one electron or to several electrons: one or several lines • Unlike in gamma decays, resulting atom is ionized
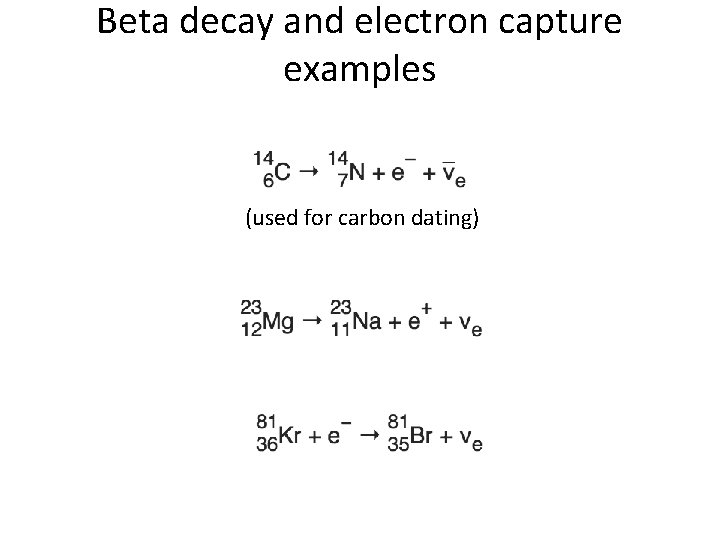
Beta decay and electron capture examples (used for carbon dating)
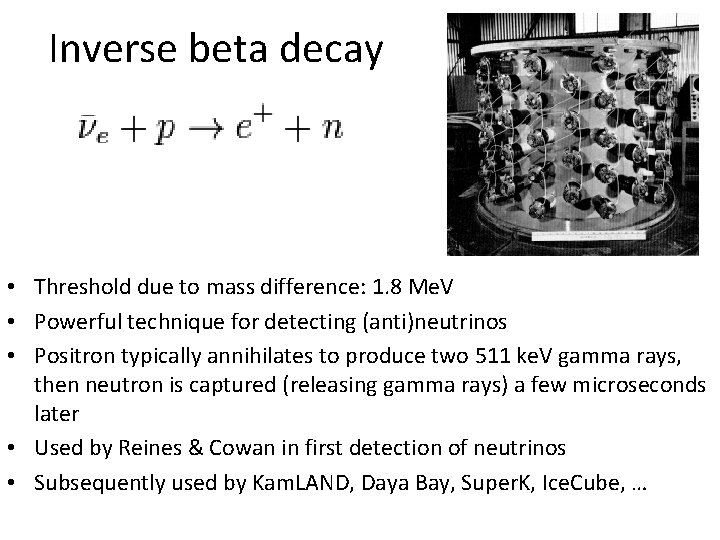
Inverse beta decay • Threshold due to mass difference: 1. 8 Me. V • Powerful technique for detecting (anti)neutrinos • Positron typically annihilates to produce two 511 ke. V gamma rays, then neutron is captured (releasing gamma rays) a few microseconds later • Used by Reines & Cowan in first detection of neutrinos • Subsequently used by Kam. LAND, Daya Bay, Super. K, Ice. Cube, …
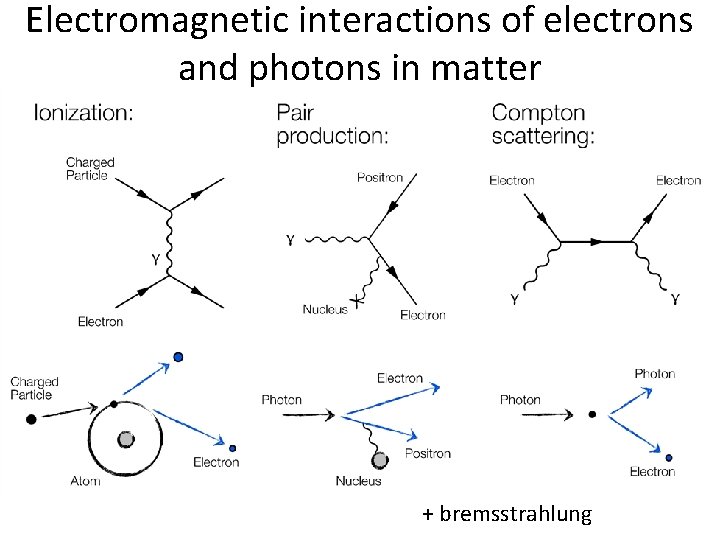
Electromagnetic interactions of electrons and photons in matter + bremsstrahlung
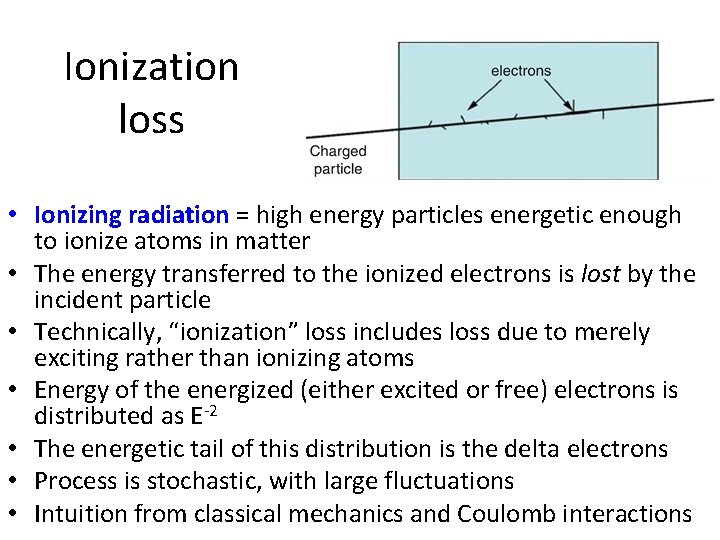
Ionization loss • Ionizing radiation = high energy particles energetic enough to ionize atoms in matter • The energy transferred to the ionized electrons is lost by the incident particle • Technically, “ionization” loss includes loss due to merely exciting rather than ionizing atoms • Energy of the energized (either excited or free) electrons is distributed as E-2 • The energetic tail of this distribution is the delta electrons • Process is stochastic, with large fluctuations • Intuition from classical mechanics and Coulomb interactions
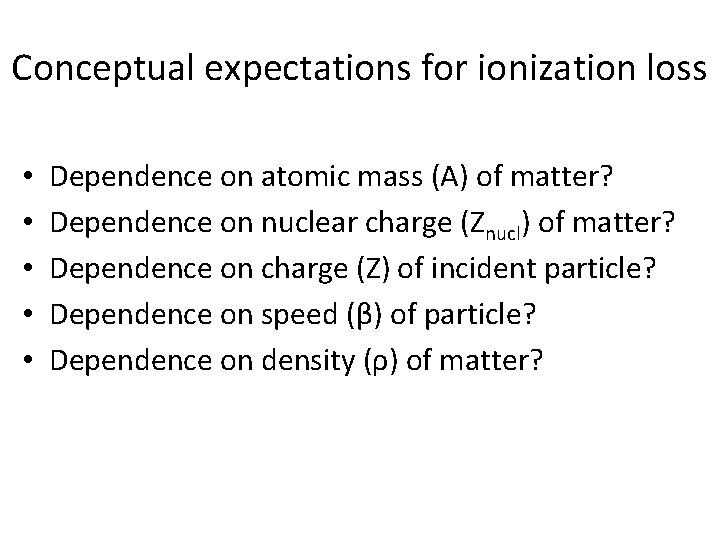
Conceptual expectations for ionization loss • • • Dependence on atomic mass (A) of matter? Dependence on nuclear charge (Znucl) of matter? Dependence on charge (Z) of incident particle? Dependence on speed (β) of particle? Dependence on density (ρ) of matter?
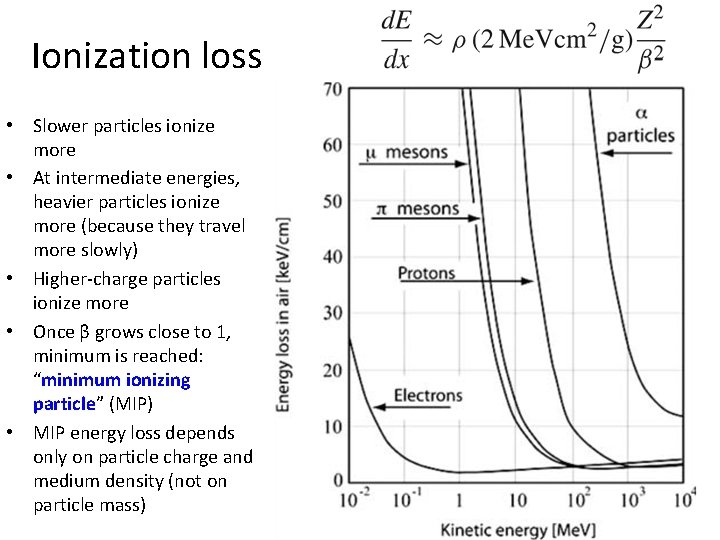
Ionization loss • Slower particles ionize more • At intermediate energies, heavier particles ionize more (because they travel more slowly) • Higher-charge particles ionize more • Once β grows close to 1, minimum is reached: “minimum ionizing particle” (MIP) • MIP energy loss depends only on particle charge and medium density (not on particle mass)
Section 736(b) payments
Experiment 736
736
Cherenkov spectrum
Lesson 15 nuclear quest nuclear reactions
Fisión nuclear vs fision nuclear
Experimental vs non experimental
Experimental research design types
Experimental vs non experimental research
Experimental vs nonexperimental
Nonexperimental study
Quantum and nuclear physics
Experimental physics and industrial control system
Experimental physics and industrial control system
Experimental physics and industrial control system
Pmt particle physics
Mark thomson modern particle physics
Particle physics
Particle physics practice quiz
Particle physics timeline
Concezio bozzi
Cern particle physics
European laboratory for particle physics
Cern particle physics