Introduction to Ultrarelativistic HeavyIon Physics at CERNSPS Lecture
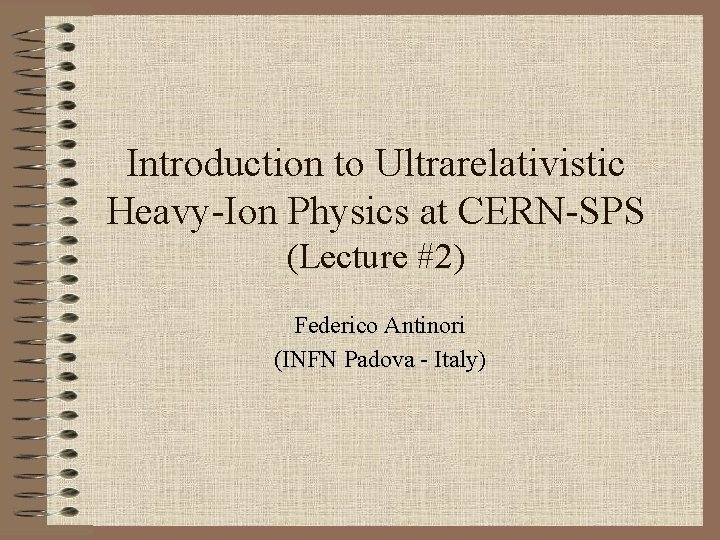
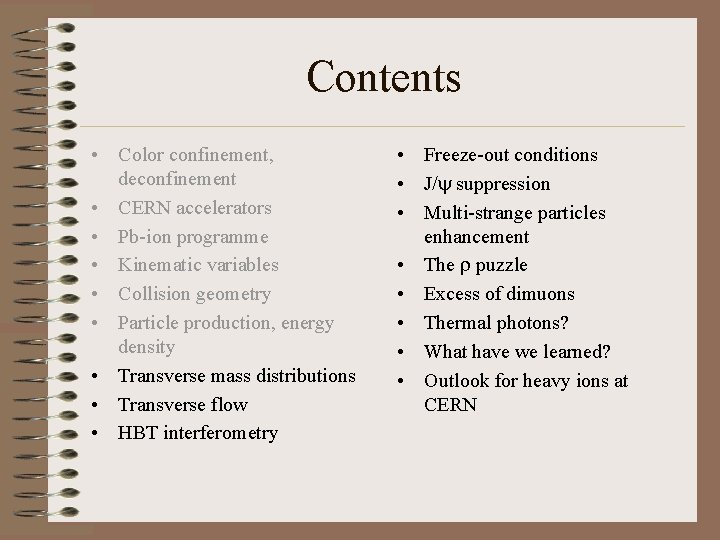
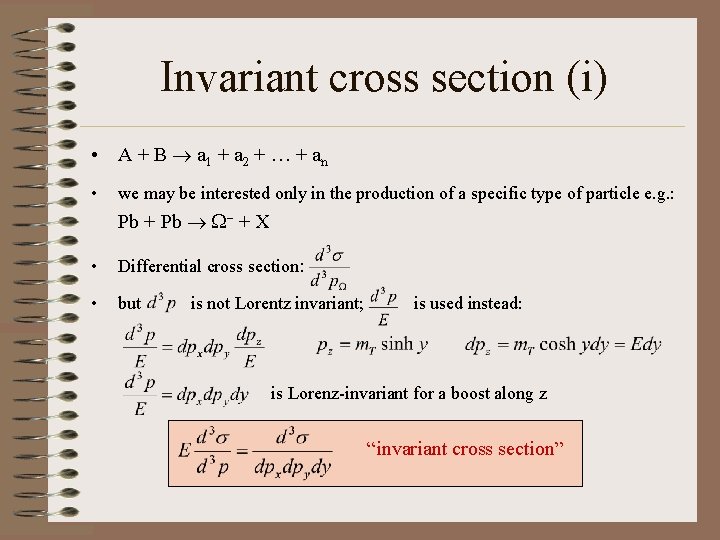
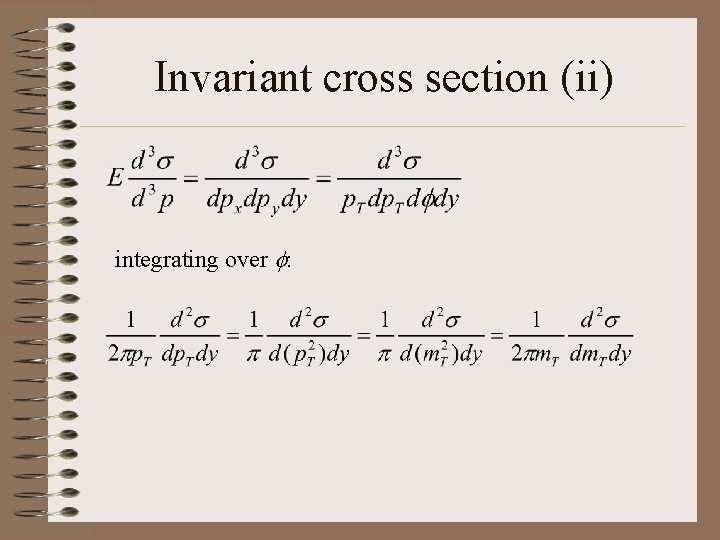
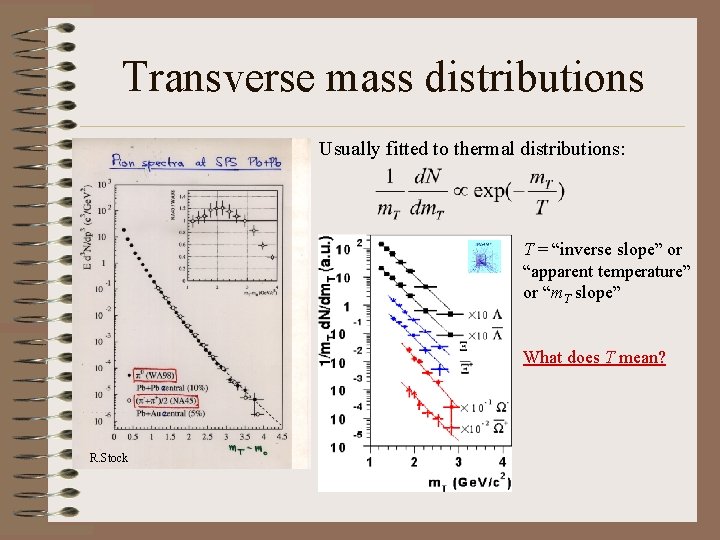
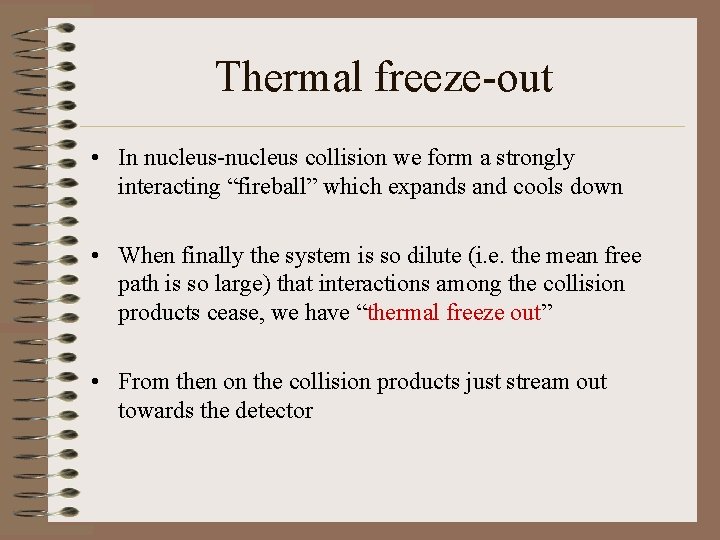
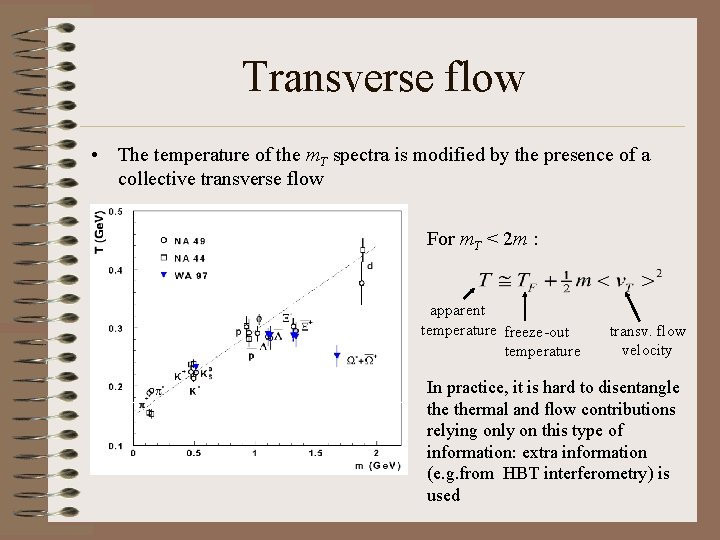
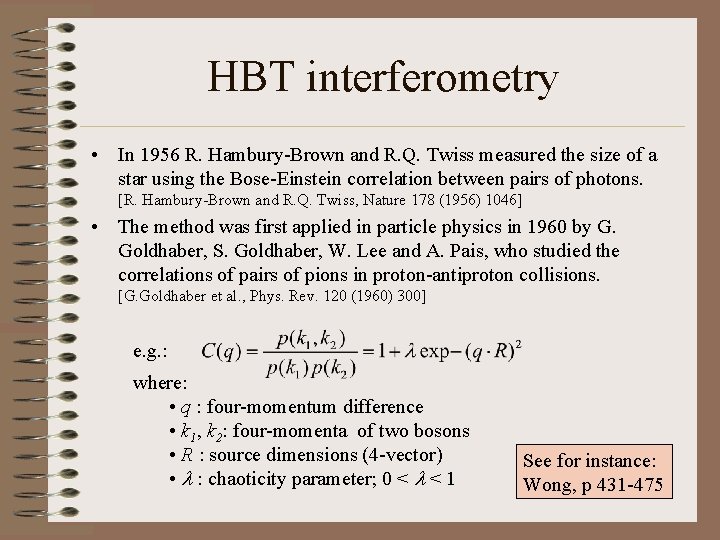
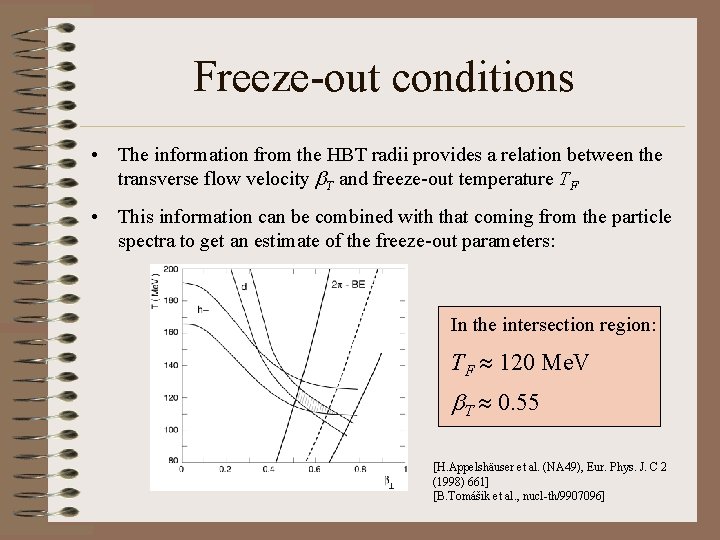
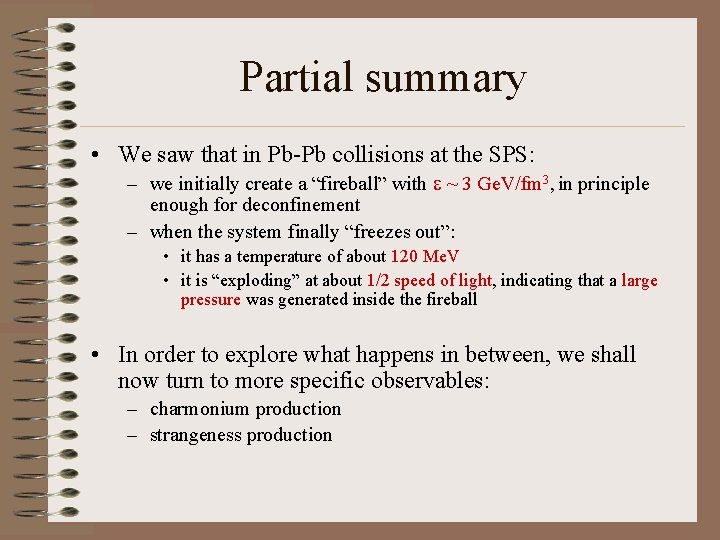
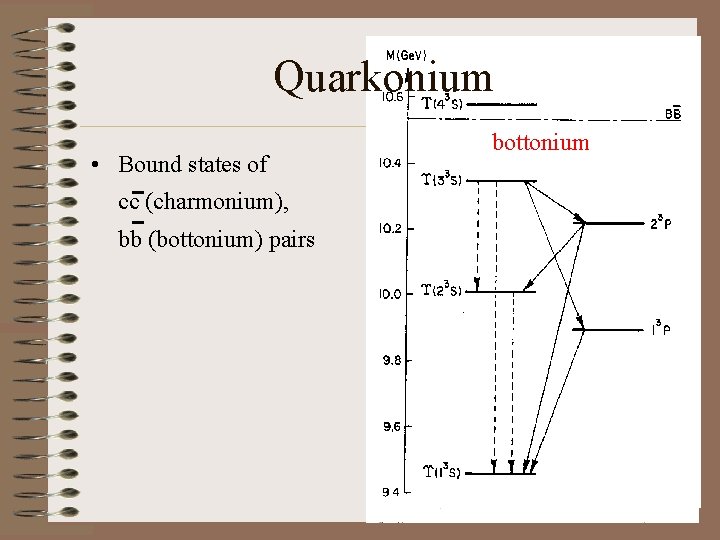
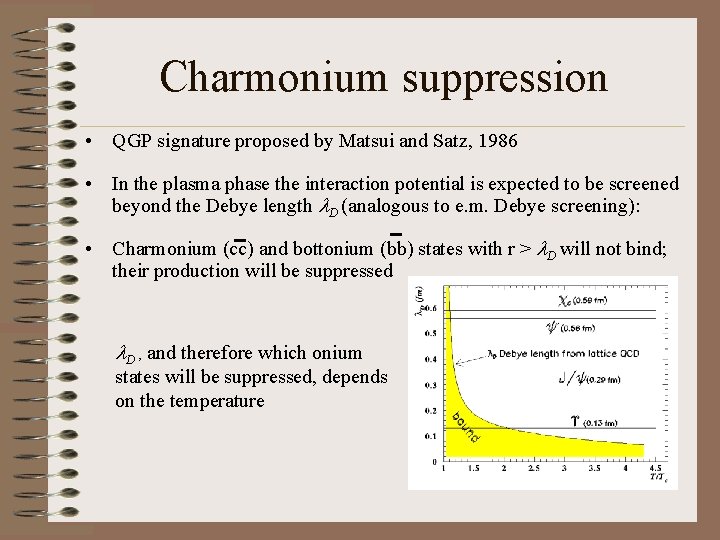
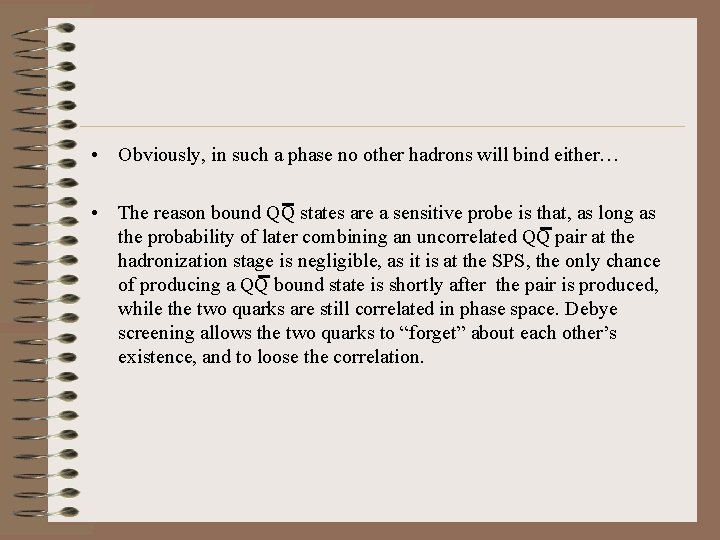
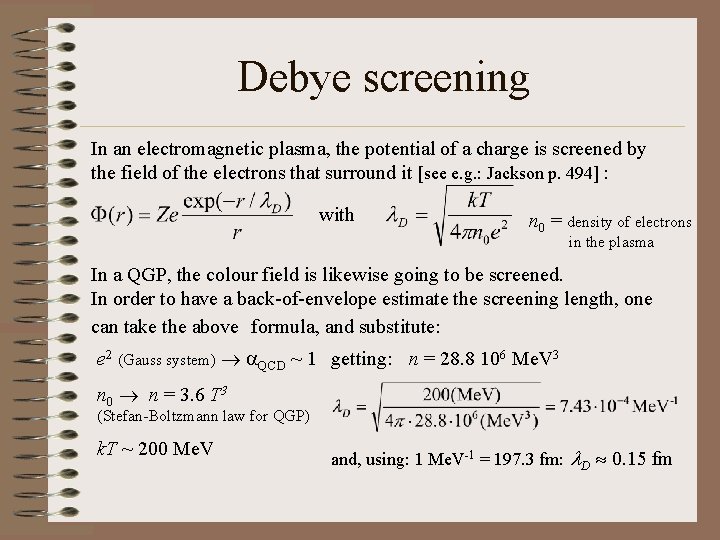
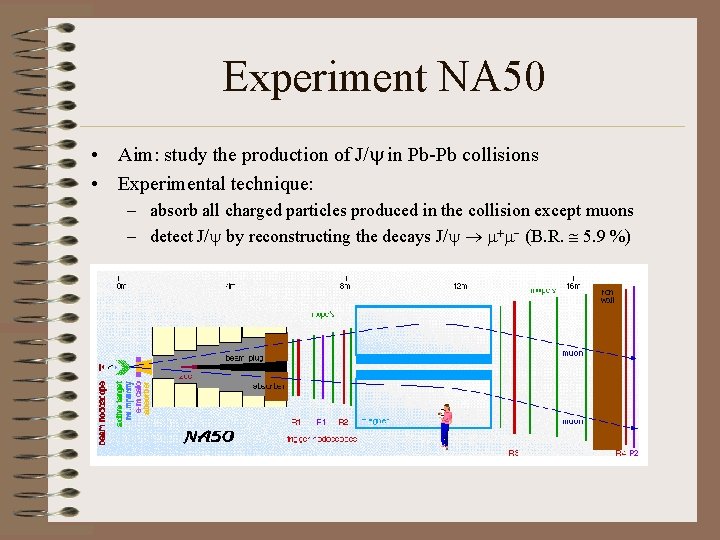
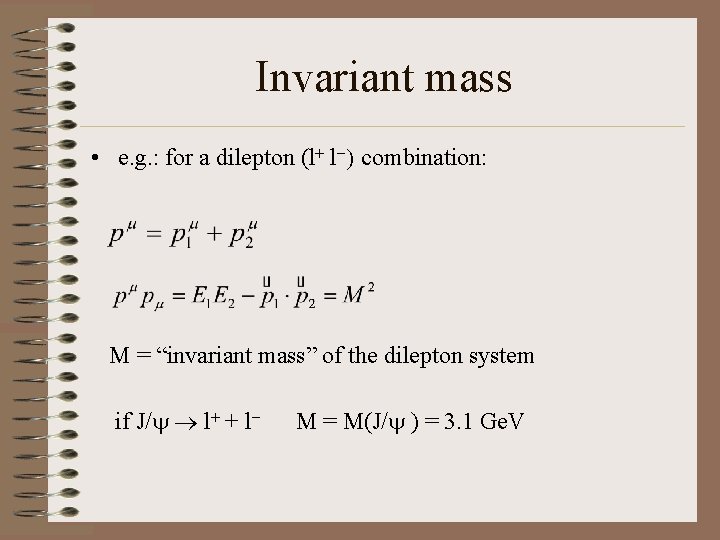
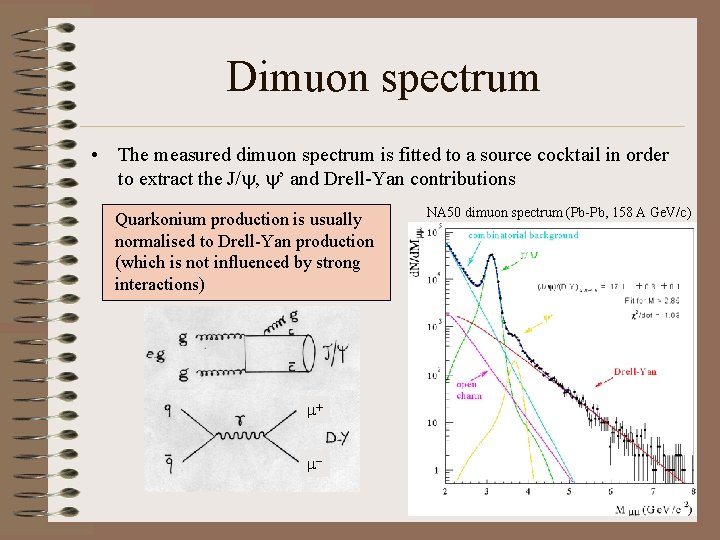
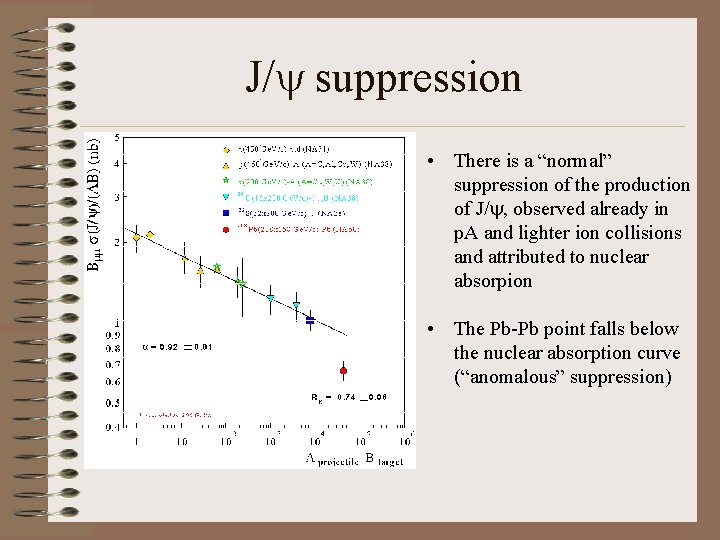
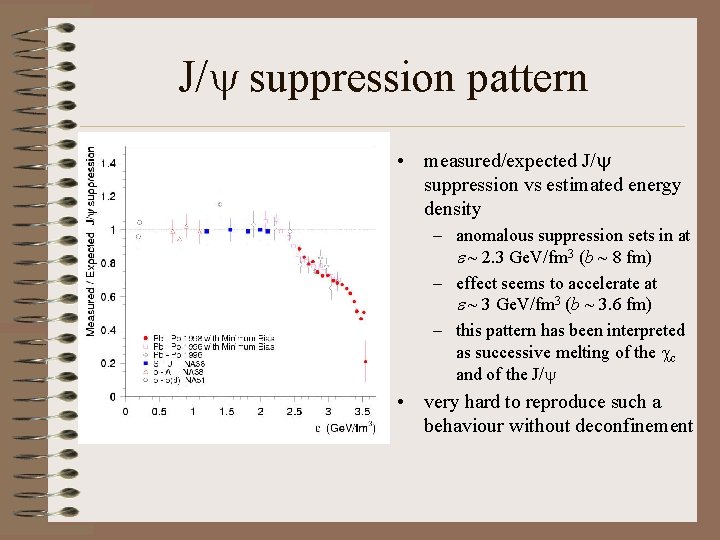
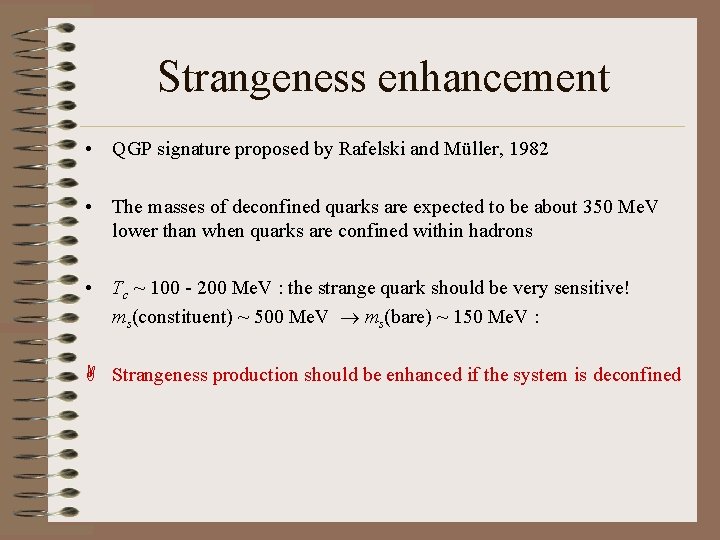
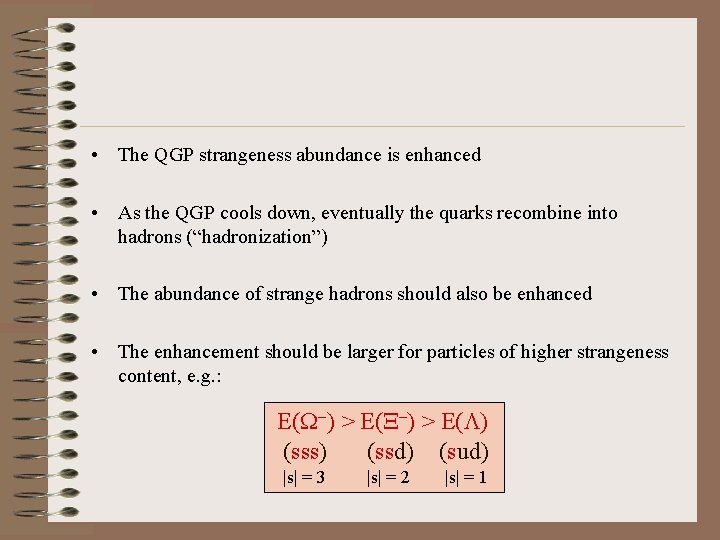
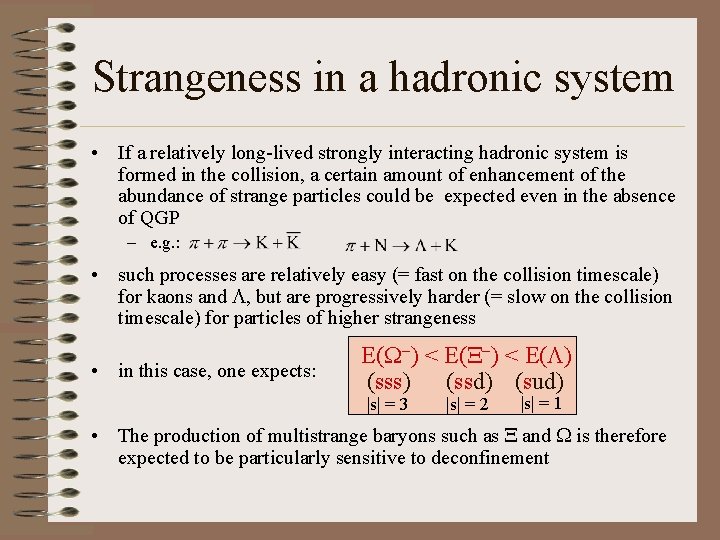
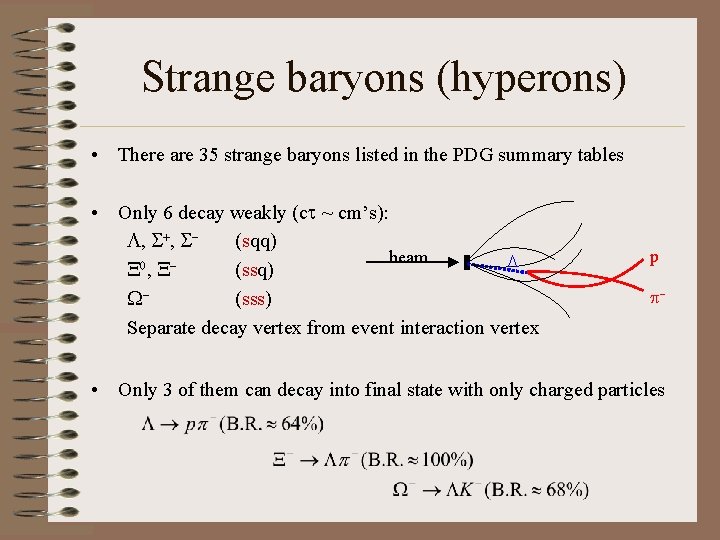
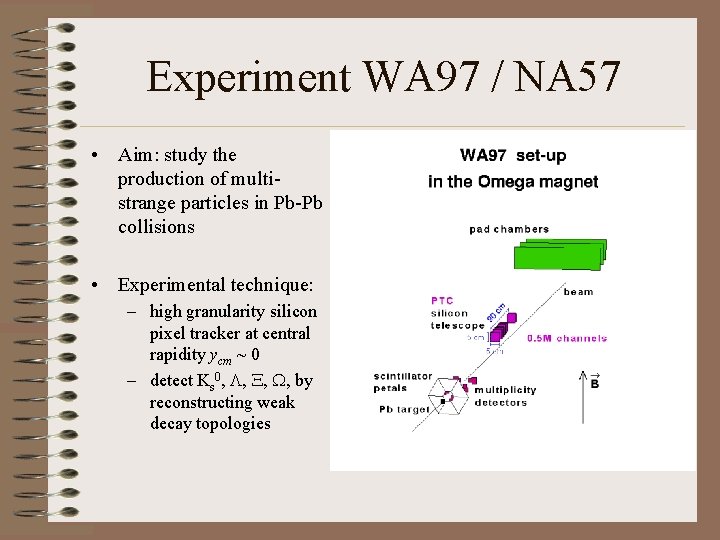
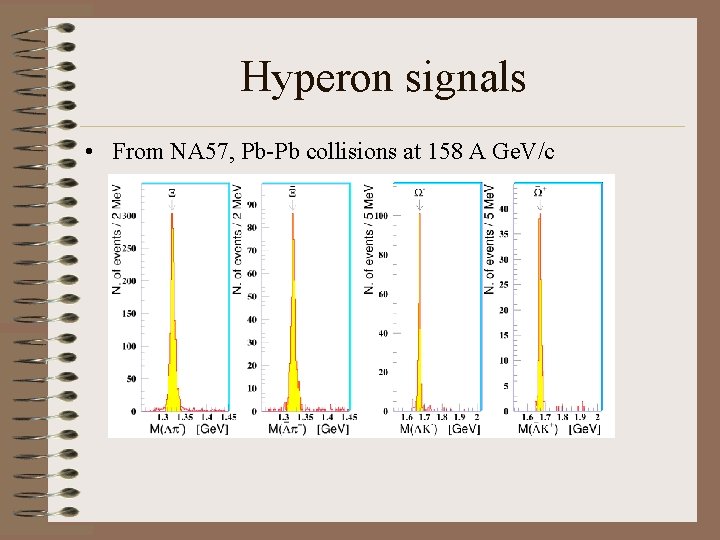
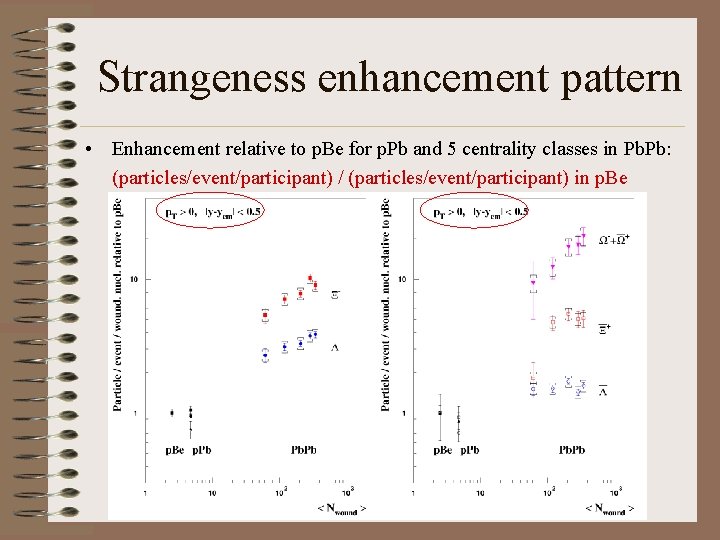
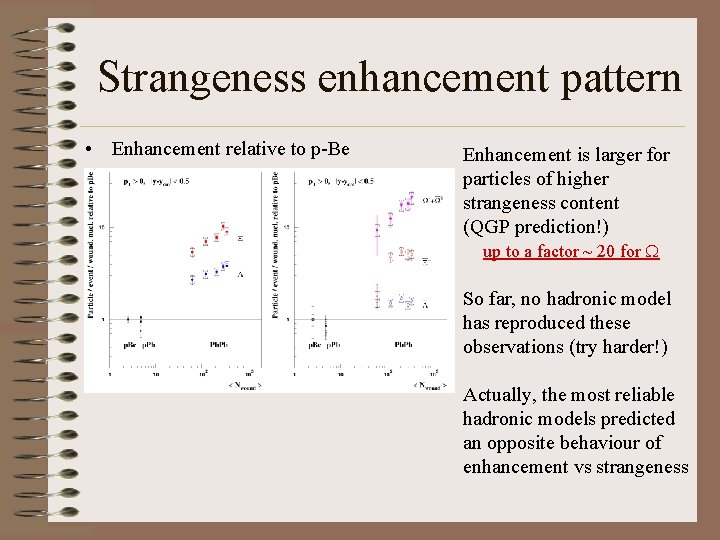
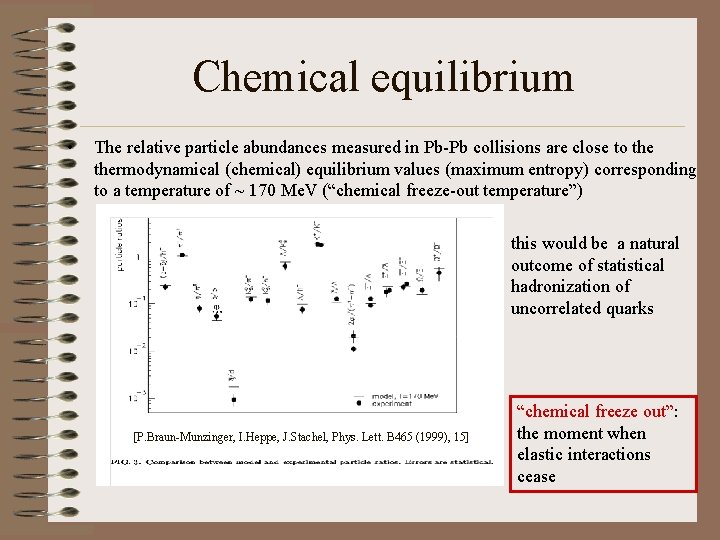
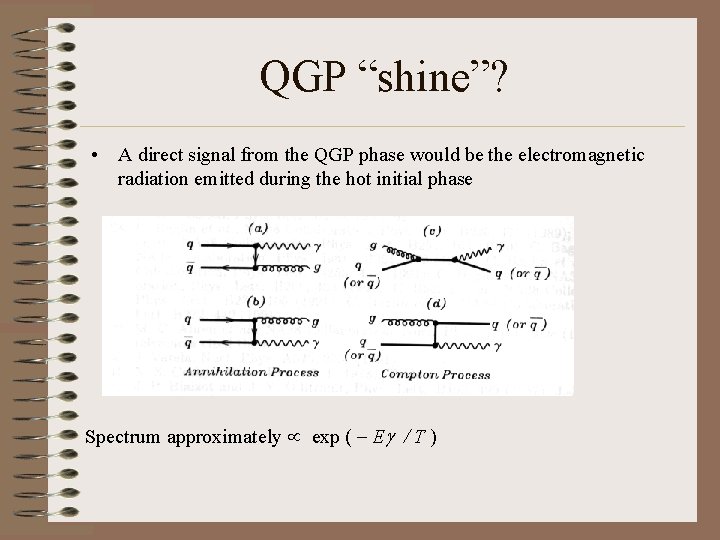
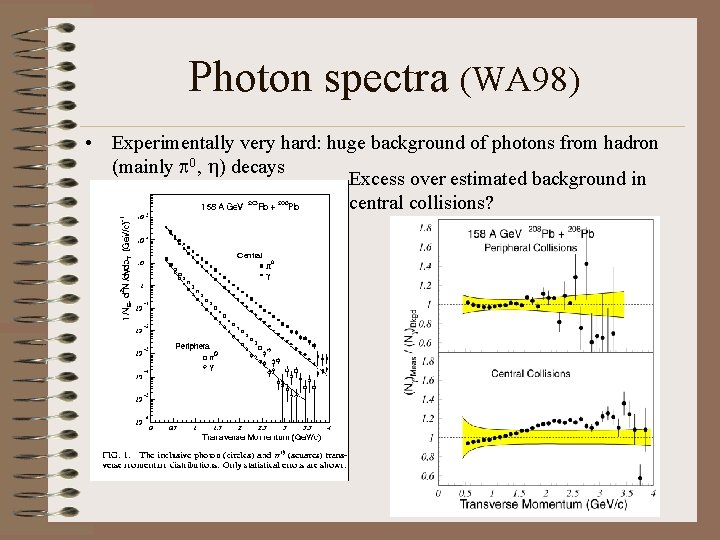
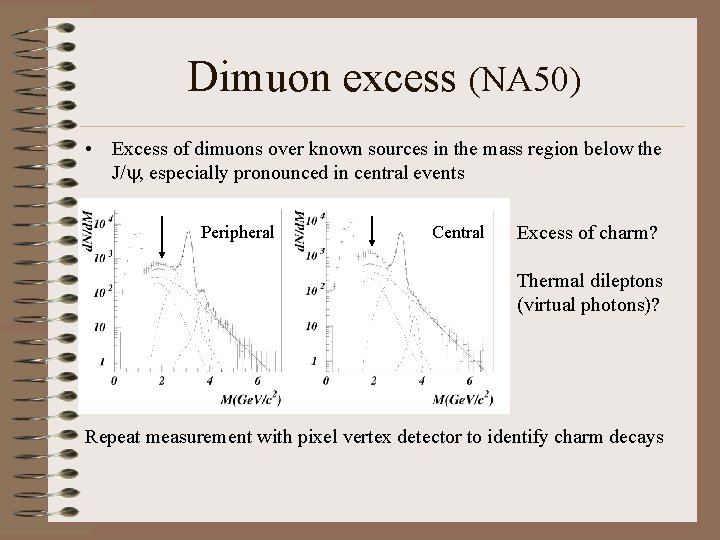
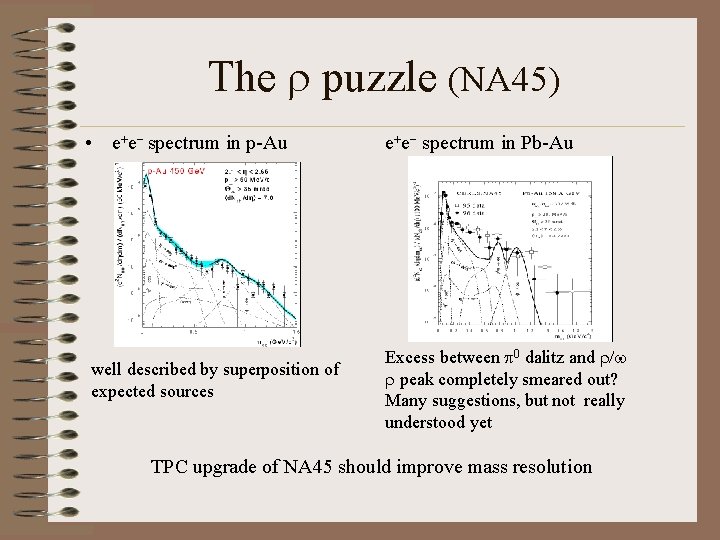
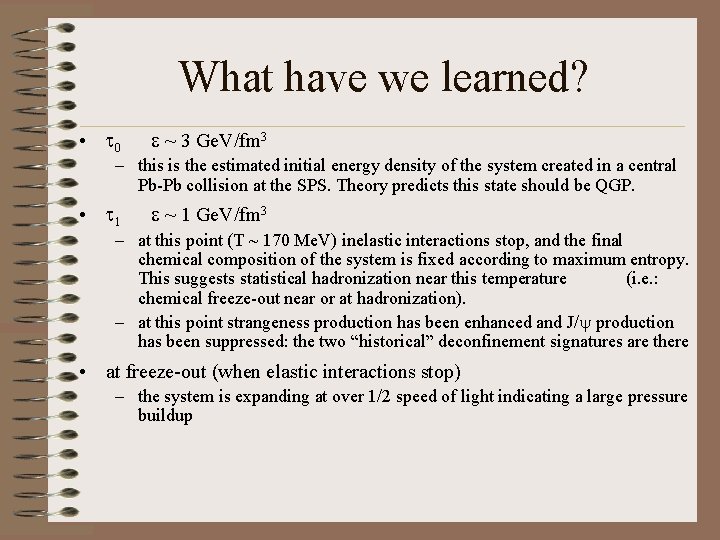
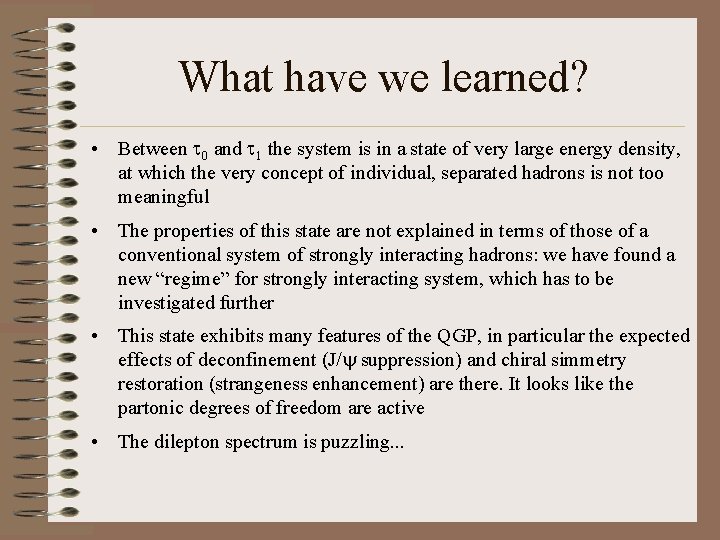
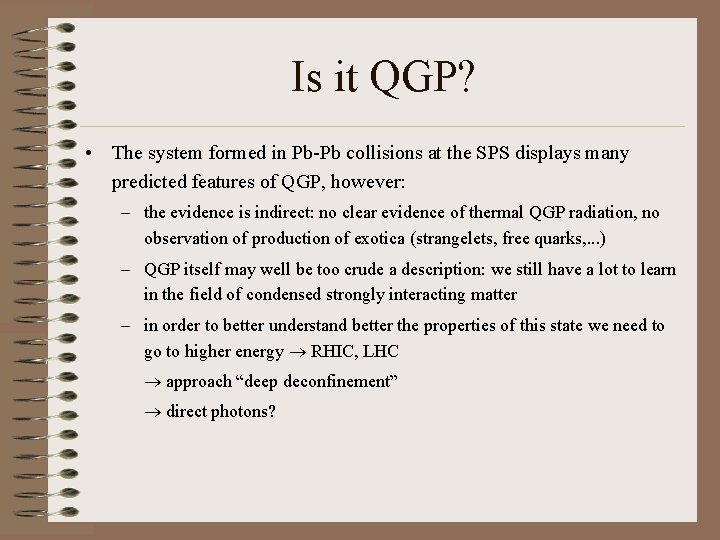
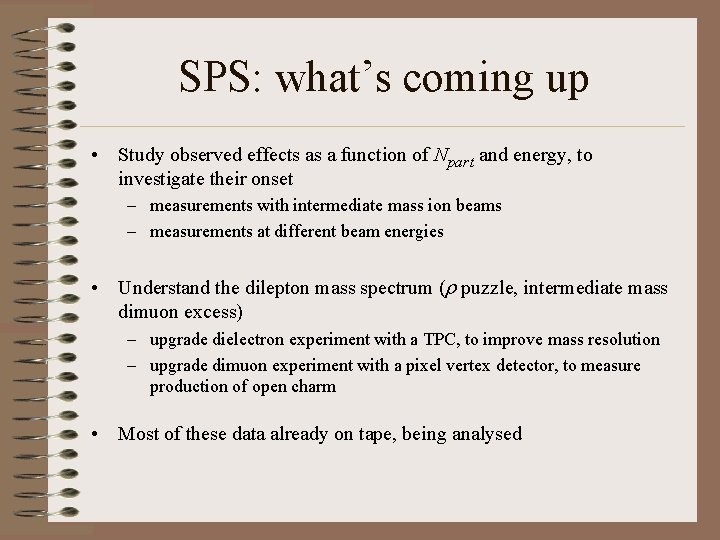
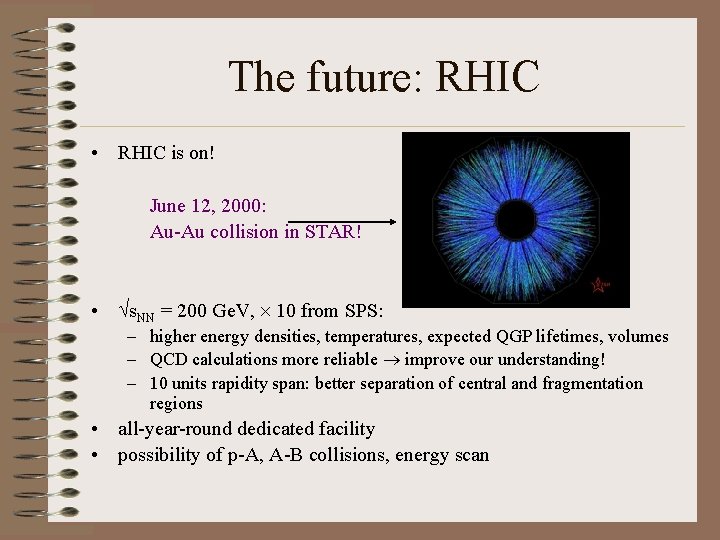
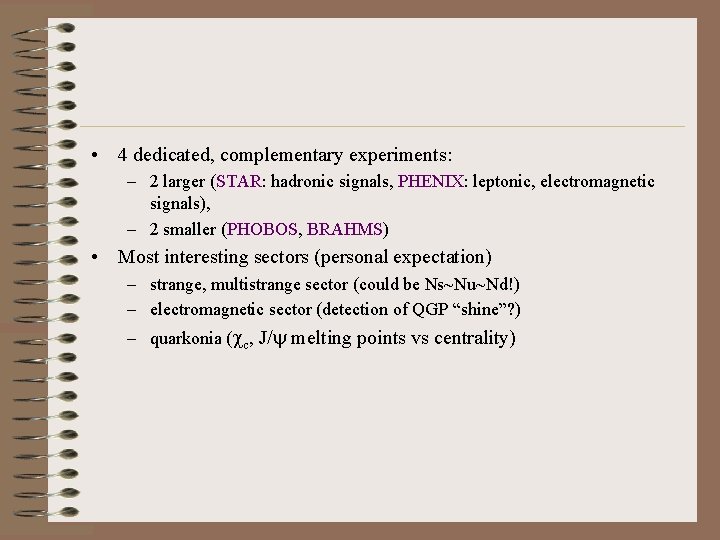
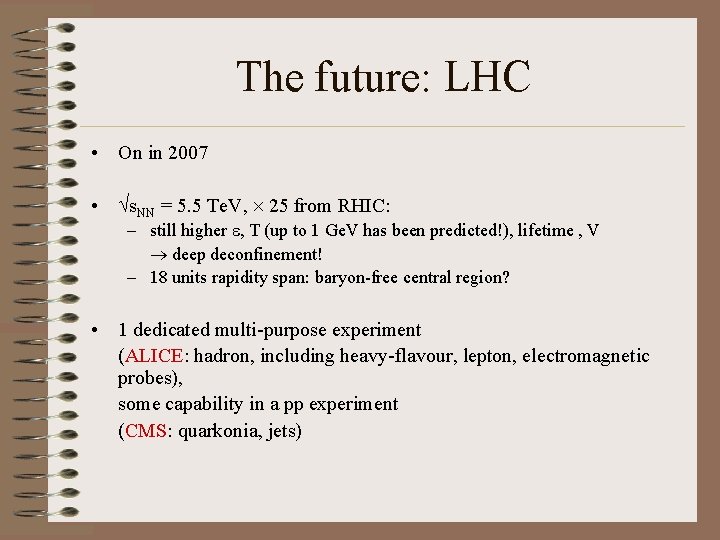
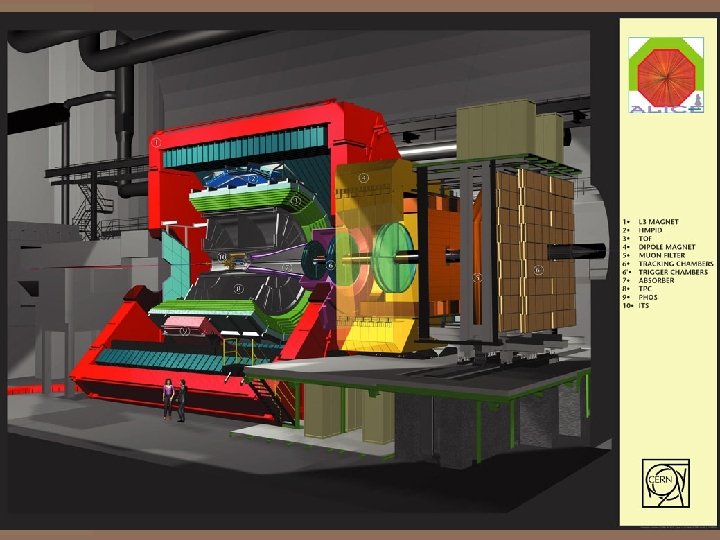
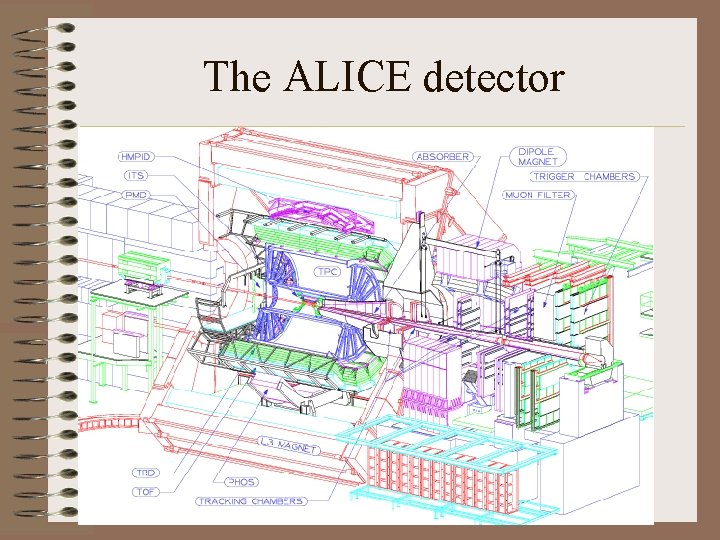
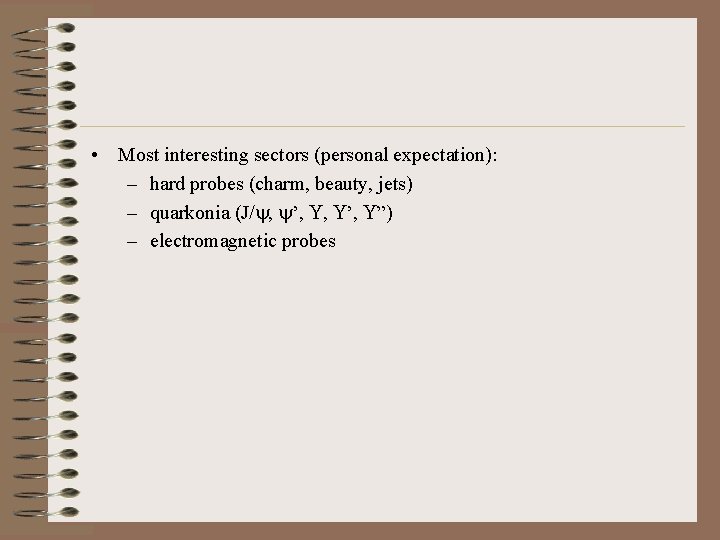
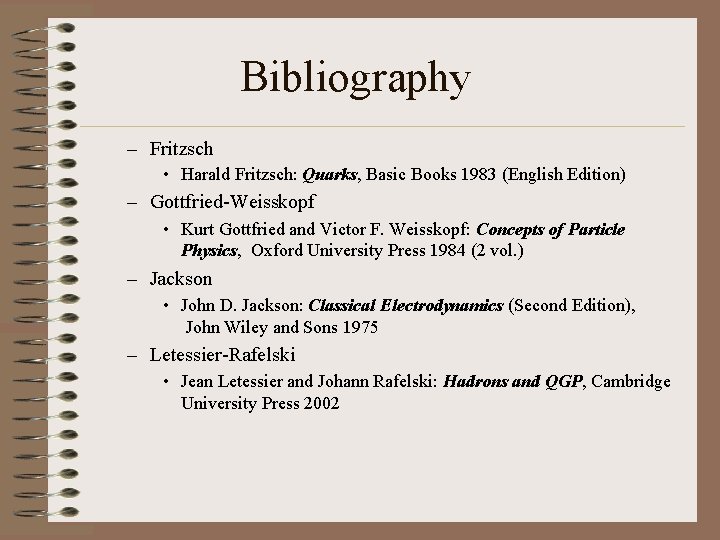
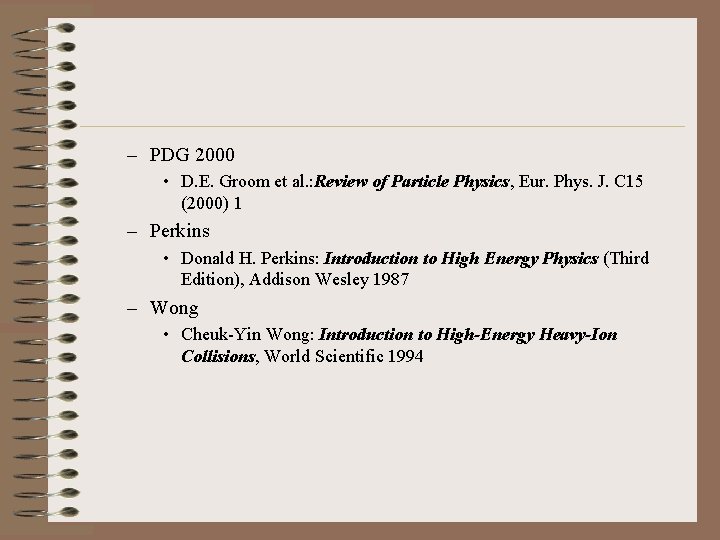
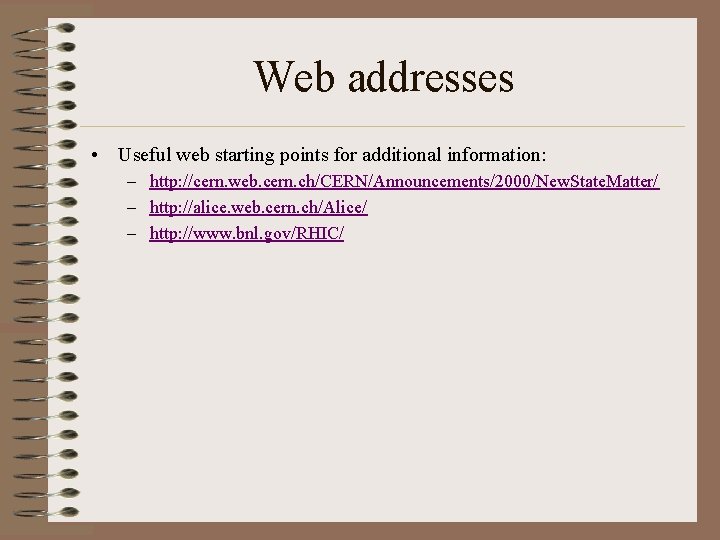
- Slides: 45
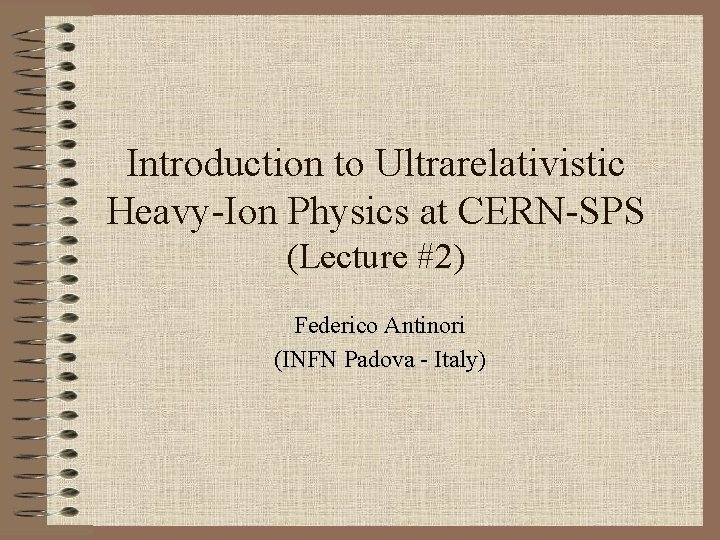
Introduction to Ultrarelativistic Heavy-Ion Physics at CERN-SPS (Lecture #2) Federico Antinori (INFN Padova - Italy)
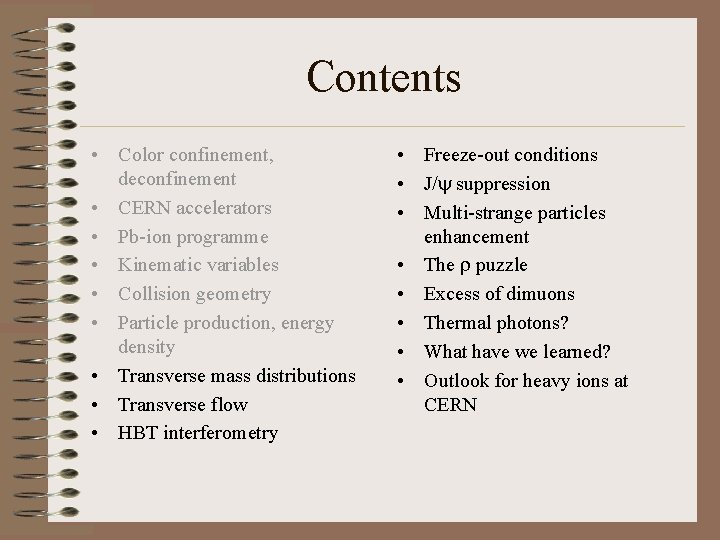
Contents • Color confinement, deconfinement • CERN accelerators • Pb-ion programme • Kinematic variables • Collision geometry • Particle production, energy density • Transverse mass distributions • Transverse flow • HBT interferometry • Freeze-out conditions • J/y suppression • Multi-strange particles enhancement • The r puzzle • Excess of dimuons • Thermal photons? • What have we learned? • Outlook for heavy ions at CERN
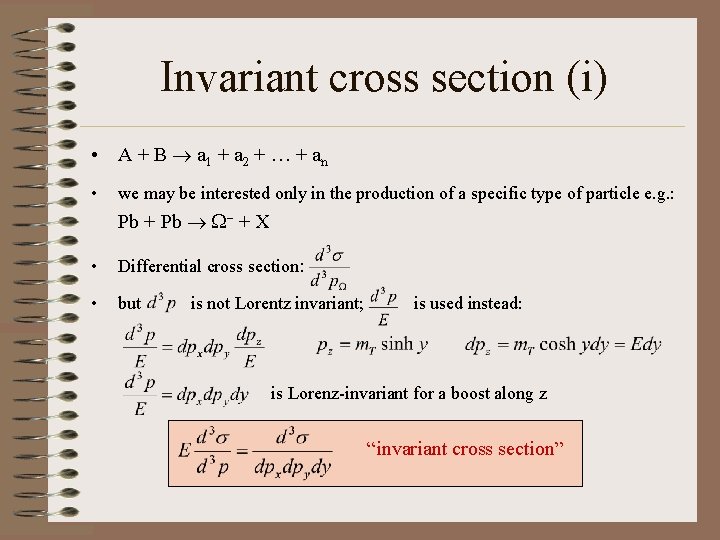
Invariant cross section (i) • A + B a 1 + a 2 + … + a n • we may be interested only in the production of a specific type of particle e. g. : Pb + Pb W- + X • Differential cross section: • but is not Lorentz invariant; is used instead: is Lorenz-invariant for a boost along z “invariant cross section”
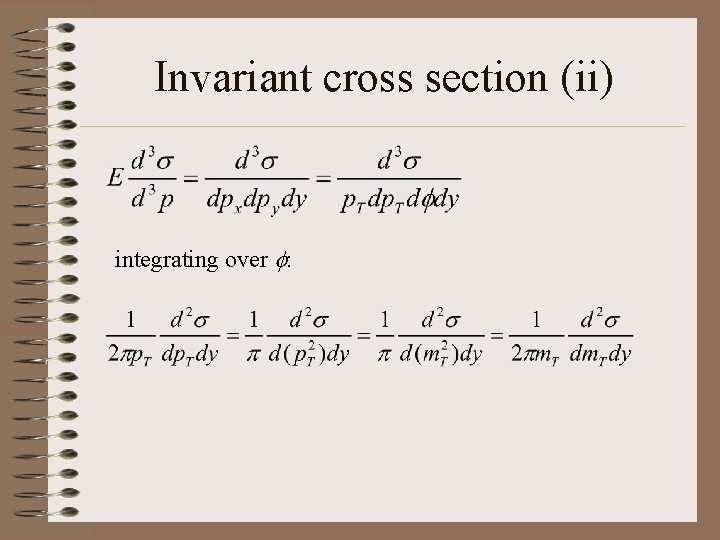
Invariant cross section (ii) integrating over f:
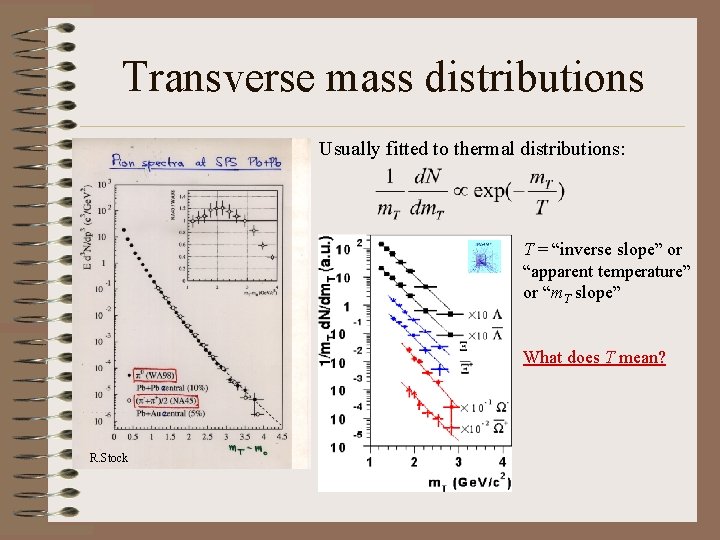
Transverse mass distributions Usually fitted to thermal distributions: T = “inverse slope” or “apparent temperature” or “m. T slope” What does T mean? R. Stock
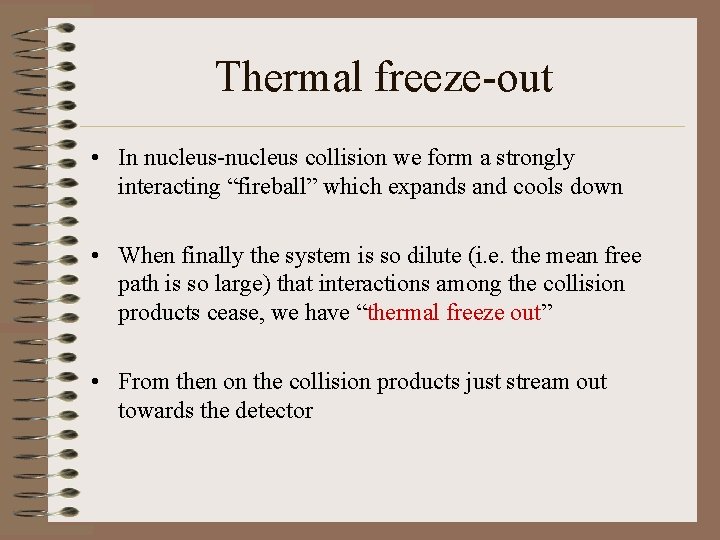
Thermal freeze-out • In nucleus-nucleus collision we form a strongly interacting “fireball” which expands and cools down • When finally the system is so dilute (i. e. the mean free path is so large) that interactions among the collision products cease, we have “thermal freeze out” • From then on the collision products just stream out towards the detector
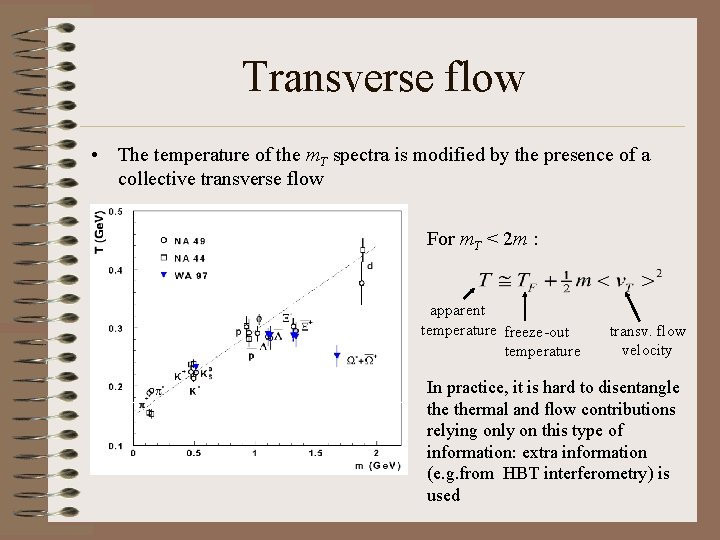
Transverse flow • The temperature of the m. T spectra is modified by the presence of a collective transverse flow For m. T < 2 m : apparent temperature freeze-out temperature transv. flow velocity In practice, it is hard to disentangle thermal and flow contributions relying only on this type of information: extra information (e. g. from HBT interferometry) is used
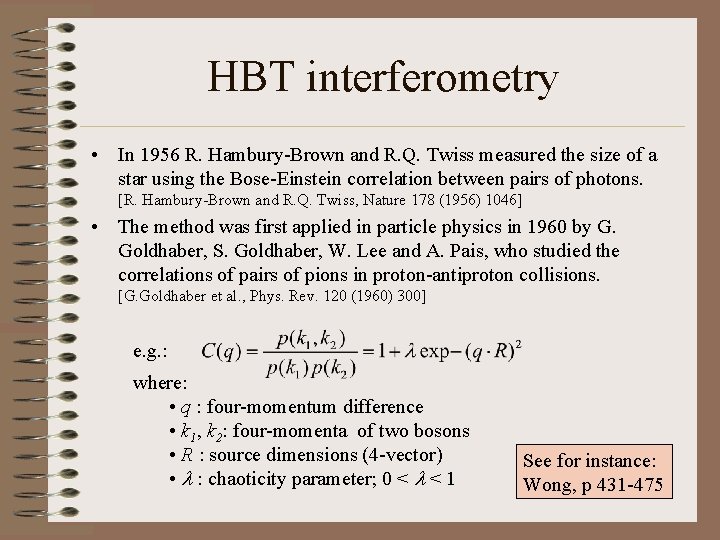
HBT interferometry • In 1956 R. Hambury-Brown and R. Q. Twiss measured the size of a star using the Bose-Einstein correlation between pairs of photons. [R. Hambury-Brown and R. Q. Twiss, Nature 178 (1956) 1046] • The method was first applied in particle physics in 1960 by G. Goldhaber, S. Goldhaber, W. Lee and A. Pais, who studied the correlations of pairs of pions in proton-antiproton collisions. [G. Goldhaber et al. , Phys. Rev. 120 (1960) 300] e. g. : where: • q : four-momentum difference • k 1, k 2: four-momenta of two bosons • R : source dimensions (4 -vector) • l : chaoticity parameter; 0 < l < 1 See for instance: Wong, p 431 -475
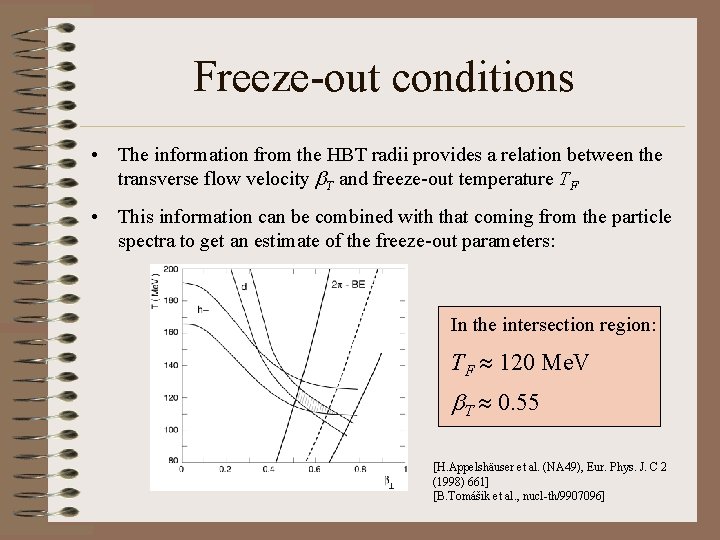
Freeze-out conditions • The information from the HBT radii provides a relation between the transverse flow velocity b. T and freeze-out temperature TF • This information can be combined with that coming from the particle spectra to get an estimate of the freeze-out parameters: In the intersection region: TF 120 Me. V b. T 0. 55 [H. Appelshäuser et al. (NA 49), Eur. Phys. J. C 2 (1998) 661] [B. Tomášik et al. , nucl-th/9907096]
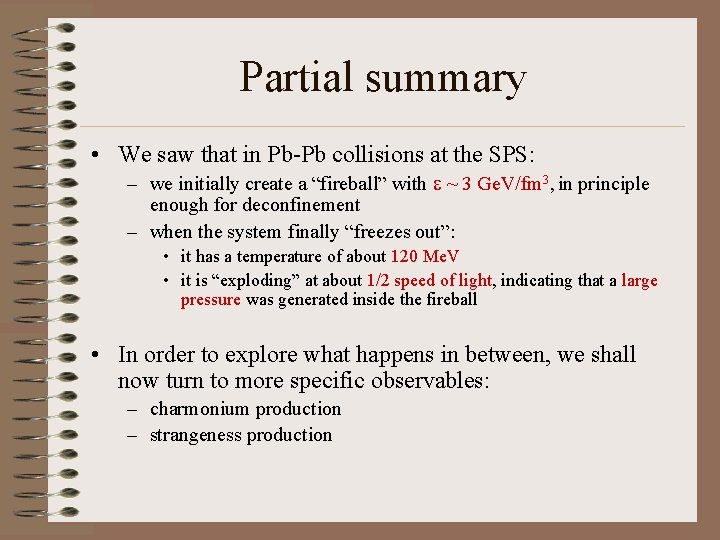
Partial summary • We saw that in Pb-Pb collisions at the SPS: – we initially create a “fireball” with e ~ 3 Ge. V/fm 3, in principle enough for deconfinement – when the system finally “freezes out”: • it has a temperature of about 120 Me. V • it is “exploding” at about 1/2 speed of light, indicating that a large pressure was generated inside the fireball • In order to explore what happens in between, we shall now turn to more specific observables: – charmonium production – strangeness production
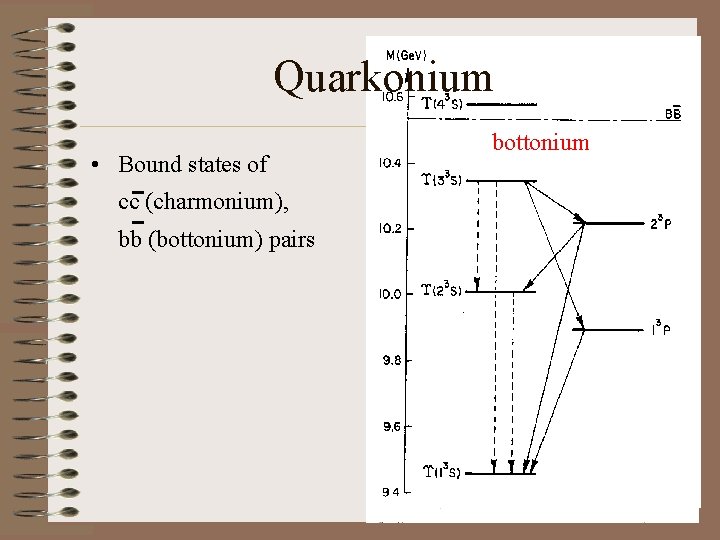
Quarkonium • Bound states of cc (charmonium), bb (bottonium) pairs bottonium charmonium
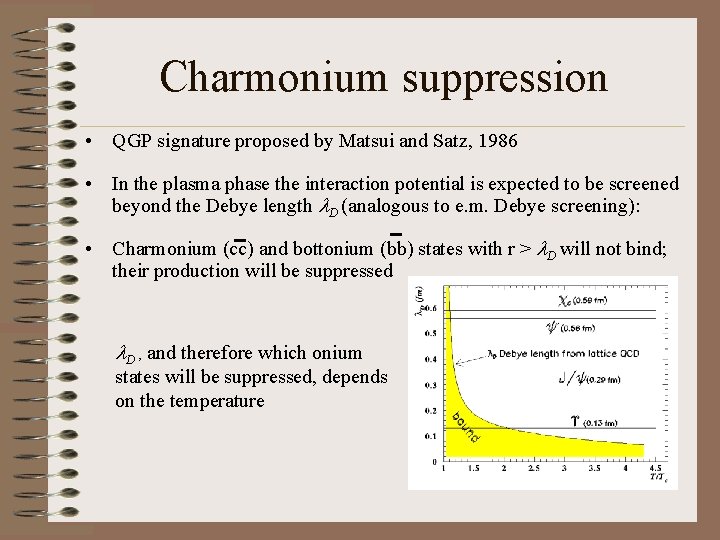
Charmonium suppression • QGP signature proposed by Matsui and Satz, 1986 • In the plasma phase the interaction potential is expected to be screened beyond the Debye length l. D (analogous to e. m. Debye screening): • Charmonium (cc) and bottonium (bb) states with r > l. D will not bind; their production will be suppressed l. D , and therefore which onium states will be suppressed, depends on the temperature
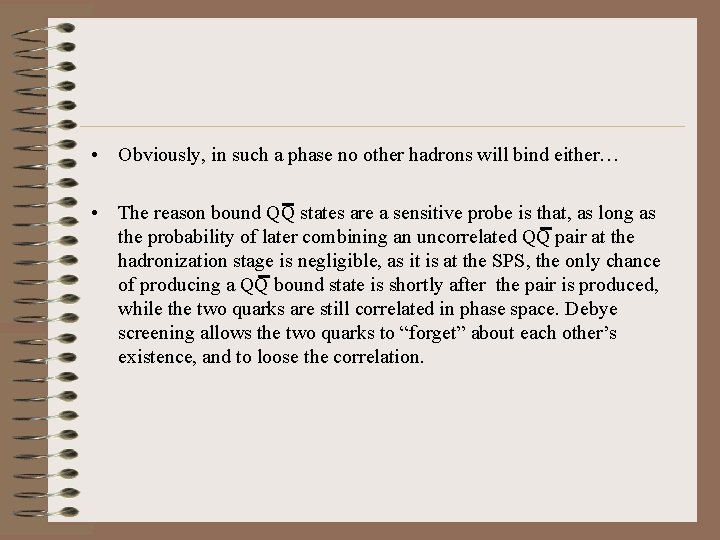
• Obviously, in such a phase no other hadrons will bind either… • The reason bound QQ states are a sensitive probe is that, as long as the probability of later combining an uncorrelated QQ pair at the hadronization stage is negligible, as it is at the SPS, the only chance of producing a QQ bound state is shortly after the pair is produced, while the two quarks are still correlated in phase space. Debye screening allows the two quarks to “forget” about each other’s existence, and to loose the correlation.
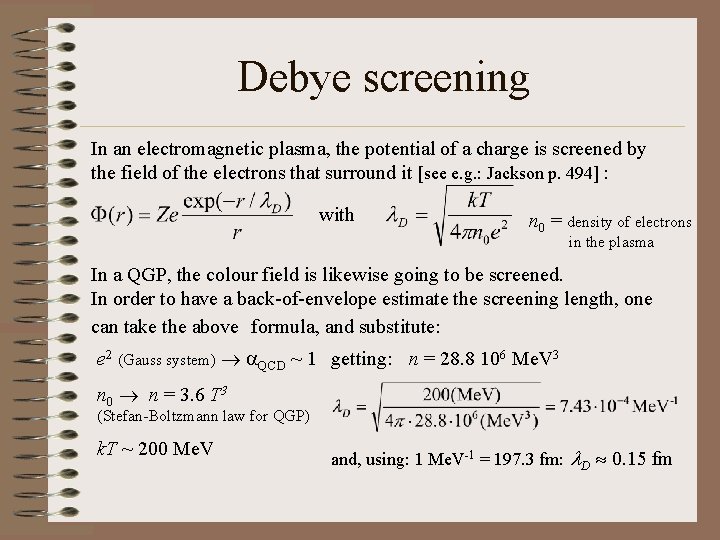
Debye screening In an electromagnetic plasma, the potential of a charge is screened by the field of the electrons that surround it [see e. g. : Jackson p. 494] : with n 0 = density of electrons in the plasma In a QGP, the colour field is likewise going to be screened. In order to have a back-of-envelope estimate the screening length, one can take the above formula, and substitute: e 2 (Gauss system) a. QCD ~ 1 getting: n = 28. 8 106 Me. V 3 n 0 n = 3. 6 T 3 (Stefan-Boltzmann law for QGP) k. T ~ 200 Me. V and, using: 1 Me. V-1 = 197. 3 fm: l. D 0. 15 fm
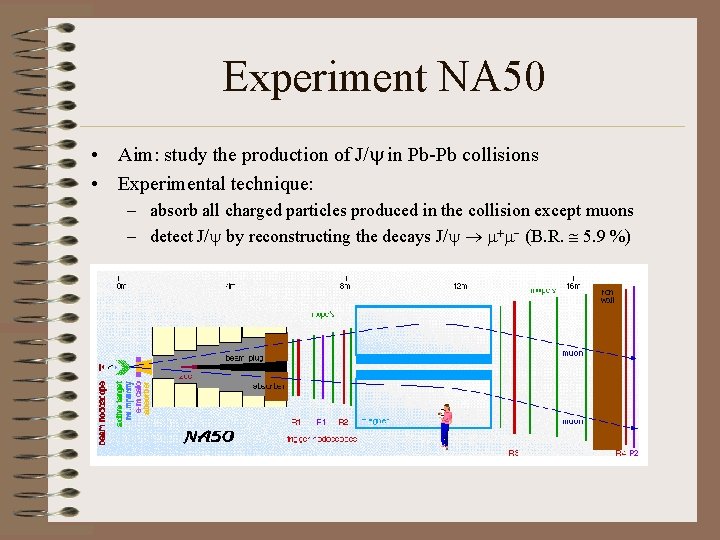
Experiment NA 50 • Aim: study the production of J/y in Pb-Pb collisions • Experimental technique: – absorb all charged particles produced in the collision except muons – detect J/y by reconstructing the decays J/y m+m- (B. R. 5. 9 %)
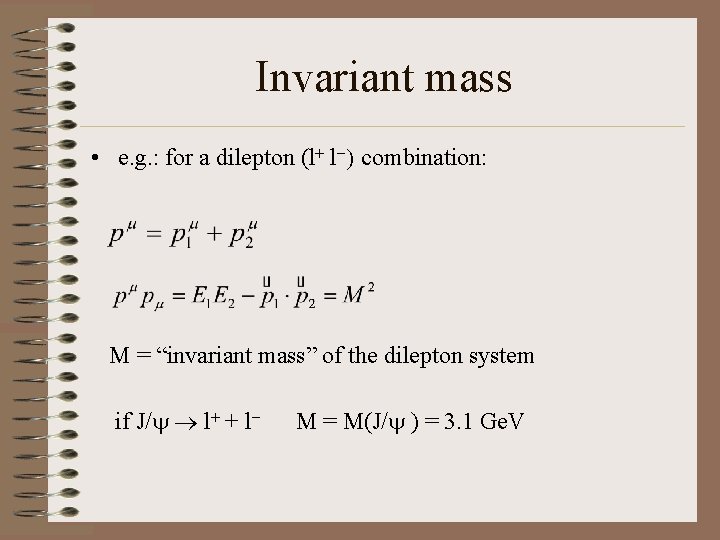
Invariant mass • e. g. : for a dilepton (l+ l-) combination: M = “invariant mass” of the dilepton system if J/y l+ + l- M = M(J/y ) = 3. 1 Ge. V
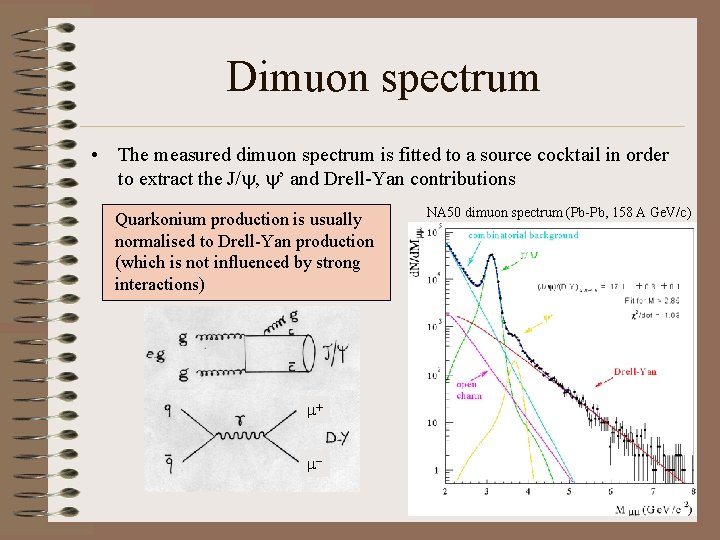
Dimuon spectrum • The measured dimuon spectrum is fitted to a source cocktail in order to extract the J/y, y’ and Drell-Yan contributions Quarkonium production is usually normalised to Drell-Yan production (which is not influenced by strong interactions) m+ m- NA 50 dimuon spectrum (Pb-Pb, 158 A Ge. V/c)
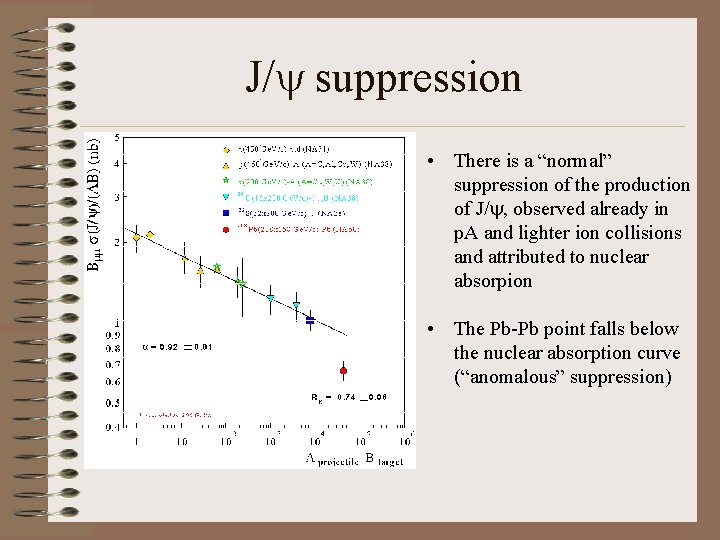
J/y suppression • There is a “normal” suppression of the production of J/y, observed already in p. A and lighter ion collisions and attributed to nuclear absorpion • The Pb-Pb point falls below the nuclear absorption curve (“anomalous” suppression)
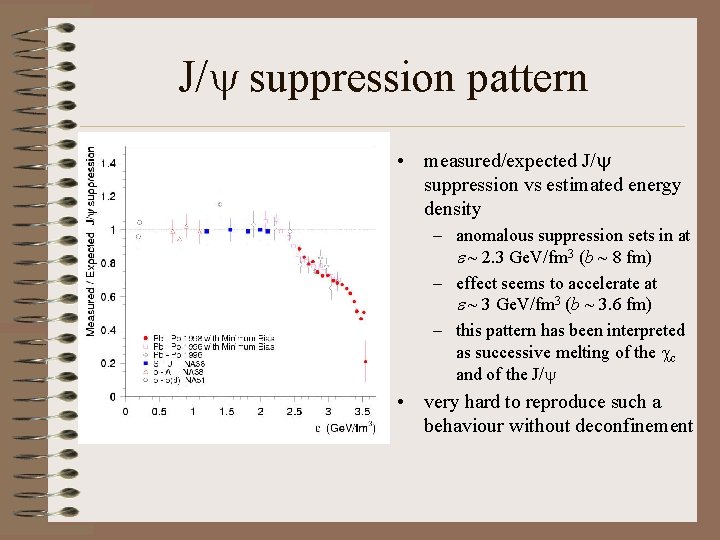
J/y suppression pattern • measured/expected J/y suppression vs estimated energy density – anomalous suppression sets in at e ~ 2. 3 Ge. V/fm 3 (b ~ 8 fm) – effect seems to accelerate at e ~ 3 Ge. V/fm 3 (b ~ 3. 6 fm) – this pattern has been interpreted as successive melting of the cc and of the J/y • very hard to reproduce such a behaviour without deconfinement
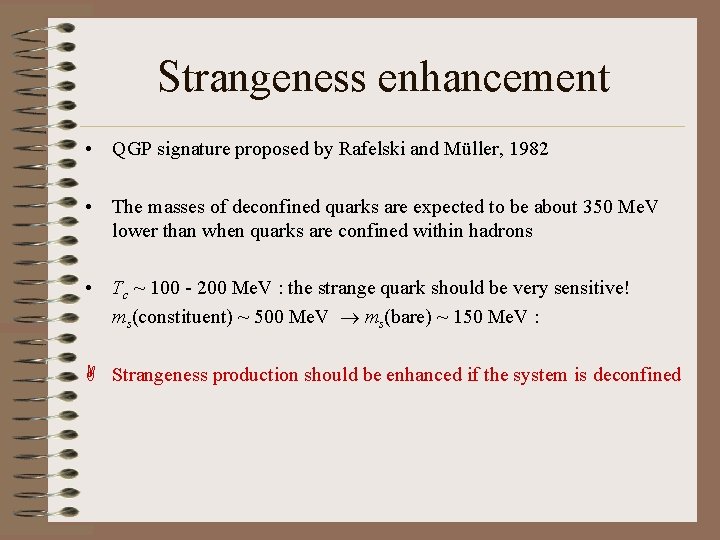
Strangeness enhancement • QGP signature proposed by Rafelski and Müller, 1982 • The masses of deconfined quarks are expected to be about 350 Me. V lower than when quarks are confined within hadrons • Tc ~ 100 - 200 Me. V : the strange quark should be very sensitive! ms(constituent) ~ 500 Me. V ms(bare) ~ 150 Me. V : Strangeness production should be enhanced if the system is deconfined
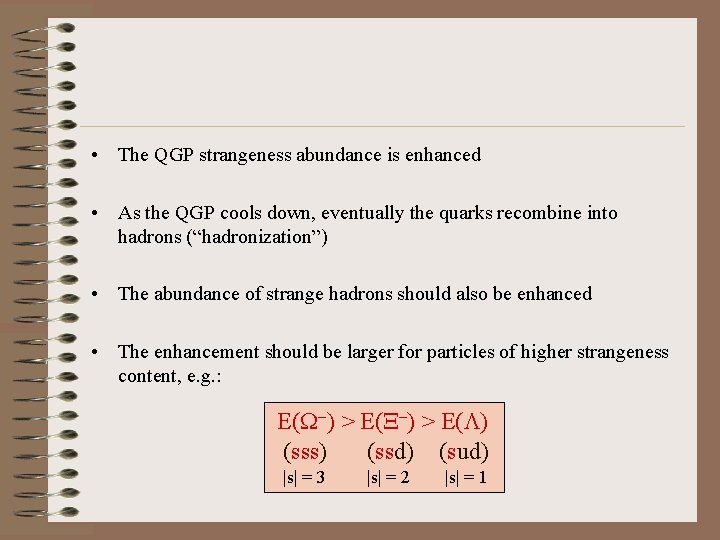
• The QGP strangeness abundance is enhanced • As the QGP cools down, eventually the quarks recombine into hadrons (“hadronization”) • The abundance of strange hadrons should also be enhanced • The enhancement should be larger for particles of higher strangeness content, e. g. : E(W-) > E(X-) > E(L) (sss) (ssd) (sud) |s| = 3 |s| = 2 |s| = 1
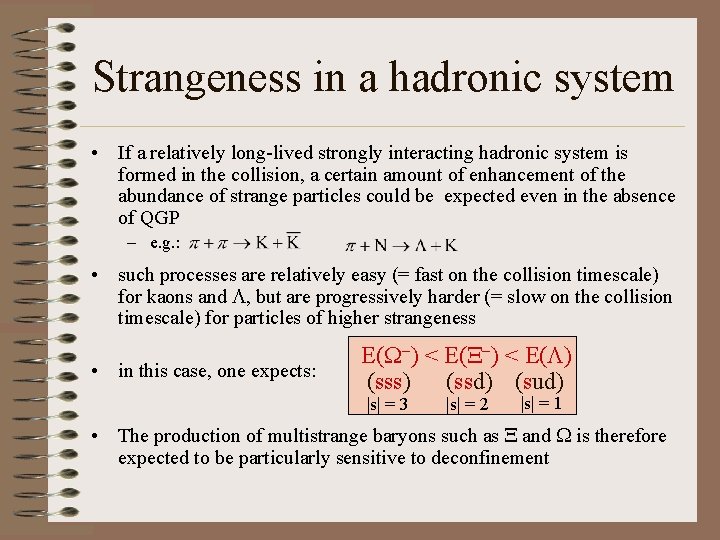
Strangeness in a hadronic system • If a relatively long-lived strongly interacting hadronic system is formed in the collision, a certain amount of enhancement of the abundance of strange particles could be expected even in the absence of QGP – e. g. : • such processes are relatively easy (= fast on the collision timescale) for kaons and L, but are progressively harder (= slow on the collision timescale) for particles of higher strangeness • in this case, one expects: E(W-) < E(X-) < E(L) (sss) (ssd) (sud) |s| = 3 |s| = 2 |s| = 1 • The production of multistrange baryons such as X and W is therefore expected to be particularly sensitive to deconfinement
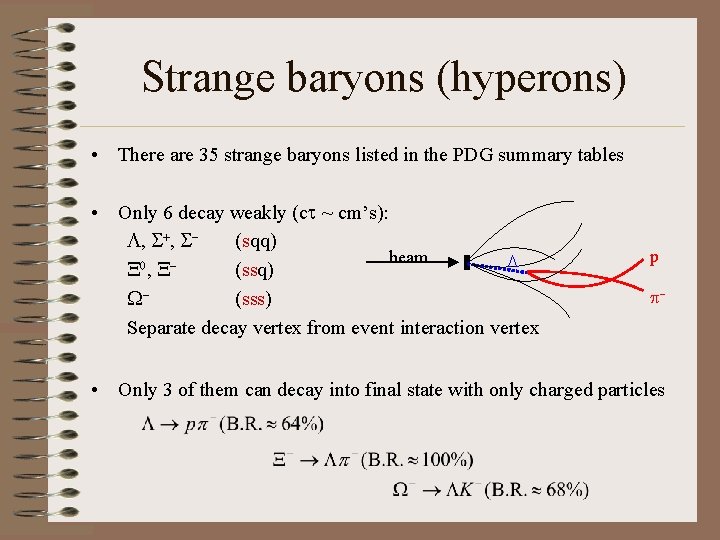
Strange baryons (hyperons) • There are 35 strange baryons listed in the PDG summary tables • Only 6 decay weakly (ct ~ cm’s): L, S+, S(sqq) beam L 0 X , X (ssq) W(sss) Separate decay vertex from event interaction vertex p p- • Only 3 of them can decay into final state with only charged particles
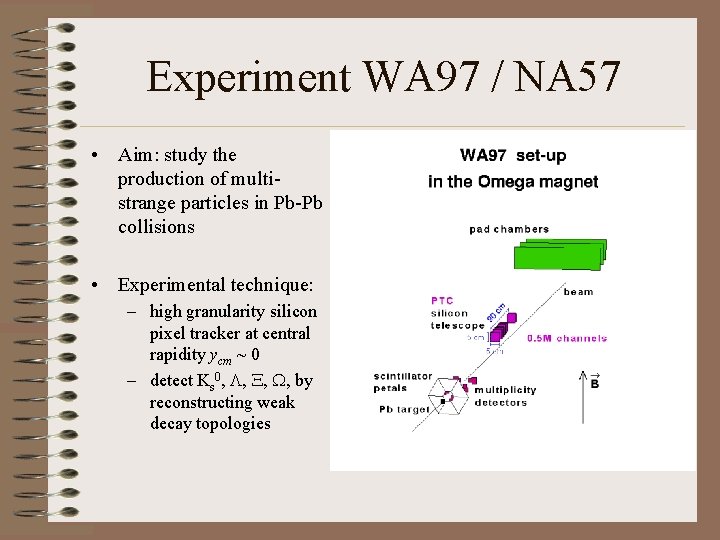
Experiment WA 97 / NA 57 • Aim: study the production of multistrange particles in Pb-Pb collisions • Experimental technique: – high granularity silicon pixel tracker at central rapidity ycm ~ 0 – detect Ks 0, L, X, W, by reconstructing weak decay topologies
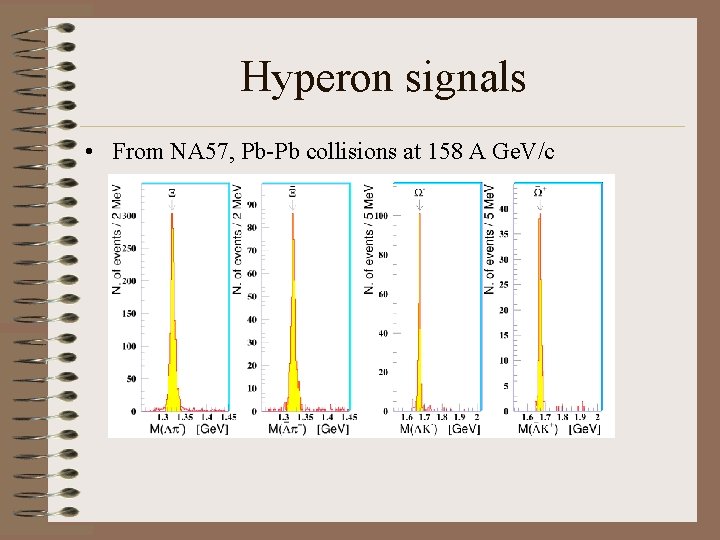
Hyperon signals • From NA 57, Pb-Pb collisions at 158 A Ge. V/c
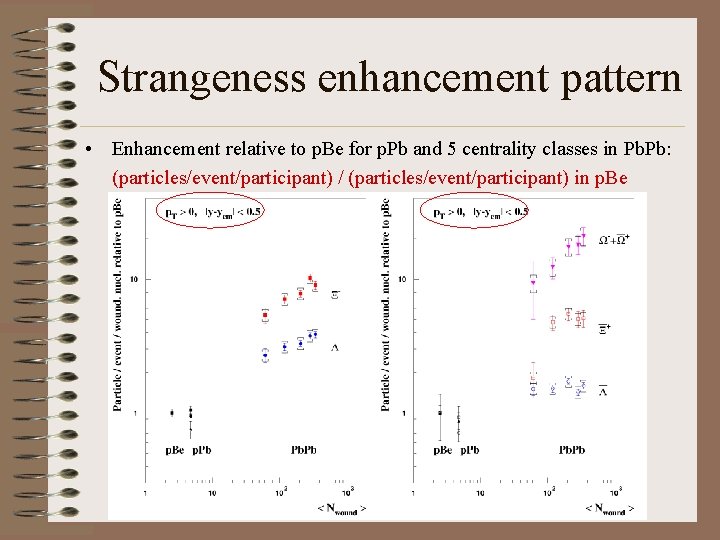
Strangeness enhancement pattern • Enhancement relative to p. Be for p. Pb and 5 centrality classes in Pb. Pb: (particles/event/participant) / (particles/event/participant) in p. Be
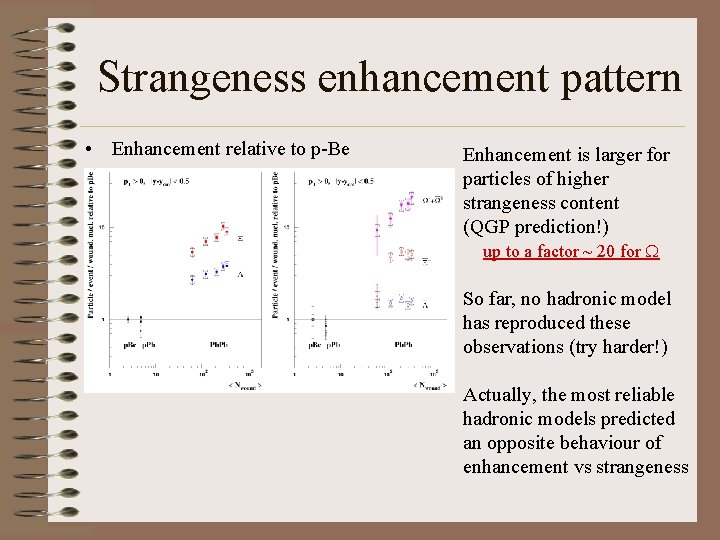
Strangeness enhancement pattern • Enhancement relative to p-Be Enhancement is larger for particles of higher strangeness content (QGP prediction!) up to a factor ~ 20 for W So far, no hadronic model has reproduced these observations (try harder!) Actually, the most reliable hadronic models predicted an opposite behaviour of enhancement vs strangeness
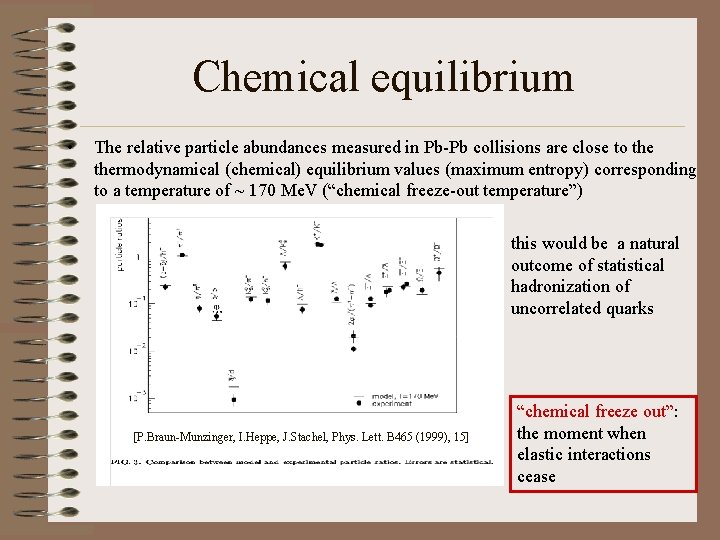
Chemical equilibrium • The relative particle abundances measured in Pb-Pb collisions are close to thermodynamical (chemical) equilibrium values (maximum entropy) corresponding to a temperature of ~ 170 Me. V (“chemical freeze-out temperature”) this would be a natural outcome of statistical hadronization of uncorrelated quarks [P. Braun-Munzinger, I. Heppe, J. Stachel, Phys. Lett. B 465 (1999), 15] “chemical freeze out”: the moment when elastic interactions cease
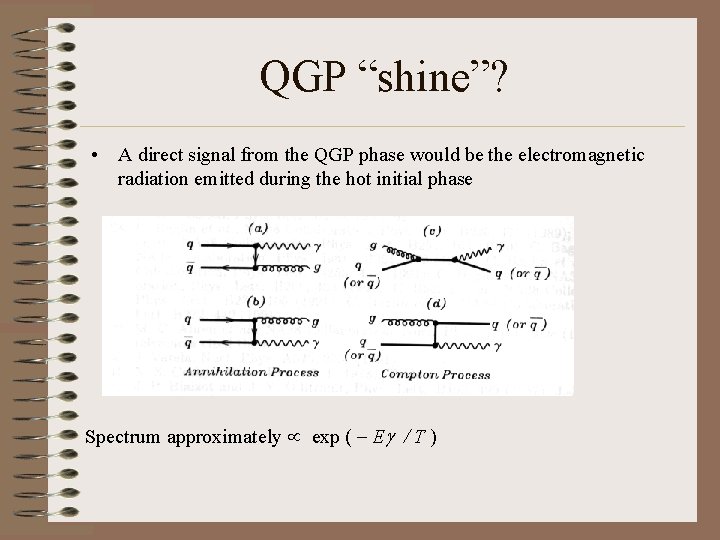
QGP “shine”? • A direct signal from the QGP phase would be the electromagnetic radiation emitted during the hot initial phase Spectrum approximately exp ( - Eg / T )
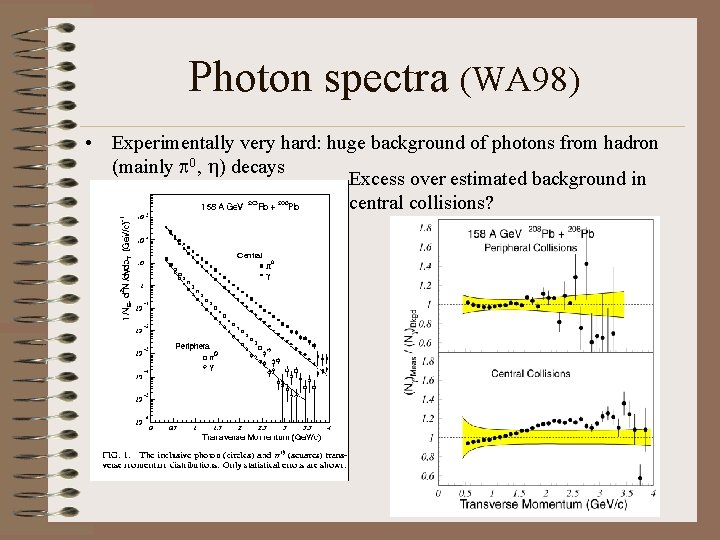
Photon spectra (WA 98) • Experimentally very hard: huge background of photons from hadron (mainly p 0, h) decays Excess over estimated background in central collisions?
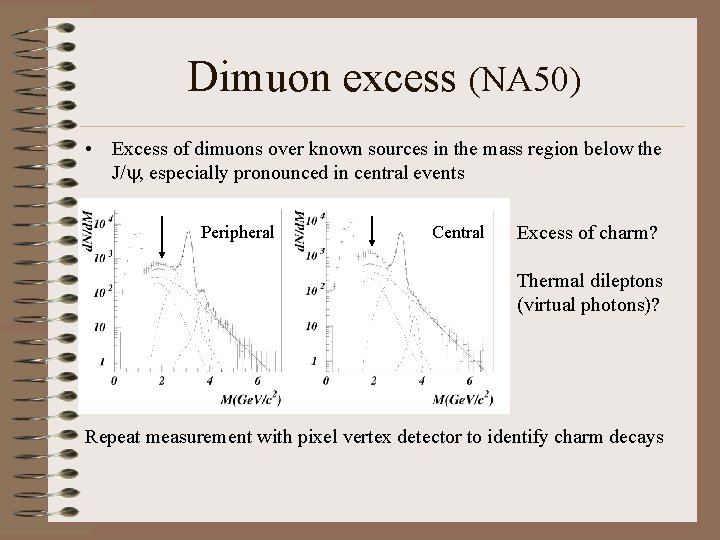
Dimuon excess (NA 50) • Excess of dimuons over known sources in the mass region below the J/y, especially pronounced in central events Peripheral Central Excess of charm? Thermal dileptons (virtual photons)? Repeat measurement with pixel vertex detector to identify charm decays
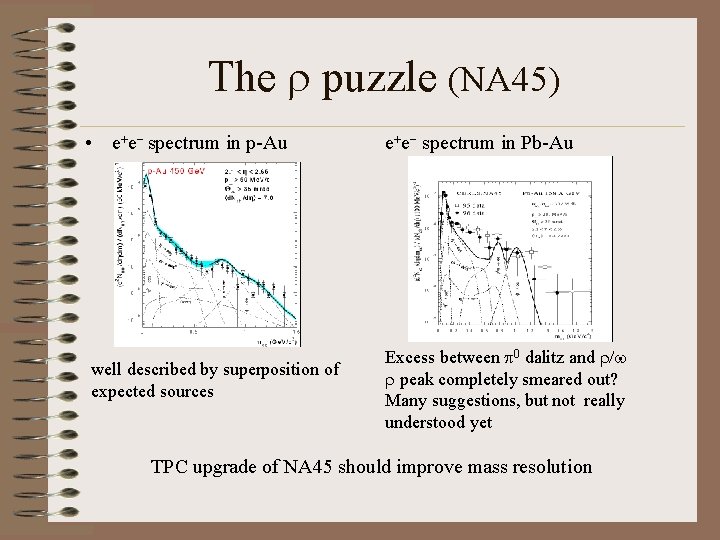
The r puzzle (NA 45) • e+e- spectrum in p-Au well described by superposition of expected sources e+e- spectrum in Pb-Au Excess between p 0 dalitz and r/w r peak completely smeared out? Many suggestions, but not really understood yet TPC upgrade of NA 45 should improve mass resolution
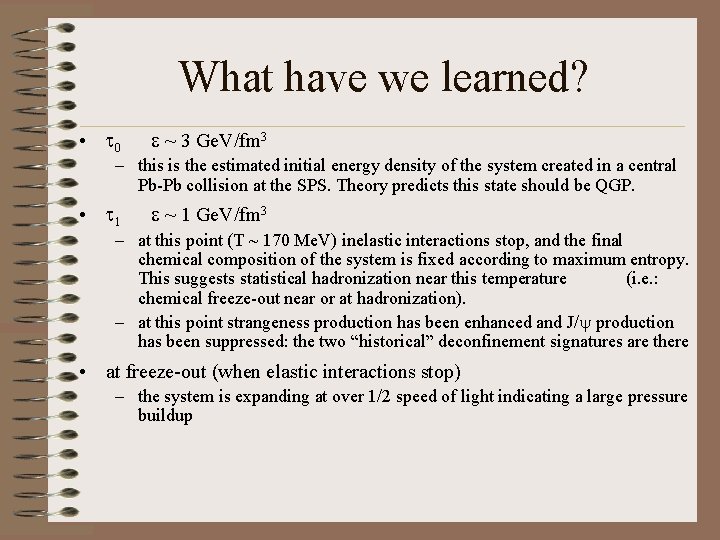
What have we learned? • t 0 e ~ 3 Ge. V/fm 3 • t 1 e ~ 1 Ge. V/fm 3 – this is the estimated initial energy density of the system created in a central Pb-Pb collision at the SPS. Theory predicts this state should be QGP. – at this point (T ~ 170 Me. V) inelastic interactions stop, and the final chemical composition of the system is fixed according to maximum entropy. This suggests statistical hadronization near this temperature (i. e. : chemical freeze-out near or at hadronization). – at this point strangeness production has been enhanced and J/y production has been suppressed: the two “historical” deconfinement signatures are there • at freeze-out (when elastic interactions stop) – the system is expanding at over 1/2 speed of light indicating a large pressure buildup
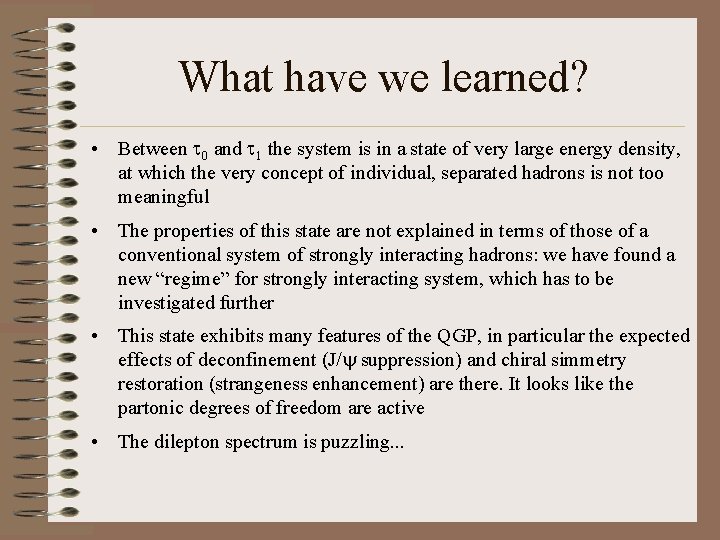
What have we learned? • Between t 0 and t 1 the system is in a state of very large energy density, at which the very concept of individual, separated hadrons is not too meaningful • The properties of this state are not explained in terms of those of a conventional system of strongly interacting hadrons: we have found a new “regime” for strongly interacting system, which has to be investigated further • This state exhibits many features of the QGP, in particular the expected effects of deconfinement (J/y suppression) and chiral simmetry restoration (strangeness enhancement) are there. It looks like the partonic degrees of freedom are active • The dilepton spectrum is puzzling. . .
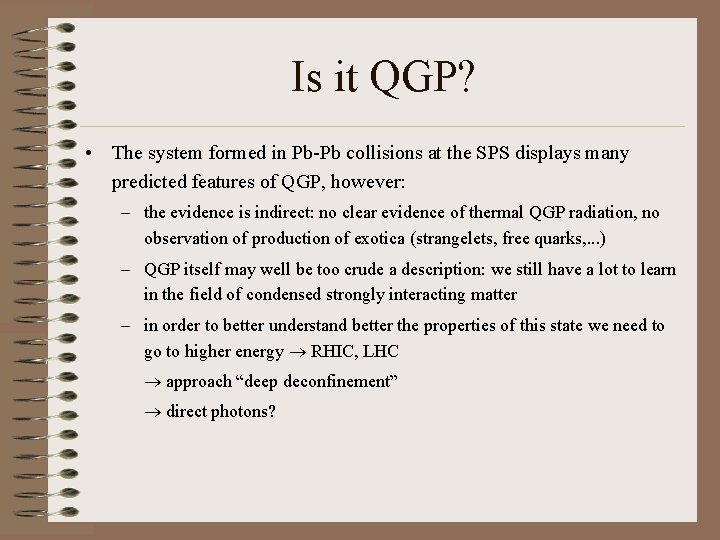
Is it QGP? • The system formed in Pb-Pb collisions at the SPS displays many predicted features of QGP, however: – the evidence is indirect: no clear evidence of thermal QGP radiation, no observation of production of exotica (strangelets, free quarks, . . . ) – QGP itself may well be too crude a description: we still have a lot to learn in the field of condensed strongly interacting matter – in order to better understand better the properties of this state we need to go to higher energy RHIC, LHC approach “deep deconfinement” direct photons?
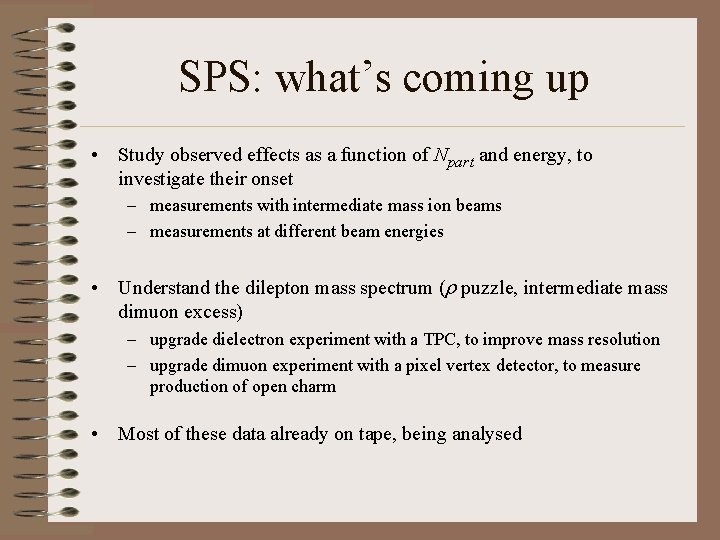
SPS: what’s coming up • Study observed effects as a function of Npart and energy, to investigate their onset – measurements with intermediate mass ion beams – measurements at different beam energies • Understand the dilepton mass spectrum (r puzzle, intermediate mass dimuon excess) – upgrade dielectron experiment with a TPC, to improve mass resolution – upgrade dimuon experiment with a pixel vertex detector, to measure production of open charm • Most of these data already on tape, being analysed
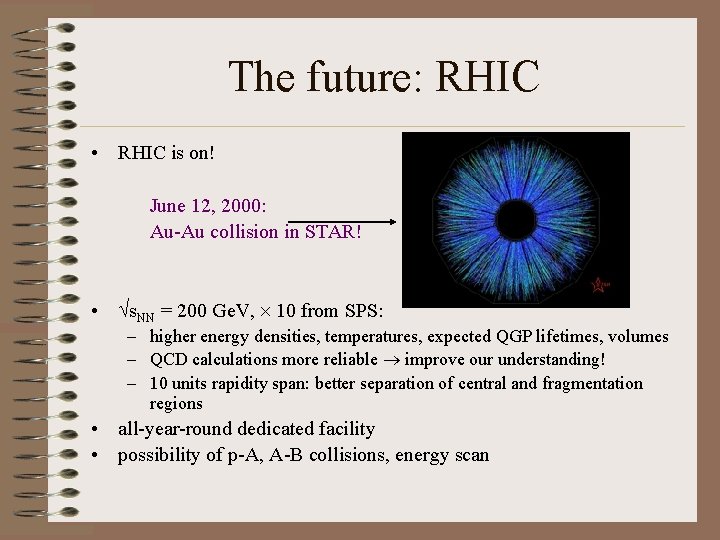
The future: RHIC • RHIC is on! June 12, 2000: Au-Au collision in STAR! • s. NN = 200 Ge. V, 10 from SPS: – higher energy densities, temperatures, expected QGP lifetimes, volumes – QCD calculations more reliable improve our understanding! – 10 units rapidity span: better separation of central and fragmentation regions • all-year-round dedicated facility • possibility of p-A, A-B collisions, energy scan
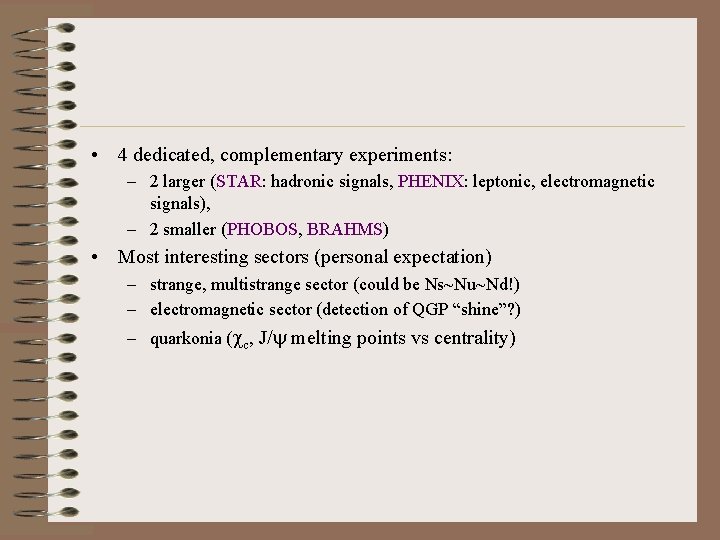
• 4 dedicated, complementary experiments: – 2 larger (STAR: hadronic signals, PHENIX: leptonic, electromagnetic signals), – 2 smaller (PHOBOS, BRAHMS) • Most interesting sectors (personal expectation) – strange, multistrange sector (could be Ns~Nu~Nd!) – electromagnetic sector (detection of QGP “shine”? ) – quarkonia (cc, J/y melting points vs centrality)
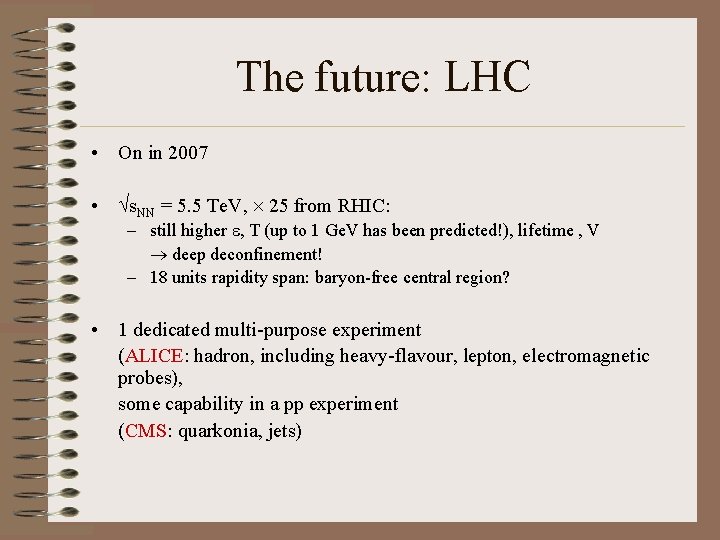
The future: LHC • On in 2007 • s. NN = 5. 5 Te. V, 25 from RHIC: – still higher e, T (up to 1 Ge. V has been predicted!), lifetime , V deep deconfinement! – 18 units rapidity span: baryon-free central region? • 1 dedicated multi-purpose experiment (ALICE: hadron, including heavy-flavour, lepton, electromagnetic probes), some capability in a pp experiment (CMS: quarkonia, jets)
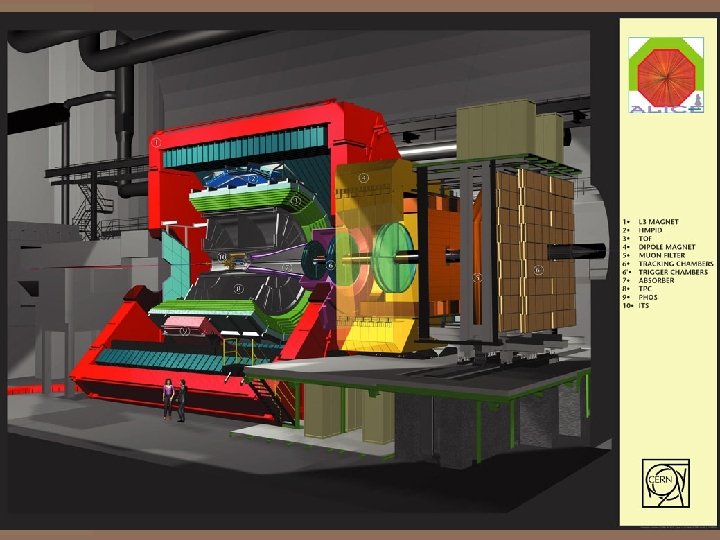
Alice
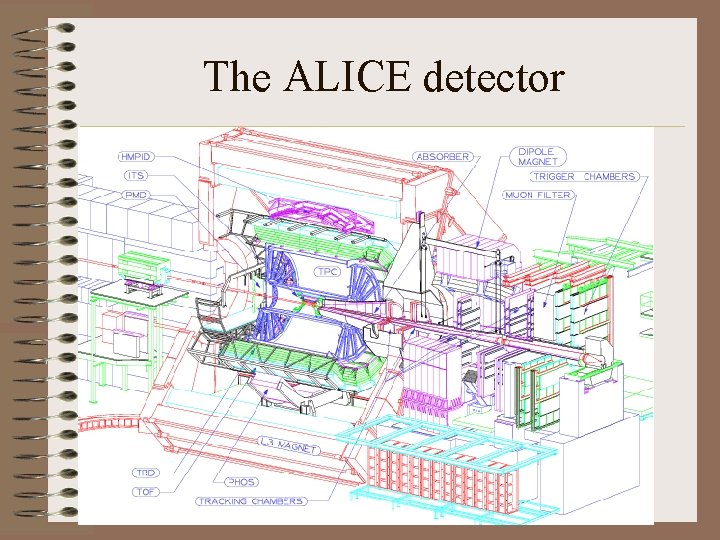
The ALICE detector
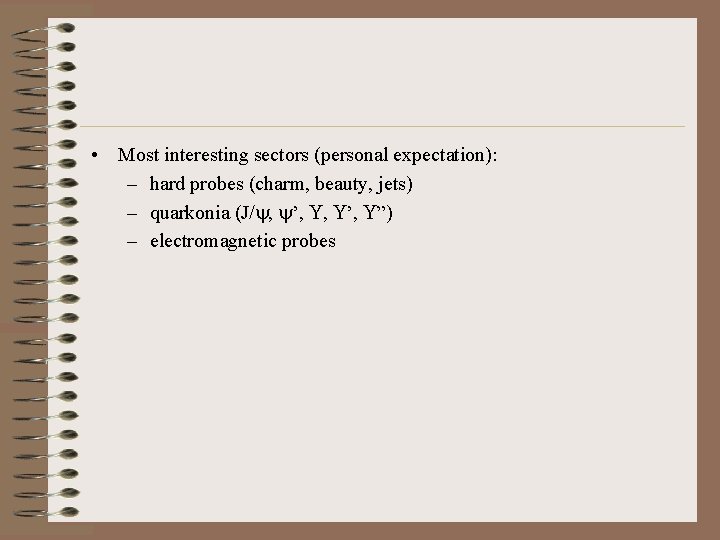
• Most interesting sectors (personal expectation): – hard probes (charm, beauty, jets) – quarkonia (J/y, y’, U, U’, U”) – electromagnetic probes
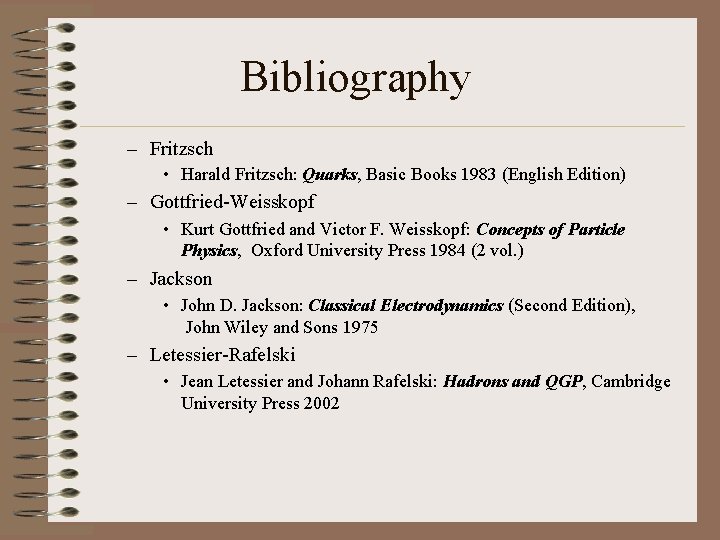
Bibliography – Fritzsch • Harald Fritzsch: Quarks, Basic Books 1983 (English Edition) – Gottfried-Weisskopf • Kurt Gottfried and Victor F. Weisskopf: Concepts of Particle Physics, Oxford University Press 1984 (2 vol. ) – Jackson • John D. Jackson: Classical Electrodynamics (Second Edition), John Wiley and Sons 1975 – Letessier-Rafelski • Jean Letessier and Johann Rafelski: Hadrons and QGP, Cambridge University Press 2002
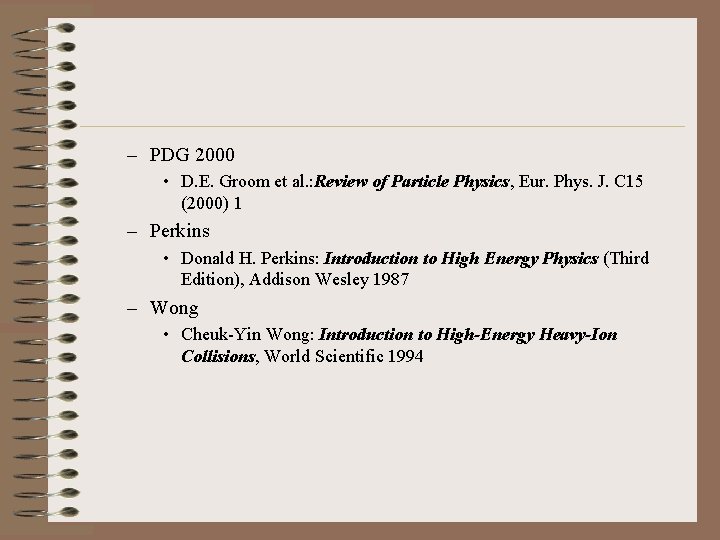
– PDG 2000 • D. E. Groom et al. : Review of Particle Physics, Eur. Phys. J. C 15 (2000) 1 – Perkins • Donald H. Perkins: Introduction to High Energy Physics (Third Edition), Addison Wesley 1987 – Wong • Cheuk-Yin Wong: Introduction to High-Energy Heavy-Ion Collisions, World Scientific 1994
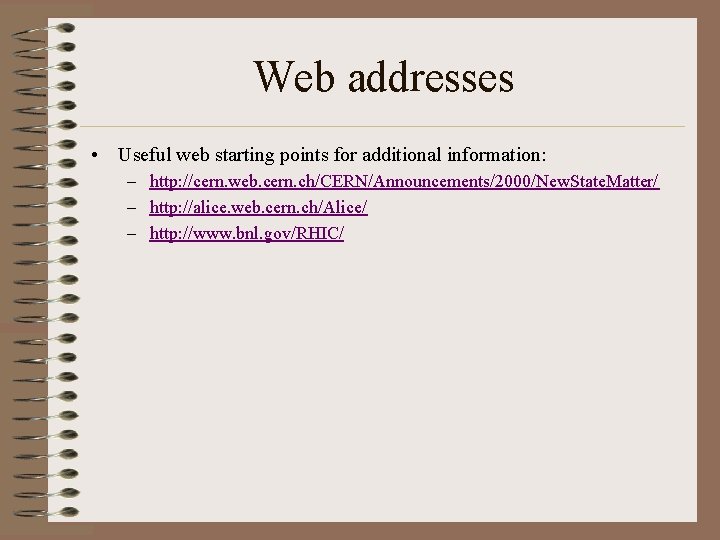
Web addresses • Useful web starting points for additional information: – http: //cern. web. cern. ch/CERN/Announcements/2000/New. State. Matter/ – http: //alice. web. cern. ch/Alice/ – http: //www. bnl. gov/RHIC/
01:640:244 lecture notes - lecture 15: plat, idah, farad
Physics 111 lecture notes
Physics 101 lecture
Physics 101 lecture 1
Physics 101 lecture notes pdf
Wave notes pdf
Atmospheric physics lecture notes
Introduction to biochemistry lecture notes
Introduction to psychology lecture
Introduction to algorithms lecture notes
Modern physics vs classical physics
University physics with modern physics fifteenth edition
Ib physics ia ideas
Introduction to static equilibrium mastering physics
Modern physics
Modern physics introduction
Modern physics introduction
Introduction to physics 110
Device physics
Webassign
Physics chapter 1 introduction and mathematical concepts
An introduction to atmospheric physics
Kinematics ppt free download
Introduction paragraph structure
Project procurement management lecture notes
Lecture about sport
Healthy lifestyle wrap up lecture
Meaning of this
Life lecture meaning
Randy pausch last lecture summary
Tensorflow lecture
Theology proper lecture notes
Strategic management lecture
Geology lecture series
Social psychology lecture
In text citation for a lecture
Public sector accounting lecture notes in kenya
4 p's of project management in software engineering
Anchorage length eurocode
Magnetism
Physical science lecture notes
Power system dynamics and stability lecture notes
Natural language processing
Microbial physiology notes
Sensors and actuators ppt
Limits fits and tolerances