Institute of UltraHigh Frequency Semiconductor Electronics Russian Academy
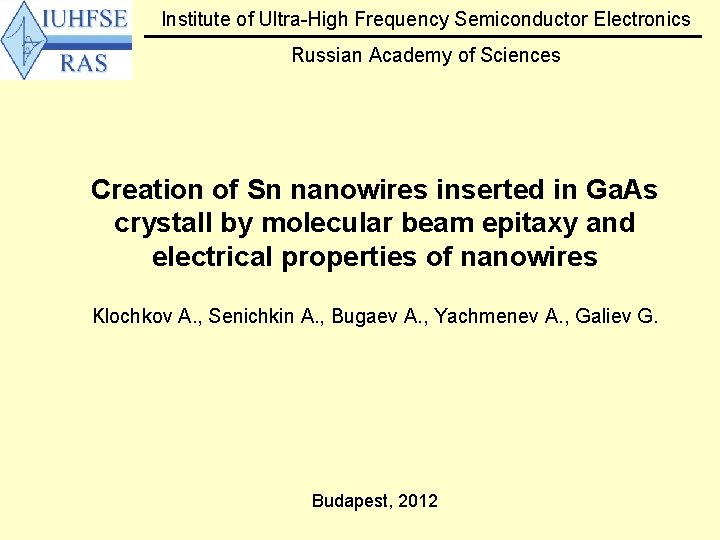
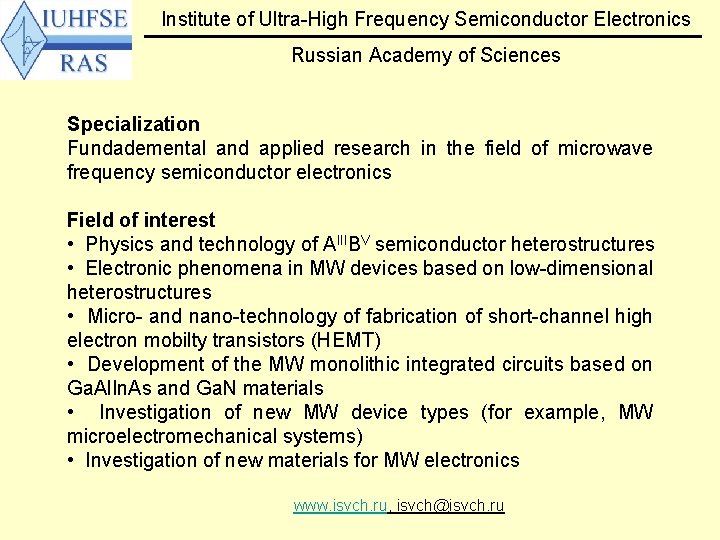
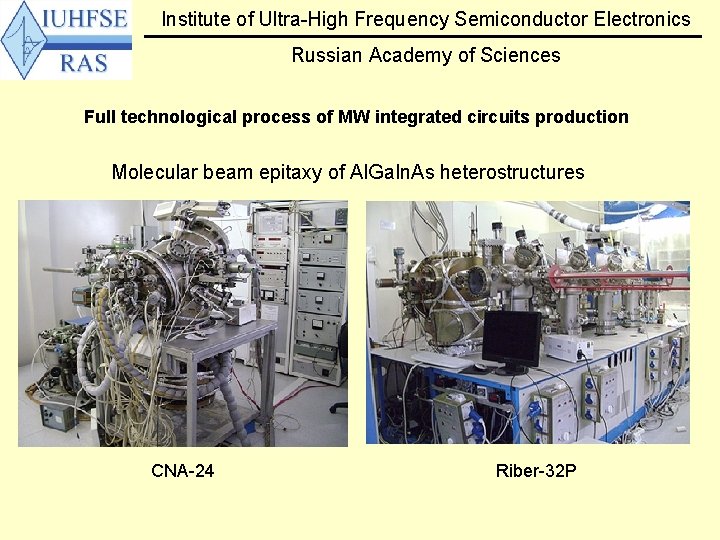
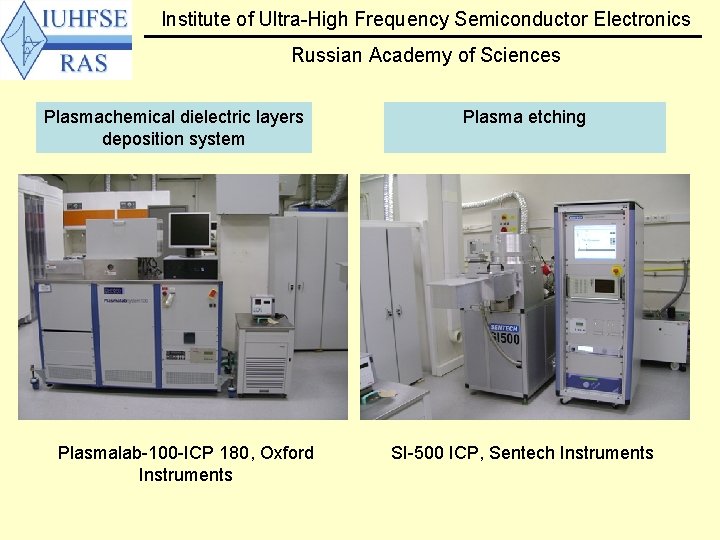
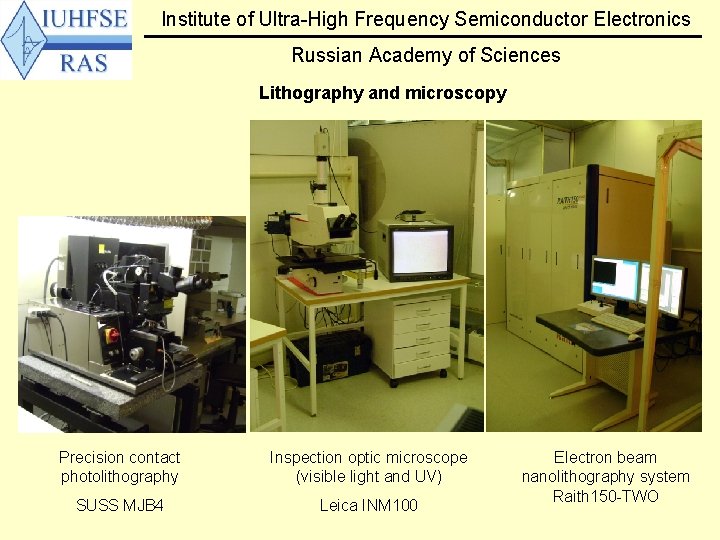
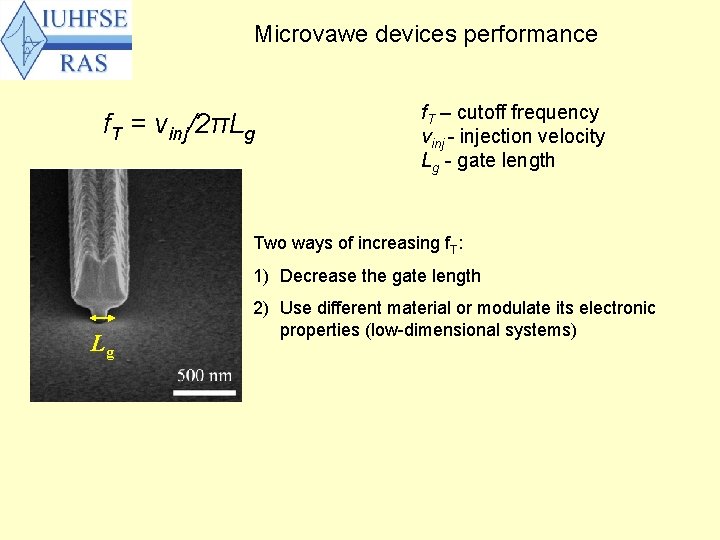
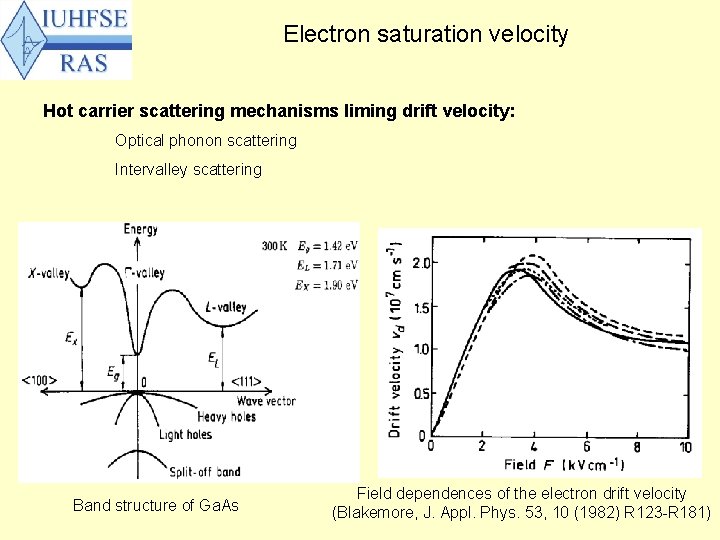
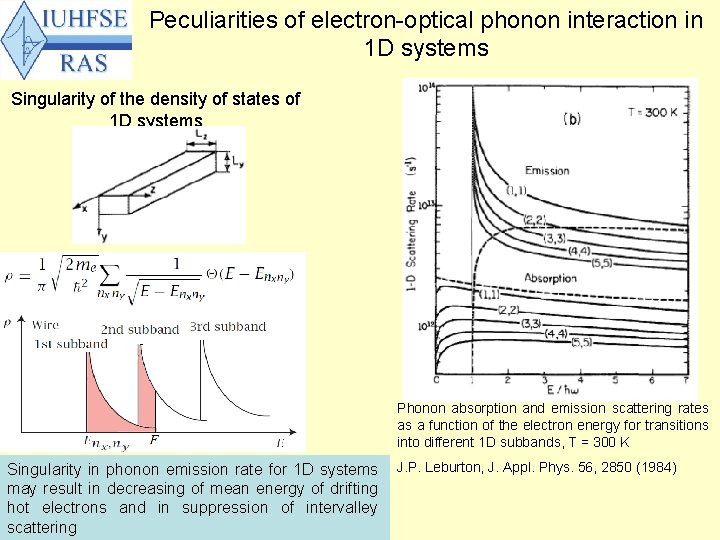
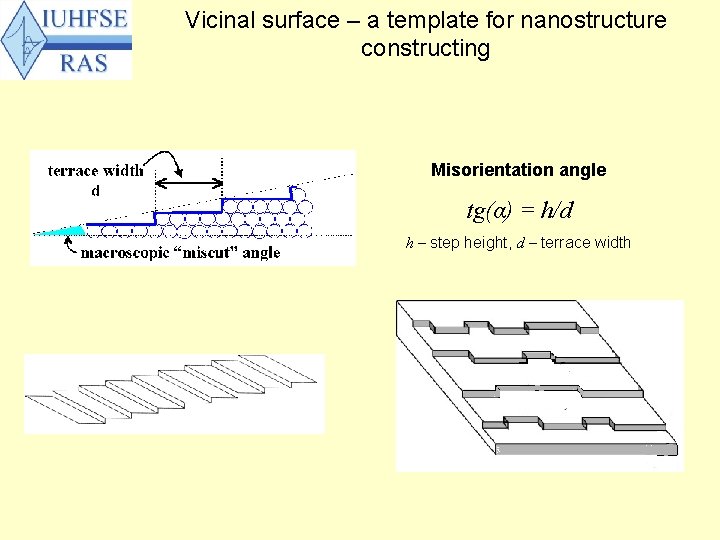
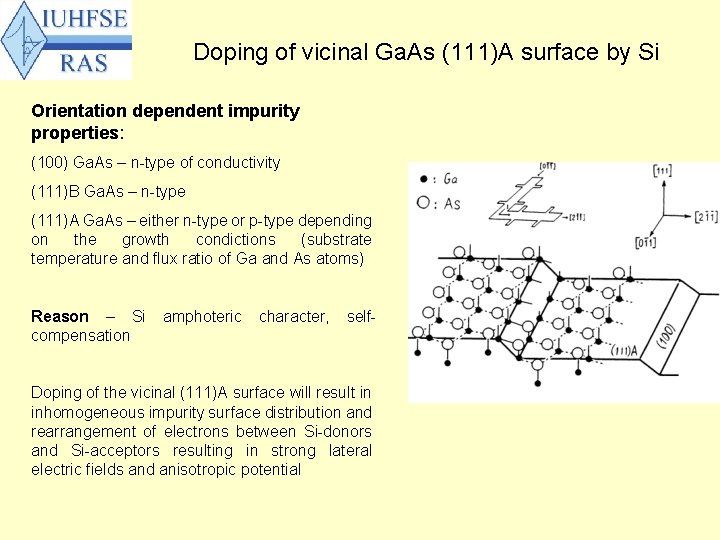
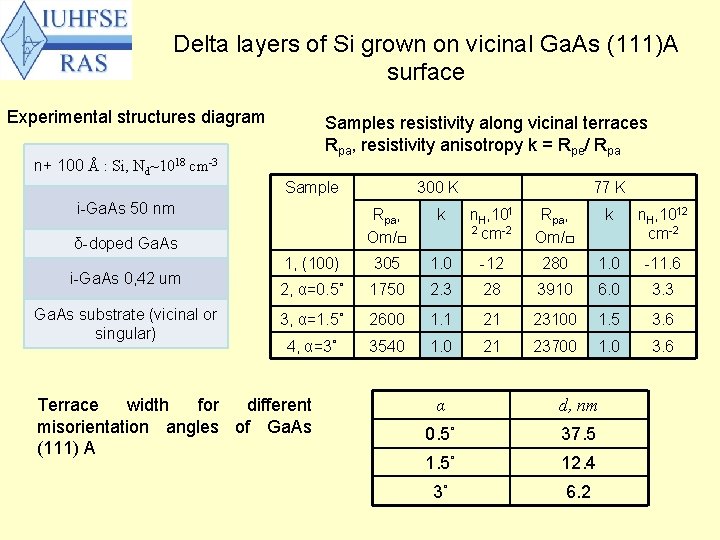
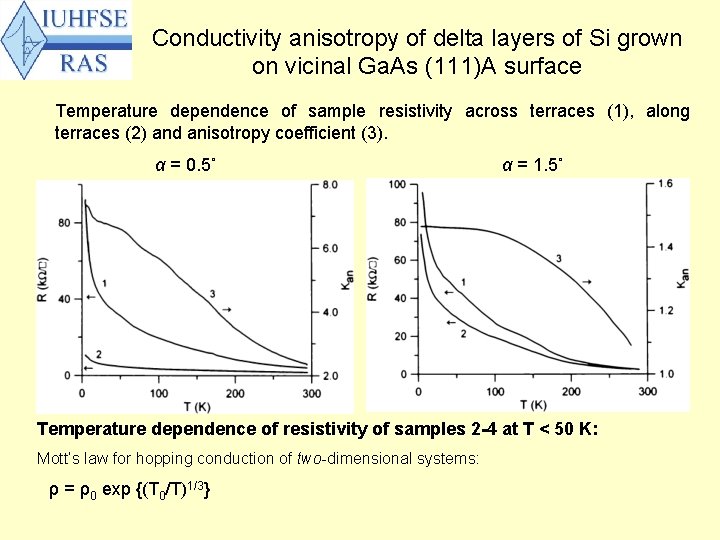
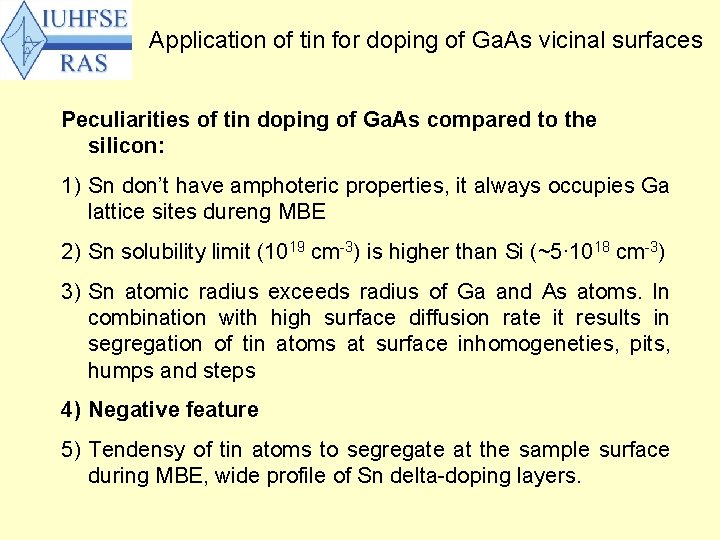
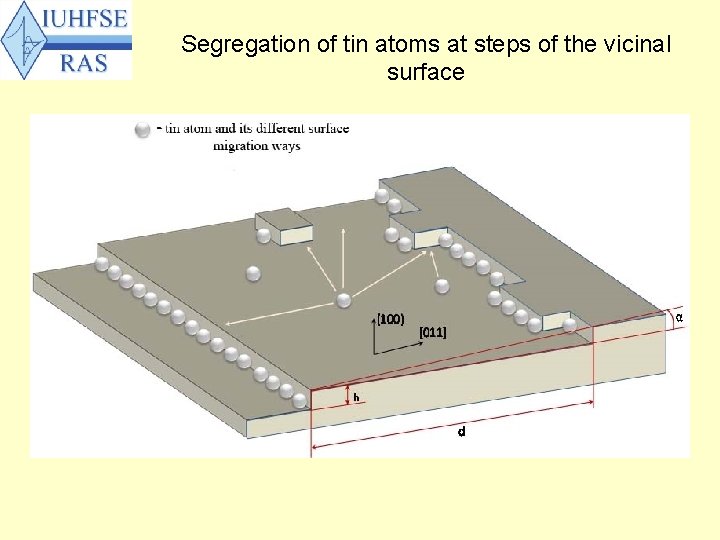
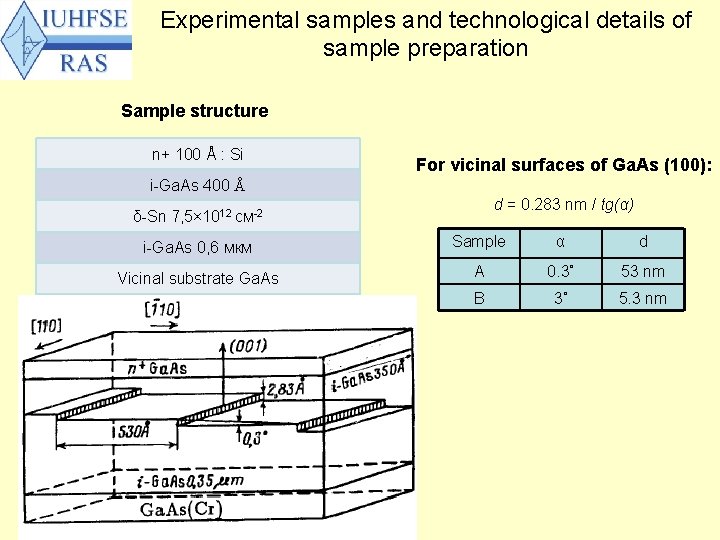
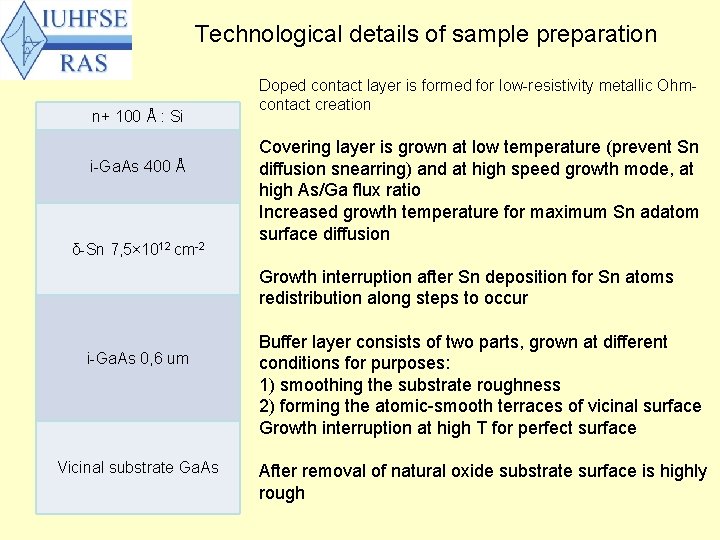
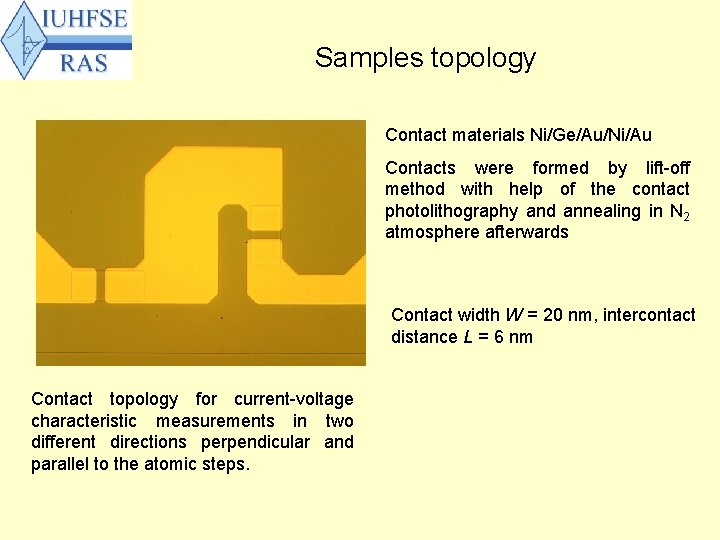
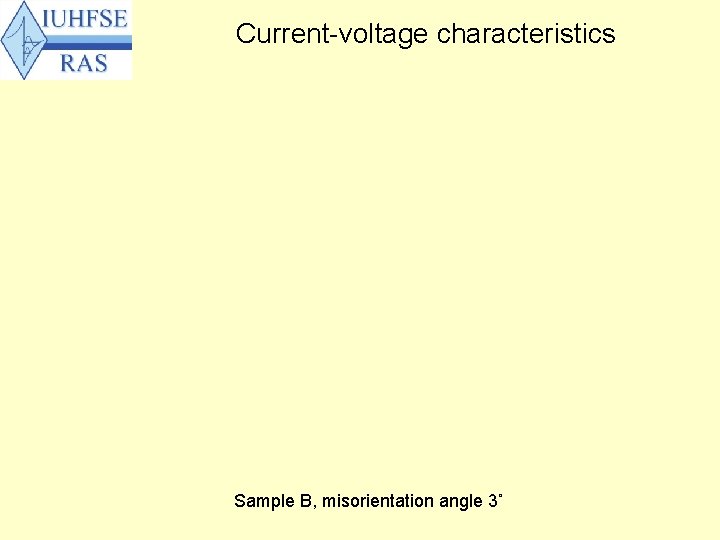
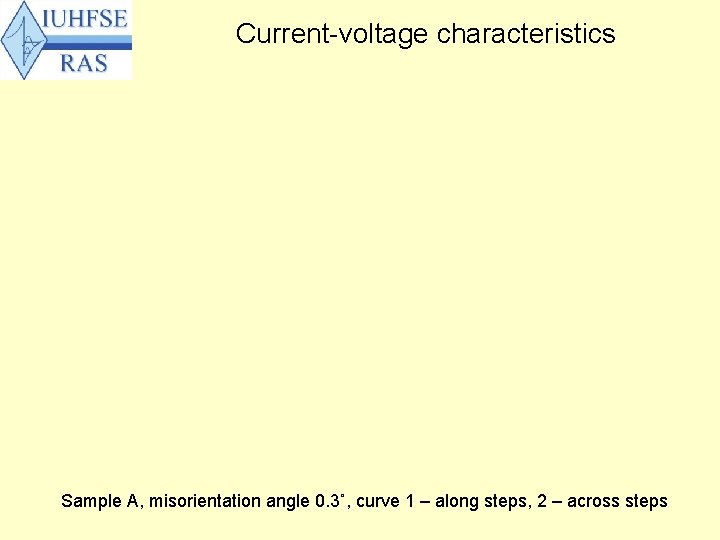
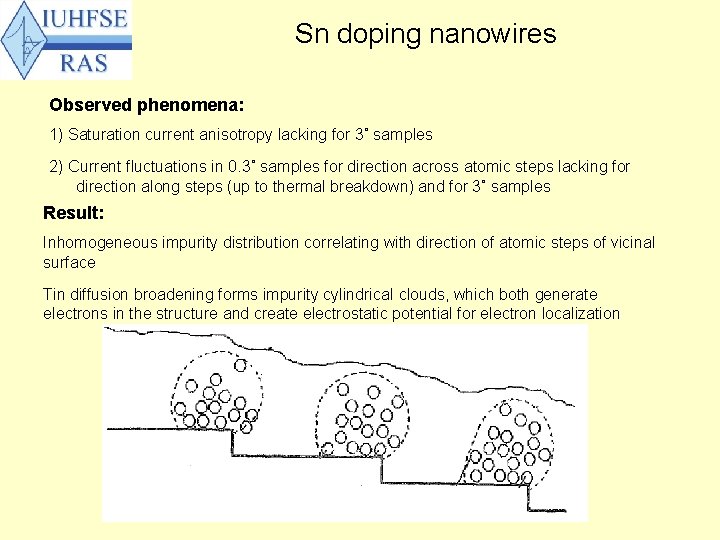
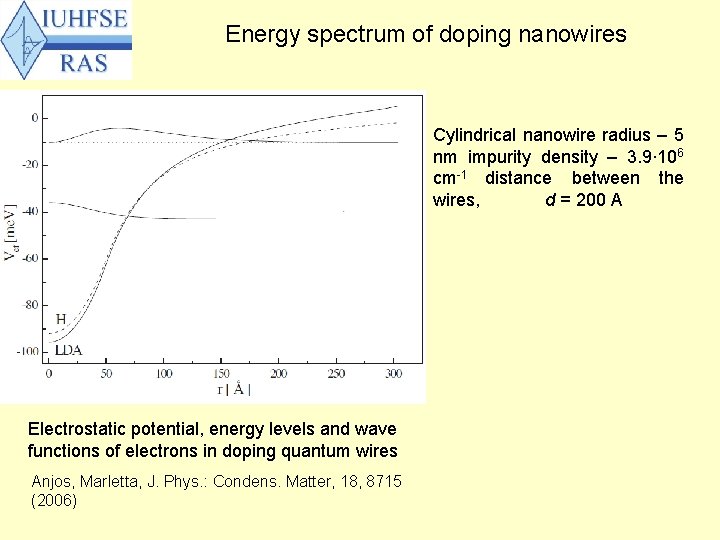
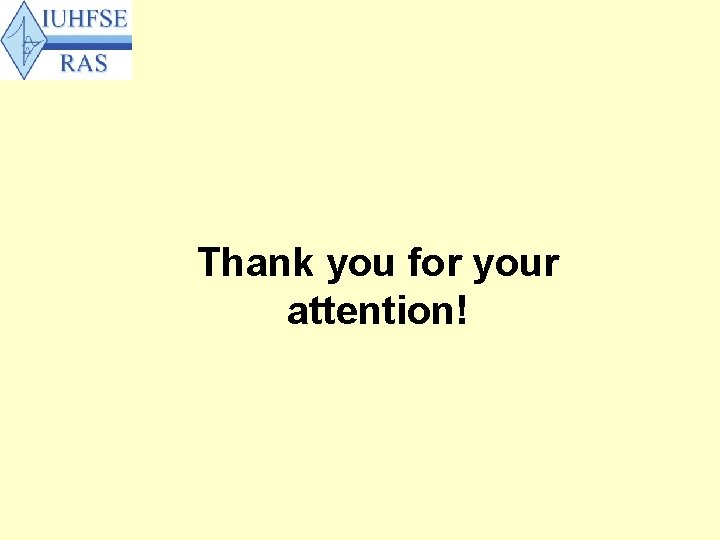
- Slides: 22
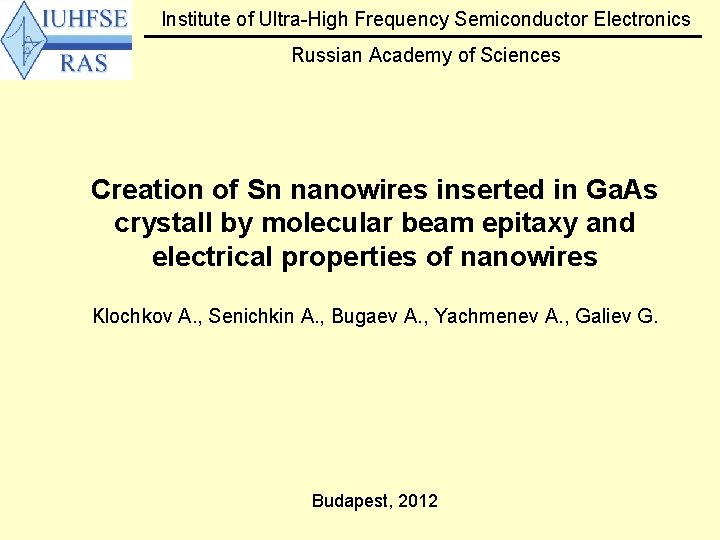
Institute of Ultra-High Frequency Semiconductor Electronics Russian Academy of Sciences Creation of Sn nanowires inserted in Ga. As crystall by molecular beam epitaxy and electrical properties of nanowires Klochkov A. , Senichkin A. , Bugaev A. , Yachmenev A. , Galiev G. Budapest, 2012
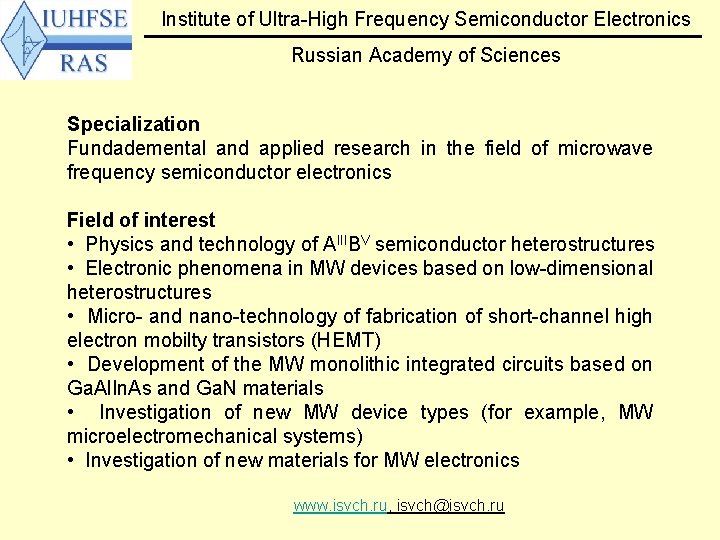
Institute of Ultra-High Frequency Semiconductor Electronics Russian Academy of Sciences Specialization Fundademental and applied research in the field of microwave frequency semiconductor electronics Field of interest • Physics and technology of AIIIBV semiconductor heterostructures • Electronic phenomena in MW devices based on low-dimensional heterostructures • Micro- and nano-technology of fabrication of short-channel high electron mobilty transistors (HEMT) • Development of the MW monolithic integrated circuits based on Ga. Al. In. As and Ga. N materials • Investigation of new MW device types (for example, MW microelectromechanical systems) • Investigation of new materials for MW electronics www. isvch. ru, isvch@isvch. ru
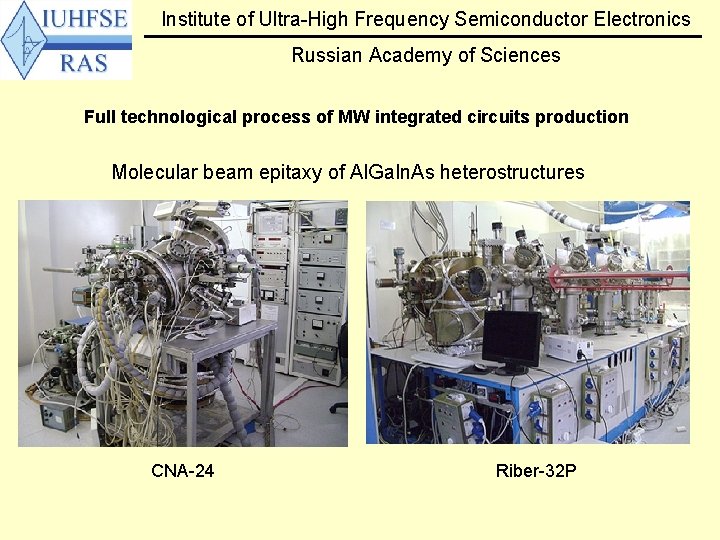
Institute of Ultra-High Frequency Semiconductor Electronics Russian Academy of Sciences Full technological process of MW integrated circuits production Molecular beam epitaxy of Al. Ga. In. As heterostructures CNA-24 Riber-32 P
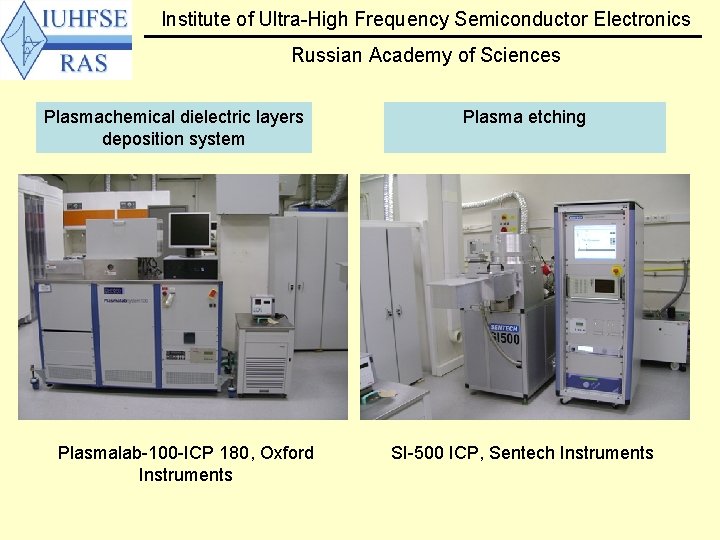
Institute of Ultra-High Frequency Semiconductor Electronics Russian Academy of Sciences Plasmachemical dielectric layers deposition system Plasmalab-100 -ICP 180, Oxford Instruments Plasma etching SI-500 ICP, Sentech Instruments
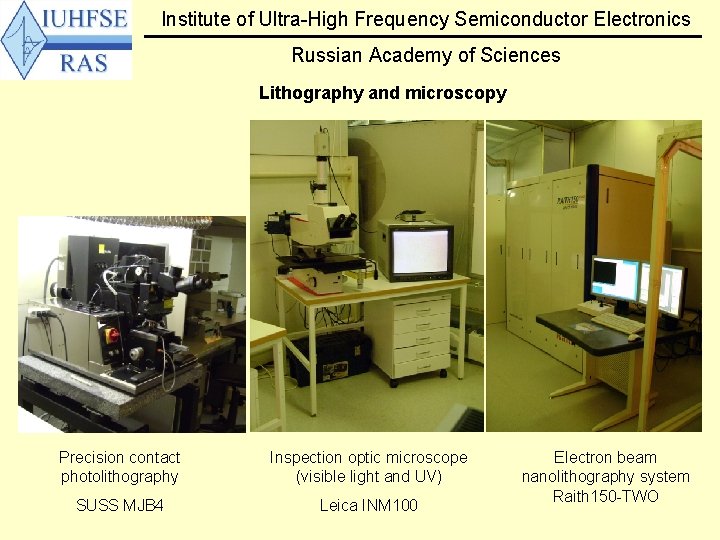
Institute of Ultra-High Frequency Semiconductor Electronics Russian Academy of Sciences Lithography and microscopy Precision contact photolithography Inspection optic microscope (visible light and UV) SUSS MJB 4 Leica INM 100 Electron beam nanolithography system Raith 150 -TWO
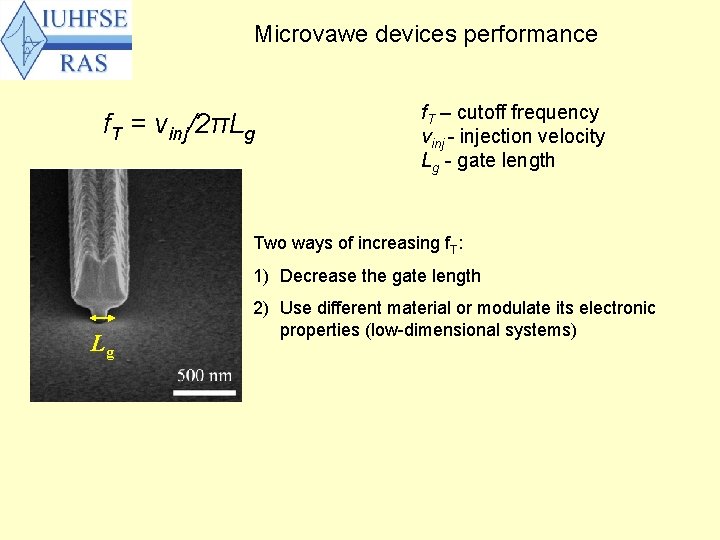
Microvawe devices performance f. T = vinj/2πLg f. T – cutoff frequency vinj - injection velocity Lg - gate length Two ways of increasing f. T: 1) Decrease the gate length Lg 2) Use different material or modulate its electronic properties (low-dimensional systems)
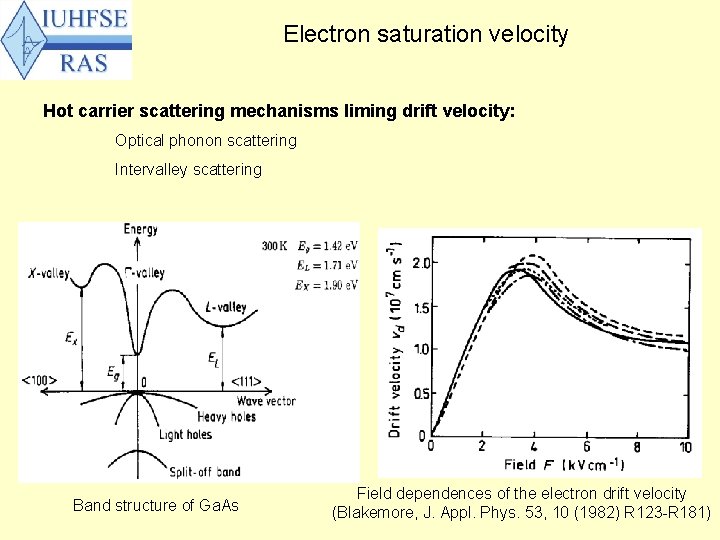
Electron saturation velocity Hot carrier scattering mechanisms liming drift velocity: Optical phonon scattering Intervalley scattering Band structure of Ga. As Field dependences of the electron drift velocity (Blakemore, J. Appl. Phys. 53, 10 (1982) R 123 -R 181)
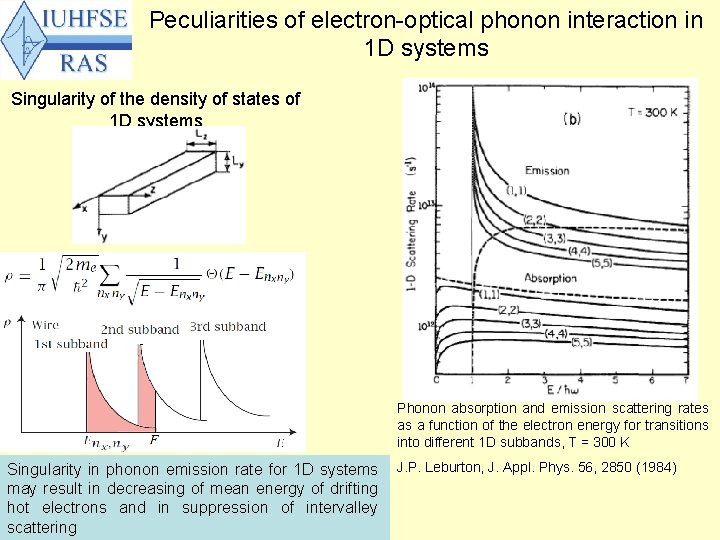
Peculiarities of electron-optical phonon interaction in 1 D systems Singularity of the density of states of 1 D systems Phonon absorption and emission scattering rates as a function of the electron energy for transitions into different 1 D subbands, T = 300 K Singularity in phonon emission rate for 1 D systems may result in decreasing of mean energy of drifting hot electrons and in suppression of intervalley scattering J. P. Leburton, J. Appl. Phys. 56, 2850 (1984)
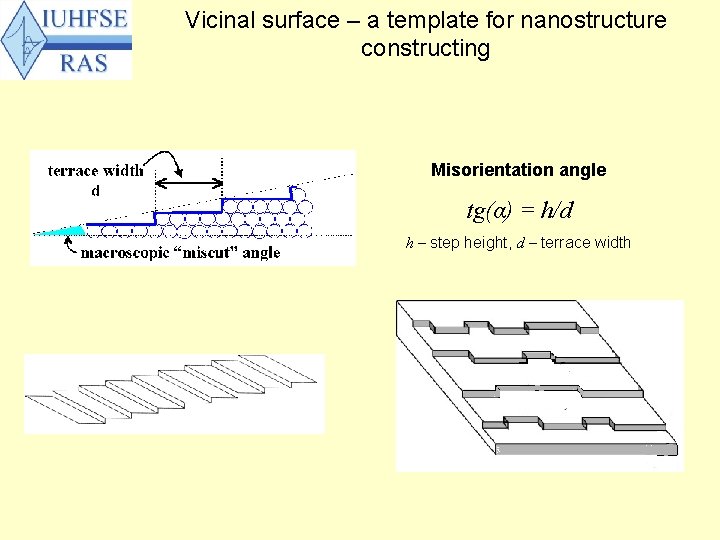
Vicinal surface – a template for nanostructure constructing Misorientation angle tg(α) = h/d h – step height, d – terrace width
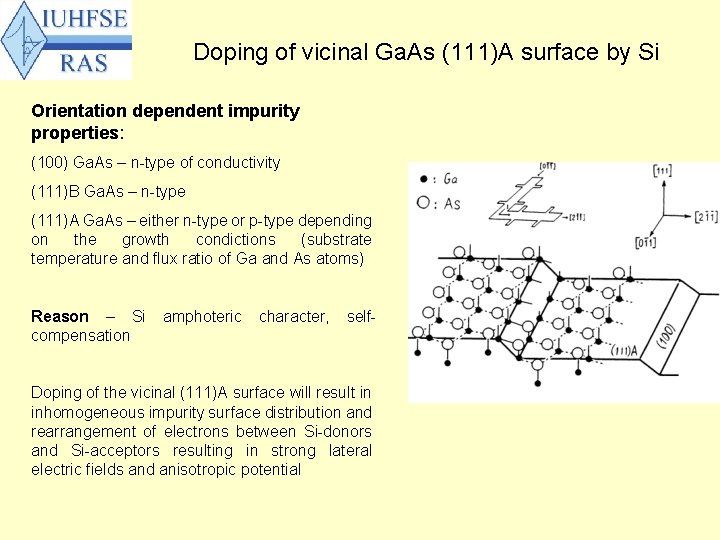
Doping of vicinal Ga. As (111)A surface by Si Orientation dependent impurity properties: (100) Ga. As – n-type of conductivity (111)B Ga. As – n-type (111)A Ga. As – either n-type or p-type depending on the growth condictions (substrate temperature and flux ratio of Ga and As atoms) Reason – Si compensation amphoteric character, self- Doping of the vicinal (111)A surface will result in inhomogeneous impurity surface distribution and rearrangement of electrons between Si-donors and Si-acceptors resulting in strong lateral electric fields and anisotropic potential
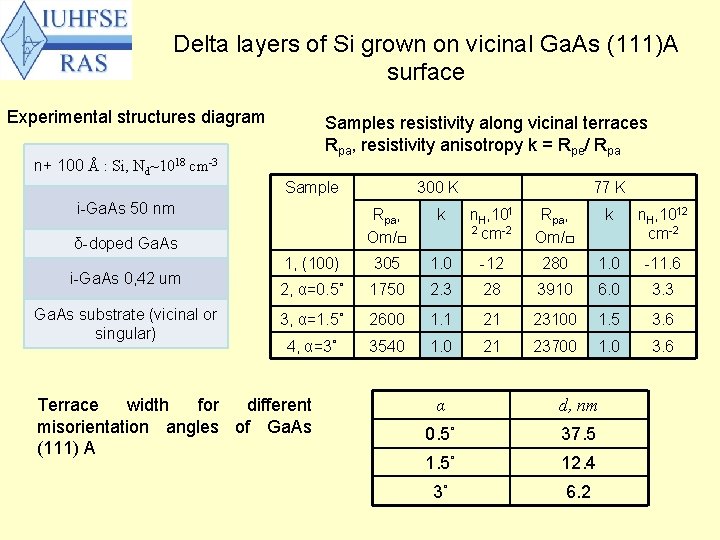
Delta layers of Si grown on vicinal Ga. As (111)A surface Experimental structures diagram Samples resistivity along vicinal terraces Rpa, resistivity anisotropy k = Rpe/ Rpa n+ 100 Å : Si, Nd~1018 cm-3 Sample i-Ga. As 50 nm Ga. As substrate (vicinal or singular) 77 K Rpa, Om/□ k n. H, 101 2 cm-2 Rpa, Om/□ k n. H, 1012 cm-2 1, (100) 305 1. 0 -12 280 1. 0 -11. 6 2, α=0. 5˚ 1750 2. 3 28 3910 6. 0 3. 3 3, α=1. 5˚ 2600 1. 1 21 23100 1. 5 3. 6 4, α=3˚ 3540 1. 0 21 23700 1. 0 3. 6 δ-doped Ga. As i-Ga. As 0, 42 um 300 K Terrace width for different misorientation angles of Ga. As (111) A α d, nm 0. 5˚ 37. 5 1. 5˚ 12. 4 3˚ 6. 2
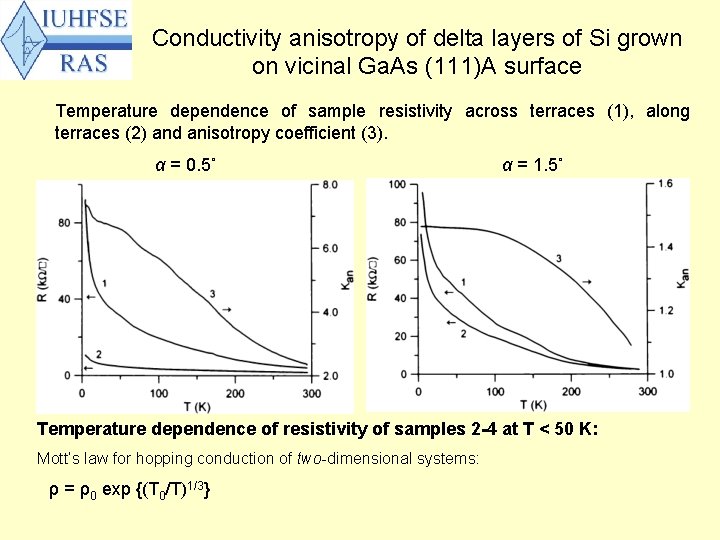
Conductivity anisotropy of delta layers of Si grown on vicinal Ga. As (111)A surface Temperature dependence of sample resistivity across terraces (1), along terraces (2) and anisotropy coefficient (3). α = 0. 5˚ α = 1. 5˚ Temperature dependence of resistivity of samples 2 -4 at T < 50 K: Mott’s law for hopping conduction of two-dimensional systems: ρ = ρ0 exp {(T 0/T)1/3}
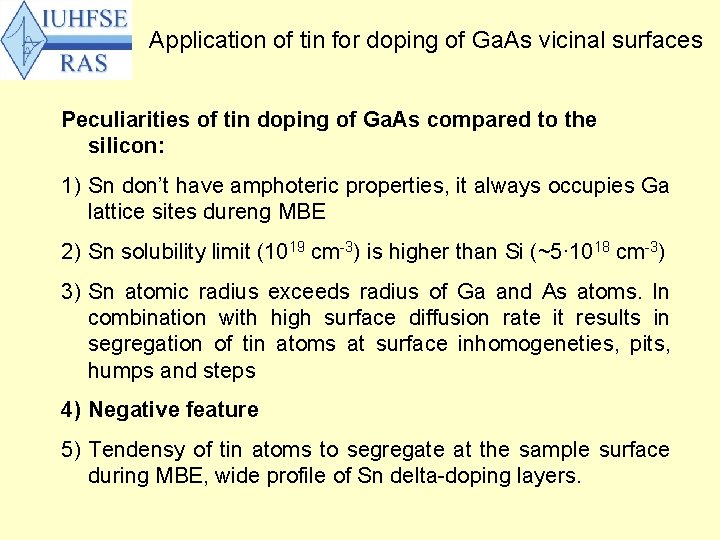
Application of tin for doping of Ga. As vicinal surfaces Peculiarities of tin doping of Ga. As compared to the silicon: 1) Sn don’t have amphoteric properties, it always occupies Ga lattice sites dureng MBE 2) Sn solubility limit (1019 cm-3) is higher than Si (~5∙ 1018 cm-3) 3) Sn atomic radius exceeds radius of Ga and As atoms. In combination with high surface diffusion rate it results in segregation of tin atoms at surface inhomogeneties, pits, humps and steps 4) Negative feature 5) Tendensy of tin atoms to segregate at the sample surface during MBE, wide profile of Sn delta-doping layers.
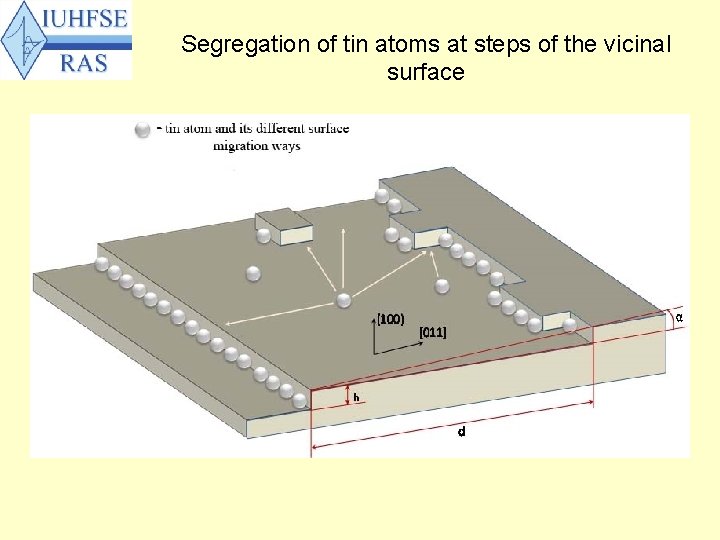
Segregation of tin atoms at steps of the vicinal surface
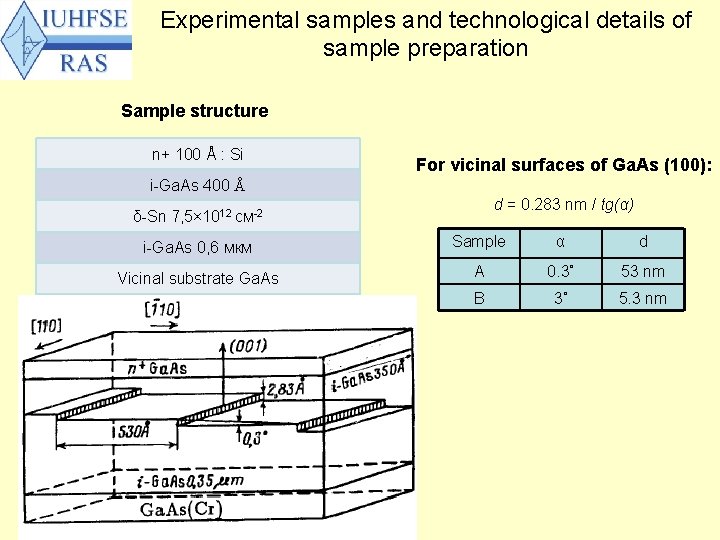
Experimental samples and technological details of sample preparation Sample structure n+ 100 Å : Si For vicinal surfaces of Ga. As (100): i-Ga. As 400 Å d = 0. 283 nm / tg(α) δ-Sn 7, 5× 1012 см-2 i-Ga. As 0, 6 мкм Sample α d Vicinal substrate Ga. As A 0. 3˚ 53 nm B 3˚ 5. 3 nm
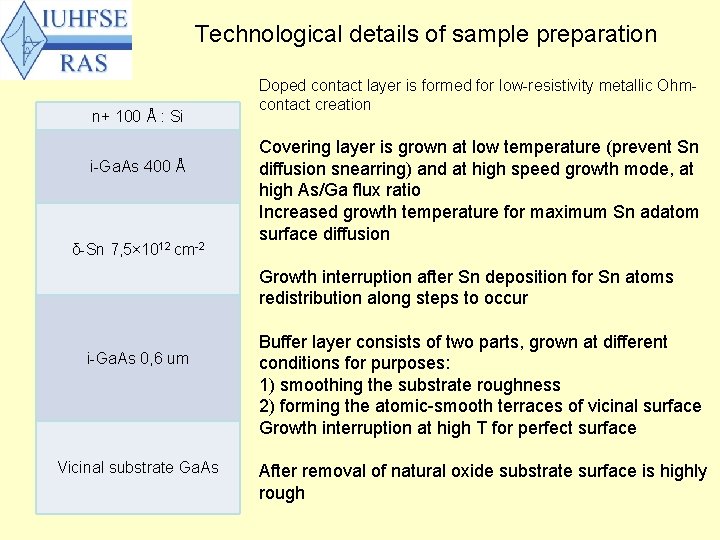
Technological details of sample preparation n+ 100 Å : Si i-Ga. As 400 Å δ-Sn 7, 5× 1012 cm-2 Doped contact layer is formed for low-resistivity metallic Ohmcontact creation Covering layer is grown at low temperature (prevent Sn diffusion snearring) and at high speed growth mode, at high As/Ga flux ratio Increased growth temperature for maximum Sn adatom surface diffusion Growth interruption after Sn deposition for Sn atoms redistribution along steps to occur i-Ga. As 0, 6 um Vicinal substrate Ga. As Buffer layer consists of two parts, grown at different conditions for purposes: 1) smoothing the substrate roughness 2) forming the atomic-smooth terraces of vicinal surface Growth interruption at high T for perfect surface After removal of natural oxide substrate surface is highly rough
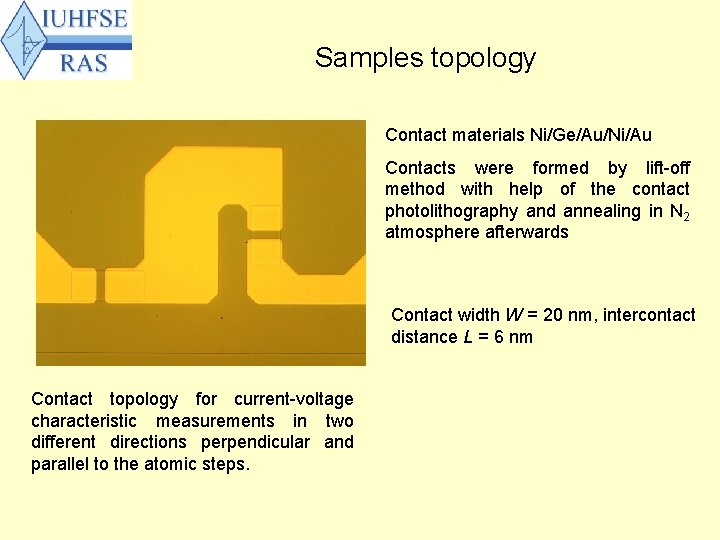
Samples topology Contact materials Ni/Ge/Au/Ni/Au Contacts were formed by lift-off method with help of the contact photolithography and annealing in N 2 atmosphere afterwards Contact width W = 20 nm, intercontact distance L = 6 nm Contact topology for current-voltage characteristic measurements in two different directions perpendicular and parallel to the atomic steps.
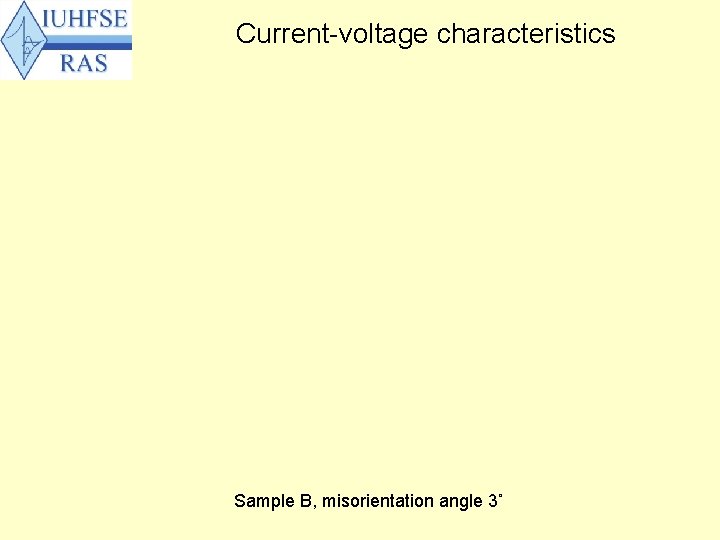
Current-voltage characteristics Sample B, misorientation angle 3˚
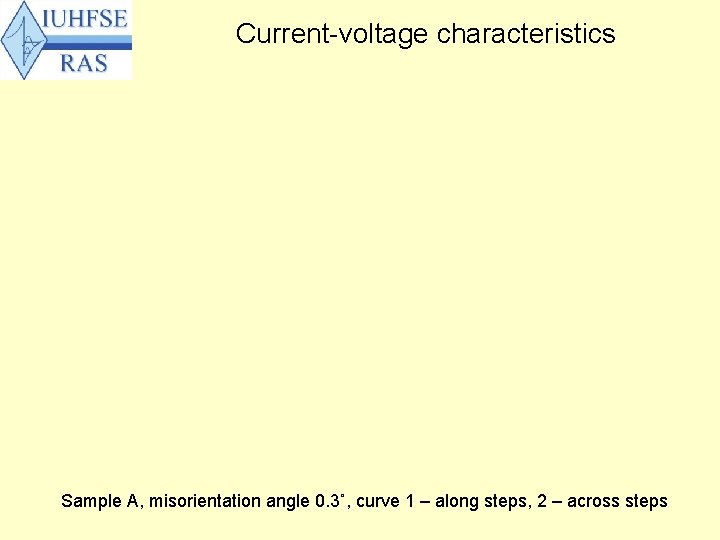
Current-voltage characteristics Sample A, misorientation angle 0. 3˚, curve 1 – along steps, 2 – across steps
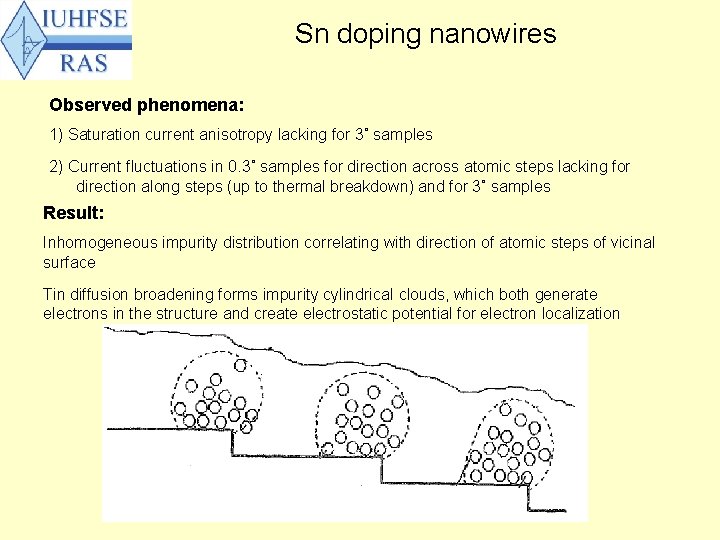
Sn doping nanowires Observed phenomena: 1) Saturation current anisotropy lacking for 3˚ samples 2) Current fluctuations in 0. 3˚ samples for direction across atomic steps lacking for direction along steps (up to thermal breakdown) and for 3˚ samples Result: Inhomogeneous impurity distribution correlating with direction of atomic steps of vicinal surface Tin diffusion broadening forms impurity cylindrical clouds, which both generate electrons in the structure and create electrostatic potential for electron localization
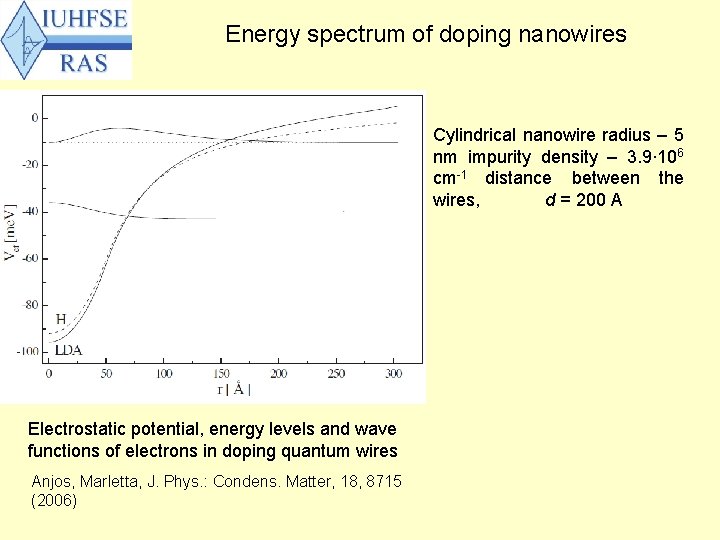
Energy spectrum of doping nanowires Cylindrical nanowire radius – 5 nm impurity density – 3. 9∙ 106 cm-1 distance between the wires, d = 200 A Electrostatic potential, energy levels and wave functions of electrons in doping quantum wires Anjos, Marletta, J. Phys. : Condens. Matter, 18, 8715 (2006)
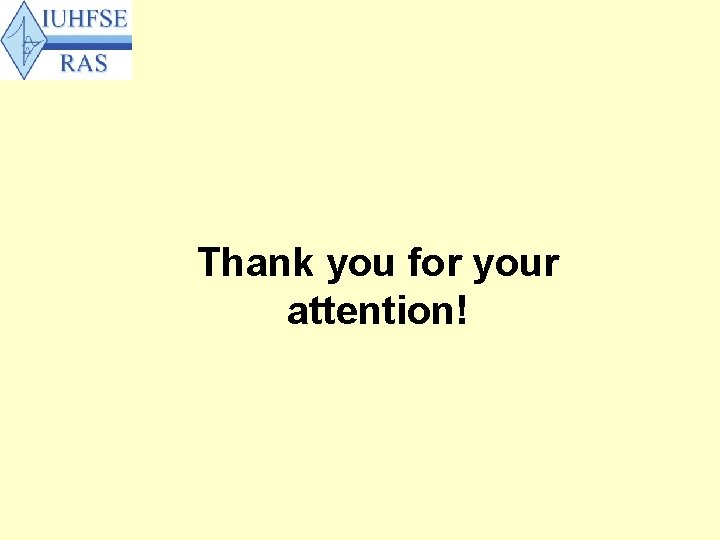
Thank you for your attention!
Nuclear safety institute of the russian academy of sciences
Difference between ram and rom in digital electronics
Graduate institute of electronics engineering
Electronics and telecommunications research institute
Graduate institute of electronics engineering
National institute of health
Graduate institute of electronics engineering
Ntu
Graduate institute of electronics engineering
Gnessin russian academy of music
How is linear frequency related to angular frequency?
Marginal relative frequency
What is a marginal frequency
Frequency vs relative frequency
Observed frequency
2 way relative frequency table
Form factor and crest factor
Draw the energy band diagram when intrinsic semiconductor
Fundamentals of semiconductor devices anderson pdf
Jan vardaman
Intrinsic semiconductor
Junction semiconductor detector
Ohmic contact