Inherent Thermometer in a Superconductor Normal metal Superconductor
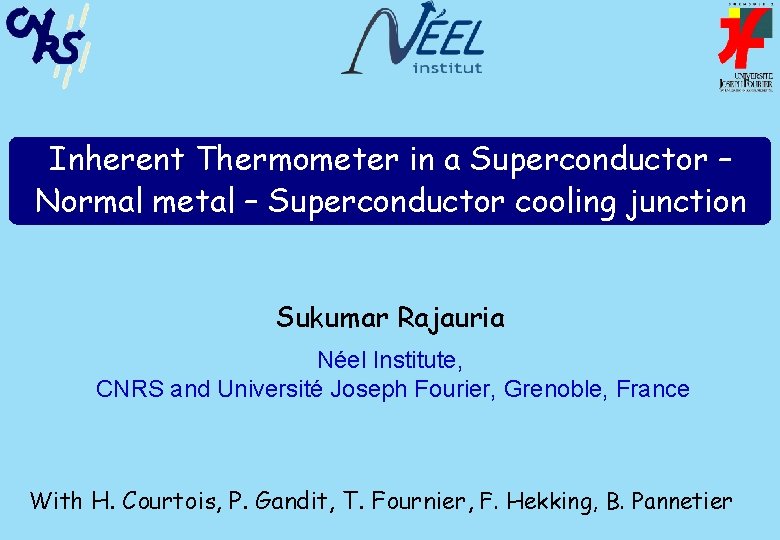
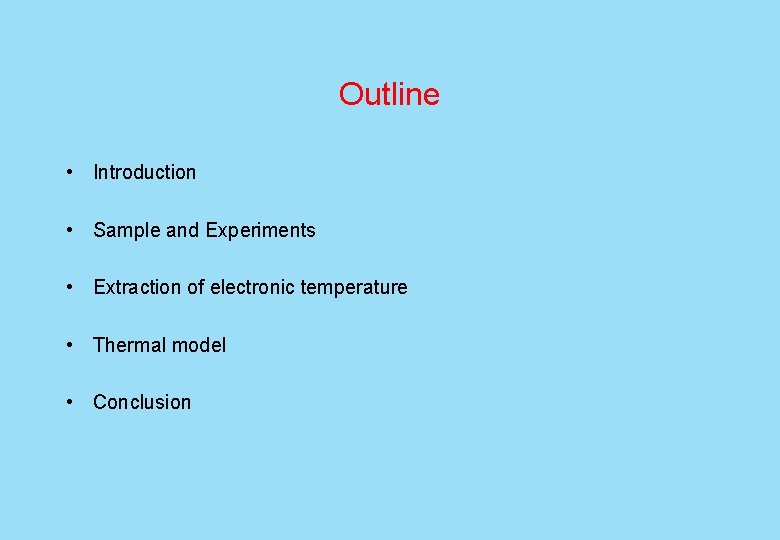
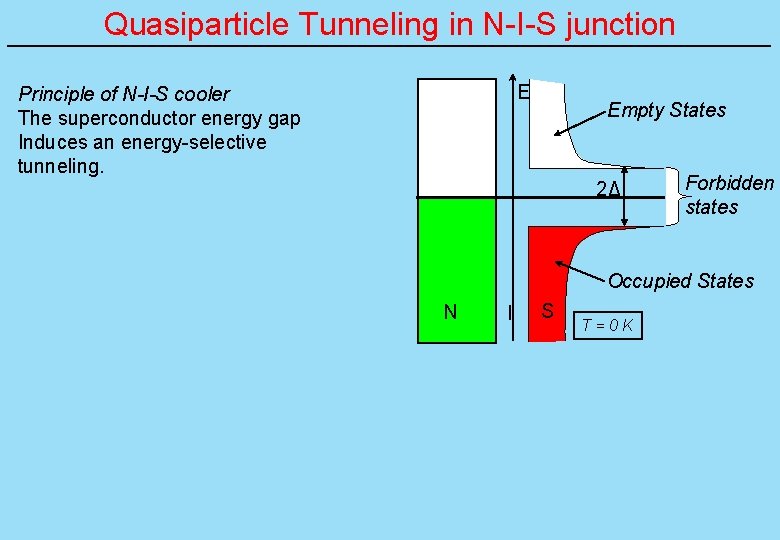
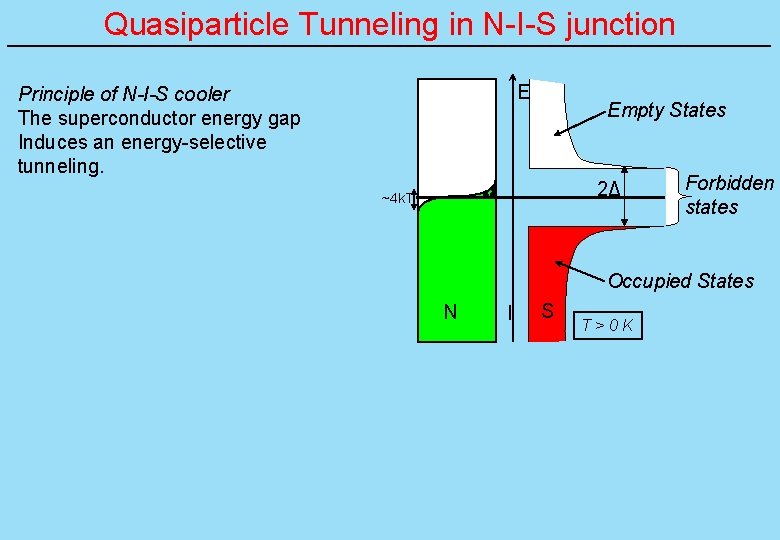
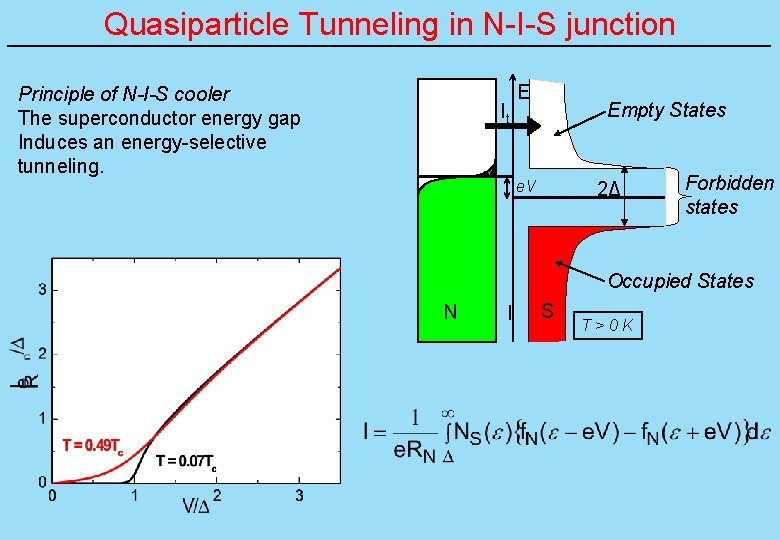
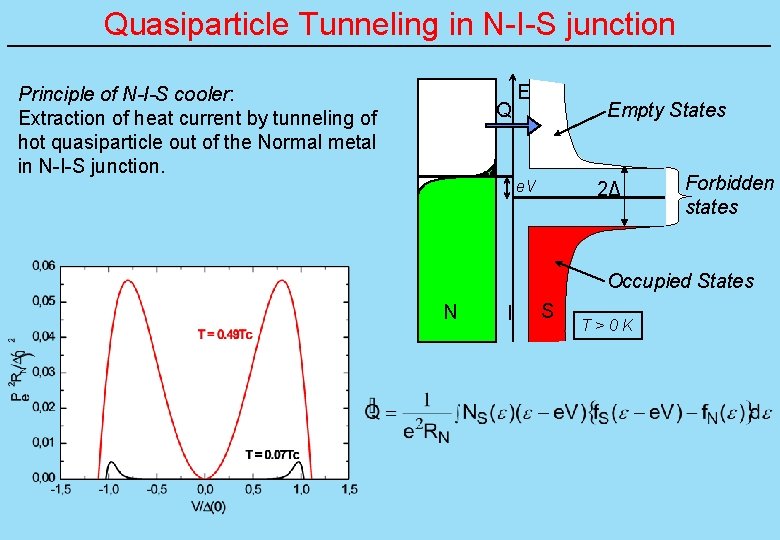
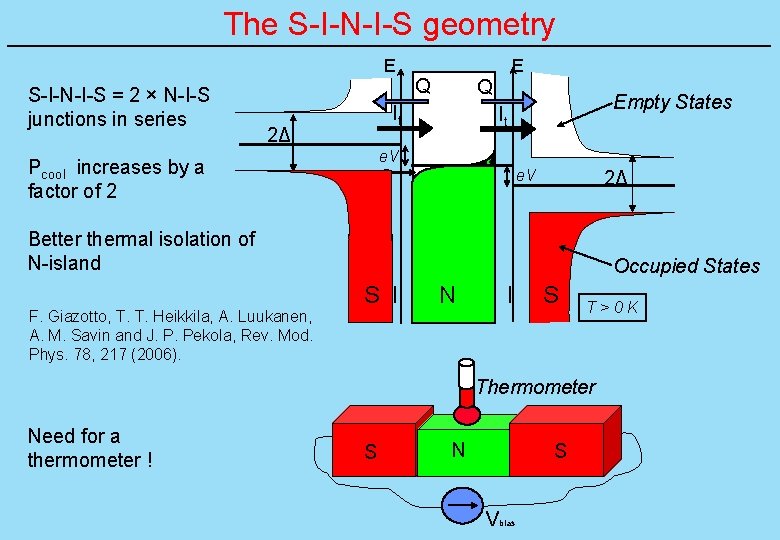
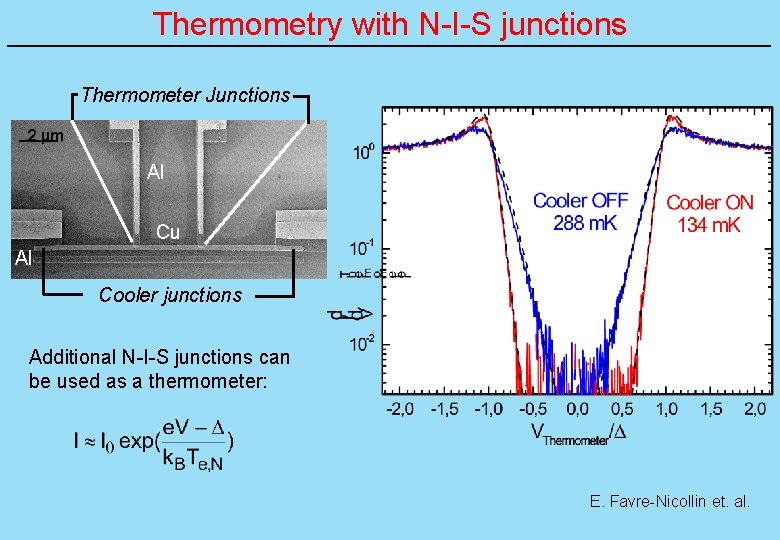
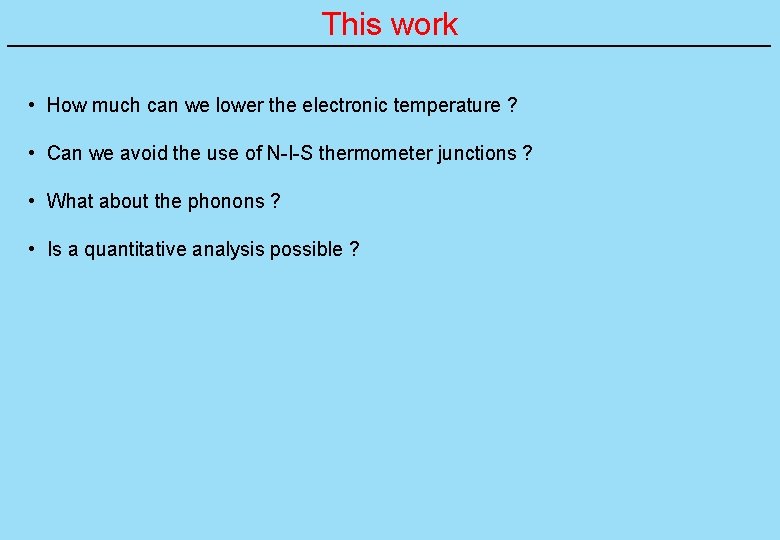
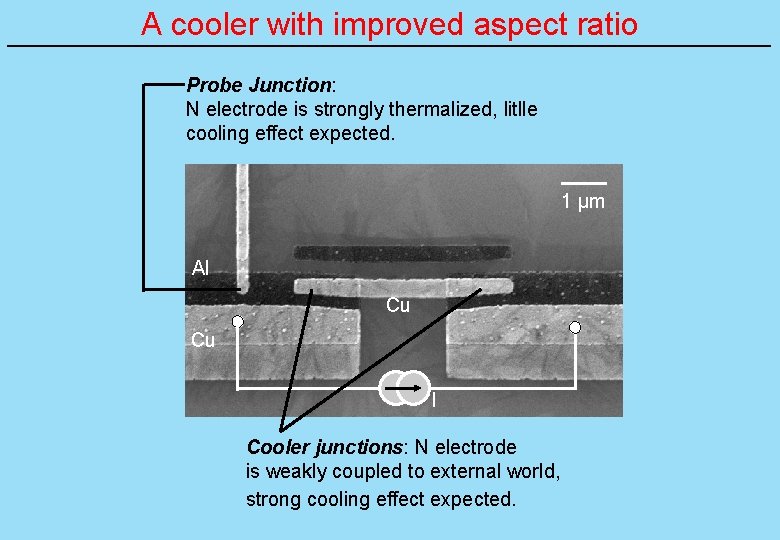
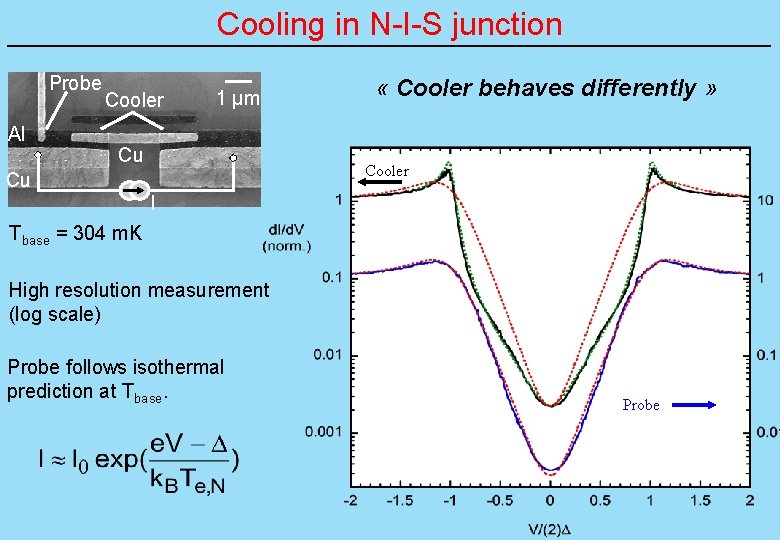
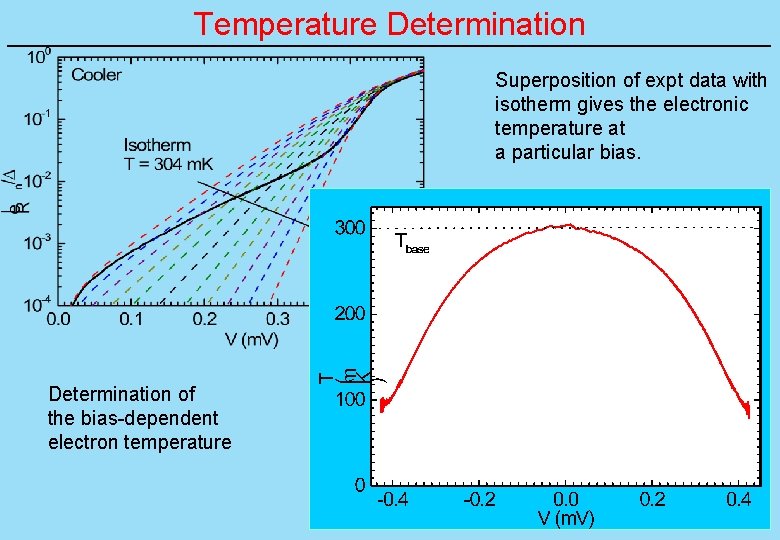
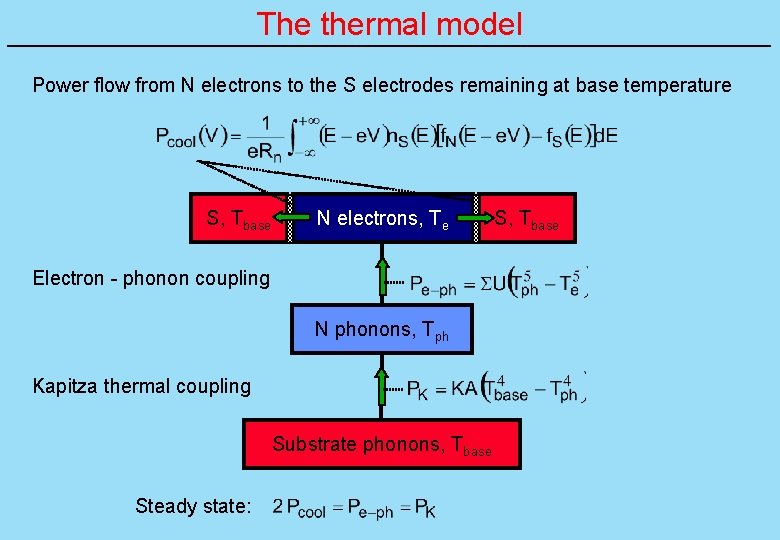
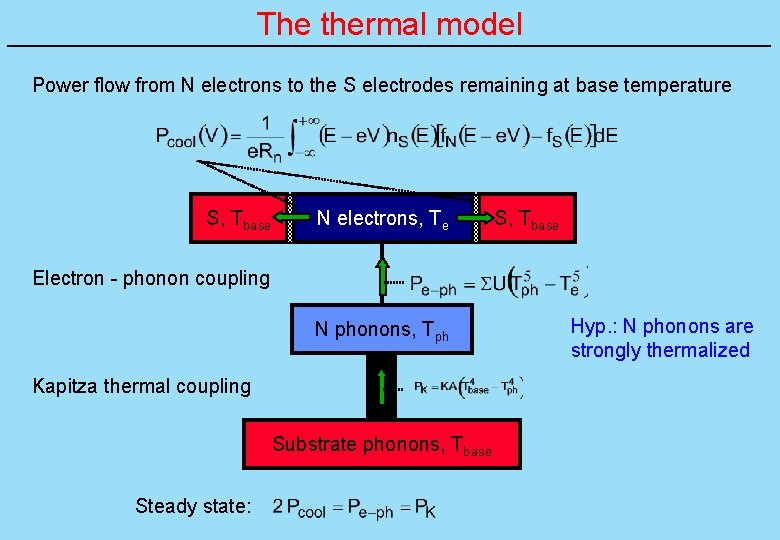
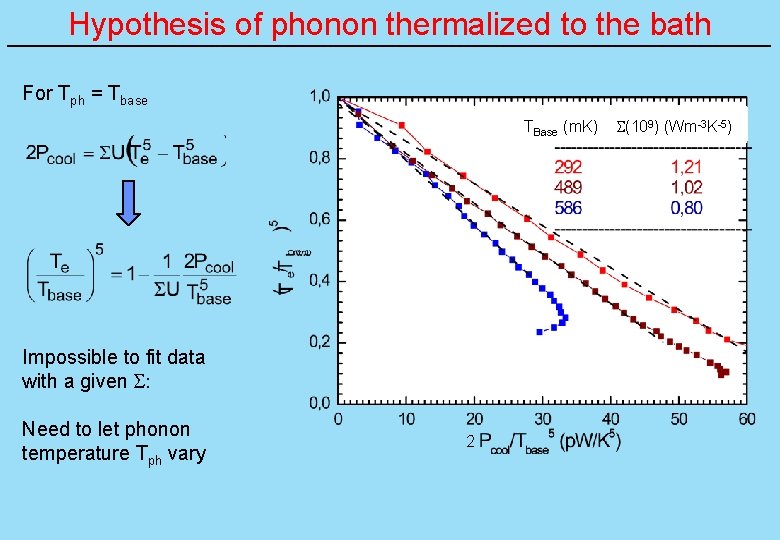
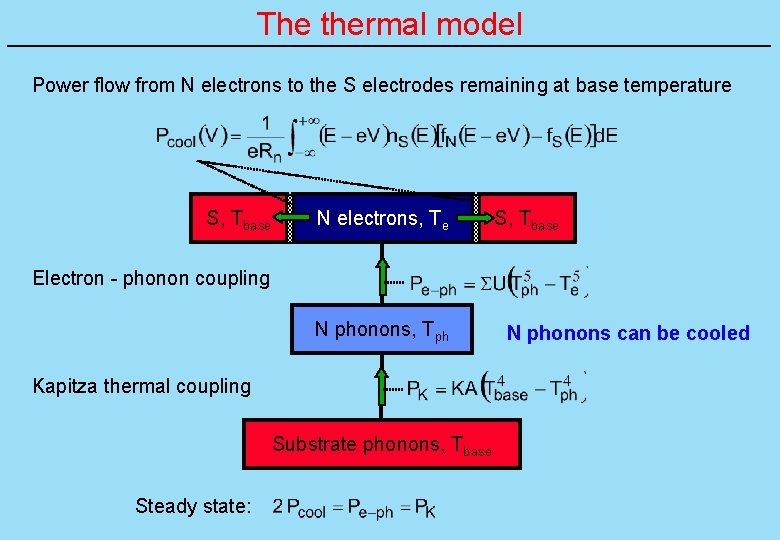
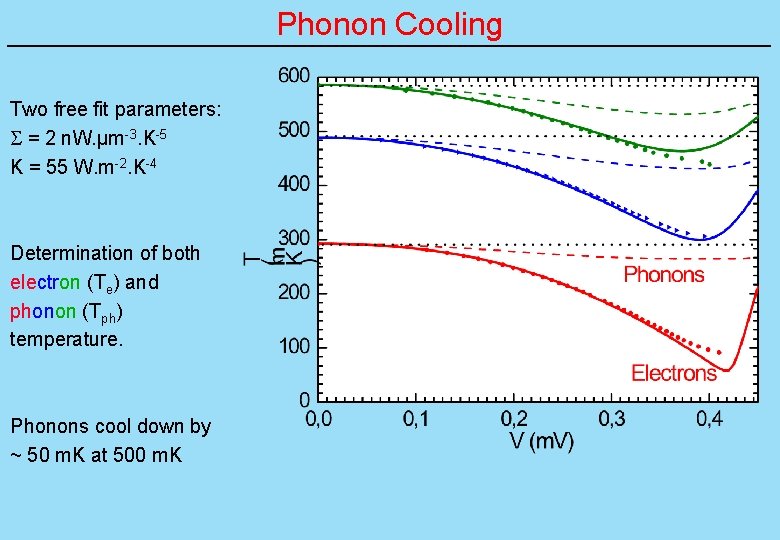
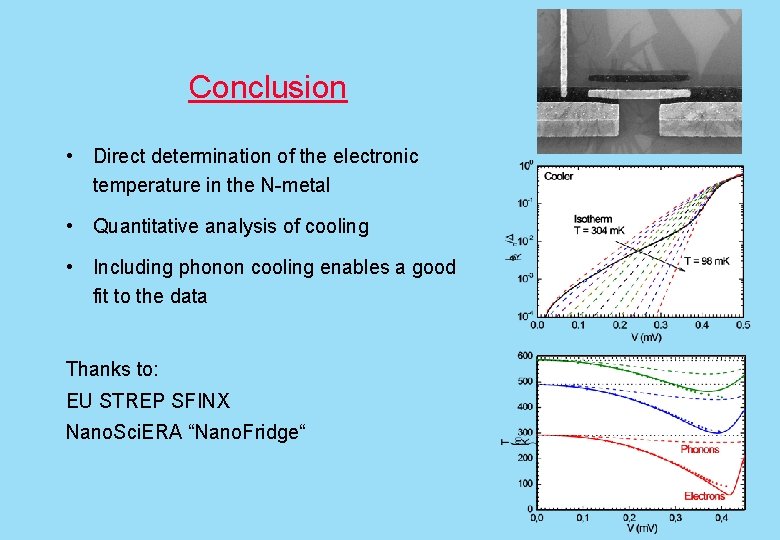
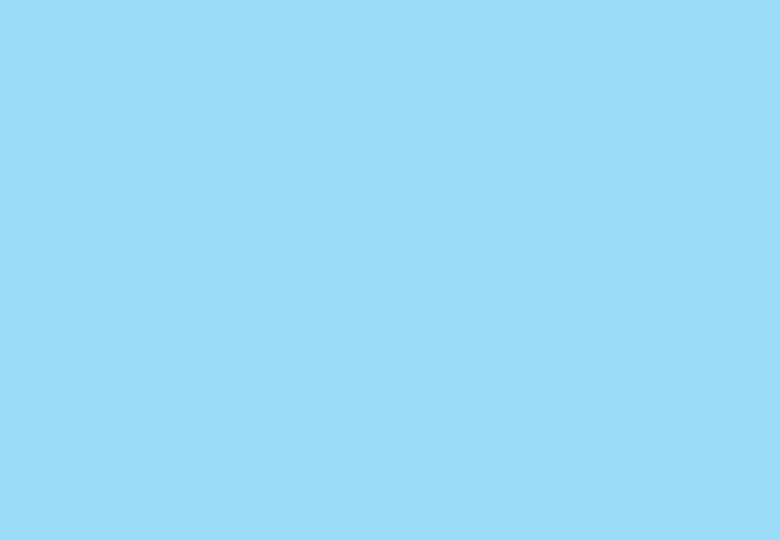
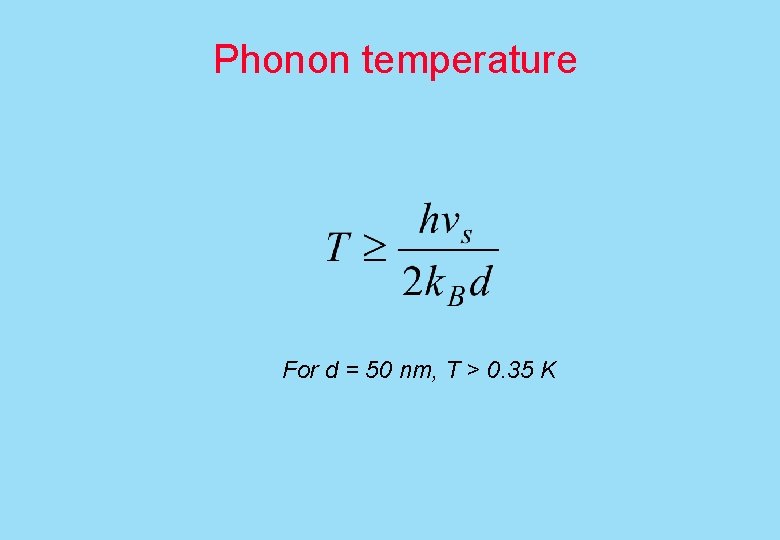
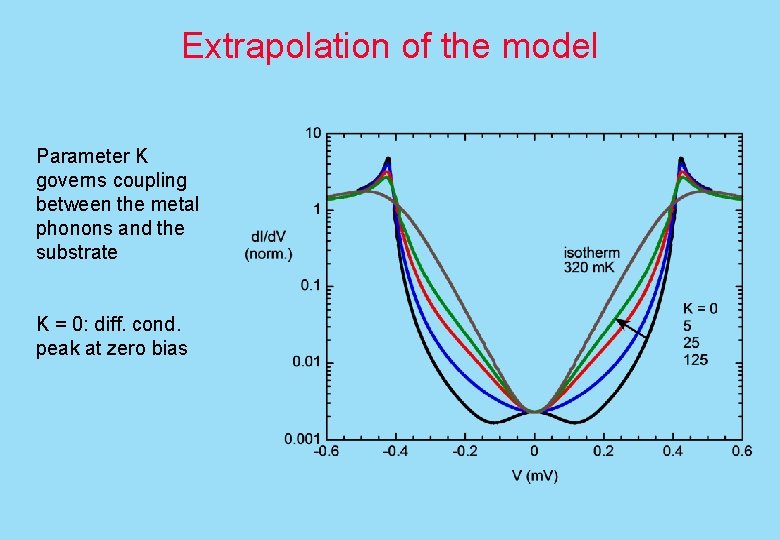
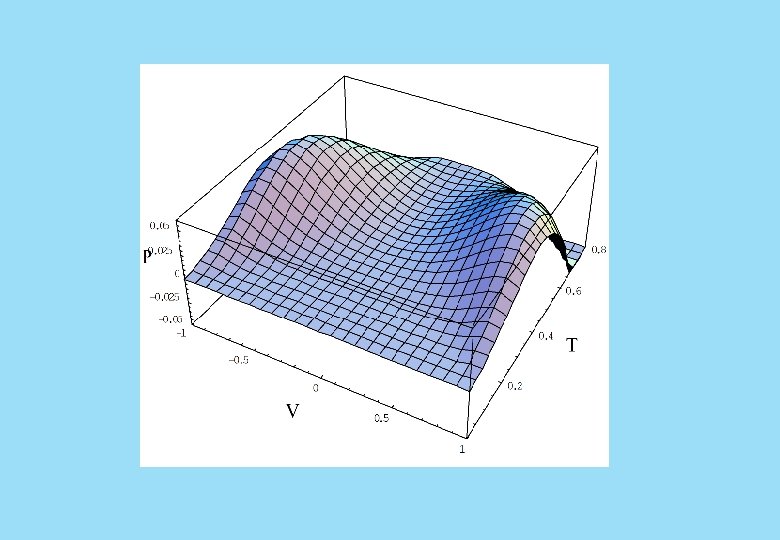
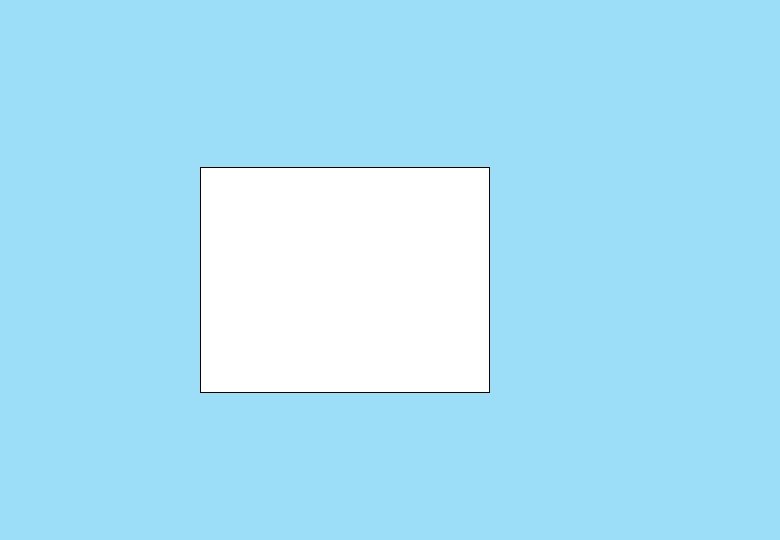
- Slides: 23
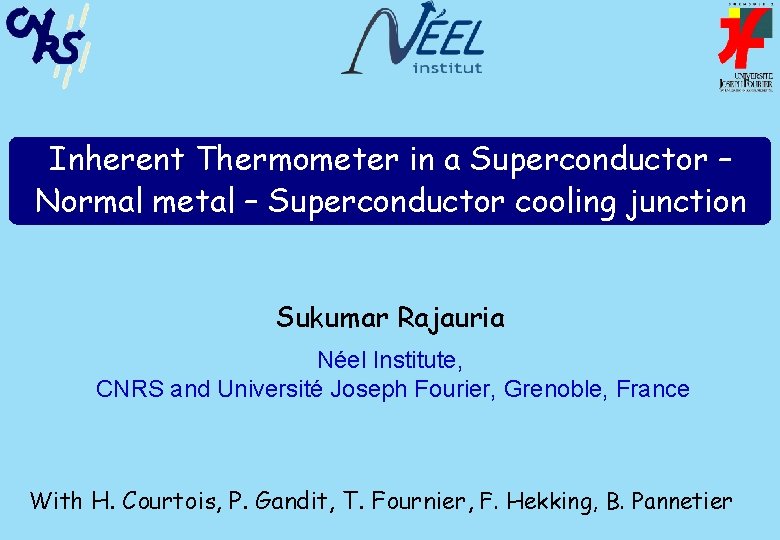
Inherent Thermometer in a Superconductor – Normal metal – Superconductor cooling junction Sukumar Rajauria Néel Institute, CNRS and Université Joseph Fourier, Grenoble, France With H. Courtois, P. Gandit, T. Fournier, F. Hekking, B. Pannetier
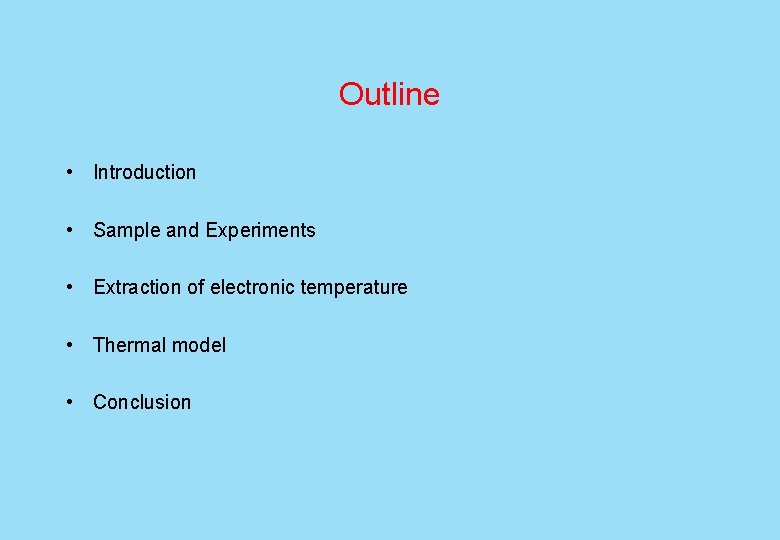
Outline • Introduction • Sample and Experiments • Extraction of electronic temperature • Thermal model • Conclusion
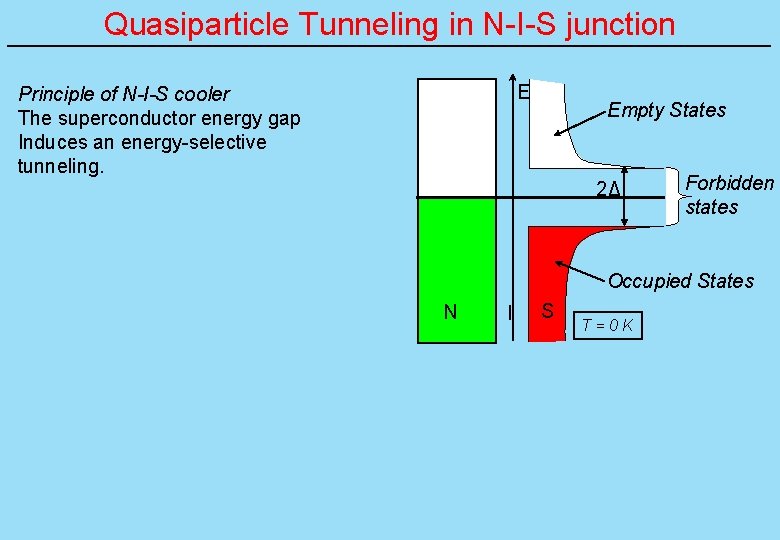
Quasiparticle Tunneling in N-I-S junction E Principle of N-I-S cooler The superconductor energy gap Induces an energy-selective tunneling. Empty States 2Δ Forbidden states Occupied States N I S T=0 K
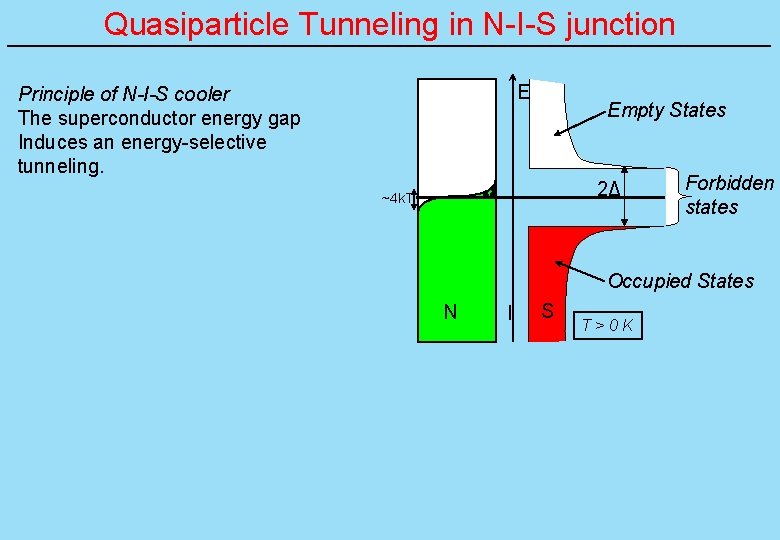
Quasiparticle Tunneling in N-I-S junction E Principle of N-I-S cooler The superconductor energy gap Induces an energy-selective tunneling. Empty States 2Δ ~4 k. T Forbidden states Occupied States N I S T>0 K
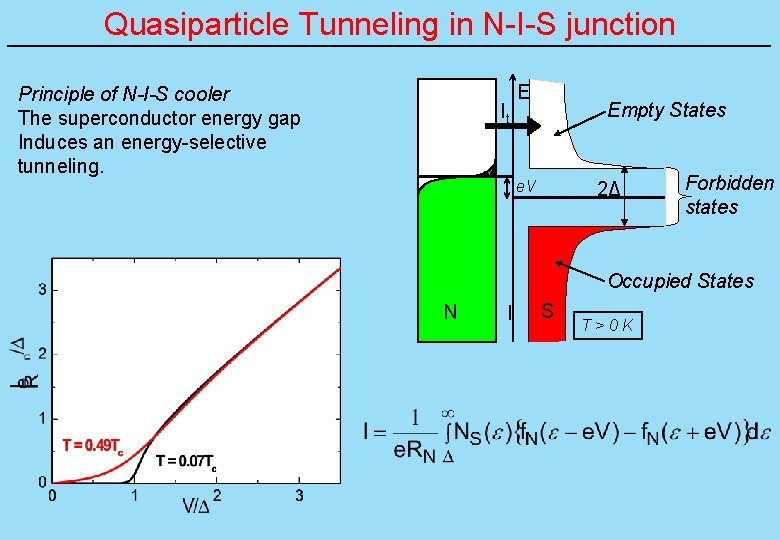
Quasiparticle Tunneling in N-I-S junction Principle of N-I-S cooler The superconductor energy gap Induces an energy-selective tunneling. It E Empty States e. V 2Δ Forbidden states Occupied States N I S T>0 K
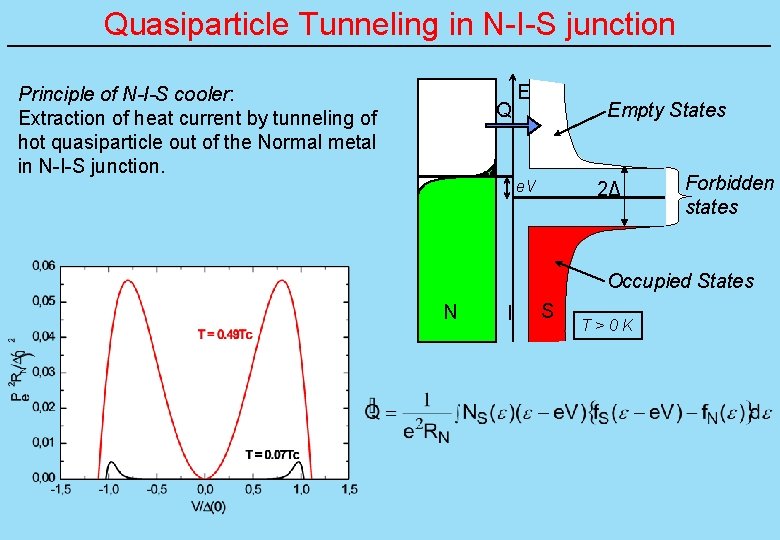
Quasiparticle Tunneling in N-I-S junction Principle of N-I-S cooler: Extraction of heat current by tunneling of hot quasiparticle out of the Normal metal in N-I-S junction. Q E Empty States e. V 2Δ Forbidden states Occupied States N I S T>0 K
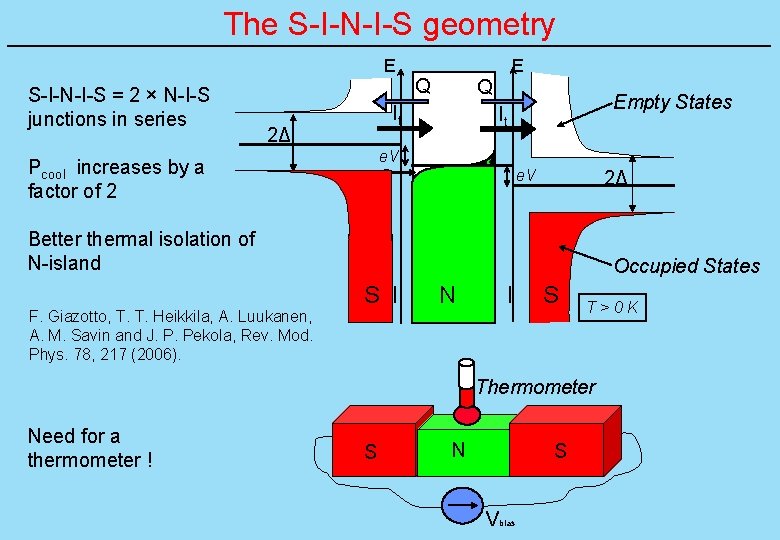
The S-I-N-I-S geometry E S-I-N-I-S = 2 × N-I-S junctions in series E Q Q It 2Δ Empty States It e. V Pcool increases by a factor of 2 e. V 2Δ Better thermal isolation of N-island F. Giazotto, T. T. Heikkila, A. Luukanen, A. M. Savin and J. P. Pekola, Rev. Mod. Phys. 78, 217 (2006). Occupied States S I N I S T>0 K Thermometer Need for a thermometer ! S N S Vbias
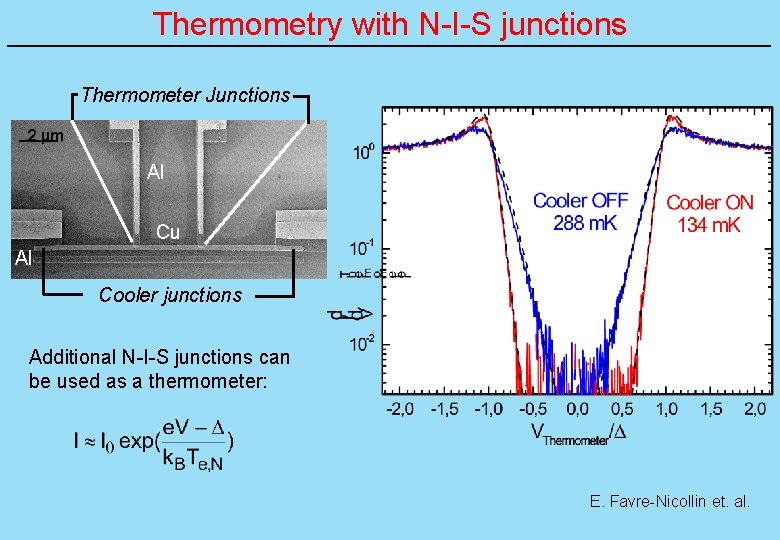
Thermometry with N-I-S junctions Thermometer Junctions 2 µm Al Cu Al Cooler junctions Additional N-I-S junctions can be used as a thermometer: E. Favre-Nicollin et. al.
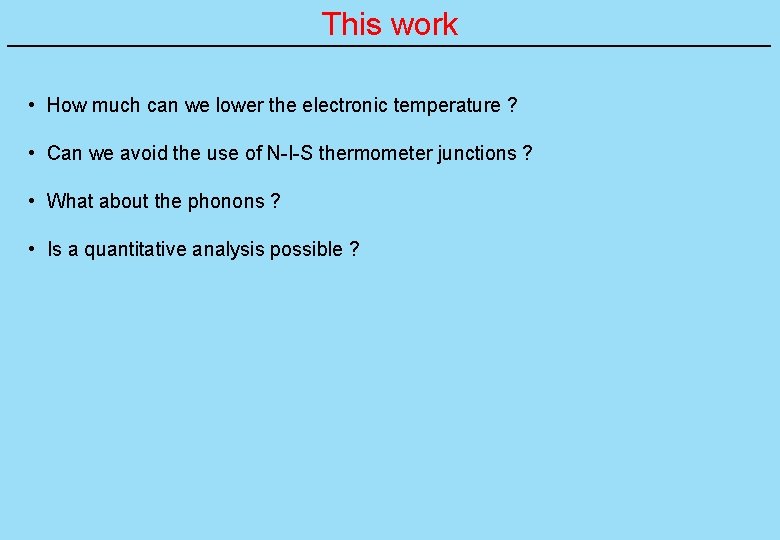
This work • How much can we lower the electronic temperature ? • Can we avoid the use of N-I-S thermometer junctions ? • What about the phonons ? • Is a quantitative analysis possible ?
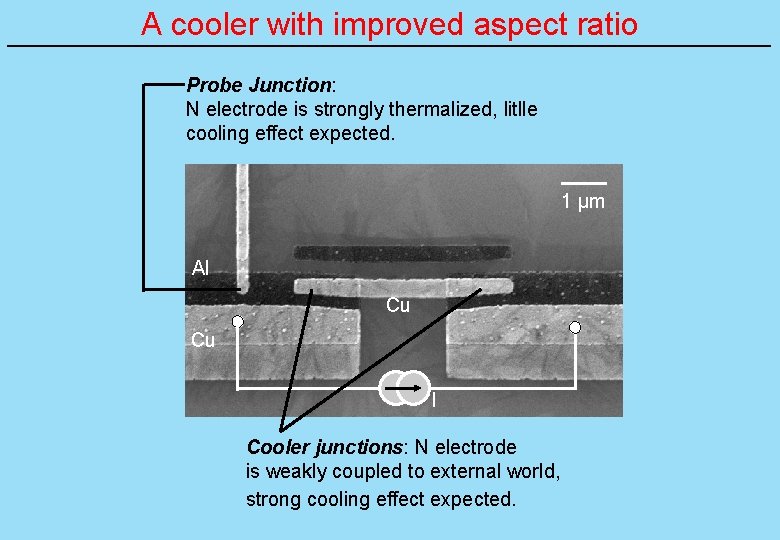
A cooler with improved aspect ratio Probe Junction: N electrode is strongly thermalized, litlle cooling effect expected. 1 µm Al Cu Cu I Cooler junctions: N electrode is weakly coupled to external world, strong cooling effect expected.
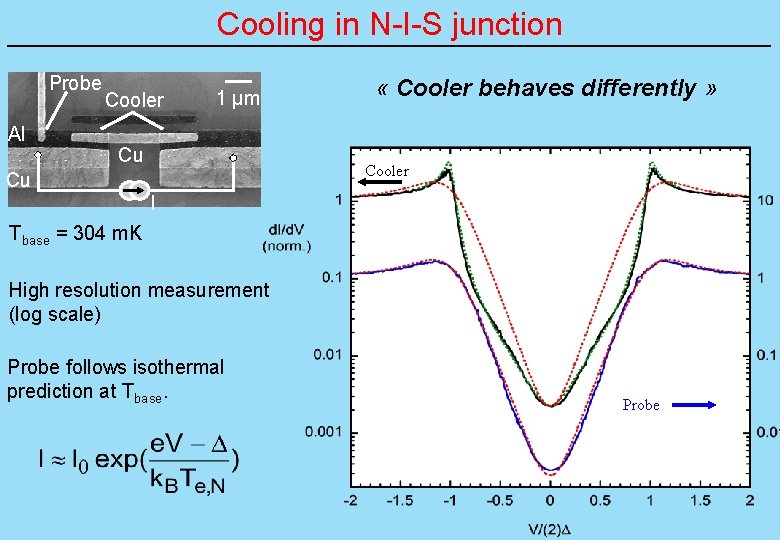
Cooling in N-I-S junction Probe Al Cooler 1 µm Cu « Cooler behaves differently » Cooler Cu I Tbase = 304 m. K High resolution measurement (log scale) Probe follows isothermal prediction at Tbase. Probe
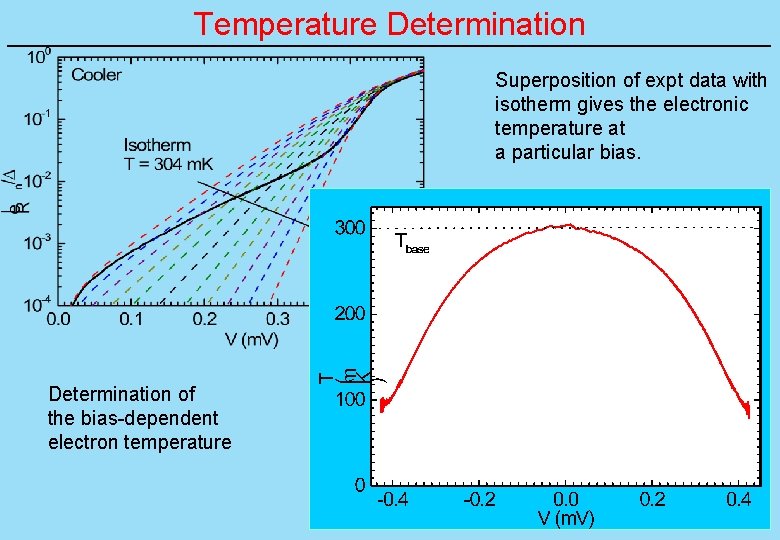
Temperature Determination Superposition of expt data with isotherm gives the electronic temperature at a particular bias. Determination of the bias-dependent electron temperature
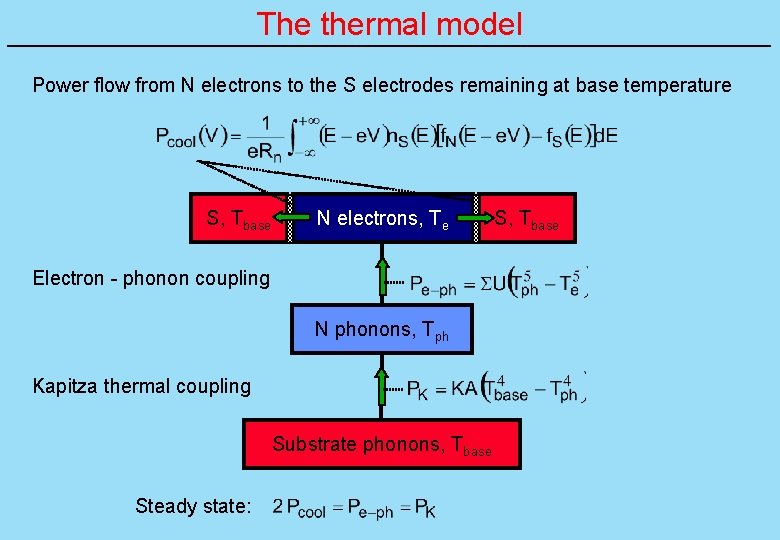
The thermal model Power flow from N electrons to the S electrodes remaining at base temperature S, Tbase N electrons, Te Electron - phonon coupling N phonons, Tph Kapitza thermal coupling Substrate phonons, Tbase Steady state: S, Tbase
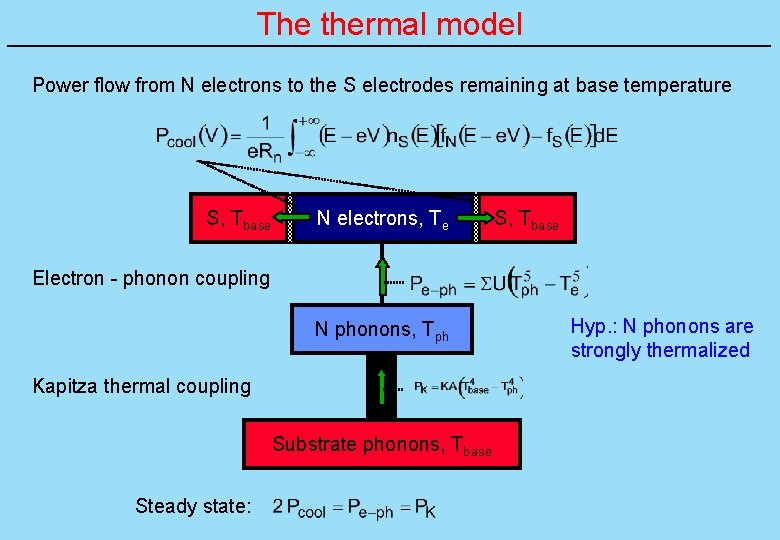
The thermal model Power flow from N electrons to the S electrodes remaining at base temperature S, Tbase N electrons, Te S, Tbase Electron - phonon coupling N phonons, Tph Kapitza thermal coupling Substrate phonons, Tbase Steady state: Hyp. : N phonons are strongly thermalized
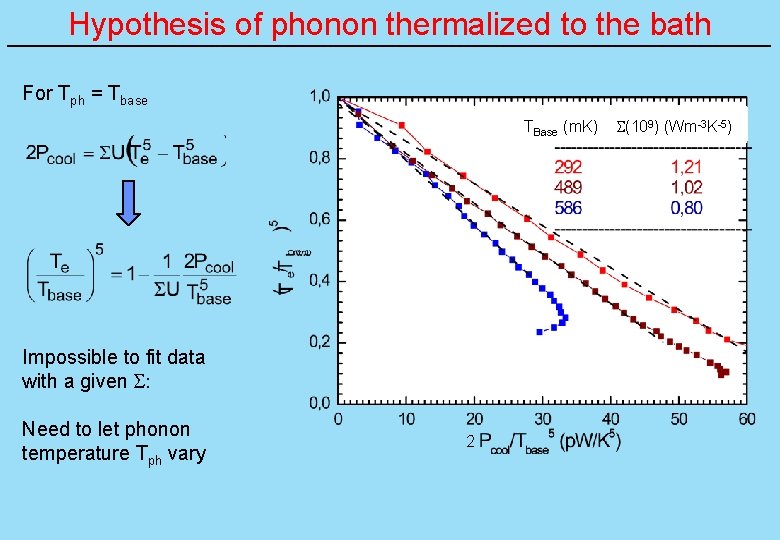
Hypothesis of phonon thermalized to the bath For Tph = Tbase TBase (m. K) Impossible to fit data with a given Need to let phonon temperature Tph vary 2 (109) (Wm-3 K-5)
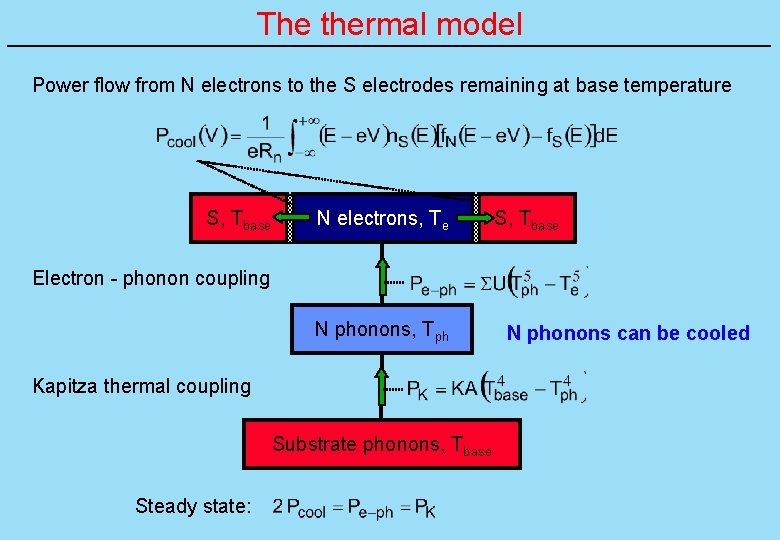
The thermal model Power flow from N electrons to the S electrodes remaining at base temperature S, Tbase N electrons, Te S, Tbase Electron - phonon coupling N phonons, Tph Kapitza thermal coupling Substrate phonons, Tbase Steady state: N phonons can be cooled
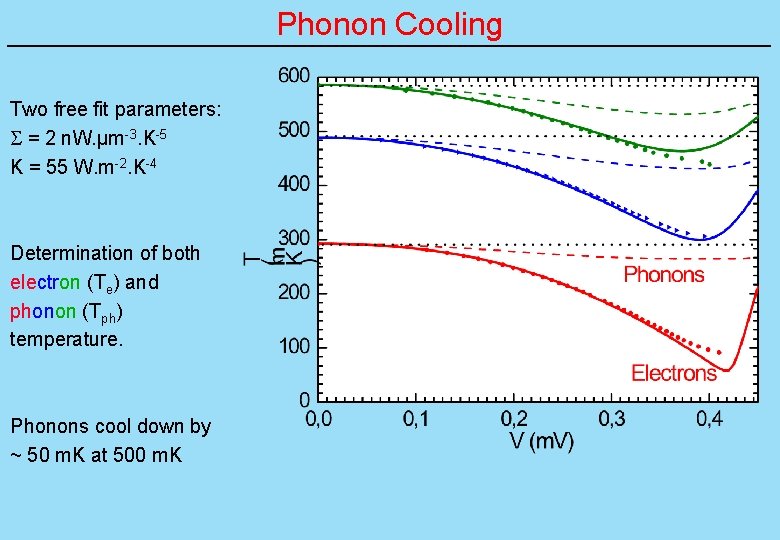
Phonon Cooling Two free fit parameters: = 2 n. W. µm-3. K-5 K = 55 W. m-2. K-4 Determination of both electron (Te) and phonon (Tph) temperature. Phonons cool down by ~ 50 m. K at 500 m. K
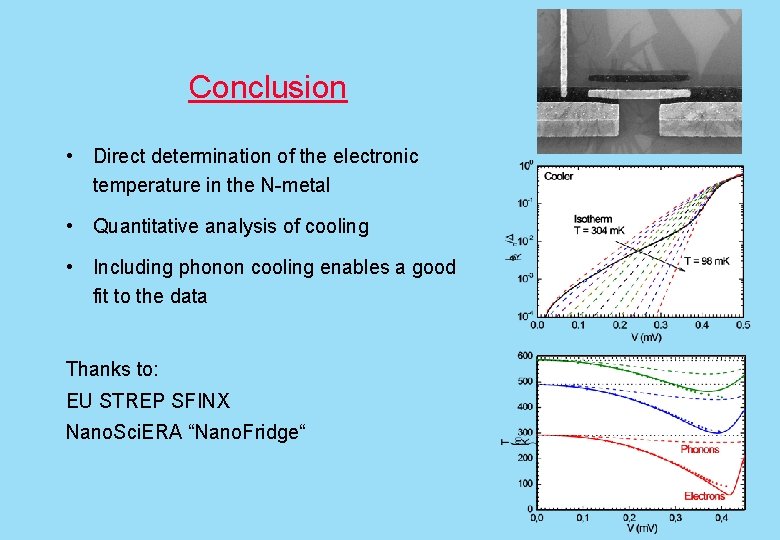
Conclusion • Direct determination of the electronic temperature in the N-metal • Quantitative analysis of cooling • Including phonon cooling enables a good fit to the data Thanks to: EU STREP SFINX Nano. Sci. ERA “Nano. Fridge“
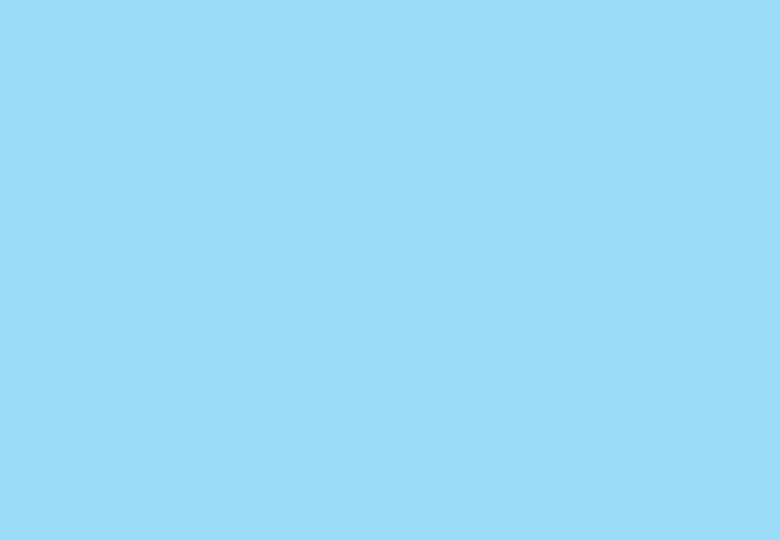
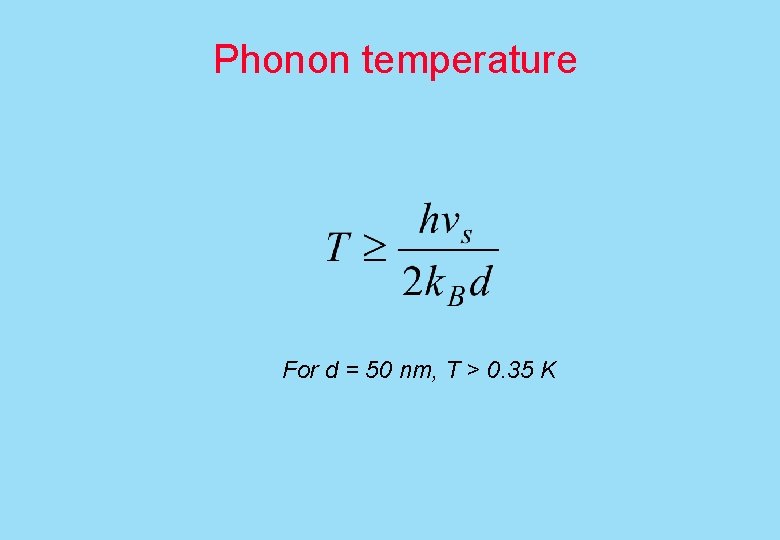
Phonon temperature For d = 50 nm, T > 0. 35 K
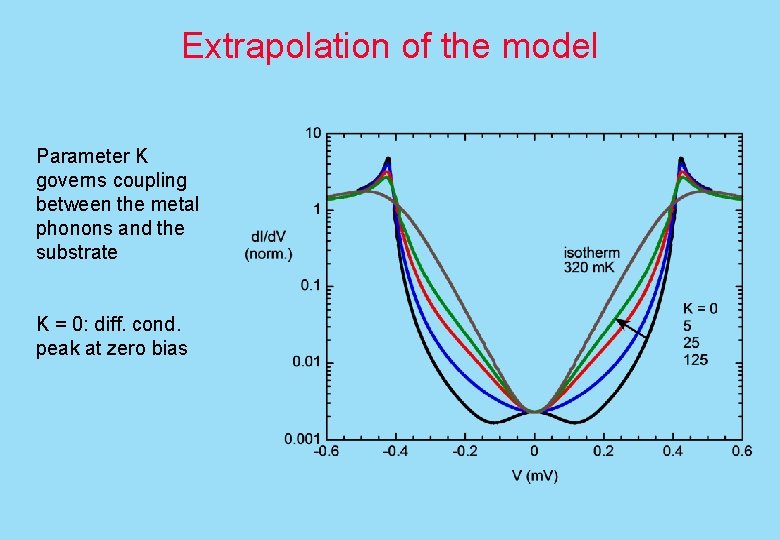
Extrapolation of the model Parameter K governs coupling between the metal phonons and the substrate K = 0: diff. cond. peak at zero bias
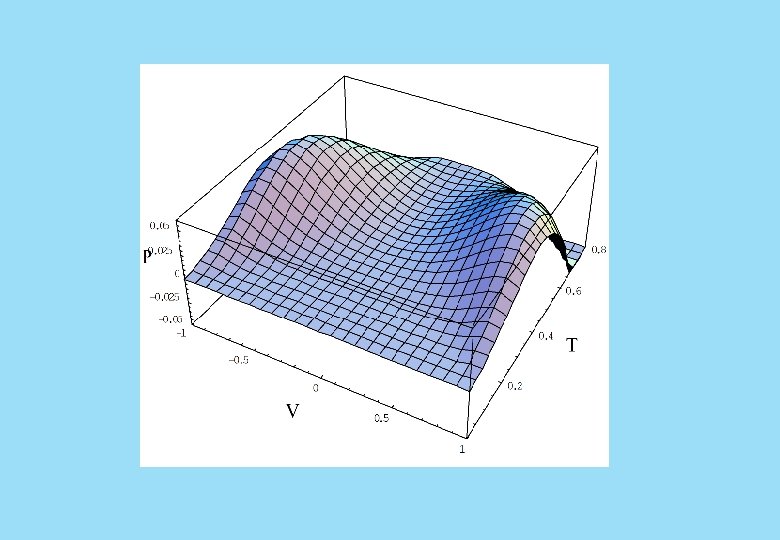
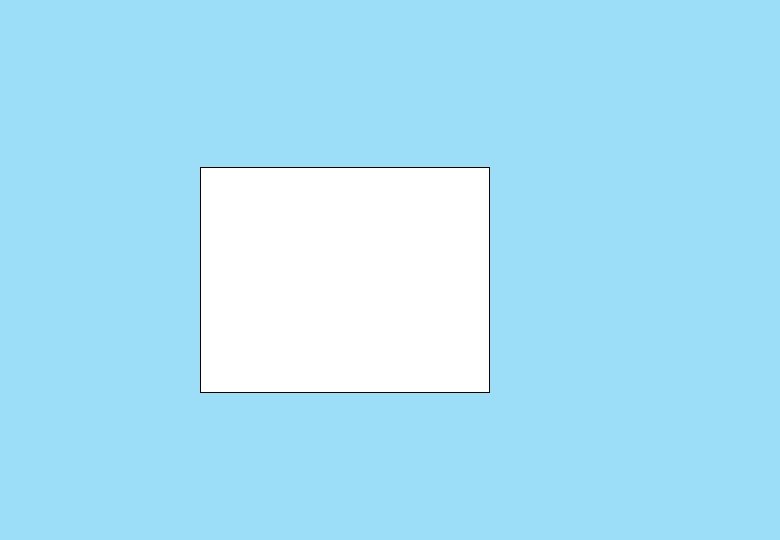
Adjectives classification
Alcohol thermometer vs mercury thermometer
Types of superconductors
Define the survey of superconductor
Superconductor
Superconductor
Superconductor examples
Non metal examples
Diamond melting point
Grupo b tabla periódica
Ionic compounds have
Venn diagram of solid liquid gas
El sodio es metal o no metal
Deep nwell
Chemical bond def
Characteristics of metal
Metals
Propiedades de los anfígenos
Acidity trends periodic table
Metal and non metal elements in periodic table
Inherent vice definition
Total hypermetropia
Ar = ir x cr x dr
Efpia