Electron transport chain and oxidative phosphorylation Chapter 17
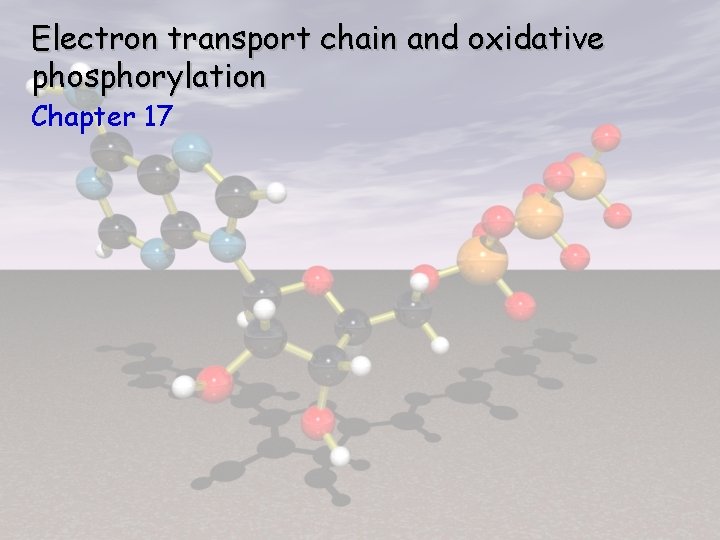
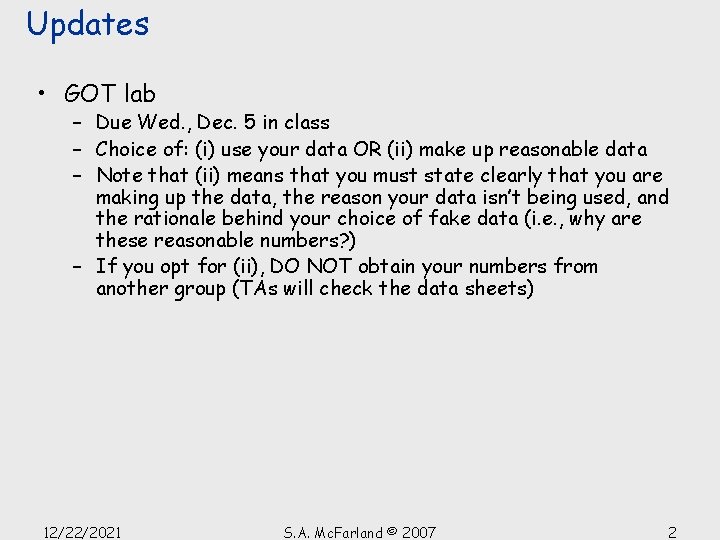
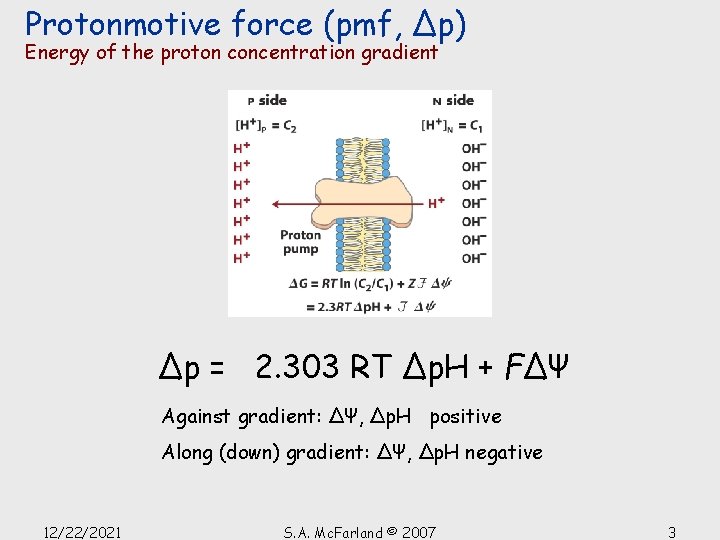
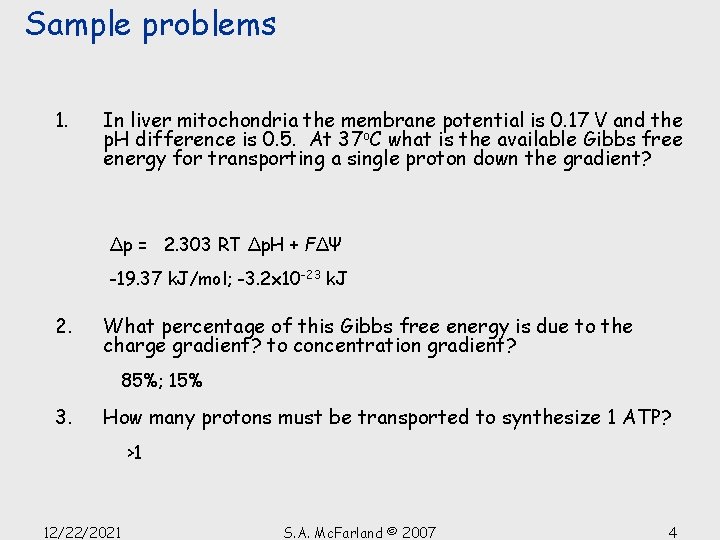
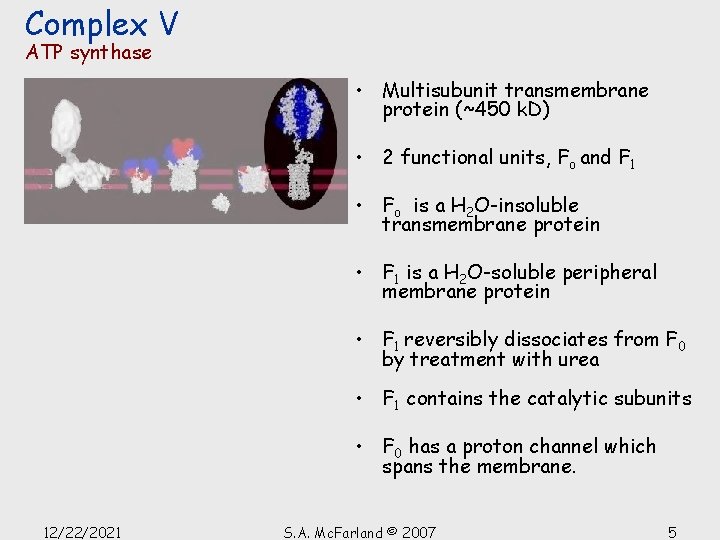
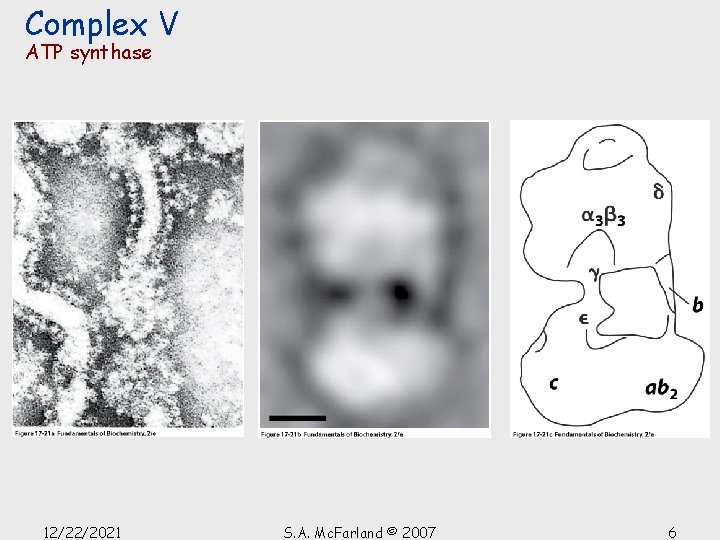
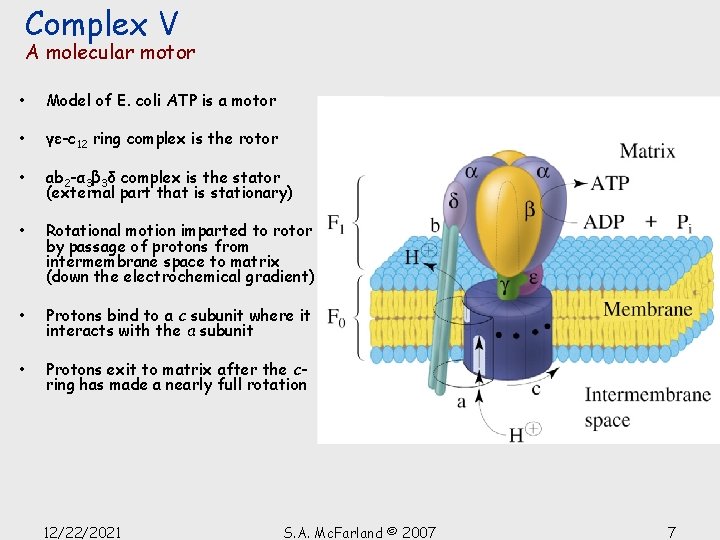
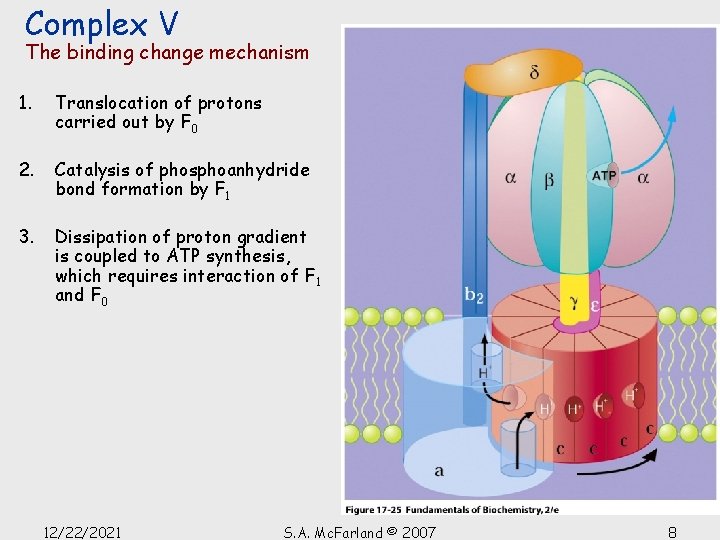
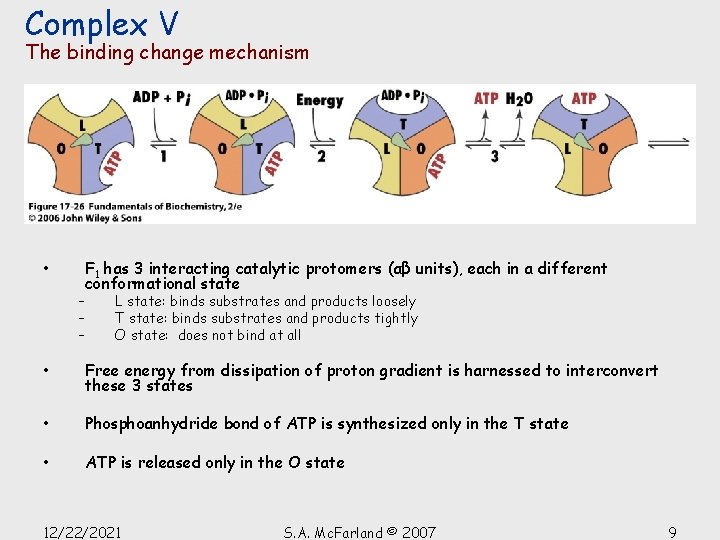
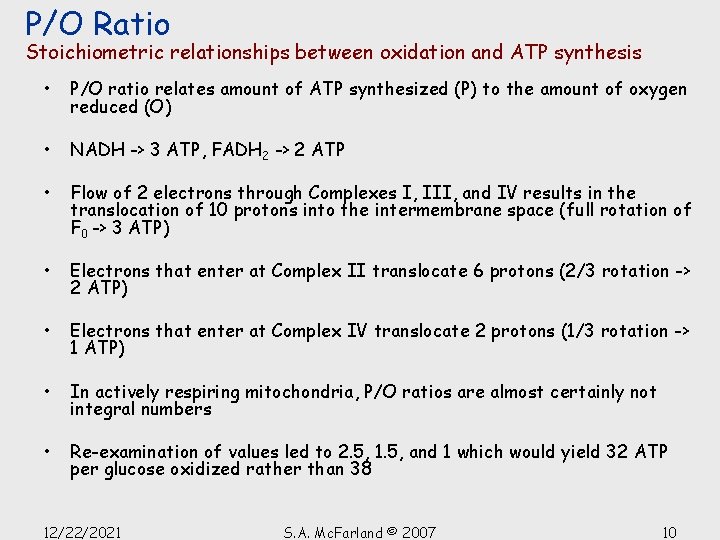
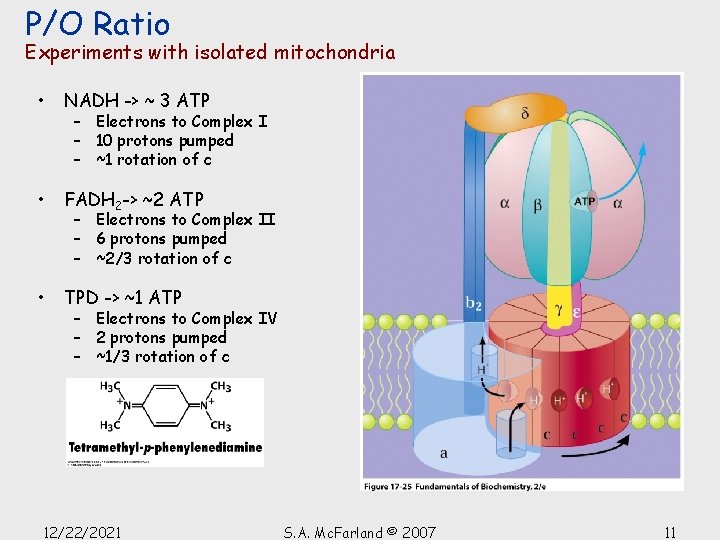
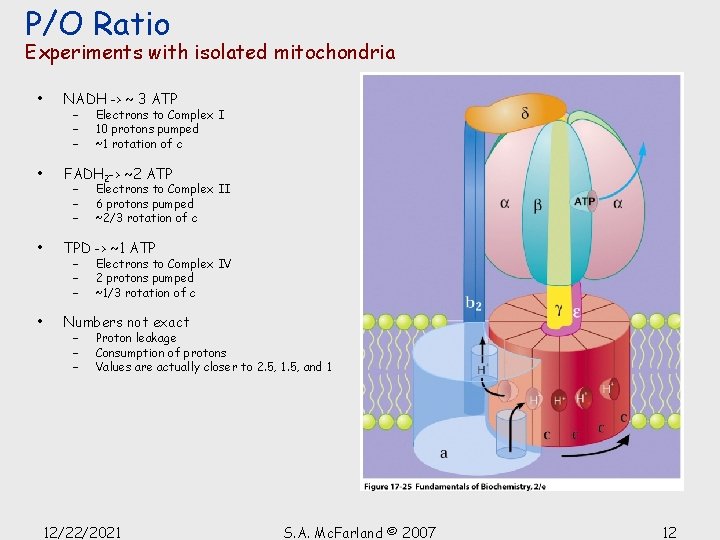
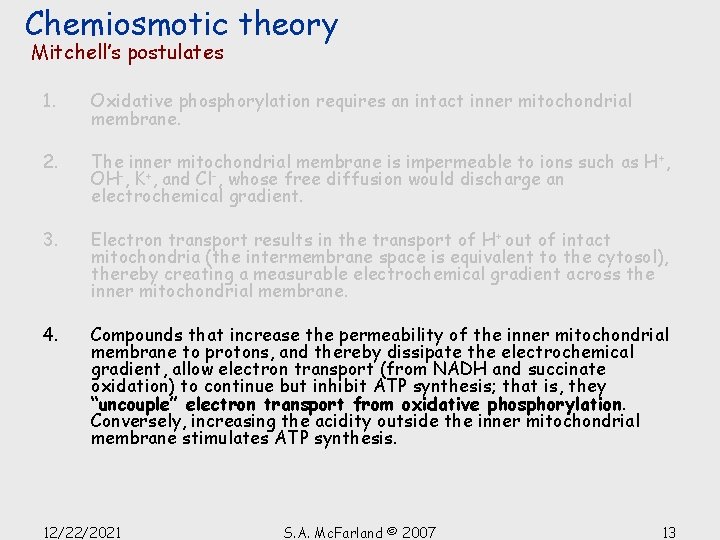
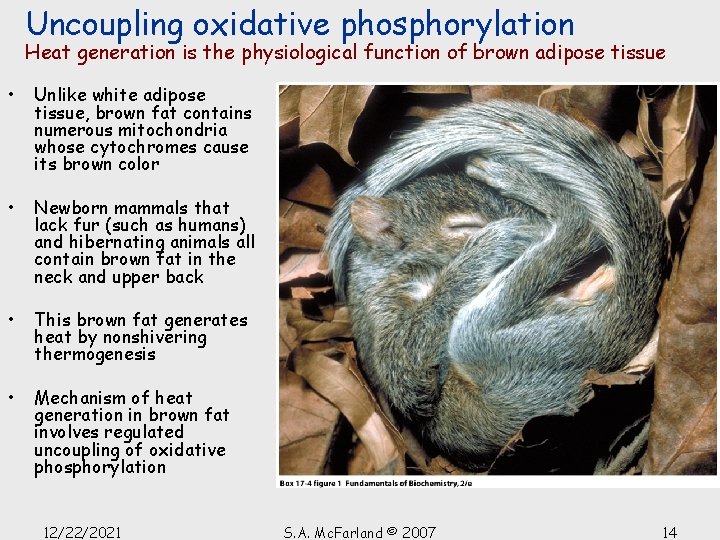
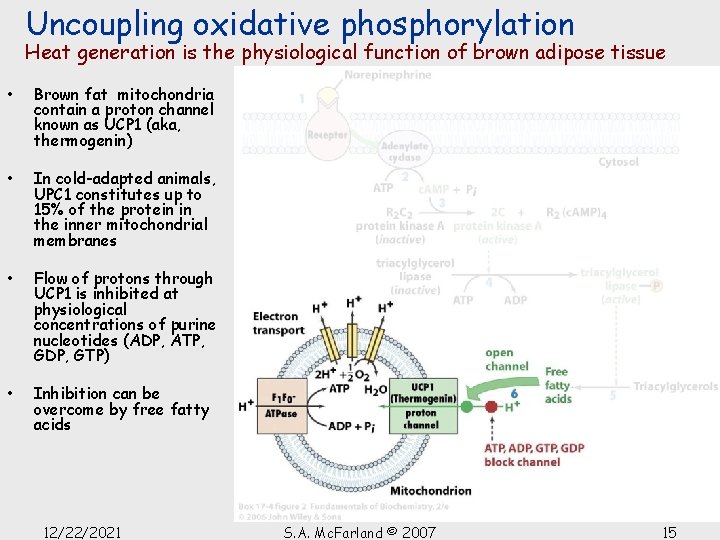
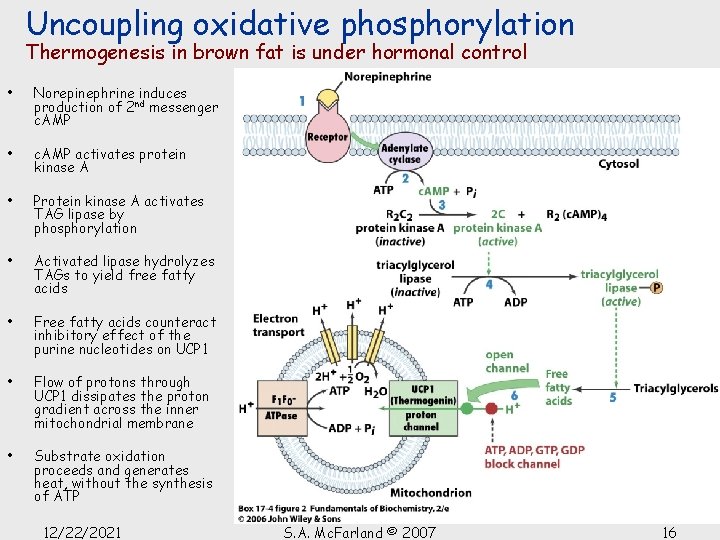
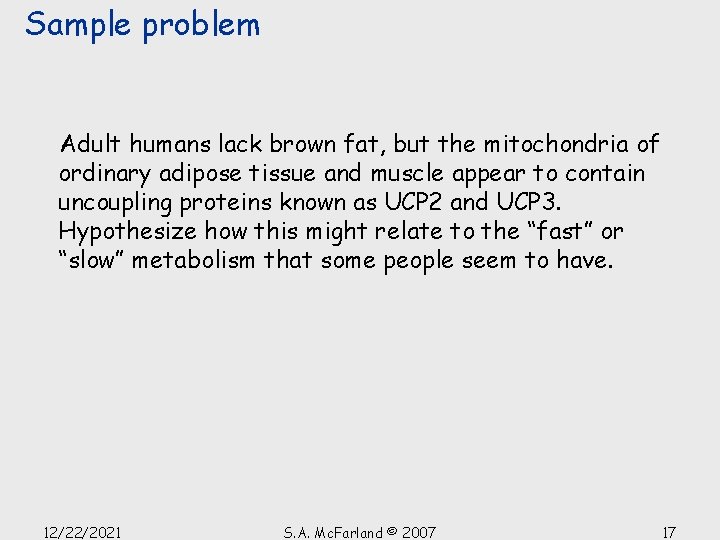
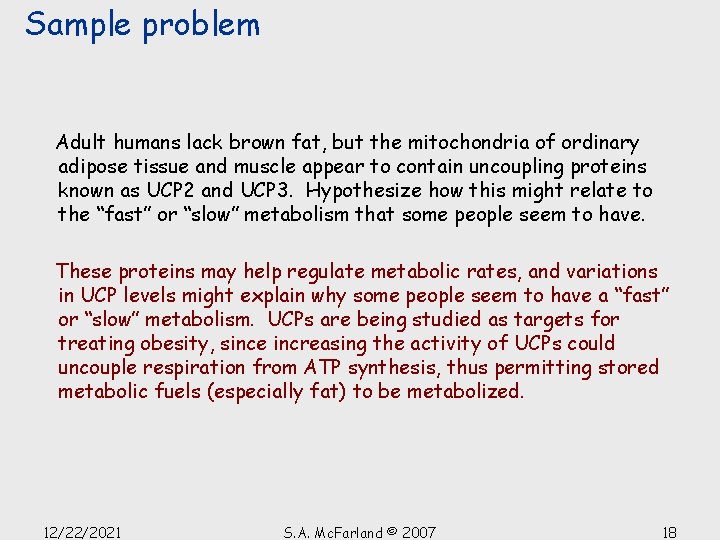
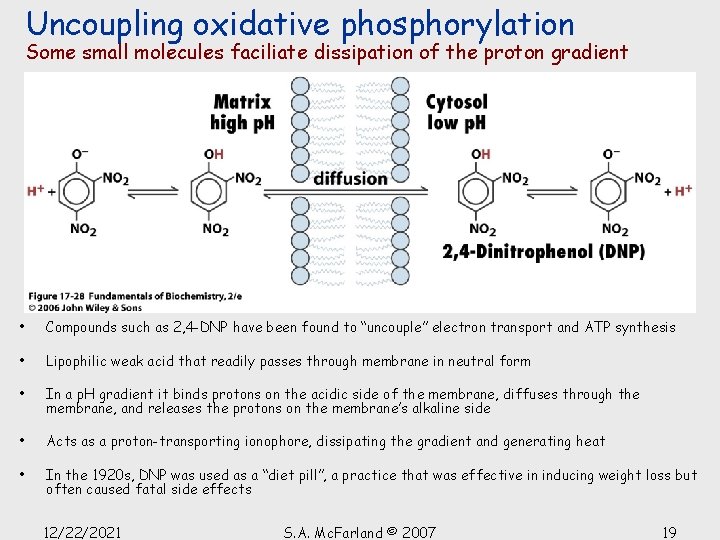
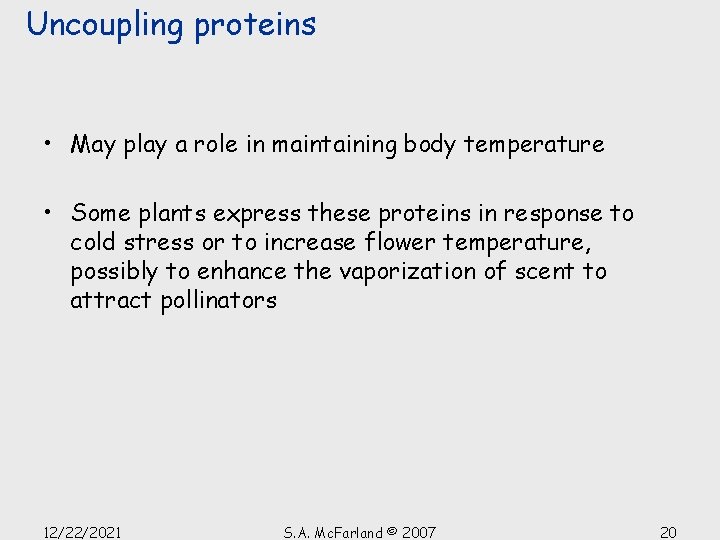
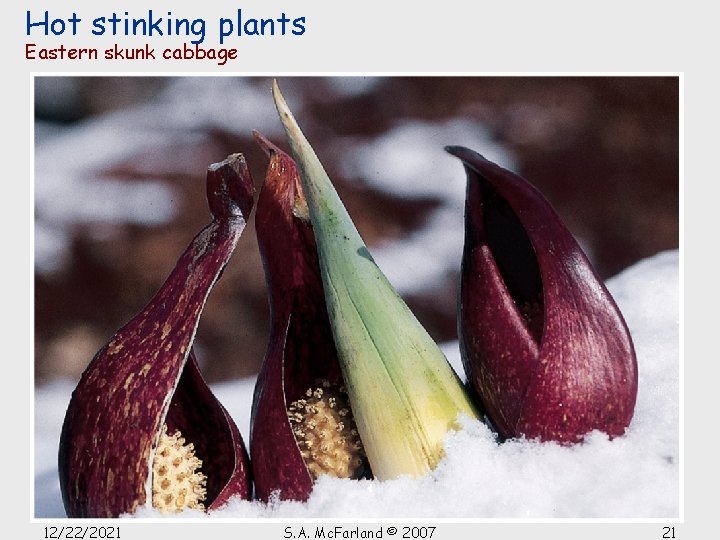
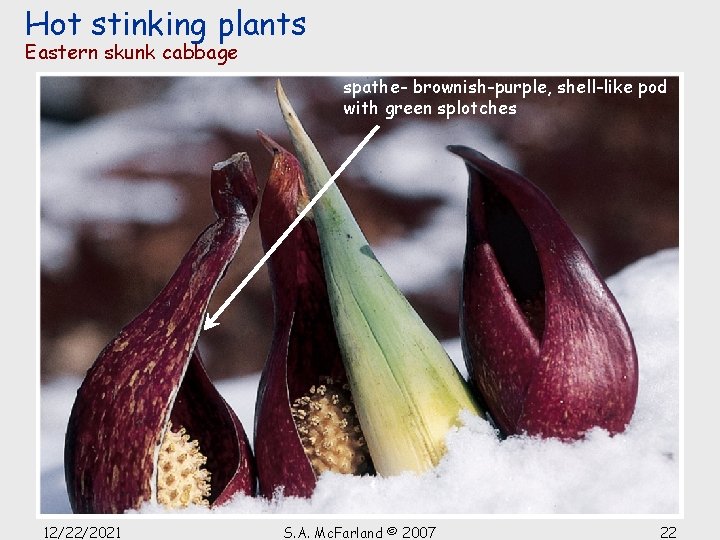
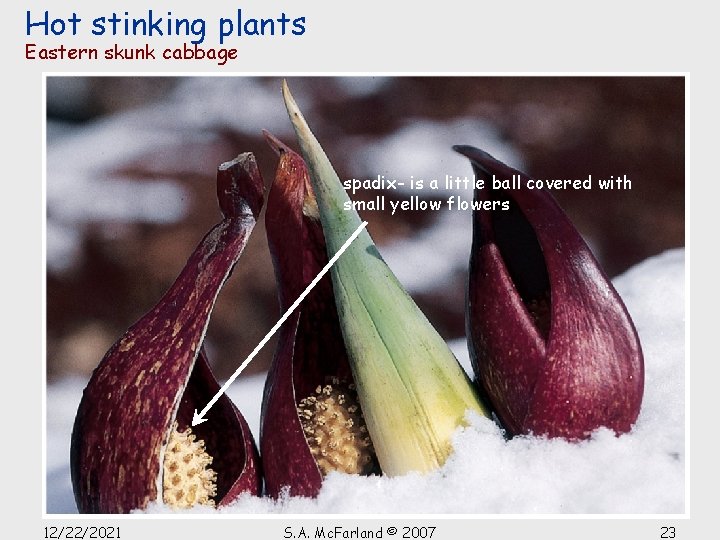
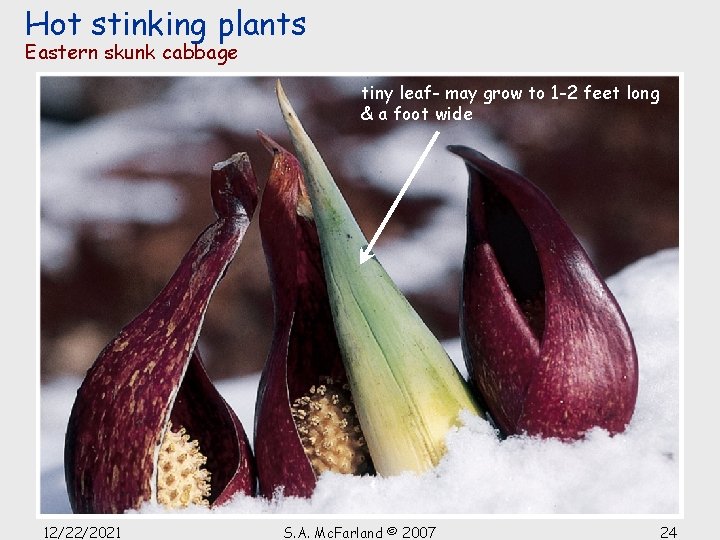
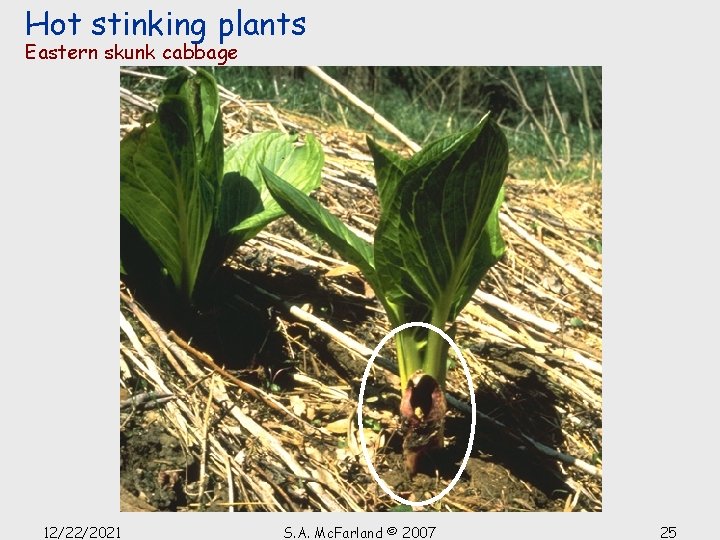
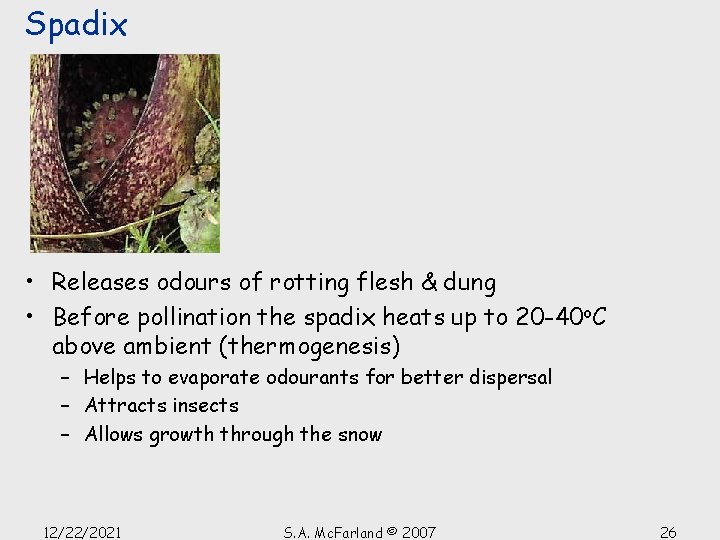
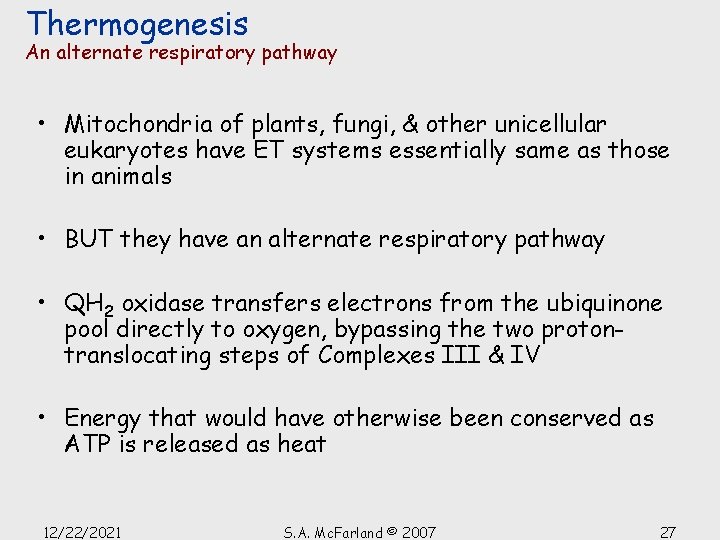
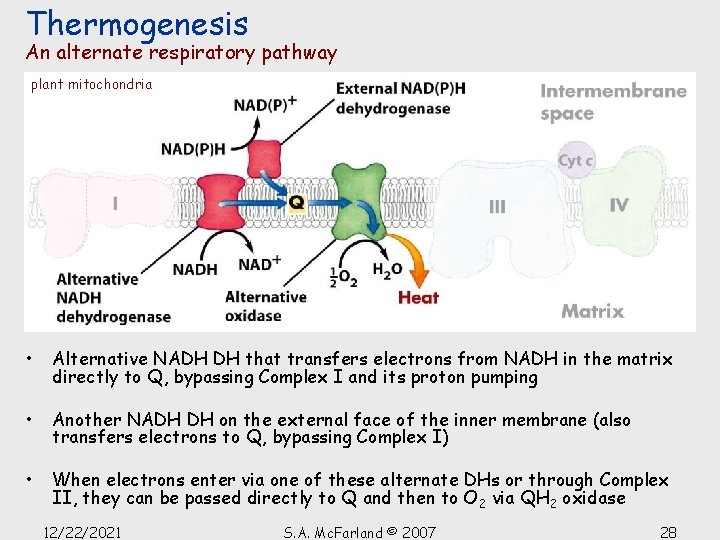
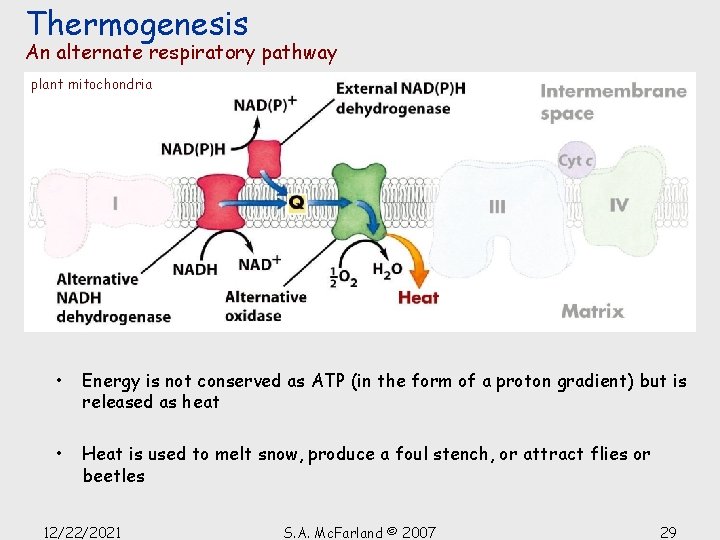
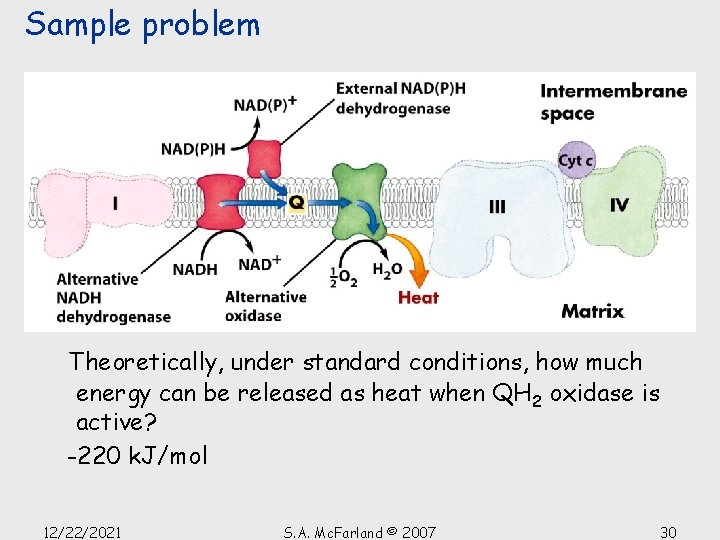
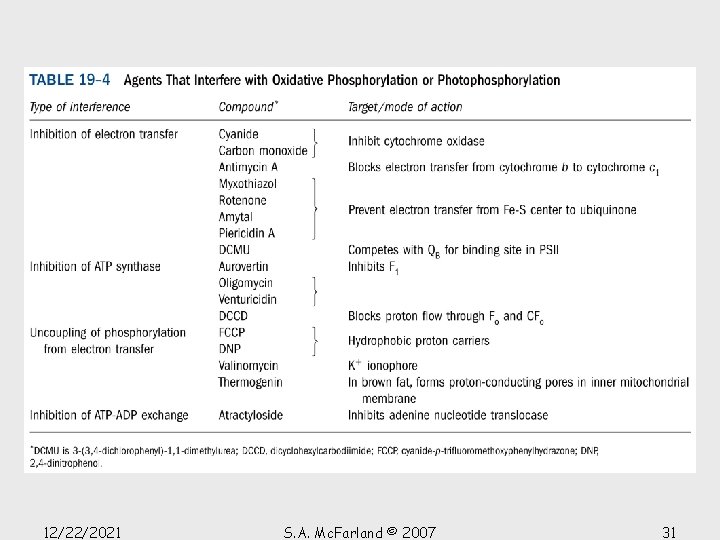
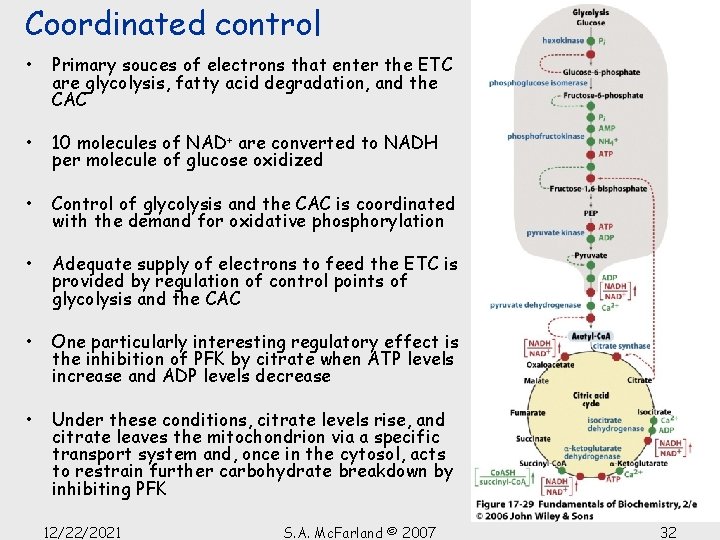
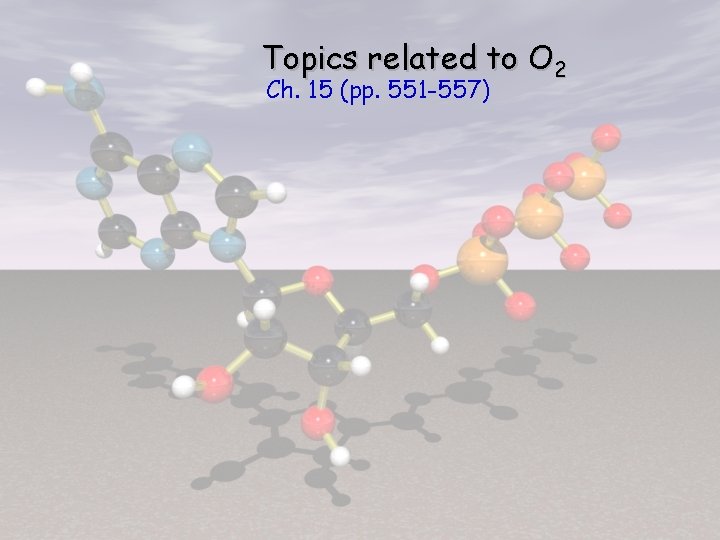
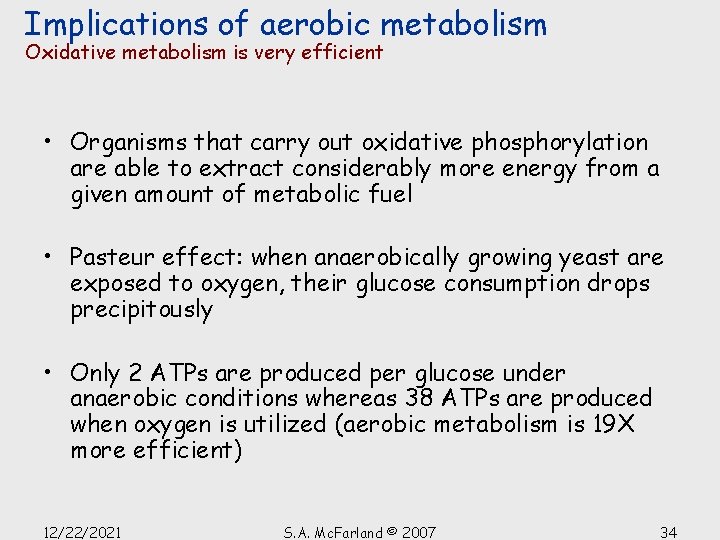
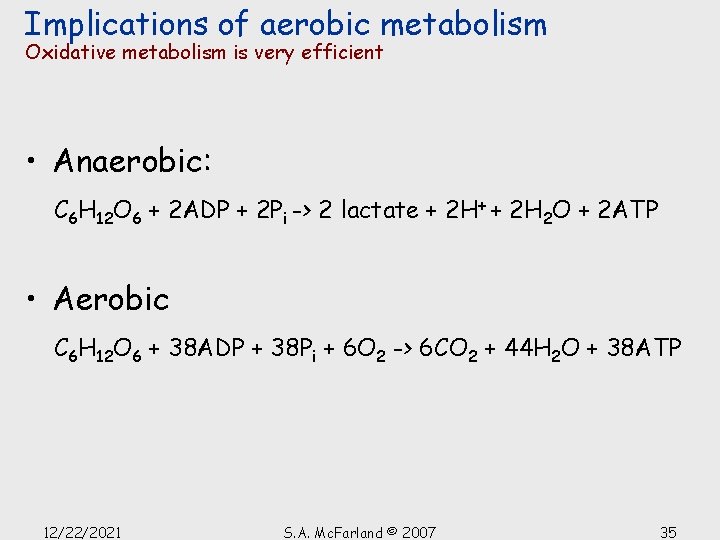
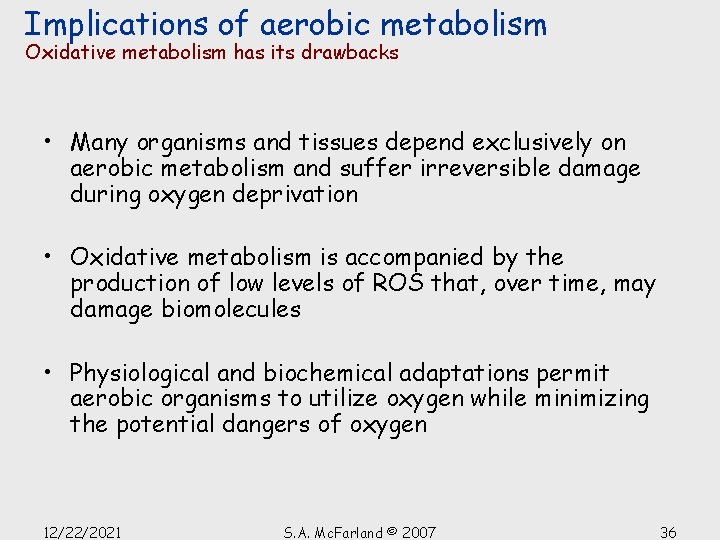
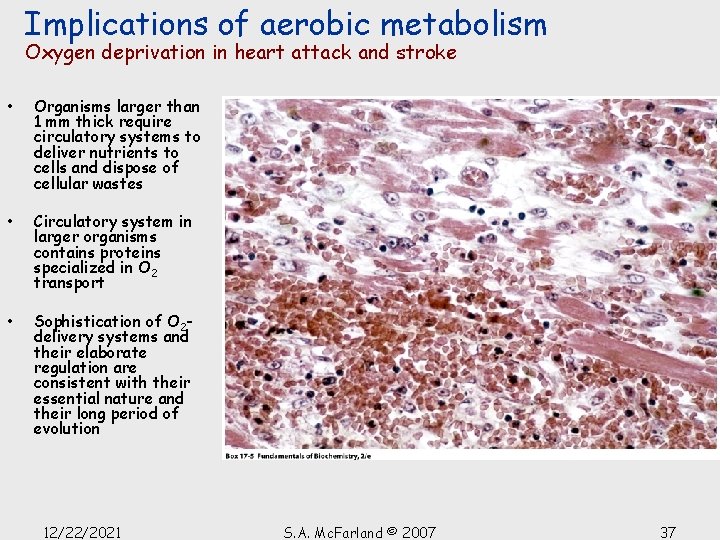
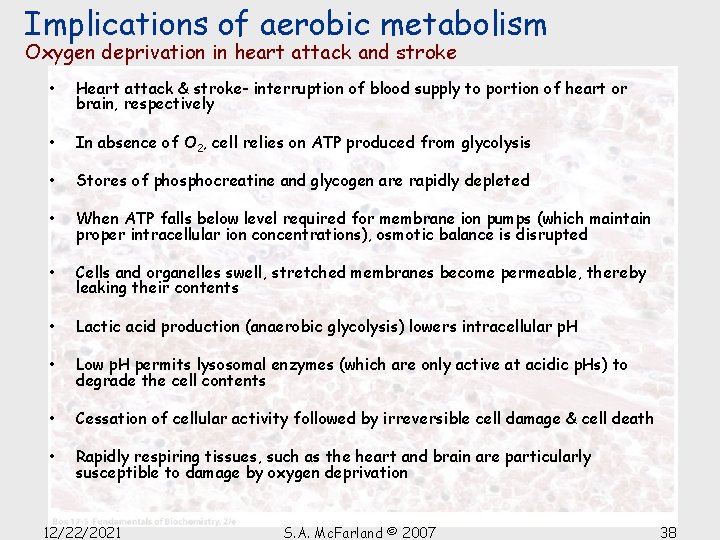
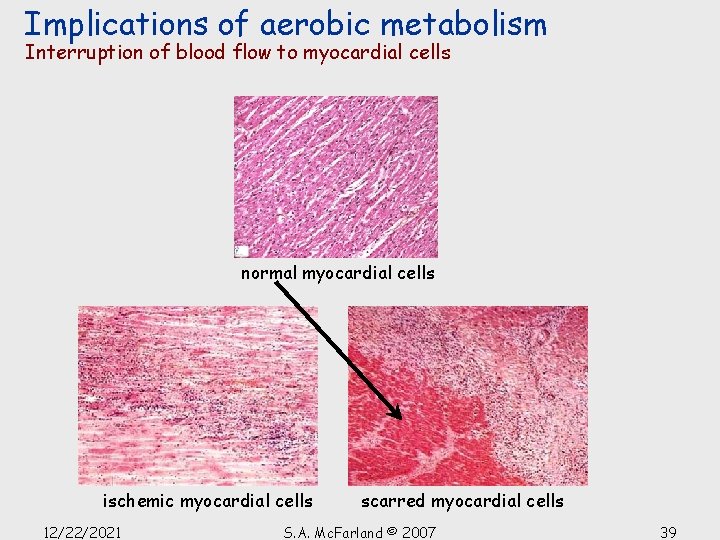
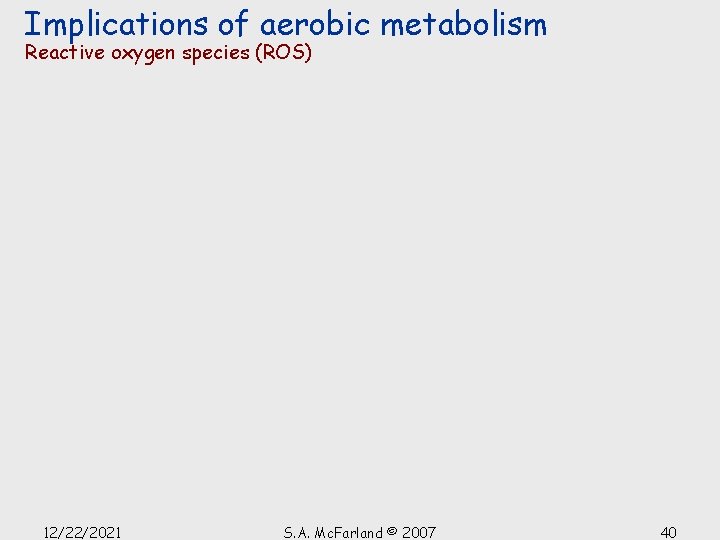
- Slides: 40
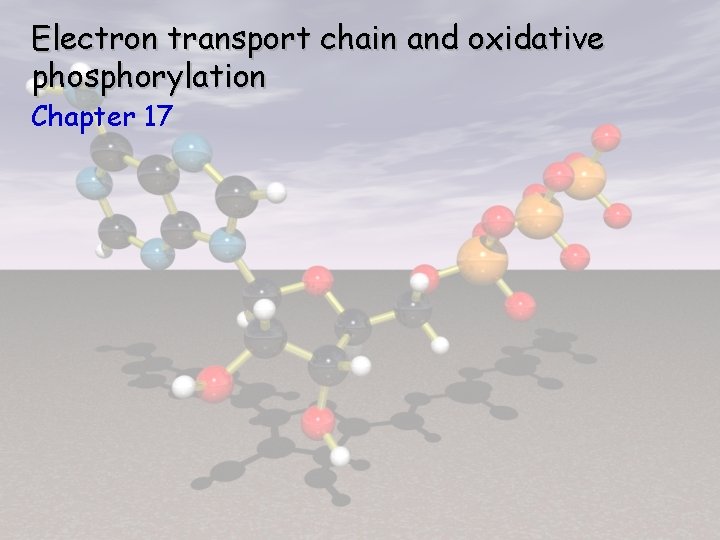
Electron transport chain and oxidative phosphorylation Chapter 17
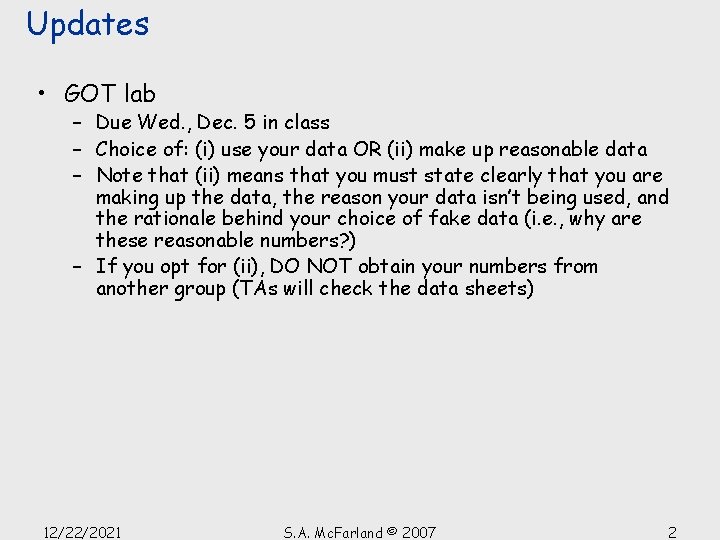
Updates • GOT lab – Due Wed. , Dec. 5 in class – Choice of: (i) use your data OR (ii) make up reasonable data – Note that (ii) means that you must state clearly that you are making up the data, the reason your data isn’t being used, and the rationale behind your choice of fake data (i. e. , why are these reasonable numbers? ) – If you opt for (ii), DO NOT obtain your numbers from another group (TAs will check the data sheets) 12/22/2021 S. A. Mc. Farland © 2007 2
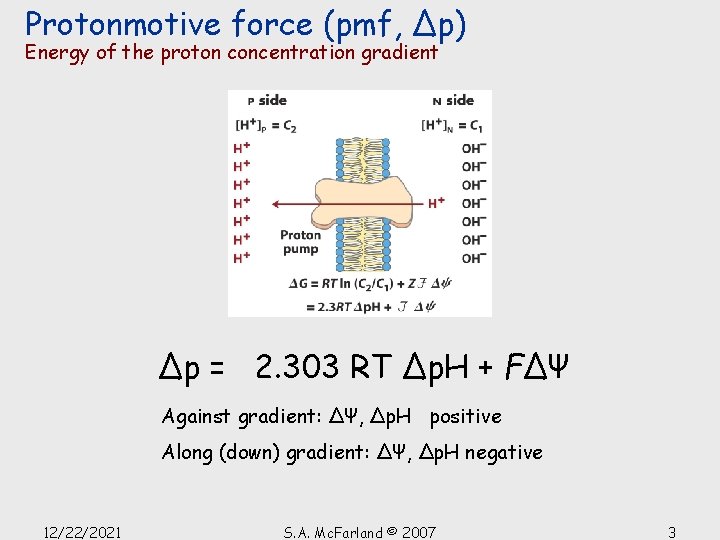
Protonmotive force (pmf, Δp) Energy of the proton concentration gradient Δp = 2. 303 RT Δp. H + FΔΨ Against gradient: ΔΨ, Δp. H positive Along (down) gradient: ΔΨ, Δp. H negative 12/22/2021 S. A. Mc. Farland © 2007 3
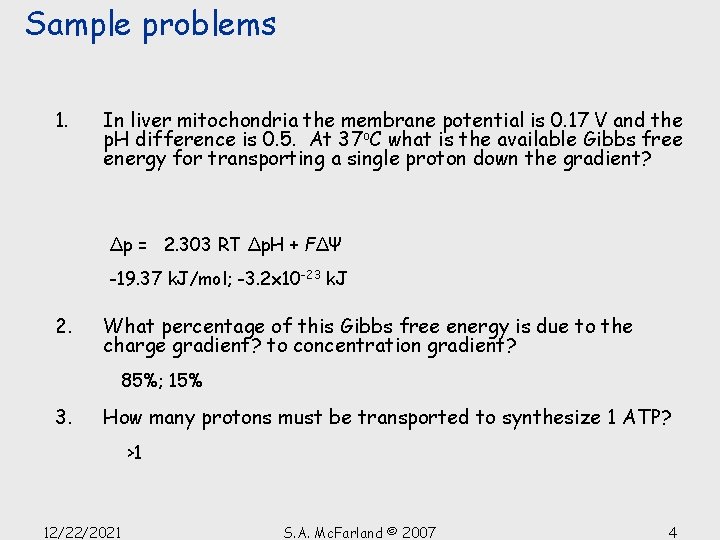
Sample problems 1. In liver mitochondria the membrane potential is 0. 17 V and the p. H difference is 0. 5. At 37 o. C what is the available Gibbs free energy for transporting a single proton down the gradient? Δp = 2. 303 RT Δp. H + FΔΨ -19. 37 k. J/mol; -3. 2 x 10 -23 k. J 2. What percentage of this Gibbs free energy is due to the charge gradient? to concentration gradient? 85%; 15% 3. How many protons must be transported to synthesize 1 ATP? >1 12/22/2021 S. A. Mc. Farland © 2007 4
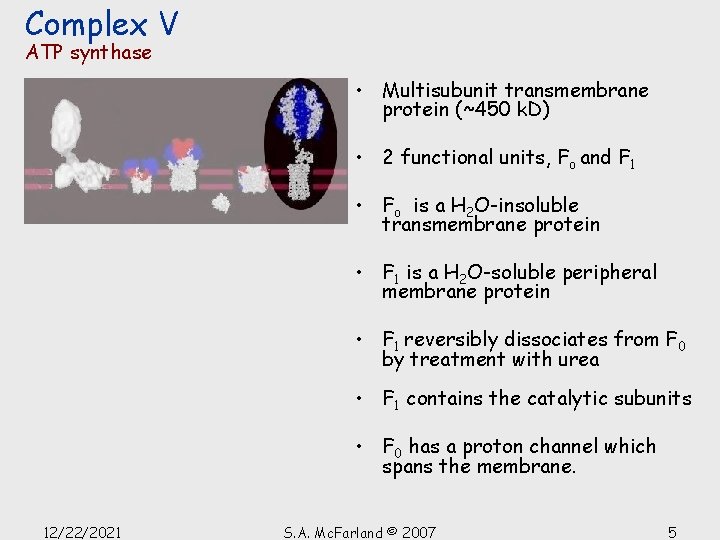
Complex V ATP synthase • Multisubunit transmembrane protein (~450 k. D) • 2 functional units, Fo and F 1 • Fo is a H 2 O-insoluble transmembrane protein • F 1 is a H 2 O-soluble peripheral membrane protein • F 1 reversibly dissociates from F 0 by treatment with urea • F 1 contains the catalytic subunits • F 0 has a proton channel which spans the membrane. 12/22/2021 S. A. Mc. Farland © 2007 5
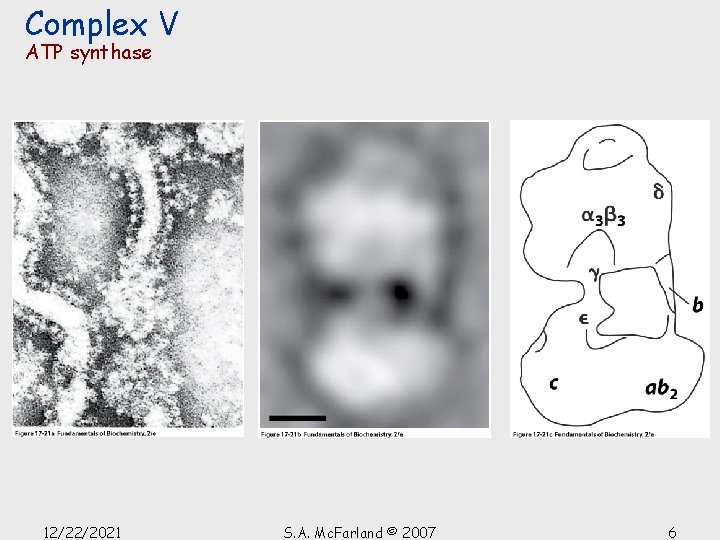
Complex V ATP synthase 12/22/2021 S. A. Mc. Farland © 2007 6
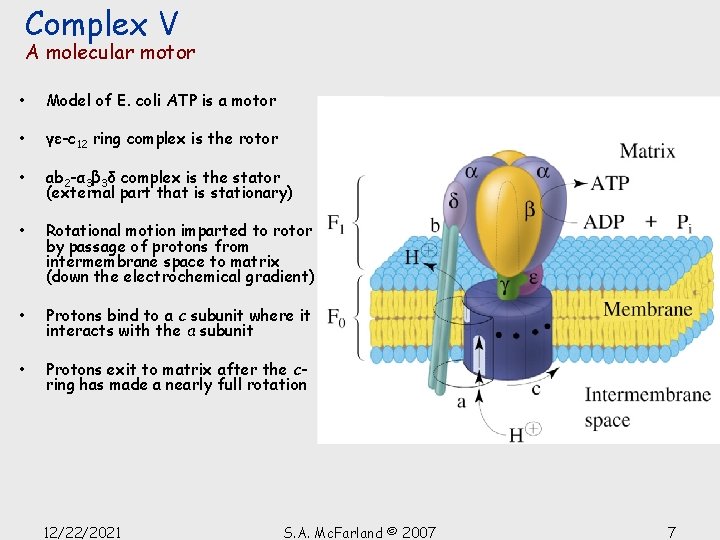
Complex V A molecular motor • Model of E. coli ATP is a motor • γε-c 12 ring complex is the rotor • ab 2 -α 3β 3δ complex is the stator (external part that is stationary) • Rotational motion imparted to rotor by passage of protons from intermembrane space to matrix (down the electrochemical gradient) • Protons bind to a c subunit where it interacts with the a subunit • Protons exit to matrix after the cring has made a nearly full rotation 12/22/2021 S. A. Mc. Farland © 2007 7
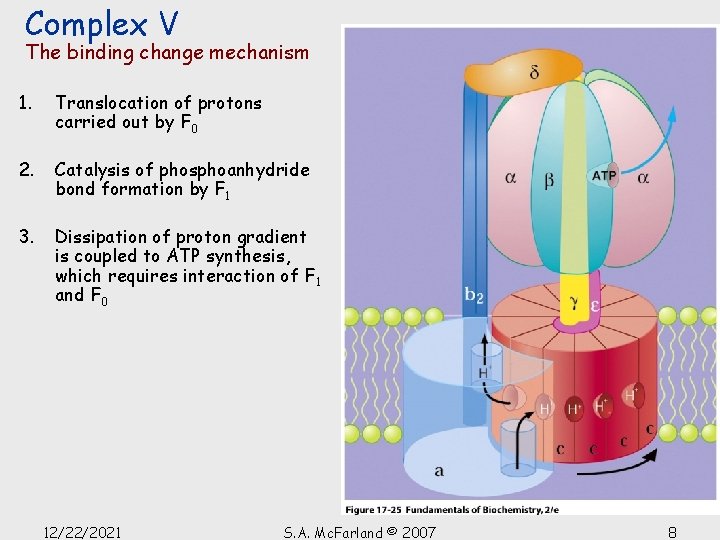
Complex V The binding change mechanism 1. Translocation of protons carried out by F 0 2. Catalysis of phosphoanhydride bond formation by F 1 3. Dissipation of proton gradient is coupled to ATP synthesis, which requires interaction of F 1 and F 0 12/22/2021 S. A. Mc. Farland © 2007 8
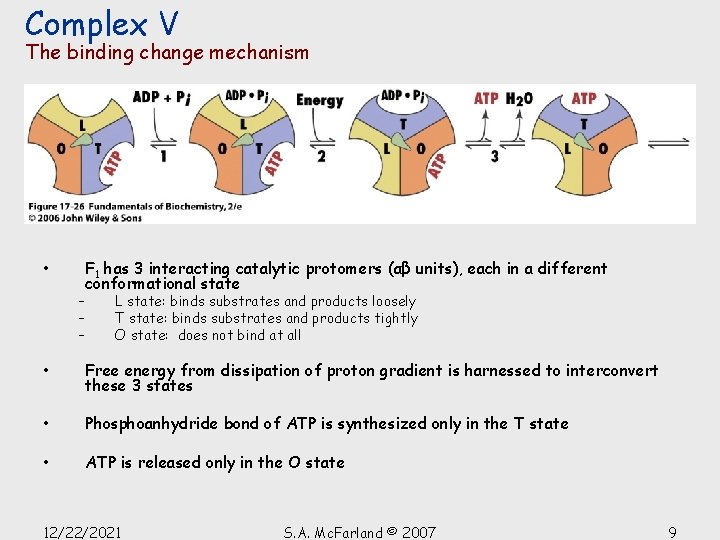
Complex V The binding change mechanism • F 1 has 3 interacting catalytic protomers (αβ units), each in a different conformational state – – – L state: binds substrates and products loosely T state: binds substrates and products tightly O state: does not bind at all • Free energy from dissipation of proton gradient is harnessed to interconvert these 3 states • Phosphoanhydride bond of ATP is synthesized only in the T state • ATP is released only in the O state 12/22/2021 S. A. Mc. Farland © 2007 9
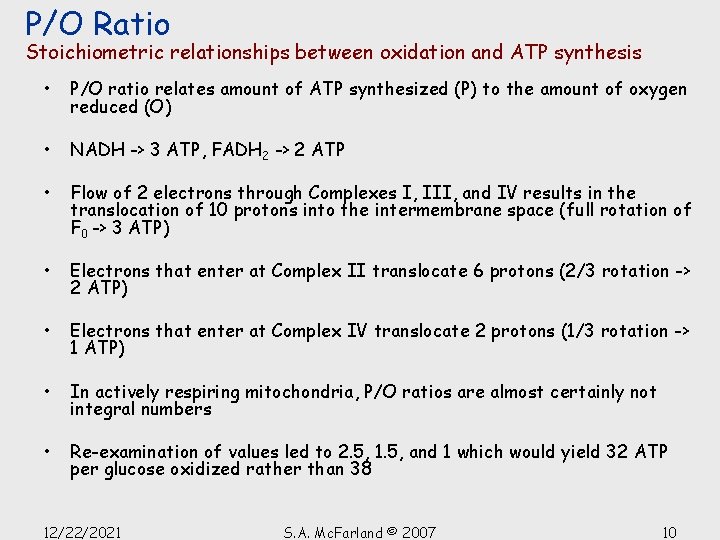
P/O Ratio Stoichiometric relationships between oxidation and ATP synthesis • P/O ratio relates amount of ATP synthesized (P) to the amount of oxygen reduced (O) • NADH -> 3 ATP, FADH 2 -> 2 ATP • Flow of 2 electrons through Complexes I, III, and IV results in the translocation of 10 protons into the intermembrane space (full rotation of F 0 -> 3 ATP) • Electrons that enter at Complex II translocate 6 protons (2/3 rotation -> 2 ATP) • Electrons that enter at Complex IV translocate 2 protons (1/3 rotation -> 1 ATP) • In actively respiring mitochondria, P/O ratios are almost certainly not integral numbers • Re-examination of values led to 2. 5, 1. 5, and 1 which would yield 32 ATP per glucose oxidized rather than 38 12/22/2021 S. A. Mc. Farland © 2007 10
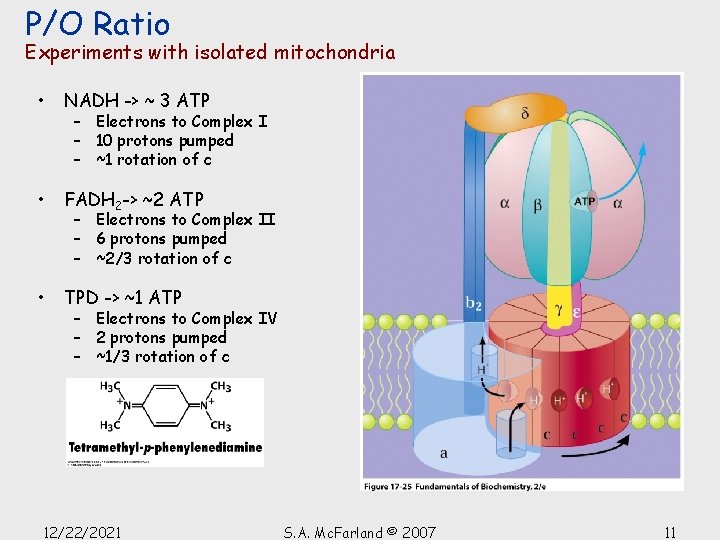
P/O Ratio Experiments with isolated mitochondria • NADH -> ~ 3 ATP • FADH 2 -> ~2 ATP • TPD -> ~1 ATP – Electrons to Complex I – 10 protons pumped – ~1 rotation of c – Electrons to Complex II – 6 protons pumped – ~2/3 rotation of c – Electrons to Complex IV – 2 protons pumped – ~1/3 rotation of c 12/22/2021 S. A. Mc. Farland © 2007 11
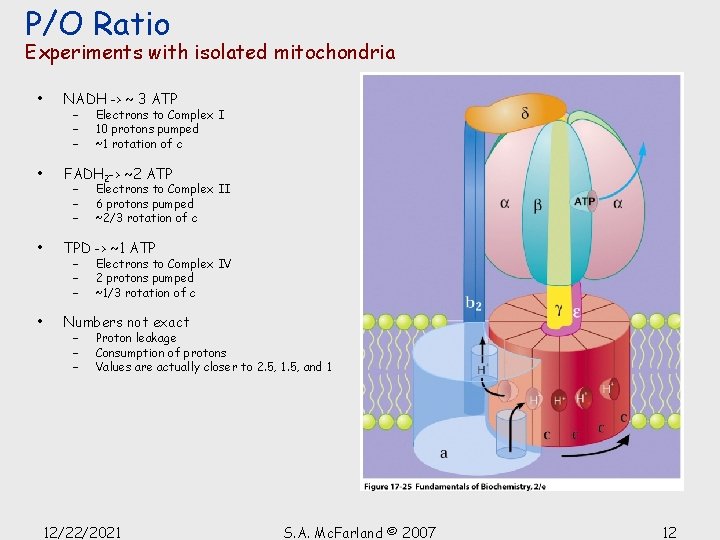
P/O Ratio Experiments with isolated mitochondria • NADH -> ~ 3 ATP • FADH 2 -> ~2 ATP • TPD -> ~1 ATP • Numbers not exact – – – Electrons to Complex I 10 protons pumped ~1 rotation of c Electrons to Complex II 6 protons pumped ~2/3 rotation of c Electrons to Complex IV 2 protons pumped ~1/3 rotation of c Proton leakage Consumption of protons Values are actually closer to 2. 5, 1. 5, and 1 12/22/2021 S. A. Mc. Farland © 2007 12
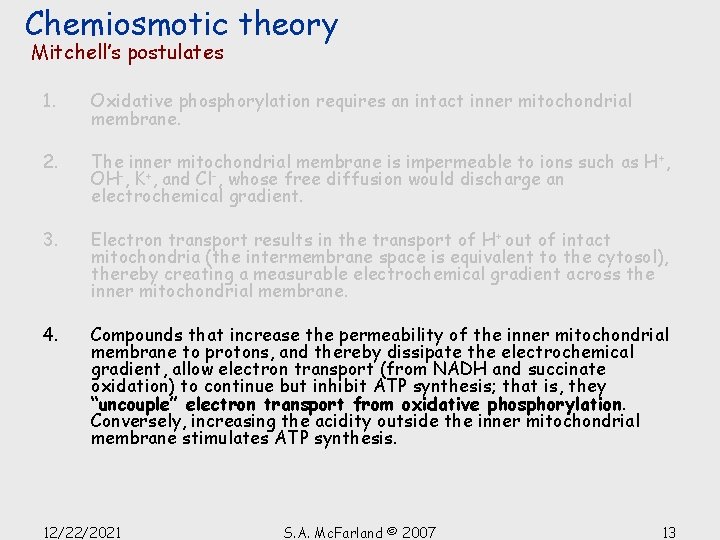
Chemiosmotic theory Mitchell’s postulates 1. Oxidative phosphorylation requires an intact inner mitochondrial membrane. 2. The inner mitochondrial membrane is impermeable to ions such as H +, OH-, K+, and Cl-, whose free diffusion would discharge an electrochemical gradient. 3. Electron transport results in the transport of H+ out of intact mitochondria (the intermembrane space is equivalent to the cytosol), thereby creating a measurable electrochemical gradient across the inner mitochondrial membrane. 4. Compounds that increase the permeability of the inner mitochondrial membrane to protons, and thereby dissipate the electrochemical gradient, allow electron transport (from NADH and succinate oxidation) to continue but inhibit ATP synthesis; that is, they “uncouple” electron transport from oxidative phosphorylation. Conversely, increasing the acidity outside the inner mitochondrial membrane stimulates ATP synthesis. 12/22/2021 S. A. Mc. Farland © 2007 13
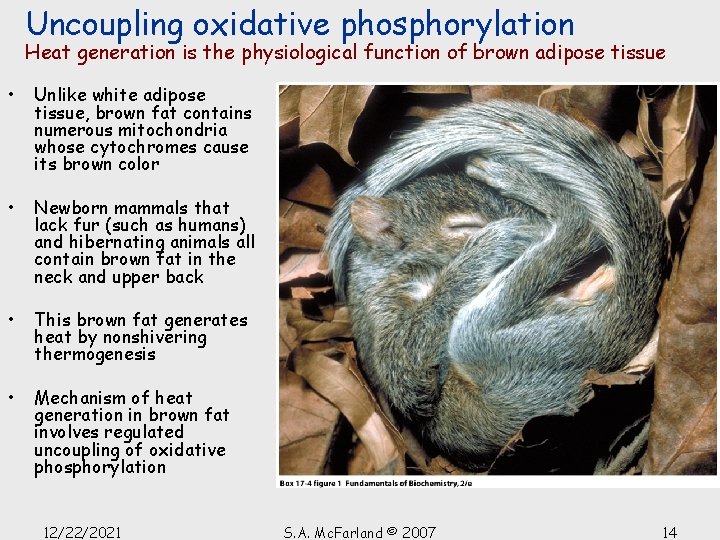
Uncoupling oxidative phosphorylation Heat generation is the physiological function of brown adipose tissue • Unlike white adipose tissue, brown fat contains numerous mitochondria whose cytochromes cause its brown color • Newborn mammals that lack fur (such as humans) and hibernating animals all contain brown fat in the neck and upper back • This brown fat generates heat by nonshivering thermogenesis • Mechanism of heat generation in brown fat involves regulated uncoupling of oxidative phosphorylation 12/22/2021 S. A. Mc. Farland © 2007 14
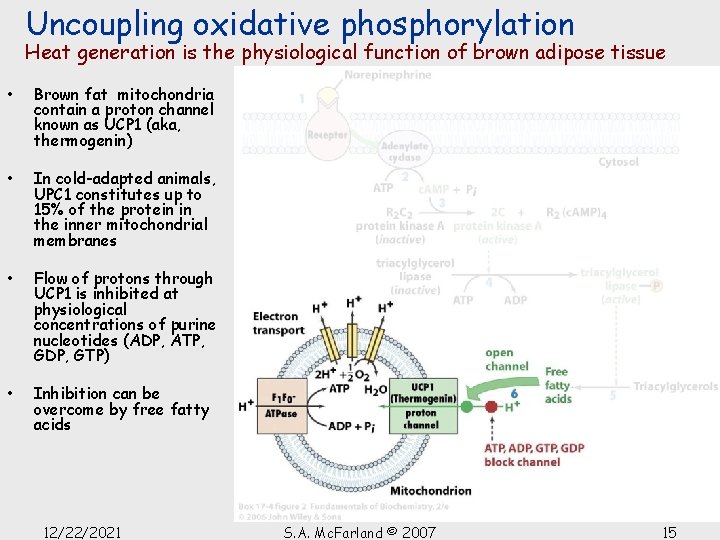
Uncoupling oxidative phosphorylation Heat generation is the physiological function of brown adipose tissue • Brown fat mitochondria contain a proton channel known as UCP 1 (aka, thermogenin) • In cold-adapted animals, UPC 1 constitutes up to 15% of the protein in the inner mitochondrial membranes • Flow of protons through UCP 1 is inhibited at physiological concentrations of purine nucleotides (ADP, ATP, GDP, GTP) • Inhibition can be overcome by free fatty acids 12/22/2021 S. A. Mc. Farland © 2007 15
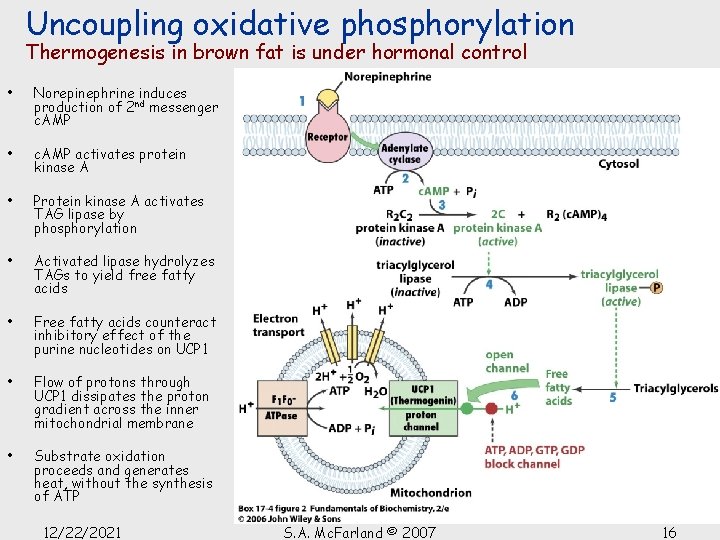
Uncoupling oxidative phosphorylation Thermogenesis in brown fat is under hormonal control • Norepinephrine induces production of 2 nd messenger c. AMP • c. AMP activates protein kinase A • Protein kinase A activates TAG lipase by phosphorylation • Activated lipase hydrolyzes TAGs to yield free fatty acids • Free fatty acids counteract inhibitory effect of the purine nucleotides on UCP 1 • Flow of protons through UCP 1 dissipates the proton gradient across the inner mitochondrial membrane • Substrate oxidation proceeds and generates heat, without the synthesis of ATP 12/22/2021 S. A. Mc. Farland © 2007 16
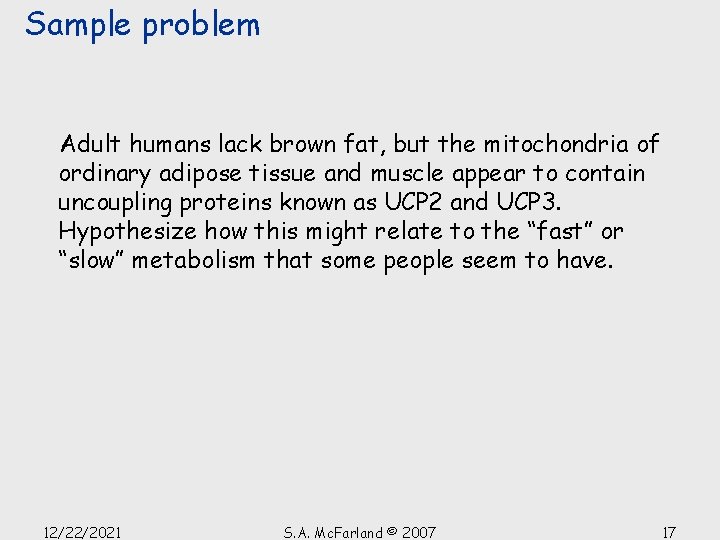
Sample problem Adult humans lack brown fat, but the mitochondria of ordinary adipose tissue and muscle appear to contain uncoupling proteins known as UCP 2 and UCP 3. Hypothesize how this might relate to the “fast” or “slow” metabolism that some people seem to have. 12/22/2021 S. A. Mc. Farland © 2007 17
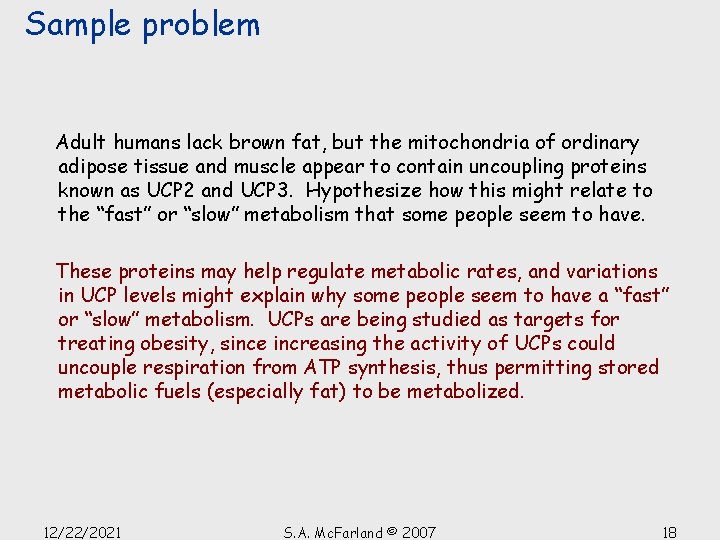
Sample problem Adult humans lack brown fat, but the mitochondria of ordinary adipose tissue and muscle appear to contain uncoupling proteins known as UCP 2 and UCP 3. Hypothesize how this might relate to the “fast” or “slow” metabolism that some people seem to have. These proteins may help regulate metabolic rates, and variations in UCP levels might explain why some people seem to have a “fast” or “slow” metabolism. UCPs are being studied as targets for treating obesity, since increasing the activity of UCPs could uncouple respiration from ATP synthesis, thus permitting stored metabolic fuels (especially fat) to be metabolized. 12/22/2021 S. A. Mc. Farland © 2007 18
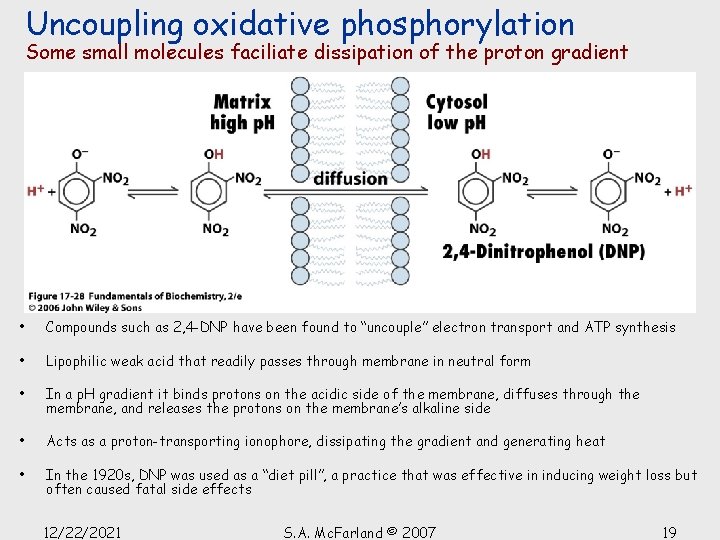
Uncoupling oxidative phosphorylation Some small molecules faciliate dissipation of the proton gradient • Compounds such as 2, 4 -DNP have been found to “uncouple” electron transport and ATP synthesis • Lipophilic weak acid that readily passes through membrane in neutral form • In a p. H gradient it binds protons on the acidic side of the membrane, diffuses through the membrane, and releases the protons on the membrane’s alkaline side • Acts as a proton-transporting ionophore, dissipating the gradient and generating heat • In the 1920 s, DNP was used as a “diet pill”, a practice that was effective in inducing weight loss but often caused fatal side effects 12/22/2021 S. A. Mc. Farland © 2007 19
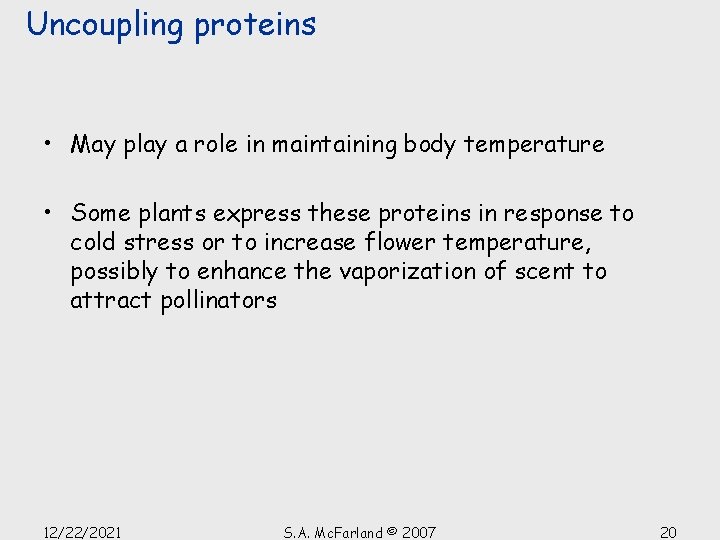
Uncoupling proteins • May play a role in maintaining body temperature • Some plants express these proteins in response to cold stress or to increase flower temperature, possibly to enhance the vaporization of scent to attract pollinators 12/22/2021 S. A. Mc. Farland © 2007 20
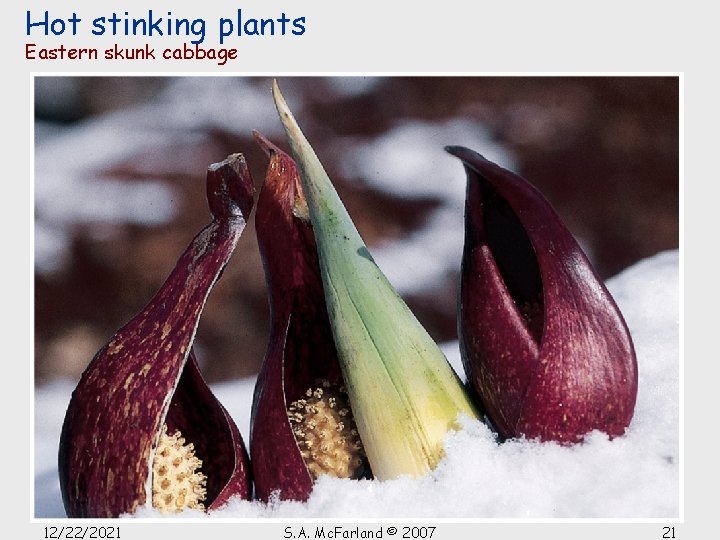
Hot stinking plants Eastern skunk cabbage 12/22/2021 S. A. Mc. Farland © 2007 21
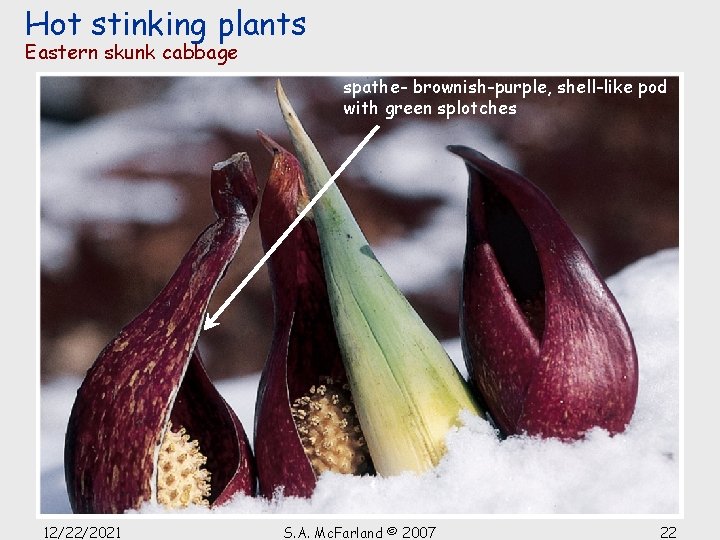
Hot stinking plants Eastern skunk cabbage spathe- brownish-purple, shell-like pod with green splotches 12/22/2021 S. A. Mc. Farland © 2007 22
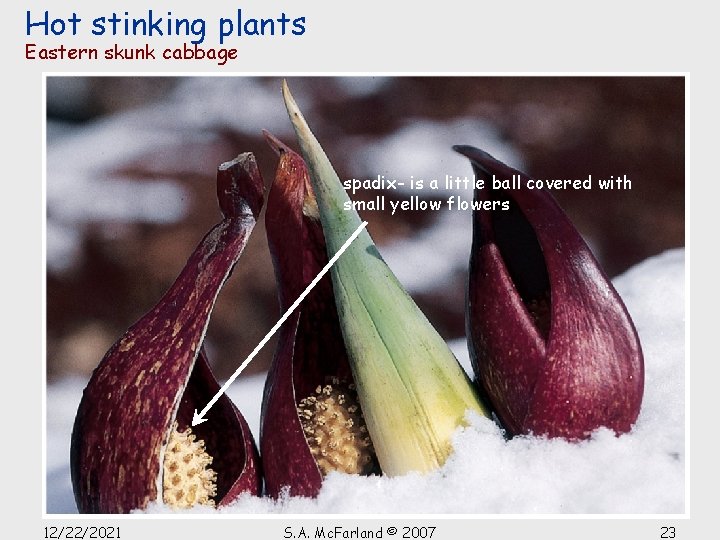
Hot stinking plants Eastern skunk cabbage spadix- is a little ball covered with small yellow flowers 12/22/2021 S. A. Mc. Farland © 2007 23
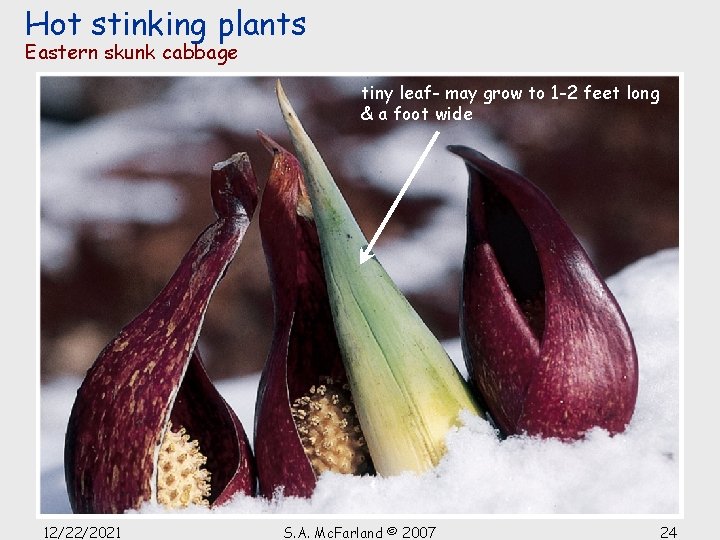
Hot stinking plants Eastern skunk cabbage tiny leaf- may grow to 1 -2 feet long & a foot wide 12/22/2021 S. A. Mc. Farland © 2007 24
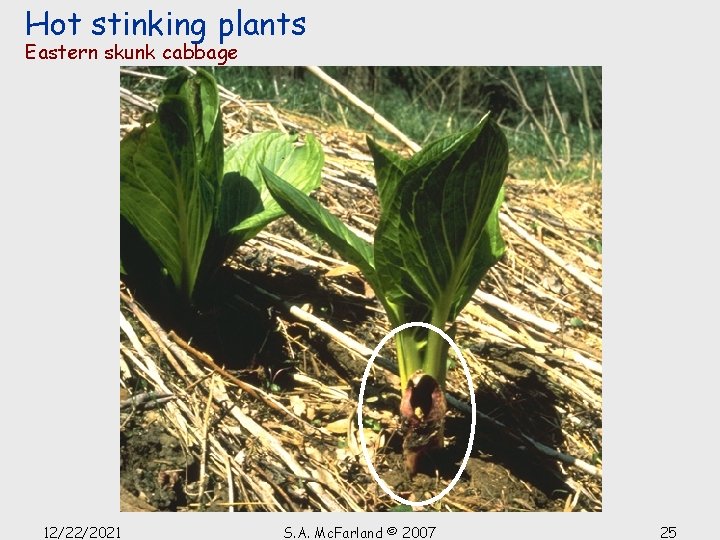
Hot stinking plants Eastern skunk cabbage 12/22/2021 S. A. Mc. Farland © 2007 25
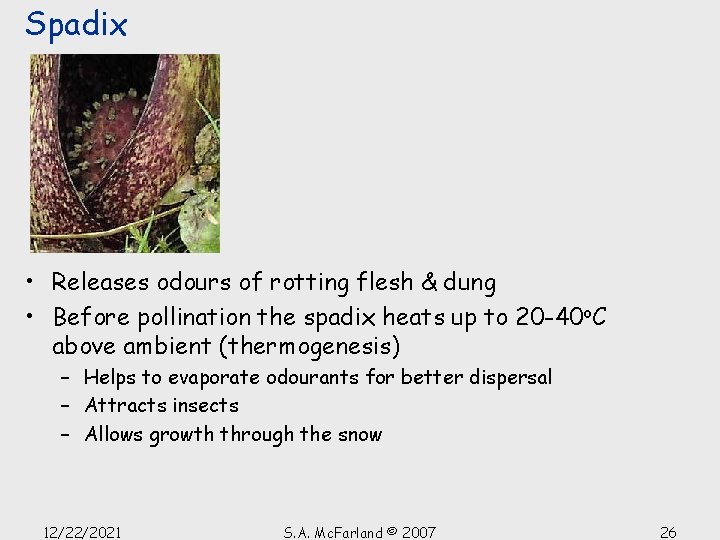
Spadix • Releases odours of rotting flesh & dung • Before pollination the spadix heats up to 20 -40 o. C above ambient (thermogenesis) – Helps to evaporate odourants for better dispersal – Attracts insects – Allows growth through the snow 12/22/2021 S. A. Mc. Farland © 2007 26
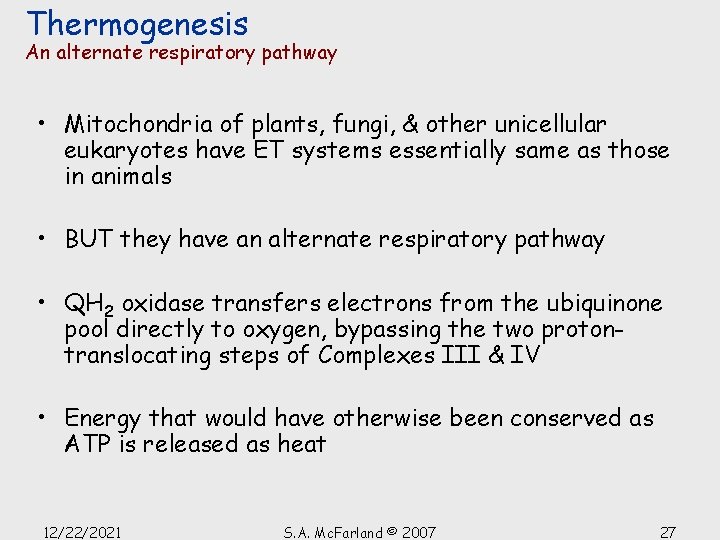
Thermogenesis An alternate respiratory pathway • Mitochondria of plants, fungi, & other unicellular eukaryotes have ET systems essentially same as those in animals • BUT they have an alternate respiratory pathway • QH 2 oxidase transfers electrons from the ubiquinone pool directly to oxygen, bypassing the two protontranslocating steps of Complexes III & IV • Energy that would have otherwise been conserved as ATP is released as heat 12/22/2021 S. A. Mc. Farland © 2007 27
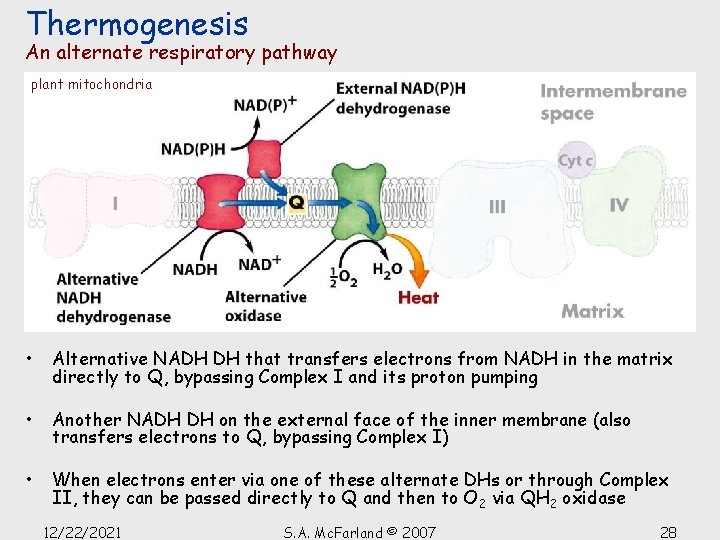
Thermogenesis An alternate respiratory pathway plant mitochondria • Alternative NADH DH that transfers electrons from NADH in the matrix directly to Q, bypassing Complex I and its proton pumping • Another NADH DH on the external face of the inner membrane (also transfers electrons to Q, bypassing Complex I) • When electrons enter via one of these alternate DHs or through Complex II, they can be passed directly to Q and then to O 2 via QH 2 oxidase 12/22/2021 S. A. Mc. Farland © 2007 28
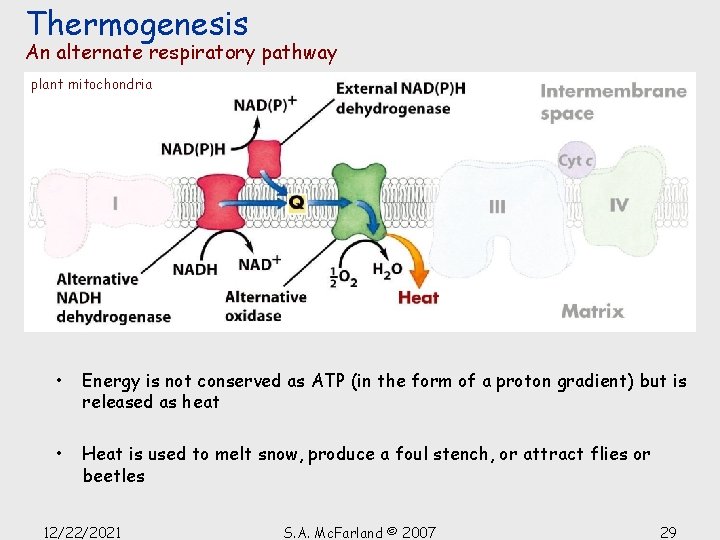
Thermogenesis An alternate respiratory pathway plant mitochondria • Energy is not conserved as ATP (in the form of a proton gradient) but is released as heat • Heat is used to melt snow, produce a foul stench, or attract flies or beetles 12/22/2021 S. A. Mc. Farland © 2007 29
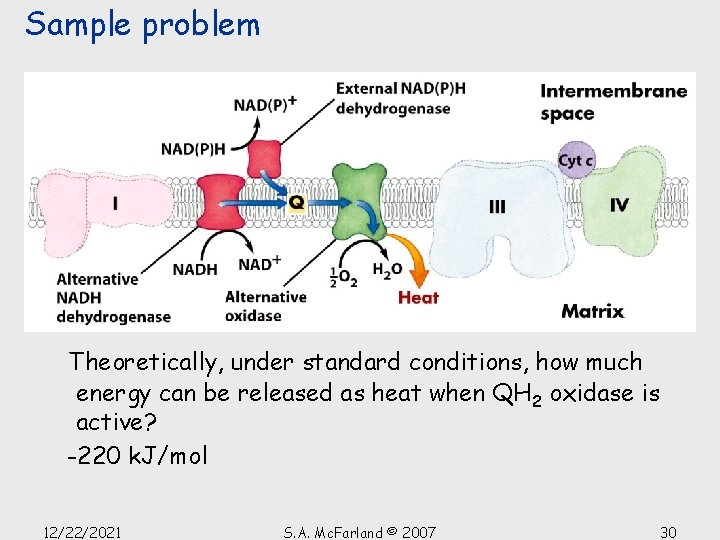
Sample problem Theoretically, under standard conditions, how much energy can be released as heat when QH 2 oxidase is active? -220 k. J/mol 12/22/2021 S. A. Mc. Farland © 2007 30
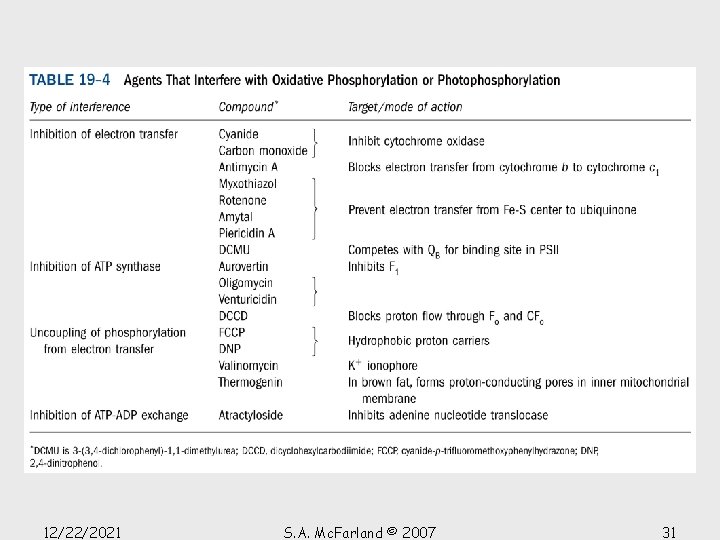
12/22/2021 S. A. Mc. Farland © 2007 31
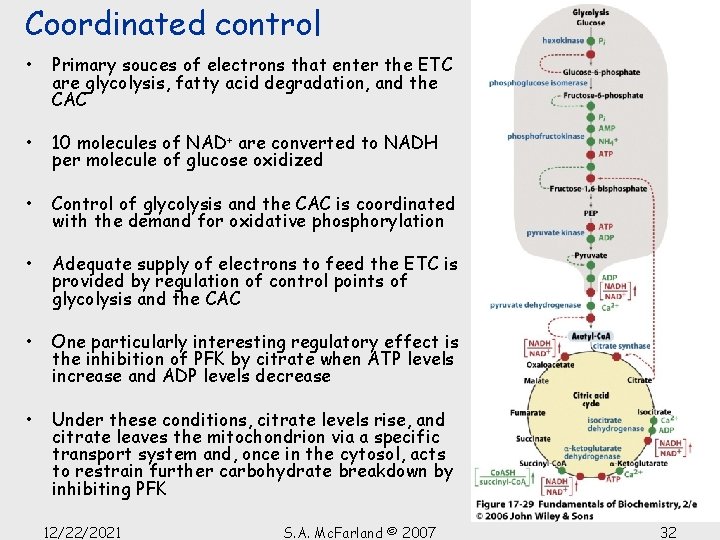
Coordinated control • Primary souces of electrons that enter the ETC are glycolysis, fatty acid degradation, and the CAC • 10 molecules of NAD+ are converted to NADH per molecule of glucose oxidized • Control of glycolysis and the CAC is coordinated with the demand for oxidative phosphorylation • Adequate supply of electrons to feed the ETC is provided by regulation of control points of glycolysis and the CAC • One particularly interesting regulatory effect is the inhibition of PFK by citrate when ATP levels increase and ADP levels decrease • Under these conditions, citrate levels rise, and citrate leaves the mitochondrion via a specific transport system and, once in the cytosol, acts to restrain further carbohydrate breakdown by inhibiting PFK 12/22/2021 S. A. Mc. Farland © 2007 32
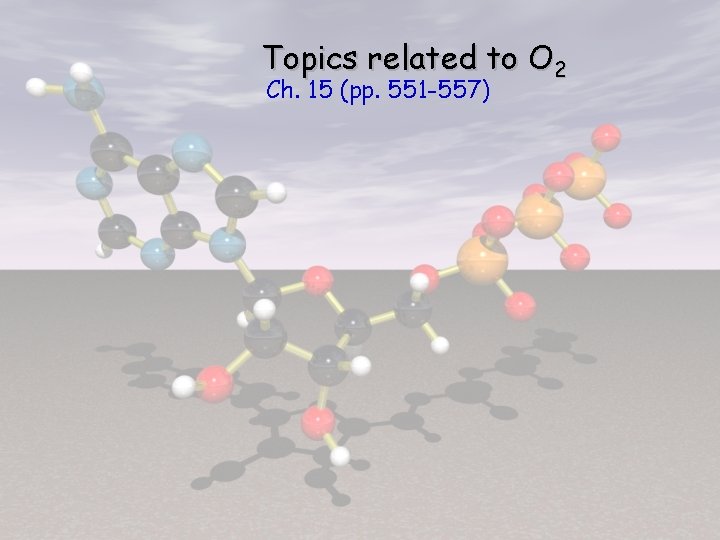
Topics related to O 2 Ch. 15 (pp. 551 -557)
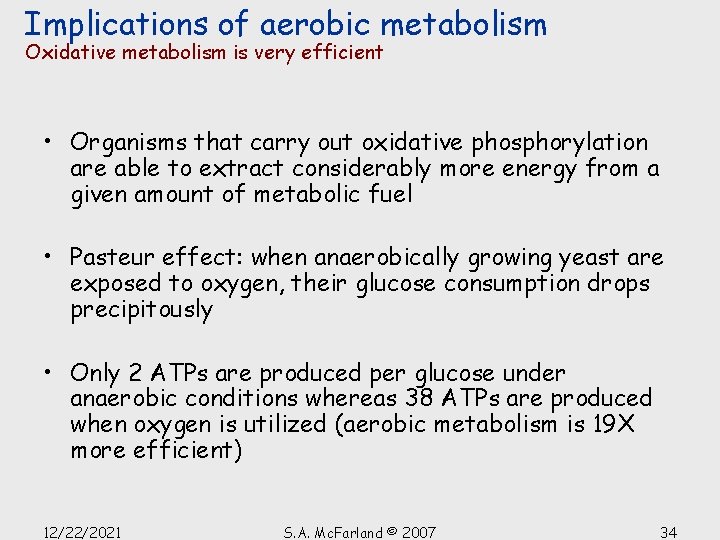
Implications of aerobic metabolism Oxidative metabolism is very efficient • Organisms that carry out oxidative phosphorylation are able to extract considerably more energy from a given amount of metabolic fuel • Pasteur effect: when anaerobically growing yeast are exposed to oxygen, their glucose consumption drops precipitously • Only 2 ATPs are produced per glucose under anaerobic conditions whereas 38 ATPs are produced when oxygen is utilized (aerobic metabolism is 19 X more efficient) 12/22/2021 S. A. Mc. Farland © 2007 34
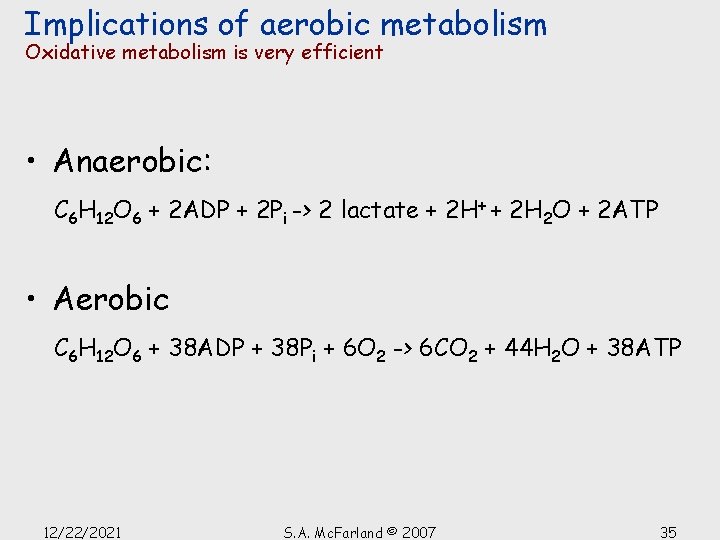
Implications of aerobic metabolism Oxidative metabolism is very efficient • Anaerobic: C 6 H 12 O 6 + 2 ADP + 2 Pi -> 2 lactate + 2 H+ + 2 H 2 O + 2 ATP • Aerobic C 6 H 12 O 6 + 38 ADP + 38 Pi + 6 O 2 -> 6 CO 2 + 44 H 2 O + 38 ATP 12/22/2021 S. A. Mc. Farland © 2007 35
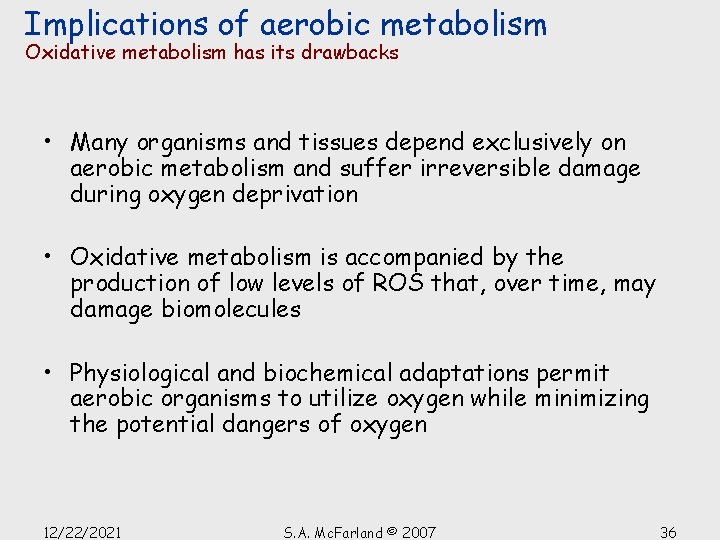
Implications of aerobic metabolism Oxidative metabolism has its drawbacks • Many organisms and tissues depend exclusively on aerobic metabolism and suffer irreversible damage during oxygen deprivation • Oxidative metabolism is accompanied by the production of low levels of ROS that, over time, may damage biomolecules • Physiological and biochemical adaptations permit aerobic organisms to utilize oxygen while minimizing the potential dangers of oxygen 12/22/2021 S. A. Mc. Farland © 2007 36
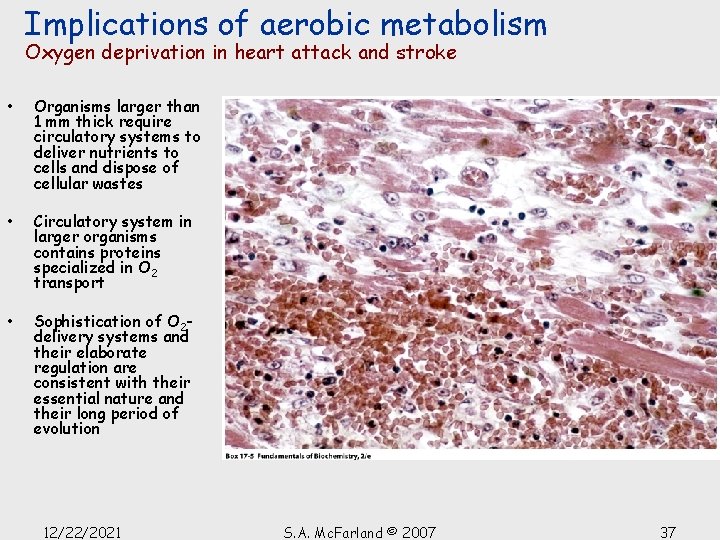
Implications of aerobic metabolism Oxygen deprivation in heart attack and stroke • Organisms larger than 1 mm thick require circulatory systems to deliver nutrients to cells and dispose of cellular wastes • Circulatory system in larger organisms contains proteins specialized in O 2 transport • Sophistication of O 2 delivery systems and their elaborate regulation are consistent with their essential nature and their long period of evolution 12/22/2021 S. A. Mc. Farland © 2007 37
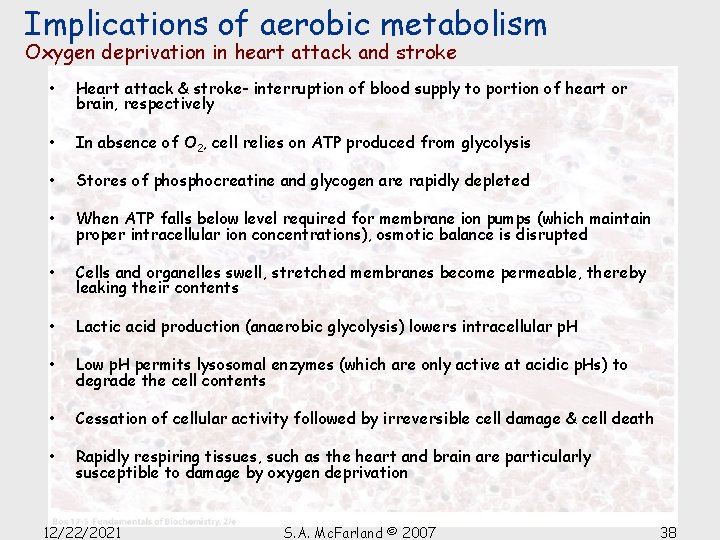
Implications of aerobic metabolism Oxygen deprivation in heart attack and stroke • Heart attack & stroke- interruption of blood supply to portion of heart or brain, respectively • In absence of O 2, cell relies on ATP produced from glycolysis • Stores of phosphocreatine and glycogen are rapidly depleted • When ATP falls below level required for membrane ion pumps (which maintain proper intracellular ion concentrations), osmotic balance is disrupted • Cells and organelles swell, stretched membranes become permeable, thereby leaking their contents • Lactic acid production (anaerobic glycolysis) lowers intracellular p. H • Low p. H permits lysosomal enzymes (which are only active at acidic p. Hs) to degrade the cell contents • Cessation of cellular activity followed by irreversible cell damage & cell death • Rapidly respiring tissues, such as the heart and brain are particularly susceptible to damage by oxygen deprivation 12/22/2021 S. A. Mc. Farland © 2007 38
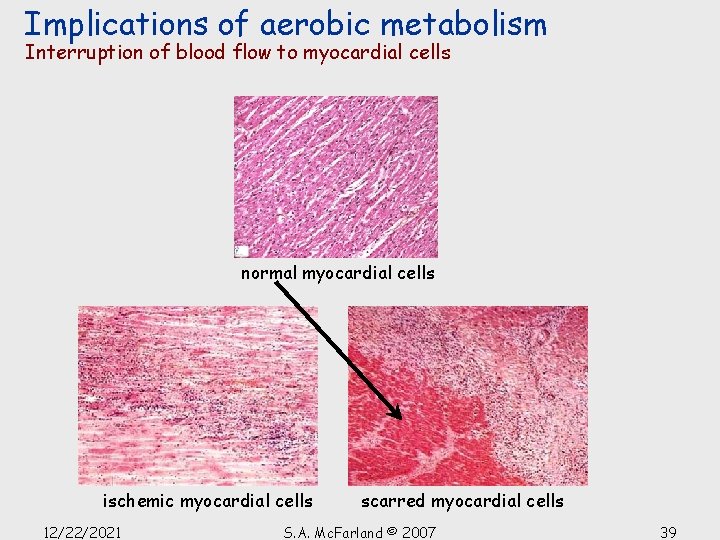
Implications of aerobic metabolism Interruption of blood flow to myocardial cells normal myocardial cells ischemic myocardial cells 12/22/2021 scarred myocardial cells S. A. Mc. Farland © 2007 39
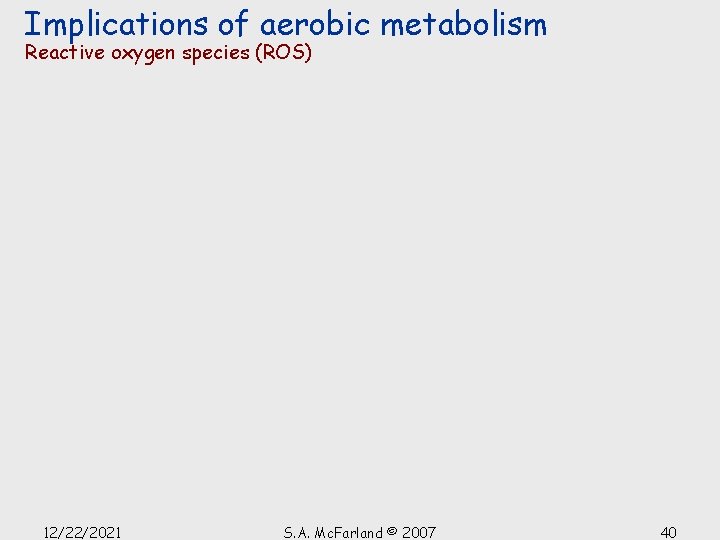
Implications of aerobic metabolism Reactive oxygen species (ROS) 12/22/2021 S. A. Mc. Farland © 2007 40
Uncouple oxidative phosphorylation
Uncouplers of oxidative phosphorylation
Oxidative phosphorylation
Substrate level phosphorylation vs oxidative
Uncouplers of oxidative phosphorylation
Concept map about oxidative phosphorylation.
Cellular respiration harvesting chemical energy
Oxidative phosphorylation energy yield
Substrate level phosphorylation
Azide electron transport chain
Inhibitors of oxidative phosphorylation
Inhibitors of oxidative phosphorylation
Uncouplers of oxidative phosphorylation
Oxidative phosphorylation
Define oxidative phosphorylation
Atp synthesis
Siklus krebs
Citric acid cycle and electron transport chain
Electron transport chain campbell
Vertical
Electron transport chain cellular respiration
Where does the electron transport chain take place
Catabolism
Electron transport chain cellular respiration
Is oxygen needed as a reactant in the krebs cycle
Electron transport chain
Electron transport chain summary
Electron transport chain
Significance of electron transport chain
Labelled
Sümbül transport
Electron transport chain animation
Respiration crash course
Food chain food chain food chain
Primary vs secondary active transport
Primary vs secondary active transport
Active transport diagram
Deamination
Transamination and oxidative deamination
Transamination and oxidative deamination
Photosynthesis reaction