DNA DAMAGE AND REPAIR DNA Damage and Repair
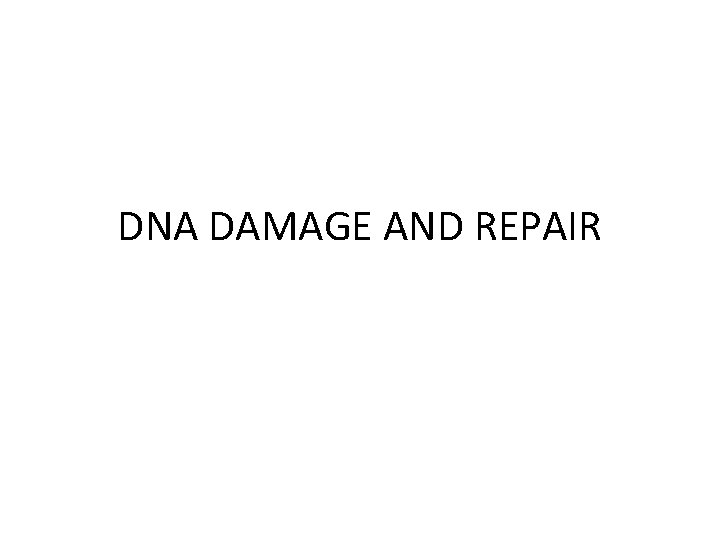
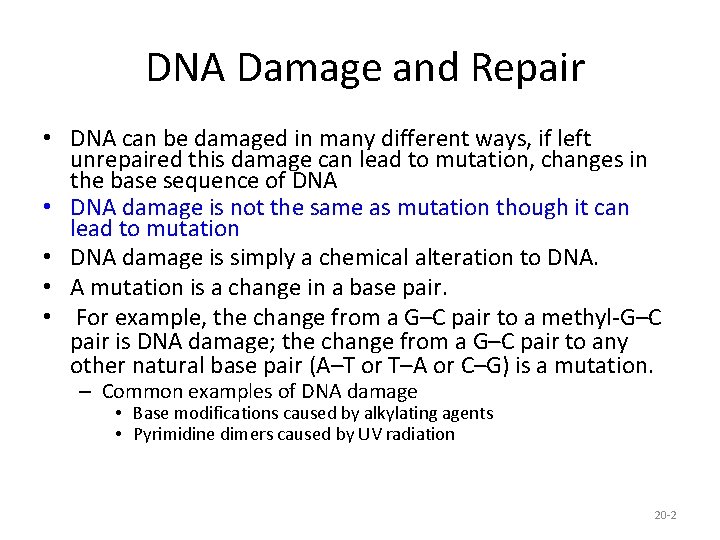
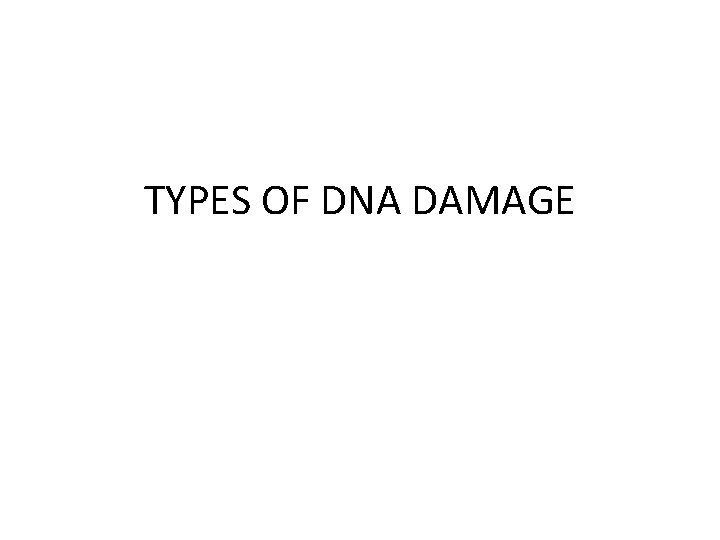
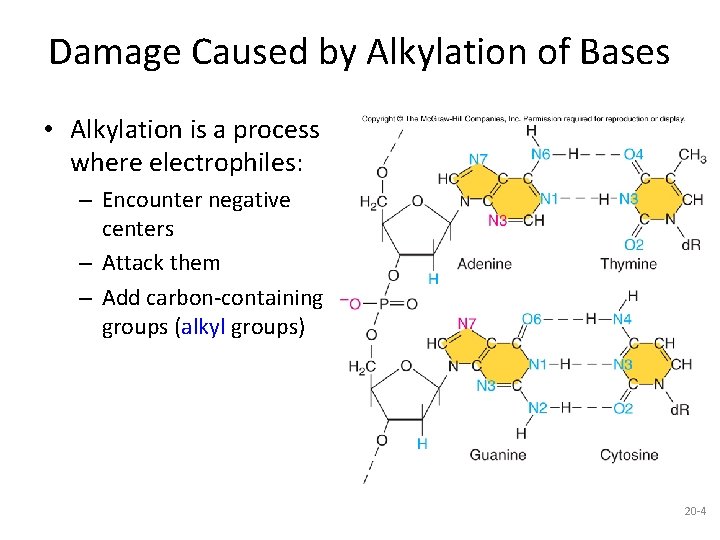
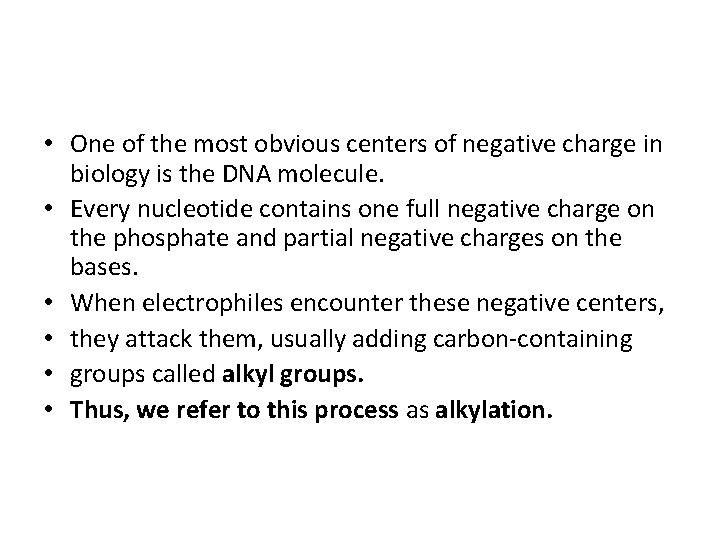
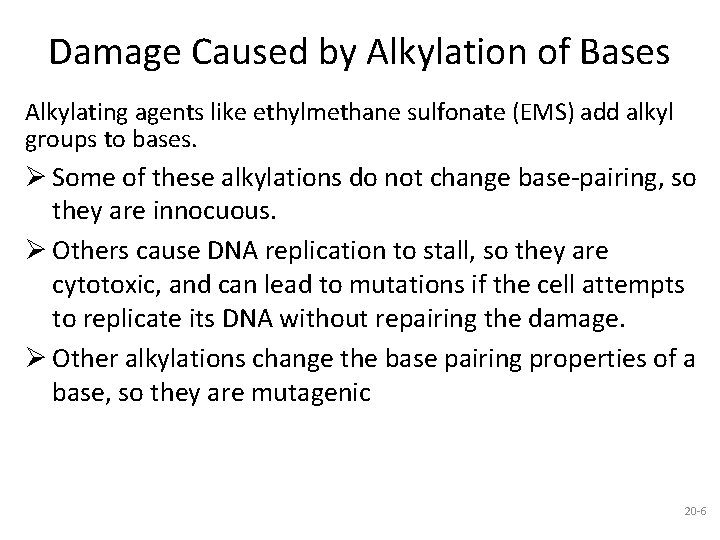
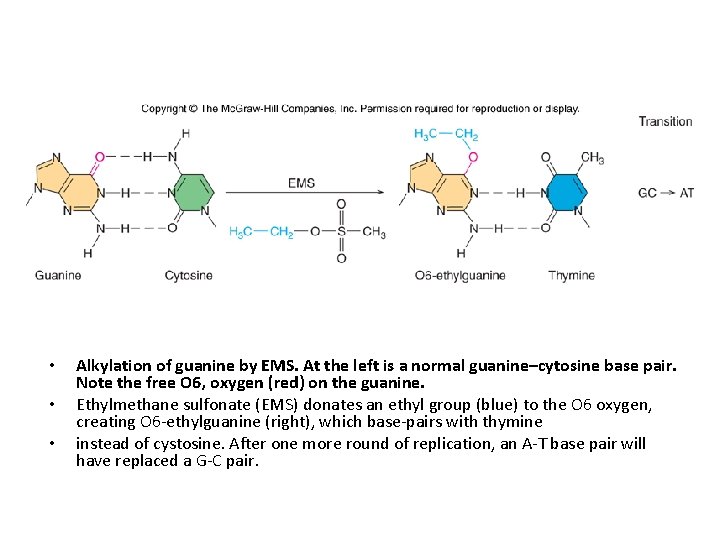
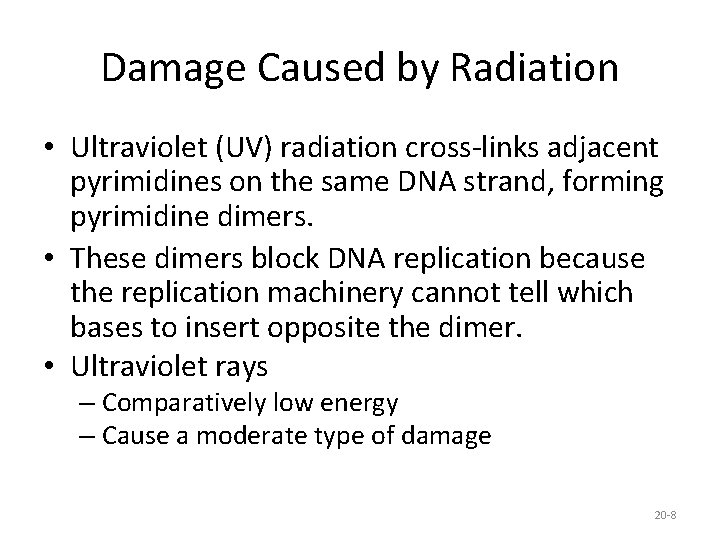
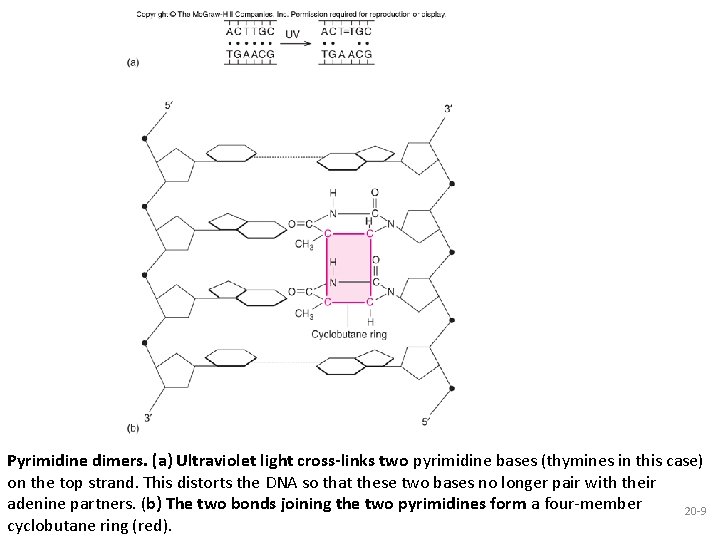
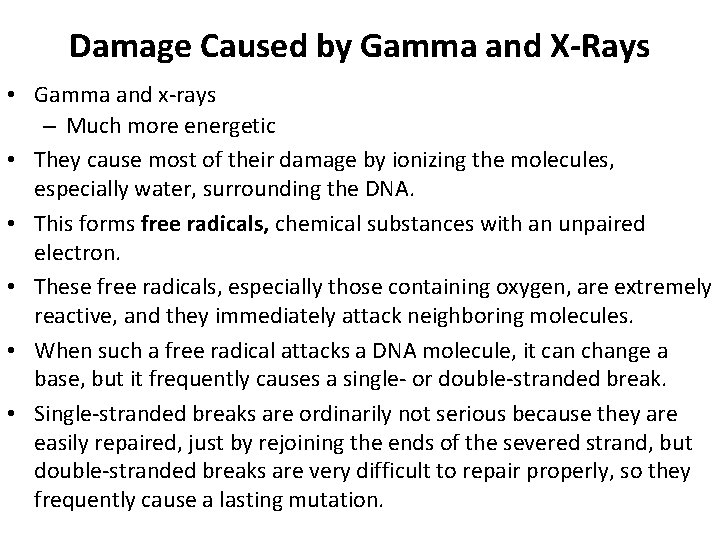
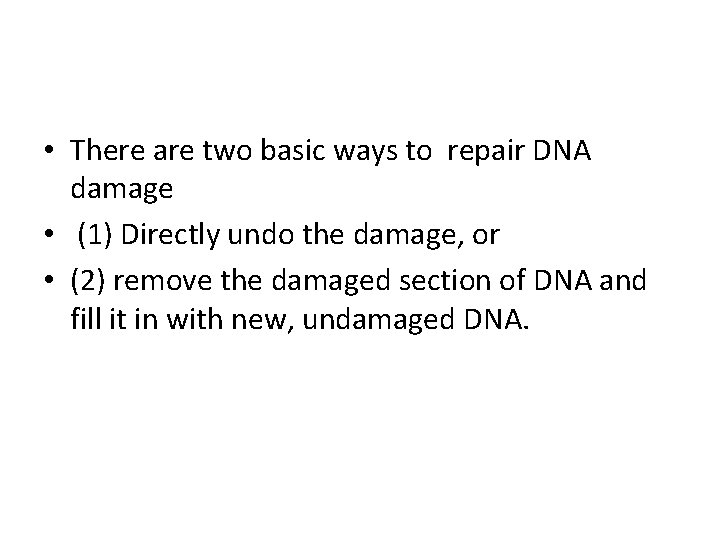
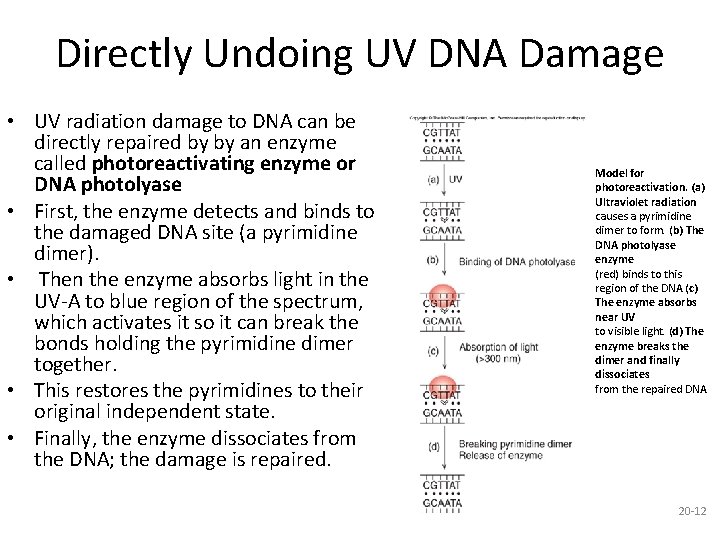
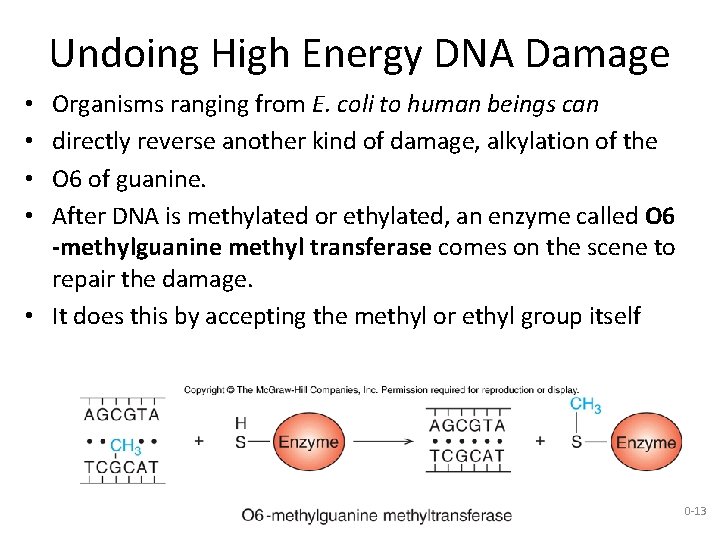
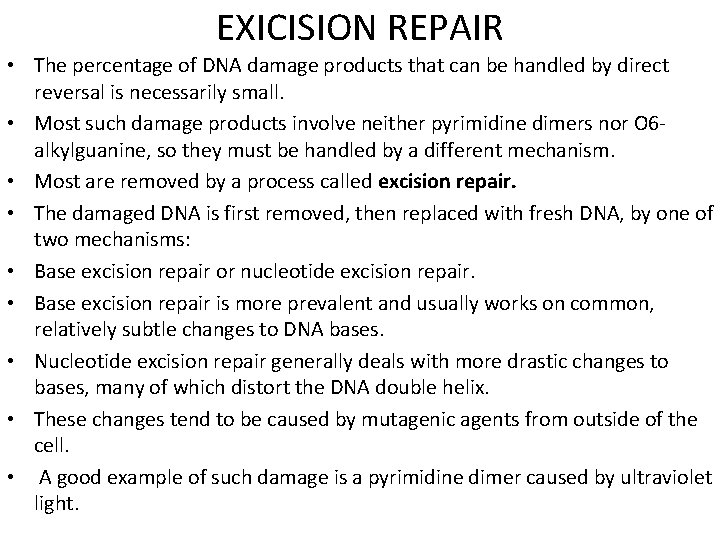
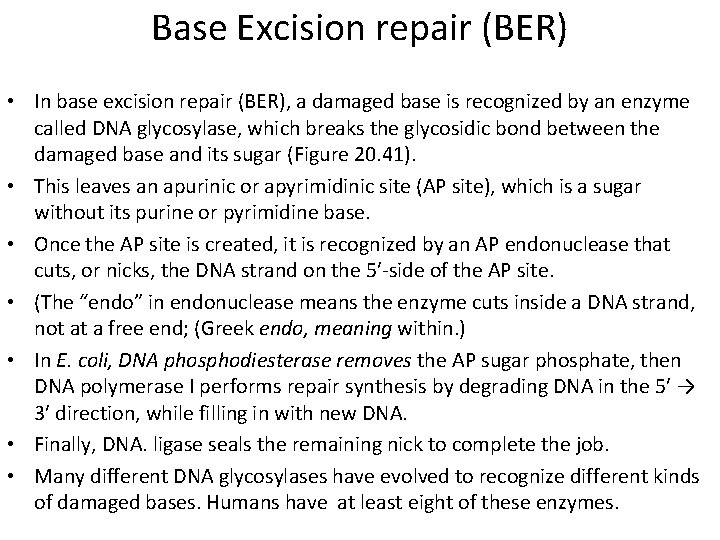
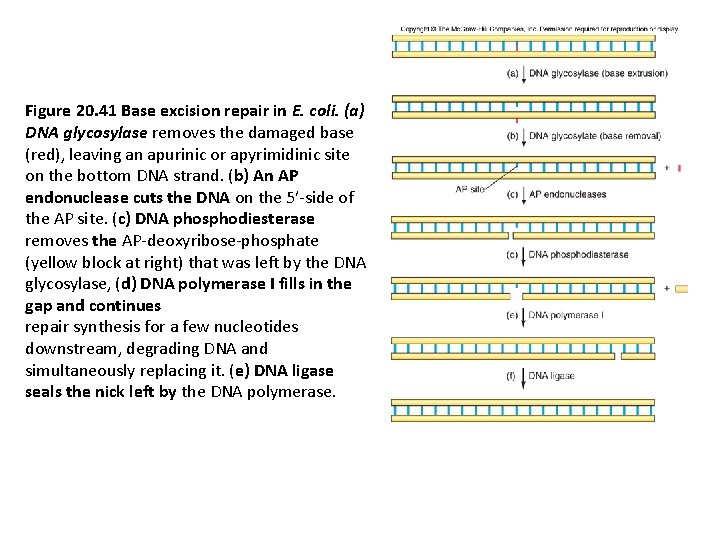
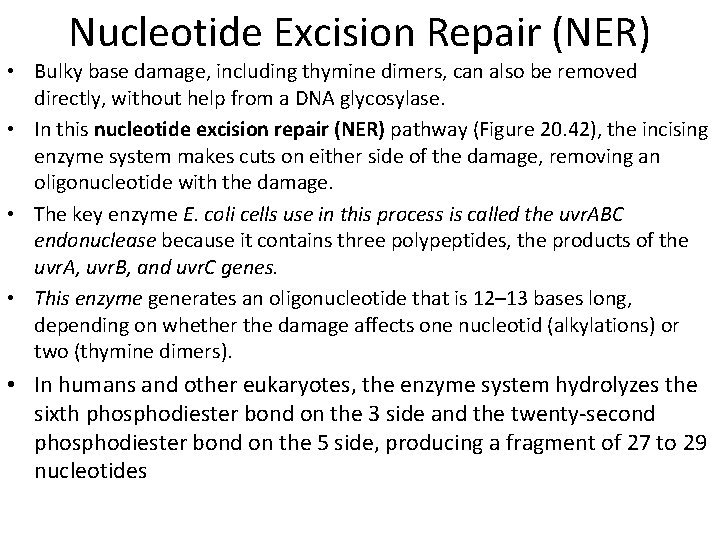
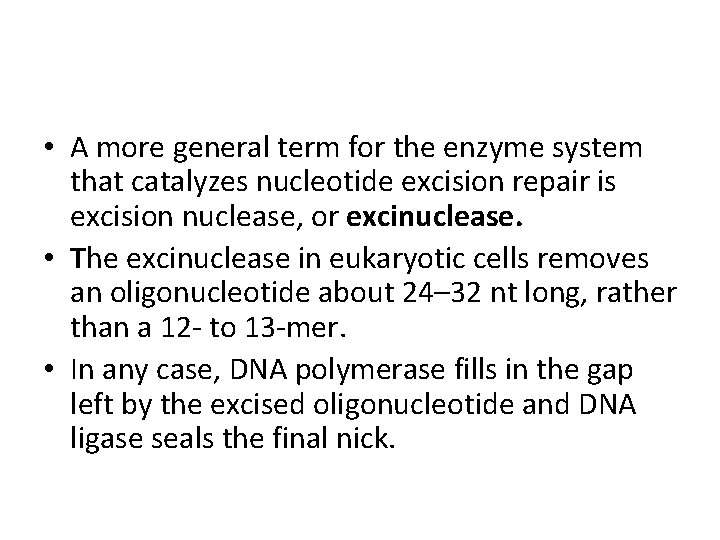
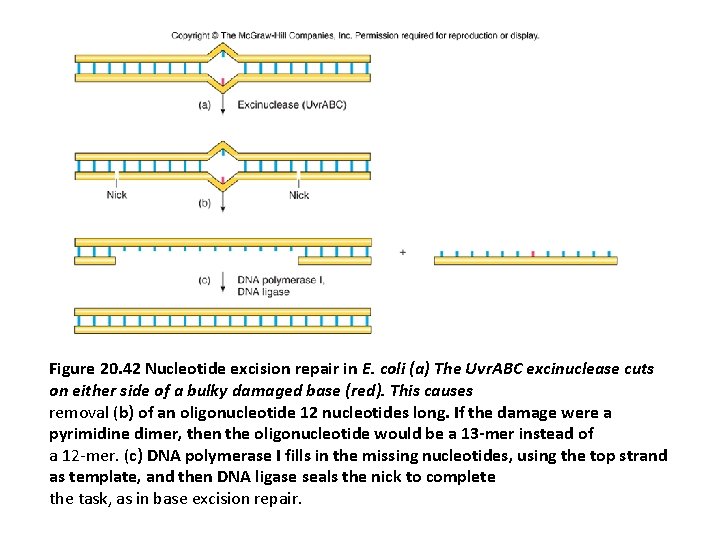
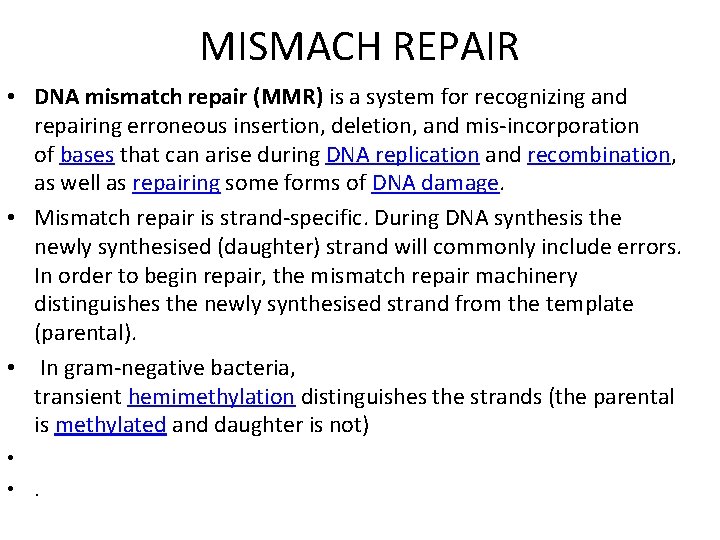
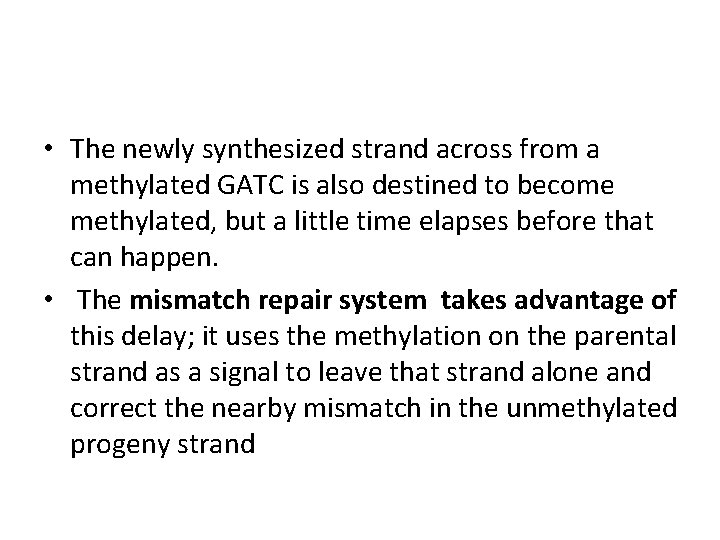
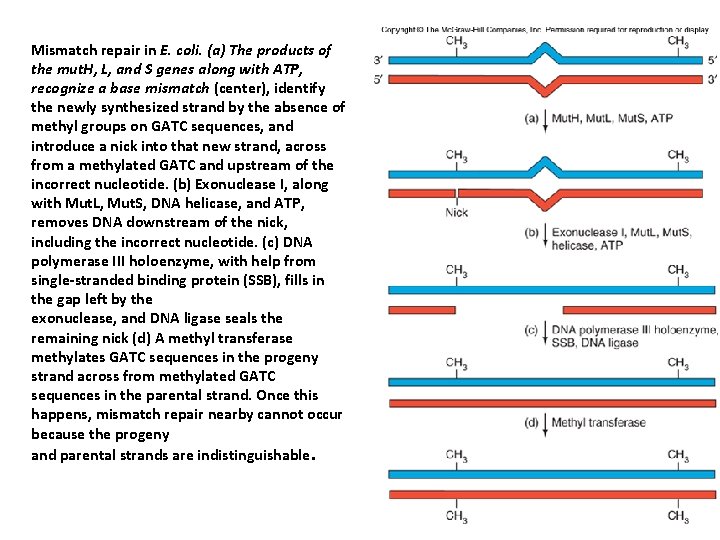
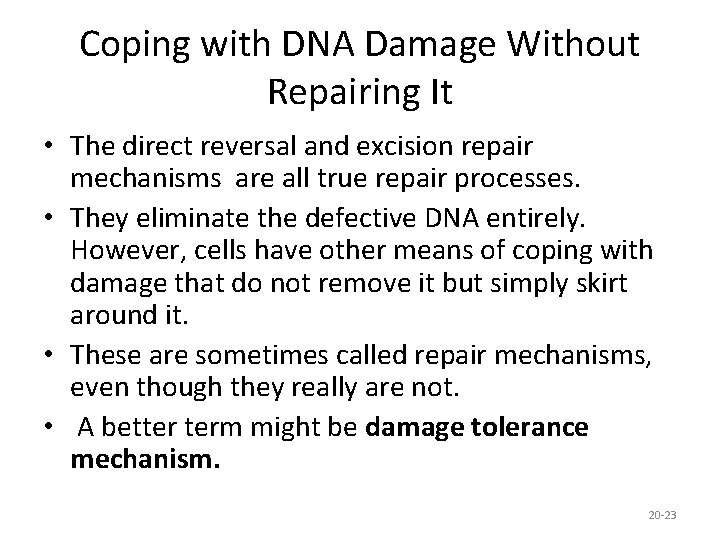
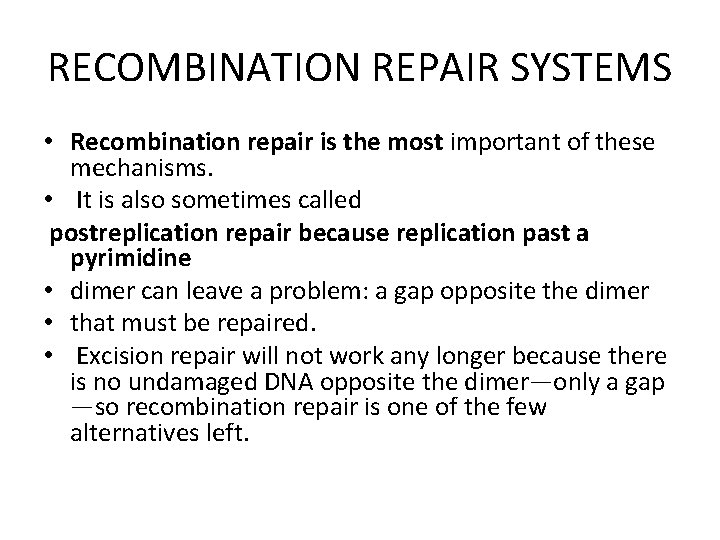
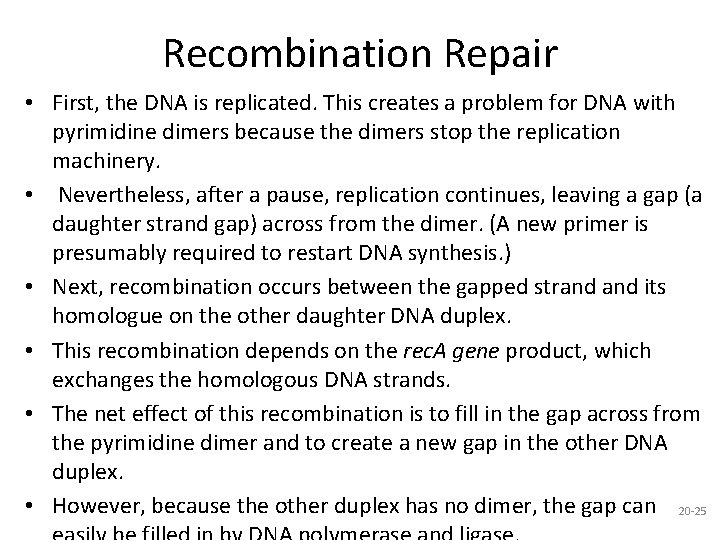
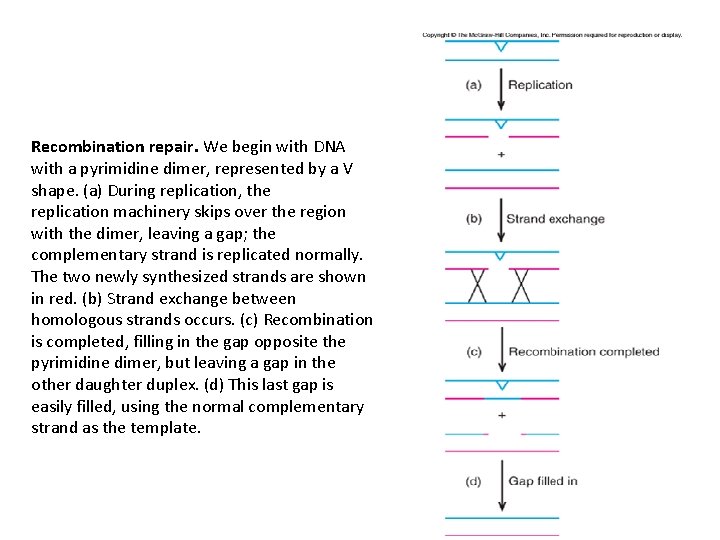
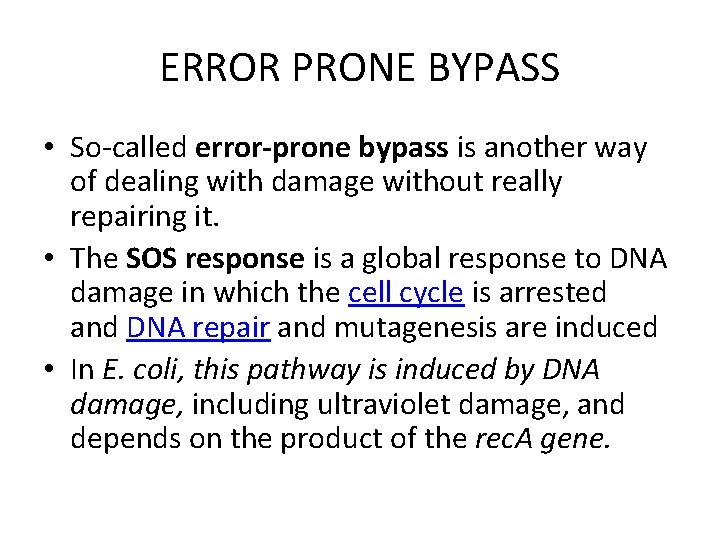
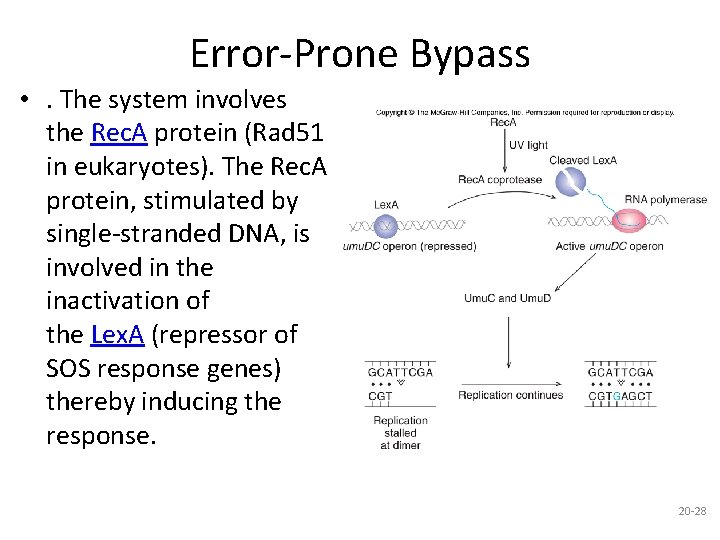
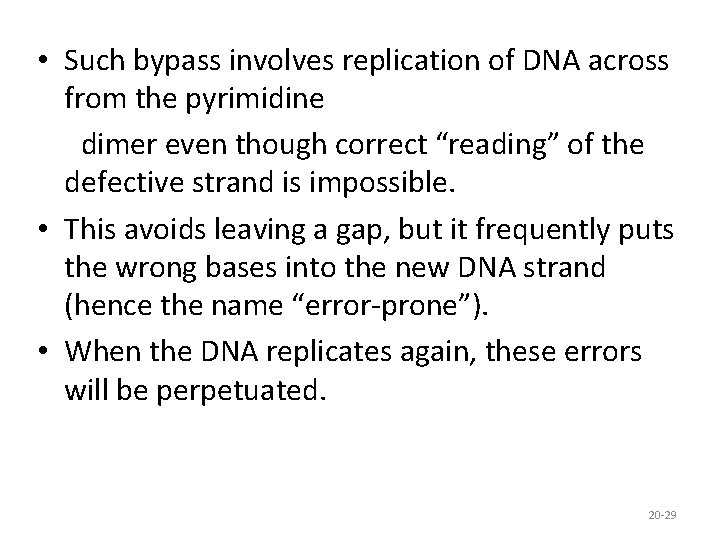
- Slides: 29
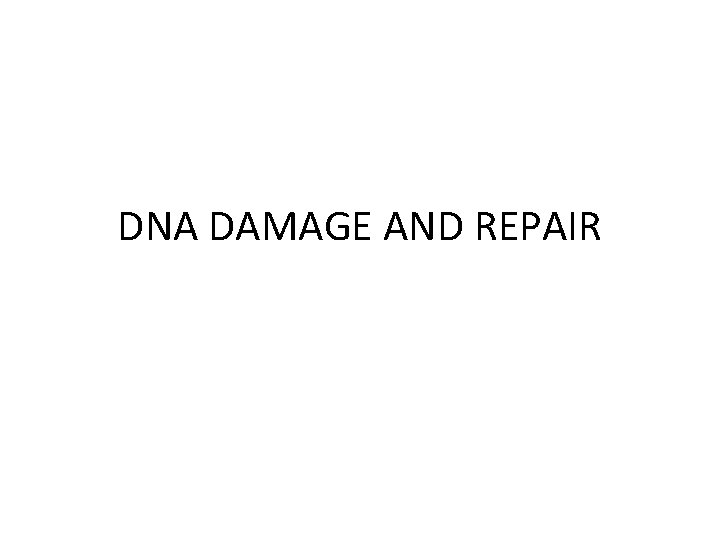
DNA DAMAGE AND REPAIR
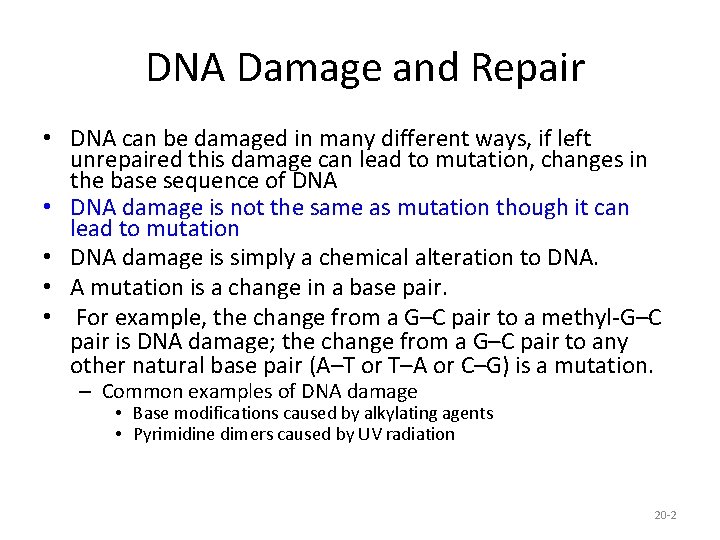
DNA Damage and Repair • DNA can be damaged in many different ways, if left unrepaired this damage can lead to mutation, changes in the base sequence of DNA • DNA damage is not the same as mutation though it can lead to mutation • DNA damage is simply a chemical alteration to DNA. • A mutation is a change in a base pair. • For example, the change from a G–C pair to a methyl-G–C pair is DNA damage; the change from a G–C pair to any other natural base pair (A–T or T–A or C–G) is a mutation. – Common examples of DNA damage • Base modifications caused by alkylating agents • Pyrimidine dimers caused by UV radiation 20 -2
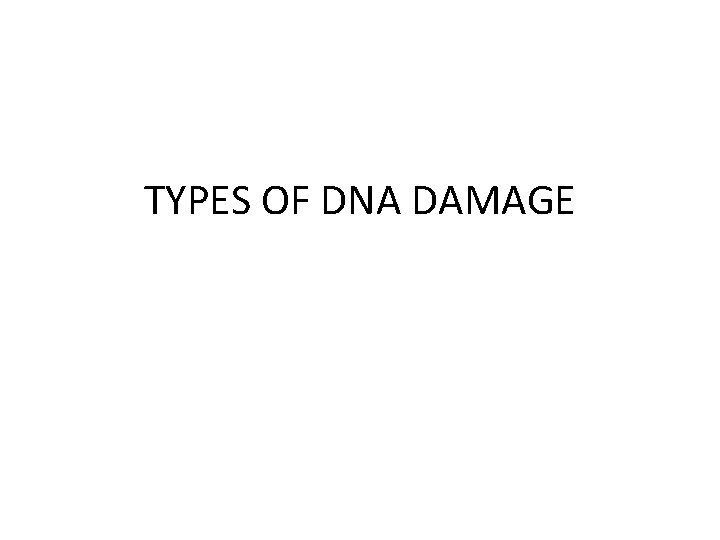
TYPES OF DNA DAMAGE
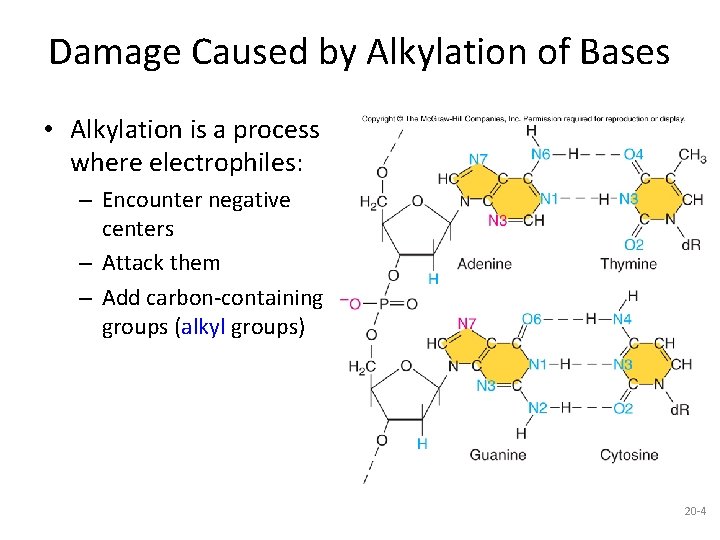
Damage Caused by Alkylation of Bases • Alkylation is a process where electrophiles: – Encounter negative centers – Attack them – Add carbon-containing groups (alkyl groups) 20 -4
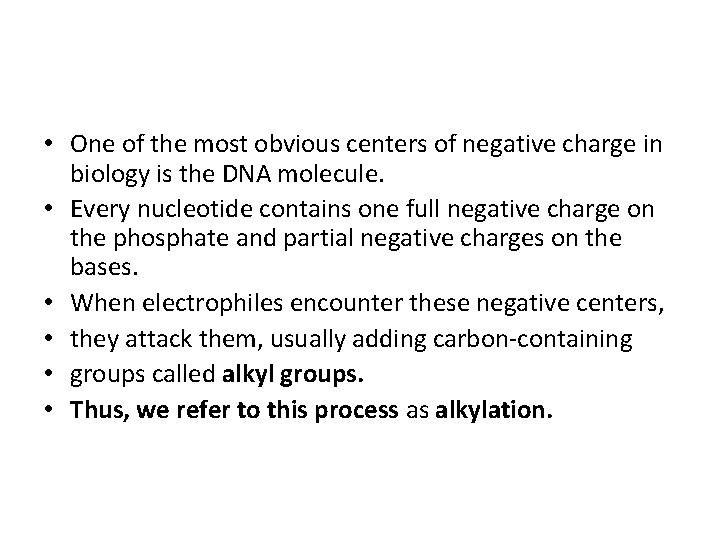
• One of the most obvious centers of negative charge in biology is the DNA molecule. • Every nucleotide contains one full negative charge on the phosphate and partial negative charges on the bases. • When electrophiles encounter these negative centers, • they attack them, usually adding carbon-containing • groups called alkyl groups. • Thus, we refer to this process as alkylation.
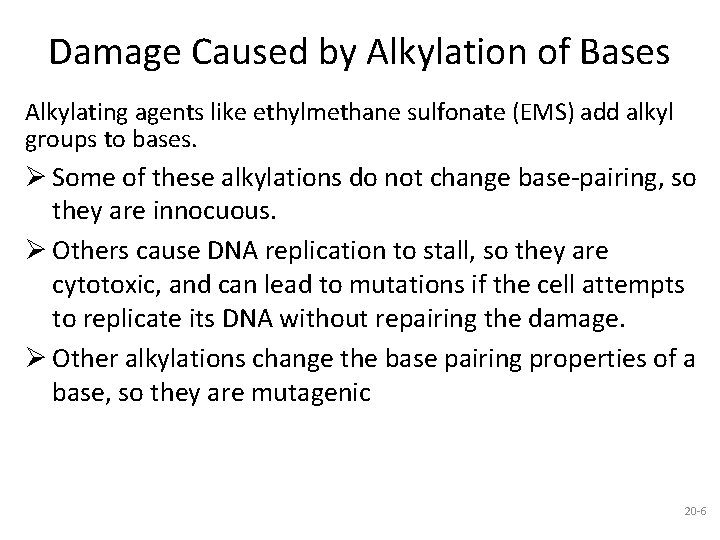
Damage Caused by Alkylation of Bases Alkylating agents like ethylmethane sulfonate (EMS) add alkyl groups to bases. Ø Some of these alkylations do not change base-pairing, so they are innocuous. Ø Others cause DNA replication to stall, so they are cytotoxic, and can lead to mutations if the cell attempts to replicate its DNA without repairing the damage. Ø Other alkylations change the base pairing properties of a base, so they are mutagenic 20 -6
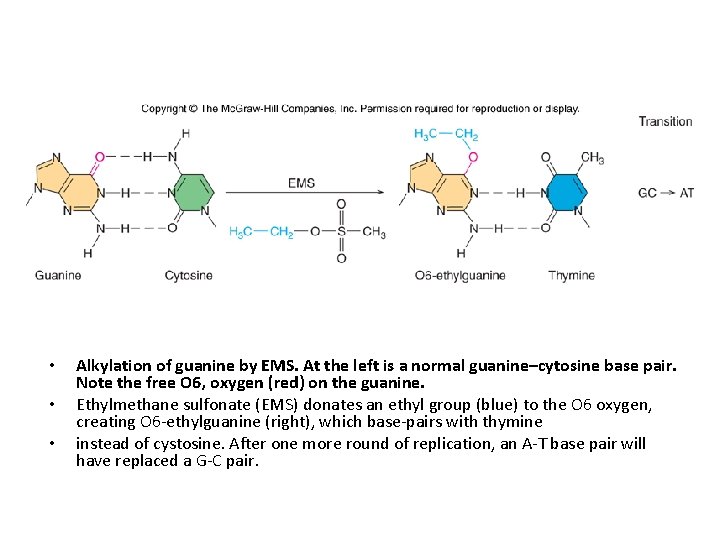
• • • Alkylation of guanine by EMS. At the left is a normal guanine–cytosine base pair. Note the free O 6, oxygen (red) on the guanine. Ethylmethane sulfonate (EMS) donates an ethyl group (blue) to the O 6 oxygen, creating O 6 -ethylguanine (right), which base-pairs with thymine instead of cystosine. After one more round of replication, an A-T base pair will have replaced a G-C pair.
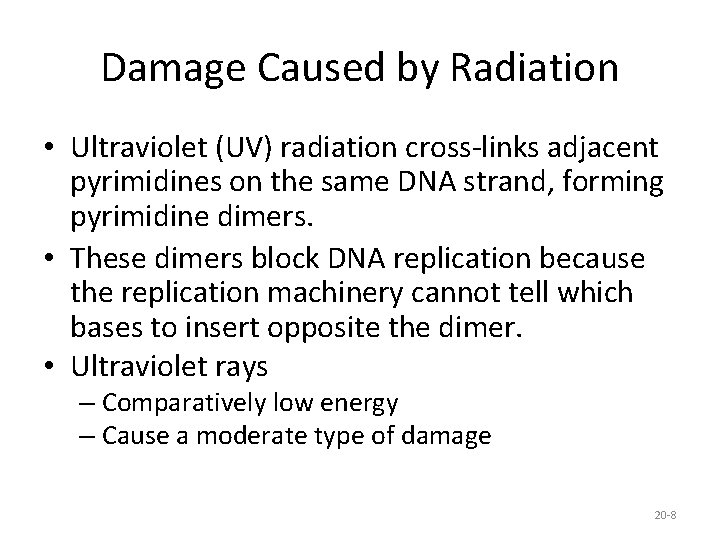
Damage Caused by Radiation • Ultraviolet (UV) radiation cross-links adjacent pyrimidines on the same DNA strand, forming pyrimidine dimers. • These dimers block DNA replication because the replication machinery cannot tell which bases to insert opposite the dimer. • Ultraviolet rays – Comparatively low energy – Cause a moderate type of damage 20 -8
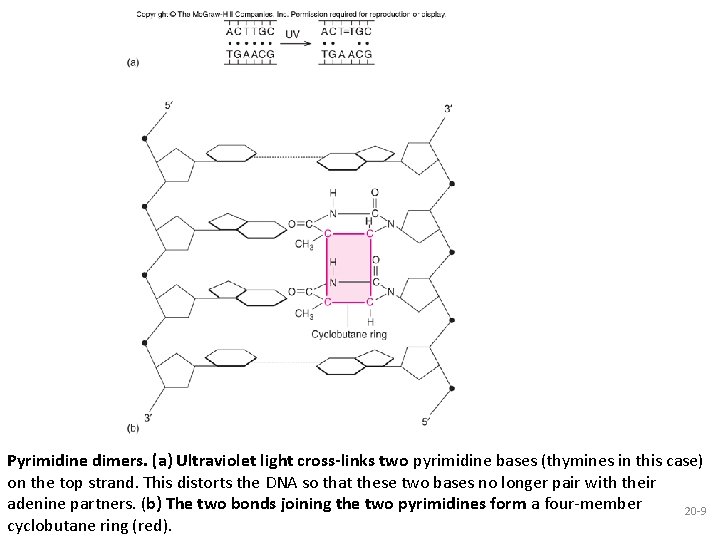
Pyrimidine dimers. (a) Ultraviolet light cross-links two pyrimidine bases (thymines in this case) on the top strand. This distorts the DNA so that these two bases no longer pair with their adenine partners. (b) The two bonds joining the two pyrimidines form a four-member 20 -9 cyclobutane ring (red).
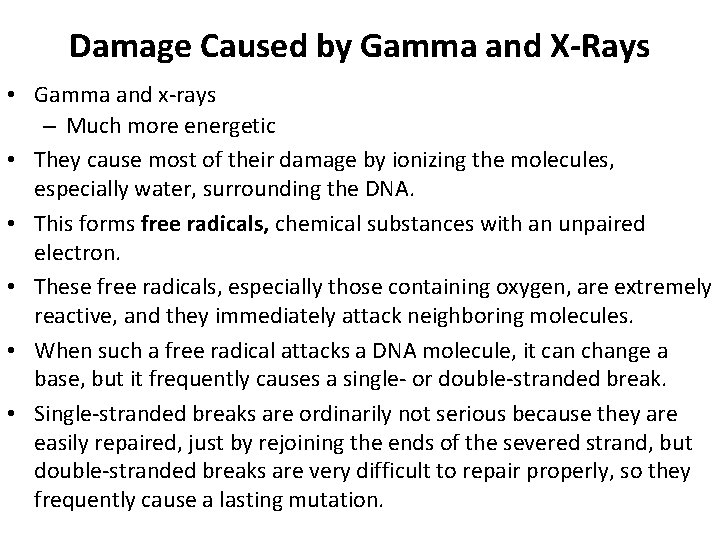
Damage Caused by Gamma and X-Rays • Gamma and x-rays – Much more energetic • They cause most of their damage by ionizing the molecules, especially water, surrounding the DNA. • This forms free radicals, chemical substances with an unpaired electron. • These free radicals, especially those containing oxygen, are extremely reactive, and they immediately attack neighboring molecules. • When such a free radical attacks a DNA molecule, it can change a base, but it frequently causes a single- or double-stranded break. • Single-stranded breaks are ordinarily not serious because they are easily repaired, just by rejoining the ends of the severed strand, but double-stranded breaks are very difficult to repair properly, so they frequently cause a lasting mutation.
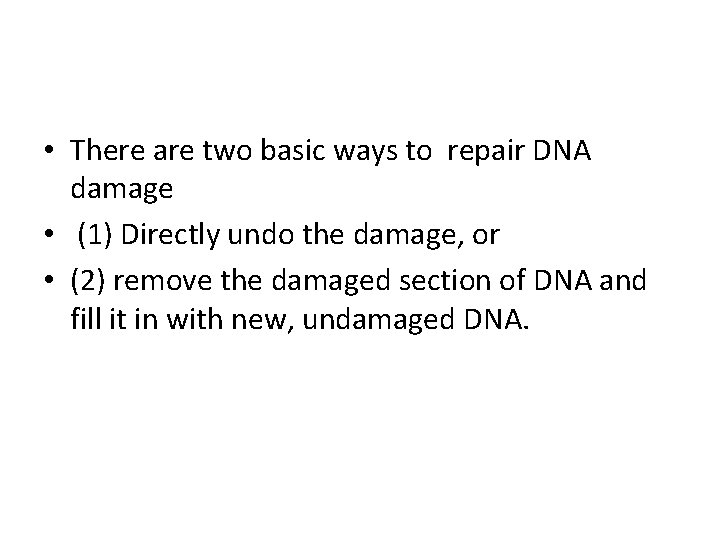
• There are two basic ways to repair DNA damage • (1) Directly undo the damage, or • (2) remove the damaged section of DNA and fill it in with new, undamaged DNA.
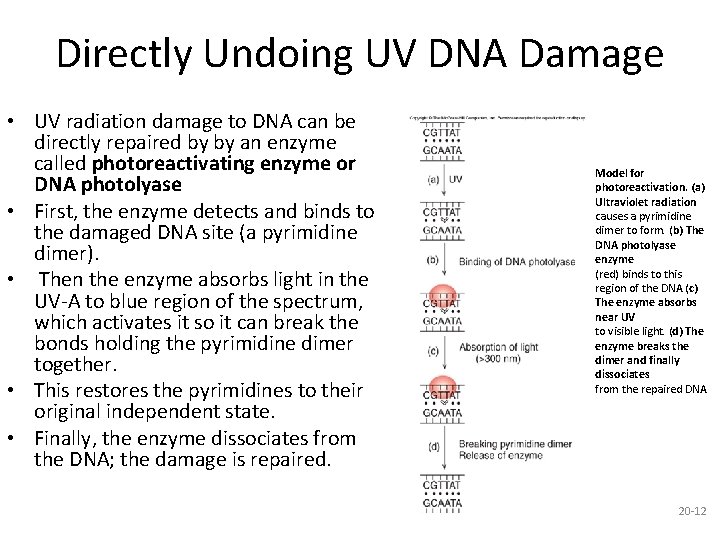
Directly Undoing UV DNA Damage • UV radiation damage to DNA can be directly repaired by by an enzyme called photoreactivating enzyme or DNA photolyase • First, the enzyme detects and binds to the damaged DNA site (a pyrimidine dimer). • Then the enzyme absorbs light in the UV-A to blue region of the spectrum, which activates it so it can break the bonds holding the pyrimidine dimer together. • This restores the pyrimidines to their original independent state. • Finally, the enzyme dissociates from the DNA; the damage is repaired. Model for photoreactivation. (a) Ultraviolet radiation causes a pyrimidine dimer to form. (b) The DNA photolyase enzyme (red) binds to this region of the DNA (c) The enzyme absorbs near UV to visible light. (d) The enzyme breaks the dimer and finally dissociates from the repaired DNA 20 -12
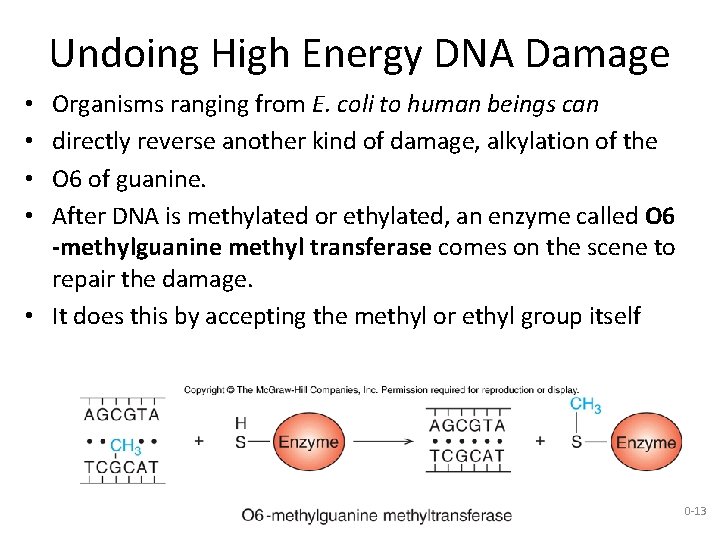
Undoing High Energy DNA Damage Organisms ranging from E. coli to human beings can directly reverse another kind of damage, alkylation of the O 6 of guanine. After DNA is methylated or ethylated, an enzyme called O 6 -methylguanine methyl transferase comes on the scene to repair the damage. • It does this by accepting the methyl or ethyl group itself • • 20 -13
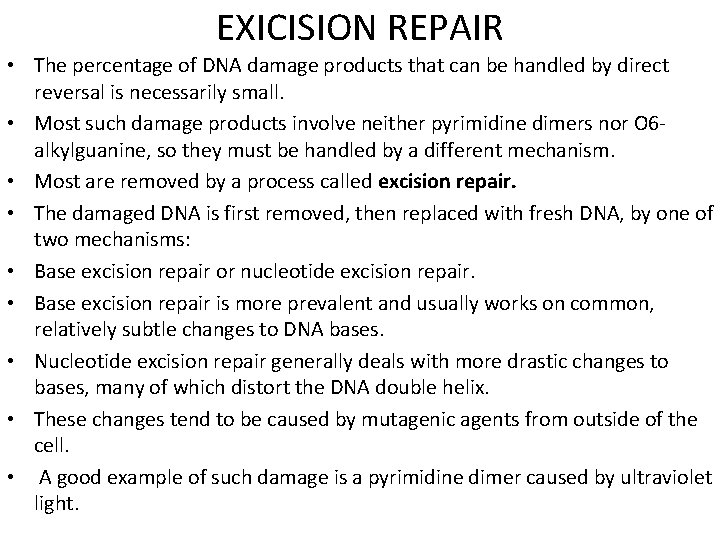
EXICISION REPAIR • The percentage of DNA damage products that can be handled by direct reversal is necessarily small. • Most such damage products involve neither pyrimidine dimers nor O 6 alkylguanine, so they must be handled by a different mechanism. • Most are removed by a process called excision repair. • The damaged DNA is first removed, then replaced with fresh DNA, by one of two mechanisms: • Base excision repair or nucleotide excision repair. • Base excision repair is more prevalent and usually works on common, relatively subtle changes to DNA bases. • Nucleotide excision repair generally deals with more drastic changes to bases, many of which distort the DNA double helix. • These changes tend to be caused by mutagenic agents from outside of the cell. • A good example of such damage is a pyrimidine dimer caused by ultraviolet light.
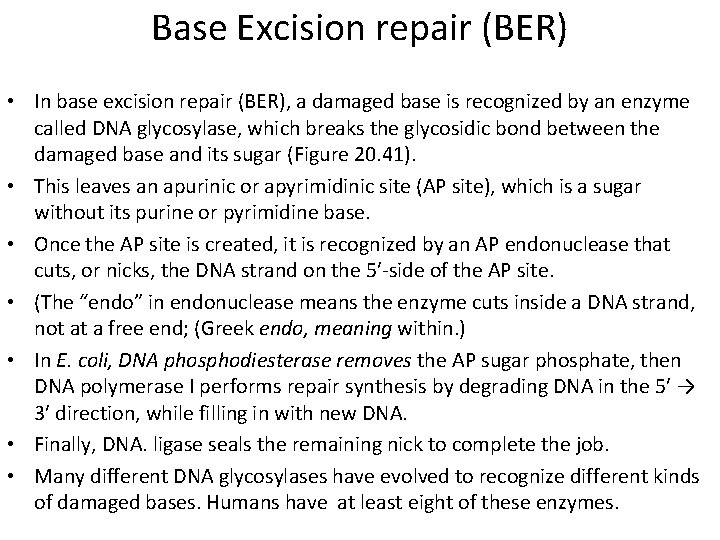
Base Excision repair (BER) • In base excision repair (BER), a damaged base is recognized by an enzyme called DNA glycosylase, which breaks the glycosidic bond between the damaged base and its sugar (Figure 20. 41). • This leaves an apurinic or apyrimidinic site (AP site), which is a sugar without its purine or pyrimidine base. • Once the AP site is created, it is recognized by an AP endonuclease that cuts, or nicks, the DNA strand on the 5′-side of the AP site. • (The “endo” in endonuclease means the enzyme cuts inside a DNA strand, not at a free end; (Greek endo, meaning within. ) • In E. coli, DNA phosphodiesterase removes the AP sugar phosphate, then DNA polymerase I performs repair synthesis by degrading DNA in the 5′ → 3′ direction, while filling in with new DNA. • Finally, DNA. ligase seals the remaining nick to complete the job. • Many different DNA glycosylases have evolved to recognize different kinds of damaged bases. Humans have at least eight of these enzymes.
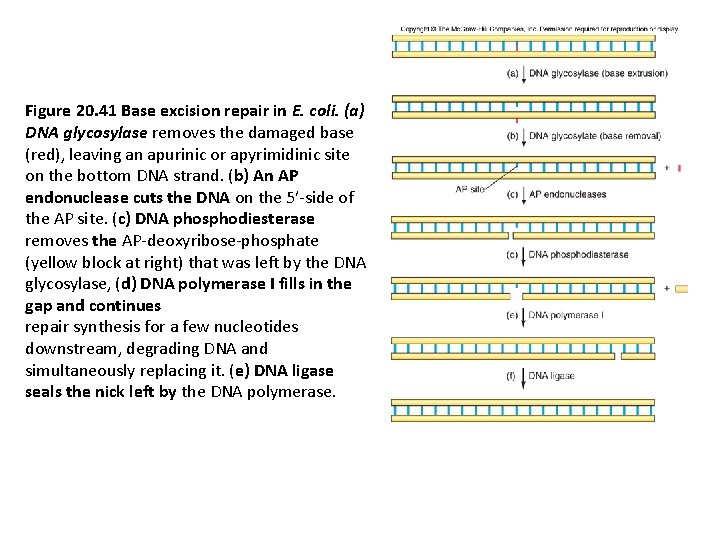
Figure 20. 41 Base excision repair in E. coli. (a) DNA glycosylase removes the damaged base (red), leaving an apurinic or apyrimidinic site on the bottom DNA strand. (b) An AP endonuclease cuts the DNA on the 5′-side of the AP site. (c) DNA phosphodiesterase removes the AP-deoxyribose-phosphate (yellow block at right) that was left by the DNA glycosylase, (d) DNA polymerase I fills in the gap and continues repair synthesis for a few nucleotides downstream, degrading DNA and simultaneously replacing it. (e) DNA ligase seals the nick left by the DNA polymerase.
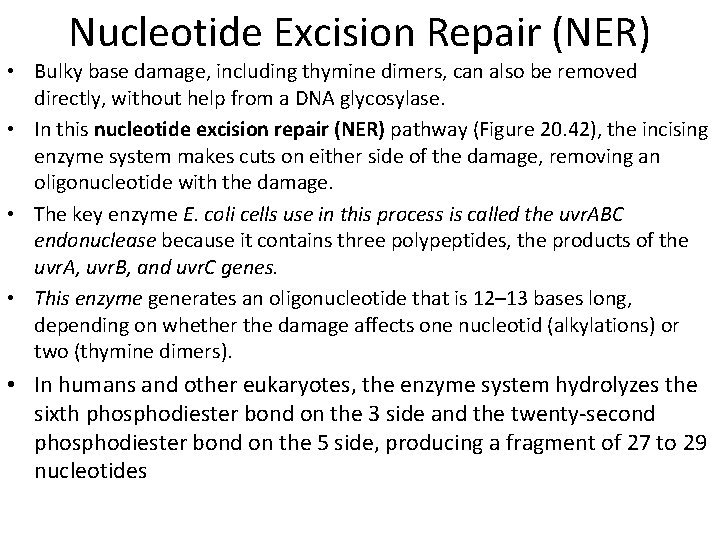
Nucleotide Excision Repair (NER) • Bulky base damage, including thymine dimers, can also be removed directly, without help from a DNA glycosylase. • In this nucleotide excision repair (NER) pathway (Figure 20. 42), the incising enzyme system makes cuts on either side of the damage, removing an oligonucleotide with the damage. • The key enzyme E. coli cells use in this process is called the uvr. ABC endonuclease because it contains three polypeptides, the products of the uvr. A, uvr. B, and uvr. C genes. • This enzyme generates an oligonucleotide that is 12– 13 bases long, depending on whether the damage affects one nucleotid (alkylations) or two (thymine dimers). • In humans and other eukaryotes, the enzyme system hydrolyzes the sixth phosphodiester bond on the 3 side and the twenty-second phosphodiester bond on the 5 side, producing a fragment of 27 to 29 nucleotides
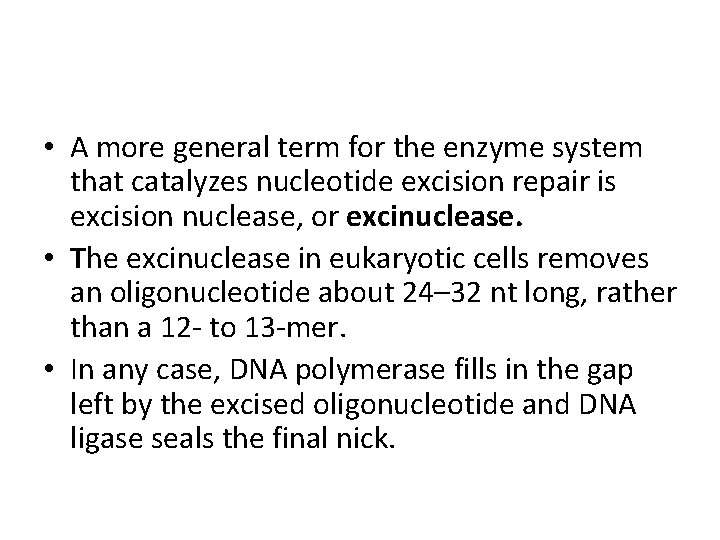
• A more general term for the enzyme system that catalyzes nucleotide excision repair is excision nuclease, or excinuclease. • The excinuclease in eukaryotic cells removes an oligonucleotide about 24– 32 nt long, rather than a 12 - to 13 -mer. • In any case, DNA polymerase fills in the gap left by the excised oligonucleotide and DNA ligase seals the final nick.
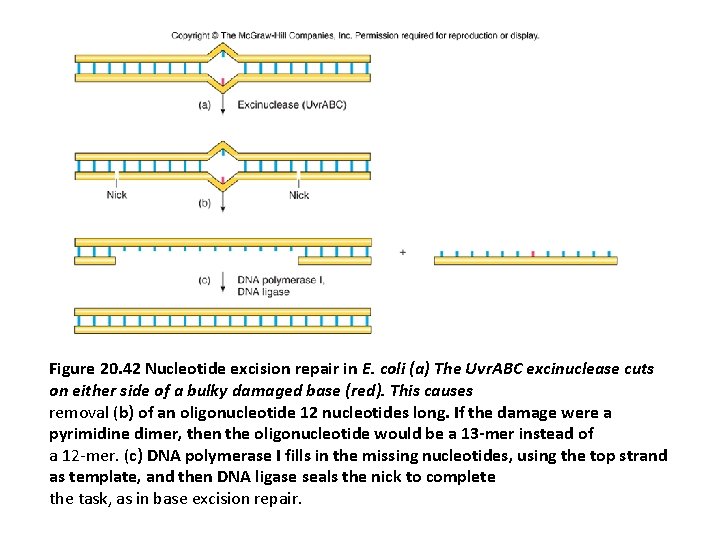
Figure 20. 42 Nucleotide excision repair in E. coli (a) The Uvr. ABC excinuclease cuts on either side of a bulky damaged base (red). This causes removal (b) of an oligonucleotide 12 nucleotides long. If the damage were a pyrimidine dimer, then the oligonucleotide would be a 13 -mer instead of a 12 -mer. (c) DNA polymerase I fills in the missing nucleotides, using the top strand as template, and then DNA ligase seals the nick to complete the task, as in base excision repair.
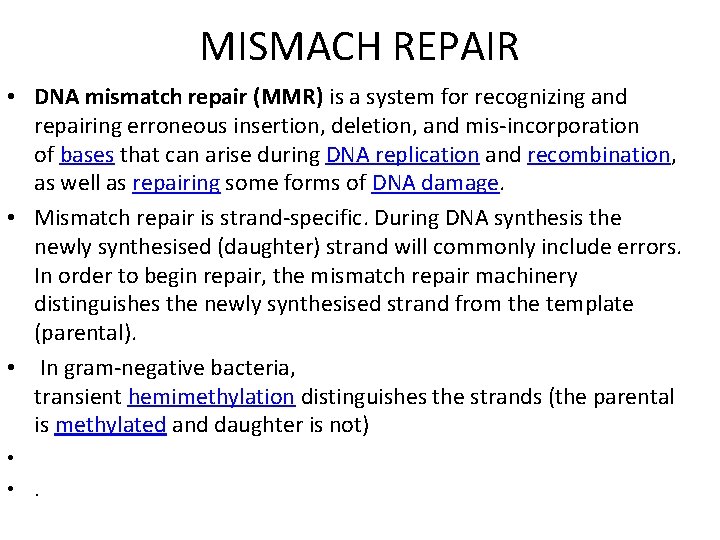
MISMACH REPAIR • DNA mismatch repair (MMR) is a system for recognizing and repairing erroneous insertion, deletion, and mis-incorporation of bases that can arise during DNA replication and recombination, as well as repairing some forms of DNA damage. • Mismatch repair is strand-specific. During DNA synthesis the newly synthesised (daughter) strand will commonly include errors. In order to begin repair, the mismatch repair machinery distinguishes the newly synthesised strand from the template (parental). • In gram-negative bacteria, transient hemimethylation distinguishes the strands (the parental is methylated and daughter is not) • • .
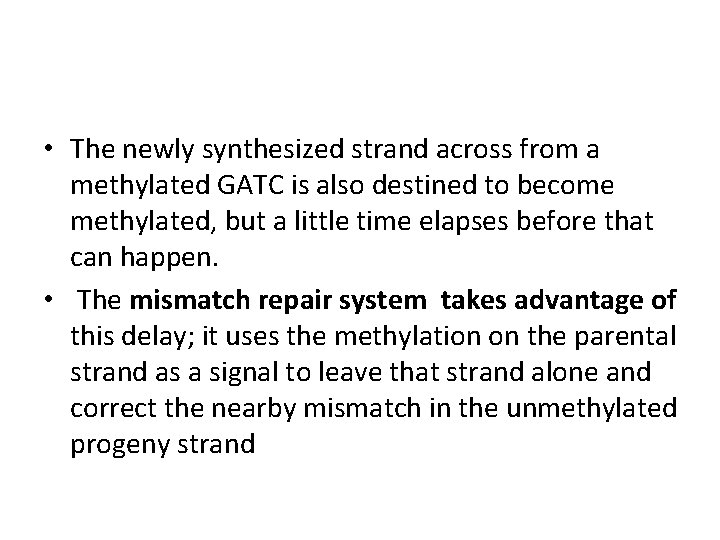
• The newly synthesized strand across from a methylated GATC is also destined to become methylated, but a little time elapses before that can happen. • The mismatch repair system takes advantage of this delay; it uses the methylation on the parental strand as a signal to leave that strand alone and correct the nearby mismatch in the unmethylated progeny strand
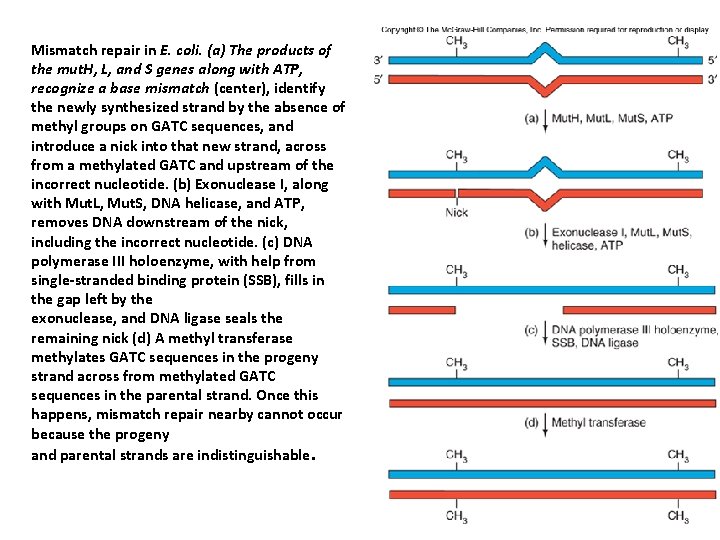
Mismatch repair in E. coli. (a) The products of the mut. H, L, and S genes along with ATP, recognize a base mismatch (center), identify the newly synthesized strand by the absence of methyl groups on GATC sequences, and introduce a nick into that new strand, across from a methylated GATC and upstream of the incorrect nucleotide. (b) Exonuclease I, along with Mut. L, Mut. S, DNA helicase, and ATP, removes DNA downstream of the nick, including the incorrect nucleotide. (c) DNA polymerase III holoenzyme, with help from single-stranded binding protein (SSB), fills in the gap left by the exonuclease, and DNA ligase seals the remaining nick (d) A methyl transferase methylates GATC sequences in the progeny strand across from methylated GATC sequences in the parental strand. Once this happens, mismatch repair nearby cannot occur because the progeny and parental strands are indistinguishable.
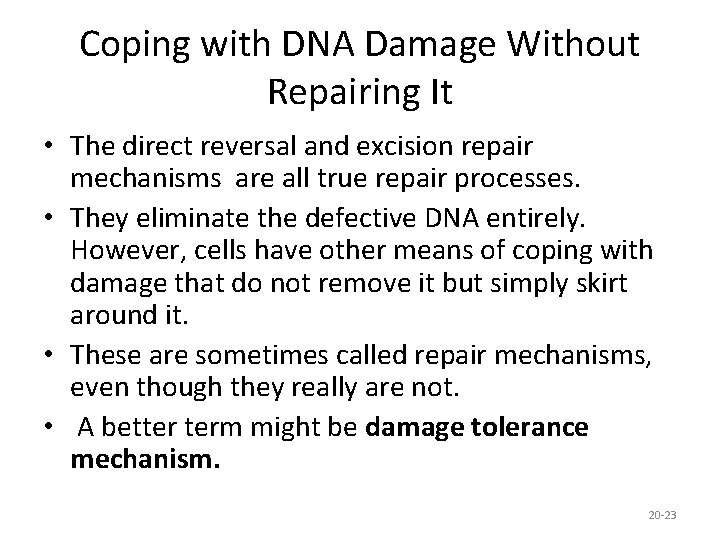
Coping with DNA Damage Without Repairing It • The direct reversal and excision repair mechanisms are all true repair processes. • They eliminate the defective DNA entirely. However, cells have other means of coping with damage that do not remove it but simply skirt around it. • These are sometimes called repair mechanisms, even though they really are not. • A better term might be damage tolerance mechanism. 20 -23
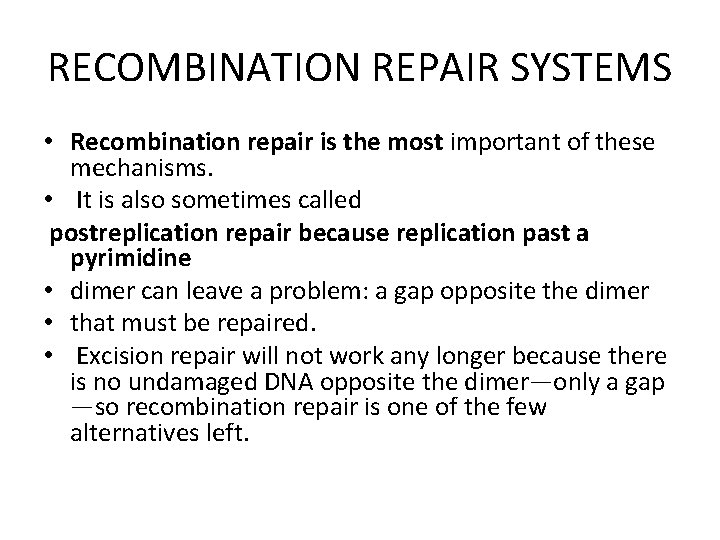
RECOMBINATION REPAIR SYSTEMS • Recombination repair is the most important of these mechanisms. • It is also sometimes called postreplication repair because replication past a pyrimidine • dimer can leave a problem: a gap opposite the dimer • that must be repaired. • Excision repair will not work any longer because there is no undamaged DNA opposite the dimer—only a gap —so recombination repair is one of the few alternatives left.
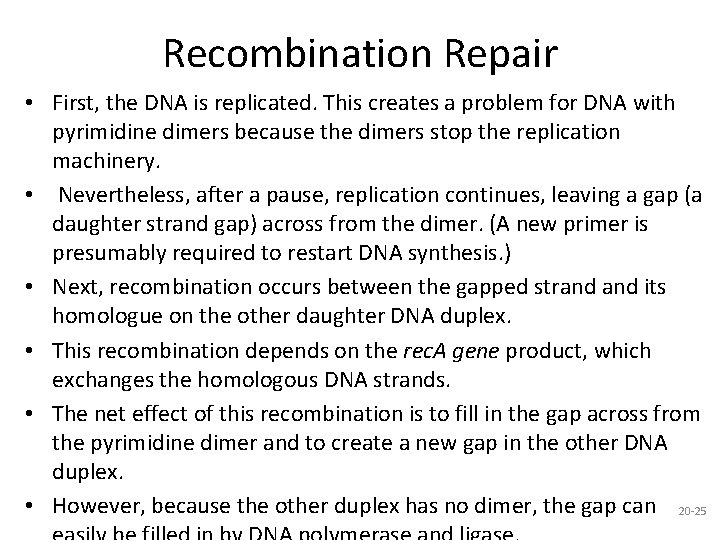
Recombination Repair • First, the DNA is replicated. This creates a problem for DNA with pyrimidine dimers because the dimers stop the replication machinery. • Nevertheless, after a pause, replication continues, leaving a gap (a daughter strand gap) across from the dimer. (A new primer is presumably required to restart DNA synthesis. ) • Next, recombination occurs between the gapped strand its homologue on the other daughter DNA duplex. • This recombination depends on the rec. A gene product, which exchanges the homologous DNA strands. • The net effect of this recombination is to fill in the gap across from the pyrimidine dimer and to create a new gap in the other DNA duplex. • However, because the other duplex has no dimer, the gap can 20 -25
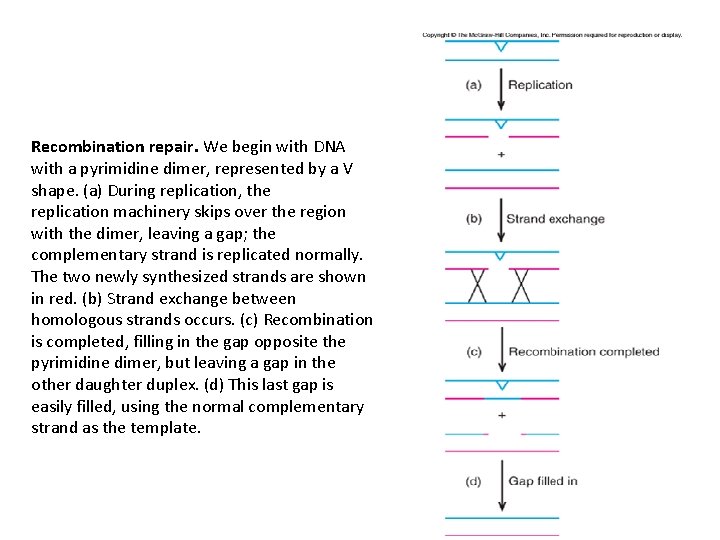
Recombination repair. We begin with DNA with a pyrimidine dimer, represented by a V shape. (a) During replication, the replication machinery skips over the region with the dimer, leaving a gap; the complementary strand is replicated normally. The two newly synthesized strands are shown in red. (b) Strand exchange between homologous strands occurs. (c) Recombination is completed, filling in the gap opposite the pyrimidine dimer, but leaving a gap in the other daughter duplex. (d) This last gap is easily filled, using the normal complementary strand as the template.
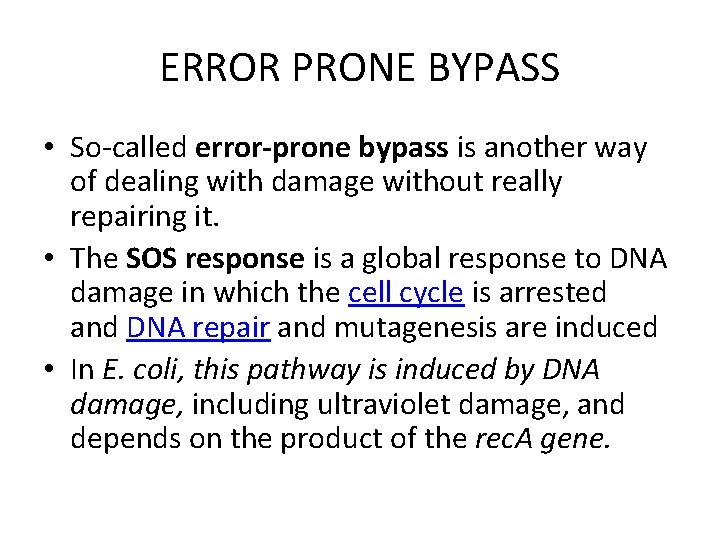
ERROR PRONE BYPASS • So-called error-prone bypass is another way of dealing with damage without really repairing it. • The SOS response is a global response to DNA damage in which the cell cycle is arrested and DNA repair and mutagenesis are induced • In E. coli, this pathway is induced by DNA damage, including ultraviolet damage, and depends on the product of the rec. A gene.
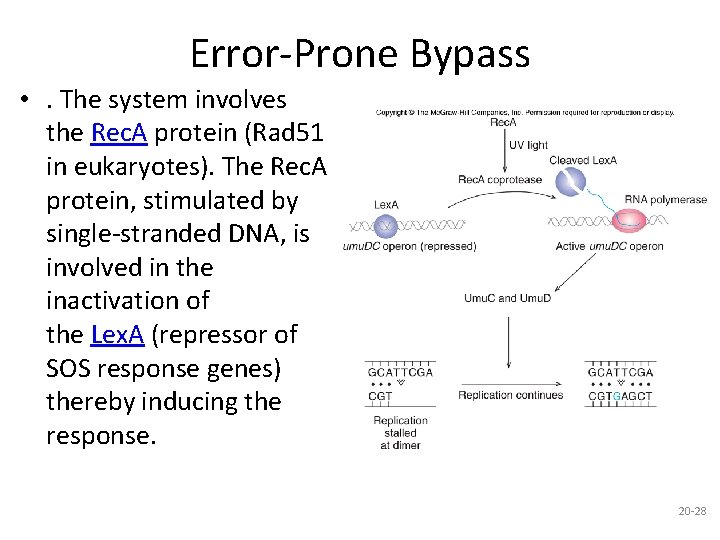
Error-Prone Bypass • . The system involves the Rec. A protein (Rad 51 in eukaryotes). The Rec. A protein, stimulated by single-stranded DNA, is involved in the inactivation of the Lex. A (repressor of SOS response genes) thereby inducing the response. 20 -28
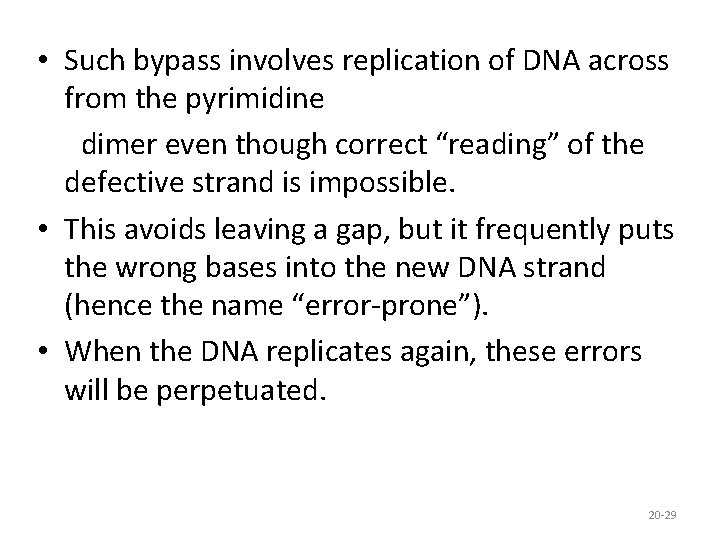
• Such bypass involves replication of DNA across from the pyrimidine dimer even though correct “reading” of the defective strand is impossible. • This avoids leaving a gap, but it frequently puts the wrong bases into the new DNA strand (hence the name “error-prone”). • When the DNA replicates again, these errors will be perpetuated. 20 -29
Nucleotide excision repair
Base excision repair
Dna polymerase proofreading
Coding dna and non coding dna
Dna polymerase function in dna replication
Bioflix activity dna replication lagging strand synthesis
Enzyme involved in dna replication
Chapter 11 dna and genes
Dana asian
Fatigue and damage tolerance course
Warsaw mechanism
Brake system diagnosis and repair
Electronic suspension system
Pressure regulator
Chapter 44 automotive wiring and wire repair
Dfd-busd
Faa 337 example
Brake system diagnosis
Auto upkeep answer key
Repair and restoration theory of sleep
Repair renovation and restoration of water bodies
Repair and restoration theory of sleep
Emotional damage pictures
Water damage somerset county
Sarasota storm damage
Which movement is the most highly organized
Paralysis of recurrent laryngeal nerve
Tetranychus urticae damage
Esd damage images
Image esd