Development of the Patientspecific Cardiovascular Modeling System using
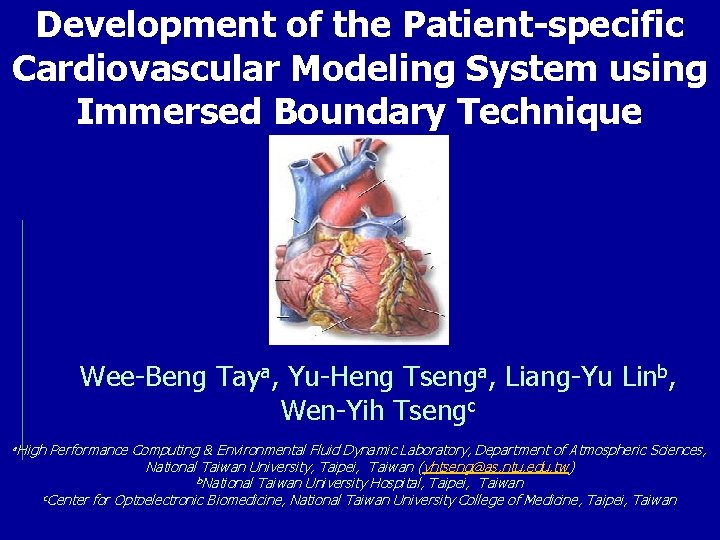
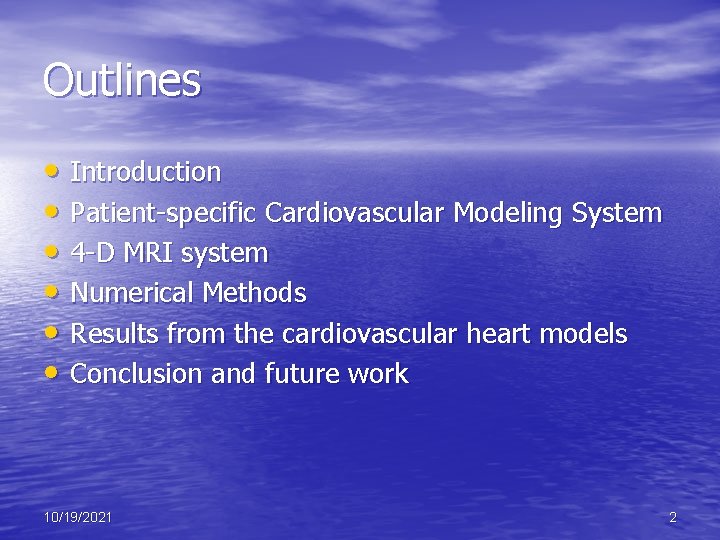
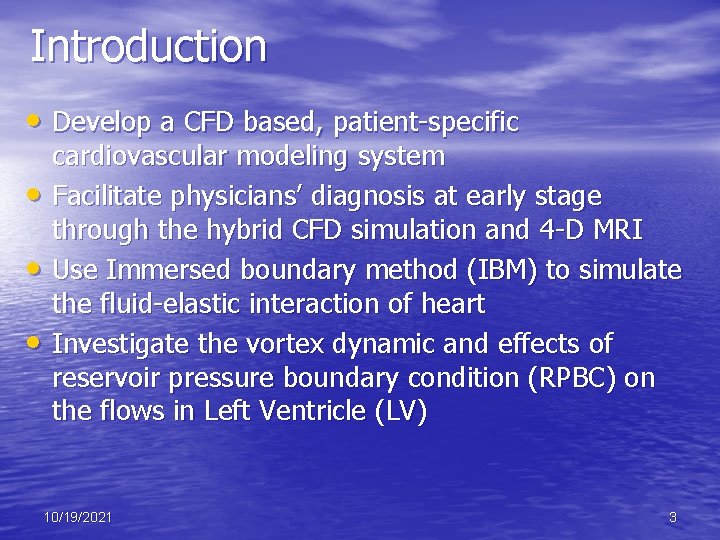
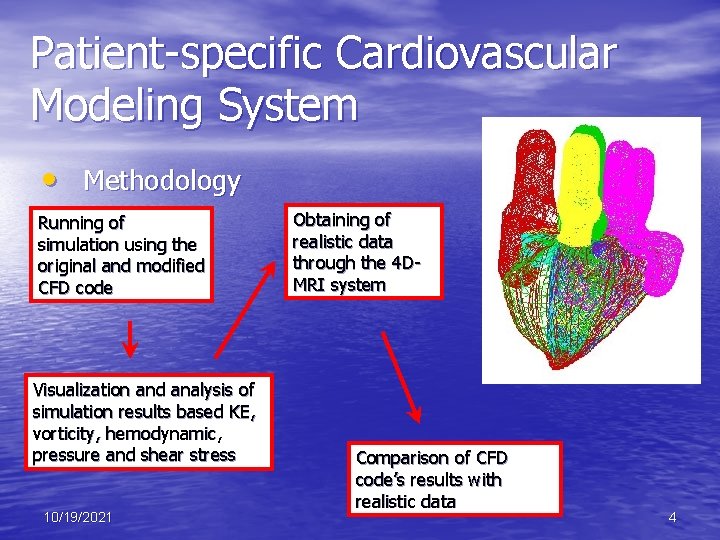
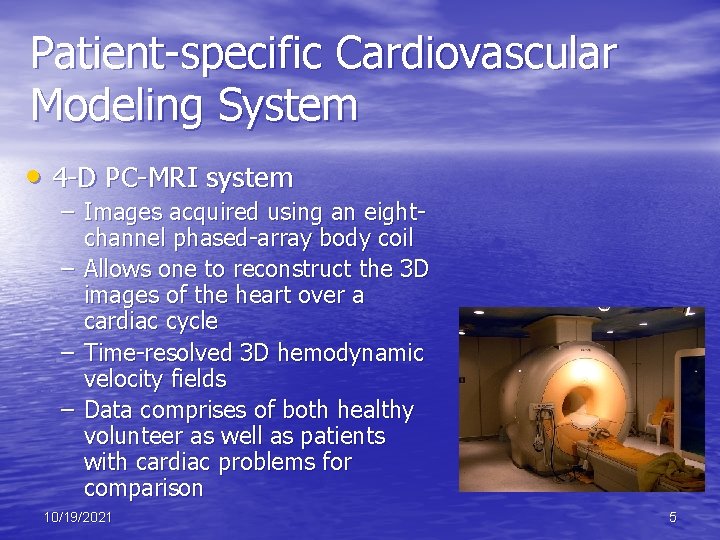
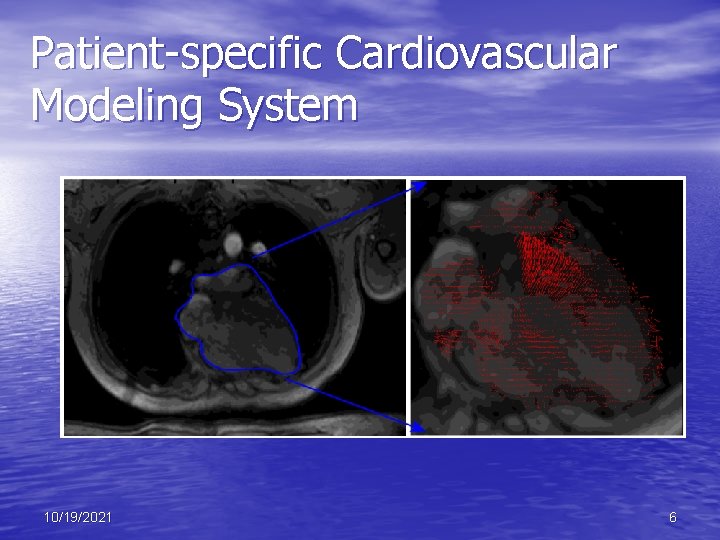
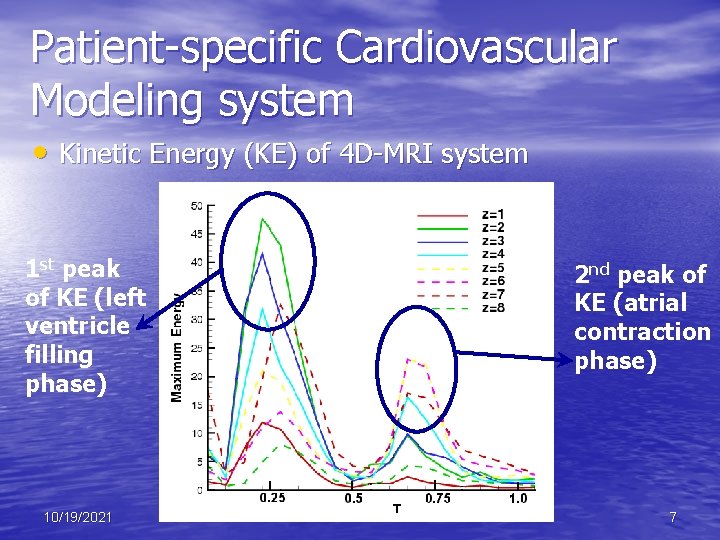
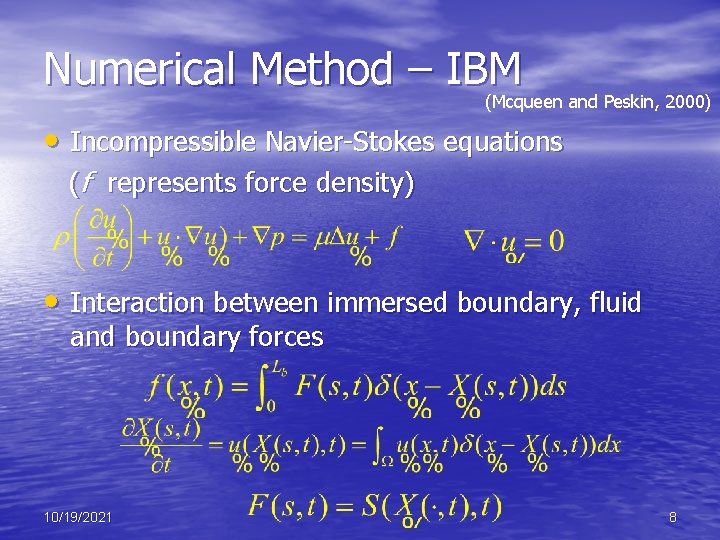
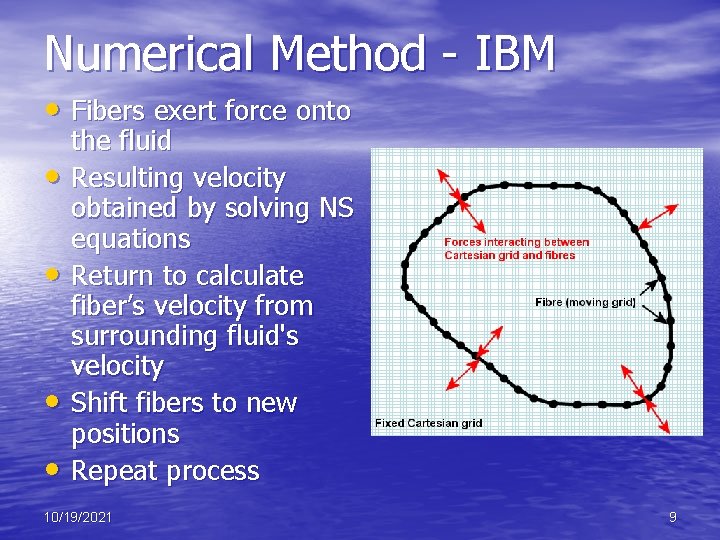
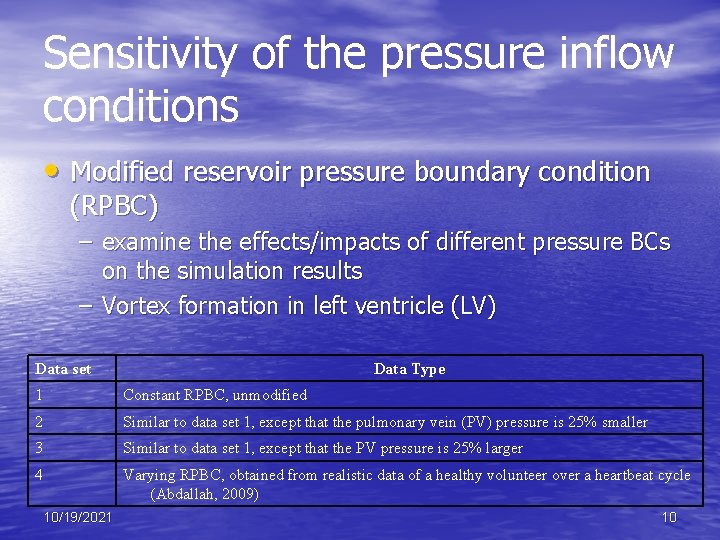
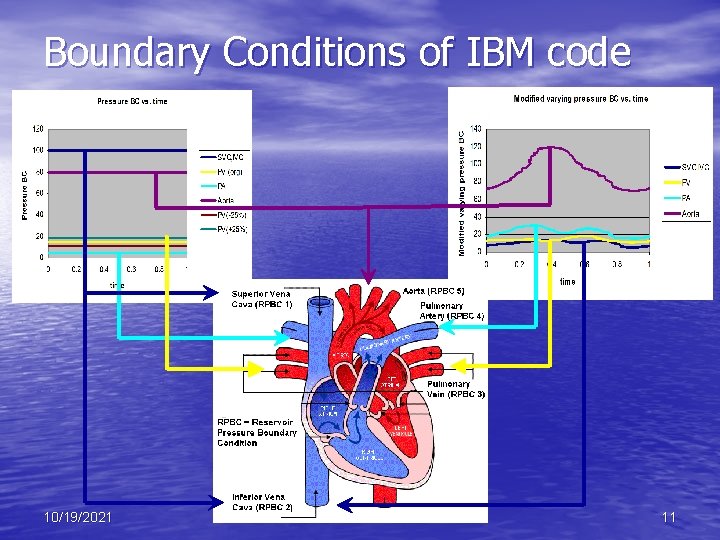
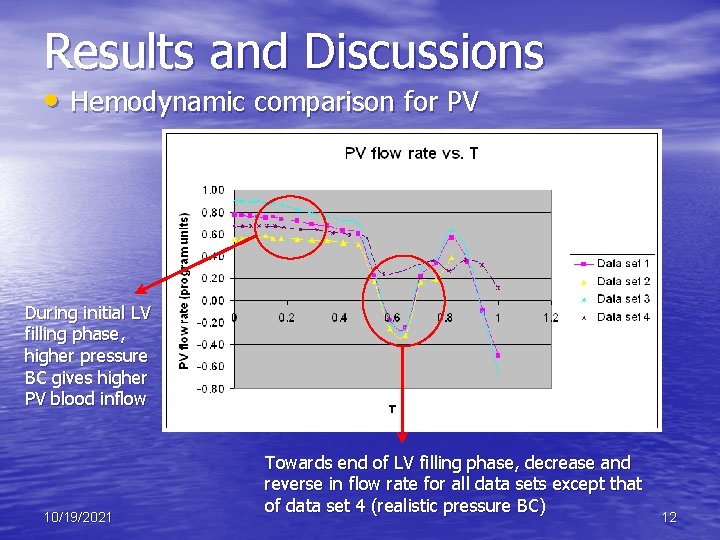
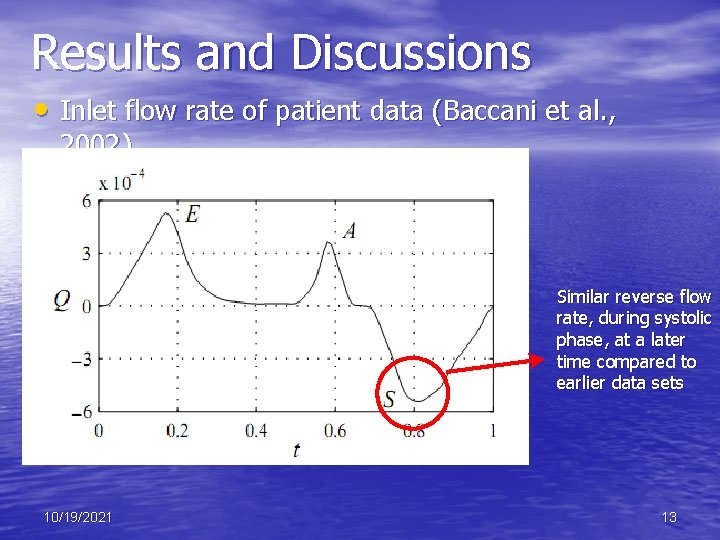
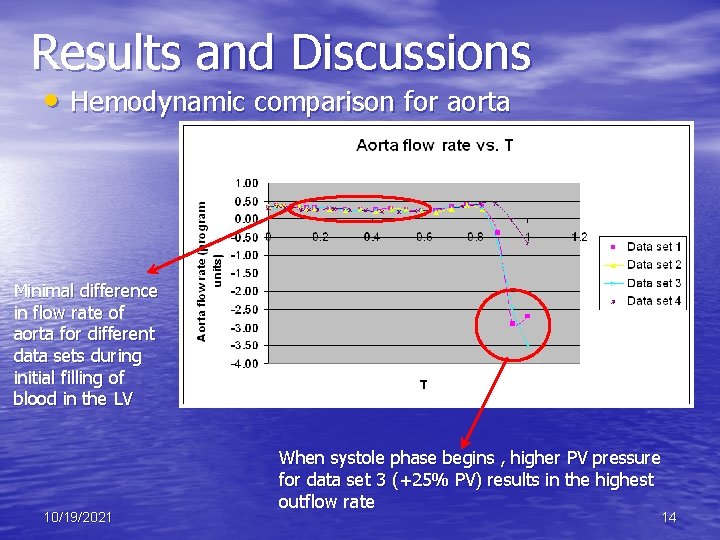
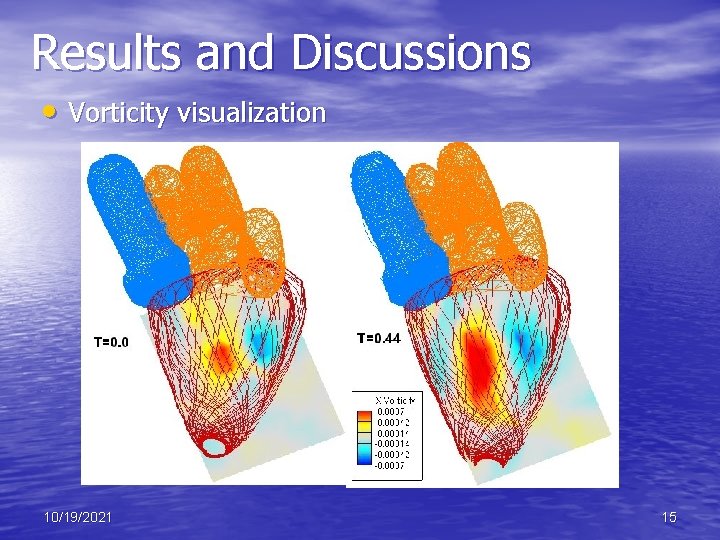
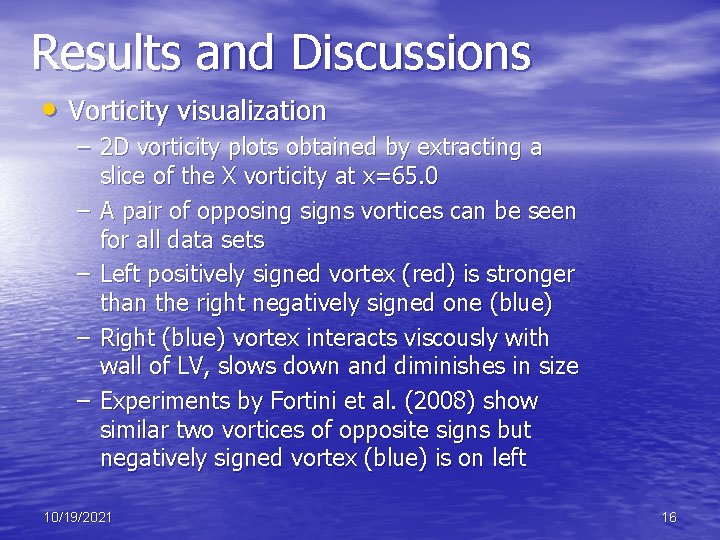
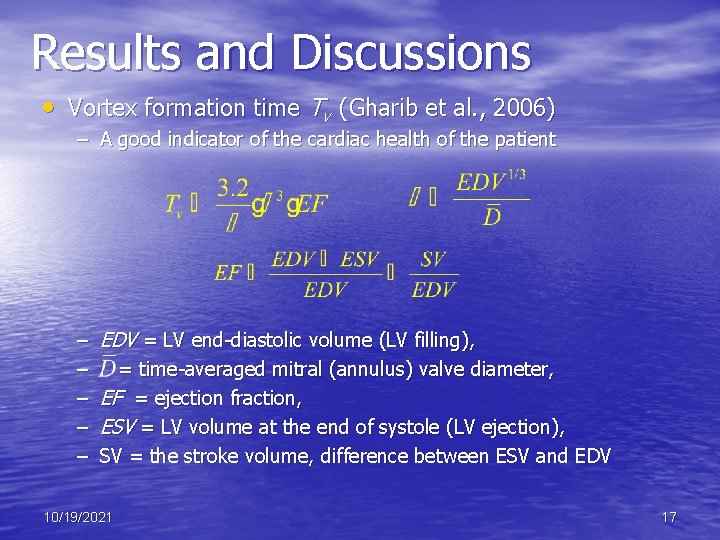
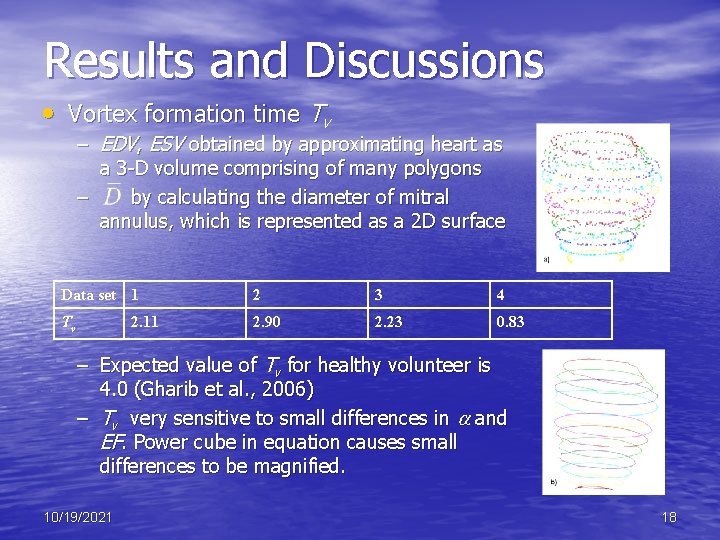
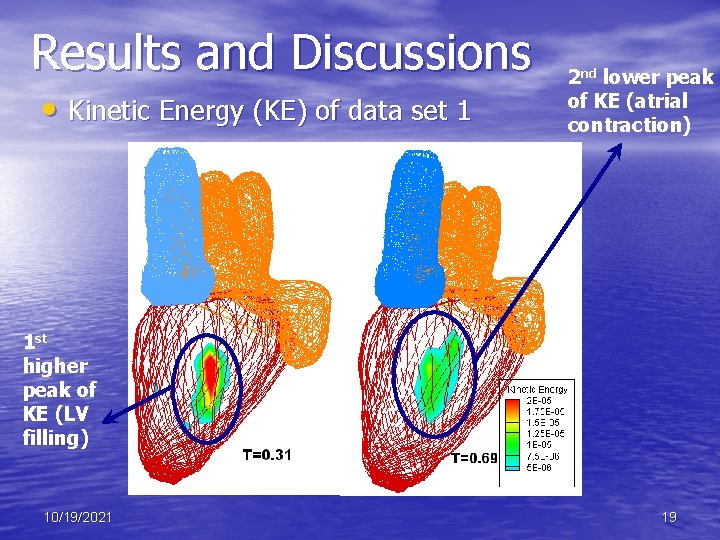
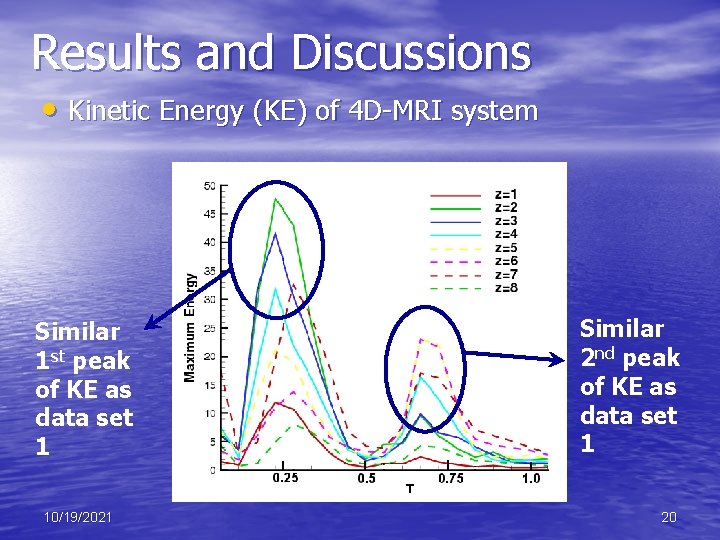
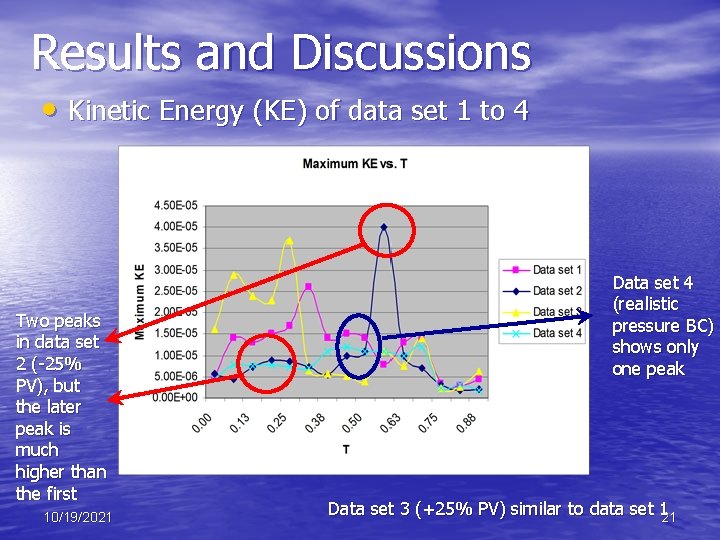
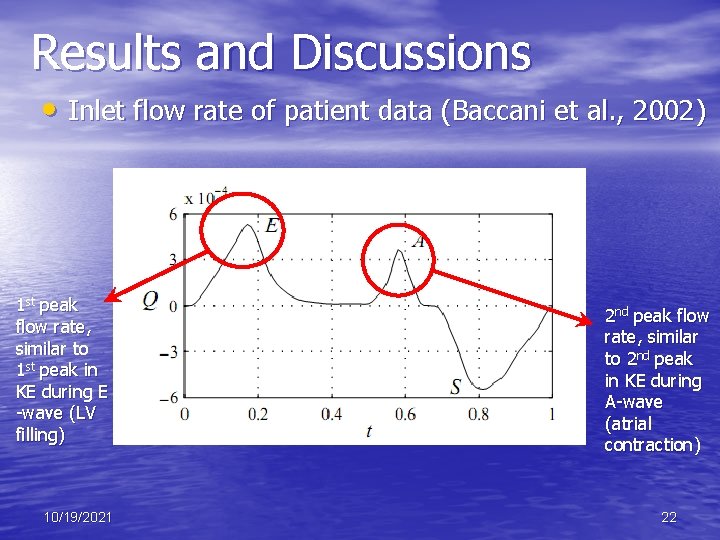
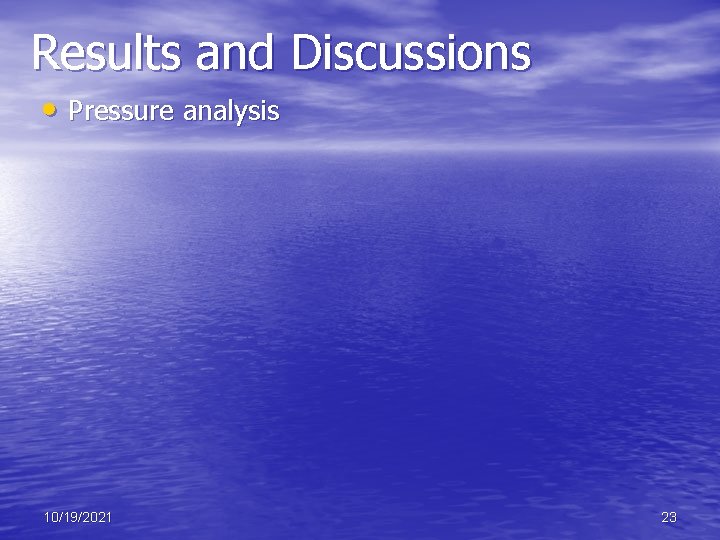
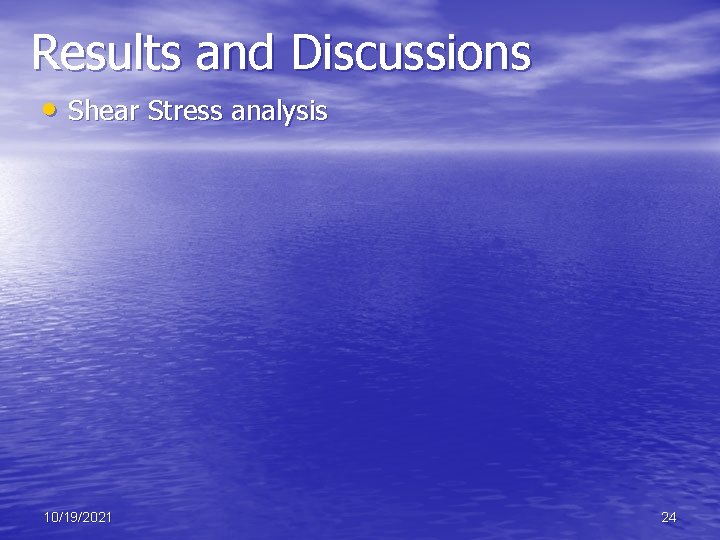
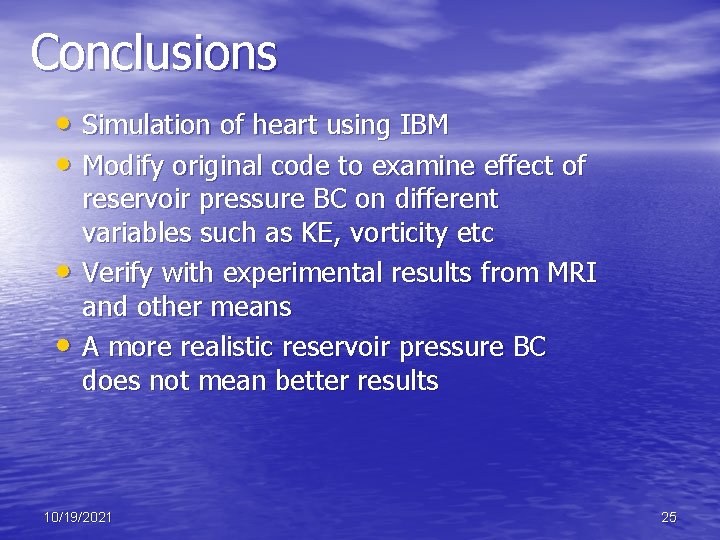
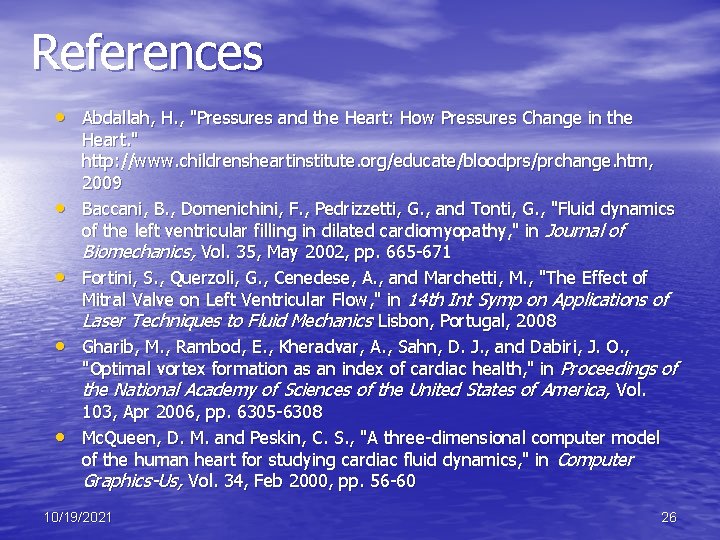
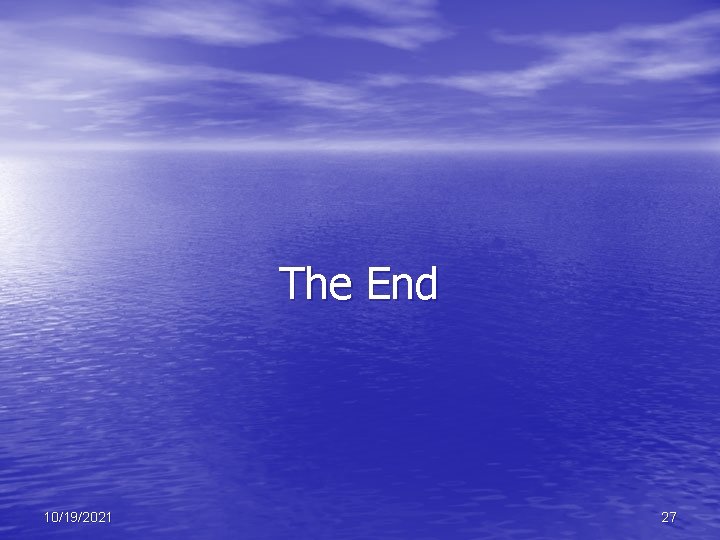
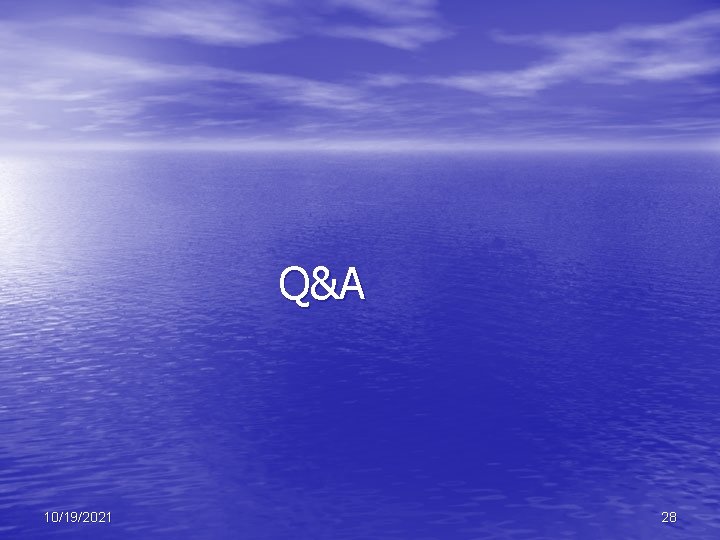
- Slides: 28
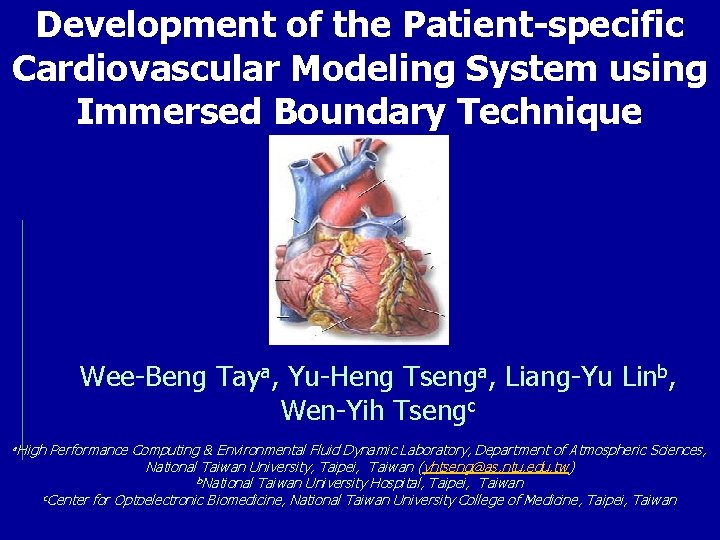
Development of the Patient-specific Cardiovascular Modeling System using Immersed Boundary Technique Wee-Beng Taya, Yu-Heng Tsenga, Liang-Yu Linb, Wen-Yih Tsengc a. High Performance Computing & Environmental Fluid Dynamic Laboratory, Department of Atmospheric Sciences, National Taiwan University, Taipei, Taiwan (yhtseng@as. ntu. edu. tw) b. National Taiwan University Hospital, Taipei, Taiwan c. Center for Optoelectronic Biomedicine, National Taiwan University College of Medicine, Taipei, Taiwan
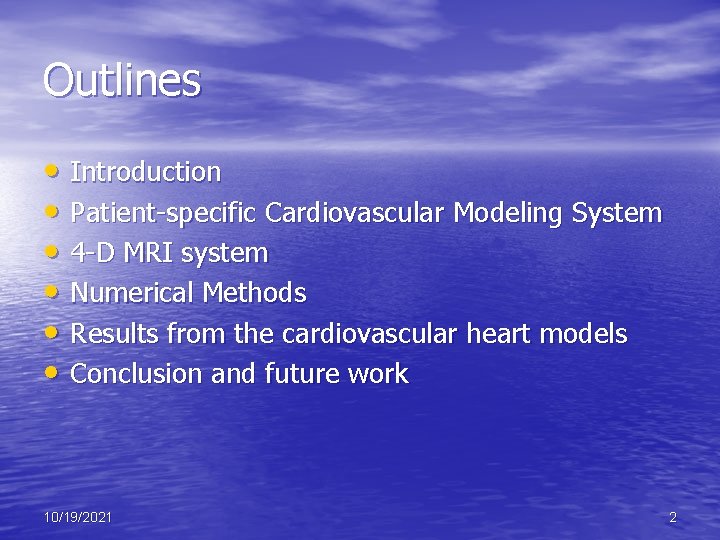
Outlines • Introduction • Patient-specific Cardiovascular Modeling System • 4 -D MRI system • Numerical Methods • Results from the cardiovascular heart models • Conclusion and future work 10/19/2021 2
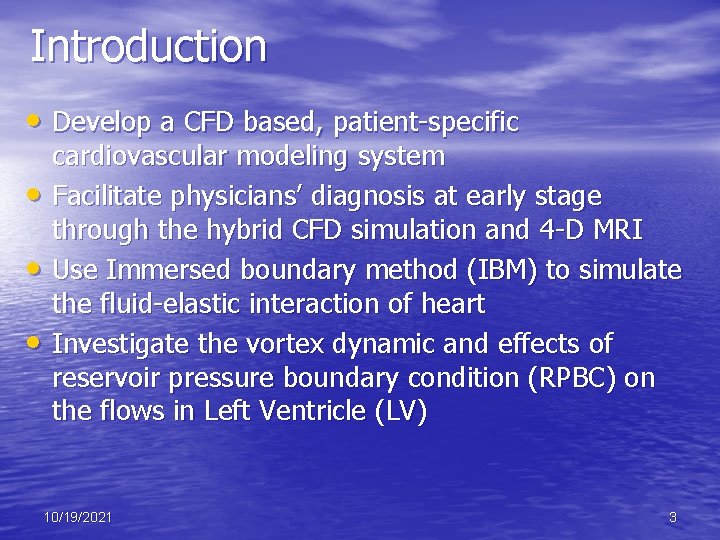
Introduction • Develop a CFD based, patient-specific • • • cardiovascular modeling system Facilitate physicians’ diagnosis at early stage through the hybrid CFD simulation and 4 -D MRI Use Immersed boundary method (IBM) to simulate the fluid-elastic interaction of heart Investigate the vortex dynamic and effects of reservoir pressure boundary condition (RPBC) on the flows in Left Ventricle (LV) 10/19/2021 3
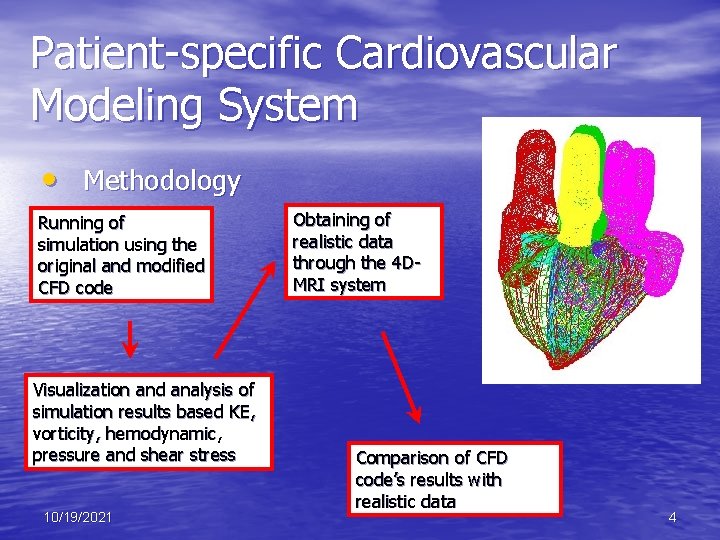
Patient-specific Cardiovascular Modeling System • Methodology Running of simulation using the original and modified CFD code Visualization and analysis of simulation results based KE, vorticity, hemodynamic, pressure and shear stress 10/19/2021 Obtaining of realistic data through the 4 DMRI system Comparison of CFD code’s results with realistic data 4
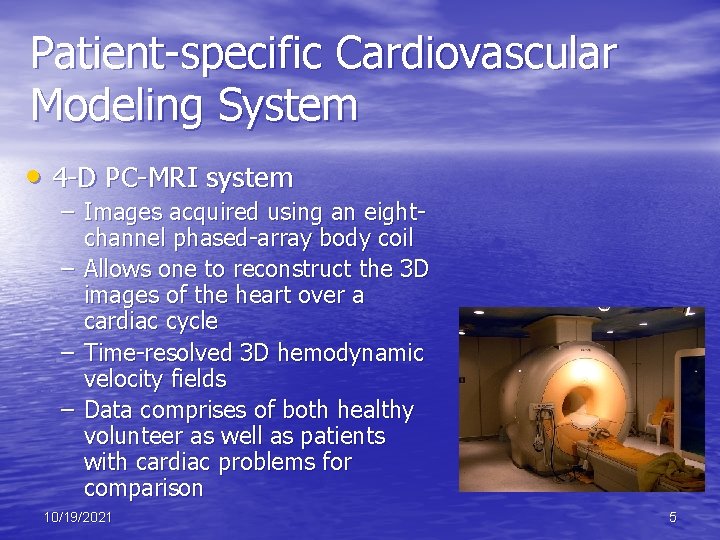
Patient-specific Cardiovascular Modeling System • 4 -D PC-MRI system – Images acquired using an eightchannel phased-array body coil – Allows one to reconstruct the 3 D images of the heart over a cardiac cycle – Time-resolved 3 D hemodynamic velocity fields – Data comprises of both healthy volunteer as well as patients with cardiac problems for comparison 10/19/2021 5
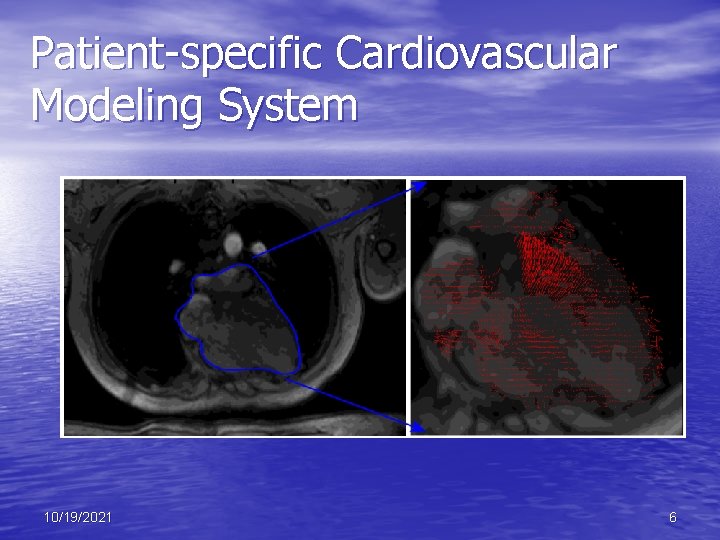
Patient-specific Cardiovascular Modeling System 10/19/2021 6
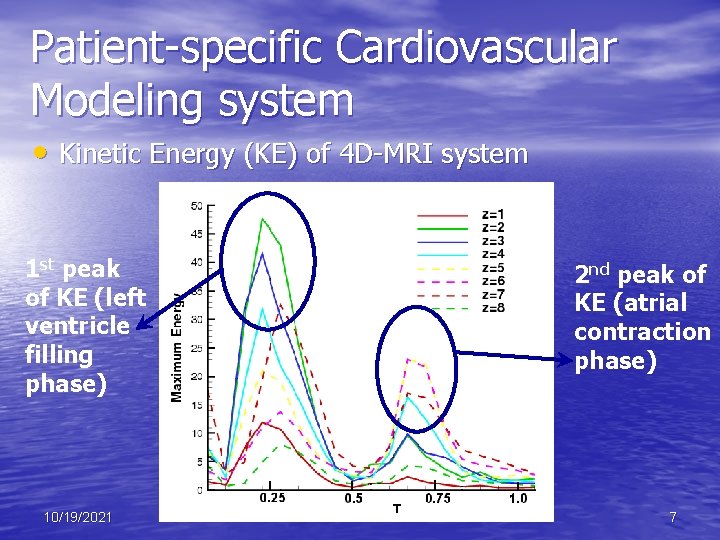
Patient-specific Cardiovascular Modeling system • Kinetic Energy (KE) of 4 D-MRI system 1 st peak of KE (left ventricle filling phase) 10/19/2021 2 nd peak of KE (atrial contraction phase) 7
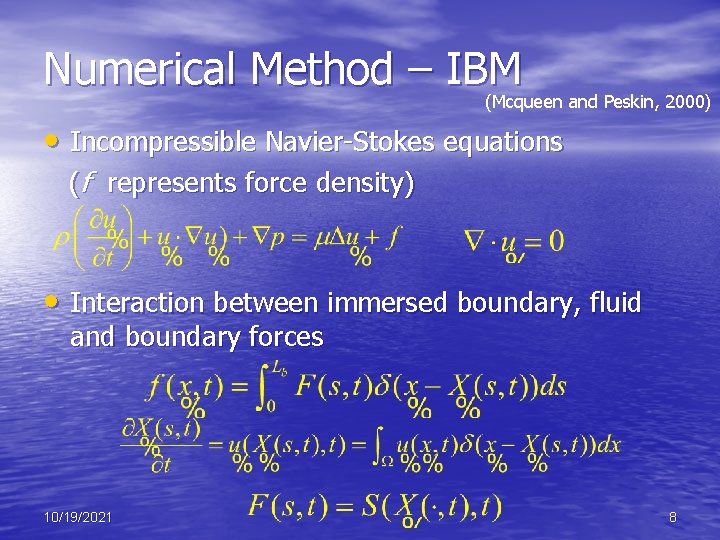
Numerical Method – IBM (Mcqueen and Peskin, 2000) • Incompressible Navier-Stokes equations (f represents force density) • Interaction between immersed boundary, fluid and boundary forces 10/19/2021 8
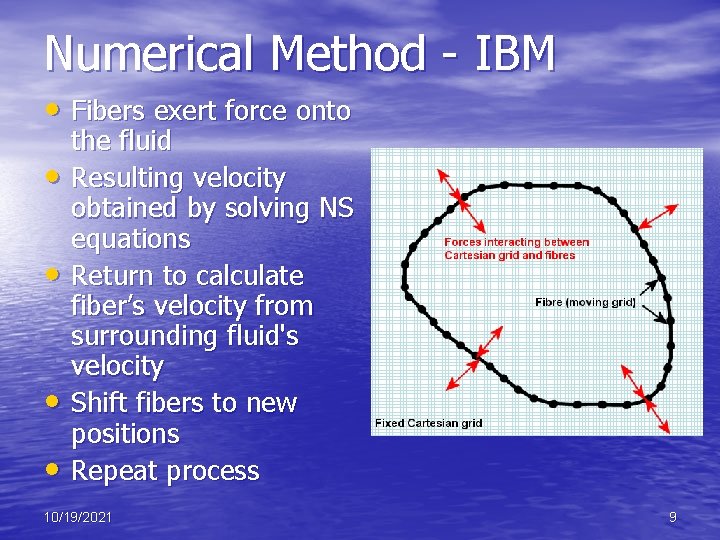
Numerical Method - IBM • Fibers exert force onto • • the fluid Resulting velocity obtained by solving NS equations Return to calculate fiber’s velocity from surrounding fluid's velocity Shift fibers to new positions Repeat process 10/19/2021 9
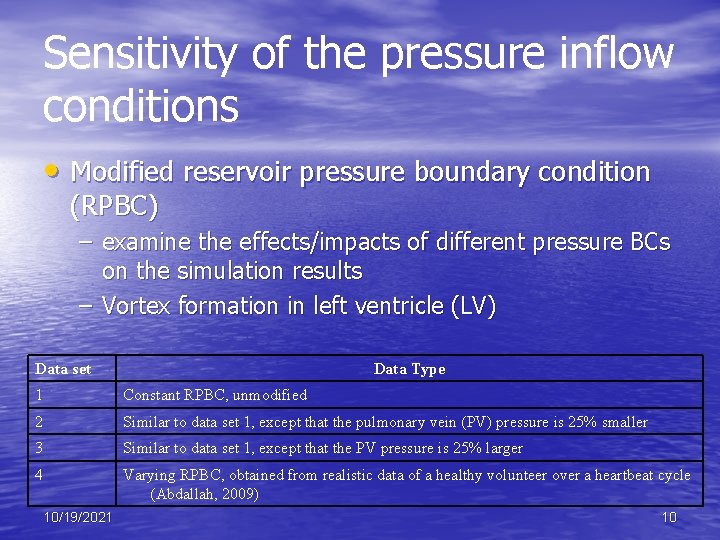
Sensitivity of the pressure inflow conditions • Modified reservoir pressure boundary condition (RPBC) – examine the effects/impacts of different pressure BCs on the simulation results – Vortex formation in left ventricle (LV) Data set Data Type 1 Constant RPBC, unmodified 2 Similar to data set 1, except that the pulmonary vein (PV) pressure is 25% smaller 3 Similar to data set 1, except that the PV pressure is 25% larger 4 Varying RPBC, obtained from realistic data of a healthy volunteer over a heartbeat cycle (Abdallah, 2009) 10/19/2021 10
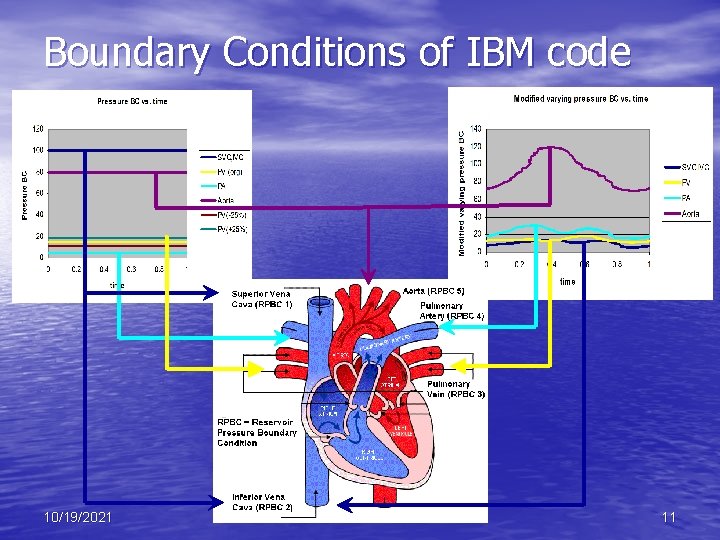
Boundary Conditions of IBM code 10/19/2021 11
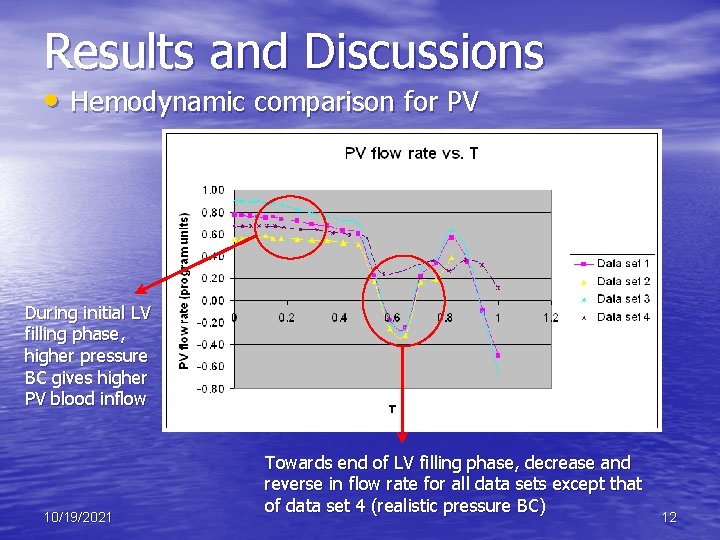
Results and Discussions • Hemodynamic comparison for PV During initial LV filling phase, higher pressure BC gives higher PV blood inflow 10/19/2021 Towards end of LV filling phase, decrease and reverse in flow rate for all data sets except that of data set 4 (realistic pressure BC) 12
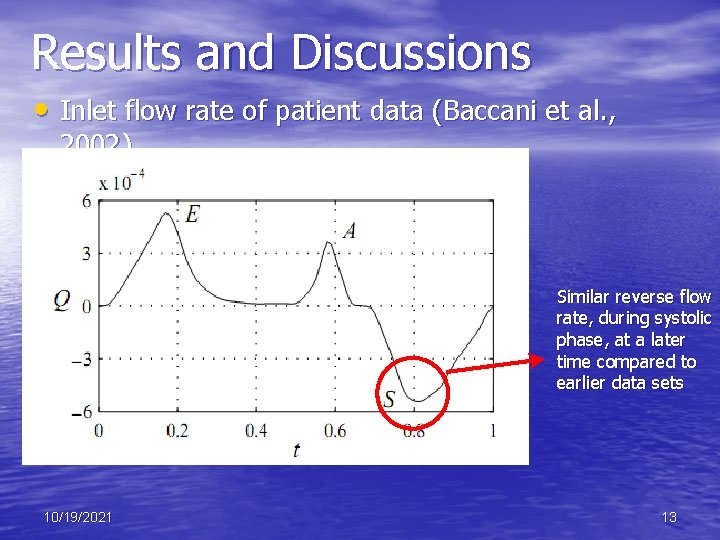
Results and Discussions • Inlet flow rate of patient data (Baccani et al. , 2002) Similar reverse flow rate, during systolic phase, at a later time compared to earlier data sets 10/19/2021 13
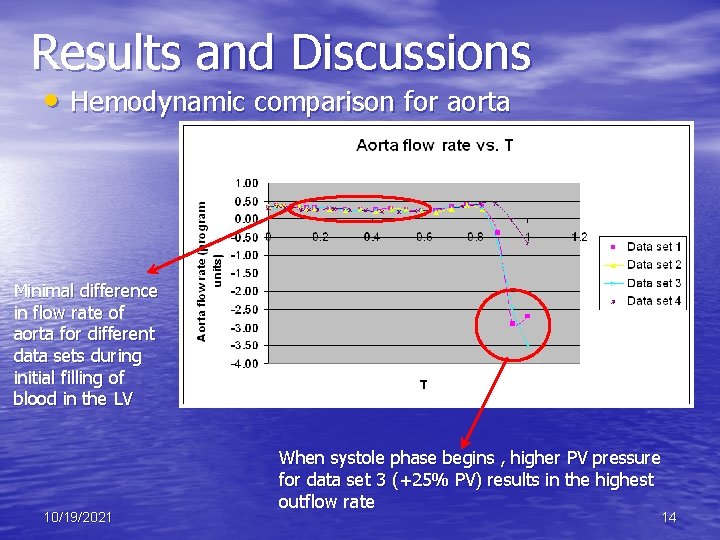
Results and Discussions • Hemodynamic comparison for aorta Minimal difference in flow rate of aorta for different data sets during initial filling of blood in the LV 10/19/2021 When systole phase begins , higher PV pressure for data set 3 (+25% PV) results in the highest outflow rate 14
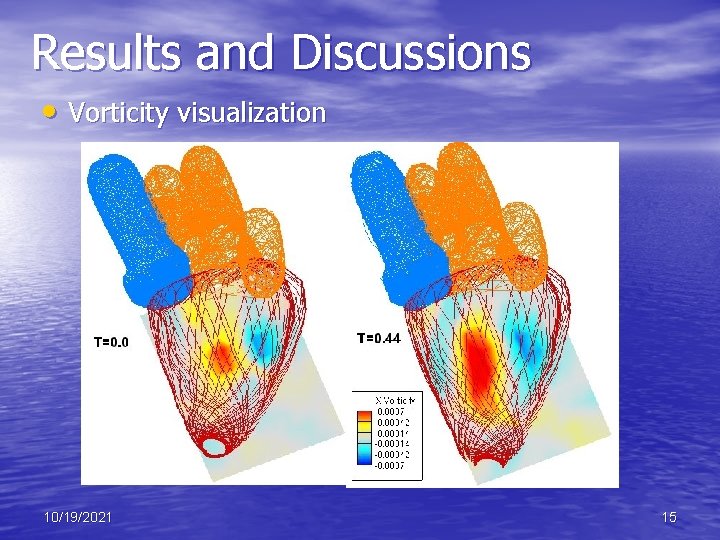
Results and Discussions • Vorticity visualization 10/19/2021 15
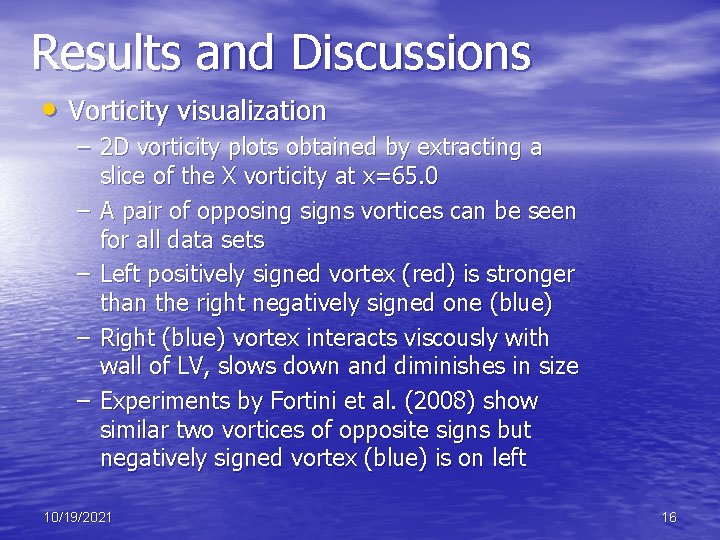
Results and Discussions • Vorticity visualization – 2 D vorticity plots obtained by extracting a slice of the X vorticity at x=65. 0 – A pair of opposing signs vortices can be seen for all data sets – Left positively signed vortex (red) is stronger than the right negatively signed one (blue) – Right (blue) vortex interacts viscously with wall of LV, slows down and diminishes in size – Experiments by Fortini et al. (2008) show similar two vortices of opposite signs but negatively signed vortex (blue) is on left 10/19/2021 16
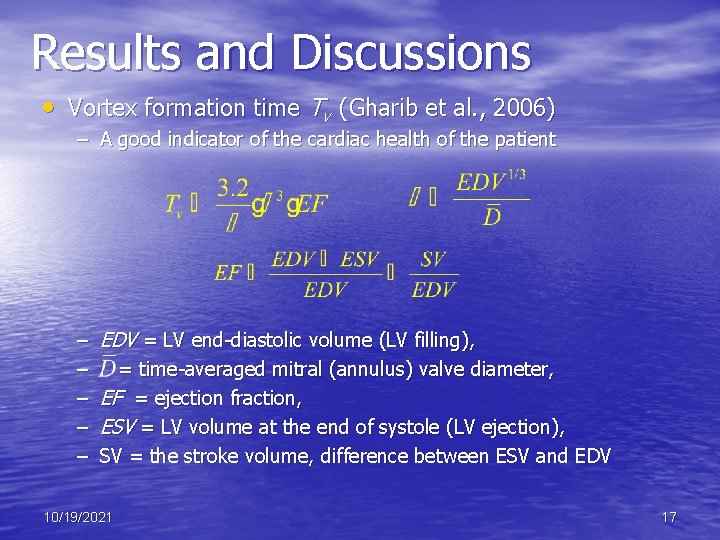
Results and Discussions • Vortex formation time Tv (Gharib et al. , 2006) – A good indicator of the cardiac health of the patient – – – EDV = LV end-diastolic volume (LV filling), = time-averaged mitral (annulus) valve diameter, EF = ejection fraction, ESV = LV volume at the end of systole (LV ejection), SV = the stroke volume, difference between ESV and EDV 10/19/2021 17
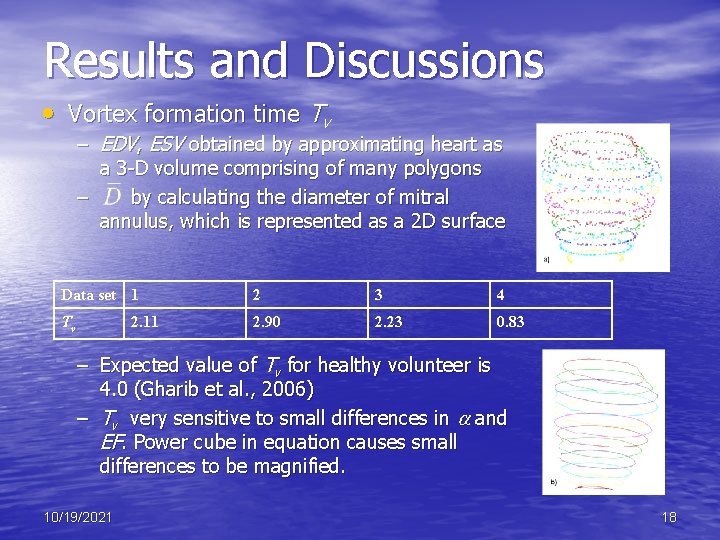
Results and Discussions • Vortex formation time Tv – EDV, ESV obtained by approximating heart as a 3 -D volume comprising of many polygons – by calculating the diameter of mitral annulus, which is represented as a 2 D surface Data set 1 2 3 4 Tv 2. 90 2. 23 0. 83 2. 11 – Expected value of Tv for healthy volunteer is 4. 0 (Gharib et al. , 2006) – Tv very sensitive to small differences in and EF. Power cube in equation causes small differences to be magnified. 10/19/2021 18
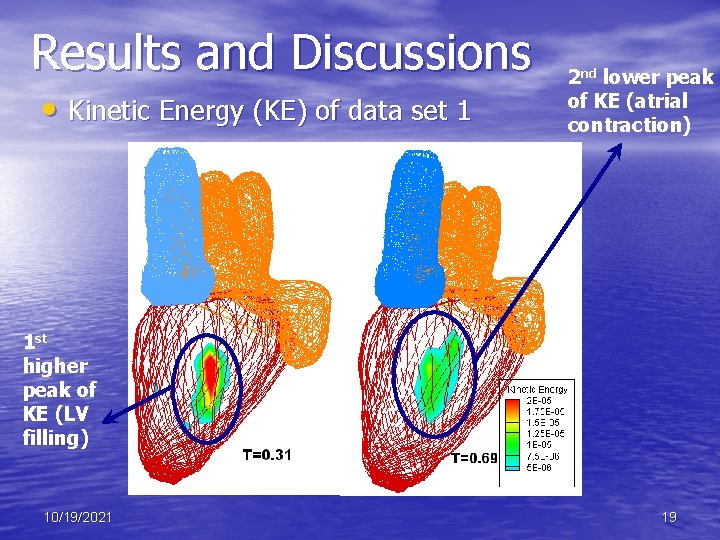
Results and Discussions • Kinetic Energy (KE) of data set 1 2 nd lower peak of KE (atrial contraction) 1 st higher peak of KE (LV filling) 10/19/2021 19
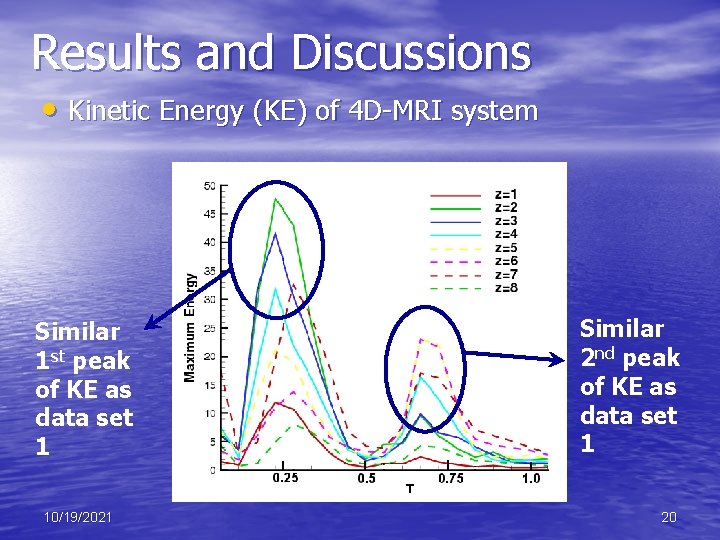
Results and Discussions • Kinetic Energy (KE) of 4 D-MRI system Similar 1 st peak of KE as data set 1 10/19/2021 Similar 2 nd peak of KE as data set 1 20
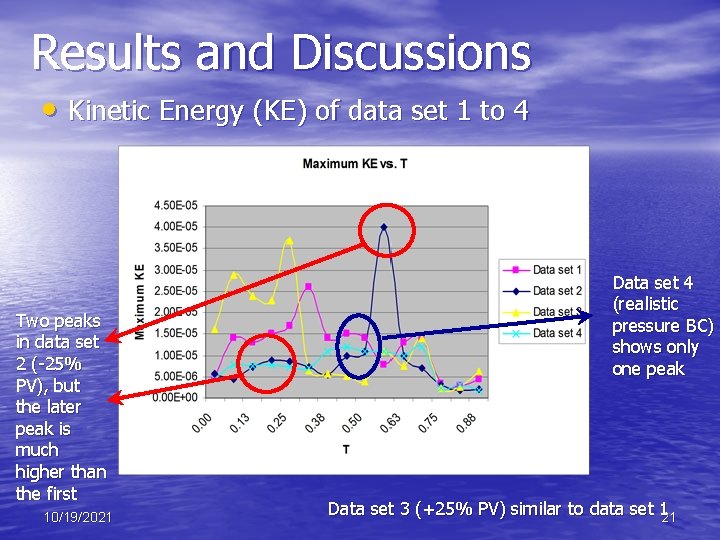
Results and Discussions • Kinetic Energy (KE) of data set 1 to 4 Two peaks in data set 2 (-25% PV), but the later peak is much higher than the first 10/19/2021 Data set 4 (realistic pressure BC) shows only one peak Data set 3 (+25% PV) similar to data set 121
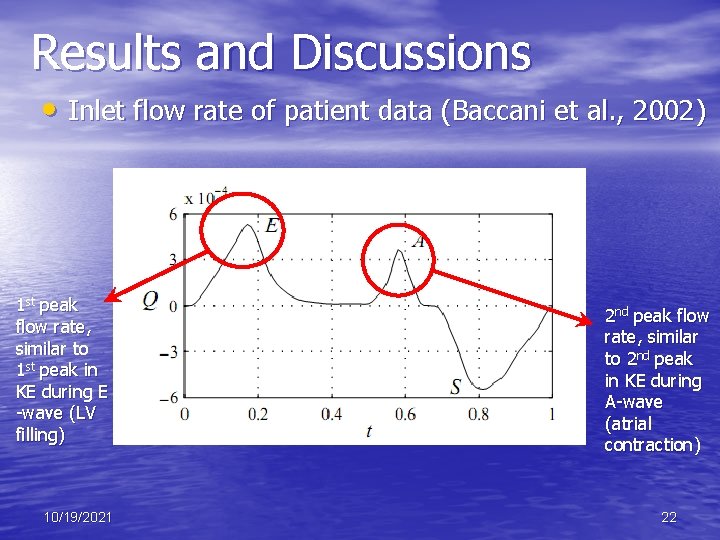
Results and Discussions • Inlet flow rate of patient data (Baccani et al. , 2002) 1 st peak flow rate, similar to 1 st peak in KE during E -wave (LV filling) 10/19/2021 2 nd peak flow rate, similar to 2 nd peak in KE during A-wave (atrial contraction) 22
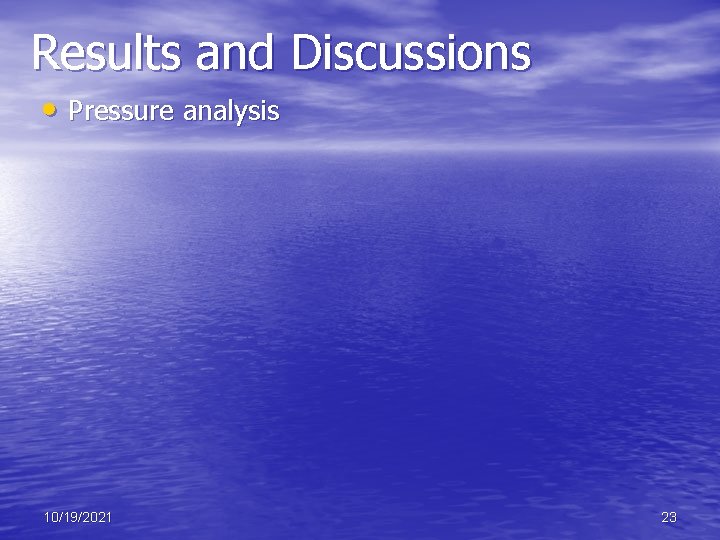
Results and Discussions • Pressure analysis 10/19/2021 23
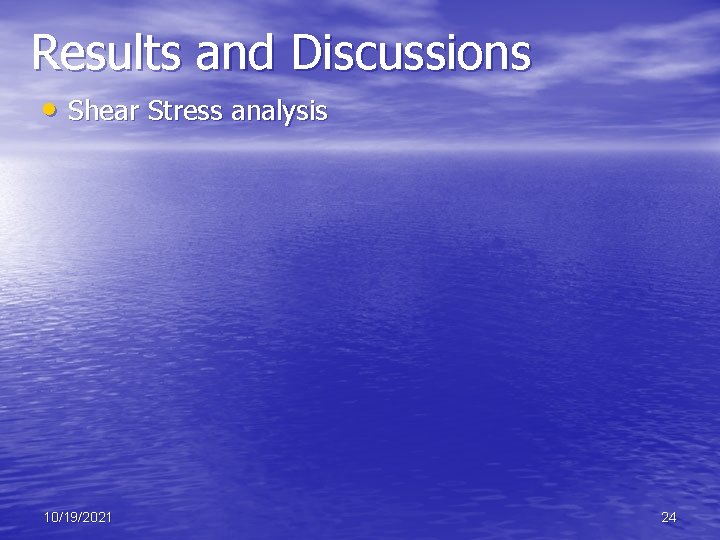
Results and Discussions • Shear Stress analysis 10/19/2021 24
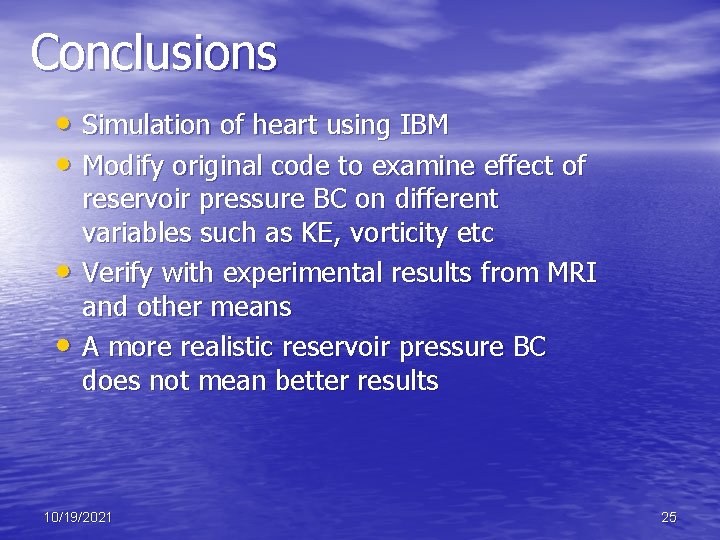
Conclusions • Simulation of heart using IBM • Modify original code to examine effect of • • reservoir pressure BC on different variables such as KE, vorticity etc Verify with experimental results from MRI and other means A more realistic reservoir pressure BC does not mean better results 10/19/2021 25
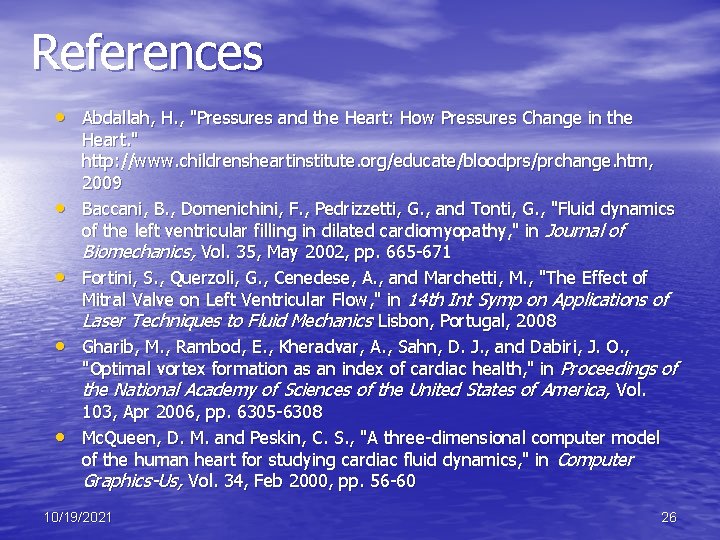
References • Abdallah, H. , "Pressures and the Heart: How Pressures Change in the • • Heart. " http: //www. childrensheartinstitute. org/educate/bloodprs/prchange. htm, 2009 Baccani, B. , Domenichini, F. , Pedrizzetti, G. , and Tonti, G. , "Fluid dynamics of the left ventricular filling in dilated cardiomyopathy, " in Journal of Biomechanics, Vol. 35, May 2002, pp. 665 -671 Fortini, S. , Querzoli, G. , Cenedese, A. , and Marchetti, M. , "The Effect of Mitral Valve on Left Ventricular Flow, " in 14 th Int Symp on Applications of Laser Techniques to Fluid Mechanics Lisbon, Portugal, 2008 Gharib, M. , Rambod, E. , Kheradvar, A. , Sahn, D. J. , and Dabiri, J. O. , "Optimal vortex formation as an index of cardiac health, " in Proceedings of the National Academy of Sciences of the United States of America, Vol. 103, Apr 2006, pp. 6305 -6308 Mc. Queen, D. M. and Peskin, C. S. , "A three-dimensional computer model of the human heart for studying cardiac fluid dynamics, " in Computer Graphics-Us, Vol. 34, Feb 2000, pp. 56 -60 10/19/2021 26
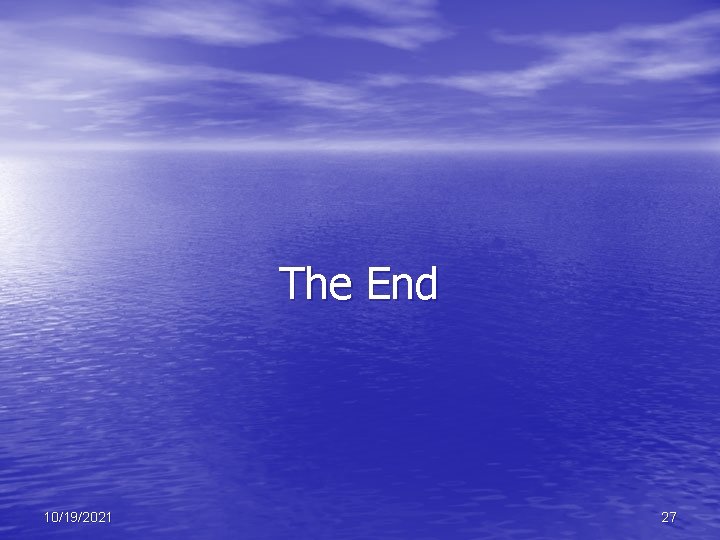
The End 10/19/2021 27
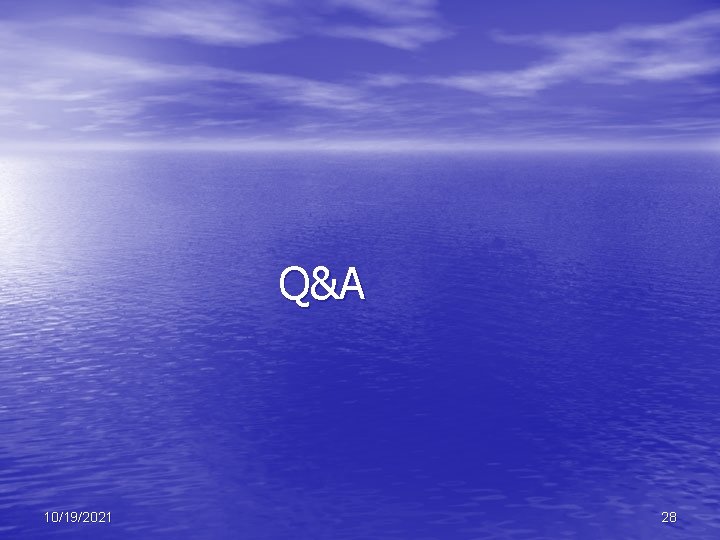
Q&A 10/19/2021 28
Helen c erickson
Dimensional modeling vs relational modeling
Using system using system.collections.generic
Blood vesel
What makes up the cardiovascular system
Pithed model
Totally tubular dude
Crash course cardiovascular system
Chapter 5 learning exercises medical terminology
Figure 11-9 is a diagram of the hepatic portal circulation
Chapter 11 the cardiovascular system figure 11-3
Lesson 11 cardiovascular system
Lesson 11 cardiovascular system
Tissue in cardiovascular system
The cardiovascular system chapter 11
Introduction of cardiovascular system
Ptca
Anatomy and physiology unit 7 cardiovascular system
Chapter 8 cardiovascular system
Chapter 13 cardiovascular system
Chapter 11 the cardiovascular system figure 11-2
The cardiovascular system includes the
Major arteries
Venule
Modeling using variation calculator
Data modeling using entity relationship model
Lesson 12 modeling using similarity
Modeling of digital communication systems using simulink
Modeling of digital communication systems using simulink