CHAPTER 2 PHARMACEUTICS PHARMACOKINETICS Part 2 CHAPTER 2
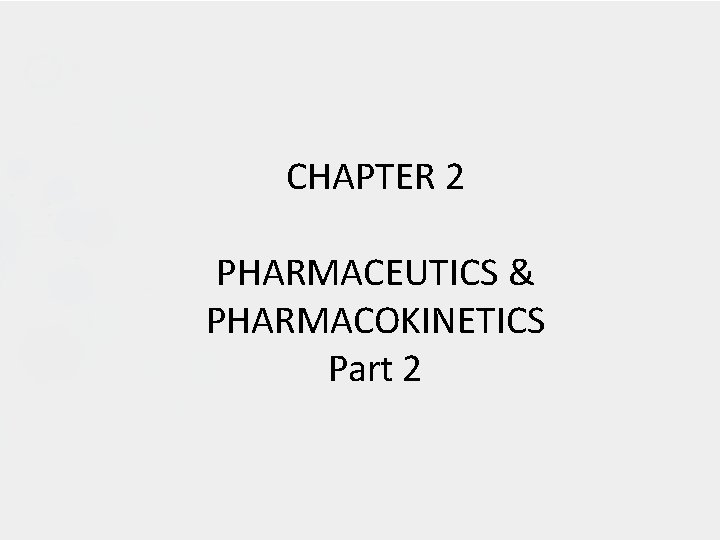
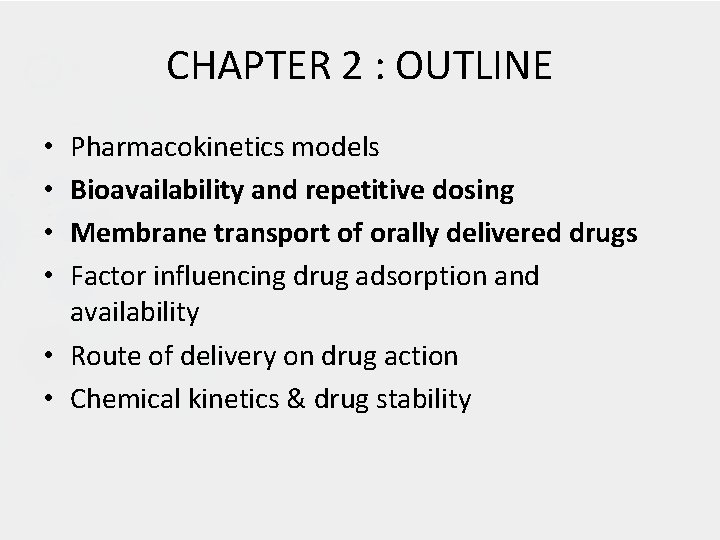
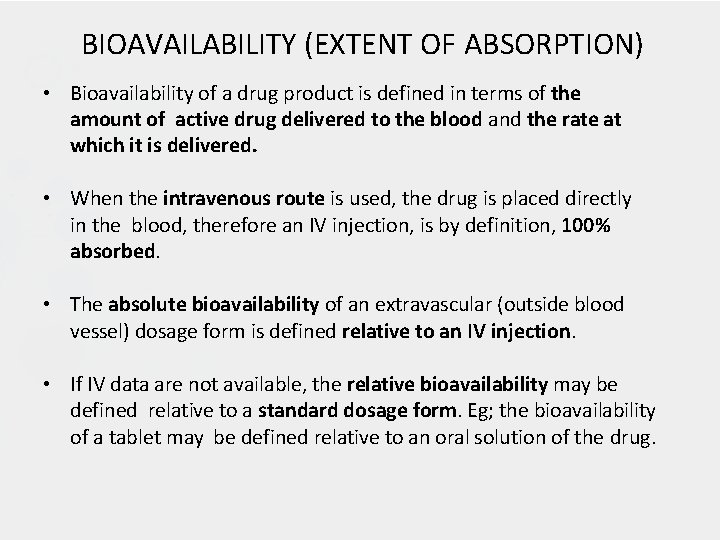
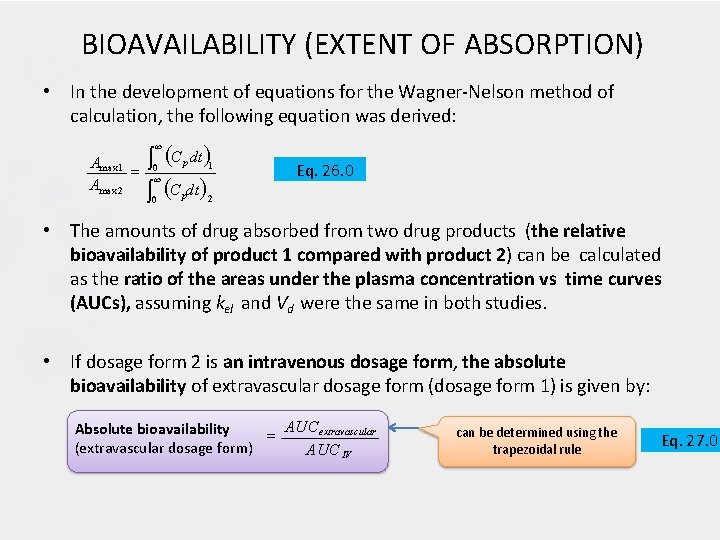
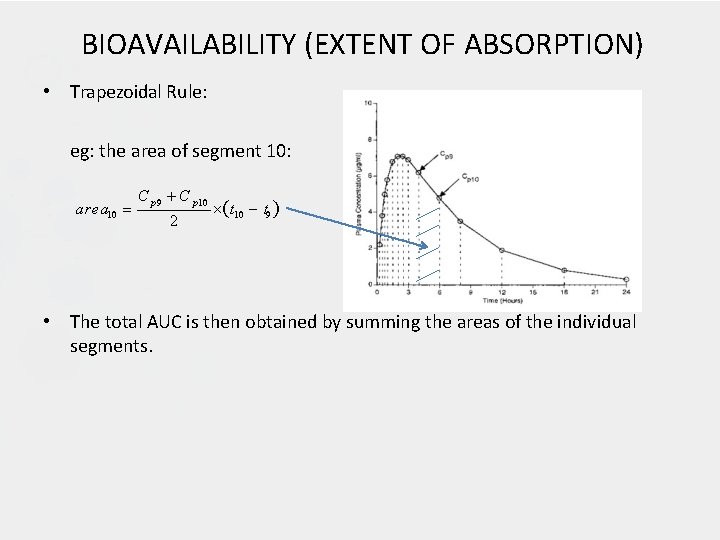
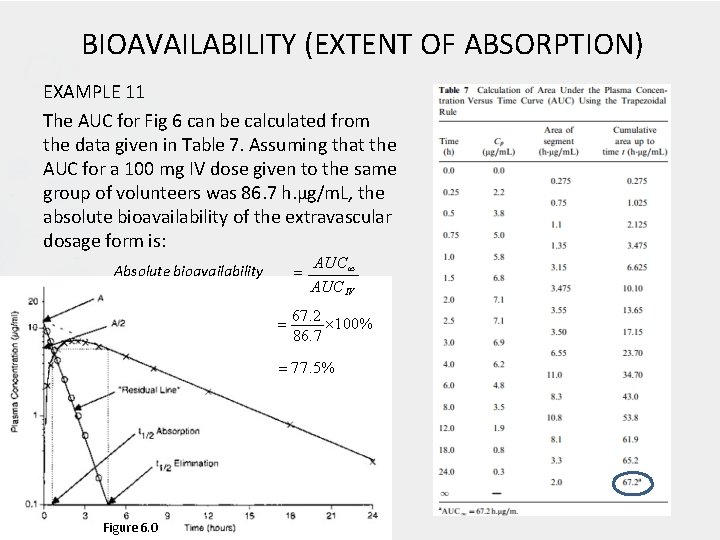
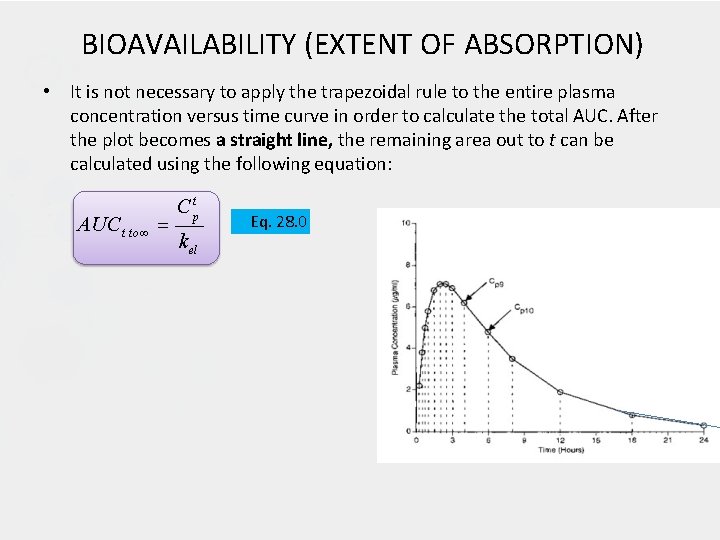
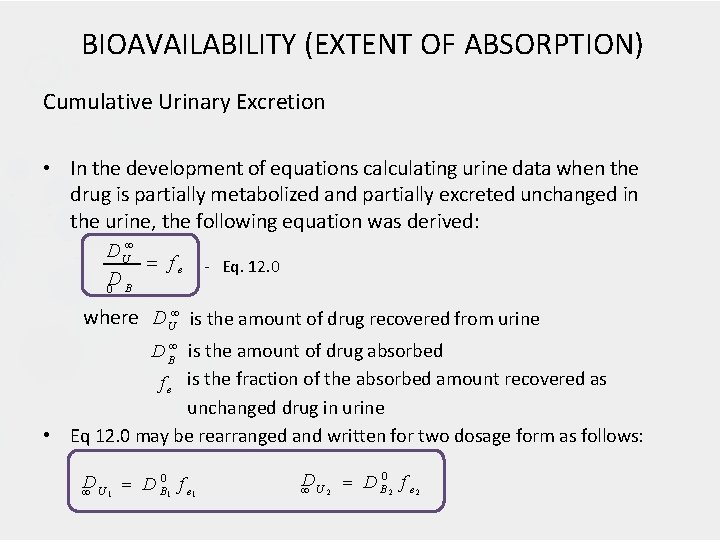
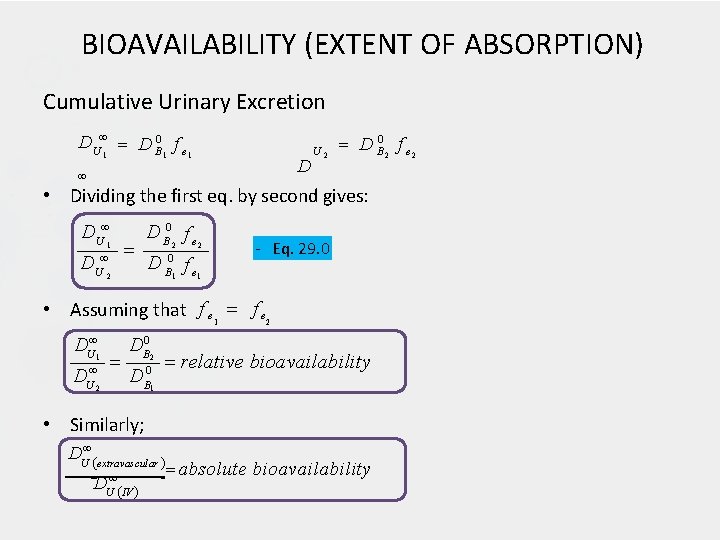
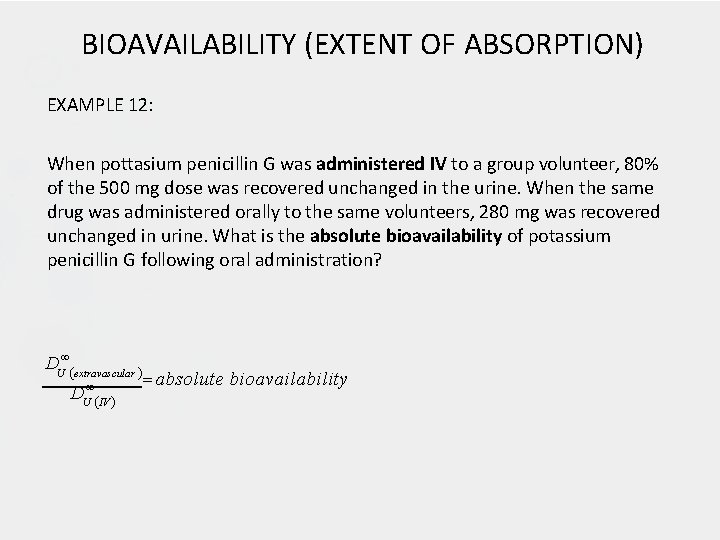
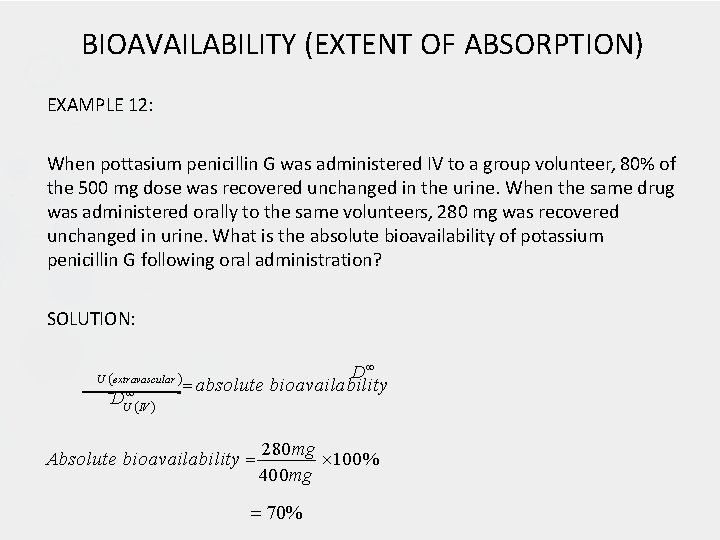
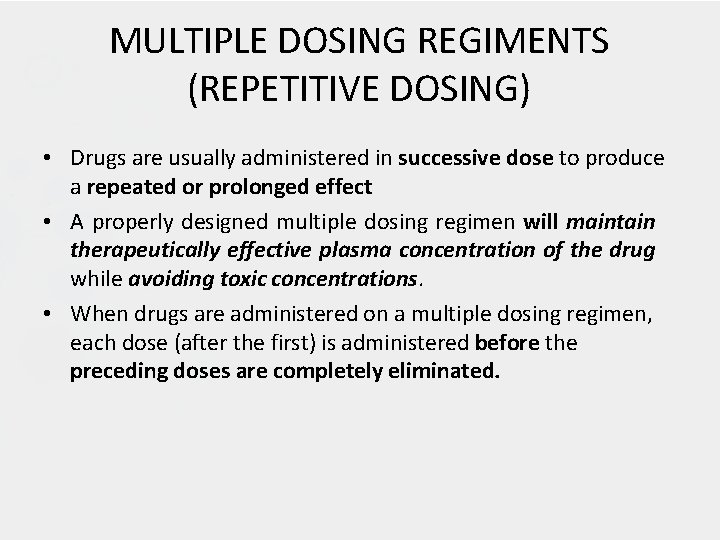
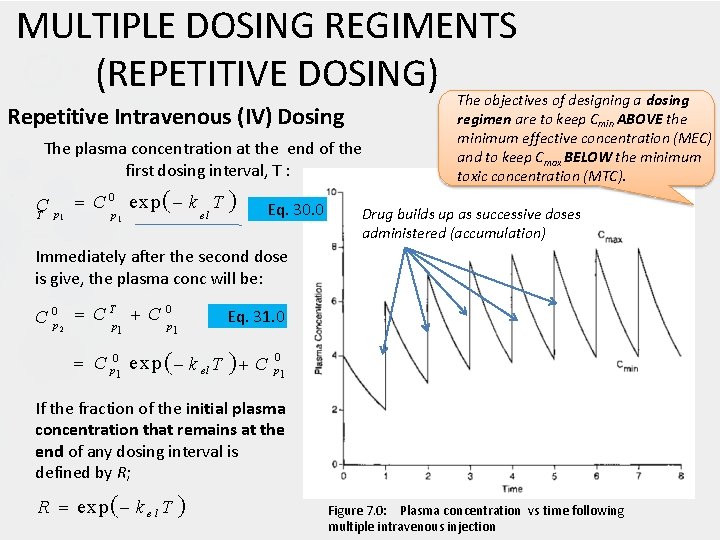
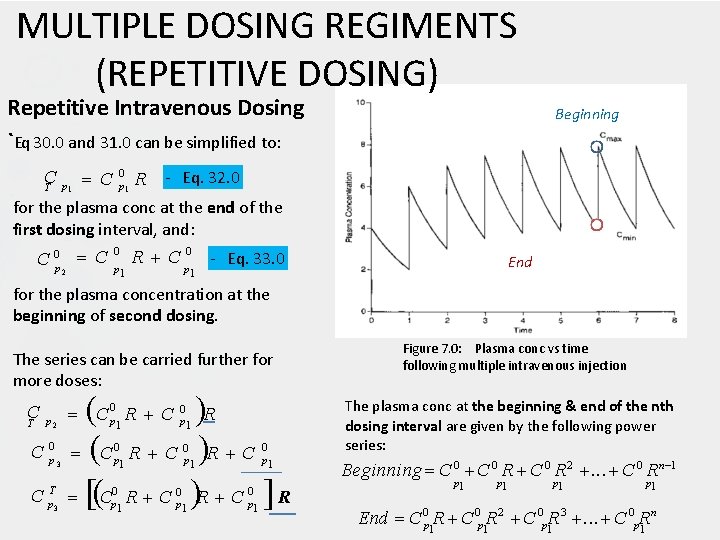
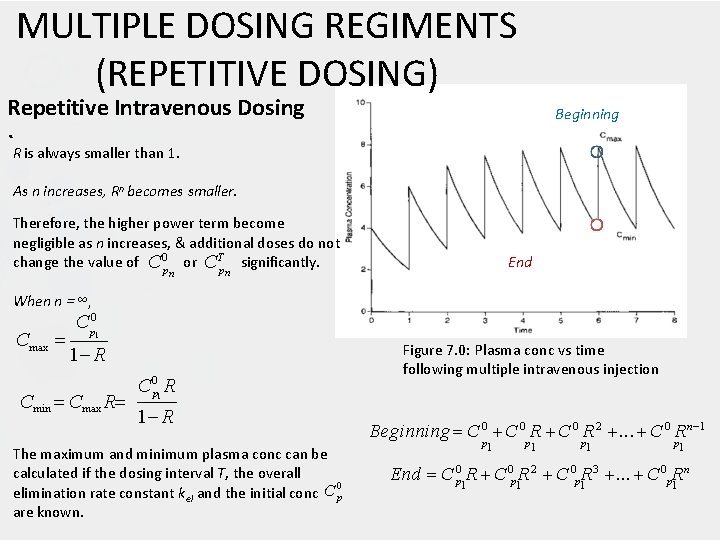
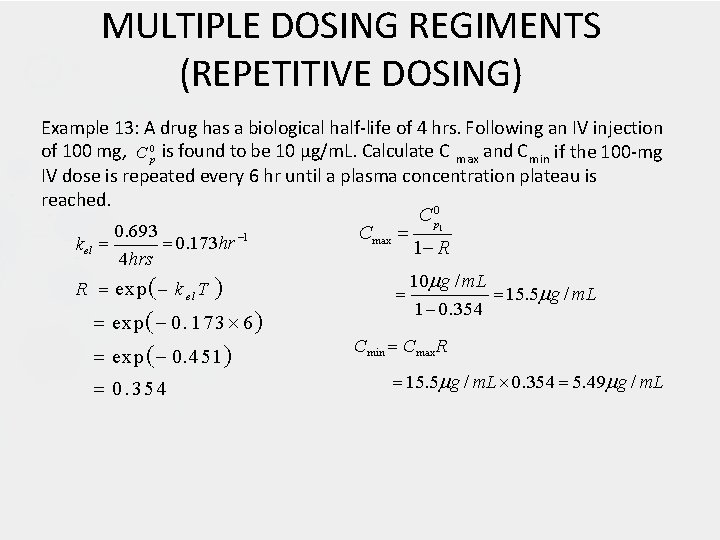
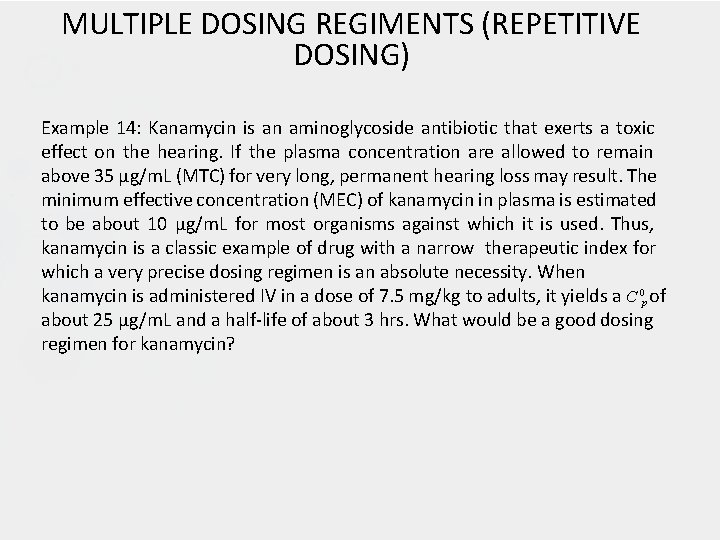
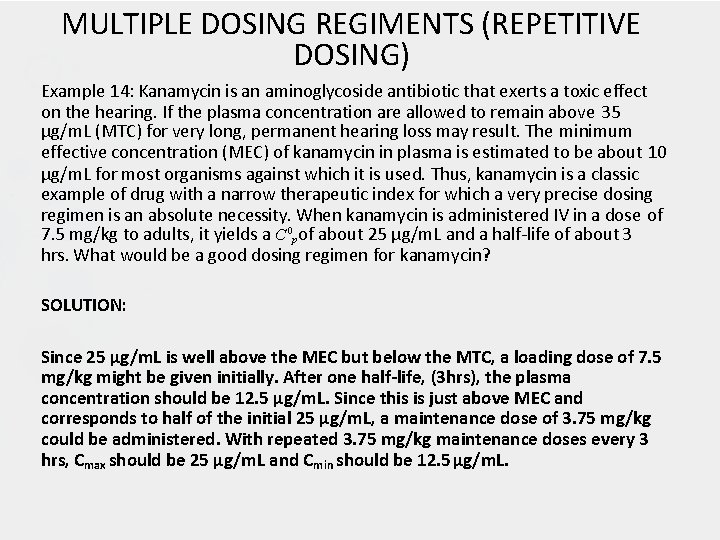
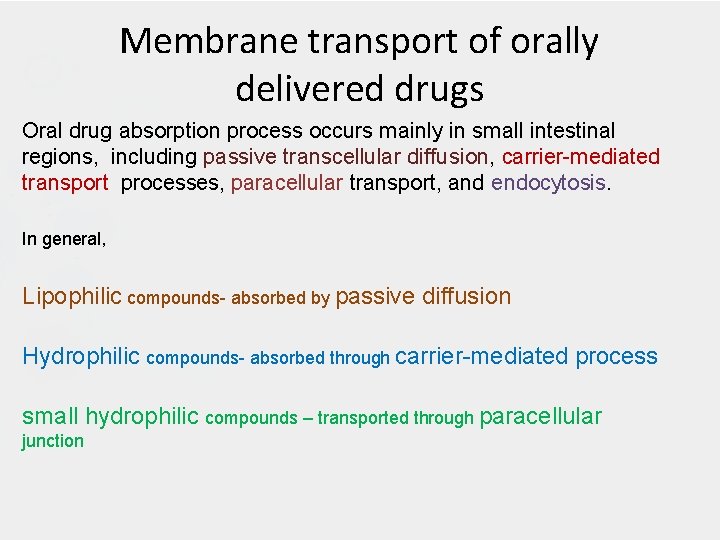
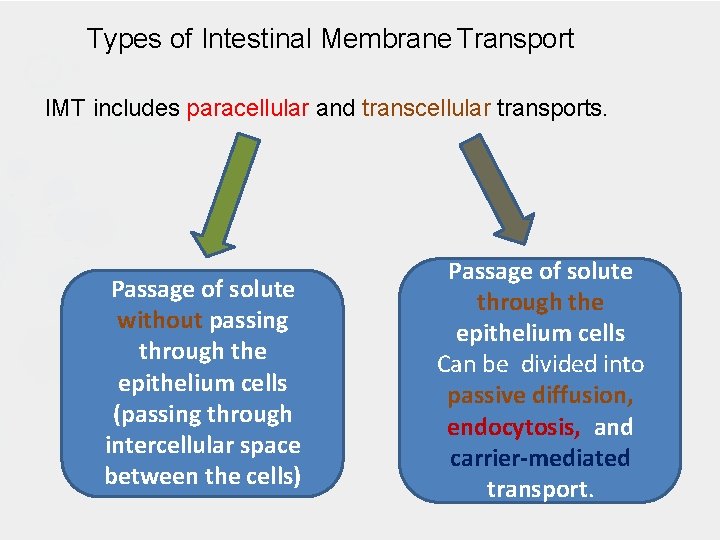
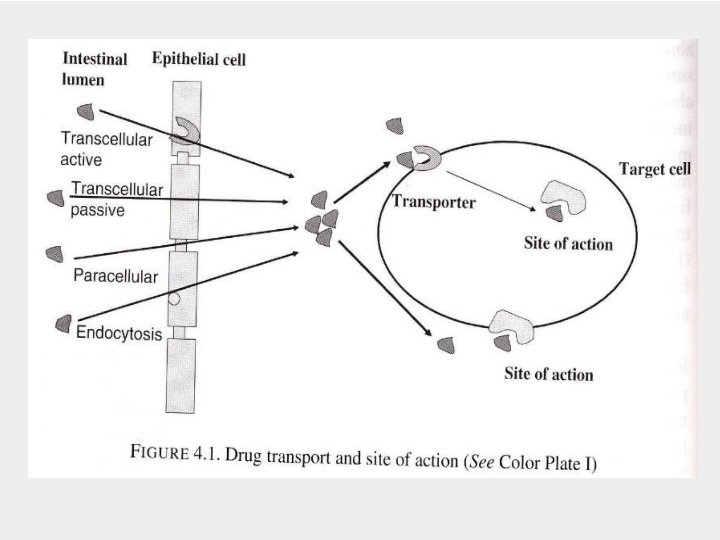
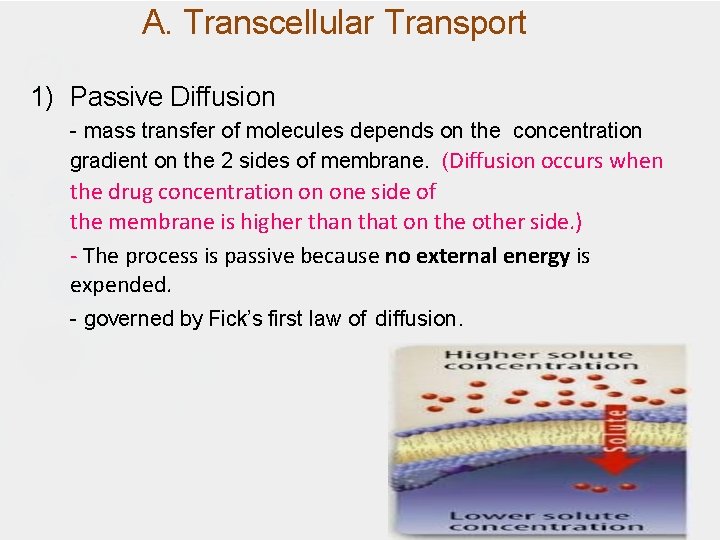
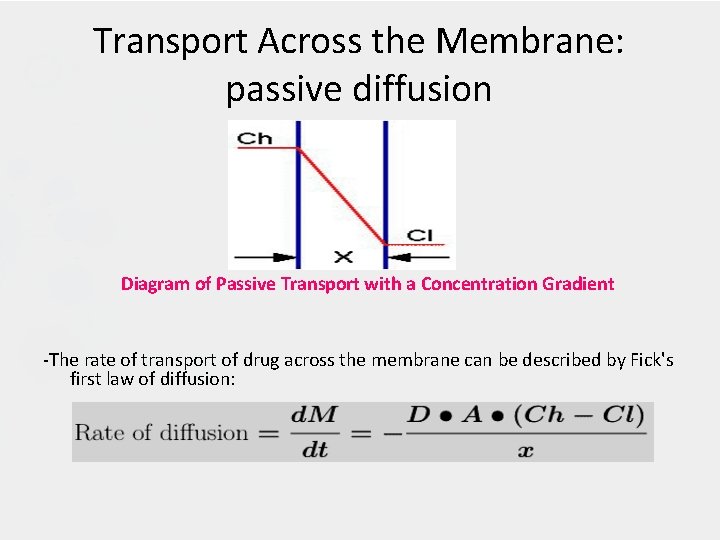
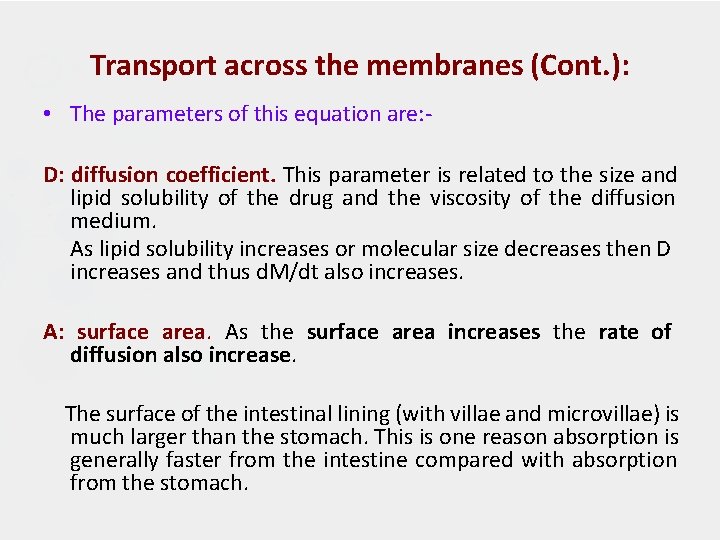
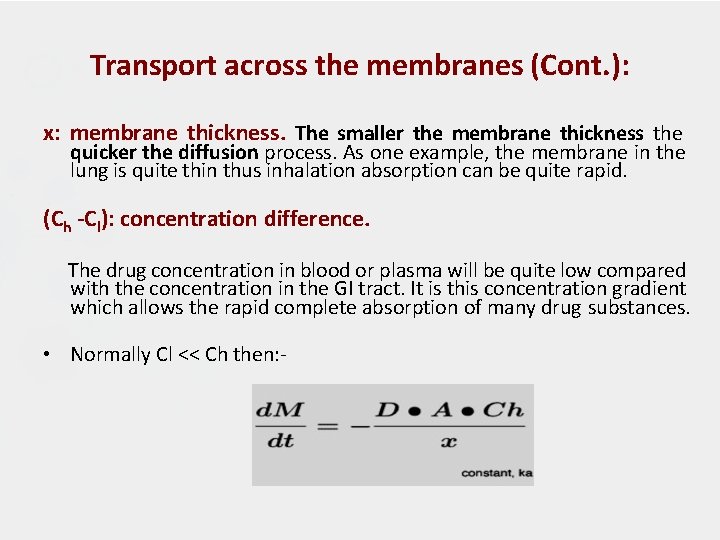
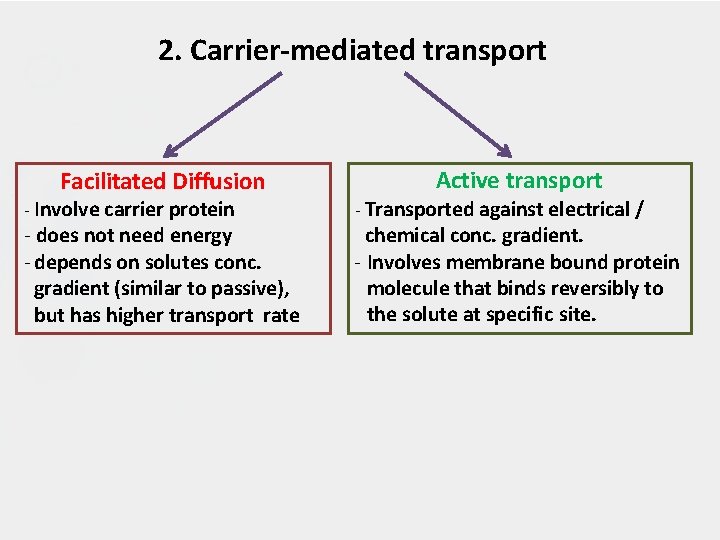
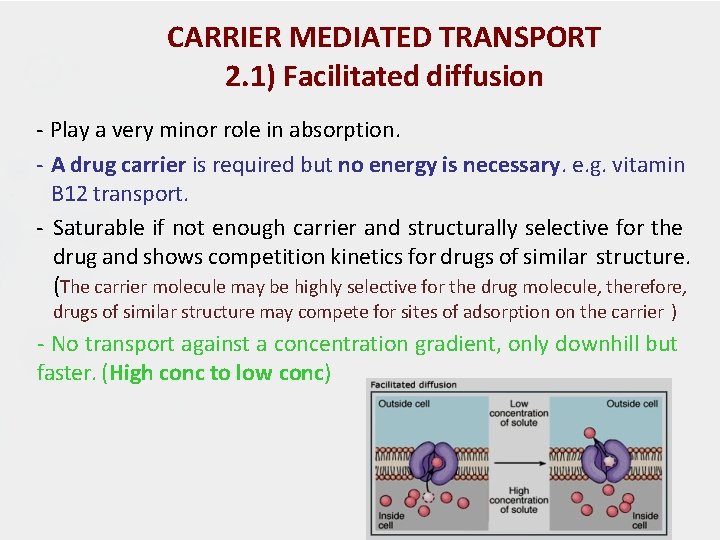
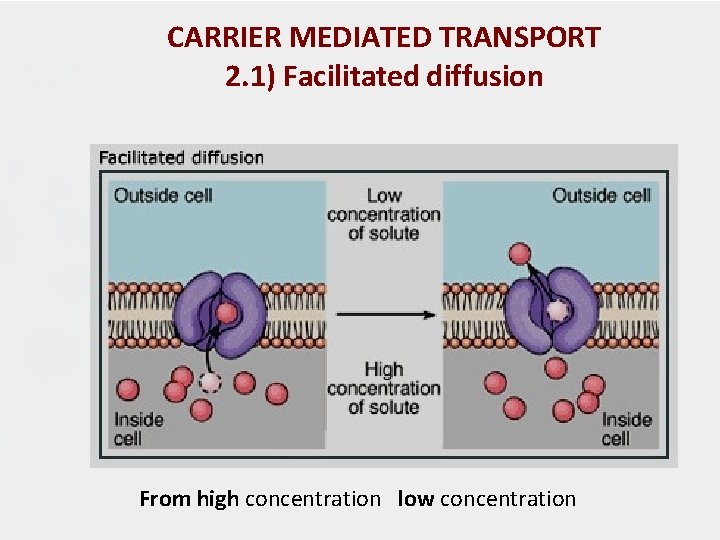
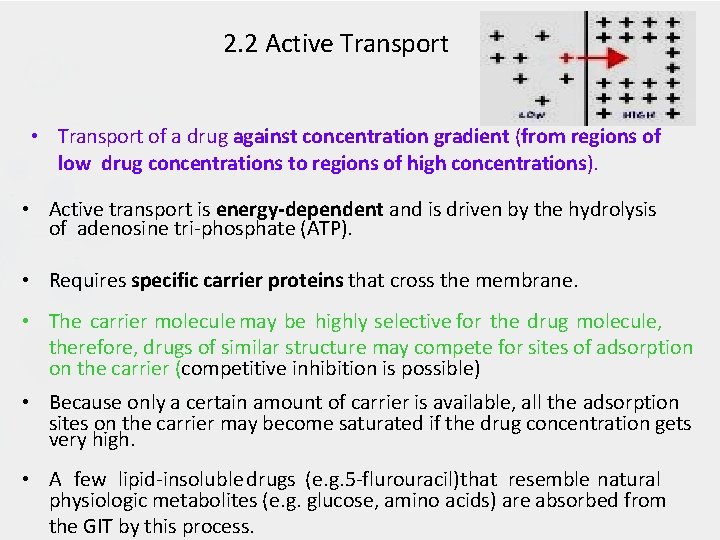
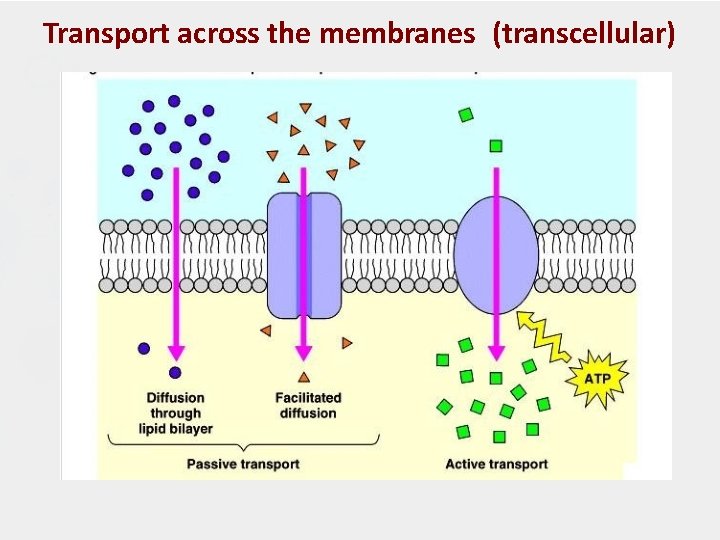
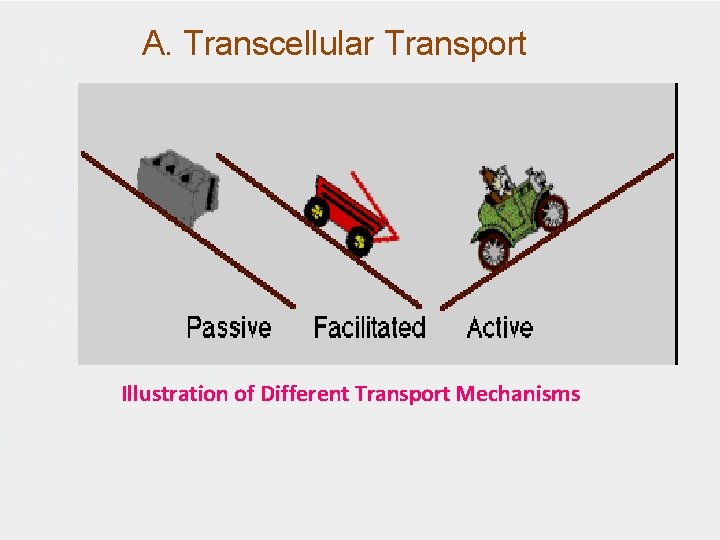
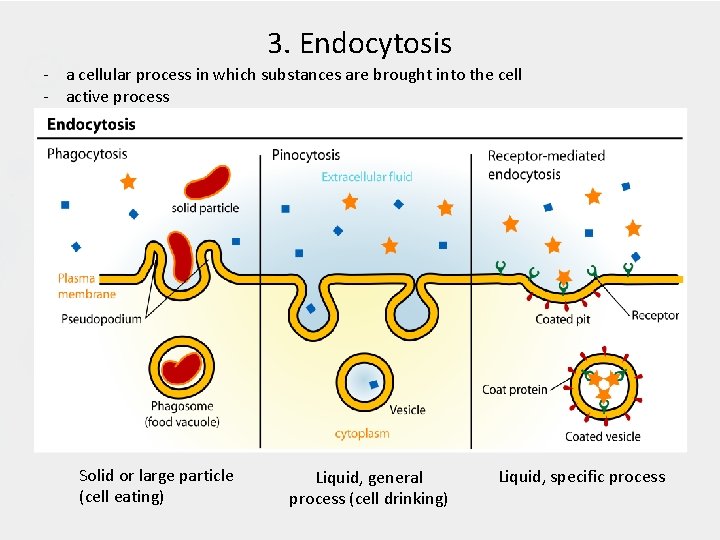
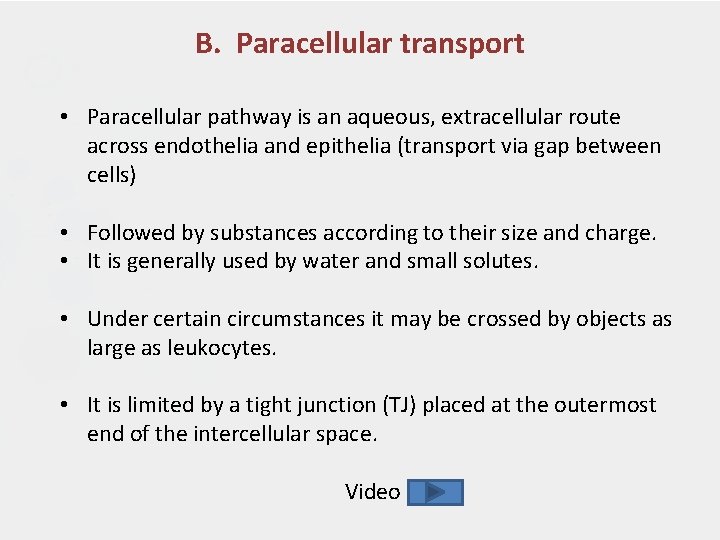
- Slides: 33
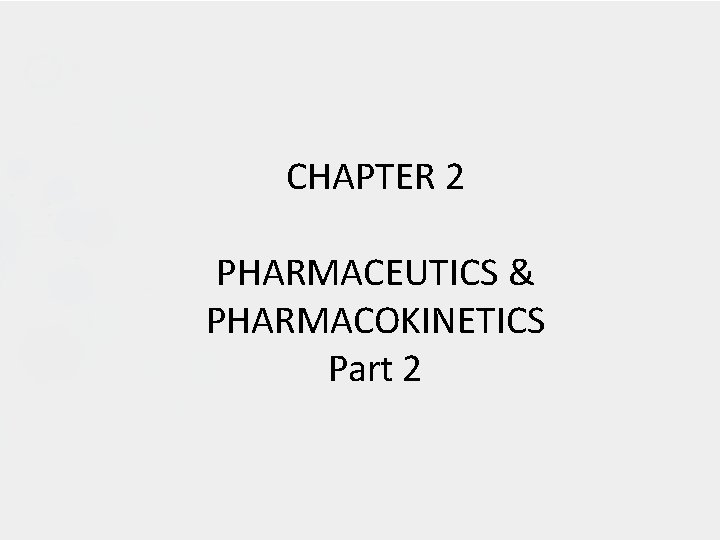
CHAPTER 2 PHARMACEUTICS & PHARMACOKINETICS Part 2
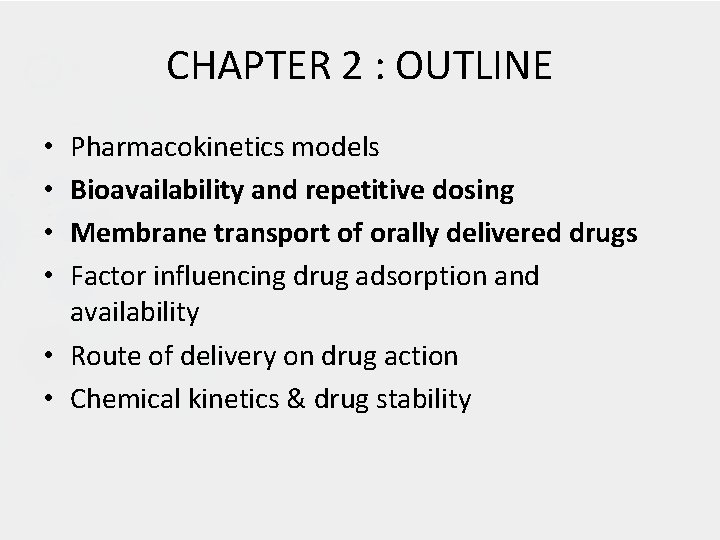
CHAPTER 2 : OUTLINE Pharmacokinetics models Bioavailability and repetitive dosing Membrane transport of orally delivered drugs Factor influencing drug adsorption and availability • Route of delivery on drug action • Chemical kinetics & drug stability • •
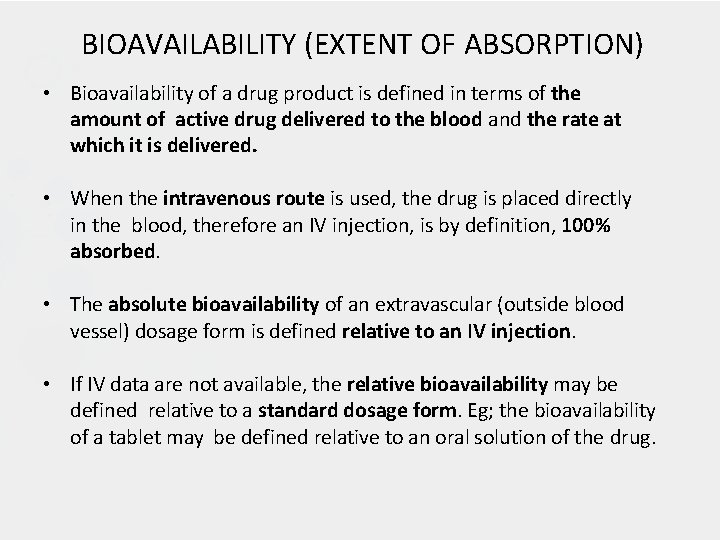
BIOAVAILABILITY (EXTENT OF ABSORPTION) • Bioavailability of a drug product is defined in terms of the amount of active drug delivered to the blood and the rate at which it is delivered. • When the intravenous route is used, the drug is placed directly in the blood, therefore an IV injection, is by definition, 100% absorbed. • The absolute bioavailability of an extravascular (outside blood vessel) dosage form is defined relative to an IV injection. • If IV data are not available, the relative bioavailability may be defined relative to a standard dosage form. Eg; the bioavailability of a tablet may be defined relative to an oral solution of the drug.
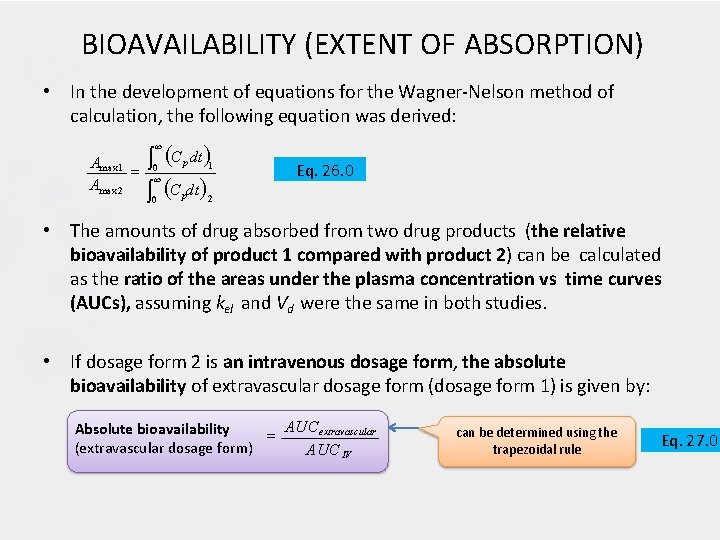
BIOAVAILABILITY (EXTENT OF ABSORPTION) • In the development of equations for the Wagner-Nelson method of calculation, the following equation was derived: C dt Amax 1 Amax 2 0 p 1 0 p 2 Eq. 26. 0 • The amounts of drug absorbed from two drug products (the relative bioavailability of product 1 compared with product 2) can be calculated as the ratio of the areas under the plasma concentration vs time curves (AUCs), assuming kel and Vd were the same in both studies. • If dosage form 2 is an intravenous dosage form, the absolute bioavailability of extravascular dosage form (dosage form 1) is given by: AUC extravascular Absolute bioavailability (extravascular dosage form) AUC IV can be determined using the trapezoidal rule Eq. 27. 0
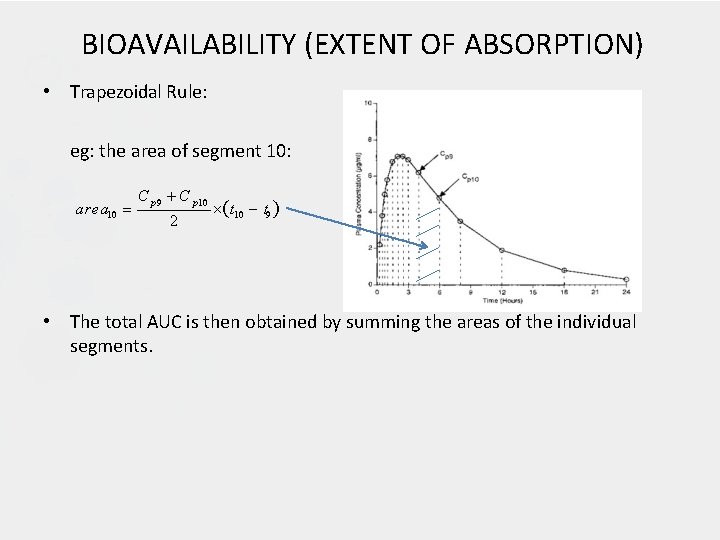
BIOAVAILABILITY (EXTENT OF ABSORPTION) • Trapezoidal Rule: eg: the area of segment 10: area 10 C p 9 C p 10 2 t 10 t 9 • The total AUC is then obtained by summing the areas of the individual segments.
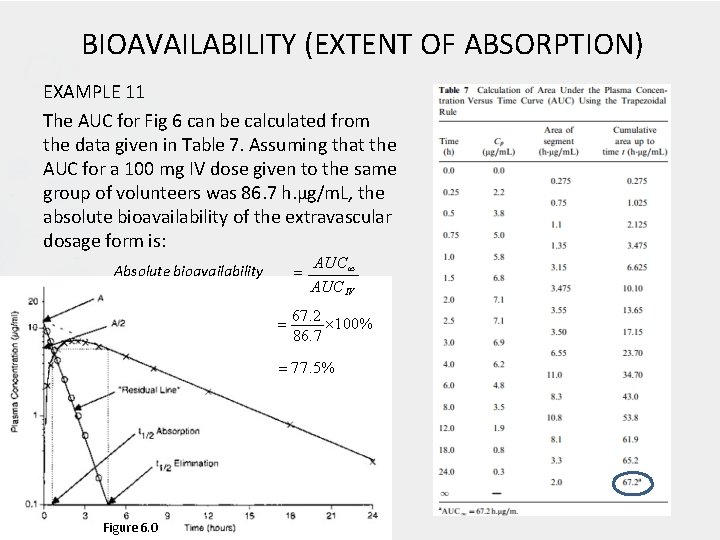
BIOAVAILABILITY (EXTENT OF ABSORPTION) EXAMPLE 11 The AUC for Fig 6 can be calculated from the data given in Table 7. Assuming that the AUC for a 100 mg IV dose given to the same group of volunteers was 86. 7 h. µg/m. L, the absolute bioavailability of the extravascular dosage form is: Absolute bioavailability AUCIV 67. 2 100% 86. 7 77. 5% Figure 6. 0
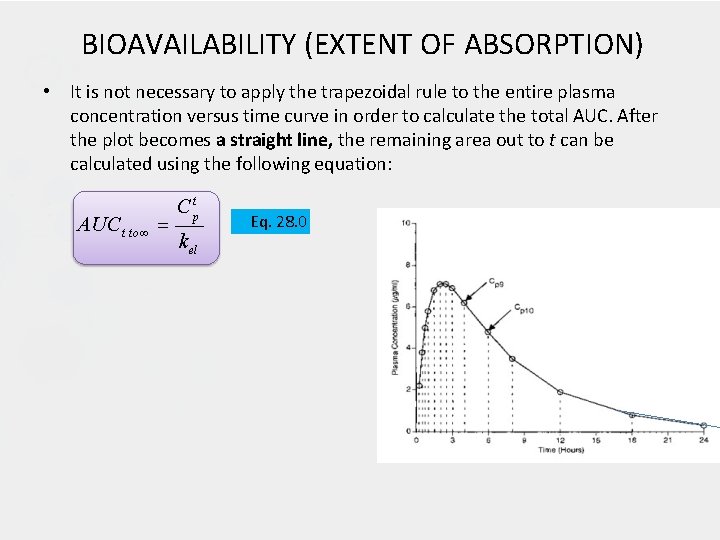
BIOAVAILABILITY (EXTENT OF ABSORPTION) • It is not necessary to apply the trapezoidal rule to the entire plasma concentration versus time curve in order to calculate the total AUC. After the plot becomes a straight line, the remaining area out to t can be calculated using the following equation: AUC t to C tp k el Eq. 28. 0
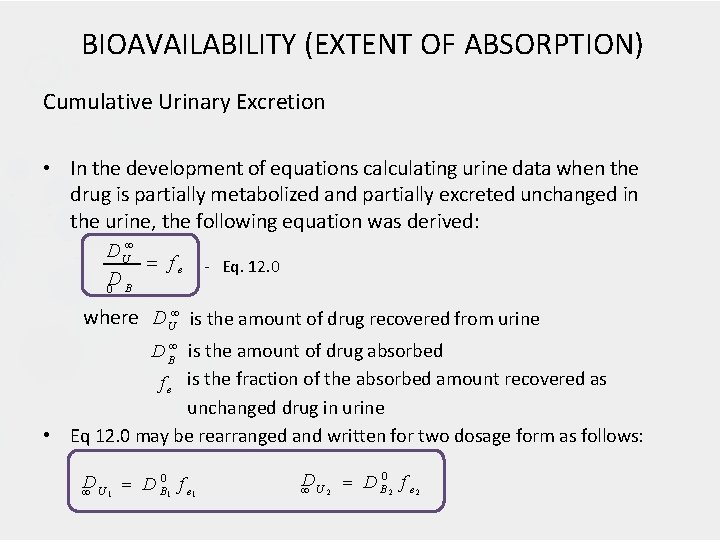
BIOAVAILABILITY (EXTENT OF ABSORPTION) Cumulative Urinary Excretion • In the development of equations calculating urine data when the drug is partially metabolized and partially excreted unchanged in the urine, the following equation was derived: D U fe D 0 B - Eq. 12. 0 where D U is the amount of drug recovered from urine • D B is the amount of drug absorbed f e is the fraction of the absorbed amount recovered as unchanged drug in urine Eq 12. 0 may be rearranged and written for two dosage form as follows: 0 D D f e 1 U B 1 1 D D B 0 2 f e 2 U 2
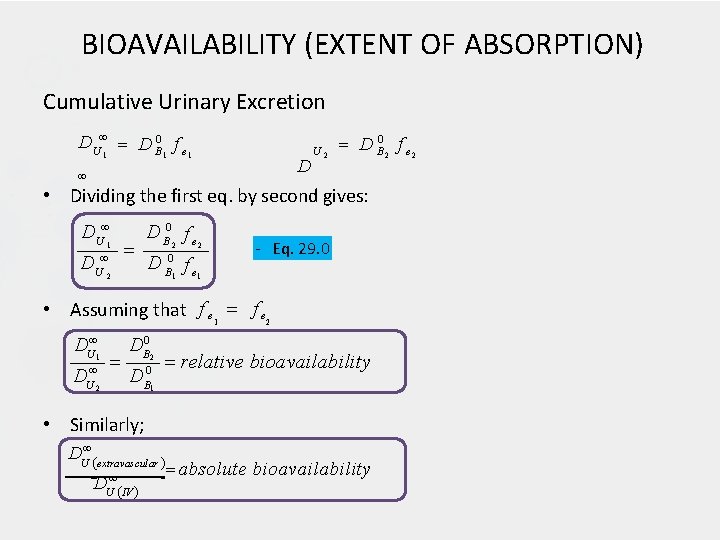
BIOAVAILABILITY (EXTENT OF ABSORPTION) Cumulative Urinary Excretion D U 1 D B 0 1 f e 1 D U 2 D B 0 2 f e 2 • Dividing the first eq. by second gives: D U 1 D U 2 D B 0 f e 2 2 1 1 - Eq. 29. 0 • Assuming that f e 1 f e 2 DU 1 U 2 D DB 0 D 2 0 B 1 relative bioavailability • Similarly; DU extravascular U IV D absolute bioavailability
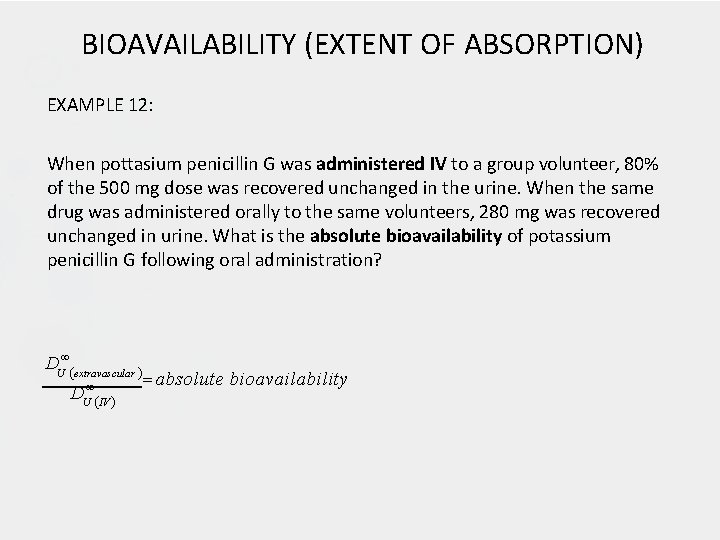
BIOAVAILABILITY (EXTENT OF ABSORPTION) EXAMPLE 12: When pottasium penicillin G was administered IV to a group volunteer, 80% of the 500 mg dose was recovered unchanged in the urine. When the same drug was administered orally to the same volunteers, 280 mg was recovered unchanged in urine. What is the absolute bioavailability of potassium penicillin G following oral administration? DU extravascular DU IV absolute bioavailability
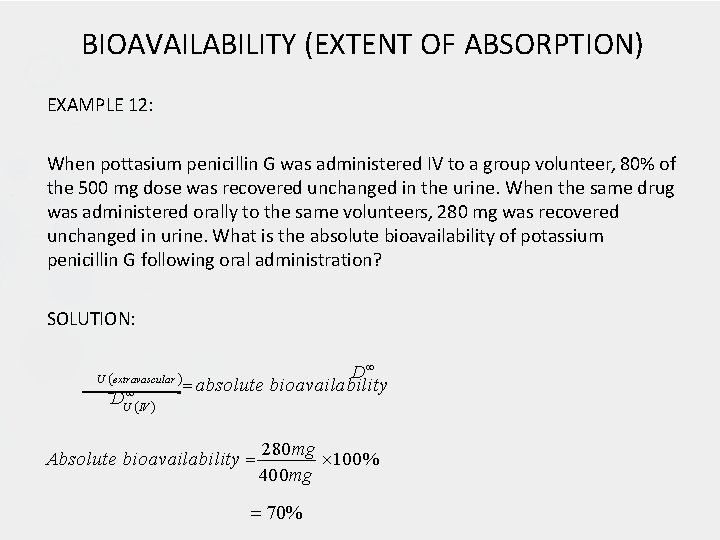
BIOAVAILABILITY (EXTENT OF ABSORPTION) EXAMPLE 12: When pottasium penicillin G was administered IV to a group volunteer, 80% of the 500 mg dose was recovered unchanged in the urine. When the same drug was administered orally to the same volunteers, 280 mg was recovered unchanged in urine. What is the absolute bioavailability of potassium penicillin G following oral administration? SOLUTION: U extravascular U IV D D absolute bioavailability Absolute bioavailability 280 mg 100% 400 mg 70%
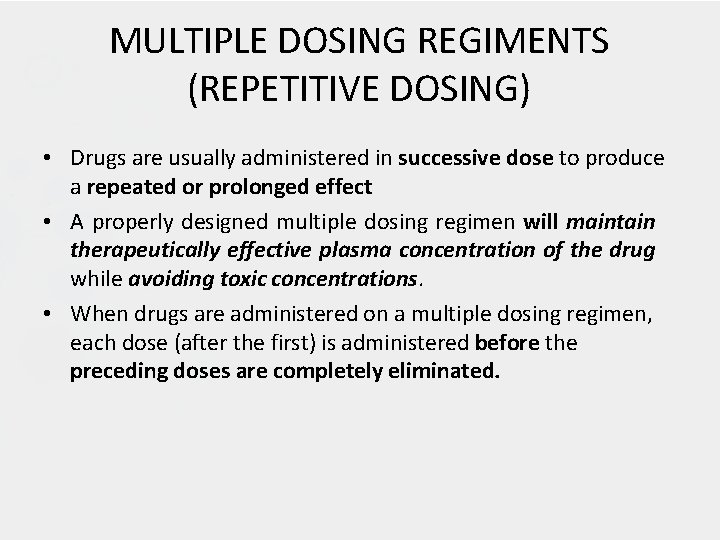
MULTIPLE DOSING REGIMENTS (REPETITIVE DOSING) • Drugs are usually administered in successive dose to produce a repeated or prolonged effect • A properly designed multiple dosing regimen will maintain therapeutically effective plasma concentration of the drug while avoiding toxic concentrations. • When drugs are administered on a multiple dosing regimen, each dose (after the first) is administered before the preceding doses are completely eliminated.
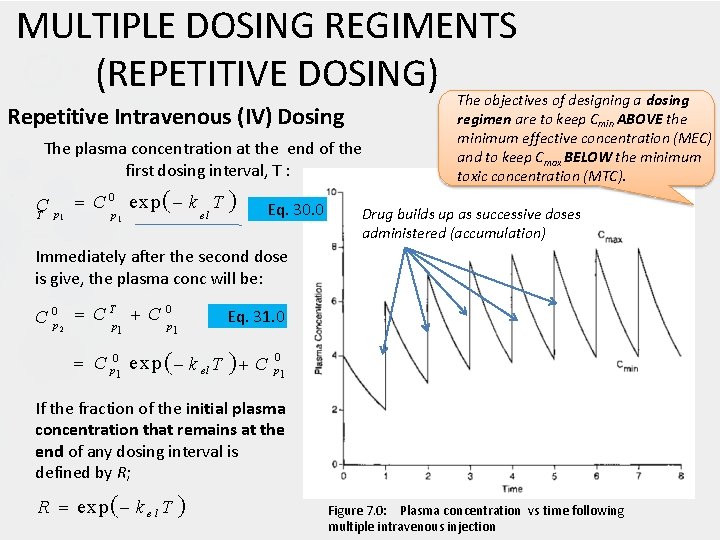
MULTIPLE DOSING REGIMENTS (REPETITIVE DOSING) Repetitive Intravenous (IV) Dosing The plasma concentration at the end of the first dosing interval, T : C 0 exp k T C p el T p 1 1 Eq. 30. 0 The objectives of designing a dosing regimen are to keep Cmin ABOVE the minimum effective concentration (MEC) and to keep Cmax BELOW the minimum toxic concentration (MTC). Drug builds up as successive doses administered (accumulation) Immediately after the second dose is give, the plasma conc will be: T 0 C 0 p 2 C p 1 1 Eq. 31. 0 C p 01 e xp k el T C 0 p 1 If the fraction of the initial plasma concentration that remains at the end of any dosing interval is defined by R; R exp k e l T Figure 7. 0: Plasma concentration vs time following multiple intravenous injection
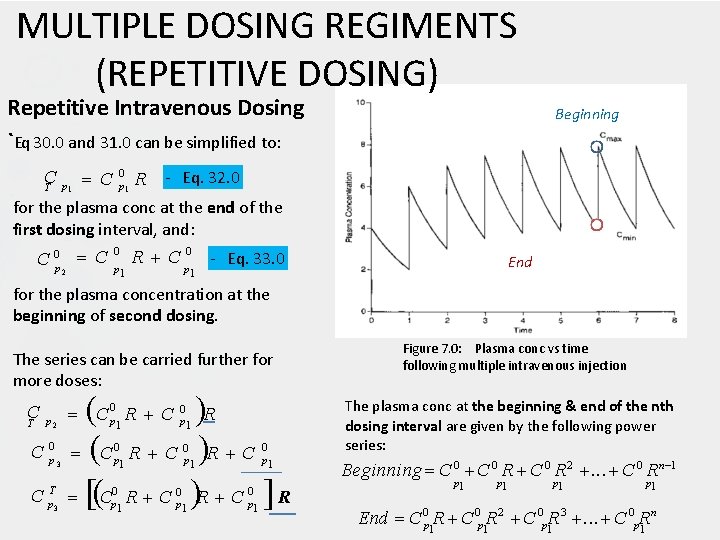
MULTIPLE DOSING REGIMENTS (REPETITIVE DOSING) Repetitive Intravenous Dosing `Eq 30. 0 and 31. 0 can be simplified to: Beginning C C 0 p 1 R - Eq. 32. 0 T p 1 for the plasma conc at the end of the first dosing interval, and: 0 0 C 0 p 2 C p R C p - Eq. 33. 0 1 End 1 for the plasma concentration at the beginning of second dosing. The series can be carried further for more doses: C C R C R 0 0 C C p 1 R T p 2 C 0 p 3 C T p 3 0 p 1 R C 0 p 1 0 p 1 R C 0 p 1 Figure 7. 0: Plasma conc vs time following multiple intravenous injection The plasma conc at the beginning & end of the nth dosing interval are given by the following power series: Beginning C 0 R 2 . . . C 0 Rn 1 p 1 p 1 n End C 0 p 1 R 2 C 0 p 1 R 3 . . . C 0 p. R 1
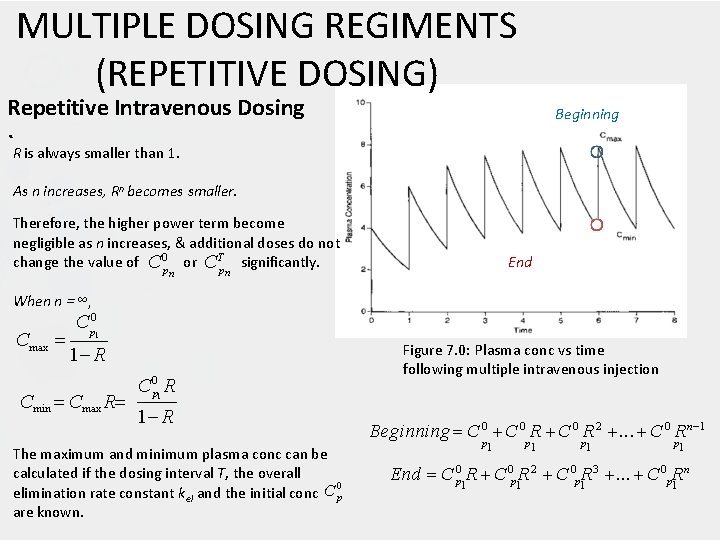
MULTIPLE DOSING REGIMENTS (REPETITIVE DOSING) Repetitive Intravenous Dosing Beginning `R is always smaller than 1. As n increases, Rn becomes smaller. Therefore, the higher power term become negligible as n increases, & additional doses do not change the value of C 0 p or CTp significantly. n End n When n = ∞, Cmax C p 0 1 1 R Cmin Cmax R C 0 p R Figure 7. 0: Plasma conc vs time following multiple intravenous injection 1 1 R The maximum and minimum plasma conc can be calculated if the dosing interval T, the overall 0 elimination rate constant kel and the initial conc C p are known. Beginning C 0 R 2 . . . C 0 Rn 1 p 1 p 1 n End C 0 p 1 R 2 C 0 p 1 R 3 . . . C 0 p. R 1
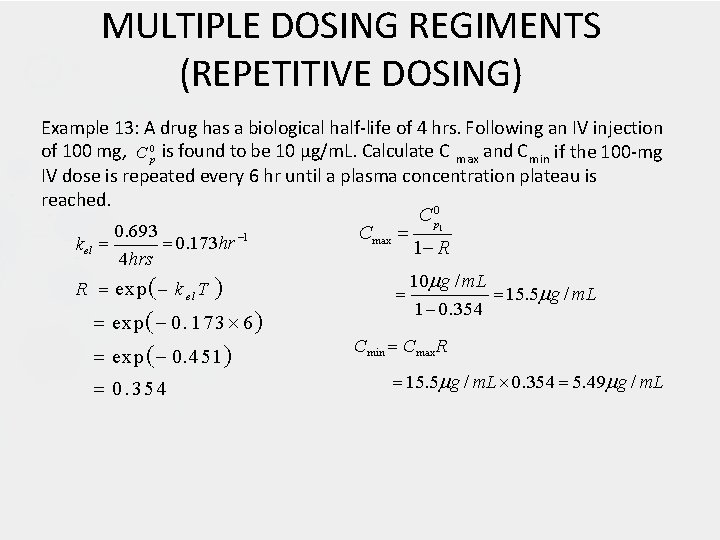
MULTIPLE DOSING REGIMENTS (REPETITIVE DOSING) Example 13: A drug has a biological half-life of 4 hrs. Following an IV injection of 100 mg, C 0 p is found to be 10 µg/m. L. Calculate C max and C min if the 100 -mg IV dose is repeated every 6 hr until a plasma concentration plateau is reached. C p 0 0. 693 Cmax 0. 173 hr 1 kel 1 R 4 hrs 10 g / m. L R exp k el T 15. 5 g / m. L 1 0. 354 exp 0. 1 7 3 6 Cmin Cmax R exp 0. 451 15. 5 g / m. L 0. 354 5. 49 g / m. L 0. 354 1
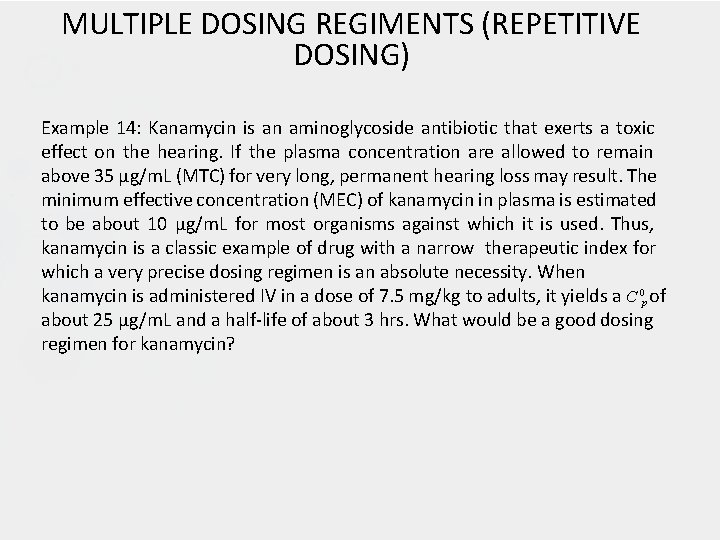
MULTIPLE DOSING REGIMENTS (REPETITIVE DOSING) Example 14: Kanamycin is an aminoglycoside antibiotic that exerts a toxic effect on the hearing. If the plasma concentration are allowed to remain above 35 µg/m. L (MTC) for very long, permanent hearing loss may result. The minimum effective concentration (MEC) of kanamycin in plasma is estimated to be about 10 µg/m. L for most organisms against which it is used. Thus, kanamycin is a classic example of drug with a narrow therapeutic index for which a very precise dosing regimen is an absolute necessity. When kanamycin is administered IV in a dose of 7. 5 mg/kg to adults, it yields a C 0 p of about 25 µg/m. L and a half-life of about 3 hrs. What would be a good dosing regimen for kanamycin?
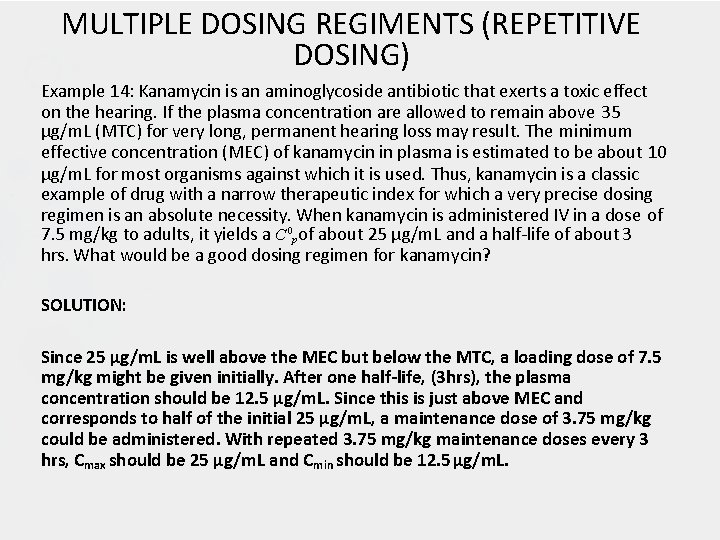
MULTIPLE DOSING REGIMENTS (REPETITIVE DOSING) Example 14: Kanamycin is an aminoglycoside antibiotic that exerts a toxic effect on the hearing. If the plasma concentration are allowed to remain above 35 µg/m. L (MTC) for very long, permanent hearing loss may result. The minimum effective concentration (MEC) of kanamycin in plasma is estimated to be about 10 µg/m. L for most organisms against which it is used. Thus, kanamycin is a classic example of drug with a narrow therapeutic index for which a very precise dosing regimen is an absolute necessity. When kanamycin is administered IV in a dose of 7. 5 mg/kg to adults, it yields a C 0 p of about 25 µg/m. L and a half-life of about 3 hrs. What would be a good dosing regimen for kanamycin? SOLUTION: Since 25 µg/m. L is well above the MEC but below the MTC, a loading dose of 7. 5 mg/kg might be given initially. After one half-life, (3 hrs), the plasma concentration should be 12. 5 µg/m. L. Since this is just above MEC and corresponds to half of the initial 25 µg/m. L, a maintenance dose of 3. 75 mg/kg could be administered. With repeated 3. 75 mg/kg maintenance doses every 3 hrs, Cmax should be 25 µg/m. L and Cmin should be 12. 5 µg/m. L.
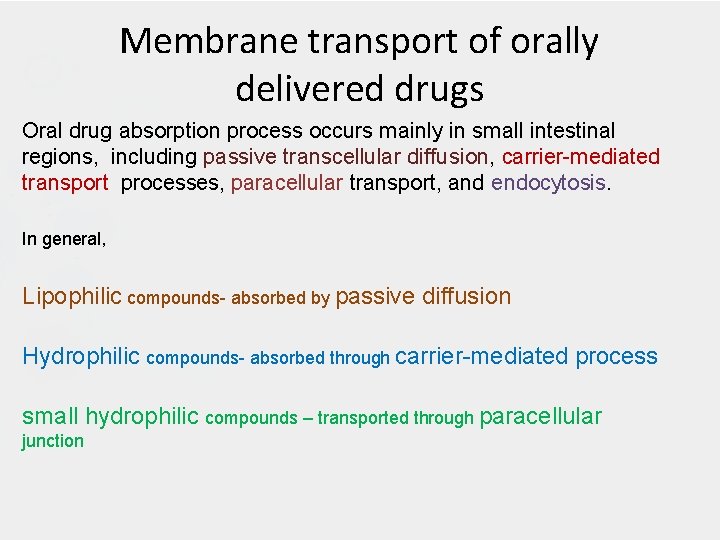
Membrane transport of orally delivered drugs Oral drug absorption process occurs mainly in small intestinal regions, including passive transcellular diffusion, carrier-mediated transport processes, paracellular transport, and endocytosis. In general, Lipophilic compounds- absorbed by passive diffusion Hydrophilic compounds- absorbed through carrier-mediated process small hydrophilic compounds – transported through paracellular junction
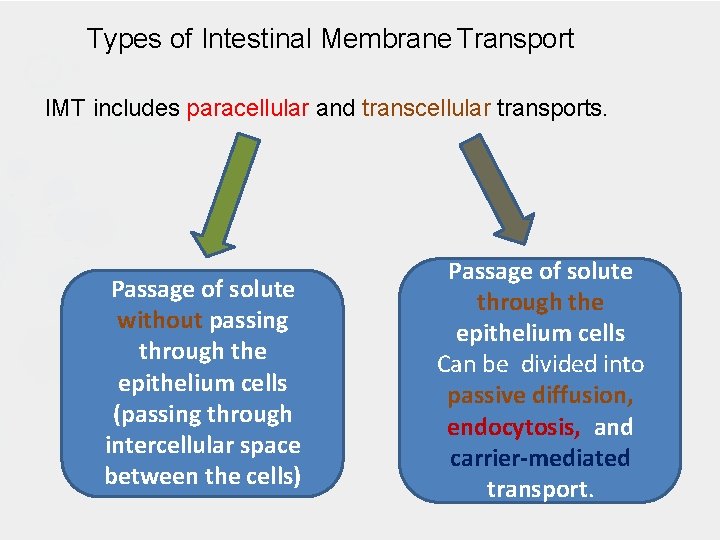
Types of Intestinal Membrane Transport IMT includes paracellular and transcellular transports. Passage of solute without passing through the epithelium cells (passing through intercellular space between the cells) Passage of solute through the epithelium cells Can be divided into passive diffusion, endocytosis, and carrier-mediated transport.
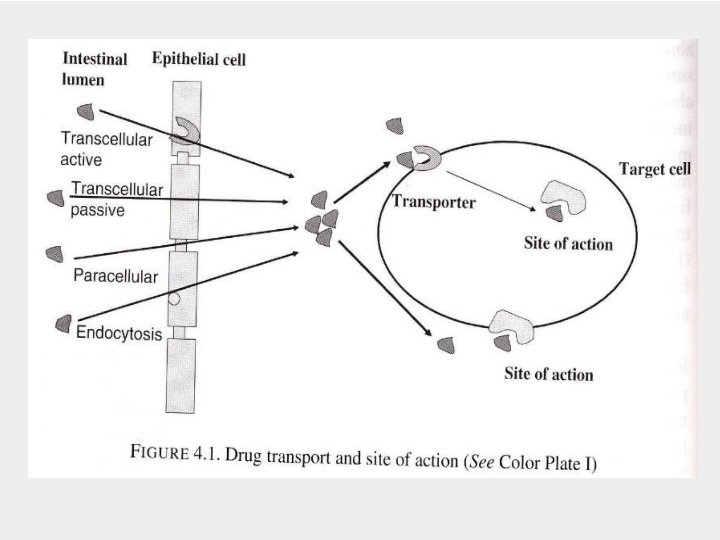
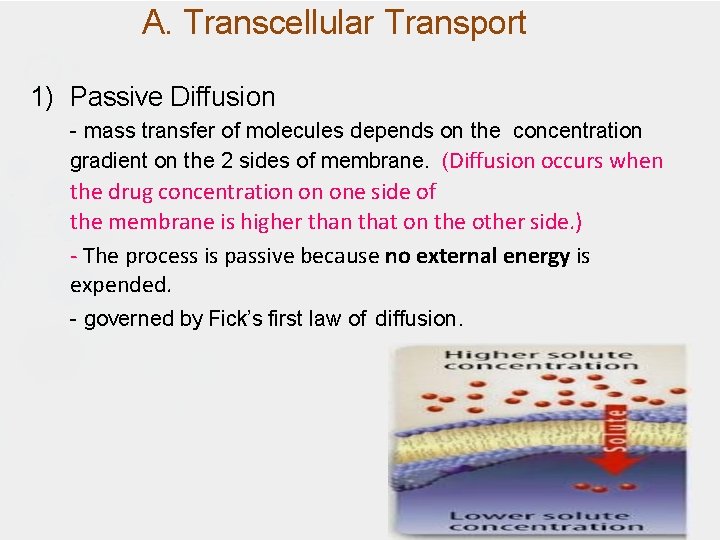
A. Transcellular Transport 1) Passive Diffusion - mass transfer of molecules depends on the concentration gradient on the 2 sides of membrane. (Diffusion occurs when the drug concentration on one side of the membrane is higher than that on the other side. ) - The process is passive because no external energy is expended. - governed by Fick’s first law of diffusion.
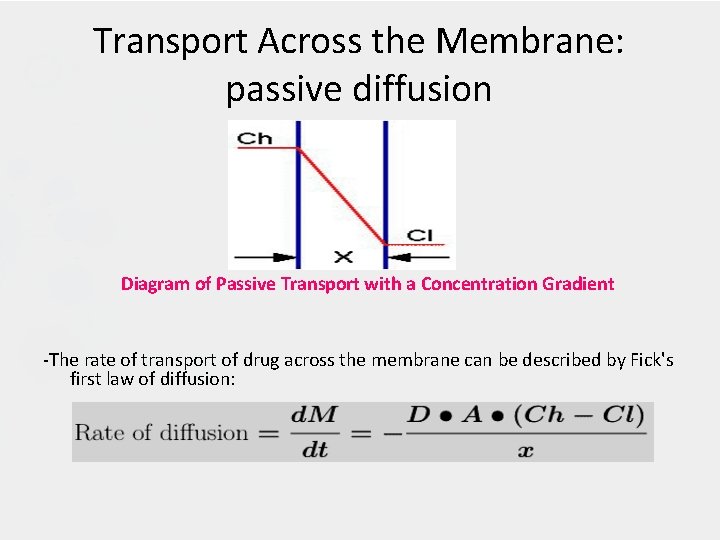
Transport Across the Membrane: passive diffusion Diagram of Passive Transport with a Concentration Gradient -The rate of transport of drug across the membrane can be described by Fick's first law of diffusion:
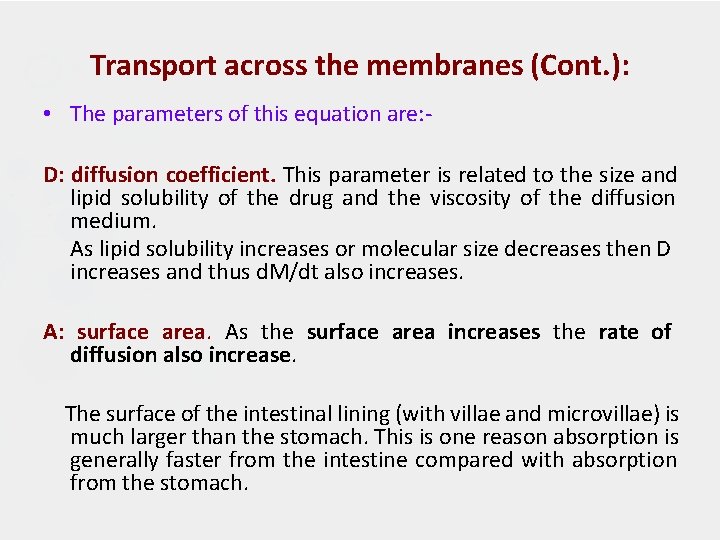
Transport across the membranes (Cont. ): • The parameters of this equation are: D: diffusion coefficient. This parameter is related to the size and lipid solubility of the drug and the viscosity of the diffusion medium. As lipid solubility increases or molecular size decreases then D increases and thus d. M/dt also increases. A: surface area. As the surface area increases the rate of diffusion also increase. The surface of the intestinal lining (with villae and microvillae) is much larger than the stomach. This is one reason absorption is generally faster from the intestine compared with absorption from the stomach.
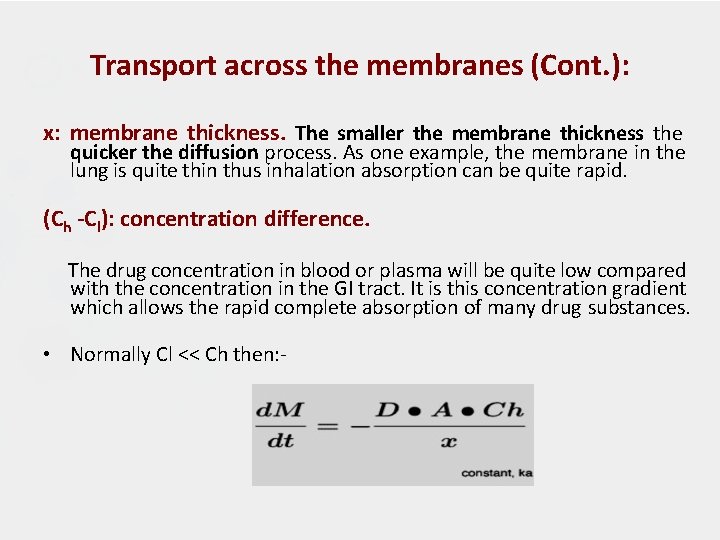
Transport across the membranes (Cont. ): x: membrane thickness. The smaller the membrane thickness the quicker the diffusion process. As one example, the membrane in the lung is quite thin thus inhalation absorption can be quite rapid. (Ch -Cl): concentration difference. The drug concentration in blood or plasma will be quite low compared with the concentration in the GI tract. It is this concentration gradient which allows the rapid complete absorption of many drug substances. • Normally Cl << Ch then: -
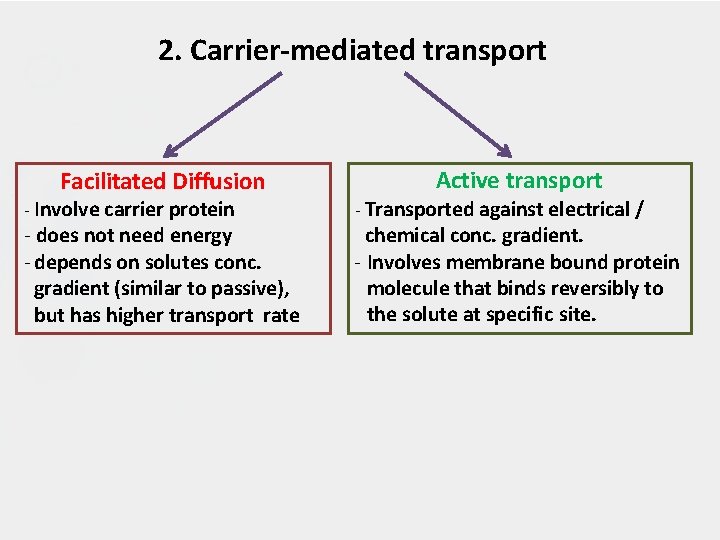
2. Carrier-mediated transport Facilitated Diffusion - Involve carrier protein - does not need energy - depends on solutes conc. gradient (similar to passive), but has higher transport rate Active transport - Transported against electrical / chemical conc. gradient. - Involves membrane bound protein molecule that binds reversibly to the solute at specific site.
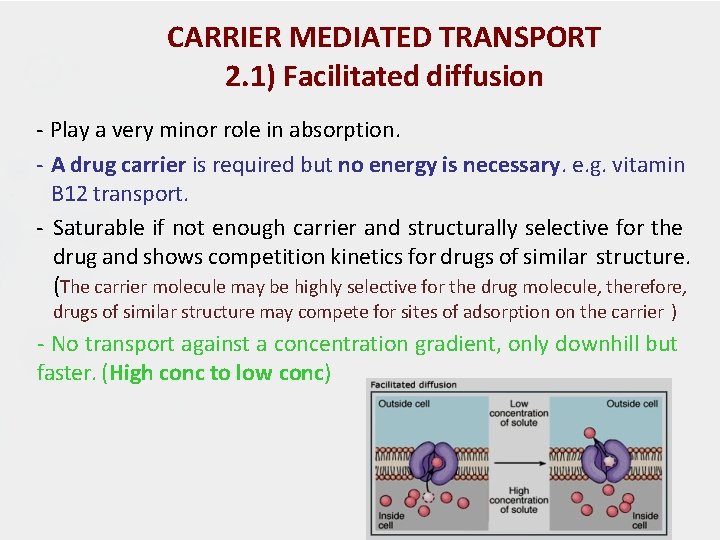
CARRIER MEDIATED TRANSPORT 2. 1) Facilitated diffusion - Play a very minor role in absorption. - A drug carrier is required but no energy is necessary. e. g. vitamin B 12 transport. - Saturable if not enough carrier and structurally selective for the drug and shows competition kinetics for drugs of similar structure. (The carrier molecule may be highly selective for the drug molecule, therefore, drugs of similar structure may compete for sites of adsorption on the carrier ) - No transport against a concentration gradient, only downhill but faster. (High conc to low conc)
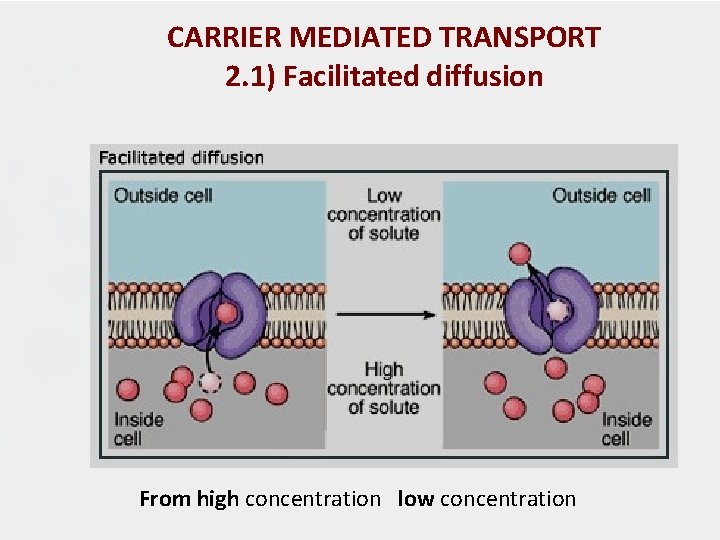
CARRIER MEDIATED TRANSPORT 2. 1) Facilitated diffusion From high concentration low concentration
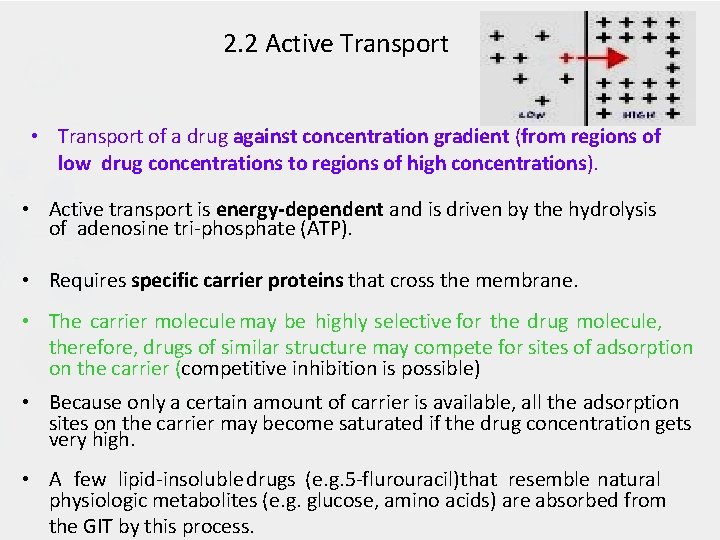
2. 2 Active Transport • Transport of a drug against concentration gradient (from regions of low drug concentrations to regions of high concentrations). • Active transport is energy-dependent and is driven by the hydrolysis of adenosine tri-phosphate (ATP). • Requires specific carrier proteins that cross the membrane. • The carrier molecule may be highly selective for the drug molecule, therefore, drugs of similar structure may compete for sites of adsorption on the carrier (competitive inhibition is possible) • Because only a certain amount of carrier is available, all the adsorption sites on the carrier may become saturated if the drug concentration gets very high. • A few lipid-insoluble drugs (e. g. 5 -flurouracil)that resemble natural physiologic metabolites (e. g. glucose, amino acids) are absorbed from the GIT by this process.
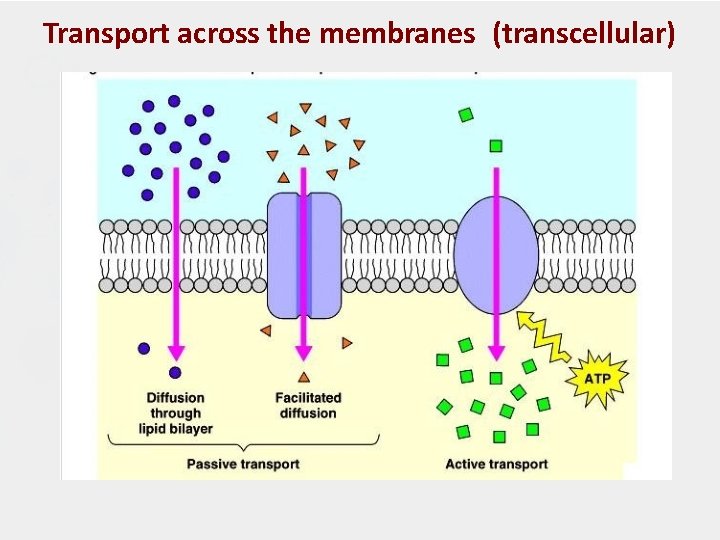
Transport across the membranes (transcellular)
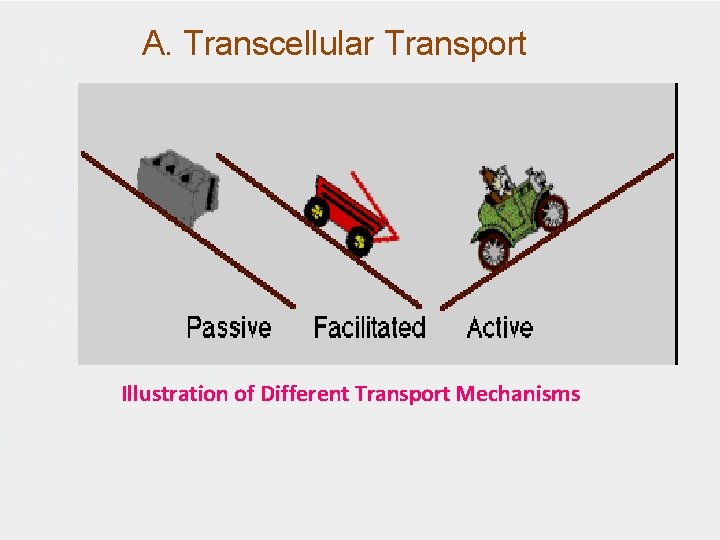
A. Transcellular Transport Illustration of Different Transport Mechanisms
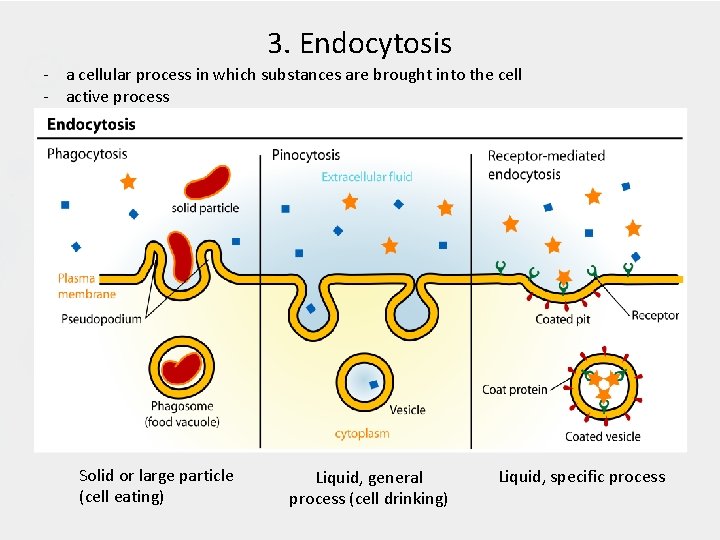
3. Endocytosis - a cellular process in which substances are brought into the cell - active process Solid or large particle (cell eating) Liquid, general process (cell drinking) Liquid, specific process
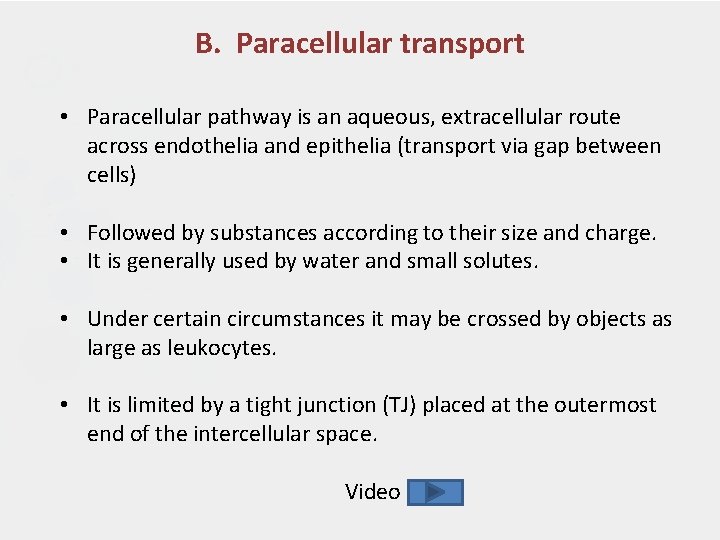
B. Paracellular transport • Paracellular pathway is an aqueous, extracellular route across endothelia and epithelia (transport via gap between cells) • Followed by substances according to their size and charge. • It is generally used by water and small solutes. • Under certain circumstances it may be crossed by objects as large as leukocytes. • It is limited by a tight junction (TJ) placed at the outermost end of the intercellular space. Video
What is pharmacokinetics
How to calculate bioavailability
Difference between absolute and relative bioavailability
Pharmacokinetics vs pharmacodynamics
"adalimumab" -"humira" -"neulasta"
Zero-order elimination
Non linear pharmacokinetics
Non linear pharmacokinetics
Multi compartment model pharmacokinetics
Difference between pharmacodynamics and pharmacokinetics
Difference between pharmacokinetics and pharmacodynamics
Nebido emc
First order elimination
Maintenance dose equation
Drug metabolism and pharmacokinetics
Alteplase pharmacokinetics
Linear pharmacokinetics
What is pharmacokinetics
Pharmacokinetics
Pharmacokinetics
Various methods of size separation
Methods of preparation of effervescent granules
The galenical prepared by extraction
Emulsifying agents examples in pharmacy
Geometric dilution helps prepare a mixture that is
Pencil shaped suppositories
What is cmc in physical pharmacy
Surface tension physical pharmacy
Example of physical incompatibility in pharmaceutics
Physical incompatibility with example
Powder flowing angle
Phase rule in physical pharmaceutics
Define levigation
Suppository types