Topic 5 Electricity and magnetism 5 2 Heating
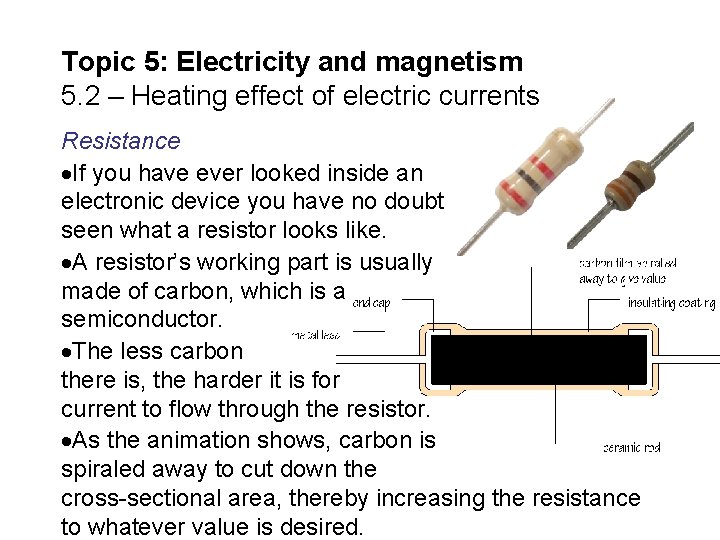
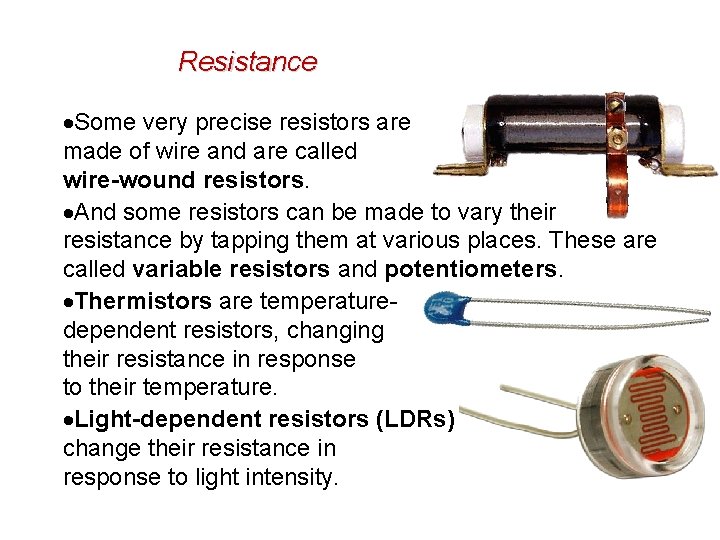
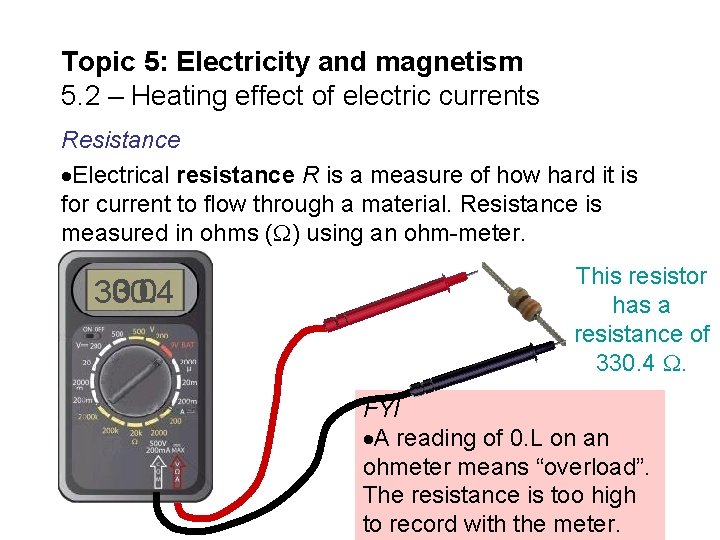
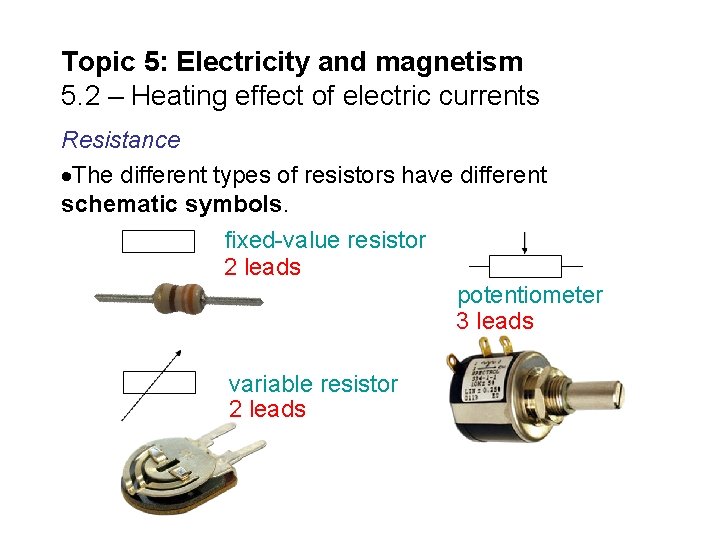
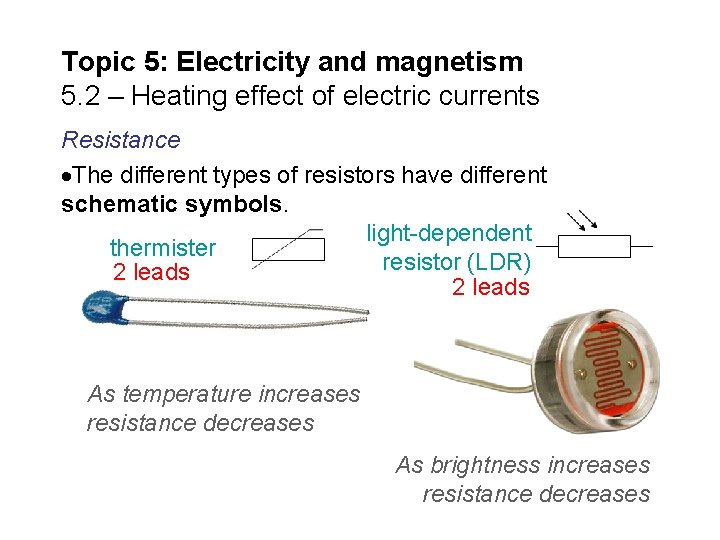
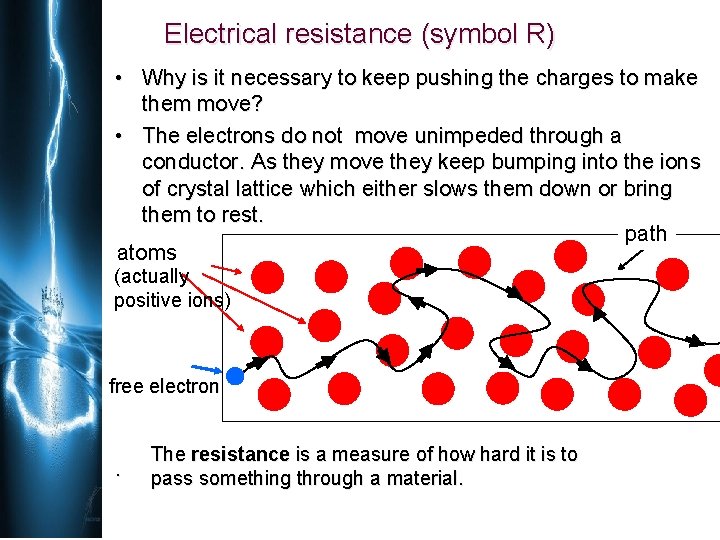
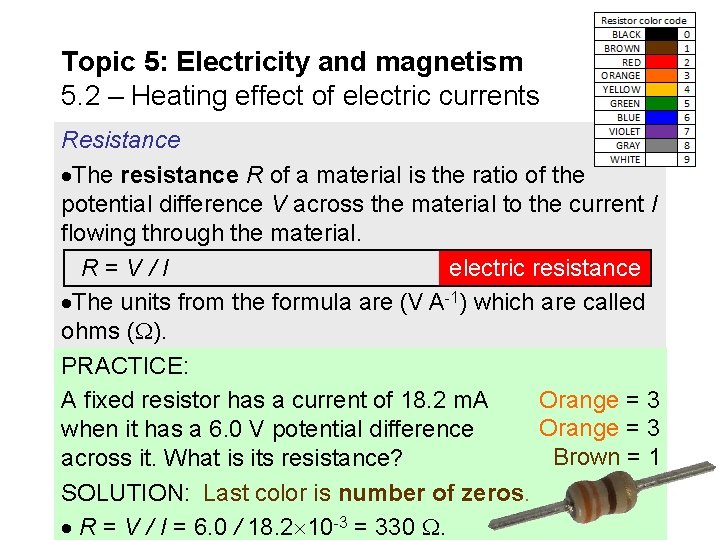
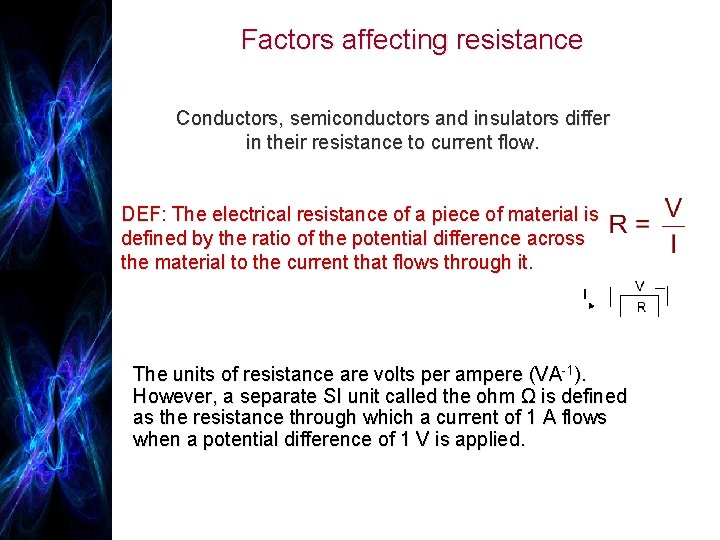
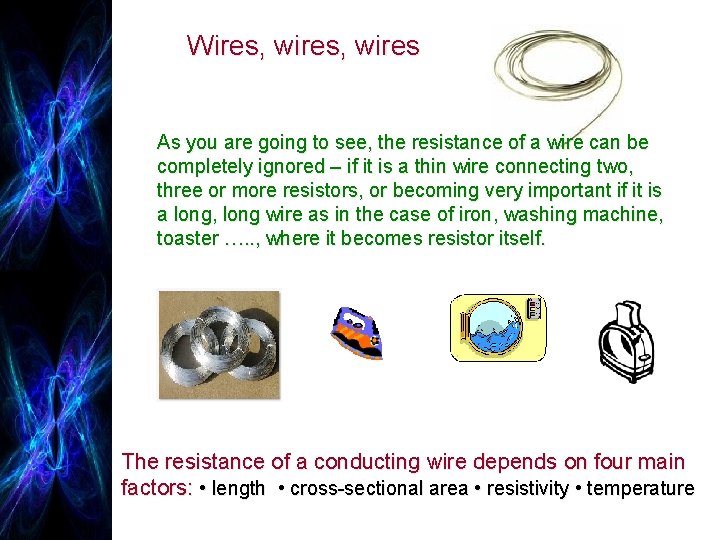
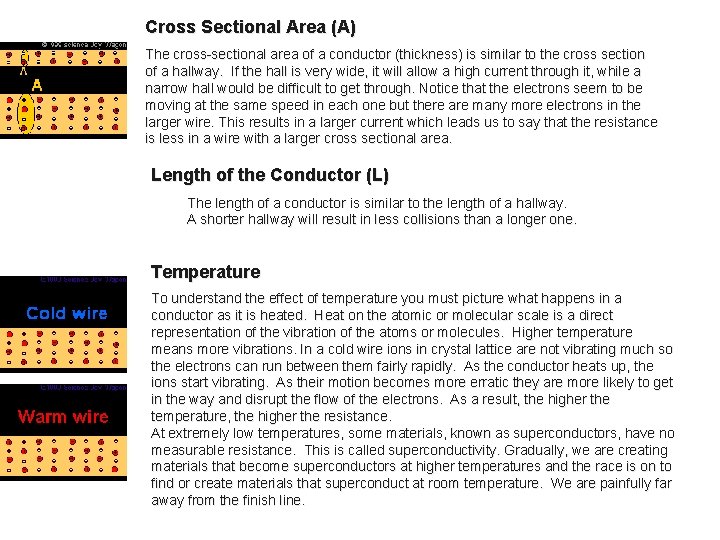
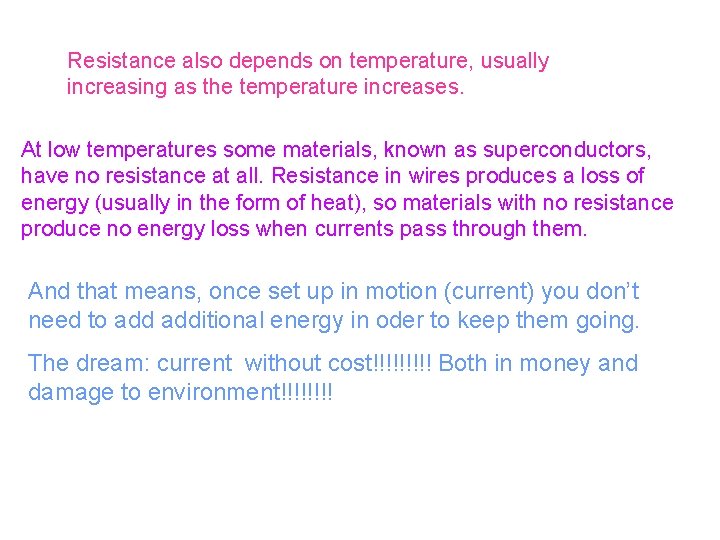
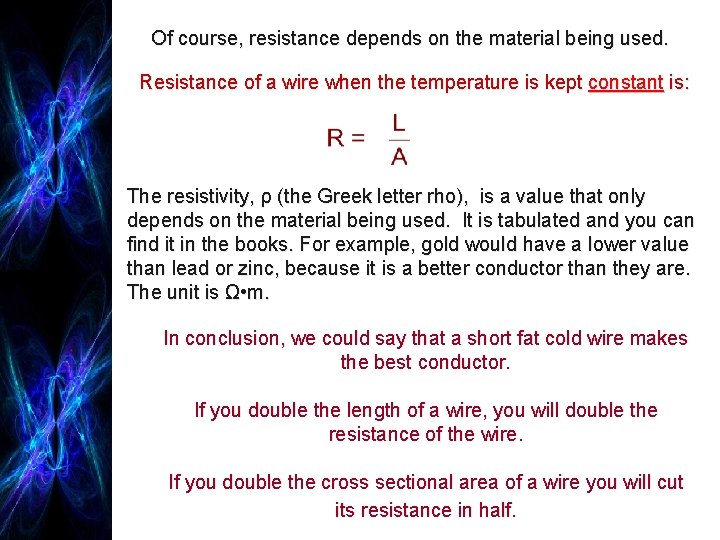
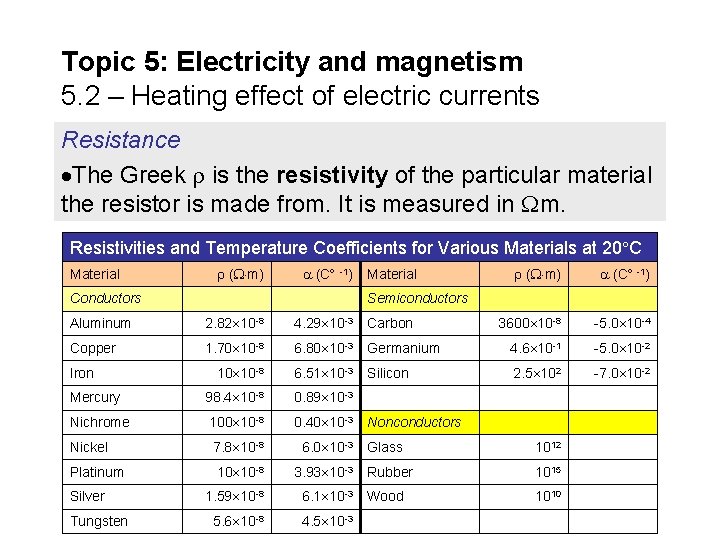
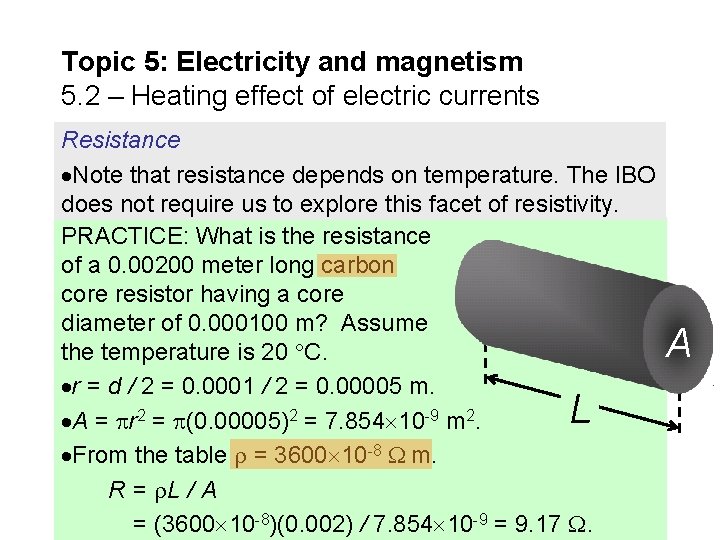
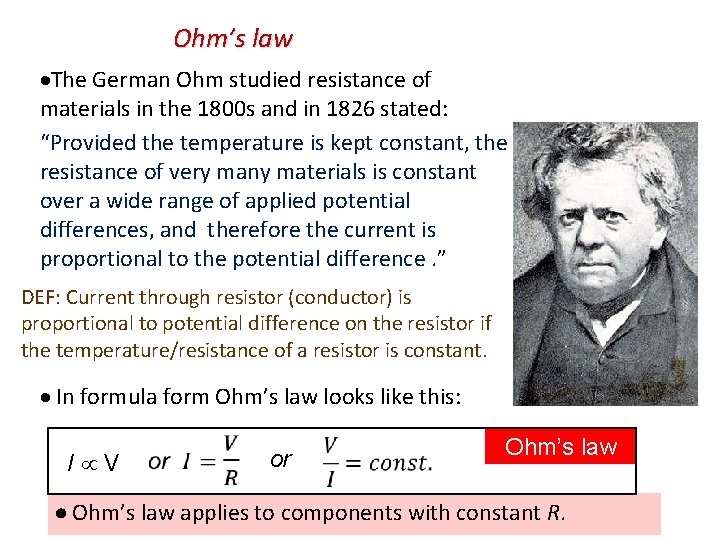
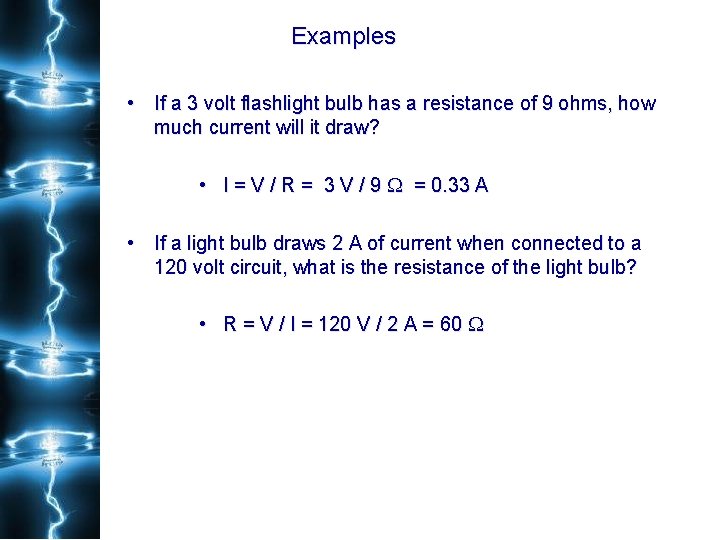
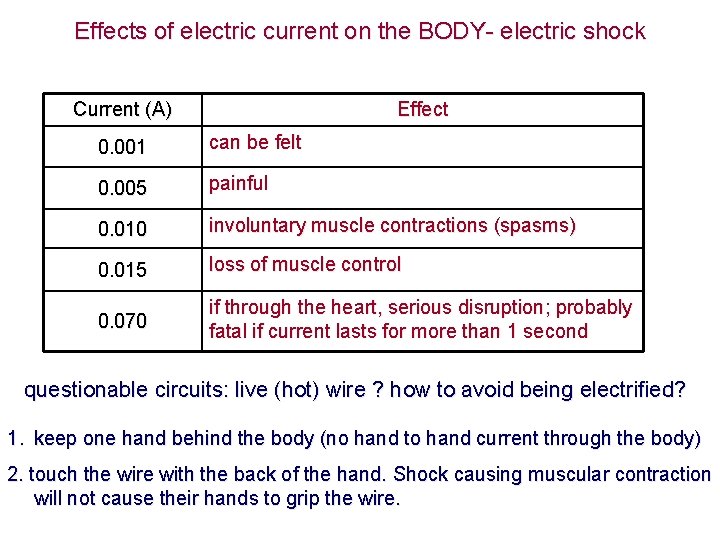
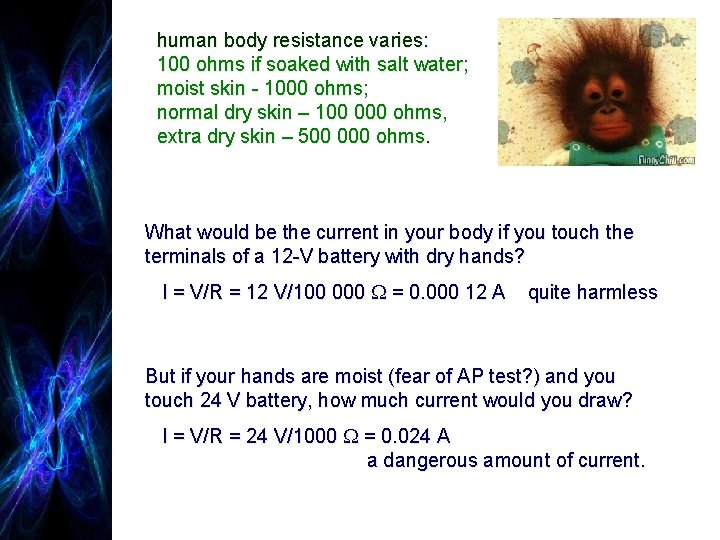
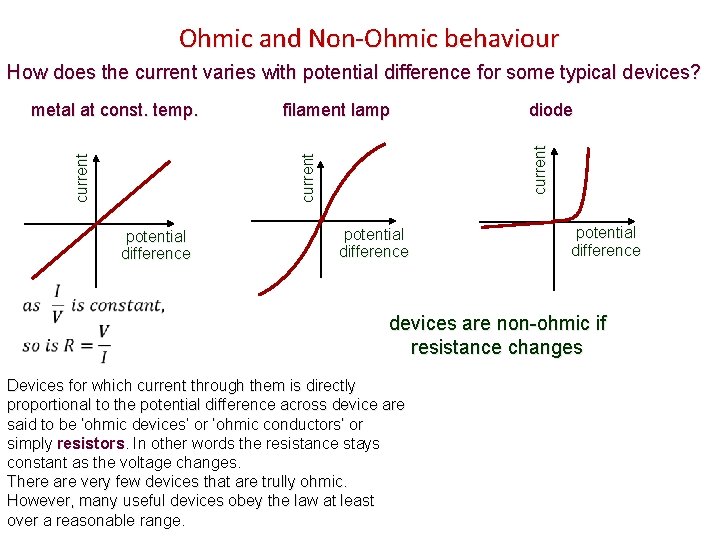
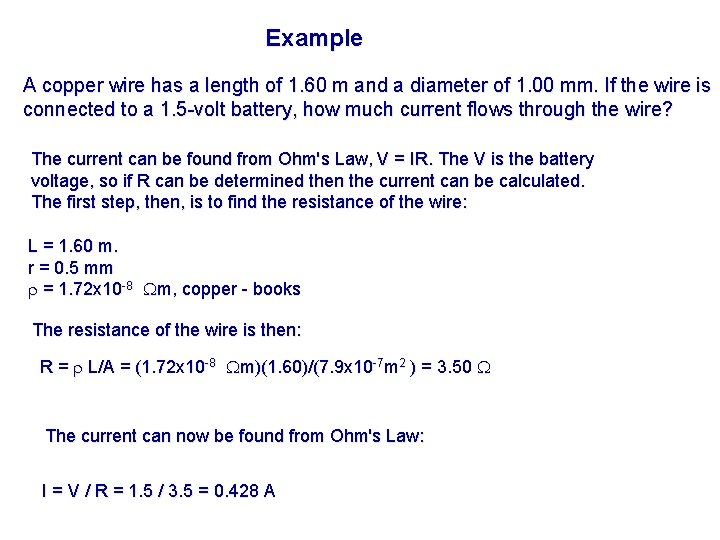
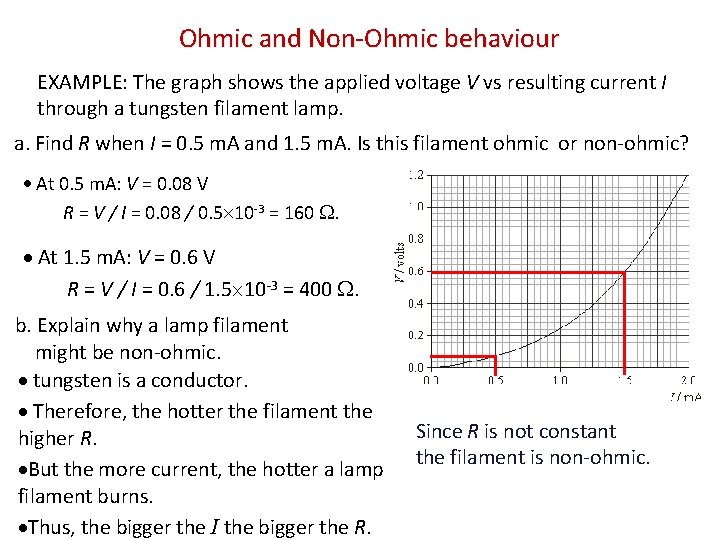
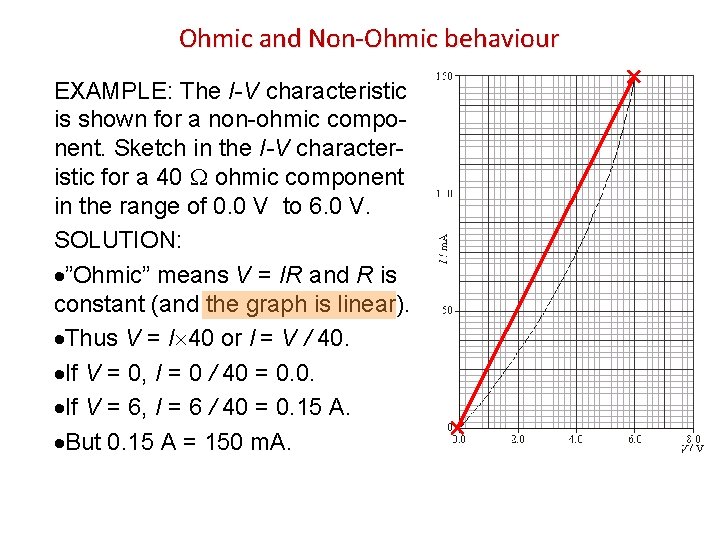
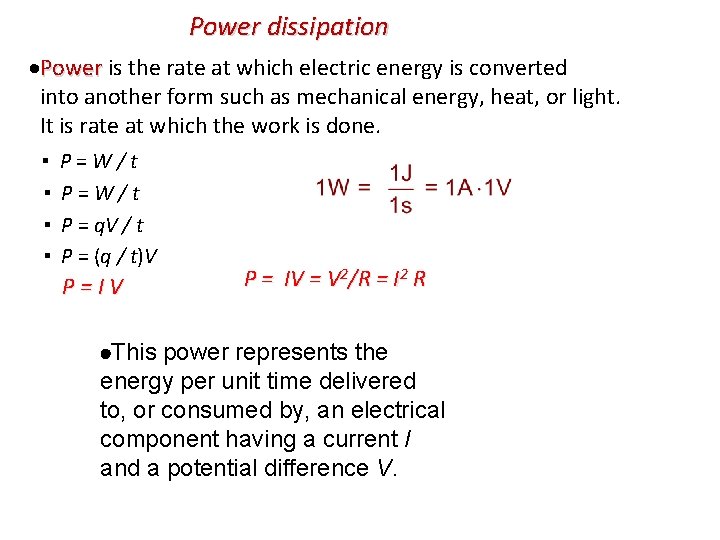
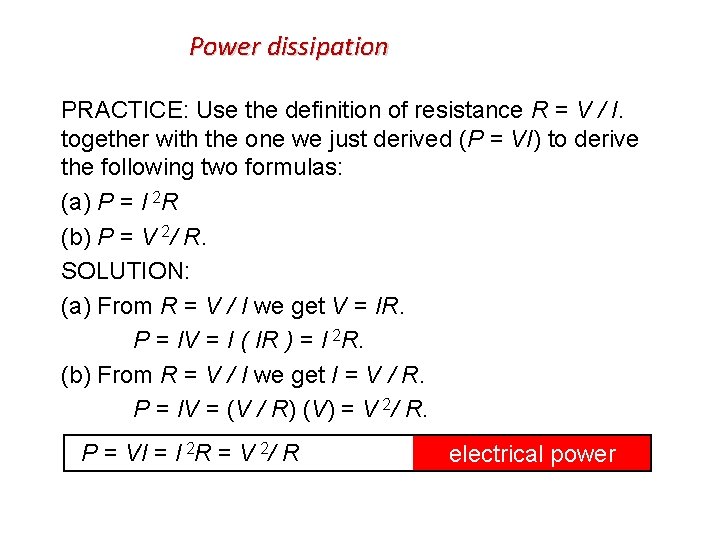
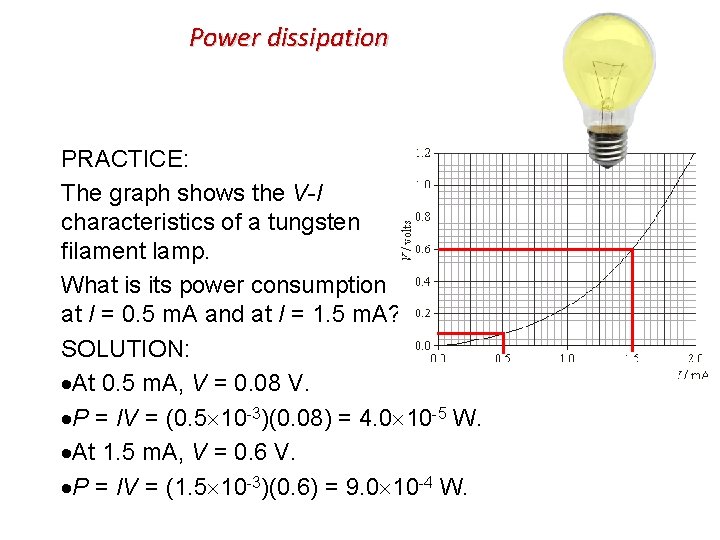
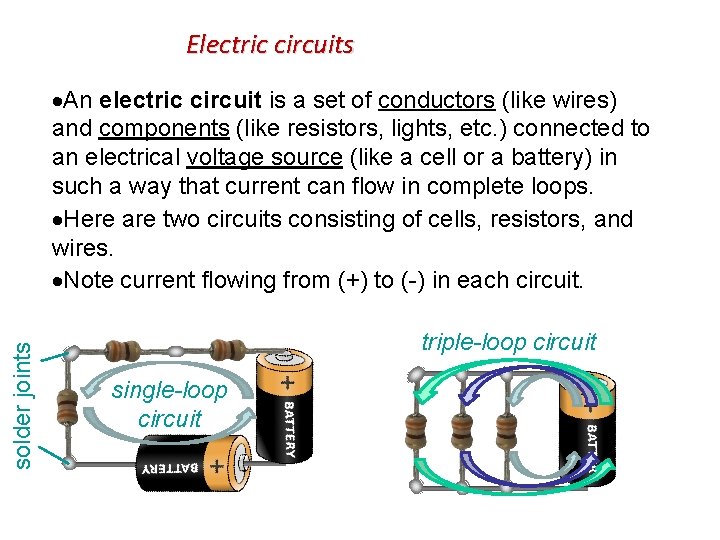
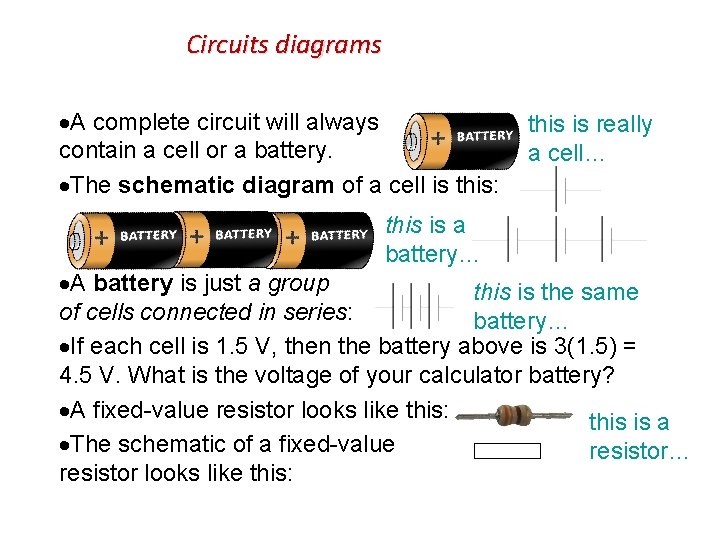
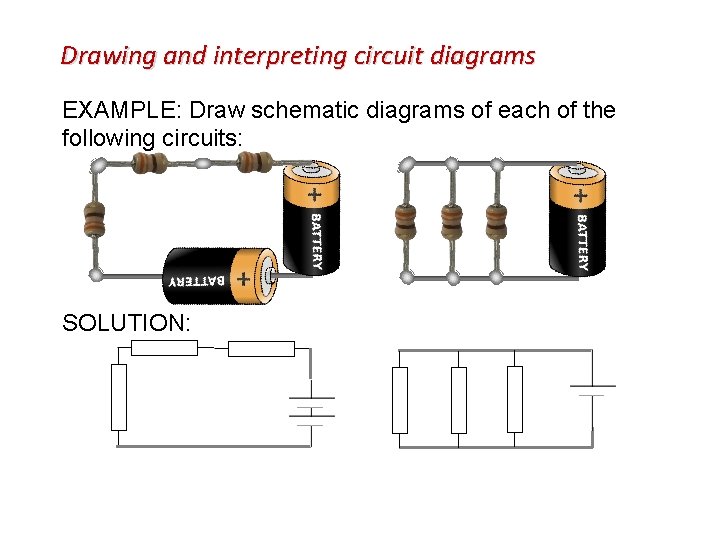
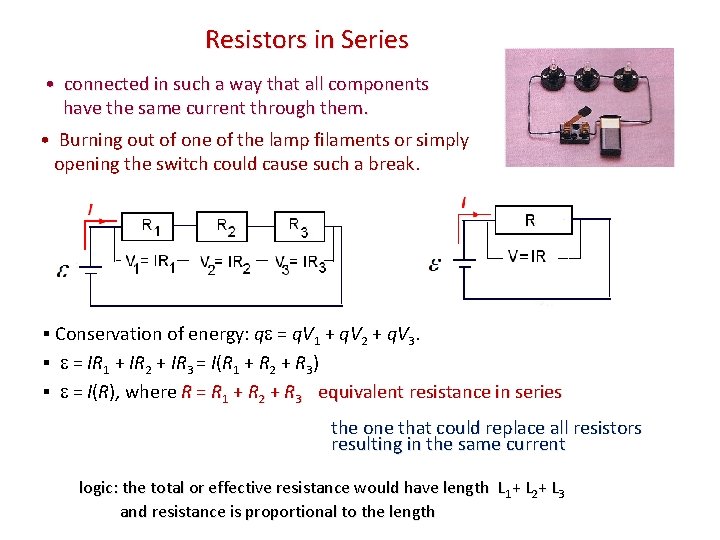
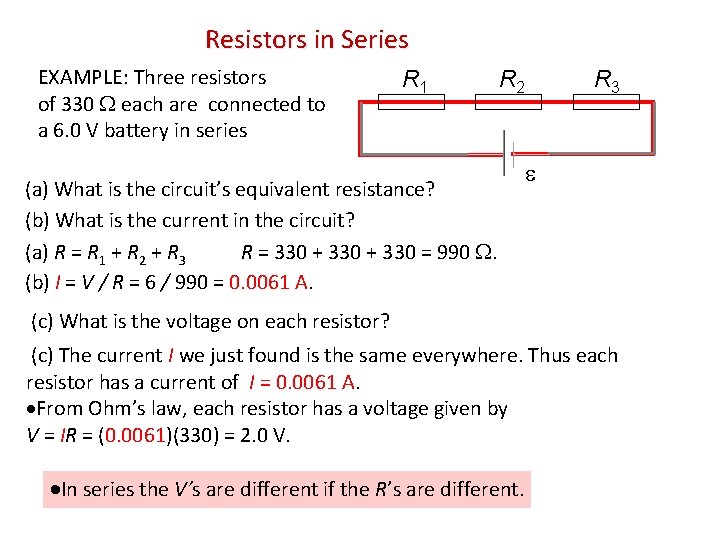
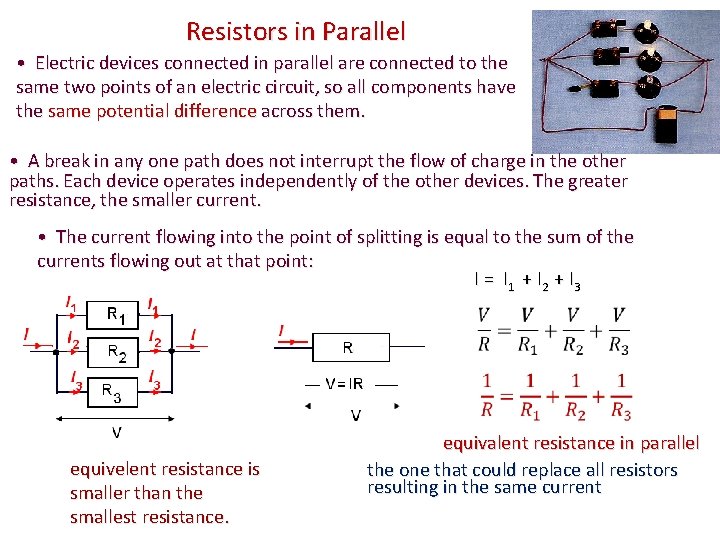
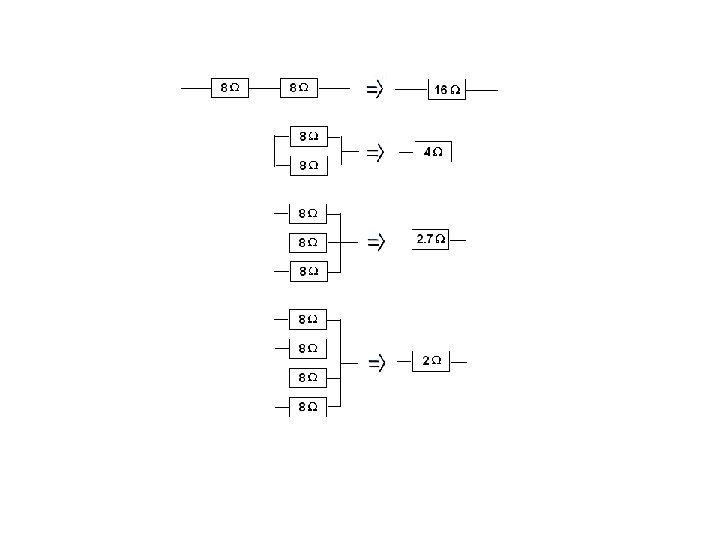
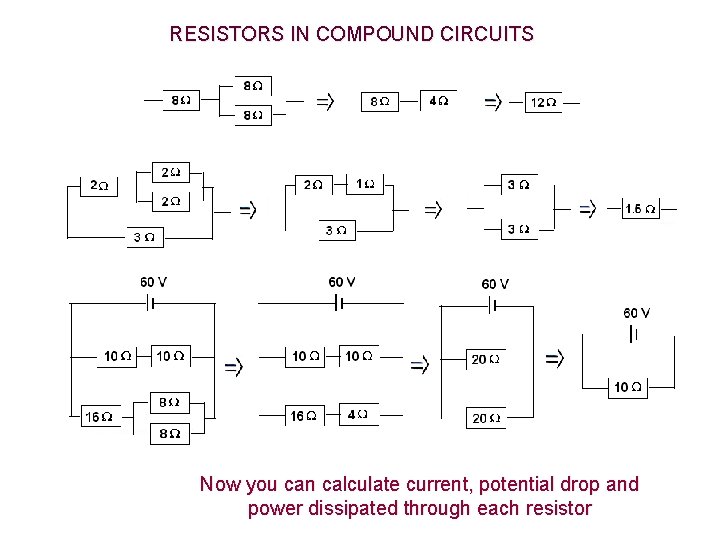
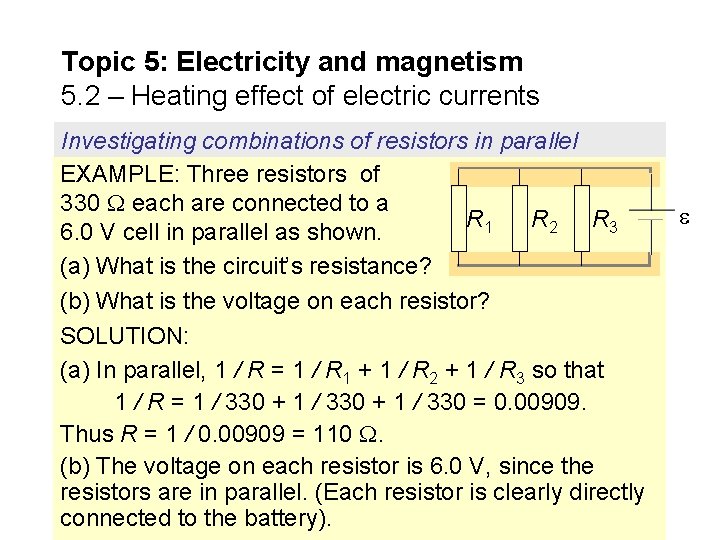
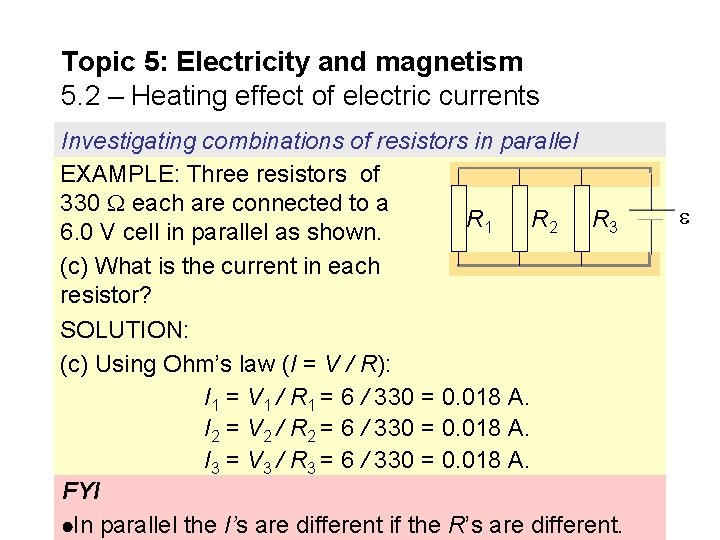
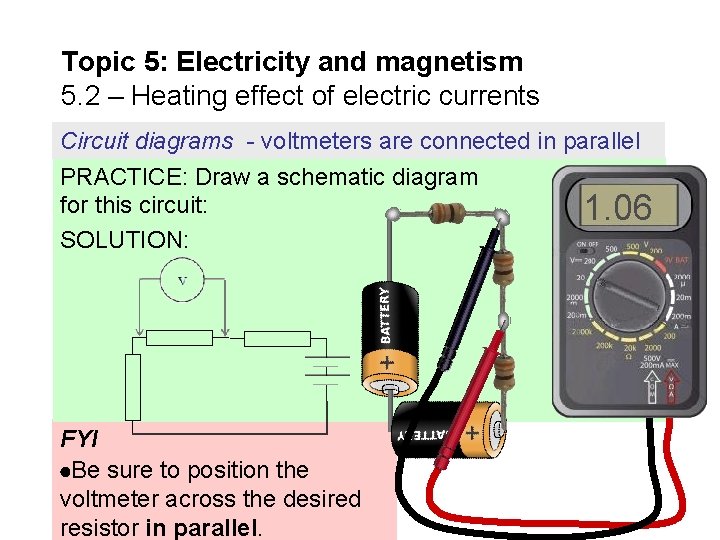
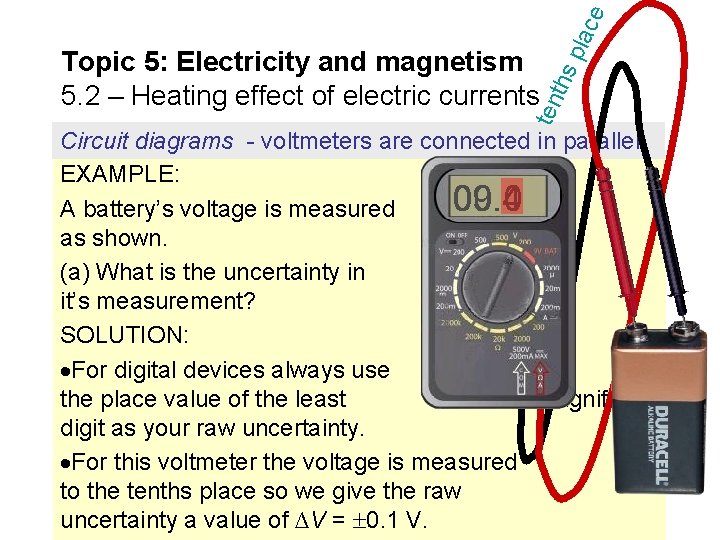
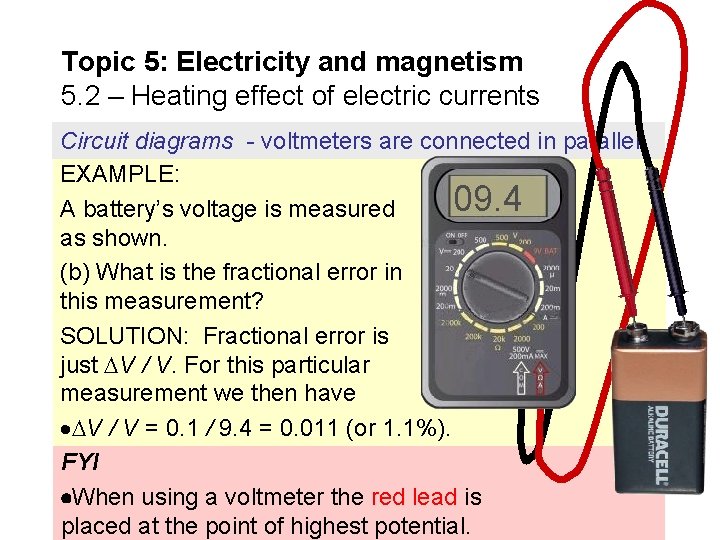
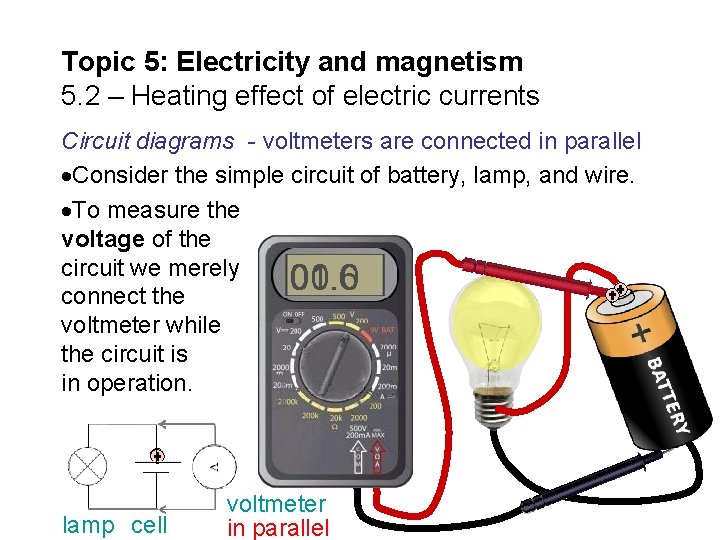
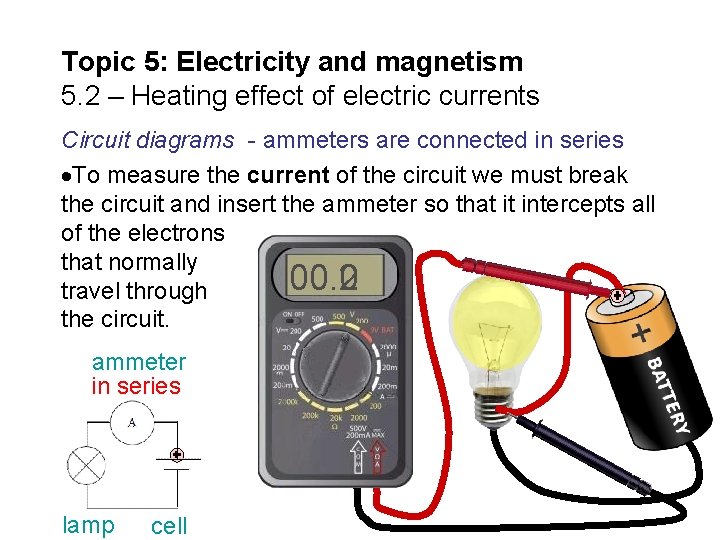
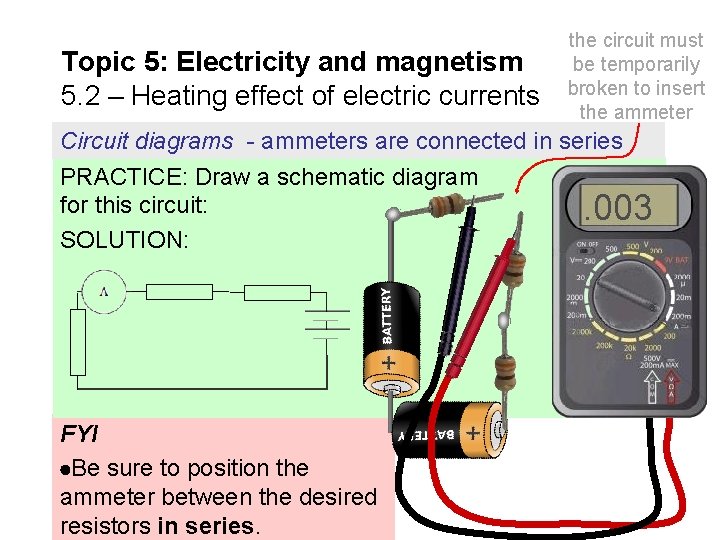
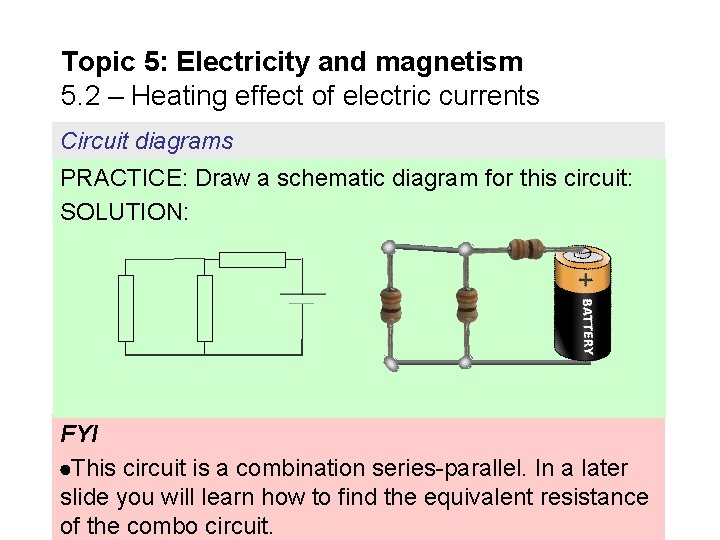
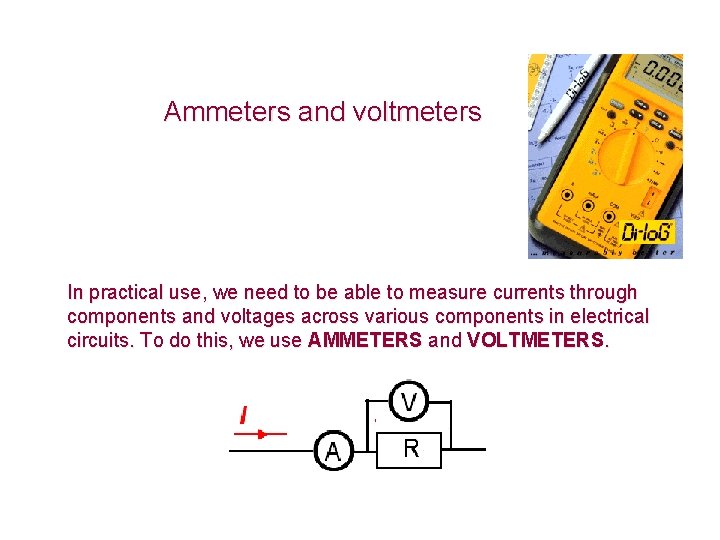
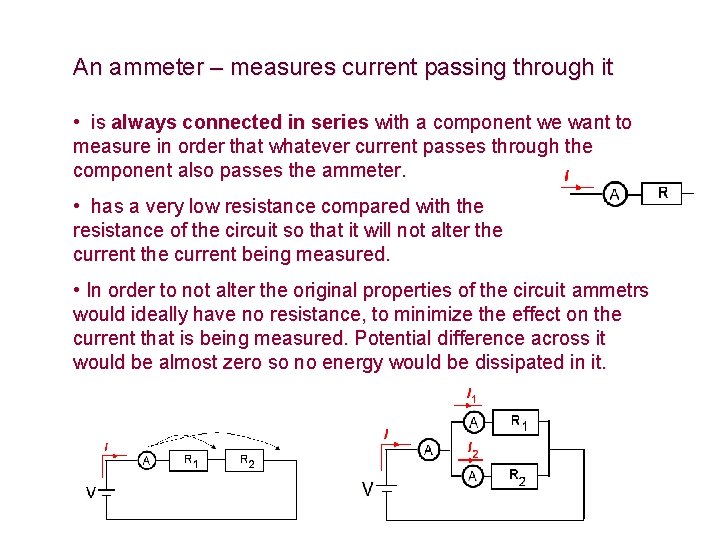
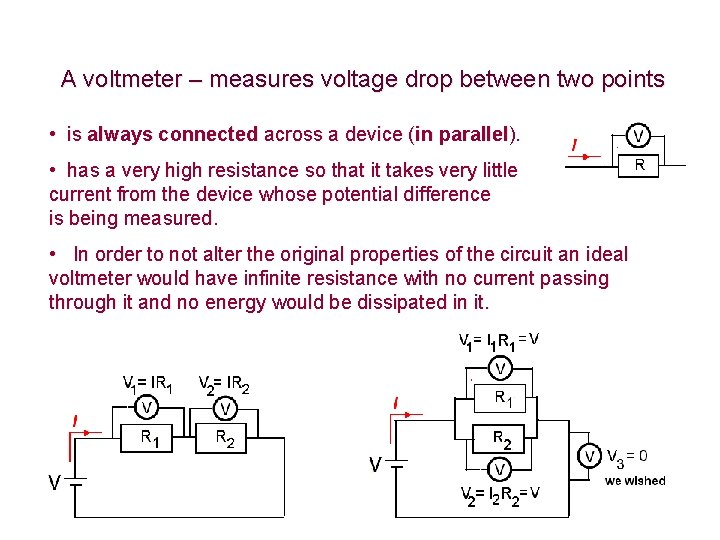
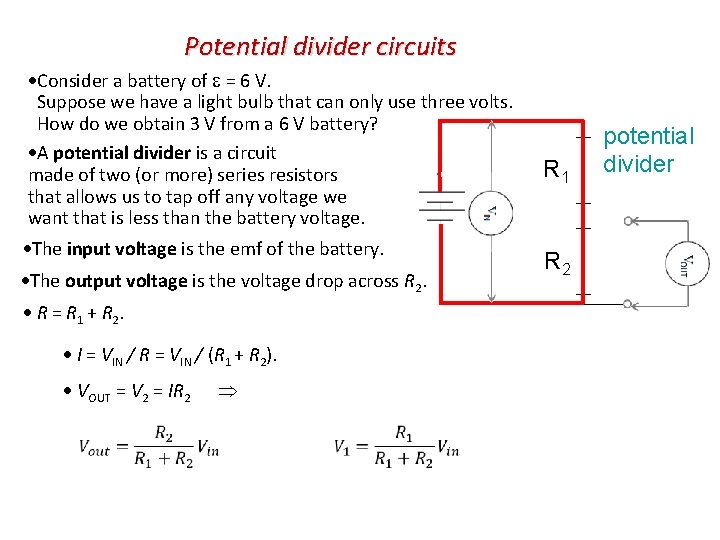
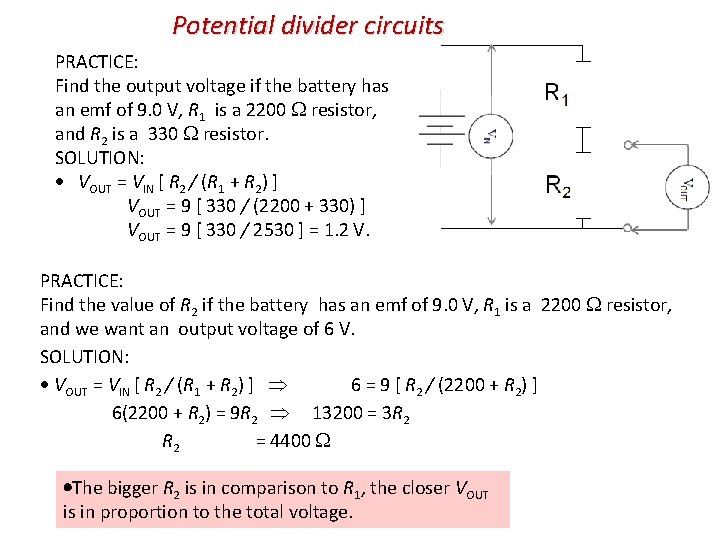
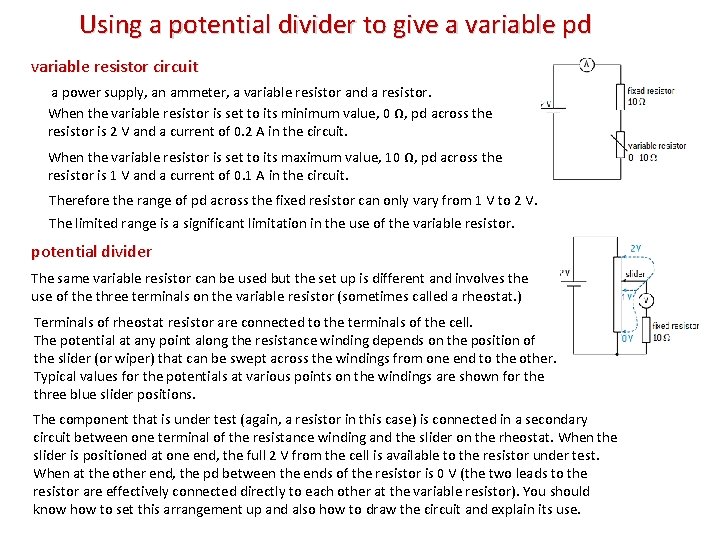
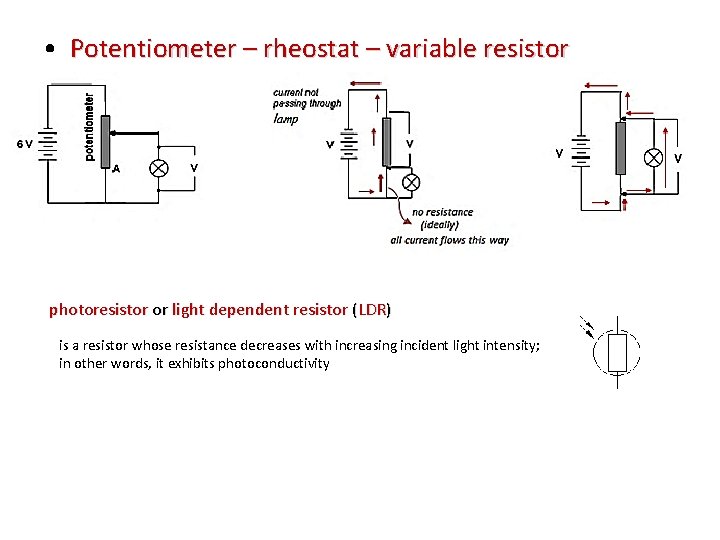
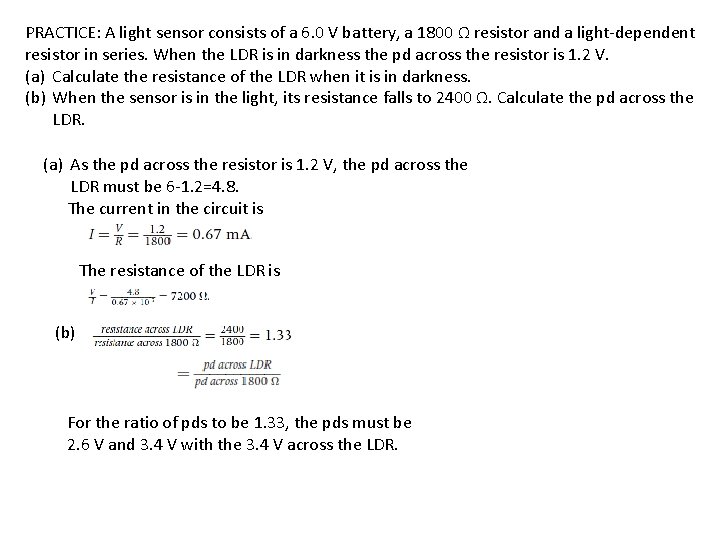
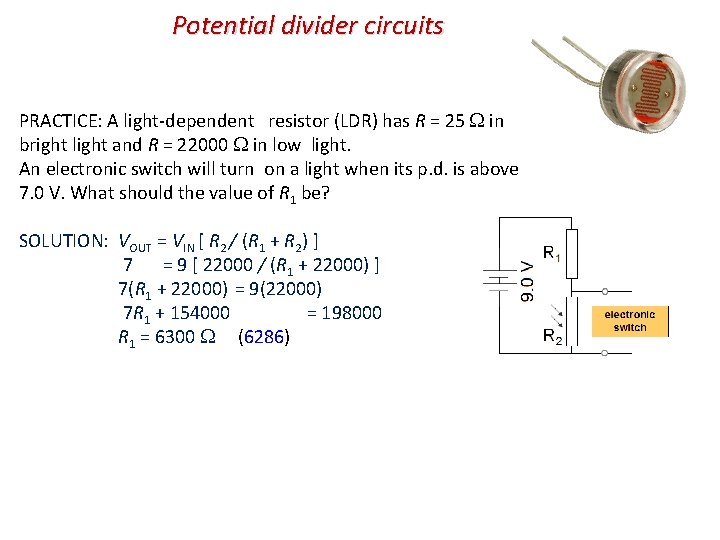
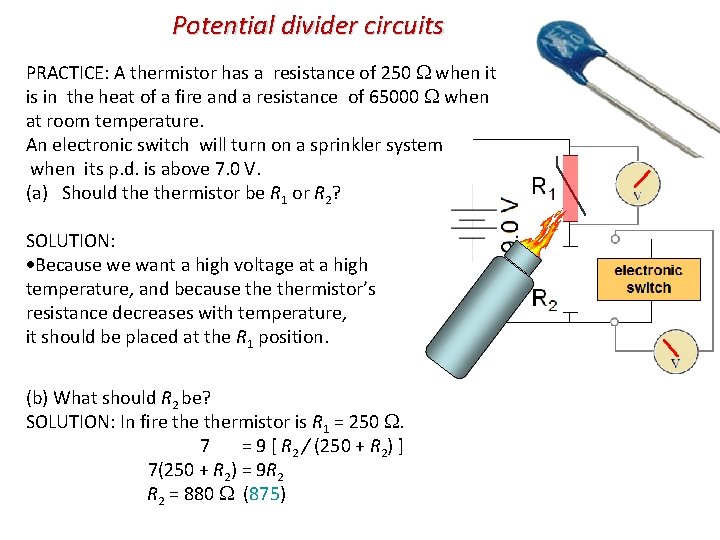
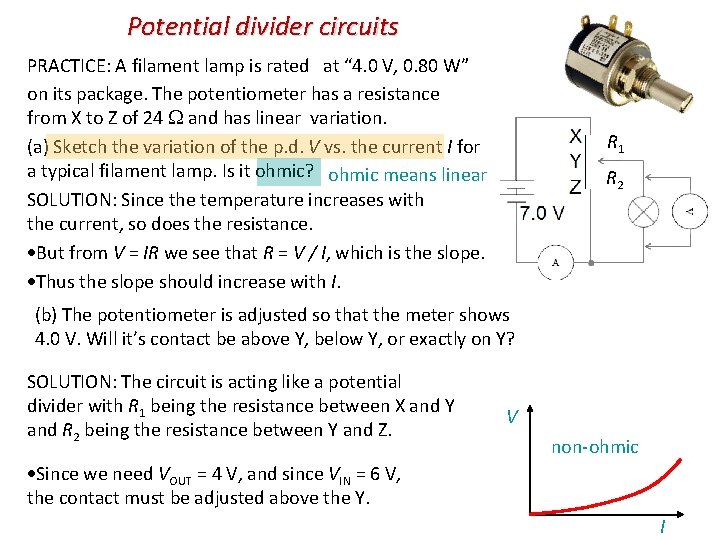
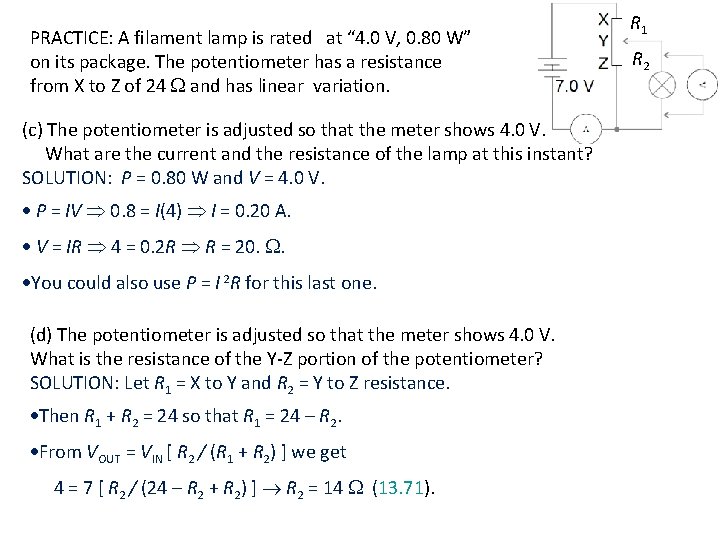
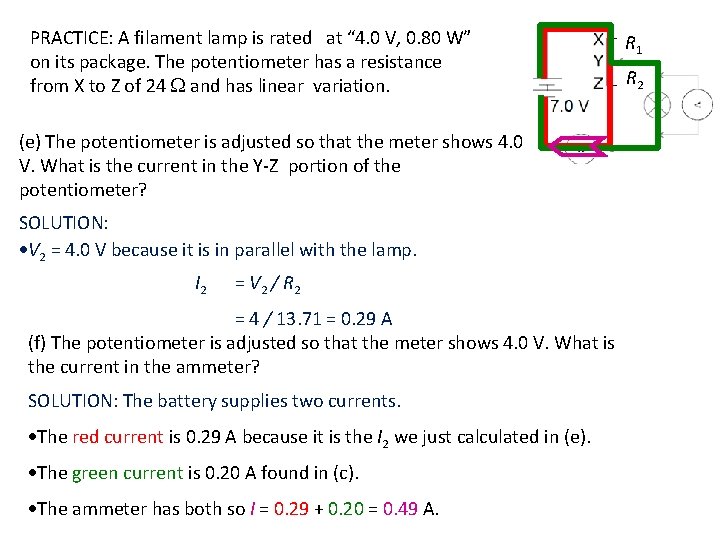
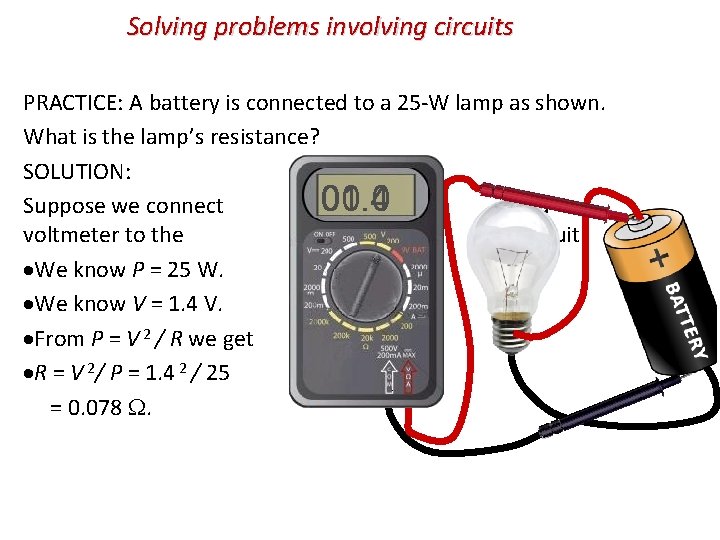
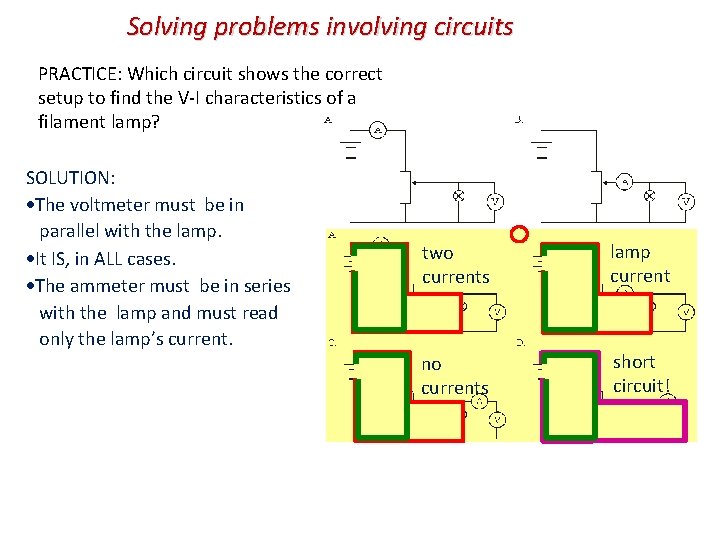
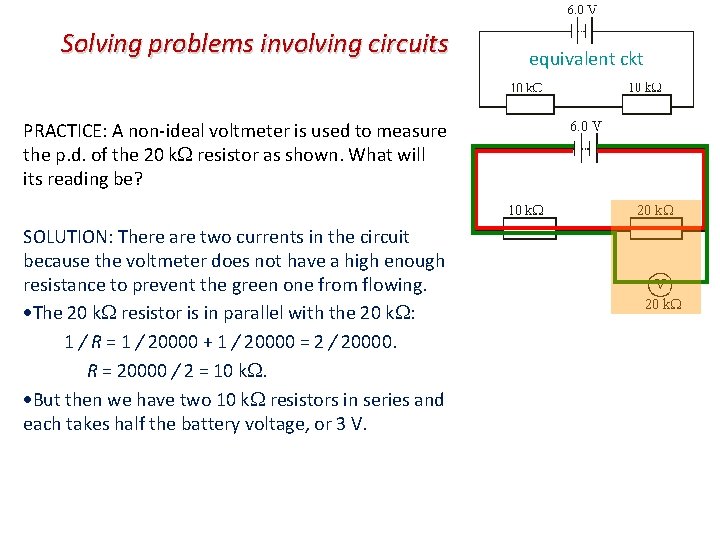
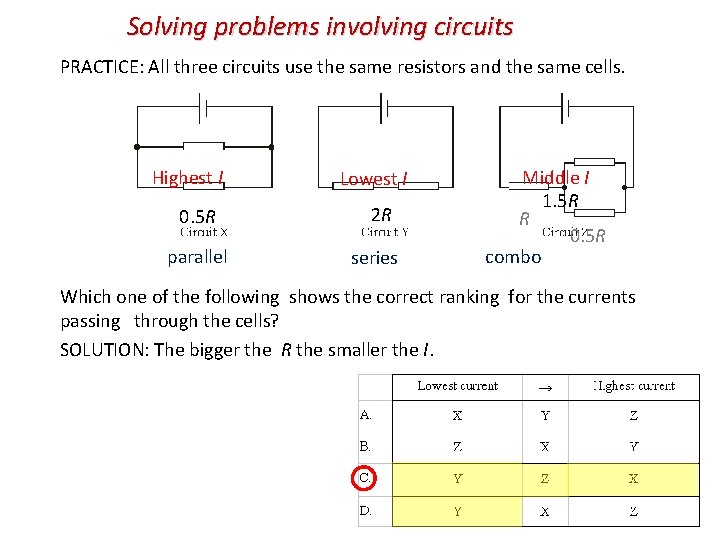
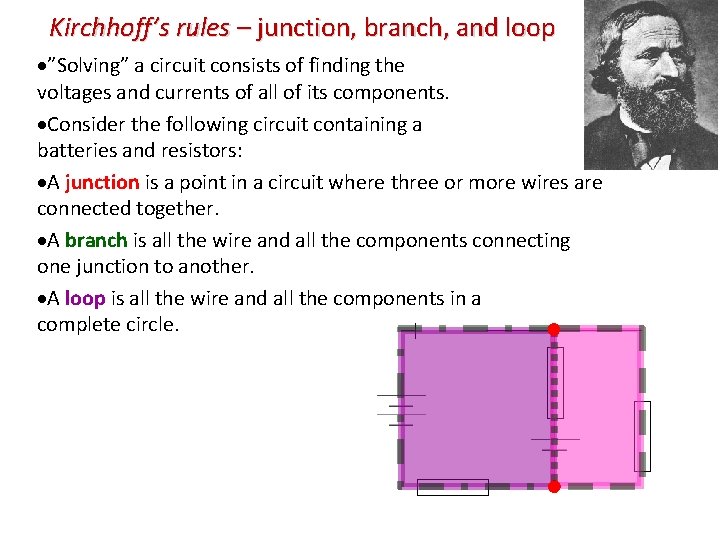
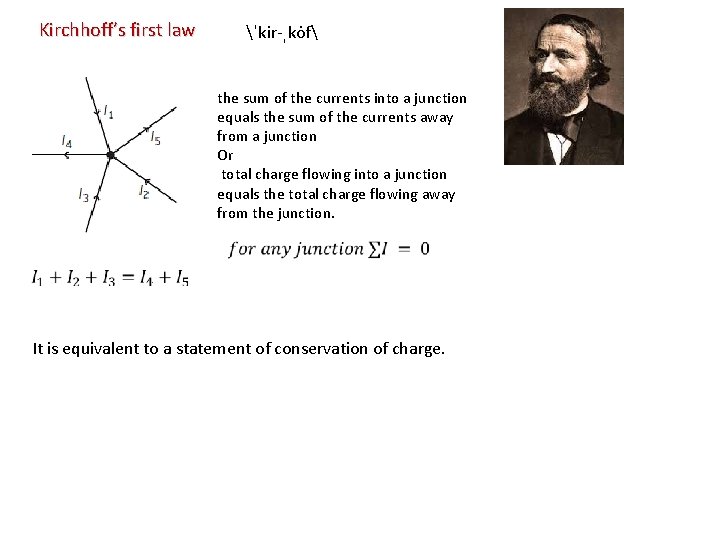
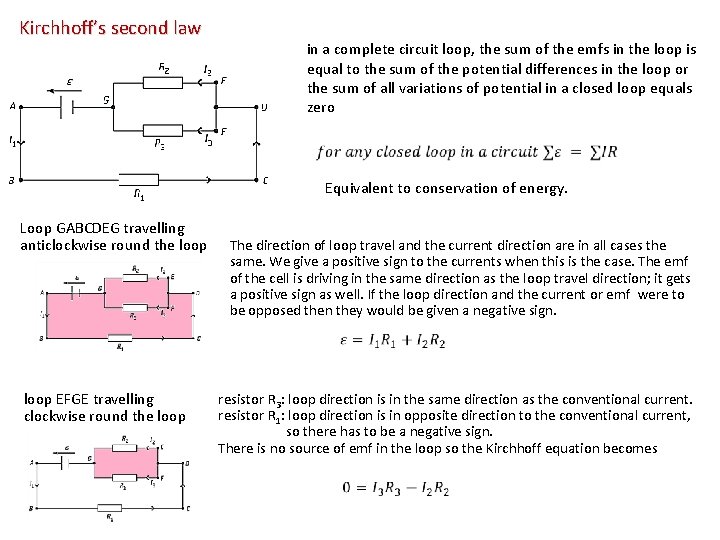
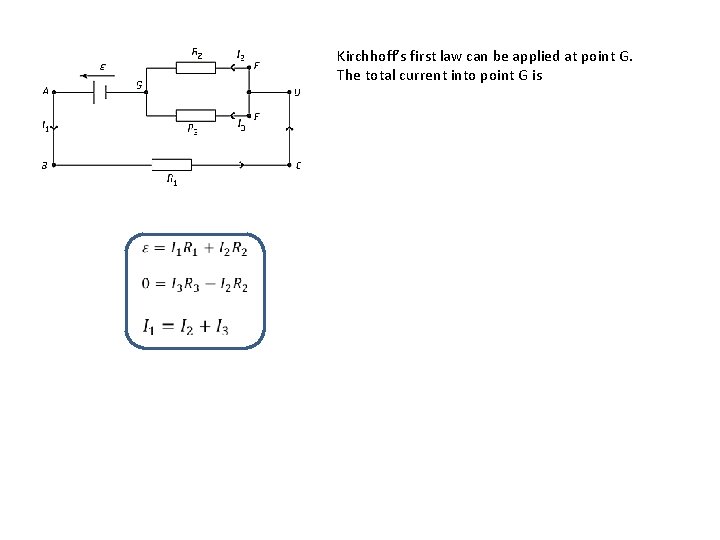
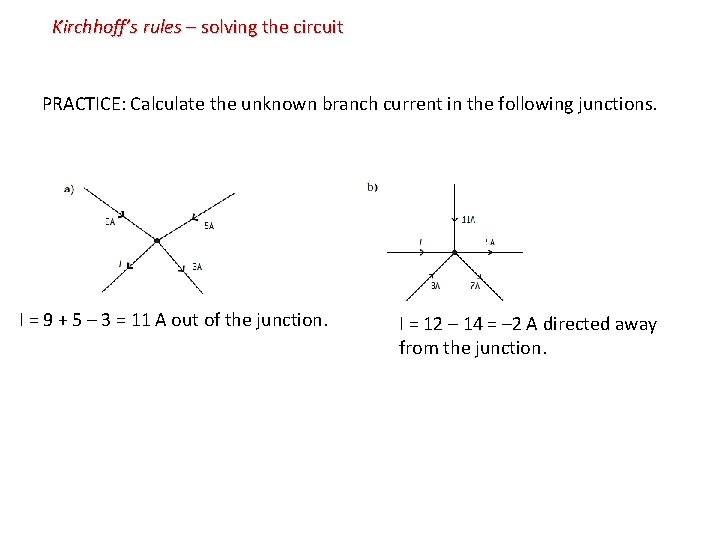
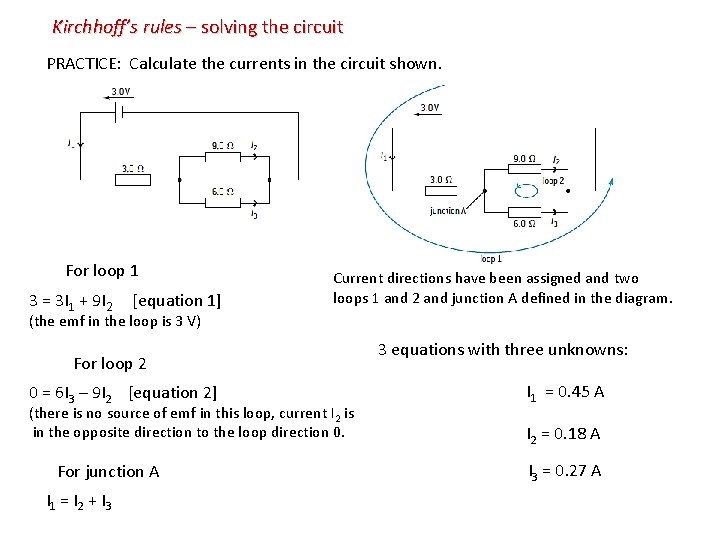
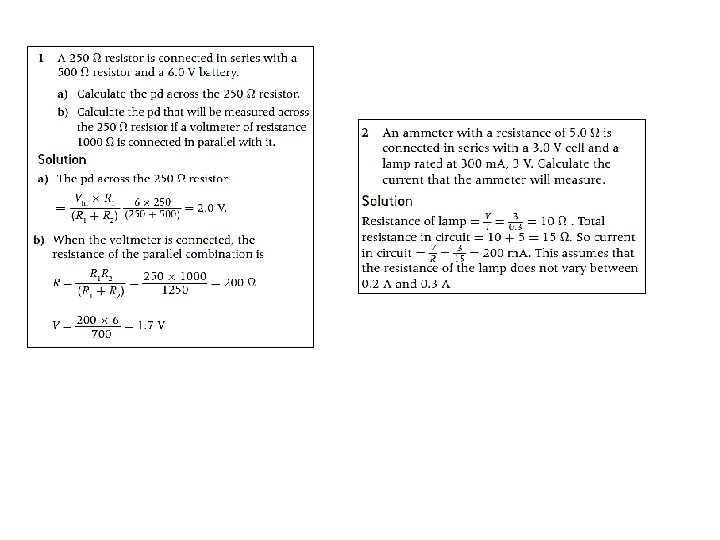
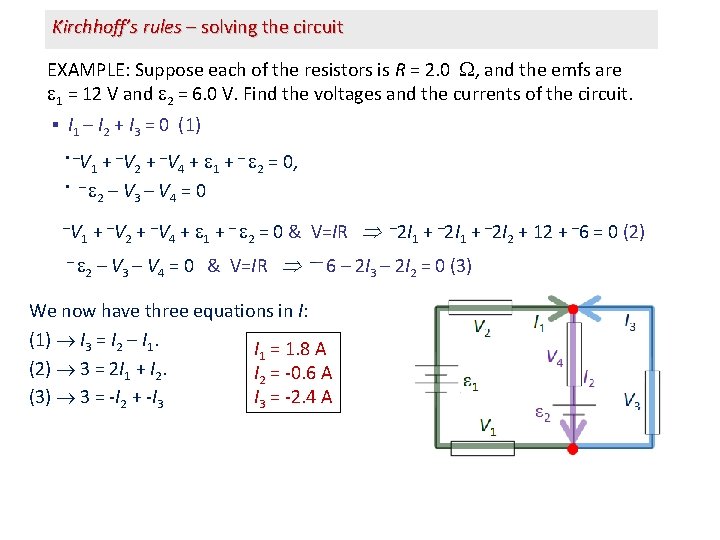
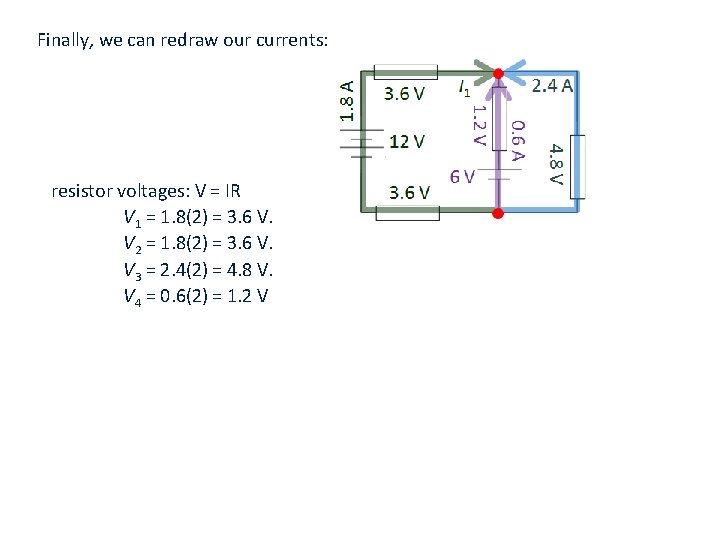
- Slides: 68
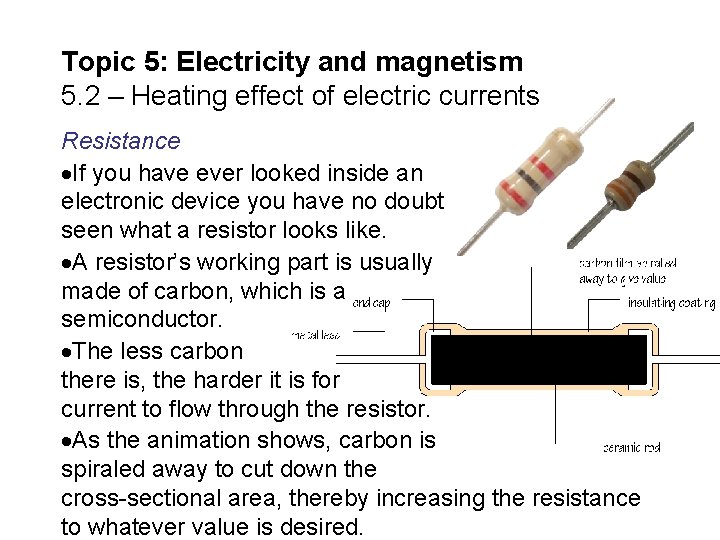
Topic 5: Electricity and magnetism 5. 2 – Heating effect of electric currents Resistance If you have ever looked inside an electronic device you have no doubt seen what a resistor looks like. A resistor’s working part is usually made of carbon, which is a semiconductor. The less carbon there is, the harder it is for current to flow through the resistor. As the animation shows, carbon is spiraled away to cut down the cross-sectional area, thereby increasing the resistance to whatever value is desired.
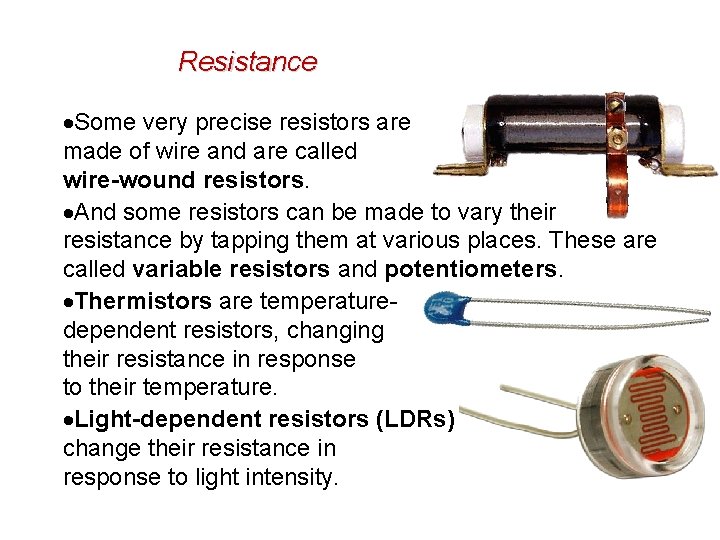
Resistance Some very precise resistors are made of wire and are called wire-wound resistors. And some resistors can be made to vary their resistance by tapping them at various places. These are called variable resistors and potentiometers. Thermistors are temperature- dependent resistors, changing their resistance in response to their temperature. Light-dependent resistors (LDRs) change their resistance in response to light intensity.
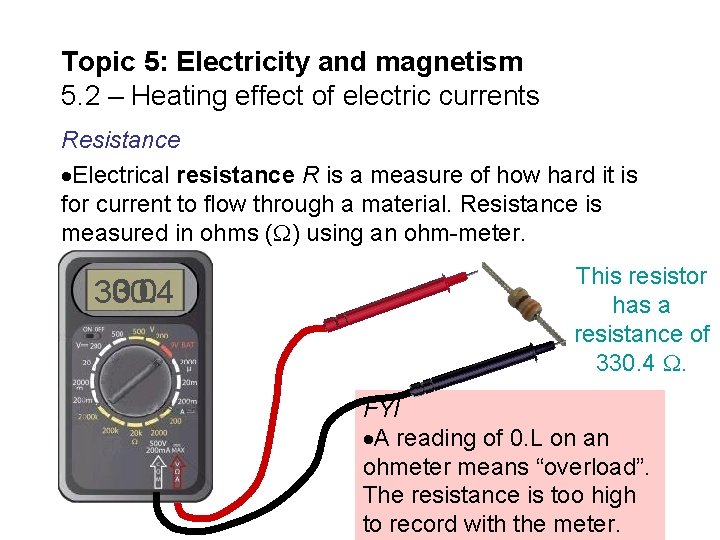
Topic 5: Electricity and magnetism 5. 2 – Heating effect of electric currents Resistance Electrical resistance R is a measure of how hard it is for current to flow through a material. Resistance is measured in ohms ( ) using an ohm-meter. 0. L 0. 0 330. 4 This resistor has a resistance of 330. 4 . FYI A reading of 0. L on an ohmeter means “overload”. The resistance is too high to record with the meter.
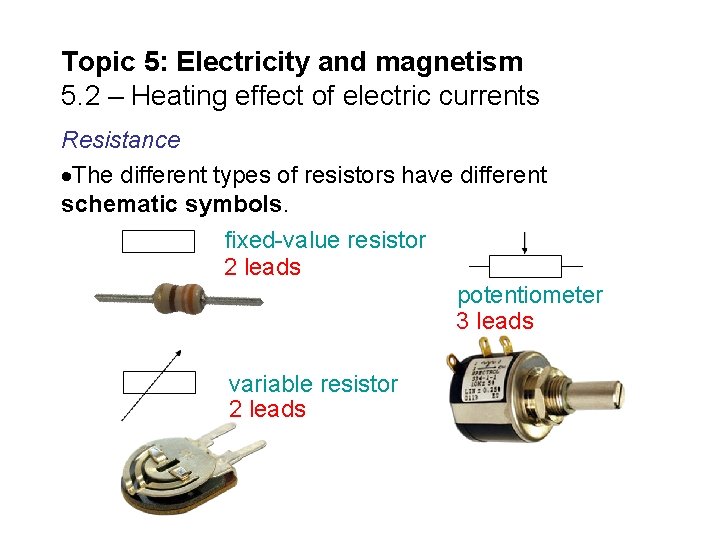
Topic 5: Electricity and magnetism 5. 2 – Heating effect of electric currents Resistance The different types of resistors have different schematic symbols. fixed-value resistor 2 leads potentiometer 3 leads variable resistor 2 leads
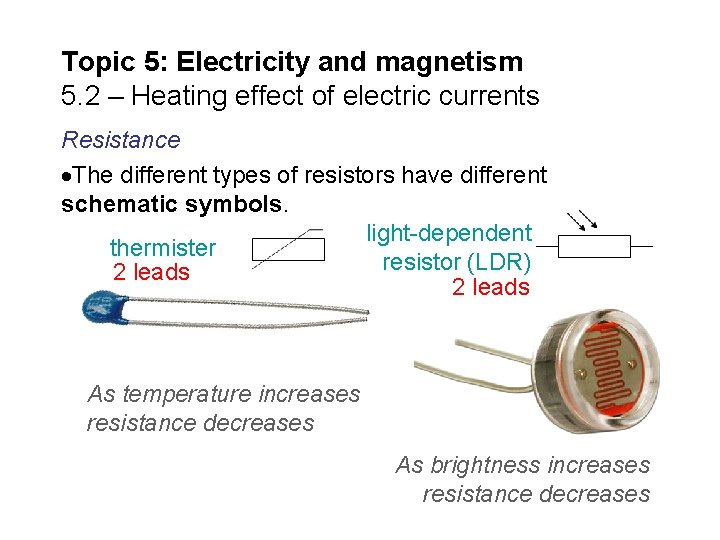
Topic 5: Electricity and magnetism 5. 2 – Heating effect of electric currents Resistance The different types of resistors have different schematic symbols. light-dependent thermister resistor (LDR) 2 leads As temperature increases resistance decreases As brightness increases resistance decreases
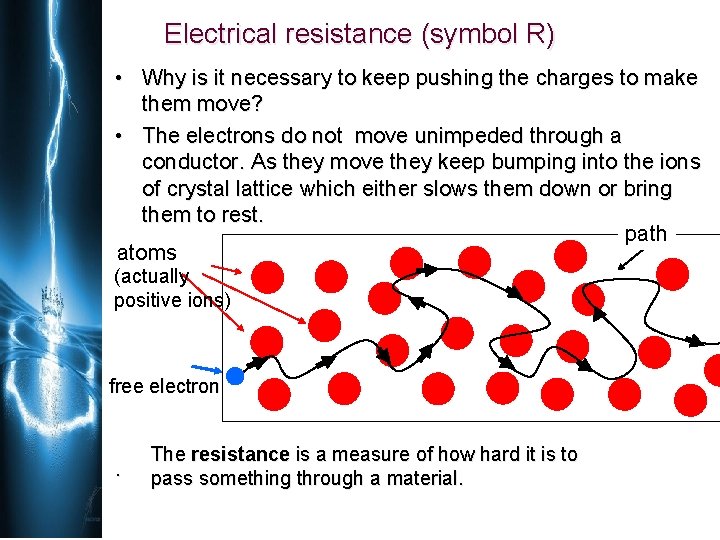
Electrical resistance (symbol R) • Why is it necessary to keep pushing the charges to make them move? • The electrons do not move unimpeded through a conductor. As they move they keep bumping into the ions of crystal lattice which either slows them down or bring them to rest. path atoms (actually positive ions) free electron . The resistance is a measure of how hard it is to pass something through a material.
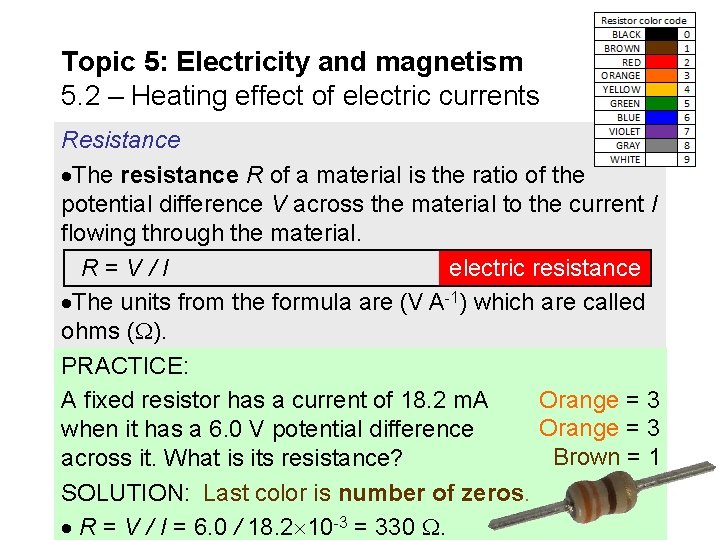
Topic 5: Electricity and magnetism 5. 2 – Heating effect of electric currents Resistance The resistance R of a material is the ratio of the potential difference V across the material to the current I flowing through the material. R = V / I electric resistance The units from the formula are (V A-1) which are called ohms ( ). PRACTICE: Orange = 3 A fixed resistor has a current of 18. 2 m. A Orange = 3 when it has a 6. 0 V potential difference Brown = 1 across it. What is its resistance? SOLUTION: Last color is number of zeros. R = V / I = 6. 0 / 18. 2 10 -3 = 330 .
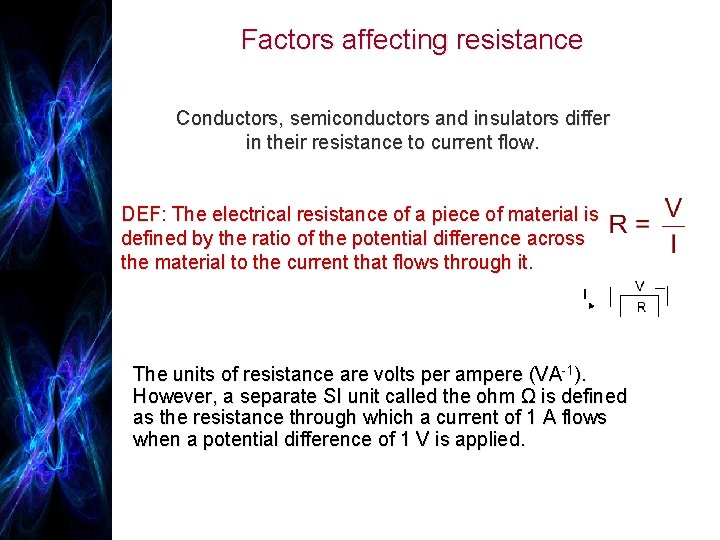
Factors affecting resistance Conductors, semiconductors and insulators differ in their resistance to current flow. DEF: The electrical resistance of a piece of material is defined by the ratio of the potential difference across the material to the current that flows through it. The units of resistance are volts per ampere (VA-1). However, a separate SI unit called the ohm Ω is defined as the resistance through which a current of 1 A flows when a potential difference of 1 V is applied.
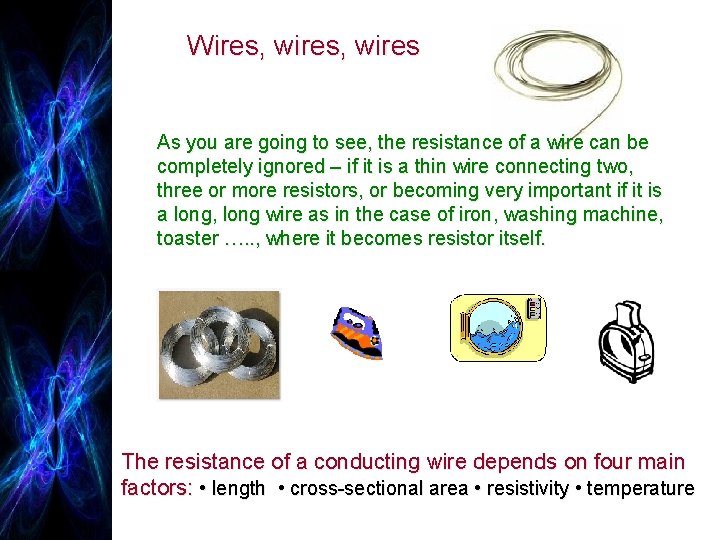
Wires, wires As you are going to see, the resistance of a wire can be completely ignored – if it is a thin wire connecting two, three or more resistors, or becoming very important if it is a long, long wire as in the case of iron, washing machine, toaster …. . , where it becomes resistor itself. The resistance of a conducting wire depends on four main factors: • length • cross-sectional area • resistivity • temperature
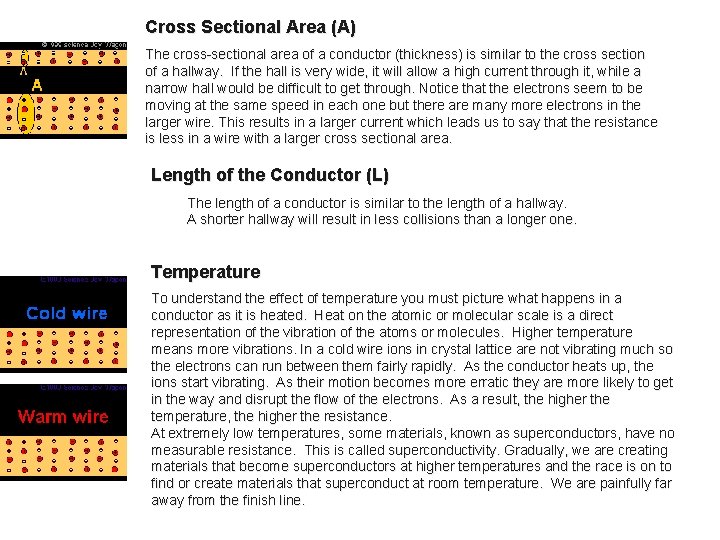
Cross Sectional Area (A) The cross-sectional area of a conductor (thickness) is similar to the cross section of a hallway. If the hall is very wide, it will allow a high current through it, while a narrow hall would be difficult to get through. Notice that the electrons seem to be moving at the same speed in each one but there are many more electrons in the larger wire. This results in a larger current which leads us to say that the resistance is less in a wire with a larger cross sectional area. Length of the Conductor (L) The length of a conductor is similar to the length of a hallway. A shorter hallway will result in less collisions than a longer one. Temperature To understand the effect of temperature you must picture what happens in a conductor as it is heated. Heat on the atomic or molecular scale is a direct representation of the vibration of the atoms or molecules. Higher temperature means more vibrations. In a cold wire ions in crystal lattice are not vibrating much so the electrons can run between them fairly rapidly. As the conductor heats up, the ions start vibrating. As their motion becomes more erratic they are more likely to get in the way and disrupt the flow of the electrons. As a result, the higher the temperature, the higher the resistance. At extremely low temperatures, some materials, known as superconductors, have no measurable resistance. This is called superconductivity. Gradually, we are creating materials that become superconductors at higher temperatures and the race is on to find or create materials that superconduct at room temperature. We are painfully far away from the finish line.
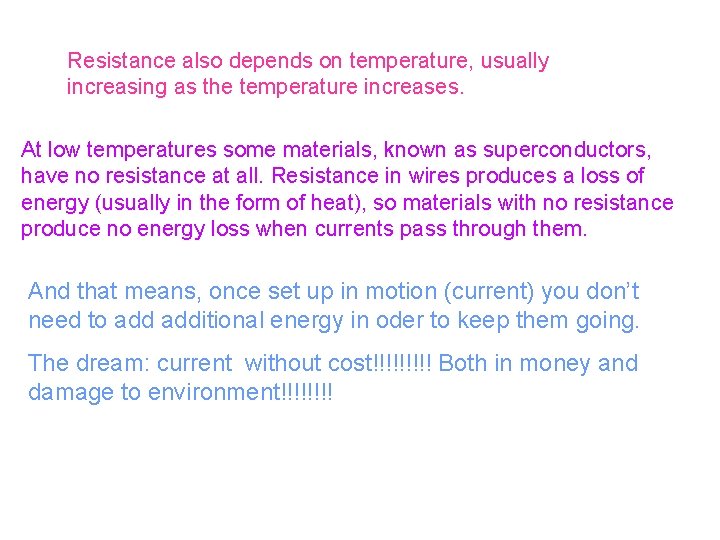
Resistance also depends on temperature, usually increasing as the temperature increases. At low temperatures some materials, known as superconductors, have no resistance at all. Resistance in wires produces a loss of energy (usually in the form of heat), so materials with no resistance produce no energy loss when currents pass through them. And that means, once set up in motion (current) you don’t need to additional energy in oder to keep them going. The dream: current without cost!!!!! Both in money and damage to environment!!!!
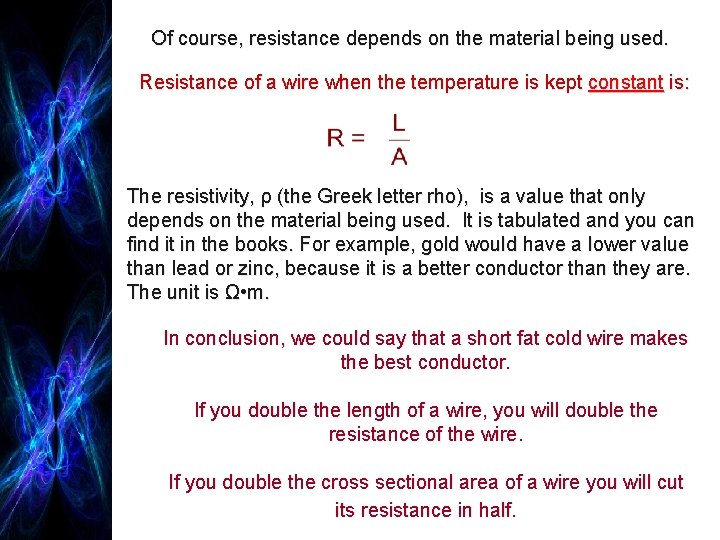
Of course, resistance depends on the material being used. Resistance of a wire when the temperature is kept constant is: The resistivity, ρ (the Greek letter rho), is a value that only depends on the material being used. It is tabulated and you can find it in the books. For example, gold would have a lower value than lead or zinc, because it is a better conductor than they are. The unit is Ω • m. In conclusion, we could say that a short fat cold wire makes the best conductor. If you double the length of a wire, you will double the resistance of the wire. If you double the cross sectional area of a wire you will cut its resistance in half.
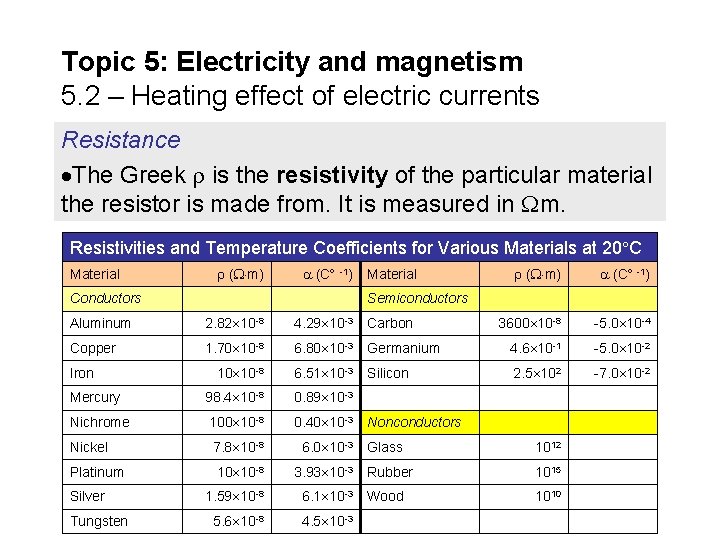
Topic 5: Electricity and magnetism 5. 2 – Heating effect of electric currents Resistance The Greek is the resistivity of the particular material the resistor is made from. It is measured in m. Resistivities and Temperature Coefficients for Various Materials at 20 C Material ( m) (C -1) Conductors Material ( m) (C -1) 3600 10 -8 -5. 0 10 -4 Semiconductors Aluminum 2. 82 10 -8 4. 29 10 -3 Carbon Copper 1. 70 10 -8 6. 80 10 -3 Germanium 4. 6 10 -1 -5. 0 10 -2 10 10 -8 6. 51 10 -3 Silicon 2. 5 102 -7. 0 10 -2 Mercury 98. 4 10 -8 0. 89 10 -3 Nichrome 100 10 -8 0. 40 10 -3 Nickel 7. 8 10 -8 6. 0 10 -3 Platinum 10 10 -8 3. 93 10 -3 1. 59 10 -8 6. 1 10 -3 5. 6 10 -8 4. 5 10 -3 Iron Silver Tungsten Nonconductors Glass 1012 Rubber 1015 Wood 1010
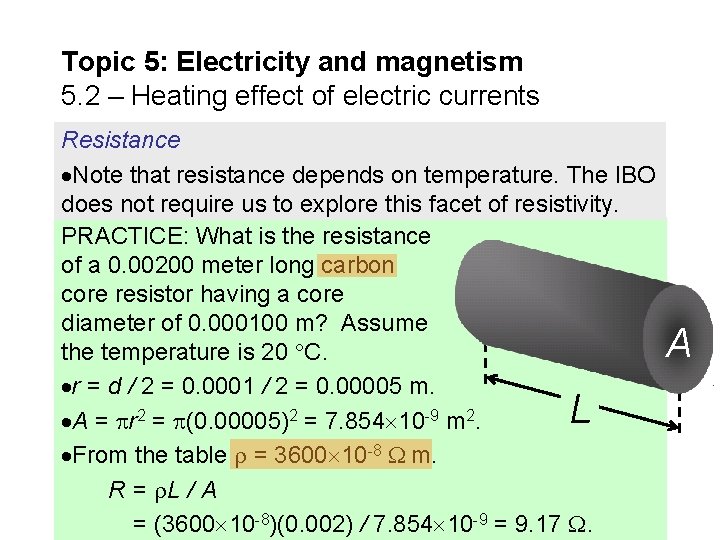
Topic 5: Electricity and magnetism 5. 2 – Heating effect of electric currents Resistance Note that resistance depends on temperature. The IBO does not require us to explore this facet of resistivity. PRACTICE: What is the resistance of a 0. 00200 meter long carbon core resistor having a core diameter of 0. 000100 m? Assume the temperature is 20 C. r = d / 2 = 0. 0001 / 2 = 0. 00005 m. A = r 2 = (0. 00005)2 = 7. 854 10 -9 m 2. From the table = 3600 10 -8 m. R = L / A = (3600 10 -8)(0. 002) / 7. 854 10 -9 = 9. 17 . A L
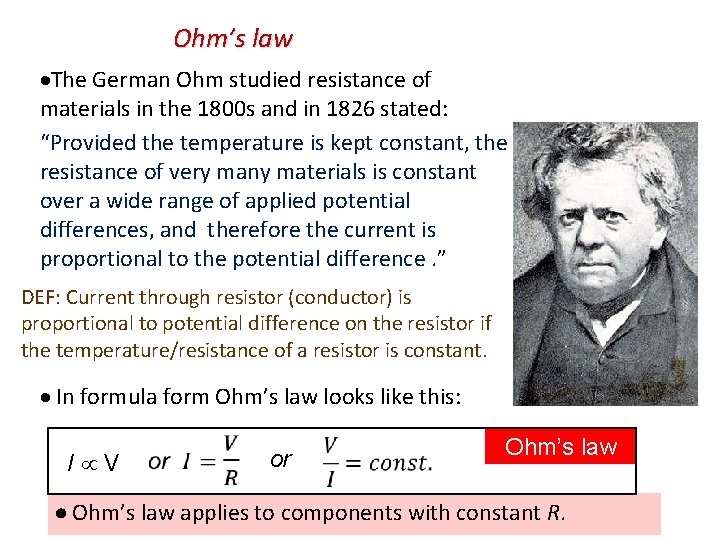
Ohm’s law The German Ohm studied resistance of materials in the 1800 s and in 1826 stated: “Provided the temperature is kept constant, the resistance of very many materials is constant over a wide range of applied potential differences, and therefore the current is proportional to the potential difference. ” DEF: Current through resistor (conductor) is proportional to potential difference on the resistor if the temperature/resistance of a resistor is constant. In formula form Ohm’s law looks like this: I V or Ohm’s law applies to components with constant R.
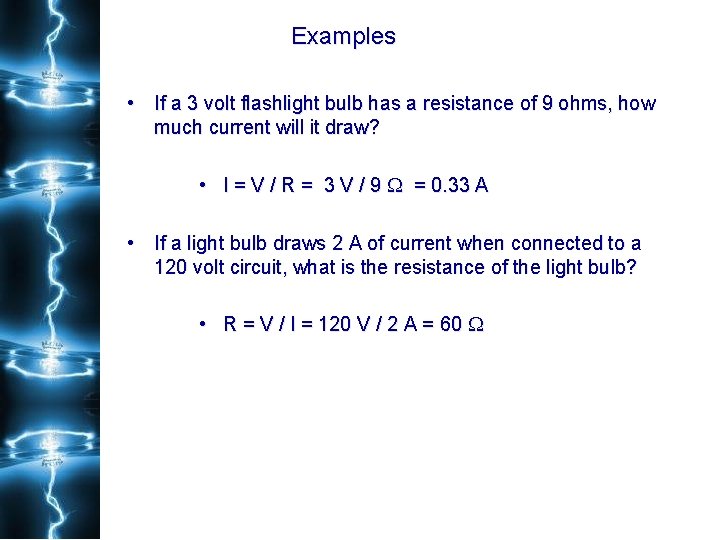
Examples • If a 3 volt flashlight bulb has a resistance of 9 ohms, how much current will it draw? • I = V / R = 3 V / 9 = 0. 33 A • If a light bulb draws 2 A of current when connected to a 120 volt circuit, what is the resistance of the light bulb? • R = V / I = 120 V / 2 A = 60
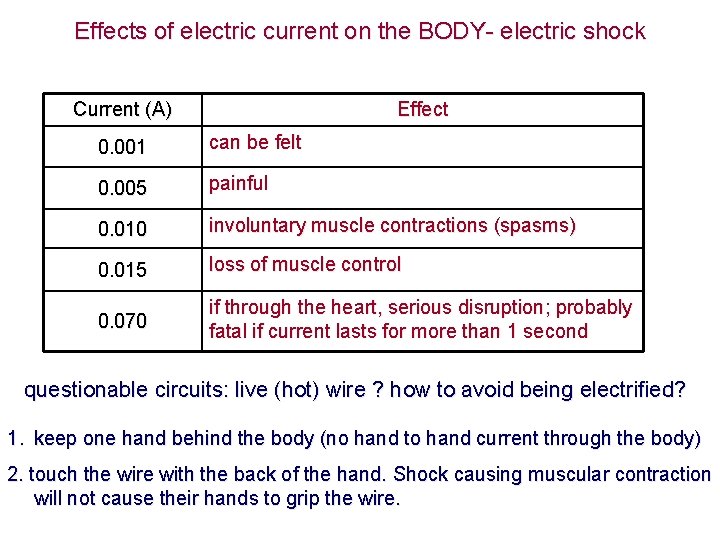
Effects of electric current on the BODY- electric shock Current (A) Effect 0. 001 can be felt 0. 005 painful 0. 010 involuntary muscle contractions (spasms) 0. 015 loss of muscle control 0. 070 if through the heart, serious disruption; probably fatal if current lasts for more than 1 second questionable circuits: live (hot) wire ? how to avoid being electrified? 1. keep one hand behind the body (no hand to hand current through the body) 2. touch the wire with the back of the hand. Shock causing muscular contraction will not cause their hands to grip the wire.
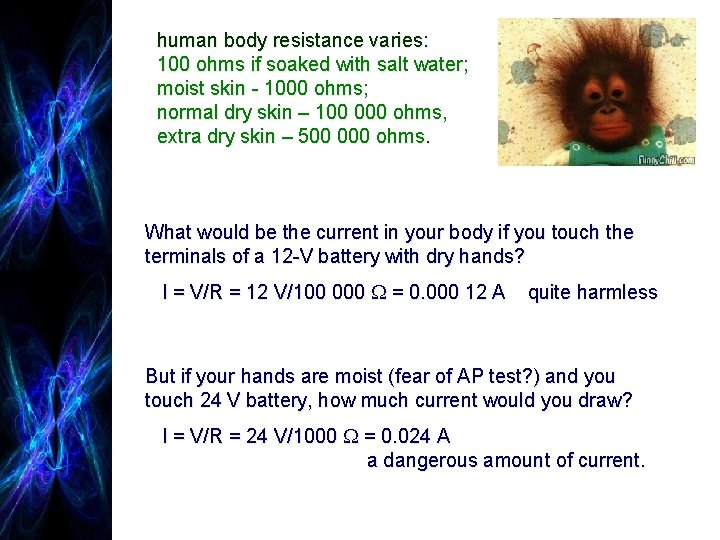
human body resistance varies: 100 ohms if soaked with salt water; moist skin - 1000 ohms; normal dry skin – 100 000 ohms, extra dry skin – 500 000 ohms. What would be the current in your body if you touch the terminals of a 12 -V battery with dry hands? I = V/R = 12 V/100 000 = 0. 000 12 A quite harmless But if your hands are moist (fear of AP test? ) and you touch 24 V battery, how much current would you draw? I = V/R = 24 V/1000 = 0. 024 A a dangerous amount of current.
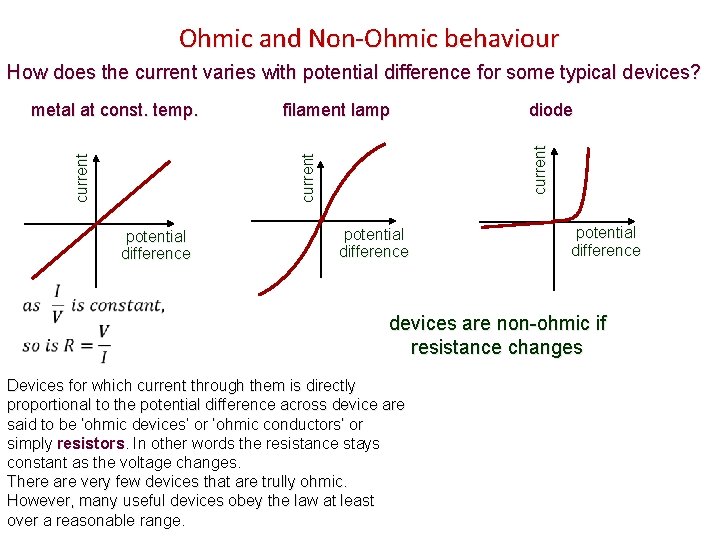
Ohmic and Non-Ohmic behaviour How does the current varies with potential difference for some typical devices? current metal at const. temp. filament lamp diode potential difference devices are non-ohmic if resistance changes Devices for which current through them is directly proportional to the potential difference across device are said to be ‘ohmic devices’ or ‘ohmic conductors’ or simply resistors. In other words the resistance stays . constant as the voltage changes. There are very few devices that are trully ohmic. However, many useful devices obey the law at least over a reasonable range.
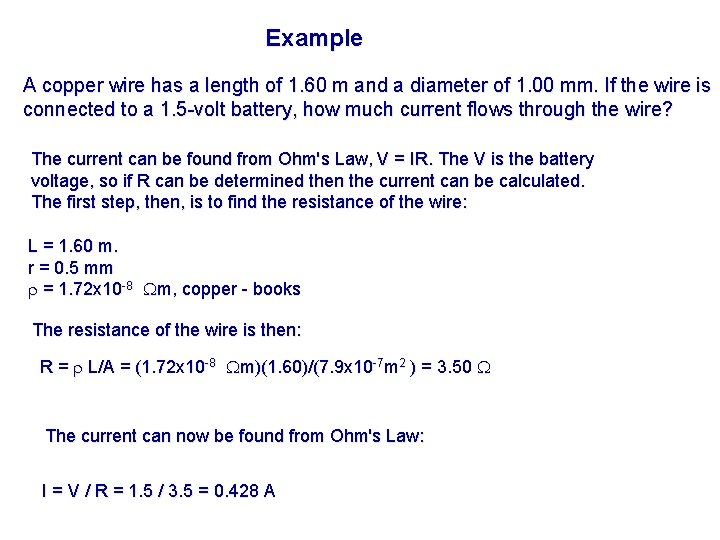
Example A copper wire has a length of 1. 60 m and a diameter of 1. 00 mm. If the wire is connected to a 1. 5 -volt battery, how much current flows through the wire? The current can be found from Ohm's Law, V = IR. The V is the battery voltage, so if R can be determined then the current can be calculated. The first step, then, is to find the resistance of the wire: L = 1. 60 m. r = 0. 5 mm = 1. 72 x 10 -8 m, copper - books The resistance of the wire is then: R = L/A = (1. 72 x 10 -8 m)(1. 60)/(7. 9 x 10 -7 m 2 ) = 3. 50 The current can now be found from Ohm's Law: I = V / R = 1. 5 / 3. 5 = 0. 428 A
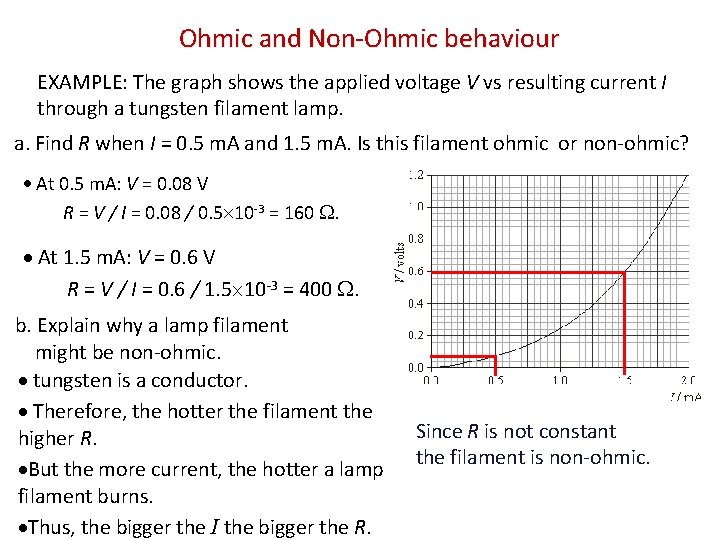
Ohmic and Non-Ohmic behaviour EXAMPLE: The graph shows the applied voltage V vs resulting current I through a tungsten filament lamp. a. Find R when I = 0. 5 m. A and 1. 5 m. A. Is this filament ohmic or non-ohmic? At 0. 5 m. A: V = 0. 08 V R = V / I = 0. 08 / 0. 5 10 -3 = 160 . At 1. 5 m. A: V = 0. 6 V R = V / I = 0. 6 / 1. 5 10 -3 = 400 . b. Explain why a lamp filament might be non-ohmic. tungsten is a conductor. Therefore, the hotter the filament the higher R. But the more current, the hotter a lamp filament burns. Thus, the bigger the I the bigger the R. Since R is not constant the filament is non-ohmic.
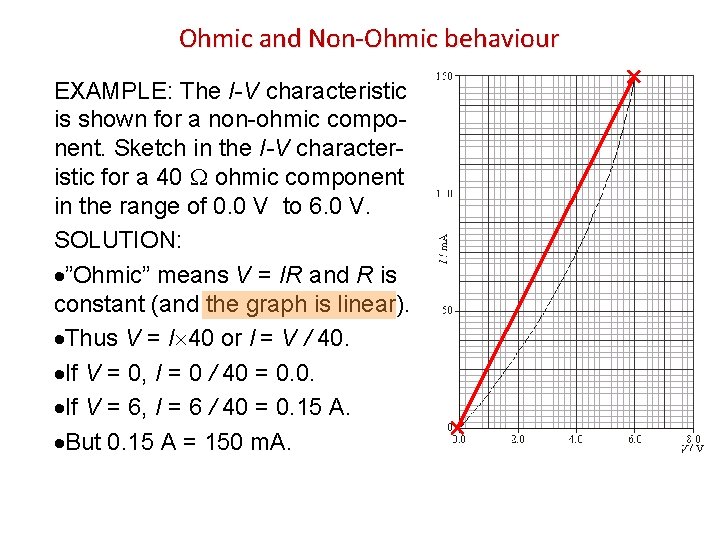
Ohmic and Non-Ohmic behaviour EXAMPLE: The I-V characteristic is shown for a non-ohmic compo- nent. Sketch in the I-V character- istic for a 40 ohmic component in the range of 0. 0 V to 6. 0 V. SOLUTION: ”Ohmic” means V = IR and R is constant (and the graph is linear). Thus V = I 40 or I = V / 40. If V = 0, I = 0 / 40 = 0. 0. If V = 6, I = 6 / 40 = 0. 15 A. But 0. 15 A = 150 m. A.
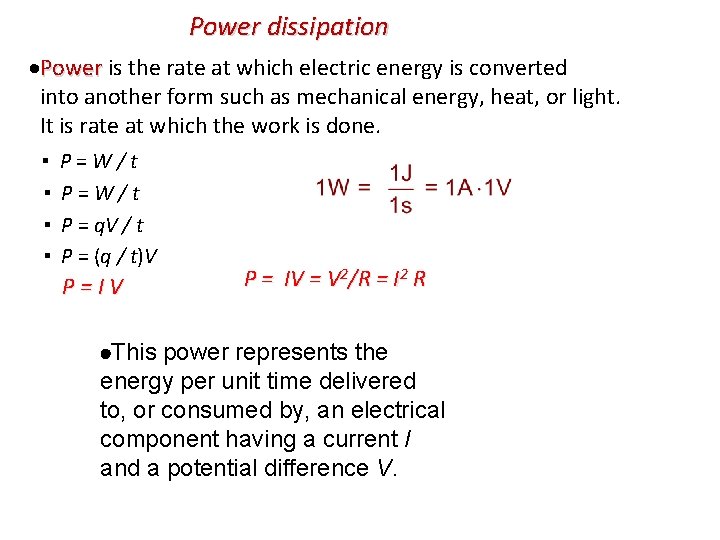
Power dissipation Power is the rate at which electric energy is converted into another form such as mechanical energy, heat, or light. It is rate at which the work is done. ▪ ▪ P=W/t P = q. V / t P = (q / t)V P=IV P = IV = V 2/R = I 2 R This power represents the energy per unit time delivered to, or consumed by, an electrical component having a current I and a potential difference V.
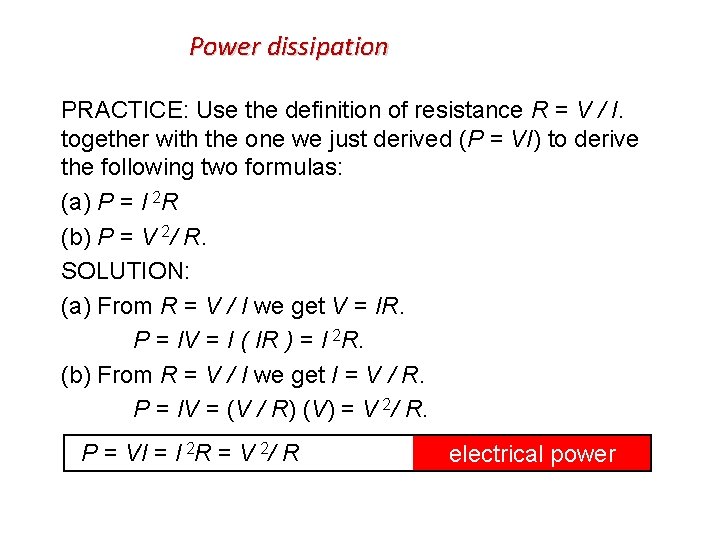
Power dissipation PRACTICE: Use the definition of resistance R = V / I. together with the one we just derived (P = VI) to derive the following two formulas: (a) P = I 2 R (b) P = V 2/ R. SOLUTION: (a) From R = V / I we get V = IR. P = IV = I ( IR ) = I 2 R. (b) From R = V / I we get I = V / R. P = IV = (V / R) (V) = V 2/ R. P = VI = I 2 R = V 2/ R electrical power
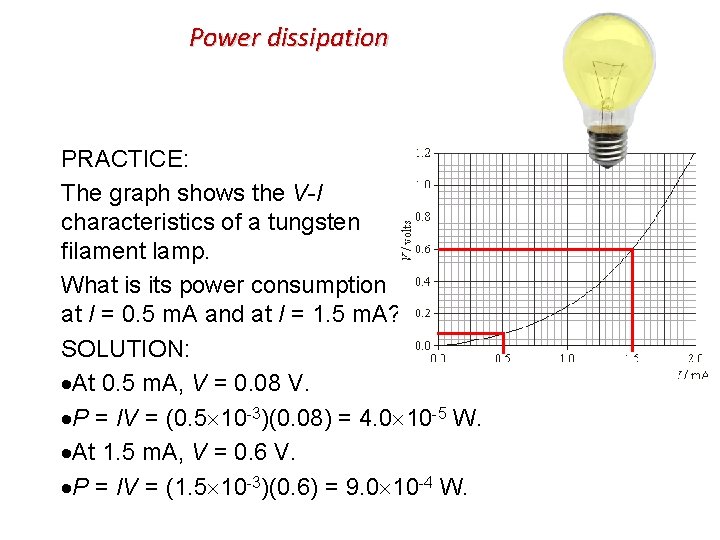
Power dissipation PRACTICE: The graph shows the V-I characteristics of a tungsten filament lamp. What is its power consumption at I = 0. 5 m. A and at I = 1. 5 m. A? SOLUTION: At 0. 5 m. A, V = 0. 08 V. P = IV = (0. 5 10 -3)(0. 08) = 4. 0 10 -5 W. At 1. 5 m. A, V = 0. 6 V. P = IV = (1. 5 10 -3)(0. 6) = 9. 0 10 -4 W.
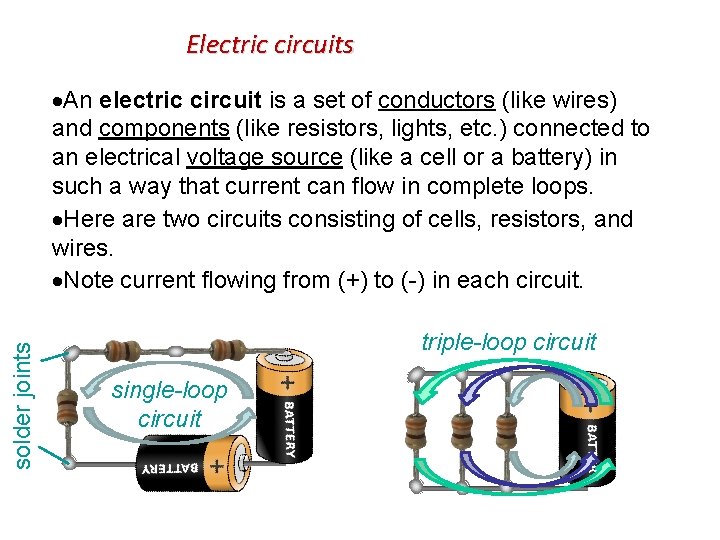
Electric circuits solder joints An electric circuit is a set of conductors (like wires) and components (like resistors, lights, etc. ) connected to an electrical voltage source (like a cell or a battery) in such a way that current can flow in complete loops. Here are two circuits consisting of cells, resistors, and wires. Note current flowing from (+) to (-) in each circuit. triple-loop circuit single-loop circuit
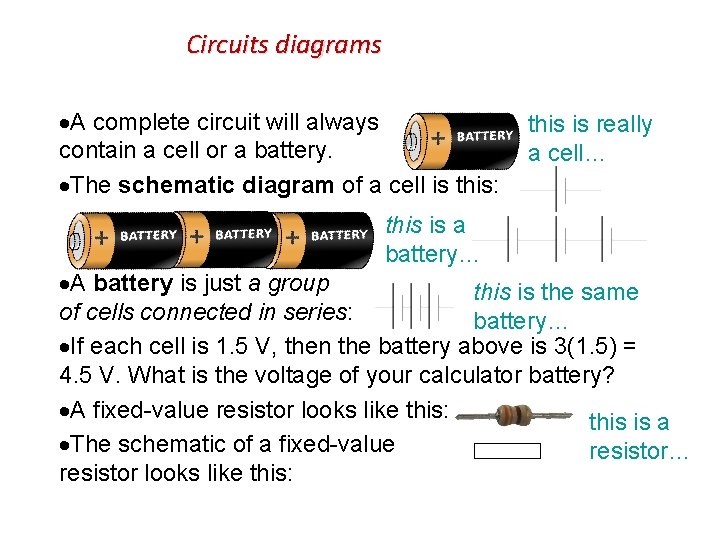
Circuits diagrams A complete circuit will always this is really contain a cell or a battery. a cell… The schematic diagram of a cell is this: this is a battery… A battery is just a group this is the same of cells connected in series: battery… If each cell is 1. 5 V, then the battery above is 3(1. 5) = 4. 5 V. What is the voltage of your calculator battery? A fixed-value resistor looks like this: this is a The schematic of a fixed-value resistor… resistor looks like this:
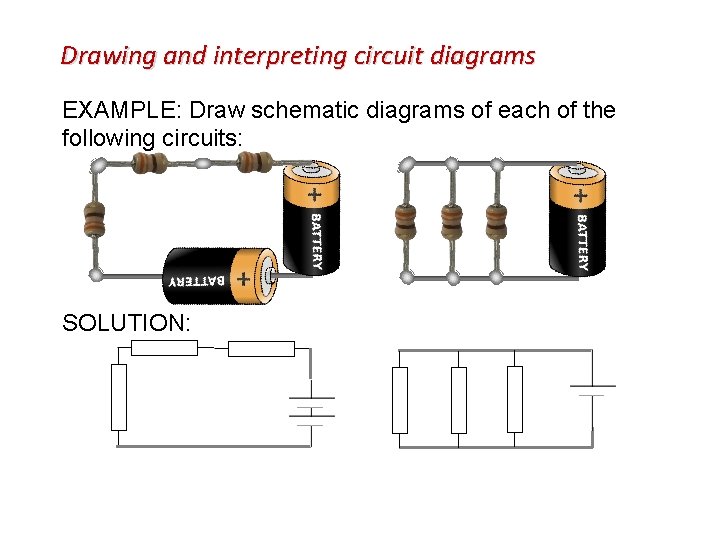
Drawing and interpreting circuit diagrams EXAMPLE: Draw schematic diagrams of each of the following circuits: SOLUTION:
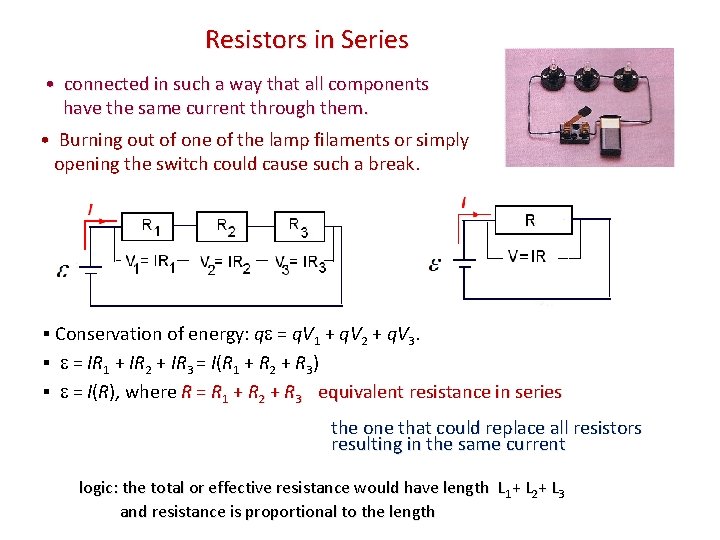
Resistors in Series • connected in such a way that all components have the same current through them. • Burning out of one of the lamp filaments or simply opening the switch could cause such a break. ▪ Conservation of energy: q = q. V 1 + q. V 2 + q. V 3. ▪ = IR 1 + IR 2 + IR 3 = I(R 1 + R 2 + R 3) ▪ = I(R), where R = R 1 + R 2 + R 3 equivalent resistance in series the one that could replace all resistors resulting in the same current logic: the total or effective resistance would have length L 1+ L 2+ L 3 and resistance is proportional to the length
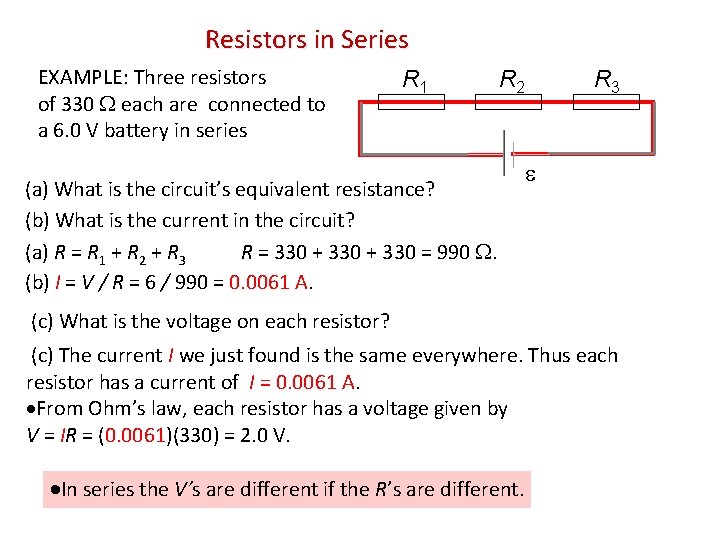
Resistors in Series EXAMPLE: Three resistors of 330 each are connected to a 6. 0 V battery in series R 1 R 2 (a) What is the circuit’s equivalent resistance? (b) What is the current in the circuit? (a) R = R 1 + R 2 + R 3 R = 330 + 330 = 990 . (b) I = V / R = 6 / 990 = 0. 0061 A. R 3 (c) What is the voltage on each resistor? (c) The current I we just found is the same everywhere. Thus each resistor has a current of I = 0. 0061 A. From Ohm’s law, each resistor has a voltage given by V = IR = (0. 0061)(330) = 2. 0 V. In series the V’s are different if the R’s are different.
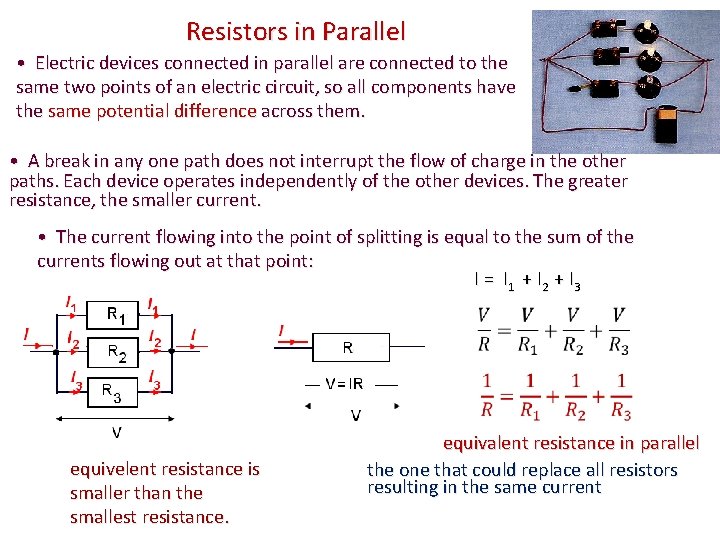
Resistors in Parallel • Electric devices connected in parallel are connected to the same two points of an electric circuit, so all components have the same potential difference across them. • A break in any one path does not interrupt the flow of charge in the other paths. Each device operates independently of the other devices. The greater resistance, the smaller current. • The current flowing into the point of splitting is equal to the sum of the currents flowing out at that point: I = I 1 + I 2 + I 3 equivelent resistance is smaller than the smallest resistance. equivalent resistance in parallel the one that could replace all resistors resulting in the same current
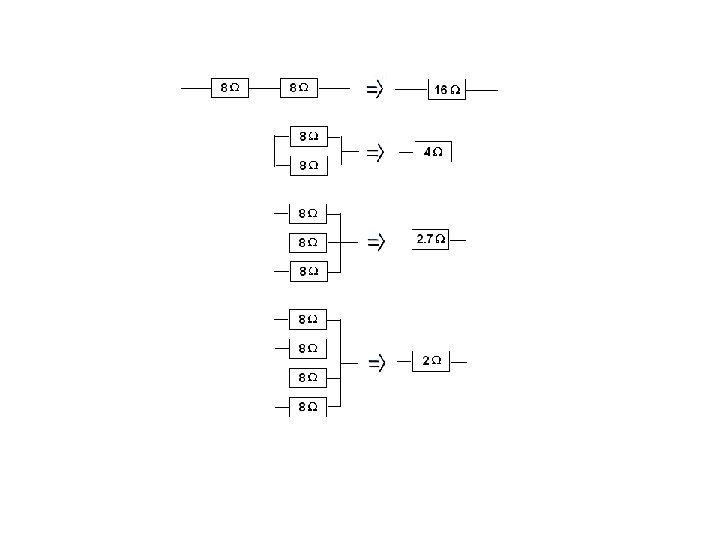
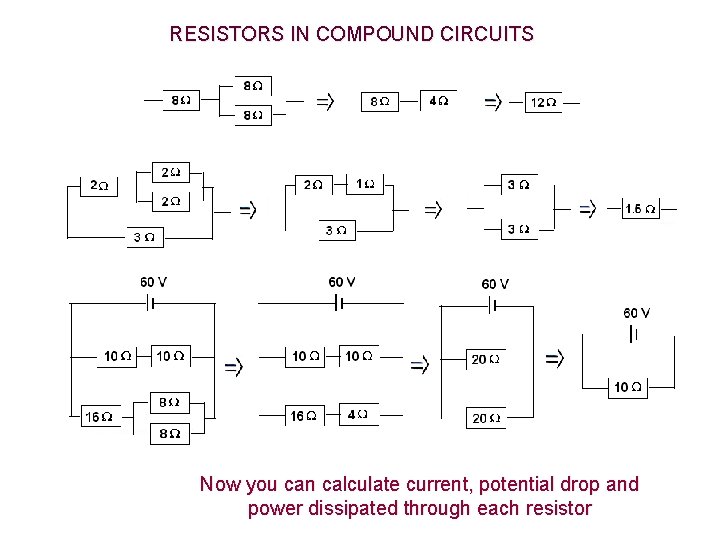
RESISTORS IN COMPOUND CIRCUITS Now you can calculate current, potential drop and power dissipated through each resistor
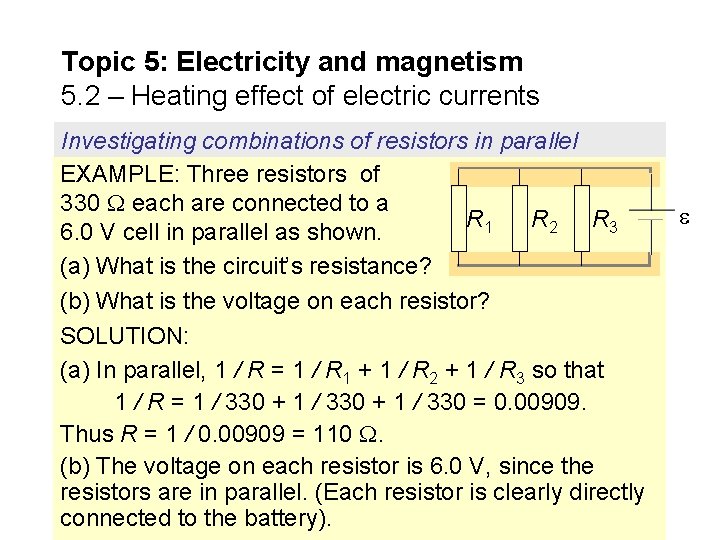
Topic 5: Electricity and magnetism 5. 2 – Heating effect of electric currents Investigating combinations of resistors in parallel EXAMPLE: Three resistors of 330 each are connected to a R 2 R 3 R 1 6. 0 V cell in parallel as shown. (a) What is the circuit’s resistance? (b) What is the voltage on each resistor? SOLUTION: (a) In parallel, 1 / R = 1 / R 1 + 1 / R 2 + 1 / R 3 so that 1 / R = 1 / 330 + 1 / 330 = 0. 00909. Thus R = 1 / 0. 00909 = 110 . (b) The voltage on each resistor is 6. 0 V, since the resistors are in parallel. (Each resistor is clearly directly connected to the battery).
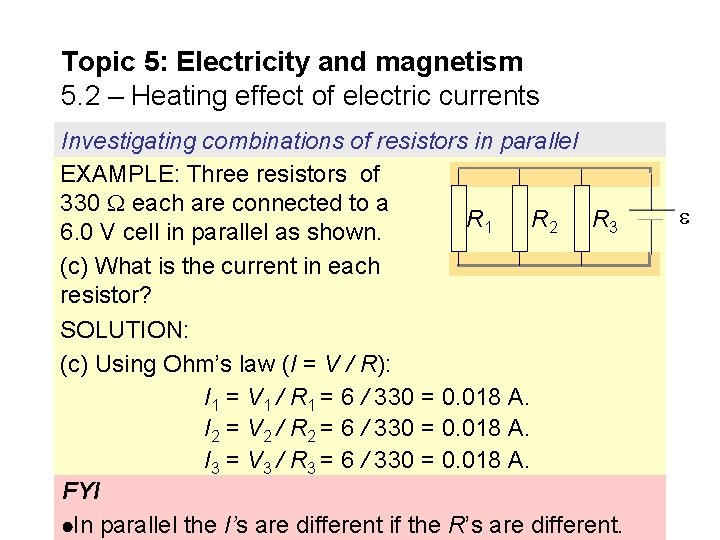
Topic 5: Electricity and magnetism 5. 2 – Heating effect of electric currents Investigating combinations of resistors in parallel EXAMPLE: Three resistors of 330 each are connected to a R 2 R 3 R 1 6. 0 V cell in parallel as shown. (c) What is the current in each resistor? SOLUTION: (c) Using Ohm’s law (I = V / R): I 1 = V 1 / R 1 = 6 / 330 = 0. 018 A. I 2 = V 2 / R 2 = 6 / 330 = 0. 018 A. I 3 = V 3 / R 3 = 6 / 330 = 0. 018 A. FYI In parallel the I’s are different if the R’s are different.
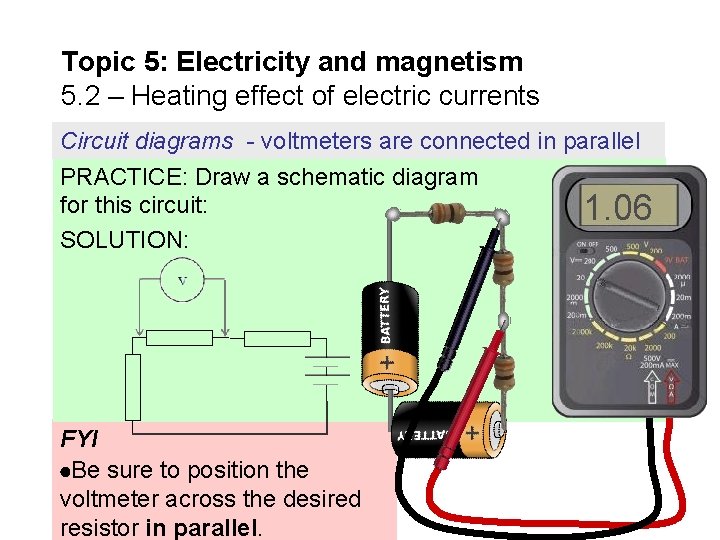
Topic 5: Electricity and magnetism 5. 2 – Heating effect of electric currents Circuit diagrams - voltmeters are connected in parallel PRACTICE: Draw a schematic diagram for this circuit: 1. 06 SOLUTION: FYI Be sure to position the voltmeter across the desired resistor in parallel.
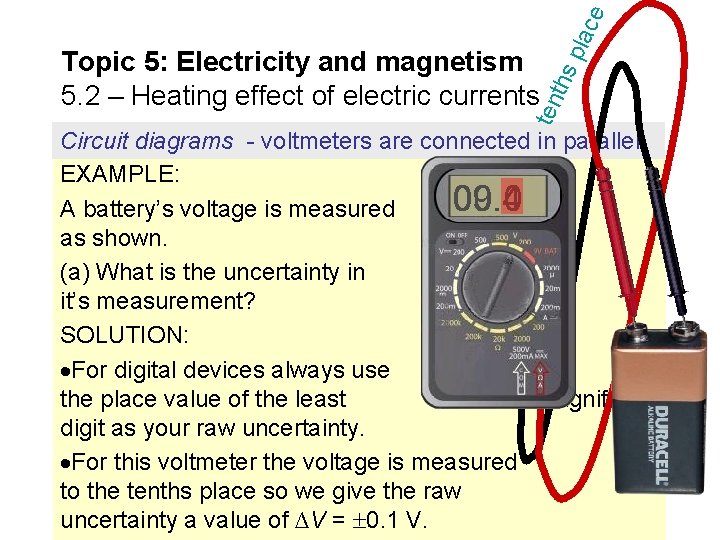
e pla c ten ths Topic 5: Electricity and magnetism 5. 2 – Heating effect of electric currents Circuit diagrams - voltmeters are connected in parallel EXAMPLE: 09. 4 00. 0 A battery’s voltage is measured as shown. (a) What is the uncertainty in it’s measurement? SOLUTION: For digital devices always use the place value of the least significant digit as your raw uncertainty. For this voltmeter the voltage is measured to the tenths place so we give the raw uncertainty a value of ∆V = 0. 1 V.
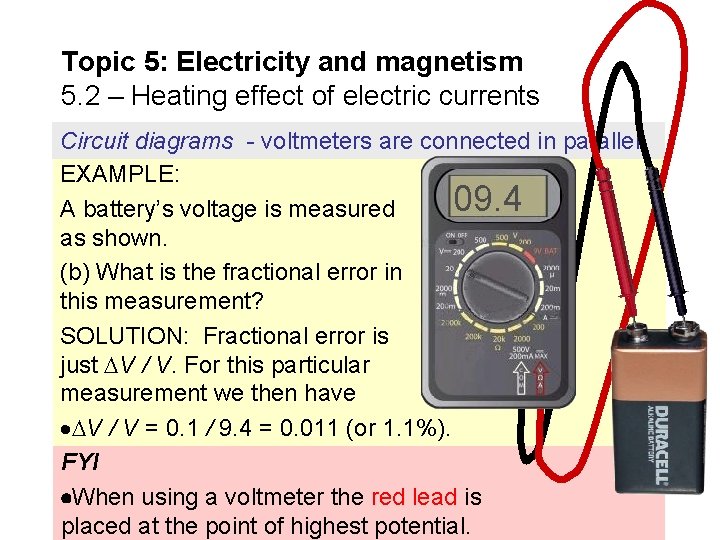
Topic 5: Electricity and magnetism 5. 2 – Heating effect of electric currents Circuit diagrams - voltmeters are connected in parallel EXAMPLE: 09. 4 A battery’s voltage is measured as shown. (b) What is the fractional error in this measurement? SOLUTION: Fractional error is just V / V. For this particular measurement we then have V / V = 0. 1 / 9. 4 = 0. 011 (or 1. 1%). FYI When using a voltmeter the red lead is placed at the point of highest potential.
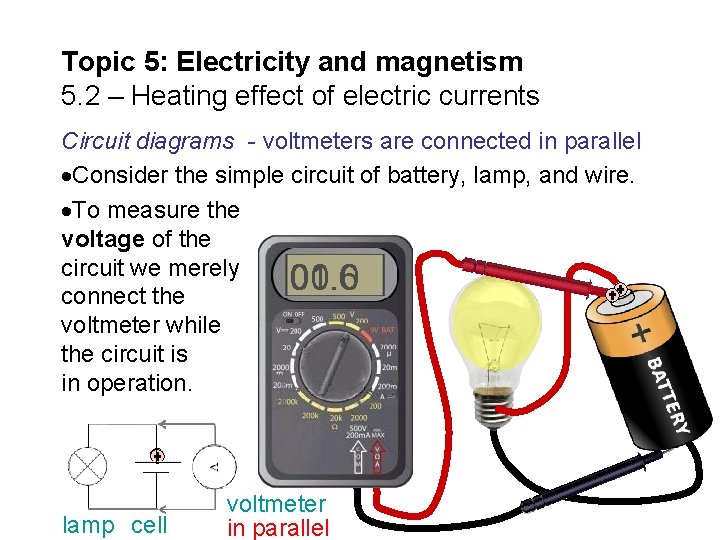
Topic 5: Electricity and magnetism 5. 2 – Heating effect of electric currents Circuit diagrams - voltmeters are connected in parallel Consider the simple circuit of battery, lamp, and wire. To measure the voltage of the circuit we merely 01. 6 00. 0 connect the voltmeter while the circuit is in operation. lamp cell voltmeter in parallel
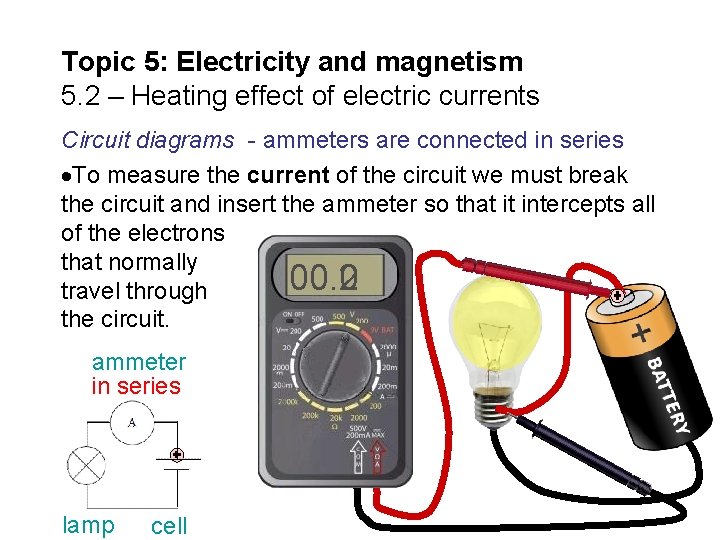
Topic 5: Electricity and magnetism 5. 2 – Heating effect of electric currents Circuit diagrams - ammeters are connected in series To measure the current of the circuit we must break the circuit and insert the ammeter so that it intercepts all of the electrons that normally 00. 2 00. 0 travel through the circuit. ammeter in series lamp cell
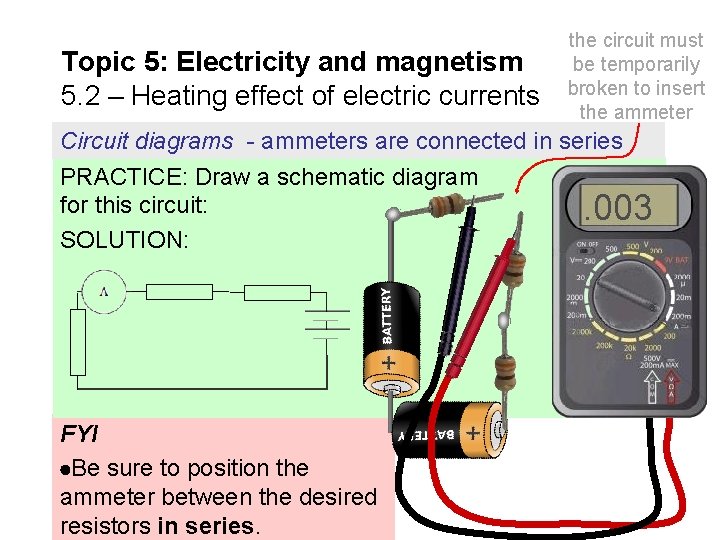
Topic 5: Electricity and magnetism 5. 2 – Heating effect of electric currents the circuit must be temporarily broken to insert the ammeter Circuit diagrams - ammeters are connected in series PRACTICE: Draw a schematic diagram for this circuit: . 003 SOLUTION: FYI Be sure to position the ammeter between the desired resistors in series.
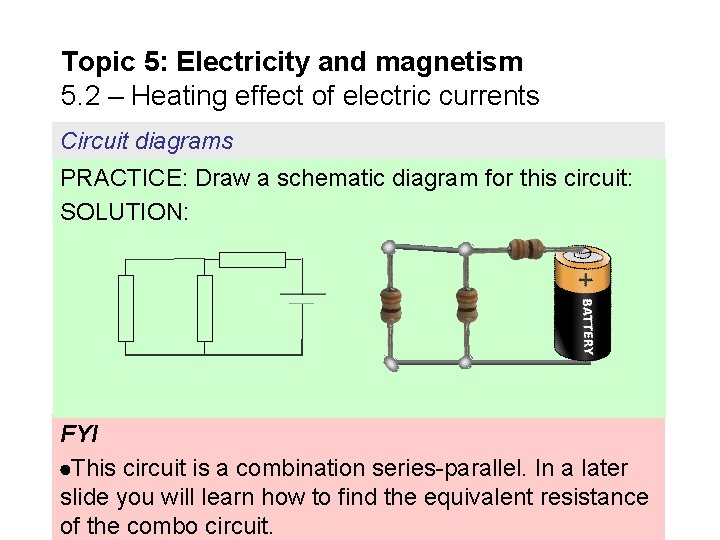
Topic 5: Electricity and magnetism 5. 2 – Heating effect of electric currents Circuit diagrams PRACTICE: Draw a schematic diagram for this circuit: SOLUTION: FYI This circuit is a combination series-parallel. In a later slide you will learn how to find the equivalent resistance of the combo circuit.
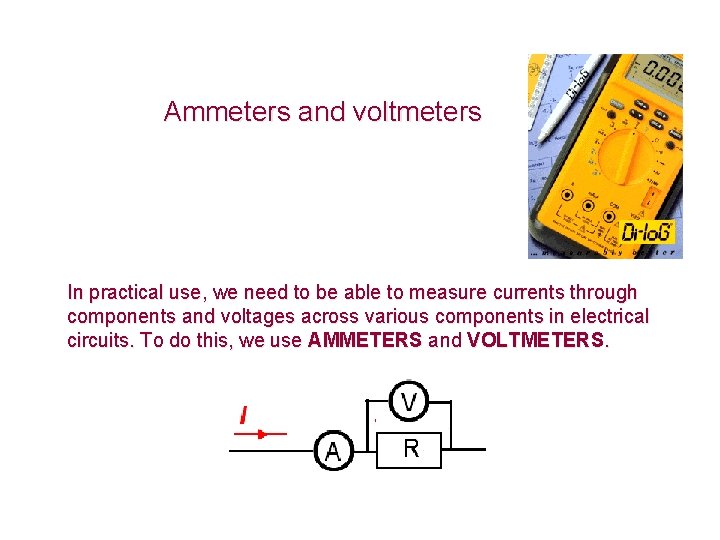
Ammeters and voltmeters In practical use, we need to be able to measure currents through components and voltages across various components in electrical circuits. To do this, we use AMMETERS and VOLTMETERS.
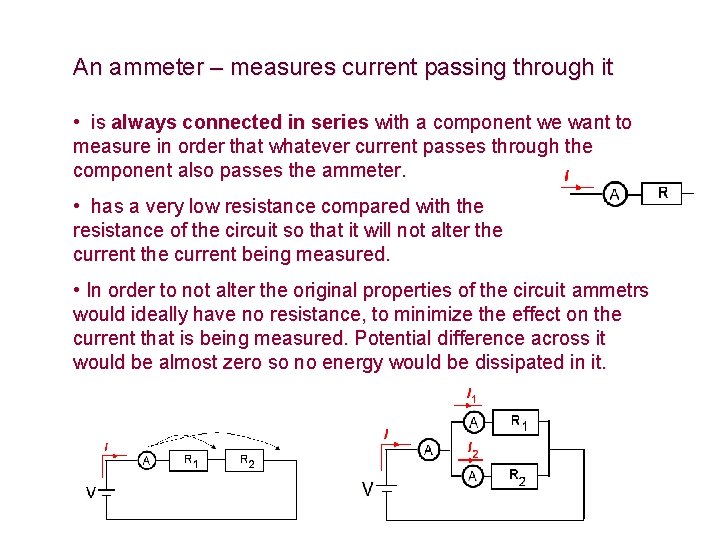
An ammeter – measures current passing through it • is always connected in series with a component we want to measure in order that whatever current passes through the component also passes the ammeter. • has a very low resistance compared with the resistance of the circuit so that it will not alter the current being measured. • In order to not alter the original properties of the circuit ammetrs would ideally have no resistance, to minimize the effect on the current that is being measured. Potential difference across it would be almost zero so no energy would be dissipated in it.
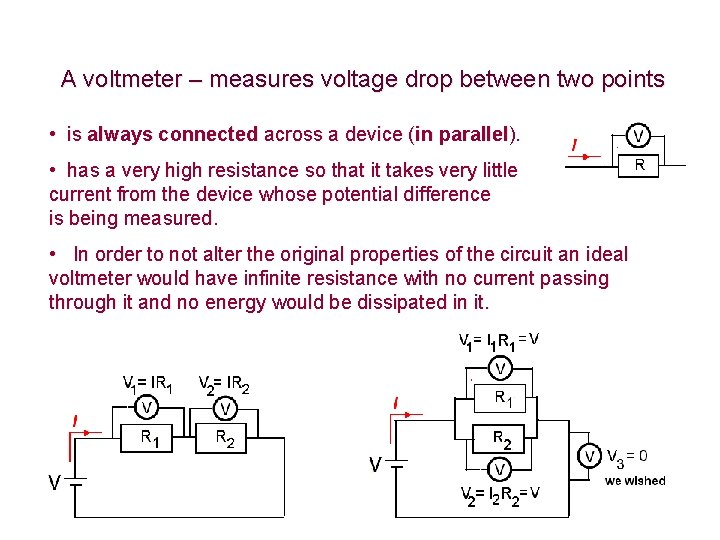
A voltmeter – measures voltage drop between two points • is always connected across a device (in parallel). • has a very high resistance so that it takes very little current from the device whose potential difference is being measured. • In order to not alter the original properties of the circuit an ideal voltmeter would have infinite resistance with no current passing through it and no energy would be dissipated in it.
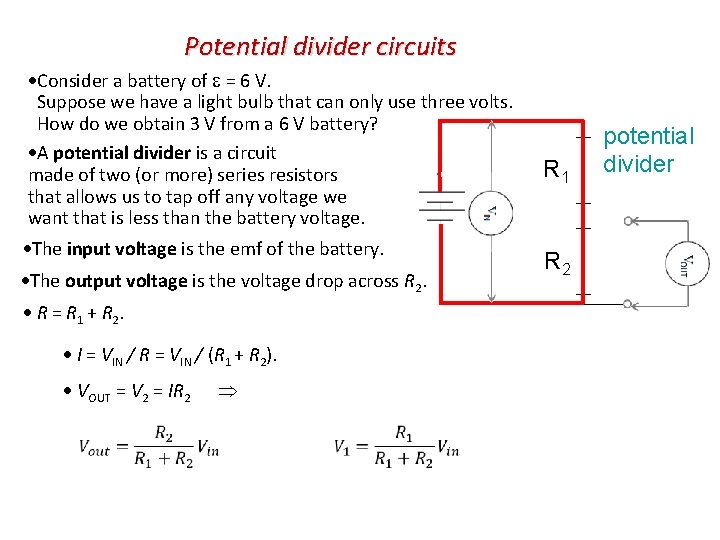
Potential divider circuits Consider a battery of = 6 V. Suppose we have a light bulb that can only use three volts. How do we obtain 3 V from a 6 V battery? A potential divider is a circuit made of two (or more) series resistors that allows us to tap off any voltage we want that is less than the battery voltage. The input voltage is the emf of the battery. The output voltage is the voltage drop across R 2. R = R 1 + R 2. I = VIN / R = VIN / (R 1 + R 2). VOUT = V 2 = IR 2 R 1 R 2 potential divider
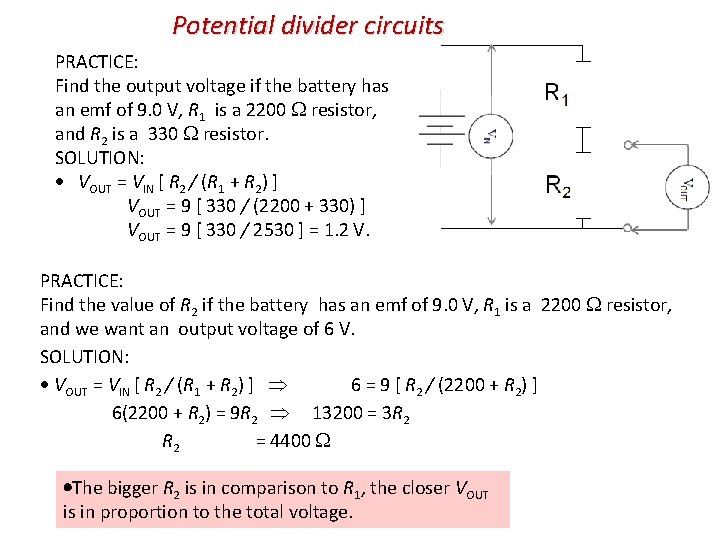
Potential divider circuits PRACTICE: Find the output voltage if the battery has an emf of 9. 0 V, R 1 is a 2200 resistor, and R 2 is a 330 resistor. SOLUTION: VOUT = VIN [ R 2 / (R 1 + R 2) ] VOUT = 9 [ 330 / (2200 + 330) ] VOUT = 9 [ 330 / 2530 ] = 1. 2 V. PRACTICE: Find the value of R 2 if the battery has an emf of 9. 0 V, R 1 is a 2200 resistor, and we want an output voltage of 6 V. SOLUTION: VOUT = VIN [ R 2 / (R 1 + R 2) ] 6 = 9 [ R 2 / (2200 + R 2) ] 6(2200 + R 2) = 9 R 2 13200 = 3 R 2 = 4400 The bigger R 2 is in comparison to R 1, the closer VOUT is in proportion to the total voltage.
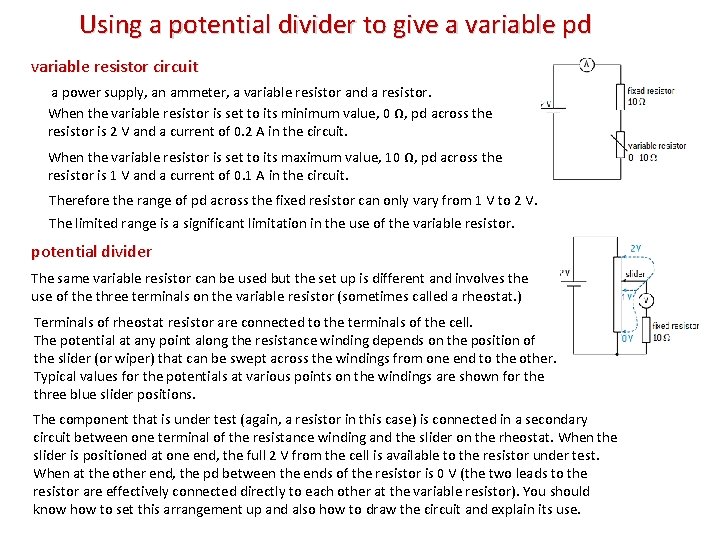
Using a potential divider to give a variable pd variable resistor circuit a power supply, an ammeter, a variable resistor and a resistor. When the variable resistor is set to its minimum value, 0 Ω, pd across the resistor is 2 V and a current of 0. 2 A in the circuit. When the variable resistor is set to its maximum value, 10 Ω, pd across the resistor is 1 V and a current of 0. 1 A in the circuit. Therefore the range of pd across the fixed resistor can only vary from 1 V to 2 V. The limited range is a significant limitation in the use of the variable resistor. potential divider The same variable resistor can be used but the set up is different and involves the use of the three terminals on the variable resistor (sometimes called a rheostat. ) Terminals of rheostat resistor are connected to the terminals of the cell. The potential at any point along the resistance winding depends on the position of the slider (or wiper) that can be swept across the windings from one end to the other. Typical values for the potentials at various points on the windings are shown for the three blue slider positions. The component that is under test (again, a resistor in this case) is connected in a secondary circuit between one terminal of the resistance winding and the slider on the rheostat. When the slider is positioned at one end, the full 2 V from the cell is available to the resistor under test. When at the other end, the pd between the ends of the resistor is 0 V (the two leads to the resistor are effectively connected directly to each other at the variable resistor). You should know how to set this arrangement up and also how to draw the circuit and explain its use.
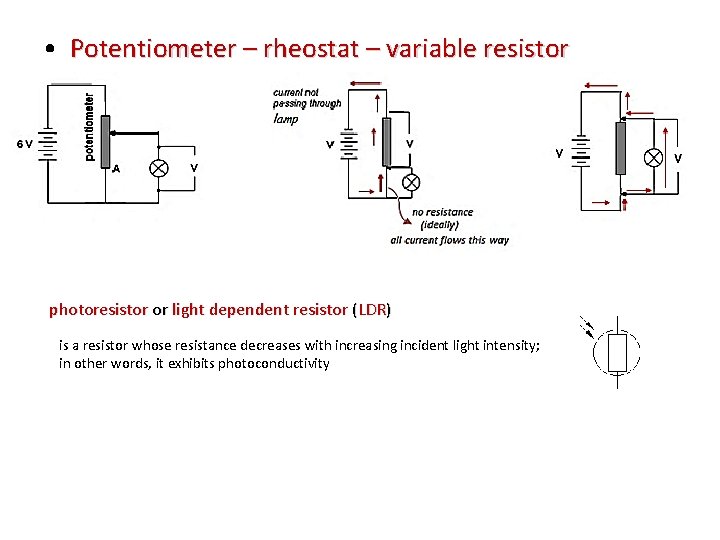
• Potentiometer – rheostat – variable resistor photoresistor or light dependent resistor (LDR) is a resistor whose resistance decreases with increasing incident light intensity; in other words, it exhibits photoconductivity
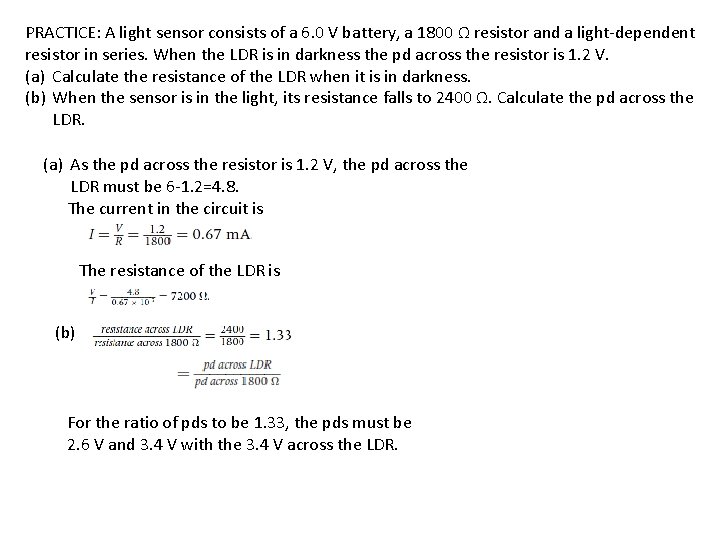
PRACTICE: A light sensor consists of a 6. 0 V battery, a 1800 Ω resistor and a light-dependent resistor in series. When the LDR is in darkness the pd across the resistor is 1. 2 V. (a) Calculate the resistance of the LDR when it is in darkness. (b) When the sensor is in the light, its resistance falls to 2400 Ω. Calculate the pd across the LDR. (a) As the pd across the resistor is 1. 2 V, the pd across the LDR must be 6 -1. 2=4. 8. The current in the circuit is The resistance of the LDR is (b) For the ratio of pds to be 1. 33, the pds must be 2. 6 V and 3. 4 V with the 3. 4 V across the LDR.
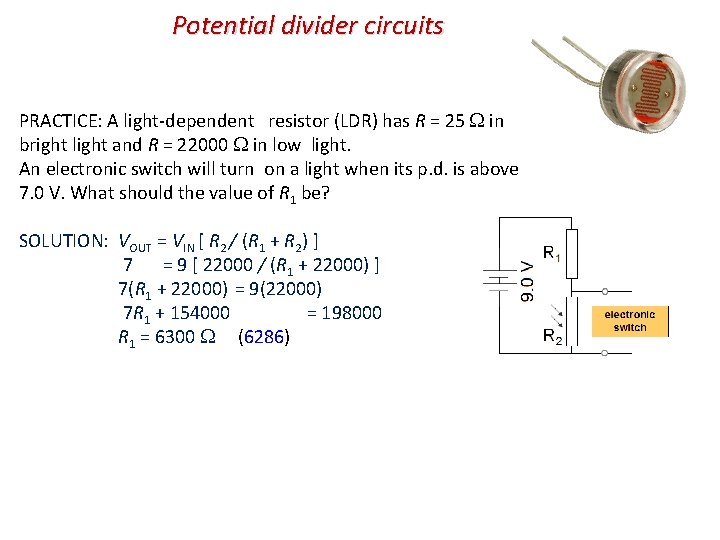
Potential divider circuits PRACTICE: A light-dependent resistor (LDR) has R = 25 in bright light and R = 22000 in low light. An electronic switch will turn on a light when its p. d. is above 7. 0 V. What should the value of R 1 be? SOLUTION: VOUT = VIN [ R 2 / (R 1 + R 2) ] 7 = 9 [ 22000 / (R 1 + 22000) ] 7(R 1 + 22000) = 9(22000) 7 R 1 + 154000 = 198000 R 1 = 6300 (6286)
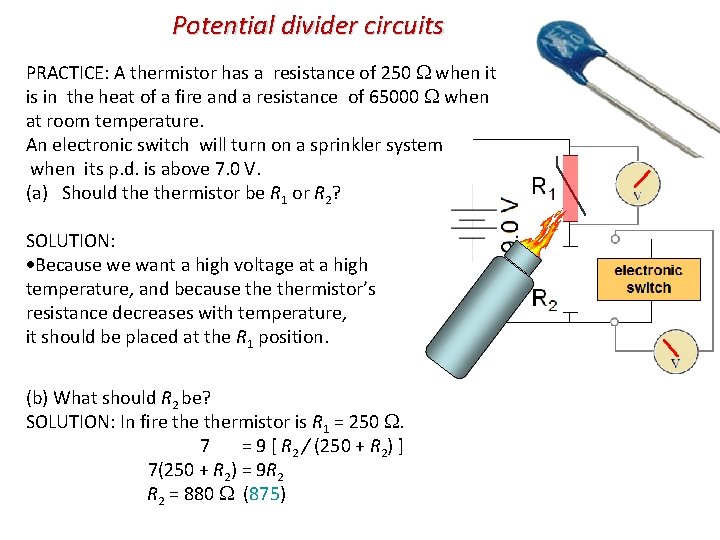
Potential divider circuits PRACTICE: A thermistor has a resistance of 250 when it is in the heat of a fire and a resistance of 65000 when at room temperature. An electronic switch will turn on a sprinkler system when its p. d. is above 7. 0 V. (a) Should thermistor be R 1 or R 2? SOLUTION: Because we want a high voltage at a high temperature, and because thermistor’s resistance decreases with temperature, it should be placed at the R 1 position. (b) What should R 2 be? SOLUTION: In fire thermistor is R 1 = 250 . 7 = 9 [ R 2 / (250 + R 2) ] 7(250 + R 2) = 9 R 2 = 880 (875)
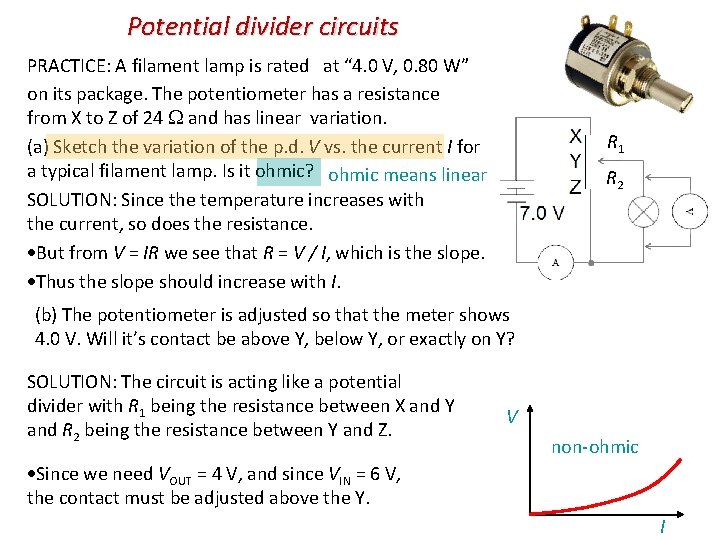
Potential divider circuits PRACTICE: A filament lamp is rated at “ 4. 0 V, 0. 80 W” on its package. The potentiometer has a resistance from X to Z of 24 and has linear variation. (a) Sketch the variation of the p. d. V vs. the current I for a typical filament lamp. Is it ohmic? ohmic means linear SOLUTION: Since the temperature increases with the current, so does the resistance. But from V = IR we see that R = V / I, which is the slope. Thus the slope should increase with I. R 1 R 2 (b) The potentiometer is adjusted so that the meter shows 4. 0 V. Will it’s contact be above Y, below Y, or exactly on Y? SOLUTION: The circuit is acting like a potential divider with R 1 being the resistance between X and Y and R 2 being the resistance between Y and Z. V non-ohmic Since we need VOUT = 4 V, and since VIN = 6 V, the contact must be adjusted above the Y. I
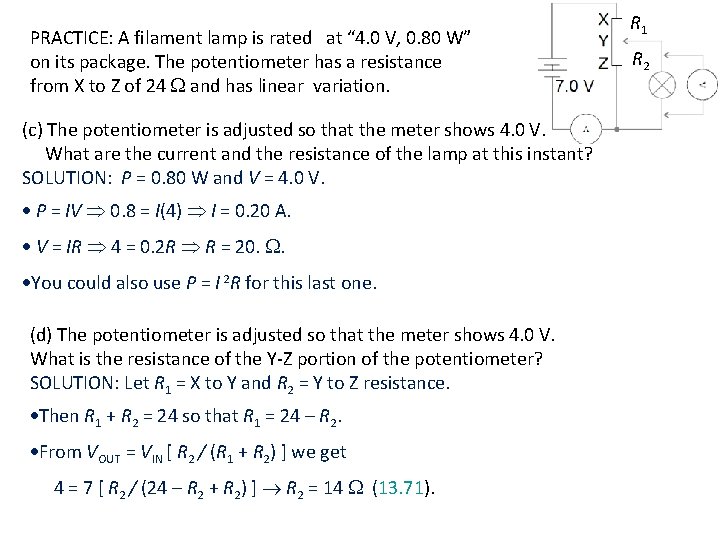
PRACTICE: A filament lamp is rated at “ 4. 0 V, 0. 80 W” on its package. The potentiometer has a resistance from X to Z of 24 and has linear variation. (c) The potentiometer is adjusted so that the meter shows 4. 0 V. What are the current and the resistance of the lamp at this instant? SOLUTION: P = 0. 80 W and V = 4. 0 V. P = IV 0. 8 = I(4) I = 0. 20 A. V = IR 4 = 0. 2 R R = 20. . You could also use P = I 2 R for this last one. (d) The potentiometer is adjusted so that the meter shows 4. 0 V. What is the resistance of the Y-Z portion of the potentiometer? SOLUTION: Let R 1 = X to Y and R 2 = Y to Z resistance. Then R 1 + R 2 = 24 so that R 1 = 24 – R 2. From VOUT = VIN [ R 2 / (R 1 + R 2) ] we get 4 = 7 [ R 2 / (24 – R 2 + R 2) ] R 2 = 14 (13. 71). R 1 R 2
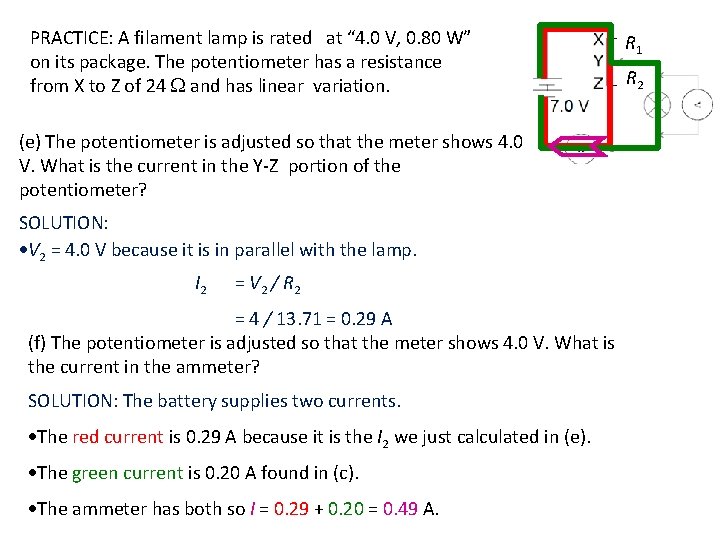
PRACTICE: A filament lamp is rated at “ 4. 0 V, 0. 80 W” on its package. The potentiometer has a resistance from X to Z of 24 and has linear variation. (e) The potentiometer is adjusted so that the meter shows 4. 0 V. What is the current in the Y-Z portion of the potentiometer? SOLUTION: V 2 = 4. 0 V because it is in parallel with the lamp. I 2 = V 2 / R 2 = 4 / 13. 71 = 0. 29 A (f) The potentiometer is adjusted so that the meter shows 4. 0 V. What is the current in the ammeter? SOLUTION: The battery supplies two currents. The red current is 0. 29 A because it is the I 2 we just calculated in (e). The green current is 0. 20 A found in (c). The ammeter has both so I = 0. 29 + 0. 20 = 0. 49 A. R 1 R 2
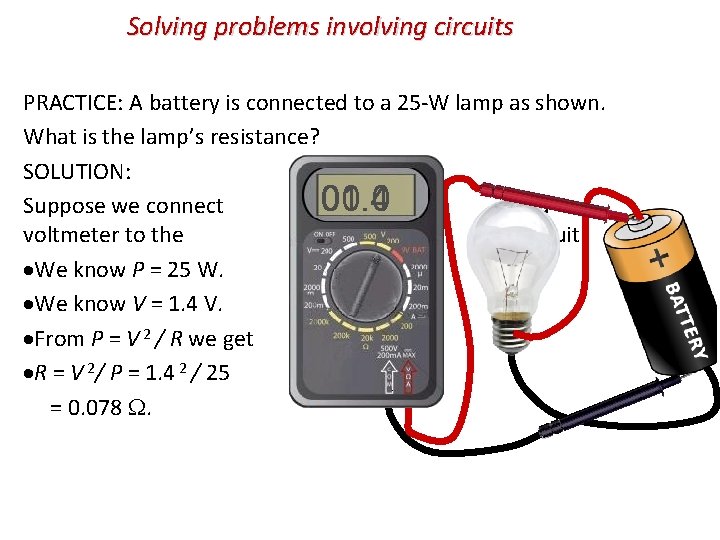
Solving problems involving circuits PRACTICE: A battery is connected to a 25 -W lamp as shown. What is the lamp’s resistance? SOLUTION: 01. 4 00. 0 Suppose we connect a voltmeter to the circuit. We know P = 25 W. We know V = 1. 4 V. From P = V 2 / R we get R = V 2/ P = 1. 4 2 / 25 = 0. 078 .
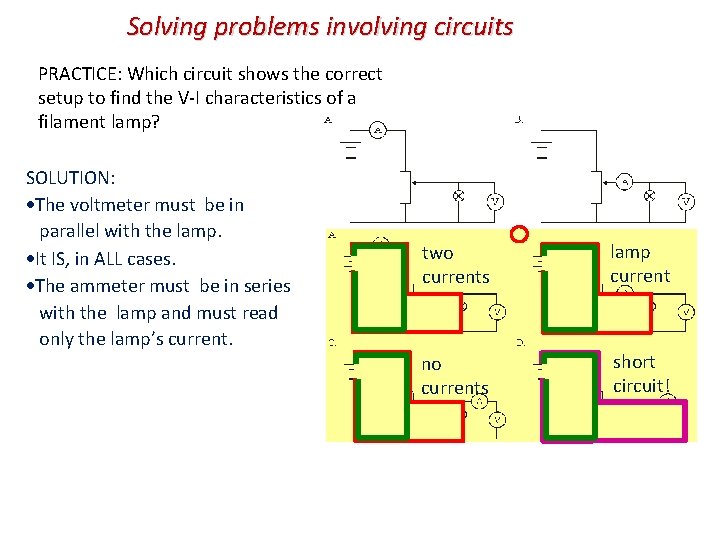
Solving problems involving circuits PRACTICE: Which circuit shows the correct setup to find the V-I characteristics of a filament lamp? SOLUTION: The voltmeter must be in parallel with the lamp. It IS, in ALL cases. The ammeter must be in series with the lamp and must read only the lamp’s current. two currents lamp current no currents short circuit!
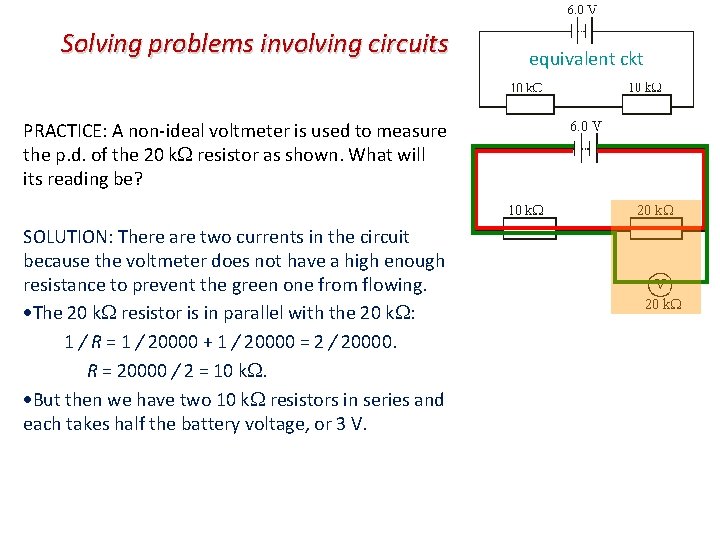
Solving problems involving circuits PRACTICE: A non-ideal voltmeter is used to measure the p. d. of the 20 k resistor as shown. What will its reading be? SOLUTION: There are two currents in the circuit because the voltmeter does not have a high enough resistance to prevent the green one from flowing. The 20 k resistor is in parallel with the 20 k : 1 / R = 1 / 20000 + 1 / 20000 = 2 / 20000. R = 20000 / 2 = 10 k. But then we have two 10 k resistors in series and each takes half the battery voltage, or 3 V. equivalent ckt
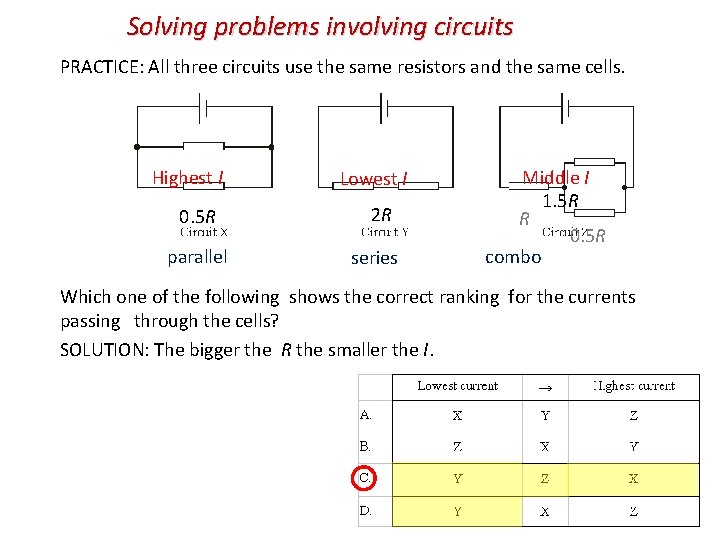
Solving problems involving circuits PRACTICE: All three circuits use the same resistors and the same cells. Highest I 0. 5 R parallel Lowest I 2 R series Middle I 1. 5 R R 0. 5 R combo Which one of the following shows the correct ranking for the currents passing through the cells? SOLUTION: The bigger the R the smaller the I.
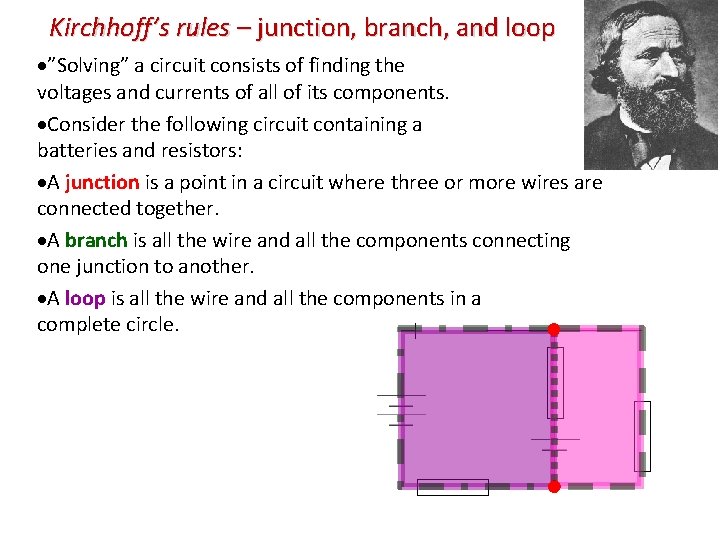
Kirchhoff’s rules – junction, branch, and loop ”Solving” a circuit consists of finding the voltages and currents of all of its components. Consider the following circuit containing a few batteries and resistors: A junction is a point in a circuit where three or more wires are connected together. A branch is all the wire and all the components connecting one junction to another. A loop is all the wire and all the components in a complete circle.
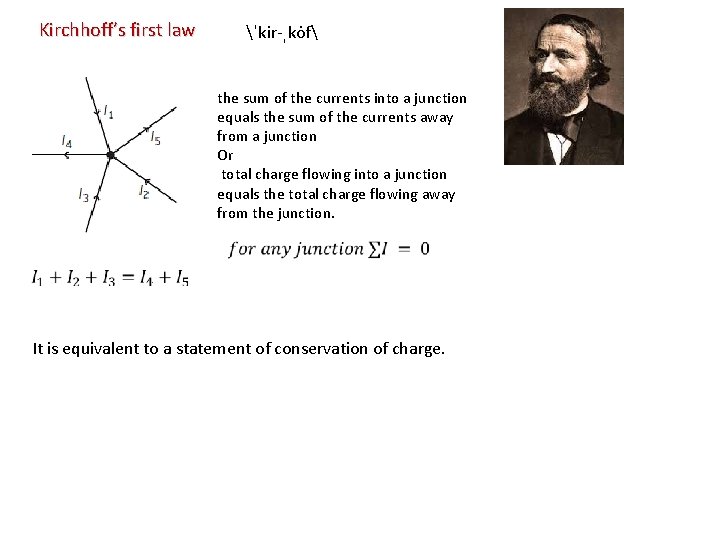
Kirchhoff’s first law ˈkir-ˌko f the sum of the currents into a junction equals the sum of the currents away from a junction Or total charge flowing into a junction equals the total charge flowing away from the junction. It is equivalent to a statement of conservation of charge.
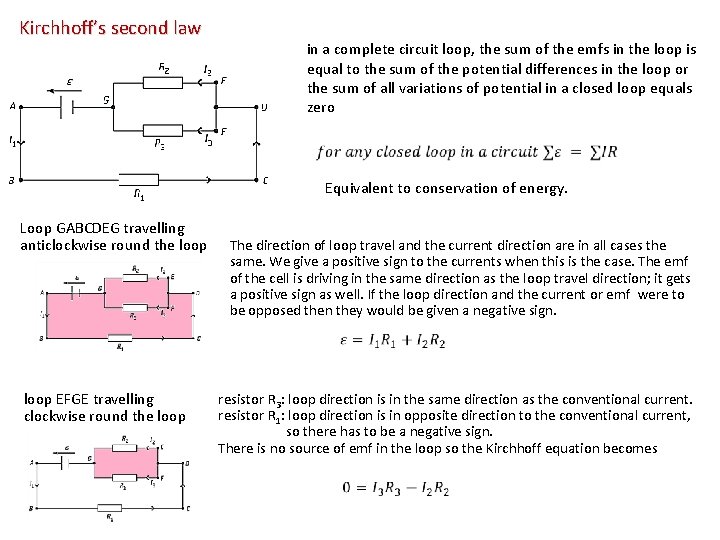
Kirchhoff’s second law in a complete circuit loop, the sum of the emfs in the loop is equal to the sum of the potential differences in the loop or the sum of all variations of potential in a closed loop equals zero Equivalent to conservation of energy. Loop GABCDEG travelling anticlockwise round the loop The direction of loop travel and the current direction are in all cases the same. We give a positive sign to the currents when this is the case. The emf of the cell is driving in the same direction as the loop travel direction; it gets a positive sign as well. If the loop direction and the current or emf were to be opposed then they would be given a negative sign. loop EFGE travelling clockwise round the loop resistor R 3: loop direction is in the same direction as the conventional current. resistor R 1: loop direction is in opposite direction to the conventional current, so there has to be a negative sign. There is no source of emf in the loop so the Kirchhoff equation becomes
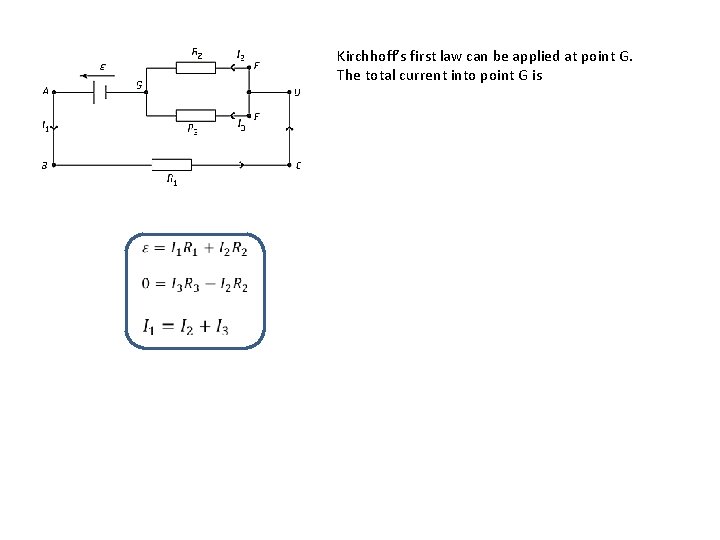
Kirchhoff’s first law can be applied at point G. The total current into point G is
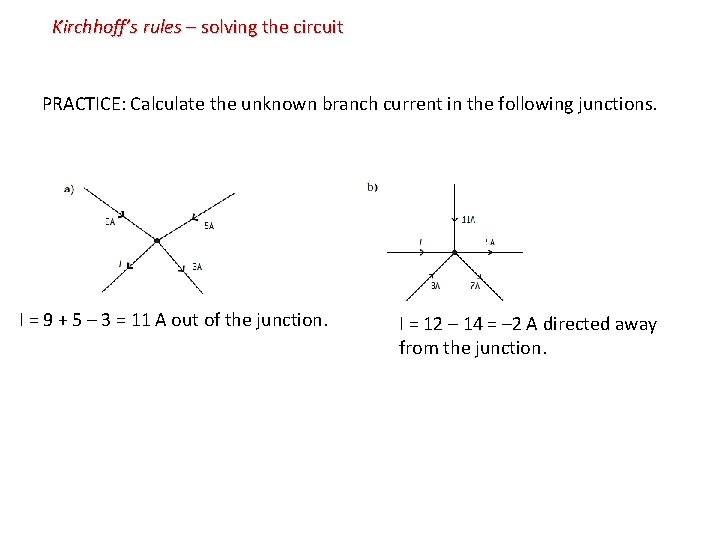
Kirchhoff’s rules – solving the circuit PRACTICE: Calculate the unknown branch current in the following junctions. I = 9 + 5 – 3 = 11 A out of the junction. I = 12 – 14 = – 2 A directed away from the junction.
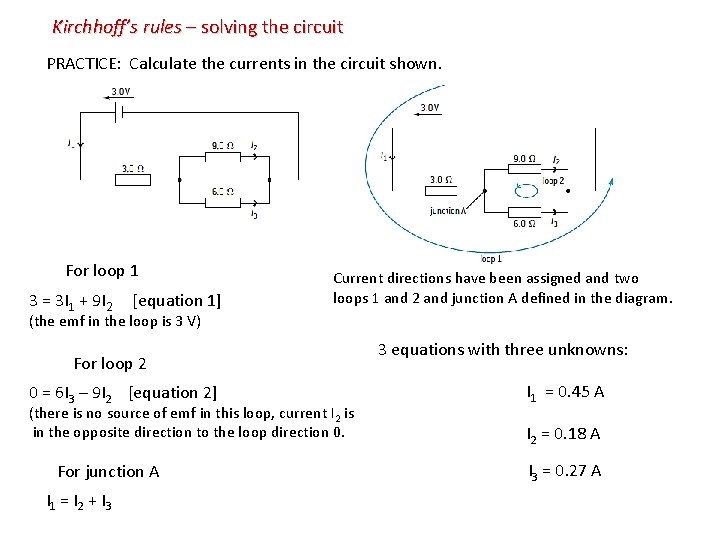
Kirchhoff’s rules – solving the circuit PRACTICE: Calculate the currents in the circuit shown. For loop 1 3 = 3 I 1 + 9 I 2 [equation 1] Current directions have been assigned and two loops 1 and 2 and junction A defined in the diagram. (the emf in the loop is 3 V) For loop 2 0 = 6 I 3 – 9 I 2 [equation 2] (there is no source of emf in this loop, current I 2 is in the opposite direction to the loop direction 0. For junction A I 1 = I 2 + I 3 3 equations with three unknowns: I 1 = 0. 45 A I 2 = 0. 18 A I 3 = 0. 27 A
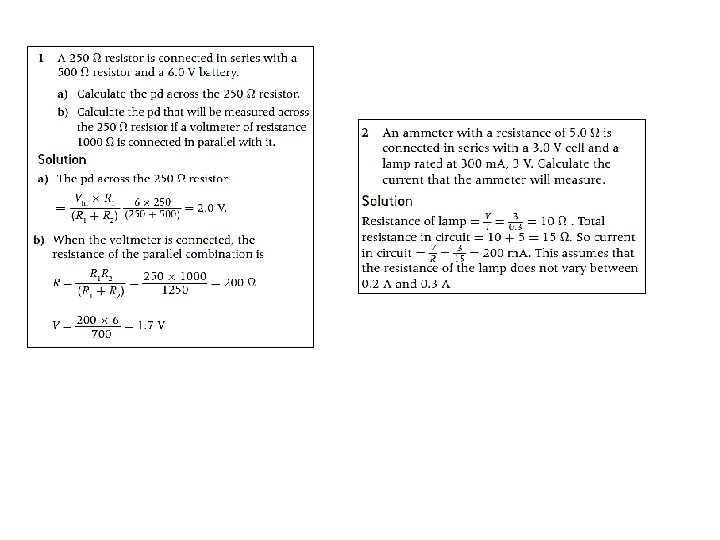
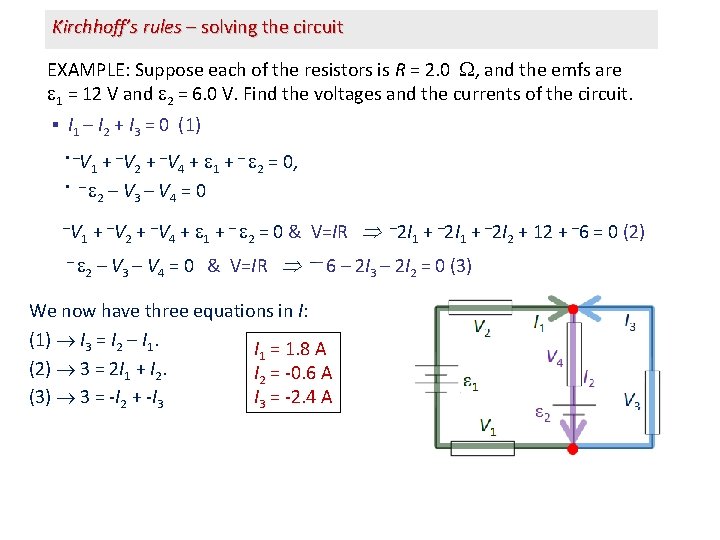
Kirchhoff’s rules – solving the circuit EXAMPLE: Suppose each of the resistors is R = 2. 0 , and the emfs are 1 = 12 V and 2 = 6. 0 V. Find the voltages and the currents of the circuit. ▪ I 1 – I 2 + I 3 = 0 (1) + –V 2 + –V 4 + 1 + – 2 = 0, – – V = 0 2 3 4 ▪ –V 1 1 – 2 + –V 4 + 1 + – 2 = 0 & V=IR – 2 I 1 + – 2 I 2 + 12 + – 6 = 0 (2) – V 3 – V 4 = 0 & V=IR –– 6 We now have three equations in I: (1) I 3 = I 2 – I 1 = 1. 8 A (2) 3 = 2 I 1 + I 2 = -0. 6 A I 3 = -2. 4 A (3) 3 = -I 2 + -I 3 – 2 I 2 = 0 (3)
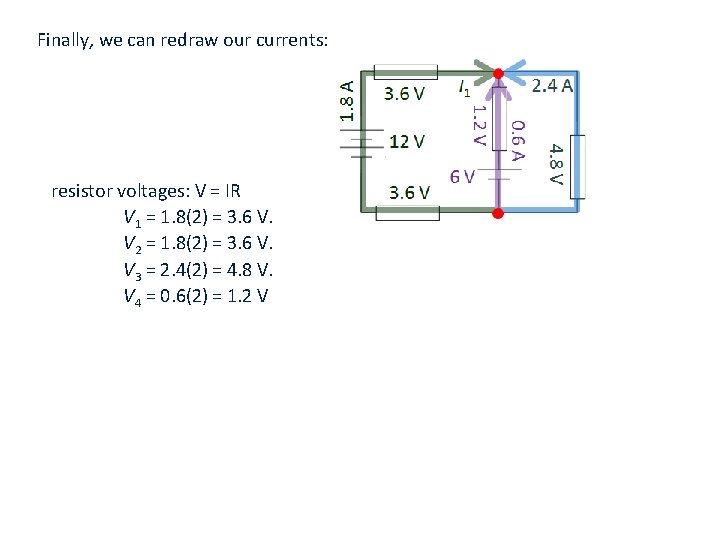
Finally, we can redraw our currents: resistor voltages: V = IR V 1 = 1. 8(2) = 3. 6 V. V 2 = 1. 8(2) = 3. 6 V. V 3 = 2. 4(2) = 4. 8 V. V 4 = 0. 6(2) = 1. 2 V
Dielectric heating
4 forces of nature
Ib physics chapter 5
Magnetism
Magnetism jeopardy
Sph3u electricity and magnetism
Relationship between electricity and magnetism
Intensity of magnetisation
Magnetism grade 5
Electricity and magnetism
Electricity and magnetism
Electricity and magnetism
Electricity and magnetism
Electricity and magnetism
Magnetism vocabulary
Static electricity and current electricity
Current electricity
Example of a clincher
Topic down
A substance that attracts pieces iron
Magnetism and electromagnetism
Magnetic field of two magnets
Para and ferro magnetism
Applications of magnetism
What is magnets
Magnetism
Definition of vocabulary
Heat load calculation software
Heating and cooling curves of water
Goddard heating and air
Sea breeze
Frazier heating and cooling
Kelvin to c
Cooling load calculation example
B&l heating and air
Evergreen plumbing and heating
Thermo bello
Hot humid
H&c heating and cooling
Principles of air conditioning ppt
Physical behavior of matter heating and cooling curves
Used for holding hot objects especially crucible and cover
Karen heating and cooling
Heating and cooling curves
Thermo bello
Schmitt heating and air
Thermal agents
Sieb plumbing and heating
Kale heating and cooling
Is vaporization endothermic or exothermic
Dsqr training
Automotive heating and air conditioning 8th edition
Magnetism love
Magnetism in solid state physics
Magnet in separating mixtures
Hans christian oersted magnetism
Magnetism is an invisible
It is an invisible force
Electromagnetic particle inspection
Hysteresis loop
Magnetismn
Magnetism equations
Lisa exposes an iron paper clip to a very strong magnet
Conceptual physics magnetism
Characteristic of magnetism
Joachim stöhr
Magnetism
Basics of magnetism
The phenomenon of magnetism is best understood in terms of