The Nervous System The nervous system is made
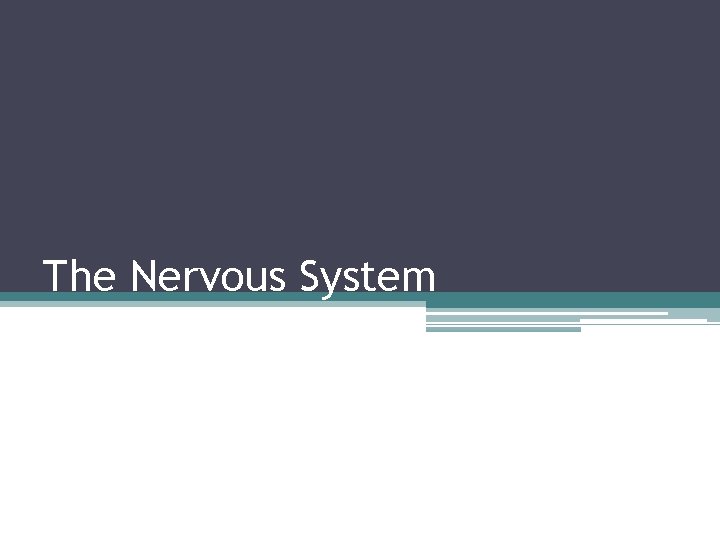
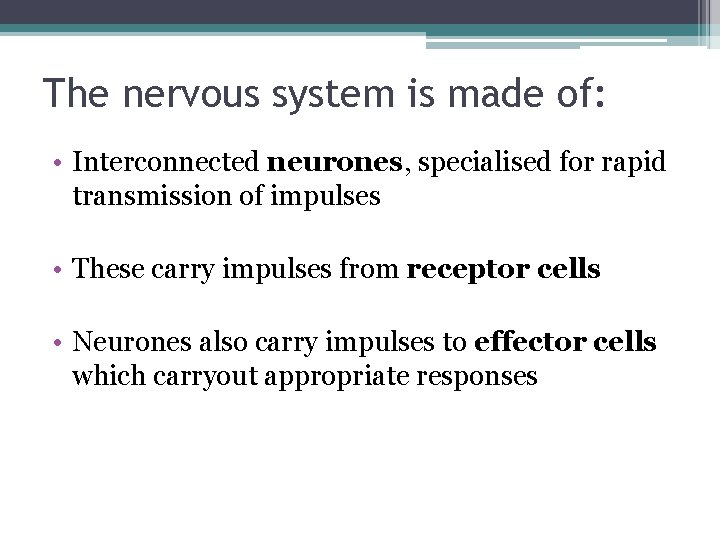
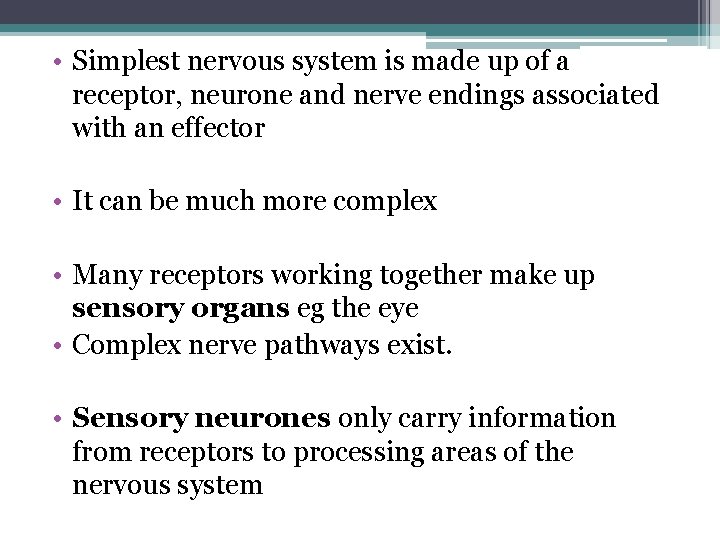
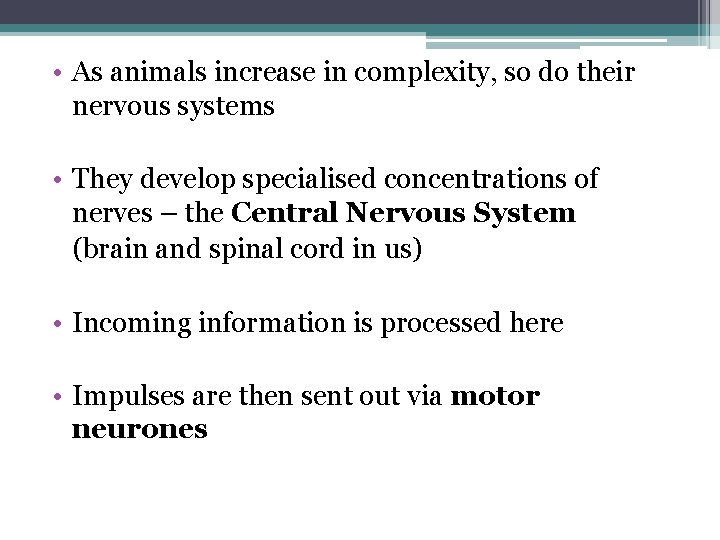
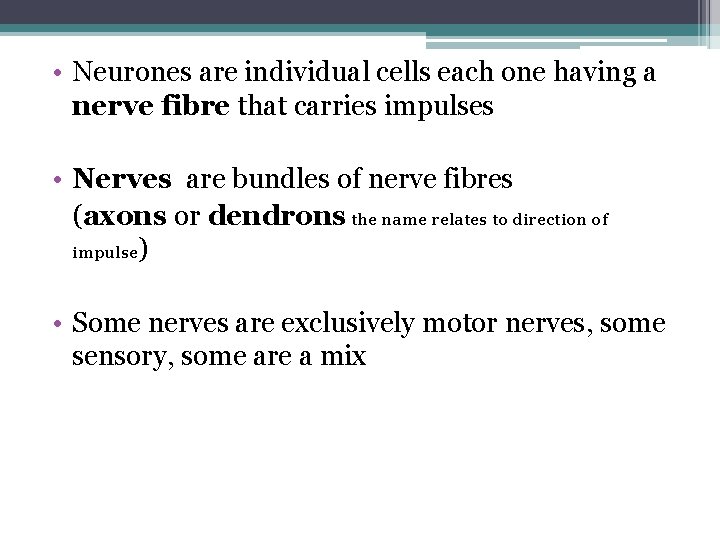
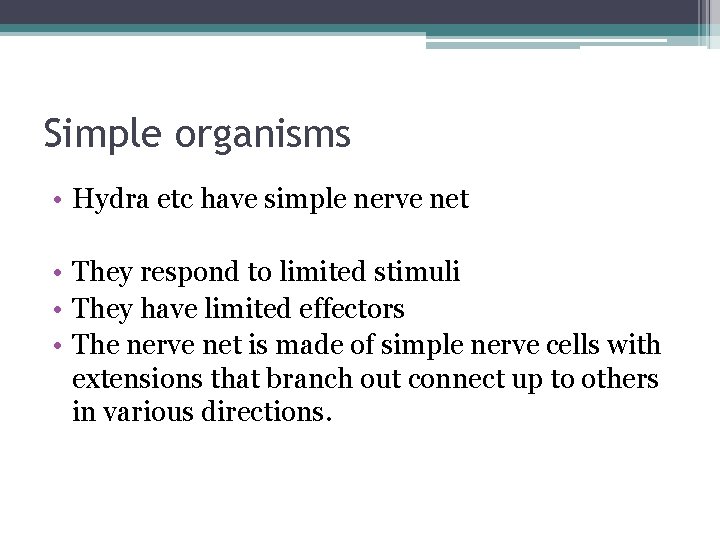
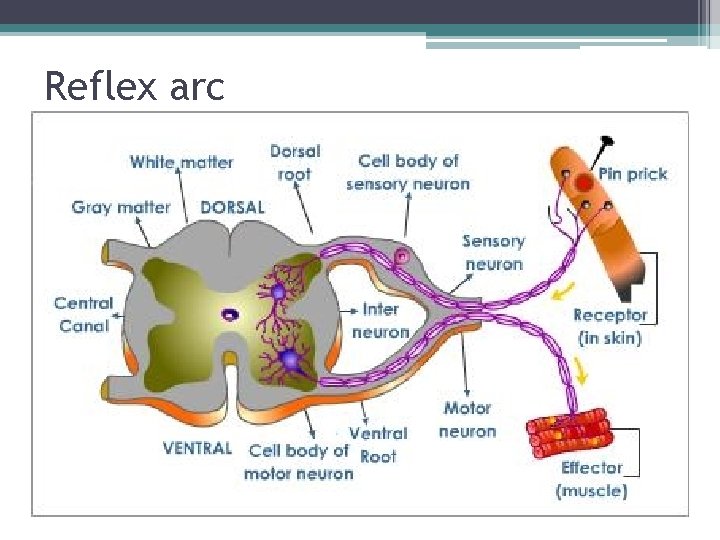
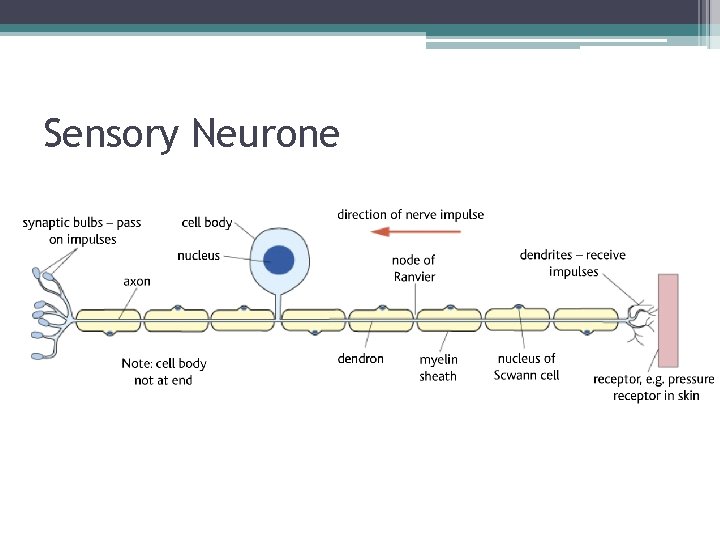
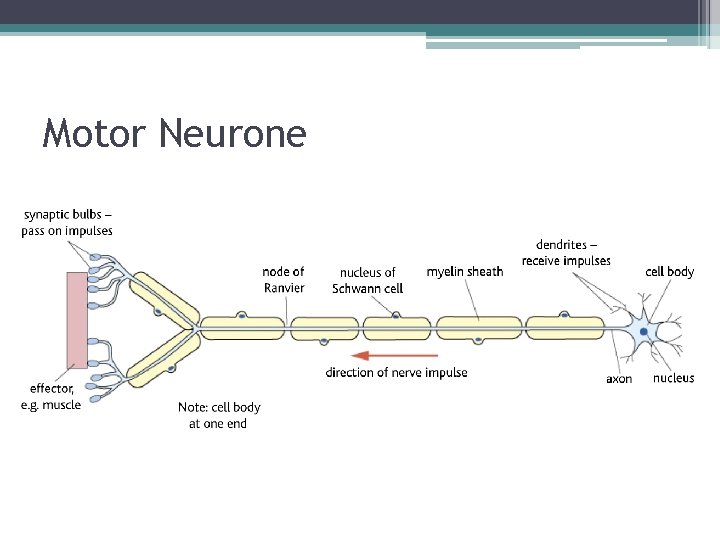
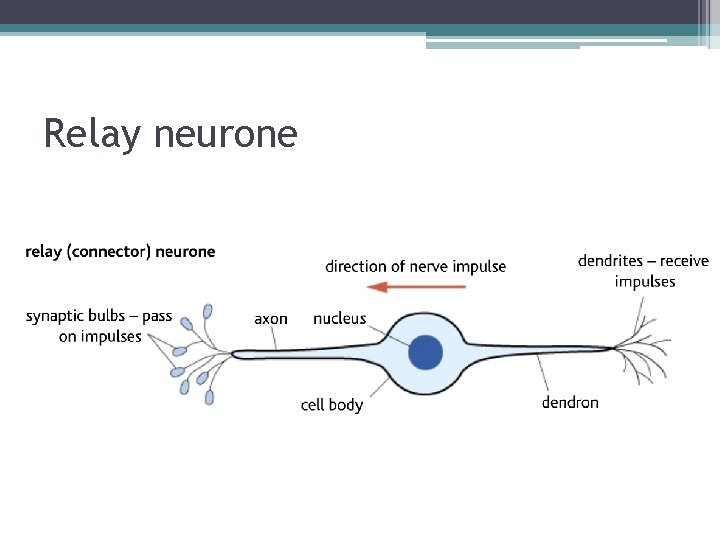
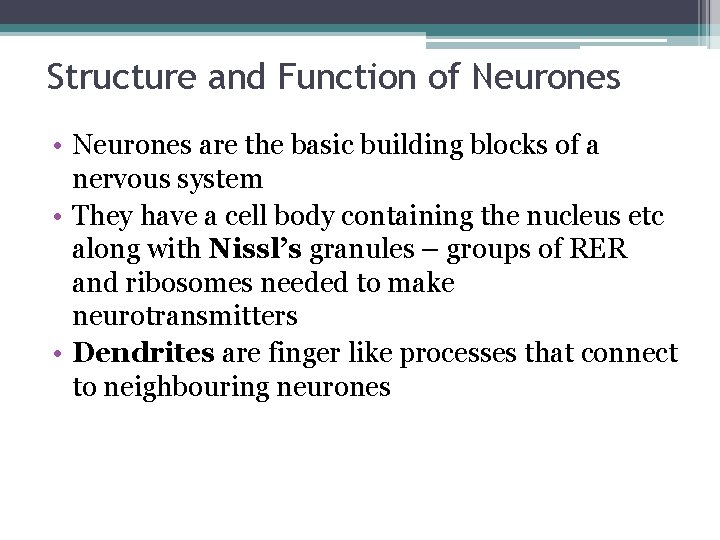
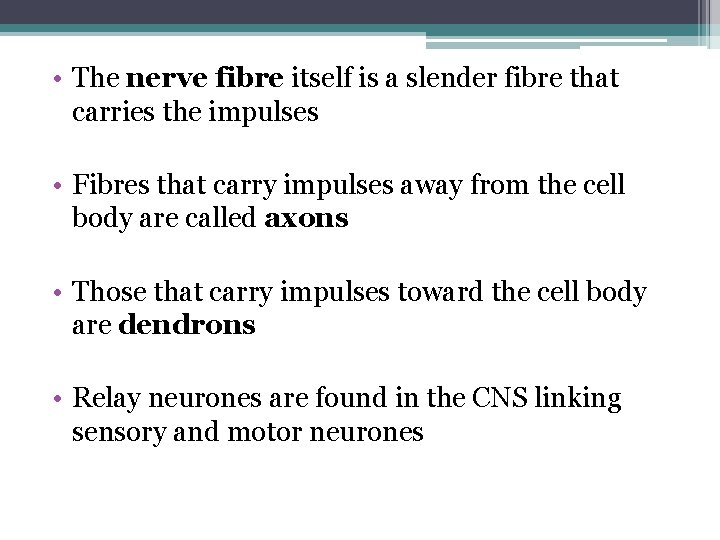
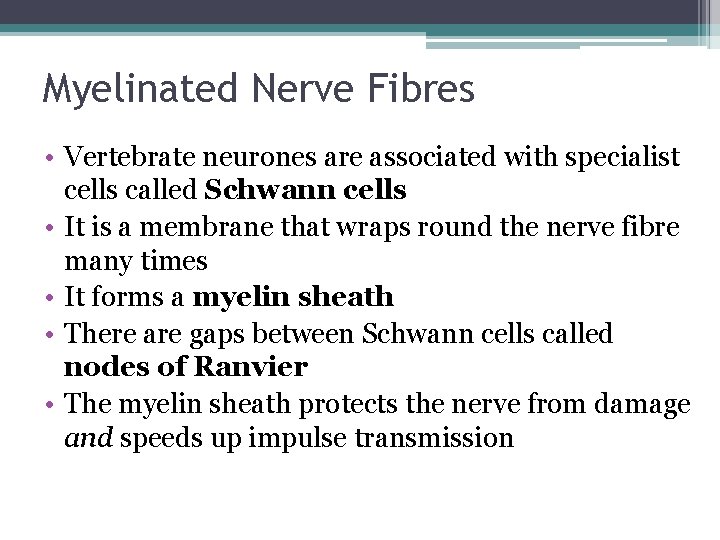
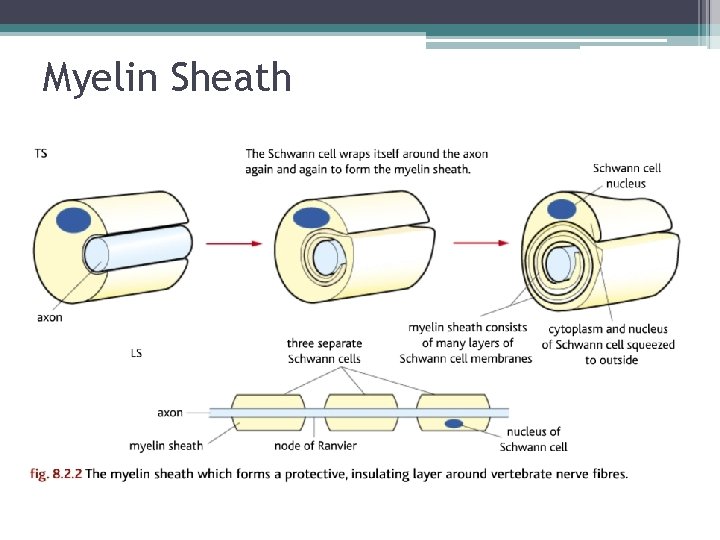
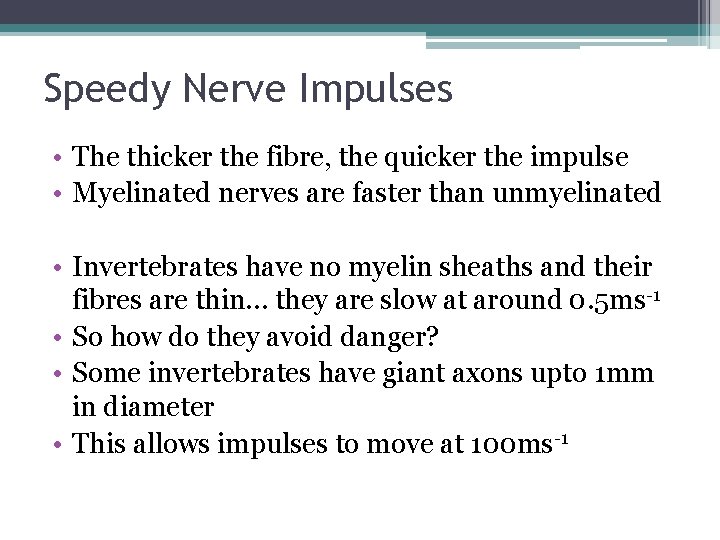
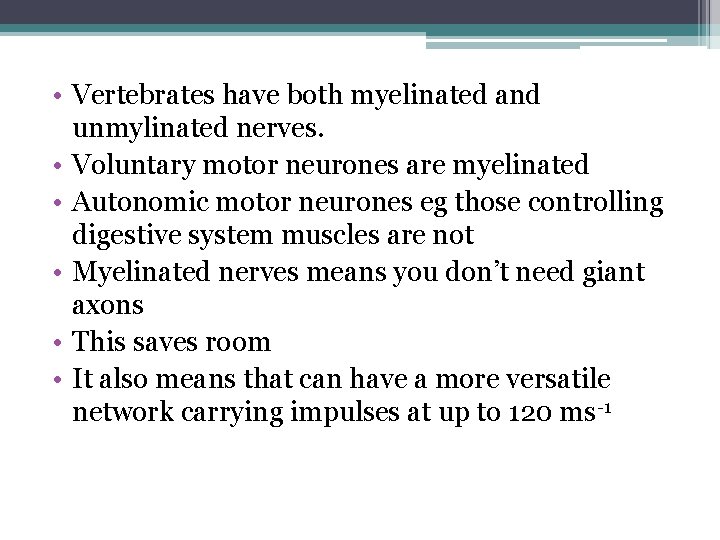
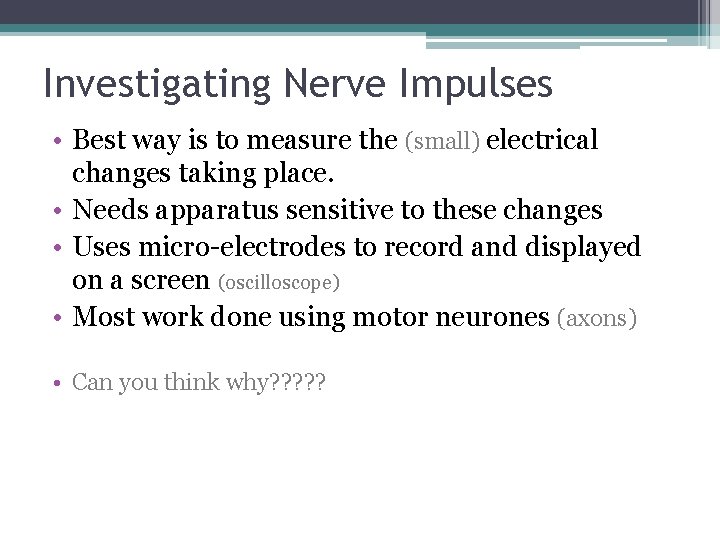
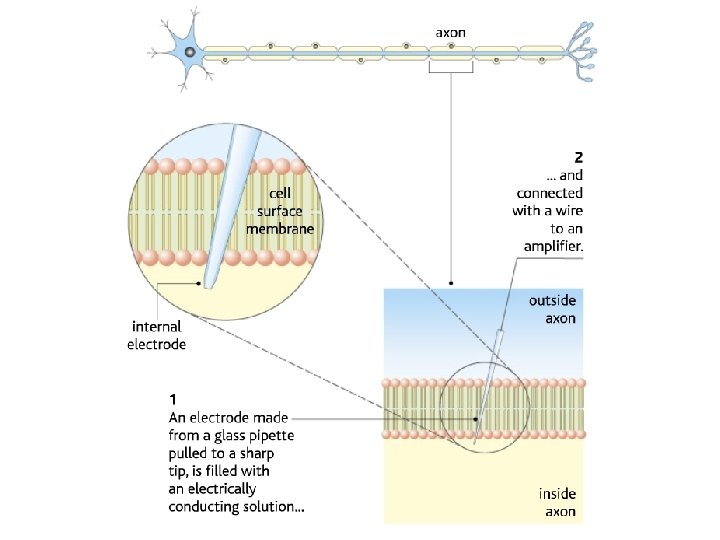
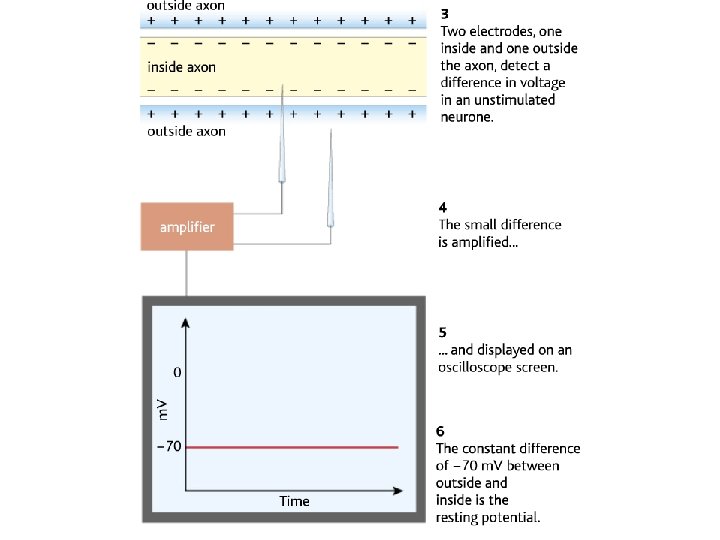
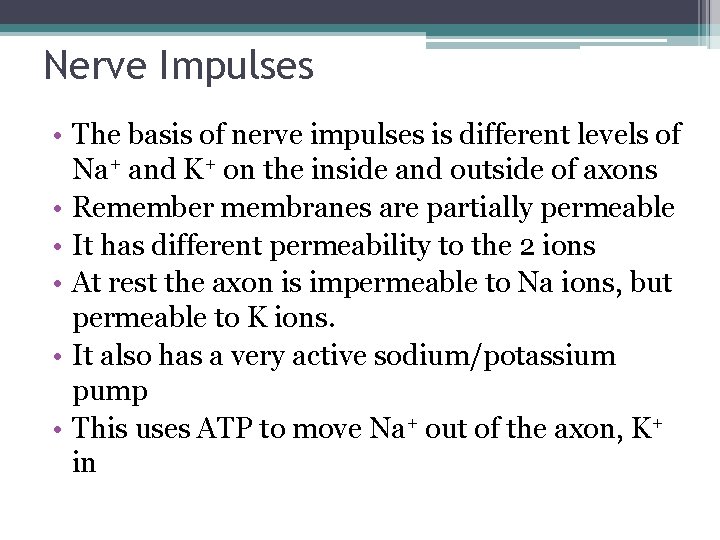
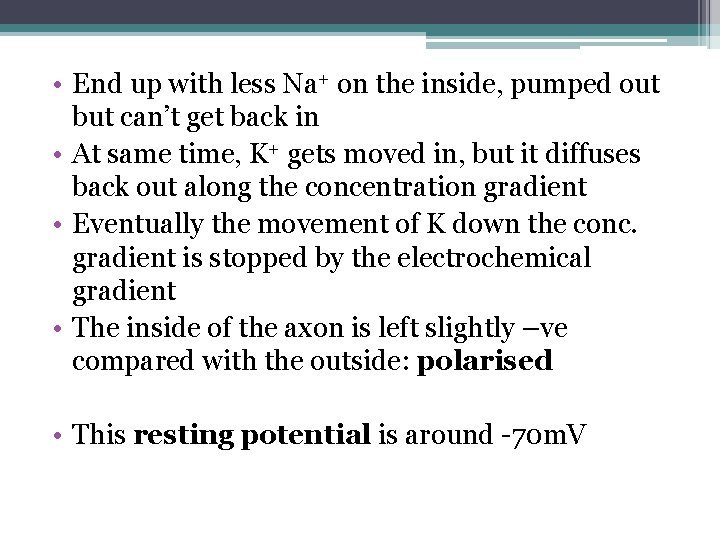
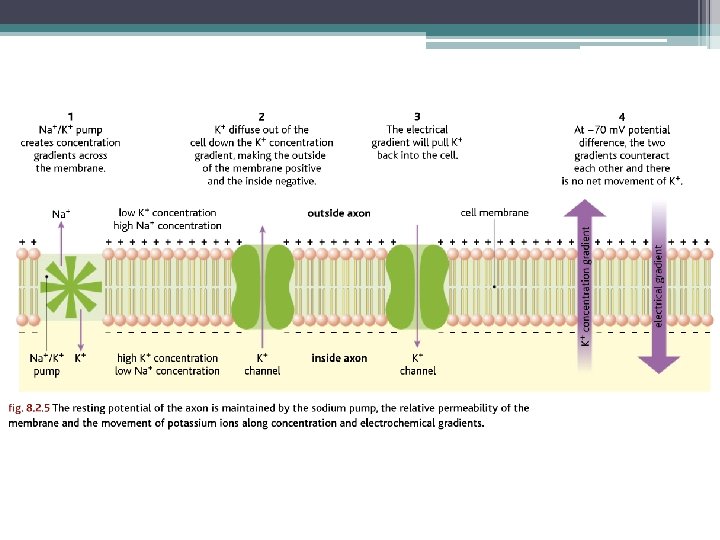
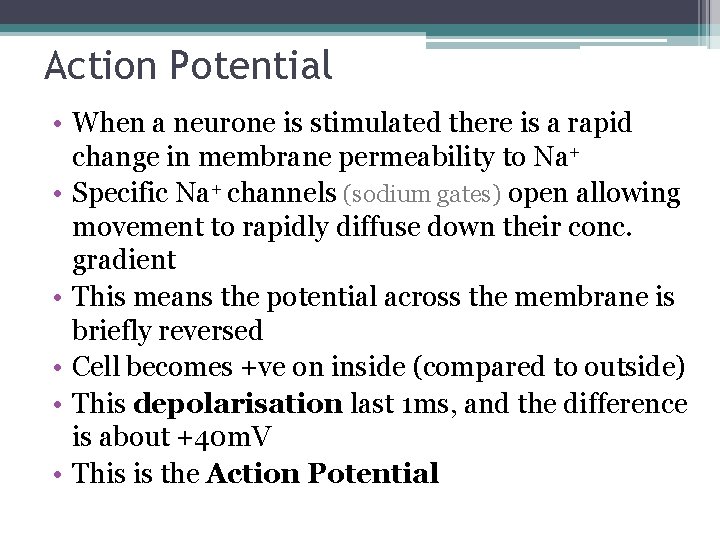
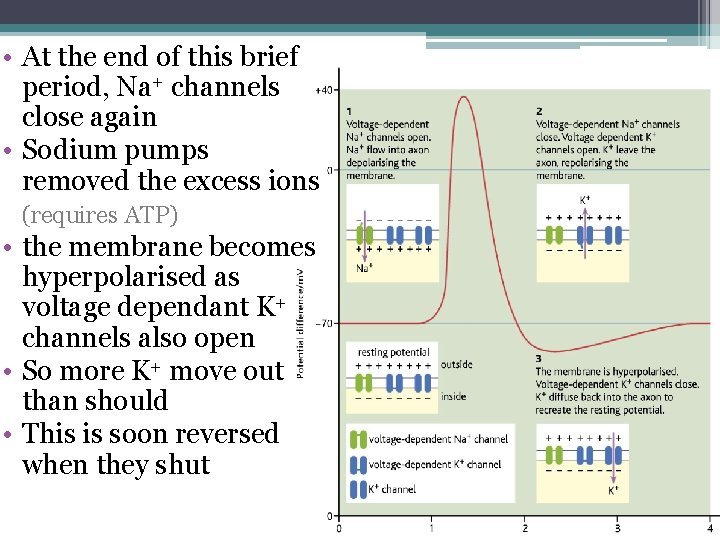
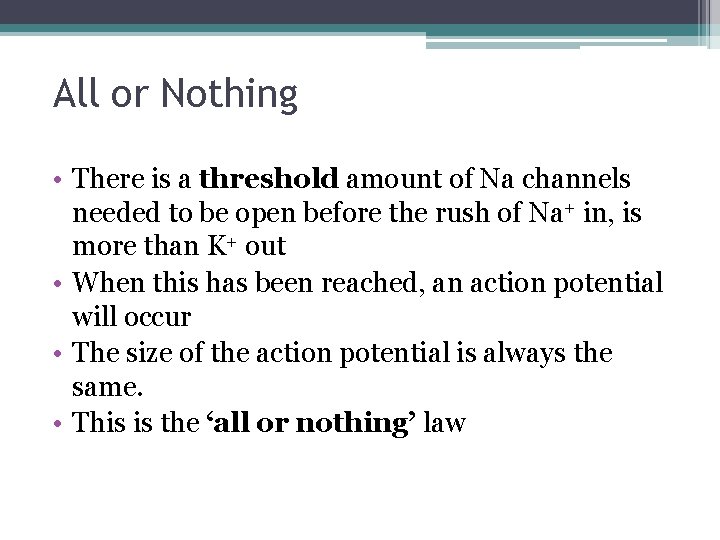
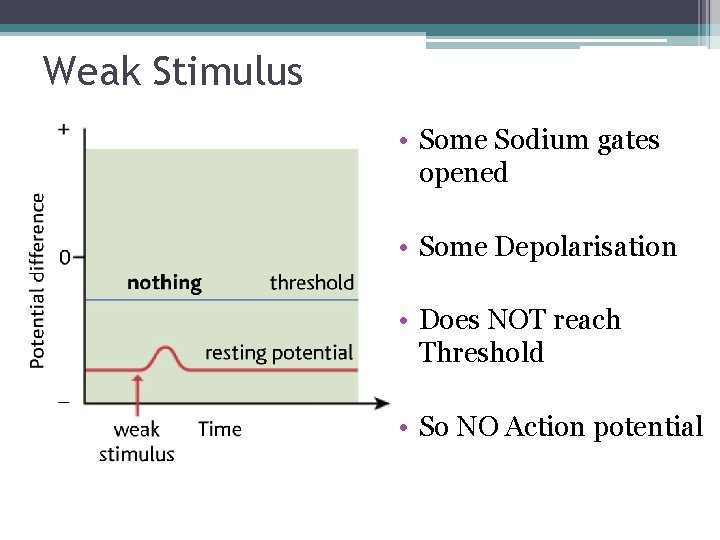
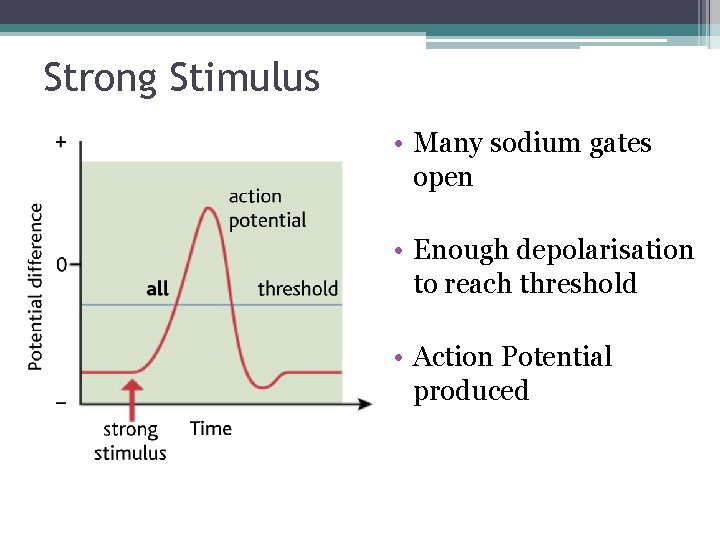
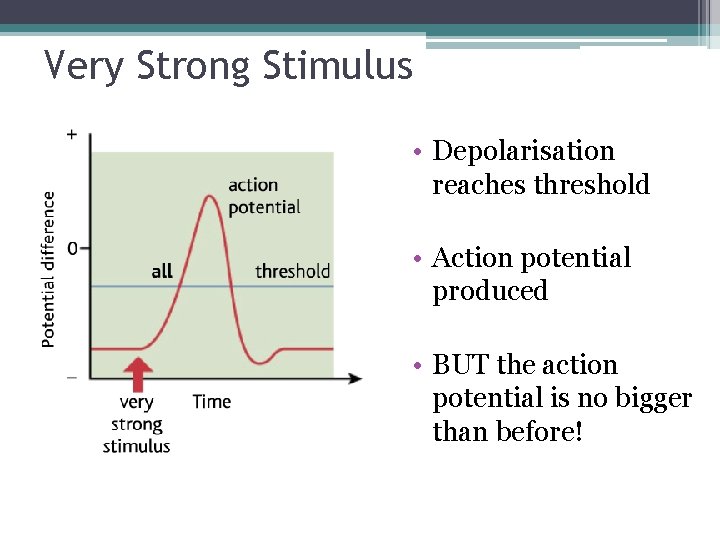
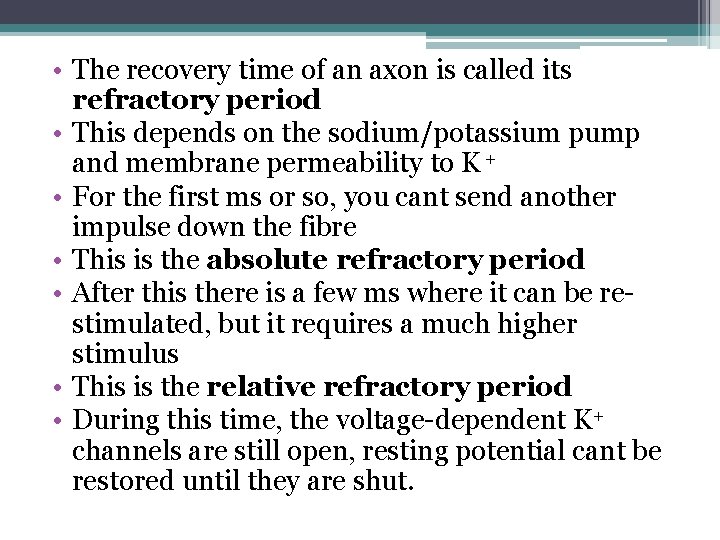
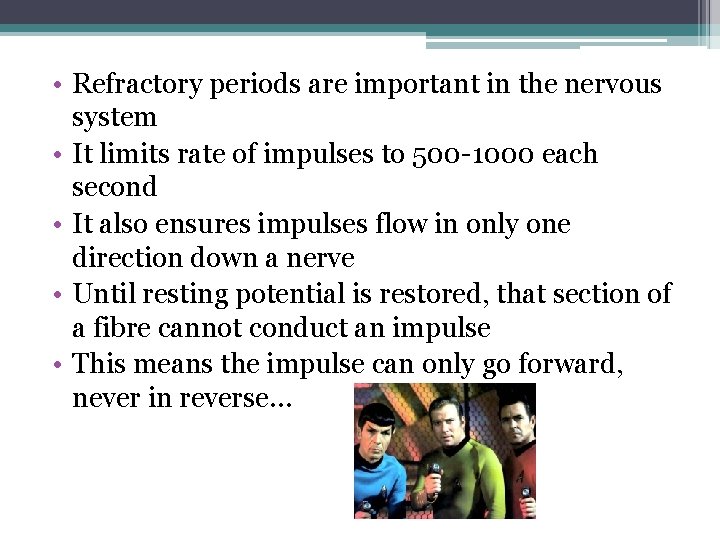
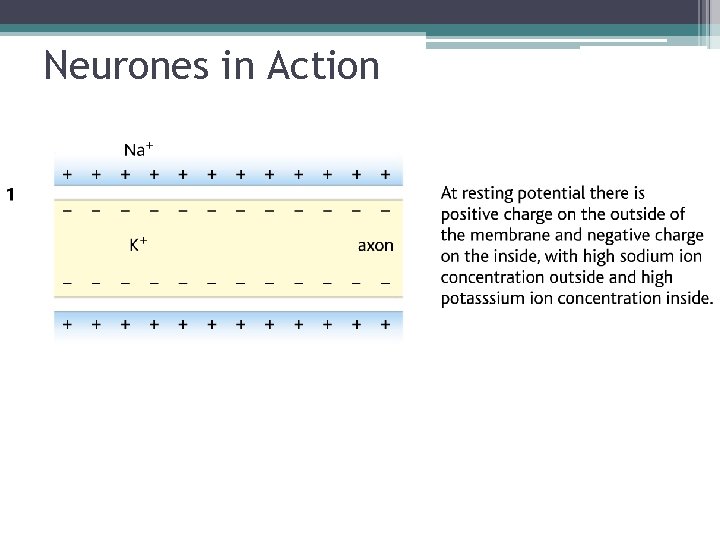
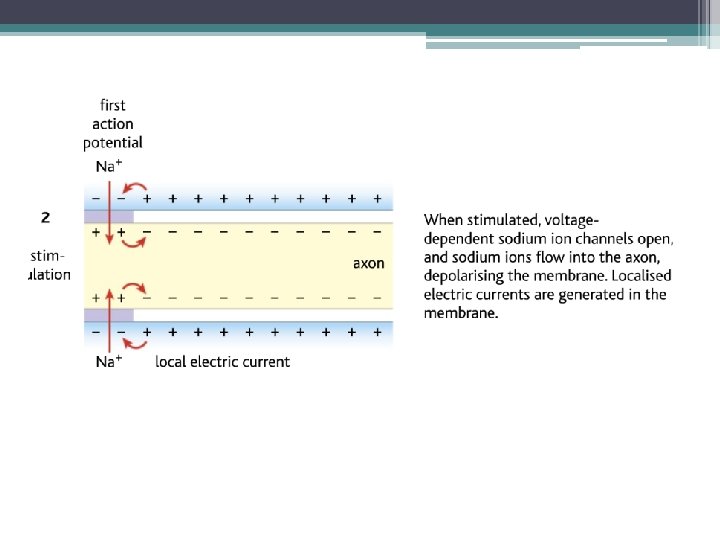
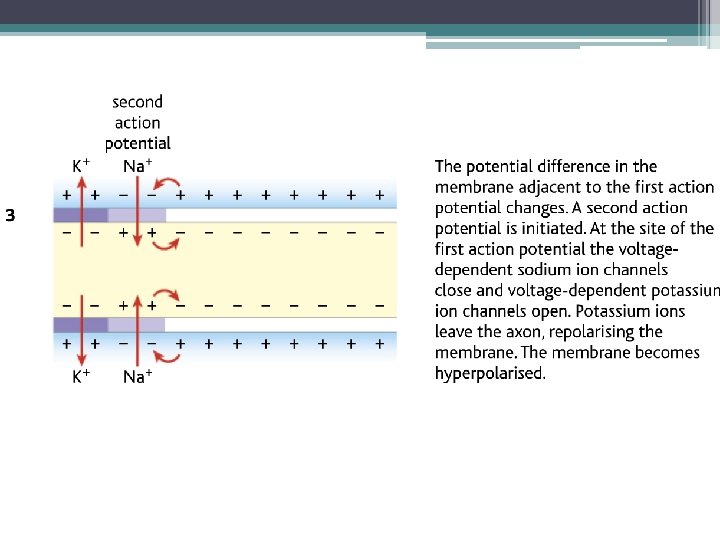
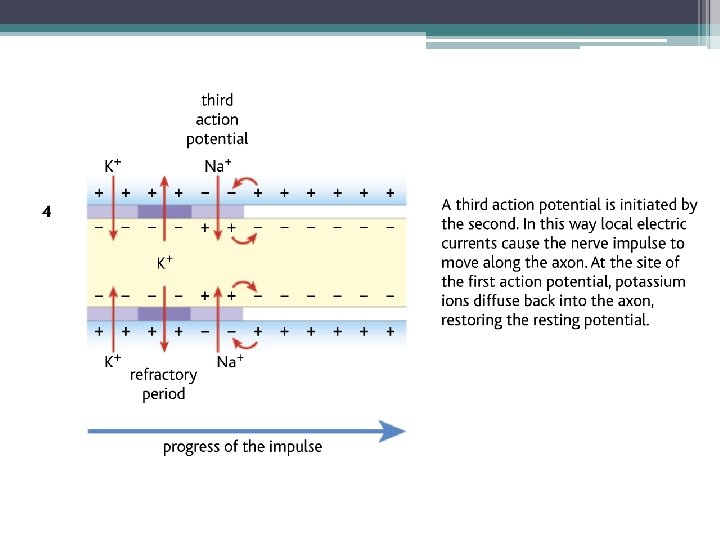
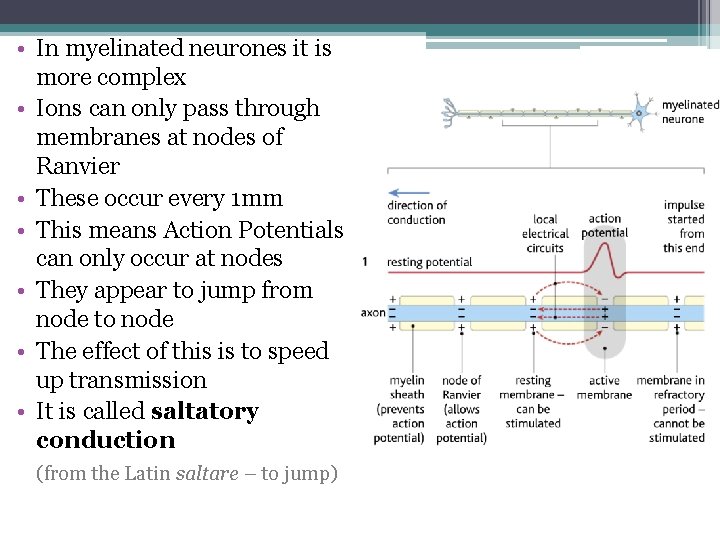
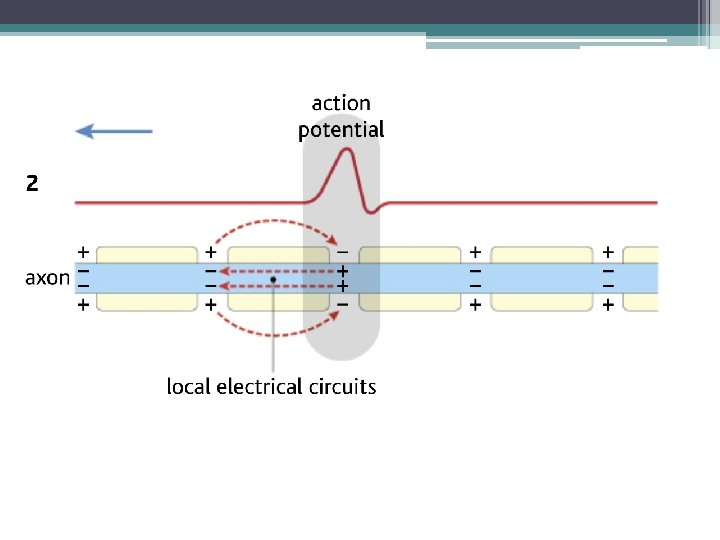
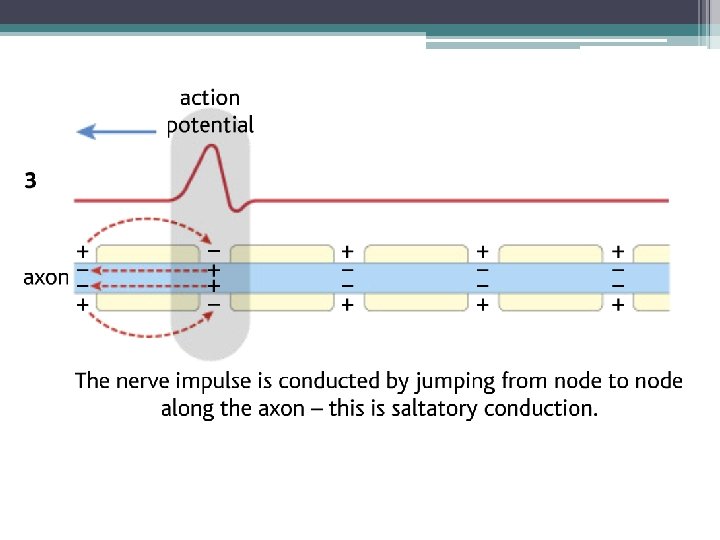
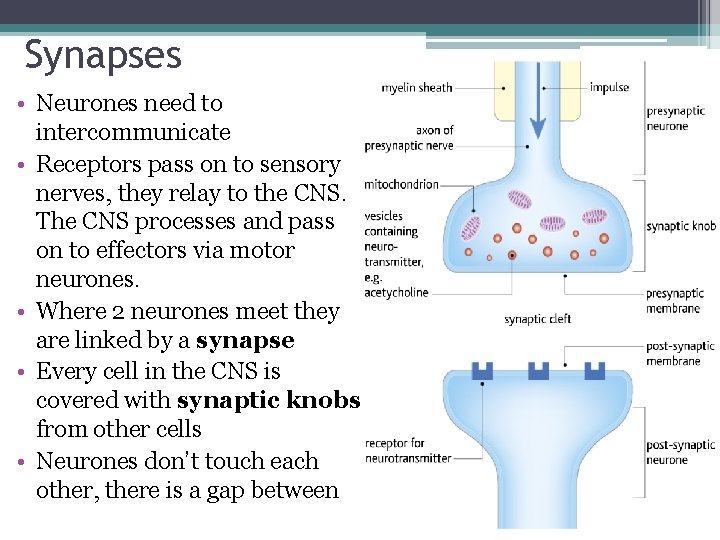
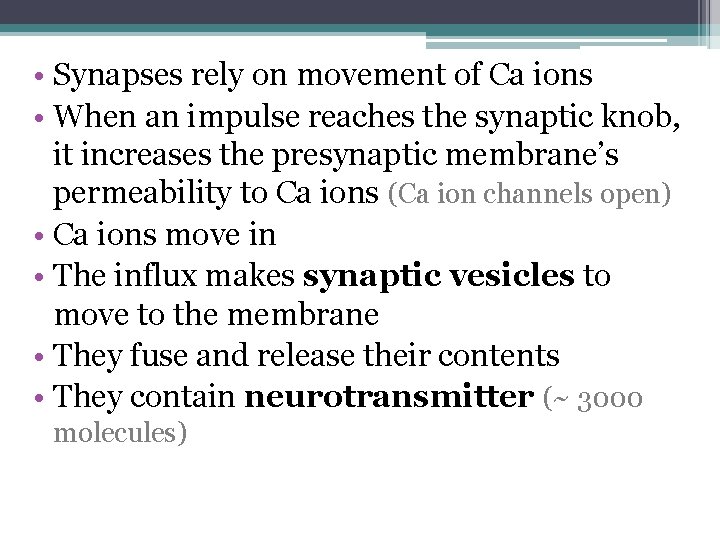
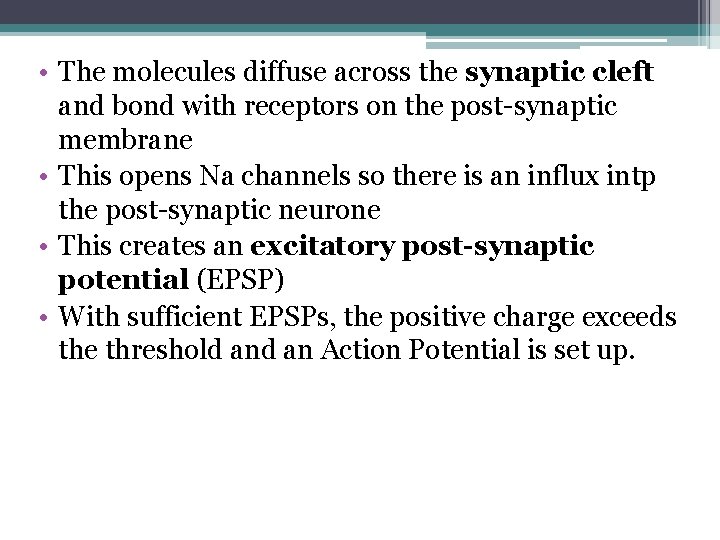
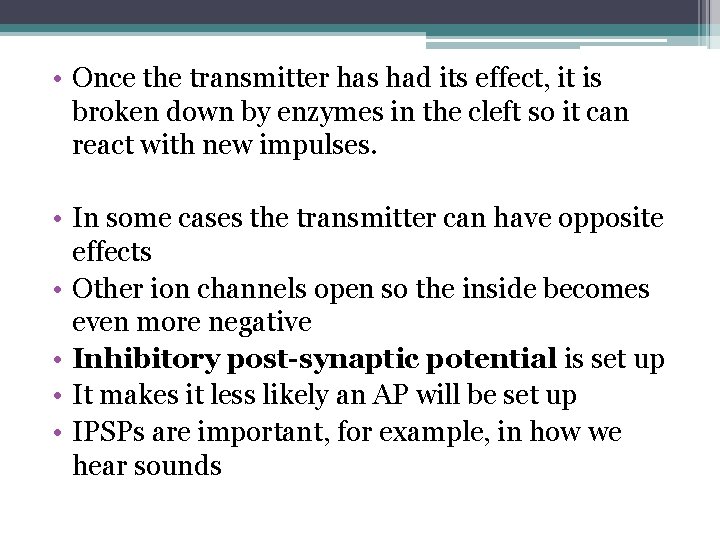
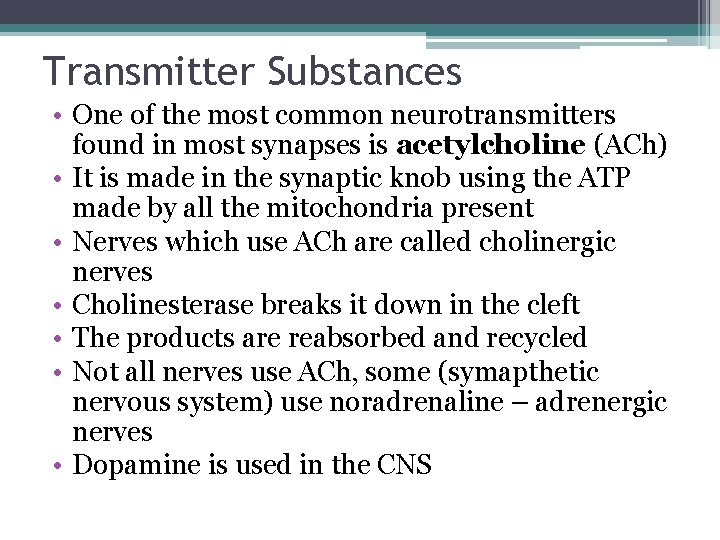
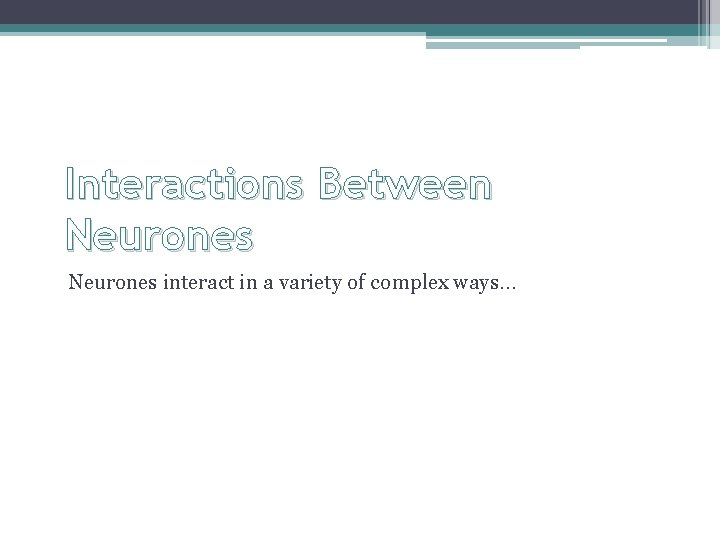
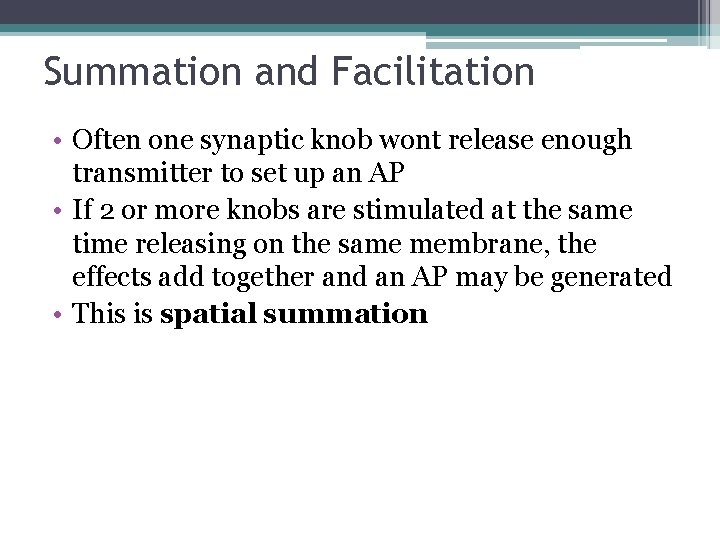
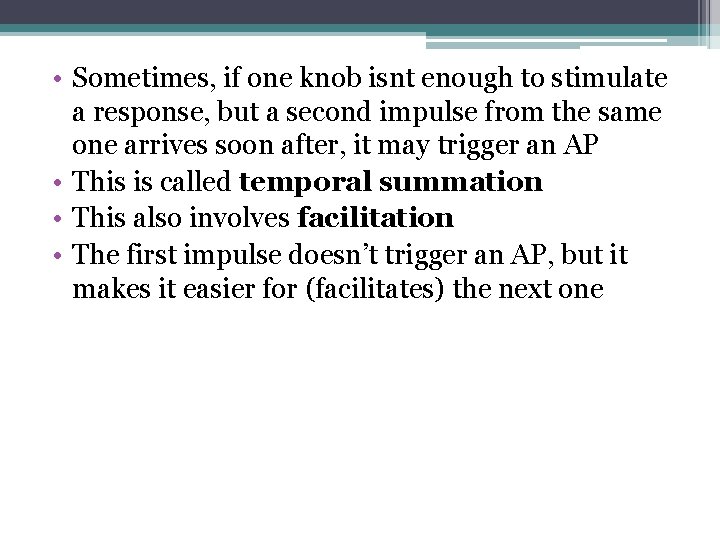
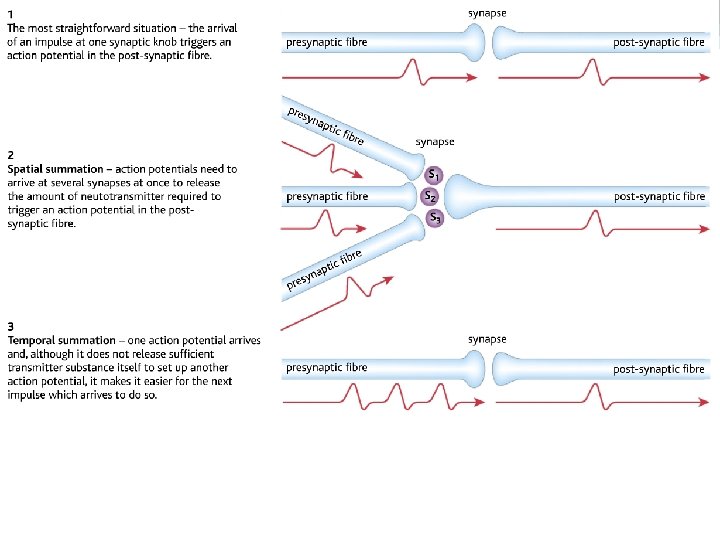
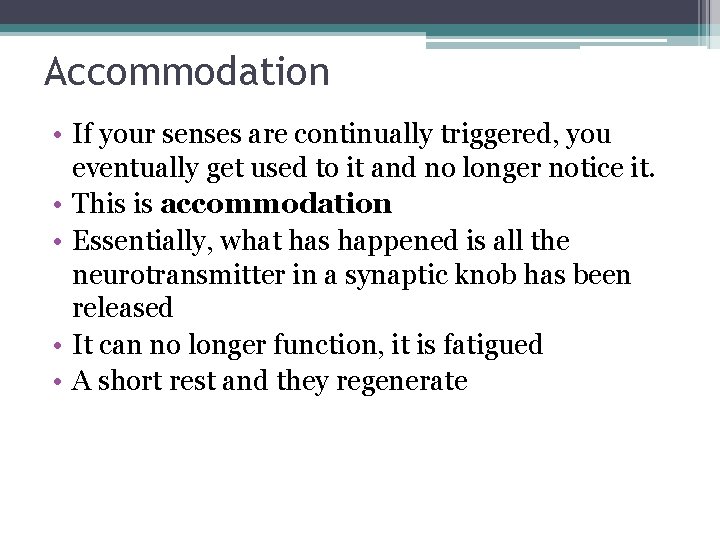
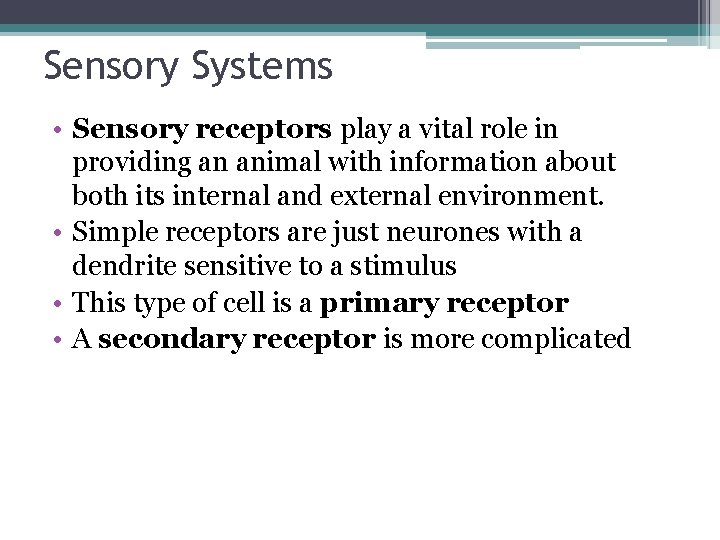
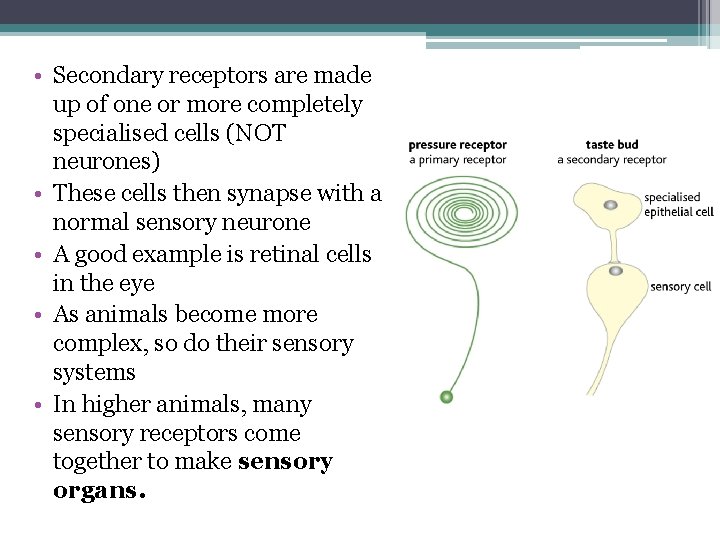
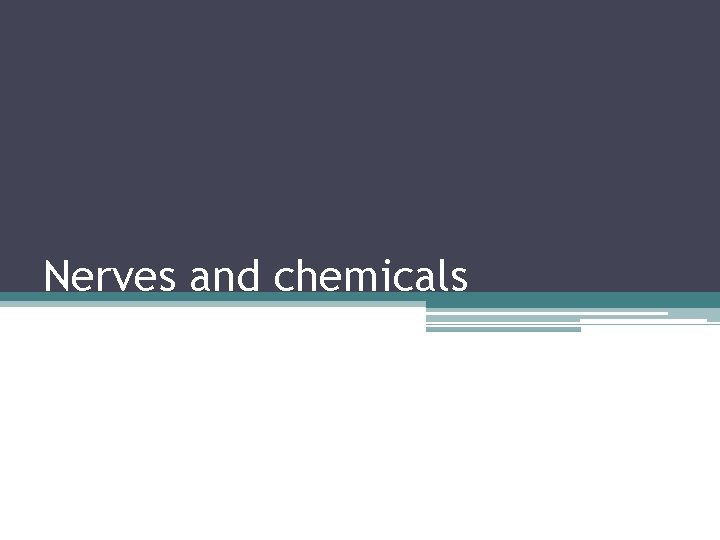
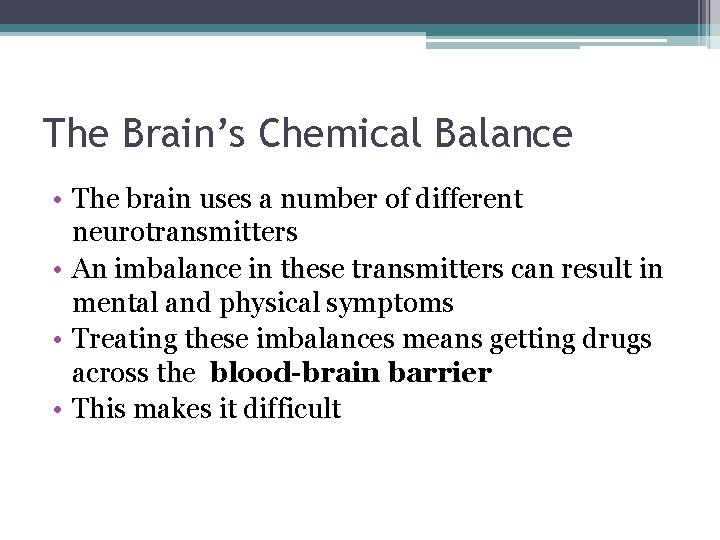
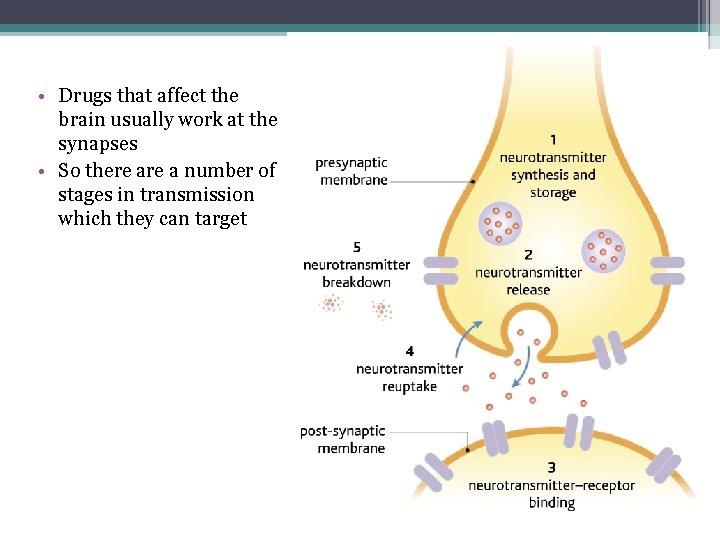
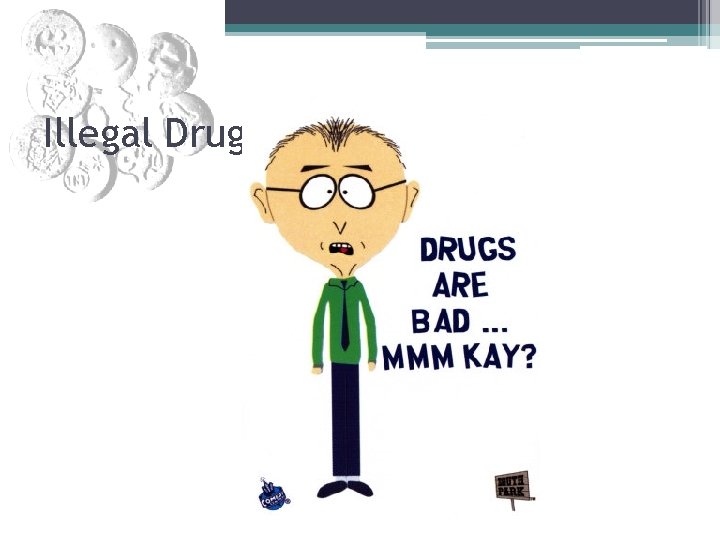
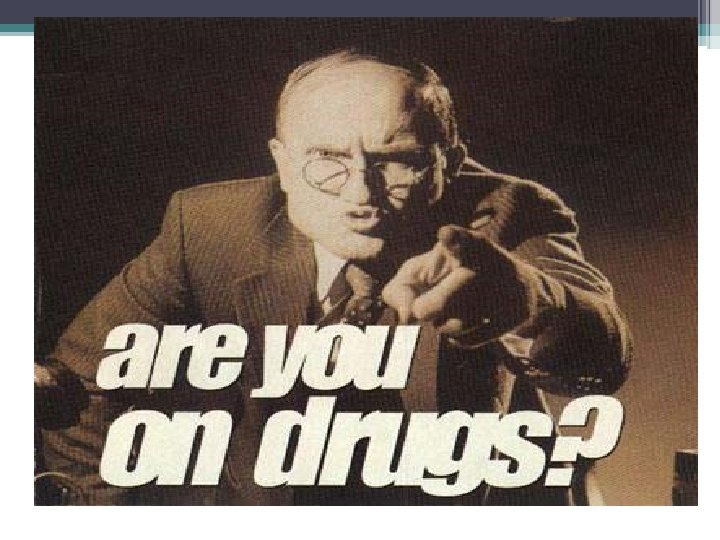
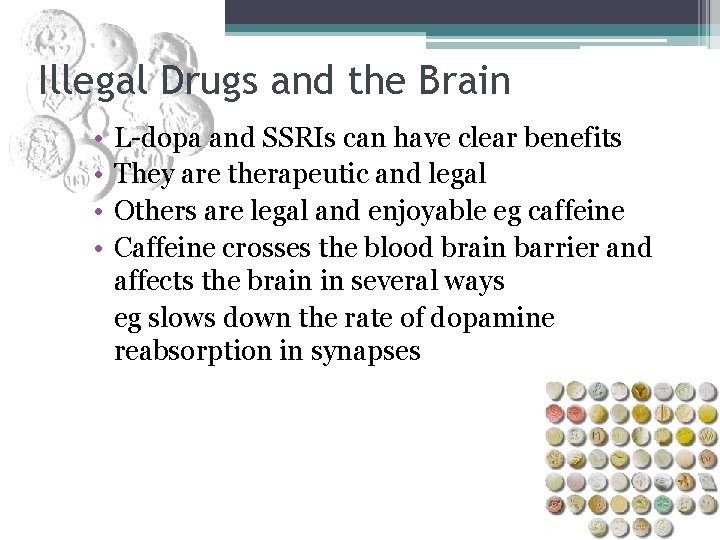
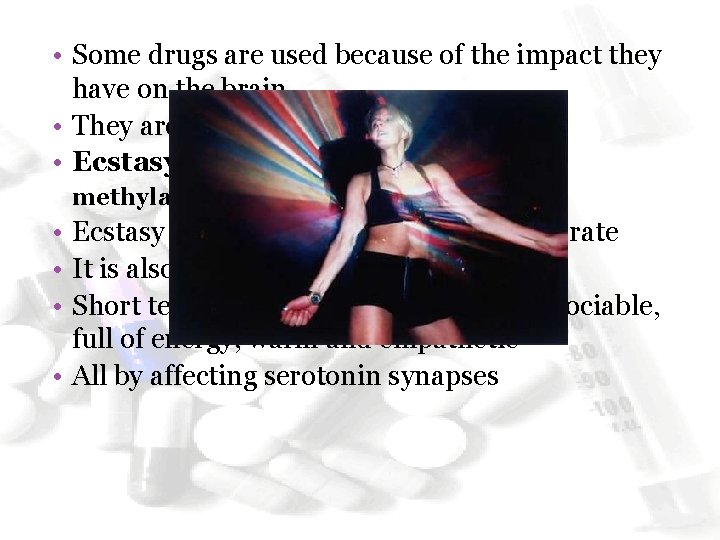
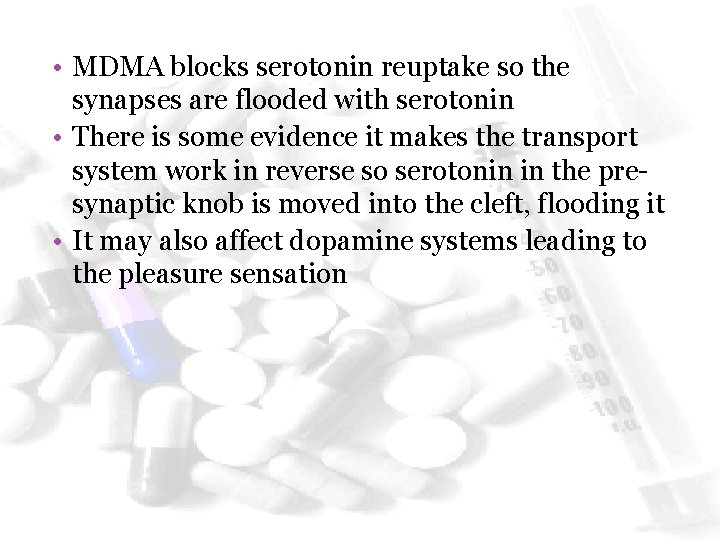
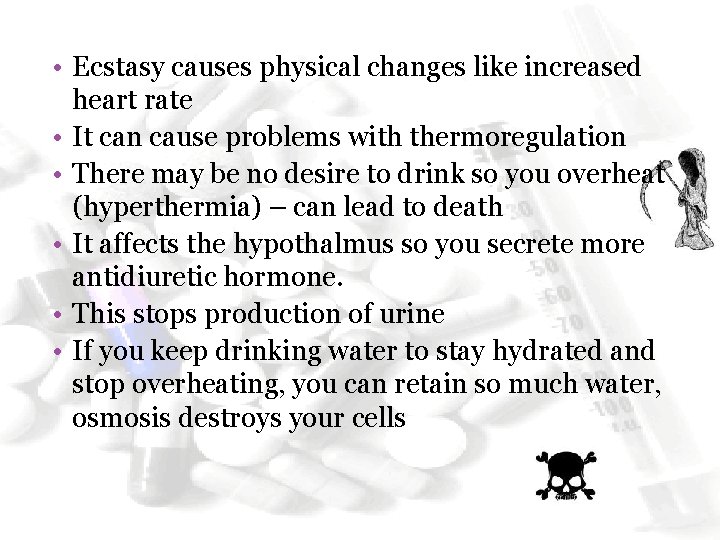
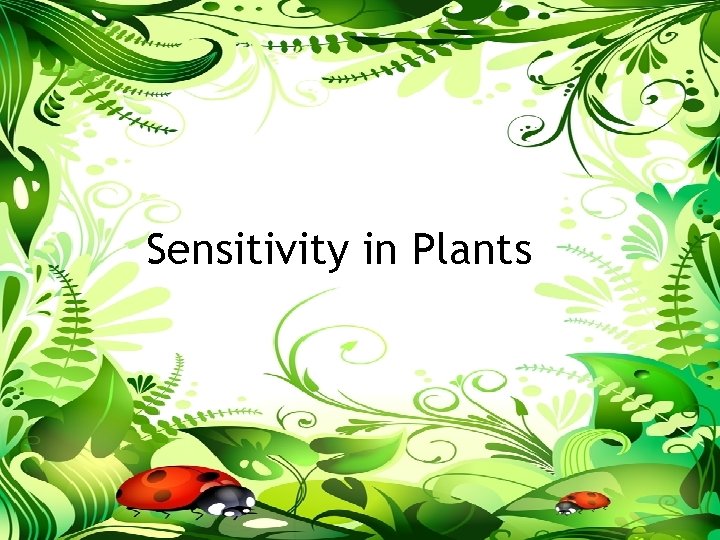
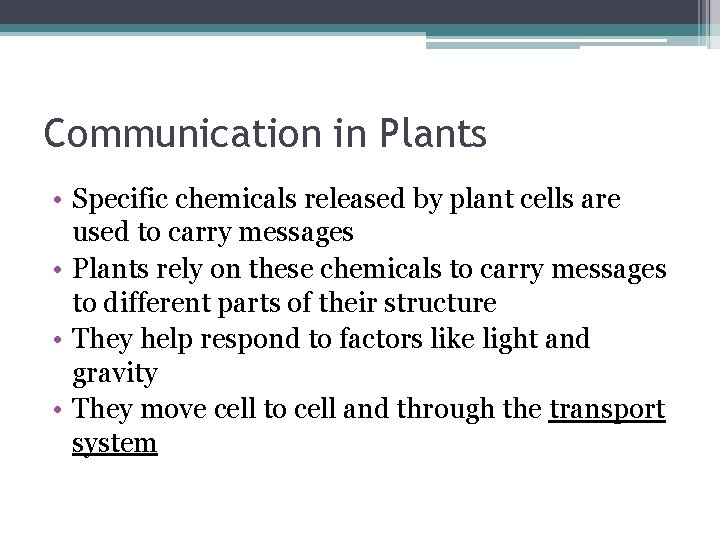
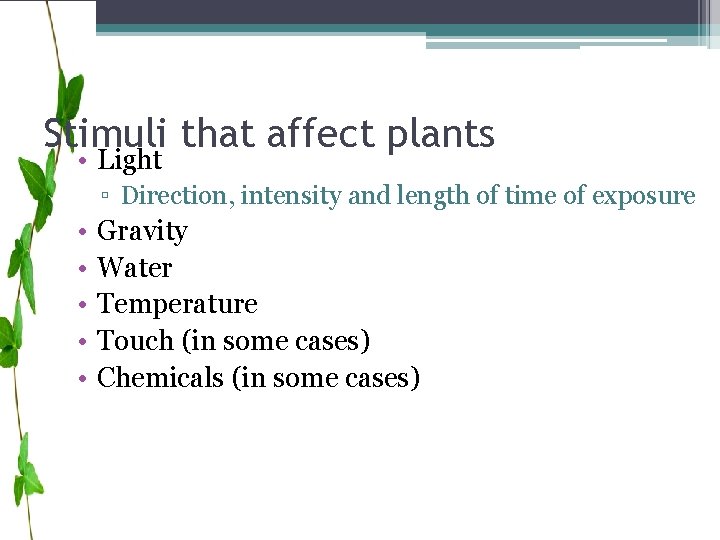
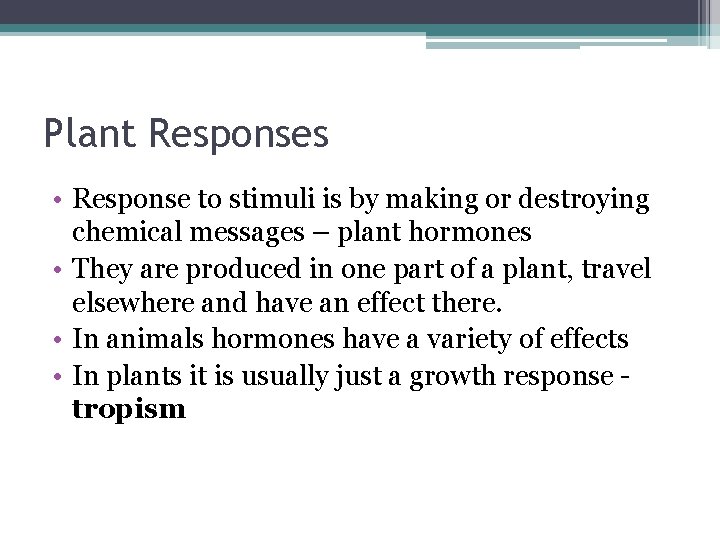
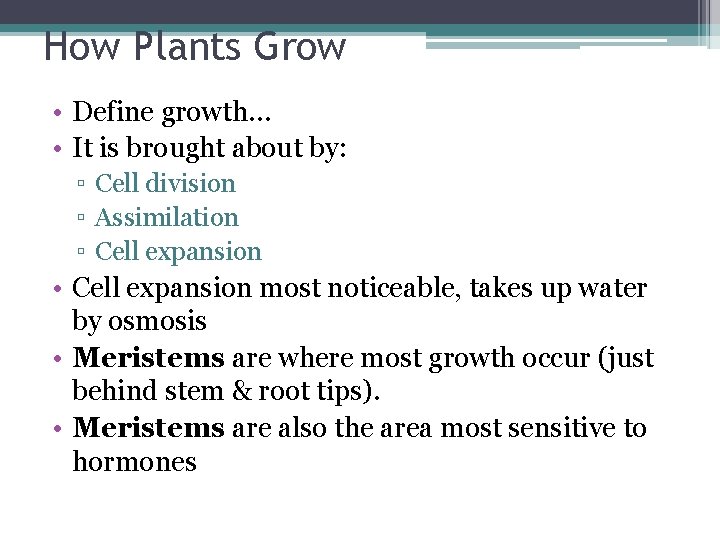
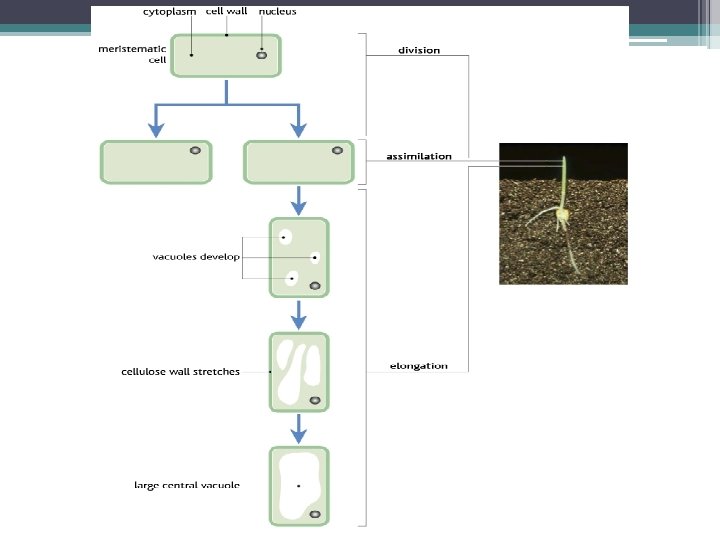
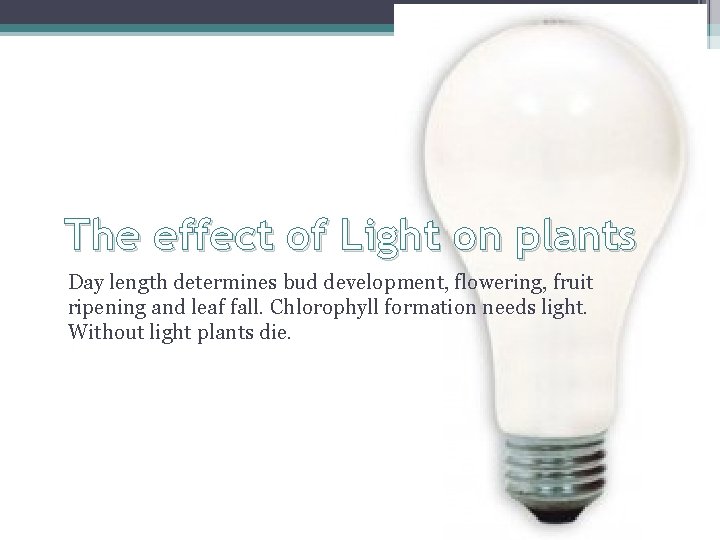
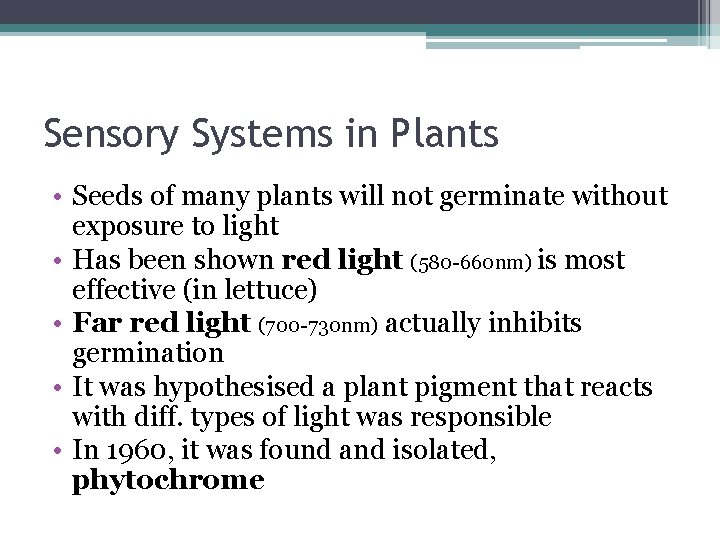
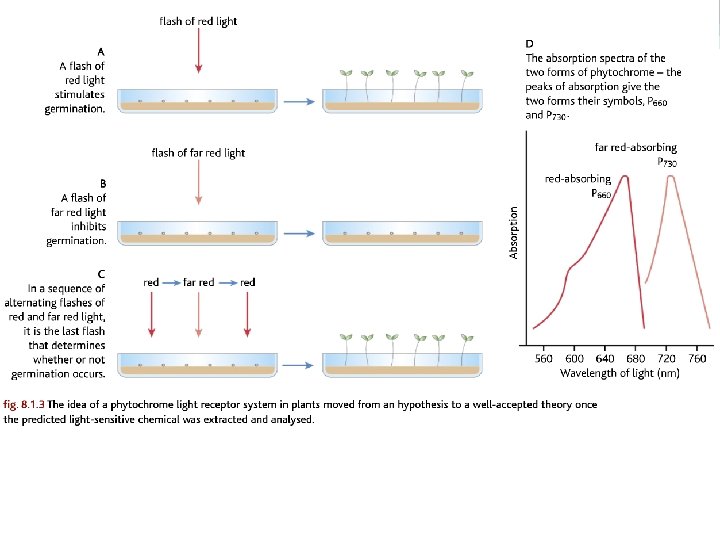
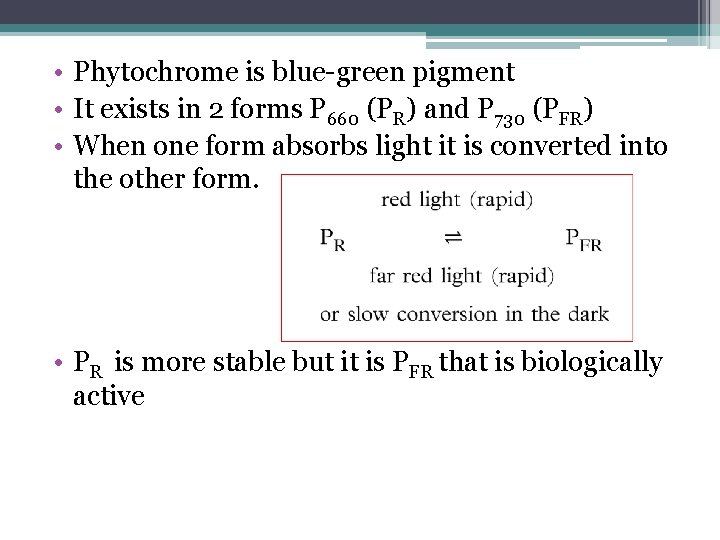
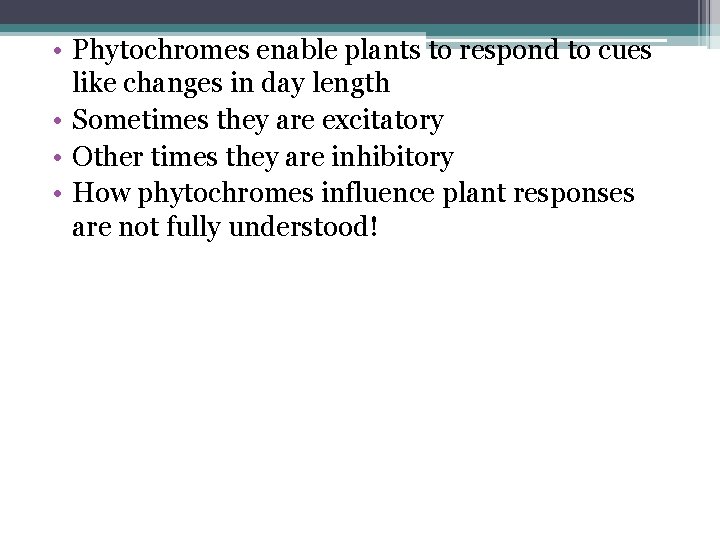
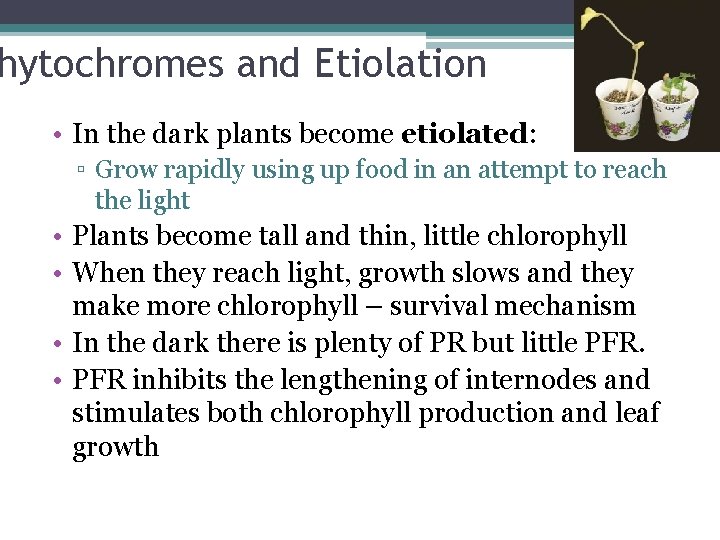
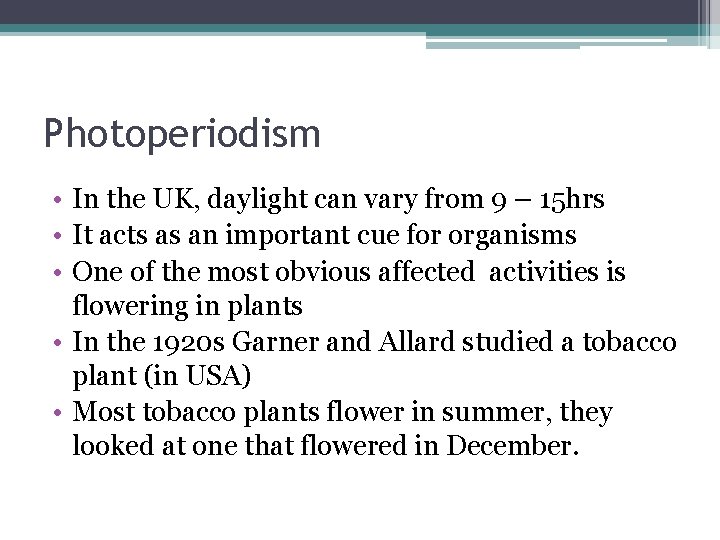
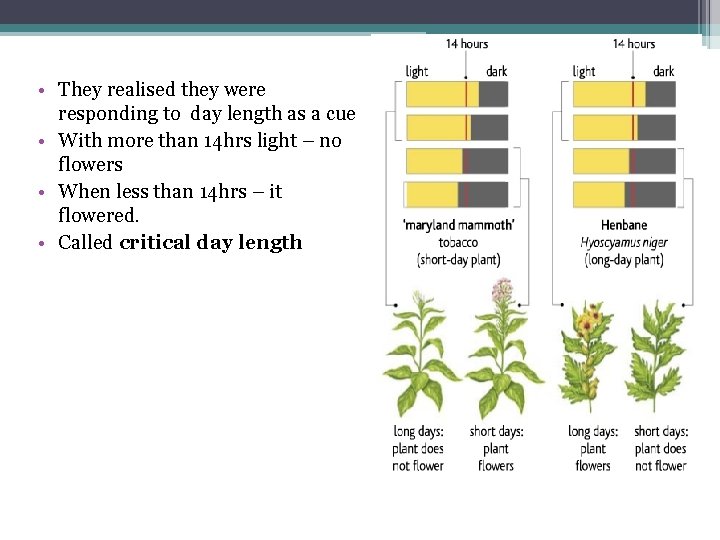
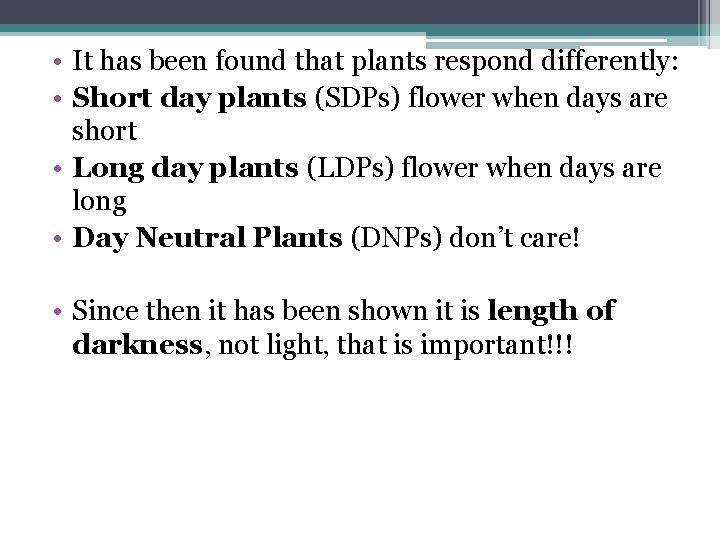
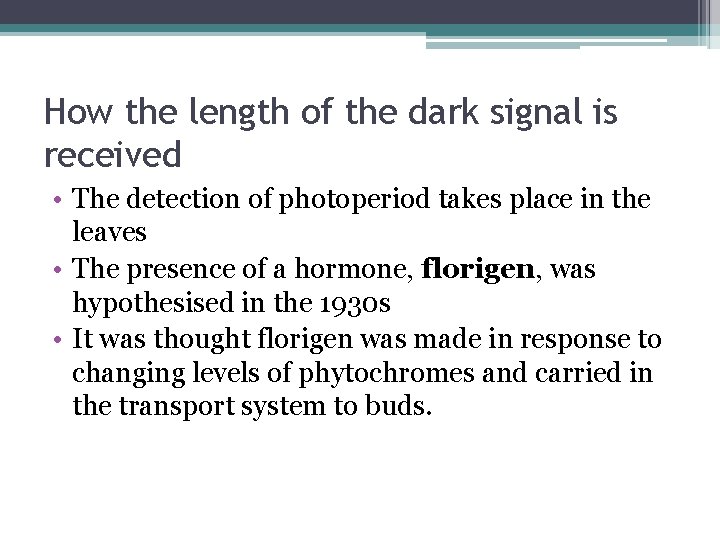
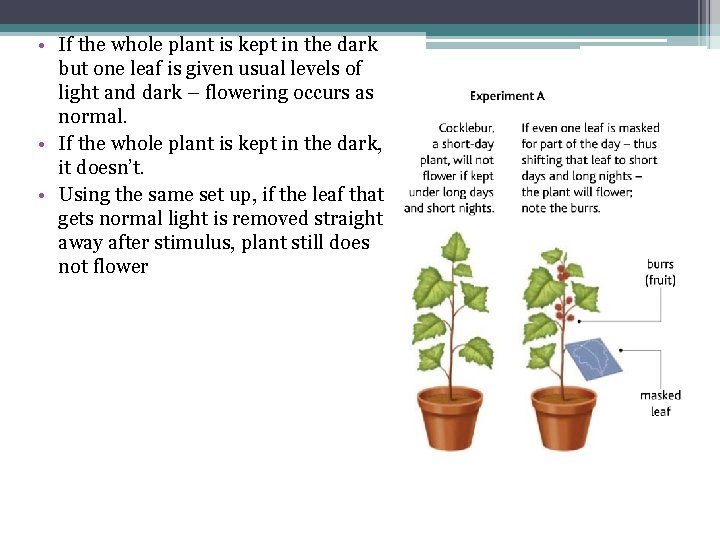
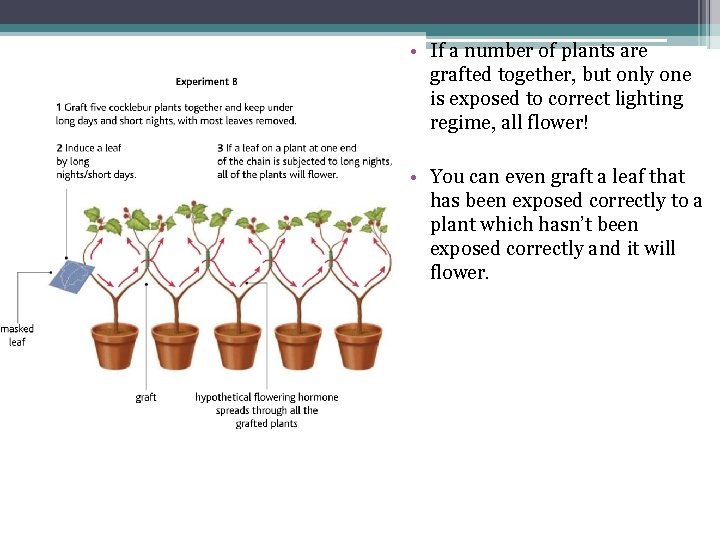
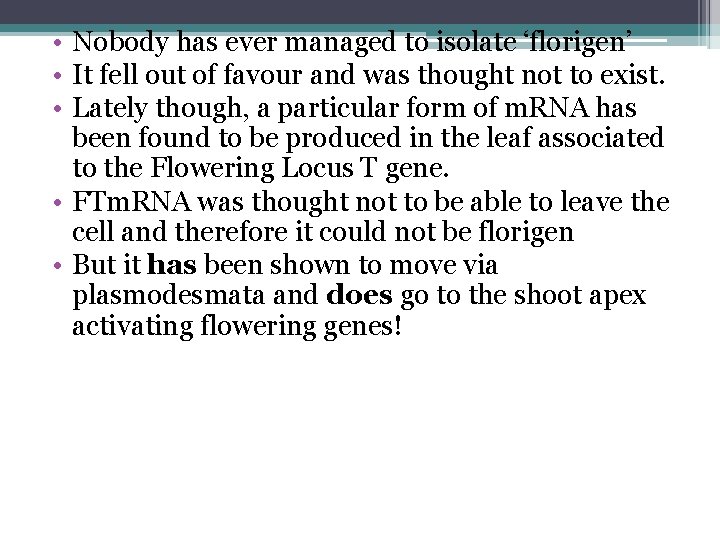
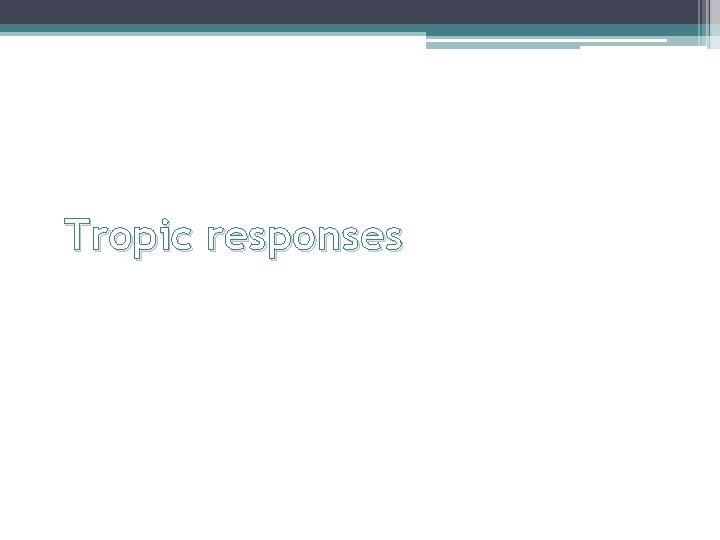
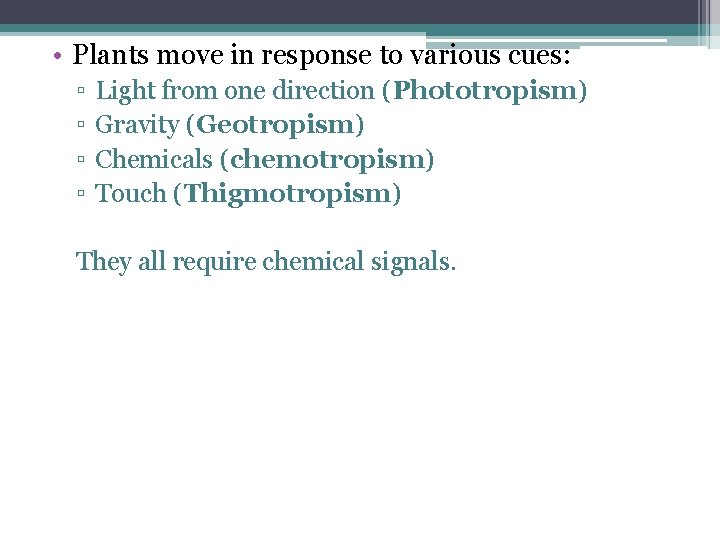
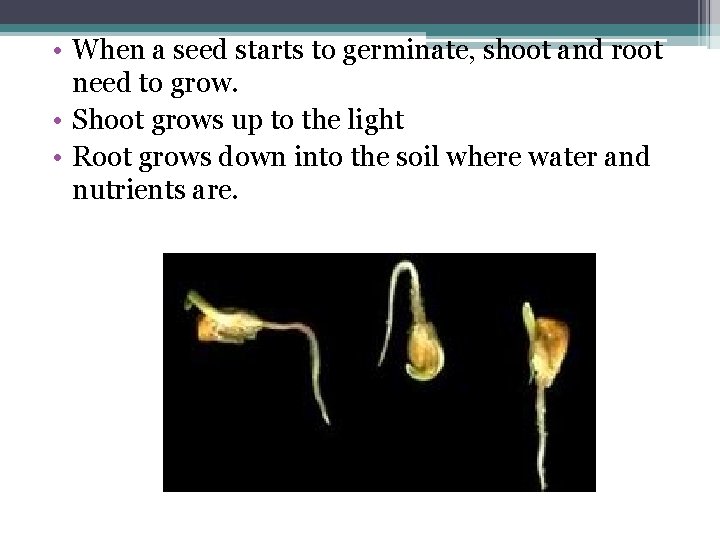
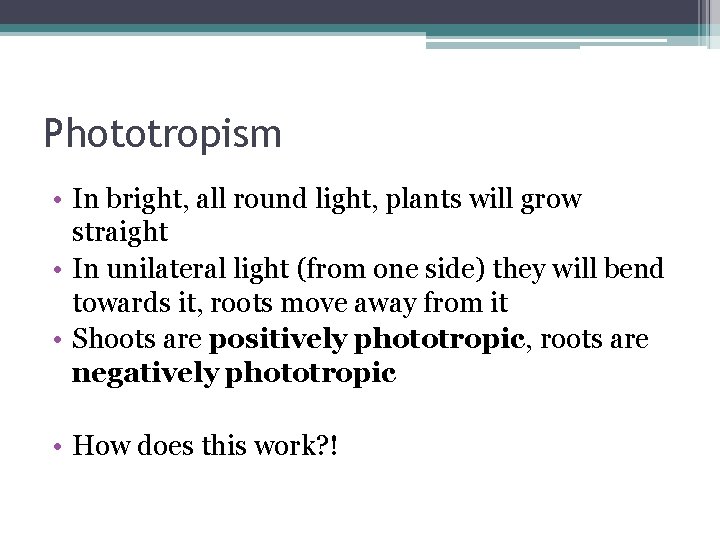
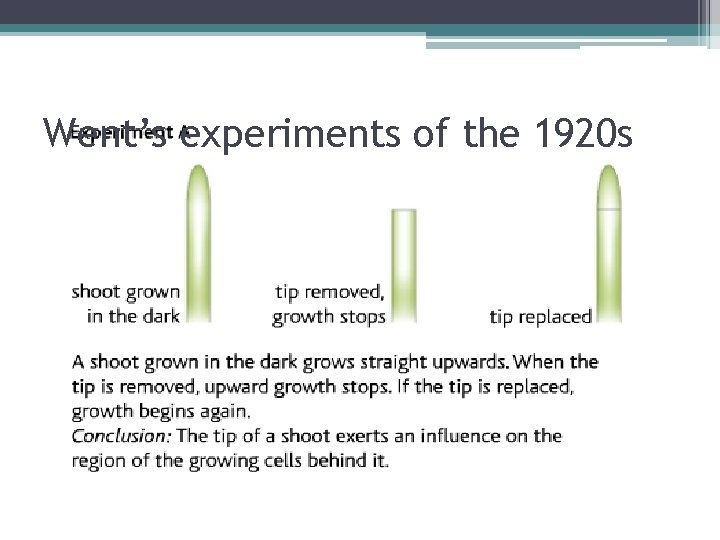
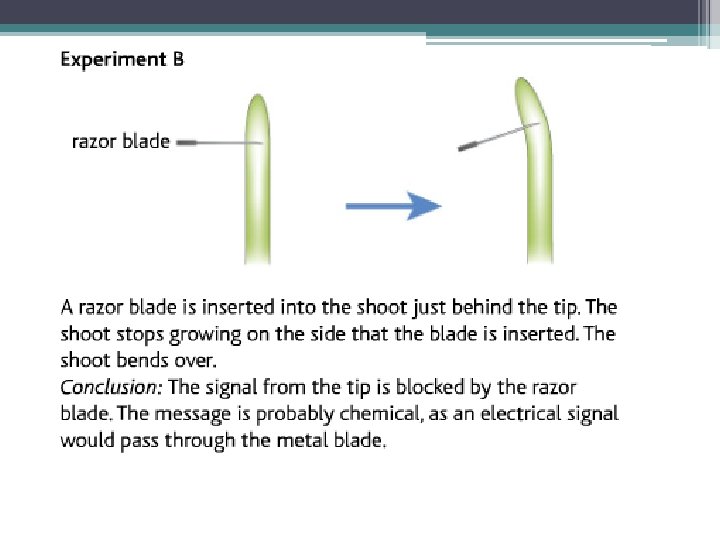
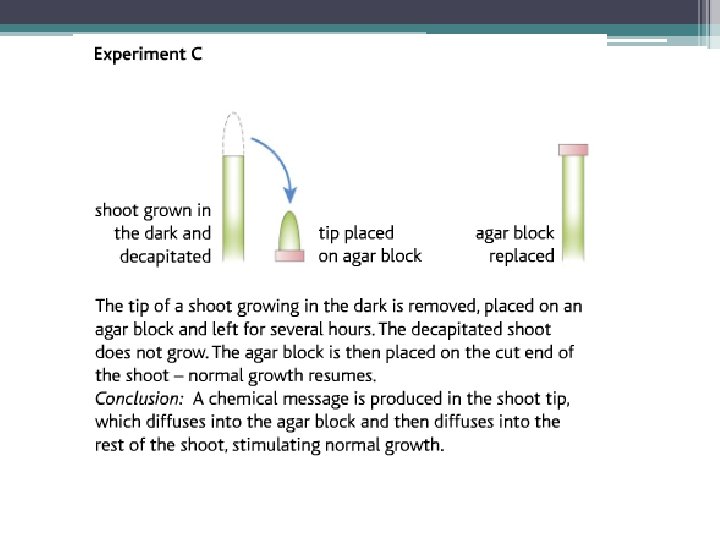
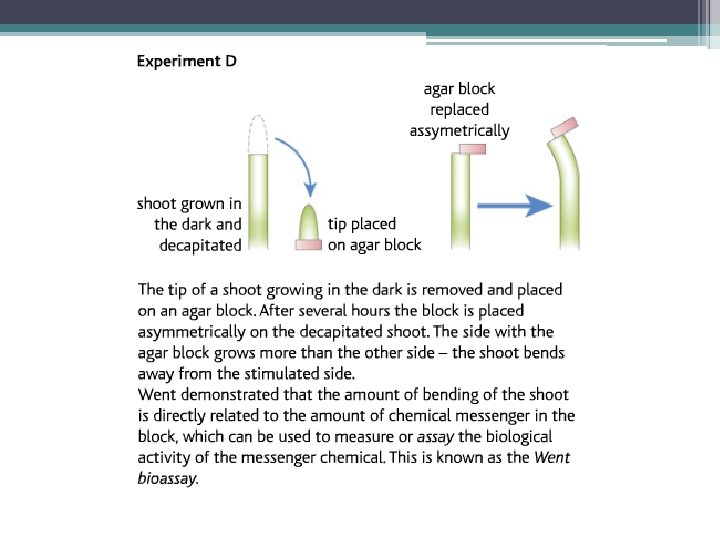
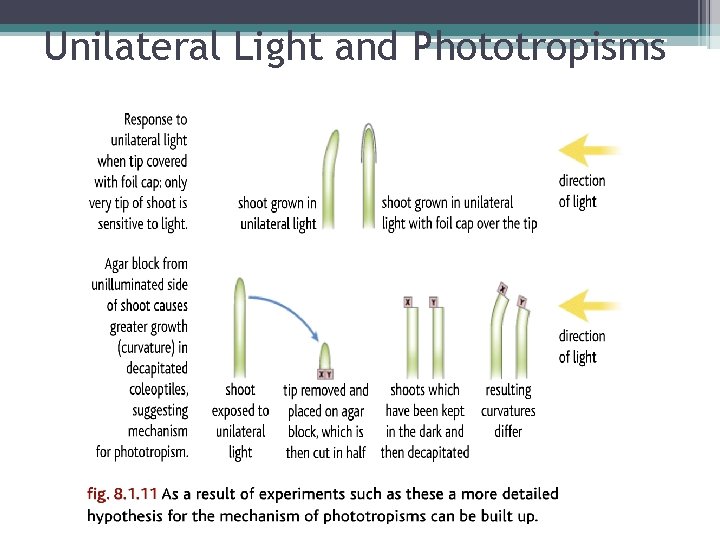
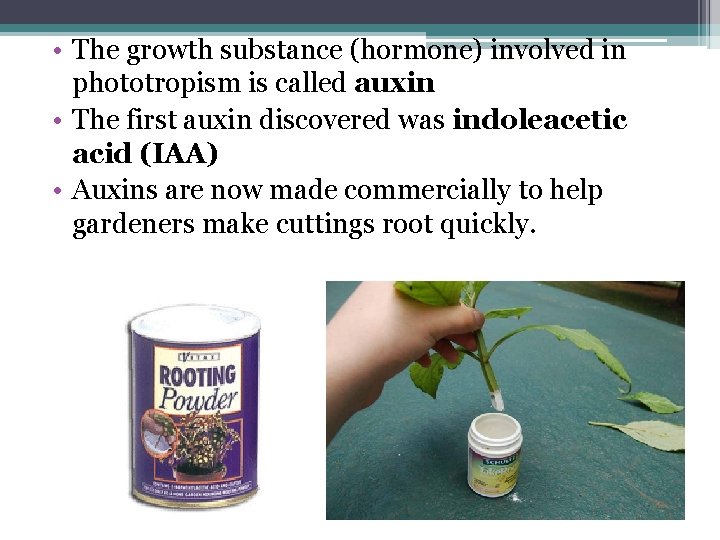
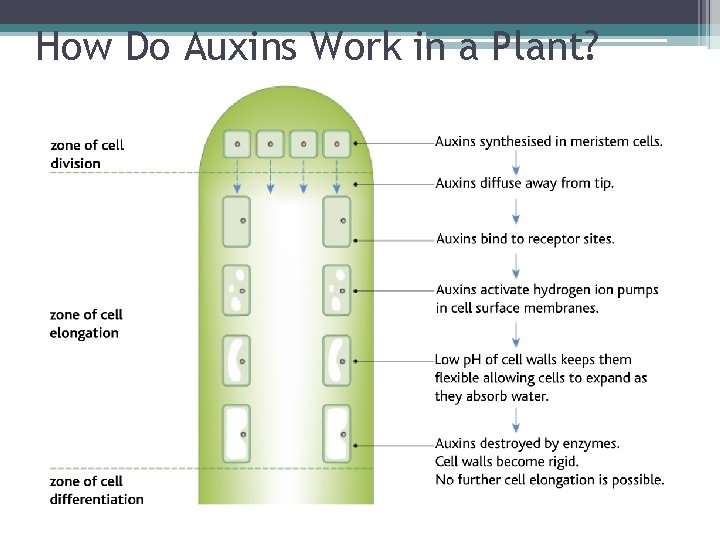
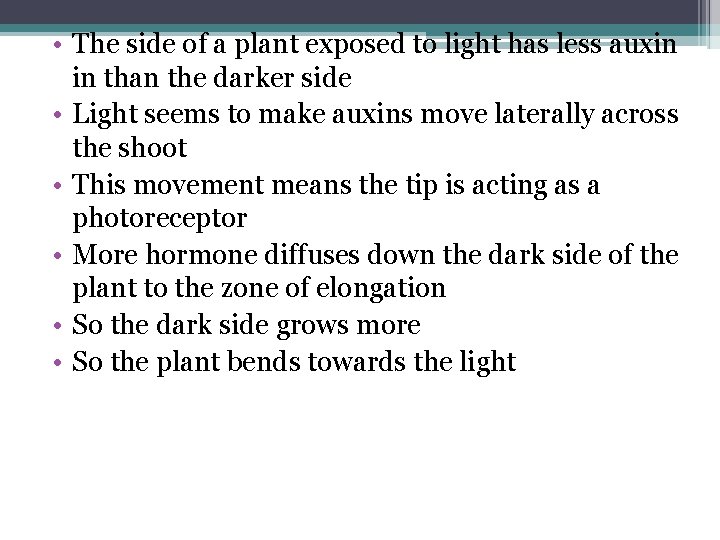
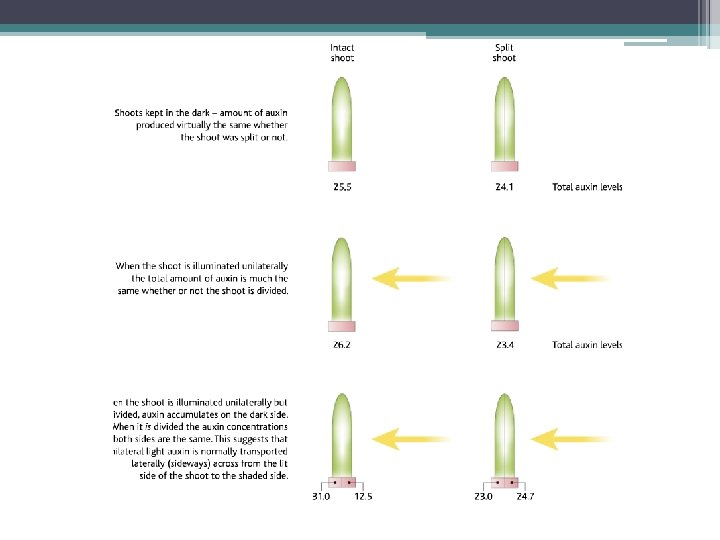
- Slides: 90
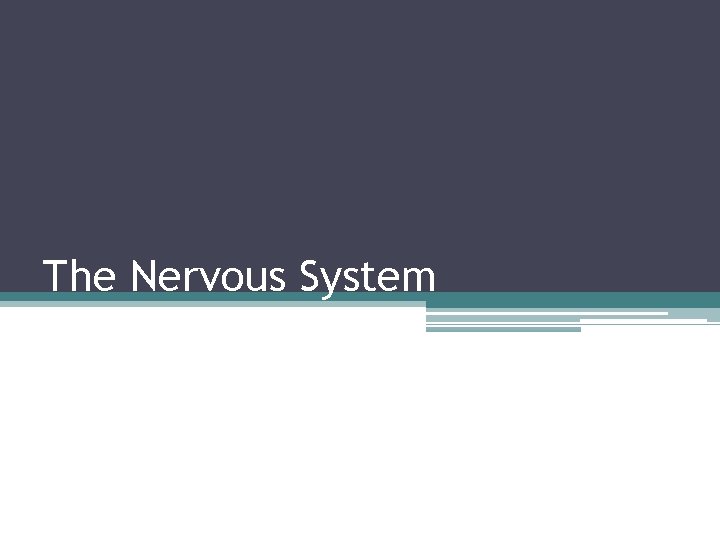
The Nervous System
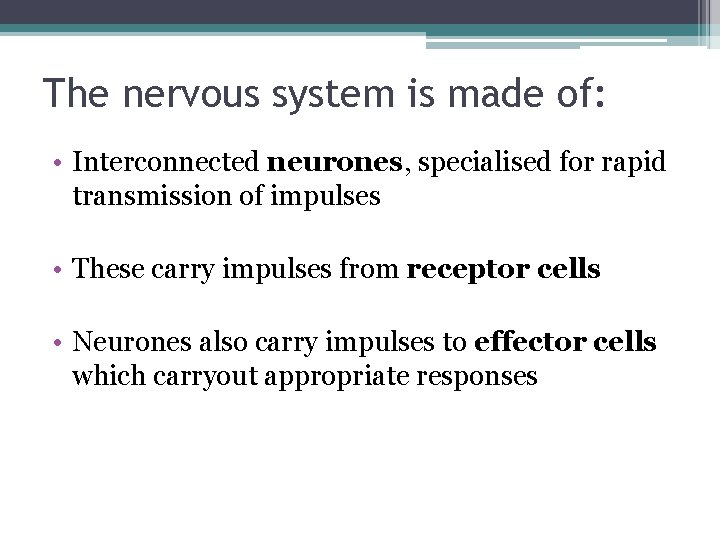
The nervous system is made of: • Interconnected neurones, specialised for rapid transmission of impulses • These carry impulses from receptor cells • Neurones also carry impulses to effector cells which carryout appropriate responses
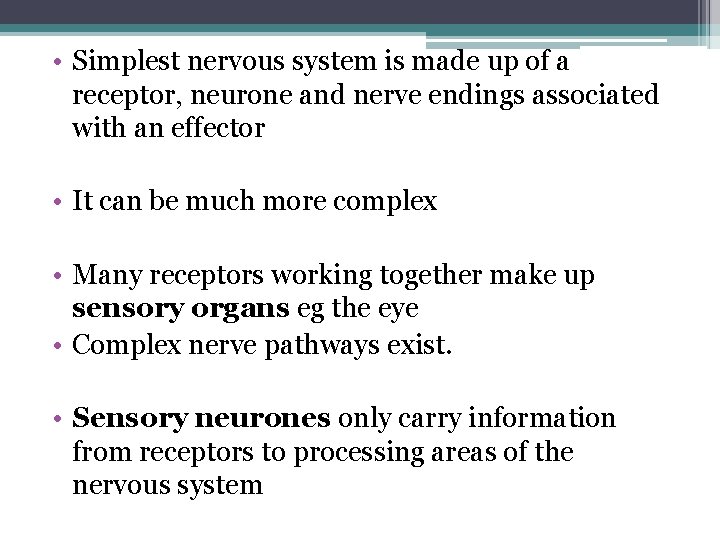
• Simplest nervous system is made up of a receptor, neurone and nerve endings associated with an effector • It can be much more complex • Many receptors working together make up sensory organs eg the eye • Complex nerve pathways exist. • Sensory neurones only carry information from receptors to processing areas of the nervous system
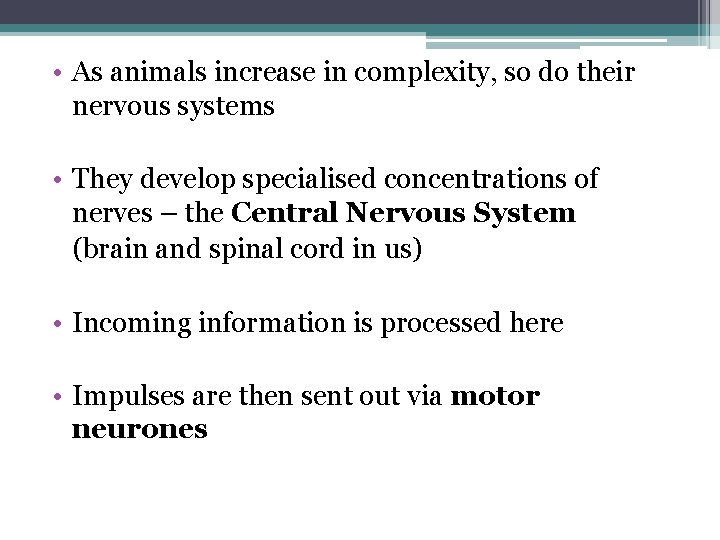
• As animals increase in complexity, so do their nervous systems • They develop specialised concentrations of nerves – the Central Nervous System (brain and spinal cord in us) • Incoming information is processed here • Impulses are then sent out via motor neurones
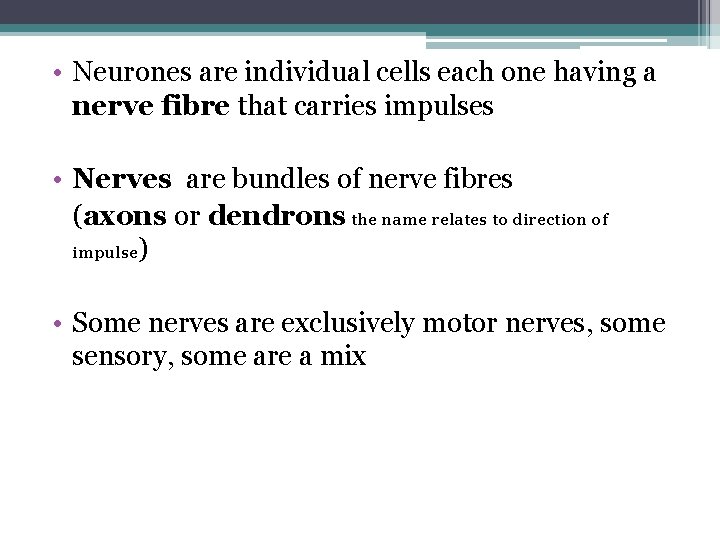
• Neurones are individual cells each one having a nerve fibre that carries impulses • Nerves are bundles of nerve fibres (axons or dendrons the name relates to direction of impulse) • Some nerves are exclusively motor nerves, some sensory, some are a mix
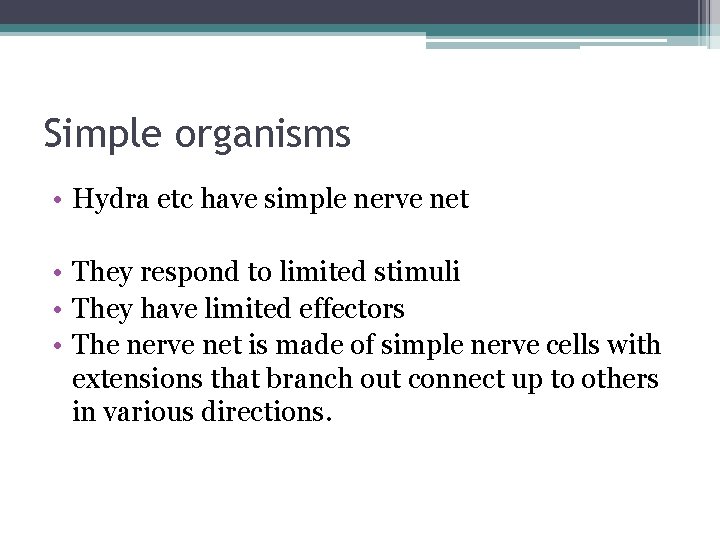
Simple organisms • Hydra etc have simple nerve net • They respond to limited stimuli • They have limited effectors • The nerve net is made of simple nerve cells with extensions that branch out connect up to others in various directions.
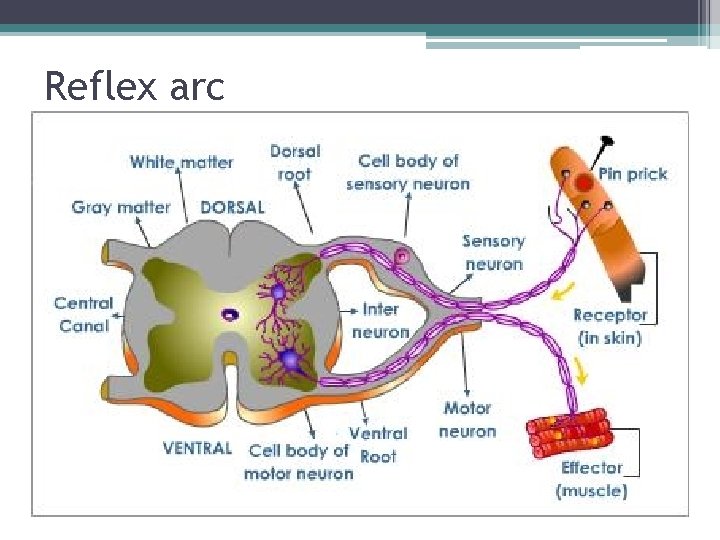
Reflex arc
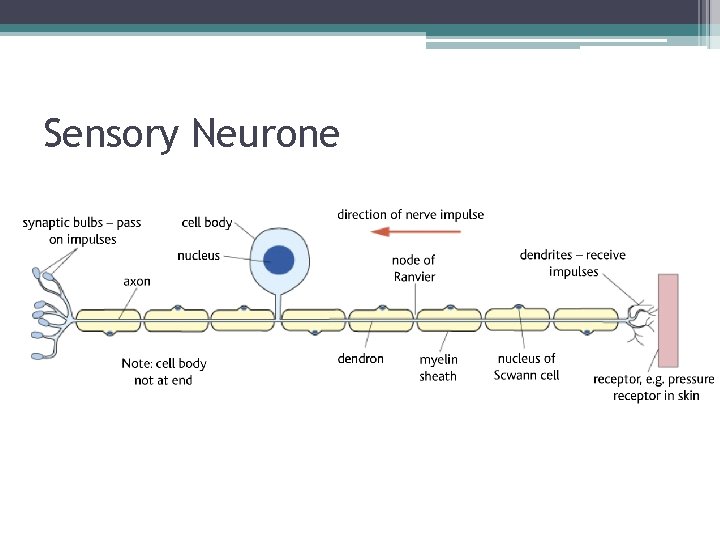
Sensory Neurone
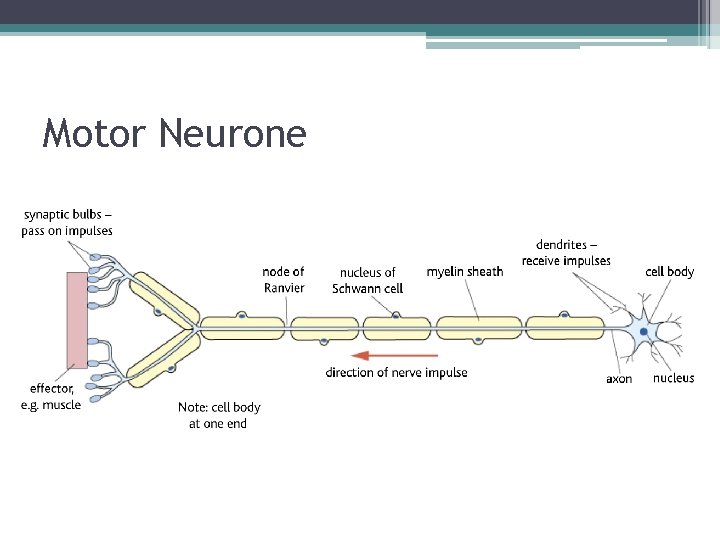
Motor Neurone
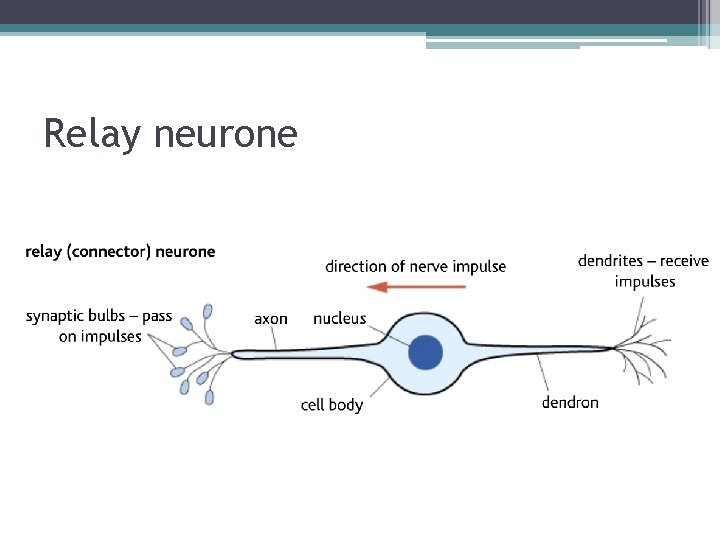
Relay neurone
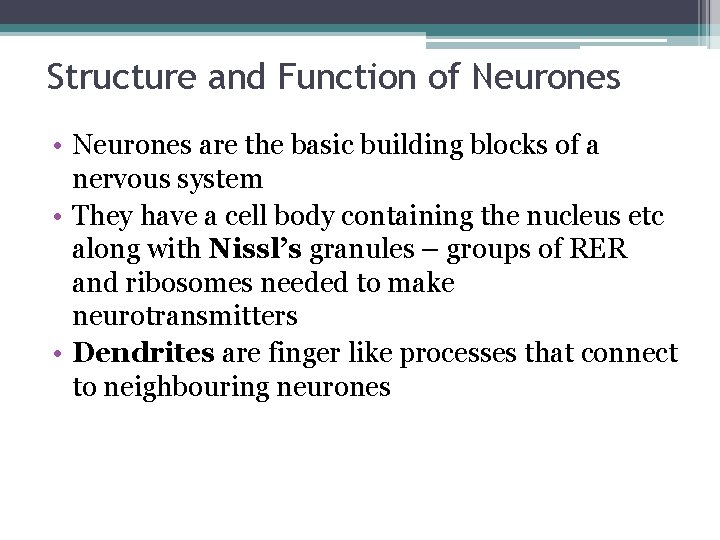
Structure and Function of Neurones • Neurones are the basic building blocks of a nervous system • They have a cell body containing the nucleus etc along with Nissl’s granules – groups of RER and ribosomes needed to make neurotransmitters • Dendrites are finger like processes that connect to neighbouring neurones
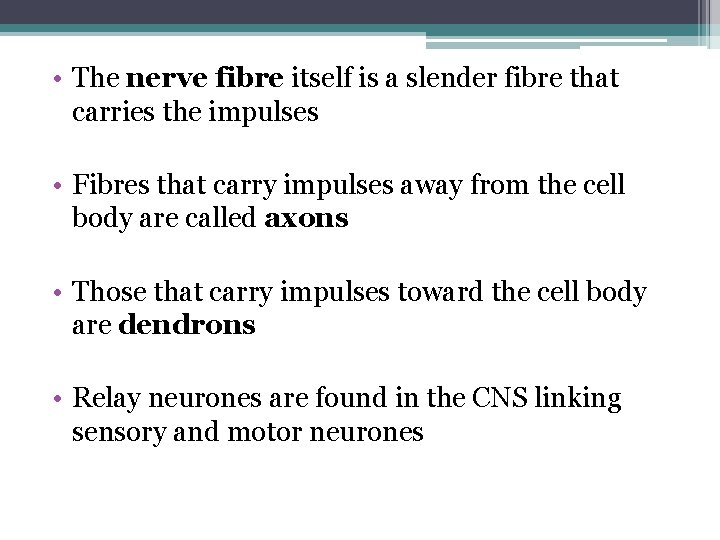
• The nerve fibre itself is a slender fibre that carries the impulses • Fibres that carry impulses away from the cell body are called axons • Those that carry impulses toward the cell body are dendrons • Relay neurones are found in the CNS linking sensory and motor neurones
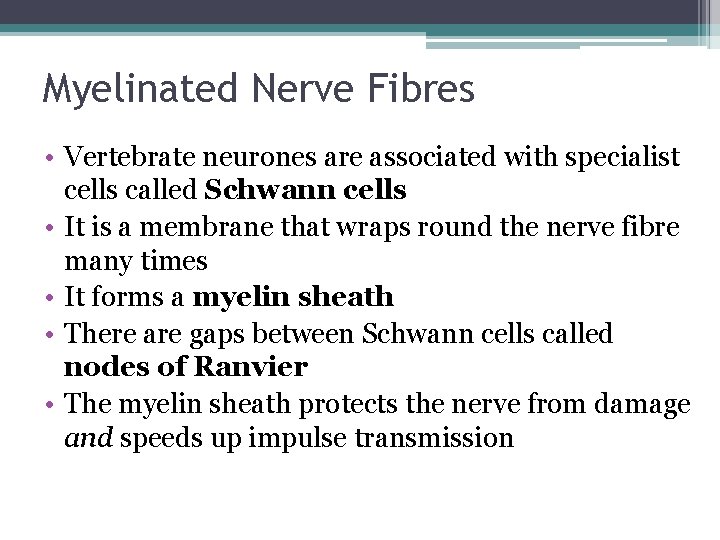
Myelinated Nerve Fibres • Vertebrate neurones are associated with specialist cells called Schwann cells • It is a membrane that wraps round the nerve fibre many times • It forms a myelin sheath • There are gaps between Schwann cells called nodes of Ranvier • The myelin sheath protects the nerve from damage and speeds up impulse transmission
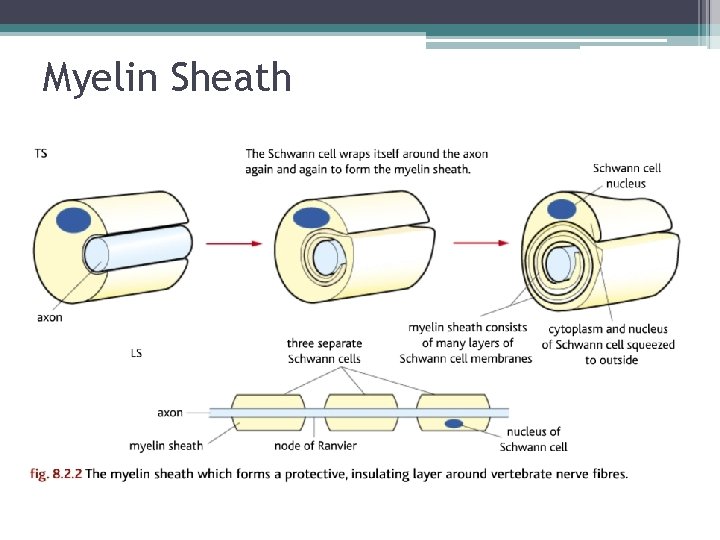
Myelin Sheath
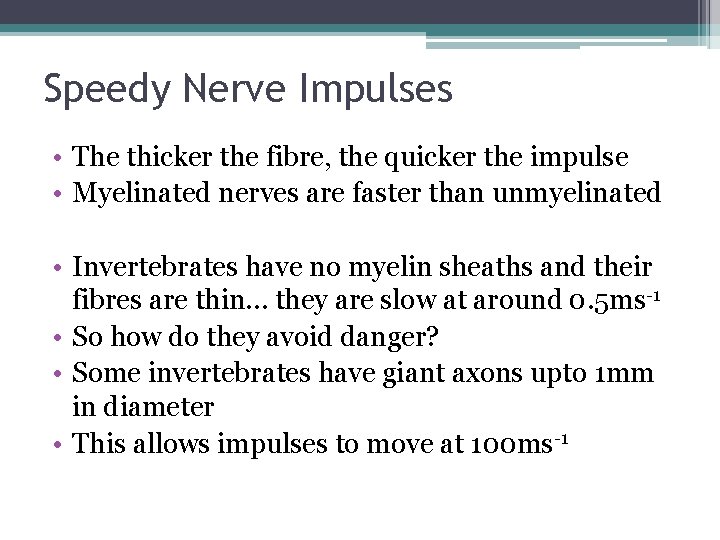
Speedy Nerve Impulses • The thicker the fibre, the quicker the impulse • Myelinated nerves are faster than unmyelinated • Invertebrates have no myelin sheaths and their fibres are thin… they are slow at around 0. 5 ms-1 • So how do they avoid danger? • Some invertebrates have giant axons upto 1 mm in diameter • This allows impulses to move at 100 ms-1
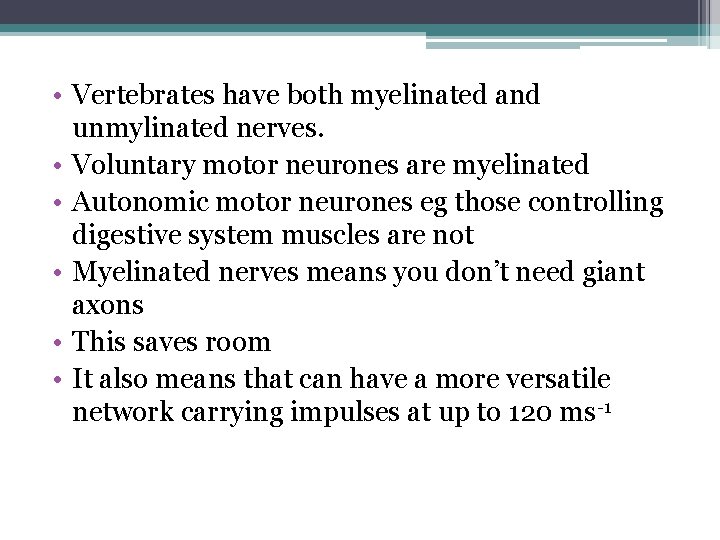
• Vertebrates have both myelinated and unmylinated nerves. • Voluntary motor neurones are myelinated • Autonomic motor neurones eg those controlling digestive system muscles are not • Myelinated nerves means you don’t need giant axons • This saves room • It also means that can have a more versatile network carrying impulses at up to 120 ms-1
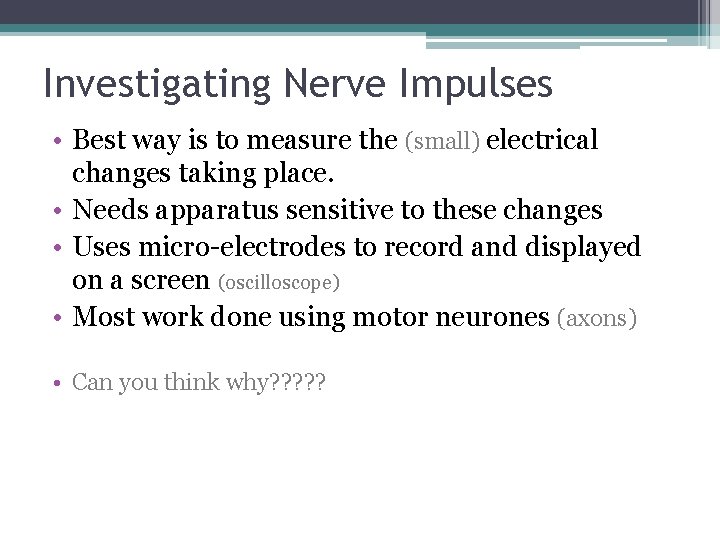
Investigating Nerve Impulses • Best way is to measure the (small) electrical changes taking place. • Needs apparatus sensitive to these changes • Uses micro-electrodes to record and displayed on a screen (oscilloscope) • Most work done using motor neurones (axons) • Can you think why? ? ?
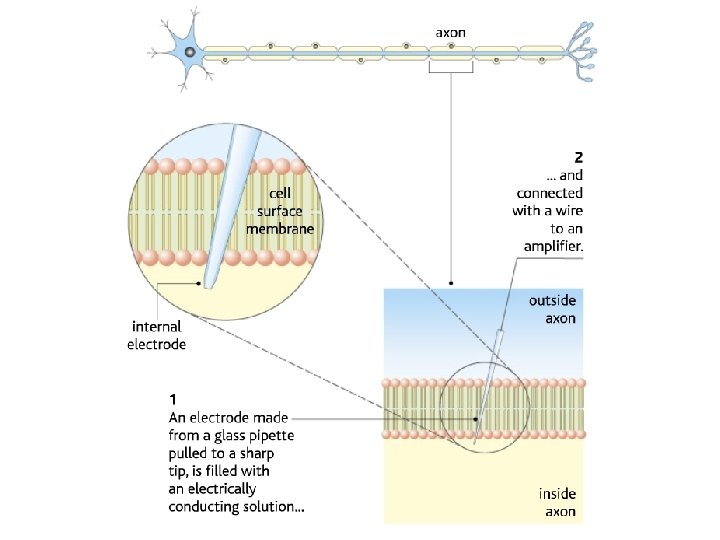
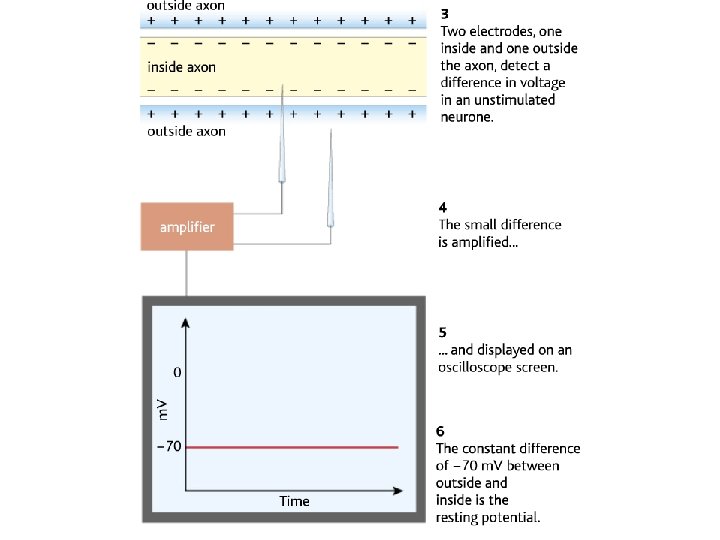
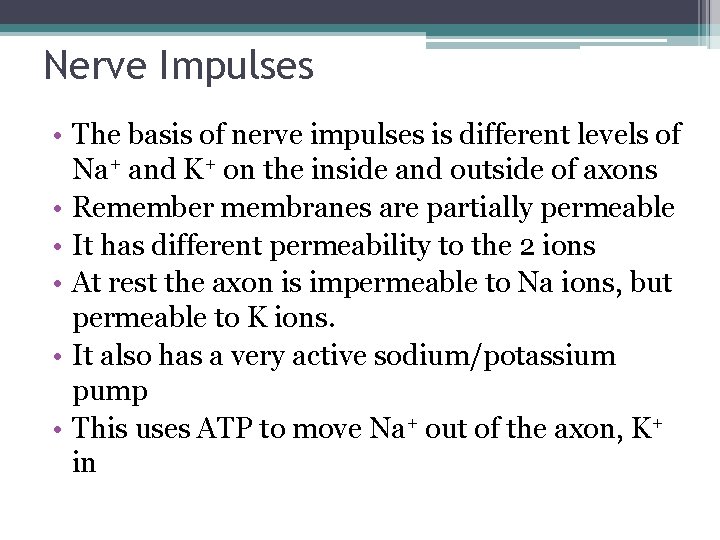
Nerve Impulses • The basis of nerve impulses is different levels of Na+ and K+ on the inside and outside of axons • Remember membranes are partially permeable • It has different permeability to the 2 ions • At rest the axon is impermeable to Na ions, but permeable to K ions. • It also has a very active sodium/potassium pump • This uses ATP to move Na+ out of the axon, K+ in
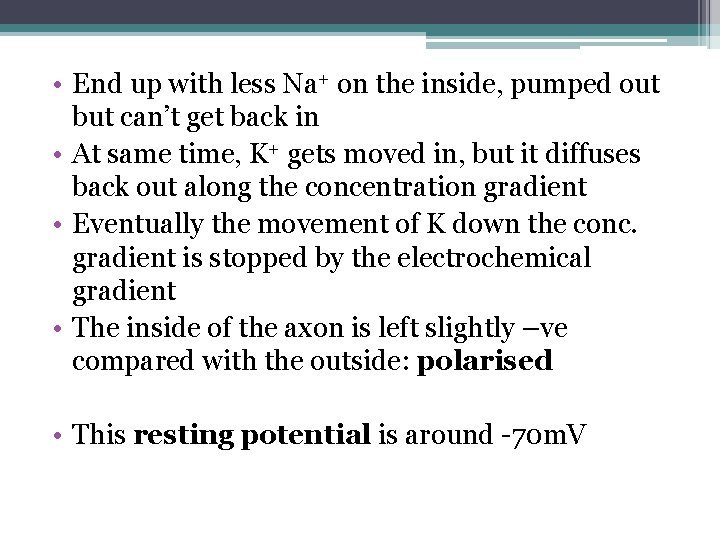
• End up with less Na+ on the inside, pumped out but can’t get back in • At same time, K+ gets moved in, but it diffuses back out along the concentration gradient • Eventually the movement of K down the conc. gradient is stopped by the electrochemical gradient • The inside of the axon is left slightly –ve compared with the outside: polarised • This resting potential is around -70 m. V
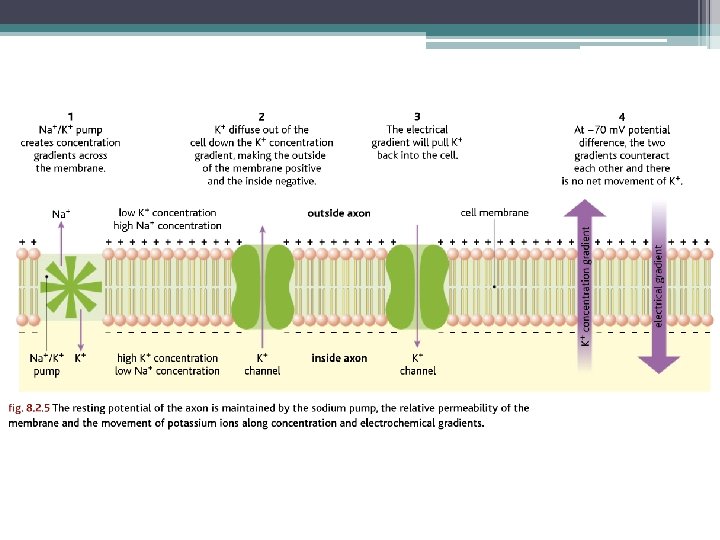
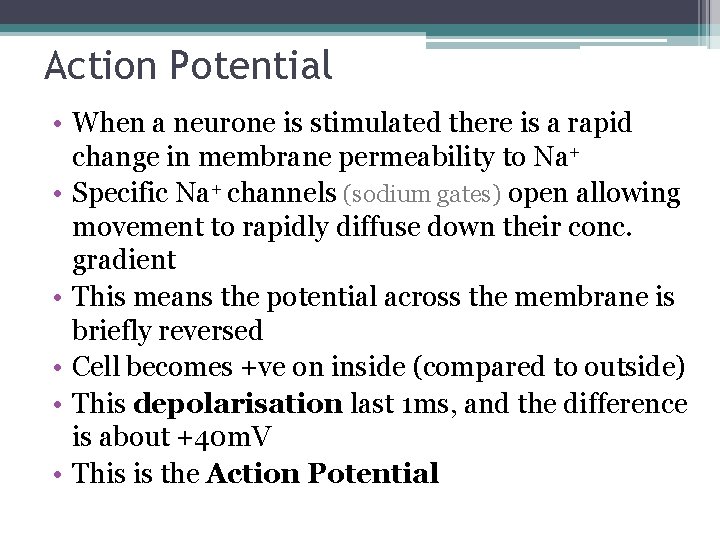
Action Potential • When a neurone is stimulated there is a rapid change in membrane permeability to Na+ • Specific Na+ channels (sodium gates) open allowing movement to rapidly diffuse down their conc. gradient • This means the potential across the membrane is briefly reversed • Cell becomes +ve on inside (compared to outside) • This depolarisation last 1 ms, and the difference is about +40 m. V • This is the Action Potential
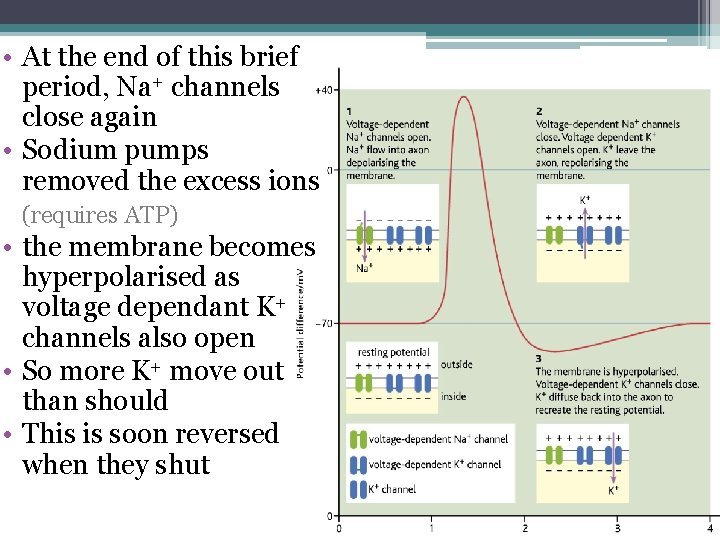
• At the end of this brief period, Na+ channels close again • Sodium pumps removed the excess ions (requires ATP) • the membrane becomes hyperpolarised as voltage dependant K+ channels also open • So more K+ move out than should • This is soon reversed when they shut
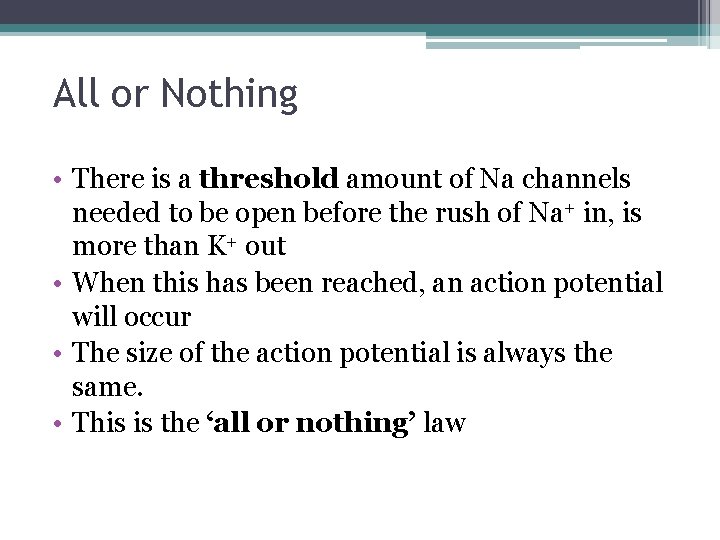
All or Nothing • There is a threshold amount of Na channels needed to be open before the rush of Na+ in, is more than K+ out • When this has been reached, an action potential will occur • The size of the action potential is always the same. • This is the ‘all or nothing’ law
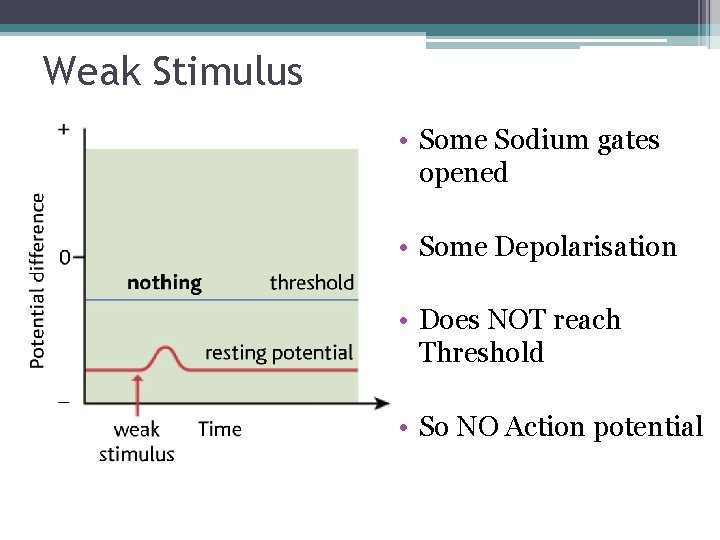
Weak Stimulus • Some Sodium gates opened • Some Depolarisation • Does NOT reach Threshold • So NO Action potential
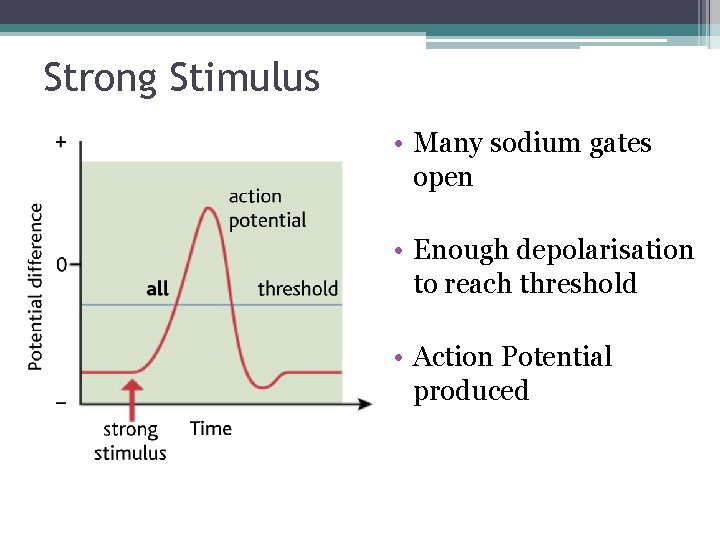
Strong Stimulus • Many sodium gates open • Enough depolarisation to reach threshold • Action Potential produced
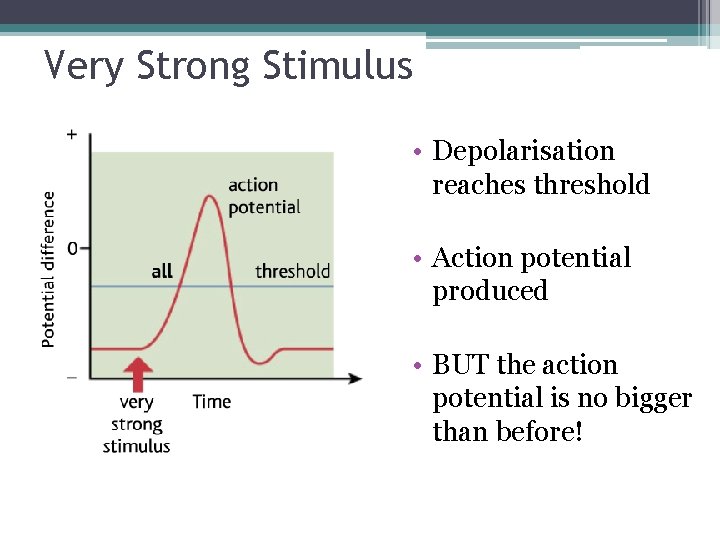
Very Strong Stimulus • Depolarisation reaches threshold • Action potential produced • BUT the action potential is no bigger than before!
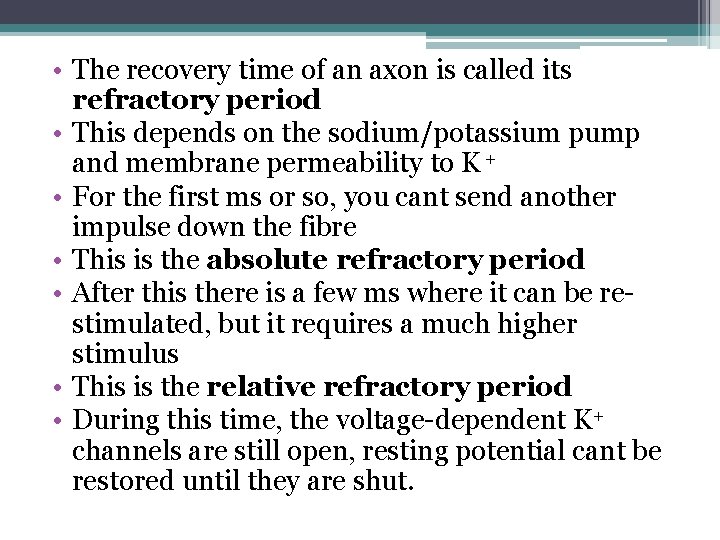
• The recovery time of an axon is called its refractory period • This depends on the sodium/potassium pump and membrane permeability to K + • For the first ms or so, you cant send another impulse down the fibre • This is the absolute refractory period • After this there is a few ms where it can be restimulated, but it requires a much higher stimulus • This is the relative refractory period • During this time, the voltage-dependent K+ channels are still open, resting potential cant be restored until they are shut.
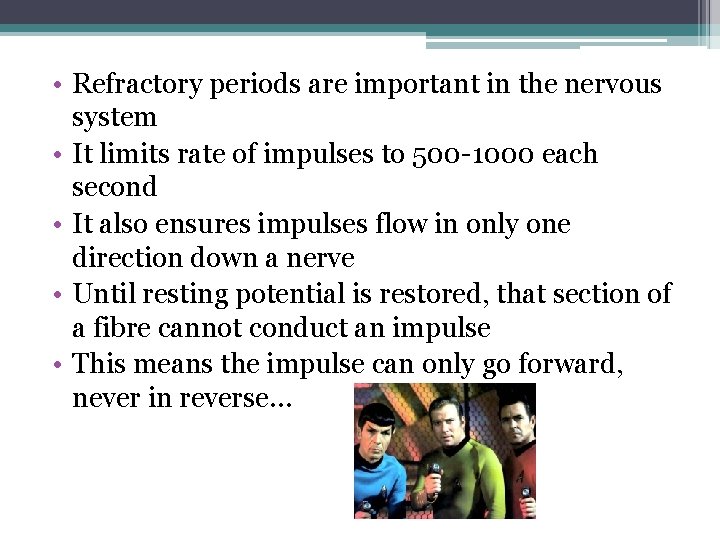
• Refractory periods are important in the nervous system • It limits rate of impulses to 500 -1000 each second • It also ensures impulses flow in only one direction down a nerve • Until resting potential is restored, that section of a fibre cannot conduct an impulse • This means the impulse can only go forward, never in reverse…
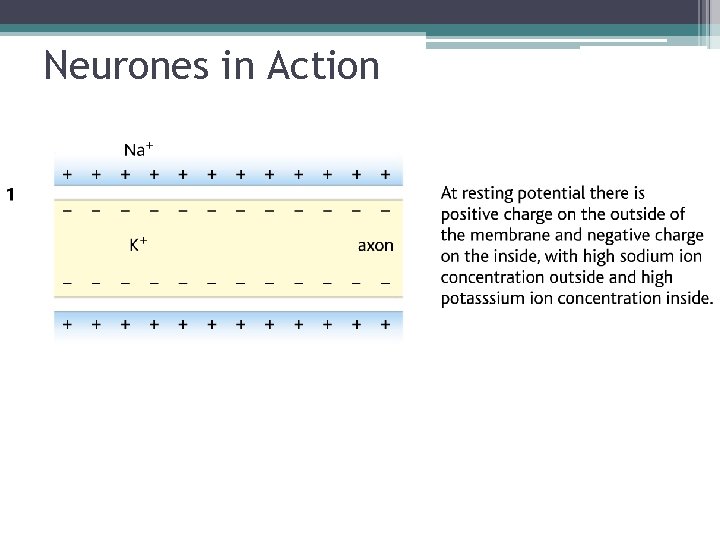
Neurones in Action
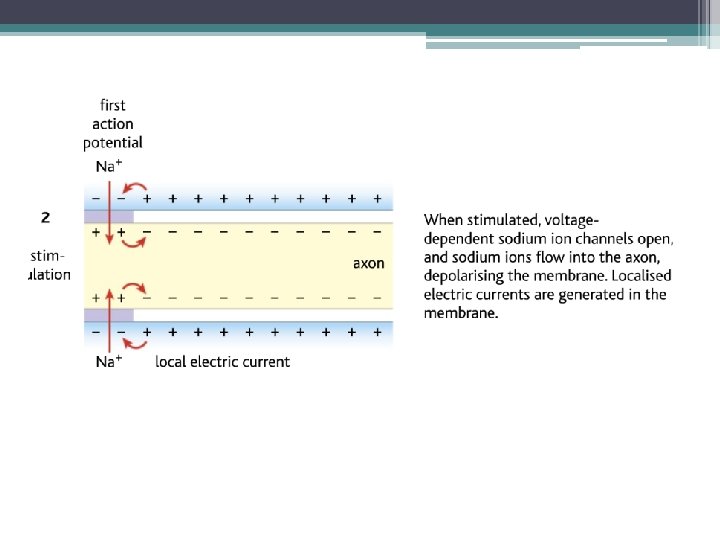
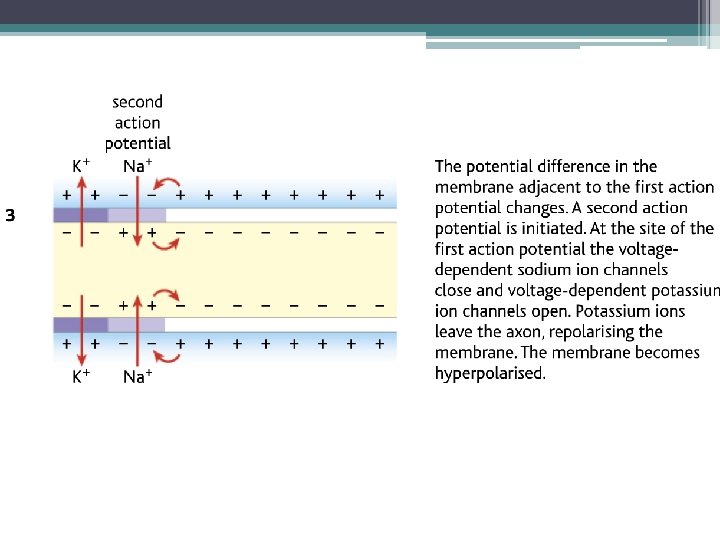
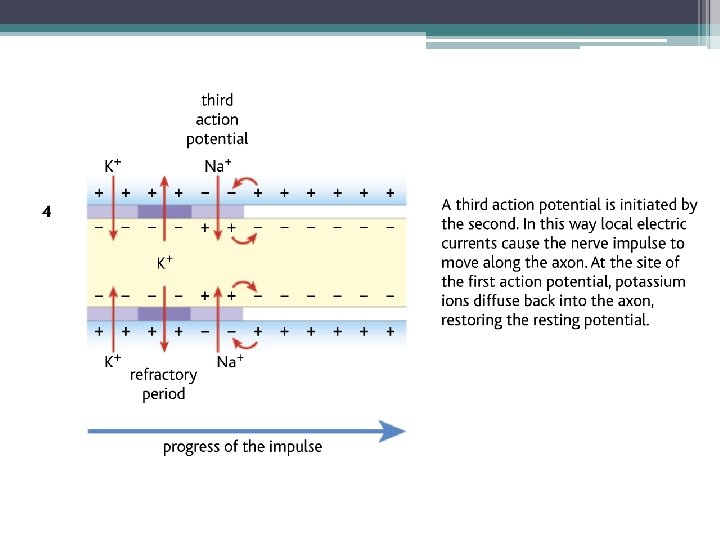
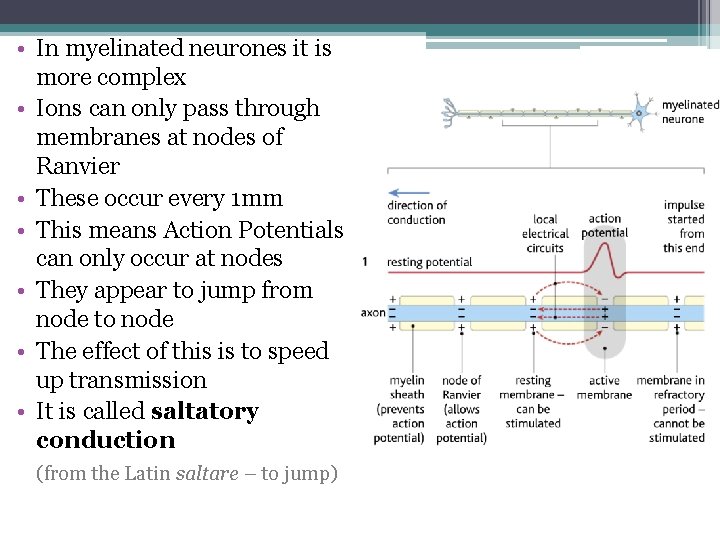
• In myelinated neurones it is more complex • Ions can only pass through membranes at nodes of Ranvier • These occur every 1 mm • This means Action Potentials can only occur at nodes • They appear to jump from node to node • The effect of this is to speed up transmission • It is called saltatory conduction (from the Latin saltare – to jump)
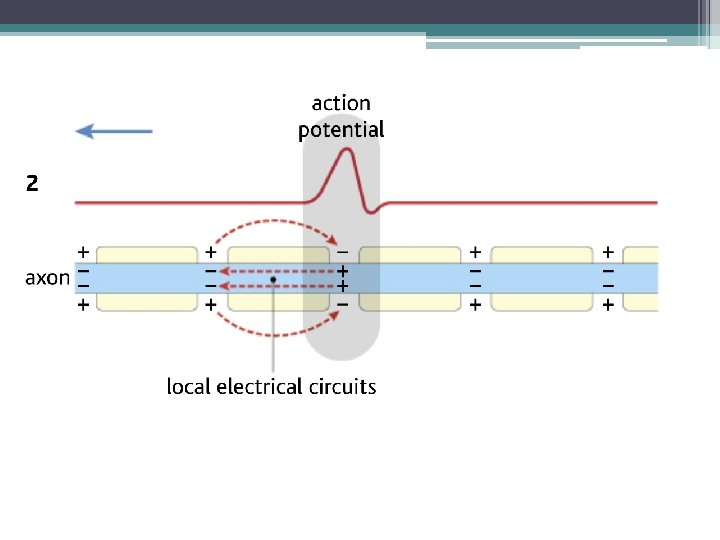
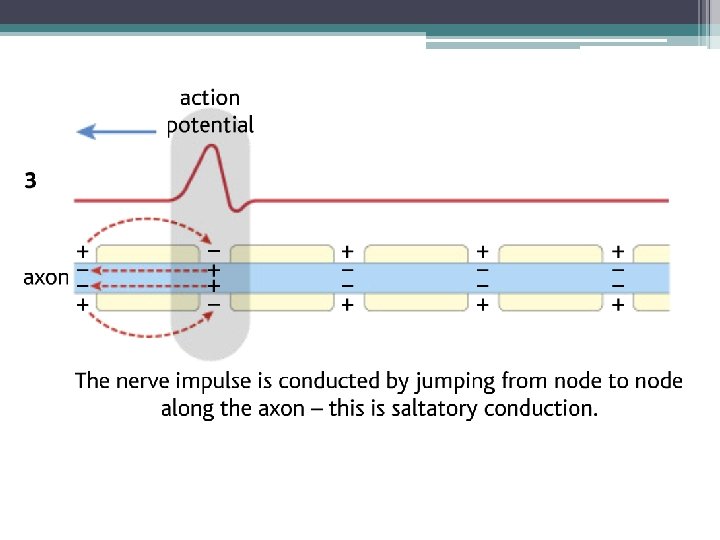
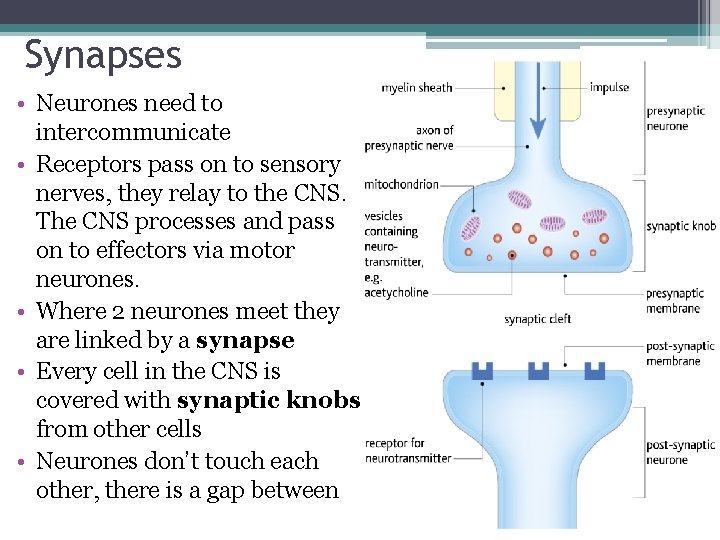
Synapses • Neurones need to intercommunicate • Receptors pass on to sensory nerves, they relay to the CNS. The CNS processes and pass on to effectors via motor neurones. • Where 2 neurones meet they are linked by a synapse • Every cell in the CNS is covered with synaptic knobs from other cells • Neurones don’t touch each other, there is a gap between
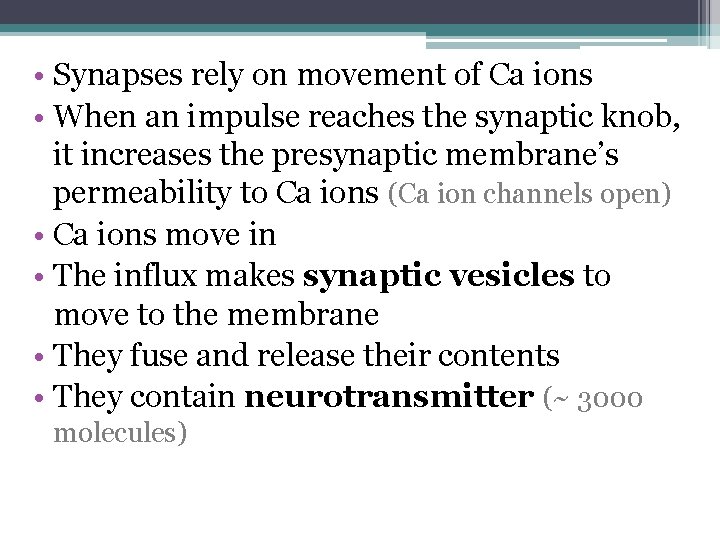
• Synapses rely on movement of Ca ions • When an impulse reaches the synaptic knob, it increases the presynaptic membrane’s permeability to Ca ions (Ca ion channels open) • Ca ions move in • The influx makes synaptic vesicles to move to the membrane • They fuse and release their contents • They contain neurotransmitter (~ 3000 molecules)
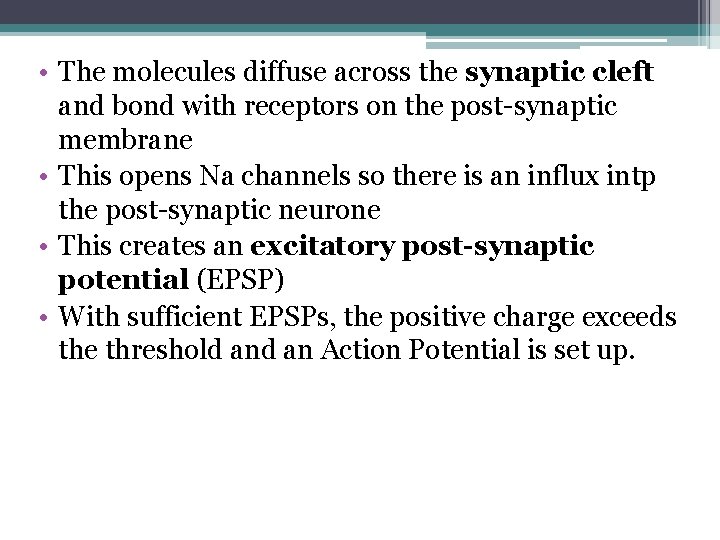
• The molecules diffuse across the synaptic cleft and bond with receptors on the post-synaptic membrane • This opens Na channels so there is an influx intp the post-synaptic neurone • This creates an excitatory post-synaptic potential (EPSP) • With sufficient EPSPs, the positive charge exceeds the threshold an Action Potential is set up.
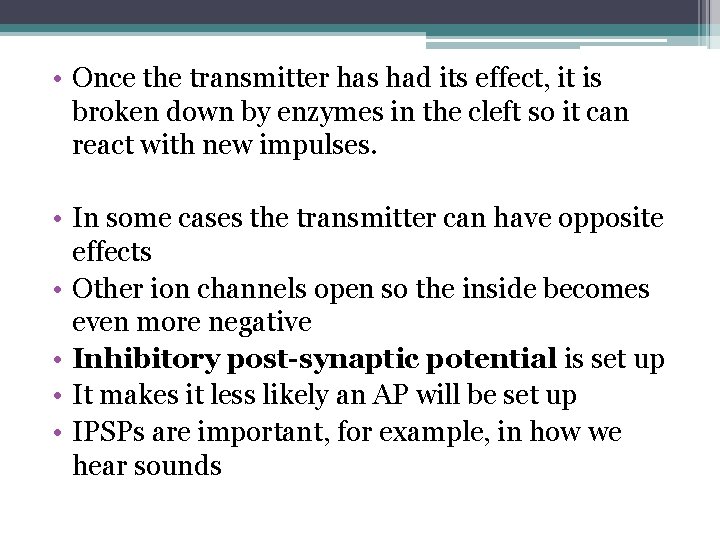
• Once the transmitter has had its effect, it is broken down by enzymes in the cleft so it can react with new impulses. • In some cases the transmitter can have opposite effects • Other ion channels open so the inside becomes even more negative • Inhibitory post-synaptic potential is set up • It makes it less likely an AP will be set up • IPSPs are important, for example, in how we hear sounds
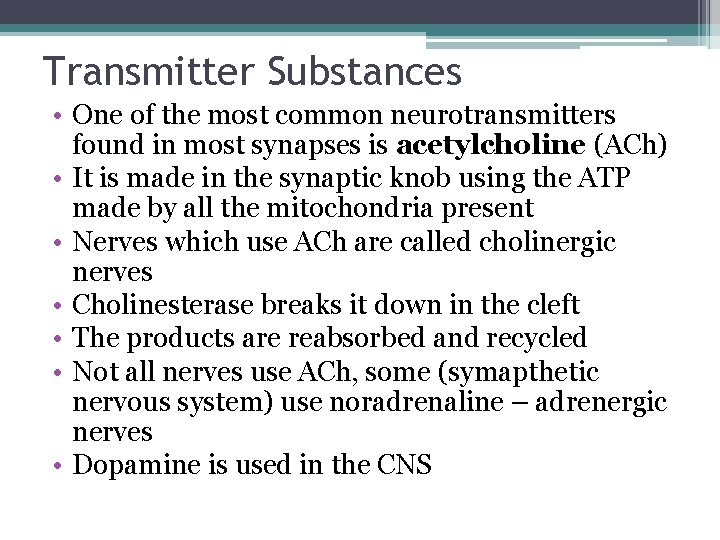
Transmitter Substances • One of the most common neurotransmitters found in most synapses is acetylcholine (ACh) • It is made in the synaptic knob using the ATP made by all the mitochondria present • Nerves which use ACh are called cholinergic nerves • Cholinesterase breaks it down in the cleft • The products are reabsorbed and recycled • Not all nerves use ACh, some (symapthetic nervous system) use noradrenaline – adrenergic nerves • Dopamine is used in the CNS
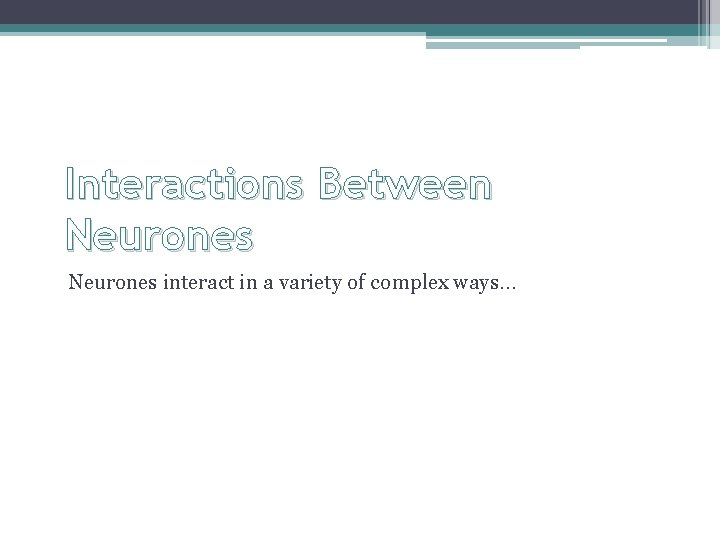
Interactions Between Neurones interact in a variety of complex ways…
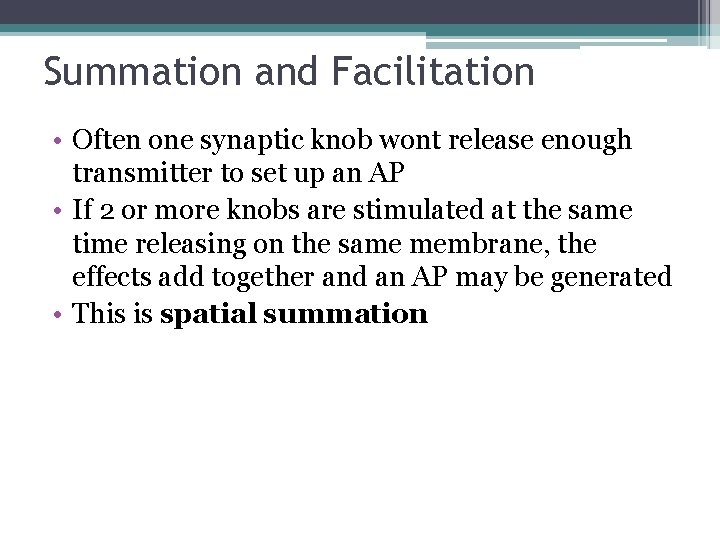
Summation and Facilitation • Often one synaptic knob wont release enough transmitter to set up an AP • If 2 or more knobs are stimulated at the same time releasing on the same membrane, the effects add together and an AP may be generated • This is spatial summation
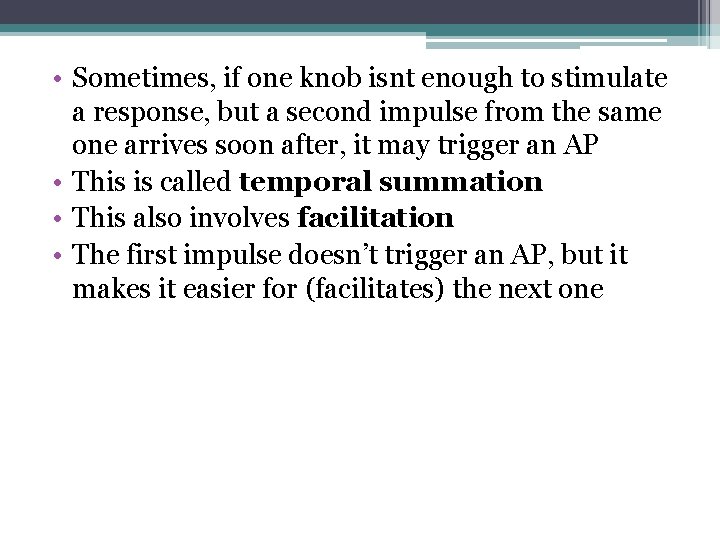
• Sometimes, if one knob isnt enough to stimulate a response, but a second impulse from the same one arrives soon after, it may trigger an AP • This is called temporal summation • This also involves facilitation • The first impulse doesn’t trigger an AP, but it makes it easier for (facilitates) the next one
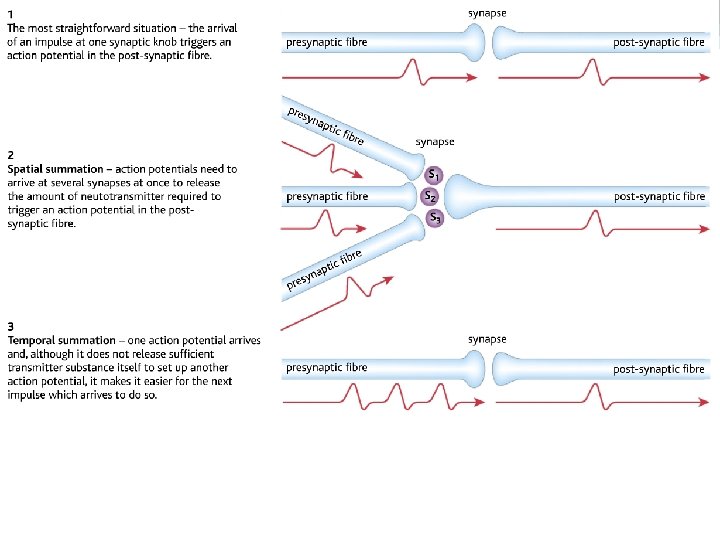
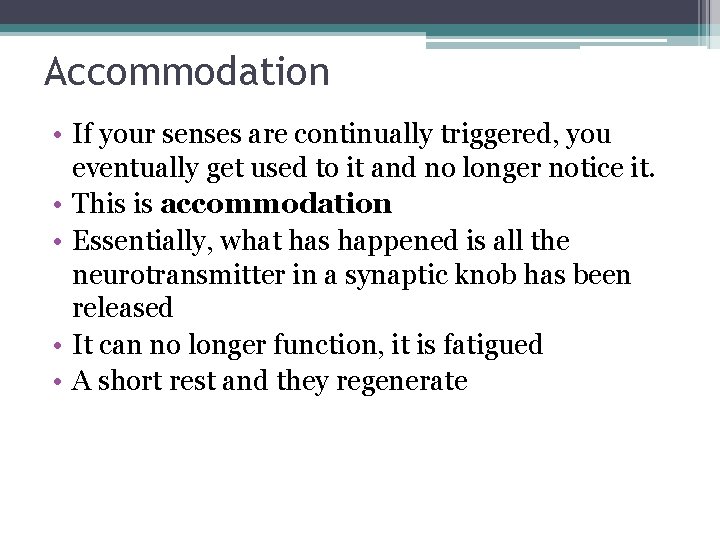
Accommodation • If your senses are continually triggered, you eventually get used to it and no longer notice it. • This is accommodation • Essentially, what has happened is all the neurotransmitter in a synaptic knob has been released • It can no longer function, it is fatigued • A short rest and they regenerate
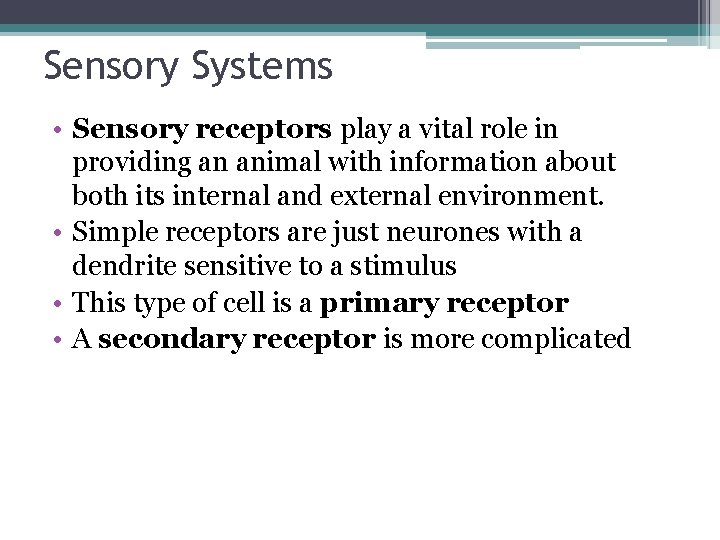
Sensory Systems • Sensory receptors play a vital role in providing an animal with information about both its internal and external environment. • Simple receptors are just neurones with a dendrite sensitive to a stimulus • This type of cell is a primary receptor • A secondary receptor is more complicated
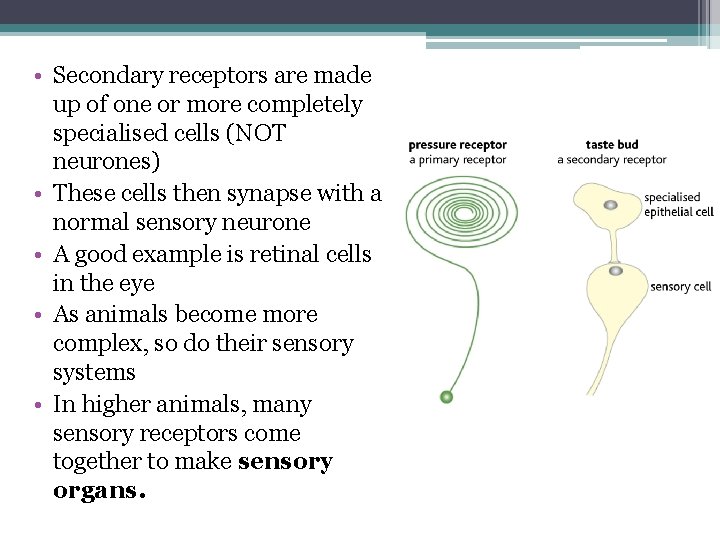
• Secondary receptors are made up of one or more completely specialised cells (NOT neurones) • These cells then synapse with a normal sensory neurone • A good example is retinal cells in the eye • As animals become more complex, so do their sensory systems • In higher animals, many sensory receptors come together to make sensory organs.
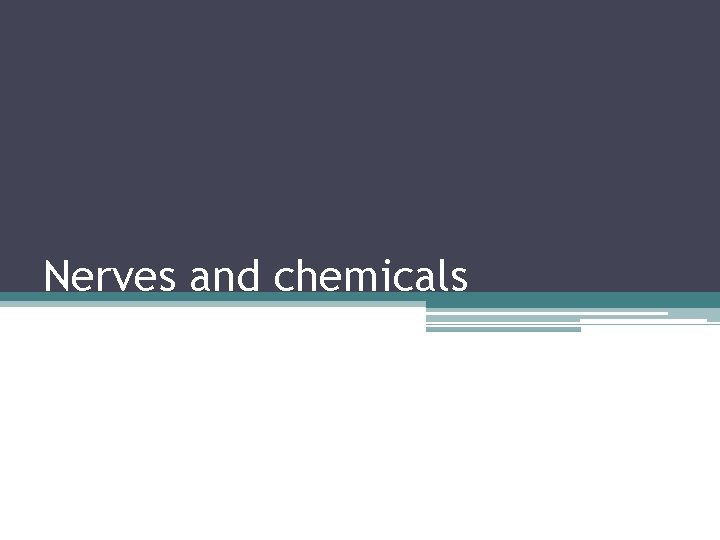
Nerves and chemicals
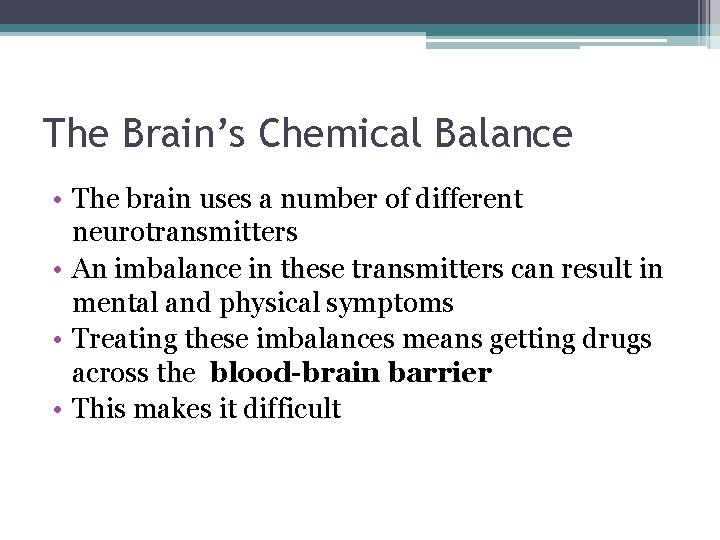
The Brain’s Chemical Balance • The brain uses a number of different neurotransmitters • An imbalance in these transmitters can result in mental and physical symptoms • Treating these imbalances means getting drugs across the blood-brain barrier • This makes it difficult
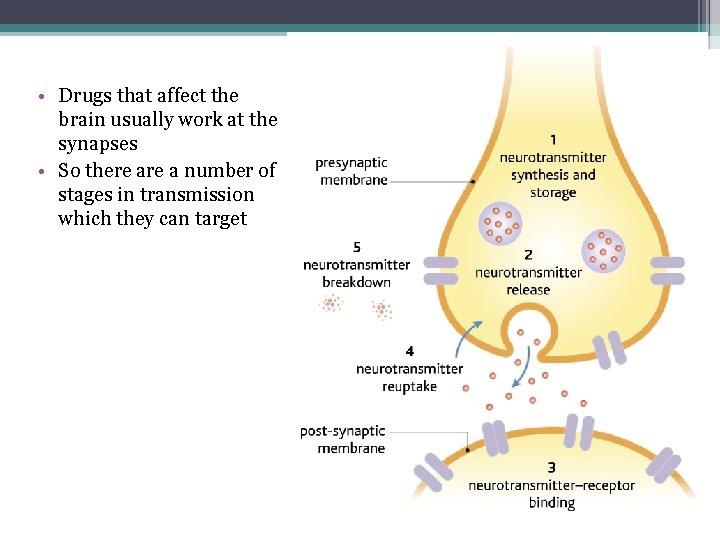
• Drugs that affect the brain usually work at the synapses • So there a number of stages in transmission which they can target
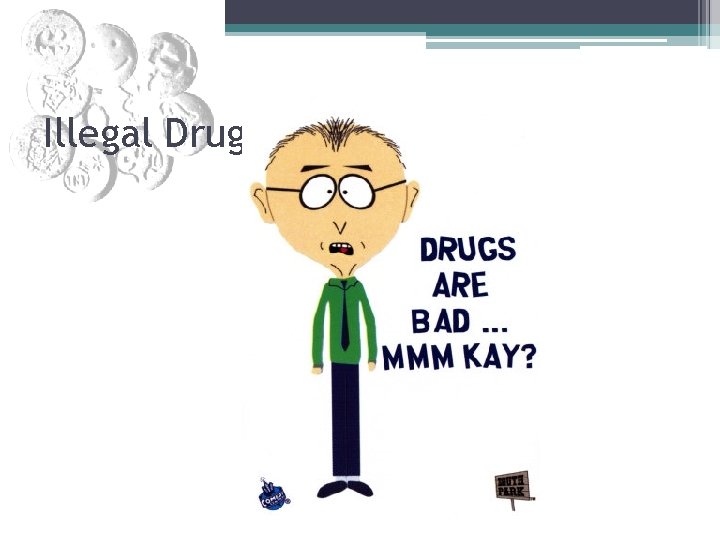
Illegal Drugs and the Brain
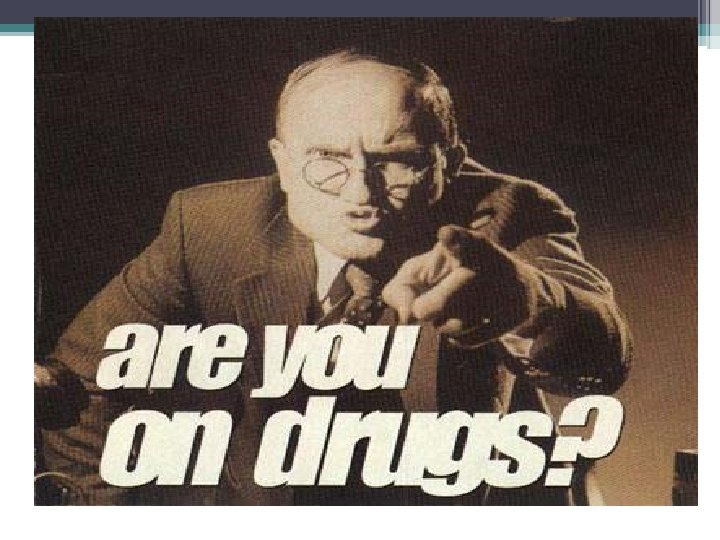
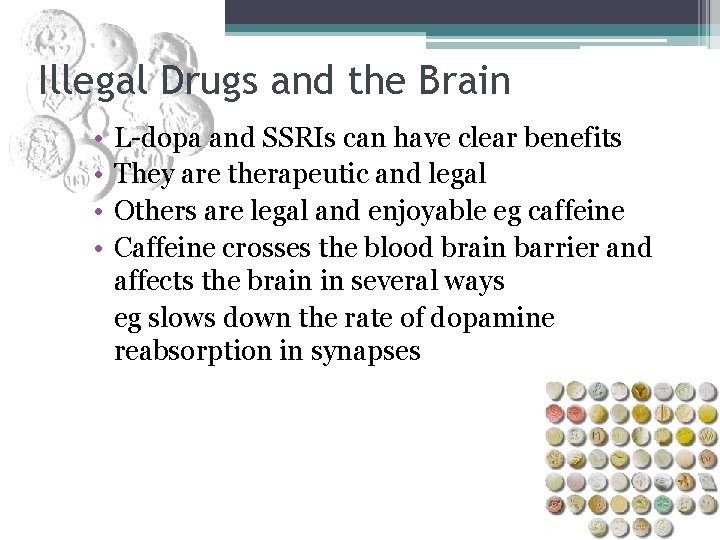
Illegal Drugs and the Brain • • L-dopa and SSRIs can have clear benefits They are therapeutic and legal Others are legal and enjoyable eg caffeine Caffeine crosses the blood brain barrier and affects the brain in several ways eg slows down the rate of dopamine reabsorption in synapses
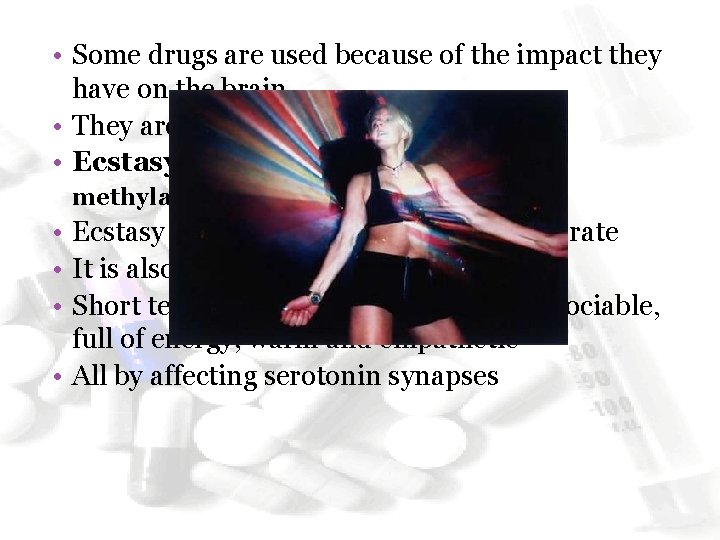
• Some drugs are used because of the impact they have on the brain • They are often illegal • Ecstasy (3, 4 -methylenedioxy-Nmethylamphetamine or MDMA) is one • Ecstasy acts as a stimulant raising heart rate • It is also psychotropic • Short term affects make people happy, sociable, full of energy, warm and empathetic • All by affecting serotonin synapses
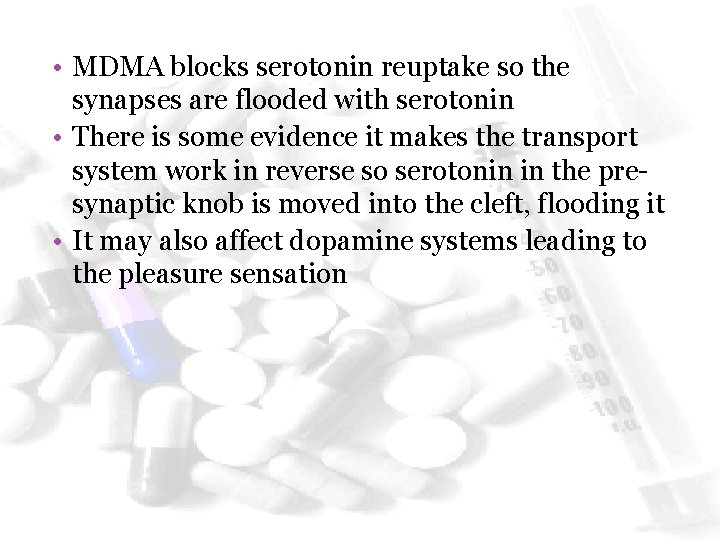
• MDMA blocks serotonin reuptake so the synapses are flooded with serotonin • There is some evidence it makes the transport system work in reverse so serotonin in the presynaptic knob is moved into the cleft, flooding it • It may also affect dopamine systems leading to the pleasure sensation
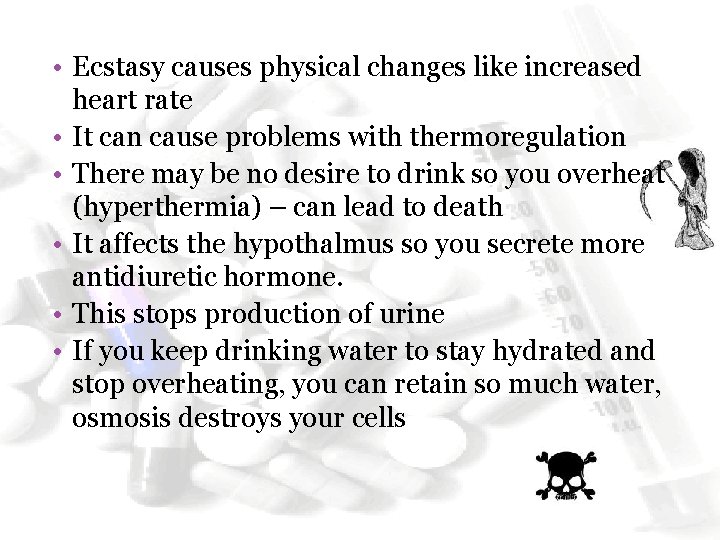
• Ecstasy causes physical changes like increased heart rate • It can cause problems with thermoregulation • There may be no desire to drink so you overheat (hyperthermia) – can lead to death • It affects the hypothalmus so you secrete more antidiuretic hormone. • This stops production of urine • If you keep drinking water to stay hydrated and stop overheating, you can retain so much water, osmosis destroys your cells
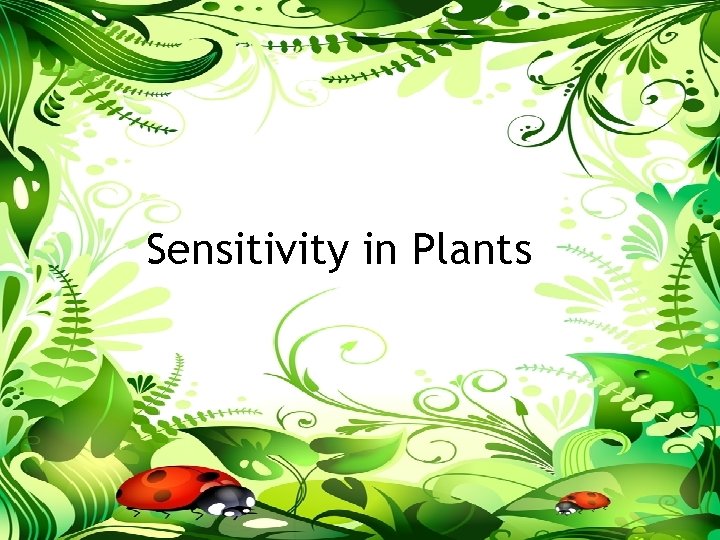
Sensitivity in Plants
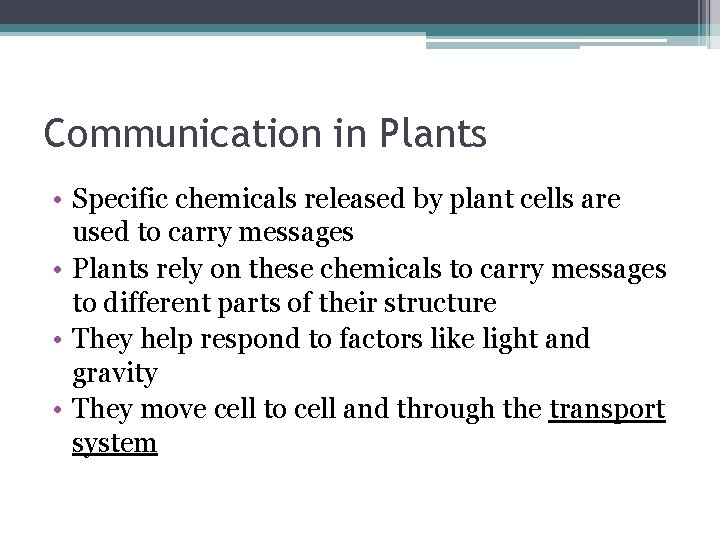
Communication in Plants • Specific chemicals released by plant cells are used to carry messages • Plants rely on these chemicals to carry messages to different parts of their structure • They help respond to factors like light and gravity • They move cell to cell and through the transport system
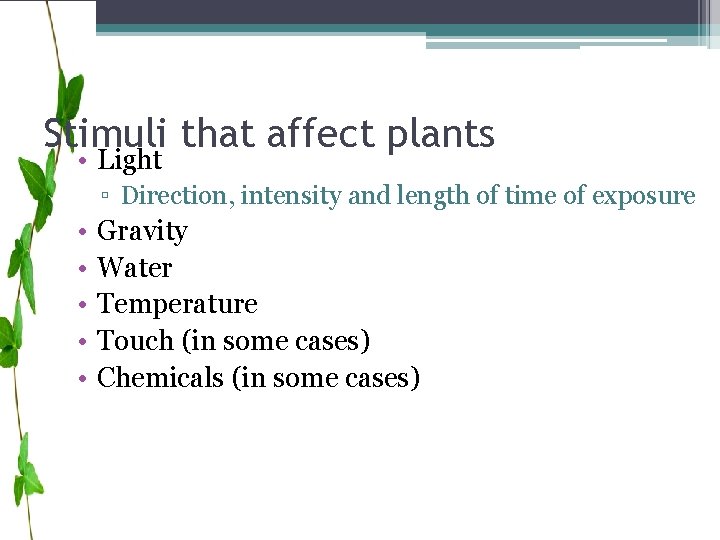
Stimuli that affect plants • Light ▫ Direction, intensity and length of time of exposure • • • Gravity Water Temperature Touch (in some cases) Chemicals (in some cases)
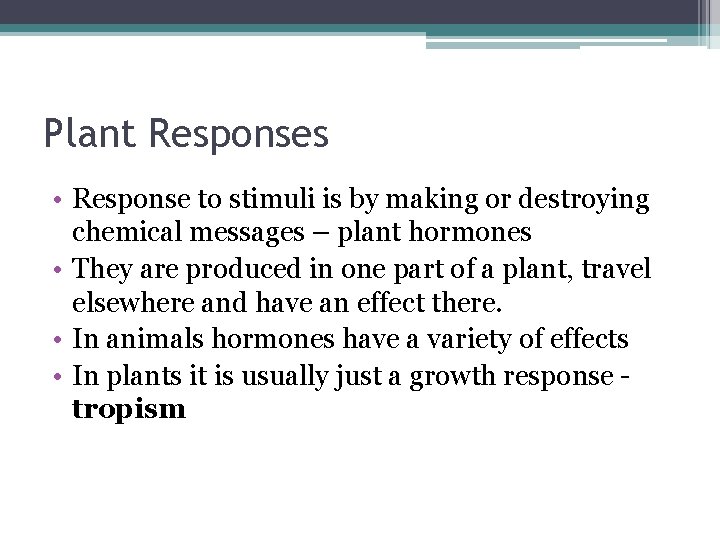
Plant Responses • Response to stimuli is by making or destroying chemical messages – plant hormones • They are produced in one part of a plant, travel elsewhere and have an effect there. • In animals hormones have a variety of effects • In plants it is usually just a growth response tropism
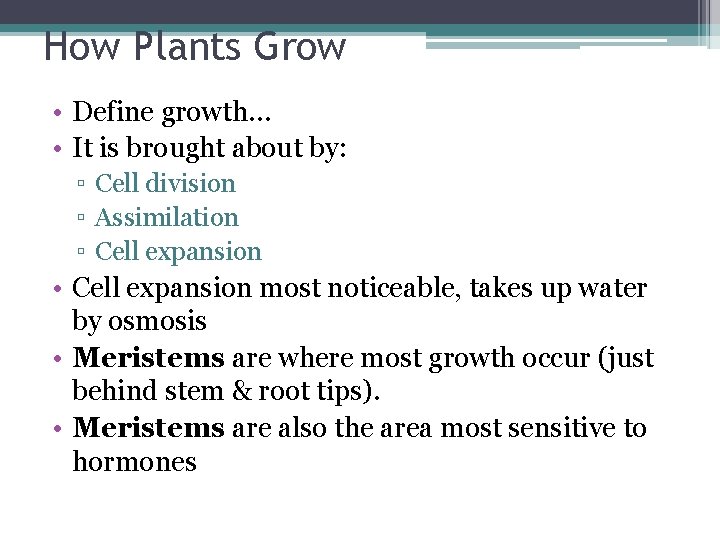
How Plants Grow • Define growth… • It is brought about by: ▫ Cell division ▫ Assimilation ▫ Cell expansion • Cell expansion most noticeable, takes up water by osmosis • Meristems are where most growth occur (just behind stem & root tips). • Meristems are also the area most sensitive to hormones
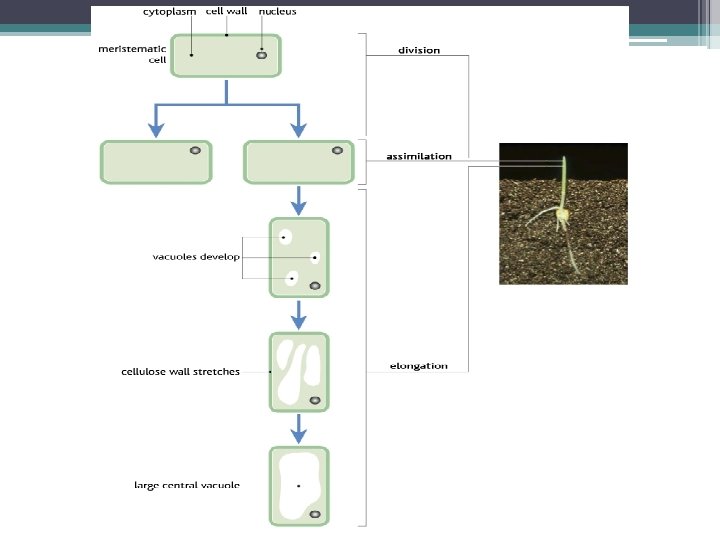
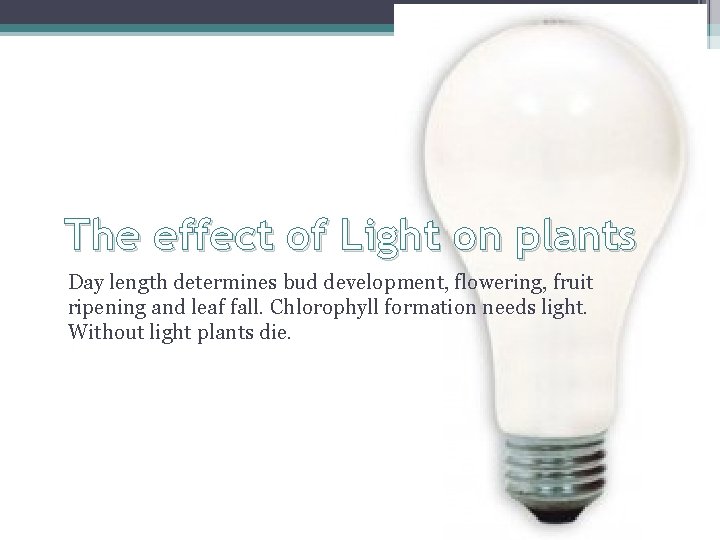
The effect of Light on plants Day length determines bud development, flowering, fruit ripening and leaf fall. Chlorophyll formation needs light. Without light plants die.
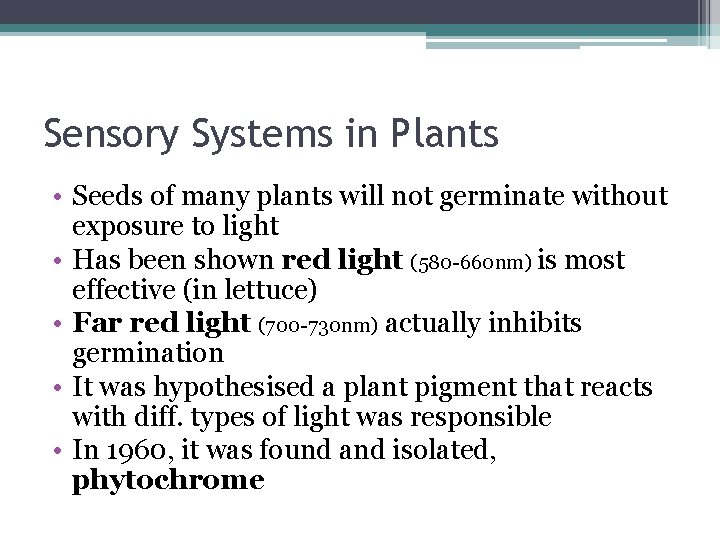
Sensory Systems in Plants • Seeds of many plants will not germinate without exposure to light • Has been shown red light (580 -660 nm) is most effective (in lettuce) • Far red light (700 -730 nm) actually inhibits germination • It was hypothesised a plant pigment that reacts with diff. types of light was responsible • In 1960, it was found and isolated, phytochrome
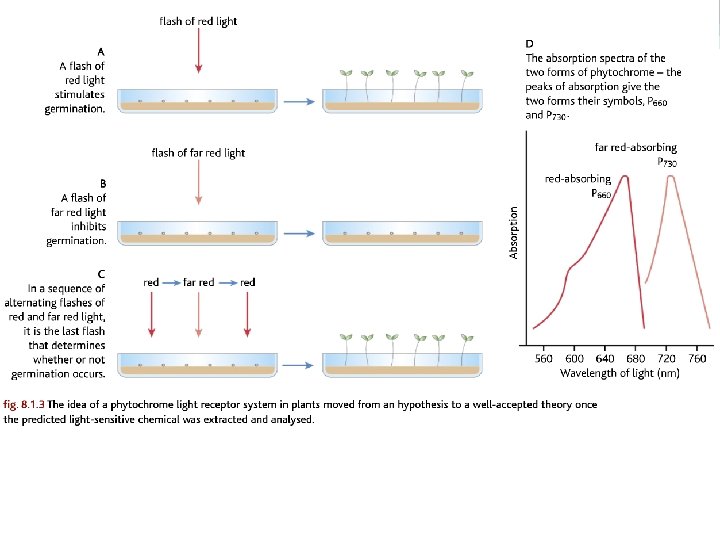
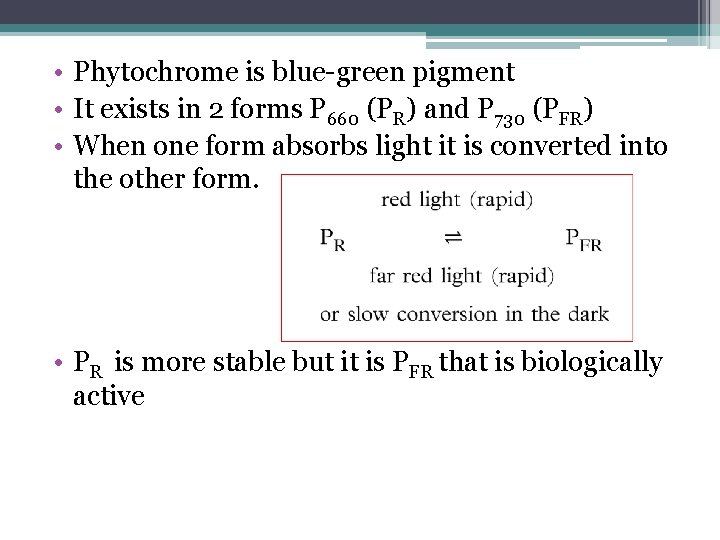
• Phytochrome is blue-green pigment • It exists in 2 forms P 660 (PR) and P 730 (PFR) • When one form absorbs light it is converted into the other form. • PR is more stable but it is PFR that is biologically active
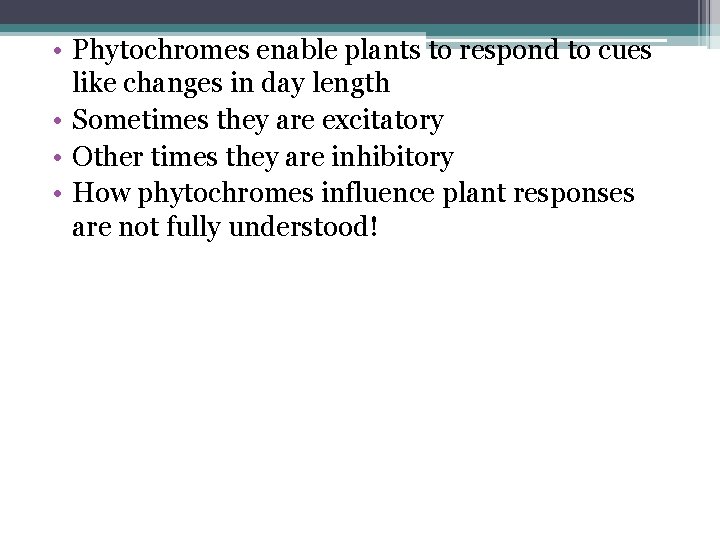
• Phytochromes enable plants to respond to cues like changes in day length • Sometimes they are excitatory • Other times they are inhibitory • How phytochromes influence plant responses are not fully understood!
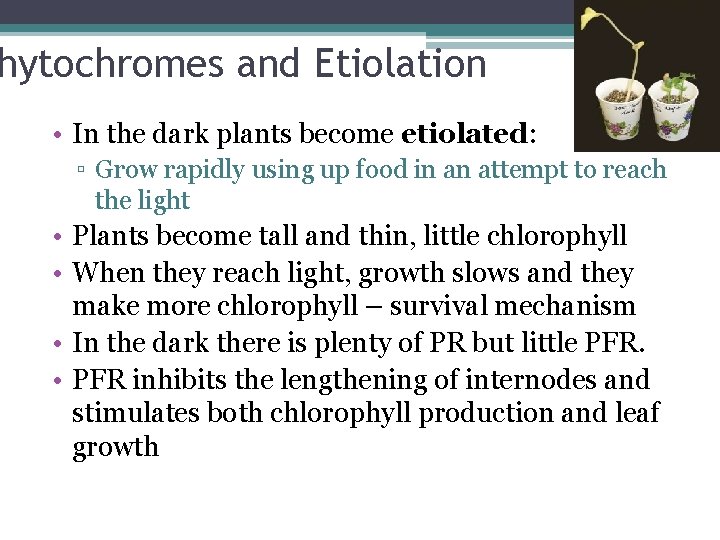
hytochromes and Etiolation • In the dark plants become etiolated: ▫ Grow rapidly using up food in an attempt to reach the light • Plants become tall and thin, little chlorophyll • When they reach light, growth slows and they make more chlorophyll – survival mechanism • In the dark there is plenty of PR but little PFR. • PFR inhibits the lengthening of internodes and stimulates both chlorophyll production and leaf growth
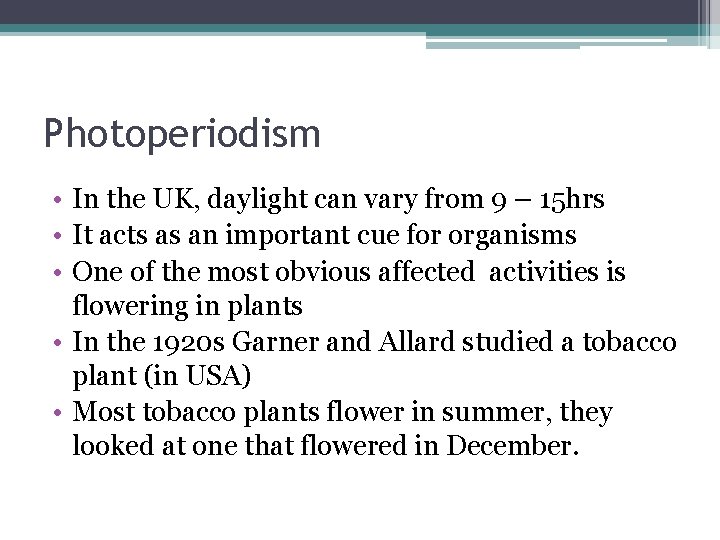
Photoperiodism • In the UK, daylight can vary from 9 – 15 hrs • It acts as an important cue for organisms • One of the most obvious affected activities is flowering in plants • In the 1920 s Garner and Allard studied a tobacco plant (in USA) • Most tobacco plants flower in summer, they looked at one that flowered in December.
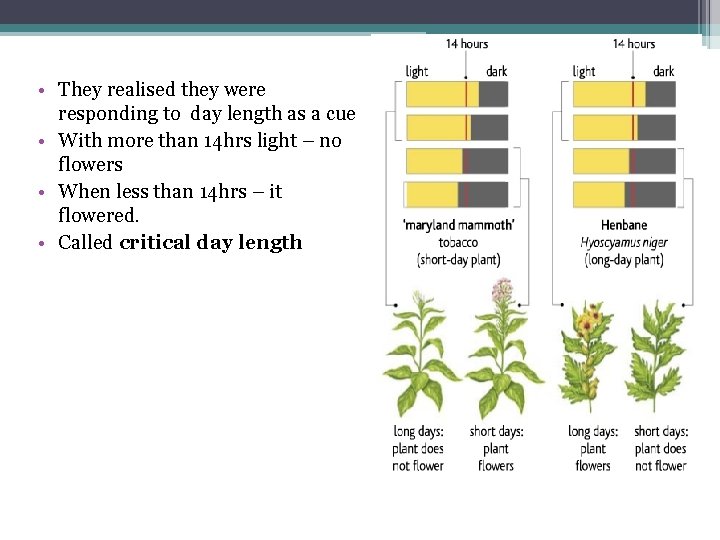
• They realised they were responding to day length as a cue • With more than 14 hrs light – no flowers • When less than 14 hrs – it flowered. • Called critical day length
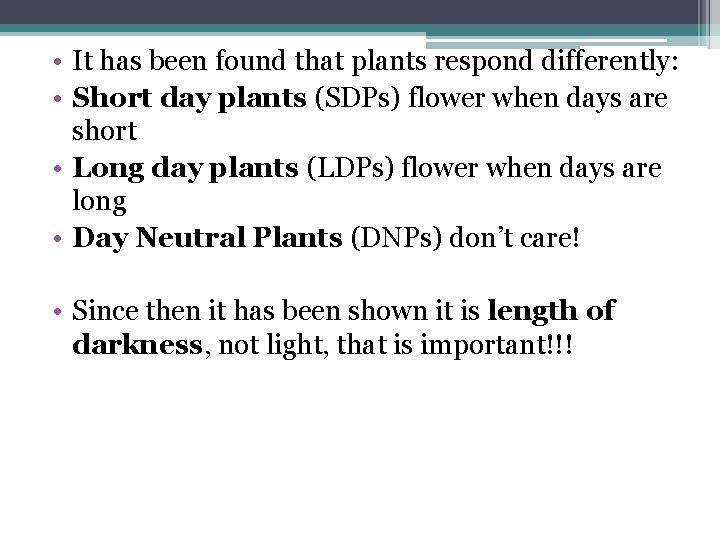
• It has been found that plants respond differently: • Short day plants (SDPs) flower when days are short • Long day plants (LDPs) flower when days are long • Day Neutral Plants (DNPs) don’t care! • Since then it has been shown it is length of darkness, not light, that is important!!!
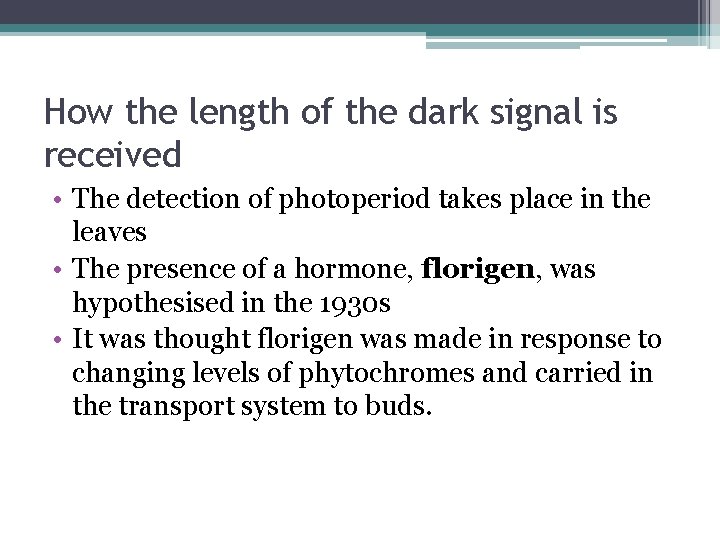
How the length of the dark signal is received • The detection of photoperiod takes place in the leaves • The presence of a hormone, florigen, was hypothesised in the 1930 s • It was thought florigen was made in response to changing levels of phytochromes and carried in the transport system to buds.
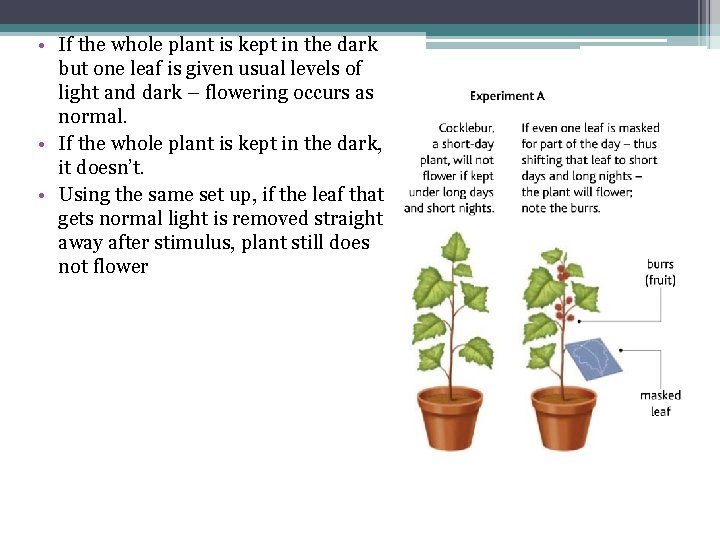
• If the whole plant is kept in the dark but one leaf is given usual levels of light and dark – flowering occurs as normal. • If the whole plant is kept in the dark, it doesn’t. • Using the same set up, if the leaf that gets normal light is removed straight away after stimulus, plant still does not flower
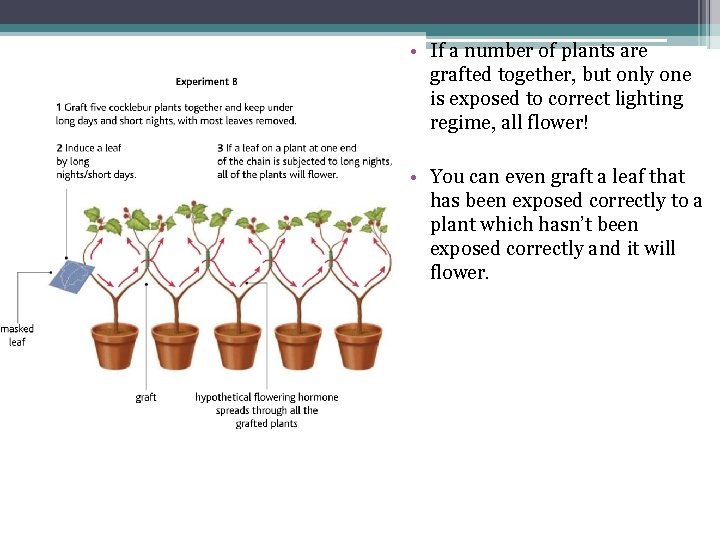
• If a number of plants are grafted together, but only one is exposed to correct lighting regime, all flower! • You can even graft a leaf that has been exposed correctly to a plant which hasn’t been exposed correctly and it will flower.
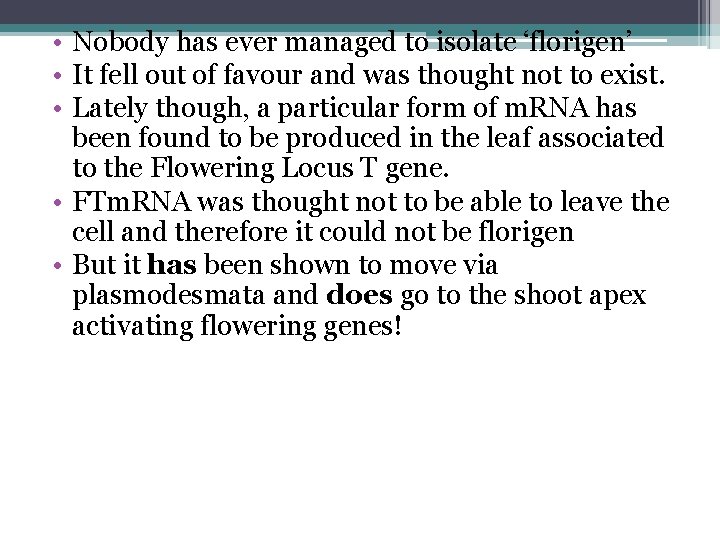
• Nobody has ever managed to isolate ‘florigen’ • It fell out of favour and was thought not to exist. • Lately though, a particular form of m. RNA has been found to be produced in the leaf associated to the Flowering Locus T gene. • FTm. RNA was thought not to be able to leave the cell and therefore it could not be florigen • But it has been shown to move via plasmodesmata and does go to the shoot apex activating flowering genes!
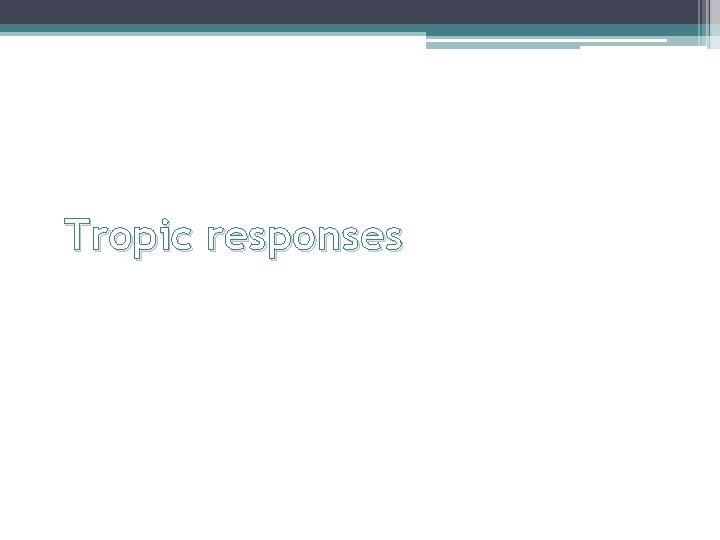
Tropic responses
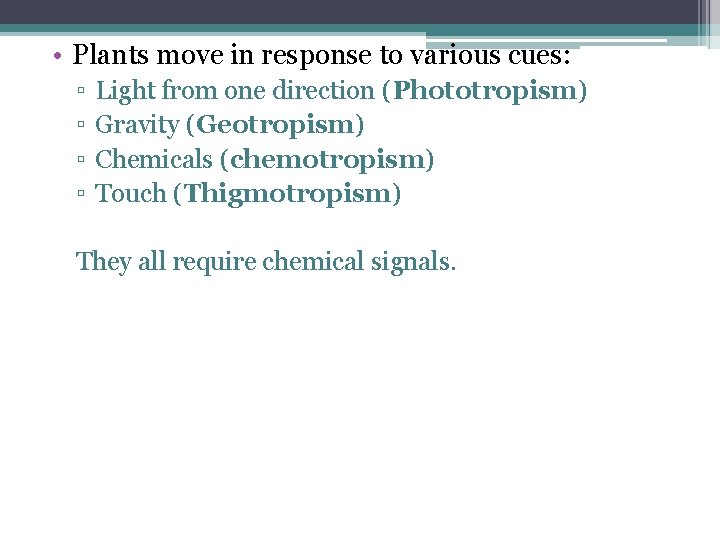
• Plants move in response to various cues: ▫ ▫ Light from one direction (Phototropism) Gravity (Geotropism) Chemicals (chemotropism) Touch (Thigmotropism) They all require chemical signals.
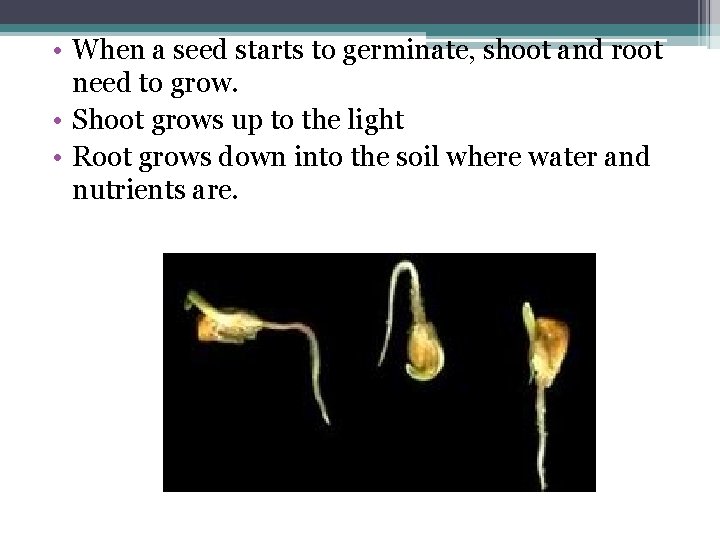
• When a seed starts to germinate, shoot and root need to grow. • Shoot grows up to the light • Root grows down into the soil where water and nutrients are.
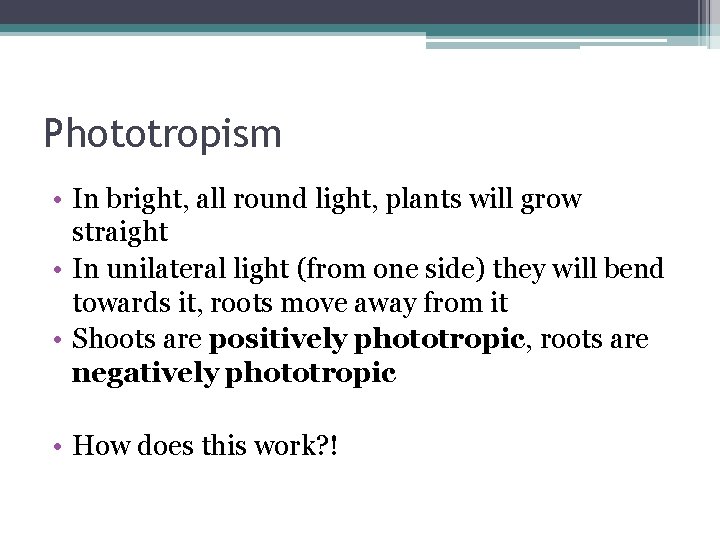
Phototropism • In bright, all round light, plants will grow straight • In unilateral light (from one side) they will bend towards it, roots move away from it • Shoots are positively phototropic, roots are negatively phototropic • How does this work? !
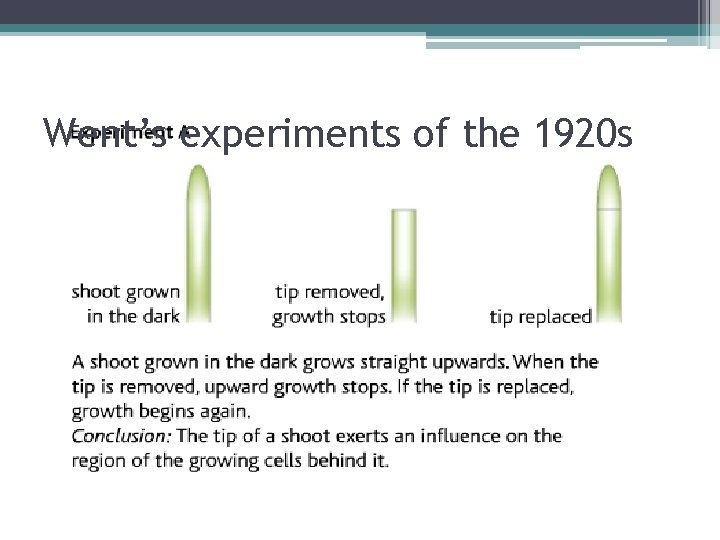
Went’s experiments of the 1920 s
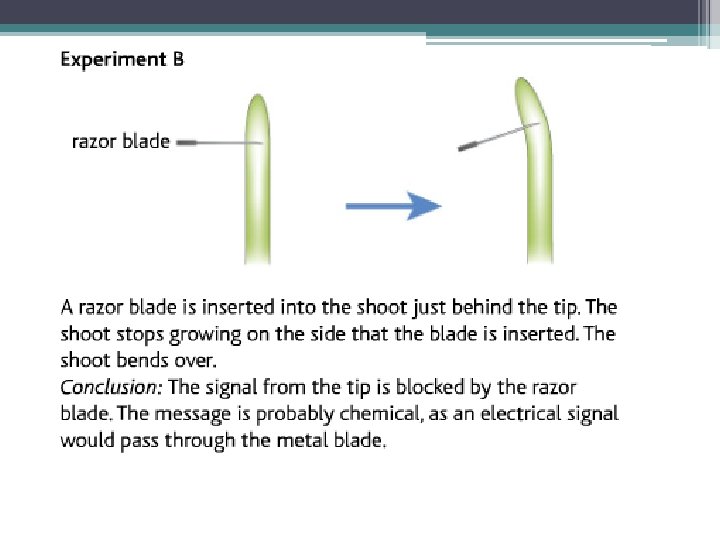
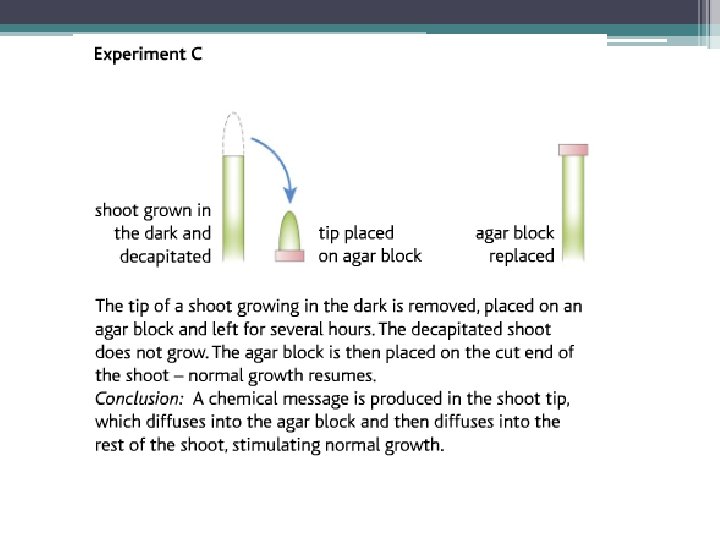
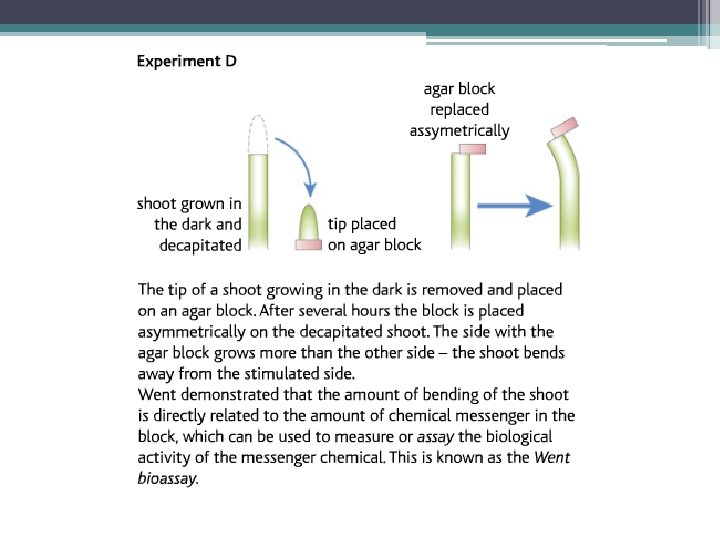
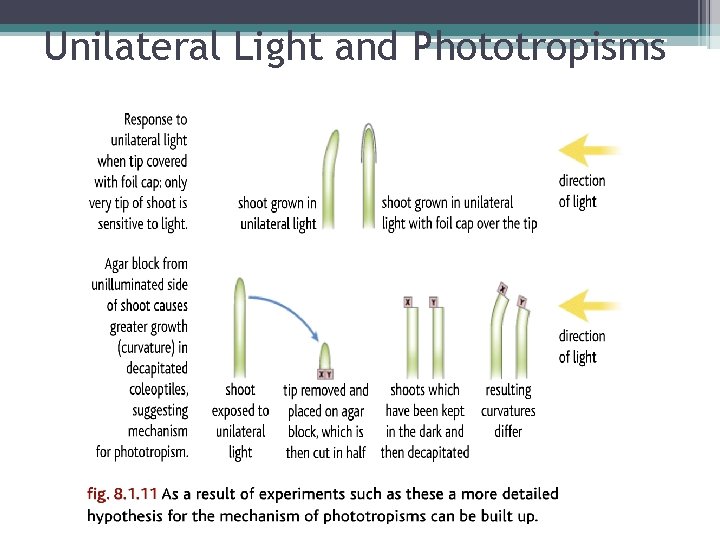
Unilateral Light and Phototropisms
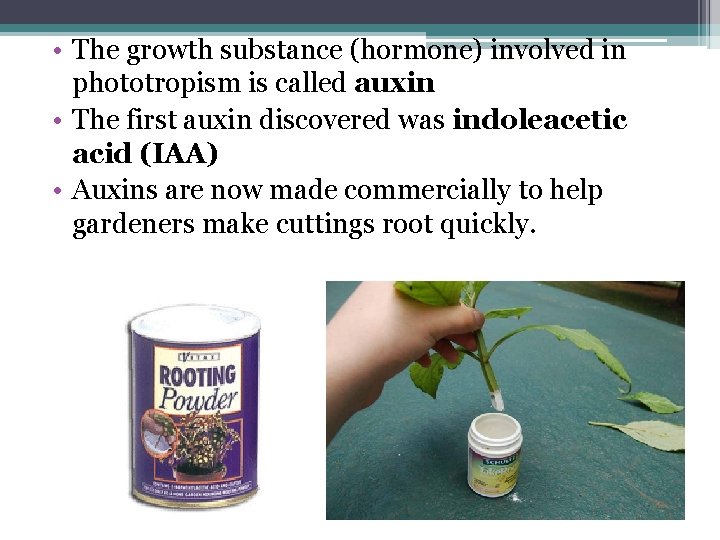
• The growth substance (hormone) involved in phototropism is called auxin • The first auxin discovered was indoleacetic acid (IAA) • Auxins are now made commercially to help gardeners make cuttings root quickly.
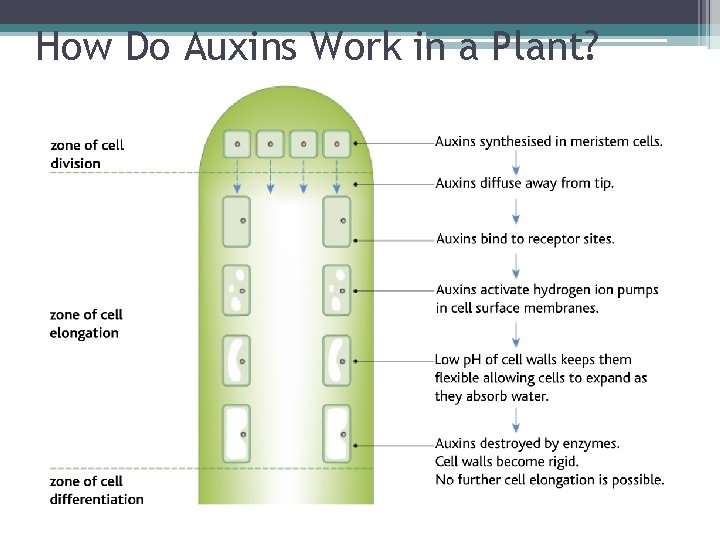
How Do Auxins Work in a Plant?
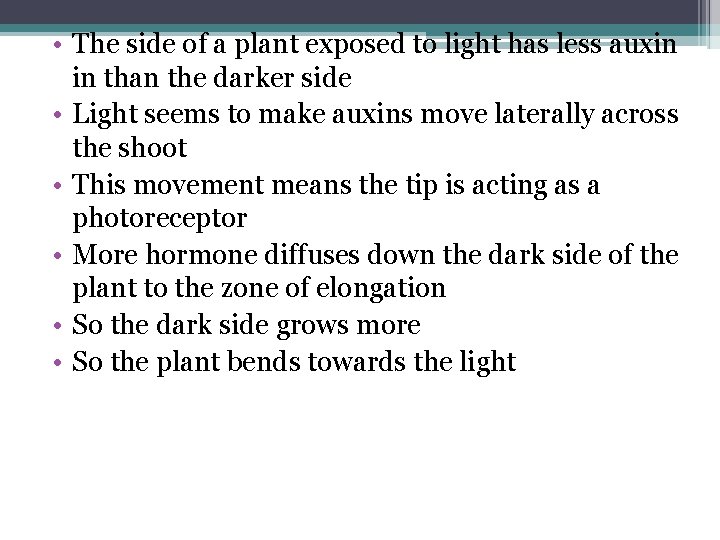
• The side of a plant exposed to light has less auxin in than the darker side • Light seems to make auxins move laterally across the shoot • This movement means the tip is acting as a photoreceptor • More hormone diffuses down the dark side of the plant to the zone of elongation • So the dark side grows more • So the plant bends towards the light
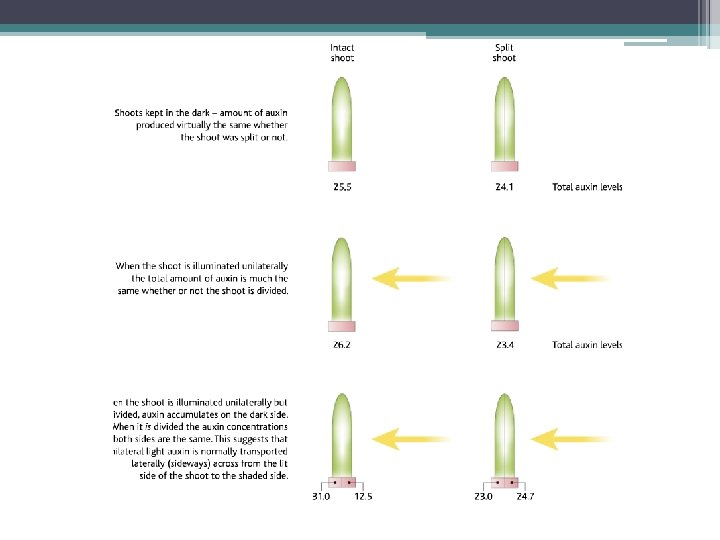
Identify each type of neuronal pool
Nervous
Neuronal pools are collections of
The nervous system is made up of
Nervous system and digestive system
Endocrine system vs nervous system
Amino acid-based hormones
Endocrine system
Chemical messengers of the nervous system
Worms phylum
Primary functions of the nervous system
Nervous system learning objectives
Componentes componentes
What is stimuli in nervous system
Spinal cord structure
Parasympathetic and sympathetic
Parasympathetic nervous system def
Spinal. nerves
Somatic nervous system (sns)
Brain scienstructable
Autonomic nervous system pathway
Nematoda
Arthropods circulatory system
Jointed foot
Nervous system of arthropods
Major divisions
Ans
Nervous system of coelenterata
Autonomic nervous system consists of
Sympathetic nervous system
Section 35-1 human body systems answer key
Somatic and autonomic nervous system
Human nervous system ppt
Basic unit of nervous system
The basic unit of the nervous system is the
Histology of nervous system ppt
What is white matter made of
Comparison of endocrine and nervous system
8 divisions of the nervous system
How to take care of your nervous system
Nervous system effector cells
Chapter 15 nervous system diseases and disorders
Endocrine and nervous system comparison
Beta-blockers for overactive sympathetic nervous system
Neurotransmitter of somatic nervous system
Ans
Anatomy of silkworm
Section 35-3 divisions of the nervous system
What is the basic building block of the nervous system
Structure of nervous system graphic organizer
Medunerve
Planaria classification
Characteristics of cnidaria
Ventral root and dorsal root
Sympathetic and parasympathetic nervous system difference
Learning objectives of nervous system
Divisions of the nervous system
Language
Nervous tissue
Disorders of the nervous system
Osteichthyes characteristics
How the nervous system works
Nervous system of computer
Enteric plexus
Adrenal nervous system
Chapter 8 the nervous system
Men in goh seel
Section 35-3 divisions of the nervous system
Autonomic nervous system
Subthalamus
Body system theme park project
The autonomic nervous system controls
Why do animals need a nervous system
Trauma splitting
Nervous system function
The human nervous system biology mad
Somatic nervous system
Sensory vs motor homunculus
Cerebral aqueduct
Phylum echinodermata introduction
Parts of the brain
Jeopardy nervous system
Oculomotor nerve
Central nervous system amusement park
Body amusement park
Brainpop nervous system
Neuroglia
Biochemistry of nervous system
What is the job of the nervous system
Peripheral nervous system
Organisation of nervous system