PLT 104 ENGINEERING SCIENCE CHAPTER 1 Electrical Properties
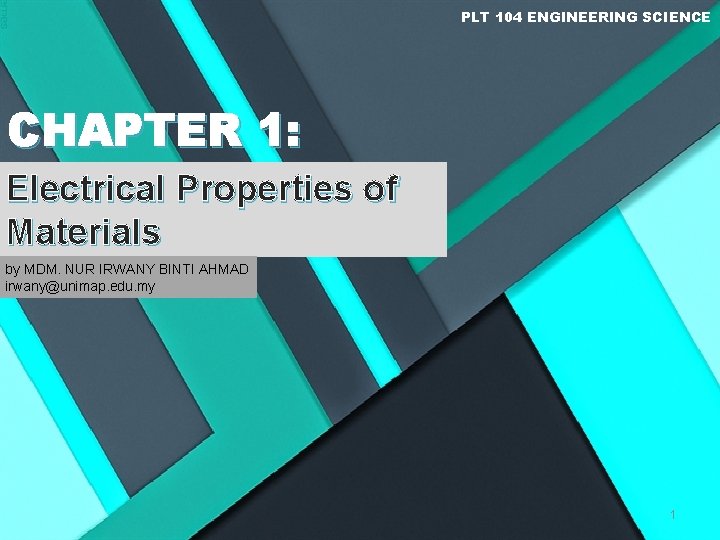
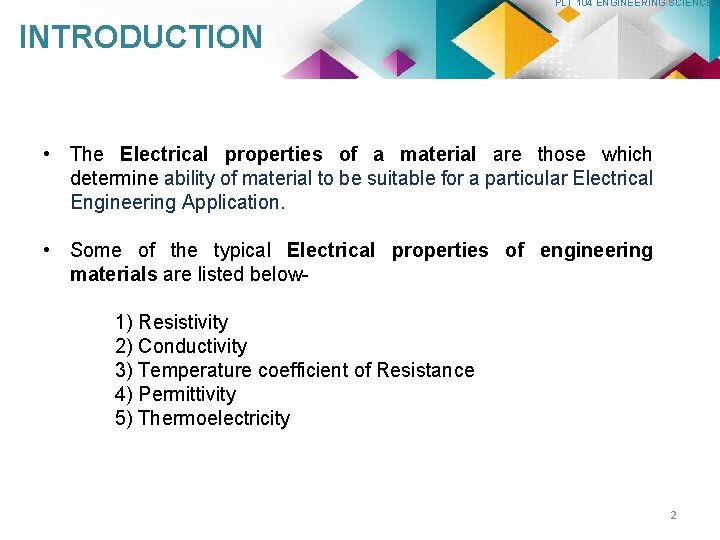
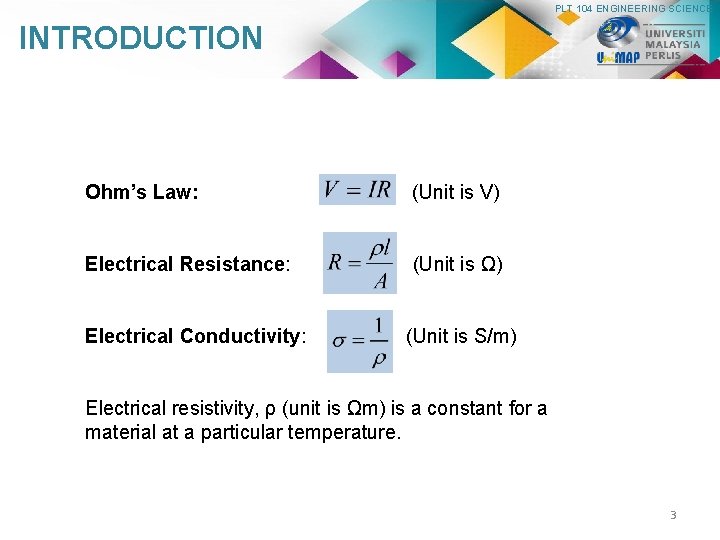
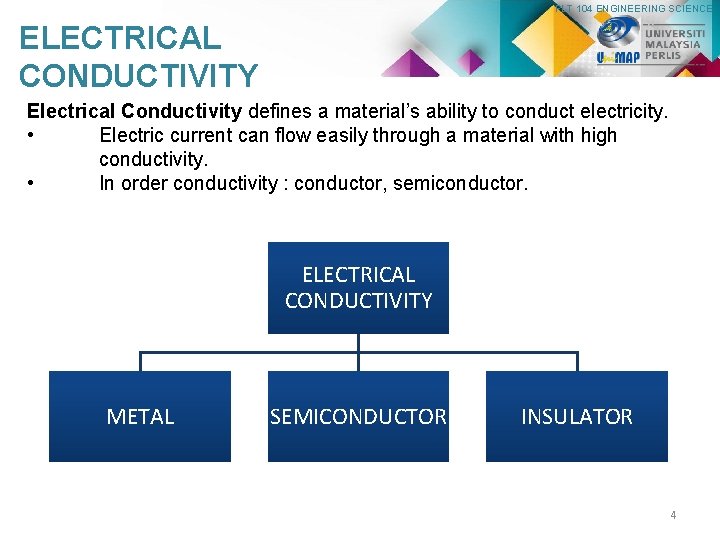
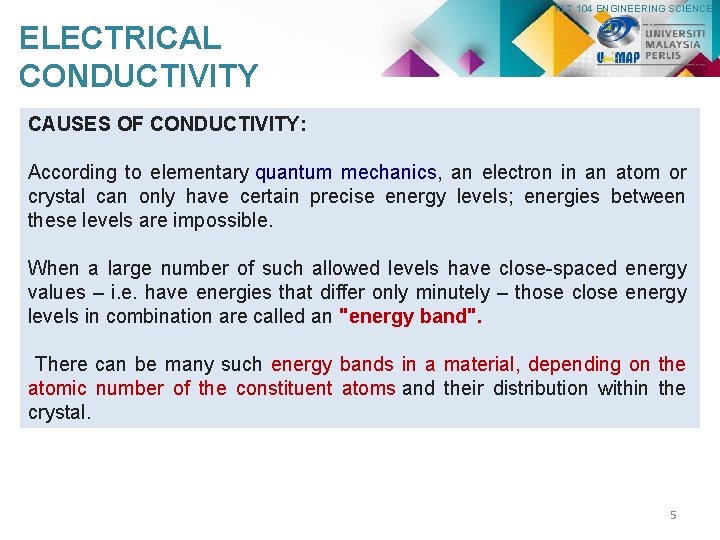
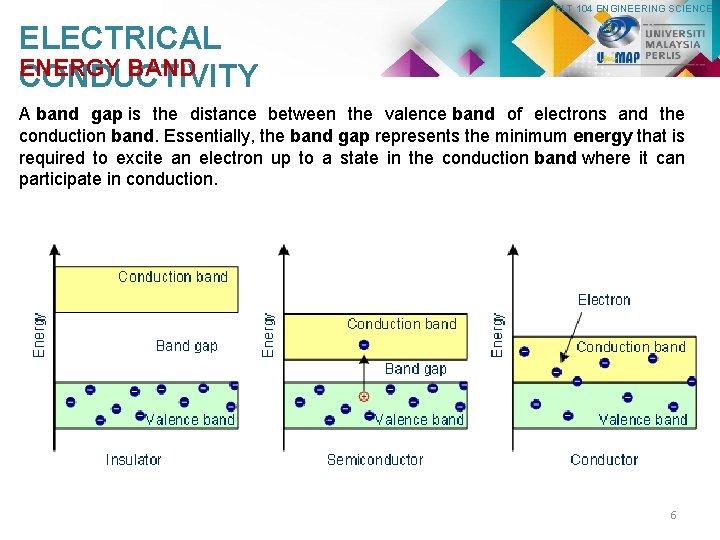
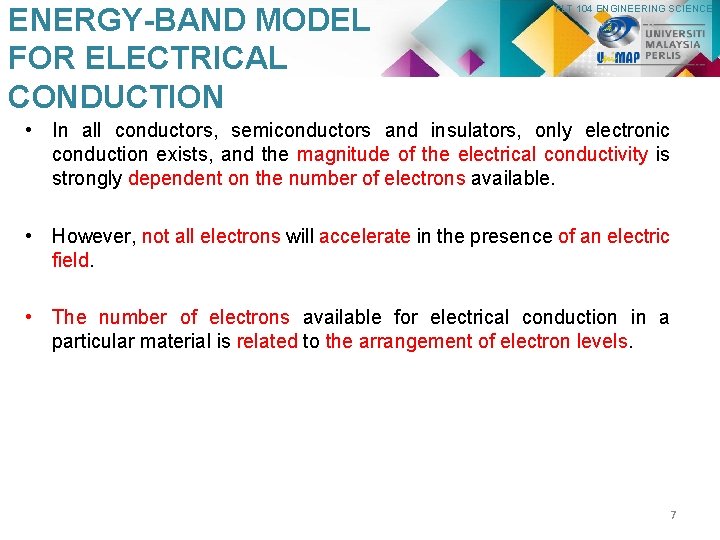
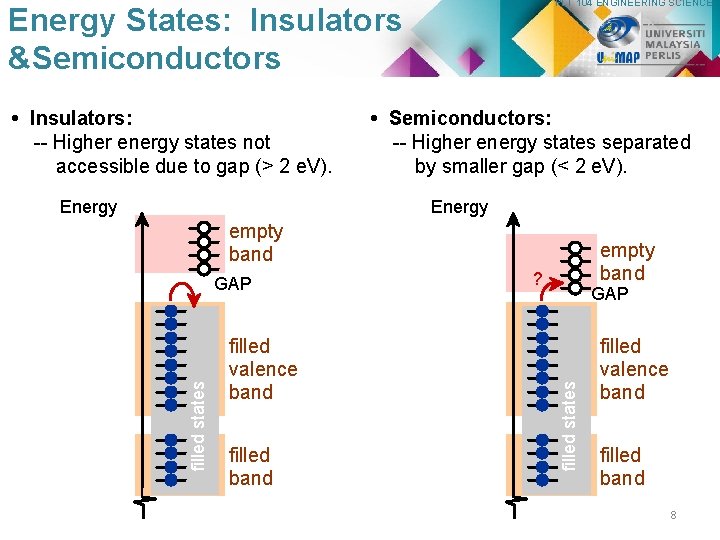
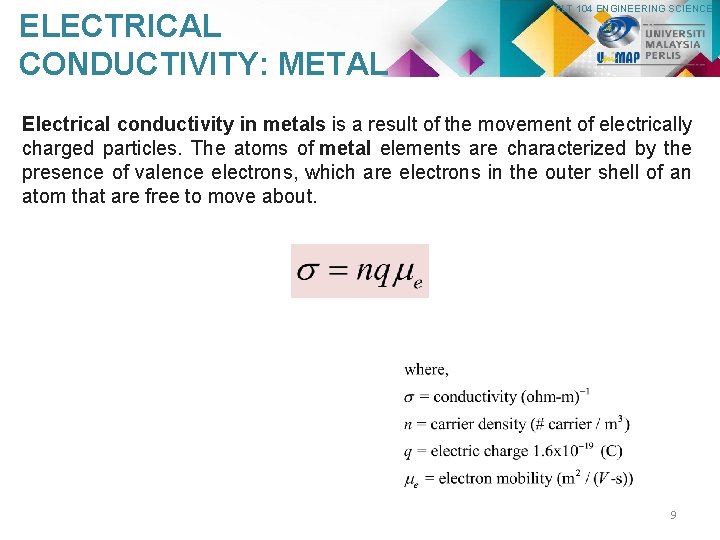
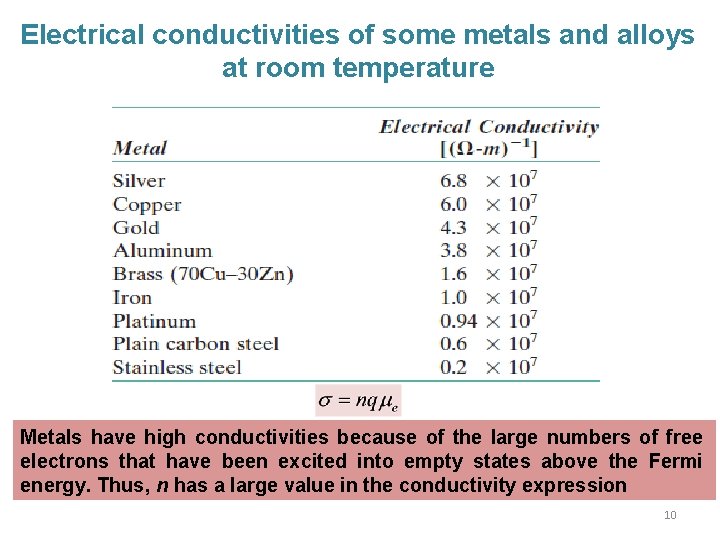
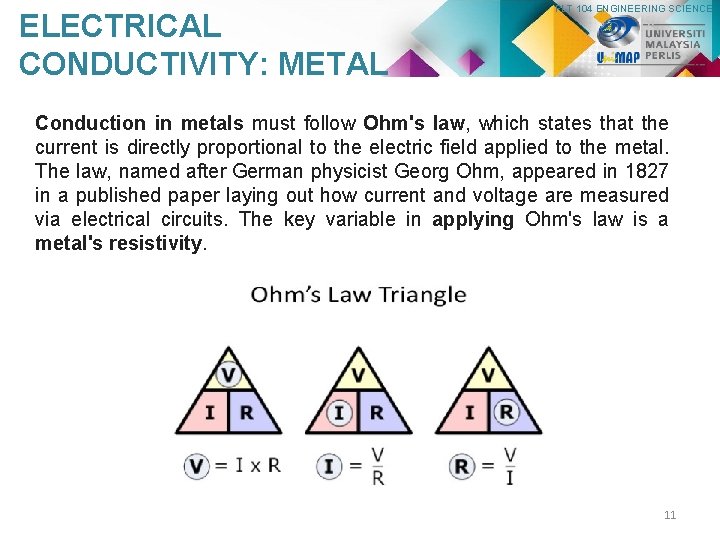
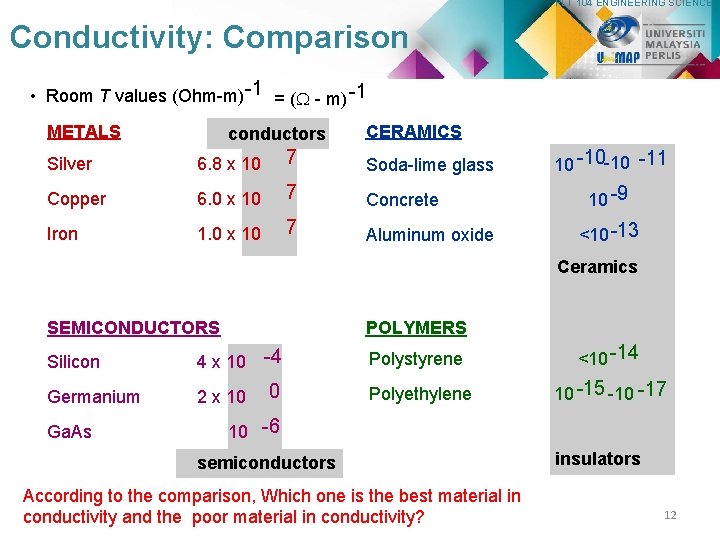
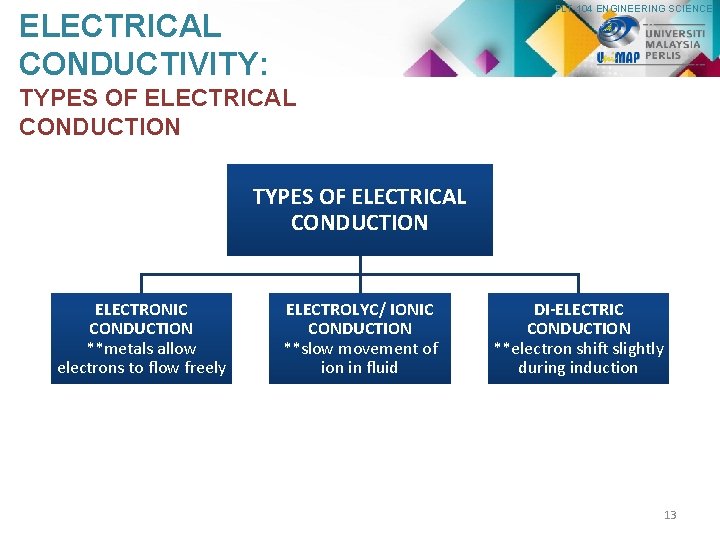
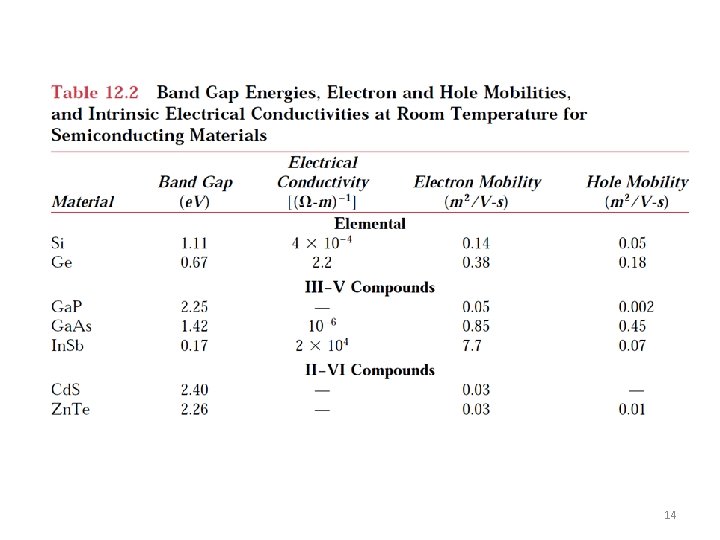
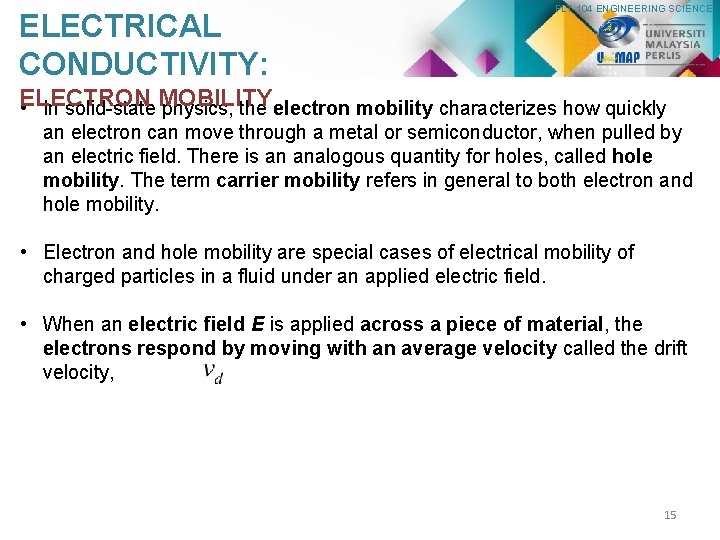
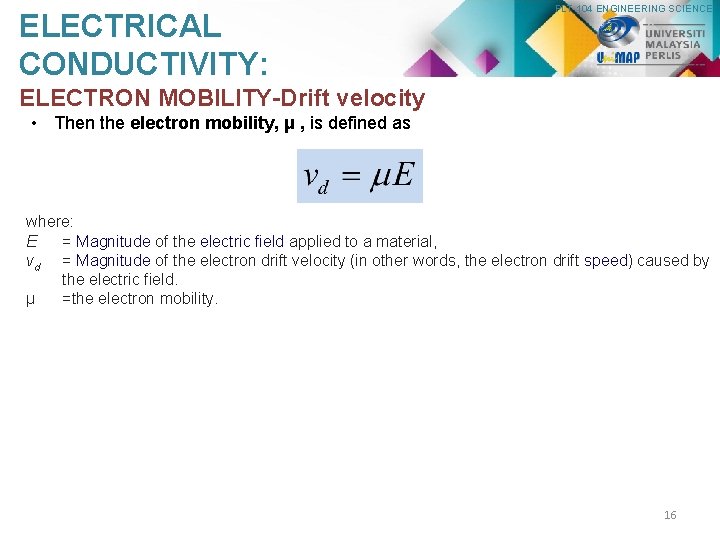
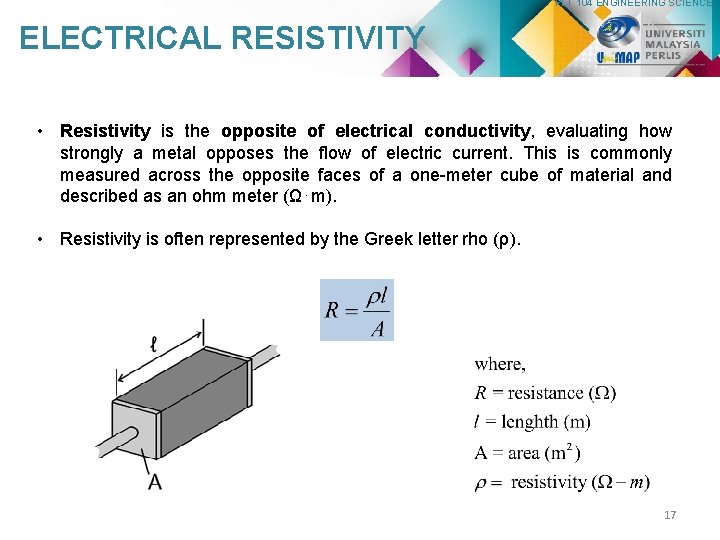
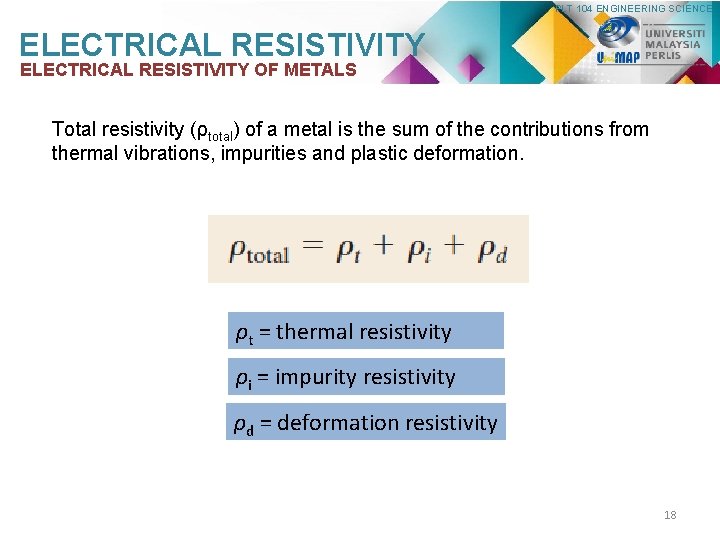
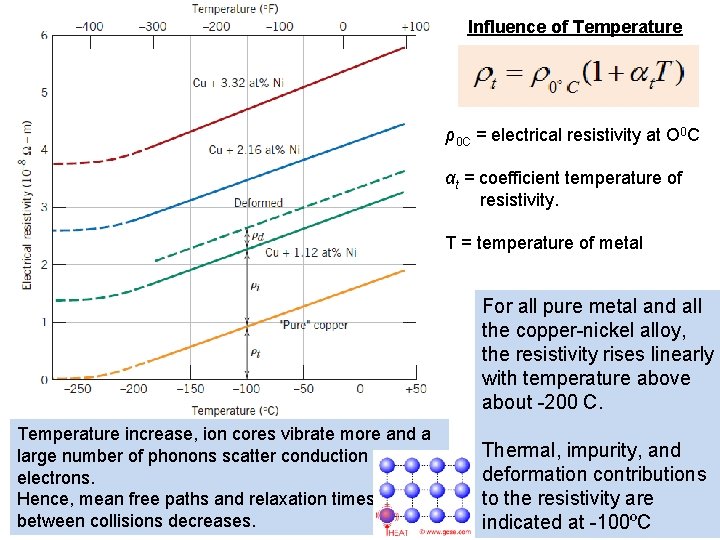
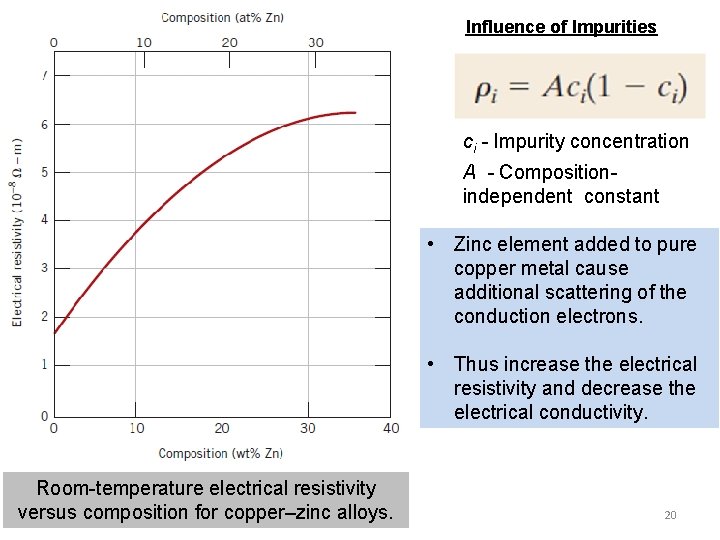
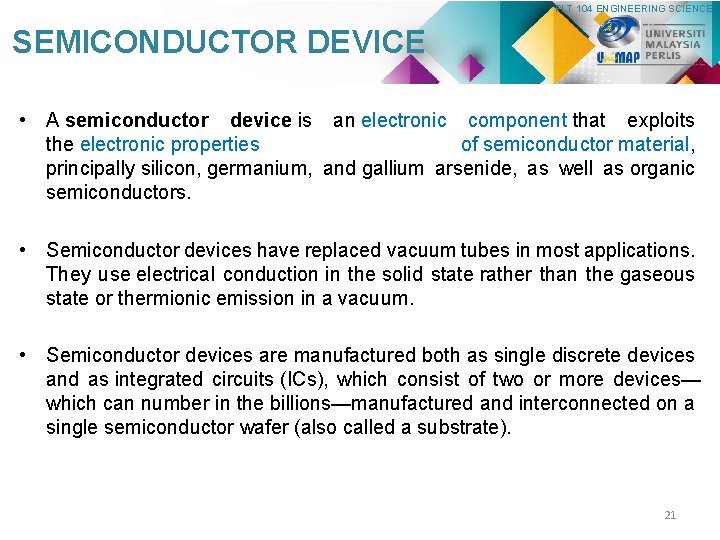
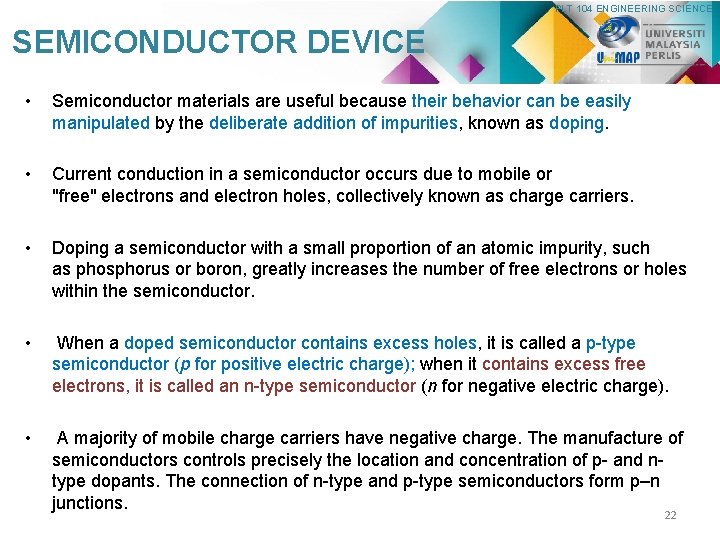
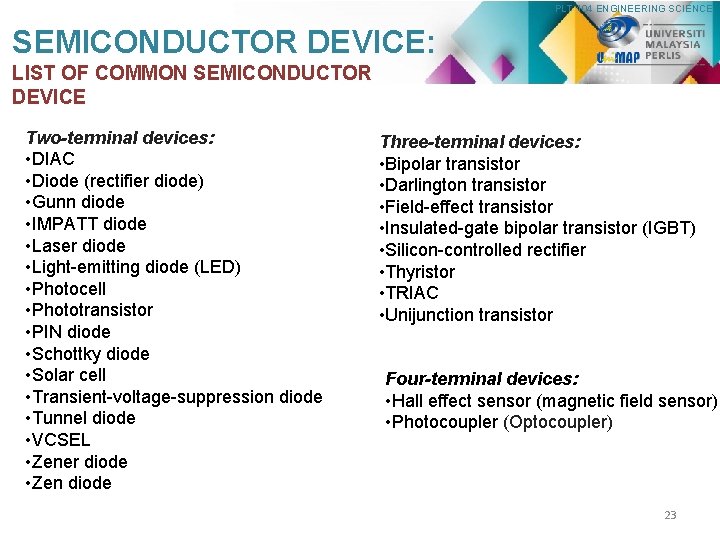
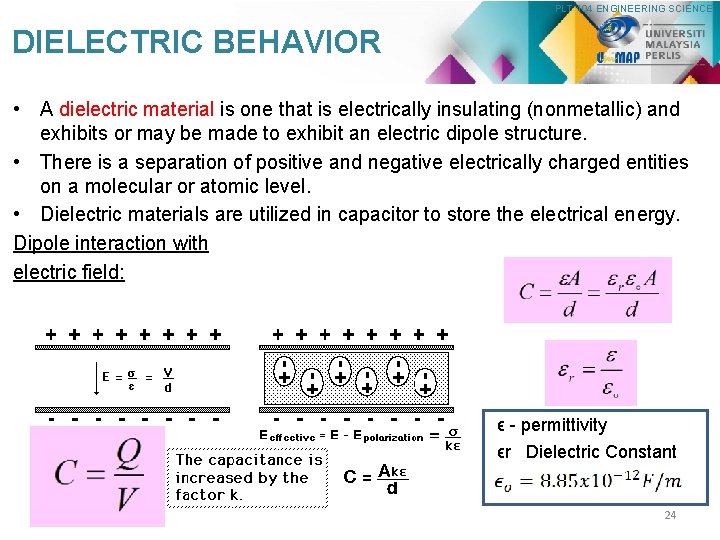
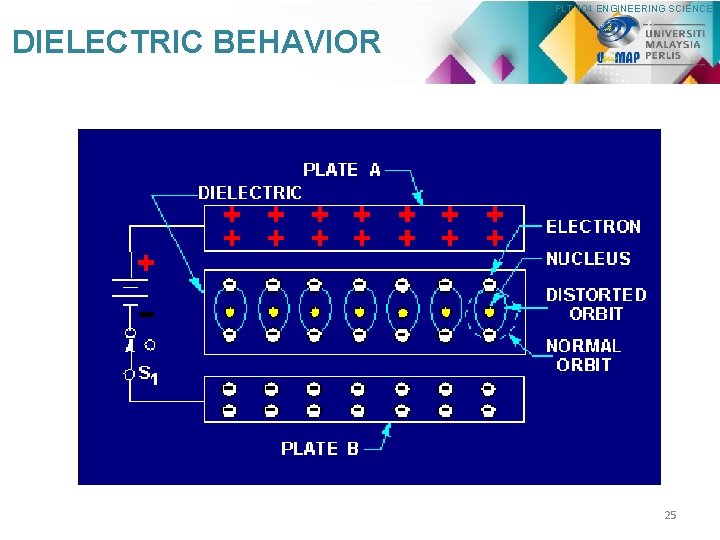
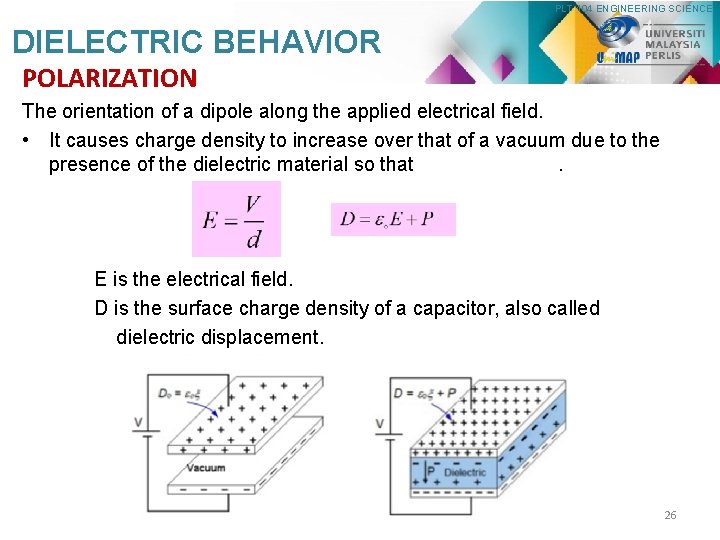
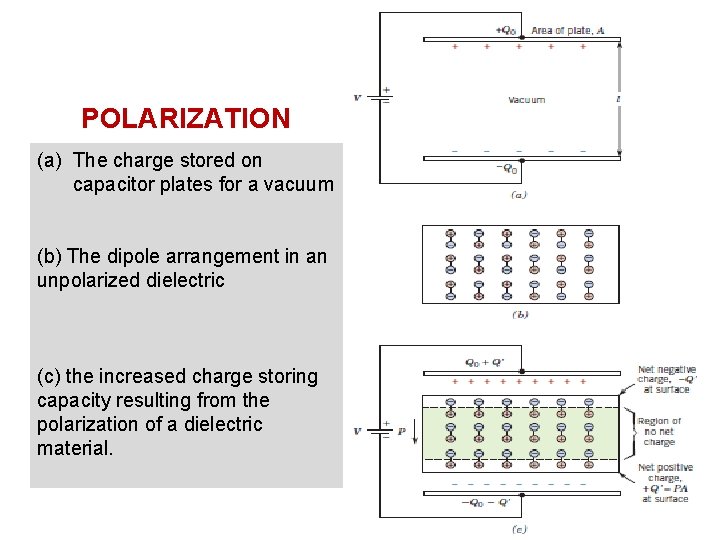
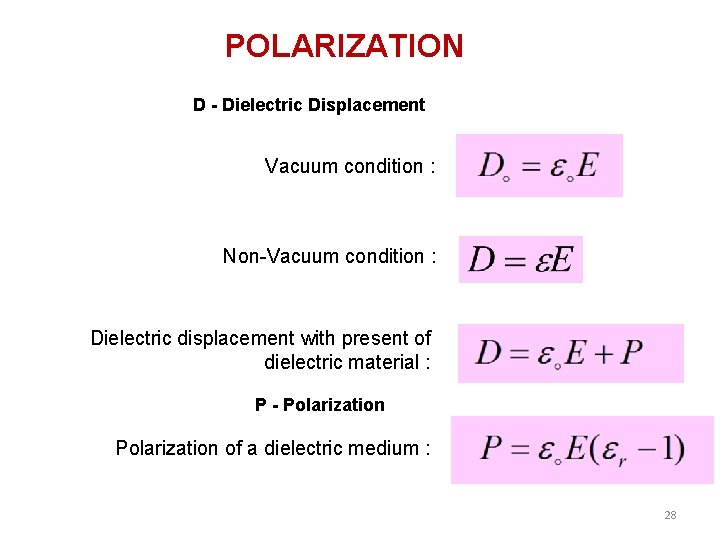
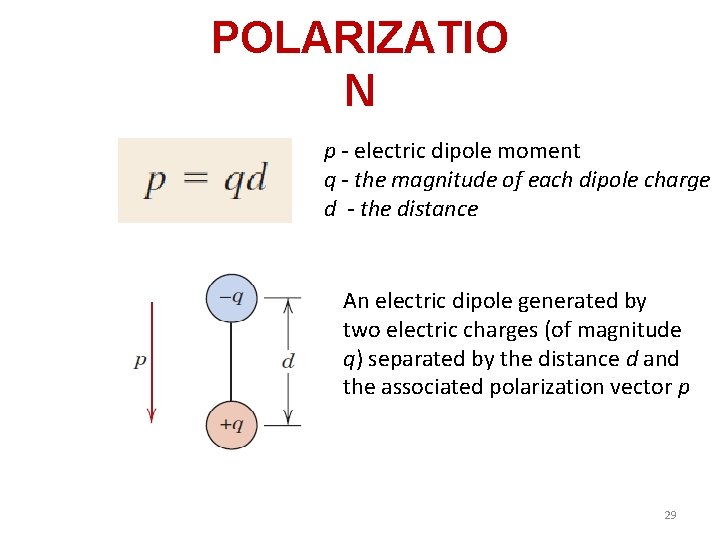
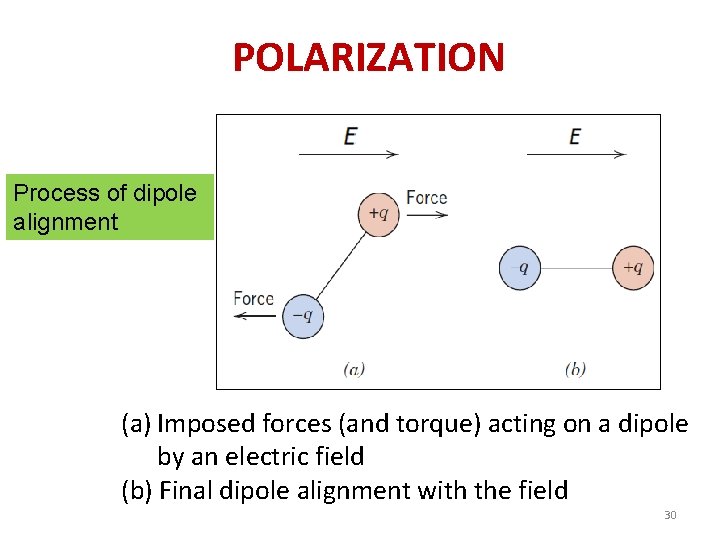
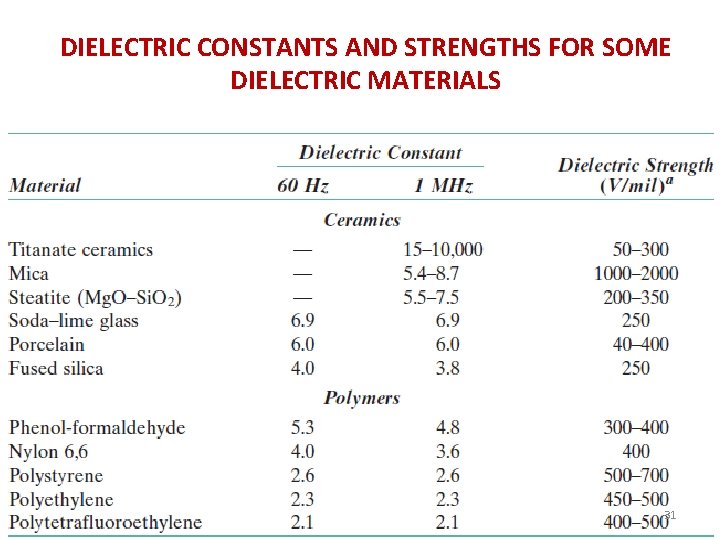
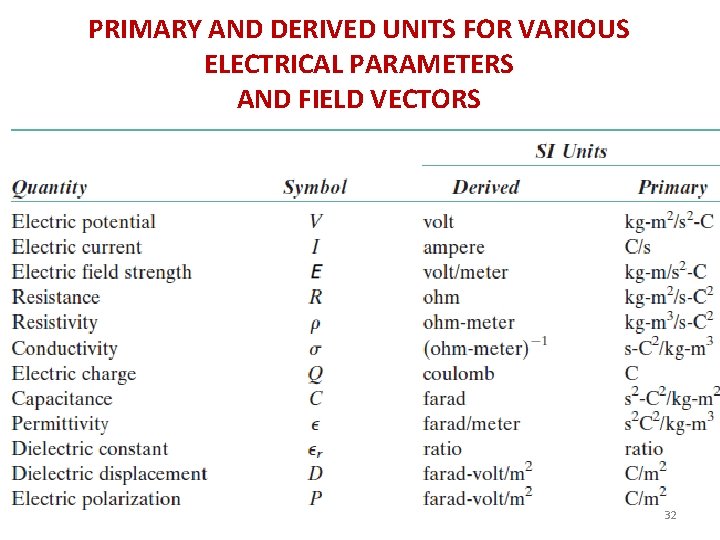
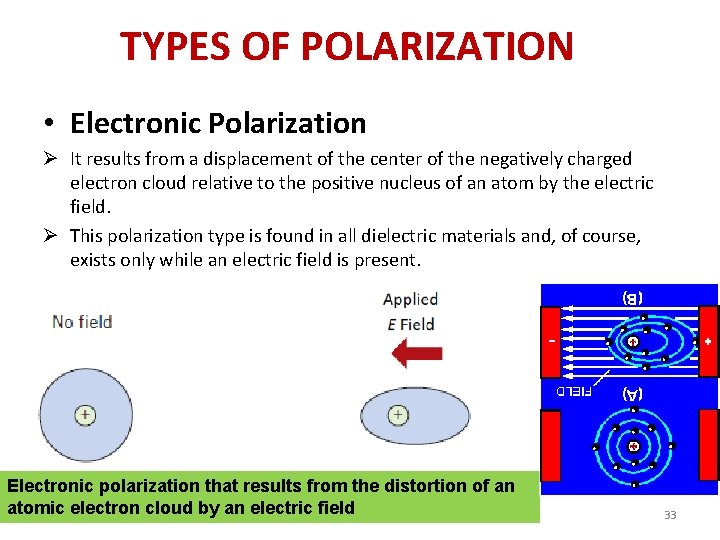
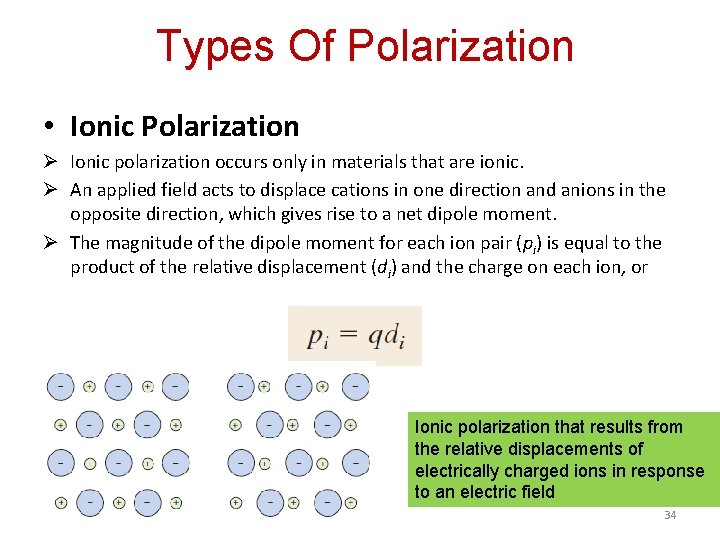
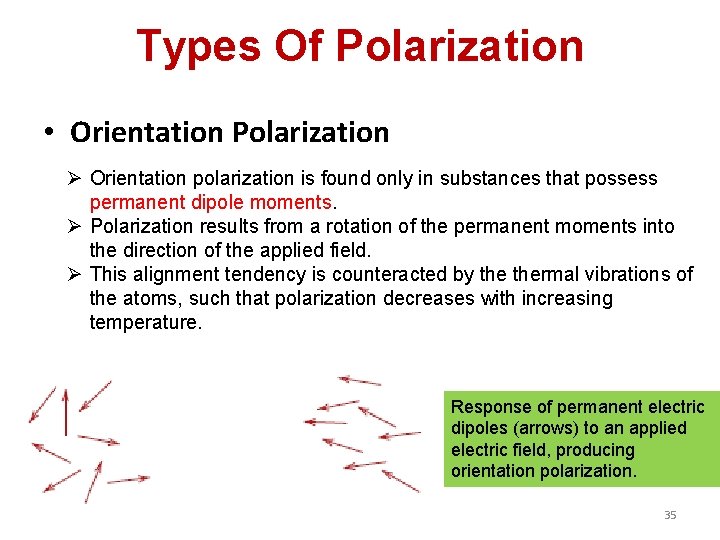
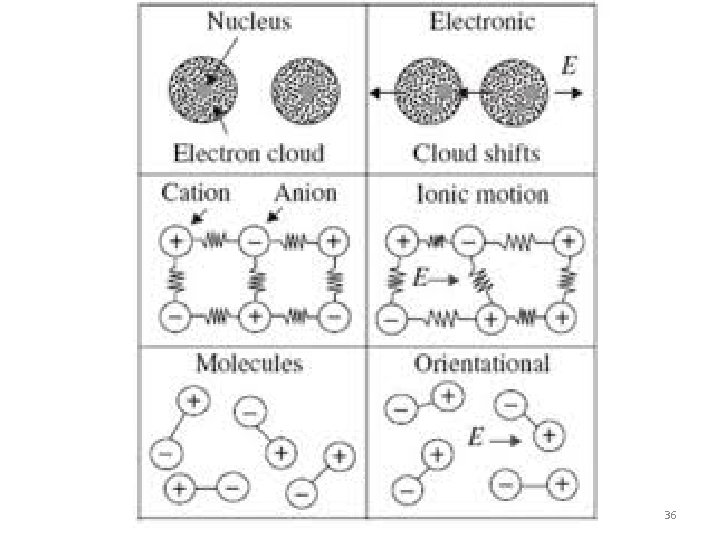
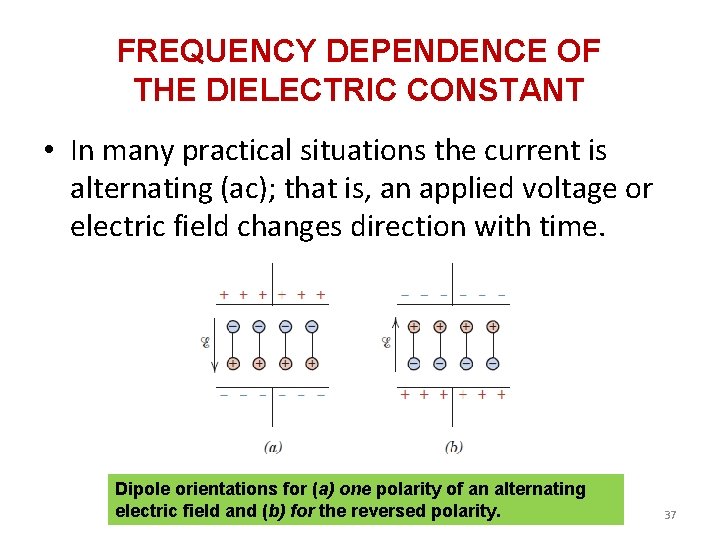
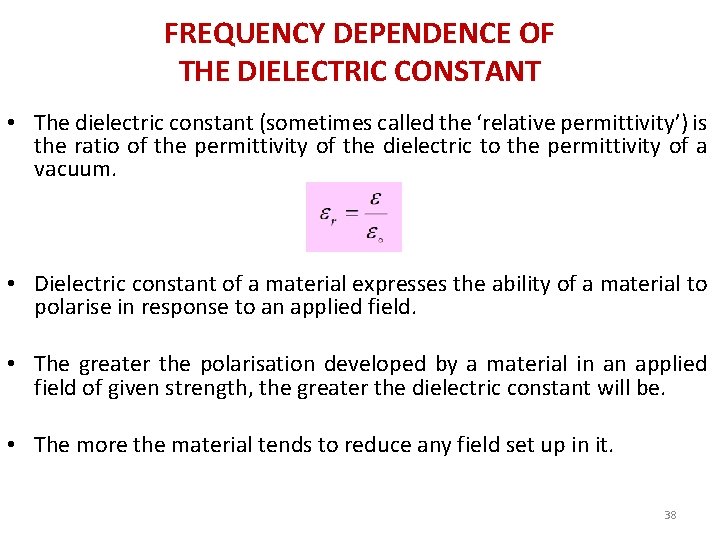
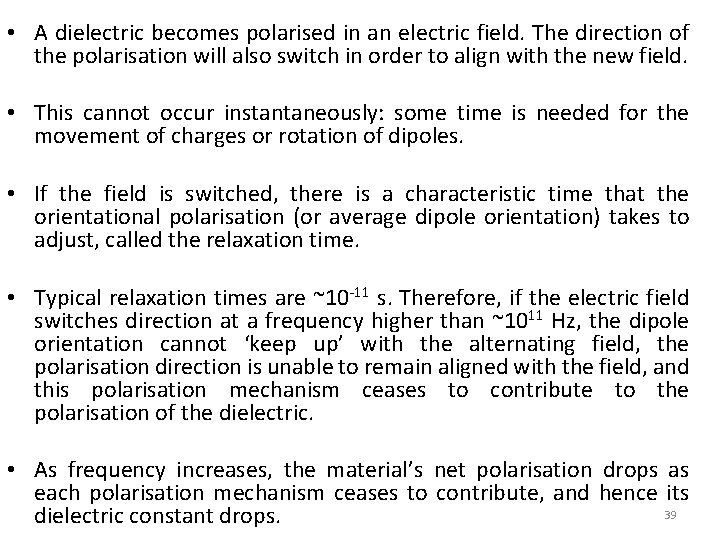
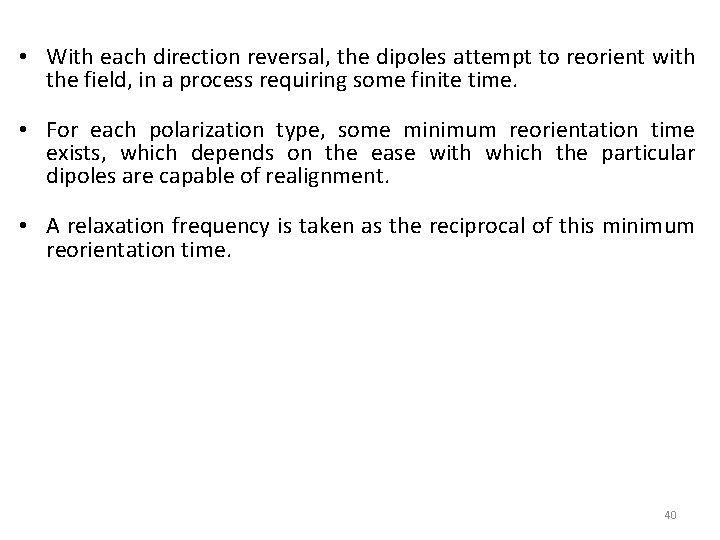
- Slides: 40
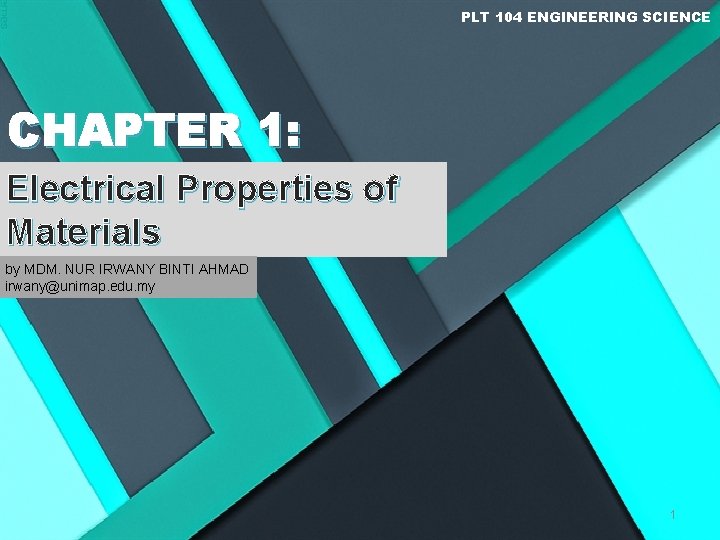
PLT 104 ENGINEERING SCIENCE CHAPTER 1: Electrical Properties of Materials by MDM. NUR IRWANY BINTI AHMAD irwany@unimap. edu. my 1
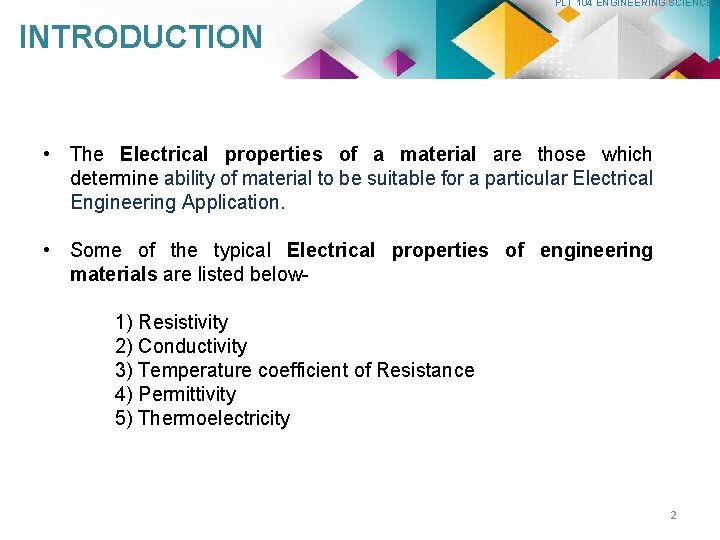
PLT 104 ENGINEERING SCIENCE INTRODUCTION • The Electrical properties of a material are those which determine ability of material to be suitable for a particular Electrical Engineering Application. • Some of the typical Electrical properties of engineering materials are listed below 1) Resistivity 2) Conductivity 3) Temperature coefficient of Resistance 4) Permittivity 5) Thermoelectricity 2
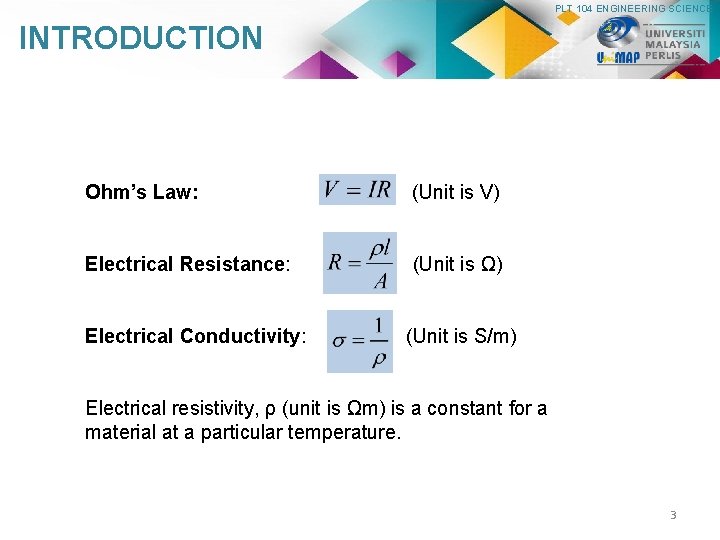
PLT 104 ENGINEERING SCIENCE INTRODUCTION Ohm’s Law: (Unit is V) Electrical Resistance: (Unit is Ω) Electrical Conductivity: (Unit is S/m) Electrical resistivity, ρ (unit is Ωm) is a constant for a material at a particular temperature. 3
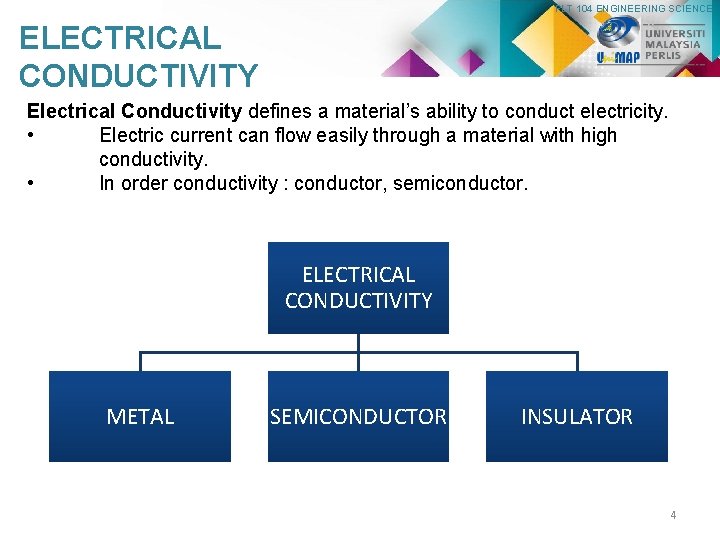
PLT 104 ENGINEERING SCIENCE ELECTRICAL CONDUCTIVITY Electrical Conductivity defines a material’s ability to conduct electricity. • Electric current can flow easily through a material with high conductivity. • In order conductivity : conductor, semiconductor. ELECTRICAL CONDUCTIVITY METAL SEMICONDUCTOR INSULATOR 4
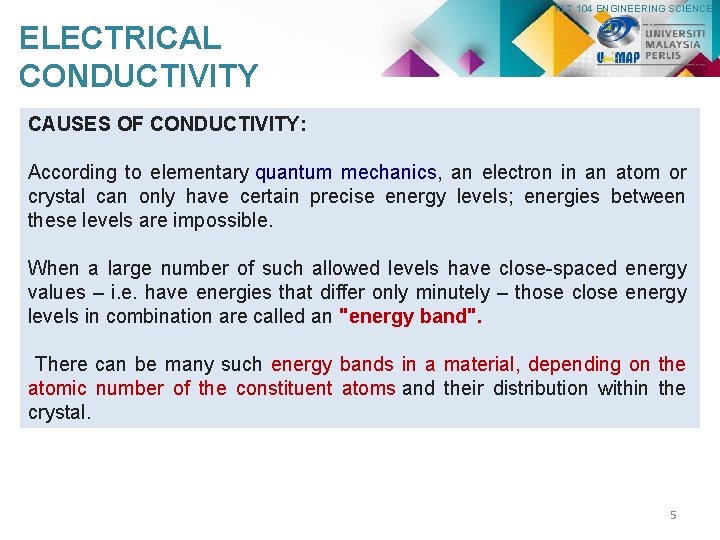
PLT 104 ENGINEERING SCIENCE ELECTRICAL CONDUCTIVITY CAUSES OF CONDUCTIVITY: According to elementary quantum mechanics, an electron in an atom or crystal can only have certain precise energy levels; energies between these levels are impossible. When a large number of such allowed levels have close-spaced energy values – i. e. have energies that differ only minutely – those close energy levels in combination are called an "energy band". There can be many such energy bands in a material, depending on the atomic number of the constituent atoms and their distribution within the crystal. 5
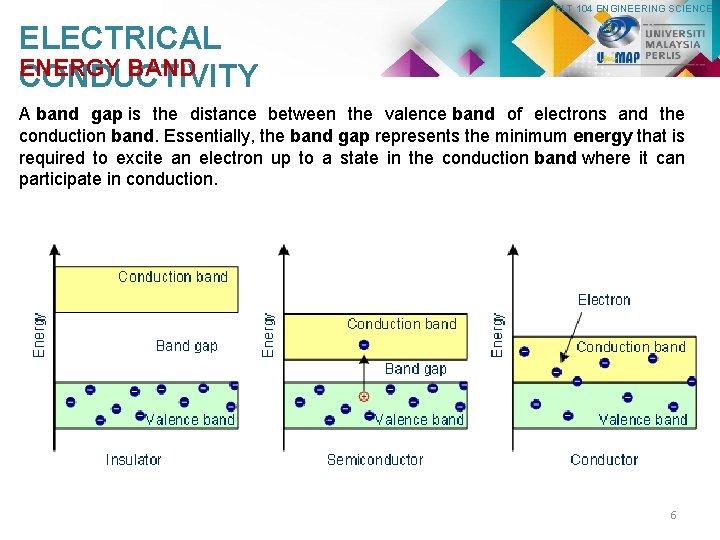
PLT 104 ENGINEERING SCIENCE ELECTRICAL ENERGY BAND CONDUCTIVITY A band gap is the distance between the valence band of electrons and the conduction band. Essentially, the band gap represents the minimum energy that is required to excite an electron up to a state in the conduction band where it can participate in conduction. 6
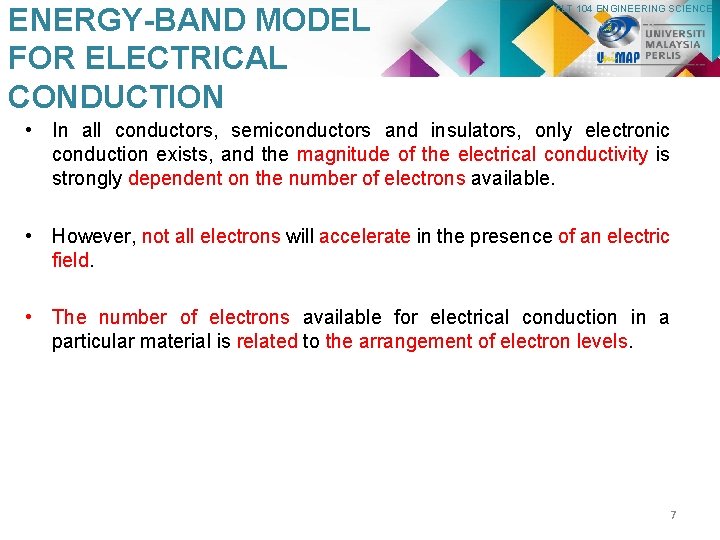
ENERGY-BAND MODEL FOR ELECTRICAL CONDUCTION PLT 104 ENGINEERING SCIENCE • In all conductors, semiconductors and insulators, only electronic conduction exists, and the magnitude of the electrical conductivity is strongly dependent on the number of electrons available. • However, not all electrons will accelerate in the presence of an electric field. • The number of electrons available for electrical conduction in a particular material is related to the arrangement of electron levels. 7
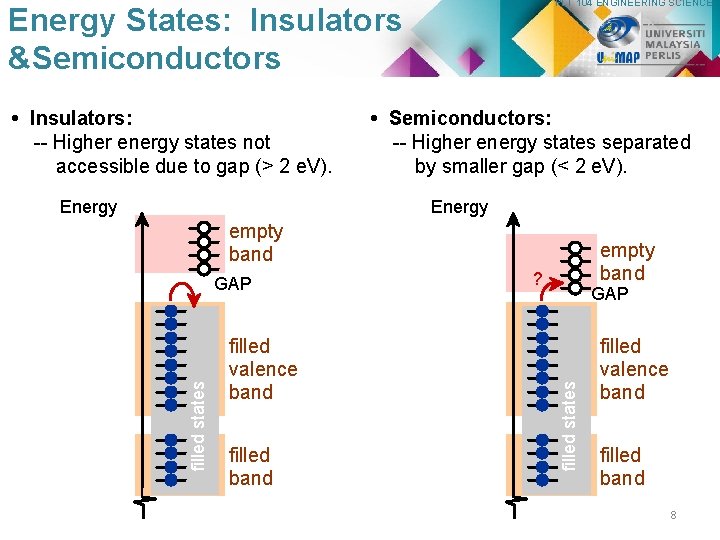
PLT 104 ENGINEERING SCIENCE Energy States: Insulators &Semiconductors • Insulators: -- Higher energy states not accessible due to gap (> 2 e. V). Energy • Semiconductors: -- Higher energy states separated by smaller gap (< 2 e. V). Energy empty band filled valence band filled band ? GAP filled states GAP empty band filled valence band filled band 8
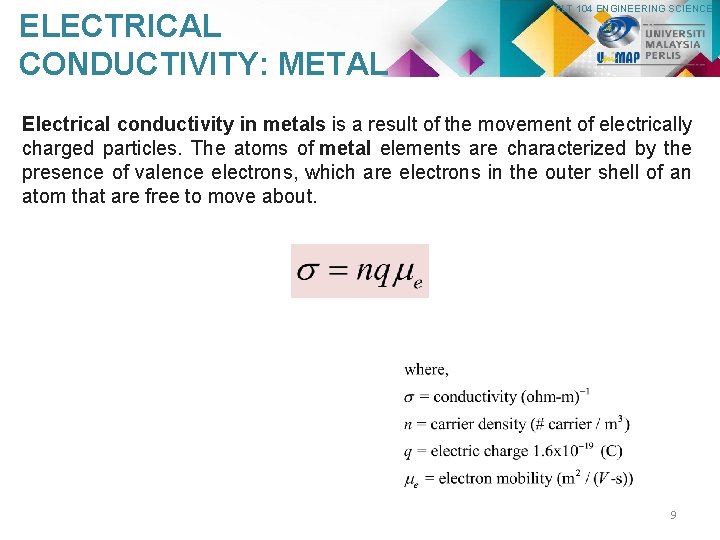
ELECTRICAL CONDUCTIVITY: METAL PLT 104 ENGINEERING SCIENCE Electrical conductivity in metals is a result of the movement of electrically charged particles. The atoms of metal elements are characterized by the presence of valence electrons, which are electrons in the outer shell of an atom that are free to move about. 9
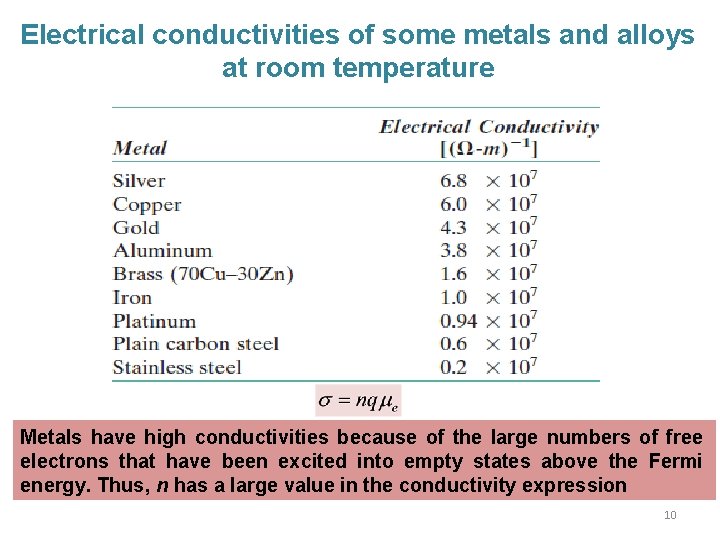
Electrical conductivities of some metals and alloys at room temperature Metals have high conductivities because of the large numbers of free electrons that have been excited into empty states above the Fermi energy. Thus, n has a large value in the conductivity expression 10
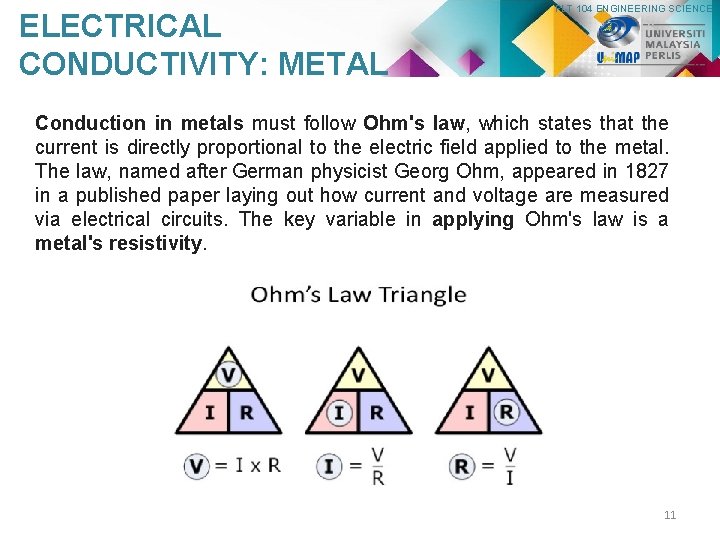
ELECTRICAL CONDUCTIVITY: METAL PLT 104 ENGINEERING SCIENCE Conduction in metals must follow Ohm's law, which states that the current is directly proportional to the electric field applied to the metal. The law, named after German physicist Georg Ohm, appeared in 1827 in a published paper laying out how current and voltage are measured via electrical circuits. The key variable in applying Ohm's law is a metal's resistivity. 11
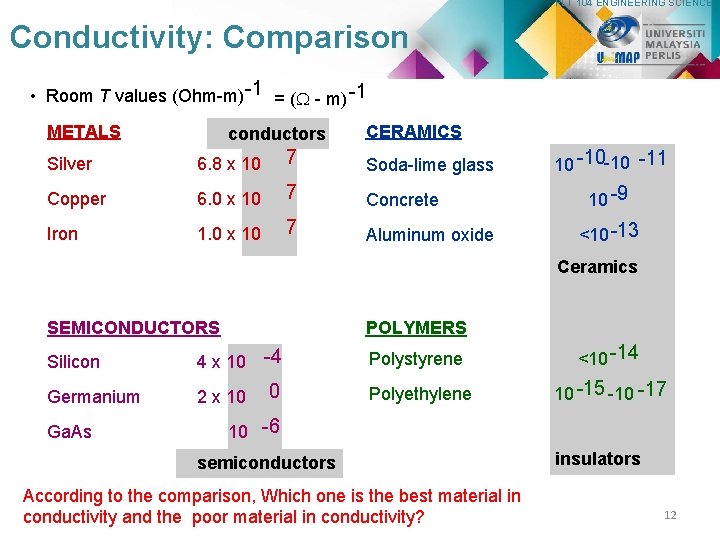
PLT 104 ENGINEERING SCIENCE Conductivity: Comparison • Room T values (Ohm-m) -1 = ( - m) -1 METALS conductors CERAMICS Silver 6. 8 x 10 7 Soda-lime glass Copper 6. 0 x 10 7 Concrete Iron 1. 0 x 10 7 Aluminum oxide 10 -10 -10 -11 10 -9 <10 -13 Ceramics SEMICONDUCTORS POLYMERS Silicon 4 x 10 -4 Germanium 2 x 10 Ga. As 0 Polystyrene <10 -14 Polyethylene 10 -15 -10 -17 10 -6 semiconductors According to the comparison, Which one is the best material in conductivity and the poor material in conductivity? insulators 12
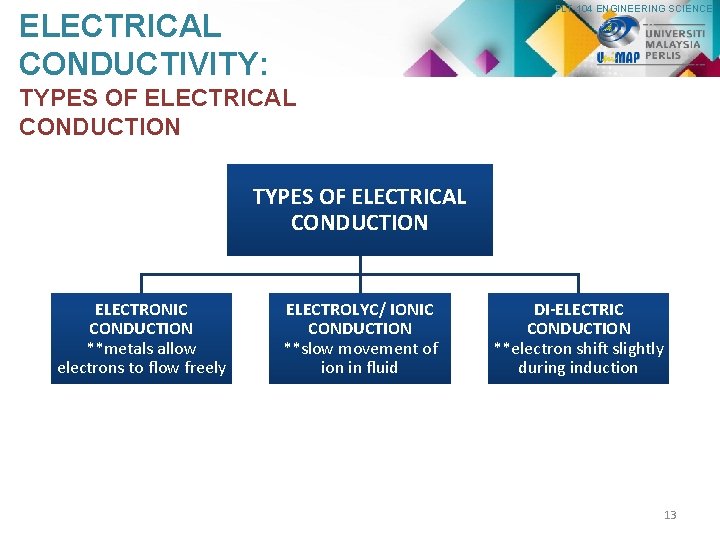
PLT 104 ENGINEERING SCIENCE ELECTRICAL CONDUCTIVITY: TYPES OF ELECTRICAL CONDUCTION ELECTRONIC CONDUCTION **metals allow electrons to flow freely ELECTROLYC/ IONIC CONDUCTION **slow movement of ion in fluid DI-ELECTRIC CONDUCTION **electron shift slightly during induction 13
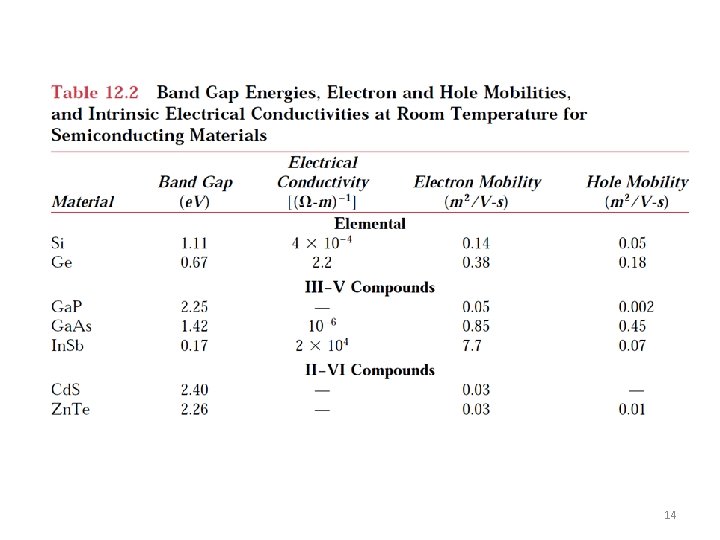
14
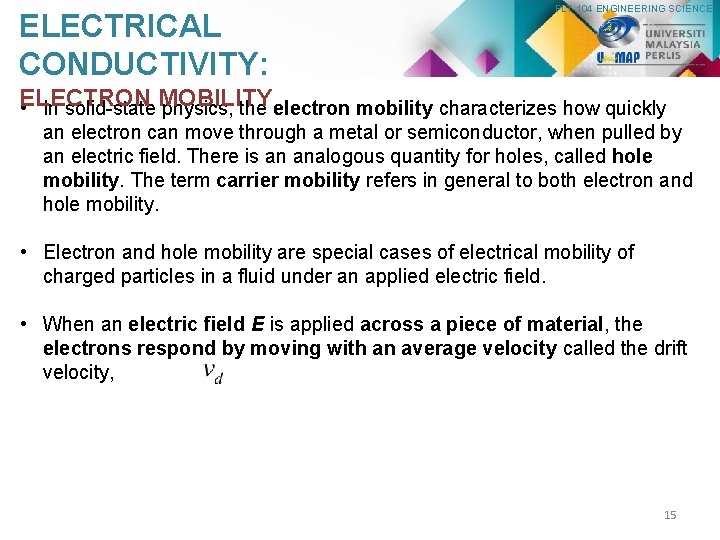
ELECTRICAL CONDUCTIVITY: PLT 104 ENGINEERING SCIENCE ELECTRON • In solid-state MOBILITY physics, the electron mobility characterizes how quickly an electron can move through a metal or semiconductor, when pulled by an electric field. There is an analogous quantity for holes, called hole mobility. The term carrier mobility refers in general to both electron and hole mobility. • Electron and hole mobility are special cases of electrical mobility of charged particles in a fluid under an applied electric field. • When an electric field E is applied across a piece of material, the electrons respond by moving with an average velocity called the drift velocity, 15
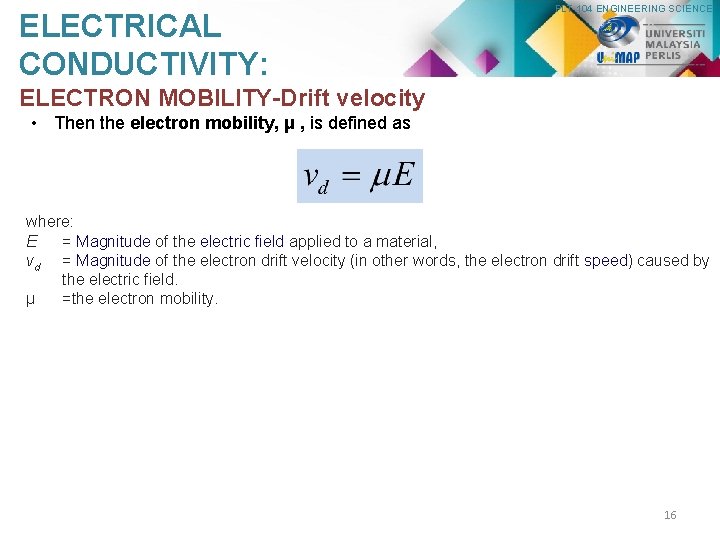
ELECTRICAL CONDUCTIVITY: PLT 104 ENGINEERING SCIENCE ELECTRON MOBILITY-Drift velocity • Then the electron mobility, μ , is defined as where: E = Magnitude of the electric field applied to a material, vd = Magnitude of the electron drift velocity (in other words, the electron drift speed) caused by the electric field. µ =the electron mobility. 16
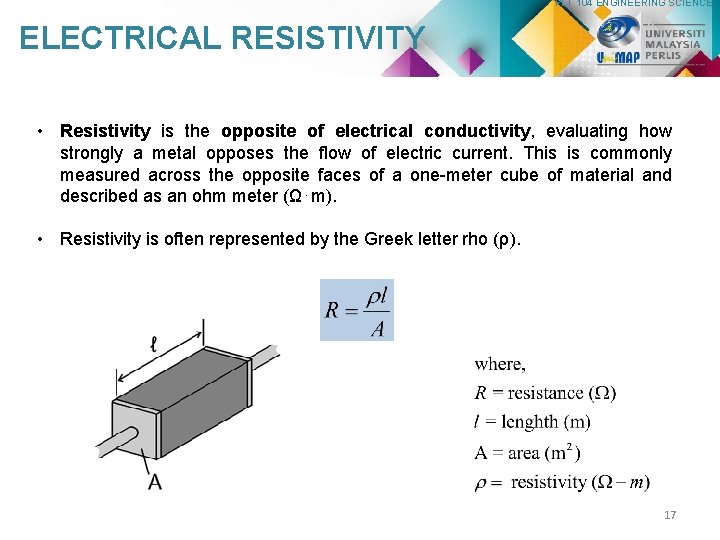
PLT 104 ENGINEERING SCIENCE ELECTRICAL RESISTIVITY • Resistivity is the opposite of electrical conductivity, evaluating how strongly a metal opposes the flow of electric current. This is commonly measured across the opposite faces of a one-meter cube of material and described as an ohm meter (Ω⋅m). • Resistivity is often represented by the Greek letter rho (ρ). 17
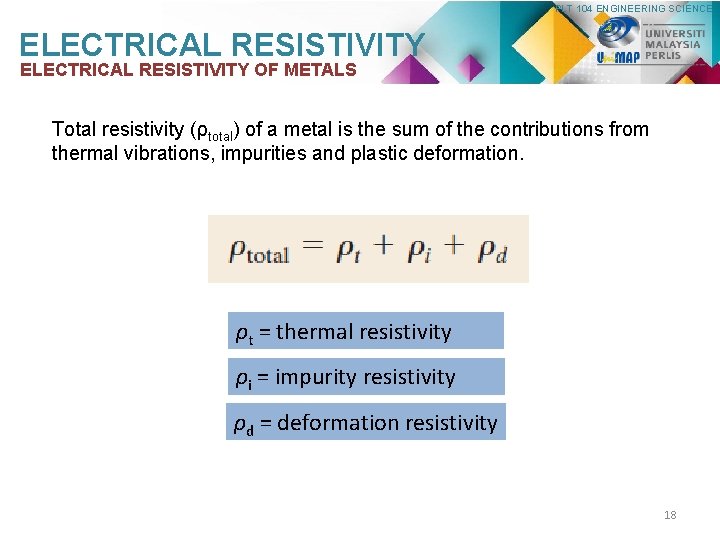
PLT 104 ENGINEERING SCIENCE ELECTRICAL RESISTIVITY OF METALS Total resistivity (ρtotal) of a metal is the sum of the contributions from thermal vibrations, impurities and plastic deformation. ρt = thermal resistivity ρi = impurity resistivity ρd = deformation resistivity 18
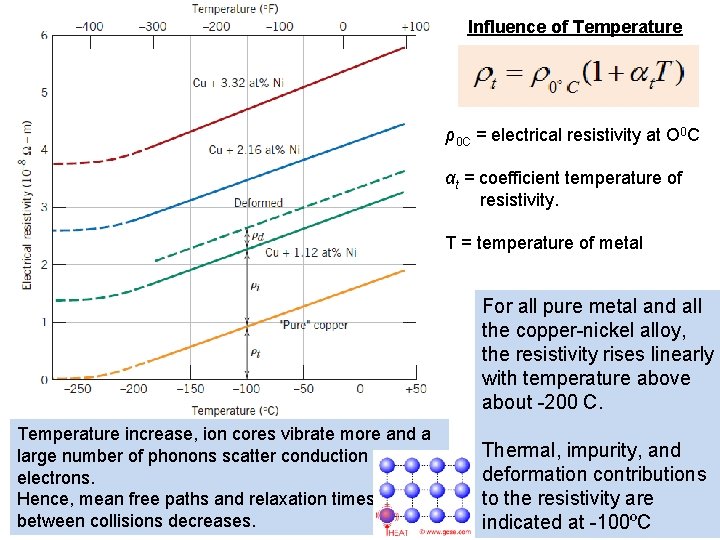
Influence of Temperature ρ0 C = electrical resistivity at O 0 C αt = coefficient temperature of resistivity. T = temperature of metal For all pure metal and all the copper-nickel alloy, the resistivity rises linearly with temperature above about -200 C. Temperature increase, ion cores vibrate more and a large number of phonons scatter conduction electrons. Hence, mean free paths and relaxation times between collisions decreases. Thermal, impurity, and deformation contributions to the resistivity are 19 indicated at -100ºC
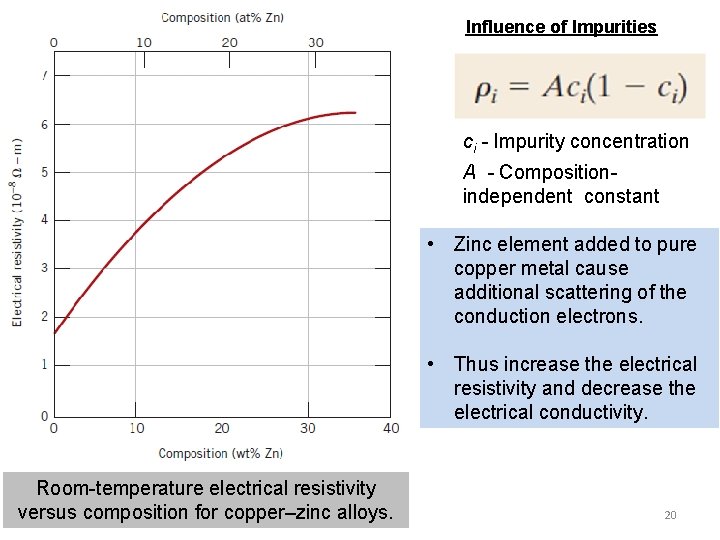
Influence of Impurities ci - Impurity concentration A - Compositionindependent constant • Zinc element added to pure copper metal cause additional scattering of the conduction electrons. • Thus increase the electrical resistivity and decrease the electrical conductivity. Room-temperature electrical resistivity versus composition for copper–zinc alloys. 20
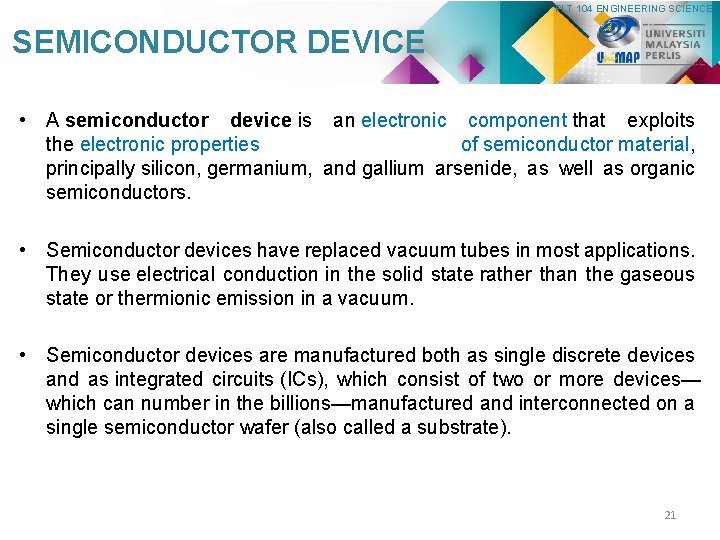
PLT 104 ENGINEERING SCIENCE SEMICONDUCTOR DEVICE • A semiconductor device is an electronic component that exploits the electronic properties of semiconductor material, principally silicon, germanium, and gallium arsenide, as well as organic semiconductors. • Semiconductor devices have replaced vacuum tubes in most applications. They use electrical conduction in the solid state rather than the gaseous state or thermionic emission in a vacuum. • Semiconductor devices are manufactured both as single discrete devices and as integrated circuits (ICs), which consist of two or more devices— which can number in the billions—manufactured and interconnected on a single semiconductor wafer (also called a substrate). 21
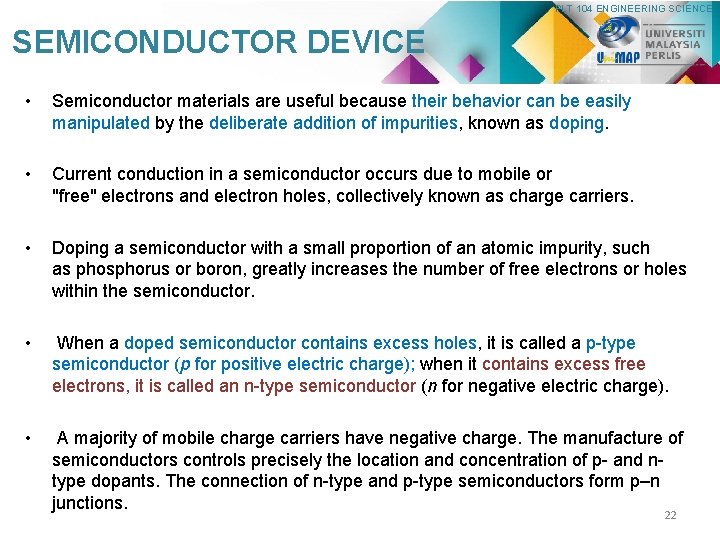
PLT 104 ENGINEERING SCIENCE SEMICONDUCTOR DEVICE • Semiconductor materials are useful because their behavior can be easily manipulated by the deliberate addition of impurities, known as doping. • Current conduction in a semiconductor occurs due to mobile or "free" electrons and electron holes, collectively known as charge carriers. • Doping a semiconductor with a small proportion of an atomic impurity, such as phosphorus or boron, greatly increases the number of free electrons or holes within the semiconductor. • When a doped semiconductor contains excess holes, it is called a p-type semiconductor (p for positive electric charge); when it contains excess free electrons, it is called an n-type semiconductor (n for negative electric charge). • A majority of mobile charge carriers have negative charge. The manufacture of semiconductors controls precisely the location and concentration of p- and ntype dopants. The connection of n-type and p-type semiconductors form p–n junctions. 22
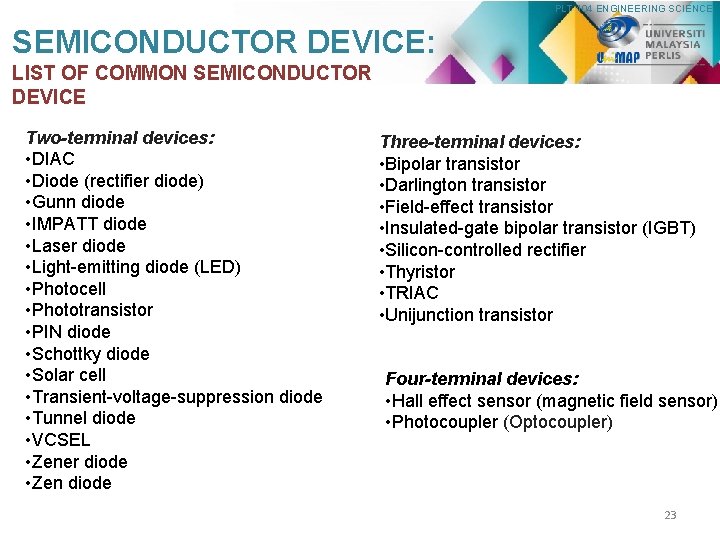
PLT 104 ENGINEERING SCIENCE SEMICONDUCTOR DEVICE: LIST OF COMMON SEMICONDUCTOR DEVICE Two-terminal devices: • DIAC • Diode (rectifier diode) • Gunn diode • IMPATT diode • Laser diode • Light-emitting diode (LED) • Photocell • Phototransistor • PIN diode • Schottky diode • Solar cell • Transient-voltage-suppression diode • Tunnel diode • VCSEL • Zener diode • Zen diode Three-terminal devices: • Bipolar transistor • Darlington transistor • Field-effect transistor • Insulated-gate bipolar transistor (IGBT) • Silicon-controlled rectifier • Thyristor • TRIAC • Unijunction transistor Four-terminal devices: • Hall effect sensor (magnetic field sensor) • Photocoupler (Optocoupler) 23
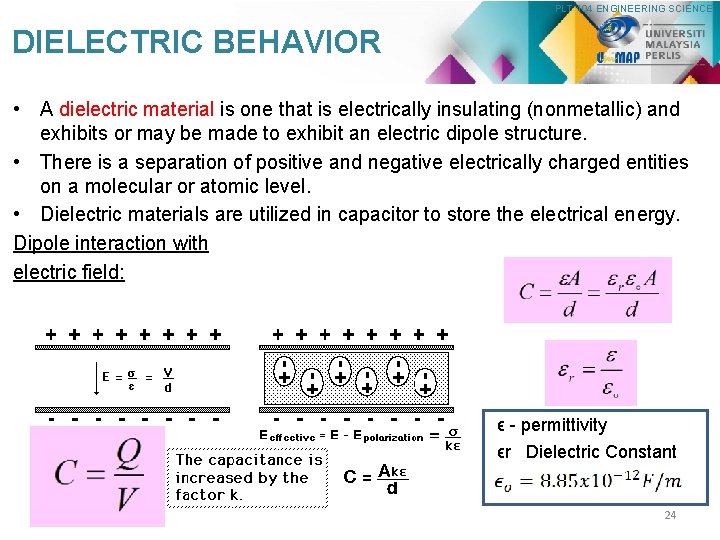
PLT 104 ENGINEERING SCIENCE DIELECTRIC BEHAVIOR • A dielectric material is one that is electrically insulating (nonmetallic) and exhibits or may be made to exhibit an electric dipole structure. • There is a separation of positive and negative electrically charged entities on a molecular or atomic level. • Dielectric materials are utilized in capacitor to store the electrical energy. Dipole interaction with electric field: ϵ - permittivity ϵr Dielectric Constant 24
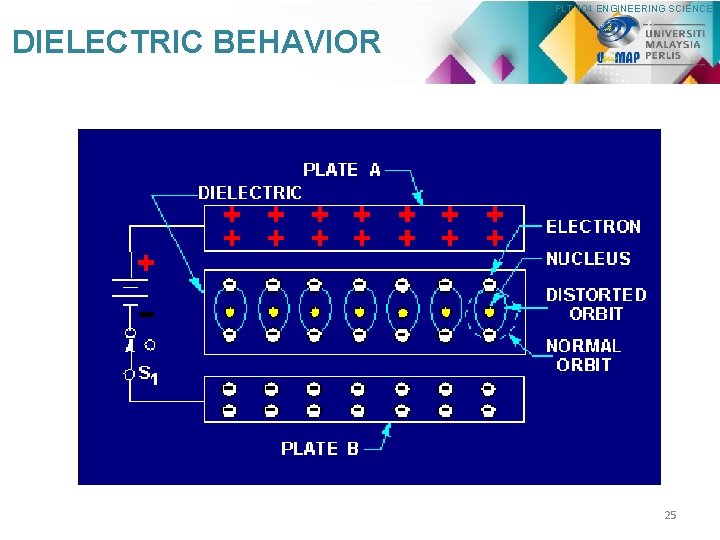
PLT 104 ENGINEERING SCIENCE DIELECTRIC BEHAVIOR 25
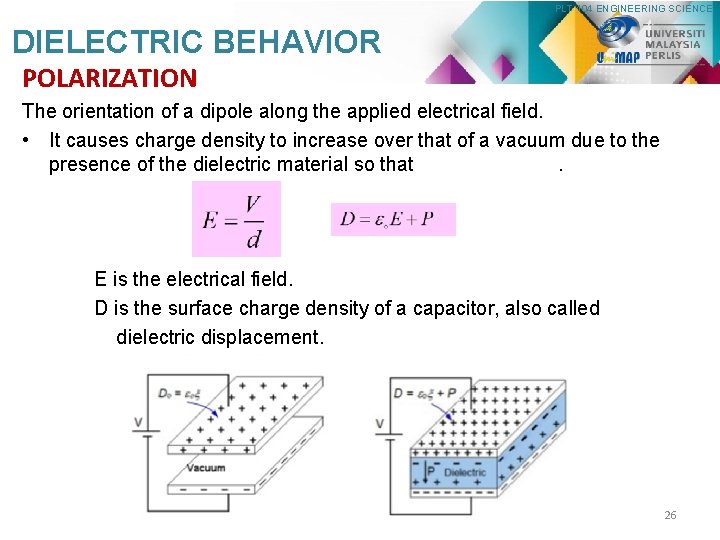
PLT 104 ENGINEERING SCIENCE DIELECTRIC BEHAVIOR POLARIZATION The orientation of a dipole along the applied electrical field. • It causes charge density to increase over that of a vacuum due to the presence of the dielectric material so that. E is the electrical field. D is the surface charge density of a capacitor, also called dielectric displacement. 26
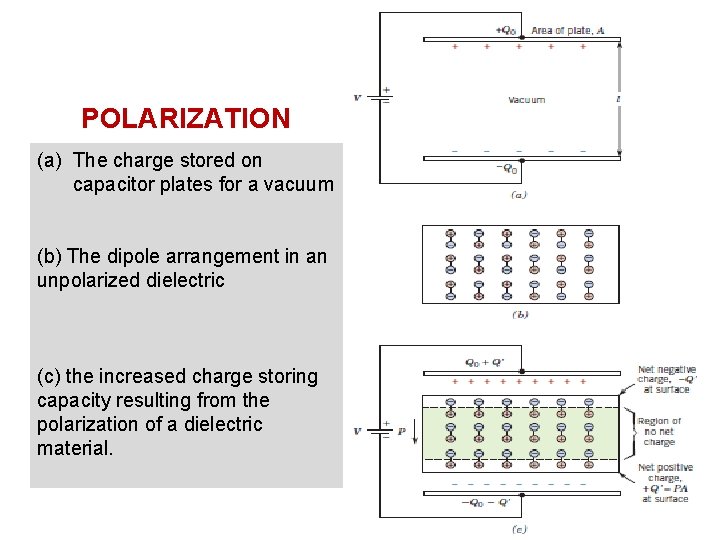
POLARIZATION (a) The charge stored on capacitor plates for a vacuum (b) The dipole arrangement in an unpolarized dielectric (c) the increased charge storing capacity resulting from the polarization of a dielectric material. 27
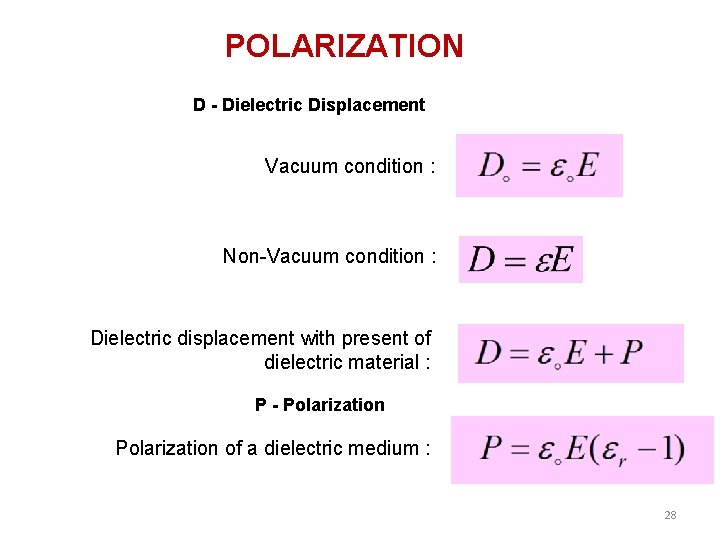
POLARIZATION D - Dielectric Displacement Vacuum condition : Non-Vacuum condition : Dielectric displacement with present of dielectric material : P - Polarization of a dielectric medium : 28
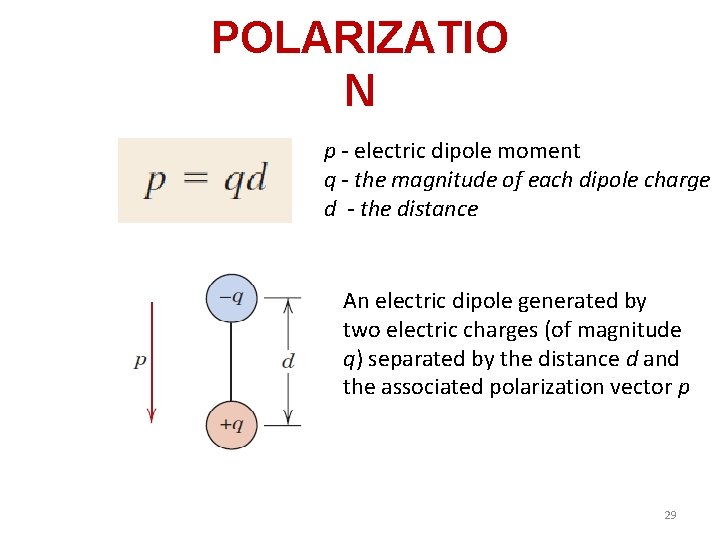
POLARIZATIO N p - electric dipole moment q - the magnitude of each dipole charge d - the distance An electric dipole generated by two electric charges (of magnitude q) separated by the distance d and the associated polarization vector p 29
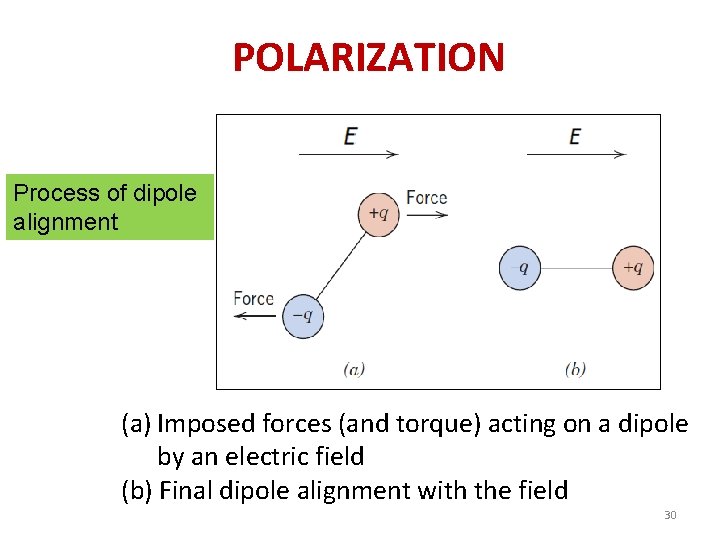
POLARIZATION Process of dipole alignment (a) Imposed forces (and torque) acting on a dipole by an electric field (b) Final dipole alignment with the field 30
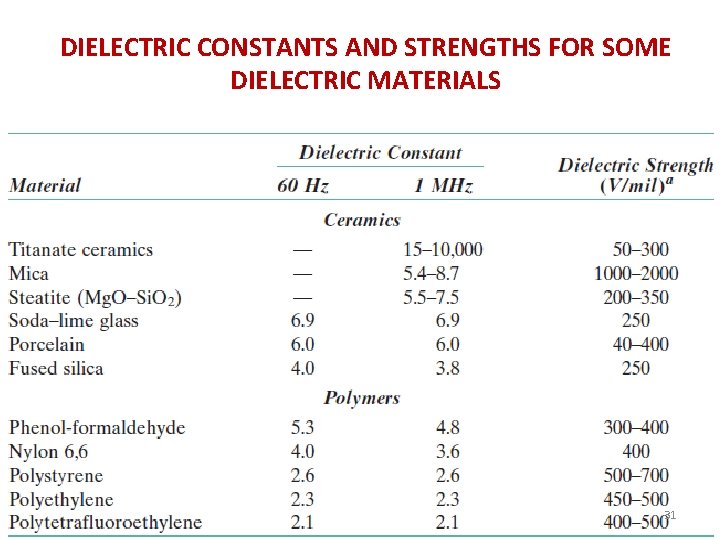
DIELECTRIC CONSTANTS AND STRENGTHS FOR SOME DIELECTRIC MATERIALS 31
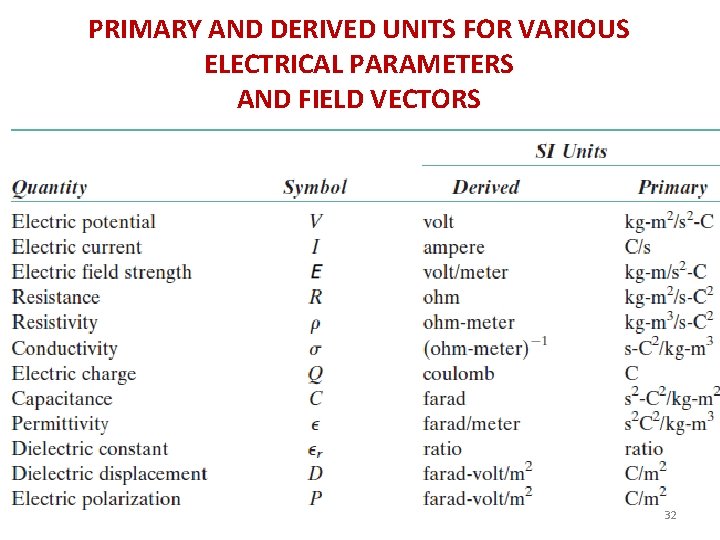
PRIMARY AND DERIVED UNITS FOR VARIOUS ELECTRICAL PARAMETERS AND FIELD VECTORS 32
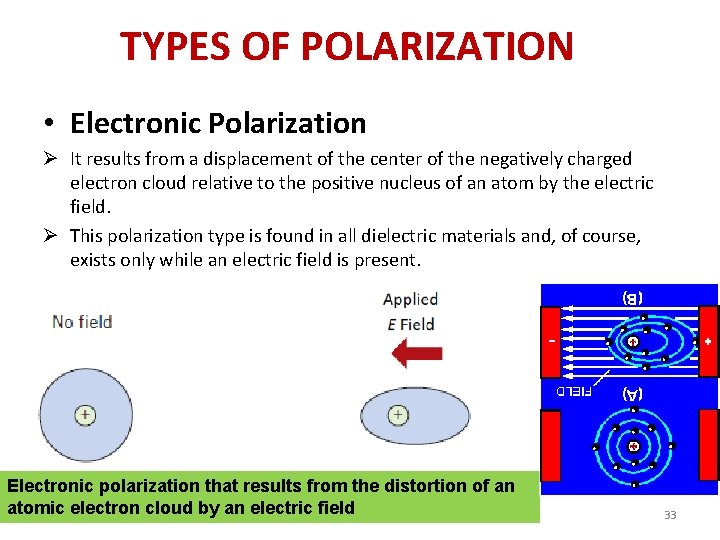
TYPES OF POLARIZATION • Electronic Polarization Ø It results from a displacement of the center of the negatively charged electron cloud relative to the positive nucleus of an atom by the electric field. Ø This polarization type is found in all dielectric materials and, of course, exists only while an electric field is present. Electronic polarization that results from the distortion of an atomic electron cloud by an electric field 33
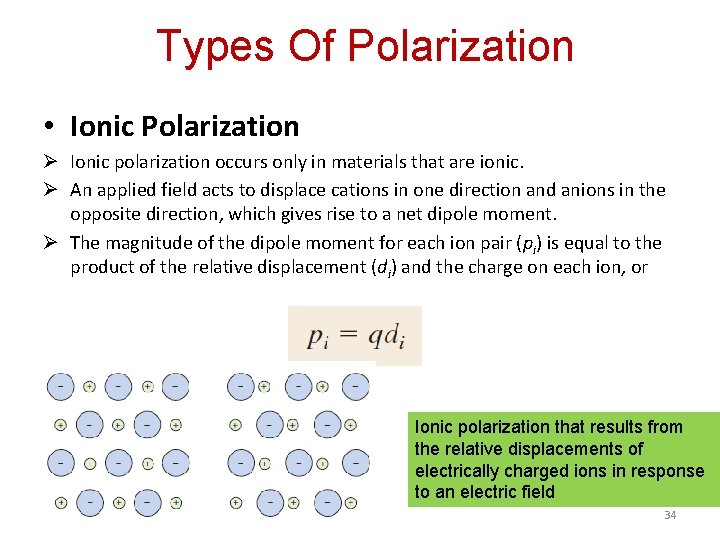
Types Of Polarization • Ionic Polarization Ø Ionic polarization occurs only in materials that are ionic. Ø An applied field acts to displace cations in one direction and anions in the opposite direction, which gives rise to a net dipole moment. Ø The magnitude of the dipole moment for each ion pair (pi) is equal to the product of the relative displacement (di) and the charge on each ion, or Ionic polarization that results from the relative displacements of electrically charged ions in response to an electric field 34
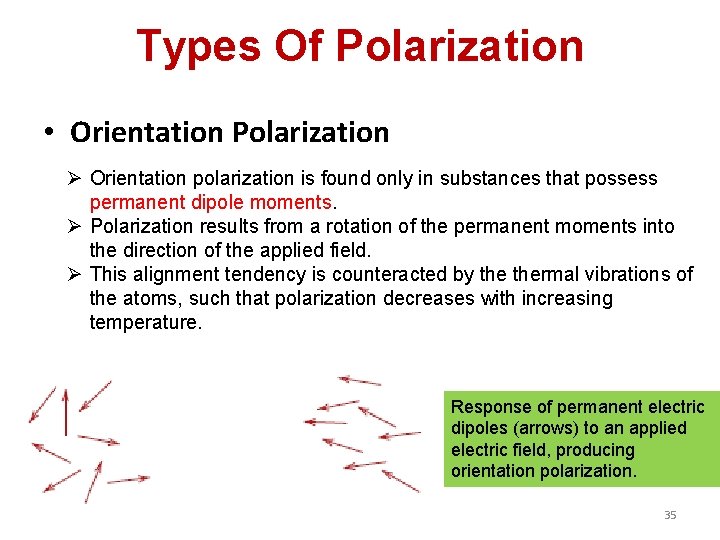
Types Of Polarization • Orientation Polarization Ø Orientation polarization is found only in substances that possess permanent dipole moments. Ø Polarization results from a rotation of the permanent moments into the direction of the applied field. Ø This alignment tendency is counteracted by thermal vibrations of the atoms, such that polarization decreases with increasing temperature. Response of permanent electric dipoles (arrows) to an applied electric field, producing orientation polarization. 35
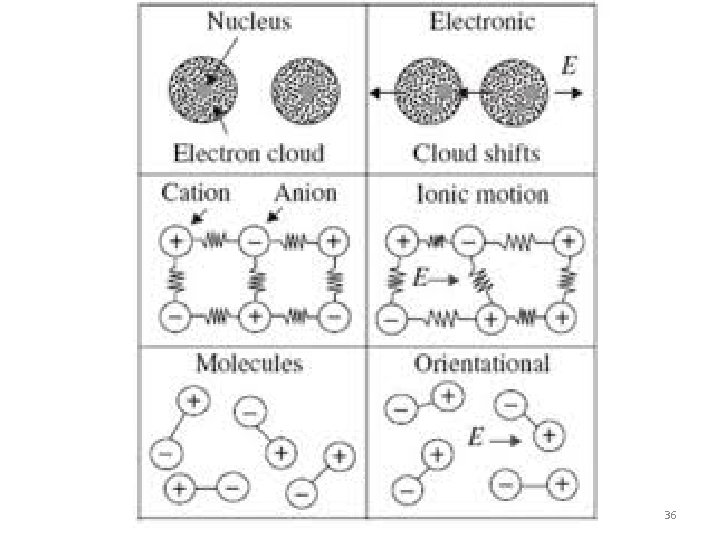
36
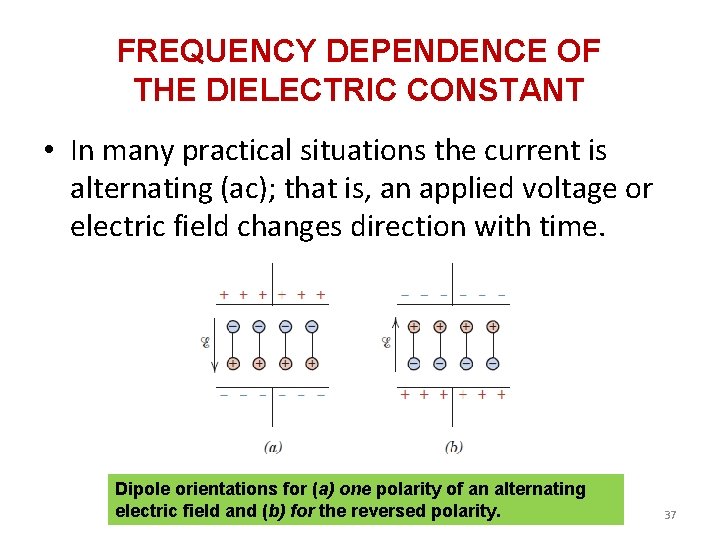
FREQUENCY DEPENDENCE OF THE DIELECTRIC CONSTANT • In many practical situations the current is alternating (ac); that is, an applied voltage or electric field changes direction with time. Dipole orientations for (a) one polarity of an alternating electric field and (b) for the reversed polarity. 37
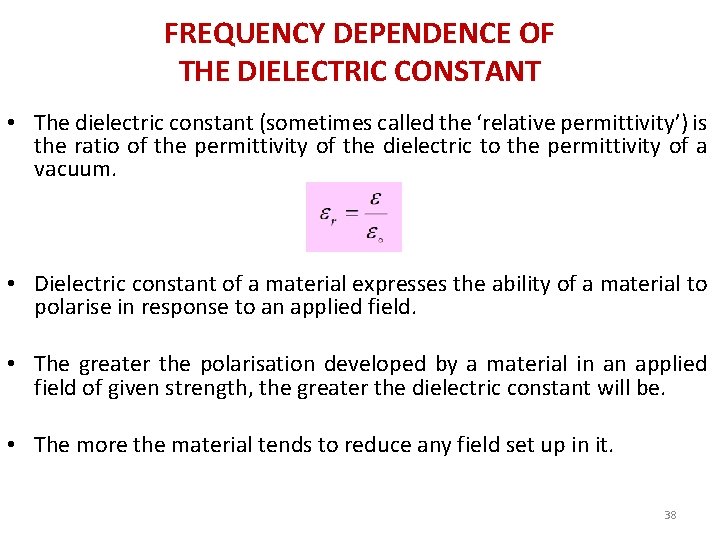
FREQUENCY DEPENDENCE OF THE DIELECTRIC CONSTANT • The dielectric constant (sometimes called the ‘relative permittivity’) is the ratio of the permittivity of the dielectric to the permittivity of a vacuum. • Dielectric constant of a material expresses the ability of a material to polarise in response to an applied field. • The greater the polarisation developed by a material in an applied field of given strength, the greater the dielectric constant will be. • The more the material tends to reduce any field set up in it. 38
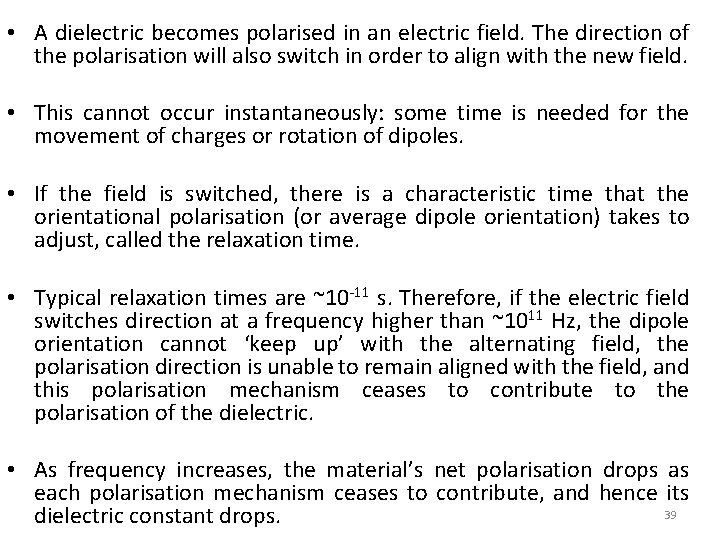
• A dielectric becomes polarised in an electric field. The direction of the polarisation will also switch in order to align with the new field. • This cannot occur instantaneously: some time is needed for the movement of charges or rotation of dipoles. • If the field is switched, there is a characteristic time that the orientational polarisation (or average dipole orientation) takes to adjust, called the relaxation time. • Typical relaxation times are ~10 -11 s. Therefore, if the electric field switches direction at a frequency higher than ~1011 Hz, the dipole orientation cannot ‘keep up’ with the alternating field, the polarisation direction is unable to remain aligned with the field, and this polarisation mechanism ceases to contribute to the polarisation of the dielectric. • As frequency increases, the material’s net polarisation drops as each polarisation mechanism ceases to contribute, and hence its 39 dielectric constant drops.
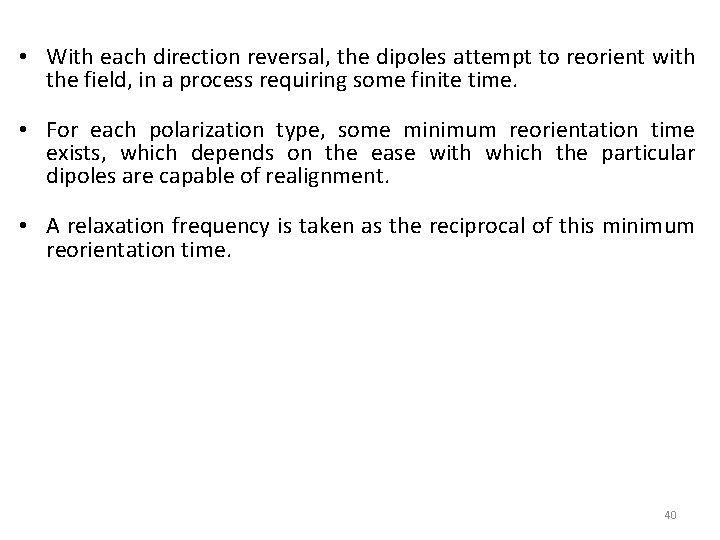
• With each direction reversal, the dipoles attempt to reorient with the field, in a process requiring some finite time. • For each polarization type, some minimum reorientation time exists, which depends on the ease with which the particular dipoles are capable of realignment. • A relaxation frequency is taken as the reciprocal of this minimum reorientation time. 40
Define electrical engineering
What is your favorite subject ?
Plt abn distribution meaning
Plt-o sysmex
Micro hydro power
Striscio di sangue
Plt scheme
Fuzzification
Plt electronics
Plt 106
Medium voltage range
Fcps my plt
Plt in cbc
Recognition of prior learning uts
คืออะไร
Plt morphology
Import numpy as np import matplotlib.pyplot as plt
Anu gdlp
Al culture
Plt.triplot
Billy b and plt
Plt skills
Plt skills
Kewenangan plt dan plh dalam aspek keuangan
Azitromisin
Protective colloid
Electrical properties of nerve ppt
Electrical properties of matter
Electrical properties of matter
Electrical properties of nerve ppt
Force engineering science
Basic of engineering
Basic engineering science
Elektro vektor
George washington university electrical engineering
Tel aviv university electrical engineering
Northwestern electrical engineering
Klipsch school of electrical and computer engineering
Electrical engineering department
Electrical engineering umd
Electrical estimating and costing