MINOS Cosmic Ray Physics Atmospheric Meson Production Ratios
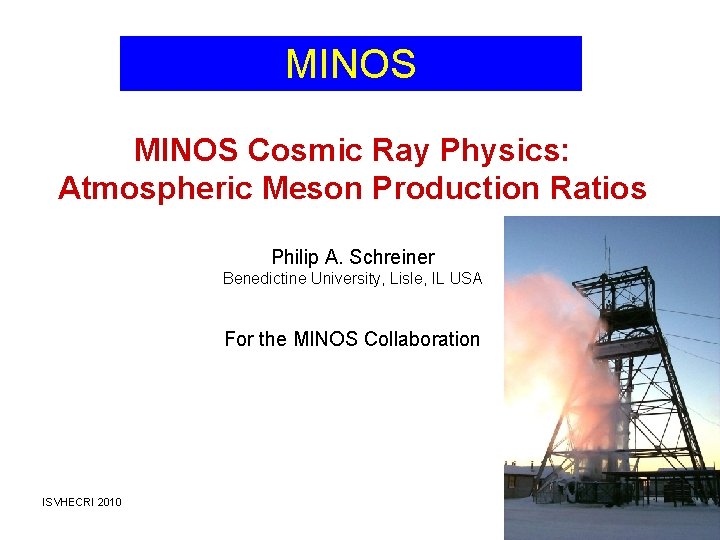
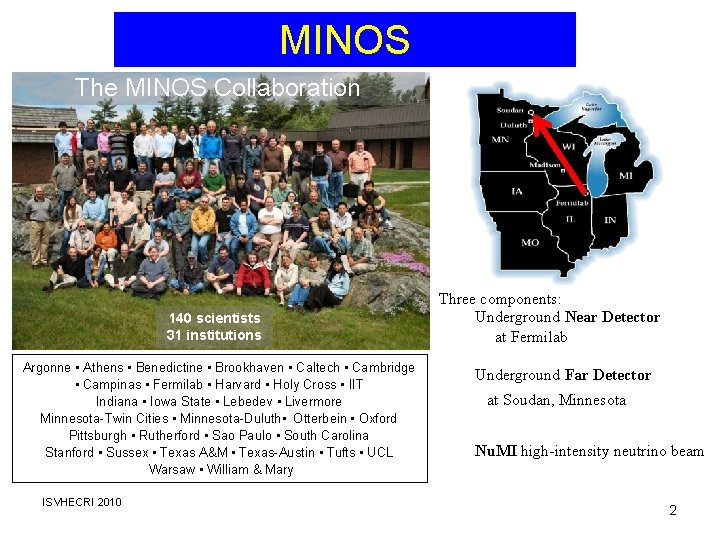
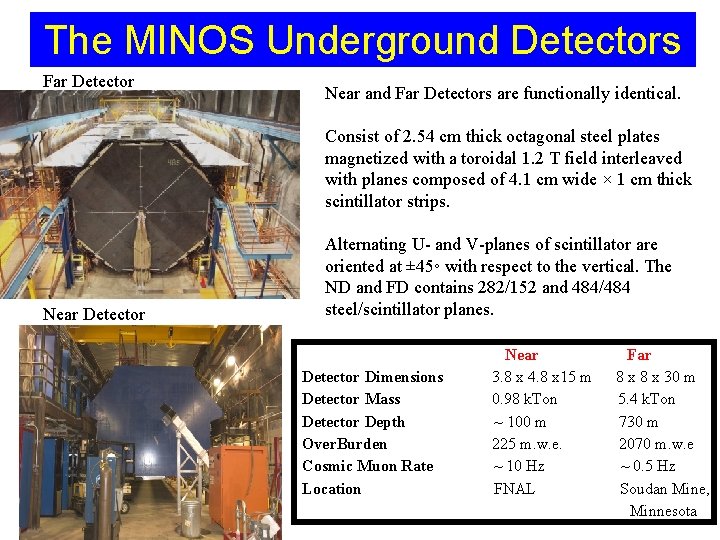
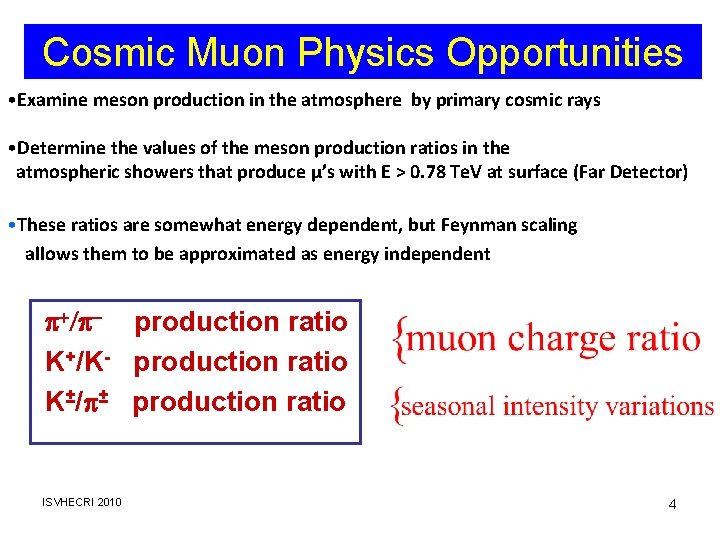
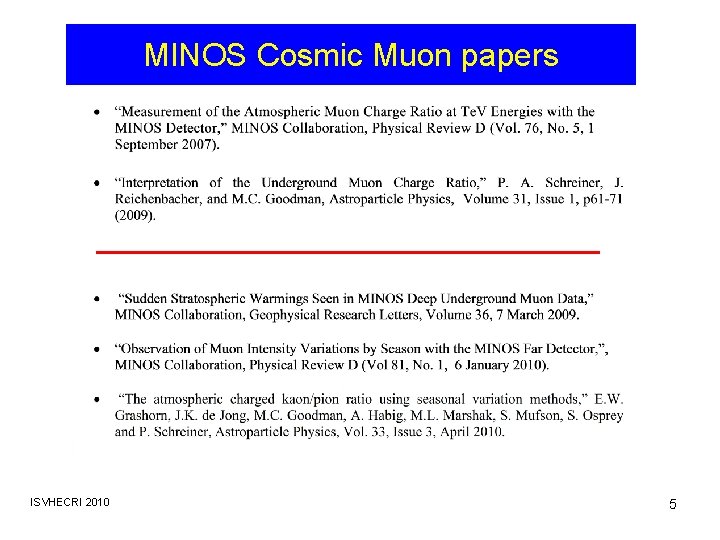
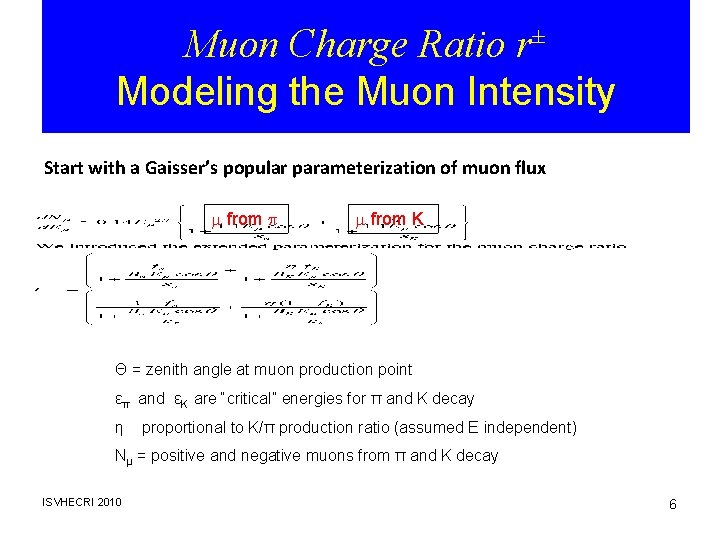
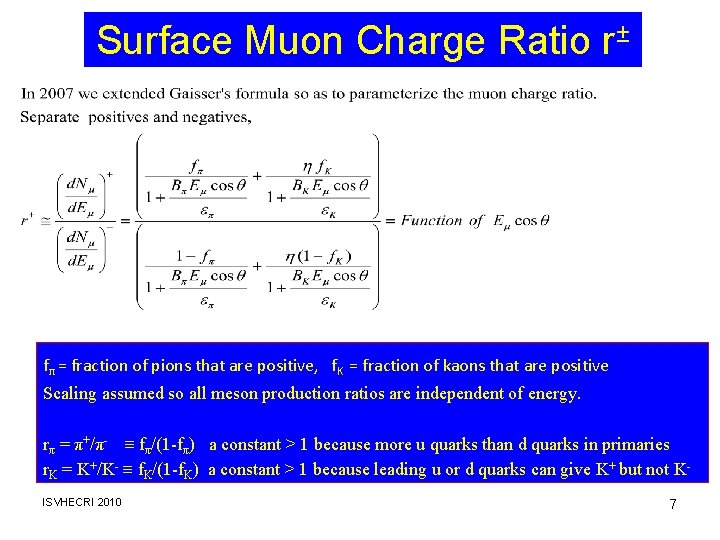
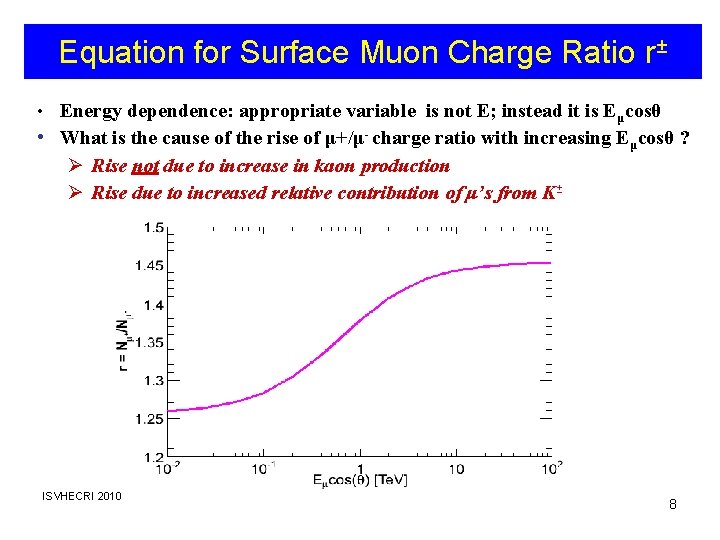
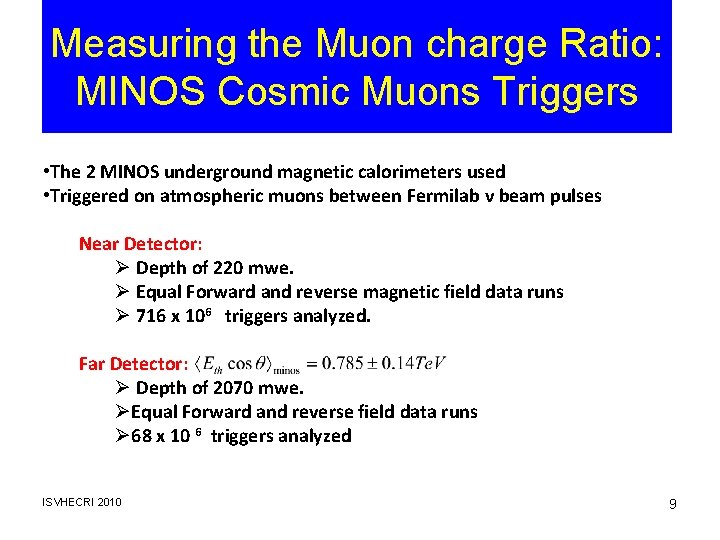
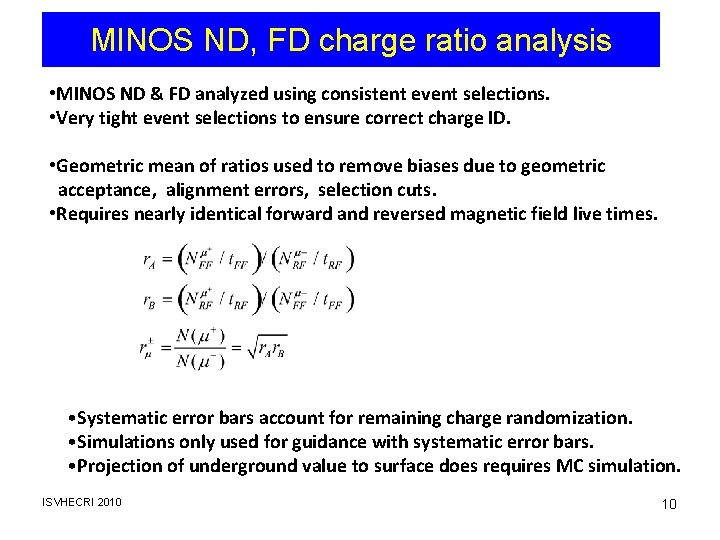
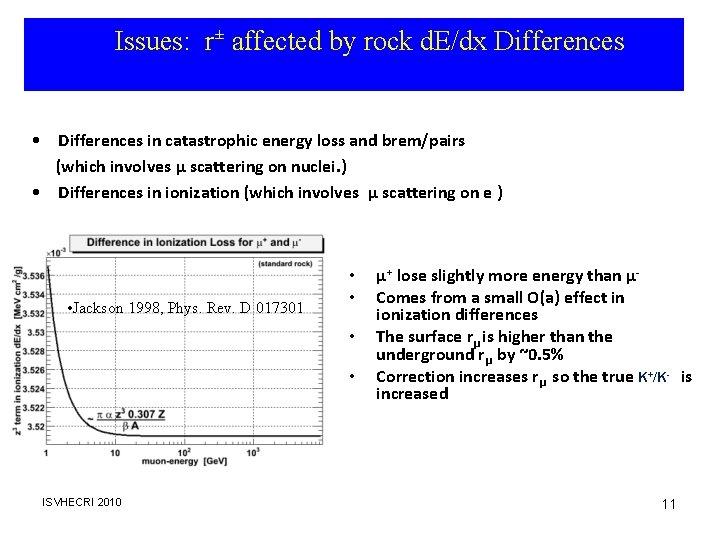
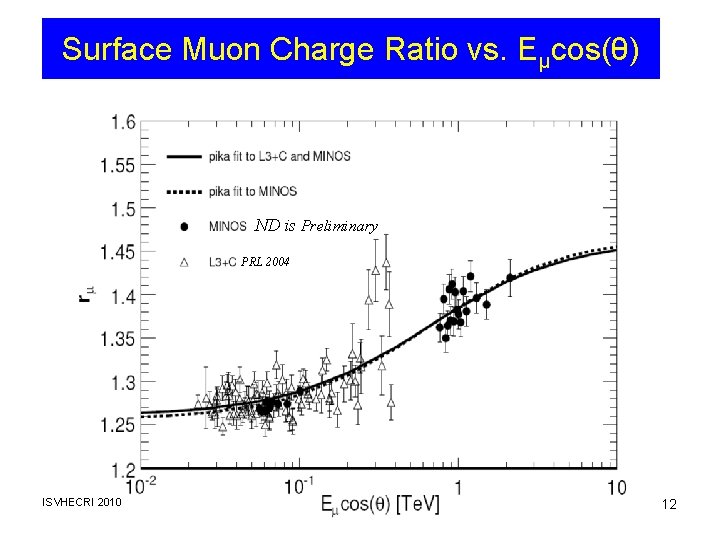
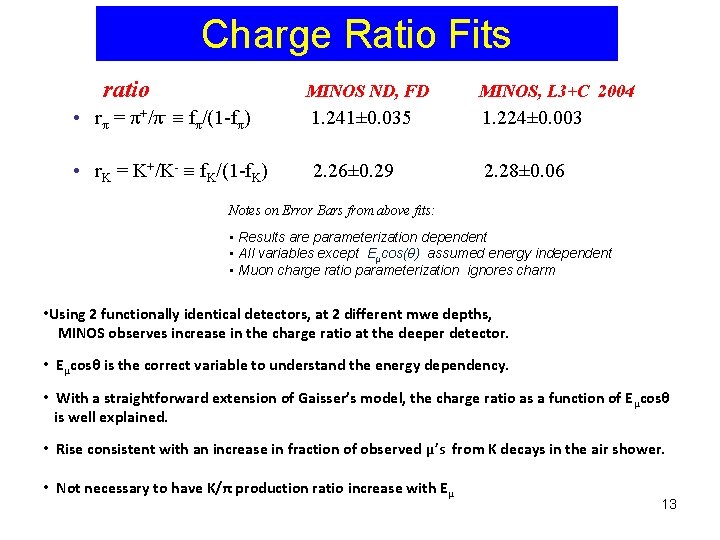
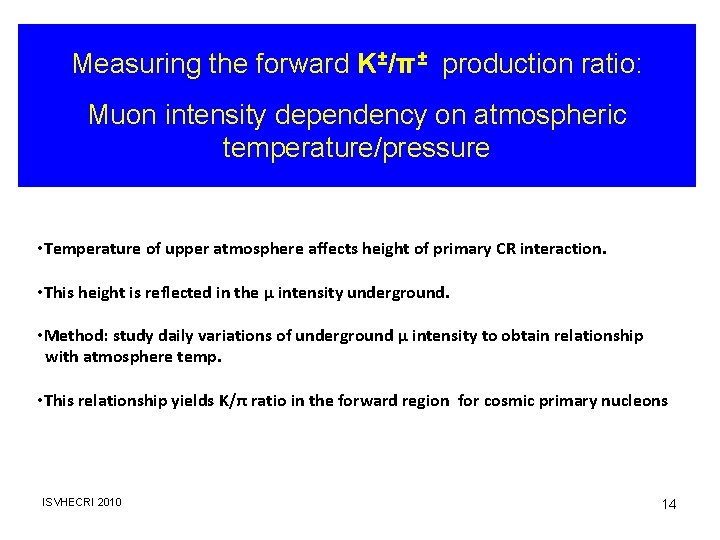
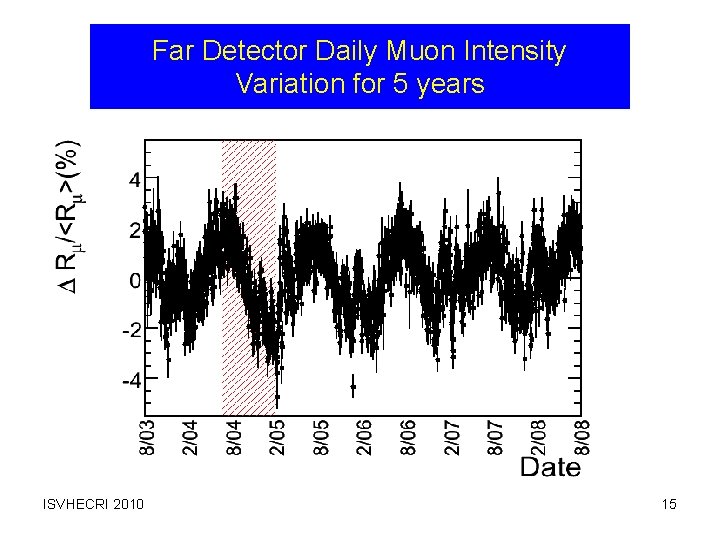
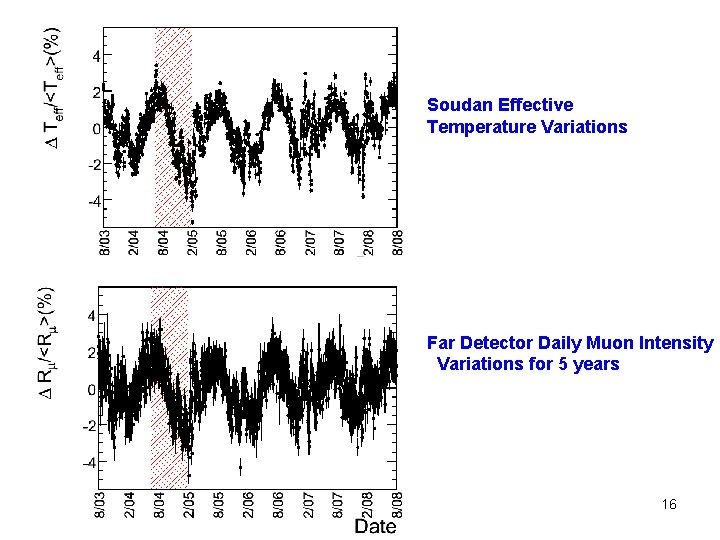
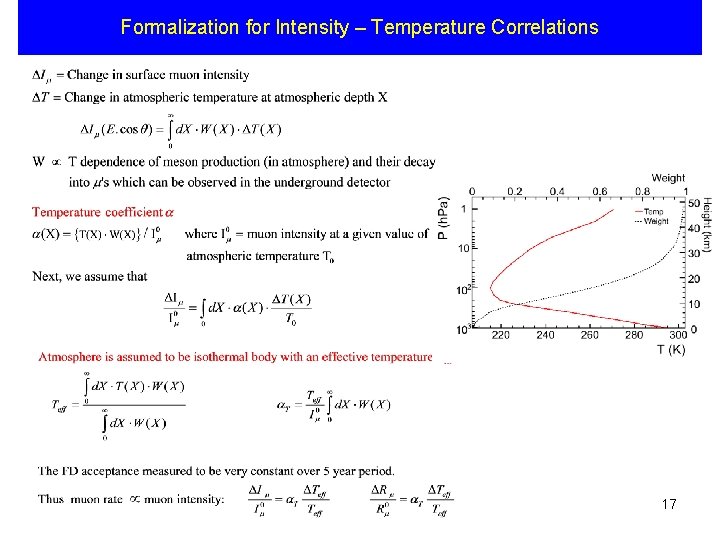
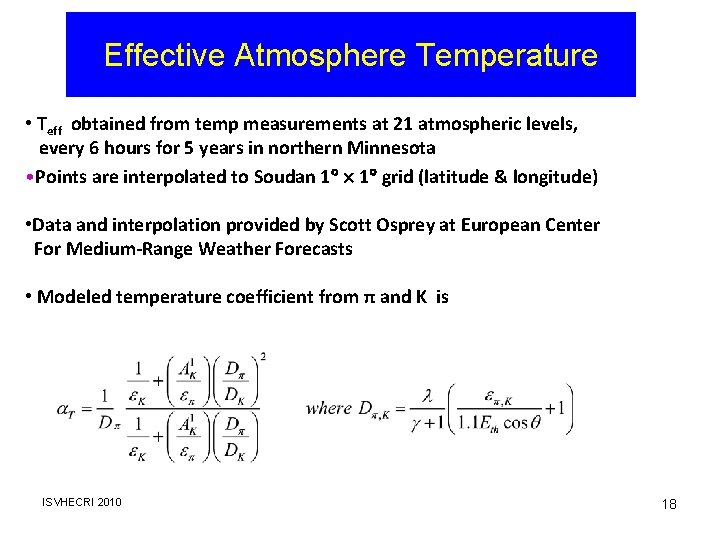
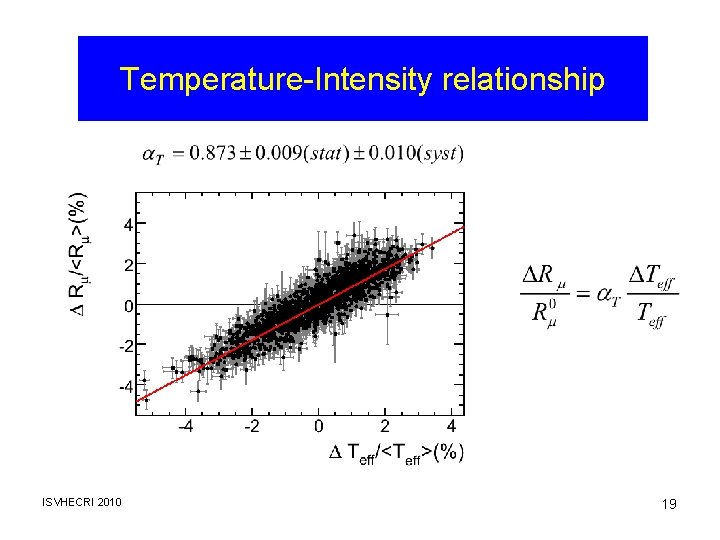
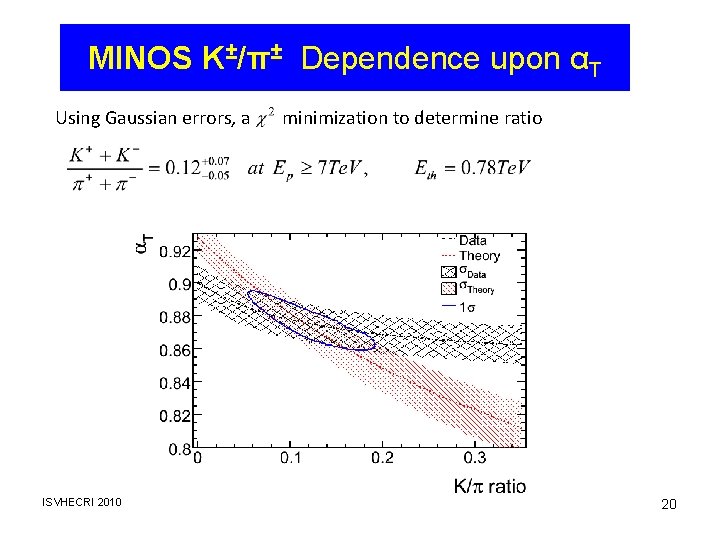
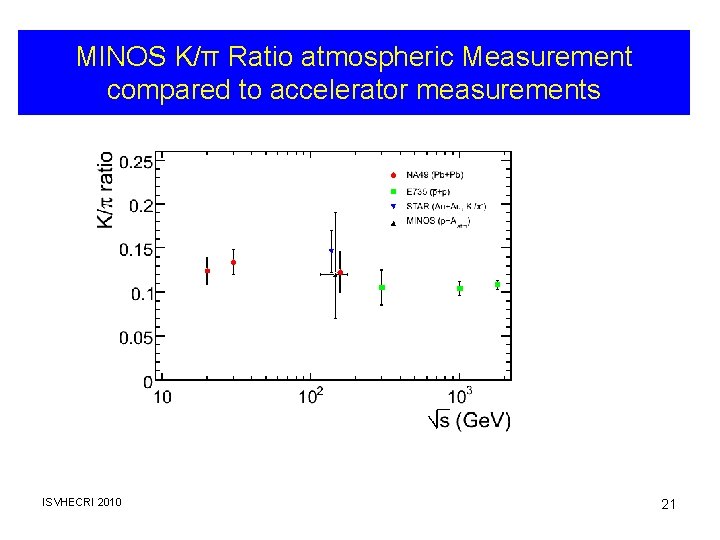
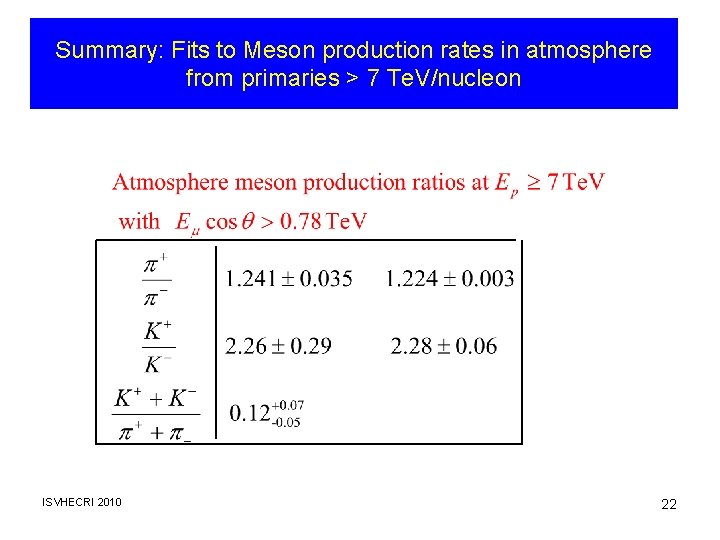
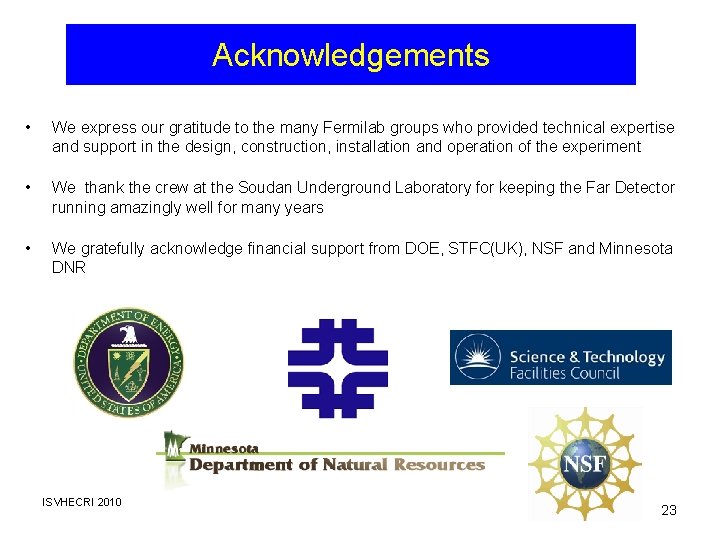
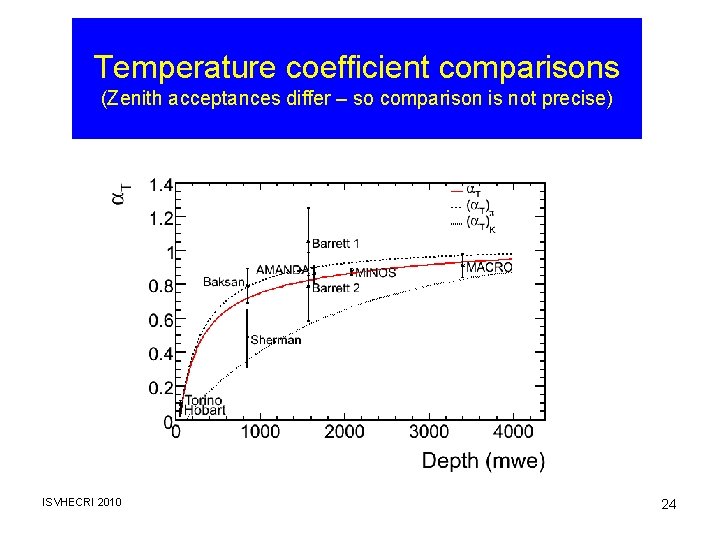
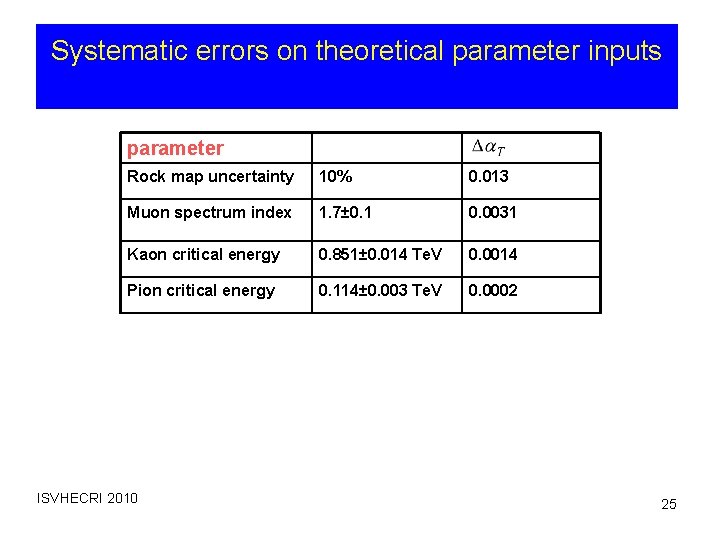
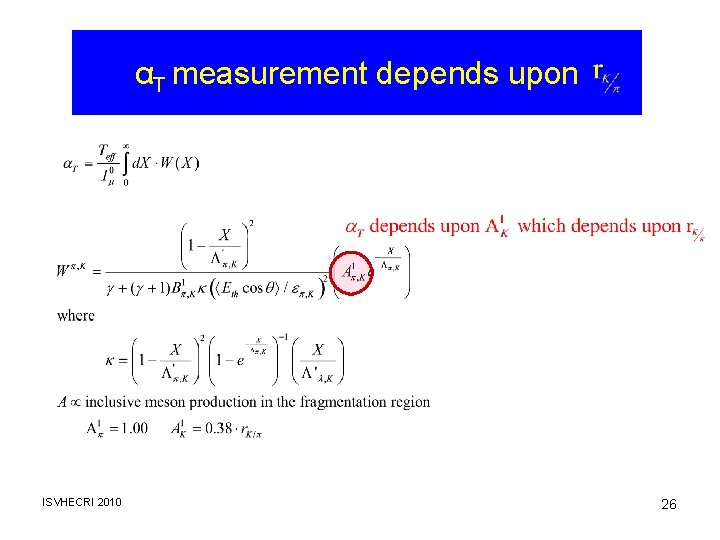
- Slides: 26
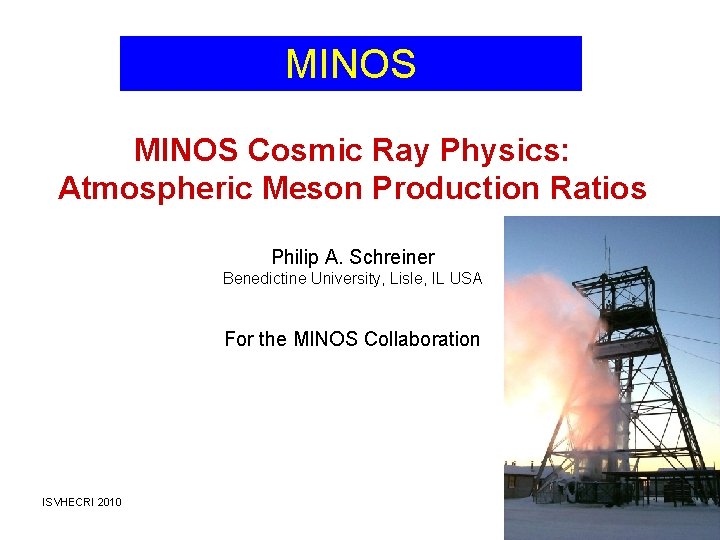
MINOS Cosmic Ray Physics: Atmospheric Meson Production Ratios Philip A. Schreiner Benedictine University, Lisle, IL USA For the MINOS Collaboration ISVHECRI 2010 1
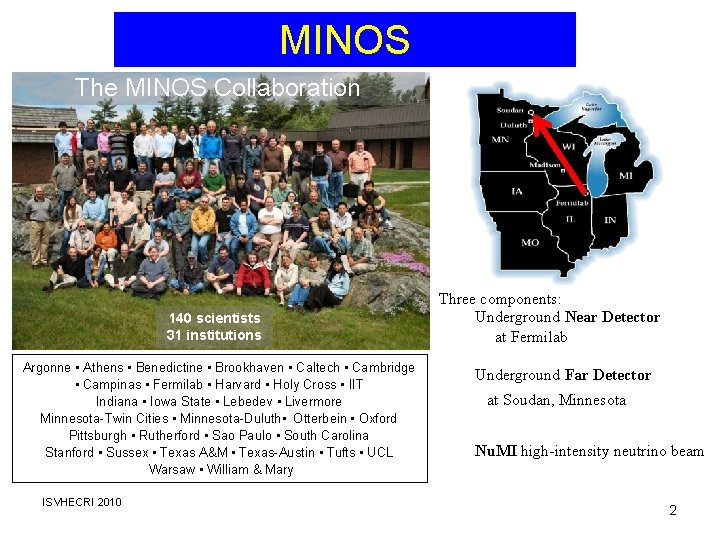
MINOS The MINOS Collaboration 140 scientists 31 institutions Argonne • Athens • Benedictine • Brookhaven • Caltech • Cambridge • Campinas • Fermilab • Harvard • Holy Cross • IIT Indiana • Iowa State • Lebedev • Livermore Minnesota-Twin Cities • Minnesota-Duluth • Otterbein • Oxford Pittsburgh • Rutherford • Sao Paulo • South Carolina Stanford • Sussex • Texas A&M • Texas-Austin • Tufts • UCL Warsaw • William & Mary ISVHECRI 2010 Three components: Underground Near Detector at Fermilab Underground Far Detector at Soudan, Minnesota Nu. MI high-intensity neutrino beam 2
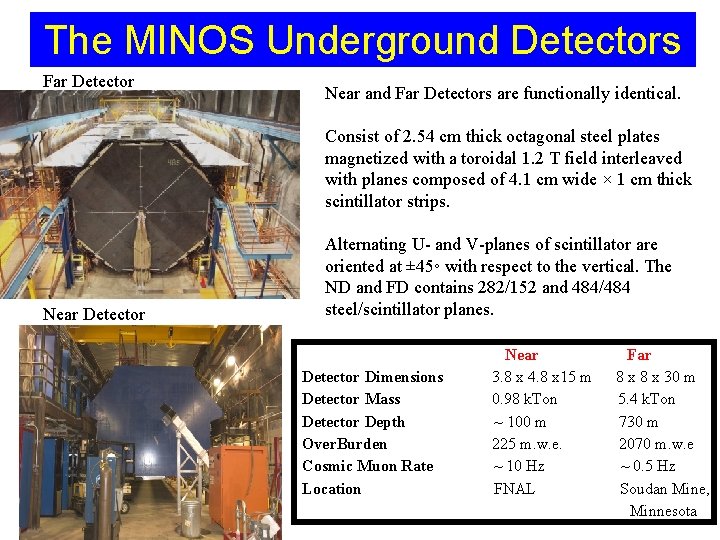
The MINOS Underground Detectors Far Detector Near and Far Detectors are functionally identical. Consist of 2. 54 cm thick octagonal steel plates magnetized with a toroidal 1. 2 T field interleaved with planes composed of 4. 1 cm wide × 1 cm thick scintillator strips. Near Detector ISVHECRI 2010 Alternating U- and V-planes of scintillator are oriented at ± 45◦ with respect to the vertical. The ND and FD contains 282/152 and 484/484 steel/scintillator planes. Detector Dimensions Detector Mass Detector Depth Over. Burden Cosmic Muon Rate Location Near 3. 8 x 4. 8 x 15 m 0. 98 k. Ton ~ 100 m 225 m. w. e. ~ 10 Hz FNAL Far 8 x 30 m 5. 4 k. Ton 730 m 2070 m. w. e ~ 0. 5 Hz Soudan Mine, Minnesota
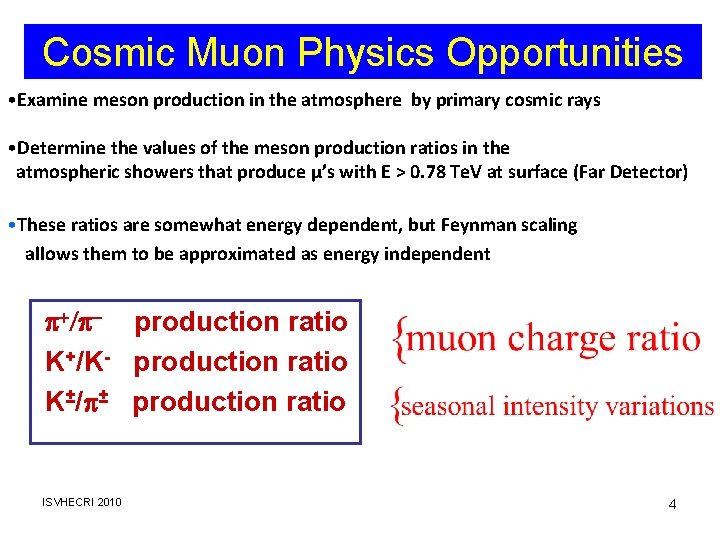
Cosmic Muon Physics Opportunities • Examine meson production in the atmosphere by primary cosmic rays • Determine the values of the meson production ratios in the atmospheric showers that produce μ’s with E > 0. 78 Te. V at surface (Far Detector) • These ratios are somewhat energy dependent, but Feynman scaling allows them to be approximated as energy independent p+/p- production ratio K+/K- production ratio K±/p± production ratio ISVHECRI 2010 4
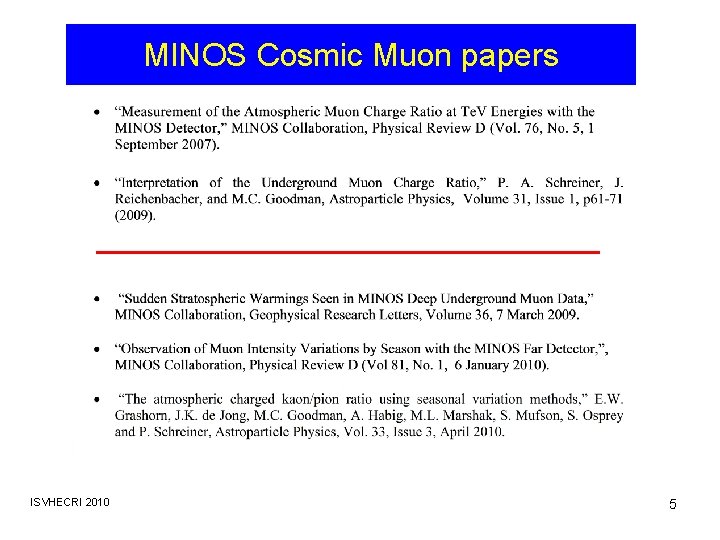
MINOS Cosmic Muon papers ISVHECRI 2010 5
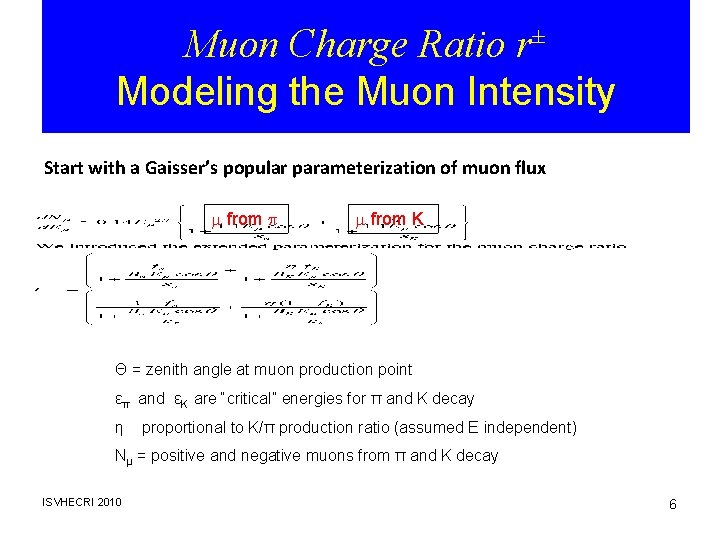
Muon Charge Ratio r± Modeling the Muon Intensity Start with a Gaisser’s popular parameterization of muon flux m from p m from K Θ = zenith angle at muon production point επ and εK are “critical” energies for π and K decay η proportional to K/π production ratio (assumed E independent) Nμ = positive and negative muons from π and K decay ISVHECRI 2010 6
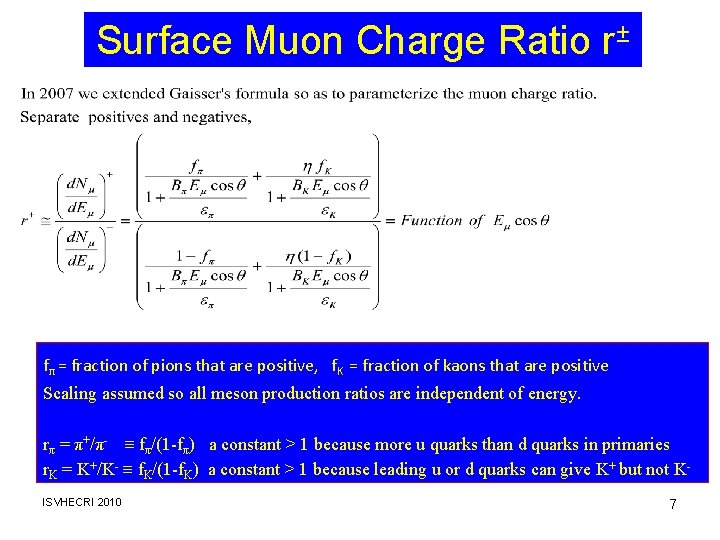
Surface Muon Charge Ratio r± fπ = fraction of pions that are positive, f. K = fraction of kaons that are positive Scaling assumed so all meson production ratios are independent of energy. rπ = π+/π- fπ/(1 -fπ) a constant > 1 because more u quarks than d quarks in primaries r. K = K+/K- f. K/(1 -f. K) a constant > 1 because leading u or d quarks can give K+ but not KISVHECRI 2010 7
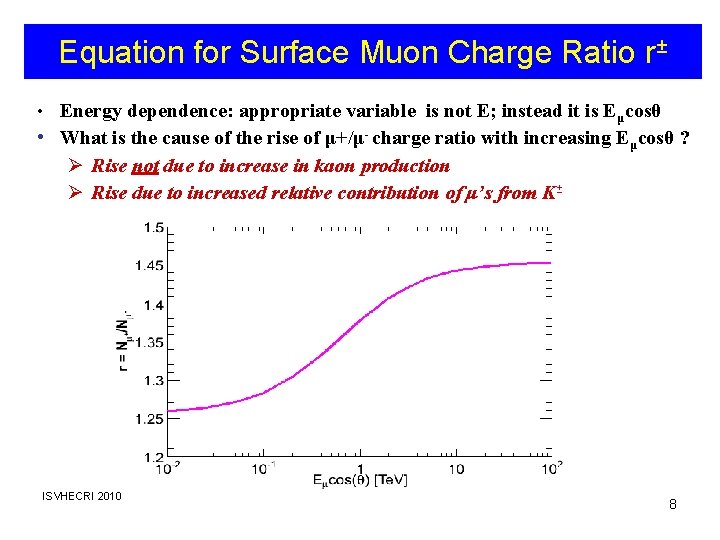
Equation for Surface Muon Charge Ratio r± • Energy dependence: appropriate variable is not E; instead it is Eμcosθ • What is the cause of the rise of μ+/μ- charge ratio with increasing Eμcosθ ? Ø Rise not due to increase in kaon production Ø Rise due to increased relative contribution of μ’s from K± ISVHECRI 2010 8
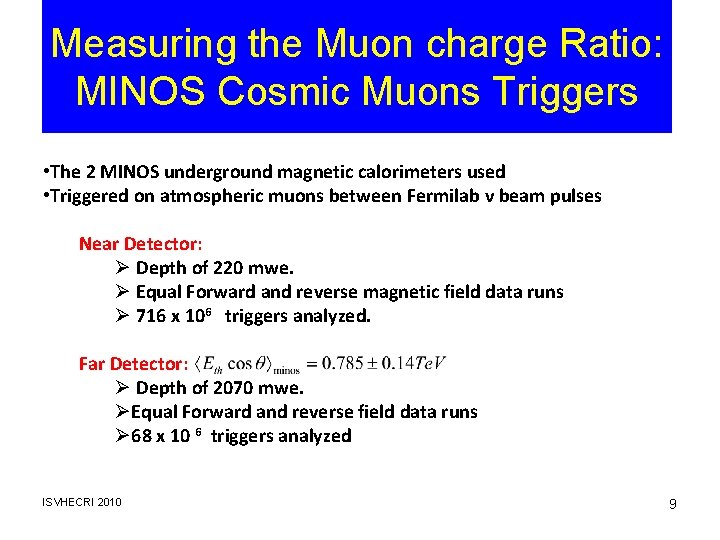
Measuring the Muon charge Ratio: MINOS Cosmic Muons Triggers • The 2 MINOS underground magnetic calorimeters used • Triggered on atmospheric muons between Fermilab ν beam pulses Near Detector: Ø Depth of 220 mwe. Ø Equal Forward and reverse magnetic field data runs Ø 716 x 106 triggers analyzed. Far Detector: Ø Depth of 2070 mwe. ØEqual Forward and reverse field data runs Ø 68 x 10 6 triggers analyzed ISVHECRI 2010 9
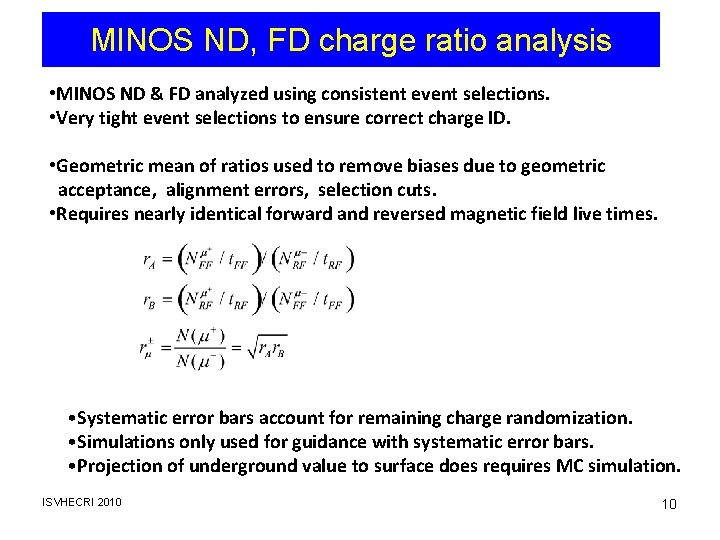
MINOS ND, FD charge ratio analysis • MINOS ND & FD analyzed using consistent event selections. • Very tight event selections to ensure correct charge ID. • Geometric mean of ratios used to remove biases due to geometric acceptance, alignment errors, selection cuts. • Requires nearly identical forward and reversed magnetic field live times. • Systematic error bars account for remaining charge randomization. • Simulations only used for guidance with systematic error bars. • Projection of underground value to surface does requires MC simulation. ISVHECRI 2010 10
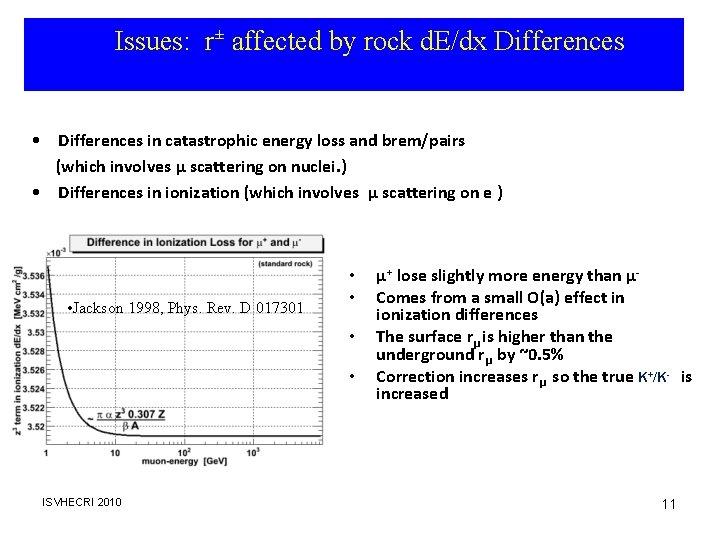
Issues: r± affected by rock d. E/dx Differences • Differences in catastrophic energy loss and brem/pairs (which involves μ scattering on nuclei. ) • Differences in ionization (which involves μ scattering on e ) • Jackson 1998, Phys. Rev. D 017301 • • ISVHECRI 2010 μ+ lose slightly more energy than μComes from a small O(a) effect in ionization differences The surface rμ is higher than the underground rμ by ~0. 5% Correction increases rμ so the true K+/K- is increased 11
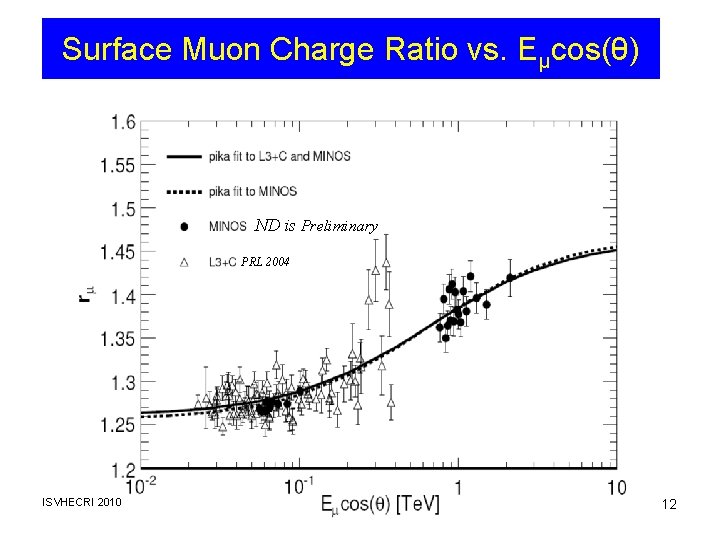
Surface Muon Charge Ratio vs. Eμcos(θ) ND is Preliminary PRL 2004 ISVHECRI 2010 12
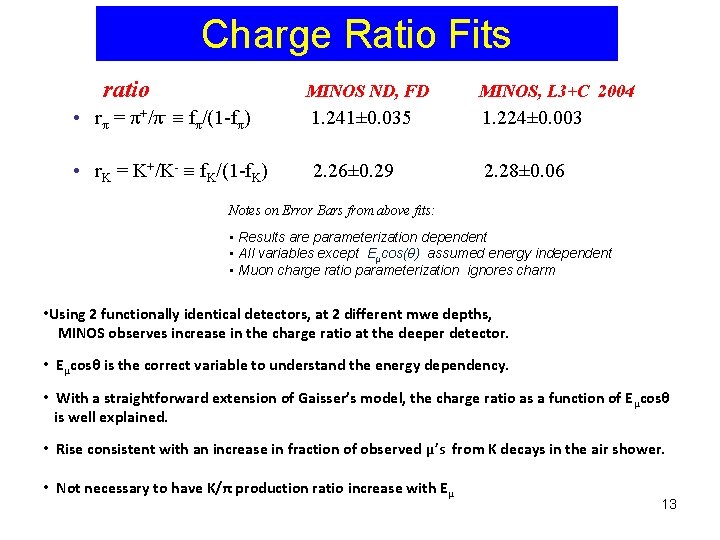
Charge Ratio Fits ratio MINOS ND, FD MINOS, L 3+C 2004 • rπ = π+/π- fπ/(1 -fπ) 1. 241± 0. 035 1. 224± 0. 003 • r. K = K+/K- f. K/(1 -f. K) 2. 26± 0. 29 2. 28± 0. 06 Notes on Error Bars from above fits: • Results are parameterization dependent • All variables except Eμcos(θ) assumed energy independent • Muon charge ratio parameterization ignores charm • Using 2 functionally identical detectors, at 2 different mwe depths, MINOS observes increase in the charge ratio at the deeper detector. • Eμcosθ is the correct variable to understand the energy dependency. • With a straightforward extension of Gaisser’s model, the charge ratio as a function of E μcosθ is well explained. • Rise consistent with an increase in fraction of observed μ’s from K decays in the air shower. • Not necessary to have K/π production ratio increase with Eμ 13
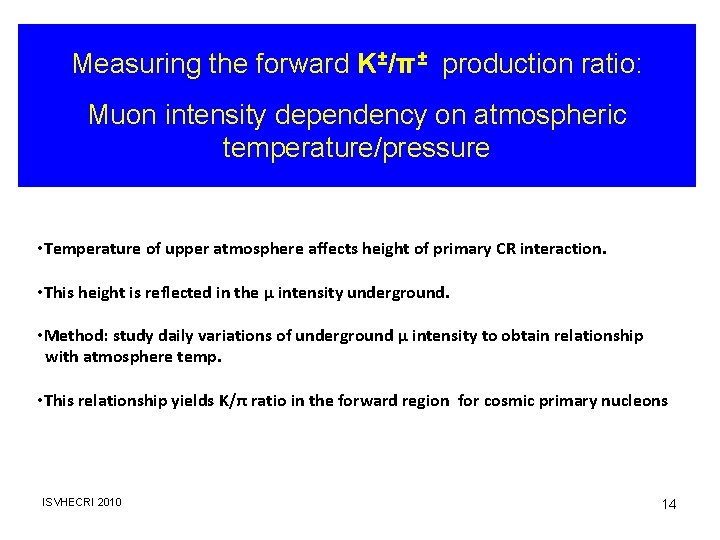
Measuring the forward K±/π± production ratio: Muon intensity dependency on atmospheric temperature/pressure • Temperature of upper atmosphere affects height of primary CR interaction. • This height is reflected in the μ intensity underground. • Method: study daily variations of underground μ intensity to obtain relationship with atmosphere temp. • This relationship yields K/π ratio in the forward region for cosmic primary nucleons ISVHECRI 2010 14
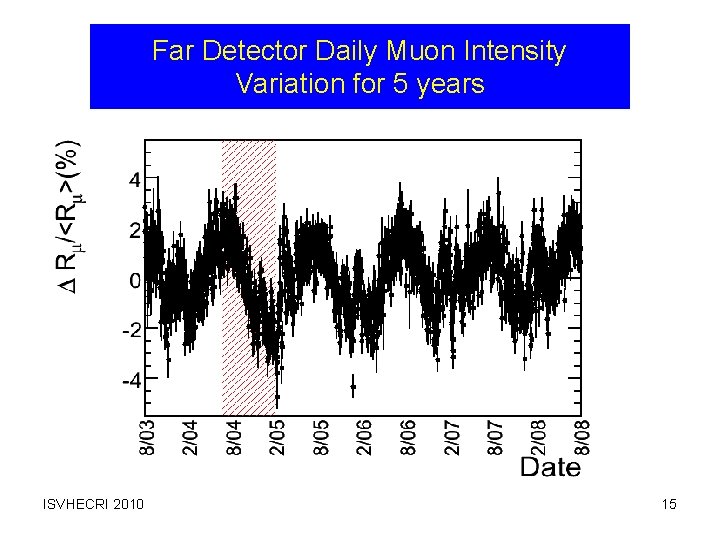
Far Detector Daily Muon Intensity Variation for 5 years ISVHECRI 2010 15
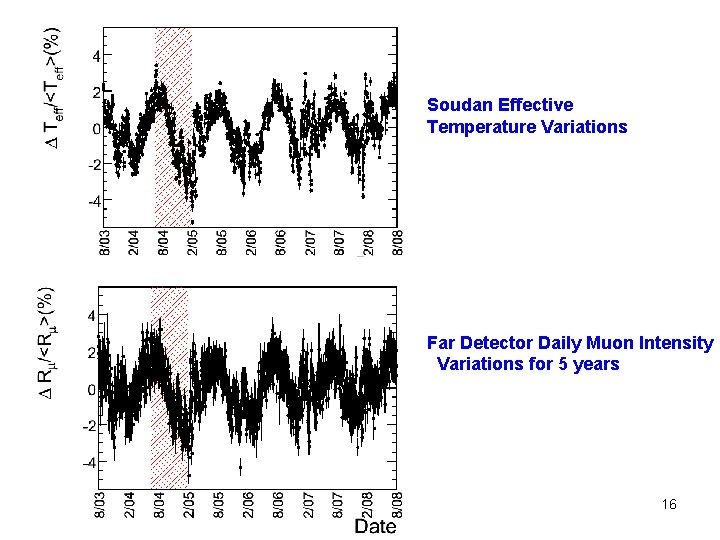
Soudan Effective Temperature Variations Far Detector Daily Muon Intensity Variations for 5 years 16
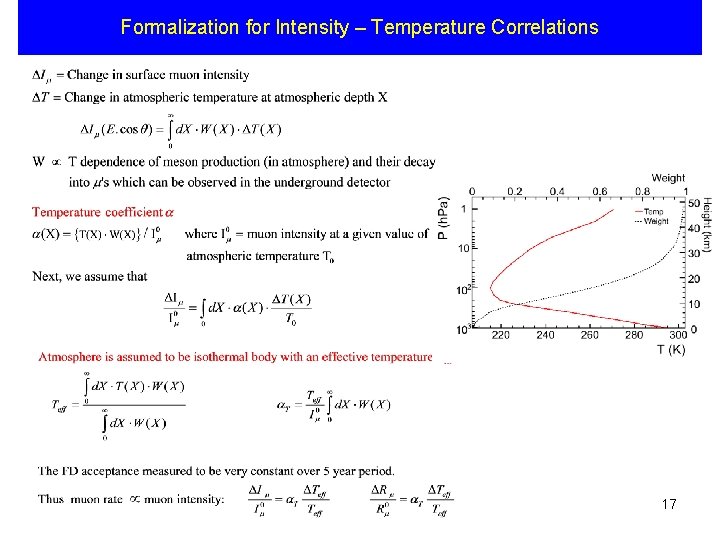
Formalization for Intensity – Temperature Correlations 17
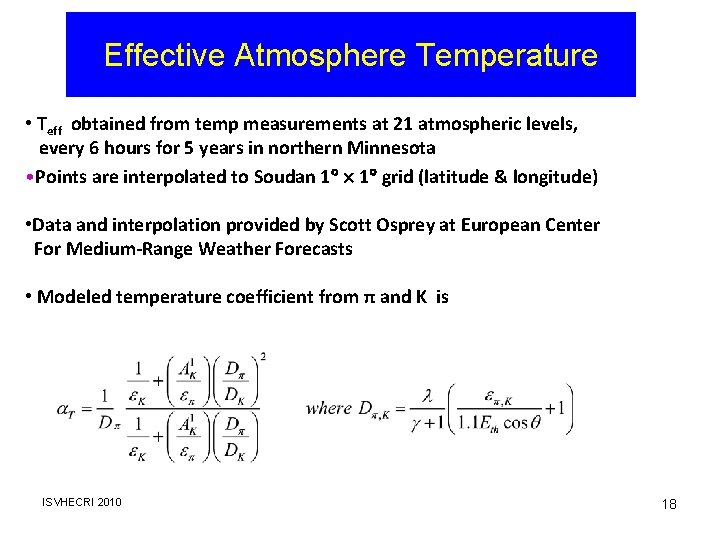
Effective Atmosphere Temperature • Teff obtained from temp measurements at 21 atmospheric levels, every 6 hours for 5 years in northern Minnesota • Points are interpolated to Soudan 1 1 grid (latitude & longitude) • Data and interpolation provided by Scott Osprey at European Center For Medium-Range Weather Forecasts • Modeled temperature coefficient from π and K is ISVHECRI 2010 18
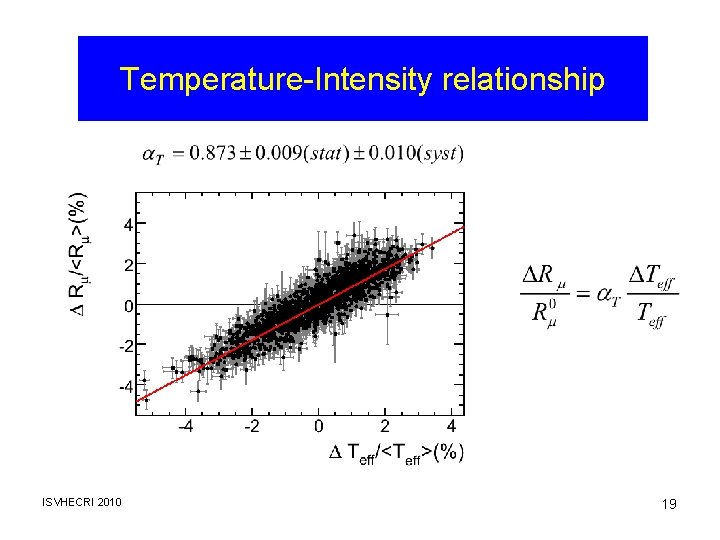
Temperature-Intensity relationship ISVHECRI 2010 19
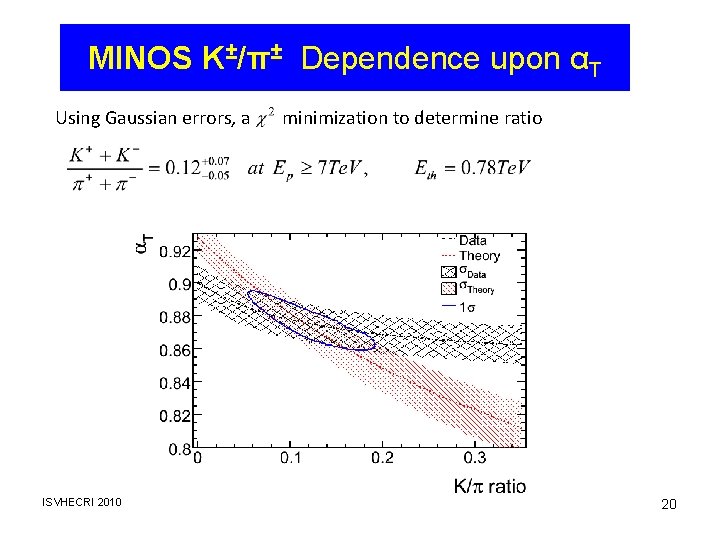
MINOS K±/π± Dependence upon αT Using Gaussian errors, a ISVHECRI 2010 minimization to determine ratio 20
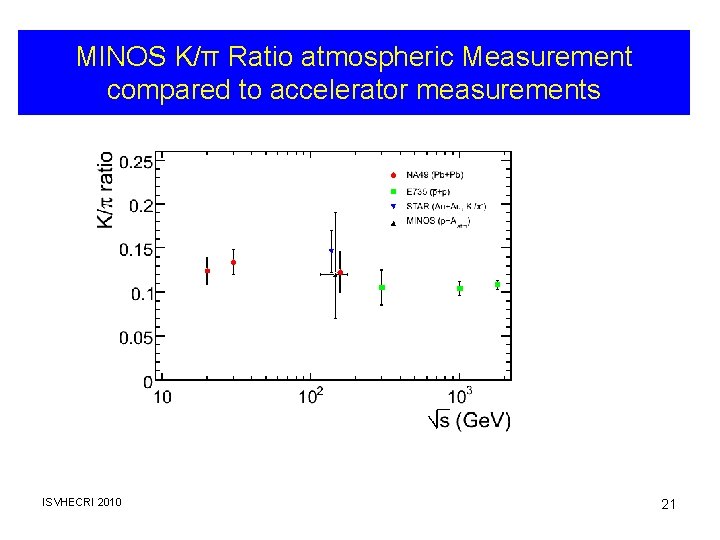
MINOS K/π Ratio atmospheric Measurement compared to accelerator measurements ISVHECRI 2010 21
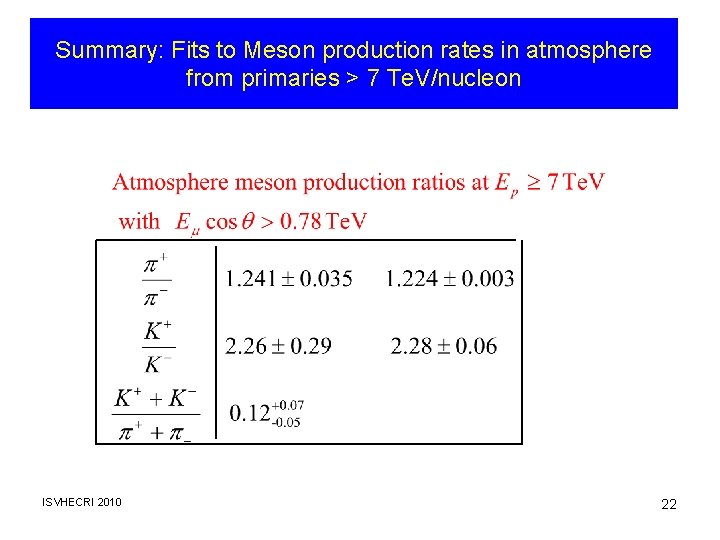
Summary: Fits to Meson production rates in atmosphere from primaries > 7 Te. V/nucleon ISVHECRI 2010 22
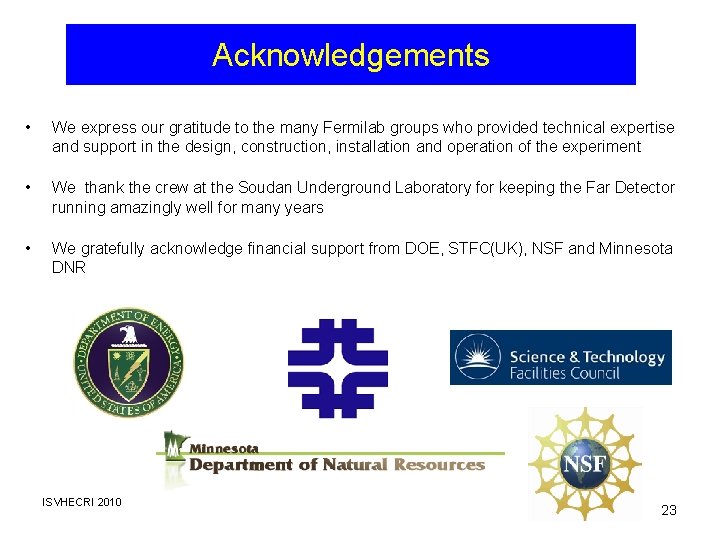
Acknowledgements • We express our gratitude to the many Fermilab groups who provided technical expertise and support in the design, construction, installation and operation of the experiment • We thank the crew at the Soudan Underground Laboratory for keeping the Far Detector running amazingly well for many years • We gratefully acknowledge financial support from DOE, STFC(UK), NSF and Minnesota DNR ISVHECRI 2010 23
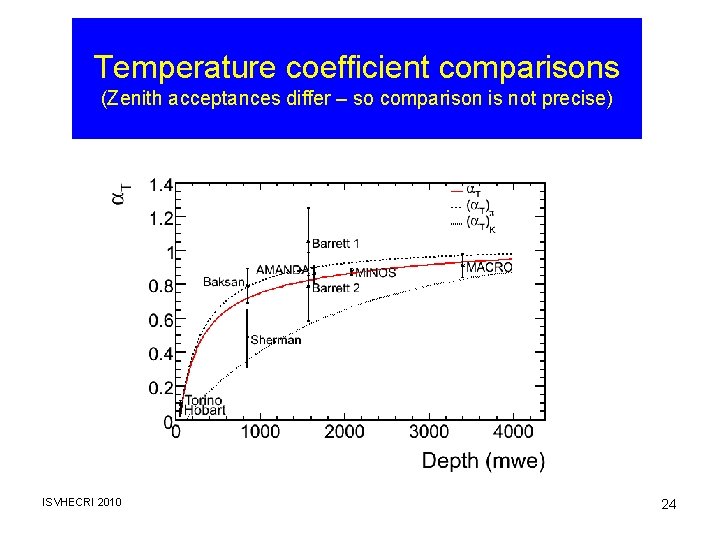
Temperature coefficient comparisons (Zenith acceptances differ – so comparison is not precise) ISVHECRI 2010 24
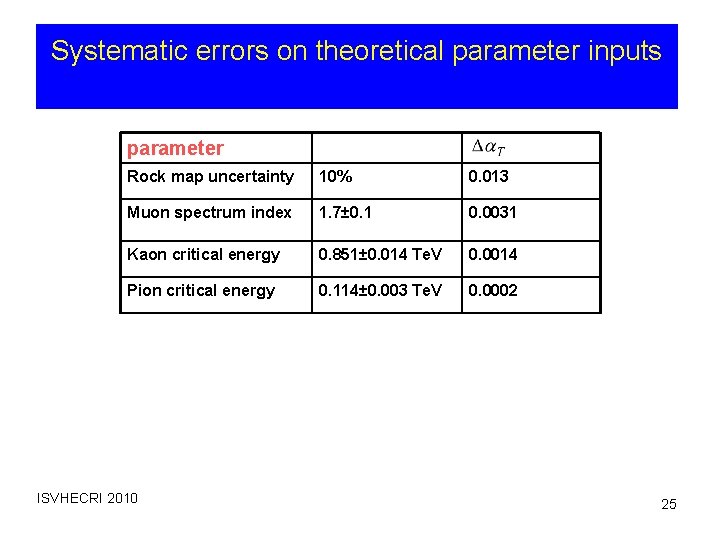
Systematic errors on theoretical parameter inputs parameter Rock map uncertainty 10% 0. 013 Muon spectrum index 1. 7± 0. 1 0. 0031 Kaon critical energy 0. 851± 0. 014 Te. V 0. 0014 Pion critical energy 0. 114± 0. 003 Te. V 0. 0002 ISVHECRI 2010 25
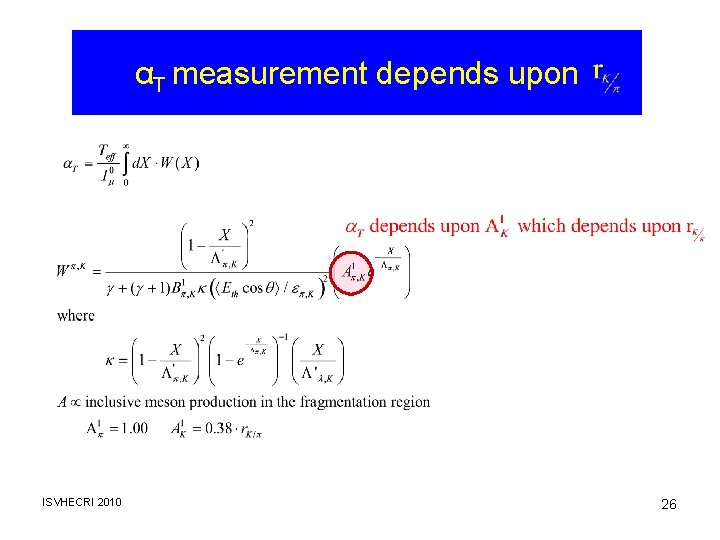
αT measurement depends upon ISVHECRI 2010 26
Produksi multimedia adalah
Cosmic ray showers
Cosmic ray elab
Cosmic ray cube
Bit flip cosmic ray
Cosmic ray
Cosmic ray spectrum
Cosmic ray
Cosmic ray
An introduction to atmospheric physics
Atmospheric physics lecture notes
Lean meson
Phi meson
Meson valves
Partikel meson
Lucas 10 30 37
K star meson
Charm
Meson alue
B meson decay
Meson
Meson
Irene mendez meson
Bc meson
Sandra malvezzi
Clockwise unit circle
Ray model of light