MICROSCOPYGreek small viewing Microscope as a tool can
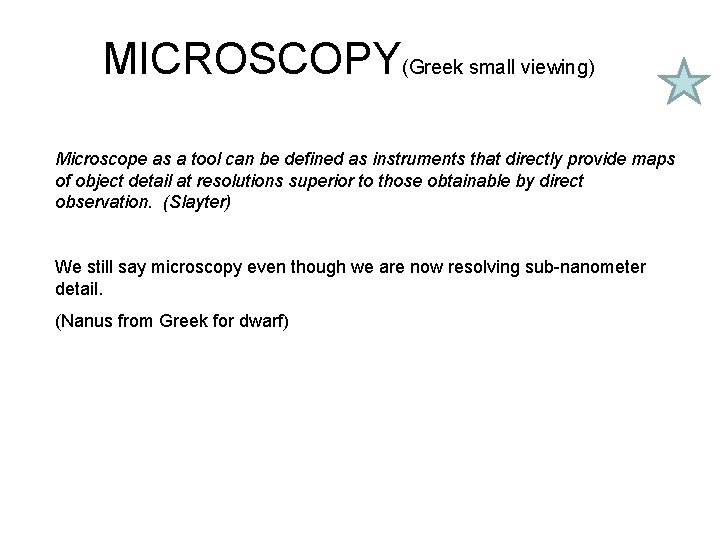
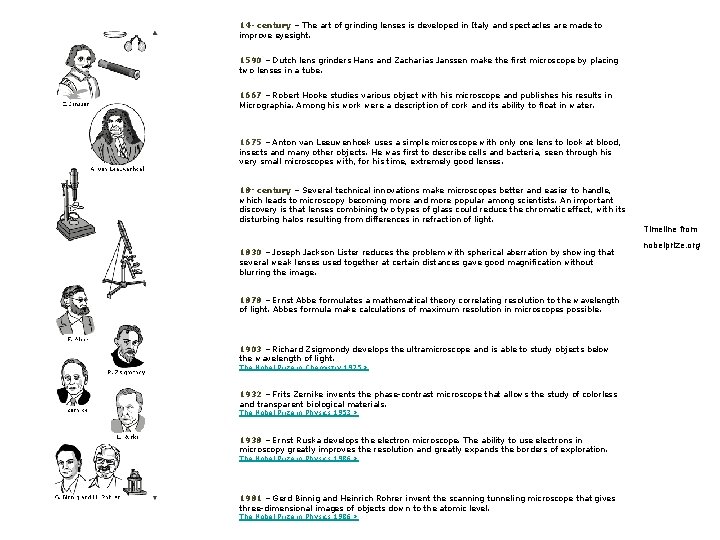
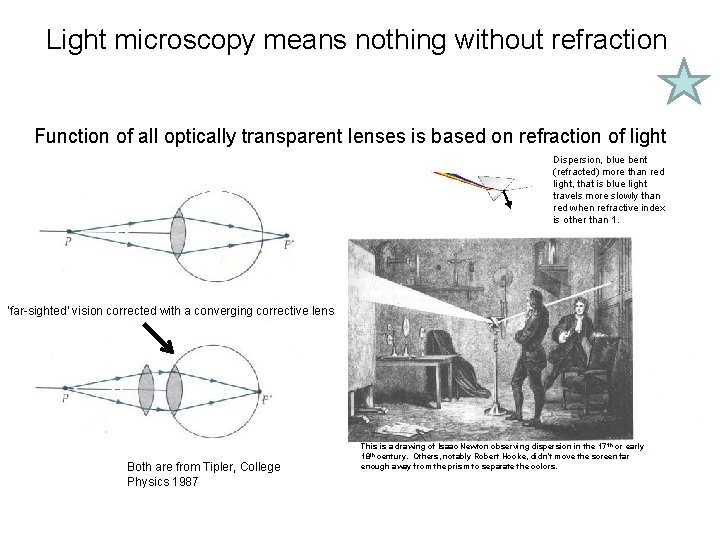
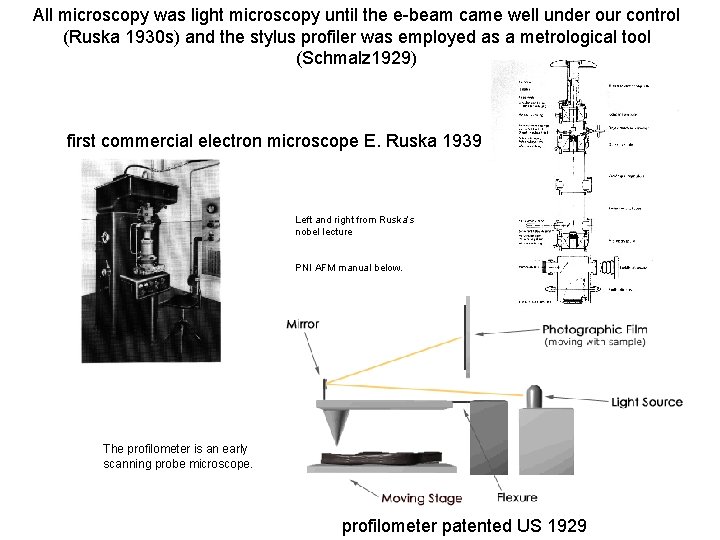
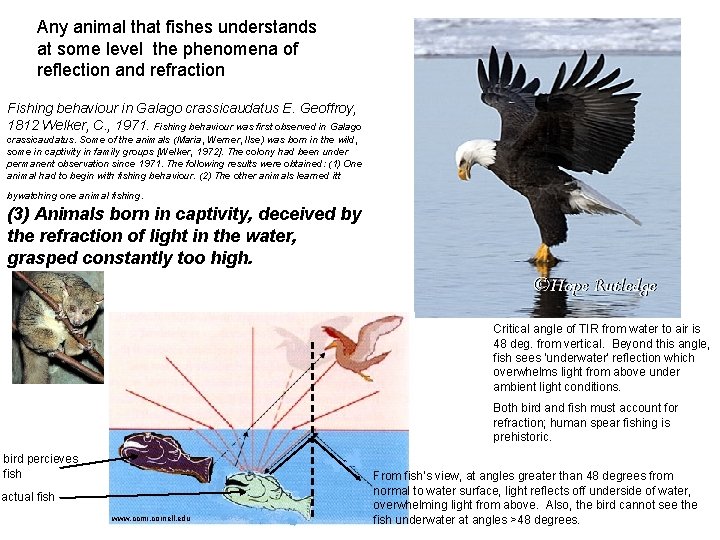
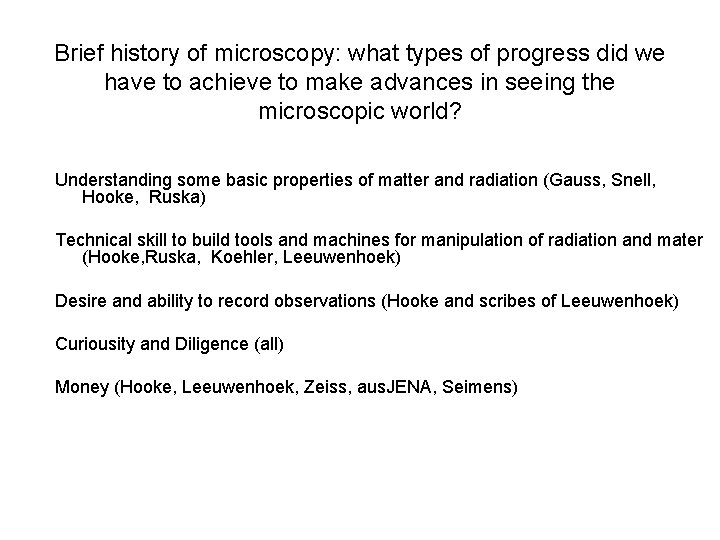
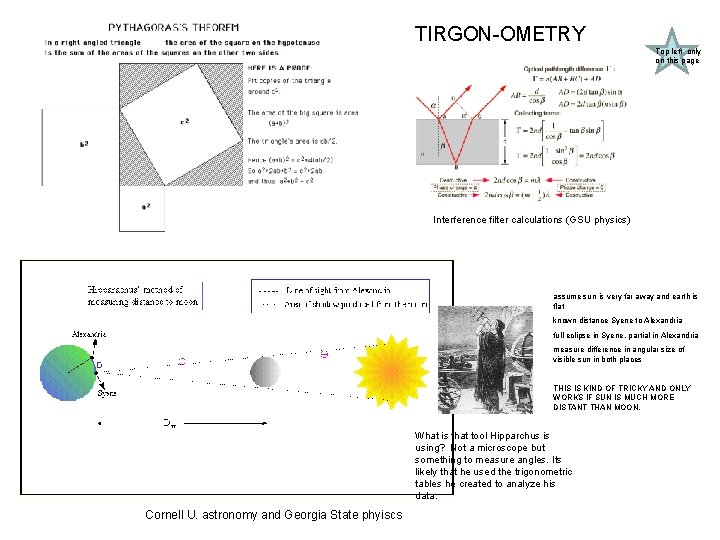
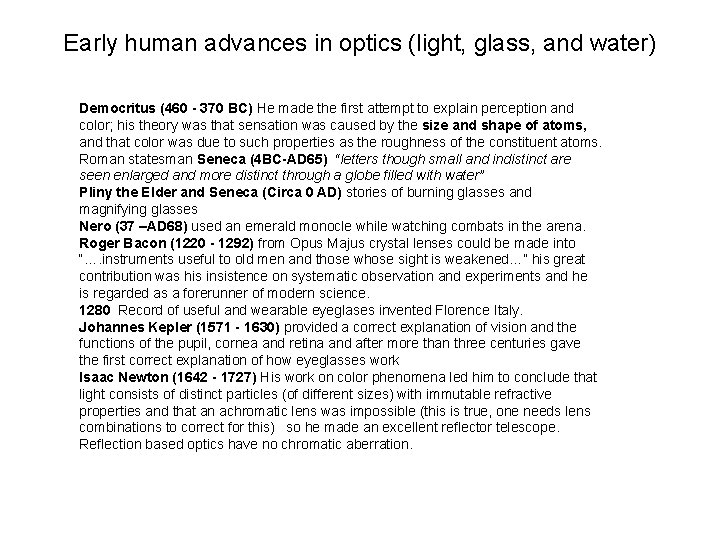
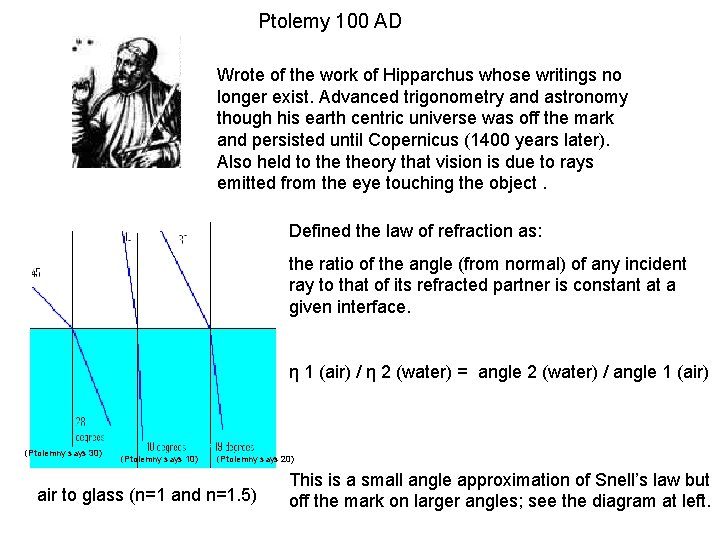
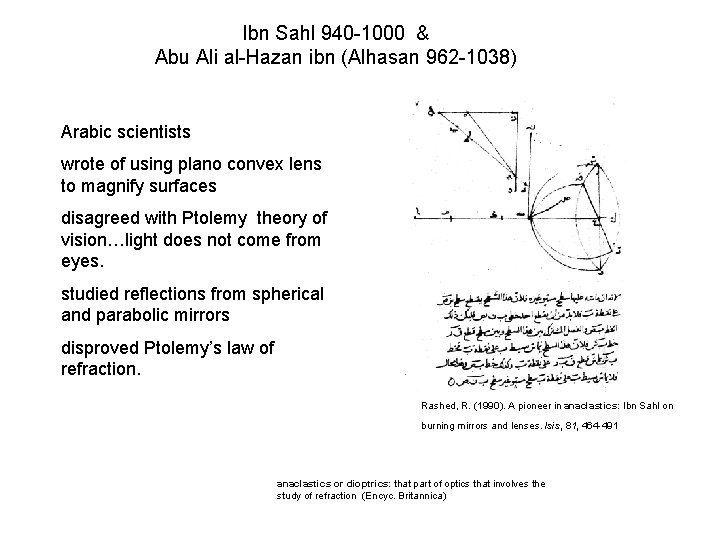
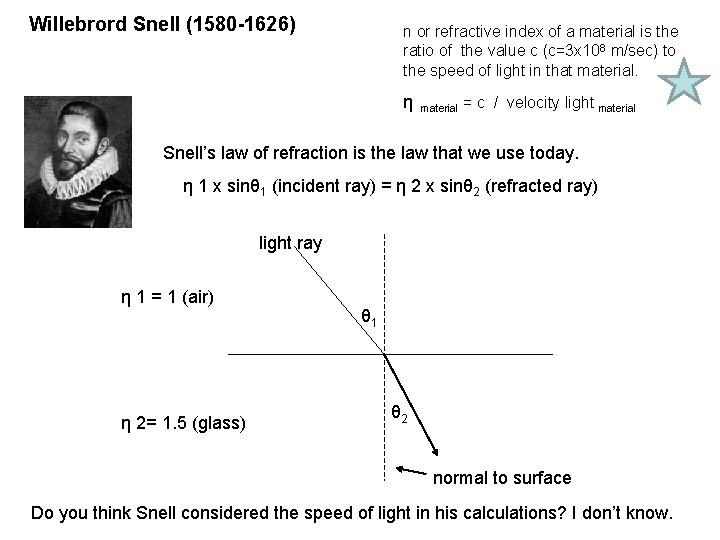
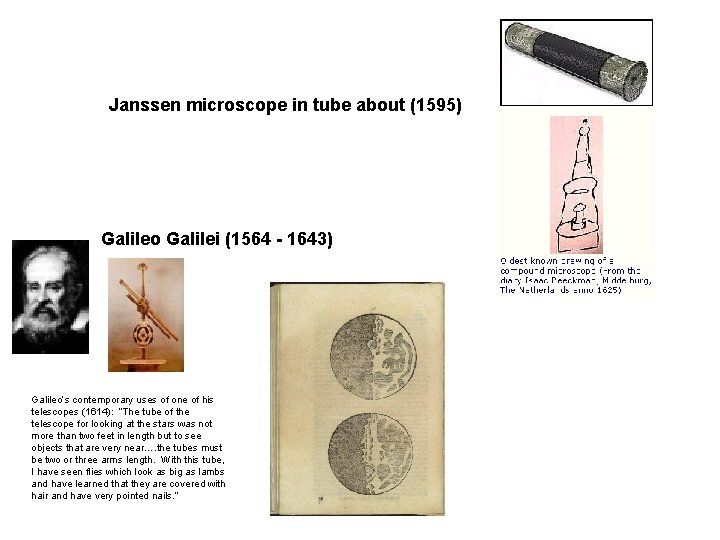
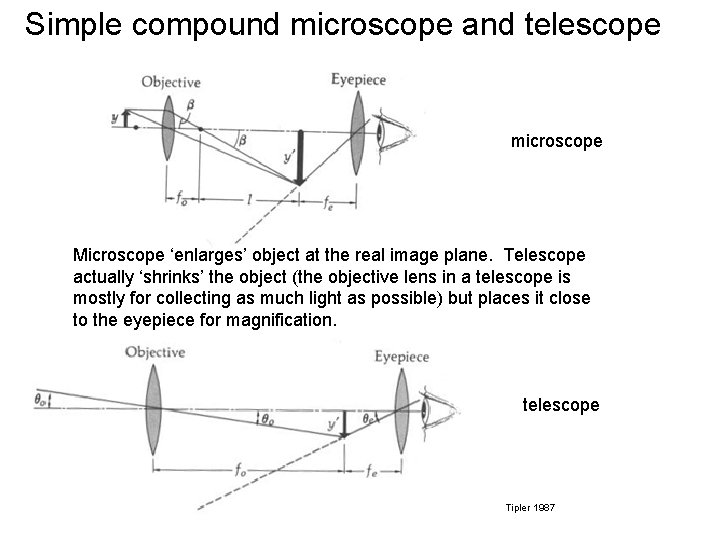
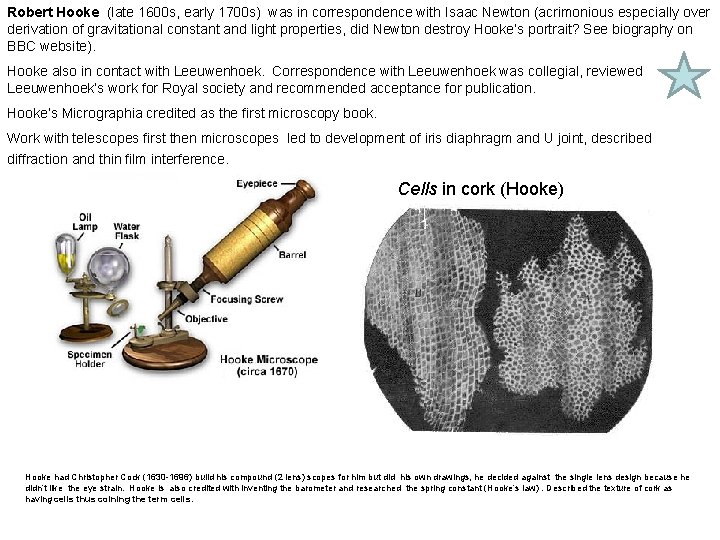
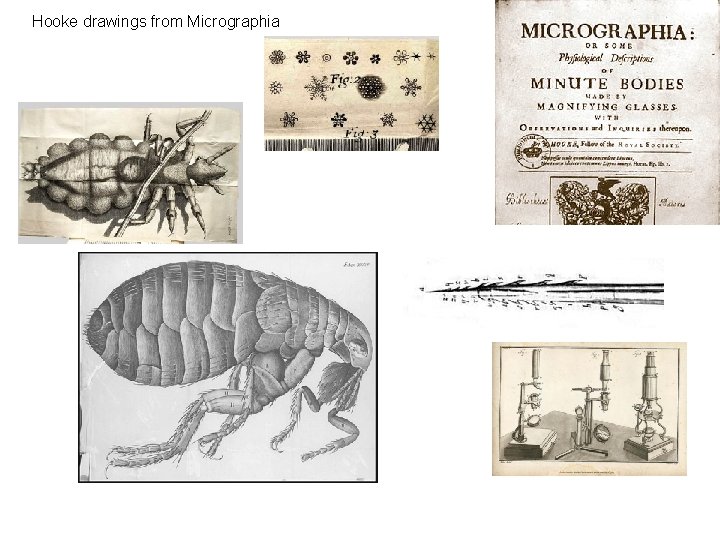
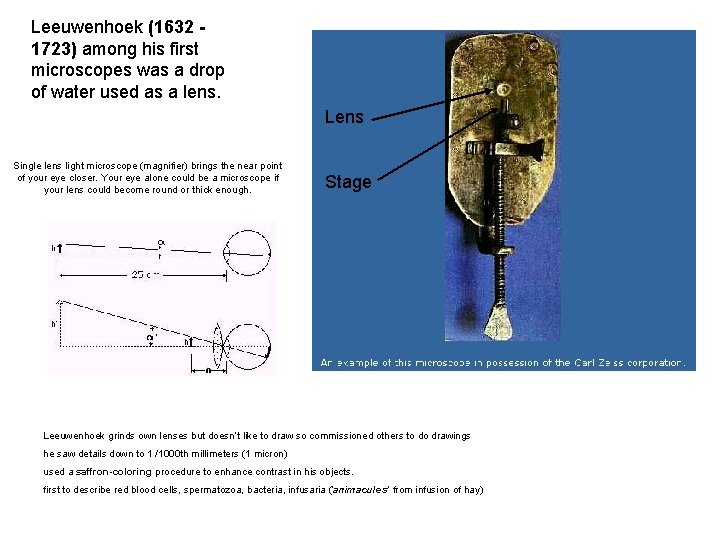
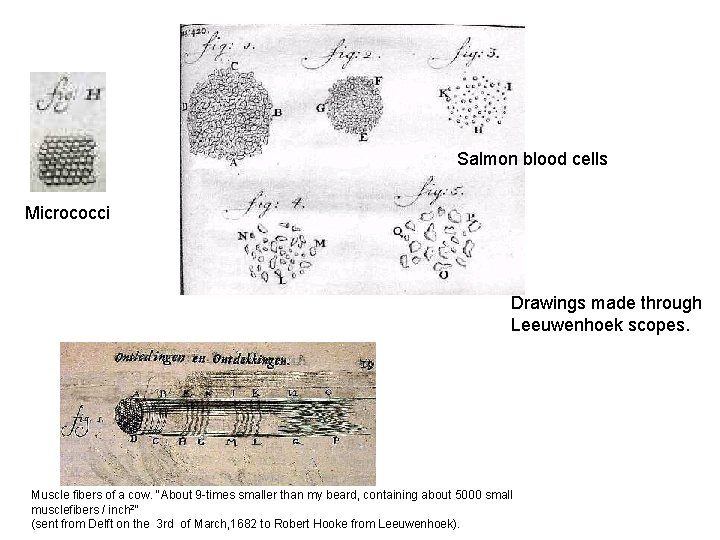
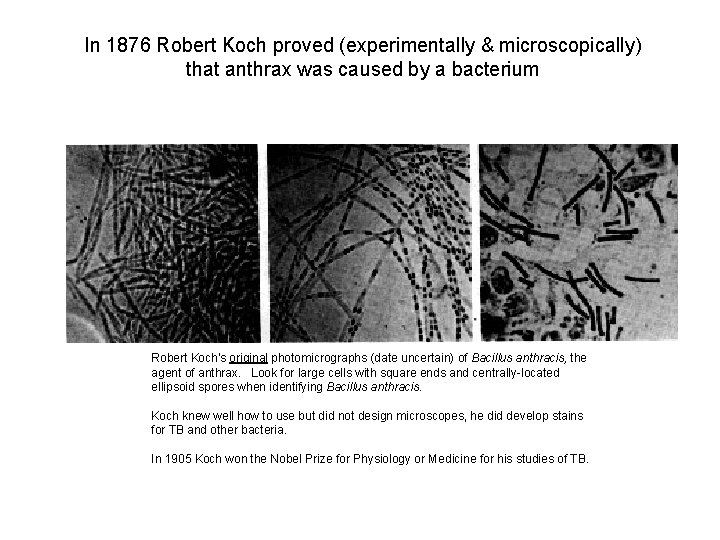
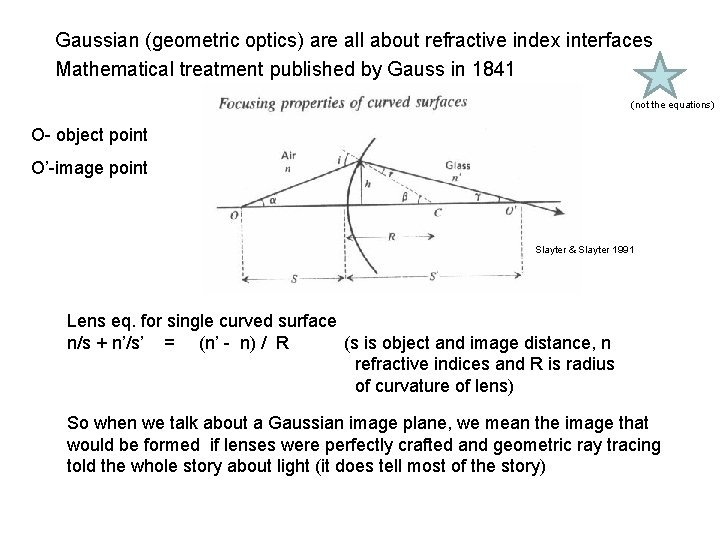
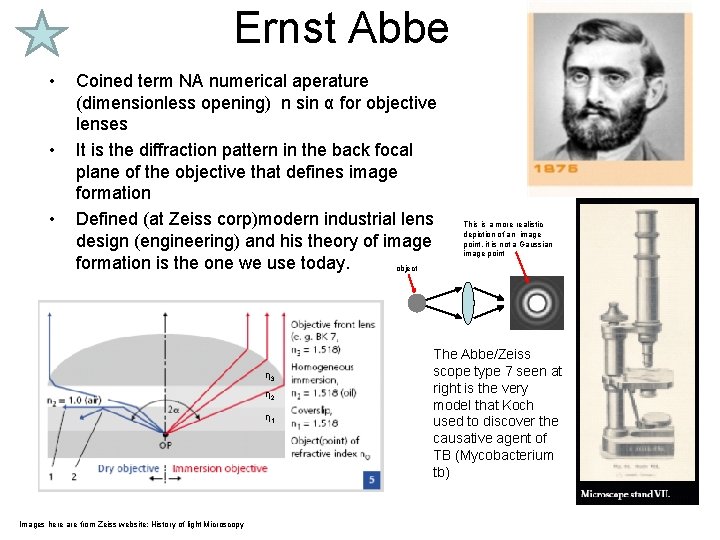
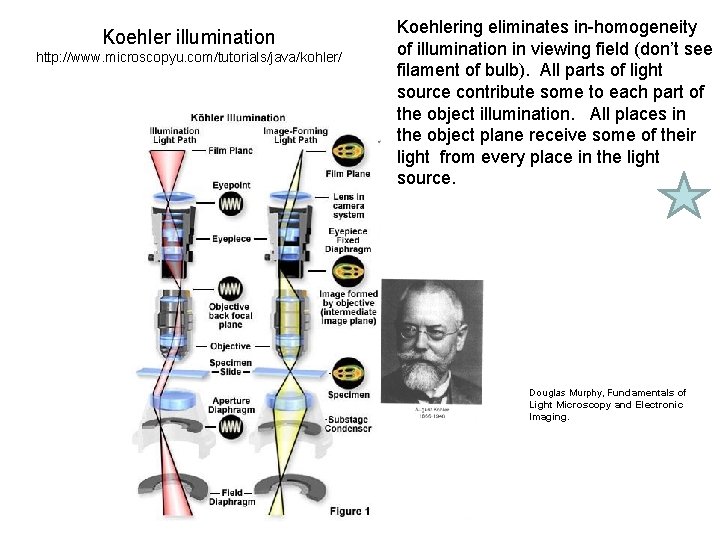
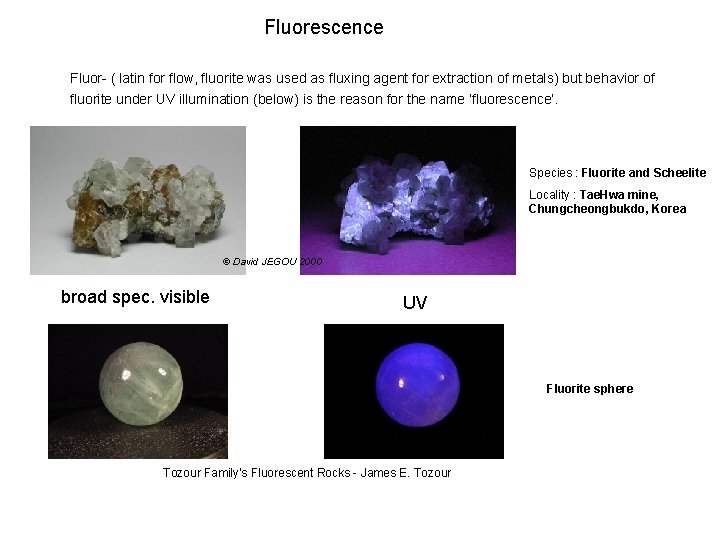
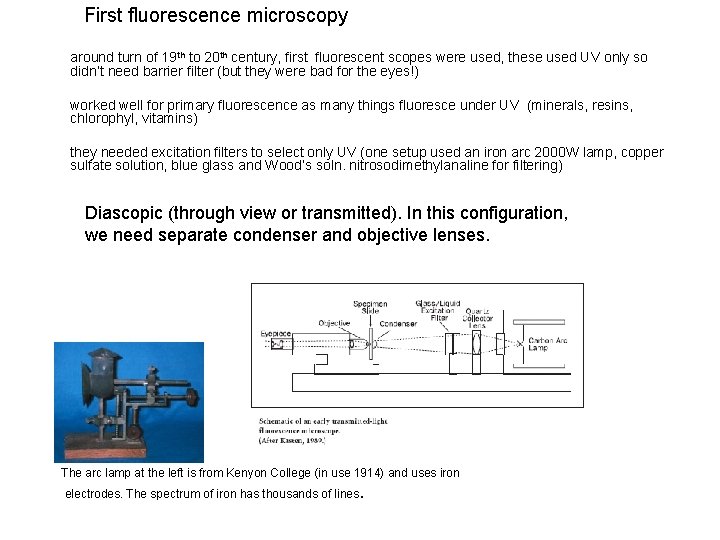
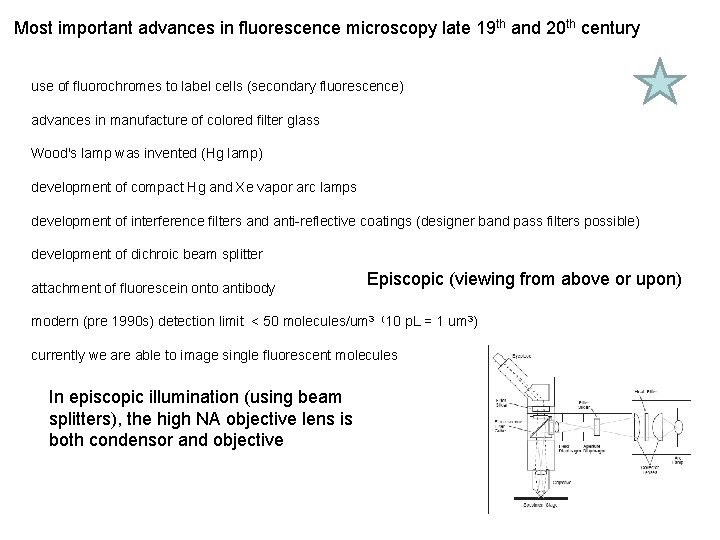
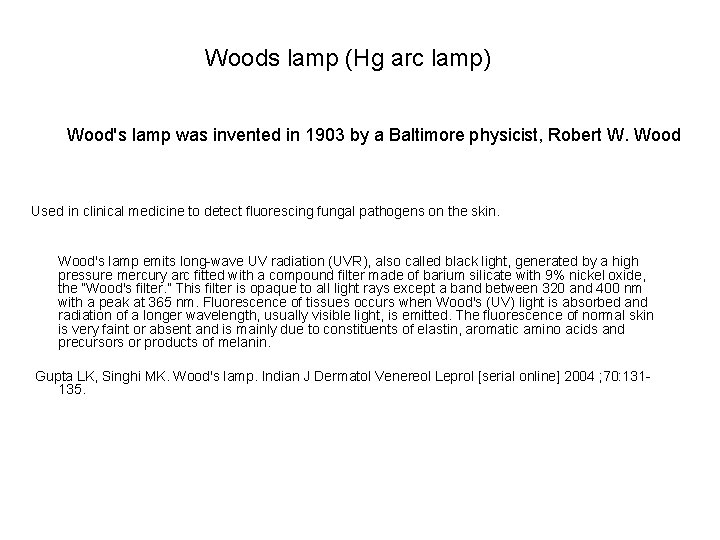
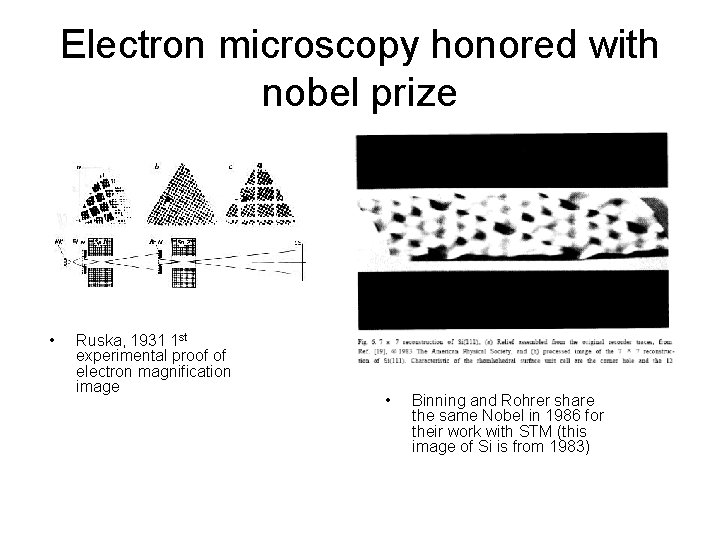
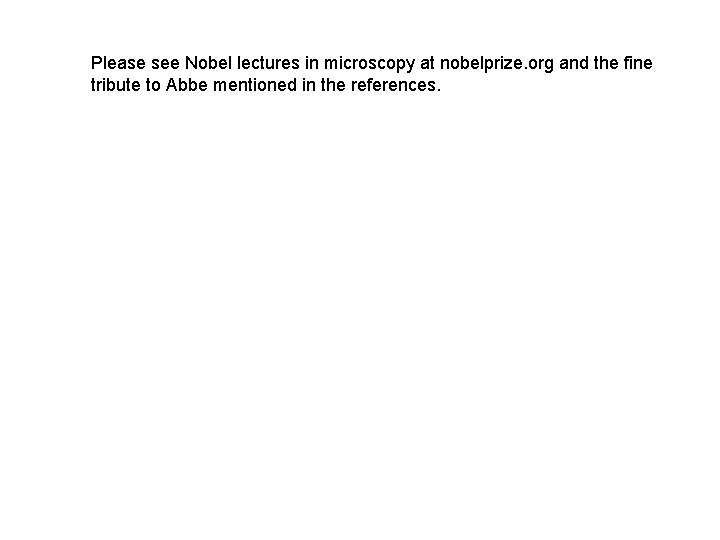
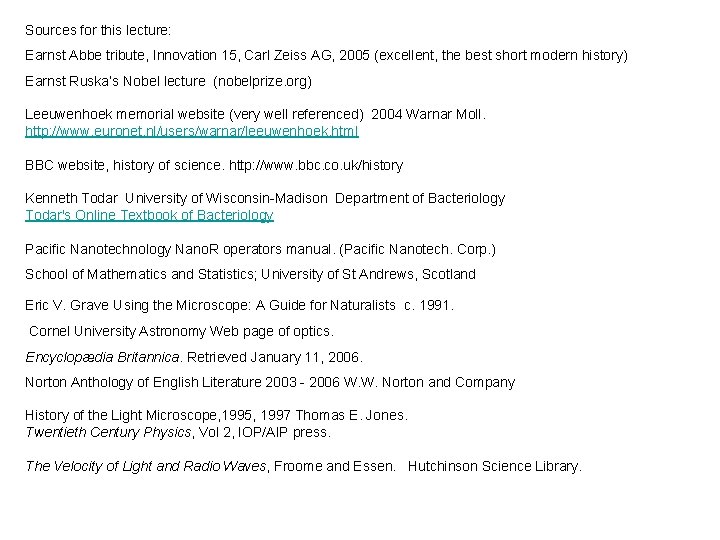
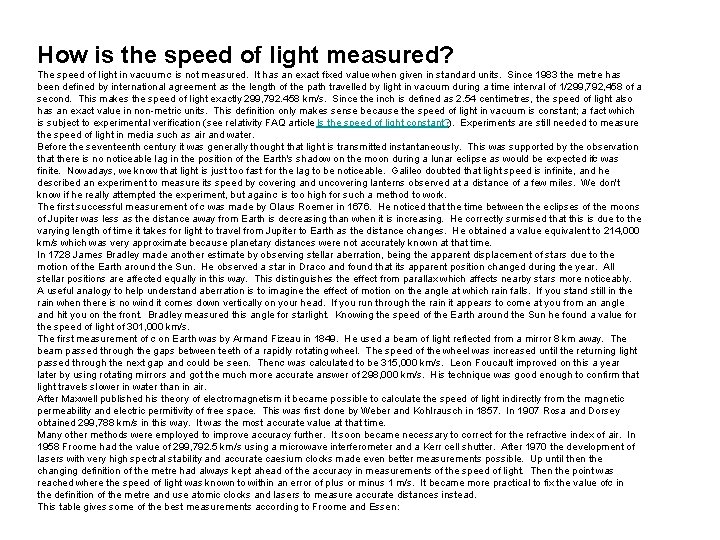
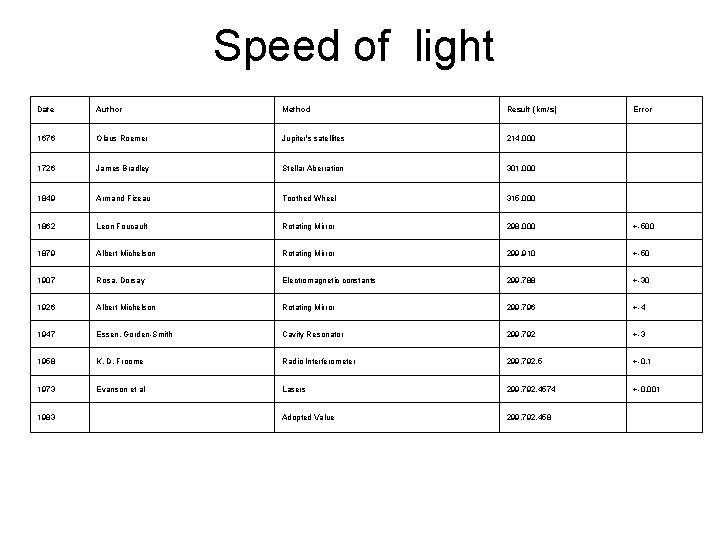
- Slides: 30
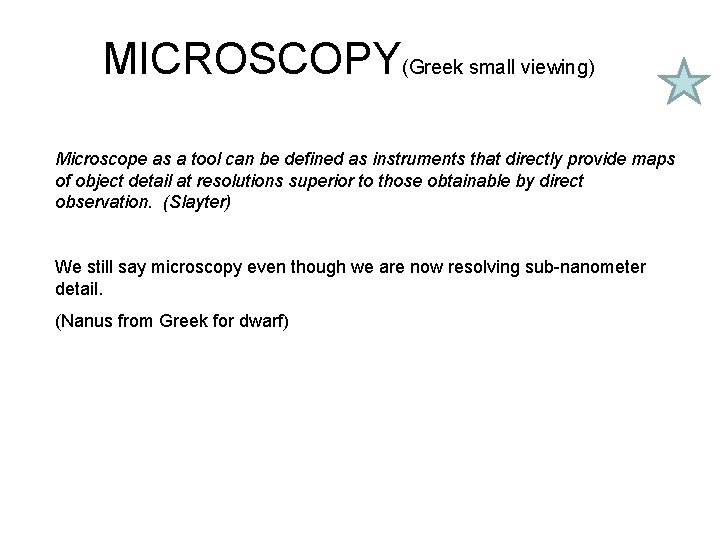
MICROSCOPY(Greek small viewing) Microscope as a tool can be defined as instruments that directly provide maps of object detail at resolutions superior to those obtainable by direct observation. (Slayter) We still say microscopy even though we are now resolving sub-nanometer detail. (Nanus from Greek for dwarf)
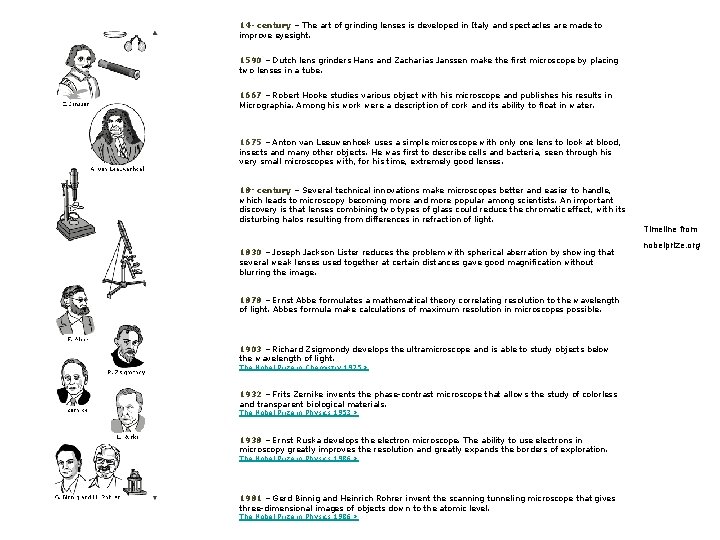
14 th century – The art of grinding lenses is developed in Italy and spectacles are made to improve eyesight. 1590 – Dutch lens grinders Hans and Zacharias Janssen make the first microscope by placing two lenses in a tube. 1667 – Robert Hooke studies various object with his microscope and publishes his results in Micrographia. Among his work were a description of cork and its ability to float in water. 1675 – Anton van Leeuwenhoek uses a simple microscope with only one lens to look at blood, insects and many other objects. He was first to describe cells and bacteria, seen through his very small microscopes with, for his time, extremely good lenses. 18 th century – Several technical innovations make microscopes better and easier to handle, which leads to microscopy becoming more and more popular among scientists. An important discovery is that lenses combining two types of glass could reduce the chromatic effect, with its disturbing halos resulting from differences in refraction of light. 1830 – Joseph Jackson Lister reduces the problem with spherical aberration by showing that several weak lenses used together at certain distances gave good magnification without blurring the image. 1878 – Ernst Abbe formulates a mathematical theory correlating resolution to the wavelength of light. Abbes formula make calculations of maximum resolution in microscopes possible. 1903 – Richard Zsigmondy develops the ultramicroscope and is able to study objects below the wavelength of light. The Nobel Prize in Chemistry 1925 » 1932 – Frits Zernike invents the phase-contrast microscope that allows the study of colorless and transparent biological materials. The Nobel Prize in Physics 1953 » 1938 – Ernst Ruska develops the electron microscope. The ability to use electrons in microscopy greatly improves the resolution and greatly expands the borders of exploration. The Nobel Prize in Physics 1986 » 1981 – Gerd Binnig and Heinrich Rohrer invent the scanning tunneling microscope that gives three-dimensional images of objects down to the atomic level. The Nobel Prize in Physics 1986 » Timeline from nobelprize. org
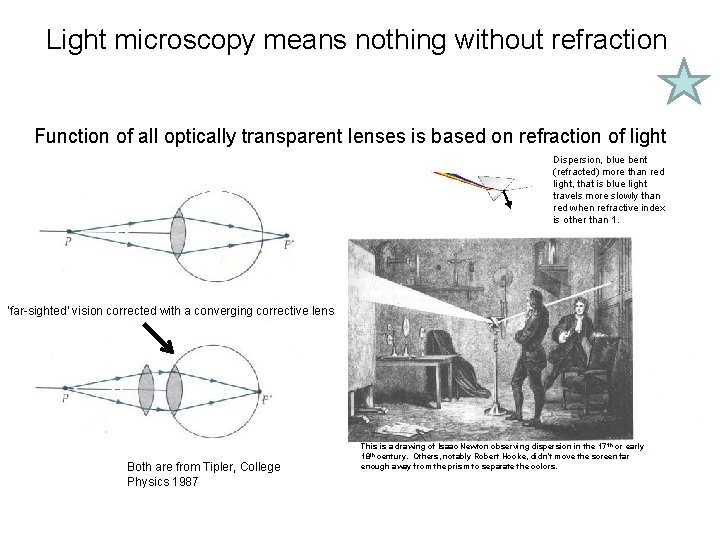
Light microscopy means nothing without refraction Function of all optically transparent lenses is based on refraction of light Dispersion, blue bent (refracted) more than red light, that is blue light travels more slowly than red when refractive index is other than 1. ‘far-sighted’ vision corrected with a converging corrective lens Both are from Tipler, College Physics 1987 This is a drawing of Isaac Newton observing dispersion in the 17 th or early 18 th century. Others, notably Robert Hooke, didn’t move the screen far enough away from the prism to separate the colors.
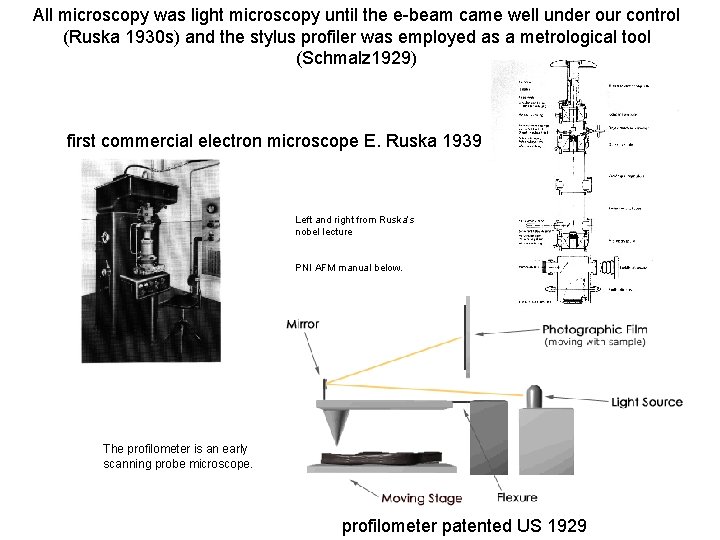
All microscopy was light microscopy until the e-beam came well under our control (Ruska 1930 s) and the stylus profiler was employed as a metrological tool (Schmalz 1929) first commercial electron microscope E. Ruska 1939 Left and right from Ruska’s nobel lecture PNI AFM manual below. The profilometer is an early scanning probe microscope. profilometer patented US 1929
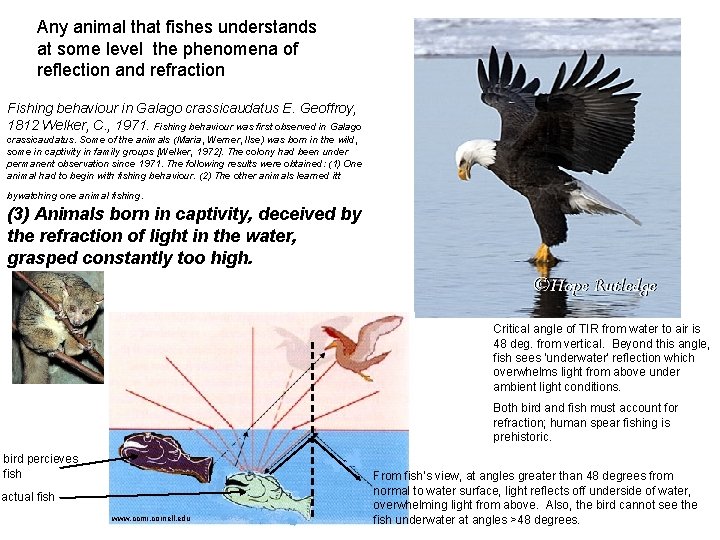
Any animal that fishes understands at some level the phenomena of reflection and refraction Fishing behaviour in Galago crassicaudatus E. Geoffroy, 1812 Welker, C. , 1971. Fishing behaviour was first observed in Galago crassicaudatus. Some of the animals (Maria, Werner, Ilse) was born in the wild, some in captivity in family groups [Welker, 1972]. The colony had been under permanent observation since 1971. The following results were obtained: (1) One animal had to begin with fishing behaviour. (2) The other animals learned itt bywatching one animal fishing. (3) Animals born in captivity, deceived by the refraction of light in the water, grasped constantly too high. Critical angle of TIR from water to air is 48 deg. from vertical. Beyond this angle, fish sees ‘underwater’ reflection which overwhelms light from above under ambient light conditions. Both bird and fish must account for refraction; human spear fishing is prehistoric. bird percieves fish actual fish www. ccmr. cornell. edu From fish’s view, at angles greater than 48 degrees from normal to water surface, light reflects off underside of water, overwhelming light from above. Also, the bird cannot see the fish underwater at angles >48 degrees.
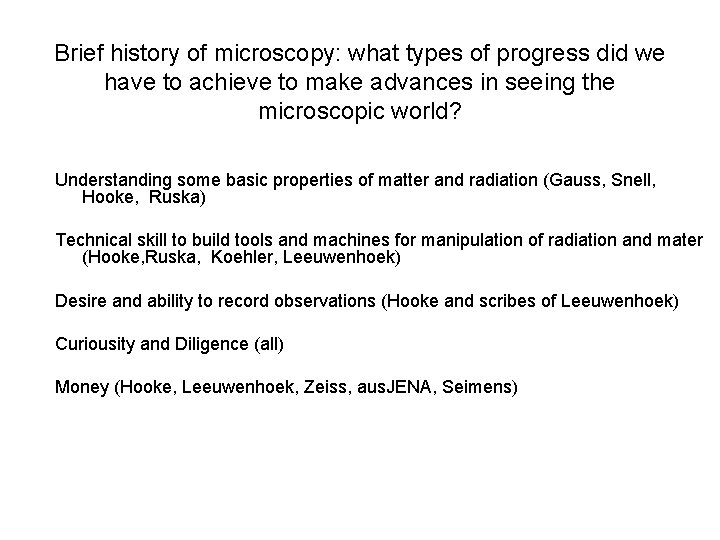
Brief history of microscopy: what types of progress did we have to achieve to make advances in seeing the microscopic world? Understanding some basic properties of matter and radiation (Gauss, Snell, Hooke, Ruska) Technical skill to build tools and machines for manipulation of radiation and mater (Hooke, Ruska, Koehler, Leeuwenhoek) Desire and ability to record observations (Hooke and scribes of Leeuwenhoek) Curiousity and Diligence (all) Money (Hooke, Leeuwenhoek, Zeiss, aus. JENA, Seimens)
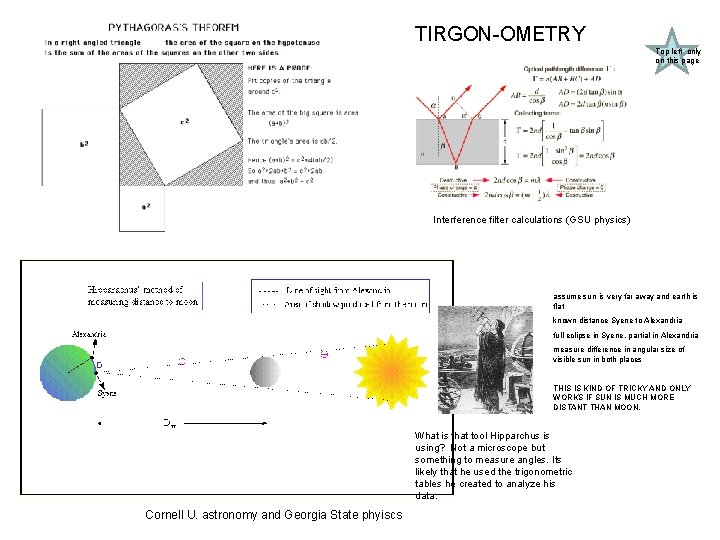
TIRGON-OMETRY Top left only on this page Interference filter calculations (GSU physics) assume sun is very far away and earth is flat known distance Syene to Alexandria full eclipse in Syene, partial in Alexandria measure difference in angular size of visible sun in both places THIS IS KIND OF TRICKY AND ONLY WORKS IF SUN IS MUCH MORE DISTANT THAN MOON. What is that tool Hipparchus is using? Not a microscope but something to measure angles. Its likely that he used the trigonometric tables he created to analyze his data. Cornell U. astronomy and Georgia State phyiscs
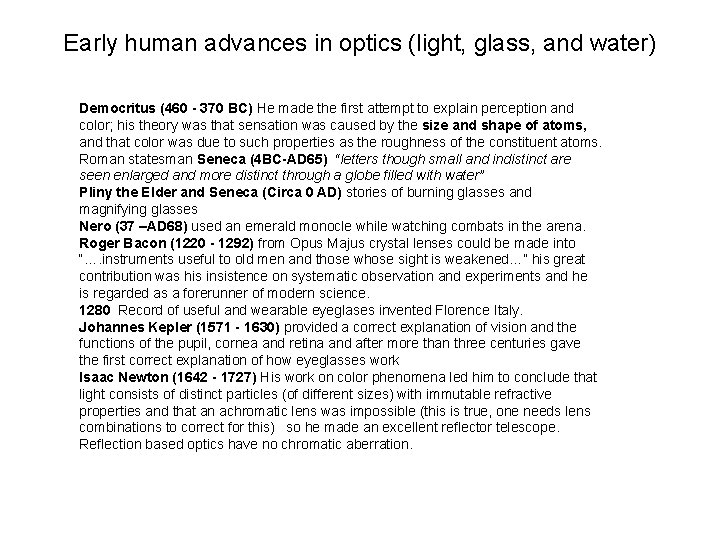
Early human advances in optics (light, glass, and water) Democritus (460 - 370 BC) He made the first attempt to explain perception and color; his theory was that sensation was caused by the size and shape of atoms, and that color was due to such properties as the roughness of the constituent atoms. Roman statesman Seneca (4 BC-AD 65) “letters though small and indistinct are seen enlarged and more distinct through a globe filled with water” Pliny the Elder and Seneca (Circa 0 AD) stories of burning glasses and magnifying glasses Nero (37 –AD 68) used an emerald monocle while watching combats in the arena. Roger Bacon (1220 - 1292) from Opus Majus crystal lenses could be made into “…. instruments useful to old men and those whose sight is weakened…” his great contribution was his insistence on systematic observation and experiments and he is regarded as a forerunner of modern science. 1280 Record of useful and wearable eyeglases invented Florence Italy. Johannes Kepler (1571 - 1630) provided a correct explanation of vision and the functions of the pupil, cornea and retina and after more than three centuries gave the first correct explanation of how eyeglasses work Isaac Newton (1642 - 1727) His work on color phenomena led him to conclude that light consists of distinct particles (of different sizes) with immutable refractive properties and that an achromatic lens was impossible (this is true, one needs lens combinations to correct for this) so he made an excellent reflector telescope. Reflection based optics have no chromatic aberration.
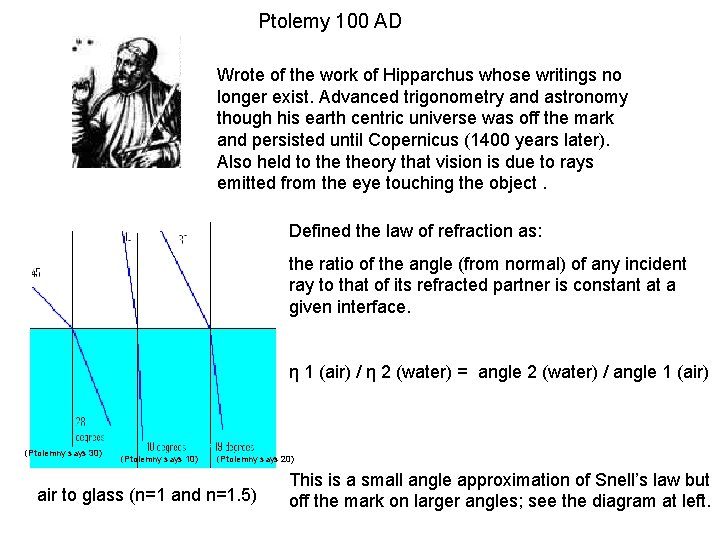
Ptolemy 100 AD Wrote of the work of Hipparchus whose writings no longer exist. Advanced trigonometry and astronomy though his earth centric universe was off the mark and persisted until Copernicus (1400 years later). Also held to theory that vision is due to rays emitted from the eye touching the object. Defined the law of refraction as: the ratio of the angle (from normal) of any incident ray to that of its refracted partner is constant at a given interface. η 1 (air) / η 2 (water) = angle 2 (water) / angle 1 (air) (Ptolemny says 30) (Ptolemny says 10) (Ptolemny says 20) air to glass (n=1 and n=1. 5) This is a small angle approximation of Snell’s law but off the mark on larger angles; see the diagram at left.
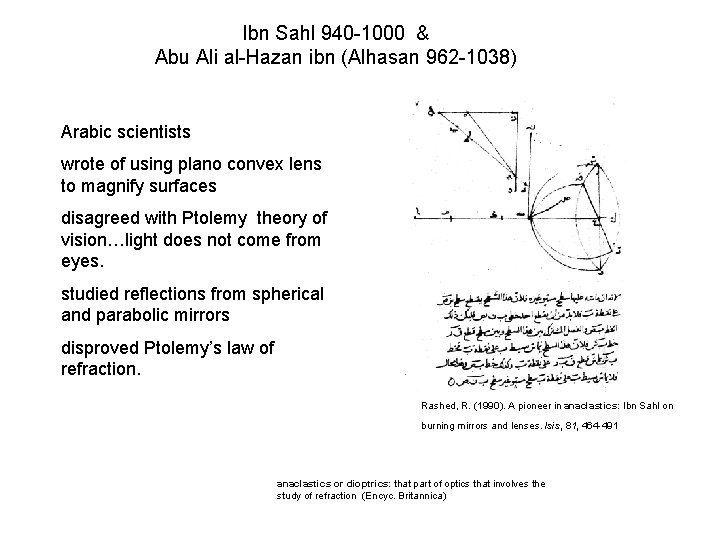
Ibn Sahl 940 -1000 & Abu Ali al-Hazan ibn (Alhasan 962 -1038) Arabic scientists wrote of using plano convex lens to magnify surfaces disagreed with Ptolemy theory of vision…light does not come from eyes. studied reflections from spherical and parabolic mirrors disproved Ptolemy’s law of refraction. Rashed, R. (1990). A pioneer in anaclastics: Ibn Sahl on burning mirrors and lenses. Isis, 81, 464 -491 anaclastics or dioptrics: that part of optics that involves the study of refraction (Encyc. Britannica)
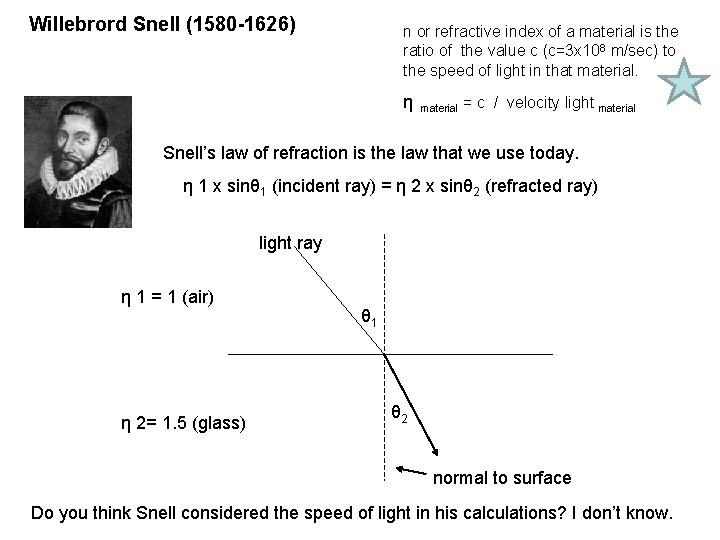
Willebrord Snell (1580 -1626) n or refractive index of a material is the ratio of the value c (c=3 x 108 m/sec) to the speed of light in that material. η material = c / velocity light material Snell’s law of refraction is the law that we use today. η 1 x sinθ 1 (incident ray) = η 2 x sinθ 2 (refracted ray) light ray η 1 = 1 (air) η 2= 1. 5 (glass) θ 1 θ 2 normal to surface Do you think Snell considered the speed of light in his calculations? I don’t know.
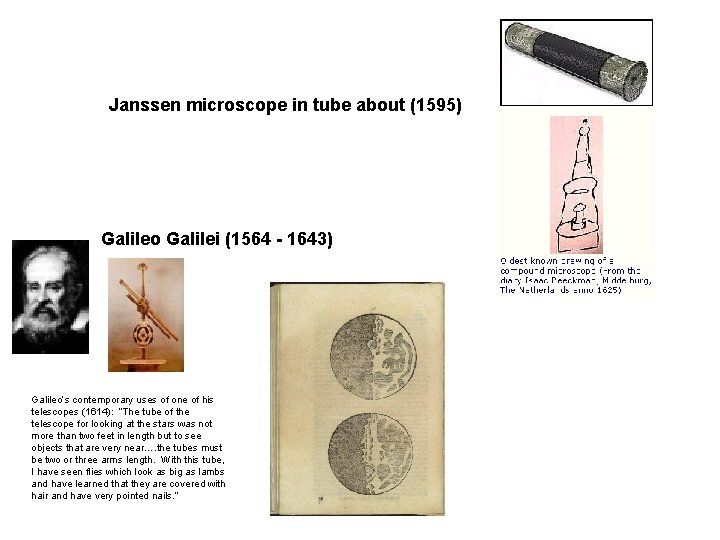
Janssen microscope in tube about (1595) Galileo Galilei (1564 - 1643) Galileo’s contemporary uses of one of his telescopes (1614): “The tube of the telescope for looking at the stars was not more than two feet in length but to see objects that are very near…. the tubes must be two or three arms length. With this tube, I have seen flies which look as big as lambs and have learned that they are covered with hair and have very pointed nails. ”
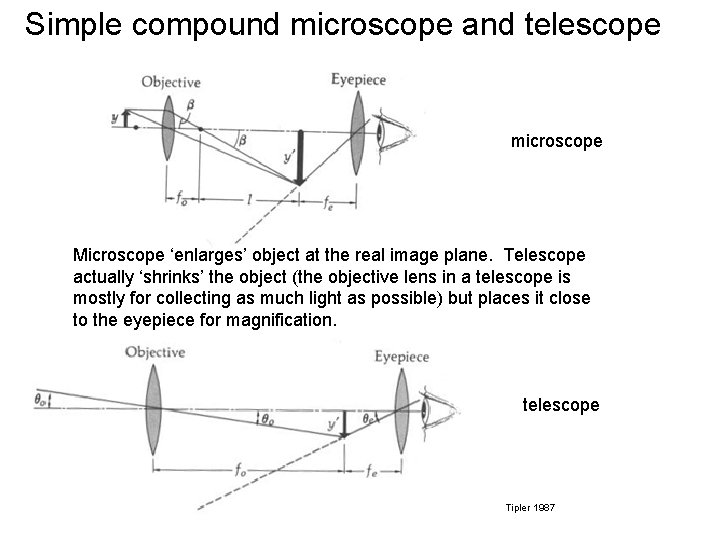
Simple compound microscope and telescope microscope Microscope ‘enlarges’ object at the real image plane. Telescope actually ‘shrinks’ the object (the objective lens in a telescope is mostly for collecting as much light as possible) but places it close to the eyepiece for magnification. telescope Tipler 1987
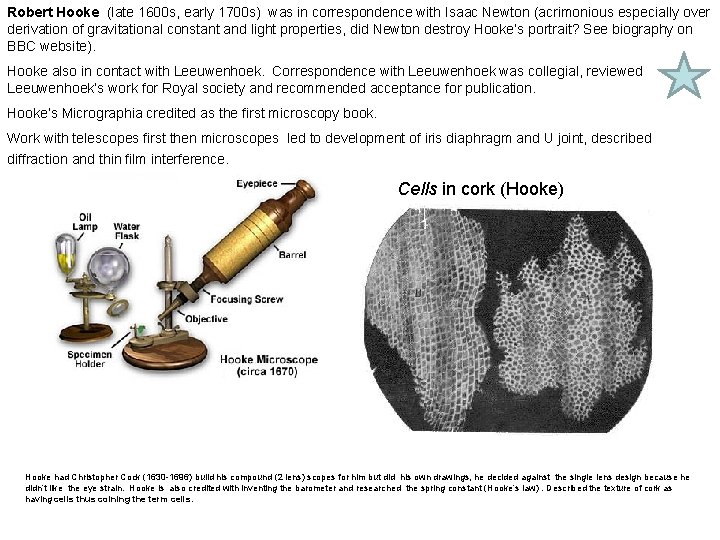
Robert Hooke (late 1600 s, early 1700 s) was in correspondence with Isaac Newton (acrimonious especially over derivation of gravitational constant and light properties, did Newton destroy Hooke’s portrait? See biography on BBC website). Hooke also in contact with Leeuwenhoek. Correspondence with Leeuwenhoek was collegial, reviewed Leeuwenhoek’s work for Royal society and recommended acceptance for publication. Hooke’s Micrographia credited as the first microscopy book. Work with telescopes first then microscopes led to development of iris diaphragm and U joint, described diffraction and thin film interference. Cells in cork (Hooke) Hooke had Christopher Cock (1630 -1696) build his compound (2 lens) scopes for him but did his own drawings, he decided against the single lens design because he didn’t like the eye strain. Hooke is also credited with inventing the barometer and researched the spring constant (Hooke’s law). Described the texture of cork as having cells thus coining the term cells.
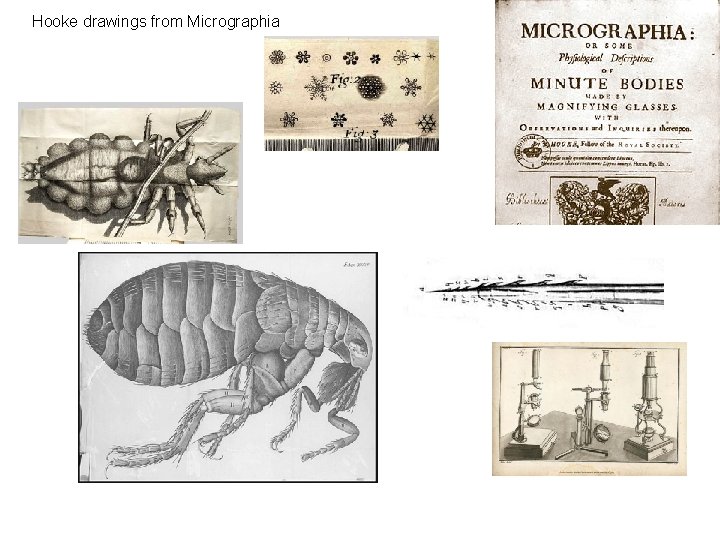
Hooke drawings from Micrographia
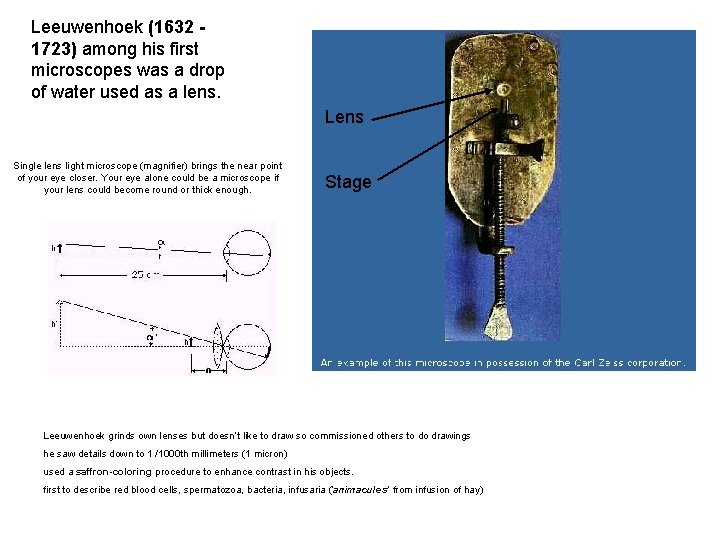
Leeuwenhoek (1632 - 1723) among his first microscopes was a drop of water used as a lens. Lens Single lens light microscope (magnifier) brings the near point of your eye closer. Your eye alone could be a microscope if your lens could become round or thick enough. Stage Leeuwenhoek grinds own lenses but doesn’t like to draw so commissioned others to do drawings he saw details down to 1 /1000 th millimeters (1 micron) used a saffron-coloring procedure to enhance contrast in his objects. first to describe red blood cells, spermatozoa, bacteria, infusaria (‘animacules’ from infusion of hay)
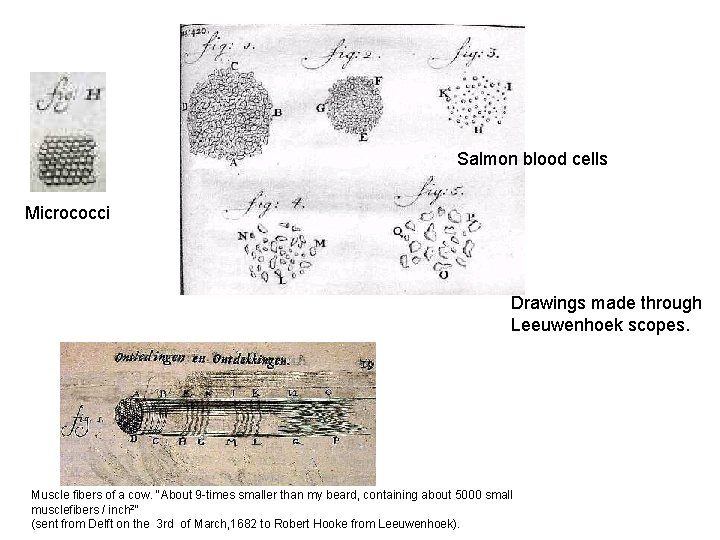
Salmon blood cells Micrococci Drawings made through Leeuwenhoek scopes. Muscle fibers of a cow. "About 9 -times smaller than my beard, containing about 5000 small musclefibers / inch 2“ (sent from Delft on the 3 rd of March, 1682 to Robert Hooke from Leeuwenhoek).
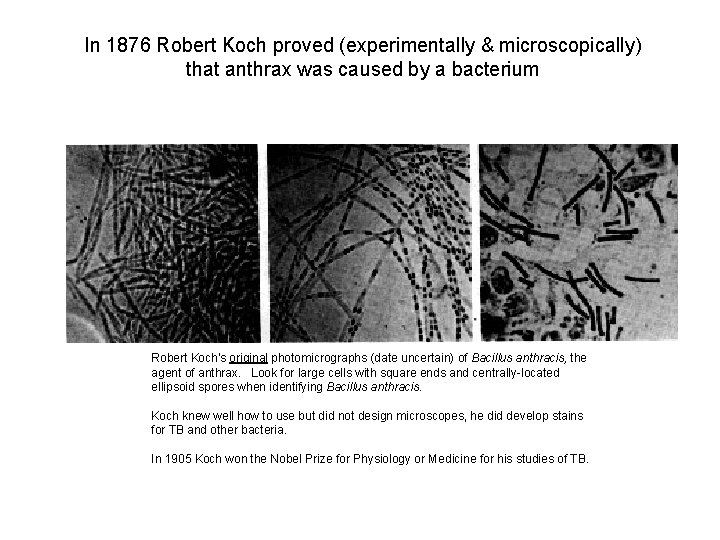
In 1876 Robert Koch proved (experimentally & microscopically) that anthrax was caused by a bacterium Robert Koch's original photomicrographs (date uncertain) of Bacillus anthracis, the agent of anthrax. Look for large cells with square ends and centrally-located ellipsoid spores when identifying Bacillus anthracis. Koch knew well how to use but did not design microscopes, he did develop stains for TB and other bacteria. In 1905 Koch won the Nobel Prize for Physiology or Medicine for his studies of TB.
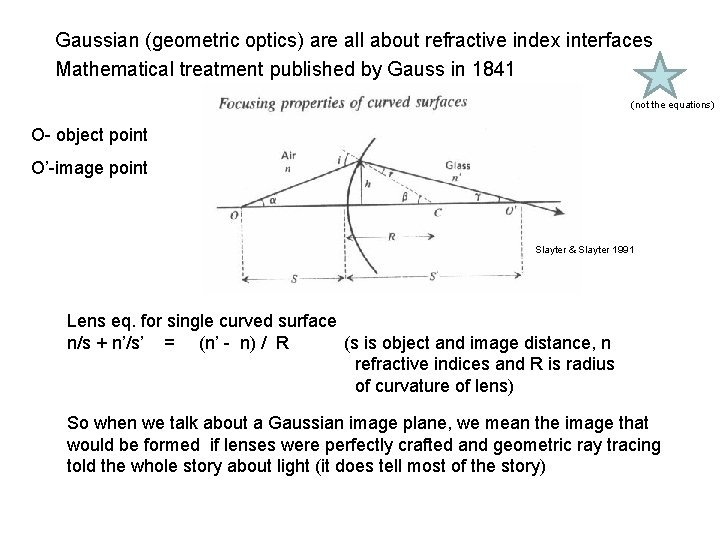
Gaussian (geometric optics) are all about refractive index interfaces Mathematical treatment published by Gauss in 1841 (not the equations) O- object point O’-image point Slayter & Slayter 1991 Lens eq. for single curved surface n/s + n’/s’ = (n’ - n) / R (s is object and image distance, n refractive indices and R is radius of curvature of lens) So when we talk about a Gaussian image plane, we mean the image that would be formed if lenses were perfectly crafted and geometric ray tracing told the whole story about light (it does tell most of the story)
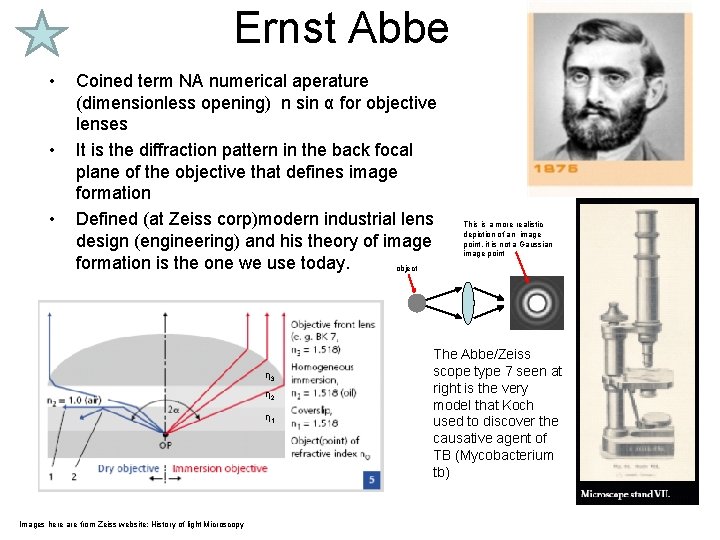
Ernst Abbe • • • Coined term NA numerical aperature (dimensionless opening) n sin α for objective lenses It is the diffraction pattern in the back focal plane of the objective that defines image formation Defined (at Zeiss corp)modern industrial lens design (engineering) and his theory of image formation is the one we use today. object η 3 η 2 η 1 Images here are from Zeiss website; History of light Microscopy This is a more realistic depiction of an image point, it is not a Gaussian image point The Abbe/Zeiss scope type 7 seen at right is the very model that Koch used to discover the causative agent of TB (Mycobacterium tb)
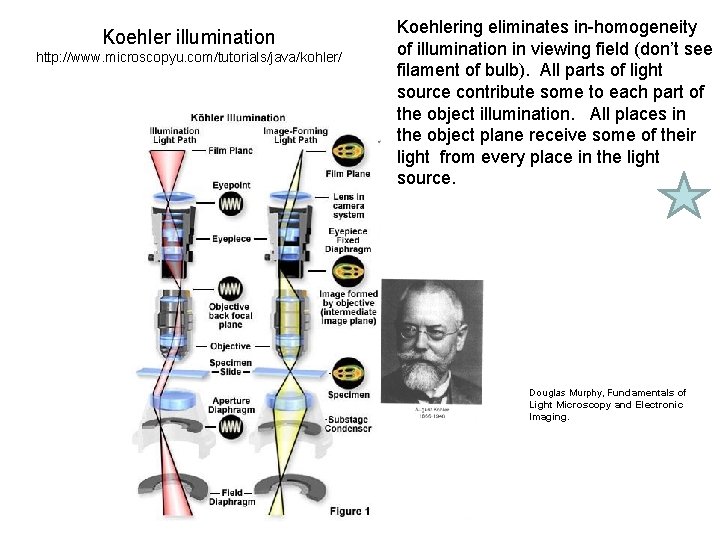
Koehler illumination http: //www. microscopyu. com/tutorials/java/kohler/ Koehlering eliminates in-homogeneity of illumination in viewing field (don’t see filament of bulb). All parts of light source contribute some to each part of the object illumination. All places in the object plane receive some of their light from every place in the light source. Douglas Murphy, Fundamentals of Light Microscopy and Electronic Imaging.
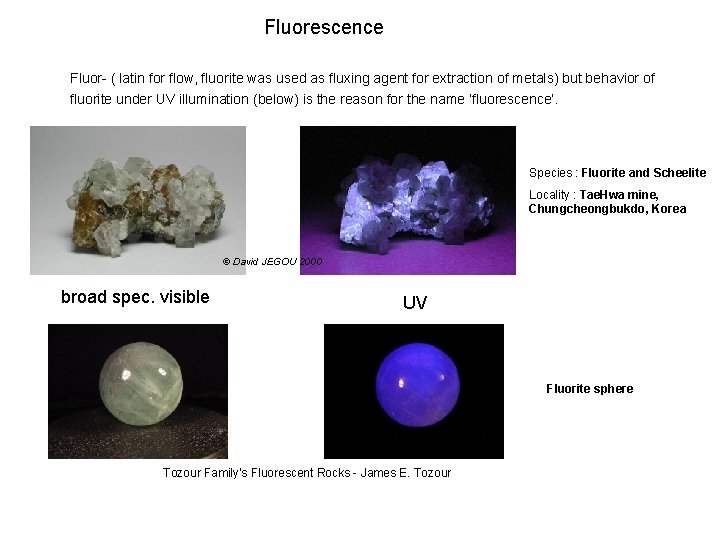
Fluorescence Fluor- ( latin for flow, fluorite was used as fluxing agent for extraction of metals) but behavior of fluorite under UV illumination (below) is the reason for the name ‘fluorescence’. Species : Fluorite and Scheelite Locality : Tae. Hwa mine, Chungcheongbukdo, Korea © David JEGOU 2000 broad spec. visible UV Fluorite sphere Tozour Family's Fluorescent Rocks - James E. Tozour
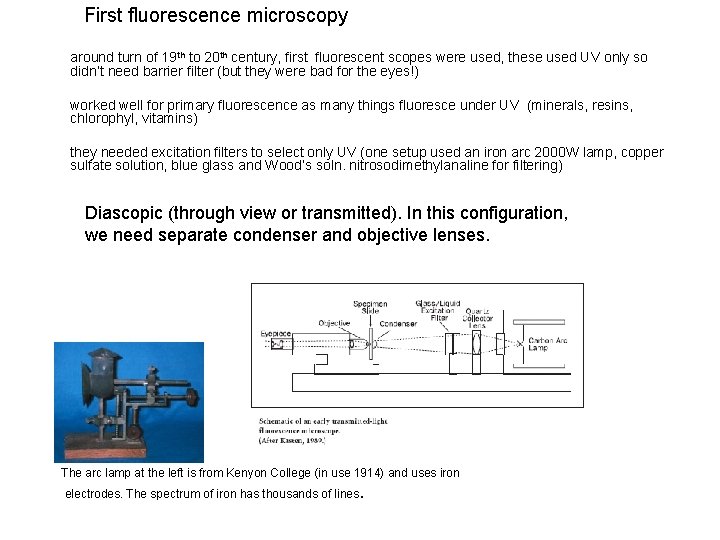
First fluorescence microscopy around turn of 19 th to 20 th century, first fluorescent scopes were used, these used UV only so didn’t need barrier filter (but they were bad for the eyes!) worked well for primary fluorescence as many things fluoresce under UV (minerals, resins, chlorophyl, vitamins) they needed excitation filters to select only UV (one setup used an iron arc 2000 W lamp, copper sulfate solution, blue glass and Wood’s soln. nitrosodimethylanaline for filtering) Diascopic (through view or transmitted). In this configuration, we need separate condenser and objective lenses. The arc lamp at the left is from Kenyon College (in use 1914) and uses iron electrodes. The spectrum of iron has thousands of lines.
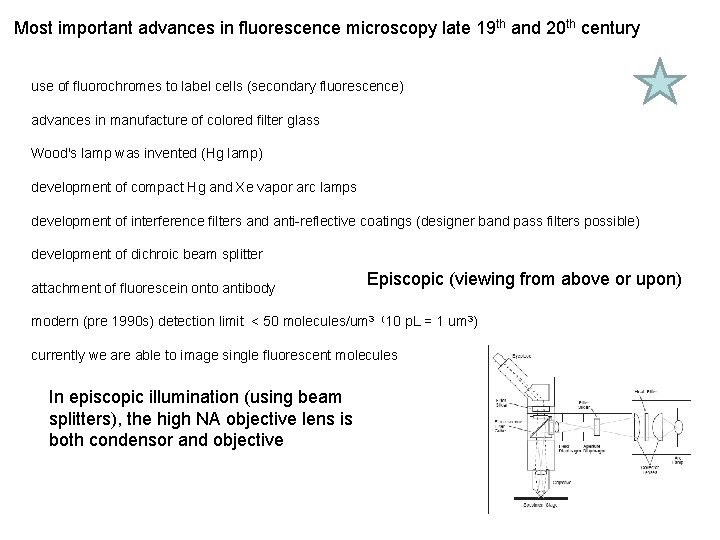
Most important advances in fluorescence microscopy late 19 th and 20 th century use of fluorochromes to label cells (secondary fluorescence) advances in manufacture of colored filter glass Wood's lamp was invented (Hg lamp) development of compact Hg and Xe vapor arc lamps development of interference filters and anti-reflective coatings (designer band pass filters possible) development of dichroic beam splitter attachment of fluorescein onto antibody Episcopic (viewing from above or upon) modern (pre 1990 s) detection limit < 50 molecules/um 3 (10 p. L = 1 um 3) currently we are able to image single fluorescent molecules In episcopic illumination (using beam splitters), the high NA objective lens is both condensor and objective
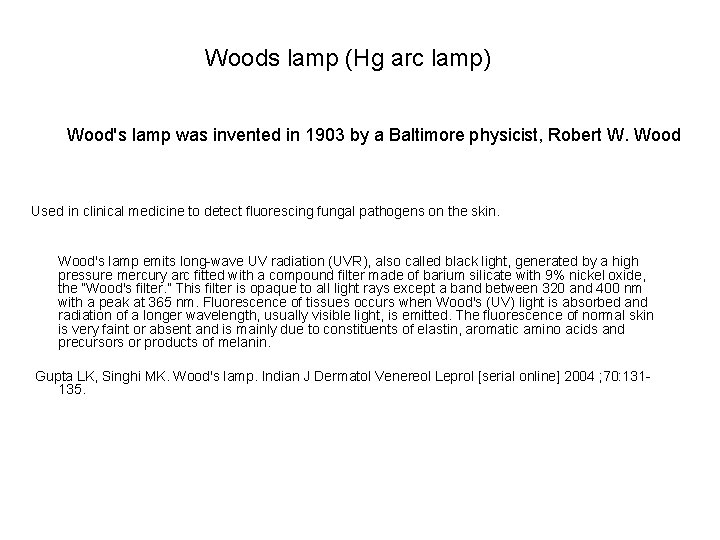
Woods lamp (Hg arc lamp) Wood's lamp was invented in 1903 by a Baltimore physicist, Robert W. Wood Used in clinical medicine to detect fluorescing fungal pathogens on the skin. Wood's lamp emits long-wave UV radiation (UVR), also called black light, generated by a high pressure mercury arc fitted with a compound filter made of barium silicate with 9% nickel oxide, the “Wood's filter. ” This filter is opaque to all light rays except a band between 320 and 400 nm with a peak at 365 nm. Fluorescence of tissues occurs when Wood's (UV) light is absorbed and radiation of a longer wavelength, usually visible light, is emitted. The fluorescence of normal skin is very faint or absent and is mainly due to constituents of elastin, aromatic amino acids and precursors or products of melanin. Gupta LK, Singhi MK. Wood's lamp. Indian J Dermatol Venereol Leprol [serial online] 2004 ; 70: 131135.
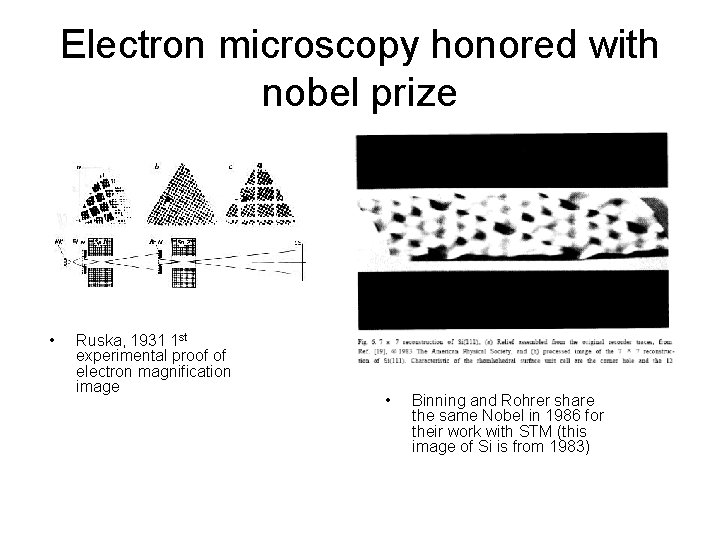
Electron microscopy honored with nobel prize • Ruska, 1931 1 st experimental proof of electron magnification image • Binning and Rohrer share the same Nobel in 1986 for their work with STM (this image of Si is from 1983)
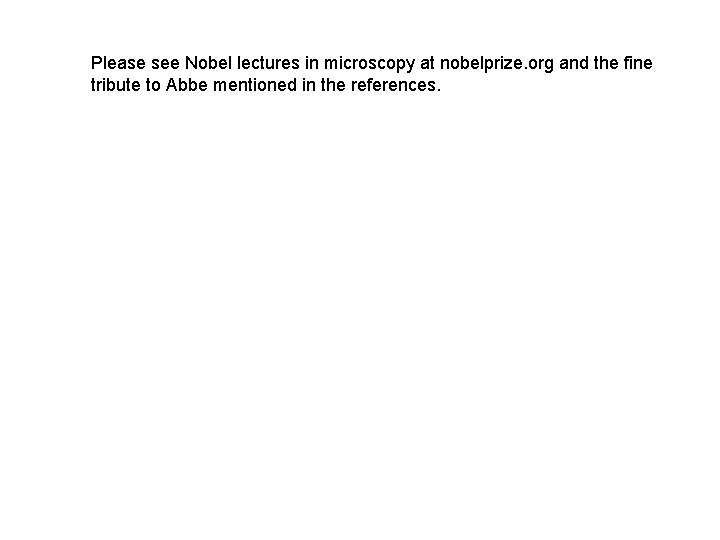
Please see Nobel lectures in microscopy at nobelprize. org and the fine tribute to Abbe mentioned in the references.
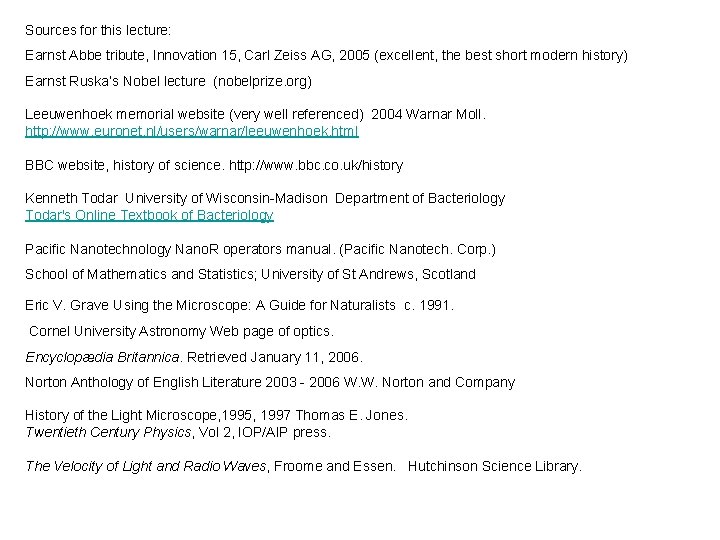
Sources for this lecture: Earnst Abbe tribute, Innovation 15, Carl Zeiss AG, 2005 (excellent, the best short modern history) Earnst Ruska’s Nobel lecture (nobelprize. org) Leeuwenhoek memorial website (very well referenced) 2004 Warnar Moll. http: //www. euronet. nl/users/warnar/leeuwenhoek. html BBC website, history of science. http: //www. bbc. co. uk/history Kenneth Todar University of Wisconsin-Madison Department of Bacteriology Todar's Online Textbook of Bacteriology Pacific Nanotechnology Nano. R operators manual. (Pacific Nanotech. Corp. ) School of Mathematics and Statistics; University of St Andrews, Scotland Eric V. Grave Using the Microscope: A Guide for Naturalists c. 1991. Cornel University Astronomy Web page of optics. Encyclopædia Britannica. Retrieved January 11, 2006. Norton Anthology of English Literature 2003 - 2006 W. W. Norton and Company History of the Light Microscope, 1995, 1997 Thomas E. Jones. Twentieth Century Physics, Vol 2, IOP/AIP press. The Velocity of Light and Radio Waves, Froome and Essen. Hutchinson Science Library.
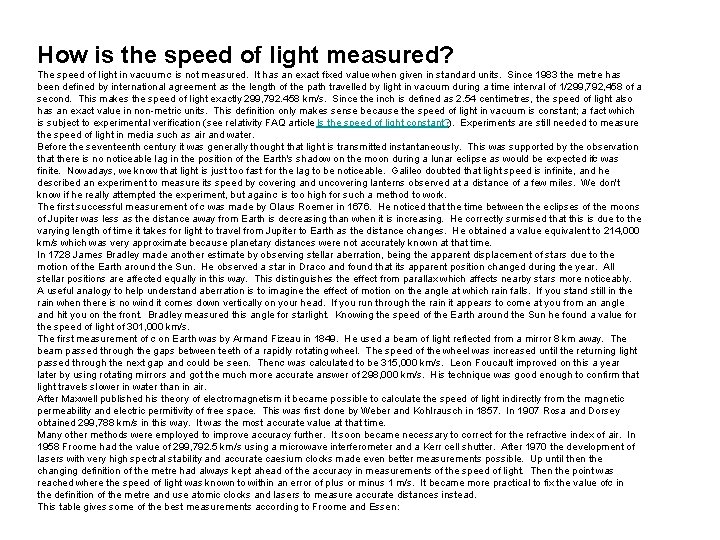
How is the speed of light measured? The speed of light in vacuum c is not measured. It has an exact fixed value when given in standard units. Since 1983 the metre has been defined by international agreement as the length of the path travelled by light in vacuum during a time interval of 1/299, 792, 458 of a second. This makes the speed of light exactly 299, 792. 458 km/s. Since the inch is defined as 2. 54 centimetres, the speed of light also has an exact value in non-metric units. This definition only makes sense because the speed of light in vacuum is constant; a fact which is subject to experimental verification (see relativity FAQ article Is the speed of light constant? ). Experiments are still needed to measure the speed of light in media such as air and water. Before the seventeenth century it was generally thought that light is transmitted instantaneously. This was supported by the observation that there is no noticeable lag in the position of the Earth's shadow on the moon during a lunar eclipse as would be expected if c was finite. Nowadays, we know that light is just too fast for the lag to be noticeable. Galileo doubted that light speed is infinite, and he described an experiment to measure its speed by covering and uncovering lanterns observed at a distance of a few miles. We don't know if he really attempted the experiment, but again c is too high for such a method to work. The first successful measurement of c was made by Olaus Roemer in 1676. He noticed that the time between the eclipses of the moons of Jupiter was less as the distance away from Earth is decreasing than when it is increasing. He correctly surmised that this is due to the varying length of time it takes for light to travel from Jupiter to Earth as the distance changes. He obtained a value equivalent to 214, 000 km/s which was very approximate because planetary distances were not accurately known at that time. In 1728 James Bradley made another estimate by observing stellar aberration, being the apparent displacement of stars due to the motion of the Earth around the Sun. He observed a star in Draco and found that its apparent position changed during the year. All stellar positions are affected equally in this way. This distinguishes the effect from parallax which affects nearby stars more noticeably. A useful analogy to help understand aberration is to imagine the effect of motion on the angle at which rain falls. If you stand still in the rain when there is no wind it comes down vertically on your head. If you run through the rain it appears to come at you from an angle and hit you on the front. Bradley measured this angle for starlight. Knowing the speed of the Earth around the Sun he found a value for the speed of light of 301, 000 km/s. The first measurement of c on Earth was by Armand Fizeau in 1849. He used a beam of light reflected from a mirror 8 km away. The beam passed through the gaps between teeth of a rapidly rotating wheel. The speed of the wheel was increased until the returning light passed through the next gap and could be seen. Then c was calculated to be 315, 000 km/s. Leon Foucault improved on this a year later by using rotating mirrors and got the much more accurate answer of 298, 000 km/s. His technique was good enough to confirm that light travels slower in water than in air. After Maxwell published his theory of electromagnetism it became possible to calculate the speed of light indirectly from the magnetic permeability and electric permitivity of free space. This was first done by Weber and Kohlrausch in 1857. In 1907 Rosa and Dorsey obtained 299, 788 km/s in this way. It was the most accurate value at that time. Many other methods were employed to improve accuracy further. It soon became necessary to correct for the refractive index of air. In 1958 Froome had the value of 299, 792. 5 km/s using a microwave interferometer and a Kerr cell shutter. After 1970 the development of lasers with very high spectral stability and accurate caesium clocks made even better measurements possible. Up until then the changing definition of the metre had always kept ahead of the accuracy in measurements of the speed of light. Then the point was reached where the speed of light was known to within an error of plus or minus 1 m/s. It became more practical to fix the value of c in the definition of the metre and use atomic clocks and lasers to measure accurate distances instead. This table gives some of the best measurements according to Froome and Essen:
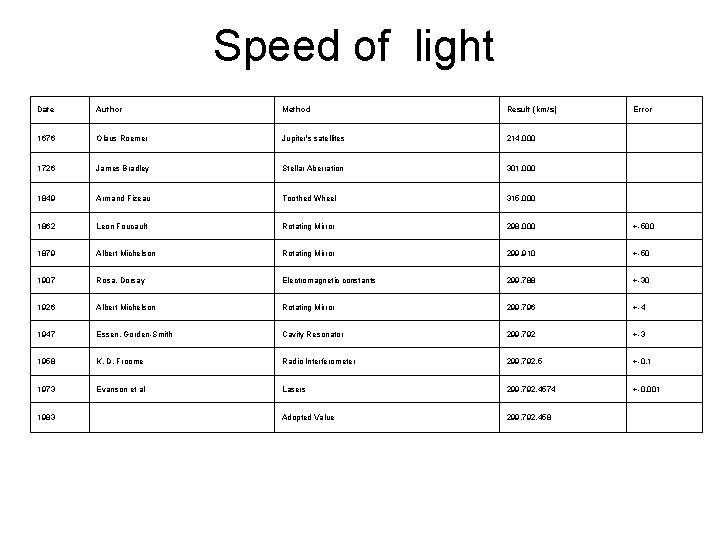
Speed of light Date Author Method Result (km/s) 1676 Olaus Roemer Jupiter's satellites 214, 000 1726 James Bradley Stellar Aberration 301, 000 1849 Armand Fizeau Toothed Wheel 315, 000 1862 Leon Foucault Rotating Mirror 298, 000 +-500 1879 Albert Michelson Rotating Mirror 299, 910 +-50 1907 Rosa, Dorsay Electromagnetic constants 299, 788 +-30 1926 Albert Michelson Rotating Mirror 299, 796 +-4 1947 Essen, Gorden-Smith Cavity Resonator 299, 792 +-3 1958 K. D. Froome Radio Interferometer 299, 792. 5 +-0. 1 1973 Evanson et al Lasers 299, 792. 4574 +-0. 001 Adopted Value 299, 792. 458 1983 Error
Slit diffraction
Difference between light microscope and electron microscope
Microscope mania compound light microscope
Microscope madness these things are crazy small answer key
It is a small round knob on the side of the microscope
Flat platform where you place the slide
Tool makers microscope diagram
Working principle of tool makers microscope
Claims of value
Section lining
Is film a text
Eccentric viewing techniques occupational therapy
Thank you for viewing my presentation
Steps in viewing
Contoh viewing 3d
The viewing pipeline
Viewing coordinate sering juga disebut
Narmeen is viewing the board in the classroom
Constant intensity shading
Types of projection in computer graphics
Introduction to computer graphics - ppt
Normalized device coordinate
Interior and exterior clipping in computer graphics
What process occurs
Orderly visual search pattern definition
Viewing angel
Potter's wheel data cleaning tool
I am hairy i like cheese and; i can chew on your things
Dissecting pins function
Original
Line/strip search pattern