Magnetic toys in the sky Yuri Levin Monash
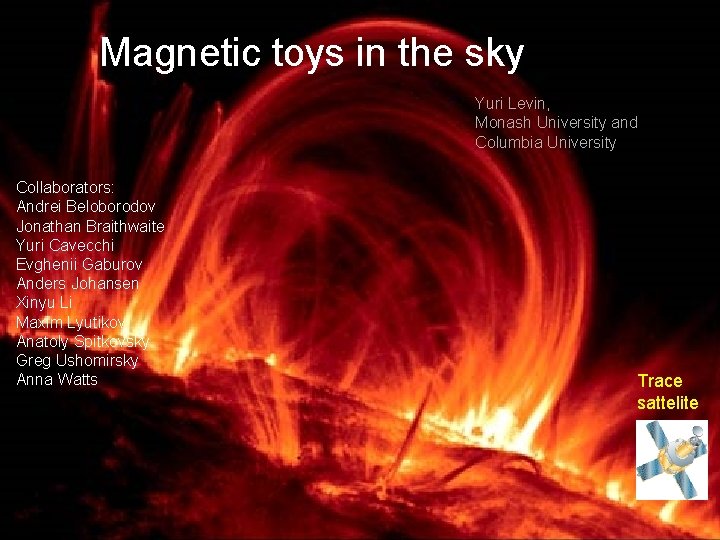
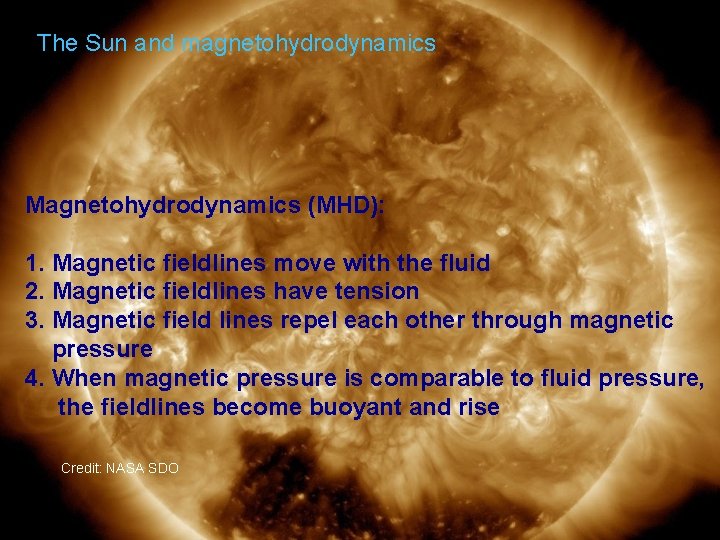
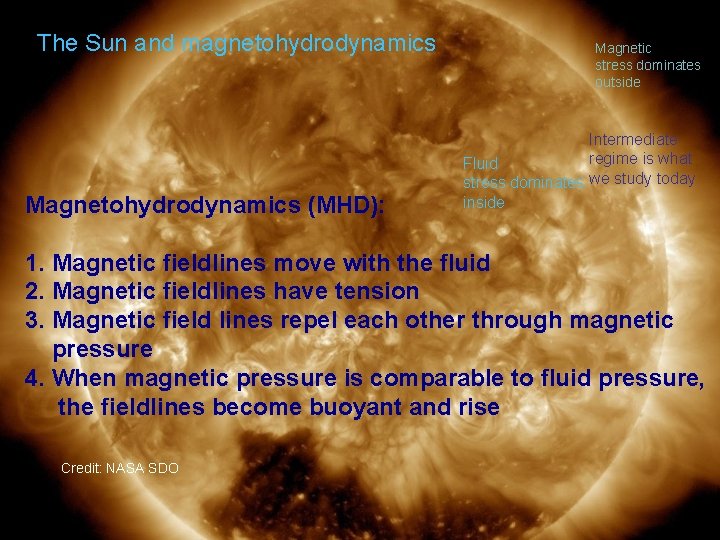
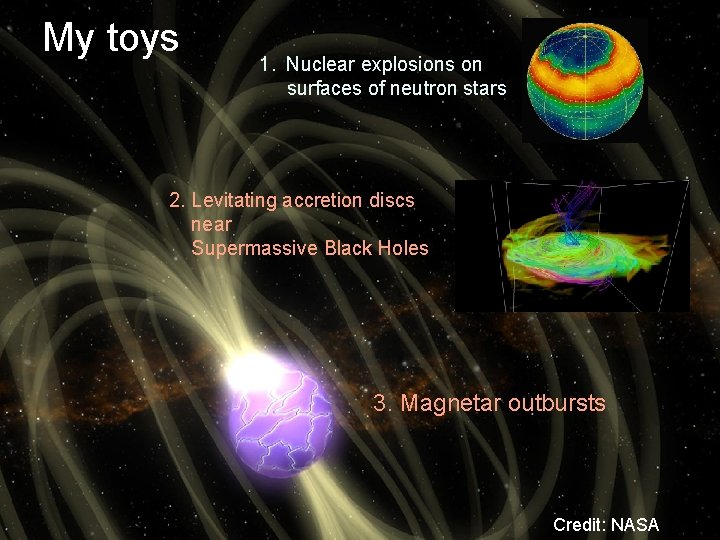
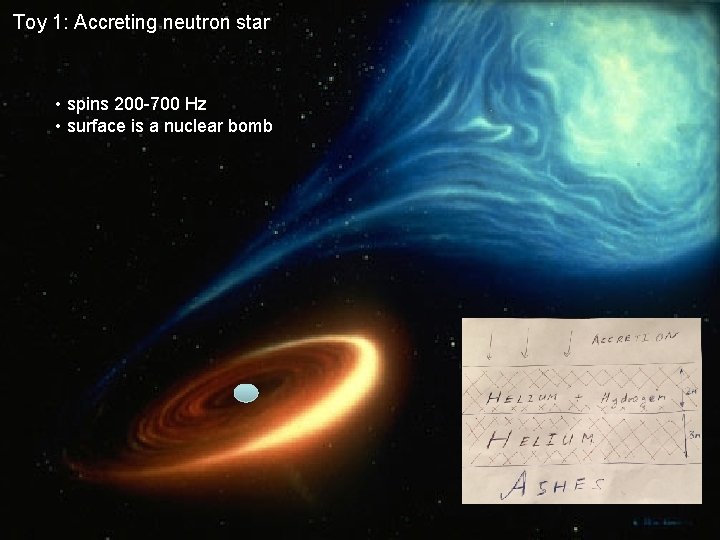
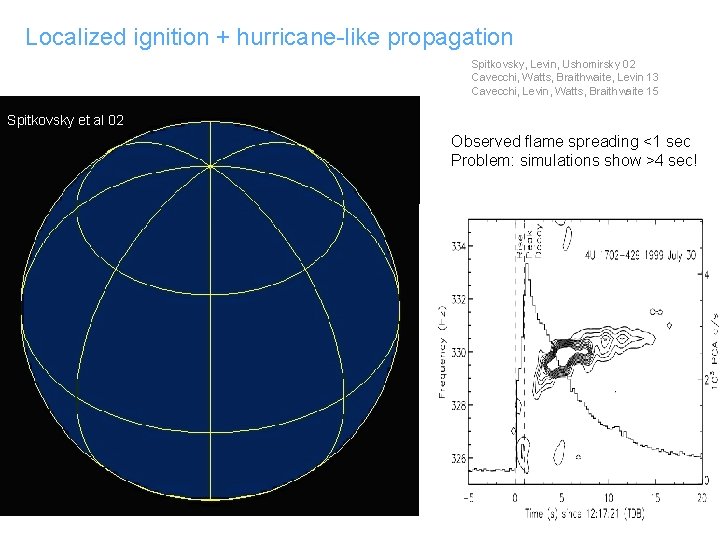
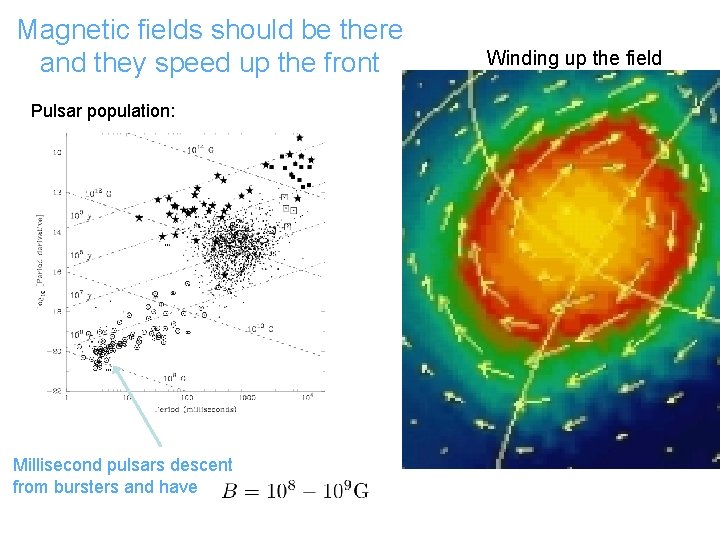
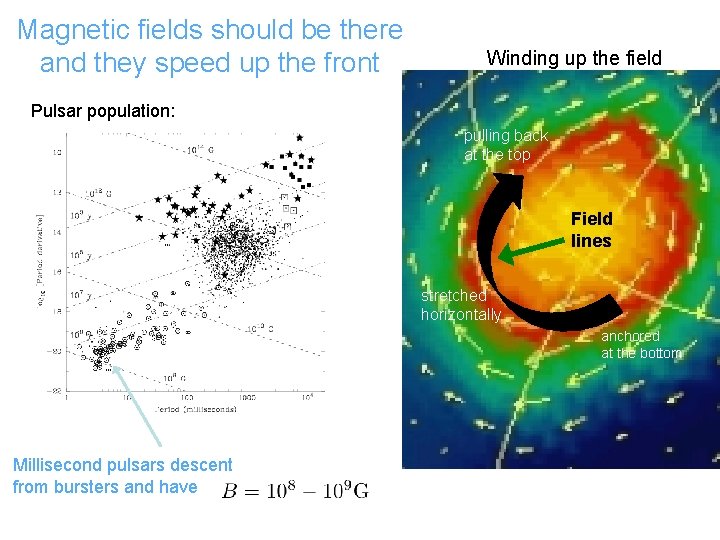
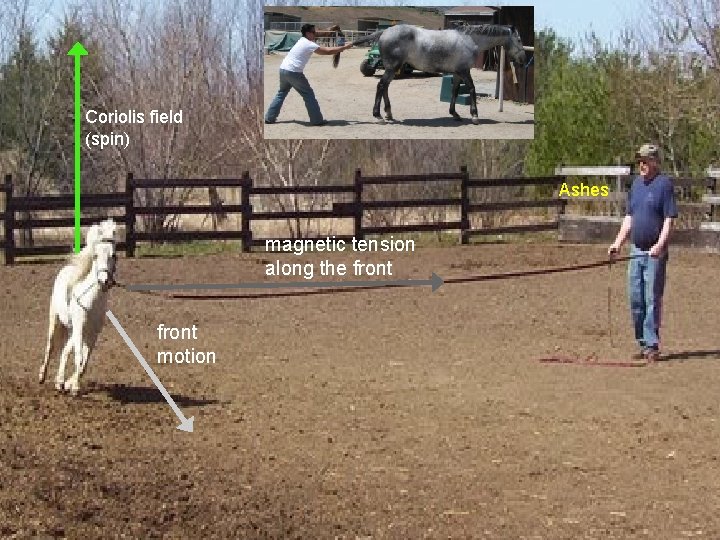
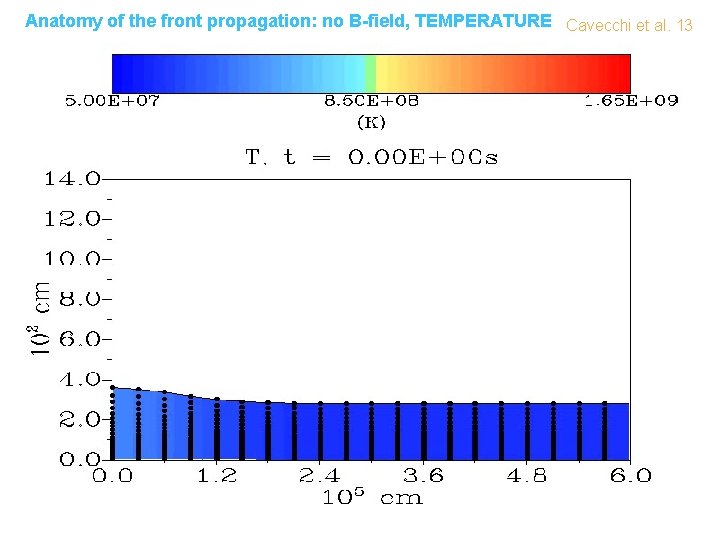
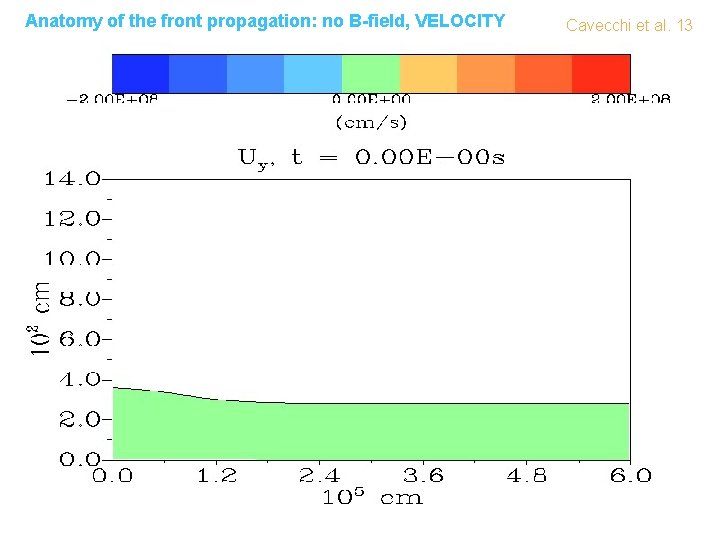
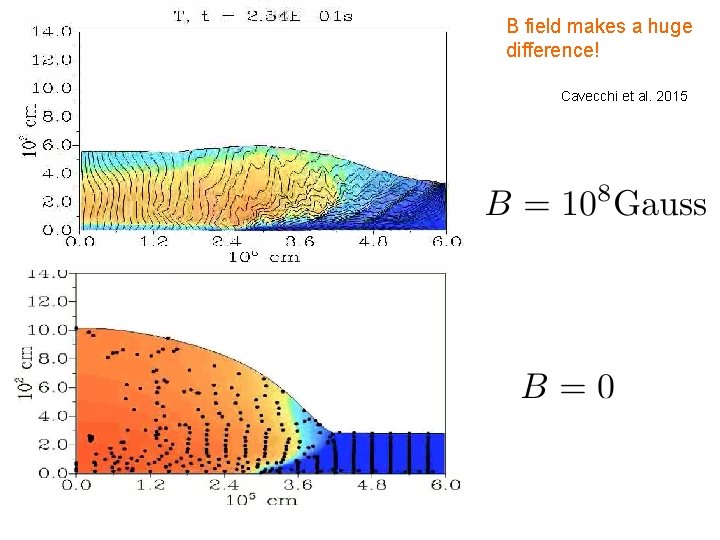
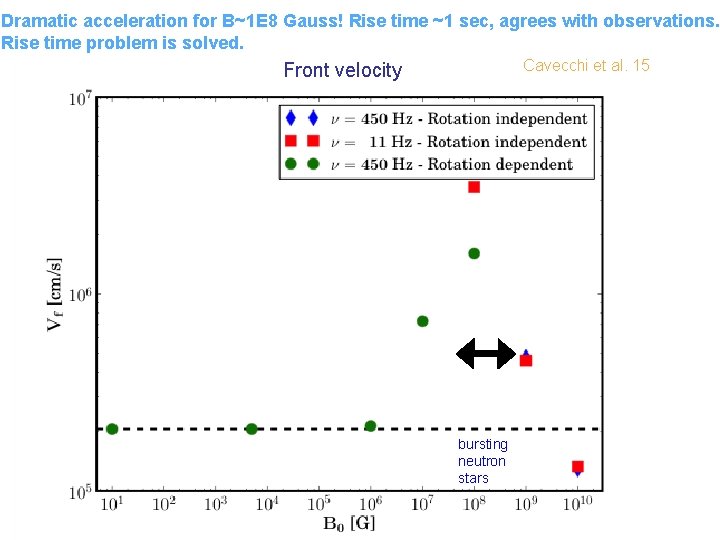
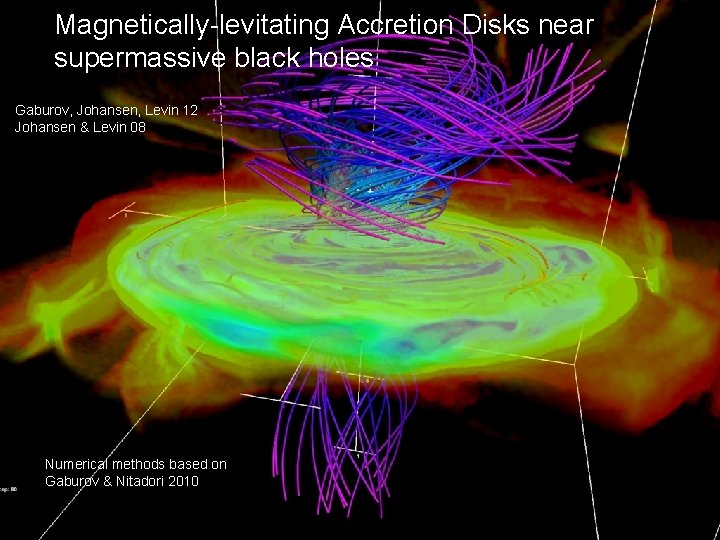
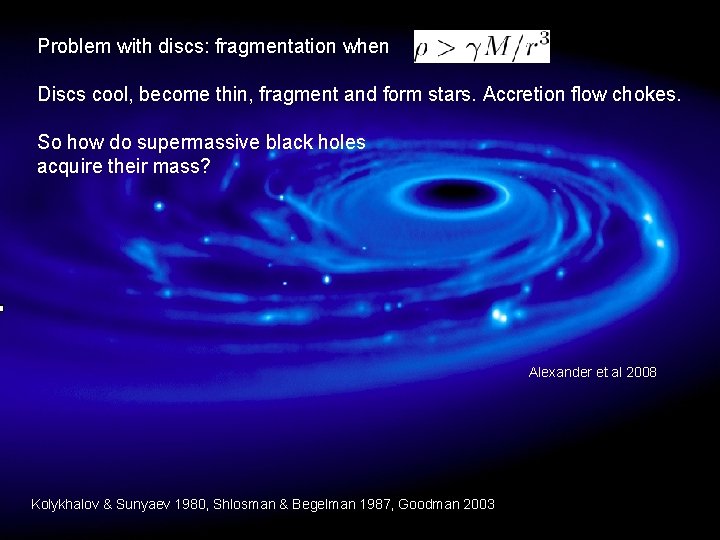
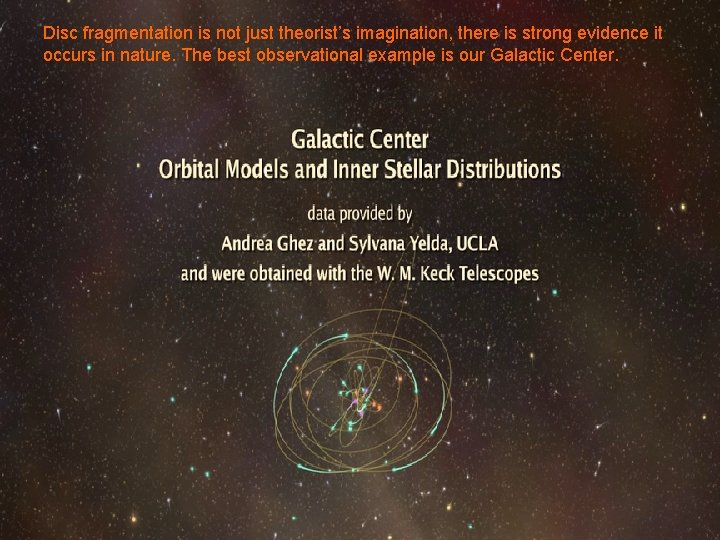
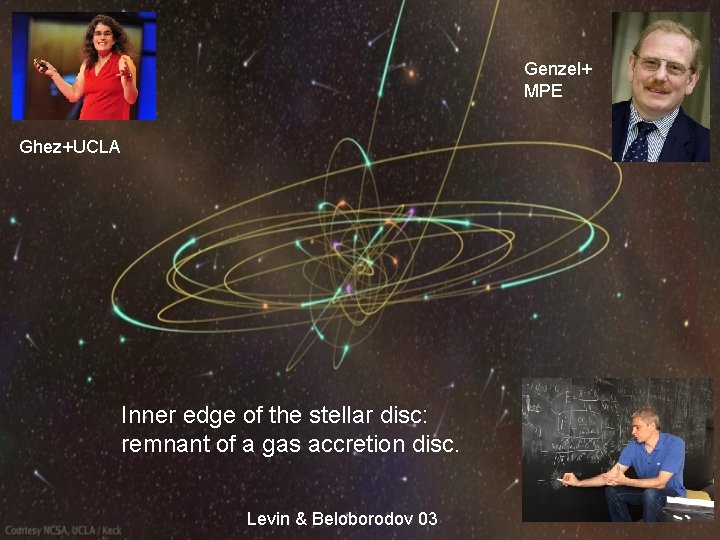
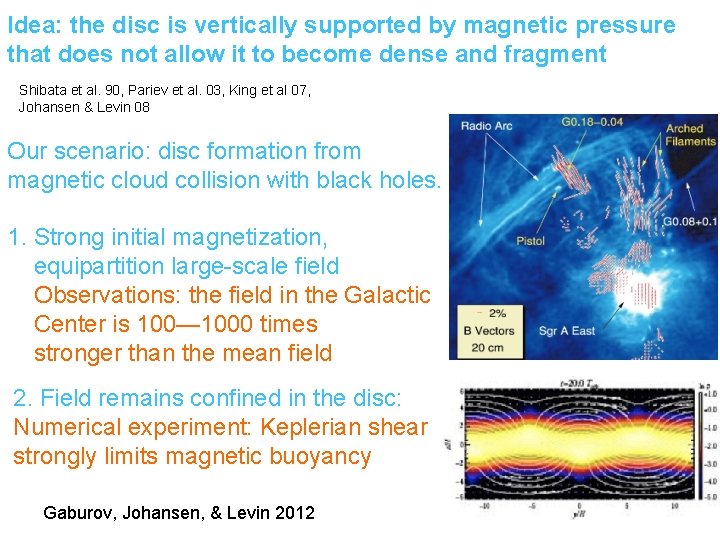
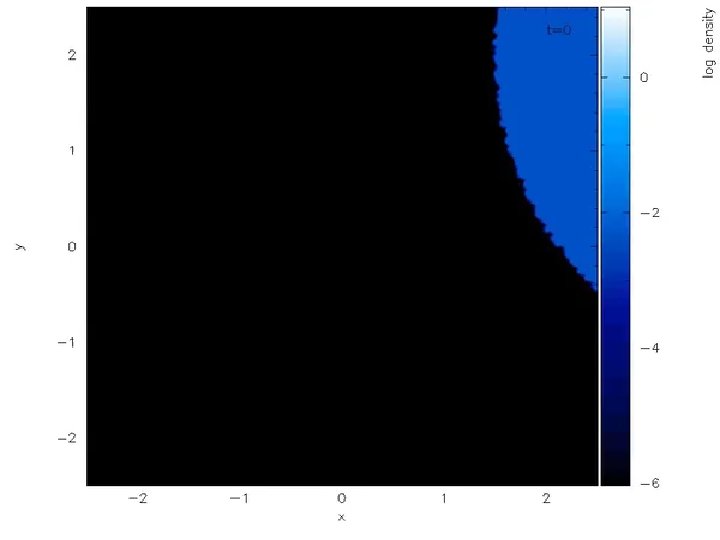
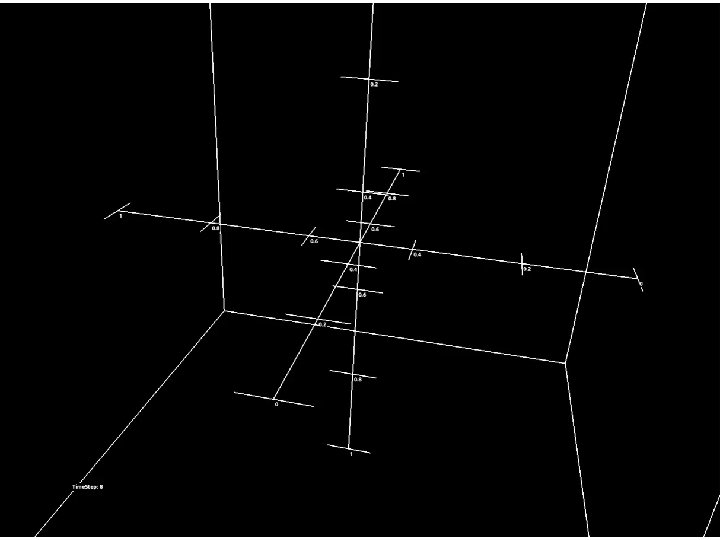
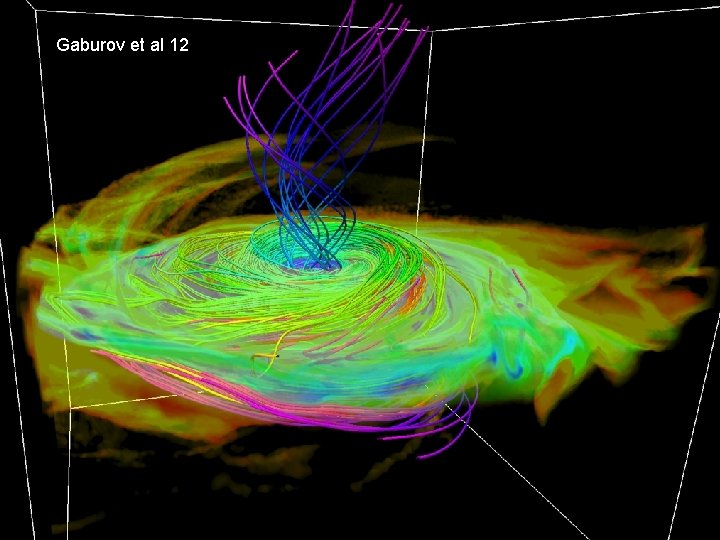
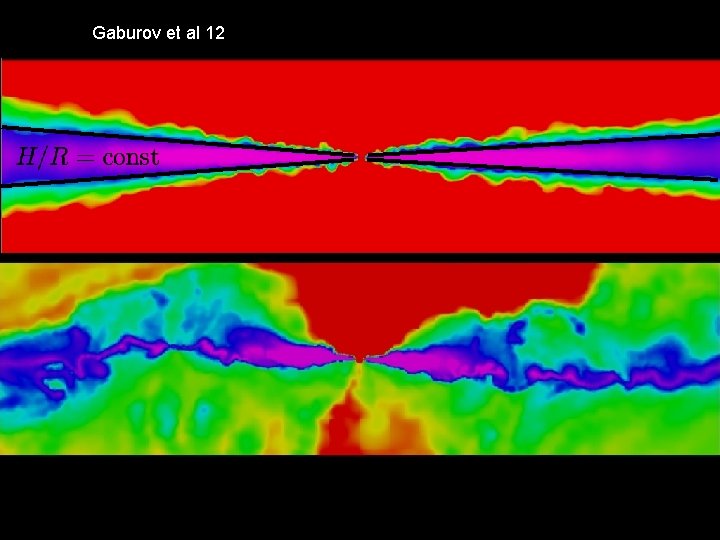
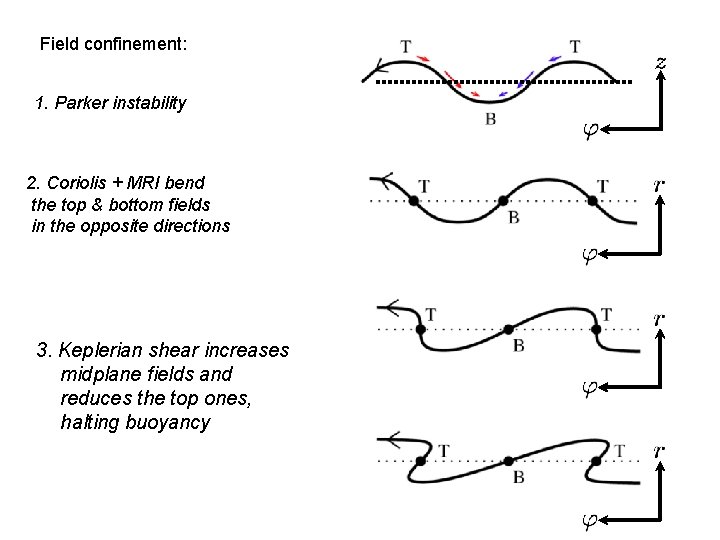
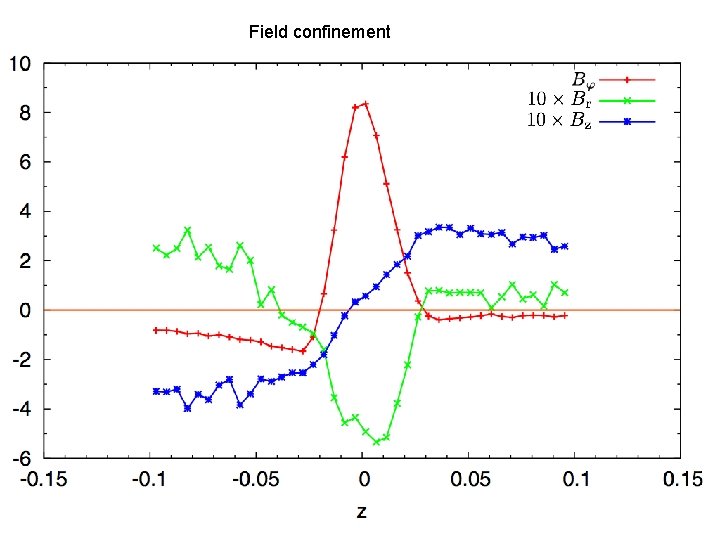
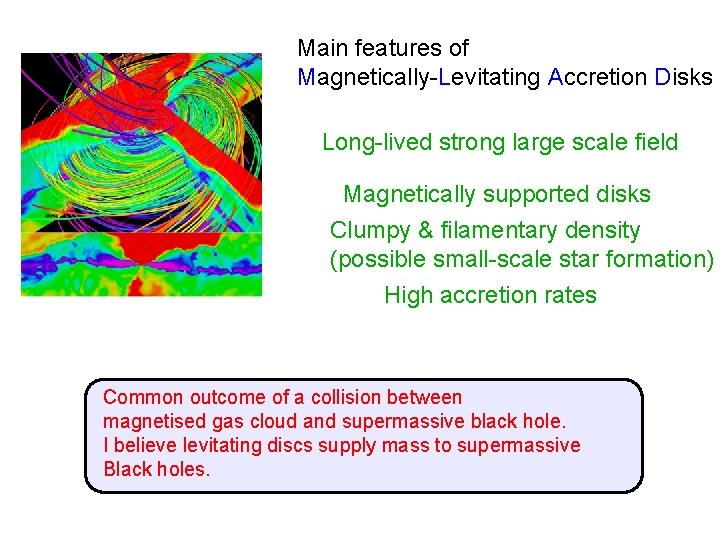
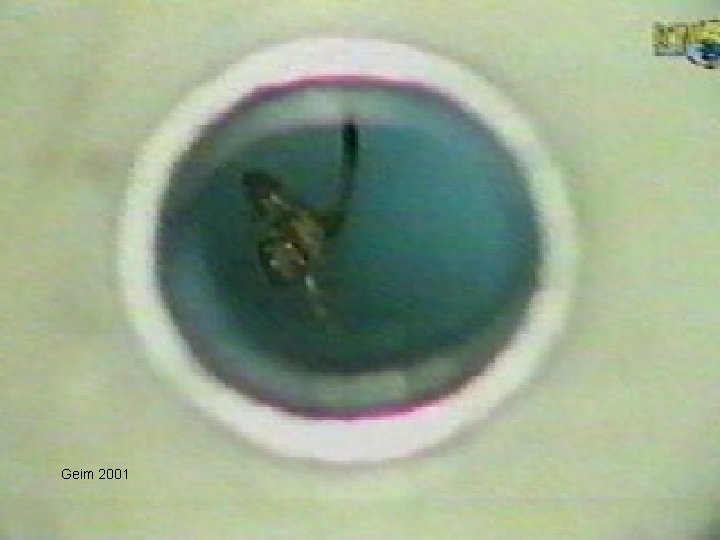
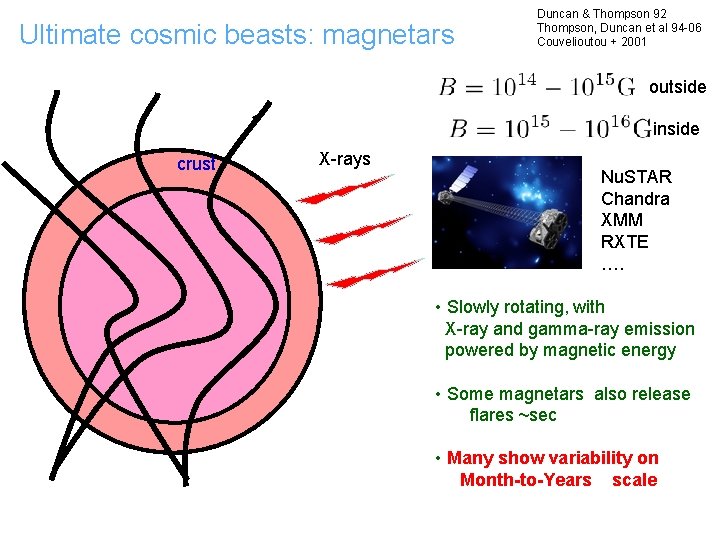
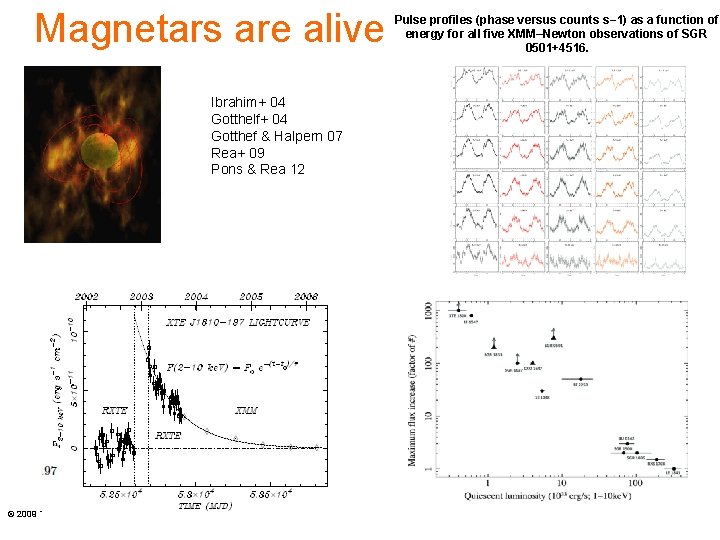
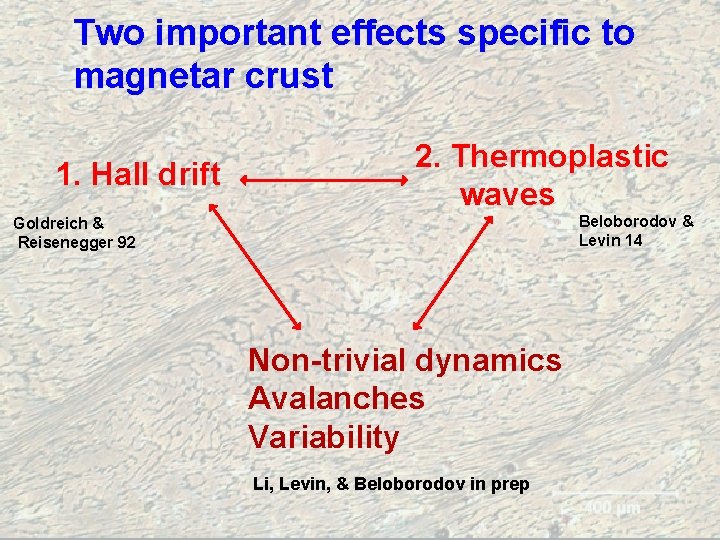
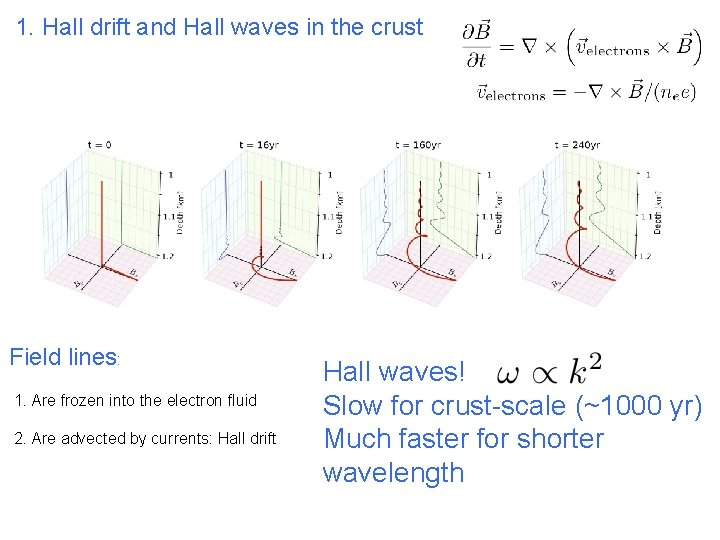
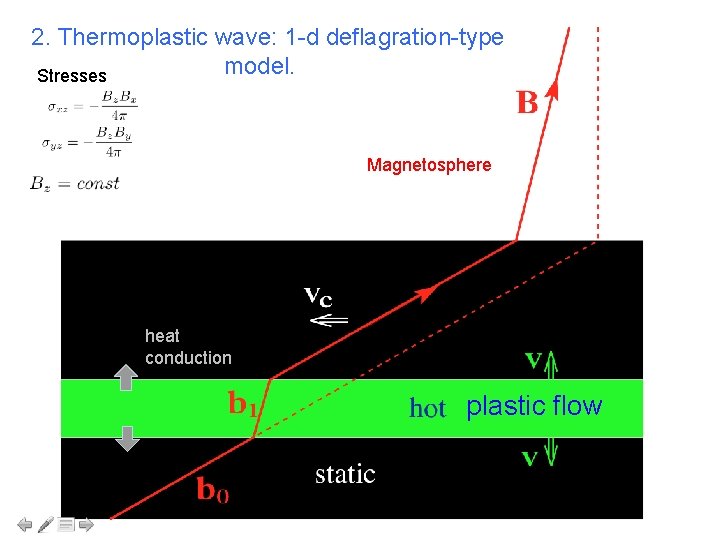
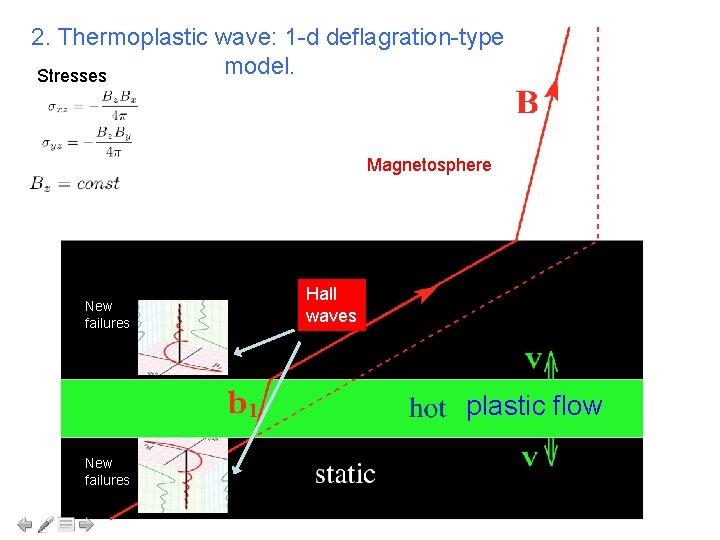
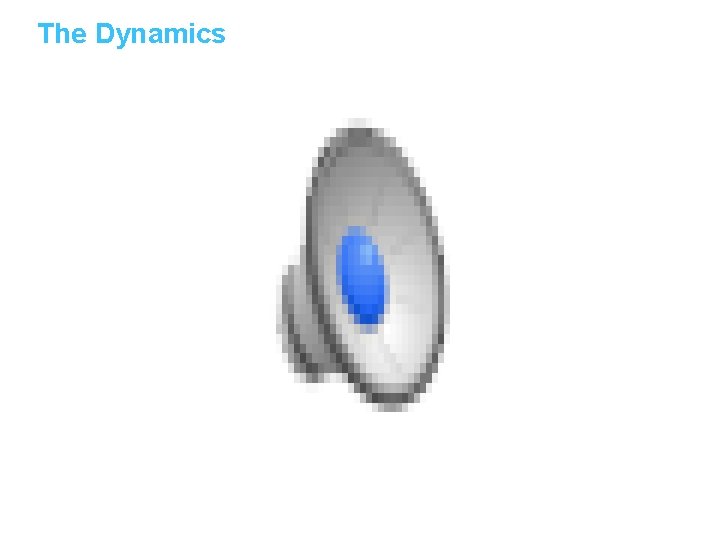
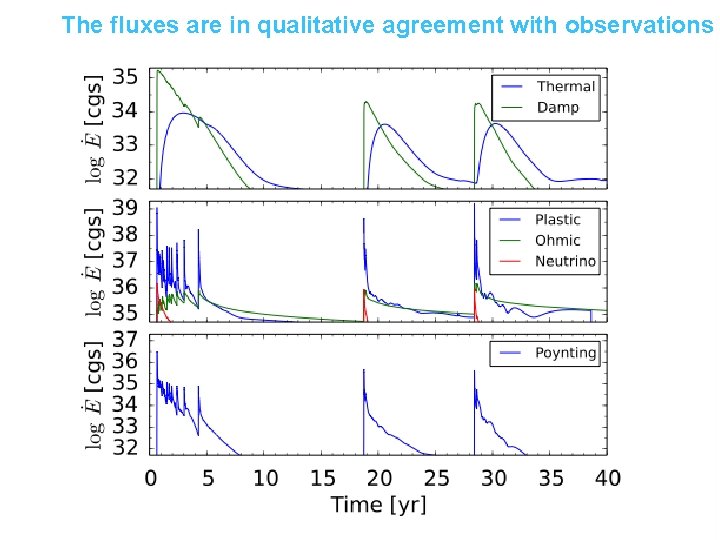
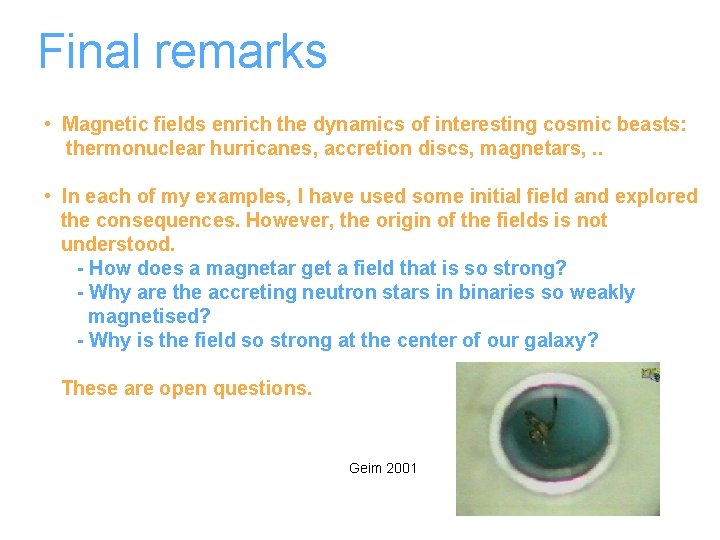
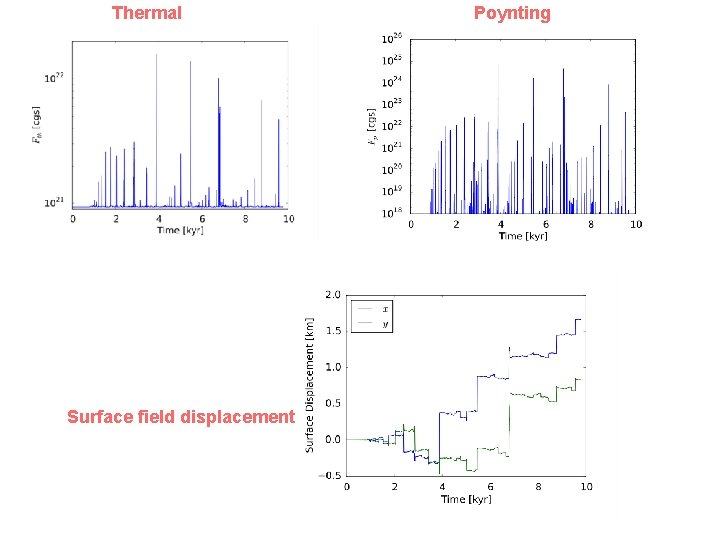
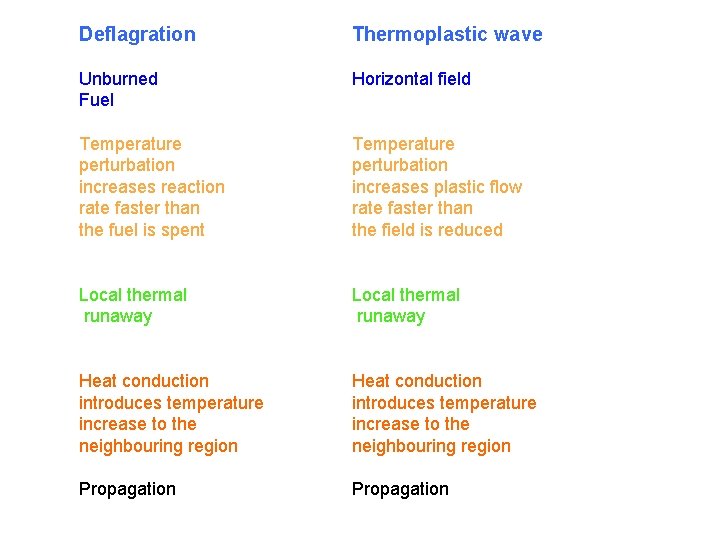
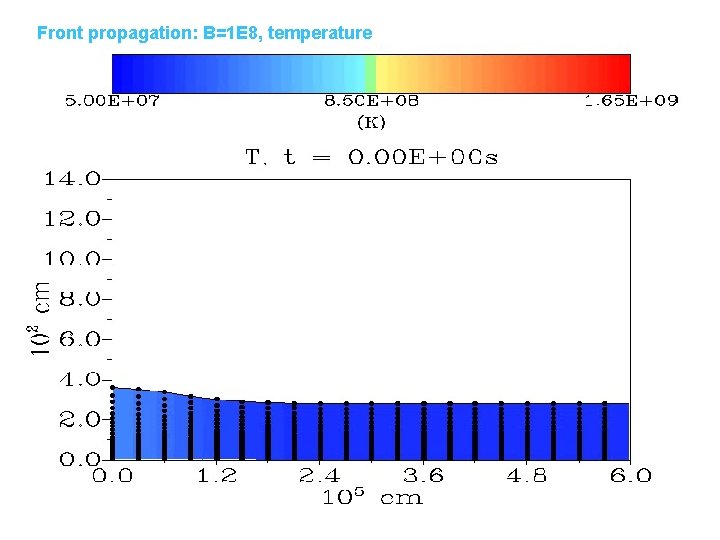
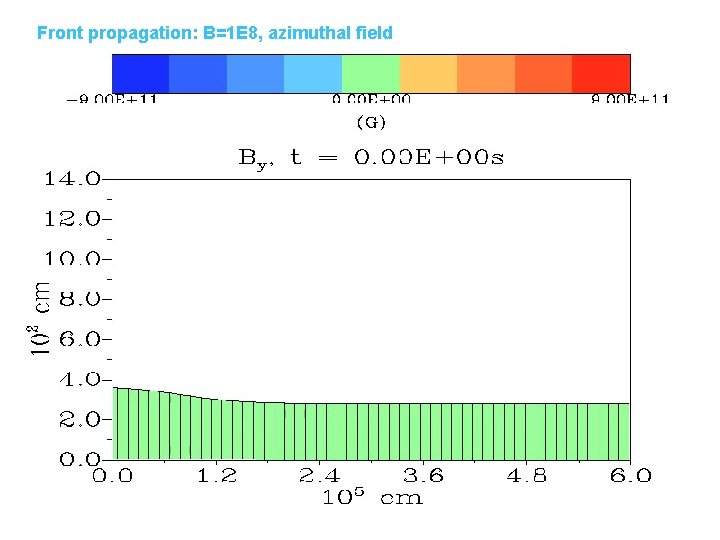
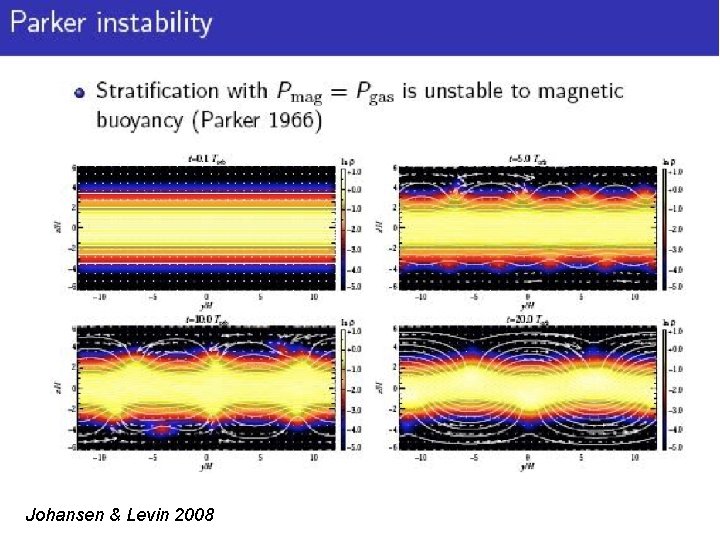
- Slides: 40
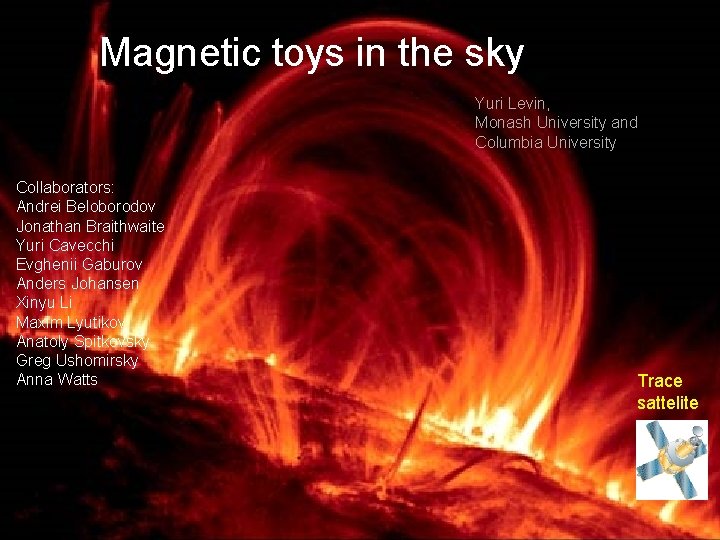
Magnetic toys in the sky Yuri Levin, Monash University and Levin, Monash Columbia Yuri University Collaborators: Andrei Beloborodov Jonathan Braithwaite Yuri Cavecchi Evghenii Gaburov Anders Johansen Xinyu Li Maxim Lyutikov Anatoly Spitkovsky Greg Ushomirsky Anna Watts Trace sattelite Credit: NASA
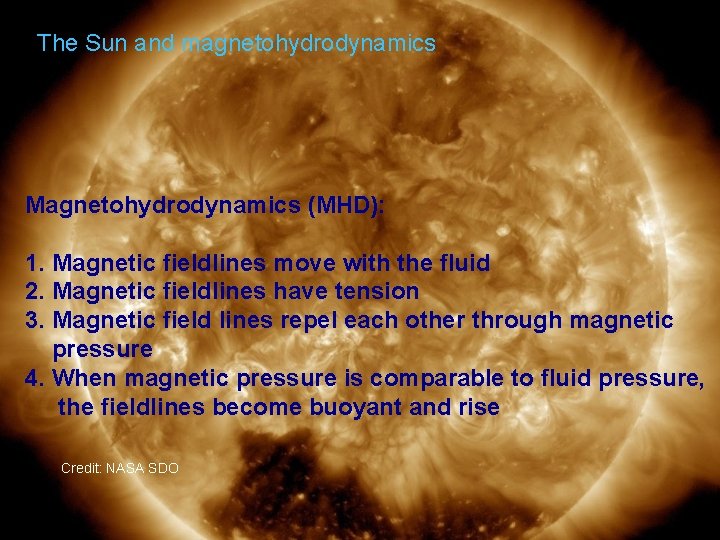
Solar convection solar corona The Sun and magnetohydrodynamics magnetic pressure and magnetic tension Magnetohydrodynamics (MHD): 1. Magnetic fieldlines move with the fluid 2. Magnetic fieldlines have tension 3. Magnetic field lines repel each other through magnetic pressure 4. When magnetic pressure is comparable to fluid pressure, the fieldlines become buoyant and rise Credit: NASA SDO
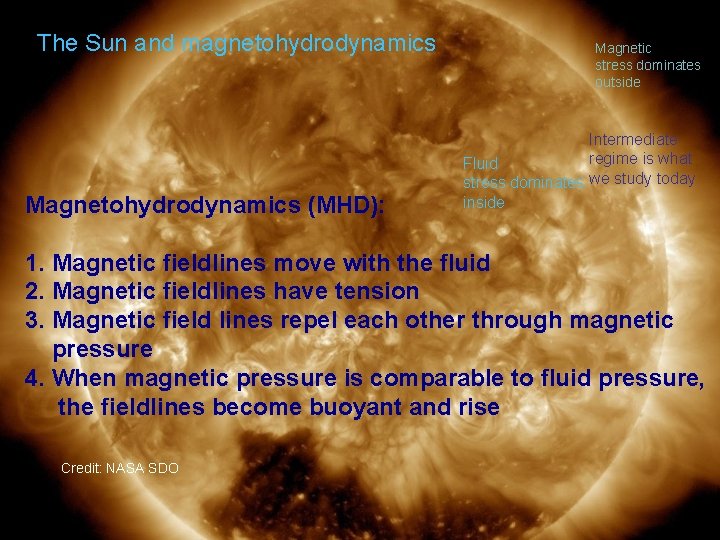
Solar convection solar corona The Sun and magnetohydrodynamics magnetic pressure and magnetic tension Magnetic stress dominates outside Magnetohydrodynamics (MHD): Intermediate regime is what Fluid stress dominates we study today inside 1. Magnetic fieldlines move with the fluid 2. Magnetic fieldlines have tension 3. Magnetic field lines repel each other through magnetic pressure 4. When magnetic pressure is comparable to fluid pressure, the fieldlines become buoyant and rise Credit: NASA SDO
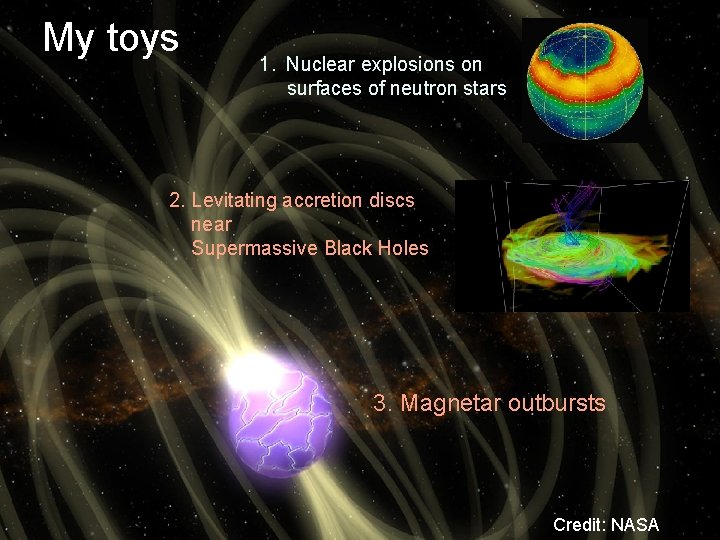
My toys 1. Nuclear explosions on surfaces of neutron stars 2. Levitating accretion discs near Supermassive Black Holes 3. Magnetar outbursts Credit: NASA
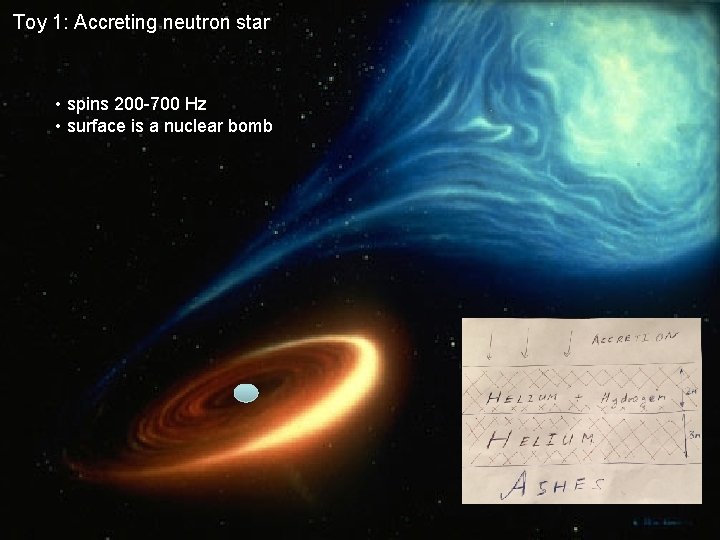
Toy 1: Accreting neutron star • spins 200 -700 Hz • surface is a nuclear bomb
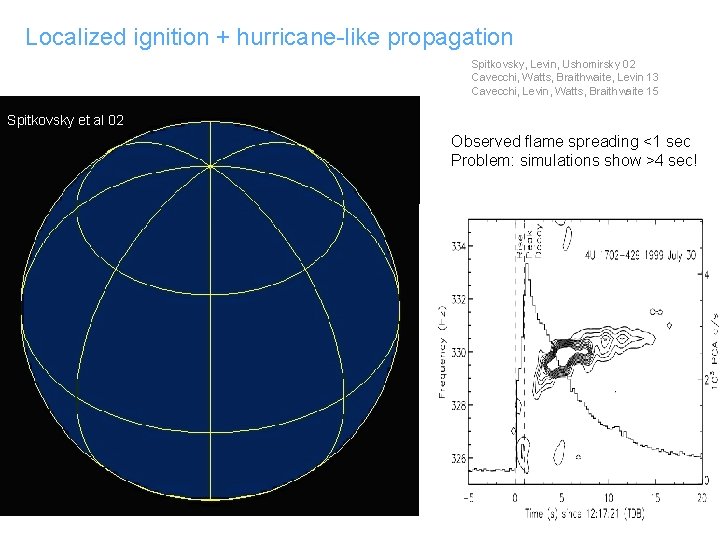
Localized ignition + hurricane-like propagation Spitkovsky, Levin, Ushomirsky 02 Cavecchi, Watts, Braithwaite, Levin 13 Cavecchi, Levin, Watts, Braithwaite 15 Spitkovsky et al 02 Observed flame spreading <1 sec Problem: simulations show >4 sec!
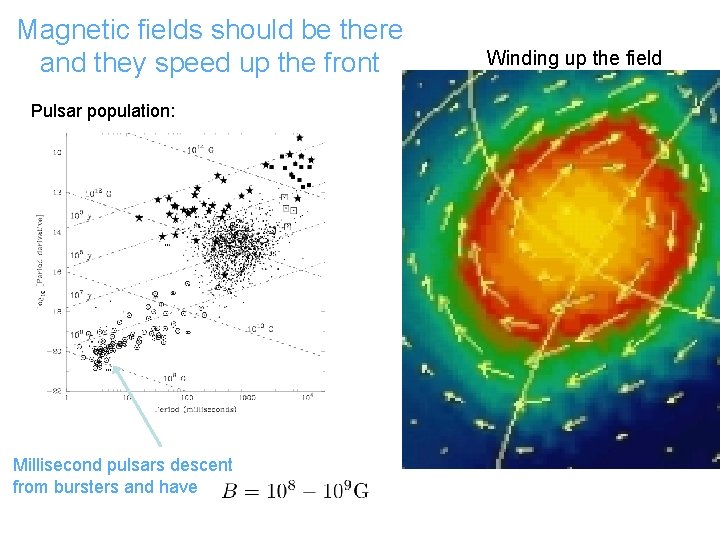
Magnetic fields should be there and they speed up the front Pulsar population: Millisecond pulsars descent from bursters and have Winding up the field
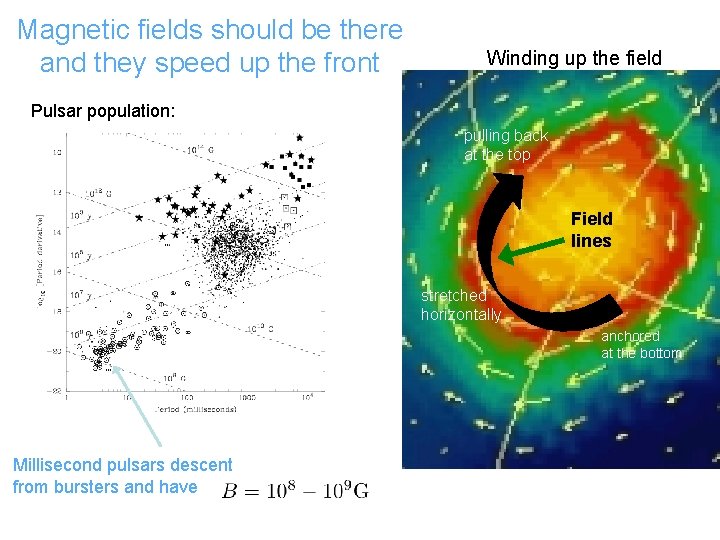
Magnetic fields should be there and they speed up the front Winding up the field Pulsar population: pulling back at the top Field lines stretched horizontally anchored at the bottom Millisecond pulsars descent from bursters and have
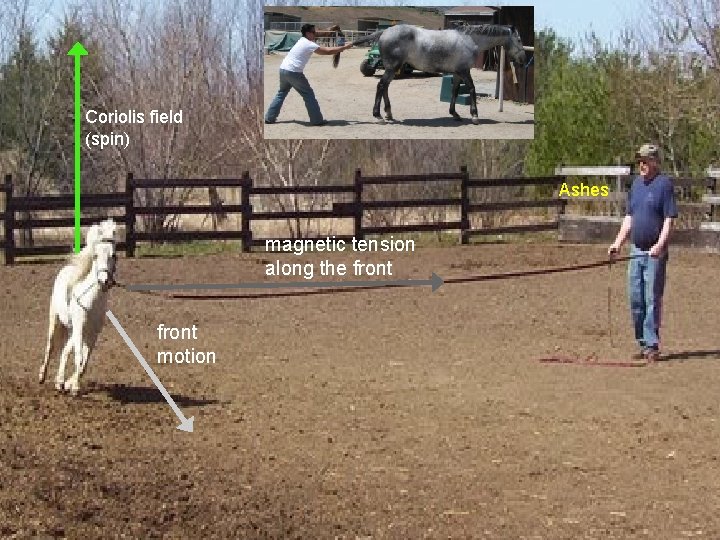
Coriolis field (spin) Ashes magnetic tension along the front motion
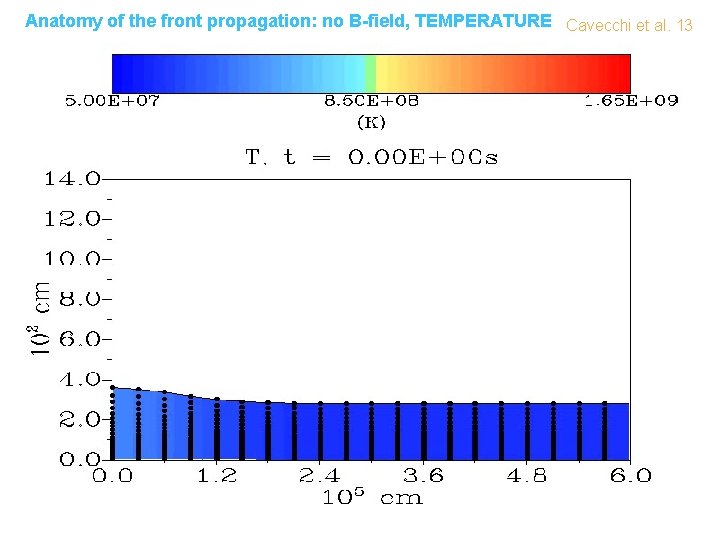
Anatomy of the front propagation: no B-field, TEMPERATURE Cavecchi et al. 13
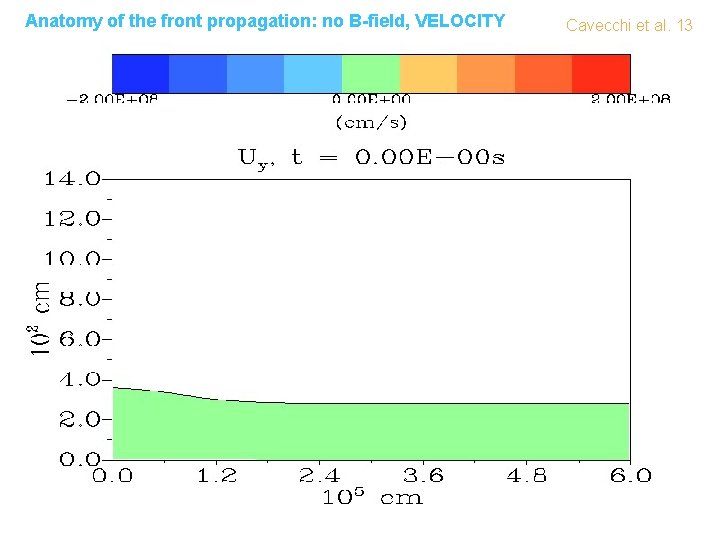
Anatomy of the front propagation: no B-field, VELOCITY Cavecchi et al. 13
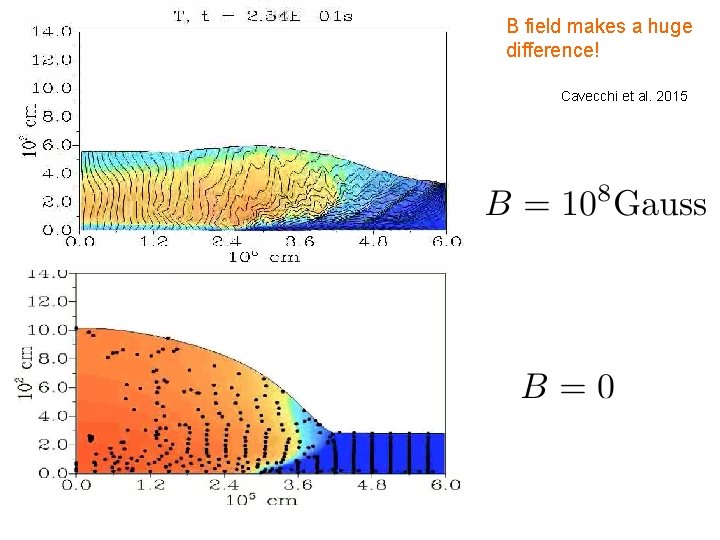
B field makes a huge difference! Cavecchi et al. 2015
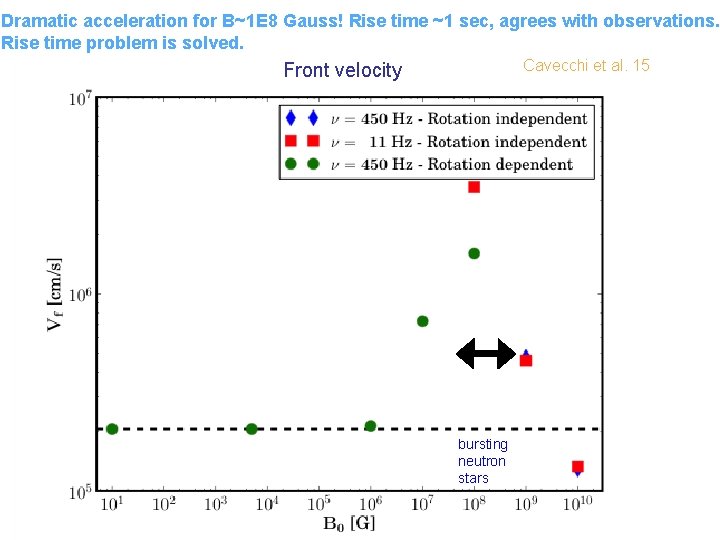
Dramatic acceleration for B~1 E 8 Gauss! Rise time ~1 sec, agrees with observations. Rise time problem is solved. Cavecchi et al. 15 Front velocity bursting neutron stars
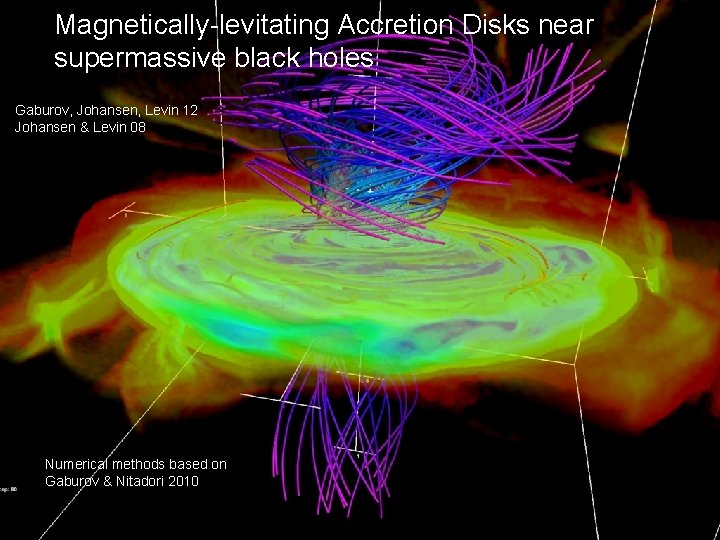
Magnetically-levitating Accretion Disks near supermassive black holes Gaburov, Johansen, Levin 12 Johansen & Levin 08 Numerical methods based on Gaburov & Nitadori 2010
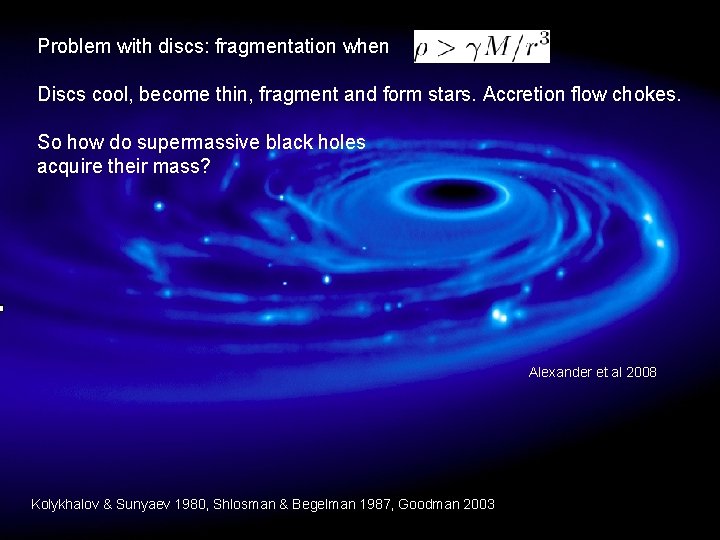
Problem with discs: fragmentation when Discs cool, become thin, fragment and form stars. Accretion flow chokes. So how do supermassive black holes acquire their mass? Alexander et al 2008 Kolykhalov & Sunyaev 1980, Shlosman & Begelman 1987, Goodman 2003
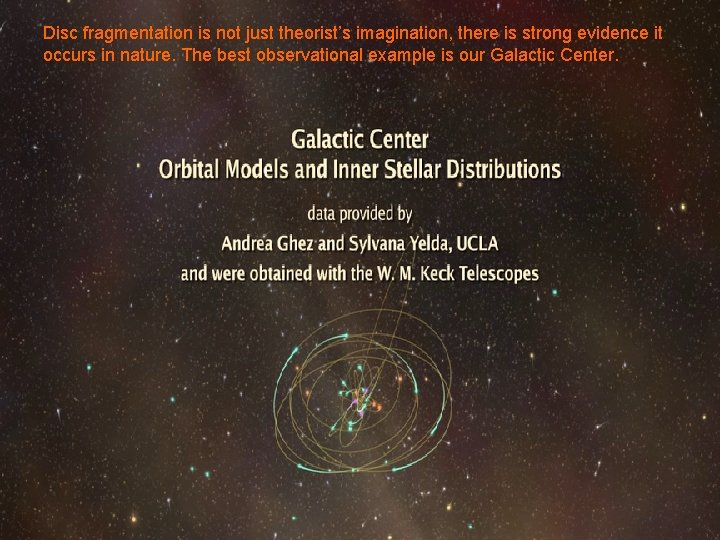
Disc fragmentation is not just theorist’s imagination, there is strong evidence it occurs in nature. The best observational example is our Galactic Center.
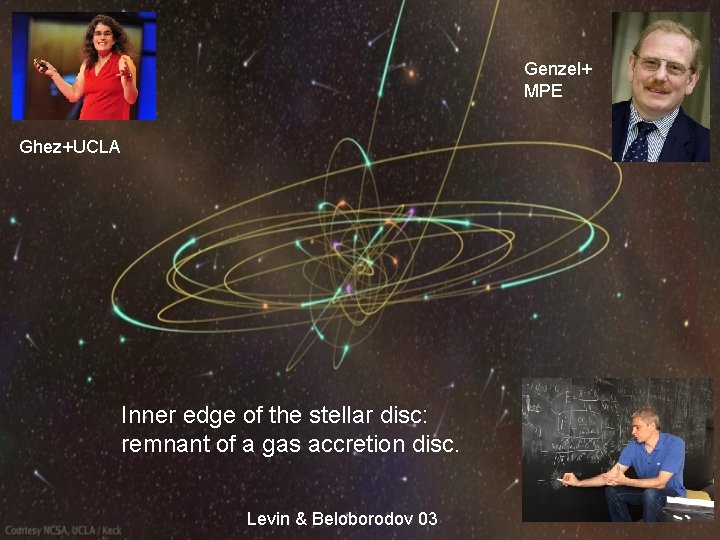
Genzel+ MPE Ghez+UCLA Inner edge of the stellar disc: remnant of a gas accretion disc. Levin & Beloborodov 03
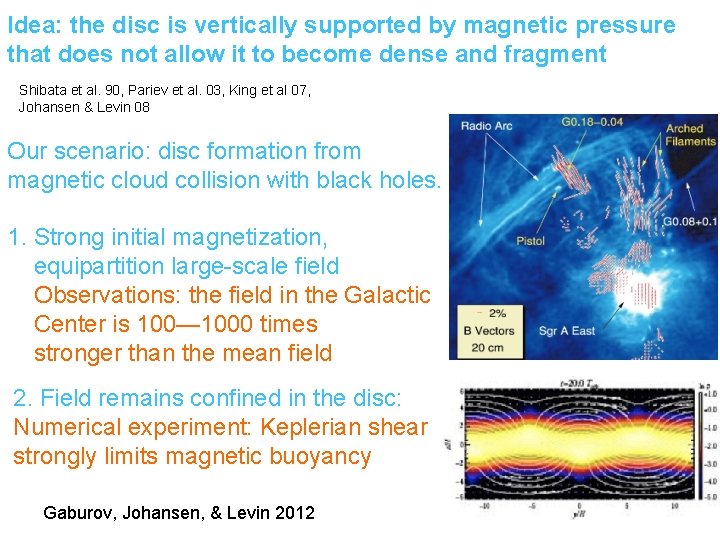
Idea: the disc is vertically supported by magnetic pressure that does not allow it to become dense and fragment Shibata et al. 90, Pariev et al. 03, King et al 07, Johansen & Levin 08 Our scenario: disc formation from magnetic cloud collision with black holes. 1. Strong initial magnetization, equipartition large-scale field Observations: the field in the Galactic Center is 100— 1000 times stronger than the mean field 2. Field remains confined in the disc: Numerical experiment: Keplerian shear strongly limits magnetic buoyancy Gaburov, Johansen, & Levin 2012
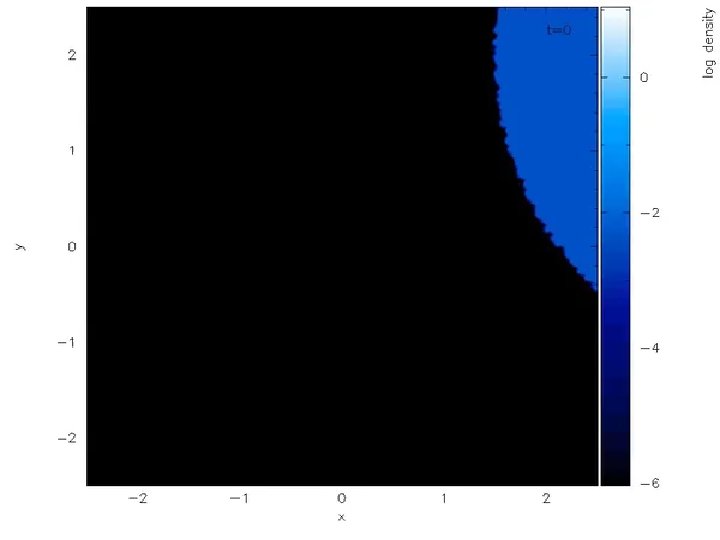
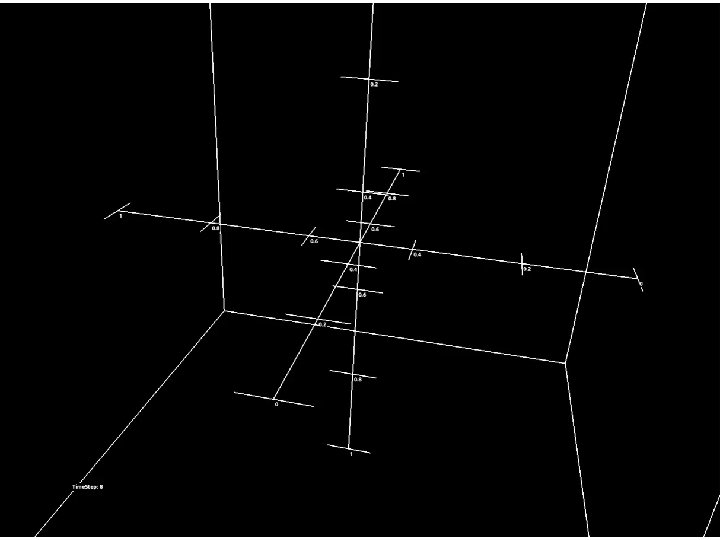
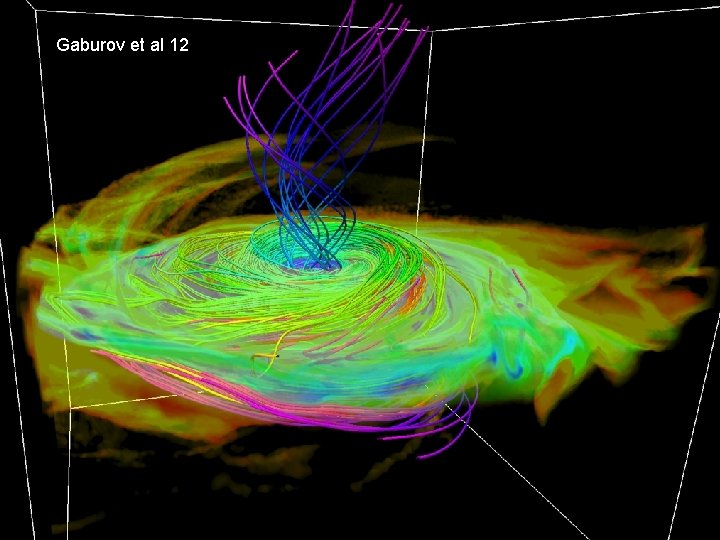
Gaburov et al 12
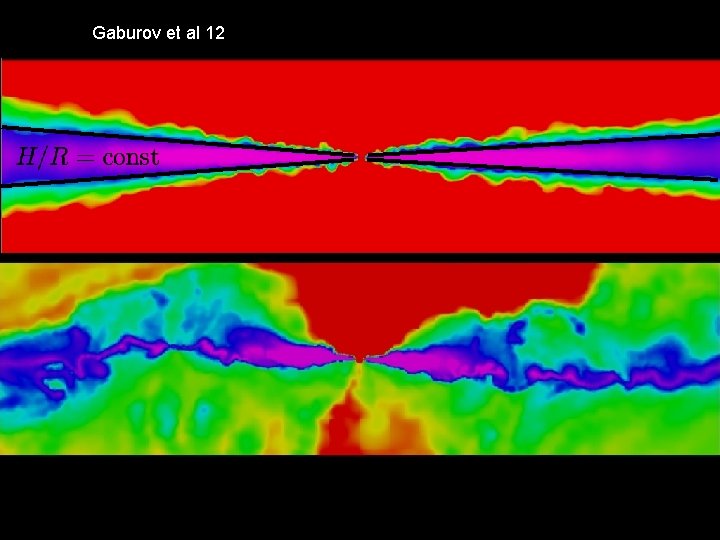
Gaburov et al 12
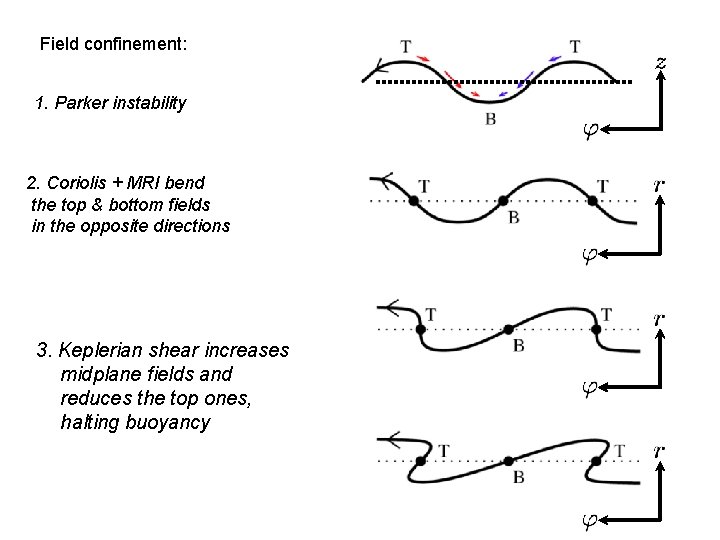
Field confinement: 1. Parker instability 2. Coriolis + MRI bend the top & bottom fields in the opposite directions 3. Keplerian shear increases midplane fields and reduces the top ones, halting buoyancy
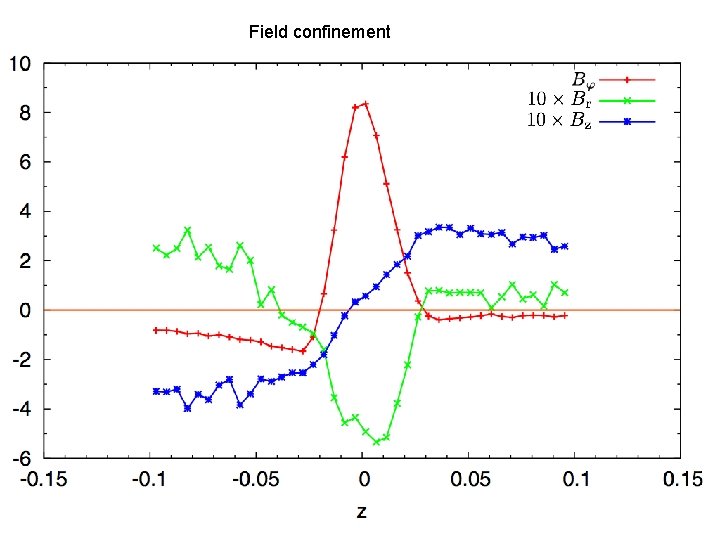
Vertical profile Field confinement
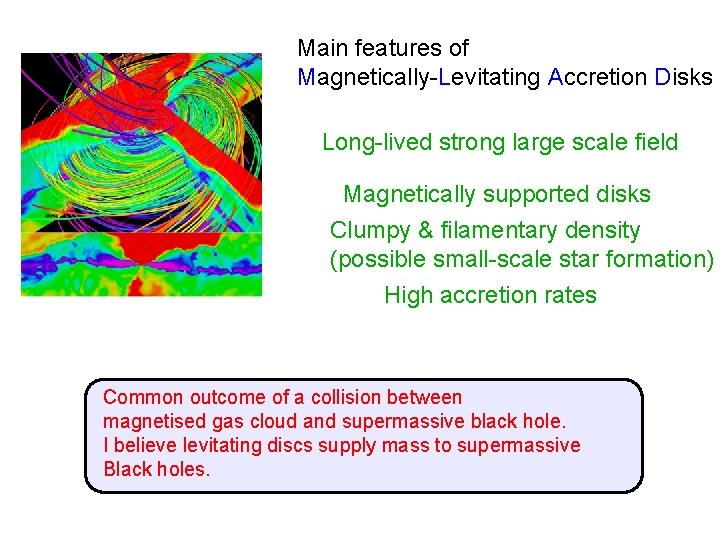
Main features of Magnetically-Levitating Accretion Disks Long-lived strong large scale field Magnetically supported disks Clumpy & filamentary density (possible small-scale star formation) High accretion rates Common outcome of a collision between magnetised gas cloud and supermassive black hole. I believe levitating discs supply mass to supermassive Black holes.
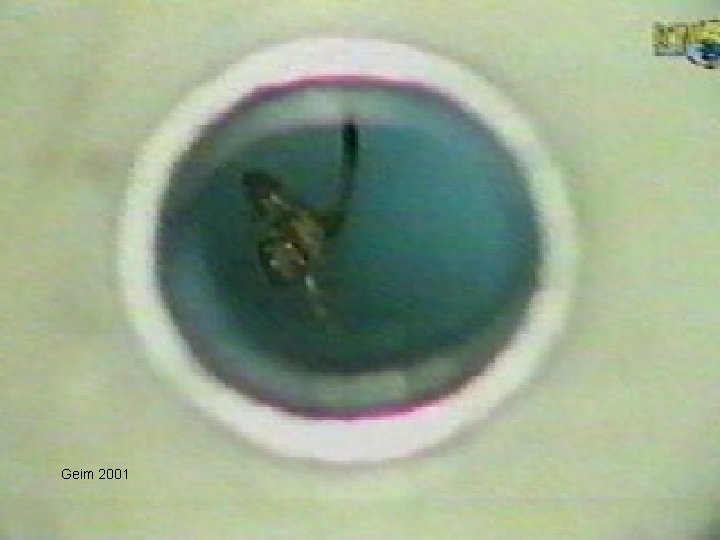
Geim 2001
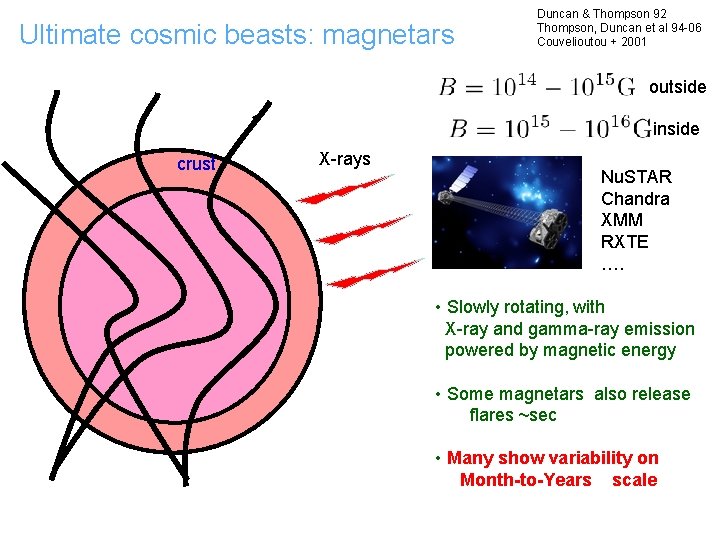
Ultimate cosmic beasts: magnetars Duncan & Thompson 92 Thompson, Duncan et al 94 -06 Couvelioutou + 2001 outside inside crust X-rays Nu. STAR Chandra XMM RXTE …. • Slowly rotating, with X-ray and gamma-ray emission powered by magnetic energy • Some magnetars also release flares ~sec • Many show variability on Month-to-Years scale
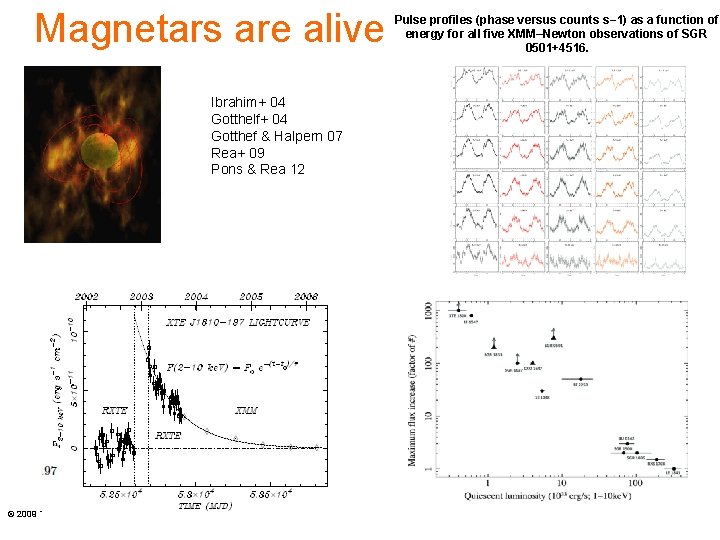
Magnetars are alive Ibrahim+ 04 Gotthelf+ 04 Gotthef & Halpern 07 Rea+ 09 Pons & Rea 12 Rea N et al. MNRAS 2009; 396: 2419 -2432 © 2009 The Authors. Journal compilation © 2009 RAS Pulse profiles (phase versus counts s− 1) as a function of energy for all five XMM–Newton observations of SGR 0501+4516.
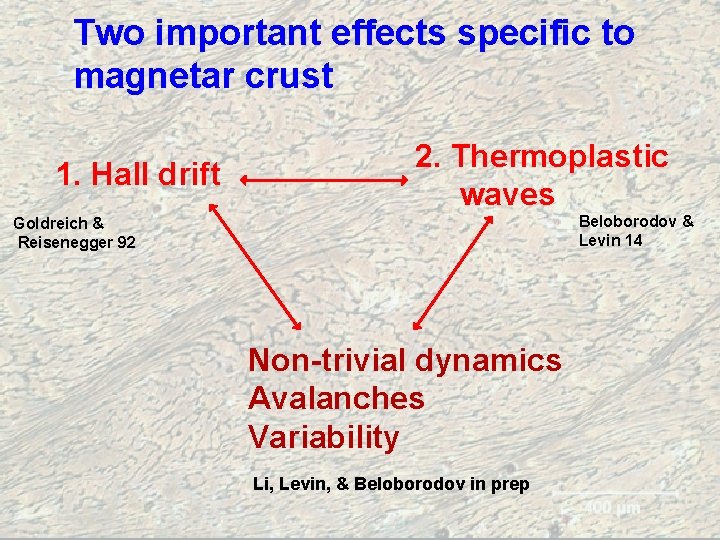
Two important effects specific to magnetar crust 1. Hall drift 2. Thermoplastic waves Beloborodov & Levin 14 Goldreich & Reisenegger 92 Non-trivial dynamics Avalanches Variability Li, Levin, & Beloborodov in prep
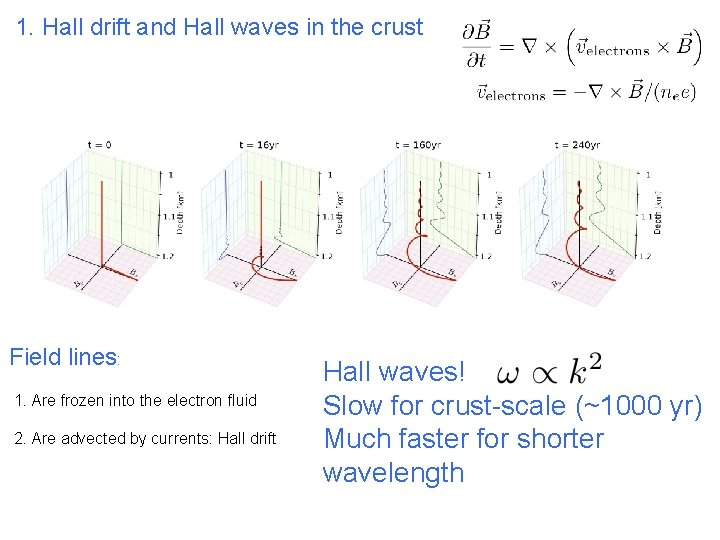
1. Hall drift and Hall waves in the crust Field lines: 1. Are frozen into the electron fluid 2. Are advected by currents: Hall drift Hall waves! Slow for crust-scale (~1000 yr) Much faster for shorter wavelength
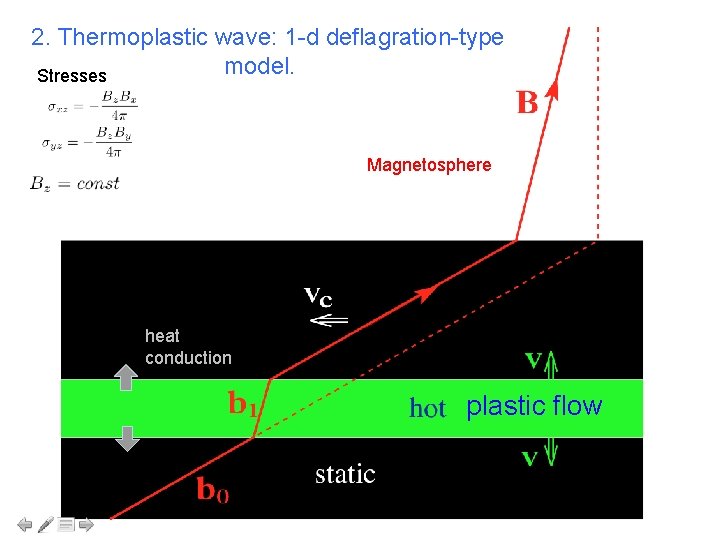
2. Thermoplastic wave: 1 -d deflagration-type model. Stresses Magnetosphere heat conduction plastic flow
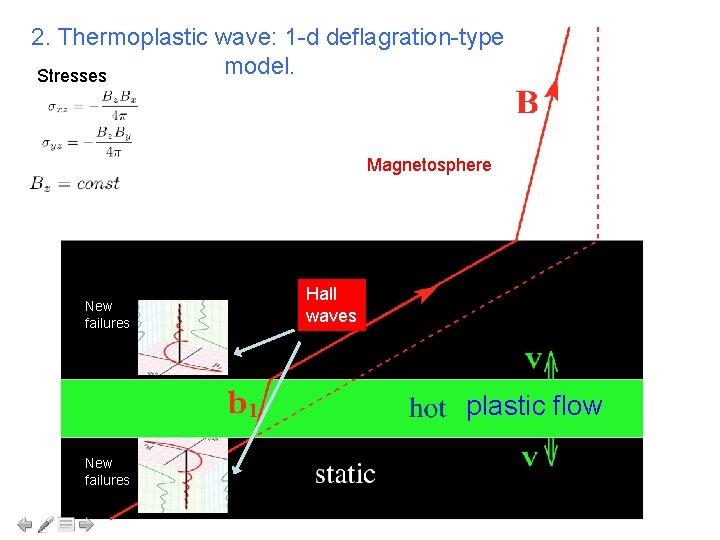
2. Thermoplastic wave: 1 -d deflagration-type model. Stresses Magnetosphere New failures Hall waves plastic flow New failures
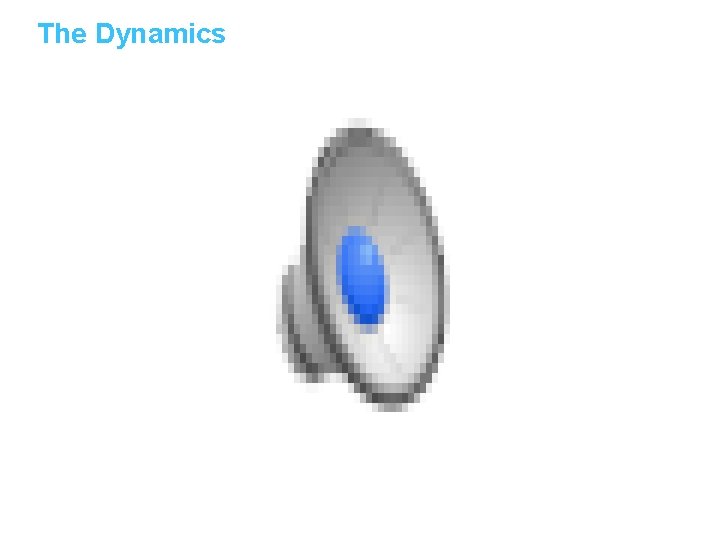
The Dynamics
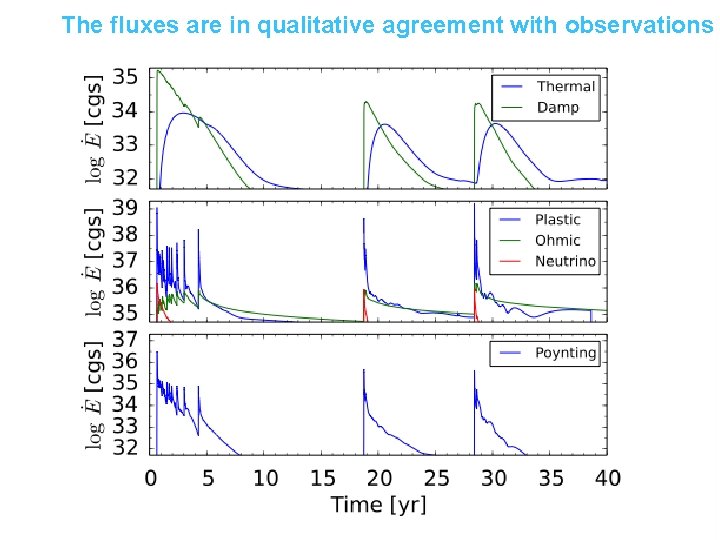
The fluxes are in qualitative agreement with observations
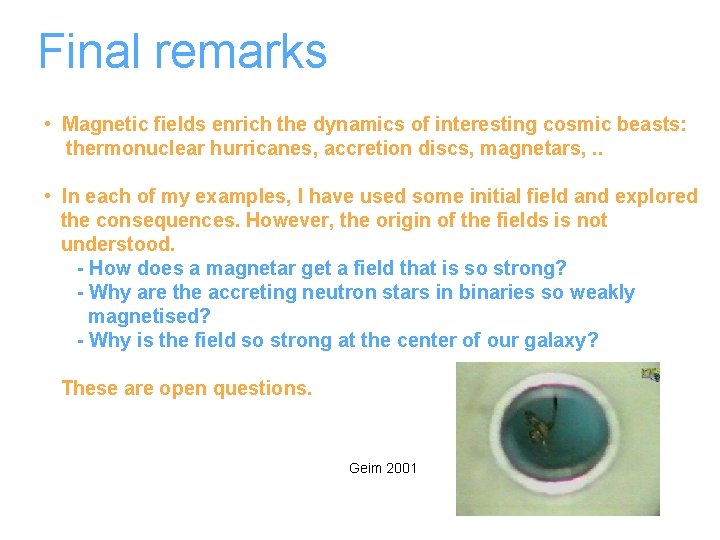
Final remarks • Magnetic fields enrich the dynamics of interesting cosmic beasts: thermonuclear hurricanes, accretion discs, magnetars, . . • In each of my examples, I have used some initial field and explored the consequences. However, the origin of the fields is not understood. - How does a magnetar get a field that is so strong? - Why are the accreting neutron stars in binaries so weakly magnetised? - Why is the field so strong at the center of our galaxy? These are open questions. Geim 2001
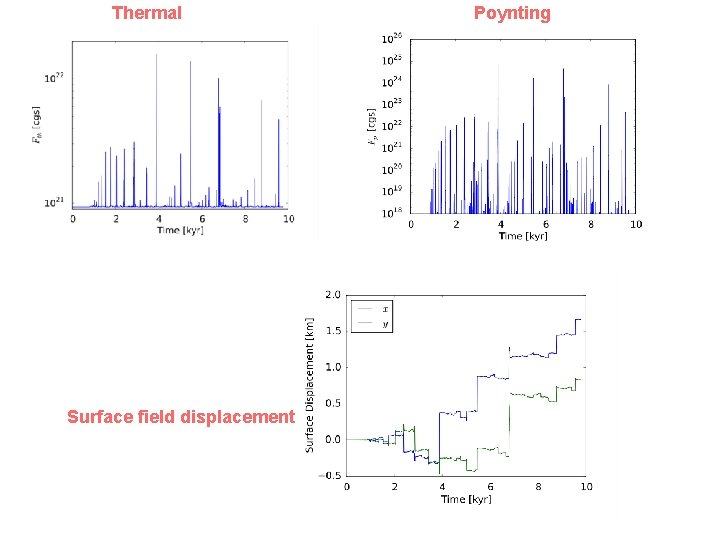
Thermal Surface field displacement Poynting
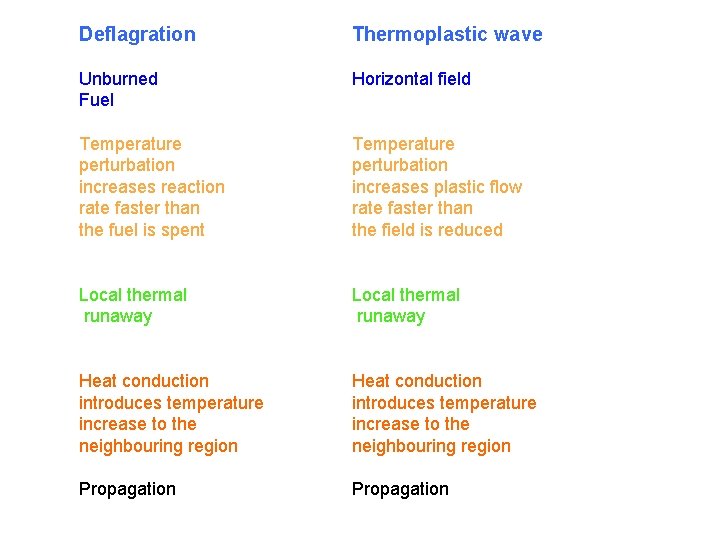
Deflagration Thermoplastic wave Unburned Fuel Horizontal field Temperature perturbation increases reaction rate faster than the fuel is spent Temperature perturbation increases plastic flow rate faster than the field is reduced Local thermal runaway Heat conduction introduces temperature increase to the neighbouring region Propagation
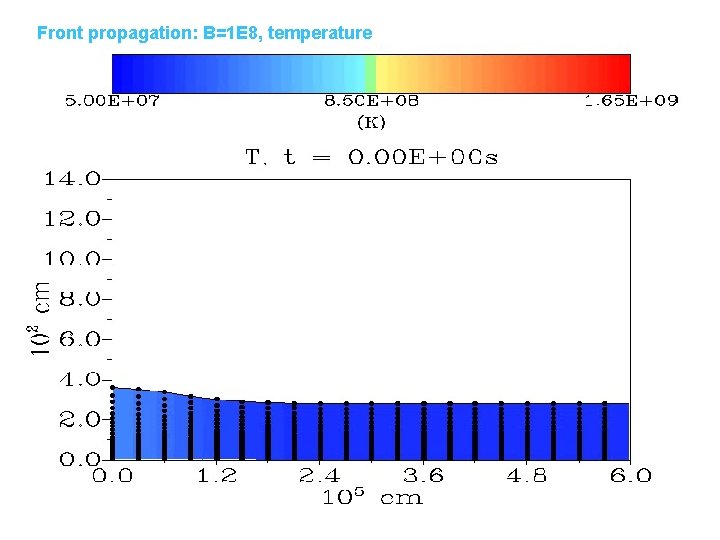
Front propagation: B=1 E 8, temperature
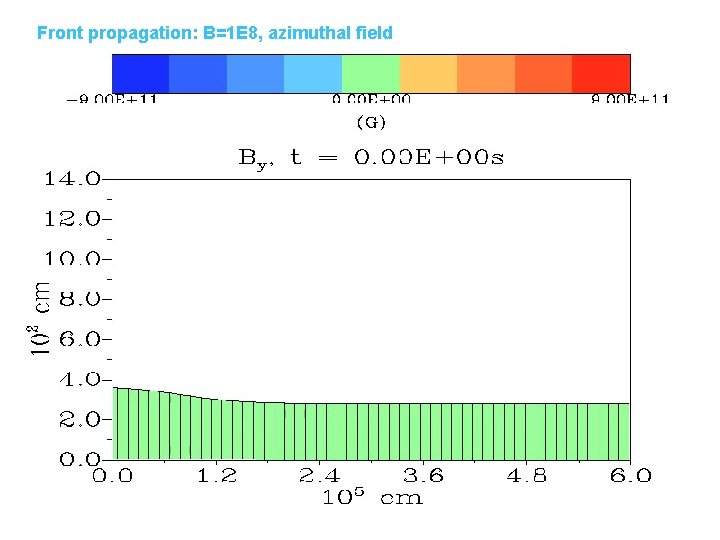
Front propagation: B=1 E 8, azimuthal field
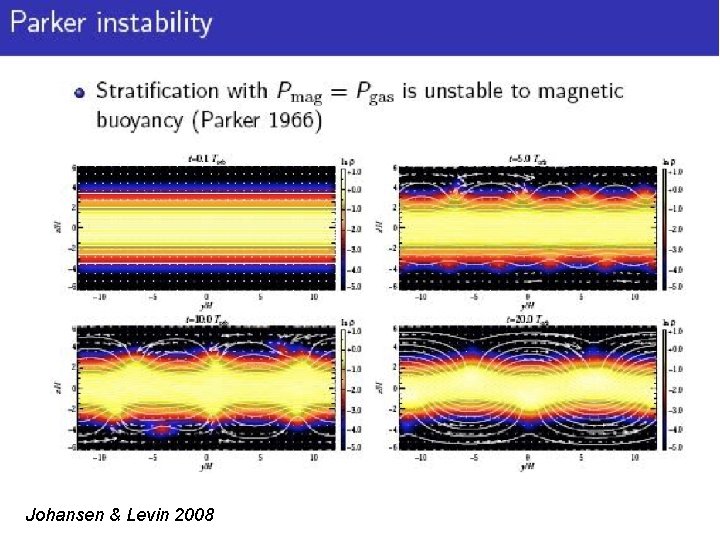
Johansen & Levin 2008
Pulsar timing
Kurt levin
Cavecchi type
Magnetic flux units
Magnetic moment and magnetic field relation
F=i(lxb)
Magnetic retentivity
Ng tube types
Estelle levin
Kurt levin
Teoria del campo di kurt lewin
Updatehal
Sonda levin y salem
Brien
Vladimir levin
Jarcho levin syndrome icd 10
Henry m levin
Sondas nasogastricas
Spectral matting
Aleksej karenin
Bogdana levin
Levin ham
Cordula luckassen
Kurt lewin espacio vital
Levin sonda
Yuri plowden
Yuri krotov
Choose the right word
Yuri gorbachev paintings
Yuri milner
Vladimir kolmogorov
Yuri bezmenov quotes
Yuri garigan
Yuri boykov
Yuri armstrong
Yuri kovalev
Yuri kovchegov
Knozorov
Yuri manin
Yuri lyubarsky
Yuri burda