Lunar Exploration Transportation System LETS MAE 491 492
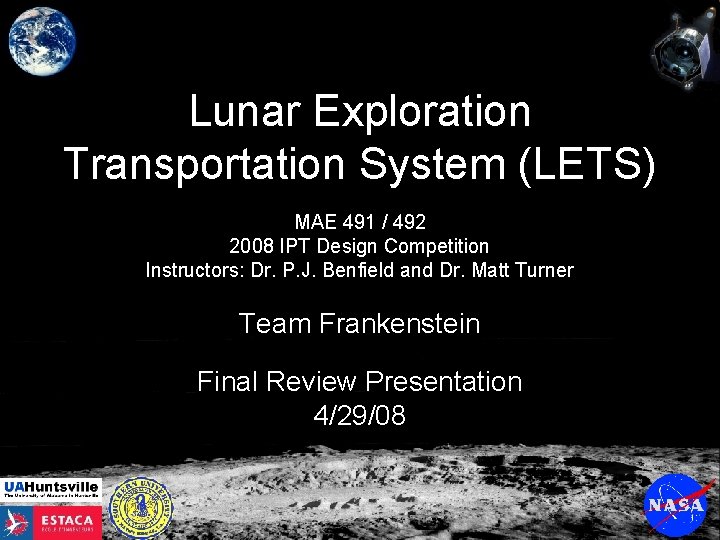
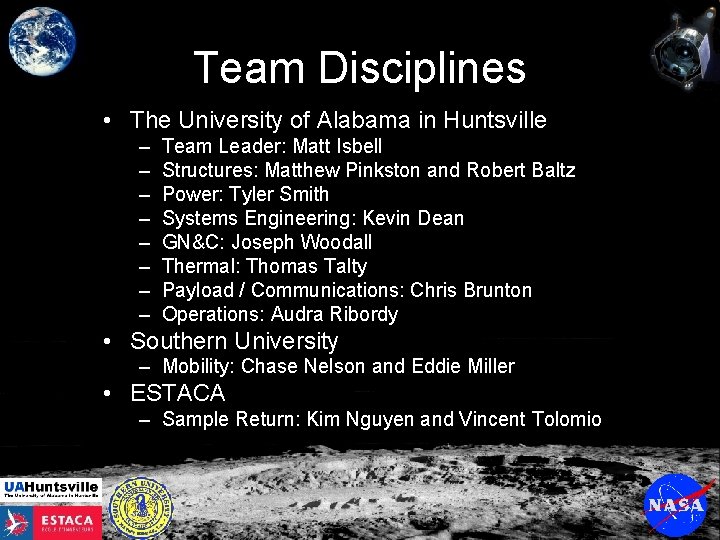
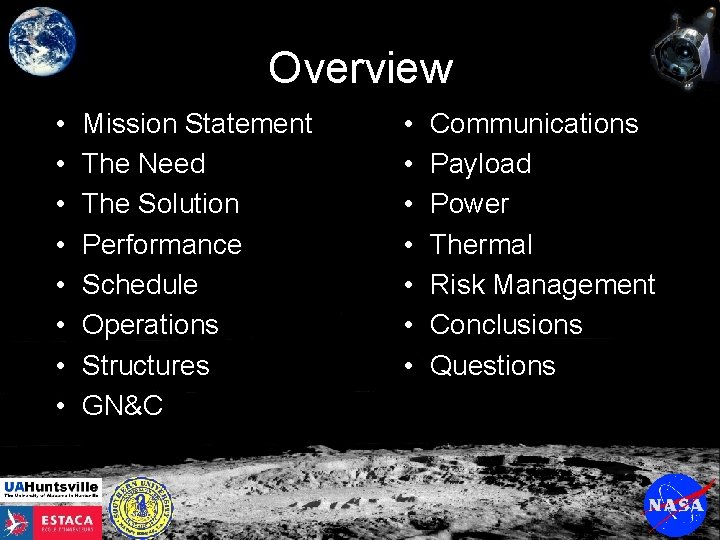
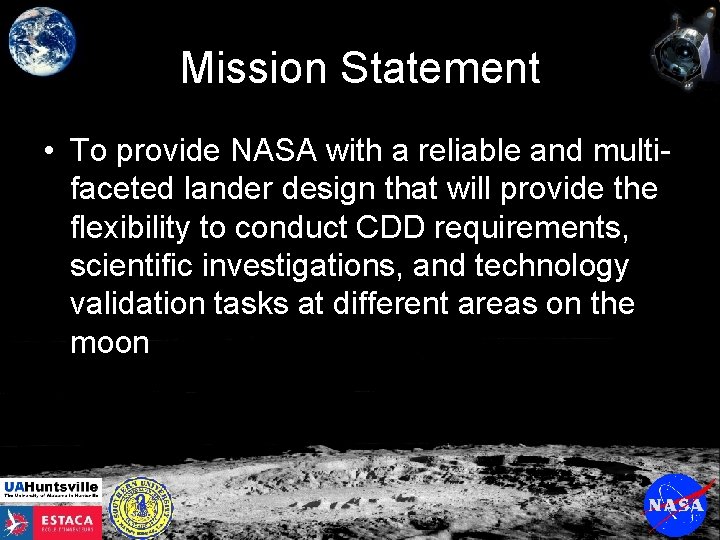
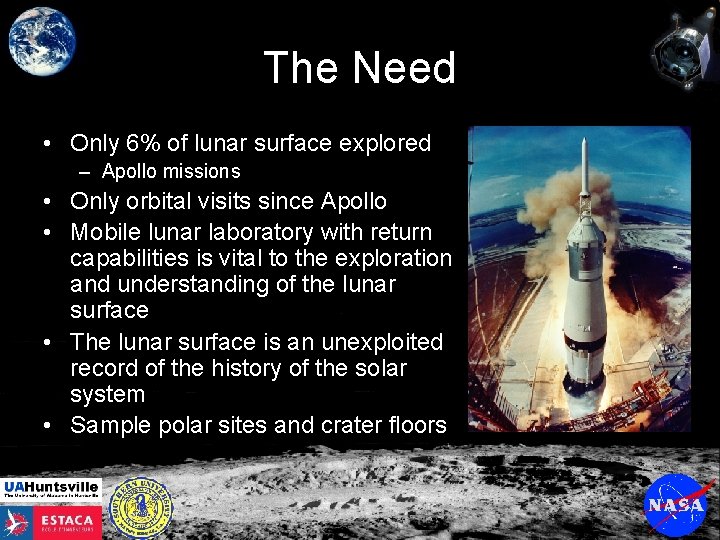
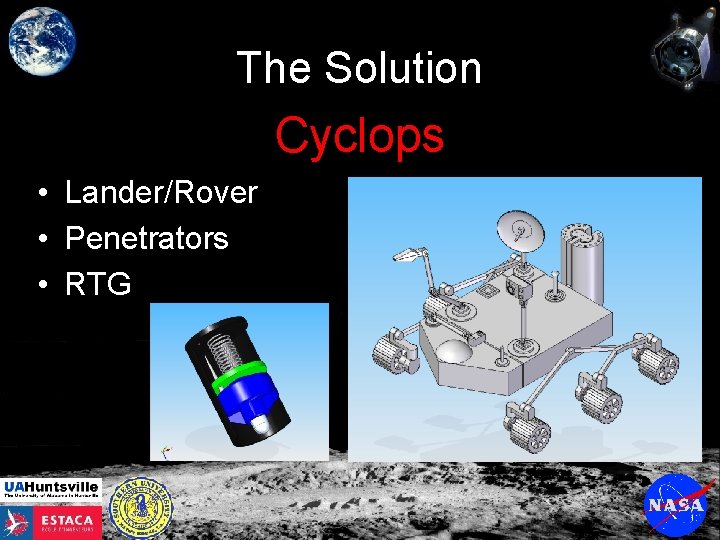
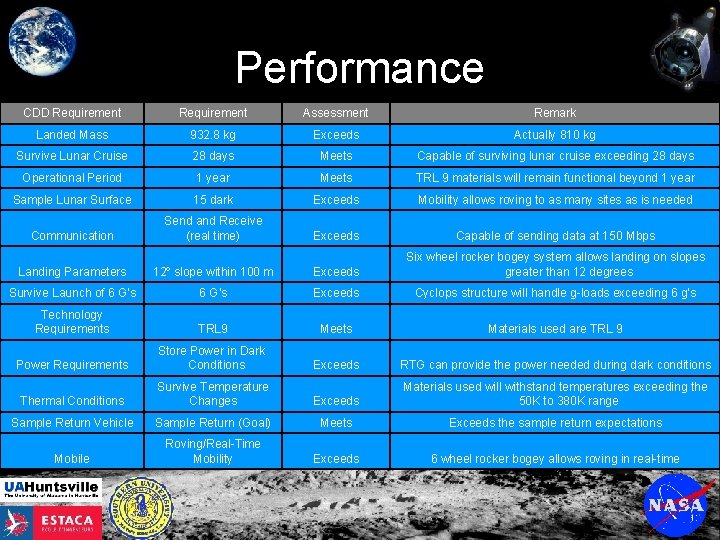
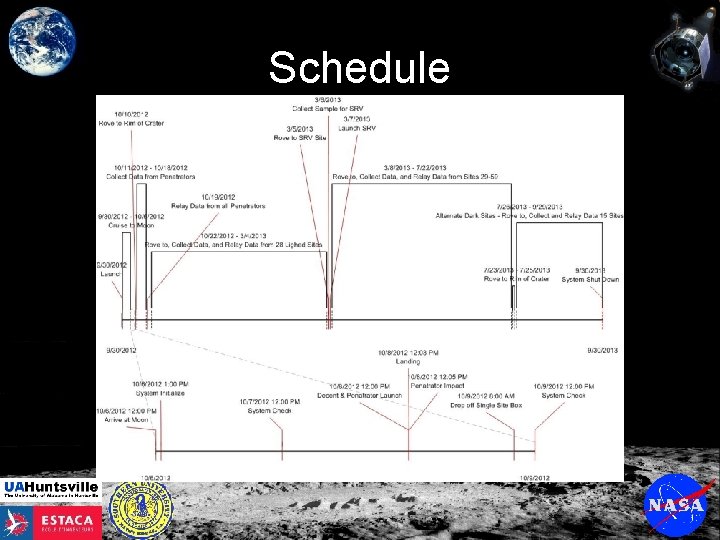
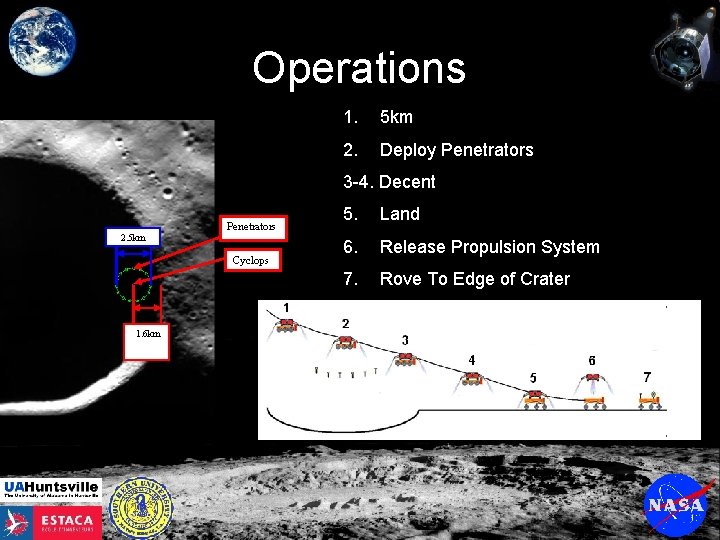
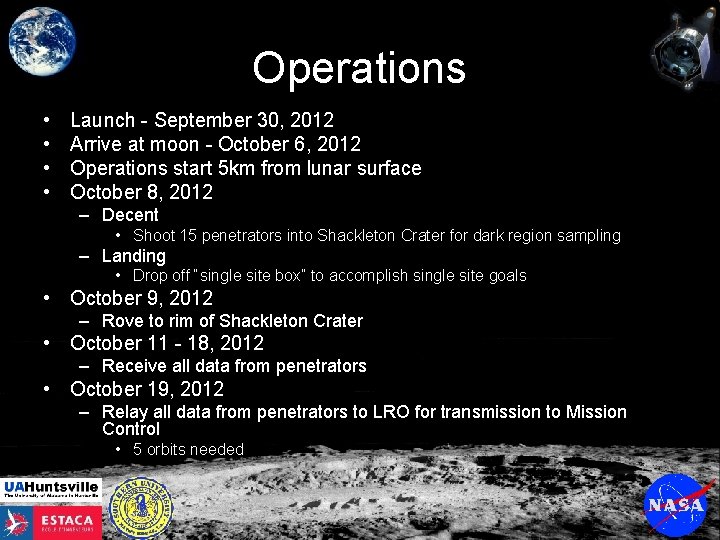
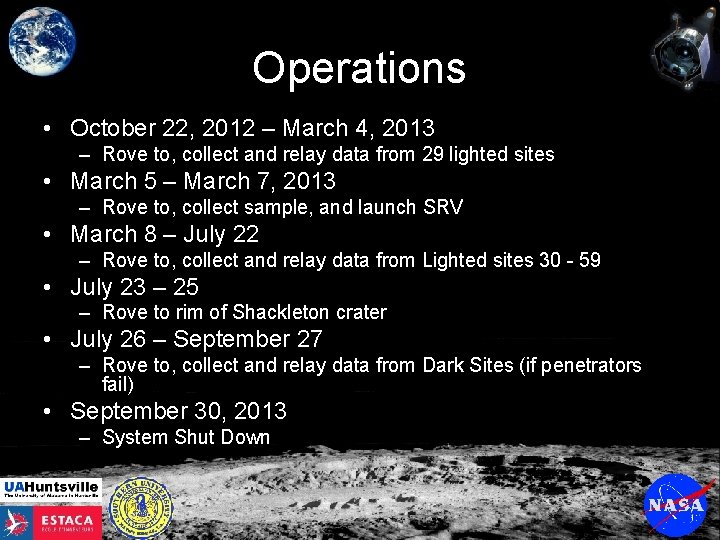
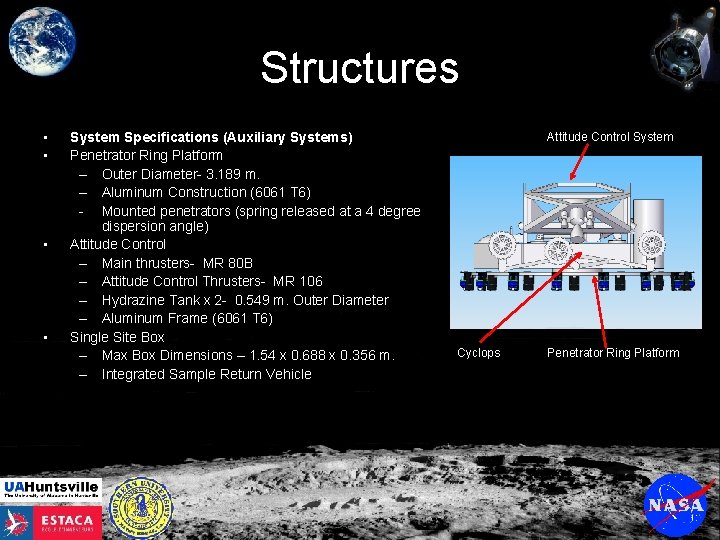
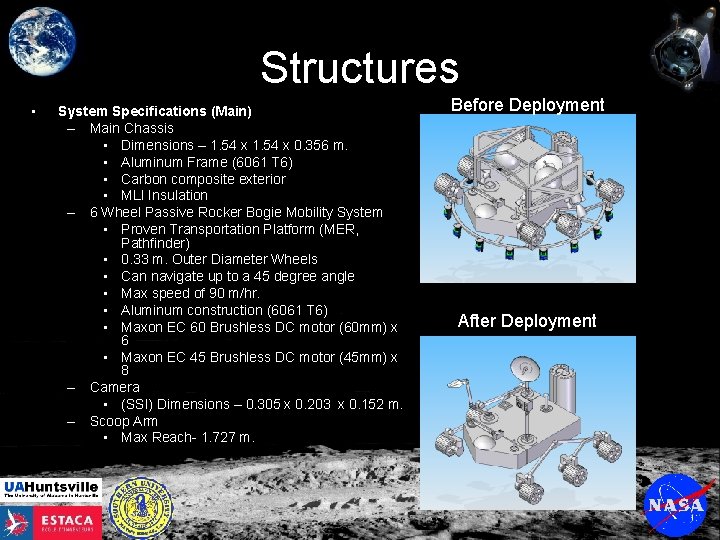
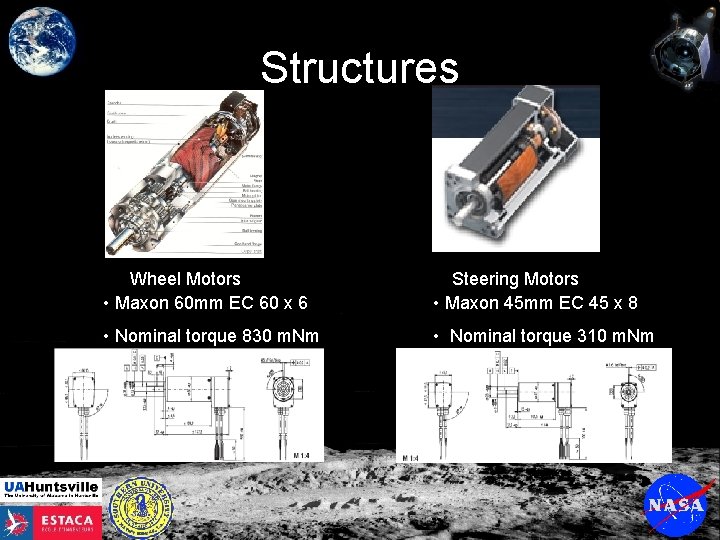
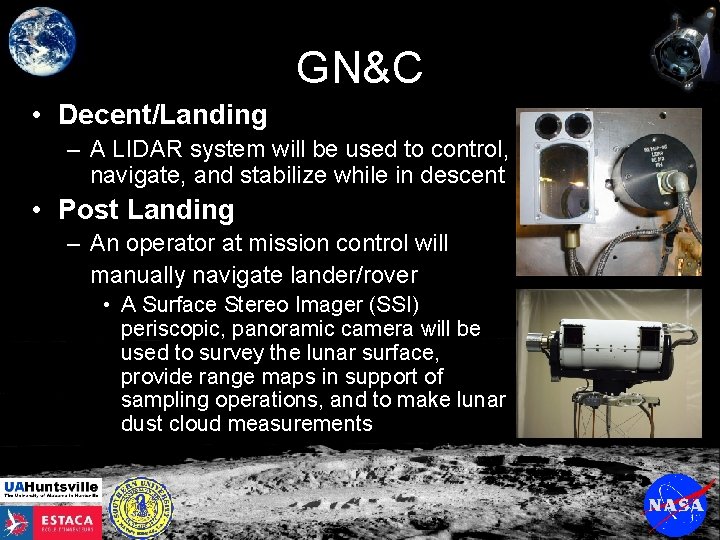
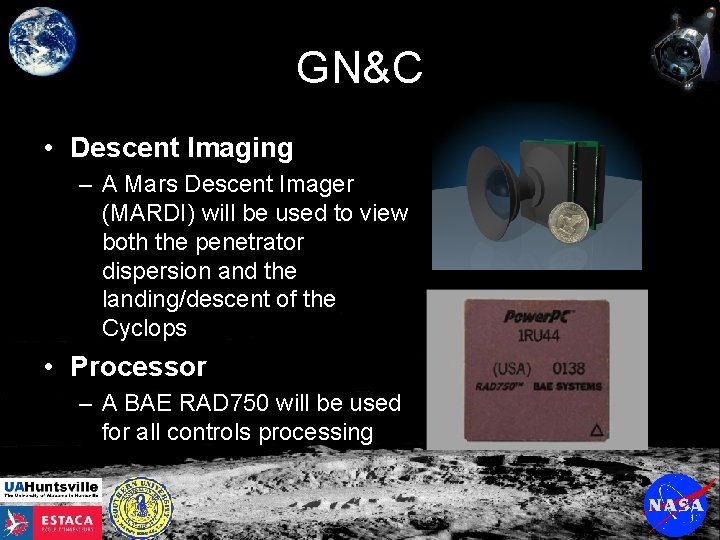
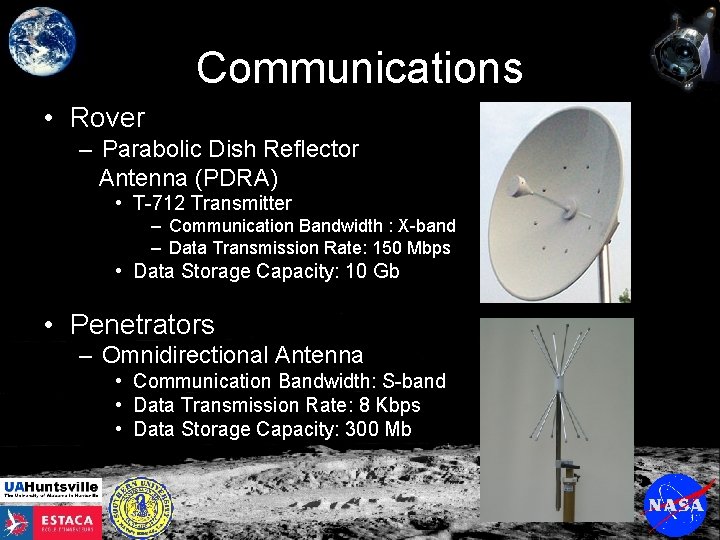
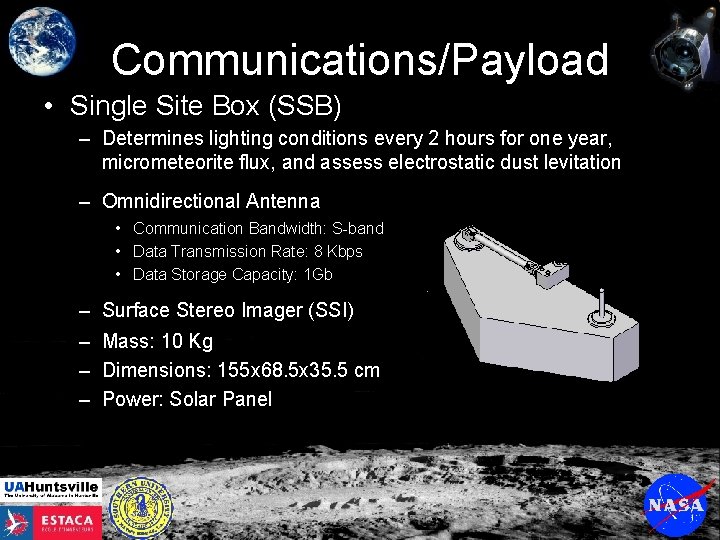
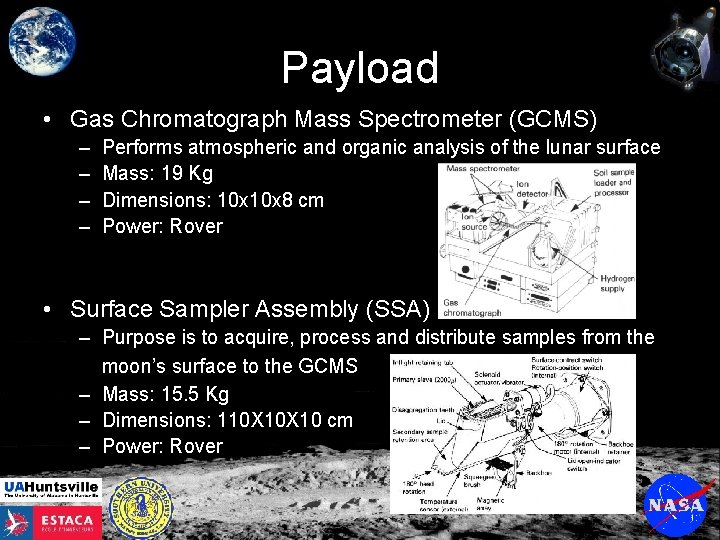
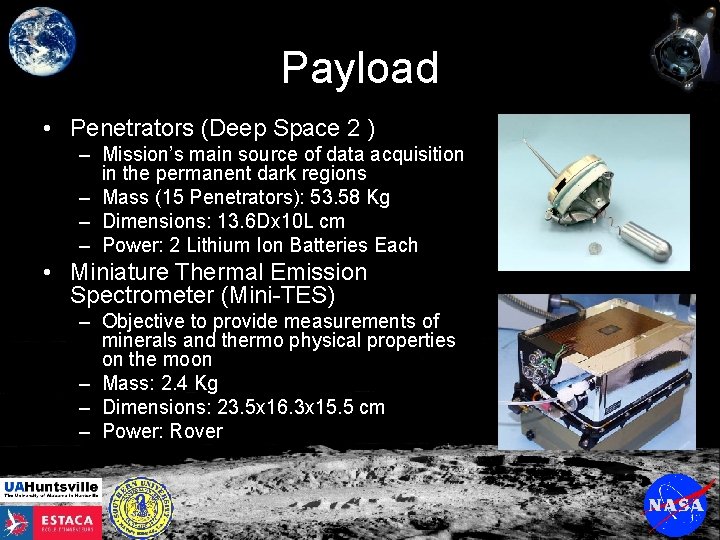
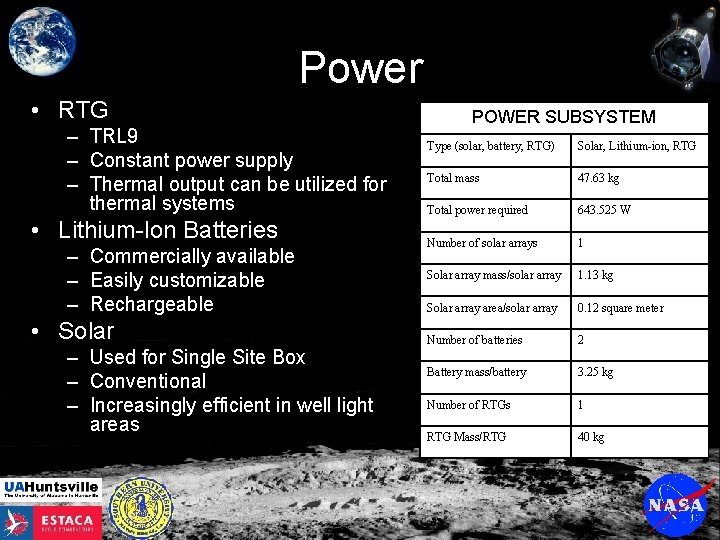
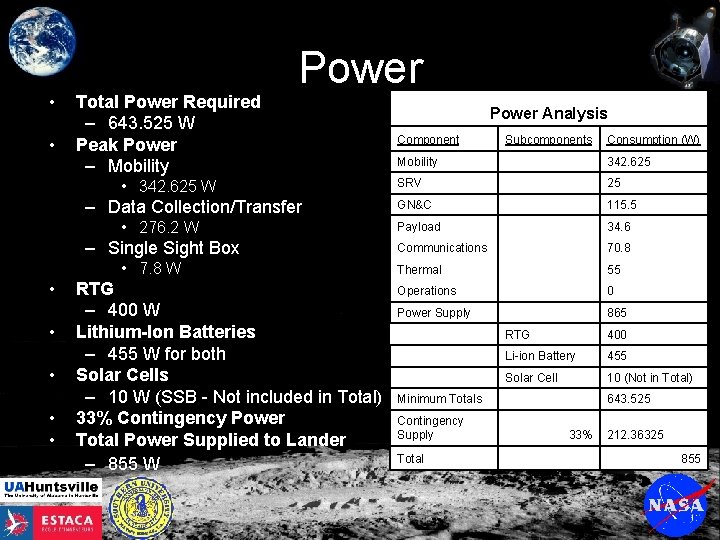
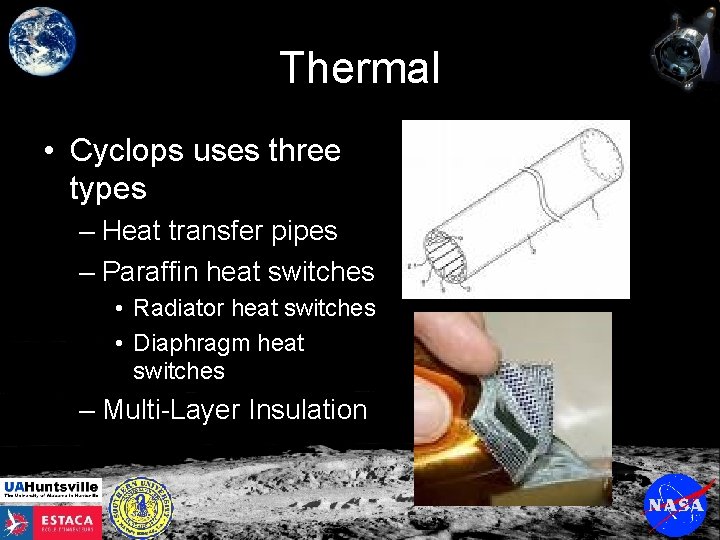
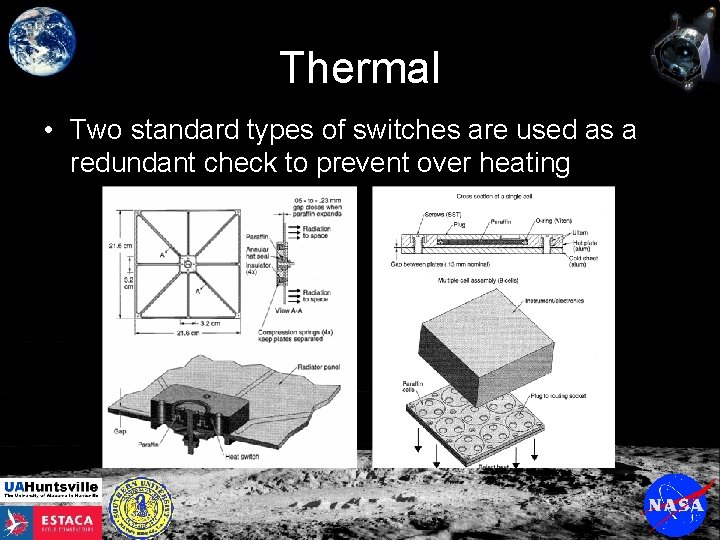
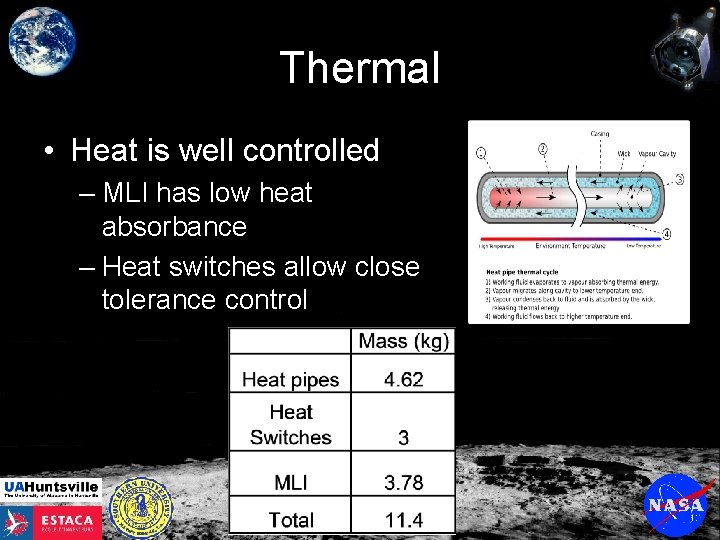
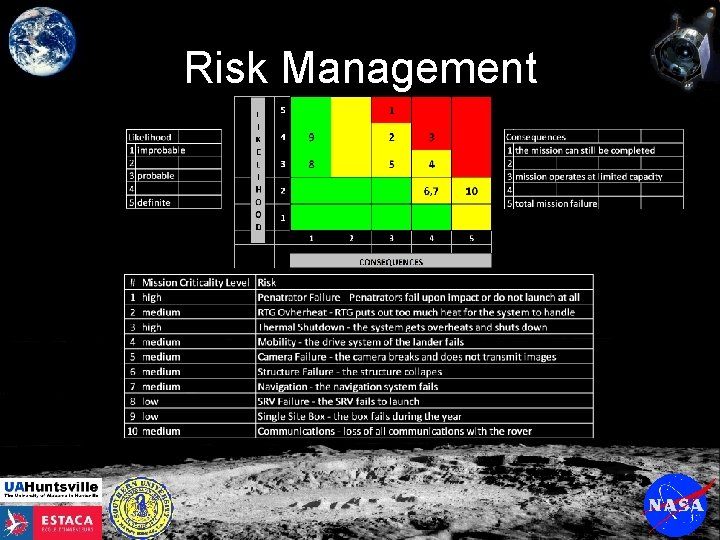
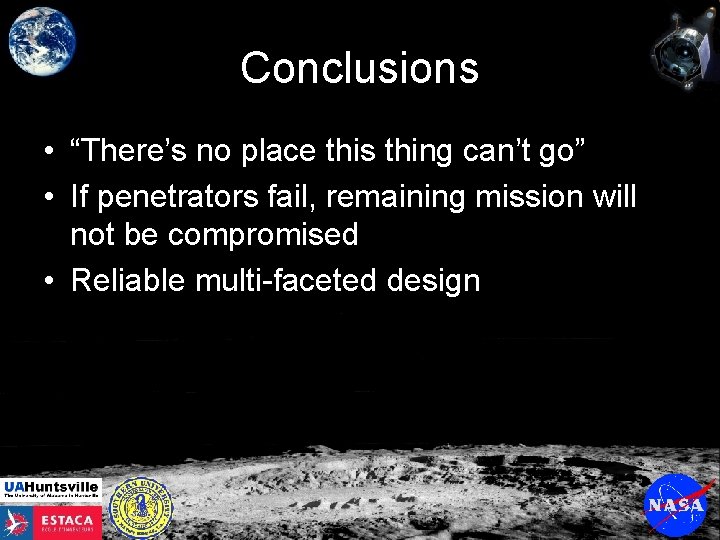
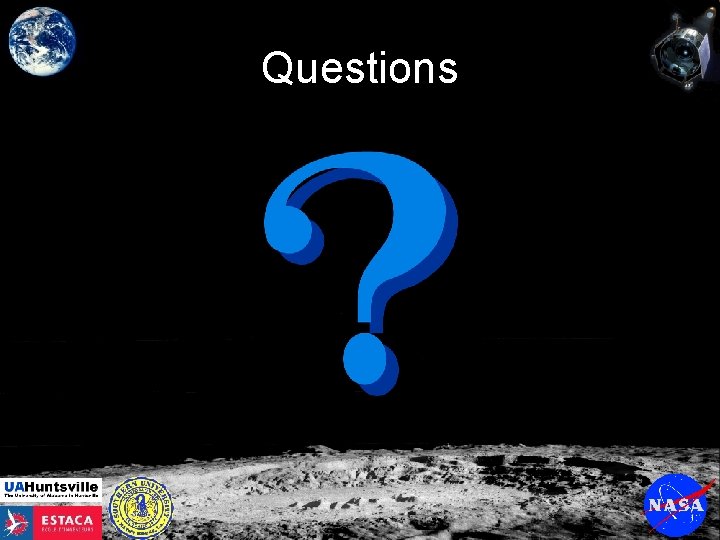
- Slides: 28
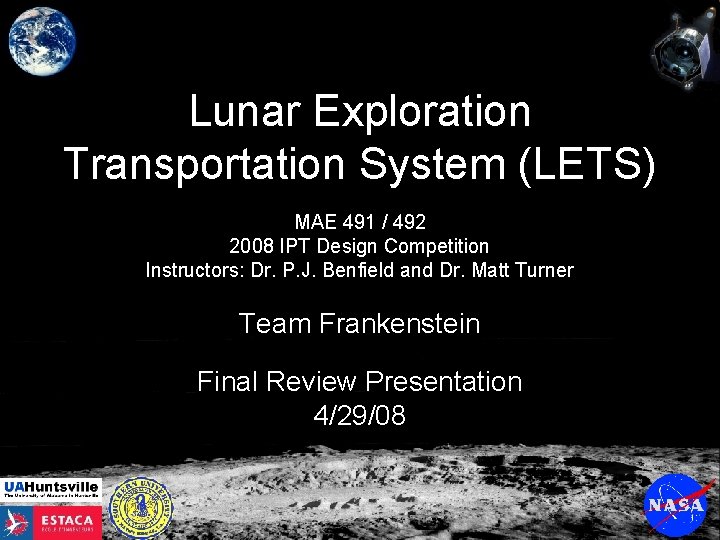
Lunar Exploration Transportation System (LETS) MAE 491 / 492 2008 IPT Design Competition Instructors: Dr. P. J. Benfield and Dr. Matt Turner Team Frankenstein Final Review Presentation 4/29/08
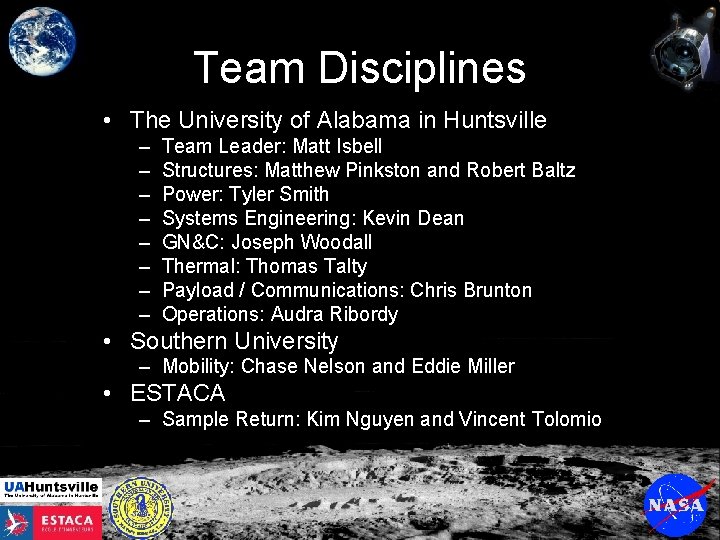
Team Disciplines • The University of Alabama in Huntsville – – – – Team Leader: Matt Isbell Structures: Matthew Pinkston and Robert Baltz Power: Tyler Smith Systems Engineering: Kevin Dean GN&C: Joseph Woodall Thermal: Thomas Talty Payload / Communications: Chris Brunton Operations: Audra Ribordy • Southern University – Mobility: Chase Nelson and Eddie Miller • ESTACA – Sample Return: Kim Nguyen and Vincent Tolomio
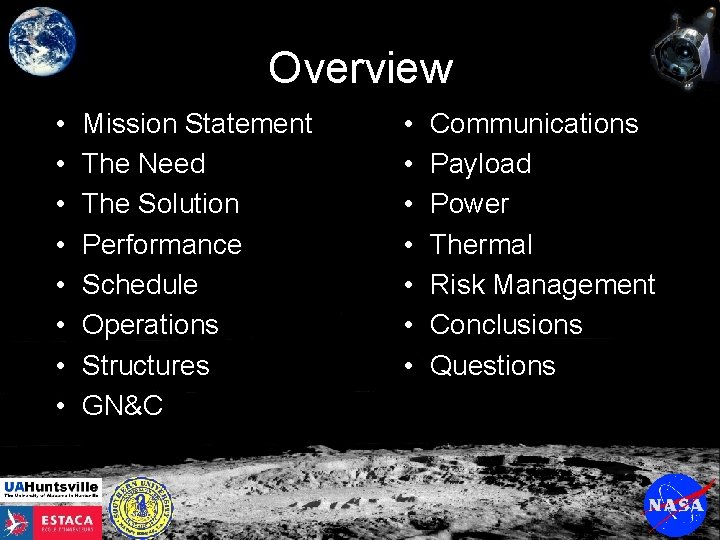
Overview • • Mission Statement The Need The Solution Performance Schedule Operations Structures GN&C • • Communications Payload Power Thermal Risk Management Conclusions Questions
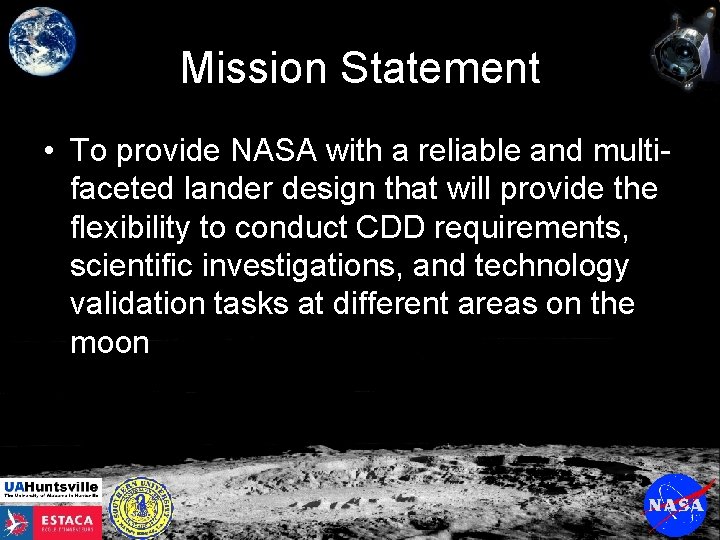
Mission Statement • To provide NASA with a reliable and multifaceted lander design that will provide the flexibility to conduct CDD requirements, scientific investigations, and technology validation tasks at different areas on the moon
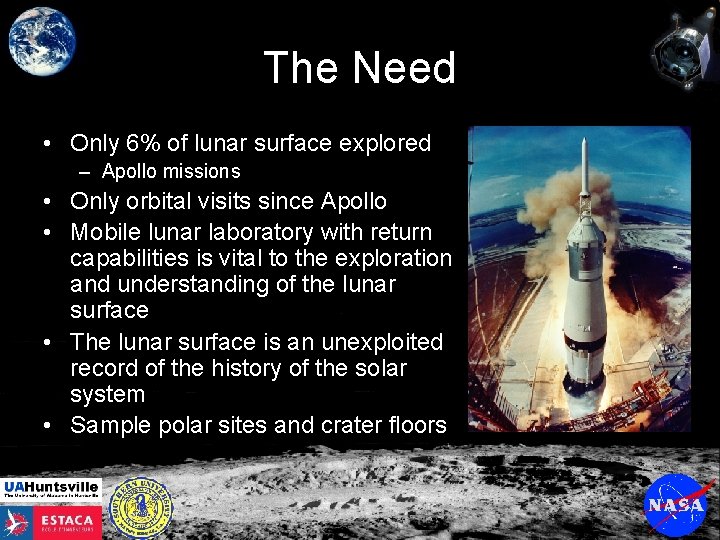
The Need • Only 6% of lunar surface explored – Apollo missions • Only orbital visits since Apollo • Mobile lunar laboratory with return capabilities is vital to the exploration and understanding of the lunar surface • The lunar surface is an unexploited record of the history of the solar system • Sample polar sites and crater floors
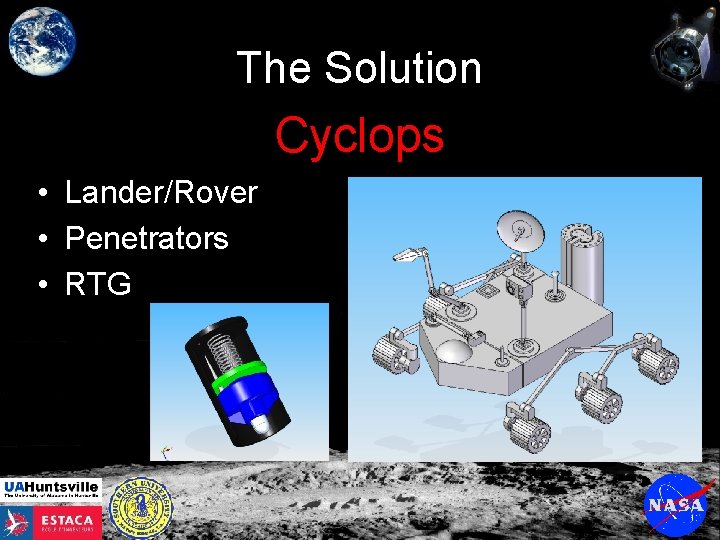
The Solution Cyclops • Lander/Rover • Penetrators • RTG
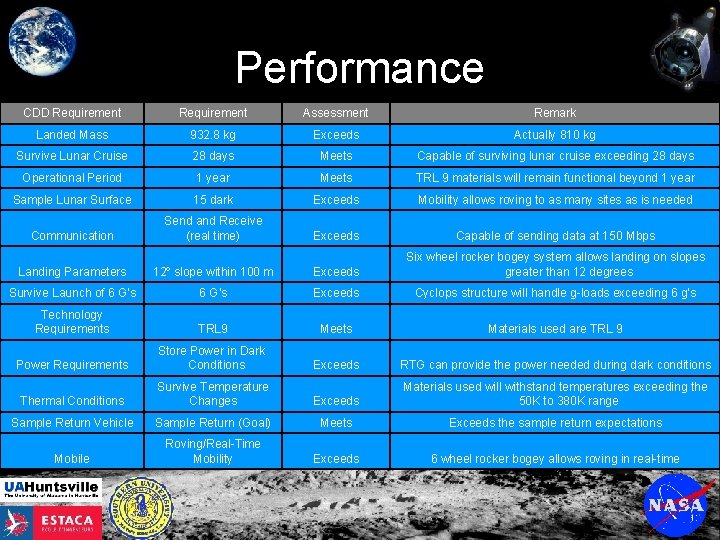
Performance CDD Requirement Assessment Remark Landed Mass 932. 8 kg Exceeds Actually 810 kg Survive Lunar Cruise 28 days Meets Capable of surviving lunar cruise exceeding 28 days Operational Period 1 year Meets TRL 9 materials will remain functional beyond 1 year Sample Lunar Surface 15 dark Exceeds Mobility allows roving to as many sites as is needed Communication Send and Receive (real time) Exceeds Capable of sending data at 150 Mbps Landing Parameters 12º slope within 100 m Exceeds Six wheel rocker bogey system allows landing on slopes greater than 12 degrees Survive Launch of 6 G's Exceeds Cyclops structure will handle g-loads exceeding 6 g’s Technology Requirements TRL 9 Meets Materials used are TRL 9 Exceeds RTG can provide the power needed during dark conditions Thermal Conditions Survive Temperature Changes Exceeds Materials used will withstand temperatures exceeding the 50 K to 380 K range Sample Return Vehicle Sample Return (Goal) Meets Exceeds the sample return expectations Mobile Roving/Real-Time Mobility Exceeds 6 wheel rocker bogey allows roving in real-time Power Requirements Store Power in Dark Conditions
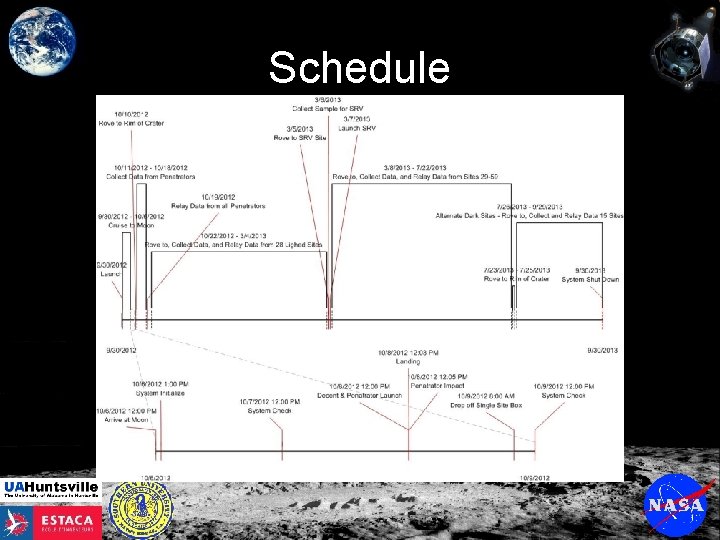
Schedule
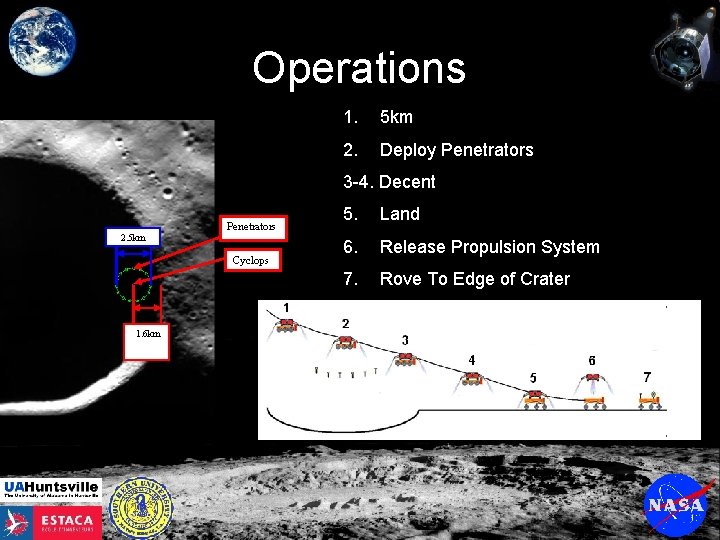
Operations 1. 5 km 2. Deploy Penetrators 3 -4. Decent 2. 5 km Penetrators Cyclops 5. Land 6. Release Propulsion System 7. Rove To Edge of Crater 1. 6 km
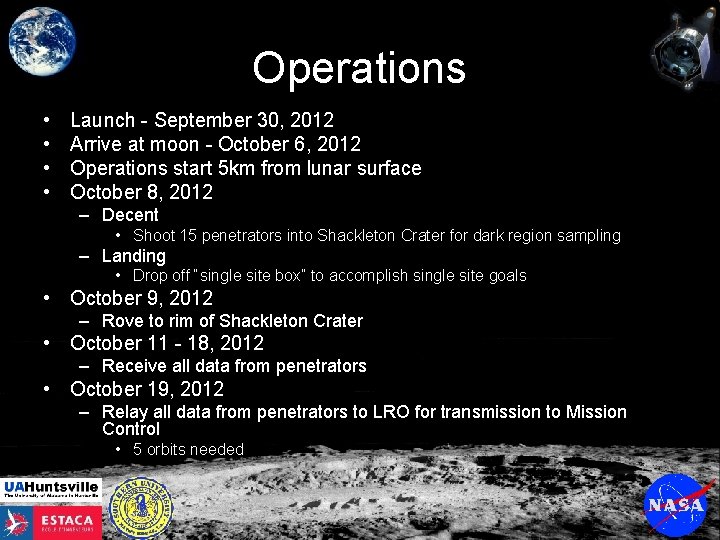
Operations • • Launch - September 30, 2012 Arrive at moon - October 6, 2012 Operations start 5 km from lunar surface October 8, 2012 – Decent • Shoot 15 penetrators into Shackleton Crater for dark region sampling – Landing • Drop off “single site box” to accomplish single site goals • October 9, 2012 – Rove to rim of Shackleton Crater • October 11 - 18, 2012 – Receive all data from penetrators • October 19, 2012 – Relay all data from penetrators to LRO for transmission to Mission Control • 5 orbits needed
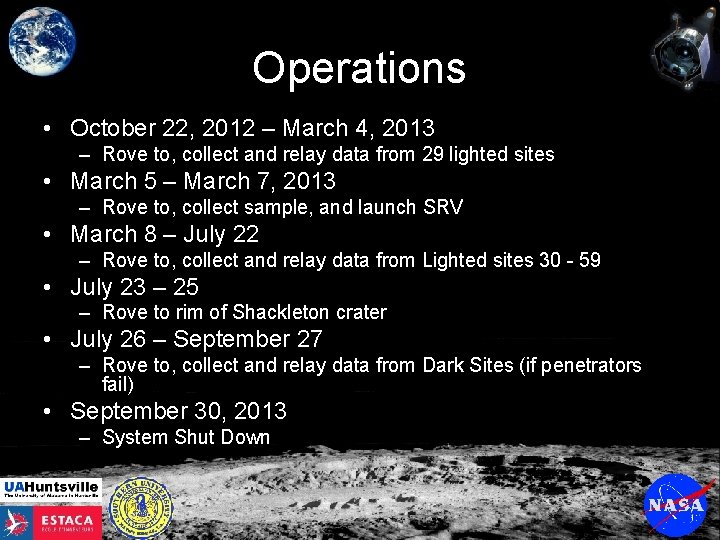
Operations • October 22, 2012 – March 4, 2013 – Rove to, collect and relay data from 29 lighted sites • March 5 – March 7, 2013 – Rove to, collect sample, and launch SRV • March 8 – July 22 – Rove to, collect and relay data from Lighted sites 30 - 59 • July 23 – 25 – Rove to rim of Shackleton crater • July 26 – September 27 – Rove to, collect and relay data from Dark Sites (if penetrators fail) • September 30, 2013 – System Shut Down
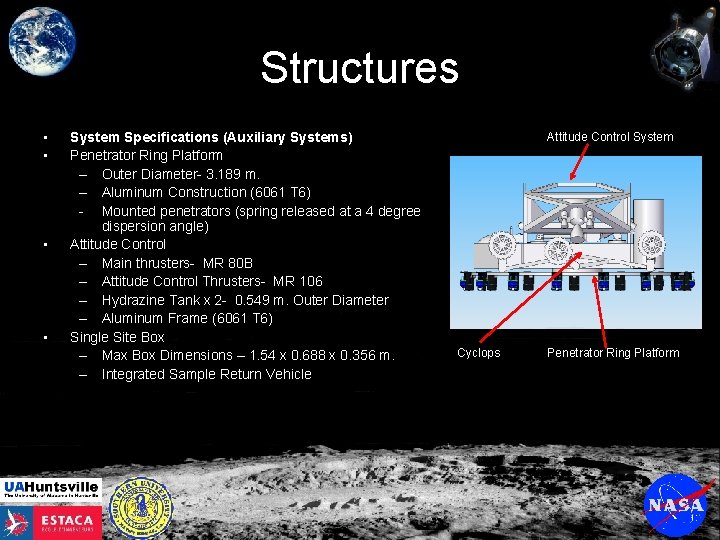
Structures • • System Specifications (Auxiliary Systems) Penetrator Ring Platform – Outer Diameter- 3. 189 m. – Aluminum Construction (6061 T 6) - Mounted penetrators (spring released at a 4 degree dispersion angle) Attitude Control – Main thrusters- MR 80 B – Attitude Control Thrusters- MR 106 – Hydrazine Tank x 2 - 0. 549 m. Outer Diameter – Aluminum Frame (6061 T 6) Single Site Box – Max Box Dimensions – 1. 54 x 0. 688 x 0. 356 m. – Integrated Sample Return Vehicle Attitude Control System Cyclops Penetrator Ring Platform
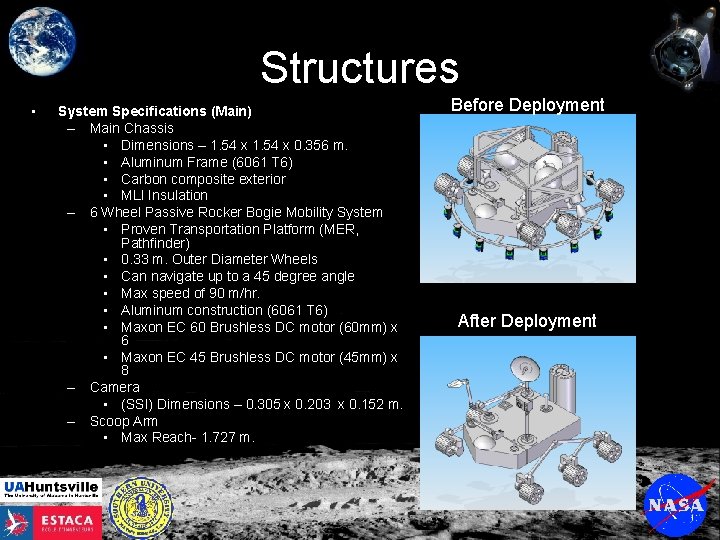
Structures • System Specifications (Main) – Main Chassis • Dimensions – 1. 54 x 0. 356 m. • Aluminum Frame (6061 T 6) • Carbon composite exterior • MLI Insulation – 6 Wheel Passive Rocker Bogie Mobility System • Proven Transportation Platform (MER, Pathfinder) • 0. 33 m. Outer Diameter Wheels • Can navigate up to a 45 degree angle • Max speed of 90 m/hr. • Aluminum construction (6061 T 6) • Maxon EC 60 Brushless DC motor (60 mm) x 6 • Maxon EC 45 Brushless DC motor (45 mm) x 8 – Camera • (SSI) Dimensions – 0. 305 x 0. 203 x 0. 152 m. – Scoop Arm • Max Reach- 1. 727 m. Before Deployment After Deployment
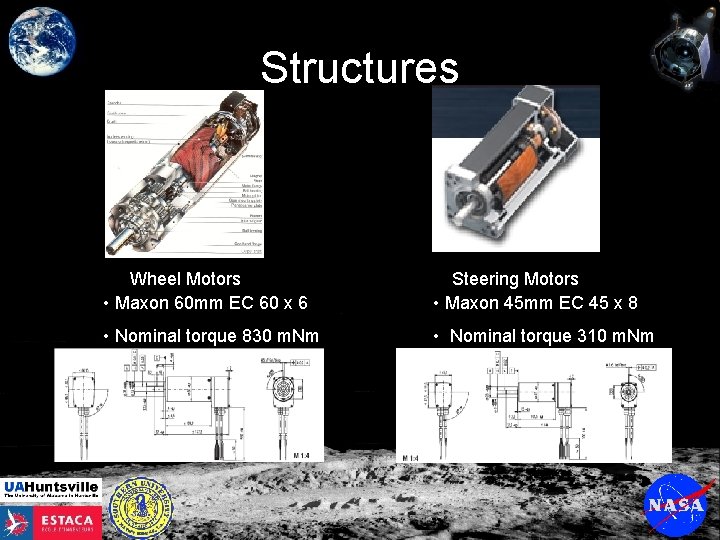
Structures Wheel Motors • Maxon 60 mm EC 60 x 6 Steering Motors • Maxon 45 mm EC 45 x 8 • Nominal torque 830 m. Nm • Nominal torque 310 m. Nm
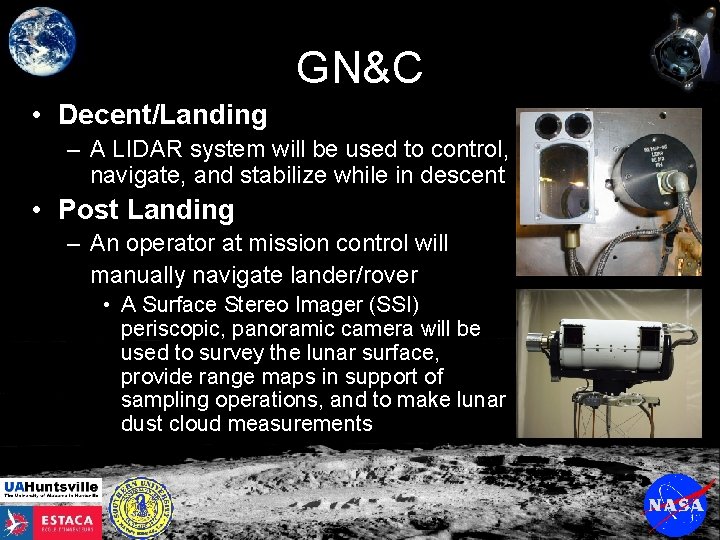
GN&C • Decent/Landing – A LIDAR system will be used to control, navigate, and stabilize while in descent • Post Landing – An operator at mission control will manually navigate lander/rover • A Surface Stereo Imager (SSI) periscopic, panoramic camera will be used to survey the lunar surface, provide range maps in support of sampling operations, and to make lunar dust cloud measurements
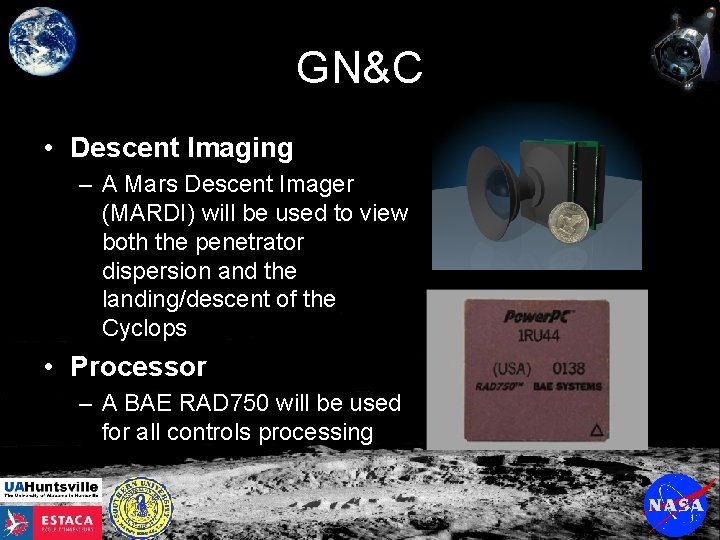
GN&C • Descent Imaging – A Mars Descent Imager (MARDI) will be used to view both the penetrator dispersion and the landing/descent of the Cyclops • Processor – A BAE RAD 750 will be used for all controls processing
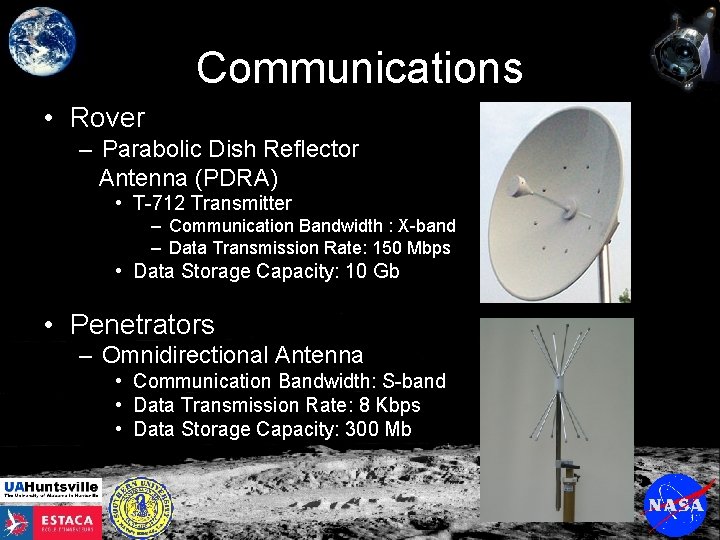
Communications • Rover – Parabolic Dish Reflector Antenna (PDRA) • T-712 Transmitter – Communication Bandwidth : X-band – Data Transmission Rate: 150 Mbps • Data Storage Capacity: 10 Gb • Penetrators – Omnidirectional Antenna • Communication Bandwidth: S-band • Data Transmission Rate: 8 Kbps • Data Storage Capacity: 300 Mb
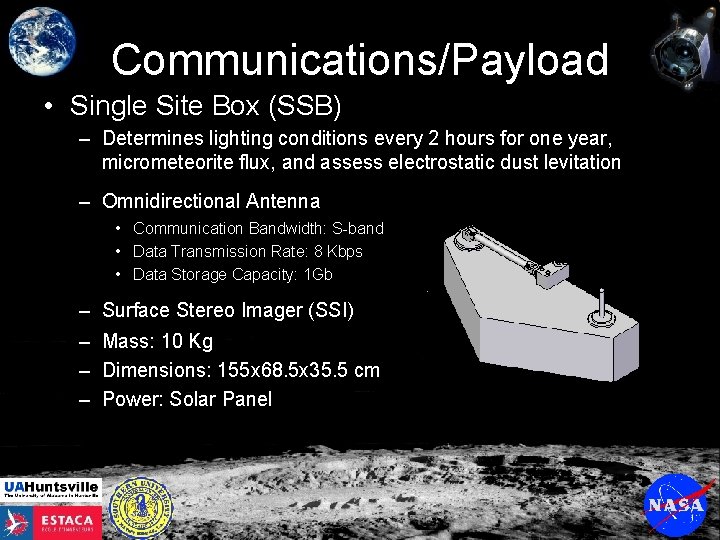
Communications/Payload • Single Site Box (SSB) – Determines lighting conditions every 2 hours for one year, micrometeorite flux, and assess electrostatic dust levitation – Omnidirectional Antenna • Communication Bandwidth: S-band • Data Transmission Rate: 8 Kbps • Data Storage Capacity: 1 Gb – – Surface Stereo Imager (SSI) Mass: 10 Kg Dimensions: 155 x 68. 5 x 35. 5 cm Power: Solar Panel
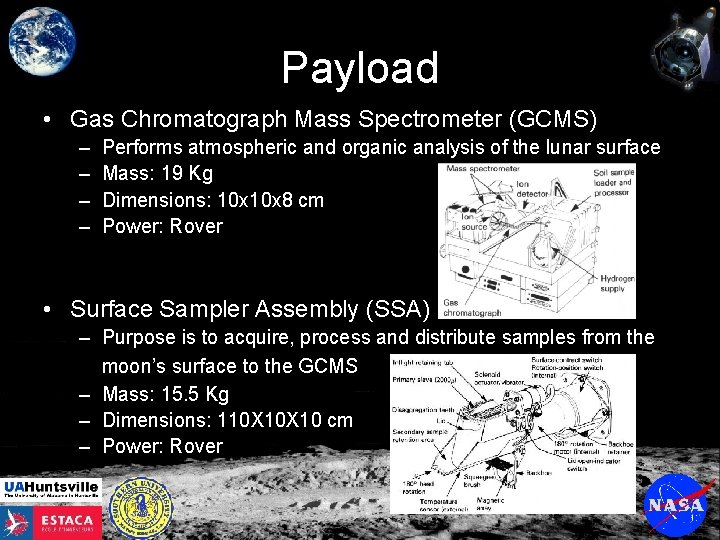
Payload • Gas Chromatograph Mass Spectrometer (GCMS) – – Performs atmospheric and organic analysis of the lunar surface Mass: 19 Kg Dimensions: 10 x 8 cm Power: Rover • Surface Sampler Assembly (SSA) – Purpose is to acquire, process and distribute samples from the moon’s surface to the GCMS – Mass: 15. 5 Kg – Dimensions: 110 X 10 cm – Power: Rover
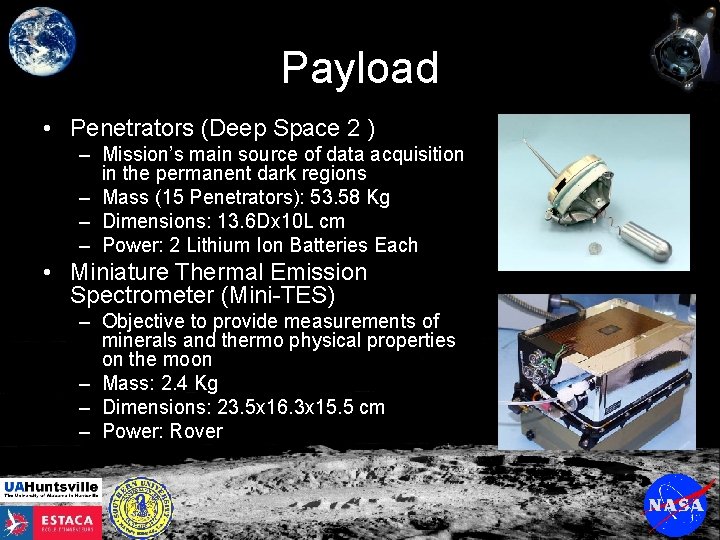
Payload • Penetrators (Deep Space 2 ) – Mission’s main source of data acquisition in the permanent dark regions – Mass (15 Penetrators): 53. 58 Kg – Dimensions: 13. 6 Dx 10 L cm – Power: 2 Lithium Ion Batteries Each • Miniature Thermal Emission Spectrometer (Mini-TES) – Objective to provide measurements of minerals and thermo physical properties on the moon – Mass: 2. 4 Kg – Dimensions: 23. 5 x 16. 3 x 15. 5 cm – Power: Rover
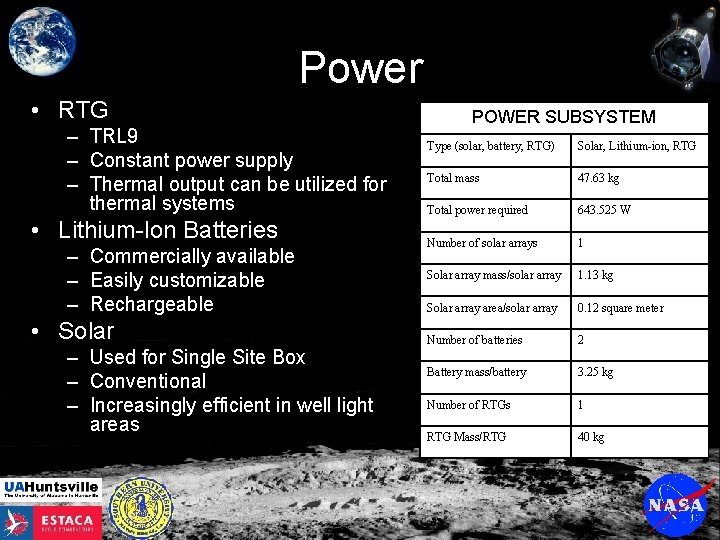
Power • RTG – TRL 9 – Constant power supply – Thermal output can be utilized for thermal systems • Lithium-Ion Batteries – Commercially available – Easily customizable – Rechargeable • Solar – Used for Single Site Box – Conventional – Increasingly efficient in well light areas POWER SUBSYSTEM Type (solar, battery, RTG) Solar, Lithium-ion, RTG Total mass 47. 63 kg Total power required 643. 525 W Number of solar arrays 1 Solar array mass/solar array 1. 13 kg Solar array area/solar array 0. 12 square meter Number of batteries 2 Battery mass/battery 3. 25 kg Number of RTGs 1 RTG Mass/RTG 40 kg
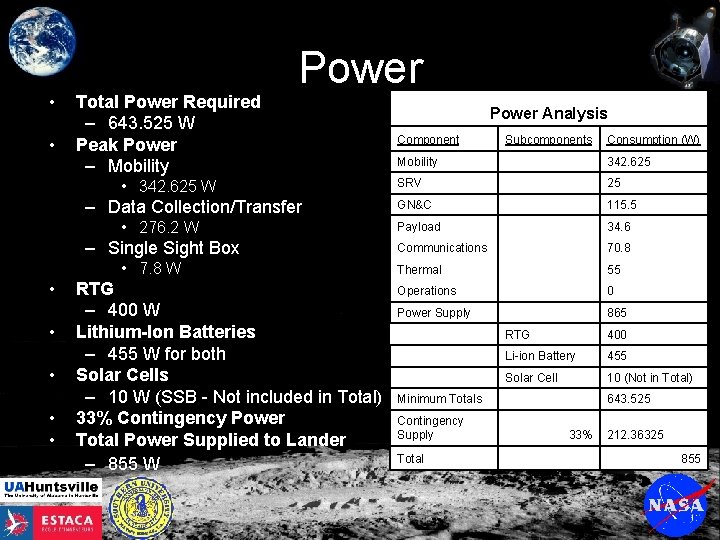
• • Total Power Required – 643. 525 W Peak Power – Mobility Power • 342. 625 W – Data Collection/Transfer • 276. 2 W – Single Sight Box • 7. 8 W • • • RTG – 400 W Lithium-Ion Batteries – 455 W for both Solar Cells – 10 W (SSB - Not included in Total) 33% Contingency Power Total Power Supplied to Lander – 855 W Power Analysis Component Subcomponents Consumption (W) Mobility 342. 625 SRV 25 GN&C 115. 5 Payload 34. 6 Communications 70. 8 Thermal 55 Operations 0 Power Supply 865 RTG 400 Li-ion Battery 455 Solar Cell 10 (Not in Total) 643. 525 Minimum Totals Contingency Supply Total 33% 212. 36325 855
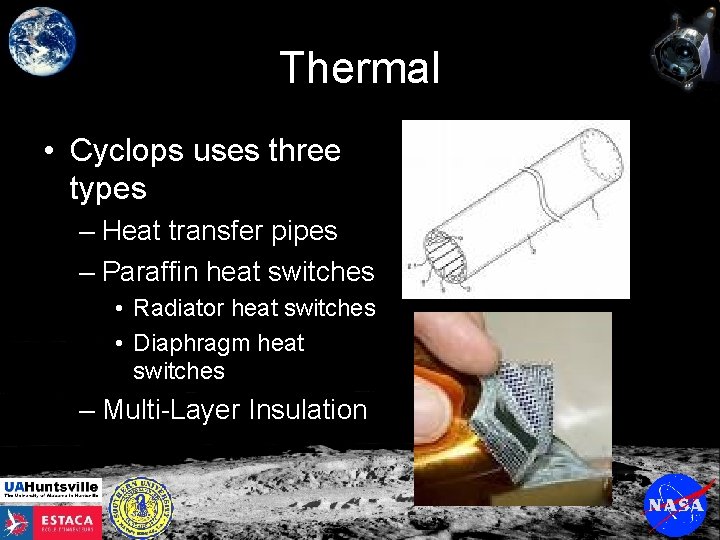
Thermal • Cyclops uses three types – Heat transfer pipes – Paraffin heat switches • Radiator heat switches • Diaphragm heat switches – Multi-Layer Insulation
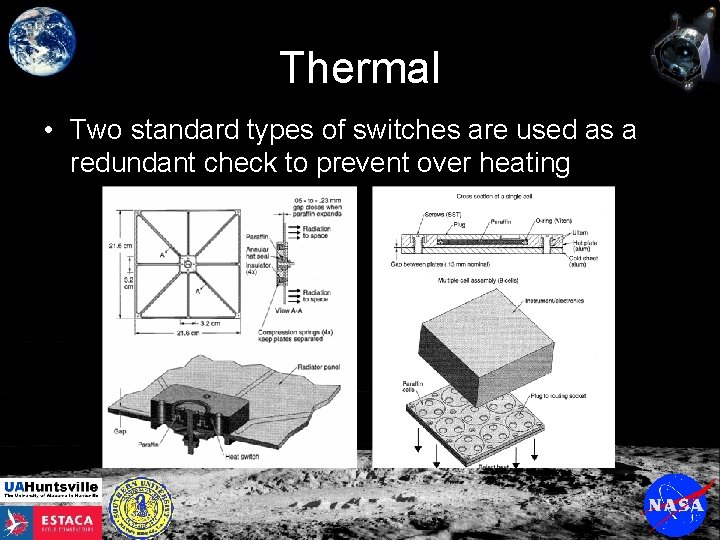
Thermal • Two standard types of switches are used as a redundant check to prevent over heating
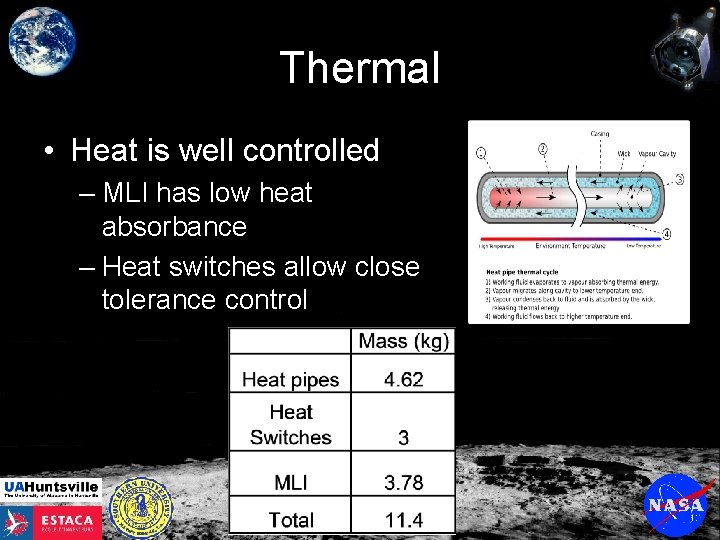
Thermal • Heat is well controlled – MLI has low heat absorbance – Heat switches allow close tolerance control
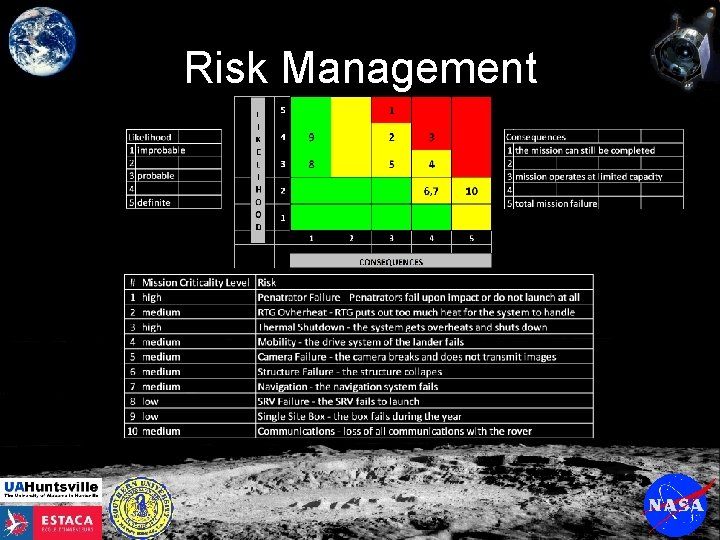
Risk Management
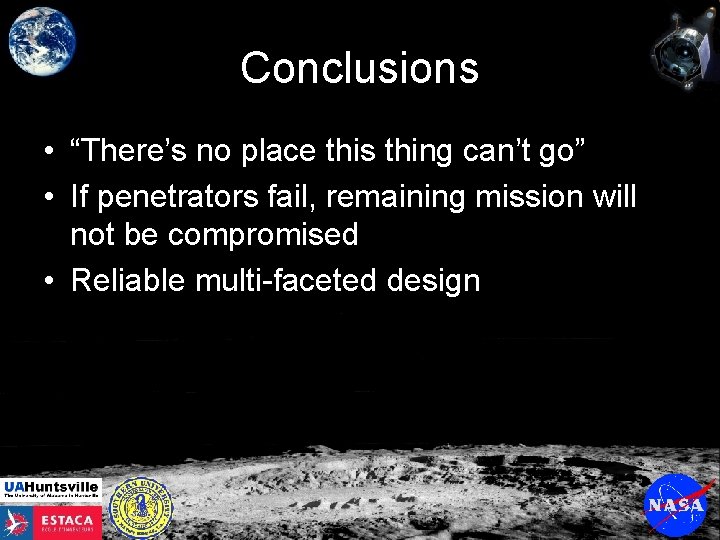
Conclusions • “There’s no place this thing can’t go” • If penetrators fail, remaining mission will not be compromised • Reliable multi-faceted design
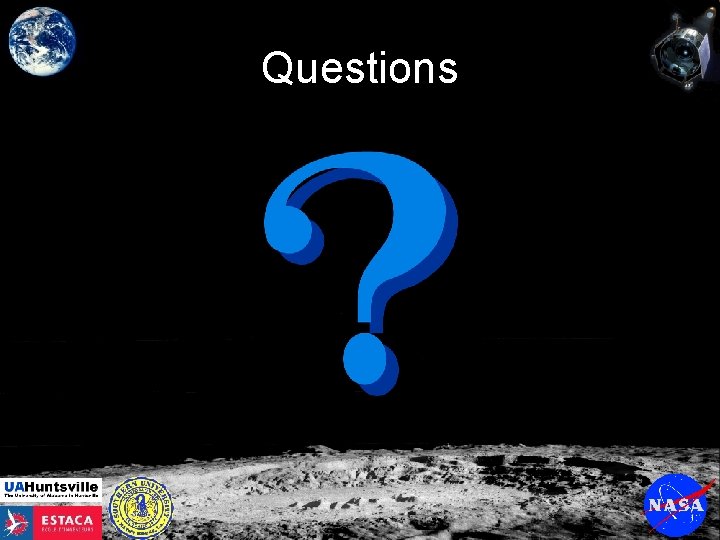
Questions
Ba 491
Ba 491
Pngpa
Cs 491
Ceng 491
Color 091807
Ece 491
Mtbf formula
Cse 491
Ece 491
Ece 491
Ee 491
Ee 491
Lunar system
Responsabilidade civil
Ece 492
Ece 492
Csce 492
Cs 492
Round 683,492 to the nearest hundred thousand
Buku daftar am 492a
492 bc
356 271 rounded to the nearest ten thousand
Ece 492
Ece 492
Cs 492
Csce 492
Lms diagram
Cs 492