Lecture 6 Signal Encoding Techniques 2 nd semester
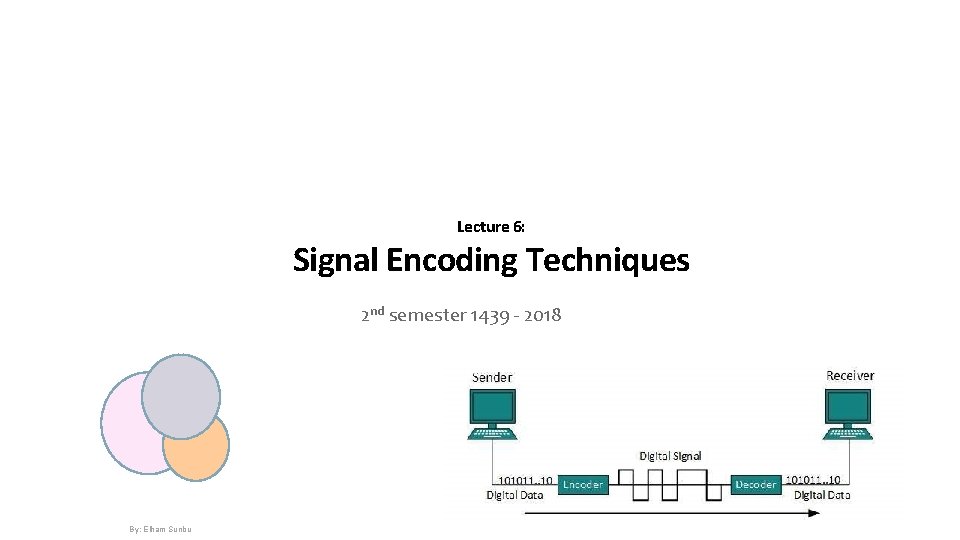
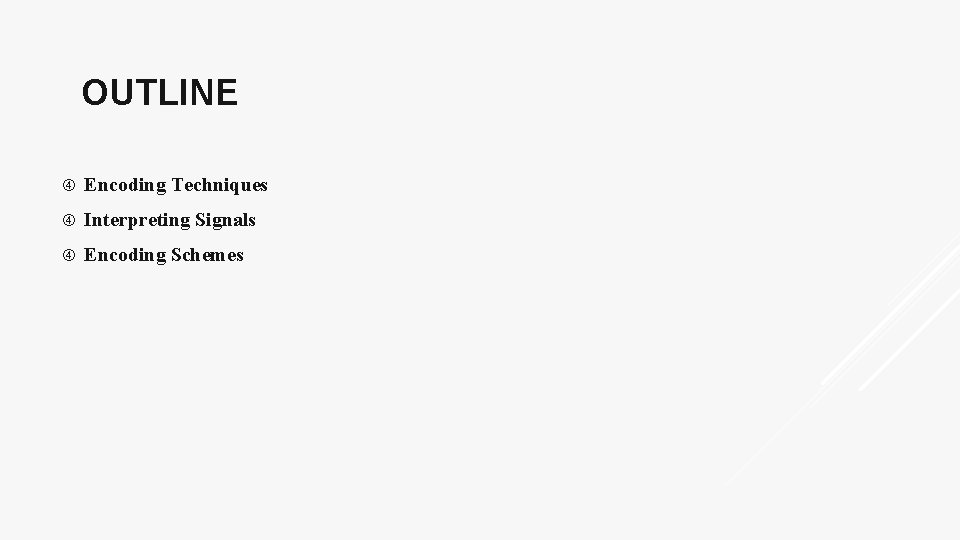
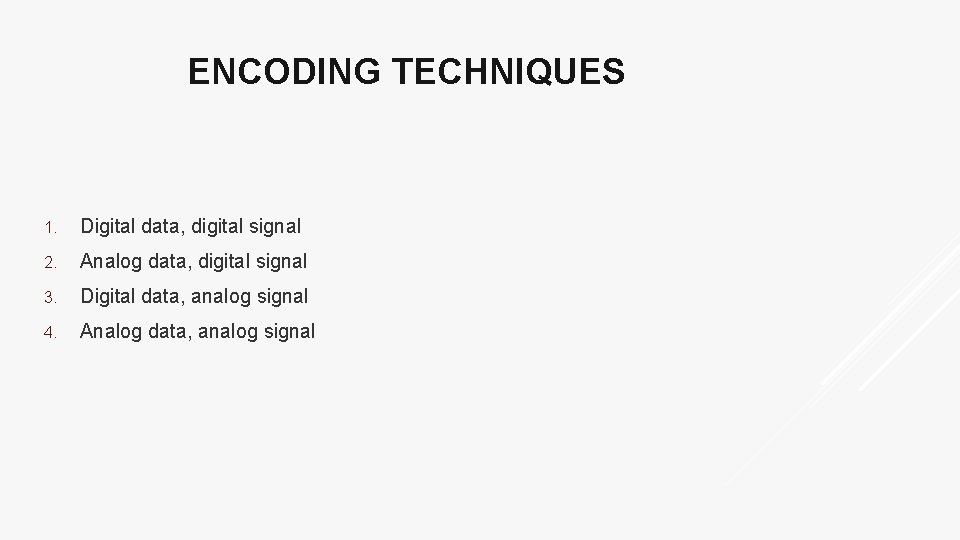
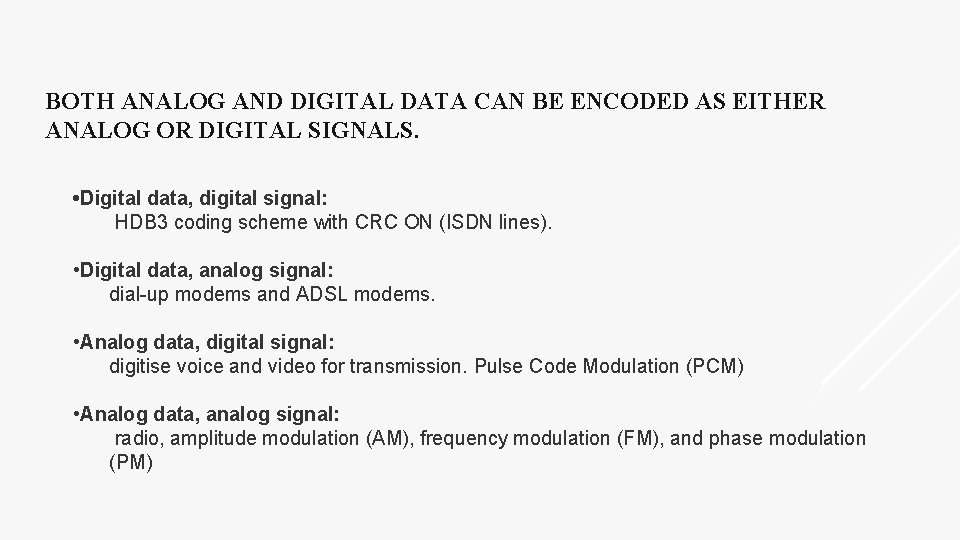
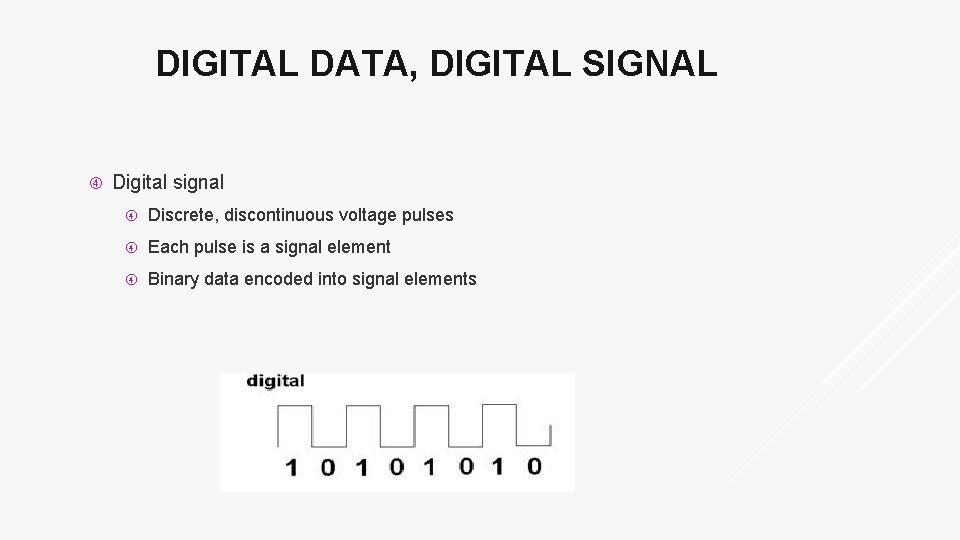
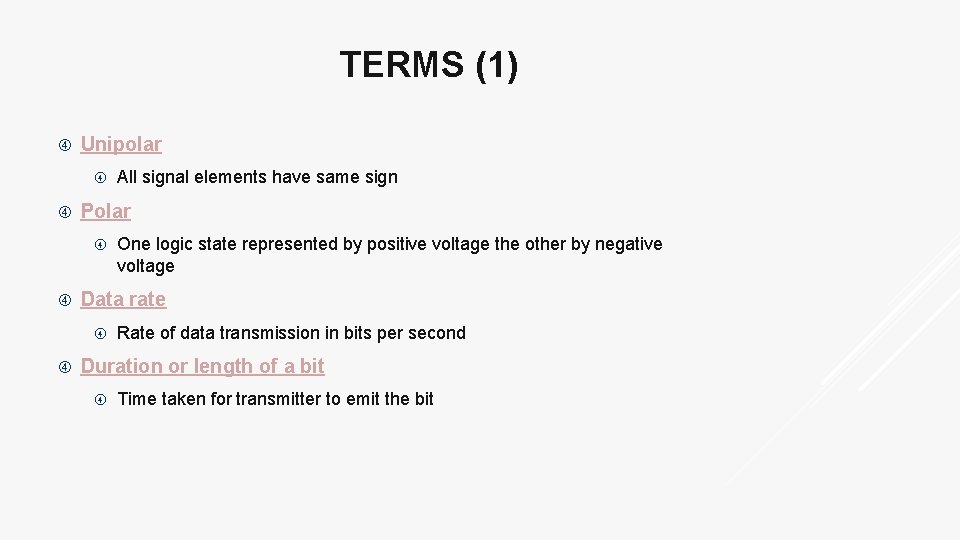
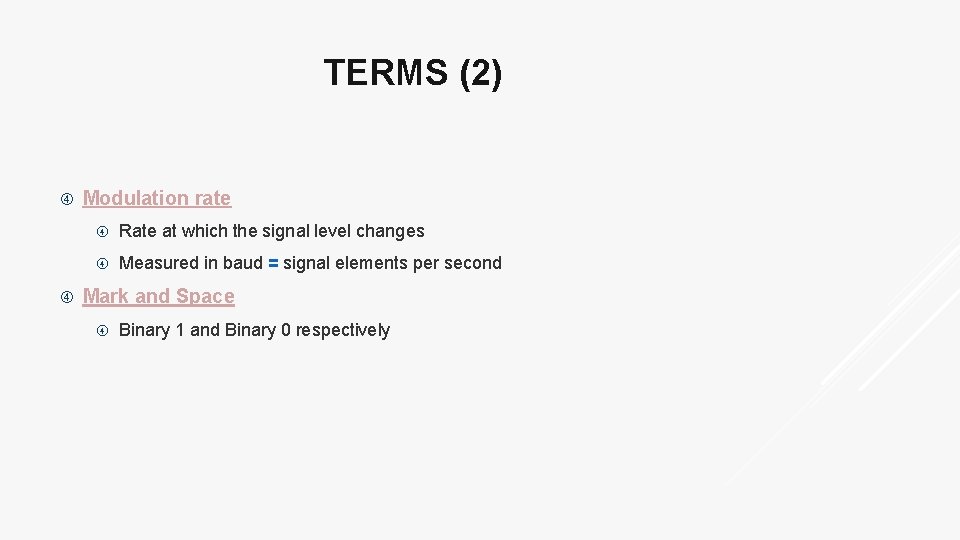
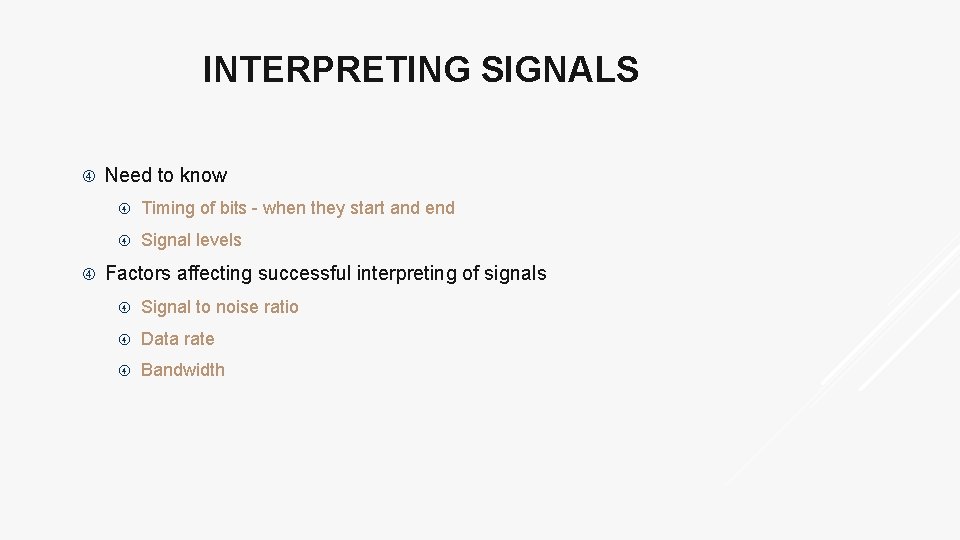
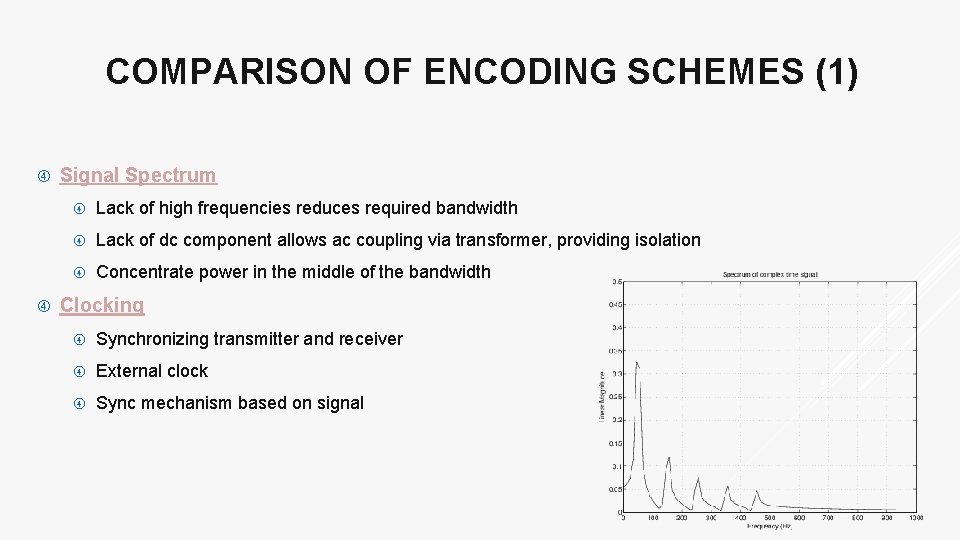
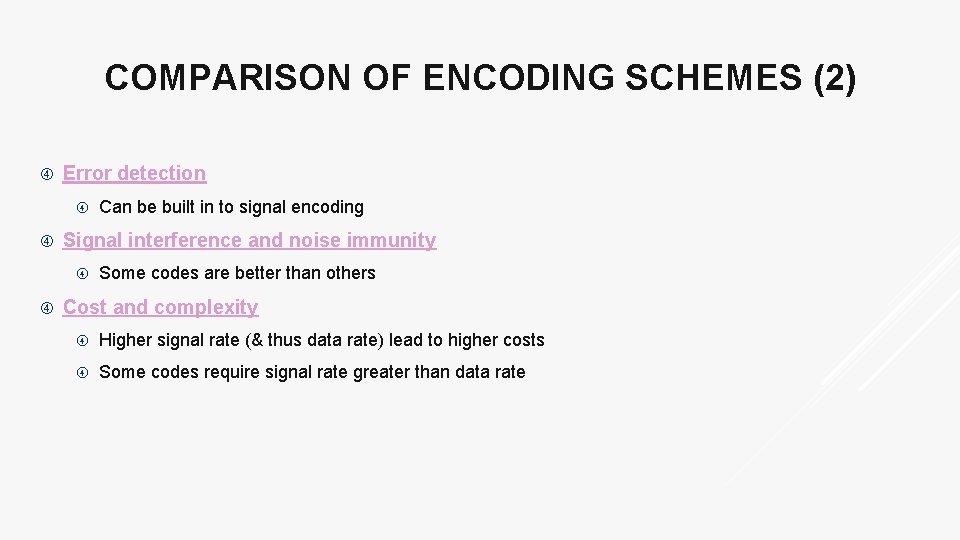
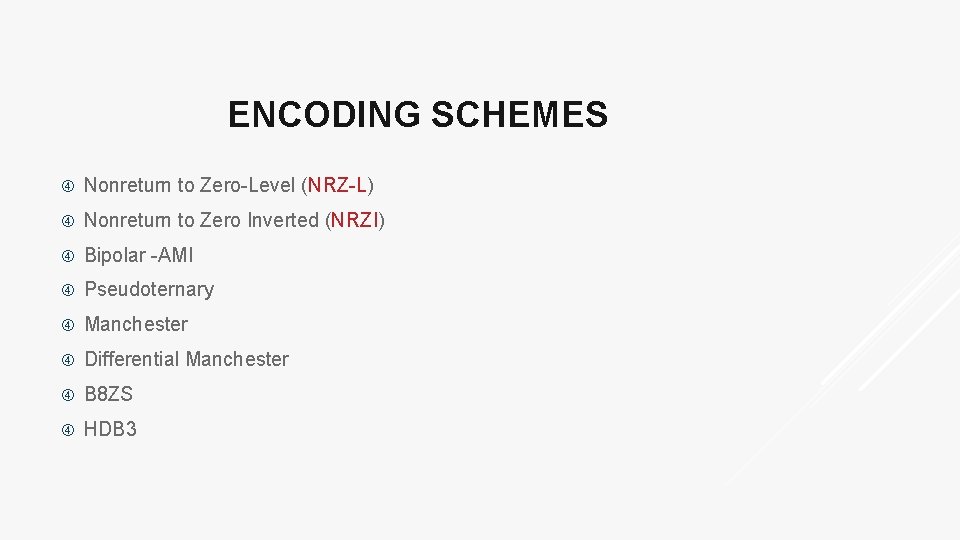
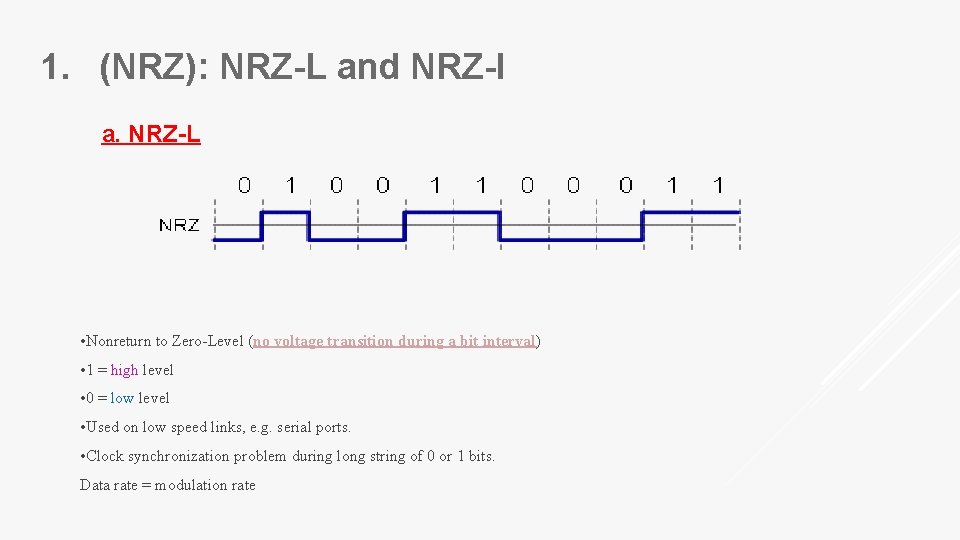
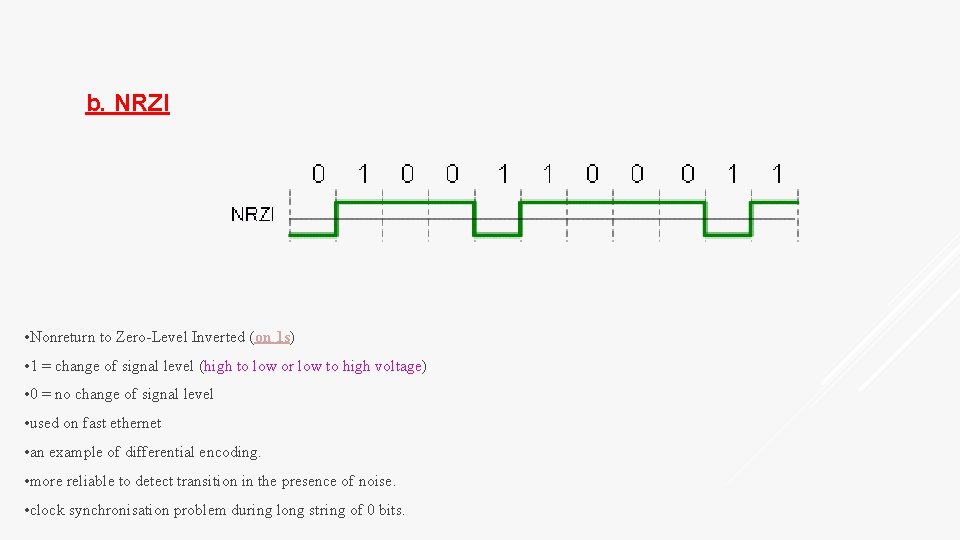
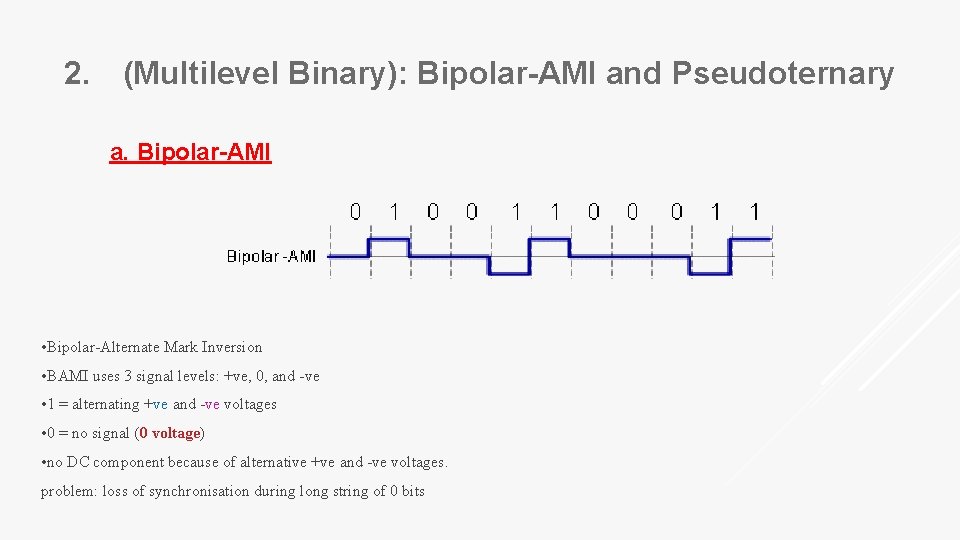
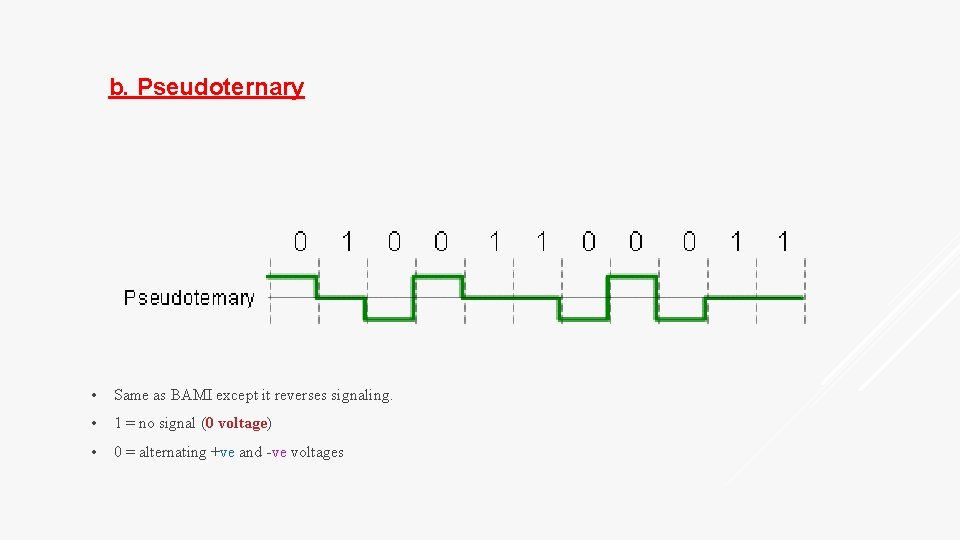
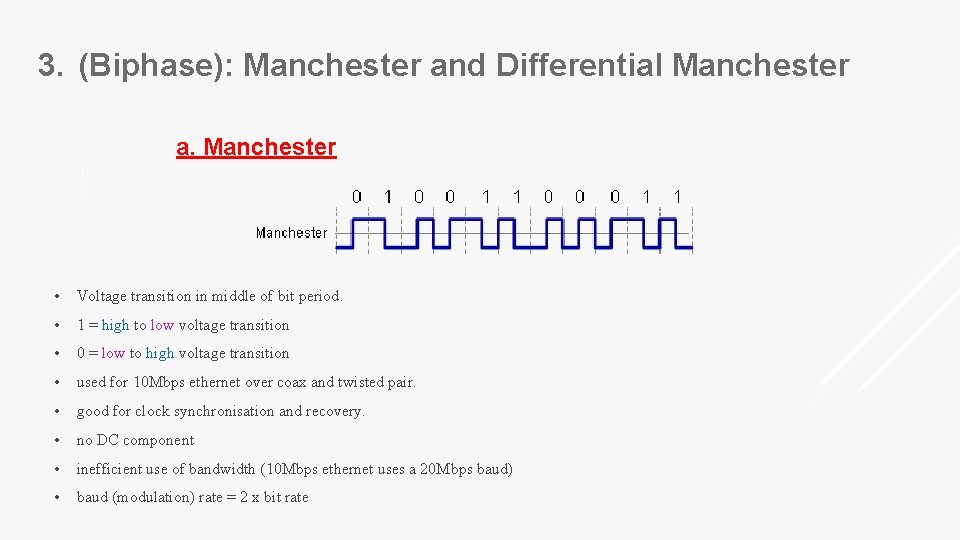
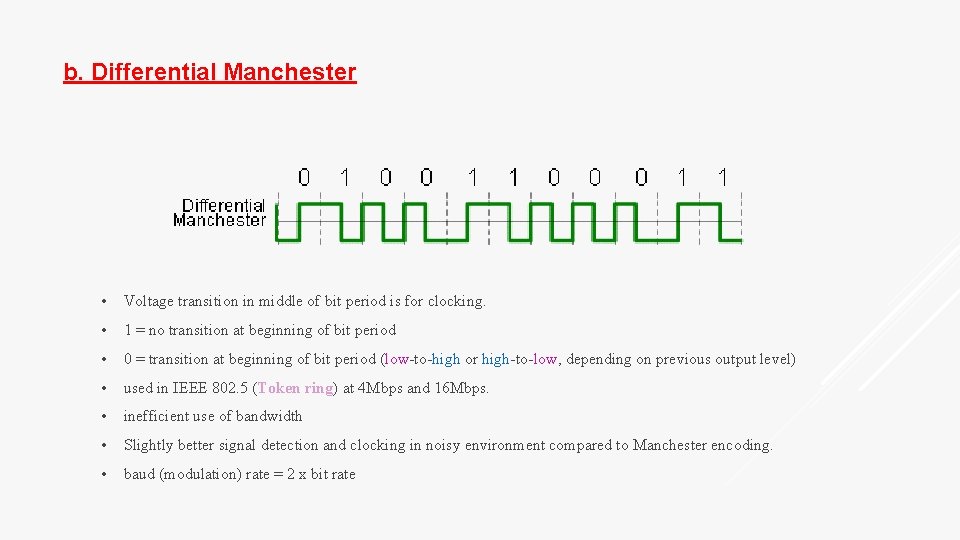
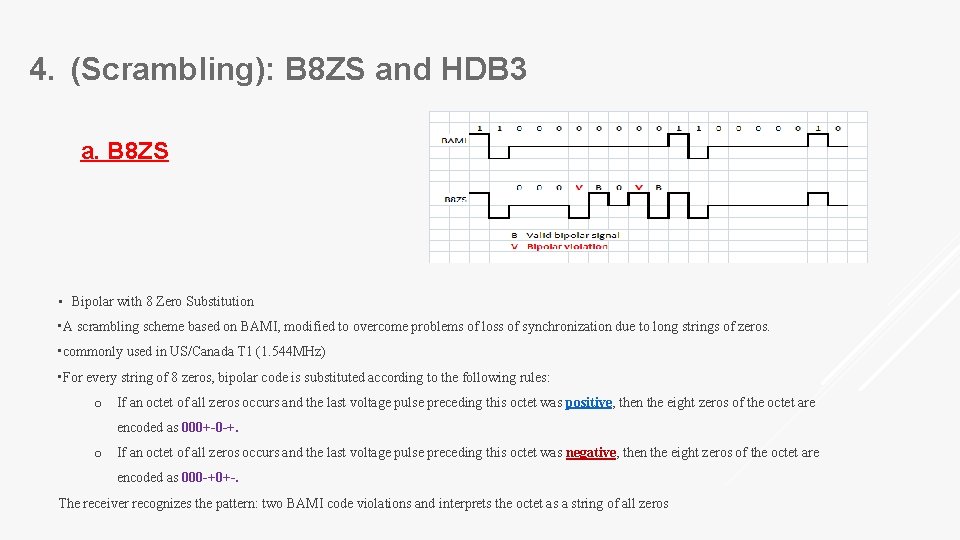
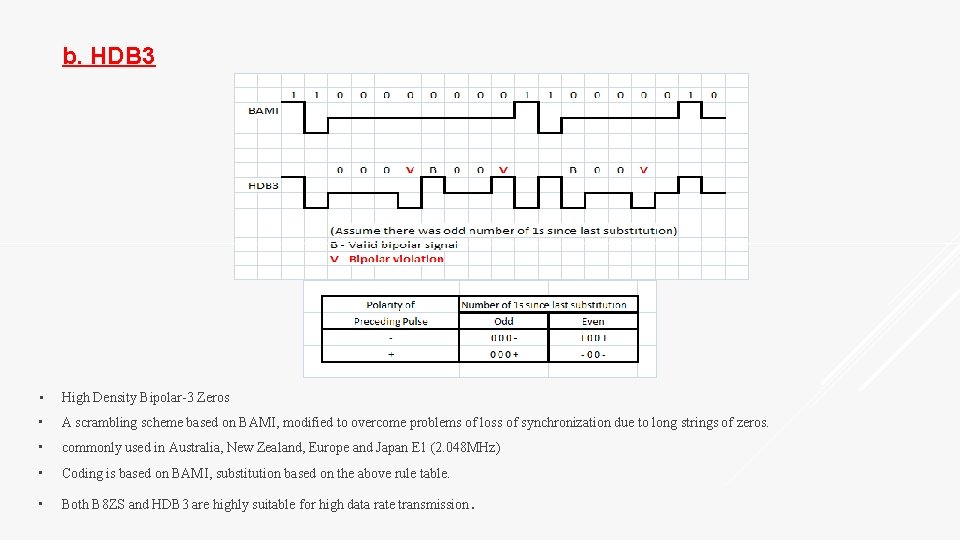
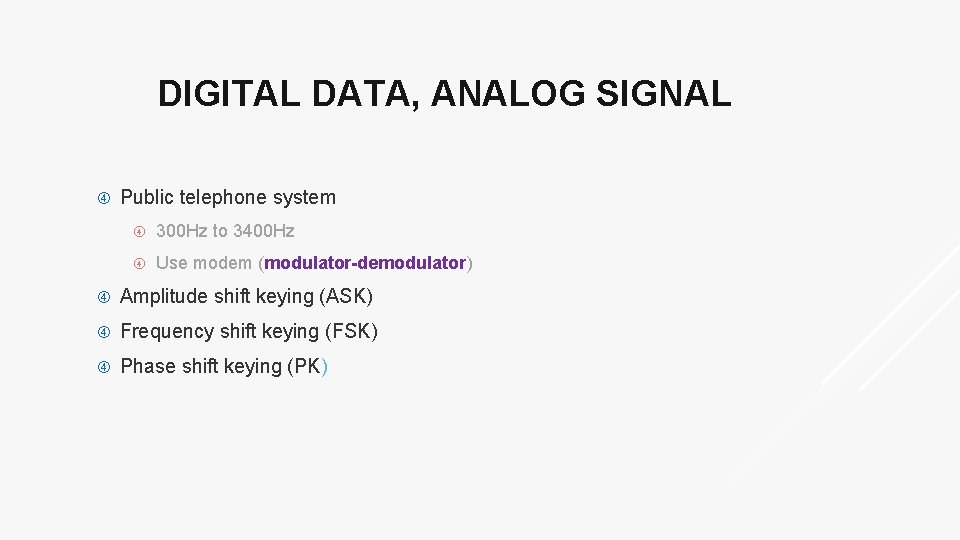
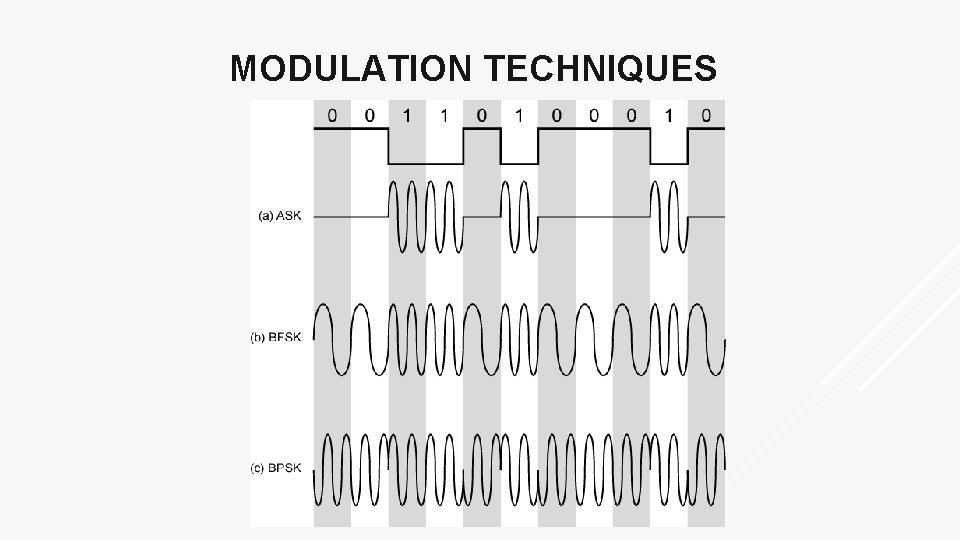
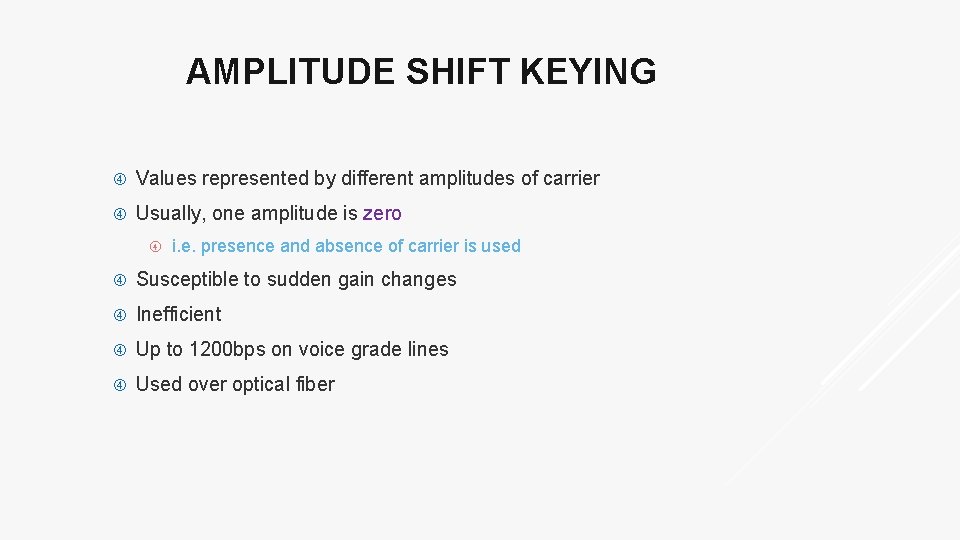
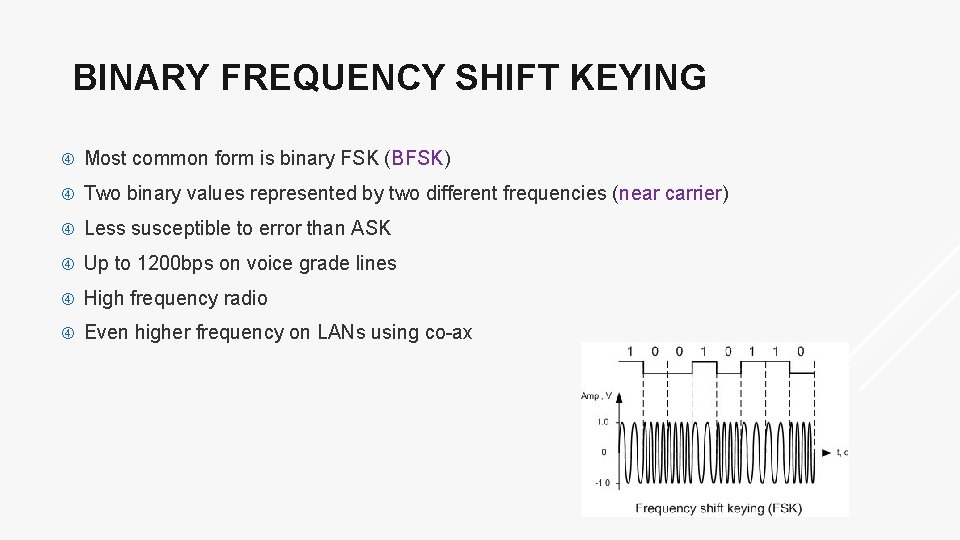
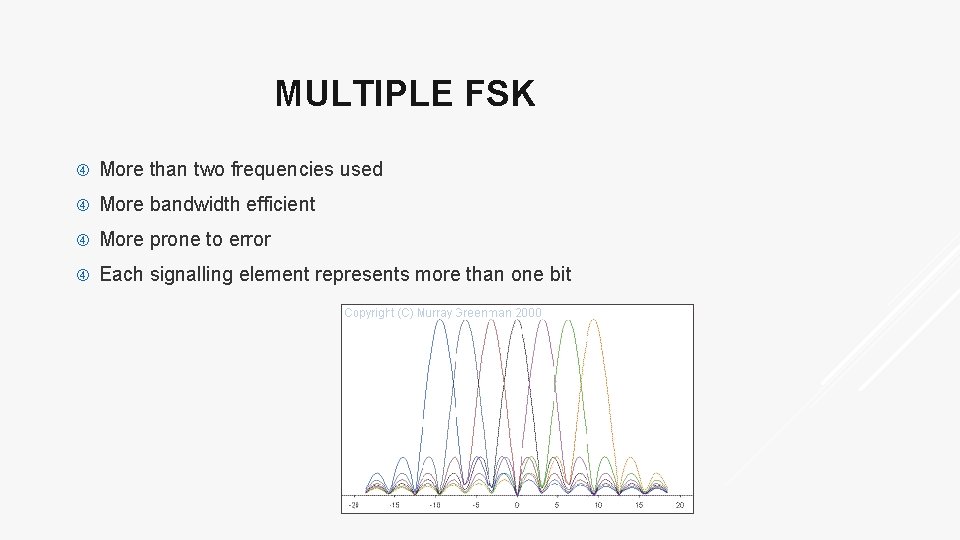
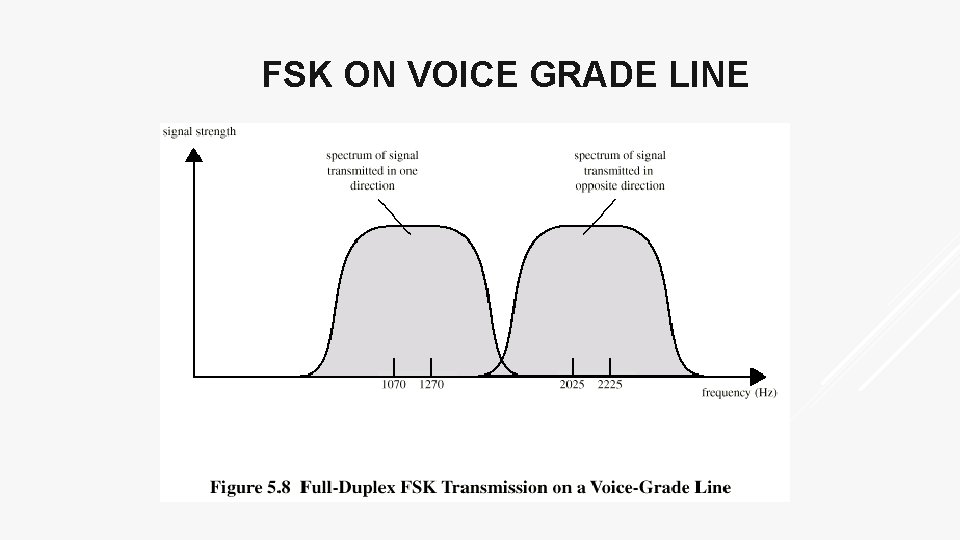
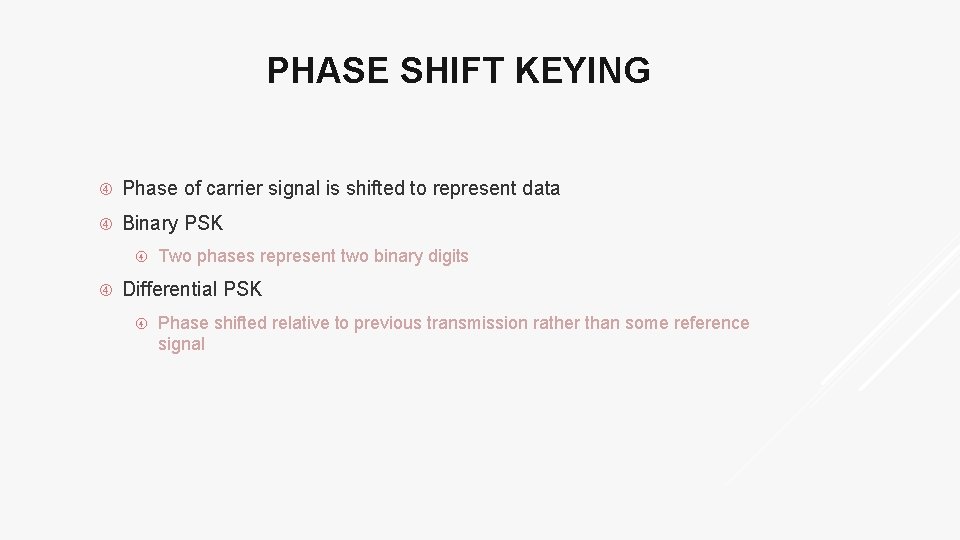
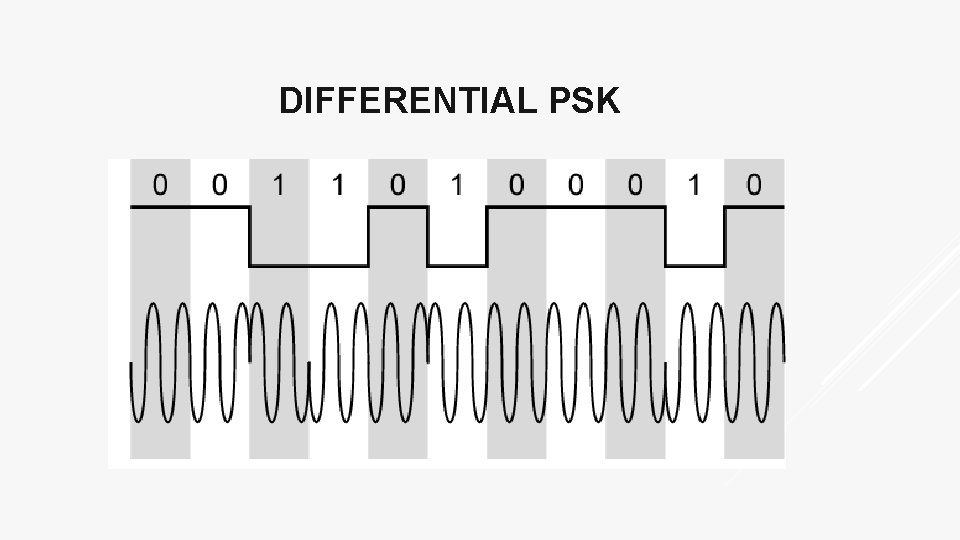
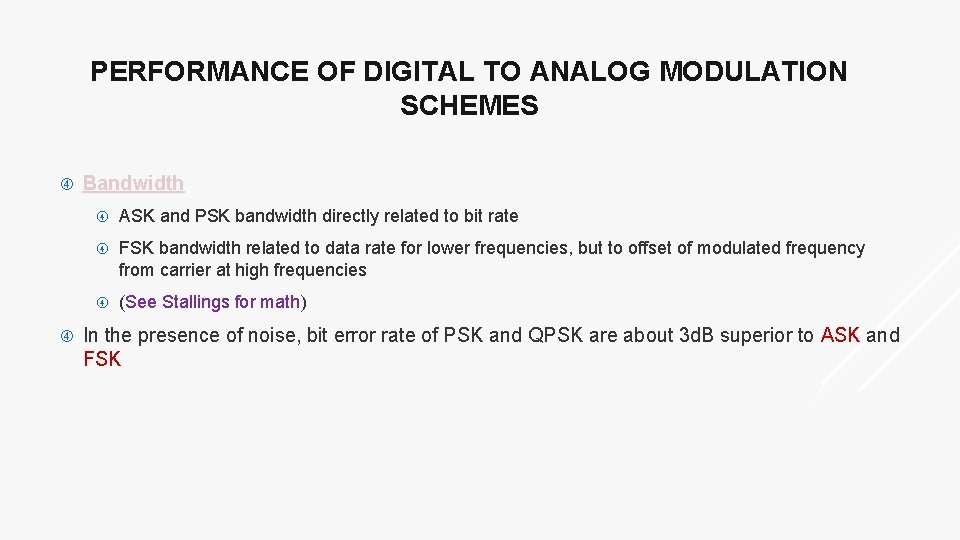
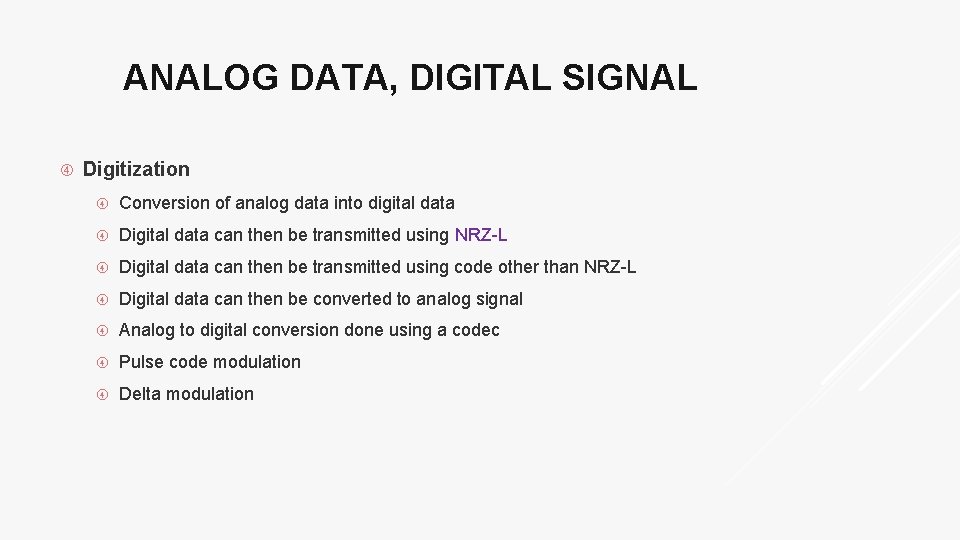
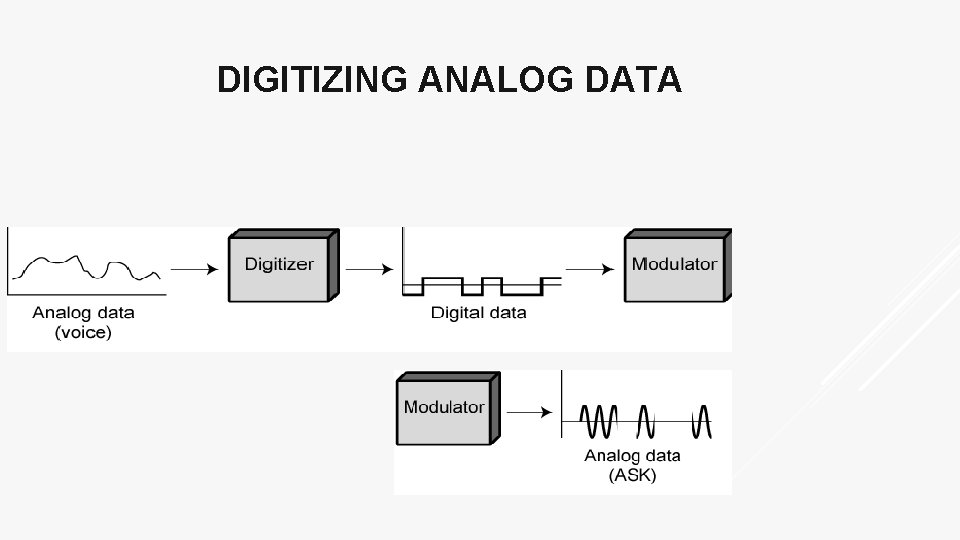
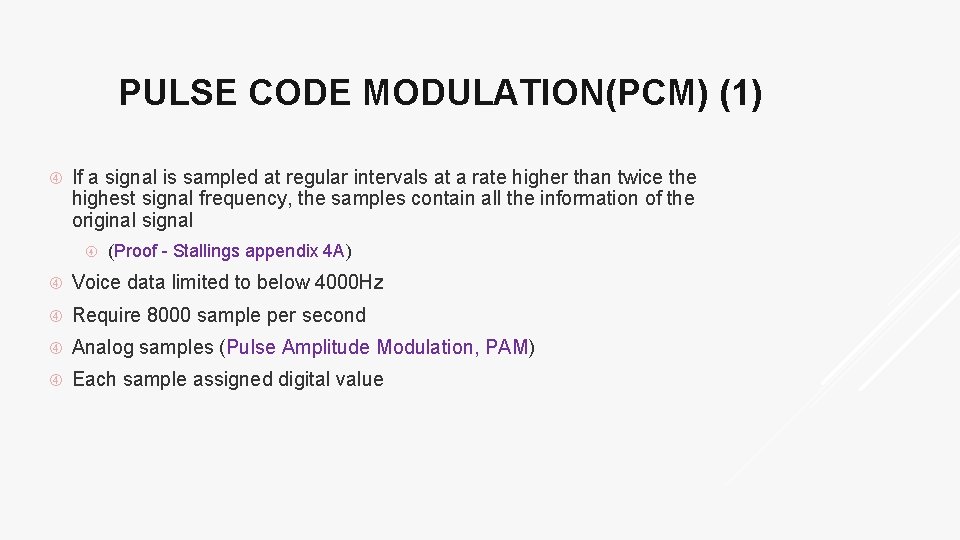
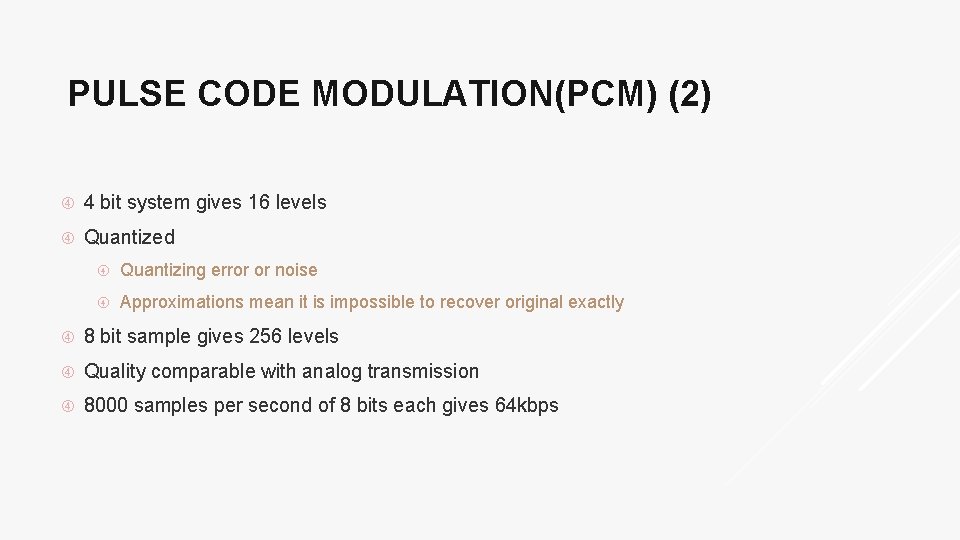
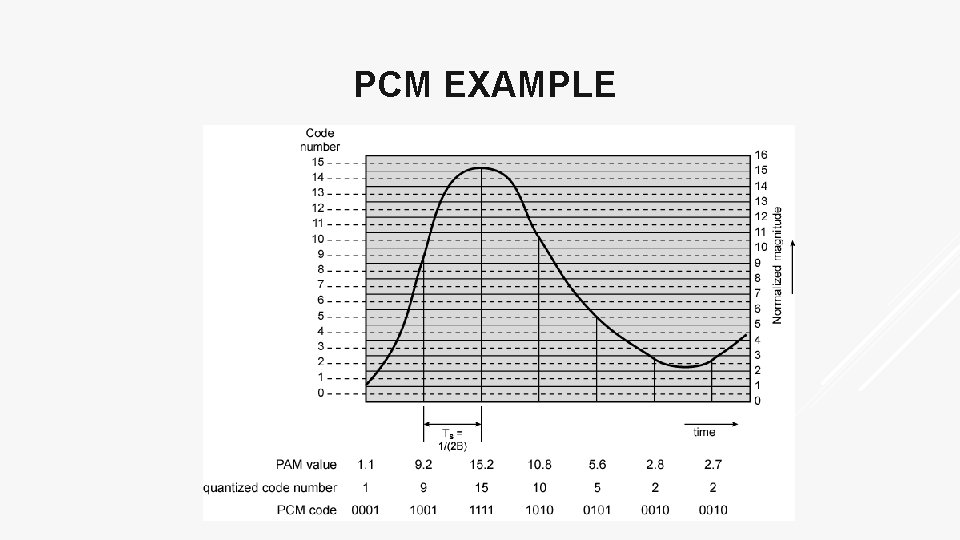
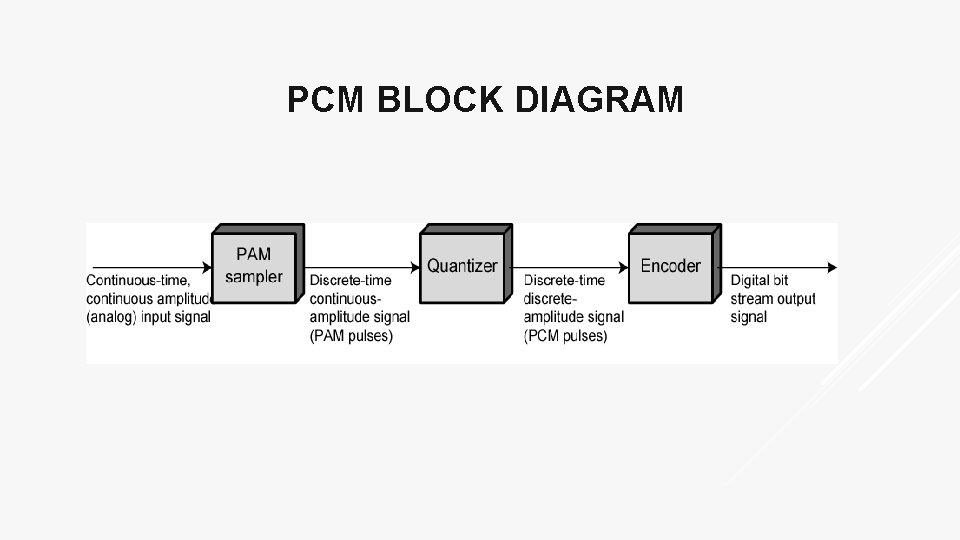
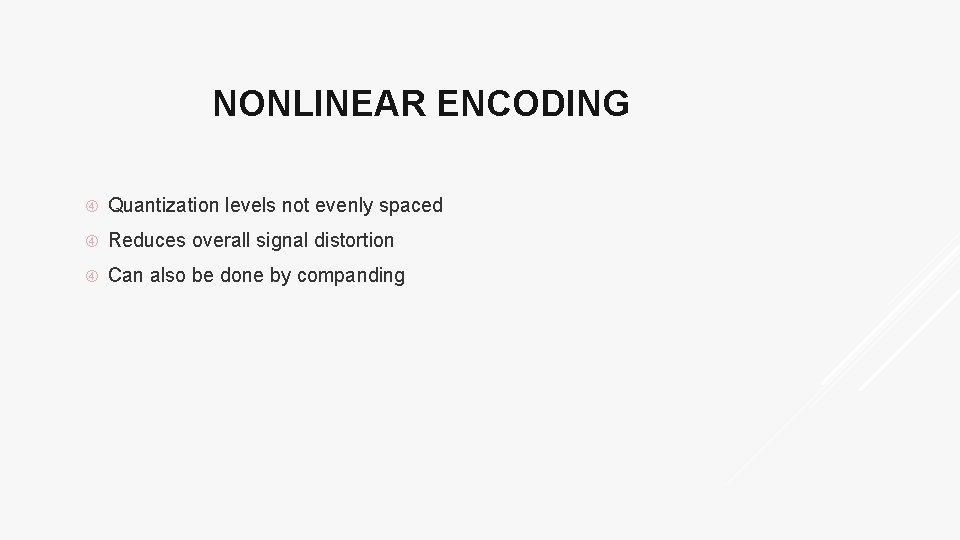
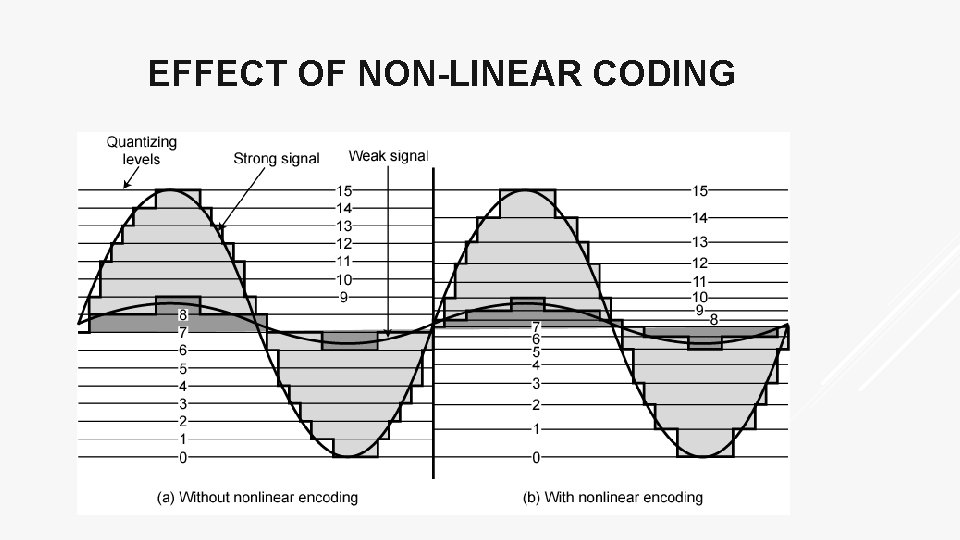
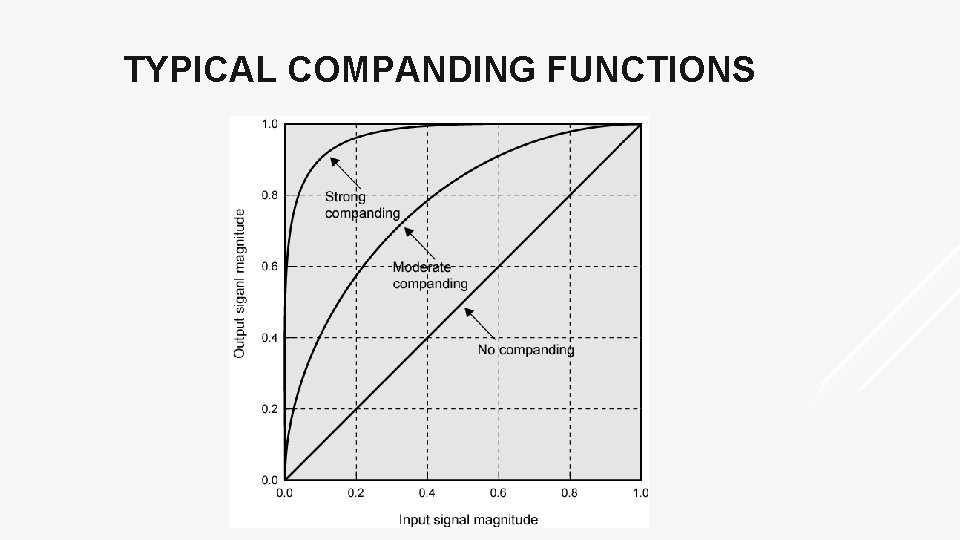
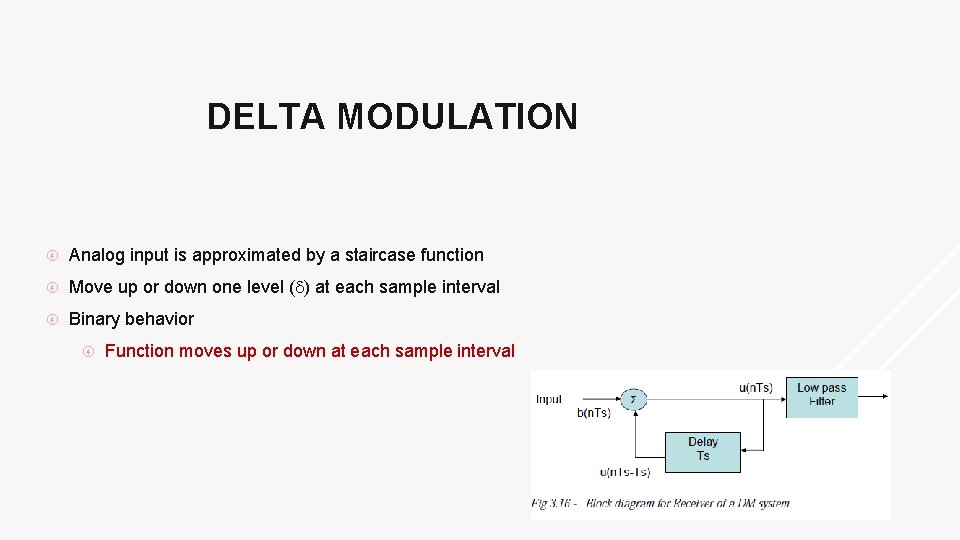
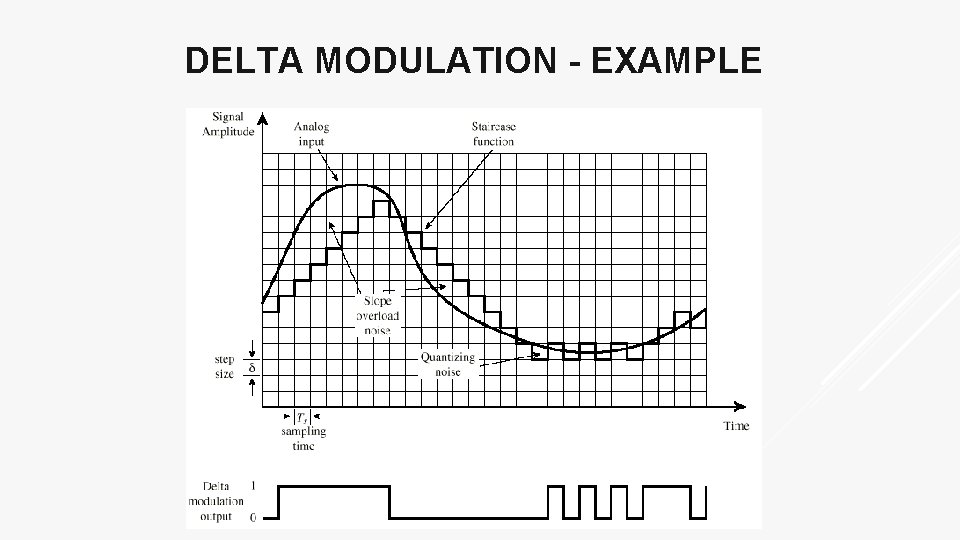
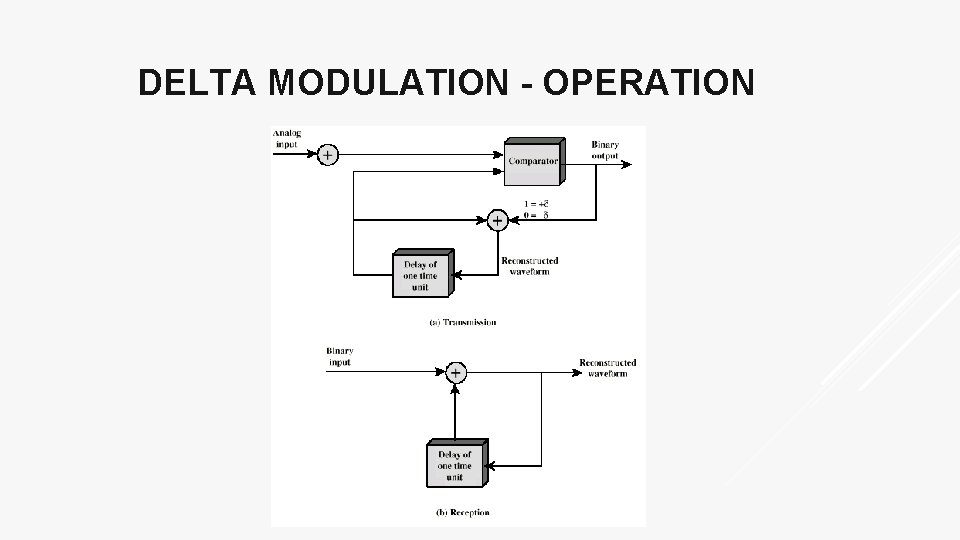
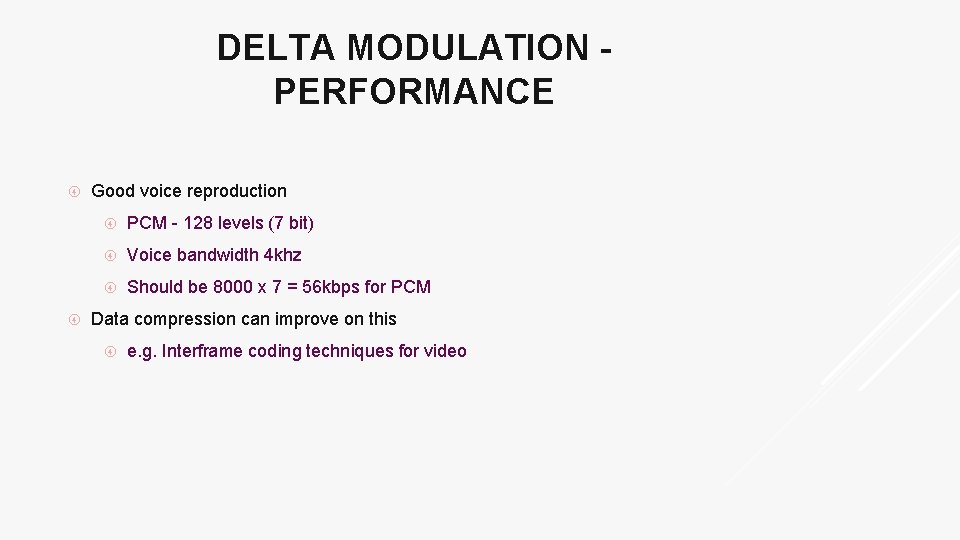
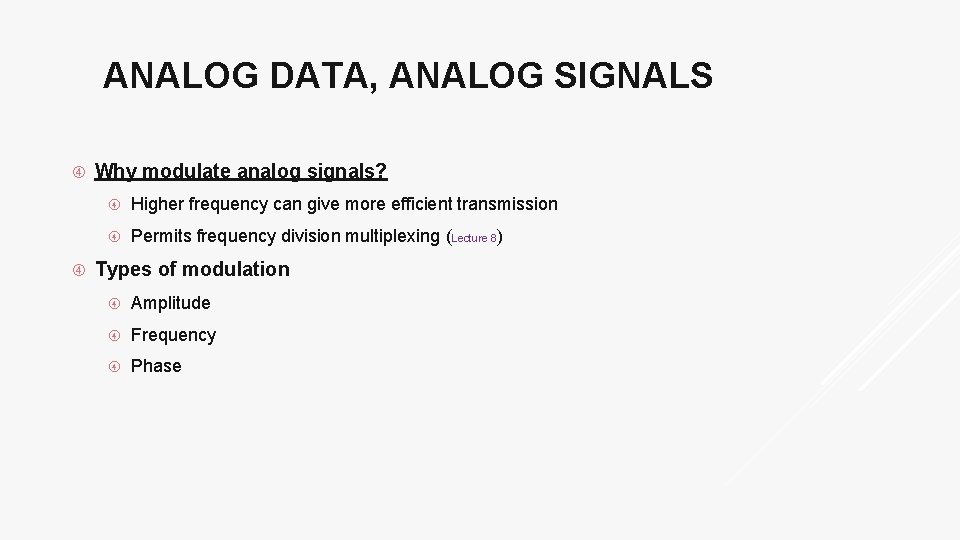
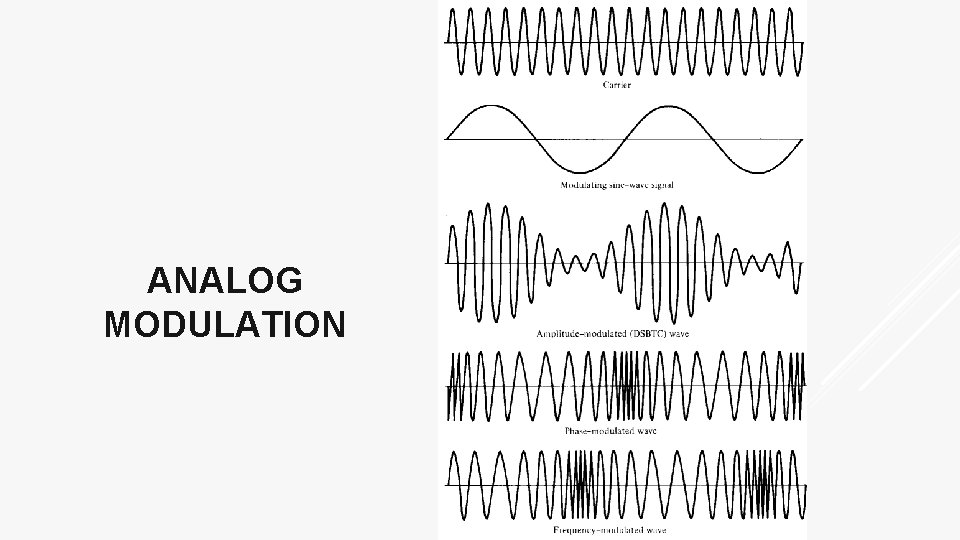
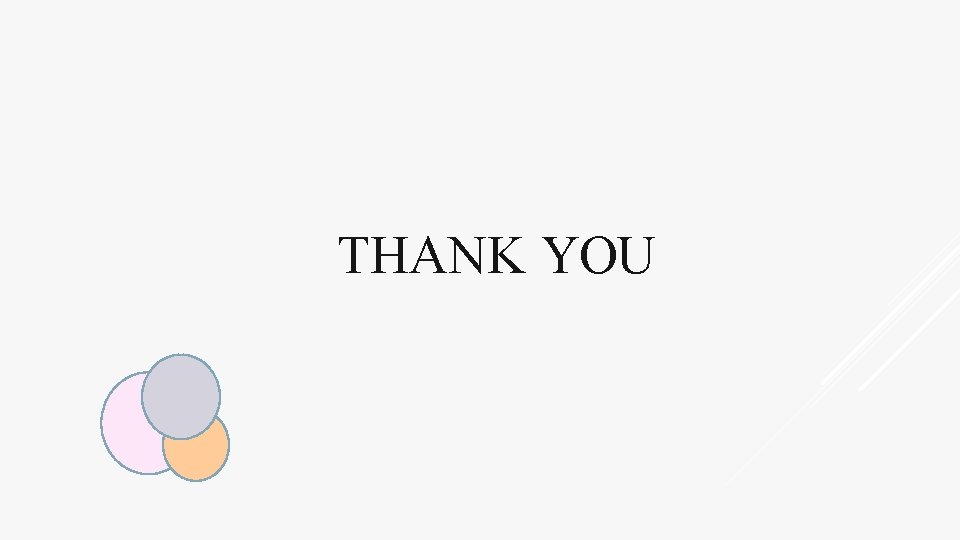
- Slides: 44
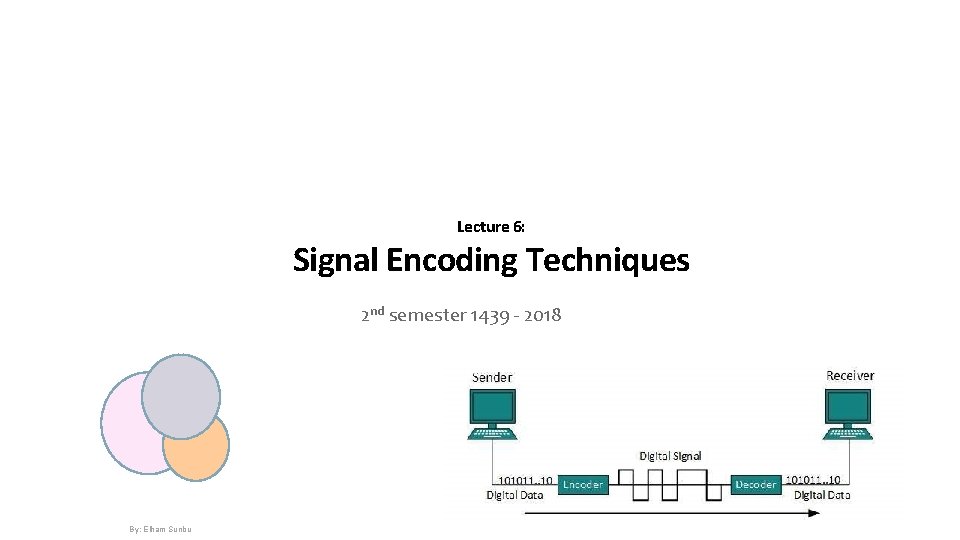
Lecture 6: Signal Encoding Techniques 2 nd semester 1439 - 2018 1 By: Elham Sunbu
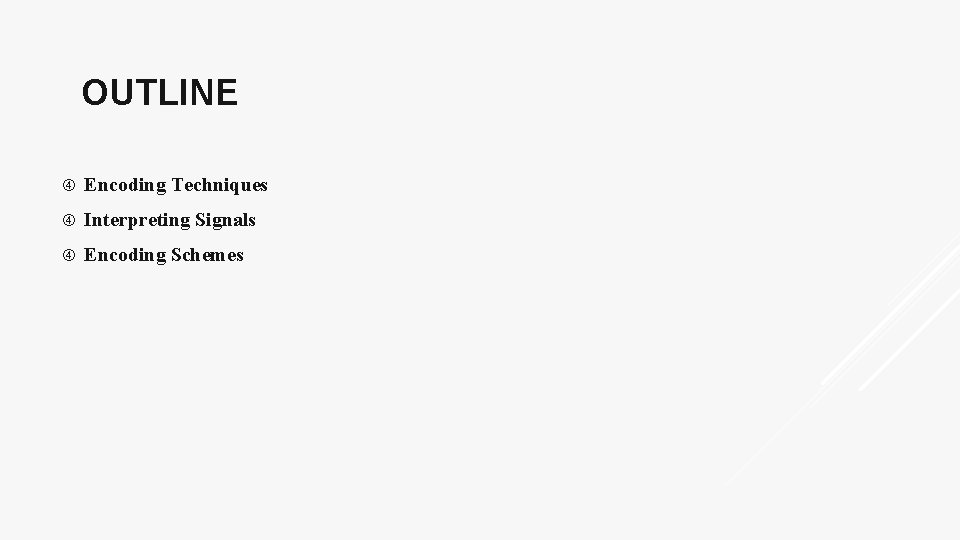
OUTLINE Encoding Techniques Interpreting Signals Encoding Schemes
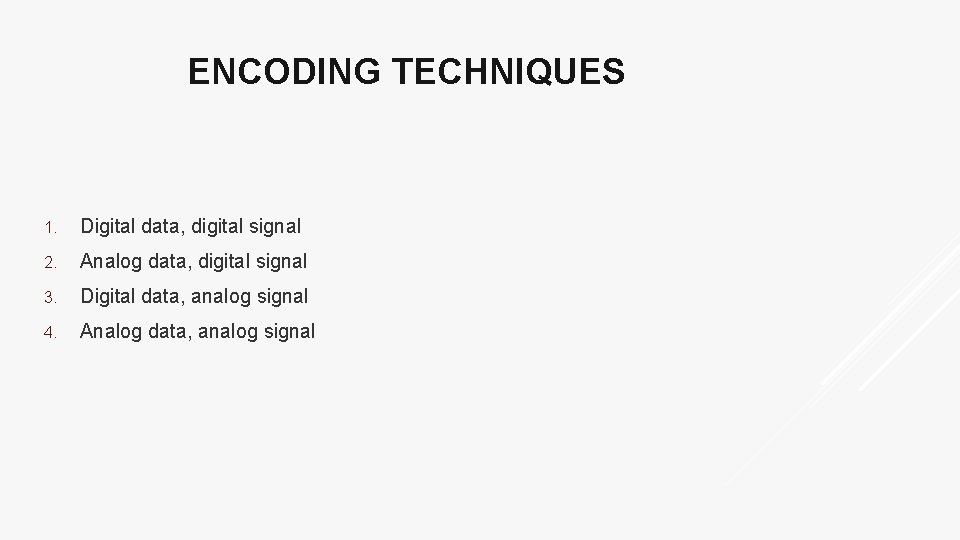
ENCODING TECHNIQUES 1. Digital data, digital signal 2. Analog data, digital signal 3. Digital data, analog signal 4. Analog data, analog signal
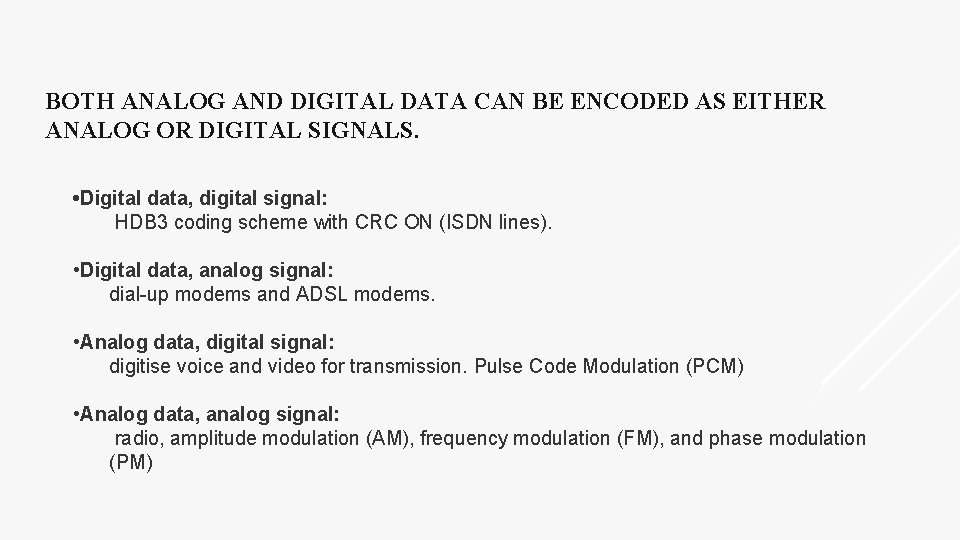
BOTH ANALOG AND DIGITAL DATA CAN BE ENCODED AS EITHER ANALOG OR DIGITAL SIGNALS. • Digital data, digital signal: HDB 3 coding scheme with CRC ON (ISDN lines). • Digital data, analog signal: dial-up modems and ADSL modems. • Analog data, digital signal: digitise voice and video for transmission. Pulse Code Modulation (PCM) • Analog data, analog signal: radio, amplitude modulation (AM), frequency modulation (FM), and phase modulation (PM)
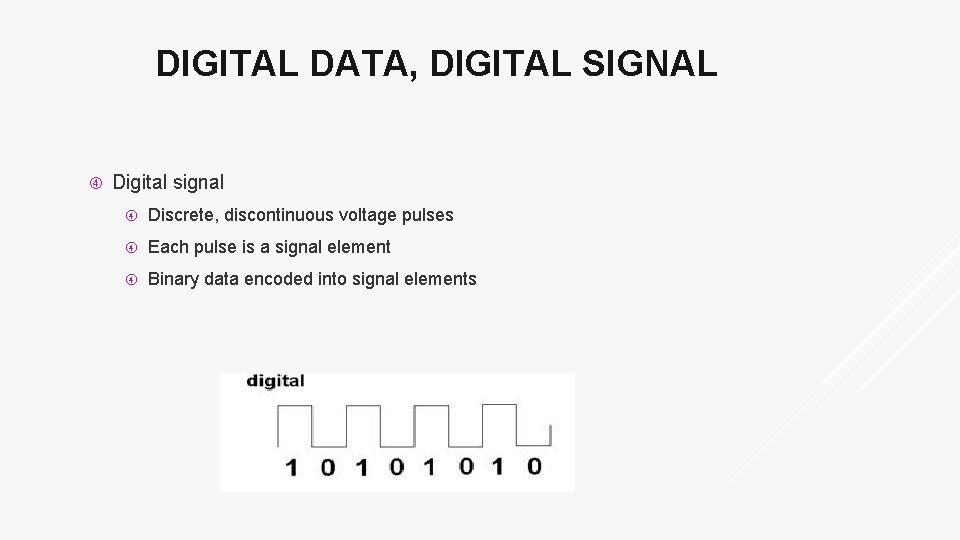
DIGITAL DATA, DIGITAL SIGNAL Digital signal Discrete, discontinuous voltage pulses Each pulse is a signal element Binary data encoded into signal elements
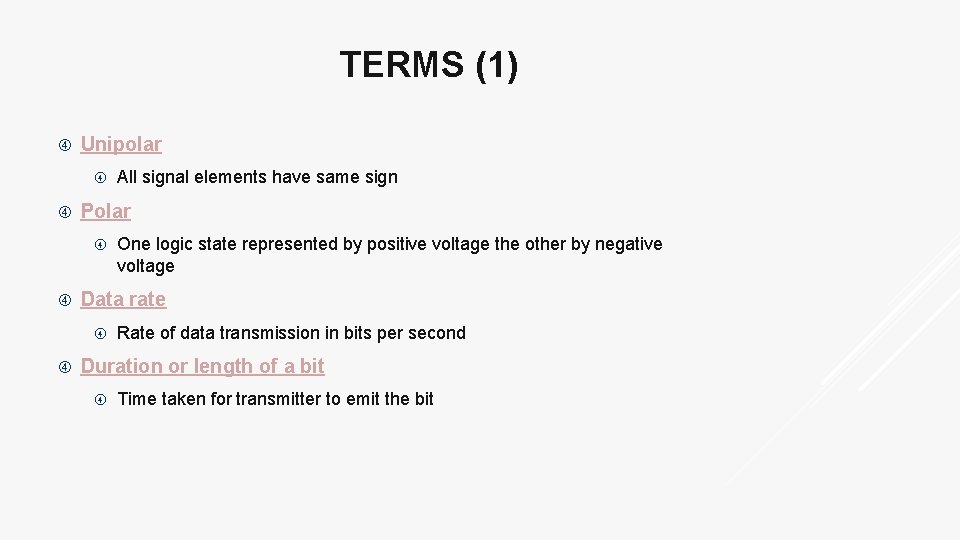
TERMS (1) Unipolar Polar One logic state represented by positive voltage the other by negative voltage Data rate All signal elements have same sign Rate of data transmission in bits per second Duration or length of a bit Time taken for transmitter to emit the bit
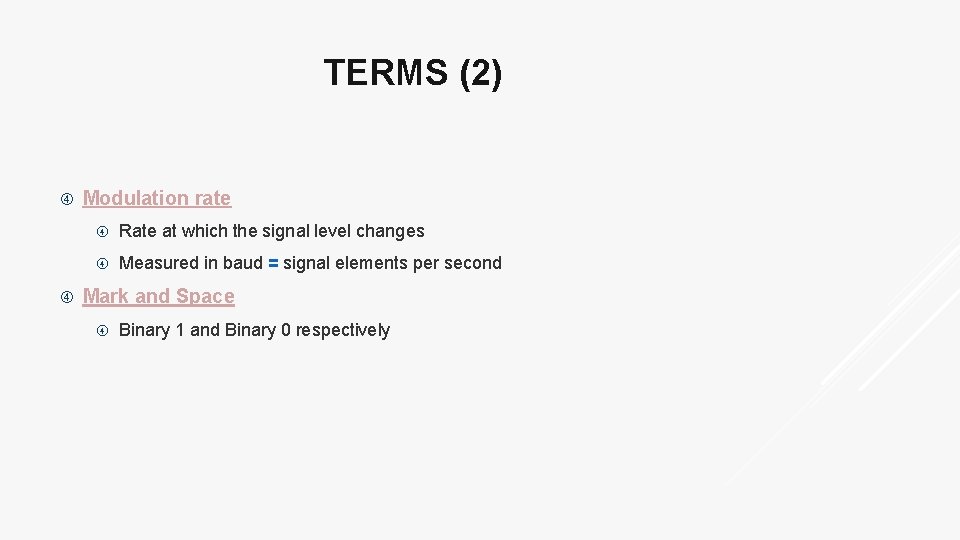
TERMS (2) Modulation rate Rate at which the signal level changes Measured in baud = signal elements per second Mark and Space Binary 1 and Binary 0 respectively
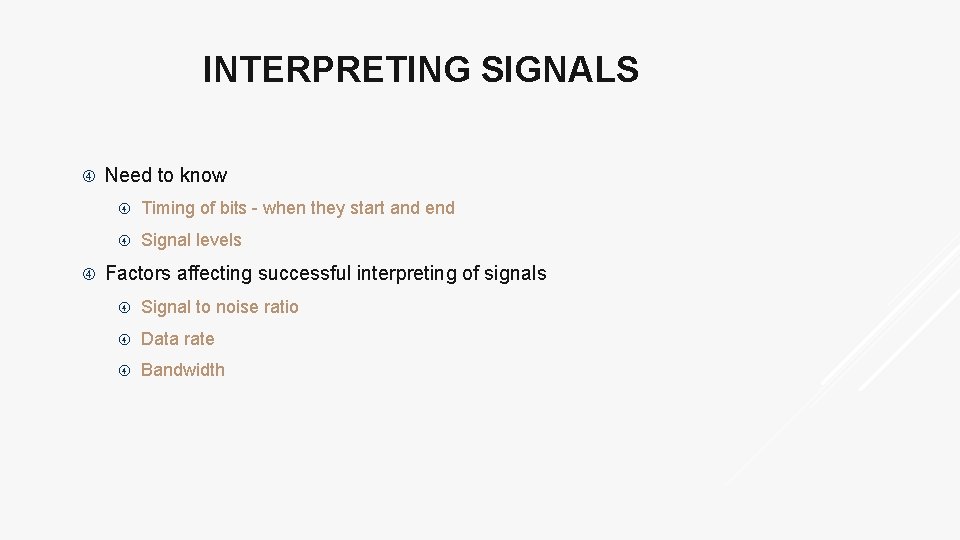
INTERPRETING SIGNALS Need to know Timing of bits - when they start and end Signal levels Factors affecting successful interpreting of signals Signal to noise ratio Data rate Bandwidth
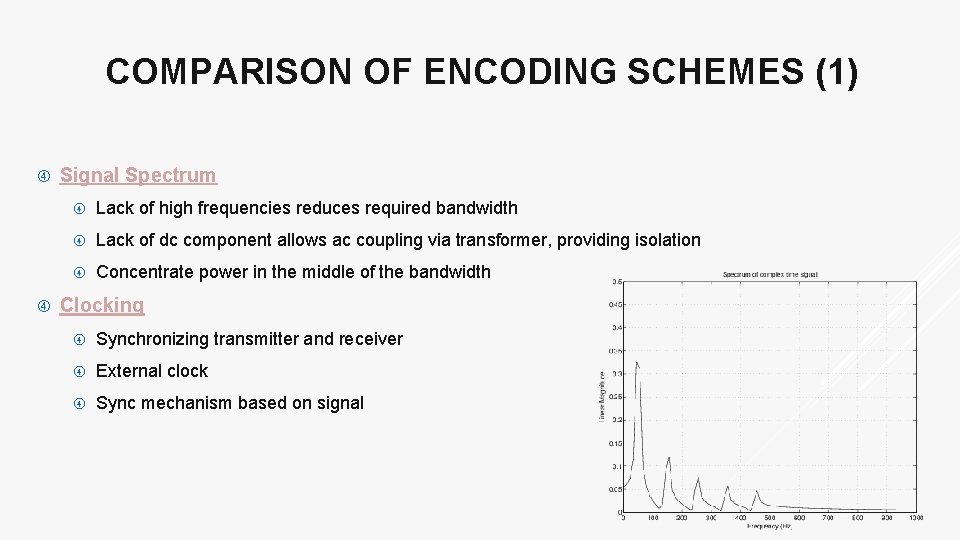
COMPARISON OF ENCODING SCHEMES (1) Signal Spectrum Lack of high frequencies reduces required bandwidth Lack of dc component allows ac coupling via transformer, providing isolation Concentrate power in the middle of the bandwidth Clocking Synchronizing transmitter and receiver External clock Sync mechanism based on signal
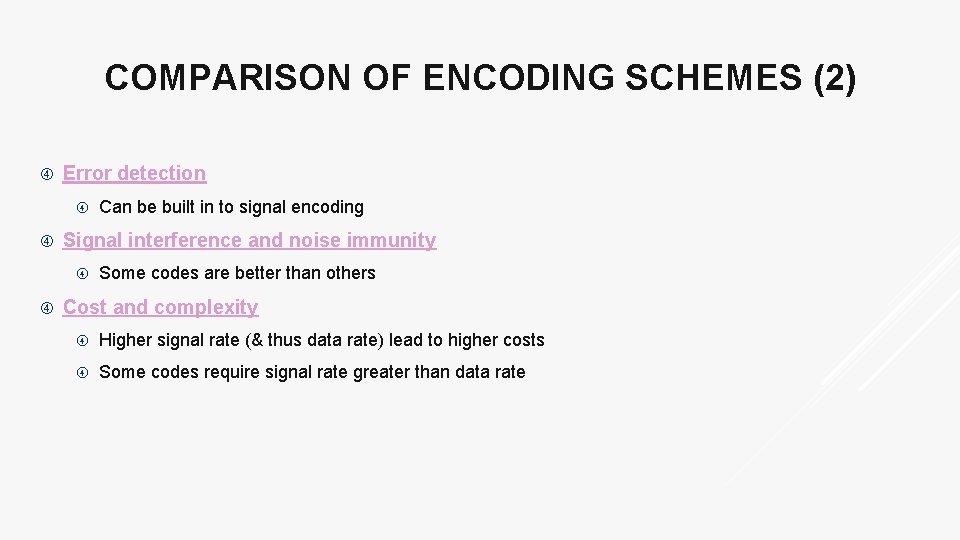
COMPARISON OF ENCODING SCHEMES (2) Error detection Signal interference and noise immunity Can be built in to signal encoding Some codes are better than others Cost and complexity Higher signal rate (& thus data rate) lead to higher costs Some codes require signal rate greater than data rate
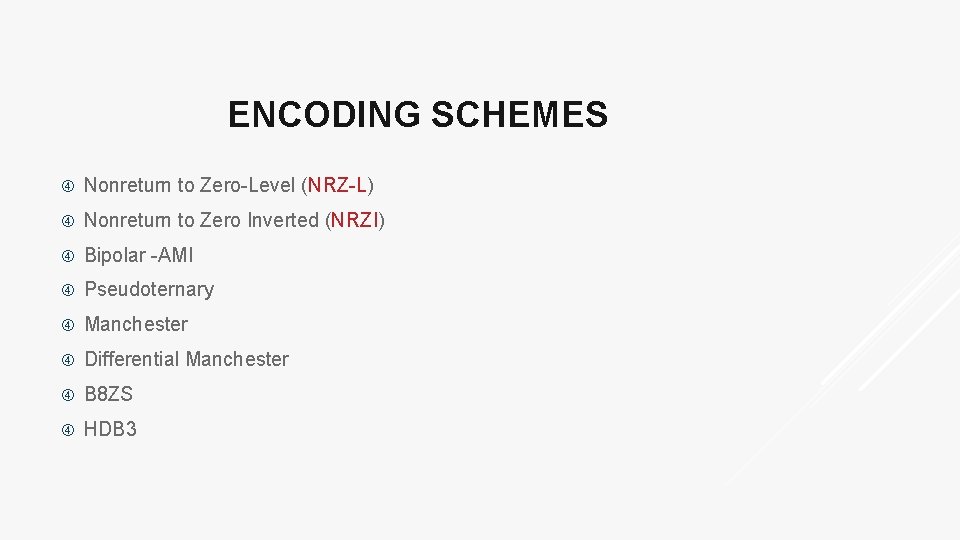
ENCODING SCHEMES Nonreturn to Zero-Level (NRZ-L) Nonreturn to Zero Inverted (NRZI) Bipolar -AMI Pseudoternary Manchester Differential Manchester B 8 ZS HDB 3
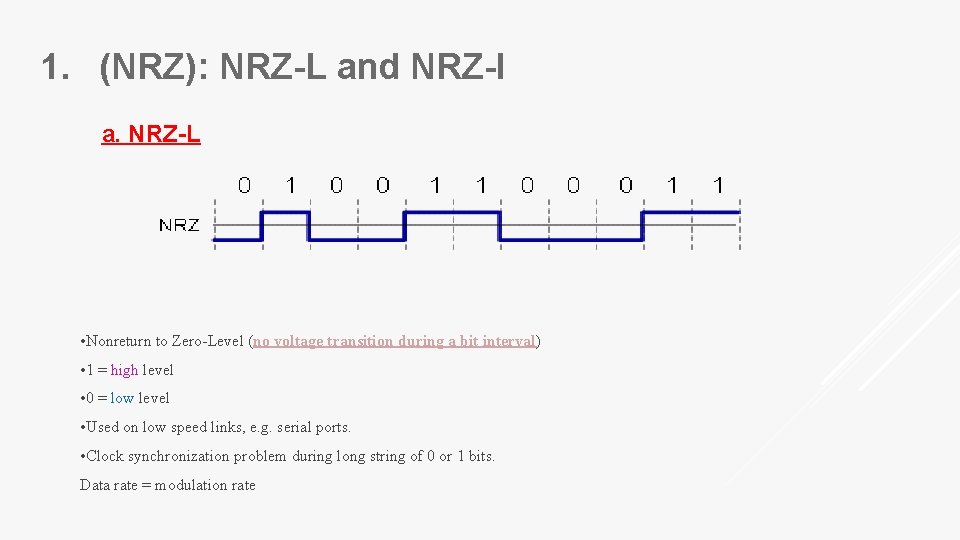
1. (NRZ): NRZ-L and NRZ-I a. NRZ-L • Nonreturn to Zero-Level (no voltage transition during a bit interval) • 1 = high level • 0 = low level • Used on low speed links, e. g. serial ports. • Clock synchronization problem during long string of 0 or 1 bits. Data rate = modulation rate
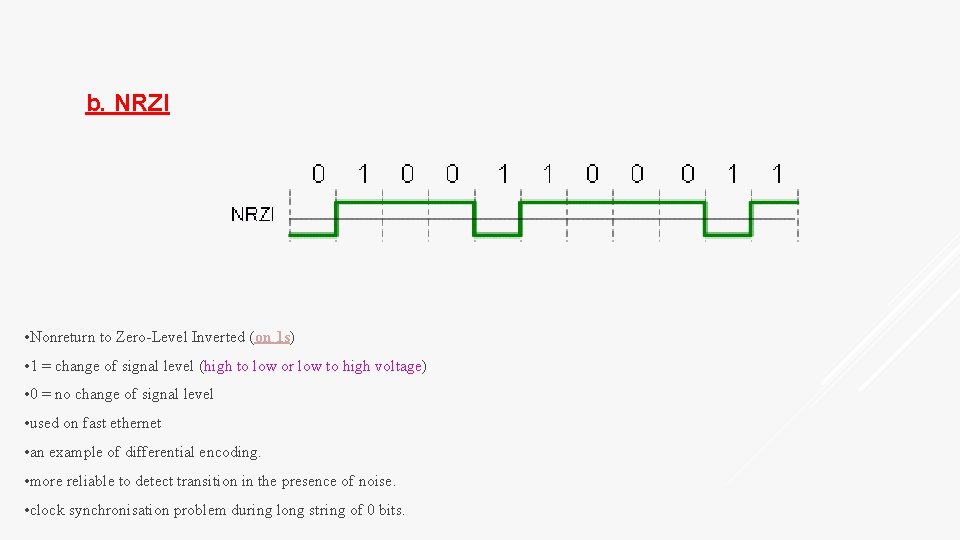
b. NRZI • Nonreturn to Zero-Level Inverted (on 1 s) • 1 = change of signal level (high to low or low to high voltage) • 0 = no change of signal level • used on fast ethernet • an example of differential encoding. • more reliable to detect transition in the presence of noise. • clock synchronisation problem during long string of 0 bits.
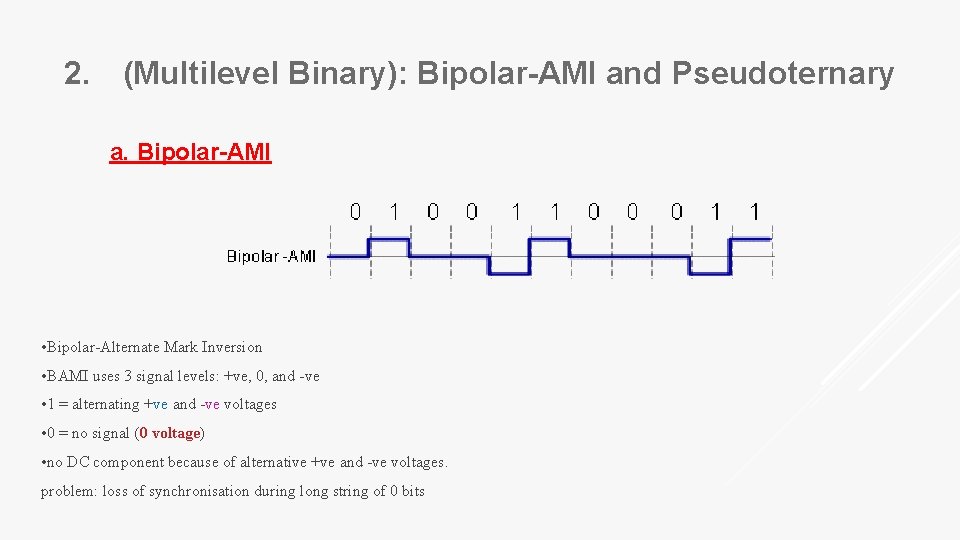
2. (Multilevel Binary): Bipolar-AMI and Pseudoternary a. Bipolar-AMI • Bipolar-Alternate Mark Inversion • BAMI uses 3 signal levels: +ve, 0, and -ve • 1 = alternating +ve and -ve voltages • 0 = no signal (0 voltage) • no DC component because of alternative +ve and -ve voltages. problem: loss of synchronisation during long string of 0 bits
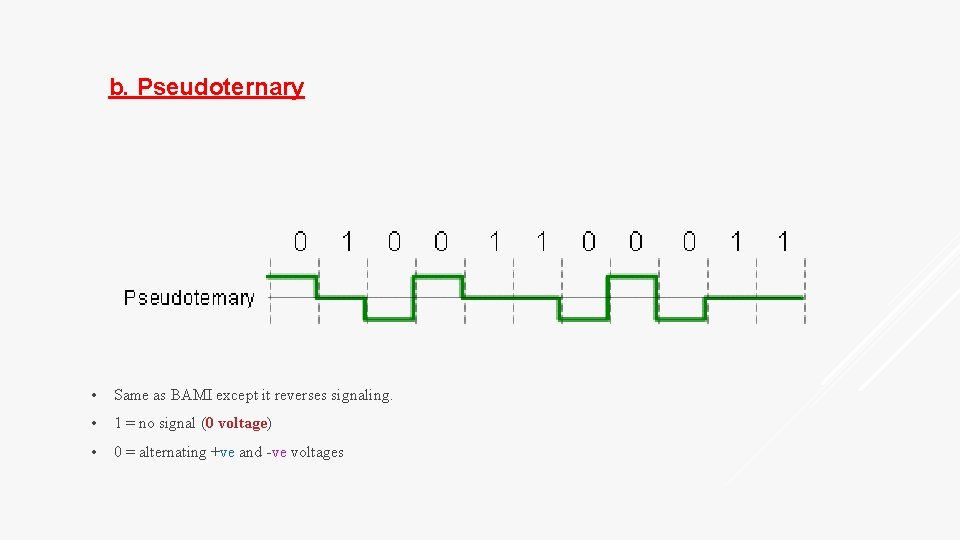
b. Pseudoternary • Same as BAMI except it reverses signaling. • 1 = no signal (0 voltage) • 0 = alternating +ve and -ve voltages
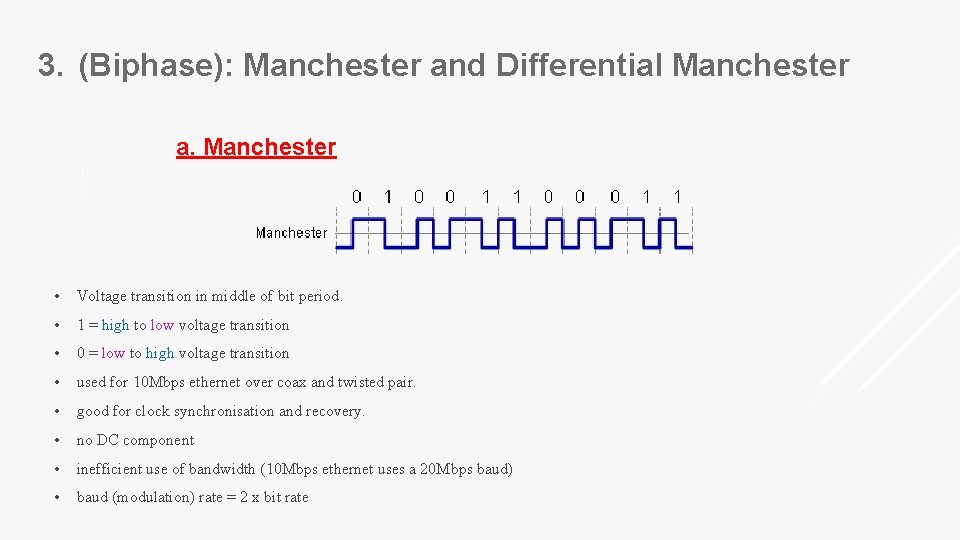
3. (Biphase): Manchester and Differential Manchester a. Manchester • Voltage transition in middle of bit period. • 1 = high to low voltage transition • 0 = low to high voltage transition • used for 10 Mbps ethernet over coax and twisted pair. • good for clock synchronisation and recovery. • no DC component • inefficient use of bandwidth (10 Mbps ethernet uses a 20 Mbps baud) • baud (modulation) rate = 2 x bit rate
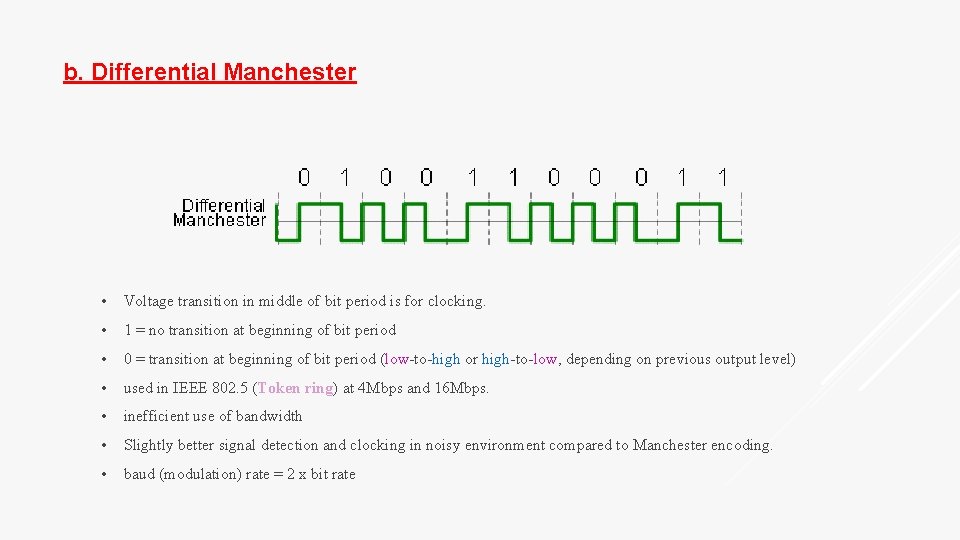
b. Differential Manchester • Voltage transition in middle of bit period is for clocking. • 1 = no transition at beginning of bit period • 0 = transition at beginning of bit period (low-to-high or high-to-low, depending on previous output level) • used in IEEE 802. 5 (Token ring) at 4 Mbps and 16 Mbps. • inefficient use of bandwidth • Slightly better signal detection and clocking in noisy environment compared to Manchester encoding. • baud (modulation) rate = 2 x bit rate
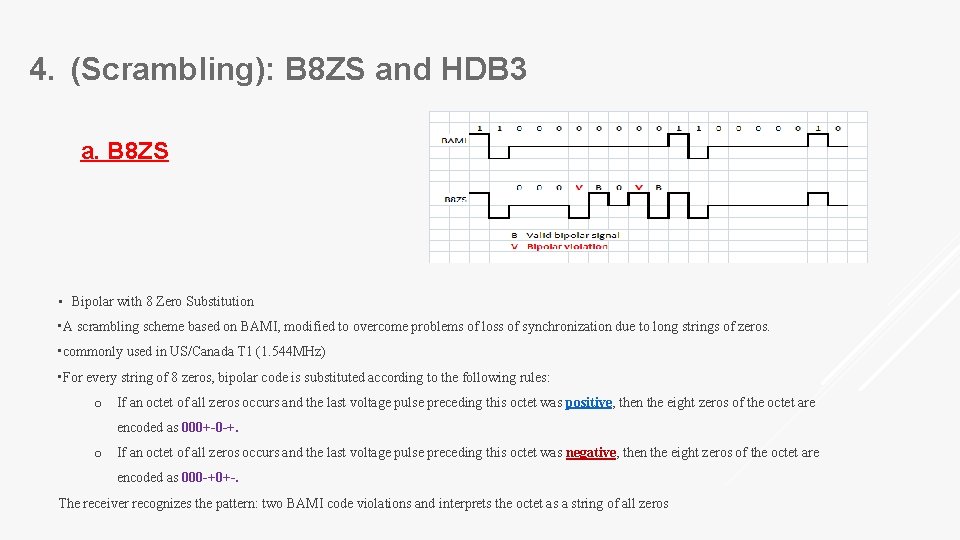
4. (Scrambling): B 8 ZS and HDB 3 a. B 8 ZS • Bipolar with 8 Zero Substitution • A scrambling scheme based on BAMI, modified to overcome problems of loss of synchronization due to long strings of zeros. • commonly used in US/Canada T 1 (1. 544 MHz) • For every string of 8 zeros, bipolar code is substituted according to the following rules: o If an octet of all zeros occurs and the last voltage pulse preceding this octet was positive, then the eight zeros of the octet are encoded as 000+-0 -+. o If an octet of all zeros occurs and the last voltage pulse preceding this octet was negative, then the eight zeros of the octet are encoded as 000 -+0+-. The receiver recognizes the pattern: two BAMI code violations and interprets the octet as a string of all zeros
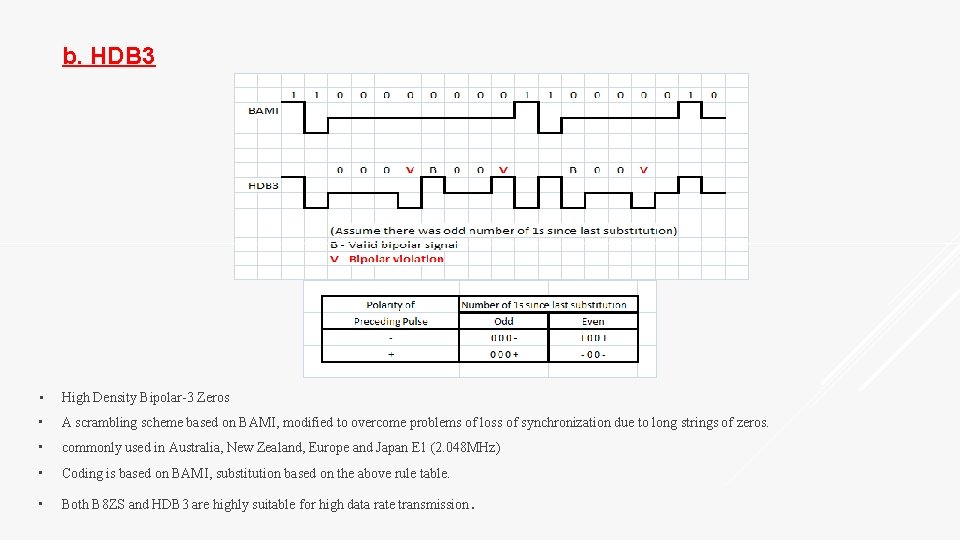
b. HDB 3 • High Density Bipolar-3 Zeros • A scrambling scheme based on BAMI, modified to overcome problems of loss of synchronization due to long strings of zeros. • commonly used in Australia, New Zealand, Europe and Japan E 1 (2. 048 MHz) • Coding is based on BAMI, substitution based on the above rule table. • Both B 8 ZS and HDB 3 are highly suitable for high data rate transmission.
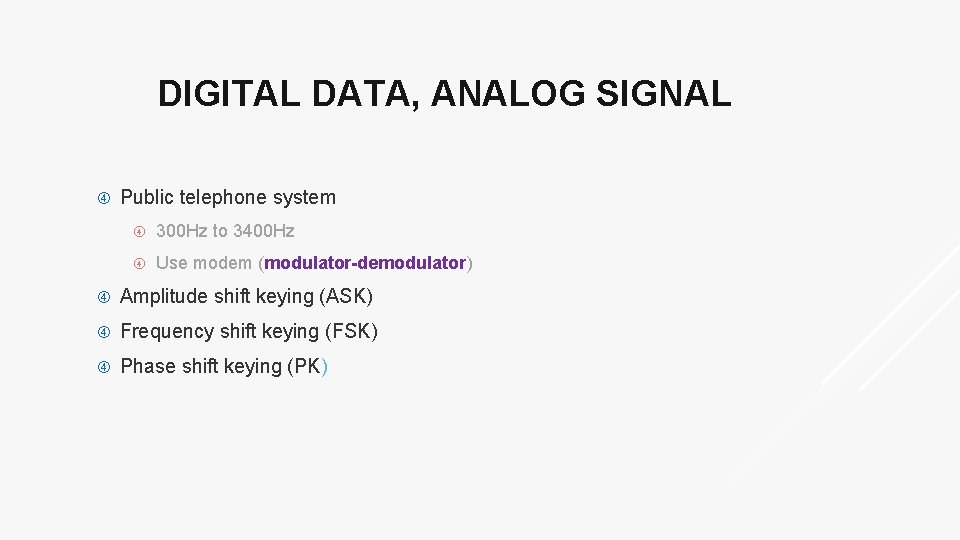
DIGITAL DATA, ANALOG SIGNAL Public telephone system 300 Hz to 3400 Hz Use modem (modulator-demodulator) Amplitude shift keying (ASK) Frequency shift keying (FSK) Phase shift keying (PK)
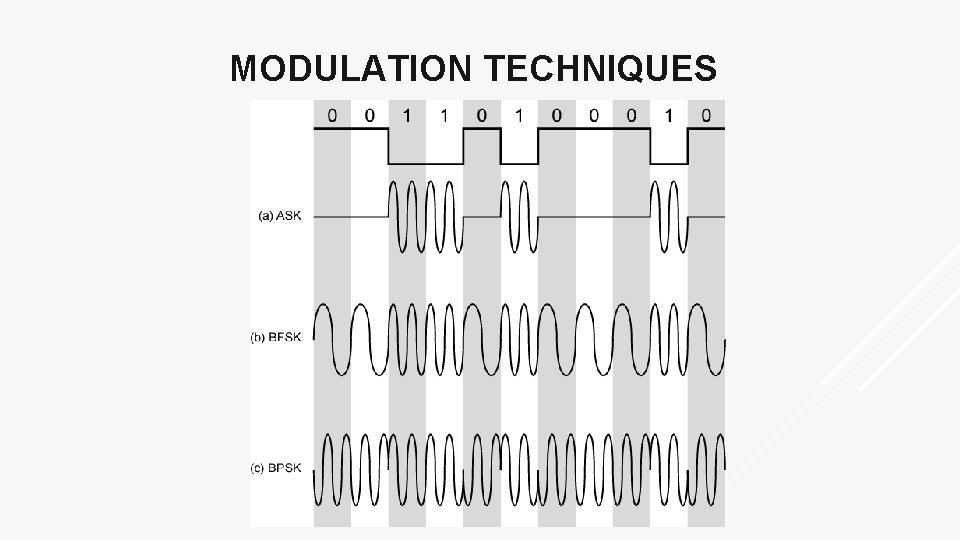
MODULATION TECHNIQUES
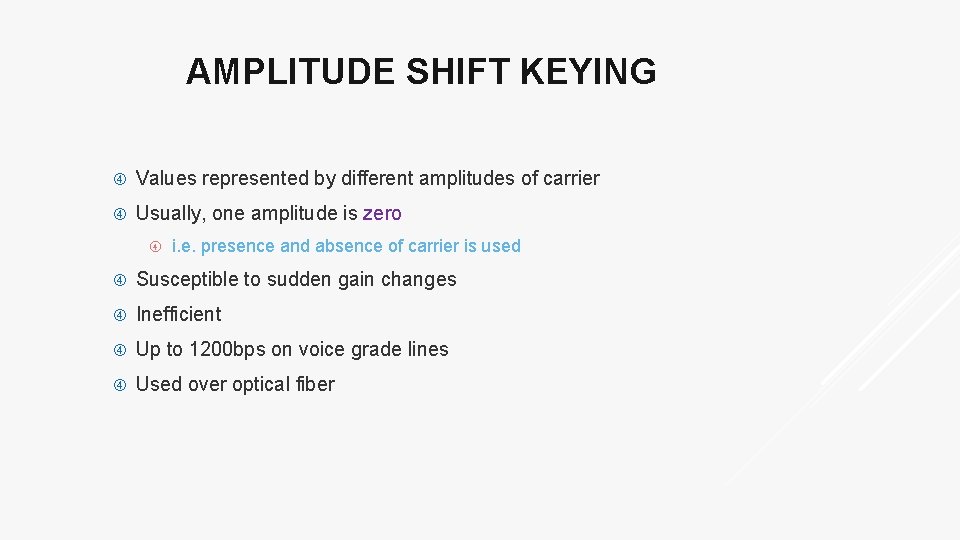
AMPLITUDE SHIFT KEYING Values represented by different amplitudes of carrier Usually, one amplitude is zero i. e. presence and absence of carrier is used Susceptible to sudden gain changes Inefficient Up to 1200 bps on voice grade lines Used over optical fiber
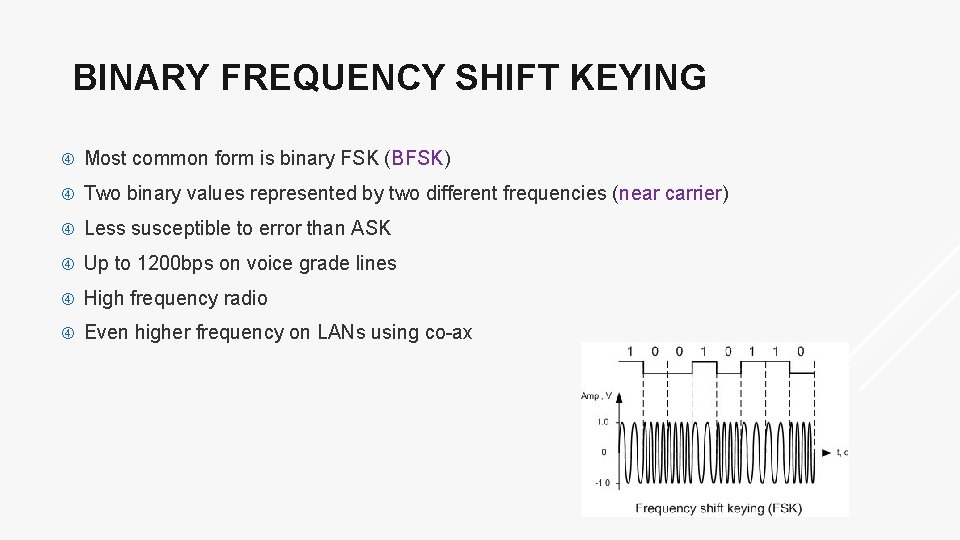
BINARY FREQUENCY SHIFT KEYING Most common form is binary FSK (BFSK) Two binary values represented by two different frequencies (near carrier) Less susceptible to error than ASK Up to 1200 bps on voice grade lines High frequency radio Even higher frequency on LANs using co-ax
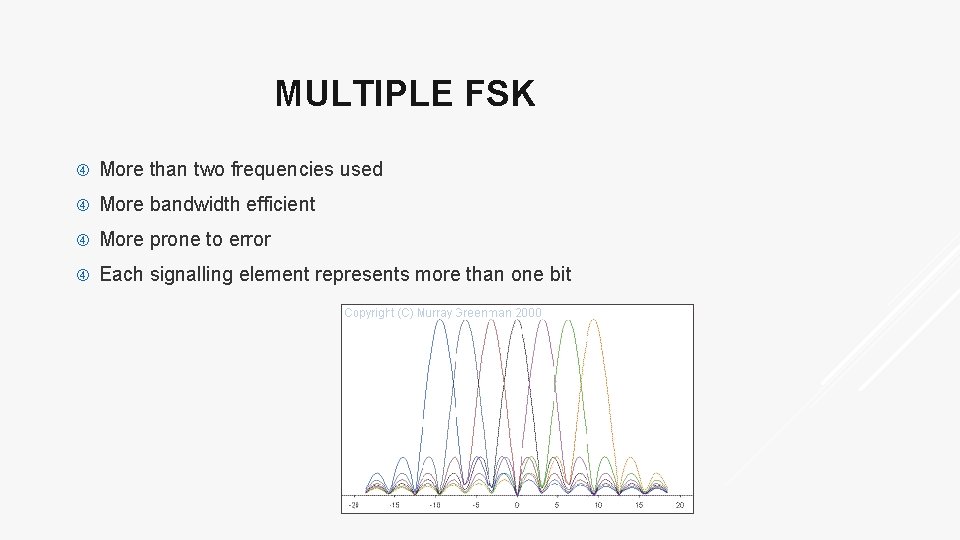
MULTIPLE FSK More than two frequencies used More bandwidth efficient More prone to error Each signalling element represents more than one bit
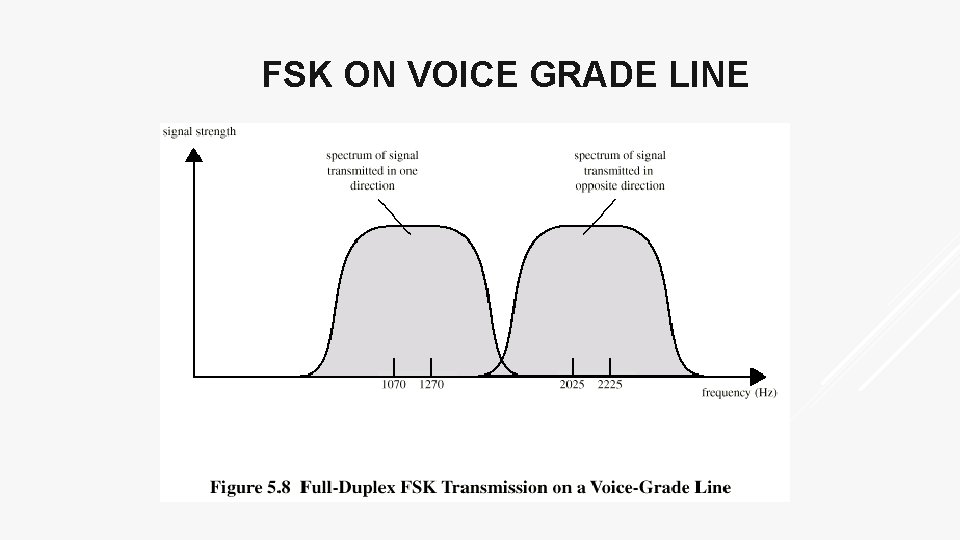
FSK ON VOICE GRADE LINE
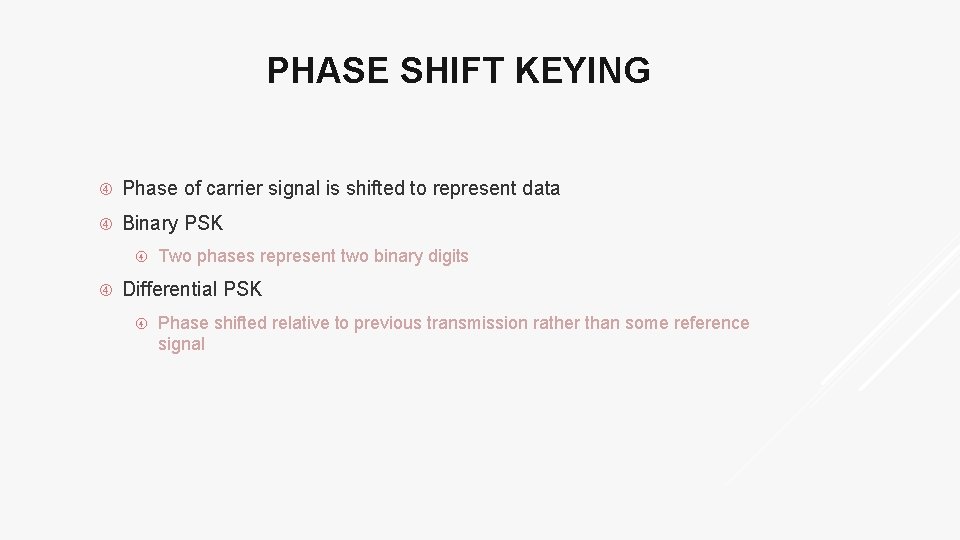
PHASE SHIFT KEYING Phase of carrier signal is shifted to represent data Binary PSK Two phases represent two binary digits Differential PSK Phase shifted relative to previous transmission rather than some reference signal
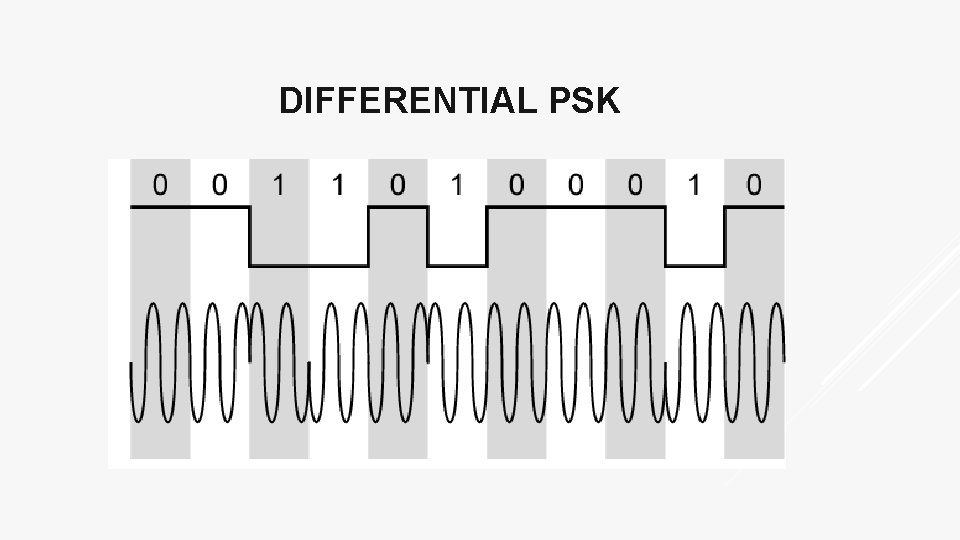
DIFFERENTIAL PSK
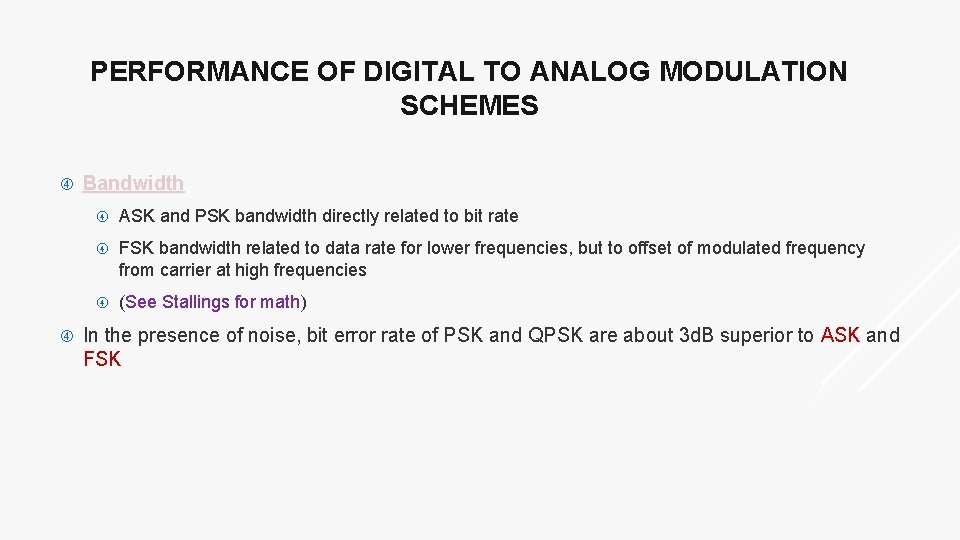
PERFORMANCE OF DIGITAL TO ANALOG MODULATION SCHEMES Bandwidth ASK and PSK bandwidth directly related to bit rate FSK bandwidth related to data rate for lower frequencies, but to offset of modulated frequency from carrier at high frequencies (See Stallings for math) In the presence of noise, bit error rate of PSK and QPSK are about 3 d. B superior to ASK and FSK
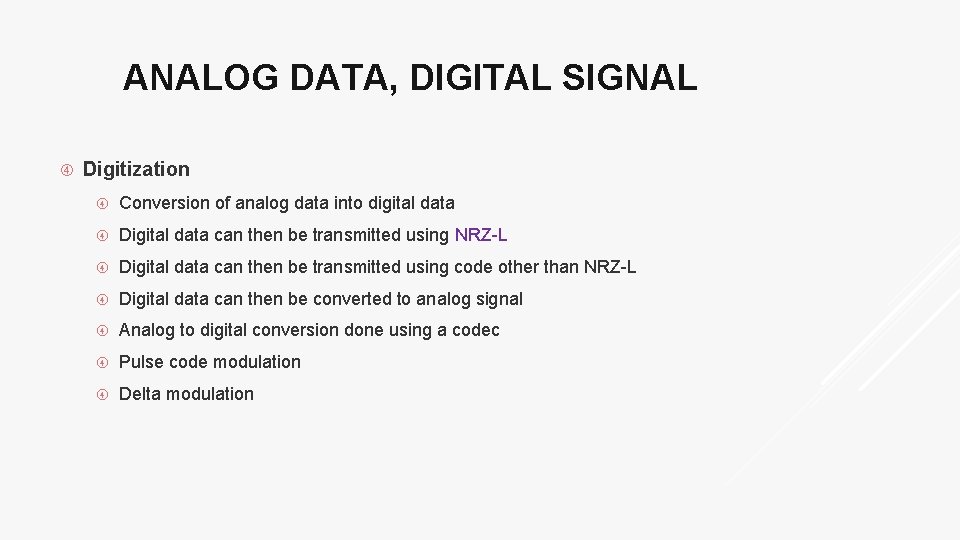
ANALOG DATA, DIGITAL SIGNAL Digitization Conversion of analog data into digital data Digital data can then be transmitted using NRZ-L Digital data can then be transmitted using code other than NRZ-L Digital data can then be converted to analog signal Analog to digital conversion done using a codec Pulse code modulation Delta modulation
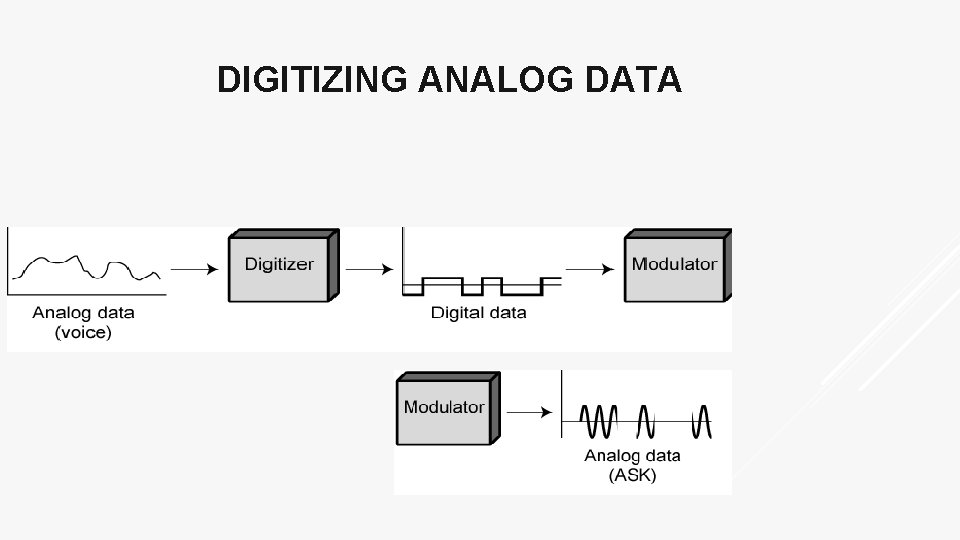
DIGITIZING ANALOG DATA
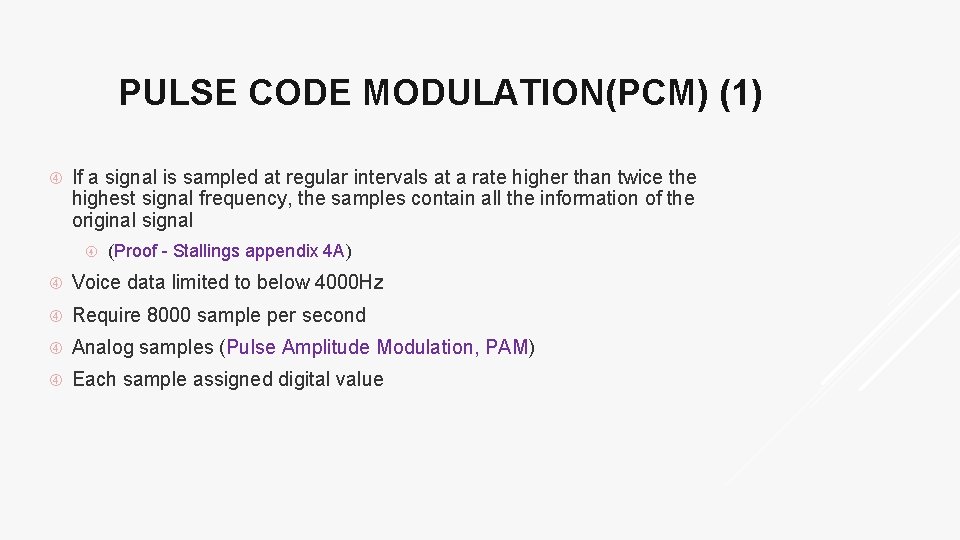
PULSE CODE MODULATION(PCM) (1) If a signal is sampled at regular intervals at a rate higher than twice the highest signal frequency, the samples contain all the information of the original signal (Proof - Stallings appendix 4 A) Voice data limited to below 4000 Hz Require 8000 sample per second Analog samples (Pulse Amplitude Modulation, PAM) Each sample assigned digital value
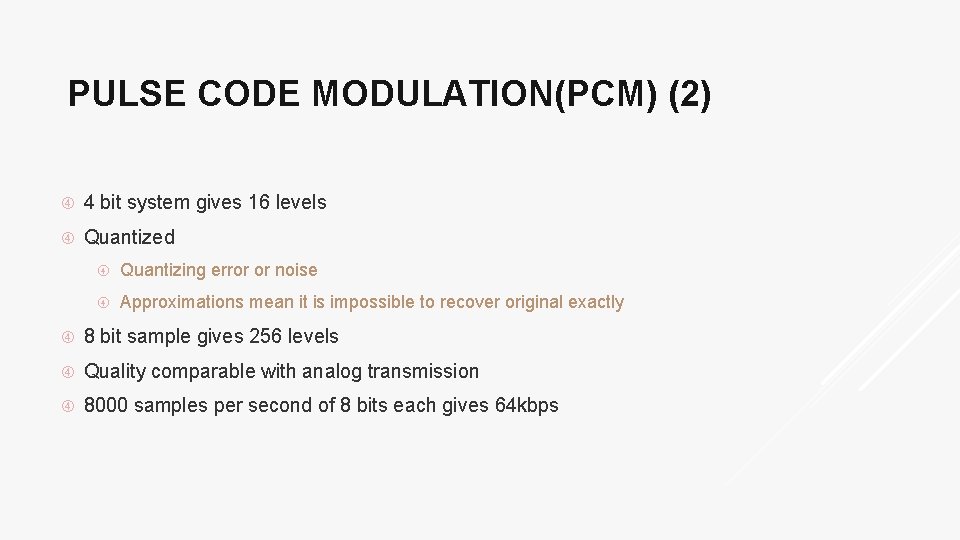
PULSE CODE MODULATION(PCM) (2) 4 bit system gives 16 levels Quantized Quantizing error or noise Approximations mean it is impossible to recover original exactly 8 bit sample gives 256 levels Quality comparable with analog transmission 8000 samples per second of 8 bits each gives 64 kbps
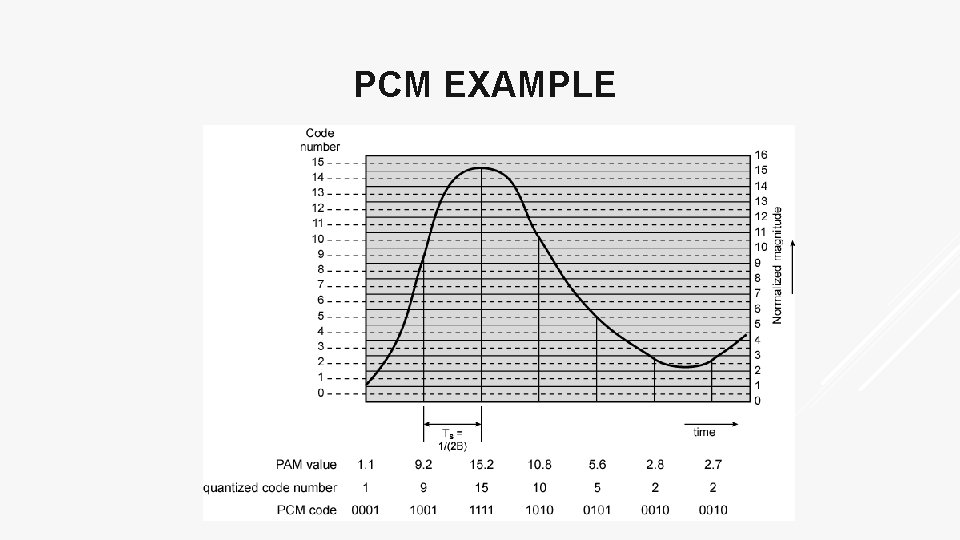
PCM EXAMPLE
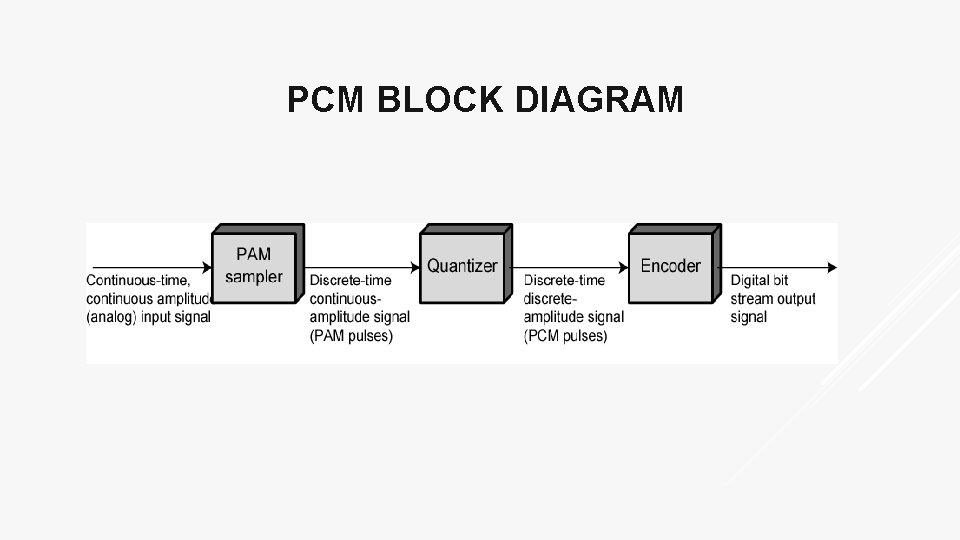
PCM BLOCK DIAGRAM
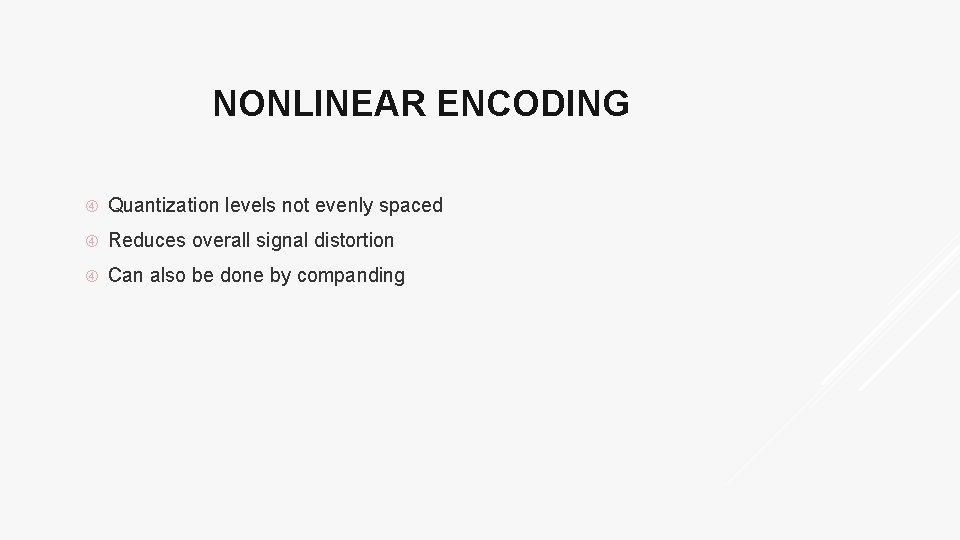
NONLINEAR ENCODING Quantization levels not evenly spaced Reduces overall signal distortion Can also be done by companding
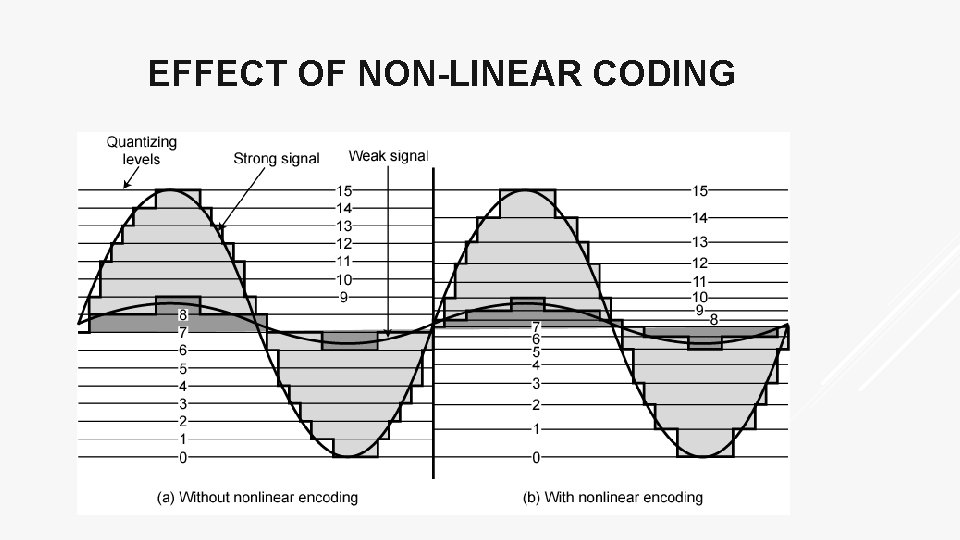
EFFECT OF NON-LINEAR CODING
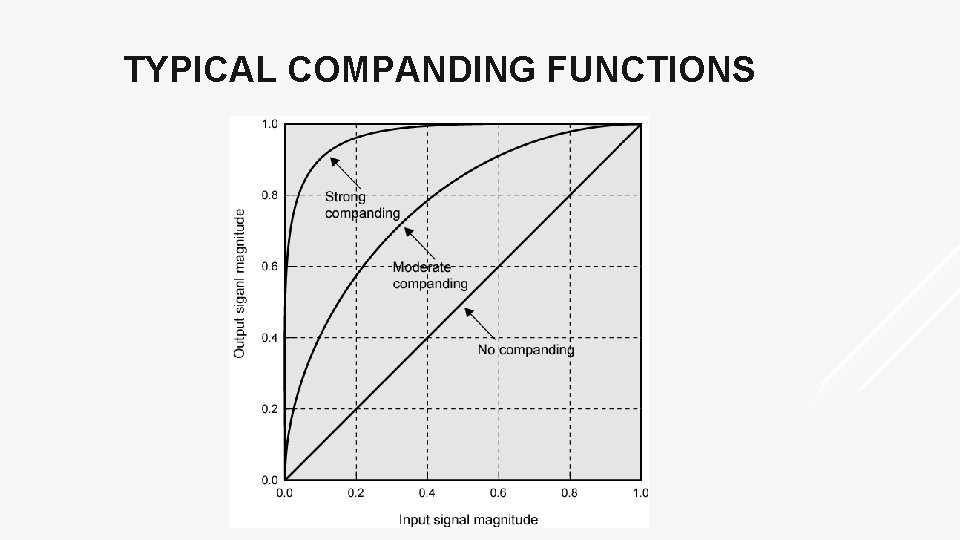
TYPICAL COMPANDING FUNCTIONS
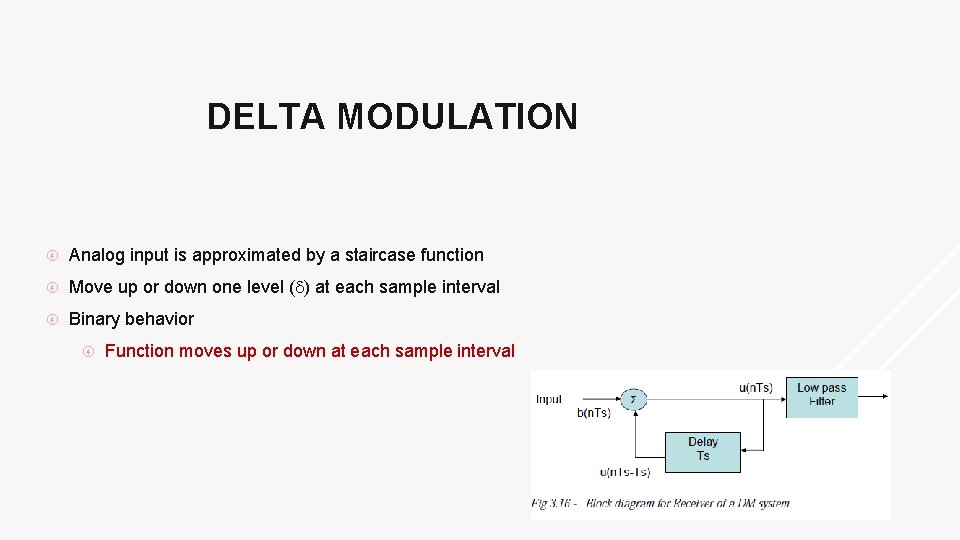
DELTA MODULATION Analog input is approximated by a staircase function Move up or down one level ( ) at each sample interval Binary behavior Function moves up or down at each sample interval
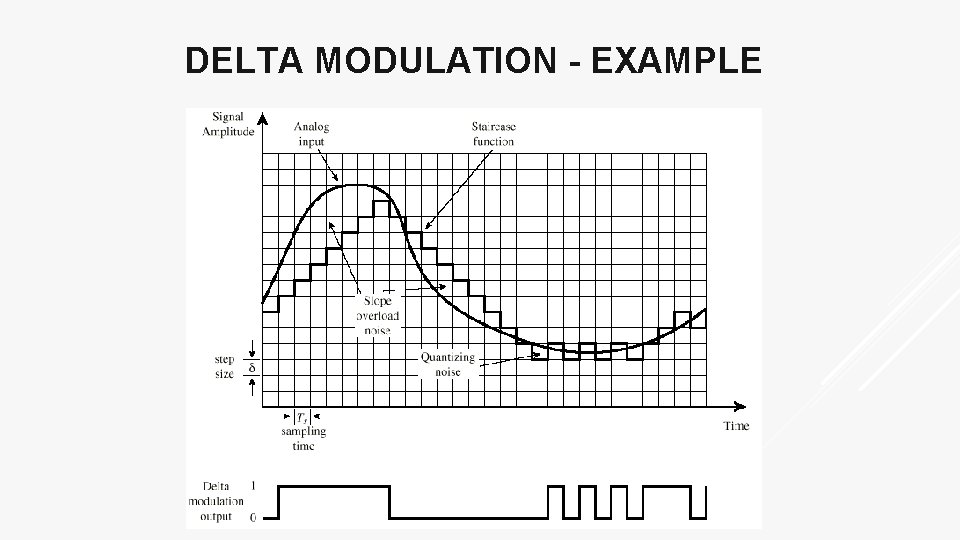
DELTA MODULATION - EXAMPLE
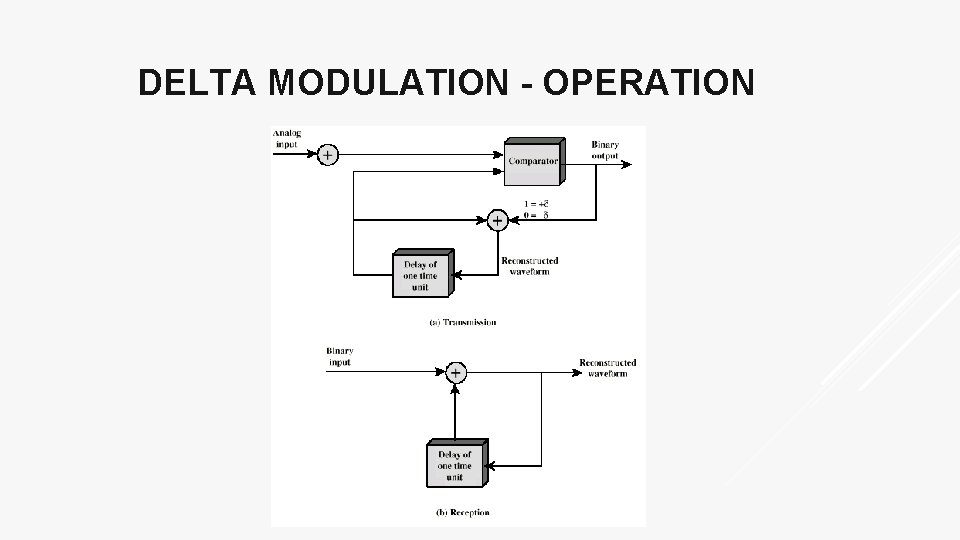
DELTA MODULATION - OPERATION
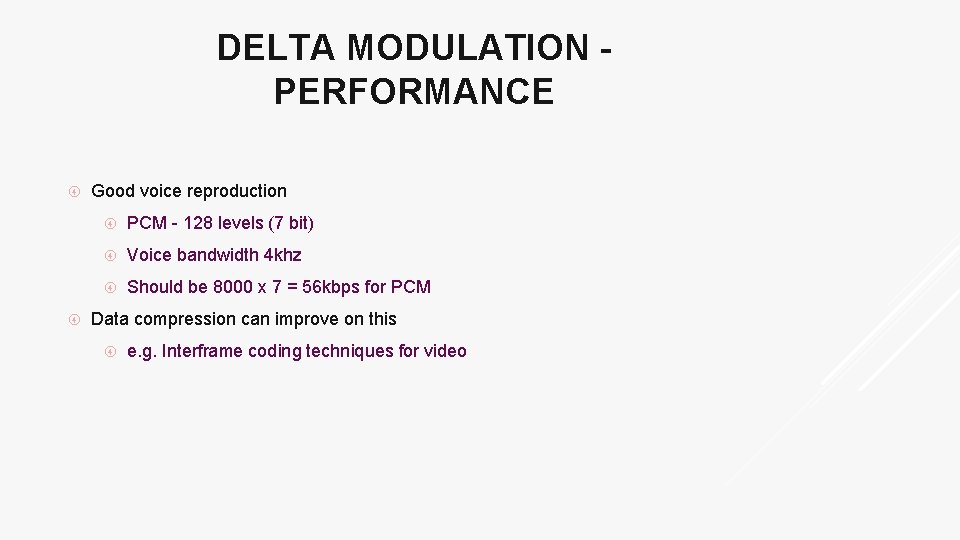
DELTA MODULATION PERFORMANCE Good voice reproduction PCM - 128 levels (7 bit) Voice bandwidth 4 khz Should be 8000 x 7 = 56 kbps for PCM Data compression can improve on this e. g. Interframe coding techniques for video
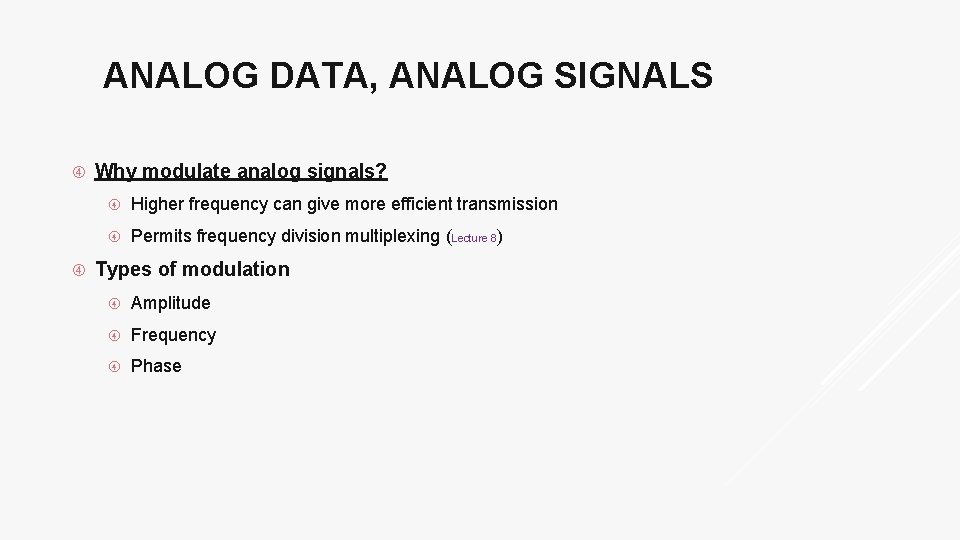
ANALOG DATA, ANALOG SIGNALS Why modulate analog signals? Higher frequency can give more efficient transmission Permits frequency division multiplexing (Lecture 8) Types of modulation Amplitude Frequency Phase
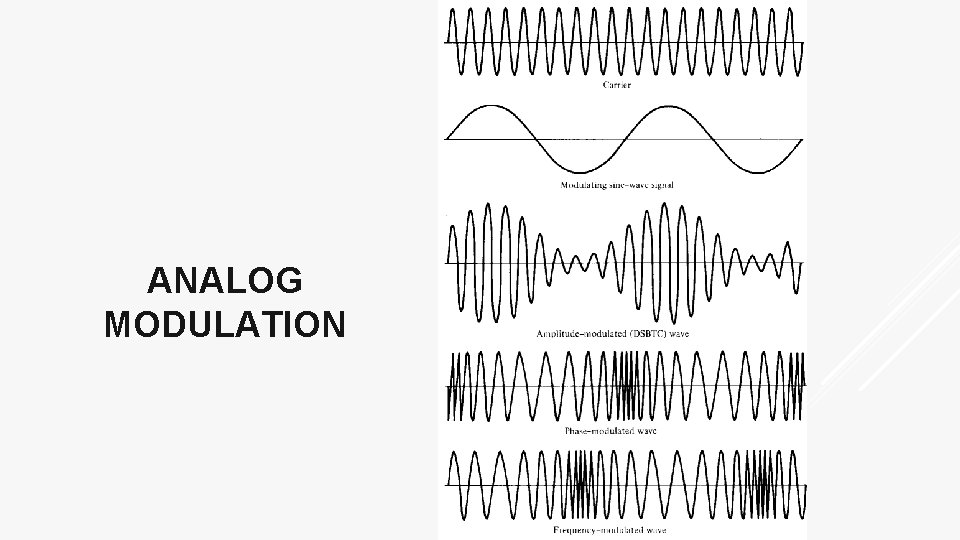
ANALOG MODULATION
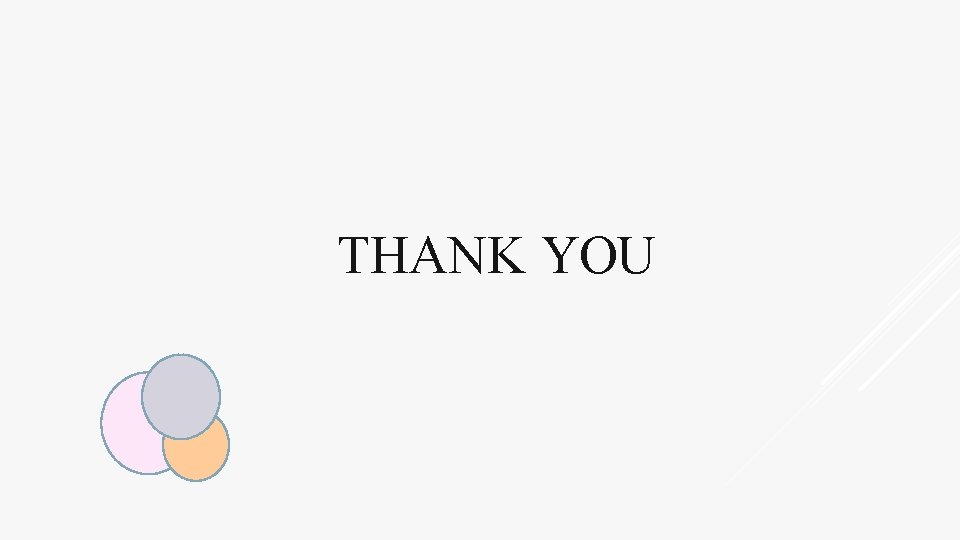
THANK YOU
Digital encoding schemes
Basic coding test
Memory encoding techniques
Coding technique
Data encoding techniques
01:640:244 lecture notes - lecture 15: plat, idah, farad
The product of two odd signals is
Baseband signal and bandpass signal
Baseband signal and bandpass signal
Digital signal as a composite analog signal
Fonction technique scooter
Manchester encoding scheme
Memory encoding
Example of encoding failure
Booth encoding
Mets metadata
Run length coding
Process of memory encoding
Arm high speed multiplier organization
Physical layer encoding
Subglottic stenosis
Source memory
Effortful processing strategies psychology
Huffman coding visualization
Decoding definition in communication
Memory encoding vs consolidation
Encoding failure
Spatial encoding
Physical layer encoding
Three-stage model of memory
Huffman encoding trie
Run-length encoding
Encoding in marketing
Memory encoding vs consolidation
Encoding
Decimal to gray
Encoding b&w images copy and paste
Levels of processing theory
Data encoding and transmission
Sender encoding message decoding receiver
Strobe of data
Elaborative encoding
Data encoding
Digital line encoding
Mips instruction encoding