Chapter Menu Chapter Introduction Genetic Variation in Populations
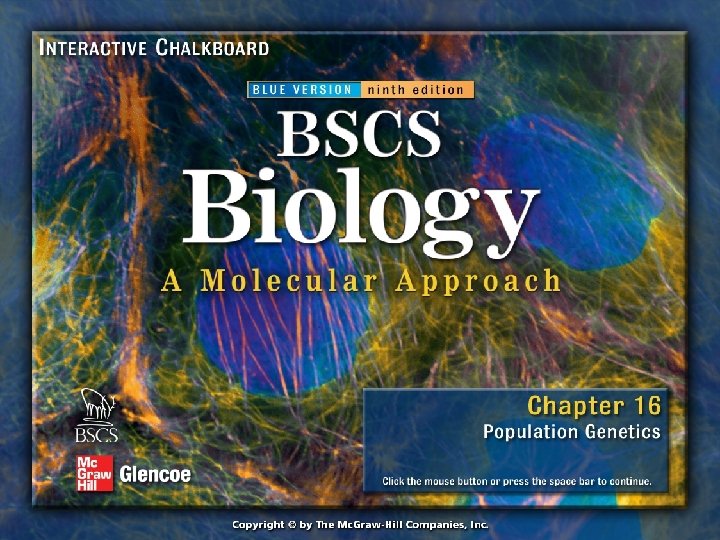
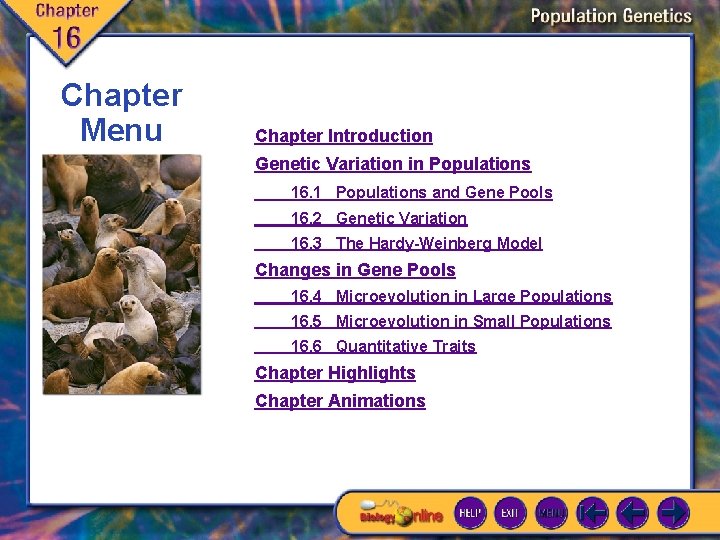
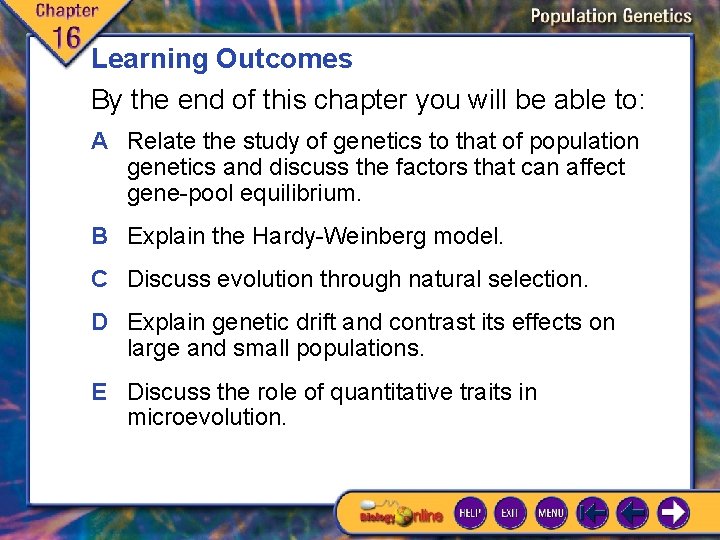
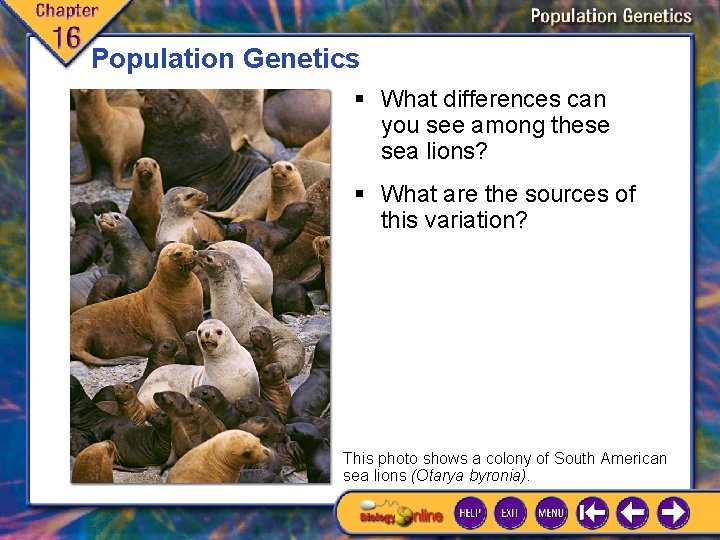
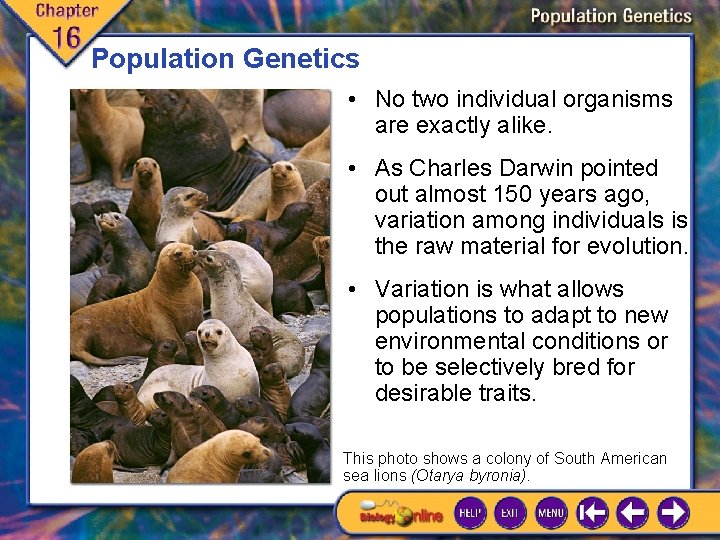
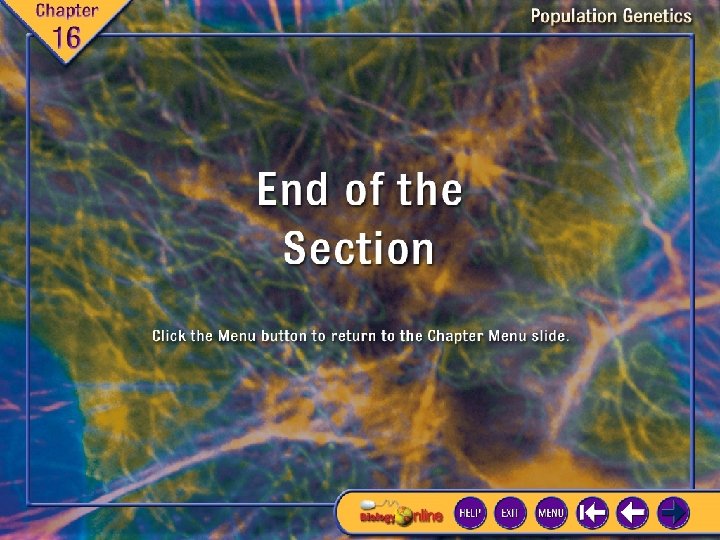
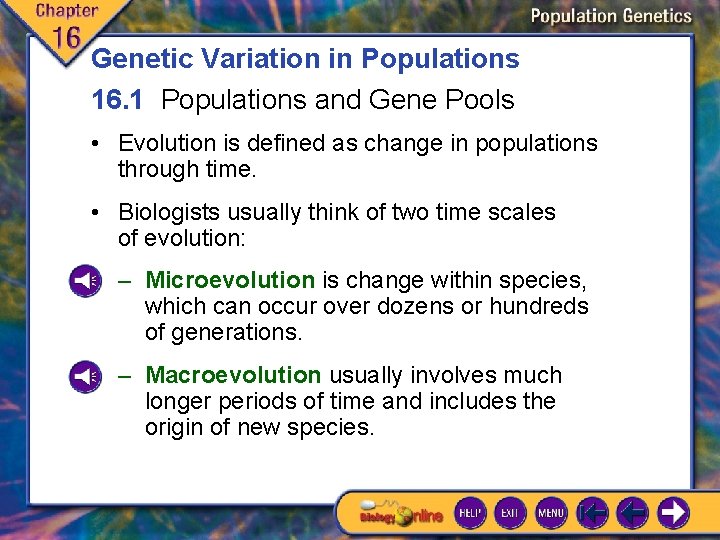
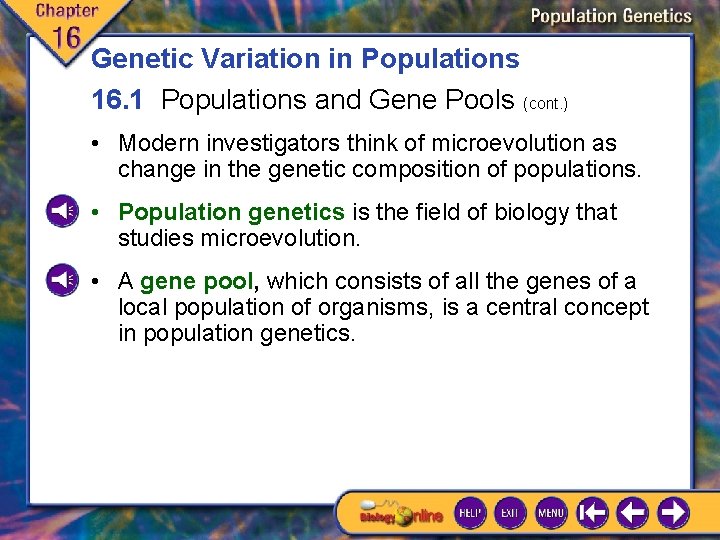
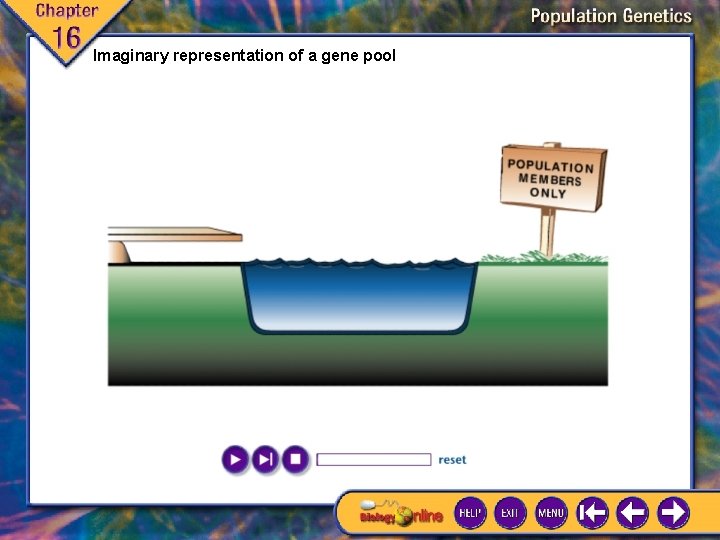
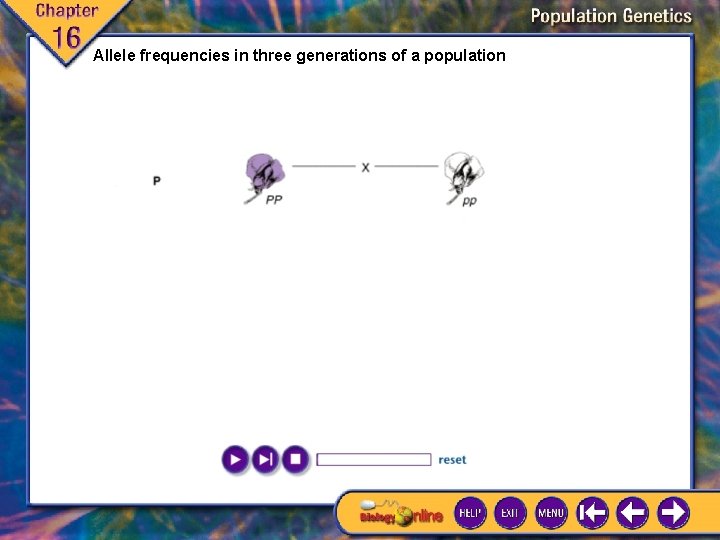
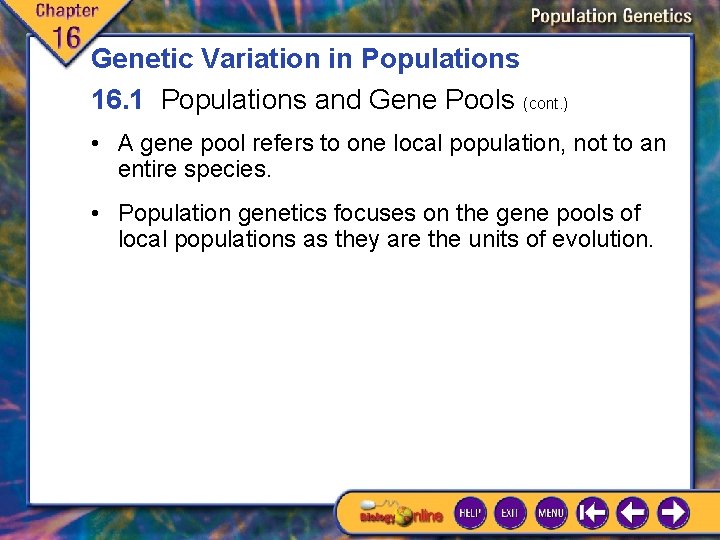
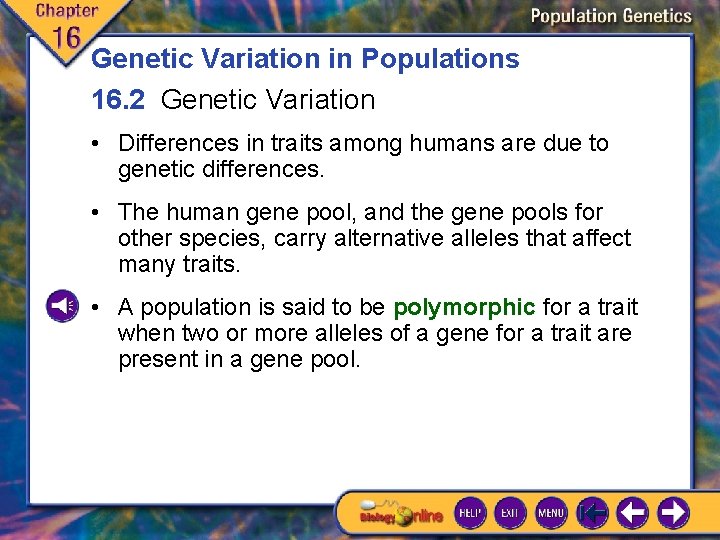
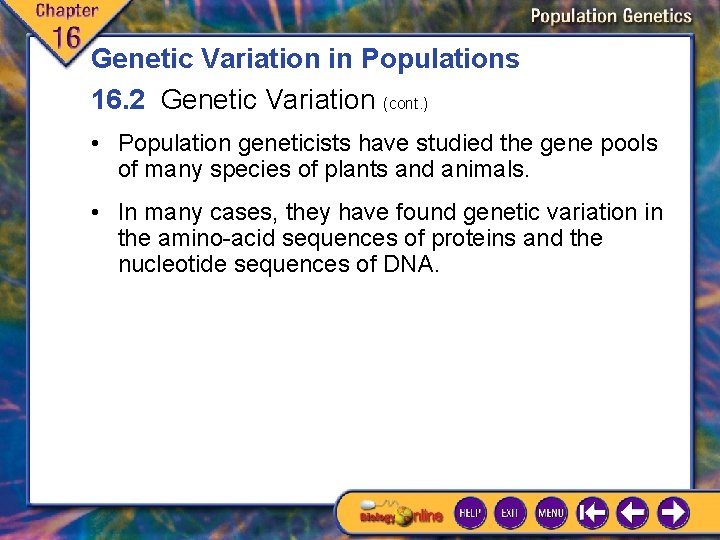
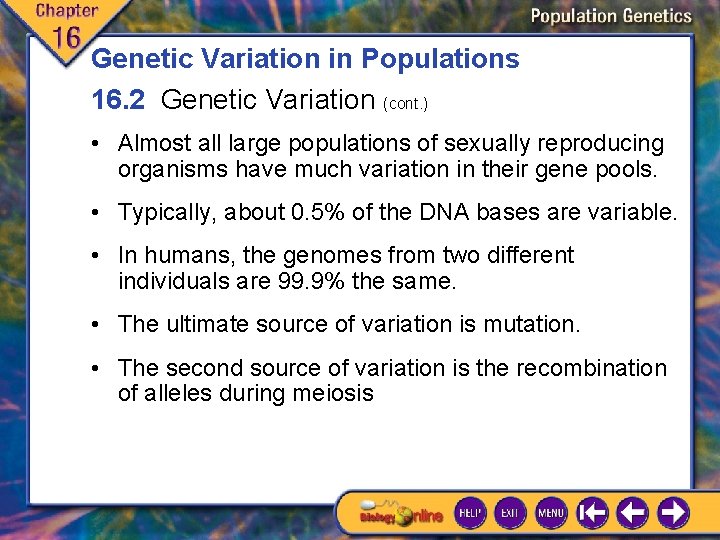
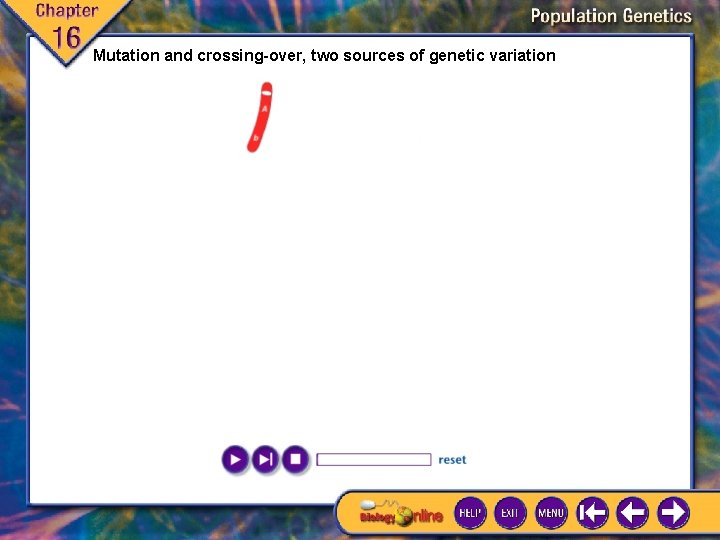
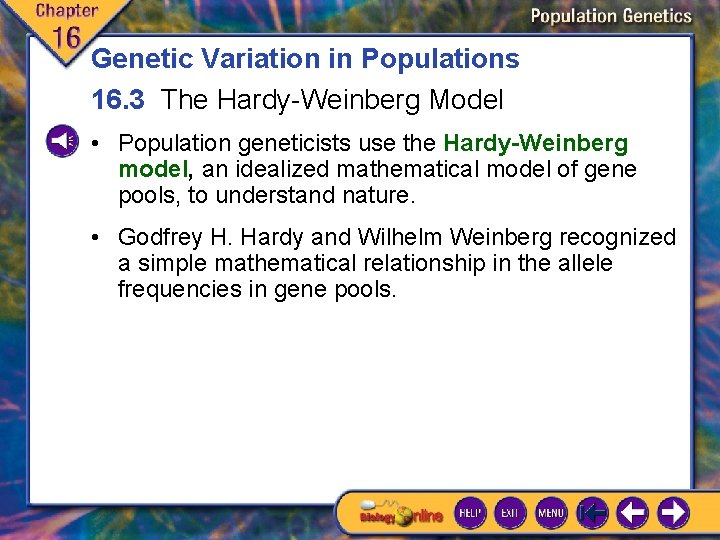
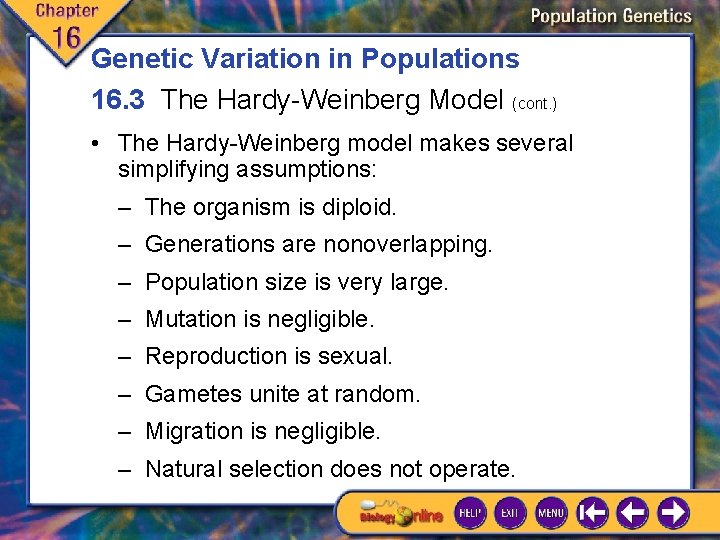
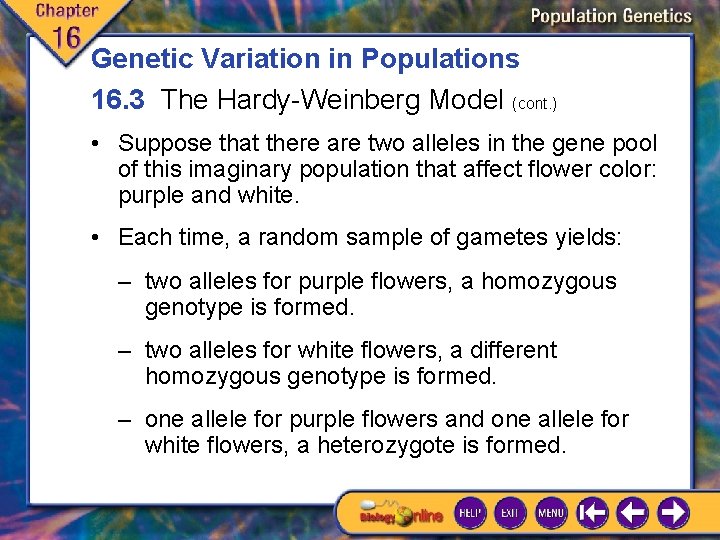
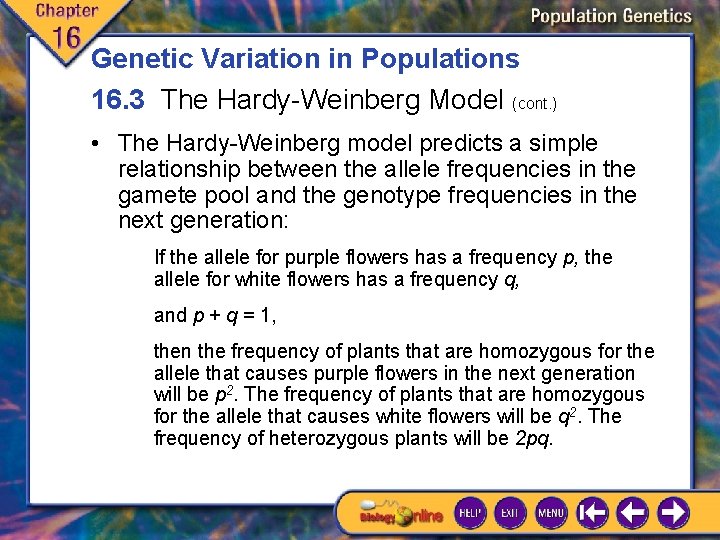
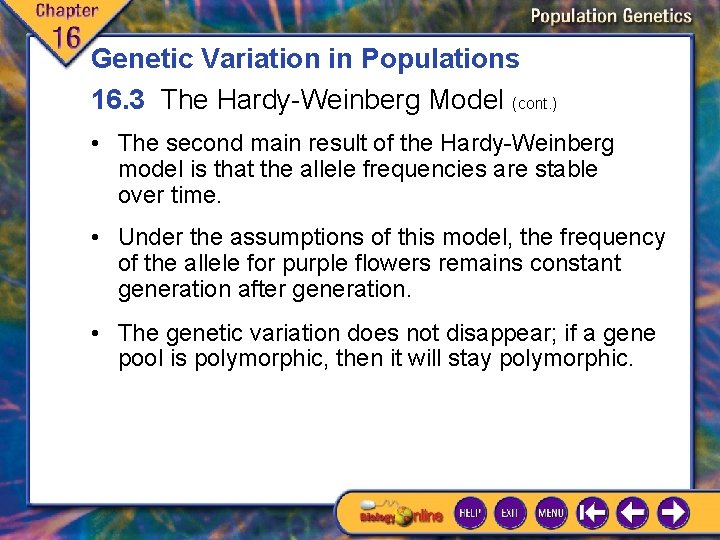
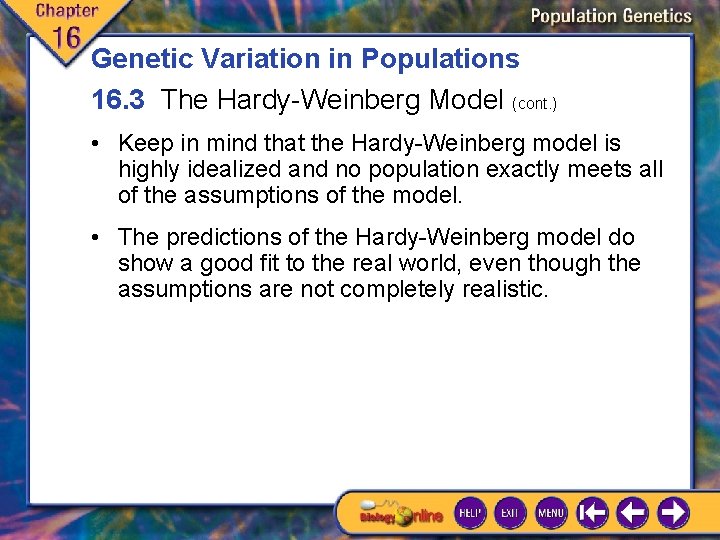
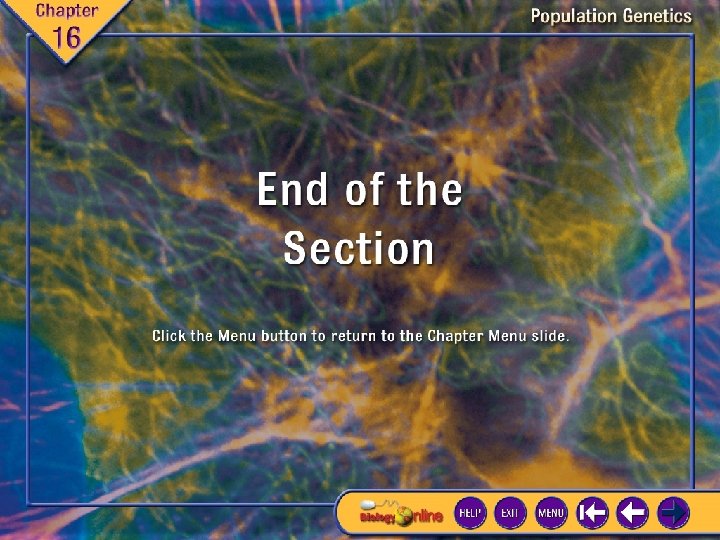
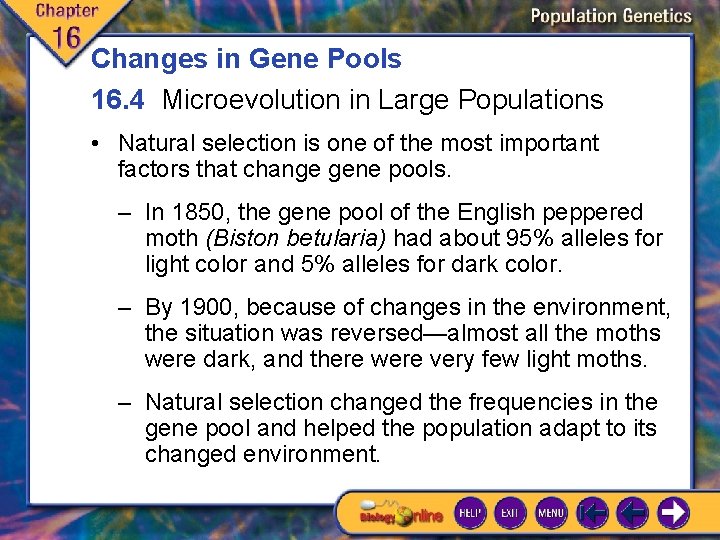
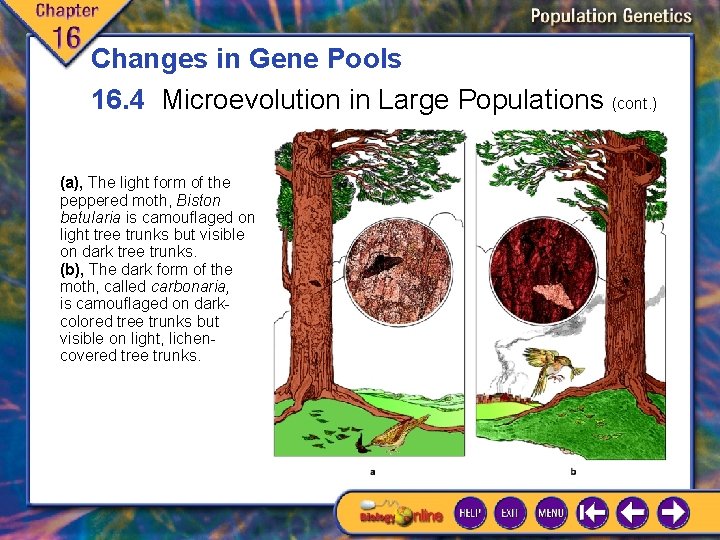
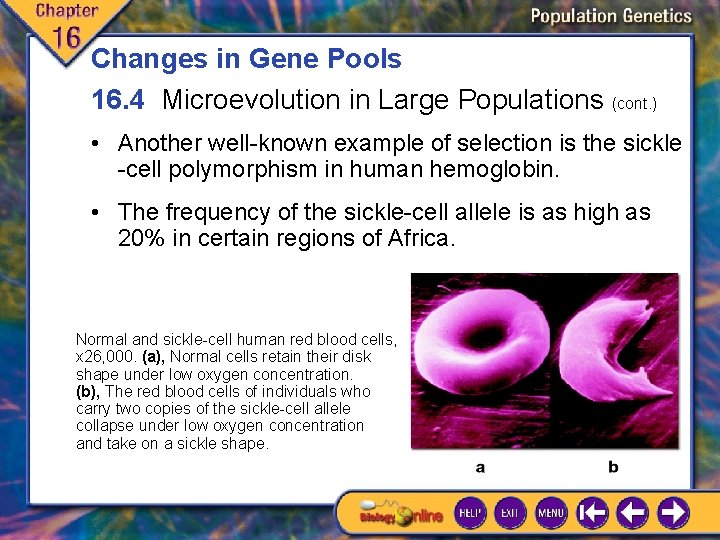
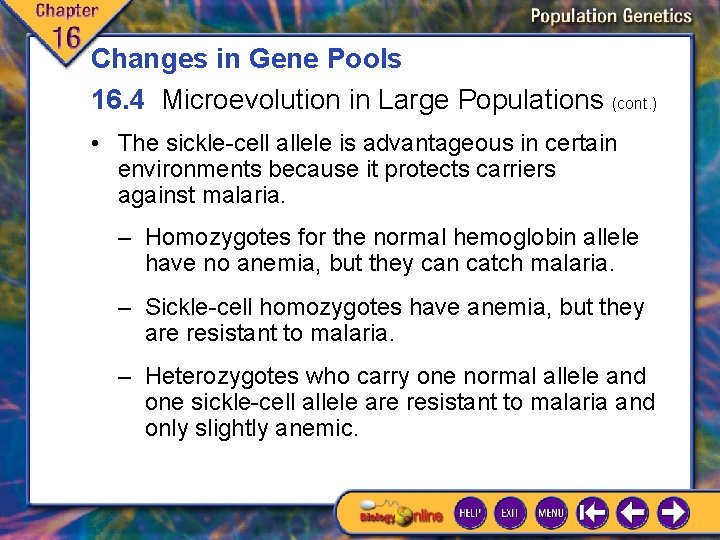
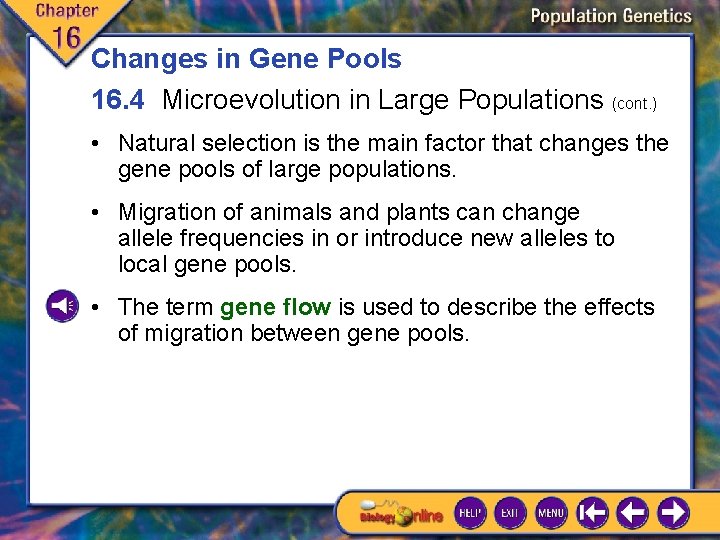
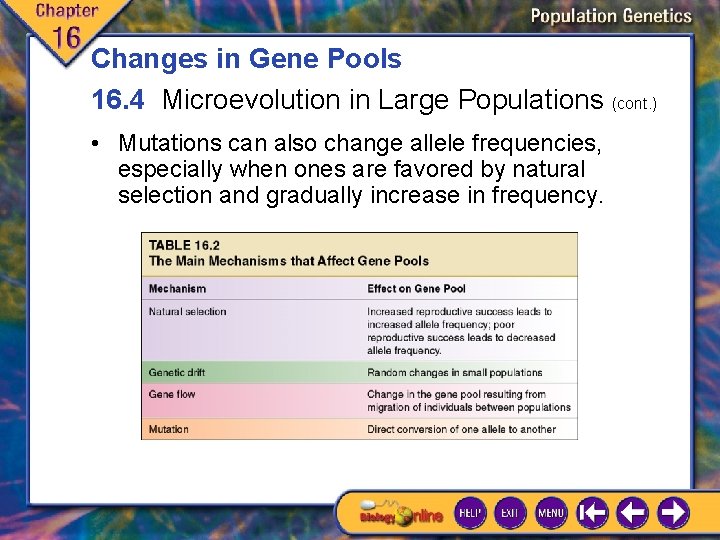
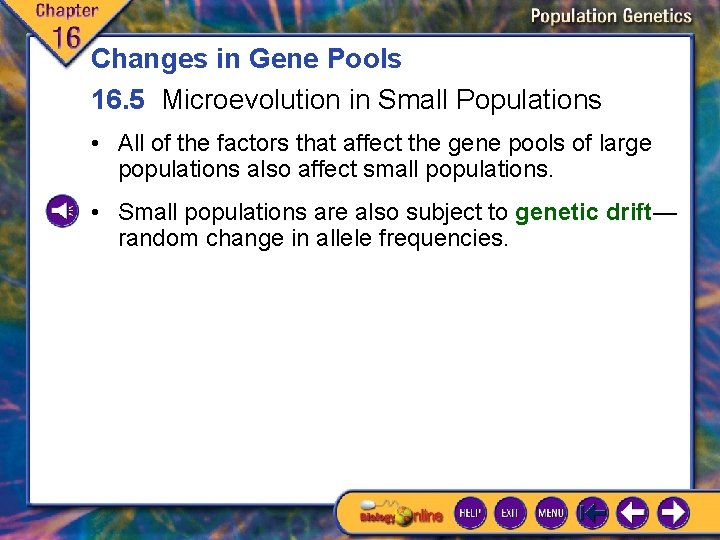
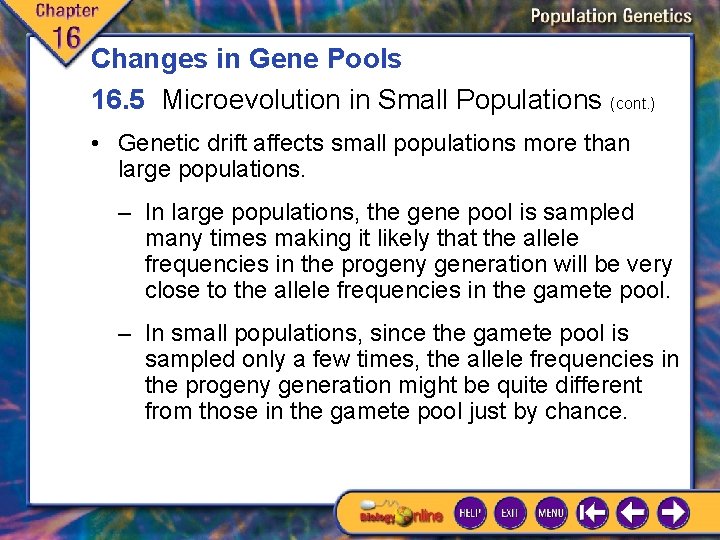
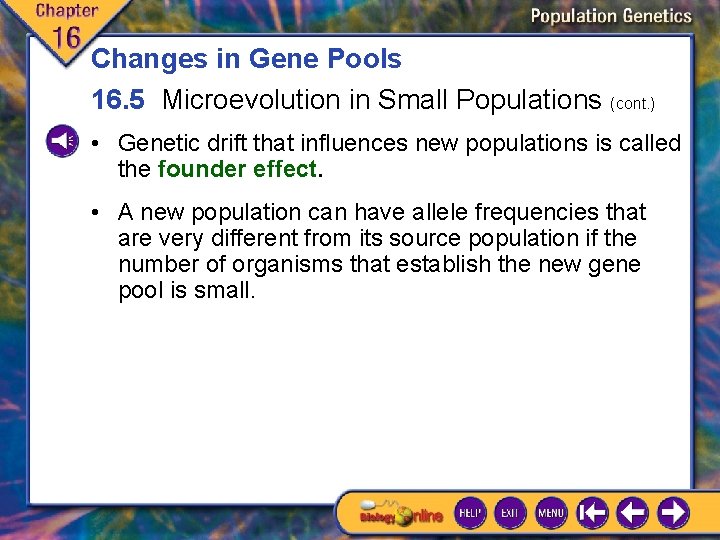
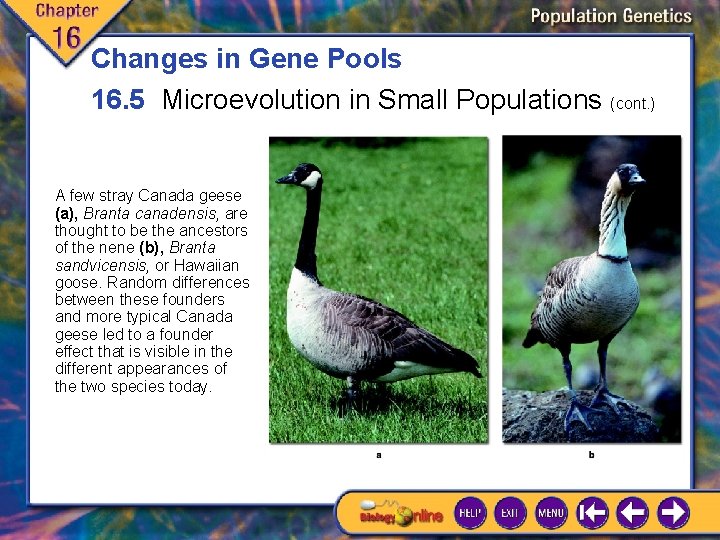
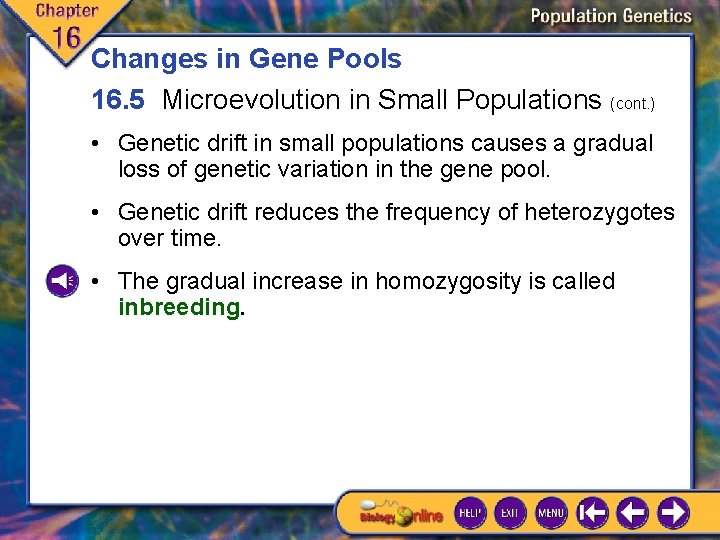
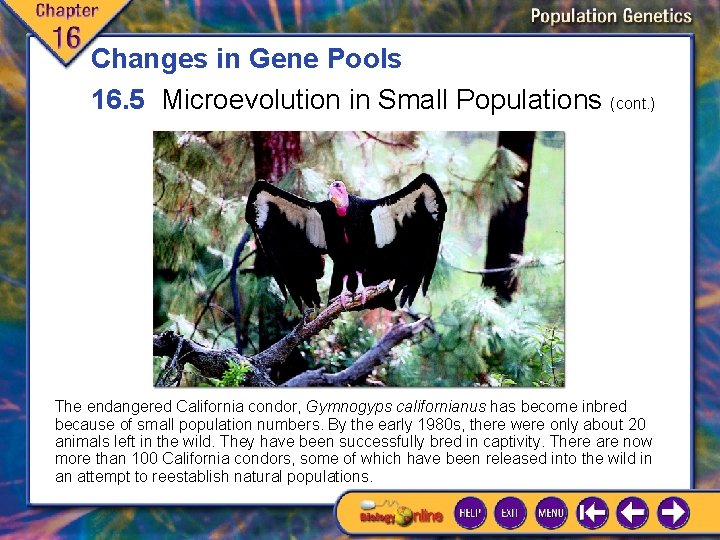
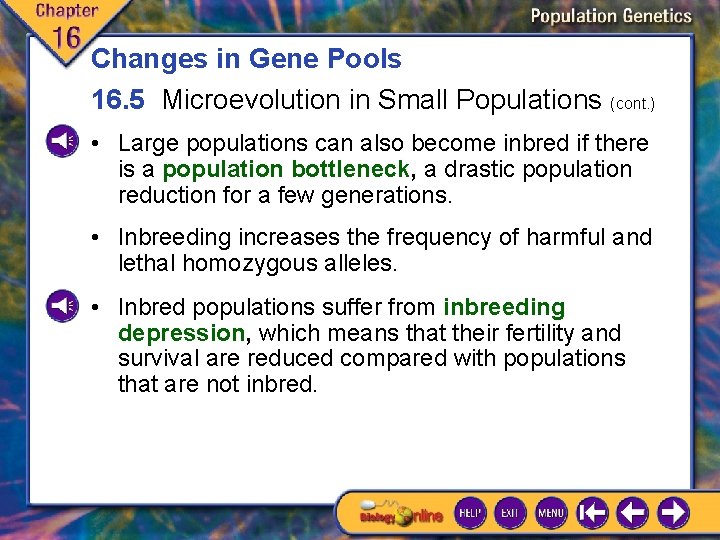
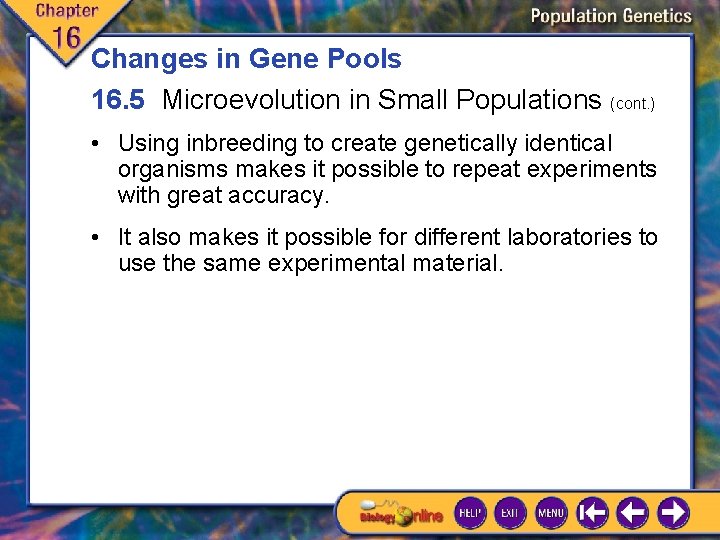
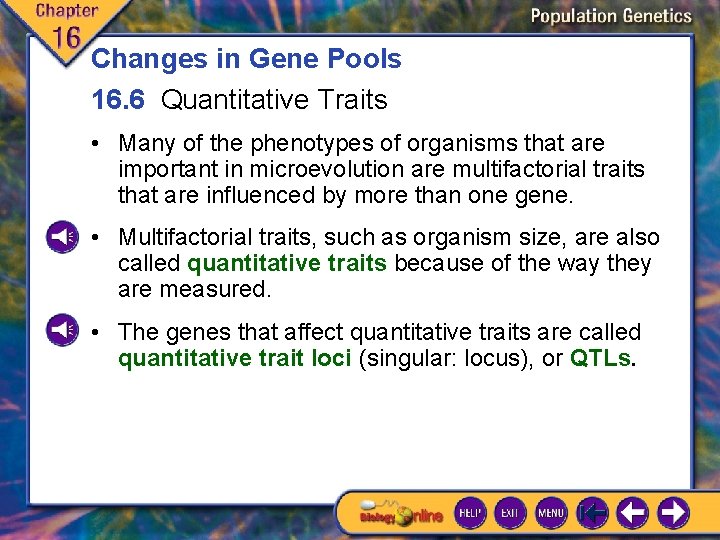
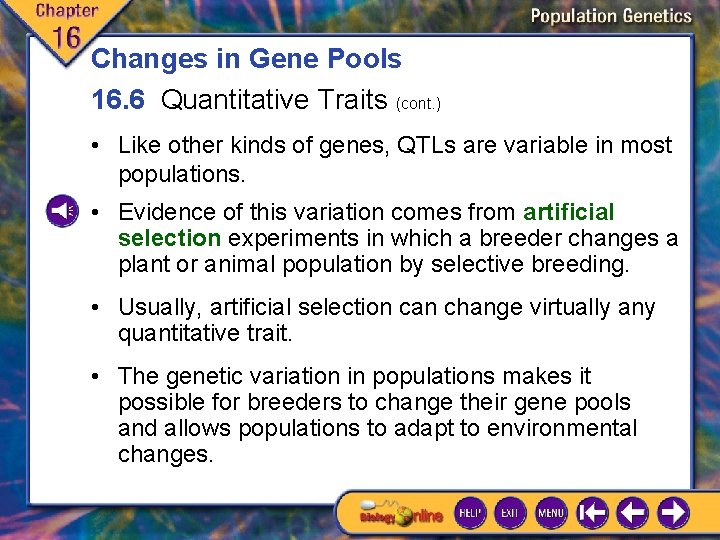
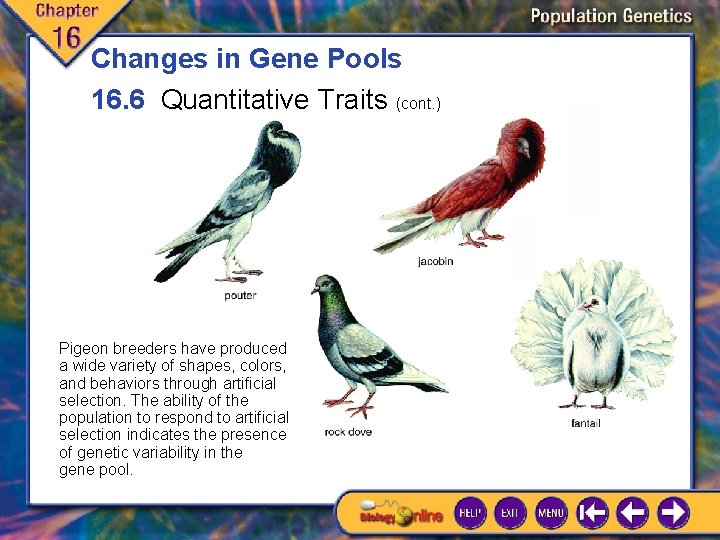
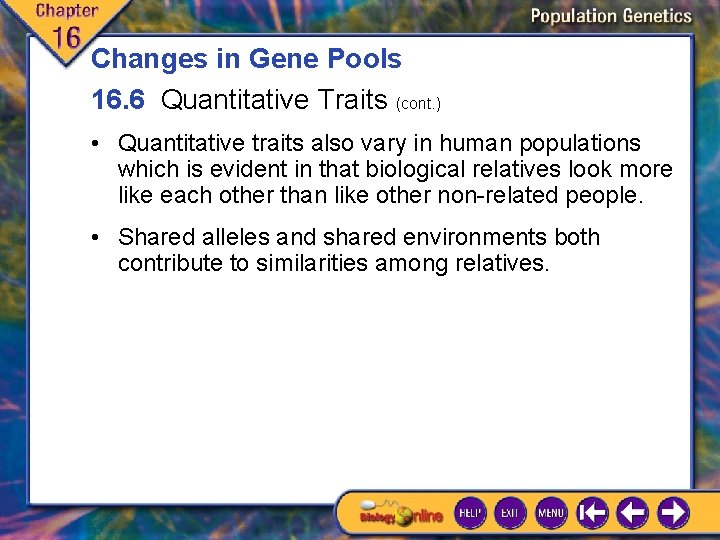
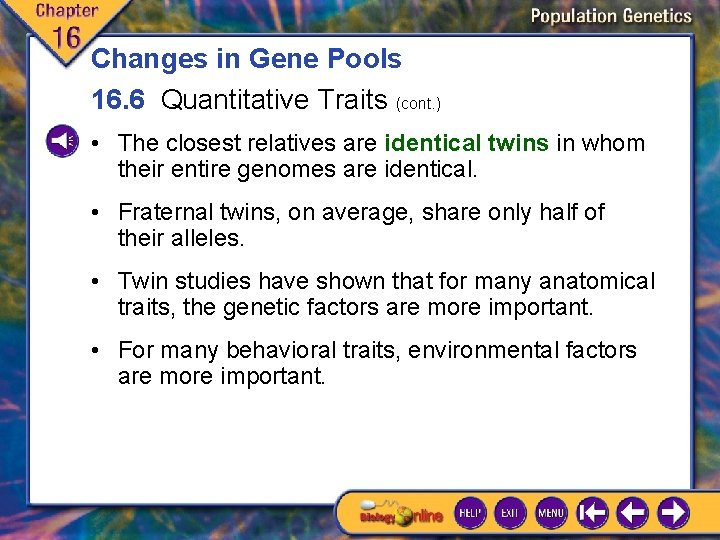
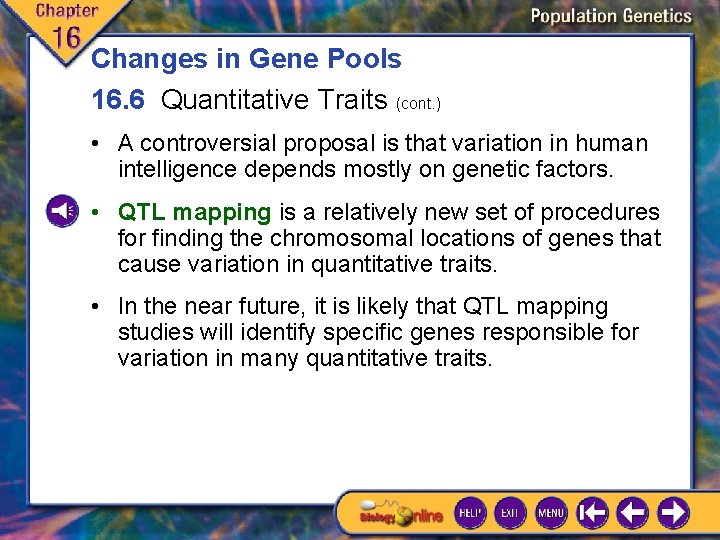
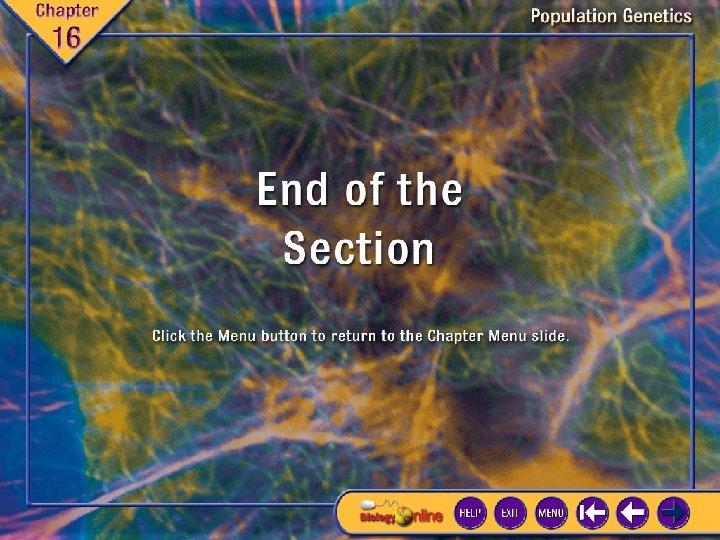
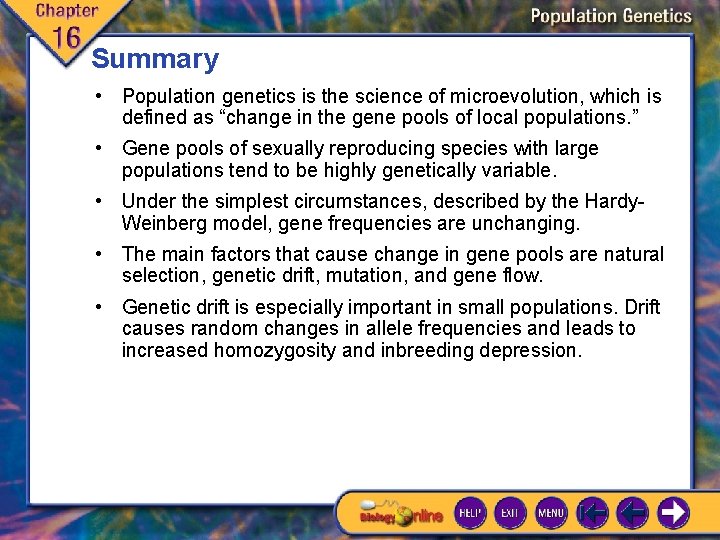
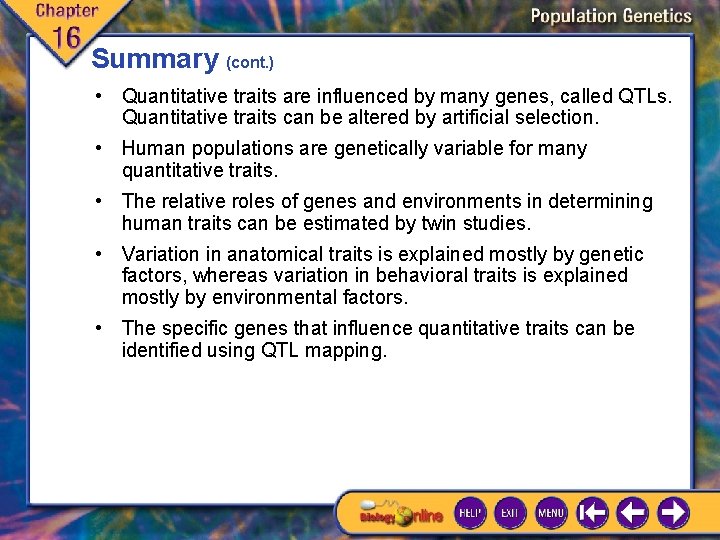
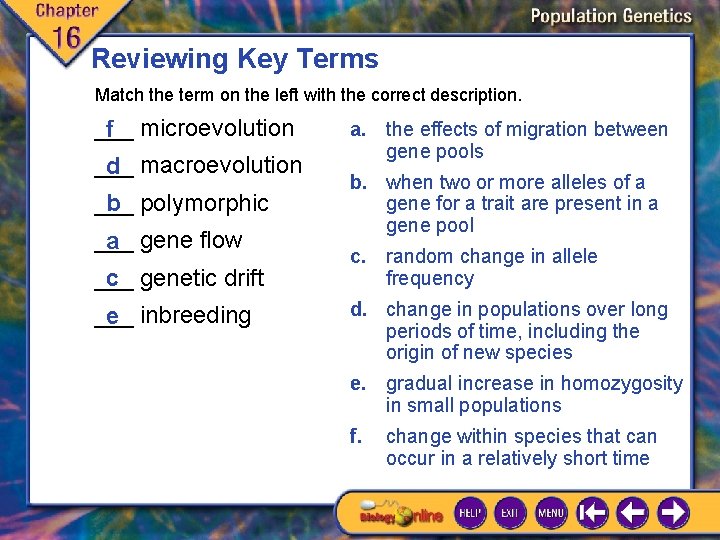
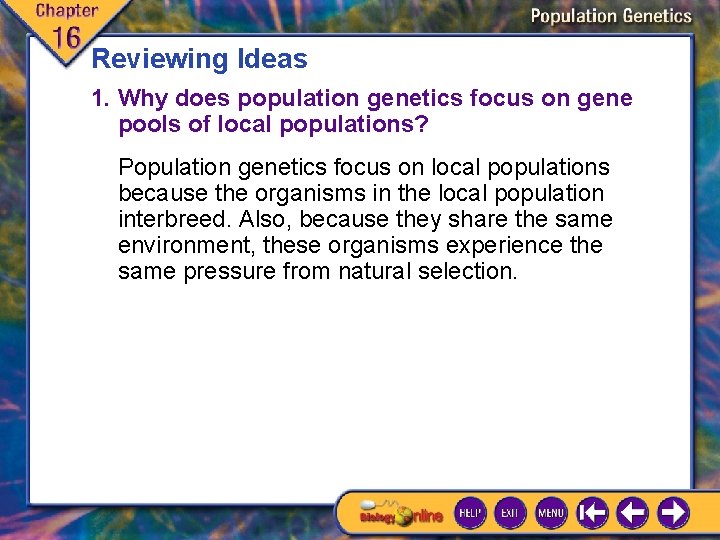
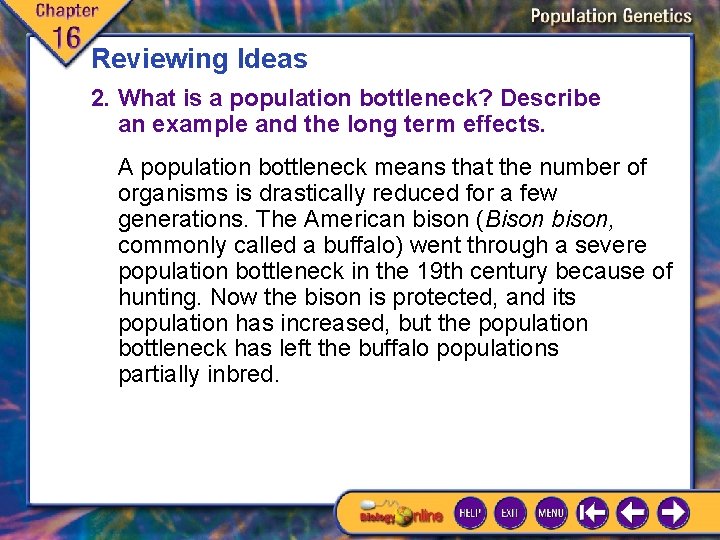
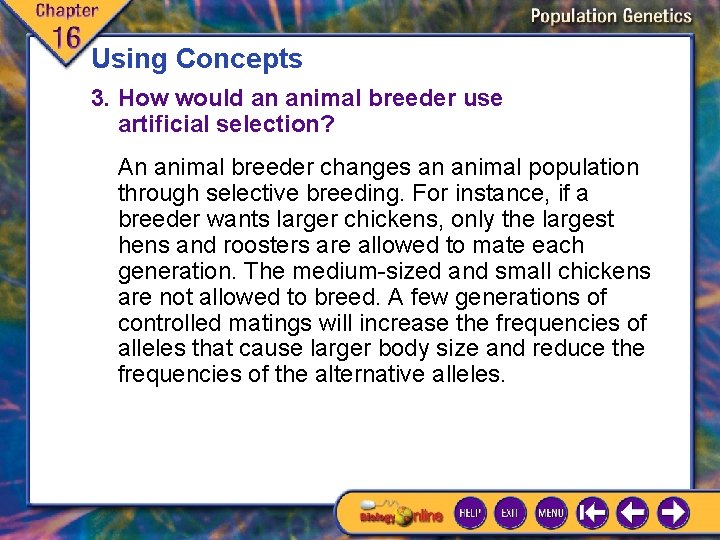
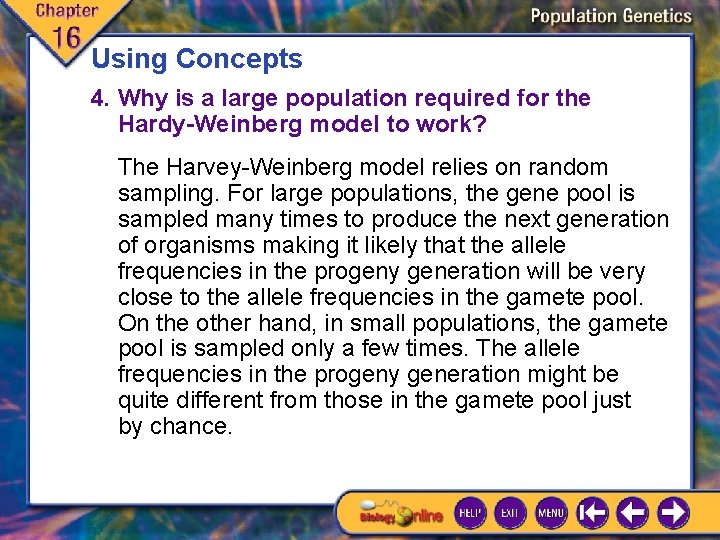
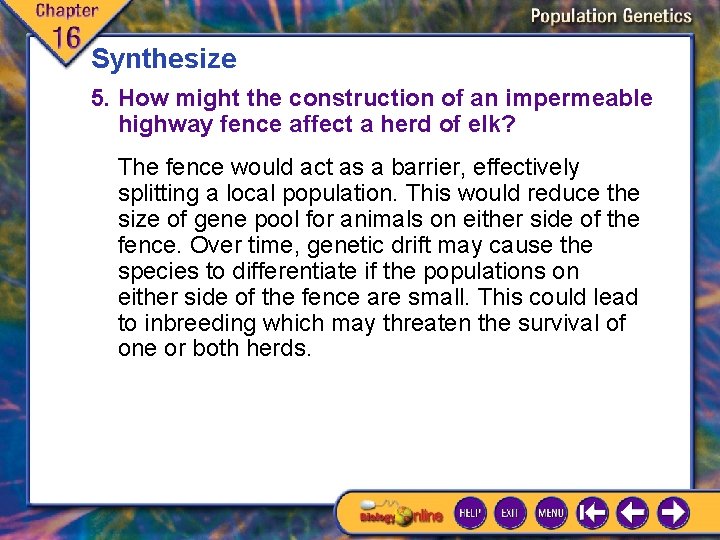
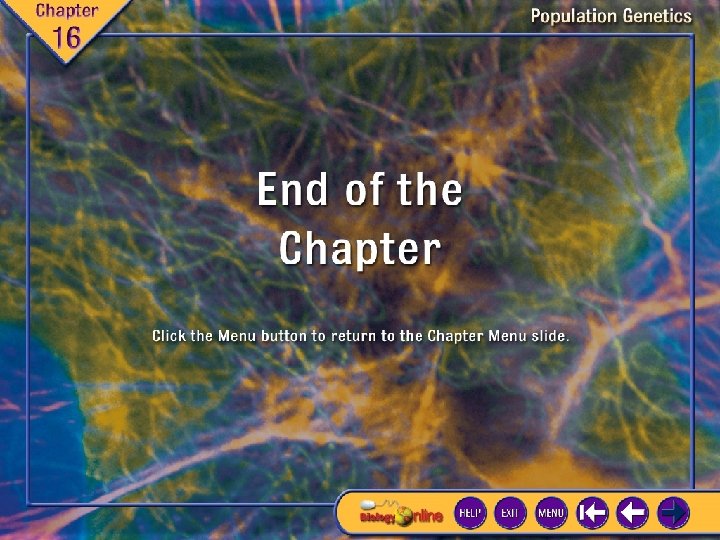
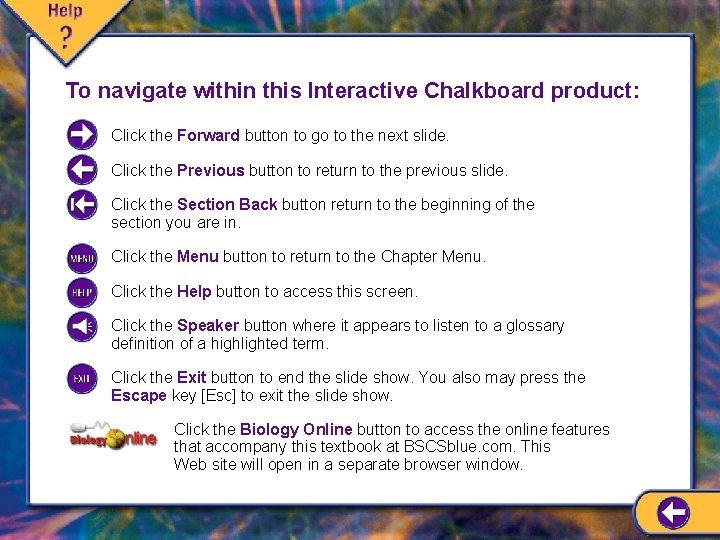
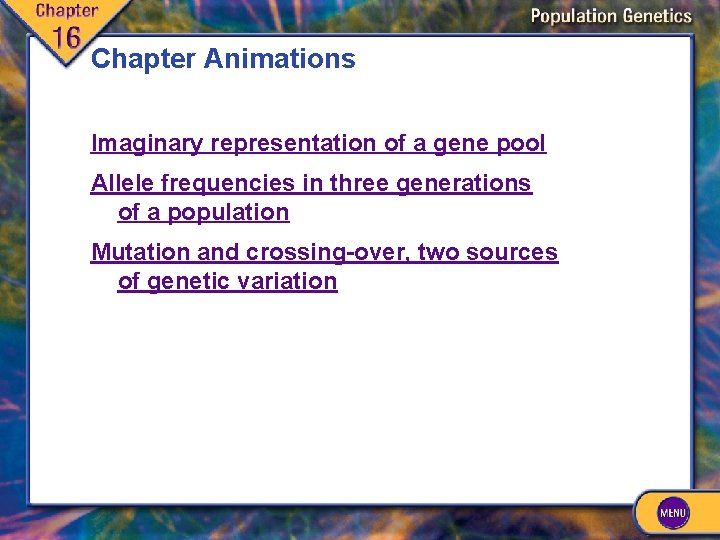
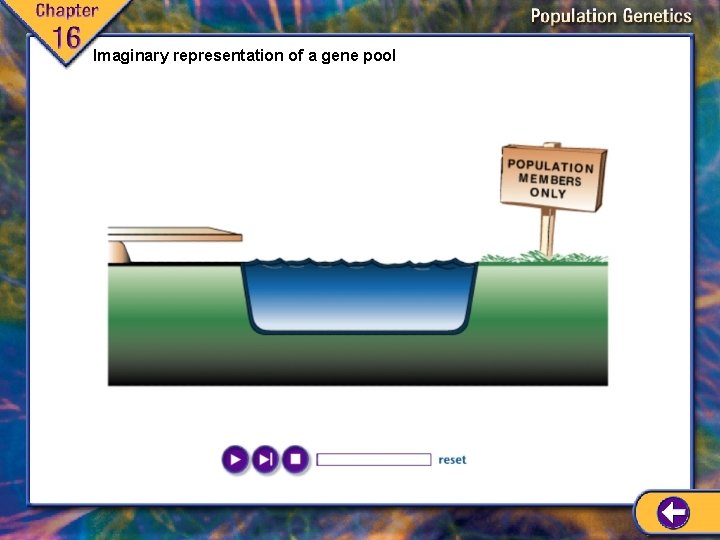
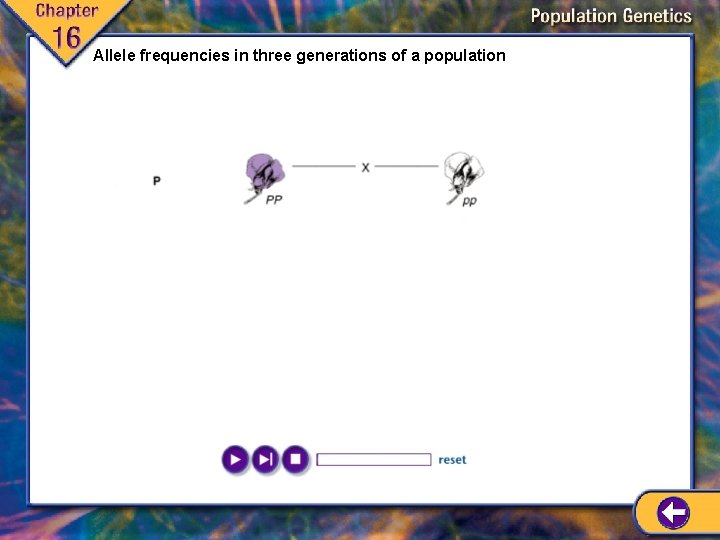
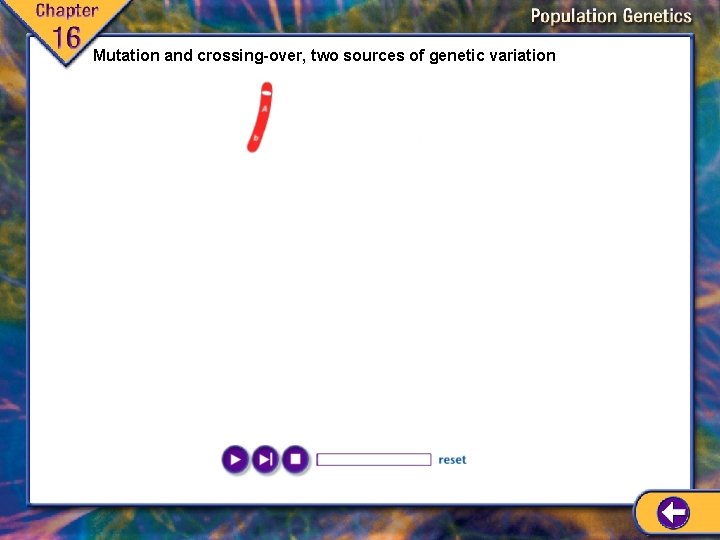
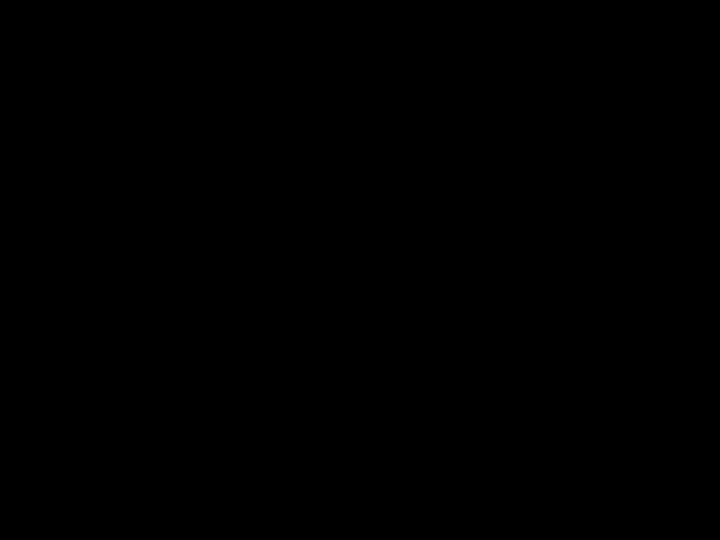
- Slides: 58
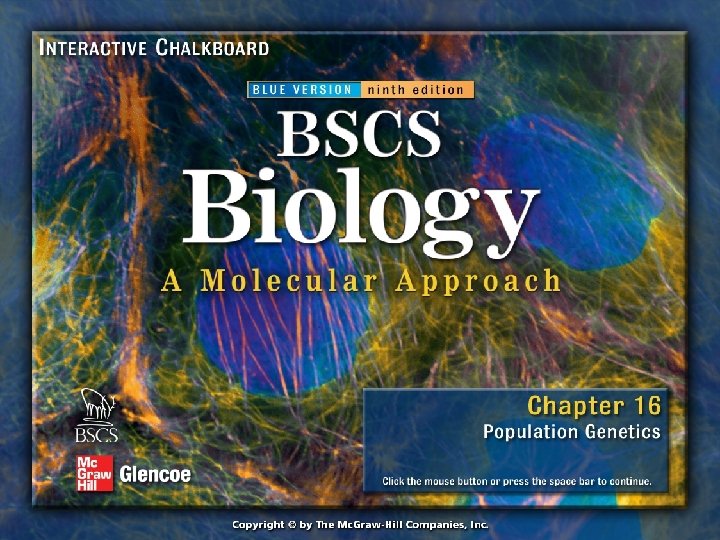
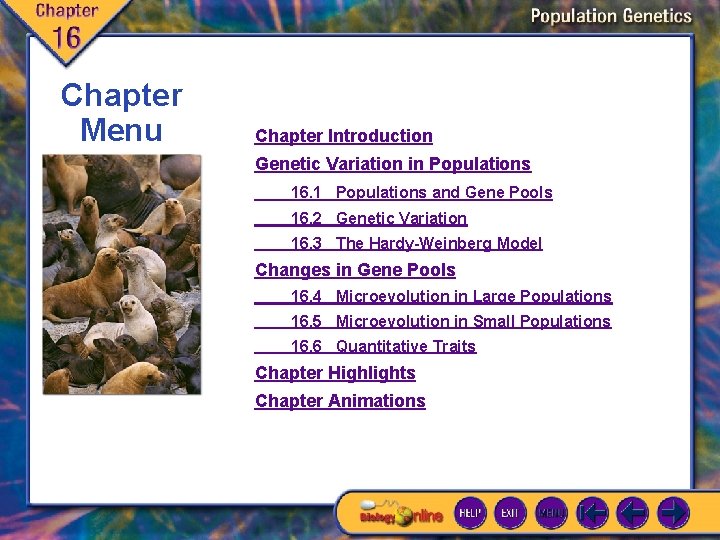
Chapter Menu Chapter Introduction Genetic Variation in Populations 16. 1 Populations and Gene Pools 16. 2 Genetic Variation 16. 3 The Hardy-Weinberg Model Changes in Gene Pools 16. 4 Microevolution in Large Populations 16. 5 Microevolution in Small Populations 16. 6 Quantitative Traits Chapter Highlights Chapter Animations
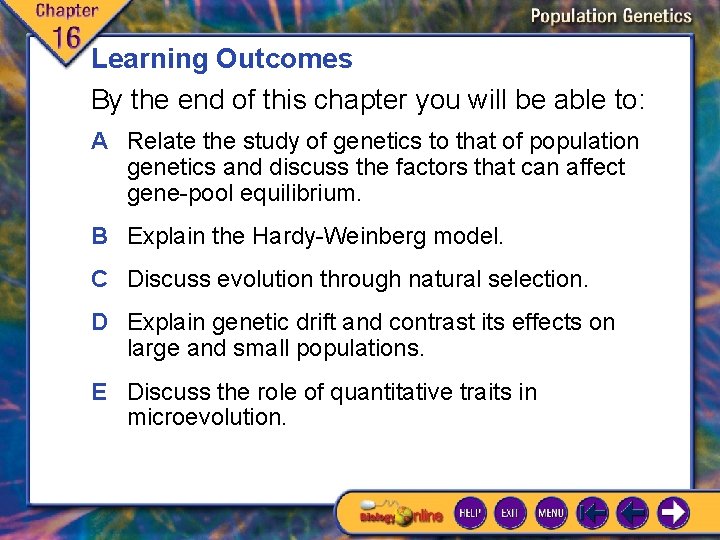
Learning Outcomes By the end of this chapter you will be able to: A Relate the study of genetics to that of population genetics and discuss the factors that can affect gene-pool equilibrium. B Explain the Hardy-Weinberg model. C Discuss evolution through natural selection. D Explain genetic drift and contrast its effects on large and small populations. E Discuss the role of quantitative traits in microevolution.
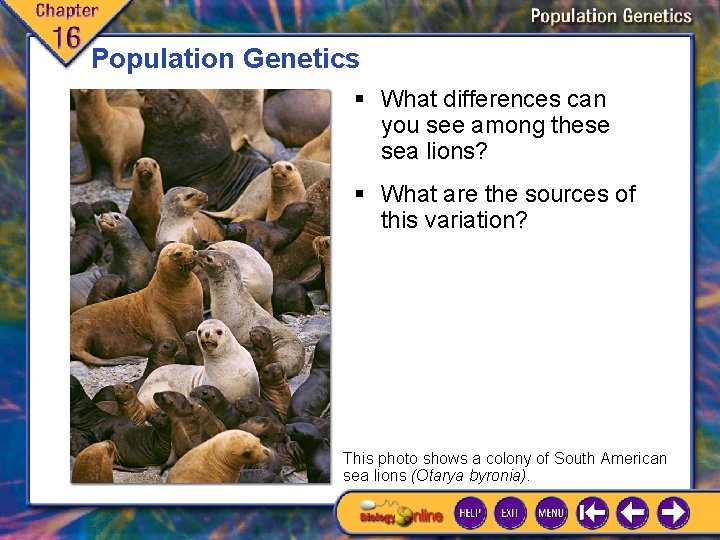
Population Genetics § What differences can you see among these sea lions? § What are the sources of this variation? This photo shows a colony of South American sea lions (Otarya byronia).
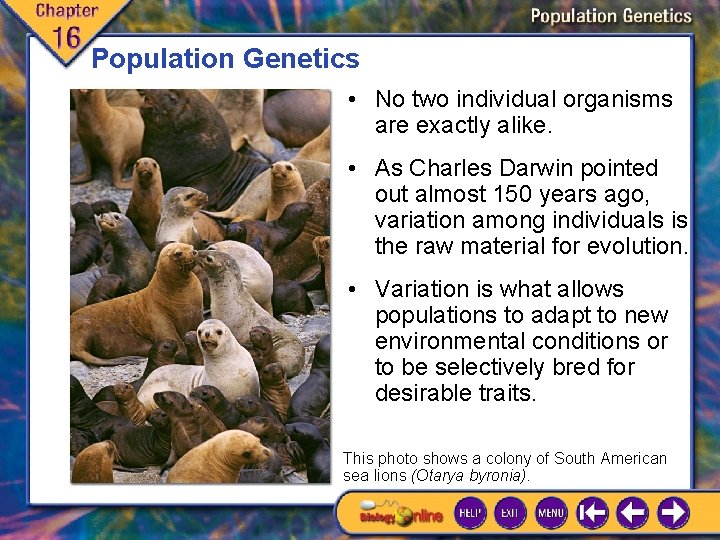
Population Genetics • No two individual organisms are exactly alike. • As Charles Darwin pointed out almost 150 years ago, variation among individuals is the raw material for evolution. • Variation is what allows populations to adapt to new environmental conditions or to be selectively bred for desirable traits. This photo shows a colony of South American sea lions (Otarya byronia).
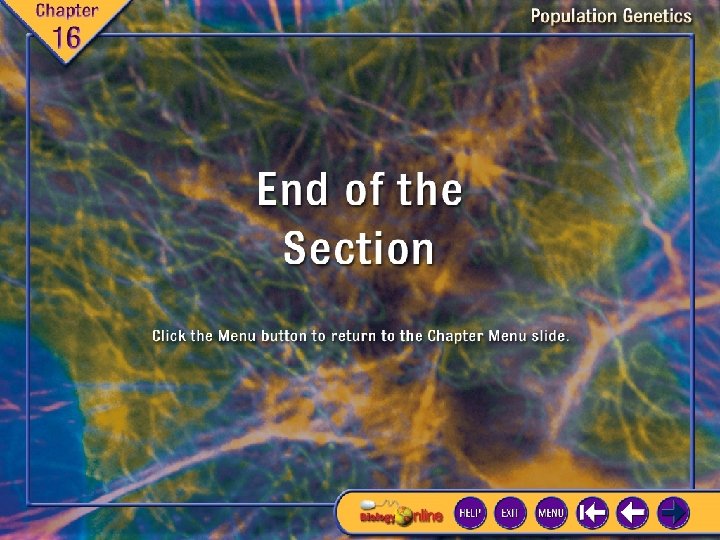
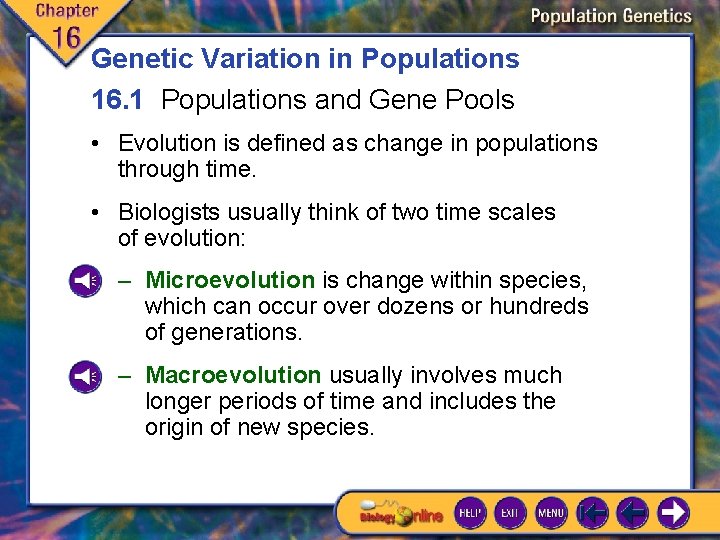
Genetic Variation in Populations 16. 1 Populations and Gene Pools • Evolution is defined as change in populations through time. • Biologists usually think of two time scales of evolution: – Microevolution is change within species, which can occur over dozens or hundreds of generations. – Macroevolution usually involves much longer periods of time and includes the origin of new species.
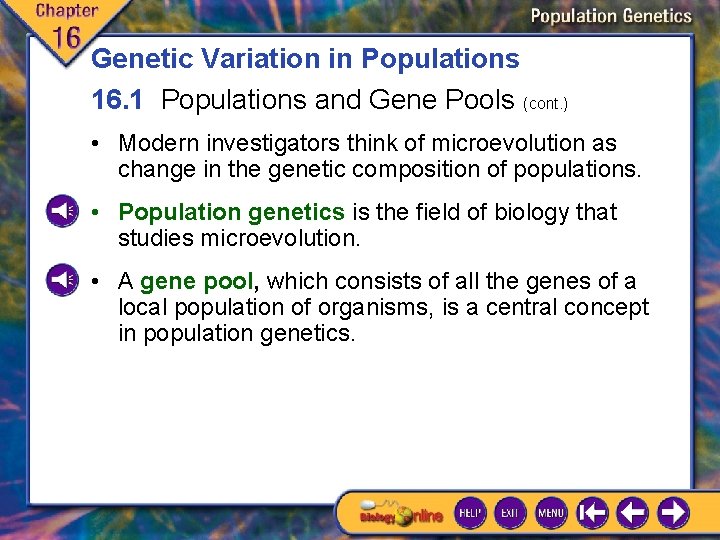
Genetic Variation in Populations 16. 1 Populations and Gene Pools (cont. ) • Modern investigators think of microevolution as change in the genetic composition of populations. • Population genetics is the field of biology that studies microevolution. • A gene pool, which consists of all the genes of a local population of organisms, is a central concept in population genetics.
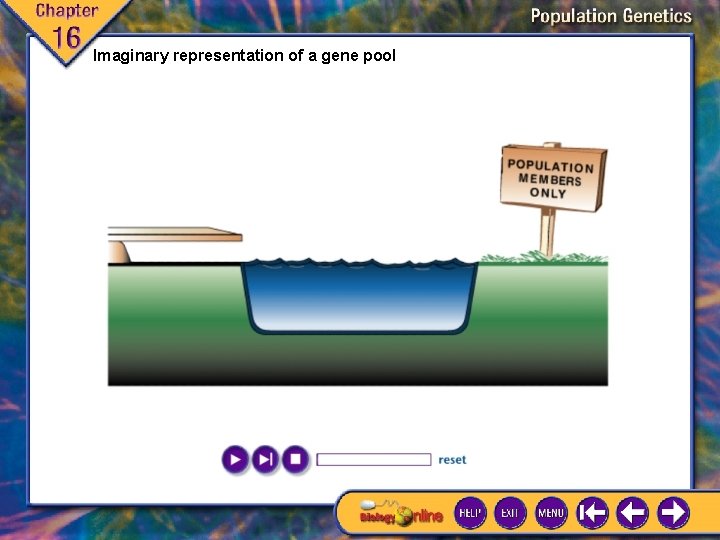
Imaginary representation of a gene pool
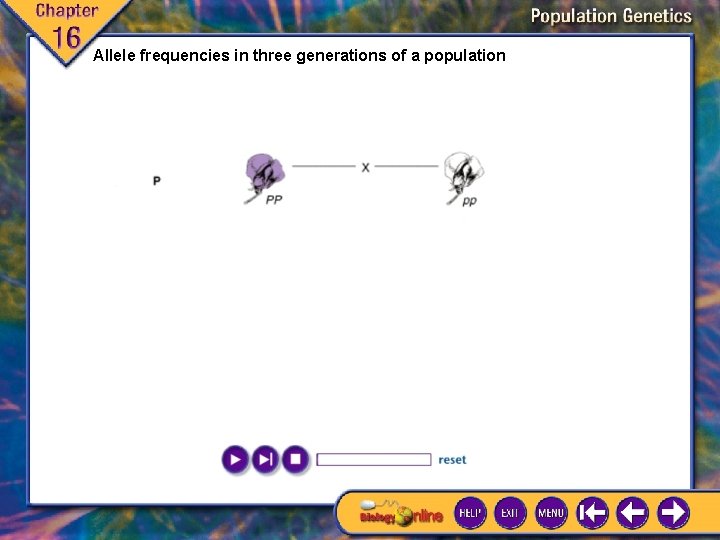
Allele frequencies in three generations of a population
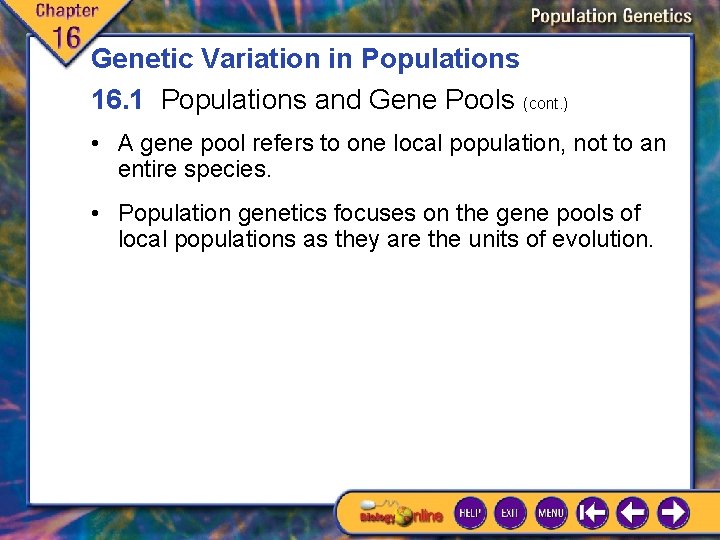
Genetic Variation in Populations 16. 1 Populations and Gene Pools (cont. ) • A gene pool refers to one local population, not to an entire species. • Population genetics focuses on the gene pools of local populations as they are the units of evolution.
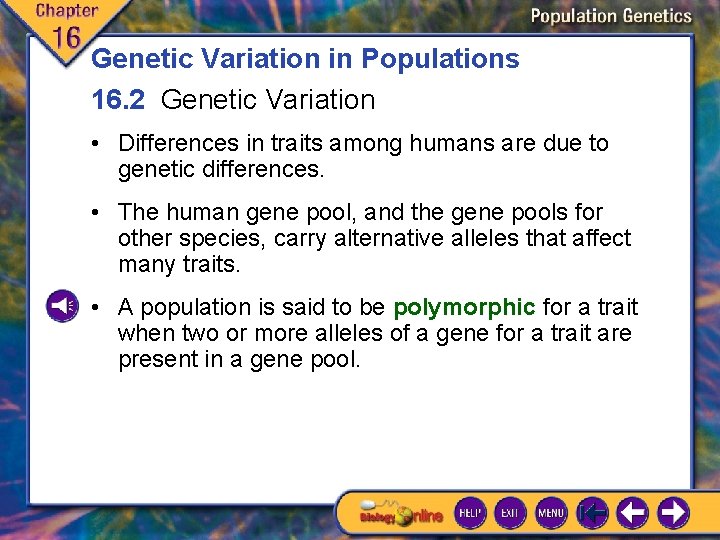
Genetic Variation in Populations 16. 2 Genetic Variation • Differences in traits among humans are due to genetic differences. • The human gene pool, and the gene pools for other species, carry alternative alleles that affect many traits. • A population is said to be polymorphic for a trait when two or more alleles of a gene for a trait are present in a gene pool.
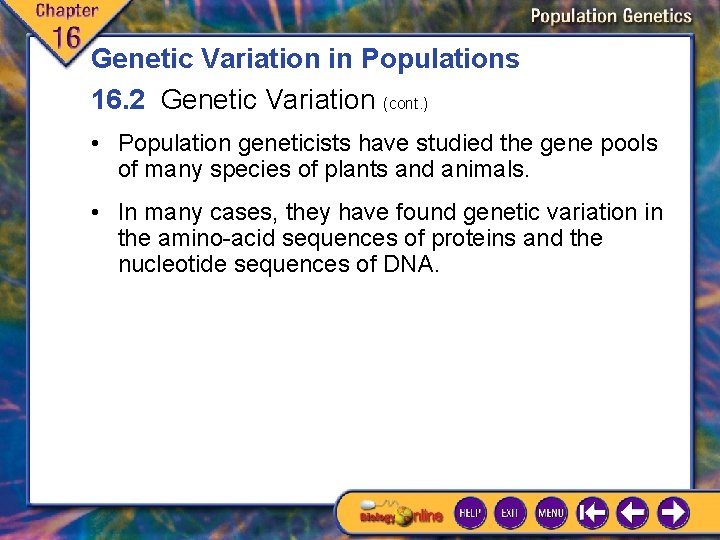
Genetic Variation in Populations 16. 2 Genetic Variation (cont. ) • Population geneticists have studied the gene pools of many species of plants and animals. • In many cases, they have found genetic variation in the amino-acid sequences of proteins and the nucleotide sequences of DNA.
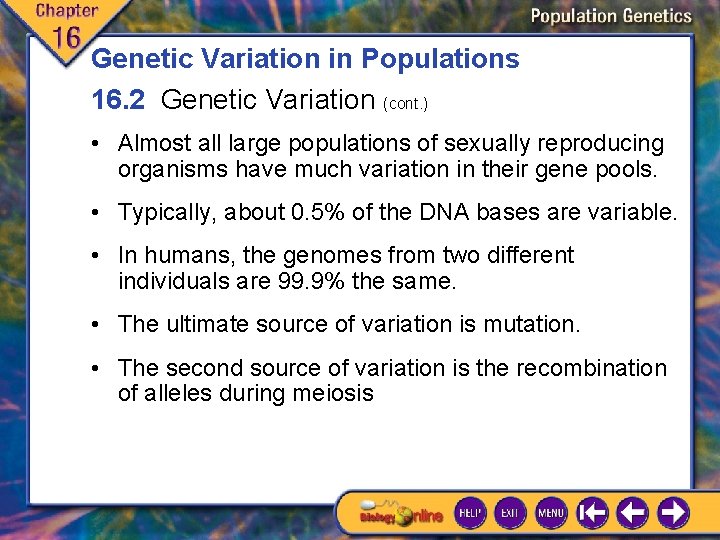
Genetic Variation in Populations 16. 2 Genetic Variation (cont. ) • Almost all large populations of sexually reproducing organisms have much variation in their gene pools. • Typically, about 0. 5% of the DNA bases are variable. • In humans, the genomes from two different individuals are 99. 9% the same. • The ultimate source of variation is mutation. • The second source of variation is the recombination of alleles during meiosis
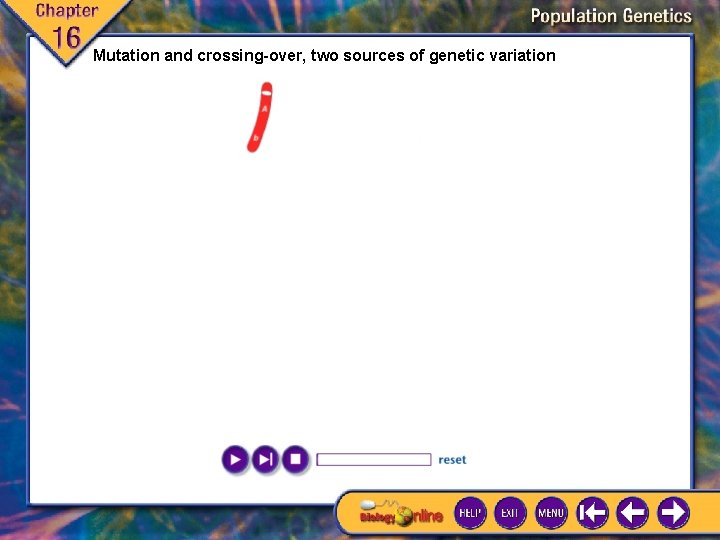
Mutation and crossing-over, two sources of genetic variation
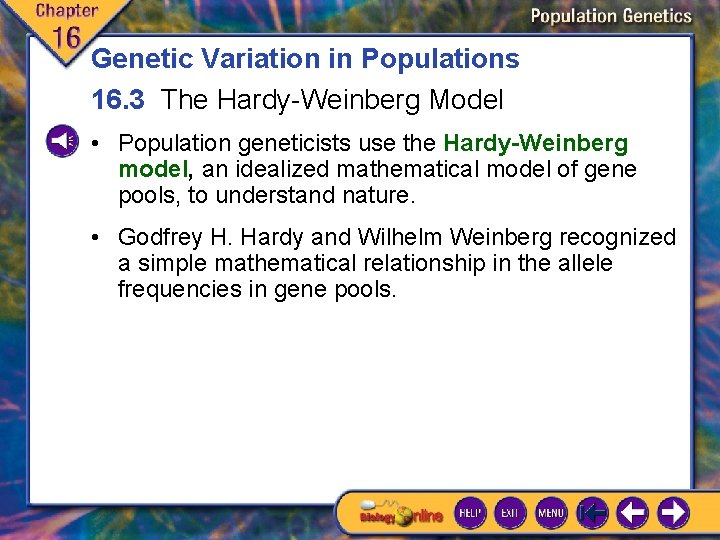
Genetic Variation in Populations 16. 3 The Hardy-Weinberg Model • Population geneticists use the Hardy-Weinberg model, an idealized mathematical model of gene pools, to understand nature. • Godfrey H. Hardy and Wilhelm Weinberg recognized a simple mathematical relationship in the allele frequencies in gene pools.
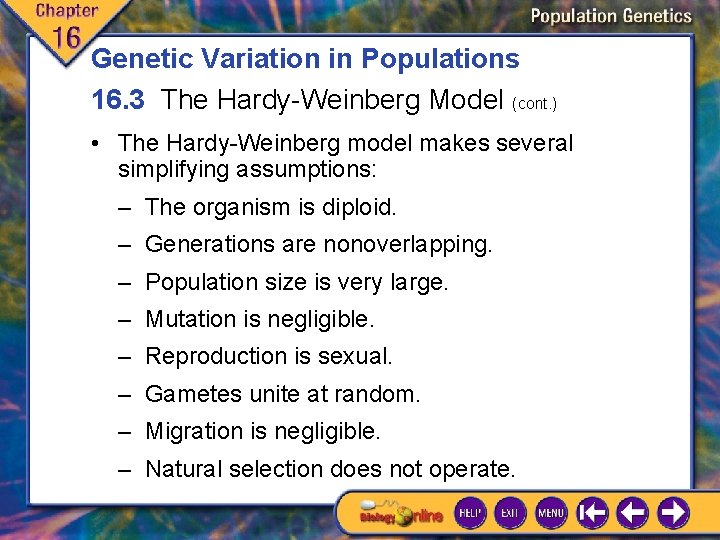
Genetic Variation in Populations 16. 3 The Hardy-Weinberg Model (cont. ) • The Hardy-Weinberg model makes several simplifying assumptions: – The organism is diploid. – Generations are nonoverlapping. – Population size is very large. – Mutation is negligible. – Reproduction is sexual. – Gametes unite at random. – Migration is negligible. – Natural selection does not operate.
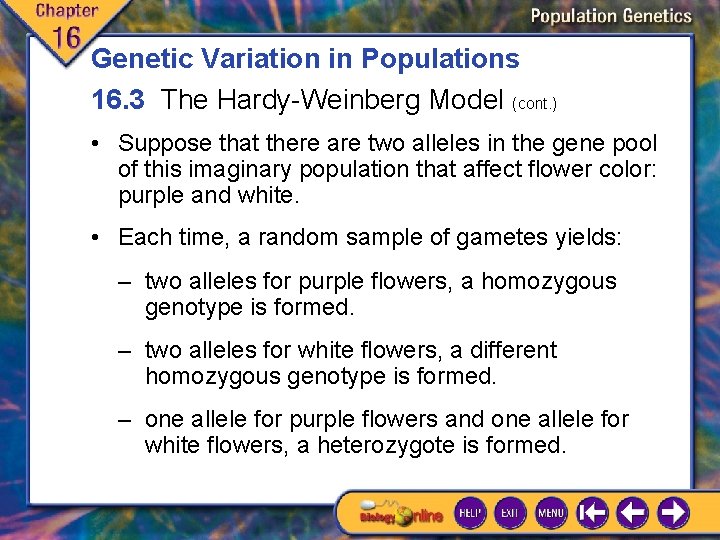
Genetic Variation in Populations 16. 3 The Hardy-Weinberg Model (cont. ) • Suppose that there are two alleles in the gene pool of this imaginary population that affect flower color: purple and white. • Each time, a random sample of gametes yields: – two alleles for purple flowers, a homozygous genotype is formed. – two alleles for white flowers, a different homozygous genotype is formed. – one allele for purple flowers and one allele for white flowers, a heterozygote is formed.
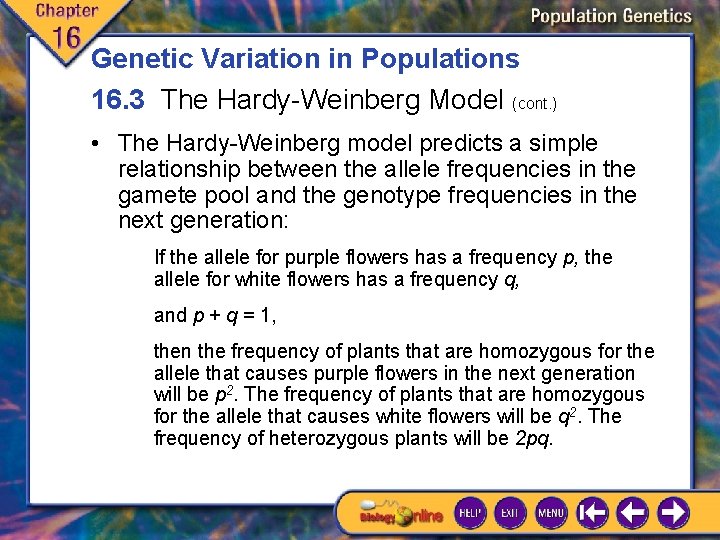
Genetic Variation in Populations 16. 3 The Hardy-Weinberg Model (cont. ) • The Hardy-Weinberg model predicts a simple relationship between the allele frequencies in the gamete pool and the genotype frequencies in the next generation: If the allele for purple flowers has a frequency p, the allele for white flowers has a frequency q, and p + q = 1, then the frequency of plants that are homozygous for the allele that causes purple flowers in the next generation will be p 2. The frequency of plants that are homozygous for the allele that causes white flowers will be q 2. The frequency of heterozygous plants will be 2 pq.
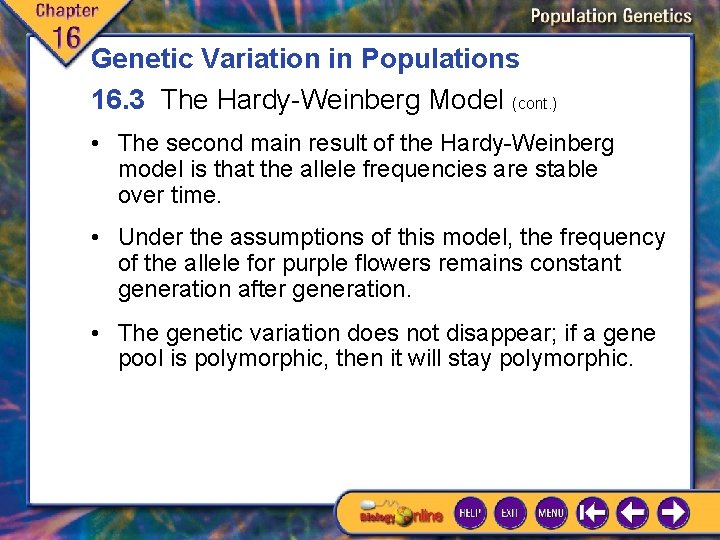
Genetic Variation in Populations 16. 3 The Hardy-Weinberg Model (cont. ) • The second main result of the Hardy-Weinberg model is that the allele frequencies are stable over time. • Under the assumptions of this model, the frequency of the allele for purple flowers remains constant generation after generation. • The genetic variation does not disappear; if a gene pool is polymorphic, then it will stay polymorphic.
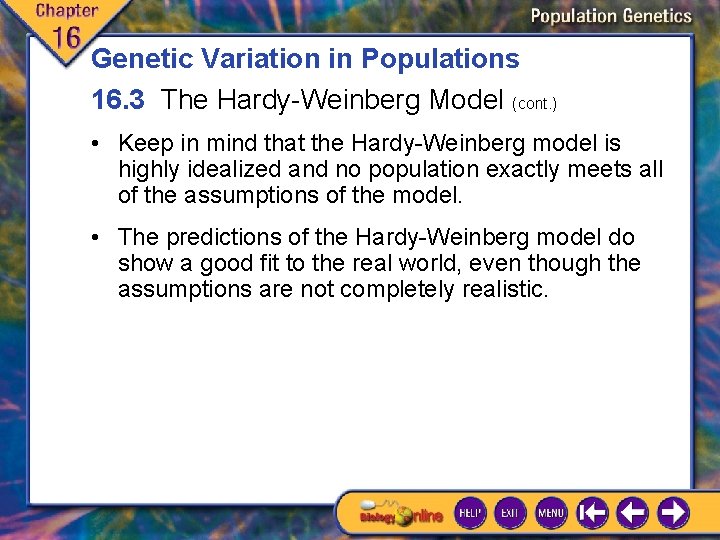
Genetic Variation in Populations 16. 3 The Hardy-Weinberg Model (cont. ) • Keep in mind that the Hardy-Weinberg model is highly idealized and no population exactly meets all of the assumptions of the model. • The predictions of the Hardy-Weinberg model do show a good fit to the real world, even though the assumptions are not completely realistic.
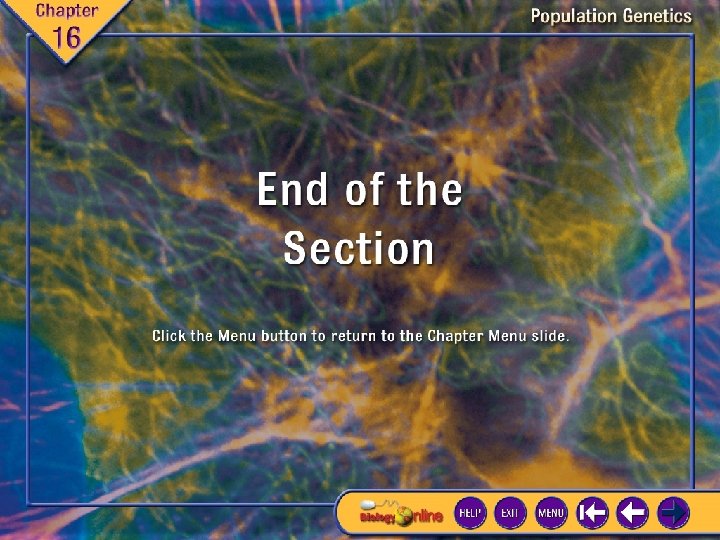
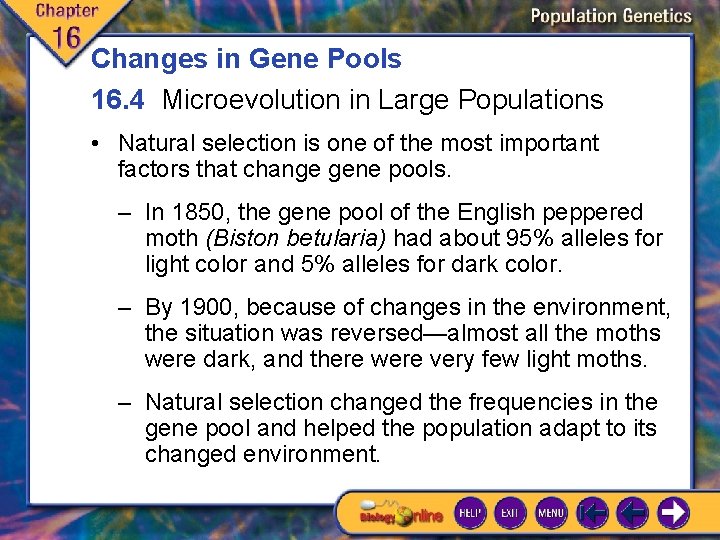
Changes in Gene Pools 16. 4 Microevolution in Large Populations • Natural selection is one of the most important factors that change gene pools. – In 1850, the gene pool of the English peppered moth (Biston betularia) had about 95% alleles for light color and 5% alleles for dark color. – By 1900, because of changes in the environment, the situation was reversed—almost all the moths were dark, and there were very few light moths. – Natural selection changed the frequencies in the gene pool and helped the population adapt to its changed environment.
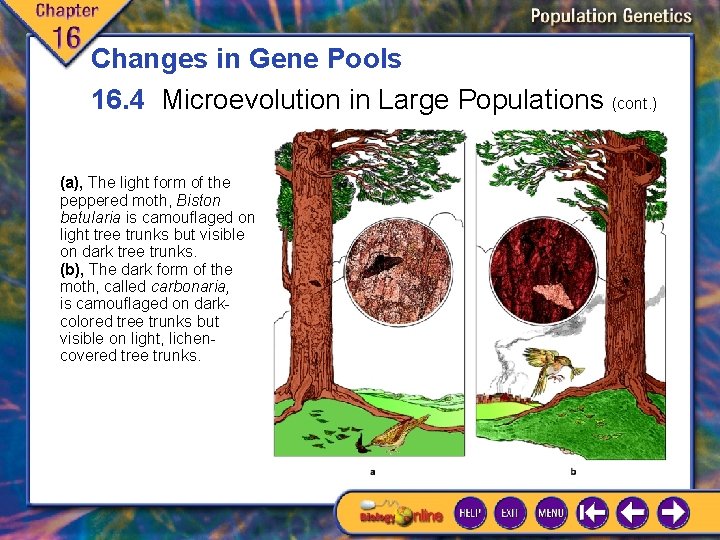
Changes in Gene Pools 16. 4 Microevolution in Large Populations (cont. ) (a), The light form of the peppered moth, Biston betularia is camouflaged on light tree trunks but visible on dark tree trunks. (b), The dark form of the moth, called carbonaria, is camouflaged on darkcolored tree trunks but visible on light, lichencovered tree trunks.
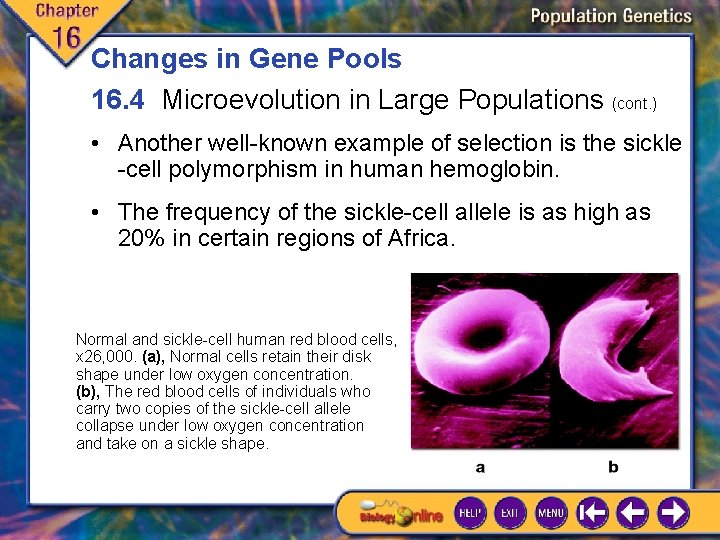
Changes in Gene Pools 16. 4 Microevolution in Large Populations (cont. ) • Another well-known example of selection is the sickle -cell polymorphism in human hemoglobin. • The frequency of the sickle-cell allele is as high as 20% in certain regions of Africa. Normal and sickle-cell human red blood cells, x 26, 000. (a), Normal cells retain their disk shape under low oxygen concentration. (b), The red blood cells of individuals who carry two copies of the sickle-cell allele collapse under low oxygen concentration and take on a sickle shape.
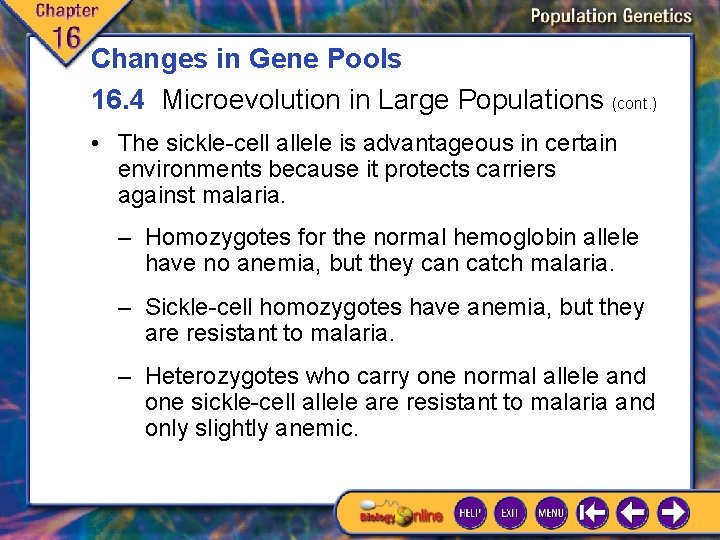
Changes in Gene Pools 16. 4 Microevolution in Large Populations (cont. ) • The sickle-cell allele is advantageous in certain environments because it protects carriers against malaria. – Homozygotes for the normal hemoglobin allele have no anemia, but they can catch malaria. – Sickle-cell homozygotes have anemia, but they are resistant to malaria. – Heterozygotes who carry one normal allele and one sickle-cell allele are resistant to malaria and only slightly anemic.
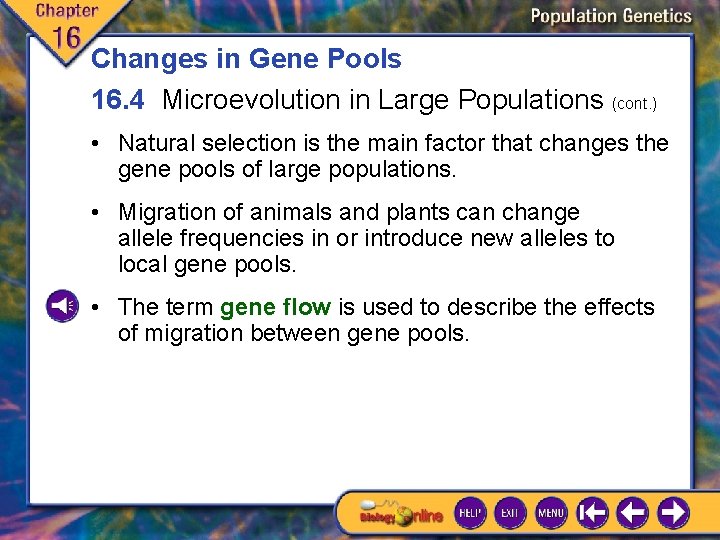
Changes in Gene Pools 16. 4 Microevolution in Large Populations (cont. ) • Natural selection is the main factor that changes the gene pools of large populations. • Migration of animals and plants can change allele frequencies in or introduce new alleles to local gene pools. • The term gene flow is used to describe the effects of migration between gene pools.
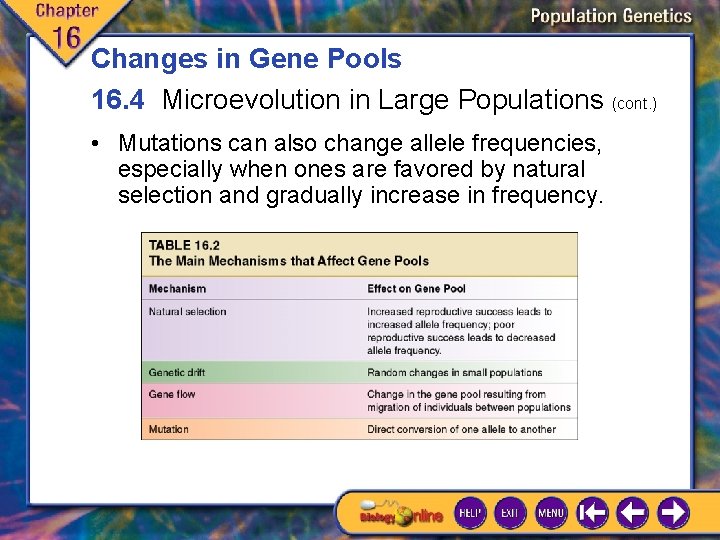
Changes in Gene Pools 16. 4 Microevolution in Large Populations (cont. ) • Mutations can also change allele frequencies, especially when ones are favored by natural selection and gradually increase in frequency.
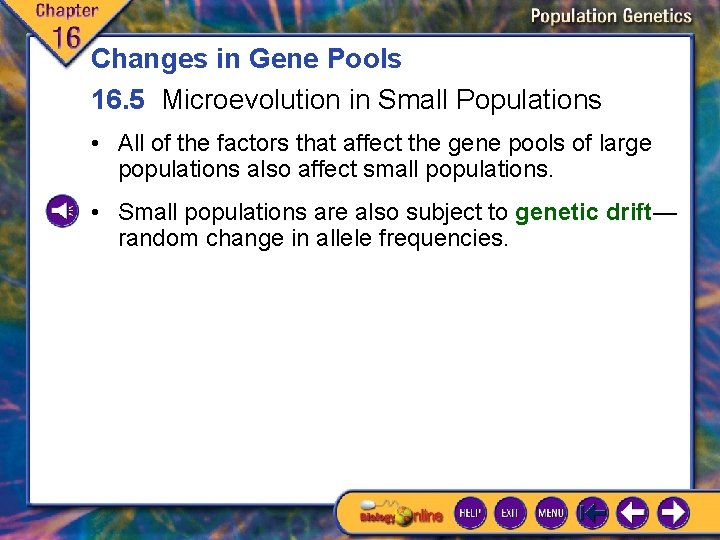
Changes in Gene Pools 16. 5 Microevolution in Small Populations • All of the factors that affect the gene pools of large populations also affect small populations. • Small populations are also subject to genetic drift— random change in allele frequencies.
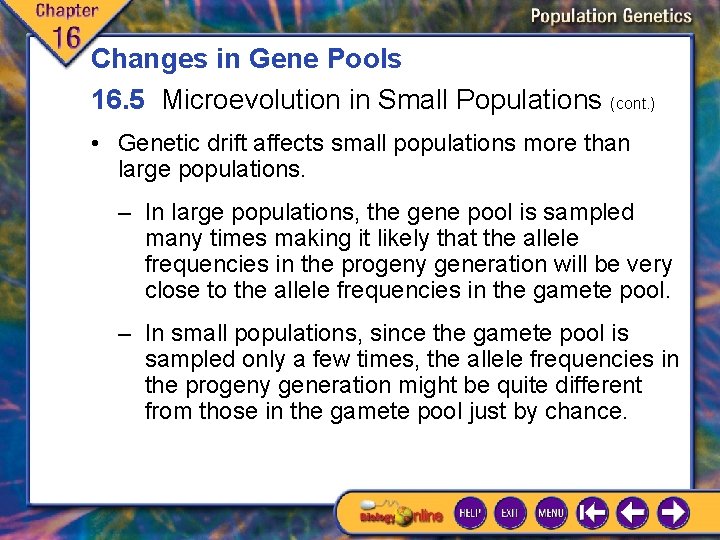
Changes in Gene Pools 16. 5 Microevolution in Small Populations (cont. ) • Genetic drift affects small populations more than large populations. – In large populations, the gene pool is sampled many times making it likely that the allele frequencies in the progeny generation will be very close to the allele frequencies in the gamete pool. – In small populations, since the gamete pool is sampled only a few times, the allele frequencies in the progeny generation might be quite different from those in the gamete pool just by chance.
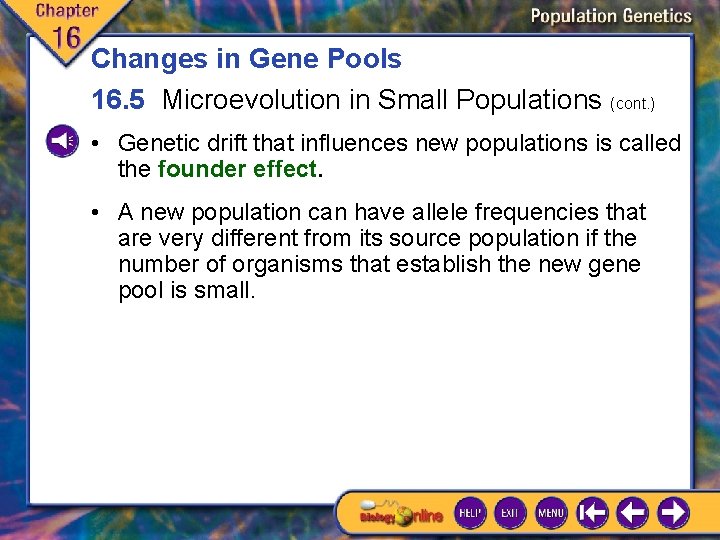
Changes in Gene Pools 16. 5 Microevolution in Small Populations (cont. ) • Genetic drift that influences new populations is called the founder effect. • A new population can have allele frequencies that are very different from its source population if the number of organisms that establish the new gene pool is small.
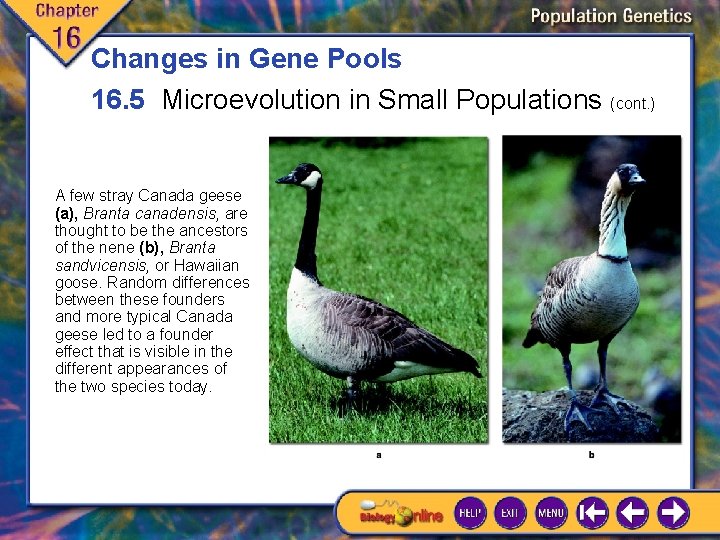
Changes in Gene Pools 16. 5 Microevolution in Small Populations (cont. ) A few stray Canada geese (a), Branta canadensis, are thought to be the ancestors of the nene (b), Branta sandvicensis, or Hawaiian goose. Random differences between these founders and more typical Canada geese led to a founder effect that is visible in the different appearances of the two species today.
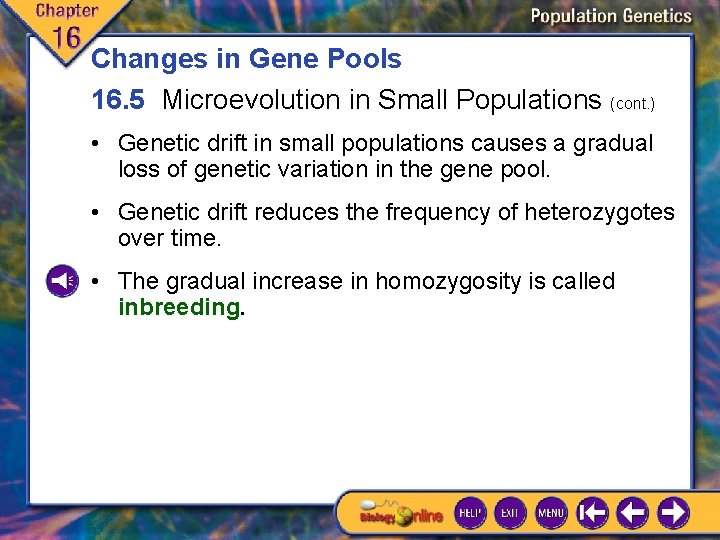
Changes in Gene Pools 16. 5 Microevolution in Small Populations (cont. ) • Genetic drift in small populations causes a gradual loss of genetic variation in the gene pool. • Genetic drift reduces the frequency of heterozygotes over time. • The gradual increase in homozygosity is called inbreeding.
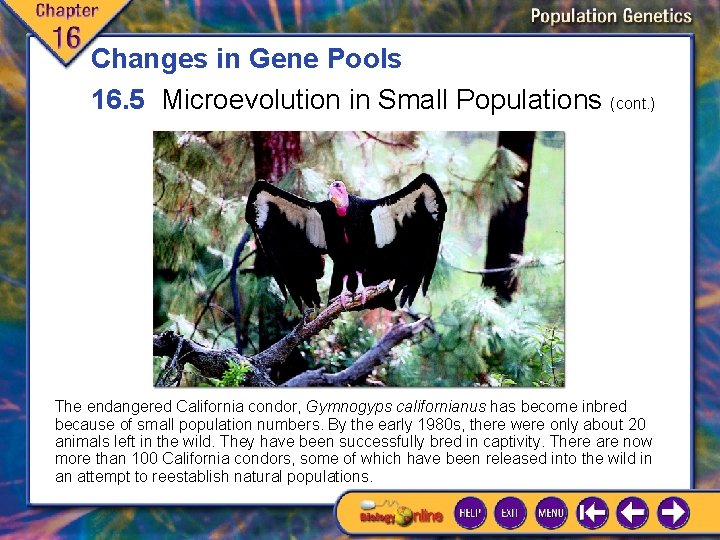
Changes in Gene Pools 16. 5 Microevolution in Small Populations (cont. ) The endangered California condor, Gymnogyps californianus has become inbred because of small population numbers. By the early 1980 s, there were only about 20 animals left in the wild. They have been successfully bred in captivity. There are now more than 100 California condors, some of which have been released into the wild in an attempt to reestablish natural populations.
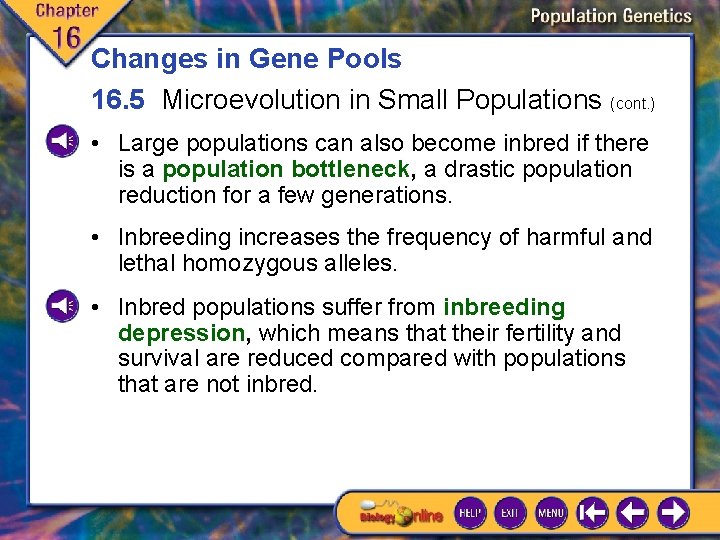
Changes in Gene Pools 16. 5 Microevolution in Small Populations (cont. ) • Large populations can also become inbred if there is a population bottleneck, a drastic population reduction for a few generations. • Inbreeding increases the frequency of harmful and lethal homozygous alleles. • Inbred populations suffer from inbreeding depression, which means that their fertility and survival are reduced compared with populations that are not inbred.
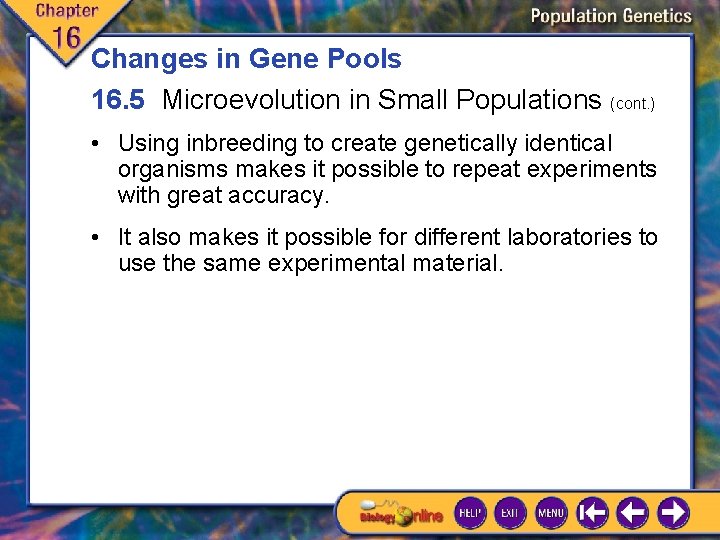
Changes in Gene Pools 16. 5 Microevolution in Small Populations (cont. ) • Using inbreeding to create genetically identical organisms makes it possible to repeat experiments with great accuracy. • It also makes it possible for different laboratories to use the same experimental material.
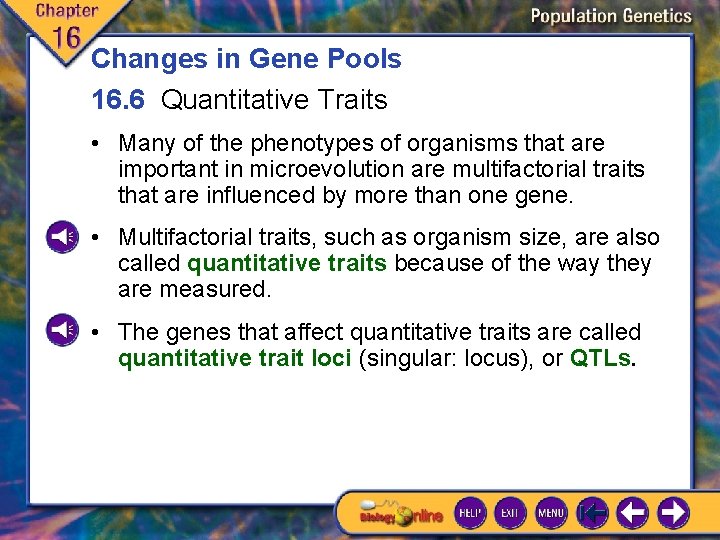
Changes in Gene Pools 16. 6 Quantitative Traits • Many of the phenotypes of organisms that are important in microevolution are multifactorial traits that are influenced by more than one gene. • Multifactorial traits, such as organism size, are also called quantitative traits because of the way they are measured. • The genes that affect quantitative traits are called quantitative trait loci (singular: locus), or QTLs.
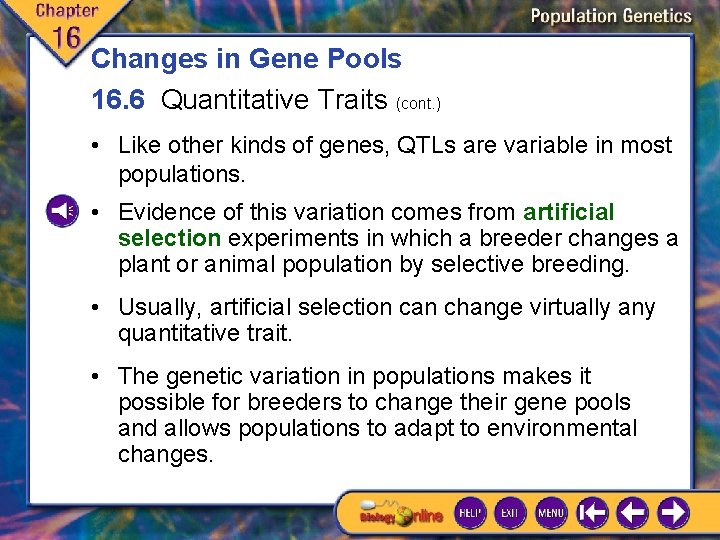
Changes in Gene Pools 16. 6 Quantitative Traits (cont. ) • Like other kinds of genes, QTLs are variable in most populations. • Evidence of this variation comes from artificial selection experiments in which a breeder changes a plant or animal population by selective breeding. • Usually, artificial selection can change virtually any quantitative trait. • The genetic variation in populations makes it possible for breeders to change their gene pools and allows populations to adapt to environmental changes.
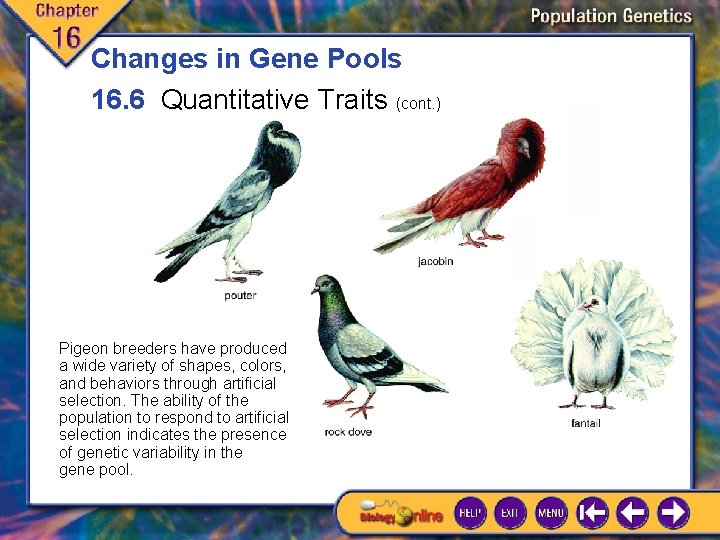
Changes in Gene Pools 16. 6 Quantitative Traits (cont. ) Pigeon breeders have produced a wide variety of shapes, colors, and behaviors through artificial selection. The ability of the population to respond to artificial selection indicates the presence of genetic variability in the gene pool.
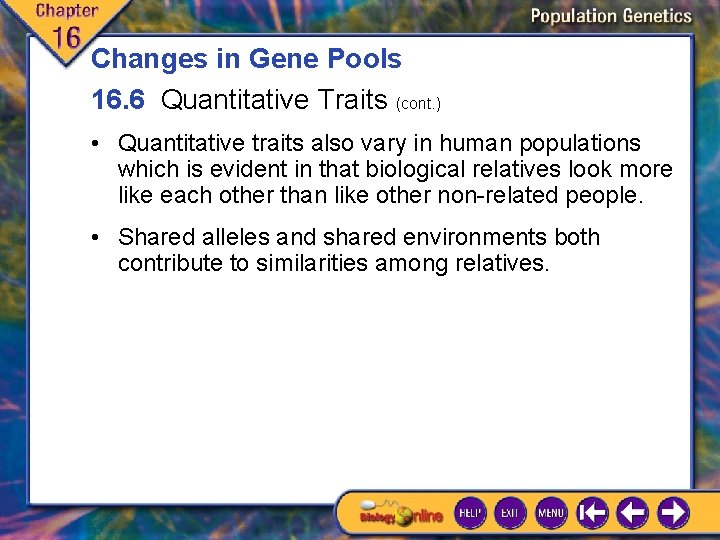
Changes in Gene Pools 16. 6 Quantitative Traits (cont. ) • Quantitative traits also vary in human populations which is evident in that biological relatives look more like each other than like other non-related people. • Shared alleles and shared environments both contribute to similarities among relatives.
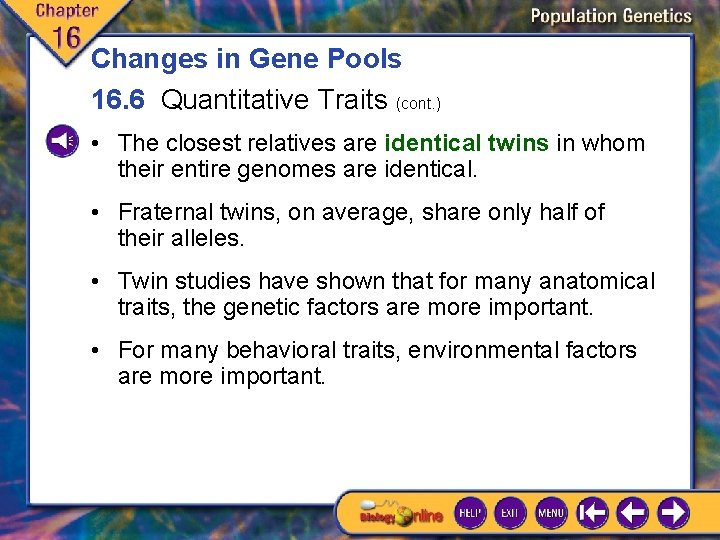
Changes in Gene Pools 16. 6 Quantitative Traits (cont. ) • The closest relatives are identical twins in whom their entire genomes are identical. • Fraternal twins, on average, share only half of their alleles. • Twin studies have shown that for many anatomical traits, the genetic factors are more important. • For many behavioral traits, environmental factors are more important.
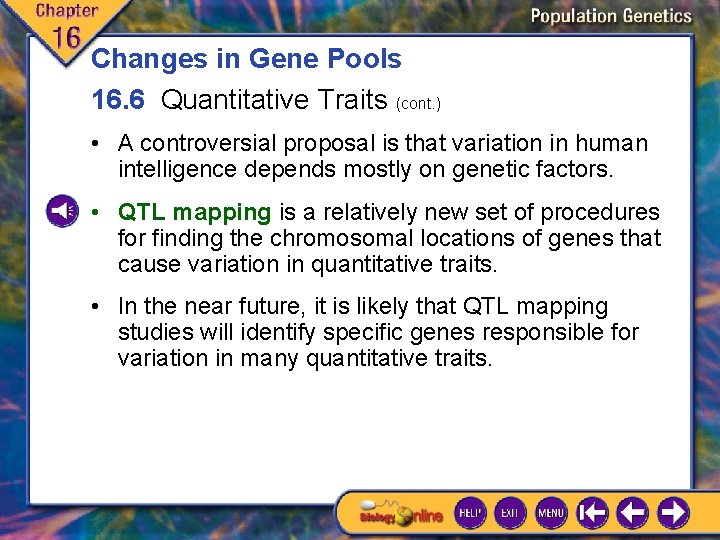
Changes in Gene Pools 16. 6 Quantitative Traits (cont. ) • A controversial proposal is that variation in human intelligence depends mostly on genetic factors. • QTL mapping is a relatively new set of procedures for finding the chromosomal locations of genes that cause variation in quantitative traits. • In the near future, it is likely that QTL mapping studies will identify specific genes responsible for variation in many quantitative traits.
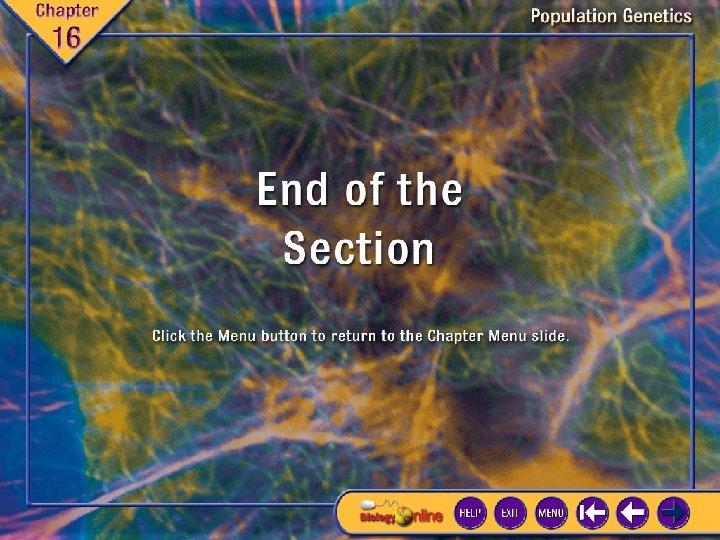
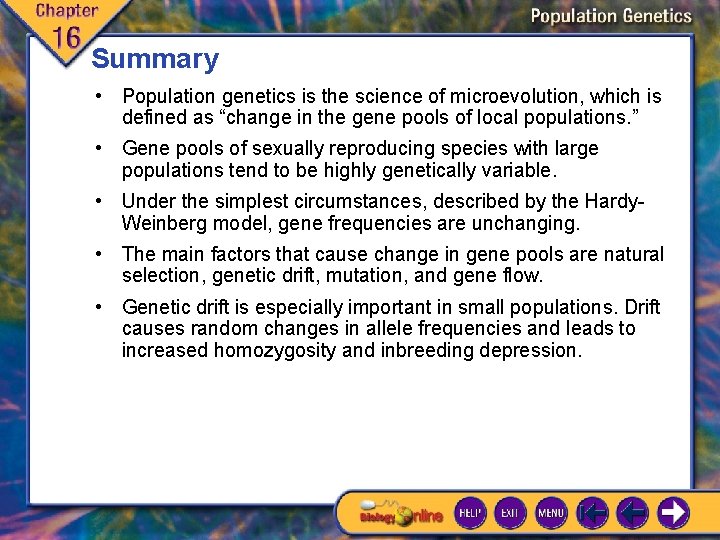
Summary • Population genetics is the science of microevolution, which is defined as “change in the gene pools of local populations. ” • Gene pools of sexually reproducing species with large populations tend to be highly genetically variable. • Under the simplest circumstances, described by the Hardy. Weinberg model, gene frequencies are unchanging. • The main factors that cause change in gene pools are natural selection, genetic drift, mutation, and gene flow. • Genetic drift is especially important in small populations. Drift causes random changes in allele frequencies and leads to increased homozygosity and inbreeding depression.
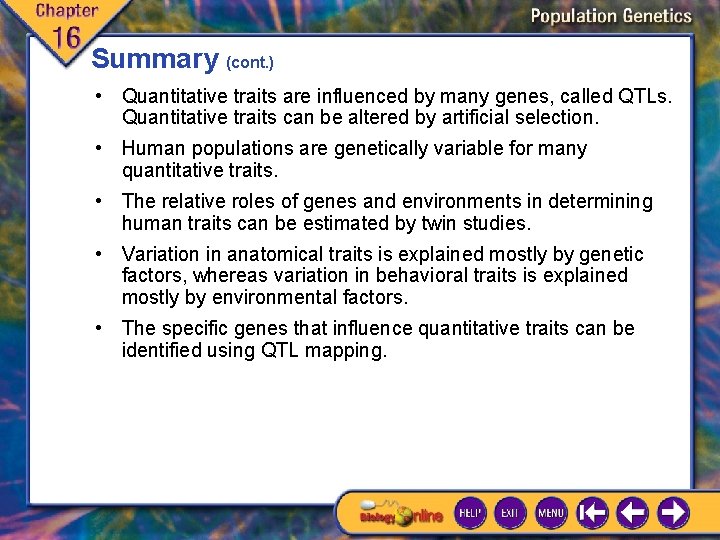
Summary (cont. ) • Quantitative traits are influenced by many genes, called QTLs. Quantitative traits can be altered by artificial selection. • Human populations are genetically variable for many quantitative traits. • The relative roles of genes and environments in determining human traits can be estimated by twin studies. • Variation in anatomical traits is explained mostly by genetic factors, whereas variation in behavioral traits is explained mostly by environmental factors. • The specific genes that influence quantitative traits can be identified using QTL mapping.
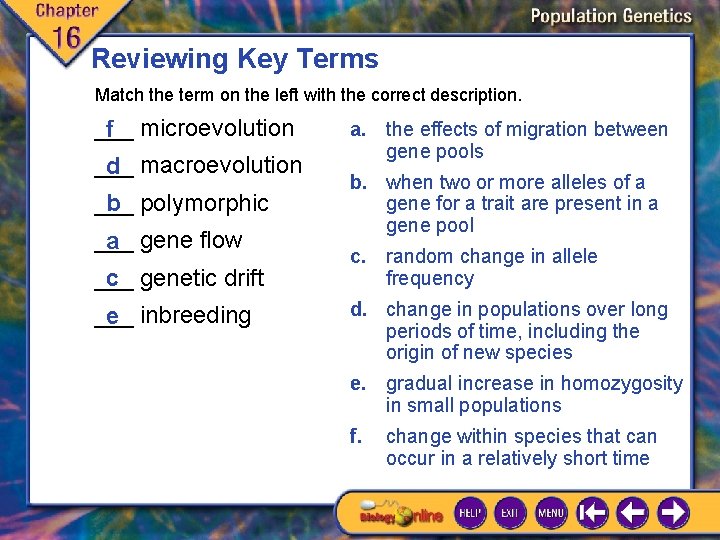
Reviewing Key Terms Match the term on the left with the correct description. ___ f microevolution ___ d macroevolution ___ b polymorphic ___ a gene flow ___ c genetic drift ___ e inbreeding a. the effects of migration between gene pools b. when two or more alleles of a gene for a trait are present in a gene pool c. random change in allele frequency d. change in populations over long periods of time, including the origin of new species e. gradual increase in homozygosity in small populations f. change within species that can occur in a relatively short time
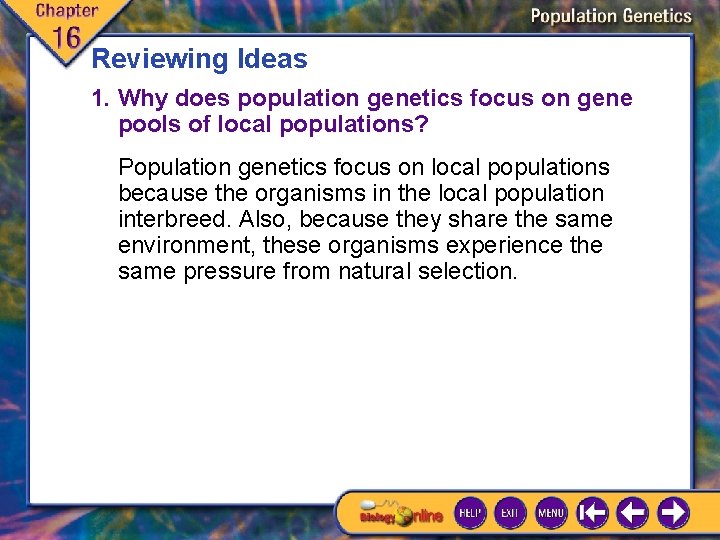
Reviewing Ideas 1. Why does population genetics focus on gene pools of local populations? Population genetics focus on local populations because the organisms in the local population interbreed. Also, because they share the same environment, these organisms experience the same pressure from natural selection.
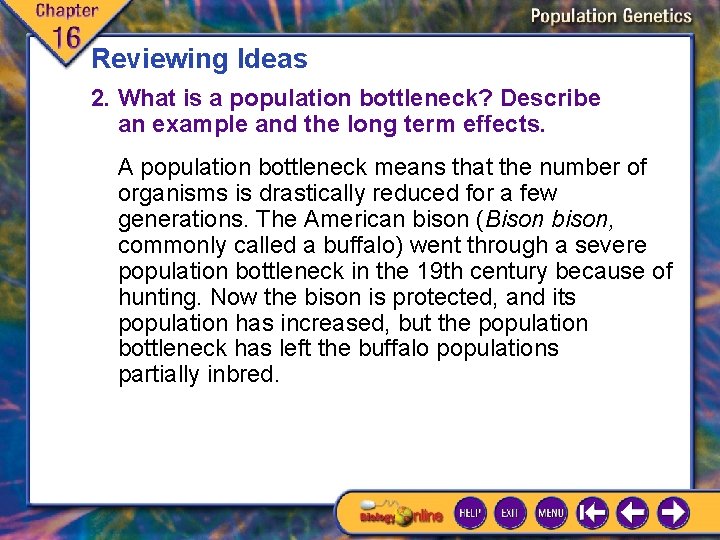
Reviewing Ideas 2. What is a population bottleneck? Describe an example and the long term effects. A population bottleneck means that the number of organisms is drastically reduced for a few generations. The American bison (Bison bison, commonly called a buffalo) went through a severe population bottleneck in the 19 th century because of hunting. Now the bison is protected, and its population has increased, but the population bottleneck has left the buffalo populations partially inbred.
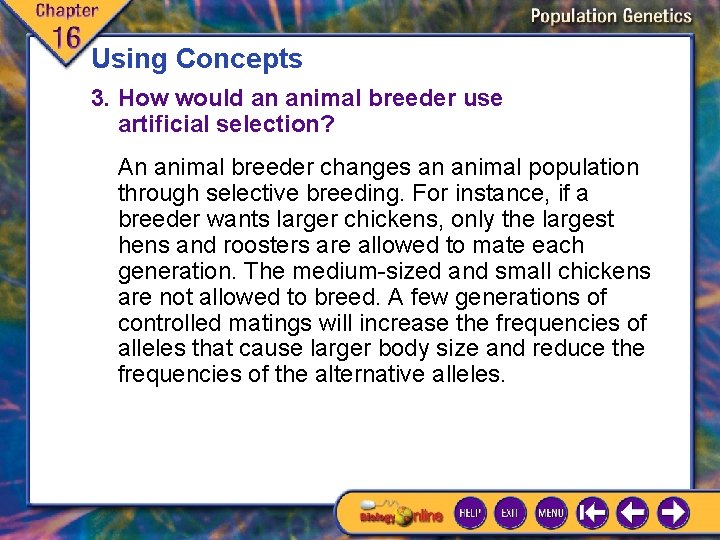
Using Concepts 3. How would an animal breeder use artificial selection? An animal breeder changes an animal population through selective breeding. For instance, if a breeder wants larger chickens, only the largest hens and roosters are allowed to mate each generation. The medium-sized and small chickens are not allowed to breed. A few generations of controlled matings will increase the frequencies of alleles that cause larger body size and reduce the frequencies of the alternative alleles.
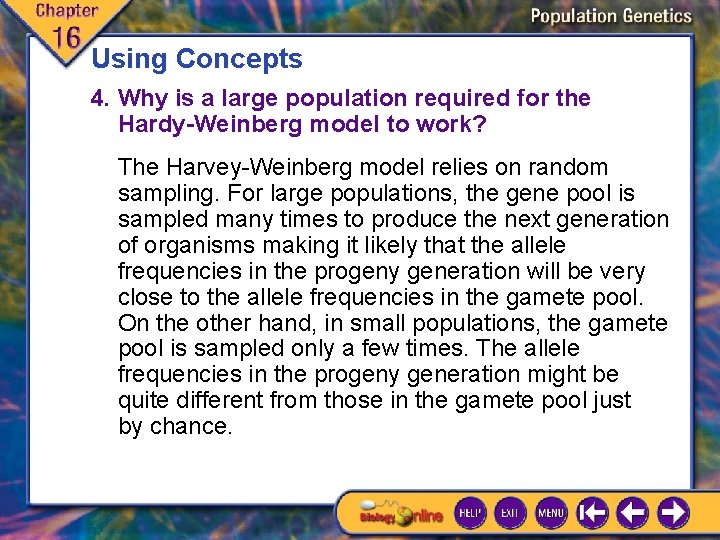
Using Concepts 4. Why is a large population required for the Hardy-Weinberg model to work? The Harvey-Weinberg model relies on random sampling. For large populations, the gene pool is sampled many times to produce the next generation of organisms making it likely that the allele frequencies in the progeny generation will be very close to the allele frequencies in the gamete pool. On the other hand, in small populations, the gamete pool is sampled only a few times. The allele frequencies in the progeny generation might be quite different from those in the gamete pool just by chance.
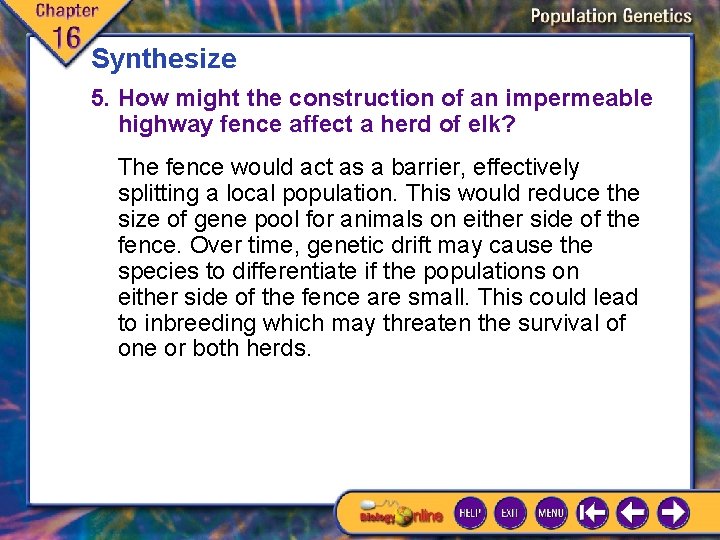
Synthesize 5. How might the construction of an impermeable highway fence affect a herd of elk? The fence would act as a barrier, effectively splitting a local population. This would reduce the size of gene pool for animals on either side of the fence. Over time, genetic drift may cause the species to differentiate if the populations on either side of the fence are small. This could lead to inbreeding which may threaten the survival of one or both herds.
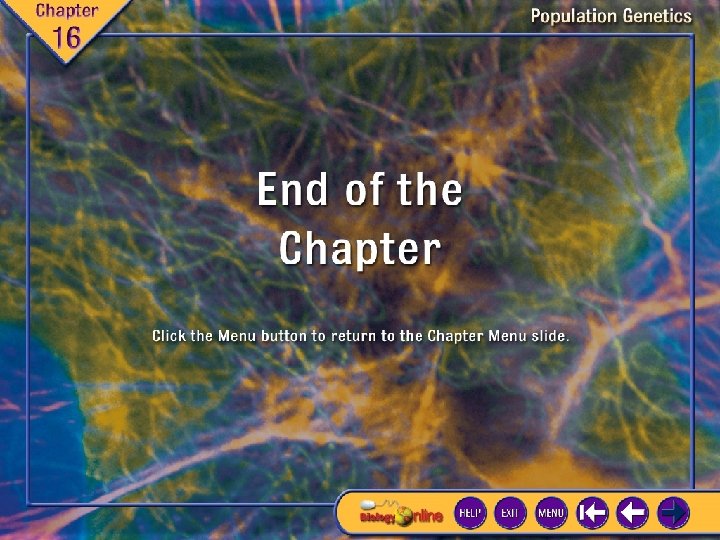
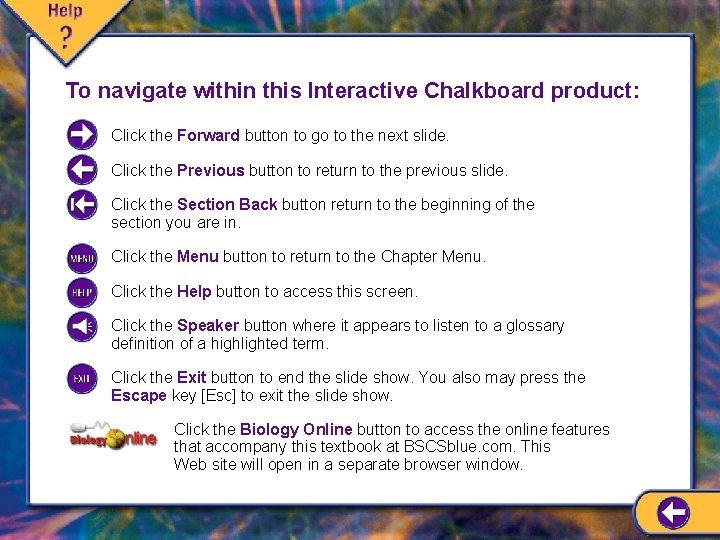
To navigate within this Interactive Chalkboard product: Click the Forward button to go to the next slide. Click the Previous button to return to the previous slide. Click the Section Back button return to the beginning of the section you are in. Click the Menu button to return to the Chapter Menu. Click the Help button to access this screen. Click the Speaker button where it appears to listen to a glossary definition of a highlighted term. Click the Exit button to end the slide show. You also may press the Escape key [Esc] to exit the slide show. Click the Biology Online button to access the online features that accompany this textbook at BSCSblue. com. This Web site will open in a separate browser window.
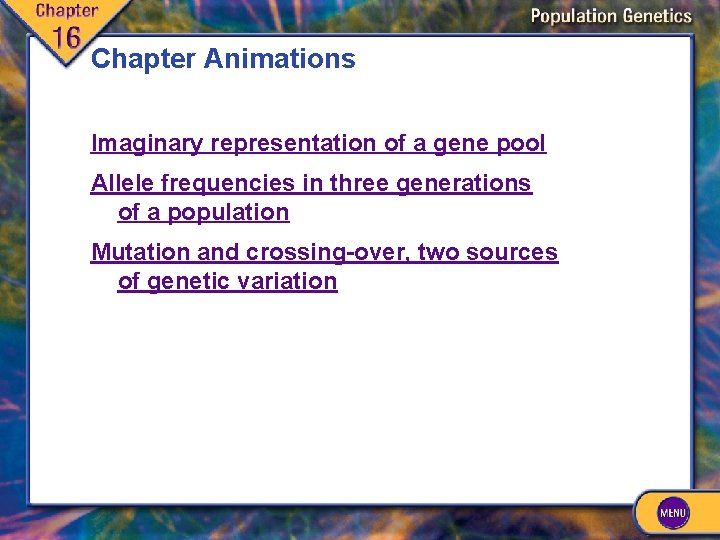
Chapter Animations Imaginary representation of a gene pool Allele frequencies in three generations of a population Mutation and crossing-over, two sources of genetic variation
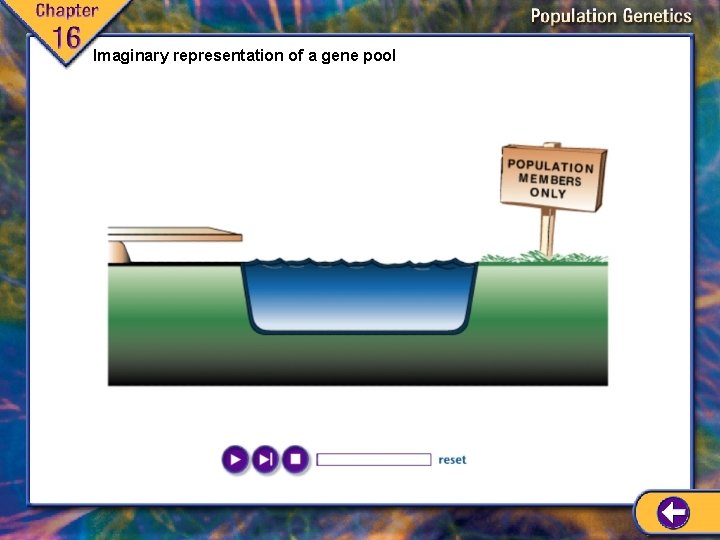
Imaginary representation of a gene pool
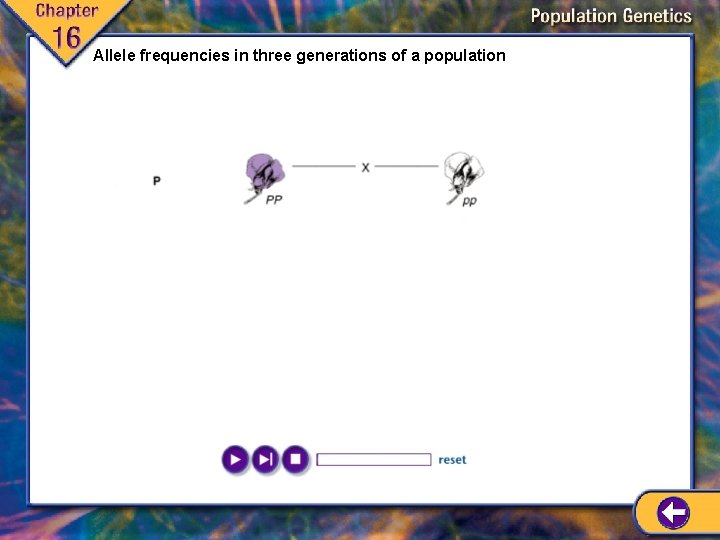
Allele frequencies in three generations of a population
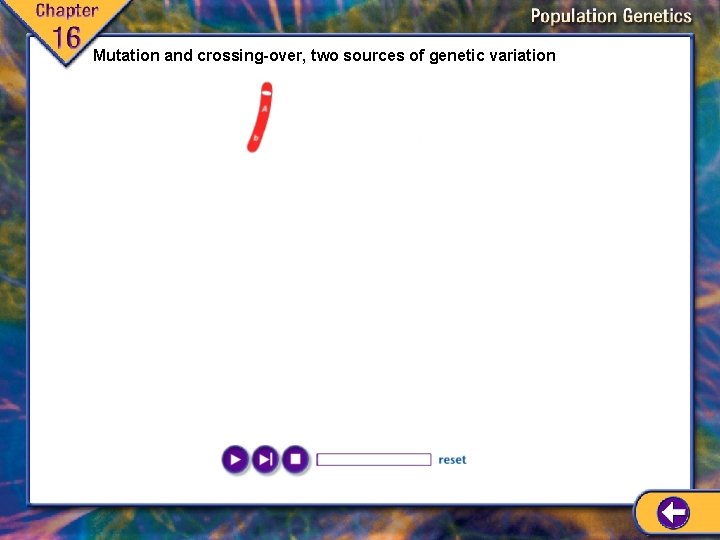
Mutation and crossing-over, two sources of genetic variation
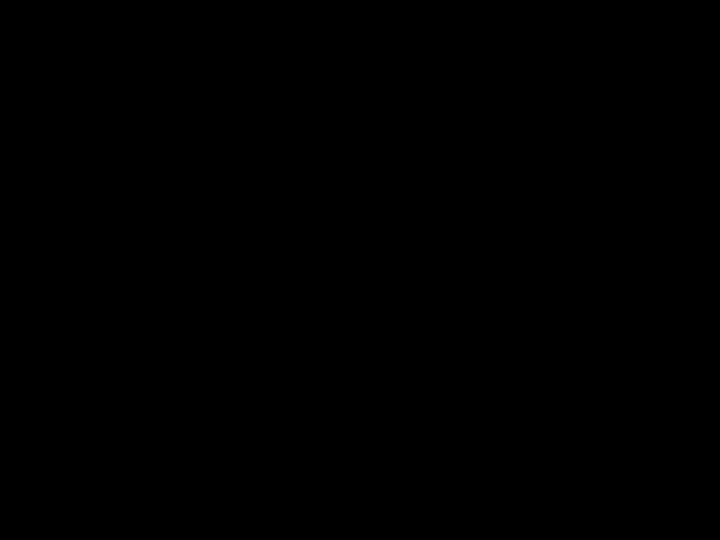
End of Custom Shows This slide is intentionally blank.
Genetic drift in small populations
Genetic drift
Genetic drift definition
Stabilizing selection human birth weight
Evolution of populations section 16-1 genes and variation
Genetic programming vs genetic algorithm
Genetic programming vs genetic algorithm
Genetic drift vs genetic flow
What is the difference between genetic drift and gene flow
Components of genetic variation
Genetic variation
Ecxed
Meiosis and genetic variation usatestprep
Dbsnp the ncbi database of genetic variation
Additive genetic variation definition
Genetic variation
Components of genetic variation
What is a direct variation
Direct variation vs inverse variation
Prediction interval formula
Human genetics concepts and applications 10th edition
Chapter 16 evolution of populations vocabulary review
Chapter 13 how populations evolve test
Chapter 17 evolution of populations answer key
5-1 how populations grow
Chapter 5 lesson 1 how populations grow
Chapter 23: the evolution of populations
Chapter 16 evolution of populations
Chapter 10 comparing two populations or groups crossword
5-1 how populations grow
Chapter 8 understanding populations
Chapter 23 the evolution of populations
Chapter 21 vulnerability and vulnerable populations
Chapter 13 how populations evolve
Chapter 10 comparing two populations or groups
Contoh siklus menu
Fungsi menu excel
Difference between classical menu and modern menu
Bagaimana cara menjalankan browser mozilla firefox
Menu pull down
Introduction to human variation
Using statistical measures to compare populations
Territoires populations et développement quels défis
A biologist discovers two populations of wolf spiders
Limitations of using a punnett square
Lesson 1 populations answer key
Section 19-1 understanding populations answer key
Gene pool
Population biology definition
Section 5-3 human population growth
Kar 5
Evolution of populations section 11 review
Gage definition
Why do mexico and peru have primarily catholic populations?
Complex patient populations
Section 5-1 how populations grow
Dynamique des populations
99image
Smallest unit of evolution