Chapter 3 Adaptation to Aquatic and Terrestrial Environments
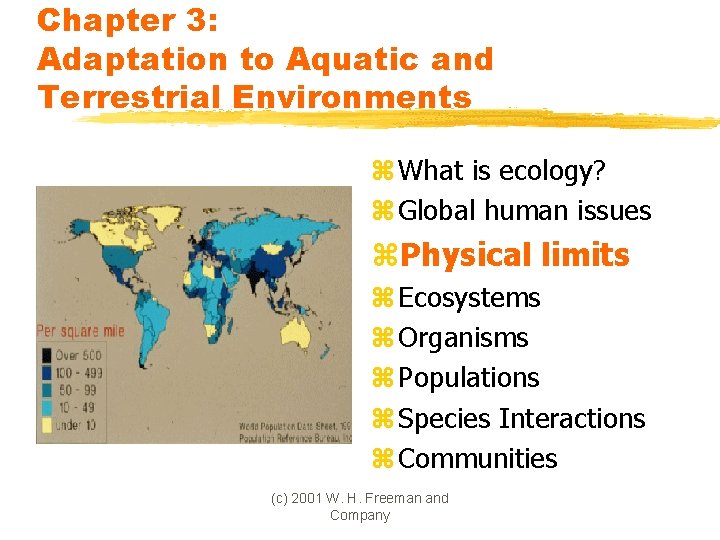
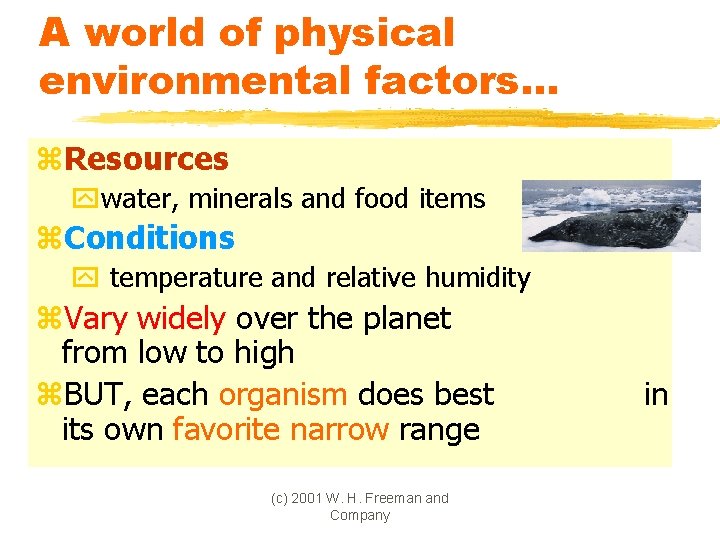
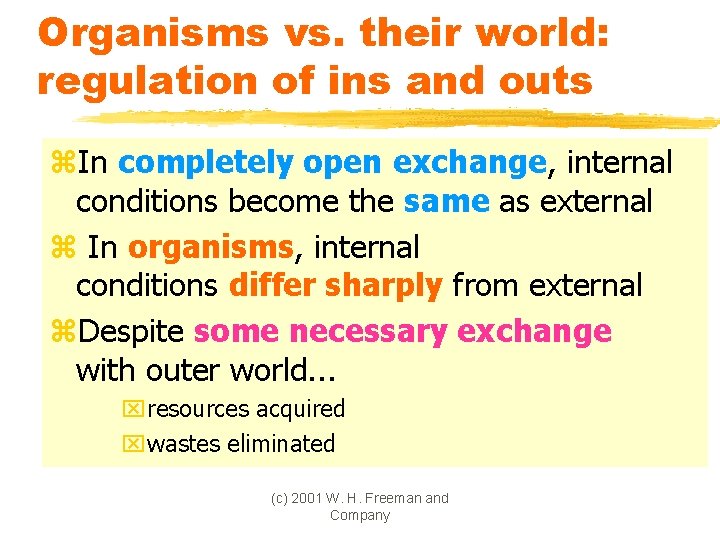
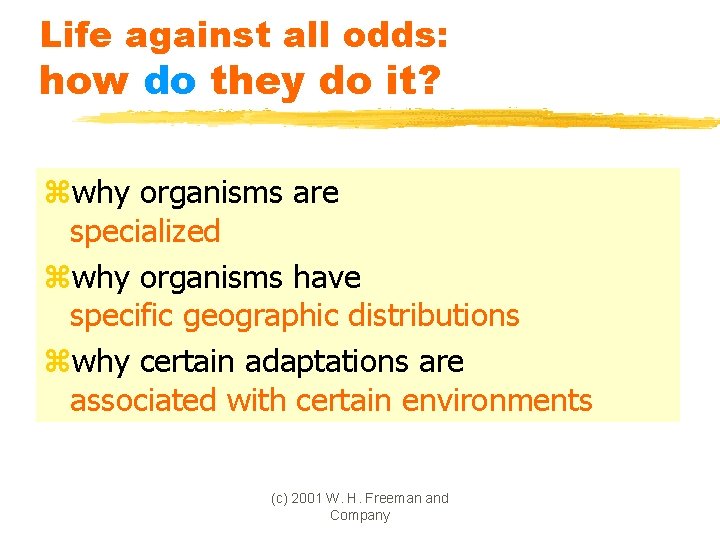
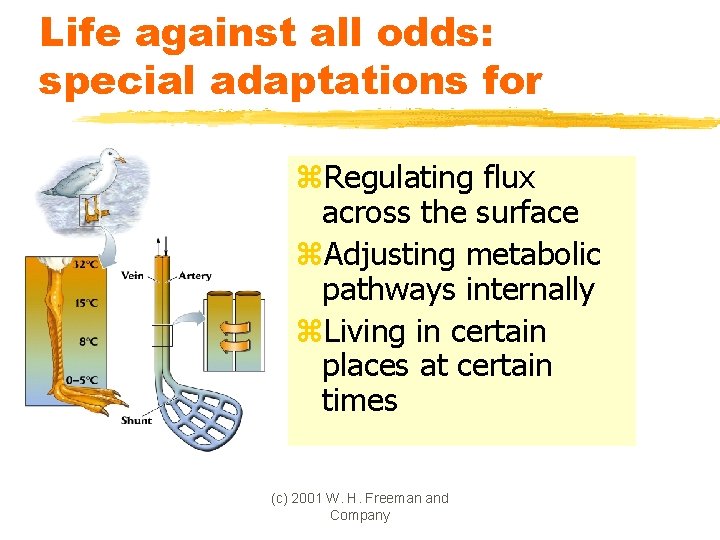
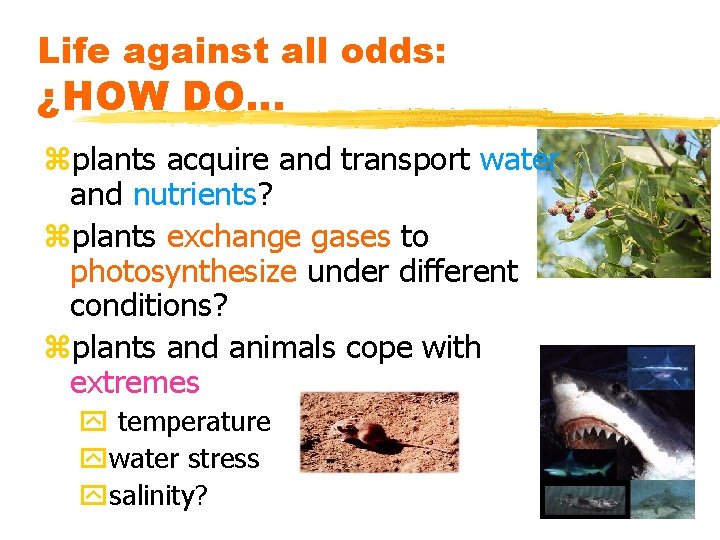
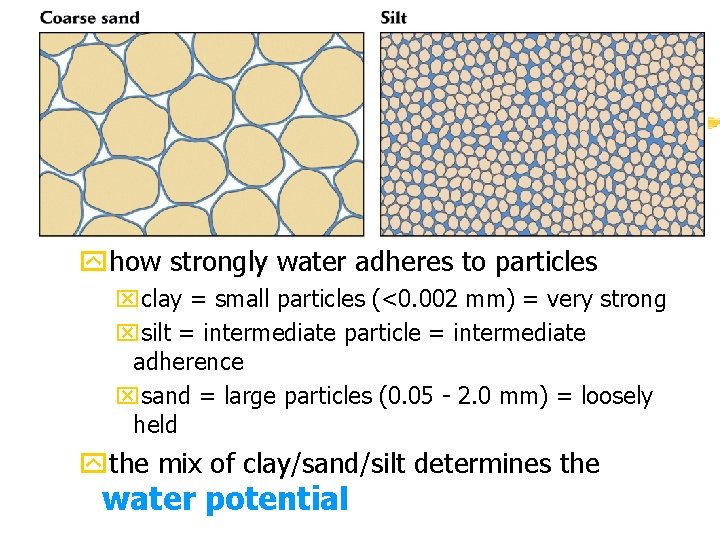
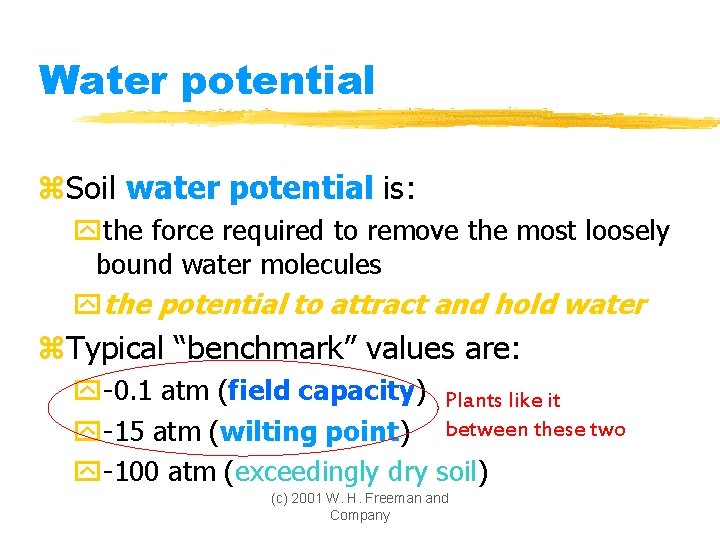
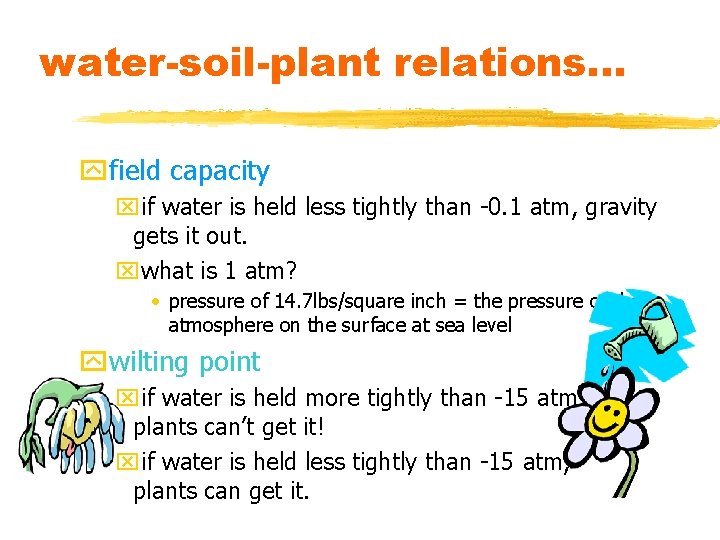
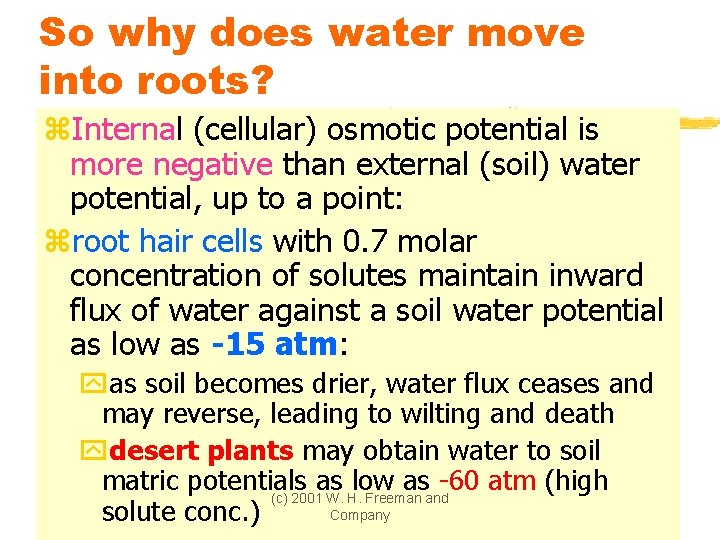
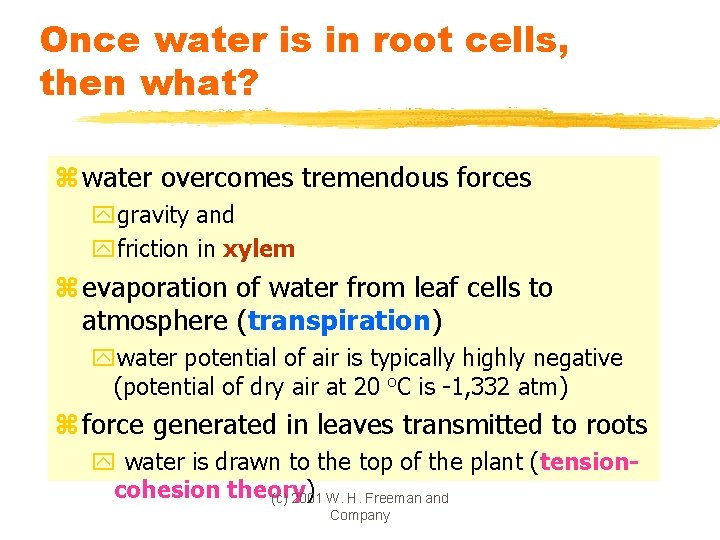
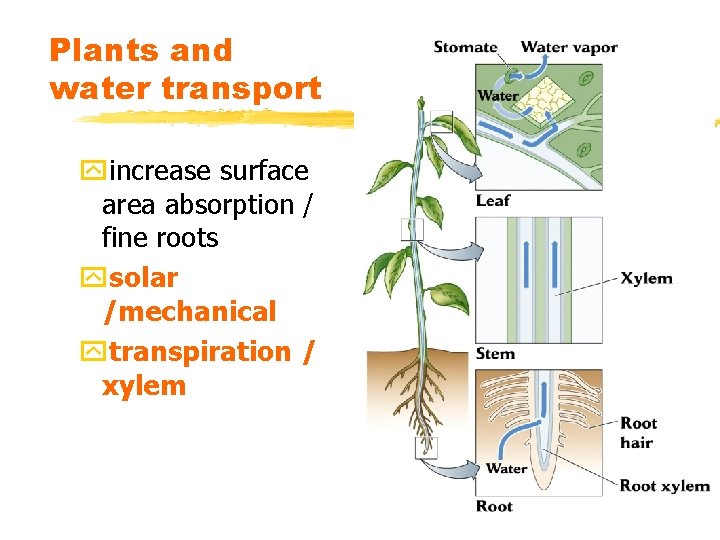
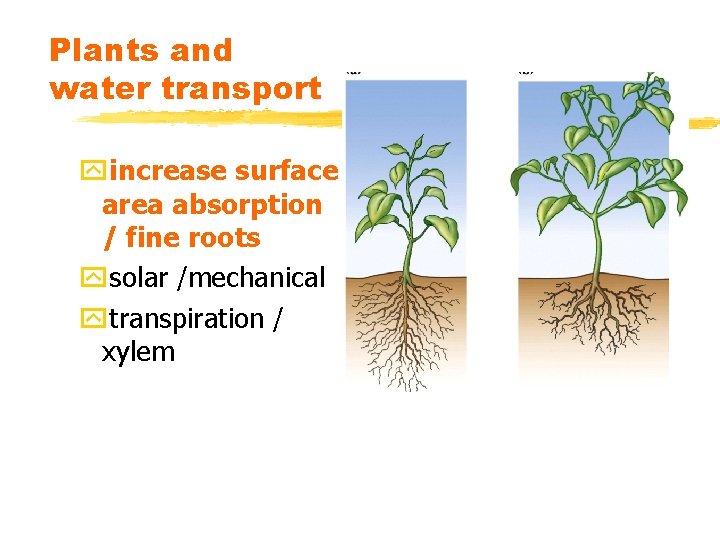
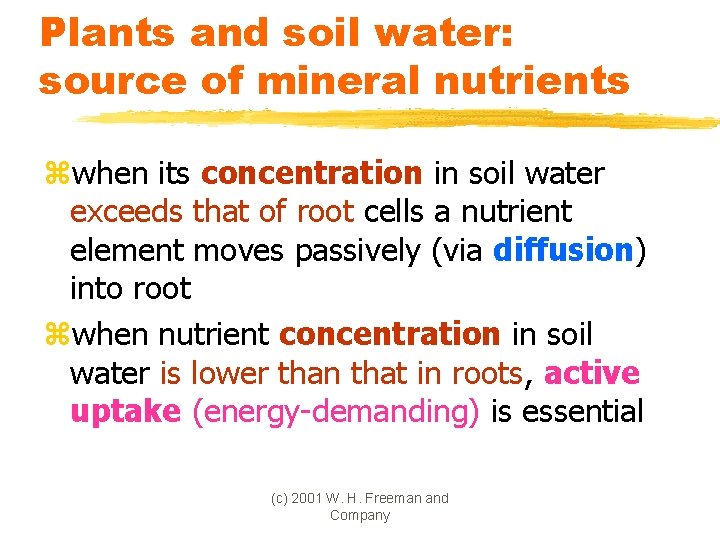
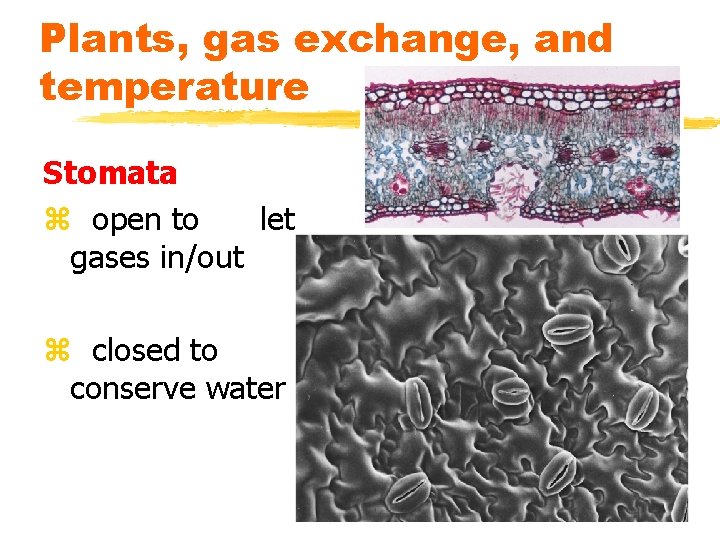
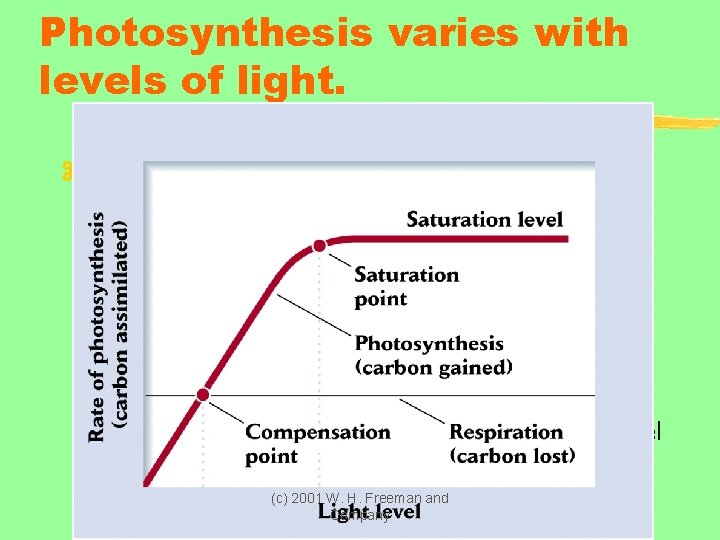
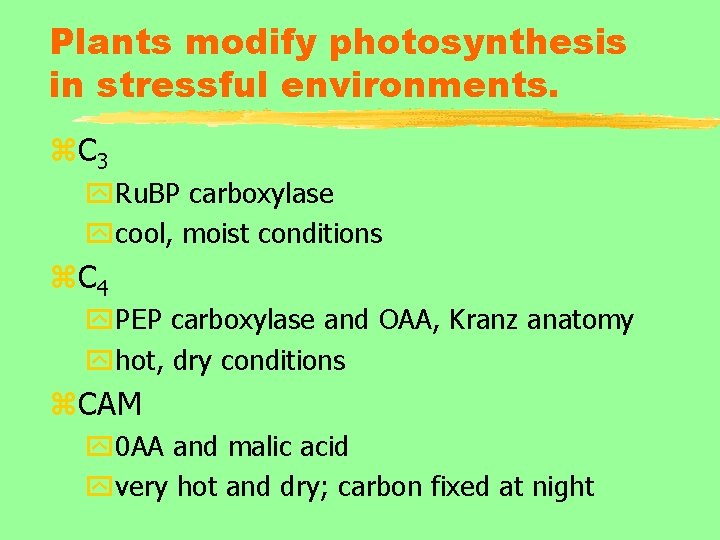
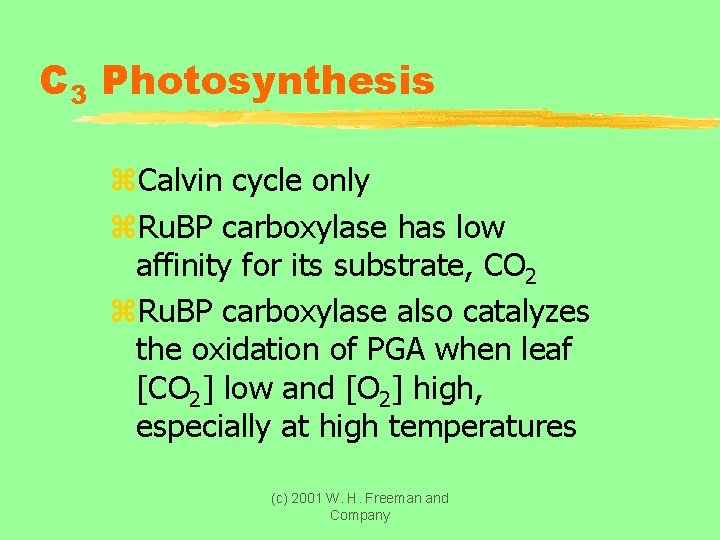
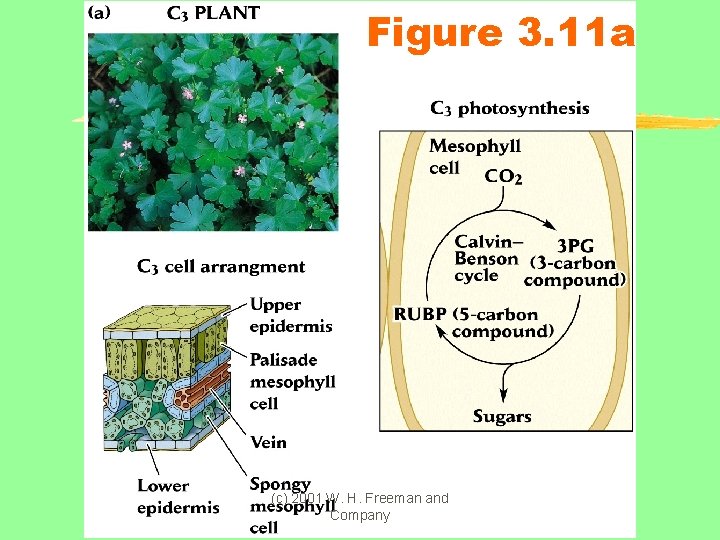
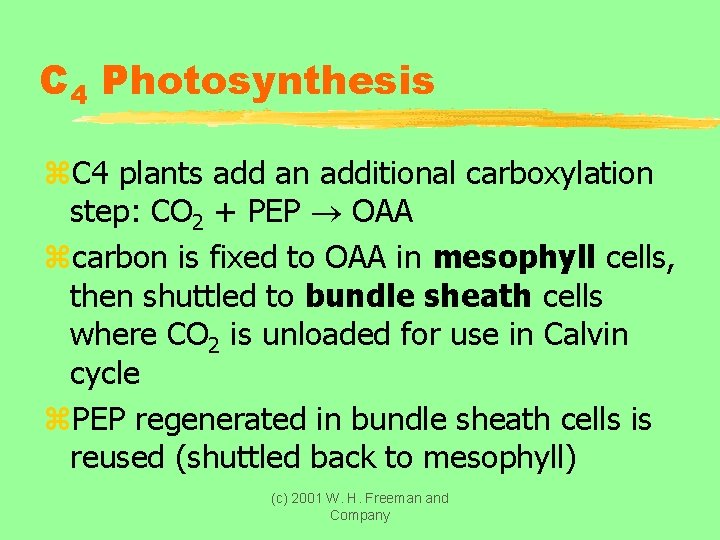
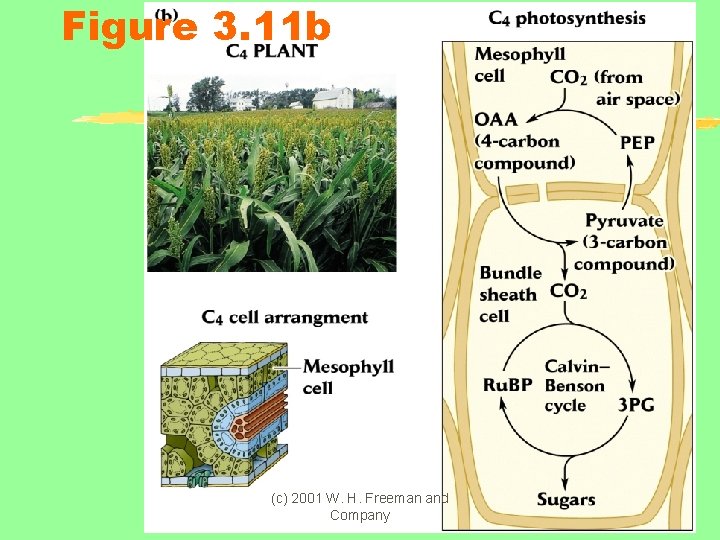
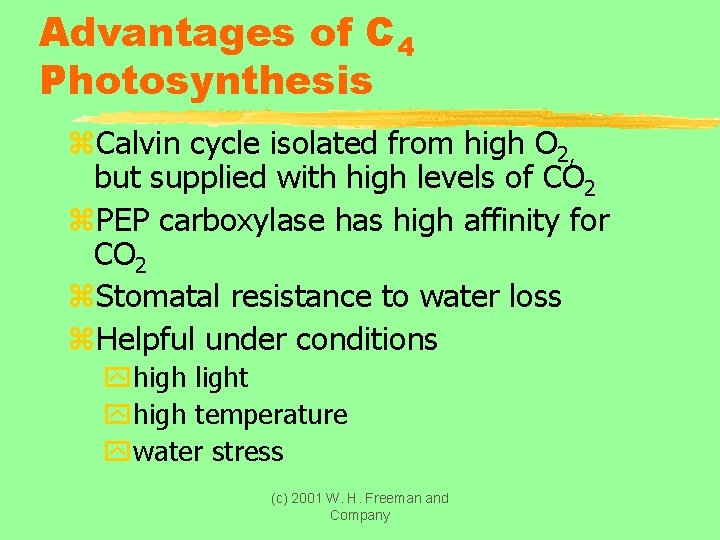
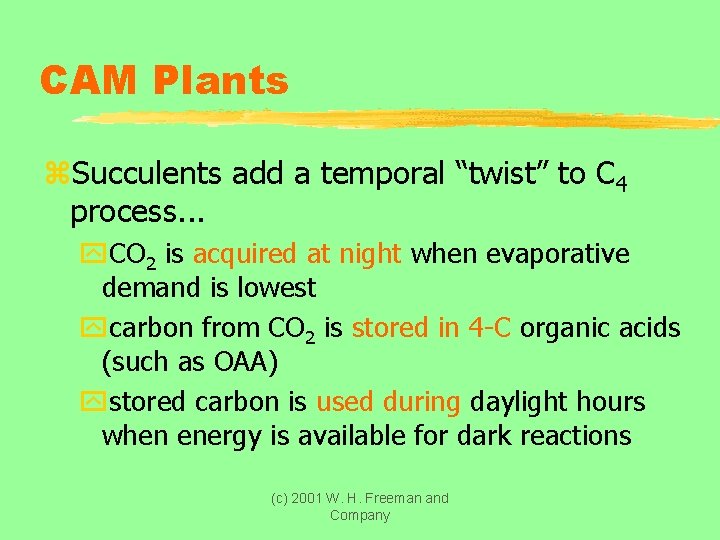
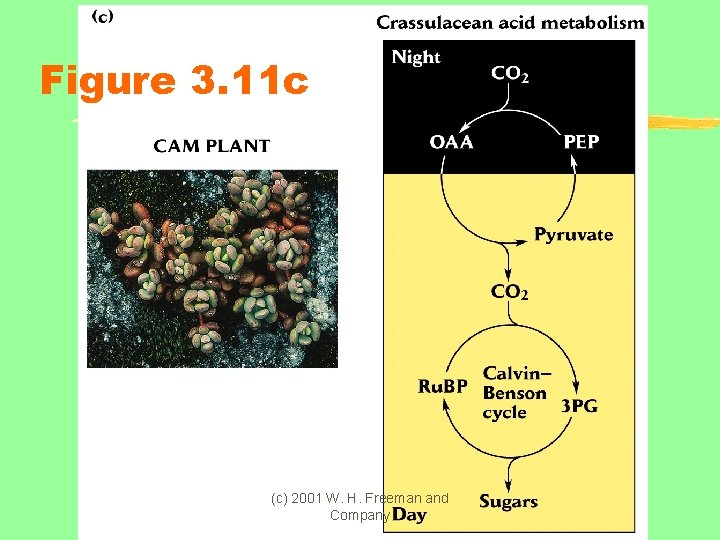
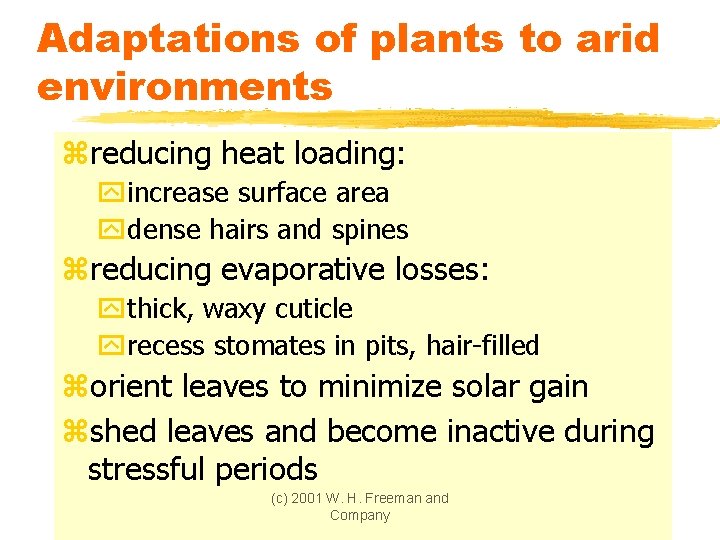
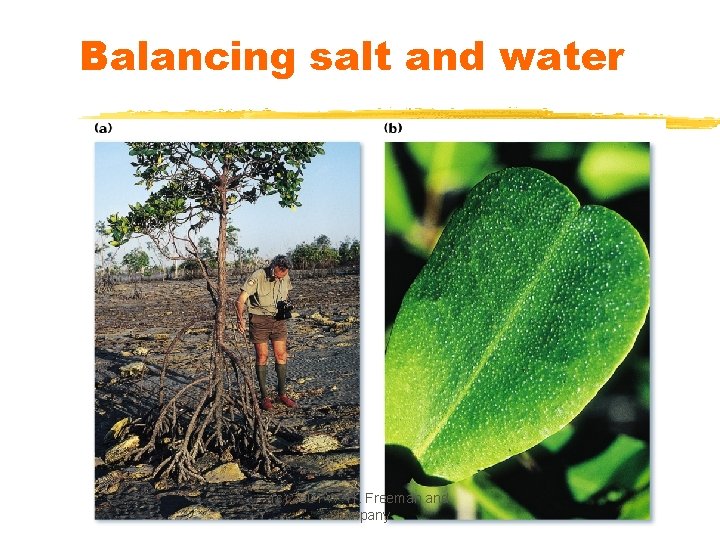
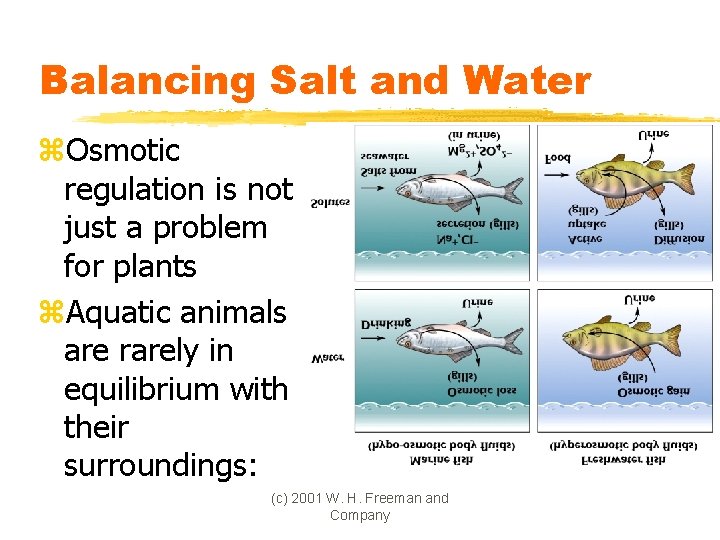
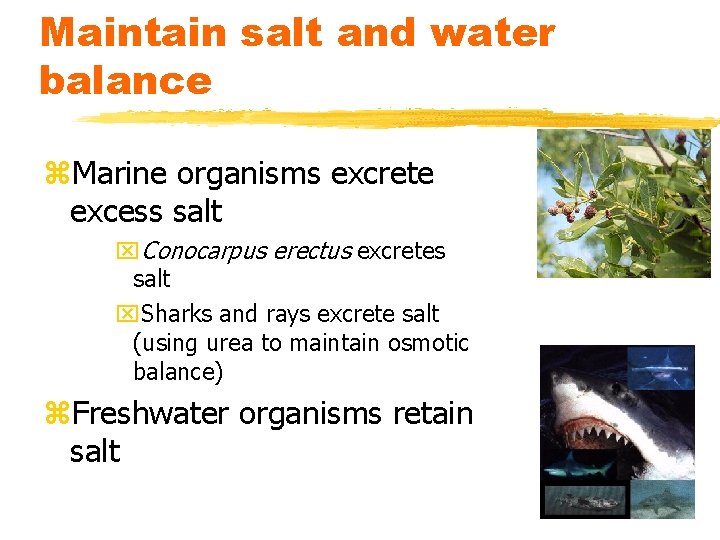
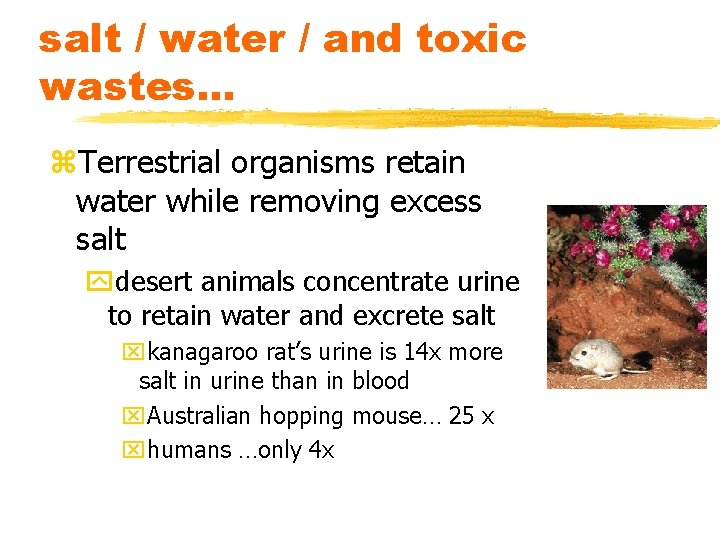
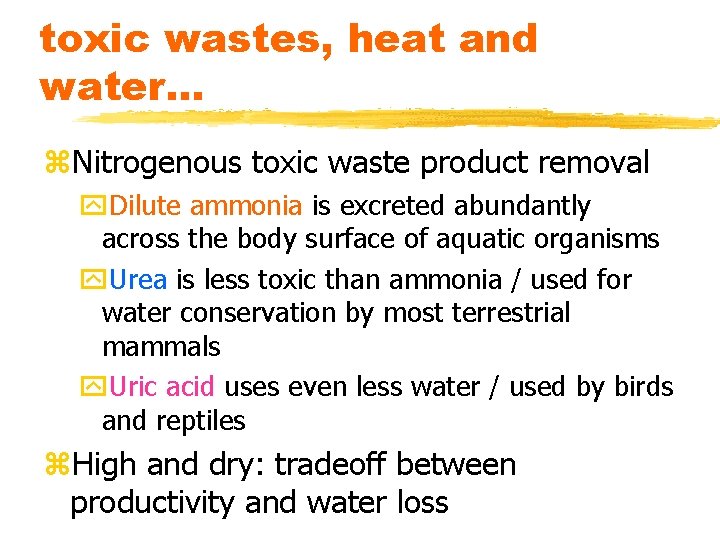
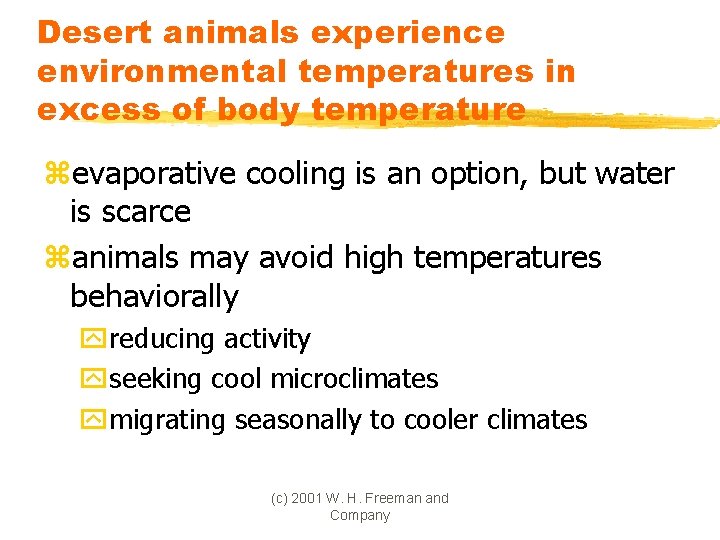
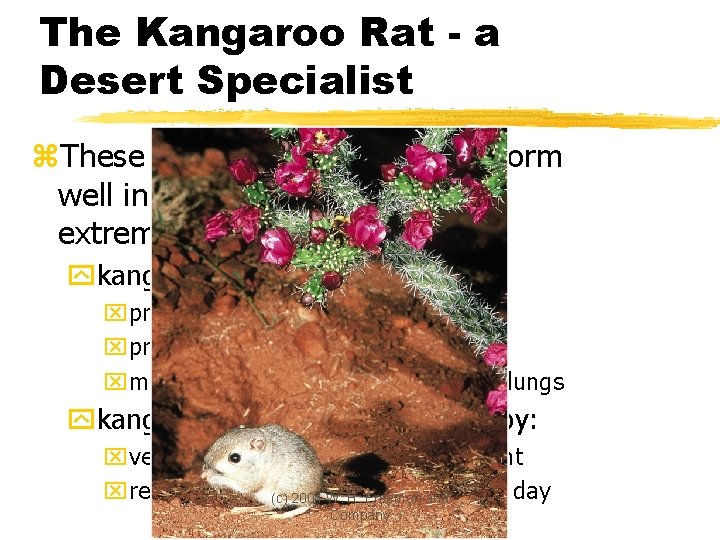
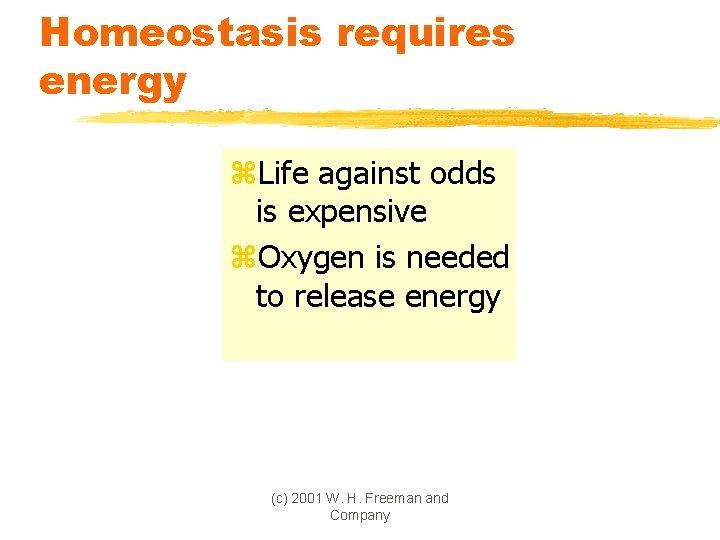
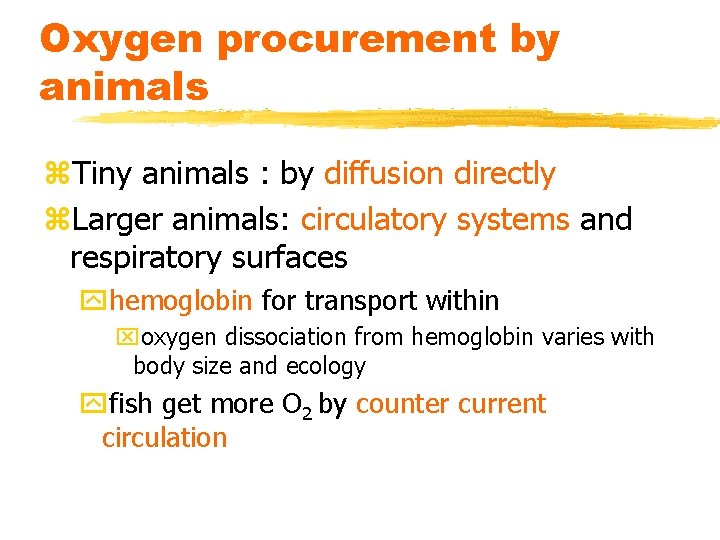
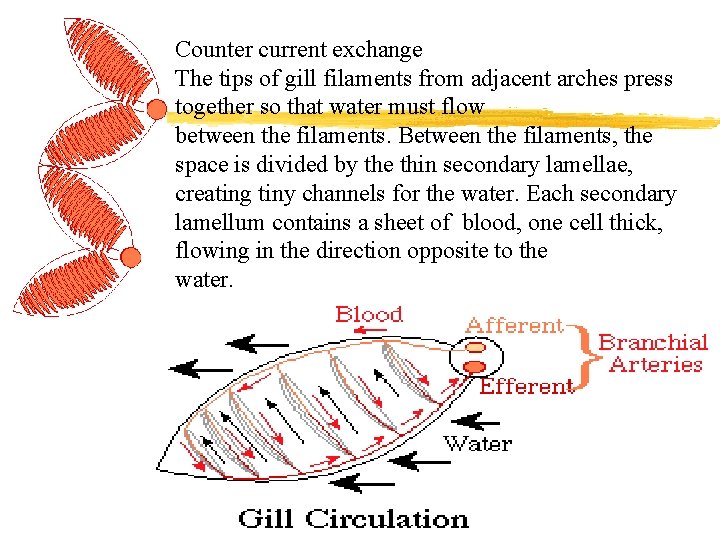
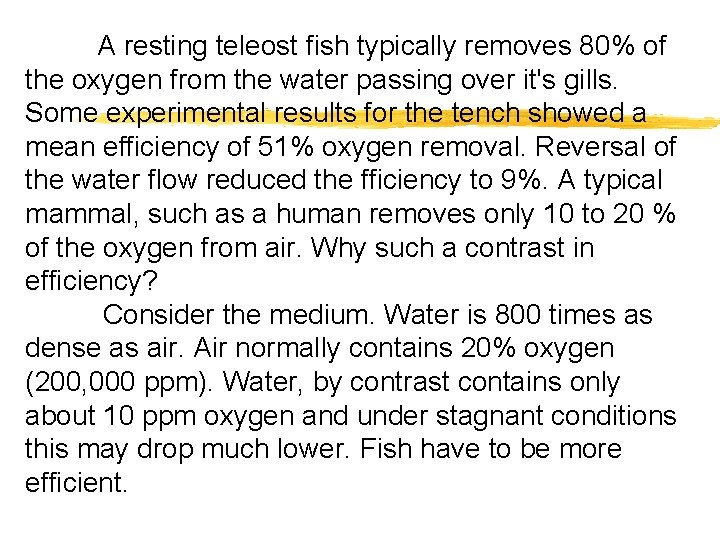
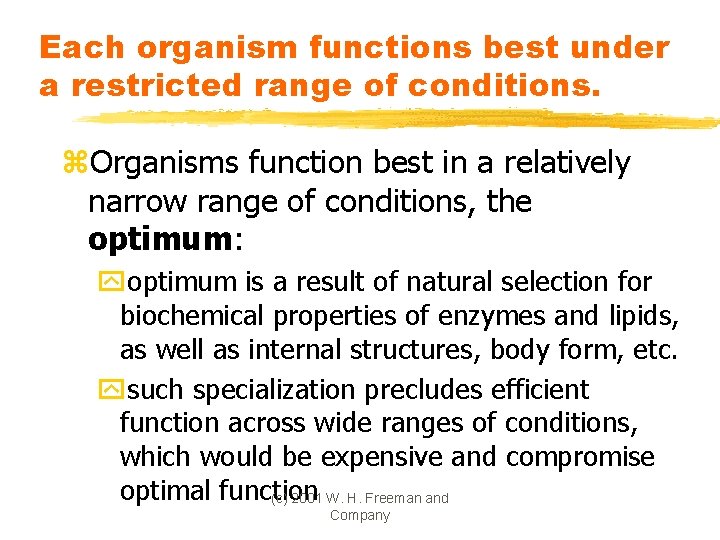
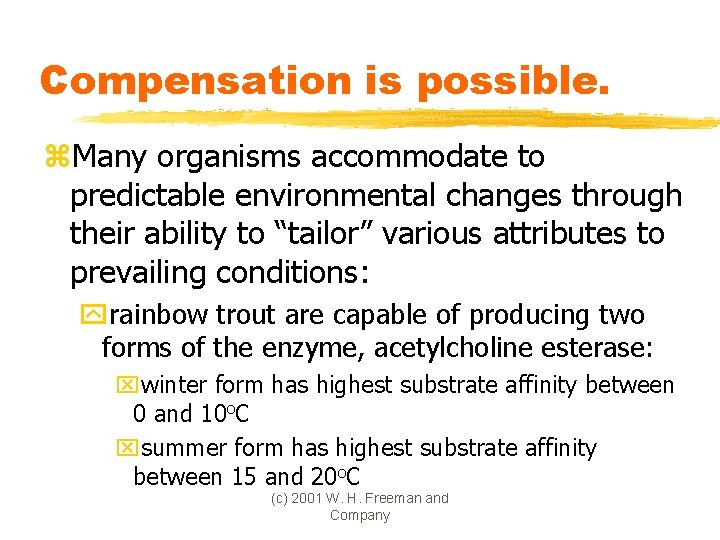
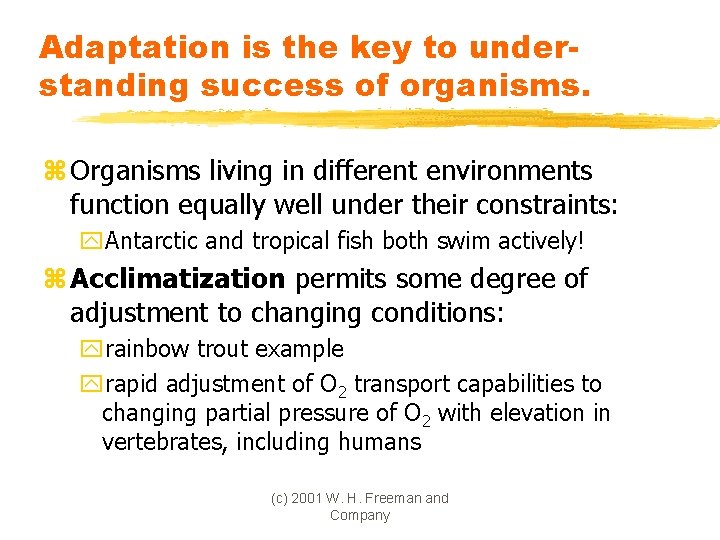
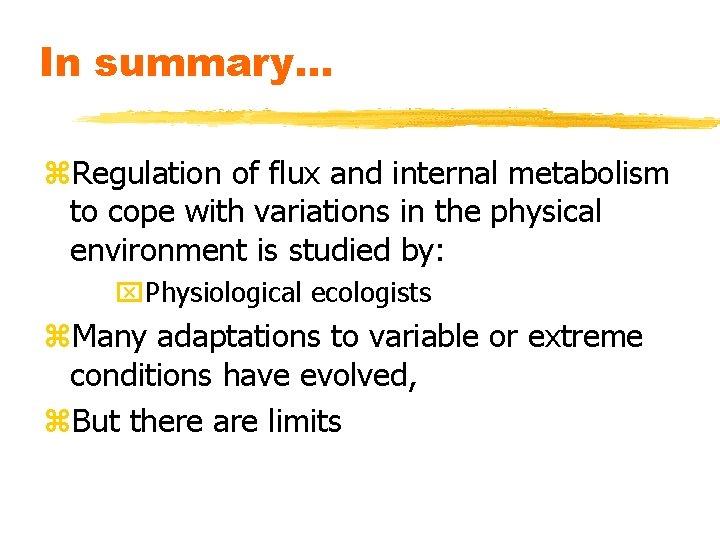
- Slides: 40
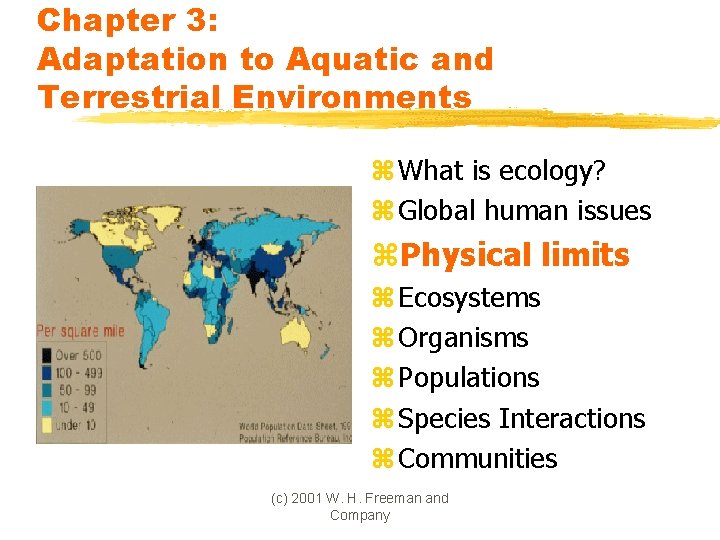
Chapter 3: Adaptation to Aquatic and Terrestrial Environments z What is ecology? z Global human issues z. Physical limits z Ecosystems z Organisms z Populations z Species Interactions z Communities (c) 2001 W. H. Freeman and Company
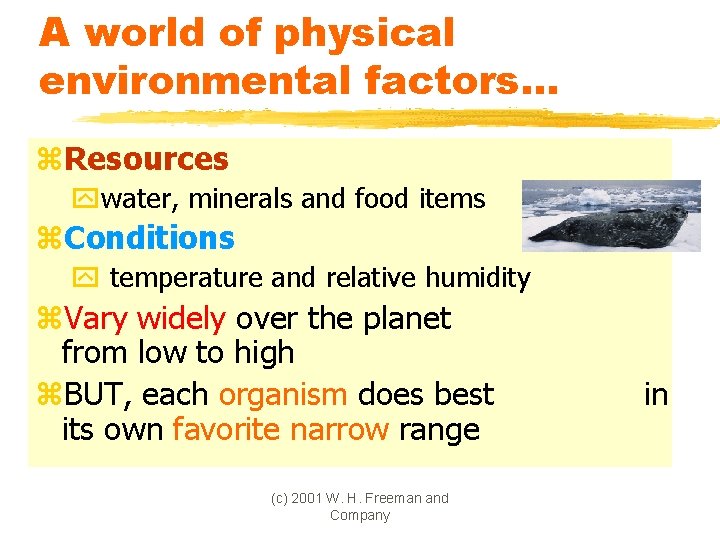
A world of physical environmental factors. . . z. Resources ywater, minerals and food items z. Conditions y temperature and relative humidity z. Vary widely over the planet from low to high z. BUT, each organism does best its own favorite narrow range (c) 2001 W. H. Freeman and Company in
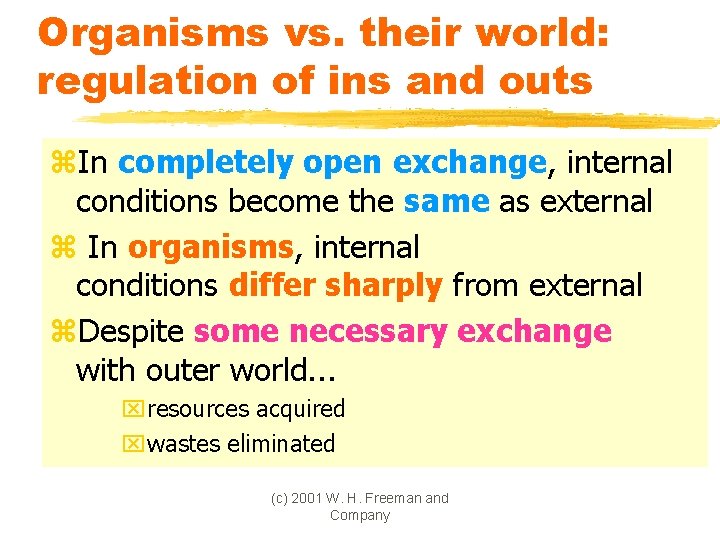
Organisms vs. their world: regulation of ins and outs z. In completely open exchange, internal conditions become the same as external z In organisms, internal conditions differ sharply from external z. Despite some necessary exchange with outer world. . . xresources acquired xwastes eliminated (c) 2001 W. H. Freeman and Company
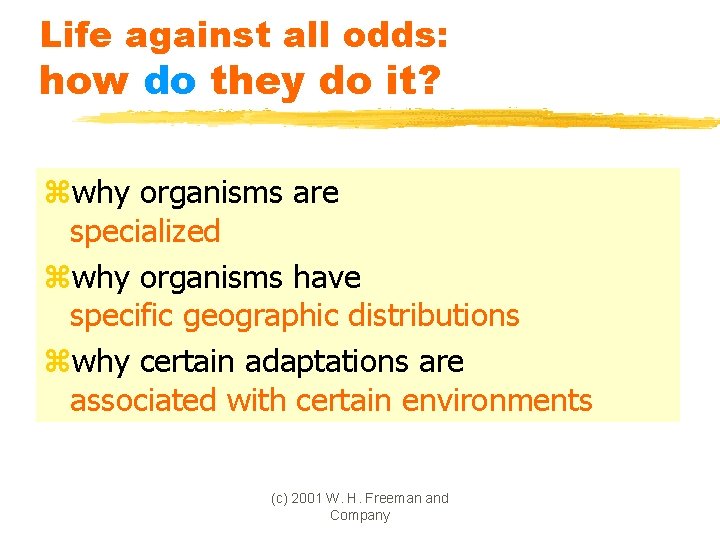
Life against all odds: how do they do it? zwhy organisms are specialized zwhy organisms have specific geographic distributions zwhy certain adaptations are associated with certain environments (c) 2001 W. H. Freeman and Company
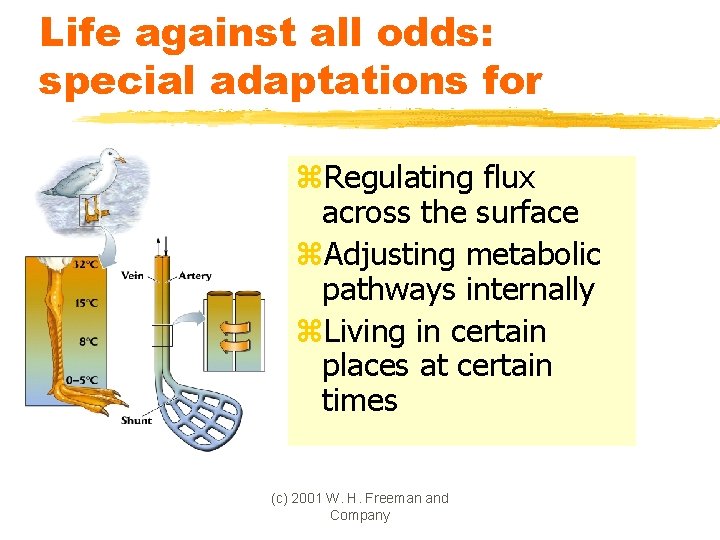
Life against all odds: special adaptations for z. Regulating flux across the surface z. Adjusting metabolic pathways internally z. Living in certain places at certain times (c) 2001 W. H. Freeman and Company
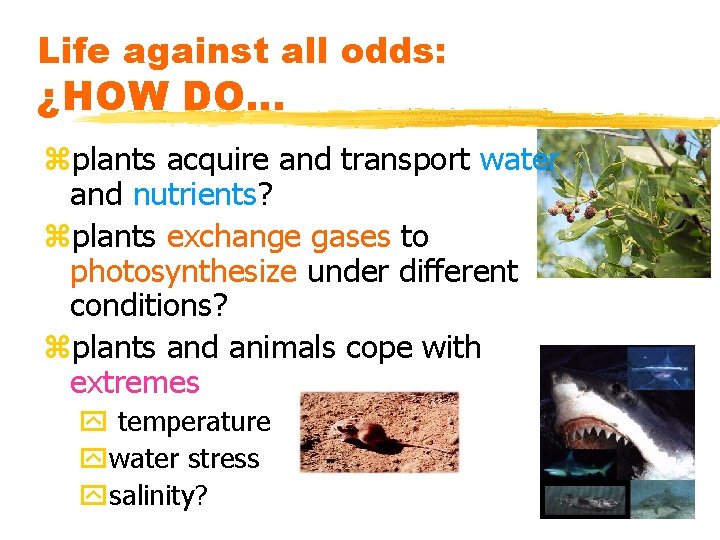
Life against all odds: ¿HOW DO. . . zplants acquire and transport water and nutrients? zplants exchange gases to photosynthesize under different conditions? zplants and animals cope with extremes y temperature ywater stress ysalinity?
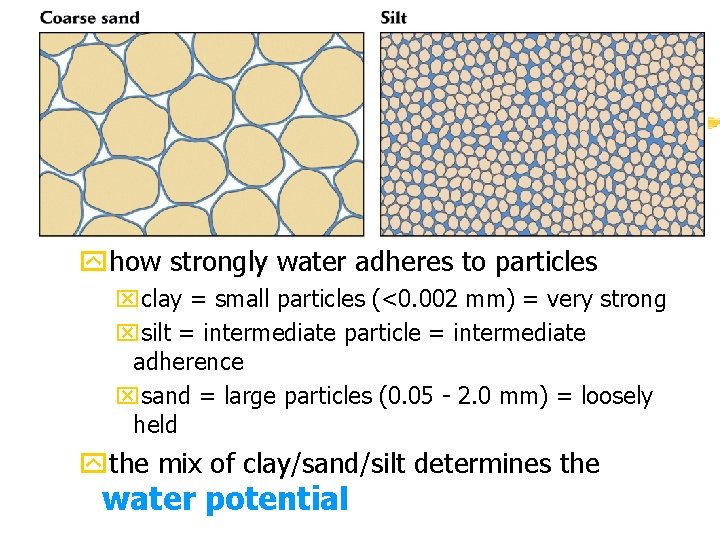
Water-soil interaction zwater potential yhow strongly water adheres to particles xclay = small particles (<0. 002 mm) = very strong xsilt = intermediate particle = intermediate adherence xsand = large particles (0. 05 - 2. 0 mm) = loosely held ythe mix of clay/sand/silt determines the water potential
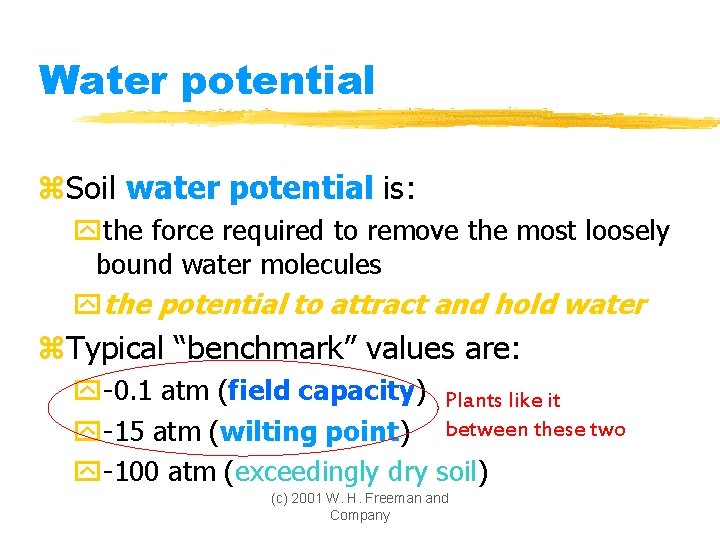
Water potential z. Soil water potential is: ythe force required to remove the most loosely bound water molecules ythe potential to attract and hold water z. Typical “benchmark” values are: y-0. 1 atm (field capacity) Plants like it y-15 atm (wilting point) between these two y-100 atm (exceedingly dry soil) (c) 2001 W. H. Freeman and Company
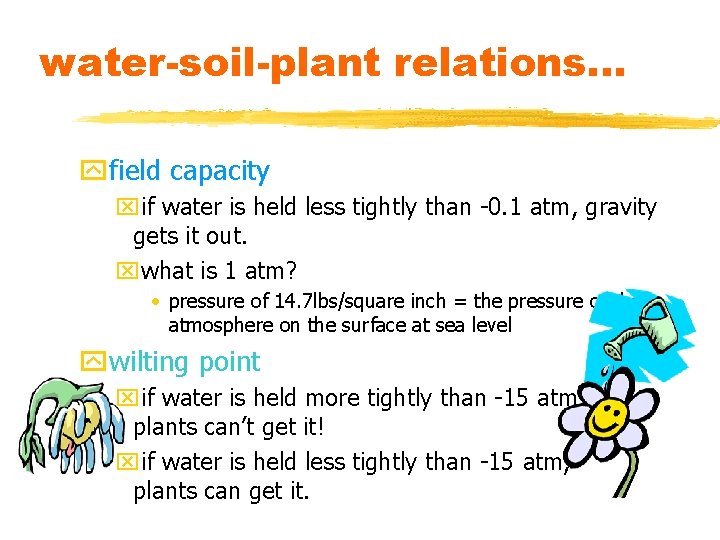
water-soil-plant relations. . . yfield capacity xif water is held less tightly than -0. 1 atm, gravity gets it out. xwhat is 1 atm? • pressure of 14. 7 lbs/square inch = the pressure of the atmosphere on the surface at sea level ywilting point xif water is held more tightly than -15 atm, plants can’t get it! xif water is held less tightly than -15 atm, plants can get it.
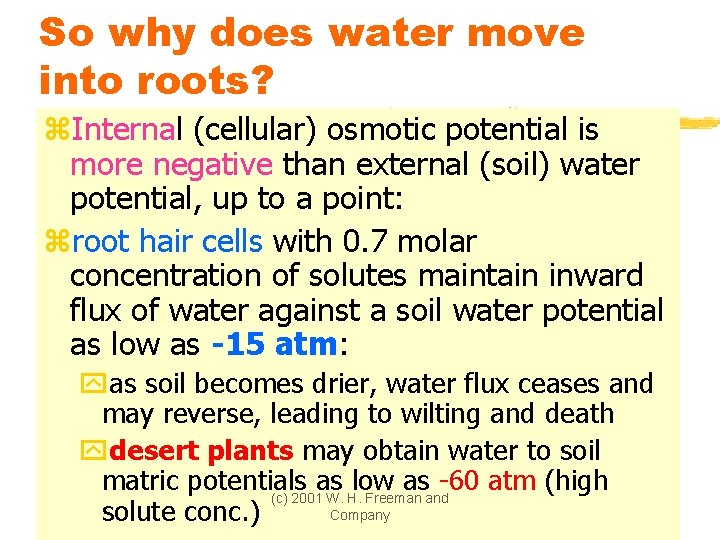
So why does water move into roots? z. Internal (cellular) osmotic potential is more negative than external (soil) water potential, up to a point: zroot hair cells with 0. 7 molar concentration of solutes maintain inward flux of water against a soil water potential as low as -15 atm: yas soil becomes drier, water flux ceases and may reverse, leading to wilting and death ydesert plants may obtain water to soil matric potentials as low as -60 atm (high (c) 2001 W. H. Freeman and Company solute conc. )
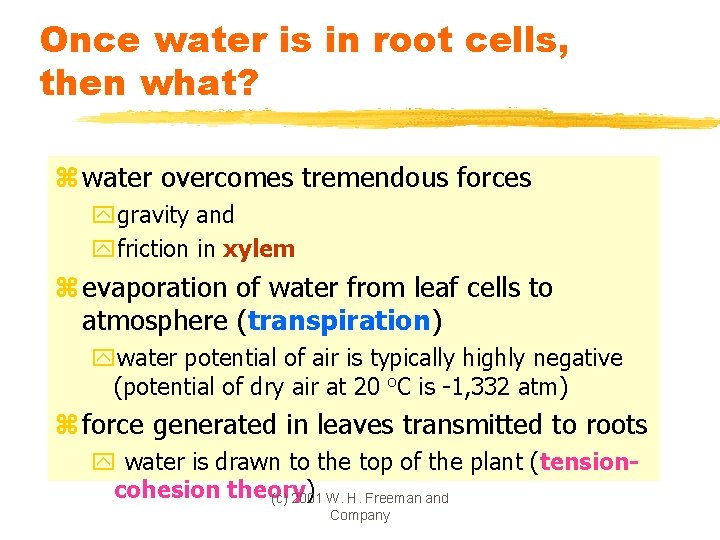
Once water is in root cells, then what? z water overcomes tremendous forces ygravity and yfriction in xylem z evaporation of water from leaf cells to atmosphere (transpiration) ywater potential of air is typically highly negative (potential of dry air at 20 o. C is -1, 332 atm) z force generated in leaves transmitted to roots y water is drawn to the top of the plant (tensioncohesion theory) (c) 2001 W. H. Freeman and Company
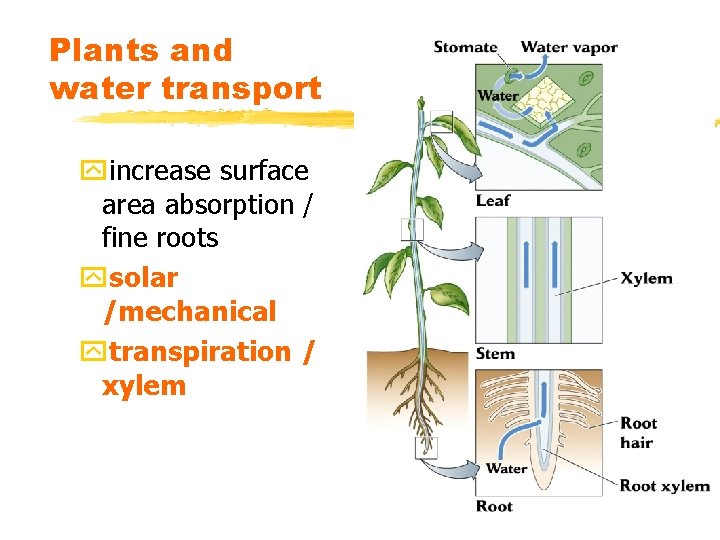
Plants and water transport yincrease surface area absorption / fine roots ysolar /mechanical ytranspiration / xylem
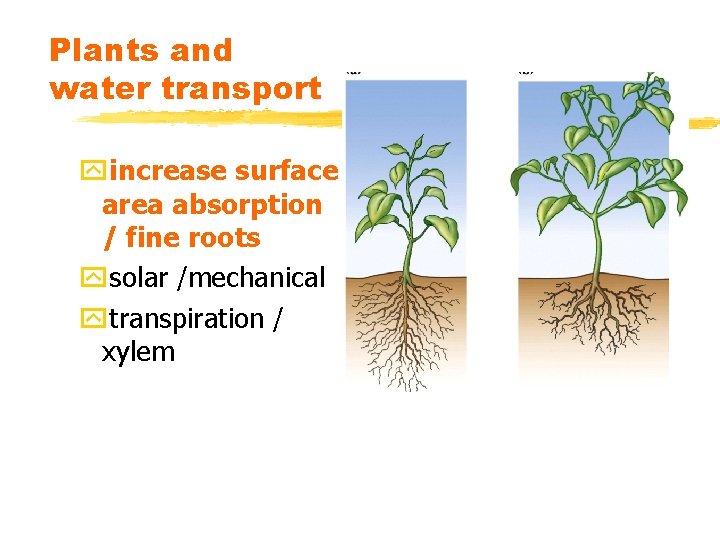
Plants and water transport yincrease surface area absorption / fine roots ysolar /mechanical ytranspiration / xylem
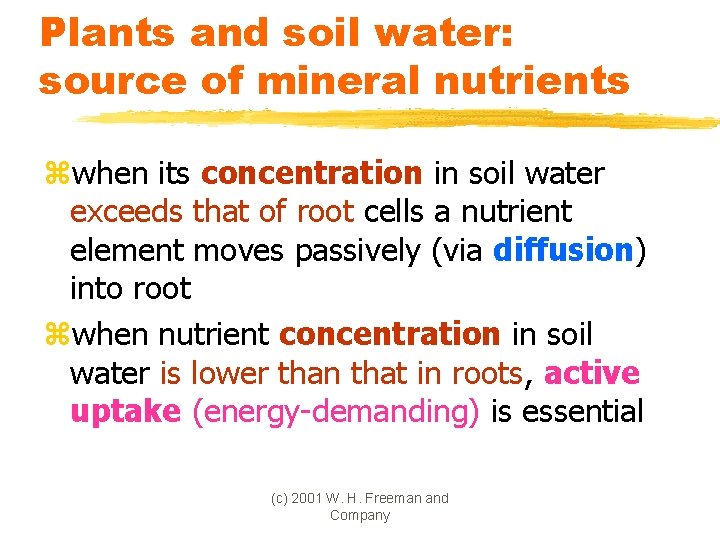
Plants and soil water: source of mineral nutrients zwhen its concentration in soil water exceeds that of root cells a nutrient element moves passively (via diffusion) into root zwhen nutrient concentration in soil water is lower than that in roots, active uptake (energy-demanding) is essential (c) 2001 W. H. Freeman and Company
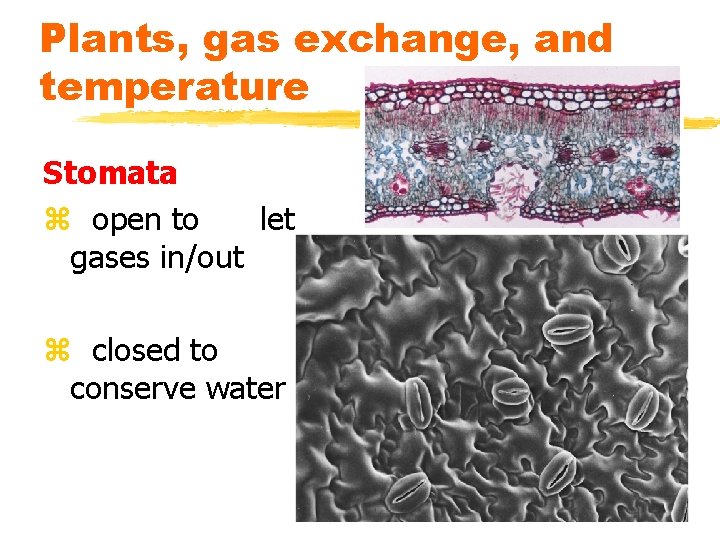
Plants, gas exchange, and temperature Stomata z open to let gases in/out z closed to conserve water
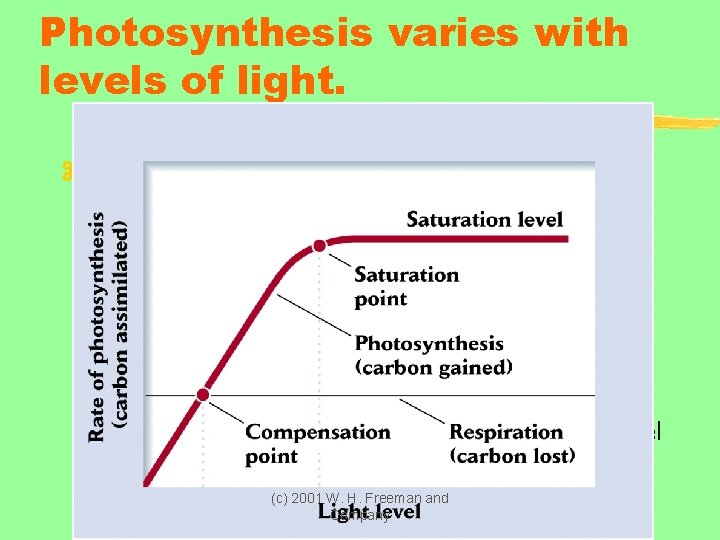
Photosynthesis varies with levels of light. z Photosynthetic rate is a function of light intensity (proportional to light intensity at low light levels, leveling off at high levels): yin dim light, plants fail to offset respiratory losses with photosynthetic gains yas light intensity increases, a break-even point (losses offset by gains) is reached, called compensation point yat saturation point, further increase in light level does not stimulate further photosynthesis (c) 2001 W. H. Freeman and Company
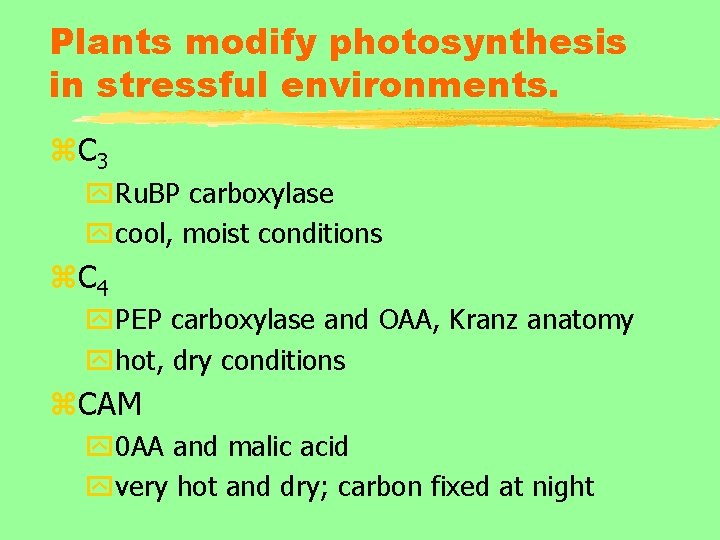
Plants modify photosynthesis in stressful environments. z. C 3 y. Ru. BP carboxylase ycool, moist conditions z. C 4 y. PEP carboxylase and OAA, Kranz anatomy yhot, dry conditions z. CAM y 0 AA and malic acid yvery hot and dry; carbon fixed at night
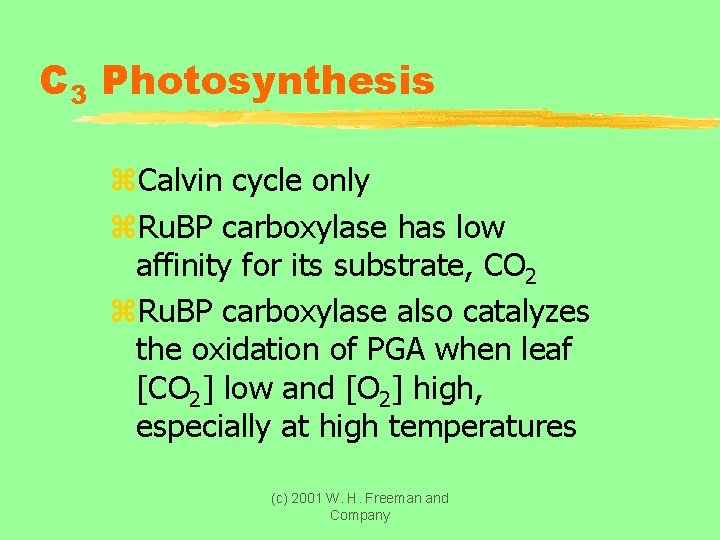
C 3 Photosynthesis z. Calvin cycle only z. Ru. BP carboxylase has low affinity for its substrate, CO 2 z. Ru. BP carboxylase also catalyzes the oxidation of PGA when leaf [CO 2] low and [O 2] high, especially at high temperatures (c) 2001 W. H. Freeman and Company
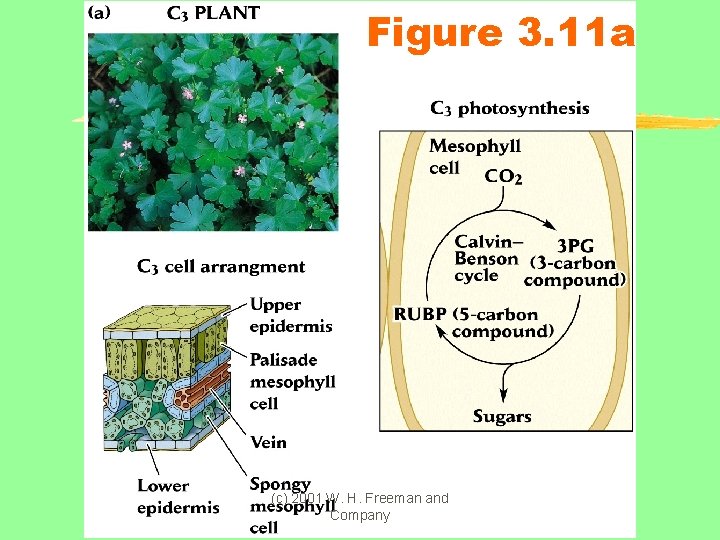
Figure 3. 11 a (c) 2001 W. H. Freeman and Company
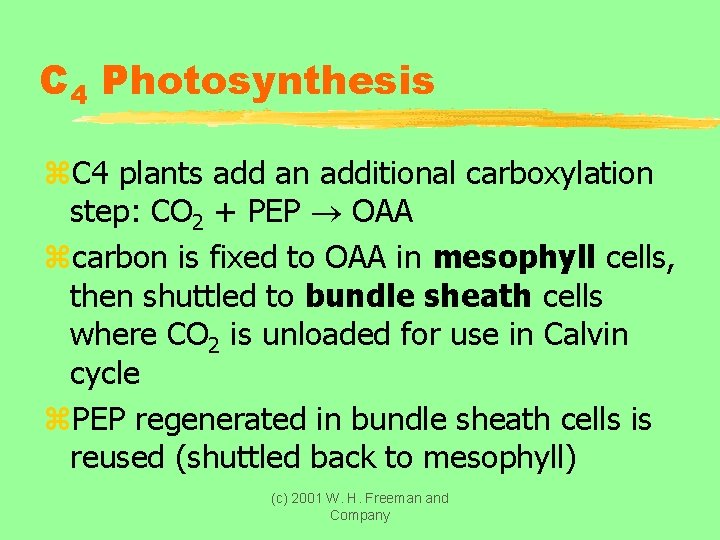
C 4 Photosynthesis z. C 4 plants add an additional carboxylation step: CO 2 + PEP OAA zcarbon is fixed to OAA in mesophyll cells, then shuttled to bundle sheath cells where CO 2 is unloaded for use in Calvin cycle z. PEP regenerated in bundle sheath cells is reused (shuttled back to mesophyll) (c) 2001 W. H. Freeman and Company
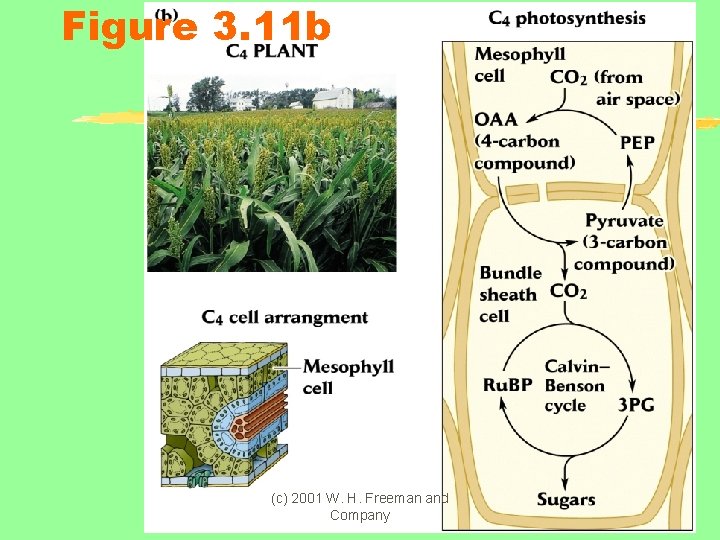
Figure 3. 11 b (c) 2001 W. H. Freeman and Company
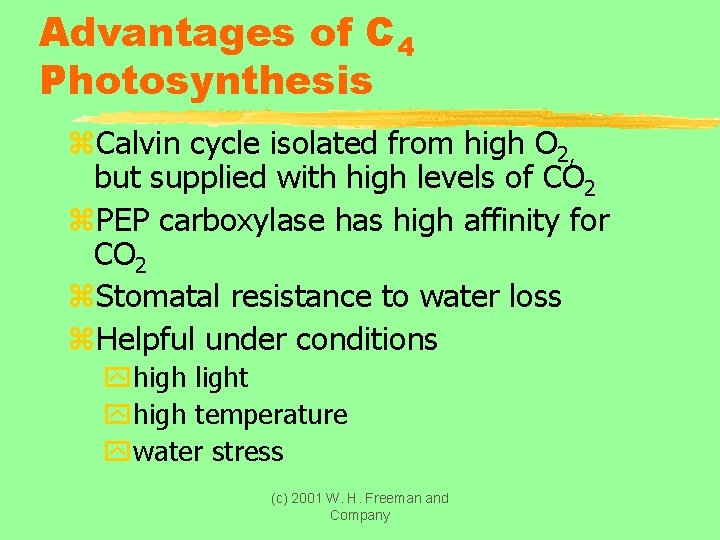
Advantages of C 4 Photosynthesis z. Calvin cycle isolated from high O 2, but supplied with high levels of CO 2 z. PEP carboxylase has high affinity for CO 2 z. Stomatal resistance to water loss z. Helpful under conditions yhigh light yhigh temperature ywater stress (c) 2001 W. H. Freeman and Company
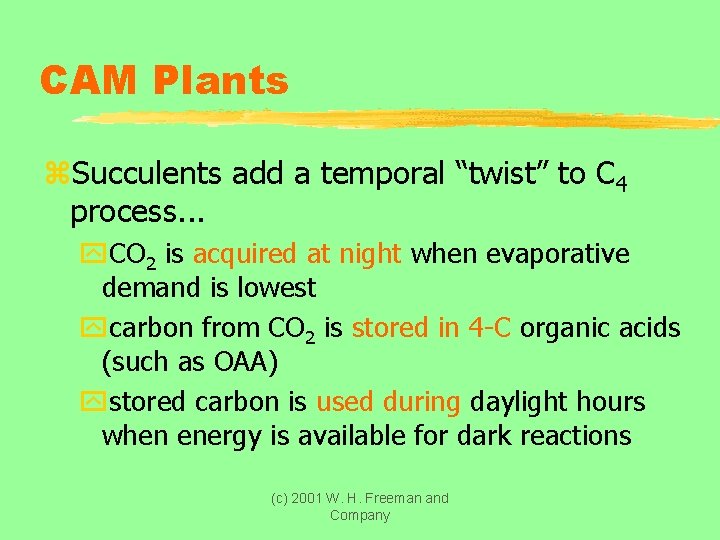
CAM Plants z. Succulents add a temporal “twist” to C 4 process. . . y. CO 2 is acquired at night when evaporative demand is lowest ycarbon from CO 2 is stored in 4 -C organic acids (such as OAA) ystored carbon is used during daylight hours when energy is available for dark reactions (c) 2001 W. H. Freeman and Company
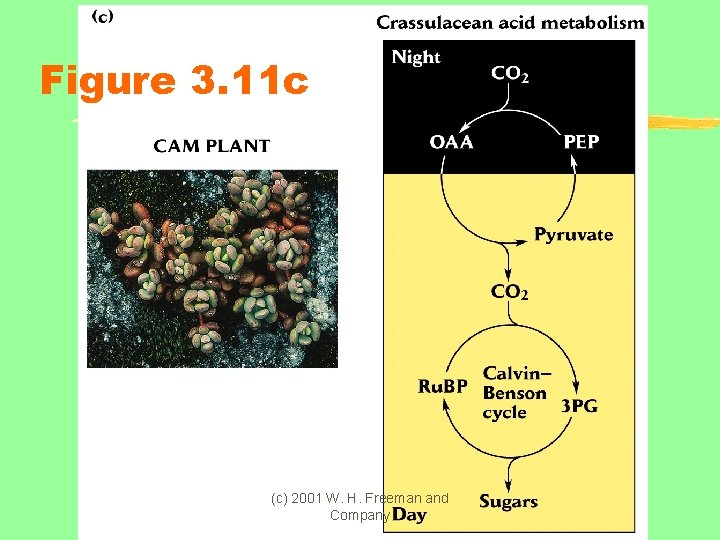
Figure 3. 11 c (c) 2001 W. H. Freeman and Company
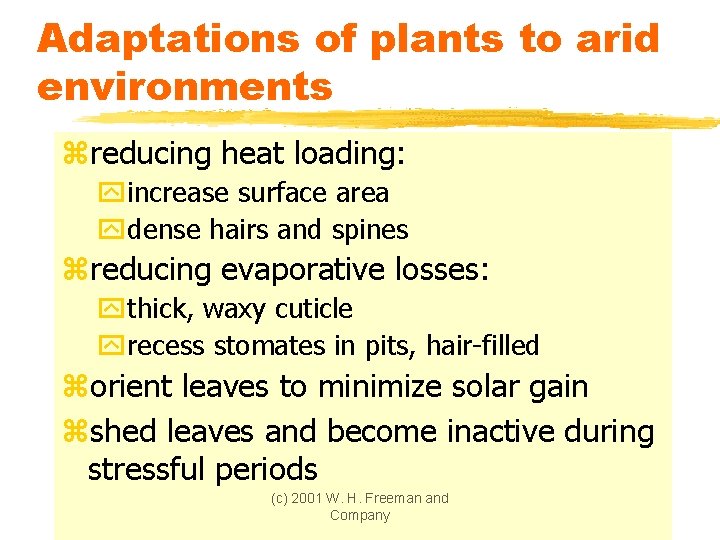
Adaptations of plants to arid environments zreducing heat loading: yincrease surface area ydense hairs and spines zreducing evaporative losses: ythick, waxy cuticle yrecess stomates in pits, hair-filled zorient leaves to minimize solar gain zshed leaves and become inactive during stressful periods (c) 2001 W. H. Freeman and Company
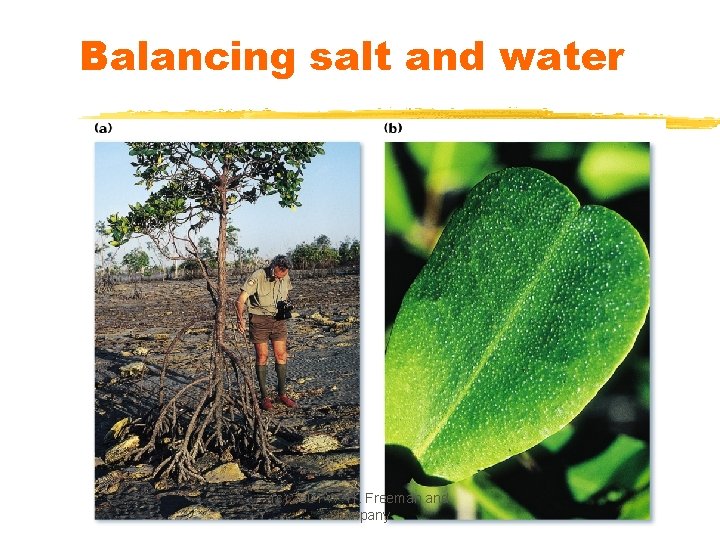
Balancing salt and water (c) 2001 W. H. Freeman and Company
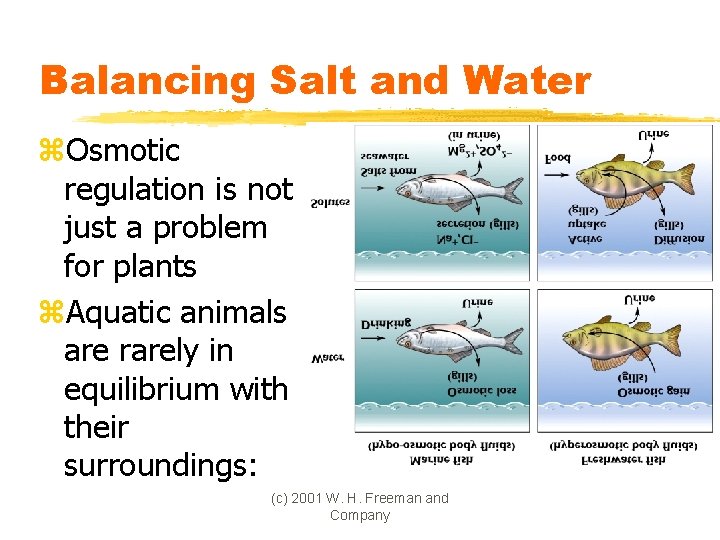
Balancing Salt and Water z. Osmotic regulation is not just a problem for plants z. Aquatic animals are rarely in equilibrium with their surroundings: (c) 2001 W. H. Freeman and Company
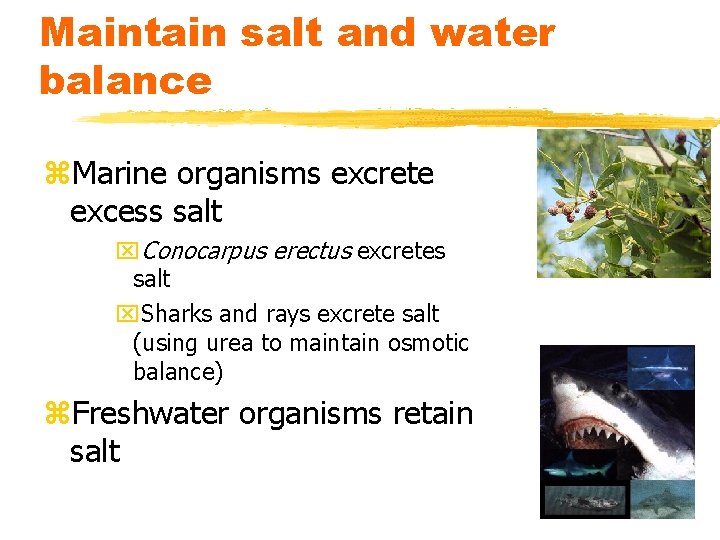
Maintain salt and water balance z. Marine organisms excrete excess salt x. Conocarpus erectus excretes salt x. Sharks and rays excrete salt (using urea to maintain osmotic balance) z. Freshwater organisms retain salt
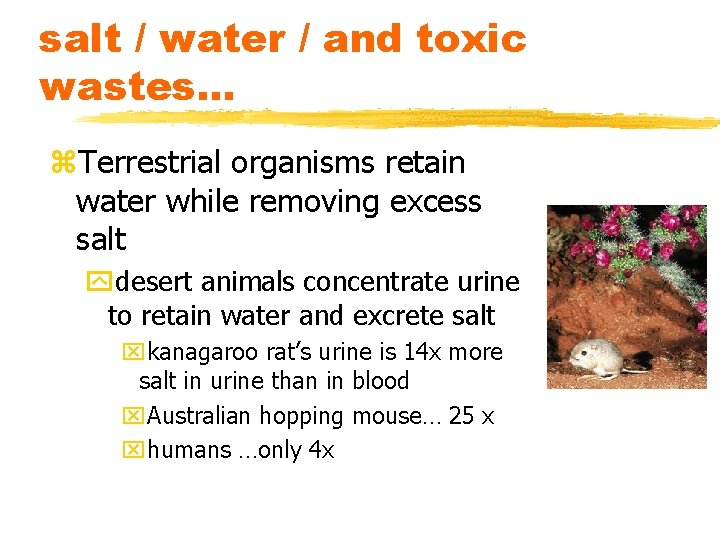
salt / water / and toxic wastes. . . z. Terrestrial organisms retain water while removing excess salt ydesert animals concentrate urine to retain water and excrete salt xkanagaroo rat’s urine is 14 x more salt in urine than in blood x. Australian hopping mouse… 25 x xhumans …only 4 x
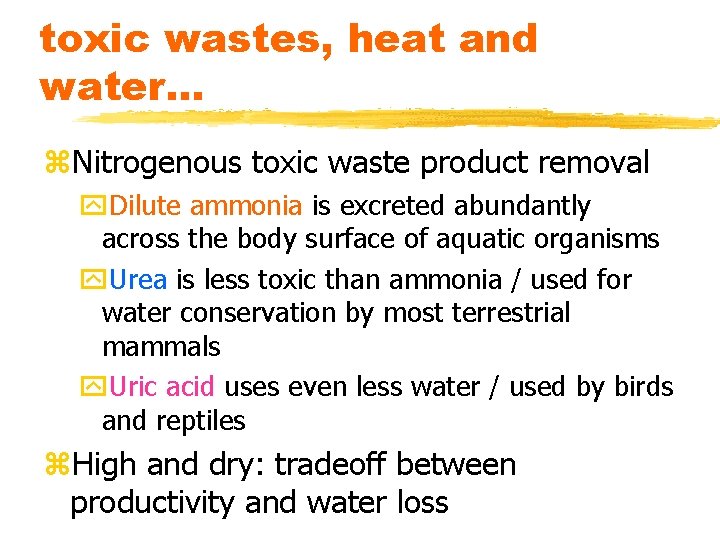
toxic wastes, heat and water. . . z. Nitrogenous toxic waste product removal y. Dilute ammonia is excreted abundantly across the body surface of aquatic organisms y. Urea is less toxic than ammonia / used for water conservation by most terrestrial mammals y. Uric acid uses even less water / used by birds and reptiles z. High and dry: tradeoff between productivity and water loss
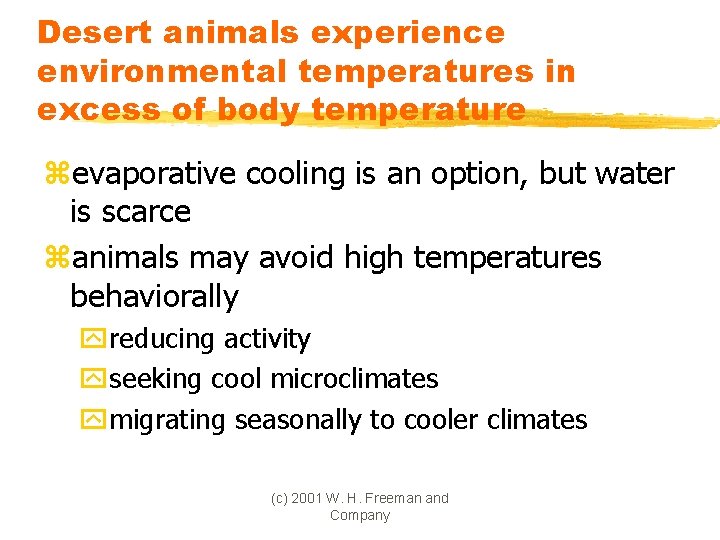
Desert animals experience environmental temperatures in excess of body temperature zevaporative cooling is an option, but water is scarce zanimals may avoid high temperatures behaviorally yreducing activity yseeking cool microclimates ymigrating seasonally to cooler climates (c) 2001 W. H. Freeman and Company
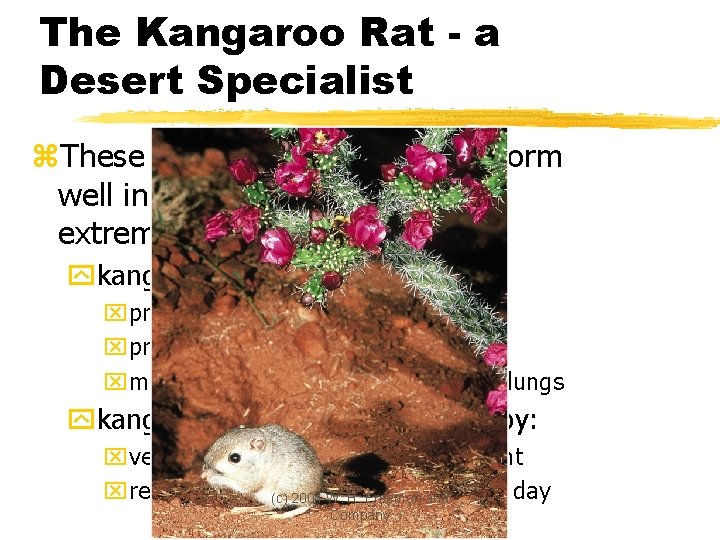
The Kangaroo Rat - a Desert Specialist z. These small desert rodents perform well in a nearly waterless and extremely hot setting. ykangaroo rats conserve water by: xproducing concentrated urine xproducing nearly dry feces xminimizing evaporative losses from lungs ykangaroo rats avoid desert heat by: xventuring above ground only at night xremaining in cool, burrow by day (c) 2001 humid W. H. Freeman and Company
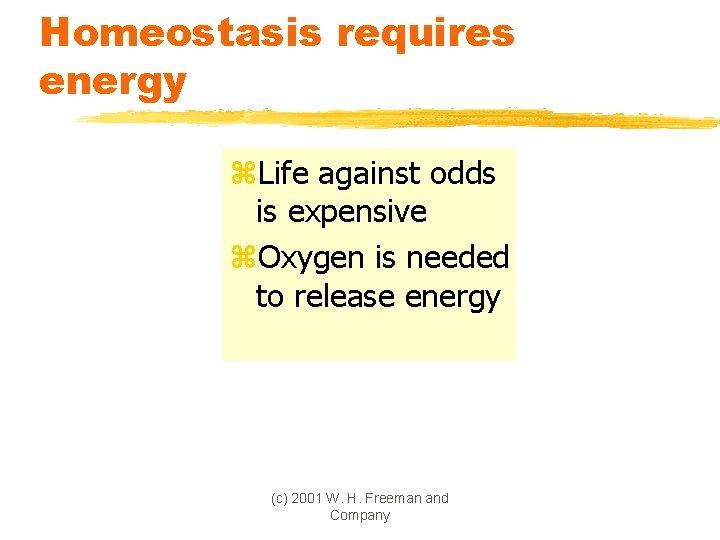
Homeostasis requires energy z. Life against odds is expensive z. Oxygen is needed to release energy (c) 2001 W. H. Freeman and Company
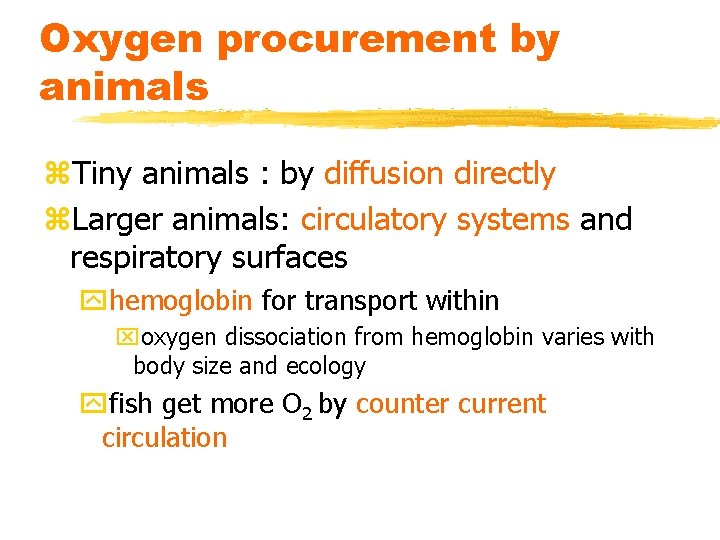
Oxygen procurement by animals z. Tiny animals : by diffusion directly z. Larger animals: circulatory systems and respiratory surfaces yhemoglobin for transport within xoxygen dissociation from hemoglobin varies with body size and ecology yfish get more O 2 by counter current circulation
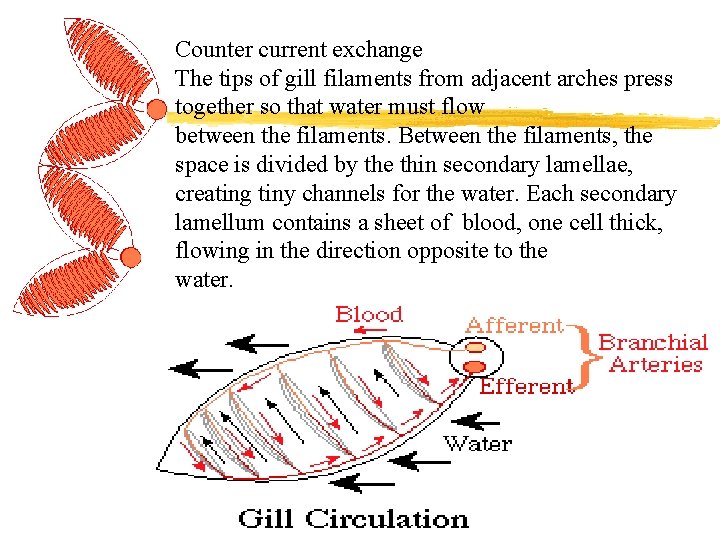
Counter current exchange The tips of gill filaments from adjacent arches press together so that water must flow between the filaments. Between the filaments, the space is divided by the thin secondary lamellae, creating tiny channels for the water. Each secondary lamellum contains a sheet of blood, one cell thick, flowing in the direction opposite to the water.
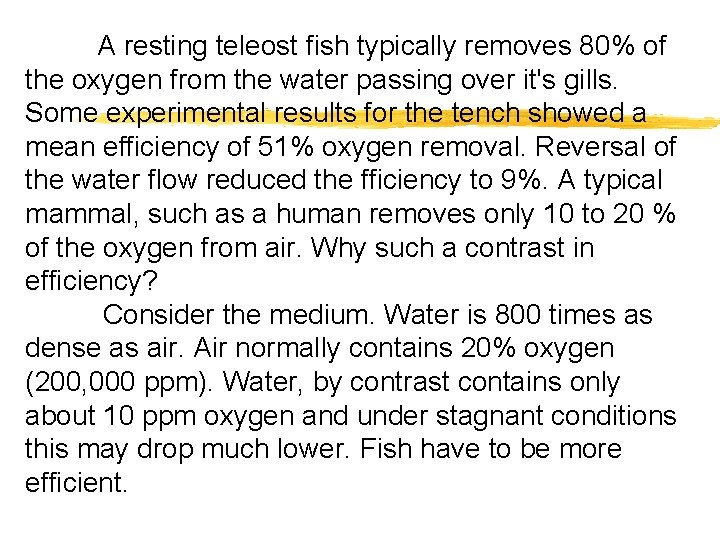
A resting teleost fish typically removes 80% of the oxygen from the water passing over it's gills. Some experimental results for the tench showed a mean efficiency of 51% oxygen removal. Reversal of the water flow reduced the fficiency to 9%. A typical mammal, such as a human removes only 10 to 20 % of the oxygen from air. Why such a contrast in efficiency? Consider the medium. Water is 800 times as dense as air. Air normally contains 20% oxygen (200, 000 ppm). Water, by contrast contains only about 10 ppm oxygen and under stagnant conditions this may drop much lower. Fish have to be more efficient.
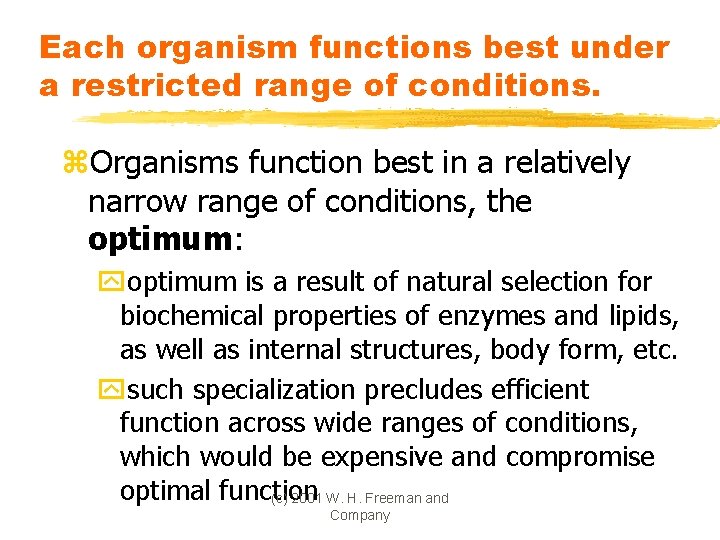
Each organism functions best under a restricted range of conditions. z. Organisms function best in a relatively narrow range of conditions, the optimum: yoptimum is a result of natural selection for biochemical properties of enzymes and lipids, as well as internal structures, body form, etc. ysuch specialization precludes efficient function across wide ranges of conditions, which would be expensive and compromise optimal function (c) 2001 W. H. Freeman and Company
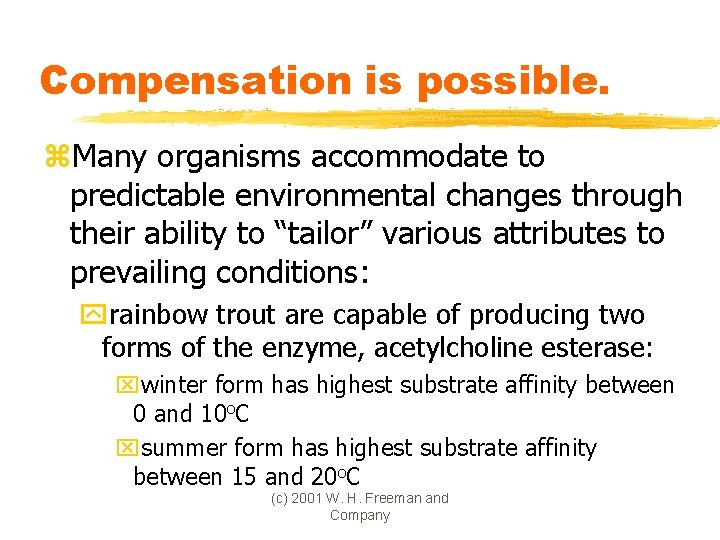
Compensation is possible. z. Many organisms accommodate to predictable environmental changes through their ability to “tailor” various attributes to prevailing conditions: yrainbow trout are capable of producing two forms of the enzyme, acetylcholine esterase: xwinter form has highest substrate affinity between 0 and 10 o. C xsummer form has highest substrate affinity between 15 and 20 o. C (c) 2001 W. H. Freeman and Company
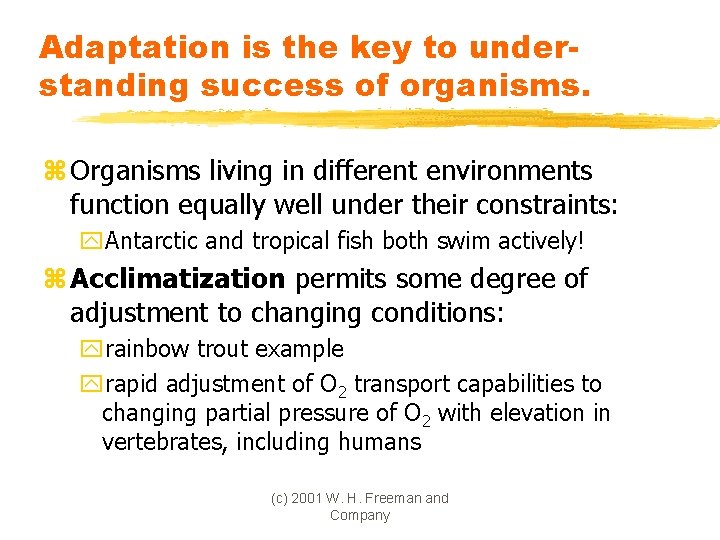
Adaptation is the key to understanding success of organisms. z Organisms living in different environments function equally well under their constraints: y. Antarctic and tropical fish both swim actively! z Acclimatization permits some degree of adjustment to changing conditions: yrainbow trout example yrapid adjustment of O 2 transport capabilities to changing partial pressure of O 2 with elevation in vertebrates, including humans (c) 2001 W. H. Freeman and Company
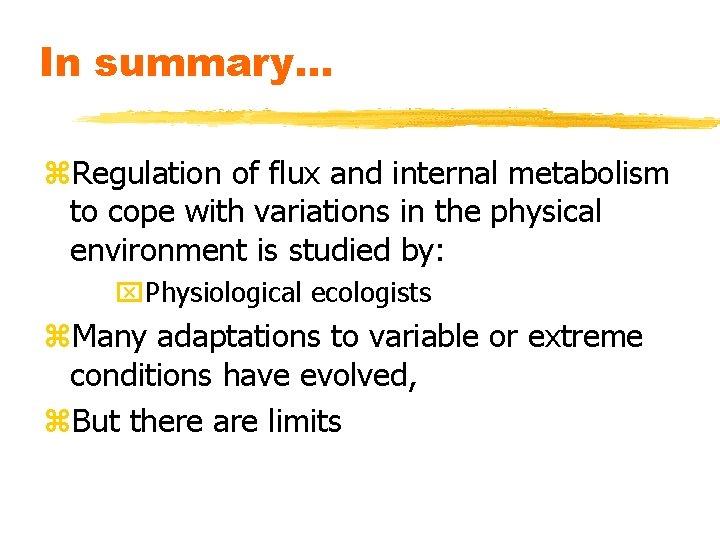
In summary. . . z. Regulation of flux and internal metabolism to cope with variations in the physical environment is studied by: x. Physiological ecologists z. Many adaptations to variable or extreme conditions have evolved, z. But there are limits
Frog life cycle
Terrestrial food production
Are protists terrestrial or aquatic
Are bacteria autotrophs or heterotrophs
Chapter 13 natural environments of europe
Chapter 13 natural environments of europe
World geography
Chapter 6 biomes and aquatic ecosystems
6 biomes and aquatic ecosystems
Chapter 3 lesson 3 biomes and aquatic ecosystems
Chapter 3 section 2 terrestrial biomes
Transitional aquatic ecosystems
Chapter 7 environmental science
Chapter 7 aquatic ecosystems test answers
International business environments and operations
External environment and accountability of schools
Every agent is rational in an unobservable environment.
Interrelationship between the micro and market environment
International business chapter 5
International business environments and operations
Exercise in hot and cold environments
International business environments and operations
Terrestrial plant
"elsevier"
Virtualization environment
Internal business environment factors
Peng cui tsinghua
Psychologically informed environments
Psychologically informed environments
Creating supportive environments smoking
High quality supportive environments
Designing constructivist learning environments
Economic activity
Spectrum protect for virtual environments
What's marketing environment
Implations
Story openers
What is global marketing environment
Diverse learning environments survey
Enabling environments eyfs
Diverse learning environments survey