Cavity Design Options N Esfahani and M Paoluzzi
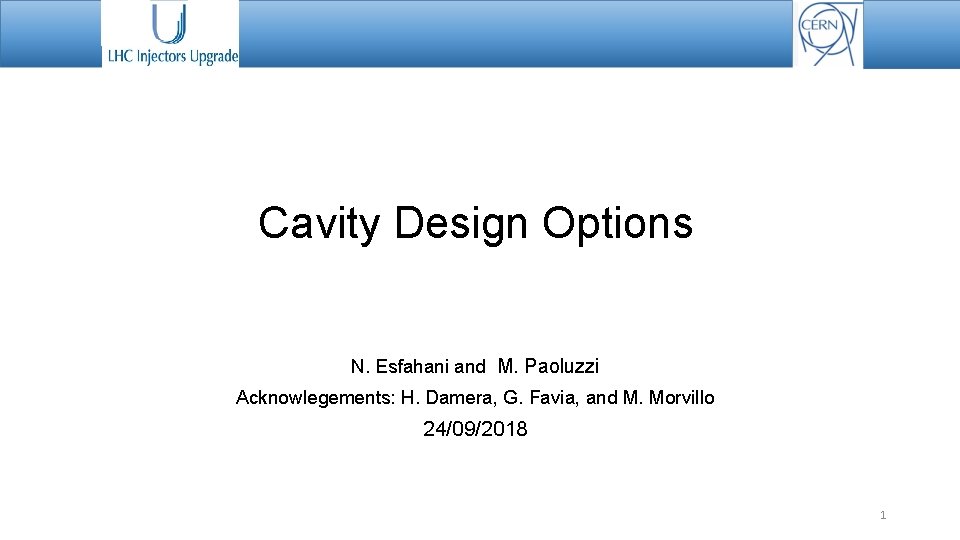
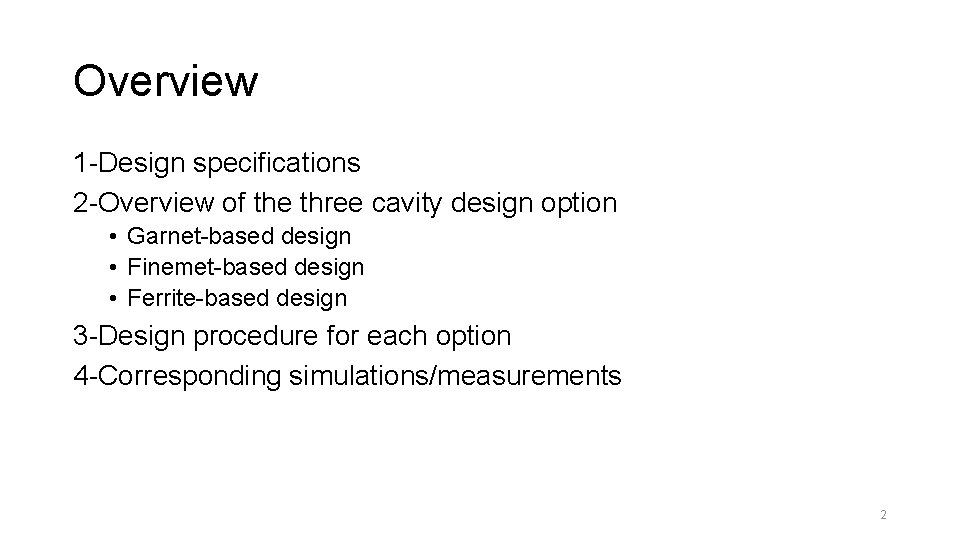
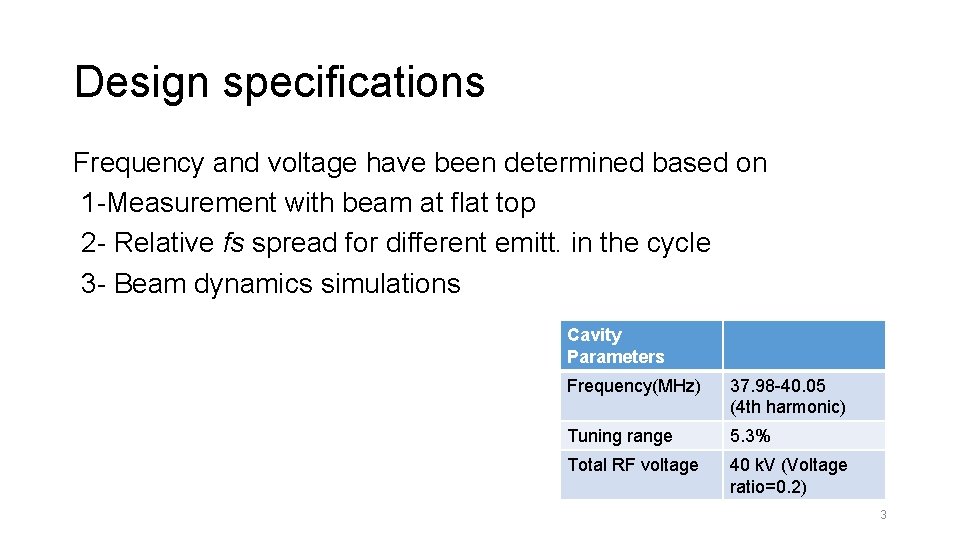
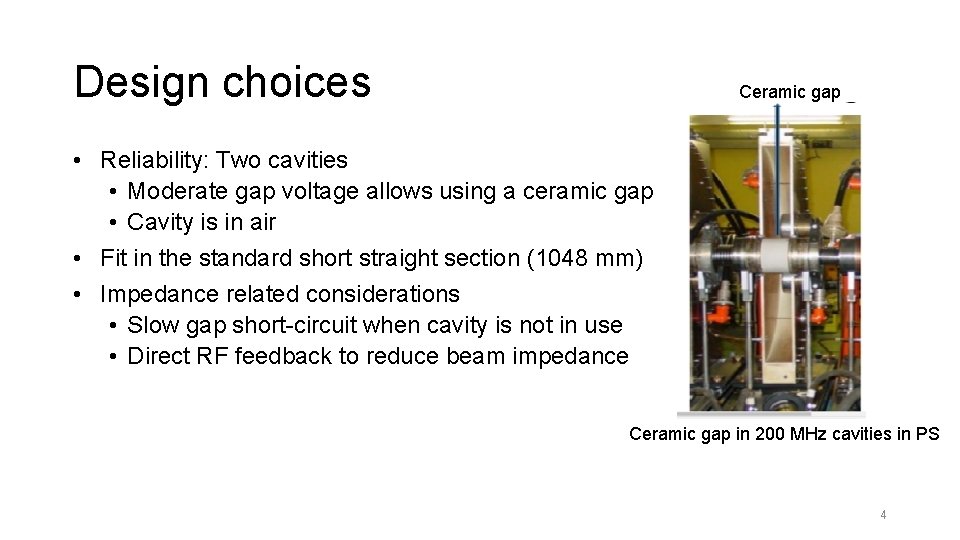
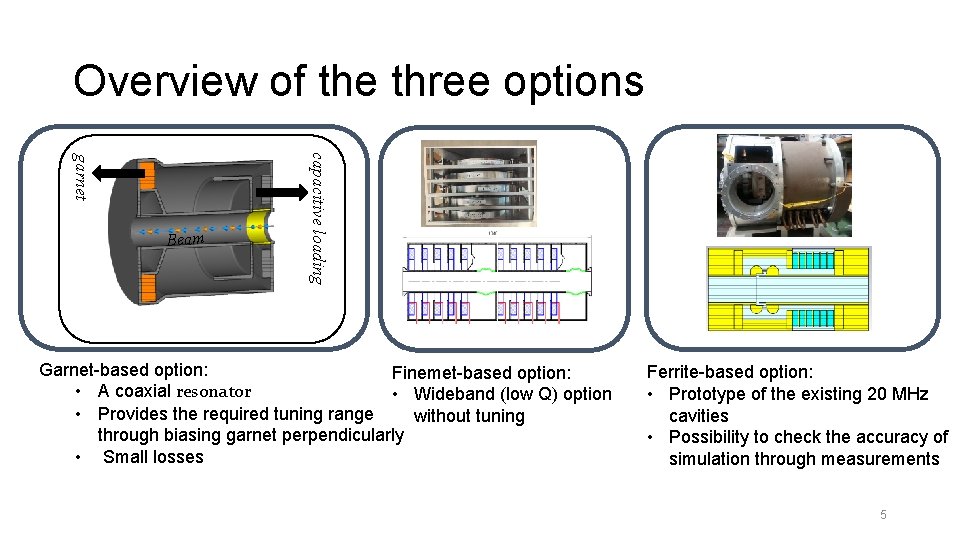
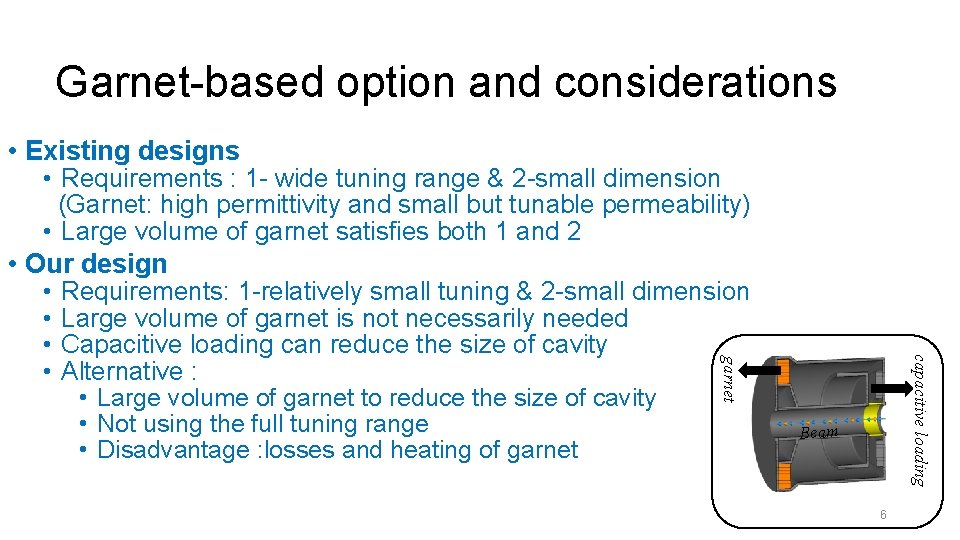
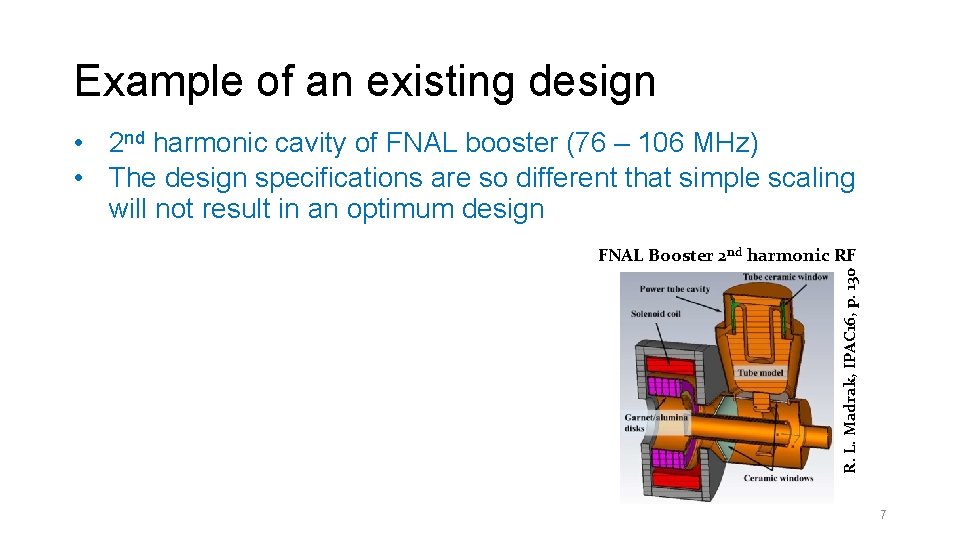
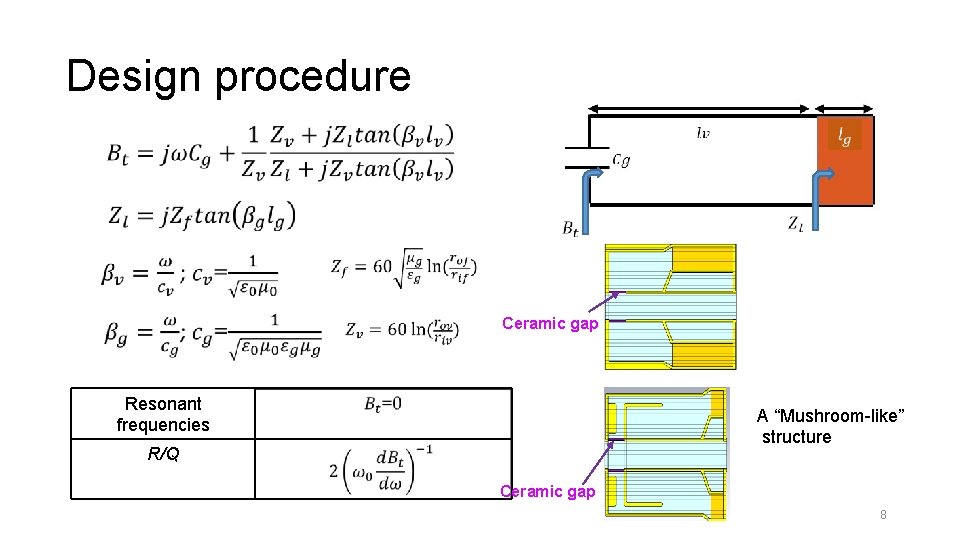
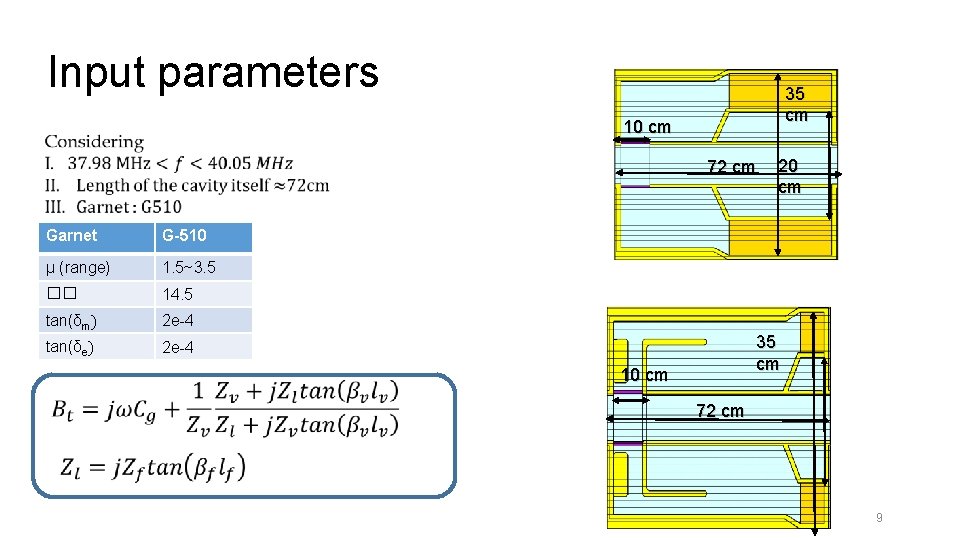
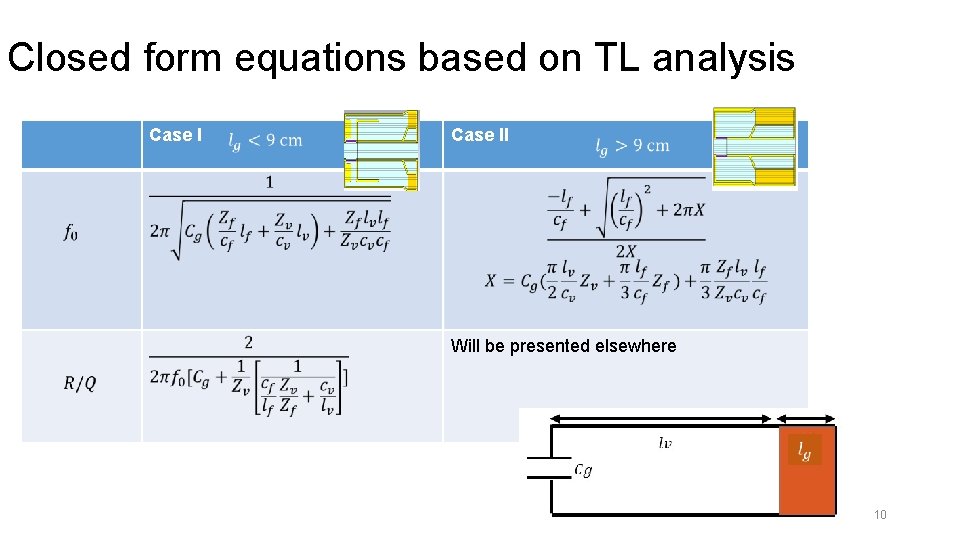
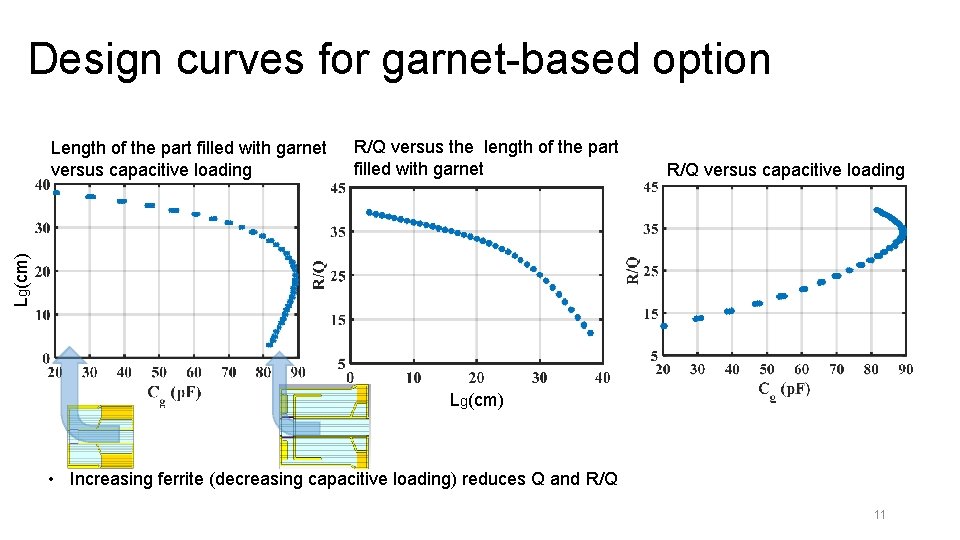
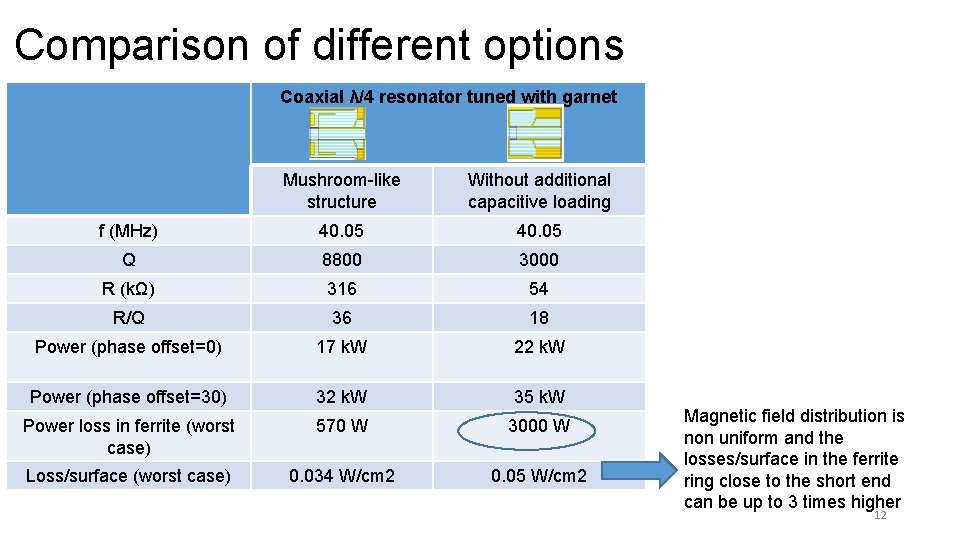
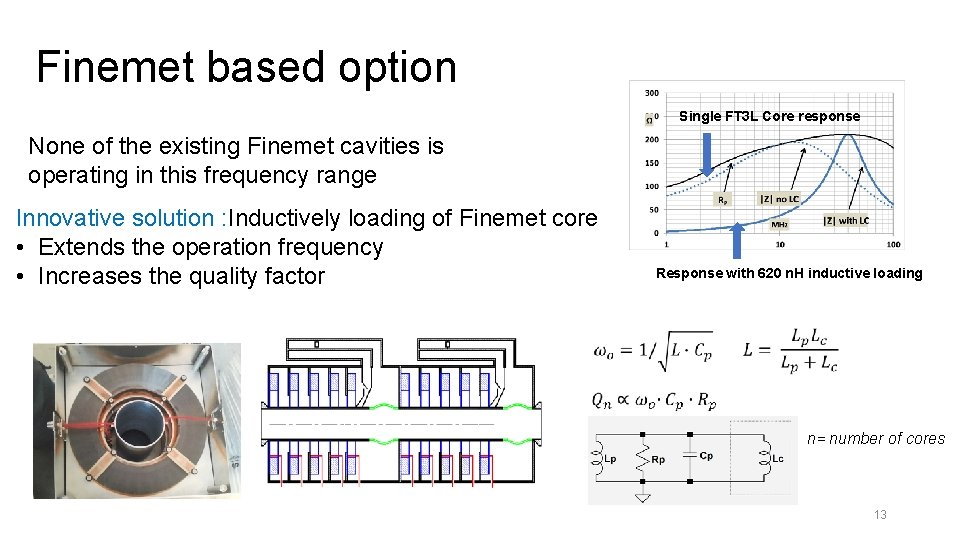
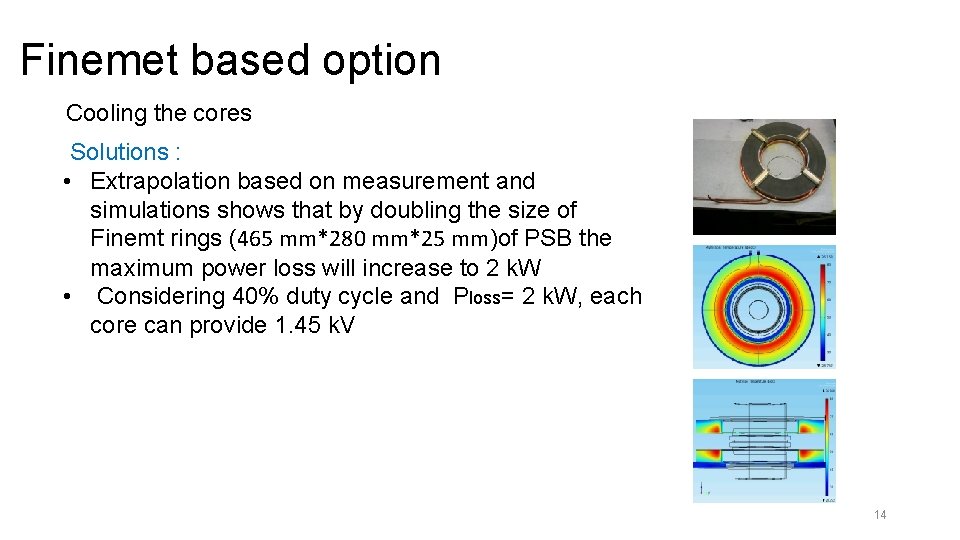
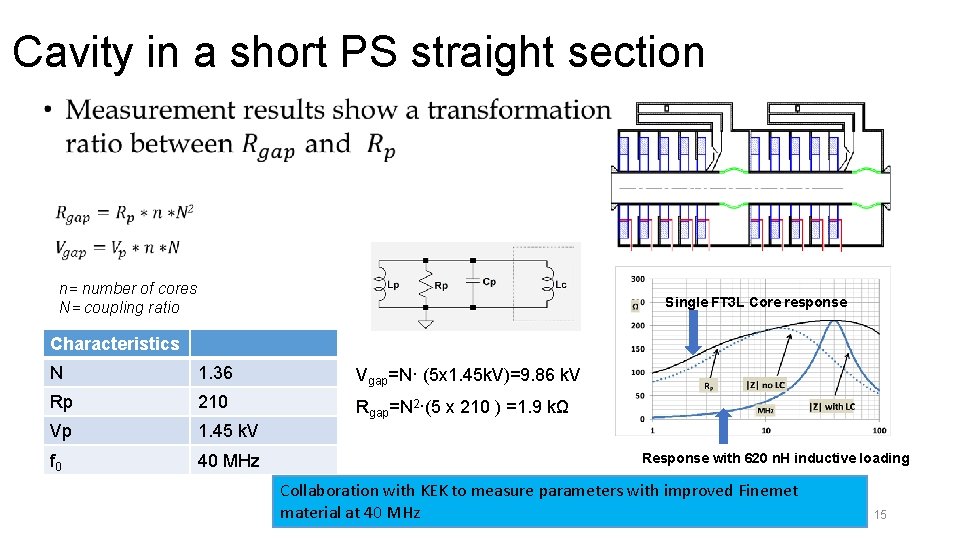
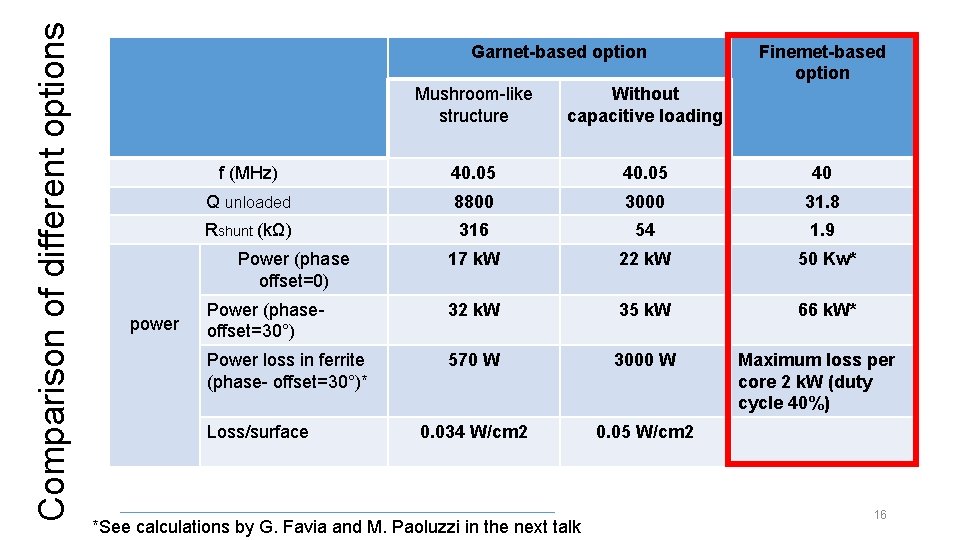
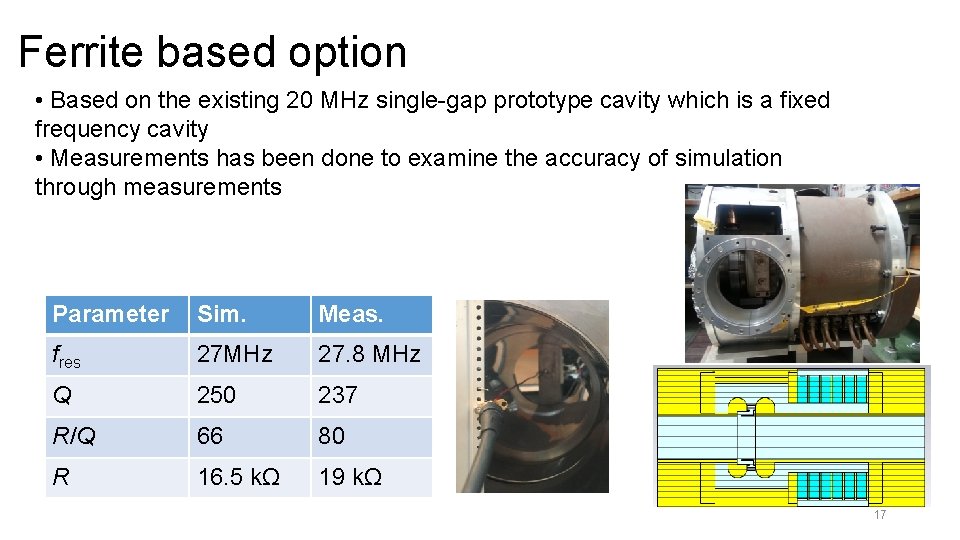
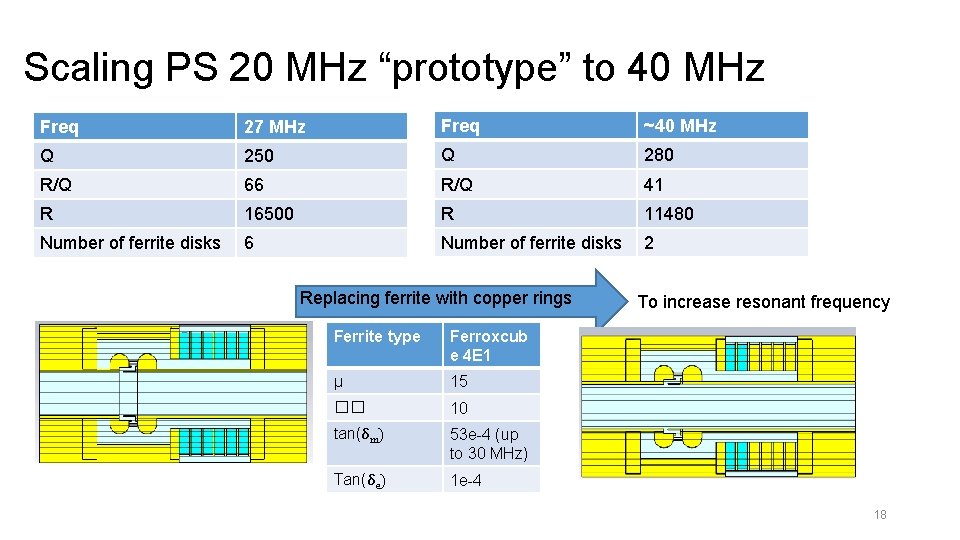
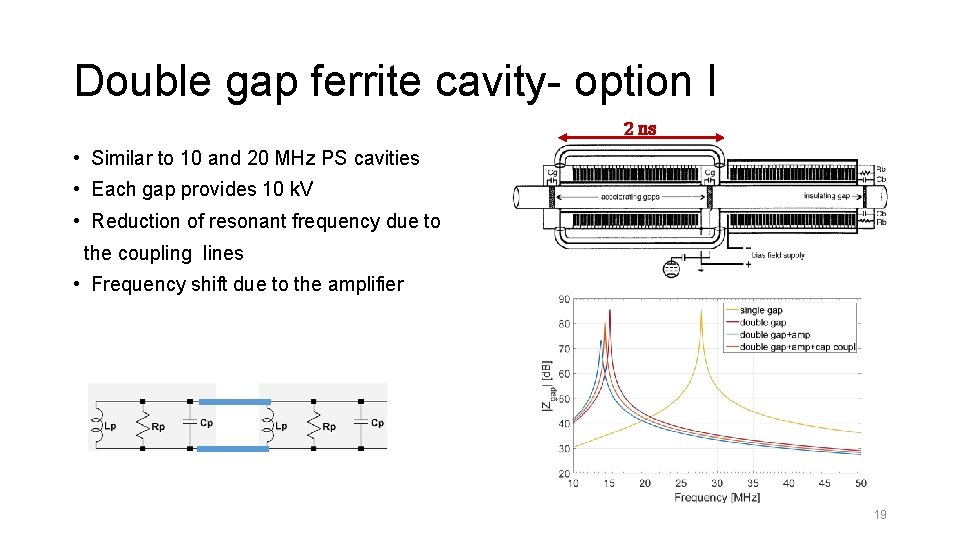
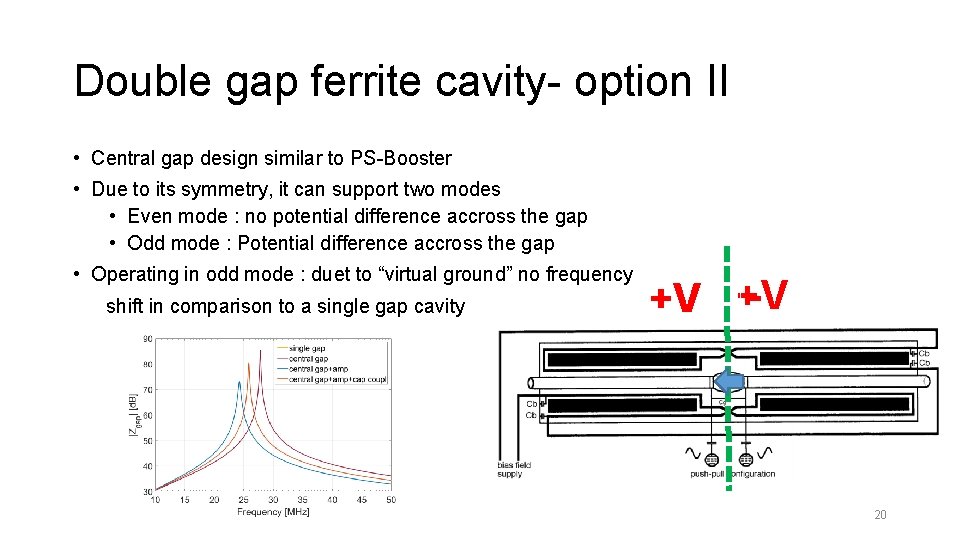
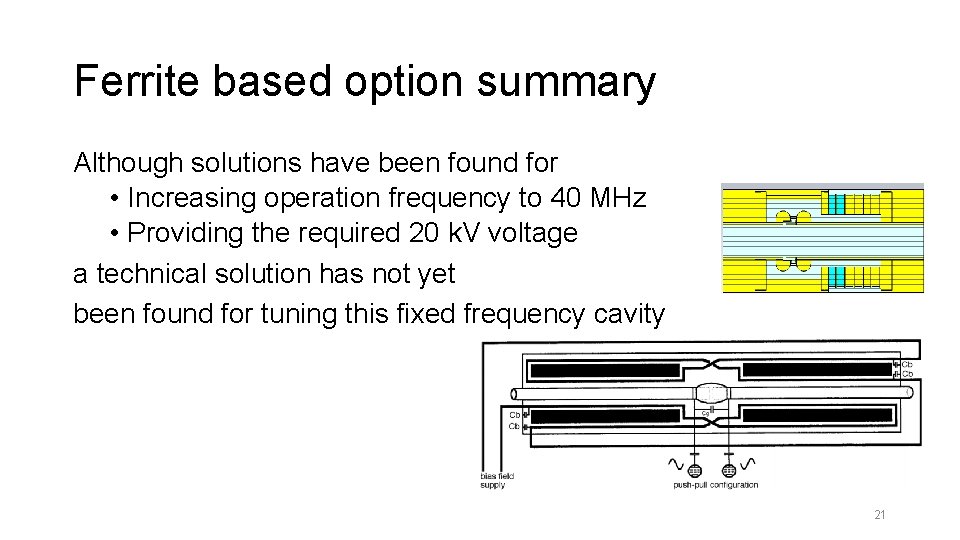
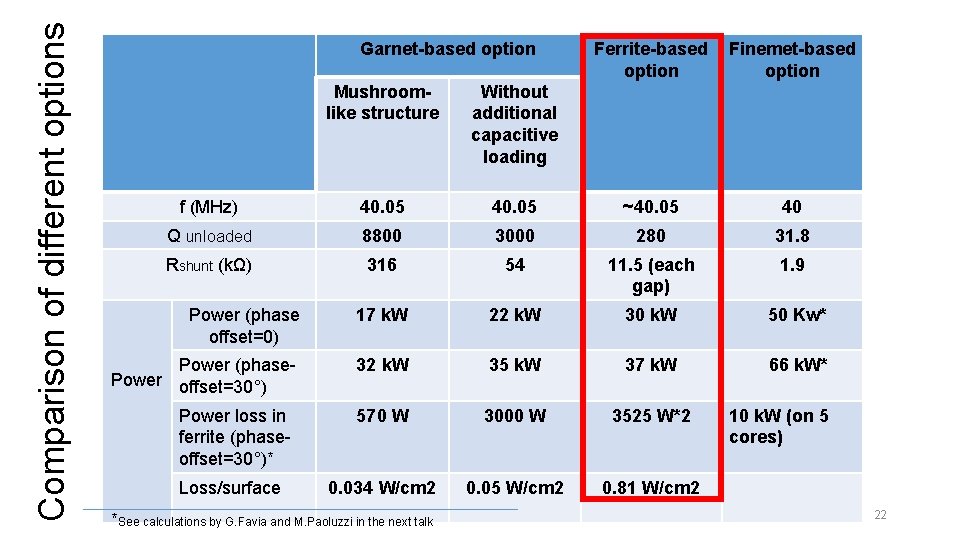
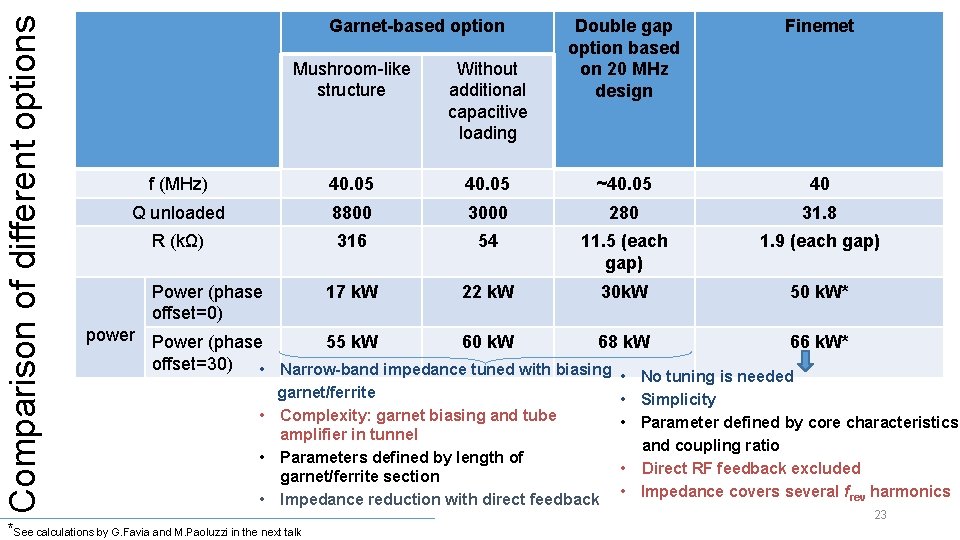
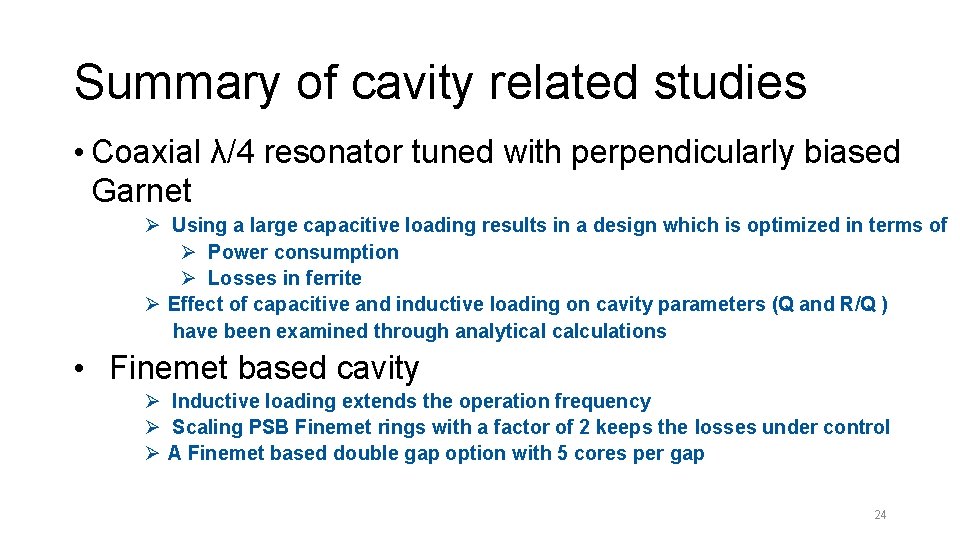
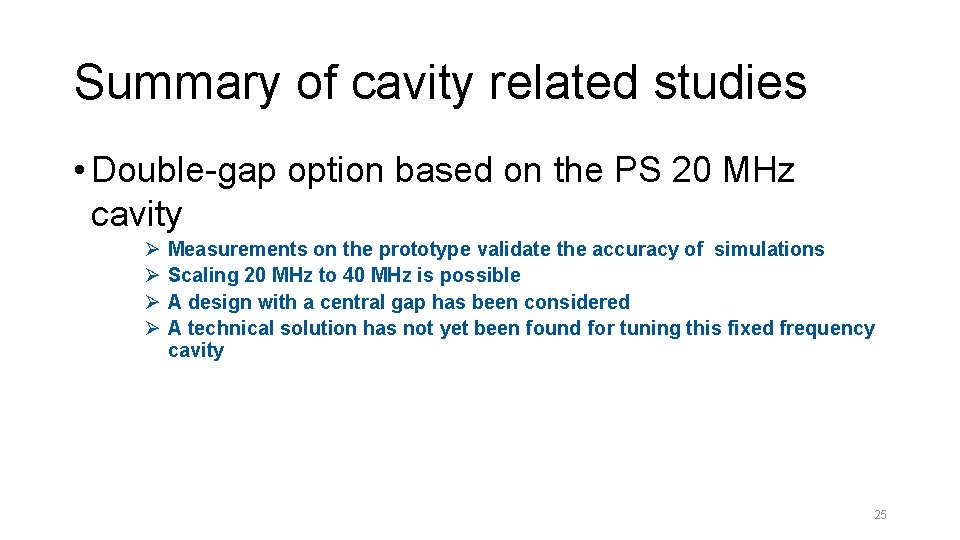
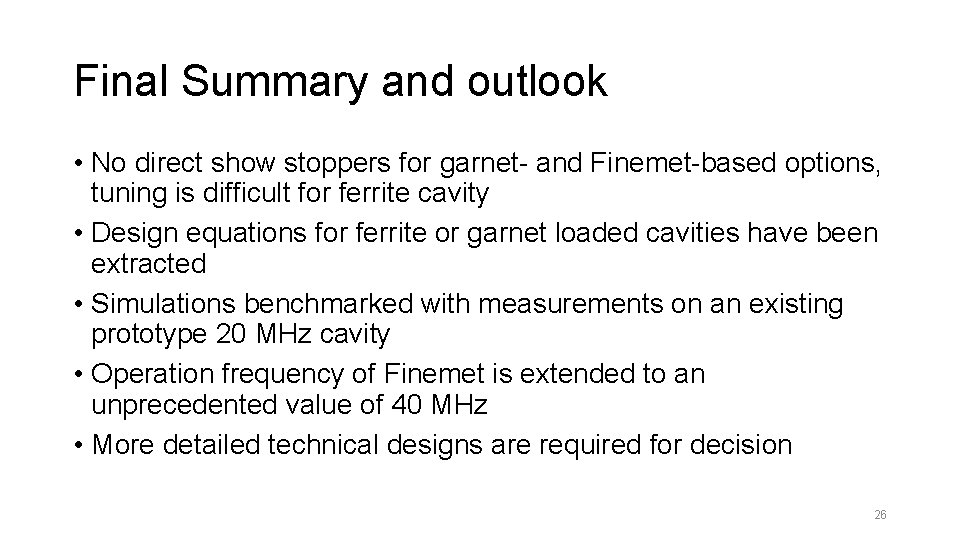
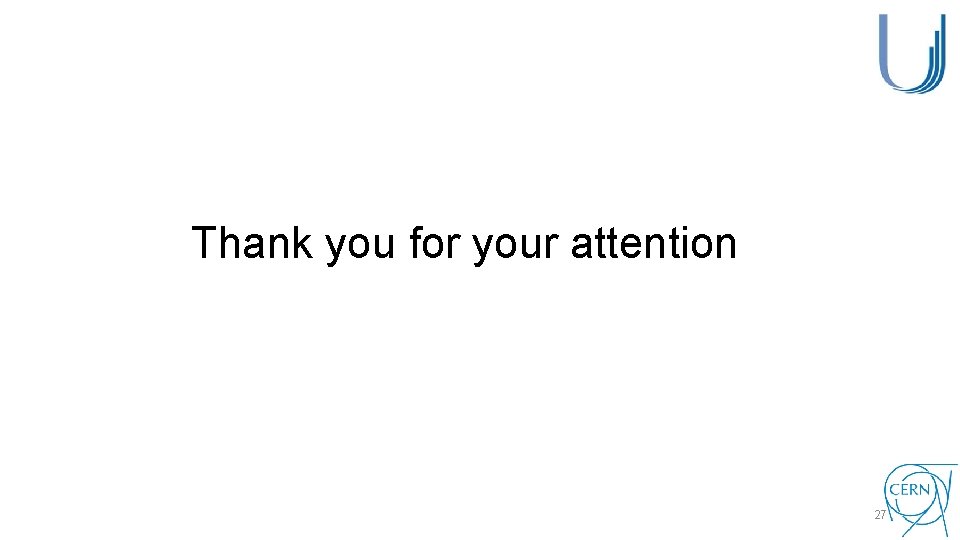
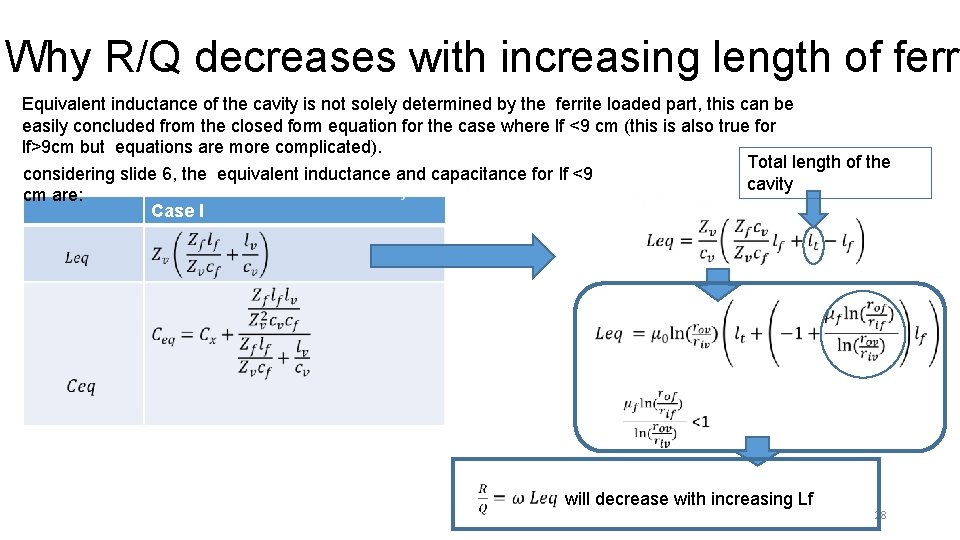
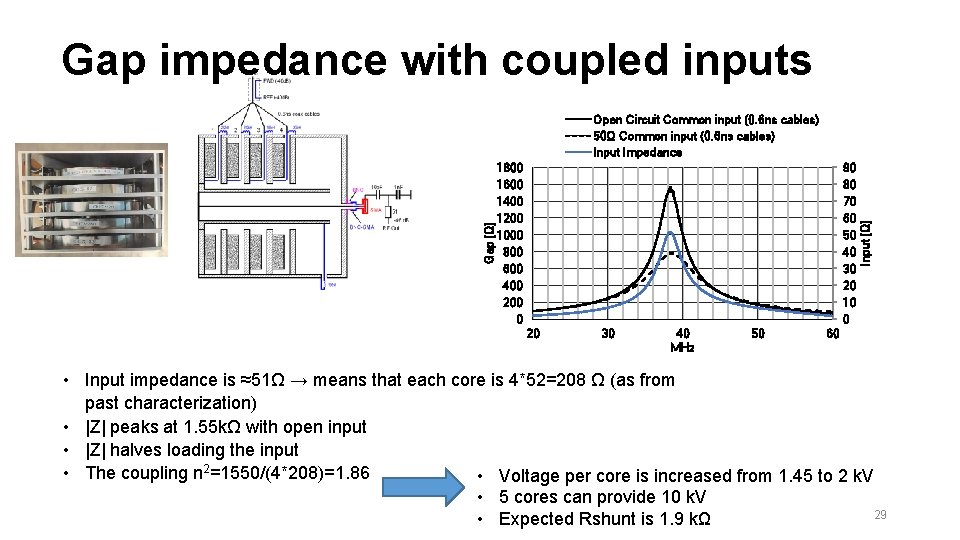
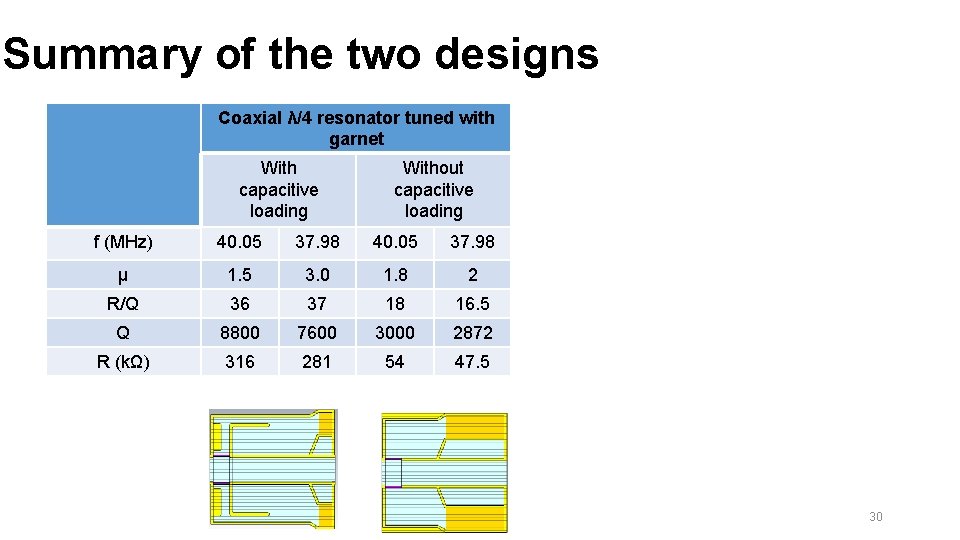
- Slides: 30
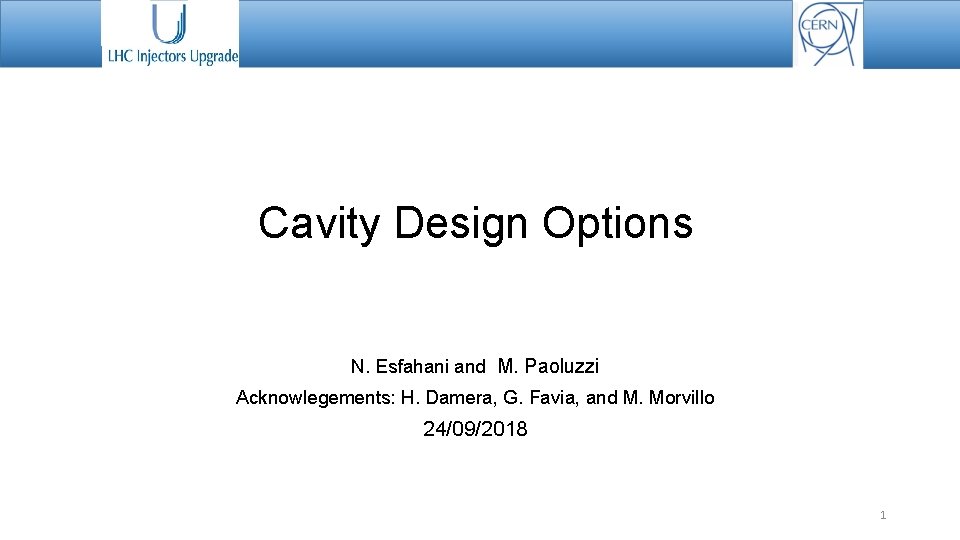
Cavity Design Options N. Esfahani and M. Paoluzzi Acknowlegements: H. Damera, G. Favia, and M. Morvillo 24/09/2018 1
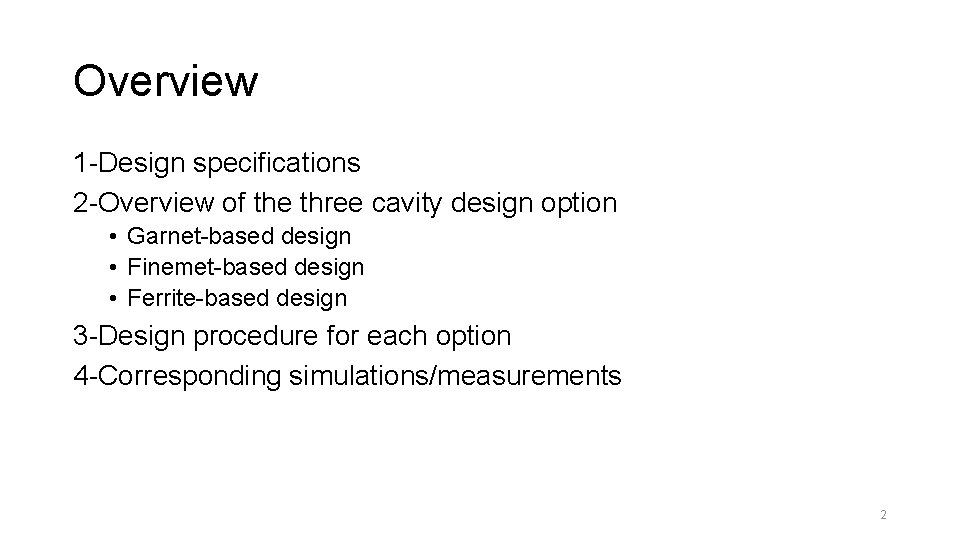
Overview 1 -Design specifications 2 -Overview of the three cavity design option • Garnet-based design • Finemet-based design • Ferrite-based design 3 -Design procedure for each option 4 -Corresponding simulations/measurements 2
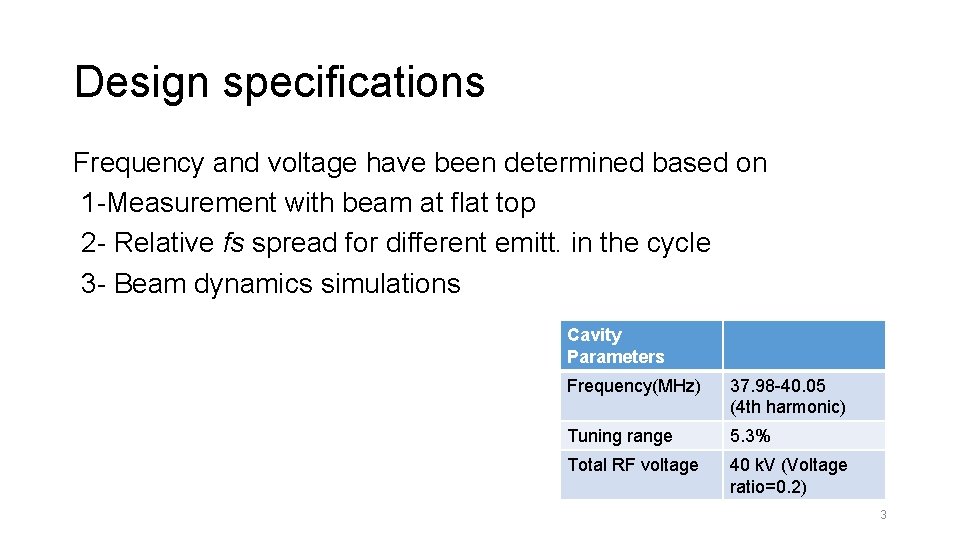
Design specifications Frequency and voltage have been determined based on 1 -Measurement with beam at flat top 2 - Relative fs spread for different emitt. in the cycle 3 - Beam dynamics simulations Cavity Parameters Frequency(MHz) 37. 98 -40. 05 (4 th harmonic) Tuning range 5. 3% Total RF voltage 40 k. V (Voltage ratio=0. 2) 3
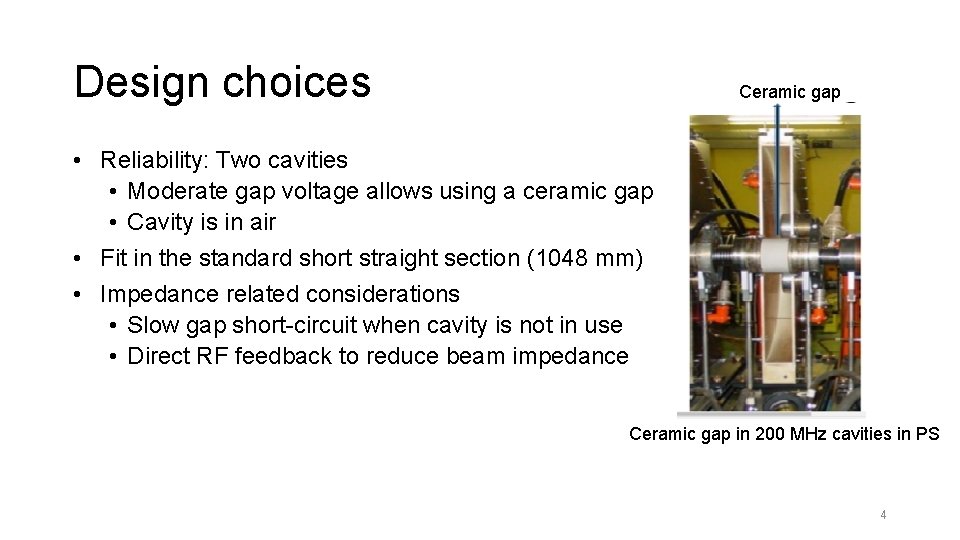
Design choices Ceramic gap • Reliability: Two cavities • Moderate gap voltage allows using a ceramic gap • Cavity is in air • Fit in the standard short straight section (1048 mm) • Impedance related considerations • Slow gap short-circuit when cavity is not in use • Direct RF feedback to reduce beam impedance Ceramic gap in 200 MHz cavities in PS 4
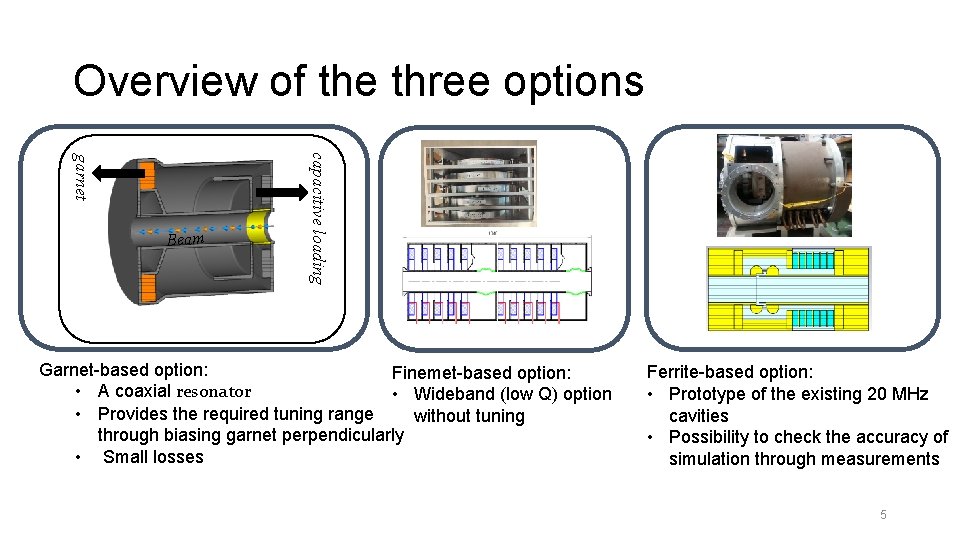
Overview of the three options capacitive loading garnet Beam Garnet-based option: Finemet-based option: • A coaxial resonator • Wideband (low Q) option • Provides the required tuning range without tuning through biasing garnet perpendicularly • Small losses Ferrite-based option: • Prototype of the existing 20 MHz cavities • Possibility to check the accuracy of simulation through measurements 5
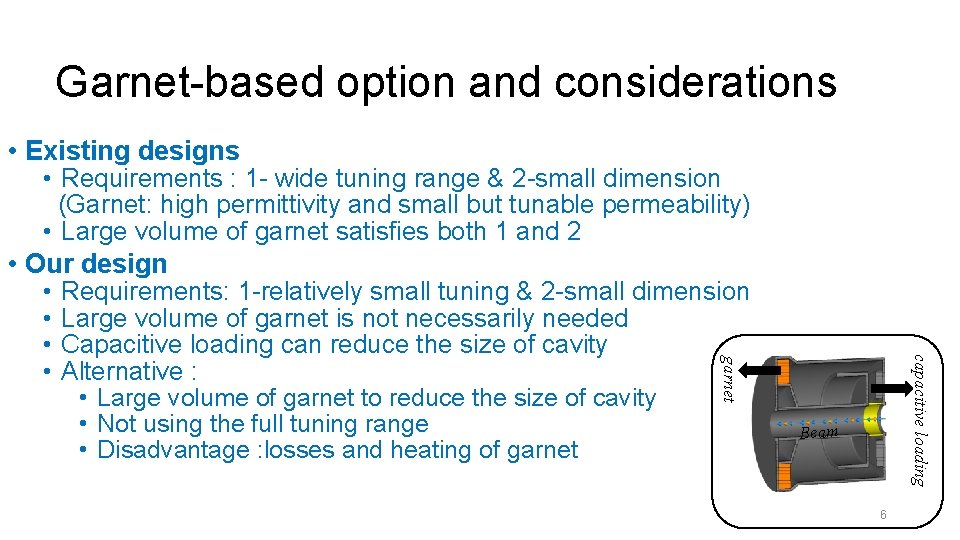
Garnet-based option and considerations • Existing designs • Requirements : 1 - wide tuning range & 2 -small dimension capacitive loading garnet (Garnet: high permittivity and small but tunable permeability) • Large volume of garnet satisfies both 1 and 2 • Our design • Requirements: 1 -relatively small tuning & 2 -small dimension • Large volume of garnet is not necessarily needed • Capacitive loading can reduce the size of cavity • Alternative : • Large volume of garnet to reduce the size of cavity • Not using the full tuning range • Disadvantage : losses and heating of garnet Beam 6
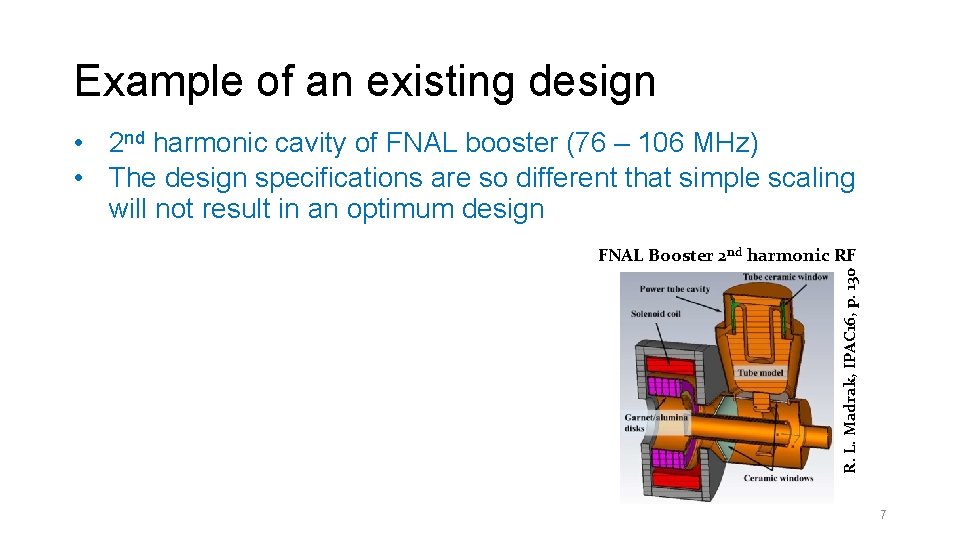
Example of an existing design • 2 nd harmonic cavity of FNAL booster (76 – 106 MHz) • The design specifications are so different that simple scaling will not result in an optimum design R. L. Madrak, IPAC 16, p. 130 FNAL Booster 2 nd harmonic RF 7
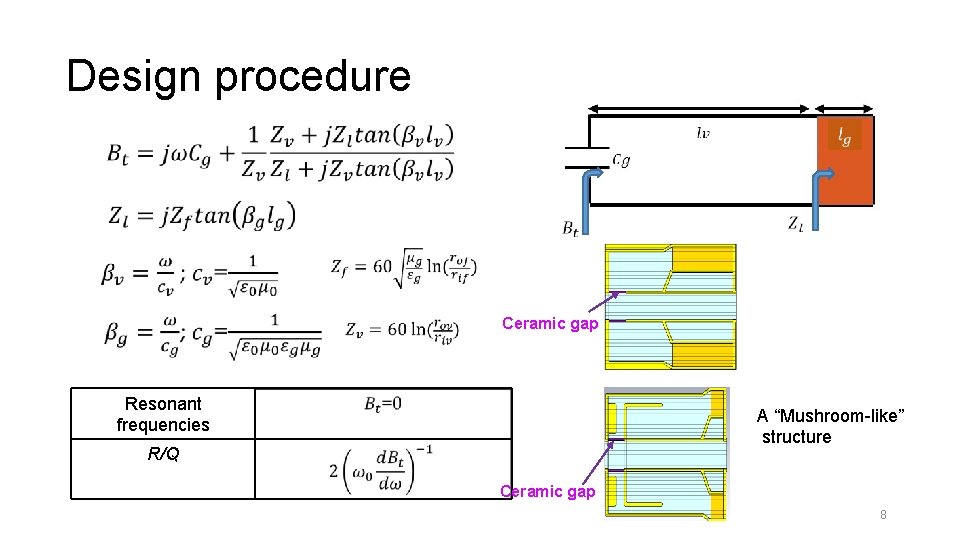
Design procedure Ceramic gap Resonant frequencies A “Mushroom-like” structure R/Q Ceramic gap 8
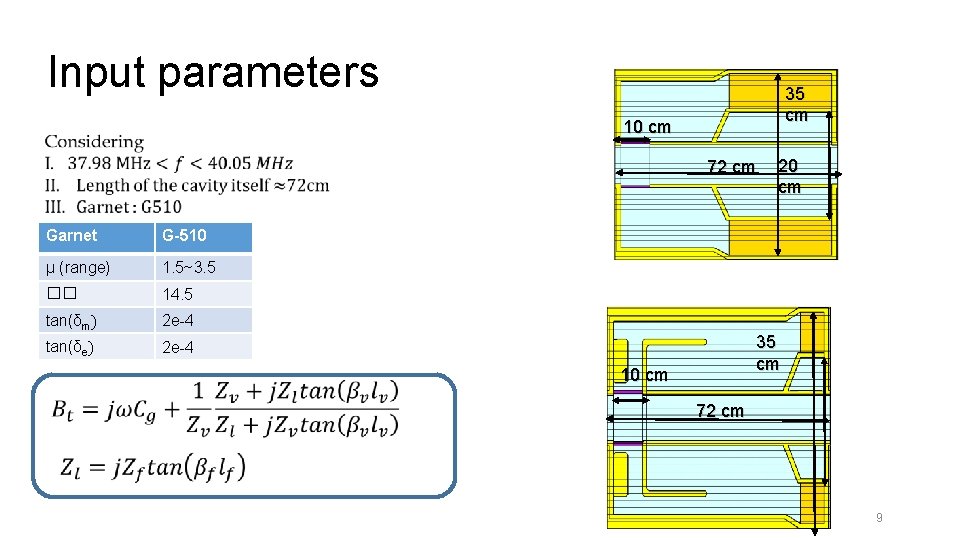
Input parameters 35 cm 10 cm 72 cm Garnet G-510 μ (range) 1. 5~3. 5 �� 14. 5 tan(δm) 2 e-4 tan(δe) 2 e-4 20 cm 35 cm 10 cm 72 cm 9
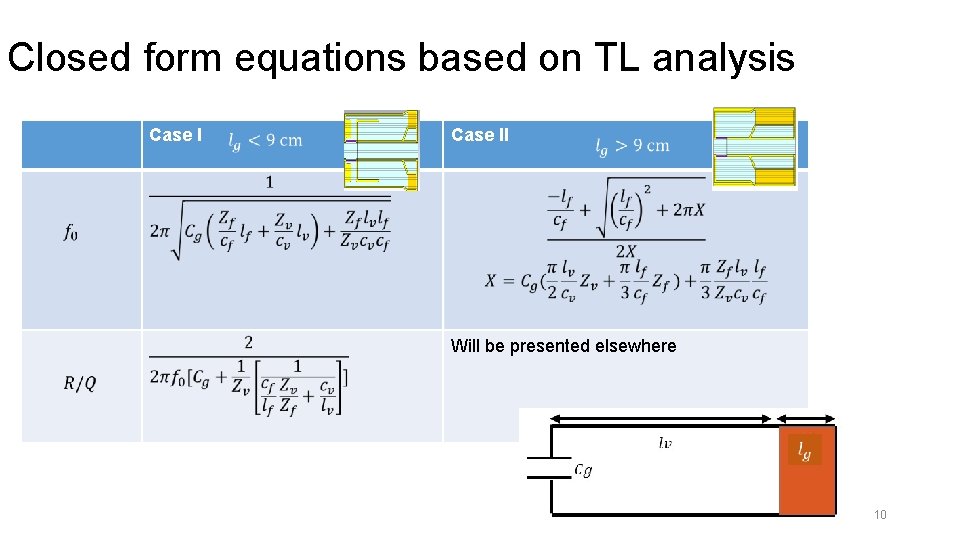
Closed form equations based on TL analysis Case II Will be presented elsewhere 10
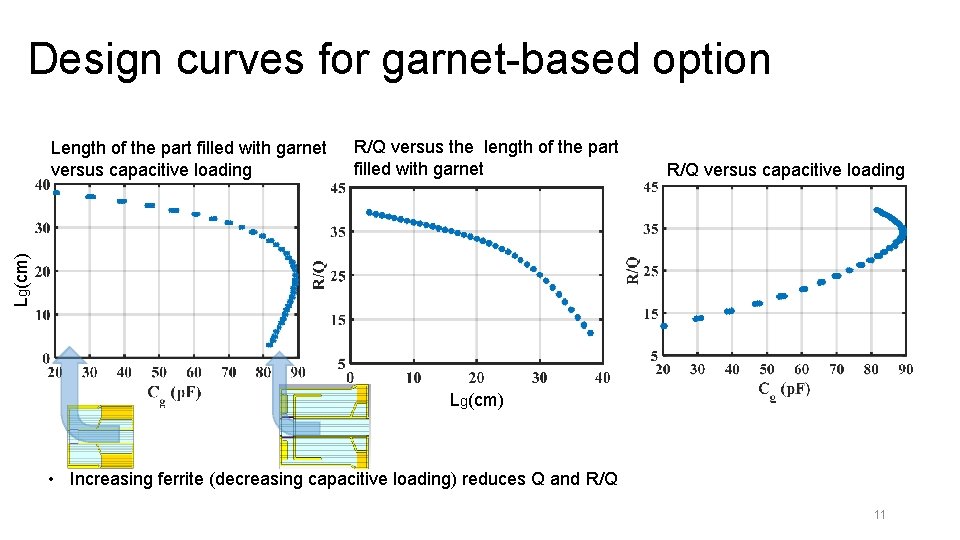
Design curves for garnet-based option R/Q versus the length of the part filled with garnet R/Q versus capacitive loading Lg(cm) Length of the part filled with garnet versus capacitive loading Lg(cm) • Increasing ferrite (decreasing capacitive loading) reduces Q and R/Q 11
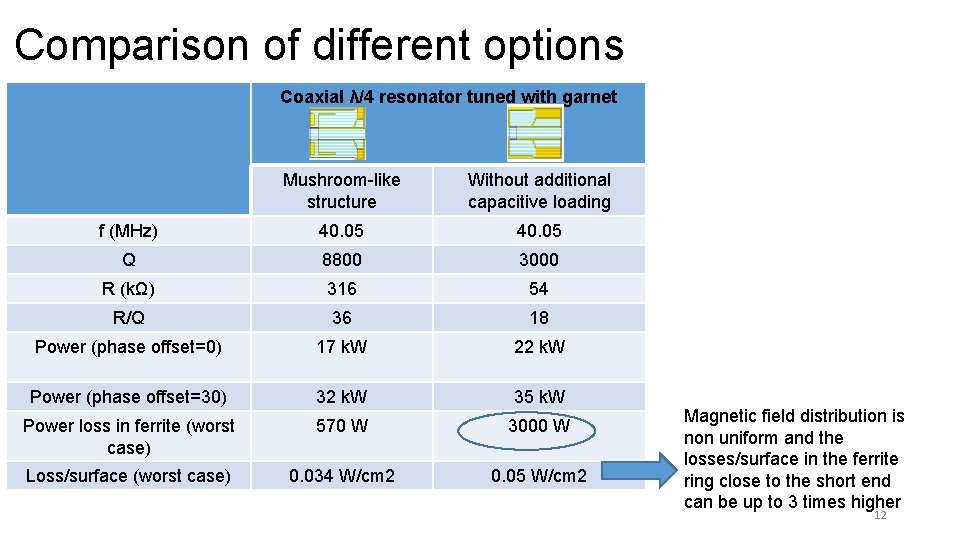
Comparison of different options Coaxial λ/4 resonator tuned with garnet Mushroom-like structure Without additional capacitive loading f (MHz) 40. 05 Q 8800 3000 R (kΩ) 316 54 R/Q 36 18 Power (phase offset=0) 17 k. W 22 k. W Power (phase offset=30) 32 k. W 35 k. W Power loss in ferrite (worst case) 570 W 3000 W Loss/surface (worst case) 0. 034 W/cm 2 0. 05 W/cm 2 Magnetic field distribution is non uniform and the losses/surface in the ferrite ring close to the short end can be up to 3 times higher 12
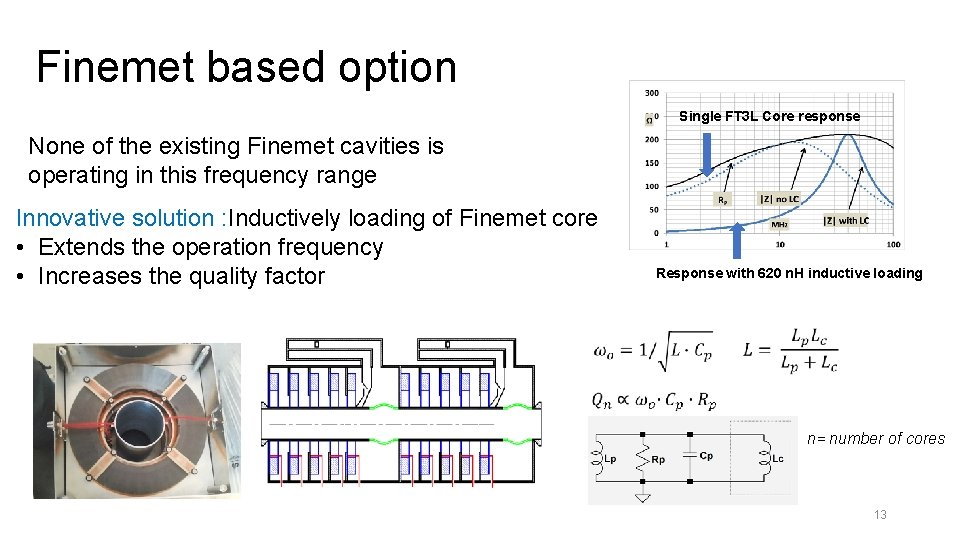
Finemet based option Single FT 3 L Core response None of the existing Finemet cavities is operating in this frequency range Innovative solution : Inductively loading of Finemet core • Extends the operation frequency • Increases the quality factor Response with 620 n. H inductive loading n= number of cores 13
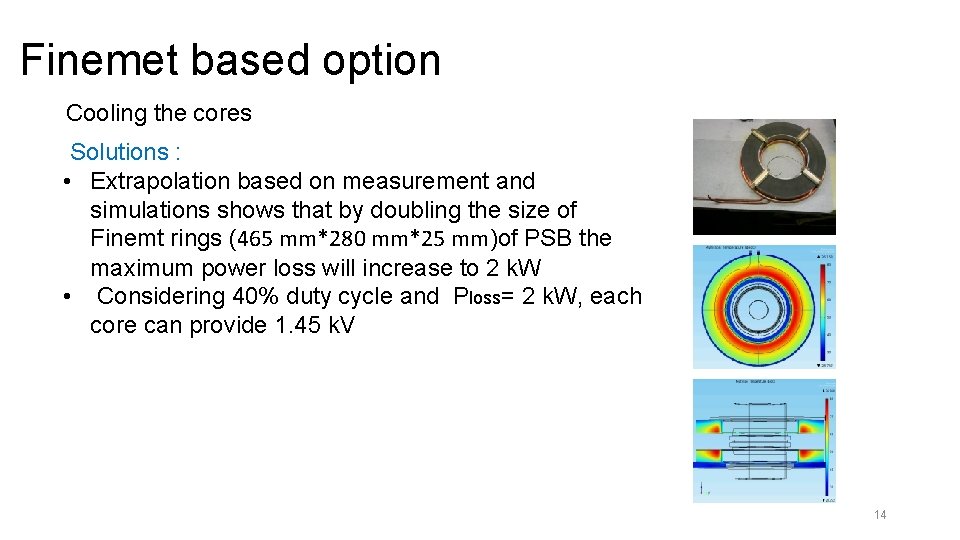
Finemet based option Cooling the cores Solutions : • Extrapolation based on measurement and simulations shows that by doubling the size of Finemt rings (465 mm*280 mm*25 mm)of PSB the maximum power loss will increase to 2 k. W • Considering 40% duty cycle and Ploss= 2 k. W, each core can provide 1. 45 k. V 14
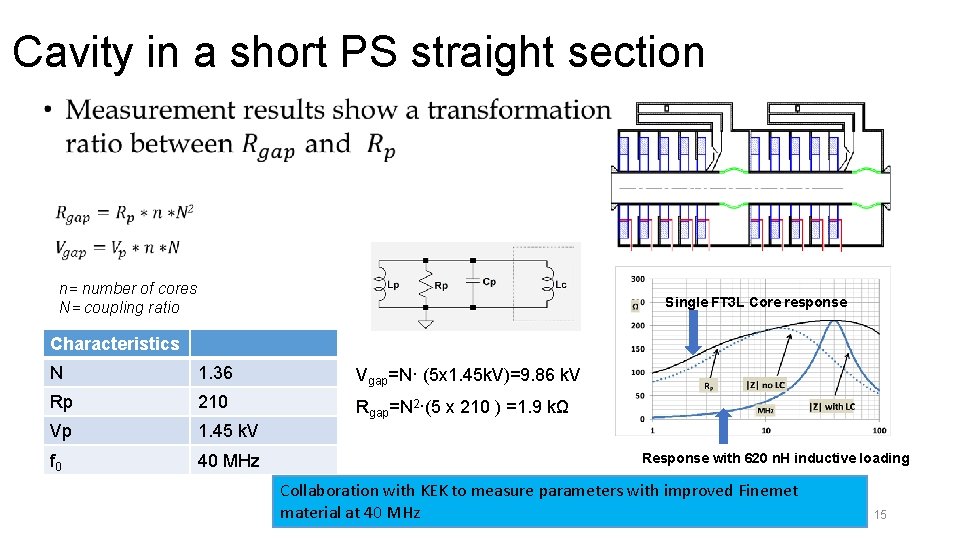
Cavity in a short PS straight section n= number of cores N= coupling ratio Single FT 3 L Core response Characteristics N 1. 36 Vgap=N· (5 x 1. 45 k. V)=9. 86 k. V Rp 210 Rgap=N 2·(5 x 210 ) =1. 9 kΩ Vp 1. 45 k. V f 0 40 MHz Response with 620 n. H inductive loading Collaboration with KEK to measure parameters with improved Finemet material at 40 MHz 15
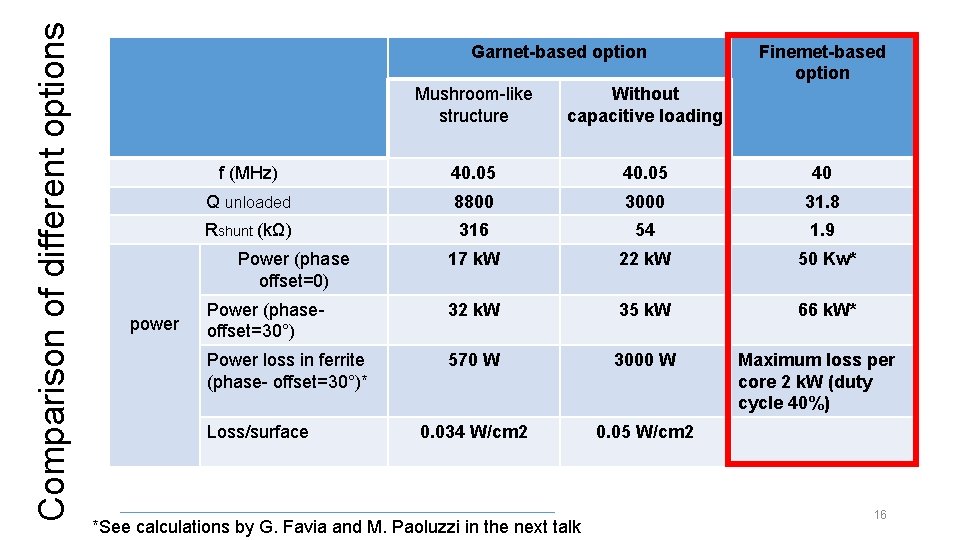
Comparison of different options Garnet-based option Mushroom-like structure Without capacitive loading f (MHz) 40. 05 40 Q unloaded 8800 3000 31. 8 Rshunt (kΩ) 316 54 1. 9 17 k. W 22 k. W 50 Kw* Power (phaseoffset=30°) 32 k. W 35 k. W 66 k. W* Power loss in ferrite (phase- offset=30°)* 570 W 3000 W 0. 034 W/cm 2 0. 05 W/cm 2 Power (phase offset=0) power Finemet-based option Loss/surface *See calculations by G. Favia and M. Paoluzzi in the next talk Maximum loss per core 2 k. W (duty cycle 40%) 16
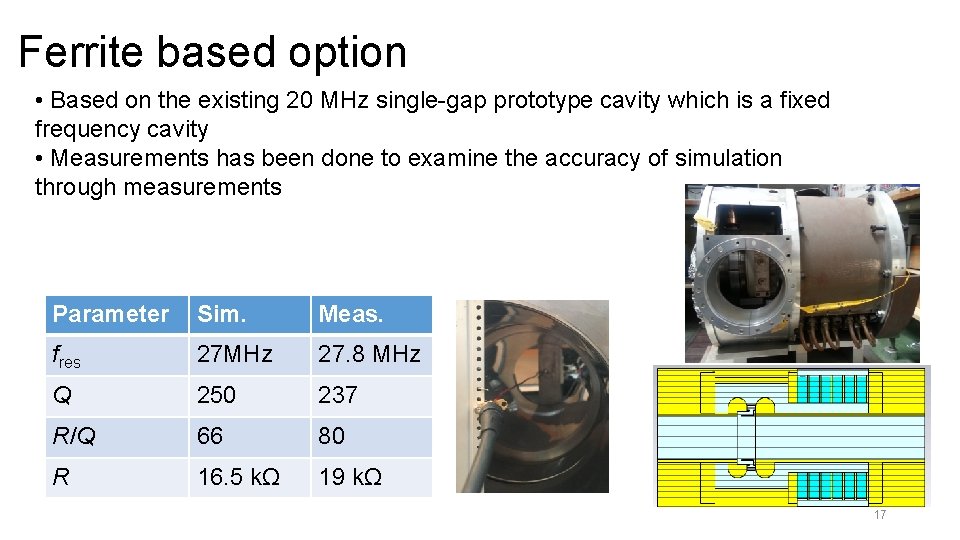
Ferrite based option • Based on the existing 20 MHz single-gap prototype cavity which is a fixed frequency cavity • Measurements has been done to examine the accuracy of simulation through measurements Parameter Sim. Meas. fres 27 MHz 27. 8 MHz Q 250 237 R/Q 66 80 R 16. 5 kΩ 19 kΩ 17
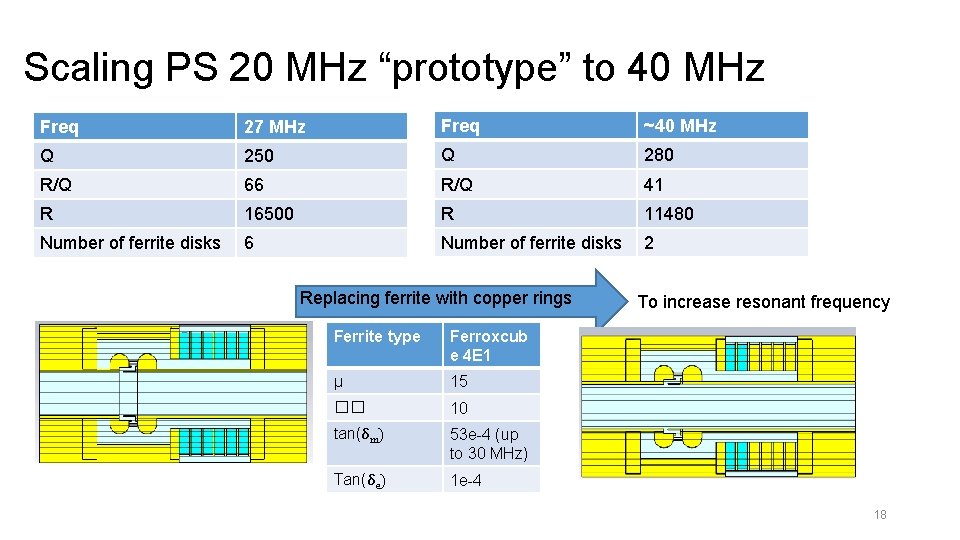
Scaling PS 20 MHz “prototype” to 40 MHz Freq 27 MHz Freq ~40 MHz Q 250 Q 280 R/Q 66 R/Q 41 R 16500 R 11480 Number of ferrite disks 6 Number of ferrite disks 2 Replacing ferrite with copper rings Ferrite type Ferroxcub e 4 E 1 μ 15 �� 10 tan(δm) 53 e-4 (up to 30 MHz) Tan(δe) 1 e-4 To increase resonant frequency 18
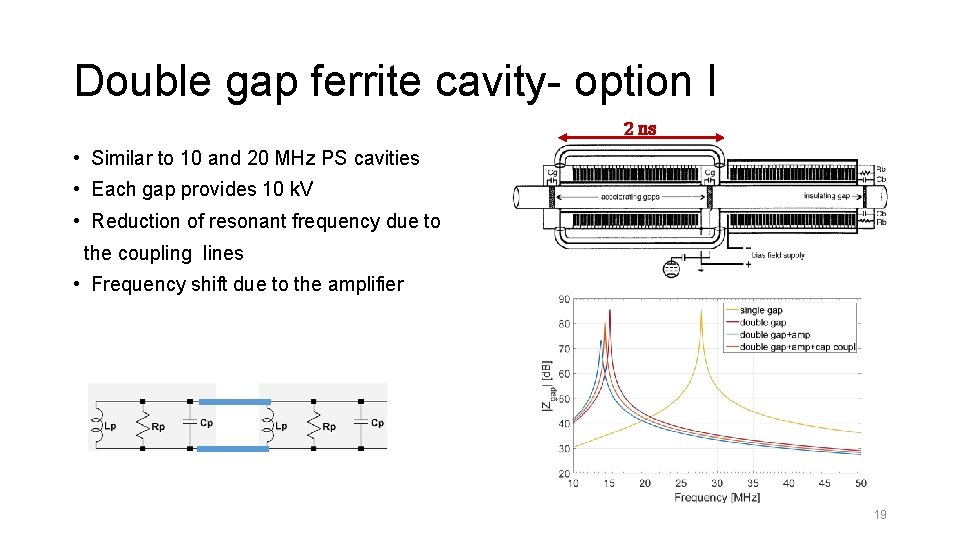
Double gap ferrite cavity- option I 2 ns • Similar to 10 and 20 MHz PS cavities • Each gap provides 10 k. V • Reduction of resonant frequency due to the coupling lines • Frequency shift due to the amplifier 19
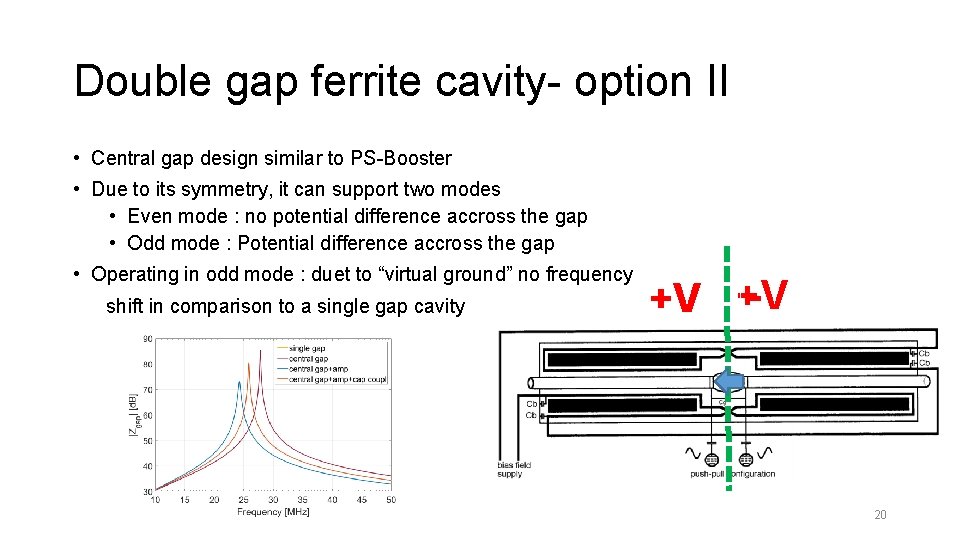
Double gap ferrite cavity- option II • Central gap design similar to PS-Booster • Due to its symmetry, it can support two modes • Even mode : no potential difference accross the gap • Odd mode : Potential difference accross the gap • Operating in odd mode : duet to “virtual ground” no frequency shift in comparison to a single gap cavity -V +V +V 20
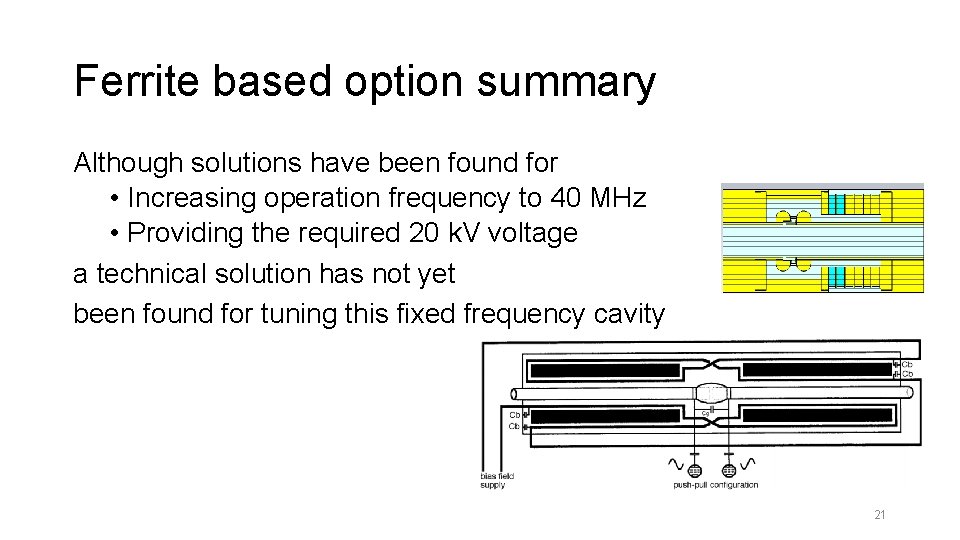
Ferrite based option summary Although solutions have been found for • Increasing operation frequency to 40 MHz • Providing the required 20 k. V voltage a technical solution has not yet been found for tuning this fixed frequency cavity 21
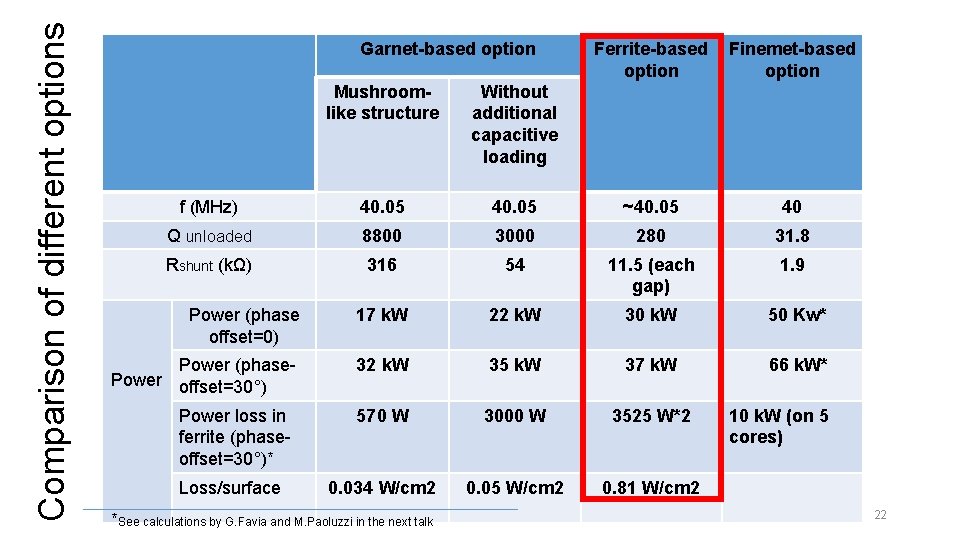
Comparison of different options Garnet-based option Ferrite-based option Finemet-based option Mushroomlike structure Without additional capacitive loading f (MHz) 40. 05 ~40. 05 40 Q unloaded 8800 3000 280 31. 8 Rshunt (kΩ) 316 54 11. 5 (each gap) 1. 9 Power (phase offset=0) 17 k. W 22 k. W 30 k. W 50 Kw* Power (phase. Power offset=30°) 32 k. W 35 k. W 37 k. W 66 k. W* Power loss in ferrite (phaseoffset=30°)* 570 W 3000 W 3525 W*2 Loss/surface 0. 034 W/cm 2 0. 05 W/cm 2 0. 81 W/cm 2 *See calculations by G. Favia and M. Paoluzzi in the next talk 10 k. W (on 5 cores) 22
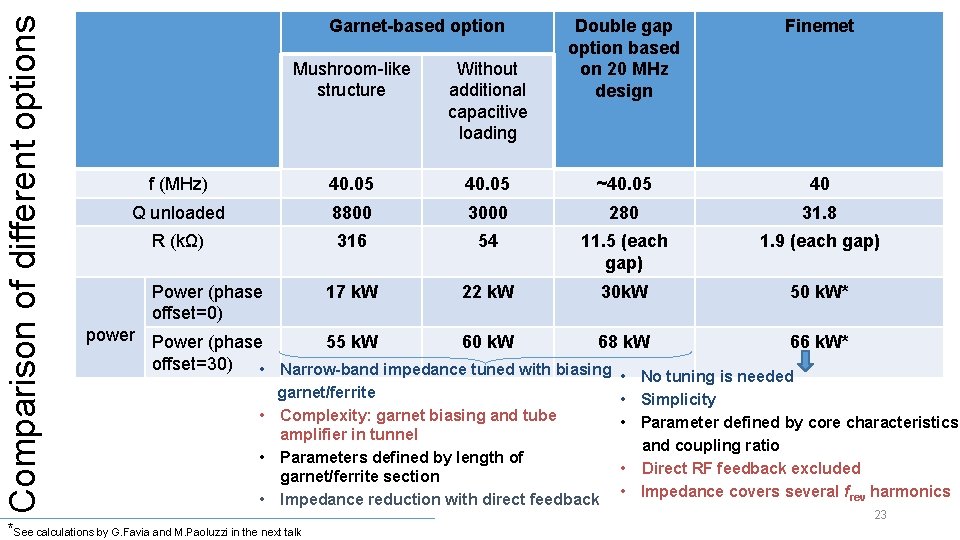
Comparison of different options Garnet-based option Double gap option based on 20 MHz design Finemet Mushroom-like structure Without additional capacitive loading f (MHz) 40. 05 ~40. 05 40 Q unloaded 8800 3000 280 31. 8 R (kΩ) 316 54 11. 5 (each gap) 1. 9 (each gap) 17 k. W 22 k. W 30 k. W 50 k. W* Power (phase offset=0) power Power (phase 55 k. W 60 k. W 68 k. W 66 k. W* offset=30) • Narrow-band impedance tuned with biasing • No tuning is needed garnet/ferrite • Complexity: garnet biasing and tube amplifier in tunnel • Parameters defined by length of garnet/ferrite section • Impedance reduction with direct feedback *See calculations by G. Favia and M. Paoluzzi in the next talk • Simplicity • Parameter defined by core characteristics and coupling ratio • Direct RF feedback excluded • Impedance covers several frev harmonics 23
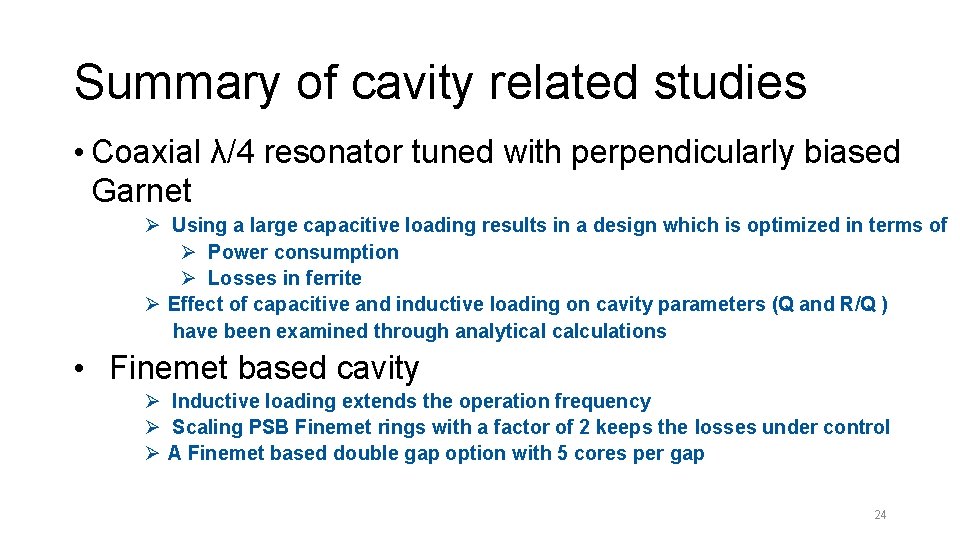
Summary of cavity related studies • Coaxial λ/4 resonator tuned with perpendicularly biased Garnet Ø Using a large capacitive loading results in a design which is optimized in terms of Ø Power consumption Ø Losses in ferrite Ø Effect of capacitive and inductive loading on cavity parameters (Q and R/Q ) have been examined through analytical calculations • Finemet based cavity Ø Inductive loading extends the operation frequency Ø Scaling PSB Finemet rings with a factor of 2 keeps the losses under control Ø A Finemet based double gap option with 5 cores per gap 24
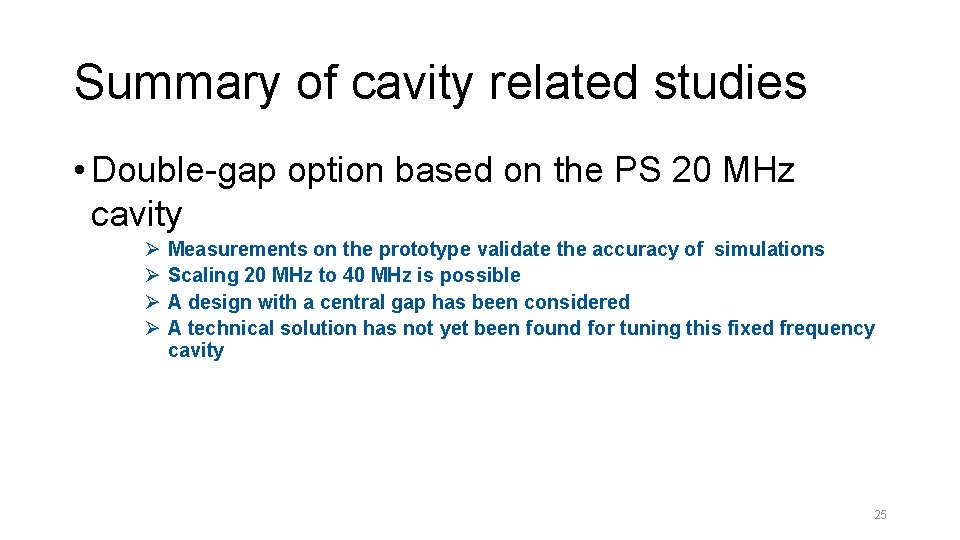
Summary of cavity related studies • Double-gap option based on the PS 20 MHz cavity Ø Ø Measurements on the prototype validate the accuracy of simulations Scaling 20 MHz to 40 MHz is possible A design with a central gap has been considered A technical solution has not yet been found for tuning this fixed frequency cavity 25
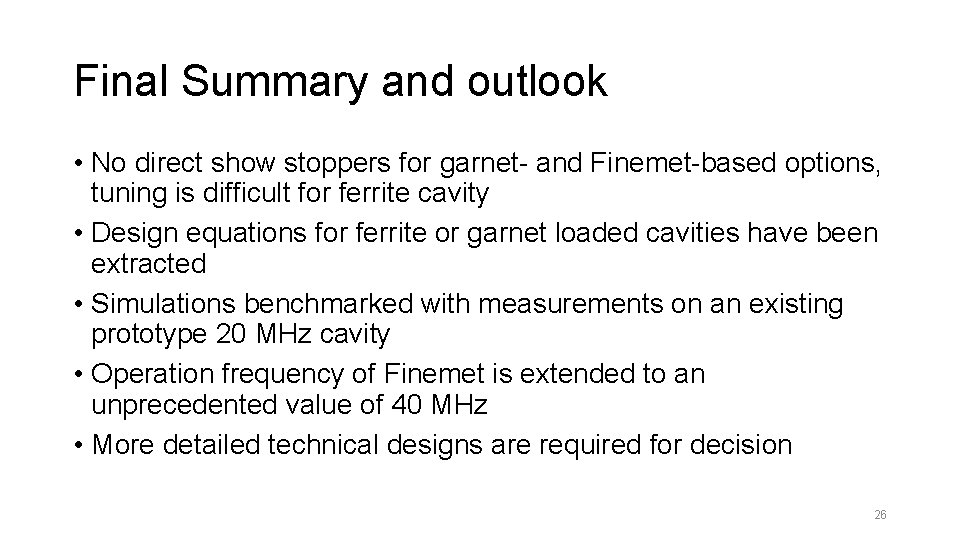
Final Summary and outlook • No direct show stoppers for garnet- and Finemet-based options, tuning is difficult for ferrite cavity • Design equations for ferrite or garnet loaded cavities have been extracted • Simulations benchmarked with measurements on an existing prototype 20 MHz cavity • Operation frequency of Finemet is extended to an unprecedented value of 40 MHz • More detailed technical designs are required for decision 26
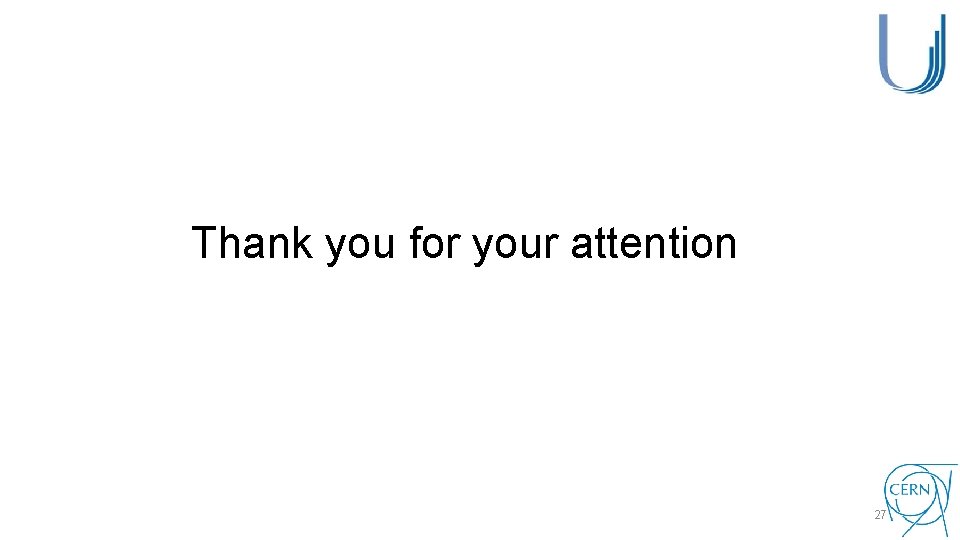
Thank you for your attention 27
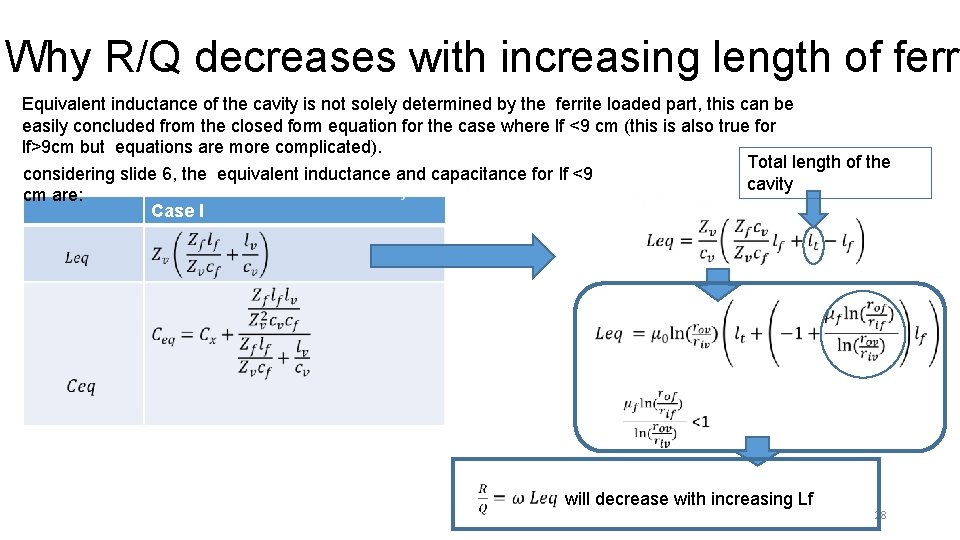
Why R/Q decreases with increasing length of ferri Equivalent inductance of the cavity is not solely determined by the ferrite loaded part, this can be easily concluded from the closed form equation for the case where lf <9 cm (this is also true for lf>9 cm but equations are more complicated). Total length of the considering slide 6, the equivalent inductance and capacitance for lf <9 cavity cm are: Case I will decrease with increasing Lf 28
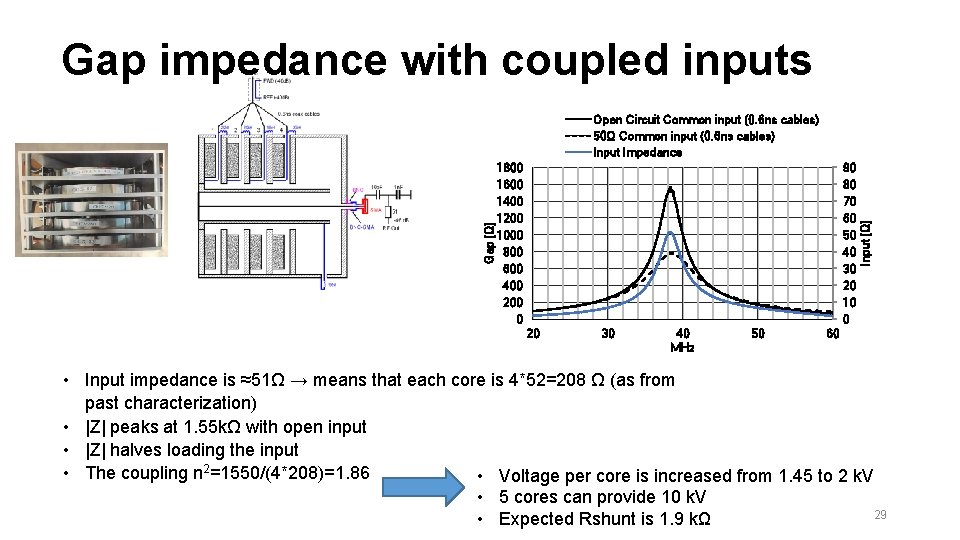
Gap impedance with coupled inputs 1800 1600 1400 1200 1000 800 600 400 200 0 90 80 70 60 50 40 30 20 10 0 20 30 40 MHz 50 Input [Ω] Gap [Ω] Open Circuit Common input (0. 6 ns cables) 50Ω Common input (0. 6 ns cables) Input Impedance 60 • Input impedance is ≈51Ω → means that each core is 4*52=208 Ω (as from past characterization) • |Z| peaks at 1. 55 kΩ with open input • |Z| halves loading the input • The coupling n 2=1550/(4*208)=1. 86 • Voltage per core is increased from 1. 45 to 2 k. V • 5 cores can provide 10 k. V 29 • Expected Rshunt is 1. 9 kΩ
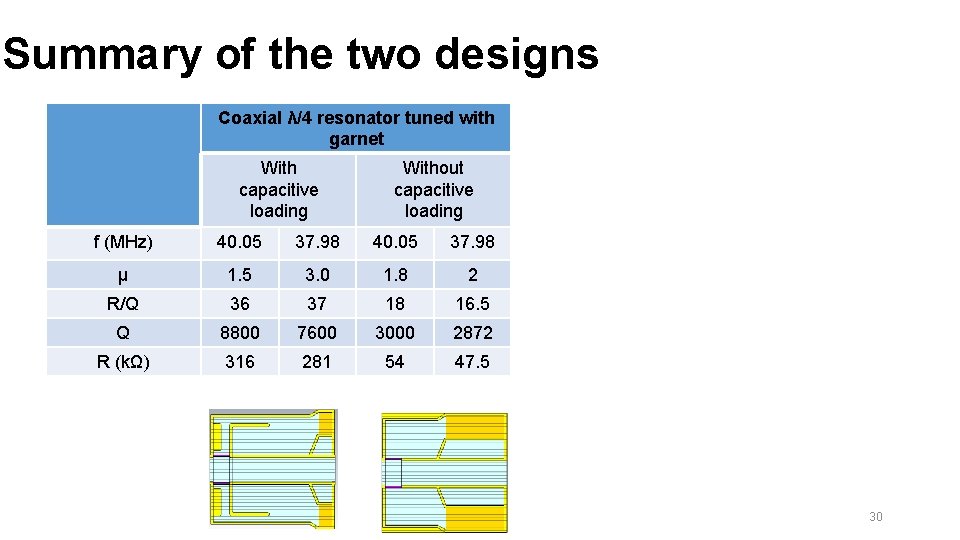
Summary of the two designs Coaxial λ/4 resonator tuned with garnet With capacitive loading Without capacitive loading f (MHz) 40. 05 37. 98 μ 1. 5 3. 0 1. 8 2 R/Q 36 37 18 16. 5 Q 8800 7600 3000 2872 R (kΩ) 316 281 54 47. 5 30
Ventral cavity
Label the abdominal regions.
Mental anatomical term
Double layer peritoneum
Epiploic foramen boundaries
Systems analysis & design in an age of options
Macro instruction arguments
Difference between linkage editor and linking loader
Factors influencing distribution network design
Design options for a distribution network
What is multi pass assembler
Distributor storage with customer pickup
Loader design options in system software
Features of loader
Isthmus tooth prep
Bevel in composite restoration
Axial wall depth in class 2 amalgam
Beveled conventional class 3
Erp selection methods and criteria
Read the paragraphs below
Options futures and risk management
Options futures and other derivatives
Forward vs option
Traders in financial futures
Currency futures
Options on stock indices and currencies
Tailing the hedge
Erp system options and selection methods
System and forms design
Tooth surfaces chart
Pharynx and oral cavity