Spontaneous Fission Properties of Superheavy Nuclei Fritz Peter
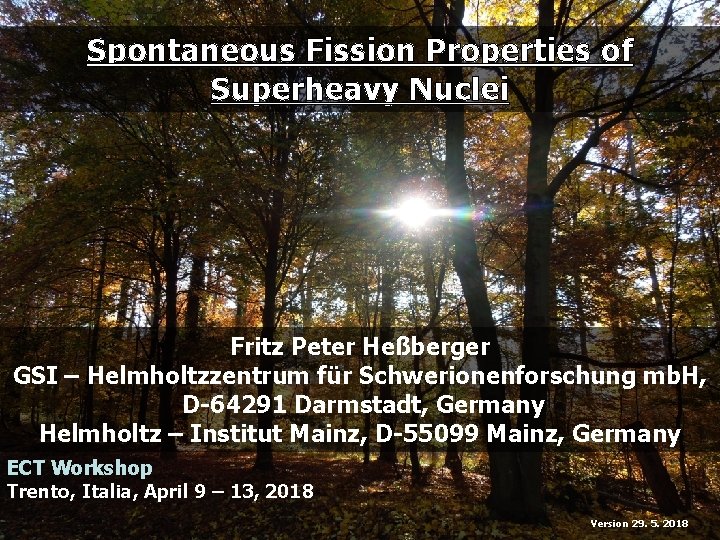
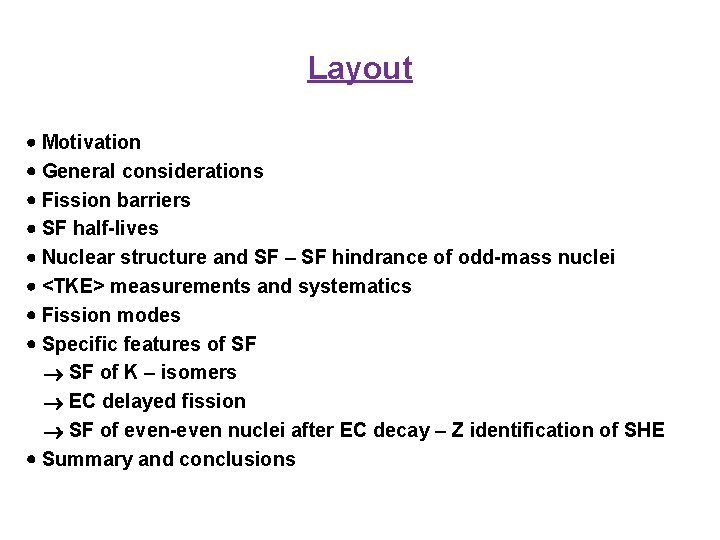
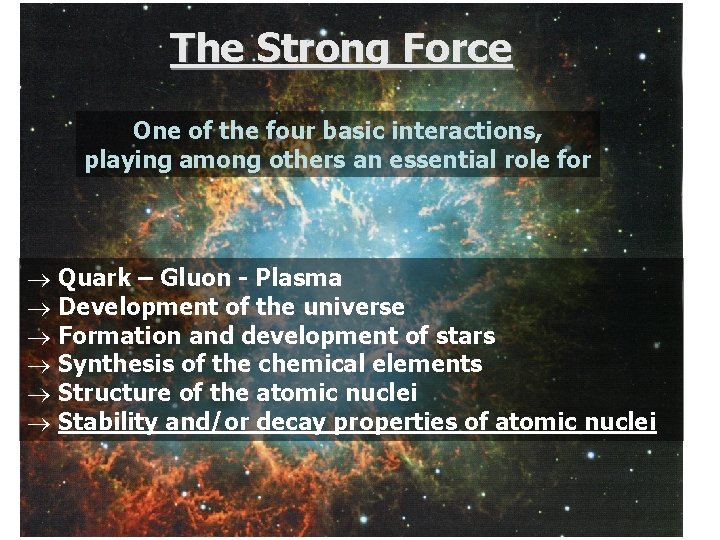
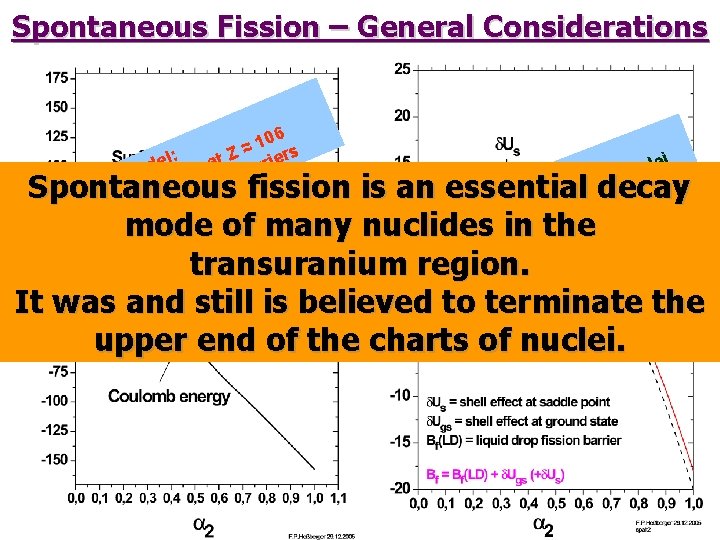
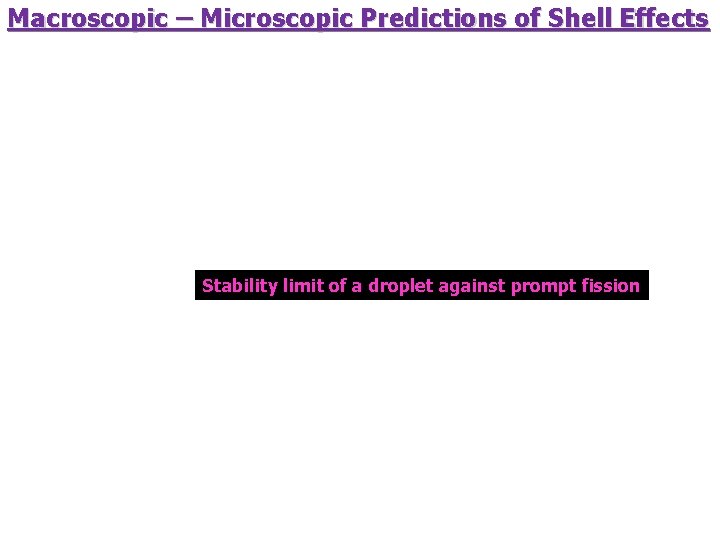
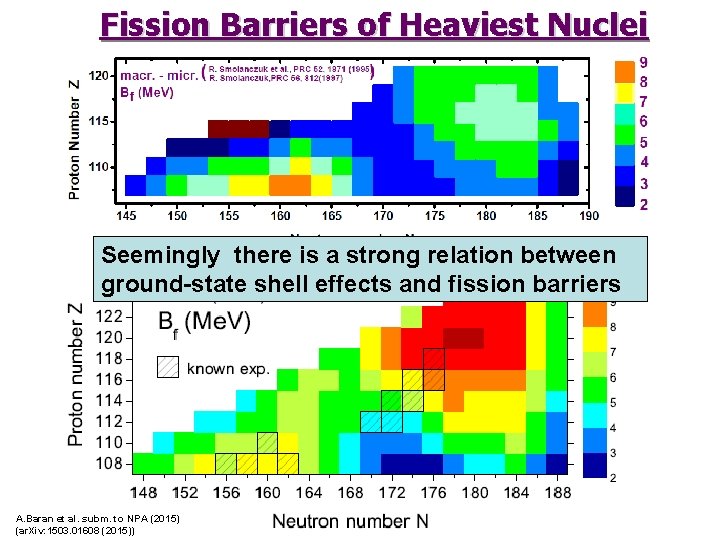
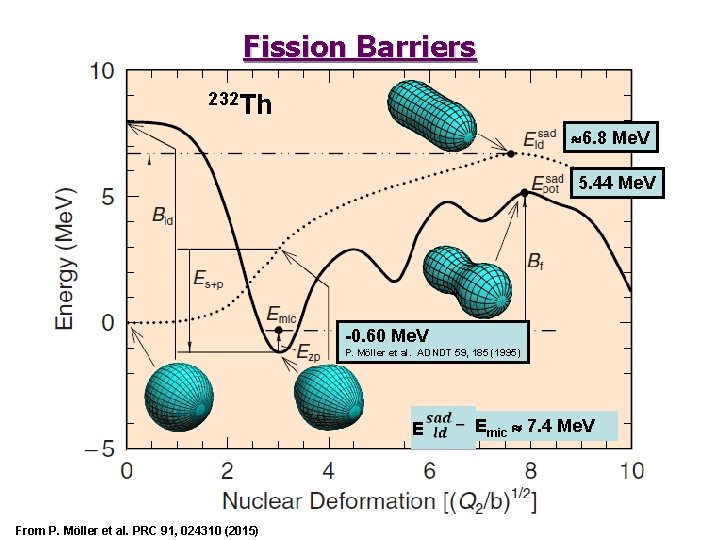
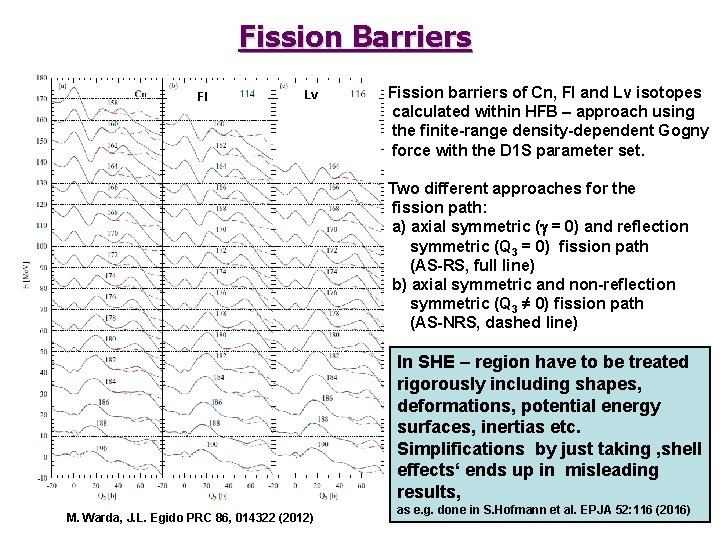
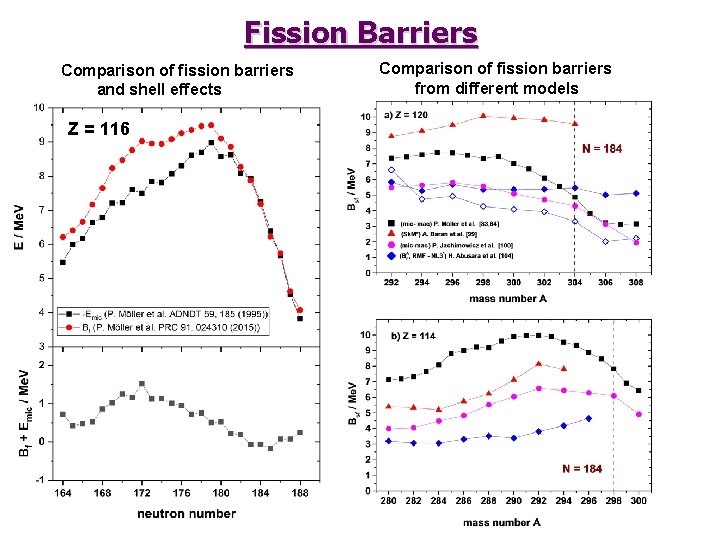
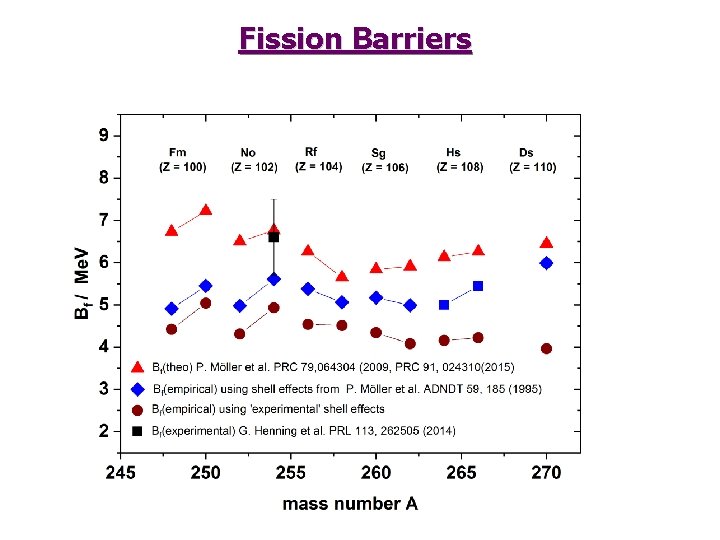
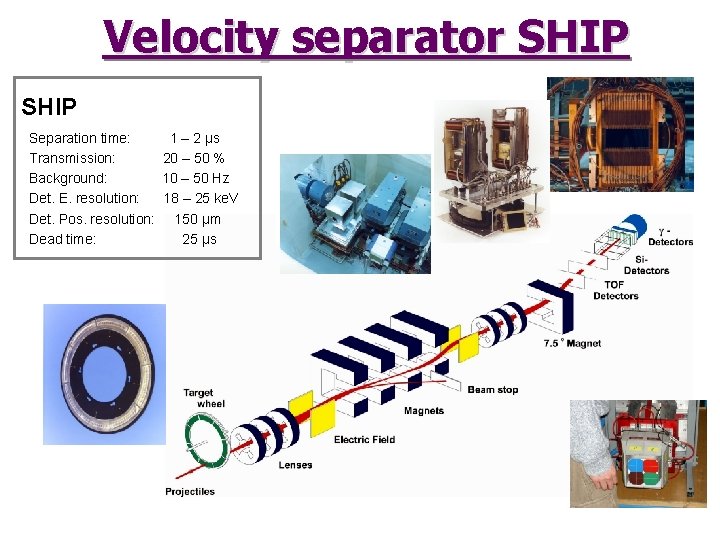
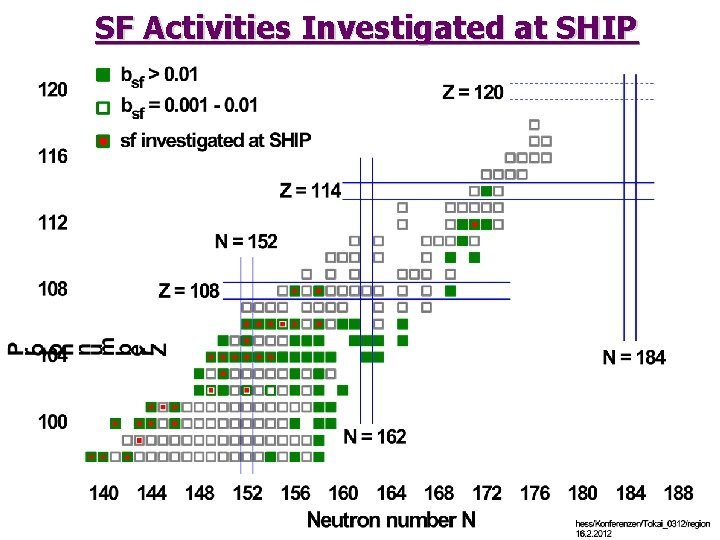
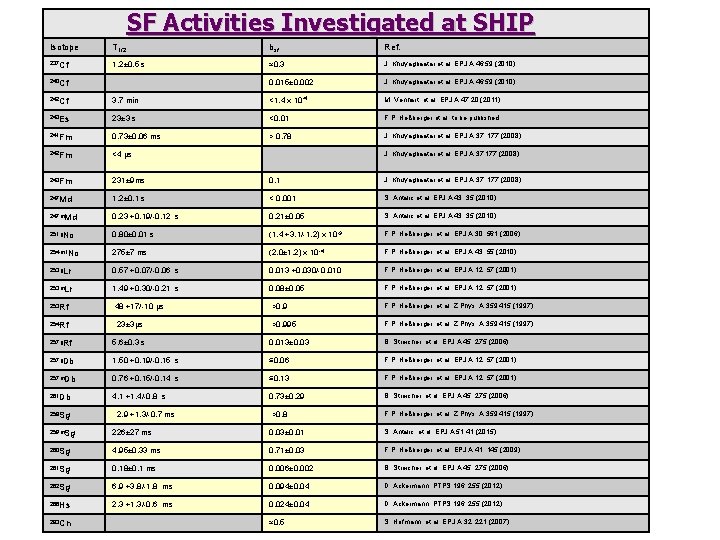
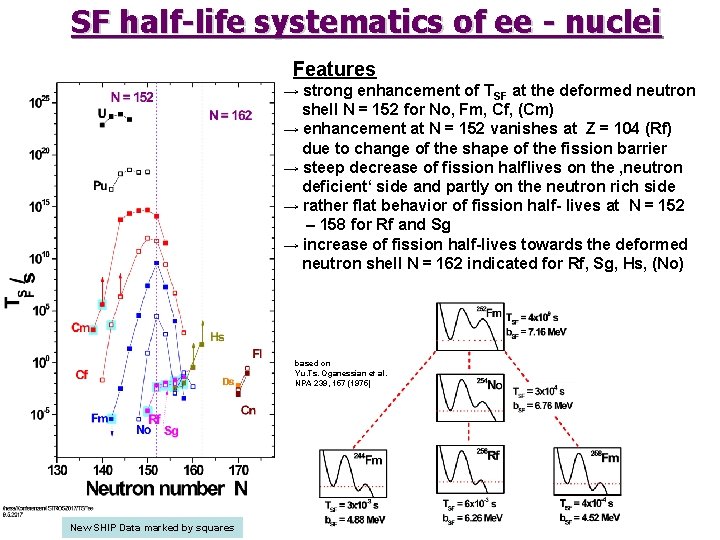
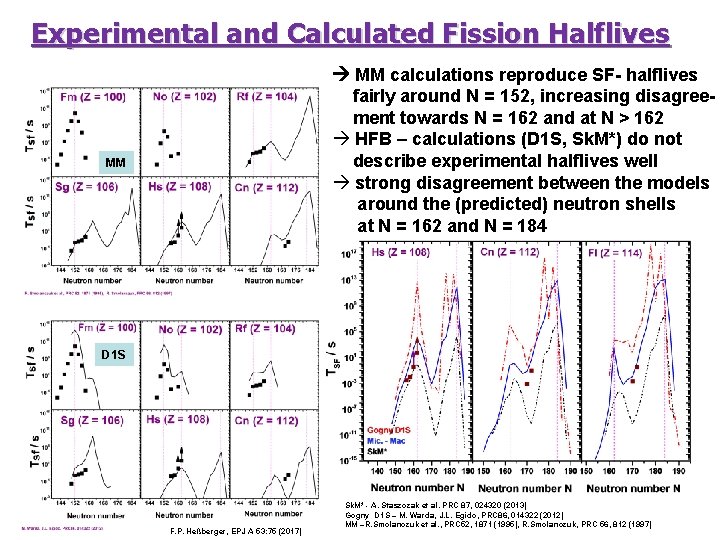
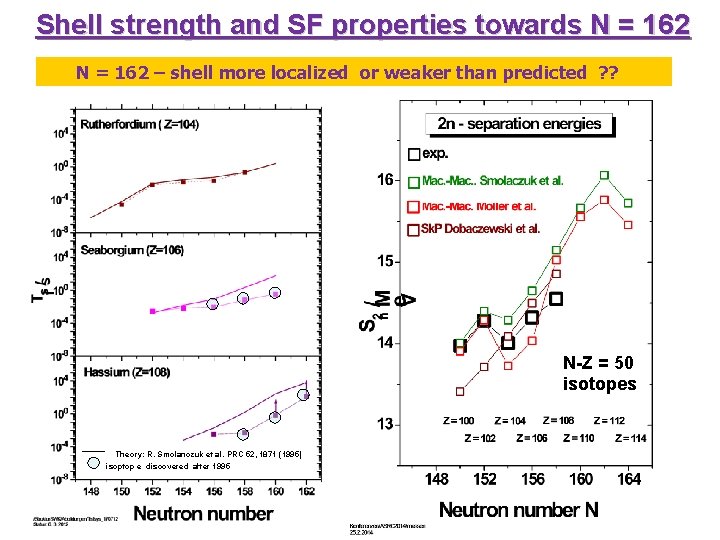
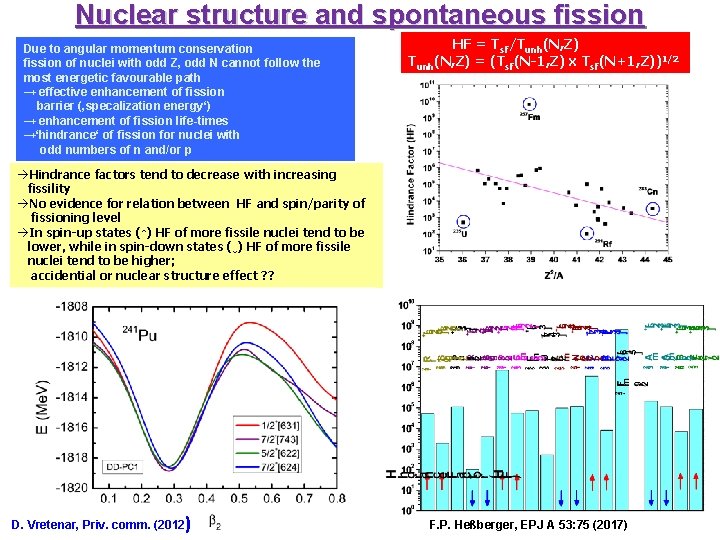
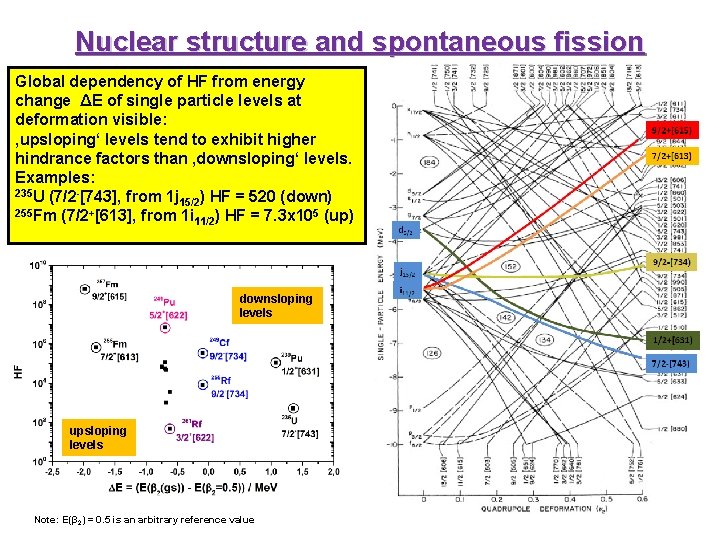
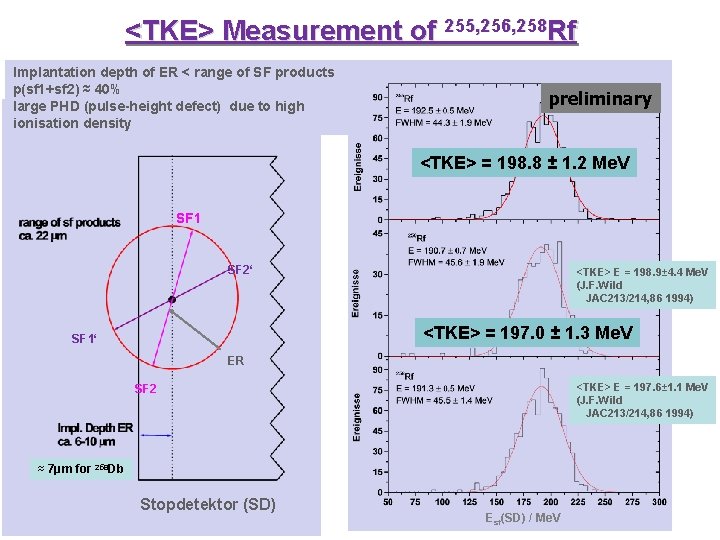
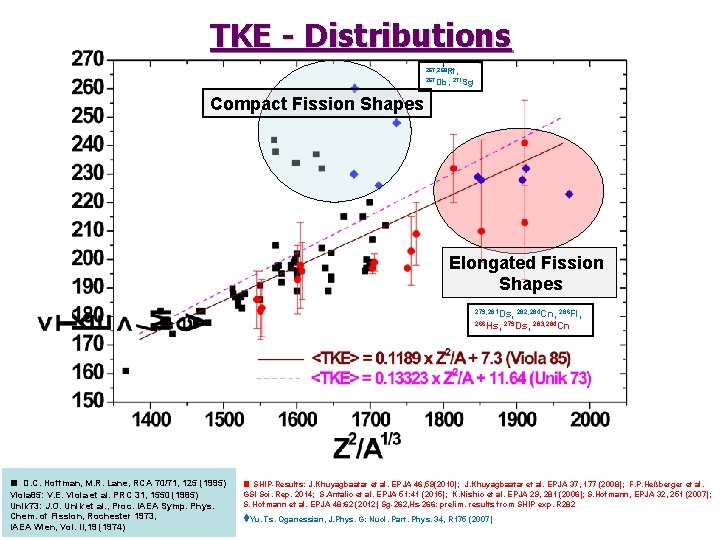
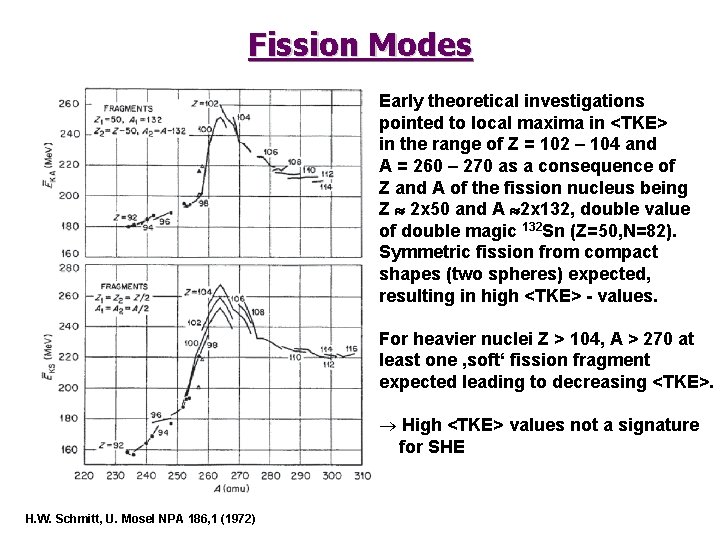
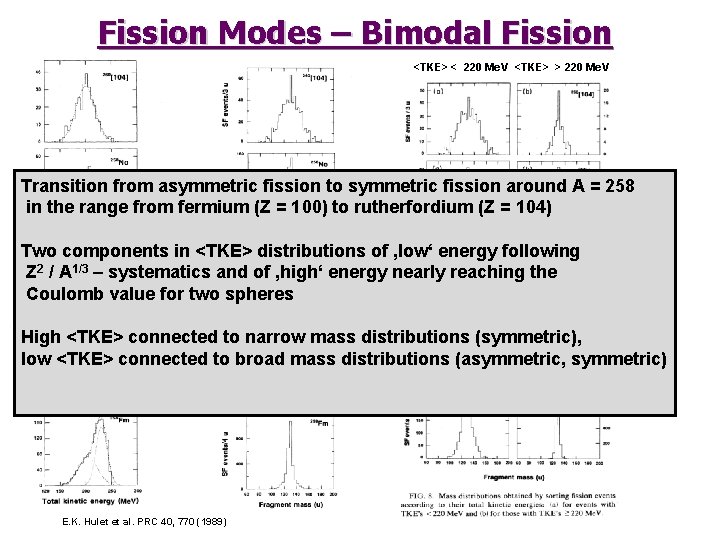
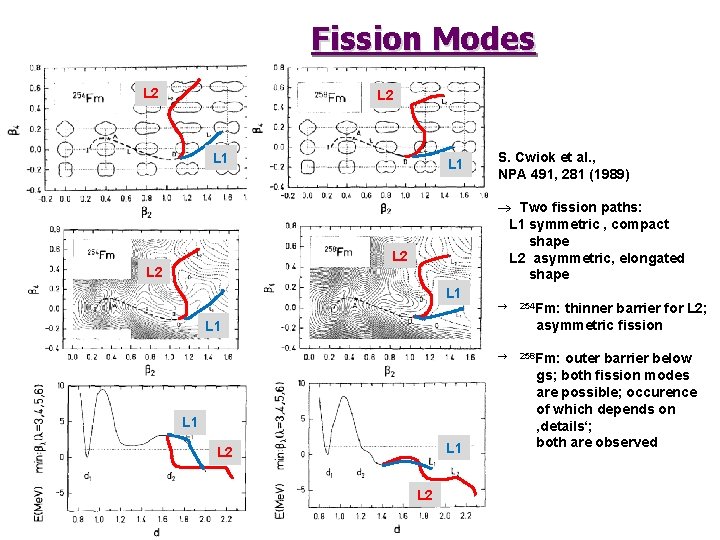
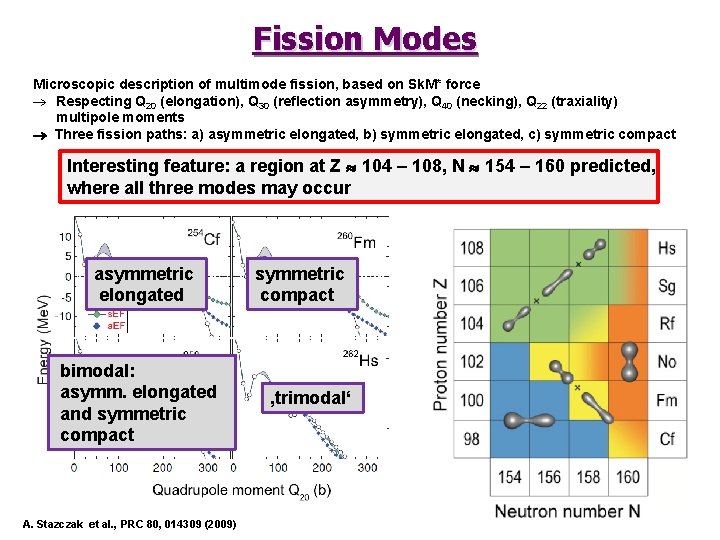
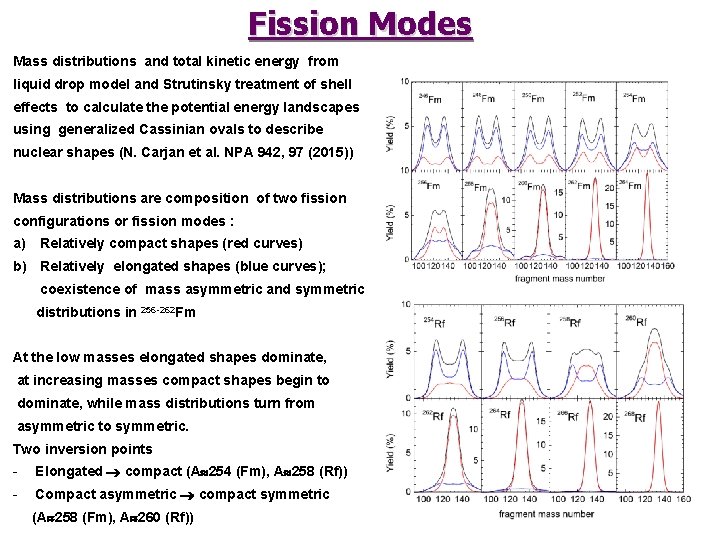
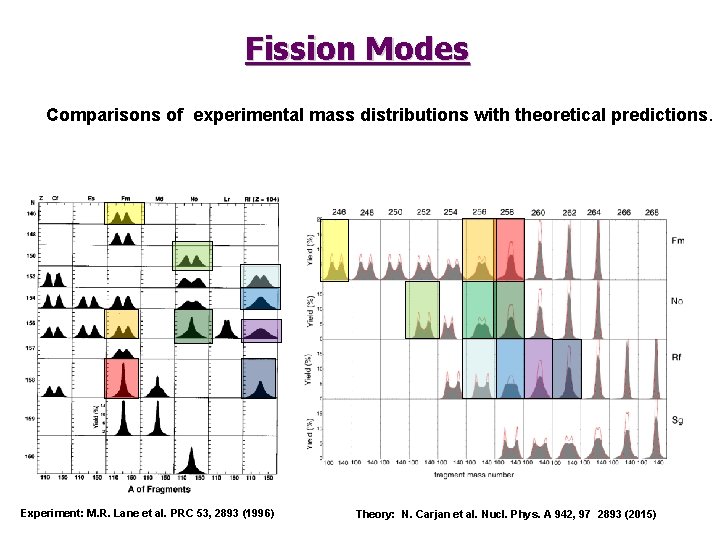
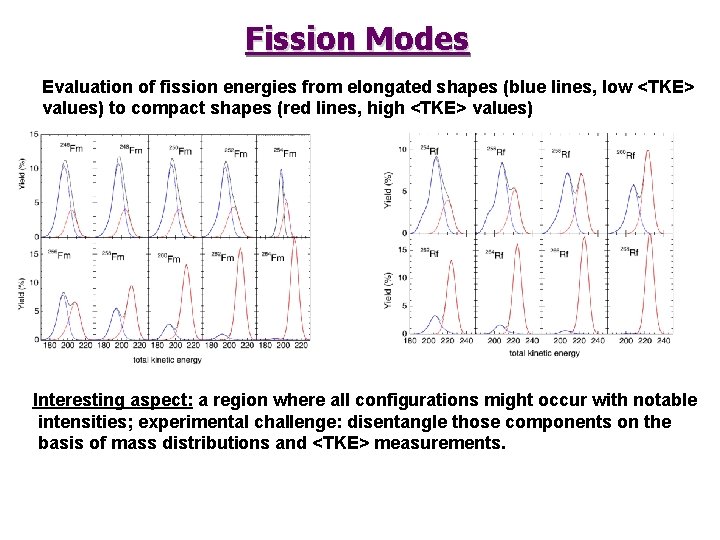
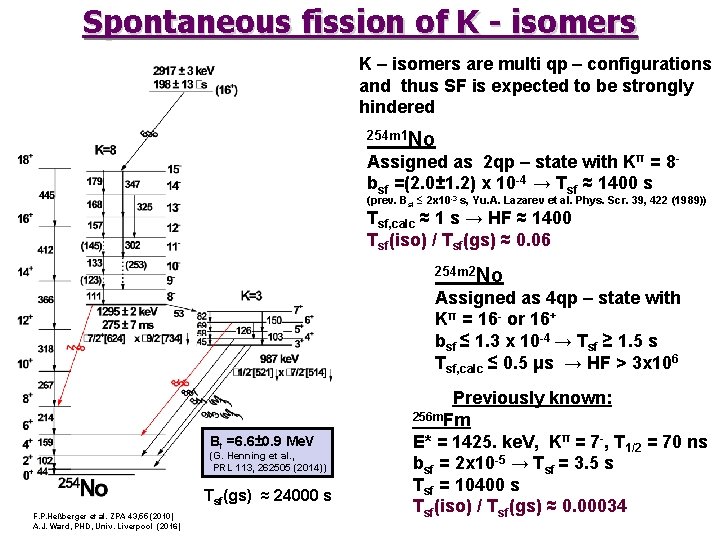
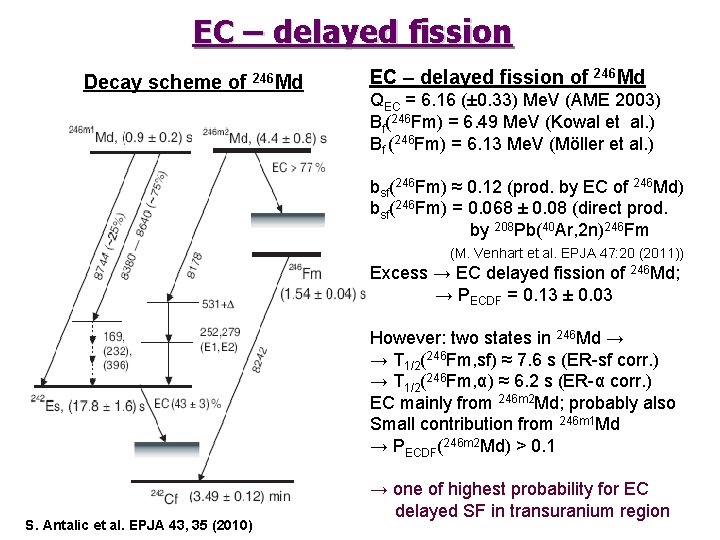
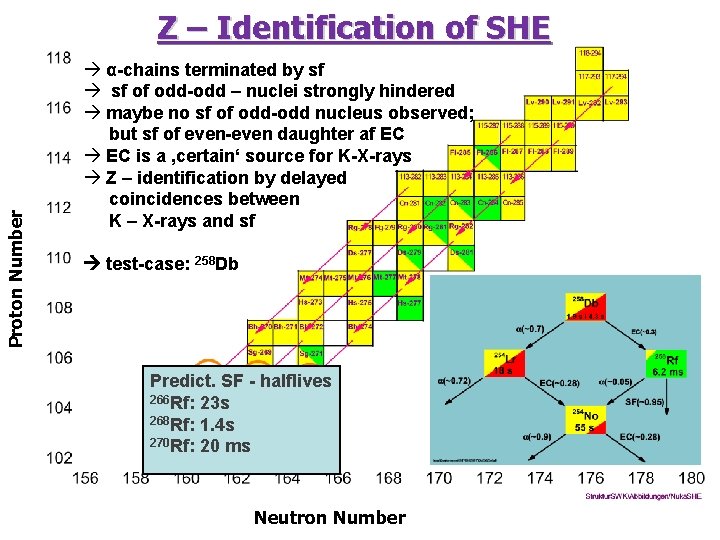
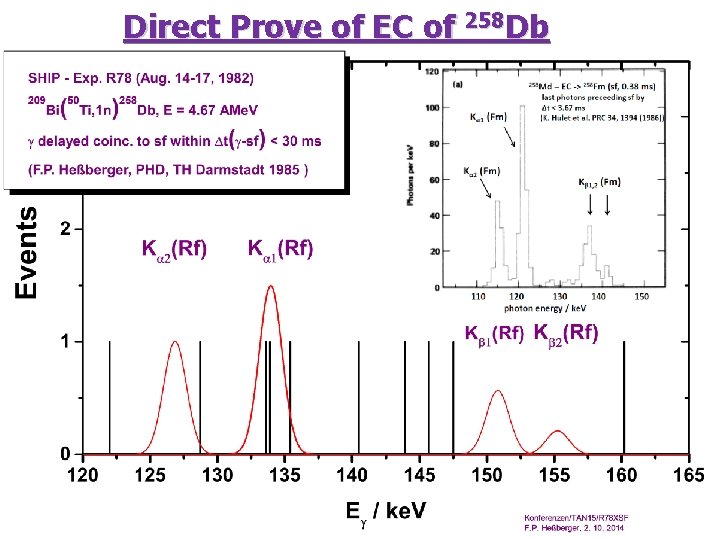
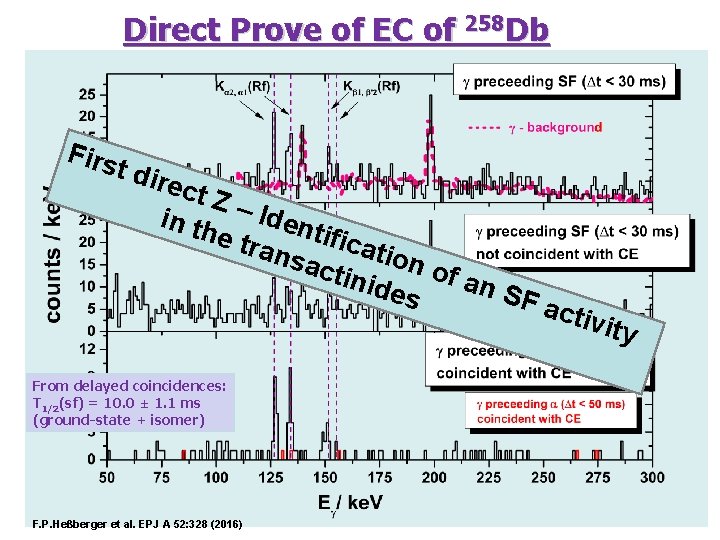
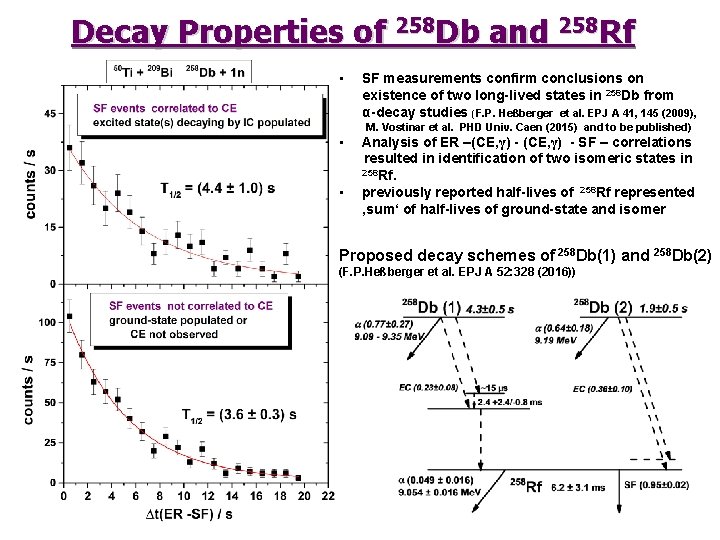
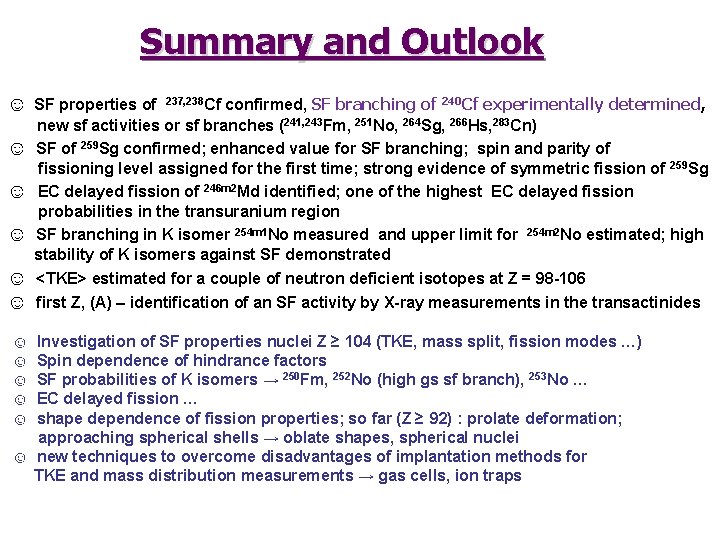
- Slides: 34
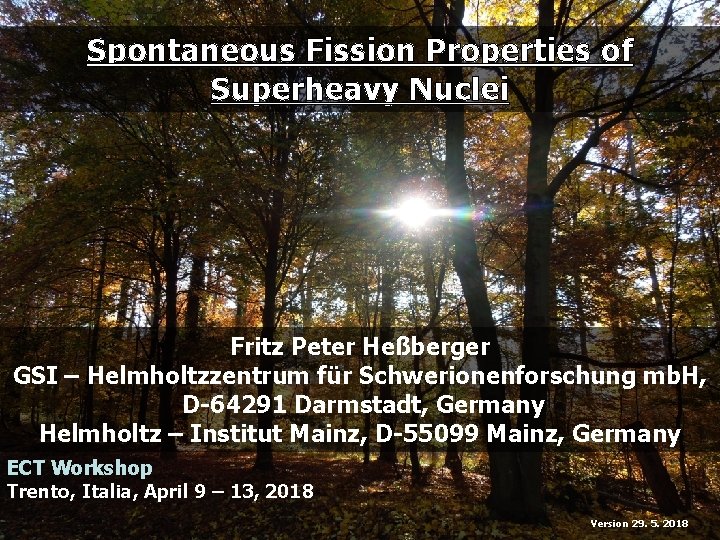
Spontaneous Fission Properties of Superheavy Nuclei Fritz Peter Heßberger GSI – Helmholtzzentrum für Schwerionenforschung mb. H, D-64291 Darmstadt, Germany Helmholtz – Institut Mainz, D-55099 Mainz, Germany ECT Workshop Trento, Italia, April 9 – 13, 2018 Version 29. 5. 2018
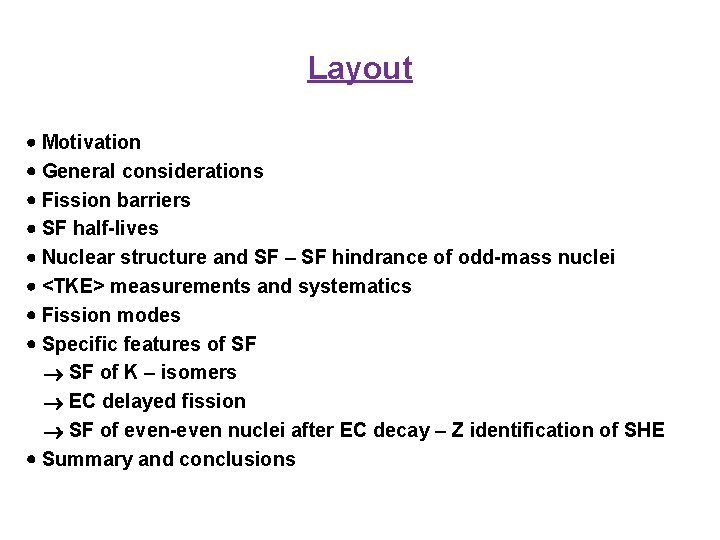
Layout Motivation General considerations Fission barriers SF half-lives Nuclear structure and SF – SF hindrance of odd-mass nuclei <TKE> measurements and systematics Fission modes Specific features of SF of K – isomers EC delayed fission SF of even-even nuclei after EC decay – Z identification of SHE Summary and conclusions
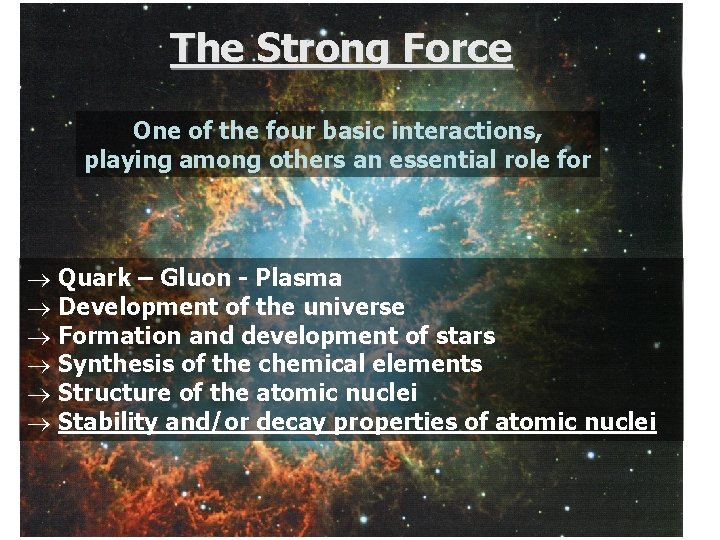
The Strong Force One of the four basic interactions, playing among others an essential role for ® Quark – Gluon - Plasma ® Development of the universe ® Formation and development of stars ® Synthesis of the chemical elements ® Structure of the atomic nuclei ® Stability and/or decay properties of atomic nuclei
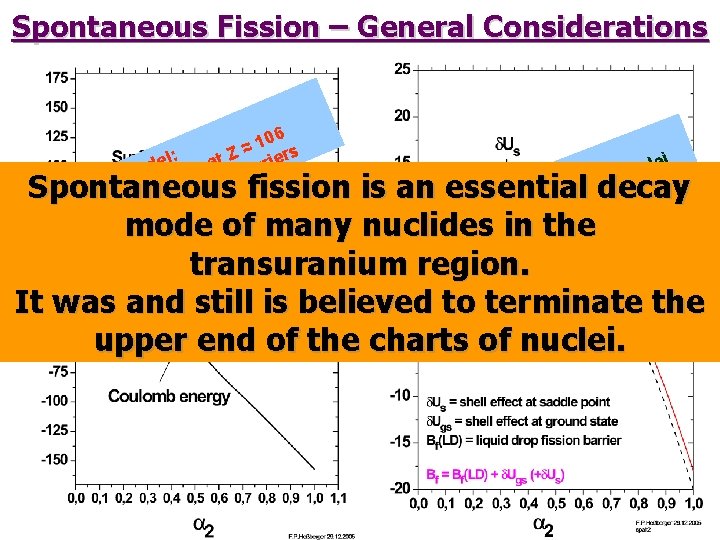
Spontaneous Fission – General Considerations 106 ≈ l: t Z rriers e a d o nds ba M n e p o ro ility issi D d ui r stab ing f q i L ea anish l c nu to v due lei c u l: t n uclei e s e d i l Mo heav cts; n l e Sh ers in ll effe r a le rri he c a s u b y N ion ned b ist s s i x i f e m r y e a det 106 m Z> Spontaneous fission is an essential decay mode of many nuclides in the transuranium region. It was and still is believed to terminate the upper end of the charts of nuclei.
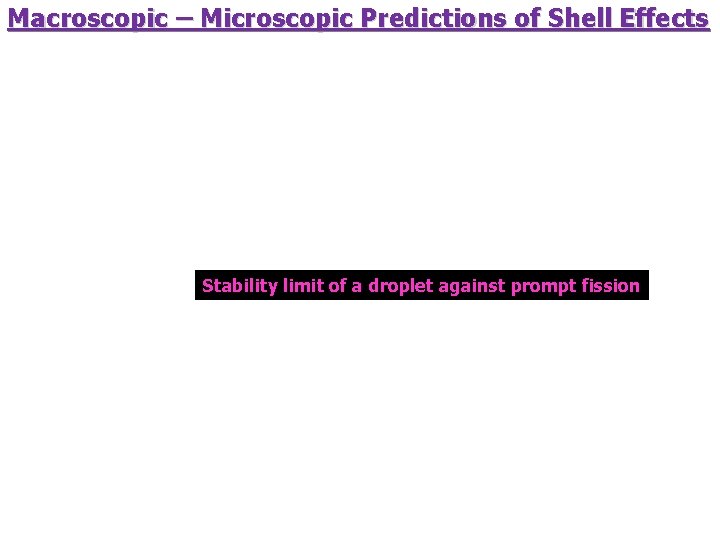
Macroscopic – Microscopic Predictions of Shell Effects Stability limit of a droplet against prompt fission
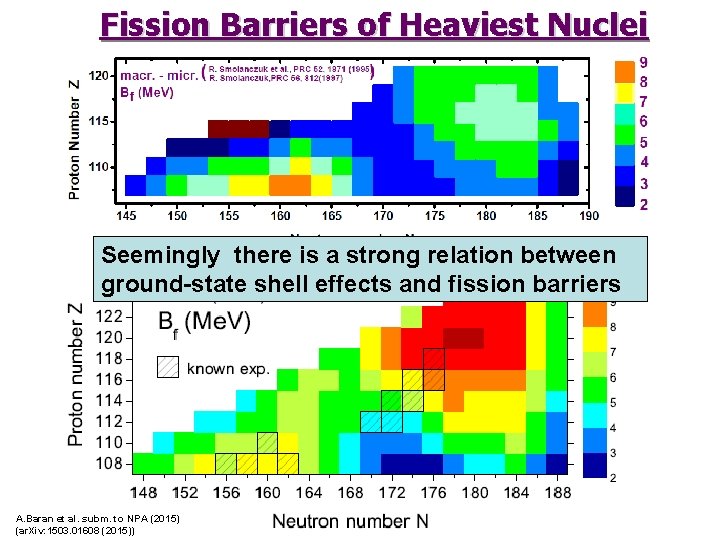
Fission Barriers of Heaviest Nuclei Seemingly there is a strong relation between ground-state shell effects and fission barriers A. Baran et al. subm. to NPA (2015) (ar. Xiv: 1503. 01608 (2015))
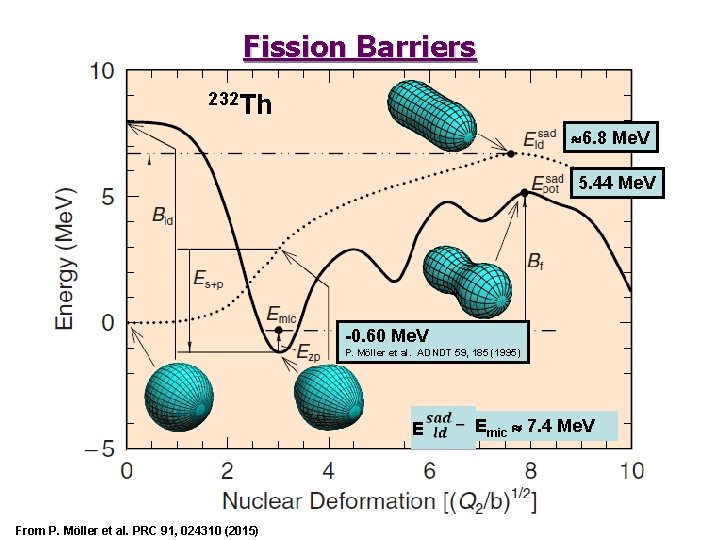
Fission Barriers 232 Th 6. 8 Me. V 5. 44 Me. V -0. 60 Me. V P. Möller et al. ADNDT 59, 185 (1995) E From P. Möller et al. PRC 91, 024310 (2015) Emic 7. 4 Me. V
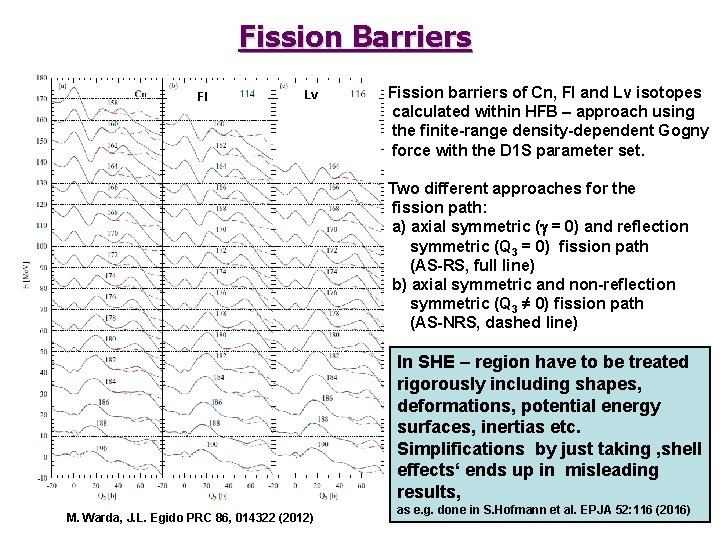
Fission Barriers Fl Lv Fission barriers of Cn, Fl and Lv isotopes calculated within HFB – approach using the finite-range density-dependent Gogny force with the D 1 S parameter set. Two different approaches for the fission path: a) axial symmetric ( = 0) and reflection symmetric (Q 3 = 0) fission path (AS-RS, full line) b) axial symmetric and non-reflection symmetric (Q 3 ≠ 0) fission path (AS-NRS, dashed line) In SHE – region have to be treated rigorously including shapes, deformations, potential energy surfaces, inertias etc. Simplifications by just taking ‚shell effects‘ ends up in misleading results, M. Warda, J. L. Egido PRC 86, 014322 (2012) as e. g. done in S. Hofmann et al. EPJA 52: 116 (2016)
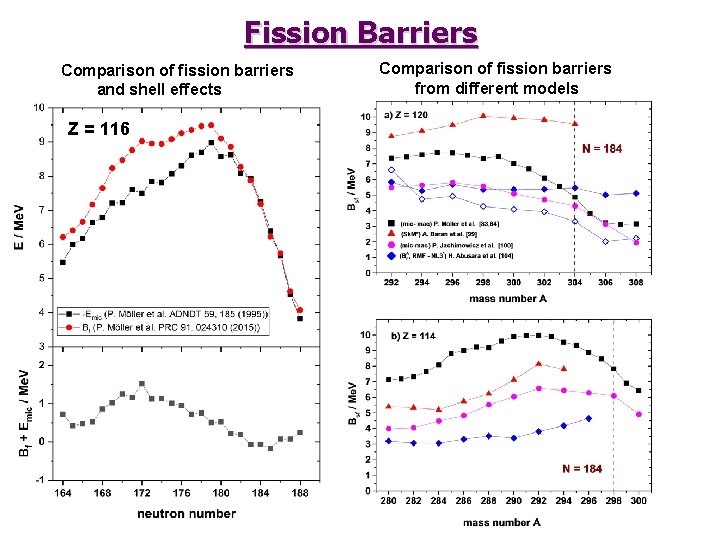
Fission Barriers Comparison of fission barriers and shell effects Z = 116 Comparison of fission barriers from different models
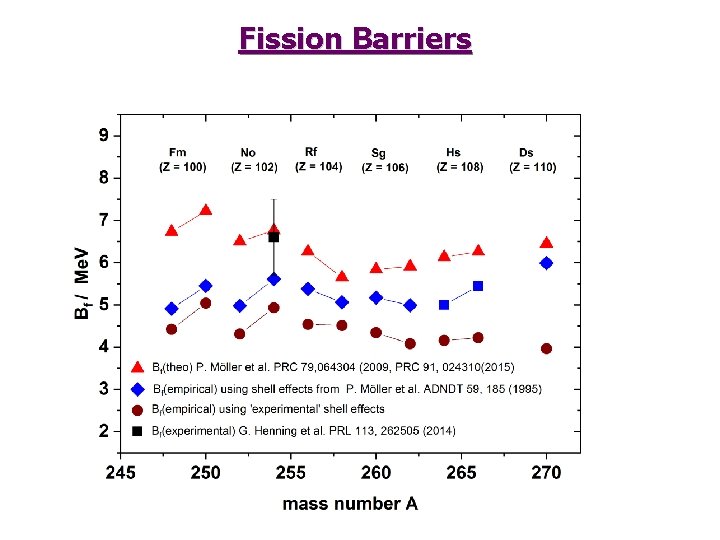
Fission Barriers
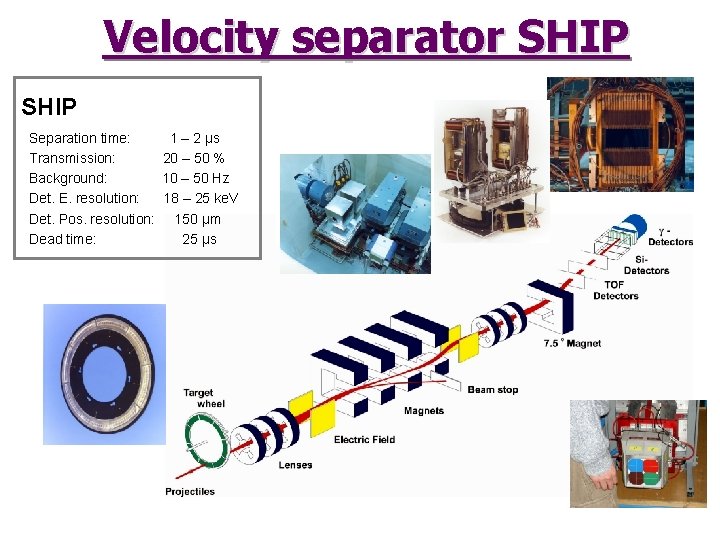
Velocity separator SHIP Separation time: 1 – 2 μs Transmission: 20 – 50 % Background: 10 – 50 Hz Det. E. resolution: 18 – 25 ke. V Det. Pos. resolution: 150 μm Dead time: 25 μs Mastertitelformat bearbeiten
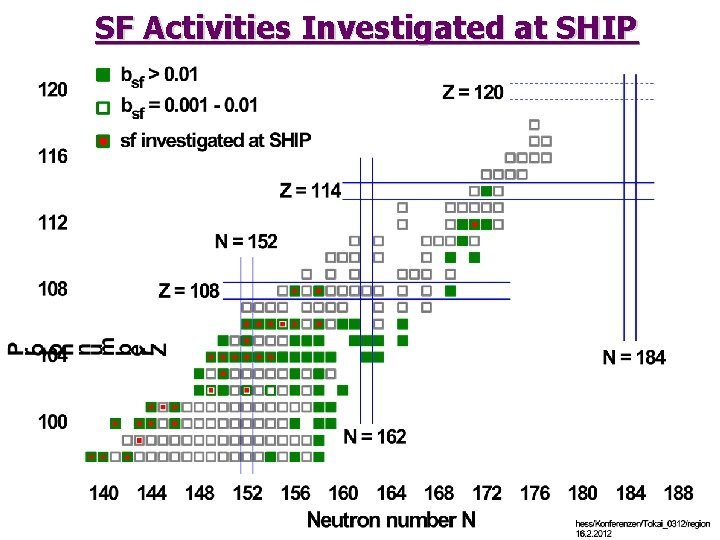
SF Activities Investigated at SHIP
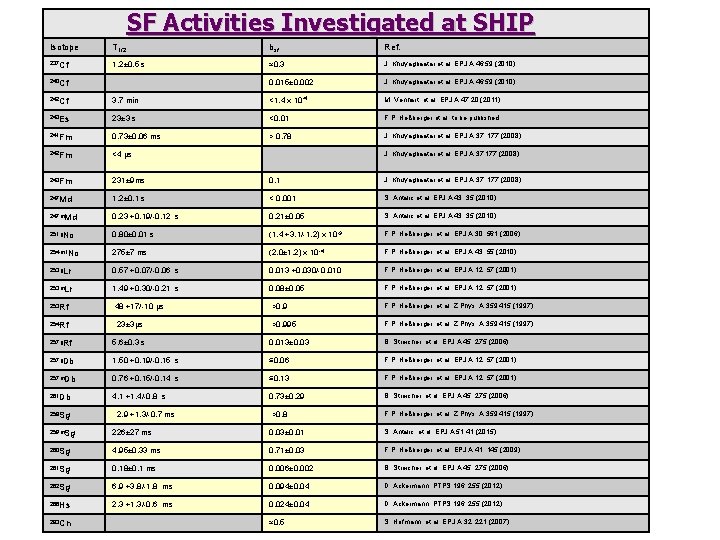
SF Activities Investigated at SHIP Isotope T 1/2 bsf Ref. 237 Cf 1. 2± 0. 5 s ≈0. 3 J. Khuyagbaatar et al. EPJ A 46, 59 (2010) 0. 015± 0. 002 J. Khuyagbaatar et al. EPJ A 46, 59 (2010) 240 Cf 242 Cf 3. 7 min <1. 4 x 10 -4 M. Venhart et al. EPJ A 47: 20 (2011) 243 Es 23± 3 s <0. 01 F. P. Heßberger et al. to be published 241 Fm 0. 73± 0. 06 ms > 0. 78 J. Khuyagbaatar et al. EPJ A 37, 177 (2008) 242 Fm <4 μs 243 Fm 231± 9 ms 0. 1 J. Khuyagbaatar et al. EPJ A 37, 177 (2008) 247 Md 1. 2± 0. 1 s < 0. 001 S. Antalic et al. EPJ A 43, 35 (2010) 247 m. Md 0. 23 +0. 19/-0. 12 s 0. 21± 0. 05 S. Antalic et al. EPJ A 43, 35 (2010) 251 g. No 0. 80± 0. 01 s (1. 4 +3. 1/-1. 2) x 10 -3 F. P. Heßberger et al. EPJ A 30, 561 (2006) 254 m 1 No 275± 7 ms (2. 0± 1. 2) x 10 -4 F. P. Heßberger et al. EPJ A 43, 55 (2010) 253 g. Lr 0. 57 +0. 07/-0. 06 s 0. 013 +0. 030/-0. 010 F. P. Heßberger et al. EPJ A 12, 57 (2001) 253 m. Lr 1. 49 +0. 30/-0. 21 s 0. 08± 0. 05 F. P. Heßberger et al. EPJ A 12, 57 (2001) 48 +17/-10 μs >0. 9 F. P. Heßberger et al. Z. Phys. A 359, 415 (1997) 254 Rf 23± 3μs >0. 995 F. P. Heßberger et al. Z. Phys. A 359, 415 (1997) 257 g. Rf 5. 6± 0. 3 s 0. 013± 0. 03 B. Streicher et al. EPJ A 45, 275 (2006) 257 g. Db 1. 50 +0. 19/-0. 15 s ≤ 0. 06 F. P. Heßberger et al. EPJ A 12, 57 (2001) 257 m. Db 0. 76 +0. 15/-0. 14 s ≤ 0. 13 F. P. Heßberger et al. EPJ A 12, 57 (2001) 261 Db 4. 1 +1. 4/-0. 8 s 0. 73± 0. 29 B. Streicher et al. EPJ A 45, 275 (2006) 253 Rf 258 Sg 2. 9 +1. 3/-0. 7 ms J. Khuyagbaatar et al. EPJ A 37, 177 (2008) >0. 8 F. P. Heßberger et al. Z. Phys. A 359, 415 (1997) 259 m. Sg 226± 27 ms 0. 03± 0. 01 S. Antalic et al. EPJ A 51: 41 (2015) 260 Sg 4. 95± 0. 33 ms 0. 71± 0. 03 F. P. Heßberger et al. EPJ A 41, 145 (2009) 261 Sg 0. 18± 0. 1 ms 0. 006± 0. 002 B. Streicher et al. EPJ A 45, 275 (2006) 262 Sg 6. 9 +3. 8/-1. 8 ms 0. 094± 0. 04 D. Ackermann, PTPS 196, 255 (2012) 266 Hs 2. 3 +1. 3/-0. 6 ms 0. 024± 0. 04 D. Ackermann, PTPS 196, 255 (2012) ≈0. 5 S. Hofmann et al. EPJ A 32, 221 (2007) 283 Cn
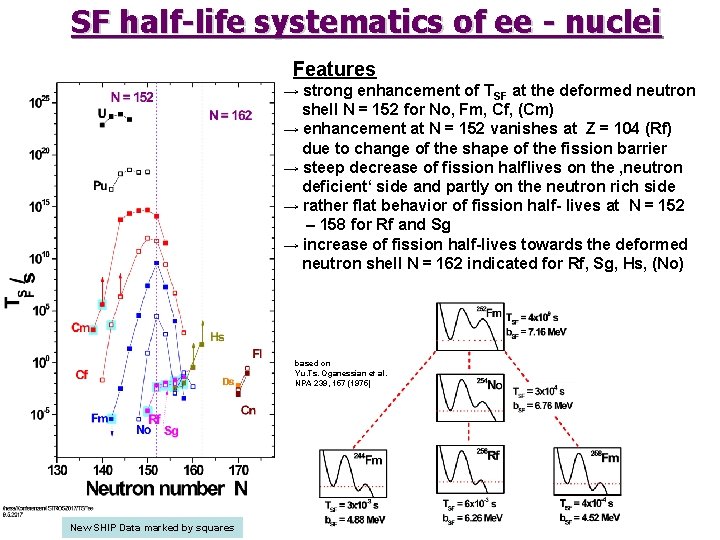
SF half-life systematics of ee - nuclei Features → strong enhancement of TSF at the deformed neutron shell N = 152 for No, Fm, Cf, (Cm) → enhancement at N = 152 vanishes at Z = 104 (Rf) due to change of the shape of the fission barrier → steep decrease of fission halflives on the ‚neutron deficient‘ side and partly on the neutron rich side → rather flat behavior of fission half- lives at N = 152 – 158 for Rf and Sg → increase of fission half-lives towards the deformed neutron shell N = 162 indicated for Rf, Sg, Hs, (No) based on Yu. Ts. Oganessian et al. NPA 239, 157 (1975) New SHIP Data marked by squares
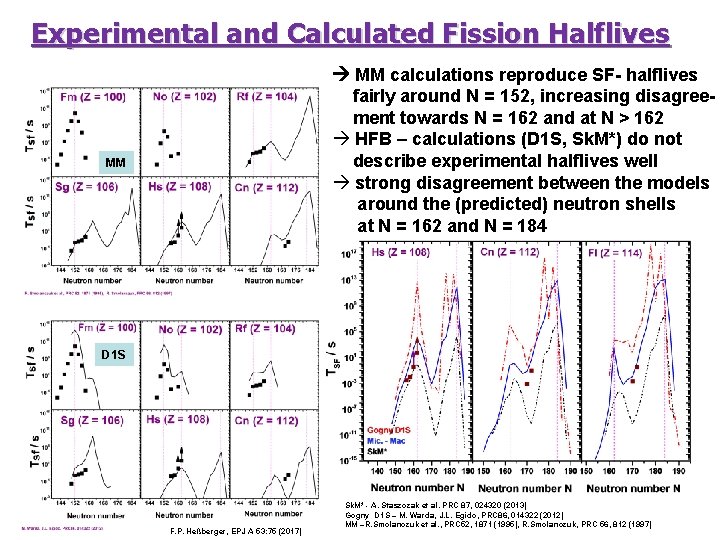
Experimental and Calculated Fission Halflives MM calculations reproduce SF- halflives fairly around N = 152, increasing disagreement towards N = 162 and at N > 162 à HFB – calculations (D 1 S, Sk. M*) do not describe experimental halflives well à strong disagreement between the models around the (predicted) neutron shells at N = 162 and N = 184 MM D 1 S F. P. Heßberger, EPJ A 53: 75 (2017) Sk. M* - A. Staszczak et al. PRC 87, 024320 (2013) Gogny D 1 S – M. Warda, J. L. Egido, PRC 86, 014322 (2012) MM –R. Smolanczuk et al. , PRC 52, 1871 (1995), R. Smolanczuk, PRC 56, 812 (1997)
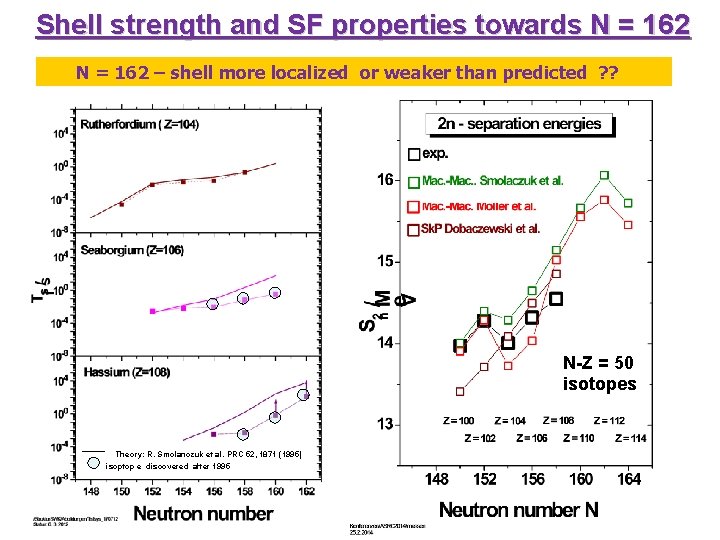
Shell strength and SF properties towards N = 162 – shell more localized or weaker than predicted ? ? N-Z = 50 isotopes Theory: R. Smolanczuk et al. PRC 52, 1871 (1995) isoptop e discovered after 1995
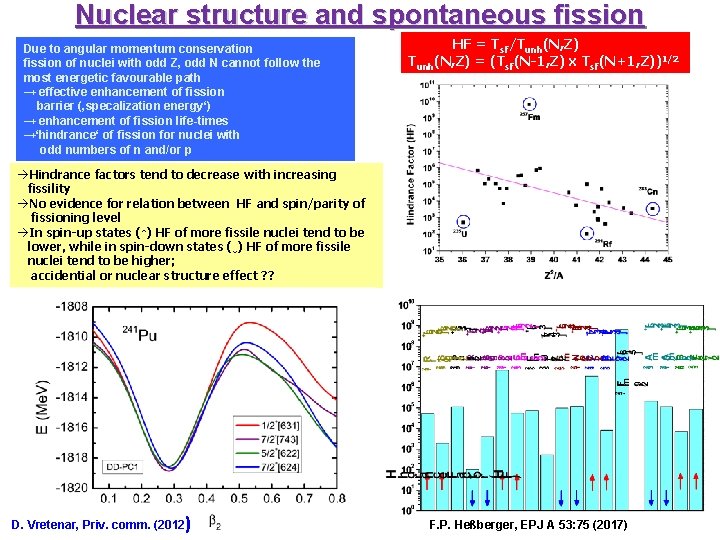
Nuclear structure and spontaneous fission Due to angular momentum conservation fission of nuclei with odd Z, odd N cannot follow the most energetic favourable path → effective enhancement of fission barrier (‚specalization energy‘) → enhancement of fission life-times →‘hindrance‘ of fission for nuclei with odd numbers of n and/or p HF = Tsf/Tunh(N, Z) = (Tsf(N-1, Z) x Tsf(N+1, Z))1/2 àHindrance factors tend to decrease with increasing fissility àNo evidence for relation between HF and spin/parity of fissioning level àIn spin-up states (↑) HF of more fissile nuclei tend to be lower, while in spin-down states (↓) HF of more fissile nuclei tend to be higher; accidential or nuclear structure effect ? ? D. Vretenar, Priv. comm. (2012) F. P. Heßberger, EPJ A 53: 75 (2017)
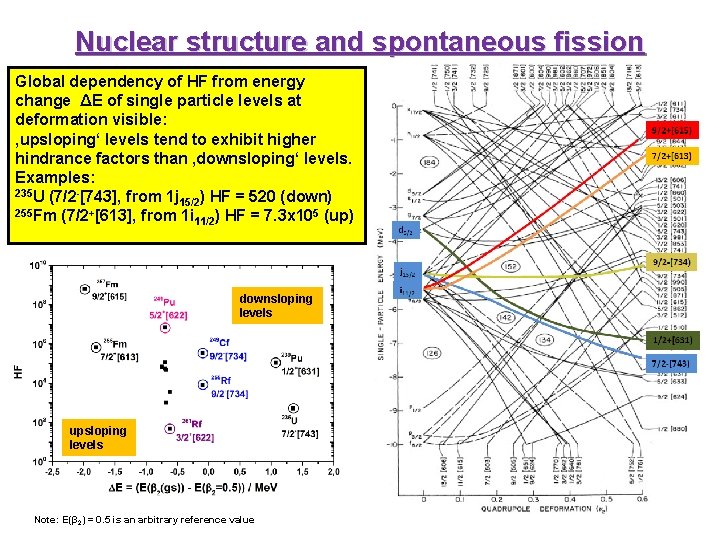
Nuclear structure and spontaneous fission Global dependency of HF from energy change ΔE of single particle levels at deformation visible: ‚upsloping‘ levels tend to exhibit higher hindrance factors than ‚downsloping‘ levels. Examples: 235 U (7/2 -[743], from 1 j 15/2) HF = 520 (down) 255 Fm (7/2+[613], from 1 i 5 11/2) HF = 7. 3 x 10 (up) downsloping levels upsloping levels Note: E(β 2) = 0. 5 is an arbitrary reference value
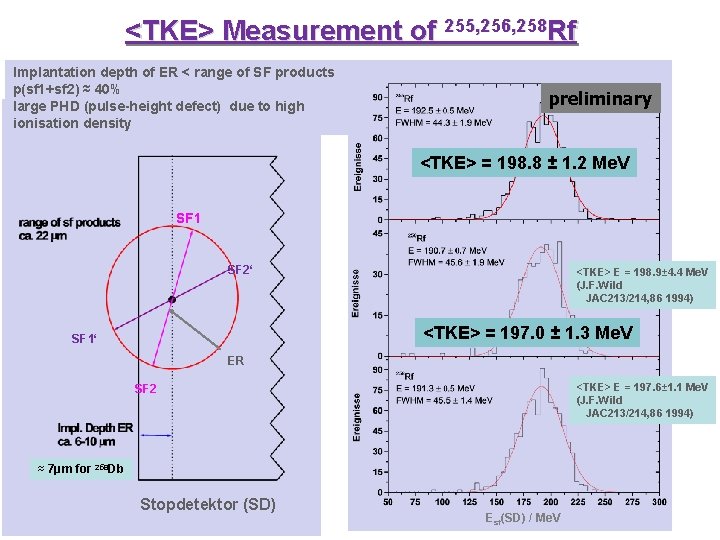
<TKE> Measurement of 255, 256, 258 Rf Implantation depth of ER < range of SF products p(sf 1+sf 2) ≈ 40% large PHD (pulse-height defect) due to high ionisation density preliminary <TKE> = 198. 8 ± 1. 2 Me. V SF 1 SF 2‘ <TKE> E = 198. 9± 4. 4 Me. V (J. F. Wild JAC 213/214, 86 1994) <TKE> = 197. 0 ± 1. 3 Me. V SF 1‘ ER <TKE> E = 197. 6± 1. 1 Me. V (J. F. Wild JAC 213/214, 86 1994) SF 2 ≈ 7μm for 258 Db Stopdetektor (SD) Esf(SD) / Me. V
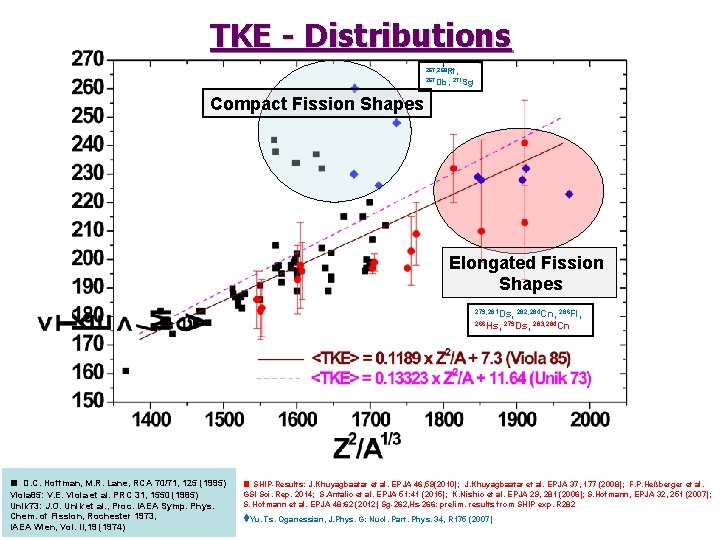
TKE - Distributions 267, 268 Rf, 267 Db, 271 Sg Compact Fission Shapes Elongated Fission Shapes 279, 281 Ds, 282, 284 Cn, 286 Fl, 266 Hs, 279 Ds, 283, 284 Cn ■ D. C. Hoffman, M. R. Lane, RCA 70/71, 125 (1995) Viola 85: V. E. Viola et al. PRC 31, 1550 (1985) Unik 73: J. O. Unik et al. , Proc. IAEA Symp. Phys. Chem. of Fission, Rochester 1973, IAEA Wien, Vol. II, 19 (1974) ■ SHIP-Results: J. Khuyagbaatar et al. EPJA 46, 59(2010); J. Khuyagbaatar et al. EPJA 37, 177 (2008); F. P. Heßberger et al. GSI Sci. Rep. 2014; S. Antalic et al. EPJA 51: 41 (2015); K. Nishio et al. EPJA 29, 281 (2006); S. Hofmann, EPJA 32, 251 (2007); S. Hofmann et al. EPJA 48: 62 (2012) Sg-262, Hs-266: prelim. results from SHIP exp. R 282 ♦Yu. Ts. Oganessian, J. Phys. G: Nucl. Part. Phys. 34, R 175 (2007)
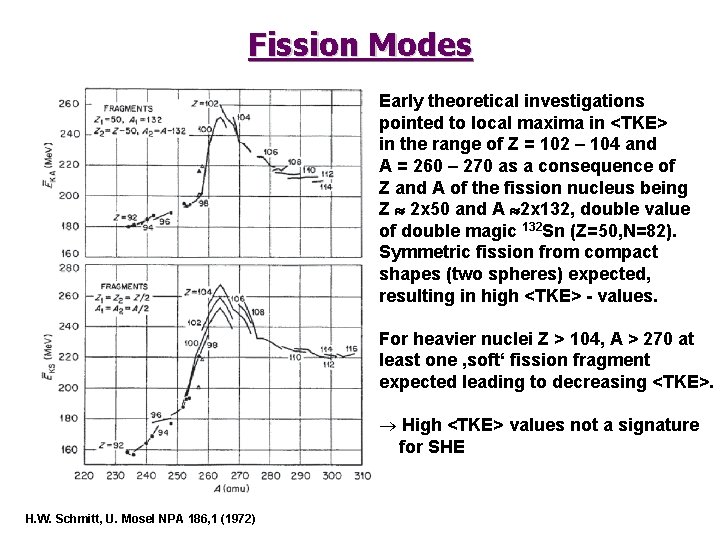
Fission Modes Early theoretical investigations pointed to local maxima in <TKE> in the range of Z = 102 – 104 and A = 260 – 270 as a consequence of Z and A of the fission nucleus being Z 2 x 50 and A 2 x 132, double value of double magic 132 Sn (Z=50, N=82). Symmetric fission from compact shapes (two spheres) expected, resulting in high <TKE> - values. For heavier nuclei Z > 104, A > 270 at least one ‚soft‘ fission fragment expected leading to decreasing <TKE>. ® High <TKE> values not a signature for SHE H. W. Schmitt, U. Mosel NPA 186, 1 (1972)
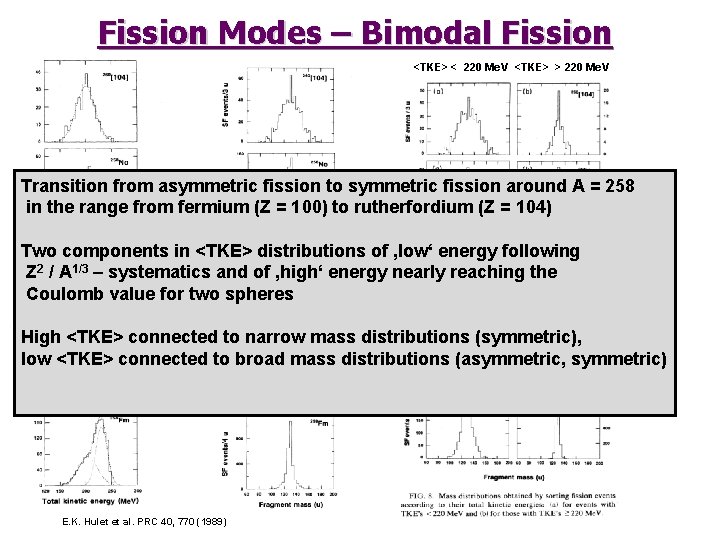
Fission Modes – Bimodal Fission <TKE> < 220 Me. V <TKE> > 220 Me. V Transition from asymmetric fission to symmetric fission around A = 258 in the range from fermium (Z = 100) to rutherfordium (Z = 104) Two components in <TKE> distributions of ‚low‘ energy following Z 2 / A 1/3 – systematics and of ‚high‘ energy nearly reaching the Coulomb value for two spheres High <TKE> connected to narrow mass distributions (symmetric), low <TKE> connected to broad mass distributions (asymmetric, symmetric) E. K. Hulet et al. PRC 40, 770 (1989)
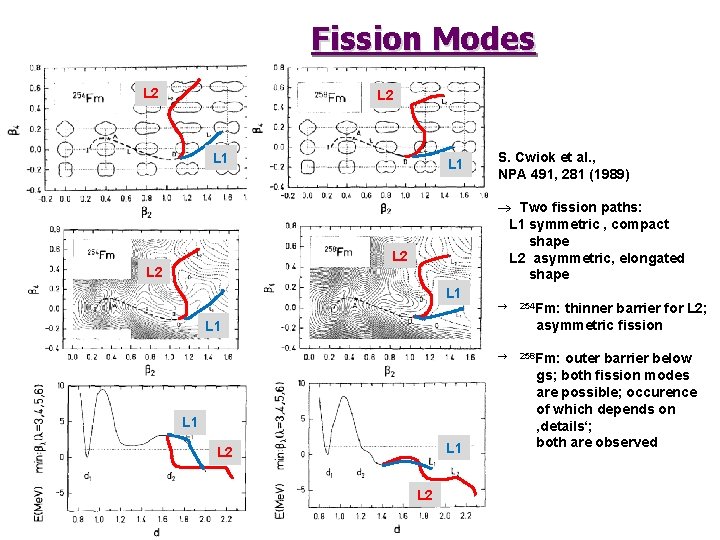
Fission Modes L 2 L 1 S. Cwiok et al. , NPA 491, 281 (1989) ® Two fission paths: L 1 symmetric , compact shape L 2 asymmetric, elongated shape L 2 L 1 ® 254 Fm: ® 258 Fm: L 1 L 1 L 2 thinner barrier for L 2; asymmetric fission outer barrier below gs; both fission modes are possible; occurence of which depends on ‚details‘; both are observed
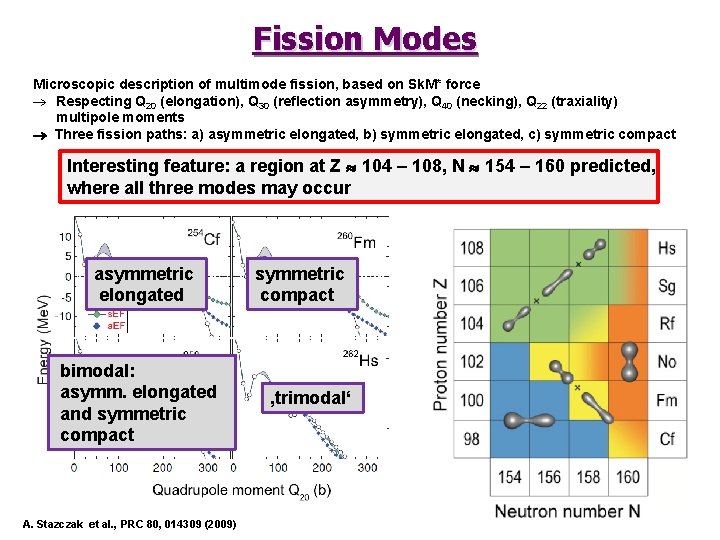
Fission Modes Microscopic description of multimode fission, based on Sk. M* force ® Respecting Q 20 (elongation), Q 30 (reflection asymmetry), Q 40 (necking), Q 22 (traxiality) multipole moments Three fission paths: a) asymmetric elongated, b) symmetric elongated, c) symmetric compact Interesting feature: a region at Z 104 – 108, N 154 – 160 predicted, where all three modes may occur asymmetric elongated bimodal: asymm. elongated and symmetric compact A. Stazczak et al. , PRC 80, 014309 (2009) symmetric compact ‚trimodal‘
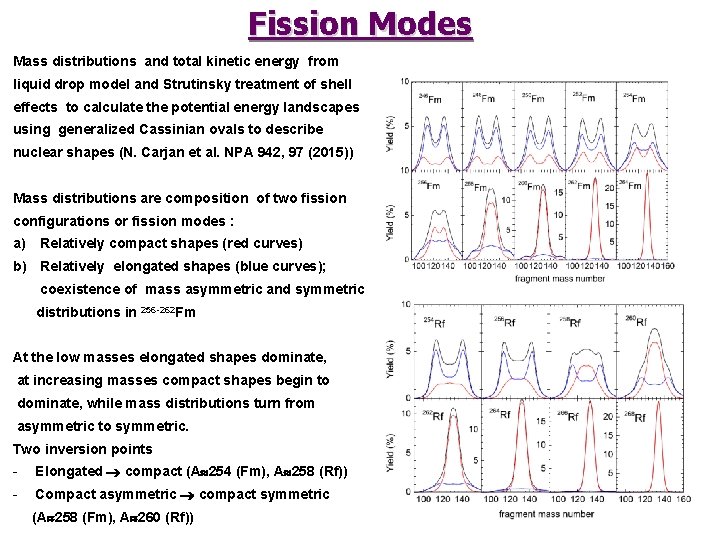
Fission Modes Mass distributions and total kinetic energy from liquid drop model and Strutinsky treatment of shell effects to calculate the potential energy landscapes using generalized Cassinian ovals to describe nuclear shapes (N. Carjan et al. NPA 942, 97 (2015)) Mass distributions are composition of two fission configurations or fission modes : a) Relatively compact shapes (red curves) b) Relatively elongated shapes (blue curves); coexistence of mass asymmetric and symmetric distributions in 256 -262 Fm At the low masses elongated shapes dominate, at increasing masses compact shapes begin to dominate, while mass distributions turn from asymmetric to symmetric. Two inversion points - Elongated compact (A 254 (Fm), A 258 (Rf)) - Compact asymmetric compact symmetric (A 258 (Fm), A 260 (Rf))
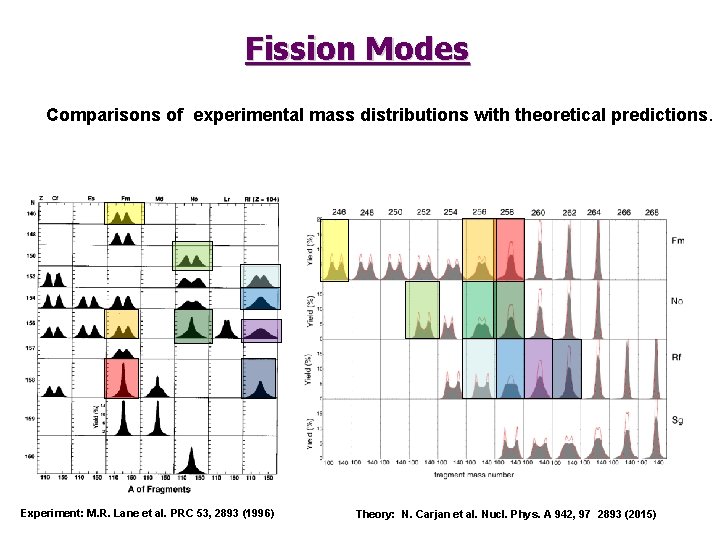
Fission Modes Comparisons of experimental mass distributions with theoretical predictions. Experiment: M. R. Lane et al. PRC 53, 2893 (1996) Theory: N. Carjan et al. Nucl. Phys. A 942, 97 2893 (2015)
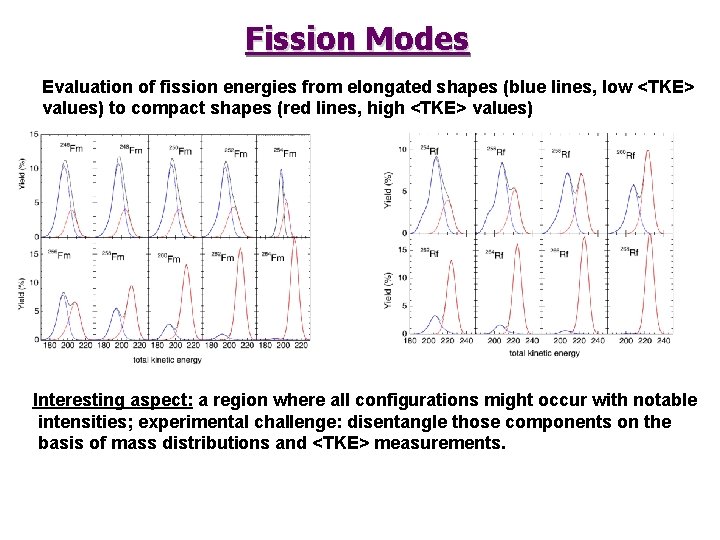
Fission Modes Evaluation of fission energies from elongated shapes (blue lines, low <TKE> values) to compact shapes (red lines, high <TKE> values) Interesting aspect: a region where all configurations might occur with notable intensities; experimental challenge: disentangle those components on the basis of mass distributions and <TKE> measurements.
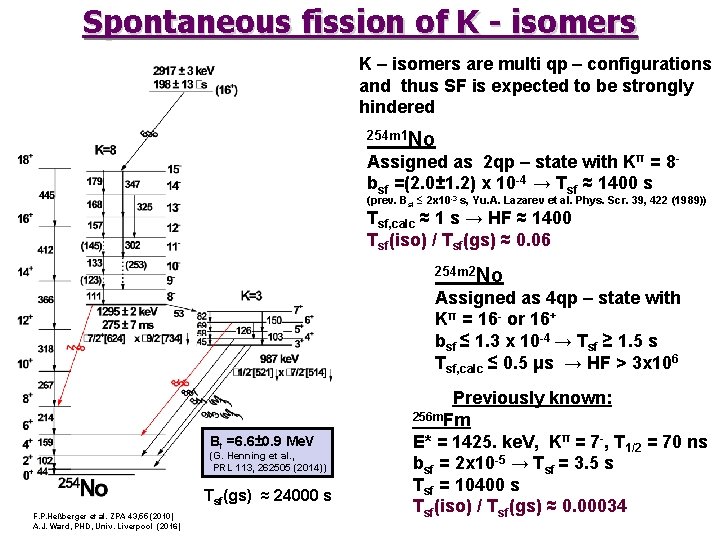
Spontaneous fission of K - isomers K – isomers are multi qp – configurations and thus SF is expected to be strongly hindered 254 m 1 No Assigned as 2 qp – state with Kπ = 8 bsf =(2. 0± 1. 2) x 10 -4 → Tsf ≈ 1400 s (prev. Bsf ≤ 2 x 10 -3 s, Yu. A. Lazarev et al. Phys. Scr. 39, 422 (1989)) Tsf, calc ≈ 1 s → HF ≈ 1400 Tsf(iso) / Tsf(gs) ≈ 0. 06 254 m 2 No Assigned as 4 qp – state with Kπ = 16 - or 16+ bsf ≤ 1. 3 x 10 -4 → Tsf ≥ 1. 5 s Tsf, calc ≤ 0. 5 μs → HF > 3 x 106 Bf =6. 6± 0. 9 Me. V (G. Henning et al. , PRL 113, 262505 (2014)) Tsf(gs) ≈ 24000 s F. P. Heßberger et al. ZPA 43, 55 (2010) A. J. Ward, PHD, Univ. Liverpool (2016) Previously known: 256 m. Fm E* = 1425. ke. V, Kπ = 7 -, T 1/2 = 70 ns bsf = 2 x 10 -5 → Tsf = 3. 5 s Tsf = 10400 s Tsf(iso) / Tsf(gs) ≈ 0. 00034
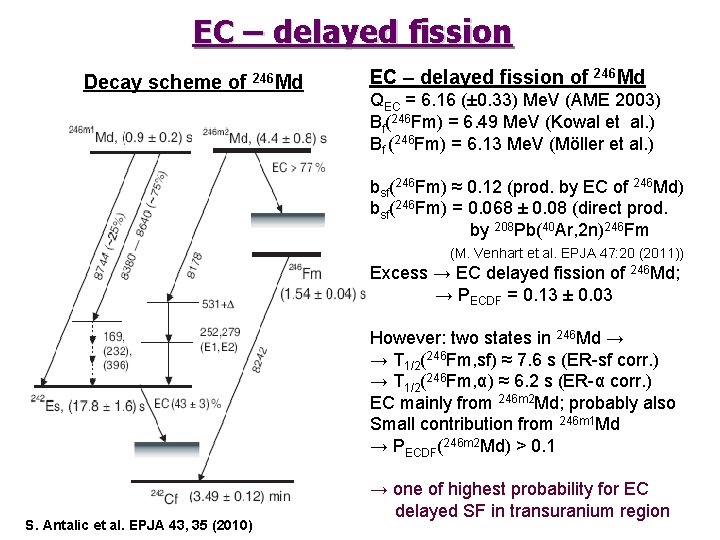
EC – delayed fission Decay scheme of 246 Md EC – delayed fission of 246 Md QEC = 6. 16 (± 0. 33) Me. V (AME 2003) Bf(246 Fm) = 6. 49 Me. V (Kowal et al. ) Bf (246 Fm) = 6. 13 Me. V (Möller et al. ) bsf(246 Fm) ≈ 0. 12 (prod. by EC of 246 Md) bsf(246 Fm) = 0. 068 ± 0. 08 (direct prod. by 208 Pb(40 Ar, 2 n)246 Fm (M. Venhart et al. EPJA 47: 20 (2011)) Excess → EC delayed fission of 246 Md; → PECDF = 0. 13 ± 0. 03 However: two states in 246 Md → → T 1/2(246 Fm, sf) ≈ 7. 6 s (ER-sf corr. ) → T 1/2(246 Fm, α) ≈ 6. 2 s (ER-α corr. ) EC mainly from 246 m 2 Md; probably also Small contribution from 246 m 1 Md → PECDF(246 m 2 Md) > 0. 1 S. Antalic et al. EPJA 43, 35 (2010) → one of highest probability for EC delayed SF in transuranium region
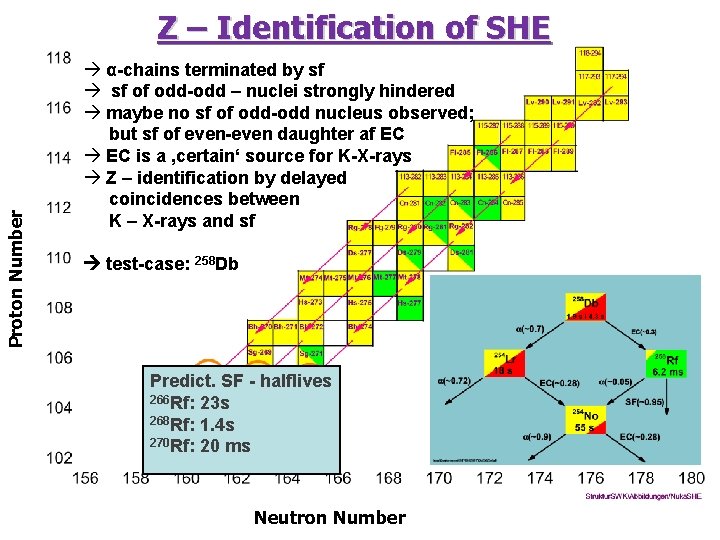
Proton Number Z – Identification of SHE à α-chains terminated by sf à sf of odd-odd – nuclei strongly hindered à maybe no sf of odd-odd nucleus observed; but sf of even-even daughter af EC à EC is a ‚certain‘ source for K-X-rays à Z – identification by delayed coincidences between K – X-rays and sf test-case: 258 Db Predict. SF - halflives 266 Rf: 23 s 268 Rf: 1. 4 s 270 Rf: 20 ms Neutron Number
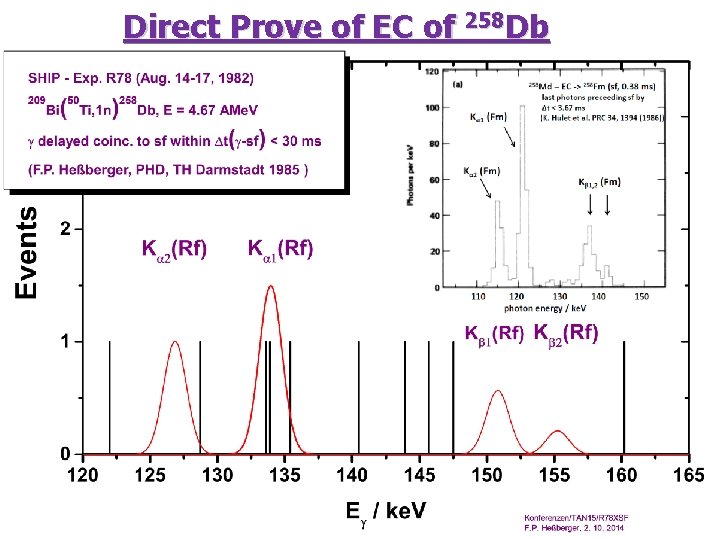
Direct Prove of EC of 258 Db From delayed coincidences T 1/2(sf) = 13 ± 11 ms
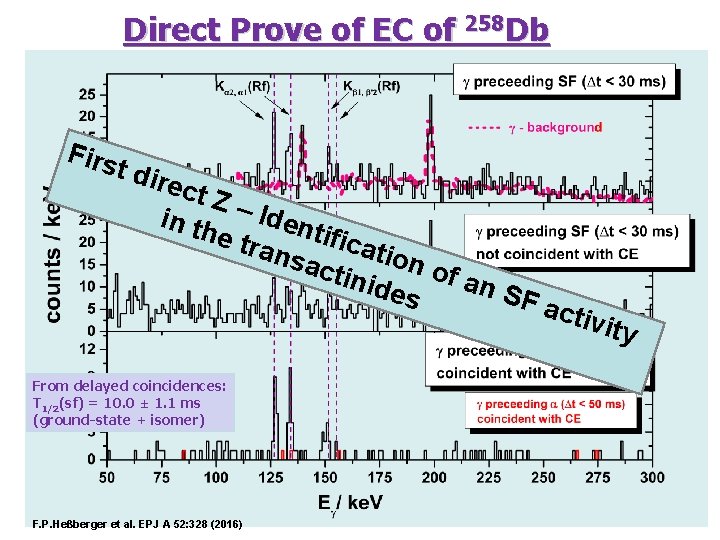
Direct Prove of EC of 258 Db Firs t dire ct Z in th – Iden e tra tifica tion nsa ctin of a ides n SF a ctiv From delayed coincidences: T 1/2(sf) = 10. 0 ± 1. 1 ms (ground-state + isomer) F. P. Heßberger et al. EPJ A 52: 328 (2016) ity
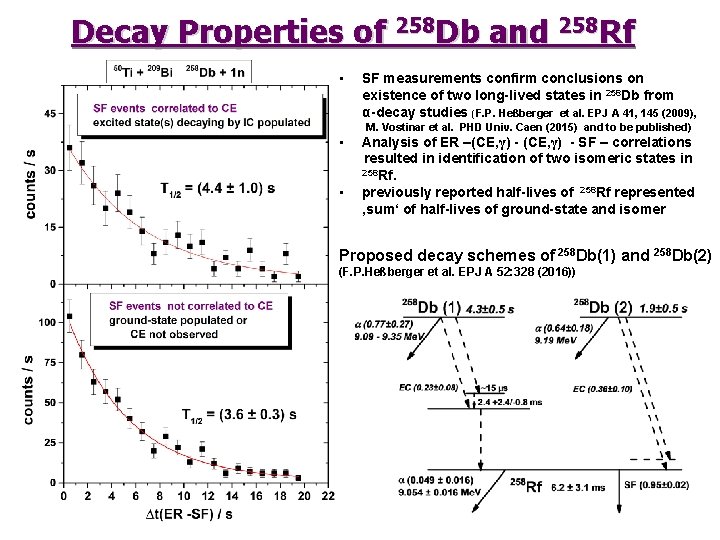
Decay Properties of 258 Db and 258 Rf • SF measurements confirm conclusions on existence of two long-lived states in 258 Db from α-decay studies (F. P. Heßberger et al. EPJ A 41, 145 (2009), M. Vostinar et al. PHD Univ. Caen (2015) and to be published) • • Analysis of ER –(CE, γ) - SF – correlations resulted in identification of two isomeric states in 258 Rf. previously reported half-lives of 258 Rf represented ‚sum‘ of half-lives of ground-state and isomer Proposed decay schemes of 258 Db(1) and 258 Db(2) (F. P. Heßberger et al. EPJ A 52: 328 (2016))
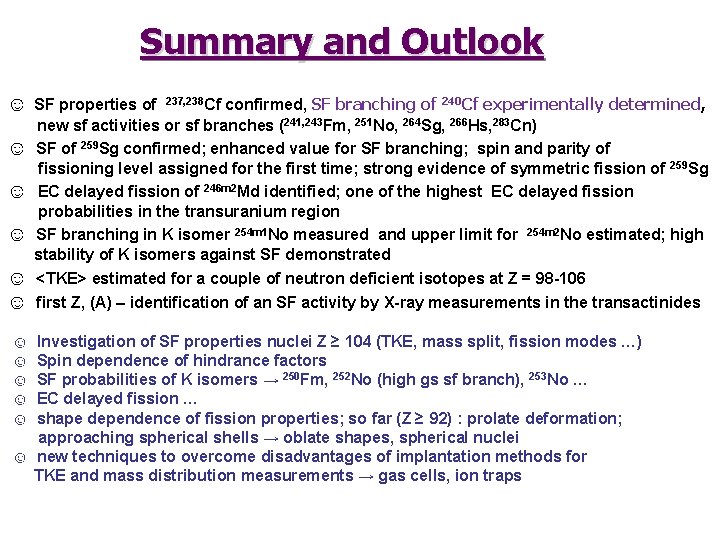
Summary and Outlook ☺ ☺ ☺ SF properties of 237, 238 Cf confirmed, SF branching of 240 Cf experimentally determined, new sf activities or sf branches (241, 243 Fm, 251 No, 264 Sg, 266 Hs, 283 Cn) SF of 259 Sg confirmed; enhanced value for SF branching; spin and parity of fissioning level assigned for the first time; strong evidence of symmetric fission of 259 Sg EC delayed fission of 246 m 2 Md identified; one of the highest EC delayed fission probabilities in the transuranium region SF branching in K isomer 254 m 1 No measured and upper limit for 254 m 2 No estimated; high stability of K isomers against SF demonstrated <TKE> estimated for a couple of neutron deficient isotopes at Z = 98 -106 first Z, (A) – identification of an SF activity by X-ray measurements in the transactinides Investigation of SF properties nuclei Z ≥ 104 (TKE, mass split, fission modes …) Spin dependence of hindrance factors SF probabilities of K isomers → 250 Fm, 252 No (high gs sf branch), 253 No … EC delayed fission … shape dependence of fission properties; so far (Z ≥ 92) : prolate deformation; approaching spherical shells → oblate shapes, spherical nuclei ☺ new techniques to overcome disadvantages of implantation methods for TKE and mass distribution measurements → gas cells, ion traps
Spontaneous fission definition
Operation fritz
Contoh teori atribusi
Sueños y existencia fritz perls
Joel rifkin
Sennheiser founder
Who is private ryan in real life
Corinne pulver germaine pulver
Heinz erhardt archimedes
Vorstreben
Fritz haselbeck
Annemarie fritz stratmann
Fritz grupe
Daniel ansorge
Fritz gerlich quotes
Neurosarcoidose
Batessche mimikry
Teoria de fritz heider
Fritz heider balance theory
Circa 1000
Fritz heider psychology
Fritz box konfiguracja
Paul fritz home instead
Fritz öhman
Fritz flanderka
Fritz reusswig
Lars fritz
Lody fritz
Codex in mir4
Fritz mackensen moormadonna
Fritz zuber-buhler
Wilitz
Fritz scholz
Srgio
Fritz caspers