SENSATION 2 Audition Audition Perception of sound Sounds
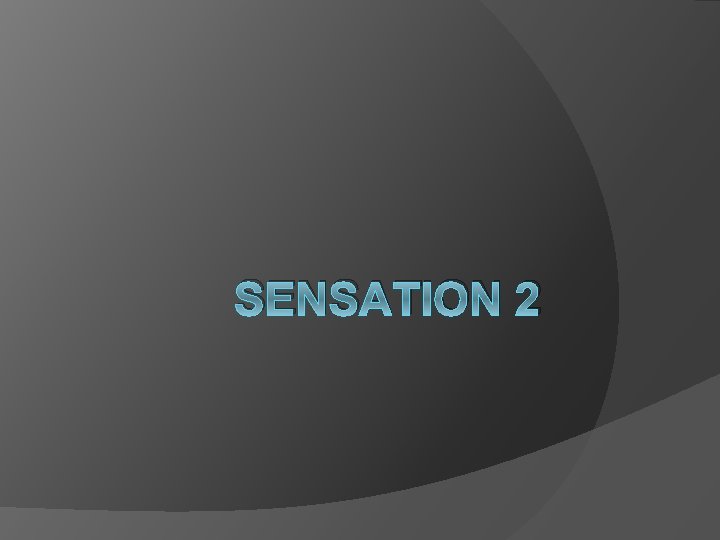
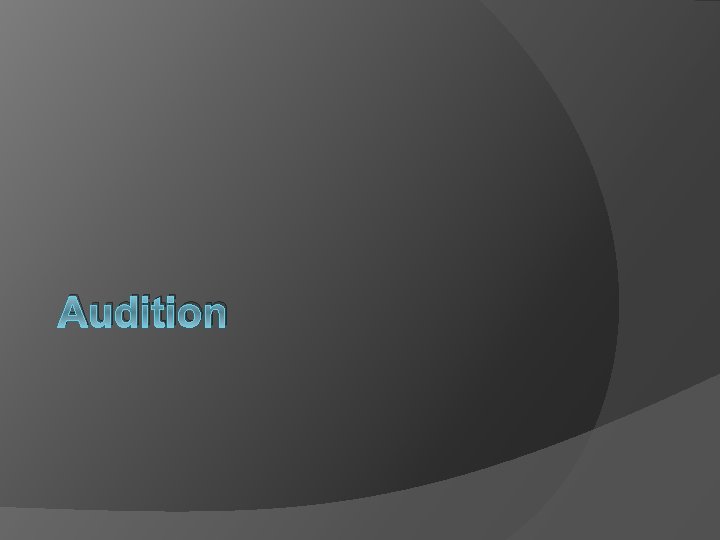
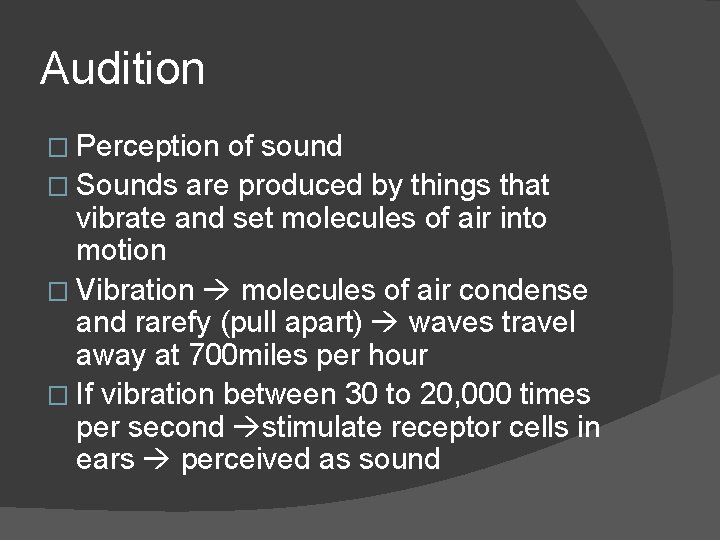
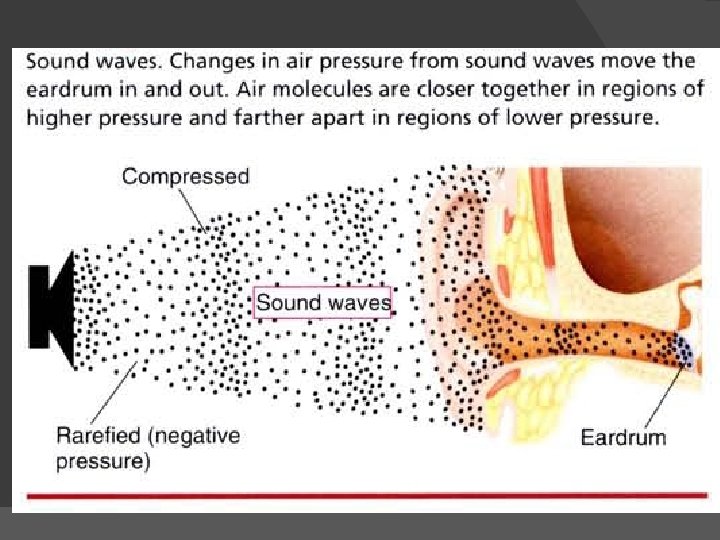
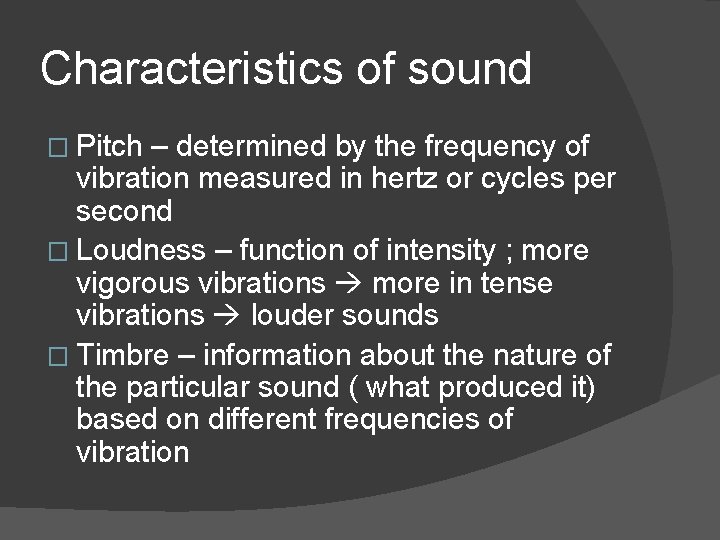
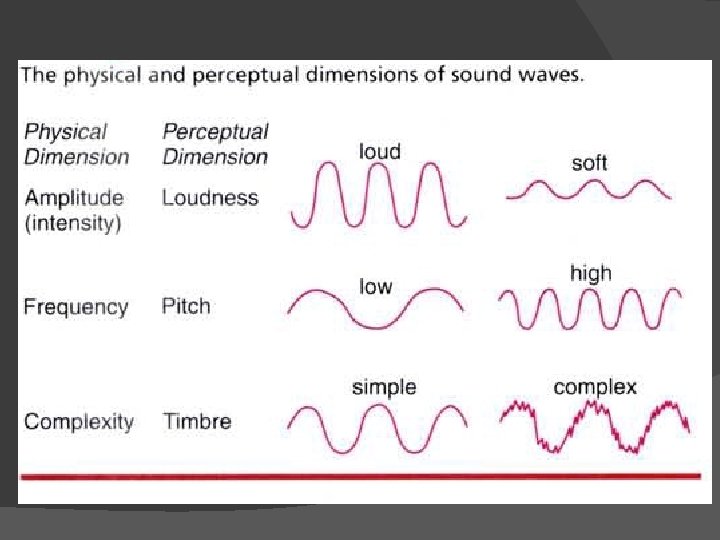
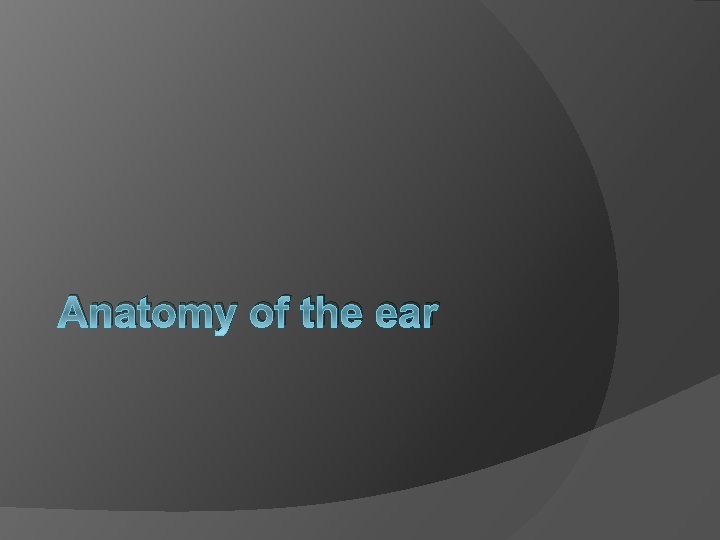
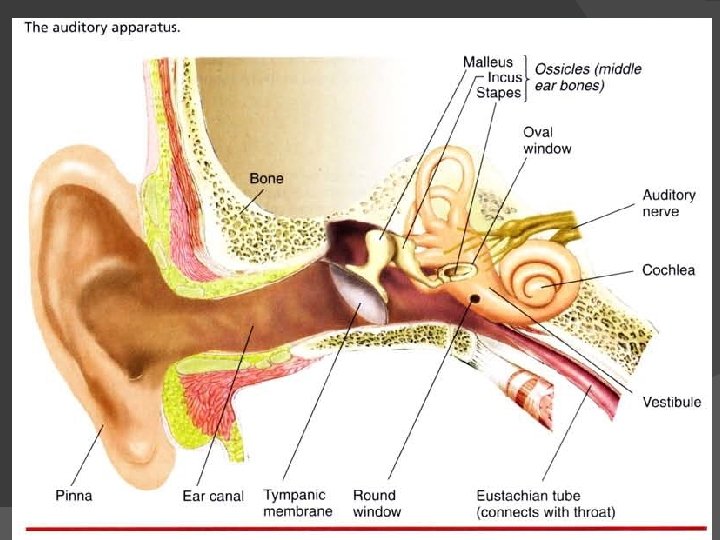
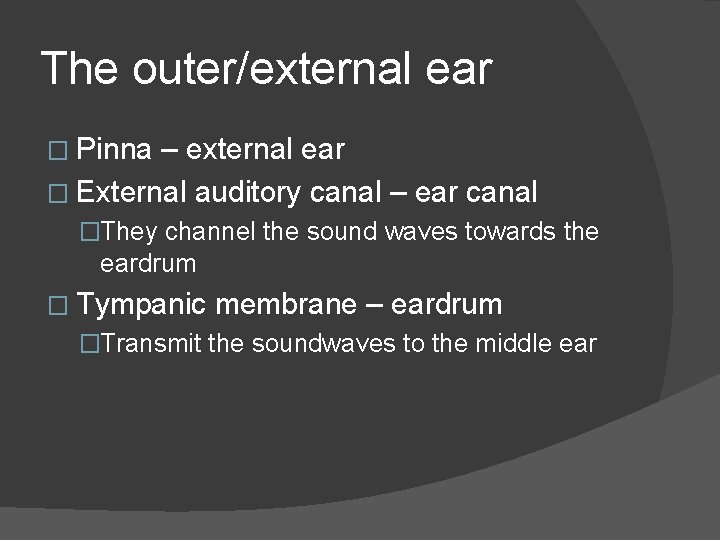
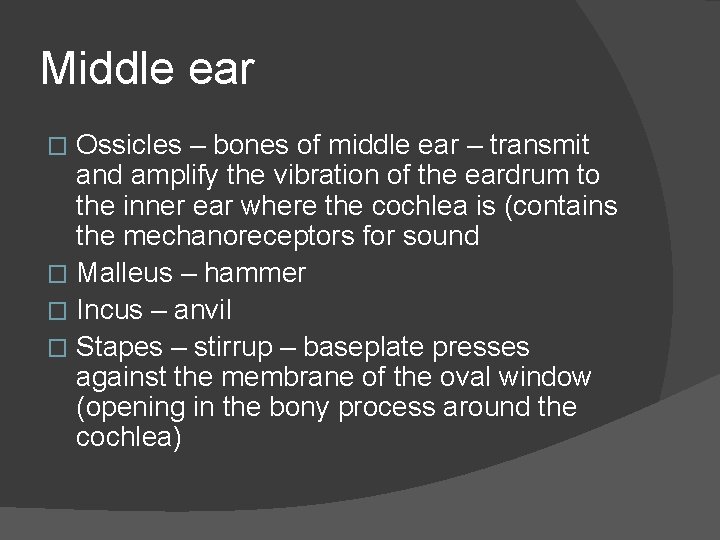
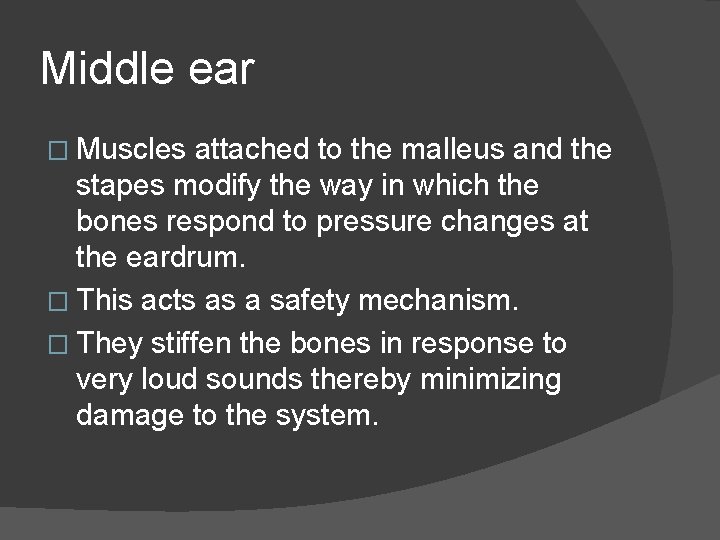
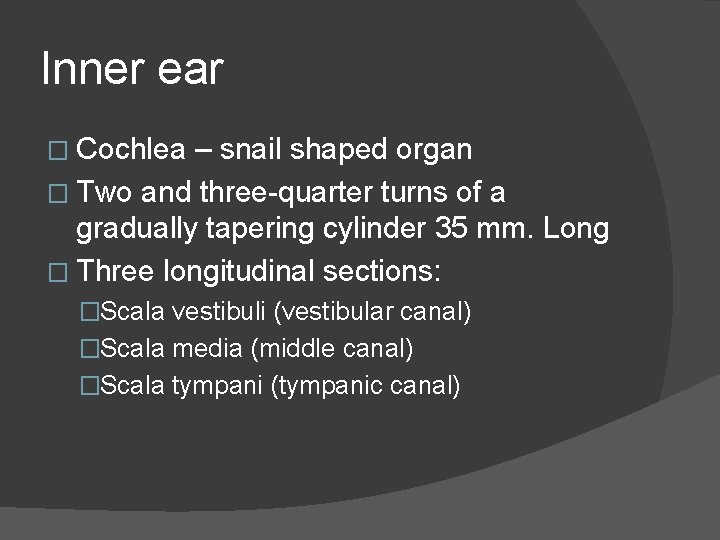
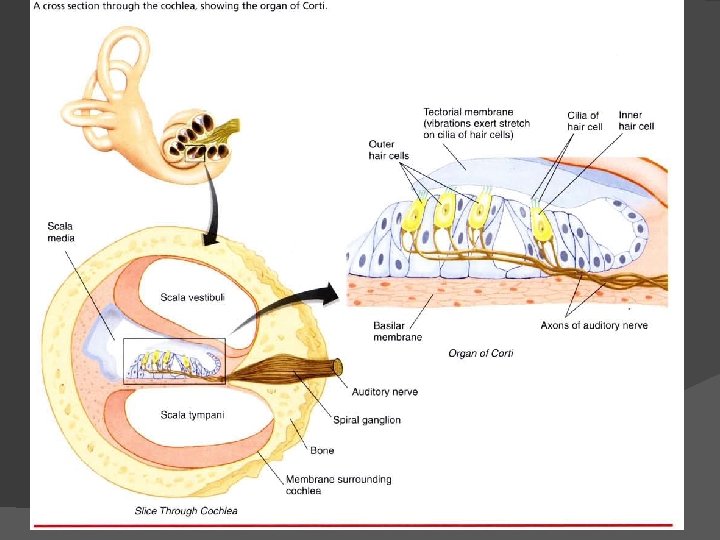
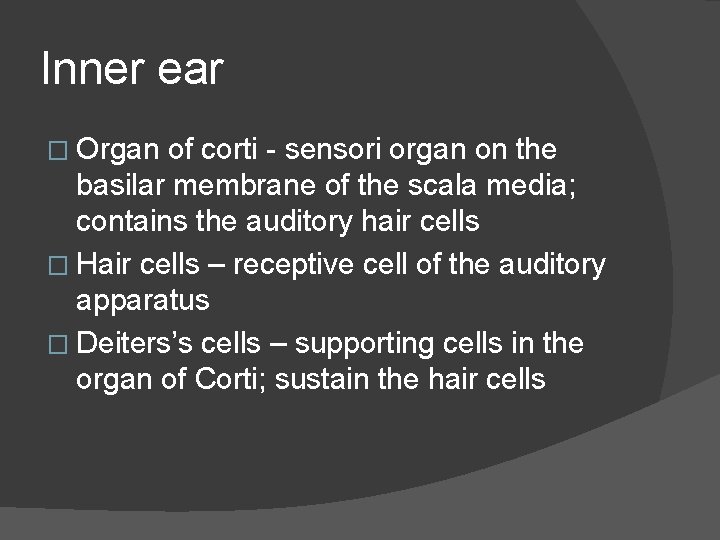
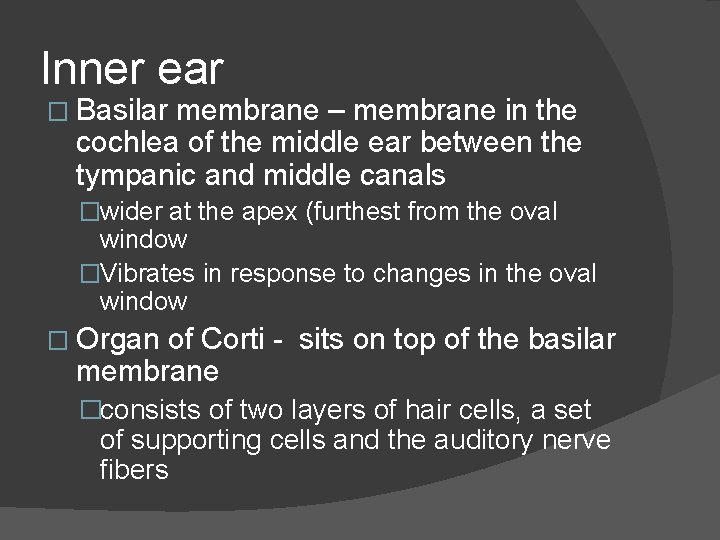
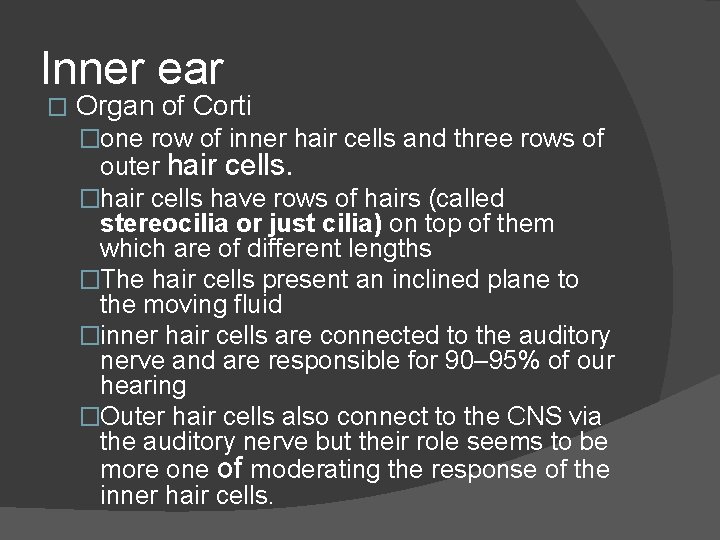
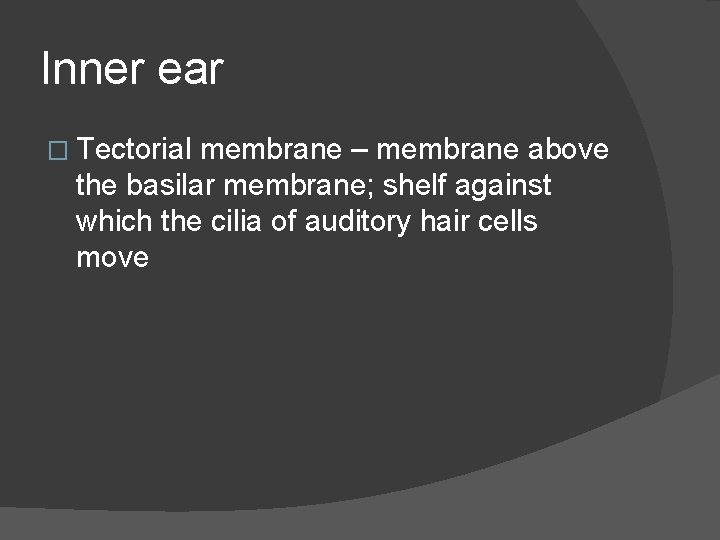
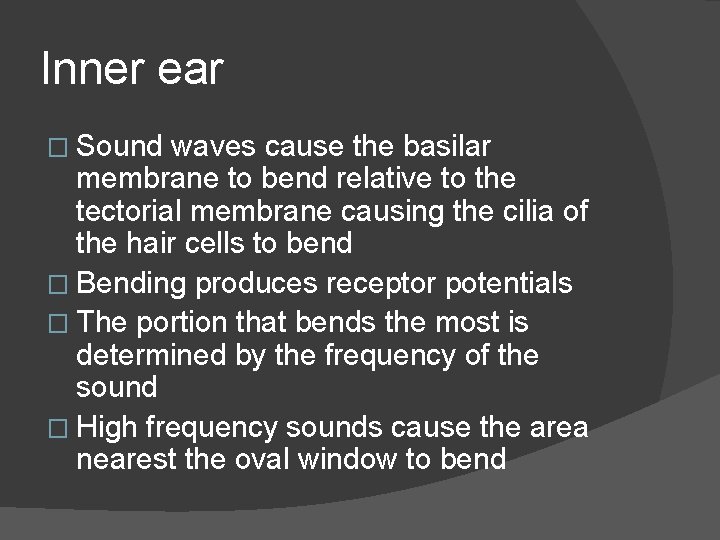
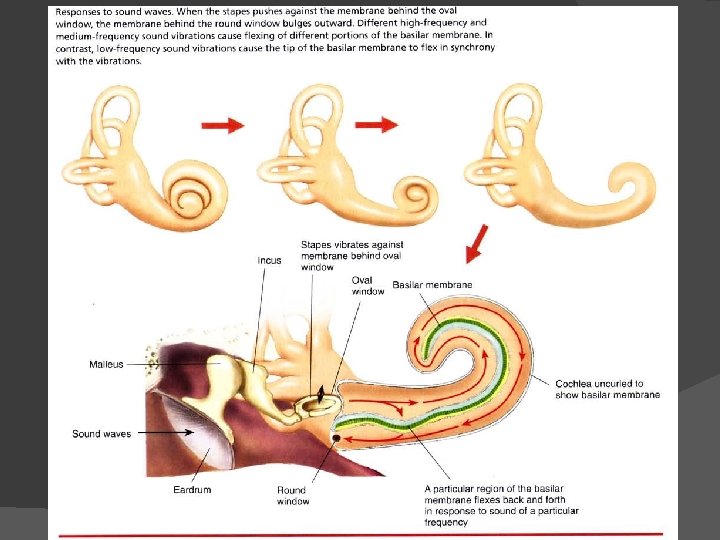
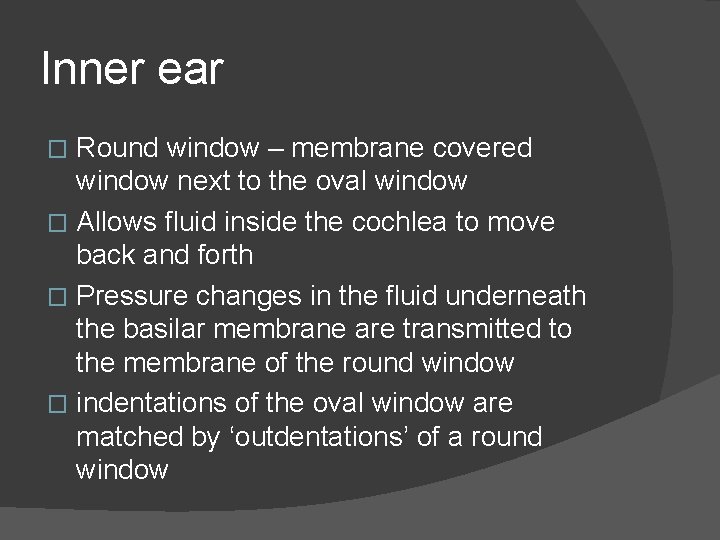
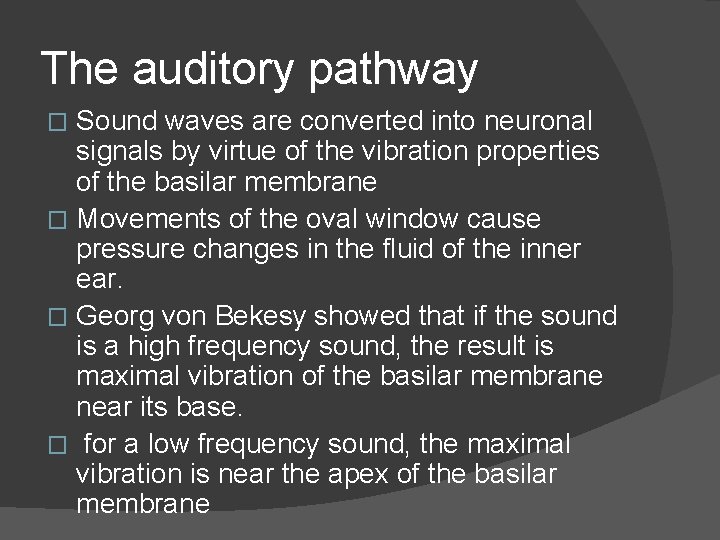
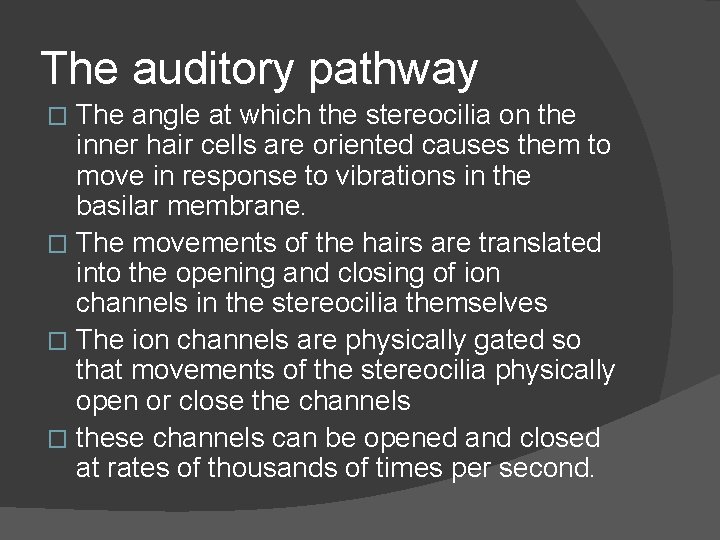
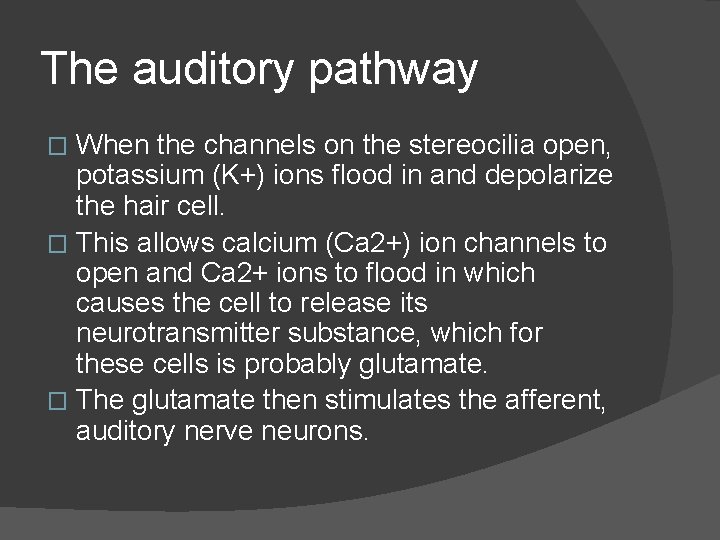
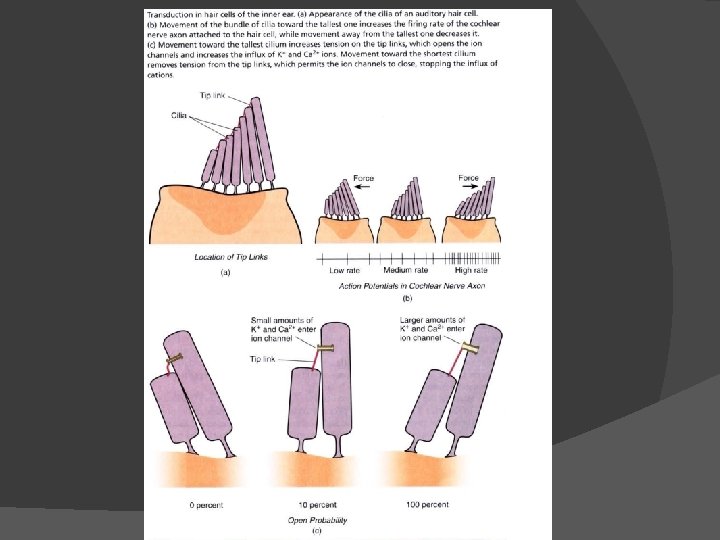
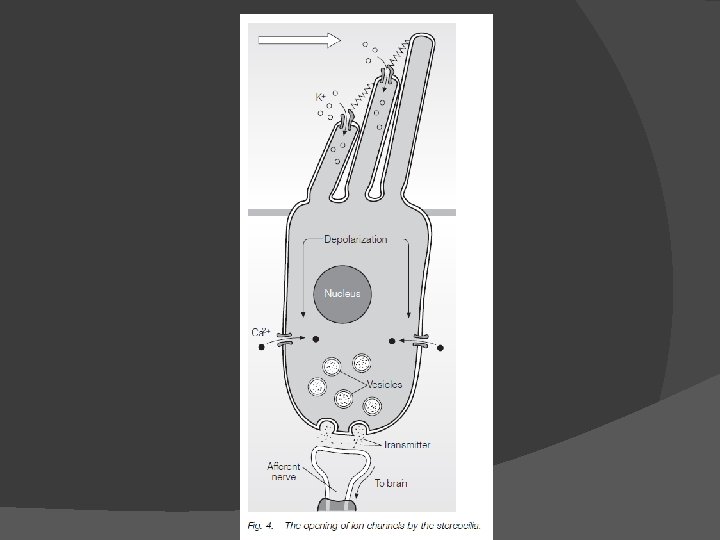
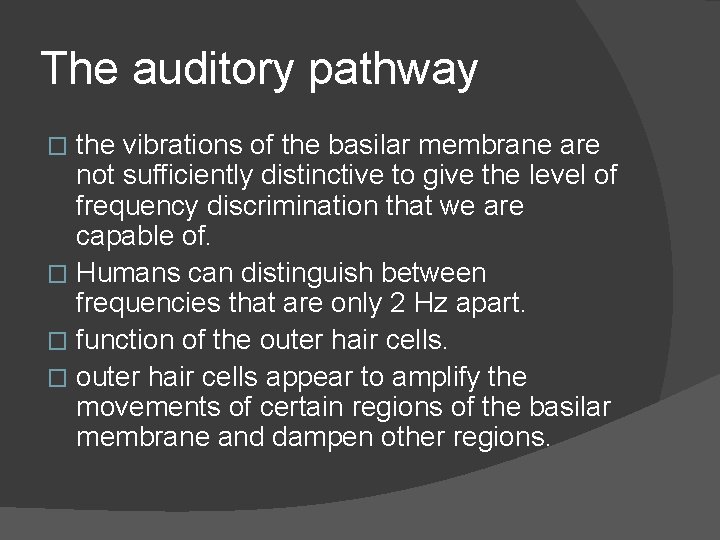
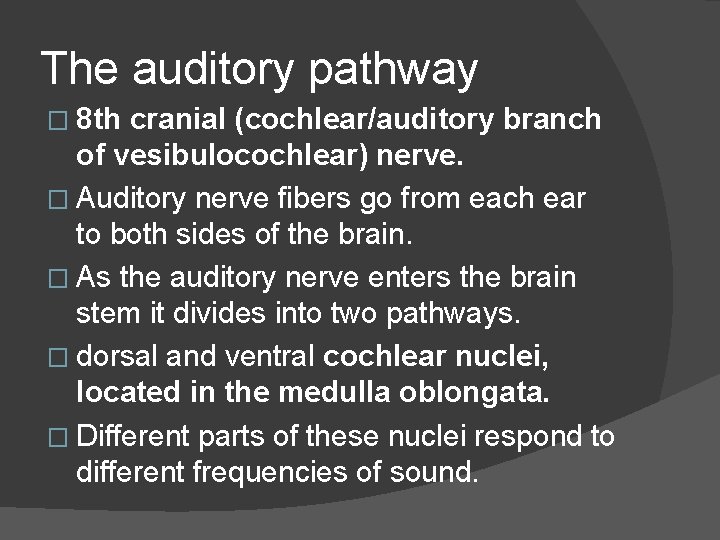
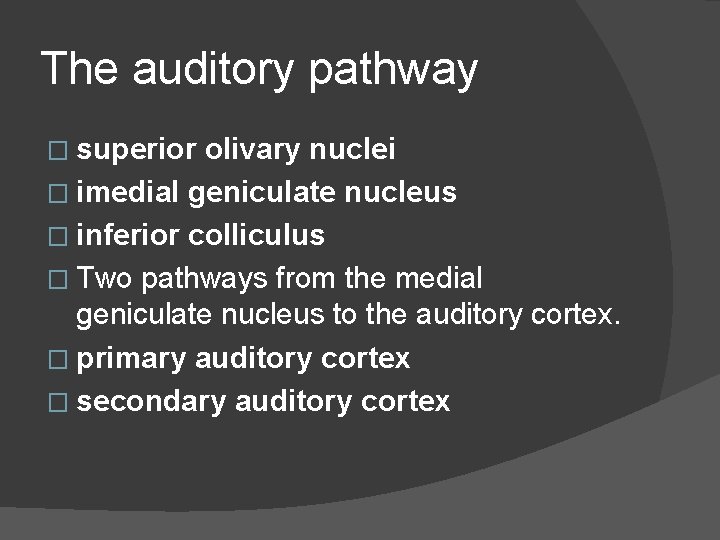
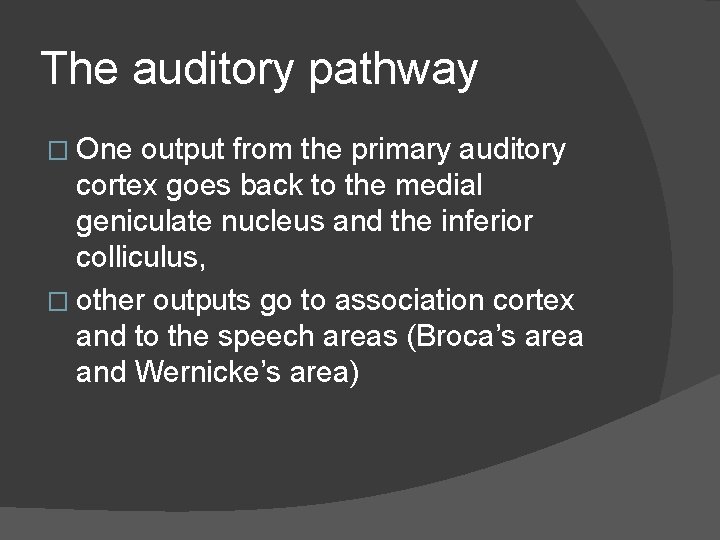
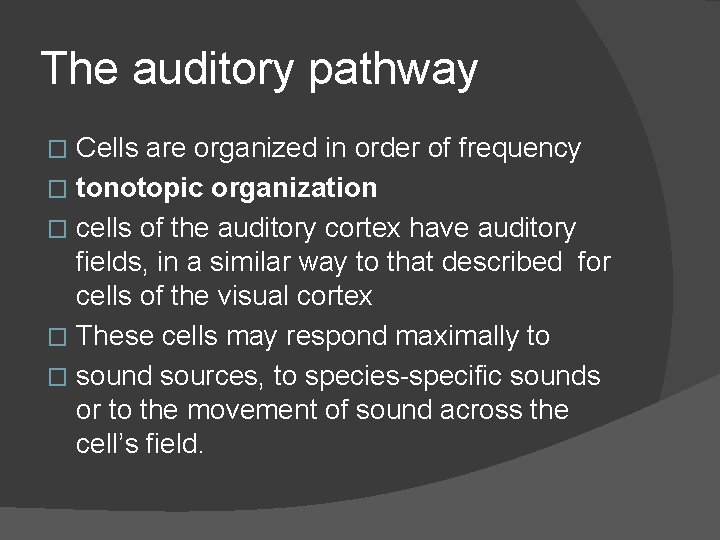
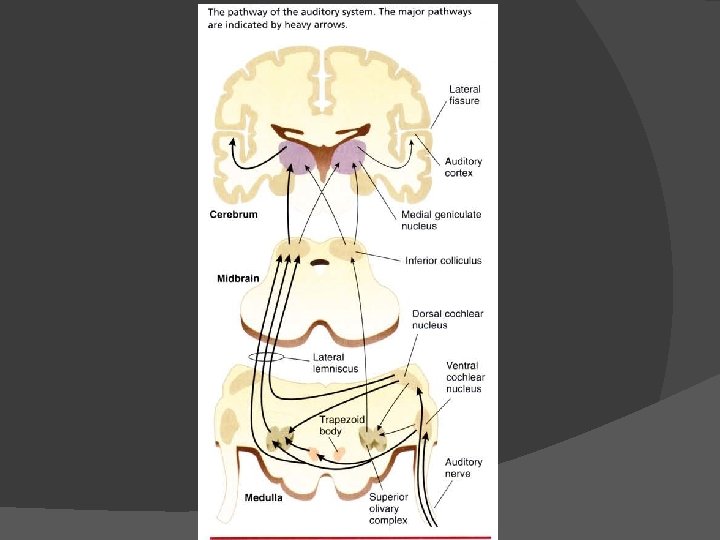
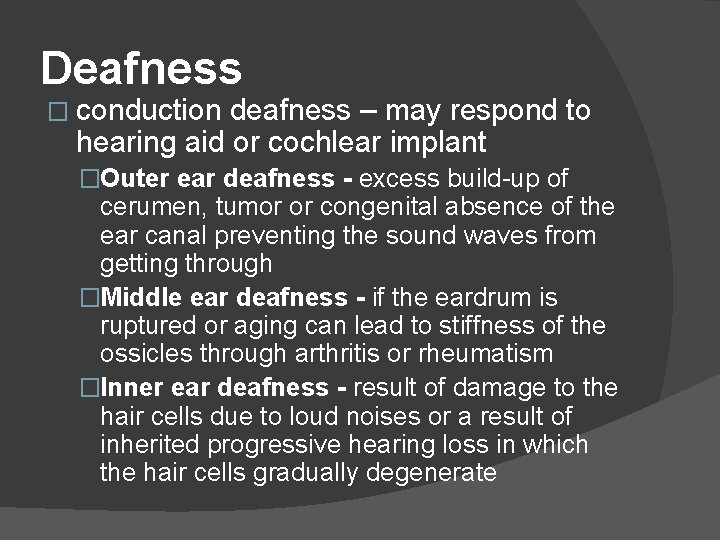
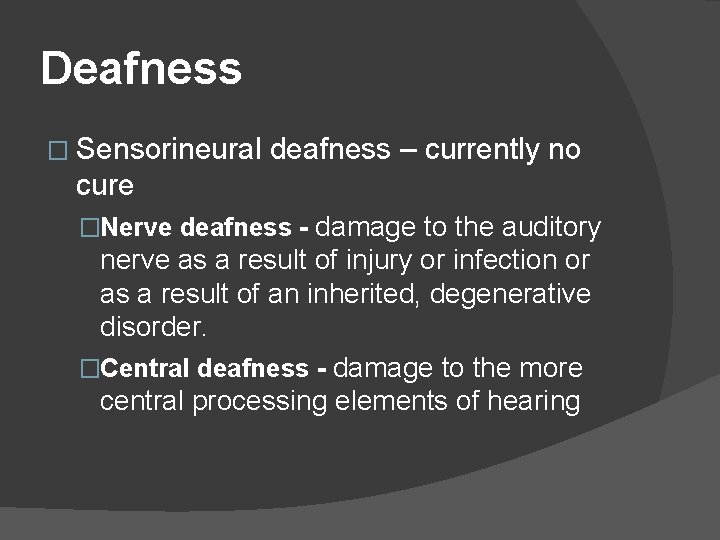
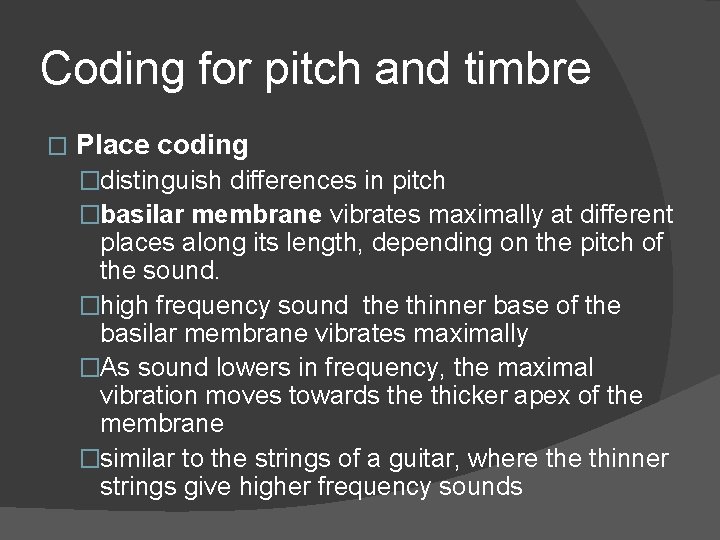
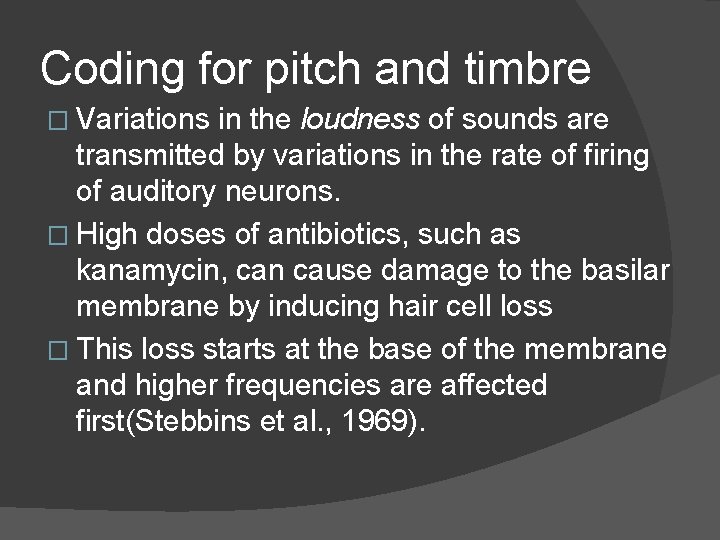
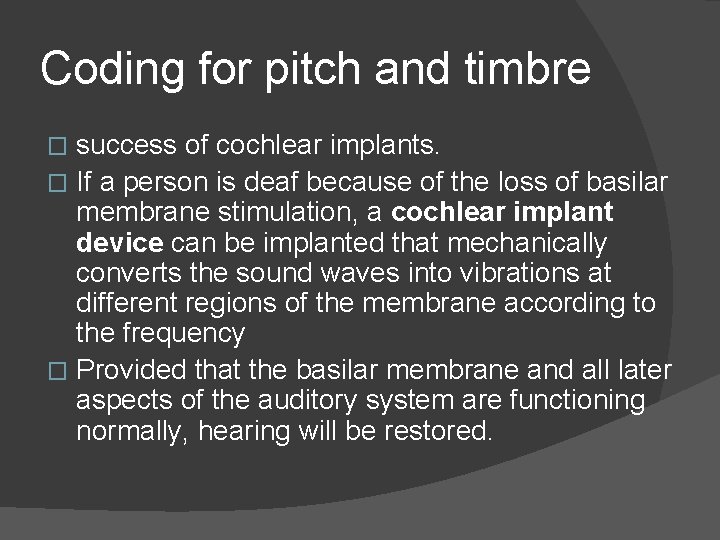
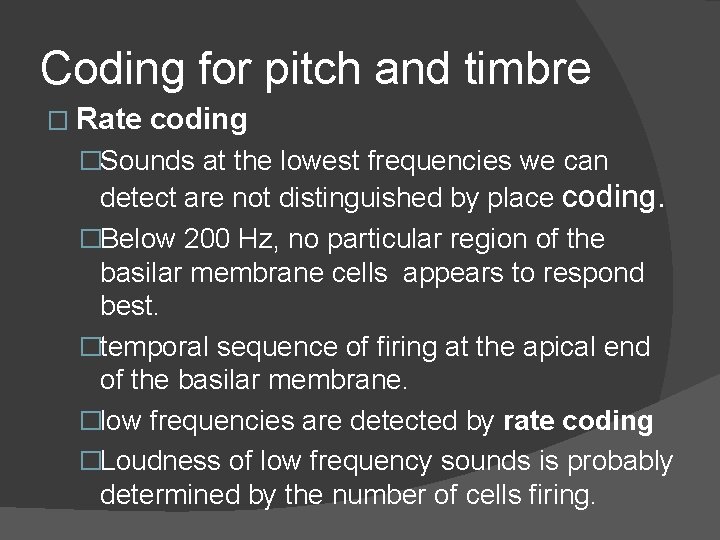
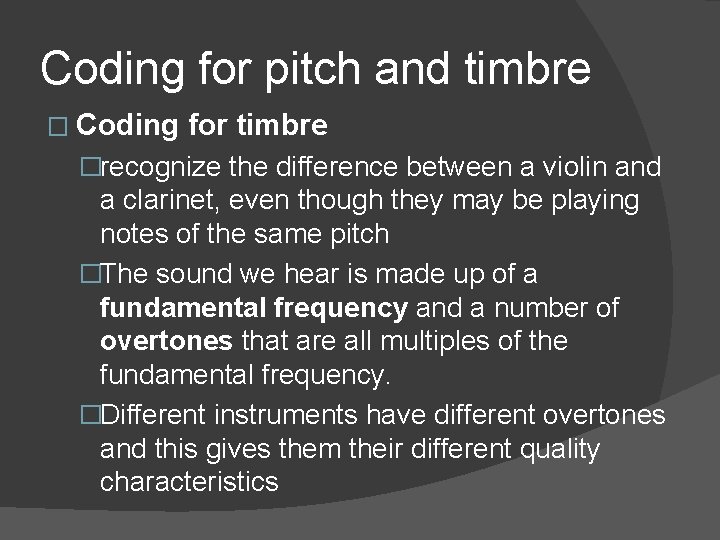
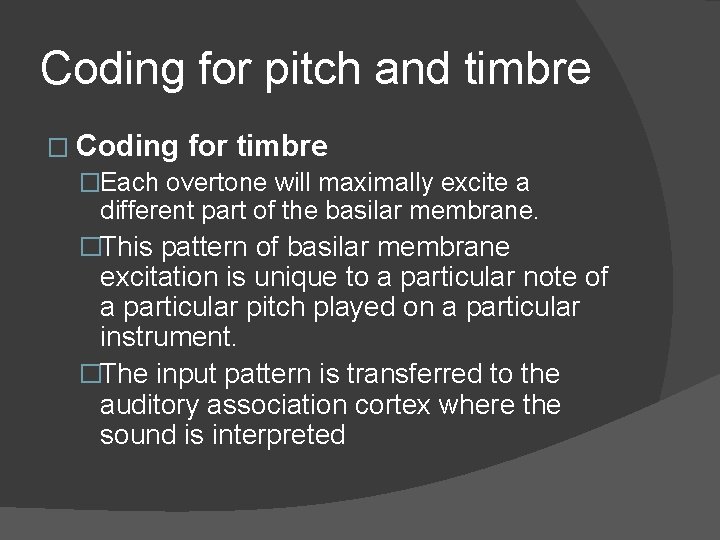
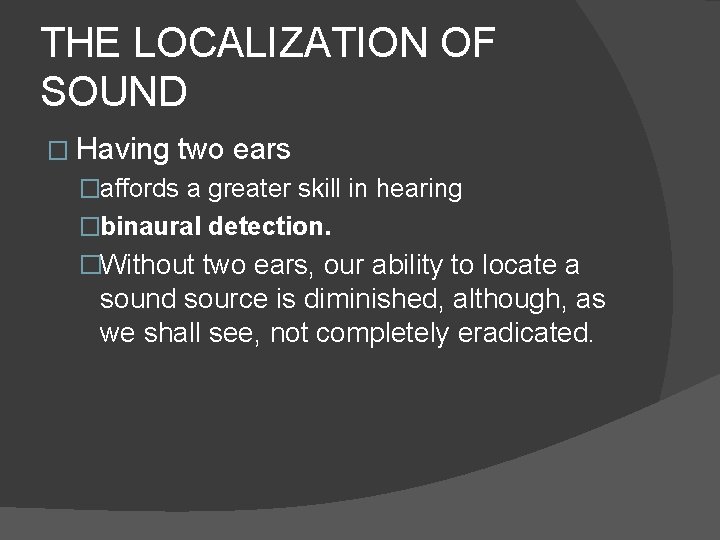
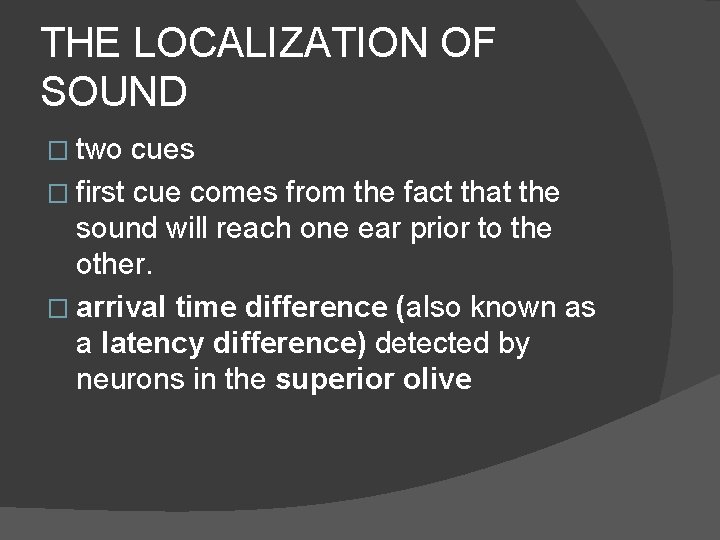
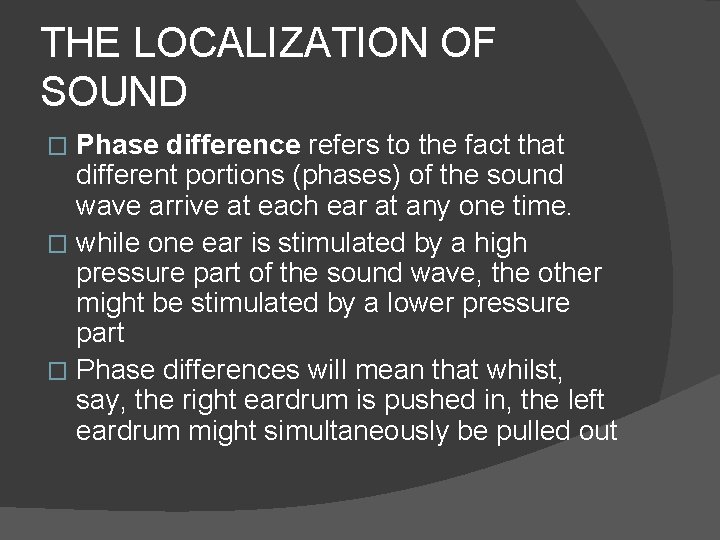
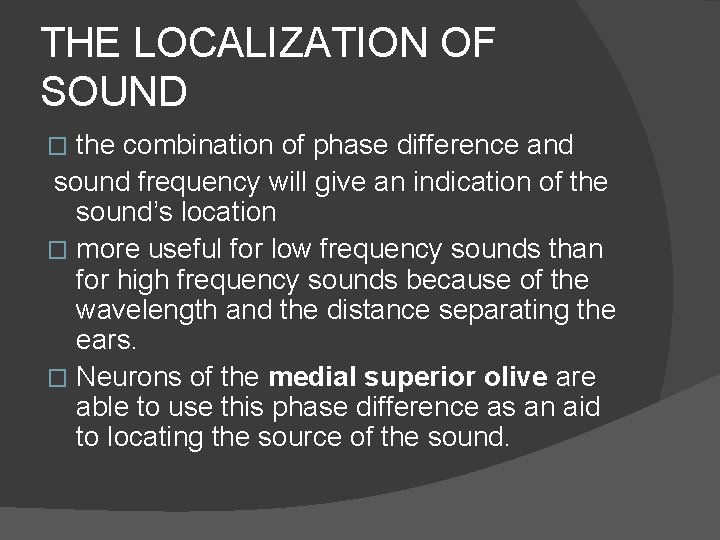
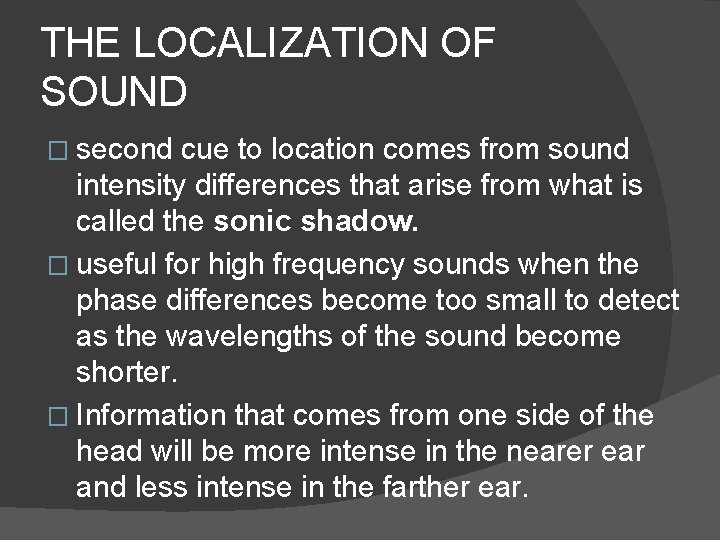
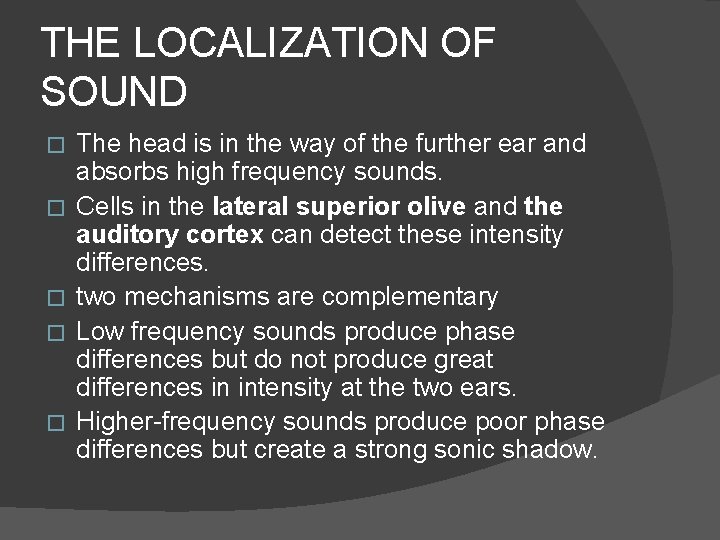
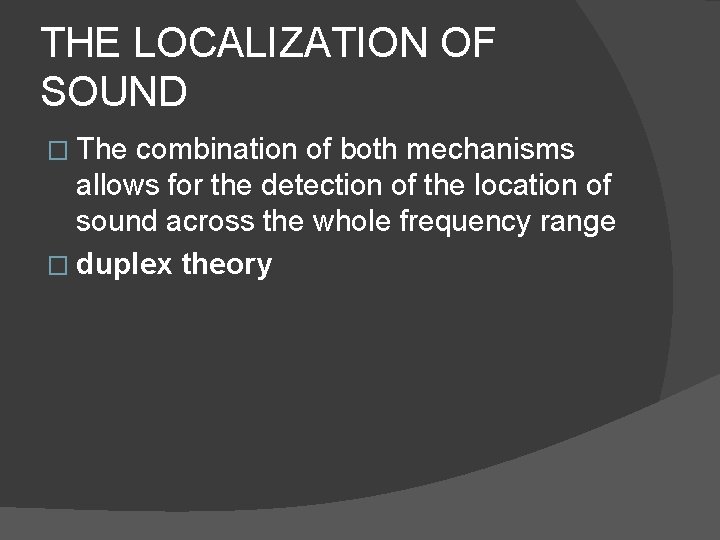
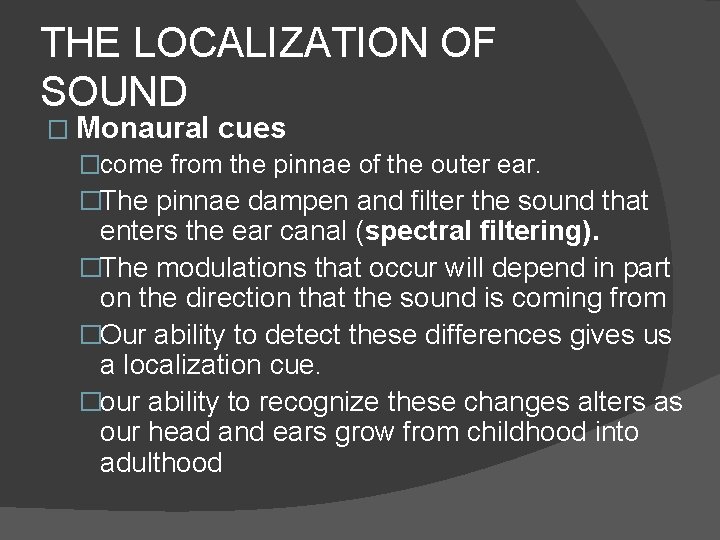
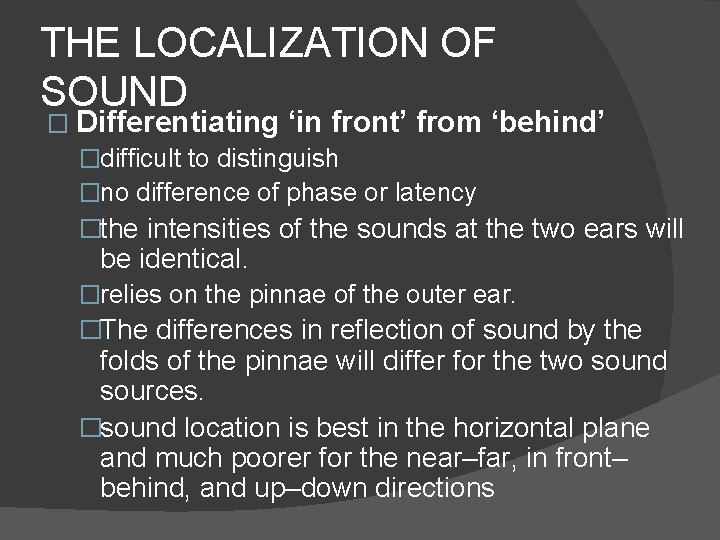
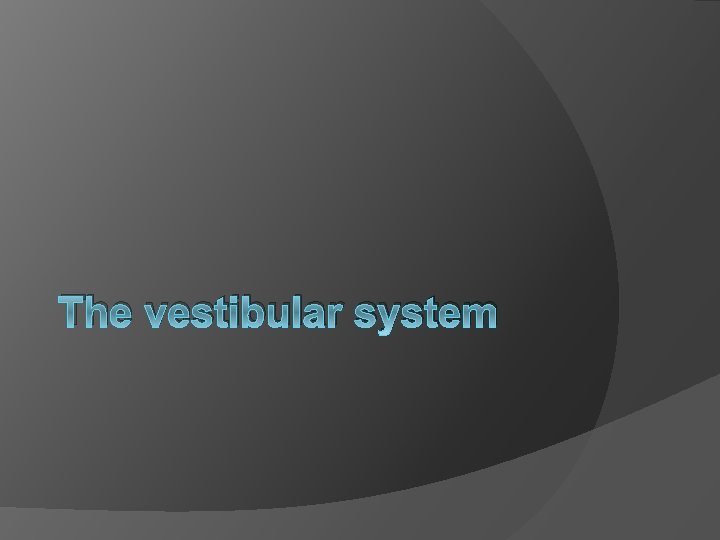
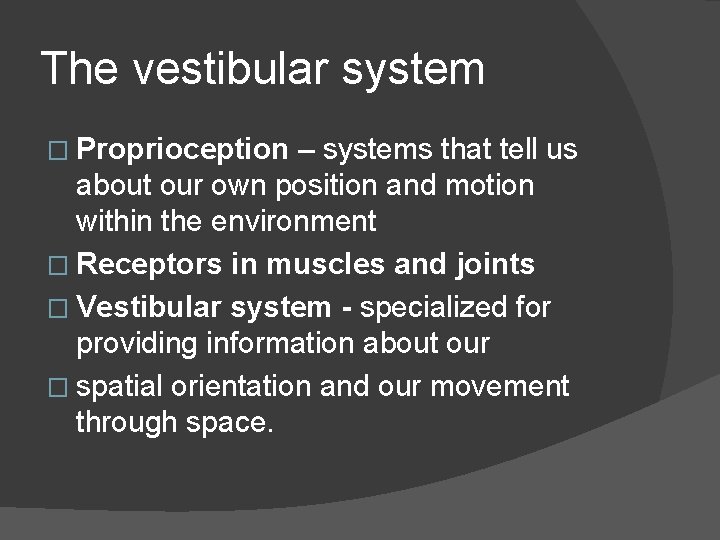
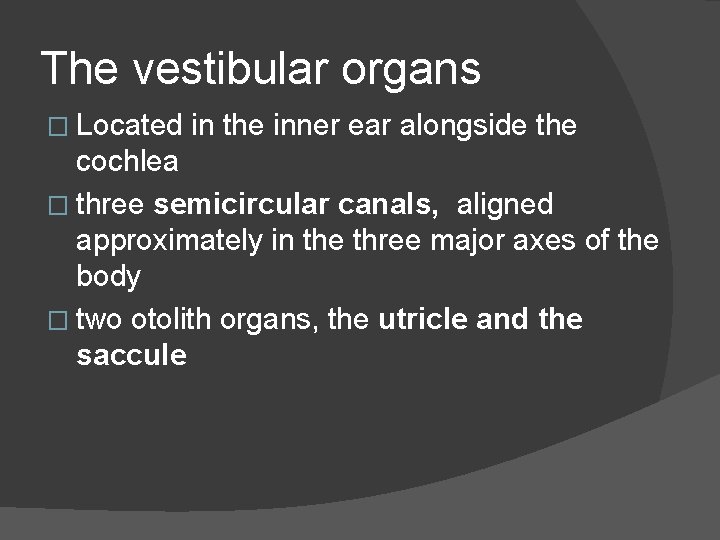
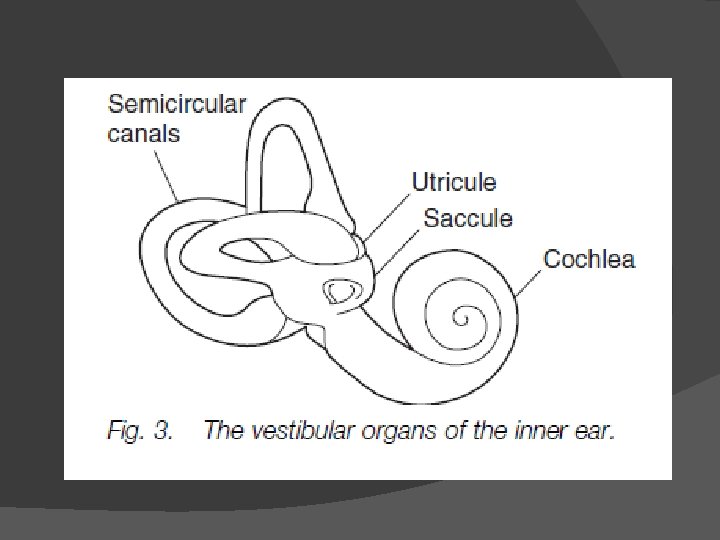
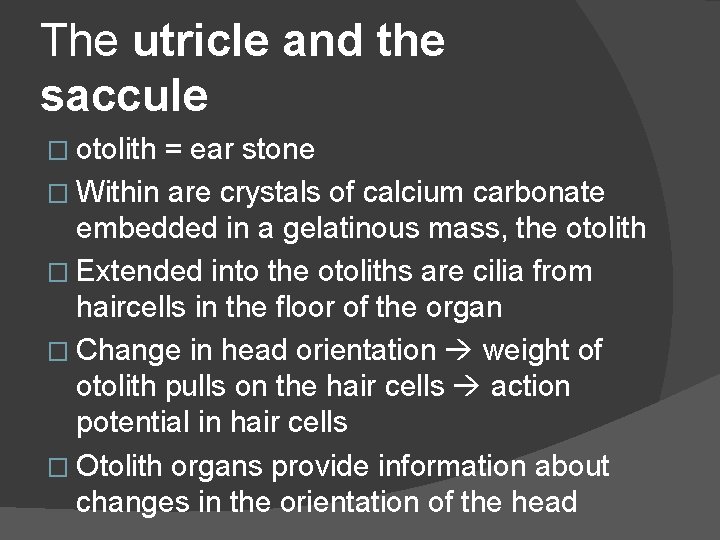
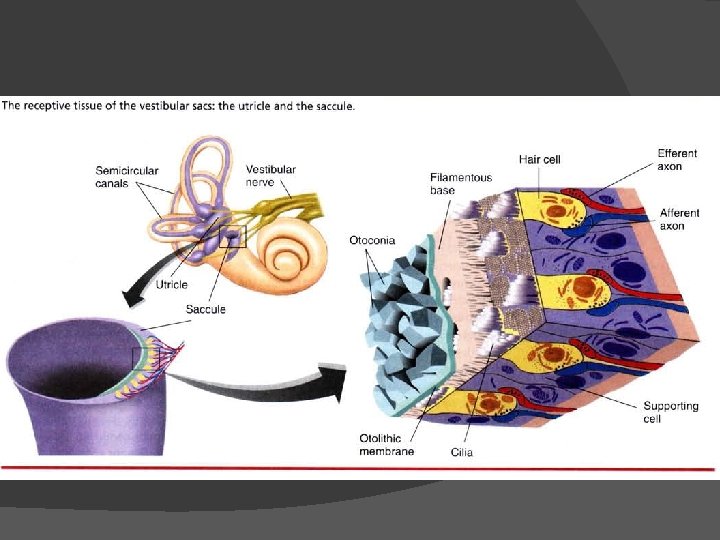
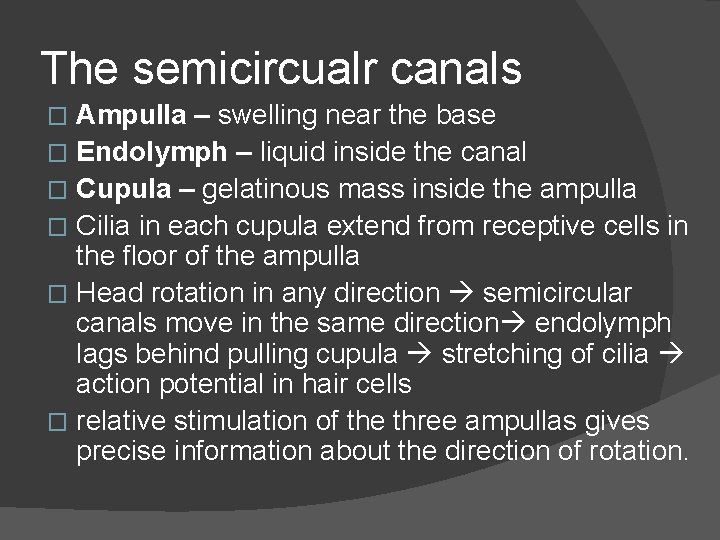
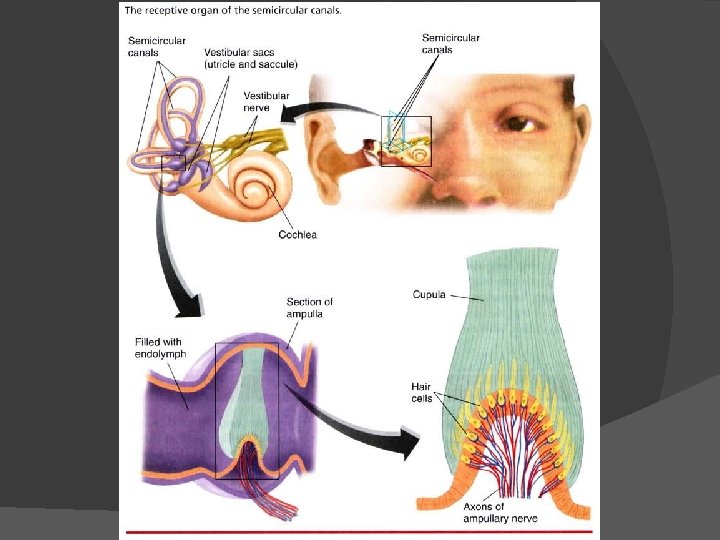
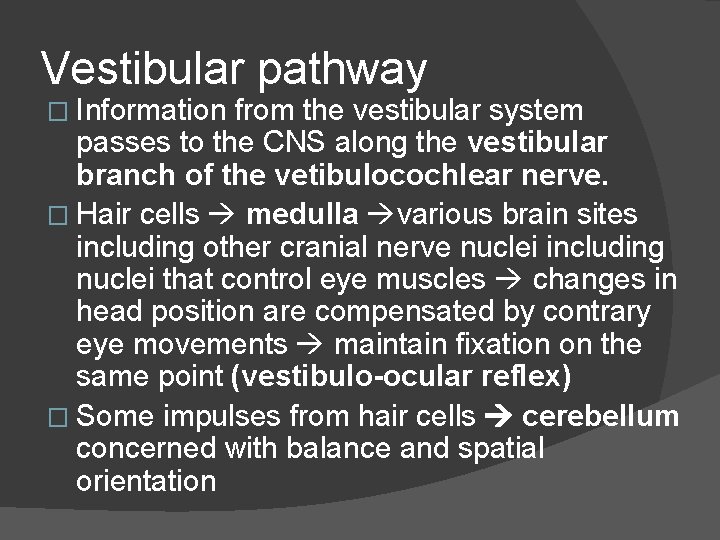
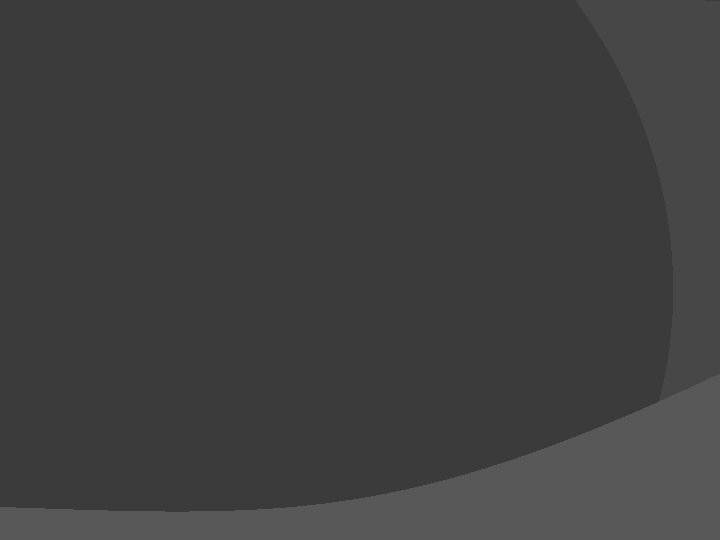
- Slides: 58
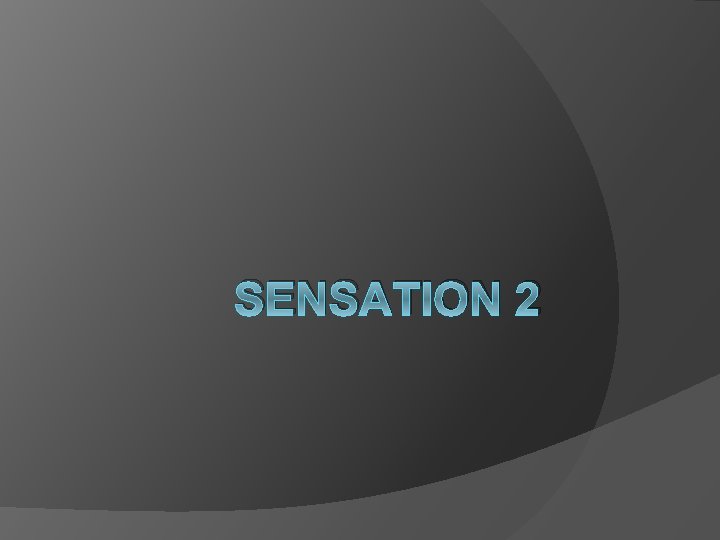
SENSATION 2
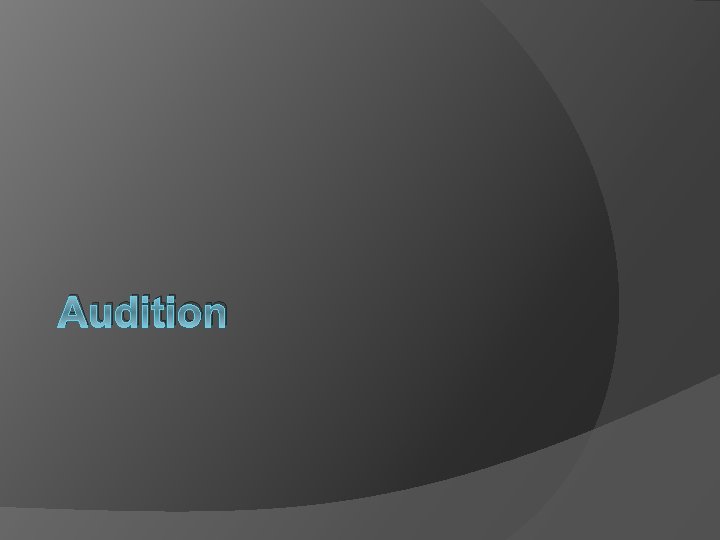
Audition
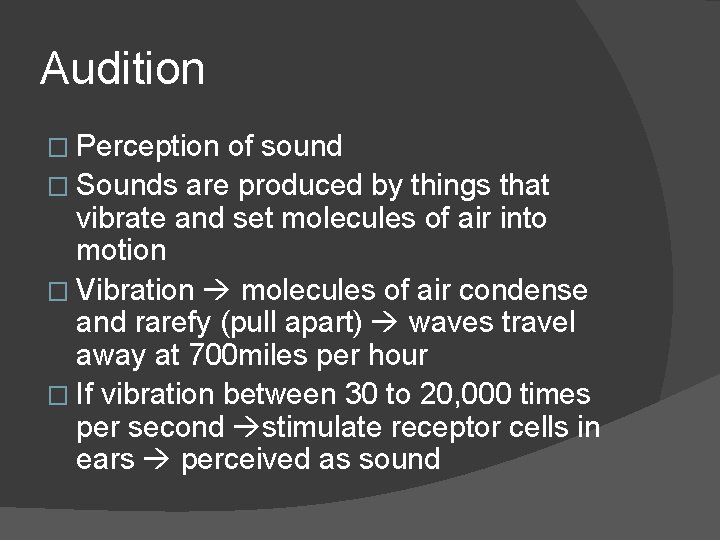
Audition � Perception of sound � Sounds are produced by things that vibrate and set molecules of air into motion � Vibration molecules of air condense and rarefy (pull apart) waves travel away at 700 miles per hour � If vibration between 30 to 20, 000 times per second stimulate receptor cells in ears perceived as sound
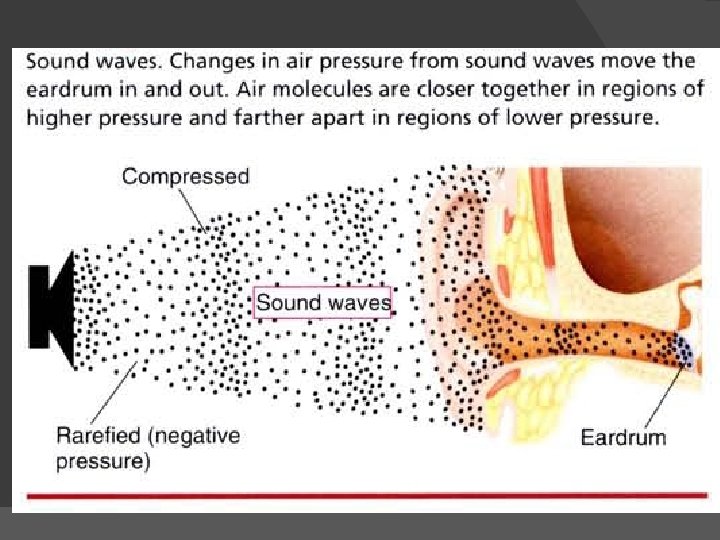
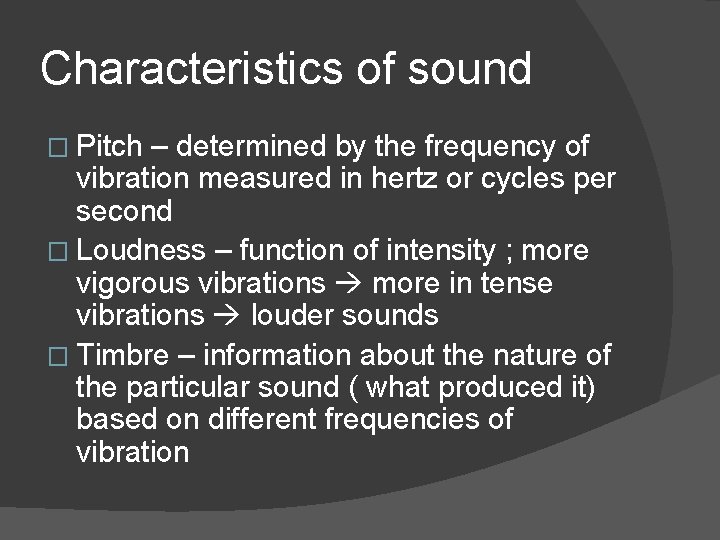
Characteristics of sound � Pitch – determined by the frequency of vibration measured in hertz or cycles per second � Loudness – function of intensity ; more vigorous vibrations more in tense vibrations louder sounds � Timbre – information about the nature of the particular sound ( what produced it) based on different frequencies of vibration
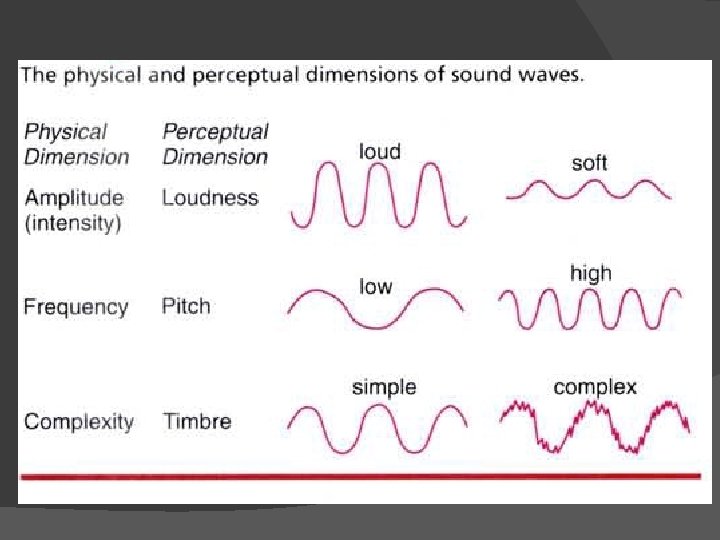
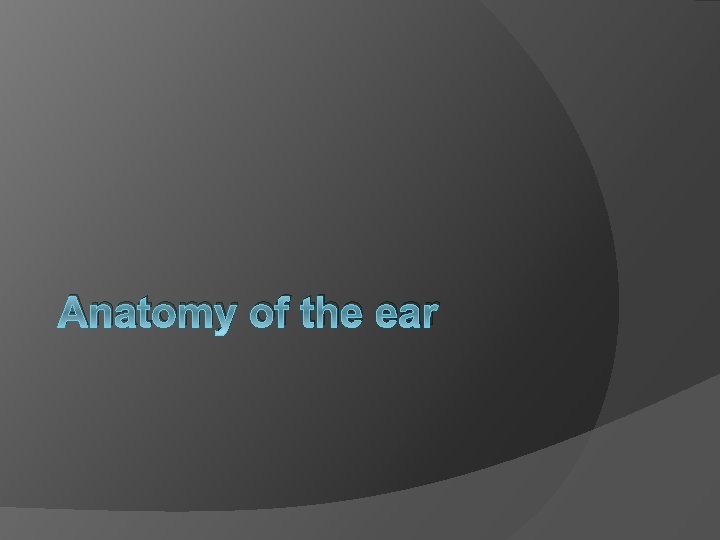
Anatomy of the ear
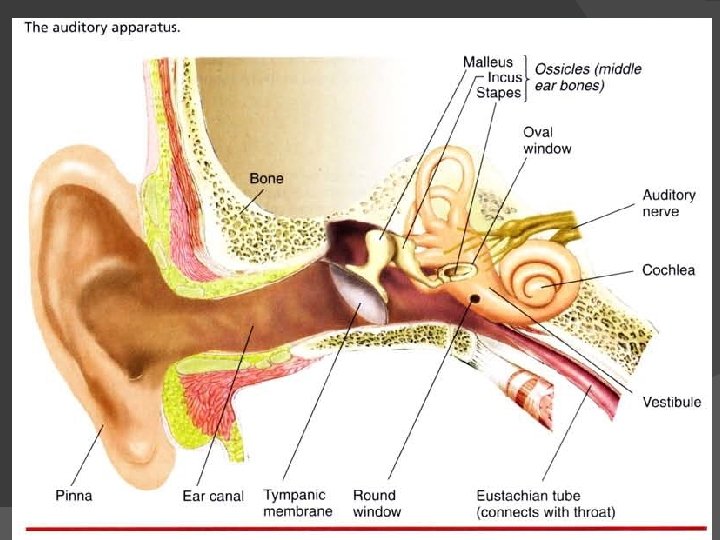
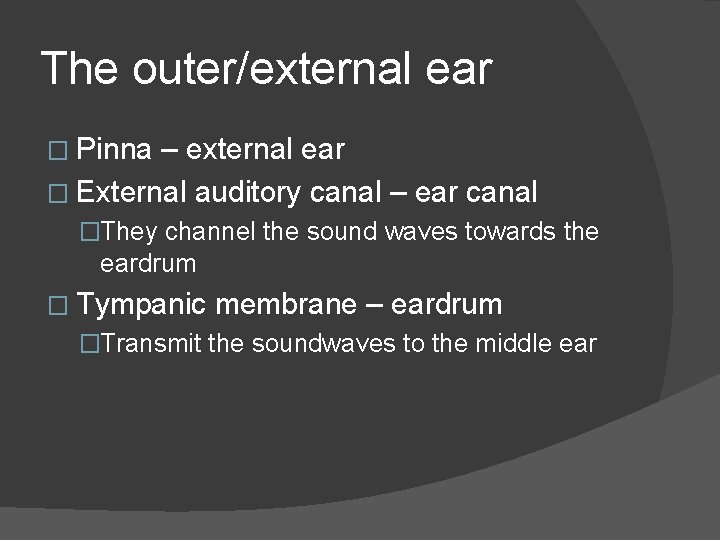
The outer/external ear � Pinna – external ear � External auditory canal – ear canal �They channel the sound waves towards the eardrum � Tympanic membrane – eardrum �Transmit the soundwaves to the middle ear
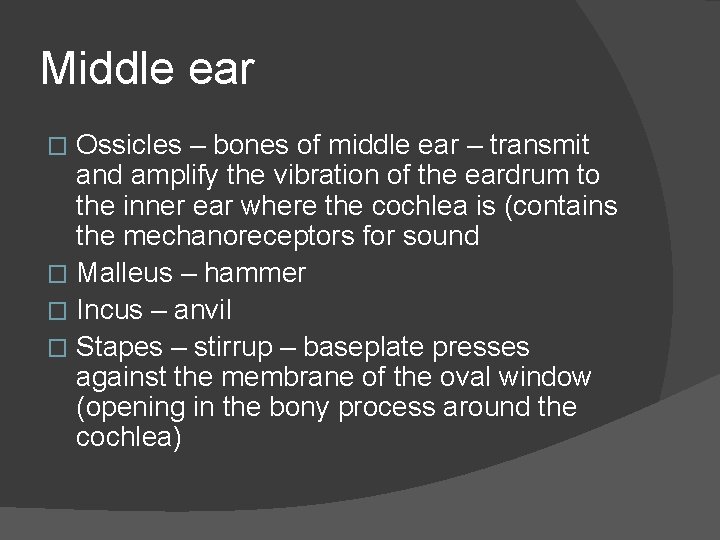
Middle ear Ossicles – bones of middle ear – transmit and amplify the vibration of the eardrum to the inner ear where the cochlea is (contains the mechanoreceptors for sound � Malleus – hammer � Incus – anvil � Stapes – stirrup – baseplate presses against the membrane of the oval window (opening in the bony process around the cochlea) �
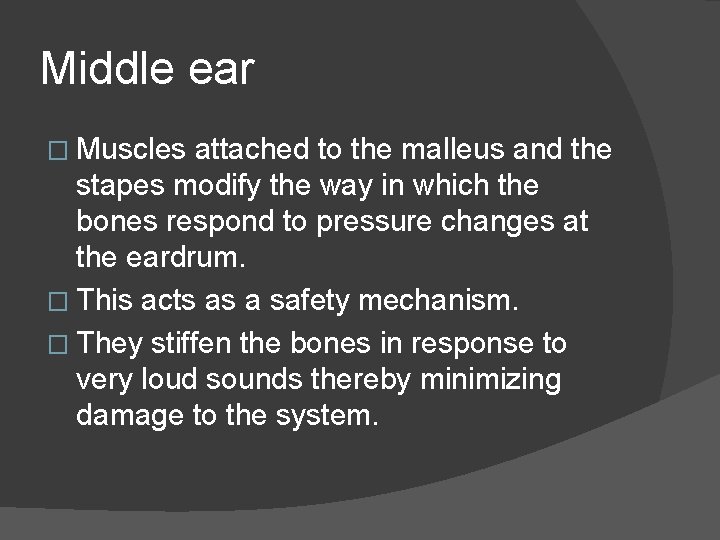
Middle ear � Muscles attached to the malleus and the stapes modify the way in which the bones respond to pressure changes at the eardrum. � This acts as a safety mechanism. � They stiffen the bones in response to very loud sounds thereby minimizing damage to the system.
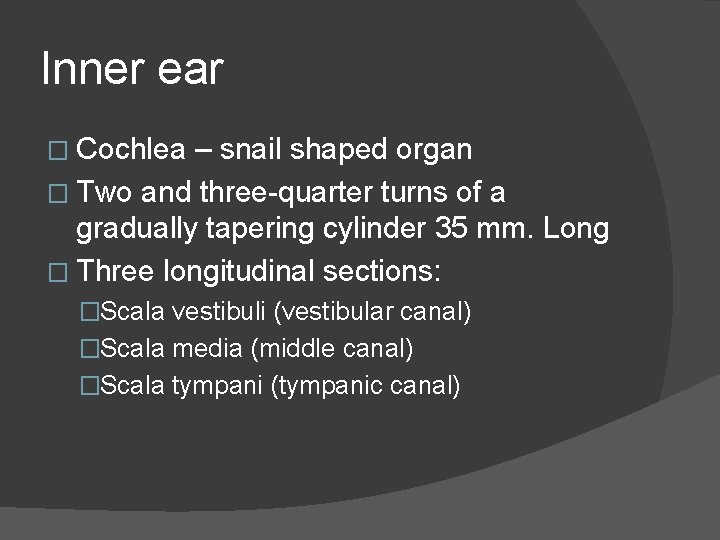
Inner ear � Cochlea – snail shaped organ � Two and three-quarter turns of a gradually tapering cylinder 35 mm. Long � Three longitudinal sections: �Scala vestibuli (vestibular canal) �Scala media (middle canal) �Scala tympani (tympanic canal)
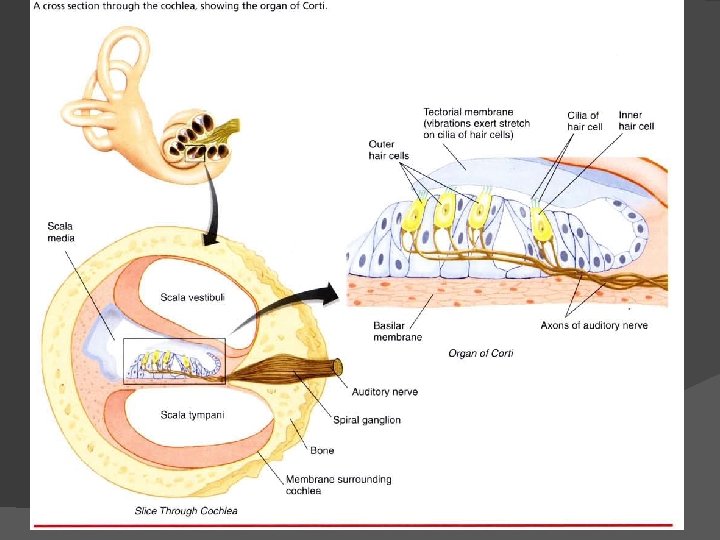
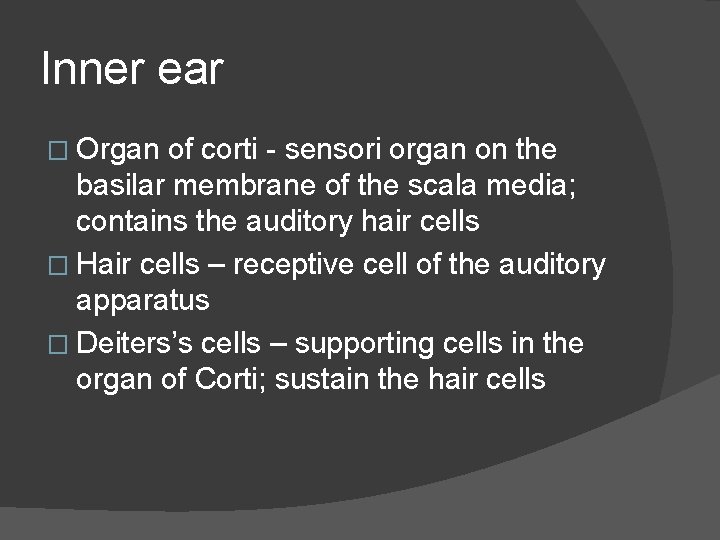
Inner ear � Organ of corti - sensori organ on the basilar membrane of the scala media; contains the auditory hair cells � Hair cells – receptive cell of the auditory apparatus � Deiters’s cells – supporting cells in the organ of Corti; sustain the hair cells
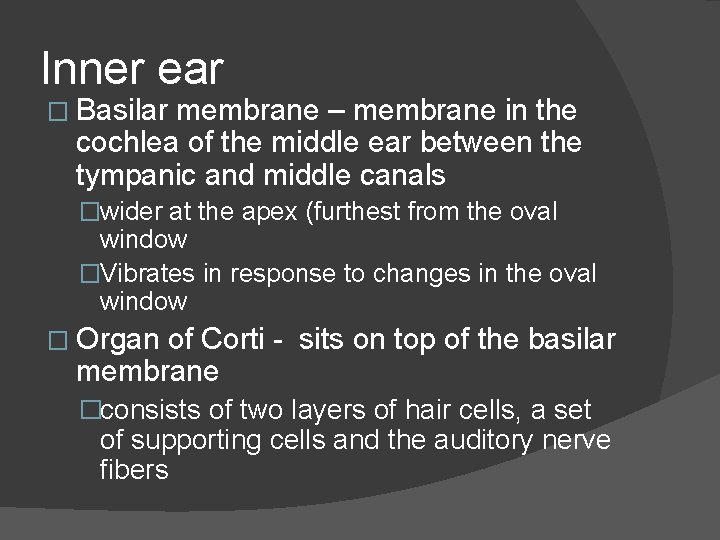
Inner ear � Basilar membrane – membrane in the cochlea of the middle ear between the tympanic and middle canals �wider at the apex (furthest from the oval window �Vibrates in response to changes in the oval window � Organ of Corti - sits on top of the basilar membrane �consists of two layers of hair cells, a set of supporting cells and the auditory nerve fibers
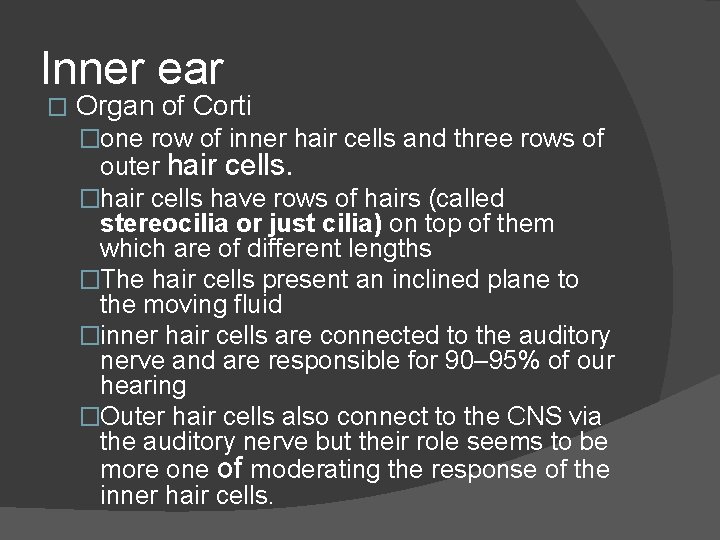
Inner ear � Organ of Corti �one row of inner hair cells and three rows of outer hair cells. �hair cells have rows of hairs (called stereocilia or just cilia) on top of them which are of different lengths �The hair cells present an inclined plane to the moving fluid �inner hair cells are connected to the auditory nerve and are responsible for 90– 95% of our hearing �Outer hair cells also connect to the CNS via the auditory nerve but their role seems to be more one of moderating the response of the inner hair cells.
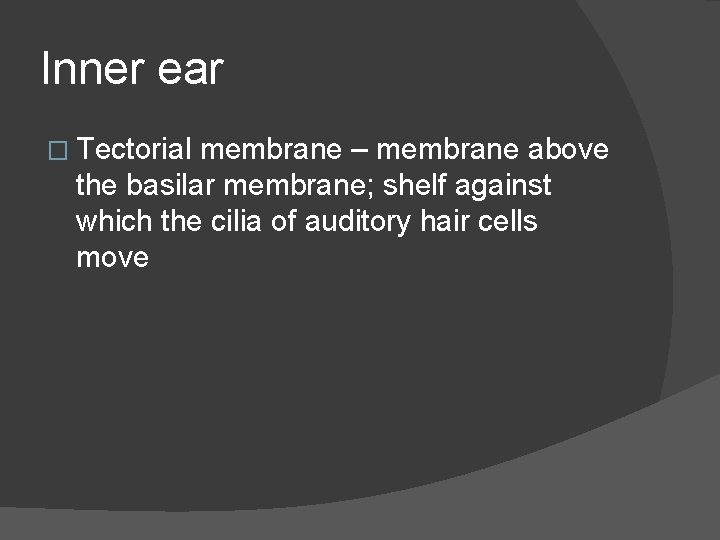
Inner ear � Tectorial membrane – membrane above the basilar membrane; shelf against which the cilia of auditory hair cells move
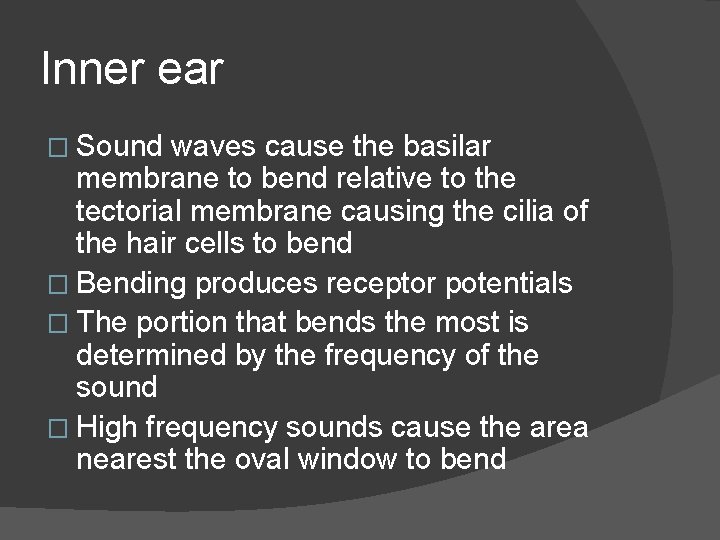
Inner ear � Sound waves cause the basilar membrane to bend relative to the tectorial membrane causing the cilia of the hair cells to bend � Bending produces receptor potentials � The portion that bends the most is determined by the frequency of the sound � High frequency sounds cause the area nearest the oval window to bend
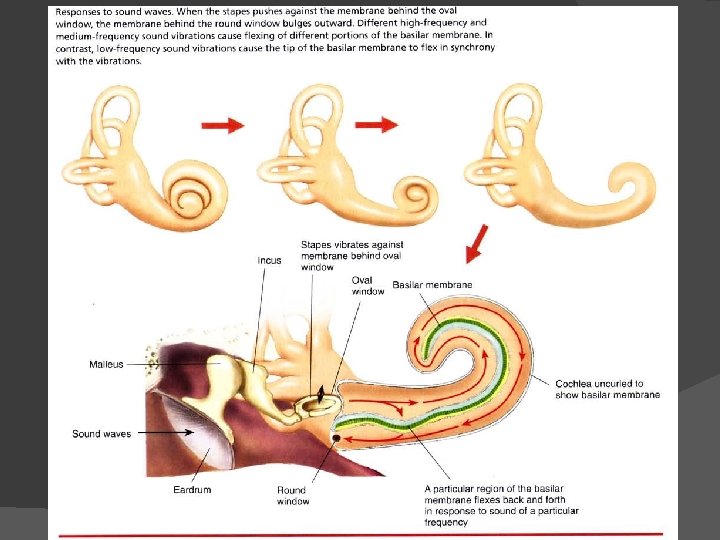
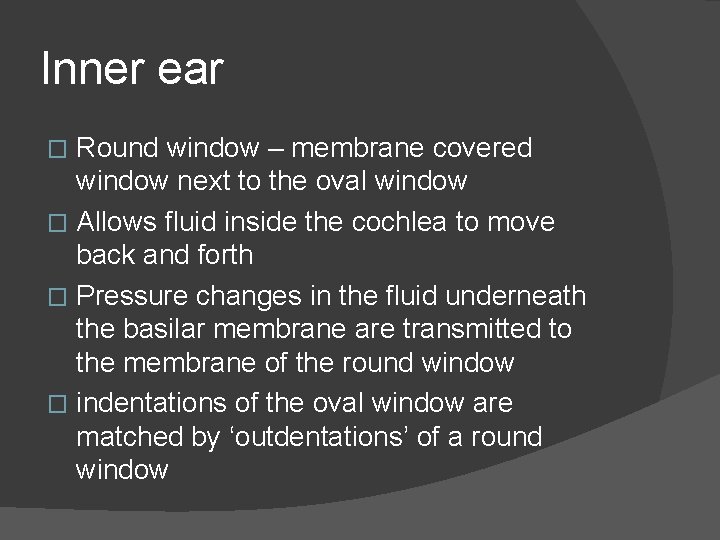
Inner ear Round window – membrane covered window next to the oval window � Allows fluid inside the cochlea to move back and forth � Pressure changes in the fluid underneath the basilar membrane are transmitted to the membrane of the round window � indentations of the oval window are matched by ‘outdentations’ of a round window �
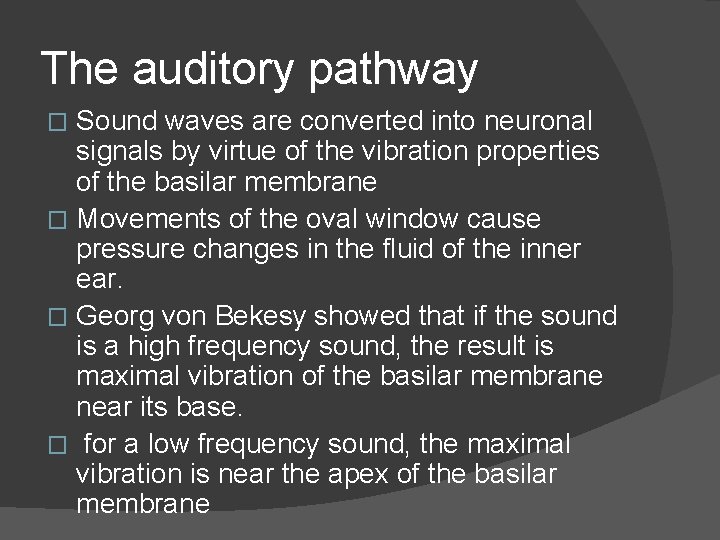
The auditory pathway Sound waves are converted into neuronal signals by virtue of the vibration properties of the basilar membrane � Movements of the oval window cause pressure changes in the fluid of the inner ear. � Georg von Bekesy showed that if the sound is a high frequency sound, the result is maximal vibration of the basilar membrane near its base. � for a low frequency sound, the maximal vibration is near the apex of the basilar membrane �
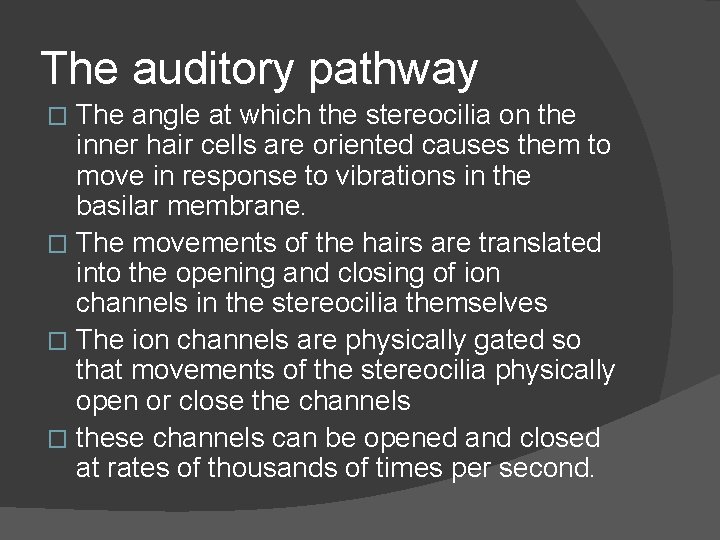
The auditory pathway The angle at which the stereocilia on the inner hair cells are oriented causes them to move in response to vibrations in the basilar membrane. � The movements of the hairs are translated into the opening and closing of ion channels in the stereocilia themselves � The ion channels are physically gated so that movements of the stereocilia physically open or close the channels � these channels can be opened and closed at rates of thousands of times per second. �
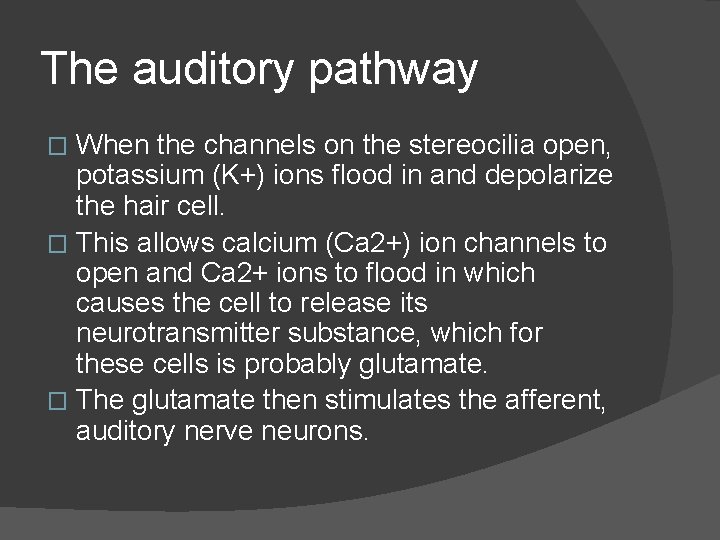
The auditory pathway When the channels on the stereocilia open, potassium (K+) ions flood in and depolarize the hair cell. � This allows calcium (Ca 2+) ion channels to open and Ca 2+ ions to flood in which causes the cell to release its neurotransmitter substance, which for these cells is probably glutamate. � The glutamate then stimulates the afferent, auditory nerve neurons. �
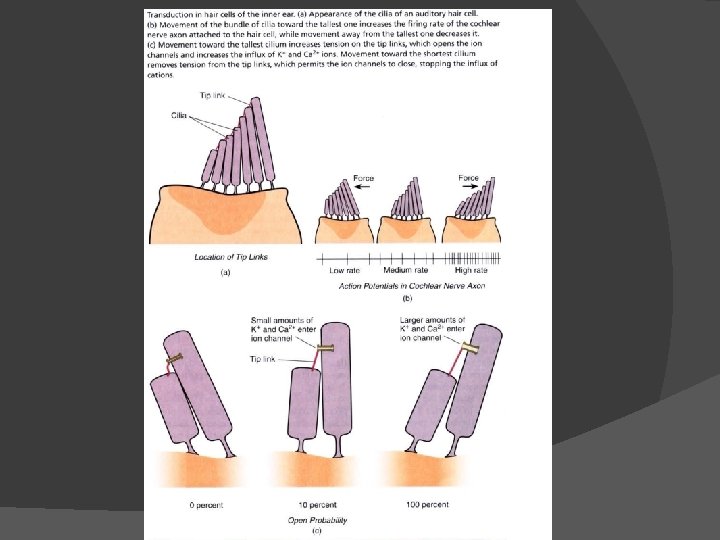
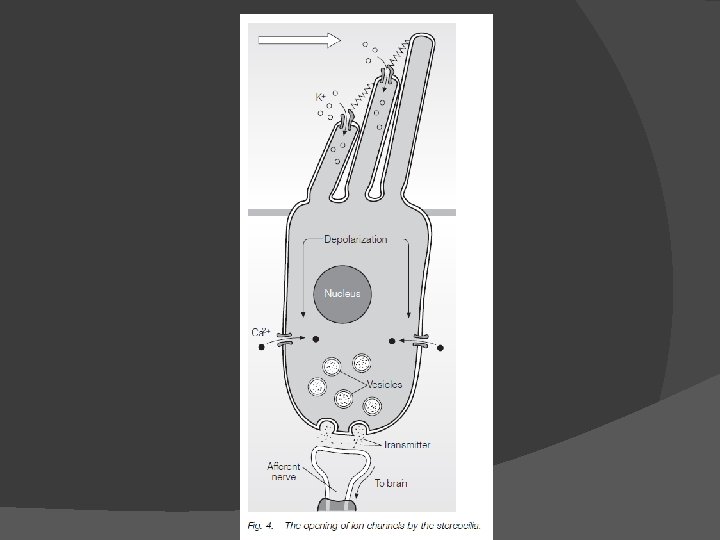
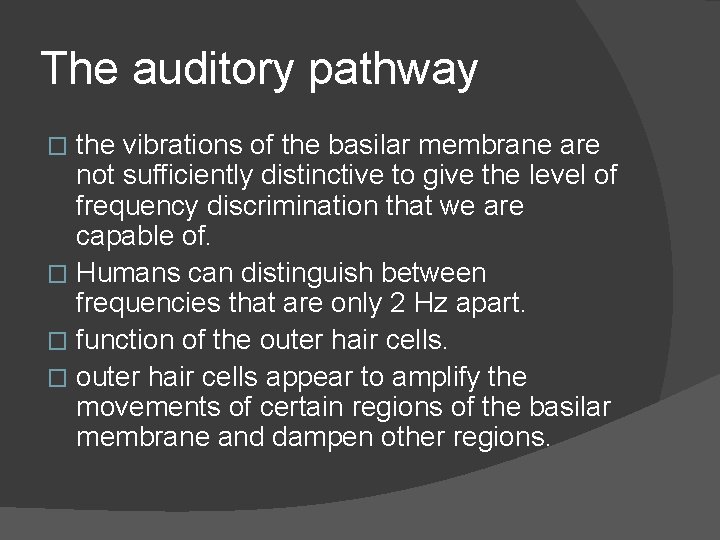
The auditory pathway the vibrations of the basilar membrane are not sufficiently distinctive to give the level of frequency discrimination that we are capable of. � Humans can distinguish between frequencies that are only 2 Hz apart. � function of the outer hair cells. � outer hair cells appear to amplify the movements of certain regions of the basilar membrane and dampen other regions. �
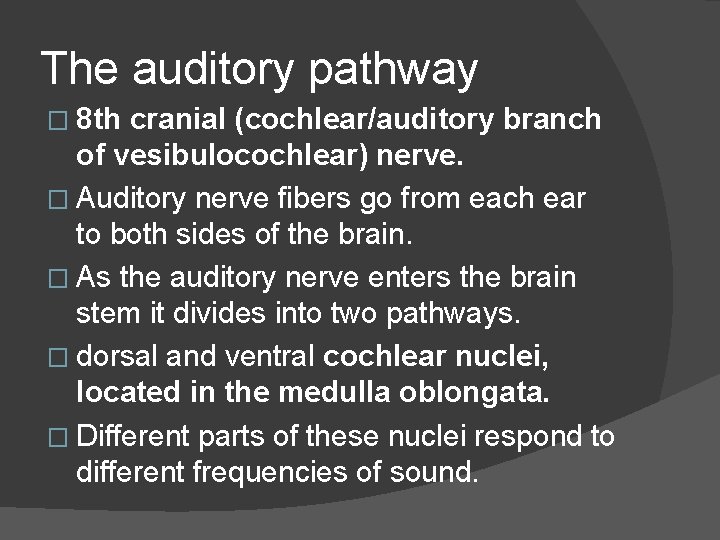
The auditory pathway � 8 th cranial (cochlear/auditory branch of vesibulocochlear) nerve. � Auditory nerve fibers go from each ear to both sides of the brain. � As the auditory nerve enters the brain stem it divides into two pathways. � dorsal and ventral cochlear nuclei, located in the medulla oblongata. � Different parts of these nuclei respond to different frequencies of sound.
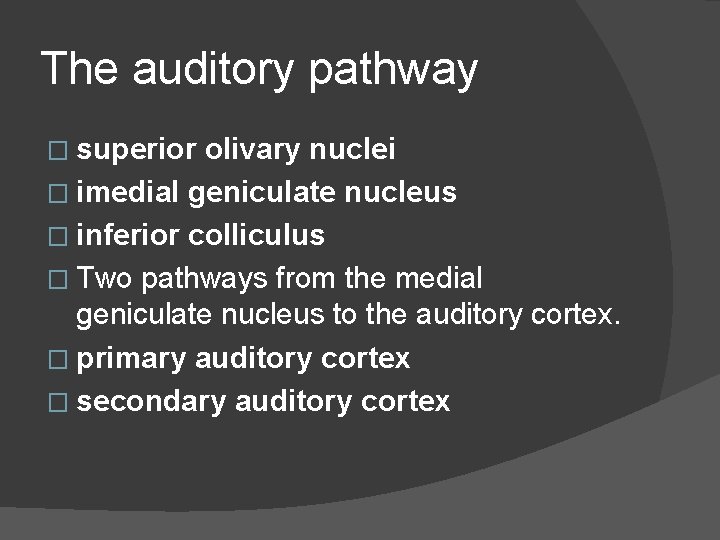
The auditory pathway � superior olivary nuclei � imedial geniculate nucleus � inferior colliculus � Two pathways from the medial geniculate nucleus to the auditory cortex. � primary auditory cortex � secondary auditory cortex
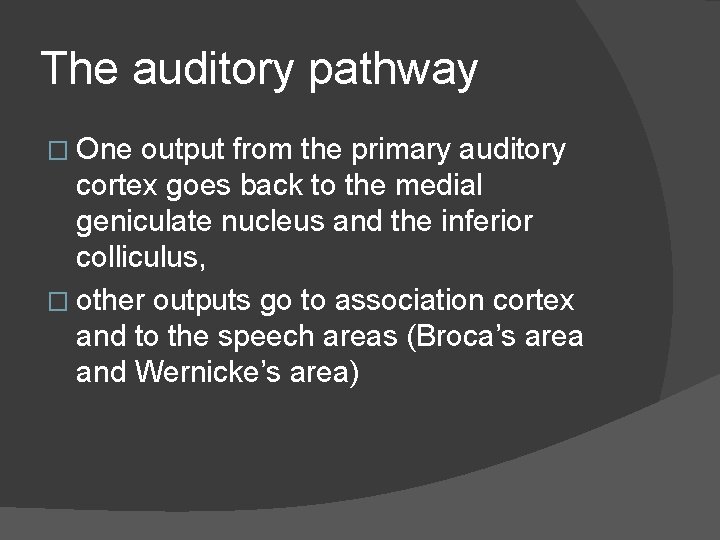
The auditory pathway � One output from the primary auditory cortex goes back to the medial geniculate nucleus and the inferior colliculus, � other outputs go to association cortex and to the speech areas (Broca’s area and Wernicke’s area)
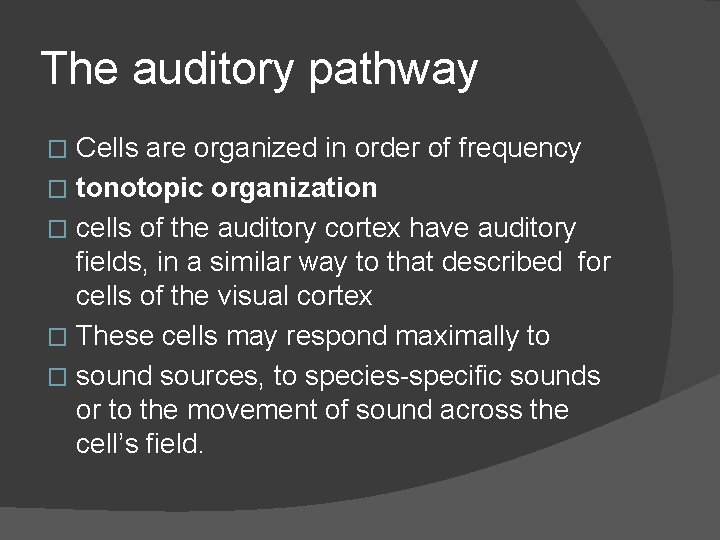
The auditory pathway Cells are organized in order of frequency � tonotopic organization � cells of the auditory cortex have auditory fields, in a similar way to that described for cells of the visual cortex � These cells may respond maximally to � sound sources, to species-specific sounds or to the movement of sound across the cell’s field. �
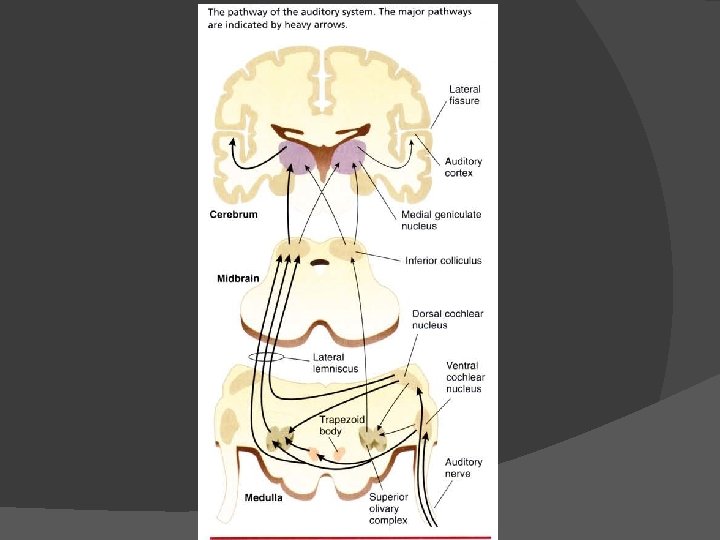
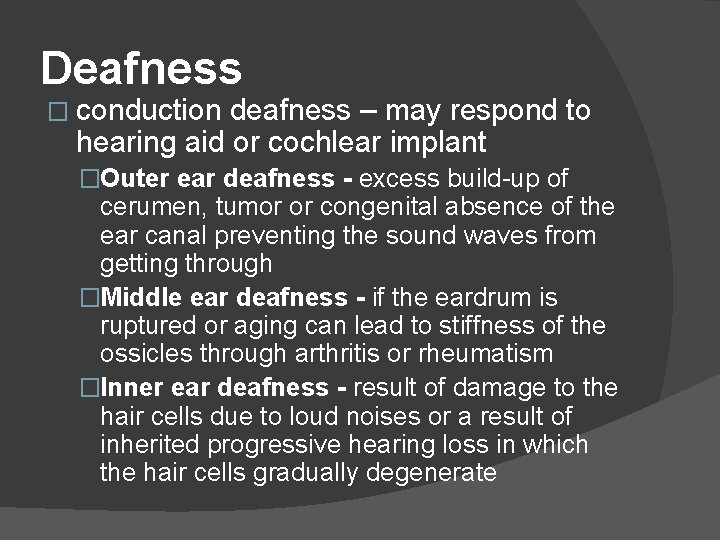
Deafness � conduction deafness – may respond to hearing aid or cochlear implant �Outer ear deafness - excess build-up of cerumen, tumor or congenital absence of the ear canal preventing the sound waves from getting through �Middle ear deafness - if the eardrum is ruptured or aging can lead to stiffness of the ossicles through arthritis or rheumatism �Inner ear deafness - result of damage to the hair cells due to loud noises or a result of inherited progressive hearing loss in which the hair cells gradually degenerate
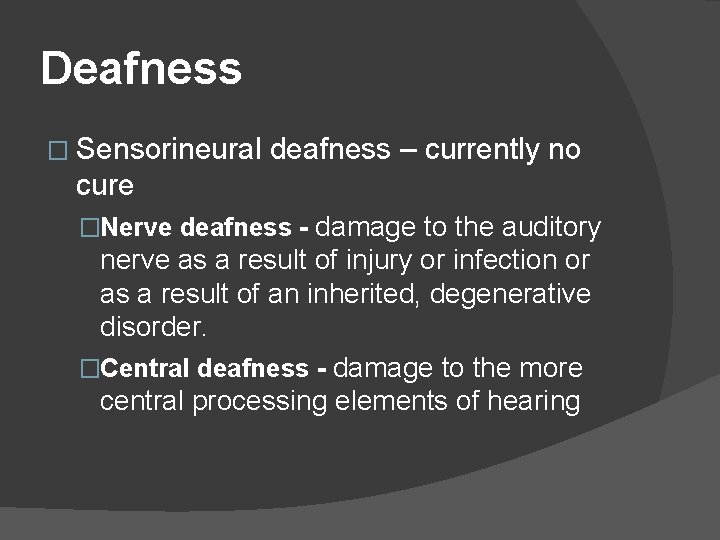
Deafness � Sensorineural deafness – currently no cure �Nerve deafness - damage to the auditory nerve as a result of injury or infection or as a result of an inherited, degenerative disorder. �Central deafness - damage to the more central processing elements of hearing
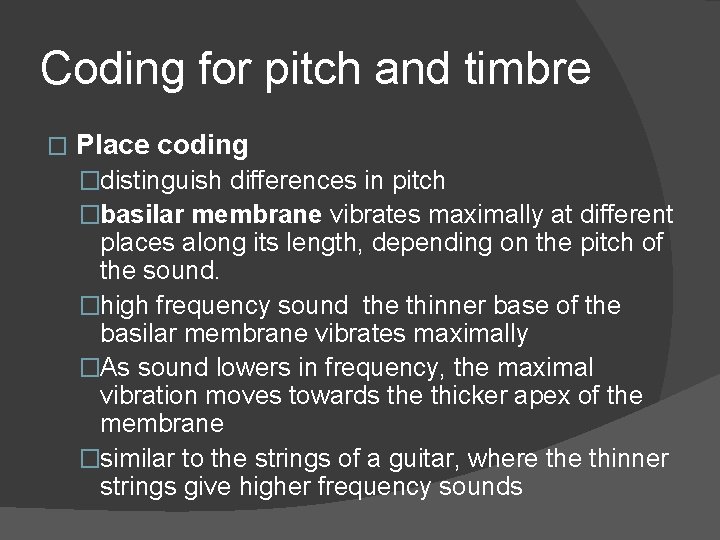
Coding for pitch and timbre � Place coding �distinguish differences in pitch �basilar membrane vibrates maximally at different places along its length, depending on the pitch of the sound. �high frequency sound the thinner base of the basilar membrane vibrates maximally �As sound lowers in frequency, the maximal vibration moves towards the thicker apex of the membrane �similar to the strings of a guitar, where thinner strings give higher frequency sounds
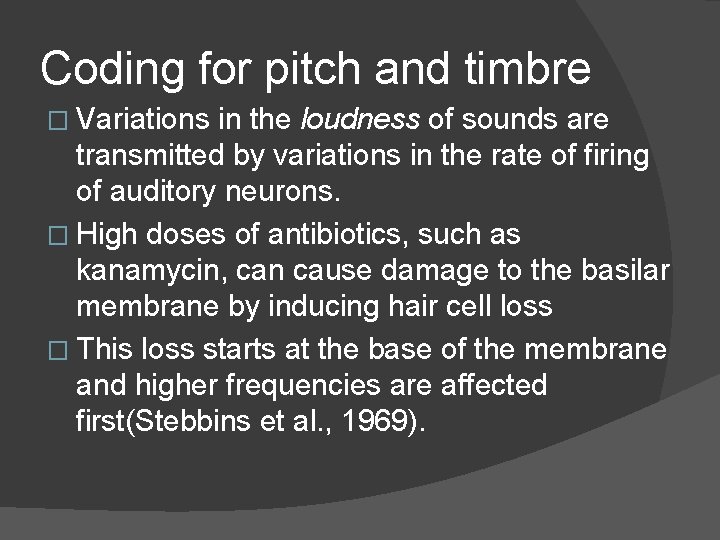
Coding for pitch and timbre � Variations in the loudness of sounds are transmitted by variations in the rate of firing of auditory neurons. � High doses of antibiotics, such as kanamycin, can cause damage to the basilar membrane by inducing hair cell loss � This loss starts at the base of the membrane and higher frequencies are affected first(Stebbins et al. , 1969).
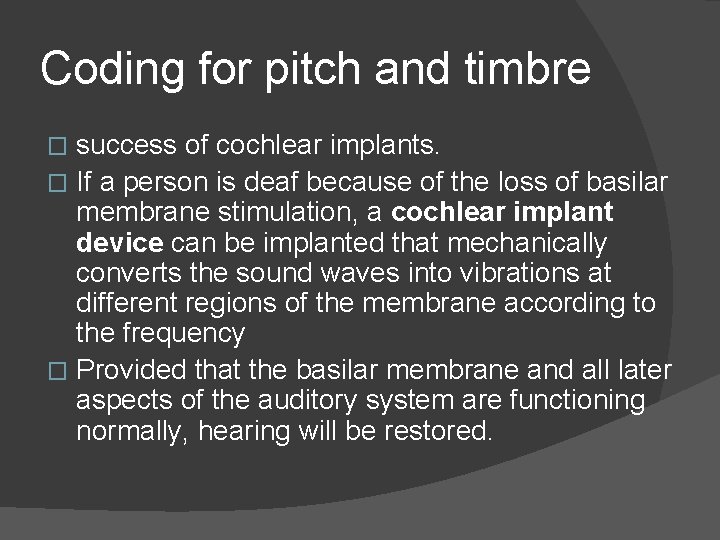
Coding for pitch and timbre success of cochlear implants. � If a person is deaf because of the loss of basilar membrane stimulation, a cochlear implant device can be implanted that mechanically converts the sound waves into vibrations at different regions of the membrane according to the frequency � Provided that the basilar membrane and all later aspects of the auditory system are functioning normally, hearing will be restored. �
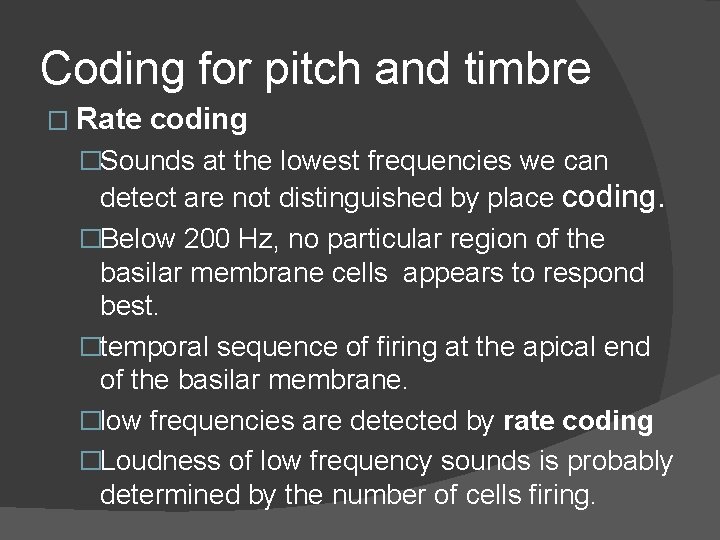
Coding for pitch and timbre � Rate coding �Sounds at the lowest frequencies we can detect are not distinguished by place coding. �Below 200 Hz, no particular region of the basilar membrane cells appears to respond best. �temporal sequence of firing at the apical end of the basilar membrane. �low frequencies are detected by rate coding �Loudness of low frequency sounds is probably determined by the number of cells firing.
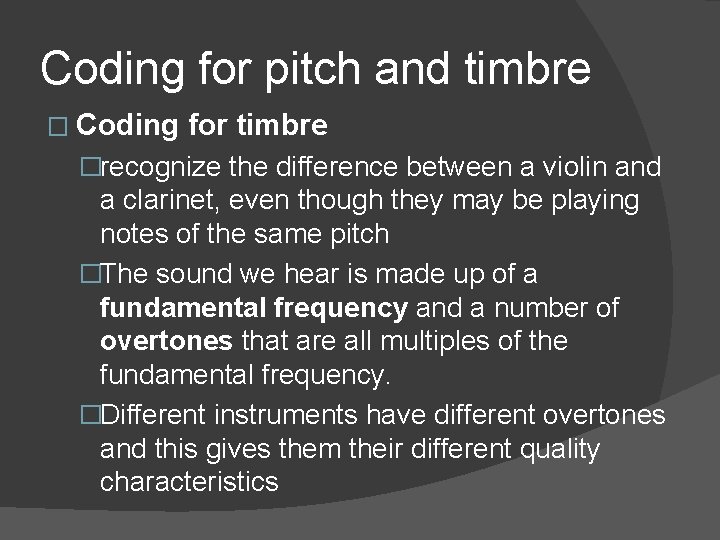
Coding for pitch and timbre � Coding for timbre �recognize the difference between a violin and a clarinet, even though they may be playing notes of the same pitch �The sound we hear is made up of a fundamental frequency and a number of overtones that are all multiples of the fundamental frequency. �Different instruments have different overtones and this gives them their different quality characteristics
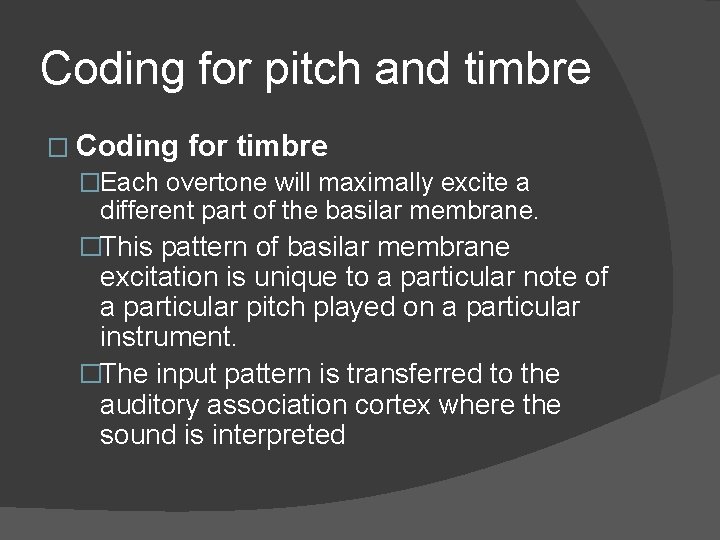
Coding for pitch and timbre � Coding for timbre �Each overtone will maximally excite a different part of the basilar membrane. �This pattern of basilar membrane excitation is unique to a particular note of a particular pitch played on a particular instrument. �The input pattern is transferred to the auditory association cortex where the sound is interpreted
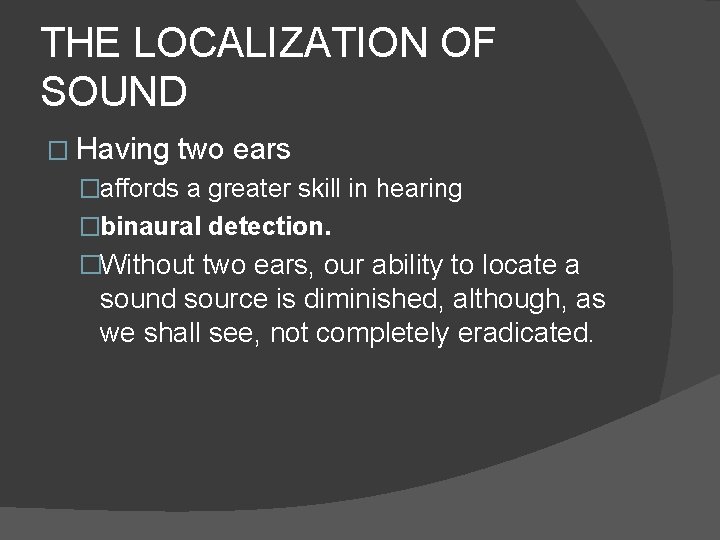
THE LOCALIZATION OF SOUND � Having two ears �affords a greater skill in hearing �binaural detection. �Without two ears, our ability to locate a sound source is diminished, although, as we shall see, not completely eradicated.
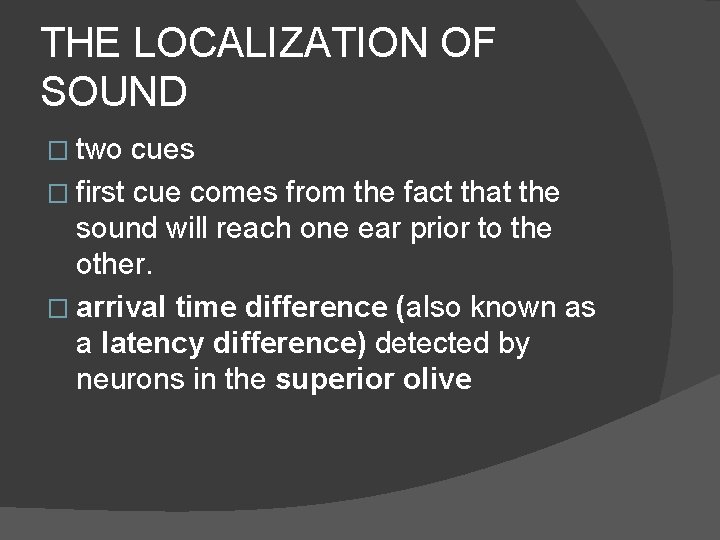
THE LOCALIZATION OF SOUND � two cues � first cue comes from the fact that the sound will reach one ear prior to the other. � arrival time difference (also known as a latency difference) detected by neurons in the superior olive
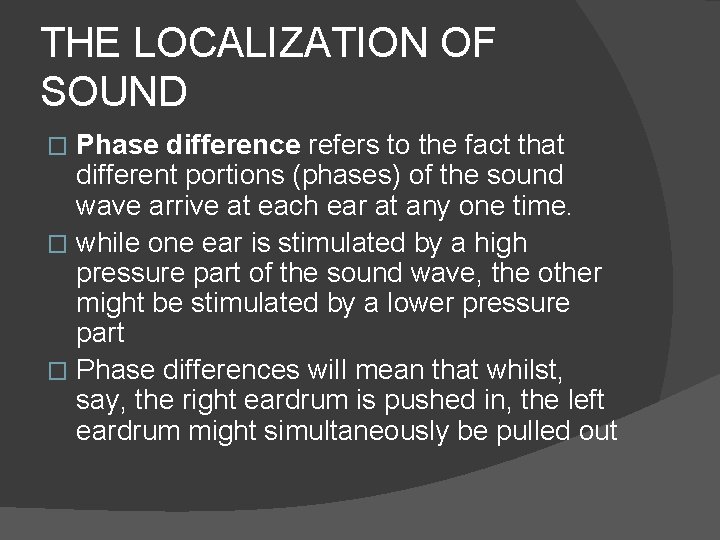
THE LOCALIZATION OF SOUND Phase difference refers to the fact that different portions (phases) of the sound wave arrive at each ear at any one time. � while one ear is stimulated by a high pressure part of the sound wave, the other might be stimulated by a lower pressure part � Phase differences will mean that whilst, say, the right eardrum is pushed in, the left eardrum might simultaneously be pulled out �
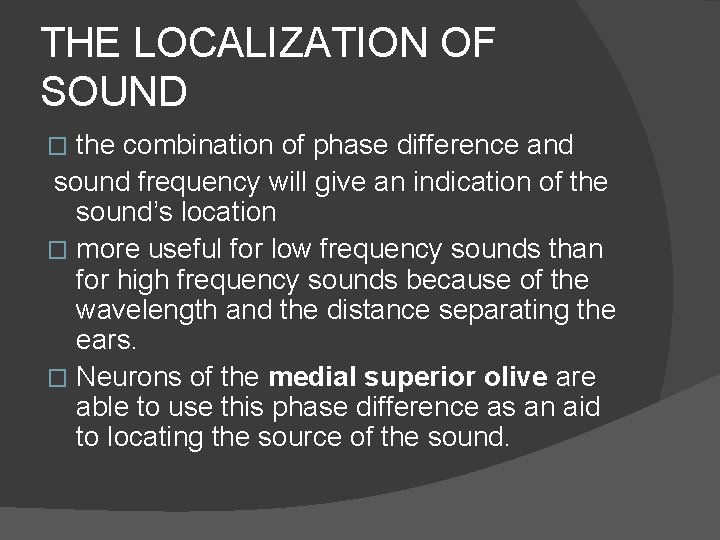
THE LOCALIZATION OF SOUND the combination of phase difference and sound frequency will give an indication of the sound’s location � more useful for low frequency sounds than for high frequency sounds because of the wavelength and the distance separating the ears. � Neurons of the medial superior olive are able to use this phase difference as an aid to locating the source of the sound. �
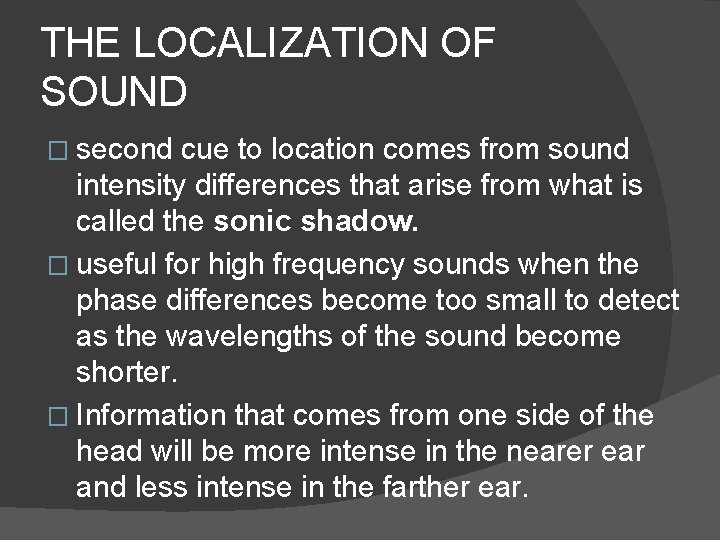
THE LOCALIZATION OF SOUND � second cue to location comes from sound intensity differences that arise from what is called the sonic shadow. � useful for high frequency sounds when the phase differences become too small to detect as the wavelengths of the sound become shorter. � Information that comes from one side of the head will be more intense in the nearer ear and less intense in the farther ear.
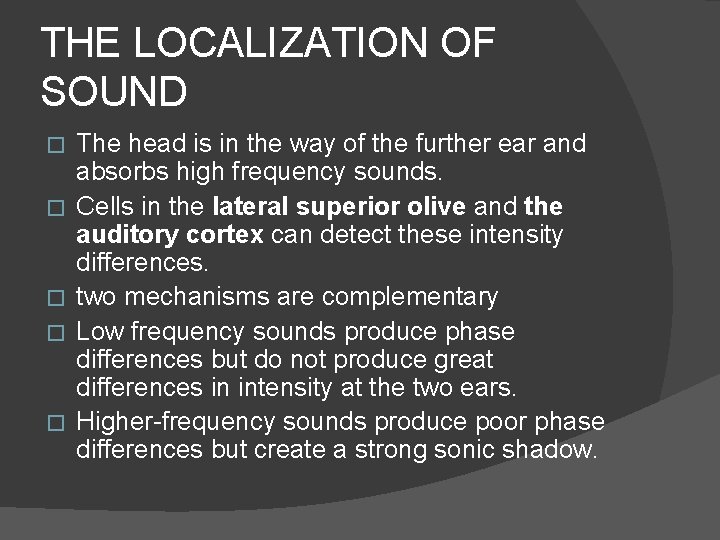
THE LOCALIZATION OF SOUND � � � The head is in the way of the further ear and absorbs high frequency sounds. Cells in the lateral superior olive and the auditory cortex can detect these intensity differences. two mechanisms are complementary Low frequency sounds produce phase differences but do not produce great differences in intensity at the two ears. Higher-frequency sounds produce poor phase differences but create a strong sonic shadow.
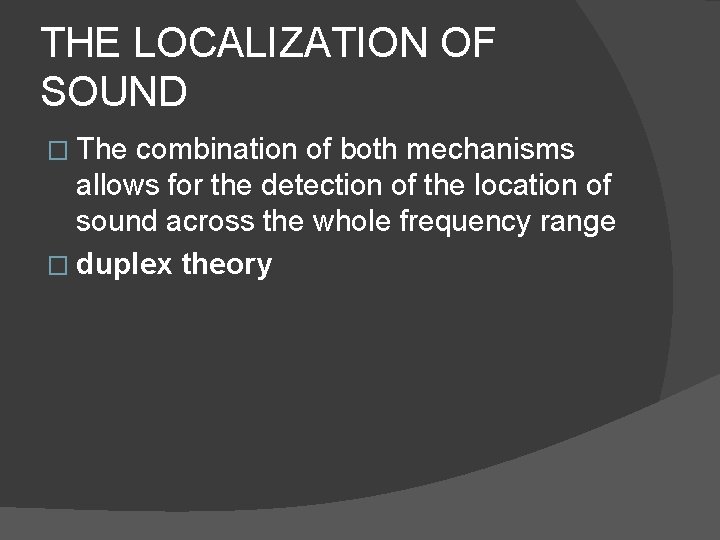
THE LOCALIZATION OF SOUND � The combination of both mechanisms allows for the detection of the location of sound across the whole frequency range � duplex theory
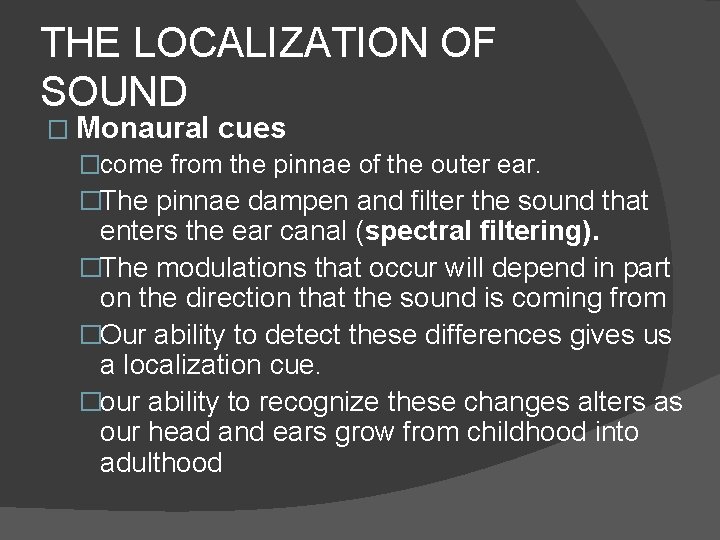
THE LOCALIZATION OF SOUND � Monaural cues �come from the pinnae of the outer ear. �The pinnae dampen and filter the sound that enters the ear canal (spectral filtering). �The modulations that occur will depend in part on the direction that the sound is coming from �Our ability to detect these differences gives us a localization cue. �our ability to recognize these changes alters as our head and ears grow from childhood into adulthood
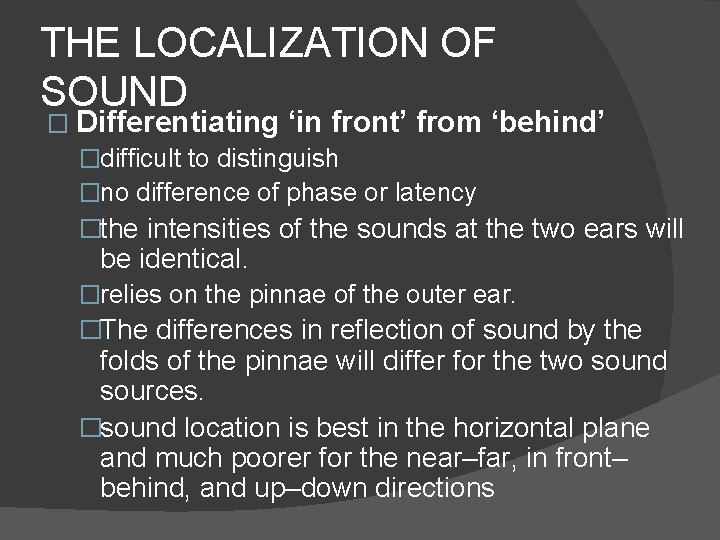
THE LOCALIZATION OF SOUND � Differentiating ‘in front’ from ‘behind’ �difficult to distinguish �no difference of phase or latency �the intensities of the sounds at the two ears will be identical. �relies on the pinnae of the outer ear. �The differences in reflection of sound by the folds of the pinnae will differ for the two sound sources. �sound location is best in the horizontal plane and much poorer for the near–far, in front– behind, and up–down directions
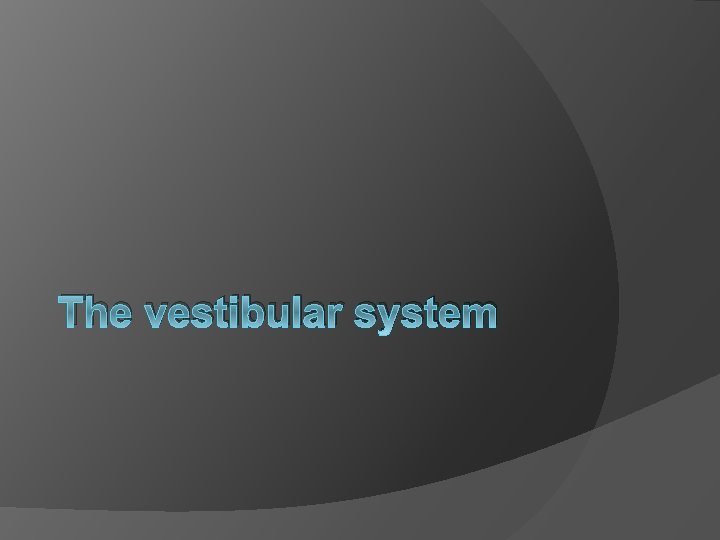
The vestibular system
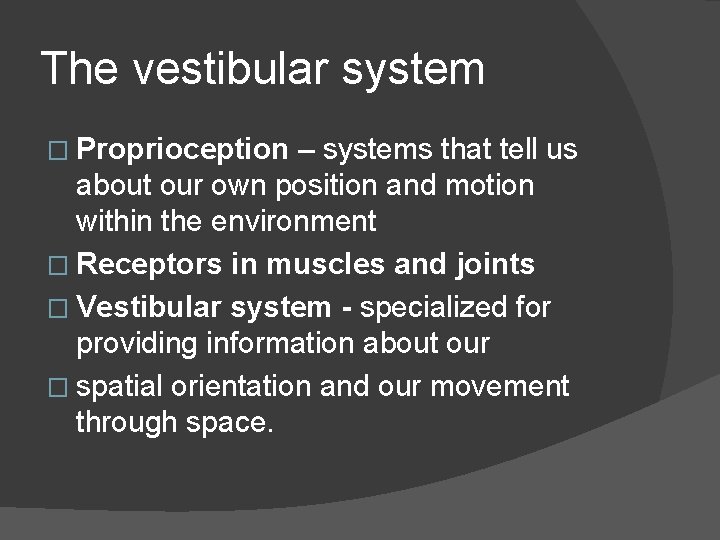
The vestibular system � Proprioception – systems that tell us about our own position and motion within the environment � Receptors in muscles and joints � Vestibular system - specialized for providing information about our � spatial orientation and our movement through space.
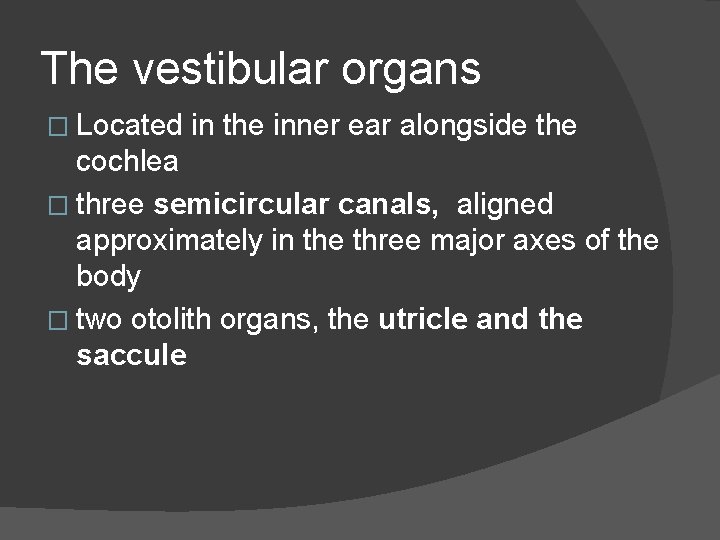
The vestibular organs � Located in the inner ear alongside the cochlea � three semicircular canals, aligned approximately in the three major axes of the body � two otolith organs, the utricle and the saccule
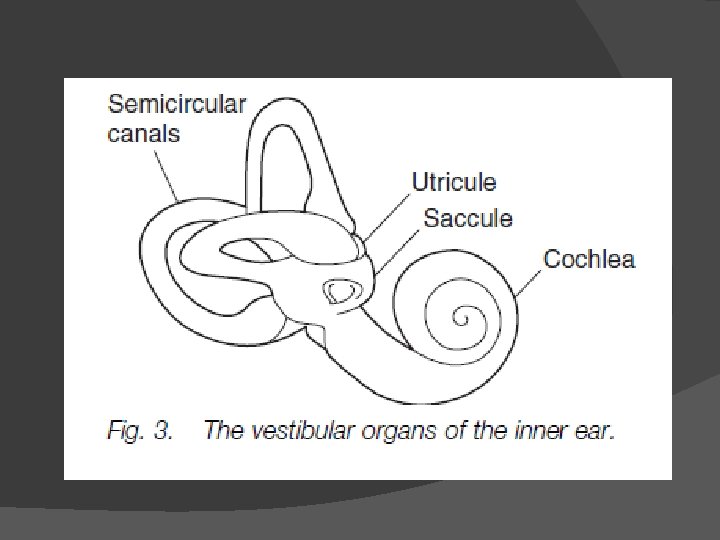
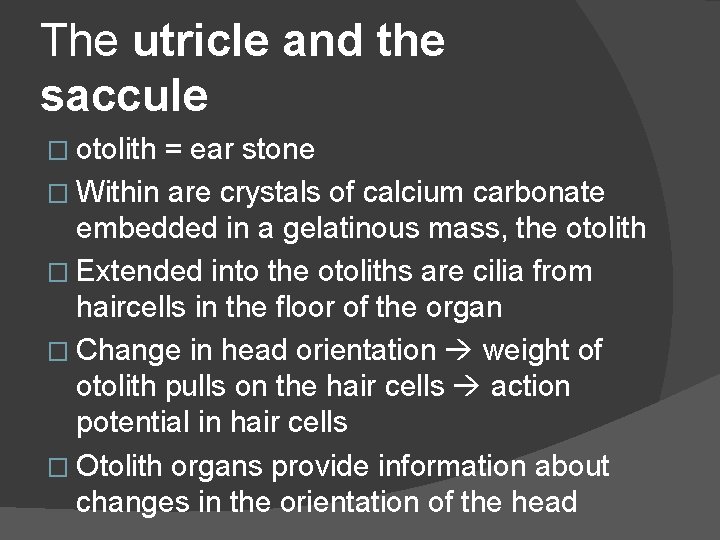
The utricle and the saccule � otolith = ear stone � Within are crystals of calcium carbonate embedded in a gelatinous mass, the otolith � Extended into the otoliths are cilia from haircells in the floor of the organ � Change in head orientation weight of otolith pulls on the hair cells action potential in hair cells � Otolith organs provide information about changes in the orientation of the head
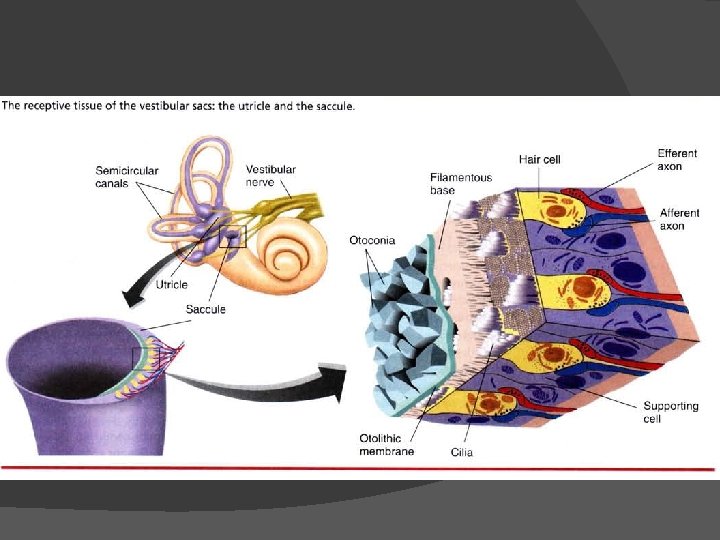
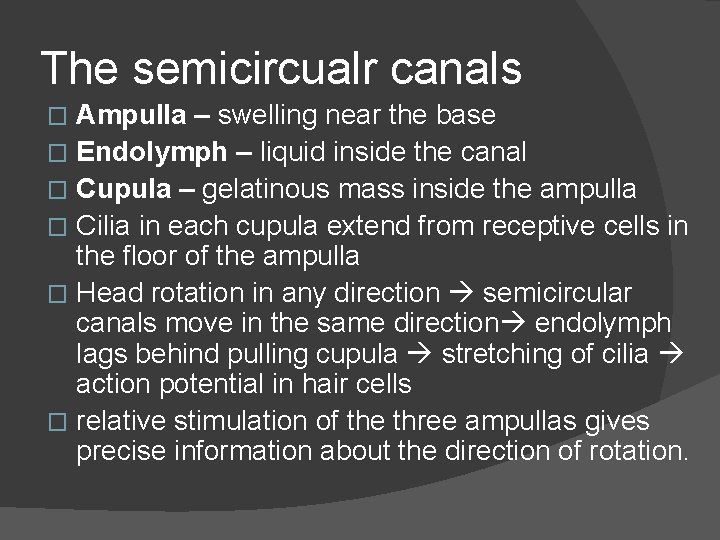
The semicircualr canals Ampulla – swelling near the base � Endolymph – liquid inside the canal � Cupula – gelatinous mass inside the ampulla � Cilia in each cupula extend from receptive cells in the floor of the ampulla � Head rotation in any direction semicircular canals move in the same direction endolymph lags behind pulling cupula stretching of cilia action potential in hair cells � relative stimulation of the three ampullas gives precise information about the direction of rotation. �
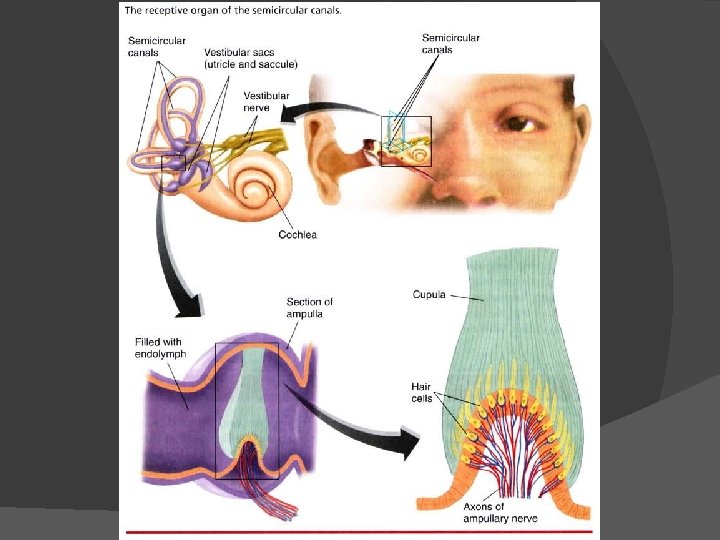
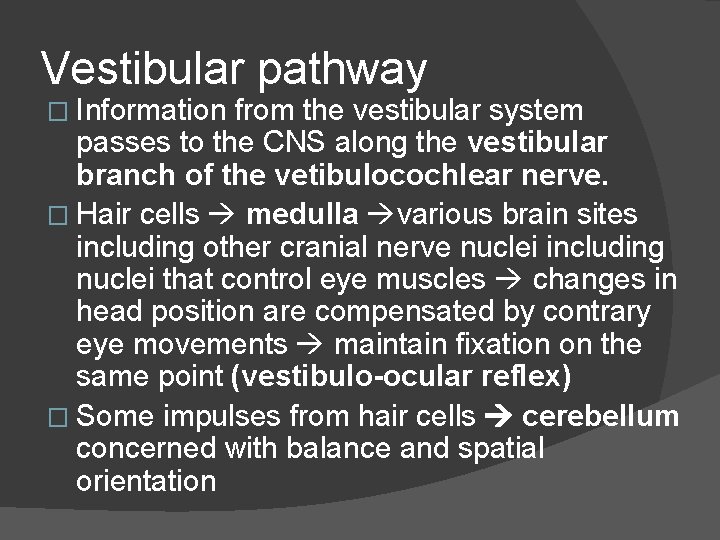
Vestibular pathway � Information from the vestibular system passes to the CNS along the vestibular branch of the vetibulocochlear nerve. � Hair cells medulla various brain sites including other cranial nerve nuclei including nuclei that control eye muscles changes in head position are compensated by contrary eye movements maintain fixation on the same point (vestibulo-ocular reflex) � Some impulses from hair cells cerebellum concerned with balance and spatial orientation
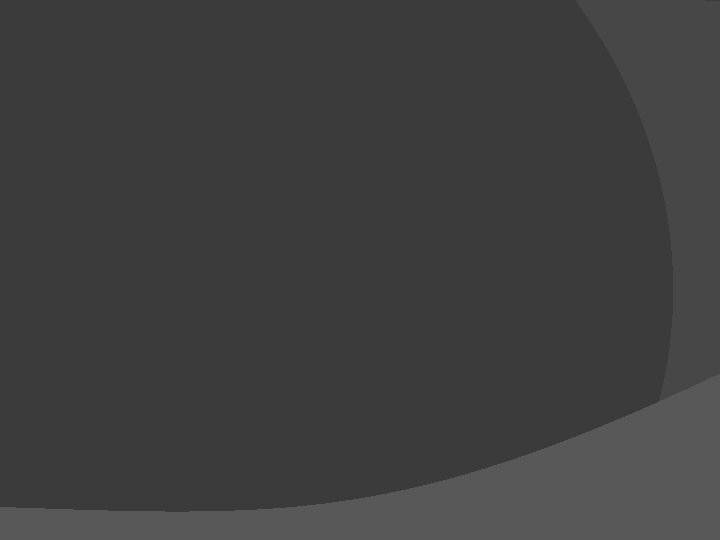
Perceptual set ap psych
Webers law
Perception vs sensation
Sensation and perception uu
Chapter 4 sensation and perception test
Perception examples
Five basic tastes
Sensation and perception
Sensation and perception
Chapter 4 sensation and perception
Chapter 5 sensation and perception
Consumer perception definition
Sensation and perception crossword review
Old woman young woman illusion
Exposé sur la sensation en psychologie
What is sensation
Gestalt approach
Perception vs sensation
Chapter 3 sensation and perception
Sensation perception
Chapter 6 sensation and perception
Closing diphthongs examples
Oral sounds and nasal sounds
“a sound mind is in a sound body”
210 decibels
Audition guideposts
Blind orchestra auditions study
Adobe audition loops
Audition by michael shurtleff summary
Script i
Audition
Mock trial audition tips
Adobe audition 4
Adobe audition for dummies
Cones are to vision as ____ are to audition
Eliminar reverberacion audition
Adobe certified associate visual design specialist
Somesthetic senses definition
Opponent process theory vs trichromatic theory
Sensation
Ditticienne base sur coute sensation
Tactile localization meaning
The touch the feel the cotton
Sensation seeking significato
Dcml sensation
Cortical sensation
Ditticienne base sur coute sensation
Nociceptive sensation
Sensation
6 principles of sensation
Thinking feeling sensing intuiting
Viscerosomatic levels
What causes the burning sensation in your muscles
Sensation seeking
Ear sensory
Sensation
Types of sensation
Cortical sensory loss
Sensation somatique