Physics at the LHC Higgs and Supersymmetry Part
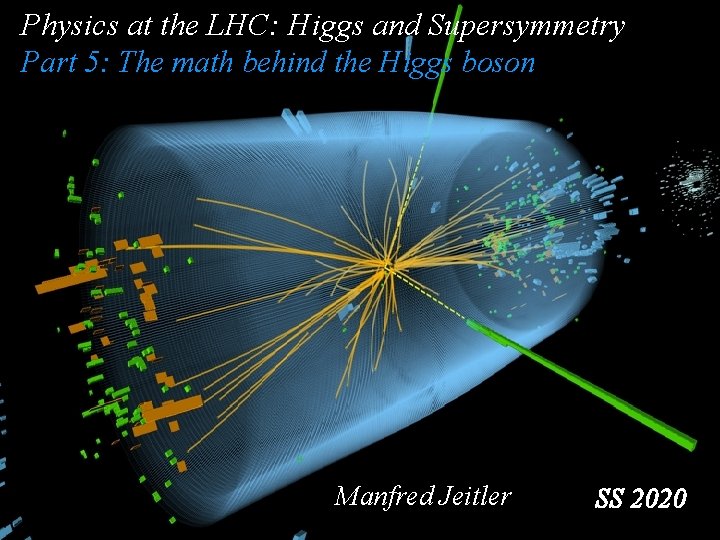
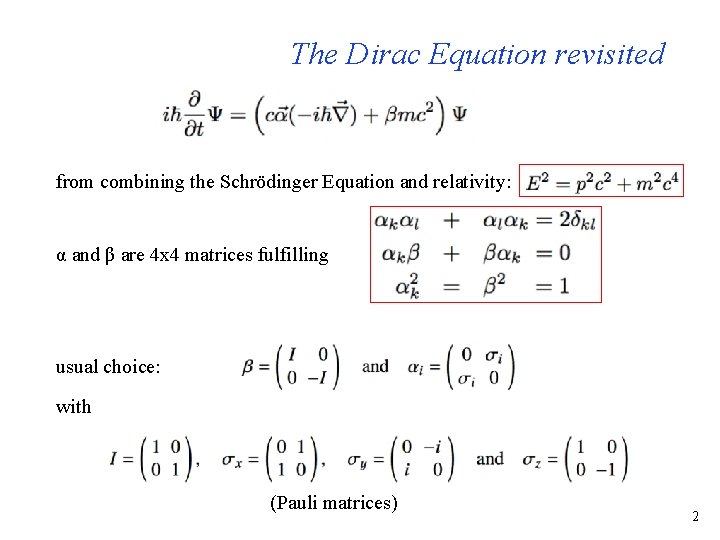
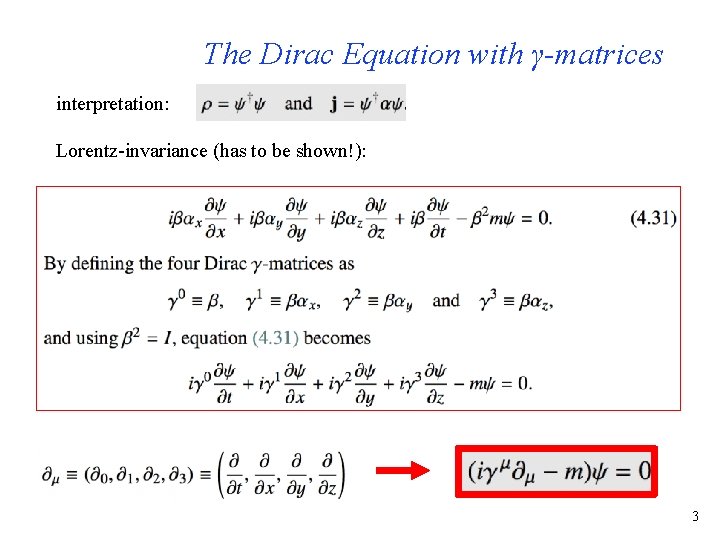
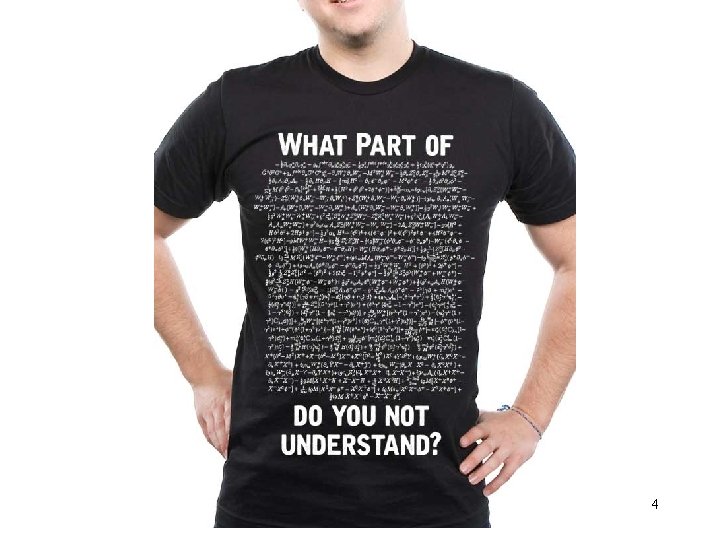
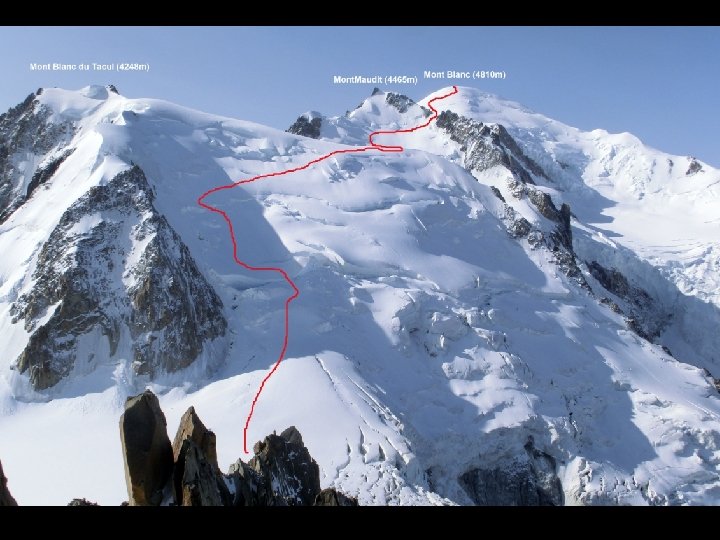
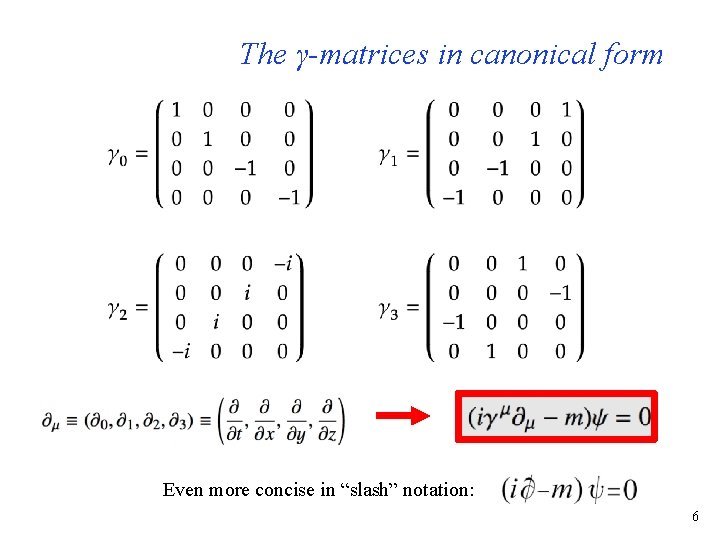
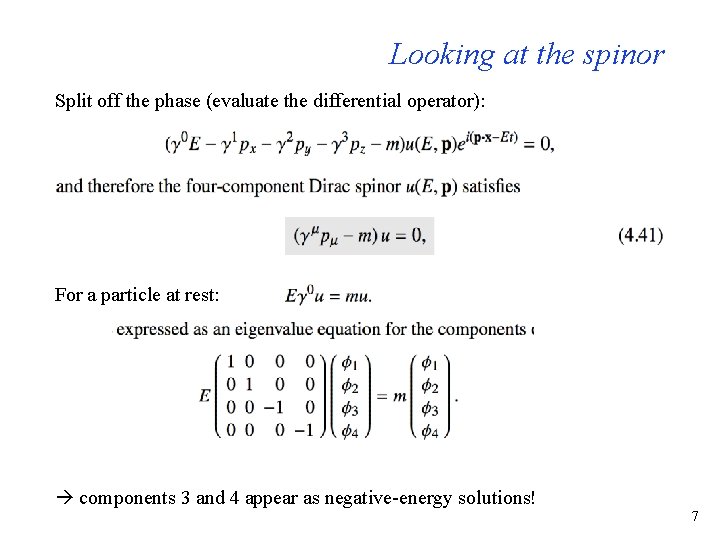
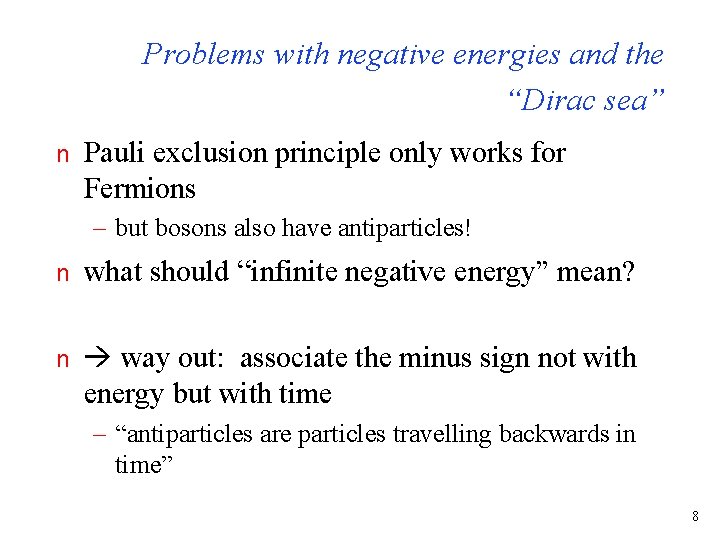
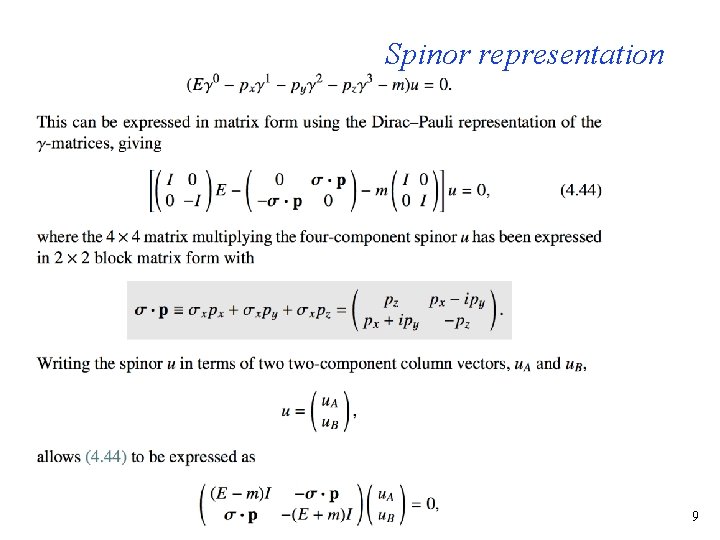
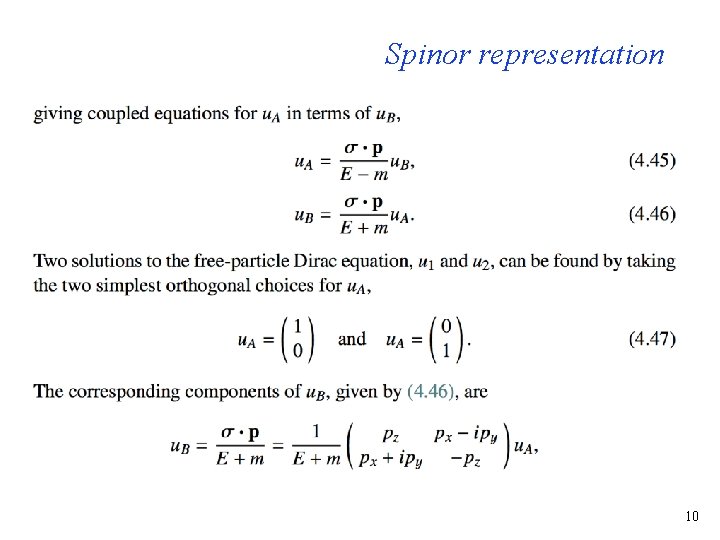
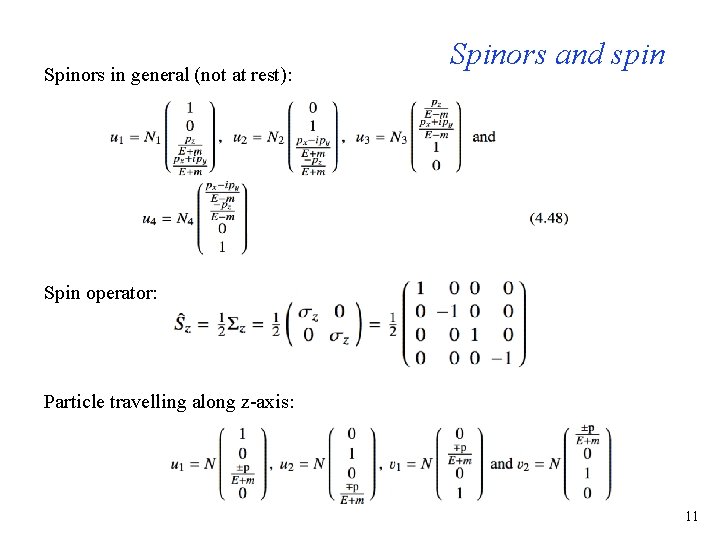
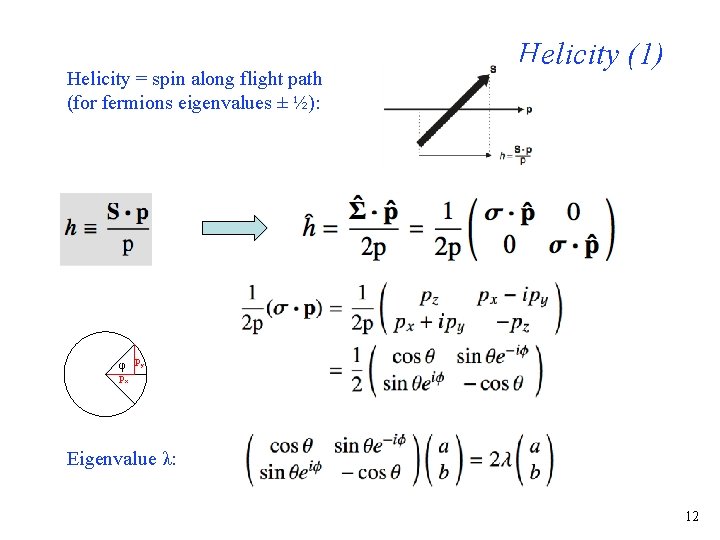
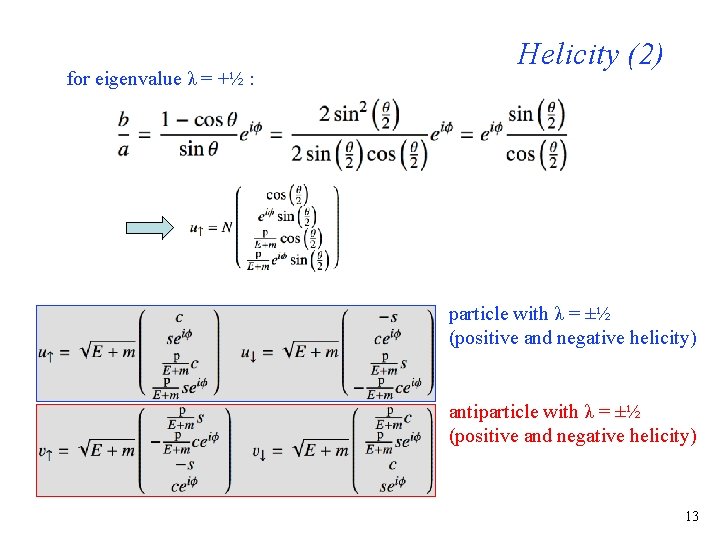
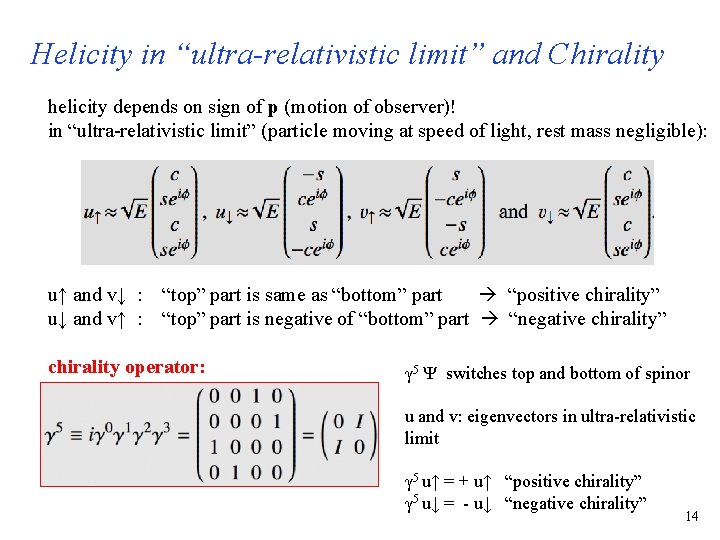
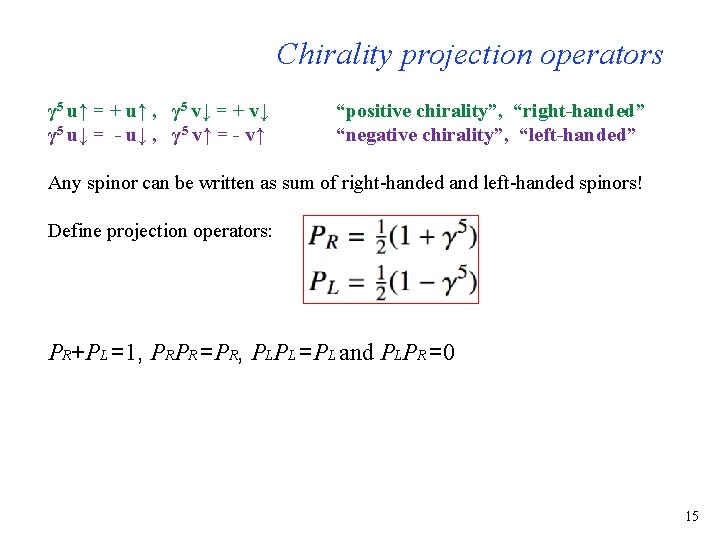
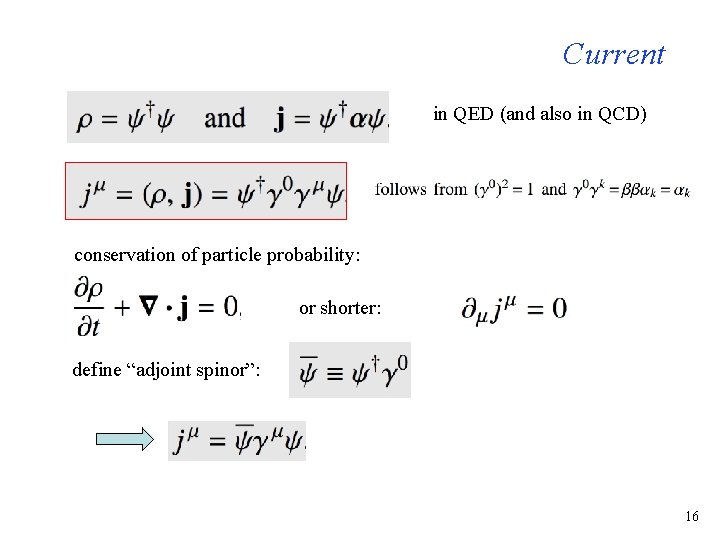
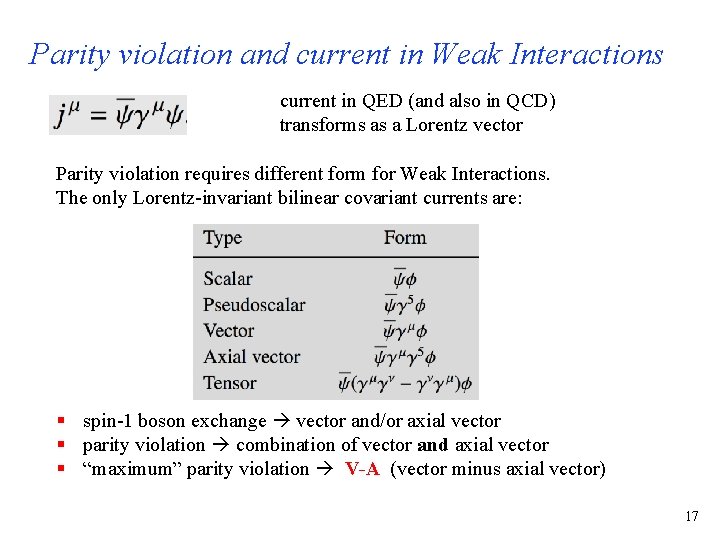
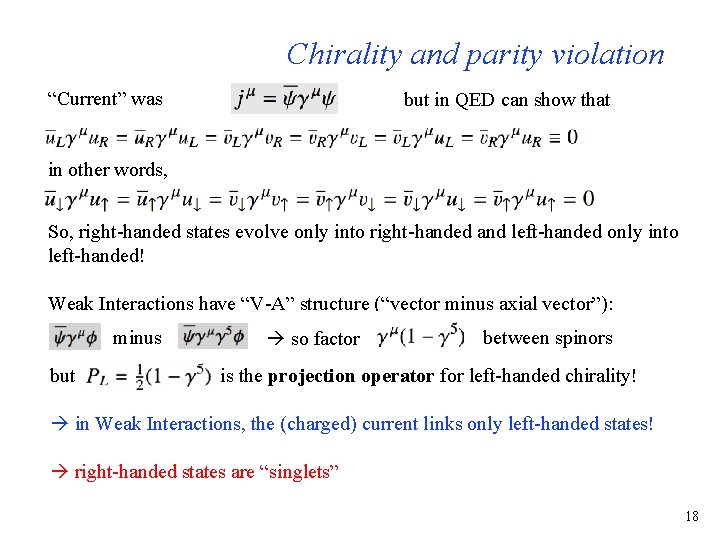
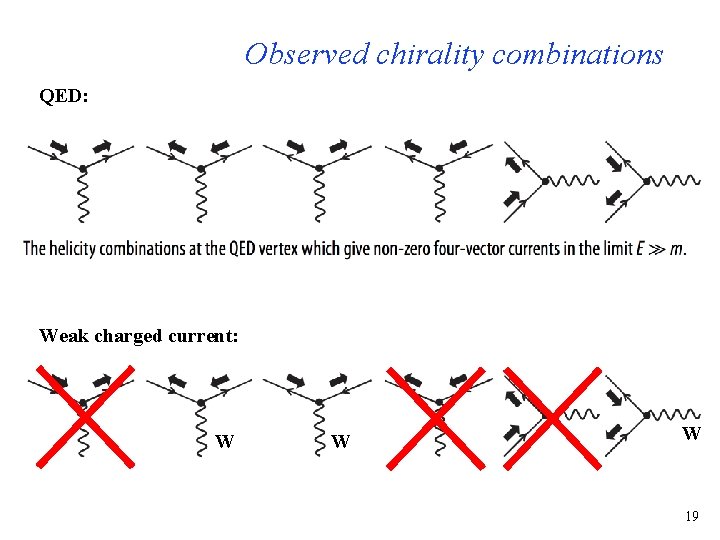
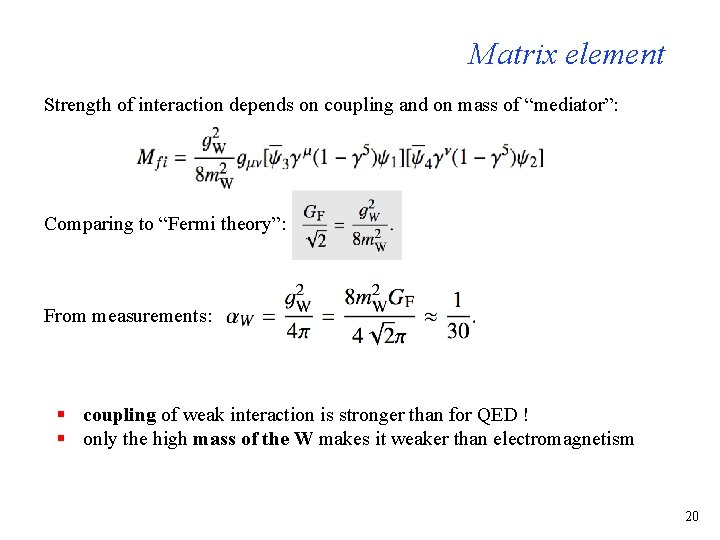
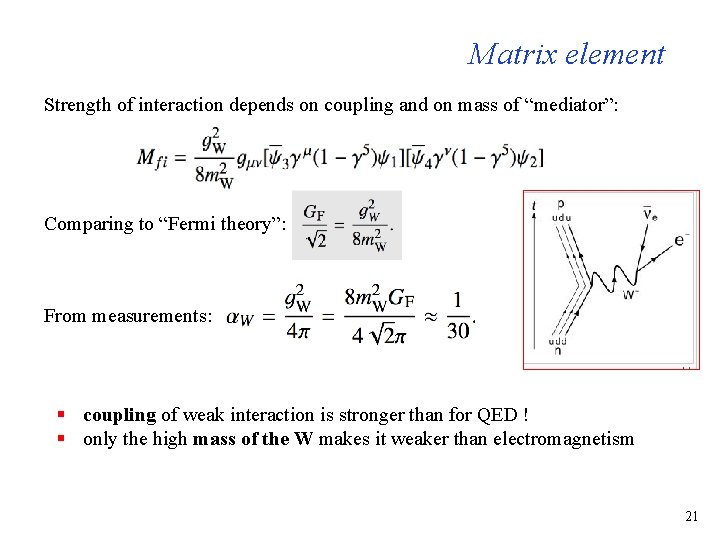
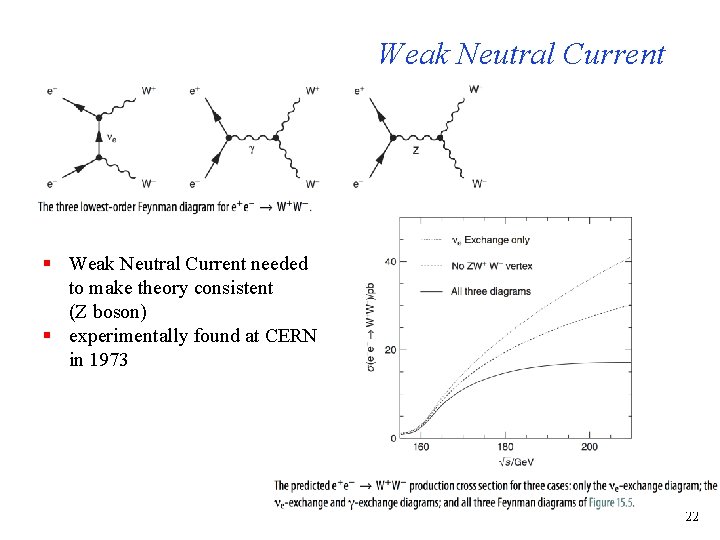
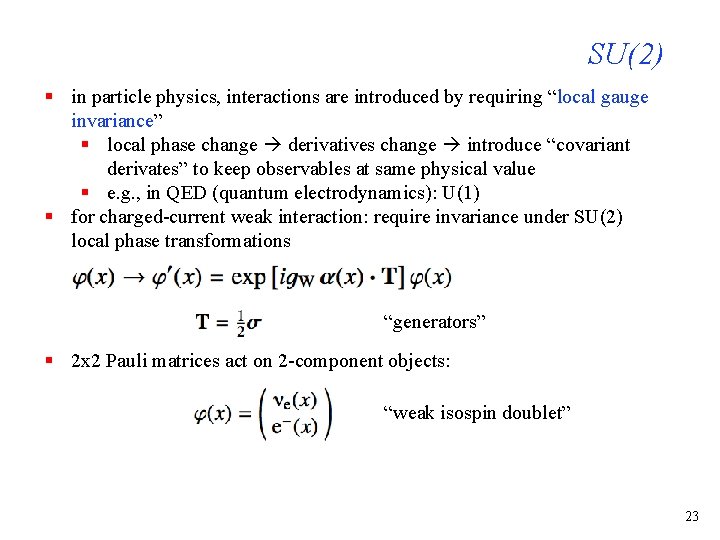
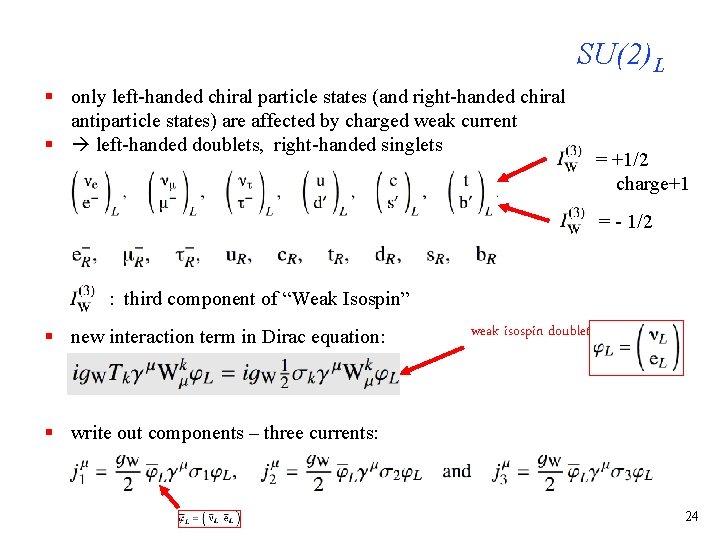
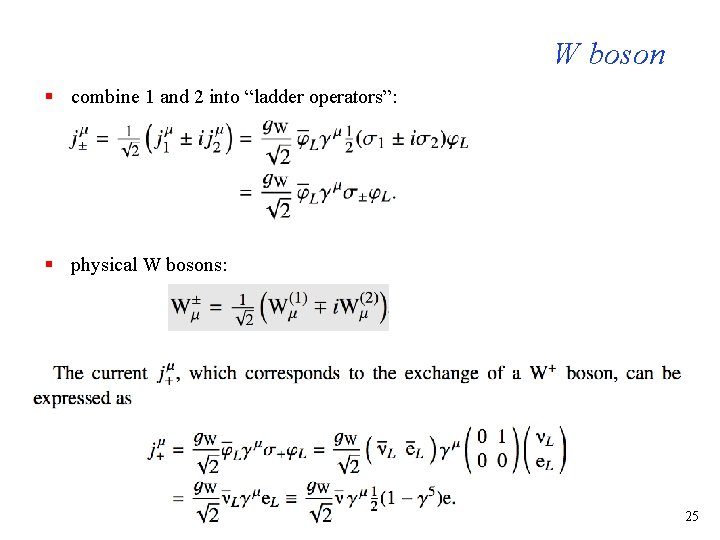
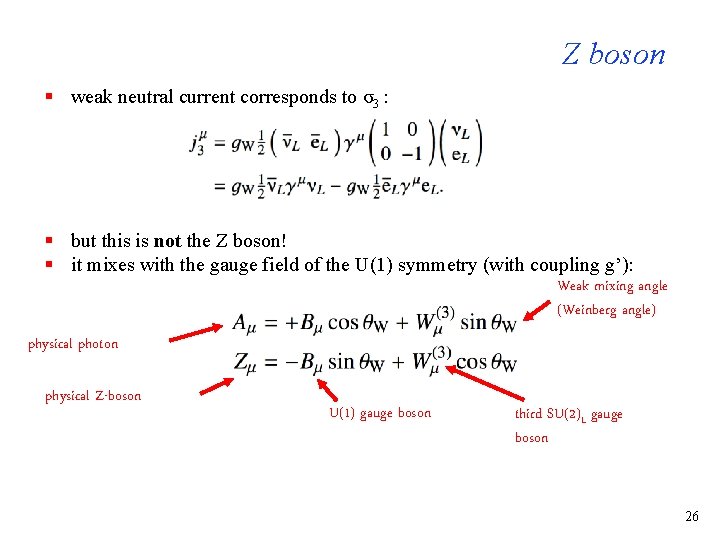
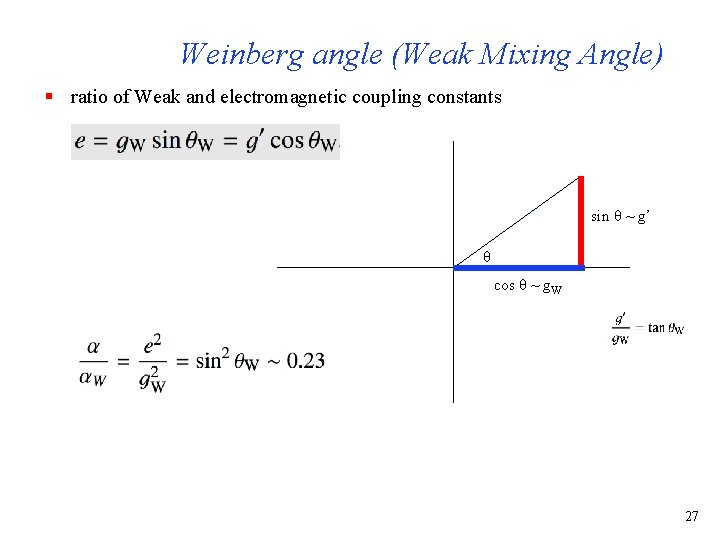
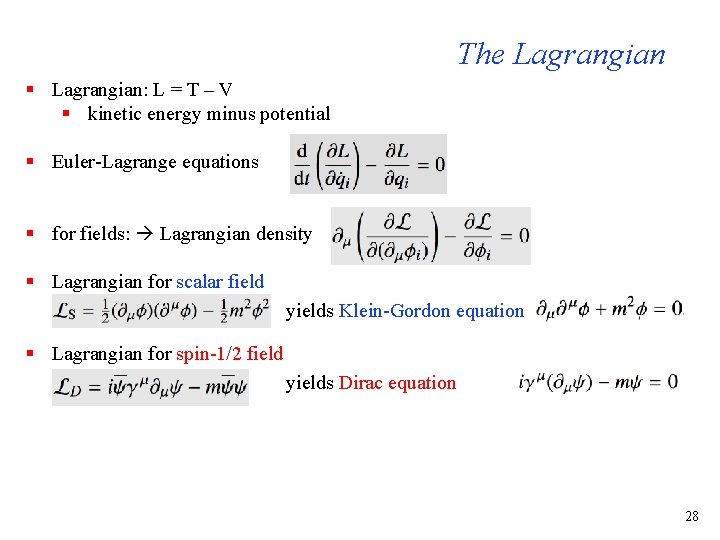
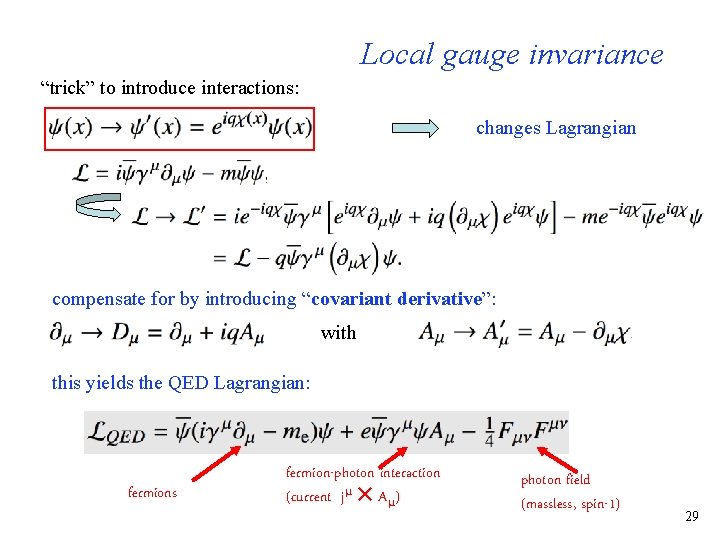
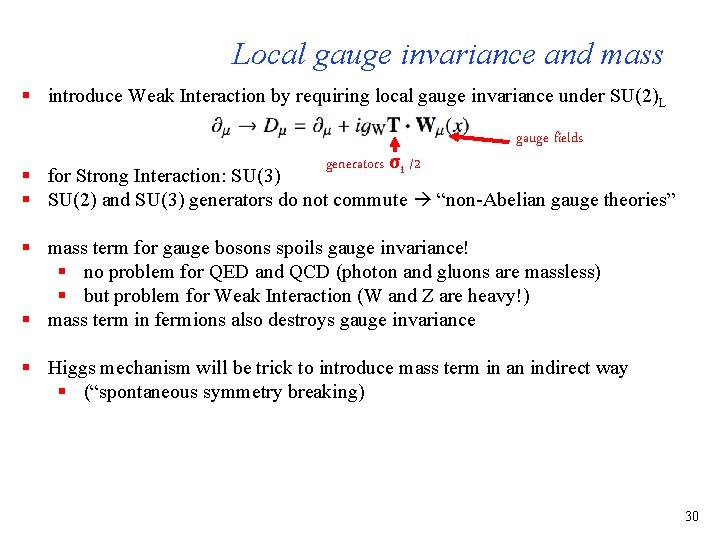
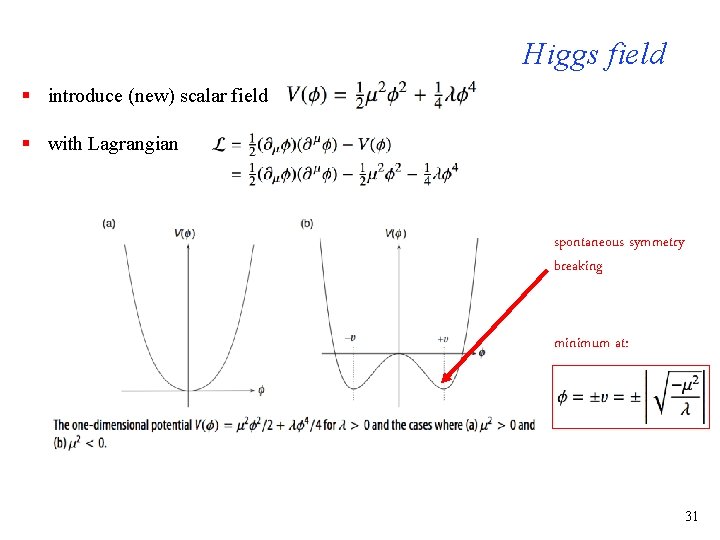
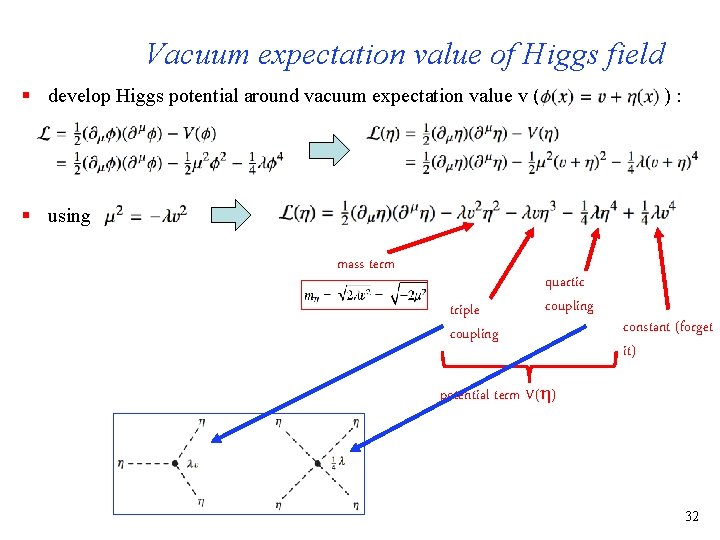
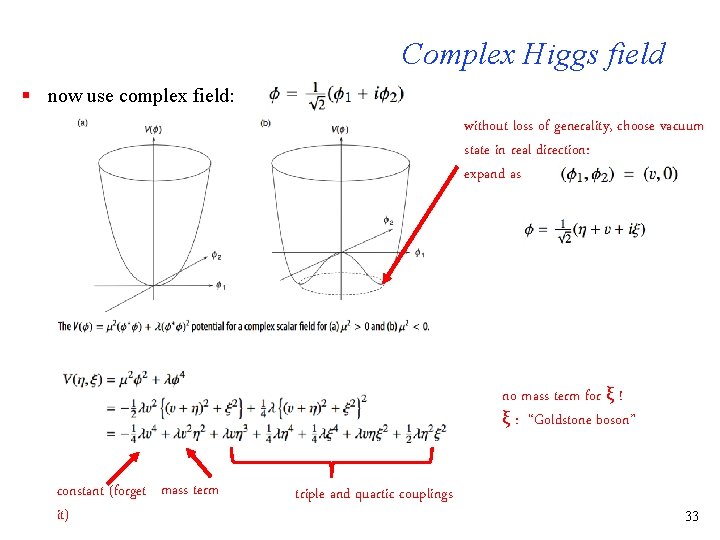
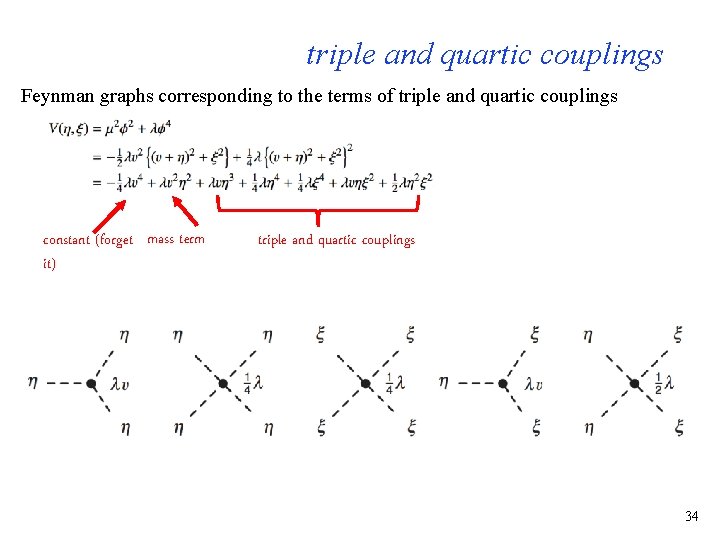
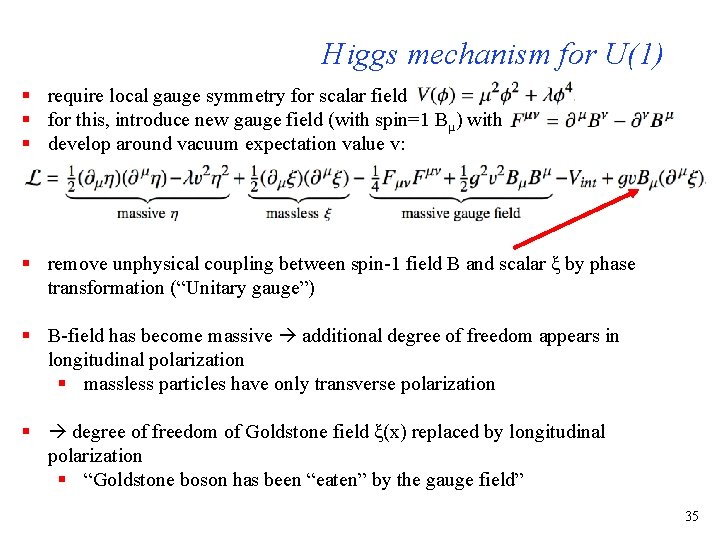
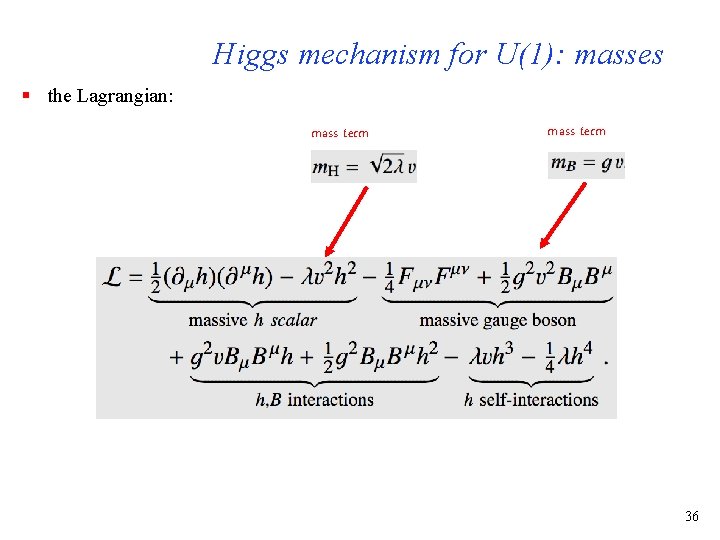
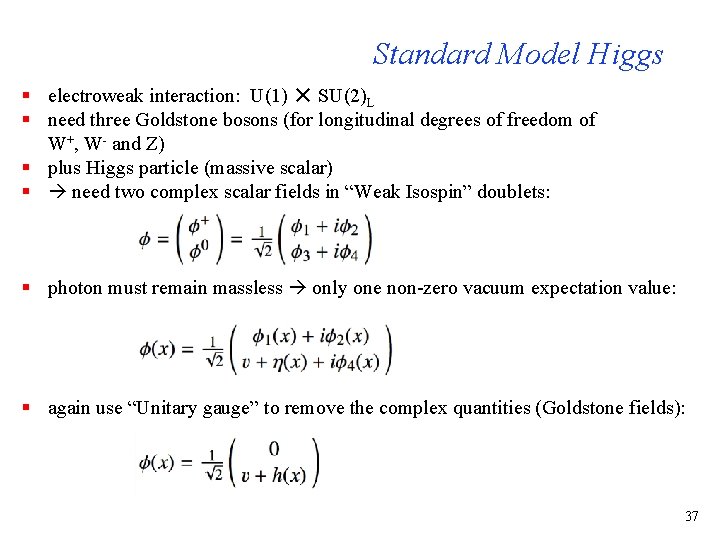
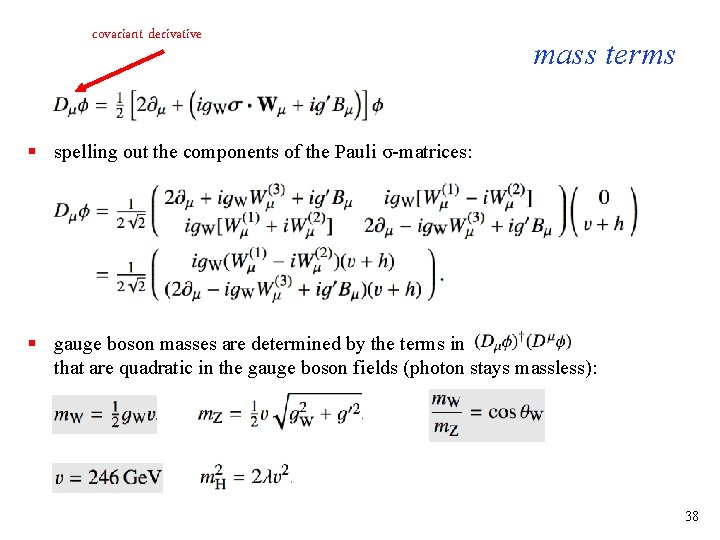
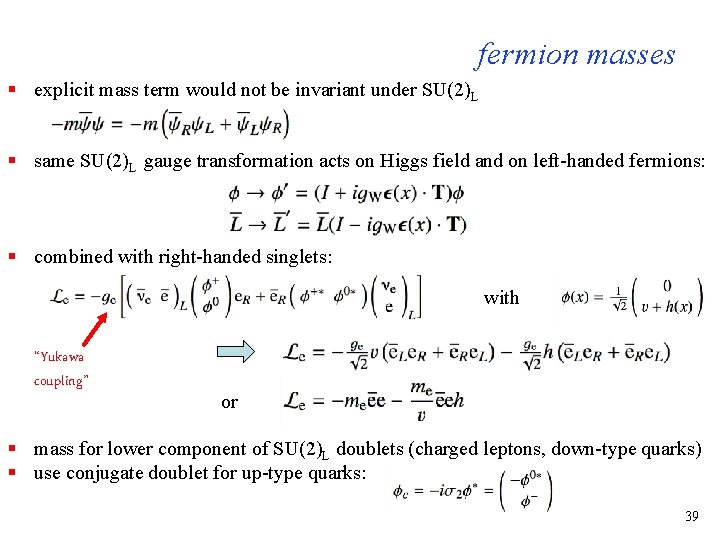
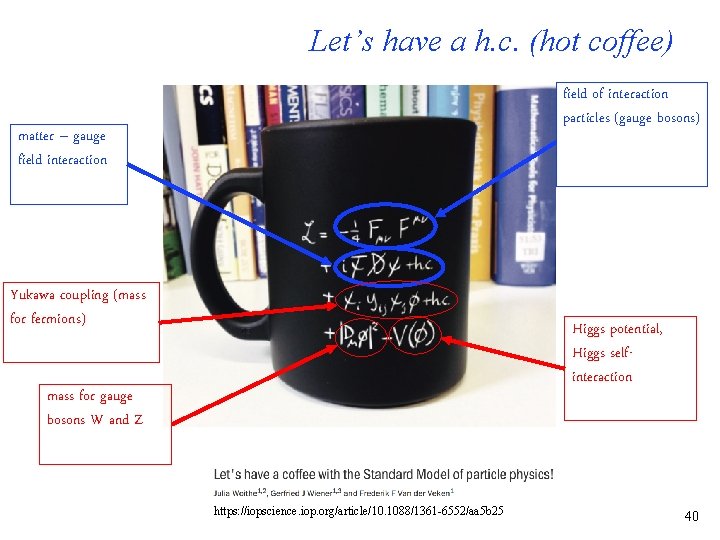
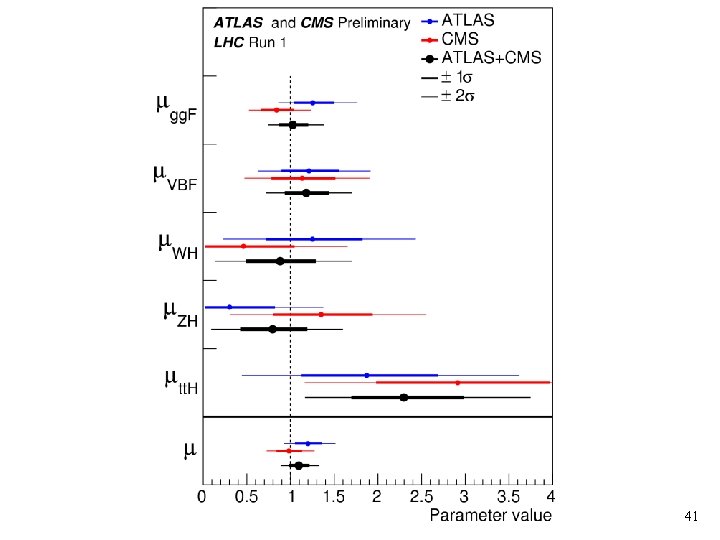
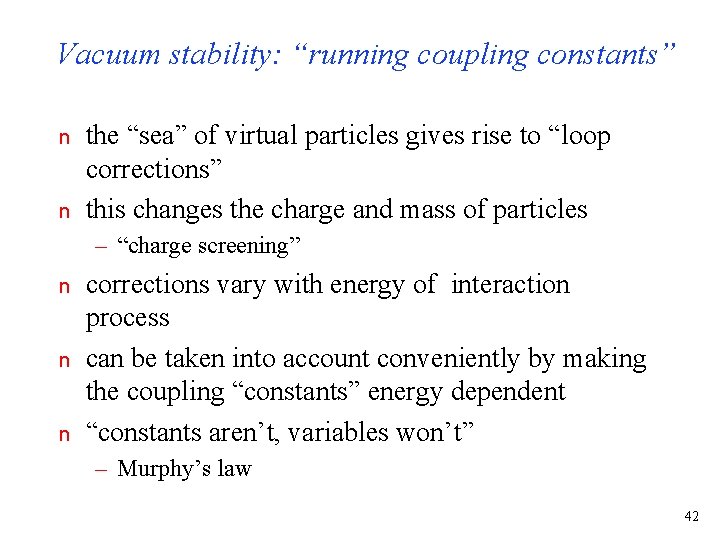
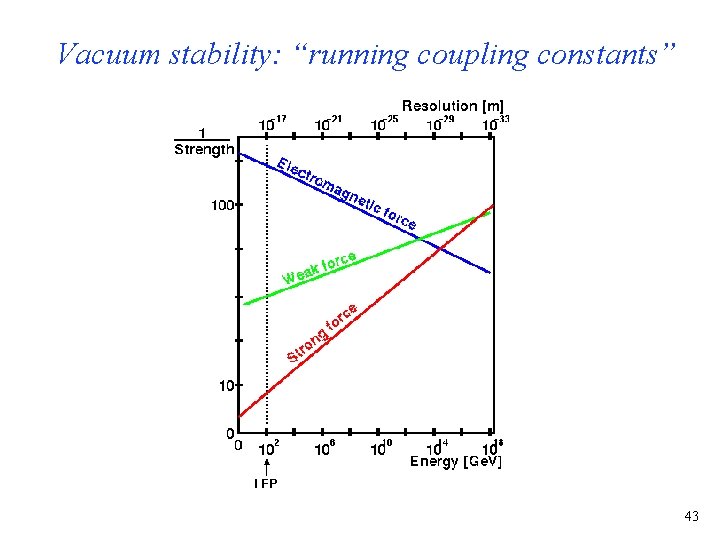
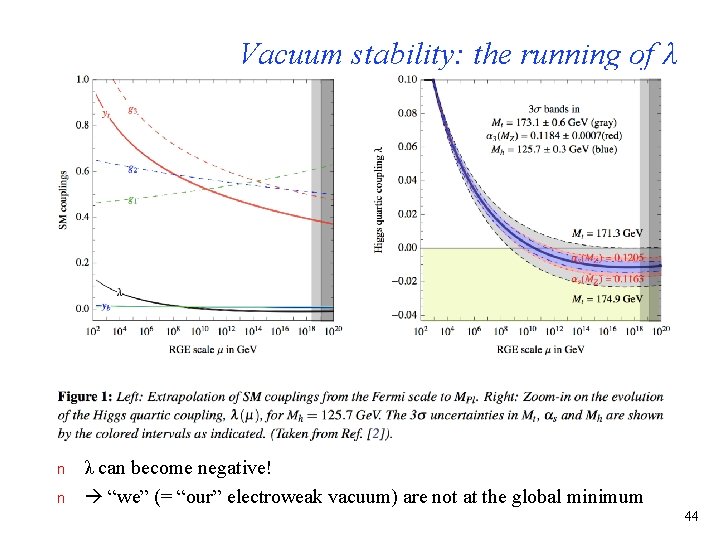
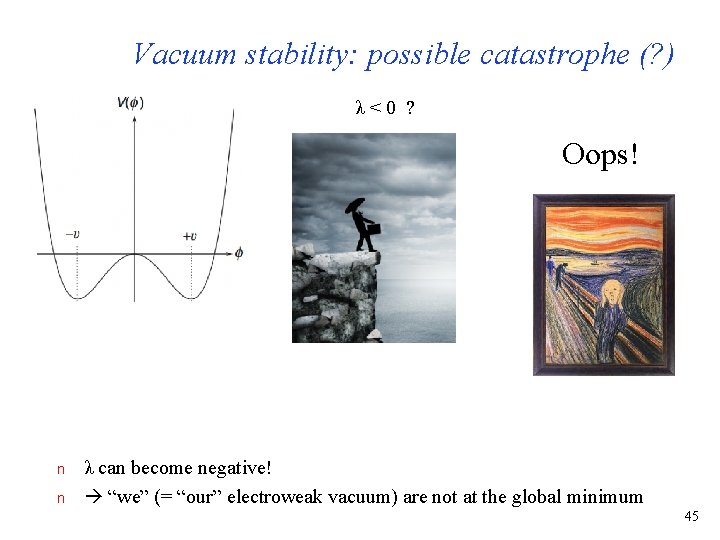
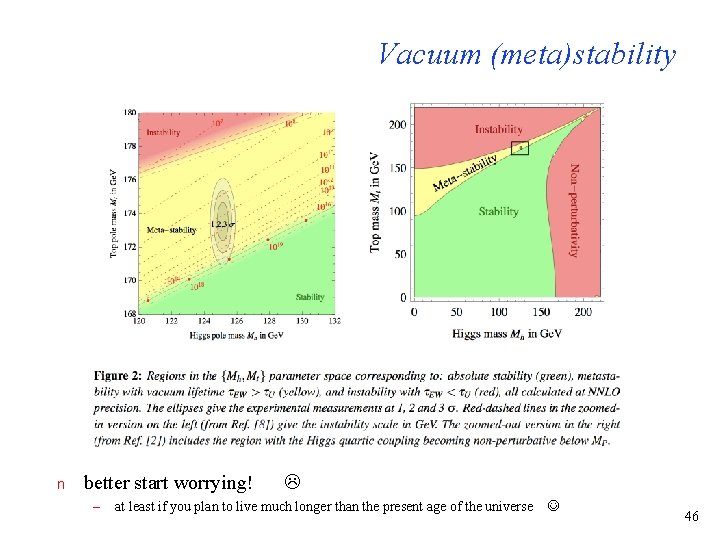
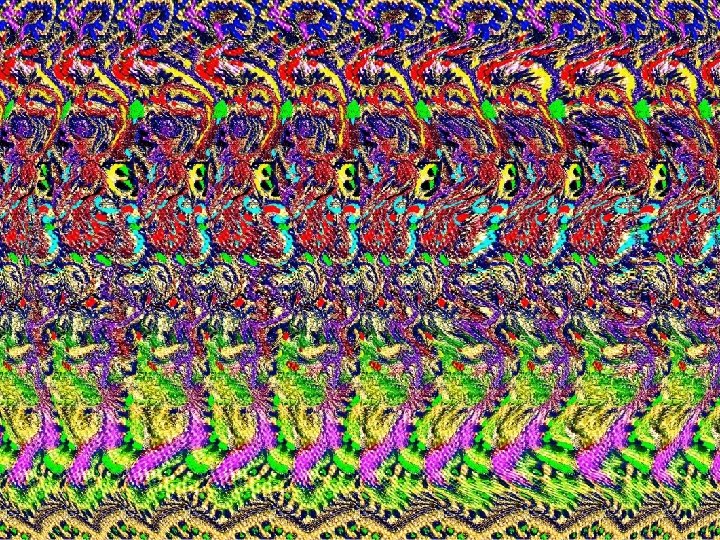
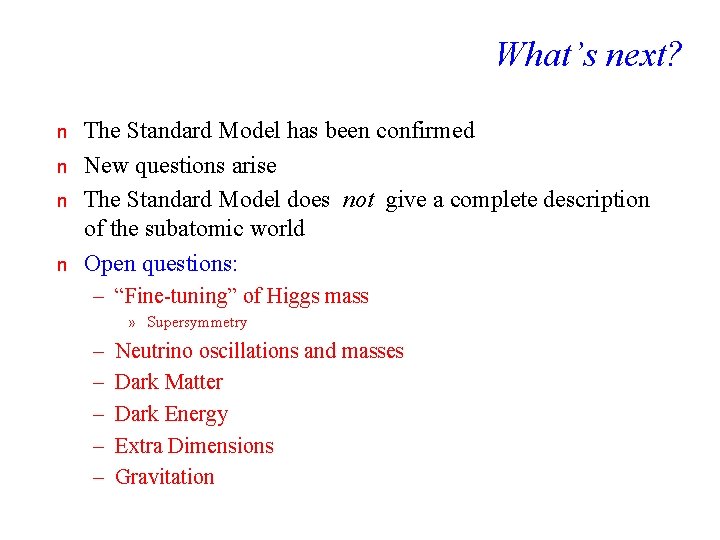
- Slides: 48
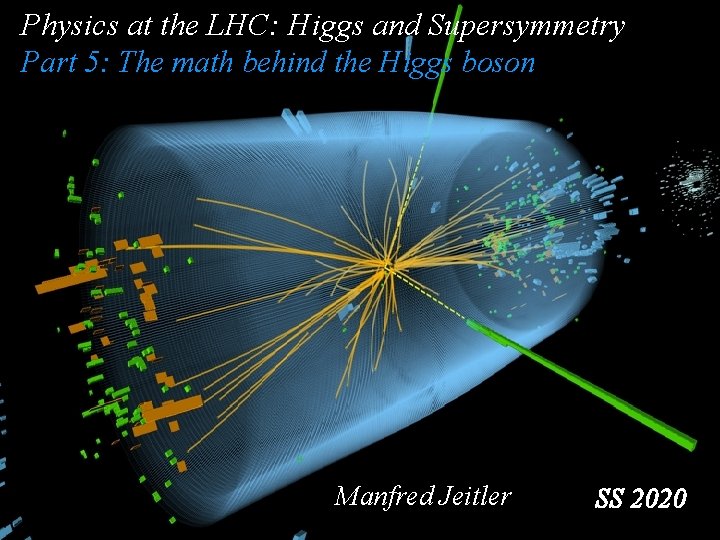
Physics at the LHC: Higgs and Supersymmetry Part 5: The math behind the Higgs boson Manfred Jeitler SS 2020
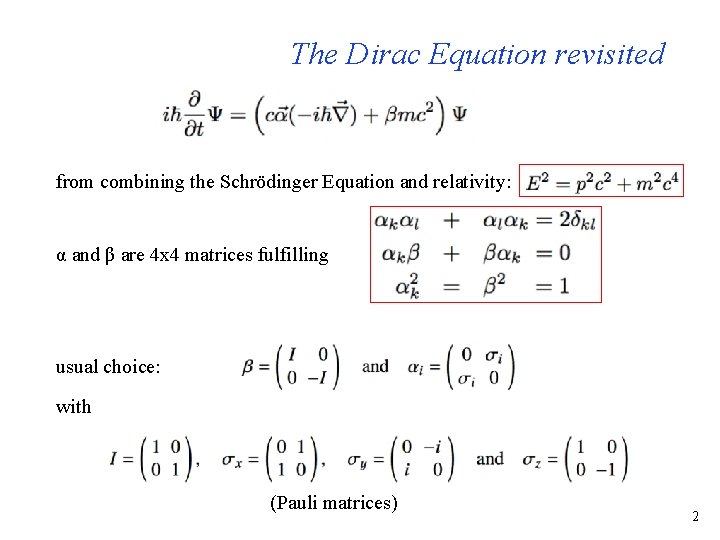
The Dirac Equation revisited from combining the Schrödinger Equation and relativity: α and β are 4 x 4 matrices fulfilling usual choice: with (Pauli matrices) 2
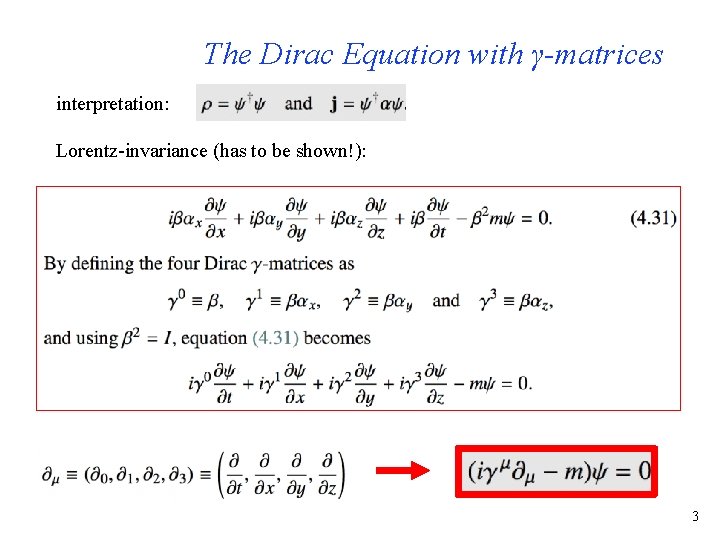
The Dirac Equation with γ-matrices interpretation: Lorentz-invariance (has to be shown!): 3
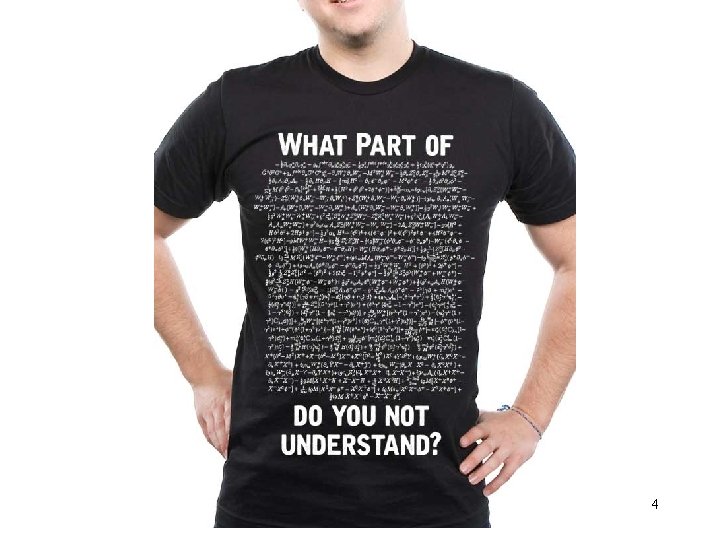
slide 4
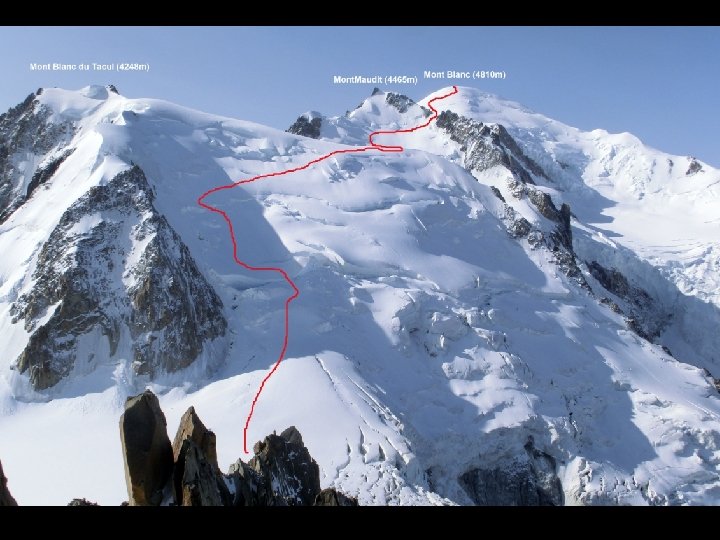
5
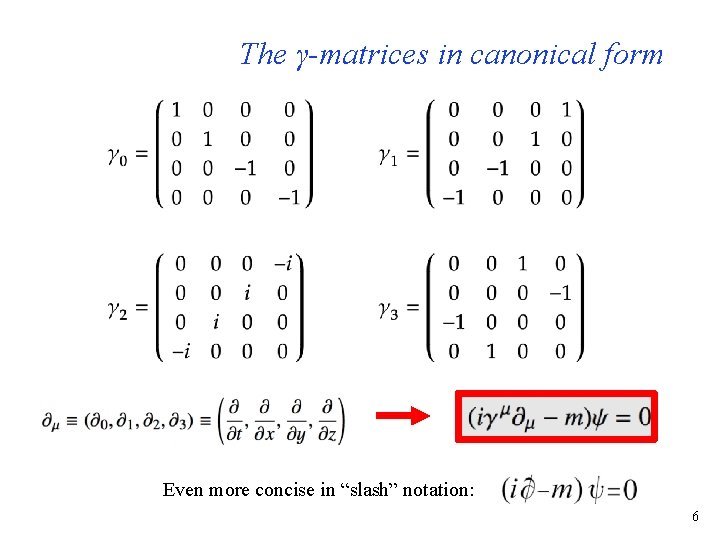
The γ-matrices in canonical form Even more concise in “slash” notation: 6
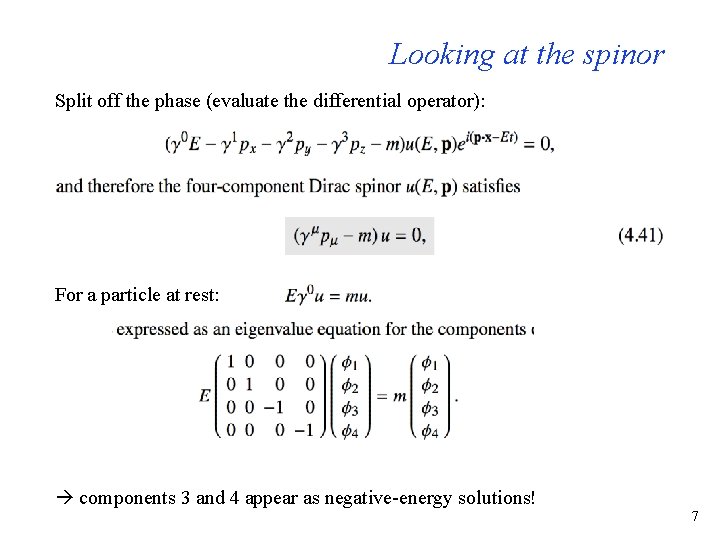
Looking at the spinor Split off the phase (evaluate the differential operator): For a particle at rest: components 3 and 4 appear as negative-energy solutions! 7
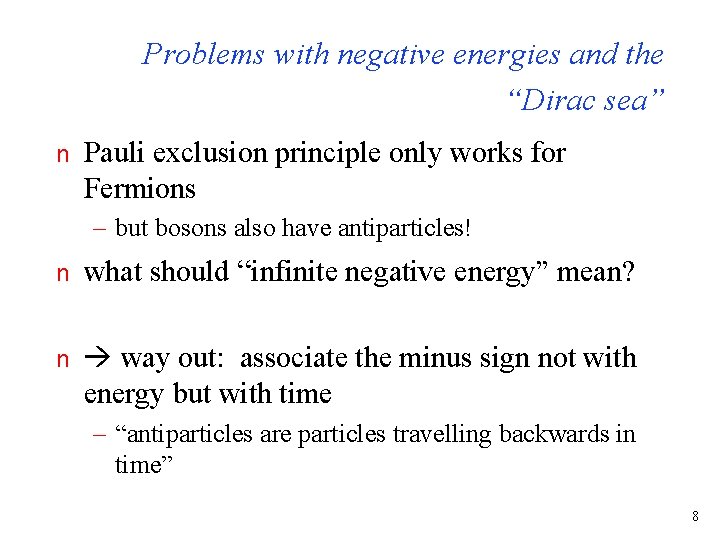
Problems with negative energies and the “Dirac sea” n Pauli exclusion principle only works for Fermions – but bosons also have antiparticles! n what should “infinite negative energy” mean? n way out: associate the minus sign not with energy but with time – “antiparticles are particles travelling backwards in time” 8
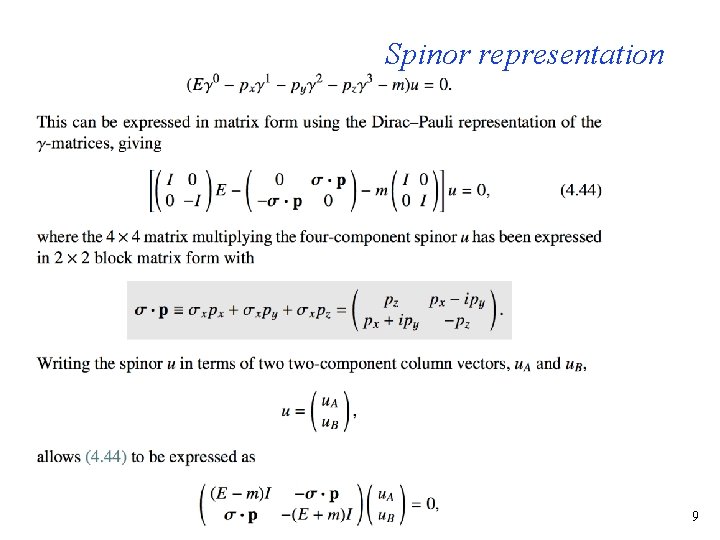
Spinor representation 9
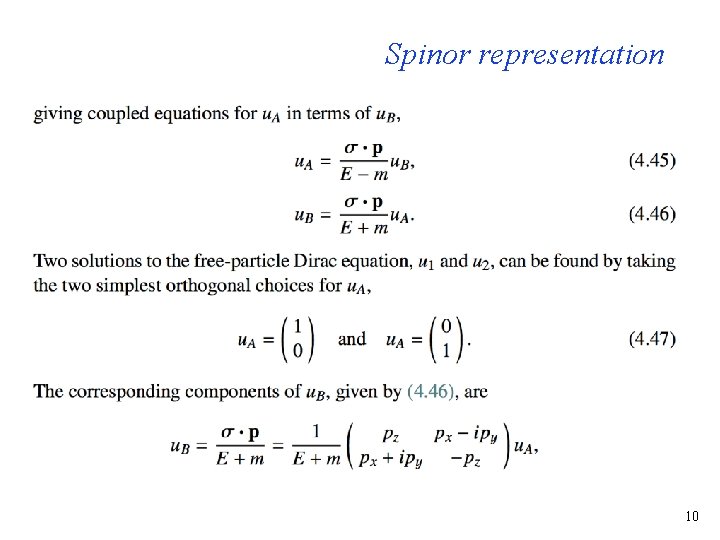
Spinor representation 10
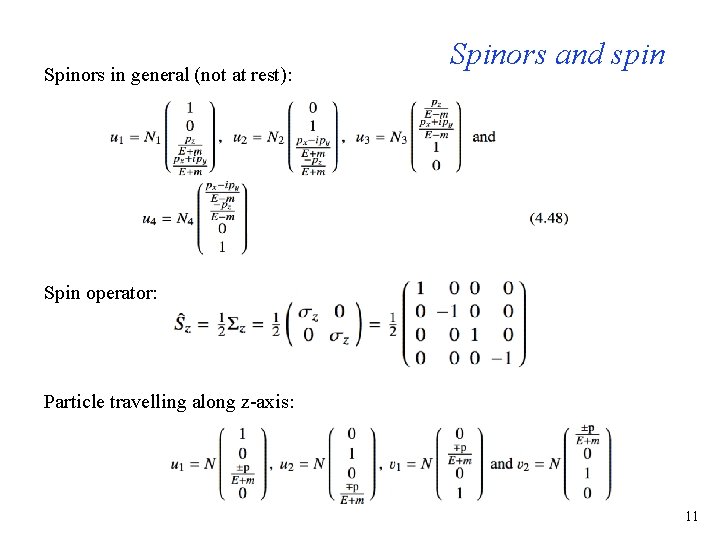
Spinors in general (not at rest): Spinors and spin Spin operator: Particle travelling along z-axis: 11
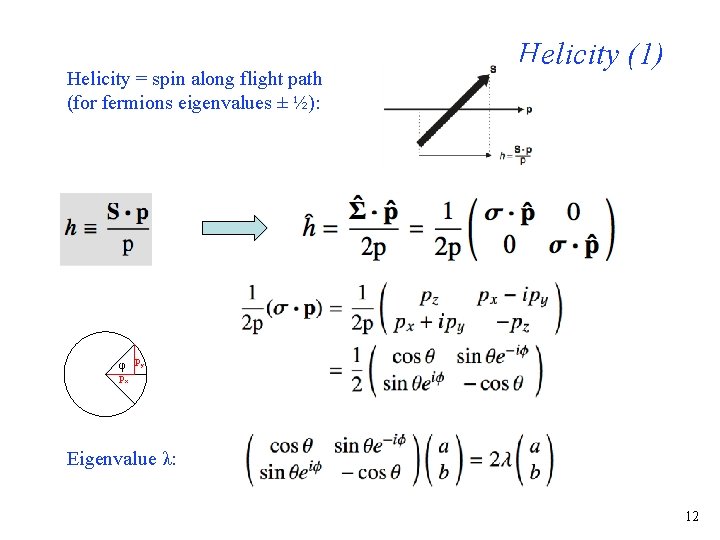
Helicity = spin along flight path (for fermions eigenvalues ± ½): Helicity (1) φ py px Eigenvalue λ: 12
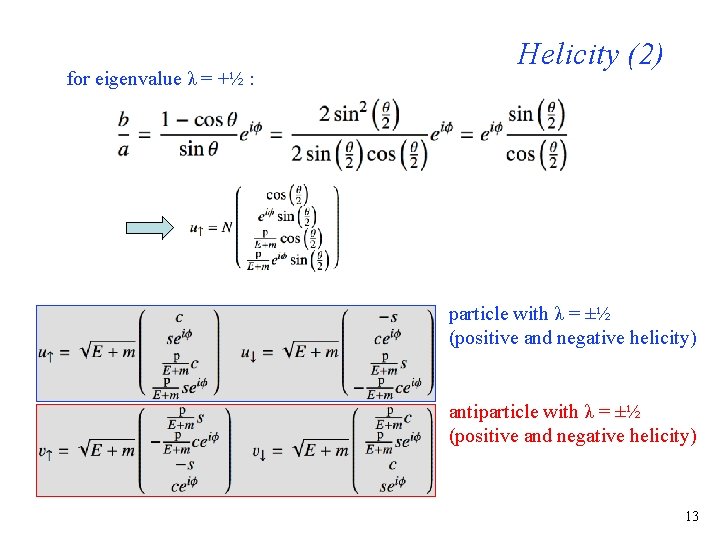
for eigenvalue λ = +½ : Helicity (2) particle with λ = ±½ (positive and negative helicity) antiparticle with λ = ±½ (positive and negative helicity) 13
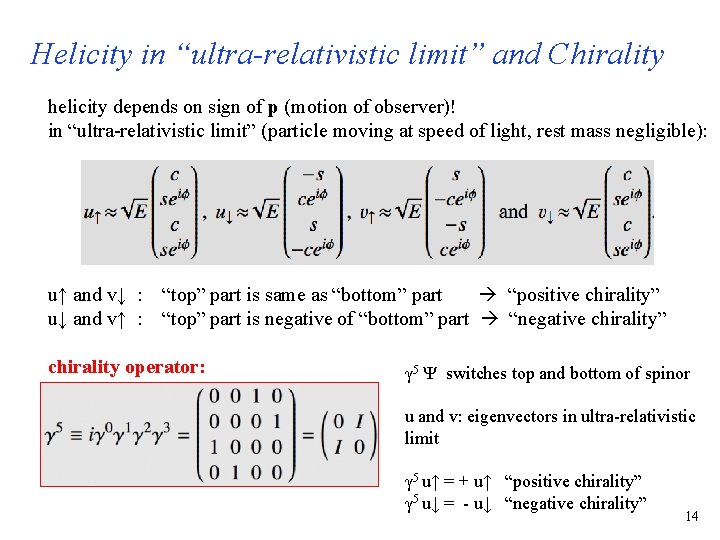
Helicity in “ultra-relativistic limit” and Chirality helicity depends on sign of p (motion of observer)! in “ultra-relativistic limit” (particle moving at speed of light, rest mass negligible): u↑ and v↓ : u↓ and v↑ : “top” part is same as “bottom” part “positive chirality” “top” part is negative of “bottom” part “negative chirality” chirality operator: γ 5 Ψ switches top and bottom of spinor u and v: eigenvectors in ultra-relativistic limit γ 5 u↑ = + u↑ “positive chirality” γ 5 u↓ = - u↓ “negative chirality” 14
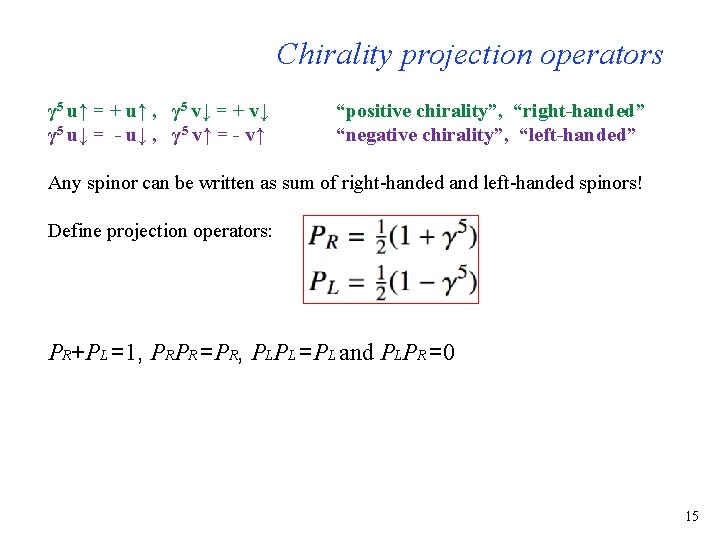
Chirality projection operators γ 5 u↑ = + u↑ , γ 5 v↓ = + v↓ γ 5 u↓ = - u↓ , γ 5 v↑ = - v↑ “positive chirality”, “right-handed” “negative chirality”, “left-handed” Any spinor can be written as sum of right-handed and left-handed spinors! Define projection operators: PR+PL =1, PRPR =PR, PLPL =PL and PLPR =0 15
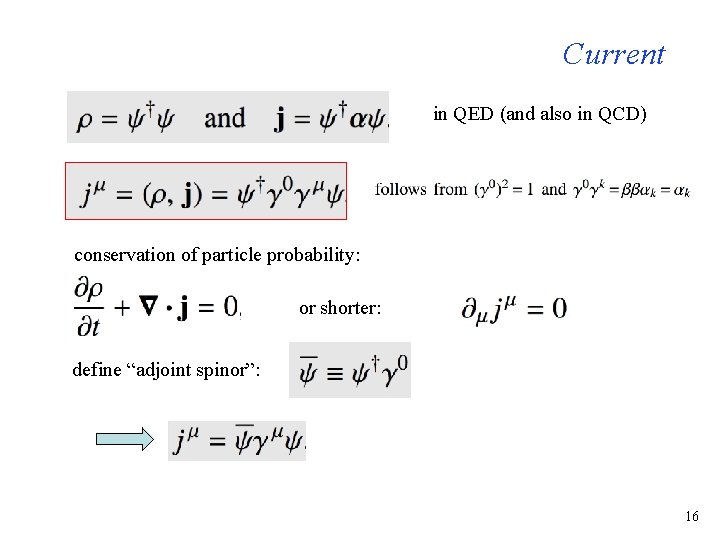
Current in QED (and also in QCD) conservation of particle probability: or shorter: define “adjoint spinor”: 16
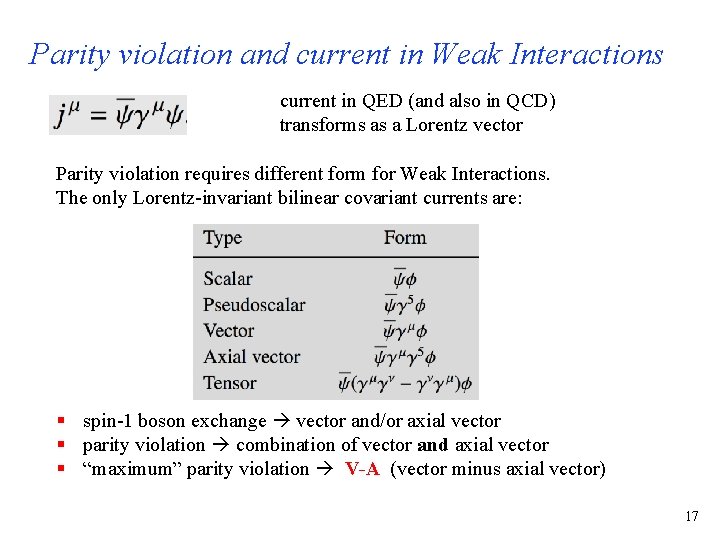
Parity violation and current in Weak Interactions current in QED (and also in QCD) transforms as a Lorentz vector Parity violation requires different form for Weak Interactions. The only Lorentz-invariant bilinear covariant currents are: § spin-1 boson exchange vector and/or axial vector § parity violation combination of vector and axial vector § “maximum” parity violation V-A (vector minus axial vector) 17
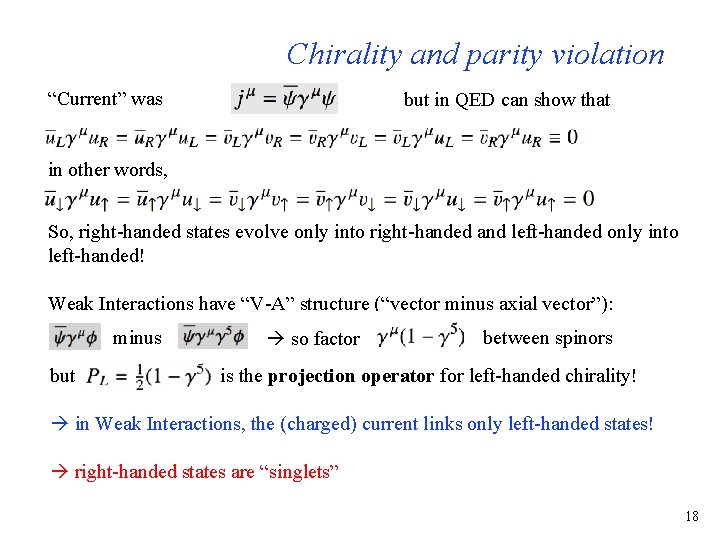
Chirality and parity violation “Current” was but in QED can show that in other words, So, right-handed states evolve only into right-handed and left-handed only into left-handed! Weak Interactions have “V-A” structure (“vector minus axial vector”): minus but so factor between spinors is the projection operator for left-handed chirality! in Weak Interactions, the (charged) current links only left-handed states! right-handed states are “singlets” 18
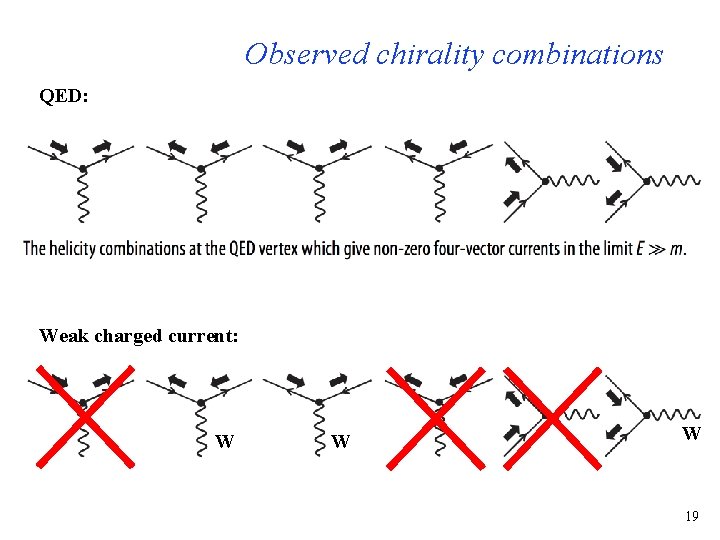
Observed chirality combinations QED: Weak charged current: W W W 19
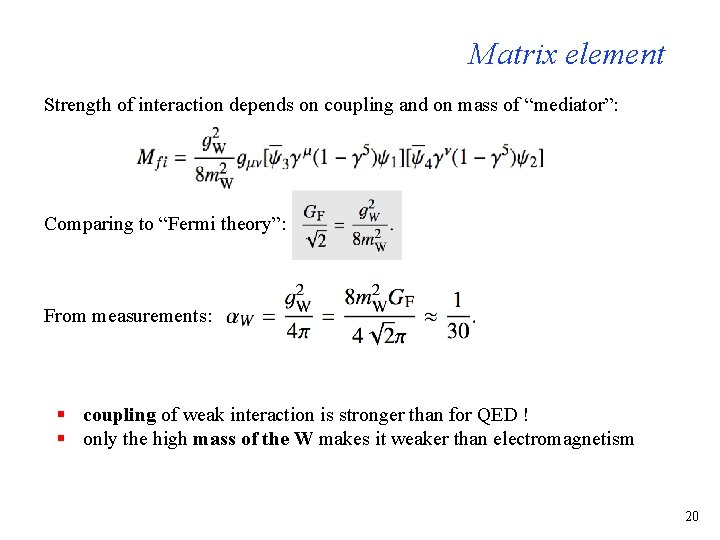
Matrix element Strength of interaction depends on coupling and on mass of “mediator”: Comparing to “Fermi theory”: From measurements: § coupling of weak interaction is stronger than for QED ! § only the high mass of the W makes it weaker than electromagnetism 20
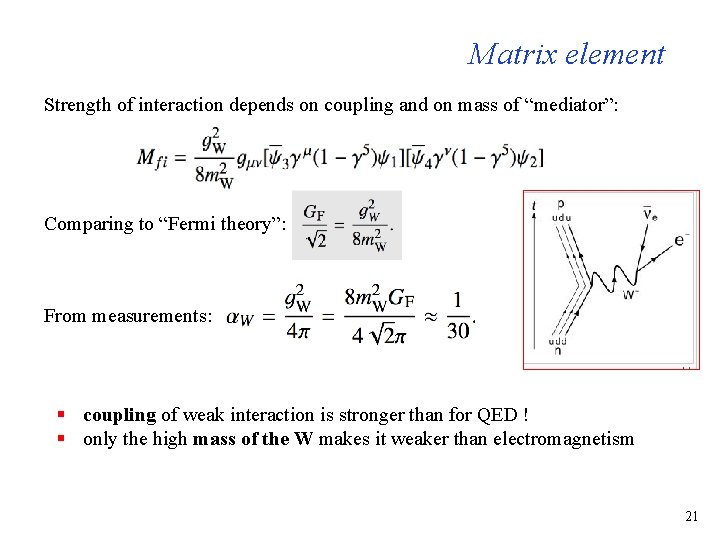
Matrix element Strength of interaction depends on coupling and on mass of “mediator”: Comparing to “Fermi theory”: From measurements: § coupling of weak interaction is stronger than for QED ! § only the high mass of the W makes it weaker than electromagnetism 21
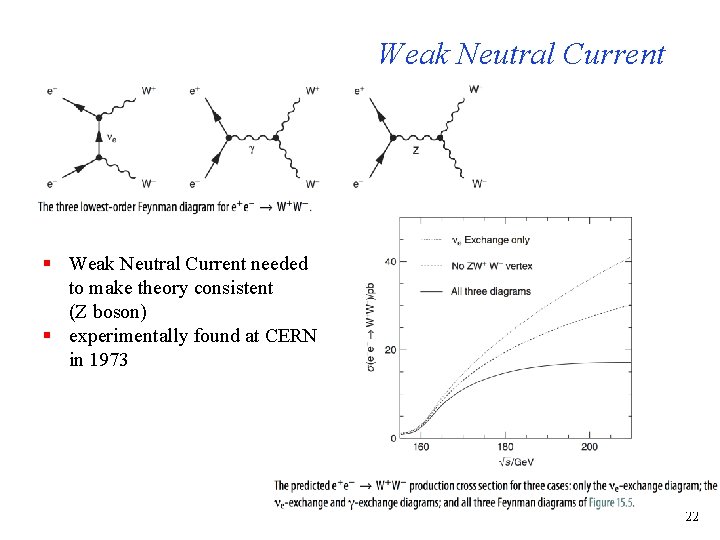
Weak Neutral Current § Weak Neutral Current needed to make theory consistent (Z boson) § experimentally found at CERN in 1973 22
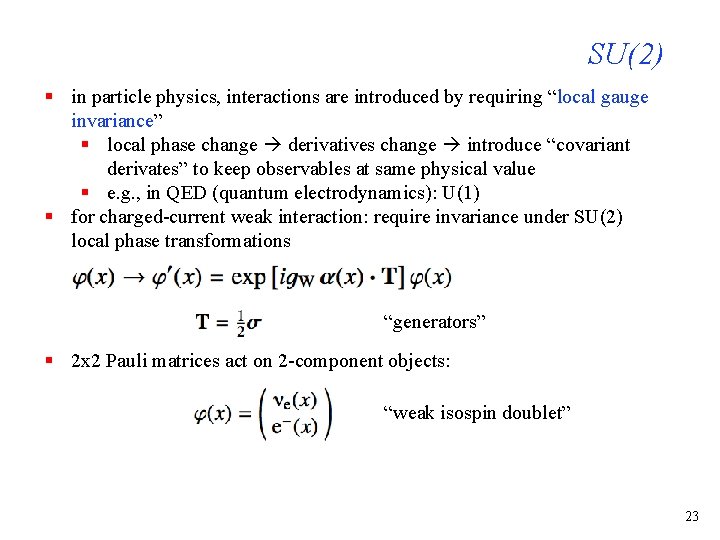
SU(2) § in particle physics, interactions are introduced by requiring “local gauge invariance” § local phase change derivatives change introduce “covariant derivates” to keep observables at same physical value § e. g. , in QED (quantum electrodynamics): U(1) § for charged-current weak interaction: require invariance under SU(2) local phase transformations “generators” § 2 x 2 Pauli matrices act on 2 -component objects: “weak isospin doublet” 23
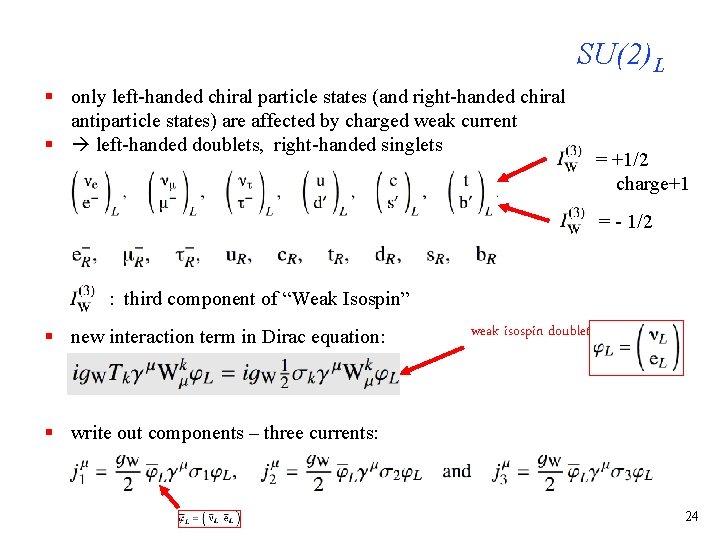
SU(2)L § only left-handed chiral particle states (and right-handed chiral antiparticle states) are affected by charged weak current § left-handed doublets, right-handed singlets = +1/2 charge+1 = - 1/2 : third component of “Weak Isospin” § new interaction term in Dirac equation: weak isospin doublet: § write out components – three currents: 24
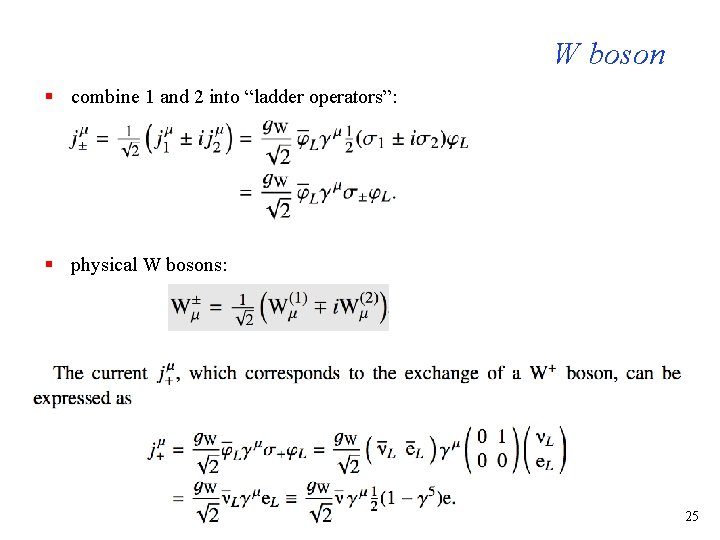
W boson § combine 1 and 2 into “ladder operators”: § physical W bosons: 25
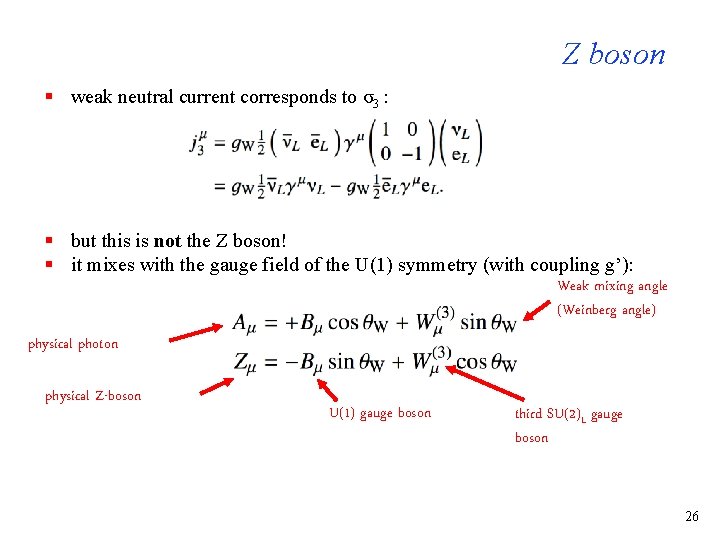
Z boson § weak neutral current corresponds to σ3 : § but this is not the Z boson! § it mixes with the gauge field of the U(1) symmetry (with coupling g’): Weak mixing angle (Weinberg angle) physical photon physical Z-boson U(1) gauge boson third SU(2)L gauge boson 26
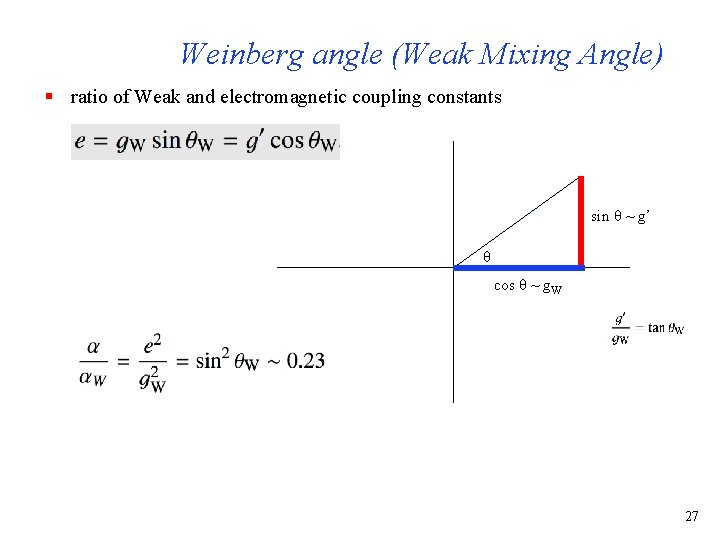
Weinberg angle (Weak Mixing Angle) § ratio of Weak and electromagnetic coupling constants sin θ ~ g’ θ cos θ ~ g. W 27
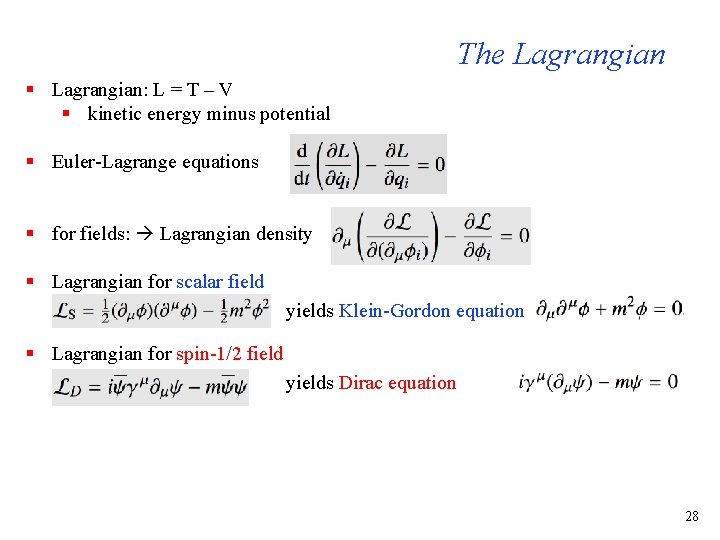
The Lagrangian § Lagrangian: L = T – V § kinetic energy minus potential § Euler-Lagrange equations § for fields: Lagrangian density § Lagrangian for scalar field yields Klein-Gordon equation § Lagrangian for spin-1/2 field yields Dirac equation 28
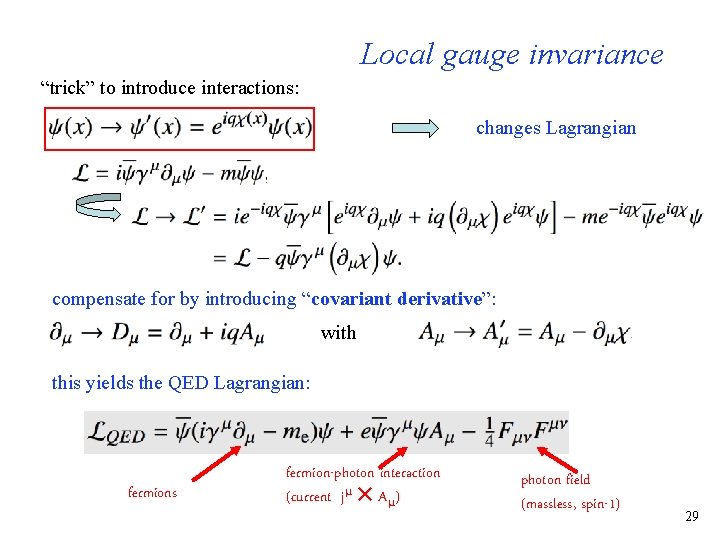
Local gauge invariance “trick” to introduce interactions: changes Lagrangian compensate for by introducing “covariant derivative”: with this yields the QED Lagrangian: fermions fermion-photon interaction (current jμ ✕ Aμ) photon field (massless, spin-1) 29
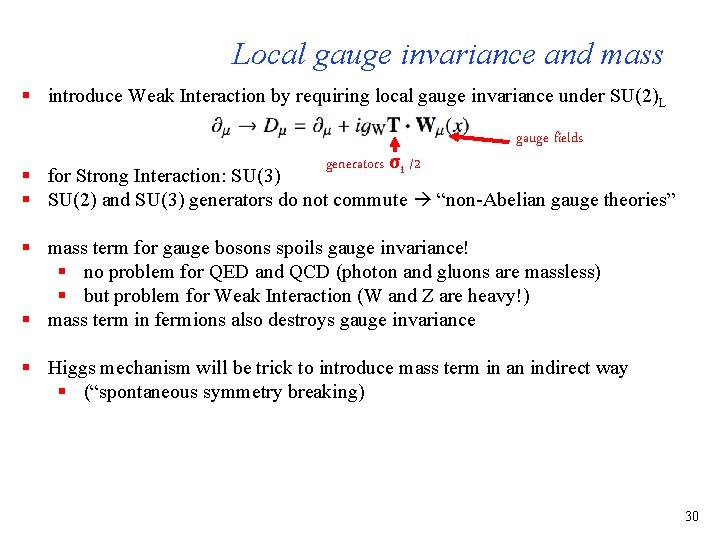
Local gauge invariance and mass § introduce Weak Interaction by requiring local gauge invariance under SU(2)L generators σi /2 gauge fields § for Strong Interaction: SU(3) § SU(2) and SU(3) generators do not commute “non-Abelian gauge theories” § mass term for gauge bosons spoils gauge invariance! § no problem for QED and QCD (photon and gluons are massless) § but problem for Weak Interaction (W and Z are heavy!) § mass term in fermions also destroys gauge invariance § Higgs mechanism will be trick to introduce mass term in an indirect way § (“spontaneous symmetry breaking) 30
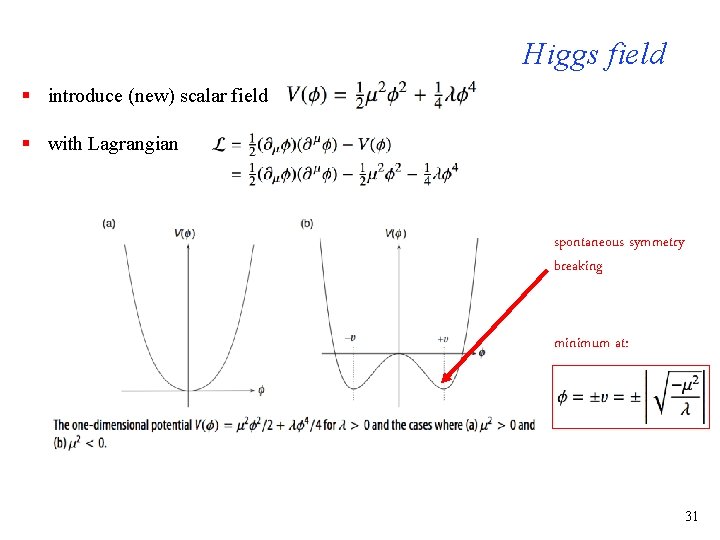
Higgs field § introduce (new) scalar field § with Lagrangian spontaneous symmetry breaking minimum at: 31
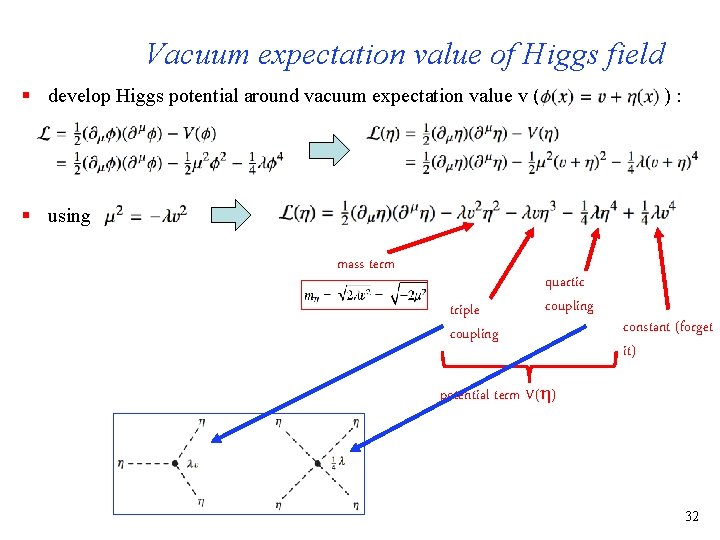
Vacuum expectation value of Higgs field § develop Higgs potential around vacuum expectation value v ( ): § using mass term triple coupling quartic coupling constant (forget it) potential term V(η) 32
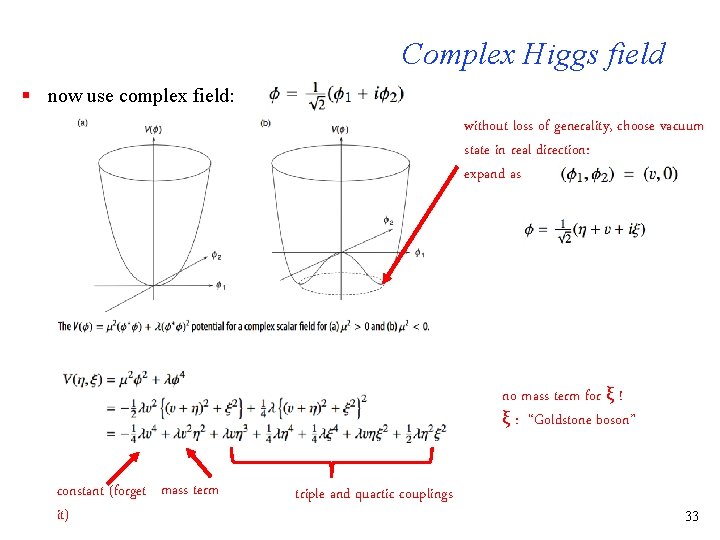
Complex Higgs field § now use complex field: without loss of generality, choose vacuum state in real direction: expand as no mass term for ξ ! ξ : “Goldstone boson” constant (forget mass term it) triple and quartic couplings 33
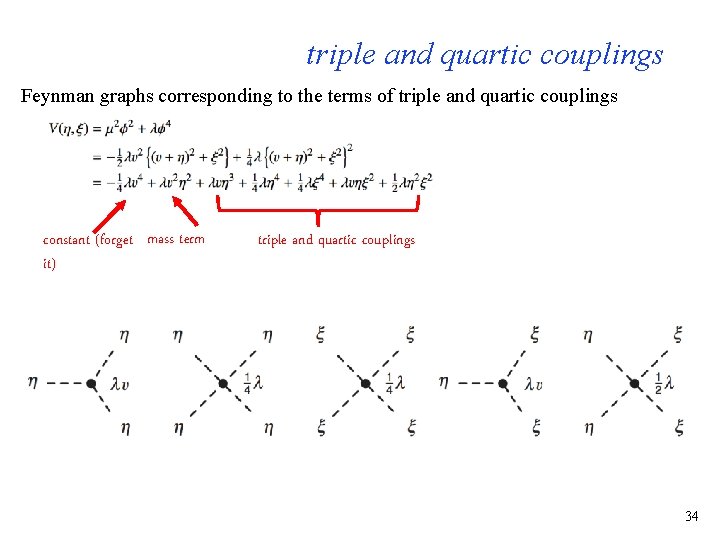
triple and quartic couplings Feynman graphs corresponding to the terms of triple and quartic couplings constant (forget mass term it) triple and quartic couplings 34
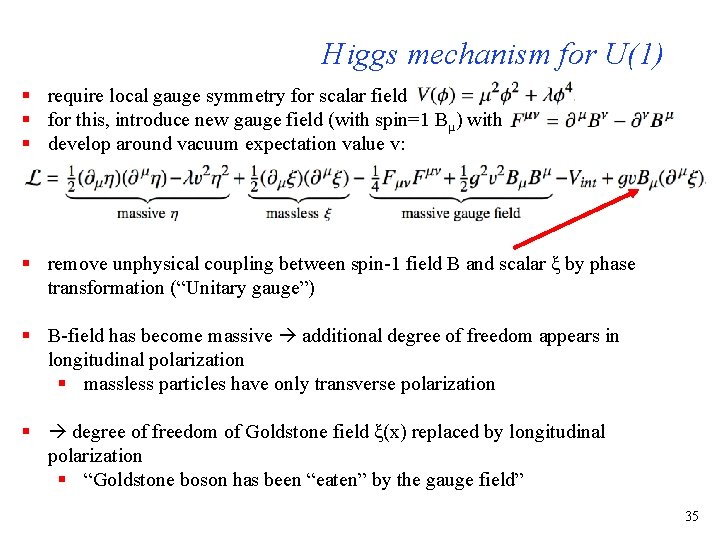
Higgs mechanism for U(1) § require local gauge symmetry for scalar field § for this, introduce new gauge field (with spin=1 Bμ) with § develop around vacuum expectation value v: § remove unphysical coupling between spin-1 field B and scalar ξ by phase transformation (“Unitary gauge”) § B-field has become massive additional degree of freedom appears in longitudinal polarization § massless particles have only transverse polarization § degree of freedom of Goldstone field ξ(x) replaced by longitudinal polarization § “Goldstone boson has been “eaten” by the gauge field” 35
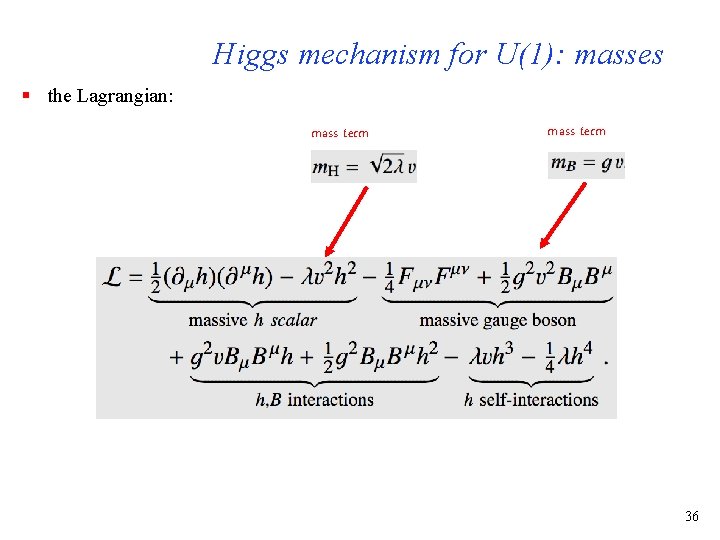
Higgs mechanism for U(1): masses § the Lagrangian: mass term 36
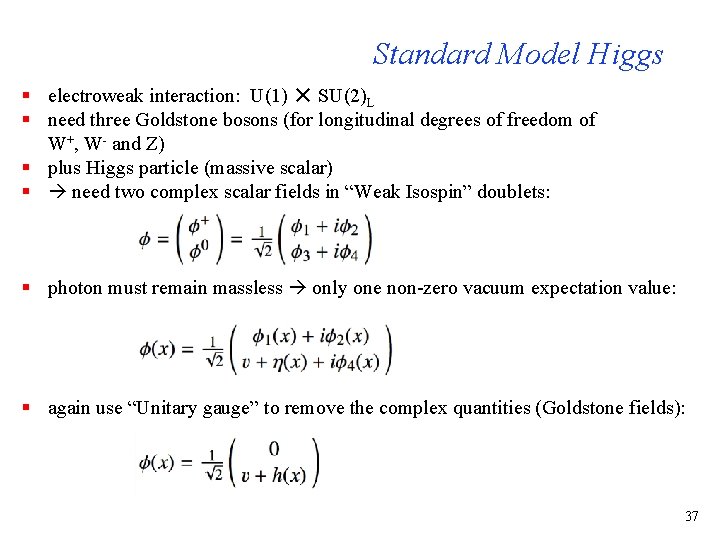
Standard Model Higgs § electroweak interaction: U(1) ✕ SU(2)L § need three Goldstone bosons (for longitudinal degrees of freedom of W+, W- and Z) § plus Higgs particle (massive scalar) § need two complex scalar fields in “Weak Isospin” doublets: § photon must remain massless only one non-zero vacuum expectation value: § again use “Unitary gauge” to remove the complex quantities (Goldstone fields): 37
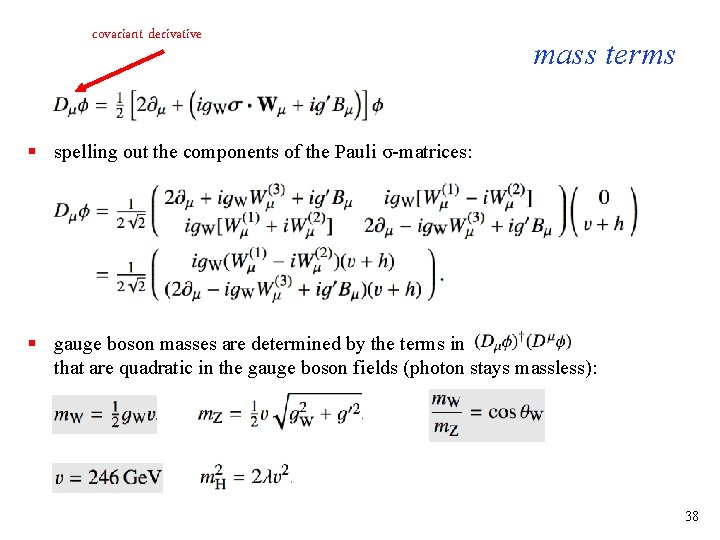
covariant derivative mass terms § spelling out the components of the Pauli σ-matrices: § gauge boson masses are determined by the terms in that are quadratic in the gauge boson fields (photon stays massless): 38
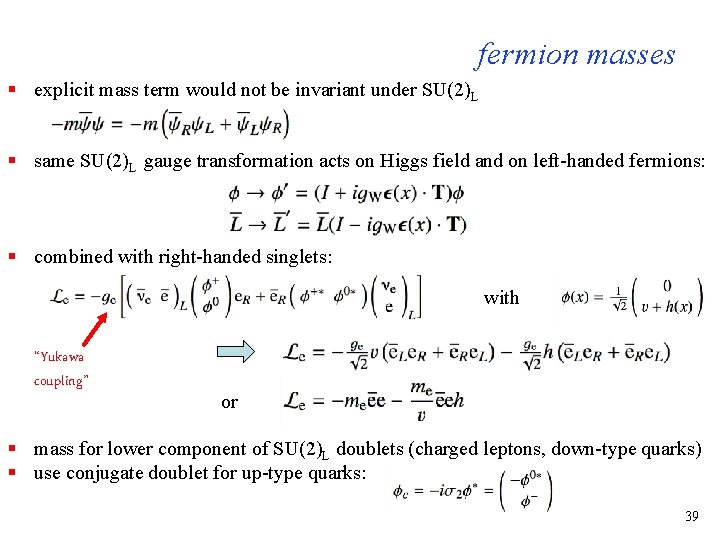
fermion masses § explicit mass term would not be invariant under SU(2)L § same SU(2)L gauge transformation acts on Higgs field and on left-handed fermions: § combined with right-handed singlets: with “Yukawa coupling” or § mass for lower component of SU(2)L doublets (charged leptons, down-type quarks) § use conjugate doublet for up-type quarks: 39
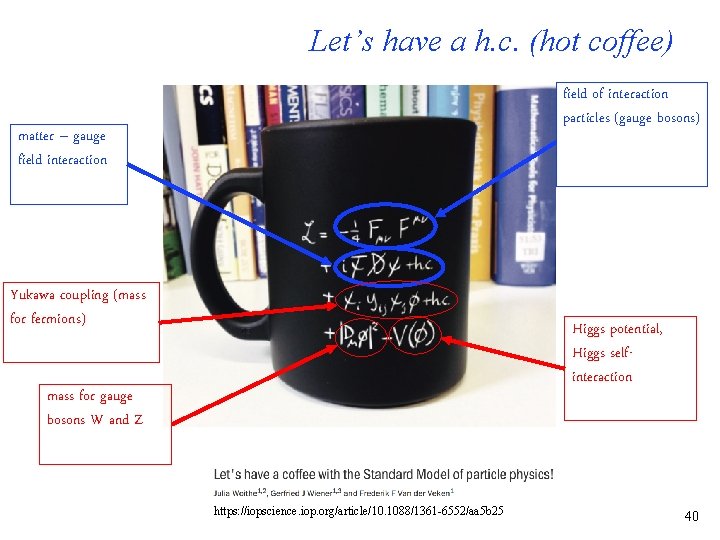
Let’s have a h. c. (hot coffee) field of interaction particles (gauge bosons) matter – gauge field interaction Yukawa coupling (mass for fermions) Higgs potential, Higgs selfinteraction mass for gauge bosons W and Z https: //iopscience. iop. org/article/10. 1088/1361 -6552/aa 5 b 25 40
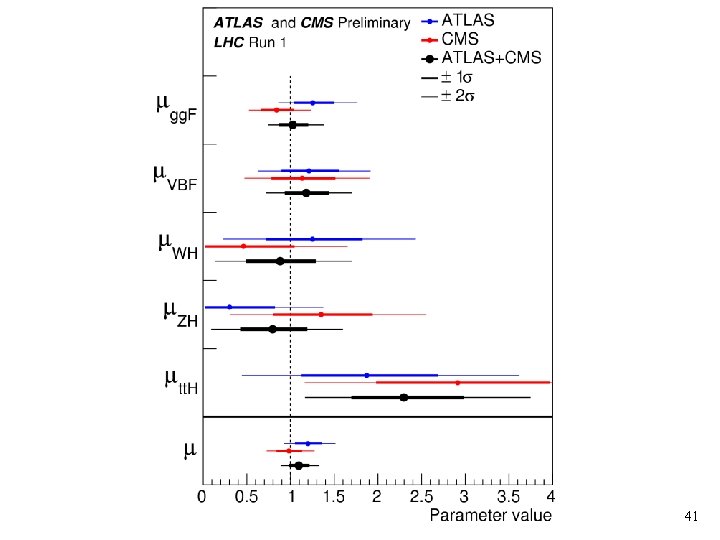
41
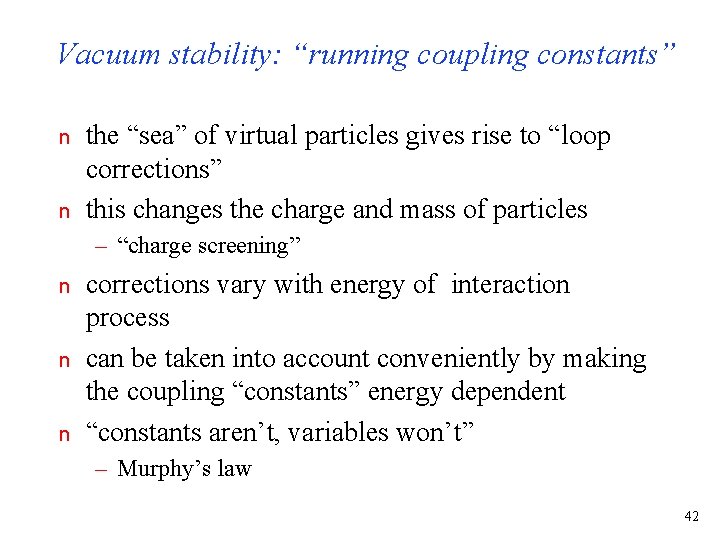
Vacuum stability: “running coupling constants” n n the “sea” of virtual particles gives rise to “loop corrections” this changes the charge and mass of particles – “charge screening” n n n corrections vary with energy of interaction process can be taken into account conveniently by making the coupling “constants” energy dependent “constants aren’t, variables won’t” – Murphy’s law 42
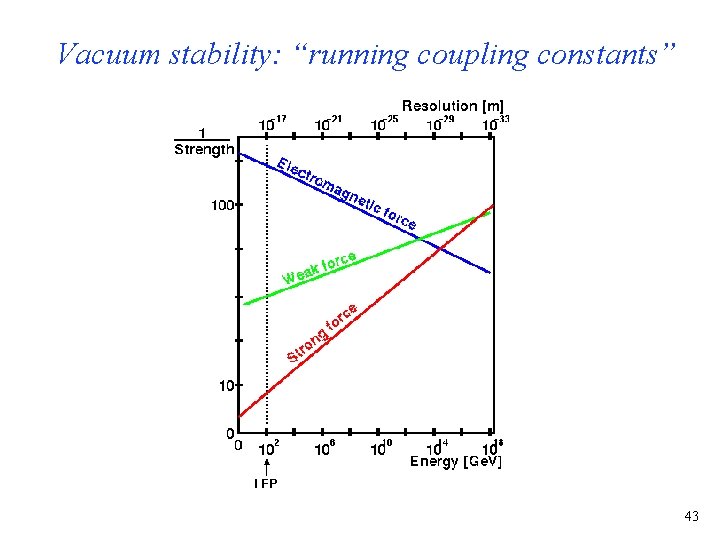
Vacuum stability: “running coupling constants” 43
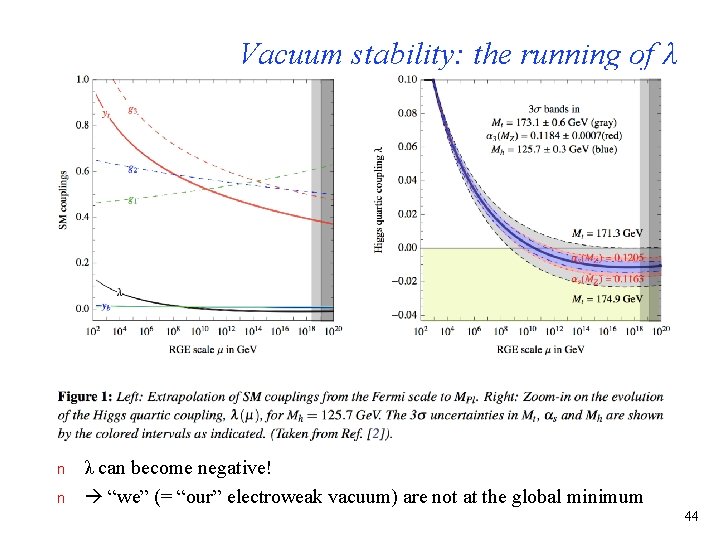
Vacuum stability: the running of λ n n λ can become negative! “we” (= “our” electroweak vacuum) are not at the global minimum 44
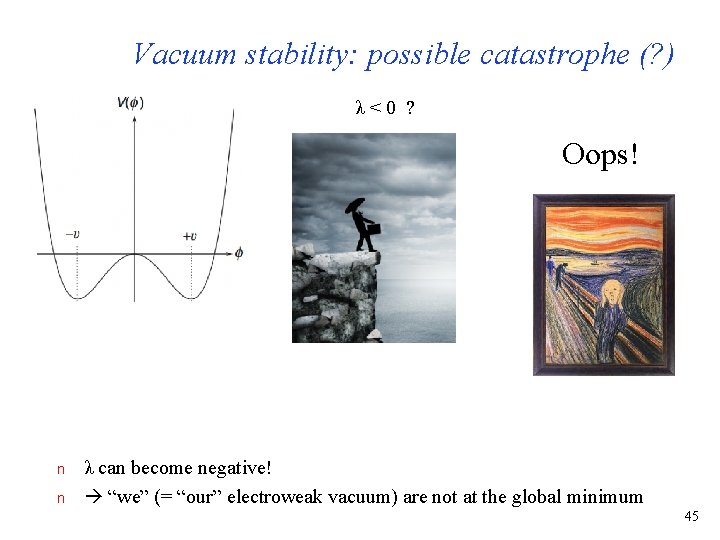
Vacuum stability: possible catastrophe (? ) λ<0 ? Oops! n n λ can become negative! “we” (= “our” electroweak vacuum) are not at the global minimum 45
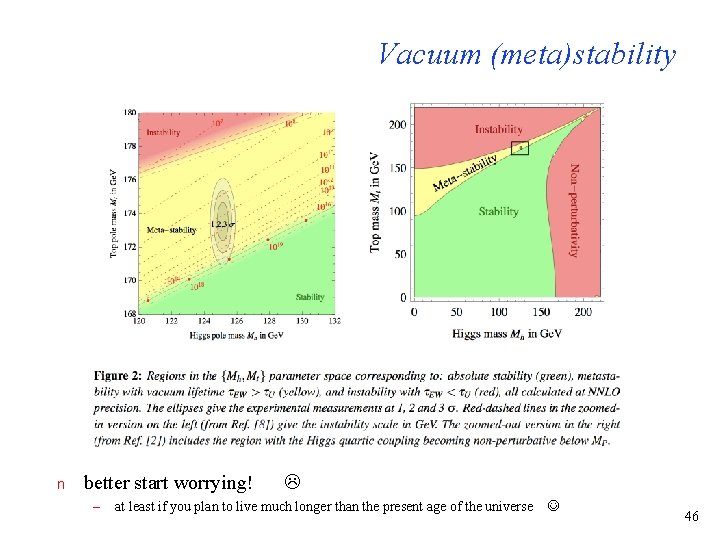
Vacuum (meta)stability n better start worrying! – at least if you plan to live much longer than the present age of the universe 46
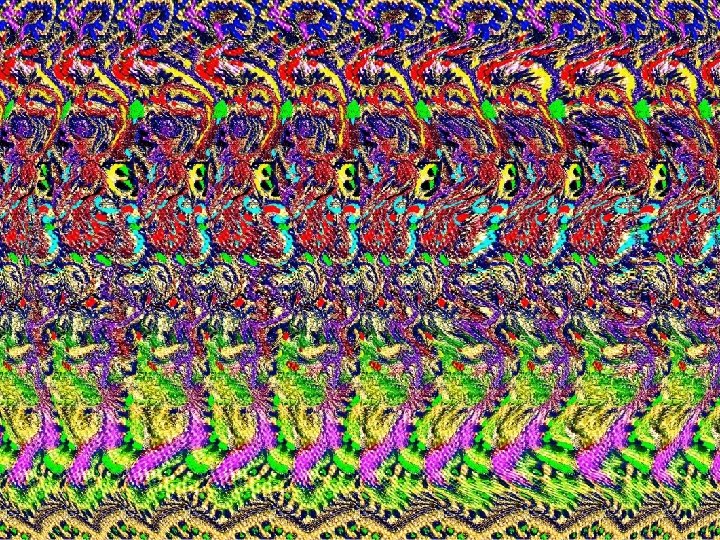
47
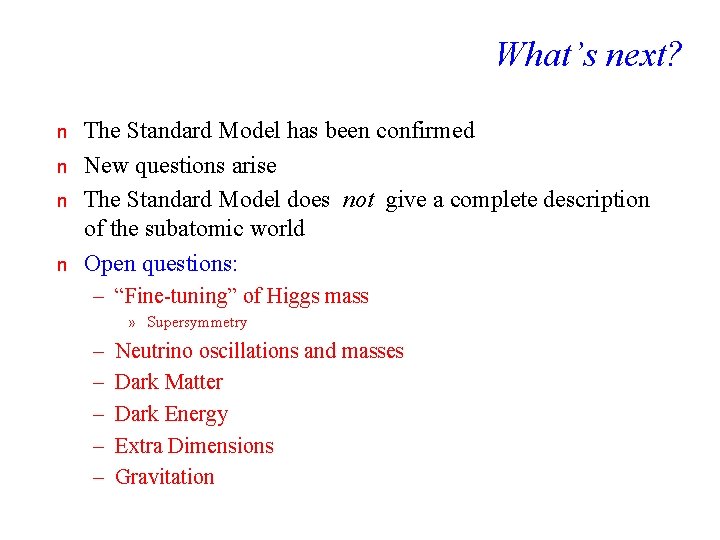
What’s next? n n The Standard Model has been confirmed New questions arise The Standard Model does not give a complete description of the subatomic world Open questions: – “Fine-tuning” of Higgs mass » Supersymmetry – – – Neutrino oscillations and masses Dark Matter Dark Energy Extra Dimensions Gravitation
Supersymmetry
A supersymmetry primer
Hadron
Higgs singlet
Scence
Cern geneva
Higgs factory
Higgs singlet
Marcela carena
Higgs doublet
Richard feynman
Chelsea higgs wise
Hungry higgs brætspil
Higgs boson
Higgs to tau tau
Higgs
Higgs boson
Higgs
Playfer
Higgs
Bellettini
Www.lhc.la.gov
Hl-lhc schedule
Hep forum
Lhc logbook
Lhc morning meeting
Worldwide lhc computing grid
Lhc budget
Lhc schedule 2022
Lhc performance workshop
Lhc performance workshop
Hl intranet
Hl-lhc schedule
Lhc performance workshop
Lhc filling scheme
Gant chart
"lhc"
Lhc
Lhc:8001692
Lhc beam dump
Lhc quench
Lhc beam dump
Forum lhc
"lhc"
"lhc"
Lhc location
Lhc schedule
Lhc technical coordination
Hl-lhc schedule