Nearinfrared NIR Single Photon Counting Detectors SPADs Chong
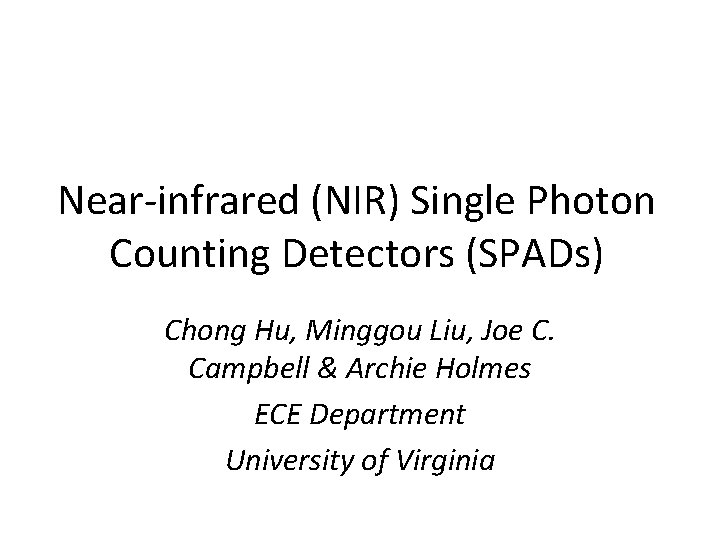
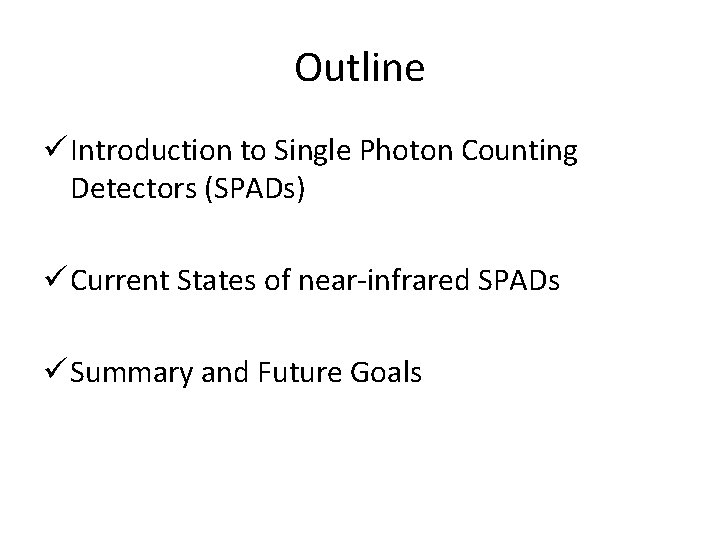
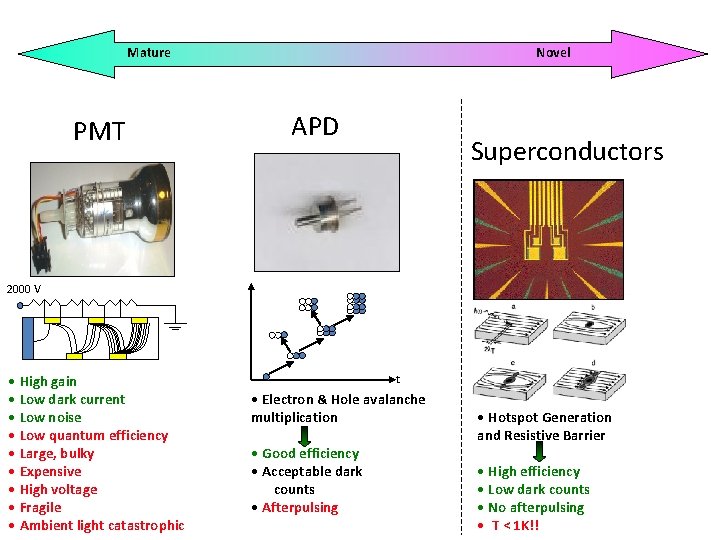
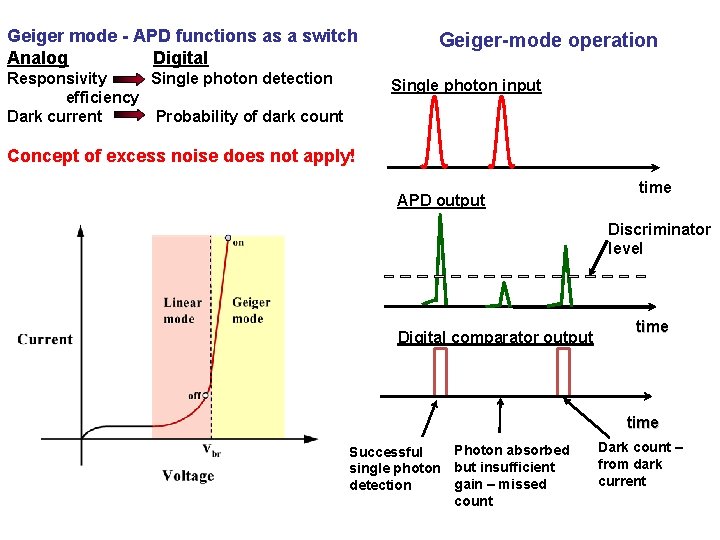
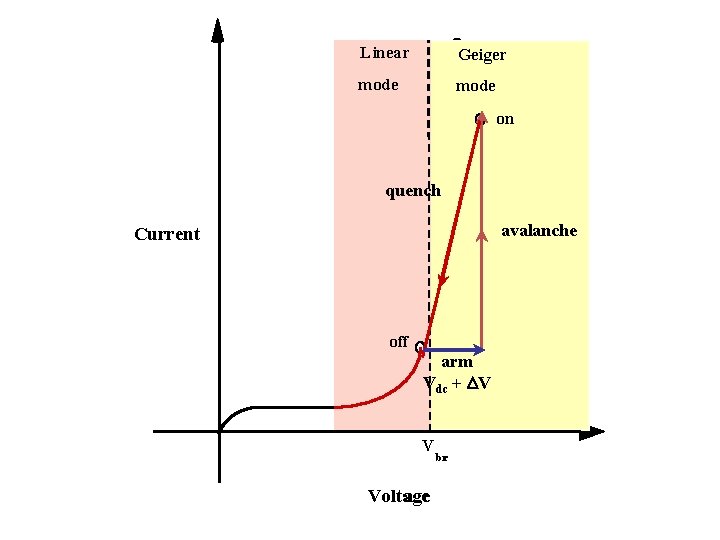
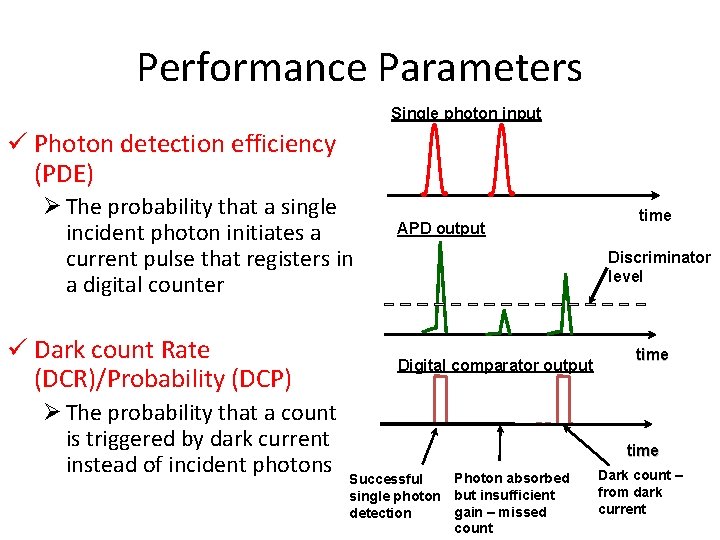
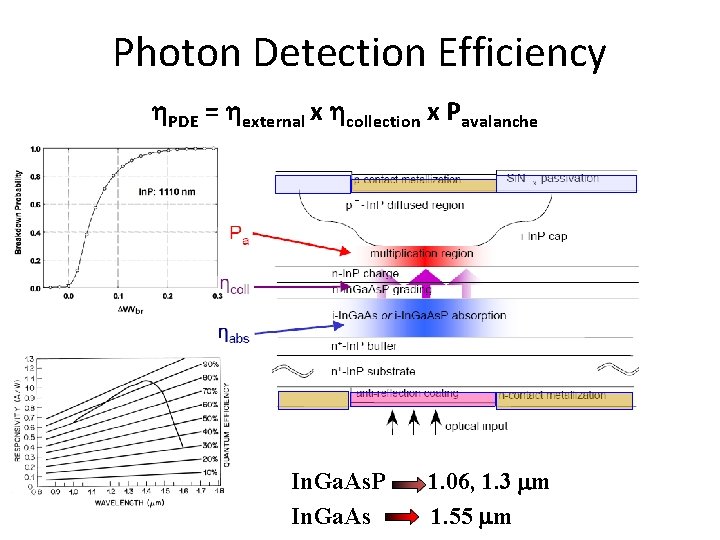
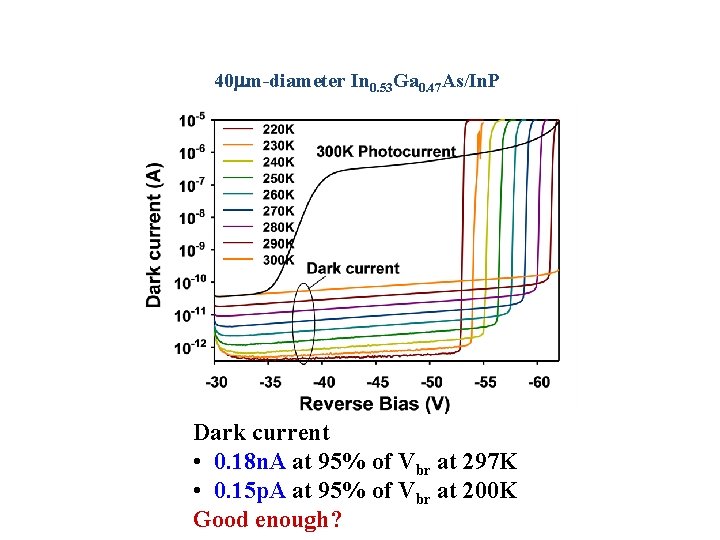
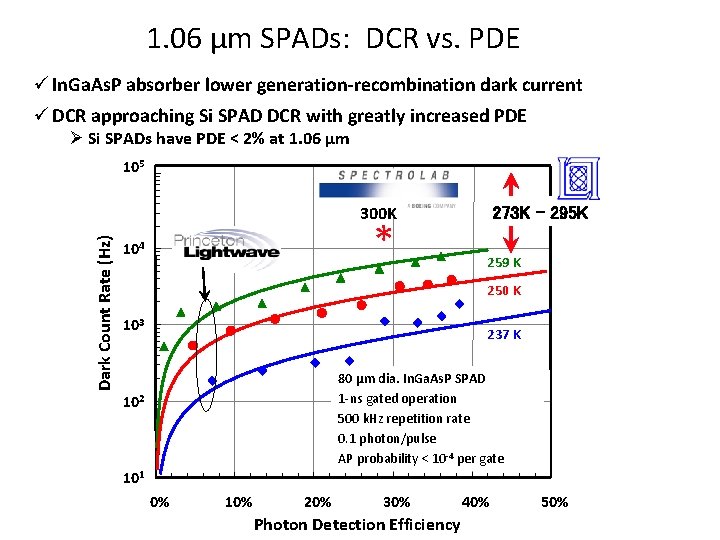
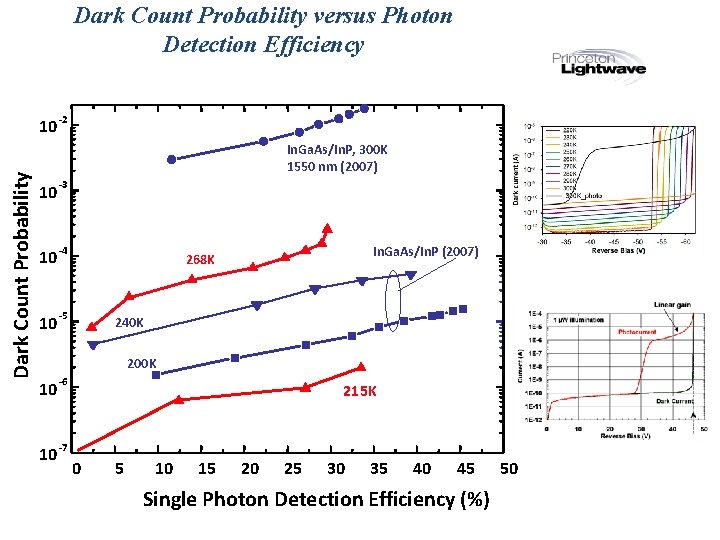
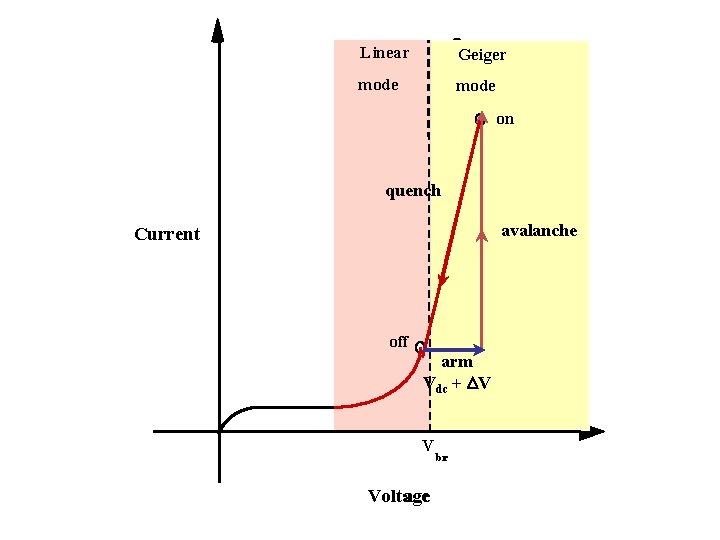
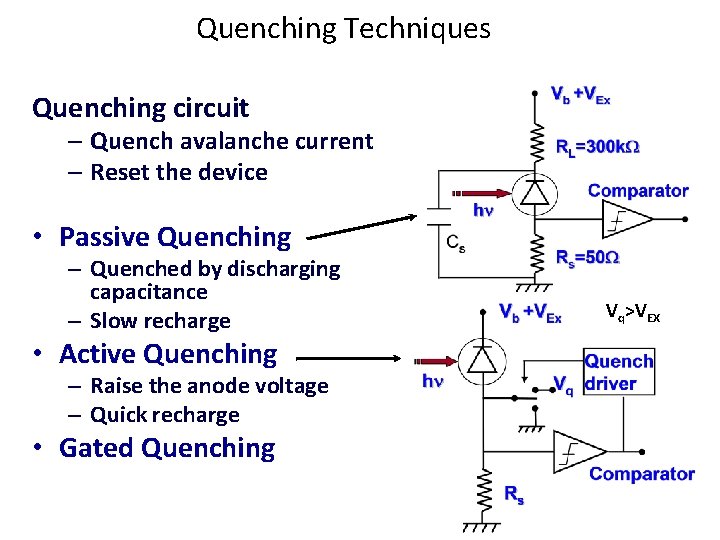
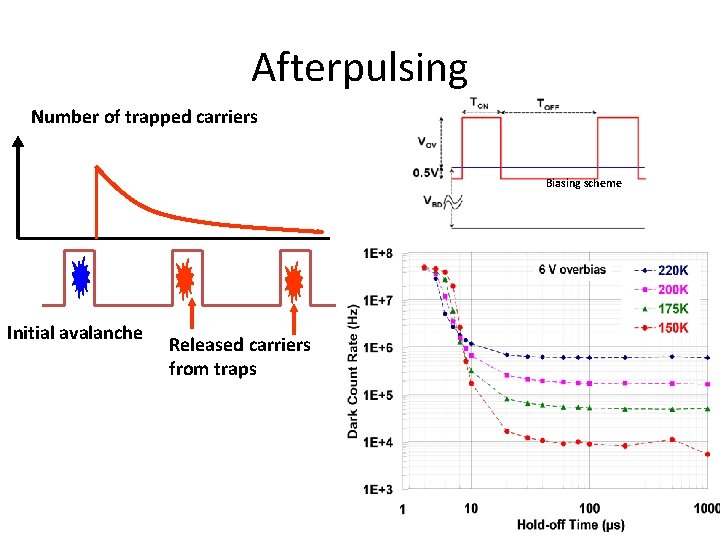
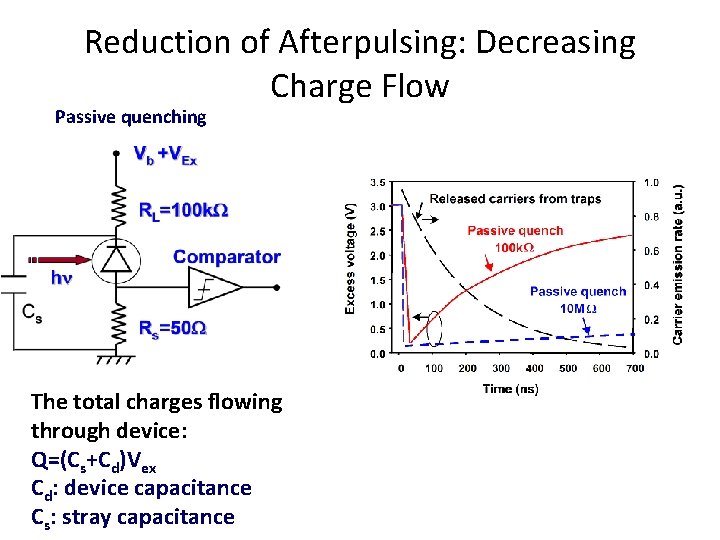
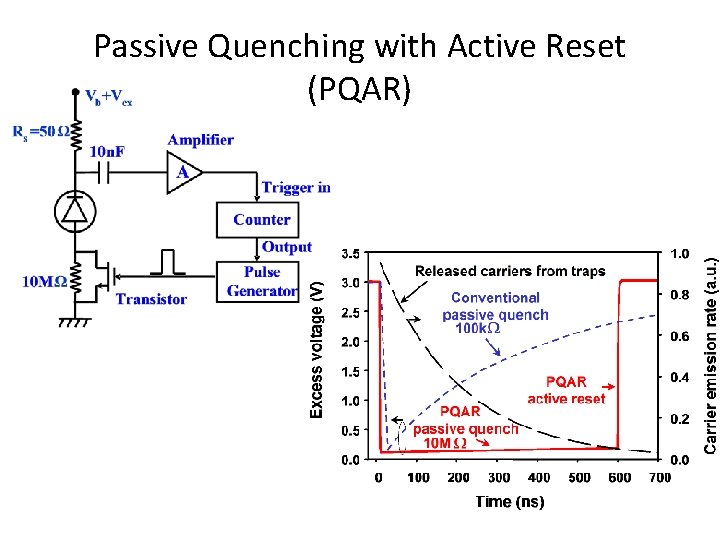
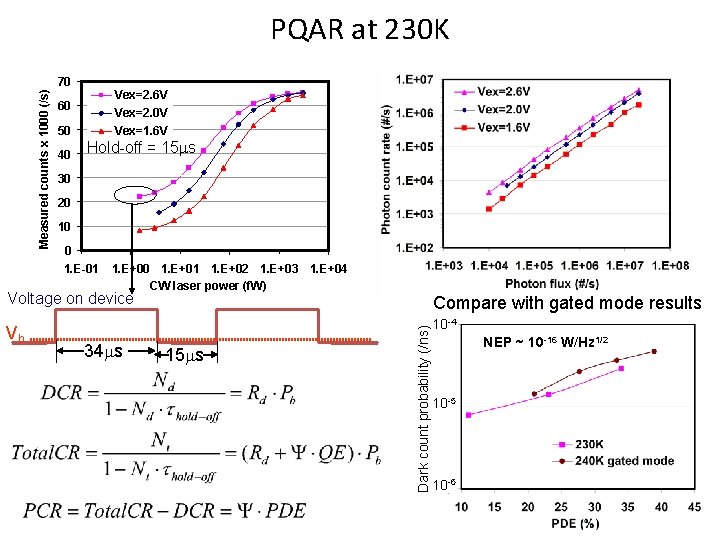
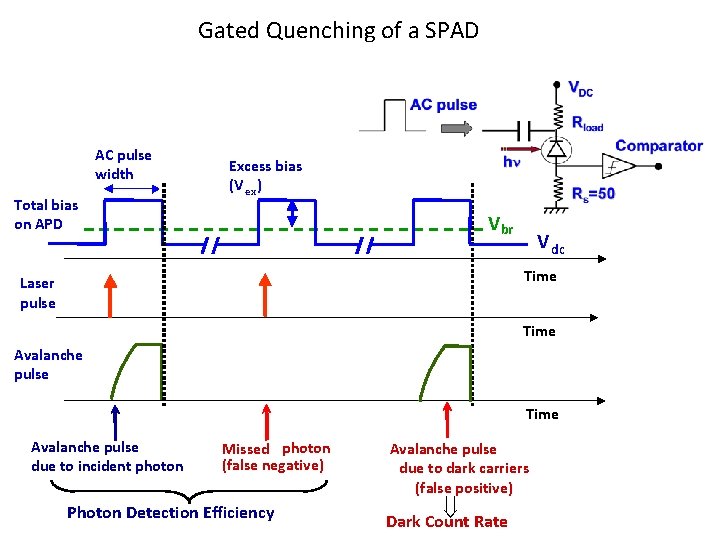
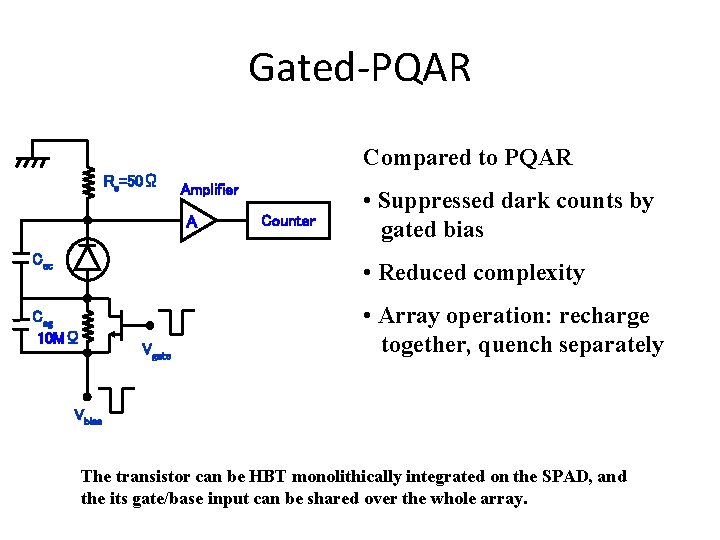
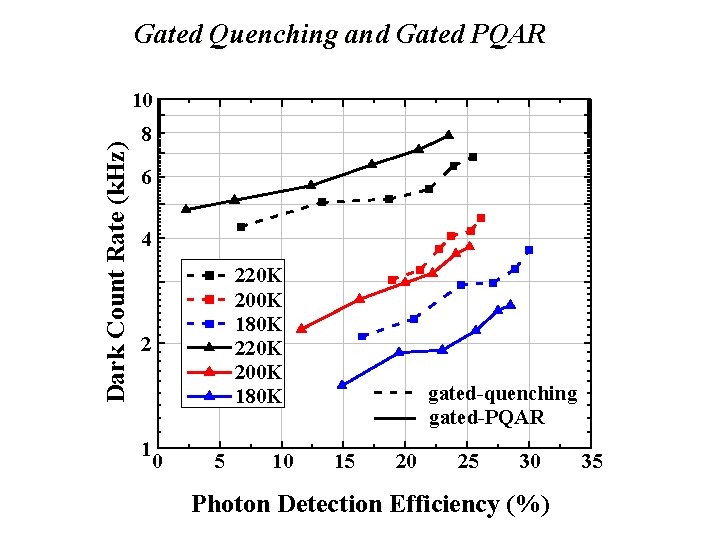
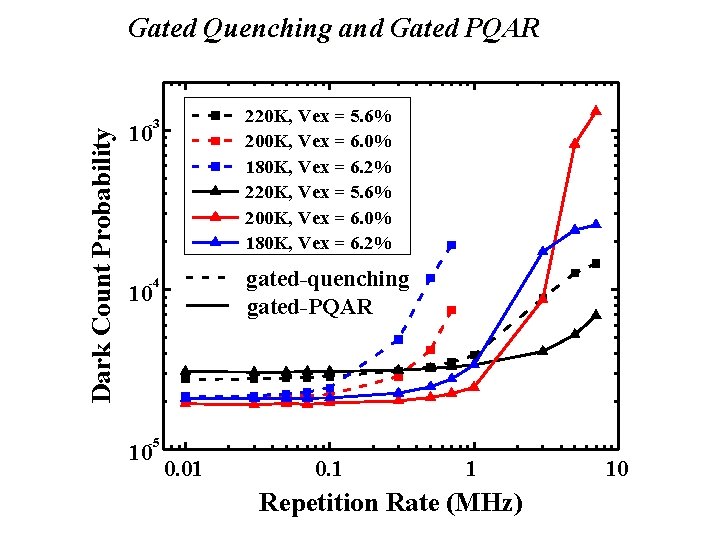
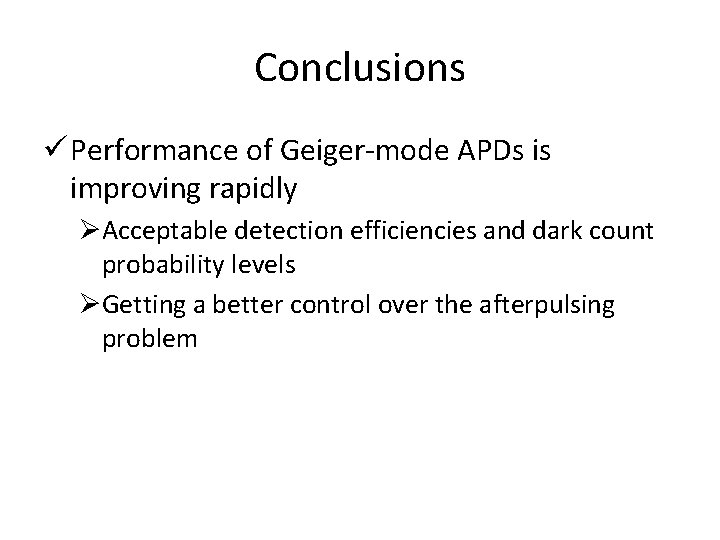
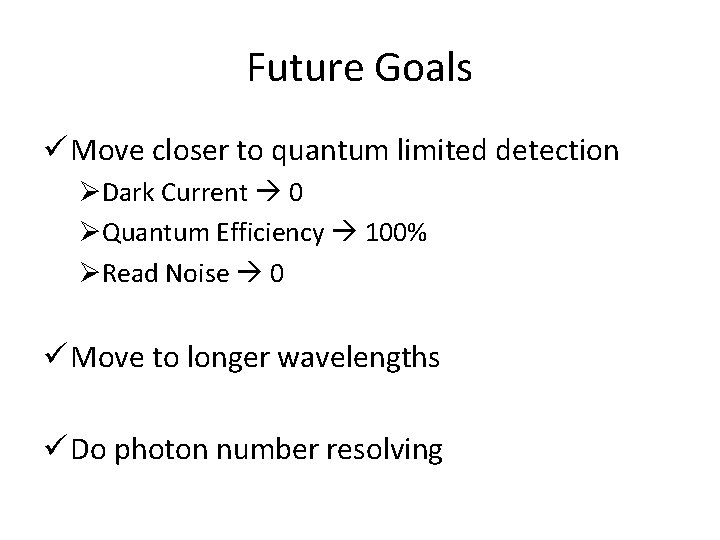
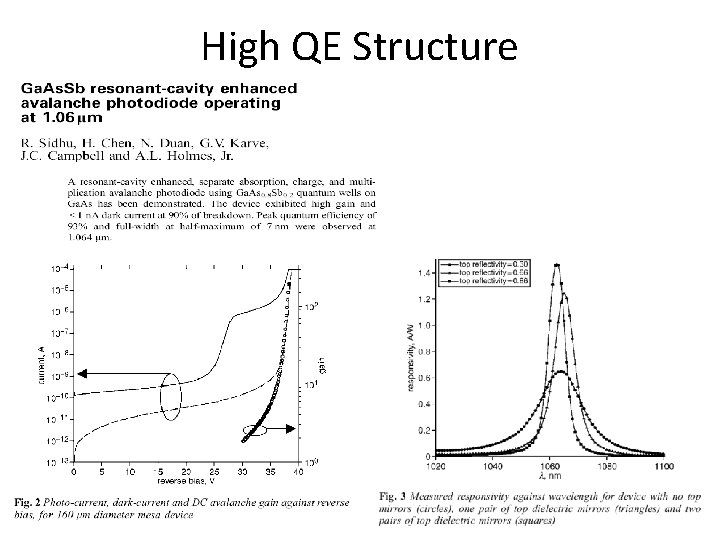
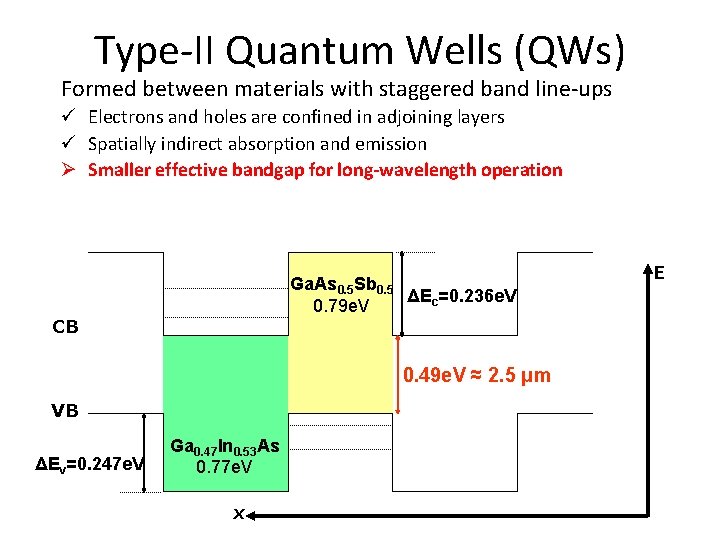
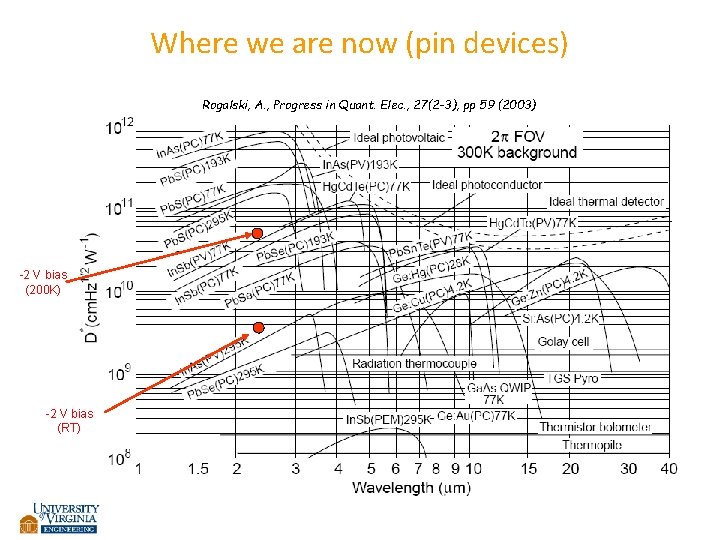
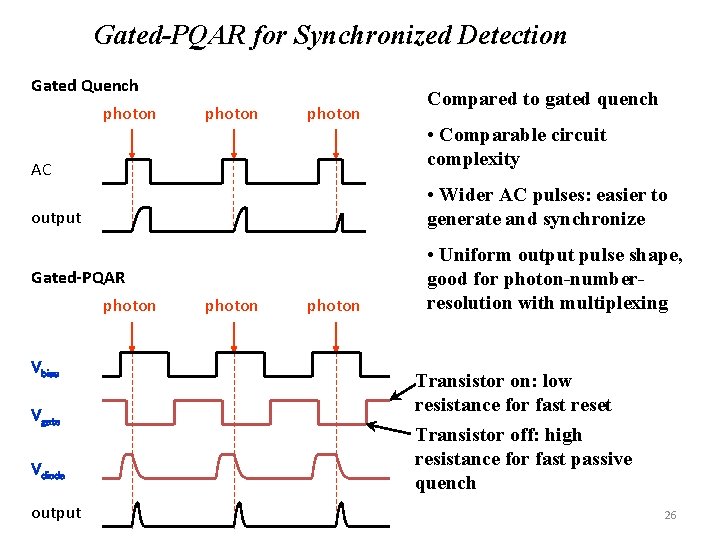
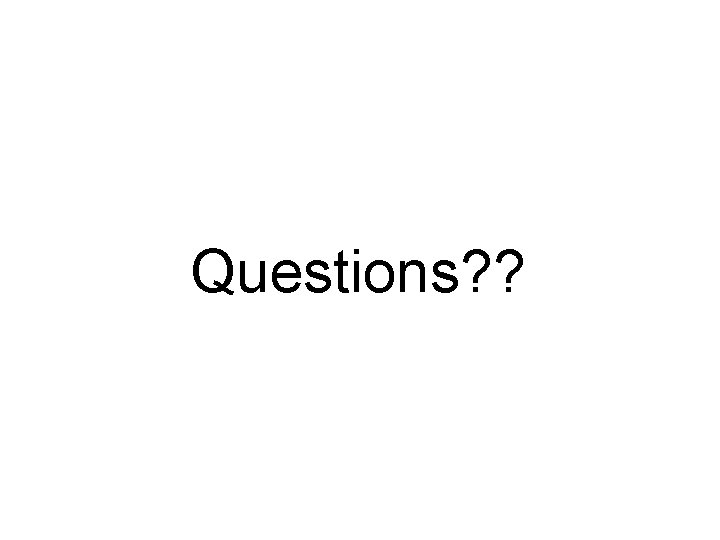
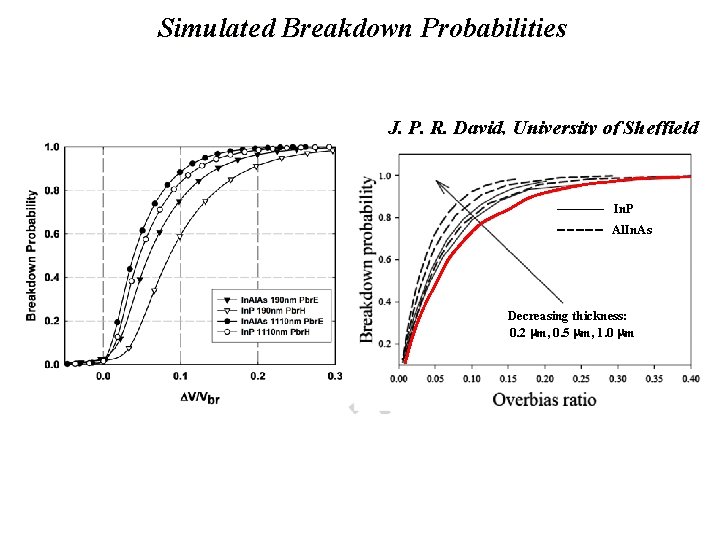
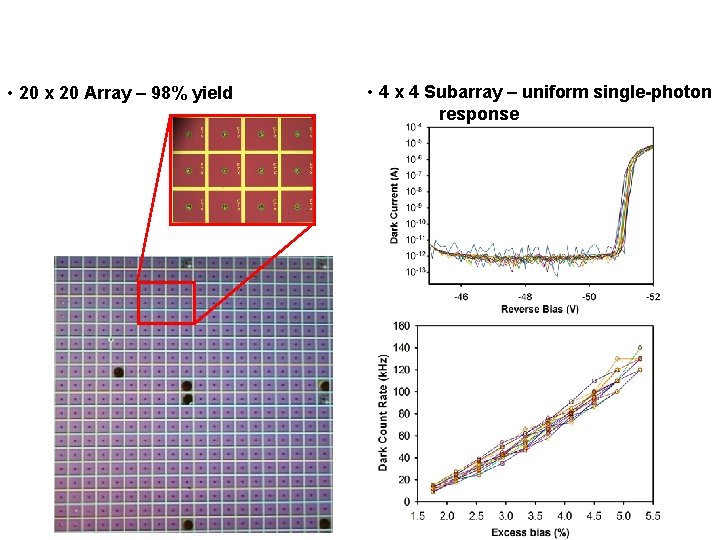
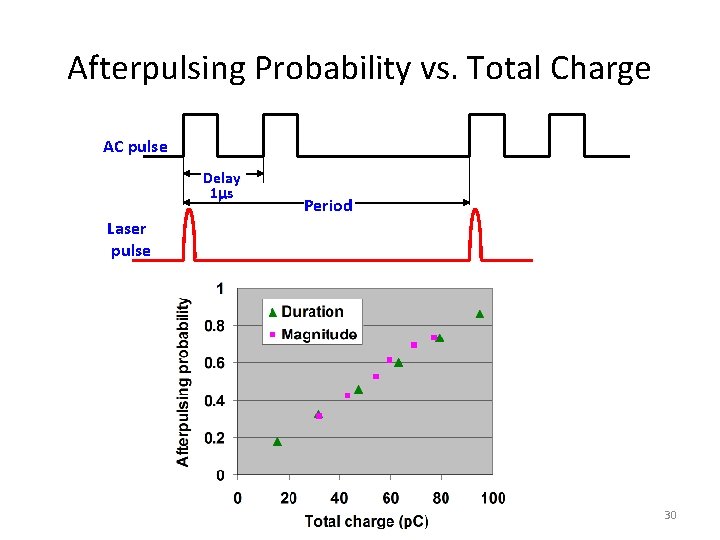
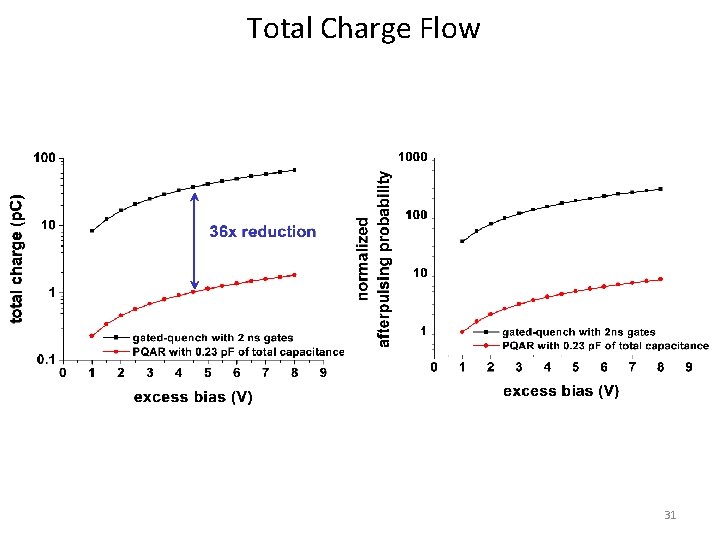
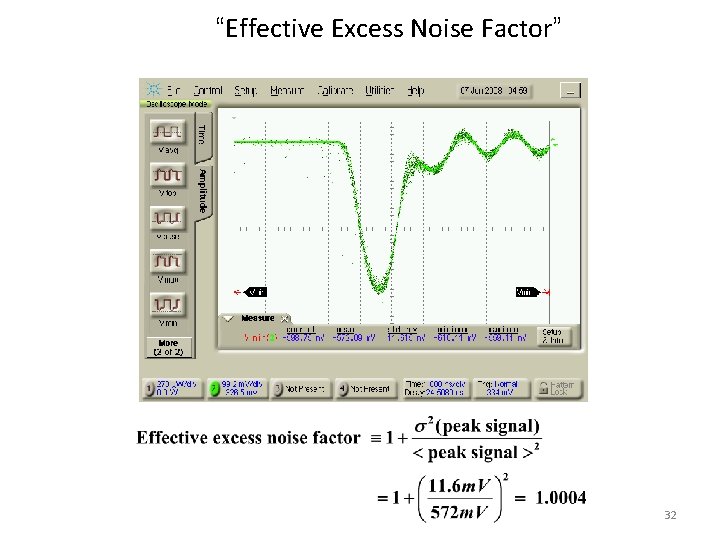
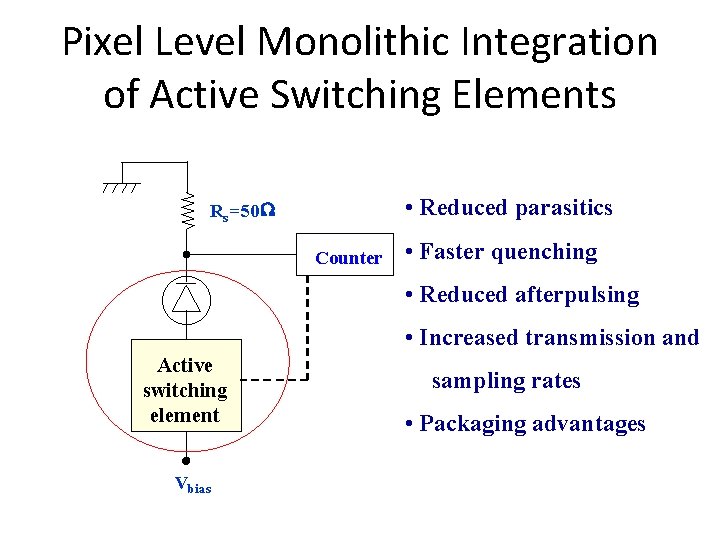
- Slides: 33
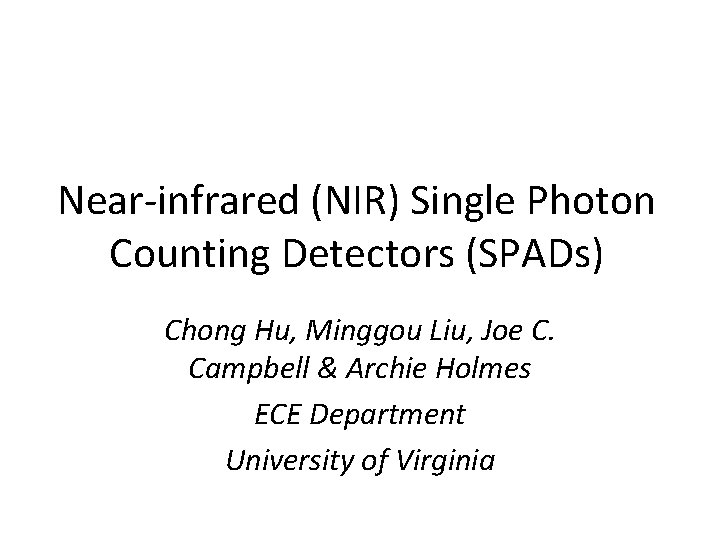
Near-infrared (NIR) Single Photon Counting Detectors (SPADs) Chong Hu, Minggou Liu, Joe C. Campbell & Archie Holmes ECE Department University of Virginia
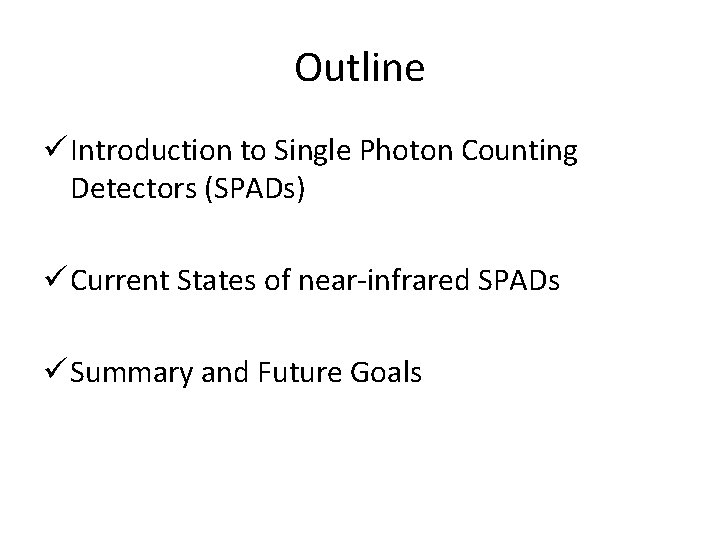
Outline ü Introduction to Single Photon Counting Detectors (SPADs) ü Current States of near-infrared SPADs ü Summary and Future Goals
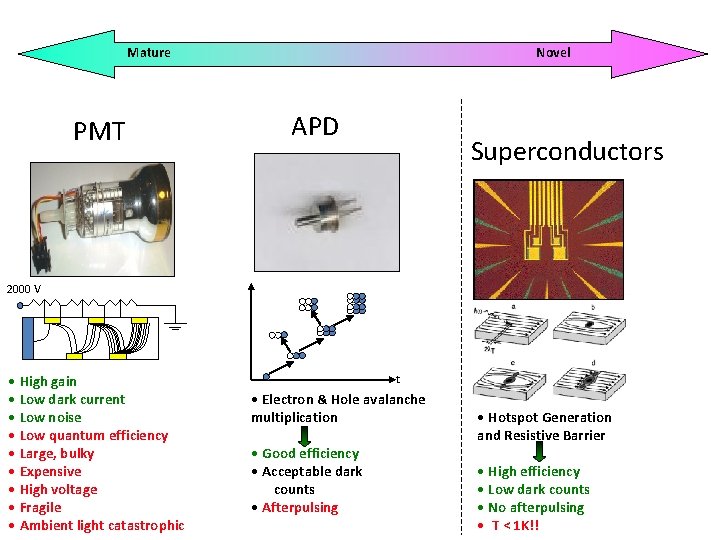
Mature PMT Novel APD Superconductors 2000 V • High gain • Low dark current • Low noise • Low quantum efficiency • Large, bulky • Expensive • High voltage • Fragile • Ambient light catastrophic t • Electron & Hole avalanche multiplication • Good efficiency • Acceptable dark counts • Afterpulsing • Hotspot Generation and Resistive Barrier • High efficiency • Low dark counts • No afterpulsing • T < 1 K!!
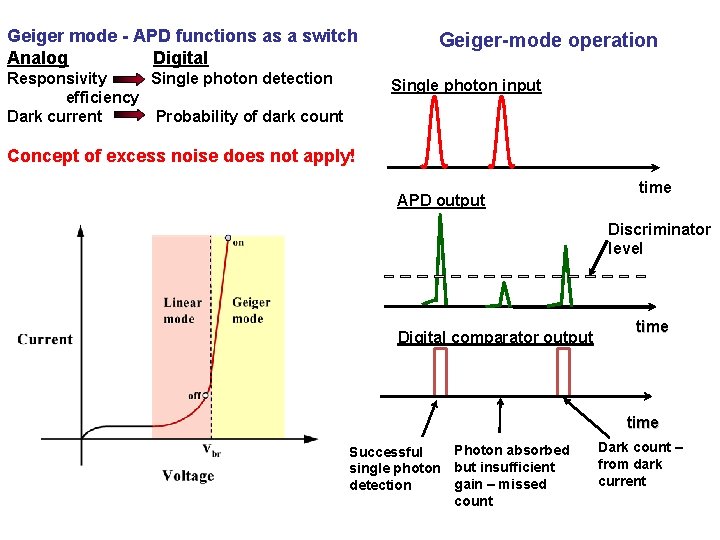
Geiger mode - APD functions as a switch Analog Digital Responsivity Single photon detection efficiency Dark current Probability of dark count Geiger-mode operation Single photon input Concept of excess noise does not apply! APD output time Discriminator level Digital comparator output time Photon absorbed Successful single photon but insufficient gain – missed detection count Dark count – from dark current
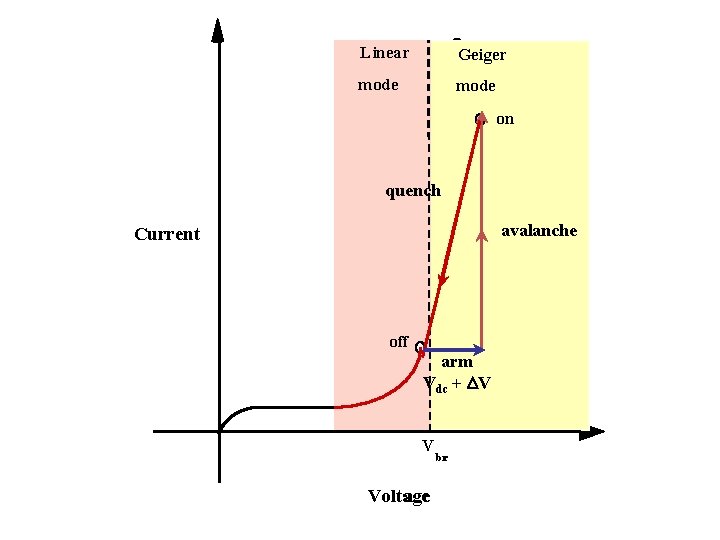
Linear on Geiger mode on Linear Geiger quench mode avalanche Current off arm Vdc + DV V Voltage br
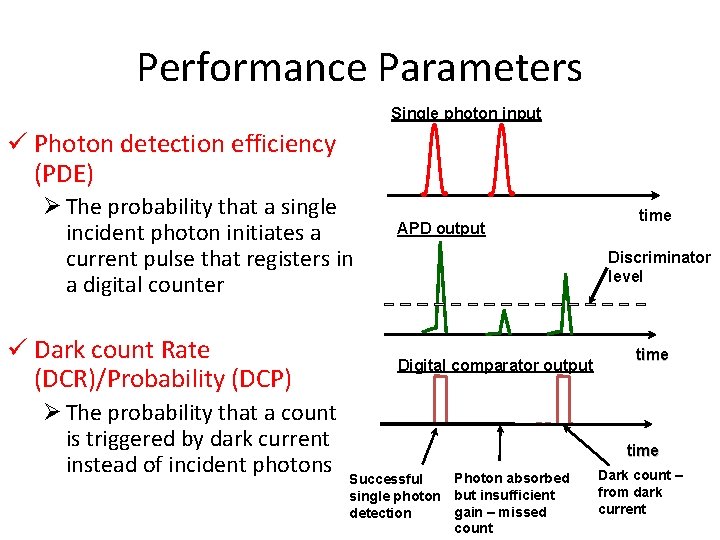
Performance Parameters Single photon input ü Photon detection efficiency (PDE) Ø The probability that a single incident photon initiates a current pulse that registers in a digital counter ü Dark count Rate (DCR)/Probability (DCP) Ø The probability that a count is triggered by dark current instead of incident photons APD output time Discriminator level Digital comparator output time Photon absorbed Successful single photon but insufficient gain – missed detection count Dark count – from dark current
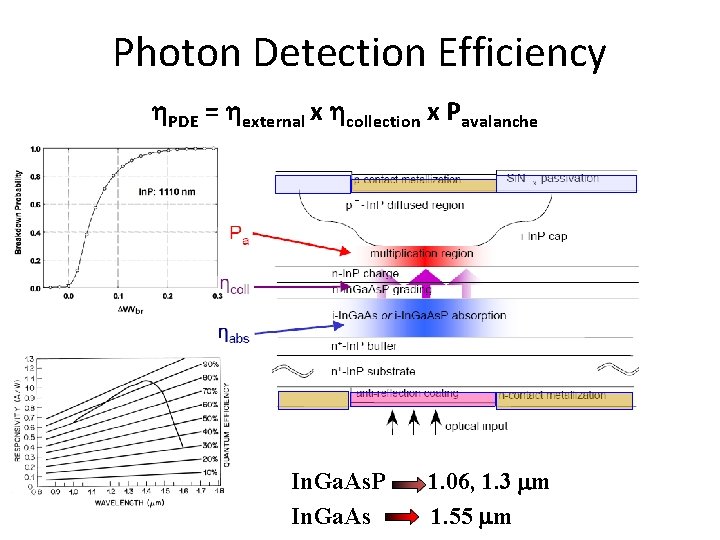
Photon Detection Efficiency h. PDE = hexternal x hcollection x Pavalanche In. Ga. As. P In. Ga. As 1. 06, 1. 3 m 1. 55 m
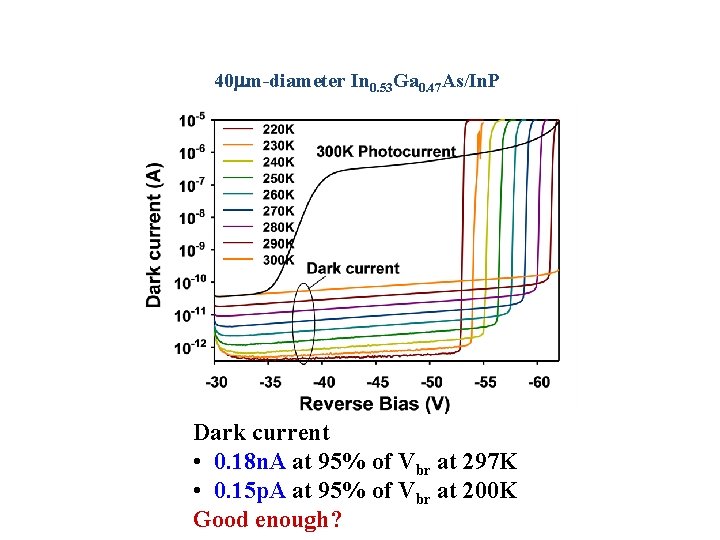
40 m-diameter In 0. 53 Ga 0. 47 As/In. P Dark current • 0. 18 n. A at 95% of Vbr at 297 K • 0. 15 p. A at 95% of Vbr at 200 K Good enough?
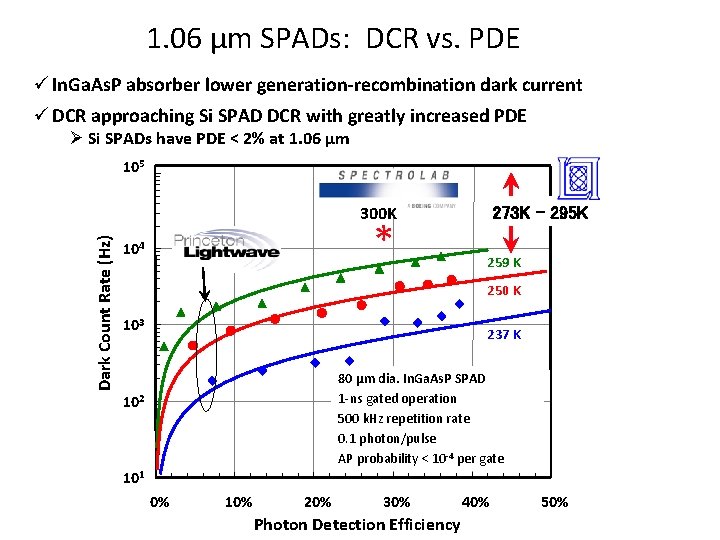
1. 06 μm SPADs: DCR vs. PDE ü In. Ga. As. P absorber lower generation-recombination dark current ü DCR approaching Si SPAD DCR with greatly increased PDE Ø Si SPADs have PDE < 2% at 1. 06 μm 105 Dark Count Rate (Hz) 300 K * 104 273 K – 295 K 259 K 250 K 103 237 K 80 μm dia. In. Ga. As. P SPAD 1 -ns gated operation 500 k. Hz repetition rate 0. 1 photon/pulse AP probability < 10 -4 per gate 102 101 0% 10% 20% 30% Photon Detection Efficiency 40% 50%
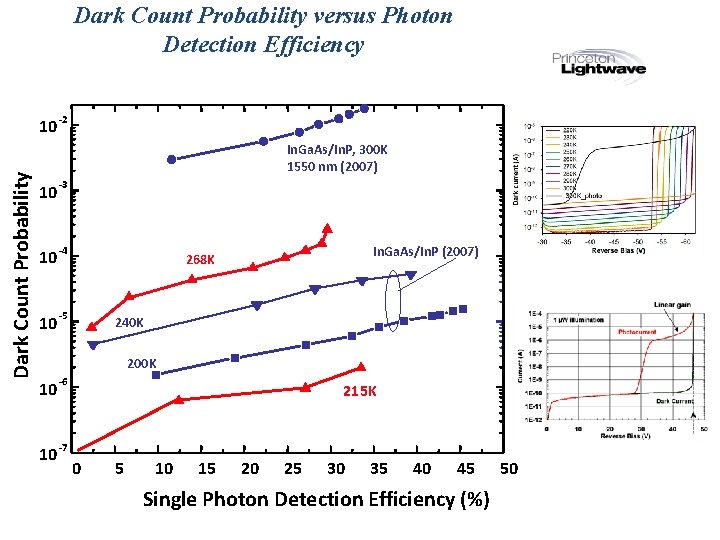
Dark Count Probability versus Photon Detection Efficiency Dark Count Probability 10 -2 In. Ga. As/In. P, 300 K 1550 nm (2007) 10 -3 10 -4 10 -5 In. Ga. As/In. P (2007) 268 K 240 K 200 K 10 -6 10 -7 215 K 0 5 10 15 20 25 30 35 40 45 Single Photon Detection Efficiency (%) 50
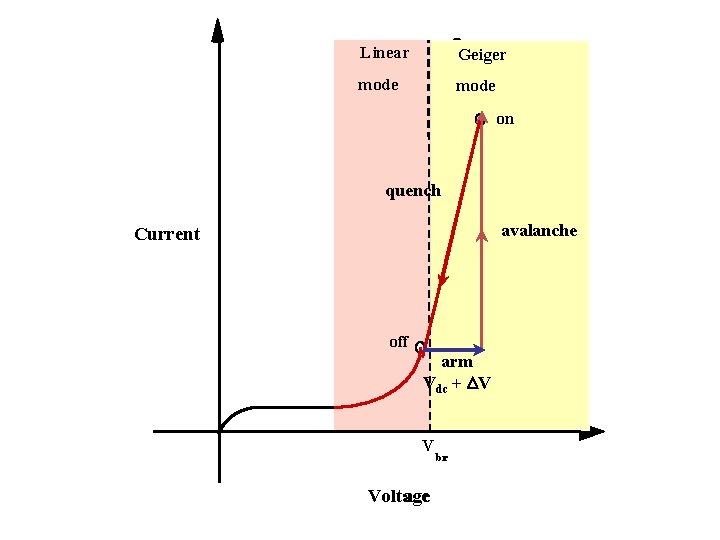
Linear on Geiger mode on Linear Geiger quench mode avalanche Current off arm Vdc + DV V Voltage br
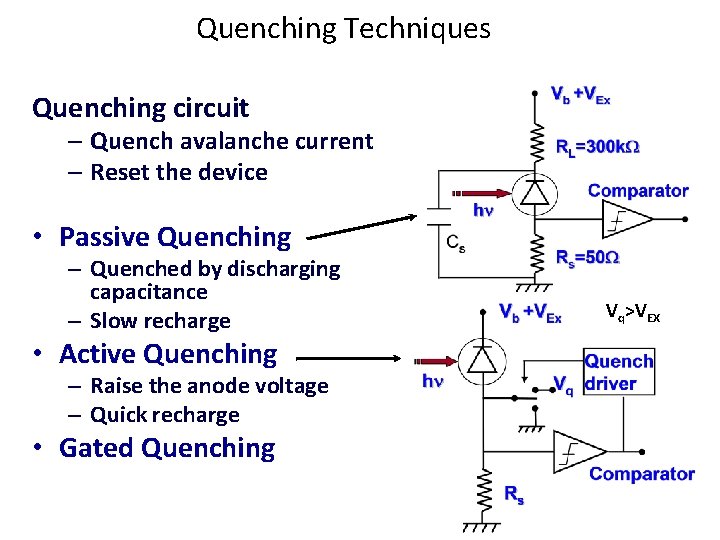
Quenching Techniques Quenching circuit – Quench avalanche current – Reset the device • Passive Quenching – Quenched by discharging capacitance – Slow recharge • Active Quenching – Raise the anode voltage – Quick recharge • Gated Quenching Vq>VEX
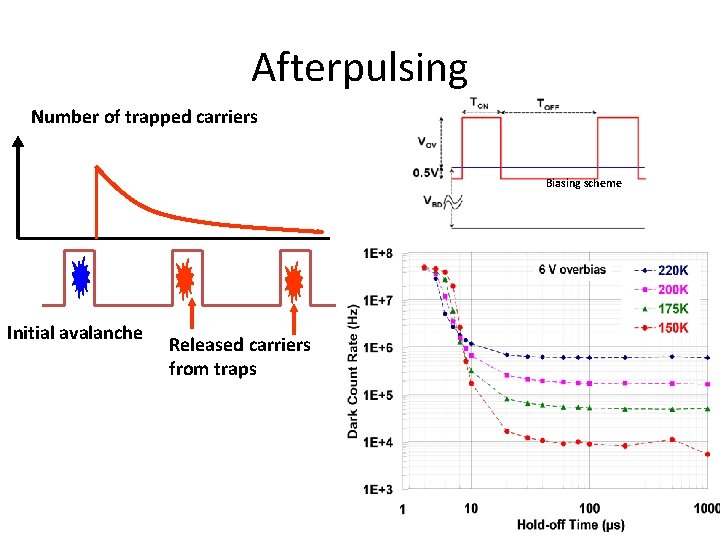
Afterpulsing Number of trapped carriers Biasing scheme Initial avalanche Released carriers from traps
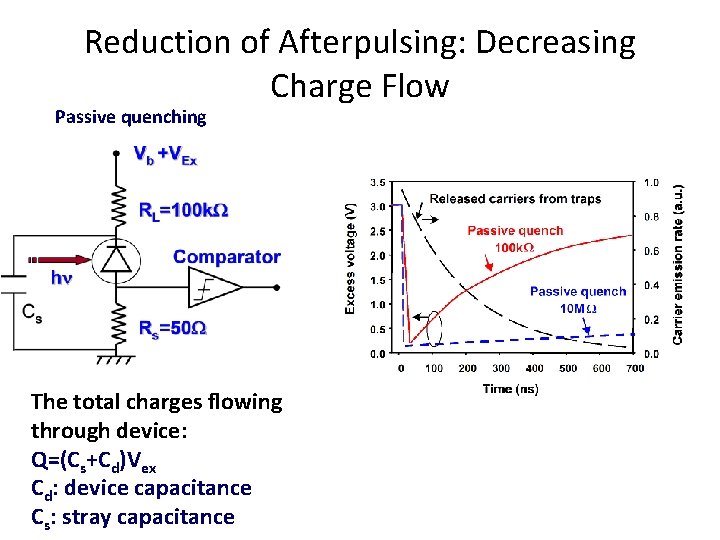
Reduction of Afterpulsing: Decreasing Charge Flow Passive quenching The total charges flowing through device: Q=(Cs+Cd)Vex Cd: device capacitance Cs: stray capacitance
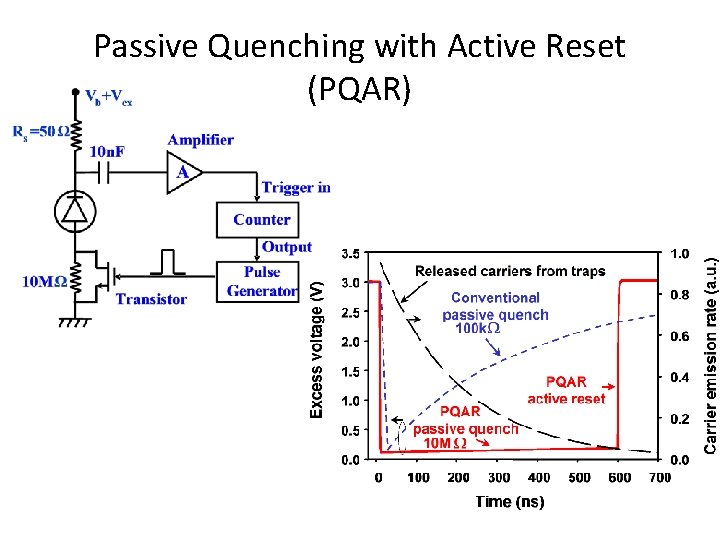
Passive Quenching with Active Reset (PQAR)
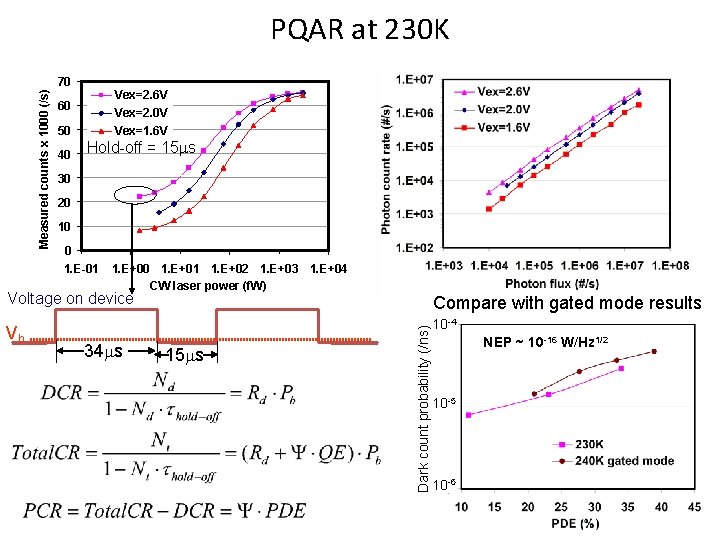
PQAR at 230 K Measured counts x 1000 (/s) 70 50 Vex=2. 6 V Vex=2. 0 V Vex=1. 6 V 40 Hold-off = 15 s 60 30 20 10 0 1. E-01 1. E+00 1. E+01 1. E+02 1. E+03 1. E+04 CW laser power (f. W) Compare with gated mode results Vb 10 -4 34 s 15 s Dark count probability (/ns) Voltage on device NEP ~ 10 -16 W/Hz 1/2 10 -5 10 -6 16
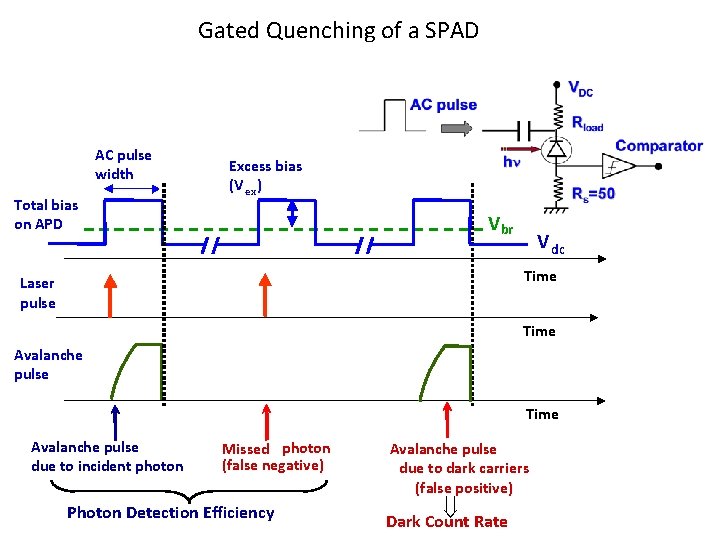
Gated Quenching of a SPAD AC pulse width Total bias on APD Excess bias (V ex ) Vbr V dc Time Laser pulse Time Avalanche pulse due to incident photon Missed photon (false negative) Photon Detection Efficiency Avalanche pulse due to dark carriers (false positive) ß Dark Count Rate
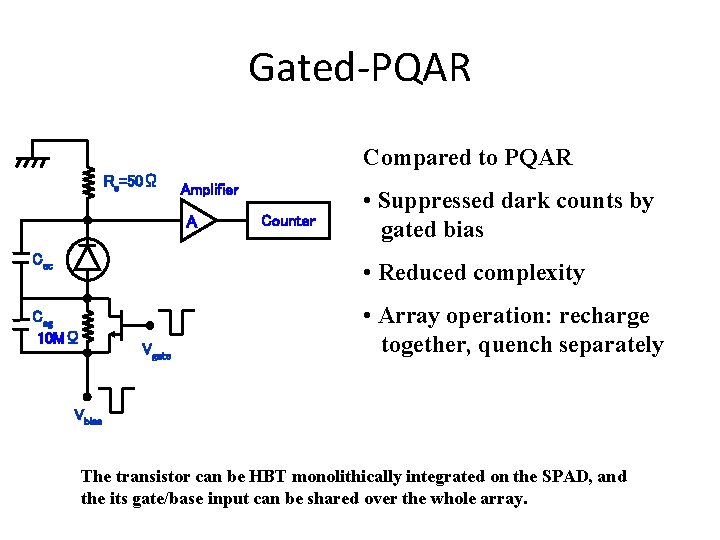
Gated-PQAR Compared to PQAR Rs=50Ω Amplifier A Counter • Suppressed dark counts by gated bias Cac • Reduced complexity Cag 10 MΩ • Array operation: recharge together, quench separately Vgate Vbias The transistor can be HBT monolithically integrated on the SPAD, and the its gate/base input can be shared over the whole array.
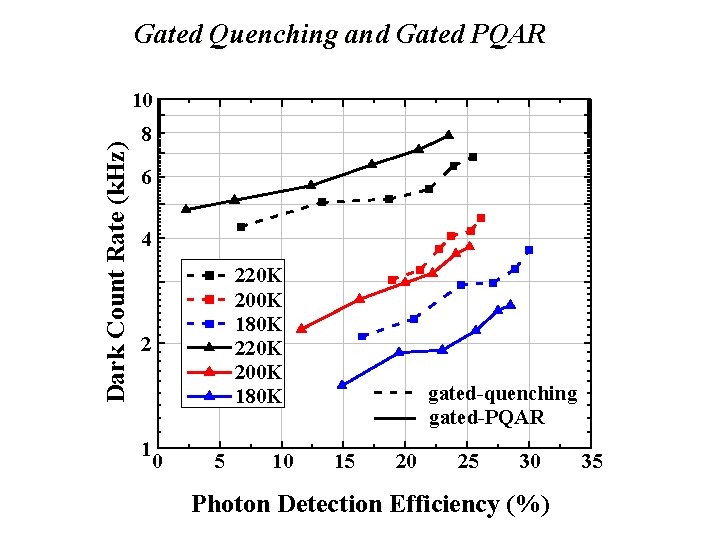
Gated Quenching and Gated PQAR Dark Count Rate (k. Hz) 10 8 6 4 220 K 200 K 180 K 2 1 0 5 10 gated-quenching gated-PQAR 15 20 25 30 Photon Detection Efficiency (%) 35
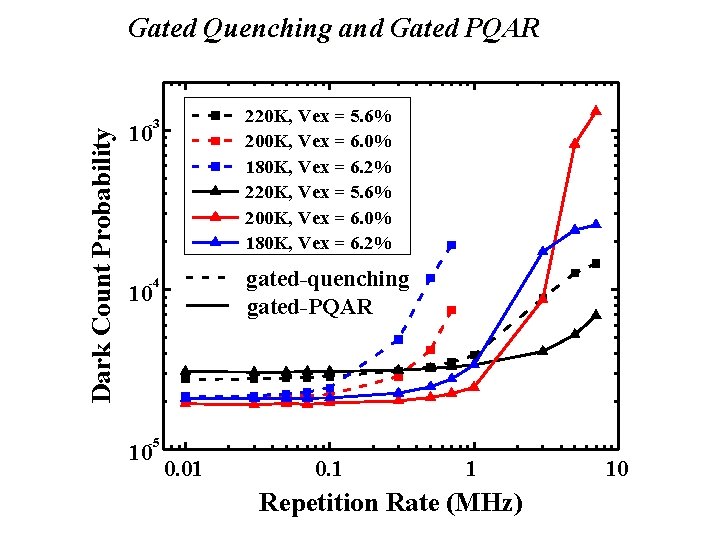
Dark Count Probability Gated Quenching and Gated PQAR 220 K, Vex = 5. 6% 200 K, Vex = 6. 0% 180 K, Vex = 6. 2% -3 10 gated-quenching gated-PQAR -4 10 -5 10 0. 01 0. 1 1 Repetition Rate (MHz) 10
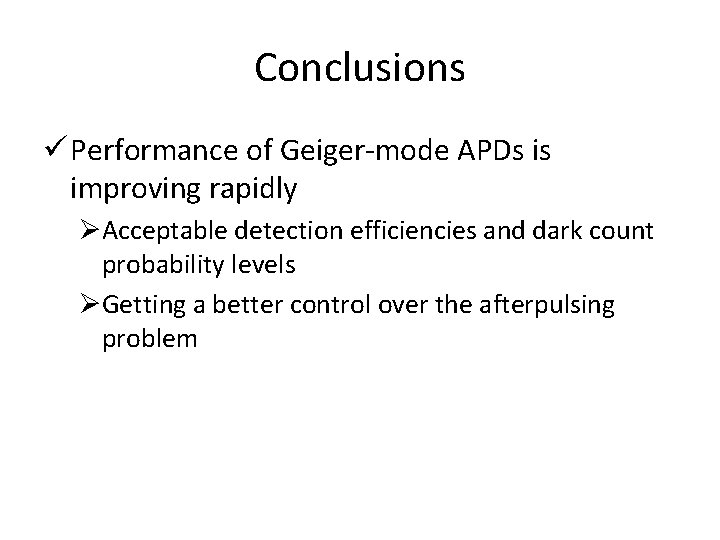
Conclusions ü Performance of Geiger-mode APDs is improving rapidly ØAcceptable detection efficiencies and dark count probability levels ØGetting a better control over the afterpulsing problem
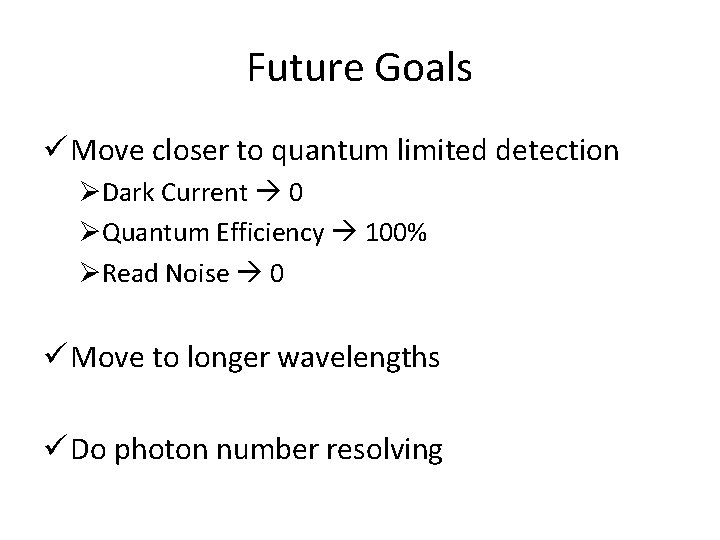
Future Goals ü Move closer to quantum limited detection ØDark Current 0 ØQuantum Efficiency 100% ØRead Noise 0 ü Move to longer wavelengths ü Do photon number resolving
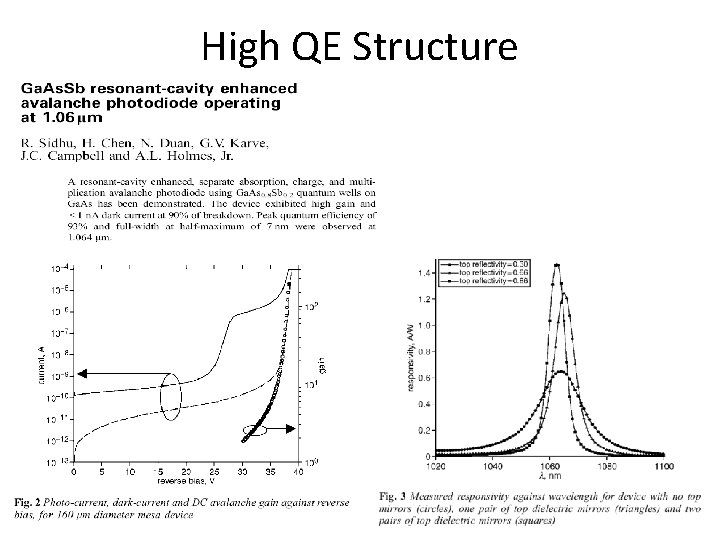
High QE Structure
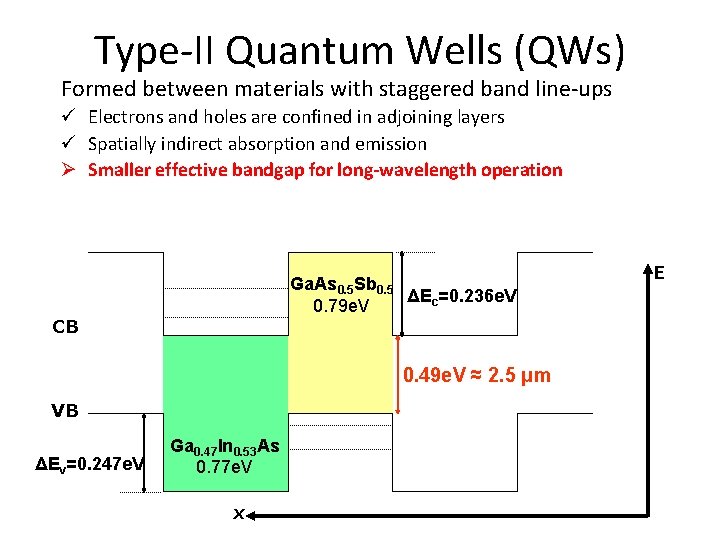
Type-II Quantum Wells (QWs) Formed between materials with staggered band line-ups ü Electrons and holes are confined in adjoining layers ü Spatially indirect absorption and emission Ø Smaller effective bandgap for long-wavelength operation Ga. As 0. 5 Sb 0. 5 ΔEc=0. 236 e. V 0. 79 e. V CB 0. 49 e. V ≈ 2. 5 μm VB ΔEv=0. 247 e. V Ga 0. 47 In 0. 53 As 0. 77 e. V x E
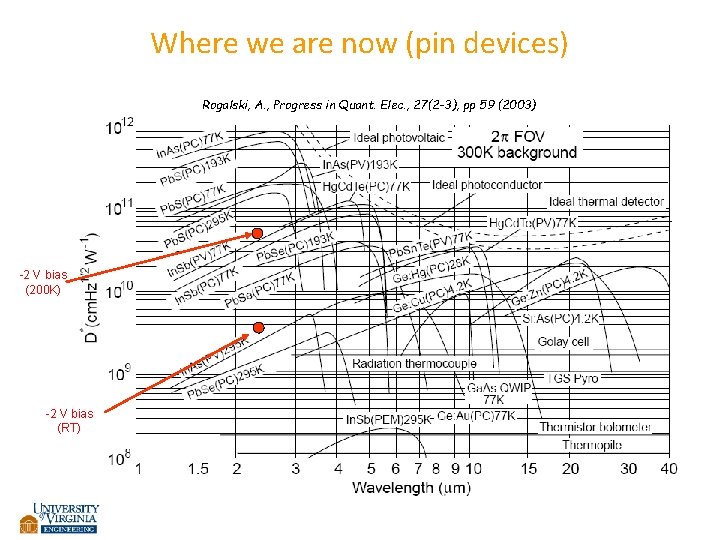
Where we are now (pin devices) Rogalski, A. , Progress in Quant. Elec. , 27(2 -3), pp 59 (2003) -2 V bias (200 K) -2 V bias (RT)
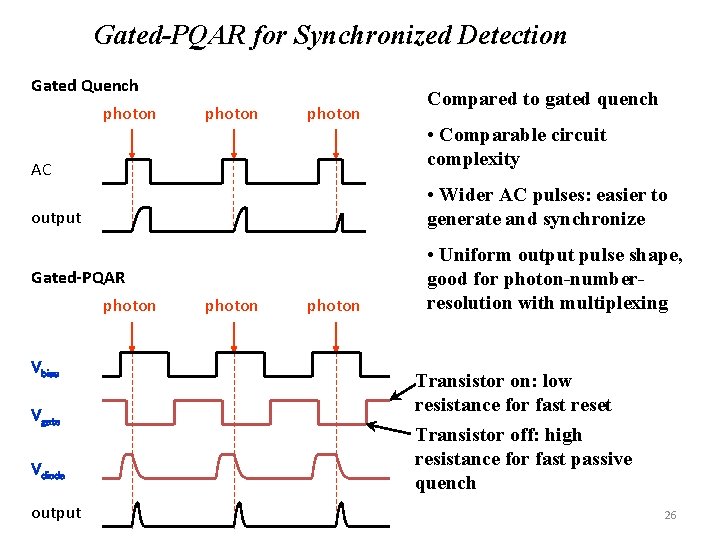
Gated-PQAR for Synchronized Detection Gated Quench photon AC Gated-PQAR photon Vgate Vdiode output • Comparable circuit complexity • Wider AC pulses: easier to generate and synchronize output Vbias Compared to gated quench photon • Uniform output pulse shape, good for photon-numberresolution with multiplexing Transistor on: low resistance for fast reset Transistor off: high resistance for fast passive quench 26
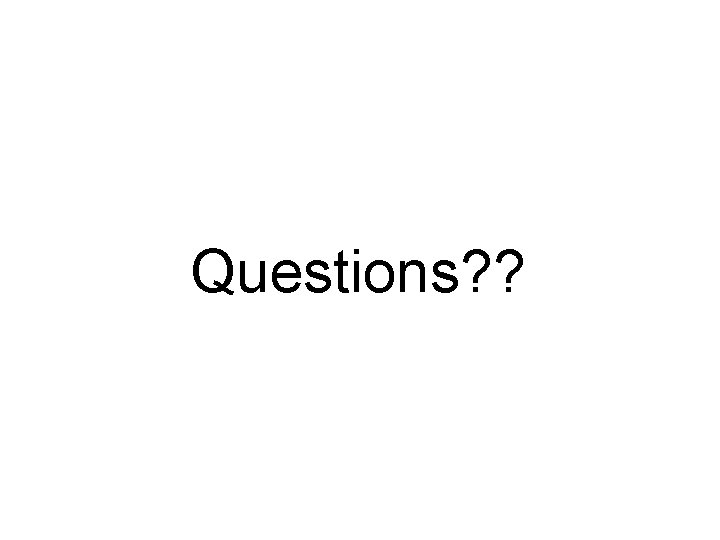
Questions? ?
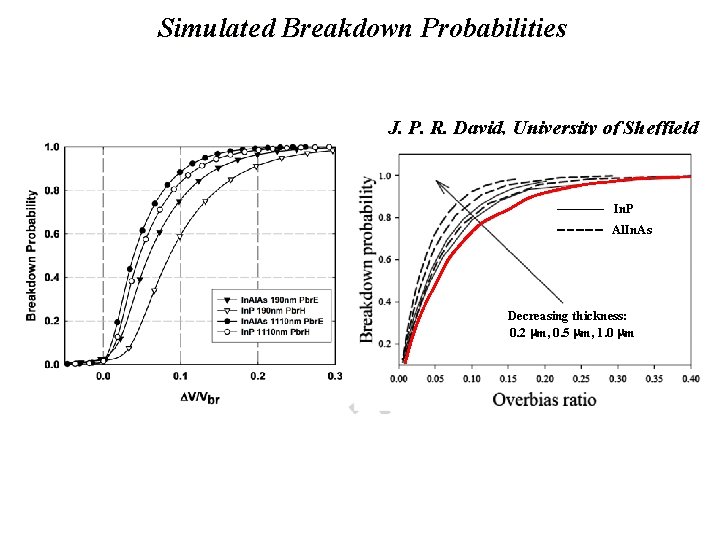
Simulated Breakdown Probabilities J. P. R. David, University of Sheffield In. P Al. In. As Decreasing thickness: 0. 2 m, 0. 5 m, 1. 0 m
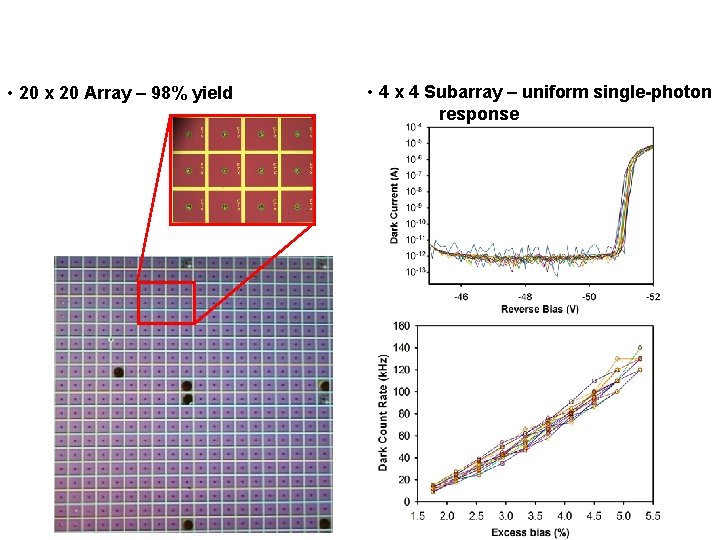
• 20 x 20 Array – 98% yield • 4 x 4 Subarray – uniform single-photon response
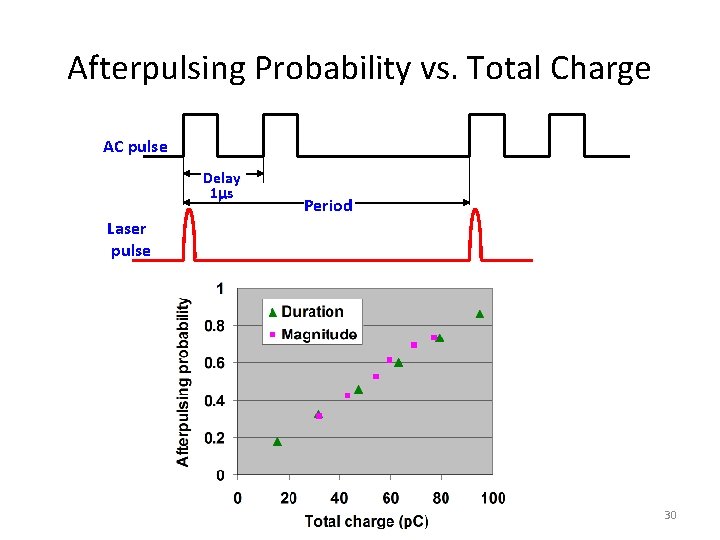
Afterpulsing Probability vs. Total Charge AC pulse Delay 1 s Period Laser pulse 30
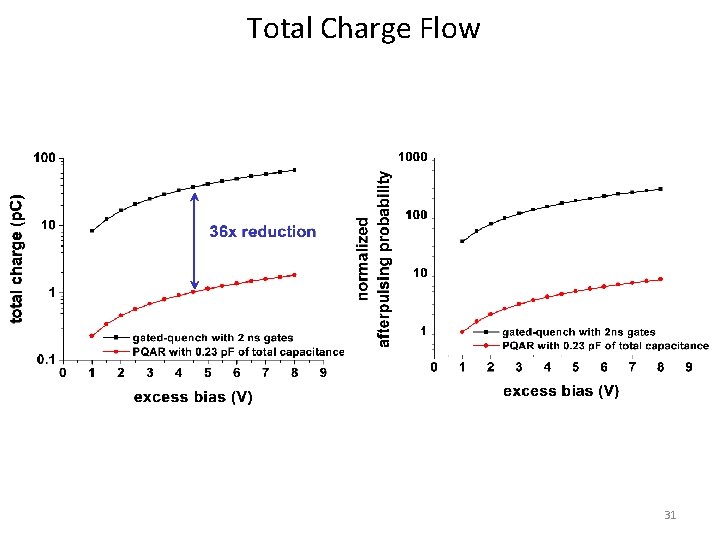
Total Charge Flow 31
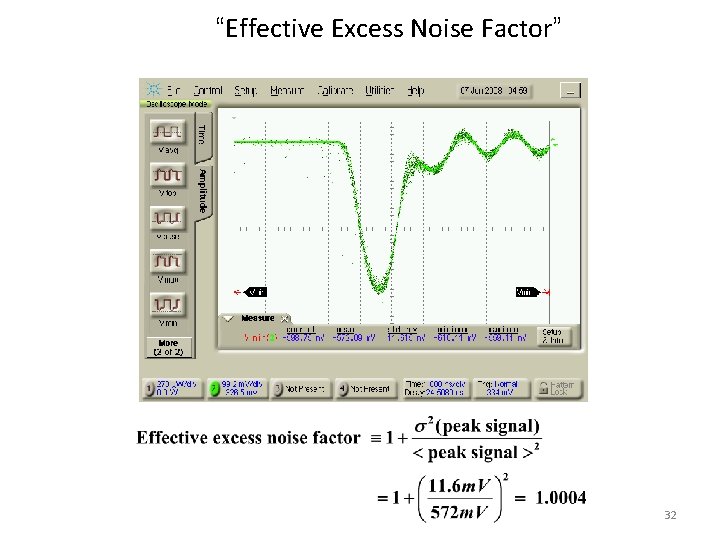
“Effective Excess Noise Factor” 32
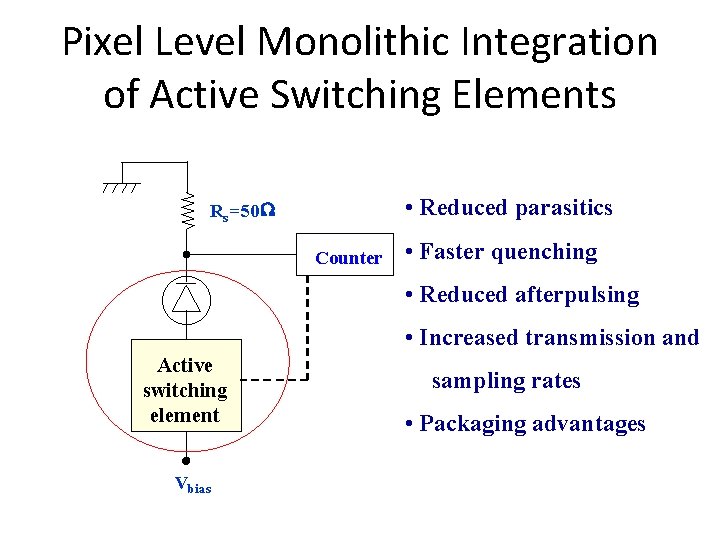
Pixel Level Monolithic Integration of Active Switching Elements • Reduced parasitics Rs =50 W Counter • Faster quenching • Reduced afterpulsing • Increased transmission and Active switching element Vbias sampling rates • Packaging advantages
"perkin elmer"
Single photon
Single photon
Aiman putra
Chong ho yu
Giant wave detectors murmurs universe
Feature vectors
Measure word
Asian porn
Hplc detector types
Where are feature detectors located
Streaming current detectors
Photo detectors
Feature detectors
Jmp pca
Photo detectors
Thế nào là sự mỏi cơ
Lim family tree
çno
Vhv voltage detectors
Hsin chong construction group ltd
Gravitational wave detectors hear murmurs from
Nuclear detectors
Diagnosing error in object detectors
Yonuk
Feature detectors ap psychology
Chúa là đấng từ bi và nhân hậu
Chong ho yu
Frontier detectors for frontier physics
Cisco network insights advisor
Nir krakowski
Nir shavit mit
Edo liberty
Nir ailon