MEIC Accelerator Design Overview Yuhong Zhang MEIC Accelerator
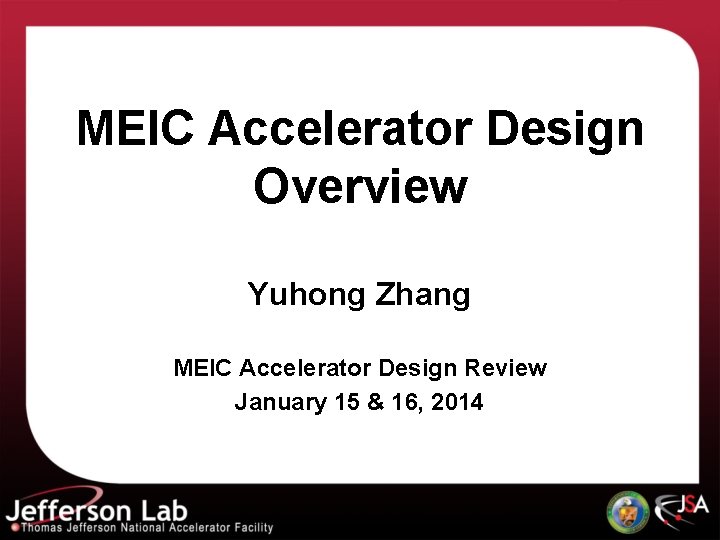
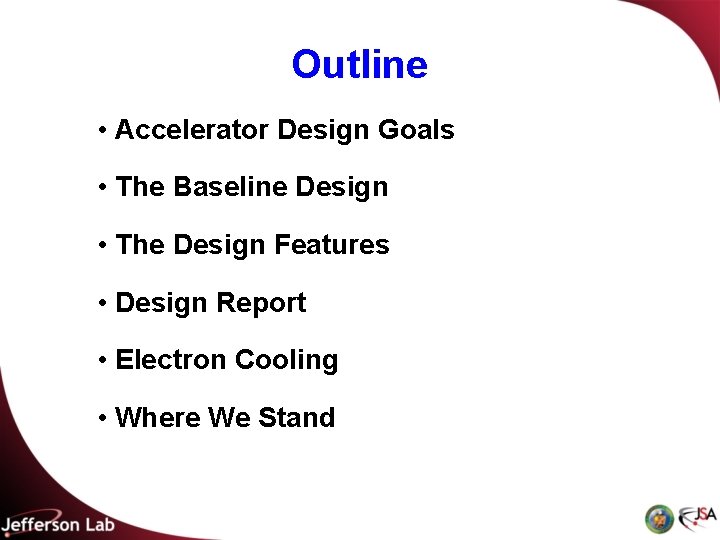
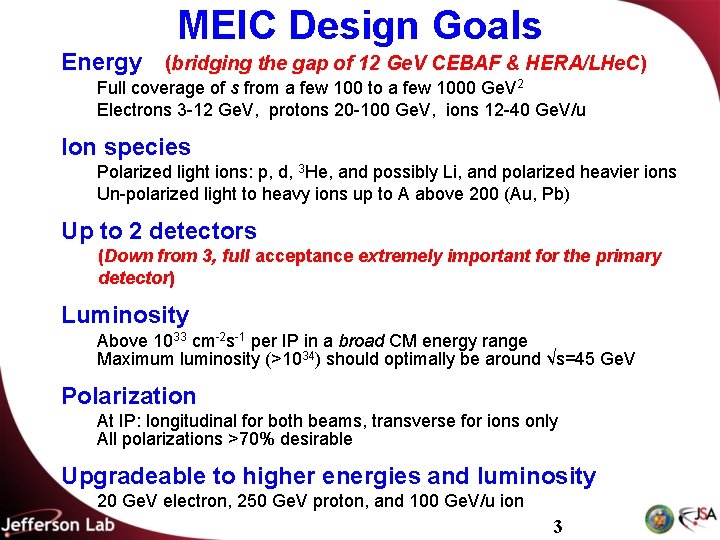
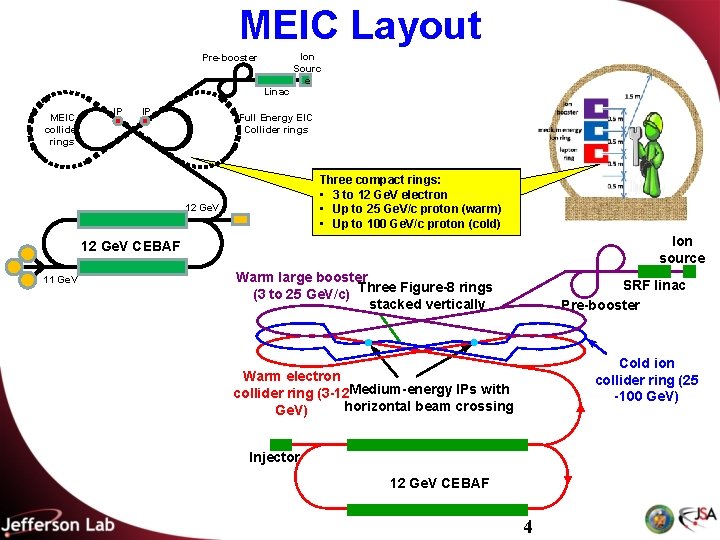
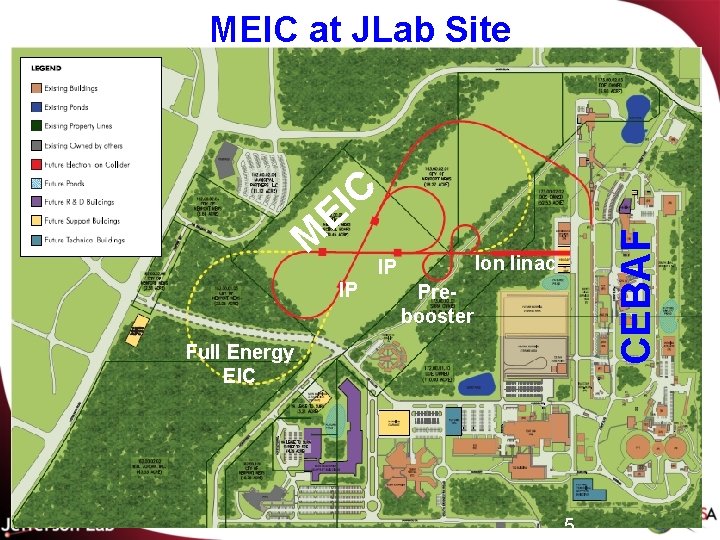
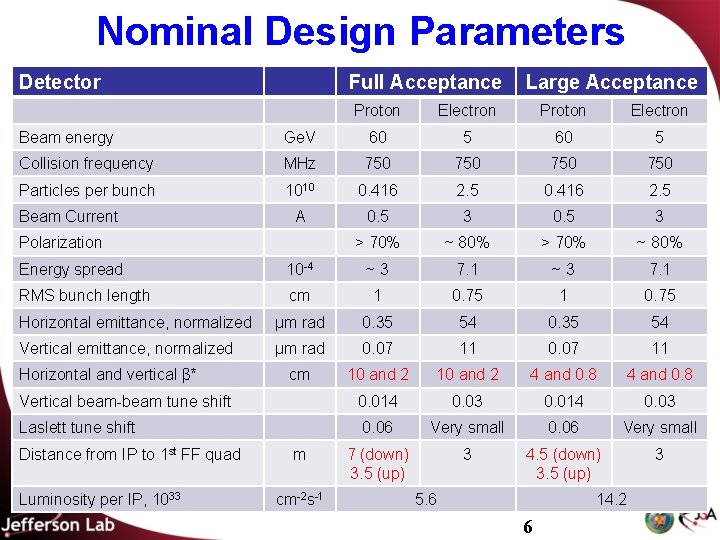
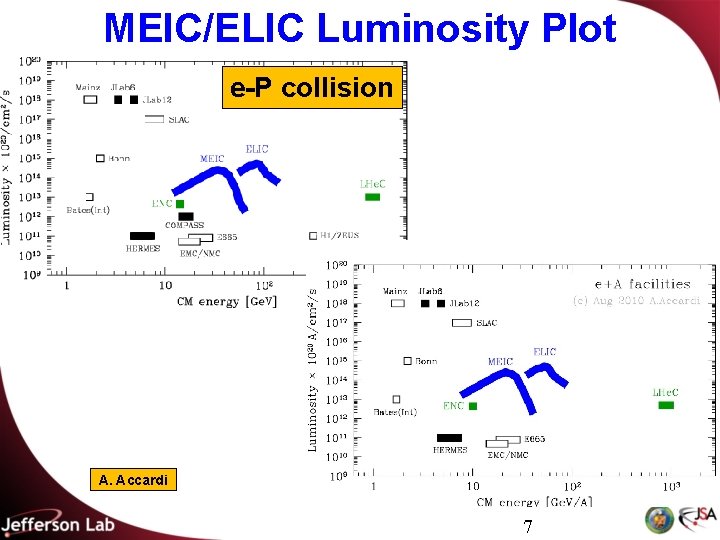
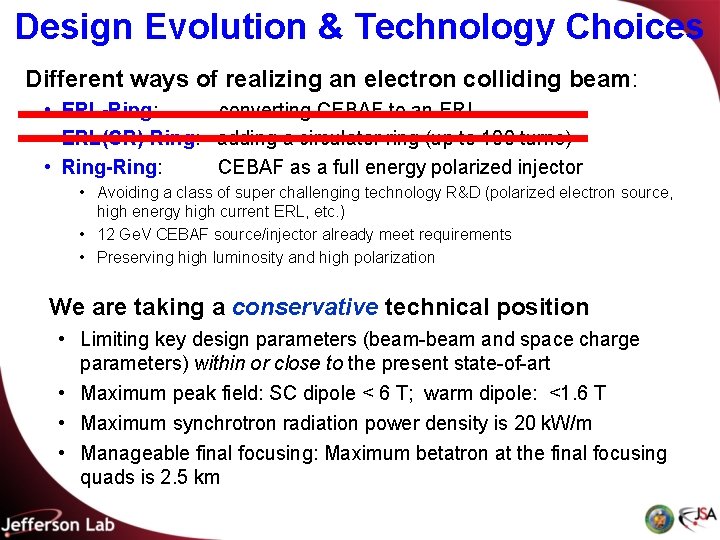
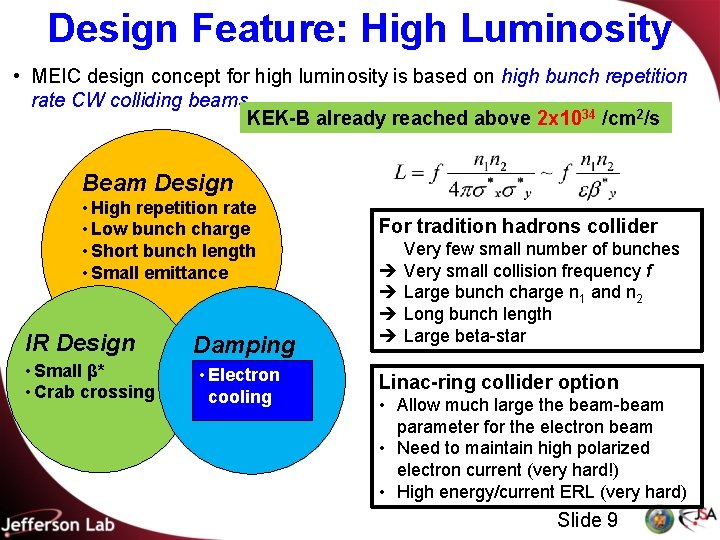
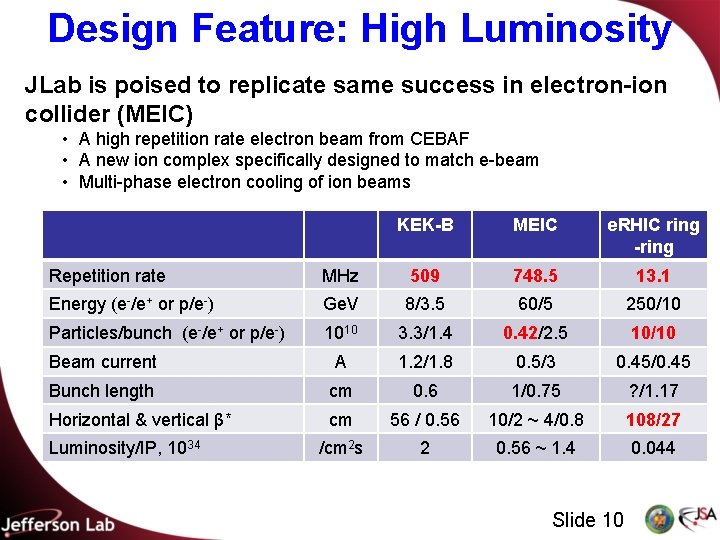
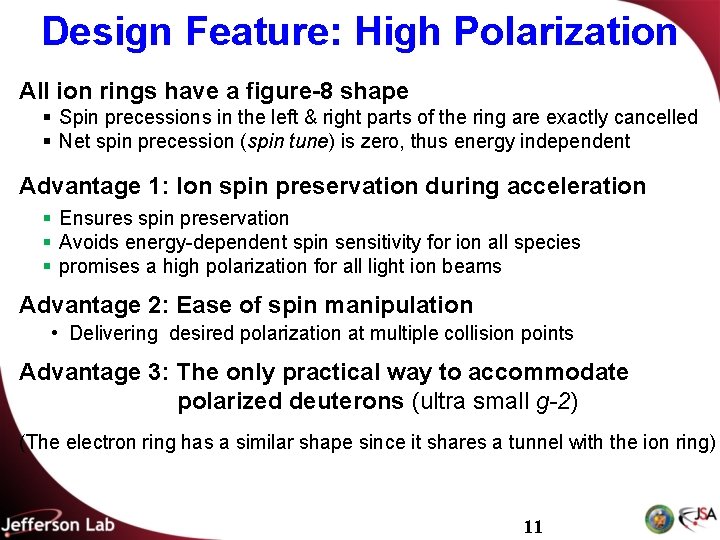
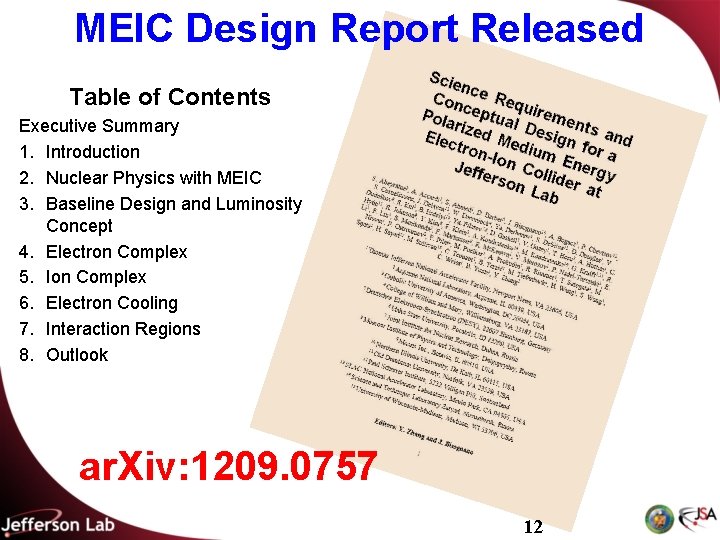
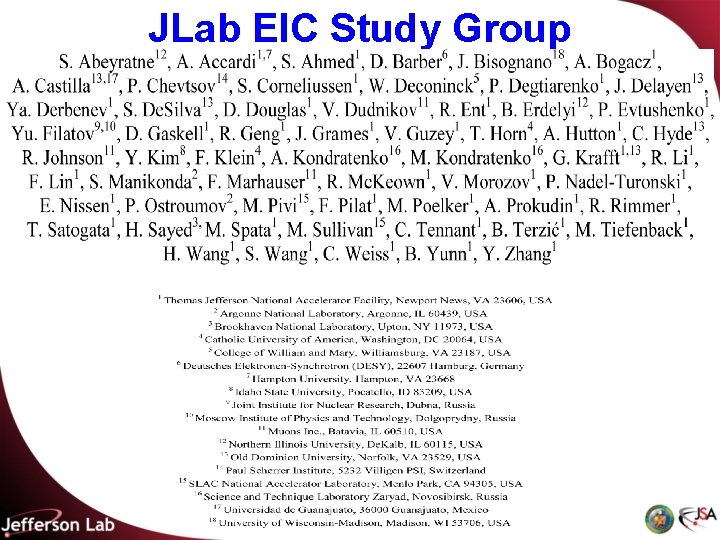
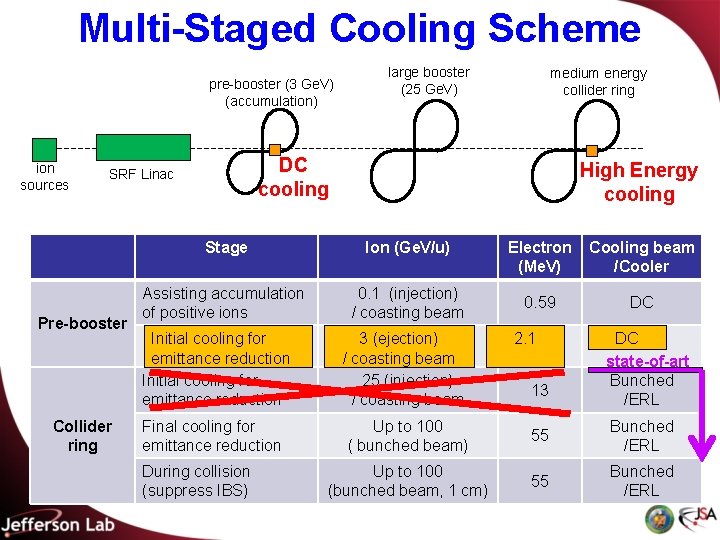
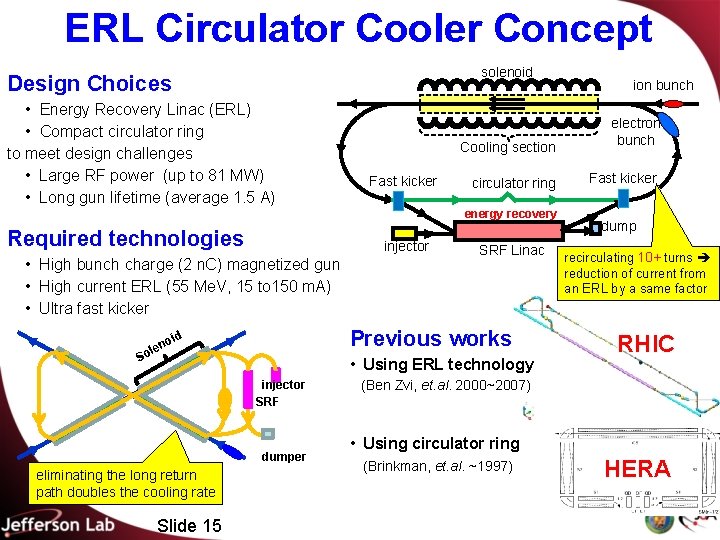
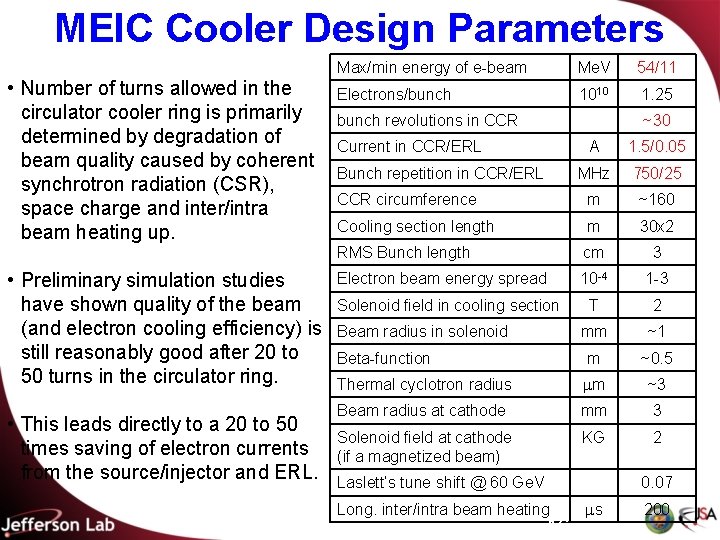
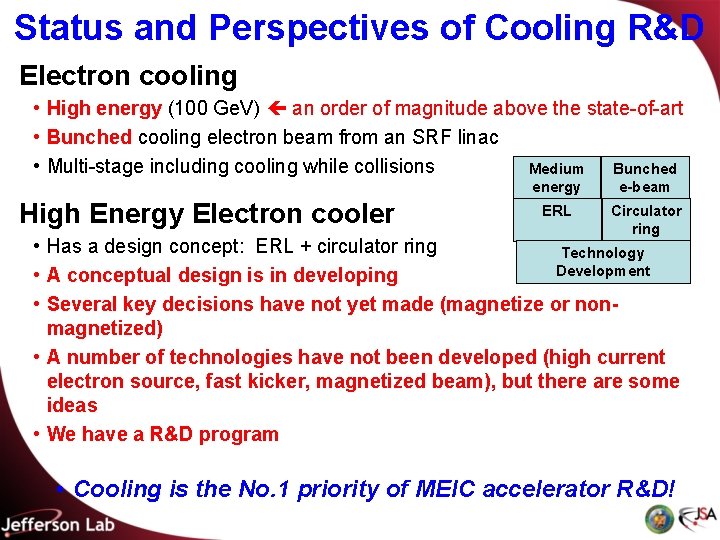
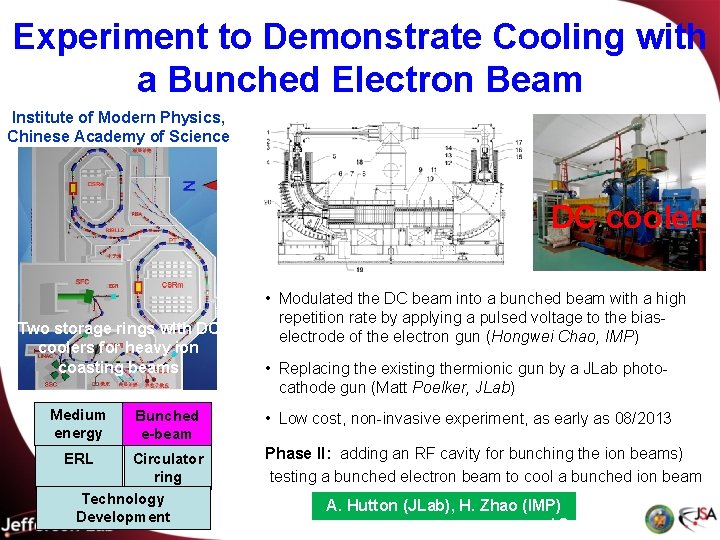
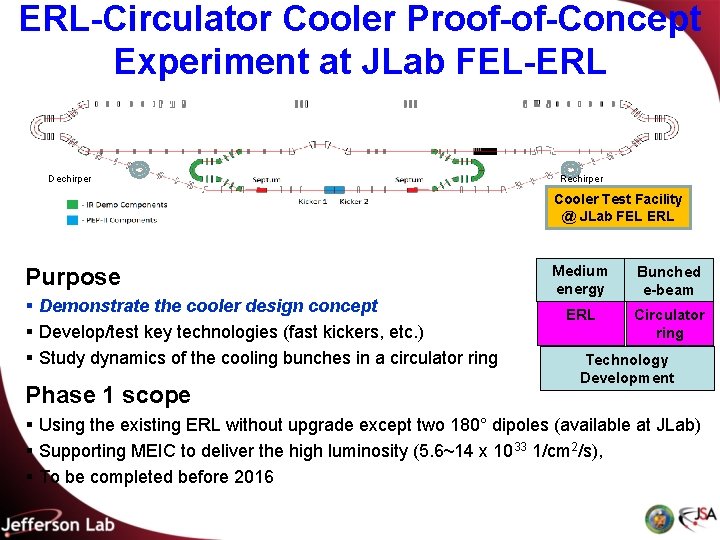
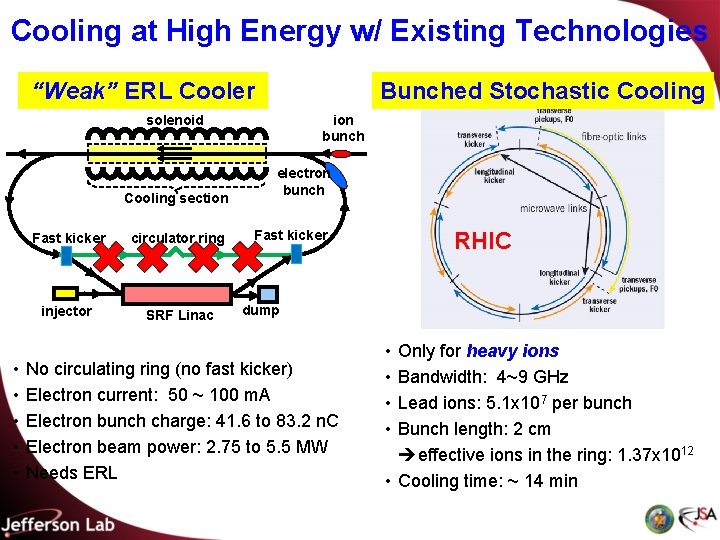
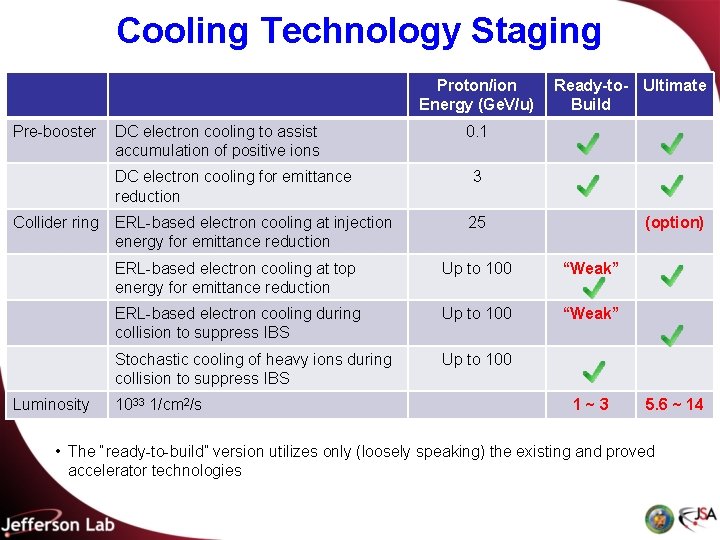
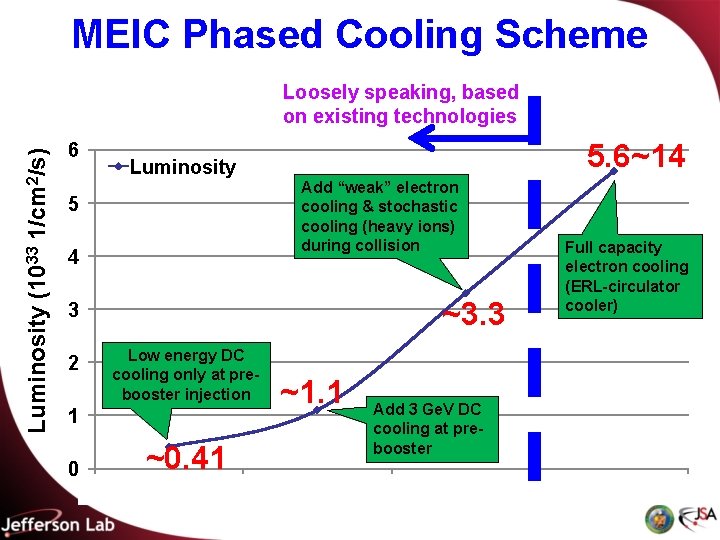
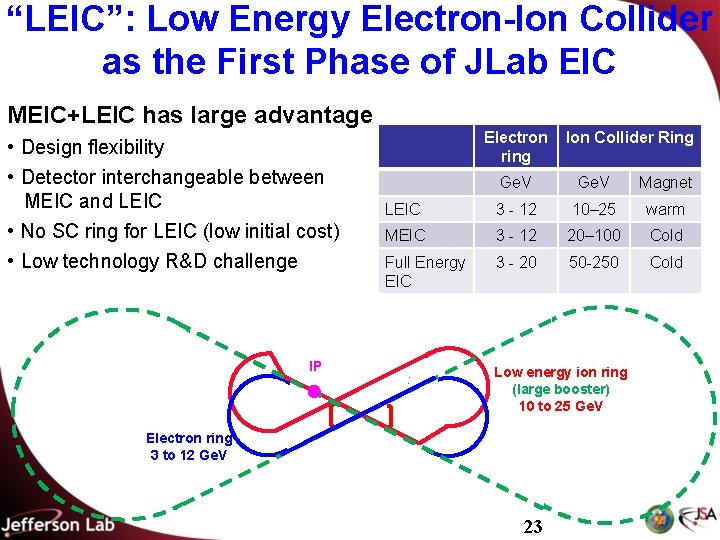
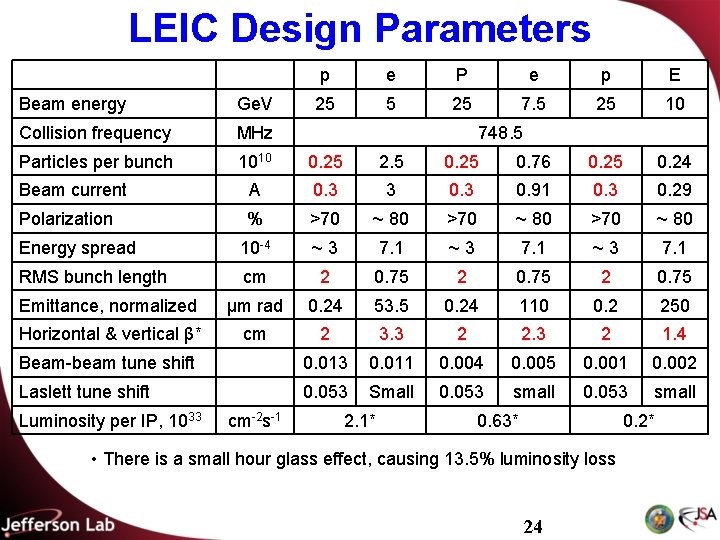
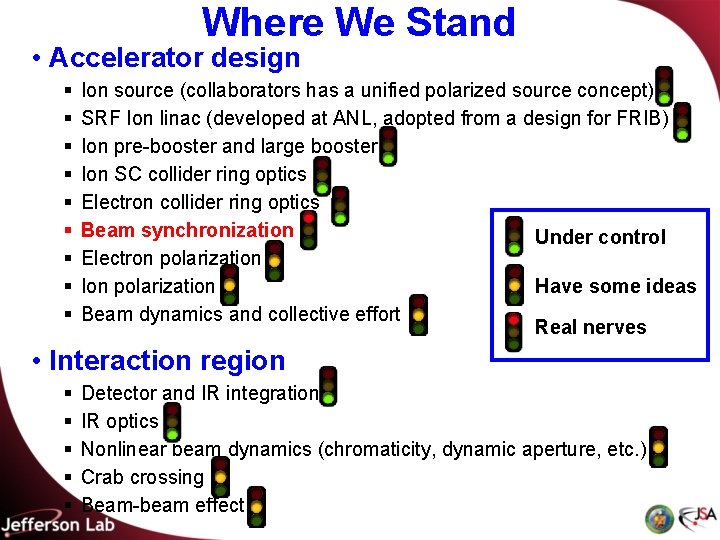
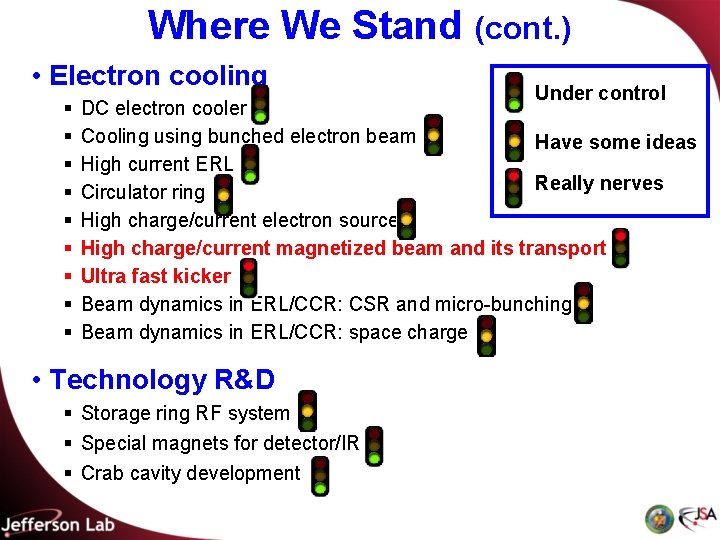
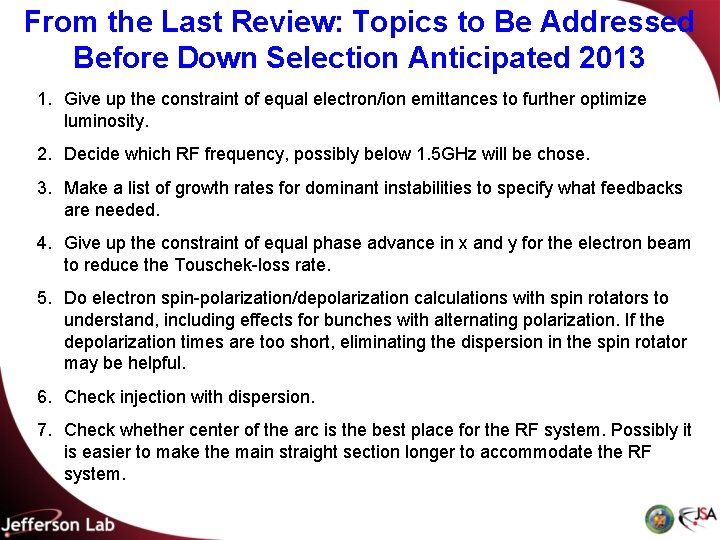
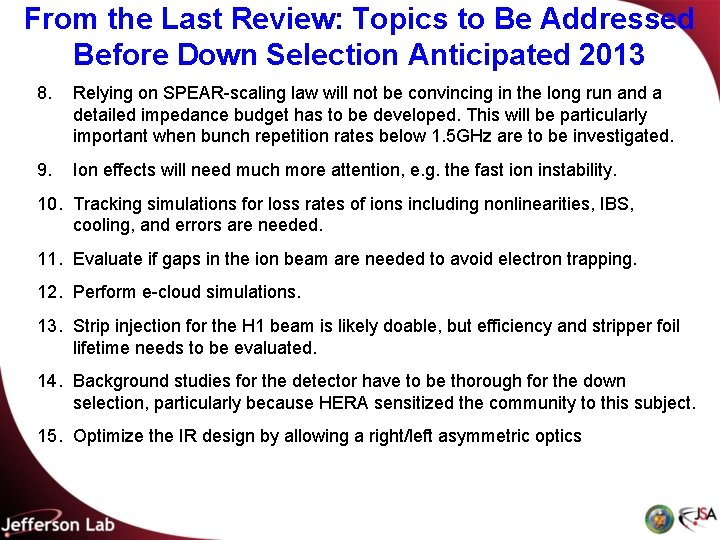
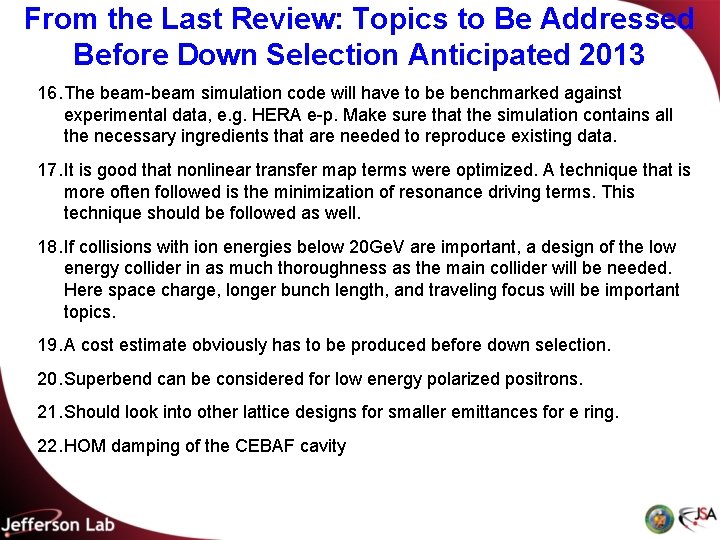
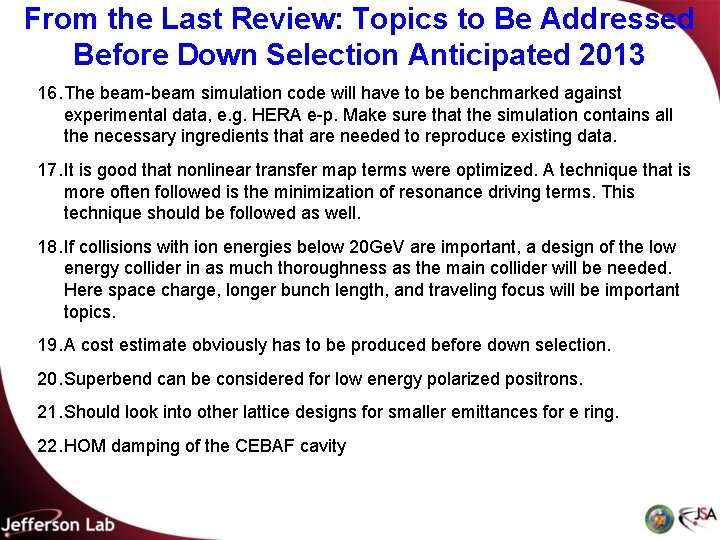
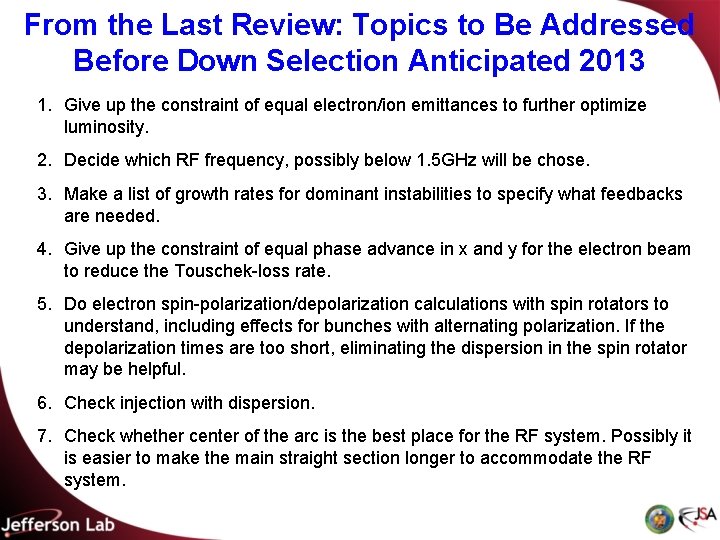
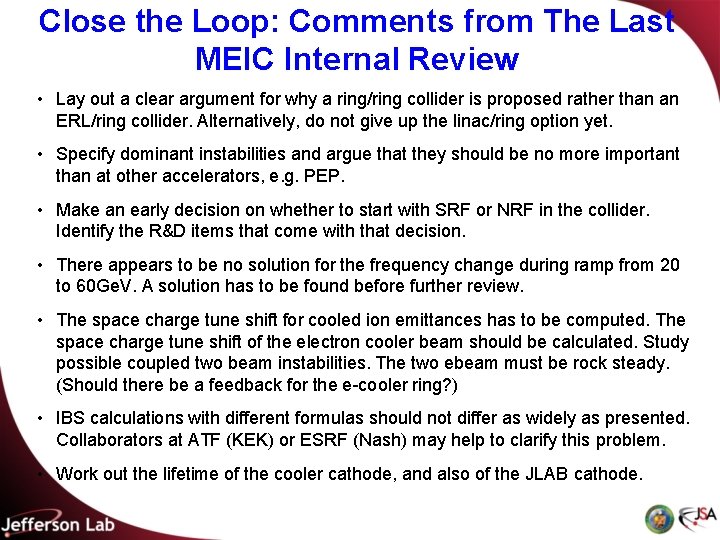
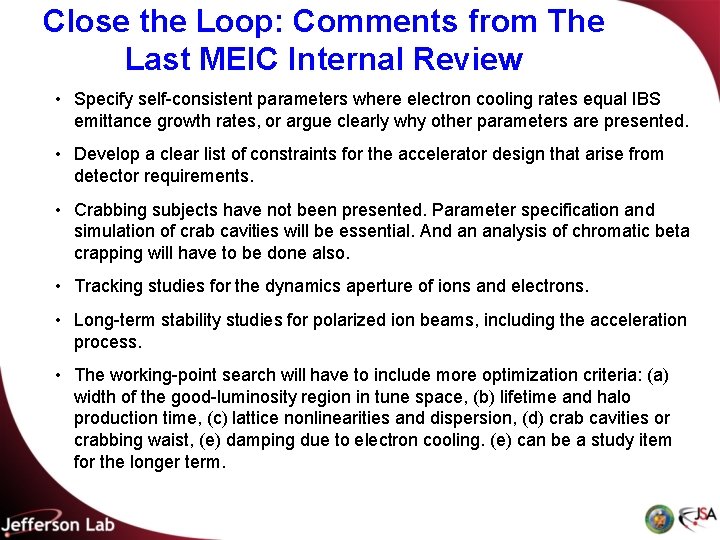
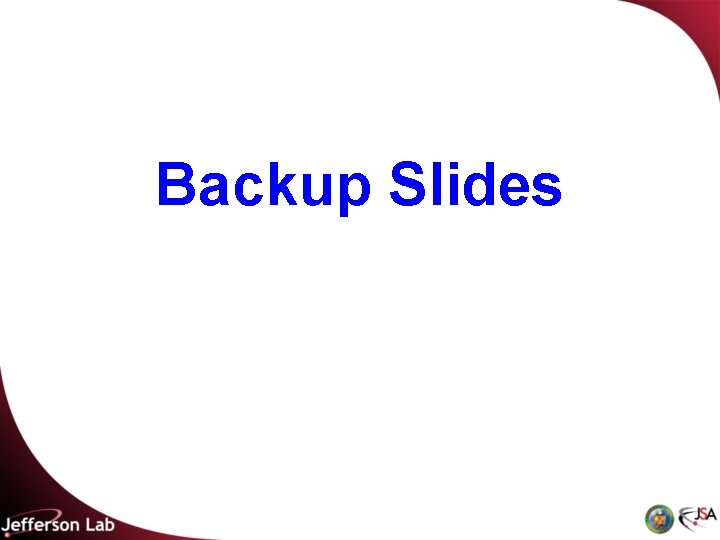
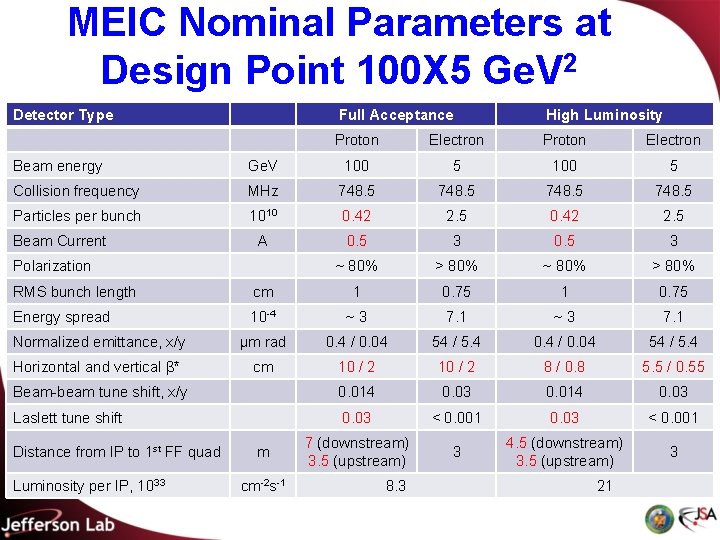
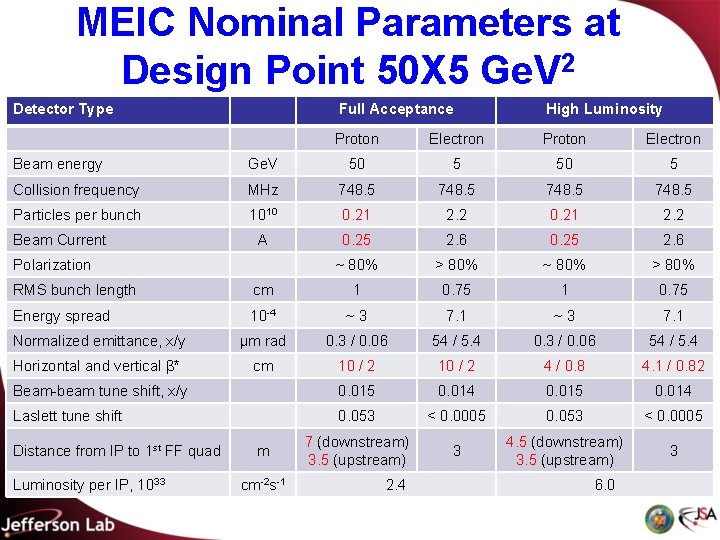
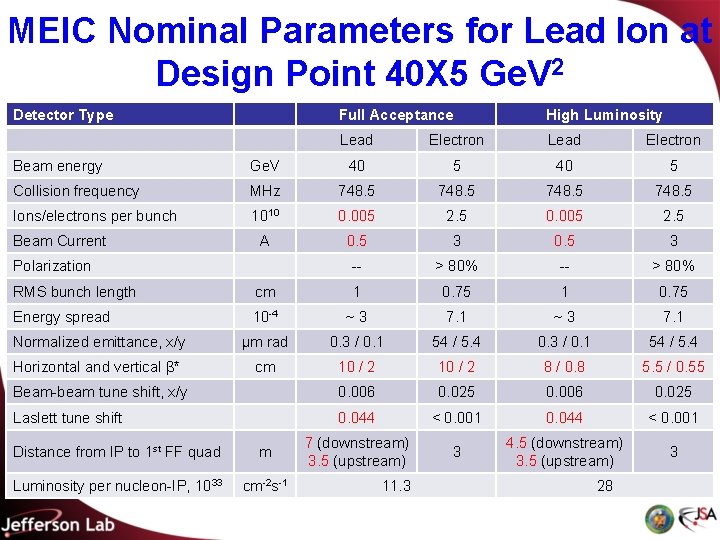
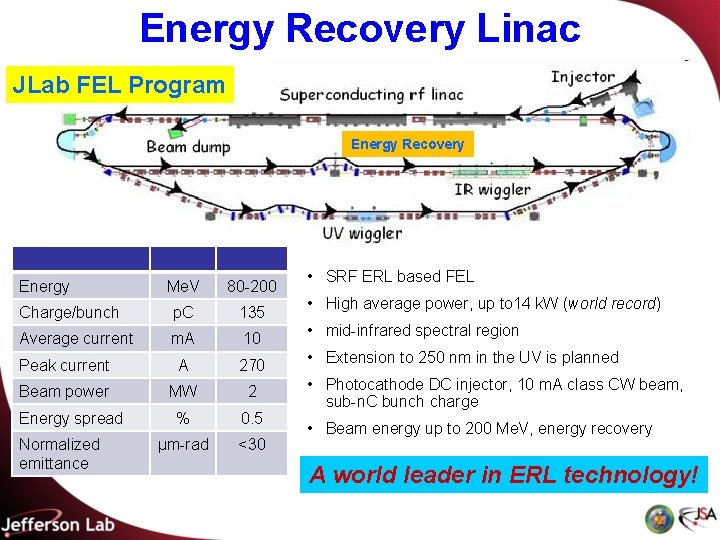
- Slides: 38
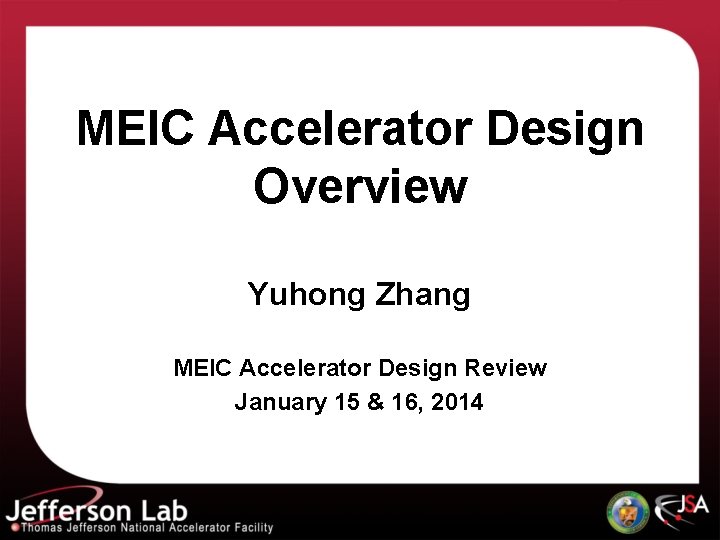
MEIC Accelerator Design Overview Yuhong Zhang MEIC Accelerator Design Review January 15 & 16, 2014
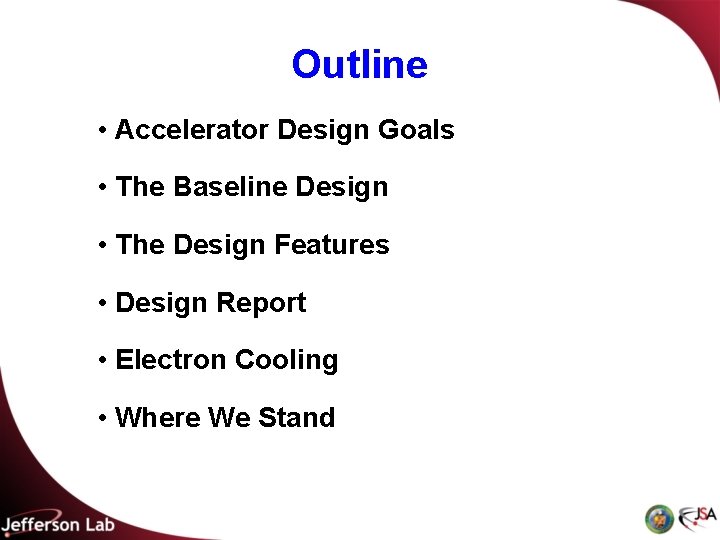
Outline • Accelerator Design Goals • The Baseline Design • The Design Features • Design Report • Electron Cooling • Where We Stand
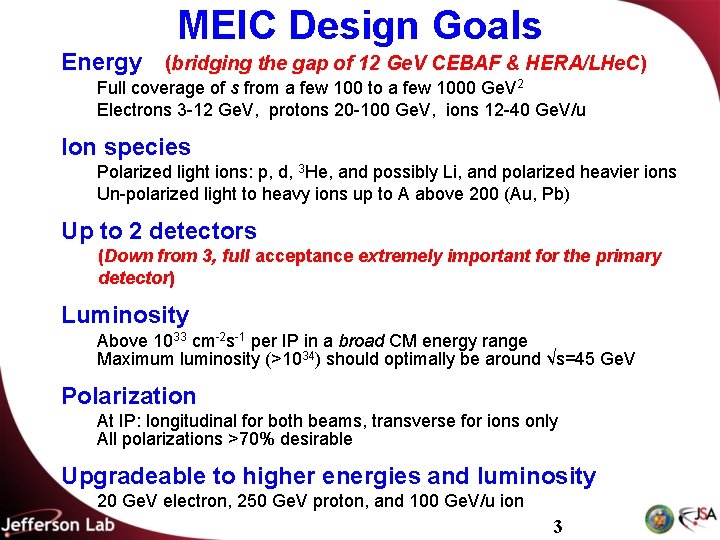
MEIC Design Goals Energy (bridging the gap of 12 Ge. V CEBAF & HERA/LHe. C) Full coverage of s from a few 100 to a few 1000 Ge. V 2 Electrons 3 -12 Ge. V, protons 20 -100 Ge. V, ions 12 -40 Ge. V/u Ion species Polarized light ions: p, d, 3 He, and possibly Li, and polarized heavier ions Un-polarized light to heavy ions up to A above 200 (Au, Pb) Up to 2 detectors (Down from 3, full acceptance extremely important for the primary detector) Luminosity Above 1033 cm-2 s-1 per IP in a broad CM energy range Maximum luminosity (>1034) should optimally be around √s=45 Ge. V Polarization At IP: longitudinal for both beams, transverse for ions only All polarizations >70% desirable Upgradeable to higher energies and luminosity 20 Ge. V electron, 250 Ge. V proton, and 100 Ge. V/u ion 3
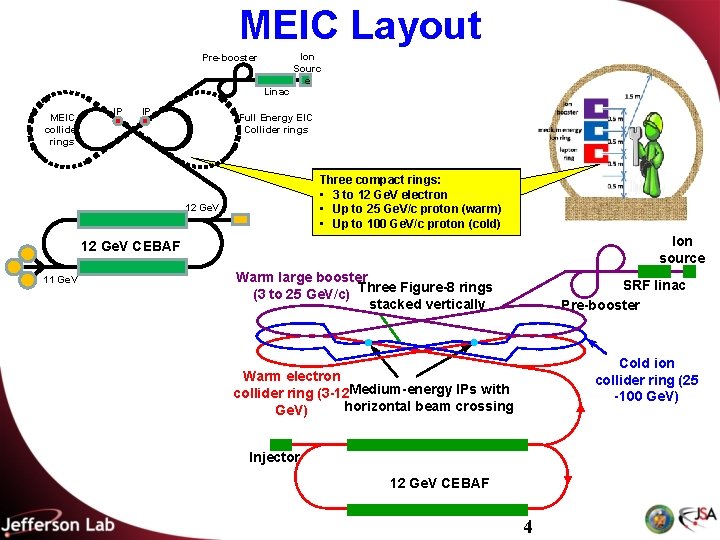
MEIC Layout Ion Sourc e Pre-booster Linac MEIC collider rings IP IP Full Energy EIC Collider rings Three compact rings: • 3 to 12 Ge. V electron • Up to 25 Ge. V/c proton (warm) • Up to 100 Ge. V/c proton (cold) 12 Ge. V Ion source 12 Ge. V CEBAF 11 Ge. V Warm large booster (3 to 25 Ge. V/c) Three Figure-8 rings stacked vertically SRF linac Pre-booster Cold ion collider ring (25 -100 Ge. V) Warm electron collider ring (3 -12 Medium-energy IPs with horizontal beam crossing Ge. V) Injector 12 Ge. V CEBAF 4
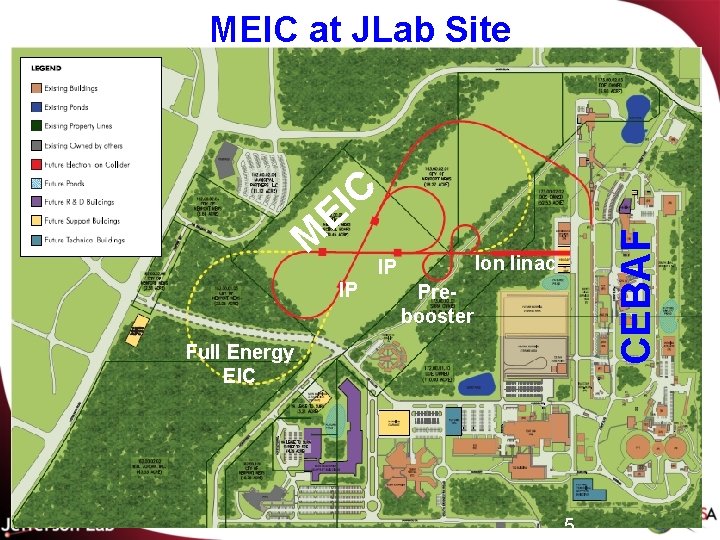
MEIC at JLab Site Ion linac IP IP CEBAF M C I E Prebooster Full Energy EIC 5
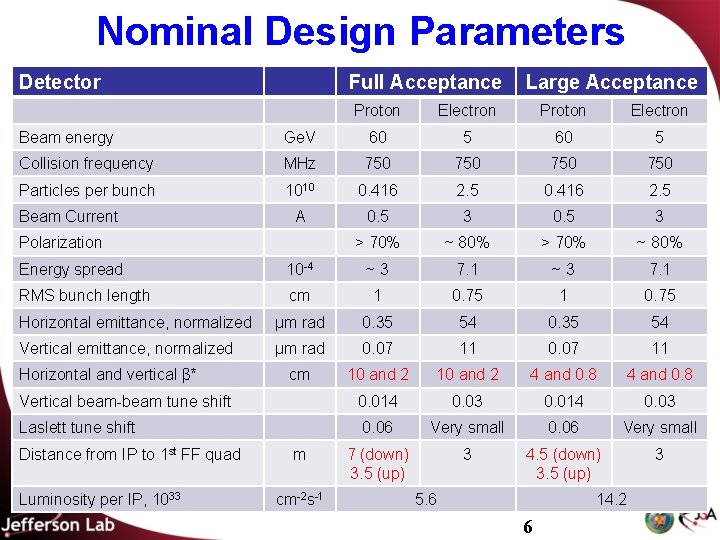
Nominal Design Parameters Detector Full Acceptance Large Acceptance Proton Electron Beam energy Ge. V 60 5 Collision frequency MHz 750 750 Particles per bunch 1010 0. 416 2. 5 A 0. 5 3 > 70% ~ 80% Beam Current Polarization Energy spread 10 -4 ~3 7. 1 RMS bunch length cm 1 0. 75 Horizontal emittance, normalized µm rad 0. 35 54 Vertical emittance, normalized µm rad 0. 07 11 cm 10 and 2 4 and 0. 8 Vertical beam-beam tune shift 0. 014 0. 03 Laslett tune shift 0. 06 Very small 7 (down) 3. 5 (up) 3 4. 5 (down) 3. 5 (up) 3 Horizontal and vertical β* Distance from IP to 1 st FF quad Luminosity per IP, 1033 m cm-2 s-1 5. 6 14. 2 6
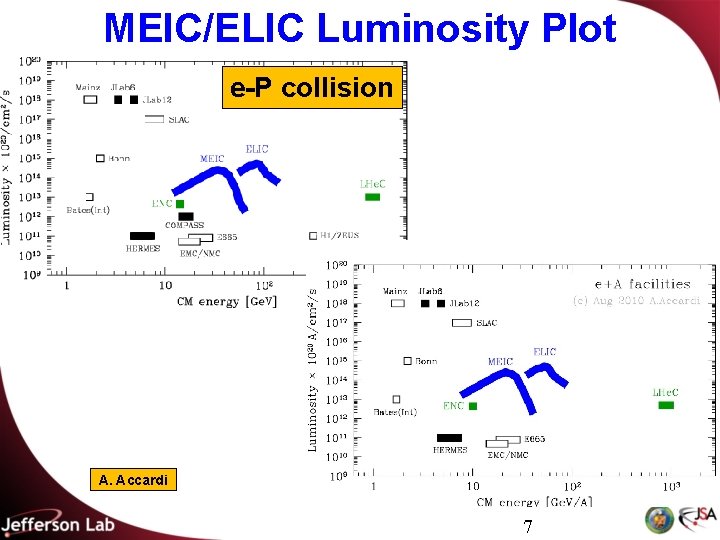
MEIC/ELIC Luminosity Plot e-P collision e-A collision A. Accardi 7
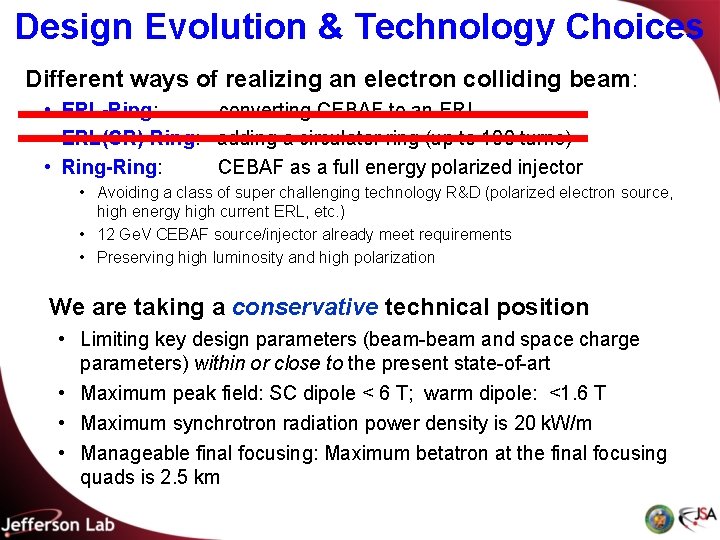
Design Evolution & Technology Choices Different ways of realizing an electron colliding beam: • ERL-Ring: converting CEBAF to an ERL • ERL(CR)-Ring: adding a circulator ring (up to 100 turns) • Ring-Ring: CEBAF as a full energy polarized injector • Avoiding a class of super challenging technology R&D (polarized electron source, high energy high current ERL, etc. ) • 12 Ge. V CEBAF source/injector already meet requirements • Preserving high luminosity and high polarization We are taking a conservative technical position • Limiting key design parameters (beam-beam and space charge parameters) within or close to the present state-of-art • Maximum peak field: SC dipole < 6 T; warm dipole: <1. 6 T • Maximum synchrotron radiation power density is 20 k. W/m • Manageable final focusing: Maximum betatron at the final focusing quads is 2. 5 km
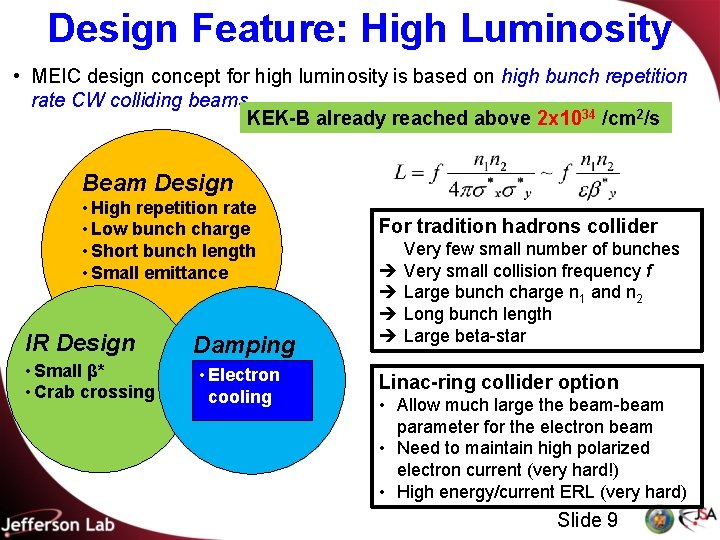
Design Feature: High Luminosity • MEIC design concept for high luminosity is based on high bunch repetition rate CW colliding beams KEK-B already reached above 2 x 1034 /cm 2/s Beam Design • High repetition rate • Low bunch charge • Short bunch length • Small emittance IR Design Damping • Small β* • Crab crossing • • Synchrotron Electron radiation cooling For tradition hadrons collider Very few small number of bunches Very small collision frequency f Large bunch charge n 1 and n 2 Long bunch length Large beta-star Linac-ring collider option • Allow much large the beam-beam parameter for the electron beam • Need to maintain high polarized electron current (very hard!) • High energy/current ERL (very hard) Slide 9
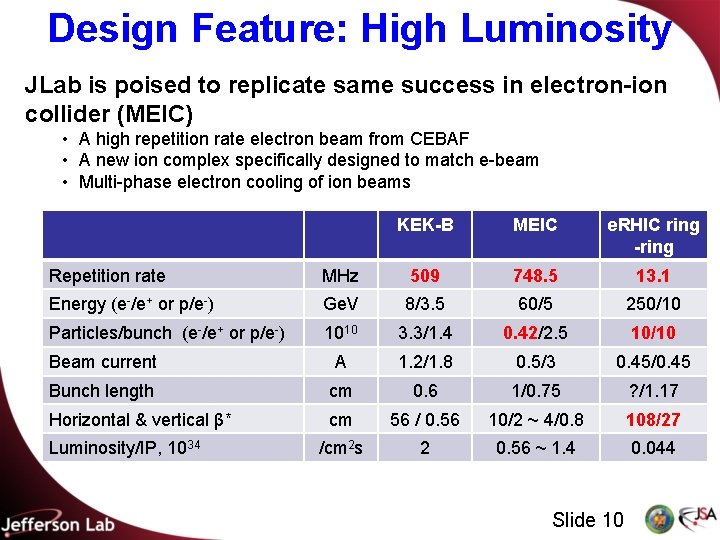
Design Feature: High Luminosity JLab is poised to replicate same success in electron-ion collider (MEIC) • A high repetition rate electron beam from CEBAF • A new ion complex specifically designed to match e-beam • Multi-phase electron cooling of ion beams KEK-B MEIC e. RHIC ring -ring Repetition rate MHz 509 748. 5 13. 1 Energy (e-/e+ or p/e-) Ge. V 8/3. 5 60/5 250/10 Particles/bunch (e-/e+ or p/e-) 1010 3. 3/1. 4 0. 42/2. 5 10/10 Beam current A 1. 2/1. 8 0. 5/3 0. 45/0. 45 Bunch length cm 0. 6 1/0. 75 ? /1. 17 Horizontal & vertical β* cm 56 / 0. 56 10/2 ~ 4/0. 8 108/27 /cm 2 s 2 0. 56 ~ 1. 4 0. 044 Luminosity/IP, 1034 Slide 10
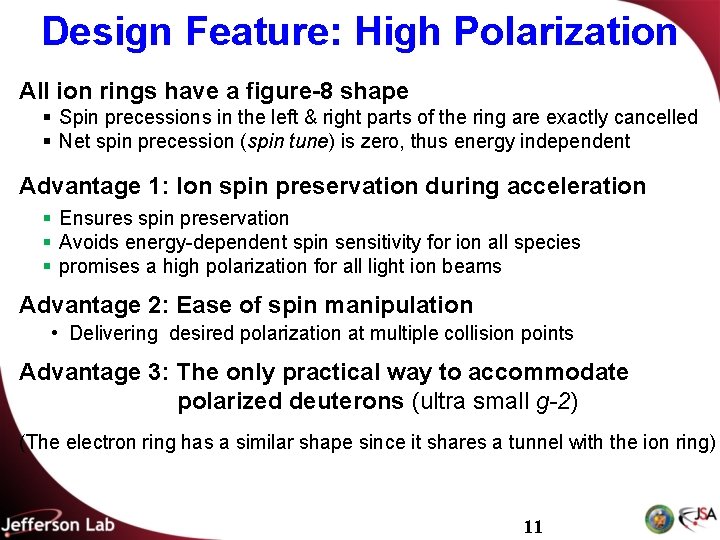
Design Feature: High Polarization All ion rings have a figure-8 shape § Spin precessions in the left & right parts of the ring are exactly cancelled § Net spin precession (spin tune) is zero, thus energy independent Advantage 1: Ion spin preservation during acceleration § Ensures spin preservation § Avoids energy-dependent spin sensitivity for ion all species § promises a high polarization for all light ion beams Advantage 2: Ease of spin manipulation • Delivering desired polarization at multiple collision points Advantage 3: The only practical way to accommodate polarized deuterons (ultra small g-2) (The electron ring has a similar shape since it shares a tunnel with the ion ring) 11
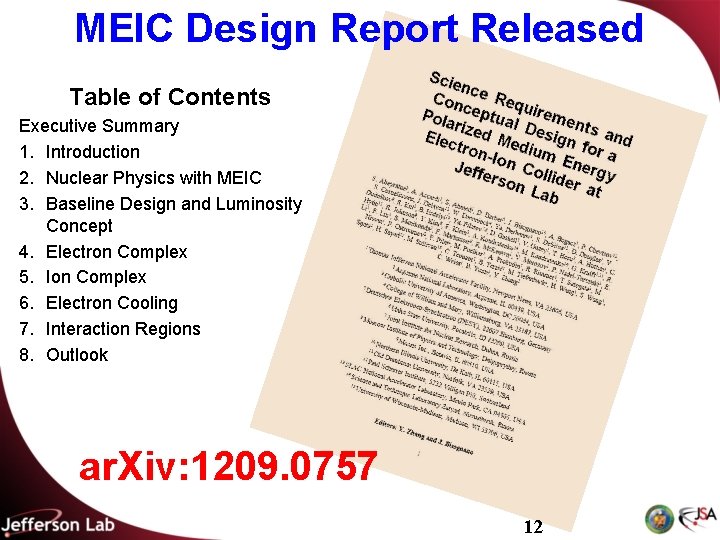
MEIC Design Report Released Table of Contents Executive Summary 1. Introduction 2. Nuclear Physics with MEIC 3. Baseline Design and Luminosity Concept 4. Electron Complex 5. Ion Complex 6. Electron Cooling 7. Interaction Regions 8. Outlook ar. Xiv: 1209. 0757 12
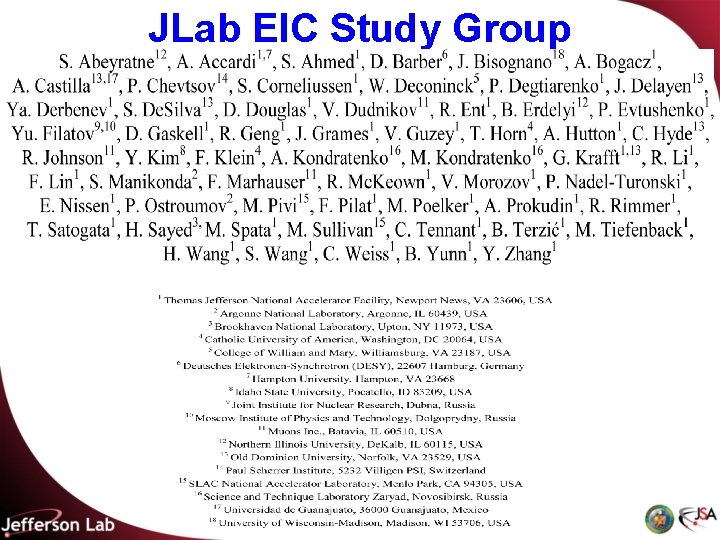
JLab EIC Study Group
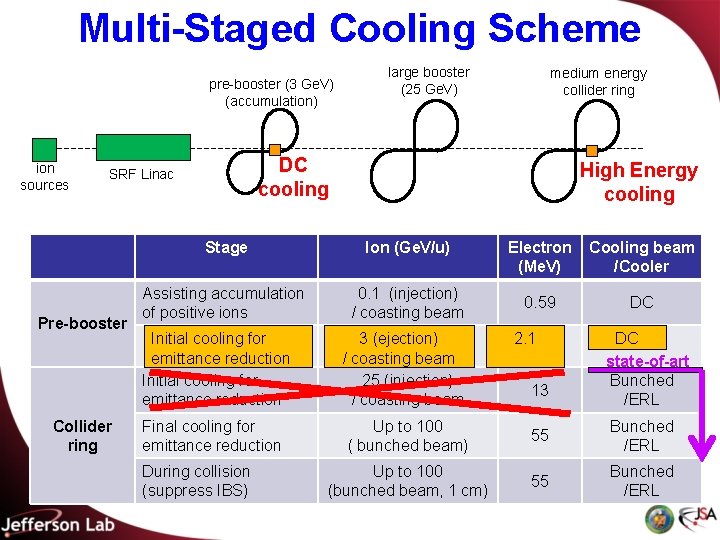
Multi-Staged Cooling Scheme pre-booster (3 Ge. V) (accumulation) ion sources Collider ring medium energy collider ring DC cooling SRF Linac Pre-booster large booster (25 Ge. V) High Energy cooling Stage Ion (Ge. V/u) Assisting accumulation of positive ions 0. 1 (injection) / coasting beam Electron (Me. V) Cooling beam /Cooler 0. 59 DC Initial cooling for emittance reduction 3 (ejection) / coasting beam 25 (injection) / coasting beam Final cooling for emittance reduction Up to 100 ( bunched beam) 55 Bunched /ERL Up to 100 (bunched beam, 1 cm) 55 Bunched /ERL During collision (suppress IBS) 2. 1 13 14 DC state-of-art Bunched /ERL
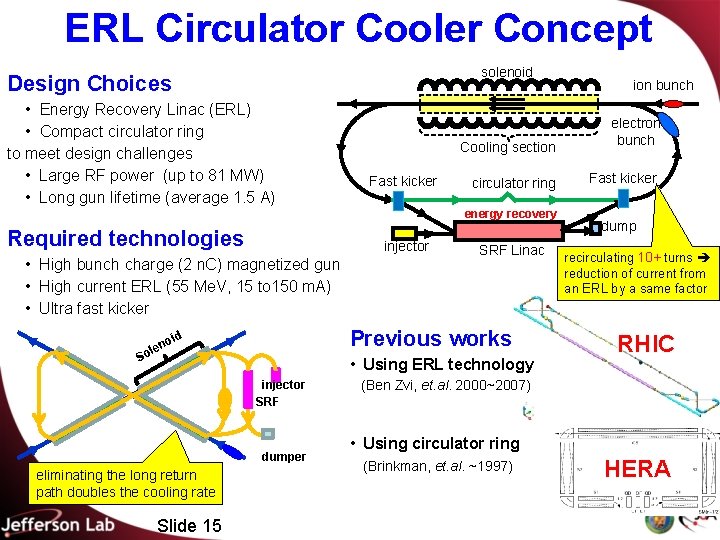
ERL Circulator Cooler Concept solenoid Design Choices • Energy Recovery Linac (ERL) • Compact circulator ring to meet design challenges • Large RF power (up to 81 MW) • Long gun lifetime (average 1. 5 A) ion bunch electron bunch Cooling section Fast kicker circulator ring energy recovery Required technologies injector • High bunch charge (2 n. C) magnetized gun • High current ERL (55 Me. V, 15 to 150 m. A) • Ultra fast kicker S RHIC • Using ERL technology injector SRF dumper Slide 15 recirculating 10+ turns reduction of current from an ERL by a same factor Previous works oid n ole eliminating the long return path doubles the cooling rate SRF Linac dump (Ben Zvi, et. al. 2000~2007) • Using circulator ring HERA (Brinkman, et. al. ~1997) 15
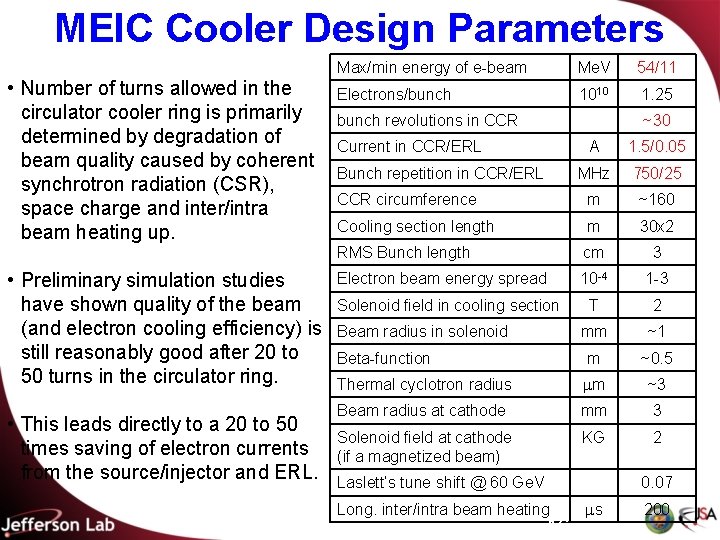
MEIC Cooler Design Parameters • Number of turns allowed in the circulator cooler ring is primarily determined by degradation of beam quality caused by coherent synchrotron radiation (CSR), space charge and inter/intra beam heating up. • Preliminary simulation studies have shown quality of the beam (and electron cooling efficiency) is still reasonably good after 20 to 50 turns in the circulator ring. • This leads directly to a 20 to 50 times saving of electron currents from the source/injector and ERL. Max/min energy of e-beam Me. V 54/11 Electrons/bunch 1010 1. 25 bunch revolutions in CCR ~30 Current in CCR/ERL A 1. 5/0. 05 MHz 750/25 CCR circumference m ~160 Cooling section length m 30 x 2 RMS Bunch length cm 3 Electron beam energy spread 10 -4 1 -3 T 2 mm ~1 Beta-function m ~0. 5 Thermal cyclotron radius m ~3 Beam radius at cathode mm 3 Solenoid field at cathode (if a magnetized beam) KG 2 Bunch repetition in CCR/ERL Solenoid field in cooling section Beam radius in solenoid Laslett’s tune shift @ 60 Ge. V 0. 07 Long. inter/intra beam heating 16 s 200
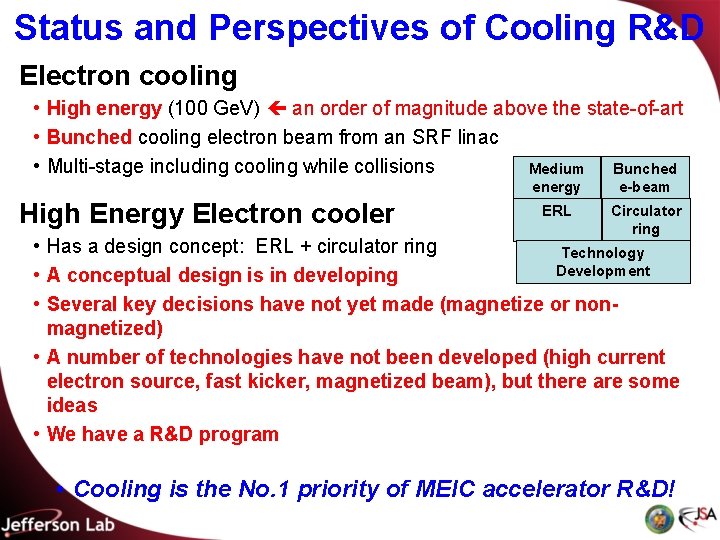
Status and Perspectives of Cooling R&D Electron cooling • High energy (100 Ge. V) an order of magnitude above the state-of-art • Bunched cooling electron beam from an SRF linac • Multi-stage including cooling while collisions Medium Bunched High Energy Electron cooler energy e-beam ERL Circulator ring • Has a design concept: ERL + circulator ring Technology Development • A conceptual design is in developing • Several key decisions have not yet made (magnetize or nonmagnetized) • A number of technologies have not been developed (high current electron source, fast kicker, magnetized beam), but there are some ideas • We have a R&D program • Cooling is the No. 1 priority of MEIC accelerator R&D! 17
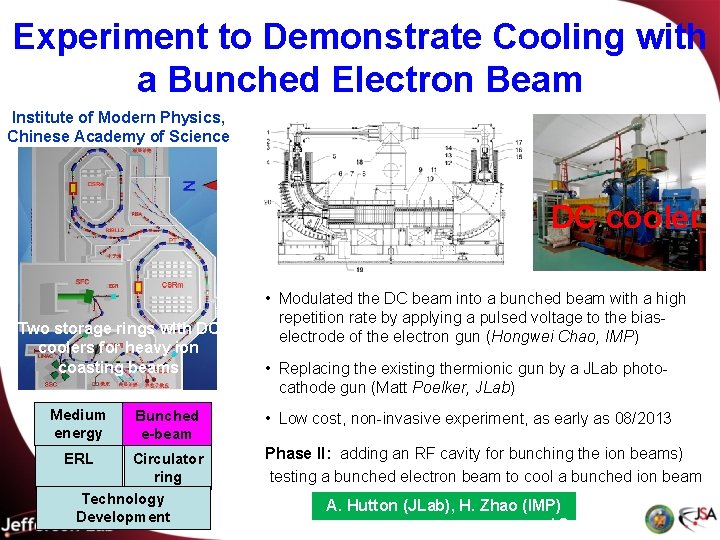
Experiment to Demonstrate Cooling with a Bunched Electron Beam Institute of Modern Physics, Chinese Academy of Science DC cooler Two storage rings with DC coolers for heavy ion coasting beams Medium energy ERL Bunched e-beam Circulator ring Technology Development • Modulated the DC beam into a bunched beam with a high repetition rate by applying a pulsed voltage to the biaselectrode of the electron gun (Hongwei Chao, IMP) • Replacing the existing thermionic gun by a JLab photocathode gun (Matt Poelker, JLab) • Low cost, non-invasive experiment, as early as 08/2013 Phase II: adding an RF cavity for bunching the ion beams) testing a bunched electron beam to cool a bunched ion beam A. Hutton (JLab), H. Zhao (IMP) 18
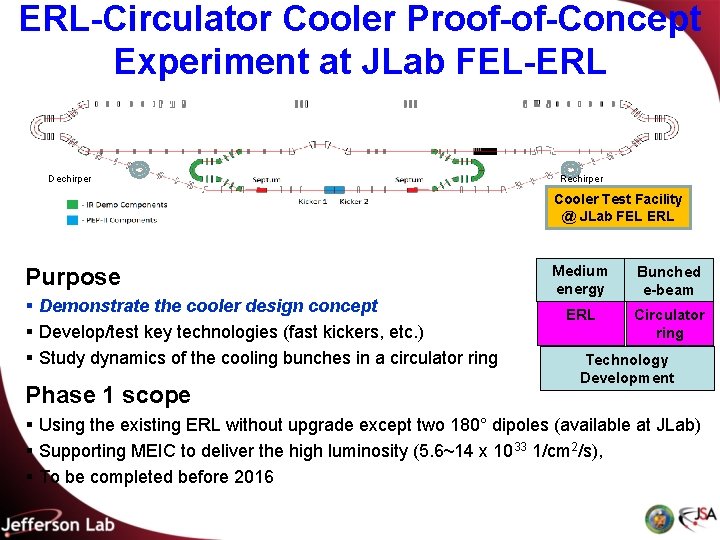
ERL-Circulator Cooler Proof-of-Concept Experiment at JLab FEL-ERL Dechirper Rechirper Cooler Test Facility @ JLab FEL ERL Purpose § Demonstrate the cooler design concept § Develop/test key technologies (fast kickers, etc. ) § Study dynamics of the cooling bunches in a circulator ring Medium energy Bunched e-beam ERL Circulator ring Technology Development Phase 1 scope § Using the existing ERL without upgrade except two 180° dipoles (available at JLab) § Supporting MEIC to deliver the high luminosity (5. 6~14 x 1033 1/cm 2/s), § To be completed before 2016 19
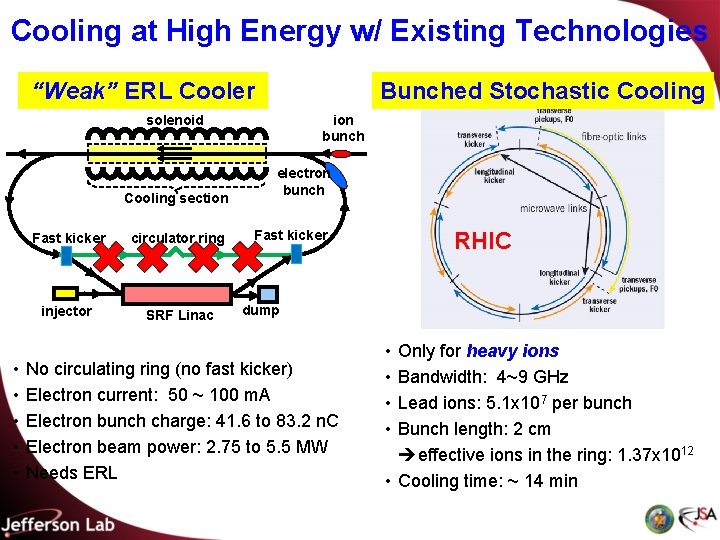
Cooling at High Energy w/ Existing Technologies “Weak” ERL Cooler Bunched Stochastic Cooling ion bunch solenoid Cooling section • • • Fast kicker circulator ring injector SRF Linac electron bunch RHIC Fast kicker dump No circulating ring (no fast kicker) Electron current: 50 ~ 100 m. A Electron bunch charge: 41. 6 to 83. 2 n. C Electron beam power: 2. 75 to 5. 5 MW Needs ERL • • Only for heavy ions Bandwidth: 4~9 GHz Lead ions: 5. 1 x 107 per bunch Bunch length: 2 cm effective ions in the ring: 1. 37 x 1012 • Cooling time: ~ 14 min
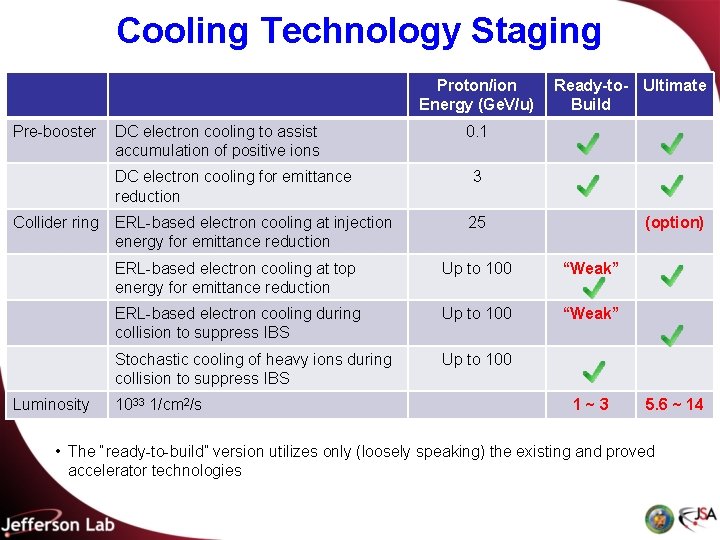
Cooling Technology Staging Proton/ion Energy (Ge. V/u) Pre-booster Collider ring Luminosity DC electron cooling to assist accumulation of positive ions Ready-to- Ultimate Build 0. 1 DC electron cooling for emittance reduction 3 ERL-based electron cooling at injection energy for emittance reduction 25 (option) ERL-based electron cooling at top energy for emittance reduction Up to 100 “Weak” ERL-based electron cooling during collision to suppress IBS Up to 100 “Weak” Stochastic cooling of heavy ions during collision to suppress IBS Up to 100 1033 1/cm 2/s 1~3 5. 6 ~ 14 • The “ready-to-build” version utilizes only (loosely speaking) the existing and proved accelerator technologies
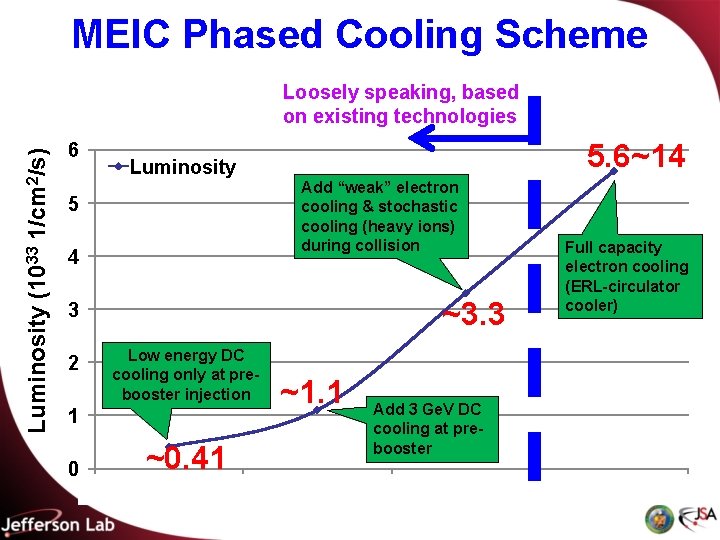
MEIC Phased Cooling Scheme Luminosity (1033 1/cm 2/s) Loosely speaking, based on existing technologies 6 5. 6~14 Luminosity Add “weak” electron cooling & stochastic cooling (heavy ions) during collision 5 4 Full capacity electron cooling (ERL-circulator cooler) ~3. 3 3 Low energy DC cooling only at prebooster injection 2 1 ~0. 41 0 0. 5 1. 5 ~1. 1 Add 3 Ge. V DC cooling at prebooster 2. 5 3. 5 4. 5
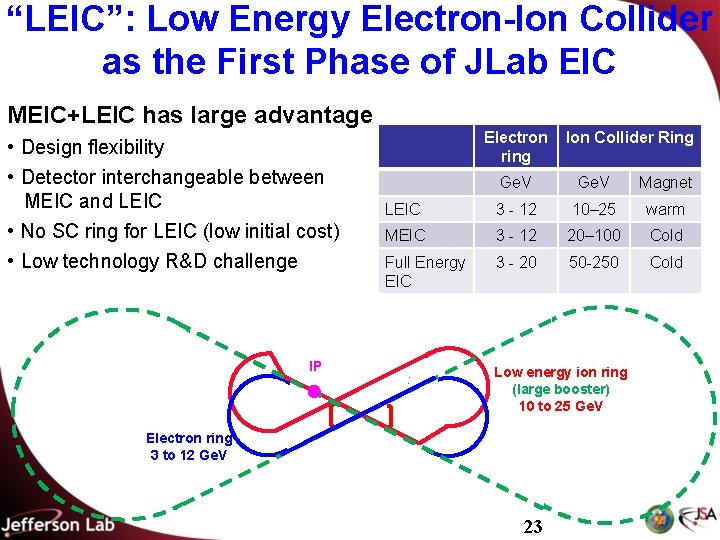
“LEIC”: Low Energy Electron-Ion Collider as the First Phase of JLab EIC MEIC+LEIC has large advantage • Design flexibility • Detector interchangeable between MEIC and LEIC • No SC ring for LEIC (low initial cost) • Low technology R&D challenge IP Electron ring Ion Collider Ring Ge. V Magnet LEIC 3 - 12 10– 25 warm MEIC 3 - 12 20– 100 Cold Full Energy EIC 3 - 20 50 -250 Cold Low energy ion ring (large booster) 10 to 25 Ge. V Electron ring 3 to 12 Ge. V 23
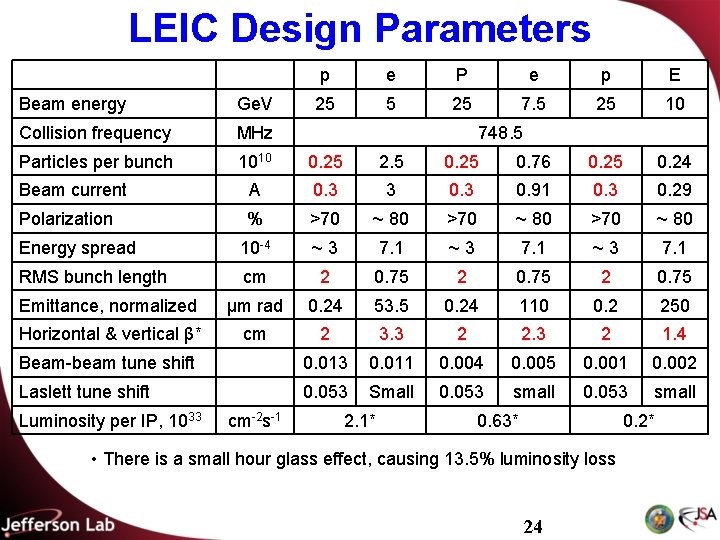
LEIC Design Parameters p e P e p E 25 5 25 7. 5 25 10 Beam energy Ge. V Collision frequency MHz Particles per bunch 1010 0. 25 2. 5 0. 25 0. 76 0. 25 0. 24 Beam current A 0. 3 3 0. 91 0. 3 0. 29 Polarization % >70 ~ 80 Energy spread 10 -4 ~3 7. 1 RMS bunch length cm 2 0. 75 Emittance, normalized µm rad 0. 24 53. 5 0. 24 110 0. 2 250 Horizontal & vertical β* cm 2 3. 3 2 2. 3 2 1. 4 Beam-beam tune shift 0. 013 0. 011 0. 004 0. 005 0. 001 0. 002 Laslett tune shift 0. 053 Small 0. 053 small Luminosity per IP, 1033 cm-2 s-1 748. 5 2. 1* 0. 63* 0. 2* • There is a small hour glass effect, causing 13. 5% luminosity loss 24
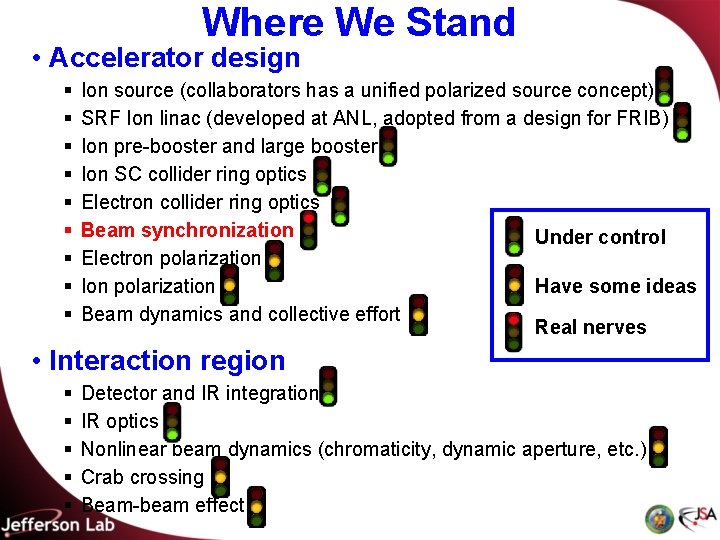
Where We Stand • Accelerator design § § § § § Ion source (collaborators has a unified polarized source concept) SRF Ion linac (developed at ANL, adopted from a design for FRIB) Ion pre-booster and large booster Ion SC collider ring optics Electron collider ring optics Beam synchronization Under control Electron polarization Ion polarization Have some ideas Beam dynamics and collective effort Real nerves • Interaction region § § § Detector and IR integration IR optics Nonlinear beam dynamics (chromaticity, dynamic aperture, etc. ) Crab crossing Beam-beam effect
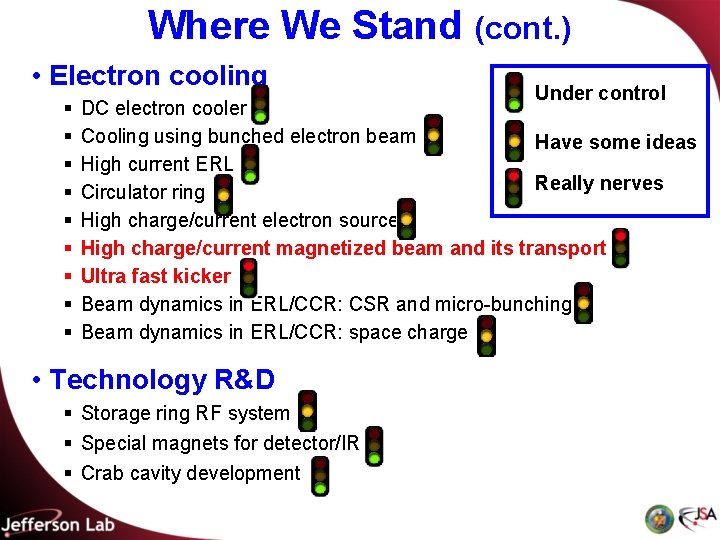
Where We Stand (cont. ) • Electron cooling § § § § § Under control DC electron cooler Cooling using bunched electron beam Have some ideas High current ERL Really nerves Circulator ring High charge/current electron source High charge/current magnetized beam and its transport Ultra fast kicker Beam dynamics in ERL/CCR: CSR and micro-bunching Beam dynamics in ERL/CCR: space charge • Technology R&D § Storage ring RF system § Special magnets for detector/IR § Crab cavity development
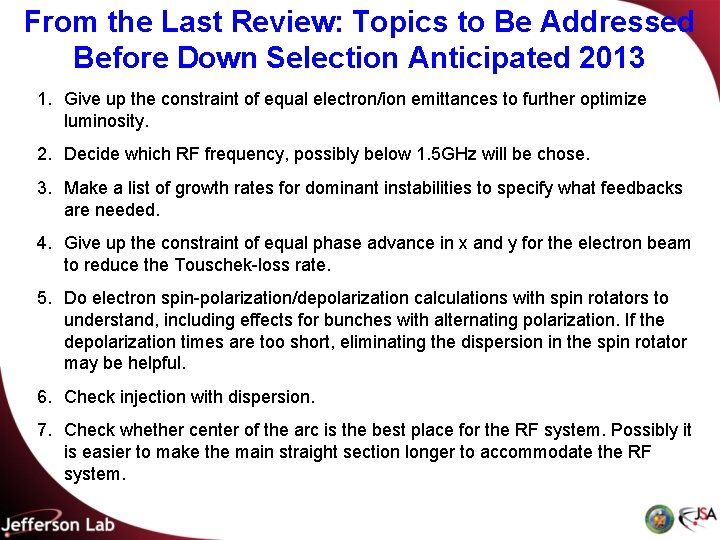
From the Last Review: Topics to Be Addressed Before Down Selection Anticipated 2013 1. Give up the constraint of equal electron/ion emittances to further optimize luminosity. 2. Decide which RF frequency, possibly below 1. 5 GHz will be chose. 3. Make a list of growth rates for dominant instabilities to specify what feedbacks are needed. 4. Give up the constraint of equal phase advance in x and y for the electron beam to reduce the Touschek-loss rate. 5. Do electron spin-polarization/depolarization calculations with spin rotators to understand, including effects for bunches with alternating polarization. If the depolarization times are too short, eliminating the dispersion in the spin rotator may be helpful. 6. Check injection with dispersion. 7. Check whether center of the arc is the best place for the RF system. Possibly it is easier to make the main straight section longer to accommodate the RF system.
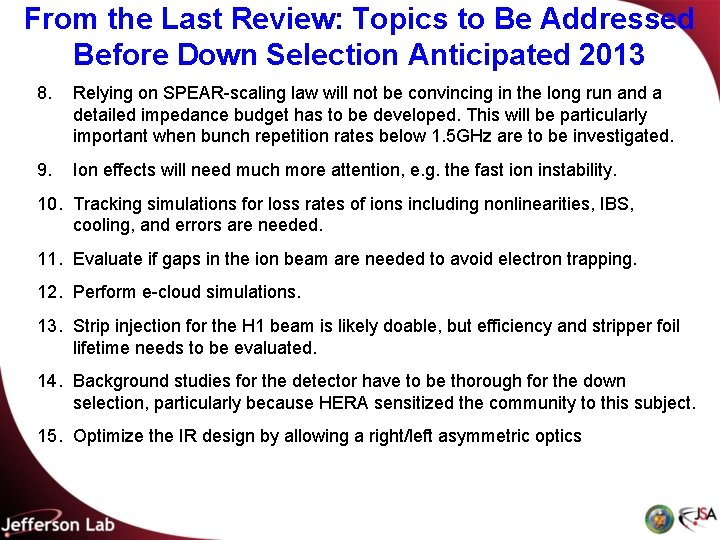
From the Last Review: Topics to Be Addressed Before Down Selection Anticipated 2013 8. Relying on SPEAR-scaling law will not be convincing in the long run and a detailed impedance budget has to be developed. This will be particularly important when bunch repetition rates below 1. 5 GHz are to be investigated. 9. Ion effects will need much more attention, e. g. the fast ion instability. 10. Tracking simulations for loss rates of ions including nonlinearities, IBS, cooling, and errors are needed. 11. Evaluate if gaps in the ion beam are needed to avoid electron trapping. 12. Perform e-cloud simulations. 13. Strip injection for the H 1 beam is likely doable, but efficiency and stripper foil lifetime needs to be evaluated. 14. Background studies for the detector have to be thorough for the down selection, particularly because HERA sensitized the community to this subject. 15. Optimize the IR design by allowing a right/left asymmetric optics
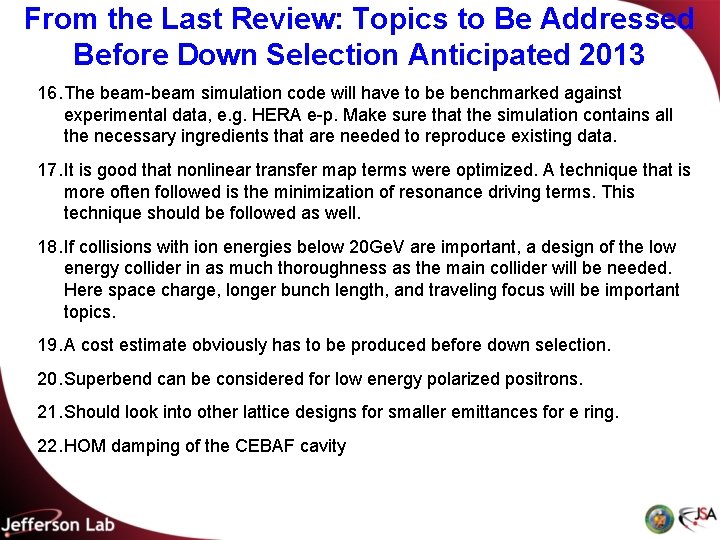
From the Last Review: Topics to Be Addressed Before Down Selection Anticipated 2013 16. The beam-beam simulation code will have to be benchmarked against experimental data, e. g. HERA e-p. Make sure that the simulation contains all the necessary ingredients that are needed to reproduce existing data. 17. It is good that nonlinear transfer map terms were optimized. A technique that is more often followed is the minimization of resonance driving terms. This technique should be followed as well. 18. If collisions with ion energies below 20 Ge. V are important, a design of the low energy collider in as much thoroughness as the main collider will be needed. Here space charge, longer bunch length, and traveling focus will be important topics. 19. A cost estimate obviously has to be produced before down selection. 20. Superbend can be considered for low energy polarized positrons. 21. Should look into other lattice designs for smaller emittances for e ring. 22. HOM damping of the CEBAF cavity
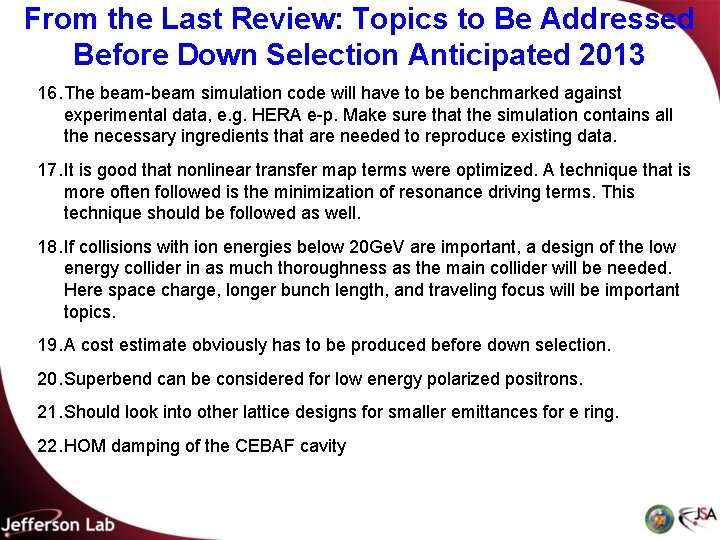
From the Last Review: Topics to Be Addressed Before Down Selection Anticipated 2013 16. The beam-beam simulation code will have to be benchmarked against experimental data, e. g. HERA e-p. Make sure that the simulation contains all the necessary ingredients that are needed to reproduce existing data. 17. It is good that nonlinear transfer map terms were optimized. A technique that is more often followed is the minimization of resonance driving terms. This technique should be followed as well. 18. If collisions with ion energies below 20 Ge. V are important, a design of the low energy collider in as much thoroughness as the main collider will be needed. Here space charge, longer bunch length, and traveling focus will be important topics. 19. A cost estimate obviously has to be produced before down selection. 20. Superbend can be considered for low energy polarized positrons. 21. Should look into other lattice designs for smaller emittances for e ring. 22. HOM damping of the CEBAF cavity
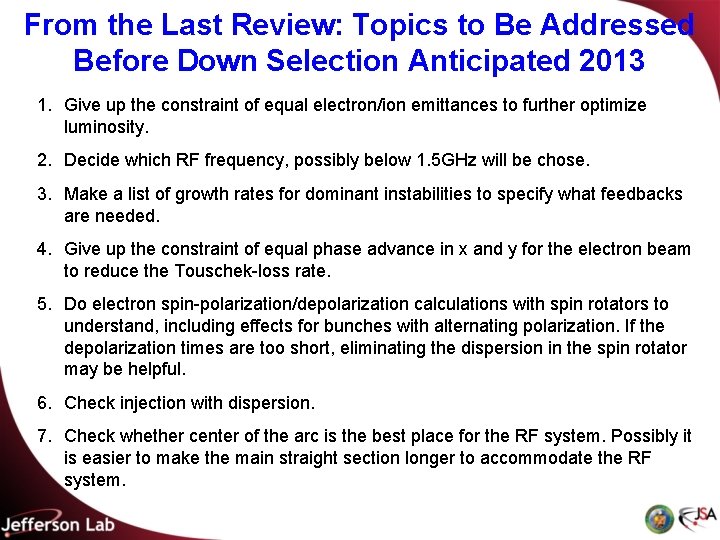
From the Last Review: Topics to Be Addressed Before Down Selection Anticipated 2013 1. Give up the constraint of equal electron/ion emittances to further optimize luminosity. 2. Decide which RF frequency, possibly below 1. 5 GHz will be chose. 3. Make a list of growth rates for dominant instabilities to specify what feedbacks are needed. 4. Give up the constraint of equal phase advance in x and y for the electron beam to reduce the Touschek-loss rate. 5. Do electron spin-polarization/depolarization calculations with spin rotators to understand, including effects for bunches with alternating polarization. If the depolarization times are too short, eliminating the dispersion in the spin rotator may be helpful. 6. Check injection with dispersion. 7. Check whether center of the arc is the best place for the RF system. Possibly it is easier to make the main straight section longer to accommodate the RF system.
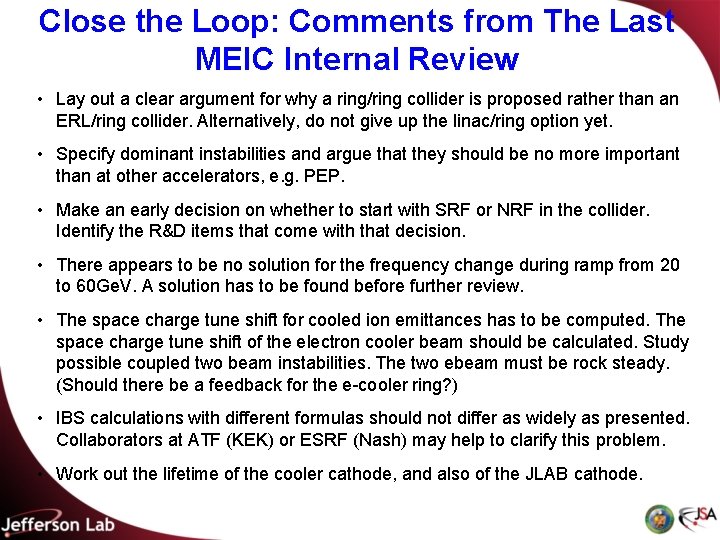
Close the Loop: Comments from The Last MEIC Internal Review • Lay out a clear argument for why a ring/ring collider is proposed rather than an ERL/ring collider. Alternatively, do not give up the linac/ring option yet. • Specify dominant instabilities and argue that they should be no more important than at other accelerators, e. g. PEP. • Make an early decision on whether to start with SRF or NRF in the collider. Identify the R&D items that come with that decision. • There appears to be no solution for the frequency change during ramp from 20 to 60 Ge. V. A solution has to be found before further review. • The space charge tune shift for cooled ion emittances has to be computed. The space charge tune shift of the electron cooler beam should be calculated. Study possible coupled two beam instabilities. The two ebeam must be rock steady. (Should there be a feedback for the e-cooler ring? ) • IBS calculations with different formulas should not differ as widely as presented. Collaborators at ATF (KEK) or ESRF (Nash) may help to clarify this problem. • Work out the lifetime of the cooler cathode, and also of the JLAB cathode.
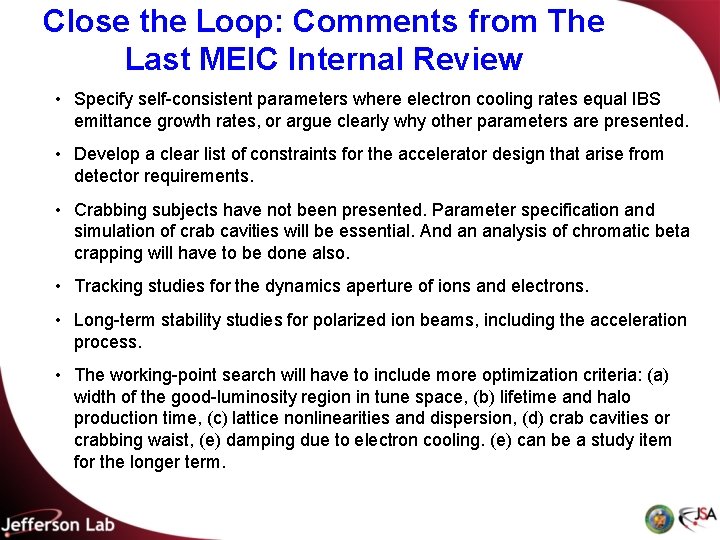
Close the Loop: Comments from The Last MEIC Internal Review • Specify self-consistent parameters where electron cooling rates equal IBS emittance growth rates, or argue clearly why other parameters are presented. • Develop a clear list of constraints for the accelerator design that arise from detector requirements. • Crabbing subjects have not been presented. Parameter specification and simulation of crab cavities will be essential. And an analysis of chromatic beta crapping will have to be done also. • Tracking studies for the dynamics aperture of ions and electrons. • Long-term stability studies for polarized ion beams, including the acceleration process. • The working-point search will have to include more optimization criteria: (a) width of the good-luminosity region in tune space, (b) lifetime and halo production time, (c) lattice nonlinearities and dispersion, (d) crab cavities or crabbing waist, (e) damping due to electron cooling. (e) can be a study item for the longer term.
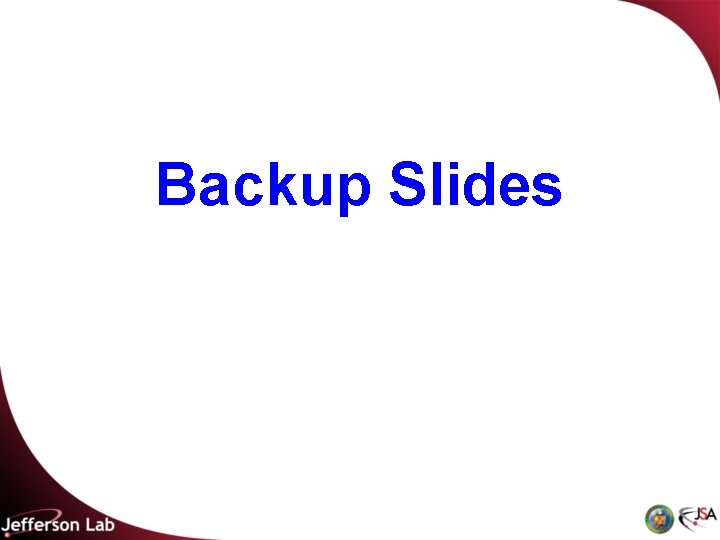
Backup Slides
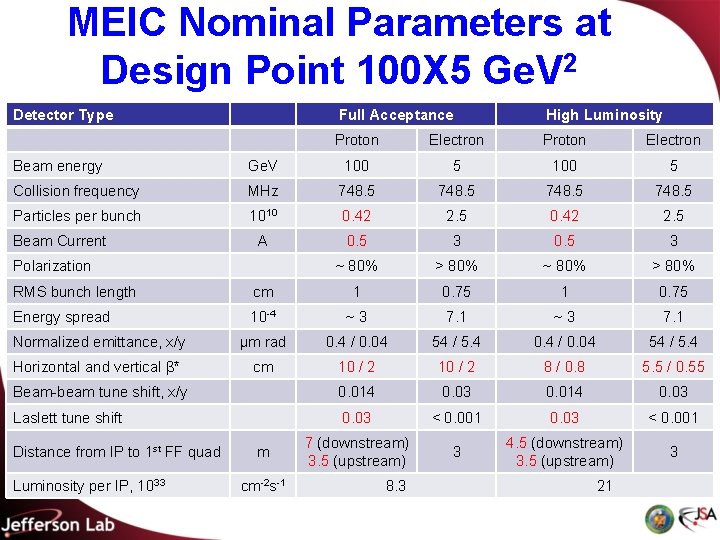
MEIC Nominal Parameters at Design Point 100 X 5 Ge. V 2 Detector Type Full Acceptance High Luminosity Proton Electron Beam energy Ge. V 100 5 Collision frequency MHz 748. 5 Particles per bunch 1010 0. 42 2. 5 A 0. 5 3 ~ 80% > 80% Beam Current Polarization RMS bunch length cm 1 0. 75 Energy spread 10 -4 ~3 7. 1 Normalized emittance, x/y µm rad 0. 4 / 0. 04 54 / 5. 4 Horizontal and vertical β* cm 10 / 2 8 / 0. 8 5. 5 / 0. 55 Beam-beam tune shift, x/y 0. 014 0. 03 Laslett tune shift 0. 03 < 0. 001 7 (downstream) 3. 5 (upstream) 3 4. 5 (downstream) 3. 5 (upstream) 3 Distance from IP to 1 st FF quad Luminosity per IP, 1033 m cm-2 s-1 8. 3 21
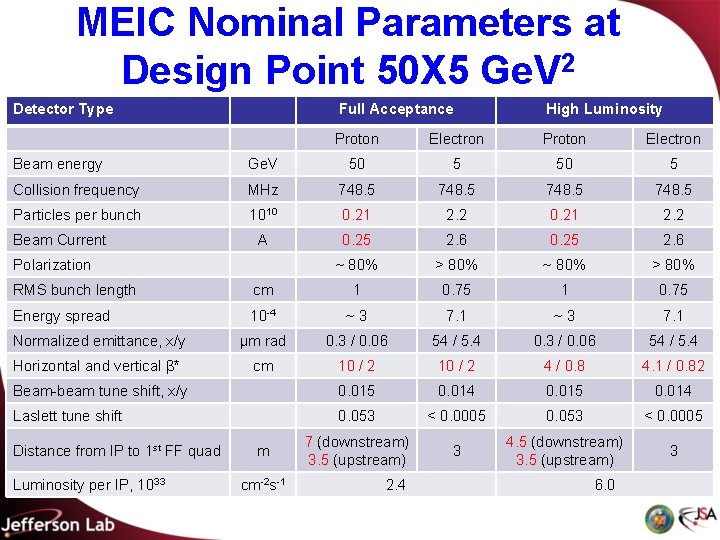
MEIC Nominal Parameters at Design Point 50 X 5 Ge. V 2 Detector Type Full Acceptance High Luminosity Proton Electron Beam energy Ge. V 50 5 Collision frequency MHz 748. 5 Particles per bunch 1010 0. 21 2. 2 A 0. 25 2. 6 ~ 80% > 80% Beam Current Polarization RMS bunch length cm 1 0. 75 Energy spread 10 -4 ~3 7. 1 Normalized emittance, x/y µm rad 0. 3 / 0. 06 54 / 5. 4 Horizontal and vertical β* cm 10 / 2 4 / 0. 8 4. 1 / 0. 82 Beam-beam tune shift, x/y 0. 015 0. 014 Laslett tune shift 0. 053 < 0. 0005 7 (downstream) 3. 5 (upstream) 3 4. 5 (downstream) 3. 5 (upstream) 3 Distance from IP to 1 st FF quad Luminosity per IP, 1033 m cm-2 s-1 2. 4 6. 0
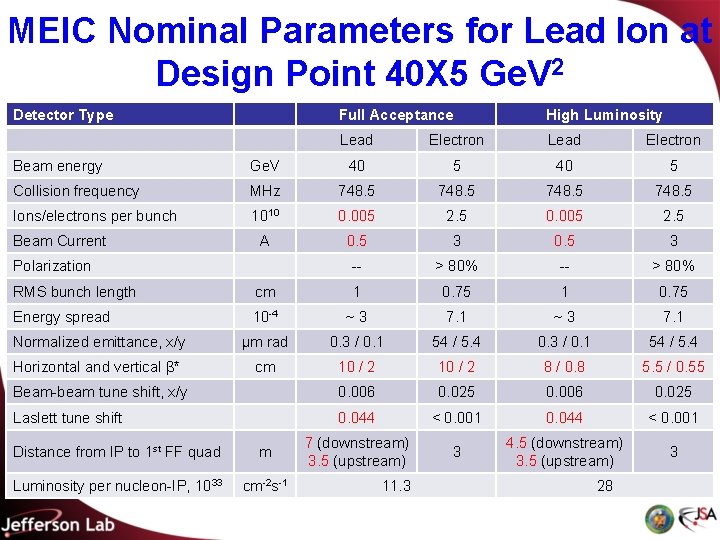
MEIC Nominal Parameters for Lead Ion at Design Point 40 X 5 Ge. V 2 Detector Type Full Acceptance High Luminosity Lead Electron Beam energy Ge. V 40 5 Collision frequency MHz 748. 5 Ions/electrons per bunch 1010 0. 005 2. 5 A 0. 5 3 -- > 80% Beam Current Polarization RMS bunch length cm 1 0. 75 Energy spread 10 -4 ~3 7. 1 Normalized emittance, x/y µm rad 0. 3 / 0. 1 54 / 5. 4 Horizontal and vertical β* cm 10 / 2 8 / 0. 8 5. 5 / 0. 55 Beam-beam tune shift, x/y 0. 006 0. 025 Laslett tune shift 0. 044 < 0. 001 7 (downstream) 3. 5 (upstream) 3 4. 5 (downstream) 3. 5 (upstream) 3 Distance from IP to 1 st FF quad m Luminosity per nucleon-IP, 1033 cm-2 s-1 11. 3 28
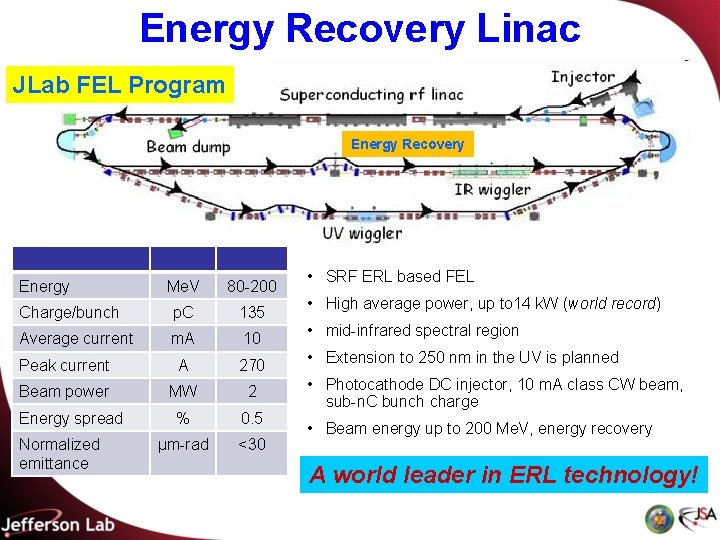
Energy Recovery Linac JLab FEL Program Energy Recovery Energy Me. V 80 -200 Charge/bunch p. C 135 Average current m. A 10 Peak current A 270 Beam power MW 2 % 0. 5 µm-rad <30 Energy spread Normalized emittance • SRF ERL based FEL • High average power, up to 14 k. W (world record) • mid-infrared spectral region • Extension to 250 nm in the UV is planned • Photocathode DC injector, 10 m. A class CW beam, sub-n. C bunch charge • Beam energy up to 200 Me. V, energy recovery A world leader in ERL technology! 38
System design
System design overview
Qirun zhang
Hui zhang cmu
Zuofeng zhang
Zuofeng zhang
Dr ming zhang
Zhang fei mask
Local lymph node assay
Dong pyou han
Mocha.jl
Grace zhang morgan stanley
Dr howard zhang
Xiaolan zhang fordham
Yunquan zhang
Ikaye zhang
Shengyu zhang
Jinghe zhang
Nikki zhang
Yunxi zhang
Wuyang zhang
Ruiliang zhang
Zfs dnode
Intellectual giftedness
Yongchao zhang
Sinan xiao
Squid
Daya zhang
Zhifei zhang
Jingxuan zhang
Yanci zhang
X. zhang os
Rui zhang unimelb
Hello edge
Zhang et al
Zhang jinan
Deep learning for dummies
Bitcoin
Tongli zhang