Leakage in MOS devices Mohammad Sharifkhani Reading Text
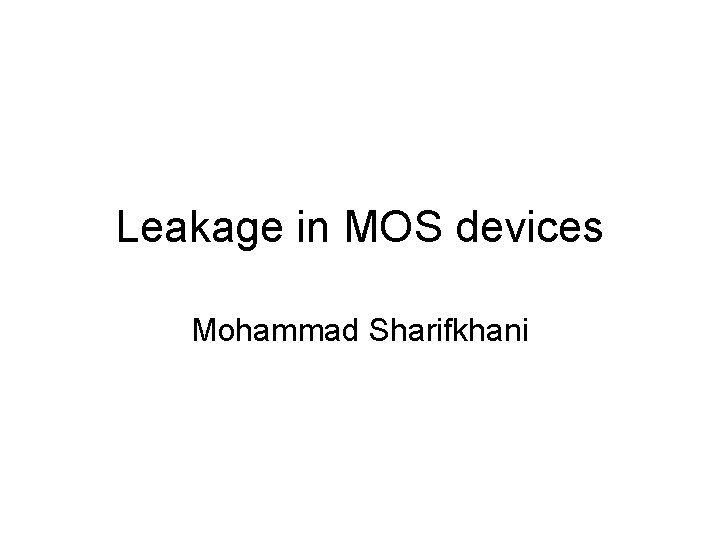
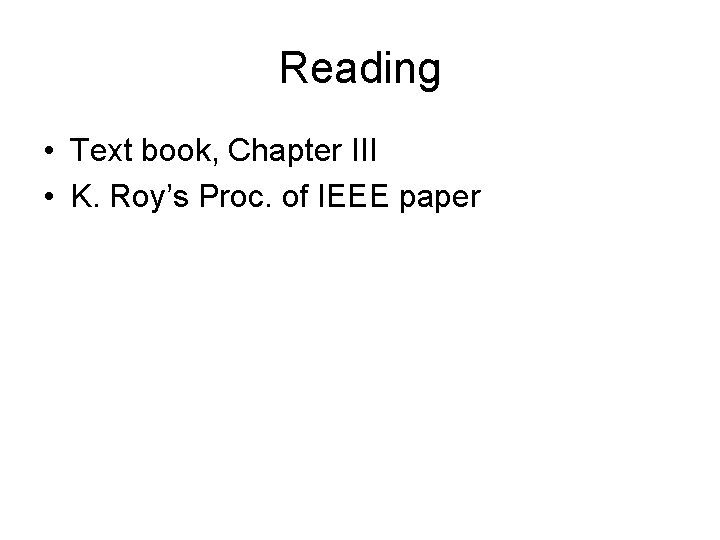
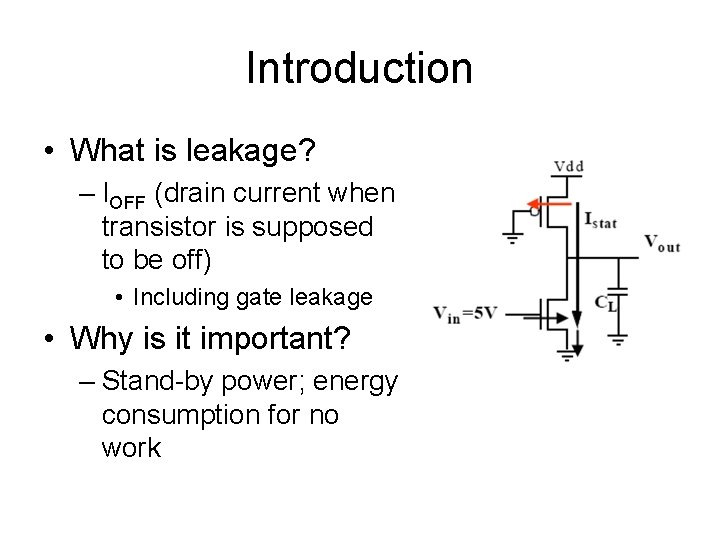
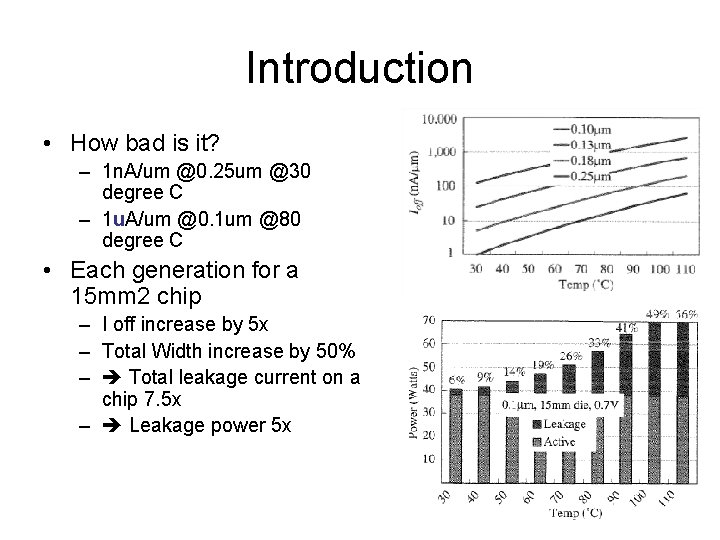
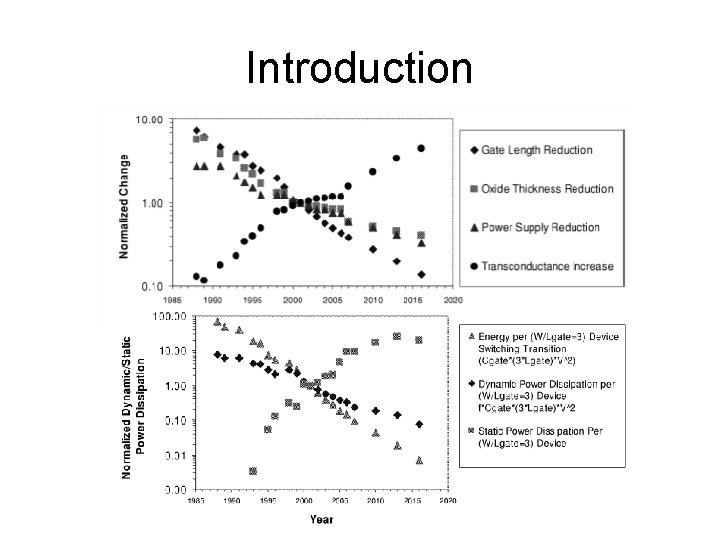
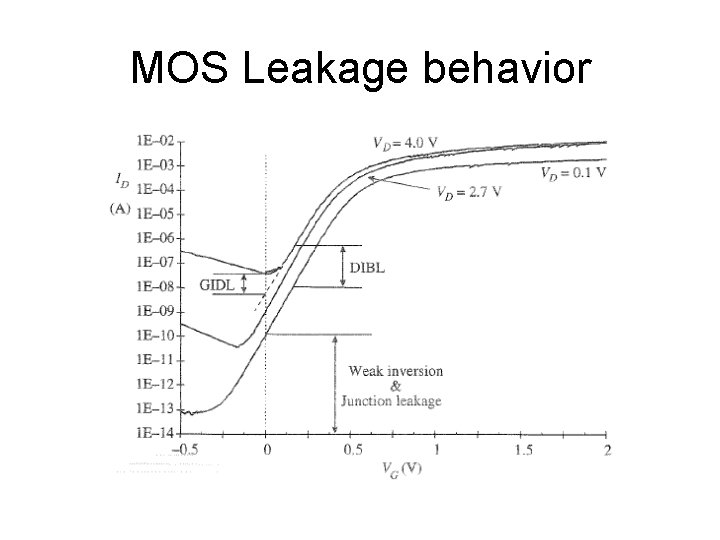
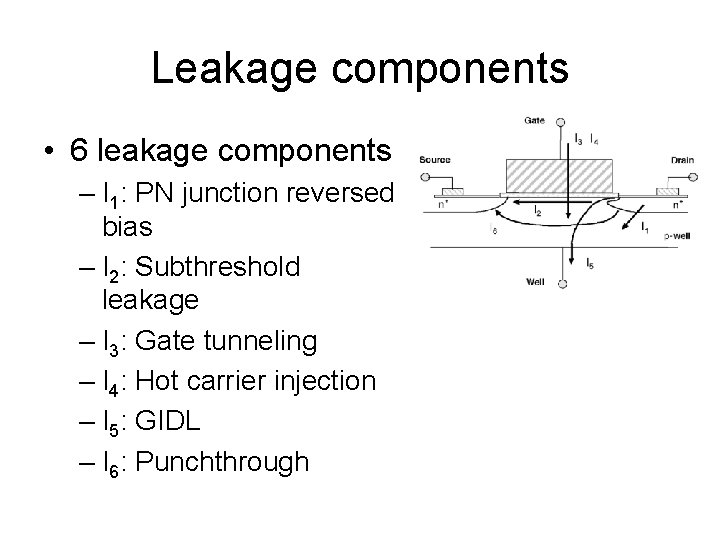
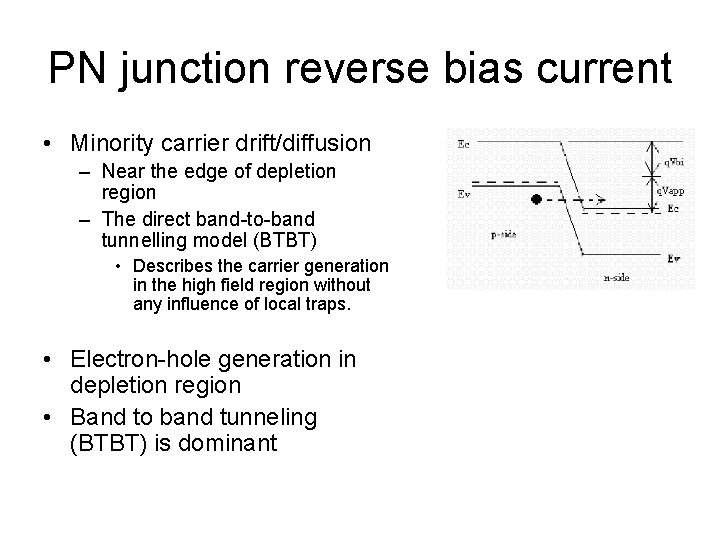
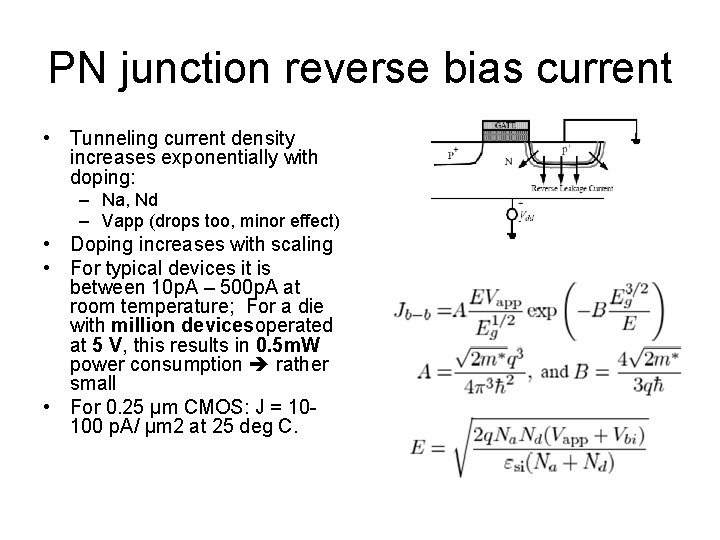
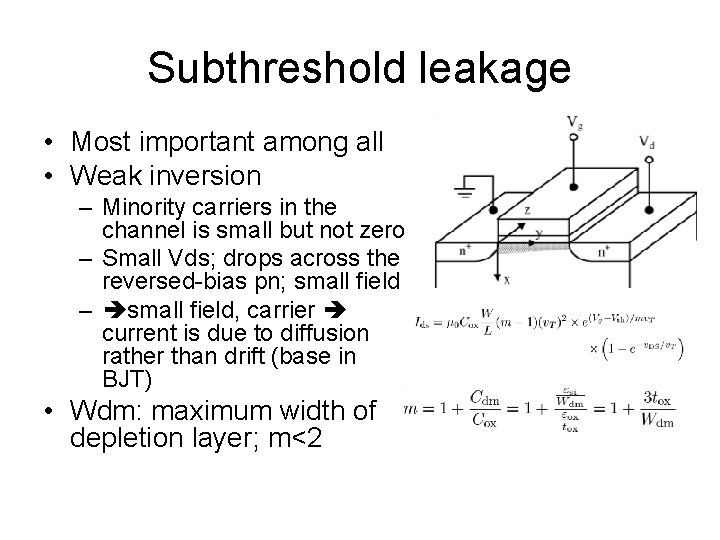
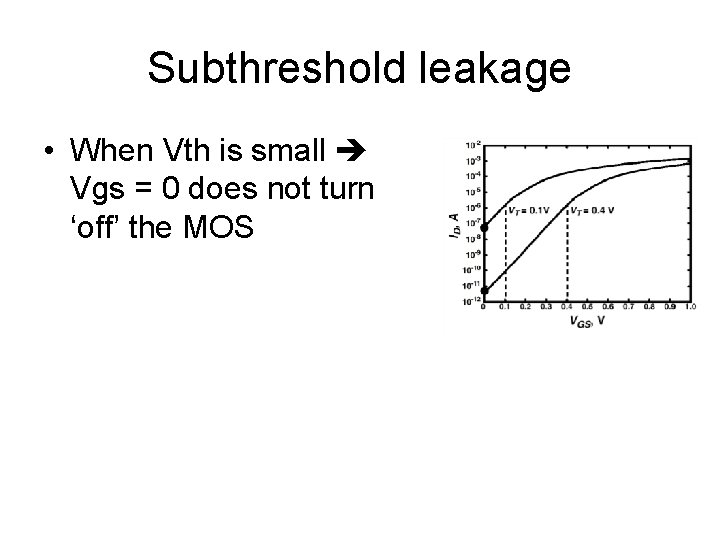
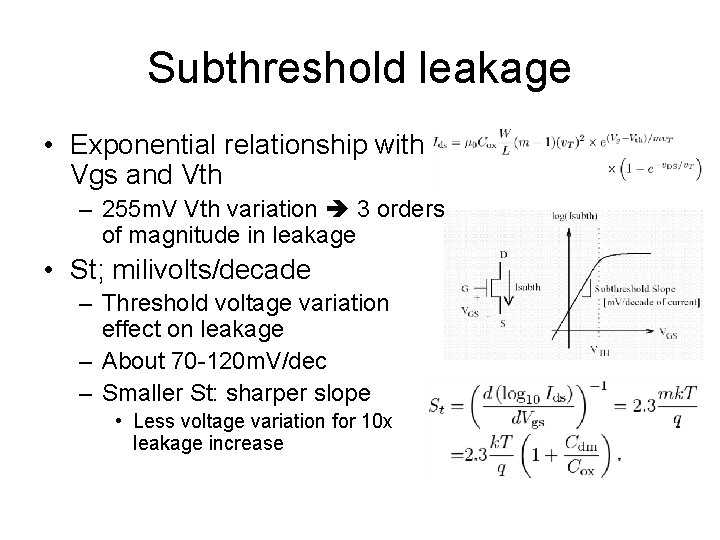
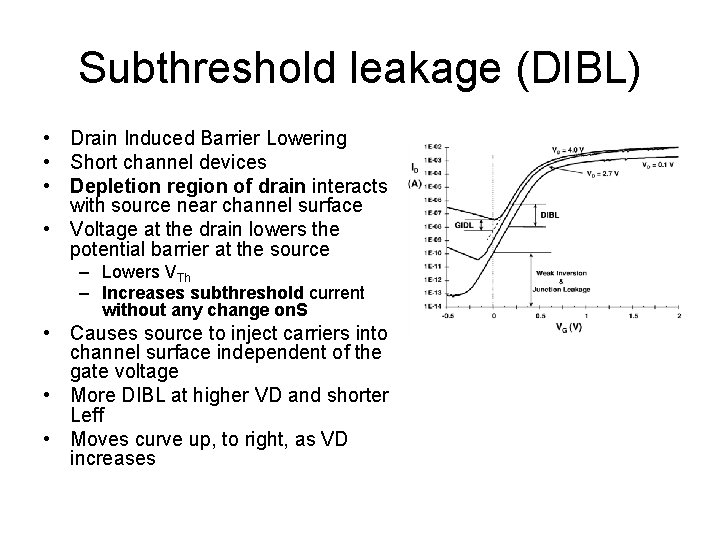
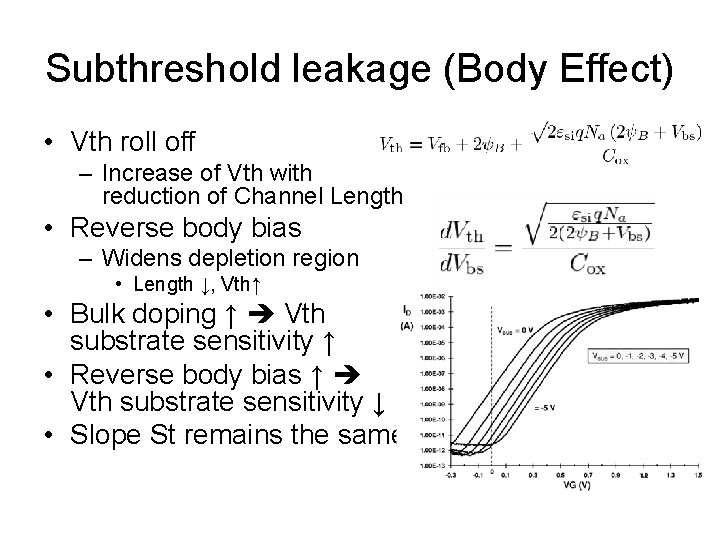
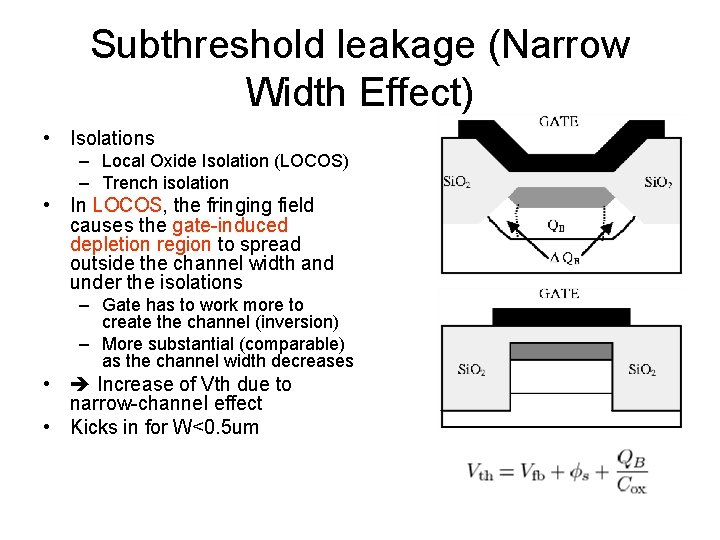
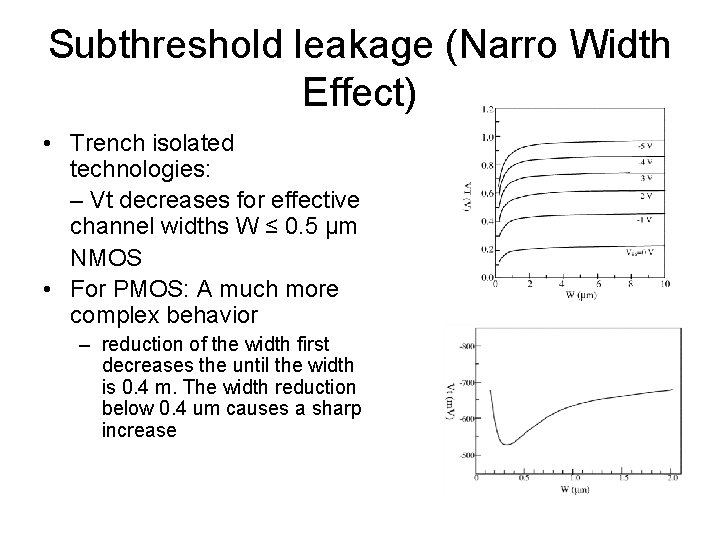
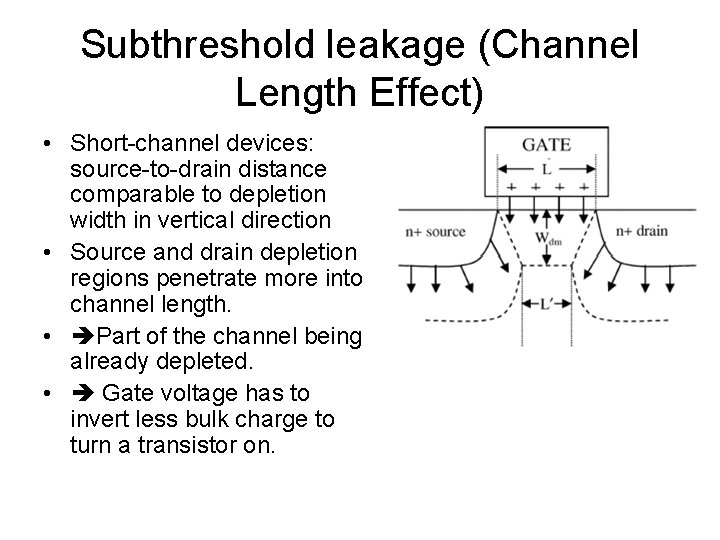
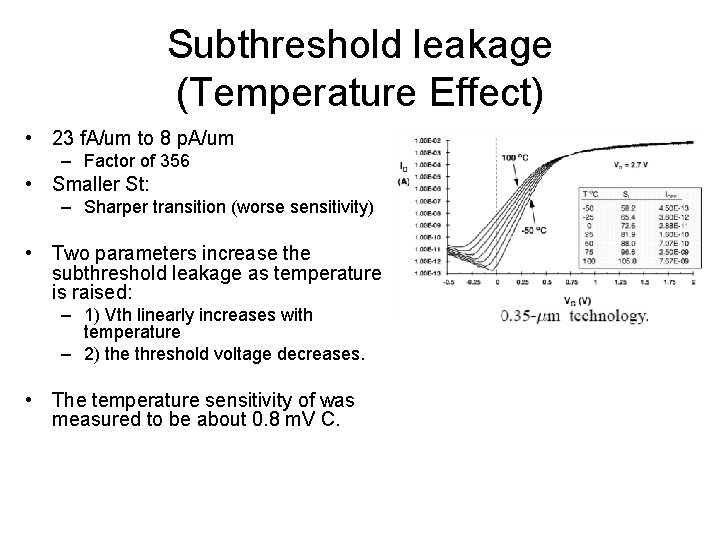
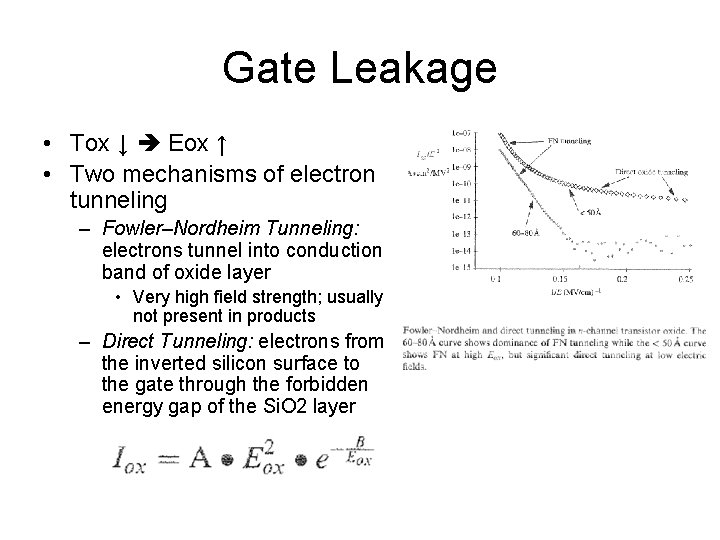
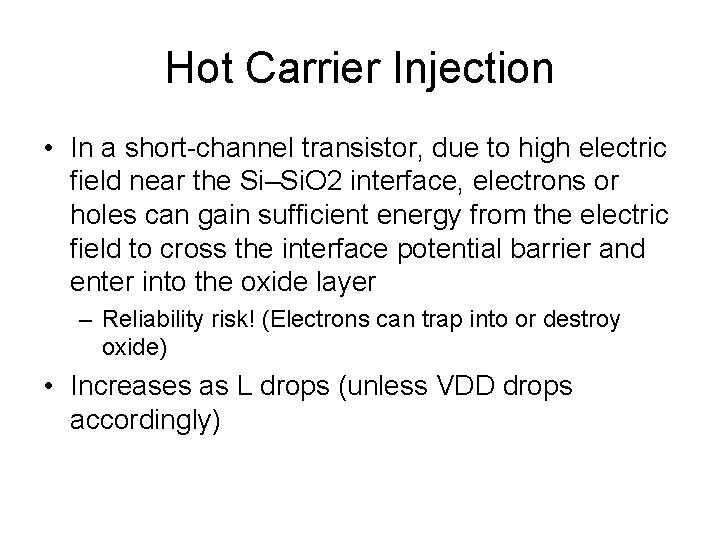
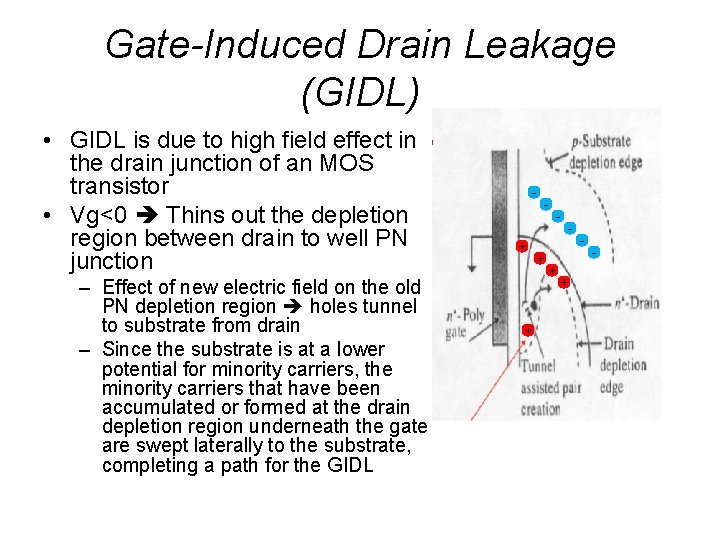
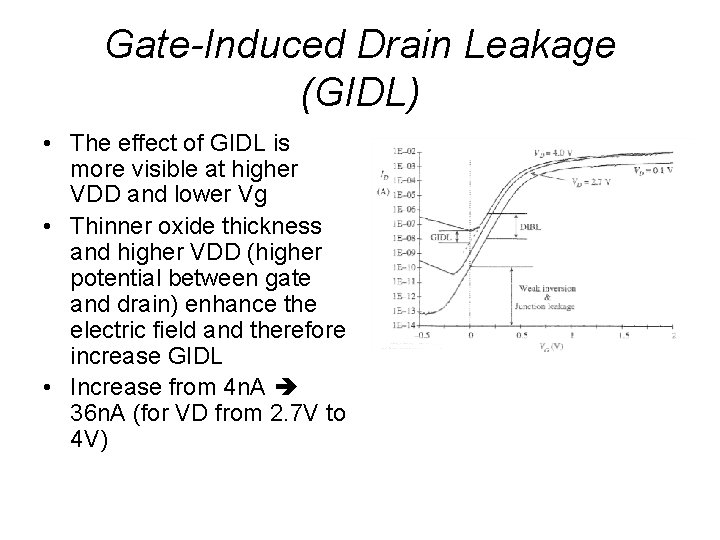
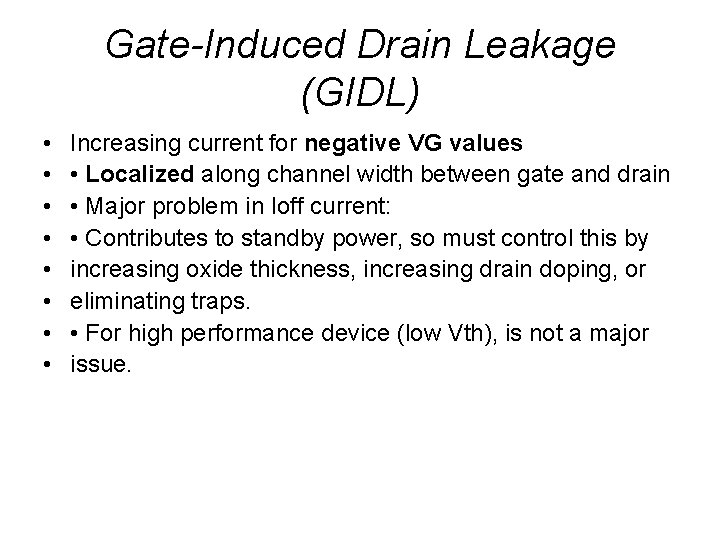
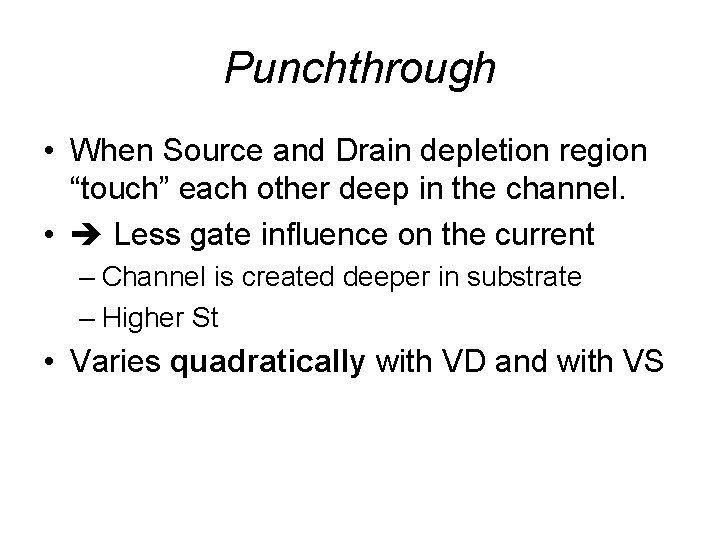
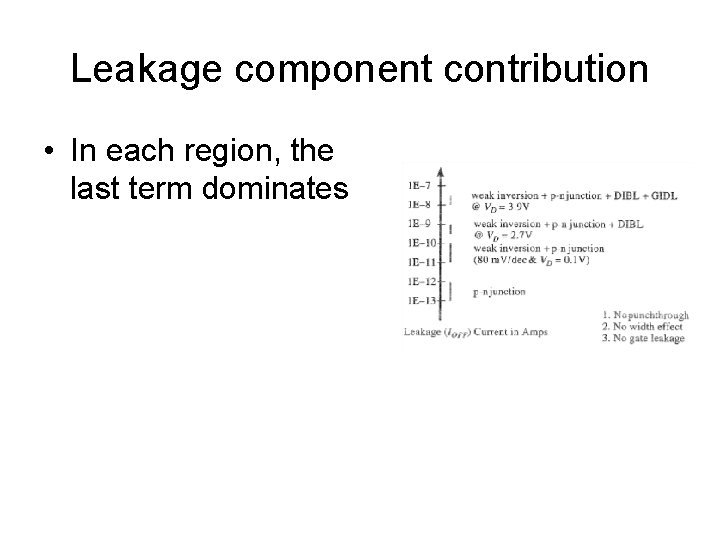
- Slides: 25
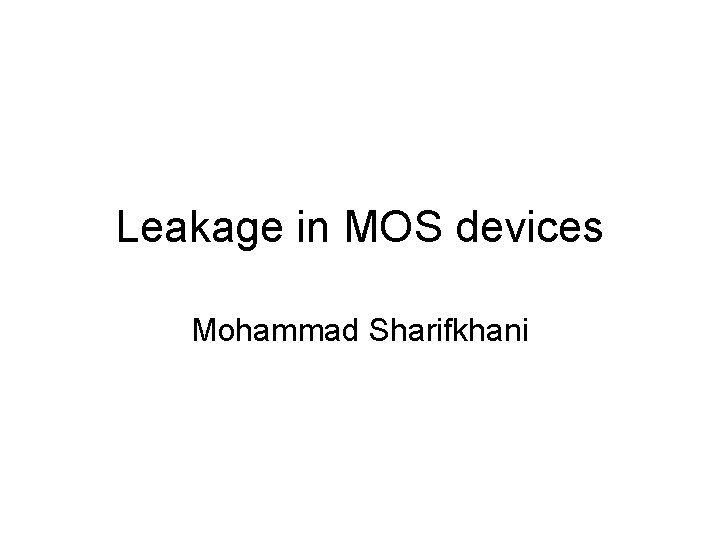
Leakage in MOS devices Mohammad Sharifkhani
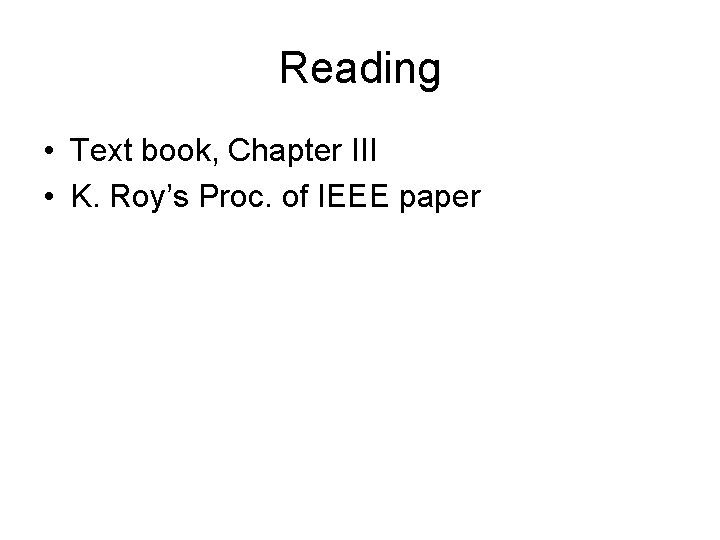
Reading • Text book, Chapter III • K. Roy’s Proc. of IEEE paper
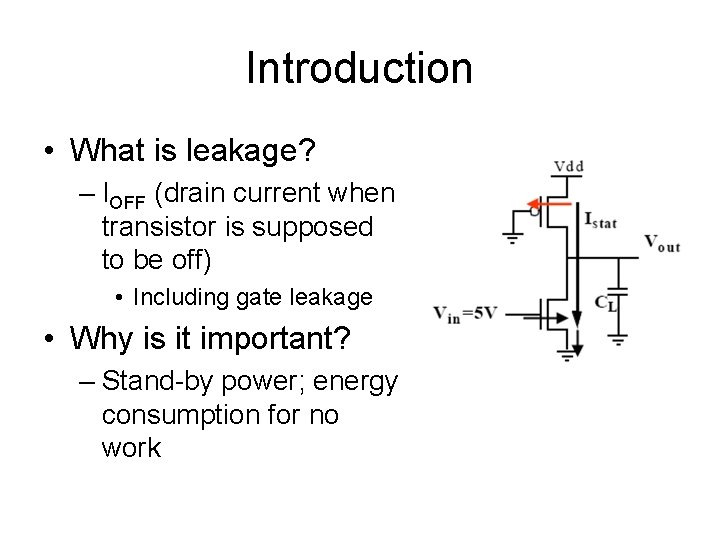
Introduction • What is leakage? – IOFF (drain current when transistor is supposed to be off) • Including gate leakage • Why is it important? – Stand-by power; energy consumption for no work
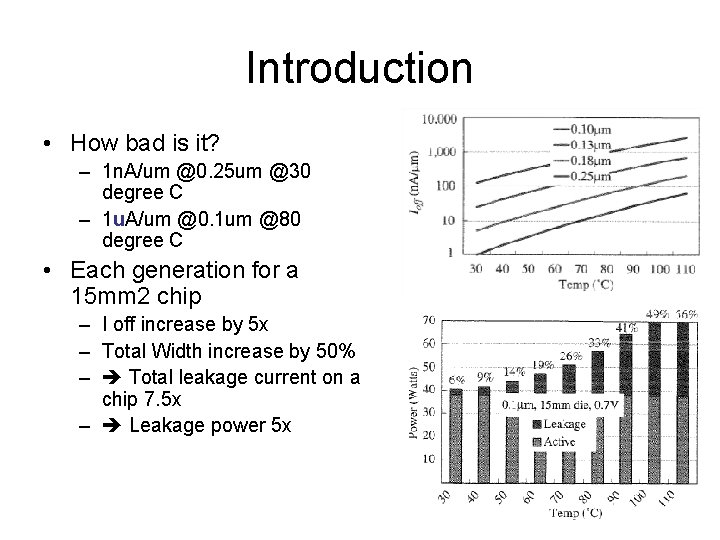
Introduction • How bad is it? – 1 n. A/um @0. 25 um @30 degree C – 1 u. A/um @0. 1 um @80 degree C • Each generation for a 15 mm 2 chip – I off increase by 5 x – Total Width increase by 50% – Total leakage current on a chip 7. 5 x – Leakage power 5 x
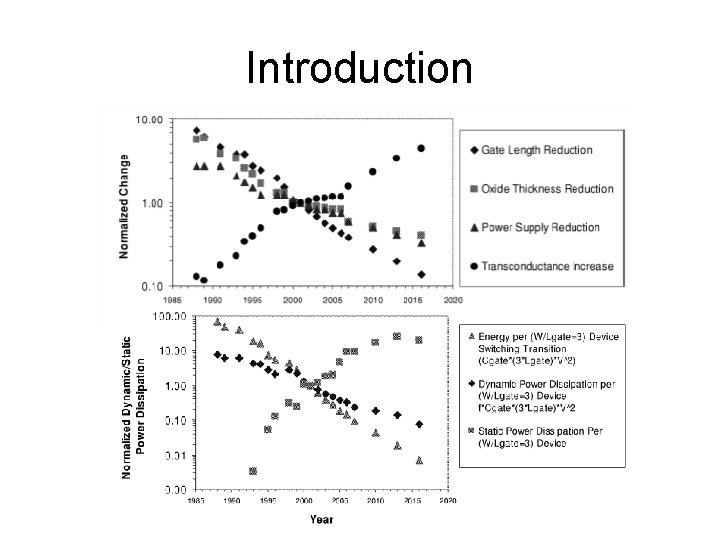
Introduction
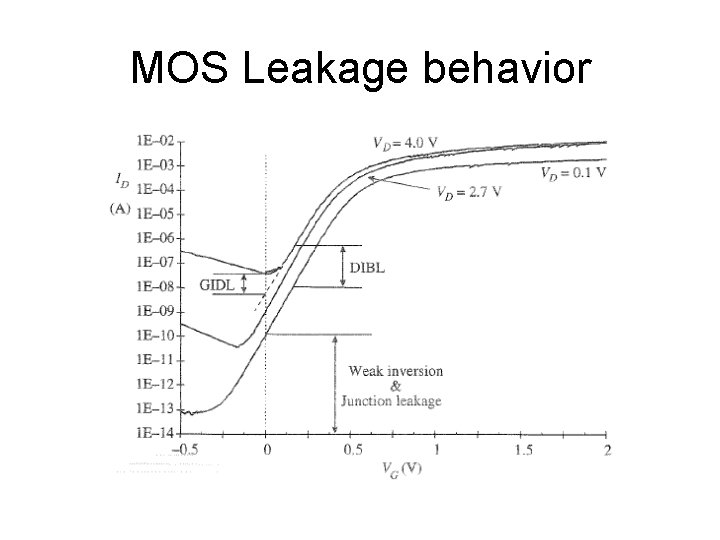
MOS Leakage behavior
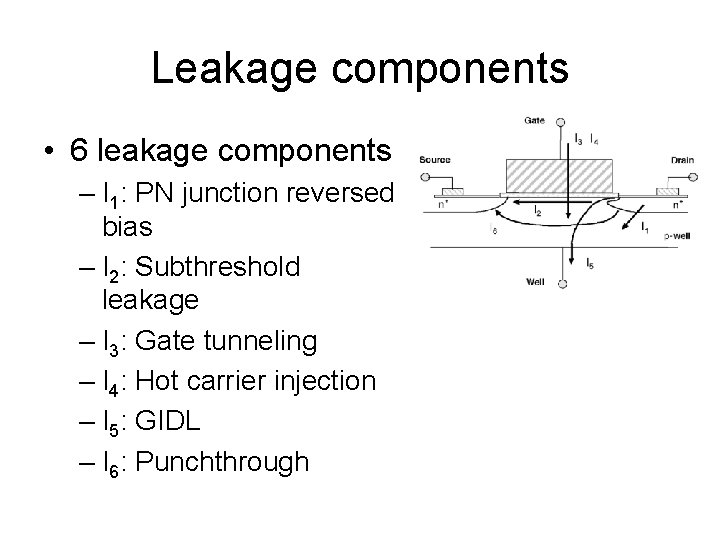
Leakage components • 6 leakage components – I 1: PN junction reversed bias – I 2: Subthreshold leakage – I 3: Gate tunneling – I 4: Hot carrier injection – I 5: GIDL – I 6: Punchthrough
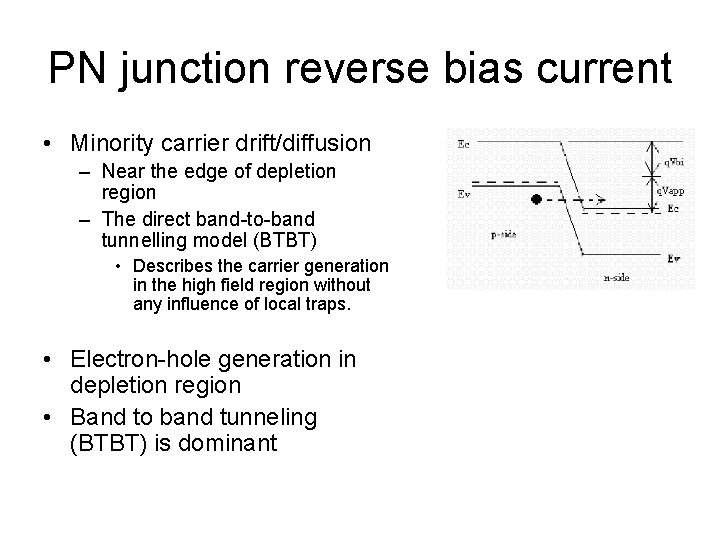
PN junction reverse bias current • Minority carrier drift/diffusion – Near the edge of depletion region – The direct band-to-band tunnelling model (BTBT) • Describes the carrier generation in the high field region without any influence of local traps. • Electron-hole generation in depletion region • Band to band tunneling (BTBT) is dominant
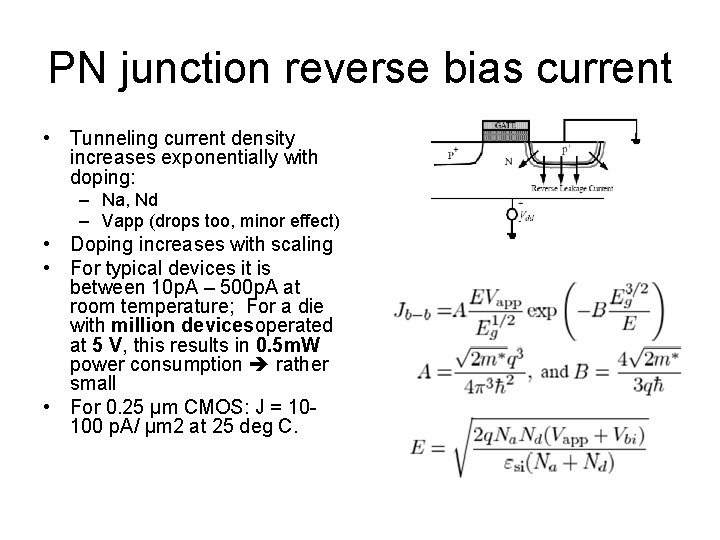
PN junction reverse bias current • Tunneling current density increases exponentially with doping: – Na, Nd – Vapp (drops too, minor effect) • Doping increases with scaling • For typical devices it is between 10 p. A – 500 p. A at room temperature; For a die with million devicesoperated at 5 V, this results in 0. 5 m. W power consumption rather small • For 0. 25 μm CMOS: J = 10100 p. A/ μm 2 at 25 deg C.
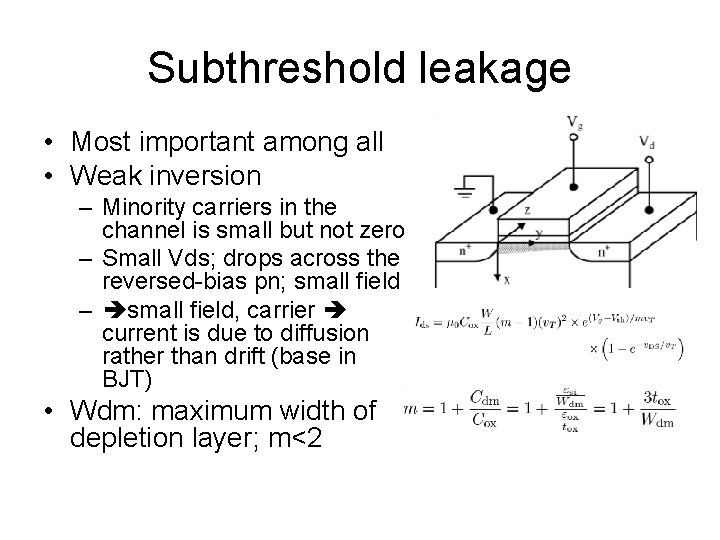
Subthreshold leakage • Most important among all • Weak inversion – Minority carriers in the channel is small but not zero – Small Vds; drops across the reversed-bias pn; small field – small field, carrier current is due to diffusion rather than drift (base in BJT) • Wdm: maximum width of depletion layer; m<2
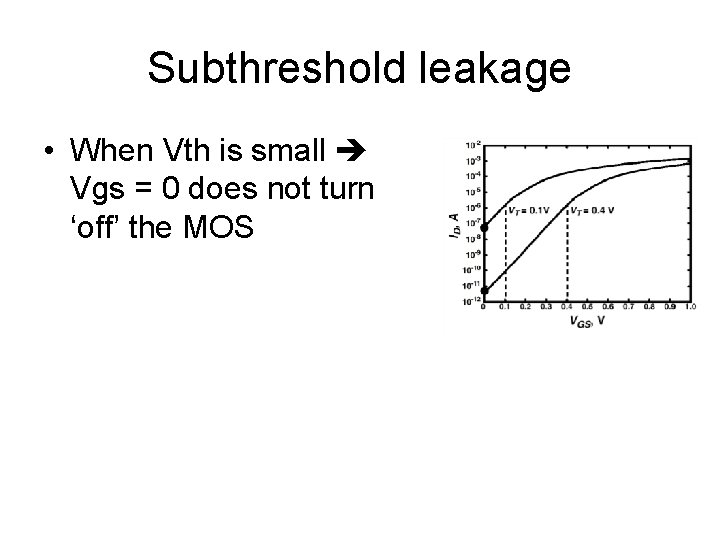
Subthreshold leakage • When Vth is small Vgs = 0 does not turn ‘off’ the MOS
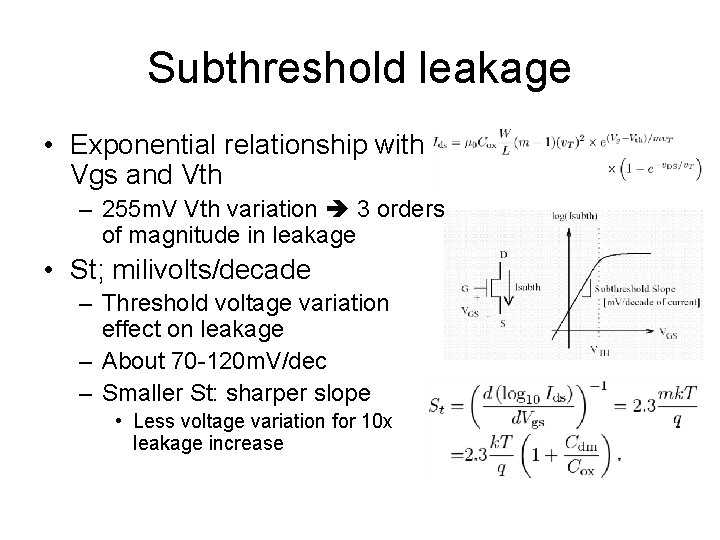
Subthreshold leakage • Exponential relationship with Vgs and Vth – 255 m. V Vth variation 3 orders of magnitude in leakage • St; milivolts/decade – Threshold voltage variation effect on leakage – About 70 -120 m. V/dec – Smaller St: sharper slope • Less voltage variation for 10 x leakage increase
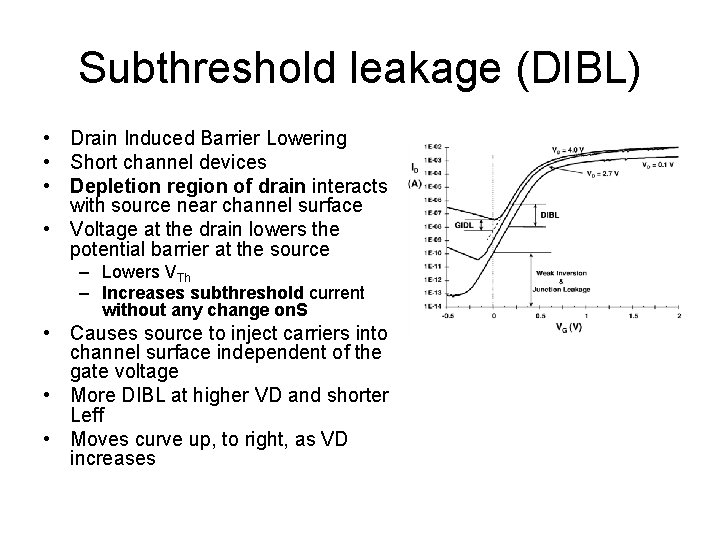
Subthreshold leakage (DIBL) • Drain Induced Barrier Lowering • Short channel devices • Depletion region of drain interacts with source near channel surface • Voltage at the drain lowers the potential barrier at the source – Lowers VTh – Increases subthreshold current without any change on. S • Causes source to inject carriers into channel surface independent of the gate voltage • More DIBL at higher VD and shorter Leff • Moves curve up, to right, as VD increases
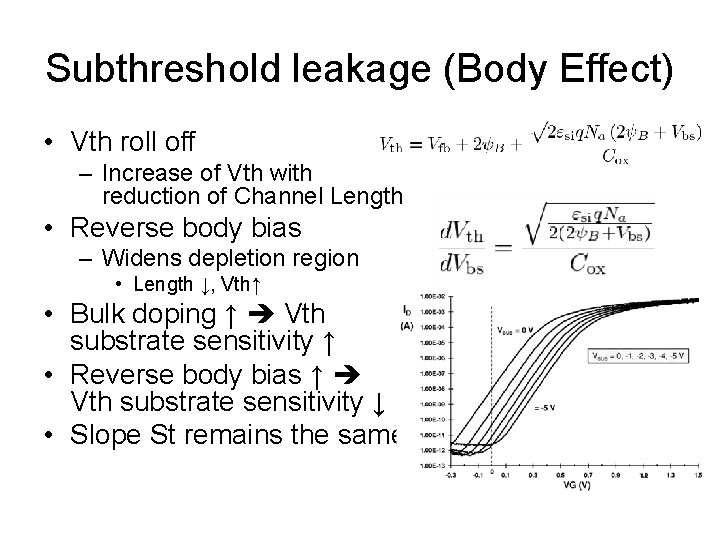
Subthreshold leakage (Body Effect) • Vth roll off – Increase of Vth with reduction of Channel Length • Reverse body bias – Widens depletion region • Length ↓, Vth↑ • Bulk doping ↑ Vth substrate sensitivity ↑ • Reverse body bias ↑ Vth substrate sensitivity ↓ • Slope St remains the same
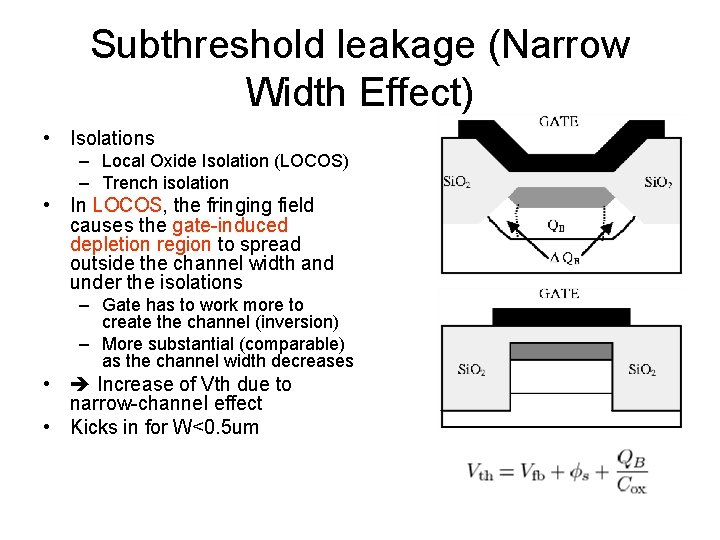
Subthreshold leakage (Narrow Width Effect) • Isolations – Local Oxide Isolation (LOCOS) – Trench isolation • In LOCOS, the fringing field causes the gate-induced depletion region to spread outside the channel width and under the isolations – Gate has to work more to create the channel (inversion) – More substantial (comparable) as the channel width decreases • Increase of Vth due to narrow-channel effect • Kicks in for W<0. 5 um
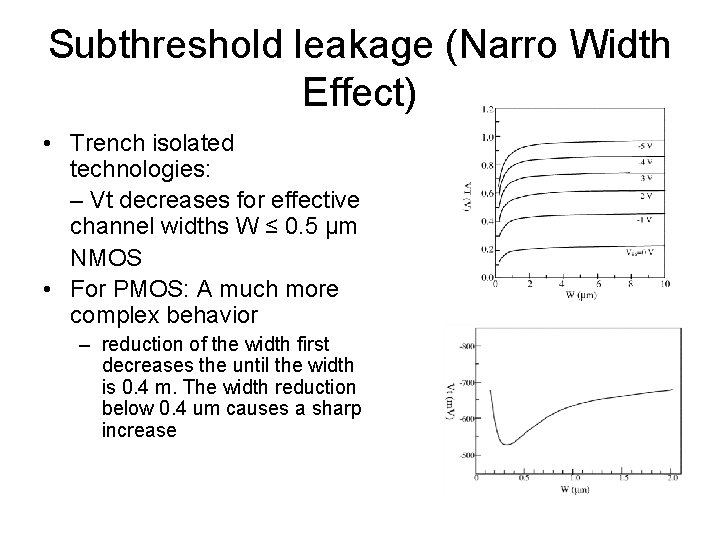
Subthreshold leakage (Narro Width Effect) • Trench isolated technologies: – Vt decreases for effective channel widths W ≤ 0. 5 μm NMOS • For PMOS: A much more complex behavior – reduction of the width first decreases the until the width is 0. 4 m. The width reduction below 0. 4 um causes a sharp increase
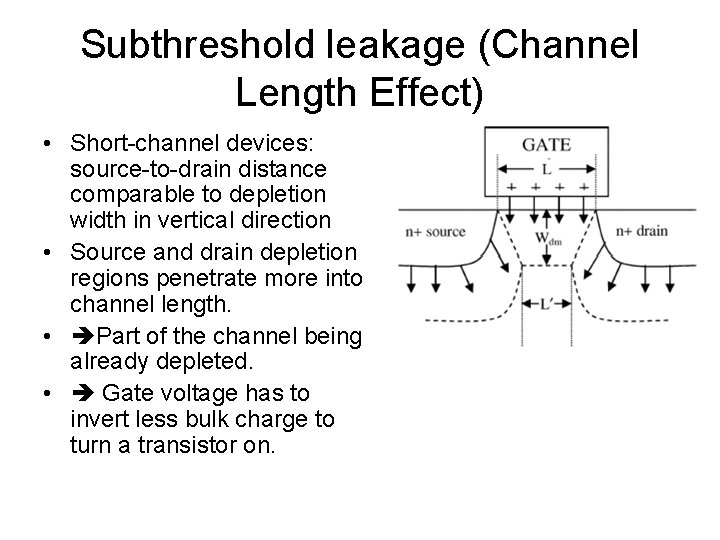
Subthreshold leakage (Channel Length Effect) • Short-channel devices: source-to-drain distance comparable to depletion width in vertical direction • Source and drain depletion regions penetrate more into channel length. • Part of the channel being already depleted. • Gate voltage has to invert less bulk charge to turn a transistor on.
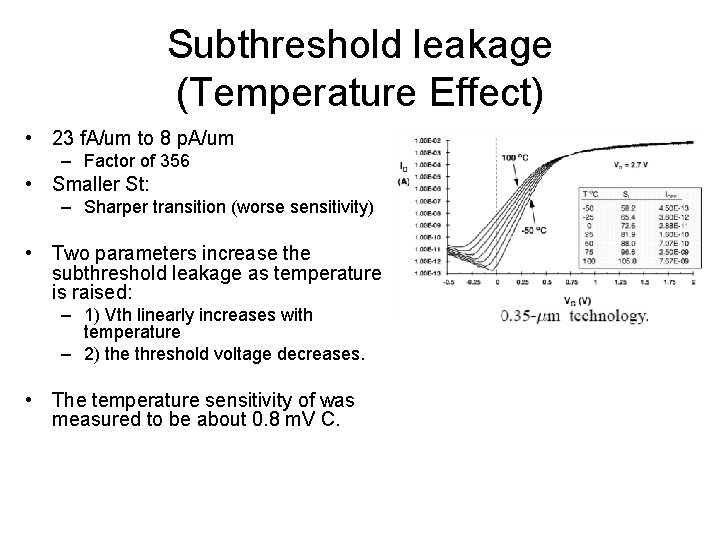
Subthreshold leakage (Temperature Effect) • 23 f. A/um to 8 p. A/um – Factor of 356 • Smaller St: – Sharper transition (worse sensitivity) • Two parameters increase the subthreshold leakage as temperature is raised: – 1) Vth linearly increases with temperature – 2) the threshold voltage decreases. • The temperature sensitivity of was measured to be about 0. 8 m. V C.
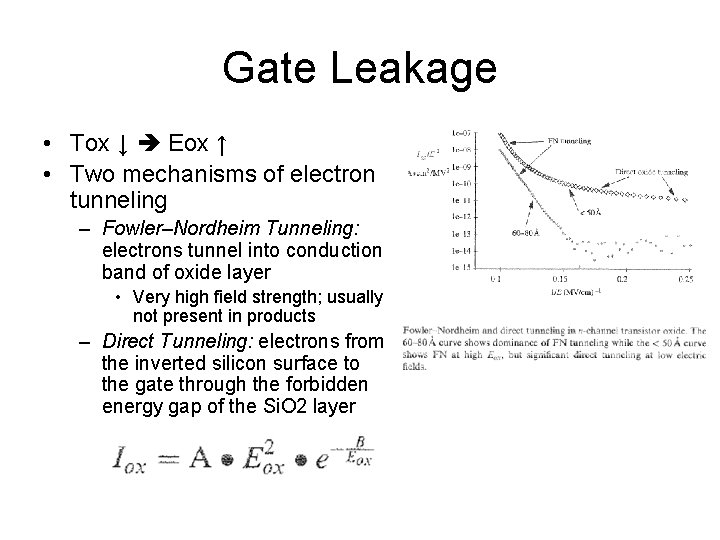
Gate Leakage • Tox ↓ Eox ↑ • Two mechanisms of electron tunneling – Fowler–Nordheim Tunneling: electrons tunnel into conduction band of oxide layer • Very high field strength; usually not present in products – Direct Tunneling: electrons from the inverted silicon surface to the gate through the forbidden energy gap of the Si. O 2 layer
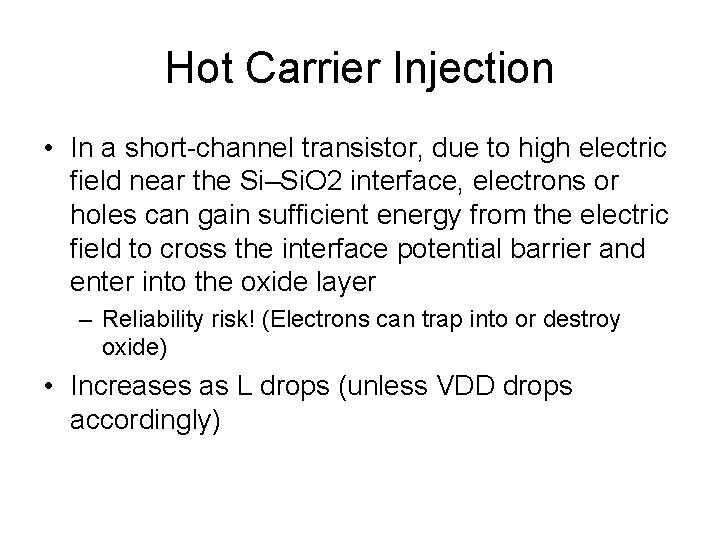
Hot Carrier Injection • In a short-channel transistor, due to high electric field near the Si–Si. O 2 interface, electrons or holes can gain sufficient energy from the electric field to cross the interface potential barrier and enter into the oxide layer – Reliability risk! (Electrons can trap into or destroy oxide) • Increases as L drops (unless VDD drops accordingly)
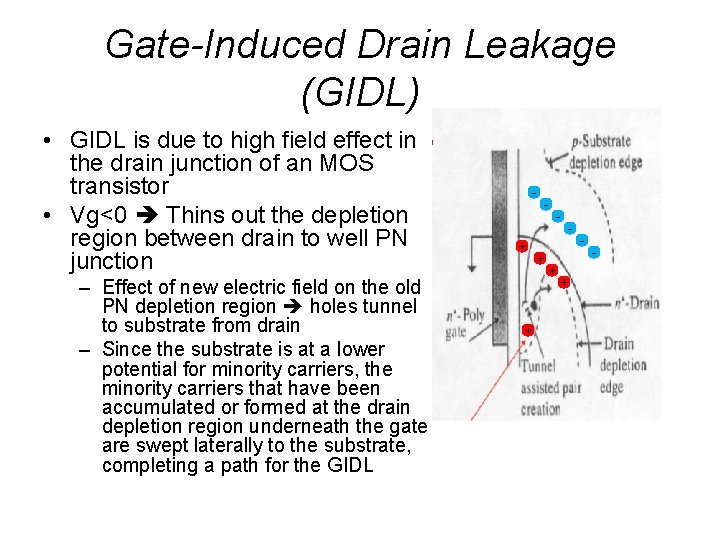
Gate-Induced Drain Leakage (GIDL) • GIDL is due to high field effect in the drain junction of an MOS transistor • Vg<0 Thins out the depletion region between drain to well PN junction – Effect of new electric field on the old PN depletion region holes tunnel to substrate from drain – Since the substrate is at a lower potential for minority carriers, the minority carriers that have been accumulated or formed at the drain depletion region underneath the gate are swept laterally to the substrate, completing a path for the GIDL - + + - + - -
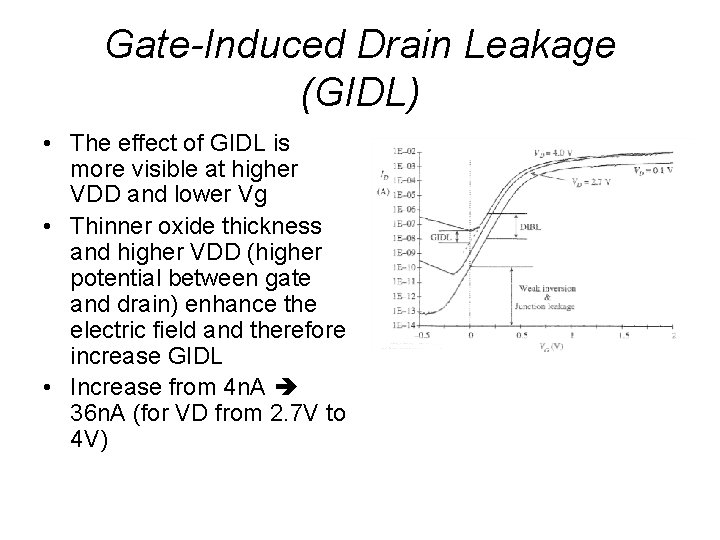
Gate-Induced Drain Leakage (GIDL) • The effect of GIDL is more visible at higher VDD and lower Vg • Thinner oxide thickness and higher VDD (higher potential between gate and drain) enhance the electric field and therefore increase GIDL • Increase from 4 n. A 36 n. A (for VD from 2. 7 V to 4 V)
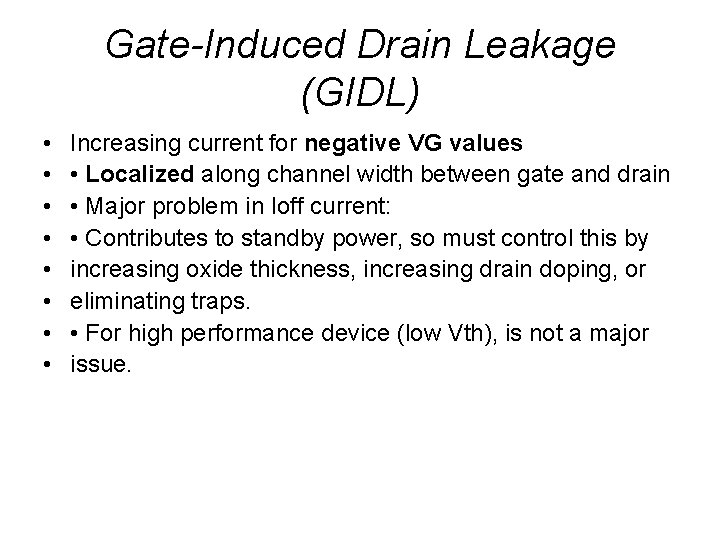
Gate-Induced Drain Leakage (GIDL) • • Increasing current for negative VG values • Localized along channel width between gate and drain • Major problem in Ioff current: • Contributes to standby power, so must control this by increasing oxide thickness, increasing drain doping, or eliminating traps. • For high performance device (low Vth), is not a major issue.
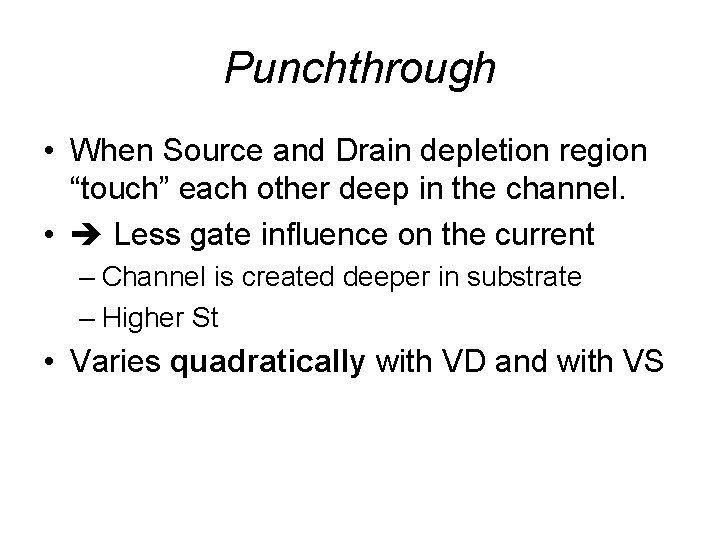
Punchthrough • When Source and Drain depletion region “touch” each other deep in the channel. • Less gate influence on the current – Channel is created deeper in substrate – Higher St • Varies quadratically with VD and with VS
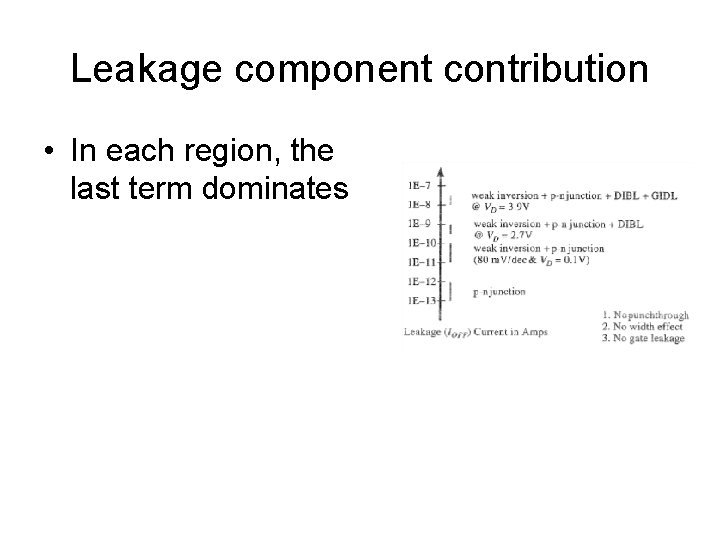
Leakage component contribution • In each region, the last term dominates
Mohammad sharifkhani
Mohammad sharifkhani
Mohammad sharifkhani
Ali sharifkhani
Ali sharifkhani
Making connections images
While reading activities
Mos text bulletins
Shaik mohammad tajuddin
Mohammad vesal
Mohammad ali javidian
Dr nur mohammad hadi zahalan
Pearo-nn
Dr mohammad khan
Dr mohammad aman
Dr mohammad aman
Mohammad ghoreishi
Wali mohammad md
Mohammad diab md
Alaa mohammad fouad
Vlsi
Mohammad arjomand
Mohammad ridwan bkn
Mohammad davidson
Mohammad sadegh rasooli
Mohammad alipour