Glycolysis Gluconeogenesis 1 G RT ln QK RT
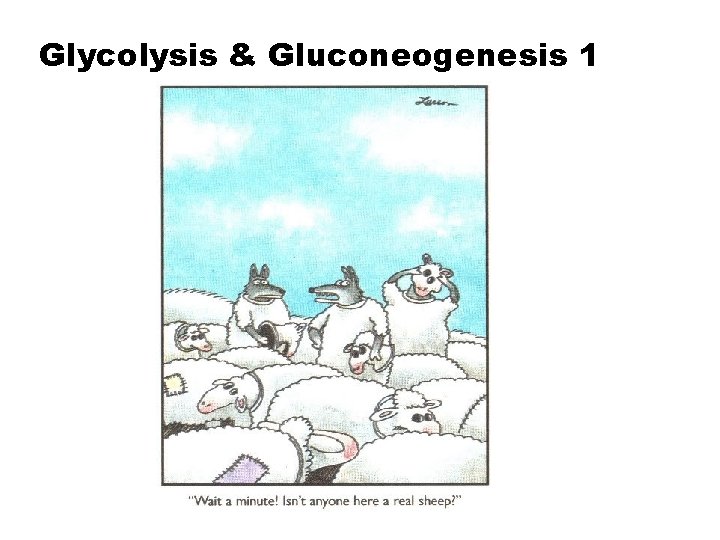
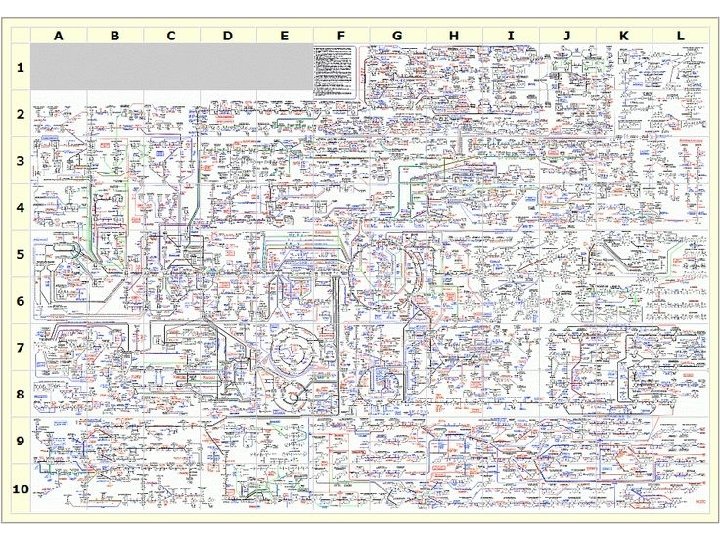
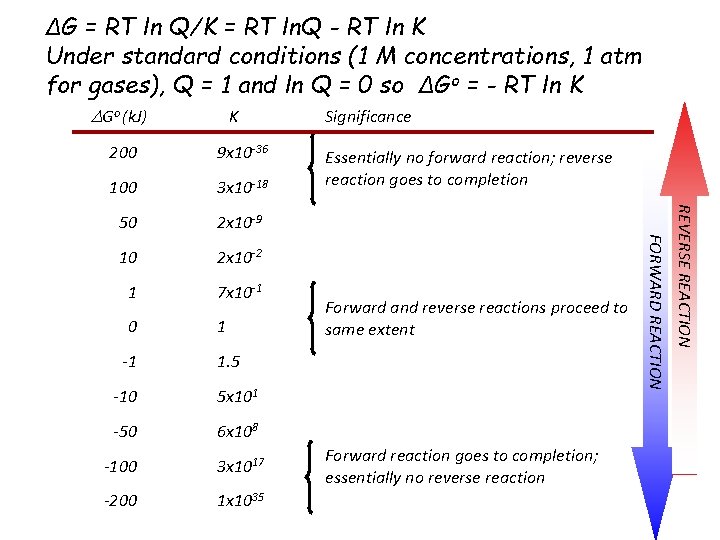
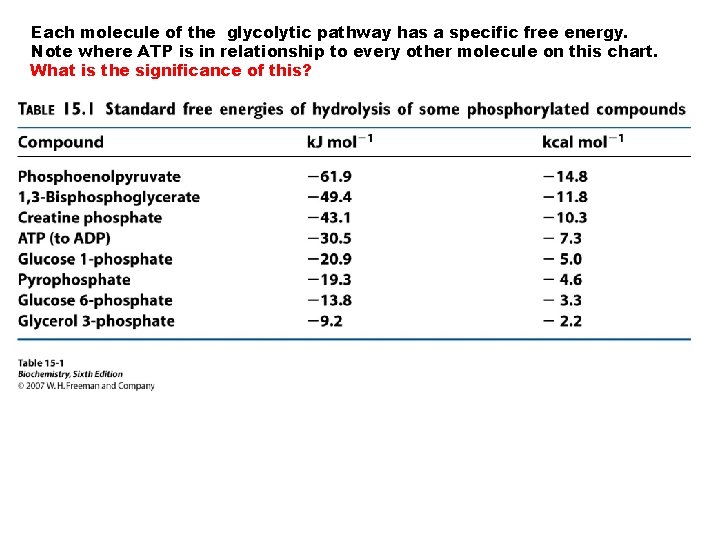
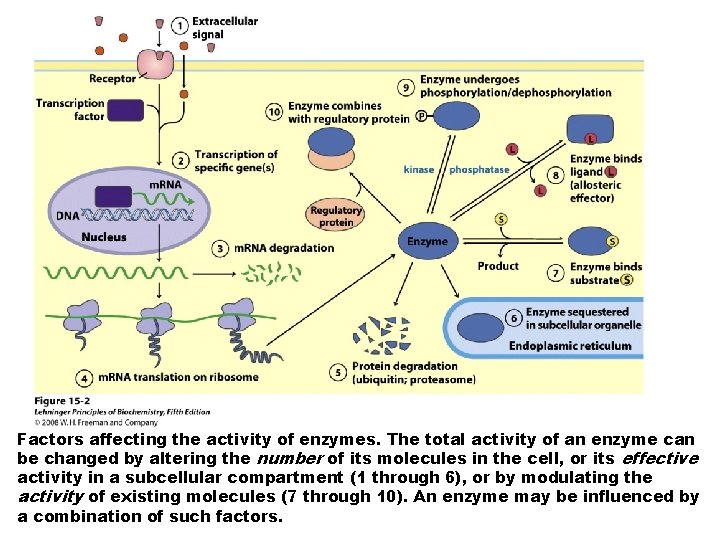
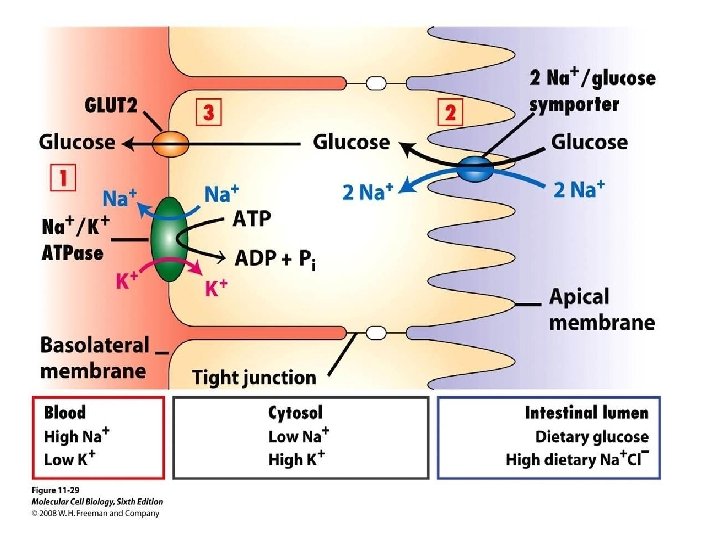
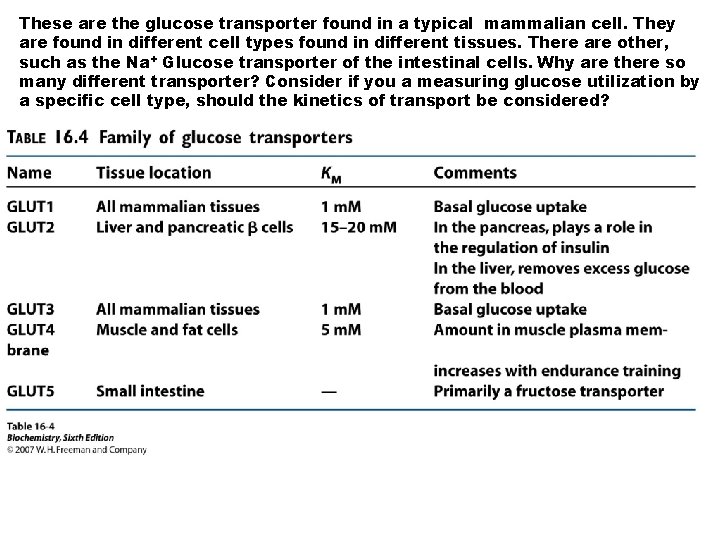
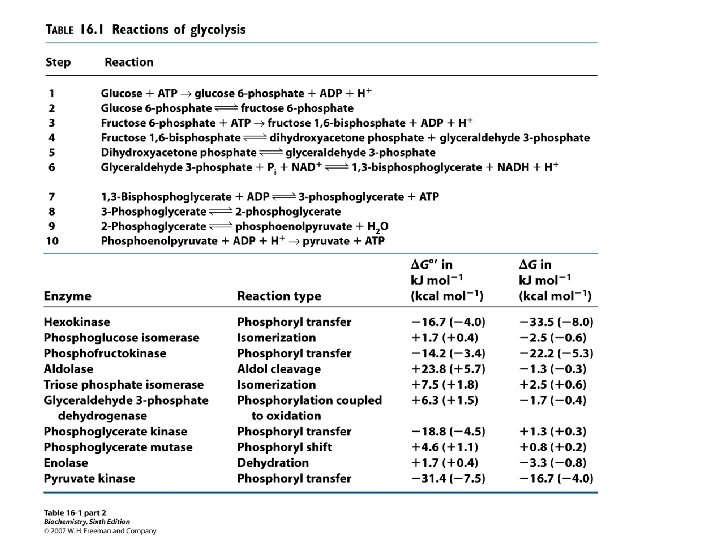
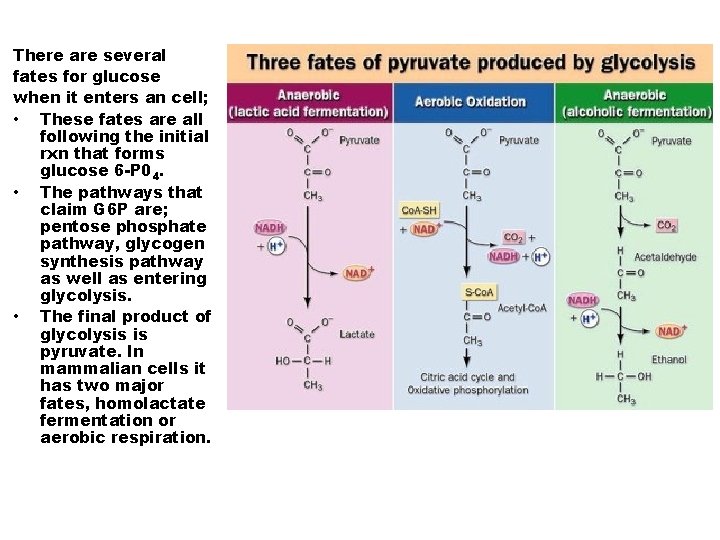
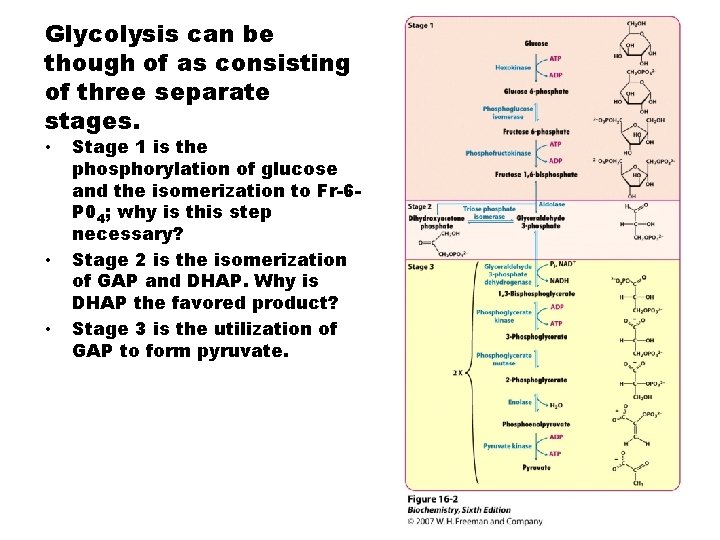
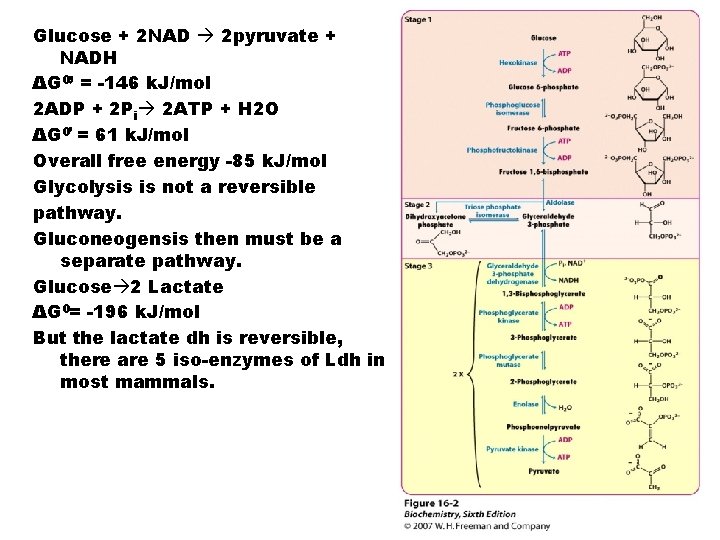
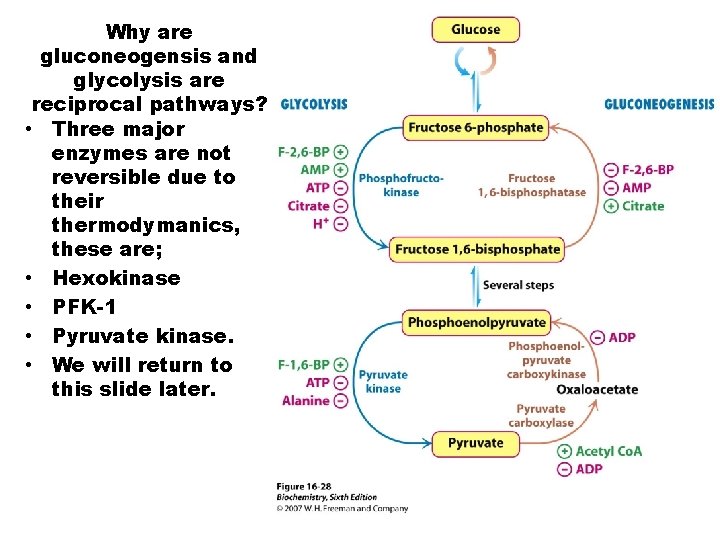
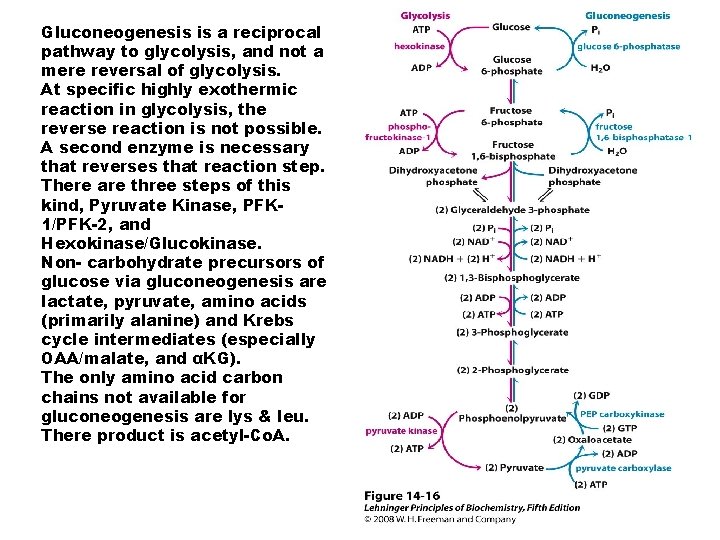
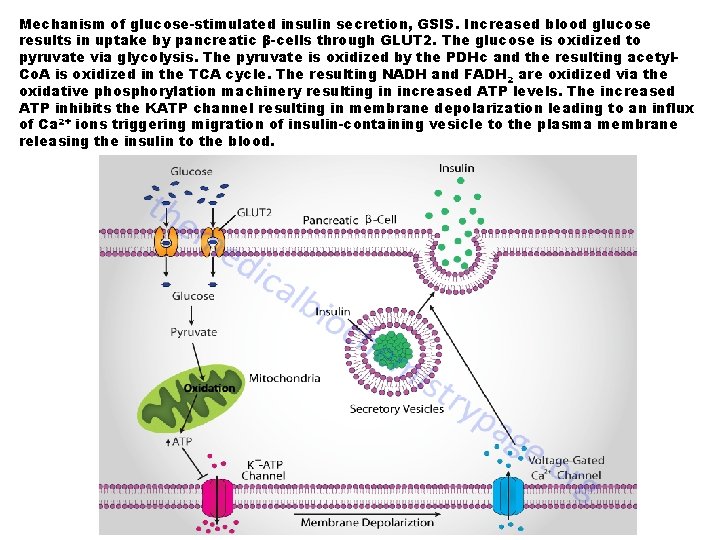
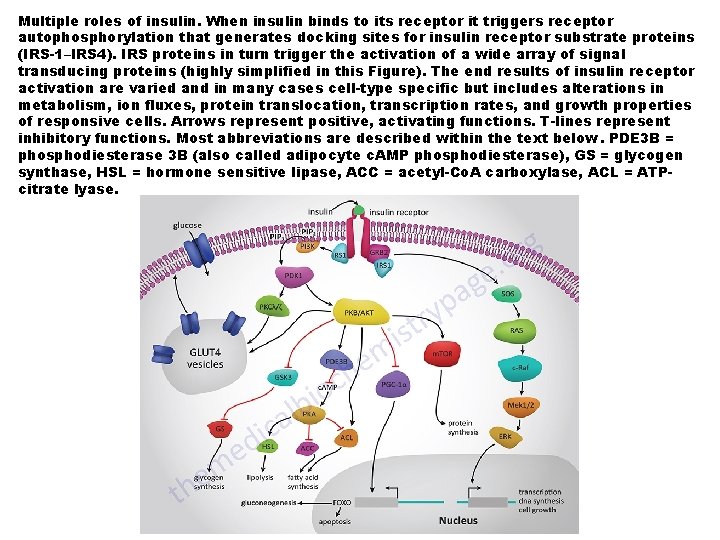
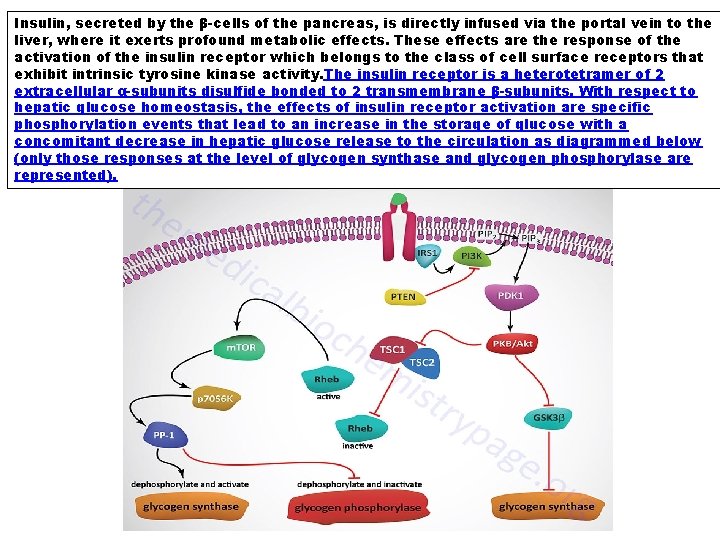
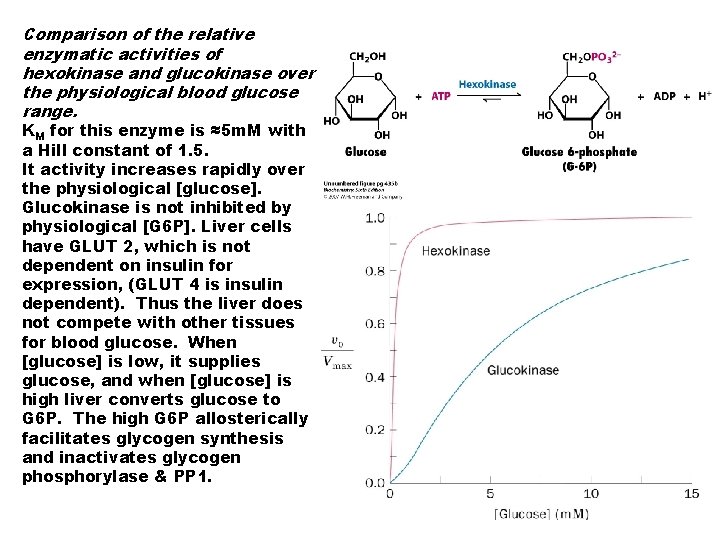
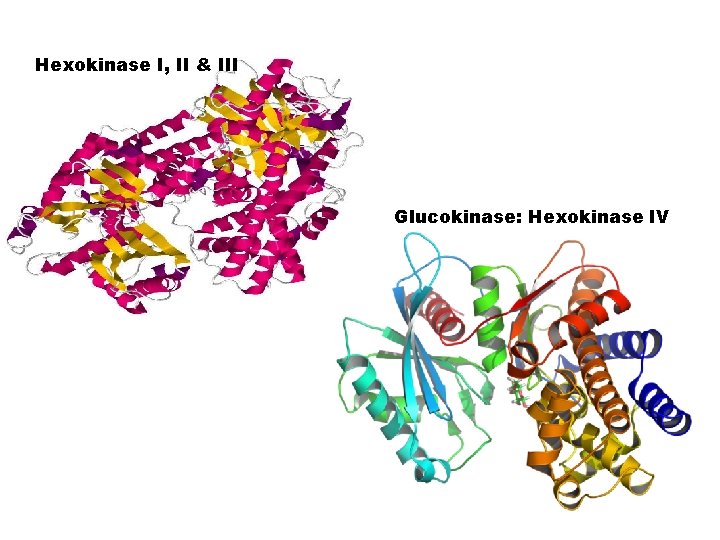
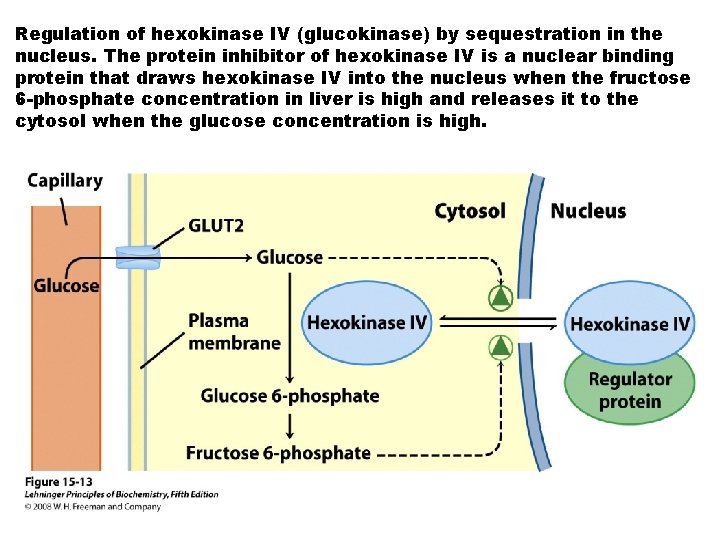
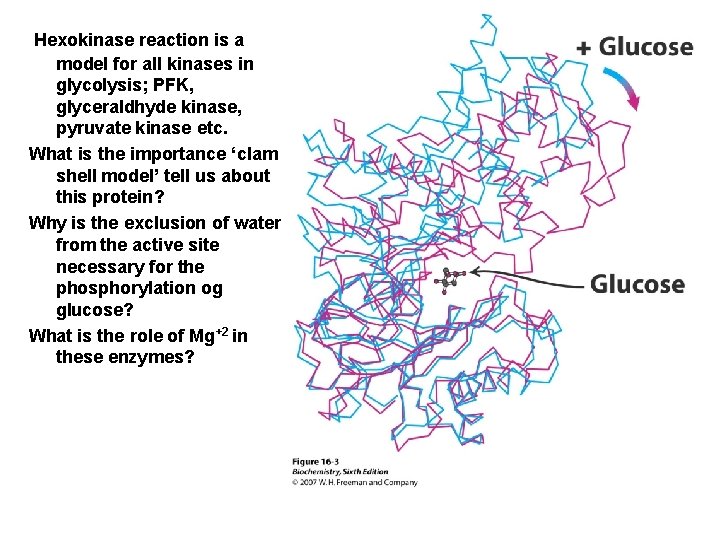
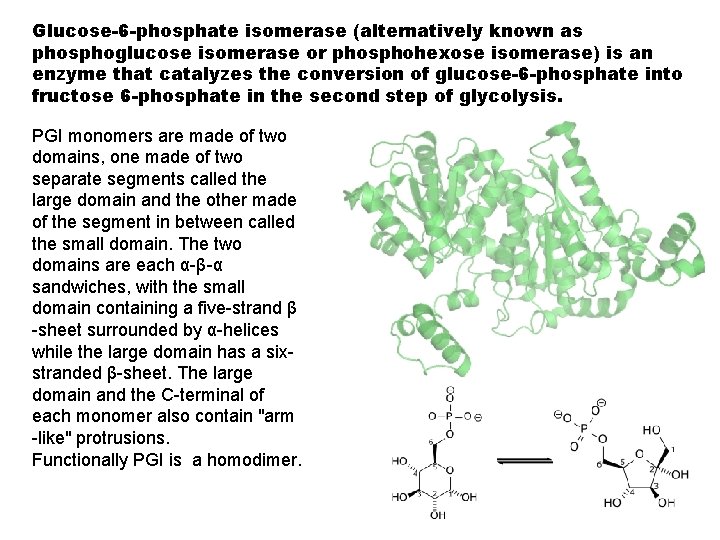
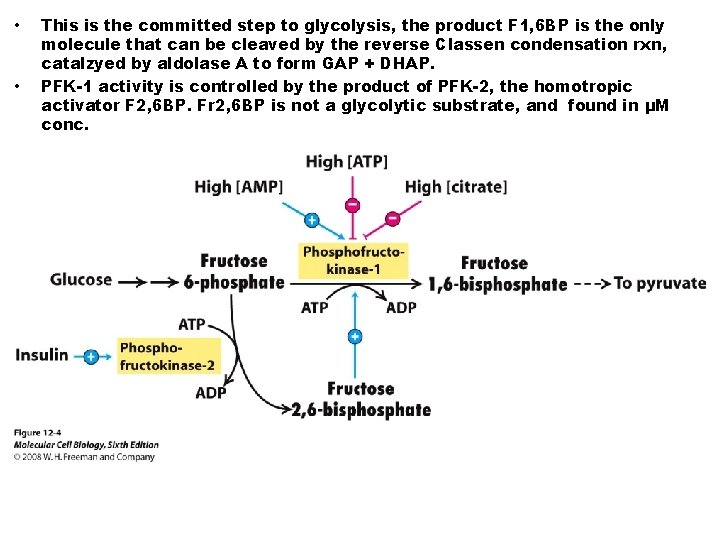
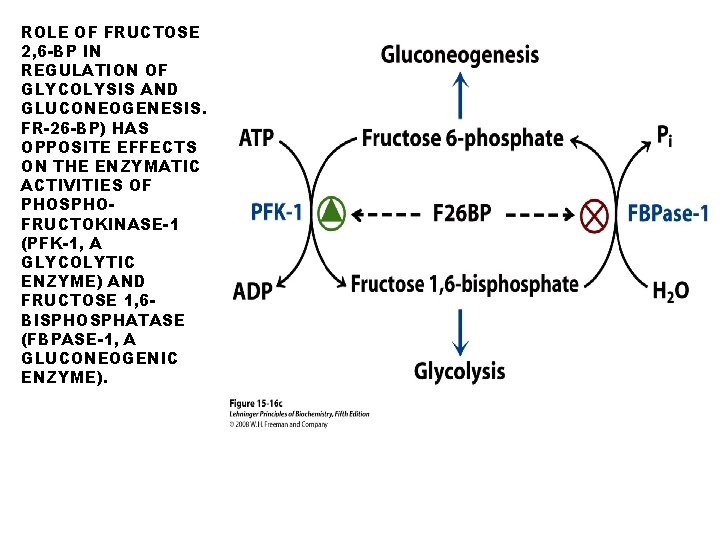
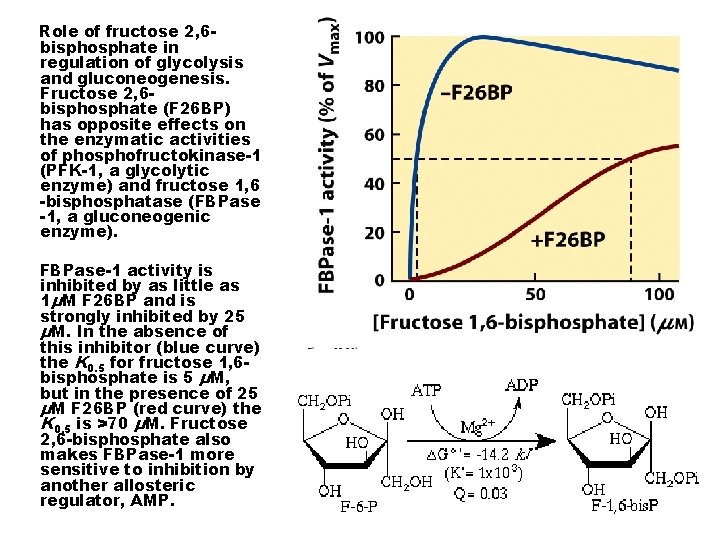
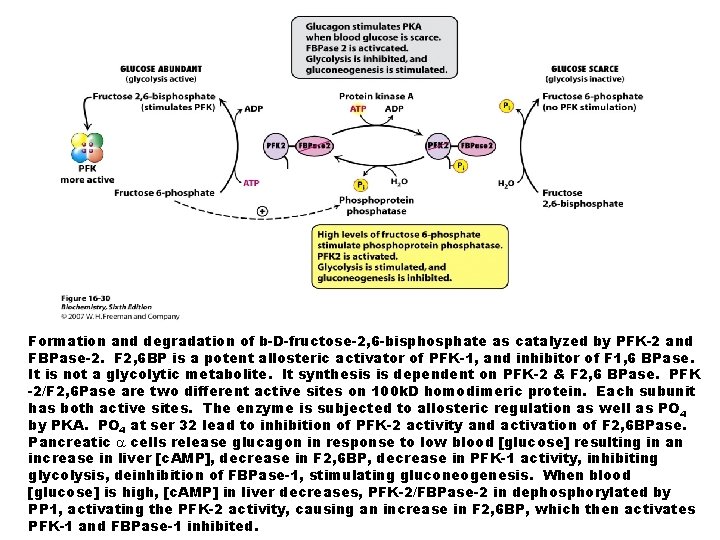
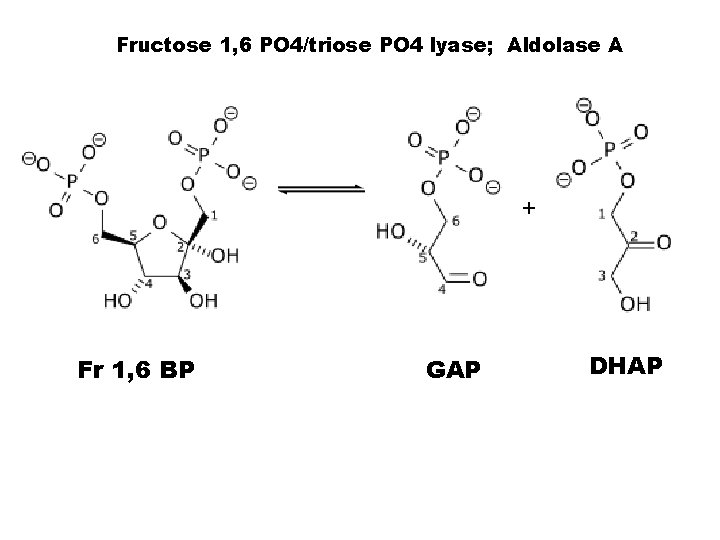
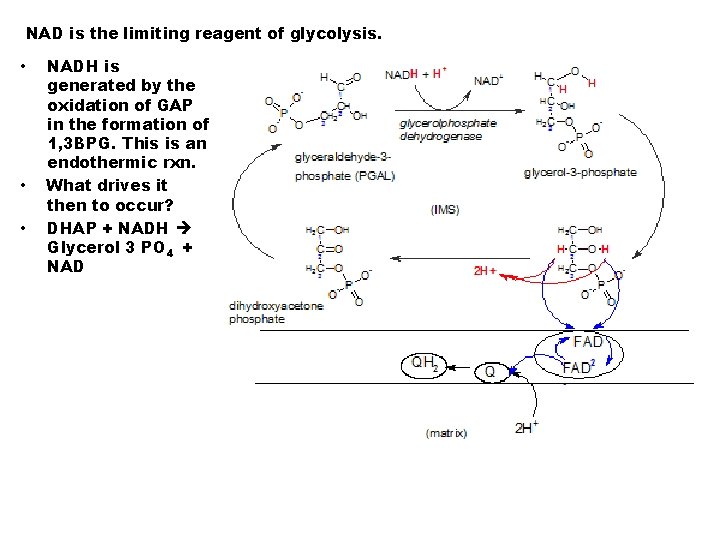
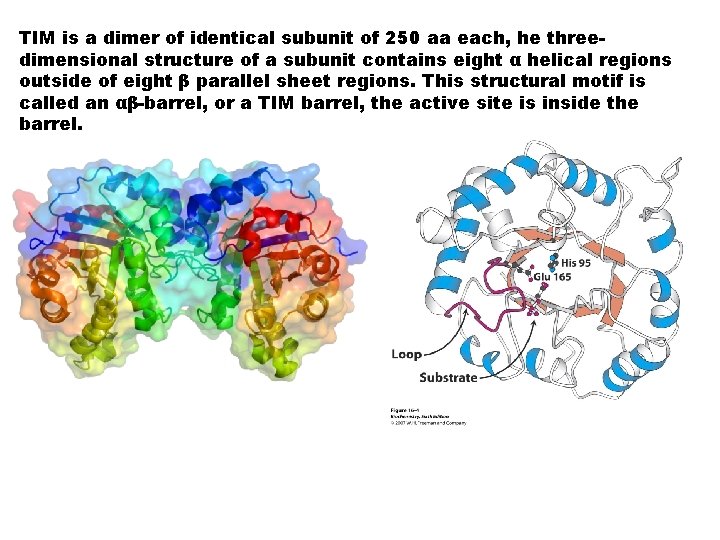
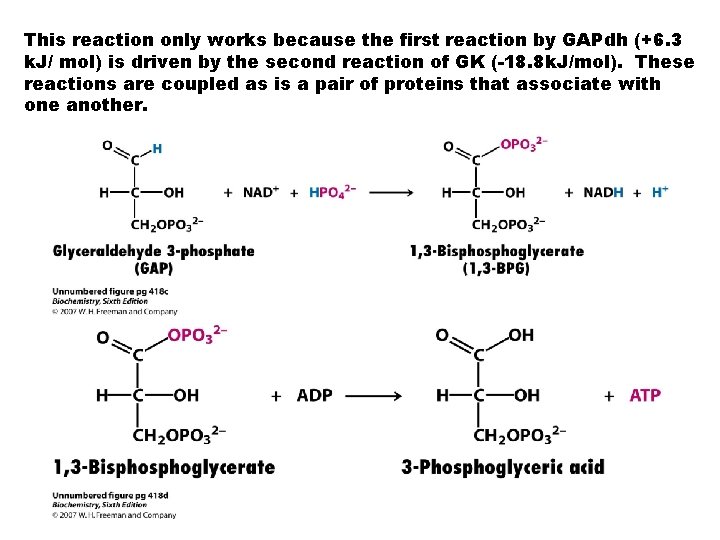
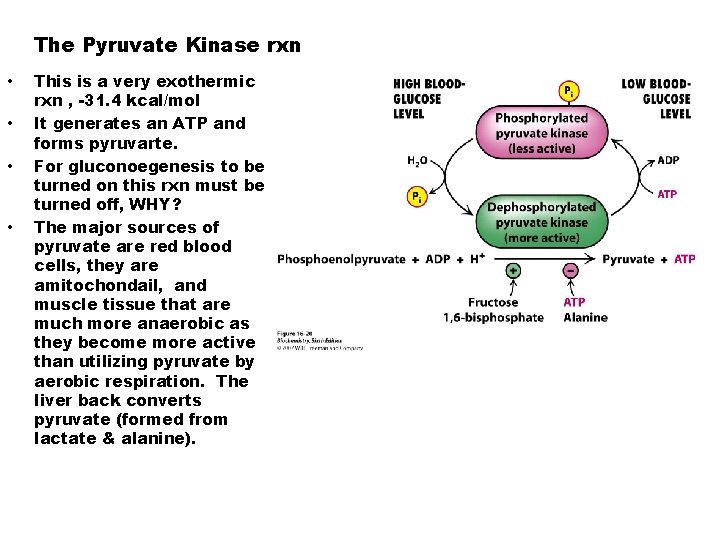
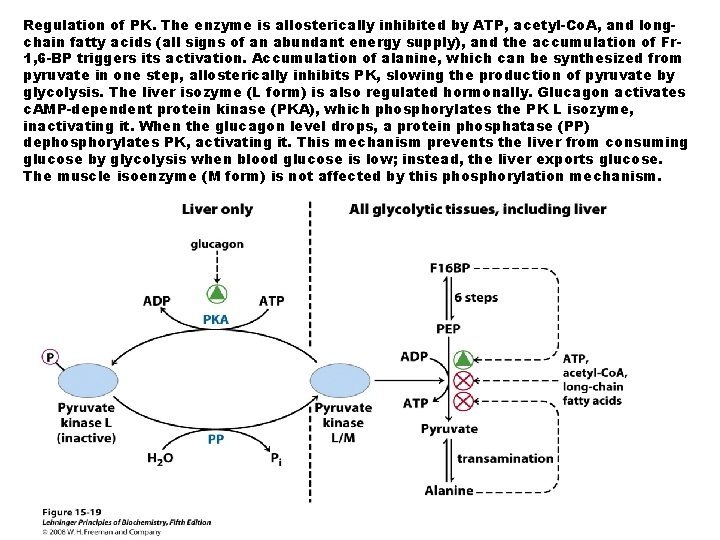
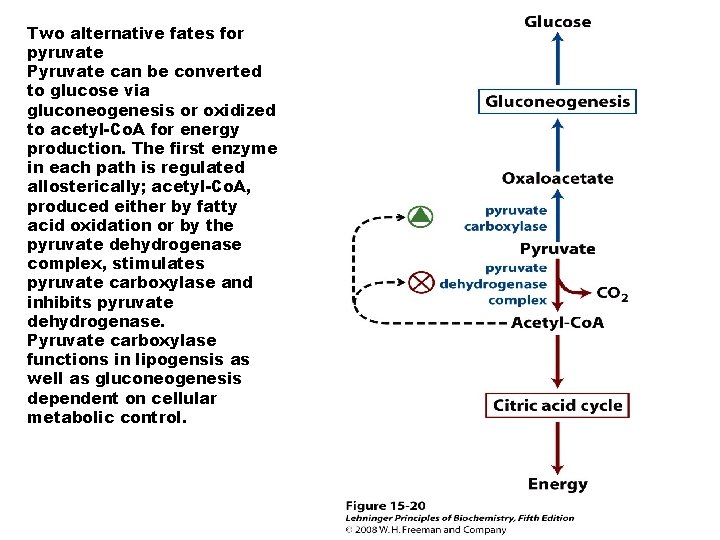
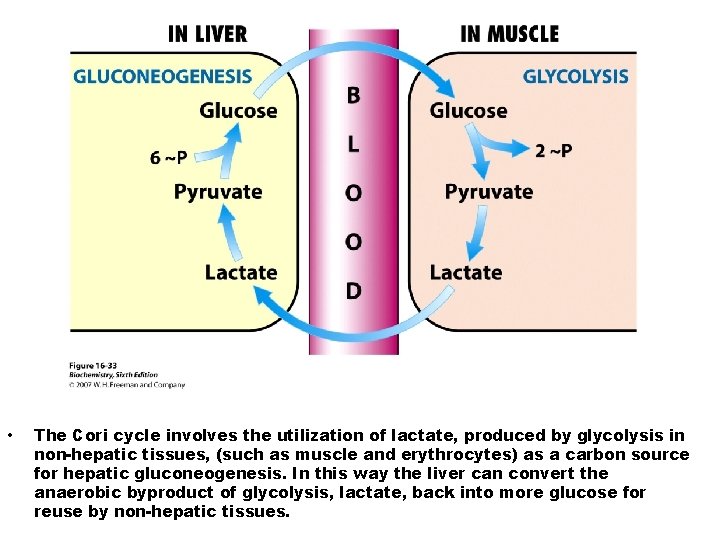
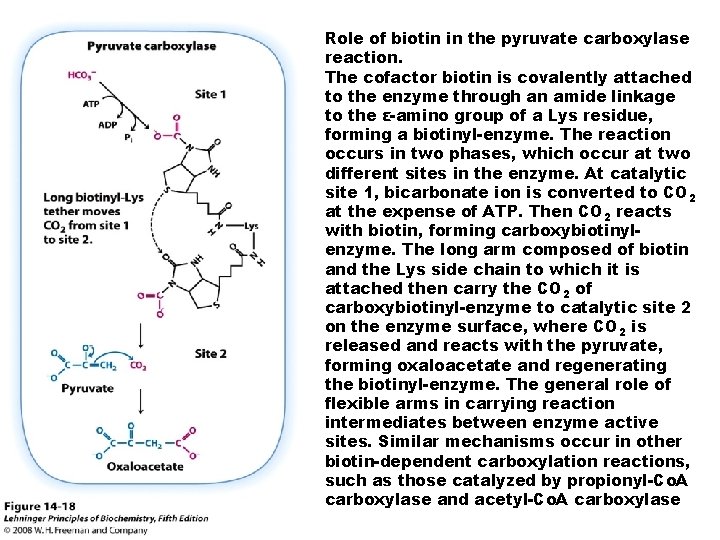
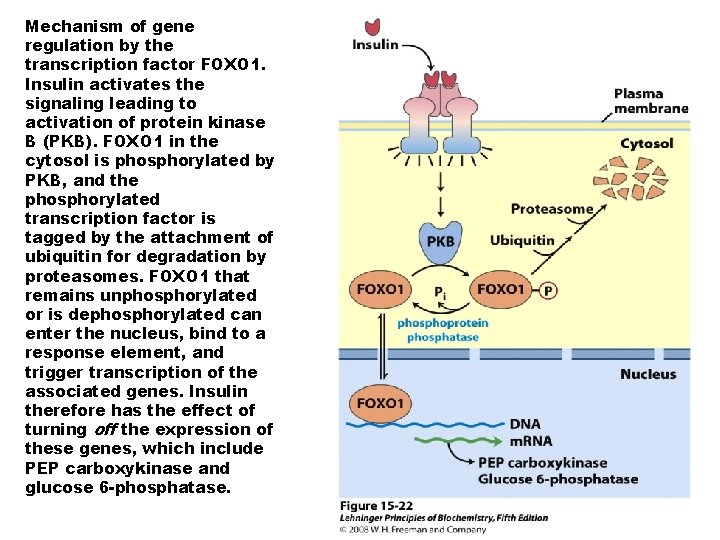
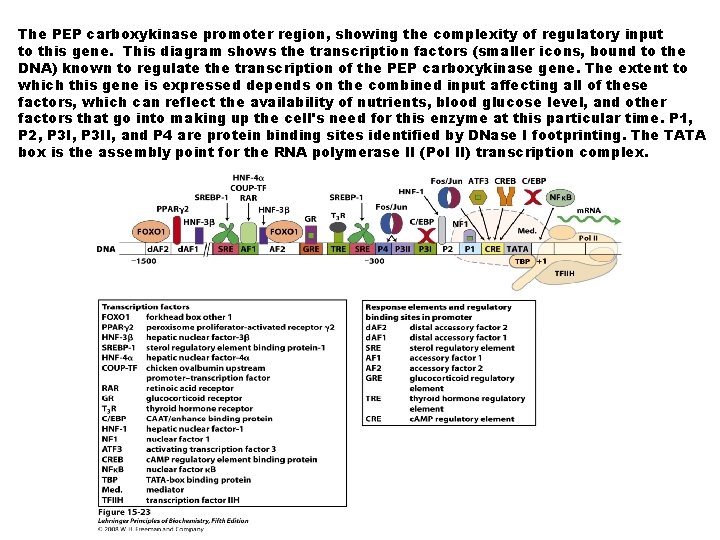
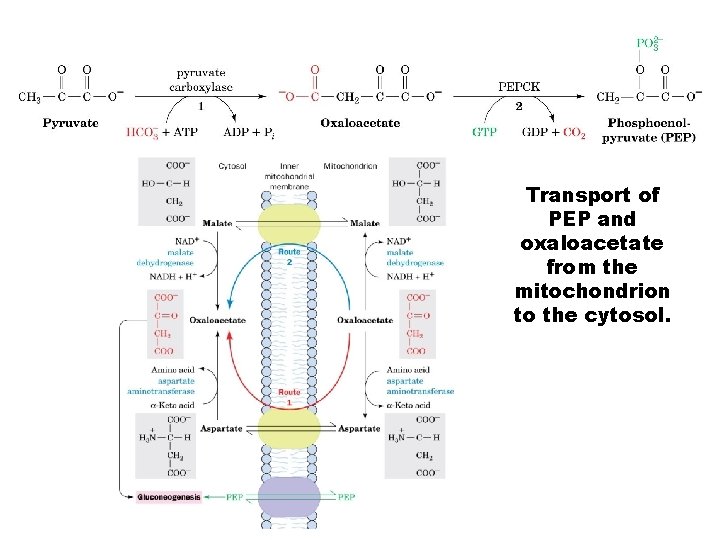
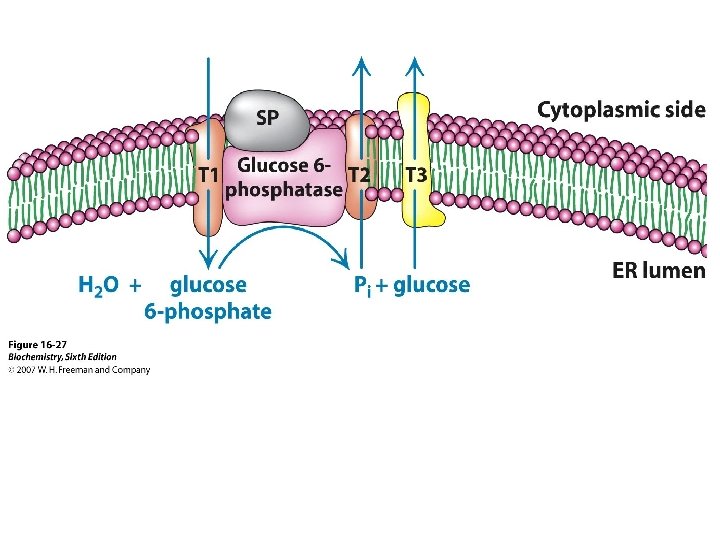
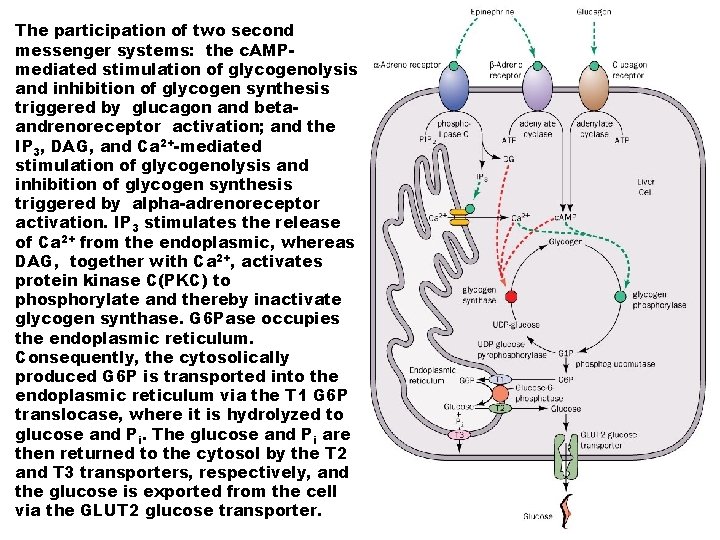
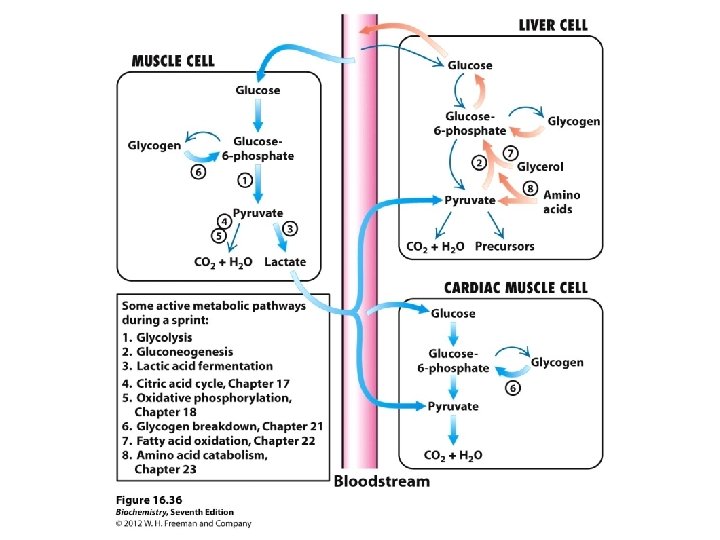
- Slides: 40
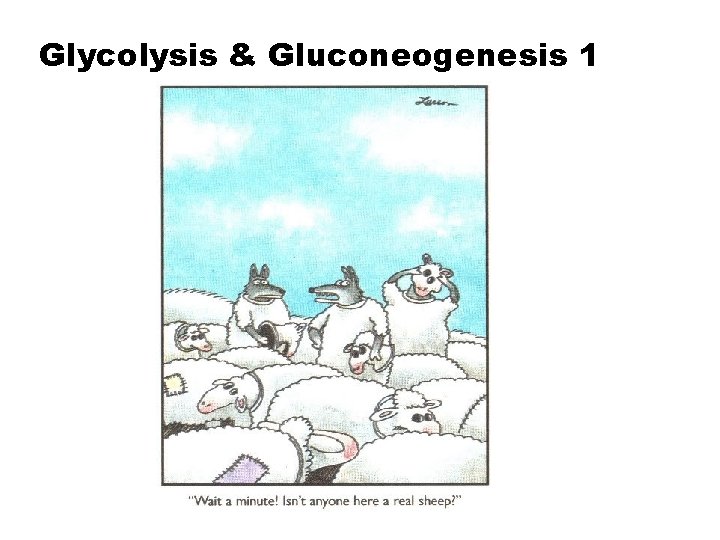
Glycolysis & Gluconeogenesis 1
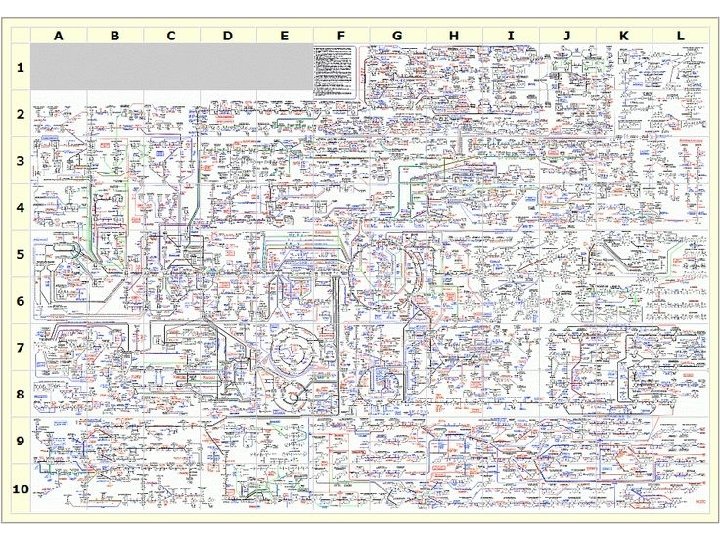
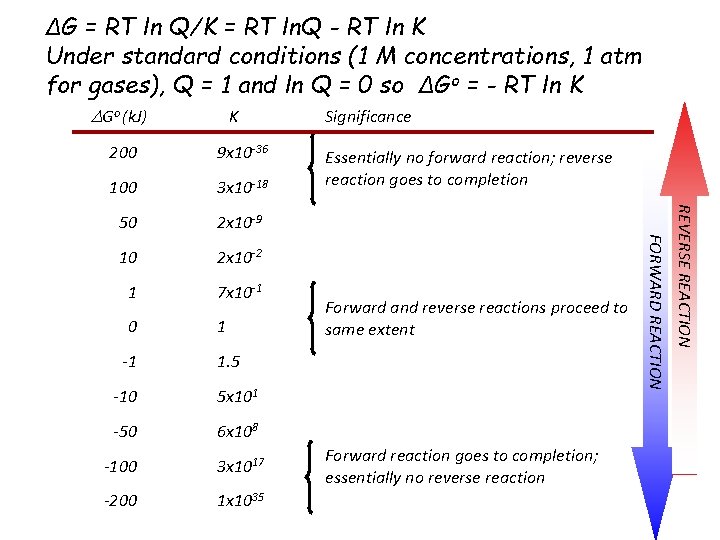
ΔG = RT ln Q/K = RT ln. Q - RT ln K Under standard conditions (1 M concentrations, 1 atm for gases), Q = 1 and ln Q = 0 so ΔGo = - RT ln K DGo (k. J) K 100 3 x 10 -18 50 2 x 10 -9 10 2 x 10 -2 1 7 x 10 -1 0 1 -1 Essentially no forward reaction; reverse reaction goes to completion Forward and reverse reactions proceed to same extent 1. 5 -10 5 x 101 -50 6 x 108 -100 3 x 1017 -200 1 x 1035 Forward reaction goes to completion; essentially no reverse reaction REVERSE REACTION 9 x 10 -36 FORWARD REACTION 200 Significance
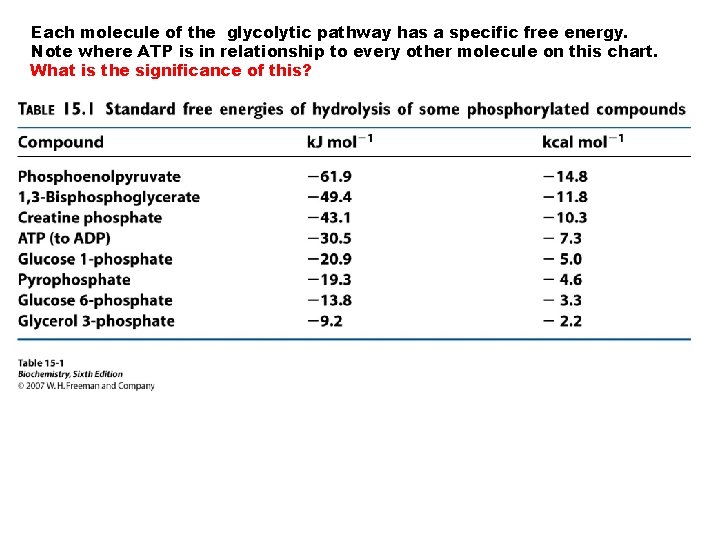
Each molecule of the glycolytic pathway has a specific free energy. Note where ATP is in relationship to every other molecule on this chart. What is the significance of this?
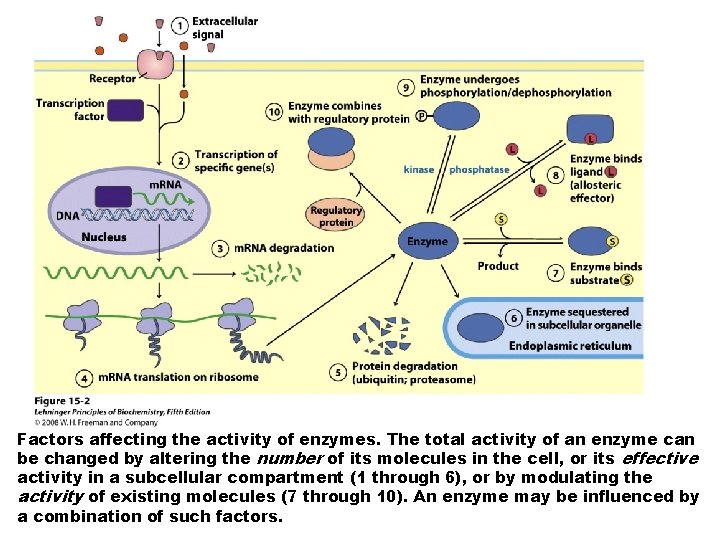
Factors affecting the activity of enzymes. The total activity of an enzyme can be changed by altering the number of its molecules in the cell, or its effective activity in a subcellular compartment (1 through 6), or by modulating the activity of existing molecules (7 through 10). An enzyme may be influenced by a combination of such factors.
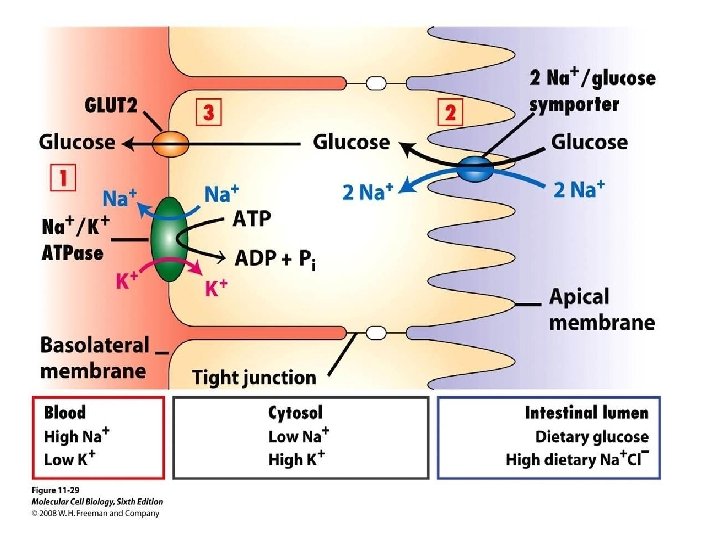
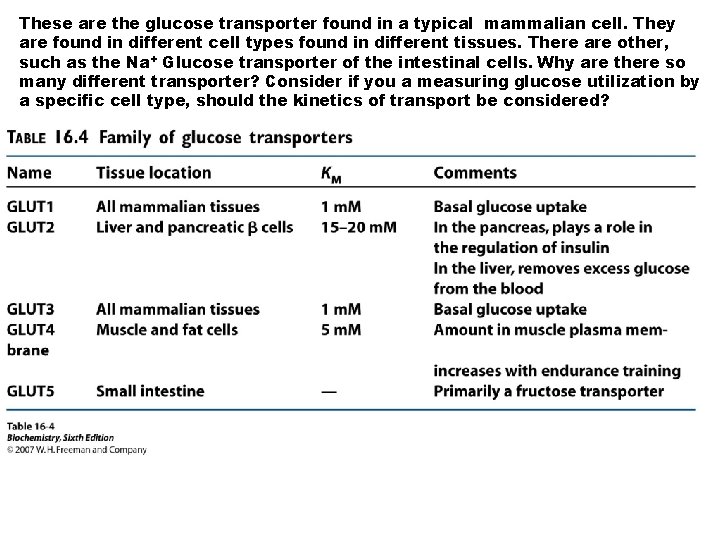
These are the glucose transporter found in a typical mammalian cell. They are found in different cell types found in different tissues. There are other, such as the Na+ Glucose transporter of the intestinal cells. Why are there so many different transporter? Consider if you a measuring glucose utilization by a specific cell type, should the kinetics of transport be considered?
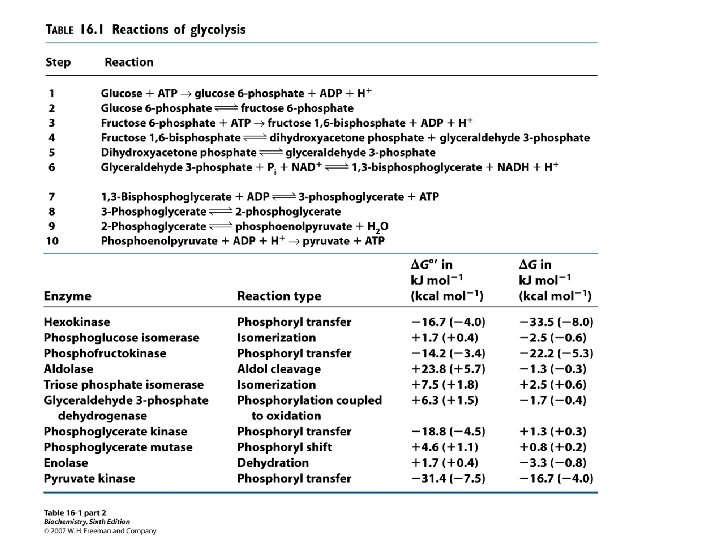
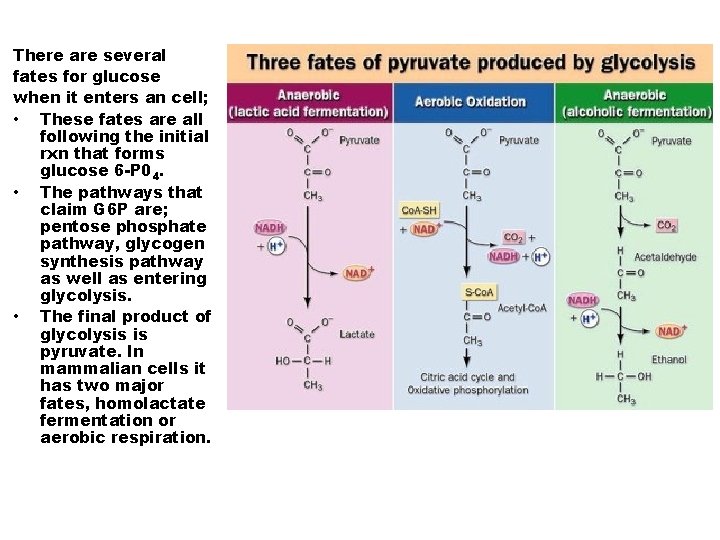
There are several fates for glucose when it enters an cell; • These fates are all following the initial rxn that forms glucose 6 -P 04. • The pathways that claim G 6 P are; pentose phosphate pathway, glycogen synthesis pathway as well as entering glycolysis. • The final product of glycolysis is pyruvate. In mammalian cells it has two major fates, homolactate fermentation or aerobic respiration.
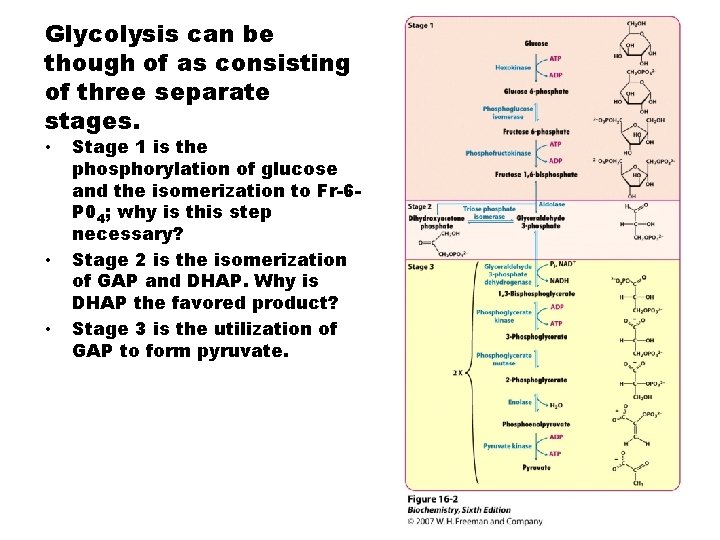
Glycolysis can be though of as consisting of three separate stages. • • • Stage 1 is the phosphorylation of glucose and the isomerization to Fr-6 P 04; why is this step necessary? Stage 2 is the isomerization of GAP and DHAP. Why is DHAP the favored product? Stage 3 is the utilization of GAP to form pyruvate.
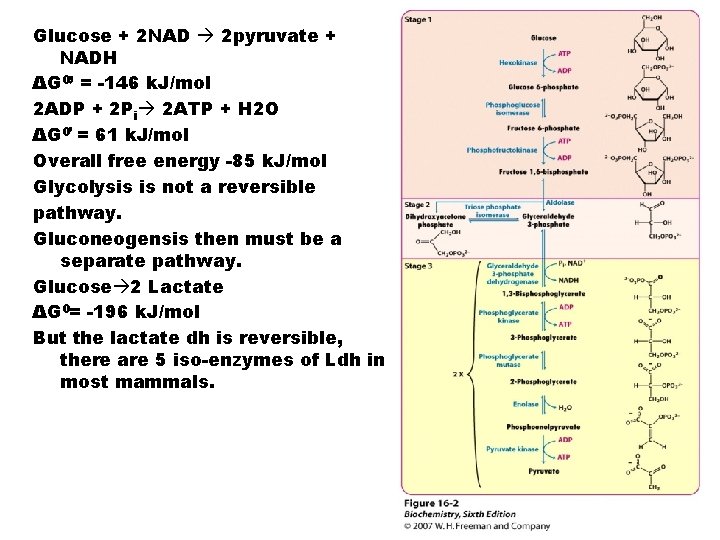
Glucose + 2 NAD 2 pyruvate + NADH ΔG 0’ = -146 k. J/mol 2 ADP + 2 Pi 2 ATP + H 2 O ΔG 0’ = 61 k. J/mol Overall free energy -85 k. J/mol Glycolysis is not a reversible pathway. Gluconeogensis then must be a separate pathway. Glucose 2 Lactate ΔG 0= -196 k. J/mol But the lactate dh is reversible, there are 5 iso-enzymes of Ldh in most mammals.
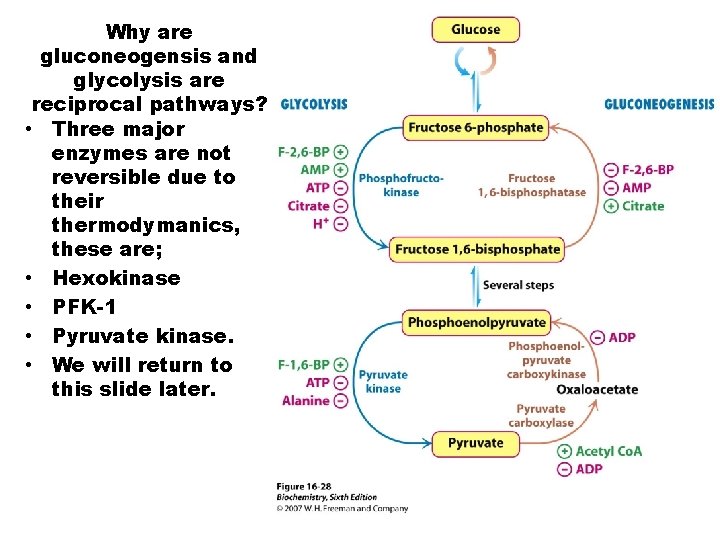
Why are gluconeogensis and glycolysis are reciprocal pathways? • Three major enzymes are not reversible due to their thermodymanics, these are; • Hexokinase • PFK-1 • Pyruvate kinase. • We will return to this slide later.
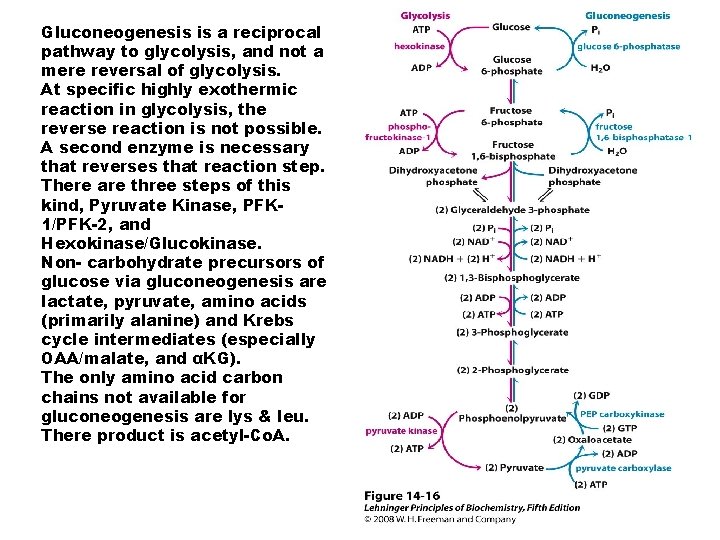
Gluconeogenesis is a reciprocal pathway to glycolysis, and not a mere reversal of glycolysis. At specific highly exothermic reaction in glycolysis, the reverse reaction is not possible. A second enzyme is necessary that reverses that reaction step. There are three steps of this kind, Pyruvate Kinase, PFK 1/PFK-2, and Hexokinase/Glucokinase. Non- carbohydrate precursors of glucose via gluconeogenesis are lactate, pyruvate, amino acids (primarily alanine) and Krebs cycle intermediates (especially OAA/malate, and αKG). The only amino acid carbon chains not available for gluconeogenesis are lys & leu. There product is acetyl-Co. A.
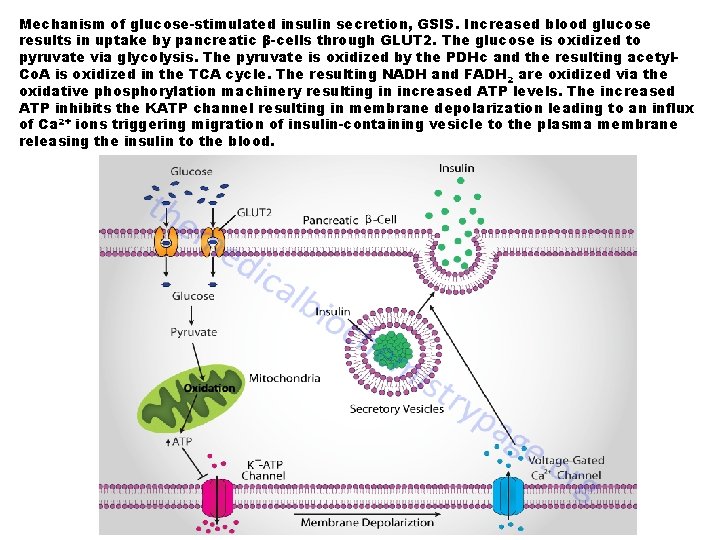
Mechanism of glucose-stimulated insulin secretion, GSIS. Increased blood glucose results in uptake by pancreatic β-cells through GLUT 2. The glucose is oxidized to pyruvate via glycolysis. The pyruvate is oxidized by the PDHc and the resulting acetyl. Co. A is oxidized in the TCA cycle. The resulting NADH and FADH 2 are oxidized via the oxidative phosphorylation machinery resulting in increased ATP levels. The increased ATP inhibits the KATP channel resulting in membrane depolarization leading to an influx of Ca 2+ ions triggering migration of insulin-containing vesicle to the plasma membrane releasing the insulin to the blood.
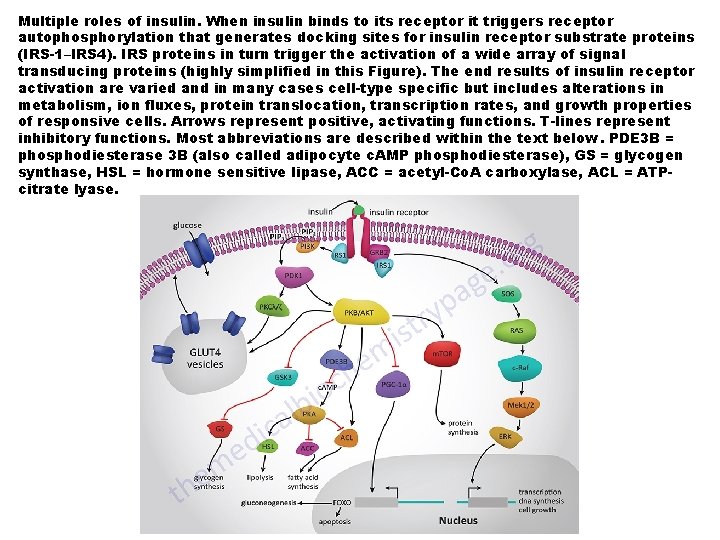
Multiple roles of insulin. When insulin binds to its receptor it triggers receptor autophosphorylation that generates docking sites for insulin receptor substrate proteins (IRS-1–IRS 4). IRS proteins in turn trigger the activation of a wide array of signal transducing proteins (highly simplified in this Figure). The end results of insulin receptor activation are varied and in many cases cell-type specific but includes alterations in metabolism, ion fluxes, protein translocation, transcription rates, and growth properties of responsive cells. Arrows represent positive, activating functions. T-lines represent inhibitory functions. Most abbreviations are described within the text below. PDE 3 B = phosphodiesterase 3 B (also called adipocyte c. AMP phosphodiesterase), GS = glycogen synthase, HSL = hormone sensitive lipase, ACC = acetyl-Co. A carboxylase, ACL = ATPcitrate lyase.
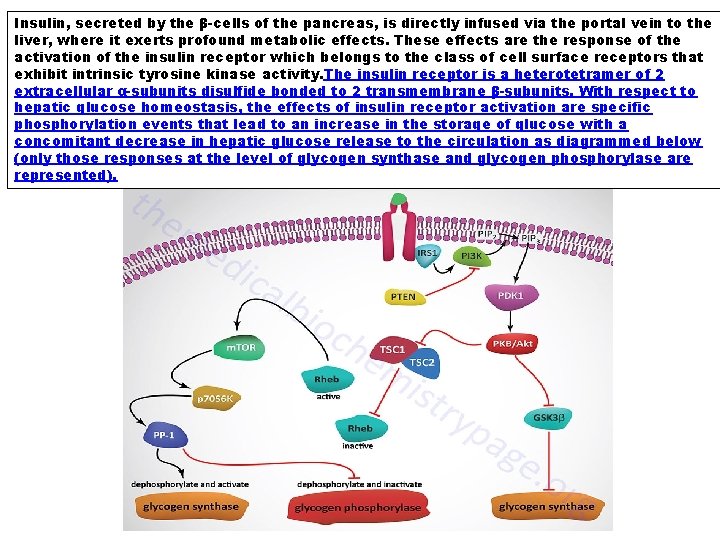
Insulin, secreted by the β-cells of the pancreas, is directly infused via the portal vein to the liver, where it exerts profound metabolic effects. These effects are the response of the activation of the insulin receptor which belongs to the class of cell surface receptors that exhibit intrinsic tyrosine kinase activity. The insulin receptor is a heterotetramer of 2 extracellular α-subunits disulfide bonded to 2 transmembrane β-subunits. With respect to hepatic glucose homeostasis, the effects of insulin receptor activation are specific phosphorylation events that lead to an increase in the storage of glucose with a concomitant decrease in hepatic glucose release to the circulation as diagrammed below (only those responses at the level of glycogen synthase and glycogen phosphorylase are represented).
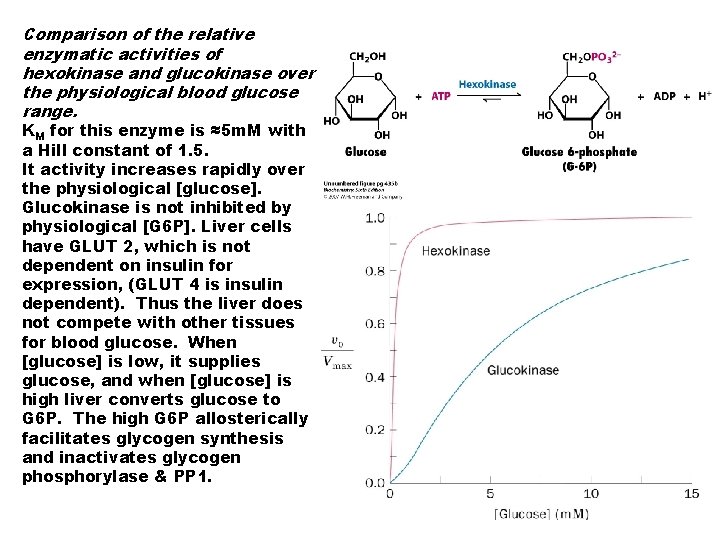
Comparison of the relative enzymatic activities of hexokinase and glucokinase over the physiological blood glucose range. KM for this enzyme is ≈5 m. M with a Hill constant of 1. 5. It activity increases rapidly over the physiological [glucose]. Glucokinase is not inhibited by physiological [G 6 P]. Liver cells have GLUT 2, which is not dependent on insulin for expression, (GLUT 4 is insulin dependent). Thus the liver does not compete with other tissues for blood glucose. When [glucose] is low, it supplies glucose, and when [glucose] is high liver converts glucose to G 6 P. The high G 6 P allosterically facilitates glycogen synthesis and inactivates glycogen phosphorylase & PP 1.
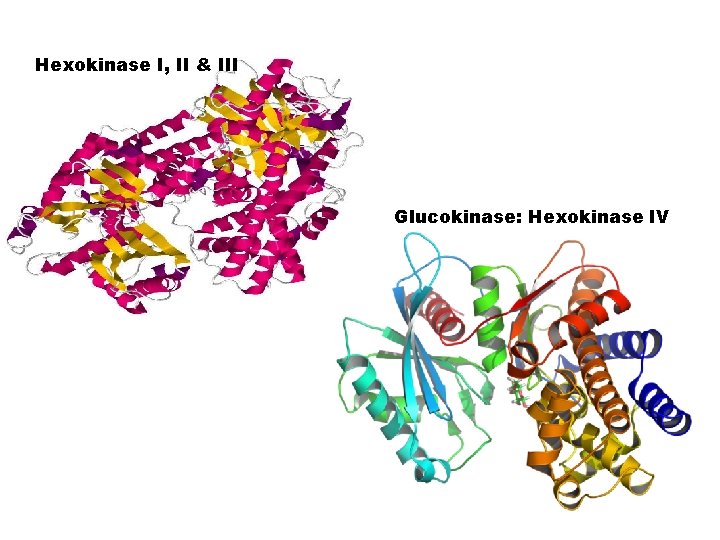
Hexokinase I, II & III Glucokinase: Hexokinase IV
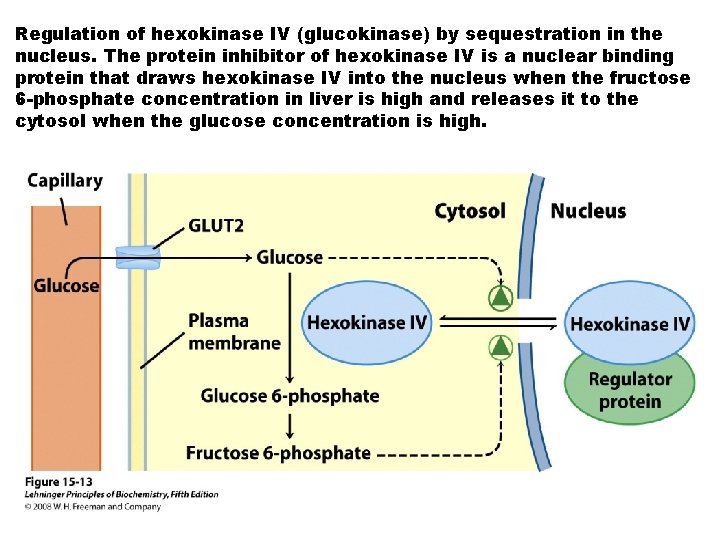
Regulation of hexokinase IV (glucokinase) by sequestration in the nucleus. The protein inhibitor of hexokinase IV is a nuclear binding protein that draws hexokinase IV into the nucleus when the fructose 6 -phosphate concentration in liver is high and releases it to the cytosol when the glucose concentration is high.
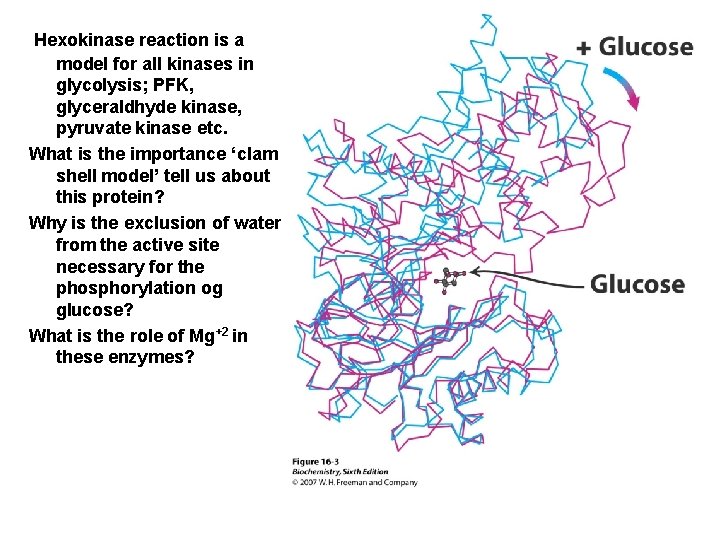
Hexokinase reaction is a model for all kinases in glycolysis; PFK, glyceraldhyde kinase, pyruvate kinase etc. What is the importance ‘clam shell model’ tell us about this protein? Why is the exclusion of water from the active site necessary for the phosphorylation og glucose? What is the role of Mg+2 in these enzymes?
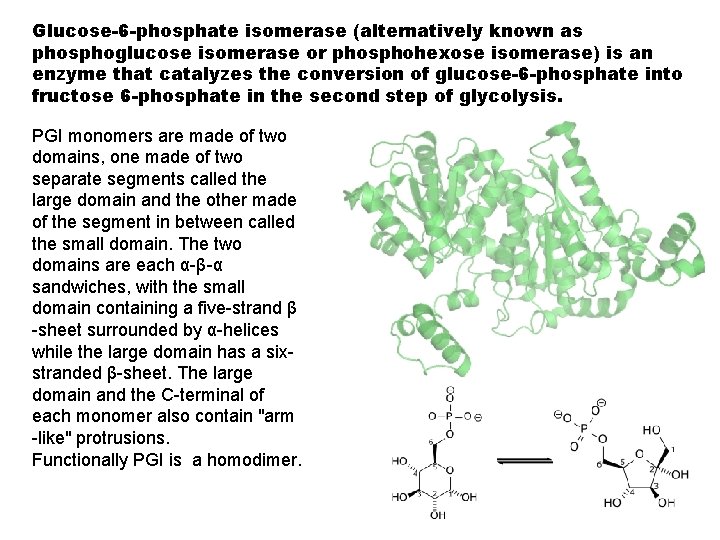
Glucose-6 -phosphate isomerase (alternatively known as phosphoglucose isomerase or phosphohexose isomerase) is an enzyme that catalyzes the conversion of glucose-6 -phosphate into fructose 6 -phosphate in the second step of glycolysis. PGI monomers are made of two domains, one made of two separate segments called the large domain and the other made of the segment in between called the small domain. The two domains are each α-β-α sandwiches, with the small domain containing a five-strand β -sheet surrounded by α-helices while the large domain has a sixstranded β-sheet. The large domain and the C-terminal of each monomer also contain "arm -like" protrusions. Functionally PGI is a homodimer.
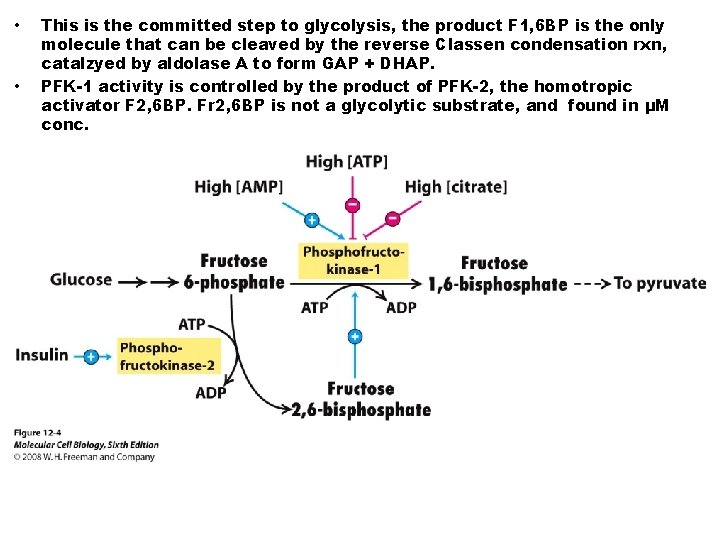
• • This is the committed step to glycolysis, the product F 1, 6 BP is the only molecule that can be cleaved by the reverse Classen condensation rxn, catalzyed by aldolase A to form GAP + DHAP. PFK-1 activity is controlled by the product of PFK-2, the homotropic activator F 2, 6 BP. Fr 2, 6 BP is not a glycolytic substrate, and found in μM conc.
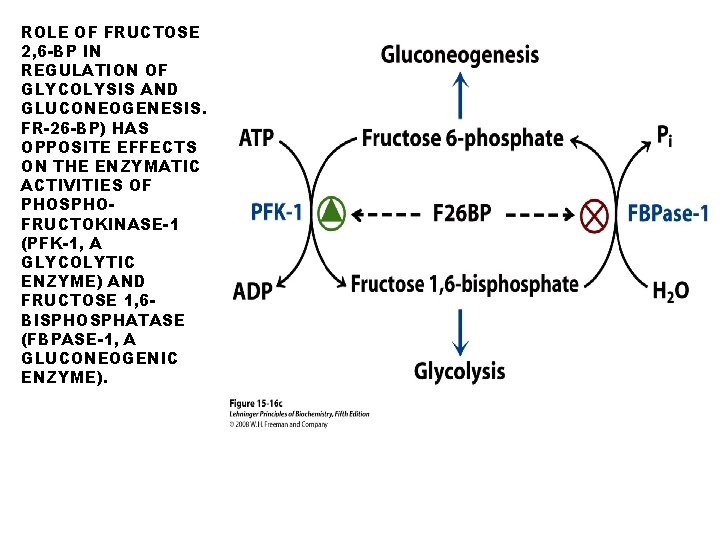
ROLE OF FRUCTOSE 2, 6 -BP IN REGULATION OF GLYCOLYSIS AND GLUCONEOGENESIS. FR-26 -BP) HAS OPPOSITE EFFECTS ON THE ENZYMATIC ACTIVITIES OF PHOSPHOFRUCTOKINASE-1 (PFK-1, A GLYCOLYTIC ENZYME) AND FRUCTOSE 1, 6 BISPHOSPHATASE (FBPASE-1, A GLUCONEOGENIC ENZYME).
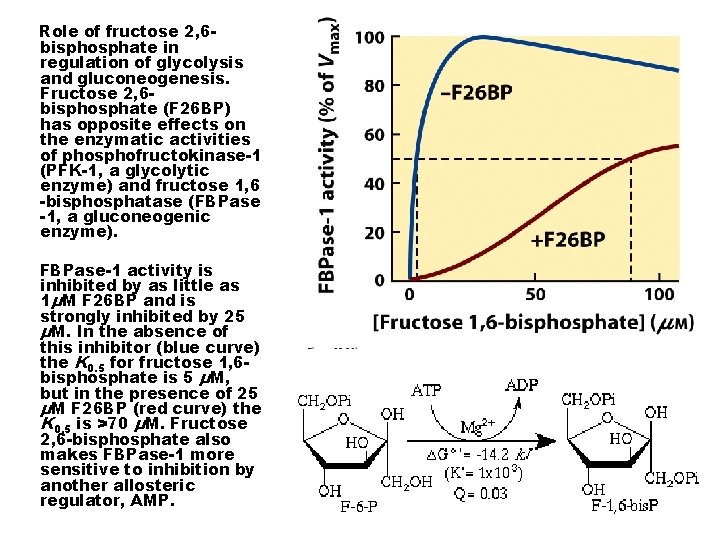
Role of fructose 2, 6 bisphosphate in regulation of glycolysis and gluconeogenesis. Fructose 2, 6 bisphosphate (F 26 BP) has opposite effects on the enzymatic activities of phosphofructokinase-1 (PFK-1, a glycolytic enzyme) and fructose 1, 6 -bisphosphatase (FBPase -1, a gluconeogenic enzyme). FBPase-1 activity is inhibited by as little as 1μM F 26 BP and is strongly inhibited by 25 μM. In the absence of this inhibitor (blue curve) the K 0. 5 for fructose 1, 6 bisphosphate is 5 μM, but in the presence of 25 μM F 26 BP (red curve) the K 0. 5 is >70 μM. Fructose 2, 6 -bisphosphate also makes FBPase-1 more sensitive to inhibition by another allosteric regulator, AMP.
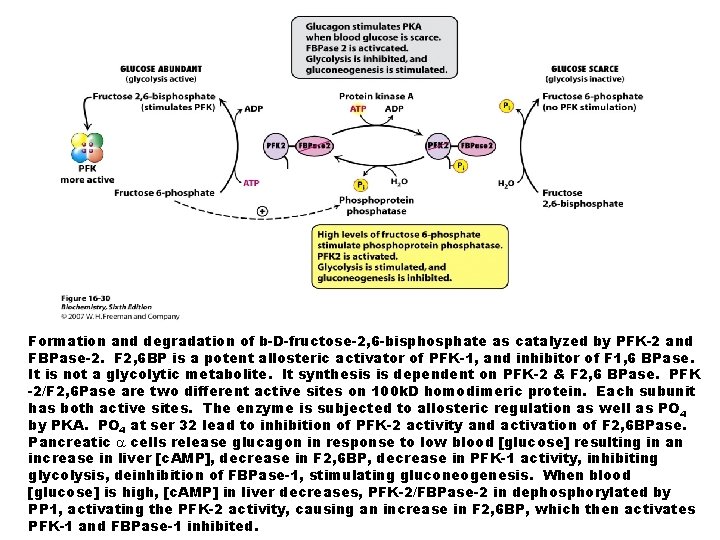
Formation and degradation of b-D-fructose-2, 6 -bisphosphate as catalyzed by PFK-2 and FBPase-2. F 2, 6 BP is a potent allosteric activator of PFK-1, and inhibitor of F 1, 6 BPase. It is not a glycolytic metabolite. It synthesis is dependent on PFK-2 & F 2, 6 BPase. PFK -2/F 2, 6 Pase are two different active sites on 100 k. D homodimeric protein. Each subunit has both active sites. The enzyme is subjected to allosteric regulation as well as PO 4 by PKA. PO 4 at ser 32 lead to inhibition of PFK-2 activity and activation of F 2, 6 BPase. Pancreatic cells release glucagon in response to low blood [glucose] resulting in an increase in liver [c. AMP], decrease in F 2, 6 BP, decrease in PFK-1 activity, inhibiting glycolysis, deinhibition of FBPase-1, stimulating gluconeogenesis. When blood [glucose] is high, [c. AMP] in liver decreases, PFK-2/FBPase-2 in dephosphorylated by PP 1, activating the PFK-2 activity, causing an increase in F 2, 6 BP, which then activates PFK-1 and FBPase-1 inhibited.
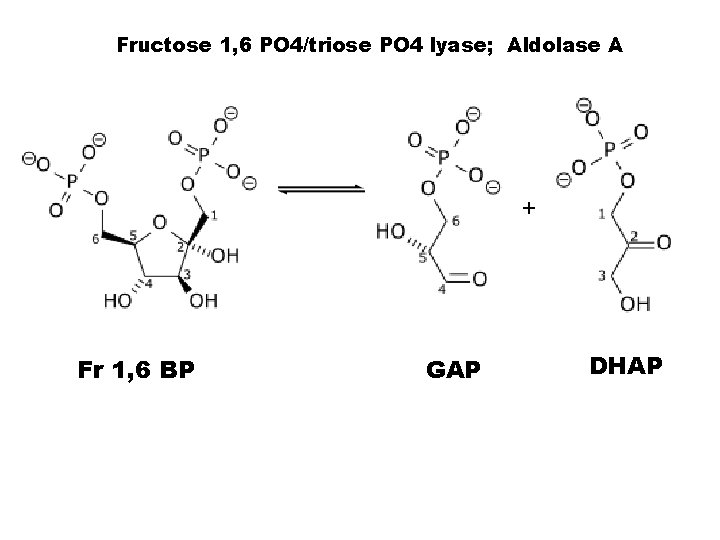
Fructose 1, 6 PO 4/triose PO 4 lyase; Aldolase A + Fr 1, 6 BP GAP DHAP
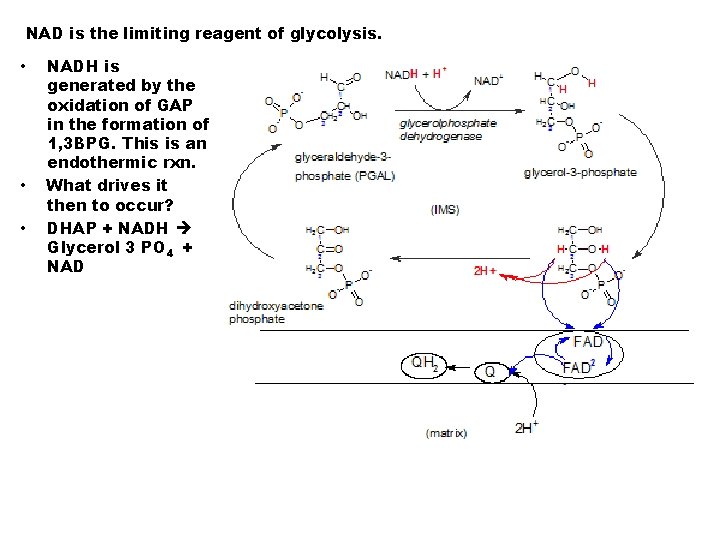
NAD is the limiting reagent of glycolysis. • • • NADH is generated by the oxidation of GAP in the formation of 1, 3 BPG. This is an endothermic rxn. What drives it then to occur? DHAP + NADH Glycerol 3 PO 4 + NAD
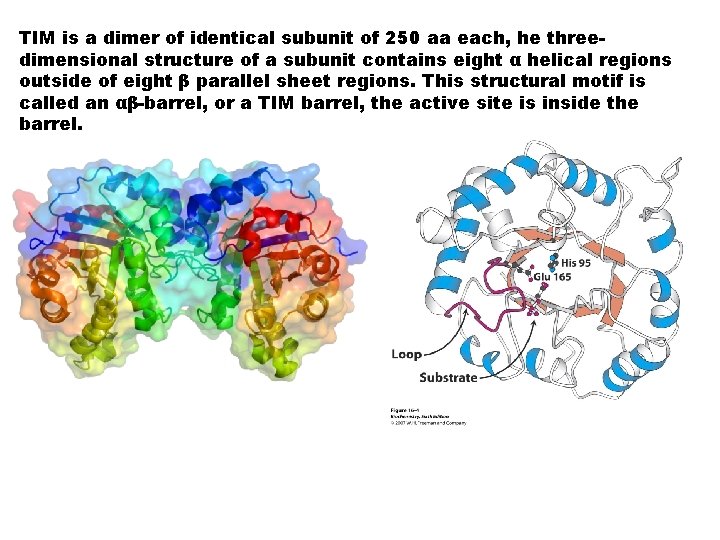
TIM is a dimer of identical subunit of 250 aa each, he threedimensional structure of a subunit contains eight α helical regions outside of eight β parallel sheet regions. This structural motif is called an αβ-barrel, or a TIM barrel, the active site is inside the barrel.
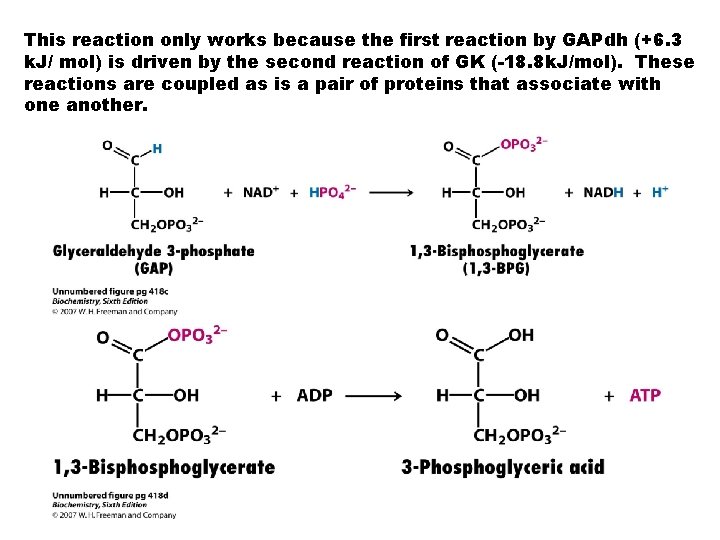
This reaction only works because the first reaction by GAPdh (+6. 3 k. J/ mol) is driven by the second reaction of GK (-18. 8 k. J/mol). These reactions are coupled as is a pair of proteins that associate with one another.
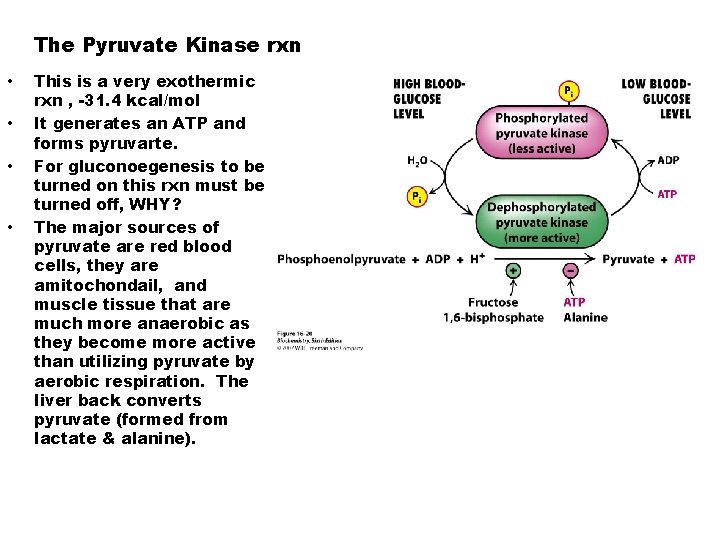
The Pyruvate Kinase rxn • • This is a very exothermic rxn , -31. 4 kcal/mol It generates an ATP and forms pyruvarte. For gluconoegenesis to be turned on this rxn must be turned off, WHY? The major sources of pyruvate are red blood cells, they are amitochondail, and muscle tissue that are much more anaerobic as they become more active than utilizing pyruvate by aerobic respiration. The liver back converts pyruvate (formed from lactate & alanine).
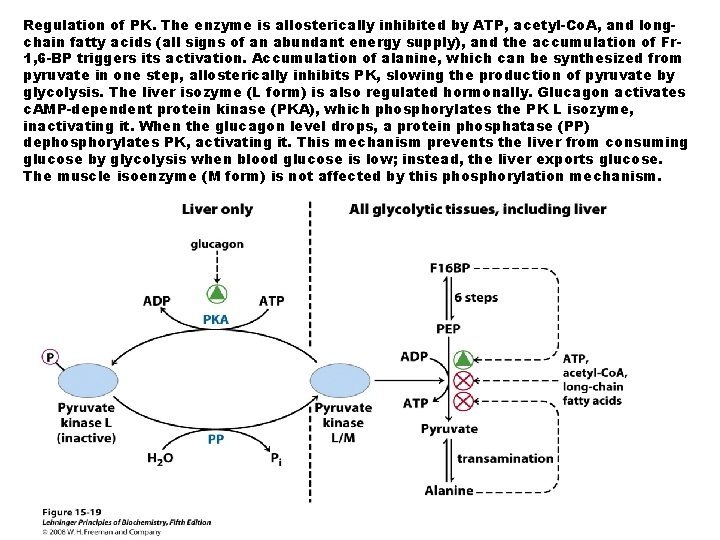
Regulation of PK. The enzyme is allosterically inhibited by ATP, acetyl-Co. A, and longchain fatty acids (all signs of an abundant energy supply), and the accumulation of Fr 1, 6 -BP triggers its activation. Accumulation of alanine, which can be synthesized from pyruvate in one step, allosterically inhibits PK, slowing the production of pyruvate by glycolysis. The liver isozyme (L form) is also regulated hormonally. Glucagon activates c. AMP-dependent protein kinase (PKA), which phosphorylates the PK L isozyme, inactivating it. When the glucagon level drops, a protein phosphatase (PP) dephosphorylates PK, activating it. This mechanism prevents the liver from consuming glucose by glycolysis when blood glucose is low; instead, the liver exports glucose. The muscle isoenzyme (M form) is not affected by this phosphorylation mechanism.
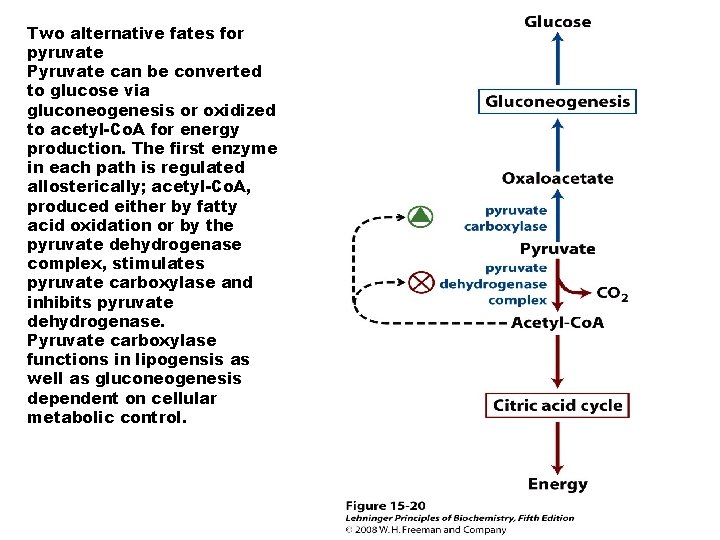
Two alternative fates for pyruvate Pyruvate can be converted to glucose via gluconeogenesis or oxidized to acetyl-Co. A for energy production. The first enzyme in each path is regulated allosterically; acetyl-Co. A, produced either by fatty acid oxidation or by the pyruvate dehydrogenase complex, stimulates pyruvate carboxylase and inhibits pyruvate dehydrogenase. Pyruvate carboxylase functions in lipogensis as well as gluconeogenesis dependent on cellular metabolic control.
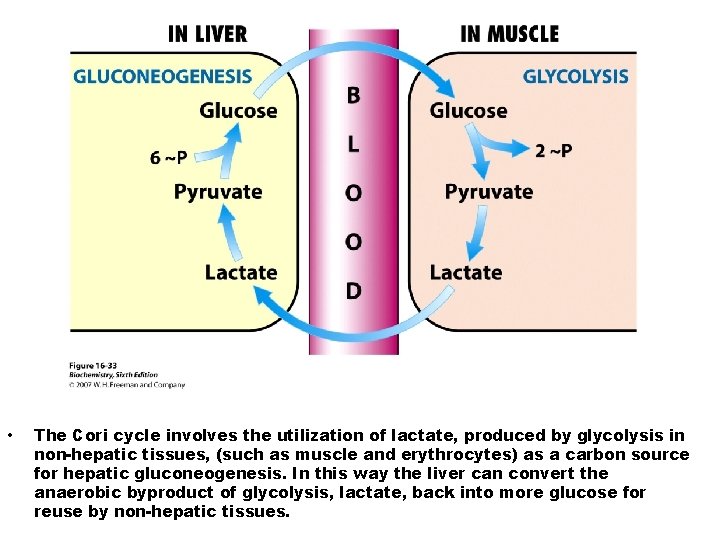
• The Cori cycle involves the utilization of lactate, produced by glycolysis in non-hepatic tissues, (such as muscle and erythrocytes) as a carbon source for hepatic gluconeogenesis. In this way the liver can convert the anaerobic byproduct of glycolysis, lactate, back into more glucose for reuse by non-hepatic tissues.
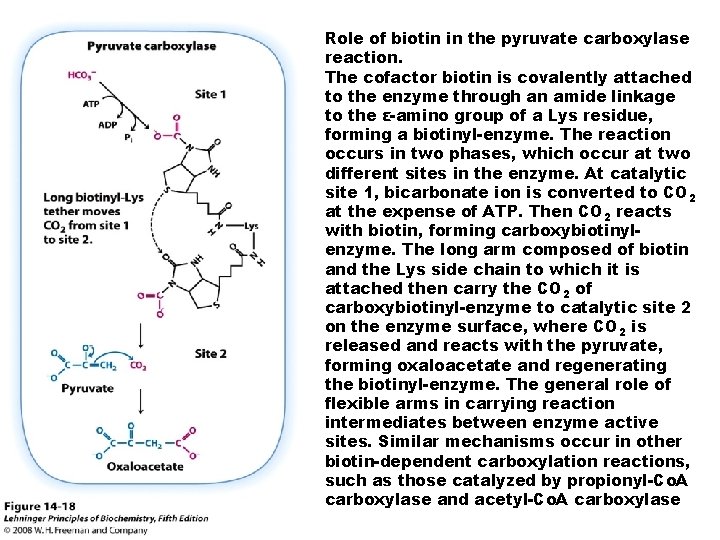
Role of biotin in the pyruvate carboxylase reaction. The cofactor biotin is covalently attached to the enzyme through an amide linkage to the ε-amino group of a Lys residue, forming a biotinyl-enzyme. The reaction occurs in two phases, which occur at two different sites in the enzyme. At catalytic site 1, bicarbonate ion is converted to CO 2 at the expense of ATP. Then CO 2 reacts with biotin, forming carboxybiotinylenzyme. The long arm composed of biotin and the Lys side chain to which it is attached then carry the CO 2 of carboxybiotinyl-enzyme to catalytic site 2 on the enzyme surface, where CO 2 is released and reacts with the pyruvate, forming oxaloacetate and regenerating the biotinyl-enzyme. The general role of flexible arms in carrying reaction intermediates between enzyme active sites. Similar mechanisms occur in other biotin-dependent carboxylation reactions, such as those catalyzed by propionyl-Co. A carboxylase and acetyl-Co. A carboxylase
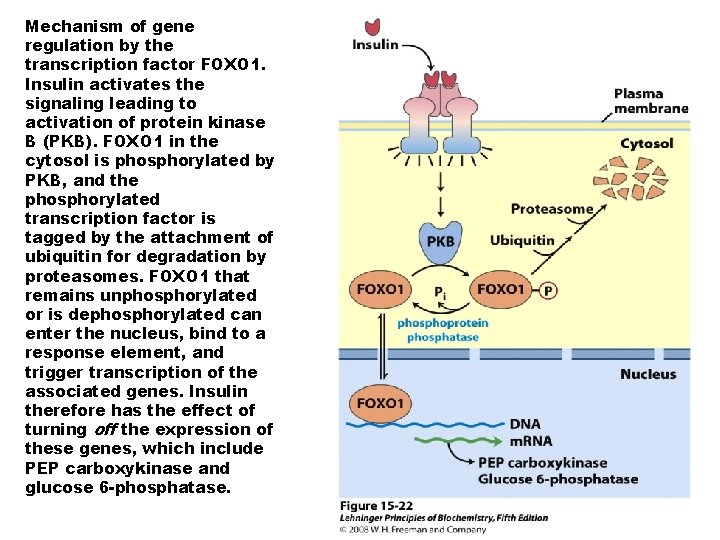
Mechanism of gene regulation by the transcription factor FOXO 1. Insulin activates the signaling leading to activation of protein kinase B (PKB). FOXO 1 in the cytosol is phosphorylated by PKB, and the phosphorylated transcription factor is tagged by the attachment of ubiquitin for degradation by proteasomes. FOXO 1 that remains unphosphorylated or is dephosphorylated can enter the nucleus, bind to a response element, and trigger transcription of the associated genes. Insulin therefore has the effect of turning off the expression of these genes, which include PEP carboxykinase and glucose 6 -phosphatase.
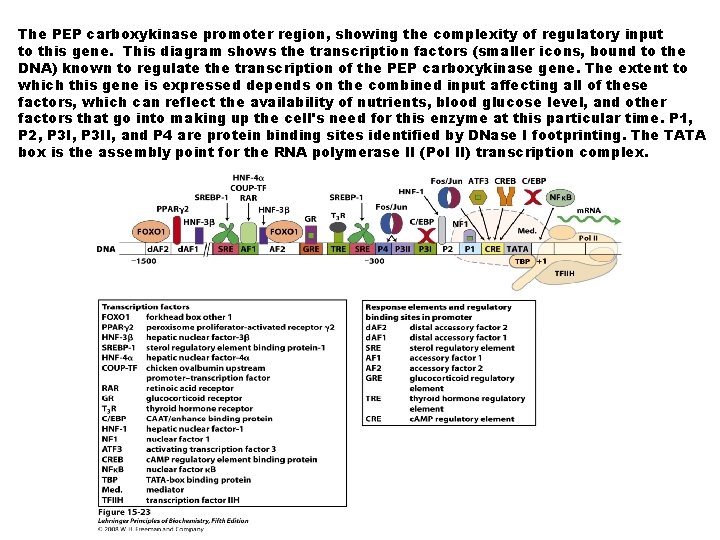
The PEP carboxykinase promoter region, showing the complexity of regulatory input to this gene. This diagram shows the transcription factors (smaller icons, bound to the DNA) known to regulate the transcription of the PEP carboxykinase gene. The extent to which this gene is expressed depends on the combined input affecting all of these factors, which can reflect the availability of nutrients, blood glucose level, and other factors that go into making up the cell's need for this enzyme at this particular time. P 1, P 2, P 3 II, and P 4 are protein binding sites identified by DNase I footprinting. The TATA box is the assembly point for the RNA polymerase II (Pol II) transcription complex.
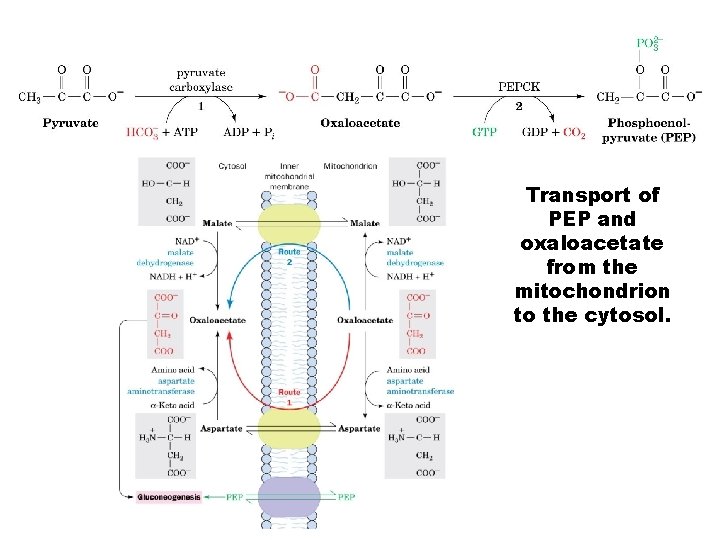
Transport of PEP and oxaloacetate from the mitochondrion to the cytosol.
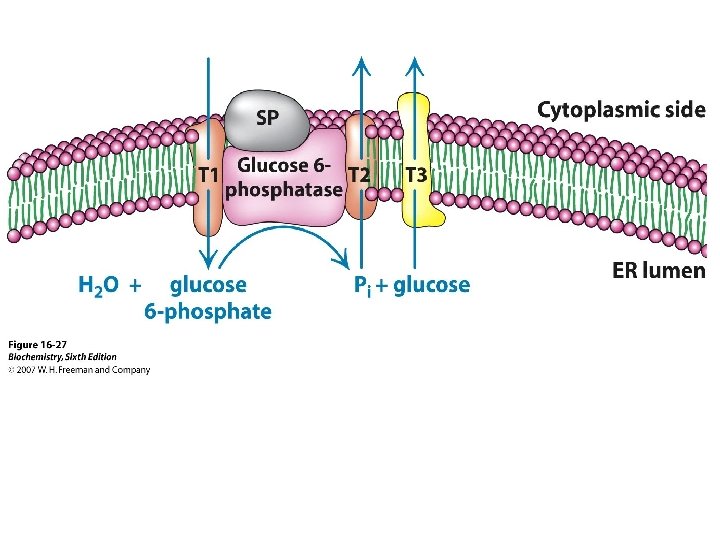
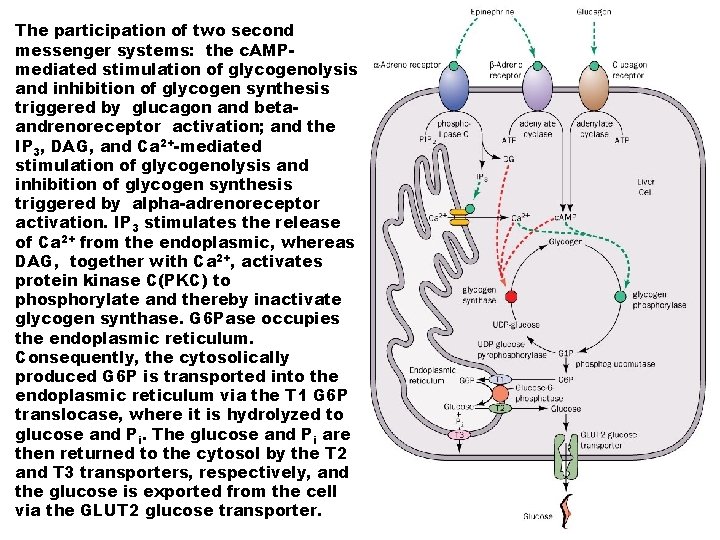
The participation of two second messenger systems: the c. AMPmediated stimulation of glycogenolysis and inhibition of glycogen synthesis triggered by glucagon and betaandrenoreceptor activation; and the IP 3, DAG, and Ca 2+-mediated stimulation of glycogenolysis and inhibition of glycogen synthesis triggered by alpha-adrenoreceptor activation. IP 3 stimulates the release of Ca 2+ from the endoplasmic, whereas DAG, together with Ca 2+, activates protein kinase C(PKC) to phosphorylate and thereby inactivate glycogen synthase. G 6 Pase occupies the endoplasmic reticulum. Consequently, the cytosolically produced G 6 P is transported into the endoplasmic reticulum via the T 1 G 6 P translocase, where it is hydrolyzed to glucose and Pi. The glucose and Pi are then returned to the cytosol by the T 2 and T 3 transporters, respectively, and the glucose is exported from the cell via the GLUT 2 glucose transporter.
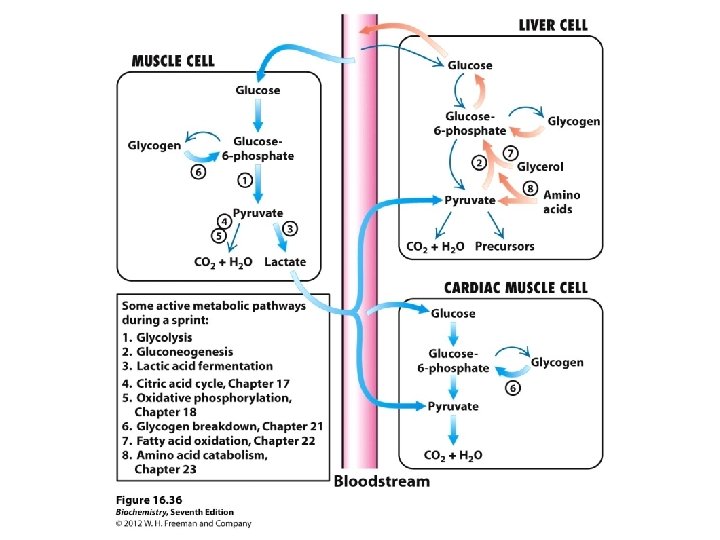
Glycogen storage disease table
Phosphoenolpyruvate
Glycogenolysis and gluconeogenesis
Cori döngüsü
Gluconeogenesis from lactate
Hexokinase phosphofructokinase and pyruvate kinase
Gluconeogenesis nedir
Net reaction of gluconeogenesis
Gluconeogenesis regulacion
Sustratos gluconeogenicos
Cori cycle
Energetics of gluconeogenesis
Precursor of gluconeogenesis
Precursores de gluconeogenesis
Glucogenolisis y gluconeogenesis
Glycogenolysis and gluconeogenesis
Gluconeogenesis definition
Glycogen metabolism
Pyruvate carboxylase gluconeogenesis
Pyruvate dehydrogenase
Gluconeognesis
Gluconeogenesis of amino acids
Pasteur effect
Net reaction of gluconeogenesis
Converting amino acids to glucose
Gluconeogenesis purpose
Krebs cycle and gluconeogenesis
Formation of glucose from noncarbohydrate sources
Glycolysis occurs in the
Glycolysis reactants
Glycolysis
Fructose 1 phosphate glycolysis
Glucose + atp
Glycolysis animation gif
Pyruvate to lactate
Glycolysis inputs and outputs
Creatine phosphate energy system
Siklus krebs
How many atp in glycolysis
Anaerobic glycolysis in red blood cells
Atp glycolysis